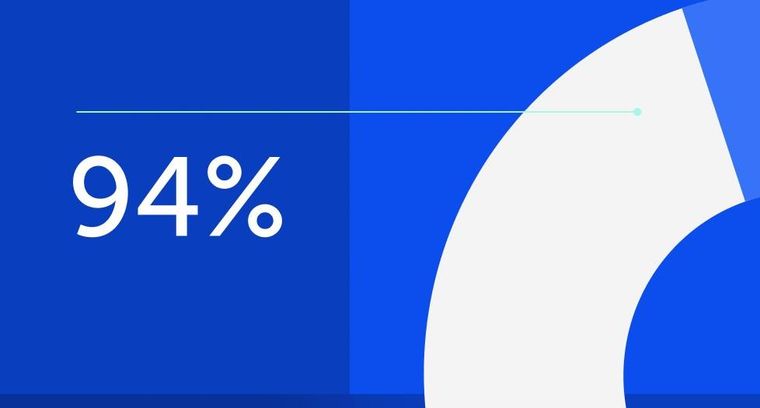
94% of researchers rate our articles as excellent or good
Learn more about the work of our research integrity team to safeguard the quality of each article we publish.
Find out more
ORIGINAL RESEARCH article
Front. Mar. Sci., 18 November 2021
Sec. Marine Megafauna
Volume 8 - 2021 | https://doi.org/10.3389/fmars.2021.744202
This article is part of the Research TopicMovement and Connectivity of Large Pelagic SharksView all 22 articles
Understanding how mobile, marine predators use three-dimensional space over time is central to inform management and conservation actions. Combining tracking technologies can yield powerful datasets over multiple spatio-temporal scales to provide critical information for these purposes. For the white shark (Carcharodon carcharias), detailed movement and migration information over ontogeny, including inter- and intra-annual variation in timing of movement phases, is largely unknown in the western North Atlantic (WNA), a relatively understudied area for this species. To address this need, we tracked 48 large juvenile to adult white sharks between 2012 and 2020, using a combination of satellite-linked and acoustic telemetry. Overall, WNA white sharks showed repeatable and predictable patterns in horizontal movements, although there was variation in these movements related to sex and size. While most sharks undertook an annual migratory cycle with the majority of time spent over the continental shelf, some individuals, particularly adult females, made extensive forays into the open ocean as far east as beyond the Mid-Atlantic Ridge. Moreover, increased off-shelf use occurred with body size even though migration and residency phases were conserved. Summer residency areas included coastal Massachusetts and portions of Atlantic Canada, with individuals showing fidelity to specific regions over multiple years. An autumn/winter migration occurred with sharks moving rapidly south to overwintering residency areas in the southeastern United States Atlantic and Gulf of Mexico, where they remained until the following spring/summer. While broad residency and migration periods were consistent, migratory timing varied among years and among individuals within years. White sharks monitored with pop-up satellite-linked archival tags made extensive use of the water column (0–872 m) and experienced a broad range of temperatures (−0.9 – 30.5°C), with evidence for differential vertical use based on migration and residency phases. Overall, results show dynamic inter- and intra-annual three-dimensional patterns of movements conserved within discrete phases. These results demonstrate the value of using multiple tag types to track long-term movements of large mobile species. Our findings expand knowledge of the movements and migration of the WNA white shark population and comprise critically important information to inform sound management strategies for the species.
The movements of individual animals over time and space have profound impacts on animal ecology at all levels from the individual to the ecosystem (Nathan et al., 2008; Earl and Zollner, 2017). For highly mobile species, horizontal movement patterns can be predictable, composed of residency areas connected by migration corridors, with population-level movements in response to regional biotic and abiotic drivers (Bowlin et al., 2010; Shaw, 2016). Migrations between distant residency regions commonly occur in response to maintaining optimal thermal envelopes (Kessel et al., 2014; Payne et al., 2016, 2018), but are often associated with seeking out highly productive areas (i.e., high prey availability) (e.g., Jorgensen et al., 2010; Barnett et al., 2011) and areas for reproduction (Chapman et al., 2015). While population-level movement may appear predictable, certain species can show variability in migration timing among individuals and across years as a result of the dynamic environment they inhabit and their individual physiological needs (Brodersen et al., 2012; Fraser et al., 2019; Bauer et al., 2020). Defining animal residency and migration routes and variation in timing of movements consequently is essential to accurately delineate core space use for wildlife management. Moreover, vertical movements of susceptible aquatic animals can reveal regions where likelihood of negative interactions can occur, for example, in depths occupied relative to fishing effort and potential for capture (Coelho et al., 2015; Tolotti et al., 2015; Hutchinson et al., 2019). Vertical movements can also be predictable with species exhibiting specific behaviors during residency vs. migration phases (Bonfil et al., 2005; Francis et al., 2012). Gaining a thorough understanding of a species’ ecology therefore requires multi-faceted long-term telemetry datasets from individual animals across and within multiple life stages (Speed et al., 2010; Hussey et al., 2015). Importantly, this assessment requires data over multiple years to capture individual and population-level variation in animal movements, to accurately inform measures for spatial and temporal management.
Contemporary research using multiple tag technologies to obtain longer-term datasets has yielded key insights into how aquatic animals interact with their environment (e.g., Vaudo et al., 2017; Braun et al., 2019; Cochran et al., 2019; Hoffmayer et al., 2021), potential drivers of vertical and horizontal movement (e.g., Coffey et al., 2017; Gaube et al., 2018; Lee et al., 2021), and how space use changes through ontogeny (e.g., Skomal et al., 2017; Ajemian et al., 2020), while also providing critical information for the implementation of effective management and conservation strategies (e.g., Acuña-Marrero et al., 2017; White et al., 2017; Bangley et al., 2020). In recent years, studies using pop-up satellite-linked archival tags (PSATs) on sharks have revealed long-term movement and migration routes as well as habitat use patterns (e.g., Weng et al., 2007a, b; Pade et al., 2009; Comfort and Weng, 2015). Additionally, this type of archival tag, if physically recovered, can deliver high resolution time series data on ambient temperature and depth, enhancing our knowledge of the complex temporal and behavioral dynamics of marine predators (Sims, 2010). While PSATs yield important information related to movements and behavior, deployments are usually short-term (<12 months) and light-based geolocations exhibit relatively high uncertainty (root mean square errors within ∼80–150 km; Braun et al., 2018). Incorporating multiple tag types, such as satellite-linked smart position and temperature transmitting (SPOT) and ultrasonic acoustic tags, on individual animals can both substantially increase the temporal scale of tracks (5–10 years) and also can provide increased accuracy of locations [LC = 3 SPOT error <250 m; CLS (Collecte Localisation Satellites), 2016]. Finally, higher-accuracy geolocations derived from SPOT and acoustic detections can be incorporated into PSAT geolocation algorithms to improve track estimation from these tags.
The white shark (Carcharodon carcharias) is a wide-ranging, apex predator distributed globally in temperate to sub-tropical waters (Bruce, 2008). The species is described to occur in seven general regions: southern Africa, Australia/New Zealand, the western North Atlantic (WNA), the southwest Atlantic, the Mediterranean, and the northwest and northeast Pacific (NEP) (Compagno et al., 1997). While population structure is not clearly defined within and among all regions, genetically distinct groups exist at the regional level such as in the WNA and southern Africa (O’Leary et al., 2015) and at finer scales such as in southern-western and eastern Australia/New Zealand (Blower et al., 2012; Gubili et al., 2015; Hillary et al., 2018). As a highly migratory species, the white shark has been shown to undertake long-distance movements along continental shelves, forays into pelagic waters, and infrequently across ocean basins (Bonfil et al., 2005; Weng et al., 2007a; Domeier and Nasby-Lucas, 2008; Duffy et al., 2012), with no evidence of trans-equatorial movements (Jorgensen et al., 2010).
Studies from various regions around the world show that most white sharks exhibit migratory and residency behaviors (Bruce et al., 2006; Duffy et al., 2012; Skomal et al., 2017; Lee et al., 2021) and in many instances these movement phases are predictable (Weng et al., 2007a; Jorgensen et al., 2010; Duffy et al., 2012). Drivers of these movements have been suggested to be abiotic factors including temperature or currents as well as biotic factors such as mating, pupping, prey availability, or predation risk (Duffy et al., 2012; Domeier and Nasby-Lucas, 2013; Hoyos-Padilla et al., 2016; Skomal et al., 2017; Jorgensen et al., 2019). White sharks from multiple ocean basins have been shown to spend considerable time in coastal over-shelf waters with regular offshore, pelagic phases (Bonfil et al., 2005; Jorgensen et al., 2010; Domeier, 2012; Duffy et al., 2012; Bradford et al., 2020). At times, these pelagic phases can coincide in latitude or longitude with a typical population-level seasonal migration pattern (Weng et al., 2007a; Domeier and Nasby-Lucas, 2008, 2013) or in contrast to the typical seasonal pattern (Bonfil et al., 2010; Skomal et al., 2017; Bradford et al., 2020; Spaet et al., 2020). Clarifying these movement phases in understudied white shark populations is critical given their designation as ‘Vulnerable’ by the IUCN (Rigby et al., 2019), their propensity to occupy coastal waters where there is potential for human-shark conflicts, and their naturally low population sizes as apex predators in marine food webs (Huveneers et al., 2018; Kock et al., 2018; Colefax et al., 2020).
Aspects of the biology, ecology, and status of the white shark population in the WNA have been documented in only a handful of studies, beginning with Casey and Pratt (1985), and updated and expanded on in Curtis et al. (2014). The WNA population is considered to have declined in abundance in the 1970s and 1980s, with evidence of a population bottleneck (O’Leary et al., 2015), followed by an apparent recovery beginning in the 1990s and continuing to the present day (Skomal et al., 2012; Curtis et al., 2014). There is a general paucity of data regarding large juvenile to adult white sharks in the WNA compared to other geographical regions (e.g., southern Africa, Australia/New Zealand, northeast Pacific), including limited data regarding their core movement phases. More specifically, information related to the residency, migration, and variation in timing of individuals when in and transitioning between phases is lacking for the WNA. Through a combination of satellite telemetric methods, Skomal et al. (2017) documented more extensive ranges of white sharks in the WNA than previously described, and confirmed a seasonal, predominantly latitudinal migration. In addition, these results demonstrated an ontogenetic shift in horizontal movements from predominantly shelf-oriented to more pelagic with increasing body size. Deep dives to mesopelagic zones during pelagic phases have been proposed as a foraging strategy (Gaube et al., 2018) and/or sexual segregation by pregnant females (Skomal et al., 2017). The New York Bight was proposed (Casey and Pratt, 1985) and subsequently confirmed (Curtis et al., 2018) to be a summer nursery area for the population with young-of-year (YOY) sharks demonstrating relatively narrow depth and temperature preferences (Shaw et al., 2021) while in the region. Additionally, Curtis et al. (2018) demonstrated a similar but much less extensive latitudinal migration for YOY sharks with individuals moving to shelf waters off the Carolinas in autumn/winter before subsequently returning north in late spring/early summer. More recently, Bastien et al. (2020) showed that movements of white sharks into Atlantic Canada were more common than previously thought, potentially as a result of population recovery and/or a northward range expansion. SPOT tags further revealed repeated seasonal movements into the region suggesting evidence for philopatry (Bastien et al., 2020).
Expanding on previous work on white shark movements in the WNA (Skomal et al., 2017; Curtis et al., 2018; Bastien et al., 2020; Winton et al., 2021), the overall objective of the current study was to broadly examine individual- and population-level variation in white shark spatial ecology across the region. Specifically, through the use of long-term SPOTs coupled with PSATs and acoustic telemetry, multi-year data were collected to examine and provide an overview of both inter- and intra-individual variation in movement behaviors in both horizontal and vertical planes. The specific aims of the study were to: (1) quantify residency and migratory phases of WNA white sharks over an annual cycle; (2) compare movement and migration patterns by size and sex while proposing potential drivers of movement; (3) examine variation in individual-level movement patterns across multiple years and determine the extent of fidelity or philopatric behavior to identified residency sites; (4) describe vertical behavior and variation associated with residency and migratory phases; and (5) propose a model of white shark population-level movements in the WNA that is consistent with their life history and accounts for ontogenetic and sex-specific differences in movement. Identifying size-based critical habitat variation in white shark movement behaviors for the WNA population has been identified as a research priority for this species (Huveneers et al., 2018).
Large juvenile and adult white sharks (n = 48) were captured by the fishing crew of the M/V OCEARCH at multiple locations along the Atlantic coast of the United States and Canada from 2012 to 2020 (Figures 1, 2). Sampling areas included waters around the southeastern United States (South Carolina to Florida), Massachusetts, and Nova Scotia. Methods of shark capture and processing followed procedures described in Domeier and Nasby-Lucas (2012) and Bastien et al. (2020). In brief, sharks were captured using a modified, break-away drumline technique or by rod-and-reel with 20/0 circle hooks deployed from a V-hull center console boat. Drumline leaders were rigged with buoys, weights, and/or bamboo crosses to prevent hook ingestion. Cable leaders were threaded through polypropylene rope to reduce the possibility of wire damaging the animal. If a large shark was sighted (>4 m total length, TL), a 27/0 circle hook was sometimes used to target that specific animal. All fishing lines and gear were monitored continuously while deployed. Once a shark was hooked, the shark was controlled with additional buoys as needed and guided onto the OCEARCH ship’s research platform submerged below the water’s surface. The platform was then raised, the shark was secured, and sampling and tag attachment procedures were initiated. While the shark was on the platform, the hook was removed, a wet towel was placed over the eyes and gill slits, and a ventilation hose providing free-flowing seawater was placed into the mouth to maintain gas exchange and reduce physiological stress. Ambient seawater was also regularly poured over the shark to keep the skin moist and the animal’s temperature stable. Beginning in 2016, an experienced aquatic veterinarian was present on the ship and monitored the health of all animals during capture, handling, and release. As a precautionary measure and in response to a recommendation by permitting agencies, a rating score to assess factors such as handling time and associated physical condition of the animal was introduced in 2019. These scores were assigned prior to bringing sharks to the platform and during work-up procedures to determine the extent of research procedures to be conducted on each animal. The rating score was developed using Hueter et al. (2006) as a framework and modified to account for differences in the species and capture/handling methods used in this study.
Figure 1. Frequency-size distribution for 48 white sharks caught and tagged in the western North Atlantic between 2012 and 2020.
Figure 2. Daily hSSM-derived locations (A) and an overall estimated kernel density (B) for all white sharks tagged between 2012 and 2020. Yellow stars in panel (A) represent general locations of tagging in NE Florida, Georgia/South Carolina, Massachusetts, and two locations in Nova Scotia.
While sharks were on the platform, work-ups were completed in a two-stage process with measurements taken, samples collected, examinations performed, and tags attached simultaneously. Sharks were equipped with one (n = 5), two (n = 22), or three (n = 21) types of transmitters, which varied in transmission type, battery longevity, accuracy, and data parameters. These comprised externally attached real-time SPOTs and PSATs (Wildlife Computers Inc., Redmond, WA, United States) and internally implanted coded acoustic tags (Vemco/InnovaSea, Bedford, NS, Canada). Length of time for sharks on the platform ranged 15–25 min ( = 17.6; SD = 3.7), after which the platform was lowered allowing the animal to swim off following a brief recovery period of usually 1–2 min. Post-release behavior was monitored visually from a small boat and/or with drone cameras, followed by data received from the tags.
Knowledge of white shark life-stage characteristics, particularly size-at-maturity for both sexes, remains uncertain, particularly in the WNA. Reported or suggested size-at-maturity in the white shark ranges from 3.5 to 3.8 m TL for males and 4.5 to 5.0 m TL for females (Francis, 1996; Pratt, 1996; Compagno, 2001; Tanaka et al., 2011), with more recent studies using a narrower range of >3.5–3.8 m TL for males and >4.5–4.8 m TL for females (Weng et al., 2007a; Bruce and Bradford, 2012; Curtis et al., 2014; Skomal et al., 2017). It has been suggested, however, based on unconfirmed evidence, that maturity may be reached at smaller sizes: 2.7–3.1 m for males and 3.9–4.5 m for females (Francis, 1996; Pratt, 1996; Malcolm et al., 2001). In the current study, maturity was directly (through physical examination and morphometrics) and indirectly (through analyses of ultrasound exams, hormone levels, and sperm collected) assessed for each captured shark. Based on this assessment, male and female sharks in our sample were classified into three and four life stages, respectively. Male groups comprised large juvenile (≤2.80 m TL), subadult (2.81–3.45 m TL for all but one animal), and adult (≥3.46 m TL for all but one animal). One male shark (3.32 m TL) smaller than 3.46 m TL was classified as an adult based on clasper condition. Female groups comprised large juvenile (≤3.30 m TL), subadult (3.31–3.79 m TL), maturing (3.80–4.19 m TL), and mature (≥4.20 m TL). The distinction of “maturing” and “mature” groups within female sharks was determined based on measured length/girth ratios and estradiol levels measured in blood collected from the sharks during the work-up procedures (Gelsleichter, unpublished data). We acknowledge the term “subadult” is often not well defined with quantitative criteria. Here the terms distinguish the difference between juvenile and subadult stages with subadults being nearer to sexual maturity. This is deemed necessary given the propensity for ontogenetic shifts in movements and diet, whereby white shark behavior and physiology are undergoing change through growth during this life stage transition period. Consequently, we classified juvenile males ≥2.8 m TL but not yet mature as subadult males and juvenile females ≥3.3 m TL but not yet maturing as subadult females.
The first stage of research procedures on the ship’s platform was conducted while the shark was in lateral recumbency to expose the abdomen and one lateral surface. An acoustic transmitter was surgically implanted into the coelomic cavity (Vemco model V16-6x, lifespan = 3,540 days, n = 40; or Vemco model V16-4x, lifespan = 2,435 days, n = 1; 85.4% of total individuals sampled). Acoustic transmitters and surgical tools were sterilized in a bath of benzalkonium chloride prior to use. The surgical procedure consisted of a 4–6 cm incision made with a sterile scalpel blade through the epidermis, muscle wall, and peritoneum using forceps to hold the body wall, to ensure internal organs were protected. Following incision, the transmitter was inserted into the coelomic cavity, and the incision was closed using 2–4 interrupted or cruciate sutures (Ethicon PDS, absorbable monofilament, Ethicon Inc., Somerville, NJ, United States).
For the second stage of procedures, sharks were rolled upright to rest on their ventral surface and SPOT tags (Wildlife Computers model SPOT-257 or SPOT-258) were attached to the first dorsal fin. The SPOT tags, which transmit messages via the Argos satellite network when the tag breaks the water–air interface, were attached to the leading edge of the first dorsal fin and located to maximize antenna height while also ensuring secure attachment to the fin. Tags were secured with four surgical-grade nylon bolts, plastic spacers, and stainless-steel locknuts inserted through holes in the fin made with a cordless electric drill. The hardware was designed to fail to allow the tag to detach from the fin after battery life has been expended. Observations from other regions suggest this attachment method results in tag shedding 1–6 years after tag deployment, with little effect on health and vitality of the shark (Jewell et al., 2011). Tags were coated with antifouling paint to reduce interference in tag function by bio-fouling organisms. A total of 44 sharks received the larger SPOT-257 tags with an estimated 5-year battery life, three sharks received a smaller SPOT-258 tag with an estimated 1-year battery life, and one shark did not receive a SPOT tag due to permit restrictions at the time on tag limits related to shark body length. All SPOT tags were programmed to provide horizontal location estimates at a repetition rate of 15 seconds when the upper wet/dry sensor detected the tag had broken the surface of the water.
For 21 sharks (large juvenile [n = 6], subadult [n = 6], maturing [n = 5], and mature [n = 4]), PSATs (Wildlife Computers MiniPAT) were attached into the dorsal musculature just below the first dorsal fin. Tags were coated with antifouling paint as above. A stainless steel or titanium dart attached to a 15-cm stainless cable tether that was protected with heat-shrink tubing (3M, Two Harbors, MN, United States) was used to anchor the device. Tags were programmed to detach after periods ranging from 180 to 365 days, float to the sea surface, and transmit a summary of their archived data via the Argos satellite system until battery failure. The PSATs archived measurements of ambient light, temperature, and pressure every 5–15 s that were summarized into depth/temperature time series at intervals ranging from 75 to 600 s for satellite transmission. Time-at-depth and time-at-temperature histogram data were summarized into 12- or 24-h periods.
All geolocations derived from sharks outfitted with SPOT tags included error estimates in the form of a location class (3,2,1,0,A,B,Z) and/or Kalman-derived error ellipses. While most tags deployed in this study are still active and providing data, a cut-off date of 30 November 2020 was established for all SPOT data used in the analyses here. Data were filtered in three steps to remove spurious locations as follows: (i) locations with no error estimate (i.e., Z class geolocations); (ii) locations on land; and (iii) by applying a speed filter (3 ms–1), unrealistic locations from previous reliable locations based on animal swimming speed. Where available, acoustic detections for each SPOT-tagged shark were included to improve geolocation estimates. While some detections were obtained from our own acoustic receivers deployed in the NE Florida region, the majority were obtained through the major acoustic collaborative networks in the WNA including the Florida Atlantic Coast Telemetry (FACT), the Atlantic Coast Telemetry (ACT), and the Ocean Tracking Network (OTN) nodes. These data-sharing networks include receiver arrays that span coastal waters from Nova Scotia to the Gulf of Mexico (GOM) and detection data are distributed three to four times per year among acoustic telemetry researchers who participate in the networks. For each shark, when consecutive acoustic detections were provided on a single receiver over a day, one random time during that detection block was chosen to incorporate into the dataset for that shark.
Raw, filtered SPOT and acoustic locations were first presented as abacus plots to define broad-scale movements into regions over time and to assess multi-year fidelity to specific residence areas. Regularized tracks were then constructed using combined SPOT and acoustic tag locations. Joint estimation of behavioral state over multiple individual movement datasets is known to improve parameter estimates (Jonsen, 2016), consequently, a hierarchical (i.e., joint) state-space model (hSSM) was fitted to the combined data using the ‘bsam’ package in the R Statistical Environment (R Core Team, 2020). hSSMs provide both daily regularized locations and an estimate of the behavioral state at each location (Jonsen et al., 2007, 2013; Acuña-Marrero et al., 2017; Bastien et al., 2020). Behavioral states in the model are estimated using a combination of turn angle and move persistence as defined in Jonsen (2016). Resultant behavioral state values ranged from 1 to 2, representing transient (relatively fast and more directional movement paths) to area-restricted (relatively slow and more meandering movement paths) behaviors, respectively (Jonsen et al., 2007). Behaviors were classified as transient when scores were ≤1.25 and resident when ≥1.75. As SPOT tag transmissions can be irregular in time, complete tracks for individuals were segmented for any time gaps >20 days (Bastien et al., 2020) prior to model fitting. Resulting segments of less than 10 locations or shorter than 5 days were not included in subsequent analyses. Daily, regularized locations were then processed for three key analyses. To identify on/off shelf movements, locations were clipped to the continental shelf (∼<200 m depth contour with 50 km buffer) using ArcGIS 10.8 and mapped by individual and defined groups (i.e., maturity state and sex). Using linear regression, ontogenetic differences within sexes were examined to test for increasing use of off-shelf waters by size class. To identify core-area use relative to season and by sex and life stage, kernel density estimates were calculated using the Geostatistical and Spatial Analyst extensions in ArcGIS 10.8. Finally, to examine annual seasonality and inter- and intra-annual variability in residence and migration timing, regularized locations were assessed by plotting latitudinal location over time for all years combined and for each year independently. Visual examination of plots of spatial distributions of sharks during each time period, patterns of latitudinal shifts in movements, and hSSM-derived behavioral scores were used to define behavioral seasons to include the bulk of individuals tracked during each respective time period. Migration corridors were defined using a combination of timing and speed of movements, the behavioral score assigned in the hSSM, along with horizontal locations during each track segment. Migration corridors and timing of migration were only utilized for track segments in which both clear departure/arrival locations and dates to and from residency areas were identifiable.
Transmitted PSAT data were decoded with manufacturer software (WC-DAP 3.0, Wildlife Computers, Inc.), and light-based geolocations were estimated using GPE3 software (Wildlife Computers, Inc.) with the animal speed parameter set at 2 ms–1 (based on empirical data from Watanabe et al., 2019). This speed parameter is used in the geolocation framework to construct a Gaussian kernel that represents allowable daily diffusion over a model grid and thus differed from the 3 ms–1 threshold that was used above as a max daily displacement filter for the SPOT-derived data. The GPE3 model is based, in part, on previous work by Pedersen et al. (2008) that used hidden Markov models to geolocate Atlantic cod. The approach uses the archived tag data (light level, depth, and temperature) and corresponding sea surface temperature (SST) and bathymetry reference data in a gridded hidden Markov model to generate a most probable track (MPT) and associated uncertainty. Double tagging of a subset of individual sharks allowed improvement of track estimation through incorporating SPOT locations into the GPE3 processing as “known” locations. Prior to track estimation, the raw SPOT locations were first filtered using the ‘foieGras’ package in R (Jonsen et al., 2020) with the speed filter set for 3 ms–1. Obvious outliers (e.g., locations on land) were excluded. If available, acoustic detections (maximum of one per day) were similarly used in GPE3 to supplement satellite-transmitted data to further optimize the derived geolocation estimates.
The 48 large juvenile to adult white sharks were caught and tagged between September 2012 and October 2020 (Table 1). Locations of capture/tagging included sites along the coast of the SE United States (n = 7), Massachusetts (n = 15), and Nova Scotia (n = 26). Sharks ranged in size from 2.00 m to 5.01 m TL and comprised 27 females (9 large juvenile, 6 subadult, 5 maturing, and 7 mature) and 21 males (5 large juvenile, 6 subadult, and 10 mature) (Figure 1).
Of the 48 sharks, 47 were equipped with SPOT tags, 24 with PSATs, and 41 with acoustic transmitters. Of the 47 SPOT-tagged sharks, all provided locations (mean locations provided = 281; 16–2,791) with track durations ranging from 38 to 2,647 days ( = 511) (Table 1). Additionally, 23 of 41 sharks with acoustic transmitters provided 2,837 detections ( = 123 per shark) between 2016 and 2020, with the majority received through the regional data-sharing collaborative networks (FACT, ACT, OTN). For the 18 individuals that did not provide acoustic detections, 5 were tagged in autumn 2019 and 12 in autumn 2020, consequently data were not yet available for most of these animals due to the time lag associated with receiver downloads and data sharing. Down-sampling to one location and associated date/time per detection event resulted in the incorporation of 378 acoustic detections into tracking datasets with assigned Argos Location Class = 1 (500–1500 m accuracy) for track modeling. A total of 46 sharks provided at least one track segment for the hSSM analysis based on the predefined criteria (See SPOT Data Processing and Analyses). The total number of daily locations generated with the hSSM model was 10,367 with an average of 225 days per shark that provided at least one segment (15–1,291 d). The resulting percentage of tracking deployment duration with regularized daily locations ranged from 2 to 100% ( = 60%).
White sharks in the WNA were tracked over a wide latitudinal and longitudinal range (23.31° to 53.73° N and 91.51° to 27.48° W), from the Straits of Florida to north of Newfoundland and the Grand Banks, and from the central GOM to east of the Mid-Atlantic Ridge (Figure 2A). Overall, there were four focal areas used by tagged sharks: (1) a large area on the shelf from the SE United States and into the GOM; (2) the waters off Massachusetts; (3) the waters surrounding Nova Scotia including the Bay of Fundy and into the Gulf of St. Lawrence; and (4) the shelf break around the Grand Banks (Figure 2B). Across all sharks, a total of 82% of daily location estimates occurred over the continental shelf in water with a depth of <200 m.
Of 21 PSATs deployed on white sharks between 2016 and 2020, nine reported and transmitted usable data, four failed to report, and eight are still pending following deployments in 2020. For the objectives of this study, we report only the results from PSAT tags programmed with standardized parameters, which comprises tags deployed on animals captured in the SE United States and Nova Scotia in 2018–2019 (n = 4). Two of the tags deployed off Nova Scotia in 2018 were physically recovered yielding complete year-long high-resolution datasets (e.g., depth/temp every 15 s). Combined, these PSAT data recorded a maximum dive depth of 872 m and water temperatures ranging from −0.9 to 30.5°C.
Larger sharks of both sexes showed a trend of increasing use of pelagic waters with a higher proportion of locations off the continental shelf relative to smaller individuals, although this trend was much stronger and only significant for female sharks (R2 = 0.433, p = 0.006) when compared to males (R2 = 0.077, p = 0.339) (Figure 3). For female sharks, there was a marked difference in the spatial movements of larger (mostly adult; >3.5 m) female sharks versus other life stages (Figures 3, 4A,C). Pooling hSSM-derived daily locations within each age class showed that adult females had a higher proportion of days in off-shelf waters (31–77%; = 61% of locations on-shelf) compared to juvenile (93–99%; = 98% of locations on-shelf), subadult (69–100%; = 90% of locations on-shelf), and maturing (63–100%; = 91% of locations on-shelf) females (Figures 3, 4A,C). When examining behavioral state for these adult females, a higher proportion of off-shelf daily locations were classified as transient behavior (b = 1.0–1.25; 32.5%) compared to on-shelf locations (3.2%) (Supplementary Figure 1). This indicated that these animals were predominantly undertaking more directed movements when in offshore habitats versus area-restricted movements. These movements, however, were not uniform in terms of destination, spatial extent, or timing, with forays as far as the Mid-Atlantic Ridge and beyond (Figure 4C). Specifically, for three mature females (WS12-17, WS13-01, and WS13-03) with >3 years of SPOT data, forays were generally made in the overwintering period, but consistent periodicity in these offshore, open-ocean forays was not observed. For one female, offshore forays were made in consecutive years (4.4 m TL, WS13-01), for another there was a 2-year interval between forays (4.9 m TL, WS12-17), and for a third there was a 3-year interval (4.3 m TL, WS13-03) (Supplementary Figures 2–4). In general, male sharks were more shelf-oriented (80–99% of locations on-shelf) than females (31–99% of locations on-shelf). Within male sharks, subadult (80–98%; = 88%) and adult (83–97%; = 91%) groups spent proportionally less time in shelf waters when compared to large juveniles (89–99%; = 95%; Figures 3, 4B,D).
Figure 3. Proportion of on-shelf locations for all white sharks with a tracking duration longer than 200 days based on regularized daily locations provided by the hSSM derived from SPOT and acoustic locations. Size ranges for life stages are found in the Section “Life Stage Classification.” Lines are best fit regression lines.
Figure 4. Kernel density plots generated using pooled locations from all female white sharks (A) and all male white sharks (B). Underlying daily hSSM-derived locations for each life stage for female (C) and male (D) sharks with color scaled by life stage from large juvenile to adult.
When considering all white sharks with daily location estimates, a clear seasonal cycle in their latitudinal movement patterns was observed. These movements comprised two distinct residency phases and two migratory phases. Residency phases included: (1) an overwintering period (1 December – 15 May) when sharks primarily occupied shelf waters from Cape Hatteras to the GOM; and (2) a late summer/early autumn period (1 July – 15 October), when sharks were generally clustered in northern waters either off Massachusetts (Cape Cod and Nantucket) or Atlantic Canada (Nova Scotia, Prince Edward Island, New Brunswick, and Newfoundland) (Figures 5, 6). The two migration phases were in late spring/early summer (16 May – 30 June) and late autumn (16 October – 30 November), when animals were transiting between their southern overwintering and northern summer regions (Figures 5A,C, 6B,D). Generally, regularized locations in the migration corridor between Long Island, NY and Cape Hatteras, NC yielded a relatively higher proportion of behavioral scores indicating transient or directed movements (<1.25 = 22.1%; >1.74 = 44.3%) while behavioral scores north and south of these regions in the residence areas were more indicative of area-restricted movements (<1.25 = 6.4%; >1.74 = 68.6%) (Figure 7). During both northern and southern migration phases, however, some white sharks demonstrated a degree of stop-over behavior in the region around Cape Hatteras, with some individuals remaining in the area between North and South Carolina during their overwintering phase while others moving farther south at varying rates into Georgia/Florida and around the Florida peninsula into the GOM. While most tracked sharks followed this seasonal movement pattern there were exceptions in terms of timing of movements, as well as differential space use relative to the conserved population pattern detailed above. This was particularly evident in the three large adult females that undertook extensive off-shelf movements in varying years and an inverse latitudinal shift in movements, with higher latitudes occupied in the late summer/early autumn and lower latitudes during warmer months in some years (Figures 5B,D and Supplementary Figures 2–4). Notably, of the five sharks tagged prior to 2016, these three large females provided most of the locations from that time period.
Figure 5. Box-and-whisker plots (A,B), summarized for all years, all sharks; and means of monthly latitude (C,D) within each year of all regularized tracks from white sharks tracked between 2013 and 2020 (A,C = males of all life stages and females of all non-adult life stages; B,D = adult females). Latitudes of regularized daily locations for individual sharks (E) tracked over a 2-year period (November 2018 – November 2020) to show individual variability in movements. In panels (A,B) boxes represent interquartile range, whiskers are 1.5 × IQR, asterisks are outliers, and numbers above each month represent # of individuals (top number) and total number of locations (bottom number) in each respective month. Lines in panels (C,D) represent mean latitude during each month and broken lines in panel (D) connect gaps within years with no data during those months. Colors and symbols in panel (E) denote individual sharks with locations during that period. Dotted horizontal lines in each figure represent latitudes from north to south of Halifax, Nova Scotia; Cape Cod, Massachusetts; Cape Hatteras, NC, and Cape Canaveral, FL as labeled to the right of figures.
Figure 6. Kernel density distributions by behavioral season of white sharks in the western North Atlantic. Interpolated kernel density distributions are derived from regularized tracks built from SPOT and acoustic locations. Data is pooled among all years, sexes, and age classes except for adult female sharks. Panels are segmented by behavioral state/season: Late summer/early autumn (A: 1 July – 15 October), southern migration (B: 16 October – 30 November), overwintering (C: 1 December – 15 May), northern migration (D: 16 May – 30 June).
Figure 7. Proportions of white shark locations across range of behavioral scores derived from the hSSM from each of three regions: North of Long Island, NY (A); south of Cape Hatteras, NC including the Gulf of Mexico (C); and the migration corridor between these two (B). Utilizing hSSM-derived behavioral scores, behavior is classified as resident (b = 1.75–2.00), transient (b = 1.00–1.25), or unclassified (b = 1.26–1.74) The basemap represents a simple point density of all daily locations. Three adult, female sharks with significant off-shelf locations were not included.
While a large proportion of WNA white sharks undertook rapid, directed shifts in latitude during the migratory phases, the initiation dates of both northern and southern migrations were variable among individual sharks and within/across years (Figures 5C–E). For example, the initiation of northward migrations (defined as movements departing 35.2N following residency behavior off Cape Hatteras; Figures 5, 6) ranged from early May to mid-August ( = 66.8 ± 21.6 days) across all sharks and years where data were available (2017–2020). The shortest interval in any year between the day that the first and the last sharks began their northward migration was 33 days (2018) and the longest interval was 92 days (2019). The timing of the initiation of the southward migration was equally variable among individuals within years ranging from mid/late September to early December ( = 53.8 ± 8.4 days) for the period 2016–2020. The shortest interval in any year between when the first and last sharks began their southward migrations was 42 days (2017) and the longest was 66 days (2018). Moreover, interannual variability in migration timing was evident (Figures 5C,D). White sharks generally arrived at and departed from their northern summer/autumn residence areas slightly earlier in 2019 and 2020 ( = 26 June arrived, = 11 September departed; passing 41.6°N [∼Nantucket, MA]) when compared to 2017 and 2018 ( = 22 July arrived, = 14 October departed; passing 41.6°N) (Figure 5C). Model-derived daily locations allowed for migration timespans to be calculated for 48 migration paths (30 southward and 11 northward migrations). While southward migrations were slightly faster on average ( = 19.7 d, SD = 11.7) than northward migrations ( = 23.1 d, SD = 16.7), there was no significant difference in the length of time of the migration (Mood’s Median Test, p > 0.1).
Summarized time-at-temperature data (n = 4 PSATs) indicated that WNA white sharks spent the largest proportion of their time in temperatures ranging between 15–18°C (27.1%) and 78.6% of their time in 12–24°C temperatures (Figure 8 and Supplementary Figure S5). Temperature time series data (n = 4; recorded at 600 s intervals) provided further insight into seasonal differences. Variation in temperatures occupied during the two seasonal residency phases was observed with sharks occupying slightly warmer average temperatures during the overwintering period off the southern United States (19.0°C; SD = 3.84) when compared to the summer residency period off Nova Scotia and Massachusetts (14.7°C; SD = 3.89; Figures 8A,C and Supplementary Figures 5A,C). During northern and southern migration phases, average temperatures occupied were similar (16.5°C [SD = 5.80] and 16.3°C [SD = 4.18], respectively) (Figures 8B,D), but the largest variation recorded occurred during the northern migration period (Figure 8D and Supplementary Figure 5D). For one 3.8 m TL female (SE2019-03), evidence of occupying extremely cold waters was recorded between mid-July to early August while the animal was near the Southeast Shoal (SES) of the Grand Banks of Newfoundland (Figure 9B). On at least four different days, this shark spent periods of time (as much as 2.1% during a single 12-h period [15.1 min]) in water < 0°C (min. −0.9°C). Significant time was also spent in the 0–3°C range during this period (as much as 35.7% during a 12-h period [4.3 h]). These cold temperatures were not associated with extreme depths, but largely occurred at high latitudes in depths of 50–100 m. For example, a minimum temperature of −0.2°C was recorded on 4 August 2019 when the day’s maximum depth was 56 m. This tag’s temperature thermistor is rated to −40°C and was calibrated down to 2°C by the manufacturer prior to shipping. We assessed the sensor’s accuracy post deployment by comparing SST recorded by the tag on the day of release with nearby (<300 m distance) remotely sensed high resolution SST values. There was <0.5°C difference in these values indicating the temperature sensor was functioning within normal parameters during deployment.
Figure 8. Aggregated temperature-at-depth profile from time series data of four PSAT-tagged white sharks demonstrating vertical habitat utilization across seasons. (A) Late summer/early autumn. (B) Southern migration. (C) Overwintering. (D) Northern migration. While depth data did extend below 250 m, this represented <1% of overall time, thus for clarity, y-axes represent time between 0 and 250 m depth.
Figure 9. Most probable tracks (MPTs) and depth-temperature profiles derived from PSATs from white sharks NS2018-06 (A) and SE2019-03 (B). The MPT is a light-based track that incorporated SST, bathymetry, and known locations from SPOT and acoustic tags into the model. The white sharks were tagged off Nova Scotia (A) and South Carolina (B). The approximate location of the Southeast Shoal is shown as a red box on the Grand Banks of Newfoundland.
High resolution data from the two recovered PSATs were used to detect changes in patterns of vertical habitat use dependent on the movement phase of the individual (i.e., transient or resident). During periods of transient or more directed horizontal movements as determined by MPTs, a shift to a larger proportion of near-surface swimming was recorded (Supplementary Figure 6). For example, shark NS2018-06 (2.9 m TL female) spent 73.7% of time in sub-surface waters (1–2 m) during the 3-day period of transient behavior compared to 19.6% of time at that depth range in the 3 days prior, when movements were indicative of more resident behavior (29 October – 5 November 2018; Supplementary Figure 6). This 3-day period of near-surface time was punctuated by occasional dives to mostly moderate depths (∼10–90 m) and one deep dive to 460 m. These relatively fast-paced dives showed a pattern of faster descents than ascents and minimal time spent at the maximum depth, a pattern consistent with a gliding descent and powered ascent (Gleiss et al., 2011) that commonly correlate with directed movements by marine species. A broader comparison of time-at-depth data from full PSAT tracks (n = 4) showed a similar pattern with 38.0% of time spent in near-surface waters (0–5 m) on days identified as transient (>60 km d–1 traveled) compared to 22.8% of time spent near surface during days identified as resident (<60 km d–1 traveled).
Overall, sharks showed coarse-scale fidelity to specific regions, most notably during the July–November residency phase at higher latitudes (Figure 10 and Supplementary Figure 7). Of six sharks tagged with SPOT tags in Atlantic Canada in 2018, all individuals returned to Atlantic Canada waters in 2019 (year 2) and three individuals were present in the region in 2020 (Figure 10B). Similarly, of 11 SPOT-tagged sharks tagged in Atlantic Canada in 2019, six provided data in the subsequent summer period and five of those six moved back into Atlantic Canada waters (Figure 10B). The shark that did not provide data (2.5 m TL male, NS2019-04) was only located during the month of October the following year off Massachusetts heading south. Consequently, its movements in Atlantic Canada may not have been detected. Several sharks tagged in Nova Scotia were tracked near and around the Massachusetts region, but these movements were typically short stop-overs either before entering or after leaving Canadian waters, indicating a preference for more northern residency areas (Figure 10B). Conversely, of the 11 sharks tagged in waters near Massachusetts in the summer period between 2012 and 2019, eight provided locations in the second summer period, seven in the third summer period, and three in the fourth and fifth summer periods (Figure 10C). There was no clear pattern for the subsequent late summer/early autumn period habitat used, but six were detected in Massachusetts waters in following years and only two sharks of the 11 [a 3.0 m TL male (ACK2016-04) and a 3.8 m TL female (WS13-02)] showed movements into Atlantic Canada waters (Figure 10C). Of the seven sharks tagged off the SE United States during the overwintering period, six provided locations in their first late summer/early autumn residency period and four in the second residency period (Figure 10D). Of these, one shark resided in waters around Massachusetts in the two summer periods it was tracked and was not detected in Atlantic Canada waters; one shark was tracked moving through Massachusetts waters and into Atlantic Canada in the first period, then was located in waters off Newfoundland in the second period; three sharks inhabited Atlantic Canada waters in both subsequent late summer/early autumn periods and were not detected in Massachusetts waters; and one was tracked into Massachusetts waters in 1 year and Atlantic Canada in two other years (Figure 10D). Regional fidelity was also observed during the overwintering residency phase. Of the 36 sharks tagged between 2013 and 2019, 14 spent time in waters around the Florida Keys or in the GOM during the overwintering period and nine of those returned to the GOM in subsequent overwintering periods (Figures 10B–D).
Figure 10. Abacus plots of raw SPOT and acoustic locations of all white sharks colored by region of location or detection (A: Map of regions denoting color of region; B: Nova Scotia caught sharks; C: Massachusetts caught sharks; D: SE United States caught sharks). On-shelf regions (all except offshelf/offshore) extend from the shoreline to the shelf break with a 50 km buffer. Regions include: Newfoundland and Grand Banks (purple), Nova Scotia and Gulf of St. Lawrence (blue), Massachusetts and north to Canadian EEZ (green), mid-Atlantic (gold), southeastern United States (brown), Gulf of Mexico and Florida Keys (red), and offshelf/offshore (black). Sharks captured/tagged in Nova Scotia (B) were less likely to be detected in subsequent years in waters near Massachusetts (green) and more likely to be detected in Canadian waters (blue or purple). Conversely, sharks captured/tagged in Massachusetts (C) were less likely to be detected in subsequent years in Canadian waters (blue or purple) and more likely to be detected back in waters near Massachusetts (green). Of the Southeastern shelf caught sharks (D), there were preferences to utilize one or the other summer/autumn region (blue/purple or green) in subsequent years of tracking but usually not both. During overwintering periods, sharks with detections in the Gulf of Mexico (red) were likely to have detections in the Gulf of Mexico in subsequent overwintering periods.
While there were migrations on- and off-shelf, this varied both among and within individuals. In all sex/size classes except adult females, there were 30 southward migrations where model-derived daily locations generated clear departure and arrival dates from pinniped foraging areas at northern latitudes to the Outer Banks region in North Carolina, the northern limit of the overwintering area. Of these 30 southward migrations, 17 occurred entirely over shelf waters (e.g., Figure 9A), three entirely in offshore waters (e.g., Figure 9B and Supplementary Figure 8A), and ten in which sharks had portions of their migration in off-shelf waters (i.e., partially off-shelf). Additionally, there were 11 northward migrations where model-derived daily locations generated clear departure and arrival dates from the Outer Banks to pinniped foraging areas. Of these 11 northward migrations, nine were over shelf waters, one was clearly in offshore waters, and one showed the shark moving into off-shelf waters during a portion of the migration. There was no clear pattern of individual sharks showing fidelity to a particular migratory pathway across sex or size (e.g., Figure 9 and Supplementary Figure 8; excluding adult females). In the adult female class, north-south migratory patterns could be resolved for four southward migrations (two over-shelf, one offshore, and one partially off-shelf) and three northward migrations (two offshore and one partially off-shelf). While sample size for this group is low, females were more likely to undertake migrations in offshore waters when compared to other groups.
For sharks with multi-year datasets, fine-scale fidelity was evident with multiple sharks returning to specific sites within residency regions in subsequent years (Figures 10, 11 and Supplementary Figures 8B,C). A 3.9 m TL male shark (NS2018-03), tagged in Nova Scotia in autumn 2018, subsequently returned in 2019 and 2020 to <2 km from the tagging location (Supplementary Figure 8C). For the overwintering residency phase, a 4.9 m TL female shark (WS12-17) was tracked during parts of five annual cycles and showed strong fidelity by returning in 4 of 5 years to a site on the shelf off the Georgia coast (Figure 11).
Figure 11. Kernel density distributions of daily locations between October 16 and June 30 for a 4.9 m TL female white shark (WS12-17) for year 1 (A: 2012–2013), year 2 (B: 2013–2014), year 3 (C: 2014–2015), year 4 (D: 2015–2016), year 5 (E: 2016–2017). This shark showed strong fidelity to an area on the shelf off the coast of Georgia in 4 of 5 years tracked.
Through a combination of satellite and acoustic telemetry, our 8 years of data provide the first comprehensive assessment of white shark movement behavior for individuals tagged at multiple locations in the WNA. Using multiple tag types and integrating resulting datasets allowed us to generate robust measures of movements over considerable spatial and temporal scales. Derived data reveal detailed insights into the broad-scale movements of large juvenile to adult life stages of both sexes. White sharks in the WNA are predominantly shelf-oriented with a repeated and conserved seasonal latitudinal migration evident in all size/sex classes except for large mature females. Residency areas of high use in northern latitudes include the waters off Massachusetts, Atlantic Canada, and the Grand Banks. While migration windows for most individuals are within the same 1.5-month period, variation in the timing of migration among individuals within years and across years is evident. Individual white sharks show fidelity to regions across years, revisiting the same general areas of residence over a multi-year period, and fine-scale fidelity is seen in multiple individuals returning to the same locations over 3+ years. Vertical diving behavior is variable but broadly corresponds to shallower occurrence during directed movements and deeper diving when resident. Our data significantly expand current knowledge of the spatio-temporal movements of white sharks to inform transboundary management of this threatened species in the WNA.
White sharks in the WNA show variation in movement patterns by both life stage and sex, similar to trends observed for other geographically isolated populations worldwide (Jorgensen et al., 2010; Kock et al., 2013; Bruce and Bradford, 2015; Bruce et al., 2019). Large juveniles of both sexes are primarily coastal, occurring on the continental shelf throughout residency and migratory phases. While larger individuals exhibit similar seasonality in migration and residency phases, a trend of greater use of off-shelf waters with increasing body size was found in both sexes, with the ontogenetic shift being considerably more pronounced and statistically significant in female sharks. These findings are in agreement with previous work on WNA white sharks (Skomal et al., 2017) highlighting the proximity of this species to regions of high human activity (Winton et al., 2021), in terms of both recreational water use and fisheries as well as anthropogenic disturbance along the entire Atlantic coast of the United States and Canada. Given YOY white sharks tagged in their primary nursery area in the New York Bight showed more restricted latitudinal movements during 1 and 2 years of age (Curtis et al., 2018; Shaw et al., 2021), it is apparent that expansion of activity space and extension of residency and migration phases occur relatively quickly, likely between ages 3 and 4. The fact that multiple sharks ≤2.5 m TL were captured and tagged in Massachusetts and Atlantic Canada supports this rapid range expansion with body size that is conserved through to adulthood, with ontogenetic increases in range being mostly longitudinal off-shelf. Evidence from acoustic telemetry data for individuals captured and tagged as YOY animals also shows movements into Florida and Massachusetts by age 3 and Canada to the GOM by age 4 (Curtis and Franks, unpublished data; Curtis et al., 2018; Shaw et al., 2021). Consequently, white sharks in the WNA are utilizing near the maximum latitudinal range (∼25°) observed in the population by approximately age 4 and a size of 2.5 m TL. Range expansion in juvenile white sharks from other regions has been shown (NEP: Weng et al., 2007b; eastern Australia: Spaet et al., 2020) but the age/size and magnitude of expansion differs from our study. This may be due to shorter track durations for other regions (Weng et al., 2007b) or physical and/or biological differences between regions, such as prey availability, predation pressures, or bathymetry (Spaet et al., 2020). In the WNA there is also a consistent shift in increased proportion of time spent in offshore waters (longitudinal expansion) with increasing body size, similar to that observed for the white shark in Australia (Bruce et al., 2019; Lee et al., 2021) and the NEP (Jorgensen et al., 2010; Domeier, 2012; Domeier and Nasby-Lucas, 2012; Hoyos-Padilla et al., 2016). These longitudinal range expansions may reflect a reduction in predation risk after threshold sizes are reached (Skov et al., 2011; Hussey et al., 2017; Stump et al., 2017); improved access to biological or physical features to meet changing physiological demands (food sources, temperature profiles) (Ford, 1983; Breau et al., 2011; Braun et al., 2019); and/or in the case of off-shelf movements, to use alternate migratory pathways that reduce travel time to/from residency areas, or to select or avoid particular features such as the Gulf Stream current or associated habitats (Block et al., 2011; Dodson et al., 2013; Chambault et al., 2017; Gaube et al., 2018).
While adult females in our study did not show a clear cycle (1–3 years) in their extensive offshore movements in the WNA, these movements are potentially linked to reproductive behavior (Skomal et al., 2017; note five sharks included in their study are also incorporated into our dataset, including the three females discussed below), during pregnancy. In the NEP, adult females are thought to be on a biennial cycle in which they move into pelagic waters for periods of up to 16 months, then undertake directed movements to coastal regions where pupping is suspected to occur (i.e., presence of newborn sharks; Domeier and Nasby-Lucas, 2013). Bowlby and Gibson (2020) suggested that the reproductive cycle for white sharks in the WNA is >2 years, which could explain the inconsistent movement cycles observed here. Given the limited data available for adult females (3 individuals), we can neither confirm nor rule out a potential 2-year reproductive cycle as proposed in the NEP (Domeier and Nasby-Lucas, 2013), but the observed inconsistent periodicity of movement patterns may reflect variability in reproductive dynamics among regional white shark populations that requires further investigation. Further data for this life stage in the WNA will provide improved resolution on the timing and duration of this offshore pattern.
The majority of geolocations of white sharks on the Grand Banks southeast of Newfoundland were from adult females, suggesting this area may play an important role for that life-stage. The region of the Grand Banks and Flemish Cap is known to be highly dynamic with the confluence of the Labrador Current and the Gulf Stream/North Atlantic Current driving areas of upwelling along with strong eddy fields (Anderson and Gardner, 1986; Zhao et al., 2013), that results in increased primary and secondary production (Anderson and Gardner, 1986; Pepin et al., 2011). Potentially abundant prey in the region includes large bony fishes, other elasmobranchs, and marine mammals such as harp (Pagophilus groenlandicus) and hooded seals (Cystophora cristata) that are present in winter to late spring (Stenson and Sjare, 1997; Coughlan, 2002; Epperly et al., 2012; Andersen et al., 2013). This region may provide high prey abundance and consequently a high-energy foraging refuge, isolated from coastal pinniped colonies, where white shark abundance is higher. Variation in diet between sexes, including adult females having a more generalized diet, has been suggested for white sharks in other regions (French et al., 2018) and may reflect broader niche utilization as a result of expanded space use.
Currently, there is no direct evidence of mating activity when sharks are present in late summer/autumn residency areas in the WNA, similar to other regions (Jorgensen et al., 2012). However, indirect evidence (i.e., location/timing of departures from shelf waters) suggests these adult females undertake protracted off-shelf forays in the WNA in late winter/early spring following mating. The timing of these offshore movements post-mating is supported by an estimated 15–20 months gestation period (Bruce, 2008; Christiansen et al., 2014; Bowlby and Gibson, 2020) and an early summer (May–June) pupping season (Curtis et al., 2018; Santana-Morales et al., 2020). If females in the WNA are gestating during these offshore forays, reasons could include physical and/or thermal refuging, or a foraging habitat geographically separated from male conspecifics, to avoid harassment by males while females are pregnant (Jorgensen et al., 2012; Sulikowski et al., 2016). These results demonstrate the importance of multi-year telemetry datasets to explain the scale and pattern of individual movements during the gestation period for long-lived species (Edwards et al., 2019).
Similar to several other elasmobranch species (Biais et al., 2017; Nosal et al., 2021; reviewed in Chapman et al., 2015), white sharks undergo predictable migrations and residency periods in the WNA on an annual cycle. For most sharks tracked in the WNA over multiple years, the annual pattern is conserved but with unequal periodicity. While most sharks in our study undertook migrations during similar time periods (May–June and October–November), the timing of migration varied among individuals both within years and across years. Individual intra-annual and inter-annual variation in migration timing leads to questions of cues and triggers of this movement behavior. Observed inter-annual variability suggests flexibility in migration cues where environmental stochasticity likely plays a role. Similar to other migratory species, there are likely multiple interacting factors impacting migration, including static, fixed cues such as photoperiod that may trigger physiological or behavioral changes as an ultimate factor along with more dynamic, local proximal factors such as temperature, or prey distribution/abundance (Bauer et al., 2011; Winkler et al., 2014). Further, intra-annual variation among individuals may reflect inherent variability, i.e., phenotypic plasticity (Pulido, 2007; Hayes et al., 2012) or variability in predation success during the preceding residency period and associated body condition and/or reproductive status.
Evidence suggests that white sharks deplete energy reserves during their migrations (Del Raye et al., 2013) and forage at a lower rate when away from coastal pinniped colonies (Carlisle et al., 2012). Moreover, white sharks are known to demonstrate a shift in diet through ontogeny, with increases in mammal prey and decreases in teleost and elasmobranch prey with increasing size (Hussey et al., 2012; Grainger et al., 2020). In the context of WNA white sharks, the 3–4 month period when sharks are present near pinniped colonies in Massachusetts and Canada likely provides a critical time for energy acquisition, whereby enhanced feeding opportunities may play a key role in the balance of annual energy budgets. Captured sharks off Nova Scotia in 2018–2020 showed direct (substantial amounts of seal fur in fecal samples and fresh seal scratch wounds around the head and mouth) and indirect (large girths and distended abdominal areas) evidence of substantial feeding on seals (Newton and Franks, pers. obs., Fotso Tagne and Hussey, unpublished data). This is further supported by observations of seal corpses bearing bite wounds attributed to white sharks around Sable Island off the coast of Nova Scotia (Lucas and Natanson, 2010). Of these corpses, the vast majority (99%) were observed from July to October and were primarily seal pups (73%) although gray seals of all ages showed evidence of white shark predation (Lucas and Natanson, 2010). Gray seal populations in the waters around Massachusetts (Wood et al., 2020) and Atlantic Canada (Hammill et al., 2017) have increased in recent years with current estimates placing the total population in Atlantic Canada at 400,000–500,000 individuals with pup production increasing at a higher rate in more southern areas of their range (NE United States and SW Nova Scotia) (den Heyer et al., 2021). Given shark foraging activity and movements in this region are likely driven not just by pinniped density but also variation in pinniped behavior through ontogeny (Brodie and Beck, 1983; Moxley et al., 2020), future work should focus on an examination of fine-scale space use by white sharks and how it relates to pinniped size, abundance, reproductive cycles, foraging behavior, and seasonal movements.
In the overwintering residency period, it is thought white sharks may be feeding on whales off the SE United States coast and in the GOM (Skomal et al., 2017) in addition to squids, teleost fishes, and other elasmobranchs. While these feeding opportunities may provide important required energy during this time, they are likely to be sporadic and less reliable, in contrast to the high availability of pinniped prey during summer/autumn. Consequently, behaviors are likely selected for those that will maximize caloric consumption during the late summer/early autumn periods near expanding pinniped colonies, while exploiting sporadic feeding opportunities, such as whale carcasses or transient concentrations of other prey, during the overwintering period. This is evident across species and habitat types, where predator search patterns shift based on prey availability (Sims et al., 2012). White sharks, as endothermic predators, likely feed frequently to maintain energetic needs (Semmens et al., 2013) and energetic costs of migration must be accounted for through gains during time spent in other portions of their range. It is possible that the consistent, energy-rich food source of seal colonies in the northern portion of their range in summer/early autumn may provide a net energy gain during this period, providing an energetic surplus for somatic and reproductive growth. In contrast, in the overwintering areas, more diffuse feeding opportunities may only provide energy to meet metabolic needs for maintenance and migration. Additionally, mean water temperatures experienced in these southern overwintering areas are warmer than that of the northern summer regions, consequently the energetic costs of endothermy may be reduced during winter.
During periods of directed horizontal travel a white shark (2.9 m TL large, juvenile female, NS2018-06) was shown to shift to a pattern of increased near-surface swimming punctuated by occasional deep dives. A number of white shark tracking studies have reported a similar pattern of increased surface swimming (Bonfil et al., 2005; Weng et al., 2007a; Domeier and Nasby-Lucas, 2008) and increased swimming speed (Bonfil and O’Brien, 2015; Watanabe et al., 2019) during traveling or oceanic phases. However, white sharks moving directly at the surface incur wave drag, which is energetically costly (Watanabe et al., 2019). Wave drag can be avoided by swimming deeper than ∼2.5 body diameters (Alexander, 2013), which would be ∼2 m for white sharks (Watanabe et al., 2019). This is consistent with our observations for shark NS2018-06, which displayed surface swimming during the transient phase predominantly in the 1–2 m subsurface range (73.7% of time). The occasional dives during these periods of near-surface swimming could play a navigational (Keller et al., 2021) and/or thermoregulatory role (Domeier and Nasby-Lucas, 2008) or represent energy-efficient foraging forays (Gleiss et al., 2011; Watanabe et al., 2019). Overall, these results suggest that white sharks can select depths and speeds depending on their behavioral state that optimize energy expenditure.
In the WNA and other regions, a combination of thermal regime, foraging opportunities, and reproduction have been proposed as reasons driving white shark seasonal migrations (Casey and Pratt, 1985; Jorgensen et al., 2010; Carlisle et al., 2012; Duffy et al., 2012; Curtis et al., 2014; Bradford et al., 2020). Our data are consistent with these interacting factors driving migration of WNA white sharks, which spend more than 75% of their time in 12–24°C waters and the largest proportion of their time (27%) in the 15–18°C range. The most likely factor driving these sharks to leave late summer/autumn foraging areas in northern latitudes to overwinter in lower latitudes is cooling water temperatures in early winter, as suggested by Casey and Pratt (1985). This is supported by our data as the timing of departure from northern latitudes during late autumn/early winter (October–November) corresponds to average daily water temperatures of ∼9–15°C. Departure from these regions is unlikely to relate to decreased regional prey availability, given that pinniped abundance in Atlantic Canada is higher during winter and spring periods (Hammill et al., 2017). While both depth (0–872 m) and temperature (−0.9 – 30.5°C) ranged considerably for white sharks in this study, sharks spent the majority of time in much narrower ranges, consistent with previous studies within the WNA and other regions.
One PSAT-tagged subadult female in our study, a 3.8 m TL maturing female (SE2019-03), spent portions of four different days in water with subzero temperatures (min. −0.9°C) during the summer (2019 15 July – 4 August) while in continental slope waters east of the Grand Banks of Newfoundland. To our knowledge, this is the coldest temperature on record for a white shark, less than the minimum temperature of 1.6°C reported by Skomal et al. (2017), or for any other lamnid species. Porbeagles (Lamna nasus) in the WNA, for example, have been shown to occupy a temperature range of 2–26°C (Pade et al., 2009; Saunders et al., 2011; Skomal et al., 2021), while salmon sharks (L. ditropis) in the NEP occur in 2–24°C (Goldman et al., 2004; Weng et al., 2005). Only the non-endothermic Greenland shark (Somniosus microcephalus) has been shown to frequent colder waters with temperatures documented as low as −1.7°C (Skomal and Benz, 2004). The subzero temperatures experienced by this female white shark occurred in the vicinity of the SES, an area with the highest benthic biomass on the Grand Banks (Hutcheson et al., 1981) and a known offshore spawning site for capelin (Mallotus villosus) (Carscadden et al., 1989). The SES has relatively warm July–August bottom water (∼2–4°C) that is well-suited for the demersal spawning capelin. In contrast, the nearby waters immediately east of the shelf are considerably colder (∼−1.5 – 0.7°C) as depths exceed 50 m (Templeman, 1975; Whitehead and Glass, 1985; Loder, 1991). During the prespawning period, capelin form relatively large and compact schools that are heavily preyed upon by humpback whales (Megaptera novaeangliae) as well as a number of other cetacean species including finback whales (Balaenoptera physalus), minke whales (Balaenoptera acutorostrata), white-beaked dolphins (Lagenorhynchus albirostris), and short-beaked saddleback dolphins (Delphinus delphis) (Whitehead and Glass, 1985). The white shark’s ability to maintain a warmer-than-ambient body temperature likely enables expansion of its thermal niche and access to habitats largely limited to other top predators, accepting that regional endothermy is more associated with higher swim speeds rather than broader thermal niches (Harding et al., 2021). It is likely the white sharks are drawn to the abundant food supply of the SES during this period and at times may actively hunt or scavenge on cetaceans in the subzero waters just off the slope of the shelf, though we cannot rule out the possibility of white sharks foraging on the spawning capelin while on the shoal.
The durations of residency and migratory periods in WNA white sharks are consistent with sharks tracked in other geographical regions (Francis et al., 2015; Spaet et al., 2020), but the seasonal patterns, irrespective of hemisphere, differ slightly among regions. This is likely due to variations in physical factors such as water temperatures and currents or biological factors such as prey behavior and reproductive cycles. The latitudinal scope of the migration of white sharks in the WNA can be attributed to the type of available winter habitat, the location of productive foraging habitats, and how the sharks move between these two. In New Zealand and the NEP, available winter habitat is mostly oceanic in off-shelf waters whereas productive foraging areas are coastal. In the WNA, Australia, and for the most part southern Africa, both residency phases generally occur in on-shelf habitat. The predominantly longitudinal migration found in NEP white sharks is likely reflective of the eastern boundary current (California Current) in the region, resulting in cooler temperatures and productive foraging habitats (i.e., pinniped colonies) being located nearer to the equator. In other areas where white shark movements and migration have been studied (eastern Australia, New Zealand, and southern Africa), the coasts are generally associated with western boundary currents, where productive foraging habitats are found at higher latitudes and overwintering habitats are nearer to the equator, either on-shelf (southern Africa, and eastern Australia) or oceanic (New Zealand). Although no comprehensive telemetry study of white sharks has been conducted in the northwest Pacific, capture and sighting data have reported that white sharks are present there in northern latitudes in most months except winter and present in southern latitudes in most months except summer (Nakaya, 1994; Christiansen et al., 2014). This trend is consistent with the migration phases observed in the WNA and demonstrates how major surface currents such as western boundary currents and variable thermal conditions interact to shape latitudinal migrations.
The variability in migration seen among individuals and years demonstrates the importance of a thorough, long-term understanding of these movement patterns, to accurately characterize spatial distribution for management and conservation. A changing climate, particularly ocean warming, may not only affect the distribution of marine species such as sharks (Bangley et al., 2018; Tanaka et al., 2021) but may also impact timing of movements and migration. Ongoing, long-term monitoring of the WNA white shark population is essential, therefore, given the observed increase in water temperature of 0.41°C between 1950 and 2009 (IPCC, 2014) in the Atlantic Ocean, the finding that some areas of the WNA white shark range (Gulf of Maine) have warmed at a faster rate than 99% of the world’s oceans (Pershing et al., 2015), and projected temperature increases of up to 3°C in the northwest Atlantic Ocean (35–45°N) by the end of this century (Saba et al., 2016).
White sharks tracked in the WNA demonstrate inter-annual fidelity to particular regions both during their overwintering and summer residency phases. In particular, most WNA sharks show a preference for one of the two known late summer/early autumn residency areas, namely Massachusetts and Nova Scotia, with relatively little overlap between the two. Individual sharks also showed fine-scale site fidelity returning to specific areas within these regions over multiple years, in some cases within 1–3 km for up to three consecutive years. Site fidelity, whereby individual animals repeatedly re-use specific areas within their range (Piper, 2011), is part of an overall behavioral pattern of animals returning to specific “homes” within their ranges, termed philopatry (Hueter et al., 2005). Philopatric behavior likely confers multiple benefits to individuals including increased familiarity with the physical and biological parameters in their range, thereby increasing biological and ecological efficiencies of behaviors such as prey capture, movement and migration, mating, and physiology, contributing to increases in fitness (Switzer, 1993; Merkle et al., 2014; Madigan et al., 2015). Similar to the results for the WNA white sharks, site fidelity has been shown for white sharks in the NEP where individuals returned to the same coastal sites over multi-year periods (Jorgensen et al., 2010, 2012; Domeier and Nasby-Lucas, 2012), with little overlap between the two pinniped foraging areas in the NEP (Central California vs. Guadalupe Island; Jorgensen et al., 2012) and evidence of some sharks returning to the same site in 14 of 22 years (Anderson et al., 2011). Major geographical differences between the residency areas in the NEP and the WNA, however, are evident. In the NEP, sharks move eastward from offshore, open-ocean areas to reach coastal sites, whereas in the WNA, sharks move north from southern overwintering regions along the continental shelf. As a result, there is a greater likelihood of WNA sharks visiting both northern latitude regions, particularly for sharks that must transit through Massachusetts waters to reach Atlantic Canada. Despite this, many tracked sharks, particularly those tagged in Canada, show strong fidelity to that region, returning in each subsequent late summer/autumn season. This provides strong evidence that a subset of animals actively selects locations in Atlantic Canada for the residency period. Fidelity to specific foraging sites while migrating through and bypassing potentially suitable forage habitat has been shown in other migratory species (Bonadonna et al., 2001; Shimada et al., 2020) where familiarity likely plays a key role. This fidelity would be enhanced in areas or with species where forage is predictable such as benthic foraging sea turtles (Shimada et al., 2020), herbivorous grazers (Merkle et al., 2015), front-associated predators (Lowther et al., 2011), or, in the case of white sharks, foraging around concentrated pinniped colonies (e.g., Robbins et al., 2015; Hewitt et al., 2018). These data provide evidence that there is delineation of foraging areas within the northern latitude residency phase (Atlantic Canada vs. Massachusetts), suggesting the possibility for subpopulation structure in the WNA, with implications for regional management. Continued long-term tracking coupled with genetics will be required to confirm this.
While there was evidence of site fidelity during the overwintering residency period, including fine-scale fidelity for some sharks (Figures 10, 11), the general pattern was not as clear as compared to the northern late summer/early autumn residency period. For instance, individuals that migrated to the GOM typically returned to that location in subsequent years, but this was not consistent for all sharks tracked over multiple years. One potential reason for this pattern may relate to prey availability as potential prey resources are more diffuse in the southern portions of their annual range during the winter period. If foraging opportunities and ultimately success are more reliable within specific areas, then it follows that fidelity to those regions may be more pronounced. With scant, direct evidence for specific forage in this overwintering region and during migrations, sharks may be foraging opportunistically in more productive regions or potentially exploiting other prey sources that are consistent, e.g., large schooling fishes or invertebrates (Murphy et al., 1998; Galuardi et al., 2010; Braun et al., 2019) or whale calves (Gowan and Ortega-Ortiz, 2014; Kennedy et al., 2014).
Strong, multi-year fidelity to specific regions demonstrates that white sharks are likely using complex navigational cues during both migratory and residency phases to return to preferred areas. White sharks around aggregation sites in California (Goldman and Anderson, 1999) and in South Africa (Jewell et al., 2013) have been shown to become more selective in space use as they increase in size. This suggests a refinement of space use over time, possibly as a result of experience and the building of memory maps (Fagan et al., 2013) that could result in increased foraging efficiency. The late summer/autumn residency phase of white sharks in the WNA is likely a strategy to maximize energy intake during this period potentially through optimal foraging, yielding net-positive energy budgets during this period. Increased familiarity, via fidelity, potentially reduces feeding search times and increases prey capture and handling efficiency, and thus increases energy inputs during this important summer foraging period when prey is abundant, driving overall annual growth. Additionally, with seal colonies relatively diffuse in the WNA region (Massachusetts to Newfoundland) when compared to other areas (Ferreira and Ferreira, 1996; Bradshaw et al., 2000), it may allow for individual sharks to establish specific areas to revisit each year to minimize intraspecific, competitive interactions and thus alleviate density-dependent effects.
While fidelity provides benefits to animals in the form of increased efficiency and fitness, there are potential implications to the animal or population if conditions change. Habitat degradation, climate change, and increased potential for localized depletion can all disproportionately impact species or populations that are philopatric or return to the same areas over time (Hueter et al., 2005). Alternatively, intra- and inter-annual variation in movement dynamics (migration timing and duration of residency phases) observed in sharks tagged for multiple years in the current study suggests a degree of flexibility to change. If true, then white sharks could adapt to modified ecosystems under climate change but may still face the threat of other natural or anthropogenic disturbances.
White sharks in the WNA are dynamic in their use of space, yet trends in their space use are consistent across age classes, with a rapid expansion of latitudinal range early in life that is conserved through to adulthood. Although overall migration and residency patterns are consistent, there is considerable variability both among groups, such as life stage and sex, as well as within groups when comparing individual space use over a multi-year period. Generally, WNA white sharks show a series of regular movements and migrations, utilizing three regions in northern latitudes (Massachusetts, Nova Scotia and the Gulf of St. Lawrence, and the Grand Banks) in late summer/early autumn and one expansive shared area along the shelf off the SE United States and in the GOM in winter and spring seasons. These patterns are consistent with the exception of some use of offshore areas, particularly forays into far offshore waters by a subset of large females.
In conclusion, with our findings presented here, we propose the following hypotheses and paradigms explaining the movement ecology of the white shark in the WNA and other regions (Figure 12):
Figure 12. Summary and conceptual framework for general movement patterns by white sharks in the western North Atlantic. Size of shark silhouette corresponds to broad age/size classes while seal silhouettes represent areas of known pinniped foraging areas by white sharks. Areas noted are based on a combination of previously published studies (Casey and Pratt, 1985; Curtis et al., 2014, 2018; Skomal et al., 2017; Gaube et al., 2018; Bastien et al., 2020; Shaw et al., 2021), data from this study, and proposed hypotheses for mating and gestation areas. Proposed mating area was derived from general locations of sharks during the late winter to early summer period of each year with the assumption of a ∼12–18 month gestation period, as well as anecdotal evidence of a large, female white shark observed with mating wounds off the shelf break of the Georgia coast in June of 2018. Proposed gestation area was derived from general locations of adult, female white sharks during these expansive offshore movement periods every 1–3 years.
(1) Strong site fidelity in WNA white sharks suggests subpopulation structure that may be detectable with additional telemetry data from individuals with 10-year acoustic tags and from parallel genetic analyses, particularly when considering Massachusetts and Atlantic Canada-oriented sharks.
(2) Western North Atlantic white sharks generally show a north-south coastal range that expands rapidly within the first few years of life with consistent seasonal migrations that expand off-shelf with ontogeny. This general pattern is broken by post-mated adult females as they move far offshore during pregnancy, potentially to avoid interactions with other sharks and/or to exploit specific biological or physical features. As we do not see evidence of mating during the late summer/early autumn period around pinniped colonies and adult females initiate offshore forays in late winter and spring, we hypothesize mating is likely occurring offshore from the coast of North Carolina to northern Florida in the late winter to early summer (Figure 12).
(3) The world’s regional populations of white sharks assort themselves into groupings sharing common patterns of movement ecology and habitat use. These patterns are likely driven by a combination of biological needs such as foraging, reproduction, and temperature constraints along with physical parameters such as shelf structure and width, major current characteristics, and landmass size/orientation. These patterns serve to connect foraging areas to meet energetic requirements for physiological and reproductive needs. With this paradigm, broad-scale movements of populations globally can be classified into general patterns: (1) major migratory routes are in the latitudinal plane (western North Atlantic, New Zealand, eastern Australia, northwest Pacific, southern Africa) or the longitudinal plane (northeast Pacific, southern-western Australia); and (2) overwintering areas are either mostly over-shelf (western North Atlantic, eastern Australia, southern Africa) with some time in oceanic waters or offshore, oceanic (northeast Pacific, New Zealand). Further refinement of this paradigm will be revealed through continued long-term behavioral and ecological research such as multi-year telemetry.
Future research will test these hypotheses and evaluate our paradigms for white shark ecology. Defining the movement patterns of migratory, wide-ranging, long-lived predators such as the white shark presents challenges related to the limitations of current telemetry technology. By deploying multiple tag types that provide data over periods of up to a decade, we can gain deeper insights into their movements and migration over multiple spatial and temporal scales (Edwards et al., 2019). Only through long-term telemetry datasets on individual animals in conjunction with other biological studies will it be possible to resolve critical questions related to the biology, ecology, conservation, and management of the white shark.
Datasets from SPOT tags used in this study are housed in the Movebank.org online repository. Data from all tags can be made available upon reasonable request to the corresponding author.
The animal study was reviewed and approved by Jacksonville University’s Institutional Animal Care and Use Committee (IACUC).
GF and BM led the fieldwork. All authors conducted fieldwork and contributed to data collection. BF, JT, CB, NH, and RH conceived the manuscript. BF analyzed the data with assistance from JT and contributions from CB and NH. BF drafted the manuscript with assistance from JT, CB, NH, and RH along with input from all the other co-authors. All authors read and approved the final manuscript.
Primary funding for this work, including research shiptime, fishing operations, and acoustic and SPOT tags, was provided by OCEARCH and its sponsors. Funding for PSATs was provided by the Shark Foundation (Hai Siftung) and the Disney Conservation Fund. PSAT data analysis was supported by a grant from NOAA/National Sea Grant to RH. Support for RH’s contributions was provided by the Perry W. Gilbert Chair in Shark Research at Mote Marine Laboratory, NOAA, and OCEARCH.
The authors declare that the research was conducted in the absence of any commercial or financial relationships that could be construed as a potential conflict of interest.
The handling editor declared a past co-authorship with one of the authors CB.
All claims expressed in this article are solely those of the authors and do not necessarily represent those of their affiliated organizations, or those of the publisher, the editors and the reviewers. Any product that may be evaluated in this article, or claim that may be made by its manufacturer, is not guaranteed or endorsed by the publisher.
We are most grateful to the Captain and entire crew and staff of the M/V OCEARCH for their unique skills in capturing and handling white sharks for this study. This work would not have been possible without their expertise and hard work. We also thank all the collaborating scientists associated with the WNA white shark project for their contributions. Special thanks are extended to Mike James of Fisheries and Oceans Canada, for his substantial efforts to recover two of our PSATs on Nova Scotia shores.
The Supplementary Material for this article can be found online at: https://www.frontiersin.org/articles/10.3389/fmars.2021.744202/full#supplementary-material
Supplementary Figure 1 | Proportions of locations across range of behavioral scores from the hSSM from locations over the continental shelf and off-the-shelf. Locations are color coded by behavioral score (1.00–1.25 = transient, 1.25–1.75, 1.75–2.00 = resident).
Supplementary Figure 2 | Daily regularized locations for WS-13-01, a 4.4 m TL adult female white shark tracked for 1,473 days.
Supplementary Figure 3 | Daily regularized locations for WS12-17, a 4.9 m TL adult female white shark tracked for 1,734 days.
Supplementary Figure 4 | Daily regularized locations for WS-13-03, a 4.3 m TL adult female white shark tracked for 2,647 days.
Supplementary Figure 5 | Time-at-temperature for four PSAT-tagged white sharks across behavioral seasons. (A) Late summer/early autumn; (B) southern migration; (C) overwintering; (D) northern migration.
Supplementary Figure 6 | Week-long depth profile from recovered PSAT of white shark NS2018-06 demonstrating contrasting vertical movement between resident and transient phases. (A) High resolution depth data from October 29 to November 5. Mean daily distance traveled for the resident (red) and transient (green) phases is shown as a colored trace (right axis). (B) Inset map of the mid Atlantic coast showing the most probable track (MPT) for this period. (C) Time-at-depth for the resident phase. (D) Time-at-depth for the transient phase.
Supplementary Figure 7 | Daily regularized locations classified as resident behavior (b > 1.75) for sharks captured in Massachusetts and Nova Scotia showing fidelity to summer/early autumn residency areas.
Supplementary Figure 8 | Migration pathways from regularized daily locations for a 3.8 m TL adult male white shark (LC2017-01) (A) over a 2-year cycle (2017–2018) demonstrating both on-shelf and off-shelf migrations. Tracks are color-coded by year and season. Northern migrations represented by red hues, southern migrations by green, and residency locations by blue. This shark migrated on-shelf during both northward migrations but migrated off-shelf during one of two southward migrations during its 2-year tracking period. Raw SPOT and acoustic locations for a 3.7 m TL subadult female (ACK2016-02) in the region around Massachusetts over a 5-year period (B) and 3.9 m TL adult male (NS2018-03) in the region around Nova Scotia over a 3-year period (C) showing fine-scale fidelity to specific areas within the summer/autumn foraging areas. In panels (B,C), common months share symbols and common years share color.
Acuña-Marrero, D., Smith, A. N. H., Hammerschlag, N., Hearn, A., Anderson, M. J., Calich, H., et al. (2017). Residency and movement patterns of an apex predatory shark (Galeocerdo cuvier) at the Galapagos Marine Reserve. PLoS One 12:e0183669. doi: 10.1371/journal.pone.0183669
Ajemian, M. J., Drymon, J. M., Hammerschlag, N., Wells, R. J. D., Street, G., Falterman, B., et al. (2020). Movement patterns and habitat use of tiger sharks (Galeocerdo cuvier) across ontogeny in the Gulf of Mexico. PLoS One 15:e02348680. doi: 10.1371/journal.pone.0234868
Alexander, R. M. (2013). Principles of Animal Locomotion. Princeton, NJ: Princeton University Press.
Andersen, J. M., Wiersma, Y. F., Stenson, G. B., Hammill, M. O., Rosing-Asvid, A., and Skern-Maurizen, M. (2013). Habitat selection by hooded seals (Cystophora cristata) in the Northwest Atlantic Ocean. ICES J. Mar. Sci. 70, 173–185. doi: 10.1093/icesjms/fss133
Anderson, J. T., and Gardner, G. A. (1986). Plankton communities and physical oceanography observed on the Southeast Shoal region, Grand Bank of Newfoundland. J. Plankton Res. 8, 1111–1135. doi: 10.1093/plankt/8.6.1111
Anderson, S. D., Chapple, T. K., Jorgensen, S. J., Klimley, A. P., and Block, B. A. (2011). Long-term individual identification and site fidelity of white sharks, Carcharodon carcharias, off California using dorsal fins. Mar. Biol. 158, 1233–1237. doi: 10.1007/s00227-011-1643-5
Bangley, C. W., Curtis, T. H., Secor, D. H., Latour, R. J., and Ogburn, M. B. (2020). Identifying important juvenile dusky shark habitat in the Northwest Atlantic Ocean using acoustic telemetry and spatial modeling. Mar. Coast. Fish. 12, 348–363. doi: 10.1002/mcf2.10120
Bangley, C. W., Paramore, L., Shiffman, D. S., and Rulifson, R. A. (2018). Increased abundance and nursery habitat use of the bull shark (Carcharhinus leucas) in response to a changing environment in a warm-temperate estuary. Sci. Rep. 8:6018. doi: 10.1038/s41598-018-24510-z
Barnett, A., Abrantes, K. G., Stevens, J. D., and Semmens, J. M. (2011). Site fidelity and sex-specific migration in a mobile apex predator: implications for conservation and ecosystem dynamics. Anim. Behav. 81, 1039–1048. doi: 10.1016/j.anbehav.2011.02.011
Bastien, G., Barkley, A., Chappus, J., Heath, V., Popov, S., Smith, R. A., et al. (2020). Inconspicuous, recovering, or northward shift: Status and management of the white shark (Carcharodon carcharias) in Atlantic Canada. Can. J. Fish. Aquat. Sci. 77, 1666–1677. doi: 10.1139/cjfas-2020-0055
Bauer, S., McNamara, J. M., and Barta, Z. (2020). Environmental variability, reliability of information and the timing of migration. Proc. R. Soc. B 287:20200622. doi: 10.1098/rspb.2020.0622
Bauer, S., Nolet, B. A., Giske, J., Chapman, J. W., Åkesson, S., Hedenström, A., et al. (2011). “Cues and decision rules in animal migration,” in Animal Migration: A Synthesis, eds E. J. Milner-Gulland, J. M. Fryxell, and A. R. E. Sinclair (New York, NY: Oxford University Press), 68–88. doi: 10.1093/acprof:oso/9780199568994.003.0006
Biais, G., Coupeau, Y., Séret, B., Calmettes, B., Lopez, R., Hetherington, S., et al. (2017). Return migration patterns of porbeagle shark (Lamna nasus) in the Northeast Atlantic: implications for stock range and structure. ICES J. Mar. Sci. 74, 1268–1276. doi: 10.1093/icesjms/fsw233
Block, B. A., Jonsen, I. D., Jorgensen, S. J., Winship, A. J., Shaffer, S. A., Bograd, S. J., et al. (2011). Tracking apex marine predator movements in a dynamic ocean. Nature 475, 86–90. doi: 10.1038/nature10082
Blower, D. C., Pandolfi, J. M., Bruce, B. D., Gomez-Cabrera, M. D. C., and Ovenden, J. R. (2012). Population genetics of Australian white sharks reveals fine-scale spatial structure, transoceanic dispersal events and low effective population sizes. Mar. Ecol. Prog. Ser. 455, 229–244. doi: 10.3354/meps09659
Bonadonna, F., Lea, M. A., Dehorter, O., and Guinet, C. (2001). Foraging ground fidelity and route-choice tactics of a marine predator: the Antarctic fur seal Arctocephalus gazella. Mar. Ecol. Prog. Ser. 223, 287–297. doi: 10.3354/meps223287
Bonfil, R., and O’Brien, S. (2015). Strongly directional and differential swimming behavior of an adult female white shark, Carcharodon carcharias (Chondrichthyes: Lamnidae) from Guadalupe Island, Mexico. Lat. Am. J. Aquat. Res. 43, 267–273. doi: 10.3856/vol43-issue1-fulltext-24
Bonfil, R., Francis, M. P., Duffy, C., Manning, M. J., and O’Brien, S. (2010). Large-scale tropical movements and diving behavior of white sharks Carcharodon carcharias tagged off New Zealand. Aquat. Biol. 8, 115–123. doi: 10.3354/ab00217
Bonfil, R., Meÿer, M., Scholl, M. C., Johnson, R., O’Brien, S., Oosthuizen, H., et al. (2005). Transoceanic migration, spatial dynamics, and population linkages of white sharks. Science 310, 100–103. doi: 10.1126/science.1114898
Bowlby, H. D., and Gibson, A. J. F. (2020). Implications of life history uncertainty when evaluating status in the Northwest Atlantic population of white shark (Carcharodon carcharias). Ecol. Evol. 10, 4990–5000. doi: 10.1002/ece3.6252
Bowlin, M. S., Bisson, I. A., Shamoun-Baranes, J., Reichard, J. D., Sapir, N., Marra, P. P., et al. (2010). Grand challenges in migration biology. Integr. Comp. Biol. 50, 261–279. doi: 10.1093/icb/icq013
Bradford, R., Patterson, T. A., Rogers, P. J., McAuley, R., Mountford, S., Huveneers, C., et al. (2020). Evidence of diverse movement strategies and habitat use by white sharks, Carcharodon carcharias, off southern Australia. Mar. Biol. 167, 1–12. doi: 10.1007/s00227-020-03712-y
Bradshaw, C. J., Davis, L. S., Lalas, C., and Harcourt, R. G. (2000). Geographic and temporal variation in the condition of pups of the New Zealand fur seal (Arctocephalus forsteri): evidence for density dependence and differences in the marine environment. J. Zool. 252, 41–51. doi: 10.1111/j.1469-7998.2000.tb00818.x
Braun, C. D., Galuardi, B., and Thorrold, S. R. (2018). HMMoce: an R package for improved geolocation of archival-tagged fishes using a hidden Markov method. Methods Ecol. Evol. 9, 1212–1220. doi: 10.1111/2041-210X.12959
Braun, C. D., Gaube, P., Sinclair-Taylor, T. H., Skomal, G. B., and Thorrold, S. R. (2019). Mesoscale eddies release pelagic sharks from thermal constraints to foraging in the ocean twilight zone. Proc. Natl. Acad. Sci. U.S.A. 116, 17187–17192. doi: 10.1073/pnas.1903067116
Breau, C., Cunjak, R. A., and Peake, S. J. (2011). Behaviour during elevated water temperatures: can physiology explain movement of juvenile Atlantic salmon to cool water? J. Anim. Ecol. 80, 844–853. doi: 10.1111/j.1365-2656.2011.01828.x
Brodersen, J., Nilsson, P. A., Chapman, B. B., Skov, C., Hansson, L. A., and Brönmark, C. (2012). Variable individual consistency in timing and destination of winter migrating fish. Biol. Lett. 8, 21–23. doi: 10.1098/rsbl.2011.0634
Brodie, P., and Beck, B. (1983). Predation by sharks on the grey seal (Haliochoerus grypus) in Eastern Canada. Can. J. Fish. Aquat. Sci. 40, 267–271. doi: 10.1139/f83-040
Bruce, B. D. (2008). “The biology and ecology of the white shark, Carcharodon carcharias,” in Sharks of the Open Ocean: Biology, Fisheries, and Conservation, eds M. D. Camhi, E. K. Pikitch, and E. A. Babcock (Oxford: Blackwell Publishing), 69–81. doi: 10.1002/9781444302516.ch5
Bruce, B. D., and Bradford, R. W. (2012). “Habitat use and spatial dynamics of juvenile white sharks Carcharodon carcharias,” in Eastern Australia, in Global Perspectives on the Biology and Life History of the White Shark, ed. M. L. Domeier (Boca Raton, FL: CRC Press), 225–254.
Bruce, B. D., Harasti, D., Lee, K., Gallen, C., and Bradford, R. (2019). Broad-scale movements of juvenile white sharks Carcharodon carcharias in eastern Australia from acoustic and satellite telemetry. Mar. Ecol. Prog. Ser. 619, 1–15. doi: 10.3354/meps12969
Bruce, B. D., Stevens, J. D., and Malcolm, H. (2006). Movements and swimming behaviour of white sharks (Carcharodon carcharias) in Australian waters. Mar. Biol. 150, 161–172. doi: 10.1007/s00227-006-0325-1
Bruce, B., and Bradford, R. (2015). Segregation or aggregation? Sex-specific patterns in the seasonal occurrence of white sharks Carcharodon carcharias at the Neptune Islands, South Australia. J. Fish Biol. 87, 1355–1370. doi: 10.1111/jfb.12827
Carlisle, A. B., Kim, S. L., Semmens, B. X., Madigan, D. J., Jorgensen, S. J., Perle, C. R., et al. (2012). Using stable isotope analysis to understand the migration and trophic ecology of northeastern Pacific white sharks (Carcharodon carcharias). PLoS One 7:e30492. doi: 10.1371/journal.pone.0030492
Carscadden, J. E., Frank, K. T., and Miller, D. S. (1989). Capelin (Mallotus villosus) spawning on the Southeast Shoal: influence of physical factors past and present. Can. J. Fish. Aquat. Sci. 46, 1743–1754. doi: 10.1139/f89-221
Casey, J. G., and Pratt, H. L. Jr. (1985). Distribution of the white shark, Carcharodon carcharias, in the Western North Atlantic. Mem. South. Calif. Acad. Sci. 9, 2–14.
Chambault, P., Roquet, F., Benhamou, S., Baudena, A., Pauthenet, E., de Thoisy, B., et al. (2017). The Gulf Stream frontal system: a key oceanographic feature in the habitat selection of the leatherback turtle? Deep Res. Part I Oceanogr. Res. Pap. 123, 35–47. doi: 10.1016/j.dsr.2017.03.003
Chapman, D. D., Feldheim, K. A., Papastamatiou, Y. P., and Hueter, R. E. (2015). There and back again: a review of residency and return migrations in sharks, with implications for population structure and management. Annu. Rev. Mar. Sci. 7, 547–570. doi: 10.1146/annurev-marine-010814-015730
Christiansen, H. M., Lin, V., Tanaka, S., Velikanov, A., Mollet, H. F., Wintner, S. P., et al. (2014). The last frontier: catch records of white sharks (Carcharodon carcharias) in the Northwest Pacific Ocean. PLoS One 9:e94407. doi: 10.1371/journal.pone.0094407
CLS (Collecte Localisation Satellites) (2016). Argos User’s Manual. Available online at: http://www.argos-system.org (accessed July 09, 2021)
Cochran, J. E. M., Braun, C. D., Fernando Cagua, E., Campbell, M. F., Hardenstine, R. S., Kattan, A., et al. (2019). Multi-method assessment of whale shark (Rhincodon typus) residency, distribution, and dispersal behavior at an aggregation site in the Red Sea. PLoS One 4:e0222285. doi: 10.1371/journal.pone.0222285
Coelho, R., Fernandez-Carvalho, J., and Santos, M. N. (2015). Habitat use and diel vertical migration of bigeye thresher shark: overlap with pelagic longline fishing gear. Mar. Environ. Res. 112, 91–99. doi: 10.1016/j.marenvres.2015.10.009
Coffey, D. M., Carlisle, A. B., Hazen, E. L., and Block, B. A. (2017). Oceanographic drivers of the vertical distribution of a highly migratory, endothermic shark. Sci. Rep. 7:10434. doi: 10.1038/s41598-017-11059-6
Colefax, A. P., Kelaher, B. P., Pagendam, D. E., and Butcher, P. A. (2020). Assessing white shark (Carcharodon carcharias) behavior along coastal beaches for conservation-focused shark mitigation. Front. Mar. Sci. 7:268. doi: 10.3389/fmars.2020.00268
Comfort, C. M., and Weng, K. C. (2015). Vertical habitat and behaviour of the bluntnose sixgill shark in Hawaii. Deep Sea Res. Part II 115, 116–126. doi: 10.1016/j.dsr2.2014.04.005
Compagno, L. J. (2001). Sharks of the World: An Annotated and Illustrated Catalogue of Shark Species Known to Date (No. 1). Rome: Food & Agriculture Organization, 269.
Compagno, L. J. V., Marks, M. A., and Fergusson, I. K. (1997). Threatened fishes of the world: Carcharodon carcharias (Linnaeus, 1758) (Lamnidae). Environ. Biol. Fish. 50, 61–62. doi: 10.1023/A:1007308406137
Coughlan, G. (2002). The Southeast Shoal Area of the Grand Banks of Newfoundland Potential as a Marine Protected Area: A Biophysical and Socio-Economic Area Examination. [Master’s thesis]. Calgary, AB: University of Calgary.
Curtis, T. H., McCandless, C. T., Carlson, J. K., Skomal, G. B., Kohler, N. E., Natanson, L. J., et al. (2014). Seasonal distribution and historic trends in abundance of white sharks, Carcharodon carcharias, in the western North Atlantic ocean. PLoS One 9:e99240. doi: 10.1371/journal.pone.0099240
Curtis, T. H., Metzger, G., Fischer, C., McBride, B., McCallister, M., Winn, L. J., et al. (2018). First insights into the movements of young-of-the-year white sharks (Carcharodon carcharias) in the western North Atlantic Ocean. Sci. Rep. 8:10794. doi: 10.1038/s41598-018-29180-5
Del Raye, G., Jorgensen, S. J., Krumhansl, K., Ezcurra, J. M., and Block, B. A. (2013). Travelling light: white sharks (Carcharodon carcharias) rely on body lipid stores to power ocean-basin scale migration. Proc. R. Soc. B Biol. Sci. 280:20130836. doi: 10.1098/rspb.2013.0836
den Heyer, C. E., Don Bowen, W., Dale, J., Gosselin, J., Hammill, M. O., Johnston, D. W., et al. (2021). Contrasting trends in gray seal (Haliochoerus grypus) pup production throughout the increasing northwest Atlantic metapopulation. Mar. Mamm. Sci. 37, 611–630. doi: 10.1111/mms.12773
Dodson, J. J., Aubin-Horth, N., Thériault, V., and Páez, D. J. (2013). The evolutionary ecology of alternative migratory tactics in salmonid fishes. Biol. Rev. 88, 602–625. doi: 10.1111/brv.12019
Domeier, M. L. (2012). “A new life-history hypothesis for white sharks, Carcharodon carcharias,” in The Northeastern Pacific, in Global Perspectives on the Biology and Life History of the White Shark, ed. M. L. Domeier (Boca Raton, FL: CRC Press), 199–224. doi: 10.1201/b11532-21
Domeier, M. L., and Nasby-Lucas, N. (2008). Migration patterns of white sharks Carcharodon carcharias tagged at Guadalupe Island, Mexico, and identification of an eastern Pacific shared offshore foraging area. Mar. Ecol. Prog. Ser. 370, 221–237. doi: 10.3354/meps07628
Domeier, M. L., and Nasby-Lucas, N. (2012). “Sex-specific migration patterns and sexual segregation of adult white sharks, Carcharodon carcharias,” in The Northeastern Pacific, in Global Perspectives on the Biology and Life History of the White Shark, ed. M. L. Domeier (Boca Raton, FL: CRC Press), 133–146.
Domeier, M. L., and Nasby-Lucas, N. (2013). Two-year migration of adult female white sharks (Carcharodon carcharias) reveals widely separated nursery areas and conservation concerns. Anim. Biotelem. 1, 1–10. doi: 10.1186/2050-3385-1-2
Duffy, C. A. J., Francis, M. P., Manning, M. J., and Bonfil, R. (2012). “Regional population connectivity, oceanic habitat, and return migration revealed by satellite tagging of white sharks, Carcharodon carcharias, at New Zealand aggregation sites,” in Global Perspectives on the Biology and Life History of the White Shark, ed. M. L. Domeier (Boca Raton, FL: CRC Press), 301–318.
Earl, J. E., and Zollner, P. A. (2017). Advancing research on animal-transported subsidies by integrating animal movement and ecosystem modelling. J. Anim. Ecol. 86, 987–997. doi: 10.1111/1365-2656.12711
Edwards, J. E., Hiltz, E., Broell, F., Bushnell, P. G., Campana, S. E., Christiansen, J. S., et al. (2019). Advancing research for the management of long-lived species: a case study on the Greenland shark. Front. Mar. Sci. 6:87. doi: 10.3389/fmars.2019.00087
Epperly, S. P., Watson, J. W., Foster, D. G., and Shah, A. K. (2012). Anatomical hooking location and condition of animals captured with pelagic longlines: the Grand Banks experiments 2002–2003. Bull. Mar. Sci. 88, 513–527. doi: 10.5343/bms.2011.1083
Fagan, W. F., Lewis, M. A., Auger-Méthé, M., Avgar, T., Benhamou, S., Breed, G., et al. (2013). Spatial memory and animal movement. Ecol. Lett. 16, 1316–1329. doi: 10.1111/ele.12165
Ferreira, C. A., and Ferreira, T. P. (1996). “Population dynamics of white sharks in South Africa,” in Great White Sharks: The Biology of Carcharodon carcharias, eds A. P. Klimley and D. G. Ainley (San Diego, CA: Academic Press), 381–391. doi: 10.1016/B978-012415031-7/50036-7
Ford, R. G. (1983). Home range in a patchy environment: optimal foraging predictions. Am. Zool. 23, 315–326. doi: 10.1093/icb/23.2.315
Francis, M. P. (1996). “Observations on a pregnant white shark with a review of reproductive biology,” in Great White Sharks: The Biology of Carcharodon carcharias, eds A. P. Klimley and D. G. Ainley (San Diego, CA: Academic Press), 0157–172. doi: 10.1016/B978-012415031-7/50016-1
Francis, M. P., Duffy, C. A. J., Bonfil, R., and Manning, M. J. (2012). “The third dimension. Vertical habitat use by white sharks, Carcharodon carcharias,” in New Zealand and in Oceanic and Tropical Waters of the Southwest Pacific Ocean, in Global Perspectives on the Biology and Life History of the White Shark, ed. M. L. Domeier (Boca Raton, FL: CRC Press), 319–342. doi: 10.1201/b11532-25
Francis, M. P., Duffy, C., and Lyon, W. (2015). Spatial and temporal habitat use by white sharks (Carcharodon carcharias) at an aggregation site in southern New Zealand. Mar. Freshw. Res. 66, 900–918. doi: 10.1071/MF14186
Fraser, K. C., Shave, A., de Greef, E., Siegrist, J., and Garroway, C. J. (2019). Individual variability in migration timing can explain long-term, population-level advances in a songbird. Front. Ecol. Evol. 7:324. doi: 10.3389/fevo.2019.00324
French, G. C. A., Rizzuto, S., Stürup, M., Inger, R., Barker, S., van Wyk, J. H., et al. (2018). Sex, size, and isotopes: cryptic trophic ecology of an apex predator, the white shark Carcharodon carcharias. Mar. Biol. 165, 1–11. doi: 10.1007/s00227-018-3343-x
Galuardi, B., Royer, F., Golet, W., Logan, J., Neilson, J., and Lutcavage, M. (2010). Complex migration routes of Atlantic bluefin tuna (Thunnus thynnus) question current population structure paradigm. Can. J. Fish. Aquat. Sci. 67, 966–976. doi: 10.1139/F10-033
Gaube, P., Braun, C. D., Lawson, G. L., McGillicuddy, D. J., Della Penna, A., Skomal, G. B., et al. (2018). Mesoscale eddies influence the movements of mature female white sharks in the Gulf Stream and Sargasso Sea. Sci. Rep. 8:7363. doi: 10.1038/s41598-018-25565-8
Gleiss, A. C., Jorgensen, S. J., Liebsch, N., Sala, J. E., Norman, B., Hays, G. C., et al. (2011). Convergent evolution in locomotory patterns of flying and swimming animals. Nat. Commun. 2:352. doi: 10.1038/ncomms1350
Goldman, K. J., and Anderson, S. D. (1999). Space utilization and swimming depth of white sharks, Carcharodon carcharias, at the South Farallon Islands, central California. Environ. Biol. Fish. 56, 351–364. doi: 10.1023/A:1007520931105
Goldman, K. J., Anderson, S. D., Latour, R. J., and Musick, J. A. (2004). Homeothermy in adult salmon sharks, Lamna ditropis. Environ. Biol. Fish. 71, 403–411. doi: 10.1007/s10641-004-6588-9
Gowan, T. A., and Ortega-Ortiz, J. G. (2014). Wintering habitat model for the North Atlantic right whale (Eubalaena glacialis) in the southeastern United States. PLoS One 9:e95126. doi: 10.1371/journal.pone.0095126
Grainger, R., Peddemors, V. M., Raubenheimer, D., and Machovsky-Capuska, G. E. (2020). Diet composition and nutritional niche breadth variability in juvenile white sharks (Carcharodon carcharias). Front. Mar. Sci. 7:422. doi: 10.3389/fmars.2020.00422
Gubili, C., Robinson, C. E. C., Cliff, G., Wintner, S. P., de Sabata, E., De Innocentiis, S. D., et al. (2015). DNA from historical and trophy samples provides insights into white shark population origins and genetic diversity. Endanger. Species Res. 27, 233–241. doi: 10.3354/esr00665
Hammill, M. O., den Heyer, C. E., Bowen, W. D., and Lang, S. L. C. (2017). Grey seal population trends in canadian waters, 1960–2016 and harvest advice. DFO Can. Sci. Advis. Sec. Res. Doc. 2017/052. v + 30 p.
Harding, L., Jackson, A., Barnett, A., Donohue, I., Halsey, L., Huveneers, C., et al. (2021). Endothermy makes fishes faster but does not expand their thermal niche. Funct. Ecol. 35, 1951–1959. doi: 10.1111/1365-2435.13869
Hayes, S. A., Hanson, C. V., Pearse, D. E., Bond, M. H., Garza, J. C., and MacFarlane, R. B. (2012). Should I stay or should I go? The influence of genetic origin on emigration behavior and physiology of resident and anadromous juvenile Oncorhynchus mykiss. N. Am. J. Fish. Manage. 32, 772–780. doi: 10.1080/02755947.2012.686953
Hewitt, A. M., Kock, A. A., Booth, A. J., and Griffiths, C. L. (2018). Trends in sightings and population structure of white sharks, Carcharodon carcharias, at Seal Island, False Bay, South Africa, and the emigration of subadult female sharks approaching maturity. Environ. Biol. Fish. 101, 39–54. doi: 10.1007/s10641-017-0679-x
Hillary, R. M., Bravington, M. V., Patterson, T. A., Grewe, P., Bradford, R., Feutry, P., et al. (2018). Genetic relatedness reveals total population size of white sharks in eastern Australia and New Zealand. Sci. Rep. 8:2661. doi: 10.1038/s41598-018-20593-w
Hoffmayer, E. R., McKinney, J. A., Franks, J. S., Hendon, J. M., Driggers, W. B., Falterman, B. J., et al. (2021). Seasonal occurrence, horizontal movements, and habitat use patterns of whale sharks (Rhincodon typus) in the Gulf of Mexico. Front. Mar. Sci. 7:1070. doi: 10.3389/fmars.2020.598515
Hoyos-Padilla, E. M., Klimley, A. P., Galván-Magaña, F., and Antoniou, A. (2016). Contrasts in the movements and habitat use of juvenile and adult white sharks (Carcharodon carcharias) at Guadalupe Island, Mexico. Anim. Biotelem. 4, 1–14. doi: 10.1186/s40317-016-0106-7
Hueter, R. E., Heupel, M. R., Heist, E. J., and Keeney, D. B. (2005). Evidence of philopatry in sharks and implications for the management of shark fisheries. J. Northwest Atl. Fish. Sci. 35, 239–247. doi: 10.2960/J.v35.m493
Hueter, R. E., Manire, C. A., Tyminski, J. P., Hoenig, J. M., and Hepworth, D. A. (2006). Assessing mortality of released or discarded fish using a logistic model of relative survival derived from tagging data. Trans. Am. Fish. Soc. 135, 500–508. doi: 10.1577/T05-065.1
Hussey, N. E., DiBattista, J. D., Moore, J. W., Ward, E. J., Fisk, A. T., Kessel, S., et al. (2017). Risky business for a juvenile marine predator? Testing the influence of foraging strategies on size and growth rate under natural conditions. Proc. R. Soc. B 284:20170166. doi: 10.1098/rspb.2017.0166
Hussey, N. E., Kessel, S. T., Aarestrup, K., Cooke, S. J., Cowley, P. D., Fisk, A. T., et al. (2015). Aquatic animal telemetry: a panoramic window into the underwater world. Science 348:6240. doi: 10.1126/science.1255642
Hussey, N. E., McCann, H. M., Cliff, G., Dudley, S. F. J., Wintner, S. P., and Fisk, A. T. (2012). “Size-based analysis of diet and trophic position of the white shark, Carcharodon carcharias,” in South African Waters, in Global Perspectives on the Biology and Life History of the White Shark, ed. M. L. Domeier (Boca Raton, FL: CRC Press), 27–50. doi: 10.1201/b11532-5
Hutcheson, M. S., Stewart, P. L., and Spry, J. M. (1981). The Biology of Benthic Communities of the Grand Banks of Newfoundland (Including the Hibernia Area). Grand Banks Oceanographic Study, Consultants Report to Mobil Oil Canada Limited, Vol. 7. St. John’s, NL: MacLaren Plansearch Limited, 138.
Hutchinson, M., Coffey, D. M., Holland, K., Itano, D., Leroy, B., Kohin, S., et al. (2019). Movements and habitat use of juvenile silky sharks in the Pacific Ocean inform conservation strategies. Fish. Res. 210, 131–142. doi: 10.1016/j.fishres.2018.10.016
Huveneers, C., Apps, K., Becerril-García, E. E., Bruce, B., Butcher, P. A., Carlisle, A. B., et al. (2018). Future research directions on the “elusive” white shark. Front. Mar. Sci. 5:455. doi: 10.3389/fmars.2018.00455
Jewell, O. J. D., Wcisel, M. A., Gennari, E., Towner, A. V., Bester, M. N., Johnson, R. L., et al. (2011). Effects of smart position only (SPOT) tag deployment on white sharks Carcharodon carcharias in South Africa. PLoS One 6:e27242. doi: 10.1371/journal.pone.0027242
Jewell, O. J., Johnson, R. L., Gennari, E., and Bester, M. N. (2013). Fine scale movements and activity areas of white sharks (Carcharodon carcharias) in Mossel Bay, South Africa. Environ. Biol. Fish. 96, 881–894. doi: 10.1007/s10641-012-0084-4
Jonsen, I. (2016). Joint estimation over multiple individuals improves behavioural state inference from animal movement data. Sci. Rep. 6:20625. doi: 10.1038/srep20625
Jonsen, I. D., Basson, M., Bestley, S., Bravington, M. V., Patterson, T. A., Pedersen, M. W., et al. (2013). State-space models for bio-loggers: a methodological road map. Deep Sea Res. Part II 88–89, 34–46. doi: 10.1016/j.dsr2.2012.07.008
Jonsen, I. D., Myers, R. A., and James, M. C. (2007). Identifying leatherback turtle foraging behaviour from satellite telemetry using a switching state-space model. Mar. Ecol. Prog. Ser. 337, 255–264. doi: 10.3354/meps337255
Jonsen, I. D., Patterson, T. A., Costa, D. P., Doherty, P. D., Godley, B. J., Grecian, W. J., et al. (2020). A continuous-time state-space model for rapid quality-control of Argos locations from animal-borne tags. Mov. Ecol. 8, 1–13. doi: 10.1186/s40462-020-00217-7
Jorgensen, S. J., Anderson, S., Ferretti, F., Tietz, J. R., Chapple, T., Kanive, P., et al. (2019). Killer whales redistribute white shark foraging pressure on seals. Sci. Rep. 9:6153. doi: 10.1038/s41598-019-39356-2
Jorgensen, S. J., Chapple, T. K., Anderson, S., Hoyos, M., Reeb, C., and Block, B. A. (2012). “Connectivity among white shark coastal aggregation areas in the Northeastern Pacific,” in Global Perspectives on the Biology and Life History of the White Shark, ed. M. L. Domeier (Boca Raton, FL: CRC Press), 159–168.
Jorgensen, S. J., Reeb, C. A., Chapple, T. K., Anderson, S., Perle, C., Van Sommeran, S. R., et al. (2010). Philopatry and migration of Pacific white sharks. Proc. R. Soc. B Biol. Sci. 277, 679–688. doi: 10.1098/rspb.2009.1155
Keller, B. A., Putman, N. F., Grubbs, R. D., Portnoy, D. S., and Murphy, T. P. (2021). Map-like use of Earth’s magnetic field in sharks. Curr. Biol. 31, 2881–2886. doi: 10.1016/j.cub.2021.03.103
Kennedy, A. S., Zerbini, A. N., Vásquez, O. V., Gandilhon, N., Clapham, P. J., and Adam, O. (2014). Local and migratory movements of humpback whales (Megaptera novaeangliae) satellite-tracked in the North Atlantic Ocean. Can. J. Zool. 92, 9–18. doi: 10.1139/cjz-2013-0161
Kessel, S. T., Chapman, D. D., Franks, B. R., Gedamke, T., Gruber, S. H., Newman, J. M., et al. (2014). Predictable temperature-regulated residency, movement, and migration in a large, highly mobile marine predator (Negaprion brevirostris). Mar. Ecol. Prog. Ser. 514, 175–190. doi: 10.3354/meps10966
Kock, A. A., Photopoulou, T., Durbach, I., Mauff, K., Meyer, M., Kotze, D., et al. (2018). Summer at the beach: spatio-temporal patterns of white shark occurrence along the inshore areas of False Bay, South Africa. Mov. Ecol. 6:7. doi: 10.1186/s40462-018-0125-5
Kock, A., O’Riain, M. J., Mauff, K., Meÿer, M., Kotze, D., and Griffiths, C. (2013). Residency, habitat use and sexual segregation of white sharks, Carcharodon carcharias in False Bay, South Africa. PLoS One 8:e55048. doi: 10.1371/journal.pone.0055048
Lee, K., Butcher, P., Harcourt, R., Patterson, T., Peddemors, V., Roughan, M., et al. (2021). Oceanographic conditions associated with white shark (Carcharodon carcharias) habitat use along eastern Australia. Mar. Ecol. Prog. Ser. 659, 143–159. doi: 10.3354/meps13572
Loder, J. W. (1991). Summertime bottom temperatures on the Southeast Shoal of the Grand Bank, and implications for exchange rates. Can. J. Fish. Aquat. Sci. 48, 1316–1325. doi: 10.1139/f91-158
Lowther, A. D., Harcourt, R. G., Hamer, D. J., and Goldsworthy, S. D. (2011). Creatures of habit: foraging habitat fidelity of adult female Australian sea lions. Mar. Ecol. Prog. Ser. 443, 249–263. doi: 10.3354/meps09392
Lucas, Z. N., and Natanson, L. J. (2010). Two shark species involved in predation on seals at Sable Island, Nova Scotia, Canada. Proc. N. S. Inst. Sci. 45, 64–88.
Madigan, D. J., Brooks, E. J., Bond, M. E., Gelsleichter, J., Howey, L. A., Abercrombie, D. L., et al. (2015). Diet shift and site-fidelity of oceanic whitetip sharks Carcharhinus longimanus along the Great Bahama Bank. Mar. Ecol. Prog. Ser. 529, 185–197. doi: 10.3354/meps11302
Malcolm, H., Bruce, B. D., and Stevens, J. D. (2001). A Review of the Biology and Status of White Sharks in Australian Waters. Report to Environment Australia, Marine Species Protection Program. Hobart, TAS: CSIRO Marine Research, 113.
Merkle, J. A., Cherry, S. G., and Fortin, D. (2015). Bison distribution under conflicting foraging strategies: site fidelity vs. Energy maximization. Ecology 96, 1793–1801. doi: 10.1890/14-0805.1
Merkle, J. A., Fortin, D., and Morales, J. M. (2014). A memory-based foraging tactic reveals an adaptive mechanism for restricted space use. Ecol. Lett. 17, 924–931. doi: 10.1111/ele.12294
Moxley, J. H., Skomal, G., Chisholm, J., Halpin, P., and Johnston, D. W. (2020). Daily and seasonal movements of Cape Cod gray seals vary with predation risk. Mar. Ecol. Prog. Ser. 644, 215–228. doi: 10.3354/meps13346
Murphy, M. D., Adams, D. H., Tremain, D. M., and Winner, B. L. (1998). Direct validation of ages determined for adult black drum, Pogonias cromis, in east-central Florida, with notes on black drum migration. Fish. Bull. 96, 382–387.
Nakaya, K. (1994). Distribution of white shark in Japanese waters. Fish. Sci. 60, 515–518. doi: 10.2331/fishsci.60.515
Nathan, R., Getz, W. M., Revilla, E., Holyoak, M., Kadmon, R., Saltz, D., et al. (2008). A movement ecology paradigm for unifying organismal movement research. Proc. Natl. Acad. Sci. U.S.A. 105, 19052–19059. doi: 10.1073/pnas.0800375105
Nosal, A. P., Cartamil, D. P., Ammann, A. J., Bellquist, L. F., Ben-Aderet, N. J., Blincow, K. M., et al. (2021). Triennial migration and philopatry in the critically endangered soupfin shark Galeorhinus galeus. J. Appl. Ecol. 58, 1570–1582. doi: 10.1111/1365-2664.13848
O’Leary, S. J., Feldheim, K. A., Fields, A. T., Natanson, L. J., Wintner, S., Hussey, N., et al. (2015). Genetic diversity of white sharks, Carcharodon carcharias, in the Northwest Atlantic and Southern Africa. J. Hered. 106, 258–265. doi: 10.1093/jhered/esv001
Pade, N. G., Queiroz, N., Humphries, N. E., Witt, M. J., Jones, C. S., Noble, L. R., et al. (2009). First results from satellite-linked archival tagging of porbeagle shark, Lamna nasus: area fidelity, wider-scale movements and plasticity in diel depth changes. J. Exp. Mar. Biol. Ecol. 370, 64–74. doi: 10.1016/j.jembe.2008.12.002
Payne, N. L., Meyer, C. G., Smith, J. A., Houghton, J. D., Barnett, A., Holmes, B. J., et al. (2018). Combining abundance and performance data reveals how temperature regulates coastal occurrences and activity of a roaming apex predator. Glob. Change Biol. 24, 1884–1893. doi: 10.1111/gcb.14088
Payne, N. L., Smith, J. A., van der Meulen, D. E., Taylor, M. D., Watanabe, Y. Y., Takahashi, A., et al. (2016). Temperature dependence of fish performance in the wild: links with species biogeography and physiological thermal tolerance. Funct. Ecol. 30, 903–912. doi: 10.1111/1365-2435.12618
Pedersen, M. W., Righton, D., Thygesen, U. H., Andersen, K. H., and Madsen, H. (2008). Geolocation of North Sea cod (Gadus morhua) using hidden Markov models and behavioural switching. Can. J. Fish. Aquat. Sci. 65, 2367–2377. doi: 10.1139/F08-144
Pepin, P., Colbourne, E., and Maillet, G. (2011). Seasonal patterns in zooplankton community structure on the Newfoundland and Labrador Shelf. Prog. Oceanogr. 91, 273–285. doi: 10.1016/j.pocean.2011.01.003
Pershing, A. J., Alexander, M. A., Hernandez, C. M., Kerr, L. A., Le Bris, A., Mills, K. E., et al. (2015). Slow adaptation in the face of rapid warming leads to collapse of the Gulf of Maine cod fishery. Science 350, 809–812. doi: 10.1126/science.aac9819
Piper, W. H. (2011). Making habitat selection more “familiar”: a review. Behav. Ecol. Sociobiol. 65, 1329–1351. doi: 10.1007/s00265-011-1195-1
Pratt, H. L. (1996). “Reproduction in the male white shark,” in Great White Sharks: The Biology of Carcharodon carcharias, eds A. P. Klimley and D. G. Ainley (San Diego, CA: Academic Press), 131–138. doi: 10.1016/B978-012415031-7/50014-8
Pulido, F. (2007). Phenotypic changes in spring arrival: evolution, phenotypic plasticity, effects of weather and condition. Clim. Res. 35, 5–23. doi: 10.3354/cr00711
R Core Team (2020). R: A Language and Environment for Statistical Computing. Vienna: R Foundation for Statistical Computing.
Rigby, C. L., Barreto, R., Carlson, J., Fernando, D., Fordham, S., Francis, M., et al. (2019). Carcharodon carcharias. IUCN Red List Threat. Species 2019:e.T3855A2878674.
Robbins, R. L., Enarson, M., Bradford, R. W., Robbins, W. D., and Fox, A. G. (2015). Residency and local connectivity of white sharks at Liguanea Island: a second aggregation site in South Australia? Open Fish Sci. J. 8, 23–29. doi: 10.2174/1874401X01508010023
Saba, V. S., Griffies, S. M., Anderson, W. G., Winton, M., Alexander, M. A., Delworth, T. L., et al. (2016). Enhanced warming of the Northwest Atlantic Ocean under climate change. J. Geophys. Res.-Oceans 121, 118–132. doi: 10.1002/2015JC011346
Santana-Morales, O., Abadía-Cardoso, A., Hoyos-Padilla, M., Naylor, G. J., Corrigan, S., Malpica-Cruz, L., et al. (2020). The smallest known free-living white shark Carcharodon carcharias (Lamniformes: Lamnidae): ecological and management implications. Copeia 108, 39–46. doi: 10.1643/OT-19-233
Saunders, R. A., Royer, F., and Clarke, M. W. (2011). Winter migration and diving behaviour of porbeagle shark, Lamna nasus, in the Northeast Atlantic. ICES J. Mar. Sci. 68, 166–174. doi: 10.1093/icesjms/fsq145
Semmens, J. M., Payne, N. L., Huveneers, C., Sims, D. W., and Bruce, B. D. (2013). Feeding requirements of white sharks may be higher than originally thought. Sci. Rep. 3:1471. doi: 10.1038/srep01471
Shaw, A. K. (2016). Drivers of animal migration and implications in changing environments. Evol. Ecol. 30, 991–1007. doi: 10.1007/s10682-016-9860-5
Shaw, R. L., Curtis, T. H., Metzger, G., McCallister, M. P., Newton, A., Fischer, G. C., et al. (2021). Three-dimensional movements and habitat selection of young white sharks (Carcharodon carcharias) across a temperate continental shelf ecosystem. Front. Mar. Sci. 8:151. doi: 10.3389/fmars.2021.643831
Shimada, T., Limpus, C. J., Hamann, M., Bell, I., Esteban, N., Groom, R., et al. (2020). Fidelity to foraging sites after long migrations. J. Anim. Ecol. 89, 1008–1016. doi: 10.1111/1365-2656.13157
Sims, D. W. (2010). “Tracking and analysis techniques for understanding free-ranging shark movements and behavior,” in Sharks and Their Relatives II: Biodiversity, Adaptive Physiology, and Conservation, eds J. C. Carrier, J. A. Musick, and M. R. Heithaus (Boca Raton, FL: CRC Press), 351–392. doi: 10.1201/9781420080483-c8
Sims, D. W., Humphries, N. E., Bradford, R. W., and Bruce, B. D. (2012). Lévy flight and Brownian search patterns of a free-ranging predator reflect different prey field characteristics. J. Anim. Ecol. 81, 432–442. doi: 10.1111/j.1365-2656.2011.01914.x
Skomal, G. B., and Benz, G. W. (2004). Ultrasonic tracking of Greenland sharks, Somniosus microcephalus, under Arctic ice. Mar. Biol. 145, 489–498. doi: 10.1007/s00227-004-1332-8
Skomal, G. B., Braun, C. D., Chisholm, J. H., and Thorrold, S. R. (2017). Movements of the white shark Carcharodon carcharias in the North Atlantic Ocean. Mar. Ecol. Prog. Ser. 580, 1–16. doi: 10.3354/meps12306
Skomal, G. B., Chisholm, J., and Correia, S. J. (2012). “Implications of increasing pinniped populations on the diet and abundance of white sharks off the coast of Massachusetts,” in Global Perspectives on the Biology and Life History of the White Shark, ed. M. L. Domeier (Boca Raton, FL: CRC Press), 405–418.
Skomal, G., Marshall, H., Galuardi, B., Natanson, L., Braun, C., and Bernal, D. (2021). Horizontal and vertical movements patterns and habitat use of juvenile porbeagles (Lamna nasus) in the western north Atlantic. Front. Mar. Sci. 8:624158. doi: 10.3389/fmars.2021.624158
Skov, C., Aarestrup, K., Baktoft, H., Brodersen, J., Brönmark, C., Hansson, L. A., et al. (2011). Sizing up your enemy: individual predation vulnerability predicts migratory probability. Proc. R. Soc. B Biol. Sci. 278, 1414–1418. doi: 10.1098/rspb.2010.2035
Spaet, J. L. Y., Patterson, T. A., Bradford, R. W., and Butcher, P. A. (2020). Spatiotemporal distribution patterns of immature Australasian white sharks (Carcharodon carcharias). Sci. Rep. 10:10169. doi: 10.1038/s41598-020-66876-z
Speed, C. W., Field, I. C., Meekan, M. G., and Bradshaw, C. J. (2010). Complexities of coastal shark movements and their implications for management. Mar. Ecol. Prog. Ser. 408, 275–293. doi: 10.3354/meps08581
Stenson, G. B., and Sjare, B. (1997). “Seasonal distribution of harp seals, (Phoca groenlandica),” in The Northwest Atlantic, 23. ICES C. M. 1997/CC 10:23.
Stump, K. L., Crooks, C. J., Fitchett, M. D., Gruber, S. H., and Guttridge, T. L. (2017). Hunted hunters: an experimental test of the effects of predation risk on juvenile lemon shark habitat use. Mar. Ecol. Prog. Ser. 574, 85–95. doi: 10.3354/meps12187
Sulikowski, J. A., Wheeler, C. R., Gallagher, A. J., Prohaska, B. K., Langan, J. A., and Hammerschlag, N. (2016). Seasonal and life-stage variation in the reproductive ecology of a marine apex predator, the tiger shark Galeocerdo cuvier, at a protected female-dominated site. Aquat. Biol. 24, 175–184. doi: 10.3354/ab00648
Switzer, P. V. (1993). Site fidelity in predictable and unpredictable habitats. Evol. Ecol. 7, 533–555. doi: 10.1007/BF01237820
Tanaka, K. R., Van Houtan, K. S., Mailander, E., Dias, B. S., Galginaitis, C., O’Sullivan, J., et al. (2021). North Pacific warming shifts the juvenile range of a marine apex predator. Sci. Rep. 11:3373. doi: 10.1038/s41598-021-82424-9
Tanaka, S., Kitamura, T., Mochizuki, T., and Kofuji, K. (2011). Age, growth and genetic status of the white shark (Carcharodon carcharias) from Kashima-nada, Japan. Mar. Freshw. Res. 62, 548–556. doi: 10.1071/MF10130
Templeman, W. (1975). Comparison of temperatures in July-August hydrographic sections of the eastern Newfoundland area in 1972 and 1973 with those from 1951 to 1971. Int. Comm. Northwest Atl. Fish. Spec. Publ. 10, 17–31.
Tolotti, M. T., Bach, P., Hazin, F., Travassos, P., and Dagorn, L. (2015). Vulnerability of the oceanic whitetip shark to pelagic longline fisheries. PLoS One 10:e0141396. doi: 10.1371/journal.pone.0141396
Vaudo, J. J., Byrne, M. E., Wetherbee, B. M., Harvey, G. M., and Shivji, M. S. (2017). Long-term satellite tracking reveals region-specific movements of a large pelagic predator, the shortfin mako shark, in the western North Atlantic Ocean. J. Appl. Ecol. 54, 1765–1775. doi: 10.1111/1365-2664.12852
Watanabe, Y. Y., Payne, N. L., Semmens, J. M., Fox, A., and Huveneers, C. (2019). Swimming strategies and energetics of endothermic white sharks during foraging. J. Exp. Biol. 222:jeb185603. doi: 10.1242/jeb.185603
Weng, K. C., Castilho, P. C., Morrissette, J. M., Landeira-Fernandez, A. M., Holts, D. B., Schallert, R. J., et al. (2005). Satellite tagging and cardiac physiology reveal niche expansion in salmon sharks. Science 310, 104–106. doi: 10.1126/science.1114616
Weng, K. C., Boustany, A. M., Pyle, P., Anderson, S. D., Brown, A., and Block, B. A. (2007a). Migration and habitat of white sharks (Carcharodon carcharias) in the eastern Pacific Ocean. Mar. Biol. 152, 877–894. doi: 10.1007/s00227-007-0739-4
Weng, K. C., O’Sullivan, J. B., Lowe, C. G., Winkler, C. E., Dewar, H., and Block, B. A. (2007b). Movements, behavior and habitat preferences of juvenile white sharks Carcharodon carcharias in the eastern Pacific. Mar. Ecol. Prog. Ser. 338, 211–224. doi: 10.3354/meps338211
White, T. D., Carlisle, A. B., Kroodsma, D. A., Block, B. A., Casagrandi, R., De Leo, G. A., et al. (2017). Assessing the effectiveness of a large marine protected area for reef shark conservation. Biol. Conserv. 207, 64–71. doi: 10.1016/j.biocon.2017.01.009
Whitehead, H., and Glass, C. (1985). The significance of the Southeast Shoal of the Grand Bank to humpback whales and other cetacean species. Can. J. Zool. 63, 2617–2625. doi: 10.1139/z85-391
Winkler, D. W., Jørgensen, C., Both, C., Houston, A. I., McNamara, J. M., Levey, D. J., et al. (2014). Cues, strategies, and outcomes: how migrating vertebrates track environmental change. Mov. Ecol. 2, 1–15. doi: 10.1186/2051-3933-2-10
Winton, M. V., Sulikowski, J., and Skomal, G. B. (2021). Fine-scale vertical habitat use of white sharks at an emerging aggregation site and implications for public safety. Wildl. Res. 48, 345–360. doi: 10.1071/WR20029
Wood, S. A., Murray, K. T., Josephson, E., and Gilbert, J. (2020). Rates of increase in gray seal (Halichoerus grypus atlantica) pupping at recolonized sites in the United States, 1988–2019. J. Mammal. 101, 121–128. doi: 10.1093/jmammal/gyz184
Keywords: white shark, western North Atlantic, telemetry, migration, fidelity
Citation: Franks BR, Tyminski JP, Hussey NE, Braun CD, Newton AL, Thorrold SR, Fischer GC, McBride B and Hueter RE (2021) Spatio-Temporal Variability in White Shark (Carcharodon carcharias) Movement Ecology During Residency and Migration Phases in the Western North Atlantic. Front. Mar. Sci. 8:744202. doi: 10.3389/fmars.2021.744202
Received: 19 July 2021; Accepted: 13 October 2021;
Published: 18 November 2021.
Edited by:
Yannis Peter Papastamatiou, Florida International University, United StatesReviewed by:
Charlie Huveneers, Flinders University, AustraliaCopyright © 2021 Franks, Tyminski, Hussey, Braun, Newton, Thorrold, Fischer, McBride and Hueter. This is an open-access article distributed under the terms of the Creative Commons Attribution License (CC BY). The use, distribution or reproduction in other forums is permitted, provided the original author(s) and the copyright owner(s) are credited and that the original publication in this journal is cited, in accordance with accepted academic practice. No use, distribution or reproduction is permitted which does not comply with these terms.
*Correspondence: Bryan R. Franks, YmZyYW5rc0BqdS5lZHU=
Disclaimer: All claims expressed in this article are solely those of the authors and do not necessarily represent those of their affiliated organizations, or those of the publisher, the editors and the reviewers. Any product that may be evaluated in this article or claim that may be made by its manufacturer is not guaranteed or endorsed by the publisher.
Research integrity at Frontiers
Learn more about the work of our research integrity team to safeguard the quality of each article we publish.