- 1Millennium Nucleus for Ecology and Sustainable Management of Oceanic Islands, Departamento de Biología Marina, Facultad de Ciencias del Mar, Universidad Católica del Norte, Coquimbo, Chile
- 2Sala de Colecciones Biológicas, Facultad de Ciencias del Mar, Universidad Católica del Norte, Coquimbo, Chile
- 3School of Earth, Environmental and Marine Sciences, University of Texas Rio Grande Valley, South Padre Island, TX, United States
- 4Conservation International, Center for Oceans, Arlington, VA, United States
- 5Oceana Inc. Chile, Santiago, Chile
One of the main priorities of marine scientific research is to provide practical information and guidance for biodiversity conservation and management. In this context, the identification of key and fragile habitat-forming species is particularly important. Despite their ecological importance, whip corals in the order Antipatharia remain poorly studied around the world, and especially in the South Pacific. During recent expeditions to explore mesophotic and deep benthic habitats around Rapa Nui (Easter Island), dense assemblages of whip black corals were observed in situ. We here report the first detailed description of the distribution and abundance of these black coral assemblages and their associated fauna and habitat. These descriptions were based on analysis of video surveys collected on 67 remotely operated vehicle dives conducted in 2014 through 2019 at three sites (Pukao seamount, Apolo peak, and Rapa Nui Island shelf and slope) within the Easter Island Ecoregion at depths between 50 and 330 m. At least four morphotypes (yellow, red, white/orange, and Pukao) of unbranched black corals were detected. Each morphotype had polyps arranged in a single row on only one side of the corallum, a diagnostic morphological character currently assigned to the antipatharian genus Stichopathes. Samples of the yellow and red morphotypes were collected and studied using morphological and molecular techniques. These analyses indicate that the genus Stichopathes is not monophyletic and requires further revisions, a conclusion that is consistent with previous studies. Extensive fields of whip black corals, primarily of the yellow and red morphotype, were present at 28 sites from 60 to 245 m on rocky substrates, coarse sand, or rhodoliths. Densities of these assemblages per frame were up to 58.2 ± 5.2 colonies m–2 (average: 13.2 ± 0.8), which represent some of the highest reported densities for black corals to date. Numerous faunal groups, including ophiuroids, echinoids, and fishes, were associated with these whip black coral assemblages. Our observations should be considered in future management and conservation efforts around Rapa Nui, where a multi-use marine protected area was established in 2018, as well as in adjacent international waters that remain unprotected but likely harbor similar assemblages.
Introduction
Antipatharians, commonly known as black corals, comprise approximately 279 species in seven families (Molodtsova and Opresko, 2021). Black corals are distributed from polar to tropical seas and have a wide bathymetric distribution ranging from 2 to 8,600 m (Opresko, 2006; Wagner et al., 2012; Gorny et al., 2018). Over 75% of described species are found in deeper waters (>50 m), primarily on hard substrates with a few species occurring on soft sediments (Wagner et al., 2012; Molodtsova and Opresko, 2017; Horowitz et al., 2018). Antipatharians have an important ecological role, as they provide crucial habitat for many associated species, several of which are adapted to live exclusively on black corals (Marin, 2007; Wagner et al., 2012; Terrana and Eeckhaut, 2019). Black corals are some of the longest living and slowest growing animals on Earth, which makes them and the animals that depend on them particularly vulnerable to disturbances of the seafloor and water column (Wagner et al., 2012). All black corals are protected under the Convention on International Trade in Endangered Species of Wild Fauna and Flora (CITES) and are also considered vulnerable marine ecosystem indicator species by several regional fishery management organizations (Wagner et al., 2012).
Despite their ecologic importance, they remain poorly studied worldwide and especially in the South Pacific (Molodtsova, 2005; Yesson et al., 2017). This underrepresentation is due to the elevated costs of studying deep and remote environments as well as the scarcity of taxonomic experts working on this taxon (Wagner et al., 2012; Wagner, 2015). Further, the lack of well-preserved tissue samples has greatly limited the scope of molecular analyses of black corals. As a result of the lack of available samples, surveys, and molecular data, the true species diversity of antipatharians is likely underestimated worldwide (Barrett et al., 2020).
Records of black corals in Chilean waters are scarce, with only 20 species in four families reported in the literature (Häussermann and Försterra, 2007; Alarcón, 2009; Araya et al., 2018; Gorny et al., 2018; Easton et al., 2019). Of these, 11 species are distributed along the continental shelf between the Peruvian and Magellan Province (18°–56°S) and the rest correspond to records from the continental slope or seamounts (Alarcón, 2009; Araya et al., 2018). Few antipatharians have been reported from offshore Chilean islands (Juan Fernández Archipelago, Desventuradas Islands, Rapa Nui [Easter Island], and Salas y Gómez Island) and colonies are often not identified to species level (Araya et al., 2018; Easton et al., 2019) because records are based on video observations or samples in poor condition that were inadvertently collected by fishers. In the Juan Fernández and Desventuradas Ecoregion (Spalding et al., 2007), a total of seven antipatharian species have been reported; six from the Juan Fernández Archipelago (Plumapathes fernandezi, Plumapathes cf. pennacea, Trissopathes sp., Leiopathes spp., Aphanipathes sp. and Antipathes sp.) and one from the Desventuradas Islands (cf. Stichopathes sp.) (Häussermann and Försterra, 2007; Alarcón, 2009; National Geographic and Oceana, 2013; Araya et al., 2018; Tapia-Guerra et al., 2021). Due to the paucity of data, the actual distribution of black corals around these islands is largely unknown (Araya et al., 2018).
Unfortunately, data on the geographic distribution or abundance of black corals from the Easter Island Ecoregion (EIE) (Spalding et al., 2007), which encompasses the islands of Rapa Nui and Salas y Gómez, are largely absent or unreliable. Only two antipatharian taxa in the family Antipathidae (Antipathes and Stichopathes) have been recorded from Rapa Nui but none were identified to species level (Disalvo et al., 1988; Fernández et al., 2014; Araya et al., 2018). Reports arising from preliminary analyses of video surveys and opportunistic samples from Rapa Nui and nearby seamount Pukao suggested the presence of at least two whip black coral species in the genus Stichopathes (Easton et al., 2019; Shepherd et al., 2020), which is characterized by having whip-like colonies and polyps that are arranged in a single row on only one side of the colony (Bo et al., 2012). However, the Stichopathes taxonomy is not yet resolved, in part because of phenotypic plasticity of diagnostic characters and the close phylogenetic relationship between species in the families Antipathidae (especially Stichopathes and Antipathes) and Aphanipathidae (Bo et al., 2012; Wagner et al., 2012; Barrett et al., 2020). For example, several previous studies that combined morphological and molecular data noted that caution should be used when using external morphology to identify black corals (Bo et al., 2012) because several colony morphologies including whip corals are not monophyletic (Bo et al., 2012; Brugler et al., 2013; Terrana et al., 2021). These taxonomic difficulties, along with the lack of historical surveys of the antipatharian fauna around Rapa Nui, highlight the need to conduct detailed studies focusing on the taxonomy and ecology of antipatharians from this region. The creation of the Rapa Nui Multiple-Use Marine Protected Area (MUMPA) in 2018 has provided the marine fauna of this region some protection as industrial fishing and bottom trawling are prohibited within its limits (Easton et al., 2019; Paredes et al., 2019; Wagner et al., 2021). However, the effectiveness of these protections is still largely unknown because critical baseline information about deep-water ecosystems in the region are largely missing (Easton et al., 2019).
To help fill this knowledge gap, we characterized assemblages of whip black corals in the EIE by combining in situ observations with an examination of collected specimens using morphological and molecular techniques. This study is the first to characterize the abundance, distribution, morphology, and phylogenetics of whip black corals in the Rapa Nui MUMPA and on the Salas y Gómez Ridge, which with the adjacent Nazca Ridge are two seamount chains that span across 2,900 km and include numerous seamounts with summits extending into mesophotic depths.
Materials and Methods
Study Area and Remotely Operated Vehicle Data Collection
Underwater video surveys with a remotely operated vehicle (ROV) were conducted on the summit of Pukao seamount (26.92°S; 110.26°W) in 2016; on a subsurface peak along the southwestern slope of Rapa Nui (Easter Island) known as Apolo peak (27.23°S; 109.48°W) in 2014, and on multiple locations around the island of Rapa Nui between 2017 and 2019 (Figure 1). Benthic surveys were conducted from local fishing boats with a Commander MK2 ROV (Mariscope Meerestechnik, Kiel, Germany) driven slowly about 50 cm above the seafloor and equipped with a high-definition camcorder (Panasonic SD 909, resolution 1920 × 1010, 30 fps), a GoPro Hero 4 camera (resolution 2704 × 1520, 60 fps), and laser pointers spaces 10 cm apart for scale. A total of 67 ROV dives were conducted to bottom depths ranging between 50 and 330 m, capturing more than 50 h of bottom video (Table 1) on six dives at Pukao seamount (∼4 h of total bottom time), three dives at Apolo peak (∼1 h of total bottom time), and 58 dives around Rapa Nui (∼45 h of total bottom time). Bottom time for each dive survey ranged from 15 to 40 min (Table 1).
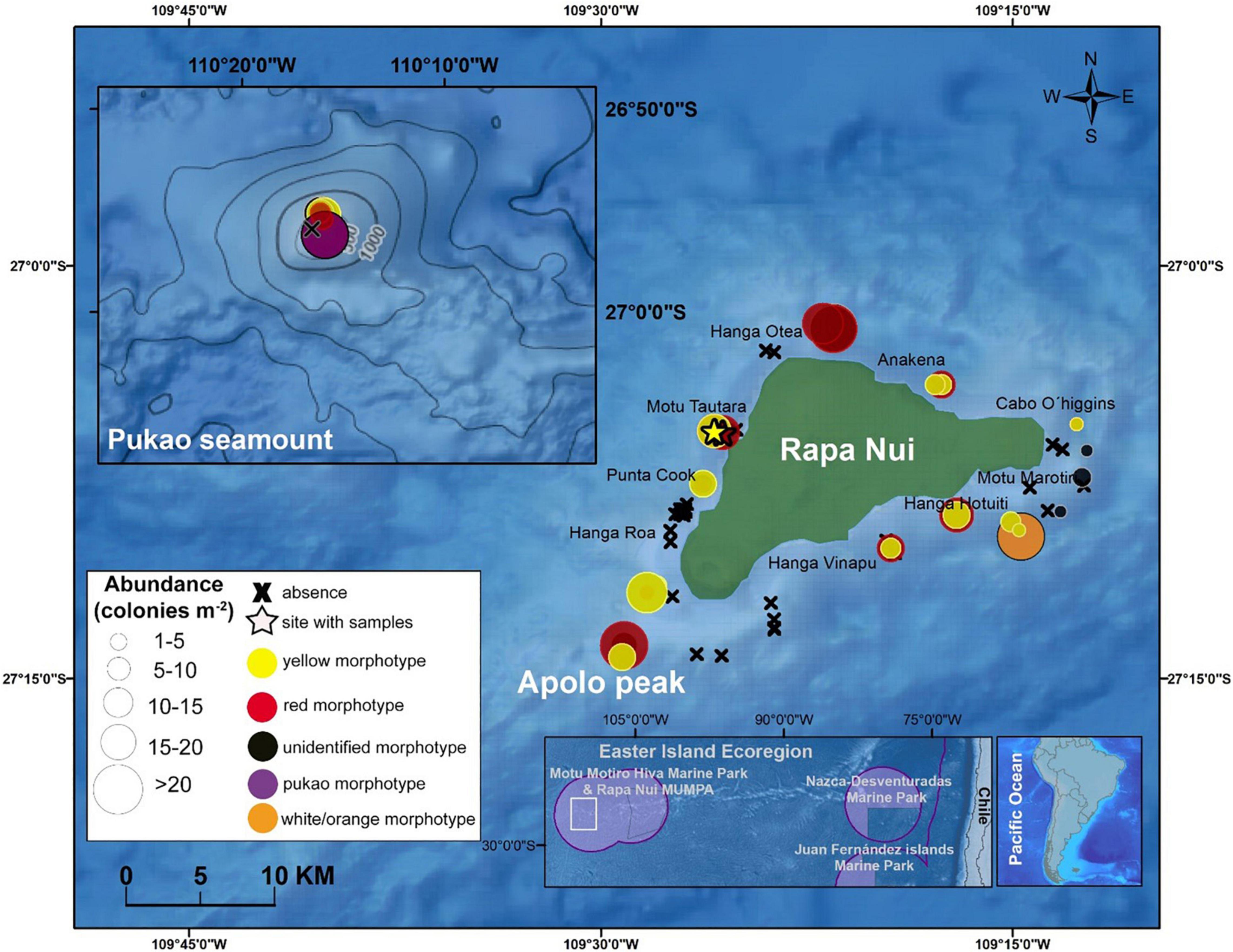
Figure 1. Whip black coral abundance and ROV survey locations (2014–2019) on the Apolo peak, the summit of Pukao seamount, and around Rapa Nui (Easter Island) at 50–330 m. X = absence of whip corals, stars = stations where specimens were collected, purple line = Chilean Economic Exclusive Zone, pink area = Chilean Marine Parks. Map generated using ArcMap (version 10.8) and GEBCO and NCEI base maps (British Oceanographic Data Centre, 2008; NCEI, 2021). Note that when multiple morphotypes were recorded during a survey, points overlap.
Sample Collections and Morphological Analyses
During two ROV dives in April 2017 at Rapa Nui, two distinct morphotypes (yellow and red) of whip black corals were inadvertently sampled when they became entangled in the ROV chassis. The yellow morphotype was sampled at station T17 (27.100°S; 109.431°W) between 120 and 180 m, and the red morphotype was sampled at station T19 (27.101°S; 109.426°W) at 180 m (Table 1). The collected specimens were stored in 95% ethanol and deposited in the Sala de Colecciones Biológicas, Universidad Católica del Norte (SCBUCN), Coquimbo, Chile under catalog numbers SCBUCN 8849 (yellow morphotype) and SCBUCN 8850 (red morphotype). Samples of each morphotype were prepared for scanning electron microscopy (SEM). To remove the tissue from the corallum, specimen clippings were placed in sodium hypochlorite (household bleach) for 5–10 s and rinsed in 70% ethanol. This process was repeated until all tissue was removed, then the corallum was placed in 95% ethanol for 5–10 s before it was dried in a Tousimis, Samdri-780A critical-point dryer using CO2, mounted on bronze stubs, and coated with gold in a JEOL JFC-100 evaporator. Coated samples were examined under a Hitachi SU3500 SEM at the Microscopy Laboratory of the Facultad de Ciencias del Mar, Universidad Católica del Norte, Coquimbo, Chile.
Morphometric measurements were taken using IC Measure software following Wagner et al. (2010). Measurements of the stem length were taken from underwater images, whereas measurements of the polyps and spines were taken from images of preserved material of each morphotype. Sample collections were performed under permits Res. Ext N°41/2016 and N°3314/2017 from SUBPESCA (National Fishing Authority of Chile) to the Universidad Católica del Norte. This project was also presented to the local “Consejo del Mar de Rapa Nui” (Council of the Sea of Rapa Nui), which allowed underwater footage and sampling around the island.
DNA Extraction, Amplification, and Sequencing
Whole genomic DNA was isolated from a single polyp of each specimen with the GeneJET Genomic DNA Purification Kit (Thermo Fisher Scientific Waltham, MA, United States) per manufacture’s protocol and submitted to the Bio-polymers Facility at Harvard Medical School for library preparation and next-generation sequencing (NextSeq 500). Reads were trimmed with BBDuk2 v. 38.84 (Bushnell, 2021) with the following settings: ktrimright = t, k = 27, hdist = 1, edist = 0, qtrim = rl, trimq = 6, minlength = 10, ordered = t, and qin = 33. Trimmed reads were mapped to Antipathidae reference sequences that extended from the 18SrRNA gene through ITS1 to the 5.8S or 28S rRNA genes in Geneious Prime 2021.1.11 with the following Geneious mapper settings: Sensitivity = Medium-Low Sensitivity/Fast and Fine Tuning = Iterate up to five times. The reads belonging to the yellow morphotype (SCBUCN 8849) were successfully mapped with good quality (>75% threshold) and coverage values (>100 reads) to four reference sequences: Cirrhipathes spiralis (AM404320), Cirrhipathes sp. GB-2006 (AM404319), Antipathes grandis (GU296494), and Cirrhipathes anguina (HM060622). The reads belonging to the red morphotype (SCBUCN 8850) were successfully mapped with good quality (>75% threshold) and coverage values (>100 reads) to three reference sequences: Antipathes griggi (KF054602), Antipathes sp.2 GB-2006 (AM404315), Antipathes elegans (AM404317). The resulting consensus sequences were aligned with default MAFFT v7.450 (Katoh et al., 2002; Katoh and Standley, 2013) settings in Geneious Prime for each species. The resulting consensus sequences were extracted at a threshold of 90% for each morphotype and were subsequently used as a reference sequence to which the trimmed reads were mapped as described above to generate the 1,682- and 1,899-bp consensus sequences extending from 18S through 28S respectively for the yellow (Stichopathes sp. SCBUCN 8849, MZ450123) and red (Stichopathes sp. SCBUCN 8850, MZ450124) morphotypes. Stichopathes sp. SCBUCN 8849 and Stichopathes sp. SCBUCN 8850 sequences were annotated manually based on an alignment with Antipathes cf. dichotoma NB-2020 (MT318863) and Tylopathes sp. NB-2020 (MT318885). Details of the quality control assessment and coverage of each consensus sequence are described in the Supplementary Material. The ITS1 region was extracted from these sequences and aligned with most of the Antipatharia ITS sequences available in GenBank and a maximum-likelihood tree was constructed (Supplementary Figure 1). Because the topology of this reconstructed phylogeny was consistent with the phylogenetic reconstructions in Bo et al. (2012), we trimmed the dataset by randomly selecting three representatives of each genus per clade. The trimmed dataset included the two Rapa Nui Stichopathes species, 35 other antipatharian sequences from GenBank (Supplementary Table 1), and one scleractinian (outgroup) ITS1 sequence (Supplementary Table 1) that were aligned with default MUSCLE 3.8.425 (Edgar, 2004) parameters in Geneious Prime. The alignment was trimmed to the extent of the shortest sequences, resulting in a 343-bp alignment. ModelTest-NG 0.1.6 (Darriba et al., 2019) was used to select the substitution models applicable to PhyML phylogenetic reconstructions with the following settings: Data type = DNA, Templates = PhyML, models = 11, Rate variation = uniform/gamma/pinv/+I+G, and Frequencies = Equal frequencies/ML frequencies. A maximum-likelihood, phylogenetic tree was constructed with PhyML 3.3.20180621 (Guindon et al., 2010) plugin of Geneious Prime with the following settings: substitution model = TPM3 + G4, bootstrap = 1000, and optimize = topology/length/rate. The scleractinian coral Porites lutea (LT558237) was used as the outgroup.
Analysis of in situ Observations
The characteristics of habitat associated with the whip black coral assemblages were described based on ROV video data for each site. The habitat was described by substrate type (i.e., rock, silty sediments, mixed substrate, coarse sand) and “modifier” elements (i.e., biological communities, sedimentation, and bio-perturbation) following Tapia-Guerra et al. (2021).
Videos for each dive were analyzed in a time-lapse mode at half normal speed in VLC Media Player (Version 3.0.12; VideoLan, 2006). The number of whip black coral colonies was estimated from ROV video surveys following Bo et al. (2009a) and Cau et al. (2015). In brief, the ROV videos were divided into short video sequences (2 min on average) from which frames were extracted every 30 s using DVDVIDEOSOFT software (version 5.0.101). Only video sequences where the ROV was near the bottom (within 0.5–1.0 m) were analyzed; video sequences with poor visibility were not considered. The field of view per frame was estimated using the laser pointers of the ROV and standardized to m2 using IC Measure software (version 2.0.0.245). Colony count per frame was estimated using ImageJ software (version 1.53e). Position data of ROV deployments were used to create distribution maps using General Bathymetric Chart of the Oceans (British Oceanographic Data Centre, 2008) and NOAA National Centers for Environmental Information (NCEI, 2021) base maps and were plotted using ArcMap software 10.82. To assess differences in abundance as a function of depth at each study site (i.e., Pukao seamount, Apolo peak, and Rapa Nui), non-parametric univariate analyses (Unpaired Two-Samples Wilcoxon Test, Kruskal–Wallis and Mann–Whitney pairwise comparisons) were conducted. All statistical analyses were performed using R software (version 4.0.5) (R Core Team, 2021) using the “car” (Fox and Weisberg, 2018), “plyr” (Wickham, 2011) and “Rmisc” (Hope, 2013) packages and were graphed using the library ggplot2 (version 3.3.2) (Wickham, 2009).
Results
Diversity and Morphology
Based on video surveys, we recorded four whip black corals morphotypes: yellow, red, white/orange, and Pukao (a small red morphotype). All morphotypes observed were characterized by a monopodial corallum, not ramified nor pinnulated, straight or slightly coiled, especially in the distal portion (Figure 2 and Supplementary Video 1). The total length of the colonies differed between morphotypes; the largest morphotype was the yellow one with heights ranging between 80 cm and 350 cm (Figure 2A), followed by the red morphotype (100–180 cm, Figure 2B), the white/orange morphotype (20–30 cm, Figure 2C), and the small-red morphotype (15–28 cm, Figure 2D). Polyps on all morphotypes were arranged in a single row on only one side of the corallum, which is a diagnostic morphological character currently assigned to the genus Stichopathes (Brook, 1889; Bo and Opresko, 2015). Therefore, all morphotypes were assigned to the genus Stichopathes. No further descriptions of the white/orange and the Pukao morphotype are possible at this time because samples of these morphotypes were not collected.
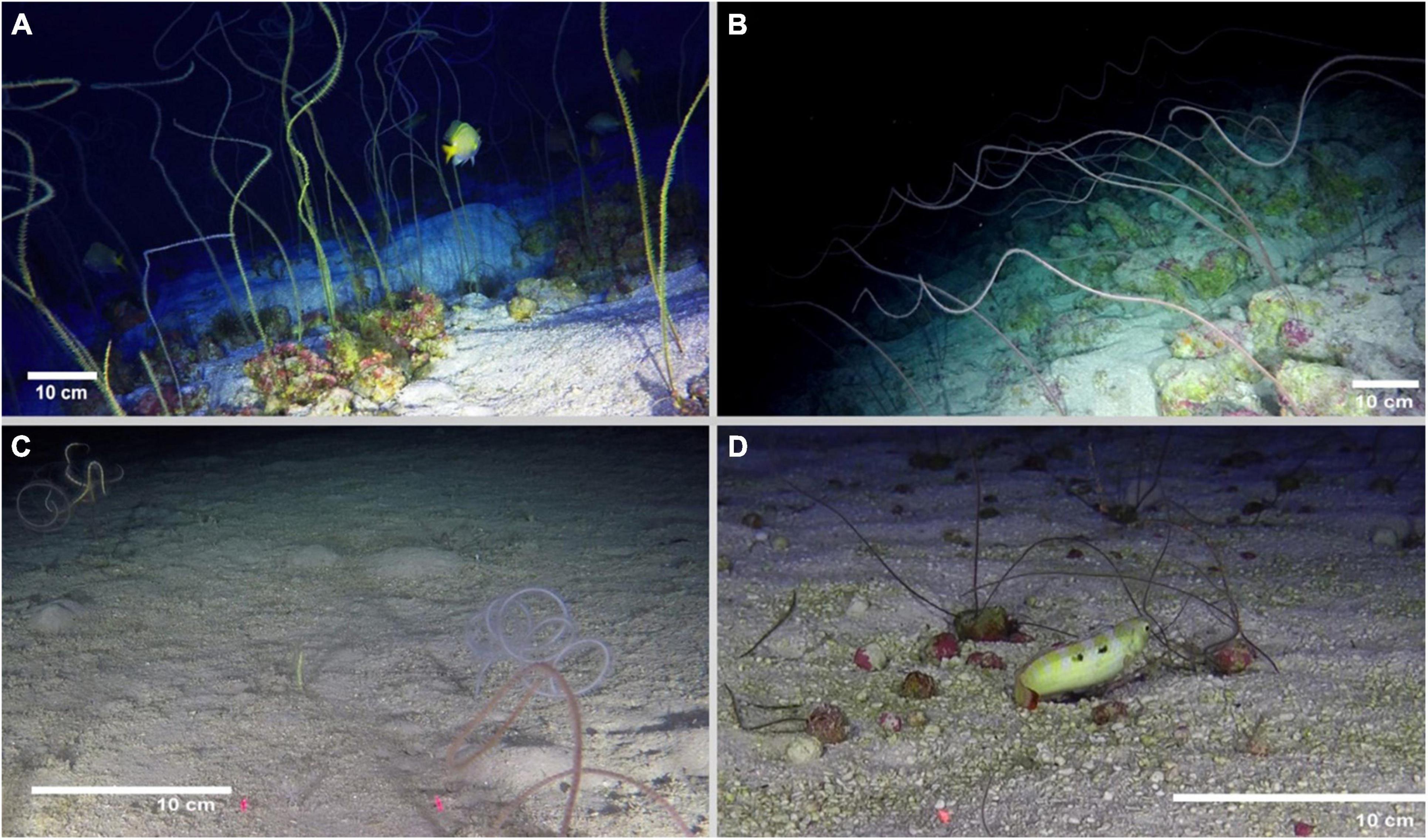
Figure 2. Photographs of whip coral morphotypes of the Easter Island Ecoregion: (A) yellow morphotype (Stichopathes sp. SCBUCN 8849), (B) red morphotype (Stichopathes sp. SCBUCN 8850), (C) white/orange morphotype, (D) Pukao morphotype (red-small). Scale bars = 10 cm. Image credit: Matthias Gorny (OCEANA) and ESMOI.
The stem of the collected colony of the yellow morphotype (Stichopathes sp. SCBUCN 8849) was 1 mm in diameter in the apical portion (Figures 3A,E). The first part of the corallum is in a straight and upright shape and the apical part in a spiral shape (Figure 2A). The skeletal spines are in the shape of an acute triangle, flattened dorsally, and covered with elongated papillae toward their distal half, with 2–3 apical lobes on some spines (Figures 3A–D). The polypar spines are generally 80–180 μm tall and spaced ∼0.8 mm apart (range = 0.6–1.3 mm in each row) (Figures 3B–D). No secondary spines are present on the corallum. On average, polyps are 1.96 mm in transverse diameter (range = 1.73–2.09 mm), are spaced 2.4 mm apart (range = 2.23–2.55 mm), and have long sagittal tentacles (up to 12 mm in living specimens) (Figure 3F). Living colonies are yellow or light brown in coloration (Figure 2A).
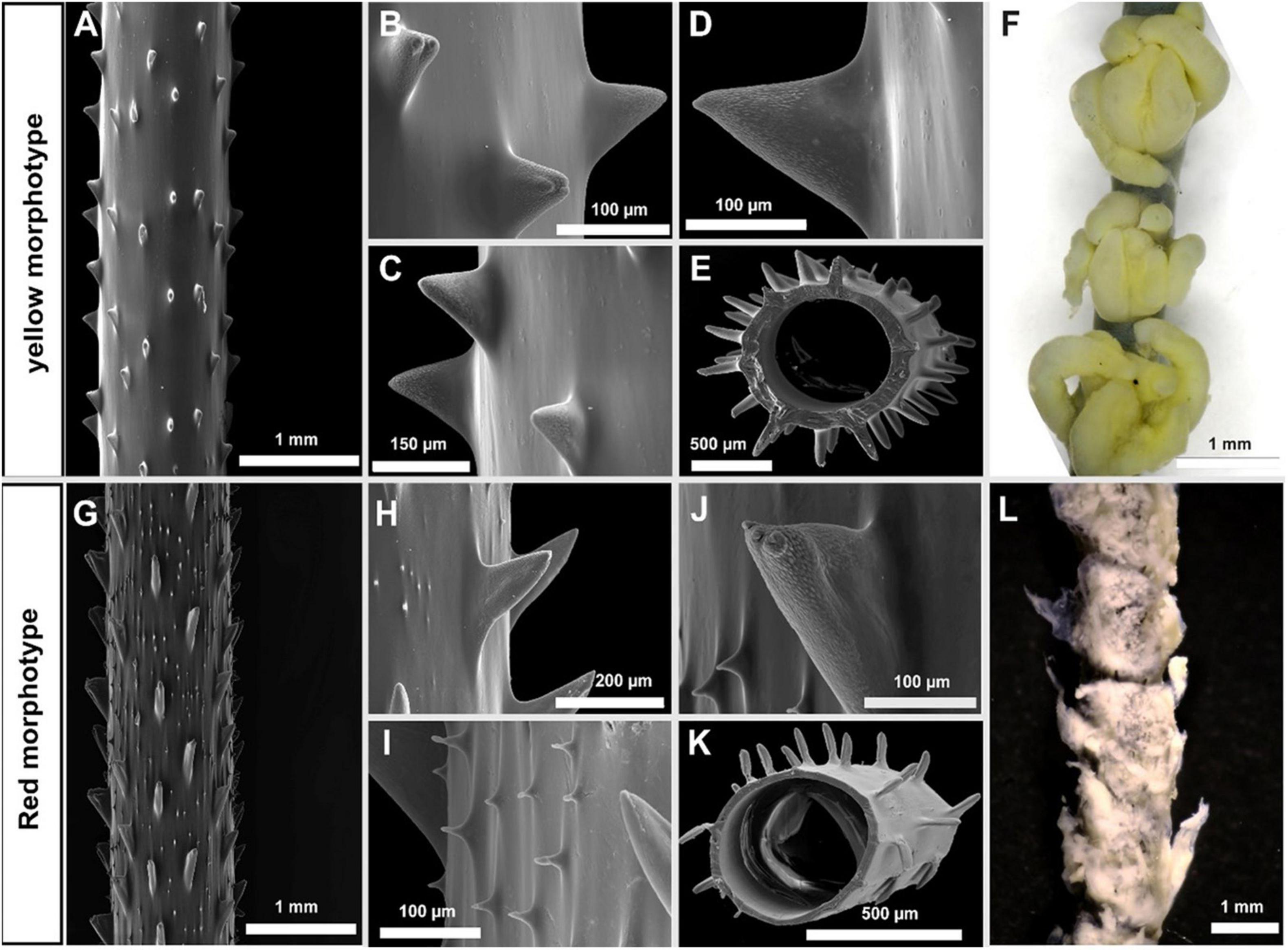
Figure 3. Morphological characteristics of stem, spines, and polyps in the yellow morphotype (Stichopathes sp. SCBUCN-8849) (A–F) and red morphotype (Stichopathes sp. SCBUCN-8850) (G–L). (A,G) Skeletal spines on terminal branch; (B,C,H,I) close-up view of the stem; (D,J) close-up view of polypar spine; (E,K) diameter of the central canal; (F) polyps on terminal branch under light microscopy; (L) polyps in poor condition under light microscopy.
The red morphotype was characterized by unbranched colonies that are up to 1.8 m in height (Figure 2B). The stem of the collected colony (Stichopathes sp. SCBUCN 8850) was ∼560 μm in diameter in the apical portion (Figures 3G,K). Colonies are coiled throughout (Figure 2B). The skeletal spines are in the shape of a right triangle, flattened dorsally and covered with elongated papillae toward their distal half, with three apical lobes on some spines (Figures 3G–J). The polypar spines are generally 155–250 μm tall and spaced 0.7 mm apart (range = 0.4–0.9 mm in each row) (Figures 3B–D). The secondary spines are triangular, without apical papillae (Figure 3I), and are much smaller in size than the primary spines at ∼20–50 μm in height. On average, polyps are 1.7 mm in transverse diameter (range = 1.6–1.9 mm, Figure 3L), with long sagittal tentacles (up to 6 mm in living specimens). Living colonies are pink or red in coloration (Figure 2B).
ITS1 Phylogenetic Relationships
The sequences obtained for the full-length ITS1 were 263 bp for the yellow morphotype (Stichopathes sp. SCBUCN 8849) and 245 bp for the red morphotype (Stichopathes sp. SCBUCN 8850). The uncorrected divergence between the yellow and red morphotypes was 38.27%. In the reconstructed maximum-likelihood phylogenetic tree based in the ITS1 alignment, the yellow morphotype (Stichopathes sp. SCBUCN 8849) is within a clade that is composed of Cirrhipathes species and Antipathes curvata and is sister to the Antipathes-Stichopathes clades recognized in Bo et al. (2012; Figure 4). Stichopathes sp. SCBUCN-8849 ITS1 is 95.51–99.23% similar to the species within that clade. The red morphotype (Stichopathes sp. SCBUCN 8850) was within Bo et al. (2012) clade C, a clade composed of Antipathes species and an unidentified Stichopathes sp. (Figure 4). Stichopathes sp. SCBUCN-8850 ITS1 is 95.08–99.16% similar to the species within clade C. Both clades are composed of species whose origin is the tropical western Pacific (Indonesia, the China Sea or Hawaii) (Supplementary Table 1). All distal clades in which Stichopathes species were included were moderately (bootstrap > 65) or well supported (bootstrap > 90); however, Antipathidae was polyphyletic with generally poor support (bootstrap < 65) at basal nodes.
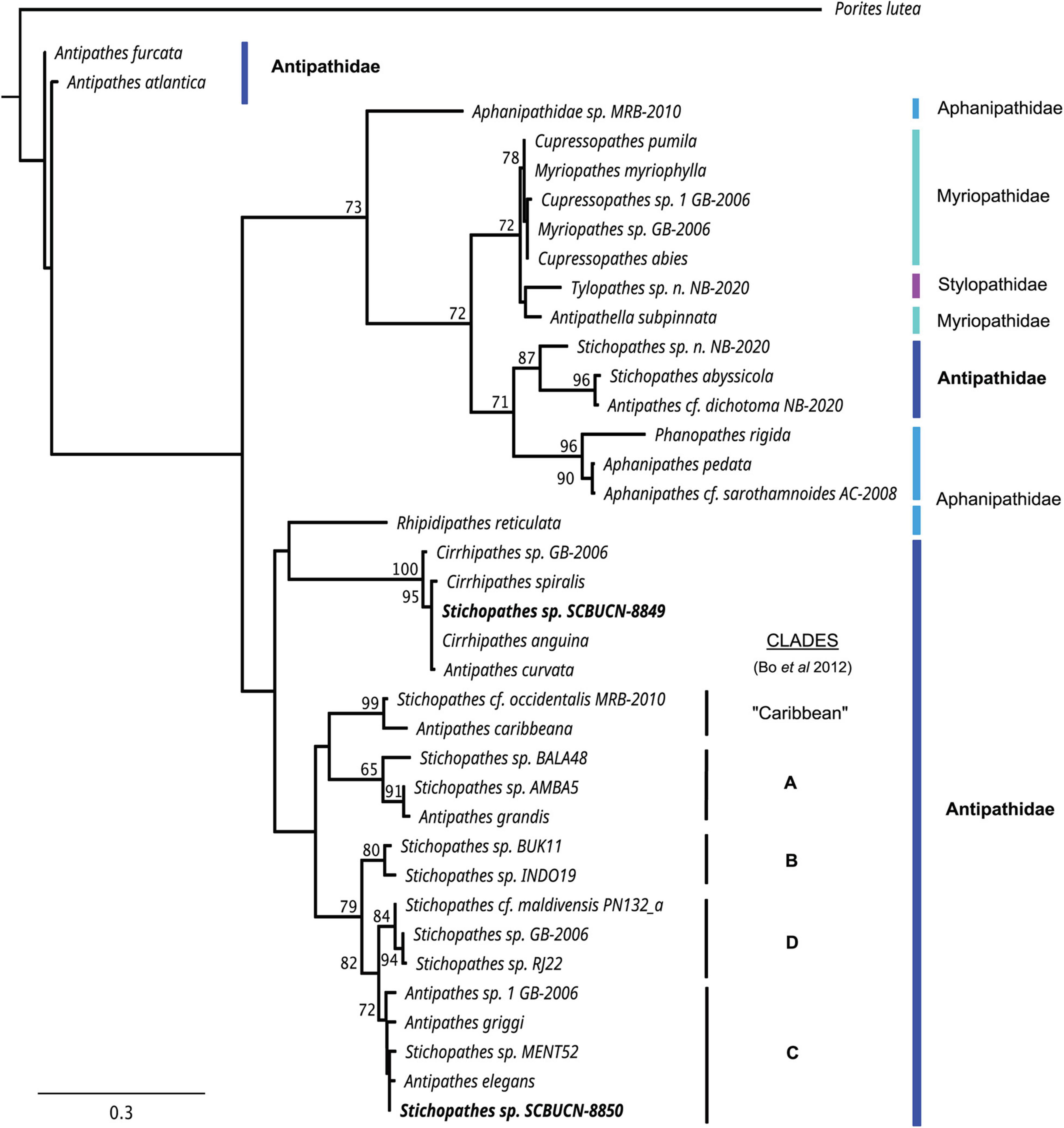
Figure 4. Phylogenetic tree obtained by maximum-likelihood analysis based on internal transcribed spacer 1 rDNA (ITS1) of 37 antipatharian specimens of four families and the scleractinian coral Porites lutea as an outgroup. The two black coral specimens collected as part of this study are in bold. The numbers at nodes are the bootstrap estimated values (>65%). Antipathes-Stichopathes clades recognized in Bo et al. (2012) are indicated. See Supplementary Table 1 for GenBank accessions and localities of specimens.
Abundance and Distribution
Whip black corals were observed on 28 (42%) of the 67 ROV dives (Apolo peak = 2 dives, Pukao seamount = 5 dives, and Rapa Nui = 21 dives) at 60–240 m (Table 1). In total, ∼7,160 whip black coral colonies were counted, with an average abundance of 13.2 ± 0.8 col m–2 across all frames in which whip black coral colonies were present (Table 1). The average total coral abundance was highest on Pukao seamount with 26.8 ± 1.4 col m–2, followed by Rapa Nui (10.6 ± 0.5 col m–2), and Apolo peak (8.0 ± 1.4 col m–2) (Figure 5A). The highest densities reported per frame were 58.2 col m–2 at Pukao seamount, 23.1 col m–2 at Rapa Nui, and 11.0 col m–2 and Apolo peak. The total abundances of whip corals at Rapa Nui and Apolo peak were not significantly different (Wilcoxon test, W = 7906, P = 0.442), whereas the abundances at Pukao seamount differed significantly from those of both Rapa Nui (Wilcoxon test, W = 27268, P < 0.001) and Apolo peak (Wilcoxon test, W = 1581, P = 0.00226). The total abundances of whip black corals also differed with depth (Figures 2B,D). Densities were generally higher at greater depths (Figure 5B) except for Apolo peak (not enough data). At Pukao seamount, intermediate (160–180 m) and deeper stations (200–220 m) had significantly greater densities (P < 0.001, H = 74.44; Figure 5C) that exceeded 20 colonies m–2 (Table 1 and Figure 1). Apolo peak also had densities exceeding 20 colonies m–2 (Table 1 and Figure 1). At Rapa Nui, deeper stations (120–240 m) had significantly greater densities (P < 0.001, H = 99.13; Figure 5D). Whip black corals were widely distributed around Rapa Nui (Figure 1). The largest assemblage of whip corals (15–20 colonies m–2, Table 1 and Figure 1) were concentrated on the western side of the island between Hanga Roa and Anakena at depths between 120 and 240 m (Figure 2D) and the southeastern coast of Hanga Hotuiti at depths between 130 and 280 m. The lowest abundance of whip black corals (1–5 colonies m–2) occurred on southeast-facing shores of Rapa Nui between Hanga Hotuiti and Cabo O’Higgins at 60–120 m. No whip black corals were observed in the southwestern sector of Rapa Nui between Punta Cook and northeast Hanga Roa or the southeastern coast of Hanga Vinapu.
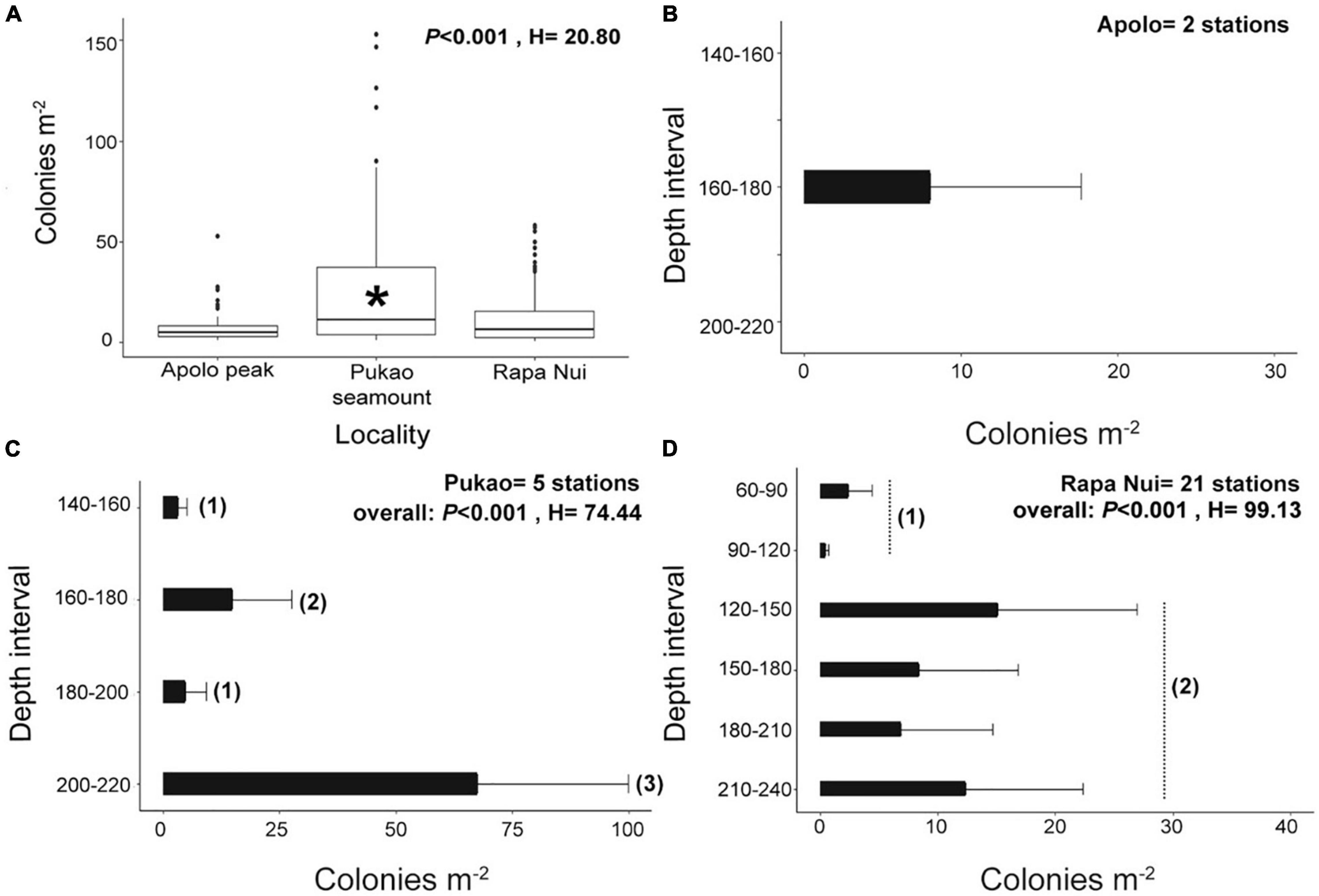
Figure 5. (A) Box plots of total coral abundance (colonies m–2) at each site. Box and whisker plots represent the 95% confidence interval. *Presents significant differences between the groups (P < 0.001). (B–D) Total abundance (colonies m–2 ± SE) of the whip black corals per depth interval on (B) Apolo peak, (C) Pukao seamount, and (D) around Rapa Nui.
The red (2,511 verifiable colonies, ∼35% of the total) and yellow (2,142 verifiable colonies, ∼30% of the total) morphotypes were the most abundant and widely distributed in the area (Figure 1). A total of 1,421 colonies (∼20% of the total) could not be assigned to morphotype because color and most diagnostic characters were not visible in the images. All of these unidentified colonies were inconsistent with orange/white or Pukao morphotypes but could be representatives of the yellow or red morphotypes based on colony length. There were no significant differences between the abundances of the yellow and red morphotypes at the different sites (Pukao seamount: P = 0.07, H = 3.34; Apolo peak: P = 0.2, H = 1.55; Rapa Nui: P = 0.3, H = 1.05; Figure 6). The yellow morphotype was observed infrequently at the shallowest depths (60–90 m), reached a maximum at 150–180 m, and then declined below 180–210 m (Figure 6). The red morphotype was absent from the shallowest stations (60–90 m); density reached a maximum at 120–150 m and decreased at the deeper stations. The two less abundant morphotypes were observed at deeper depths at a single site. The white/orange morphotype (349 colonies, ∼5% of the total) was exclusively recorded at 260 m at Rapa Nui station T14. The Pukao morphotype (734 colonies, ∼10% of the total) was exclusively recorded at Pukao seamount station T19 at 230 m.
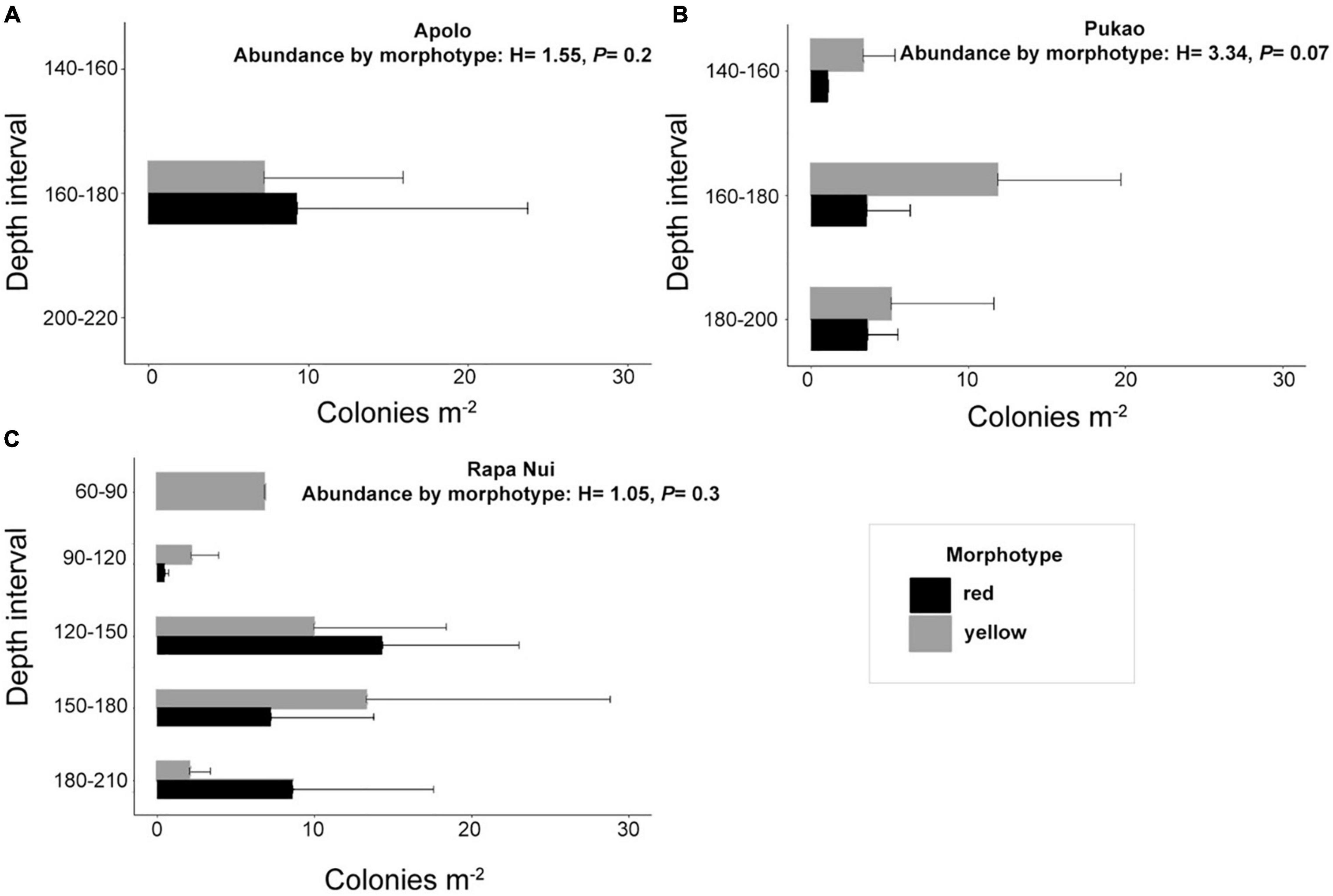
Figure 6. Abundance (colonies m–2 ± SE) of the yellow and red morphotypes by depth interval on (A) Apolo peak, (B) summit of Pukao seamount, and (C) around Rapa Nui.
Habitat
Whip black coral assemblages at Apolo peak and Rapa Nui (∼60 to 240 m depth) were associated with three types of habitats (Figure 7): (i) small reef patches of irregular rock substrate, surrounded by large areas of sand separating exposed rocks inhabited by numerous adult and juvenile fishes (e.g., Chromis mamatapara, Prognathodes sp.) and invertebrates (e.g., the ophiurids Ophiocoma spp., the echinoids Diadema spp. and the sea stars Anthenoides sp. and cf. Sclerasterias) (Figures 7A,B), (ii) hard substrates, including those interspersed with patches of coarse sand and large rock formations, dominated by incrusting red algae, with a steep slope in which the presence of cracks provided shelter to numerous fishes (e.g., Pseudolabrus semifasciatus, Synodus cf. doaki) (Figure 7C), and (iii) homogeneous bottom with little relief, coarse sand, and thanatocoenosis (foraminifera deposits), that was inhabited by ophiurids and numerous fishes (e.g., Antigonia cf. capros) (Figure 7D). The white/orange morphotype was exclusively associated with this third habitat type (i.e., coarse sand) at 260 m (Figure 7D and Supplementary Video 1). On the summit of Pukao seamount (∼160 to 220 m depth), whip black coral assemblages were associated with (i) irregular rocky protrusions interspersed with sandy areas (Figure 7E) and (ii) rhodolith beds on coarse sand with a few inhabited by some fishes (Suezichthys sp. and gobiids) (Figure 7F). The Pukao morphotype was exclusively found on this second habitat type (i.e., rhodoliths) at 230 m (Figure 7E and Supplementary Video 1).
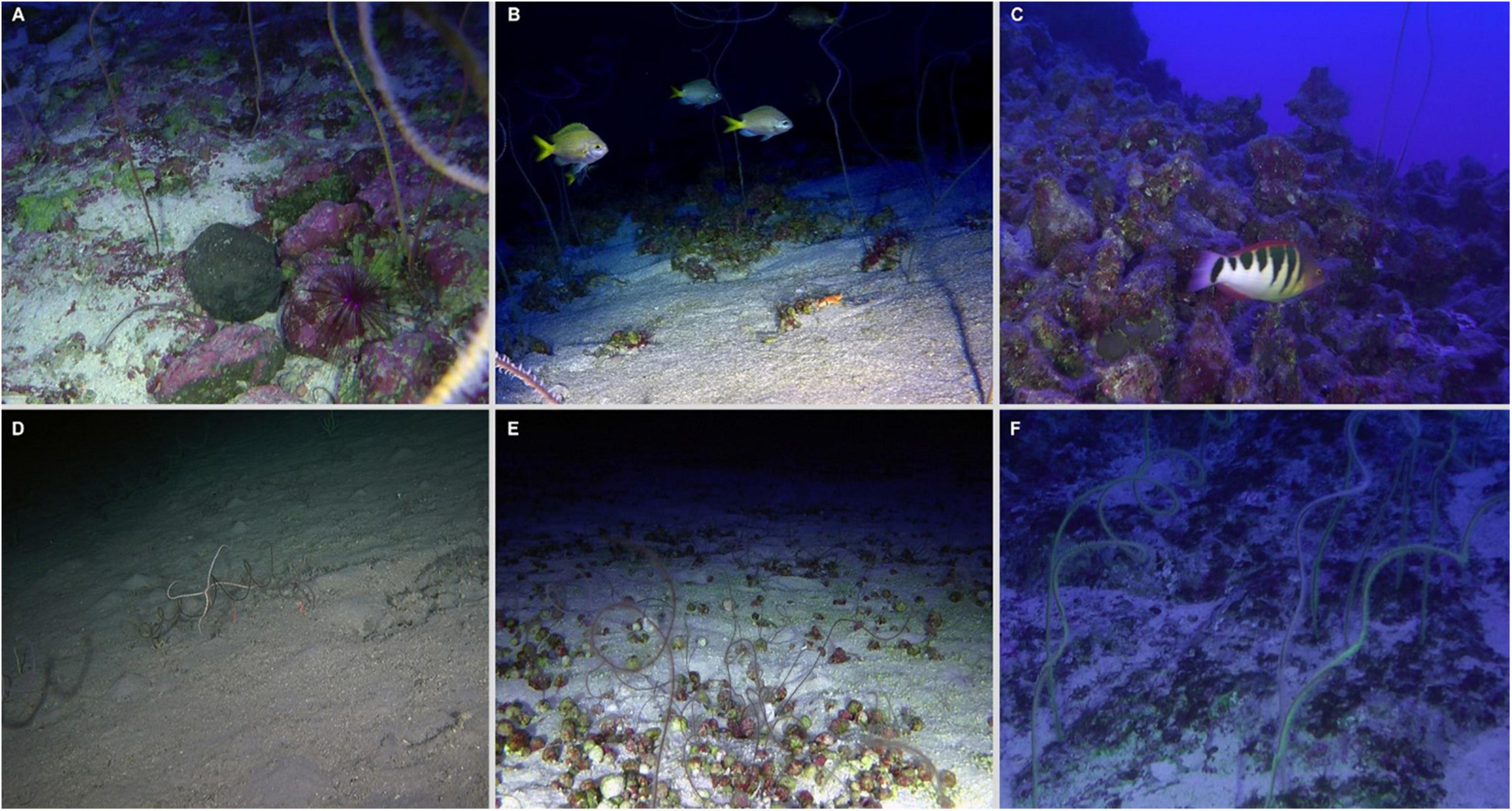
Figure 7. Benthic habitat types on Apolo peak (A), the upper slope of Rapa Nui (Easter Island) (B–D) and the summit of Pukao seamount (E,F). (A,B,F) Small patches of irregular rock substrate, with large areas of sand, inhabited by some fishes (Chromis mamatapara). (C) Irregular rock bottom with fractures and faults that are dominated by incrusting red algae and are used as habitats for small fishes (Pseudolabrus semifasciatus). (D) Regular continuous homogeneous bottom with coarse sand. (E) Regular continuous homogeneous bottom with coarse sand, rhodoliths, and little relief. Image credit: Matthias Gorny (OCEANA) and ESMOI.
Discussion
Historic information about whip black corals within the EIE is based largely on opportunistic observations included sporadically in descriptions of other taxonomic groups (e.g., echinoderms: Mecho et al., 2019 and Mah, 2021; fishes: Easton et al., 2017 and Shepherd et al., 2020). There have been no previous studies focusing on the taxonomy, distribution, or abundance of antipatharians in the EIE (Easton et al., 2019). This first systematic study based on in situ observations revealed the presence of at least four distinct morphotypes of unbranched black corals that could clearly be differentiated in the videos based on differences in coloration and overall appearance of the colonies (Figure 3). Based on morphological differences in colony length, color, and external characteristics of the polyps (sagittal tentacles), we believe these four morphotypes to be distinct species. However, we were only able to assess this conclusion for the yellow and red morphotypes because species-level diagnostic characters (e.g., DNA markers, size and shape of the primary spines, presence of secondary spines) can often only be assessed by examining collected specimens. The yellow and red morphotypes differed in colony length, color, and skeletal spines characters, which are considered diagnostic for antipatharian species. Further support them being distinct species includes ITS1 divergence values (uncorrected p values) >30% compared to ∼3% reported for within-species divergences in Stichopathes maldivensis and S. variabilis (Terrana et al., 2021), phylogenetic reconstruction based on ITS (Figure 4), phylogenetic reconstruction based on partial 18S rRNA gene, internal transcribed spacer 1 rDNA (ITS1), 5.8S rRNA gene, internal transcribed spacer 2 (ITS2), and partial 28S rRNA gene (Supplementary Figure 2), and genetic divergence (1.31%) and phylogenetic reconstructions based on alignment of the mitochondrial protein-coding genes (Asorey et al., 2021). Our observations of at least four morphotypes with two being confirmed as distinct species corroborate field identifications made in 2017, which suggested that at least two species of Stichopathes are present in the EIE (Easton et al., 2019). Without specimens, we cannot assess whether the unbranched Antipathes reported from the EIE in previous studies (Disalvo et al., 1988) were mistakenly assigned to Antipathes or whether they are one of the species reported in this study. Misidentifications of antipatharian species have been reported in many locations around the world when unbranched forms (e.g., Stichopathes or Cirrhipathes) are incorrectly assigned to Antipathes (Molodtsova, 2005), which is considered a taxonomic dumping ground that is morphologically and phylogenetically heterogeneous (Daly et al., 2007; Bo et al., 2008; Brugler et al., 2013). Even though many species have been removed from Antipathes on the basis of branching pattern and added to other genera, the genus still groups together many uncertain species and requires revision (Bo, 2008).
There are currently three described genera of antipatharian corals with a whip-like or unbranched corallum: Cirrhipathes, Pseudocirrhipathes and Stichopathes. In both Cirrhipathes and Pseudocirrhipathes, polyps are arranged irregularly on all sides of the corallum, with the distinguishing factor being that Pseudocirrhipathes has skeletal spines arranged in verticills (Bo et al., 2009b). In contrast to Cirrhipathes and Pseudocirrhipathes, the genus Stichopathes is characterized by colonies that have polyps that are arranged in a single row on only one side of the corallum (Brook, 1889; Bo and Opresko, 2015). Polyp arrangement on all four EIE morphotypes was in a single row on only one side of the corallum, so all morphotypes were assigned to the genus Stichopathes (Opresko, 2006; Bo and Opresko, 2015). However, several authors have questioned the validity of polyp arrangement and corallum branching as diagnostic external morphological differences between some antipatharian genera (Van Pesch, 1914; Pax, 1918; Pasternak, 1977; Bo, 2008). Evidence that supports the questionable utility of these diagnostic characters is mounting as phylogenetic studies reveal that several antipatharian genera are polyphyletic. For example, recent phylogenetic studies have shown that not only are antipatharian genera, such as Stichopathes and Cirrhipathes, not monophyletic but neither are some antipatharian families (Bo, 2008; Bo et al., 2012; Brugler et al., 2013; Wagner, 2015; Barrett et al., 2020). These studies found that species with polyps arranged in a single row (Stichopathes spp.) and those with polyps arranged irregularly on all sides of the corallum (e.g., Cirrhipathes spp.) are commonly found in the same clade, as are species with unbranched (e.g., Stichopathes spp.) and branched corallum (e.g., Antipathes spp.). Our ITS1-based phylogenetic reconstructions (Figure 4) are consistent with these patterns. For example, Stichopathes sp. SCBUCN-8849 is in a clade with Antipathes curvata and Cirrhipathes spp., whereas Stichopathes sp. SCBUCN-8850 is in Bo et al. (2012) clade C, which is composed mainly of Antipathes species. Morphologically, Stichopathes sp. SCBUCN-8849 and the Cirrhipathes species in that clade share a tissue coloration that ranges between white and brown, and an unbranched corallum whose length can reach more than 3 m in height (Lapian et al., 2007; Terrana and Eeckhaut, 2019). Other morphological characters of Stichopathes sp. SCBUCN-8849 that have been reported in these Cirrhipathes spp. (Lapian et al., 2007) include a spiral corallum, a triangular-shaped skeletal spine with the presence of papillae (Figure 3D), and the presence of a few lobes on the apex of the primary skeletal spines (Figure 3B). However, Stichopathes sp. SCBUCN-8849 only has polyps on one side of the corallum arranged in a single row unlike the irregular polyp arrangement characteristic of Cirrhipathes (Figure 3F). Therefore, these skeletal characteristics may be diagnostic for this clade, whereas polyp arrangement and branching pattern are not. In contrast, no consistent morphological characteristics were recognized among Stichopathes sp. SCBUCN-8850 and other species in clade C. These species have a variety of the primary spine shapes (Lapian et al., 2007; Opresko, 2009; Wagner et al., 2010; Bo et al., 2012; Wagner, 2015), which may present a few apical tubercles as in some of the triangular spines of Stichopathes sp. SCBUCN-8850 (Figure 3G). Some of these species, including Stichopathes sp. SCBUCN-8850 (Figure 3I), have small interspersed secondary spines (Lapian et al., 2007; Bo et al., 2012) but not all of them have these secondary spines (Lapian et al., 2007; Wagner et al., 2010).
Collectively, these observations highlight the need for further taxonomic revisions within the order Antipatharia. Pending those revisions, we continue to use the name Stichopathes for unbranched black corals with polyps on only one side of the corallum. Greater sampling effort of antipatharians and the use of phylogenetic reconstructions and morphological analyses will be critical for revising antipatharian taxonomy. For example, Bo et al. (2012) suggested that Antipathes and Stichopathes could be considered as a single genus based on phylogenetic reconstructions (ITS1) and the similarity among Antipathes-Stichopathes clades (maximum internal divergence 11.13%). The placement of some Antipathes and Stichopathes species within other clades with representatives predominantly from other genera or families that differ by >30% (uncorrected) is indicative that those species may need to be reassigned to other taxonomic groups. For example, the ITS1 sequence of Stichopathes sp. SCBUCN-8849 is ∼99% similar to several Cirrhipathes species but only ∼62% similar to Antipathes-Stichopathes clades from Bo et al. (2012; Figure 4).
Our records of 7,160 colonies at 60–245 m indicate that dense aggregations of whip black corals are present and widely distributed on Pukao seamount, around Apolo peak, and around Rapa Nui, especially along the west-facing shore. The population density of EIE whip black corals (average across all frames: 13.2 ± 0.8 col m–2, Table 1) is considerably higher than that reported for Stichopathes spp. in most other geographic locations, including the Colombian Caribbean (<7.32 m–2), deep North Pacific seamounts (<1 m–2), Puerto Rico (<0.5 m–2), and Mexican waters of the Gulf of Mexico (<0.5 m–2) (reviewed in Wagner et al., 2012). The only location with higher reported population densities is for Stichopathes spiessi on Jasper seamount in the North Pacific (<20 m–2; Genin et al., 1986). The highest whip coral densities in our study were observed between 120 and 240 m (Figure 2). Unlike the high densities values in the west-facing shore of Rapa Nui, whip corals were not observed in the southeast-facing Hanga Vinapu sector, probably because the southeast-facing shelf is a barren and eroded lava pavement that may not be favorable to colonization by corals (Hubbard and Garcia, 2003).
Given the scarcity of quantitative data on whip corals globally (Wagner et al., 2012; Yesson et al., 2017), comparative analyses of distribution by morphotype cannot be performed. However, the yellow and red morphotypes had generally similar densities (<15 col m–2) and were commonly observed at Pukao seamount, Apolo peak, and Rapa Nui on hard substrates (Figure 6). The other morphotypes (i.e., white/orange and Pukao), were found to be quite abundant (>20 col m–2) in unique habitats (i.e., sandy and rhodoliths bottoms) and at depths that were not surveyed as frequently (≥230 m) (Table 1). These observations suggest that these corals appear to have very specific habitat requirements. Further studies at these depths are needed to understand their true distribution. Nevertheless, this first characterization presents a fundamental basic knowledge of the whip corals and environment in this relatively poorly studied region of the Pacific Ocean, with high conservation value (Friedlander et al., 2021; Georgian et al., 2021; Wagner et al., 2021).
Surveys within the EIE have revealed the presence of a variety of often heterogenous substrates (i.e., rocky, sandy and rhodoliths bottoms) (Easton et al., 2017, 2019; Mecho et al., 2019; Shepherd et al., 2020) that provide a wide variety of habitats for black whip corals. The presence of these whip coral gardens seems to contribute to increasing the availability of habitat and resources for other species. Our study supports previous reports that whip black coral populations within the EIE provide important habitat and nursery grounds for a wide variety of organisms (e.g., ophiuroids, echinoids, and potentially new species of fishes). Easton et al. (2017, 2018) and Shepherd et al. (2020) documented the presence of yellowfin soldierfish (Myripristis chryseres) and juveniles of Chromis mamatapara and butterflyfishes (Prognathodes sp.) in association with whip black corals, highlighting the potential habitat preference of these species in whip coral gardens of Pukao seamount and Rapa Nui at 90–230 m. In our study, some invertebrates (e.g., sea star: Goniasteridae and gastropod: unidentified Coralliophilinae) were observed preying on the tissues of whip corals. In a recent description of sea stars from the South Pacific, Mah (2021) mentioned that a sea star (cf. Ceramaster australis) preyed on fallen antipatharian colonies off Rapa Nui. In situ observations show that this sea star is only present in fields of whip coral of the yellow morphotype at ∼130–140 m on Rapa Nui (Supplementary Video 1) (F. Valdes, personal communication). Other potential predators, muricid gastropods, were observed on red morphotype whip corals at ∼180–200 m off Rapa Nui (Supplementary Figure 3). The portion of the stem of corals where muricids were present were denuded or did not contain any tissue. This observation suggests that these gastropods are preying on whip corals as has been reported in other regions (reviewed in Wagner et al., 2012), highlighting that this gastropod family may be specialized to prey on black corals. These observations provide further reinforcement that black corals are ecologically important because they enhance local biodiversity of other taxa (Tazioli et al., 2007; Bo et al., 2012; Wagner et al., 2012; Deidun et al., 2015). Our observations provide important new information on the ecology of antipatharians and their associates, a group for which very limited information is available globally (see Wagner et al., 2012).
While our surveys all occurred in the Rapa Nui MUMPA within 100 km of Rapa Nui, it is likely that similar habitat also occurs on other MUMPA seamounts (e.g., Moai seamount near Rapa Nui, see in Haase et al., 1997) and seamounts in adjacent international waters. Seafloor topography data and seamount databases (British Oceanographic Data Centre, 2008; Yesson et al., 2011, 2020; Harris et al., 2014) indicate that there are over 50 seamounts with summits shallower than 250 m in international waters of the Salas y Gómez and Nazca ridges to the east of our study areas (see Figure 1 inset). While most of these seamounts remain unexplored, they likely harbor similar habitats as those documented during this study. Furthermore, recently developed habitat-suitability models indicate that these high seas seamounts provide highly suitable habitat for various deep-sea corals and sponges (Georgian et al., 2021). Given the slow recovery rates of these taxa, they should be proactively protected from destructive practices such as bottom trawling and seabed mining (Rogers, 2018; Friedlander et al., 2021; Wagner et al., 2021).
Conclusion
The diversity of antipatharians for the EIE is much higher than that reported in previous studies and includes at least four distinct morphologies assigned to the genus Stichopathes based on the diagnostic characters currently assigned to the genus: unbranched corallum with polyps arranged in a single row on only one side of the corallum. The true diversity of whip black corals within the EIE remains unknown due to logistical challenges of surveying the region and taxonomic uncertainties within this group. Our ROV surveys showed that whip black corals in the EIE can occur in very high densities and across at least three distinct habitats (rocky, sandy, and rhodoliths bottoms). These black coral aggregations enhance biodiversity by providing suitable habitat for many associated species, including ophiuroids, echinoids, and fishes. As such, these communities should be prioritized for conservation. The presence of dense corals gardens with many associated species should be considered in a management plan to monitor ecosystem health within the Rapa Nui MUMPA as well as in guiding future conservations efforts in adjacent regions that have not yet been protected but likely include similar habitats.
Data Availability Statement
The datasets presented in this study can be found in online repositories. The names of the repository/repositories and accession number(s) can be found below: NCBI (accession: MZ450123 and MZ450124).
Author Contributions
JT-G analyzed the ROV data, assisted in species identification prepared figures and/or tables, conducted the statistical analysis and wrote the manuscript. JS, EE, and MG developed the proposal and secured funding for participation in the Easter Island cruises and conducted fieldwork. DW secured funding for the development of the manuscript and subsequent analyzes. CA analyzed the molecular data, assisted in species identification, prepared figures and/or tables and wrote the manuscript. EE, DW, and JS assisted in species identification, analyzed the data, contributed to the concept, writing, and editing of the manuscript. MG generated the ROV data, contributed to writing and editing the manuscript. All authors reviewed the final version of the manuscript.
Funding
The present study was funded in part by ANID – Millennium Science Initiative ESMOI, OCEANA, FONDEQUIP EQM 150109 and 160085, FONDECYT 1181153 and 1180694 grants to JS, Beca Magister Nacional CONICYT # 22190560 (now ANID) to JT-G, and Conservation International grants to CA and JT-G. Additional support was provided by the Paul M. Angell Foundation, Alan Eustace, Tom and Currie Barron through the Coral Reefs of the High Seas Coalition. OCEANA funded part of the expeditions and provided the ROV and the operator.
Conflict of Interest
The authors declare that the research was conducted in the absence of any commercial or financial relationships that could be construed as a potential conflict of interest.
Publisher’s Note
All claims expressed in this article are solely those of the authors and do not necessarily represent those of their affiliated organizations, or those of the publisher, the editors and the reviewers. Any product that may be evaluated in this article, or claim that may be made by its manufacturer, is not guaranteed or endorsed by the publisher.
Acknowledgments
We thank Poky Tane Haoa, Ricardo Hito, Enrique Pate, and Enrique Hey from the Rapa Nui community for their collaboration during field work. Special thanks go to Sergio Rapu and the Rapa Nui Heritage Foundation for providing land and facilities for our on-island laboratory and Iván Hinojosa, Ariadna Mechó, Maria Valladares, and Germán Zapata-Hernández for assistance in the field.
Supplementary Material
The Supplementary Material for this article can be found online at: https://www.frontiersin.org/articles/10.3389/fmars.2021.755898/full#supplementary-material
Footnotes
References
Alarcón, M. A. (2009). Biodiversidad de Corales (Cnidaria: Anthozoa) en Chile. Ph.D. thesis. Valparaíso: Pontificia Universidad Católica Valparaíso.
Araya, J. F., Aliaga, J. A., and Araya, M. E. (2018). First record of Lillipathes ritamariae Opresko and Breedy, 2010 (Cnidaria: Antipatharia) from the southeastern Pacific Ocean. Mar. Biodivers. 48, 1601–1605. doi: 10.1007/s12526-016-0591-1
Asorey, C. M., Sellanes, J., Wagner, D., and Easton, E. E. (2021). Complete mitochondrial genomes of two species of Stichopathes Brook, 1889 (Hexacorallia: Antipatharia: Antipathidae) from Rapa Nui (Easter Island). Mitochondrial DNA B Resour. 6, 3226–3228. doi: 10.1080/23802359.2021.1990150
Barrett, N. J., Hogan, R. I., Allcock, A. L., Molodtsova, T., Hopkins, K., Wheeler, A. J., et al. (2020). Phylogenetics and mitogenome organisation in black corals (Anthozoa: Hexacorallia: Antipatharia): an order-wide survey inferred from complete mitochondrial genomes. Front. Mar. Sci. 7:440. doi: 10.3389/fmars.2020.00440
Bo, M. (2008). Taxonomy and Ecology of Antipatharians. Ph.D. thesis. Italy: Universita Politecnica Delle.
Bo, M., Barucca, M., Biscotti, M. A., Canapa, A., Lapian, H. F. N., Olmo, E., et al. (2009a). Description of pseudocirrhipathes (Cnidaria: Anthozoa: Hexacorallia: Antipathidae), a new genus of whip black corals from the Indo-Pacific. Ital. J. Zool. 76, 392–402. doi: 10.1080/11250000802684104
Bo, M., Bavestrello, G., Barucca, M., Makapedua, D. M., Poliseno, A., Forconi, M., et al. (2012). Morphological and molecular characterization of the problematic whip black coral genus Stichopathes (hexacorallia: Antipatharia) from Indonesia (north sulawesi, celebes sea). Zool. J. Linn. Soc. 166, 1–13. doi: 10.1111/j.1096-3642.2012.00834.x
Bo, M., Bavestrello, G., Canese, S., Giusti, M., Salvati, E., Angiolillo, M., et al. (2009b). Characteristics of a black coral meadow in the twilight zone of the central Mediterranean Sea. Mar. Ecol. Prog. Ser. 397, 53–61. doi: 10.3354/meps08185
Bo, M., and Opresko, D. M. (2015). Redescription of stichopathes pourtalesi brook, 1889 (Cnidaria: Anthozoa: Antipatharia: Antipathidae). Breviora 540, 1–18. doi: 10.3099/mcz16.1
Bo, M., Tazioli, S., Spanò, N., and Bavestrello, G. (2008). Antipathella subpinnata (Antipatharia, Myriopathidae) in Italian seas. Ital. J. Zool. 75, 185–195. doi: 10.1080/11250000701882908
British Oceanographic Data Centre (2008). Gridded Bathymetry Data (General Bathymetric Chart of the Oceans). 2008. Available online at: http://www.bodc.ac.uk/data/online_delivery/gebco/ (accessed January 13, 2021).
Brook, G. (1889). Report on the antipatharia. report of the scientific results of the voyage of the H.M.S. Challenger. Zoology 32, 1–222.
Brugler, M. R., Opresko, D., and France, S. C. (2013). The evolutionary history of the order Antipatharia (Cnidaria: Anthozoa: Hexacorallia) as inferred from mitochondrial and nuclear DNA: implications for black coral taxonomy and systematics. Zool. J. Linn. Soc. 169, 312–361. doi: 10.1111/zoj.12060
Bushnell, B. (2021). BBDuk. Jt Genome Inst. Available online at: https://jgi.doe.gov/data-and-tools/bbtools/bb-tools-user-guide/bbduk-guide/ (accessed October 15, 2021).
Cau, A., Follesa, M. C., Moccia, D., Alvito, A., Bo, M., Angiolillo, M., et al. (2015). Deepwater corals biodiversity along roche du large ecosystems with different habitat complexity along the south Sardinia continental margin (CW Mediterranean Sea). Mar. Biol. 162, 1865–1878. doi: 10.1007/s00227-015-2718-5
Daly, M., Brugler, M. R., Cartwright, P., Collins, A. G., Dawson, M. N., Fautin, D. G., et al. (2007). The phylum Cnidaria: a review of phylogenetic patterns and diversity 300 years after Linnaeus. Zootaxa 1668, 127–182. doi: 10.11646/zootaxa.1668.1.11
Darriba, D., Posada, D., Kozlov, A. M., Stamatakis, A., Morel, B., and Flouri, T. (2019). ModelTest-NG: a new and scalable tool for the selection of DNA and protein evolutionary models. Biorxiv [Preprint] doi: 10.1101/612903
Deidun, A., Andaloro, F., Bavestrello, G., Canese, S., Consoli, P., Micallef, A., et al. (2015). First characterisation of a Leiopathes glaberrima (Cnidaria: Anthozoa: Antipatharia) forest in Maltese exploited fishing grounds. Ital. J. Zool. 82, 271–280. doi: 10.1080/11250003.2014.986544
Disalvo, L., Randall, J., and Cea, A. C. (1988). Ecological reconnaissance of the easter island south pacific sublittoral marine environment. Natl. Geogr. Res. 4, 451–473.
Easton, E. E., Gaymer, C. F., Sellanes, J., Morales, N., Berkenpas, E., and Gorny, M. (2017). Diversity of deep-sea fishes of the Easter Island Ecoregion. Deep Sea Res. 2 Top. Stud. Oceanogr. 137, 78–88. doi: 10.1016/j.dsr2.2016.12.006
Easton, E. E., Gorny, M., Mecho, A., Sellanes, J., Gaymer, C. F., Spalding, H. L., et al. (2019). “Chile and the salas y gómez ridge,” in Mesophotic Coral Ecosystems, eds Y. Loya, K. Puglise, and T. Bridge (Cham: Springer), 477–490.
Easton, E. E., Sellanes, J., and Gorny, M. (2018). First record of the yellowfin soldierfish, myripristis chryseres jordan & evermann, 1903, in the Easter Island Ecoregion. Pacific Sci. 72, 143–148. doi: 10.2984/72.1.9
Edgar, R. C. (2004). MUSCLE: Multiple sequence alignment with high accuracy and high throughput. Nucleic Acids Res. 32, 1792–1797. doi: 10.1093/nar/gkh340
Fernández, M., Pappalardo, P., Rodríguez-Ruiz, M. C., and Castilla, J. C. (2014). Síntesis del estado del conocimiento sobre la riqueza de especies de macroalgas, macroinvertebrados y peces en aguas costeras y oceánicas de isla de pascua e isla salas y gómez. Lat. Am. J. Aquat. Res. 42, 760–802. doi: 10.3856/vol42-issue4-fulltext-7
Fox, J., and Weisberg, S. (2018). An {R} Companion to Applied Regression, 3rd Edn. Thousand Oaks CA: Sage Publishing. Available online at: https://socialsciences.mcmaster.ca/jfox/Books/Companion/
Friedlander, A. M., Goodell, W., Giddens, J., Easton, E. E., and Wagner, D. (2021). Deep-sea biodiversity at the extremes of the salas y gómez and nazca ridges with implications for conservation. PLoS One 16:e0253213. doi: 10.1371/journal.pone.0253213
Genin, A., Dayton, P. K., Lonsdale, P. F., and Spiess, F. N. (1986). Corals on seamount peaks provide evidence of current acceleration over deep-sea topography. Nature 322, 59–61. doi: 10.1038/322059a0
Georgian, S. E., Morgan, L., and Wagner, D. (2021). The modeled distribution of corals and sponges surrounding the salas y gómez and nazca ridges with implications for high seas conservation. PeerJ 9:e11972. doi: 10.7717/peerj.11972
Gorny, M., Easton, E. E., and Sellanes, J. (2018). First record of black corals (Antipatharia) in shallow coastal waters of northern Chile by means of underwater video. Lat. Am. J. Aquat. Res. 46, 457–460. doi: 10.3856/vol46-issue2-fulltext-20
Guindon, S., Dufayard, J. F., Lefort, V., Anisimova, M., Hordijk, W., and Gascuel, O. (2010). New algorithms and methods to estimate maximum-likelihood phylogenies: assessing the performance of PhyML 3.0. Syst. Biol. 59, 307–321. doi: 10.1093/sysbio/syq010
Haase, K. M., Stoffers, P., and Garbe-Schönberg, C. D. (1997). The petrogenetic evolution of lavas from Easter Island and neighbouring seamounts, near-ridge hotspot volcanoes in the SE Pacific. J. Petrol. 38, 785–813. doi: 10.1093/petrology/38.6.785
Harris, P. T., Macmillan-Lawler, M., Rupp, J., and Baker, E. K. (2014). Geomorphology of the oceans. Mar. Geol. 352, 4–24. doi: 10.1016/j.margeo.2014.01.011
Häussermann, V., and Försterra, G. (2007). Large assemblages of cold-water corals in Chile: a summary of recent findings and potential impacts. Bull. Mar. Sci. 81, 195–207.
Hope, R. M. (2013). Rmisc: Ryan Miscellaneous. R Package Version 1.5. Available online at: https://cran.r-project.org/web/packages/Rmisc/index.html (accessed October 15, 2021).
Horowitz, J., Opresko, D. M., and Bridge, T. C. L. (2018). Black corals (Anthozoa: Antipatharia) from the deep (916 m–2542 m) Coral Sea, north-eastern Australia. Zootaxa 4472, 307–326. doi: 10.11646/zootaxa.4472.2.5
Hubbard, D. K., and Garcia, M. (2003). “The corals and coral reefs of easter island — a preliminary look,” in Easter Island, eds J. Loret and J. T. Tanacredi (Boston, MA: Springer), 53–77. doi: 10.1007/978-1-4615-0183-1_5
Katoh, K., Misawa, K., Kuma, K. I., and Miyata, T. (2002). MAFFT: A novel method for rapid multiple sequence alignment based on fast Fourier transform. Nucleic Acids Res. 30, 3059–3066. doi: 10.1093/nar/gkf436
Katoh, K., and Standley, D. M. (2013). MAFFT multiple sequence alignment software version 7: improvements in performance and usability. Mol. Biol. Evol. 30, 772–780. doi: 10.1093/molbev/mst010
Lapian, H. F. N., Barucca, M., Bavestrello, G., Biscotti, M. A., Bo, M., Canapa, A., et al. (2007). A systematic study of some black corals species (Antipatharia, Hexacorallia) based on rDNA internal transcribed spacers sequences. Mar. Biol. 151, 785–792. doi: 10.1007/s00227-006-0525-8
Mah, C. L. (2021). The East Pacific/South Pacific boundary: new taxa and occurrences from Rapa Nui (Easter Island), New Caledonia and adjacent regions. Zootaxa 4980, 401–450. doi: 10.11646/zootaxa.4980.3.1
Marin, I. N. (2007). The coral-associated shrimp genus Pontonides (Caridea, Palaemonidae, Pontoniinae) in Nhatrang Bay, Vietnam, with description of two new species. Zootaxa 1635, 1–21. doi: 10.11646/zootaxa.1635.1.1
Mecho, A., Easton, E. E., Sellanes, J., Gorny, M., and Mah, C. (2019). Unexplored diversity of the mesophotic echinoderm fauna of the Easter Island ecoregion. Mar. Biol. 166:91. doi: 10.1007/s00227-019-3537-x
Molodtsova, T. N. (2005). A new species of Saropathes (Cnidaria, Anthozoa, Antipatharia) from the Norfolk Ridge (south- west Pacific, New Caledonia). Zoosystema 27, 699–70718.
Molodtsova, T. N., and Opresko, D. M. (2017). Black corals (Anthozoa: Antipatharia) of the Clarion-Clipperton Fracture Zone. Mar. Biodivers. 47, 349–365. doi: 10.1007/s12526-017-0659-6
Molodtsova, T. N., and Opresko, D. M. (2021). World List of Antipatharia. Accessed through: World Register of Marine Species. Availble online at: www.marinespecies.org/ (accessed October 15, 2021).
National Geographic and Oceana (2013). Islas Desventuradas. Biodiversidad Marina y Propuesta de Conservación. Washington, DC: National Geographic, and Oceana.
NCEI. (2021). NOAA National Centers For Environmental Information- Bathymetric Data Viewer. Available online at: https://maps.ngdc.noaa.gov/viewers/bathymetry/?layers=dem (accessed January 13, 2021).
Opresko, D. (2006). Revision of the Antipatharia (Cnidaria: Anthozoa). Part V. establishment of a new family, Stylopathidae. Zool. Meded. 80, 109–138.
Opresko, D. M. (2009). A new name for the hawaiian antipatharian coral formerly known as Antipathes dichotoma (Cnidaria: Anthozoa: Antipatharia). Pacific Sci. 63, 277–291. doi: 10.2984/049.063.0209
Paredes, F., Flores, D., Figueroa, A., Gaymer, C. F., and Aburto, J. A. (2019). Science, capacity building and conservation knowledge: the empowerment of the local community for marine conservation in Rapa Nui. Aquat. Conserv. Mar. Freshw. Ecosyst. 29, 130–137. doi: 10.1002/aqc.3114
Pasternak, F. A. (1977). Antipatharia. Scientific results of the Danish Deep-Sea Expedition Round the World 1950-1952. Galathea Report. Copenhagen: Seandinavian Science, 57–164.
Pax, F. (1918). Die Antipatharien. Zool. Jahrbuücher. Abteilung für Anat. und Ontog. der Thiere 41, 419–479.
R Core Team (2021). R: A Language and Environment for Statistical Computing. Version 4.0.5. R Foundation for Statistical Computing. Vienna: R Core Team.
Shepherd, B., Pinheiro, H. T., Phelps, T. A. Y., Easton, E. E., Pérez-Matus, A., and Rocha, L. A. (2020). A new species of Chromis (Teleostei: Pomacentridae) from mesophotic coral ecosystems of rapa nui (Easter Island) and Salas y Gómez, Chile. Copeia 108, 326–332. doi: 10.1643/CI-19-294
Spalding, M. D., Fox, H. E., Allen, G. R., Davidson, N., Ferdaña, Z. A., Finlayson, M., et al. (2007). Marine ecoregions of the world: a bioregionalization of coastal and shelf areas. Bioscience 57, 573–583. doi: 10.1641/B570707
Tapia-Guerra, J. M., Mecho, A., Easton, E. E., Gallardo, M. D. L. A., and Sellanes, J. (2021). First characterization of deep benthic habitats and communities of oceanic islands and seamounts of the Nazca-Desventuradas Marine Park, Chile. Sci. Rep. 11:6209. doi: 10.1038/s41598-021-85516-8
Tazioli, S., Bo, M., Boyer, M., Rotinsulu, H., and Bavestrello, G. (2007). Ecological observations of some common antipatharian corals in the marine park of Bunaken (North Sulawesi, Indonesia). Zool. Stud. 46, 227–241.
Terrana, L., and Eeckhaut, I. (2019). Sexual reproduction of the shallow-water black coral Cirrhipathes anguina (Dana, 1846) from Madagascar. Mar. Biol. Res. 15, 410–423. doi: 10.1080/17451000.2019.1662444
Terrana, L., Flot, J. F., and Eeckhaut, I. (2021). ITS1 variation among Stichopathes cf. maldivensis (Hexacorallia: Antipatharia) whip black corals unveils conspecificity and population connectivity at local and global scales across the Indo-Pacific. Coral Reefs 40, 521–533. doi: 10.1007/s00338-020-02049-8
Van Pesch, A. J. (1914). The Antipatharians of the Siboga Expedition. Siboga-Expeditie, 17. Leyden, IL: E.J. Brill.
VideoLan. (2006). VLC Media Player - Open Source Multimedia Framework and Player. Available online at: http://www.videolan.org/vlc/ (accessed October 15, 2021).
Wagner, D. (2015). A taxonomic survey of the shallow-water (<150 m) black corals (Cnidaria: Antipatharia) of the Hawaiian Islands. Front. Mar. Sci. 2:24. doi: 10.3389/fmars.2015.00024
Wagner, D., Brugler, M. R., Opresko, D. M., France, S. C., Montgomery, A. D., and Toonen, R. J. (2010). Using morphometrics, in situ observations and genetic characters to distinguish among commercially valuable Hawaiian black coral species; a redescription of Antipathes grandis Verrill, 1928 (Antipatharia: Antipathidae). Invertebr. Syst. 24, 271–290. doi: 10.1071/IS10004
Wagner, D., Luck, D. G., and Toonen, R. J. (2012). The Biology and Ecology of Black Corals (Cnidaria: Anthozoa: Hexacorallia: Antipatharia), 1st Edn. Amsterdam: Elsevier Ltd.
Wagner, D., van der Meer, L., Gorny, M., Sellanes, J., Gaymer, C. F., Soto, E. H., et al. (2021). The Salas y Gómez and Nazca ridges: a review of the importance, opportunities and challenges for protecting a global diversity hotspot on the high seas. Mar. Policy 126:104377. doi: 10.1016/j.marpol.2020.104377
Yesson, C., Bedford, F., Rogers, A. D., and Taylor, M. L. (2017). The global distribution of deep-water Antipatharia habitat. Deep. Res. 2 Top. Stud. Oceanogr. 145, 79–86. doi: 10.1016/j.dsr2.2015.12.004
Yesson, C., Clark, M. R., Taylor, M. L., and Rogers, A. D. (2011). The global distribution of seamounts based on 30 arc seconds bathymetry data. Deep. Res. 1 Oceanogr. Res. Pap. 58, 442–453. doi: 10.1016/j.dsr.2011.02.004
Keywords: Antipathidae, Stichopathes, mesophotic coral ecosystems, ITS1, marine protected area, biodiversity
Citation: Tapia-Guerra JM, Asorey CM, Easton EE, Wagner D, Gorny M and Sellanes J (2021) First Ecological Characterization of Whip Black Coral Assemblages (Hexacorallia: Antipatharia) in the Easter Island Ecoregion, Southeastern Pacific. Front. Mar. Sci. 8:755898. doi: 10.3389/fmars.2021.755898
Received: 09 August 2021; Accepted: 19 October 2021;
Published: 05 November 2021.
Edited by:
Elva G. Escobar-Briones, National Autonomous University of Mexico, MexicoReviewed by:
Anna Maria Addamo, European Commission, Joint Research Centre (JRC), ItalyHanzhi Lin, Science Systems and Applications, Inc., United States
Copyright © 2021 Tapia-Guerra, Asorey, Easton, Wagner, Gorny and Sellanes. This is an open-access article distributed under the terms of the Creative Commons Attribution License (CC BY). The use, distribution or reproduction in other forums is permitted, provided the original author(s) and the copyright owner(s) are credited and that the original publication in this journal is cited, in accordance with accepted academic practice. No use, distribution or reproduction is permitted which does not comply with these terms.
*Correspondence: Erin E. Easton, erin.easton@utrgv.edu