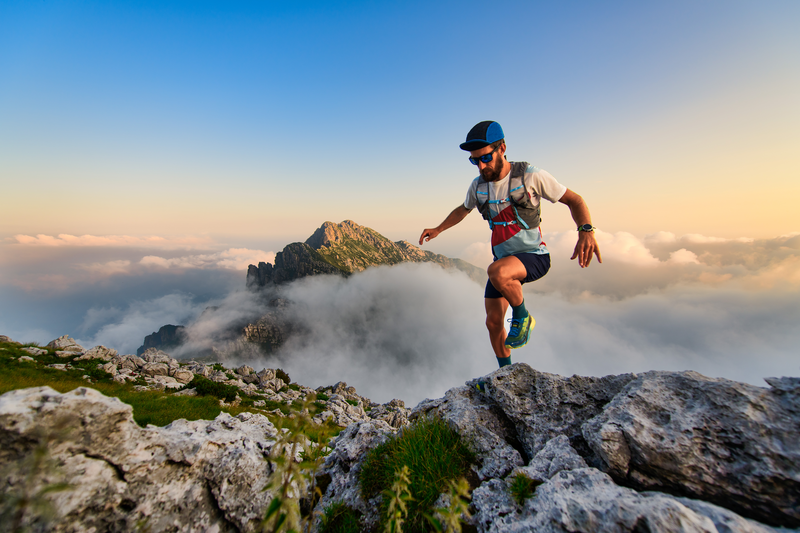
94% of researchers rate our articles as excellent or good
Learn more about the work of our research integrity team to safeguard the quality of each article we publish.
Find out more
ORIGINAL RESEARCH article
Front. Mar. Sci. , 05 January 2022
Sec. Marine Megafauna
Volume 8 - 2021 | https://doi.org/10.3389/fmars.2021.758435
This article is part of the Research Topic Small Cetacean Conservation: Current Challenges and Opportunities View all 51 articles
For long-lived species such as marine mammals, having sufficient data on ranging patterns and space use in a timescale suitable for population management and conservation can be difficult. Yawuru Nagulagun/Roebuck Bay in the northwest of Western Australia supports one of the largest known populations of Australian snubfin dolphins (Orcaella heinsohni)—a species with a limited distribution, vulnerable conservation status, and high cultural value. Understanding the species’ use of this area will inform management for the long-term conservation of this species. We combined 11 years of data collected from a variety of sources between 2007 and 2020 to assess the ranging patterns and site fidelity of this population. Ranging patterns were estimated using minimum convex polygons (MCPs) and fixed kernel densities (weighted to account for survey effort) to estimate core and representative areas of use for both the population and for individuals. We estimated the population to range over a small area within the bay (103.05 km2). The Mean individual representative area of use (95% Kernel density contour) was estimated as 39.88 km2 (± 32.65 SD) and the Mean individual core area of use (50% Kernel density contour) was estimated as 21.66 km2 (±18.85 SD) with the majority of sightings located in the northern part of the bay less than 10 km from the coastline. Most individuals (56%) showed moderate to high levels of site fidelity (i.e., part-time or long-term residency) when individual re-sight rates were classified using agglomerative hierarchical clustering (AHC). These results emphasize the importance of the area to this vulnerable species, particularly the area within the Port of Broome that has been identified within the population’s core range. The pressures associated with coastal development and exposure to vessel traffic, noise, and humans will need to be considered in ongoing management efforts. Analyzing datasets from multiple studies and across time could be beneficial for threatened species where little is known on their ranging patterns and site fidelity. Combined datasets can provide larger sample sizes over an extended period of time, fill knowledge gaps, highlight data limitations, and identify future research needs to be considered with dedicated studies.
As top predators, marine mammals play important ecological roles in marine ecosystems (Bowen, 1997; Heithaus et al., 2008) and are often considered indicators of ecosystem health (Bossart, 2011; Nelms et al., 2021). Many species and populations, including coastal dolphins, are experiencing cumulative anthropogenic pressures such as habitat degradation and modification (Parra et al., 2017; Kreb et al., 2020), increasing vessel traffic and noise (Bejder et al., 2006; Jensen et al., 2009a; Marley et al., 2017a; Erbe et al., 2019), and pollution (Cagnazzi et al., 2013a; Parra et al., 2017). Direct impacts may come from incidental entanglement in fishing gear (Laist, 1997; Jensen et al., 2009b; Reeves et al., 2013) and vessel strike (Wells and Scott, 1997; Thiele, 2010; Peel et al., 2018; Schoeman et al., 2020), whilst habitat loss, reduced prey from fishing (recreational, commercial and aquaculture activities) and increasing tourism have indirect impacts often resulting in disturbance, avoidance, and displacement from critical habitats (Paiva et al., 2015; Strickland-Munro et al., 2016; Karczmarski et al., 2017; Wang et al., 2017; Clarkson et al., 2020; Kassamali-Fox et al., 2020; Lin et al., 2021). Managers rely on science to better understand these impacts to wildlife populations and, in particular, how to mitigate these. This includes understanding how animals use space and resources over time to identify areas of overlap or conflict with human activity (Lotze et al., 2017), which can then be applied through environmental impact assessments, species conservation assessments, protected area design, and marine spatial planning.
Animal movement can influence the dynamics of a population, including abundance, distribution, habitat selection, species interactions, and social and population structure, all of which, in turn, can affect the fitness of an individual and population (Nathan et al., 2008; Börger, 2016). Ranging patterns, (i.e., the size of the area used by each individual and population within the confines of a study area), and site fidelity, as defined by Switzer (1993), are components of animal movement that are thought to be driven by changes in an individual’s needs and the distribution of its conspecifics, predators, and resources (Switzer, 1993, 1997; Nathan et al., 2008). Areas of high-quality habitat, for example, areas with abundant resources and shelter from predators, are likely to be associated with individuals that exhibit high site fidelity and a small range (Knip et al., 2012; Habel et al., 2016; Passadore et al., 2018). However, populations with high site fidelity and limited ranges can potentially be more vulnerable to anthropogenic impacts, stochastic events, and population declines, particularly where there is limited connectivity with other populations as they may have limited resilience or adaptability to local impacts. Information on ranging and residency patterns can contribute to existing knowledge of the species’ ecology and lead to a better understanding of overlap with pressures which, in turn, can inform management (Worton, 1989; Flores and Bazzalo, 2004; Sprogis et al., 2016).
For long-lived species such as marine mammals, having sufficient data that can identify population dynamics and needs in a timescale suitable for population management and conservation can be difficult. In some cases, particularly for vulnerable and data deficient species, sightings from multiple sources can be pooled together to increase the sample size and ability to estimate ranging patterns and detect site fidelity over a broader range and timescale (Maes et al., 2015; Molinari-Jobin et al., 2018). A similar approach could be used for cryptic species to study population ranging patterns, where individual range estimation is not possible, and tagging is not an ethical or feasible option due to the technology available or inability to capture the animals, however, where it is possible to identify individuals through natural marks (Andrews et al., 2019).
The Australian snubfin dolphin (Orcaella heinsohni, hereafter, snubfin dolphin) was described as a separate species in 2005 and is endemic to the tropical waters of northern Australia and southern New Guinea (Beasley et al., 2005). Snubfin dolphins are typically found in shallow coastal waters (<20 m) and usually in proximity (<15 km) to freshwater inputs (Parra et al., 2002, 2006b; Parra, 2006; Bouchet et al., 2021). Due to small population sizes and ongoing cumulative impacts across their coastal habitat, snubfin dolphins were assigned a conservation status of Vulnerable to extinction by the International Union for Conservation of Nature (IUCN) (Parra et al., 2017). Previous research suggests they are discontinuously distributed across their range as small local populations of 50–200 individuals (Parra et al., 2006a; Palmer et al., 2014b; Brown et al., 2016; Brooks et al., 2017; Bouchet et al., 2021) that exhibit site fidelity (Parra, 2006; Brown et al., 2016), and limited gene flow between populations (Brown et al., 2014b,2017). A recent study by Bouchet et al. (2021) found that snubfin dolphins occupy a relatively small area within their distribution in the Kimberley, further highlighting the limited range and vulnerability of the species. Due to their low numbers, limited resilience, and apparent reliance upon shallow waters (Parra et al., 2006b; Bejder et al., 2012; Brown et al., 2016; Bouchet et al., 2021), combined with their geographic isolation and long-lived characteristics (e.g., late maturation and low reproductive rates), snubfin dolphins are increasingly vulnerable to anthropogenic impacts, as reflected by their IUCN status. Furthermore, the importance of conserving snubfin dolphins has not only been identified at the global level but is also a priority at local, state, and national levels (DoE, 2015; Department of Parks and Wildlife, 2016a; Parra et al., 2017; Waples and Raudino, 2018).
Yawuru Nagulagun, also known as Roebuck Bay (hereafter, Yawuru Nagulagun, meaning sea country of the Yawuru people), in the northwest of Western Australia is part of Yawuru Country — the land and sea cared for by Yawuru people for thousands of years (Department of Parks and Wildlife, 2016a). In recognition of the unique and rich habitat known for its biodiversity and cultural values, Yawuru Nagulagun was partially gazetted as a marine park in 2016 under the Conservation and Land Management Act 1984 (CALM Act) and is jointly managed by the Yawuru Registered Native Title Prescribed Body Corporate (Yawuru P.B.C) and the Department of Biodiversity, Conservation and Attractions (DBCA). The northern half of Yawuru Nagulagun represents the highest density of snubfin dolphins among any population yet studied (∼130 individuals in a 100 km2 study area) (Brown et al., 2016; Raudino et al., 2020). Consequently, snubfin dolphins are considered a key biodiversity asset of the marine park and are the focal point for a small tourism industry in the area. While this population has been the subject of several studies on population abundance and connectivity across the region (Brown et al., 2014b,2016; Raudino et al., 2020), there is still limited understanding of how the population uses the bay both spatially and temporally.
On a global scale, the northern waters of Australia are relatively undisturbed (Halpern et al., 2008) partly due to their remoteness. However, usage of the region is predicted to increase (Palmer et al., 2014b; Department of Parks and Wildlife, 2016a), raising concerns about increasing pressures within Yawuru Nagulagun from population growth and development (Boschetti et al., 2020) and associated increases in recreational use and tourism (Allen et al., 2012; Bejder et al., 2012; Department of Parks and Wildlife, 2016a). More specifically, current pressures include development associated with the Port of Broome (Bejder et al., 2012), shipping traffic (Beckley, 2015), interactions with commercial tour vessels and interactions with recreational vessels including recreational fishing (Thiele, 2010; Department of Parks and Wildlife, 2016a). These pressures, along with effects of climate change, are predicted to impact the conservation of snubfin and other dolphin populations in the region (Boschetti et al., 2020).
Our study aimed to improve current knowledge on snubfin dolphin ranging patterns and site fidelity to Yawuru Nagulagun with a focus on identifying areas of high use and importance. To fulfill these aims, our study: (1) estimated range size and locations at the individual and population levels and (2) classified site fidelity at the individual and population levels. Data collected over 11 years by a variety of sources (i.e., researchers, rangers, managers, and citizen scientists) were used to allow the exploration of space use and site fidelity over an extended timeframe (2007–2019). Collating data from different programs is becoming increasingly common in ecological research (Fletcher et al., 2019; Isaac et al., 2020) as it offers a cost-effective means of increasing sample size over space and time for long-lived and wide-ranging species. The approach taken in this study allowed for the first assessment of individual ranging patterns for snubfin dolphins, and one of very few on site fidelity for this species to be produced and published.
Yawuru Nagulagun is a large tropical embayment (∼500 km2) near the township of Broome in north-western Australia (Figure 1). The area is subject to a large tidal range of up to 10 m during peak spring tides (Cresswell et al., 2011), resulting in 200 km2 of exposed mud flats at low tide that become unavailable to dolphins (Brown et al., 2014a; Raudino et al., 2020). The benthic substrate consists of mud and silt with seagrass and mangroves lining the coastal edge of the bay. The waters are commonly turbid, due to the predominantly shallow bathymetry of the bay, strong tidal flow, wind action, fine sediments, and organic matter in the water column from fringing mangrove systems. Water movement and mixing patterns in the nearshore zone are thought to be driven by large tidal ranges, seabed topography, and local winds (Department of Parks and Wildlife, 2016b).
Figure 1. (Left) Yawuru Nagulagun with boundaries of the Yawuru Nagulagun Roebuck Bay Marine Park (YNRBMP) illustrated, including zonation. (Right) Map of Australia illustrating the location of Yawuru Nagulagun (pictured inside box).
Data used in this project were collected through a range of research and citizen science projects, including dolphin surveys using standard survey techniques, as well as opportunistic sightings (see Supplementary Table 1). The majority of surveys were designed to cover the northern part of the Bay (∼100 km2). A full description of methods for individual surveys are available from the references listed in Supplementary Table 1 and a brief description is provided here. Each data source defined a dolphin group as “individuals within 100 m of each other engaging in the same or similar behavioral activity” (Irvine et al., 1981; Wells et al., 1987; Parra et al., 2006a). Age class was defined by comparing size and position in relation to an assumed adult: Adults appear fully grown relative to others in the group and are ∼2 m long; juveniles are around 2/3 the length of an adult and appear to be independent of an adult and calves are around 1/2 the length of an adult or smaller and swim in close association with an adult (Parra et al., 2006a; Jefferson et al., 2015). During systematic surveys, when a dolphin group was sighted, information collected on dolphin sightings included species present, number of individuals, behavior, and location. Photographs of individual snubfin dolphins were taken for identification. Sighting information included identification of individual dolphins, allowing for the compilation of individual sighting histories, including when, where and with whom they were sighted. The same information was recorded during opportunistic sightings, though photographs were not always available for all individuals.
Individuals were identified in photographs based on the natural marks present on their body and dorsal fins (Würsig and Jefferson, 1990). Adults and juveniles were included in analysis where they could be reliably identified through photo-identification (photo-ID). Calves were excluded from analysis. Evaluating images according to photographic quality and distinctiveness in a consistent manner is a critical step for all photo-ID studies, as this helps minimize bias and prevent misidentification of individuals (Urian et al., 1999, 2015; Rosel et al., 2011). Previous studies have successfully photo-identified individual snubfin dolphins in northern Australia and used protocols described by Urian et al. (1999) to study these populations (Parra et al., 2006a; Cagnazzi et al., 2013b; Palmer et al., 2014a; Brooks and Pollock, 2015; Brown et al., 2016; Raudino et al., 2020). In Yawuru Nagulagun, studies using photo-ID of individual snubfin dolphins have been conducted over many years (see Supplementary Table 1) to develop information on the population. To ensure consistency across datasets, the protocol developed by Urian et al. (1999, 2014) was applied to all photo-ID images used in this study to ensure the photo quality and distinctiveness of individual dolphins was standardized for all data (see Supplementary Appendix 1). An assumption of this study is accurate identification of individuals across time and space (Stolar and Nielsen, 2015), and the methods described here used to standardize image analysis from each data source minimizes potential mis-identification that could introduce such bias.
We used two methods to improve our understanding of the animals’ use of space: (i) minimum convex polygons (MCPs), which contain all points where an individual has been recorded (Mohr, 1947); and (ii) kernel density estimation (KDE) to measure the utilization distribution, which examines the intensity of space use to describe an animal’s range (Jennrich and Turner, 1969; Worton, 1989; Kie et al., 2010). While the MCP method can provide a representation of the overall range, it is known to be biased and can introduce intraspecific variation when small sample sizes are used (Nilsen et al., 2008). Therefore, we also used KDE to estimate the use of space within the species’ range. Individuals with a minimum of 10 sightings were used for this analysis as per Bräger et al. (2002) and Rayment et al. (2009). To minimize temporal and spatial autocorrelation, when an individual was sighted more than once within a day, only one randomly chosen sighting of an individual on that day was used. Methods for individual range analysis were repeated to estimate the population range using one randomly chosen dolphin group sighting per day.
The MCP is widely recognized and commonly used in the estimation of ranging patterns due to its validity for comparison to previous studies and simple calculation methods (Seaman et al., 1999; Flores and Bazzalo, 2004). MCPs provide a maximum representation of a species’ range based on the data available which can provide useful information for spatial risk assessments (Rayment et al., 2009; Sprogis et al., 2016). To estimate range on an individual level, MCPs were used to delineate the boundaries of the ranging patterns of individual snubfin dolphins by connecting the outermost sighting points (White and Garrott, 2012). For every individual, cumulative polygonal areas for each new sighting were calculated using R v 1.2.5042 (R Core Team, 2020) to determine if any individuals reached a maximum range size (Cobarrubia-Russo et al., 2020). The tool set Home Range Tools (MacLeod, 2013) in ArcMap v 10.7 (ESRI, 2019) was used to calculate MCPs.
One of the most common methods used to measure utilization distribution is KDE, which estimates the intensity of use throughout the spatial range using a density based statistical approach (Silverman, 1986). KDE is a non-parametric method and has the potential to accurately estimate densities of any shape when the most appropriate level of smoothing is selected (Seaman and Powell, 1996). The width of the kernel, also known as “bandwidth” or “smoothing parameter,” determines the relationship between the distance of a location from which a species has been sighted and the contribution of the location to the density estimate at that point (Gitzen et al., 2006). For this study, several methods were considered (see Supplementary Table 2) and trialed for the individual and population home range estimates. The likelihood cross-validation (CVh) method was chosen as it produced relatively consistent density estimates and density contours that were the least fragmented, despite the relatively small number of sightings. Smoothing parameters using the CVh method were calculated via Animal Space Use v 1.3 (Horne and Garton, 2009).
The tool set Home Range Tools (MacLeod, 2013) in ArcMap v 10.7 (ESRI, 2019) was used to estimate the utilization distribution using fixed kernel densities. Fixed kernels (i.e., where the bandwidth remains constant) were used rather than adaptive kernels (i.e., where the bandwidth varies depending on nearby observations) as they generally have lower bias at outer contours of the home range (Seaman et al., 1999; Gitzen et al., 2006). The “Kernel Density Estimate with Barriers” tool was chosen to ensure that land was excluded and would not bias the density estimates. Using the Spatial Analyst and Data Management toolbox, the kernel density values were extracted and then used to produce percentage density contours (PDC) at 50% and 95% levels to indicate core and representative areas for individual dolphins, respectively (Gill et al., 2011; Brough et al., 2019).
A consequence of using data from multiple sources (including opportunistic data) in this study was the lack of equal survey coverage across the study area. To account for non-uniform survey effort, each dolphin sighting was assigned a weight based on the probability of obtaining that sighting (Fieberg, 2007; Rayment et al., 2009; Brough et al., 2019). Survey tracks were extracted from each dataset where available (see Supplementary Table 1) and imported into ArcMap v 10.7 (ESRI, 2019). Using data from 2014 (the year with the highest survey intensity), the maximum detection distance (distance from the survey vessel where dolphins were detected) was estimated as 450 m. A buffer of 450 m was added to both sides of each survey track to include the estimated detection range in the surveyed area. As this study aimed to obtain minimum ranging estimates, a uniform key function was applied using the maximum distance that groups were sighted within. This approach aimed to reduce large spatial scale biases caused by survey effort varying significantly over the broad study area, and not detection bias. The potential bias of reduced detection range from the vessel over several hundred meters from the transect was considered to be smaller in scale, and relatively small in its impact on gaining insight into broader scale ranging patterns, which was the main aim of the study. A grid with a cell size of 0.01 km2 was applied to the survey area and the number of times a grid cell was surveyed and the number of sightings per grid cell were calculated. Different grid cell sizes ranging from 0.01 to 1 km2 were trialed to determine the most suitable size for the analysis. A cell size of 0.01 km2 was used as it produced density estimates at a high resolution with the least amount of fragmentation and over-smoothing (Powell, 2000; Gregory, 2016). Grid cells were then weighted using the following equation modified from Rayment et al. (2009):
where, Ai = the area of cell i covered by a survey, Vi = the number of visits to cell i during the time the dolphin was known to be alive, and T = the total number of cells surveyed. The scaled weight for each sighting (), such that all the scaled weights for an individual would sum to one and the resulting distribution would be a true probability density function, was calculated using the following equation from Rayment et al. (2009):
This weighting process assigns greater weight to sightings in areas that received less survey effort. Some assumptions for this study that were not validated included: (1) detection within the buffer area (450 m) was uniform and (2) that the individuals were in the study area and therefore available for detection. Weighting for survey effort with the assumptions given above reduced spatial bias in the kernel density estimates toward areas that received more survey effort.
To investigate the tendency of individual snubfin dolphins to return to or remain in the study area, three measures of site fidelity using re-sighting rates were calculated for individual dolphins: (1) monthly sighting rate (number of months a dolphin was identified in as a proportion of the total number of months over which surveys took place), (2) yearly sighting rate (the number of calendar years a dolphin was identified in as a proportion of the total number of calendar years surveyed) and (3) site fidelity indices (SFIs) (Parra et al., 2006a; Zanardo et al., 2016; Hunt et al., 2017; Passadore et al., 2018; Haughey et al., 2020). SFIs were calculated as the ratio between the number of re-sightings for each individual and the number of survey days (defined as the number of survey days from an individual’s first sighting to its last re-sighting) (Simpfendorfer et al., 2011; Daly et al., 2014). Individuals that were first sighted in the last survey period (2019) were excluded from the analysis. To reduce temporal autocorrelation, only one randomly chosen sighting per day of an individual was included.
Due to variation in sampling effort over the study period (2007–2019), minimum sampling times were implemented for the site fidelity measures to ensure that the months and years included had sufficient survey effort to reliably identify all snubfin dolphins present at that time. Months with a minimum of two survey days were included for the monthly sighting rate calculation. Years with surveys in at least 2 months were included for the yearly sighting rate calculation. These minimum sampling periods were chosen to eliminate months and years with potentially insufficient sampling coverage whilst aiming to maximize the ability to detect site fidelity (Vermeulen et al., 2017).
To determine if there were groups or “clusters” of individuals with similar levels of site fidelity, an agglomerative hierarchical clustering (AHC) analysis was run using the monthly and yearly sighting rates (Zanardo et al., 2016; Hunt et al., 2017; Passadore et al., 2018; Haughey et al., 2020). AHC is a clustering method that builds a dendrogram beginning with each observation as an individual cluster. These clusters are then successively combined based on similarity until all clusters have been combined into one (Legendre and Legendre, 2012). While several methods were considered, the Euclidean distance and Ward’s method (minimum variance) were used as the dissimilarity measure and the clustering algorithm, respectively, as these are considered suitable approaches (Ward, 1963; Singh et al., 2011) and comparable to past research (Zanardo et al., 2016; Hunt et al., 2017; Passadore et al., 2018; Haughey et al., 2020).
To assess the strength of each cluster identified, approximately unbiased (AU) probability values (i.e., p-values) were calculated by generating 1,000 bootstrap resampling replications per cluster (Suzuki and Shimodaira, 2006; Singh et al., 2011; Zanardo et al., 2016). The AU—p-values were also used to define a dissimilarity threshold to determine the most suitable number of clusters. The validity of dissimilarities among observations was assessed using the cophenetic correlation coefficient (CCC). The CCC measures the relationship between the original dissimilarity matrix and the cophenetic matrix, which is obtained after the dissimilarities are recalculated by the clustering algorithm (Sokal and Rohlf, 1962; Passadore et al., 2018). A CCC-value greater than 0.8 can be considered a reliable representation of the data (Bridge and Fry, 1993). The AHC analysis was performed using the “pvclust” package (Suzuki and Shimodaira, 2006) in R v 1.2.5042 (R Core Team, 2020).
To investigate site fidelity at the population level, indicators such as permanence and periodicity as defined by Tschopp et al. (2018) were calculated as part of a standardized site fidelity index (SSFI) using the following equations:
and
followed by:
where IT (Permanence) is the average amount of time spent in the study area expressed as the number of days between the first and final sighting of each individual as a proportion of the total number of days from the beginning to the end of sampling (non-constant effort). It (Periodicity) is the average recurrence of an individual, expressed as the number of days between an individual’s first, and final sighting as a proportion of the individual’s total number of sightings minus one.
A total of 147 surveys were conducted between 2007 and 2019 (see Supplementary Figure 1). Surveys were usually conducted in the dry season (April-October) with few surveys conducted in the wet season (November-March). The most intensive survey periods included October/November in 2013, April/May in 2014 and July 2014 (see Supplementary Figure 1). During the study period, there were 688 group sightings of snubfin dolphins in Yawuru Nagulagun (see Supplementary Figure 2 Left). Effort was unevenly distributed throughout the bay, with the northern area of the bay (∼100 km2) receiving notably more effort in comparison to the southern end of the bay (see Supplementary Figure 2 Right). There were 2,306 images processed, with 1,390 (60.28%) classified as excellent/good quality of a distinctive individual (D1 or D2) of a total of 268 unique individuals.
For ranging pattern analyses of individuals, there were 24 individuals with 10 or more sightings that qualified for range analysis and the number of sightings per individual ranged from 10–17 (see Supplementary Table 3 and Supplementary Figures 3.1–3.6). The cumulative range area curve (Figure 2) indicated only four individuals (oh307, oh340, rb069oh and rb078oh) reached a stable asymptote at 14 sightings; however, the area for these individuals began to increase slightly after 14 sightings. The majority of individuals did not reach a stable asymptote, indicating that their full range size is likely underestimated.
Figure 2. Cumulative range area (MCP in km2) for each individual (with at least 10 sightings) as a function of the number of times sighted.
The core and representative areas for the 24 individuals were located in the northern part of the bay where the majority of survey effort occurred (see Supplementary Figures 3.1–3.6). Three individuals (rb077oh, rb041oh and rb095oh) also had core areas located in the southern part of the bay (∼20 km between areas) (see Supplementary Figure 3.2; rb077oh, Supplementary Figure 3.3; rb041oh and Supplementary Figure 3.5; rb095oh). The MCP for the 24 individuals ranged from 4.3 to 124.57 km2 with a mean of 29.30 km2 (± 31.73 SD) (see Supplementary Table 3 and Supplementary Figures 3.1–3.6). The 50% PDC ranged from 6.51 to 90.09 km2 with a mean of 21.66 km2 (± 18.85 SD) and the 95% PDC ranged from 12.27 to 157.52 km2 with a mean of 39.88 km2 (± 32.65 SD) (see Supplementary Table 3 and Supplementary Figures 3.1–3.6).
For population level ranging pattern analyses, 134 sightings were used. The core and representative areas were in both the northern and southern areas in the bay and within 5–10 km of the coastline (Figure 3, Right). The population MCP area was 215.05 km2 (Figure 3, Left). The 50% PDC was 54.15 km2 and the 95% PDC was 103.05 km2 (Figure 3, Right).
Figure 3. Ranging pattern estimations for the Yawuru Nagulagun snubfin dolphin population using the minimum convex polygon (MCP) method and a kernel density estimate (KDE) of effort-weighted group dolphin sightings showing 50% and 95% percentage density contours (PDC) (i.e., core and representative area, respectively).
For the site fidelity analyses, 20 months (out of 30) and six years (out of 11) were included. Site fidelity analyses indicated that 24.10% of individuals (n = 47) were sighted only once, 23.59% (n = 46) were sighted two or three times, and 52.31% (n = 102) were sighted four or more times (based on 195 individuals that could be included in the analyses). The average monthly re-sight rate was (±SD) 0.13 ± 0.09 (95% CI: 0.12–0.14) indicating individuals were usually sighted in two or three out of the 20 months surveyed. Site fidelity analyses indicated 34.36% of individuals (n = 67) were sighted during only one month, while three individuals (rb025oh, rb078oh and rb080oh) were seen in eight survey months and had the highest monthly re-sighting rate (0.40). The average yearly re-sighting rate was 0.31 ± 0.17 (95% CI: 0.30–0.32) indicating that individuals were typically sighted in one or two years out of the six included in the site fidelity measures. In total, 43.59% of individuals were sighted in one year (n = 85), 50.26% sighted in two or three years (n = 98), and 6.15% individuals (n = 12) sighted in four or more years. One individual was sighted in all six years (rb107oh). The longest period between first and last re-sighting was 11 years (n = 2) followed by 10 years (n = 3). The average SFI was 0.10 ± 0.08 (95% CI: 0.09–0.11), however, this includes the 24.10% of individuals (n = 47) that were sighted only once (SFI = 0).
Individuals were separated into three clusters based on the AHC analysis (dissimilarity threshold = 12.5) (Figure 4) using monthly and yearly re-sighting rates. Clusters in the dendrogram were considered a reasonable representation [the cophenetic correlation coefficient (CCC) = 0.67 and the AU—p-values (0.91–0.95)]. Cluster 1 consisted of 43.59% of individuals (n = 85) that were sighted in one out of the six years and can be considered as “non-residents” with no site fidelity to the area. Cluster 2 consisted of 44.62% of individuals (n = 87) that were sighted in two or three years and can be considered as “part-time residents” with moderate site fidelity. Cluster 3 consisted of 11.79% of individuals (n = 23) that were sighted in either three or four of the six years and can be considered as “long-term residents” who displayed a high level of site fidelity (Table 1). This finding was further supported by the primary cluster for clusters 2 and 3 being derived from the same “root” in the dendrogram tree (AU p-value = 0.95; Figure 4).
Figure 4. Agglomerative hierarchical clustering (AHC) dendrogram of snubfin dolphins in Yawuru Nagulagun based on monthly and yearly sighting rates. The rectangles (dissimilarity threshold = 12.5) indicate three clusters with cluster 1 (“non-residents”) with 85 individuals (43.59% of the population), cluster 2 (“part-time residents”) with 87 individuals (44.62% of the population) and cluster 3 (“long-term residents”) with 23 individuals (11.79% of the population). The approximately unbiased (AU) probability values of these three clusters are shown in the dendrogram.
Table 1. Average monthly and yearly resight rates and average site fidelity indices (SFI) of the three clusters of snubfin dolphins in Yawuru Nagulagun identified through an agglomerative hierarchical clustering analysis.
The standardized site fidelity estimate for the population (SSFI) overall was low (0.19) in Yawuru Nagulagun, given that an SSFI of zero suggests low site fidelity for the population and one suggests high site fidelity for the population (Tschopp et al., 2018).
Our approach of analyzing datasets from multiple sources collected over an 11-year period has contributed to our current understanding of the ranging patterns and site fidelity of snubfin dolphins and have provided further evidence of the importance of Yawuru Nagulagun to this species. The importance of the nearby un-surveyed areas remains unknown and will be a priority for future research to compare with Yawuru Nagulagun. In terms of sightings, our combined dataset was over four and a half times larger than the single largest component study (Brown et al., 2016). This study collated data from multiple sources and produced results that could not have been obtained with the data from any single study alone. However, as indicated here, using data opportunistically from multiple studies can present biases in spatial and temporal coverage and may be subject to other limitations (Isaac and Pocock, 2015). The results in this study have been interpreted taking these limitations into account and reducing biases where possible and have provided information that can be used by natural resource managers. As such, we recommend that consideration be given to the use of multiple data sources and datasets, particularly when evaluating long-lived and data deficient species. Strategic planning of research should also be supported where possible to augment existing datasets. For example, a strategic research program should aim to produce comprehensive spatial and temporal data on populations, individuals and groups over decadal time frames that will support research questions relevant to long-lived animals such as snubfin dolphins. Often this is only possible through integration of data collected over multiple individual studies that collectively span time periods relevant to long-lived species. This approach will also help detect any shifts or patterns (e.g., in population size or distribution) over a finer resolution and will benefit on-going management planning and implementation.
The ranging patterns of individuals that could be evaluated (∼20% of the estimated local population) demonstrated some variability; however, all included shallow (<20 m), inshore waters in the northern area of the bay (see Supplementary Figures 3.1–3.6), although this could be a result of the northern area receiving the most survey effort. Range estimates from this study illustrate the importance of relatively shallow waters for snubfin dolphins, as has been reported for the species elsewhere throughout their range (Parra et al., 2006b; Cagnazzi et al., 2013b; Palmer et al., 2014b; Bouchet et al., 2021), providing further evidence for their dependency on these habitats.
Notably, three individuals (rb077oh, rb041oh and rb095oh) sighted in the northern area were also recorded in the southern area of the bay (∼20 km between areas), indicating that at least some dolphins in this population use a larger proportion of the bay (see Supplementary Figure 3.2; rb077oh, Supplementary Figure 3.3; rb041oh and Supplementary Figure 3.5; rb095oh). The weighted kernel densities for these sightings, however, are potentially over-inflated in the southern area of the bay due to relatively low survey effort (see areas with high kernel densities in Supplementary Figure 3.2; rb077oh, Supplementary Figure 3.3; rb041oh and Supplementary Figure 3.5; rb095oh); therefore, there is greater uncertainty in the estimated levels of use in the south of the bay which would also apply to the population home range estimate. Despite this uncertainty, the results confirm that the southern part of the bay is within the populations’ known range in Yawuru Nagulagun and may include important habitat. Full range sizes, as compared to the kernel densities, are expected to be larger for individuals and the population, which should be considered when comparing results with those from studies elsewhere, when applying these results to management decisions, and when designing future studies of ranging patterns (i.e., surveying a larger area than those covered by existing studies).
The range estimate for the snubfin dolphin population in Yawuru Nagulagun calculated using KDE (95% PDC = 103 km2) is considerably smaller than the estimates for two populations on the east coast of Australia (Parra, 2006; Cagnazzi et al., 2013b). Parra (2006) estimated the area (95% kernel range) for a population of 64–76 snubfin dolphins in Cleveland Bay (Queensland) as 197 km2 using data collected from surveys over a 4-year period covering an approximate area of 310 km2. Cagnazzi et al. (2013b) estimated the area (95% kernel range) for a population of approximately 100 snubfin dolphins in Keppel Bay on the Queensland coast as 349 km2 over a 6-year period within a study area of 4,000 km2. Whilst there may be true differences in the ranges between these populations, it is likely that differences in the size of area surveyed between the studies is a key influence, with most effort in the current study focusing on 100 km2. Further, our estimate of the representative area of use with MCP was similar to the 95% kernel range estimates of Parra (2006) (215.05 km2; Figure 3). MCPs are considered less conservative than KDEs and are thought to be a measure of maximum range, while 95% kernel range is considered a representative range. The estimates from this study reflect minimum range size; we suggest that the larger MCP estimate is likely closer to the true range than that estimated by KDE, albeit still an under-estimate due to survey effort not encompassing the entire range of the population. The relatively smaller population ranging pattern, coupled with the high density for this population, may also be a reflection of the rich and productive habitat of Yawuru Nagulagun (Department of Parks and Wildlife, 2016a).
In this study, range sizes represent minimum estimates, with a greater number of sightings (>10) than available here required to estimate the full ranges for these dolphins (Figure 2; Owen et al., 2002). Future studies should aim for a larger sample size of individuals and more sightings per individual to estimate the representative range of the population (Börger et al., 2006). Due to the limited sample size, it was not possible to investigate seasonal or age and sex differences in dolphin ranging patterns (e.g., Sprogis et al., 2016). Future dedicated studies are needed to assess if the ranging patterns presented here are representative across seasons and age/sex classes. This would require increasing survey effort across the entire bay and throughout the year and would yield a larger sample size with more even spatial coverage that could be used to investigate these questions as well as how ranging patterns change over time.
Yawuru Nagulagun is a large embayment covering ∼500 km2, and much of the survey effort was concentrated in the northern part of the bay (∼100 km2). To reduce the uncertainty around range estimates and to optimize management, greater effort over the entire embayment and beyond the extent of existing sightings is required. An additional approach that may be more accessible in future studies, is to optimize results from analyses of existing datasets by accounting for autocorrelation in analyses rather than removing autocorrelated temporally proximal observations (as done here), which reduces the overall available sample size. Using a subset of data with reduced autocorrelation is statistically sound (Noonan et al., 2019); however, the resulting estimate could be considered a conservative home range estimate. Seasonal autocorrelation should be considered in future analyses, as this was not accounted for due to the nature of the dataset and the uneven sampling effort over the study period (2007–2019). Future studies on ranging patterns should aim for year-round survey effort encompassing several seasons.
The individual ranging patterns presented here are the first for this species. An application of these data includes informing the area of occupancy (AOO) (i.e., area occupied within their distribution), which reflects the species vulnerability due to a restricted range, as part of the IUCN conservation assessments. The AOO is calculated by superimposing a grid over the extent of occurrence (EOO) of the species and summing the area of cells occupied by the species. The quantification of the area they occupy through home range estimates will be useful for selecting an appropriate grid cell size used to calculate the AOO for future IUCN conservation assessments (Bouchet et al., 2021). While the estimates we present provide a baseline, given that ten sightings proved inadequate to capture full range size, the estimates of ranging patterns reported here should be re-evaluated when more sightings per individual become available and the survey area has been expanded.
Findings regarding the importance of Yawuru Nagulagun to the snubfin dolphin based on ranging patterns are supported by our results relating to residency and site fidelity. The majority of individuals (56%) in the area demonstrated some level of residency, either long-term (years) or short-term (months), emphasizing the use of the area across multiple years for a species whose life expectancy is ∼28–30 years (Parra et al., 2017).
While results suggested a large proportion of the population (44%) demonstrated no site fidelity to the area, this is likely to be influenced by the restrictions placed on the data for site fidelity analyses (i.e., minimum number of months/days required to be considered a sampling period), and/or the overall relatively low intensity of sampling which may have limited the number of resightings of individuals. It is also possible that these “non-resident” individuals exhibit a home range with less overlap to the survey area than individuals classified as exhibiting greater residency, therefore only occasionally visiting the area of greatest survey effort in the northern half of the bay where the probability of being sighted was greatest.
Brown et al. (2016) suggested there was preliminary evidence of site fidelity within Yawuru Nagulagun, where a majority of individuals were re-sighted between two relatively short study periods (albeit of high survey effort) separated by 5 months (Oct/Nov 2013 and April 2014). The current study has confirmed these findings based on an assessment of 195 individuals in the bay and, further, shown evidence of site fidelity over longer time periods. Future surveys designed to include Yawuru Nagulagun and surrounding areas would clarify the question of whether these snubfin dolphins have core home ranges outside the study area or if these “non-resident” individuals are an artifact of sampling bias. Future studies could survey a broader area, including increasing the survey effort in the southern section of the bay as well as further offshore. This additional information will better inform site fidelity across the entire bay and core areas of use. Another potential bias that could explain these results would be the mis-identification of dolphins due to changes in dorsal fin markings as a result of injury (Smith et al., 2018). This source of bias has been noted for this population, where natural identifying marks on individuals (e.g., resulting from injuries) evolve rapidly and their original identity may not be apparent due to the modifications to their fin and thus they are given a new identification code.
Whilst the estimated site fidelity index for the Yawuru Nagulagun snubfin dolphin population (SSFI = 0.19) was lower than expected given the individual site fidelity results, it indicates some residency. The SSFI is a relatively new measure for site fidelity at the population level (Tschopp et al., 2018) with only one published study available for comparison. Haughey et al. (2020) measured site fidelity for the Indo-pacific bottlenose dolphin (Tursiops aduncus) population on the North-West Cape in Western Australia as 0.019, indicating lower levels of site fidelity. Due to inconsistent sampling effort and survey intensity across months and years, our results should be interpreted cautiously and investigated further using systematic sampling across a defined study period before robust conclusions on the site fidelity of the population can be drawn.
Studies have found that ranging patterns and site fidelity are driven by several extrinsic factors including environmental conditions, quality of habitat, distribution of food resources, population density, and potential mates or predators as well as intrinsic factors such as body size, sex, and age (Switzer, 1993; McLoughlin and Ferguson, 2000; Duncan et al., 2015). Food availability and population density are thought to have the strongest influence in determining the size, shape, and location of ranging patterns (Mitchell and Powell, 2012; Duncan et al., 2015; Schoepf et al., 2015). Studies have also demonstrated that increasing population density decreases range size because individuals’ space use patterns are constrained by competitive interactions with neighboring individuals. Parra (2006) investigated resource partitioning between snubfin and humpback dolphins on the east coast of Australia and suggested the main driving factors of habitat selection included diet and inter-specific agonistic interactions. Previous studies in Yawuru Nagulagun have confirmed that other dolphin species (e.g., Australian humpback dolphins and Indo-Pacific bottlenose dolphins) use the area, although they have been recorded in lower numbers than snubfin dolphins (Brown et al., 2016; Raudino et al., 2020). The results from this study can guide future studies investigating driving factors of range and site fidelity patterns in Yawuru Nagulagun (e.g., examining inter-specific interactions or prey availability).
Data on ranging patterns is limited for most cetacean species, however, several studies have reported a strong correlation between cetacean movement patterns, patterns of distribution, and abundance of food (Stevick et al., 2002; Silva et al., 2008). In marine mammal populations with high levels of site fidelity and residency, it is likely that there is high food availability and low predation risk (Gubbins, 2002; Gowans et al., 2007; Fury and Harrison, 2008; Zanardo et al., 2016; Passadore et al., 2018). Alternatively, other populations with large ranging patterns in areas where food resources are more dispersed, are required to travel further in search of food (Ballance, 1992; Gowans et al., 2007; Silva et al., 2008). Sprogis et al. (2016) also found that home range size can differ between and within sex classes within a dolphin population potentially due to differences in diet, amongst other factors. Based on previous studies which analyzed gut content from a small sample size of bycaught snubfin dolphins, it is thought they are opportunistic-generalist feeders, preying on a variety of fish and cephalopods, which are available in shallow coastal/estuarine environments (Parra, 2006; Parra and Jedensjö, 2014). Given that Yawuru Nagulagun is a highly biodiverse environment and one of the most productive tropical intertidal areas globally (Department of Parks and Wildlife, 2016a), there is likely to be a large variety of food sources available for snubfin dolphins. Further research using a combination of direct observation, stable isotope analysis and gut analysis of hard parts recovered opportunistically from carcasses would help to determine diet preferences (McCluskey et al., 2021) for the Yawuru Nagulagun population, and may help better understand whether the distribution of prey drives dolphin spatial distribution.
Based on the results from this study as well as those conducted on the east coast of Australia, home range and site fidelity for snubfin dolphins vary depending on the attributes of the region (Parra, 2006; Cagnazzi et al., 2013b), and investigating and estimating these parameters at each location is key to effective management, particularly for the identification of high use or important areas and potential overlap with anthropogenic activities. We found that some dolphin group sightings occurred in a deep channel (10–50 m) (see Supplementary Figure 2) in an area that lies within the Port of Broome tenure, which is excised from the marine park, and experiences a high density of vessel traffic (Beckley, 2015). Not only does the population range overlap with the Port of Broome, but this study also provides evidence of site fidelity, further emphasizing the importance of the area to snubfin dolphins. Overlap with this area can lead to greater exposure for dolphins to pressures such as vessel disturbance (Bejder et al., 2006; Jensen et al., 2009a; Marley et al., 2017b), noise (Jensen et al., 2009a; Erbe et al., 2019), vessel strikes (Thiele, 2010; Schoeman et al., 2020), and potential exposure to contaminants (Cagnazzi et al., 2013a). Given that this area is not within the marine park, dolphin use of the Port of Broome should be noted and considered in management decisions regarding port development activities.
Beckley (2015) found that use of recreational vessels peaked during the dry season, but remained consistent throughout the wet season; therefore, potential pressures on snubfin dolphins such as boat strike, entanglement in fishing gear, increased exposure to underwater noise (and its potential impacts on intra-specific communication and in elevating stress), and general disturbance will occur year-round. Recreational activity likely occurs throughout the representative home range area. The likelihood of impacts in these areas should be evaluated so that measures could be taken to mitigate them if required, particularly if these pressures increase as predicted. Wildlife tourism (i.e., dolphin watching) represents another potential pressure on snubfin dolphins in the bay. Future research, including a spatial risk assessment, could evaluate the distribution of pressures (i.e., vessel traffic and areas used by tour operators) relative to dolphin high use areas to further inform management. For example, the findings from this study on range patterns could be compared with areas identified in the spatial risk assessment to quantify the spatial overlap. To minimize potential impacts from the tourism industry, managers should understand the basic interactions between marine mammals and tourism vessels, including the timeframe and locations where these occur and the potential for displacement or disruption of activities so that appropriate management strategies can be considered (Waples, 2003; Tyne et al., 2018).
This study provides the first insights into ranging patterns and site fidelity of a significant population of snubfin dolphins and confirms that there is a resident community of dolphins that use Yawuru Nagulagun. These important insights into the local population contribute new information for continued conservation and management of snubfin dolphins at the broader species level as well as more specifically for the local population. Where there is a data deficiency for a species or where a single standardized long-term dataset does not exist, we recommend combining data from separate sources as we have shown this can significantly extend the current knowledge on the species’ and could potentially improve the power to detect changes in the population and response to pressures over decadal timeframes. This approach could be applied to improve conservation management strategies for other cryptic, data deficient, vulnerable, and long-lived species.
All sightings compiled in this study have been previously published, with the exception of observations collected by Rangers from the Yawuru Native Title Prescribed Body Corporate (Yawuru P.B.C) and Dolphin Watch citizen scientists. Available data can be accessed through the State-wide catalogue (Dolfin database) hosted and managed by DBCA (see https://dolfin.dbca.wa.gov.au). Requests to access these datasets should be directed to HR, aG9sbHkucmF1ZGlub0BkYmNhLndhLmdvdi5hdQ==.
Ethical review and approval was not required for the animal study because this study collated data from six different projects over 11 years. Each research project had animal ethics approval.
HR, KW, and CSK conceived the study. Data was collected by many contributors (see Supplementary Table 1). ADC processed and analyzed the data with advice and contributions to data analysis from CSK and HR. ADC wrote the first draft of the manuscript with contributions to drafting, critical review, and editorial input from HR, KW, CSK, AB, SM, and Yawuru PBC. All authors designed the study.
Projects from which data were collated from were supported by the following organizations: Australian National University, WWF-Australia, The Australian Marine Mammal Centre (Commonwealth Government), Murdoch University, Western Australian Marine Science Institution, Nyamba Buru Yawuru, Dolphin Watch and the Department of Biodiversity, Conservation and Attractions (DBCA). This project was also supported by Edith Cowan University.
Authors from Yawuru PBC were employed by the Yawuru Native Title Prescribed Body Corporate.
The remaining authors declare that the research was conducted in the absence of any commercial or financial relationships that could be construed as a potential conflict of interest.
All claims expressed in this article are solely those of the authors and do not necessarily represent those of their affiliated organizations, or those of the publisher, the editors and the reviewers. Any product that may be evaluated in this article, or claim that may be made by its manufacturer, is not guaranteed or endorsed by the publisher.
We would like to thank the Traditional Owners of the land and sea on which this study was based on, the Yawuru people, and pay our respects to elders past, present and emerging. We would also like to thank all those involved with data collection (See Supplementary Table 1). We would also further like to thank Kathryn McMahon and Danielle Cagnazzi for providing feedback on an earlier draft of the manuscript.
The Supplementary Material for this article can be found online at: https://www.frontiersin.org/articles/10.3389/fmars.2021.758435/full#supplementary-material
Allen, S. J., Cagnazzi, D. D., Hodgson, A. J., Loneragan, N. R., and Bejder, L. (2012). Tropical inshore dolphins of north-western Australia: unknown populations in a rapidly changing region. Pac. Conserv. Biol. 18, 56–63. doi: 10.1071/PC120056
Andrews, R. D., Baird, R. W., Calambokidis, J., Goertz, C. E., Gulland, F. M., Heide-Jorgensen, M. P., et al. (2019). Best practice guidelines for cetacean tagging. J. Cetacean Res. Manage. 20, 27–66. doi: 10.47536/jcrm.v20i1.237
Ballance, L. T. (1992). Habitat use patterns and ranges of the bottlenose dolphin in the Gulf of California, Mexico. Mar. Mamm. Sci. 8, 262–274. doi: 10.1111/j.1748-7692.1992.tb00408.x
Beasley, I., Robertson, K. M., and Arnold, P. (2005). Description of a new dolphin, the Australian snubfin dolphin Orcaella heinsohni sp. n.(Cetacea, Delphinidae). Mar. Mamm. Sci. 21, 365–400. doi: 10.1111/j.1748-7692.2005.tb01239.x
Beckley, L. E. (2015). Human Use Patterns and Impacts for Coastal Waters of the Kimberley. Final Report of Project 2.1.1 of the Kimberley. Perth, WA: Marine Research Program of WAMSI.
Bejder, L., Hodgson, A., Loneragan, N., and Allen, S. (2012). Coastal dolphins in north-western Australia: the need for re-evaluation of species listings and short-comings in the Environmental Impact Assessment process. Pac. Conserv. Biol. 18, 22–25. doi: 10.1071/PC120022
Bejder, L., Samuels, A., Whitehead, H., Gales, N., Mann, J., Connor, R., et al. (2006). Decline in relative abundance of bottlenose dolphins exposed to long-term disturbance. Conserv. Biol. 20, 1791–1798. doi: 10.1111/j.1523-1739.2006.00540.x
Börger, L. (2016). Stuck in motion? Reconnecting questions and tools in movement ecology. J. Anim. Ecol. 85, 5–10. doi: 10.1111/1365-2656.12464
Börger, L., Franconi, N., De Michele, G., Gantz, A., Meschi, F., Manica, A., et al. (2006). Effects of sampling regime on the mean and variance of home range size estimates. J. Anim. Ecol. 75, 1393–1405. doi: 10.1111/j.1365-2656.2006.01164.x
Boschetti, F., Lozano-Montes, H., and Stelfox, B. (2020). Modelling regional futures at decadal scale: application to the Kimberley region. Sci. Rep. 10:849. doi: 10.1038/s41598-019-56646-x
Bossart, G. D. (2011). Marine mammals as sentinel species for oceans and human health. Vet. Pathol. 48, 676–690. doi: 10.1177/0300985810388525
Bouchet, P. J., Thiele, D., Marley, S. A., Waples, K., Weisenberger, F., Rangers, B., et al. (2021). Regional assessment of the conservation status of snubfin dolphins (Orcaella heinsohni) in the Kimberley region, Western Australia. Front. Mar. Sci. 7:614852. doi: 10.3389/fmars.2020.614852
Bowen, W. (1997). Role of marine mammals in aquatic ecosystems. Mar. Ecol. Prog. Ser. 158, 267–274. doi: 10.3354/meps158267
Bräger, S., Dawson, S. M., Slooten, E., Smith, S., Stone, G. S., and Yoshinaga, A. (2002). Site fidelity and along-shore range in Hector’s dolphin, an endangered marine dolphin from New Zealand. Biol. Conserv. 108, 281–287. doi: 10.1016/S0006-3207(02)00124-6
Bridge, P., and Fry, J. (1993). Classification. Biological Data Analysis: A Practical Approach. Oxford: IRL Press.
Brooks, L., Palmer, C., Griffiths, A. D., and Pollock, K. H. (2017). Monitoring variation in small coastal dolphin populations: an example from Darwin, Northern Territory, Australia. Front. Mar. Sci. 4:94. doi: 10.3389/fmars.2017.00094
Brooks, L., and Pollock, K. (2015). The Darwin Dolphin Monitoring Program. Abundance, Apparent Survival, Movements and Habitat Use of Humpback, Bottlenose and Snubfin Dolphins in the Darwin Area. Final Report. Woodburn, NSW: Statplan Consulting Pty Ltd, 51.
Brough, T., Rayment, W., Slooten, E., and Dawson, S. (2019). Fine scale distribution for a population of New Zealand’s only endemic dolphin (Cephalorhynchus hectori) shows long-term stability of coastal hotspots. Mar. Mamm. Sci. 35, 140–163. doi: 10.1111/mms.12528
Brown, A. M., Kopps, A. M., Allen, S. J., Bejder, L., Littleford-Colquhoun, B., Parra, G. J., et al. (2014b). Population differentiation and Hybridisation of Australian snubfin (Orcaella heinsohni) and indo-pacific humpback (Sousa chinensis) Dolphins in North-Western Australia. PLoS One 9:e101427. doi: 10.1371/journal.pone.0101427
Brown, A. M., Bejder, L., Pollock, K. H., and Allen, S. J. (2014a). Abundance of Coastal Dolphins in Roebuck Bay, Western Australia: Updated Results from 2013 and 2014 Sampling Periods. Report to WWF-Australia. Perth, WA: Murdoch University.
Brown, A. M., Bejder, L., Pollock, K. H., and Allen, S. J. (2016). Site-specific assessments of the abundance of three inshore dolphin species to inform conservation and management. Front. Mar. Sci. 3:4. doi: 10.3389/fmars.2016.00004
Brown, A. M., Smith, J., Allen, S. J., Bejder, L., Chabanne, D. Salgado-Kent, C., et al. (2017). Relative Abundance, Population Genetic Structure and Passive Acoustic Monitoring of Australian Snubfin and Humpback Dolphins in Regions Within the Kimberley. Final Report of Project 1.2.4 of the Kimberley. Perth, WA: Marine Research Program of WAMSI.
Cagnazzi, D., Fossi, M. C., Parra, G. J., Harrison, P. L., Maltese, S., Coppola, D., et al. (2013a). Anthropogenic contaminants in Indo-Pacific humpback and Australian snubfin dolphins from the central and southern Great Barrier Reef. Environ. Pollut. 182, 490–494. doi: 10.1016/j.envpol.2013.08.008
Cagnazzi, D., Parra, G. J., Westley, S., and Harrison, P. L. (2013b). At the heart of the industrial boom: Australian snubfin dolphins in the Capricorn Coast, Queensland, need urgent conservation action. PLoS One 8:e56729. doi: 10.1371/journal.pone.0056729
Clarkson, J., Christiansen, F., Awbery, T., Abbiss, L., Nikpaljevic, N., and Akkaya, A. (2020). Non-targeted tourism affects the behavioural budgets of bottlenose dolphins Tursiops truncatus in the South Adriatic (Montenegro). Mar. Ecol. Prog. Ser. 638, 165–176. doi: 10.3354/meps13257
Cobarrubia-Russo, S. E., Barreto-Esnal, G. R., Molero-Lizarraga, A. E., and Mariani-Di Lena, M. A. (2020). Individual home ranges of Tursiops truncatus and their overlap with ranges of Stenella frontalis and fishermen in Aragua, Venezuela, South Caribbean. J. Mar. Biolog. Assoc. 100, 857–866. doi: 10.1017/S0025315420000557
Cresswell, I., Bridgewater, P., and Semeniuk, V. (2011). The coastal habitats and vegetation of the Kimberley region. J R Soc West Aust 94:197.
Daly, R., Smale, M. J., Cowley, P. D., and Froneman, P. W. (2014). Residency patterns and migration dynamics of adult bull sharks (Carcharhinus leucas) on the east coast of southern Africa. PLoS One 9:e109357. doi: 10.1371/journal.pone.0109357
Department of Parks and Wildlife (2016a). Yawuru Nagulagun/Roebuck Bay Marine Park Indicative Joint Management Plan 2016. Perth, WA: Department of Parks and Wildlife.
Department of Parks and Wildlife (2016b). Yawuru Nagulagun/Roebuck Bay Marine Park Supporting Information: Bioregional and Social Setting. Perth, WA: Department of Parks and Wildlife.
DoE (2015). A Coordinated National Research Framework to Inform the Conservation and Management of Australia’s Tropical Inshore Dolphins: The Australian Snubfin Dolphin, Orcaella heinsohni, the Australian Humpback Dolphin, Sousa sahulensis, and the Indo-Pacific Bottlenose Dolphin, Tursiops aduncus. Canberra, ACT: The Australian Government Department of the Environment.
Duncan, C., Nilsen, E. B., Linnell, J. D., and Pettorelli, N. (2015). Life-history attributes and resource dynamics determine intraspecific home-range sizes in Carnivora. Remote Sens. Ecol. Conserv. 1, 39–50. doi: 10.1002/rse2.6
Erbe, C., Marley, S. A., Schoeman, R. P., Smith, J. N., Trigg, L. E., and Embling, C. B. (2019). The effects of ship noise on marine mammals—a review. Front. Mar. Sci. 6:606. doi: 10.3389/fmars.2019.00606
ESRI (2019). ArcGIS Desktop version 10.7. [Online]. Redlands, CA: Environmental Research Systems Institute.
Fieberg, J. (2007). Kernel density estimators of home range: smoothing and the autocorrelation red herring. Ecology 88, 1059–1066. doi: 10.1890/06-0930
Fletcher, R. J. Jr., Hefley, T. J., Robertson, E. P., Zuckerberg, B., McCleery, R. A., and Dorazio, R. M. (2019). A practical guide for combining data to model species distributions. Ecology 100:e02710. doi: 10.1002/ecy.2710
Flores, P. A., and Bazzalo, M. (2004). Home ranges and movement patterns of the marine tucuxi dolphin, Sotalia fluviatilis, in Baía Norte, Southern Brazil. Latin Am. J. Aquat. Mamm. 3, 37–52. doi: 10.5597/lajam00047
Fury, C. A., and Harrison, P. L. (2008). Abundance, site fidelity and range patterns of Indo-Pacific bottlenose dolphins (Tursiops aduncus) in two Australian subtropical estuaries. Mar. Freshw. Res 59, 1015–1027. doi: 10.1071/MF08109
Gill, P. C., Morrice, M. G., Page, B., Pirzl, R., Levings, A. H., and Coyne, M. (2011). Blue whale habitat selection and within-season distribution in a regional upwelling system off southern Australia. Mar. Ecol. Prog. Ser. 421, 243–263. doi: 10.3354/meps08914
Gitzen, R. A., Millspaugh, J. J., and Kernohan, B. J. (2006). Bandwidth selection for fixed-kernel analysis of animal utilization distributions. J.Wildl. Manag. 70, 1334–1344. doi: 10.2193/0022-541X(2006)70[1334:BSFFAO]2.0.CO;2
Gowans, S., Würsig, B., and Karczmarski, L. (2007). The social structure and strategies of delphinids: predictions based on an ecological framework. Adv. Mar. Biol. 53, 195–294. doi: 10.1016/S0065-2881(07)53003-8
Gregory, T. (2016). “Home range estimation,” in The International Encyclopedia of Primatology (Atlanta, GA: American Cancer Society), 1–4. doi: 10.1002/9781119179313.wbprim0177
Gubbins, C. (2002). Use of home ranges by resident bottlenose dolphins (Tursiops truncatus) in a South Carolina estuary. J. Mammal. 83, 178–187. doi: 10.1644/1545-1542(2002)083<0178:UOHRBR>2.0.CO;2
Habel, J. C., Hillen, J., Schmitt, T., and Fischer, C. (2016). Restricted movements and high site fidelity in three East African cloud-forest birds. J. Trop. Ecol. 32, 83–87. doi: 10.1017/S0266467415000516
Halpern, B. S., Walbridge, S., Selkoe, K. A., Kappel, C. V., Micheli, F., D’Agrosa, C., et al. (2008). A global map of human impact on marine ecosystems. Science 319, 948–952. doi: 10.1126/science.1149345
Haughey, R., Hunt, T., Hanf, D., Rankin, R. W., and Parra, G. J. (2020). Photographic capture-recapture analysis reveals a large population of Indo-Pacific bottlenose dolphins (Tursiops aduncus) with low site fidelity off the North West Cape, Western Australia. Front. Mar. Sci. 6:781. doi: 10.3389/fmars.2019.00781
Heithaus, M. R., Frid, A., Wirsing, A. J., and Worm, B. (2008). Predicting ecological consequences of marine top predator declines. Trends. Ecol. Evol. 23, 202–210. doi: 10.1016/j.tree.2008.01.003
Horne, J. S., and Garton, E. O. (2009). Animal Space Use 1.3. Available online at: http://www.cnr.uidaho.edu/population_ecology/animal_space_use.htm
Hunt, T. N., Bejder, L., Allen, S. J., Rankin, R. W., Hanf, D., and Parra, G. J. (2017). Demographic characteristics of Australian humpback dolphins reveal important habitat toward the southwestern limit of their range. Endanger. Species Res. 32, 71–88. doi: 10.3354/esr00784
Irvine, A. B., Scott, M. D., Wells, R. S., and Kaufmann, H. (1981). Movements and activities of the Atlantic bottlenose dolphin, Tursiops truncatus, near Sarasota, Florida. Fish. Bull. U.S. 79, 671–688.
Isaac, N. J., Jarzyna, M. A., Keil, P., Dambly, L. I., Boersch-Supan, P. H., Browning, E., et al. (2020). Data integration for large-scale models of species distributions. Trends. Ecol. Evol. 35, 56–67. doi: 10.1016/j.tree.2019.08.006
Isaac, N. J., and Pocock, M. J. (2015). Bias and information in biological records. Biol. J. Linn. 115, 522–531. doi: 10.1111/bij.12532
Jefferson, T. A., Webber, M. A., and Pitman, R. L. (2015). Marine Mammals of the World A Comprehensive Guide to Their Identification, 2nd Edn. London: Elsevier, 180.
Jensen, F. H., Bejder, L., Wahlberg, M., Soto, N. A., Johnson, M., and Madsen, P. T. (2009a). Vessel noise effects on delphinid communication. Mar. Ecol. Prog. Ser. 395, 161–175. doi: 10.3354/meps08204
Jensen, A., Williams, M., and Jemison, L. Raum-Suryan, K. (2009b). “Somebody untangle me! Taking a closer look at marine mammal entanglement in marine debris,” in Proceedings of the Marine Debris in Alaska: Coordinating Our Efforts, (Fairbanks, AK: Alaska Sea Grant College Program, University of Alaska).
Jennrich, R., and Turner, F. (1969). Measurement of non-circular home range. J. Theor. Biol 22, 227–237. doi: 10.1016/0022-5193(69)90002-2
Karczmarski, L., Huang, S.-L., Wong, W.-H., Chang, W.-L., Chan, S. C., and Keith, M. (2017). Distribution of a coastal delphinid under the impact of long-term habitat loss: Indo-Pacific humpback dolphins off Taiwan’s west coast. Estuaries Coasts 40, 594–603. doi: 10.1007/s12237-016-0146-5
Kassamali-Fox, A., Christiansen, F., May-Collado, L. J., Ramos, E. A., and Kaplin, B. A. (2020). Tour boats affect the activity patterns of bottlenose dolphins (Tursiops truncatus) in Bocas del Toro, Panama. PeerJ 8:e8804. doi: 10.7717/peerj.8804
Kie, J. G., Matthiopoulos, J., Fieberg, J., Powell, R. A., Cagnacci, F., Mitchell, M. S., et al. (2010). The home-range concept: are traditional estimators still relevant with modern telemetry technology? Philos. Trans. Biol. Sci. 365, 2221–2231. doi: 10.1098/rstb.2010.0093
Knip, D. M., Heupel, M. R., and Simpfendorfer, C. A. (2012). To roam or to home: site fidelity in a tropical coastal shark. Mar. Biol. Int. J. Life Oceans Coast. Waters 159, 1647–1657. doi: 10.1007/s00227-012-1950-5
Kreb, D., Lhota, S., Porter, L., Redman, A., Susanti, I., and Lazecky, M. (2020). Long-term population and distribution dynamics of an endangered irrawaddy dolphin population in Balikpapan Bay, Indonesia in response to coastal development. Front. Mar. Sci. 7:533197. doi: 10.3389/fmars.2020.533197
Laist, D. W. (1997). “Impacts of marine debris: entanglement of marine life in marine debris including a comprehensive list of species with entanglement and ingestion records,” in Marine Debris, eds J. M. Coe and D. B. Rogers (New York, NY: Springer), 99–139. doi: 10.1007/978-1-4613-8486-1_10
Lin, W., Karczmarski, L., Zhou, R., Mo, Y., Guo, L., Yiu, S. K. F., et al. (2021). Prey decline leads to diet shift in the largest population of Indo-Pacific humpback dolphins? Integr. Zool. 16, 548–574. doi: 10.1111/1749-4877.12548
Lotze, H. K., Flemming, J. M., and Magera, A. M. (2017). Critical factors for the recovery of marine mammals marine-mammal recovery. Conserv. Biol. 31, 1301–1311. doi: 10.1111/cobi.12957
MacLeod, C. D. (2013). Home Range Tools Toolbox. Available online at: GISInEcology.com/Home_Range_Tools.zip
Maes, D., Isaac, N. J., Harrower, C. A., Collen, B., Van Strien, A. J., and Roy, D. B. (2015). The use of opportunistic data for IUCN Red List assessments. Biol. J. Linn 115, 690–706. doi: 10.1111/bij.12530
Marley, S. A., Kent, C. P. S., Erbe, C., and Parnum, I. M. (2017b). Effects of vessel traffic and underwater noise on the movement, behaviour and vocalisations of bottlenose dolphins in an urbanised estuary. Sci. Rep. 7:13437. doi: 10.1038/s41598-017-13252-z
Marley, S. A., Salgado Kent, C. P., Erbe, C., and Thiele, D. (2017a). A Tale of two soundscapes: comparing the acoustic characteristics of urban versus pristine coastal dolphin habitats in Western Australia. Acoust. Aust. 45, 159–178. doi: 10.1007/s40857-017-0106-7
McCluskey, S., Sprogis, K., London, J., Bejder, L., and Loneragan, N. (2021). Foraging preferences of an apex marine predator revealed through stomach content and stable isotope analyses. Glob. Ecol. Conserv. 25:e01396. doi: 10.1016/j.gecco.2020.e01396
McLoughlin, P. D., and Ferguson, S. H. (2000). A hierarchical pattern of limiting factors helps explain variation in home range size. Ecoscience 7, 123–130. doi: 10.1080/11956860.2000.11682580
Mitchell, M. S., and Powell, R. A. (2012). Foraging optimally for home ranges. J. Mamm. 93, 917–928. doi: 10.1644/11-MAMM-S-157.1
Mohr, C. O. (1947). Table of equivalent populations of North American small mammals. Am. Midl. Nat. 37, 223–249. doi: 10.2307/2421652
Molinari-Jobin, A., Kéry, M., Marboutin, E., Marucco, F., Zimmermann, F., Molinari, P., et al. (2018). Mapping range dynamics from opportunistic data: spatiotemporal modelling of the lynx distribution in the Alps over 21 years. Anim. Conserv. 21, 168–180. doi: 10.1111/acv.12369
Nathan, R., Kadmon, R., Getz, W. M., Revilla, E., Holyoak, M., Saltz, D., et al. (2008). A movement ecology paradigm for unifying organismal movement research. Proc. Natl. Acad. Sci. U.S.A. 105, 19052–19059. doi: 10.1073/pnas.0800375105
Nelms, S. E., Alfaro-Shigueto, J., Arnould, J. P., Avila, I. C., Nash, S. B., Campbell, E., et al. (2021). Marine mammal conservation: over the horizon. Endanger. Species Res. 44, 291–325. doi: 10.3354/esr01115
Nilsen, E. B., Pedersen, S., and Linnell, J. D. C. (2008). Can minimum convex polygon home ranges be used to draw biologically meaningful conclusions? Ecol. Res. 23, 635–639. doi: 10.1007/s11284-007-0421-9
Noonan, M. J., Tucker, M. A., Fleming, C. H., Akre, T. S., Alberts, S. C., Ali, A. H., et al. (2019). A comprehensive analysis of autocorrelation and bias in home range estimation. Ecol. Monogr. 89:e01344. doi: 10.1002/ecm.1344
Owen, E. C., Wells, R. S., and Hofmann, S. (2002). Ranging and association patterns of paired and unpaired adult male Atlantic bottlenose dolphins, Tursiops truncatus, in Sarasota, Florida, provide no evidence for alternative male strategies. Can. J. Zool. 80, 2072–2089. doi: 10.1139/z02-195
Paiva, E. G., Salgado Kent, C. P., Gagnon, M. M., McCauley, R., and Finn, H. (2015). Reduced detection of Indo-Pacific bottlenose dolphins (Tursiops aduncus) in an inner harbour channel during pile driving activities. Aquat. Mamm. 41, 455–468. doi: 10.1578/AM.41.4.2015.455
Palmer, C., Woinarski, J., Parra, G. J., and Rogers, T. (2014b). Collation and review of sightings and distribution of three coastal dolphin species in waters of the Northern Territory, Australia. Pac. Conserv. Biol. 20, 116–125. doi: 10.1071/PC140116
Palmer, C., Brooks, L., Parra, G. J., Rogers, T., Glasgow, D., and Woinarski, J. C. (2014a). Estimates of abundance and apparent survival of coastal dolphins in Port Essington harbour, Northern Territory, Australia. Wildl. Res. 41, 35–45. doi: 10.1071/WR14031
Parra, G. J., Azuma, C., Preen, A. R., Corkeron, P. J., and Marsh, H. (2002). Distribution of Irrawaddy dolphins, Orcaella brevirostris, in Australian waters. Raffles Bull. Zool. 10, 141–154.
Parra, G., Cagnazzi, D., and Beasley, I. (2017). Orcaella heinsohni (Errata Version Published in 2018). IUCN Red List Threat. Species 2017 e.T136315A123793740. Available online at: https://www.iucnredlist.org/species/136315/123793740 (accessed November 20, 2020).
Parra, G. J. (2006). Resource partitioning in sympatric delphinids: space use and habitat preferences of Australian snubfin and Indo-Pacific humpback dolphins. J. Anim. Ecol. 75, 862–874. doi: 10.1111/j.1365-2656.2006.01104.x
Parra, G. J., Schick, R., and Corkeron, P. J. (2006b). Spatial distribution and environmental correlates of Australian snubfin and Indo-Pacific humpback dolphins. Ecography 29, 396–406. doi: 10.1111/j.2006.0906-7590.04411.x
Parra, G. J., Corkeron, P. J., and Marsh, H. (2006a). Population sizes, site fidelity and residence patterns of Australian snubfin and Indo-Pacific humpback dolphins: implications for conservation. Biol. Conserv. 129, 167–180. doi: 10.1016/j.biocon.2005.10.031
Parra, G. J., and Jedensjö, M. (2014). Stomach contents of Australian snubfin (Orcaella heinsohni) and Indo-Pacific humpback dolphins (Sousa chinensis). Mar. Mamm. Sci. 30, 1184–1198. doi: 10.1111/mms.12088
Passadore, C., Möller, L., Diaz-Aguirre, F., and Parra, G. J. (2018). High site fidelity and restricted ranging patterns in southern Australian bottlenose dolphins. Ecol. Evol. 8, 242–256. doi: 10.1002/ece3.3674
Peel, D., Smith, J. N., and Childerhouse, S. (2018). Vessel strike of whales in Australia: the challenges of analysis of historical incident data. Front. Mar. Sci. 5:69. doi: 10.3389/fmars.2018.00069
Powell, R. A. (2000). Animal home ranges and territories and home range estimators. Res. Techn. Anim. Ecol. 442, 65–110.
R Core Team (2020). R: A Language and Environment for Statistical Computing [Online]. Vienna: R Foundation for Statistical Computing.
Raudino, H., D’Cruz, E., Waples, K., Menzies, J., Murdoch, J., Quartermaine, T., et al. (2020). Dry season dreaming: snubfin census on Yawuru sea country. Landscope 36, 41–44.
Rayment, W., Dawson, S., Slooten, E., Bräger, S., Fresne, S. D., and Webster, T. (2009). Kernel density estimates of alongshore home range of Hector’s dolphins at Banks Peninsula, New Zealand. Mar. Mamm. Sci. 25, 537–556. doi: 10.1111/j.1748-7692.2008.00271.x
Reeves, R. R., McClellan, K., and Werner, T. B. (2013). Marine mammal bycatch in gillnet and other entangling net fisheries, 1990 to 2011. Endanger. Species Res. 20, 71–97. doi: 10.3354/esr00481
Rosel, P. E., Hohn, A. A., Mullin, K., Garrison, L. P., Schwacke, L. H., Adams, J., et al. (2011). Photo-Identification Capture-Mark-Recapture Techniques for Estimating Abundance of Bay, Sound and Estuary Populations of Bottlenose Dolphins Along the US East Coast and Gulf of Mexico, A Workshop Report. Washington, DC: NOAA.
Schoeman, R. P., Patterson-Abrolat, C., and Plön, S. (2020). A global review of vessel collisions with marine animals. Front. Mar. Sci. 7:292. doi: 10.3389/fmars.2020.00292
Schoepf, I., Schmohl, G., König, B., Pillay, N., and Schradin, C. (2015). Manipulation of population density and food availability affects home range sizes of African striped mouse females. Anim. Behav. 99, 53–60. doi: 10.1016/j.anbehav.2014.10.002
Seaman, D. E., Millspaugh, J. J., Kernohan, B. J., Brundige, G. C., Raedeke, K. J., and Gitzen, R. A. (1999). Effects of sample size on kernel home range estimates. J.Wildl. Manag. 63, 739–747. doi: 10.2307/3802664
Seaman, D. E., and Powell, R. A. (1996). An evaluation of the accuracy of kernel density estimators for home range analysis. Ecology 77, 2075–2085. doi: 10.2307/2265701
Silva, M. A., Prieto, R., Magalhães, S., Seabra, M. I., Santos, R. S., and Hammond, P. S. (2008). Ranging patterns of bottlenose dolphins living in oceanic waters: implications for population structure. Mar. Biol. 156, 179–192. doi: 10.1007/s00227-008-1075-z
Silverman, B. W. (1986). Density Estimation for Statistics and Data Analysis. Boca Raton, FL: CRC press.
Simpfendorfer, C. A., Yeiser, B. G., Wiley, T. R., Poulakis, G. R., Stevens, P. W., and Heupel, M. R. (2011). Environmental influences on the spatial ecology of juvenile smalltooth sawfish (Pristis pectinata): results from acoustic monitoring. PLoS One 6:e16918. doi: 10.1371/journal.pone.0016918
Singh, W., Hjorleifsson, E., and Stefansson, G. (2011). Robustness of fish assemblages derived from three hierarchical agglomerative clustering algorithms performed on Icelandic groundfish survey data. ICES J. Mar. Sci. 68, 189–200. doi: 10.1093/icesjms/fsq144
Smith, F., Allen, S. J., Bejder, L., and Brown, A. M. (2018). Shark bite injuries on three inshore dolphin species in tropical northwestern Australia. Mar. Mamm. Sci. 34, 87–99. doi: 10.1111/mms.12435
Sokal, R. R., and Rohlf, F. J. (1962). The comparison of dendrograms by objective methods. Taxon 11, 33–40. doi: 10.2307/1217208
Sprogis, K. R., Raudino, H. C., Rankin, R., MacLeod, C. D., and Bejder, L. (2016). Home range size of adult Indo-Pacific bottlenose dolphins (Tursiops aduncus) in a coastal and estuarine system is habitat and sex-specific. Mar. Mamm. Sci. 32, 287–308. doi: 10.1111/mms.12260
Stevick, P. T., McConnell, B. J., and Hammond, P. S. (2002). “Patterns of movement,” in Marine Mammal Biology: An Evolutionary Approach, ed. A. R. Hoelzel (Oxford: Blackwell), 185–216.
Stolar, J., and Nielsen, S. E. (2015). Accounting for spatially biased sampling effort in presence-only species distribution modelling. Divers. Distrib. 21, 595–608. doi: 10.1111/ddi.12279
Strickland-Munro, J., Kobryn, H., Moore, S., and Brown, G. (2016). Valuing the wild, remote and beautiful: using public participation GIS to inform tourism planning in the Kimberley, Western Australia. Int. J. Sustain. Dev. Plan. 11, 355–364. doi: 10.2495/SDP-V11-N3-355-364
Suzuki, R., and Shimodaira, H. (2006). Pvclust: an R package for assessing the uncertainty in hierarchical clustering. Bioinformatics 22, 1540–1542. doi: 10.1093/bioinformatics/btl117
Switzer, P. V. (1993). Site fidelity in predictable and unpredictable habitats. Evol. Ecol. 7, 533–555. doi: 10.1007/BF01237820
Switzer, P. V. (1997). Factors affecting site fidelity in a territorial animal, Perithemis tenera. Anim. Behav. 53, 865–877. doi: 10.1006/anbe.1996.0352
Thiele, D. (2010). Collision Course: Snubfin Dolphin Injuries in Roebuck Bay. Report to WWF-Australia. Broome, WA: WWF-Australia, 15.
Tschopp, A., Ferrari, M. A., Crespo, E. A., and Coscarella, M. A. (2018). Development of a site fidelity index based on population capture-recapture data. PeerJ 6:e4782. doi: 10.7717/peerj.4782
Tyne, J. A., Christiansen, F., Heenehan, H. L., Johnston, D. W., and Bejder, L. (2018). Chronic exposure of Hawaii Island spinner dolphins (Stenella longirostris) to human activities. R. Soc. Open Sci. 5:171506. doi: 10.1098/rsos.171506
Urian, K., Gorgone, A., Read, A., Balmer, B., Wells, R. S., Berggren, P., et al. (2015). Recommendations for photo-identification methods used in capture-recapture models with cetaceans. Mar. Mamm. Sci. 31, 298–321. doi: 10.1111/mms.12141
Urian, K. W., Hohn, A. A., and Hansen, L. J. (1999). Status of the Photo-Identification Catalog of Coastal Bottlenose Dolphins of the Western North Atlantic: Report of a Workshop of Catalog Contributors. Beaufort, NC: US Department of Commerce, National Oceanic and Atmospheric Administration.
Urian, K. W., Waples, D. M., Tyson, R. B., Hodge, L. E., and Read, A. J. (2014). Abundance of bottlenose dolphins (Tursiops truncatus) in estuarine and near-shore waters of North Carolina, USA. J. N. C. Acad. Sci. 129, 165–171.
Vermeulen, E., Balbiano, A., Belenguer, F., Colombil, D., Failla, M., Intrieri, E., et al. (2017). Site-fidelity and movement patterns of bottlenose dolphins (Tursiops truncatus) in central Argentina: essential information for effective conservation. Aquat. Conserv. 27, 282–292. doi: 10.1002/aqc.2618
Wang, X., Wu, F., Zhu, Q., and Huang, S. L. (2017). Long-term changes in the distribution and core habitat use of a coastal delphinid in response to anthropogenic coastal alterations. Aquat. Conserv. 27, 643–652. doi: 10.1002/aqc.2720
Waples, K. (2003). “The role of research in the management of whale watching,” in Conserving Marine Environments: Out of Sight Out of Mind, eds P. Hutchings and D. Lunney (Mosman, NSW: Royal Toological Society of New South Wales), 62–72.
Waples, K., and Raudino, H. (2018). Setting a course for marine mammal research in Western Australia. Pac. Conserv. Biol. 24, 289–303. doi: 10.1071/PC18014
Ward, J. H. Jr. (1963). Hierarchical grouping to optimize an objective function. J. Am. Stat. Assoc. 58, 236–244. doi: 10.1080/01621459.1963.10500845
Wells, R. S., and Scott, M. D. (1997). Seasonal incidence of boat strikes on bottlenose dolphins near Sarasota, Florida. Mar. Mamm. Sci. 13, 475–480. doi: 10.1111/j.1748-7692.1997.tb00654.x
Wells, R. S., Scott, M. D., and Irvine, A. B. (1987). “The social structure of free-ranging bottlenose dolphins,” in Current Mammalogy, ed. H. H. Genoways (London: Plenum Press), 247–305. doi: 10.1007/978-1-4757-9909-5_7
White, G. C., and Garrott, R. A. (2012). Analysis of Wildlife Radio-Tracking Data. Amsterdam: Elsevier.
Worton, B. J. (1989). Kernel methods for estimating the utilization distribution in home-range studies. Ecology 70:164. doi: 10.2307/1938423
Würsig, B., and Jefferson, T. (1990). “Methods of photo-identification for small cetaceans,” in Individual Recognition of Cetaceans: Use of Photo-Identification and Other Techniques to Estimate Population Parameters, eds P. S. Hammond, S. A. Mizroch, and G. P. Donovan (Cambridge: International Whaling Commission), 43–52.
Keywords: ranging patterns, site fidelity, snubfin dolphin, Orcaella heinsohni, Yawuru Nagulagun, Roebuck Bay
Citation: D’Cruz A, Salgado Kent C, Waples K, Brown AM, Marley SA, Thiele D, Yawuru PBC and Raudino HC (2022) Ranging Patterns and Site Fidelity of Snubfin Dolphins in Yawuru Nagulagun/Roebuck Bay, Western Australia. Front. Mar. Sci. 8:758435. doi: 10.3389/fmars.2021.758435
Received: 13 August 2021; Accepted: 23 November 2021;
Published: 05 January 2022.
Edited by:
Jeremy Kiszka, Florida International University, United StatesReviewed by:
Danielle Kreb, Conservation Foundation for Rare Aquatic Species of Indonesia, IndonesiaCopyright © 2022 D’Cruz, Salgado Kent, Waples, Brown, Marley, Thiele, Yawuru PBC and Raudino. This is an open-access article distributed under the terms of the Creative Commons Attribution License (CC BY). The use, distribution or reproduction in other forums is permitted, provided the original author(s) and the copyright owner(s) are credited and that the original publication in this journal is cited, in accordance with accepted academic practice. No use, distribution or reproduction is permitted which does not comply with these terms.
*Correspondence: Alexandra D’Cruz, YWxleHRkY3J1ekBnbWFpbC5jb20=
Disclaimer: All claims expressed in this article are solely those of the authors and do not necessarily represent those of their affiliated organizations, or those of the publisher, the editors and the reviewers. Any product that may be evaluated in this article or claim that may be made by its manufacturer is not guaranteed or endorsed by the publisher.
Research integrity at Frontiers
Learn more about the work of our research integrity team to safeguard the quality of each article we publish.