- 1Institut de Biologie Intégrative et des Systèmes, Université Laval, Quebec, QC, Canada
- 2Département de Biologie, Faculté des Sciences et de Génie, Université Laval, Quebec, QC, Canada
- 3Département de Microbiologie-Infectiologie et d’Immunologie, Faculté de Médecine, Université Laval, Quebec, QC, Canada
- 4Department of Microbiology and Immunology, College of Veterinary Medicine, Cornell University, Ithaca, NY, United States
- 5Département de Biochimie, de Microbiologie et de Bio-Informatique, Faculté des Sciences et de Génie, Université Laval, Quebec, QC, Canada
- 6Centre de Recherche de l’Institut Universitaire de Cardiologie et de Pneumologie de Québec, Université Laval, Quebec, QC, Canada
Aeromonas salmonicida subsp. salmonicida is a Gram-negative bacterium that causes furunculosis, a fish disease claiming substantial economic losses in the aquaculture industry. Major challenges exist in monitoring and controlling fish infections in aquaculture farms. Development of management practices to improve the sustainability of fish farming with disease prevention necessitates studies using well-defined systems and well-characterized bacterial isolates. Even though several A. salmonicida subsp. salmonicida genomes have been completely assembled and thoroughly annotated, in vivo pathogenicity data are lacking. Here we present A. salmonicida subsp. salmonicida 890054 as a prototype strain for standardized furunculosis challenges with survival data. Computational analysis of sequencing results provided a complete circular genome with annotations of plasmids carrying virulence factors, antimicrobial resistance, and secondary metabolite coding genes. The analysis also revealed the presence of an IncU plasmid distinct from other IncU plasmids previously associated with Aeromonas.
Introduction
The Gram-negative bacterium Aeromonas salmonicida subsp. salmonicida is the etiologic agent of furunculosis, a disease affecting salmonids and being a substantial burden in the aquaculture industry (Bartkova et al., 2017). Monitoring and controlling fish infections is a critical management aspect in fisheries and aquaculture farms (FAO, 2020). Well-defined systems are required for the study of freshwater fish culture and disease prevention. A collection of A. salmonicida subsp. salmonicida isolates have been used in various in vivo pathogenicity studies especially in vaccine development (Marquis and Lallier, 1989; Garduño et al., 1993; Marsden et al., 1996; Marana et al., 2017). However, most of those studies used different strains of A. salmonicida subsp. salmonicida for infection challenges. This may lead to difficulties in repeating and generalizing results, in part due to the tremendous genomic diversity within the accessory genome of this subspecies (Attéré et al., 2017; Massicotte et al., 2019; Vincent et al., 2020).
To better understand and define the underlying mechanisms of fish microbial pathogenesis, experimental protocols defining conditions mimicking natural infections and the use of microbial pathogens characterized at both phenotypic and genomic levels need to be adopted. For this purpose, we used A. salmonicida subsp. salmonicida strain 890054, a strain isolated from a diseased brook charr (Gansevoort, NY, United States) in 1989 by Dr. Paul Bowser of the Fish Disease Diagnostic Laboratory of Cornell University (Ithaca, NY, United States). Recently, we tested a tailor-made infection challenge protocol for brook charr (Salvelinus fontinalis) using a combination of intraperitoneal (IP) injection and cross-contamination methods that are reminiscent of a progressive natural outbreak (Figure 1A).
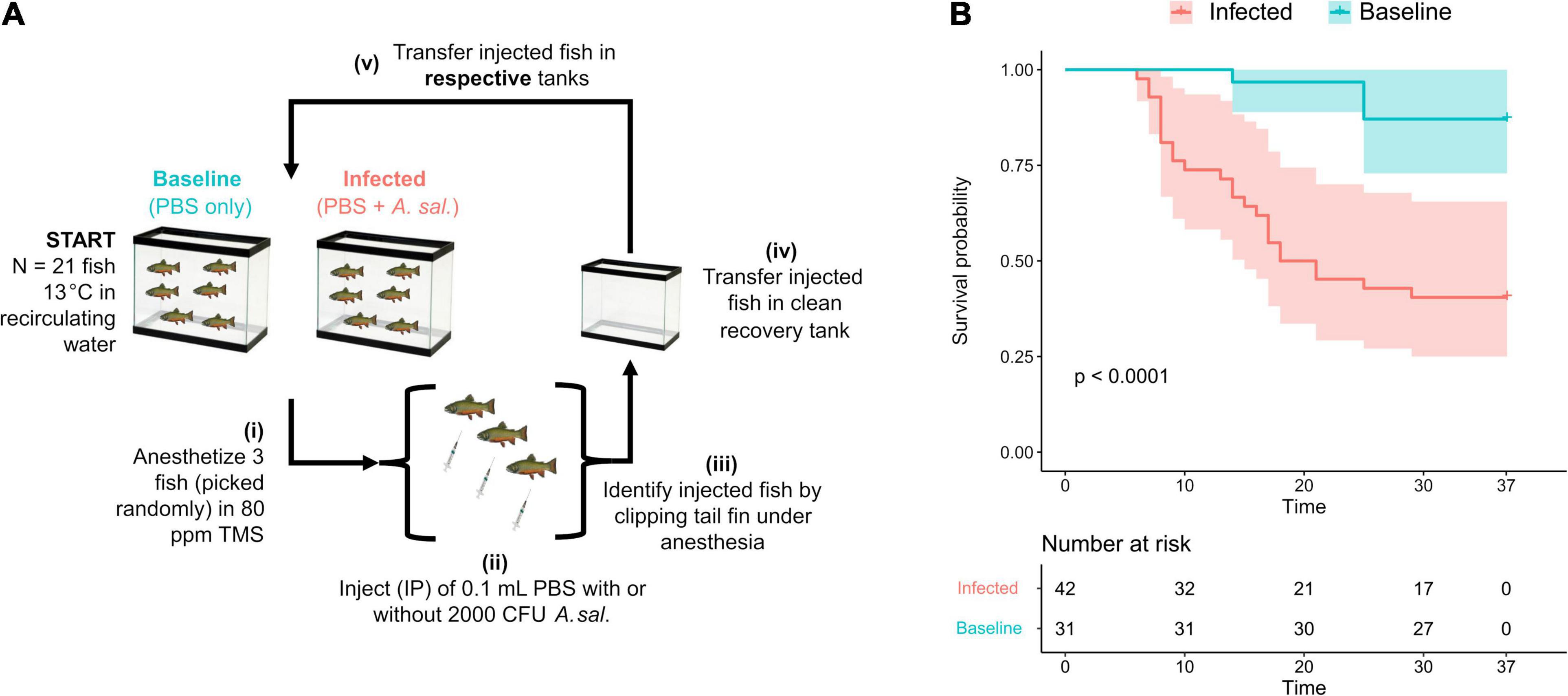
Figure 1. A model system for pathogenicity studies involving A. salmonicida subsp. salmonicida. (A) Cohabitation strategy to experimentally infect brook charr with A. salmonicida subsp. salmonicida strain 890054 as the infectious agent. Even though three replicate tanks per group are required in this protocol, they were not shown here for simplicity. Infected fish and Baseline fish are kept in separate sets of three tanks to avoid cross-contamination. (B) Survival probability of brook charr infected with A. salmonicida subsp. salmonicida 890054 during a 37-day cohabitation protocol. The p-value reported in this figure is from a log-rank test of the Kaplan–Meier statistic. The colored areas represent confidence intervals (significance threshold = 0.01). Injected fish (N = 18) were discarded from the survival analysis, hence the number of 73 individuals at risk at time T = 0. Number at risk represents the number of individuals within each group that may or may not develop furunculosis at a given time.
Here, in this short perspective article, we present A. salmonicida subsp. salmonicida strain 890054 as a prototype strain for standardized furunculosis challenges with characterization at the genomic level with complete chromosome assembly and plasmid content, including annotations for virulence factors (VFs), and potential of antimicrobial resistance (AMR) and secondary metabolite production. We entertain the possibility that standardized animal infection models using prototype isolates well-defined at the genomic and annotation levels will prove useful in the development of drugs or alternative treatments, especially through the added knowledge of VFs, drug resistance, and secondary metabolite production.
Materials and Methods
In vivo Furunculosis Model
Animal Ethics Statement
The in vivo furunculosis challenge was performed at the Veterinary Medical Center Aquatic Facility, Cornell University (Ithaca, NY, United States). Fish were maintained and euthanized prior to measurements and samplings in compliance with standard operating procedures of Cornell University’s Institutional Animal Care and Use Committee (IACUC, protocol number 2017-0033).
Brook Charr and Rearing Conditions
Brook charr fingerlings (weight ∼5 g, length ∼5 cm) were obtained from the Aquaculture Center of the State University of New York in Morrisville, NY, United States. Fish were acclimated in 40 L tanks with recirculation system for a period of 30 days before being assigned to an experimental group (Infected, Baseline). For either the “Infected” or “Baseline” group, three independent recirculating aquariums were set up, with each one containing 21 fish in 40 L dechlorinated freshwater maintained at 13.6 ± 0.2°C, pH 7.2, throughout the experiment (i.e., three biological replicates per treatment for a total of six independent aquariums). Dissolved oxygen was maintained above 8 mg/L with air bubbling stones running at maximum flow (1 per tank). Fish were fed 1% body weight daily with 1.5 mm Bio-Vita pellets (Bio-Oregon).
Pathogenic Strain Selection
Aeromonas salmonicida subsp. salmonicida strain 890054 was selected from a collection of seven A. salmonicida subsp. salmonicida strains isolated in fish from the New York state to assess a set of genomic and proteomic factors associated with virulence.
i) The integrity of the type three secretion system (T3SS) on the plasmid pAsa5 was tested by PCR amplification of the ati2 and resD genes as described by Daher et al. (2011);
ii) Protease activity was tested on skimmed milk agar medium (Rasch et al., 2007);
iii) The presence of the A-layer protein was tested on Coomassie Brilliant Blue agar (Cipriano and Bertolini, 1988).
Strain 890054 met all three virulence criteria in addition of having been isolated from brook charr. Then, its virulence was verified in a preliminary experiment using adult brook charr ranging from 160 to 260 g body weight. Doses of 100–10,000 CFU were injected intraperitoneally in anesthetized fish. Fish receiving a dose of ≈1,000 and 2,000 CFU showed signs of infection 4–10 days post-infection (dpi).
Furunculosis Challenge
For each replicate tank, three randomly selected fish (average 7–8 g body weight) received 2,000 CFU of A. salmonicida subsp. salmonicida 890054 suspended in 0.1 mL 1× PBS, pH 7.4 (Infected group) or sterile PBS (Baseline group) by IP injection. A small segment of the caudal fin was clipped for identifying injected fish (Axelsson et al., 2020). Fish were anesthetized in 80 mg/L tricaine methanesulfonate (TMS) during the procedure. Fish were returned to their respective units after recovery from anesthesia.
Statistical Analysis
Survival probability was assessed throughout the 37 days of experiment using R (R Core Team, 2019) and RStudio (RStudio Team, 2016). Survival fits were calculated from mortality counts with package survival (Therneau, 2015). Package survminer (Kassambara and Kosinski, 2018) was used for plotting Kaplan–Meier survival curves. To verify that each death event was caused by furunculosis, kidney stabs from dead or moribund individuals were streaked on Brain–Heart Infusion (BHI) agar (BD) and incubated at 18°C for 3 days. When no colonies producing dark brown pigments [a key phenotype of A. salmonicida subsp. salmonicida (Austin and Austin, 2012)] were found on agar, death events were censored (i.e., not caused by furunculosis). Only one such event occurred out of all 91 recorded mortality events over the whole experiment (event #24, see Supplementary Table 1).
Genome Analysis of Aeromonas salmonicida subsp. salmonicida 890054
DNA Library Preparation and Sequencing
First, the 890054 strain was grown in pure culture (in LB broth) for 24 h at 18°C and then adjusted to an OD600 of ∼1.0. A volume of 1 mL of this culture was used for DNA extraction using the DNeasy Blood and Tissue Kit (QIAGEN) with the recommended protocol for Gram-negative bacteria. Genomic DNA was sequenced in short, paired-end reads (2 × 300 bp) with the Illumina MiSeq technology at the IBIS Genomic Analysis Platform (Université Laval, Quebec, QC, Canada). Long reads were obtained with MinION sequencing (Oxford Nanopore); input DNA was prepared using the Ligation Sequencing Kit (SQK-LSK109). The resulting DNA library was analyzed on a R9.5 (FLO-MIN106) flow cell and sequenced on a MinION Mk1B apparatus.
Hybrid Assembly
The sequencing effort resulted in 1,177,819 raw short read pairs and 346,938 raw long reads. Short reads with mean Phred quality score greater than 20 were selected with seqtk1 and adapters were trimmed with Trimmomatic v0.36,2 resulting in 1,070,840 filtered short reads. Long reads were basecalled and adapters were trimmed with Guppy v4.2.2-GPU.3 Long reads with mean Phred quality score over 10 were selected with NanoFilt v2.3.0.4 An additional filtering step was applied in which Nanopore reads matching with MiSeq reads were enriched with Filtlong v0.2.0,5 with the –keep_percent option set to 90 and –min_length set to 1,000. A total of 500,000,000 bases from long reads were retained.
Short and long filtered reads were then assembled with Unicycler v0.4.86 using the default parameters for hybrid genome assembly. Briefly, Unicycler assembled short reads into contigs and bridged those contigs with long reads, and then circularized the complete chromosome and or plasmid sequences when possible.
Taxonomic Assessment
The taxonomy of 890054 was confirmed with an average nucleotide identity (ANI) analysis using pyani v0.2 (Pritchard et al., 2016). In addition to 890054, a total of 88 representative A. salmonicida genomes spanning across all five known subspecies (achromogenes, masoucida, pectinolytica, salmonicida, and smithia) was included in this analysis. The representative genomes formed clusters that correlated with their subspecies above 99.99% ANI. Therefore, this threshold was used to verify 890054’s belonging to a subspecies.
Annotation and Visualization
The final assembly was then annotated with RAST v2.07 using the “RASTtk” algorithm with default parameters. AMR genes were specifically annotated with Resistance Gene Identifier v5.1.18 using the CARD v3.1.1 database. VF subsystems were retrieved from RAST annotations and compared with the ones of reference strain A449 (also annotated for this purpose with RAST to limit annotation bias). Secondary metabolite production was predicted from genome data using antiSMASH v6.0 alpha 1.9 Plasmid maps were drawn with SnapGene v5.210 and pairwise alignments were drawn with EasyFig v2.2.5.11
Small Plasmid Wet Lab Analysis
The presence of three small cryptic plasmids (pAsa1, pAsa2, and pAsa3) as well as and pAsal1 in 890054 was confirmed by PCR and plasmid profiling using agarose gel electrophoresis as previously described (Boyd et al., 2003; Attéré et al., 2015). For the needs of this study, an agarose gel electrophoresis was performed to visualize small cryptic plasmids (4–6 kb range) in the 890054 strain as well as in three control strains: 01-B526, which is known to bear all four small plasmids, and strains SHY13-2317 and SHY13-3795 which lack pAsa3 and pAsal1, respectively (Attéré et al., 2015). For each strain, plasmid extractions were made by resuspended one quarter of a TSB petri dish culture directly in the first buffer from the QIAprep Spin Miniprep Kit (QIAGEN). After DNA quantitation with a NanoDrop 2000 spectrophotometer (Thermo Fisher), 1 μg of each plasmid DNA extraction was digested for 2 h at 37°C with restriction enzyme EcoRI-HF from New England Biolabs. The DNA fragments were separated on a 0.7%-agarose gel for 90 min at 90 V in 1× tris-borate-EDTA (TBE) buffer. The Quick-load 2-log DNA ladder from New England Biolabs was used as a molecular weight marker. The agarose gel was stained in a bath of ethidium bromide (0.5 μg/mL) after migration to visualize DNA under UV illumination.
Small Plasmid Reference-Based Assembly
pAsa3 and pAsal1 were not detected in the hybrid assembly described above even though they were detected by the wet lab analysis. In order to detect plasmids pAsa3 and pAsal1 in the sequence data, these plasmids were assembled by using Minimap2 v2.2012 to align Oxford Nanopore long reads to reference sequences (GenBank accessions NC_004924 and NC_004338, respectively). Minimap2 assemblies were then converted from SAM to FASTA format with Samtools13 and underwent two rounds of polishing. The first one was performed with Racon v1.4.1014 using long reads as input to fill gaps and correct base ambiguities; the second round was performed with Pilon v1.2415 on the Racon-polished assembly with short Illumina reads (aligned to draft assembly in BAM format) as input, mostly to improve consensus accuracy and correct artifactual indels that could cause frameshifts in gene annotations.
Results and Discussion
In vivo Furunculosis Model
The hybrid infection protocol using A. salmonicida subsp. salmonicida strain 890054 led to daily mortalities without depleting fish stocks; the mortality rate was roughly 50% 37 dpi (Figure 1B). The Baseline control group, which received a placebo, had a 95% overall survival rate and significantly differed from the Infected group (Figure 1B). A single death event in the Baseline control group could not be linked to a positive A. salmonicida subsp. salmonicida culture from the kidney stab and was censored prior to plotting survival curves. All other mortality events were associated with a positive culture from the kidney stab, suggesting that fish were dying of furunculosis (Supplementary Table 1). Note that bacteria other than A. salmonicida may also produce brown-red pigments on agar, meaning either bacteria other than A. salmonicida infected those fish, or endemic strains of A. salmonicida were already present within their microbiota, and that other organs, e.g., gills, spleen, intestine, heart, or brain could have been sampled for supplemental validation and gained knowledge on the infection process.
The Kaplan–Meier survival fits and risk tables presented in Figure 1B also showed that the infection stops spreading circa 30 dpi, with no more additional deaths until the end of the experiment at 37 dpi (Figure 1B). There were significant differences between the survival curves of infected fish relative to the baseline supported by non-overlapping 99% confidence intervals. The major differences in terms of survival allowed rigorous pathogenicity assays where there was a clear distinction between infected and control groups.
Genome Analysis of Aeromonas salmonicida 890054
Accessory Genome
We obtained a complete genome assembly that includes one 4.7 Mbp circular chromosome and eight circular plasmids from ∼5.2 to 157 Kbp (Supplementary Table 2). Seven of these plasmids are typical for a strain isolated in North America (pAsa1, pAsa2, pAsa3, pAsal1, pRAS3.3, pAsa9-like, and pAsa5) (Vincent et al., 2014; Attéré et al., 2015; Tanaka et al., 2017). The genome of 890054 contains the typical prophages 1 and 2 found in virtually all A. salmonicida subsp. salmonicida strains as well as AsaGEI1a, a genomic island, identical to the one found in 01-B526 (Reith et al., 2008; Emond-Rheault et al., 2015).
A novel 46.7 kb plasmid (contig 4) with no known equivalent identified in the A. salmonicida plasmidome (Vincent et al., 2020) was compared to sequences from the chromosome and the pAsa5 and pAsa9-like plasmids to rule out potential misassembly from other genetic elements. No significant alignments between contig 4, the chromosome or other plasmids were found (Supplementary Figure 1). This data indicated that contig 4 is a novel plasmid in A. salmonicida subsp. salmonicida that we hereby refer to as p890054-1.
Novel Plasmid p890054-1
Plasmid p890054-1 is roughly divided into three main regions: a conjugal transfer system, a Vir-like type IV secretion system gene cluster and a putative composite transposon containing several AMR genes (Figure 2A). It is highly similar in structure with pRA3 (Figure 2B), a IncU plasmid from A. hydrophila, a mesophilic Aeromonas species also known for causing infections in fish, amphibians, and even humans (Janda and Abbott, 2010). Globally, p890054-1 and pRA3 share a similar backbone, but a different transposon. It is the second IncU plasmid recently identified in A. salmonicida subsp. salmonicida strains. The first one, pRAS1b (Vaillancourt et al., 2021), shares backbone similarity with p890054-1 except major differences in their transposon (Supplementary Figure 2A). However, unlike pRA3 and pRAS1b, an IS5195 family transposase, flanked by 23 bp inverted repeats, is inserted in a DNA topoisomerase I (topA) gene within the IncU backbone of p890054-1 (Supplementary Figure 2B). This insertion appears to truncate topA in two open reading frames, potentially leading to a non-functional TopA protein. The exact function of this topA gene in p890054-1 is unknown as well as the impact of the inserted transposase gene within this sequence.
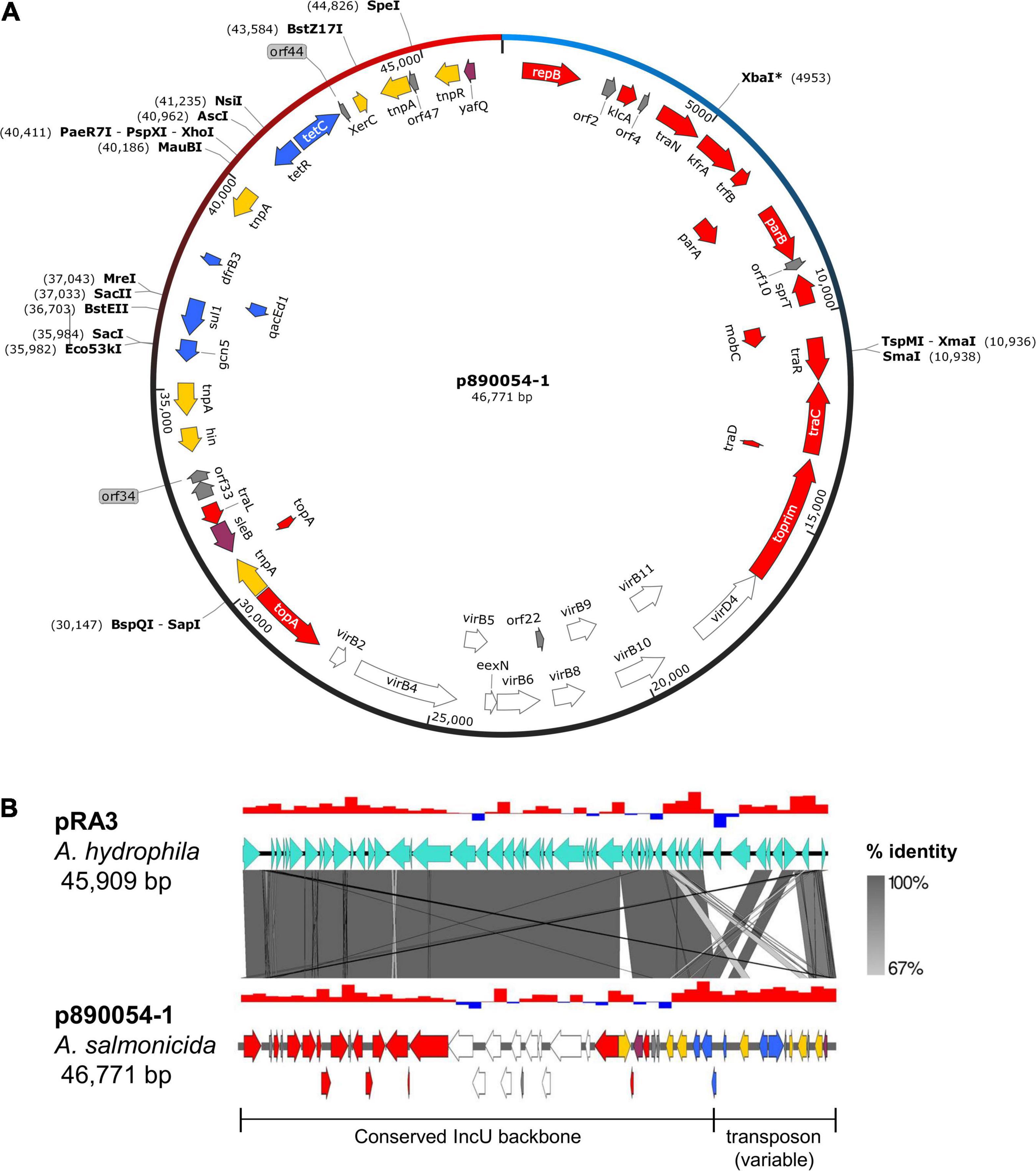
Figure 2. Genetic map of p890054-1 (contig 4). (A) Circular map including coding DNA sequences (arrows) and unique restriction sites. Red arrows: conjugative transfer and partitioning genes. White arrows: Vir-like type IV secretion system genes. Yellow arrows: site-specific recombinases (transposase, resolvase, and invertase). Blue arrows: AMR genes. Purple arrows: Virulence and toxin/antitoxin genes. Gray arrows: genes encoding hypothetical proteins. (B) Pairwise alignment with pRA3 (A. hydrophila), a highly similar IncU family plasmid sharing the same mobility genes and Vir-like system gene cluster. Percentages indicate sequence identity while red/blue bars above sequences represent GC skew.
Small Cryptic Plasmids pAsa3 and pAsal1
Despite being undetected in the Unicycler hybrid assembly, the sequence of plasmids pAsa3 and pAsal1 could be retrieved, each in a single contig, with a reference-based approach, either with Illumina short DNA reads or with Oxford Nanopore long DNA reads (Supplementary Figure 3). Both plasmids were also visible in agarose gels within expected sizes as in other strains known to possess those plasmids (Supplementary Figure 4).
Antibiotic Resistance
The CARD AMR database identified a series of AMR genes in the chromosome, in the pRAS3.3 plasmid and in the novel p890054-1 plasmid (Supplementary Table 3). More specifically, p890054-1 encodes the following genes: gcn5, sul1, qacEdelta1, dfrB3, and tet(A) (Supplementary Table 3 and Supplementary Figure 5). Based on this analysis, 890054 contains AMR genes against several families of antibiotics: fluoroquinolone, tetracycline, carbapenems, cephalosporin, elfamycin, sulfonamides, acridine, polymyxin, and diaminopyrimidine.
Secondary Metabolites
An antiSMASH analysis detected five antimicrobial and secondary metabolite biosynthetic gene clusters in 890054’s chromosome (Supplementary Figure 6A), but none in its plasmids. Among those five, a biosynthesis cluster for pseudomonine, a siderophore usually found in Pseudomonas species. This cluster had 100% similarity with the pseudomonine siderophore of Pseudomonas fluorescens WCS374 (Supplementary Figure 6B), which is a well-known strain used in agriculture against plant pathogens such as pear and apple blight (Erwinia spp.) (Mercado-Blanco et al., 2001). Therefore, using P. fluorescens WCS374 as a candidate to control an outbreak of furunculosis might prove unsuccessful, considering that A. salmonicida subsp. salmonicida strain 890054 possesses its own pseudomonine iron uptake system.
Virulence Factors
The VF profile of 890054 was similar to reference strain A449 with a few exceptions (Supplementary Table 4). Notably, a Vir-like type IV secretion system (located on p890054-1) was absent in A449, whereas the type VI secretion system (T6SS) genes located on the pAsa4 plasmid in A449 was absent in 890054. However, the A449 T6SS is unlikely to be functional because of several truncated T6SS genes (Reith et al., 2008). Whether this Vir-like system (if active) is involved in conjugation of secretion of effector protein remains to be determined. Also notable was the absence of mannose-sensitive fimbriae and colicin-like bacteriocin production cluster genes, all present in A449 (Supplementary Table 4). Note that the presence of one or more genes does not necessarily imply that a given cluster is active.
Relationship With Other Aeromonas salmonicida Strains
Strain 890054 was initially selected as a representative virulent A. salmonicida subsp. salmonicida strain because it possesses its hallmarks of virulence, namely T3SS, protease activity and A-layer (see section “Materials and Methods” “Pathogenic Strain Selection”). We have verified our taxonomic assessment for 890054 by analyzing its complete genome sequence. First, an ANI analysis has clearly situated 890054 within subspecies salmonicida (summarized in Supplementary Table 5; see full table with 88 representative genomes in Supplementary Data Sheet 2). Indeed, 890054 and other genomes from subspecies salmonicida form a subspecies group with less than 0.01% dissimilarity, which is 10 times less dissimilarity than with genomes from other subspecies (i.e., achromogenes, masoucida, pectinolytica, and smithia). In addition, 890054 possesses all four small cryptic plasmids (Supplementary Table 6) from the core A. salmonicida subsp. salmonicida plasmidome proposed by Vincent et al. (2020) as well as AsaGEI1a which is typically found in about 1/3 of the North American A. salmonicida subsp. salmonicida strains (Emond-Rheault et al., 2015). Therefore, we conclude that 890054 is indeed a representative virulent A. salmonicida subsp. salmonicida strain.
Conclusion
The bacterial pathogen A. salmonicida subsp. salmonicida creates havoc in salmonid fisheries by causing furunculosis. Several studies have initiated the genomic and plasmid content description of A. salmonicida subsp. salmonicida, but not necessarily in the context of studying furunculosis in vivo. A better understanding of fish microbial diseases requires defined environmental conditions, hosts, and well-defined microbial pathogens for laboratory experiments.
This short perspective article is a first step in resolving these issues. Here we present a well-defined in vivo furunculosis infection model using brook charr, a salmonid fish highly sensitive to furunculosis, and a virulent A. salmonicida subsp. salmonicida strain whose genome sequence is complete and annotated, including plasmid content, VFs, antibiotic resistance genes and metabolite biosynthesis gene clusters.
We expect that this prototype fish infection model will prove useful as a standard method for studying the genotype–phenotype interactions and evolutive aspects of A. salmonicida subsp. salmonicida pathogenicity in vivo. We do, however, recognize the role of A. salmonicida biogeography and evolution in its pathogenicity, with novel strains causing outbreaks annually (Burr et al., 2005; Emond-Rheault et al., 2015; Trudel et al., 2016). Nevertheless, the methods proposed herein could also apply to novel A. salmonicida strains for standardizing in vivo assessment of novel strategies against this ravaging disease.
Data Availability Statement
The genome sequence of A. salmonicida subsp. salmonicida 890054 and raw sequencing reads have been deposited under NCBI BioProject PRJNA773379. The infection challenge data and source code for statistical analyses is available at https://github.com/jeffgauthier/furunculosis-model.
Ethics Statement
The animal study was reviewed and approved by the Comité de Protection des Animaux de l’Université Laval (CPAUL), Laval University, Quebec, QC, Canada and the Institutional Animal Care and Use Committee (IACUC) of Cornell University College of Veterinary Medicine.
Author Contributions
JG performed the sequencing and assembly and drafted the manuscript. HM provided the strain and facilities for in vivo furunculosis challenge, worked on planning the experimental design, and reviewed the manuscript along with VP, SC, RL, and ND. VP performed the wet lab plasmid analysis. RL provided the Nanopore sequencing expertise and manuscript concept, and funded the sequencing project. All authors contributed to the article and approved the submitted version.
Funding
This work was funded by NSERC Discovery and MAPAQ Innovamer grants (to ND and SC), CIHR and Genome Canada grants (to RL), and a NSF grant (to HM). JG received a FRQNT student mobility grant during his work at HM’s Laboratory in Cornell University.
Conflict of Interest
The authors declare that the research was conducted in the absence of any commercial or financial relationships that could be construed as a potential conflict of interest.
Publisher’s Note
All claims expressed in this article are solely those of the authors and do not necessarily represent those of their affiliated organizations, or those of the publisher, the editors and the reviewers. Any product that may be evaluated in this article, or claim that may be made by its manufacturer, is not guaranteed or endorsed by the publisher.
Acknowledgments
We express our gratitude to the team of the IBIS Genomics analysis platform (Université Laval) for excellent work and collaborations (http://www.ibis.ulaval.ca/en/services-2/genomic-analysis-platform/).
Supplementary Material
The Supplementary Material for this article can be found online at: https://www.frontiersin.org/articles/10.3389/fmars.2021.744052/full#supplementary-material
Footnotes
- ^ https://github.com/lh3/seqtk
- ^ https://github.com/timflutre/trimmomatic
- ^ https://nanoporetech.com/nanopore-sequencing-data-analysis
- ^ https://github.com/wdecoster/nanofilt
- ^ https://github.com/rrwick/filtlong
- ^ https://www.github.com/rrwick/unicycler
- ^ https://rast.nmpdr.org
- ^ http://arpcard.mcmaster.ca
- ^ http://antismash.secondarymetabolites.org
- ^ https://www.snapgene.com/
- ^ https://mjsull.github.io/Easyfig/
- ^ https://github.com/lh3/minimap2
- ^ https://github.com/samtools/
- ^ https://github.com/isovic/racon
- ^ https://github.com/broadinstitute/pilon
References
Attéré, S. A., Vincent, A. T., Paccaud, M., Frenette, M., and Charette, S. J. (2017). The role for the small cryptic plasmids as moldable vectors for genetic innovation in aeromonas salmonicida subsp. salmonicida. Front. Genet. 8:211. doi: 10.3389/fgene.2017.00211
Attéré, S. A., Vincent, A. T., Trudel, M. V., Chanut, R., and Charette, S. J. (2015). Diversity and homogeneity among small plasmids of aeromonas salmonicida subsp. salmonicida linked with geographical origin. Front. Microbiol. 6:1274. doi: 10.3389/fmicb.2015.01274
Austin, B., and Austin, D. A. (2012). Aeromonadaceae Representative (Aeromonas salmonicida). New York, NY: Springer.
Axelsson, M., Peterson, E., Gräns, A., and Ljung, P. E. (2020). Fish identification: marking and tagging methods. Jönköping: Swedish Board of Agriculture.
Bartkova, S., Leekitcharoenphon, P., Aarestrup, F. M., and Dalsgaard, I. (2017). Epidemiology of Danish Aeromonas salmonicida subsp. salmonicida in fish farms using whole genome sequencing. Front. Microbiol. 8:2411. doi: 10.3389/fmicb.2017.02411
Boyd, J., Williams, J., Curtis, B., Kozera, C., Singh, R., and Reith, M. (2003). Three small, cryptic plasmids from Aeromonas salmonicida subsp. salmonicida A449. Plasmid 50, 131–144. doi: 10.1016/s0147-619x(03)00058-1
Burr, S. E., Pugovkin, D., Wahli, T., Segner, H., and Frey, J. (2005). Attenuated virulence of an Aeromonas salmonicida subsp. salmonicida type III secretion mutant in a rainbow trout model. Microbiology 151, 2111–2118. doi: 10.1099/mic.0.27926-0
Cipriano, R. C., and Bertolini, J. (1988). Selection for virulence in the fish pathogen Aeromonas salmonicida, using Coomassie Brilliant Blue agar. J. Wildlife Dis. 24, 672–678. doi: 10.7589/0090-3558-24.4.672
Daher, R. K., Filion, G., Tan, S. G. E., Dallaire-Dufresne, S., Paquet, V. E., and Charette, S. J. (2011). Alteration of virulence factors and rearrangement of pAsa5 plasmid caused by the growth of Aeromonas salmonicida in stressful conditions. Vet. Microbiol. 152, 353–360. doi: 10.1016/j.vetmic.2011.04.034
Emond-Rheault, J.-G., Vincent, A. T., Trudel, M. V., Brochu, F., Boyle, B., Tanaka, K. H., et al. (2015). Variants of a genomic island in Aeromonas salmonicida subsp. salmonicida link isolates with their geographical origins. Vet. Microbiol. 175, 68–76. doi: 10.1016/j.vetmic.2014.11.014
Garduño, R. A., Thornton, J. C., and Kay, W. W. (1993). Fate of the fish pathogen Aeromonas salmonicida in the peritoneal cavity of rainbow trout. Can. J. Microbiol. 39, 1051–1058.
Janda, J. M., and Abbott, S. L. (2010). The genus aeromonas: taxonomy, pathogenicity, and infection. Clin. Microbiol. Rev. 23, 35–73. doi: 10.1128/cmr.00039-09
Kassambara, A., and Kosinski, M. (2018). survminer: Drawing Survival Curves using “ggplot2.” Available online at: https://CRAN.R-project.org/package=survminer
Marana, M. H., Jørgensen, L., von, G., Skov, J., Chettri, J. K., Mattsson, A. H., et al. (2017). Subunit vaccine candidates against Aeromonas salmonicida in rainbow trout Oncorhynchus mykiss. Plos One 12:e0171944. doi: 10.1371/journal.pone.0171944
Marquis, H., and Lallier, R. (1989). Efficacy studies of passive immunization against Aeromonas salmonicida infection in brook trout, Salvelinus fontinalis (Mitchill). J. Fish Dis. 12, 233–240. doi: 10.1111/j.1365-2761.1989.tb00307.x
Marsden, M. J., Devoy, A., Vaughan, L. M., Foster, T. J., and Secombes, C. J. (1996). Use of a genetically attenuated strain of Aeromonas salmonicida to vaccinate salmonid fish. Aquacult. Int. 4, 55–66. doi: 10.1007/bf00175221
Massicotte, M.-A., Vincent, A. T., Schneider, A., Paquet, V. E., Frenette, M., and Charette, S. J. (2019). One Aeromonas salmonicida subsp. salmonicida isolate with a pAsa5 variant bearing antibiotic resistance and a pRAS3 variant making a link with a swine pathogen. Sci. Total Environ. 690, 313–320. doi: 10.1016/j.scitotenv.2019.06.456
Mercado-Blanco, J., Drift, K. M. G. M., van der Olsson, P. E., Thomas-Oates, J. E., Loon, L. C., and Bakker, P. A. H. M. (2001). Analysis of the pmsCEAB gene cluster involved in biosynthesis of salicylic acid and the siderophore pseudomonine in the biocontrol strain pseudomonas fluorescens WCS374. J. Bacteriol. 183, 1909–1920. doi: 10.1128/jb.183.6.1909-1920.2001
Pritchard, L., Glover, R. H., Humphris, S., Elphinstoneb, J. G., and Tothc, I. K. (2016). Genomics and taxonomy in diagnostics for food security: soft-rotting enterobacterial plant pathogens. Anal. Methods 8, 12–24 doi: 10.1039/C5AY02550H
R Core Team (2019). R: A Language and Environment for Statistical Computing. Vienna: Foundation for Statistical Computing. Available online at: https://www.R-project.org/
RStudio Team (2016). RStudio: Integrated Development Environment for R. Available online at: http://www.rstudio.com/
Rasch, M., Kastbjerg, V., Bruhn, J., Dalsgaard, I., Givskov, M., and Gram, L. (2007). Quorum sensing signals are produced by Aeromonas salmonicida and quorum sensing inhibitors can reduce production of a potential virulence factor. Dis. Aquat. Organ. 78, 105–113. doi: 10.3354/dao01865
Reith, M. E., Singh, R. K., Curtis, B., Boyd, J. M., Bouevitch, A., Kimball, J., et al. (2008). The genome of Aeromonas salmonicida subsp. salmonicida A449: insights into the evolution of a fish pathogen. BMC Genomics 9:427. doi: 10.1186/1471-2164-9-427
Tanaka, K. H., Vincent, A. T., Emond-Rheault, J.-G., Adamczuk, M., Frenette, M., and Charette, S. J. (2017). Plasmid composition in Aeromonas salmonicida subsp. salmonicida 01-B526 unravels unsuspected type three secretion system loss patterns. BMC Genomics 18:528. doi: 10.1186/s12864-017-3921-1
Therneau, T. M. (2015). A Package for Survival Analysis in S. New York, NY: Springer. Available online at: https://CRAN.R-project.org/package=survival
Trudel, M. V., Vincent, A. T., Attéré, S. A., Labbé, M., Derome, N., Culley, A., et al. (2016). Diversity of antibiotic-resistance genes in Canadian isolates of Aeromonas salmonicida subsp. salmonicida: dominance of pSN254b and discovery of pAsa8. Sci. Rep. 6:35617. doi: 10.1038/srep35617
Vaillancourt, K. C., Paquet, V. E., Vincent, A. T., Schneider, A., Thompson, C., Laurent, M., et al. (2021). Draft genome sequence of an aeromonas salmonicida subsp. salmonicida strain from the canadian pacific coast bearing a variant of pRAS1. Microbiol. Resour. Announc. 10:21. doi: 10.1128/mra.00291-21
Vincent, A. T., Hosseini, N., and Charette, S. J. (2020). The Aeromonas salmonicida plasmidome: a model of modular evolution and genetic diversity. Ann. Ny. Acad. Sci. 2020:14503. doi: 10.1111/nyas.14503
Vincent, A. T., Trudel, M. V., Paquet, V. E., Boyle, B., Tanaka, K. H., Dallaire-Dufresne, S., et al. (2014). Detection of Variants of the pRAS3, pAB5S9, and pSN254 Plasmids in Aeromonas salmonicida subsp. salmonicida: multidrug resistance, interspecies exchanges, and plasmid reshaping. Antimicrob. Agents Ch. 58, 7367–7374. doi: 10.1128/aac.03730-14
Keywords: Aeromonas salmonicida, host–pathogen models, salmonid, fish, genome
Citation: Gauthier J, Marquis H, Paquet VE, Charette SJ, Levesque RC and Derome N (2021) Genomic Perspectives on Aeromonas salmonicida subsp. salmonicida Strain 890054 as a Model System for Pathogenicity Studies and Mitigation of Fish Infections. Front. Mar. Sci. 8:744052. doi: 10.3389/fmars.2021.744052
Received: 23 July 2021; Accepted: 21 October 2021;
Published: 10 November 2021.
Edited by:
Ciro Rico, Institute of Marine Sciences of Andalusia (ICMAN), SpainReviewed by:
Javier Santander, Memorial University of Newfoundland, CanadaSimona Bartkova, Tallinn University of Technology, Estonia
Copyright © 2021 Gauthier, Marquis, Paquet, Charette, Levesque and Derome. This is an open-access article distributed under the terms of the Creative Commons Attribution License (CC BY). The use, distribution or reproduction in other forums is permitted, provided the original author(s) and the copyright owner(s) are credited and that the original publication in this journal is cited, in accordance with accepted academic practice. No use, distribution or reproduction is permitted which does not comply with these terms.
*Correspondence: Jeff Gauthier, amVmZi5nYXV0aGllci4xQHVsYXZhbC5jYQ==
†These authors have contributed equally to this work