- 1Norwegian Polar Institute, Fram Centre, Tromsø, Norway
- 2Institute of Marine Research, Fram Centre, Tromsø, Norway
- 3Department of Arctic and Marine Biology, Faculty of Biosciences, Fisheries and Economics, UiT The Arctic University of Norway, Tromsø, Norway
- 4P. P. Shirshov Institute of Oceanology, Moscow, Russia
Sea-ice macrofauna includes ice amphipods and benthic amphipods, as well as mysids. Amphipods are important components of the sympagic food web, which is fuelled by the production of ice algae. Data on the diversity of sea-ice biota have been collected as a part of scientific expeditions over decades, and here we present a pan-Arctic analysis of data on ice-associated amphipods and mysids assimilated over 35 years (1977–2012). The composition of species differed among the 13 locations around the Arctic, with main differences between basins and shelves and also between communities in drift ice and landfast sea ice. The sea ice has been dramatically reduced in extent and thickness during the recorded period, which has resulted in reduced abundance of ice amphipods as well as benthic amphipods in sea ice from the 1980’s to the 2010’s. The decline mainly involved Gammarus wilkitzkii coinciding with the disappearance of much of the multiyear sea ice, which is an important habitat for this long-lived species. Benthic amphipods were most diverse, and also showed a decline over the time-span. They had higher abundance closer to land where they are associated with landfast ice. However, they also occurred in the Central Arctic Ocean, which is likely related to the origin of sea ice over shallow water and subsequent transport in the transpolar ice drift. Recent sampling in the waters east and north of Svalbard has found continued presence of Apherusa glacialis, but almost no G. wilkitzkii. Monitoring by standardized methods is needed to detect further changes in community composition of ice amphipods related to reductions in sea-ice cover and ice type.
Introduction
Sea ice in the Arctic provides a habitat for a range of biota that provide organic carbon and energy to Arctic marine ecosystems (Horner et al., 1992; Kohlbach, 2016, 2021; Flores et al., 2019). The spatial distribution of sea-ice biota (hereafter referred to as sympagic or ice biota, cf. Legendre et al., 1992) is shaped by dynamic properties of both sea ice and the underlying water column. The origin and age of Arctic sea ice as well as water depths or distance from land are important factors which impact the resulting community composition of sea-ice biota, with pronounced differences among biota and living conditions in annual coastal landfast sea ice, and off-shore drift ice, which is composed of first-year and multiyear ice. The sea-ice fauna inhabits a wide range of microhabitats inside the brine channel system, in melt ponds on top of the ice, and immediately underneath the ice at the ice-water boundary including pressure ridges (Hop and Pavlova, 2008; Gradinger et al., 2010).
Based on size, sea-ice fauna can be separated into microfauna, which includes single-celled organisms (generally <62 μm; Carey, 1985), meiofauna, which comprises multi-cellular organisms (20–500 μm; Bluhm et al., 2018) and larger-bodied macrofauna (>500 μm; Hop and Pavlova, 2008). Sea-ice macrofauna comprises mainly ice amphipods, some species of benthic amphipods, mysids (Mysis polaris and M. litoralis), polychaetes (Harmathoinae indet.) as well as the copepods Jaschnovia brevis and Tisbe furcata (Melnikov and Kulikov, 1980; Gulliksen and Lønne, 1991; Lønne and Gulliksen, 1991b; Scott et al., 2002). The autochthonous macrofauna spends their entire life cycle in association with sea ice, although recent studies have indicated that the ice amphipod Apherusa glacialis also occurs pelagically (Berge et al., 2012; Kunisch et al., 2020); it may leave the ice during winter for reproduction. Dozens of other taxa, such as copepods, pteropods and ctenophores inhabit the under-ice realm (Arndt and Swadling, 2006). Gelatinous zooplankton (ctenophores and jellyfish) have been observed at high densities just below the ice by remotely operated vehicles and scuba divers (Raskoff et al., 2005, 2010; Purcell et al., 2010; H. Hop, dive observations), where they congregate in turbulence areas created by ridges. Winged snails, particularly Clione limacina, are also commonly observed below sea ice (Böer et al., 2005; H. Hop, dive observations).
Ice amphipods feed on ice algae, sympagic, and pelagic fauna, as well as detritus (Werner, 1997; Poltermann, 2001). The small gadid, polar cod (Boreogadus saida), is often associated with sea ice, where it feeds on ice-amphipods as well as pelagic amphipods and copepods (Lønne and Gulliksen, 1989; Gradinger and Bluhm, 2004; Hop and Gjøsæter, 2013). This species receives much of its carbon from the production by ice algae (Kohlbach et al., 2017). Ice algal carbon has been traced to the sea-ice macrofauna as well as pelagic and benthic fauna, including marine mammals, in different regions of the Arctic (Wang et al., 2015; Kohlbach et al., 2016, 2019, 2021; Brown et al., 2017, Koch et al., 2021).
Higher trophic levels in the Arctic Ocean and its marginal seas are directly or indirectly supported by organisms that are associated with sea ice. Ice amphipods and polar cod, particularly the younger year-classes of polar cod, which can be found in drifting pack-ice (Lønne and Gulliksen, 1989; Gradinger and Bluhm, 2004; David et al., 2016), are important prey for Arctic marine mammals and seabirds (Bradstreet and Cross, 1982; Lønne and Gabrielsen, 1992; Welch et al., 1992; Bluhm and Gradinger, 2008).
The hallmark of climate change is the drastic decline (over 30%) in the sea-ice cover over the past 40 years since the satellite record has allowed accurate observation and interannual comparisons (e.g., Polyakov et al., 2017; Perovich et al., 2018). The Arctic ice cover has declined during all seasons of the year (Nghiem et al., 2007; Barber et al., 2015). The average age of individual Arctic ice floes has decreased from multiyear to mainly first- and second-year sea ice, generally decreasing the thickness of Arctic sea ice by 0.5 m or more from the 1980’s to 2010’s (Bi et al., 2016; Perovich et al., 2018). Multiyear sea ice, which used to cover about 75% of the Arctic seas in 1983, is currently limited to the areas north of Greenland, the central Arctic and parts of the Canadian Arctic Archipelago, with as little as 62% of the summer ice cover remaining compared to 1978–1988 values (Maslanik et al., 2007; Stroeve et al., 2012; Meier et al., 2014). First-year ice has concomitantly increased in relative proportion and importance, although dates of its freeze-up and break-up have also shifted substantially (Markus et al., 2009), leaving large parts of the Arctic without sea ice for an increasing part of the summer-autumn seasons (Arrigo et al., 2011; Renaut et al., 2018).
Data on sea-ice biota diversity have been collected as part of scientific expeditions over many years, yet here we present data on amphipods and mysids in a pan-Arctic context based on a comprehensive approach of data assimilation and integration. Under-ice crustaceans have been reviewed in Arndt and Swadling (2006), and estimated with regard to density (references in Table 1), but no numerical attempt has so far been made to synthesize data on macrofauna in a pan-Arctic context in a statistically rigorous manner.
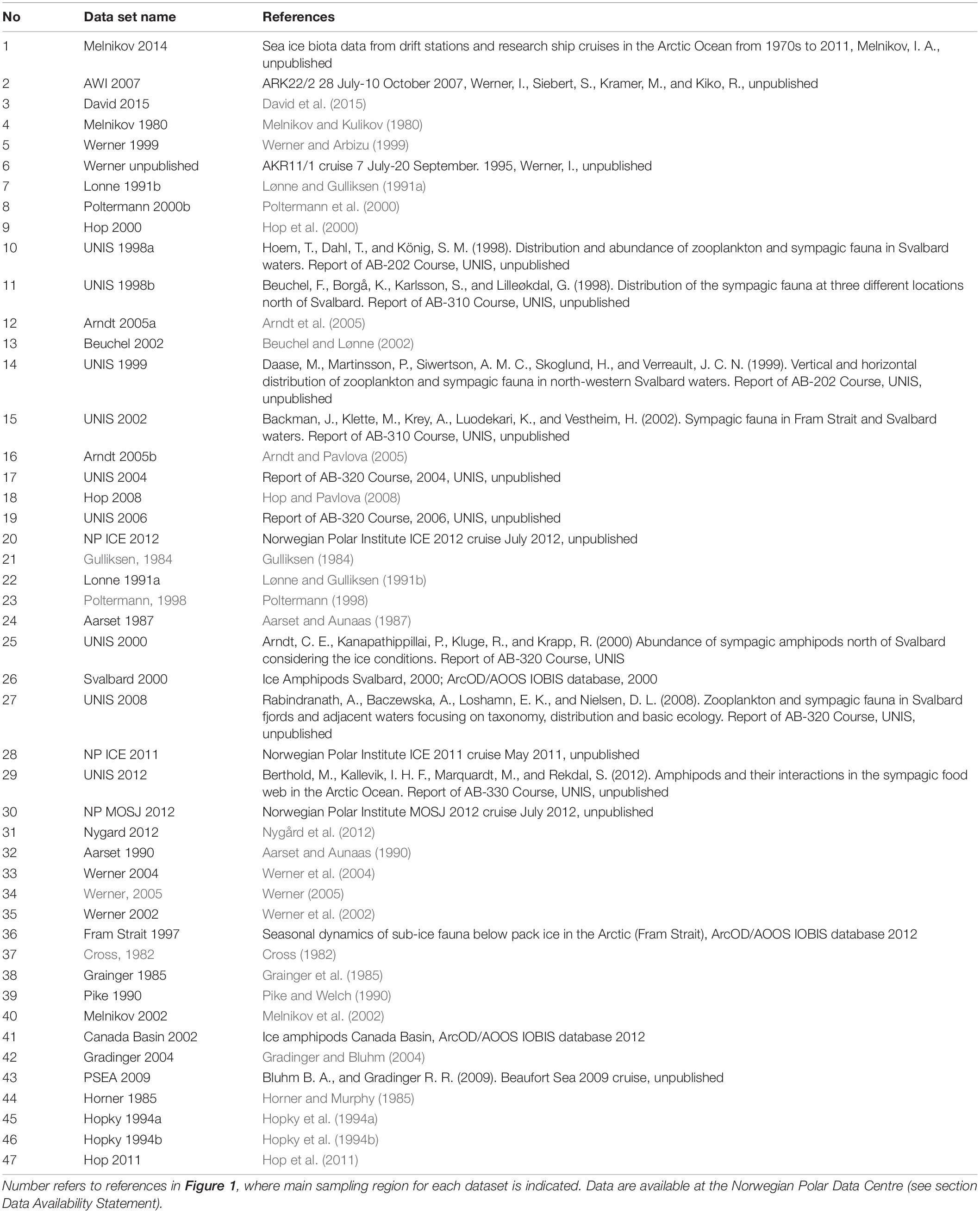
Table 1. Published and unpublished data sources on Arctic sea ice amphipods and mysids included in the present study.
Here, we summarize sea-ice macrofauna species (juvenile-adult size range 3–50 mm) composition and distribution patterns on a pan-Arctic scale, and analyze which predictor variables best explain variability in abundance of different ice amphipods species. More specifically, we determine how coastal regions compare to off-shore regions in terms of amphipod composition and distribution by comparing the frequency of sympagic and benthic amphipods in coastal regions versus off-shore regions with regard to distance from land and depth. Furthermore, we address the question whether there has been a change in amphipod occurrence during 35-years (1977–2012) of quantitative sampling. Based on researcher observations and memory, the abundances of ice amphipods have decreased in response to sea-ice decline. This question has already been partly answered in Barber et al. (2015) and Bluhm et al. (2017), but neither of these studies applied rigorous statistics to test trends. We here address this question statistically, with regard to changes over years and seasons. However, main challenges for analyses, such as differences in sampling effort and methods over years and regions, needed to be accounted for in the analyses.
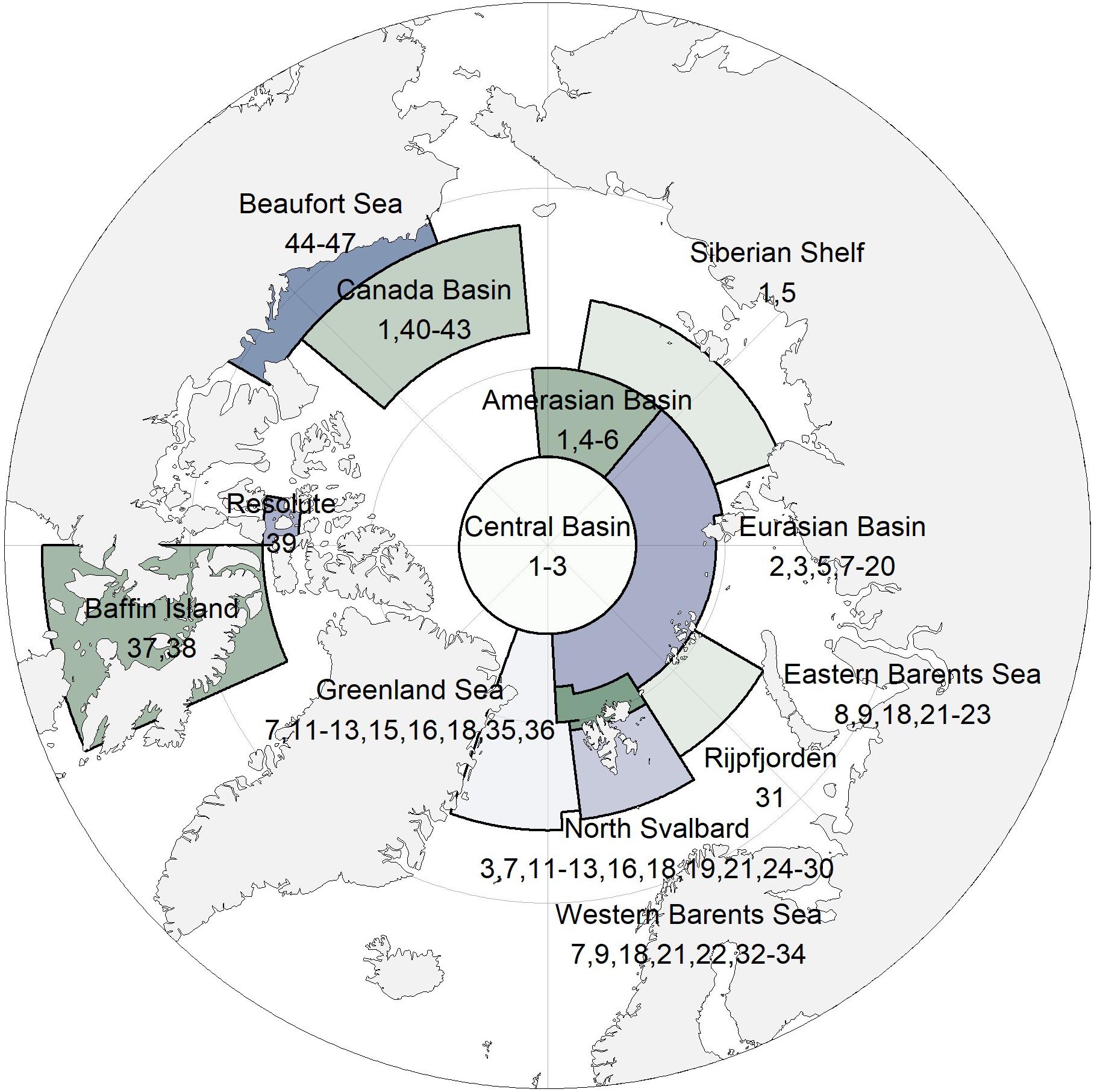
Figure 1. Pan-Arctic map with study regions. Numbers refer to data sets in Table 1.
Consequences of extensive sea-ice loss for its resident biota – from bacteria to polar bears – seem inevitable, but are largely undocumented and poorly monitored (CAFF, 2017). Shifts are expected in ice-associated biota composition, abundance, biomass and the timing of the seasonal development (Gradinger et al., 2010; Leu et al., 2015). Documentation of ice biota composition, abundance and natural variability is critical for evaluating responses to the decline in Arctic sea ice. However, without sufficient monitoring, such changes will be impossible, or at best difficult to detect until effects are dramatic, e.g., disappearance of certain species, or until effects have been detected in other parts of the ecosystem due to the coupled processes between sea ice, water column and benthic biota. We are then left with the option of amalgamating and analyzing composite data sets, such as this 35-year dataset on ice macrofauna, in a pan-Arctic perspective. Results are discussed in a scenario of declining sea ice, which may seasonally completely remove the sympagic habitat and its ecosystem within decades (Stroeve et al., 2012; Overland and Wang, 2013).
Materials and Methods
Data Sources
We synthesized sea-ice amphipod data from 47 sources from 13 locations across the Arctic for this study (Table 1 and Figure 1). The temporal coverage was 35 years, with samples taken at different intervals. Sample is here defined as a separate sampling event. The methods for amphipod collection varied as did the reported units (presence, catch per unit of effort, counts as ind. m–2, counts as ind. m–3, wet or dry weight in g m–2 or g m–3). Samples were mostly collected by scuba divers using suction pumps (Lønne, 1988) and frames for quantitative sampling (Hop et al., 2000), or nets operated by divers or pulled below the sea ice with ropes (Melnikov, 2019). For specific methods, see references listed in Table 1.
Data Processing
Data were compiled to presence-absence, absolute, and relative abundance datasets. Binary presence/absence information on ice-amphipods was extracted from all data sources regardless of the original unit. Ice-amphipod abundances (ind. m–2 or ind. m–3) were extracted from data sources that reported them. The abundances of each species were summed up for individual sampling efforts and used in further analyses. Individuals per cubic meter values were directly converted to ind. m–2 values assuming that all amphipods sampled using methods reporting m–3 values were located directly under the ice (<0.2 m, based on vertical dimension of sampling net). Percentage contribution of ice-amphipod species to a sample were calculated from other units than presence. A contribution of a single species was divided by the summed contribution of all species within a sample.
The estimates and comparisons include sampling bias due to different sampling methods (e.g., nets vs. suction pumps). Most datasets reported amphipod presences when at least one ice amphipod individual was present, which implied that we could not use the binary dataset as presence/absence data, but rather as relative species occurrence data. There were exceptions, such as the data from Rijpfjorden, Svalbard (Nygård et al., 2012), recorded as catch per baited-trap day including sampling events without sympagic amphipods. Most samples were taken during the summer when ice was present (Figure 2). Data were collected from different regions of the Arctic in different years. Each region, no matter how large, did not contain data from similar range of years, methods, and sample sizes. Data were collected during varying clusters of years leaving gaps in the temporal dataset (Figure 2). This, together with varying sampling methods, locations, years and times led to unbalanced data. Juveniles were counted as individuals in some data sets, but not in all (or it was not specified if they had been included), increasing the abundances in certain samples by several orders of magnitude. Excluding these samples as outliers was considered, but would have been difficult to do consistently for all datasets.
Some of the metrics used here are abundance (contribution of species by number or weight), occurrence (how often species occurred in the dataset), frequency of occurrence (proportion of samples containing one or more specimens of a given species mostly, but not always, when at least one sympagic amphipod was present), and aggregate percentage (AP; mean percentage contribution of a species to the total abundance of a sample, calculated as an arithmetic mean of percentages, see Martin et al., 1946; Bluhm et al., 2018).
Statistical Methods
We used the statistical techniques found most appropriate, although the assumptions were sometimes not valid. This also makes it difficult to draw firm conclusions on the effect of temporal changes in sea ice on its inhabitants. To examine the long-term changes in sea-ice crustaceans (sympagic amphipods and Mysis polaris) abundance over time, predictor variables were categorized (year, data source, distance from land, month, latitude, day length, region, solar angle, and ice type) and their relative importance was examined using R2 values adjusted to number of groups in ANOVA models with logarithm-transformed abundance (ind. m–2) as response variable and groups for a single explanatory factor as predictors (Table 2). The most important factor, data source (i.e., reference), also produced the lowest Akaike’s Information Criterion (AIC) in consequent general additive mixed models [GAMMs; log(total) ∼ s(year, by = data source) with AIC of 1368, followed by an AIC of 1426 of log(total) ∼ s(year, by = month)]. Consequently, data source was used as a random intercept in a GAMM, which was formulated as log(total) ∼ s(year), random = list(data source = ∼1), where total is the total abundance, year the year as an integer, and data source a categorical variable, using the mgcv package for R (Wood, 2017). Species trends were further examined using similar models with log +1 transformed species abundances to account for zero values. Monthly abundances were examined using similar GAMMs with s(month) as the predictor variable, where months were handled as integers. The occurrences of sympagic (A. glacialis, G. wilkitzkii, and Onisimus spp.) and benthic amphipods at distances from land (<850 km) were examined using GAMMs with binomial logit link function, data source as random intercept, smooth term of distance from land as predictor, and presence/absence as response variable. The analysis was run using binary data to increase number of observations (n = 600). All data were examined together using non-metric multidimensional scaling (nMDS) with a Bray-Curtis dissimilarity matrix calculated from the percentage data. Species scores were fitted using square-root transformation and Wisconsin double standardization using the function metaMDS from the vegan package for R (Oksanen et al., 2020). Environmental variables were fitted to this ordination using the envfit and ordisurf functions. Factors were data source, year, ice type, month and region, and vectors included depth, distance from land, Photosynthetic Active Radiation (PAR) and solar angle at the time of sampling. Only predictor variables that fitted the ordination with p < 0.05 were included.
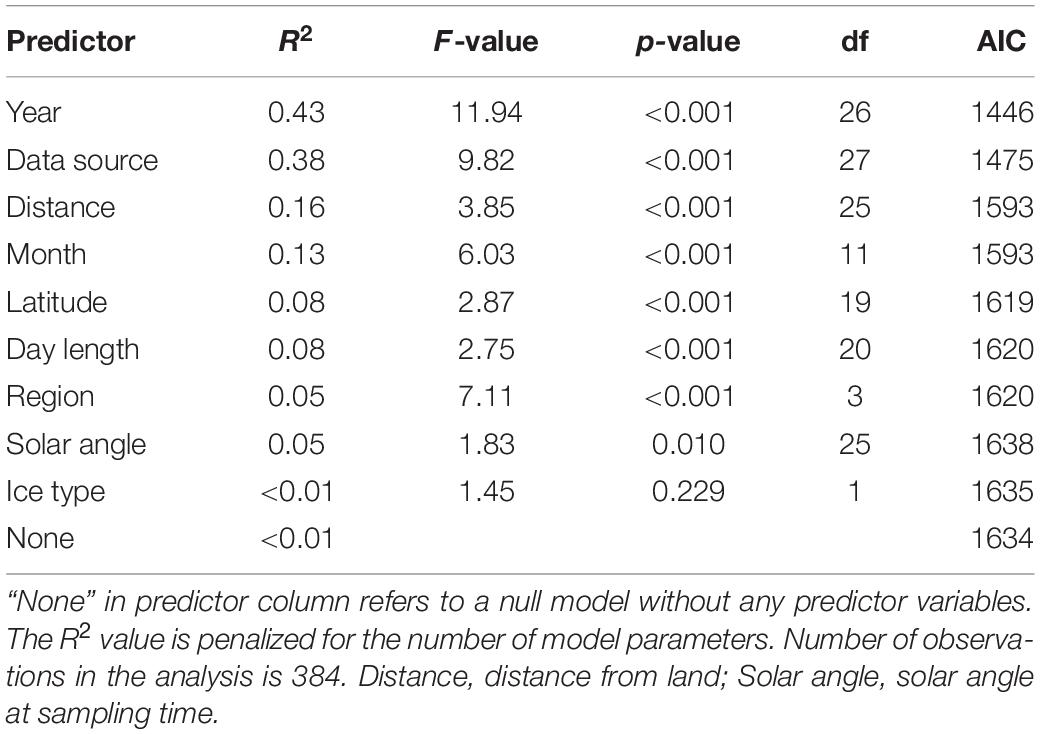
Table 2. Relative importance of categorized predictor variables in explaining the variability in sea ice macrofauna abundance measured using ANOVAs.
Results
Pan-Arctic Distribution
We recorded a total of 16 amphipod species (and a few taxa identified to genera) and one mysid species in 600 samples from 13 locations across the Arctic Ocean and over the 35-year period considered (Table 3). Of the ice amphipods recorded, Apherusa glacialis was the most frequently occurring amphipod encountered across the Arctic sea ice and the most abundant ice amphipod (Table 3). Apherusa glacialis occurred in high abundances both in shelf and off-shelf areas, but lower frequency of occurrence (%) in the landfast ice of Rijpfjorden (Figure 3). The second-most frequently occurring species, and third-most abundant ice amphipod, was Gammarus wilkitzkii (Table 3) which occurred in all sampling areas (Figure 3), although in <50% frequency of occurrence in landfast ice areas. Onisimus spp. was the second-most abundant amphipod and present in all types of ice and regions, albeit in lower abundances when juveniles were not included (Table 3 and Figure 3). Eusirus holmii occurred rarely and was mostly present in the central Arctic Ocean, but could also occur on shelves (Figure 3). Amphipods of benthic origin had a higher species richness than ice-endemic taxa, with at least 14 species (in some cases only identified to genus; Table 3) and were most frequent in sea ice close to land, but were also observed in the Central Arctic Ocean. The most frequently occurring benthic amphipods were Anonyx nugax, Gammaracanthus loricatus, Neopleustes sp., and Gammarus setosus. Onisimus litoralis was rare, but very abundant when present (Table 3). The highest percentage values for benthic amphipods were from coastal waters in Resolute (Canada), the Siberian shelf and Rijpfjorden, although they also occurred in the Central Basin and Amerasian Basin (Figure 3). The mysid Mysis polaris was only reported with quantitative values from Baffin Island sea ice.
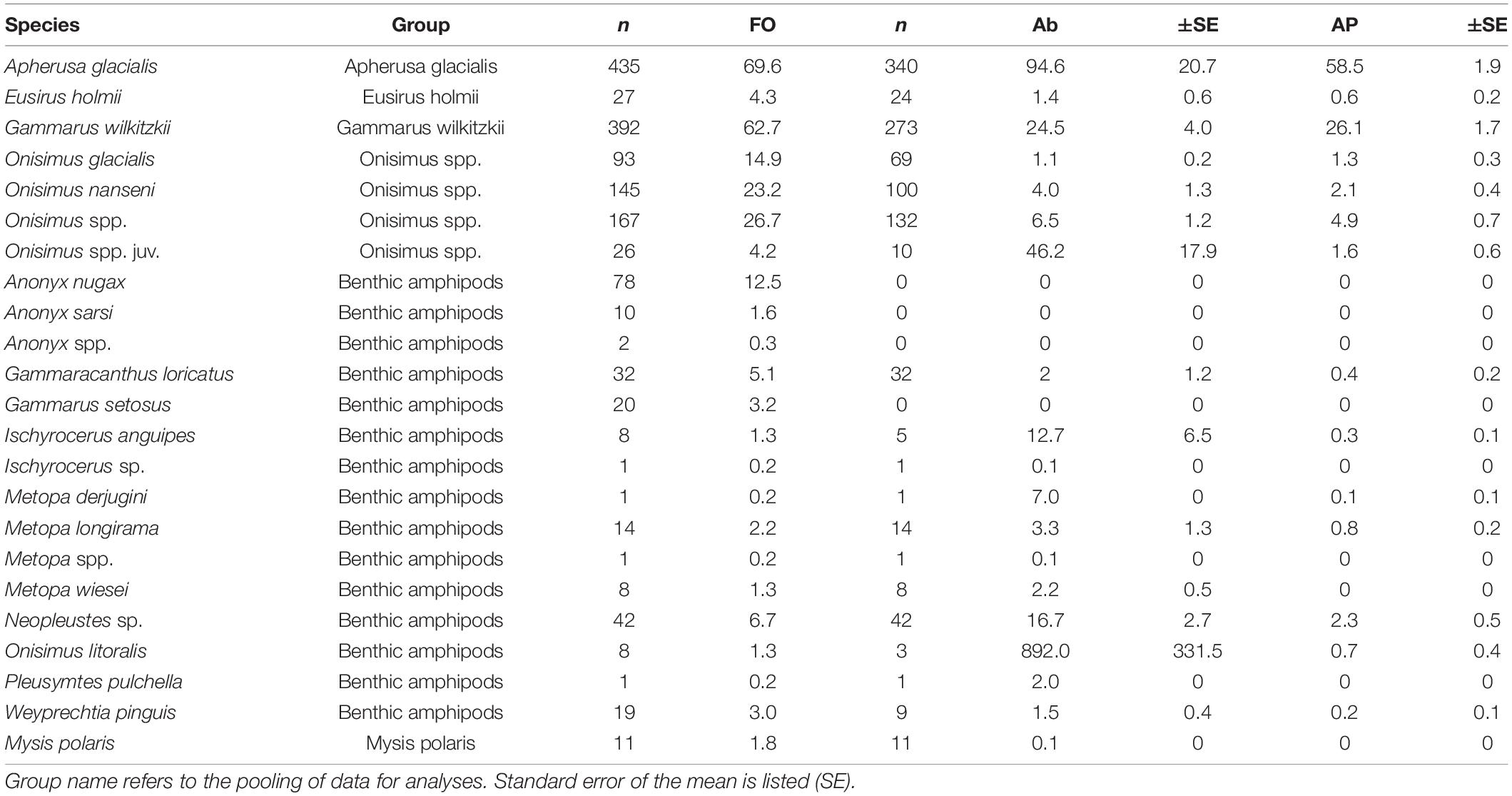
Table 3. Species associated with sea ice, by their frequency of occurrence (FO, % samples), abundance (Ab, ind. m–2) and aggregate percentage (AP).
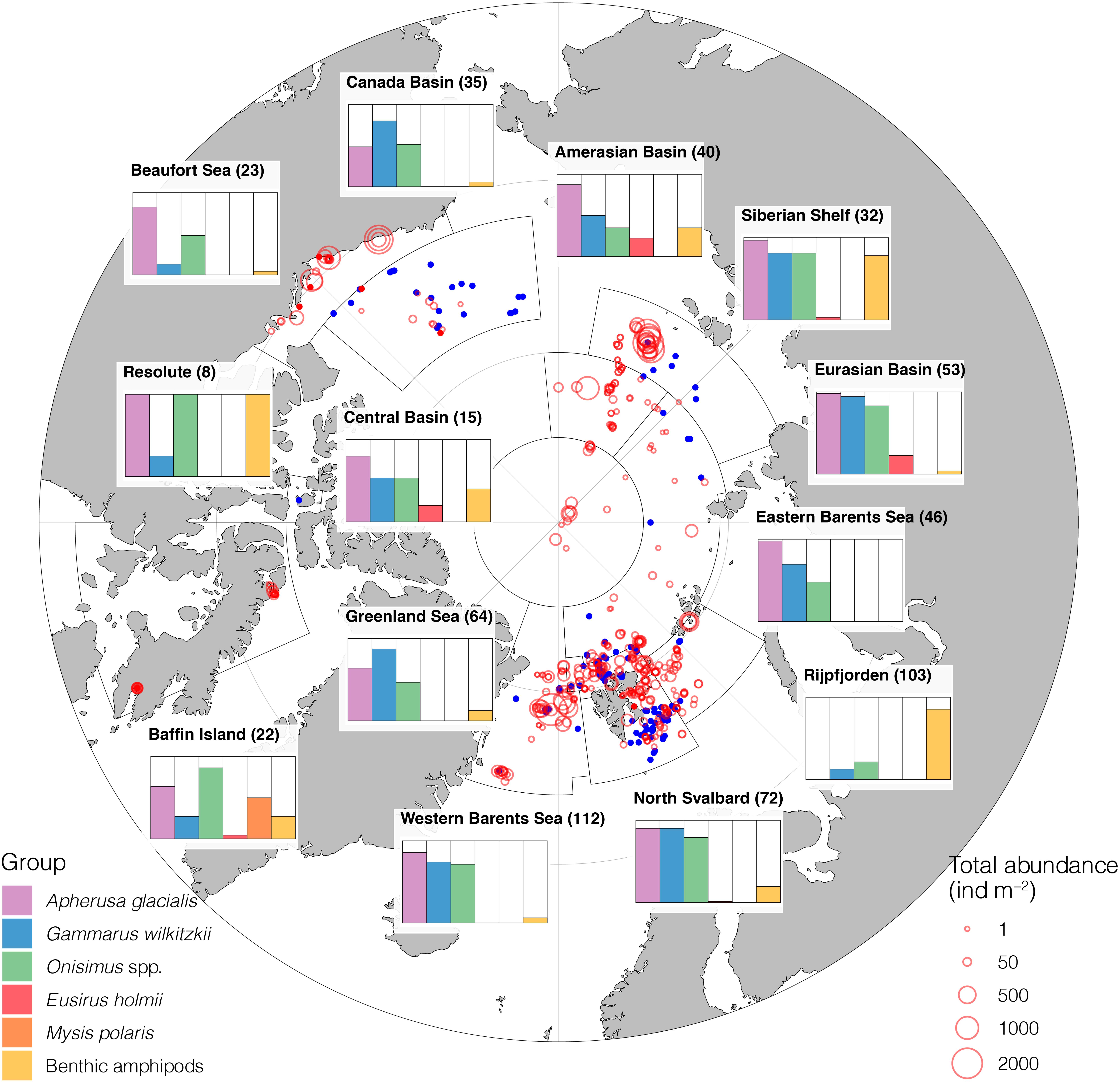
Figure 3. Pan-Arctic distribution of sea-ice macrofauna (ice amphipods and mysids). Distribution and abundance across the Arctic were aggregated from 47 sources (Table 1) between 1977 and 2012. Bar graphs illustrate the frequency of occurrence (%) of macrofauna in samples that contained at least one ice-associated crustacean. Red circles illustrate the total abundances of all ice-associated crustaceans in quantitative samples (ind. m–2) at locations of sampling. Number of sampling efforts for each region is given in parenthesis after region name. Blue dots represent samples where only presence data were available.
Inter-Annual Changes in Abundance
The variation in abundance data was best explained by year as categorical variable followed by data source (Table 2). Distance from land and month explained 16 and 13% of variation in the abundance data, respectively. The combined sympagic amphipod and Mysis polaris abundance had no meaningful linear trends (Figure 4A), although the values appeared higher in the 1980’s than the 2000’s. Apherusa glacialis abundance data showed no trend over time. However, the abundance trends were negative for Gammarus wilkitzkii, Onisimus spp. and benthic amphipods (Figure 4B).
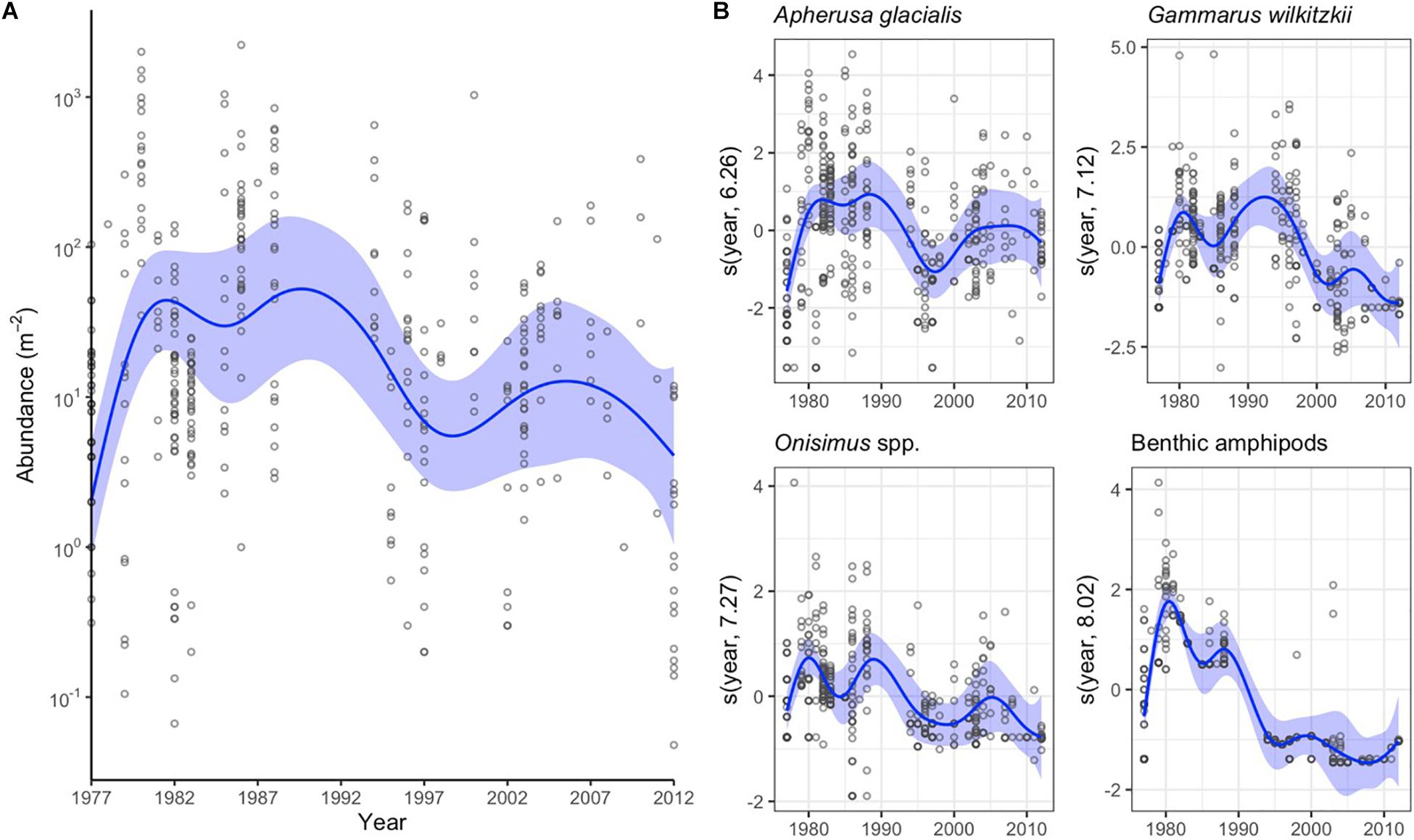
Figure 4. Annual changes in sympagic crustacean abundance as indicated by the general additive mixed models (GAMM). (A) Changes in total abundance of sympagic crustaceans (excluding benthic amphipods). Blue line indicates the GAMM model, blue shading the 95% confidence intervals and dots actual data. Note the logarithm scale on y-axis. (B) GAMM models for the abundance of four amphipod taxa. The values are presented as the centered covariate scale of the annual smoother. Dots indicate residuals.
Monthly Changes
The abundance of sympagic amphipods was higher during winter months, January–April, than in May–October (Figure 5A). The difference was mainly caused by A. glacialis and G. wilkitzkii (Figure 5B). Also benthic amphipods were more abundant under sea ice during winter, although they were not included in the total abundance estimate in Figure 5A.
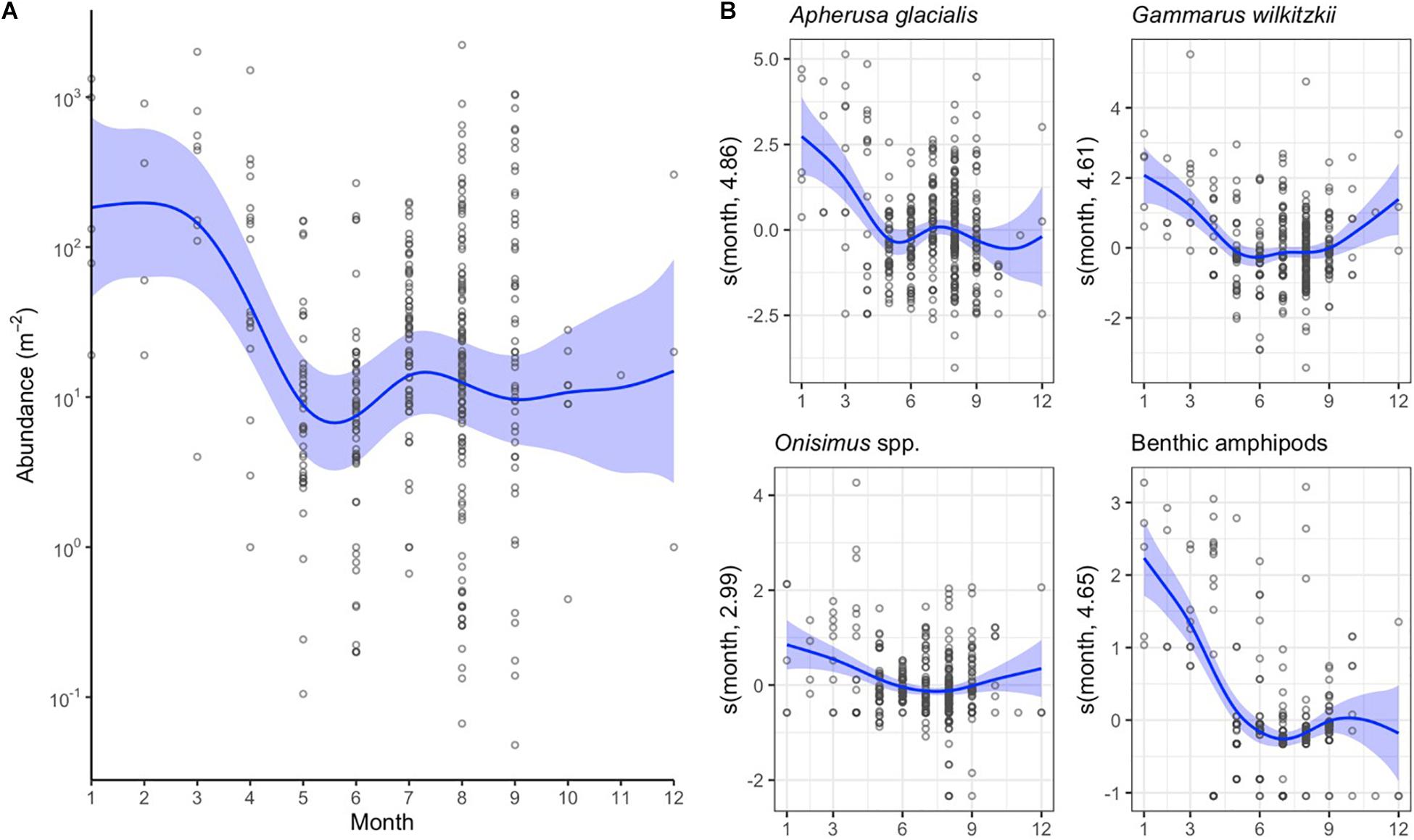
Figure 5. Monthly changes in sympagic crustacean abundance as indicated by the general additive mixed models (GAMM). (A) Changes in total abundance of sympagic crustaceans (excluding benthic amphipods). (B) GAMM models for abundance of four amphipod taxa. See Figure 4 for additional explanation.
Distribution Related to Distance From Land and Ice Type
Ice amphipods (G. wilkitzkii, A. glacialis, and Onisimus spp.) were rare but present adjacent to land and were most frequently found approximately 100–200 km from land (Figure 6). The occurrence of these amphipods was relatively stable >200 km from land, with an increase in G. wilkitzkii in the central basin. Onisimus spp. and benthic amphipods, in contrast, were most frequently present in ice close to land, but benthic amphipods somewhat surprisingly also occurred in drift ice far away (>600 km) from land (Figure 6). The overall abundance of sea-ice macrofauna declined after 200 km from land potentially indicating a sampling bias (Supplementary Figure 1A).
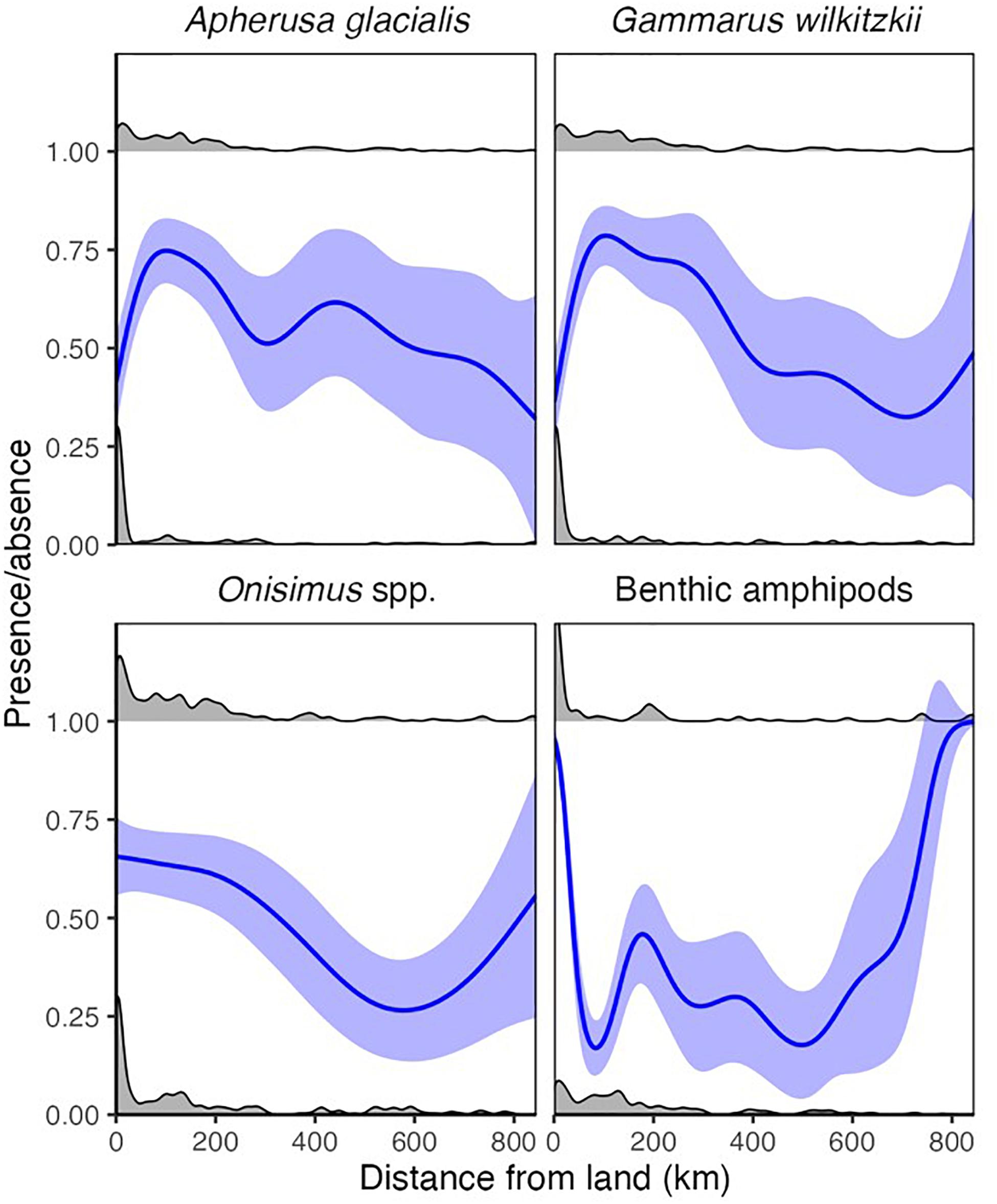
Figure 6. Distance from land for binary (occurrence) data. The lines represent binomial GAMM models for each species. The gray density distributions indicate presences (1) and absences (0).
Community Composition
Ice amphipod composition was clearly separated by dominant species, depth and sea-ice type in the nMDS of which the latter two drove the separation along nMDS1 (Figure 7). Gammarus wilkitzkii occurred predominately in drift ice over deep water in the Beaufort Gyre and the Transpolar Drift area into Fram Strait. Apherusa glacialis was also mostly associated with drift ice over deep water during summers and was still abundant during later years (2009–2012) in the time series. Eusirus holmii and Onisimus spp. were predominately associated with shelf areas, although 7.4% were recorded from Basins. Eusirus holmii was only represented by few individuals, and did not contribute to region centroids (and its placement in Figure 7). Benthic amphipods were associated with shallow depths near landfast ice.
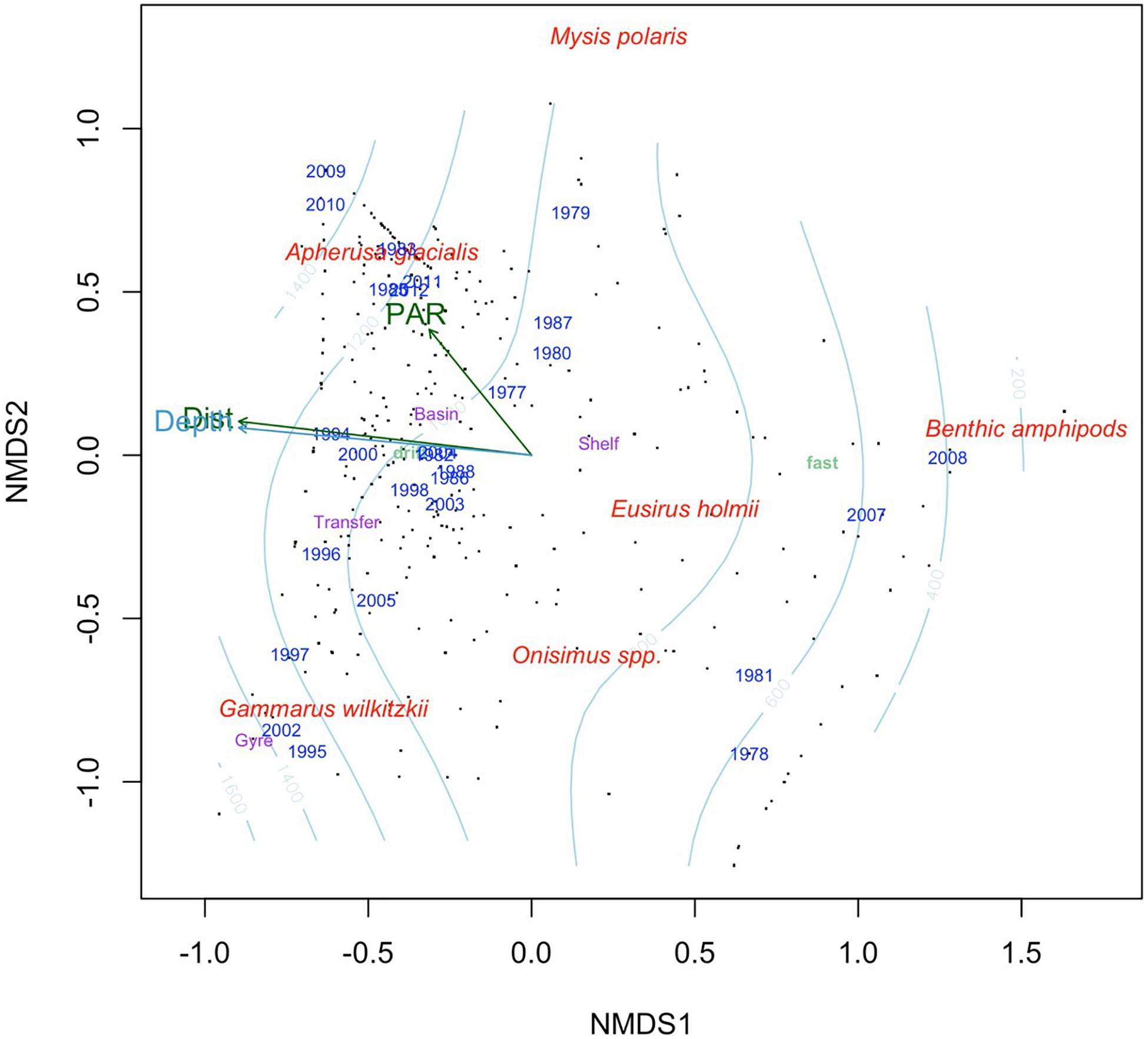
Figure 7. Non-metric multidimensional scaling using Bray-Curtis dissimilarity matrix calculated from the percentage data. Black dots indicate samples, red text is species scores fitted using square-root transformation and Wisconsin double standardization, the light blue contour indicates bottom depth, light green text is ice type (landfast ice and drift ice) and purple text indicates region. The arrows represent continuous variables fitted to the ordination, while text indicates categorical variables. Dist, distance from land; PAR, photosynthetic active radiation.
Data source was the best explanatory factor of categorical variables (Table 4). This is not a surprise, because it had most levels (and therefore centroids) and most studies were conducted within a limited time-frame and region. Year contained the second-most levels and therefore explained the ordination second best. Ice type and month had R2 values of approximately 0.3, whereas region had the least explanatory power in the nMDS ordination. Bottom depth, which was highly correlated with distance from land, explained the ordination best of the continuous variables with R2 = 0.12 (Table 4). Solar angle at the time of sampling and PAR, available for a subset of samples below sea ice, explained minor fractions of the ordination (Table 4); PAR was included in Figure 7 to show its potential relation to Apherusa glacialis.
Discussion
Pan-Arctic Distribution Patterns of Ice Amphipods
Under-ice amphipod distribution and abundance demonstrated large spatial, ice-related, and temporal (seasonal and interannual) variability. Despite this variability, our compilation confirmed the previously stated pan-Arctic occurrence of sympagic amphipods as well as the widespread association of various benthic amphipods with sea ice across ice types and regions (Arndt and Swadling, 2006).
Apherusa glacialis was found in all but one region (Rijpfjorden with newly formed fast ice), and its occurrence was highest 100 km from the coast, but high occurrence was also found further into the Central Basins. Earlier studies have stated that this short-lived (2-year life span) species tends to be most associated with first-year drift ice (Beuchel and Lønne, 2002). Some studies have indicated that these amphipods are capable of inhabiting the water column in absence of sea ice, at least for part of the year. Berge et al. (2012) suggested that A. glacialis in the Atlantic gateway may overwinter at depth within the Atlantic-water inflow near Svalbard, thereby avoiding being exported out of the Arctic Ocean through western Fram Strait. Kunisch et al. (2020) collated pelagic occurrence records over a 71-year period and found that A. glacialis was indeed consistently found away from its presumed sea-ice habitat on a pan-Arctic scale, albeit at different depths and water masses. In the Svalbard region, these authors demonstrated that A. glacialis was found in Atlantic Water both during summer and winter.
Gammarus wilkitzkii is an autochthonous sympagic species, which is known to spend all of its life cycle in association with sea ice (Melnikov, 1997). Even though this long-lived (6–7 years) species typically thrives in multiyear sea ice (Lønne and Gulliksen, 1991b; Hop et al., 2000; Beuchel and Lønne, 2002), it can also occur in other ice types (Poltermann, 1998; this study), as well as in planktonic or benthic habitats for some parts of the year (Weslawski, 1994; Werner et al., 1999). Consistent with the records of few individuals in benthic samples (Weslawski et al., 2010), the data synthesized here showed that the distribution of G. wilkitzkii included coastal areas, which would facilitate a sympagic-benthic coupling for part of their life cycle when the drift ice disappears during the summer. However, the mortality is likely high for individuals that lose their sympagic habitat, and this becomes a large annual drain on the population (Werner et al., 1999; Hop and Pavlova, 2008).
The two ice endemic Onisimus species, O. nanseni and O. glacialis, seem to follow a similar distribution pattern as Apherusa glacialis, but at much lower abundance. Their increase closer to the coast could be because of the inclusion of Onisimus spp. juveniles, which were abundant, may also have included juveniles of the benthic Onisimus litoralis. This benthic amphipod is common along the coast where it uses sea-ice algae as food when present (Gradinger and Bluhm, 2010).
Eusirus holmii, a large amphipod of similar adult size to G. wilkitzkii, is ice-associated (Macnaughton et al., 2007; this study), but also has a bathypelagic distribution (Barnard and Karaman, 1991) and was even found at the deep-sea floor in the Chukchi Borderland (Zhulay et al., 2019). Different colorations and eye characteristics are recognized in different habitats raising the possibility of cryptic species being present (Macnaughton et al., 2007). The signal of the ice-algal trophic marker IP25 in this species indicates that sympagic individuals obtain their organic carbon in part from sources other than ice algae, even though most of the carbon in this amphipod as well as other ice-associated amphipods was of sympagic origin in the studied individuals (Brown et al., 2017). This species has increasingly been reported from sea ice in recent years, with recorded densities of 0.3 ind. m–2 during sampling in the Nansen Basin in 2012 (Brown et al., 2017).
Benthic amphipods included 14 species (Table 2), but likely more since some were only classified to genus. We cannot exclude the potential presence of Pleusymptes karstensi, which was recently reported as ice-associated (Macnaughton et al., 2007), since other similar Pleustidae were reported in our dataset. Benthic ice-associated amphipods are mostly found in coastal areas below landfast sea ice or in shallow regions with drift ice (e.g., Pike and Welch, 1990; Werner and Arbizu, 1999; Nygård et al., 2012). They were more abundant closer to the coast, but, surprisingly, also abundant off-shore. Because of ice formation in shelf areas and vertical migration of certain organisms, the inclusion of benthic species into sea ice can happen over shallow water, and the benthic fauna is then subsequently transported with the sea ice into the Central Arctic Basin. The benthic amphipod species were often not separated into adults and juveniles, and the abundance could include high numbers of juveniles, which may settle and develop as the ice drifts around. The mysid Mysis polaris is considered to be part of the autochthonous ice fauna (Melnikov, 1984). It is listed as rare in Arndt and Swadling (2006), but likely underreported since our dataset only contained abundance values from Baffin Island sea ice. Based on its occurrence at several locations in the Arctic Ocean, it has been suggested that it lives toward the edge of the continental shelf and is carried from there by currents into oceanic areas (Geiger, 1969).
Temporal and Ice-Related Trends
The community composition changed with environmental variables as well as year of collection, which includes variability due to sampling gear and effort. Monthly variability in ice amphipod abundance indicated higher abundance during winter and autumn. The high abundance during the winter months is difficult to explain. A study of seasonal abundance of sympagic amphipods in Fram Strait found both A. glacialis and G. wilkitzkii during the winter, but at lower abundances (Werner and Auel, 2005). Young A. glacialis, however, appear in high proportions in March–April (Kunisch et al., 2020) and may have contributed to the pattern for those months, given that this species is the most abundant ice amphipod. In the late autumn, juveniles released from brood pouches during summer are likely increasing the counts when they are caught in sampling gear (Poltermann et al., 2000).
The main explanatory vectors in the multivariate similarity pattern of ice amphipod stations were depth below sea ice and distance from land, which were highly correlated. Landfast ice and drift ice are also related to depth and distance from land, since the fast ice is attached to land mostly over shallow water, whereas drift ice is further off-shore. The multivariate analysis (Figure 7) showed separation between the ice amphipod taxa, with A. glacialis being off-shore and most related to PAR reflecting the production season for ice algae, a seasonal food item for A. glacialis (Iken et al., 2005; Brown et al., 2017). Gammarus wilkitzkii was positively related to distance from land and depth, because as a species with a multiyear life cycle it is associated with older ice which is generally found in drift ice off-shore (Lønne and Gulliksen, 1991a; Hop et al., 2000). This ice amphipod separated from the benthic amphipods dominating closer to shore, with E. holmii placed somewhere on the shelf, although this species was also found at low abundances in basins.
Under-ice amphipod abundance overall demonstrated large seasonal and interannual variability, partly due to the patchiness of their habitats and heterogeneous distribution below ice floes (Lønne and Gulliksen, 1991a,b; Werner and Gradinger, 2002). Ice amphipods are able to withstand large fluctuations in environmental factors. They can reside inside brine channels as well as in the thin water layer (<0.3 m) with strongly reduced salinity that is typically formed under Arctic sea ice during the summer melt period (Eicken, 1994; Melnikov, 1997). As was shown by Aarset and Aunaas (1990), individuals of G. wilkitzkii subjected to such conditions show much higher energy expenses caused by osmotic stress. They may therefore prefer to stay associated with ridges or more complex multiyear ice floes (Hop et al., 2000; Gradinger et al., 2010). Thus, ice-amphipod abundance seems to be connected to both sea-ice morphology and age. Because multiyear ice has been severely reduced in the Arctic Ocean (Kwok and Untersteiner, 2011; Perovich et al., 2018), one would expect that this species has declined, and composite data from the Svalbard area have indeed indicated a drop in G. wilkitzkii abundance and biomass over time (Hop et al., 2013). Quantitative collections of ice amphipods are no longer possible at many ice stations in that area because of extremely low abundances of these crustaceans, with typical catches of <1 ind. m–2 (H. Hop pers. obs.; Ehrlich et al., 2020). Gammarus wilkitzkii abundances have been reduced to very low abundance levels (Hop et al., 2013) or complete absence from lists of under-ice fauna (e.g., Ehrlich et al., 2020). During recent expeditions (May–June 2021) to the northern Barents Sea and Nansen Basin of the Arctic Ocean in the Nansen Legacy Project1 and the NPI Microplastic Cruise, scientific divers found only two individuals of G. wilkitzkii below ridged first-year ice at seven ice stations (H. Hop, pers. obs.). A similar trend has been independently observed in the central Arctic (I. A. Melnikov pers. obs.). During the recent (2021) expeditions, Eusirus holmii was the only large amphipod present below sea ice, typically with few individuals sampled at each ice station. The smaller A. glacialis was still abundant (not quantified) and collected by scientific divers at most ice stations. This species is less likely to be reduced in abundance with changing ice cover, including seasonal ice loss. Regular observations of A. glacialis in the pelagic realm suggest that this species may be less dependent on sea ice as a habitat (Kunisch et al., 2020), thus being more flexible and able to adapt to changing ice cover. Benthic amphipods also showed a decline, which may be related to less sea ice over the shelves of the Arctic Ocean, where these organisms are incorporated, and seasonal melt of the first-year pack ice which drops them to the sea floor.
Temporal trends in sea-ice biota diversity and/or abundance/biomass are very challenging to detect for two main reasons: the large natural variability within the sea-ice system and the lack of systematic and consistent monitoring of sea-ice biota. The community structure of ice algae, with dominance of diatoms, seems to have prevailed in the central Arctic from the 1980s to the 2010s, and reduction in sea-ice protist diversity was coincident with a shift in the regions sampled and decreased sampling effort (Hop et al., 2020). However, these authors showed that multiyear sea ice contained 39% more diatom species than first-year ice, which explained the previously reported decreases in sea-ice protist diversity (Bluhm et al., 2017). The meiofauna community in sea ice has changed, with reductions in biodiversity noted in studies conducted in the 1990’s compared to the 1970’s (Melnikov et al., 2002). However, analysis of longer time series, 1979–2015, showed that region and local environmental factors (e.g., distance from land and depth) were most important for the observed variability (Bluhm et al., 2018). Declines in sea ice also have negative consequences for some species or populations of marine mammals and seabirds (Laidre et al., 2015; Descamps and Strøm, 2021), particularly ice-dependent species, such as ringed seal (Pusa hispida), polar bear (Ursus maritimus) and ivory gull (Pagophila eburnea) (Strøm et al., 2020; Florko et al., 2021). Cetaceans, on the other hand, have generally increased from 2002 to 2014 in waters around the Svalbard archipelago because of ocean warming, increase in temperate prey species and decline in sea ice (Storrie et al., 2018).
Conclusion and Outlook
Sea ice is a species-rich habitat, with ice amphipods as important components that constitute a direct link to higher trophic levels. In the current study, we expected reductions in ice amphipod abundance over time based on our composite 35-year dataset. Some of us have worked a lifetime in the Arctic, as researchers and scientific divers, and certainly have this impression from in situ observations. While we were not able to detect meaningful linear reductions in the overall abundance, most likely due to biases and problems with the compiled dataset, G. wilkitzkii did demonstrate a negative and significant linear trend from the mid 1990’s, and negative trends were also apparent for Onisimus spp. and benthic amphipods.
The diversity of amphipods associated with sea ice was higher close to land because of higher abundances of benthic amphipods, but these were also found in the Central Arctic Ocean reflecting the drift of sea ice. The autochthonous amphipods were most abundant in the off-shore regions at distances >200 km from shore, but varied among regions, partly because of sampling bias.
Future projections for the under-ice associated fauna are uncertain. The multiyear ice ecosystem is capable of supporting a relatively constant species composition of permanent ice biota, while the species composition of the biota of the seasonal sea-ice ecosystem of first-year ice largely depends on ridges as complex structures for protection and the biota of the water column for recruitment. Thus, first-year ice would be sufficient for short-lived species like A. glacialis, and Onisimus spp. with a 3-year life span (Arndt and Beuchel, 2006), but not for G. wilkitzkii. In the current sea-ice cover situation in the Arctic, these two ice situations co-exist (Figure 8). However, the future pan-Arctic scenario, which needs to be consistently monitored, will include predominately first-year sea ice and more open water. Thus, large changes are still expected in the sympagic ecosystem.
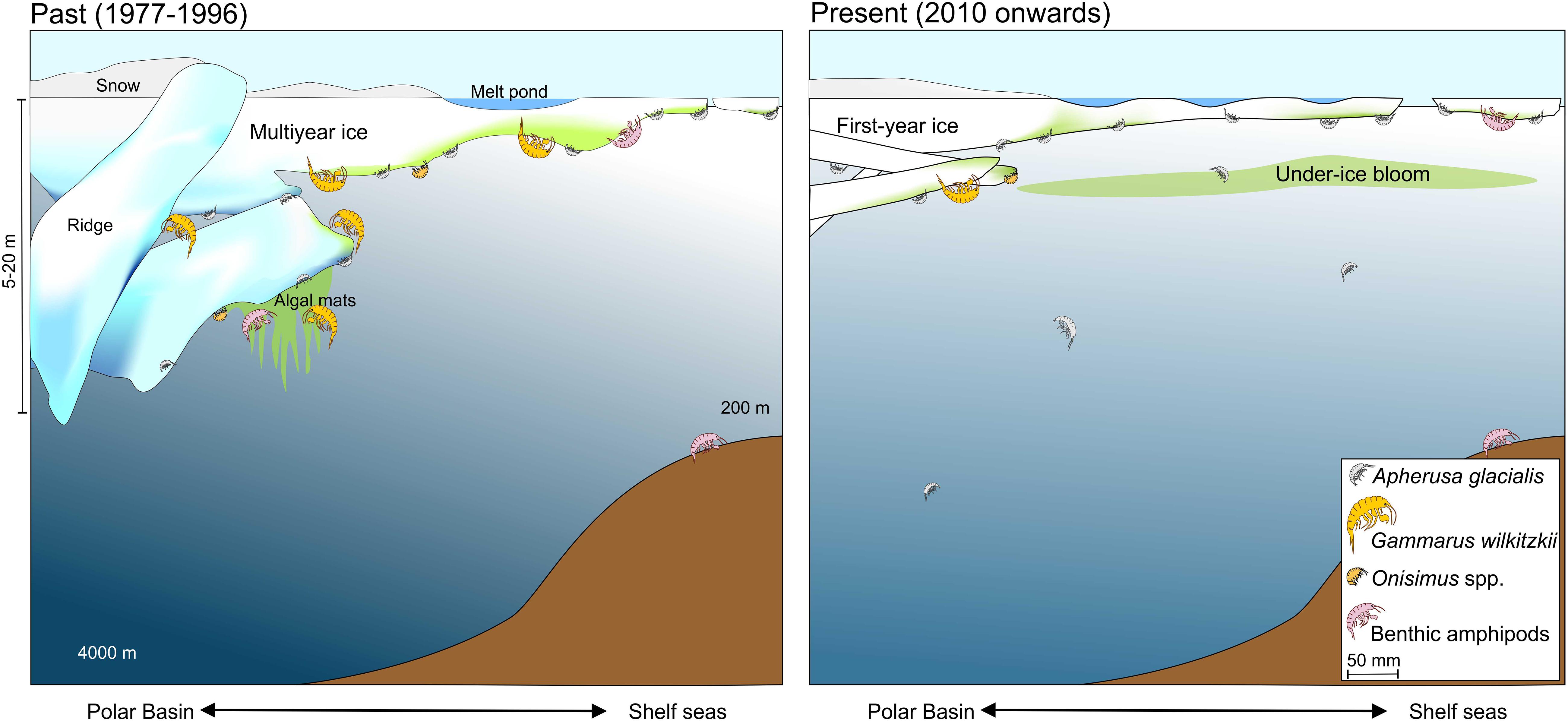
Figure 8. Ice-amphipods below sea ice during spring-summer in past and present scenarios of declining sea ice over the Polar Basin and shelf seas.
Data Availability Statement
Data are available at the Norwegian Polar Data Centre (doi: 10.21334/npolar.2014.777e66b3).
Author Contributions
Contributed datasets are according to Table 1. MV performed the data analysis. MV and MD prepared figures. HH led the project and wrote the first draft of the manuscript together with MV, with subsequent input and revisions from all co-authors. All authors contributed to the article and approved the submitted version.
Funding
Funding for data analysis and synthesis was provided by the former Centre for Ice, Climate and Ecosystems at the Norwegian Polar Institute, Norwegian Environment Agency, as part of the CBMP-Marine project, and the Ministry of Foreign Affairs, Norway (project ID Arctic). This work was supported by grants of the RF #0149-2018-0009, RFBR #18-05-00099 and RFBR/RGS #17-05-41197, and Research Council of Norway (projects #244646 and #268286). Digitization of the Russian data records was financed by CBMP. BB, RG, and HH were supported by the Arctic SIZE project co-funded by UiT – The Arctic University of Norway and the Tromsø Research Foundation (project number 01vm/h15).
Conflict of Interest
The authors declare that the research was conducted in the absence of any commercial or financial relationships that could be construed as a potential conflict of interest.
Publisher’s Note
All claims expressed in this article are solely those of the authors and do not necessarily represent those of their affiliated organizations, or those of the publisher, the editors and the reviewers. Any product that may be evaluated in this article, or claim that may be made by its manufacturer, is not guaranteed or endorsed by the publisher.
Acknowledgments
This study builds upon the State of the Arctic Marine Biodiversity Report (SAMBR 2017) developed by the Circumpolar Biodiversity Monitoring Program (CBMP), under the Conservation of Arctic Flora and Fauna (CAFF) Arctic Council working Group. Samples were provided from expeditions to the Arctic Ocean by institutions and research projects listed in Table 1.
Supplementary Material
The Supplementary Material for this article can be found online at: https://www.frontiersin.org/articles/10.3389/fmars.2021.743152/full#supplementary-material
Supplementary Figure 1 | Distance from land for abundance data as indicated by the general additive mixed models (GAMM). (A) Changes in total abundance of sympagic crustaceans (excluding benthic amphipods). Blue line indicates the GAMM model, blue shading the 95% confidence intervals and dots the actual data. Note the logarithm scale on y-axis. (B) GAMM models for the abundance of four amphipod taxa. The values are presented as the centered covariate scale of the annual smoother. Dots indicate residuals.
Footnotes
References
Aarset, A. V., and Aunaas, T. (1987). Physiological adaptations to low temperature and brine exposure in the circumpolar amphipod Gammarus wilkitzkii. Polar Biol. 8, 129–133. doi: 10.1007/bf00297067
Aarset, A. V., and Aunaas, T. (1990). Effects of osmotic stress on oxygen consumption and ammonia excretion of the Arctic sympagic amphipod Gammarus wilkitzkii. Mar. Ecol. Prog. Ser. 58, 217–224. doi: 10.3354/meps058217
Arndt, C. E., Berge, J., and Brandt, A. (2005). Mouthpart-atlas of Arctic sympagic amphipods—trophic niche separation based on mouthpart morphology and feeding ecology. J. Crust. Biol. 25, 401–412. doi: 10.1651/c-2544
Arndt, C. E., and Beuchel, F. (2006). Life cycle and population dynamics of the Arctic sympagic amphipods Onisimus nanseni SARS and O. glacialis SARS (Grammaridea: Lysianassidae). Polar Biol. 29, 239–248. doi: 10.1007/s00300-005-0045-x
Arndt, C. E., and Pavlova, O. (2005). Origin and fate of ice fauna in the Fram Strait and Svalbard area. Mar. Ecol. Prog. Ser. 301, 55–66. doi: 10.3354/meps301055
Arndt, C. E., and Swadling, K. M. (2006). Crustacea in Arctic and Antarctic sea ice: distribution, diet and life history strategies. Adv Mar Biol. 51, 197–315. doi: 10.1016/s0065-2881(06)51004-1
Arrigo, K. R., Matrai, P. A., and van Dijken, G. L. (2011). Primary productivity in the Arctic Ocean: impacts of complex optical properties and subsurface chlorophyll maxima on large-scale estimates. J. Geophys. Res. 116:C11022. doi: 10.1029/2011JC007273
Barber, D. G., Hop, H., Mundy, C. J., Else, B., Dmitrenko, I. A., Tremblay, J.-É., et al. (2015). Selected physical, biological and biogeochemical implications of a rapidly changing Arctic Marginal Ice Zone. Progr. Oceanogr. 139, 122–150. doi: 10.1016/j.pocean.2015.09.003
Barnard, J. L., and Karaman, G. S. (1991). The families and genera of marine Gammaridean Amphipoda (except marine Gammaroids). Parts 1 & 2. Rec. Aus. Mus. Suppl. 13, 1–866. doi: 10.3853/j.0812-7387.13.1991.91
Berge, J., Varpe, Ø, Moline, M. A., Wold, A., Renaud, P. E., Daase, M., et al. (2012). Retention of amphipods: possible consequences for an ice-free Arctic Ocean. Biol. Lett. 8, 10–13.
Beuchel, F., and Lønne, O. J. (2002). Population dynamics of the sympagic amphipods Gammarus wilkitzkii and Apherusa glacialis in sea ice north of Svalbard. Polar Biol. 25, 241–250. doi: 10.1007/s00300-001-0329-8
Bi, H., Fu, M., Sun, K., Liu, Y., Xu, X., and Huang, H. (2016). Arctic sea ice thickness changes in terms of sea ice age. Acta Oceanol. Sin. 35, 1–10. doi: 10.1007/s13131-016-0922-x
Bluhm, B. A., and Gradinger, R. (2008). Regional variability in food availability for arctic marine mammals. Ecol. Appl. 18, S77–S96.
Bluhm, B. A., Hop, H., Melnikov, I. A., Poulin, M., Vihtakari, M., Collins, E., et al. (2017). “Sea ice biota,” in State of the Arctic Marine Biodiversity Report, eds T. Barry, C. Price, M. Olsen, T. Christensen, and M. Fredriksen (Akureyri: Conservation of Arctic Flora and Fauna International Secretariat), 33–61.
Bluhm, B. A., Hop, H., Vihtakari, M., Gradinger, R., Iken, K., Melnikov, I. A., et al. (2018). Sea ice meiofauna distribution on local to pan-Arctic scales. Ecol. Evol. 8, 2350–2364. doi: 10.1002/ece3.3797
Böer, M., Gannefors, C., Kattner, G., Graeve, M., Hop, H., and Falk-Petersen, S. (2005). The Arctic pteropod Clione limacina: seasonal lipid dynamics and life strategy. Mar. Biol. 147, 707–717. doi: 10.1007/s00227-005-1607-8
Bradstreet, M. S. W., and Cross, W. E. (1982). Trophic relationships at High Arctic ice edges. Arctic 35, 1–12.
Brown, T. A., Assmy, P., Hop, H., Wold, A., and Belt, S. T. (2017). Transfer of ice algae carbon to ice-associated amphipods in the high-Arctic pack ice environment. J. Plankton Res. 39, 664–674. doi: 10.1093/plankt/fbx030
CAFF (2017). State of the Arctic Marine Biodiversity Report. Akureyri: Conservation of Arctic Flora and Fauna International Secretariat.
Cross, W. E. (1982). Under-ice biota at the Pond Inlet ice edge and in adjacent fast ice areas during spring. Arctic 35, 13–27.
David, C., Lange, B., Krumpen, T., Schaafsma, F., van Franeker, J. A., and Flores, H. (2016). Under-ice distribution of polar cod Boreogadus saida in the central Arctic Ocean and their association with sea-ice habitat properties. Polar Biol. 39, 981–994. doi: 10.1007/s00300-015-1774-0
David, C., Lange, B., Rabe, B., and Flores, H. (2015). Community structure of under-ice fauna in the Eurasian central Arctic Ocean in relation to environmental properties of sea-ice habitats. Mar. Ecol. Prog. Ser. 522, 15–32. doi: 10.3354/meps11156
Descamps, S., and Strøm, H. (2021). As the Arctic becomes boreal: ongoing shifts in a high-Arctic seabird community. Ecology. doi: 10.1002/ecy.3485 [Epub ahead of print].
Ehrlich, J., Schaafsma, F. L., Bluhm, B. A., Peeken, I., Castellani, G., Brandt, A., et al. (2020). Sympagic fauna in and under Arctic pack ice in the annual sea ice system of the new Arctic. Front. Mar. Sci. 7:452.
Eicken, H. (1994). Structure of under-ice melt ponds in the central Arctic and their effect on the sea-ice cover. Limnol. Oceanogr. 39, 682–694. doi: 10.4319/lo.1994.39.3.0682
Flores, H., David, C., Ehrlich, J., Hardge, J., Kohlbach, D., Lange, B. A., et al. (2019). Sea-ice properties and nutrient concentration as drivers of the taxonomic and trophic structure of high-Arctic protist and metazoan communities. Polar Biol. 42, 1377–1395. doi: 10.1007/s00300-019-02526-z
Florko, K. R. N., Thiemann, G. W., Bromaghin, J. F., and Richardson, E. S. (2021). Diet composition and body condition of polar bears (Ursus maritimus) in relation to sea ice habitat in the Canadian High Arctic. Polar Biol. 44, 1445–1456. doi: 10.1007/s00300-021-02891-8
Geiger, S. R. (1969). Distribution and development of mysids (Crustacea, Mysidacea) from the Arctic Ocean and confluent seas. Bull. Sth. Calif. Acad. Sci. 68, 13–111.
Gradinger, R., Bluhm, B., and Iken, K. (2010). Arctic sea-ice ridges–Safe heavens for sea-ice fauna during periods of extreme ice melt? Deep Sea Res. II 57, 86–95. doi: 10.1016/j.dsr2.2009.08.008
Gradinger, R., and Bluhm, B. A. (2010). Timing of ice algal grazing by the Arctic nearshore benthic amphipod Onisimus litoralis. Arctic 63, 355–358.
Gradinger, R. R., and Bluhm, B. A. (2004). In-situ observations on the distribution and behavior of amphipods and Arctic cod (Boreogadus saida) under the sea ice of the High Arctic Canada Basin. Polar Biol. 27, 595–603.
Grainger, E. H., Mohammed, A. A., and Lovrity, J. E. (1985). The sea ice fauna of Frobisher Bay, Arctic Canada. Arctic 38, 23–30.
Gulliksen, B. (1984). Under-ice fauna from Svalbard waters. Sarsia 69, 17–23. doi: 10.1080/00364827.1984.10420585
Gulliksen, B., and Lønne, O. J. (1991). Sea ice macrofauna in the Antarctic and Arctic. J. Mar. Syst. 2, 53–61. doi: 10.1016/0924-7963(91)90013-k
Hop, H., Bluhm, B. A., Gradinger, R., and Poulin, M. (2013). Arctic Sea Ice Biota. Arctic Report Cards, NOAA. Available online at: https://arctic.noaa.gov/Report-Card/Report-Card-Archive (accessed September 23, 2021).
Hop, H., and Gjøsæter, H. (2013). Polar cod (Boreogadus saida) and capelin (Mallotus villosus) as key species in marine food webs of the Arctic and the Barents Sea. Mar. Biol. Res. 9, 878–894. doi: 10.1080/17451000.2013.775458
Hop, H., Mundy, C. J., Gosselin, M., Rossnagel, A. L., and Barber, D. G. (2011). Zooplankton boom and ice amphipod bust below melting sea ice in the Amundsen Gulf, Arctic Canada. Polar Biol. 34, 1947–1958. doi: 10.1007/s00300-011-0991-4
Hop, H., and Pavlova, O. (2008). Distribution and biomass transport of ice amphipods in drifting sea ice around Svalbard. Deep Sea Res. II 55, 2292–2307. doi: 10.1016/j.dsr2.2008.05.023
Hop, H., Poltermann, M., Lønne, O. J., Falk-Petersen, S., Korsnes, R., and Budgell, W. P. (2000). Ice amphipod distribution relative to ice density and under-ice topography in the northern Barents Sea. Polar Biol. 23, 357–367. doi: 10.1007/s003000050456
Hop, H., Vihtakari, M., Bluhm, B. A., Assmy, P., Poulin, M., Gradinger, R., et al. (2020). Changes in sea-ice protist diversity with declining sea ice in the Arctic Ocean from the 1980s to 2010s. Front. Mar. Sci. 7:243.
Hopky, G. E., Lawrence, M. J., and Chiperzak, D. B. (1994a). NOGAP 82; Zooplankton data from the Canadian Beaufort Sea Shelf, 1984 and 1985. Canadian Data Report of Fisheries and Aquatic Sciences 922. Winnipeg: Department of Fisheries and Oceans.
Hopky, G. E., Lawrence, M. J., and Chiperzak, D. B. (1994b). NOGAP 82; Zooplankton data from the Canadian Beaufort Sea Shelf, 1986. Canadian Data Report of Fisheries and Aquatic Sciences 923. Winnipeg: Department of Fisheries and Oceans.
Horner, R., Ackley, S., Dieckmann, S., Gulliksen, B., Hoshiai, T., Legendre, L., et al. (1992). Ecology of the sea ice biota. 1. Habitat, terminology, and methodology. Polar Biol. 12, 417–427.
Horner, R., and Murphy, D. (1985). Species composition and abundance of zooplankton in the nearshore Beaufort Sea in winter-spring. Arctic 38, 201–209.
Iken, K., Bluhm, B. A., and Gradinger, R. (2005). Food web structure in the High Arctic Canada basin: evidence from δ13C and δ15N analysis. Polar Biol. 28, 238–249. doi: 10.1007/s00300-004-0669-2
Koch, C. W., Cooper, L. W., Woodland, R. J., Grebmeier, J. M., Frey, K. E., Stimmelmayr, R., et al. (2021). Female Pacific walruses (Odobenus rosmarus divergens) show greater partitioning of sea ice organic carbon than males: evidence from ice algae trophic markers. PLoS One 16:e0255686. doi: 10.1371/journal.pone.0255686
Kohlbach, D., Ferguson, S. H., Brown, T. A., and Michel, C. (2019). Landfast sea ice-benthic coupling during spring and potential impacts of system changes on food web dynamics in Eclipse Sound, Canadian Arctic. Mar. Ecol. Prog. Ser. 627, 33–48. doi: 10.3354/meps13071
Kohlbach, D., Graeve, M., Lange, B. A., David, C., Peeken, I., and Flores, H. (2016). The importance of ice algae-produced carbon in the central Arctic Ocean ecosystem: food web relationships revealed by lipid and stable isotope analyses. Limnol. Oceanogr. 61, 2027–2044. doi: 10.1002/lno.10351
Kohlbach, D., Hop, H., Wold, A., Schmidt, K., Smik, L., Belt, S. T., et al. (2021). Multiple trophic markers trace dietary carbon sources in Barents Sea zooplankton during late summer. Front. Mar. Sci. 7:610248. doi: 10.3389/fmars.2020.610248
Kohlbach, D., Schaafsma, F. L., Graeve, M., Lebreton, B., Lange, B. A., David, C., et al. (2017). Strong linkage of polar cod (Boreogadus saida) to sea ice algae-produced carbon: evidence from stomach content, fatty acid and stable isotope analyses. Progr. Oceanogr. 152, 62–74. doi: 10.1016/j.pocean.2017.02.003
Kunisch, E. H., Bluhm, B. A., Daase, M., Gradinger, R., Hop, H., Melnikov, I. A., et al. (2020). Pelagic occurrences of the ice amphipod Apherusa glacialis throughout the Arctic. J. Plankton Res. 42, 73–86. doi: 10.1093/plankt/fbz072
Laidre, K. L., Stern, H., Kovacs, K. M., Lowry, L., Moore, S. E., Regehr, E. V., et al. (2015). Arctic marine mammal population status, sea ice habitat loss, and conservation recommendations for the 21st century. Cons. Biol. 29, 724–737. doi: 10.1111/cobi.12474
Legendre, L., Ackley, S. F., Dieckmann, G. S., Gulliksen, B., Horner, R., Hoshiai, T., et al. (1992). Ecology of sea ice biota. 2. Global significance. Polar Biol. 12, 429–444.
Leu, E., Mundy, C. J., Assmy, P., Campbell, K., Gabrielsen, T. M., Gosselin, M., et al. (2015). Arctic spring awakening – Steering principles behind the phenology of vernal ice algal blooms. Progr. Oceanogr. 139, 151–170. doi: 10.1016/j.pocean.2015.07.012
Lønne, O. J. (1988). A diver-operated electric suction sampler for sympagic (= under-ice) invertebrates. Polar Res. 6, 135–136. doi: 10.3402/polar.v6i1.6854
Lønne, O. J., and Gabrielsen, G. W. (1992). Summer diet of seabirds feeding in sea-ice-covered waters near Svalbard. Polar Biol. 12, 685–692.
Lønne, O. J., and Gulliksen, B. (1989). Size, age and diet of polar cod, Boreogadus saida (Lepechin 1773), in ice covered waters. Polar Biol. 9, 187–191. doi: 10.1007/bf00297174
Lønne, O. J., and Gulliksen, B. (1991b). On the distribution of sympagic macro-fauna in the seasonally ice covered Barents Sea. Polar Biol. 11, 457–469.
Lønne, O. J., and Gulliksen, B. (1991a). Sympagic macro-fauna from multiyear sea-ice near Svalbard. Polar Biol. 11, 471–477.
Macnaughton, M. O., Thormar, J., and Berge, J. (2007). Sympagic amphipods in the Arctic pack ice: redescriptions of Eusirus holmii Hansen, 1887 and Pleusymtes karstensi (Barnard, 1959). Polar Biol. 30, 1013–1025. doi: 10.1007/s00300-007-0260-8
Markus, T., Stroeve, J. C., and Miller, J. (2009). Recent changes in Arctic sea ice melt onset, freezeup, and melt season length. J. Geophys. Res. Oceans 114, 1–14.
Martin, A., Gensch, R., and Brown, C. (1946). Alternative methods in upland gamebird food analysis. J. Wildl. Manage. 10, 8–12. doi: 10.2307/3795806
Maslanik, J. A., Fowler, C., Stroeve, J., Drobot, S., Zwally, J., Yi, D., et al. (2007). A younger, thinner Arctic ice cover: increased potential for rapid, extensive sea-ice loss. Geophys. Res. Lett. 34:L24501.
Meier, W. N., Hovelsrud, G. K., van Oort, B. E. H., Key, J. R., Kovacs, K. M., Michel, C., et al. (2014). Arctic sea ice in transformation: a review of recent observed changes and impacts on biology and human activity. Rev. Geophys. 52, 185–217. doi: 10.1002/2013rg000431
Melnikov, I. A. (1984). Distribution and behaviour of the common species of cryopelagic fauna under drifting Arctic ice. Zool. Zh. 63, 16–21.
Melnikov, I. A. (2019). “Comparative evaluation of the effectiveness of methods for collecting cryofauna under Arctic sea ice,” in Proceedings of the 2019 XVI International Scientific and Technical Conference “Modern Methods and Means of Oceanological Research”, IO RAS, Pskov, 263–267.
Melnikov, I. A., Kolosova, E. G., Welch, H. E., and Zhitina, L. S. (2002). Sea ice biological communities and nutrient dynamics in the Canada Basin of the Arctic Ocean. Deep Sea Res. I 49, 1623–1649. doi: 10.1016/s0967-0637(02)00042-0
Melnikov, I. A., and Kulikov, A. S. (1980). “«Cryopelagic fauna of the Central Arctic Basin,” in Biology of the Central Arctic Basin, eds M. E. Vinogradov and I. A. Melnikov (Moscow: Nauka), 97–111.
Nghiem, S. V., Rigor, I. G., Perovich, D. K., Clemente-Colón, P., Weatherly, J. W., and Neumann, G. (2007). Rapid reduction of Arctic perennial sea ice. J. Geophys. Res. Lett. 34:L19504. doi: 10.1029/2007GL031138
Nygård, H., Berge, J., Søreide, J. E., Vihtakari, M., and Falk-Petersen, S. (2012). The amphipod scavenging guild in two Arctic fjords: seasonal variations, abundance and trophic interactions. Aquat. Biol. 14, 247–264. doi: 10.3354/ab00394
Oksanen, J., Guillaume Blanchet, F., Friendly, M., Kindt, R., Legendre, P., McGlinn, D., et al. (2020). vegan: Community Ecology Package. R package version 2.5-7. Available online at: https://CRAN.R-project.org/package=vegan (accessed September 23, 2021).
Overland, J. E., and Wang, M. (2013). When will the summer Arctic be nearly sea ice free? Geophys. Res. Lett. 40, 2097–2101. doi: 10.1002/grl.50316
Perovich, D., Meier, W. N., Tschudi, M., Farrell, S., Hendricks, S., Gerland, S., et al. (2018). Sea ice. Arctic Report Cards. Available online at: https://arctic.noaa.gov/Report-Card/Report-Card-Archive (accessed September 23, 2021).
Pike, D. G., and Welch, H. E. (1990). Spatial and temporal distribution of sub-ice macrofauna in the Barrow Strait Area, Northwest Territories. Can. J. Fish. Aquat. Sci. 47, 81–91. doi: 10.1139/f90-008
Poltermann, M. (1998). Abundance, biomass and small-scale distribution of cryopelagic amphipods in the Franz Josef Land area (Arctic). Polar Biol. 20, 134–138. doi: 10.1007/s003000050287
Poltermann, M. (2001). Arctic sea ice as feeding ground for amphipods – food sources and strategies. Polar Biol. 24, 89–96. doi: 10.1007/s003000000177
Poltermann, M., Hop, H., and Falk-Petersen, S. (2000). Life under Arctic sea ice - reproduction strategies of two sympagic (ice-associated) amphipod species, Gammarus wilkitzkii and Apherusa glacialis. Mar. Biol. 136, 913–920. doi: 10.1007/s002270000307
Polyakov, I. V., Pnyushkov, A. V., Alkire, M. B., Ashik, I. M., Baumann, T. M., Carmack, E. C., et al. (2017). Greater role for Atlantic inflows on sea-ice loss in the Eurasian Basin of the Arctic Ocean. Science 356, 285–291. doi: 10.1126/science.aai8204
Purcell, J. P., Hopcroft, R. R., Kosobokova, K. N., and Whitledge, T. E. (2010). Distribution, abundance, and predation effects of epipelagic ctenophores and jellyfish in the western Arctic Ocean. Deep Sea Res. II 57, 127–135. doi: 10.1016/j.dsr2.2009.08.011
Raskoff, K. A., Hopcroft, R. R., Kosobokova, K. N., Purcell, J. E., and Youngbluth, M. (2010). Jellies under ice: ROV observations from the Arctic 2005 hidden ocean expedition. Deep Sea Res. II 57, 111–126. doi: 10.1016/j.dsr2.2009.08.010
Raskoff, K. A., Purcell, J. E., and Hopcroft, R. R. (2005). Gelatinous zooplankton of the Arctic Ocean: in situ observations under the ice. Polar Biol. 28, 207–217. doi: 10.1007/s00300-004-0677-2
Renaut, S., Devred, E., and Babin, M. (2018). Northward expansion and intensification of phytoplankton growth during the early ice-free season in Arctic. Geophys. Res. Lett. 45, 10,590–10,598. doi: 10.1029/2018GL078995
Scott, C. L., Kwasniewski, S., Falk-Petersen, S., and Sargent, J. R. (2002). Lipids and fatty acids in the copepod Jaschnovia brevis (Jaschnov) and in particulates from Arctic waters. Polar Biol. 25, 65–71. doi: 10.1007/s003000100312
Storrie, L., Lydersen, C., Andersen, M., Wynn, R. B., and Kovacs, K. M. (2018). Determining the species assemblage and habitat use of cetaceans in the Svalbard Archipelago, based on observations from 2002 to 2014. Polar Res. 37:1463065. doi: 10.1080/17518369.2018.1463065
Stroeve, J. C., Serreze, M. C., Holland, M. M., Kay, J. E., Malanik, J., and Barrett, A. P. (2012). The Arctic’s rapidly shrinking sea ice cover: a research synthesis. Clim. Change 110, 1005–1027.
Strøm, H., Bakken, V., Skoglund, A., Descamps, S., Fjeldheim, V. B., and Steen, H. (2020). Population status and trend of the threatened ivory gull Pagophila eburnea in Svalbard. Endang. Spec. Res. 43, 435–445. doi: 10.3354/esr01081
Wang, S. W., Budge, S. M., Iken, K., Gradinger, R. R., Springer, A. M., and Wooller, M. J. (2015). Importance of sympagic production to Bering Sea zooplankton as revealed from fatty acid-carbon stable isotope analyses. Mar. Ecol. Prog. Ser. 518, 31–50. doi: 10.3354/meps11076
Welch, H. E., Bergmann, M. A., Siferd, T. D., Martin, K. A., Curtis, M. F., Crawford, R. E., et al. (1992). Energy flow through the marine ecosystem of the Lancaster Sound region, Arctic Canada. Arctic 45, 343–357.
Werner, I. (1997). Grazing of Arctic under-ice amphipods on sea-ice algae. Mar. Ecol. Prog. Ser. 160, 93–99. doi: 10.3354/meps160093
Werner, I. (2005). Living conditions, abundance and biomass of under-ice fauna in the Storfjord area (western Barents Sea, Arctic) in late winter (March 2003). Polar Biol. 28, 311–318. doi: 10.1007/s00300-004-0678-1
Werner, I., and Arbizu, P. M. (1999). The sub-ice fauna of the Laptev Sea and the adjacent Arctic Ocean in summer 1995. Polar Biol. 21, 71–79. doi: 10.1007/s003000050336
Werner, I., and Auel, H. (2005). Seasonal variability in abundance, respiration and lipid composition of Arctic under-ice amphipods. Mar. Ecol. Prog. Ser. 292, 251–262. doi: 10.3354/meps292251
Werner, I., Auel, H., and Friedrich, C. (2002). Carnivorous feeding and respiration of the Arctic under-ice amphipod Gammarus wilkitzkii. Polar Biol. 25, 523–530. doi: 10.1007/s00300-002-0376-9
Werner, I., Auel, H., Garrity, C., and Hagen, W. (1999). Pelagic occurrence of the sympagic amphipod Gammarus wilkitzkii in ice-free waters of the Greenland Sea – dead end or part of life cycle? Polar Biol. 22, 56–60. doi: 10.1007/s003000050390
Werner, I., Auel, H., and Kiko, R. (2004). Occurrence of Anonyx sarsi (Amphipoda: Lysianassoidea) below Arctic pack ice: An example for cryo-benthic coupling? Polar Biol. 27, 474–481.
Werner, I., and Gradinger, R. (2002). Under-ice amphipods in the Greenland Sea and Fram Strait (Arctic): environmental controls and seasonal patterns below the pack ice. Mar. Biol. 140, 317–326. doi: 10.1007/s00227-001-0709-1
Weslawski, J. M. (1994). Gammarus (Crustacea, Amphipoda) from Svalbard and Franz Josef Land. Distribution and density. Sarsia 79, 145–150. doi: 10.1080/00364827.1994.10413553
Weslawski, J. M., Wiktor, J. Jr., and Kotwicki, L. (2010). Increase in biodiversity in the arctic rocky littoral, Sorkappland, Svalbard, after 20 years of climate warming. Mar. Biodivers. 40, 123–130. doi: 10.1007/s12526-010-0038-z
Wood, S. N. (2017). Generalized Additive Models: An introduction with R, 2nd Edn. Boca Raton, FL: CRC Press.
Keywords: ice amphipods, sympagic, time-series, climate change, Arctic Ocean
Citation: Hop H, Vihtakari M, Bluhm BA, Daase M, Gradinger R and Melnikov IA (2021) Ice-Associated Amphipods in a Pan-Arctic Scenario of Declining Sea Ice. Front. Mar. Sci. 8:743152. doi: 10.3389/fmars.2021.743152
Received: 19 July 2021; Accepted: 16 September 2021;
Published: 13 October 2021.
Edited by:
Paolo Magni, National Research Council (CNR), ItalyReviewed by:
Alexander Tzetlin, Lomonosov Moscow State University, RussiaSarat Chandra Tripathy, National Centre for Polar and Ocean Research (NCPOR), India
Copyright © 2021 Hop, Vihtakari, Bluhm, Daase, Gradinger and Melnikov. This is an open-access article distributed under the terms of the Creative Commons Attribution License (CC BY). The use, distribution or reproduction in other forums is permitted, provided the original author(s) and the copyright owner(s) are credited and that the original publication in this journal is cited, in accordance with accepted academic practice. No use, distribution or reproduction is permitted which does not comply with these terms.
*Correspondence: Haakon Hop, aGFha29uLmhvcEBucG9sYXIubm8=