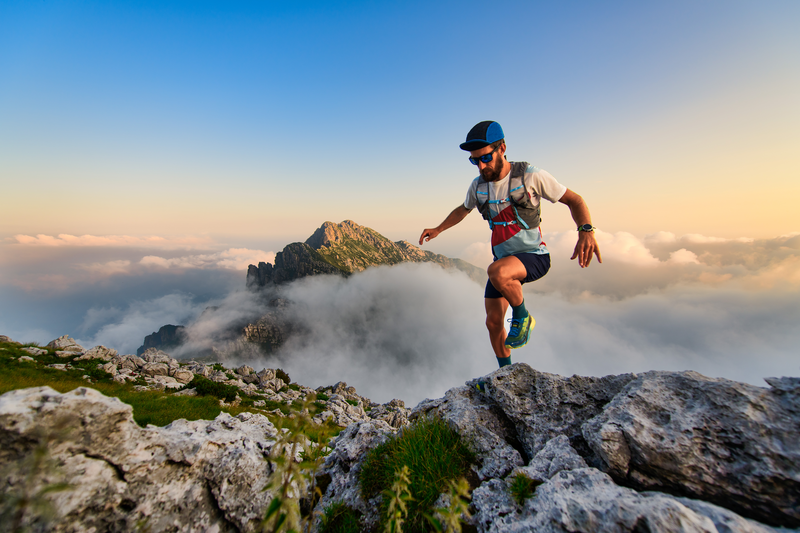
94% of researchers rate our articles as excellent or good
Learn more about the work of our research integrity team to safeguard the quality of each article we publish.
Find out more
POLICY AND PRACTICE REVIEWS article
Front. Mar. Sci. , 27 October 2021
Sec. Ocean Observation
Volume 8 - 2021 | https://doi.org/10.3389/fmars.2021.760840
This article is part of the Research Topic Best Practices in Ocean Observing View all 84 articles
Offshore wind energy development is rapidly ramping up in United States (U.S.) waters in order to meet renewable energy goals. With a diverse suite of endangered large whale species and a multitude of other protected marine species frequenting these same waters, understanding the potential consequences of construction and operation activities is essential to advancing responsible offshore wind development. Passive acoustic monitoring (PAM) represents a newer technology that has become one of several methods of choice for monitoring trends in the presence of species, the soundscape, mitigating risk, and evaluating potential behavioral and distributional changes resulting from offshore wind activities. Federal and State regulators, the offshore wind industry, and environmental advocates require detailed information on PAM capabilities and techniques needed to promote efficient, consistent, and meaningful data collection efforts on local and regional scales. PAM during offshore wind construction and operation may be required by the National Oceanic and Atmospheric Administration and Bureau of Ocean Energy Management through project-related permits and approvals issued pursuant to relevant statutes and regulations. The recommendations in this paper aim to support this need as well as to aid the development of project-specific PAM Plans by identifying minimum procedures, system requirements, and other important components for inclusion, while promoting consistency across plans. These recommendations provide an initial guide for stakeholders to meet the rapid development of the offshore wind industry in United States waters. Approaches to PAM and agency requirements will evolve as future permits are issued and construction plans are approved, regional research priorities are refined, and scientific publications and new technologies become available.
Rapid global economic growth has contributed to today’s increasing demand for energy. The development of alternative renewable and clean energy sources, such as solar, wind, and hydrogen energy, has become a priority as countries seek to expand their use of renewable energy sources and meet goals to reduce greenhouse gas emissions (Leung and Yang, 2012). Among these many renewable resources, offshore wind energy development offers rapidly evolving technological approaches, promising commercial prospects, and large-scale electricity generation such as in Europe. The speed and manner in which many coastal nations pursue offshore renewable energy development has varied dramatically in the past (Portman et al., 2009), and the United States (U.S.) is now poised to rapidly develop offshore wind leases throughout the Atlantic Outer Continental Shelf (OCS), as well as the Pacific and Gulf of Mexico.
A recent White House statement announced that in order to position the domestic offshore wind industry to meet its target of deploying 30 gigawatts of offshore wind by 2030, the Department of the Interior’s Bureau of Ocean Energy Management (BOEM) plans to advance new lease sales and complete review of at least 16 Construction and Operations Plans (COPs) by 2025, representing more than 19 GW of new clean energy (Office of the Press Secretary, 2021). The main environmental concerns related to offshore wind development for marine animals are primarily focused around construction and operations through increased noise levels, behavioral changes, displacement from important biological areas such as feeding grounds, risk of vessel collisions, changes to benthic and pelagic habitats, alterations to food webs, and pollution from increased vessel traffic or release of contaminants from seabed sediments (e.g., Tougaard et al., 2009; Bailey et al., 2014).
The potential effects of offshore wind energy on protected marine species are regulated primarily by National Oceanic and Atmospheric Administration (NOAA) under the Endangered Species Act, Marine Mammal Protection Act, National Environmental Policy Act, National Marine Sanctuary Act, and the Energy Policy Act and by BOEM through issuance of leases and approval of COPs. During activities where potential adverse effects may occur to marine species, including marine mammals, a combination of visual surveying and passive acoustic monitoring (PAM) may be required to record information on species presence and behavior to inform BOEM and NOAA mitigation requirements measures aimed at minimizing potential effects. PAM may also be required during operations to record ambient noise levels and to monitor noise impacts on marine species. In addition, PAM is a proven method for monitoring calling species to gain ecological context before, during, and after offshore wind development activities (site characterization, construction, operations, and decommissioning).
The inclusion of PAM alongside visual data collection is valuable to provide the most accurate record of species presence as possible. In the case of data collection for mitigation, visual observers will add to the detection probability of the species of interest, which is ideal when aiming for a 100% detection rate. While in the case of data collection for monitoring visual surveys, PAM can be seen as orthogonal and complementary methods. PAM provides long continuous time series with low spatial resolution, while visual surveys provide snapshots with low temporal resolution but high spatial resolution. Just as visual observations can be limited by poor weather and light conditions, PAM systems also have limitations, such as when animals are not calling. Visual and PAM approaches are well understood to provide best results when combined together (e.g., Barlow and Taylor, 2005; Clark et al., 2010; Gerrodette et al., 2011). However, in these recommendations we just focus on the applications and uses of PAM.
Passive acoustic monitoring encompasses a functional suite of technologies that can answer scientific questions and inform management and/or mitigation decisions over long temporal and large spatial scales (Rountree et al., 2006; Van Parijs et al., 2009, 2015; Marques et al., 2013; Gibb et al., 2019). The tools that are available to acquire and analyze passive acoustic data have undergone a revolutionary change over the last couple of decades and have substantially increased our ability to both collect extensive time series and apply PAM as a functional management tool (e.g., Mellinger et al., 2007; Luczkovich et al., 2008; Van Opzeeland et al., 2008; Zimmer, 2011; Sugai et al., 2019; Desjonquères et al., 2020). PAM platforms include moored recording buoys, autonomous underwater or surface vehicles (Autonomous Underwater Vehicles/Autonomous Underwater Vehicles), profile drifters, and towed hydrophone arrays (Figure 1), which can be strategically located to provide real-time information for immediate mitigative decision-making, monitor or assess the effects from specific activities, and gather continuous archival recordings for long-term monitoring, periodic evaluation, and adaptive management (Van Parijs and Southall, 2007; Van Parijs et al., 2009). PAM allows a broad spectrum of data to be collected, including all calling marine animal species within recording range, different call types, distributions and occupancy, individual calling locations, and abundance of some species, as well as anthropogenic and other natural sounds, collectively known as an underwater “soundscape” (e.g., Van Parijs et al., 2009; Marques et al., 2013; Mooney et al., 2013; Baumgartner et al., 2018; Figure 2).
Figure 1. The illustration shows examples of different types of acoustic technologies. From left to right, the illustration shows a moored surface buoy, wave glider, SoundTrap on the seafloor, bottom-mounted acoustic recorder (High-frequency Acoustic Recording Package [HARP]), Slocum glider, NOAA ship towing a hydrophone array, tagged Atlantic cod, humpback whale with an archival tag, drop hydrophone deployed from a small boat, and autonomous, free-floating acoustic recorder (Drifting Acoustic Spar Buoy Recorder [DASBR]). The different technologies are highlighted with colored circles that show a zoomed-in view of the instruments and colors that represent the type of data collected: green for real-time data capabilities, orange for archival data, and blue for active acoustics.
Figure 2. This conceptual illustration shows images of anthropogenic (human-created), biological (marine animal), and abiotic (environmental) sources of sound and approximately proportional sound waves, making up an ocean soundscape. The sound sources include weather, earthquakes, snapping shrimp, harbor seal, Atlantic cod, right whale, sperm whale, common dolphins, fishing vessel, shipping vessel, seismic survey ship, and wind farm development. The sound waves are represented by overlapping colored circles that indicate the type of sounds: human-made sounds are orange, animal sounds are light blue, and environmental sounds are light green. The circles increase in size to show the approximate magnitude of sound waves and distances noise travels underwater.
Although our primary focus is on marine mammal mitigation and monitoring for offshore wind applications, the PAM techniques mentioned here can also be used to characterize soundscapes, monitor ambient noise levels, and provide essential information on other soniferous species such as fishes (e.g., Zemeckis et al., 2019; Caiger et al., 2020). As offshore wind development expands across regions, PAM data can increase in utility when collected in a standardized method and analyzed using similar techniques. Given the value of PAM data, especially for future permit requests, authorizations, and research, these recommendations also contain information on standardizing data collection methods, processing and analyses, archiving acoustic recordings, and data products, as well as steps to making these products publicly available. Several previous workshops have started the discussion to improve standards for PAM data collection, data analyses, and archiving (BOEM, 2018; Gulka and Williams, 2018; Kraus et al., 2019; POWER-US, 2019; NYSERDA, 2020; BOEM, 2021; WCS, 2021), and standards are increasingly being documented in the Oceans Best Practices Repository1. Our recommendations build on these previous efforts.
Project-specific PAM Plans, developed by project proponents and approved by Federal agencies, should include descriptions of equipment, procedures (deployment, retrieval, detection, and analyses), ISO data quality standards and protocols that will be used for monitoring and mitigation. In the United States, PAM specifications for inclusion in a PAM Plan will need to be developed in consultation with NOAA and other permitting agencies, such as BOEM. To design a PAM Plan, the following six topics need to be included and addressed: species of interest, PAM system types, PAM recording technologies, PAM study design, PAM system requirements, and PAM data archiving and reporting.
Prior to designing any PAM Plan, it is essential to identify and understand the acoustic frequency ranges of the sound sources that are of interest and in need of monitoring (Figure 3). Unlike in southern North Sea waters, where only a handful of marine mammal species require consideration, most United States waters are frequented by a large number of protected species (Jefferson et al., 2011). In the case of the Atlantic OCS, where offshore wind energy development will initially occur, the primary baleen whale (mysticetes) species of concern include: North Atlantic right whales (North Atlantic right whales; Eubalaena glacialis), humpback whales (Megaptera novaeangliae), minke whales (Balaenoptera acutorostrata), fin whales (Balaenoptera physalus), blue whales (Balaenoptera musculus), and sei whales (Balaenoptera borealis). These species are low-frequency sound producers (i.e., most of the acoustic energy is below 1 kilohertz [kHz]), and therefore all PAM recording technologies, PAM system requirements, and PAM designs need to be constructed with these frequency requirements and specific call types in mind (Table 1). Other species of interest for this region are the higher frequency producing toothed whale (odontocetes) species, such as sperm whales (Physeter macrocephalus), beaked whales (Ziphiidae), pilot whales (Globicephala spp.), dolphins (Delphinidae), and ultra-high-frequency harbor porpoises (Phocoena phocoena). The frequency ranges for these species and the need for additional PAM recording technology, PAM system requirements and PAM design needs to be considered when creating a PAM Plan (Table 2). Additional species of interest are acoustically active fishes or invertebrates, for which a combination of PAM and acoustic telemetry can be used to delineate the temporal and spatial extent of spawning grounds (e.g., Ingram et al., 2019; Zemeckis et al., 2019). Fish are generally low-frequency sound producers with most species’ core frequency occurring below 1 kHz (Figure 3).
Figure 3. This figure shows the frequency ranges over which groups of marine animals call (top) and the primary frequency ranges over which some anthropogenic sound sources (bottom) overlap. The frequency scale is on a logarithmic scale, expanding from 1 to 200,000 Hz. Mysticetes (baleen whales), such as the North Atlantic right whale, fin, sei, blue, humpback, and minke whale, produce low-frequency sounds, while odontocetes (toothed whales), such as the sperm whale, produce higher frequency sounds. Anthropogenic sound sources and primary frequency ranges are displayed on the bottom of the figure, with the bracketed boxes corresponding to the following high resolution geophysical (HRG) equipment sound sources: (A) Sparkers/boomers; (B) Non-parametric sub-bottom profilers; (C) Acoustic corers; (D) Acoustic positioning systems; (E) Parametric sub-bottom profilers; (F) Multibeam echosounders and sidescan sonar.
Table 1. A list of the primary baleen whale species found along the Atlantic Outer Continental Shelf and their primary call type, call frequency range, and core detection bandwidth.
Table 2. A list of the primary odontocete species found along the Atlantic Outer Continental Shelf and their primary call type, frequency range, and core detection bandwidth.
Here, we divide the PAM approaches into two different system types of data collection, archival and real-time data collection (Figure 1). Both the scientific objectives and the specifications for data management differ depending on the data collection methods. These approaches may have distinct applications for either mitigation or long-term monitoring, or have utility for both applications.
Archival PAM recordings are primarily used for long-term monitoring, with capabilities for recording durations ranging from several weeks up to several years (Sousa-lima et al., 2013). Continuous recordings provide an uninterrupted record of species’ acoustic presence, allowing investigators to evaluate species distribution and occurrence, and changes in animal calls, which provide information on behavioral state (e.g., foraging, reproduction, socializing) in a given area or region. In addition they allow for the evaluation of seasonal, inter and intra annual variation in species presence and occurrence over time. Alternatively, recordings can be duty-cycled (defined as the fraction of time that a PAM system is actively recording) to maximize recording duration at sea while limiting equipment interactions (e.g., retrieval to swap out hard drives or batteries). Duty-cycled data is less preferred, as inevitably some information is lost, and biases are introduced by using a reduced recording schedule. If the duty-cycle listening period and recording interval are not appropriately matched to the duration and timing of animal calls, potential detections may be missed and species occurrence underestimated (Miksis-Olds et al., 2010; Sousa-lima et al., 2013; Thomisch et al., 2015; Stanistreet et al., 2016). For example, in Thomisch et al. (2015), duty-cycling at 50 and 2% showed a decrease in accuracy in both acoustic presence and call rate estimates. If it is necessary to duty-cycle, frequent and shorter recording periods may improve accuracy of daily acoustic presence. Duty-cycling effects are most pronounced for species with low and/or temporally clustered calling activity (Thomisch et al., 2015)—i.e., non-song call types such as the North Atlantic right whale—and are less pronounced for species that click over long time intervals, such as beaked whales (Stanistreet et al., 2016). For higher frequency species, a PAM click detector recorder can be an efficient method for data collection (e.g., Bailey et al., 2010a; Temple et al., 2016; Wingfield et al., 2017). The click detector recorder stores continuous higher frequency clicks of delphinids, harbor porpoise, and other high-frequency odontocetes but does not provide a PAM sound record as is the case for most other recorders.
Archival PAM systems are often moored near the seabed with no surface expression and are returned to the surface by divers or by using an acoustic release mechanism. Acoustic data are therefore only recovered and analyzed at the end of the recorder deployment. Consequently, the analyses that are conducted will be retrospective and not real time. However, the need to wait for data records until retrieval can be resolved by using archival PAM systems with surface expression such as those used by Brandt et al. (2018) allow for more frequent data collection. Archival data can be useful to build long-term monitoring records of the presence of sound producing species, both temporally (seasonal and yearly occurrence) as well as spatially (occurrence in and across different regions). These data can also be valuable for evaluating potential effects of construction as changes in species presence and behavior can be correlated with construction activities.
Real-time PAM systems that enable rapid detection and recognition of marine mammal calls are invaluable for monitoring but are essential components for mitigating potential effects from wind energy development. Real-time acoustic alerts can be used to respond quickly to the presence of protected species in a construction area (e.g., during impact pile driving as long as the presence of multiple construction noise sources do not mask their presence) or in the vicinity of transiting vessels, thereby reducing the risk of vessel strike (e.g., Spaulding et al., 2009; Baumgartner et al., 2019, 2020, 2021; Norris et al., 2019; Kowarski et al., 2020; Wood et al., 2020). Real time is defined here as the relay of PAM data (processed or raw) within an operationally usable time span (e.g., data relay frequency may range from every minute, hour, to daily, depending on how quickly the information is needed for decision-making). In effect, any data from the acoustic detection can be used to optimize, or at least provide, timely information to help direct current operations and/or tracking of a species (Klinck et al., 2012; Baumgartner et al., 2013, 2019, 2020; Kowarski et al., 2020). Real-time PAM can also be used to improve and adjust to noise produced by pile driving, by offering real time feedback on the noise produced by the hammer and the capacity to adjust this if needed. Real-time PAM can be conducted from a variety of platforms, including vessels, surface buoys, autonomous vehicles such as gliders, and drifting buoys. The PAM data will travel from a PAM recording sensor to the receiving station on shore/vessel at regular time intervals agreed upon in the PAM Plan. The frequency of data relay is constrained by the type and cost of data upload. Cell phone towers can be used for data relay if sensors are located close to land, as reception tends to be lost beyond 15 miles offshore. Iridium satellite data transmission (currently the most common type) costs are based on the quantity of data and frequency at which you want to upload, and which data service plan you select. Another possible option is cabled arrays laid out on the seafloor, which can also provide a real-time data feed straight to shore where such an installation is feasible [e.g., Lindsey et al. (2019)].
There was an ever-increasing number of PAM technologies, varying in recording and data collection capabilities, available to broader science, management, and industry communities (Figure 1). The following represent several of the general categories of recording technologies currently available. The considerations and recommendations provided in the PAM System Types section (“PAM System Types”) above should be evaluated based on the chosen recording technology.
Passive acoustic monitoring fixed, bottom-mounted archival recorders are moored on or near the ocean floor for several weeks to months (many recorders can now record continuously for 4 to 6 months), and up to several years. They should be spaced at distances that encompass the estimated calling/detection radius of the species of interest when they are within, and in the vicinity of, the operating area. The detection radius will vary depending on a number of factors, including those influencing signal propagation (i.e., water depth and temperature, substrate type, noise levels), as well as source level and directivity differences in calls between individuals and species. An estimate of the minimum number of hydrophones needed for detection of the call types of the species of interest should be made and hydrophones placed with these considerations in mind to minimize potential missed portions of the operating or surrounding areas (Figure 4; Table 3). The percentage of area that is desirable or required to be covered by the hydrophone spacing needs to be considered. An example of such a design can be seen in Supplemental Information I, where we aim to have 100% coverage of the acoustic radius of a calling North Atlantic right whale individual within the lease block areas and 50% coverage outside of the areas. In addition, where required, multi-element bottom-mounted hydrophone arrays can be used to track the movements of calling individuals (e.g., Stanistreet et al., 2013).
Figure 4. This figure provides a stylized example of the average acoustic detection ranges of varying categories (A–E) over which different species groups and representative call types for each can be heard. Detection range A covers 0.1 km and includes sounds such as Atlantic cod (Gadus morhua) grunts. Detection range B covers up to 0.5 km and includes harbor porpoise (Phocoena phocoena) clicks and other fishes. Detection range C covers up to 6 km and generally includes dolphin species whistles. Detection range D covers up to 10 km and includes vocalizations of baleen whale species such as North Atlantic right whale (Eubalaena glacialis) upcalls and sperm whale (Physeter macrocephalus) clicks. Detection range E covers a range from 20–200 km and represents vocalizations of other baleen whale species such as blue whale (Balaenoptera musculus) and fin whale (Balaenoptera physalus) song. Circles sizes are not to scale.
Table 3. A list of the PAM platform types that are currently used for the collection of data for monitoring or mitigation purposes, the spatial scale over which they can collect data, the format and type of data collected, and the current applications in which they have been used to date.
Passive acoustic monitoring surface buoys are a valuable technology used for both relaying real-time information at regular intervals as well as collecting long-term archival data from a single location. They have been used effectively for monitoring and for mitigation purposes, spaced at appropriate listening distances for the species of interest, in busy shipping lanes, as well as in numerous other areas, including prospective wind leases (Table 3; Supplementary Information II). As there is a connection to the surface, noise produced by the mooring/floatation system can add unwanted noise and must be carefully considered in the design phase. In the cases where these buoys have been used successfully, the surface recorders were anchored using a special mooring system constructed to reduce noise (e.g., Baumgartner et al., 2019). PAM moored surface buoys are essential for the purpose of real-time mitigation, but they are also extensively used for long-term monitoring of species presence. PAM real-time buoy systems should be placed with similar listening distances in mind as for PAM bottom-mounted recorders. However, depending on the use of the platform and requirements, the number of moored surface buoys may differ. If the intent is to minimize ship strikes in a high vessel transit area, the PAM moored surface buoys should cover the vessel transit lanes (see example in Table 3 and Supplementary Information II). However, if the intent is to listen for the presence of endangered species in the vicinity and within the wind lease construction area, then the number and spacing of buoys should reflect the effective listening area that needs to be covered (see Supplemental Information II for an example).
An Autonomous Underwater Vehicle is a robot that travels underwater without requiring input from an operator. Underwater gliders are a subclass of Autonomous Underwater Vehicles and can have a single PAM recorder or a PAM array placed inside or strapped to the outside of the vehicle. Autonomous Underwater Vehicles are boats or ships that operate on the surface of the water without a crew and can similarly be equipped with PAM recording equipment. PAM Autonomous Underwater Vehicles are very versatile and increasingly used to monitor large spatial areas [with the capacity to cover 10 to 1000 s of kilometers (Baumgartner et al., 2020)] over long time periods (generally from 3 to 6 months) and to relay back information either in real time or through archival recordings. They can be programmed to follow tracklines and navigate to new positions throughout a deployment, either diving up and down the water column to collect oceanographic data or following a straight path at a set depth. Gliders can be categorized into battery-operated gliders and wave-propelled gliders. The former have been demonstrated to be highly effective for real-time monitoring and mitigation for North Atlantic right whales and other baleen whale species (Table 3; Baumgartner et al., 2014, 2020; Kowarski et al., 2020). They have also been shown to be valuable in understanding the spatial distribution of toothed whales, such as beaked whales (Klinck et al., 2012). Wave gliders have had some success in archival monitoring of toothed whales (Küsel et al., 2017) but still require further development. In particular, the self-noise produced by the wave glider continues to limit the detection capability of low-frequency baleen whales (Baumgartner et al., 2021). Further technological development may be able to shield this noise in the future. Similarly, Autonomous Underwater Vehicle technologies, such as sail drones and self-navigating vessels, are in development and will likely present new and innovative solutions to add to the current suite of real-time and archival solutions for PAM in the near future (Klinck et al., 2009; Mordy et al., 2017).
Autonomous Underwater Vehicles are ideally suited for monitoring an area to inform BOEM and NOAA mitigation requirements. Their tracklines can be remotely piloted or redirected if needed while the instrument is out at sea. For example, if the glider locates an area of high vocal activity, it can be instructed to stay in place and only move on once the activity has decreased. Currents and wind can influence coverage of tracklines, and this needs to be taken into consideration during the design process (Figure 5). Again, tracklines should be designed with consideration of the listening radius of the focal species and the total detection radius of the mitigation and monitoring area that is required. Gliders are slow moving instruments (∼ 3 knots) that provide point sample data. If the aim is to understand a small area in great detail so as to not miss the presence of the species of interest, then dense tracklines that are surveyed frequently are recommended. If the intention is to cover a large area to detect individuals before an activity requiring mitigation occurs or to inform BOEM and NOAA mitigation decisions over larger areas, then broader tracklines are needed to be able to cover the detection area in question. This will result in less frequent coverage of a trackline, unless several gliders are used. The detection radius and duration it would take a glider to cover the entire desired mitigation area would need to be considered in determining the confidence in monitoring of the detection area for broadly spaced tracklines over a set time period, for example over 24-hour segments. In contrast to fixed recorders, the information that gliders can provide is sparse but spatially broad (Table 3).
Figure 5. Example of (A) proposed glider tracklines compared to (B) actual tracklines traveled from a real-time slocum glider deployment off Cox’s Ledge in 2019. This design was used both for detecting spawning Atlantic cod as well as North Atlantic right whales and other baleen whale species.
Passive acoustic monitoring drifters involve the deployment of acoustic recorders that have a single hydrophone and/or a vertical array suspended in the water column, depending on their design. They typically have a surface expression that allows for satellite tracking capabilities for subsequent recovery. Due to their surface expression, they can provide either real-time information or archival recordings, depending on what is needed. Their location and movement patterns are dependent on currents and wind. Unlike gliders, they cannot be repositioned or redirected remotely. This technology has potential, but has not yet been extensively used or tested in many studies, although it shows promise for certain applications such as monitoring species presence, estimating abundance and measuring ocean noise metrics (e.g., Barlow et al., 2014; Griffiths and Barlow, 2016; Fregosi et al., 2018). When incorporating vertical line arrays in PAM drifters, it can provide information on vertical bearing angles, thus obtaining the estimates on the depth of vocalizing animals (e.g., Griffiths and Barlow, 2016).
Passive acoustic monitoring drifter placement and density can vary greatly depending on the monitoring goal and the local oceanic conditions. Currents and wind direction would need to be well understood because they will affect each drifter independently. It is important to determine how the oceanographic conditions of the area will affect coverage of the monitoring region using these types of platforms. For example, if the site is near areas of high current, such as the Gulf Stream, the recorders may be quickly displaced when they reach that current. However, in a more sheltered, less dynamic environment, PAM drifters could provide good coverage. Drifters can be outfitted with satellite transmitters and so can be retrieved once their data collection period is over. In contrast to fixed recorders, the information that drifters can provide is sparse but spatially broad; however, unlike Autonomous Underwater Vehicles, they are constrained in the areas they cover by ocean currents, tides, and wind (Table 3).
When it is necessary to know the range, bearing, location, or depth of a vocalizing marine mammal (e.g., for real-time tracking or estimating abundance of individuals), an array of time-synchronized hydrophones is required (Thode, 2004; von Benda-Beckmann et al., 2010; Gillespie et al., 2013; DeAngelis et al., 2017). PAM arrays may be towed behind a vessel that is underway, but in this case, flow noise can obstruct low-frequency sounds from being heard. Generally, lower speeds produce lower flow noise; however, speed must also be considered to maintain horizontal orientation of the towed array. This generally prevents towed arrays from being useful to monitor for low-frequency baleen whale species unless the ability to record low-frequency sounds over the noise is clearly demonstrated. The Acoustical Society of America (ASA) is currently working to develop an American National Standards Institute-approved standard for towed hydrophone arrays. The fundamental goal of this ASA standard is to reduce situations where background noise levels prevent effective PAM. To achieve this goal, the standard employs a suite of strategies to standardize how acoustic measurements are logged, reported, and evaluated (Thode and Guan, 2019). Typical PAM arrays for monitoring purposes can be towed behind a vessel (or Autonomous Underwater Vehicle), in which case the tracklines to be covered by the vessel (or Autonomous Underwater Vehicle) with the array need to be designed with the listening radius of the system and species in mind (Table 3). Other types of arrays, such as bottom-mounted cabled arrays, are not discussed here because they are less frequently used, given the cost and infrastructure needed to lay and maintain them.
The study design is a critical component to any monitoring program and needs to be carefully defined. Both the study objectives and the capacity to address these objectives need careful consideration. Three basic questions need to be asked and addressed in any study design: Why monitor? What needs to be monitored? How should monitoring be carried out? (Yoccoz et al., 2001). PAM technology can be used to satisfy a wide range of monitoring and mitigation requirements (e.g., Bailey et al., 2010b; Forney et al., 2017; Brandt et al., 2018). In relation to wind energy development, there are several questions that most monitoring and mitigation programs need to address: does wind energy activity within and across multiple lease areas affect marine animal distribution, behavior, and communication space, and how can we reduce vessel strike risk and prevent exposure of marine animals to loud sounds during construction activities in the wind energy lease areas?
To answer the first question, baseline acoustic data collection is essential in order to build an understanding of the inter- and intra- year variability of species presence in an area. Robust baseline monitoring allows for inference to be drawn as to the cause of any observed changes and whether they are a result of oceanographic, ecological, or climatological factors, or due to anthropogenic effects. Both large-scale and small-scale trends and changes in species distribution, occurrence, calling behavior (e.g., foraging, socializing, reproduction), and movements can be derived from archival PAM data collection. For examples of large-scale monitoring studies for baleen whales see Davis et al. (2017, 2020), toothed whales see Barlow and Taylor (2005), Verfuß et al. (2007), Stanistreet et al. (2017), Carlén et al. (2018), Stanistreet et al. (2018), and fishes see Wall et al. (2012), Wall et al. (2013). For small-scale regional or area specific monitoring studies for baleen whales see Parks et al. (2007), Morano et al. (2012a), and Charif et al. (2019); toothed whales see Lewis et al. (2007), Johnston et al. (2008), and Bailey and Thompson (2010), and fishes see Rowell et al. (2015), Zemeckis et al. (2019) and Caiger et al. (2020).
Passive acoustic monitoring archival recordings are also increasingly being used to monitor the long-term ambient noise and communication space available to marine animals is a given area, in addition to the composition and health of marine soundscapes e.g., the prevalence of non-biological or anthropogenic sound sources (Clark et al., 2009; Hatch et al., 2012; Staaterman et al., 2014; Erbe et al., 2016; Merchant et al., 2016; Haver et al., 2018). Potential effects of anthropogenic activities on marine species can be evaluated through applying the collected data to analytical frameworks, such as Before-After-Control-Impact (BACI) and Beyond-BACI designs (e.g., Underwood, 1992, 1994), or Before-After-Gradient (BAG) analyses (Ellis and Schneider, 1997; Brandt et al., 2011; Methratta, 2020). Some of the first applications of BACI to offshore wind development evaluation for marine mammals are Carstensen et al. (2006) and Scheidat et al. (2011).
To answer the mitigation question on reducing vessel strikes and preventing exposure to loud construction sounds, robust real-time monitoring needs to be established throughout the impacted area and the area directly in the vicinity. The timing of data reporting and the subsequent actions taken need to clearly show how it will be effective at minimizing risk. In Supplemental Information I we outline and map a regional PAM monitoring design approach for long term monitoring focused primarily on baleen whales. In Supplemental II, we discuss design approaches and considerations for mitigation of vessel strike risk, while in Supplemental III we provide ISO data templates which serve as guidelines for consistent and standardized data collection.
When PAM is utilized, the design intent may be to:
(1) Understand distribution of species.
This involves monitoring a given area prior to, during, and after the construction period in order to understand the presence and distribution of species of interest. The duration of this monitoring can vary, but in order to capture variation in movement patterns, data collection is recommended at least 3 to 5 years prior to construction, during construction, and at least 3 to 5 years during wind farm operation. It would be a best practice to continuously collect the data during these time periods was continuous through the three phases (pre-construction, construction, and operations). Multiple years of data from the same area are needed in order to understand the inter-annual variability in species movement. These data provide an understanding of the annual presence, occupancy, and distribution of a species; help discern the potential impact of other factors, such as climate change, that may influence distribution; and help determine the likelihood of the species being in the area during construction and/or during subsequent long-term operation. They can also help understand any changes, or lack thereof, in species’ acoustic presence related to construction activities or turbine operation (e.g., Carstensen et al., 2006; Brandt et al., 2011; Scheidat et al., 2011; Dähne et al., 2013, 2017). For example, Brandt et al. (2011) were able to demonstrate a decreasing effect of construction noise on harbor porpoise acoustic activity with distance using a BAG design. Seasonal and annual variations in presence and distribution can also be analyzed with respect to oceanographic conditions (e.g., DNV KEMA Renewables Inc, 2018). Paired with metocean monitoring and visual survey data, these efforts can add meaning as both methodologies can complement each other and reduce their biases (e.g., when a marine mammal is not calling or not visible at the surface). Supplemental Information I provides an example of a proposed United States East Coast PAM design for understanding distributional changes of species with regards to offshore wind development.
(2) Monitoring to reduce effects on species during construction.
This focuses on monitoring a given area during the construction period of a wind development area to inform mitigation actions, such as delaying, ceasing, or proceeding with pile driving when a protected species is confirmed acoustically within a relevant impact zone (i.e., Shutdown or Clearance Zone). Rules for defining which acoustic information triggers this decision should be established in advance and in concert with visual monitoring. Applying any mitigating action based on PAM needs to be clearly thought through with consideration to limitations of each type of system and thoroughly described in the PAM Plan. Supplemental Information II provides an example of a proposed United States East Coast PAM design for monitoring in order to reduce effects on species during construction.
(3) Monitoring for reducing risk of vessel strike.
In order to monitor for species presence to reduce vessel strike risk, the design of the PAM system must be able to reliably detect the presence of the species of interest. Additionally, a thorough decision-making and communication process when a detection is made is needed to ensure that vessels are alerted and slow down to reduce vessel strike risk. An example of this decision-making process is the triggering of NOAA’s Slow Zones2. These Slow Zones are established when North Atlantic right whales are detected both visually (i.e., Dynamic Management Area) and acoustically (i.e., Acoustic Slow Zone). A Dynamic Management Area is triggered when 3 or more North Atlantic right whales are sighted within 3–5 miles of one another. This criteria emerged from Clapham and Pace (2001), which showed an aggregation of three or more whales is likely to remain in the area for several days, in contrast to an aggregation of fewer whales. Given that visual and acoustic data differ, where the number of individual North Atlantic right whales cannot yet be derived from acoustic data alone, an Acoustic Slow Zone is established when three or more upcall detections from an acoustic system occur within an evaluation period (e.g., 15 min), an acoustic equivalent determined by NOAA NEFSC acoustic experts. To trigger an Acoustic Slow Zone, an acoustic system must meet the following criteria: (1) evaluation of the system has been published in the peer-reviewed literature, (2) false detection rate is 10% or lower over daily time scales, and (3) missed detection rate is 50% or lower over daily time scales. Once triggered, Slow Zones are set up as a rectangular area encompassing a circle of 15 (for Dynamic Management Areas) or 20 (for Acoustic Slow Zones) nautical miles around the core sightings (Dynamic Management Area) or recorder location at the time of detection (Acoustic Slow Zone). The Slow Zone lasts for 15 days and can be extended with additional sightings or acoustic detections. Supplemental Information II provides a more detailed example of a United States East Coast PAM design for reducing vessel strike risk.
All real-time PAM designs need a clear and well thought out and consistent process, including PAM placement and technology type, species detection, integration with other visual data observations, communication of information to Protected Species Operators (PSOs)/shoreside operators, and response of the information/detection. Limitations of each real-time PAM system should be well understood and considered in detail in any PAM Plan. Efficacy of the system used and its capacity to detect the signal/species of interest is essential to developing a successful and credible PAM Plan. Lastly, it is important to note that no one PAM system is capable of answering all needs and that frequently a mixture of PAM systems, technologies and designs are likely needed to address all monitoring and mitigation requirements.
In this section, we present some broad PAM system requirements both in terms of hardware needed and automated software for analysis of calls and ambient noise metric measurement. Standards and guidelines are increasing in availability (e.g., Robinson et al., 2014; van der Schaar et al., 2017; Ainslie et al., 2019), through projects such as ADEON (Atlantic Deep Water Sea Ecosystem Observatory Network3), JONAS (Joint Framework for Ocean Noise in the Atlantic Seas4), JOMOPANS (Joint Monitoring Programme for Ambient Noise North Sea5), additional existing practices on PAM such as the International Quiet Ocean Experiment can also be found through the Ocean Best Practices Repository6.
For all PAM technologies, the hydrophones and related hardware need to be calibrated (every 3 to 5 years) and their performance systematically measured and optimized within frequency bandwidths of interest for the particular activity, species, and environment. Calibration data, and relevant settings and sensitivities should be noted for all hardware used in recording/monitoring to ensure consistency among measurements for particular hardware and software [more detail can be found in Biber et al. (2018)]. Array synchronization information (where relevant) should also be documented. This information should be permanently associated with the recordings as metadata.
All hardware should be tested and optimized for low self-noise, including the mooring system. In addition to calibration, the system should be fully tested to ensure adequate sensitivity in the area where it will be deployed and with the type of signals it would receive. Additional environmental data will need to be collected to allow for adequate system evaluation. If this cannot be done at the project site, the system should be fully tested in a comparable location (i.e., an area exhibiting similar depth, temperature, substrate, current, acoustic propagation, and ambient noise, with relevant sound sources).
At a minimum, the following specifications should be measured and reported on:
• Bandwidth and frequency response (i.e., 10 to 2000 kHz)
• System sensitivity (i.e., −207 dB re 1 V/μPa @ 1 kHz) and dynamic range (dB)
• System self-noise (i.e., the equivalent bandwidth noise pressure level)
• Gain (dB)
• Directional response (i.e., omnidirectional or angular dependent)
• Sample rate (kHz)
• Sample resolution (i.e., 12 Bits)
• Recording schedule (i.e., recording duration/interval).
The system needs to be designed, installed, and operated by those having expertise with the specific PAM technology, including placement in the water, attachment of cables to reduce strumming and noise, acoustic release, suitable anchorage for the conditions, software use, etc., Knowledgeable and experienced personnel should operate the units in all situations.
Ideally, the PAM technology used should have been used for the same purpose in other field efforts and have clear and detailed information available about its previous performance and reliability for PAM purposes. If this is not the case, this information needs to be gathered and provided in publicly available documentation as part of the PAM Plan.
Passive acoustic monitoring data analyses for species presence should occur through either a) visual processing of data by an acoustic expert familiar with the call types of the species of interest, or b) by using comprehensively tested PAM software detector(s) for which performance has been documented, and the performance metrics are publicly available for outside evaluation and have been reviewed and deemed acceptable by a panel or group of experts. Visual review will likely be required to some degree when dealing with acoustic detection of rare species such as North Atlantic right whales and/or for ensuring data quality. Standard performance metrics require evaluation and reporting, such as precision, recall, and accuracy, as well as false detection, false positive, false omission and missed detection rates (Figure 6) (e.g., Baumgartner et al., 2019; Kirsebom et al., 2020; Madhusudhana et al., 2020; Gervaise et al., 2021). PAM software detectors comprise a wide range of custom-built computer programs, aimed at automating the process of detecting target species’ calls in a dataset [see review in Bittle and Duncan (2013), Shiu et al. (2020), Gervaise et al. (2021)]. In both PAM archival and real-time data analysis, in addition to any software detector(s) used, some level of visual confirmation by an acoustic expert often still remains essential to improve accuracy and minimize error in call type reporting.
Figure 6. Definitions of performance metrics for comparing occurrence estimates from the near real–time and audio analyses. A good detection process minimizes the quantities in red and maximizes the quantities in black. Replicate of Figure 4 in Baumgartner et al. (2019).
For PAM archival data analysis, where the objectives tend to focus on retrospective understanding of species presence, movements, or behavior, daily or hourly reporting of species detections are the time frames most frequently used (e.g., Davis et al., 2017, 2020; Stanistreet et al., 2017; Halliday et al., 2019). This method can speed up the process of data analysis by only requiring a positive species confirmation at the hourly or daily level. For example, when evaluating the presence of North Atlantic right whales along the United States East Coast, the presence of three upcalls within a 24-hour time period serves as the determination that at least one whale is present during that day (Davis et al., 2017). Three upcalls, rather than a single upcall, are used in order to decrease the likelihood of incorrect species determination given that other baleen whales can produce similar calls to North Atlantic right whale upcalls (Davis et al., 2017). However, each species and call type will require different levels of additional verification as needed (if any) and decisions made as to what level of certainty is acceptable. For example, automated detectors for 20 Hz fin whales have a high detection accuracy (e.g., Morano et al., 2012b), and, in this case, it may be reasonable to simply take the detector output with no further evaluation.
For PAM real-time data analysis, additional visual verification of the detected sound is likely to be needed since the occurrence of a given call type may influence whether operations are able to continue or are required to shut down (in the situations where shut down is possible). The ability to satisfy this confidence metric can be achieved by carrying out PAM training for operators and through evaluation of detections by analysis experts. A PAM expert is defined as a scientist who has 6 months or more of experience working with the call types of the species of interest, who can distinguish between confounding sounds (Kowarski et al., 2020), and who has experience working with the relevant detection software. Additionally, established and publicly available protocols on how a species is determined present for the specific PAM system and software must be documented in the PAM Plan.; for an example, see DeAngelis et al. (2016).
All PAM Plans should provide clear documentation of the efficacy of their detection capabilities and classification software for the specific signals of interest. Examples of comprehensive testing for real-time and archival PAM can be found in Baumgartner et al. (2019, 2020), Kowarski et al. (2020), and for PAM towed arrays can be found in Gillespie et al. (2013).
The PAM Plan should demonstrate for all PAM systems and moorings that (a) the species’ signal of interest can be heard reliably beyond the self-noise, and (b) any detection and classification software that is used has (1) been tested; (2) clearly documented reliability in detecting a given species; and (3) software performance metrics that are openly available.
Localizing calling species during the construction phase of offshore wind projects would be very useful for satisfying mitigation requirements regarding the location and distance of the species in question, relative to the sound source (e.g., pile driving location). Localization can be carried out by the placement of multiple fixed or mobile omnidirectional hydrophones arranged in a configuration that allows for localization of the vocalizing animal using the difference in the time of arrival of a call (or calls) on multiple time-synchronized sound recorders (e.g., Stanistreet et al., 2013; Hastie et al., 2014; Risch et al., 2014; Gillespie et al., 2020; Gervaise et al., 2021). It can also be achieved by using multiple sensors that can calculate bearing [e.g., directional autonomous seafloor acoustic recorders (DASARs); Greene et al., 2004; Blackwell et al., 2007; Mathias et al., 2012; Blackwell et al., 2013]. For stationary systems, a minimum of three hydrophones placed within a range that guarantees overlapping receptivity (i.e., multiple arrivals) is necessary to localize the positions of vocalizing animals using time-of-arrival methods (e.g., Stanistreet et al., 2013; Tremblay et al., 2019). Sensor positional, timing, and speed accuracy all need to be considered, as well as sensor configuration (geometric dilution of precision). However, for mobile platforms, such as a ship with a linear towed hydrophone array, two or more hydrophones can be used for the calculation of bearings; sequential bearing calculated as the platform moves can be used to estimate the location of calling animals (i.e., time-motion analysis, typically with left-right ambiguity) (e.g., von Benda-Beckmann et al., 2010; von Benda-Beckmann et al., 2013). In most cases, decisions on mitigation measures (e.g., pile driving shutdown when feasible or vessel speed reduction) can be made simply based on the range of calling animals from the noise source, without resolving the left-right ambiguity issues or knowing the bearing of the calling animal. However, under certain situations with anisotropic noise propagations, it would be necessary to know the location of the calling animal. Under such situations, localization and methodology should be included in the PAM Plan to approximate locations of the animal or sound source for purposes of taking action. Bottom-mounted recorders, real-time systems, towed and vertical arrays, and drifters can all be used for localization purposes, depending on the accuracy needed and the species of interest. Determining which system and technology to use requires careful consideration and supporting evidence to demonstrate that the design is appropriate. The analytical component of localization can be highly time consuming, which can be costly, and each array design requires careful documentation of the localization errors of the system.
The measurement of background sound levels—i.e., ambient noise metrics—is an additional and important dataset that can be obtained from acoustic recordings made on any platform. Although the primary focus of this effort is to document the vocalizations of marine mammals, additional acoustic analyses of abiotic acoustic sources in the same recordings can reveal temporal patterns in distinct frequency bands that correlate with other factors such as wind speed. Ambient noise metrics could also document the level of potential increases in ambient noise due to wind farms. Metrics for the coincidental recording of ambient noise should be included in PAM Plans and include factors anticipated to be associated with offshore wind development such as vessel traffic and operational noise. These kinds of ambient noise metrics provide a record of acoustic conditions in a given environment and are essential for understanding changes in the sound levels across different regions and time (Dekeling et al., 2014). Currently, available standards and those in development can be found by searching the Ocean Best Practices Repository (see foot note 1). Measurements of ambient noise metrics can be carried out using a number of open source programs such as PAMGUIDE (Merchant et al., 20157) or MANTA8. These programs provide a standard series of measurements at the decidecadal level that can be replicated across projects. An ongoing framework inventory on existing standards for observations of sound in the ocean can be found in the Ocean Best Practices Repository (International Quiet Ocean Experiment WG on Standardization, 2018).
Here we define PAM archiving as (1) the storage of recordings in a publicly accessible location; (2) PAM reporting as the reporting of data outputs such as detections, locations, or bearing of species-specific calls in a structured and publicly available venue; and (3) PAM visualization as the representation of these data outputs on a publicly available website.
Passive acoustic monitoring archiving is essential in order to provide a long-lasting record of the efforts invested in PAM data collection. PAM archival and real-time datasets require the archiving of several items:
• The acoustic sound recordings, which are the raw sound recordings made using the PAM technology, should be compressed into a standardized lossless format such as FLAC for archiving.
• The associated metadata, which is the information associated with the deployment and retrieval of the PAM technology at sea (e.g., recorder type, depth, location, and functionality) and information on the recording settings, such as the sampling rate and recording schedule.
• Derived analytical products, such as the software program used and evaluation of efficacy of species detection, number of hourly or daily species detections, sound source levels, and other relevant measured sound parameters.
Archiving of acoustic sound recordings is encouraged through NOAA’s National Center for Environmental Information (NCEI) archiving service9. PAM metadata are required criteria for archiving at NCEI. The process and metadata details can be provided upon request. These should be used as a guide in PAM Plans for documenting relevant information regarding the field recording effort (deployment and retrieval information), as well as resulting analyses (such as species detections or noise metrics).
All confirmed passive acoustic detections of target species/species, whether from archival or real-time data, must be archived in a publicly accessible location. For the United States East Coast, all species detection data and ambient noise metrics should be reported to the Northeast Passive Acoustic Reporting System via bm1mcy5wYWNtZGF0YUBub2FhLmdvdg==. Formatted spreadsheets that follow ISO standards with required detection, measurement, and metadata information are available for submission purposes (see Supplemental Information III for details). When real-time PAM is used during construction for mitigation purposes, a subset of the information required on species detections is expected to be provided and uploaded no later than 24 after the detection. Full acoustic detection data, metadata, and GPS data records must be submitted within 48 h via the formatted spreadsheets. When PAM is used for long-term monitoring, all data (detection data, metadata, GPS data, and ambient noise data) should be provided via the formatted spreadsheets and uploaded within 90 days of the retrieval of the recorder or data collection. The spreadsheets can be downloaded from https://www.fisheries.noaa.gov/resource/document/passive-acoustic-reporting-system-templates. For further assistance, contact bm1mcy5wYWNtZGF0YUBub2FhLmdvdg==.
All PAM detections and metadata submitted to the Northeast Passive Acoustic Reporting System are visualized on the Passive Acoustic Cetacean Map10. We encourage PAM detections to be shared across widely used and recognized platforms and regional web portals; for the United States East Coast, some of these standardized efforts are the Passive Acoustic Cetacean Map (see foot note 10), WhaleMap11, and WhaleAlert12.
These PAM recommendations provide a guide to understanding the various aspects required for designing and conducting PAM for both monitoring and mitigation. While the PAM Plans approved by agencies will ultimately determine full requirements, this six-step process provides a holistic look at each of the components that are needed when considering the development of a PAM Plan as well as long-term baseline monitoring. PAM technologies are a rapidly developing area, and new technologies and applications are likely to be available in the near future. Similarly, the data collection, analysis, and archiving of these data is ever evolving as the needs and applications grow; therefore, new developments will emerge as offshore wind development gets underway. These NOAA and BOEM recommendations will be updated and improved as new information and guidance becomes available.
All authors listed have made a substantial, direct and intellectual contribution to the work, and approved it for publication.
NOAA’s National Marine Fisheries Service and BOEM provided salary time for each of the co-authors to provide input into these recommendations.
The authors declare that the research was conducted in the absence of any commercial or financial relationships that could be construed as a potential conflict of interest.
All claims expressed in this article are solely those of the authors and do not necessarily represent those of their affiliated organizations, or those of the publisher, the editors and the reviewers. Any product that may be evaluated in this article, or claim that may be made by its manufacturer, is not guaranteed or endorsed by the publisher.
We would like to thank the large number of people who were provided the opportunity to review and provided support, insights, input and/or comments on these recommendations, including Shannon Bettridge, Danielle Cholewiak, Mary Cody, Julie Crocker, Sam Denes, Caroline Good, Sean Hayes, Allison Hernandez, Jolie Harrison, Brian Hooker, Stan Labak, Benjamin Laws, Jill Lewandowski, Andy Lipsky, Michelle Morin, Laura Morse, Kelsey Potluck, Kate McLellan-Press, Chris Orphanides, Ruth Perry, Cynthia Pyc, Emily Schumchenia, and Trevor Spradlin.
The Supplementary Material for this article can be found online at: https://www.frontiersin.org/articles/10.3389/fmars.2021.760840/full#supplementary-material
Ainslie, M., de Jong, C., and Miksis-Olds, J. (2019). IQOE Workshop Report: Guidelines for Observation of Ocean Sound, 13 July 2019, Park Hotel, Den Haag, Netherlands. Den Haag: International Quiet Ocean Experiment.
Ansmann, I. C., Goold, J. C., Evans, P. G. H., Simmonds, M., and Keith, S. G. (2007). Variation in the whistle characteristics of short-beaked common dolphins, Delphinus delphis, at two locations around the British Isles. J. Mar. Biol. Assoc. U. K. 87, 19–19. doi: 10.1017/S0025315407054963
Au, W. W., Kastelein, R. A., Rippe, T., and Schooneman, N. M. (1999). Transmission beam pattern and echolocation signals of a harbor porpoise (Phocoena phocoena). J. Acoust. Soc. Am. 106, 3699–3705. doi: 10.1121/1.428221
Au, W. W. L., and Herzing, D. L. (2003). Echolocation signals of wild Atlantic spotted dolphin (Stenella frontalis). J. Acoust. Soc. Am. 113, 598–604. doi: 10.1121/1.1518980
Au, W. W. L., Penner, R. H., and Kadane, J. (1982). Acoustic behavior of echolocating Atlantic Bottlenose Dolphins. J. Acoust. Soc. Am. 71, 1269–1275. doi: 10.1121/1.387733
Bailey, H., Brookes, K. L., and Thompson, P. M. (2014). Assessing environmental impacts of offshore wind farms: lessons learned and recommendations for the future. Aquat. Biosyst. 10:13. doi: 10.1186/2046-9063-10-8
Bailey, H., Clay, G., Coates, E. A., Lusseau, D., Senior, B., and Thompson, P. M. (2010a). Using T-PODs to assess variations in the occurrence of coastal bottlenose dolphins and harbour porpoises. Aquat. Conserv. Mar. Freshw. Ecosyst. 20, 150–158. doi: 10.1002/aqc.1060
Bailey, H., Senior, B., Simmons, D., Rusin, J., Picken, G., and Thompson, P. M. (2010b). Assessing underwater noise levels during pile-driving at an offshore windfarm and its potential effects on marine mammals. Mar. Pollut. Bull. 60, 888–897. doi: 10.1016/j.marpolbul.2010.01.003
Bailey, H., and Thompson, P. (2010). Effect of oceanographic features on fine-scale foraging movements of bottlenose dolphins. Mar. Ecol. Prog. Ser. 418, 223–233. doi: 10.3354/meps08789
Barlow, J., Griffiths, E., and Rankin, S. (2014). The use of passively drifting acoustic recorders for bioacoustic sensing. J. Acoust. Soc. Am. 136:2117. doi: 10.1121/1.4899625
Barlow, J., and Taylor, B. L. (2005). Estimates of sperm whale abundance in the northeastern temperate Pacific from a combined acoustic and visual survey. Mar. Mamm. Sci. 21, 429–445. doi: 10.1111/j.1748-7692.2005.tb01242.x
Baron, S. C., Martinez, A., Garrison, L. P., and Keith, E. O. (2008). Differences in acoustic signals from Delphinids in the western North Atlantic and northern Gulf of Mexico. Mar. Mamm. Sci. 24, 42–56. doi: 10.1111/j.1748-7692.2007.00168.x
Baumann-Pickering, S., McDonald, M. A., Simonis, A. E., Solsona Berga, A., Merkens, K. P. B., Oleson, E. M., et al. (2013). Species-specific beaked whale echolocation signals. J. Acoust. Soc. Am. 134, 2293–2301. doi: 10.1121/1.4817832
Baumann-Pickering, S., Simonis, A. E., Oleson, E. M., Baird, R. W., Roch, M. A., and Wiggins, S. M. (2015). False killer whale and short-finned pilot whale acoustic identification. Endang. Species Res. 28, 97–108. doi: 10.3354/esr00685
Baumgartner, M. F., Ball, K., Partan, J., Pelletier, L. P., Bonnell, J., Hotchkin, C., et al. (2021). Near real-time detection of low-frequency baleen whale calls from an autonomous surface vehicle: implementation, evaluation, and remaining challenges. J. Acoust. Soc. Am. 149:2950. doi: 10.1121/10.0004817
Baumgartner, M. F., Bonnell, J., Corkeron, P. J., Van Parijs, S. M., Hotchkin, C., Hodges, B. A., et al. (2020). Slocum gliders provide accurate near real-time estimates of baleen whale presence from human-reviewed passive acoustic detection information. Front. Mar. Sci. 7:100. doi: 10.3389/fmars.2020.00100
Baumgartner, M. F., Bonnell, J., Van Parijs, S. M., Corkeron, P. J., Hotchkin, C., Ball, K., et al. (2019). Persistent near real-time passive acoustic monitoring for baleen whales from a moored buoy: system description and evaluation. Methods Ecol. Evol. 10, 1476–1489. doi: 10.1111/2041-210X.13244
Baumgartner, M. F., Fratantoni, D. M., Hurst, T. P., Brown, M. W., Cole, T. V. N., Van Parijs, S. M., et al. (2013). Real-time reporting of baleen whale passive acoustic detections from ocean gliders. J. Acoust. Soc. Am. 134, 1814–1823. doi: 10.1121/1.4816406
Baumgartner, M. F., Stafford, K. M., and Latha, G. (2018). “Near real-time underwater passive acoustic monitoring of natural and anthropogenic sounds,” in Observing the Oceans in Real Time, eds R. Venkatesan, A. Tandon, E. D’Asaro, and M. A. Atmanand (Cham: Springer International Publishing), 203–226. doi: 10.1007/978-3-319-66493-4_10
Baumgartner, M. F., Stafford, K. M., Winsor, P., Statscewich, H., and Fratantoni, D. M. (2014). Glider-based passive acoustic monitoring in the Arctic. Mar. Technol. Soc. J. 48, 40–51. doi: 10.4031/MTSJ.48.5.2
Baumgartner, M. F., Van Parijs, S. M., Wenzel, F. W., Tremblay, C. J., Esch, H. C., and Warde, A. M. (2008). Low frequency vocalizations attributed to sei whales (Balaenoptera borealis). J. Acoust. Soc. Am. 124, 1339–1349. doi: 10.1121/1.2945155
Berchok, C. L., Bradley, D. L., and Gabrielson, T. B. (2006). St. Lawrence blue whale vocalizations revisited: characterization of calls detected from 1998 to 2001. J. Acoust. Soc. Am. 120, 2340–2354. doi: 10.1121/1.2335676
Biber, A., Çorakçı, A. C., Golick, A., Robinson, S., Hayman, G., Ablitt, J., et al. (2018). Calibration standards for hydrophones and autonomous underwater recorders for frequencies below 1 kHz: current activities of “UNAC-LOW” project. Acta IMEKO 7, 32–38. doi: 10.21014/acta_imeko.v7i2.542
Bittle, M., and Duncan, A. (2013). “A review of current marine mammal detection and classification algorithms for use in automated passive acoustic monitoring,” in Proceedings of the Acoustics. Australian Acoustical Society, Victor Harbor, SA.
Blackwell, S. B., Nations, C. S., McDonald, T. L., Greene, C. R., Thode, A. M., Guerra, M., et al. (2013). Effects of airgun sounds on bowhead whale calling rates in the Alaskan Beaufort Sea. Mar. Mamm. Sci. 29, E342–E365. doi: 10.1111/mms.12001
Blackwell, S. B., Richardson, W. J., Greene, C. R. Jr., and Streever, B. (2007). Bowhead whale (Balaena mysticetus) migration and calling behaviour in the Alaskan Beaufort Sea, autumn 2001–04: an acoustic localization study. Arctic 60, 255–270. doi: 10.14430/arctic218
BOEM (2018). Summary Report: Best Management Practices Workshop for Atlantic Offshore Wind Facilities and Marine Protected Species (2017). Washington, DC: US Department of the Interior, Bureau of Ocean Energy Management, Atlantic OCS Region.
BOEM (2021). Improving Monitoring, Data Consistency, Archiving, and Access for Improved Regional Integration of Renewable Energy Projects and Science: Passive Acoustic Monitoring and Marine Mammals (Workshop Summary). Stirling, VA: Bureau of Ocean Energy Management.
Brandt, M. J., Diederichs, A., Betke, K., and Nehls, G. (2011). Responses of harbour porpoises to pile driving at the Horns Rev II offshore wind farm in the Danish North Sea. Mar. Ecol. Prog. Ser. 421, 205–216. doi: 10.3354/meps08888
Brandt, M. J., Dragon, A. C., Diederichs, A., Bellmann, M. A., Wahl, V., Piper, W., et al. (2018). Disturbance of harbour porpoises during construction of the first seven offshore wind farms in Germany. Mar. Ecol. Prog. Ser. 596, 213–232. doi: 10.3354/meps12560
Caiger, P. E., Dean, M. J., DeAngelis, A. I., Hatch, L. T., Rice, A. N., Stanley, J. A., et al. (2020). A decade of monitoring Atlantic cod Gadus morhua spawning aggregations in Massachusetts Bay using passive acoustics. Mar. Ecol. Prog. Ser. 635, 89–103. doi: 10.3354/meps13219
Carlén, I., Thomas, L., Carlström, J., Amundin, M., Teilmann, J., Tregenza, N., et al. (2018). Basin-scale distribution of harbour porpoises in the Baltic Sea provides a basis for effective conservation actions. Biol. Conserv. 226, 42–53. doi: 10.1016/j.biocon.2018.06.031
Carstensen, J., Henriksen, O. D., and Teilmann, J. (2006). Impacts of offshore wind farm construction on harbour porpoises: acoustic monitoring of echolocation activity using porpoise detectors (T-PODs). Mar. Ecol. Prog. Ser. 321, 295–308. doi: 10.3354/meps321295
Charif, R. A., Shiu, Y., Muirhead, C. A., Clark, C. W., Parks, S. E., and Rice, A. N. (2019). Phenological changes in North Atlantic right whale habitat use in Massachusetts Bay. Glob. Change Biol. 26, 734–745. doi: 10.1111/gcb.14867
Cholewiak, D., Clark, C. W., Ponirakis, D., Frankel, A., Hatch, L. T., Risch, D., et al. (2018). Communicating amidst the noise: modeling the aggregate influence of ambient and vessel noise on baleen whale communication space in a national marine sanctuary. Endang. Species Res. 36, 59–75. doi: 10.3354/esr00875
Clapham, P. J., and Pace, R. M. (2001). Defining Triggers for Temporary Area Closures to Protect Right Whales from Entanglements: Issues and Options. Washington, DC: NOAA.
Clark, C. W., Brown, M. W., and Corkeron, P. (2010). Visual and acoustic surveys for North Atlantic right whales, Eubalaena glacialis, in Cape Cod Bay, Massachusetts, 2001-2005: management implications. Mar. Mamm. Sci. 26, 837–854. doi: 10.1111/j.1748-7692.2010.00376.x
Clark, C. W., Ellison, W. T., Southall, B. L., Hatch, L. T., Van Parijs, S. M., Frankel, A. S., et al. (2009). Acoustic masking in marine ecosystems: intuitions, analysis, and implication. Mar. Ecol. Prog. Ser. 395, 201–222. doi: 10.3354/meps08402
Clark, C. W., Gillespie, D., Nowacek, D. P., and Parks, S. E. (2007). “Listening to their world: acoustics for monitoring and protecting right whales in an urbanized ocean,” in The Urban Whale: North Atlantic Rights Whales at the Crossroads, eds S. D. Kraus and R. M. Rolland (Cambridge, MA: Harvard University Press), 333–357. doi: 10.2307/j.ctv1pnc1q9.16
Clarke, E. D., Feyrer, L. J., Moors-Murphy, H., and Stanistreet, J. E. (2019). Click characteristics of northern bottlenose whales (Hyperoodon ampullatus) and Sowerby’s beaked whales (Mesoplodon bidens) off eastern Canada. J. Acoust. Soc. Am. 147, 307–315. doi: 10.1121/1.5111336
Dähne, M., Gilles, A., Lucke, K., Peschko, V., Adler, S., Krügel, K., et al. (2013). Effects of pile-driving on harbour porpoises (Phocoena phocoena) at the first offshore wind farm in Germany. Environ. Res. Lett. 8:025002. doi: 10.1088/1748-9326/8/2/025002
Dähne, M., Tougaard, J., Carstensen, J., Rose, A., and Nabe-Nielsen, J. (2017). Bubble curtains attenuate noise from offshore wind farm construction and reduce temporary habitat loss for harbour porpoises. Mar. Ecol. Prog. Ser. 580, 221–237. doi: 10.3354/meps12257
Davis, G. E., Baumgartner, M. F., Bonnell, J. M., Bell, J., Berchok, C., Bort Thornton, J., et al. (2017). Long-term passive acoustic recordings track the changing distribution of North Atlantic right whales (Eubalaena glacialis) from 2004 to 2014. Sci. Rep. 7:13460. doi: 10.1038/s41598-017-13359-3
Davis, G. E., Baumgartner, M. F., Corkeron, P. J., Bell, J., Berchok, C., Bonnell, J. M., et al. (2020). Exploring movement patterns and changing distributions of baleen whales in the western North Atlantic using a decade of passive acoustic data. Glob. Chang. Biol. 26:29. doi: 10.1111/gcb.15191
DeAngelis, A., Wilder, J., Davis, G., Cholewiak, D., Gerlach, D., Van Parijs, S., et al. (2016). Guide to Monitoring Real-time Marine Mammal Detections using Autonomous Platforms. Washington, DC: NOAA.
DeAngelis, A. I., Stanistreet, J. E., Baumann-Pickering, S., and Cholewiak, D. M. (2018). A description of echolocation clicks recorded in the presence of true’s beaked whale (Mesoplodon mirus). J. Acoust. Soc. Am. 144, 2691–2700. doi: 10.1121/1.5067379
DeAngelis, A. I., Valtierra, R., Van Parijs, S. M., and Cholewiak, D. (2017). Using multipath reflections to obtain dive depths of beaked whales from a towed hydrophone array. J. Acoust. Soc. Am. 142, 1078–1087. doi: 10.1121/1.4998709
Dekeling, R. P. A., Tasker, M. L., Van der Graaf, A. J., Ainslie, M. A., Andersson, M. H., André, M., et al. (2014). Monitoring Guidance for Underwater Noise in European Seas, Part II: Monitoring Guidance Specifications. A Guidance Document within the Common Implementation Strategy for the Marine Strategy Framework Directive by MSFD Technical Subgroup on Underwater Noise. Luxembourg: Publications Office of the European Union.
Delarue, J., Todd, S. K., Van Parijs, S. M., and Di Iorio, L. (2009). Geographic variation in Northwest Atlantic fin whale (Balaenoptera physalus) song: implications for stock structure assessment. J. Acoust. Soc. Am. 125, 1774–1782. doi: 10.1121/1.3068454
Desjonquères, C., Gifford, T., and Linke, S. (2020). Passive acoustic monitoring as a potential tool to survey animal and ecosystem processes in freshwater environments. Freshw. Biol. 65, 7–19. doi: 10.1111/fwb.13356
DNV KEMA Renewables Inc (2018). Metocean Characterization Recommended Practices for U.S. Offshore Wind Energy. BOEM 2018-057. Report 10039663-HOU-01. Washington, DC: Bureau of Ocean Energy Management (BOEM).
Ellis, J. I., and Schneider, D. C. (1997). Evaluation of a gradient sampling design for environmental impact assessment. Environ. Monitor. Assess. 48, 157–172. doi: 10.1023/A:1005752603707
Erbe, C., McCauley, R., and Gavrilov, A. (2016). “Characterizing marine soundscapes,” in The Effects of Noise on Aquatic Life II. Advances in Experimental Medicine and Biology, eds A. Popper and A. Hawkins (New York, NY: Springer). doi: 10.1007/978-1-4939-2981-8_31
Eskesen, I. G., Wahlberg, M., Simon, M., and Larsen, O. N. (2011). Comparison of echolocation clicks from geographically sympatric killer whales and long-finned pilot whales (L). J. Acoust. Soc. Am. 130, 9–12. doi: 10.1121/1.3583499
Forney, K. A., Southall, B. L., Slooten, E., Dawson, S., Read, A. J., Baird, R. W., et al. (2017). Nowhere to go: noise impact assessments for marine mammal populations with high site fidelity. Endang. Spec. Res. 32, 391–413. doi: 10.3354/esr00820
Fregosi, S., Harris, D., Mellinger, D. K., Barlow, J., Thomas, L., and Klinck, H. (2018). Detection probability of Cuvier’s beaked whale clicks from a glider and a deep-water float. J. Acoust. Soc. Am. 144:1849. doi: 10.1121/1.5068141
Gerrodette, T., Taylor, B. L., Swift, R., Rankin, S., Jaramillo-Legorreta, A. M., and Rojas-Bracho, L. (2011). A combined visual and acoustic estimate of 2008 abundance, and change in abundance since 1997, for the vaquita, Phocoena sinus. Mar. Mamm. Sci. 27, 1–22. doi: 10.1111/j.1748-7692.2010.00438.x
Gervaise, C., Simard, Y., Aulanier, F., and Roy, N. (2021). Optimizing passive acoustic systems for marine mammal detection and localization: application to real-time monitoring North Atlantic right whales in Gulf of St. Lawrence. Appl. Acoust. 178:107949. doi: 10.1016/j.apacoust.2021.107949
Gibb, R., Browning, E., Glover-Kapfer, P., Jones, K. E., and Börger, L. (2019). Emerging opportunities and challenges for passive acoustics in ecological assessment and monitoring. Methods Ecol. Evol. 10, 169–185. doi: 10.1111/2041-210X.13101
Gillespie, D., Caillat, M., Gordon, J., and White, P. (2013). Automatic detection and classification of odontocete whistles. J. Acoust. Soc. Am. 134, 2427–2437. doi: 10.1121/1.4816555
Gillespie, D., Palmer, L., Macaulay, J., Sparling, C., and Hastie, G. (2020). Passive acoustic methods for tracking the 3D movements of small cetaceans around marine structures. PLoS One 15:e0229058. doi: 10.1371/journal.pone.0229058
Greene, C. R., McLennan, M. W., Norman, R. G., McDonald, T. L., Jakubczak, R. S., and Richardson, W. J. (2004). Directional frequency and recording (DIFAR) sensors in seafloor recorders to locate calling bowhead whales during their fall migration. J. Acoust. Soc. Am. 116, 799–813. doi: 10.1121/1.1765191
Griffiths, E. T., and Barlow, J. (2016). Cetacean acoustic detections from free-floating vertical hydrophone arrays in the southern California Current. J. Acoust. Soc. Am. 140:EL399. doi: 10.1121/1.4967012
Gulka, J. G., and Williams, K. A. (2018). “The state of the science on wildlife and offshore wind energy development,” in Proceedings of the Workshop Held November 13-14, 2018 (Woodbury, NY: New York State Energy Research and Development Authority (NYSERDA)), 84.
Halliday, W. D., Pine, M. K., Insley, S. J., Soares, R. N., Kortsalo, P., and Mouy, X. (2019). Acoustic detections of Arctic marine mammals near Ulukhaktok, Northwest Territories, Canada. Can. J. Zool. 97, 72–80. doi: 10.1139/cjz-2018-0077
Hastie, G. D., Gillespie, D. M., Gordon, J. C. D., Macaulay, J. D. J., McConnell, B. J., and Sparling, C. E. (2014). “Tracking technologies for quantifying marine mammal interactions with tidal turbines: pitfalls and possibilities,” in Marine Renewable Energy Technology and Environmental Interactions, eds M. A. Shields and A. I. L. Payne (Dordrecht: Springer), 127–139. doi: 10.1007/978-94-017-8002-5_10
Hatch, L. T., Clark, C. W., Van Parijs, S. M., Frankel, A. S., and Ponirakis, D. W. (2012). Quantifying loss of acoustic communication space for right whales in and around a U.S. National Marine Sanctuary. Conserv. Biol. 26, 983–994. doi: 10.1111/j.1523-1739.2012.01908.x
Haver, S. M., Gedamke, J., Hatch, L. T., Dziak, R. P., Van Parijs, S., McKenna, M. F., et al. (2018). Monitoring long-term soundscape trends in U.S. waters: the NOAA/NPS ocean noise reference station network. Mar. Pollut. 90, 6–13. doi: 10.1016/j.marpol.2018.01.023
Ingram, E. C., Cerrato, R. M., Dunton, K. J., and Frisk, M. G. (2019). Endangered Atlantic sturgeon in the New York wind energy area: implications of future development in an offshore wind energy site. Sci. Rep. 9:12432. doi: 10.1038/s41598-019-48818-6
International Quiet Ocean Experiment WG on Standardization (2018). IQOE Inventory of Existing Standards for Observations of Sound in the Ocean. Draft. Version 06 April 2018. Minnesota: International Quiet Ocean Experiment.
Jefferson, T. A., Webber, M. A., and Pitman, R. L. (2011). Marine Mammals of the World: A Comprehensive Guide to Their Identification. Amsterdam: Elsevier.
Johnston, D. W., McDonald, M., Polovina, J., Domokos, R., Wiggins, S., and Hildebrand, J. (2008). Temporal patterns in the acoustic signals of beaked whales at Cross Seamount. Biol. Lett. 4, 208–211. doi: 10.1098/rsbl.2007.0614
Kirsebom, O. S., Frazao, F., Simard, Y., Roy, N., Matwin, S., and Giard, S. (2020). Performance of a deep neural network at detecting North Atlantic right whale upcalls. J. Acoust. Soc. Am. 147, 2636–2646. doi: 10.1121/10.0001132
Klinck, H., Mellinger, D. K., Klinck, K., Bogue, N. M., Luby, J. C., Jump, W. A., et al. (2012). Near-real-time acoustic monitoring of beaked whales and other cetaceans using a SeagliderTM. PLoS One 7:e36128. doi: 10.1371/journal.pone.0036128
Klinck, H., Stelzer, R., Jafarmadar, K., and Mellinger, D. K. (2009). “AAS endurance: an autonomous acoustic sailboat for marine mammal research,” in Proceedings of the International Robotic Sailing Conference, Matosinhos.
Kowarski, K., Moors-Murphy, H., Maxner, E., and Cerchio, S. (2019). Western North Atlantic humpback whale fall and spring acoustic repertoire: insight into onset and cessation of singing behavior. J. Acoust. Soc. Am. 145:2305. doi: 10.1121/1.5095404
Kowarski, K. A., Gaudet, B. J., Cole, A. J., Maxner, E. E., Turner, S. P., Martin, S. B., et al. (2020). Near real-time marine mammal monitoring from gliders: practical challenges, system development, and management implications. J. Acoust. Soc. Am. 148:1215. doi: 10.1121/10.0001811
Kraus, S. D., Kenney, R. D., and Thomas, L. (2019). A Framework for Studying the Effects of Offshore Wind Development on Marine Mammals and Turtles. Report Prepared for the Massachusetts Clean Energy Center, Boston MA 02110, and the Bureau of Ocean Energy Management. Boston, MA: Bureau of Ocean Energy Management.
Küsel, E. T., Munoz, T., Siderius, M., Mellinger, D. K., and Heimlich, S. (2017). Marine mammal tracks from two-hydrophone acoustic recordings made with a glider. Ocean Sci. 13, 273–288.
Leung, D. Y. C., and Yang, Y. (2012). Wind energy development and its environmental impact: a review. Renew. Sustain. Energy Rev. 16, 1031–1039.
Lewis, T., Gillespie, D., Lacey, C., Matthews, J., Danbolt, M., Leaper, R., et al. (2007). Sperm whale abundance estimates from acoustic surveys of the Ionian sea and straits of Sicily in 2003. J. Mar. Biol. Assoc. U. K. 87, 353–353.
Lindsey, N. J., Dawe, T. C., and Ajo-Franklin, J. B. (2019). Illuminating seafloor faults and ocean dynamics with dark fiber distributed acoustic sensing. Science 366, 1103–1107. doi: 10.1126/science.aay5881
Luczkovich, J. J., Mann, D. A., and Rountree, R. A. (2008). Passive acoustics as a tool in fisheries science. Trans. Am. Fish. Soc. 137, 533–541. doi: 10.1577/T06-258.1
Madhusudhana, S., Murray, A., and Erbe, C. (2020). Automatic detectors for low-frequency vocalizations of Omura’s whales, Balaenoptera omurai: a performance comparison. J. Acoust. Soc. Am. 147, 3078–3090. doi: 10.1121/10.0001108
Madsen, P. T., Payne, R., Kristiansen, N. U., Wahlberg, M., Kerr, I., and Møhl, B. (2002). Sperm whale sound production studied with ultrasound time/depth-recording tags. J. Exp. Biol. 205, 1899–1906. doi: 10.1242/jeb.205.13.1899
Marques, T. A., Thomas, L., Martin, S. W., Mellinger, D. K., Ward, J. A., Moretti, D. J., et al. (2013). Estimating animal population density using passive acoustics. Biol. Rev. Cambr. Philos. Soc. 88, 287–309. doi: 10.1111/brv.12001
Mathias, D., Thode, A. M., Kim, K. H., Blackwell, S. B., Greene, C. R., and Macrander, M. A. (2012). Long-range tracking of bowhead whale calls using directional autonomous seafloor acoustic recorders. J. Acoust. Soc. Am. 132:1950. doi: 10.1121/1.4755174
May-Collado, L. J., and Wartzok, D. (2008). A comparison of bottlenose dolphin whistles in the Atlantic Ocean: factors promoting whistle variation. J. Mammal. 89, 1229–1240. doi: 10.1644/07-MAMM-A-310.1
Mellinger, D. K., and Clark, C. W. (2003). Blue whale (Balaenoptera musculus) sounds from the North Atlantic. J. Acoust. Soc. Am. 114, 1108–1119. doi: 10.1121/1.1593066
Mellinger, D. K., Stafford, K. M., Moore, S. E., Dziak, R. P., and Matsumoto, H. (2007). An overview of fixed passive acoustic observation methods for cetaceans. Oceanography 20, 36–45. doi: 10.5670/oceanog.2007.03
Merchant, N. D., Brookes, K. L., Faulkner, R. C., Bicknell, A. W., Godley, B. J., and Witt, M. J. (2016). Underwater noise levels in UK waters. Sci. Rep. 6:36942. doi: 10.1038/srep36942
Merchant, N. D., Fristrup, K. M., Johnson, M. P., Tyack, P. L., Witt, M. J., Blondel, P., et al. (2015). Measuring acoustic habitats. Methods Ecol. Evol. 6, 257–265. doi: 10.1111/2041-210X.12330
Merkens, K., Mann, D., Janik, V. M., Claridge, D., Hill, M., and Oleson, E. (2018). Clicks of dwarf sperm whales (Kogia sima). Mar. Mamm. Sci. 34, 963–978. doi: 10.1111/mms.12488
Methratta, E. T. (2020). Monitoring fisheries resources at offshore wind farms: BACI vs. BAG designs. ICES J. Mar. Sci. 77, 890–900. doi: 10.1093/icesjms/fsaa026
Miksis-Olds, J. L., Nystuen, J. A., and Parks, S. E. (2010). Detecting marine mammals with an adaptive sub-sampling recorder in the Bering Sea. Appl. Acoust. 71, 1087–1092. doi: 10.1016/j.apacoust.2010.05.010
Mooney, T. A., Kaplan, M. B., Lamoni, L., Boucher, A., and Sayigh, L. S. (2013). Passive acoustic monitoring of biological and anthropogenic sounds at America’s first offshore wind farm. J. Acoust. Soc. Am. 134:4148. doi: 10.1121/1.4831206
Morano, J. L., Rice, A. N., Tielens, J. T., Estabrook, B. J., Murray, A., Roberts, B. L., et al. (2012a). Acoustically detected year-round presence of right whales in an urbanized migration corridor. Conserv. Biol. 26, 698–707. doi: 10.1111/j.1523-1739.2012.01866.x
Morano, J. L., Salisbury, D. P., Rice, A. N., Conklin, K. L., Falk, K. L., and Clark, C. W. (2012b). Seasonal and geographical patterns of fin whale song in the western North Atlantic Ocean. J. Acoust. Soc. Am. 132, 1207–1212. doi: 10.1121/1.4730890
Mordy, C. W., Cokelet, E. D., De Robertis, A., Jenkins, R., Kuhn, C. E., Lawrence-Slavas, N., et al. (2017). Advances in ecosystem research: Saildrone surveys of oceanography, fish, and marine mammals in the bering sea. Oceanography 30, 113–115. doi: 10.5670/oceanog.2017.230
Nemiroff, L., and Whitehead, H. (2009). Structural characteristics of pulsed calls of long-finned pilot whales (Globicephala melas). Bioacoustics 19, 67–92. doi: 10.1080/09524622.2009.9753615
Norris, T. F., Riddoch, N., Küsel, E. T., Doniol-Valcroze, T., Abernethy, R., and Nichol, L. (2019). Real-time passive acoustic monitoring results of the 2018 Pacific region international survey of marine megafauna off Western Canada. J. Acoust. Soc. Am. 146, 2805–2805. doi: 10.1121/1.5136717
NYSERDA (2020). State of the Science Workshop on Wildlife and Offshore Wind Energy 2020: Cumulative Impacts. Albany, NY: New York State Energy Research and Development Authority (NYSERDA).
Office of the Press Secretary (2021). FACT SHEET: Biden Administration Jumpstarts Offshore Wind Energy Projects to Create Jobs. Washington, DC: The White House.
Parks, S. E., Clark, C. W., and Tyack, P. L. (2007). Short- and long-term changes in right whale calling behavior: the potential effects of noise on acoustic communication. J. Acoust. Soc. Am. 122, 3725–3731. doi: 10.1121/1.2799904
Parks, S. E., Hamilton, P. K., Kraus, S. D., and Tyack, P. L. (2005). The gunshot sound produced by male North Atlantic right whales (Eubalaena glacialis) and its potential function in reproductive advertisement. Mar. Mamm. Sci. 21, 458–475. doi: 10.1111/j.1748-7692.2005.tb01244.x
Parks, S. E., Searby, A., Célérier, A., Johnson, M. P., Nowacek, D. P., and Tyack, P. L. (2011). Sound production behavior of individual North Atlantic right whales: implications for passive acoustic monitoring. Endang. Species Res. 15, 63–76.
Parks, S. E., and Tyack, P. L. (2005). Sound production by North Atlantic right whales (Eubalaena glacialis) in surface active groups. J. Acoust. Soc. Am. 117, 3297–3306.
Portman, M. E., Duff, J. A., Köppel, J., Reisert, J., and Higgins, M. E. (2009). Offshore wind energy development in the exclusive economic zone: legal and policy supports and impediments in Germany and the US. Energy Policy 37, 3596–3607. doi: 10.1016/j.enpol.2009.04.023
POWER-US (2019). POWER-US Workshop Final Report on Offshore Wind Energy and Passive Acoustic Monitoring: Establishing Standards for Operational Real-time Systems. Woods Hole, MA: Woods Hole Oceanographic Institution. doi: 10.1575/1912/25140
Risch, D., Clark, C. W., Dugan, P. J., Popescu, M., Siebert, U., and Van Parijs, S. M. (2013). Minke whale acoustic behavior and multi-year seasonal and diel vocalization patterns in Massachusetts Bay, USA. Mar. Ecol. Prog. Ser. 489, 279–295. doi: 10.3354/meps10426
Risch, D., Siebert, U., and Parijs, S. M. V. (2014). Individual calling behaviour and movements of North Atlantic minke whales (Balaenoptera acutorostrata). Behaviour 151, 1335–1360. doi: 10.1163/1568539X-00003187
Robinson, S. P., Lepper, P. A., and Hazelwood, R. A. (2014). Good Practice Guide for Underwater Noise Measurement. London: The Crown Estate.
Rountree, R. A., Gilmore, R. G., Goudey, C. A., Hawkins, A. D., Luczkovich, J. J., and Mann, D. A. (2006). Listening to fish: applications of passive acoustics to fisheries science. Fisheries 31, 433–446. doi: 10.1577/1548-8446(2006)31[433:LTF]2.0.CO;2
Rowell, T. J., Nemeth, R. S., Schärer, M. T., and Appeldoorn, R. S. (2015). Fish sound production and acoustic telemetry reveal behaviors and spatial patterns associated with spawning aggregations of two Caribbean groupers. Mar. Ecol. Prog. Ser. 518, 239–254. doi: 10.3354/meps11060
Scheidat, M., Tougaard, J., Brasseur, S., Carstensen, J., van Polanen Petel, T., Teilmann, J., et al. (2011). Harbour porpoises (Phocoena phocoena) and wind farms: a case study in the Dutch North Sea. Environ. Res. Lett. 6: 025102.
Shiu, Y., Palmer, K. J., Roch, M. A., Fleishman, E., Liu, X., Nosal, E. M., et al. (2020). Deep neural networks for automated detection of marine mammal species. Sci. Rep. 10:607. doi: 10.1038/s41598-020-67560-y
Sousa-lima, R. S., Norris, T. F., Oswald, J. N., and Fernandes, D. P. (2013). A review and inventory of fixed autonomous recorders for passive acoustic monitoring of marine mammals. Aquat. Mamm. 39, 23–53.
Spaulding, E., Robbins, M., Calupca, T., Clark, C. W., Tremblay, C., Waack, A., et al. (2009). An autonomous, near-real-time buoy system for automatic detection of North Atlantic right whale calls. Proc. Meet. Acoust. 6:010001. doi: 10.1121/1.3340128
Staaterman, E., Paris, C. B., DeFerrari, H. A., Mann, D. A., Rice, A. N., and D’Alessandro, E. K. (2014). Celestial patterns in marine soundscapes. Mar. Ecol. Prog. Ser. 508, 17–32. doi: 10.3354/meps10911
Stanistreet, J. E., Nowacek, D. P., Baumann-Pickering, S., Bell, J. T., Cholewiak, D. M., Hildebrand, J. A., et al. (2017). Using passive acoustic monitoring to document the distribution of beaked whale species in the western North Atlantic Ocean. Can. J. Fish. Aquat. Sci. 74, 2098–2109.
Stanistreet, J. E., Nowacek, D. P., Bell, J. T., Cholewiak, D. M., Hildebrand, J. A., Hodge, L. E. W., et al. (2018). Spatial and seasonal patterns in acoustic detections of sperm whales Physeter macrocephalus along the continental slope in the western North Atlantic Ocean. Endang. Species Res. 35, 1–13. doi: 10.3354/esr00867
Stanistreet, J. E., Nowacek, D. P., Read, A. J., Baumann-Pickering, S., Moors-Murphy, H. B., and Van Parijs, S. M. (2016). Effects of duty-cycled passive acoustic recordings on detecting the presence of beaked whales in the northwest Atlantic. J. Acoust. Soc. Am. 140:EL31.
Stanistreet, J. E., Risch, D., and Van Parijs, S. M. (2013). Passive acoustic tracking of singing humpback whales (Megaptera novaeangliae) on a northwest Atlantic feeding ground. PLoS One 8:e61263. doi: 10.1371/journal.pone.0061263
Sugai, L. S. M., Silva, T. S. F., Ribeiro, J. W., and Llusia, D. (2019). Terrestrial passive acoustic monitoring: review and perspectives. Bioscience 69, 15–25. doi: 10.1093/biosci/biy147
Temple, A. J., Tregenza, N., Amir, O. A., Jiddawi, N., and Berggren, P. (2016). Spatial and temporal variations in the occurrence and foraging activity of coastal dolphins in Menai Bay, Zanzibar, Tanzania. PLoS One 11:e0148995. doi: 10.1371/journal.pone.0148995
Thode, A. (2004). Tracking sperm whale (Physeter macrocephalus) dive profiles using a towed passive acoustic array. J. Acoust. Soc. Am. 116, 245–253. doi: 10.1121/1.1758972
Thode, A., and Guan, S. (2019). Achieving consensus and convergence on a towed array passive acoustic monitoring standard for marine mammal monitoring. J. Acoust. Soc. Am. 146, 2934–2934.
Thomisch, K., Boebel, O., Zitterbart, D. P., Samaran, F., Van Parijs, S., and Van Opzeeland, I. (2015). Effects of subsampling of passive acoustic recordings on acoustic metrics. J. Acoust. Soc. Am. 138, 267–278. doi: 10.1121/1.4922703
Tougaard, J., Carstensen, J., Teilmann, J., Skov, H., and Rasmussen, P. (2009). Pile driving zone of responsiveness extends beyond 20 km for harbor porpoises (Phocoena phocoena (L.)). J. Acoust. Soc. Am. 126, 11–14. doi: 10.1121/1.3132523
Tremblay, C. J., Van Parijs, S. M., and Cholewiak, D. (2019). 50 to 30-Hz triplet and singlet down sweep vocalizations produced by sei whales (Balaenoptera borealis) in the western North Atlantic Ocean. J. Acoust. Soc. Am. 145, 3351–3358.
Underwood, A. J. (1992). Beyond BACI: the detection of environmental impacts on populations in the real, but variable, world. J. Exp. Mar. Biol. Ecol. 161, 145–178. doi: 10.1016/0022-0981(92)90094-Q
Underwood, A. J. (1994). On beyond BACI: sampling designs that might reliably detect environmental disturbances. Ecol. Appl. 4, 4–15. doi: 10.2307/1942110
van der Schaar, M., Andre, M., Delory, E., Gillespie, D., and Rolin, J.-F. (2017). Passive Acoustic Monitoring from Fixed Platform Observatories. Deliverable 12.6, FixO3. Brest: IFREMER for FixO3.
Van Opzeeland, I. C., Kindermann, L., Boebel, O., and Van Parijs, S. M. (2008). “Insights into the acoustic behaviour of polar pinnipeds–current knowledge and emerging techniques of study,” in Animal Behavior: New Research, eds L. H. Krause and E. A. Weber (New York, NY: Nova Science Publishers, Inc).
Van Parijs, S. M., Baumgartner, M., Cholewiak, D., Davis, G., Gedamke, J., Gerlach, D., et al. (2015). NEPAN: a U. S. northeast passive acoustic sensing network for monitoring, reducing threats and the conservation of marine animals. Mar. Technol. Soc. J. 49, 70–86. doi: 10.4031/MTSJ.49.2.16
Van Parijs, S. M., Clark, C. W., Sousa-lima, R. S., Parks, S. E., Rankin, S., Risch, D., et al. (2009). Management and research applications of real-time and archival passive acoustic sensors over varying temporal and spatial scales. Mar. Ecol. Prog. Ser. 395, 21–36.
Van Parijs, S. M., and Southall, B. L. (2007). Report of the 2006 NOAA National Passive Acoustics Workshop – Developing a Strategic Program Plan for NOAA’s Passive Acoustics Ocean Observing System (PAOOS). Woods Hole, MA: NOAA Technical Memorandum. NMFS-F/SPO-81, 64.
Verfuß, U. K., Honnef, C. G., Meding, A., Dähne, M., Mundry, R., and Benke, H. (2007). Geographical and seasonal variation of harbour porpoise (Phocoena phocoena) presence in the German Baltic Sea revealed by passive acoustic monitoring. J. Mar. Biol. Assoc. U. K. 87, 165–176.
von Benda-Beckmann, A. M., Beerens, S. P., and van Ijsselmuide, S. P. (2013). Effect of towed array stability on instantaneous localization of marine mammals. J. Acoust. Soc. Am. 134, 2409–2417.
von Benda-Beckmann, A. M., Lam, F. P. A., Moretti, D. J., Fulkerson, K., Ainslie, M. A., van Ijsselmuide, S. P., et al. (2010). Detection of Blainville’s beaked whales with towed arrays. Appl. Acoust. 71, 1027–1035. doi: 10.1016/j.apacoust.2010.05.015
Wall, C. C., Lembke, C., and Mann, D. A. (2012). Shelf-scale mapping of sound production by fishes in the eastern Gulf of Mexico, using autonomous glider technology. Mar. Ecol. Prog. Ser. 449, 55–64.
Wall, C. C., Simard, P., Lembke, C., and Mann, D. A. (2013). Large-scale passive acoustic monitoring of fish sound production on the West Florida Shelf. Mar. Ecol. Prog. Ser. 484, 173–188. doi: 10.3354/meps10268
Watkins, W. A. (1981). Activities and underwater sounds of fin whales (Balaenoptera physalus). Sci. Rep. Whales Res. Inst. 33, 83–117.
WCS (2021). New York Bight Passive Acoustic Monitoring Data Synthesis Workshop (October 2020) Summary Report. Albany, NY: New York State Energy Research and Development Authority (NYSERDA).
Weilgart, L., and Whitehead, H. (1990). Vocalizations of the North Atlantic pilot whale (Globicephala melas) as related to behavioral contexts. Behav. Ecol. Sociobiol. 26, 399–402. doi: 10.1007/BF00170896
Wingfield, J. E., O’Brien, M., Lyubchich, V., Roberts, J. J., Halpin, P. N., Rice, A. N., et al. (2017). Year-round spatiotemporal distribution of harbour porpoises within and around the Maryland wind energy area. PLoS One 12:e0176653. doi: 10.1371/journal.pone.0176653
Winn, H. E., and Winn, L. K. (1978). The song of the humpback whale Megaptera novaeangliae in the West Indies. Mar. Biol. 47, 97–114.
Wood, M., Baumgartner, M., Visalli, M., and Širović, A. (2020). Near real time passive acoustic monitoring in the Santa Barbara Channel. J. Acoust. Soc. Am. 148:2773. doi: 10.1121/1.5147720
Yoccoz, N. G., Nichols, J. D., and Boulinier, T. (2001). Monitoring of biological diversity in space and time. Trends Ecol. Evol. 16, 446–453. doi: 10.1016/S0169-5347(01)02205-4
Zemeckis, D. R., Dean, M. J., DeAngelis, A. I., Van Parijs, S. M., Hoffman, W. S., Baumgartner, M. F., et al. (2019). Identifying the distribution of Atlantic cod spawning using multiple fixed and glider-mounted acoustic technologies. ICES J. Mar. Sci. 76, 1610–1625.
Keywords: passive acoustic monitoring, offshore wind energy, baleen whales, recommendations, marine mammal monitoring and mitigation
Citation: Van Parijs SM, Baker K, Carduner J, Daly J, Davis GE, Esch C, Guan S, Scholik-Schlomer A, Sisson NB and Staaterman E (2021) NOAA and BOEM Minimum Recommendations for Use of Passive Acoustic Listening Systems in Offshore Wind Energy Development Monitoring and Mitigation Programs. Front. Mar. Sci. 8:760840. doi: 10.3389/fmars.2021.760840
Received: 18 August 2021; Accepted: 04 October 2021;
Published: 27 October 2021.
Edited by:
Johannes Karstensen, GEOMAR Helmholtz Centre for Ocean Research Kiel, GermanyReviewed by:
Maria Boethling, Federal Maritime and Hydrographic Agency, GermanyCopyright © 2021 Van Parijs, Baker, Carduner, Daly, Davis, Esch, Guan, Scholik-Schlomer, Sisson and Staaterman. This is an open-access article distributed under the terms of the Creative Commons Attribution License (CC BY). The use, distribution or reproduction in other forums is permitted, provided the original author(s) and the copyright owner(s) are credited and that the original publication in this journal is cited, in accordance with accepted academic practice. No use, distribution or reproduction is permitted which does not comply with these terms.
*Correspondence: Sofie M. Van Parijs, c29maWUudmFucGFyaWpzQG5vYWEuZ292
Disclaimer: All claims expressed in this article are solely those of the authors and do not necessarily represent those of their affiliated organizations, or those of the publisher, the editors and the reviewers. Any product that may be evaluated in this article or claim that may be made by its manufacturer is not guaranteed or endorsed by the publisher.
Research integrity at Frontiers
Learn more about the work of our research integrity team to safeguard the quality of each article we publish.