- Marine Biodiversity and Environmental Assessment Research Center, Research Institute for Global Change (RIGC), Japan Agency for Marine-Earth Science and Technology (JAMSTEC), Yokosuka, Japan
Marine ecosystems are continuously subjected to anthropogenic environmental pollution. Understanding the spread of pollution and the potential risks it poses to deep-sea ecosystems is important for developing better conservation measures. Here, we identified non-negligible levels of persistent organic pollutants in deep-sea chemosynthetic bivalves with limited or no filter feeding. The bivalves were collected from two sites: one located near a highly populated region and the other located relatively far from human activity. Analyses of samples collected nearly every decade in a period of 30 years suggested that environmental policy restrictions might be effective in reducing chemical pollution. However, the detection of contamination in deep-sea chemosynthetic animals suggests that the pollution could be spreading globally to chemosynthetic organisms with limited or no feeding. To protect these highly endemic and vulnerable deep-sea chemosynthetic ecosystems, our findings indicate that further research on chemical contamination and its effects on these ecosystems is required.
Introduction
Some organic compounds are resistant to environmental degradation and can accumulate in organisms, causing harmful effects. These chemicals are called persistent organic pollutants (POPs) and they have been of great academic and social interest since the late 20th century. Polychlorinated biphenyls (PCBs) are a group of substances obtained by the chlorination of biphenyls; they have 10 homologues and 209 congeners, theoretically, depending on the number and position of the chlorine atoms (Yuan et al., 2015). Additionally, coplanar PCBs are known to be extremely toxic (Safe, 1994). Owing to their advantageous electrical insulating, chemical resistant, and non-flammable properties, PCBs were manufactured globally during the mid-20th century for numerous applications including insulating oils for electrical transformers and capacitors, as well as additives in paints and plastics (Erickson and Kaley, 2011). However, large-scale PCB contamination of cooking oil eventually drew attention to their toxicity and potential for environmental pollution. The manufacturing of PCBs was banned in closed systems in the United States in 1979 and in the United Kingdom in 1981, but was not phased out until 1987 in European countries that border the Mediterranean Sea (Jepson et al., 2016). The international Stockholm Convention (2001), which aimed to eliminate all designated POPs, prohibited the manufacture of PCBs and severely restricted the use of remaining PCB stocks, and a number of East Asian countries have signed the agreement. In Japan, The Chemical Substance Control Law (CSCL) was enacted in 1974 to prohibit the production, use, and import of PCBs (Koshiba et al., 2019). However, the disposal of PCB-containing products has not progressed sufficiently. The PCB Special Measures Law was enacted in 2001 for the secure and appropriate disposal of PCB waste by 2016 (later extended to 2027) based on the international Stockholm Convention. Additionally, polybrominated diphenyl ethers (PBDEs), which are brominated aromatic hydrocarbons heavily used as flame-retardants in plastics, possess structural variations similar to PCBs (Talsness, 2008). Due to their persistence in the human body, tetra-, penta-, hexa-, and hepta-BDEs were listed in the Stockholm Convention in 2009. In Japan, the use of PBDEs (other than deca-BDE) was stopped since the 1990s, owing to self-restrictions by the manufacturers. Additionally, deca-BDE was listed in the Stockholm Convention in 2017 and was banned from import and use in Japan in 2018. However, regardless of these efforts, environmental pollution caused by these substances continues to be detected in a wide range of biota collected worldwide (Domingo and Bocio, 2007; Lee and Kim, 2015).
Due to their hydrophobic and lipophilic natures, PCBs and PBDEs are only slightly soluble in water and readily associate with organic particles in aquatic environments, including algae, suspended sediment, and micro- or nano-plastics (Ghosh et al., 2003; Olenycz et al., 2015; Yamashita et al., 2019). Aquatic animals can be exposed to PCBs and PBDEs through two routes: uptake across the body surface (e.g., gills and/or epidermis), and dietary uptake through the digestive tract (Bjork, 1995). These pollutants can enter the adipose tissue and liver and bioaccumulate through the food chain (Matthews and Dedrick, 1984). Bivalve mollusks are generally filter feeders and are considered to be good indicators of environmental pollution because they accumulate various POPs from the surrounding water (Chiesa et al., 2018). There have been many reports of PCB and PBDE contamination in coastal bivalves worldwide, including in Japan (Ramu et al., 2007; Supplementary Table 1). These pollutants are transported for long distances by ocean currents, and therefore lead to transboundary problems that require the special attention and coordination of international efforts (Dewailly et al., 2007).
Persistent organic pollutants are considered to collect in the deep ocean via vertical transport through the various biogeochemical processes (Takahashi et al., 2014). Examining the behaviors of POPs provides insight into the extent of anthropogenic impacts on deep-sea ecosystems, in which organisms have long lifespans, slow growth, and late maturity, and are therefore particularly vulnerable to environmental disturbances. Recovery from such disturbances can be a long process, and in some cases can lead to the extinction of some organisms (Llodra and Billett, 2006). However, little is known about the distribution of POPs in deep-sea animals, except for fish (Takahashi et al., 2009). A recent study quantified PCBs and PBDEs in scavenging amphipods collected from the Kermadec and Mariana trenches at depths of more than 10,000 m (Jamieson et al., 2017; Supplementary Table 1). Information on other benthic animals is limited; however, the PCB contents in epibenthic deep-sea invertebrates (sea anemones, sea cucumbers, sea pens, and sea lilies) from the northern Gulf of Mexico have been reported (Lawson et al., 2021; Supplementary Table 1).
Deep-sea endobenthic vesicomyid clams, including those of the genus Phreagena (formerly Calyptogena), and deep-sea epibenthic mussels belonging to the genus Bathymodiolus are endemic and dominant members of deep-sea chemosynthetic communities (Fisher, 1990). They harbor symbiotic chemosynthetic bacteria (gram-negative Gammaproteobacteria) in the epithelial cells of their gills (Kuwahara et al., 2007). The vesicomyid clams depend on these symbionts for nutrition, as their digestive tracts are non-functional (Childress and Fisher, 1992; Le Pennec et al., 1995). Bacterial symbionts are also the primary nutritional source for Bathymodiolus mussels, although they possess a functional gut (Page et al., 1991; Ponnudurai et al., 2017). Assuming that the contamination of bivalve mollusks by highly hydrophobic pollutants such as PCBs is predominantly due to dietary uptake through filter feeding, the pollution of chemosynthetic bivalves (that perform limited to no filter feeding) is expected to be negligible. However, as the non-dietary uptake of hydrophobic organic contaminants has been documented in bivalve filter feeders (Bjork, 1995), uptake across the body surface likely occurs, even in animals with limited filter feeding. In this study, we hypothesized that PCB contamination might be detected in chemosynthetic animals, and that the level of contamination would reflect the proximity of human activity. To test this and to understand the spread of POP pollution in marine ecosystems, we measured the concentrations of PCBs and PBDEs in deep-sea chemosynthetic Phreagena clams and Bathymodiolus mussels from Sagami Bay, which is located near a highly populated region that includes Tokyo (Statistics Bureau of Japan [SBJ], 2020; Nakajima et al., 2021; Figures 1A,B). For the Phreagena clams, we used 30 years of archived samples (collected from Sagami Bay in 1989, 1998, 2010, and newly in 2019) to investigate the changes in contamination over time. For comparison, we also analyzed Bathymodiolus mussels that inhabit Myojin Knoll, which is a relatively remote area located far from dense human activity (Nakajima et al., 2021; Figures 1A,C).
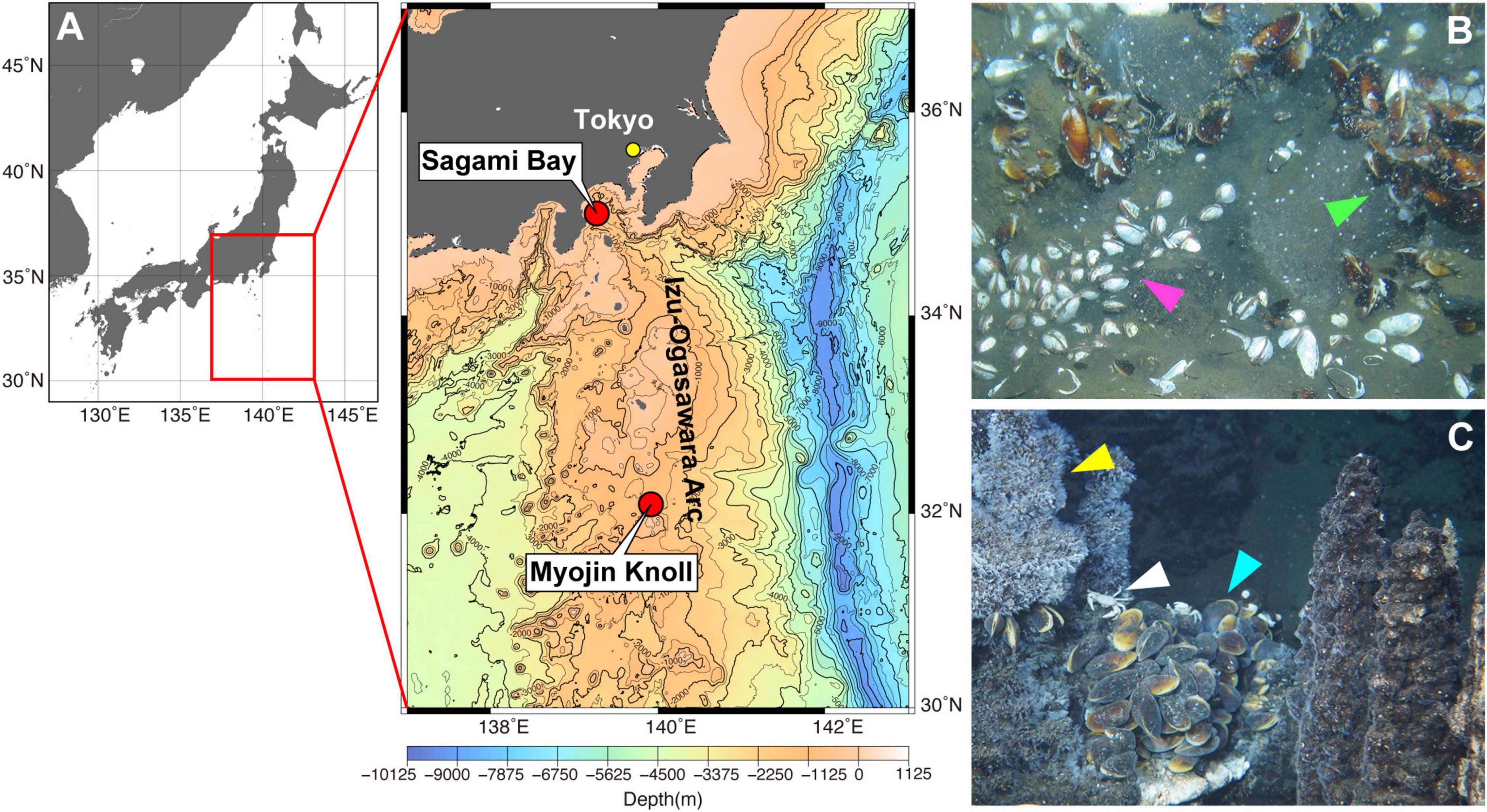
Figure 1. Sampling sites in this study. (A) Maps of the sampling sites in this study. The red box in the left panel [generated using Ocean Data View (https://odv.awi.de)] corresponds to the area in the right panel [generated using Generic Mapping Tools (Wessel et al., 2019) by Japan Oceanographic Data Center, with 500 m mesh water depth data (J-EGG500)]. (B) Phreagena clams and Bathymodiolus japonicus in Sagami Bay. The clams burrow into the sediment (magenta arrowhead), while the mussels attach to rocks on the bottom (light green arrowhead). (C) Bathymodiolus septemdierum at Myojin Knoll. The mussels form a colony near the chimneys (light blue arrowhead). Bythograeid crabs (white arrowhead) and vent barnacles (yellow arrowhead) are also observed on the chimneys.
Methods
Faunal Sampling
Phreagena clams were collected from the seep site off Hatsushima Island in Sagami Bay, Japan, at a depth of 857 m (Dive #6K1557) during cruise YK19-11 (August 28–September 14, 2019) using the HOV Shinkai 6500, operated by the R/V Yokosuka (Japan Agency of Marine-Earth Science and Technology; JAMSTEC). We also used the archived clams collected at a depth of 1,171 m (Dive #1074) during cruise NT10-01 (January 12–18, 2010) using the ROV Hyper-Dolphin operated by the R/V Natsushima (JAMSTEC), as well as at depths of 1205 m (Dive #2K0998) and 1,195 m (Dive #2K0450) during cruises NT98-06 (April 2–26, 1998) and N89-08 (October 7–29, 1989), respectively, using the HOV Shinkai 2000 operated by the R/V Natsushima (Table 1). These clams were preserved as whole at JAMSTEC at −80°C. At the seep site, two morphologically similar clam species (Phreagena okutanii and Phreagena soyoae) are known to form a mixed colony (Ikuta et al., 2018); however, we did not distinguish between these two species in this study. Bathymodiolus japonicus mussels were also collected from the seep site off Hatsushima Island at a depth of 901 m (Dive #6K1557), while Bathymodiolus septemdierum mussels were collected from hydrothermal vent sites at Myojin Knoll, Japan, at a depth of 1235 m (Dive #6K1556). All of the Bathymodiolus specimens were collected during cruise YK19-11 (Table 1). The bivalves were frozen and stored at −80°C. For each bivalve species and collection year, three individuals with a shell length of ∼100 mm were used for subsequent analyses (Table 1).
The samples were processed on a clean bench. For dissection, the bivalve specimens were thawed in a dry-heat sterilized stainless steel tray at room temperature for several hours. Sterilized stainless steel scalpels were used to remove the shells. The entire soft bodies of the specimens were placed directly in sterile glass jars, covered with combusted aluminum foil, and stored at −30°C until analysis.
Chemical Analyses
Polychlorinated biphenyl and PBDE analyses were conducted in the laboratory of IDEA Consultants (Tokyo, Japan). The analytical method followed the procedures described by United States Environmental Protection Agency (US EPA) (United States Environmental Protection Agency [USEPA], 2003, 2010). Briefly, after internal standard solution was spiked into the homogenized bivalve samples (approximately 4 g wet), an acetone/hexane (1:2) mixture was added and two 2-min stirred extractions were conducted using a homogenizer. The extract obtained by centrifugation was treated with sulfuric acid and purified using multi-layer silica gel column chromatography after acetonitrile/hexane partitioning. After purification using gel permeation chromatography, the extract was divided for the PCB and PBDE analyses. For the PCB analysis, the sample volume was fixed at 30 μL, and syringe spikes were added. For the PBDE measurements, the sample was further purified using activated carbon dispersed silica gel column chromatography, the sample volume was fixed at 30 μL, and syringe spikes were added. The concentrations of PCBs and PBDEs in the samples were determined using gas chromatography–mass spectrometry with an Agilent 6890 GC (Agilent Technologies, Santa Clara, CA, United States) equipped with an AutoSpec Ultima mass selective detector (Waters Corporation, Milford, MA, United States). For the PCBs, 10 homologues (mono-CBs–deca-CB) and 14 coplanar PCBs were assessed. For the PBDEs, seven homologues (tetra-BDEs–deca-BDE) and six congeners listed in Annex A by the Stockholm Convention, were assessed. Procedural blanks were run at a rate of one in every ten samples and did not affect the sample concentrations. The method detection limits (MDLs) for each congener or homologue concentration were calculated using the following equation:
where, t(n-1, 0.05) represents the Student’s t-value at an α level of 0.05 with n-1 degrees of freedom, and s represents the standard deviation of the blank measurements in seven replicates. The total lipid contents of the samples were determined using a single-step extraction method, based on the conventional chloroform-methanol extraction method (Daugherty and Lento, 1983). The results are expressed either as ng/g lipid weight (lw) or ng/g dry weight (dw) calculated based on the weight of water loss from the freeze-dried samples. The Tukey–Kramer test (P = 0.05) was performed for statistical analyses using Excel 2011 (Microsoft, Redmond, WA, United States) and the add-on software Statcel4 (OMS publishers, Tokyo, Japan).
Results
Table 1 presents the concentrations of PCBs (ng/g lw and ng/g dw) and PBDEs (ng/g lw) in Phreagena clams and Bathymodiolus mussels. PCBs were detected in all of the samples, and the total concentrations of PCBs (ΣPCBs) were 18–110 ng/g lw and 1.2–13 ng/g dw (mean = 44 ng/g lw, standard deviation, SD = 32 and mean = 4.3 ng/g dw, SD = 3.9, respectively). Among the Phreagena clams, in terms of weight per lipid, the mean ΣPCB values of individuals collected in 1989 and 1998 were significantly higher than those of individuals collected in 2010 and 2019 (Figure 2A). However, there were no significant differences in the mean values per dw. Among the three bivalve species collected in 2019, the mean ΣPCB value of B. japonicus was higher than those of the Phreagena clams and B. septemdierum, for both the lw and dw (Figure 2A). There were no statistical differences between the mean ΣPCBs of the Phreagena clams and B. septemdierum collected in 2019.
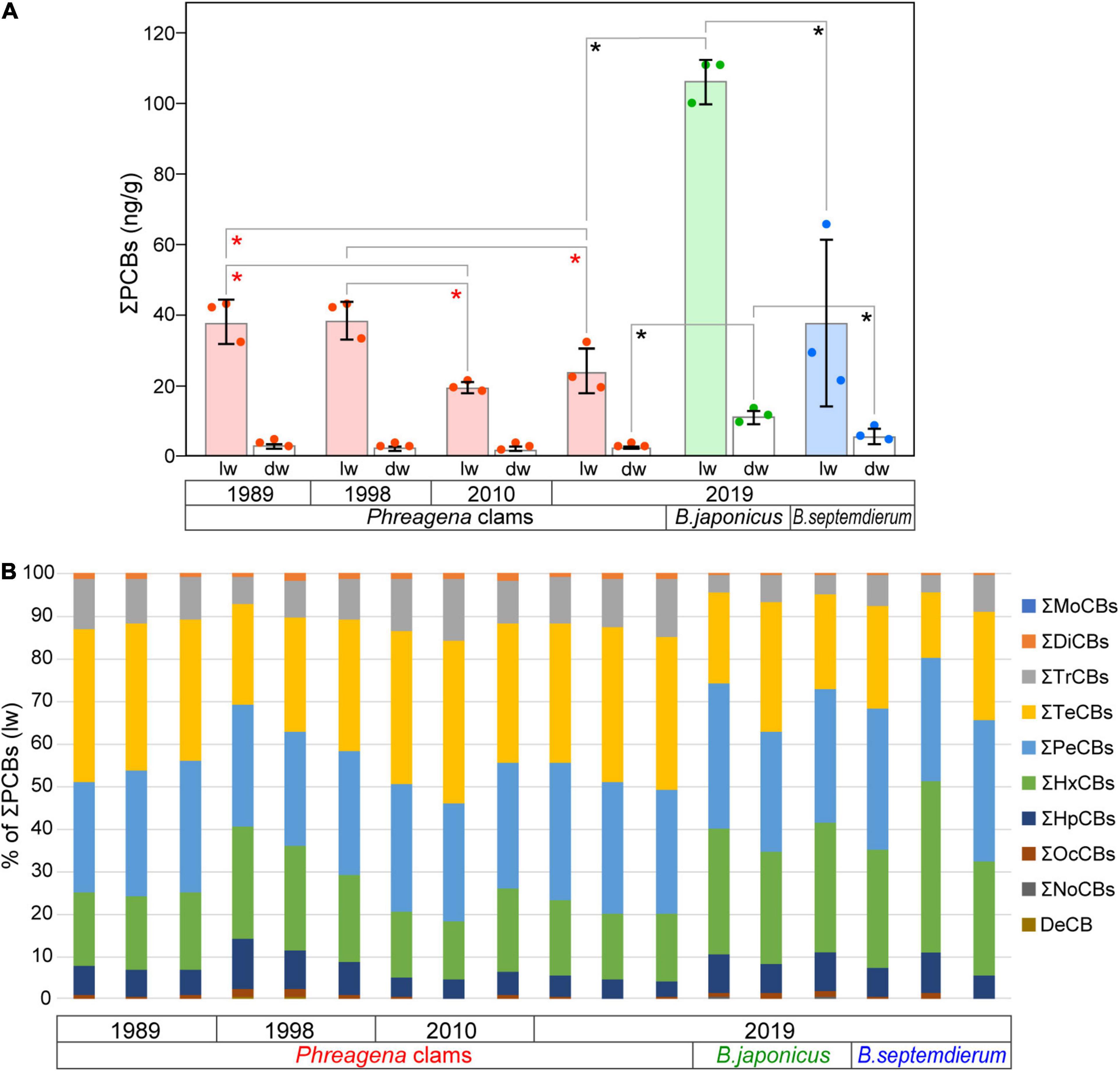
Figure 2. PCB concentrations measured in the samples. (A) Mean total PCB concentrations per g lw and dw. The mean values from three individuals are shown for each bivalve species and each collection year. Pink, light green, and light blue bars indicate mean total PCB concentrations per g lw for Phreagena clams, Bathymodiolus japonicus, and Bathymodiolus septemdierum, respectively. White bars are mean values per g dw. Red, green, and blue dots indicate the values for Phreagena clams, B. japonicus, and B. septemdierum, respectively. Red and black asterisks indicate statistically significant differences (P < 0.05) in the Phreagena clam samples and in the bivalves collected in 2019, respectively. Error bars represent the standard deviation (n = 3). (B) Congener compositions of PCBs in all samples. Concentrations are based on the weight per lipid. Mo, mono-; Di, di-; Tr, Tri-; Te, tetra-; Pe, penta-; Hx, hexa-; Hp, hepta-; Oc, octa-; No, nona-; De, deca-; PCB, polychlorinated biphenyl; lw, lipid weight; dw, dry weight.
In terms of the percentage of PCB homologues in the ΣPCBs of each individual, hexa- (14–40%, lw), penta- (25–35%, lw), and tetra-CBs (15–39%, lw) dominated the overall samples, followed by tri- (4–15%, lw) and hepta- CBs (4–12%, lw) (Figure 2B). The amounts of other homologues were notably small, and mono-CBs were not detected. Additionally, the percentages of the 14 coplanar PCBs in the ΣPCBs ranged from 8.6 to 17% (lw) for all samples (Supplementary Table 2).
No PBDEs were detected in the Phreagena clams collected in 2010 and 2019, although small amounts were detected in three clams collected in 1989 (average of total PBDEs = 0.2 ng/g lw) and in two clams collected in 1998 (average of total PBDEs = 0.9 ng/g lw) (Table 1). For the Bathymodiolus mussels, all of which were collected in 2019, small amounts of PBDEs were detected in two B. japonicus (0.2 ng/g lw) individuals and one B. septemdierum (0.3 ng/g lw) individual. Among the samples, most of the PBDEs detected were tetra-BDEs; deca-BDE was detected in one Phreagena clam individual collected in 1998 (Supplementary Table 2).
Discussion
This study is the first detailed report of PCBs and PBDEs detected in deep-sea chemosynthetic animals, with ΣPCBs of 18–110 ng/g lw and 1.2–13 ng/g dw (mean = 44 ng/g lw and 4.3 ng/g dw). This range is well below the very high concentrations of PCBs detected in shallow water mussels collected from sites near highly populated regions of Japan (3,000 ng/g lw in Tokyo Bay and 2,000 ng/g lw in Osaka Bay). However, it is comparable to levels detected at some sites in other countries (Monirith et al., 2003; Ramu et al., 2007; Kimbrough et al., 2008, 2009; Olenycz et al., 2015; Supplementary Table 1). Furthermore, this range is comparable to or slightly less than that in the other deep-sea organisms at lower trophic levels collected from Sagami Bay, including pelagic fishes, crustaceans, and zooplankton (Toyoshima et al., 2009). PCBs affect a number of biological and physiological processes in a variety of animals, and the deleterious effects of PCBs on reproduction is documented in aquatic organisms at various trophic levels, including bivalves (Chu et al., 2003; Lehmann et al., 2007). The biological and physiological effects of pollution on chemosynthetic bivalves and the amount of contamination that leads to these effects remain poorly understood; however, this study raises the potential possibility of additional anthropogenic disturbances to these highly endemic and vulnerable fauna; therefore, further attention and study are required. On a global scale, in addition to the seas around Japan, hydrothermal vents and seeps with chemosynthetic communities are distributed near relatively densely populated areas such as Monterey Bay, Tyrrhenian Sea, and the Gulf of Mexico (German et al., 2011). This study suggests that the pollution could be spreading to the non-feeding chemosynthetic organisms that inhabit these areas.
In this study, very small amounts of PBDEs were detected in the deep-sea chemosynthetic clams and mussels. In contrast, a previous study detected non-negligible amounts of PBDEs in deep-sea animals (fishes, crustaceans, and zooplankton) collected from Sagami Bay (Toyoshima et al., 2009), although in amounts less than PCBs. To understand the implications of our results, it is necessary to understand the contamination of the associated habitats, including sediments and seawater, by these pollutants.
The average total PCBs per g lw of B. japonicus was significantly higher than that in the Phreagena clams collected during the same cruise (2019) and from the same site. This could be because Bathymodiolus mussels reportedly filter feed to a slight extent (Page et al., 1991; Ponnudurai et al., 2017), and dietary uptake of the pollutant may increase the PCB contamination level. Alternatively, some biological or physiological differences between the two mollusks (e.g., differences in pumping and filtration rates, and an endobenthic or epibenthic habitat) could have affected the results. In any case, non-negligible amounts of PCBs were detected in the deep-sea chemosynthetic bivalves, which indicates that the contaminants were taken up completely or predominantly across the body surface without feeding. Lower-chlorinated PCBs (tetra-, penta-, and hexa-CBs) were dominant in all samples in this study, and similar results were obtained in previous studies of shallow-water mussels (Porte and Albaiges, 1994; Yamaguchi et al., 2000). Low-chlorinated compounds are relatively soluble in water (Bjork, 1995); therefore, it is possible that they were taken up by the Phreagena clams and Bathymodiolus mussels as dissolved materials. In contrast, there are two possible routes for the uptake of particle-bound substances from the body surface: cell membrane permeation due to the lipophilic nature of the contaminants and the direct uptake of particles into the cell. In the former route, contaminants must have sufficient time to desorb from the particles before diffusion across the body surface, such as through the gills (Klump et al., 1987; Gobas et al., 1993). The mucus present on the surface of the bivalve body may contribute to this process. The direct uptake of organic particles, such as micro- or nano-plastics, across the body surface of bivalves, has been reported previously (Al-Sid-Cheikh et al., 2018; Kolandhasamy et al., 2018). PCBs and PBDEs are associated with micro- or nano-plastics in the ocean (Galloway and Lewis, 2016; Yu et al., 2019); therefore, the possibility of the latter route should be investigated further. Complex interactions between biological and non-biological factors govern the bioavailability and uptake of hydrophobic organic contaminants in filter feeding bivalves (Bjork, 1995). Therefore, the details of the contaminant uptake pathways in deep-sea chemosynthetic bivalves should be investigated in the future.
Comparing the total amounts of PCBs per g lw in the Phreagena clams approximately every 10 years since 1989, the level of contamination in 1989 and 1998 was significantly higher than that in 2010 and 2019. It was estimated that Phreagena clams with a shell length of ∼100 mm, as collected in this study, were alive for at least 10 years (Tada, 2009). Therefore, the samples collected may reflect pollution accumulation over a period of 10 years. However, the degradation of contaminants within the organism and differences in the degradation rates between the bivalves collected from deep-sea and those from the shallow waters needs further investigation and should be taken into account in future studies. PCBs were actively manufactured since the 1960s, and their production and use in Japan were prohibited in 1974. However, PCBs continued to leak into the environment after 1974, and strict disposal measures became mandatory in 2001. Applying this to the lifespan of the Phreagena clams, the relatively low amounts of PCBs observed in individuals after 2010 might reflect the effects of changes in environmental policies. However, the concentrations of pollutants in bivalves are dependent on both the pollutant concentration in the environments and the physiology of the organisms, such as body size and species (Bjork, 1995). In the Phreagena clams (including the two species, P. okutanii and P. soyoae) used in study, older samples were collected from slightly deeper levels (Table 1). We cannot completely exclude the possibility that differences in the environmental concentrations or species variations, arising from the differences in the sampling depths, could have influenced the results.
Myojin Knoll is located relatively far from dense human activity (Statistics Bureau of Japan [SBJ], 2020). The B. septemdierum individuals collected from this site, which has a very low amount of marine macro-debris (Nakajima et al., 2021), contained PCBs in amounts comparable to that in the Phreagena clams collected from Sagami Bay. This suggests that anthropogenic environmental pollution could be spreading over a wider area than expected. Myojin Knoll is located under the main current of Kuroshio; therefore, the pollutant could have been transported from the contaminated areas lying to the southwest of Myojin Knoll (Nakajima et al., 2021). Mussels enhance PCB contamination and bioavailability in their habitat by depositing PCB-enriched pseudofeces (Prince et al., 2021); hence, there are also concerns regarding the bioaccumulation of PCBs in co-existing crustaceans and other animals. Thus, to protect fragile deep-sea chemosynthetic ecosystems, it is necessary to continue monitoring these pollutants.
Conclusion
This study has suggested that environmental policy restrictions might be effective in reducing chemical pollution. However, the detection of POP contamination in deep-sea chemosynthetic animals suggests that the pollution may be globally spreading to vulnerable chemosynthetic organisms with limited or no feeding. Although the number of samples used in this study is small, this is the first report of POPs in a deep-sea chemosynthetic organism, and we provide an important baseline for future research. This study highlights the need for further detailed investigations and urgent actions to address POP contamination in deep-sea habitats, including that in non-feeding chemosynthetic animals.
Data Availability Statement
The original contributions presented in the study are included in the article/Supplementary Material, further inquiries can be directed to the corresponding author/s.
Ethics Statement
All animal experiments were conducted in accordance with the Guidelines for Proper Conduct of Animal Experiments (Science Council of Japan).
Author Contributions
TI: conceptualization, methodology, formal analysis, investigation, writing – original draft, and visualization. RN: conceptualization, methodology, and writing – review and editing. MT: project administration and writing – review and editing. SC and KF: supervision and writing – review and editing. All authors contributed to the article and approved the submitted version.
Conflict of Interest
The authors declare that IDEA Consultants, Inc. supported and conducted the chemical analyses.
Publisher’s Note
All claims expressed in this article are solely those of the authors and do not necessarily represent those of their affiliated organizations, or those of the publisher, the editors and the reviewers. Any product that may be evaluated in this article, or claim that may be made by its manufacturer, is not guaranteed or endorsed by the publisher.
Acknowledgments
We thank IDEA Consultants, Inc., for supporting and conducting the chemical analyses. We are grateful to all the researchers on board YK19-11 cruise, the operation teams of the HOV Shinkai 6500, and the crews of the R/V Yokosuka for their assistance with sample collection, as well as Yoshihiro Fujiwara and Shinji Tsuchida (JAMSTEC) for allowing us to use the archived samples. We also thank Editage [http://www.editage.com] for editing and reviewing this manuscript for English language.
Supplementary Material
The Supplementary Material for this article can be found online at: https://www.frontiersin.org/articles/10.3389/fmars.2021.751848/full#supplementary-material
References
Al-Sid-Cheikh, M., Rowland, S. J., Stevenson, K., Rouleau, C., Henry, T. B., and Thompson, R. C. (2018). Uptake, whole-body distribution, and depuration of nanoplastics by the scallop Pecten maximus at environmentally realistic concentrations. Environ. Sci. Technol. 52, 14480–14486. doi: 10.1021/acs.est.8b05266
Bjork, M. (1995). Bioavailability and uptake of hydrophobic organic contaminants in bivalve filter-feeders. Ann. Zool. Fenn. 32, 237–245.
Chiesa, L. M., Nobile, M., Malandra, R., Pessina, D., Panseri, S., Labella, G. F., et al. (2018). Food safety traits of mussels and clams: distribution of PCBs, PBDEs, OCPs, PAHs and PFASs in sample from different areas using HRMS-Orbitrap (R) and modified QuEChERS extraction followed by GC-MS/MS. Food Addit. Contam. Part A Chem. Anal. Control Expo. Risk Assess. 35, 959–971. doi: 10.1080/19440049.2018.1434900
Childress, J. J., and Fisher, C. R. (1992). The biology of hydrothermal vent animals – physiology, biochemistry, and autotrophic symbioses. Oceanogr. Mar. Biol. 30, 337–441.
Chu, F.-L. E., Soudant, P., and Hale, R. C. (2003). Relationship between PCB accumulation and reproductive output in conditioned oysters Crassostrea virginica fed a contaminated algal diet. Aquat. Toxicol. 65, 293–307. doi: 10.1016/s0166-445x(03)00152-8
Daugherty, C. E., and Lento, H. G. (1983). Chloroform-methanol extraction method for determination of fat in foods: collaborative study. J. Assoc. Off. Anal. Chem. 66, 927–932. doi: 10.1093/jaoac/66.4.927
Dewailly, E., Pereg, D., Knap, A., Rouja, P., Galvin, J., and Owen, R. (2007). “Exposure and effects of seafood-borne contaminants in maritime populations,” in Oceans and Human Health: Risks and Remedies From the Seas, eds P. J. Walsh, S. L. Smith, L. E. Fleming, H. M. Solo-Gabriele, and W. H. Gerwick (Burlington, MA: Academic Press), 181–191.
Domingo, J. L., and Bocio, A. (2007). Levels of PCDD/PCDFs and PCBs in edible marine species and human intake: a literature review. Environ. Int. 33, 397–405. doi: 10.1016/j.envint.2006.12.004
Erickson, M. D., and Kaley, R. G. (2011). Applications of polychlorinated biphenyls. Environ. Sci. Pollut. Res. 18, 135–151. doi: 10.1007/s11356-010-0392-1
Fisher, C. R. (1990). Chemoautotrophic and methanotrophic symbioses in marine invertebrates. Rev. Aquat. Sci. 2, 399–436.
Galloway, T. S., and Lewis, C. N. (2016). Marine microplastics spell big problems for future generations. Proc. Natl. Acad. Sci. U.S.A. 113, 2331–2333. doi: 10.1073/pnas.1600715113
German, C. R., Ramirez-Llodra, E., Baker, M. C., Tyler, P. A., and ChEss Scientific Steering Committee (2011). Deep-water chemosynthetic ecosystem research during the census of marine life decade and beyond: a proposed deep-ocean road map. PLoS One 6:e23259. doi: 10.1371/journal.pone.0023259
Ghosh, U., Zimmerman, J. R., and Luthy, R. G. (2003). PCB and PAH speciation among particle types in contaminated harbor sediments and effects on PAH bioavailability. Environ. Sci. Technol. 37, 2209–2217. doi: 10.1021/es020833k
Gobas, F. A. P. C., McCorquodale, J. R., and Haffner, G. D. (1993). Intestinal absorption and biomagnification of organochlorines. Environ. Toxicol. Chem. 12, 567–576. doi: 10.1002/etc.5620120316
Ikuta, T., Sugimura, M., Nemoto, S., Aoki, Y., Tame, A., Yamamoto, M., et al. (2018). Effects of a long-term rearing system for deep-sea vesicomyid clams on host survival and endosymbiont retention. Fish. Sci. 84, 41–51. doi: 10.1007/s12562-017-1149-2
Jamieson, A. J., Malkocs, T., Piertney, S. B., Fujii, T., and Zhang, Z. L. (2017). Bioaccumulation of persistent organic pollutants in the deepest ocean fauna. Nat. Ecol. Evol. 1:51. doi: 10.1038/s41559-016-0051
Jepson, P. D., Deaville, R., Barber, J. L., Aguilar, A., Borrell, A., Murphy, S., et al. (2016). PCB pollution continues to impact populations of orcas and other dolphins in European waters. Sci. Rep. 6:18573. doi: 10.1038/srep18573
Kimbrough, K. L., Johnson, W. E., Lauenstein, G. G., Christensen, J. D., and Apeti, D. A. (2008). An Assessment of Two Decades of Contaminant Monitoring in the Nation’s Coastal Zone. NOAA Technical Memorandum NOS NCCOS 74. Silver Spring, MD: NOAA.
Kimbrough, K. L., Johnson, W. E., Lauenstein, G. G., Christensen, J. D., and Apeti, D. A. (2009). An assessment of Polybrominated Diphenyl Ethers (PBDEs) in Sediments and Bivalves of the U.S. Coastal Zone. NOAA Technical Memorandum NOS NCCOS 94. Silver Spring, MD: NOAA.
Klump, J. V., Krezoski, J. R., Smith, M. E., and Kaster, J. L. (1987). Dual tracer studies of the assimilation of an organic contaminant from sediments by deposit feeding oligochaetes. Can. J. Fish. Aquat. Sci. 44, 1574–1583. doi: 10.1139/f87-190
Kolandhasamy, P., Su, L., Li, J. N., Qu, X. Y., Jabeen, K., and Shi, H. H. (2018). Adherence of microplastics to soft tissue of mussels: a novel way to uptake microplastics beyond ingestion. Sci. Total Environ. 610–611, 635–640. doi: 10.1016/j.scitotenv.2017.08.053
Koshiba, J., Hirai, Y., and Sakai, S. (2019). Historical and future polychlorinated biphenyl emission trends in Japan. Chemosphere 232, 387–395. doi: 10.1016/j.chemosphere.2019.04.206
Kuwahara, H., Yoshida, T., Takaki, Y., Shimamura, S., Nishi, S., Harada, M., et al. (2007). Reduced genome of the thioautotrophic intracellular symbiont in a deep-sea clam Calyptogena okutanii. Curr. Biol. 17, 881–886. doi: 10.1016/j.cub.2007.04.039
Lawson, M. C., Cullen, J. A., Nunnally, C. C., Rowe, G. T., and Hala, D. N. (2021). PAH and PCB body-burdens in epibenthic deep-sea invertebrates from the northern Gulf of Mexico. Mar. Pollut. Bull. 162:111825. doi: 10.1016/j.marpolbul.2020.111825
Le Pennec, M., Beninger, P. G., and Herry, A. (1995). Feeding and digestive adaptations of bivalve molluscs to sulphide-rich habitats. Comp. Biochem. Phys. A 111, 183–189. doi: 10.1016/0300-9629(94)00211-B
Lee, H. J., and Kim, G. B. (2015). An overview of polybrominated diphenyl ethers (PBDEs) in the marine environment. Ocean Sci. J. 50, 119–142. doi: 10.1007/s12601-015-0010-8
Lehmann, D. W., Levine, J. F., and McHugh Law, J. (2007). Polychlorinated biphenyl exposure causes gonadal atrophy and oxidative stress in Corbicula fluminea clams. Toxicol. Pathol. 35, 356–365. doi: 10.1080/01926230701230288
Llodra, E. R., and Billett, D. S. M. (2006). “Deep-sea ecosystems: pristine biodiversity reservoir and technological challenges,” in The Exploration of Marine Biodiversity: Scientific and Technological Challenges, ed. C. M. Duarte (Bilbao: Fundacion BBVA), 63–92.
Matthews, H. B., and Dedrick, R. L. (1984). Pharmacokinetics of PCBs. Annu. Rev. Pharmacol. Toxicol. 24, 85–103. doi: 10.1146/annurev.pa.24.040184.000505
Monirith, I., Ueno, D., Takahashi, S., Nakata, H., Sudaryanto, A., Subramanian, A., et al. (2003). Asia-Pacific mussel watch: monitoring contamination of persistent organochlorine compounds in coastal waters of Asian countries. Mar. Pollut. Bull. 46, 281–300. doi: 10.1016/s0025-326x(02)00400-9
Nakajima, R., Tsuchiya, M., Yabuki, A., Masuda, S., Kitahashi, T., Nagano, Y., et al. (2021). Massive occurrence of benthic plastic debris at the abyssal seafloor beneath the Kuroshio Extension, the North West Pacific. Mar. Pollut. Bull. 166:112188. doi: 10.1016/j.marpolbul.2021.112188
Olenycz, M., Sokolowski, A., Niewinska, A., Wolowicz, M., Namiesnik, J., Hummel, H., et al. (2015). Comparison of PCBs and PAHs levels in European coastal waters using mussels from the Mytilus edulis complex as biomonitors. Oceanologia 57, 196–211. doi: 10.1016/j.oceano.2014.12.001
Page, H. M., Fiala-Medioni, A., Fisher, C. R., and Childress, J. J. (1991). Experimental-evidence for filter-feeding by the hydrothermal vent mussel, Bathymodiolus thermophilus. Deep Sea Res. Part I Oceanogr. Res. Pap. 38, 1455–1461. doi: 10.1016/0198-0149(91)90084-S
Ponnudurai, R., Kleiner, M., Sayavedra, L., Petersen, J. M., Moche, M., Otto, A., et al. (2017). Metabolic and physiological interdependencies in the Bathymodiolus azoricus symbiosis. ISME J. 11, 463–477. doi: 10.1038/ismej.2016.124
Porte, C., and Albaiges, J. (1994). Bioaccumulation patterns of hydrocarbons and polychlorinated biphenyls in bivalves, crustaceans, and fishes. Arch. Environ. Contam. Toxicol. 26, 273–281. doi: 10.1007/BF00203552
Prince, K. D., Crotty, S. M., Cetta, A., Delfino, J. J., Palmer, T. M., Denslow, N. D., et al. (2021). Mussels drive polychlorinated biphenyl (PCB) biomagnification in a coastal food web. Sci. Rep. 11:9180. doi: 10.1038/s41598-021-88684-9
Ramu, K., Kajiwara, N., Sudaryanto, A., Isobe, T., Takahashi, S., Subramanian, A., et al. (2007). Asian mussel watch program: contamination status of polybrominated diphenyl ethers and organochlorines in coastal waters of Asian countries. Environ. Sci. Technol. 41, 4580–4586. doi: 10.1021/es070380p
Safe, S. H. (1994). Polychlorinated-biphenyls (PCBs): environmental-impact, biochemical and toxic responses, and implications for risk assessment. Crit. Rev. Toxicol. 24, 87–149. doi: 10.3109/10408449409049308
Statistics Bureau of Japan [SBJ] (2020). Statistical Handbook of Japan 2020. Shinjuku: Statistics Bureau of Japan [SBJ].
Tada, Y. (2009). Life History Characteristics of the Cold Seep Bivalve Calyptogena by Means of In-Situ Shell Growth Experiment. Ph.D. thesis. Tokyo: The University of Tokyo.
Takahashi, S., Karri, R., and Tanabe, S. (2014). “Contamination by persistent organic pollutants and related compounds in deep-sea ecosystems along frontal zones around Japan,” in Chemical Oceanography of Frontal Zones, ed. L. M. Belkin (Berlin: Springer-Verlag), 1–36.
Takahashi, S., Oshihoi, T., Isobe, T., Ramu, K., Ohmori, K., Kubodera, T., et al. (2009). “Contamination by persistent organohalogen compounds in deep-sea fishes from off the pacific coast of Northern Japan,” in Deep-Sea Fauna and Pollutants off Pacific Coast of Northern Japan, ed. T. Fujita (Tokyo: National Museum of Nature and Science), 737–744.
Talsness, C. E. (2008). Overview of toxicological aspects of polybrominated diphenyl ethers: a flame-retardant additive in several consumer products. Environ. Res. 108, 158–167. doi: 10.1016/j.envres.2008.08.008
Toyoshima, S., Isobe, T., Ramu, K., Miyasaka, H., Omori, K., Takahashi, S., et al. (2009). “Organochlorines and brominated flame retardants in deep-sea ecosystem of Sagami Bay,” in Interdisciplinary Studies on Environmental Chemistry – Environmental Research in Asia, eds T. Oshihoi, T. Isobe, A. Subramanian, S. Suzuki, and S. Tanabe (Tokyo: Terra Pub.), 83–90.
United Nations Environment Programme [UNEP] (2001). Final Act of the Conference of Plenipotentiaries on the Stockholm Convention on Persistent Organic Pollutants. Nairobi: United Nations Environment Programme.
United States Environmental Protection Agency [USEPA] (2003). Method 1668, Revision A Chlorinated Biphenyl Congeners in Water, Soil, Sediment, Biosolids, and Tissue by HRGC/HRMS. Washington, DC: United States Environmental Protection Agency [USEPA].
United States Environmental Protection Agency [USEPA] (2010). Method 1614A, Brominated Diphenyl Ethers in Water Soil, Sediment and Tissue by HRGC/HRMS. Washington, DC: United States Environmental Protection Agency [USEPA].
Wessel, P., Luis, J. F., Uieda, L., Scharroo, R., Wobbe, F., Smith, W. H. F., et al. (2019). The generic mapping tools version 6. Geochem. Geophy. Geosy. 20, 5556–5564. doi: 10.1029/2019GC008515
Yamaguchi, Y., Sato, F., Akiyama, K., Kouno, E., Tsutsumi, S., and Takada, H. (2000). Biomonitoring of organic micropollutants in coastal zones using mussels -characteristics of accumulated pollutants and the application to Tokyo Bay-. Chikyukagaku 34, 41–57. doi: 10.14934/chikyukagaku.34.41
Yamashita, R., Tanaka, K., Yeo, B. G., Takada, H., van Franeker, J. A., Dalton, M., et al. (2019). “Hazardous cemicals in plastics in marine environments: international pellet watch,” in Handbook of Environmental Chemistry, eds H. Takada and H. Karapanagioti (Berlin: Springer Verlag), 163–183. doi: 10.1007/698_2018_299
Yu, F., Yang, C., Zhu, Z., Bai, X., and Ma, J. (2019). Adsorption behavior of organic pollutants and metals on micro/nanoplastics in the aquatic environment. Sci. Total Environ. 694:133643. doi: 10.1016/j.scitotenv.2019.133643
Yuan, X. T., Yang, X. L., Na, G. S., Zhang, A. G., Mao, Y. Z., Liu, G. Z., et al. (2015). Polychlorinated biphenyls and organochlorine pesticides in surface sediments from the sand flats of Shuangtaizi Estuary, China: levels, distribution, and possible sources. Environ. Sci. Pollut. Res. 22, 14337–14348. doi: 10.1007/s11356-015-4688-z
Keywords: persistent organic pollutant, polychlorinated biphenyl, polybrominated diphenyl ether, bioaccumulation, deep-sea chemosynthetic bivalve, Phreagena, Bathymodiolus
Citation: Ikuta T, Nakajima R, Tsuchiya M, Chiba S and Fujikura K (2021) Interdecadal Distribution of Persistent Organic Pollutants in Deep-Sea Chemosynthetic Bivalves. Front. Mar. Sci. 8:751848. doi: 10.3389/fmars.2021.751848
Received: 02 August 2021; Accepted: 29 October 2021;
Published: 22 November 2021.
Edited by:
Ilaria Corsi, University of Siena, ItalyReviewed by:
Samir R. Damare, National Institute of Oceanography, Council of Scientific and Industrial Research (CSIR), IndiaBegoña Jiménez, Consejo Superior de Investigaciones Científicas (CSIC), Spain
Ke Pan, Shenzhen University, China
Copyright © 2021 Ikuta, Nakajima, Tsuchiya, Chiba and Fujikura. This is an open-access article distributed under the terms of the Creative Commons Attribution License (CC BY). The use, distribution or reproduction in other forums is permitted, provided the original author(s) and the copyright owner(s) are credited and that the original publication in this journal is cited, in accordance with accepted academic practice. No use, distribution or reproduction is permitted which does not comply with these terms.
*Correspondence: Tetsuro Ikuta, dGVpa3V0YUBqYW1zdGVjLmdvLmpw