- 1State Key Laboratory of Estuarine and Coastal Research, East China Normal University, Shanghai, China
- 2Hong Kong Branch of the Southern Marine Science and Engineering Guangdong Laboratory (Guangzhou), State Key Laboratory of Marine Pollution and Department of Ocean Science, The Hong Kong University of Science and Technology, Hong Kong SAR, China
- 3The Southern Marine Science and Engineering Guangdong Laboratory (Guangzhou), Guangzhou, China
Estuarine sediments are increasingly contaminated by heavy metals as a result of urbanization and human activities. Continuous multi-heavy metal accumulation in the ecosystem can provoke new effects on top of the complex environmental interactions already present in estuarine ecosystems. It is important to study their integrated influence on imperative microbial communities to reflect on the environmental and ecological risks they may impose. Inductively coupled plasma optical emission spectroscopy analysis for five metals Cd, Cr, Cu, Pb, and Zn showed that Cr and Cu concentrations in intertidal sediments of the urbanized Yangtze River estuary in China have consistently exceeded respective threshold effect concentration (TEC) levels. The geo-accumulation and potential ecological risk index results of the five metals showed that all sampling sites were weakly to moderately polluted, and at considerable to high ecological risk, respectively. Redundancy and correlation analyses showed that Zn followed by Cr in the ecosystem were explanatory of the shifts in recorded microbial community structures. However, the spatial variation in metal concentrations did not correspond to the selection of metal resistance genes (MRGs). Unlike many other dominant bacterial taxa, most of the sulfate-reducing bacteria (SRB) and associated sulfate respiration as the dominant microbially contributed ecological function were found to negatively correlate with Zn and total heavy metal pollution. Zn concentration was proposed to be a potent indicator for heavy metal pollution-associated microbial community compositional shifts under urbanized estuarine conditions. The associations between heavy metals and estuarine microbial communities in this study demonstrate the influence of heavy metals on microbial community structure and adaptations that is often overshadowed by environmental factors (i.e., salinity and nutrients).
Introduction
Estuarine ecosystems are some of the most economically and ecologically important ecosystems on Earth. However, most estuaries face environmental pollution problems resulting from human industrial activities and urbanization, through routes such as urban runoff and industrial waste discharge. As the intersection between terrestrial and marine ecosystems, urbanized estuarine sediments are often found to be sinks for wide range of pollutants from land (Barletta et al., 2019). Heavy metal pollution in the estuarine environment is an increasing concern as most metals beyond threshold concentrations are capable of exerting toxicity to organisms (Prabhakaran et al., 2016). Metals in the environment can find their ways to enter the food chain. The toxicity and associated risks of individual metals are relatively well studied with established baselines. However, the joint toxicity and risks imposed by multi-heavy metal mixtures that currently co-exist in the environment have proven to be a challenge. Furthermore, estuarine environments are naturally dynamic systems that are often coupled with seawater dilution effects and various anthropogenic input. All environmental variables can then further exert knock-on effects on the interactions between heavy metal pollutants and the aquatic biota. Thus, the integrated impact of heavy metal pollution in estuarine environments cannot be easily understood in full. Currently, most existing assessments and studies cannot fully consider all major contributing factors such as different environmental variables, multi-heavy metal mixtures and their speciation, and aquatic biota as receivers (Gu and Gao, 2021), when attempting to investigate underlying relationships and mechanisms between heavy metals and the biota. Nevertheless, the need for a better understanding remains fundamental to address the joint toxicity and associated risks of multiple heavy metal-pollution issues.
The microbial communities that inhabit estuarine sediments are both highly diverse and susceptible to environmental changes (Wang et al., 2012). Shifts in microbial community structures, in response to changing environmental conditions, can be sensitive indicators to reflect and assess environmental pollution levels and risks. Previous microbial ecology studies have largely focused on investigating the impact of changing environmental factors such as salinity, pH, and nutrient concentration gradients. Yi et al. (2020) showed that sedimentary microbial communities can well reflect changes in important physicochemical properties such as pH and nutrient concentrations along spatiotemporal scale. In contrast, our current understanding of the interactions between microbial communities and other anthropogenic pollutants in the environment, such as heavy metals, is limited in comparison, but the field is rapidly advancing. Previous study by Yin H. et al. (2015) found that increasing heavy metal contamination in sediments can lead to decrease in relative abundance of major bacterial phyla such as Proteobacteria and Actinobacteria. In wetland sediments, elements Cr, Pb, and Zn were reported to associate negatively with the relative abundance of bacterial phyla Nitrospirae, Bacteroidetes, and Verrucomicrobia (Li et al., 2020). Variations in bacterial community structures in heavy metal polluted conditions have been partially attributed to the selective enrichment of metal-resistant bacteria and archaea (Sheeba et al., 2017); and metal resistance can be co-selected with antibiotic resistance genes (Zhao et al., 2019). In all cases, shifts in bacterial community structure resulting from either natural or anthropogenically induced environmental changes can have knock-on effects on their associated metabolic functions (Echavarri-Bravo et al., 2015).
Microbes play an important role in regulating almost all redox reactions and hence the biogeochemical processes of the ecosystem (Falkowski et al., 2008). In aquatic environments, the diverse group of sulfate-reducing bacteria (SRB) is a dominant and important component of the sulfur cycle (Niu et al., 2018). A shift in SRB composition due to environmental stress from heavy metals may result in ecological functional consequences. Furthermore, from a geomicrobiology perspective, microbes can facilitate the cycling of heavy metals through means such as biosorption (Jin et al., 2018). This cycling process depends on physiochemical factors including pH, metal concentration present, redox potential and chemistry of metal ions within the system (Shamim, 2018). SRB-generated sulfide can interact with many heavy metals to form low solubility metal sulfides, such as copper sulfide, zinc sulfide, and lead sulfide, which are less bio-available (Bao et al., 2018). This has implications in heavy metal removal and reduces the environmental pressure exerted by heavy metal pollution (Kiran et al., 2018). Thus, investigating the interactions and interconnected relationship between microbial functional groups and heavy metals can have great potential and implication in managing ecological health and functioning of ecosystems.
Previous studies have reported that the Yangtze River estuary in China was heavily and increasingly polluted by different heavy metals including As, Cd, Cr, Cu, Hg, Pb, and Zn (Liu et al., 2015; Fan et al., 2020). This can be largely attributed to the rapid growth in industrial activities and input over the past decades. In the Yangtze River delta, accumulating Cu, Cr, and Zn are primarily contributed by natural sources with added input from industries such as anti-fouling paints in shipping activities, agricultural fertilizers, metal mining and refinery; Cd and Pb are trace metals more commonly derived from atmospheric emission and deposition, and industrial discharges from electroplating plants (Yin S. et al., 2015; Liu et al., 2019; Hu et al., 2021). Together, excessive concentrations of these heavy metals pose a multitude of environmental health risks that destabilize an ecosystem through toxic impact on the biota, which can subsequently lead to human health risks. In Guo et al. (2019) revealed high average concentrations for four of the heavy metals Cr, Cu, Pb, and Zn (41.28, 80.54, 56.82, and 364.08mg/kg, respectively) in the intertidal surface sediments of Yangtze River estuary. The toxicity and risk of exposure to such heavy metal mixtures at increasing concentrations must be constantly examined. While monitoring programs of heavy metal pollution have seen global efforts, relatively few field studies have attempted to disentangle the potential integrated impact of multiple metals and the roles that microbial community may play.
The Yangtze estuary is bifurcated by the Chongming Island into a less disturbed northern branch of salt marshes, and a more anthropogenically disturbed southern branch of mudflats. The two branches receive similar freshwater input but differ in environmental conditions and anticipated heavy metal pollution status. Potential difference in environmental heavy metal compositions and their influence on inhabiting bacterial communities have yet to be explored. The Yangtze River estuary serves as a representative dynamic, heavy metal-contaminated study site with great ecological and economic significance. It is anticipated that the estuarine environment will be increasingly heavy metal-polluted. Comprehensive studies will be required to elucidate potential patterns and responses in estuarine microbial communities. Here in this study, environmental intensity, contamination status, and ecological risks imposed by heavy metals Cd, Cr, Cu, Pb, and Zn in sediments were first analyzed and assessed. The geo-accumulation index (Igeo) was used to evaluate the pollution risk of heavy metals in sediments, accounting for human influence and geological processes on the background value. The potential ecological risk index (RI) was then used to assess the heavy metal pollution in sediments with respect to both the toxicity of heavy metals and the response of the environment (Liu et al., 2021). We then used 16S rRNA metabarcoding data from sediment samples to analyze the diversity, relative abundance, and ecological functions of microbial communities, allowing potential influence of heavy metal pollution on estuarine microbial communities to be revealed. The reproducibility of metal-microbial interactions and potential abundance of metal resistance genes (MRGs) in sedimentary bacterial communities were also experimentally investigated. This study can help understand the role of heavy metals and the responses and potential adaptations of microbial communities to various heavy metal-polluted environments. This will improve current knowledge on the ecological risks imposed by multiple heavy metal accumulation in estuarine ecosystems and will be a beneficial reference for future metal-microbial interaction studies.
Materials and Methods
Sampling Sites and Sample Collection
Sediment samples were collected from five sampling sites at the Yangtze River estuary in October 2015 and April 2016. Sampling sites include Beibayao (BBY, 31°40′ N, 121°41′ E), Dongwangsha (DWS, 31°23′ N, 121°30′ E), Wusongkou (WSK, 31°24′ N, 121°30′ E), Shidongkou (SDK, 31°28′ N, 121°23′ E) and Liuhekou (LHK, 31°31′ N, 121°17′ E). BBY and DWS sites were salt marshes to the east of Chongming Island. WSK, SDK, and LHK sites were estuarine mudflats along the south branch of the Yangtze River estuary (Figure 1). All sediment samples were collected during the low tide period.
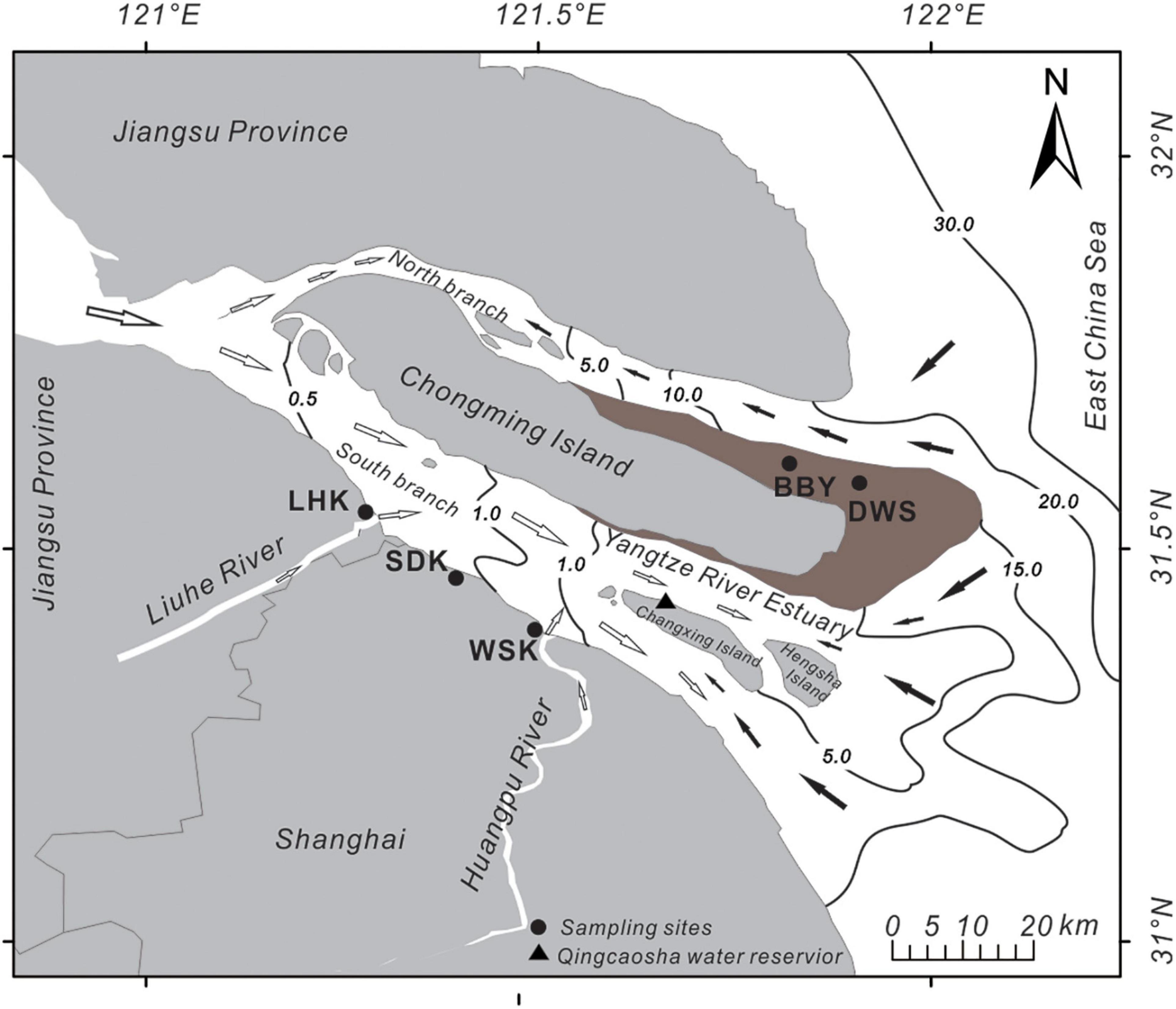
Figure 1. Map of the locations of the five sampling sites at the Yangtze River estuary. LHK, SDK, and WSK sample sites are estuarine mudflats; BBY and DWS sample sites are salt marshes. Black arrows indicate the direction of incoming saltwater; white arrows indicate the direction of downstream freshwater. Numbered black lines indicate salinity gradients.
At each sampling site, the surface sediments were sampled using a stainless-steel shovel and sealed in separate sterile plastic bags in an icebox. Triplicate samples were collected. Samples were immediately transported back to laboratory on the same day and then stored at 4°C. At the laboratory, samples were separated into two portions. One portion included 100 g of each sediment sample to be used for heavy metal characterization, stored at room temperature in sterile plastic bags. The remaining portion was stored at −80°C for DNA extraction and subsequent sequencing analysis. All samples were prepared and handled within 7 days after collection.
Characterization of Physicochemical Properties and Heavy Metals in Sediments
The physicochemical properties of sediment samples were measured in situ or in the laboratory. The salinity and pH of pore water in the sediment samples were measured in situ using a portable salinity meter (Model 30, YSI Incorporated, United States) and pH meter (Five-Go, Mettler-Toledo Instruments, Shanghai, China). Triplicate readings were taken to ensure accuracy of measurements. After transporting the samples to the laboratory, the sediments were dried at 50°C for further characterization. The sediment grain size was measured using a laser granulometer (LS13320, Beckman Coulter Corporation, CA, United States).
Five important heavy metals, copper (Cu), zinc (Zn), lead (Pb), chromium (Cr), and cadmium (Cd), were selected for analysis in this study. First, sediment samples were grinded into fine particles and sieved through a 0.15 mm sieve. Then, sediment samples were digested with 10 mL of acid solution containing HNO3: HClO4: HF at a ratio of 5:3:2 and heated on a hot plate at 120°C for 2 h then at 180°C until fully digested. After digestion, samples were filtered through a 0.45 μm filter membrane. Filtrates were prepared for detection and subjected to inductively coupled plasma optical emission spectroscopy (ICP-OES) using an iCAP 7000 Optical Emission Spectrometer (Thermo Fisher Scientific, United States) to test for Zn and Cr, and atomic absorption spectroscopy (AAS) using an AAnalyst 800 atomic absorption spectrometer (PerkinElmer, United States) to test for Cu, Pb, and Cd. Quality assurance and quality control were implemented through the use of triplicate samples, analytical blanks and standard reference materials (Yangtze River standard stream sediment sample GSD-9), subjected to the same procedure to validate the method and data. The recovery rates for the heavy metals were between 74.8 and 105.7%. The relative standard deviation between replicate samples were generally less than 5%.
Risk Assessment Methods
Geo-Accumulation Index (Igeo)
The Igeo originally defined by Muller (1969) was calculated using the following equation (Zhang et al., 2017):
Where Ci is the measured concentration of heavy metal i, and Bi is the geological background concentration of heavy metal i. The constant factor of 1.5 is used to account for variation in background values for metals. The geological background concentrations of Zn, Cu, Cr, Pb, and Cd in sediments from Yangtze River intertidal mudflats were 48.79, 17.43, 28.27, 20.14, and 0.09 mg/kg, respectively, according to an earlier study based on intertidal sediments supposedly formed between 70 and 100 years ago, before any impact imposed by human activities (Xu et al., 1997). The China National Environmental Monitoring Center (Wei et al., 1991) have also previously reported similar geological background concentrations of Zn, Cu, Cr, Pb, and Cd in soils from Shanghai to be 81.3, 27.2, 70.2, 25.0, and 0.138 mg/kg, respectively. The Igeo values of heavy metals can be classified into seven grades as follows: Igeo < 0, class 0, unpolluted; 0 < Igeo < 1, class I, weakly polluted; 1 < Igeo < 2, class II, moderately polluted; 2 < Igeo < 3, class III, moderately to heavily polluted; 3 < Igeo < 4, class IV, heavily polluted; 4 < Igeo < 5, class V, heavily to extremely polluted; and Igeo > 5, class VI, extremely polluted.
Potential Ecological Risk Index
The risk index (RI) defined by Hakanson (1980) was calculated using the following equations:
where is the contamination factor of heavy metal i and and are the measured concentration and background reference concentration of heavy metal i, respectively. is the potential ecological risk factor and is the toxic response factor of heavy metal i. The toxic response factor accounts for the threat to humans and to aquatic ecological system; the values for of Cd, Cr, Cu, Pb, and Zn are 30, 2, 5, 5, and 1, respectively (Hakanson, 1980). The RI then represents potential ecological risk considering the overall contamination. The resulting and RI values are classified into five and four modified grades (Ma and Han, 2019) as shown in Supplementary Table 1.
DNA Extraction and 16S rRNA Gene Sequencing
The total DNA was extracted and purified from each sediment sample, using the E.Z.N.A.TM Soil DNA Kit (Omega Bio-Tek, Inc., United States). The bacterial 16S rRNA gene was selected for amplification with primers 341F (5′-CCTACGGGNGGCWGCAG-3′) and 805R (5′GACTACHVGGGTATCTAATCC-3′) targeting the V3–V4 hypervariable regions (Herlemann et al., 2011). PCR was conducted following methods detailed in a previous study (Yi et al., 2020). The PCR products were paired-end sequenced using the IlluminaTM MiSeq sequencing platform (Illumina, United States), conducted at Sangon Biotech (Shanghai, China). The sequence reads generated are deposited in the National Center for Biotechnology Information (NCBI) Sequence Read Archive under the following accession numbers: SRX3855667 (BBY), SRX3855668 (DWS), SRX3855669 (WSK), SRX3855670 (SDK) and SRX3855671 (LHK) for October 2015 samples; SRX12453355 (BBY), SRX12453356 (DWS), SRX12453359 (WSK), SRX12453358 (SDK) and SRX12453357 (LHK) for April 2016 samples.
Data Analysis
Taxonomic Assignment and Functional Annotation
The Quantitative Insights Into Bacterial Ecology 2 (QIIME2; Bolyen et al., 2019) platform was used to process sequencing data and conduct subsequent analysis. In brief, raw sequencing data from samples were imported into QIIME2 and demultiplexed according to the barcode sequences. The bacterial paired-end reads were then merged using the VSEARCH pipeline (Rognes et al., 2016). After filtering chimeric and short sequences, reads were clustered into OTUs with a 97% similarity cutoff (Edgar et al., 2011; Rognes et al., 2016). The Ribosomal Database Project classifier (Wang et al., 2007) was then used to classify OTUs to taxonomy according to the Greengenes database (DeSantis et al., 2006; McDonald et al., 2012). At each taxonomic level (phylum, class, order, family), taxa were considered dominant if their average relative abundance was above 1%. The Functional Annotation of Prokaryotic Taxa (FAPROTAX) tool was then used to annotate the ecological functions of bacterial communities (Louca et al., 2016) through reference to microbial database based on cultured microorganisms and past literature.
Metal Resistance Gene Analysis
Sequencing reads were also aligned against the 16S Ribosomal RNA database at NCBI GenBank using BLAST+ 2.12.0 (Camacho et al., 2009). The taxonomic affiliations according to NCBI taxonomy database were recorded for identical hits. Candidate genomes were compiled and aligned against the BacMet 2.0 reference database of genes with experimentally confirmed metal resistance functions (Pal et al., 2014). The search was conducted using the BLASTx option of DIAMOND sequence aligner 2.0.11 (Buchfink et al., 2015) under the following criteria: e-value 10–5, amino acid identity ≥ 90%, and alignment length > 25 amino acids (Gupta et al., 2018). The relative abundance of MRGs associated to our studied metals (Cd, Cr, Cu, Pb, Zn, and multimetal-resistant) were then calculated.
Statistical Analysis
Differences in physicochemical properties and heavy metal concentrations between estuarine mudflat (WSK, SDK, and LHK) and salt marsh (BBY and DWS) sediment samples were analyzed using independent-samples t-test. Differences in microbial relative abundance were analyzed using Welch’s t-test (White et al., 2009). Spearman’s correlation was used to investigate the relationship between heavy metal concentrations, bacterial community structure and ecological functions. The SPSS Statistics Professional Version 22.0 (IBM Corp., United States) software was used to carry out the statistical analyses unless otherwise specified. Differences were considered significant when P < 0.05.
Redundancy analysis (RDA) was used to study bacterial communities in relation to the concentrations and geo-accumulation indices of heavy metals (Zhang et al., 2016), which can help to disclose the overall impact on microbial communities. For RDA, all explanatory variables were log (x + 1) transformed prior to analysis. Permutation test parameters were set to 999 permutations in each test. All RDA were conducted using Canoco5 (ter Braak and Šmilauer, 2002).
Results
Physicochemical Characteristics of Sediments
The major physicochemical characteristics of sediments at studied sampling sites are summarized in Table 1. Salinity was significantly higher in the salt marsh sediments (BBY and DWS) compared to the estuarine mudflat sediments (WSK, SDK, and LHK) (P < 0.05). The pH values of pore water in both the studied salt marsh and estuarine mudflat sediment samples were mostly slightly alkaline. But in October 2015, the pH value of pore water was significantly lower in estuarine mudflat sediments (pH values at WSK, SDK, and LHK were below 7) compared to that of the salt marsh sediments (P < 0.05). Sediment samples from most of the sampling sites were mainly composed of silt and clay (amounted to over 90% of total) with small amounts (below 10%) of sand, with the exception of WSK and SDK sediments sampled in April 2016.
Risk Assessment of Heavy Metal Concentrations in Sediments
Heavy metal concentrations in intertidal sediments sampled during October 2015 and April 2016 across the five sampling sites were examined and summarized in Supplementary Table 2. Samples from the salt marshes (BBY and DWS) had slightly lower heavy metal concentrations compared to samples from the estuarine mudflats (WSK, SDK, and LHK). The concentration values were compared with known threshold effect concentration (TEC) values from a previous study (MacDonald et al., 2000), as presented in Figure 2. Results showed that Cr and Cu concentrations in intertidal sediments across all sampling sites exceeded respective TEC levels. In contrast, the concentrations of Cd were consistently below the TEC level. A notable difference was observed for Zn concentrations, as it was recorded to be below the TEC level in the salt marsh sampling sites (BBY and DWS), and above the TEC level in estuarine mudflat sampling sites (WSK, SDK, and LHK). Similar pattern was observed but to a lesser extent after 6 months in April 2016. Pb concentrations recorded were consistently, albeit barely, under the TEC level in estuarine mudflat sampling sites, and occasionally above the TEC level in brackish marsh sites.
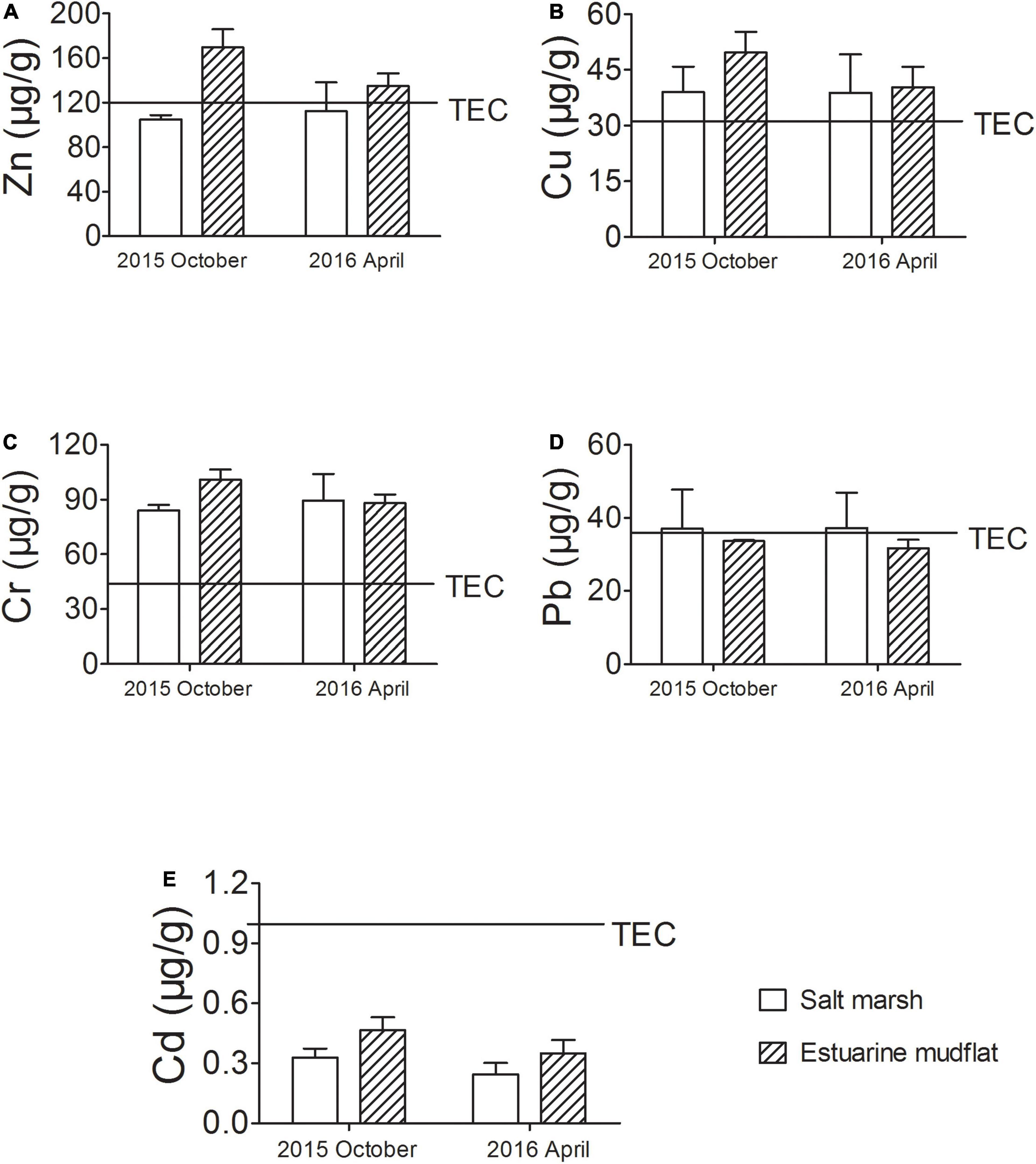
Figure 2. Concentrations of heavy metals (A) Zn, (B) Cu, (C) Cr, (D) Pb, and (E) Cd in the intertidal sediments from estuarine mudflats (WSK, SDK, and LHK) and Chongming salt marshes (BBY and DWS) collected during October 2015 and April 2016 at the Yangtze River estuary. The threshold effect concentrations (TEC) of respective heavy metals are indicated (MacDonald et al., 2000).
Geo-Accumulation Indices of Heavy Metals in Sediments
The geo-accumulation indices (Igeo) of heavy metals in the sediment samples are summarized in Figure 3. In October 2015, Zn, Cr and Cd were recorded at moderate levels in estuarine mudflat sediments; in the Chongming salt marsh sediments, only Cd was recorded at a moderate level, with Cr being very close to the moderate level at an Igeo of 0.985. In April 2016, Igeo values for Zn and Cd were classified as weakly polluted in estuarine mudflats following the decrease in Zn and Cd concentrations measured. In contrast, the IgeoCr results for salt marsh sediments showed increase and was classified as moderately polluted. While Cd concentrations recorded were below the established TEC levels for all sites consistently over two periods of time, IgeoCd suggested that the studied sediments in estuarine areas could be considered moderately polluted by Cd. Based on the Igeo of the five heavy metals studied, the cumulative Igeo total of heavy metals in the estuarine mudflat and salt marsh sediments was calculated to provide an overview of total heavy metal pollution. In 2015, at an Igeo total of 5.25 and 3.544 for estuarine mudflat and salt marsh sediments, respectively, the estuarine mudflats can be considered moderately polluted by heavy metals, while the Chongming salt marsh sediments were weakly polluted by heavy metals. In 2016 April, both salt marsh and estuarine mudflat sediments were considered weakly polluted by total heavy metals (Igeo total of 3.216 and 3.894).
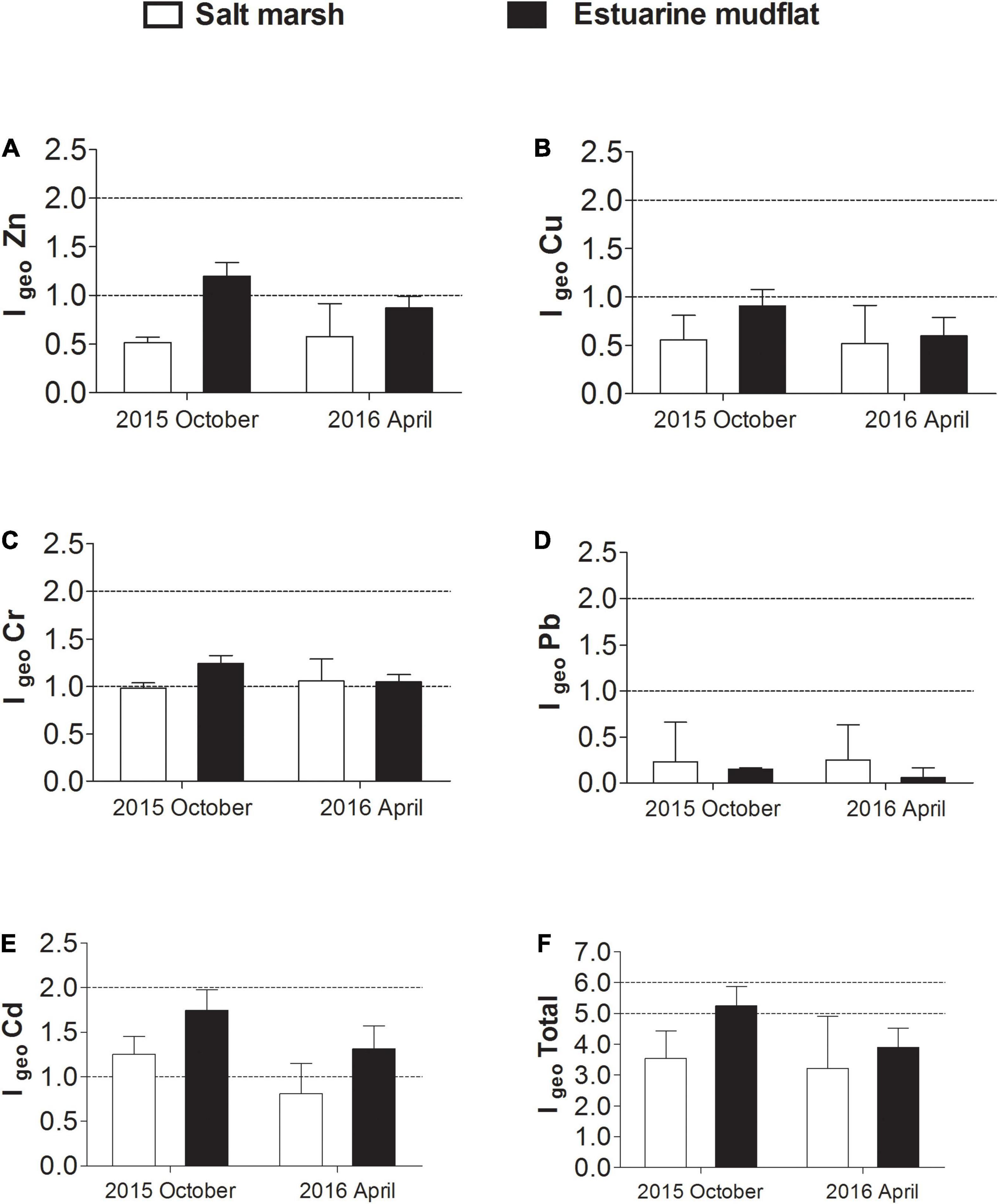
Figure 3. Geo-accumulation indices (Igeo) of heavy metals (A) Zn, (B) Cu, (C) Cr, (D) Pb, (E) Cd and their (F) Igeo total of measured heavy metals in the estuarine mudflat (WSK, SDK, and LHK) and Chongming salt marsh (BBY and DWS) sediments from the Yangtze River estuary, collected during October 2015 and April 2016. An Igeo total of less than 5 represents Class I, weakly polluted; an Igeo total between 5 and 10 represents Class II, moderately polluted.
Potential Ecological Risk Indices of Heavy Metals in Sediments
The potential ecological risk indices (RI) and risk levels of heavy metals at different sampling sites are summarized in Figure 4. As shown in Table 2, Cr, Cu, Pb, and Zn had relatively low toxicity coefficients and hence relatively low ecological risk factor contributed toward the corrected RI. The observation was consistent across all sampling sites. In contrast, the ecological risk factors for Cd were much higher for its high toxicity, ranging from considerable risk in salt marsh sites (BBY and DWS) to high risk in the estuarine mudflat sites (SDK and LHK). The presence of Cd at high concentrations above background across all sites primarily contributed to the RI and risk levels recorded, defined as the total of the potential ecological risk factors of individual heavy metals considered. As a result, almost all studied sample sites could be considered consistently exposed to at least considerable levels of ecological risk from heavy metals from October 2015 to April 2016.
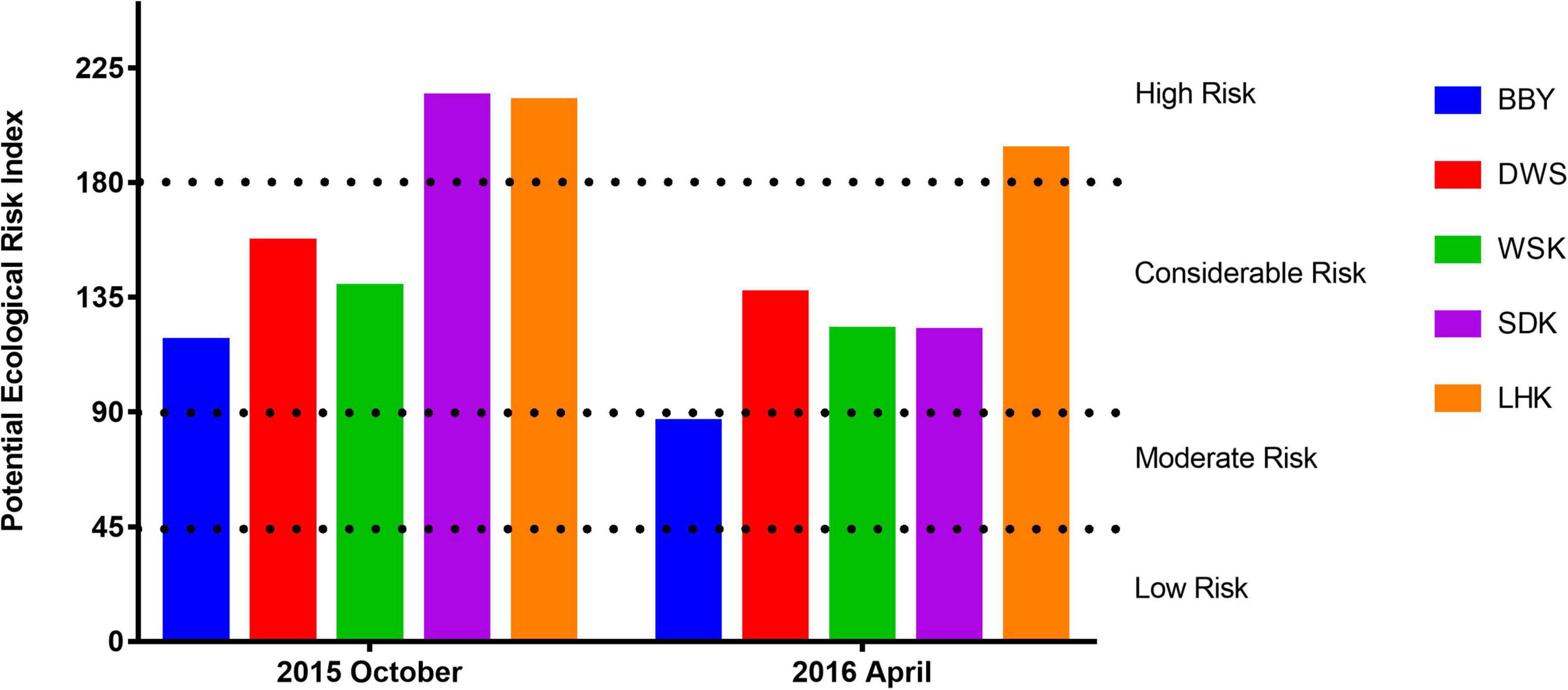
Figure 4. Potential ecological risk indices (RI) of heavy metals in intertidal sediments from various sites at the Yangtze River estuary sampled during October 2015 and April 2016. WSK, SDK, and LHK were estuarine mudflats, BBY and DWS were salt marshes. The potential ecological risk levels represented by the RI values are indicated by dotted lines.
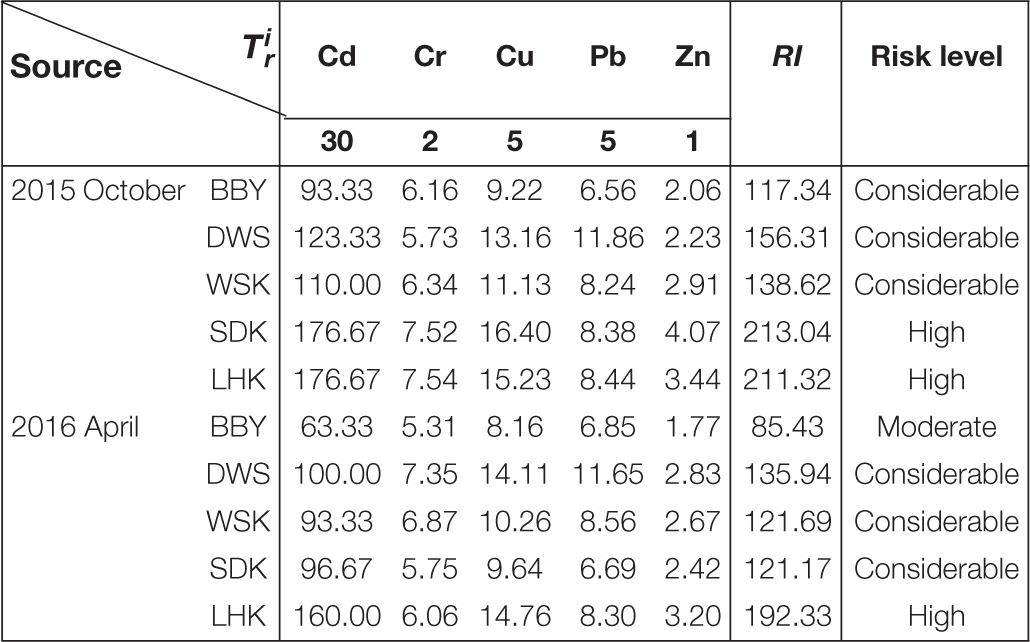
Table 2. Potential ecological risk factor (), risk indices (RI), and risk levels of heavy metals in the intertidal sediments at Yangtze River estuary.
Relative Abundance of Bacterial Taxa in Sediment Communities
The microbial community profiles of the more heavily polluted 2015 sediment samples were first analyzed and compared. The relative abundances of dominant bacterial phyla recorded from representative microbial communities sampled are summarized in Figure 5A. Bacterial phyla Proteobacteria and Actinobacteria had higher relative abundance at salt marsh (BBY) sampling site than at estuarine mudflat (SDK) sampling site, while Firmicutes, Bacteroidetes, and Acidobacteria showed the opposite. At the class level, Deltaproteobacteria, and Gammaproteobacteria, which are major subtaxa of Proteobacteria, also showed higher relative abundances in salt marsh sediments than in estuarine mudflat sediments, as shown in Figure 5B. The opposite was recorded for Betaproteobacteria. Acidimicrobiia is a major class of Actinobacteria and both the phylum and the class showed similar significant changes in relative abundance with spatial variation. The class Deltaproteobacteria, which is largely responsible for regulating the sulfur cycle, was selected to further study the impact of heavy metal pollution on bacterial functional groups. The relative abundance of genera associated with respiration of sulfur compound was examined and Desulfococcus, Desulfosarcina, and Desulfomicrobium were found to significantly differ between estuarine mudflat and salt marsh sediments, as shown in Figure 5C.
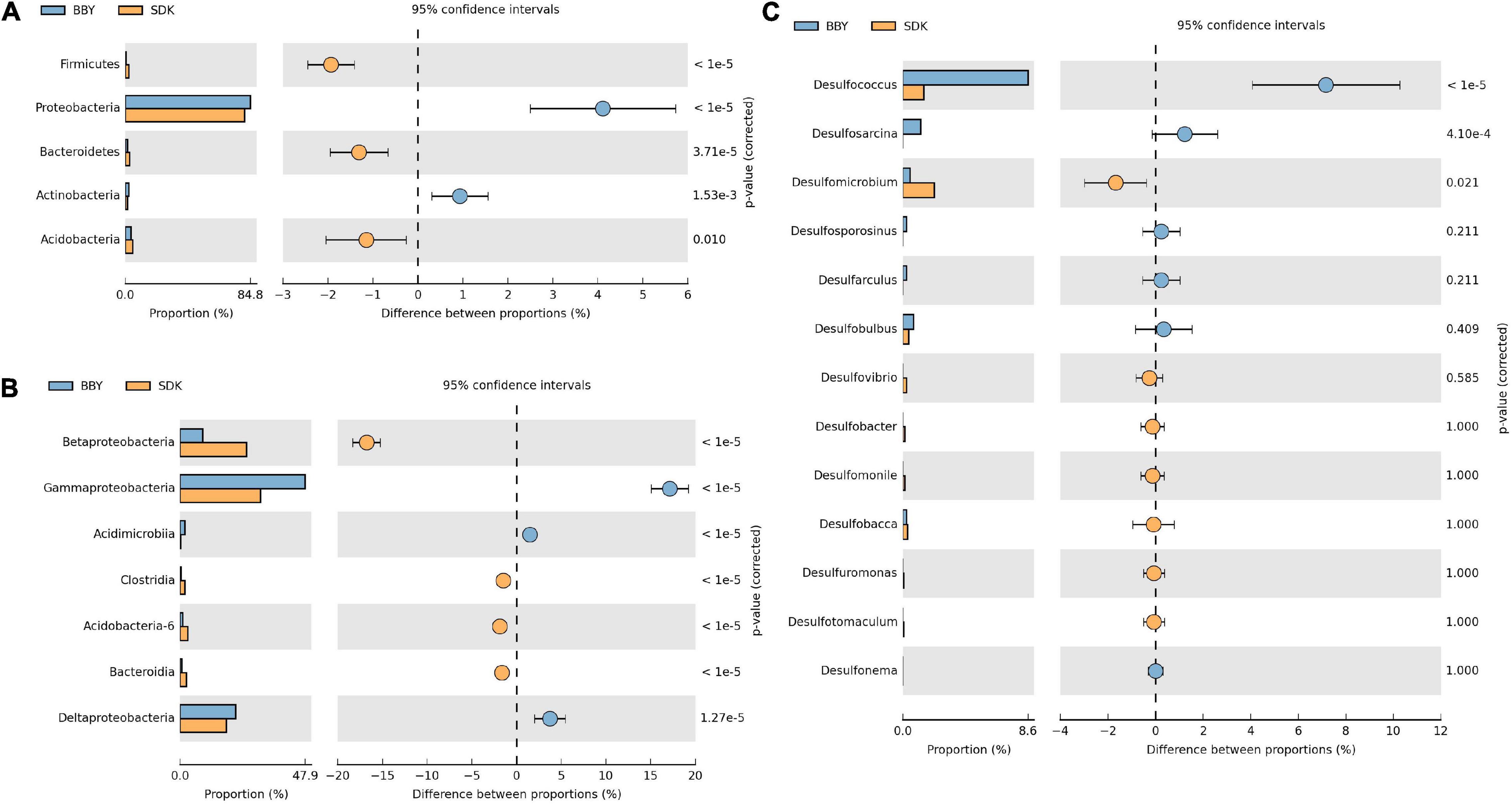
Figure 5. The difference in proportions of recorded bacterial OTUs classified by dominant (A) phyla, (B) classes, and (C) sulfur-reducing bacteria in sediment samples from representative estuarine mudflat (SDK) and the Chongming salt marsh (BBY) sites, collected in October 2015. Significant difference between proportions were analyzed using Welch’s t-test.
The Influence of Heavy Metals on Microbial Community Composition
Dominant bacteria at each taxonomic rank (relative abundance > 1%) were subjected to RDA to investigate the relationship between bacterial community structure and heavy metal concentration in the environment. Results first revealed that Cr (Explains = 40.0%, F = 2.0, P = 0.018) and Zn (Explains = 36.9%, F = 2.0, P = 0.036) were significant factors influencing microbial community composition and relative abundance at the phylum level. At lower taxonomic levels including class, order, and family, RDA revealed that only Zn remained to be a significant factor (Supplementary Tables 3–6). The concentrations of Zn were above TEC in estuarine mudflat sediments and below TEC in salt marsh sediments, supporting the spatial variation in microbial community composition being linked to Zn concentration. Zn concentration should be considered a useful indicator to be monitored to identify future community shifts. A similar set of RDA analysis was performed using Igeo indices instead of heavy metal concentrations, as summarized in Figure 6. The influence on microbial communities were overall similar. Results revealed that the IgeoCr had significant impact (Conditional Term Effects) on the dominant bacterial phyla in the communities (P = 0.022), while IgeoZn had significant impact on the dominant bacterial classes (P = 0.048), orders (P = 0.046) and families (P = 0.001) in the communities. At the genus level, variation in heavy metals had no significant impact on the dominant genera in communities.
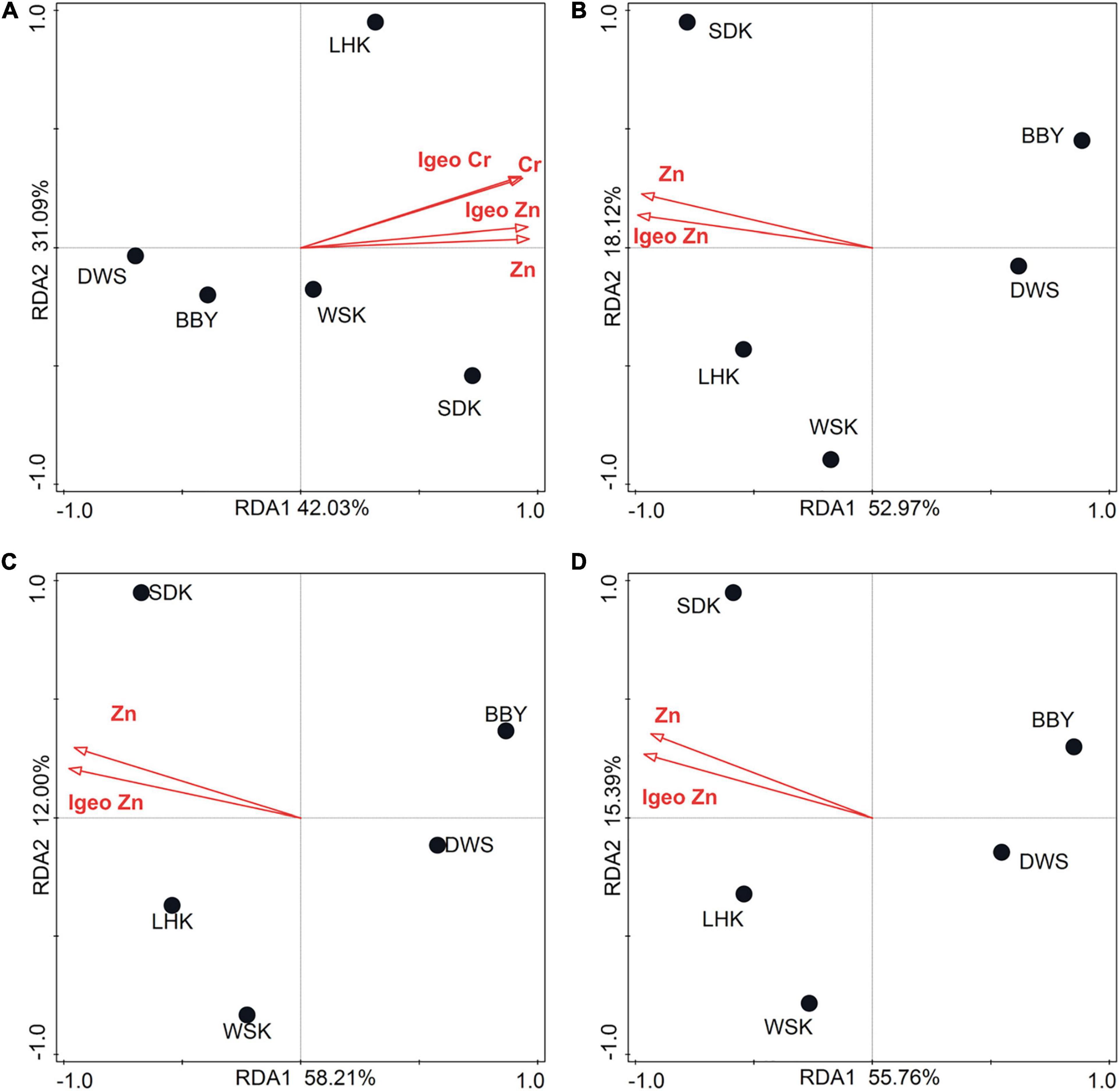
Figure 6. RDA ordination plots of the composition and abundances of dominant bacterial (A) phyla, (B) classes, (C) orders, and (D) families from sediment samples collected in October 2015 in relation to heavy metals and their Igeo indices.
Spearman’s correlation was used to further investigate how heavy metal pollution may correspond and map with the relative abundance of bacterial taxa. Significant correlations were identified for various bacterial taxa across different taxonomic ranks. Among the 10 recorded dominant phyla (relative abundance > 1%), 4 phyla showed a significant positive correlation with the various heavy metals studied. Similarly, among the 17 dominant classes, 28 dominant orders, 25 dominant families, and 16 dominant genera, results reported 5 classes, 11 orders, 9 families, and 5 genera to show significant correlation with the different heavy metals, as summarized in Figure 7A. To better understand the environmental implications of pollution, a similar analysis was conducted with Igeo indices instead, as summarized in Figure 7B. Overall, the results of the two analyses complement each other. For most of these dominant taxa, a positive correlation was identified between their relative abundance and either heavy metal concentrations or respective Igeo indices. This may be indicative of the tolerance for various heavy metals within bacterial communities if the environmental conditions are otherwise favorable. In contrast, the relative abundances of order Desulfobacterales and its subset family Desulfobulbaceae, as well as order Rhodobacterales and its subset family Rhodobacteraceae, showed significant negative correlations with heavy metals and Igeo indices. These bacterial groups may be relatively susceptible to heavy metal pollution. Subsequently, only the dominant bacterial phylum Acidobacteria, order Burkholderiales, Nitrosomonadales and family Comamonadaceae had positive correlation with combined heavy metal pollution (Igeo total).
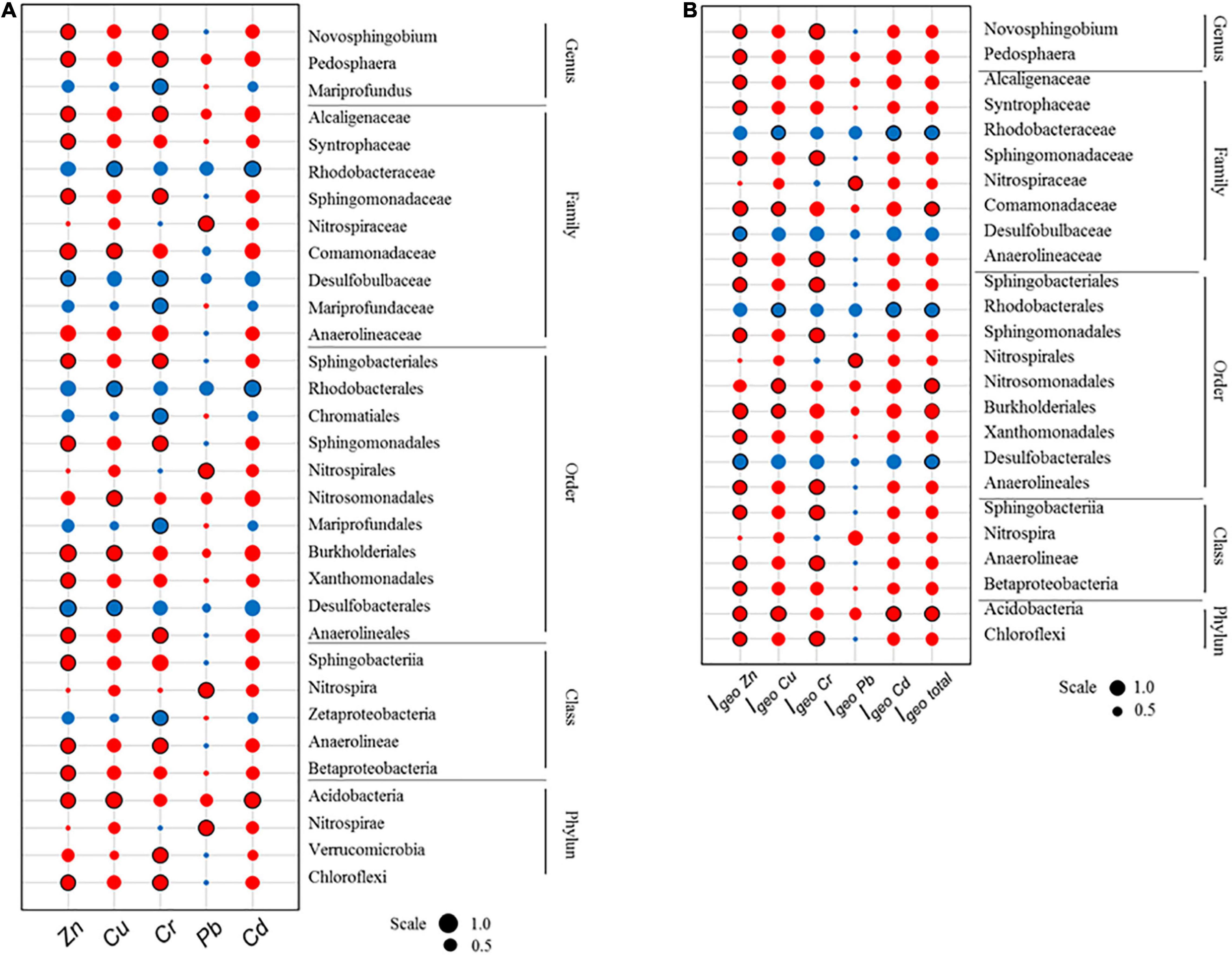
Figure 7. Spearman’s correlation between (A) heavy metal concentration or (B) heavy metal Igeo index and relative abundances of dominant bacterial taxa in microbial communities from sediment samples collected in October 2015. Red and blue colors indicate positive and negative correlations, respectively. Scale represents the numeric data of Spearman coefficients. Significant correlations (P < 0.05) are indicated by a black circular border.
The Influence of Heavy Metals on Relative Functional Contributions by Microbial Communities
Bacterial communities were functionally annotated with FAPROTAX; the relationship between heavy metals and the relative contributions to ecological functions by bacterial functional groups were subjected to analysis using Spearman’s correlation. A total of 50 annotated ecological functions was contributed by sampled microbial communities, of which 11 showed significant correlations with either Cu, Zn, Cd, and/or Cr, as summarized in Figure 8A. Among the 11, sulfate respiration was the most dominant function contributed by microbial functional groups. Similarly, the analysis was repeated with Igeo indices to indicate the relationship between heavy metal pollution and microbial community ecological functions; there were 6 ecological functions which showed significant correlations with heavy metal pollution, as summarized in Figure 8B. The Igeo of heavy metals yielded more specific results.
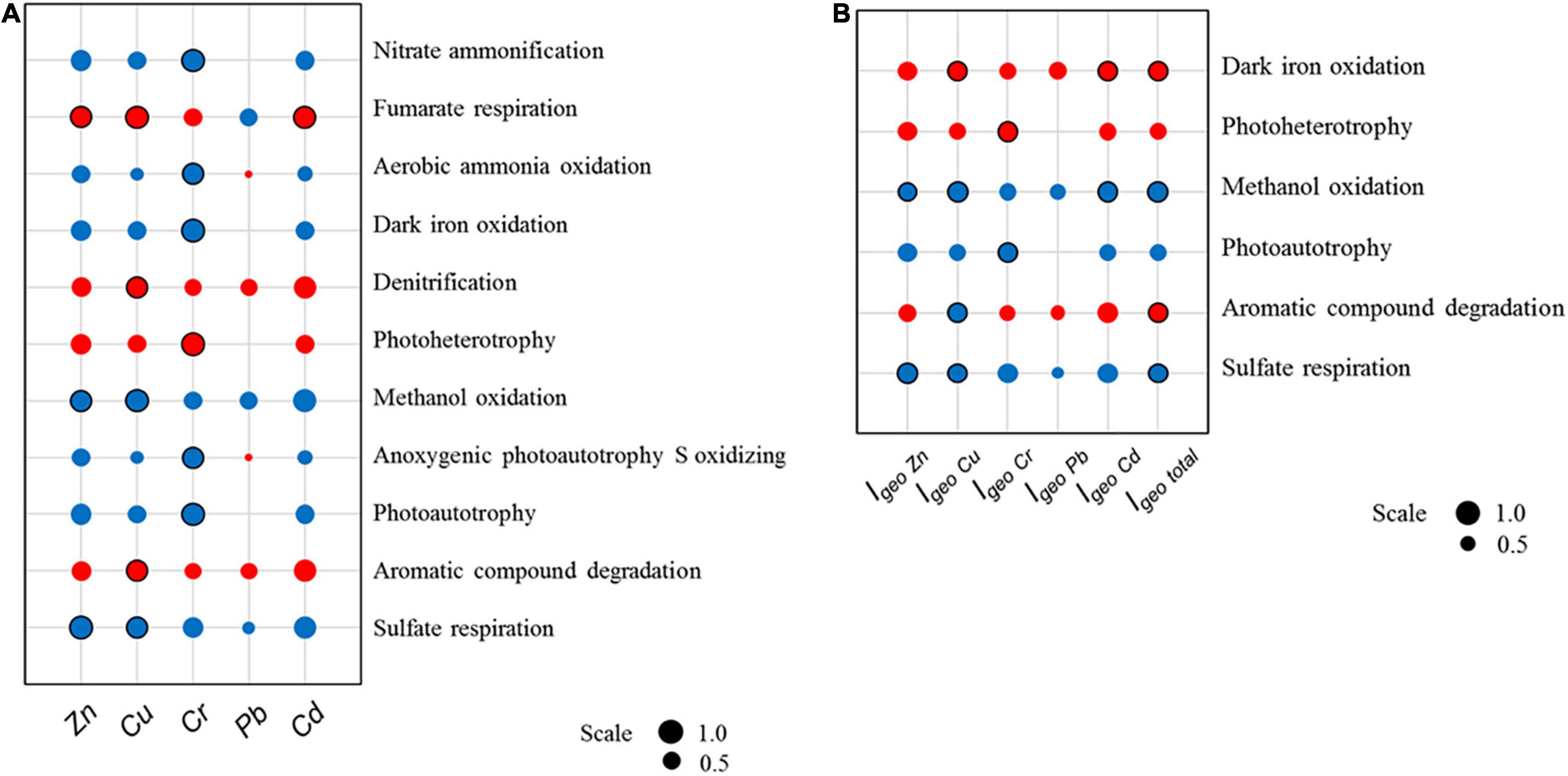
Figure 8. Spearman’s correlations between (A) heavy metal concentrations or (B) heavy metal Igeo indices and relative contributions toward ecological functions by microbial communities from sediment samples collected in October 2015. Red and blue colors indicate positive and negative correlations, respectively. Scale represents the numeric data of Spearman’s coefficients. Significant correlations (P < 0.05) are indicated by a black circular border.
Notably, sulfate respiration and methanol oxidation have significant negative correlations with total heavy metal pollution, while aromatic compound degradation and iron oxidation have significant positive correlations with heavy metal pollution. The order Desulfobacterales was largely responsible for the contributions in sulfate respiration. Based on the results from the previous section, for Desulfobacterales, both bacterial abundance and its contribution in regulating sulfate respiration showed similar negative correlations with the studied heavy metal (Zn, Cu, Cr, Pb, and Cd) and their respective Igeo indices. This consistency suggested a spatial pattern in microbial sulfate respiration processes which is linked to heavy metal pollution. Throughout the analysis, Pb was found to have negligible influence on the ecological functions of microbial communities, which is likely due to Pb having the lowest Igeo value among the five heavy metals recorded in the intertidal sediments. Microbial communities may have adapted to the gradual accumulation of Pb.
The Potential Abundance of Metal Resistance Genes in Recovered Microbial Communities
The relative abundance of MRGs corresponding to studied metals (Cd, Cr, Cu, Pb, and Zn) in microbial communities were calculated and summarized in Figure 9. Results showed that, out of the five studied heavy metals, Cu and Cr resistance genes were common among most study sites in Yangtze River estuary. Cd resistance genes were rare and only recorded in three samples at 3.69–5.53 × 10–5 copies per 16S rRNA. There are no records of Pb resistance genes. Overall, bacterial communities from the salt marshes sites (DWS and BBY) displayed a higher diversity and relative abundance in MRG types harbored for the October 2015 samples, while the opposite was recorded for April 2016.
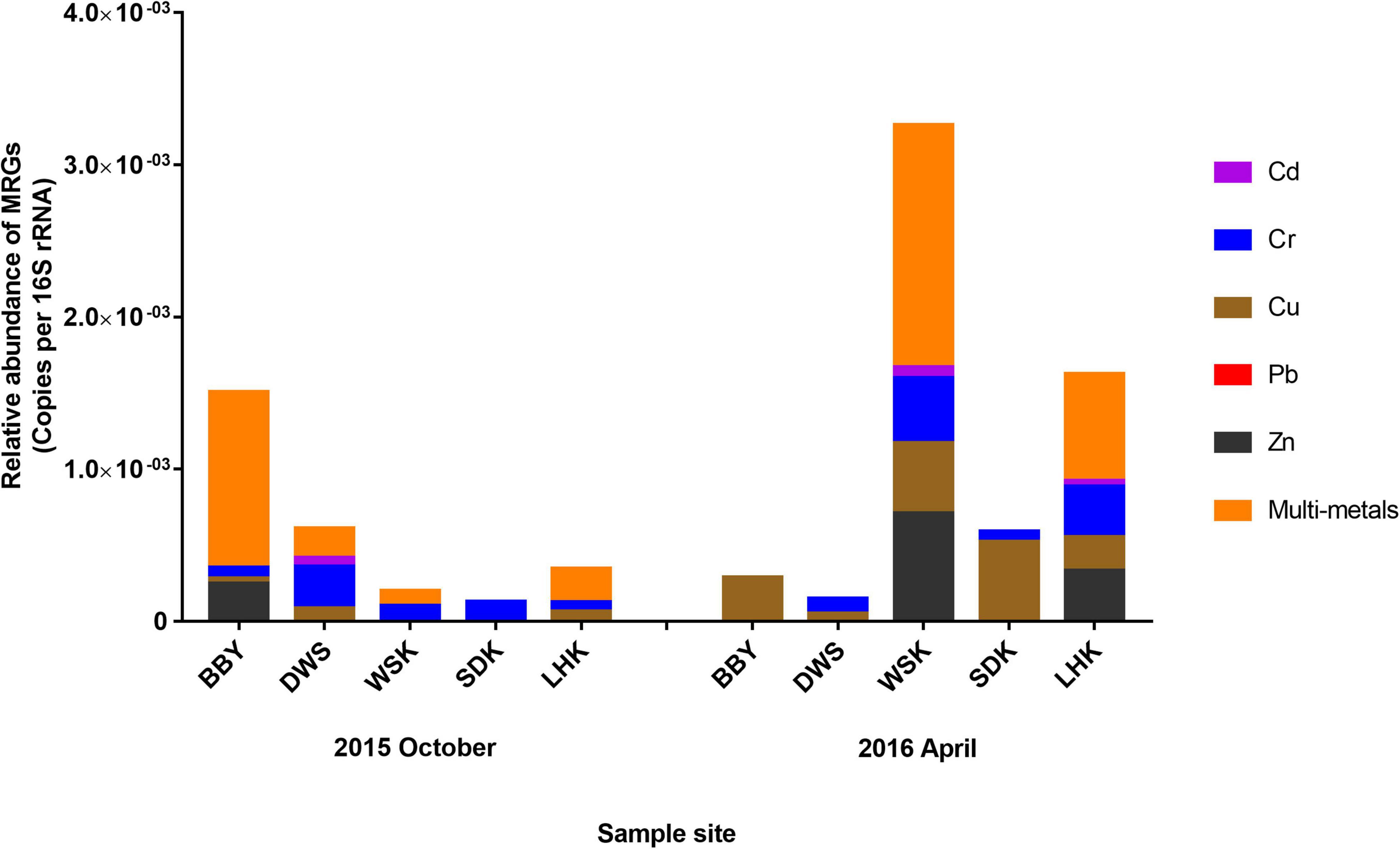
Figure 9. Relative abundance of metal resistance genes (MRGs) in bacterial community at various sites of the Yangtze River estuary sampled during October 2015 and April 2016. WSK, SDK, and LHK were estuarine mudflats; BBY and DWS were salt marshes.
The Interactions Between Heavy Metals and Microbial Communities Over Time
A similar data analysis pipeline was applied for sediment samples collected in April 2016 to investigate the identified correlation between metal concentration and estuarine microbial communities. A special focus was placed onto the dominant bacterial taxa (relative abundance > 1%) previously shown to be significantly correlated with heavy metal pollution. The change in relative abundance of identified bacterial is summarized in Figure 10. Temporal variation was observed for several bacterial taxa. Notably, at the phylum level, Verrucomicrobia and Acidobacteria significantly increased in relative abundance among all sites, whereas Nitrospirae and its subtaxa showed corresponding decrease in relative abundance (Welch’s t-test, P < 0.05). A redundant analysis was repeated to investigate the impact of heavy metal concentration and associated pollution index (Igeo) on microbial community composition at different taxonomic levels from phylum to family. Results showed that no significant impact on community composition can be linked with the minor shifts in heavy metal concentration (Supplementary Tables 7–10).
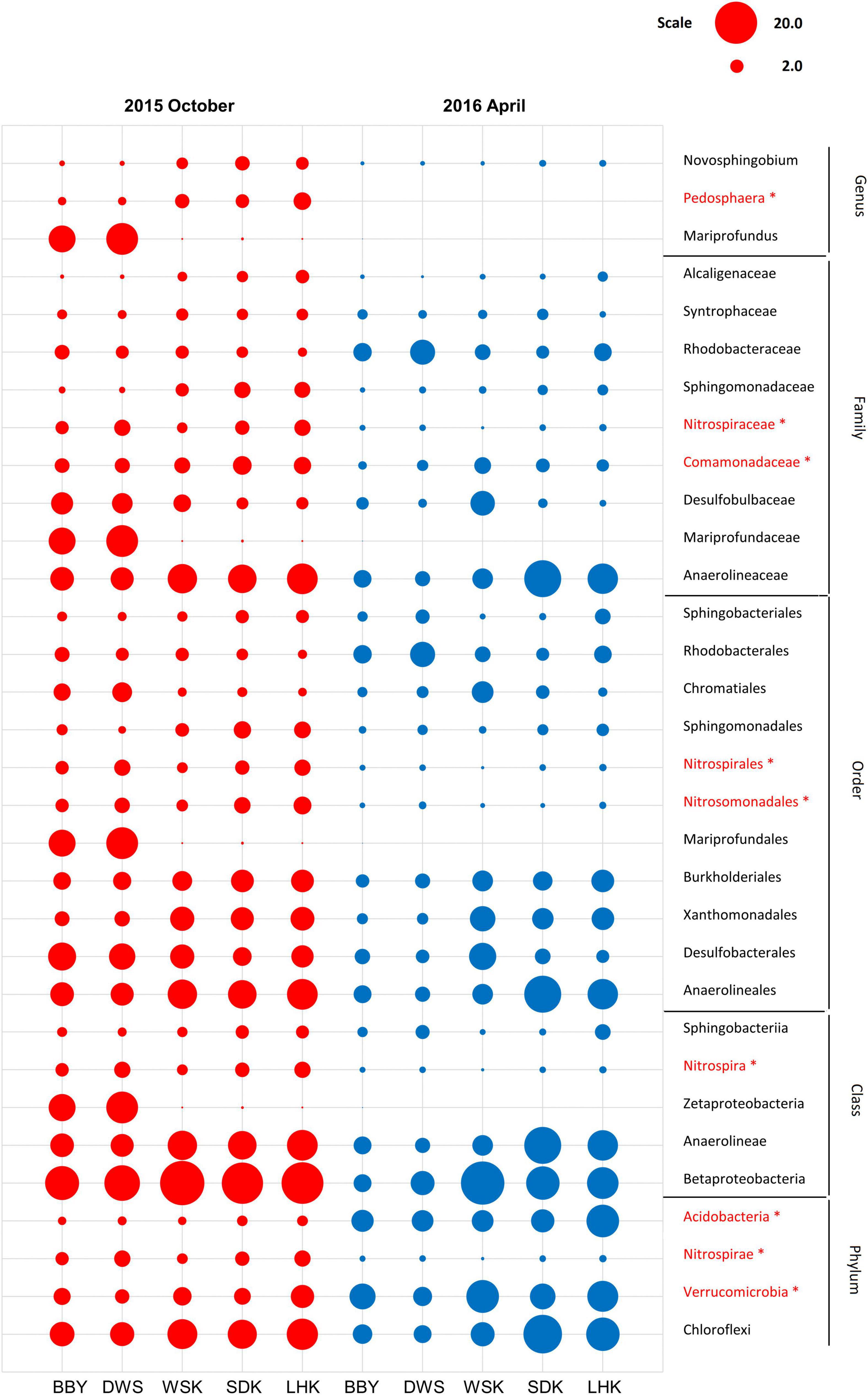
Figure 10. Relative abundances of heavy metal-associated bacterial taxa in sediments from various sites of the Yangtze River estuary (Red = October 2015, Blue = April 2016). Asterisks represent a significant difference between the 2 months according to Welch’s t-test (P < 0.05).
Discussion
Urbanized coastal estuarine ecosystems worldwide have received increasing anthropogenic input over time. Discharged multi-heavy metal pollutants accumulating in aquatic environments are indisputable environmental threats. From the present study, the urbanized Yangtze intertidal sediments were shown to contain various heavy metals at total concentrations that exceeds established individual threshold levels. Among the five studied heavy metals, four (Zn, Cu, Cr, and Pb) are of confirmed concern as they have been found to exceed the TEC by similar if not a greater margin when compared to previous literature (Wang et al., 2014; Xu et al., 2014; Liu et al., 2015). In 2015, Zn, Cu and Cr concentrations were found to be consistently higher in the estuarine mudflat sediment samples. The concentration difference shrunk in April 2016 as the average heavy metal concentrations in estuarine sediments showed uniform reduction, while those of the salt marsh remained similar. This observed fluctuation, however, could be due to seasonal variations. Precipitation may subject surface sediments in the Yangtze River estuary to constant washing effect that is difficult to consider and account for properly. There was a spatial difference in heavy metal distribution, which was anticipated with the difference in environmental conditions and potentially anthropogenic disturbance. While both southern and northern channels of the Yangtze River receive similar upstream freshwater input, the sampling sites on the southern branch may receive more local anthropogenic input such as urban runoff. Sampling sites to the east of Chongming Island are relatively distant from direct human impact and more frequently contacted by seawater and dilution effects. The current results indicated that variations in estuarine mudflat heavy metal concentration could be more associated with local impact instead of upstream input. Nevertheless, further pollution source tracking will be required to convincingly determine the nature of heavy metal distribution in the Yangtze River.
According to the Igeo and RI results, Cd and Zn were major contributors to recorded pollution levels that should be considered an environmental and ecological risk. Despite relatively low Cd concentration, Cd poses considerable to high risks for its high toxic response factor, and the ability for Cd exposure to impact microbial community structure, heavy metal (Salam et al., 2020) and antibiotic resistome (Zhao et al., 2019). Within the Yangtze River estuary, the estuarine mudflat sediments were found to be more polluted (Class II, moderately polluted) and up to high ecological risk compared to the salt marsh sediments. The ability for heavy metal concentration and pollution levels to explain and predict the response of microbial community composition was subsequently tested on the October 2015 samples. This subset was selected for downstream analysis to minimize the impact of unaccountable factors such as rainfall dilution. October samples serve as sediments representative of the dry season with low monthly precipitation and could be considered a better subset to reflect the impact of heavy metal pollution exposure on sedimentary bacterial communities. RDA results revealed Zn to be the only consistent significant factor indicative of shifts in dominant microbial community composition from the class level to the family level. Spearman’s correlation analysis further showed that many dominant taxa among all estuarine sediment samples positively correlate with Zn concentration and pollution indices.
Relatively few taxa have shown negative correlations (e.g., Order Desulfobacterales and subtaxa) or were seemingly unaffected (e.g., Class Nitrospira and subtaxa). For the April 2016 sample subset, similar explanatory effects of heavy metal Zn on microbial compositions cannot be detected. This may have indicated a dilution effect for surface sediments sampled. It is also likely that this seasonal change introduced more complex variations in dominant microbial taxa across the sediment samples. To examine this potential difference, responses of dominant taxa previously reported to significantly correlate with heavy metal concentrations were subsequently investigated. It is anticipated that the relative abundance of this group of bacteria taxa associated with heavy metals could be robust when metal concentrations do not greatly differ. Yet, a significant increase in relative abundance of Verrucomicrobia was observed between 2015 and 2016. This is because Verrucomicrobia is a key bacterial phylum reported to favor the wet season (Yi et al., 2020) and correlate negatively with heavy metals Cr, Pb, and Zn (Li et al., 2020), which may explain the observation. For most of the other previous identified heavy metal-associated taxa, it is also revealed that decreasing trends in Zn and heavy metal concentration in estuarine sediments did not significantly impact their relative abundances. Among the multi-metals studied, increased Zn concentrations may serve as an important indicator to quantify influence and stress of heavy metal pollution on microbial communities. Their potential ability to further select tolerant taxa and should be closely monitored.
Heavy metals are one of the many pollutants recorded in the environment. Although the present study has unveiled new associations between heavy metals and estuarine microbial communities, the mechanisms behind their impact, behavior and interactions with the complex ecosystem are still far from clear. A previous study has shown that Zn concentrations can alter microbial community compositions in soil and affect soil enzyme activities (Liu et al., 2020); an excess in Zn may decrease enzyme activity. A similar path of impact may have indirectly caused the recorded shifts in structure of sedimentary microbial communities. Alternatively, the ability for microbes to acquire MRGs as a response to continuous exposure should also be considered. It has been shown that exposure to heavy metals such as Cu for 21 days may induce community-wide tolerance to heavy metals at the cost of a loss in diversity with the sensitive taxa (Ahmed et al., 2020).
For the Yangtze River sedimentary microbial community, presence of Cu resistance genes was widely recorded but at relatively low abundance (between 3.50 × 10–5 and 4.61 × 10–4 copies per 16S rRNA). Cd and Zn resistance genes were rare in both occurrence and abundance, and no convincing associations could be drawn with the spatial difference in heavy metal concentrations. Further, highest diversity and abundance of MRGs were displayed in estuarine mudflat sediments from April 2016. The potential influx of microbes from land may have carried more resistance genes. This could also be a result of sediment conditions influencing the actual bioavailability of heavy metals for bacterial communities (Miranda et al., 2021). This suggested that habitat niche and microbial community characteristics may have greater influence on MRG distribution over heavy metal concentrations (Song et al., 2019). In this study, heavy metal content was dissolved and measured in total concentration. Chemical metal speciation analyses could help to identify the different chemical metal forms available as potentially better predictors. Specifically, microbial groups such as SRBs and methanotrophs can be responsible for a range of speciation transformation processes for metals (Zhao et al., 2021). Many studies have attempted to utilize specific microbial groups as a promising heavy metal bioremediation tool. However, predicting metal bioavailability and interactions with microbes at a community-level is still naturally complex. A combination of controlled laboratory exposure and transcriptomic studies will be beneficial to complement existing field results on the integrated impact of heavy metal pollution in an estuarine environment condition.
The relationship between microbial community structure and heavy metal pollution was also investigated. The relative abundance results showed that major bacterial phyla such as Proteobacteria and Actinobacteria preferred the relatively less polluted salt marsh environment. The opposite was recorded for phyla such as Firmicutes and Acidobacteria. Results at the class level greatly varied within Proteobacteria. Gammaproteobacteria and Deltaproteobacteria were major taxa found in marine sediments (Di Cesare et al., 2020) and preference was shown for the less polluted sites. In contrast, Betaproteobacteria were more abundant in the more polluted southern branch. These class level results have been consistent and are not restricted to estuarine environments as similar trends saw resemblance in microbial communities in Cd-contaminated soils (Li et al., 2017). The spatial variation is believed to stem from the adaptation by bacterial subtaxa utilizing these different biogeographical niches, alongside different sources and concentrations of nutrients such as carbon (Bouskill et al., 2010). Hence, heavy metal concentration should be considered important parameters that interact with, but is often overshadowed by, the distinct impact of environmental parameters such as salinity gradients.
Additionally, potential shifts in microbial functional groups were examined. In particular, the respiration of sulfur compound is a known major ecological function contributed by class Deltaproteobacteria. Results indicated that sulfate respiration has significant negative correlations with total heavy metal pollution, while sulfate-oxidizing processes has been found to positively correlate with heavy metals (Lu et al., 2019). This observation is closely associated to the relative abundances of order Desulfobacterales and its subset family Desulfobulbaceae, as they are major contributors to sulfate respiration. Both these taxa showed significant negative correlations with heavy metals in general. Their subset genus Desulfococcus have also been proposed as sentinel bacteria used to detect metal pollution risks (Fernández-Cadena et al., 2020). Thus, SRBs are yet another important bacteria group to investigate its interaction with the environment and potential susceptibility in the context of heavy metal pollution (Niu et al., 2018). Additionally, in the study by Zhao et al. (2018), SRBs were found to facilitate the transformation of mercury to methylmercury in paddy soils with the release of root exudates from rice cultivation. While heavy metal can influence the structure of microbial communities and functional groups, bacterial groups can also take part in converting different forms of metals through means of adsorption and chemical precipitation. SRBs were employed in treatments such as wastewater remediation to remove pollutants such as organic contaminants and heavy metals (Li et al., 2018). A better understanding of microbial interactions with exposure to heavy metals in the field is desired as microbial services can offer many great application opportunities.
Conclusion
The present study assessed concentrations, pollution statuses, and ecological risks of heavy metals in sediments of the Yangtze River estuary. Moderate levels of multi-heavy metal pollution were recorded, posing a considerable level of potential ecological risk. The integrated impact of multi-heavy metal pollution on microbial community structure and ecological functions was investigated. We revealed new associations between sedimentary microbial communities and various heavy metals under an estuarine environment condition. Results highlighted the importance of Zn among the studied metals for its potential as a stressor and indicator for heavy metal pollution-associated community shifts. Zn concentration under urbanized estuarine conditions deserves further monitoring attention. Additionally, MRGs were generally found to be present at low relative abundance with no significant associations with heavy metal concentrations. It is suggested that microbial community characteristics and sediment conditions may play more determinant roles in MRG distribution. We conclude by calling attention to the need for future studies and assessments to carefully consider the dynamic influence and interactive mechanisms between multiple heavy metals and microbial communities. This should be done in conjunction with dominant environmental factors especially when attempting to disentangle the complex nature of microbial ecotoxicology. This will benefit both our fundamental understanding of metal-biota-environment interactions and contribute to a better basis for risk assessment and ecosystem management.
Data Availability Statement
The datasets presented in this study can be found in online repositories. The name of the repository and accession numbers can be found in the article and below: National Center for Biotechnology Information (NCBI; https://www.ncbi.nlm.nih.gov); accession numbers SRX3855667-SRX3855671 and SRX12453355-SRX12453359.
Author Contributions
JY performed data curation, methodology, investigation, formal analysis, and writing of the manuscript. LL performed investigation and writing of the manuscript. HL and P-YQ provided resources and supervision, and commented on the writing of the manuscript. JC performed conceptualization, resources, supervision, funding acquisition, data curation, formal analysis, and writing of the manuscript. All authors contributed to the article and approved the submitted version.
Funding
This work was supported by the Hong Kong Branch of Southern Marine Science and Engineering Guangdong Laboratory (Guangzhou) (SMSEGL20SC01).
Conflict of Interest
The authors declare that the research was conducted in the absence of any commercial or financial relationships that could be construed as a potential conflict of interest.
Publisher’s Note
All claims expressed in this article are solely those of the authors and do not necessarily represent those of their affiliated organizations, or those of the publisher, the editors and the reviewers. Any product that may be evaluated in this article, or claim that may be made by its manufacturer, is not guaranteed or endorsed by the publisher.
Supplementary Material
The Supplementary Material for this article can be found online at: https://www.frontiersin.org/articles/10.3389/fmars.2021.741912/full#supplementary-material
References
Ahmed, A. M., Tardy, V., Bonnineau, C., Billard, P., Pesce, S., and Lyautey, É (2020). Changes in sediment microbial diversity following chronic copper-exposure induce community copper-tolerance without increasing sensitivity to arsenic. J. Hazard. Mater. 391:122197. doi: 10.1016/j.jhazmat.2020.122197
Bao, P., Li, G. X., Sun, G. X., Xu, Y. Y., Meharg, A. A., and Zhu, Y. G. (2018). The role of sulfate-reducing prokaryotes in the coupling of element biogeochemical cycling. Sci. Total Environ. 613, 398–408. doi: 10.1016/j.scitotenv.2017.09.062
Barletta, M., Lima, A. R., and Costa, M. F. (2019). Distribution, sources and consequences of nutrients, persistent organic pollutants, metals and microplastics in South American estuaries. Sci. Total Environ. 651, 1199–1218. doi: 10.1016/j.scitotenv.2018.09.276
Bolyen, E., Rideout, J. R., Dillon, M. R., Bokulich, N. A., Abnet, C. C., Al-Ghalith, G. A., et al. (2019). Reproducible, interactive, scalable and extensible microbiome data science using QIIME 2. Nat. Biotechnol. 37, 852–857. doi: 10.1038/s41587-019-0209-9
Bouskill, N. J., Barker-Finkel, J., Galloway, T. S., Handy, R. D., and Ford, T. E. (2010). Temporal bacterial diversity associated with metal-contaminated river sediments. Ecotoxicology 19, 317–328. doi: 10.1007/s10646-009-0414-2
Buchfink, B., Xie, C., and Huson, D. H. (2015). Fast and sensitive protein alignment using DIAMOND. Nat. Methods 12, 59–60. doi: 10.1038/nmeth.3176
Camacho, C., Coulouris, G., Avagyan, V., Ma, N., Papadopoulos, J., Bealer, K., et al. (2009). BLAST+: architecture and applications. BMC Bioinformatics 10:421. doi: 10.1186/1471-2105-10-421
DeSantis, T. Z., Hugenholtz, P., Larsen, N., Rojas, M., Brodie, E. L., Keller, K., et al. (2006). Greengenes, a chimera-checked 16S rRNA gene database and workbench compatible with ARB. Appl. Environ. Microbiol. 72, 5069–5072. doi: 10.1128/AEM.03006-05
Di Cesare, A., Petra, P., Ester, M. E., Neven, C., Maritina, M. Š, Gianluca, C., et al. (2020). The role of metal contamination in shaping microbial communities in heavily polluted marine sediments. Environ. Pollut. 265:114823. doi: 10.1016/j.envpol.2020.114823
Echavarri-Bravo, V., Paterson, L., Aspray, T. J., Porter, J. S., Winson, M. K., Thornton, B., et al. (2015). Shifts in the metabolic function of a benthic estuarine microbial community following a single pulse exposure to silver nanoparticles. Environ. Pollut. 201, 91–99. doi: 10.1016/j.envpol.2015.02.033
Edgar, R. C., Haas, B. J., Clemente, J. C., Quince, C., and Knight, R. (2011). UCHIME improves sensitivity and speed of chimera detection. Bioinformatics 27, 2194–2200. doi: 10.1093/bioinformatics/btr381
Falkowski, P. G., Fenchel, T., and Delong, E. F. (2008). The microbial engines that drive Earth’s biogeochemical cycles. Science 320, 1034–1039. doi: 10.1126/science.1153213
Fan, H., Chen, S., Li, Z., Liu, P., Xu, C., and Yang, X. (2020). Assessment of heavy metals in water, sediment and shellfish organisms in typical areas of the Yangtze River Estuary, China. Mar. Pollut. Bull. 151:110864. doi: 10.1016/j.marpolbul.2019.110864
Fernández-Cadena, J. C., Ruíz-Fernández, P. S., Fernández-Ronquillo, T. E., Díez, B., Trefault, N., Andrade, S., et al. (2020). Detection of sentinel bacteria in mangrove sediments contaminated with heavy metals. Mar. Pollut. Bull. 150:110701. doi: 10.1016/j.marpolbul.2019.110701
Gu, Y. G., and Gao, Y. P. (2021). A new method for estimating sedimental integrated toxicity of heavy metal mixtures to aquatic biota: a case study. Ecotoxicology 30, 373–380. doi: 10.1007/s10646-021-02346-0
Guo, X. P., Yang, Y., Niu, Z. S., Lu, D. P., Zhu, C. H., Feng, J. N., et al. (2019). Characteristics of microbial community indicate anthropogenic impact on the sediments along the Yangtze Estuary and its coastal area, China. Sci. Total Environ. 648, 306–314. doi: 10.1016/j.scitotenv.2018.08.162
Gupta, S. K., Shin, H., Han, D., Hur, H. G., and Unno, T. (2018). Metagenomic analysis reveals the prevalence and persistence of antibiotic-and heavy metal-resistance genes in wastewater treatment plant. J. Microbiol. 56, 408–415. doi: 10.1007/s12275-018-8195-z
Hakanson, L. (1980). An ecological risk index for aquatic pollution control. A sedimentological approach. Water Res. 14, 975–1001. doi: 10.1016/0043-1354(80)90143-8
Herlemann, D. P., Labrenz, M., Jürgens, K., Bertilsson, S., Waniek, J. J., and Andersson, A. F. (2011). Transitions in bacterial communities along the 2000 km salinity gradient of the Baltic Sea. ISME J. 5, 1571–1579. doi: 10.1038/ismej.2011.41
Hu, Y., He, N., Wu, M., Wu, P., He, P., Yang, Y., et al. (2021). Sources and ecological risk assessment of the seawater potentially toxic elements in Yangtze River Estuary during 2009–2018. Environ. Monit. Assess. 193, 1–14. doi: 10.1007/s10661-020-08795-0
Jin, Y., Luan, Y., Ning, Y., and Wang, L. (2018). Effects and mechanisms of microbial remediation of heavy metals in soil: a critical review. Appl. Sci. 8:1336. doi: 10.3390/app8081336
Kiran, M. G., Pakshirajan, K., and Das, G. (2018). Heavy metal removal from aqueous solution using sodium alginate immobilized sulfate reducing bacteria: mechanism and process optimization. J. Environ. Manage. 218, 486–496. doi: 10.1016/j.jenvman.2018.03.020
Li, C., Quan, Q., Gan, Y., Dong, J., Fang, J., Wang, L., et al. (2020). Effects of heavy metals on microbial communities in sediments and establishment of bioindicators based on microbial taxa and function for environmental monitoring and management. Sci. Total Environ. 749:141555. doi: 10.1016/j.scitotenv.2020.141555
Li, X., Lan, S. M., Zhu, Z. P., Zhang, C., Zeng, G. M., Liu, Y. G., et al. (2018). The bioenergetics mechanisms and applications of sulfate-reducing bacteria in remediation of pollutants in drainage: a review. Ecotoxicol. Environ. Saf. 158, 162–170. doi: 10.1016/j.ecoenv.2018.04.025
Li, X., Meng, D., Li, J., Yin, H., Liu, H., Liu, X., et al. (2017). Response of soil microbial communities and microbial interactions to long-term heavy metal contamination. Environ. Pollut. 231, 908–917. doi: 10.1016/j.envpol.2017.08.057
Liu, P., Zheng, C., Wen, M., Luo, X., Wu, Z., Liu, Y., et al. (2021). Ecological risk assessment and contamination history of heavy metals in the sediments of Chagan Lake, Northeast China. Water 13:894. doi: 10.3390/w13070894
Liu, R., Guo, L., Men, C., Wang, Q., Miao, Y., and Shen, Z. (2019). Spatial-temporal variation of heavy metals’ sources in the surface sediments of the Yangtze River Estuary. Mar. Pollut. Bull. 138, 526–533. doi: 10.1016/j.marpolbul.2018.12.010
Liu, Y. M., Cao, W. Q., Chen, X. X., Yu, B. G., Lang, M., Chen, X. P., et al. (2020). The responses of soil enzyme activities, microbial biomass and microbial community structure to nine years of varied zinc application rates. Sci. Total Environ. 737:140245. doi: 10.1016/j.scitotenv.2020.140245
Liu, Z., Pan, S., Sun, Z., Ma, R., Chen, L., Wang, Y., et al. (2015). Heavy metal spatial variability and historical changes in the Yangtze River estuary and North Jiangsu tidal flat. Mar. Pollut. Bull. 98, 115–129. doi: 10.1016/j.marpolbul.2015.07.006
Louca, S., Parfrey, L. W., and Doebeli, M. (2016). Decoupling function and taxonomy in the global ocean microbiome. Science 353, 1272–1277. doi: 10.1126/science.aaf4507
Lu, M., Luo, X., Jiao, J. J., Li, H., Wang, X., Gao, J., et al. (2019). Nutrients and heavy metals mediate the distribution of microbial community in the marine sediments of the Bohai Sea, China. Environ. Pollut. 255:113069. doi: 10.1016/j.envpol.2019.113069
Ma, L., and Han, C. (2019). “Water quality ecological risk assessment with sedimentological approach,” in Water Quality-Science, Assessments and Policy, ed. K. Summers (London: IntechOpen). doi: 10.5772/intechopen.88594
MacDonald, D. D., Ingersoll, C. G., and Berger, T. A. (2000). Development and evaluation of consensus-based sediment quality guidelines for freshwater ecosystems. Arch. Environ. Contam. Toxicol. 39, 20–31. doi: 10.1007/s002440010075
McDonald, D., Price, M. N., Goodrich, J., Nawrocki, E. P., DeSantis, T. Z., Probst, A., et al. (2012). An improved Greengenes taxonomy with explicit ranks for ecological and evolutionary analyses of bacteria and archaea. ISME J. 6, 610–618. doi: 10.1038/ismej.2011.139
Miranda, L. S., Ayoko, G. A., Egodawatta, P., Hu, W. P., Ghidan, O., and Goonetilleke, A. (2021). Physico-chemical properties of sediments governing the bioavailability of heavy metals in urban waterways. Sci. Total Environ. 763:142984. doi: 10.1016/j.scitotenv.2020.142984
Niu, Z. S., Pan, H., Guo, X. P., Lu, D. P., Feng, J. N., Chen, Y. R., et al. (2018). Sulphate-reducing bacteria (SRB) in the Yangtze Estuary sediments: abundance, distribution and implications for the bioavailibility of metals. Sci. Total Environ. 634, 296–304. doi: 10.1016/j.scitotenv.2018.03.345
Pal, C., Bengtsson-Palme, J., Rensing, C., Kristiansson, E., and Larsson, D. J. (2014). BacMet: antibacterial biocide and metal resistance genes database. Nucleic Acids Res. 42, D737–D743. doi: 10.1093/nar/gkt1252
Prabhakaran, P., Ashraf, M. A., and Aqma, W. S. (2016). Microbial stress response to heavy metals in the environment. RSC Adv. 6, 109862–109877. doi: 10.1039/C6RA10966G
Rognes, T., Flouri, T., Nichols, B., Quince, C., and Mahé, F. (2016). VSEARCH: a versatile open source tool for metagenomics. PeerJ 4:e2584. doi: 10.7717/peerj.2584
Salam, L. B., Obayori, O. S., Ilori, M. O., and Amund, O. O. (2020). Effects of cadmium perturbation on the microbial community structure and heavy metal resistome of a tropical agricultural soil. Bioresour. Bioprocess. 7:25. doi: 10.1186/s40643-020-00314-w
Sheeba, V. A., Abdulaziz, A., Gireeshkumar, T. R., Ram, A., Rakesh, P. S., Jasmin, C., et al. (2017). Role of heavy metals in structuring the microbial community associated with particulate matter in a tropical estuary. Environ. Pollut. 231, 589–600. doi: 10.1016/j.envpol.2017.08.053
Song, W., Qi, R., Zhao, L., Xue, N., Wang, L., and Yang, Y. (2019). Bacterial community rather than metals shaping metal resistance genes in water, sediment and biofilm in lakes from arid northwestern China. Environ. Pollut. 254:113041. doi: 10.1016/j.envpol.2019.113041
ter Braak, C. J. F., and Šmilauer, P. (2012). Canoco Reference Manual and User’s Guide: Software for Ordination (Version 5.0). Ithaca, NY: Microcomputer Power.
Wang, J., Liu, R., Zhang, P., Yu, W., Shen, Z., and Feng, C. (2014). Spatial variation, environmental assessment and source identification of heavy metals in sediments of the Yangtze River Estuary. Mar. Pollut. Bull. 87, 364–373. doi: 10.1016/j.marpolbul.2014.07.048
Wang, Q., Garrity, G. M., Tiedje, J. M., and Cole, J. R. (2007). Naive Bayesian classifier for rapid assignment of rRNA sequences into the new bacterial taxonomy. Appl. Environ. Microbiol. 73, 5261–5267. doi: 10.1128/AEM.00062-07
Wang, Y., Sheng, H. F., He, Y., Wu, J. Y., Jiang, Y. X., Tam, N. F. Y., et al. (2012). Comparison of the levels of bacterial diversity in freshwater, intertidal wetland, and marine sediments by using millions of illumina tags. Appl. Environ. Microbiol. 78, 8264–8271. doi: 10.1128/AEM.01821-12
Wei, F., Zheng, C., Chen, J., and Wu, Y. (1991). Study of the background contents of 61 elements of soils in China. Environ. Sci. 12, 12–19.
White, J. R., Nagarajan, N., and Pop, M. (2009). Statistical methods for detecting differentially abundant features in clinical metagenomic samples. PLoS Comput. Biol. 5:e1000352. doi: 10.1371/journal.pcbi.1000352
Xu, S., Tao, J., Chen, Z., Chen, Z., and Lu, Q. (1997). Dynamic accumulation of heavy metals in tidal flat sediments of Shanghai. Oceanol. Limnol. Sin. 28, 509–515.
Xu, X., Zhao, Y., Zhao, X., Wang, Y., and Deng, W. (2014). Sources of heavy metal pollution in agricultural soils of a rapidly industrializing area in the Yangtze Delta of China. Ecotoxicol. Environ. Saf. 108, 161–167. doi: 10.1016/j.ecoenv.2014.07.001
Yi, J., Lo, L. S. H., and Cheng, J. (2020). Dynamics of microbial community structure and ecological functions in estuarine intertidal sediments. Front. Mar. Sci. 7:585970. doi: 10.3389/fmars.2020.585970
Yin, H., Niu, J., Ren, Y., Cong, J., Zhang, X., Fan, F., et al. (2015). An integrated insight into the response of sedimentary microbial communities to heavy metal contamination. Sci. Rep. 5:14266. doi: 10.1038/srep14266
Yin, S., Feng, C., Li, Y., Yin, L., and Shen, Z. (2015). Heavy metal pollution in the surface water of the Yangtze Estuary: a 5-year follow-up study. Chemosphere 138, 718–725. doi: 10.1016/j.chemosphere.2015.07.060
Zhang, C., Nie, S., Liang, J., Zeng, G., Wu, H., Hua, S., et al. (2016). Effects of heavy metals and soil physicochemical properties on wetland soil microbial biomass and bacterial community structure. Sci. Total Environ. 557, 785–790. doi: 10.1016/j.scitotenv.2016.01.170
Zhang, G., Bai, J., Xiao, R., Zhao, Q., Jia, J., Cui, B., et al. (2017). Heavy metal fractions and ecological risk assessment in sediments from urban, rural and reclamation-affected rivers of the Pearl River Estuary, China. Chemosphere 184, 278–288. doi: 10.1016/j.chemosphere.2017.05.155
Zhao, J. Y., Ye, Z. H., and Zhong, H. (2018). Rice root exudates affect microbial methylmercury production in paddy soils. Environ. Pollut. 242, 1921–1929. doi: 10.1016/j.envpol.2018.07.072
Zhao, Q., Li, X., Xiao, S., Peng, W., and Fan, W. (2021). Integrated remediation of sulfate reducing bacteria and nano zero valent iron on cadmium contaminated sediments. J. Hazard. Mater. 406:124680. doi: 10.1016/j.jhazmat.2020.124680
Keywords: estuarine sediments, microbial communities, heavy metals, metal resistance genes, ecological functions
Citation: Yi J, Lo LSH, Liu H, Qian P-Y and Cheng J (2021) Study of Heavy Metals and Microbial Communities in Contaminated Sediments Along an Urban Estuary. Front. Mar. Sci. 8:741912. doi: 10.3389/fmars.2021.741912
Received: 15 July 2021; Accepted: 27 October 2021;
Published: 17 November 2021.
Edited by:
Allyson O’Brien, The University of Melbourne, AustraliaReviewed by:
Lucienne R. D. Human, Elwandle Coastal Node, South African Environmental Observation Network, South AfricaPrasanta Rath, Kalinga Institute of Industrial Technology Deemed to Be University, India
Wenguang Sun, University of Nebraska-Lincoln, United States
Copyright © 2021 Yi, Lo, Liu, Qian and Cheng. This is an open-access article distributed under the terms of the Creative Commons Attribution License (CC BY). The use, distribution or reproduction in other forums is permitted, provided the original author(s) and the copyright owner(s) are credited and that the original publication in this journal is cited, in accordance with accepted academic practice. No use, distribution or reproduction is permitted which does not comply with these terms.
*Correspondence: Jinping Cheng, jincheng@ust.hk