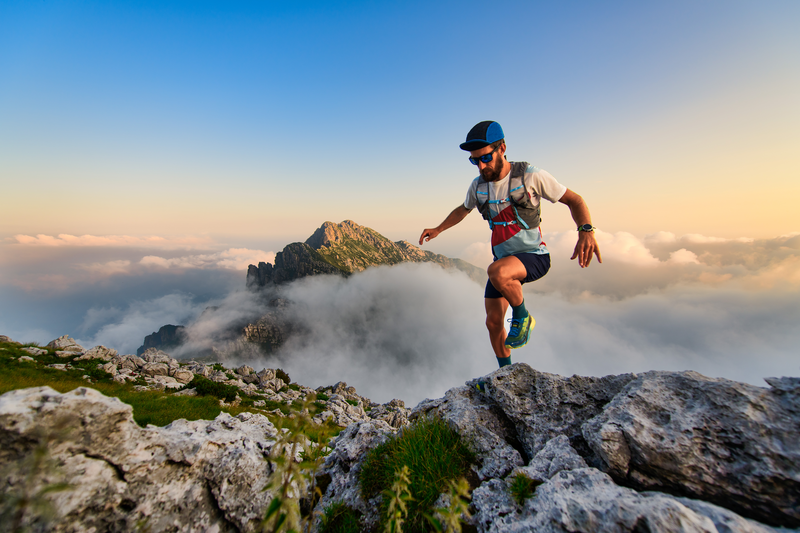
95% of researchers rate our articles as excellent or good
Learn more about the work of our research integrity team to safeguard the quality of each article we publish.
Find out more
ORIGINAL RESEARCH article
Front. Mar. Sci. , 10 November 2021
Sec. Marine Biology
Volume 8 - 2021 | https://doi.org/10.3389/fmars.2021.755275
South African coelacanths, Latimeria chalumnae, were intensively studied using submersibles in the Comoros Islands before recent progress in deep-diving techniques led to the discovery of coelacanths living at shallower depths off Sodwana Bay, South Africa, which were then studied by divers in close encounters or from underwater vehicles. However, all previous observations were made under intense human influence, so the “natural” behaviors of coelacanths have never been observed. Here we sought to record the natural behaviors of coelacanths by minimizing anthropogenic influences using trimix-gas diving and remote recording techniques. We thus set fixed camera and current/temperature recorders at 98 and 113 m to monitor the behaviors of fishes and oceanographic parameters over a 6-day period. Time-lapse video observations succeeded to record one coelacanth that entered the cave during the coldest-water period, two sand tiger sharks, and many other smaller fishes entering a cave. An extensive analysis of the footage led to an interesting discovery that the first dorsal fin angle of the coelacanth corelated with the presence-or-absence of a large shark that frequently passed through the cave. When no shark was present, the coelacanth’s dorsal fin was folded ∼3/4 of the time, but when the shark entered the cave, its dorsal fin was unfolded > 95% of the time, while no such reactions were observed with other observed fishes. The erected coelacanth first dorsal fin posture is an important part of the iconic symbol of these ancient fish, but it may be reflecting stressful situations such as responses to potential predators. Our observation clearly showed that the dorsal fin is in a folded position during a steady relaxed state of the fish and it can be unfolded in response to external stimuli. Use of non-biased observation systems is strongly recommended when observing the natural behavior of coelacanths.
Coelacanths were thought to be extinct for 65 million years after the end of the Cretaceous, until the first discovery of an extant Latimeria coelacanth was made in 1938 when one was caught by a fisherman off East London and brought to the attention of the South African ichthyologist Dr. J.L.B. Smith who named it Latimeria chalumnae (Smith, 1939). They were then found to be living in the Comoros Islands, and a few were found in by-catches of commercial fisheries off eastern Africa in Tanzania and Kenya and along southwest Madagascar (Figure 1). The first encounter with a live African coelacanth was made by Millot (1954) who observed a dying individual exhibiting feeble movements, but many live individuals were later observed from submersibles. The discovery of these ancient fish generated interest around the world that created a demand for specimens for museums, scientists, or private collectors, which then became a conservation concern for the species (Bruton and Stobbs, 1991).
Figure 1. Bathymetric map of the western Indian Ocean region showing the areas where living coelacanths, Latimeria chalumnae, have been observed by divers or underwater vehicles or caught by fishermen (red circles), the East London area where the species was first discovered in 1938 (yellow square), the most recent new area where divers observed a coelacanth (shown in Supplementary Figure 2) off the South Coast of KwaZulu-Natal 2019 (yellow star), and the inset shows a 3-D bathymetric depiction of the system of canyons that are located off the coast of Sodwana Bay in the northern area of iSimangaliso Wetland National Park in eastern South Africa. The present study was conducted June–July 2018 at the upper end of the southern branch of Jesser Canyon in the inset (blue square), which was redrawn from Ramsay and Miller (2006). The bathymetric map of the western Indian Ocean region was obtained from the NOAA National Centers for Environmental Information [NCEI; ETOPO1 Global Relief | NCEI (noaa.gov)], with color showing depth (dark blue showing deep and light blue shallow-depths). The areas where coelacanths have been found all have a narrow zone of continental shelf and steep slopes to deep depths.
In the late 1980’s, live coelacanths were filmed from a submersible in the Comoros Islands and the images of swimming coelacanths were a remarkable discovery (Fricke et al., 1987). The coelacanths living in the Comoros Islands were studied using submersibles and remote operated vehicles (ROV) between 1986 and 2009, which enabled knowledge to be acquired about various aspects of the population of about 300–400 individuals (distinguished by spot patterns) who reside in caves mostly between 150 and 250 m during the day, but they dive as deep as 600 m for feeding at night (Fricke et al., 1991, 2011; Fricke and Hissmann, 2000). More than a decade after the research began in the Comoros Islands, a few living coelacanths were seen in Jesser Canyon in Sodwana Bay, South Africa in October–November 2000 at 104–108 m in their natural environment, by Venter et al. (2000) who used specialized Trimix gas deep-diving scuba equipment. The encounter marked the first direct contact between wild coelacanths and humans. After making the initial discovery, Venter et al. (2000) made more dives that increased the number of individuals observed in various areas of Jesser Canyon.
The next stage of exploration of the coelacanths in the canyons off Sodwana Bay was when the submersible Yago made 47 dives during 2002, 2003, and 2004 and observed 21 different individual coelacanths at 16 different locations at depths of 96–144 m (Hissmann et al., 2006). The submersible survey showed the presence of multiple individuals in several submarine canyons off Sodwana Bay (Supplementary Figure 1). They were also found to be moving between Jesser and Wright canyons (Hissmann et al., 2006; Roberts et al., 2006). Subsequent diving surveys by Décamps et al. (2017) were also conducted during 2010 and 2013 to study the kinematics of fin movements in coelacanths off Sodwana Bay. An ROV survey of Jesser Canyon and other areas to the north and south was also conducted in April–May 2013 (Sink, 2013; Geldenhuys, 2015). Up to 2019, 32 individuals were recognized from the canyons in this area using the unique patterns of white body spots on the sides of their bodies (Cooke et al., 2021), and these coelacanths form a genetically different population than the Comoros and Tanzania populations (Nikaido et al., 2011; Lampert et al., 2012). Recently, an encounter with a coelacanth at a shallower (67.3 m) rocky reef was reported along the South Coast of KwaZulu-Natal, about half way between East London and Sodwana Bay (Figure 1 and Supplementary Figure 2; Fraser et al., 2020). Most recently, various aspects about the coelacanth population off southwest Madagascar were overviewed by Cooke et al. (2021).
While surveys and other types of research were ongoing to study the western Indian Ocean species, L. chalumnae, the second extant species of coelacanth, the Indonesian coelacanth, Latimeria menadoensis, was caught in 1997 near Manado of northern Sulawesi Island, Indonesia (Erdmann et al., 1998). Erdmann et al. (1999) photographed the swimming posture of a L. menadoensis that was caught as by-catch of local fisherman, but was still alive. The Indonesian coelacanth was genetically determined to be a separate species with at least 2 genetically separate populations (Holder et al., 1999; Fricke et al., 2000; Kadarusman et al., 2020), and the Indonesian and East African species appear to have diverged about 30–40 million years ago (Inoue et al., 2005). ROV and submersible surveys were conducted to learn about the newly discovered Indonesian species, which seemed generally similar in its behavior and habitat use characteristics (Iwata et al., 2019a) compared to the Indian Ocean species.
What all the types of observations of the two coelacanth species had in common was that they were made using invasive techniques that include the influences of human factors such as lights (Décamps et al., 2017), noise caused by submersibles, or the presence of large ROVs, which prevented the natural behaviors of coelacanths from being recorded. Another thing the observations had in common, was that almost all of the images of coelacanths obtained from research surveys and other sources showed the fish with their first dorsal fins fully unfolded (e.g., Supplementary Figures 1, 2). The distinctly erect, unfolded dorsal fin of coelacanths has become an iconic symbol of these ancient “living fossils,” as they have been referred to, but the behavioral significance of the erect dorsal fin has received little attention.
The objectives of our study were to test the hypothesis that previously recorded coelacanth behavior in their resting caves is strongly influenced by human observers, by using a new method of fixed camera deployments, and to determine if their use of the caves at the upper extent of their vertical distribution of habitat-use is influenced by oceanographic conditions such as water temperature and current velocity. Additionally, we aimed to learn about the natural interactions between coelacanths and the other fish species that live in and around the caves used by coelacanths.
Here we report the results of a first observation of a live coelacanth that was made in the absence of the bias of divers or underwater vehicles that includes ecologically relevant video imagery that was recorded using a fixed underwater camera placed within the natural habitats of L. chalumnae in the southern branch of upper Jesser Canyon off Sodwana Bay (Figure 1). The coelacanth that was recorded was a known individual (Supplementary Figure 3), so it was observed within its normal home range. Our study focuses on the unique observation that this coelacanth was resting during daytime within its normal home range environment and it frequently had its first dorsal fin in a folded position. But when a shark entered the cave, its dorsal fin became unfolded. An analysis of published or other video recordings found that those images predominantly show coelacanths with unfolded dorsal fins, which seems to indicate that the famous shape of the coelacanth in science, popular culture, and art with an erect unfolded dorsal fin (Fricke, 1997) is actually a behavioral response to potentially threatening external stimuli. We also conducted a survey of the fishes living around the coelacanth habitats and recorded data to understand the oceanographic conditions of the habitat to contribute to understanding the ecology of coelacanths in order to obtain information related to their conservation and environmental protection.
The survey was conducted near the iSimangaliso Wetland National Park (32°40’44″25E, 27°32’23″S) in South Africa, at a location 4 km off the coast of Sodwana Bay. The dive site was located at the southern side of the upper end of the submarine valley referred to as Jesser Canyon at depths of about 95–130 m deep (Figure 1) in the area where this population of coelacanths was first discovered and then studied (Venter et al., 2000; Hissmann et al., 2006). The various aspects of the survey were carried out between 23 June and 11 July 2018. Jesser Canyon is the southernmost of a series of canyons in that area (Ramsay and Miller, 2006; Sink et al., 2006) as shown in the inset of Figure 1. The canyons have been surveyed for coelacanths (Hissmann et al., 2006; Sink, 2013), other fishes and biodiversity (Heemstra et al., 2006a; Sink et al., 2006; Geldenhuys, 2015), or for their geological structure (Ramsay and Miller, 2006) and oceanography (Roberts et al., 2006). Jesser Canyon has octocorals and black corals as the dominant invertebrates, and gorgonians, antipatharian black corals, bryozoans and various types of sponges are also present (Sink et al., 2006).
Our study was designed around using highly technical deep-diving SCUBA techniques (Figures 2B,C) that allowed us to reach the upper depth ranges used by coelacanths in the area. All divers used trimix gas mixtures (Lang and Smith, 2006; Coenen and Zivkovic, 2015), which have been used in other scientific surveys (e.g., Wagner et al., 2013). Trimix gas was prepared that allowed the divers to reach a maximum water depth of 140 m, and the gases that adapt to each water depth were used. Two researchers used rebreather systems (Pollock et al., 2016) with no exhaust (Figures 2B,C) in consideration of the influence of noise on coelacanths. The rebreathers used were Sentinel (VMS, Vobster Marine Systems, United Kingdom) and Inspiration (AP Diving, United Kingdom) systems. Support divers used the open circuit SCUBA method. Two Zodiac (6.4 m) boats were used for transportation from the beach to the site.
Figure 2. Photographs of the cave overhang area (A) where current meters and temperature recorders (B) were set at 98 and 133 m, and camera systems (C) were placed in front and to the side (also see Supplementary Figures 4, 5A,B) of the opening of the cavern. The cameras and meters were set on the bottom by divers using a tri-mix gas system (B,C). The camera in (C) is shown with its light on, but for the observation period that camera was set to have its light off. North is approximately in the right direction in (A).
Several test dives and initial dive surveys were made to observe the fish fauna of the area, which were conducted by two researchers (each day 23–27 June 2018), two assistant divers, and six divers who supported decompression at the time of surfacing. Dives continued to be conducted to check for coelacanths, observe the fish fauna, and deploy and retrieve set-cameras and the oceanographic meters (1, 4, 6, 7, 9 10, 11 July). These observations were made to search for coelacanths and document the other fish species that use the same habitats as the coelacanths. The fish fauna around the upper part of Jesser Canyon was recorded by the divers using cameras and the observed species were compared with the list of fish species recorded by Heemstra et al. (2006a); but also see Geldenhuys (2015) who examined the fishes from a wider set of areas in the region. Some cameras were set in preliminary locations (1–4 July 2018), but their recordings are not used in the present study. We mainly focus on reporting additional species that we identified that were not listed by Heemstra et al. (2006a), and some species seen in the u-shaped cave that was occupied by the coelacanth near the end of the study are also mentioned.
The next stage of the survey was to set a fixed camera in a u-shaped cave and to deploy oceanographic measurement devices near the cave (Figure 2 and Supplementary Figures 4, 5). The study cave (or large cavity under a rock overhang) and oceanographic conditions observation period was set to be 4–12 July 2018, but it was stopped short by 1 day due to the occurrence of rough weather. The video recording was performed in time-lapse mode to conserve battery power and disc storage space at an interval of 5 s using two SonyX 3000 video cameras (Sony Corp., Japan) equipped with water-proof pressure-resistant housings (Aqua Pazza, Nagoya, Japan), that were set at the water depth of 113 m near the entrance of the cave. Depth was estimated from a dive computer during the setting of the equipment at 13:00 on 4 July, and no adjustment was made for the effect of tide. An RG-Blue System 02 LED light (AOI RGBlue, Tokyo, Japan), with an illuminance of about 17 lx was used when the camera set on the south side of the cave was recording (Supplementary Figures 5A,B), but only natural light was used for the camera in front of the cave. The battery of the side-view camera with a light died before 11 July, so the coelacanth was only recorded by the front camera.
To record the current direction, velocity, and water temperature, oceanographic recording meters (JFE Advantec, Nishinomiya, Japan) were set at 98 m on the shelf above the cave and at 113 m in front of the cave along the drop-off of the submarine canyon (Figure 2). During the oceanographic and cave observation period from 13:00 on 4 July to 13:00 on 11 July, current velocity, current direction, and water temperature were recorded by each meter every 15 s.
From the obtained images of the coelacanth, the state of spreading and folding of the first dorsal fin (DF) were analyzed to calculate the amount of time the DF was folded or spread. The angle of the DF was determined as the angle formed by a line from the base of the first dorsal spine to the tip of the spine and another line from the base to the tip of the last dorsal spine (Figure 3). Judgment criteria for the spread and folded states of the DF were that spread was more than half to a fully spread state (Figure 3C), and folded was less than half of the fully folded state (Figure 3A). In addition, when the consecutive images showed a transition from the state half spread to fully spread, the time between images was considered to be a spread state, and conversely the state from folded to half folded was considered to be folded (Figure 3). Based on the above, the amount of time the DF was spread or folded was defined as one operation from the folded state to the spread state, or from the spread state to the folded state.
Figure 3. Images of dorsal fin angle of the coelacanth, Latimeria chalumnae, that was recorded in a cave at the upper end of Jesser Canyon off of Sodwana Bay on 11 July 2018 showing the dorsal fin folded when there was no shark present (A), partly unfolded (B), and fully unfolded when a shark was present (C).
A coelacanth entered the cave on the morning of 11 July 2018 and could subsequently be clearly identified as being the frequently seen individual known as Individual no. 14 that was named “Noah” (Supplementary Figure 3). It had been previously observed 4 times in April 2003 during the submersible survey of Hissmann et al. (2006) in both Jesser and Wright canyons that are separated by about 4 km. The emergence of the coelacanth swimming into the cave in Jesser Canyon was recorded at 08:44 by the camera placed near the cave entrance and the fish left the cave at 09:13. Total appearance duration of the coelacanth was 29 min 20 s (1760 s) (Table 1).
Table 1. The amount of time that the first dorsal fin (DF) of a coelacanth, Latimeria chalumnae, was in the folded or spread states during the observations made in the cave at the top of Jesser Canyon in relation to presence or absence of a shark.
The behavior of the coelacanth was captured clearly because it moved directly in front of the camera and remained there for the duration of the observation as seen in Supplementary Video. It entered the cave from the front-left side of the camera and then moved to a location in front of the inner wall under the rock overhang, but it did not go deeper into the cave. The coelacanth remained in the same area for about 5 min before a large sand tiger shark, Carcharias taurus (see Supplementary Materials), was seen along the southern edge of the cave. Without a frame of reference to use, the size of the coelacanth and shark was difficult to estimate; but the shark appeared to be about 2 m or less in length, and the coelacanth was substantially smaller than the shark. That species is a common littoral shark found from the surf zone and shallow bays to at least 191 m along the outer continental shelf (2.3 m maximum size1; accessed 2 May 2021).
The shark first came close to the coelacanth after moving from the deeper part of the cave on the north side (Figure 4A) and then swam back around and entered the cave from the south side and passed by the coelacanth (Figure 4B) as shown in more detail in Supplementary Figure 6 and the Supplementary Video. The shark was present in the cave for all but 11.5 min of the time the coelacanth was present, and the shark swam past the coelacanth in front, behind and to the side about 25 times at various distances away. The general types of swimming routes used by the shark that appeared to be patrolling the cave are shown in Figure 5. It came quite close a few times, and in a few cases, it may have caused the coelacanth to calmly change its position. Because of the time-lapse nature of the recordings, it is unclear if direct encroachment of the shark actually caused the coelacanth to leave the cave or not at the end of their time together in the cave (09:13). The shark remained in or around the cave until 09:47 and then returned at 11:08, and was last seen in the cave at 11:27. A second shark briefly entered the cave at the same time as the other shark for about 1 min at 09:00 (Supplementary Figures 7A,B), but a dark marking on the right side of the body near the second dorsal fin of the shark that was frequently seen (this species has individual markings; Bansemer and Bennett, 2008), indicated that it was probably just the one shark that frequently moved through the cave.
Figure 4. Photographs of the coelacanth in the cave with its first dorsal fin unfolded (yellow arrow) when a shark (white arrow) was present and likely visible to the coelacanth (A,B).
Figure 5. Estimated examples of the paths of the swimming tracks of the shark that repeatedly swam through the cave while the coelacanth was present that are shown superimposed over a photograph looking down at the rock overhang that forms the cave, with the approximate extent of the cave also shown.
For the entire 1760 s observation period of the coelacanth, the DF was spread 63.6% and was folded 31.3% of the time, with 5.1% of the time being uncertain (Table 1). The largest unfolded angle obtained was 60° (Figure 3C), and the smallest folded state was 6° (Figure 3A). With its DF folded, the appearance of the coelacanth looks quite different than the classic image of those iconic ancient fishes (Figure 6). There was a clear difference in the DF posture with the shark present (DF spread 95.5% of time) and absent (DF folded 74.4% of time). The video recording suggested that the posture of the DF changed frequently as the shark approached.
Figure 6. Photographs of the coelacanth in the cave with its first dorsal fin folded when there were no sharks or other predatory fishes nearby, which appear to represent the resting state of the fish (A,B).
The observations from 4 to 11 July 2018 (192 h and 43 min) at 98 and 113 m depths resulted in 40,498 data records for each recorded parameter of water velocity, current direction, and water temperature. The average combined current direction at 98 and 113 m depths was 208.7° (∼SSW), and the average (± S.D.) current direction at 98 m was 153.9 ± 100.6° and that at 113 m was 208.7 ± 126.5°. This indicates there was generally southward flow, but there was some flow from the shelf above the cave and some into the cave from deeper water. The large S.D. values indicated frequent fluctuations in the current direction occurred.
The average water velocities above the cave (0.32 ± 0.39 km/h; or 8.9 cm/s was higher than at 113 m (0.11 ± 0.09 km/h; 3.0 cm/s), with many fluctuations (Figure 7B). The current velocity was usually relatively slow, but frequently increased briefly and sometimes spiked to very high values at 98 m, particularly in the first 4 days. The current velocity at 98 m ranged from 0.001 km/h to 2.38 km/h (1.29 kt.; 66.1 cm/s) and from 0.0 km/h to 2.29 km/h (1.24 kt.; 63.6 cm/s) at 113 m.
Figure 7. Daily fluctuations of water temperature (A) and current speed (B) that were recorded by the temperature and current meters at a location just above the cave (98 m) at the upper end of Jesser Canyon and to the side of the cave at 113 m during early July 2018.
The water temperatures also continuously fluctuated across about a 2–5°C range, with the minimum temperatures at both depths increasing from 18°C to 20–21°C after 2–3 days, and then both minimum and maximum temperatures decreased until the end of observations (Figure 7A). The maximum water temperature at 98 m was 23.5°C, the minimum value was 16.8°C, and the average was 20.8 ± 1.7°C. The maximum value at 113 m was 23.1°C, the minimum was 16.6°C, and the average was 19.7 ± 1.5°C. As can be seen in Figure 7A, the daily fluctuations appeared to be reflecting the tidal rhythm.
During the coelacanth appearance at the cave on the morning of 11 July, the average current direction at both depths was 154.6 ± 65.1°. The average current velocity while Noah was present was 0.16 ± 0.11 km/h (4.4 cm/s), which was about half of the mean for the 7 days of observations. At 113 m, the average current direction was 117.8 ± 62.8°, and the values varied slightly eastward from the mean relative to the 7 days of observations. The average current velocity of 0.11 ± 0.05 km/h and water temperature of 18.7 ± 0.1°C while the coelacanth was present were almost the lowest values throughout the observation period. The new moon (13 July) was only 2 days after the coelacanth was observed.
The divers observed a variety of fish species in the upper south-side of the Jesser Canyon study area. Our survey was not intended to be an extensive survey of the fish fauna due to the limitations on the amount of time that can be spent at the depths where coelacanths live and the limitations of making clear identifications from photographs or videos. However, we compared our observed species to those of Heemstra et al. (2006a) and also the less extensive list of Sink et al. (2006), and we may have observed more than 10 previously unreported species or taxa found below 60 m around the canyon (Supplementary Materials).
The diversity of fishes present at depths in and around the cave where the coelacanth was seen, included mobile species, small schooling species, and those that use the caves for resting or possibly feeding areas. Some of these fishes were recorded by the set cameras and this showed the species composition and behaviors of the fishes that use the same caves as the coelacanths (Figures 8, 9). A recently described species of fusiler, Caesio xanthalytos (Caesionidae) (Holleman et al., 2013) appears to be the type of small schooling fishes that frequently appeared in front of the coelacanth (Figures 8A,D and Supplementary Video), which was not reported previously. When the coelacanth was in the cave, in addition to sharks, a grouper and schools of a small apogonid cardinal fish species and the fusilers were present, and fish frequently resided near the south side of the cave (Supplementary Figure 7). The variety of species present and the small fishes appearing in front of the coelacanth at times, can also be seen in the Supplementary Video, but the coelacanth did not appear to interact with any of these fishes, and they did not seem to react to its presence in the cave.
Figure 8. Photographs of fishes that were in the cave when no coelacanth was present, showing what appears to be a species of jobfish (Aphareus sp., snappers, Lutjanidae) near the ceiling (A) above a school of fusilers, Caesio xanthalytos (Caesionidae) that have a yellowish lateral stripe that are shown in (D), 3 yellowtail blue snappers, Paracaesio xanthura, near the ceiling with a grouper farther back in the cave, and a species of hogfish with a black tail (Bodianus sp.; Labridae) in front looking at a hermit crab in a shell on the bottom (B), many small cardinal fish (possibly Ostorhinchus neotes) with a grouper near the ceiling (C), a santer seabream, Cheimerius nufar (Sparidae) (E), what appears to be a species of sandperch, Parapercis (Pinguipedidae) that was curious about the hermit crab (F), a P. xanthura (upper left) and 3 groupers (G), 2 shoulderbar soldierfish, Myripristis kuntee in the midwater of the cave (H), and a sparid cleaning its body by brushing against the bottom sand (I).
Figure 9. Photographs of the cave where the coelacanth was video recorded as seen when divers were approaching to retrieve the cameras and oceanographic meters showing a grouper, Epinephelus sp. hiding near the ceiling of the rock overhang and small red fish resting on the ceiling on the left side (A), groupers near the ceiling (B), and a close-up image of the small orange and red colored fishes that reside on the surface of the underside of the cave, along with various invertebrates attached to the cave ceiling (C).
The same types of fishes were present when the coelacanth was not in the cave, and various fish appeared and disappeared over time (Figure 8). Some species such as groupers (Figures 8B,C,G) or fishes residing deeper in the cave (Figure 8H) or living on the ceiling of the cave (Figure 9), may reside there for long periods of time. A hermit crab in its shell was observed moving on the bottom of the cave, making tracks in the sand, and it attracted the attention of 2 species of fish (Figures 8B,F). A sparid was observed brushing its body on the sand (Figure 8I), so the set camera in front of the cave recorded the interactions and natural behavioral ecology of fishes and invertebrates in the cave off Sodwana Bay in the absence of humans for the first time.
The 29 min that the coelacanth was in the cave mostly overlapped with the presence of a large sand tiger shark, Carcharias taurus, that continuously returned back to swim past the coelacanth. The coelacanth likely came to rest in the cave during the day, which is thought to be their typical daytime behavior (Fricke and Hissmann, 2000; Hissmann et al., 2006; Uken and Green, 2006; Fricke et al., 2011). Coelacanths are drift feeders that may feed at night in a vertical body position (Uyeno and Tsutsumi, 1991; Fricke and Hissmann, 2000), but they return to caves during the day. It does not appear that the coelacanth was very disturbed by the shark that passed by it about 25 times, because none of the movements of either fish appeared to be agonistic, but the changes in the coelacanth’s dorsal fin posture showed it was clearly aware of the presence or absence of the shark as discussed below.
The shark may have been foraging for other species, and frequently went in and out of the cave, while at least one other shark was briefly present in the cave. The shark swam through the location regardless of the presence coelacanth, but although it did not show any obvious aggressive behavior, there is a possibility that the shark might have ended up contributing to the coelacanth leaving the cave at the end of the video observation (Supplementary Video). However, the shark made no obvious type of agonistic display and its pectoral fins were horizontally flat and not depressed (Figure 4B), which is one type of agonistic display in some sharks (Martin, 2007).
Sand tiger sharks are present in several specific regions worldwide, and their behaviors have been studied in some locations (e.g., Haulsee et al., 2016; Coleman and Burge, 2021). They are present along the southern and eastern parts of Africa up to about 15°S, so they overlap with L. chalumnae; and they have a stable population where coelacanths are present off Sodwana Bay (Dicken et al., 2007; Klein et al., 2020). The sand tiger shark diet off eastern South Africa was found to include a wide range of species, and some prey sizes were a meter or more, but these sharks may be unlikely to feed on coelacanths (Smale, 2005). In addition, the gut contents of coelacanths suggest (Uyeno and Tsutsumi, 1991; Fricke and Hissmann, 2000) that these sharks may not be direct competitors with coelacanths for prey (Uyeno and Tsutsumi, 1991; Fricke and Hissmann, 2000). Coelacanths may detect prey using an electroreception sense (Berquist et al., 2015) or olfaction (Picone et al., 2014).
Although no direct behavioral interactions between sharks and coelacanths have been recorded in previous observations, a small cat shark, Cephaloscyllium sufflans, was found in a coelacanth stomach (Uyeno and Tsutsumi, 1991). Predation on coelacanths by larger predatory fishes was not observed during the 18-year observation period of Fricke et al. (2011) in the Comoros. Similarly, no interaction between sharks or other fishes and coelacanths, were reported, even after extensive submersible surveys in Sodwana Bay (Hissmann et al., 2006). Therefore, our observations of the interactions between a coelacanth and a large shark, appear to be unique.
The observations of Hissmann et al. (2006) suggested that coelacanths may be less affected by the presence of the submersible than some other species, and they were sometimes observed to have the DF in a folded position while showing no unusual breathing patterns; but when humans or underwater vehicles were present, coelacanths typically had their dorsal fin unfolded, which may be a form of “lateral display” with some motivational meaning (Hissmann et al., 2006). Although no further annotations of this behavior were provided in the previous studies, our observations made in the least stressful conditions compared to former studies strongly suggested that it is associated with natural defensive behaviors. We observed that the DF was transiently unfolded as a shark approached, but it became folded shortly after the shark left the cave. However, the presence of anthropogenic stress in the previous studies may have induced constant stress, causing the coelacanths to unfold their DF continuously.
Endo et al. (2012) studied the morphology of the first and second dorsal fins and concluded the first dorsal fin acts as a stabilizer and the second acts as a thruster during slow locomotion. But the present study suggests the first dorsal fin may also play an important role as a protection or defense posture. Dorsal fin spines appear to have evolved as an anti-predator mechanism, which along with overall body depth, affect the gape-limitations of some predators (Price et al., 2015). Therefore, by spreading their DF, which has 8 spinous fin rays, coelacanths would appear larger to a potential predator. Thus, the observation that the DF was unfolded 95.5% of the time with the shark present seems to indicate that the unfolded posture represents a stressed condition, and might be an anti-predator response that makes the coelacanth look larger or difficult to prey on. Unfolding the dorsal fin of ray-finned fishes has many functions however, such as in agonistic encounters or courtship (Garita-Alvarado et al., 2018), inviting cleaner fish, or in hydrodynamics (Lauder and Drucker, 2004), as illustrated by Figure 10. Except for those types of situations, usually the first dorsal or spinous dorsal is folded in ray-finned fishes. In other fishes such as sharks, their dorsal fin position is fixed, but the positions of their pectoral fins or body postures are used to express behavioral intentions (Martin, 2007). Interestingly, although their body forms have changed somewhat, the DF of coelacanths has not changed much at all across their 400-million-year history, while other animal lineages have greatly changed, including diverse fish species radiations and the occurrence of many types of fins in fishes (Casane and Laurenti, 2013; Cavin and Guinot, 2014; Larouche et al., 2017; Toriñoa et al., 2021).
Figure 10. Photographs of the dorsal fin (DF) positions of Pacific bluefin tuna, Thunnus orientalis, showing the DF unfolded (2 top fish) for hydrodynamics while turning, and folded in the lower fish (A), a bignose unicornfish, Naso vlamingii, unfolding its DF while be cleaned by a cleaner fish (B), a lined dartgoby, Ptereleotris grammica, unfolding its DF when frightened or threatened probably because of the camera flash (C) and then with its DF completely folded (F), the unfolded DF of 2 fighting sabretooth blenny, Petroscirtes xestus (D), a male pink basslet, Pseudanthias hypselosoma, unfolding its DF during courtship with a female (E), a pink-speckled shrimp goby, Cryptocentrus leptocephalus, with an unfold DF while wary of a three-striped whiptail, Pentaposus caines, whose DF is folded (G), an unfolded DF in a goby, Lubricogobius sp., whose own burrow has been occupied by a coconut octopus, Amphioctopus marginatus (H), and two different species of wrasses (blueside wrasse, Cirrhilabrus cyanopleura, upper; pink flasher, Paracheilinus carpentri, lower) that both have their DF unfolded while threatening each other (I).
In most of the photographs and videos so far reported, the first dorsal fin of coelacanths was in a fully spread state (Table 2), which suggests that the targeted individuals were exposed to stress from submersible and ROV noises, lights for filming, or the general presence of the underwater vehicles or divers. One example of very wide DF spreading (70°) is seen in the very close-up image of a juvenile coelacanth, Latimeria menadoensis (Supplementary Figure 8), that was video recorded by an ROV in a crevice in Indonesia (Iwata et al., 2019b). All of these observation tools seem to be acting as un-natural or unpleasant biases for the fish being observed. Although underwater vehicles are clearly useful tools for observations of coelacanths to detect their basic swimming style, habitat use, abundance, and the sizes of the fish (Fricke et al., 1987; Fricke and Hissmann, 2000; Hissmann et al., 2006), and for making many types of biological observations (Sward et al., 2019), it must be taken into consideration that any invasive observation method will affect the behavior of the fish being observed.
Table 2. The state of the angle of the first dorsal fins of coelacanths of Latimeria chalumnae (L. c.; East Africa region) and L. menadoensis (L. m.; Indonesia) in some selected reports or sources listing the number of angle measurements and the mean angles.
The coelacanth entered the area in front of the camera at 08:44 in the morning on 11 July, after not being seen there for the previous 6 days. Both types of oceanographic conditions had fluctuated greatly each day, and the water temperature had been decreasing for several days before the coelacanth entered the cave. The timing of entry into the cave about 2 h after sunrise (sunrise: 06:39), indicates that the coelacanth was active even when some light would be present at that depth, but its appearance at that time may still be part of its diurnal vertical movement from deeper depths at night. The lack of movement deeper into the cave, suggests the possibility that observations of coelacanths hiding in inner recesses of caves could be a result of them moving deeper into caves to avoid observers or underwater vehicles that were detected in advance by their low-light adapted eyes (Yokohama, 2000) or by their lateral line system (Hensel and Balon, 2001).
The oceanographic data recorded at 98 and 113 m near the cave where the coelacanth was observed showed that temperatures would reach 22–23°C and then drop down to 17–19°C each day, but by the last day of observations when the coelacanth was seen, the temperatures were only ranging between about 17–21°C (mostly 17–19°C). The daily fluctuations of water temperatures at both depths that are probably a result of upwelling of cooler water during the tidal cycle have been reported previously, but they only appear to occur between depths of 85–120 m (Roberts et al., 2006). Changes in the temperatures at relatively shallow depths might affect the upper depths occupied by coelacanths, because one was seen by divers at the 54 m shelf-edge on 15 February 2004 when the temperature was 17–19°C during an upwelling event, which is similar to the temperatures of 15–22°C typically observed at depths of 100–140 m (Roberts et al., 2006).
In fact, almost all of the 26 different submersible observations of coelacanths in Jesser Canyon and other areas off Sodwana Bay at depths of 96–144 m, recorded the fish at temperatures of 16–19°C (Hissmann et al., 2006). This appears to indicate that the water temperatures in the cave we observed were too high for coelacanths to be present for most of each day for the first 5 days. Coelacanths live deeper in the Comoros Islands (Fricke and Hissmann, 2000), probably because of the warmer waters in that lower latitude region; but Hissmann et al. (2000) found that acoustically tagged Comoros coelacanths spent 75% of their time at 15–19°C temperatures, so the 2 populations appear to have similar temperature preferences, despite utilizing different but overlapping depth ranges. This specific temperature range may be related to metabolism and oxygen requirement physiology, with their observed temperature ranges being optimum for oxygen uptake (Hissmann et al., 2000). The lunar cycle may also influence coelacanth activity-depth behaviors (the coelacanth appeared just before new moon in our study) and the highest-amplitude temperature changes occur during full and new moon periods (Hissmann et al., 2000).
Although the causal factors of the coelacanth occurrence in our study are unknown, a current direction change might have caused the decreasing water temperatures up until the coelacanth was seen. The current directions were frequently close to the direction of the southward flowing Agulhas Current and the velocities exceeded 33 cm/s (1.2 km/h) 8 times at 98 m during the study period and reached 50 cm/s (1.8 km/h) during each of the first 3 days; but velocities were low when the coelacanth appeared.
The strong peaks in current velocity may be related to the Agulhas Current coming closer to shore or eddies causing stronger currents. The Agulhas Current is a strong western boundary current (Imawaki et al., 2013) that forms just north of Sodwana Bay from a combination of water flowing westward past southern Madagascar and southward flowing eddies of the Mozambique Current (Roberts et al., 2006). Roberts et al. (2006) surveyed transects along the coast (including Jesser Canyon) and found strong current flows above 100 m that were up to 60–80 cm/s over the continental shelf. Cooling events in these areas may be related to the Agulhas Current being closer to shore causing upwelling of colder water (Roberts et al., 2006). These types of fluctuations may have important influences on the depth distributions and between-canyon lateral movements of the coelacanths of Sodwana Bay, and also regarding when they can be found at shallow enough depths to be reachable by trimix divers.
Our diving efforts and use of set cameras mostly in and around one cave off Sodwana Bay resulted in the observation of many fish species that are present in the upper habitats occupied by coelacanths. In comparison however, Heemstra et al. (2006a) used the observations made from 47 dives of the submersible Jago (Hissmann et al., 2006) or scuba divers and reported 54 fish species from canyons off Sodwana Bay at depths of 100–200 m. They estimated that due to the limitations of what could be seen during the submersible video recordings made while searching for coelacanths, including one dive with an expert ichthyologist (P.C. Heemstra), the 54 species recorded were probably less than a third of the total fish fauna. For example, an additional 94 species are known from depths of 100–359 m along the of northern KwaZulu-Natal coast (Smith and Heemstra, 1995). The 54 fish species seen within the habitats off Sodwana Bay included a wide range of taxa that were categorized as possible prey species for coelacanths or as potential predators (Heemstra et al., 2006a). A similar number of 52 species was recorded by an ROV survey in a wider set of areas (Geldenhuys, 2015), with only 26 overlapping species between the two studies, which likely reflects the limitations of surveying fishes with underwater vehicles. Habitats at depths of 150–400 m were surveyed by the submersibles Geo and Jago at Ngazidja Island in the Comoros Islands, because coelacanths live at slightly deeper depths there, and 89 fish taxa including 65 identifiable species were observed (Heemstra et al., 2006b).
Although we could add some species to the list of fishes known from the coelacanth habitats off Sodwana Bay, the time limitations associated with trimix diving do not make that an efficient methodology to study the fish communities that interact with coelacanths. The fixed cameras showed that several types of fish species reside in or near the caves. Some larger fish remain near the ceiling of the rock overhang, and smaller fish live on the of the outer rock overhang surfaces. A video made by Richard Pyle of a dive off Sodwana Bay in 2011 shows many of these same fish species in the caves and rock overhangs at depths including at about 110 m (see link in Table 2).
Our first attempt to place cameras to observe a daytime habitat of coelacanths provided an example of an alternative method to observe these habitats. Underwater video camera systems have been increasingly used in studies of marine fishes or marine biodiversity using baited, stationary, or moving camera systems (Mallet and Pelletier, 2014; Zarco-Perello and Enríquez, 2019; Piggott et al., 2020; Coleman and Burge, 2021). Our study suggests that fixed cameras may be a highly useful method for studying the communities of fishes that interact with coelacanths.
From the images obtained from one of the cameras (Supplementary Video), it was found that other species of fish inhabit the cave and coexist around coelacanths. Many fish were seen along the south side of the cave and many small and medium-size fishes moved in front of the camera and around the coelacanth. These fishes have not been seen together with coelacanths in existing photographs or videos. This cave seems to be a location where coelacanths appear frequently, and although it was not seen in the video when the coelacanth was in the cave, cleaning behavior of a Labroidei fish (a cleaner fish) with a grouper was observed by one of the divers. It seems possible that this place may act a cleaning station. Our observations indicate that various species use the cave for hiding during the day, many fishes are moving around or passing through the caves, and sharks sometimes move through the caves, which make them important habitats for the biodiversity of fishes, including coelacanths, living off this part of South Africa.
Their unique ecological situation of slow growth, long lives and small population sizes have made it obvious that coelacanths were vulnerable to impacts from fishing and the strong general interest in them (Bruton and Stobbs, 1991; Fricke, 1997; Benno et al., 2006; Erdmann, 2006; Hukom et al., 2020; Mahé et al., 2021), resulting in coelacanths being listed by CITES to restrict their sale for commercial gain. The western Indian Ocean species, L. chalumnae, is listed as Critically Endangered, and the Indonesian species, L. menadoensis, is listed as Vulnerable by the IUCN (Whittaker, 2014; Cooke et al., 2021). Our study suggests that fixed underwater camera deployments might be a logical new methodology for studying the behavior and ecology of coelacanths without causing disturbances to their lives and habitats, even if these camera systems might be deployed from submersibles instead of using deep-scuba methods.
The original contributions presented in the study are included in the article/Supplementary Material, further inquiries can be directed to the corresponding author/s.
JS, KHM, KT, HA, KYM, and HI planned and carried out the expedition to South Africa and made the tri-mix or support dives. JS, HA, and HI analyzed the video recordings, oceanographic data, and coelacanth fin positions in the study and from existing materials and made the fish identifications. JS, HI, MJM, and RS drafted and edited the manuscript. JS, HI, and MJM made the figures. All authors evaluated the manuscript.
The expedition to South Africa and support for publishing the study was funded by Kazuhiko Maeda. Some of the equipment used in the study was provided by SONY Co., Ltd., Tokyo Japan, KM-One Co., Ltd., AQUA LUNG Japan, Ltd., Atsugi Japan, and AOI Yokohama Japan. They were not involved in the study design, collection, analysis, interpretation of data, the writing of this article or the decision to submit it for publication.
KHM and KYM were employed by KM-One Co., Ltd.
The remaining authors declare that the research was conducted in the absence of any commercial or financial relationships that could be construed as a potential conflict of interest.
All claims expressed in this article are solely those of the authors and do not necessarily represent those of their affiliated organizations, or those of the publisher, the editors and the reviewers. Any product that may be evaluated in this article, or claim that may be made by its manufacturer, is not guaranteed or endorsed by the publisher.
We are grateful to the former Director Andrew Zaloumis and other staff of the iSimangaliso Wetland Park who gave us permission for making underwater observations at Sodwana Bay. We also are grateful to Naoto Kishi of Sun Planning Co., Ltd., Mondonishi, Hyogo, Japan, Susumu Kamakura of KEDS, Yokosuka, Kanagawa, Japan, Takeshi Okumura and the late Mitsuyoshi Tanaka of Nijusseiki Corp, Tokyo, Japan, and Naoshi Ueda of Dive Kooza, Higashimuro, Wakayama, Japan helped underwater observations. We also thank Nobina Morimoto who supported our stay at Sodwana Ryo Ochi Ryo, and Tetsuya Numata, Ysuki Ogiwara of Sony Co., Ltd., Yasunori Kuno of AOI Co., Ltd., Aqua Lung Japan, Co., Ltd., Toshiki Yamamoto of Yamamoto Industry Co., Ltd., Minoru Nomoto of Anser Co., Ltd., Nijuseiki Trade Co., Ltd., Yamamoto Optical Co., Ltd., CEO David Graham of GD Outdoor Ltd., Fish Eye Ltd., Sukeyasu Ito, and Kazuhiro Yoshikawa, because without those people our research could not have been accomplished. We thank Eve Marshall and all the staff of Triton Dive Lodge who supported our underwater observations. Director Terry Castis of DAN South Africa managed safety dives of our underwater observations in the Bay. We thank the editors of the South African Journal of Science and the Bulletin of Kitakyushu Museum of Natural History and Human History for allowing us to use published imagery of coelacanths in our Supplementary Materials.
The Supplementary Material for this article can be found online at: https://www.frontiersin.org/articles/10.3389/fmars.2021.755275/full#supplementary-material
Supplementary Video | Time-lapse video recording (1 frame every 5 s) of the 29 min when the coelacanth known as Noah was inside the cave at a depth of 113 m on the morning of 11 July 2018 while a shark swam back and forth through the cave and a second shark was also briefly in the cave. Various other fishes can be seen in the cave. The video was lightened to allow the coelacanth to be seen more clearly.
Bansemer, C.S., and Bennett, M.B. (2008). Multi-year validation of photographic identification of grey nurse sharks, Carcharias taurus, and applications for non-invasive conservation research. Mar. Freshw. Res. 59, 322–331. doi: 10.1071/MF07184
Benno, B., Verheij, E., Stapley, J., Ramisha, C., Ngatunga, B., Abdallah, A., et al. (2006). Coelacanth (Latimeria chalumnae) discoveries and conservation in Tanzania. South Afr. J. Sci. 102, 486–490.
Berquist, R. M., Galinsky, V. L., Kajiura, S. M., and Frank, L. R. (2015). The coelacanth rostral organ is a unique low-resolution electro-detector that facilitates the feeding strike. Sci. Rep. 5:8962. doi: 10.1038/srep08962
Bruton, M. N., and Stobbs, R. E. (1991). The ecology and conservation of the coelacanth Latimeria chalumnae. Environ. Biol. Fish. 32, 313–339. doi: 10.1007/BF00007464
Cavin, L., and Guinot, G. (2014). Coelacanths as “almost living fossils”. Front. Ecol. Evol. 2:49. doi: 10.3389/fevo.2014.00049
Casane, D., and Laurenti, P. (2013). Why coelacanths are not “living fossils”. Bioessays 35, 332–338. doi: 10.1002/bies.201200145
Coenen, M. J. J., and Zivkovic, J. (2015). Graphical description of trimix diving gases using ternary plots. Underw. Technol. 33, 127–131. doi: 10.3723/ut.33.127
Coleman, N. C., and Burge, E. J. (2021). Association behavior between sand tiger sharks and round scad is driven by mesopredators. PeerJ 9:e11164. doi: 10.7717/peerj.11164
Cooke, A., Bruton, M. N., and Ravololoharinjara, M. (2021). Coelacanth discoveries in Madagascar, with recommendations on research and conservation. S. Afr. J. Sci. 117, (3/4). doi: 10.17159/sajs.2021/8541
Décamps, T., Herrel, A., Ballesta, L., Holon, F., Rauby, T., Gentil, Y., et al. (2017). The third dimension: a novel set-up for filming coelacanths in their natural environment. Methods Ecol. Evol. 8, 322–328. doi: 10.1111/2041-210X.12671
Dicken, M. L., Booth, A. J., Smale, M. J., and Cliff, G. (2007). Spatial and seasonal distribution patterns of juvenile and adult raggedtooth sharks (Carcharias taurus) tagged off the east coast of South Africa. Mar. Freshw. Res. 5, 127–134. doi: 10.1071/MF06018
Endo, H., Omura, A., Sakai, T., Itou, T., Koie, H., Iwata, M., et al. (2012). The differences of the functional-morphological strategy between the first and second dorsal fins of the living coelacanth (Latimeria chalumnae). Japan. J. Zoo. Wildl. Med. 17, 79–86. doi: 10.5686/jjzwm.17.79
Erdmann, M. (2006). Lessons learned from the conservation campaign for the Indonesian coelacanth, Latimeria menadoensis. South Afr. J. Sci. 102, 501–504.
Erdmann, M. V., Caldwell, R. L., and Moosa, M. K. (1998). Indonesian ‘king of the sea’ discovered. Nature 395:335. doi: 10.1038/26376
Erdmann, M. V., Caldwell, R. L., Jewett, S. L., and Tjakrawidjaja, A. (1999). The second recorded living coelacanth from North Sulawesi. Envir. Biol. Fish 54, 445–451. doi: 10.1023/A:1007533629132
Fraser, M. D., Henderson, B. A. S., Castens, P. B., Fraser, A. D., Henderson, B. S., Dukes, M. C., et al. (2020). Live coelacanth discovered off the KwaZulu-Natal South Coast, South Africa. South Afr. Jour. Sci. 116, 1–15. doi: 10.17159/sajs.2020/7806
Fricke, F. (1997). Living coelacanths: values, eco-ethics and human responsibility. Mar. Ecol. Progr. Ser. 161, 1–15. doi: 10.3354/meps161001
Fricke, H., and Hissmann, K. (2000). Feeding ecology and evolutionary survival of the living coelacanth Latimeria chalumnae. Mar. Biol. 136, 379–386. doi: 10.1007/s002270050697
Fricke, H., Reinicke, O., Hofer, H., and Nachtigall, W. (1987). Locomotion of the coelacanth Latimeria chalumnae in its natural environment. Nature 329, 331–333. doi: 10.1038/329331a0
Fricke, H., Hissmann, K., Schauer, J., Reinicke, O., and Ksang, L. (1991). Habitat and population size of the coelacanth Latimeria chalumnae at Grand Comoro. Environ. Biol. Fish 32, 287–300. doi: 10.1007/BF00007462
Fricke, H., Hissmann, K., Schauer, J., Erdmann, M., Moosa, M. K., and Plante, R. (2000). Biogeography of the Indonesian coelacanths. Nature 403:38. doi: 10.1038/47400
Fricke, H., Hissmann, K., Froese, R., Schauer, J., Plante, R., and Fricke, S. (2011). The population biology of the living coelacanth studied over 21 years. Mar. Biol. 158, 1511–1522. doi: 10.1007/s00227-011-1667-x
Garita-Alvarado, C. A., Naranjo-Elizondo, B., and Barrantes, G. (2018). Mating and aggressive behaviour of Brachyrhaphis olomina (Cyprinodontiformes: Poeciliidae). J. Ethol. 36, 1–13. doi: 10.1007/s10164-017-0523-y
Geldenhuys, D. A. (2015). Quantitative Fish Survey of the Submarine Canyons of the iSimangaliso Wetland Park (Masters Thesis). Cape Town: University of Cape Town.
Haulsee, D. E., Fox, D. A., Breece, M. W., Brown, L. M., Kneebone, J., Skomal, G. B., et al. (2016). Social network analysis reveals potential fission-fusion behavior in a shark. Sci. Rep. 6:34087. doi: 10.1038/srep34087
Heemstra, P. C., Fricke, H., Hissmann, K., Schauer, J., Smale, M., and Sink, K. (2006a). Interactions of fishes with particular reference to coelacanths in the canyons at Sodwana Bay and the St Lucia Marine Protected Area of South Africa. South Afr. J. Sci. 102, 461–465.
Heemstra, P. C., Hissmann, K., Fricke, H., Smale, M. J., and Schauer, J. (2006b). Fishes of the deep demersal habitat at Ngazidja (Grand Comoro) Island, western Indian Ocean. South Afr. J. Sci. 102, 444–460.
Hensel, K., and Balon, E. K. (2001). The sensory canal systems of the living coelacanth, Latimeria chalumnae: a new instalment. Envir. Biol. Fish. 61, 117–124. doi: 10.1023/A:1011062609192
Hissmann, K., Fricke, and Schauer, J. (2000). Patterns of time and space utilisation in coelacanths (Latimeria chalumnae), determined by ultrasonic telemetry. Mar. Biol. 136, 943–952. doi: 10.1007/s002270000294
Hissmann, K., Fricke, H., Schauer, J., Ribbink, A. J., Roberts, M., Sink, K., et al. (2006). The South African coelacanth–an account of what is known after three submersible expeditions. S. Afr. J. Sci. 102, 491–500.
Holder, M. T., Erdmann, M. V., Wilcox, T. P., Caldwell, R. L., and Hillis, D. M. (1999). Two living species of coelacanths? Proc. Natl. Acad. Sci. U.S.A. 96, 12616–12620. doi: 10.1073/pnas.96.22.12616
Holleman, W., Connell, A. D., and Carpenter, K. E. (2013). Caesio xanthalytos, a new species of fusilier (Perciformes: Caesionidae) from the Western Indian Ocean, with records of range extensions for several species of Caesionidae. Zootaxa 3702, 262–272. doi: 10.11646/zootaxa.3702.3.4
Hukom, F. D., Iwata, M., Syahailatua, A., Peristiwady, T., Masengi, K. W. A., Dirhamsyah, et al. (2020). “History, conservation and research program of Indonesian coelacanth,” in Proceedings of the 10th International. Aquarium Congress Fukushima 2018, (Onahama), 122–126.
Imawaki, S., Bower, A. S., Beal, L., and Qiu, B. (2013). “Western boundary currents,” in Ocean Circulation and Climate - A 21st Century Perspective, Vol. 103, eds G. Siedler, S. J. Griffies, J. Gould, and J. A. Church (Cambridge, MA: Academic Press), 305–338. doi: 10.1016/B978-0-12-391851-2.00013-1
Inoue, J. G., Miya, M., Venkatesh, B., and Nishida, M. (2005). The mitochondrial genome of Indonesian coelacanth Latimeria menadoensis (Sarcopterygii: Coelacanthiformes) and divergence time estimation between the two coelacanths. Gene 349, 227–235. doi: 10.1016/j.gene.2005.01.008
Iwata, M., Yabumoto, Y., Saruwatari, T., Yamaguchi, S., Fujii, K., Ishii, R., et al. (2019a). Field surveys on the Indonesian coelacanth, Latimeria menadoensis using remotely operated vehicles from 2005 to 2015. Bull. Kitakyushu Mus. Nat. Hist. Hum. Ser. A 17, 49–56.
Iwata, M., Yabumoto, Y., Saruwatari, T., Yamaguchi, S., Fujii, K., Ishii, R., et al. (2019b). Observation of the first juvenile Indonesian coelacanth, Latimeria menadoensis from Indonesian waters with a comparison to embryos of Latimeria chalumnae. Bull. Kitakyushu Mus. Nat. Hist. Hum. Ser. A. 17, 57–65.
Kadarusman, Sugeha, H. Y., Pouyaud, L., Hocdé, R., Hismayasari, I. B., Gunaisah, E., et al. (2020). A thirteen-million-year divergence between two lineages of Indonesian coelacanths. Sci. Rep. 10:192. doi: 10.1038/s41598-020-61557-3
Klein, J. D., Bester-van der Merwe, A. E., Dicken, M. L., Emami-Khoyi, A., Mmonwa, K. L., and Teske, P. R. (2020). A globally threatened shark, Carcharias taurus, shows no population decline in South Africa. Nat. Sci. Rep. 10:17959. doi: 10.1038/s41598-020-75044-2
Larouche, O., Zelditch, M., and Cloutier, R. (2017). Fin modules: an evolutionary perspective on appendage disparity in basal vertebrates. BMC Biol. 15:32. doi: 10.1186/s12915-017-0370-x
Lampert, K. P., Fricke, H., Hissmann, K., Schauer, J., Blassmann, K., Ngatunga, B. P., et al. (2012). Population divergence in East African coelacanths. Curr. Biol. 22, R439–R440. doi: 10.1016/j.cub.2012.04.053
Lang, M. A., and Smith, N. E. (eds) (2006). Proceedings of the Advanced Scientific Diving Workshop. February 23–24, 2006. Washington, DC: Smithsonian Institution, 277.
Lauder, G. V., and Drucker, E. G. (2004). Morphology and experimental hydrodynamics of fish fin control surfaces. IEEE J. Oceanic Engineering 29, 556–571. doi: 10.1109/JOE.2004.833219
Mahé, K., Ernande, B., and Herbin, M. (2021). New scale analyses reveal centenarian African coelacanths. Current Biol. 31, 3621–3628.e4. doi: 10.1016/j.cub.2021.05.054
Mallet, D., and Pelletier, D. (2014). Underwater video techniques for observing coastal marine biodiversity: a review of sixty years of publications (1952–2012). Fish. Res. 154, 44–62. doi: 10.1016/j.fishres.2014.01.019
Martin, R. A. (2007). A review of shark agonistic displays: comparison of display features and implications for shark–human interactions. Mar. Freshw. Behav. Physiol. 40, 3–34. doi: 10.1080/10236240601154872
Millot, J. (1954). Le Triosieme Coelacanth: Historique Elements d‘Ecologie/Morphologie Extreme Documents Divers. Le naturaliste Malgache, Premier Supplement. Paris: Pierre Andre Imp., 80.
Nikaido, M., Sasaki, T., Emerson, J., Aibara, M., Mzighani, S. I., Budeba, Y. L., et al. (2011). Genetically distinct coelacanth population off the northern Tanzanian coast. Proc. Natl. Acad. Sci. U.S.A. 108, 18009–18013. doi: 10.1073/pnas.1115675108
Piggott, C. V. H., Depczynski, M., Gagliano, M., and Langlois, T. J. (2020). Remote video methods for studying juvenile fish populations in challenging environments. J. Exp. Mar. Biol. Ecol. 532:151454. doi: 10.1016/j.jembe.2020.151454
Pollock, N. W., Sellers, S. H., and Godfrey, J. M. (eds) (2016). “Rebreathers and scientific diving,” in Proceedings of NPS/NOAA/DAN/AAUS June 16–19, 2015 Workshop, (Catalina Island, CA: Wrigley Marine Science Center), 272.
Picone, B., Hesse, U., Panji, S., Van Heusden, P., Jonas, M., and Christoffels, A. (2014). Taste and odorant receptors of the coelacanth—a gene repertoire in transition. J. Exp. Zool. B Mol. Dev. Evol. 322, 403–414. doi: 10.1002/jez.b.22531
Price, S. A., Friedman, S. T., and Wainwright, P. C. (2015). How predation shaped fish: the impact of fin spines on body form evolution across teleosts. Proc. R. Soc. B 282:20151428. doi: 10.1098/rspb.2015.1428
Ramsay, P. J., and Miller, W. R. (2006). Marine geophysical technology used to define coelacanth habitats on the KwaZulu-Natal shelf, South Africa. South Afr. J. Sci. 102, 427–434.
Roberts, M. J., Ribbink, A. J., Morris, T., van den Berg, M. A., Engelbercht, D. C., and Harding, R. T. (2006). Oceanographic environment of Sodwana Bay coelacanth (Latimeria chalumnae), South Africa. South Afr. J. Sci. 102, 435–443.
Sink, K., Boshoff, W., Samaai, T., Timm, P. G., and Kerwath, S. E. (2006). Observations of the habitats and biodiversity of the submarine canyons at Sodwana Bay. South Afr. J. Sci. 102, 466–474.
Sink, K. (2013). “75th Anniversary expedition and living coelacanths of the iSimangaliso wetland park,” in Building on the South African Coelacanth Legacy African Coelacanth Ecosystem Programme 2001–2013, ed. P. Hayworth (Grahamstown: The South African Institute for Aquatic Biodiversity).
Smale, M. J. (2005). The diet of the ragged-tooth shark Carcharias taurus Rafinesque 1810 in the Eastern Cape, South Africa. Afr. J. Mar. Sci. 27, 331–335. doi: 10.2989/18142320509504091
Smith, M. M., and Heemstra, P. C. (1995). Smiths’ Sea Fishes. Johannesburg: Southern Book Publishers.
Sward, D., Monk, J., and Barret, N. (2019). A systematic review of remotely operated vehicle surveys for visually assessing fish assemblages. Front. Mar. Sci. 6:134. doi: 10.3389/fmars.2019.00134
Toriñoa, P., Soto, M., and Perea, D. (2021). A comprehensive phylogenetic analysis of coelacanth fishes (Sarcopterygii, Actinistia) with comments on the composition of the Mawsoniidae and Latimeriidae: evaluating old and new methodological challenges and constraints. Hist. Biol. 1–21. doi: 10.1080/08912963.2020.1867982
Uken, R., and Green, A. N. (2006). Coelacanth (Latimeria chalumnae) resting traces in a cave floor, Chaka Canyon, South Africa. S. Afr. J. Sci. 102, 474–475.
Uyeno, T., and Tsutsumi, T. (1991). Stomach contents of Latimeria chalumnae and further notes on its feeding habits. Environ. Biol. Fish. 32, 275–279. doi: 10.1007/BF00007460
Venter, P., Timm, P., Gunn, G., le Roux, E., Serfontein, C., Smith, P., et al. (2000). Discovery of a viable population of coelacanths (Latimeria chalumnae Smith, 1939) at Sodwana Bay, South Africa. S. Afr. J. Sci. 96, 567–568.
Wagner, D., Toonen, R. J., Papastamatiou, Y. P., Kosaki, R. K., Gleason, K. A., McFall, G. B., et al. (2013). “Mesophotic surveys of the northwestern Hawaiian Islands with records of black coral species,” in Proceedings of the Joint International Scientific Diving Symposium, American Academy of Underwater Sciences and European Scientific Diving. Curaçao AAUS/ESDP Joint International Symposium, 314–345, eds M. A. Lang and M. D. J. Sayer (Dauphin Island, AL: American Academy of Underwater Sciences).
Whittaker, K. (2014). Endangered Species Act Status Review Report for the Coelacanth. Washington, DC: NOAA, 1–47.
Yokohama, S. (2000). Color vision of the coelacanth (Latimeria chalumnae) and adaptive evolution of rhodopsin (RH1) and rhodopsin-like (RH2) pigments. J. Hered. 91, 215–220. doi: 10.1093/jhered/91.3.215
Keywords: coelacanth, Latimeria chalumnae, dorsal fin, Sodwana Bay, set cameras, trimix-diving, ocean currents, fish fauna
Citation: Sakaue J, Maeda K, Miller MJ, Sakai R, Tahara K-i, Abe H, Maeda K and Ida H (2021) New Insights About the Behavioral Ecology of the Coelacanth Latimeria chalumnae Video Recorded in the Absence of Humans Off South Africa. Front. Mar. Sci. 8:755275. doi: 10.3389/fmars.2021.755275
Received: 16 August 2021; Accepted: 12 October 2021;
Published: 10 November 2021.
Edited by:
Lionel Cavin, Natural History Museum of Geneva, SwitzerlandReviewed by:
Oliver J. D. Jewell, Murdoch University, AustraliaCopyright © 2021 Sakaue, Maeda, Miller, Sakai, Tahara, Abe, Maeda and Ida. This is an open-access article distributed under the terms of the Creative Commons Attribution License (CC BY). The use, distribution or reproduction in other forums is permitted, provided the original author(s) and the copyright owner(s) are credited and that the original publication in this journal is cited, in accordance with accepted academic practice. No use, distribution or reproduction is permitted which does not comply with these terms.
*Correspondence: Jiro Sakaue, c2FrYXVlQHNtbC1wYWxhdS5jb20=
Disclaimer: All claims expressed in this article are solely those of the authors and do not necessarily represent those of their affiliated organizations, or those of the publisher, the editors and the reviewers. Any product that may be evaluated in this article or claim that may be made by its manufacturer is not guaranteed or endorsed by the publisher.
Research integrity at Frontiers
Learn more about the work of our research integrity team to safeguard the quality of each article we publish.