- 1Department of Geology, Banaras Hindu University, Varanasi, India
- 2Department of Science and Technology-Mahamana Centre of Excellence in Climate Change Research, Institute of Environmental and Sustainable Development, Banaras Hindu University, Varanasi, India
- 3Former Scientist Council of Scientific and Industrial Research-National Institute of Oceanography, Dona Paula, India
In this study, we presented a high-resolution benthic foraminiferal assemblage record from the western Bay of Bengal (BoB) (off Krishna–Godavari Basin) showing millennial-scale variations during the last 45 ka. We studied temporal variations in benthic foraminiferal assemblages (relative abundances of ecologically sensitive groups/species, microhabitat categories, and morphogroups) to infer past changes in sea bottom environment and to understand how monsoon induced primary productivity-driven organic matter export flux and externally sourced deep-water masses impacted the deep-sea environment at the core site. Our records reveal a strong coupling between surface productivity and benthic environment on glacial/interglacial and millennial scale in concert with Northern Hemisphere climate events. Faunal data suggest a relatively oxic environment when the organic matter flux to the sea floor was low due to low primary production during intensified summer monsoon attributing surface water stratification and less nutrient availability in the mixed layer. Furthermore, records of oxygen-sensitive benthic taxa (low-oxygen vs. high-oxygen benthics) indicate that changes in deep-water circulation combined with the primary productivity-driven organic matter flux modulated the sea bottom oxygen condition over the last 45 ka. We suggest that the bottom water at the core site was well-ventilated during the Holocene (except for the period since 3 ka) compared with the late glacial period. At the millennial timescale, our faunal proxy records suggest relatively oxygen-poor condition at the sea floor during the intervals corresponding to the cold stadials and North Atlantic Heinrich events (H1, H2, H3, and H4) compared with the Dansgaard/Oeschger (D-O) warm interstadials. The study further reveals oxygen-poor bottom waters during the last glacial maximum (LGM, 19–22 ka) which is more pronounced during 21–22 ka. A major shift in sea bottom condition from an oxygenated bottom water during the warm Bølling–Allerød (B/A) (between 13 and 15 ka) to the oxygen-depleted condition during the cold Younger Dryas (YD) period (between 10.5 and 13 ka) is noticed. It is likely that the enhanced inflow of North Atlantic Deep Water (NADW) to BoB would have ventilated bottom waters at the core site during the Holocene, B/A event, and probably during the D-O interstadials of marine isotope stage (MIS) 3.
Introduction
The potentiality of fossil benthic foraminifera in deciphering past changes in sea bottom oceanographic conditions has long been recognized. Studies have shown a strong relationship between distribution patterns of benthic foraminifera and bottom water oxygen condition coupled with surface productivity-driven organic matter flux to the sea floor (e.g., Bernhard, 1986; Gooday, 1986; Lutze et al., 1986; Corliss and Chen, 1988; Altenbach and Sarnthein, 1989; Jorissen et al., 1992; Kaiho, 1999). Benthic foraminifera strongly responds to the variation in benthic environment by showing variations in their abundances, diversity, microhabitats, and adaptive morphologies (e.g., Corliss, 1985; Corliss and Chen, 1988; Corliss and Emerson, 1990; Corliss and Fois, 1990; Kaiho, 1994; Almogi-Labin et al., 1996; Gupta and Thomas, 1999; Nigam et al., 2007; Singh et al., 2015; Verma et al., 2018; Ma et al., 2019). Therefore, temporal variations in benthic foraminiferal assemblage in terms of these faunal parameters are extensively used to reconstruct past deep-sea environments (e.g., Corliss, 1986; Schnitker, 1994; Otto et al., 1995; Thomas and Gooday, 1996; Schmiedl et al., 1998; Drinia et al., 2004; Ma et al., 2019).
Arabian Sea (AS) and Bay of Bengal (BoB) are the two basins in the northern Indian Ocean with different oceanographic features. The fossil records of deep-sea benthic foraminiferal assemblages in the AS have been extensively used to reconstruct the history of past changes in the deep-water environment (primarily oxygen and trophic conditions) and to understand its linkage with monsoon and deep-water circulation (Hermelin and Shimmield, 1990; Otto et al., 1995; den Dulk et al., 1998, 2000; Schmiedl et al., 1998; Gupta and Thomas, 1999; von Rad et al., 1999; Schmiedl and Leuschner, 2005; Arumugm et al., 2014; Sarkar and Gupta, 2014). Results of earlier studies provided deep insight into the surface ocean circulation, deep-water ventilation through externally sourced water masses impacting development of oxygen minimum zone (OMZ) and benthic environment in AS and its atmosphere–ocean teleconnections with climatic fluctuations at different timescales. In recent years, significant contributions on the oxygenation history and OMZ variability at millennial timescale were made using benthic foraminiferal records from the eastern AS, a region of an intensified OMZ (Bharti and Singh, 2013; Singh et al., 2015; Verma et al., 2018).
The existing paleoceanographic reconstructions based on benthic foraminiferal assemblages in BoB are limited mainly to the longer timescales (Sharma, 1970; Singh, 1979; Srinivasan and Rajshekhar, 1981; Gupta, 1987; Raju and Mishra, 1991). The Quaternary benthic foraminiferal assemblage records on a high-resolution timescale are very sparse from the bay (Ma et al., 2019). Most of the sea bottom paleoceanographic reconstructions made earlier were based on the stable isotope records and geochemical tracers (Ahmad and Labeyrie, 1994; Piotrowski et al., 2009; Ahmad et al., 2012; Pattan et al., 2013; Raza et al., 2014; Ma et al., 2019). Here, we present a first high-resolution benthic foraminiferal assemblage record from the western BoB (off Krishna—Godavari Basin) showing millennial-scale variation during the last 45 ka. We used temporal variations in faunal proxies (abundances of ecologically sensitive groups/species, microhabitat categories, morphogroups) to decipher past changes in the benthic environment and to understand how monsoon-induced organic matter fluxes and global deep-water circulation influenced the deep-sea environments of BoB.
Materials and Methods
Core Location and Regional Oceanographic Settings
Gravity core SK 218/1 (8.2 m length) was collected from 3,307 m water depth in the western BoB (14°02′06″ N, 82°00′12″ E; ~204 km offshore) by ORV Sagar Kanya in 2005 (Figure 1).
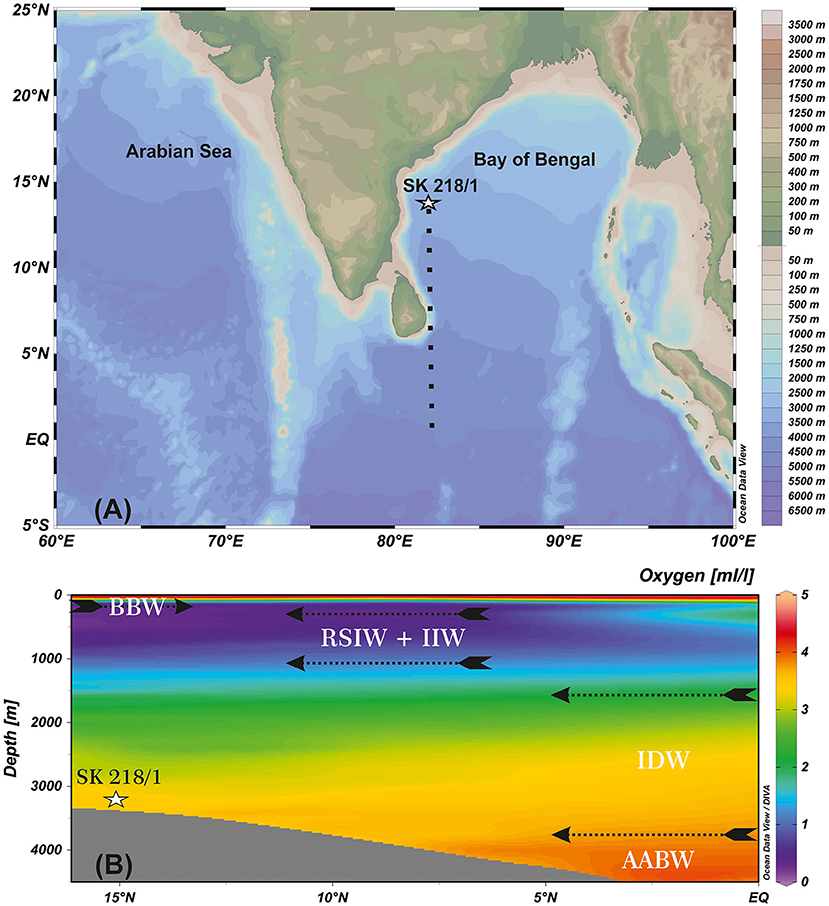
Figure 1. (A) Map showing the location of studied core (SK 218/1) in the Bay of Bengal (BoB). (B) Oxygen (ml/L, color shading) depth (m) latitude section and vertical structure of water masses in the BoB [N–S cross-section represented by black dashed line in (A)]. Map source Ocean Data View (ODV; Schlitzer, 2015). BBW, Bay of Bengal Water; RSIW, Red Sea Intermediate Water; IIW, Indonesian Intermediate Water; IDW, Indian Deep Water; AABW, Antarctic Bottom Water.
BoB is a semi-enclosed basin in the eastern part of the northern Indian Ocean. Surface hydrography of BoB is driven by seasonally reversing monsoon wind circulations. During the summer season (June–September), a huge amount of freshwater and sediments are discharged into the bay through major rivers Ganga–Brahmaputra, Irrawaddy–Salween from the Himalayas and Mahanadi, Krishna–Godavari, Cauvery rivers from the Indian peninsula (Bird et al., 2008). The freshwater discharge and overhead precipitation freshen the surface layers by 3–7 psu, inducing strong stratification in the upper water column (Prasanna Kumar et al., 2002). The surface stratification restricts the wind driven mixing to a shallow depth (<20 m), thereby, inhibits the injection of nutrients from the subsurface into the euphotic zone leading to an oligotrophic condition and low primary productivity in BoB. In the winter season (December–February), surface stratification weakens and winter monsoon winds induced mixing invokes vertical transport of nutrients to the surface, enhancing primary productivity (Kay et al., 2018).
The water masses in BoB are a combination of locally and externally sourced (Schott and McCreary, 2001). The upper 100 m of the water column consists of low-saline Bay of Bengal waters (BBW) derived mainly from river discharge into the bay. The water masses at thermocline depth up to about 1,000 m are Red Sea Intermediate Water (RSIW) and Indonesian Intermediate Water (IIW). IIW is derived from North Pacific Central Water, which enters BoB via the Indonesian Throughflow (You, 1998). The water mass between 1,500 and 3,800 m is the Indian Deep Water (IDW) comprising mainly of North Atlantic Deep Water (NADW) and Circumpolar Deep Water (You and Tomczak, 1993; Tomczak and Godfrey, 2003). The Antarctic Intermediate Water (AAIW) lies just above the NADW at 1000–1500 m depth in the Indian Ocean up to 10° S (Lynch-Stieglitz et al., 1994). The Antarctic Bottom Water (AABW) is reported from water depths below 3,800 m (Tomczak and Godfrey, 2003; Figure 1).
Benthic Foraminiferal Analysis
A total of 158 sediment samples at regular intervals of 2–4 cm were taken for benthic foraminiferal studies to obtain an average temporal resolution of ~288 years. For separation of foraminiferal tests, sediment samples were processed following the standard micropaleontological techniques (e.g., Singh et al., 2018). Census counts of benthic foraminifera were made on >125 μm size fraction. Based on census data, the relative abundances of each benthic foraminiferal species were calculated. The taxonomic classification of benthic foraminiferal species is based on Schwager (1877) revised by Srinivasan and Sharma (1980), Brady (1884) revised by Barker (1960), Loeblich and Tappan (1988, 1990), and Sen Gupta (2002).
Age Model
The age model of the core adopted in this study was established by Govil and Naidu (2011) based on 8 AMS 14C dates and δ18O record of Globigerinoides ruber correlated with δ18Oc global isostack curve of Martinson et al. (1987) (Table 1).
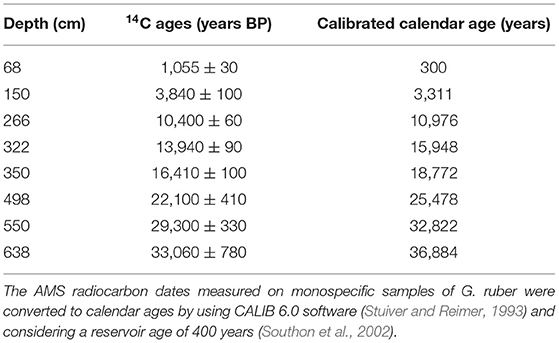
Table 1. AMS 14C dates and calibrated ages (in calendar years before present) for core SK 218/1 (Govil and Naidu, 2011).
Results
Temporal Variations and Environmental Preferences of Abundant Benthic Foraminifera
A total of 136 species representing 78 genera of benthic foraminifera is recorded (Appendix 1). Temporal variation in relative abundances of major benthic foraminiferal groups and their important constituent species are shown in Figures 2–4. Selected ecologically sensitive benthic species recorded in this study are illustrated in Plate 1.
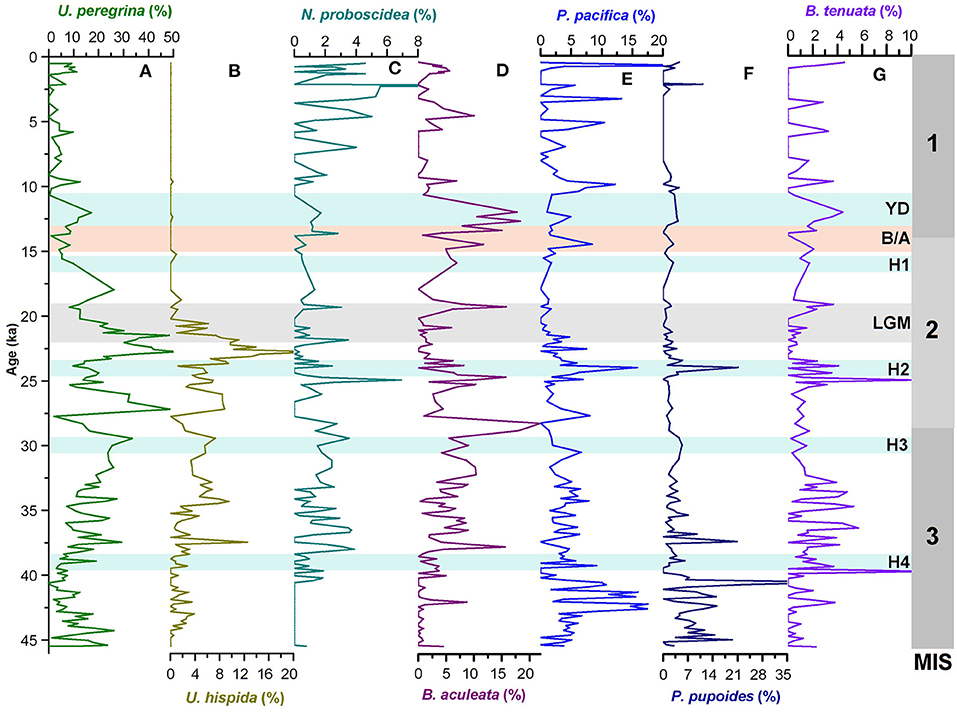
Figure 2. Temporal variations in relative abundances of (A–C) uvigerinid species (U. peregrina, U. hispida, and N. probscidea) and (D–G) buliminid species (B. aculeata, P. pacific, P. pupoides, and B. tenuata) in the core SK 218/1. MIS 1–3 denotes the Marine Isotope Stages, H1–H4, Heinrich Events; YD, Younger Dryas; B/A, Bølling/Allerød; LGM, Last Glacial Maxima.
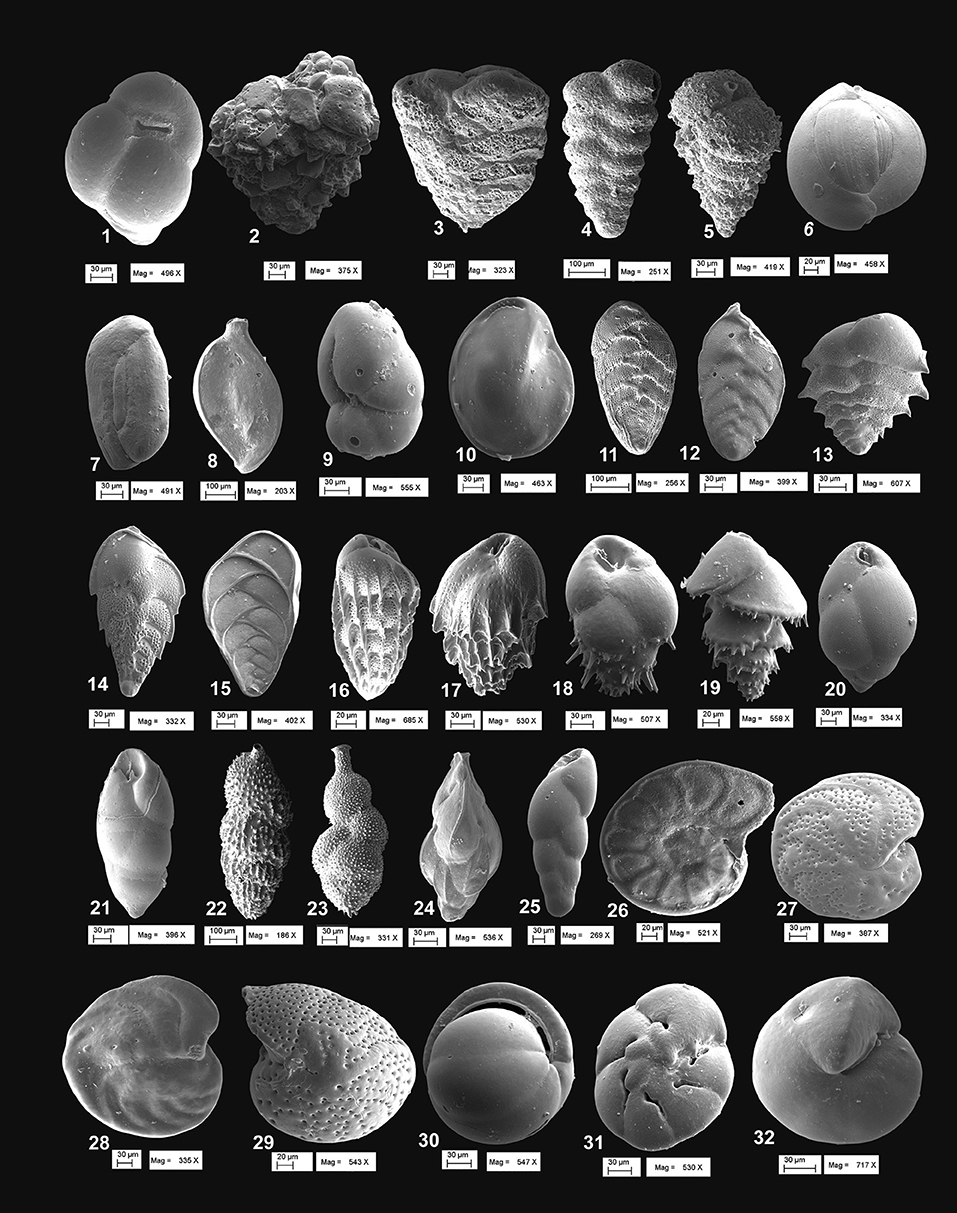
Plate 1. The benthic foraminiferal species were recorded from the western Bay of Bengal (SK 218/1). 1. Eggerella bradyi (Cushman) Apertural view: 458–460 cm, 23.67 ka; 2. Textularia lythostrota (Schwager) Agglutinated form: 282–284 cm, 12.40 ka; 3. Textularia laxata (Schwager) Front view: 282–284 cm, 12.40 ka; 4. Textularia agglutinans d'Orbigny Agglutinated form: 290–292 cm, 13.11 ka; 5. Siphotextularia bengalensis n. sp. Oblique front view: 458–460 cm, 23.67 ka; 6. Quinqueloculina seminulum (Linné) Front view: 278–280 cm, 12.04 ka; 7. Quinqueloculina oblonga (Montagu) Front view: 262–264 cm, 10.71 ka; 8. Quinqueloculina lamarckiana d'Orbigny Front view: 144–146 cm, 3.09 ka; 9. Ceratobulimina pacifica Cushman and Harris Side view: 702–704 cm, 39.84 ka; 10. Hoeglundina elegans (d'Orbigny) Front view: 702–704 cm, 39.84 ka; 11. Bolivina robusta Brady Front view: 278–280 cm, 12.04 ka; 12. Bolivina spathulata (Williamson) Front view: 188–190 cm, 5.82 ka; 13. Brizalina pygmaea Brady Front view: 730–731 cm, 41.13 ka; 14. Brizalina pseudobeyrichi Cushman Front view: 514–516 cm, 27.74 ka; 15. Bolivinita quadrilateral (Schwager) Back view: 362–364 cm, 19.32 ka; 16. Bulimina alazenensis Cushman Front view: 518–520 cm, 28.31 ka; 17. Bulimina striata d'Orbigny Front view: 214–216 cm, 7.54 ka; 18. Bulimina aculeata d'Orbigny Front view: 278–280 cm, 12.04 ka; 19. Bulimina marginata d'Orbigny Side view: 118–120 cm, 2.14 ka; 20. Protoglobobulimina pupoides (d'Orbigny) Front view: 120–122 cm, 2.21 ka; 21. Buliminella tenuata Cushman Front view: 698–690 cm, 39.65 ka; 22. Uvigerina peregrina Cushman Front view: 390–392 cm, 20.58 ka; 23. Neouvigerina proboscidea Schwager Back view: 120–122 cm, 2.21 ka; 24. Angulogerina carinata Cushman Front view: 86–88 cm, 0.96 ka; 25. Fursenkoina schreibersiana (CŽjŽek) Side view: 514–516 cm, 27.74 ka; 26. Hyalinea balthica (Schröter) Spiral view: 282–284cm, 12.40 ka; 27. Cibicides marialana gigas Keijzer Umbilical view: 92–94 cm, 1.18 ka; 28. Cibicides wuellerstorfi (Schwager) Umbilical view: 99–100 cm, 1.40 ka; 29. Cibicides lobatulus (Walker and Jacob) Umbilical view: 170–172 cm, 4.63 ka; 30. Pullenia bulloides (d'Orbigny) Umbilical view: 170–172 cm, 4.63 ka; 31. Alabaminatenui marginata (Chapman, Parr, and Collins) Umbilical view: 298–300 cm, 13.82 ka; 32. Oridorsalis tenara (Brady) Umbilical view: 118–120 cm, 2.14 ka.
The benthic foraminiferal assemblage comprises of various taxa belonging mainly to uvigerinids, buliminids, cibicidids, miliolids, cassidulinids, and bolivinids (in decreasing order of relative abundances). Other quantitatively significant taxa of the assemblage are Gyroidinoides spp., Oridorsalis spp., Chillostomella oolina, Eggrella brady, Pullenia spp., and Fursenkoina spp.
The uvigerinid group, a major component of benthic assemblages is represented mainly by Uvigerina peregrina, Uvigerina hispida, and Neouvigerina proboscidea. The relative abundance of total uvigerinids in the core varies between 0 and 76 % with maximum abundances occurring during 21–23 and 26–27 ka (Figure 4). There has been a significant reduction in the abundance of uvigerinids during 14–15, 23–24 ka, and between 38 and 40 ka. The Holocene in general is characterized by a very low abundance of the uvigerinids. The constituent species U. peregrina and U. hispida both follow a pattern similar to that of the total uvigerinids (Figures 2, 4). The uvigerinid taxa are known to be related to both the organic carbon flux and sea bottom oxygen level (Lutze et al., 1986; Hermelin and Shimmield, 1990; Jannink et al., 1998; Singh et al., 2015). The uvigerinids have preference for high organic carbon flux to the sea floor and low-to-moderate oxygen levels.
The buliminid group comprising genera Protoglobobulimina and Bulimina is the next important component of the benthic assemblages. The major constituent species of this group are Bulimina aculeata, Protoglobobulimina pacifica, Protoglobobulimina pupoides, and Buliminella tenuata. The buliminid group shows its high abundances during 12–13, 24–25, around 28 ka, between 30 and 37 ka and between 40 and 43 ka (Figure 4). Its abundance declines significantly during the Holocene (except for the period since 3 ka) and the last glacial maximum (LGM; 19–22 ka). However, B. aculeata, P. pacifica, and B. tenuata show an increase in their abundances during brief intervals within the Holocene (Figure 2). The tapered/cylindrical tests of the buliminids are suggestive of their preferences for infaunal habitat. Hence, their distribution pattern in the core is possibly controlled by the organic matter content in sediment and dissolved oxygen concentrations (e.g., Phleger and Soutar, 1973; Kaiho, 1994). B. aculeata, the major constituent of the buliminid population is considered to be a tolerant species to the low-oxygen environment (den Dulk et al., 2000).
The cibicidid group, which is represented mainly by Cibicides wuellerstorfi and Cibicides robertsonianus shows large variation in its abundance down core. The total abundance of cibicidids was very low between 16 and 45 ka, as compared with the Holocene (Figure 4). There has been a rapid increase in its abundance from 11 ka, reaching to its maximum during 6–8 ka. The variation pattern of C. robertsonianus abundance appears to be almost similar to that of the total cibicidids. The pattern of C. wuellerstorfi abundance, however, is slightly different with its abundance maxima occurring between 40 and 41, 19 and 22, 13.5 and 16 ka, and during 8–9 and 1–3 ka (Figure 3). Cibicides generally prefer epifaunal habitat (Kaiho, 1994; 1999) and are known to be associated with the high-oxygen sea bottom condition (Corliss and Fois, 1990; Singh et al., 2015; Verma et al., 2018; Verma and Singh, 2019).
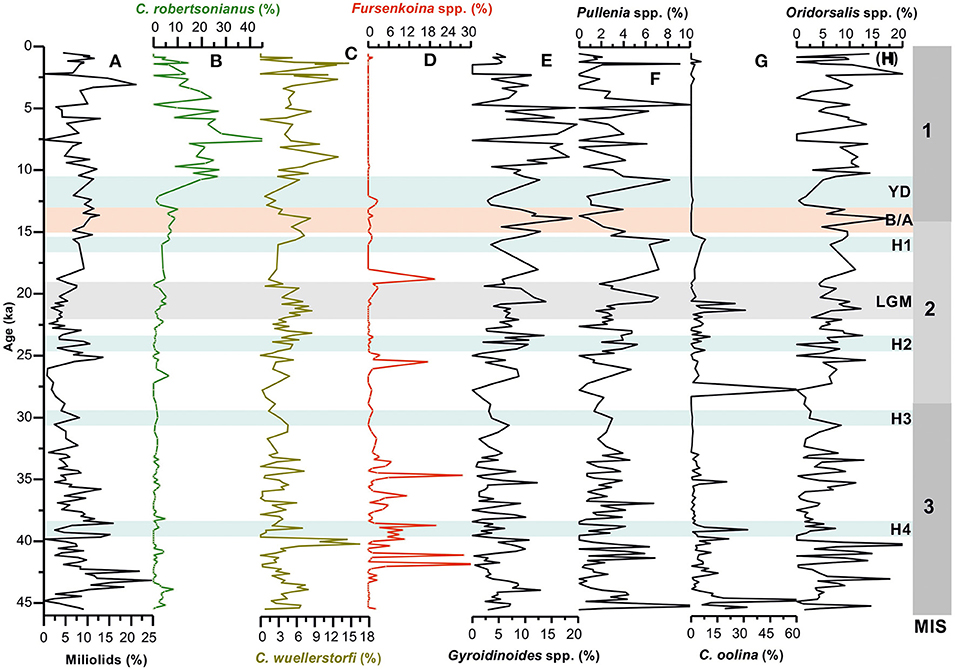
Figure 3. Temporal variations in relative abundances of (A) total miliolids; (B,C) cibicidid species (C. wuellerstorfi and C. robertsonianus); (D) Fursenkoina spp., (E) Gyroidinoides spp., (F) Pullenia spp., (G) Chillostomella oolina, and (H) Oridorsalis spp. in core SK 218/1. MIS 1–3 denotes the Marine Isotope Stages; H1–H4, Heinrich Events; YD, Younger Dryas; B/A, Bølling/Allerød; LGM, Last Glacial Maxima.
The miliolids are relatively abundant in the Holocene when compared with the late glacial period. Its high abundance is also recorded during 42–44 ka of MIS 3 (Figure 3). The miliolids are known to have epifaunal habitat and are considered to be associated with oxic environment and low organic carbon flux (Kaiho, 1994, 1999; Singh et al., 2015; Verma et al., 2018).
Temporal variations in abundance patterns of other important groups (cassidulinids and bolivinids) and Gyroidinoides spp., Pullenia spp., and Oridorsalis spp. all show significant changes in their abundances down-core (Figures 3, 4) and the interval of major changes (highs and lows) are associated possibly to variation in sea bottom condition mainly organic matter flux and oxygen level.
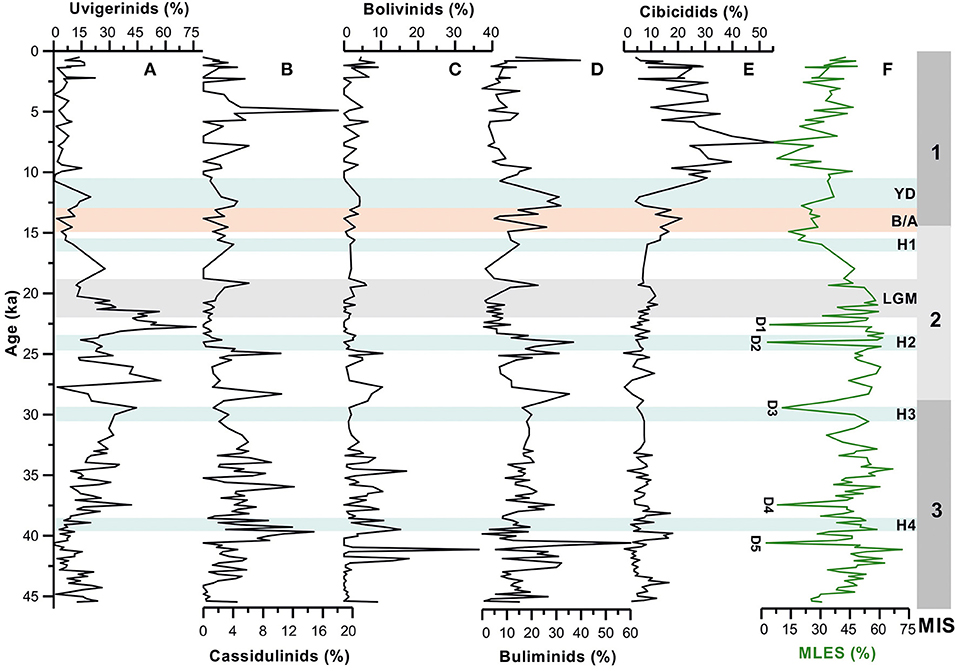
Figure 4. Temporal variations in relative abundances of major benthic foraminiferal groups [(A) uvigerinids, (B) cassidulinids, (C) bolivinids, (D) buliminids, and (E) cibicidids] compared with (F) Mixed layer eutrophic species (MLES) abundance record (surface productivity proxy). D1–D5 represents possible dissolution points following Verma et al. (under revision)1. MIS 1–3 denotes the Marine Isotope Stages; H1–H4, Heinrich Events; YD, Younger Dryas; B/A, Bølling/Allerød; LGM, Last Glacial Maxima.
The cassidulinids are known to prefer infaunal habitat, moderate to high organic matter flux, and low-oxygen environment (Kaiho, 1994; Almogi-Labin et al., 2000; den Dulk et al., 2000; Singh et al., 2015). The bolivinids being infaunal species are associated with low-oxygen condition and high organic matter content in the sediment. Pullenia prefers infaunal microhabitat in high productive regions (Gooday, 1994), suboxic condition and is less tolerant to oxic environment. Although the environmental preferences of Oridorsalis are not fully known, some studies have shown its association with the low productive condition (Kuhnt et al., 1999). Nevertheless, its abundant occurrence in high productivity areas has also been reported (Woodruff, 1985). Gyroidinoides species have a preference for shallow infaunal habitat and suboxic condition (Kaiho, 1994; Guichard, 1997). Fursenkoina and Chilostomella oolina, both are strikingly absent or rarely present in the Holocene sediment of the examined core. The abundance patterns of these taxa indicate their peak abundances during certain time intervals of the late glacial period (Figure 3). Earlier studies have shown these taxa to be associated with very low sea bottom oxygen condition (Kaiho, 1994, 1999).
Benthic Foraminiferal Morphotype and Microhabitat Categories
Benthic foraminifera depending upon their adaptation capabilities to variation in sea floor environment and microhabitat preferences develop various functional morphologies (e.g., Singh et al., 2015; Verma et al., 2018). Therefore, various morphotypes shown by benthic foraminifera and their association with specific microhabitats can be potentially used in paleoenvironmental reconstructions (e.g., Nigam et al., 2007; Singh et al., 2015). Epifaunal, shallow infaunal, and deep infaunal are the broad microhabitat categories of benthic foraminifera (e.g., Corliss and Fois, 1990; Jorissen et al., 1995). The epifauna are those living on the sediment surface, shallow infauna within sediment top 2 cm, and deep infauna below 2 cm (Buzas et al., 1993). As it is difficult to distinguish clearly the shallow infauna from epifauna, generally both together are classified as epifauna (Jorissen et al., 1995). We grouped quantitatively significant benthic foraminiferal taxa into two broad categories: epifauna and infauna based on the existing knowledge of their microhabitat preferences (Figure 5). Down core variation in abundance patterns of these two groups reflects prominent changes on glacial/interglacial scale and also on the millennial scale. The Holocene period is characterized by a high abundance of epifaunal taxa and corresponding low abundances of infaunal taxa except for the late Holocene showing an opposite pattern since 3 ka. The abundance of infaunal taxa was generally high during the late glacial period between 21 and 45 ka. At the millennial timescale, the faunal record shows a conspicuous decline in infaunal taxa during 38–38.5, 28–29, 23.5–25, and 19–21 ka. During these time intervals, the abundances of epifaunal taxa increased. Additionally, there have been periods of a major increase in epifauna and corresponding decline in infauna between 13 and 15, 40 and 41, and 43 and 44 ka. The period between 10.5 and 13 ka is characterized by a conspicuous reduction of epifauna taxa and corresponding increased abundance of infauna (Figure 5).
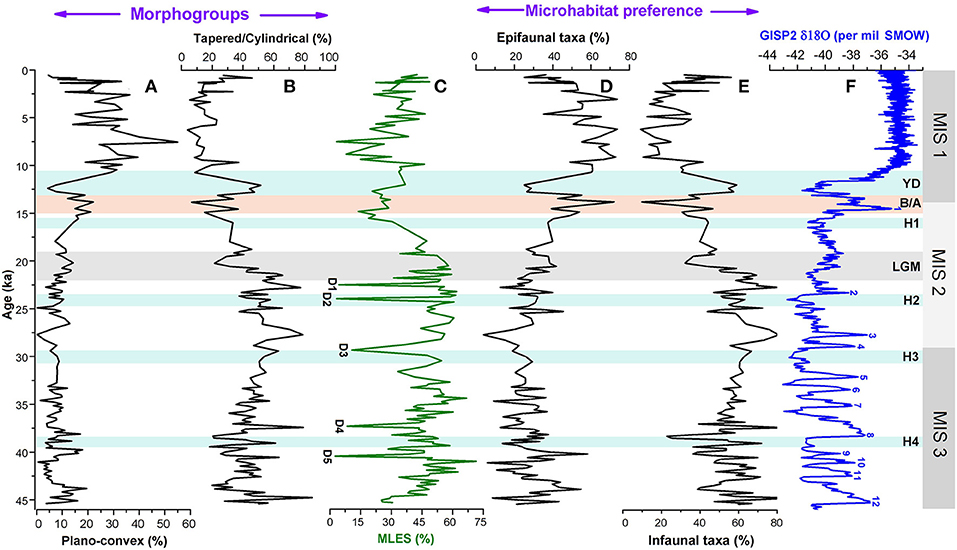
Figure 5. Comparison of relative abundances of (A,B) major morphogroups (plano-convex and tapered/cylindrical), (C) mixed layer eutrophic species (MLES), and (D,E) microhabitat categories (epifaunal and infaunal taxa) of foraminiferal assemblages with (F) GISP2 δ18O ice core record. D1–D5 represents possible dissolution points following Verma et al. (under revision). MIS 1–3 denotes the Marine Isotope Stages; H1–H4, Heinrich Events; YD, Younger Dryas; B/A, Bølling/Allerød; LGM, Last Glacial Maxima.
Epifaunal taxa generally show varying morphologies such as plano-convex, biconvex, milioline, and rounded trochospiral. The infaunal species commonly prefer to have tapered/cylindrical, flattened ovoid, spherical, or rounded plani-spiral tests (Singh et al., 2015). Singh et al. (2015) in their recent study from the AS OMZ suggested benthic foraminifera with plano-convex tests (epifaunal) and tapered/ cylindrical (infaunal) to be more sensitive to changes in bottom oxygen level coupled with organic matter flux. The relative abundance profiles of these two ecologically sensitive benthic morphotype categories in the examined core are shown in Figure 5. Abundance records of these morphogroups reveal that the pattern of variation in tapered/cylindrical category is broadly opposite to the plano-convex record. The variation pattern of tapered/cylindrical category mirrors to the pattern of infaunal abundance record both on glacial/interglacial and millennial scales. The abundance of tapered/ cylindrical forms was high during the late glacial, whereas, plano-convex tests were more abundant during the Holocene (Figure 5).
Discussion
Impact of Surface Productivity Variation on Benthic Environment
The benthic foraminiferal distribution in deep sea is primarily controlled by primary productivity-related organic matter flux to the sea floor and dissolved oxygen level (Otto et al., 1995; den Dulk et al., 1998, 2000; von Rad et al., 1999; Schmiedl and Leuschner, 2005; Bharti and Singh, 2013; Singh et al., 2015). In this study, we evaluate the benthic foraminiferal response to the past changes in surface productivity in western BoB. We compared the abundance records of major benthic foraminiferal groups (uvigerinids, buliminids, bolivinids, and cassidulinids) with the record of mixed layer eutrophic planktic foraminiferal assemblage (relative abundances of Globigerina bulloides, Globigerinita glutinata, and Globigerina falconensis), which has been suggested to be linked to the surface primary productivity (Singh et al., 2011, 2018; Verma et al. under revision); Figure 4). From the faunal abundance records, it is evident that the intervals of increased abundances of these benthic groups broadly correspond to the peak abundances of eutrophic planktic species. It is to be noted that the abundance of eutrophic planktic species is high during the late glacial period as compared with the Holocene. The millennial-scale variations in both the planktic and benthic assemblage records broadly correspond to the Northern Hemisphere cold stadials and warm interstadials (Figure 5). However, the temporal variation in abundance of uvigerinid and buliminid groups appears to be governed by the surface primary productivity-driven organic carbon flux to the sea floor. The primary productivity in BoB is mainly modulated by the summer monsoon-induced precipitation and continental runoff. Earlier studies have shown low primary productivity and thus low export flux of organic carbon to the sea floor, during the periods of intensified summer monsoon when sea surface was more stratified due to increased fluvial discharge into the bay (e.g., Narvekar and Prasanna Kumar, 2006; Da Silva et al., 2017). In contrast, the surface productivity-induced organic flux enhanced during the periods of weakened summer monsoon resulting in less stratified surface waters coupled with winter monsoon intensification invoking vertical advection of nutrient-rich waters to the surface mixed layer.
Abundances of uvigerinids and buliminids are generally low during the Holocene and warm B/A periods when summer monsoon was intensified leading to the low primary production and reduced export flux of organic matter. Conversely, the group cibicidids show its maximum abundances during the periods of low primary productivity.
It is also noticed that the patterns of temporal variation in benthic foraminiferal groups individually vary and do not always match each other. These differences in the individual pattern may be related to their differential responses to the surface productivity-related organic fluxes. Additionally, it is plausible that changes in deep-water oxygenation/ventilation through externally sourced water masses might have influenced their distribution patterns.
Changes in Deep-Water Oxygenation
Benthic foraminifera are sensitive to changes in dissolved oxygen concentration over the sea floor. They show different microhabitat preferences in response to changes in ocean-bottom oxygen level and adapt distinct morphological features (Corliss, 1985; Corliss and Chen, 1988; Corliss and Emerson, 1990). Previous studies have indicated a good correlation between microhabitat preferences and morphological characteristics of benthic foraminifera and sea bottom oxygen condition (e.g., Corliss and Fois, 1990; Kaiho, 1994). Therefore, the abundance records of different microhabitat categories and morphogroups are the potential proxies to reconstruct the deep-sea oxygenation history (Figure 5). This approach has been applied to the late Quaternary benthic foraminiferal assemblages of the AS to decipher temporal changes of the OMZ intensity in the eastern AS (e.g., Nigam et al., 2007; Mazumder and Nigam, 2014; Singh et al., 2015; Verma et al., 2018). In this study, the relative abundance records of two broad groups of microhabitat categories (epifauna and infauna) in combination with the two predominant morphogroups (tapered/cylindrical and plano-convex) (Tables 2, 3) show major changes on glacial/interglacial and millennial scales. Epifaunal taxa have a preference for high-oxygen condition over the sea floor, whereas infaunal taxa prefer a low-oxygen habitat associated with a decrease in dissolved oxygen content within the sediment (e.g., Corliss and Chen, 1988; Kaiho, 1994). High abundances of tapered/cylindrical benthic foraminiferal tests in sediment are suggestive of low bottom oxygen condition, whereas increased abundances of plano-convex tests indicate the presence of an oxygenated environment at the sea floor (Corliss and Fois, 1990; Gooday, 1994; Verma et al., 2018). The predominance of epifaunal taxa and benthic foraminifera with plano-convex tests during the Holocene suggests an oxygenated sea bottom environment compared with the late glacial period between 21 and 45 ka characterized by a general increase in abundances of infauna, tapered/cylindrical tests, and very low abundances of plano-convex tests. A rapid increase in epifaunal taxa corresponding to a significant decline of infaunal and tapered/cylindrical tests between 13 and 15 ka (equivalent to the warm B/A event) reflects a major shift in the benthic environment from a low-oxygen to the oxygenated condition. From the faunal record, it is clearly evident that the oxygenated benthic environment rapidly switched to a low-oxygen condition again during 10.5–13 ka, a period corresponding to the cold Younger Dryas (YD) event. Further, the relative abundance records of benthic microhabitat and morphotype categories for late glacial period depicts millennial-scale oscillations in the late glacial period, apparently in concert with the Northern Hemisphere Heinrich and Dansgaard–Oeschger (D-O) events (Figure 5). These short-term fluctuations in benthic records are obviously related to the changes in bottom water oxygen condition, which seems to be more pronounced between 40 and 45 ka.
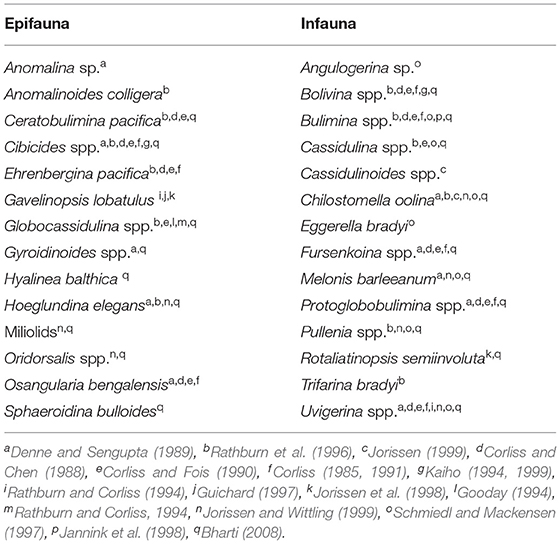
Table 3. Microhabitat preferences for quantitatively significant benthic foraminifera recorded from the core SK 218/1.
In order to have a better reconstruction of past changes in bottom oxygen condition, we used oxygen-sensitive benthic species of assemblages and classified them into low-oxygen and high-oxygen groups based on their association with changing dissolved oxygen levels (e.g., Douglas, 1981; Hermelin and Shimmield, 1990; Kaiho, 1994; Jannink et al., 1998; Singh et al., 2015) (Table 4; Figure 6). Temporal changes in relative abundances of these oxygen-sensitive benthic foraminiferal groups exhibit major variations during 3–10.5 ka when low-oxygen taxa were significantly replaced by the benthic species associated with a high-oxygen environment. The faunal proxy record, however, further suggests a relatively low-oxygen condition between 3 and 5 ka (Figure 6).
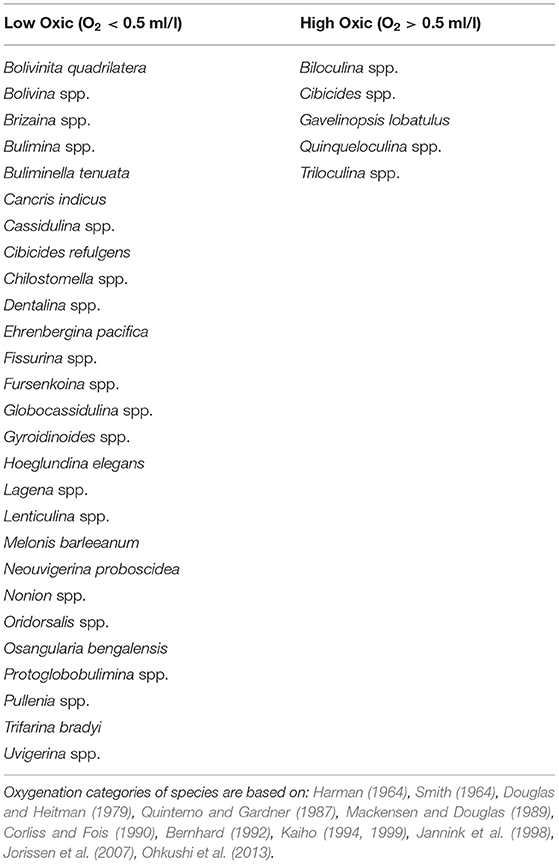
Table 4. Constituent species of low O2 (<0.5 ml/L O2) and high O2 (>0.5 ml/L O2) benthic foraminiferal assemblages.
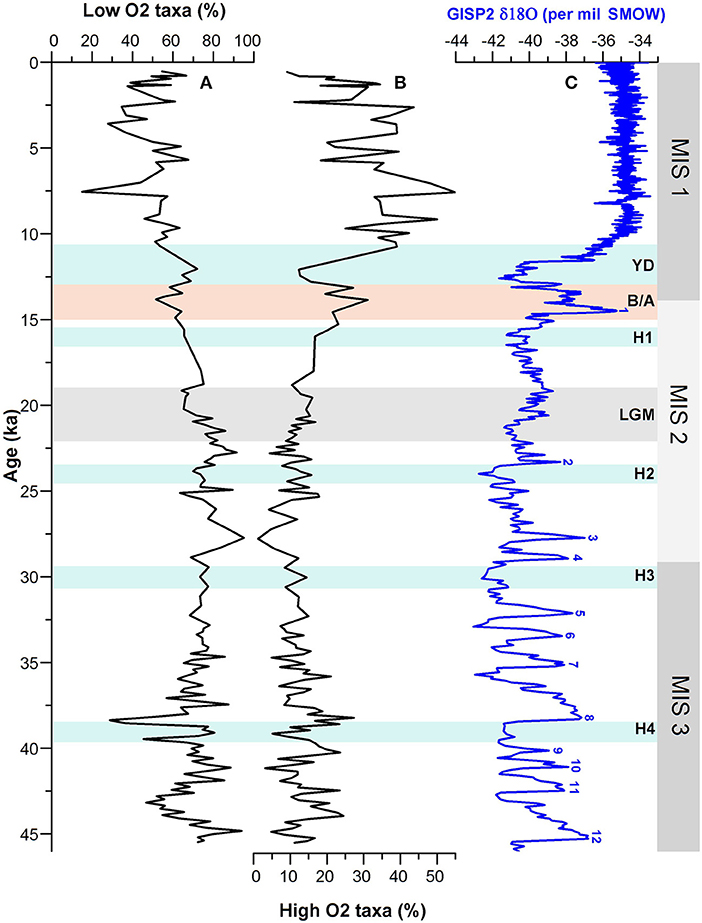
Figure 6. Comparison of (A) % low-oxygen and (B) % high-oxygen taxa of benthic foraminiferal assemblages with (C) GISP2 δ18O ice core record. MIS 1–3 denotes the Marine Isotope Stages; H1–H4, Heinrich Events; YD, Younger Dryas; B/A, Bølling/Allerød; LGM, Last Glacial Maxima.
Our study reveals that the sea bottom oxygen condition in the western BoB oscillated in concert with the Northern Hemisphere climate events. The bottom water was relatively oxygen-poor during the time intervals corresponding broadly to the North Atlantic Heinrich events H1, H2, H3, and H4 and D-O cold stadials as compared with the warm interstadials. Apparently, the change in bottom water oxygen condition during the H4 event was more pronounced than H1–H3. Furthermore, the benthic environment at the core site during the cold YD event is also characterized by low-oxygen condition. Prior to YD, the bottom water was oxygenated during the period corresponding to the warm B/A. It is also evident from the faunal record that the bottom water was relatively oxygen-poor during the LGM (19 - 22 ka). However, it appears that the bottom water during 19–21 ka was more oxygenated than during 21–22 ka suggesting two-step changes in sea floor environment within the LGM. The other most striking feature of major fluctuation in oxygen condition during MIS 3 is noticed between 35 and 45 ka. The faunal data reveal a high oxygenated environment at around 38, 40, and 42.5 ka which broadly corresponds to the D-O interstadials 8, 9, and 11, respectively (Figure 6).
The bottom water oxygen condition is driven mainly by two factors: (1) amount of organic matter to the sea floor and magnitude of its degradation and (2) deep-water circulation (Joos et al., 2003; Rathburn et al., 2018). By comparison of benthic foraminiferal record with the surface productivity record (mixed layer eutrophic planktic foraminifera abundance), it can be inferred that the bottom oxygen condition at the core site is governed primarily by the surface productivity-related export flux of organic carbon to the sea floor. The respiration of sinking organic matter and the release of CO2 lead to a low-oxygen condition on the sea floor. The benthic environment was more oxygenated (as evident by high abundances of high-oxygen taxa) when surface productivity and export flux of organic carbon was low, a condition associated with the intensified SW summer monsoon precipitation resulting in high fluvial discharge and stratified surface waters in BoB. On the other hand, the export flux of organic carbon was enhanced during the intensified NE winter monsoon wind-induced vertical mixing, coupled with a weakened summer monsoon. Additionally, the deep-water circulation might have also influenced the oxygen level of bottom water mass at the core site. Previous studies have shown that the relative proportions of NADW and southern sourced AAIW and AABW modulated the bottom water oxygen condition in the northern Indian Ocean both on the glacial/interglacial and millennial scales (e.g., Ahmad and Labeyrie, 1994; Piotrowski et al., 2009; Ma et al., 2019 and references therein). A significant reduction in NADW export to the Indian Ocean during the cold North Atlantic events (Heinrich and YD events) has been suggested (Naqvi et al., 1994; Piotrowski et al., 2009). These studies provided ample evidences of increased propagation of NADW to BoB during the Holocene (Piotrowski et al., 2009; Ma et al., 2019) compared with the late glacial period. It is plausible, therefore, that the deep water at the core site was more ventilated by NADW during the Holocene. We also suggest that the high-oxygen condition during the warm D-O interstadials of MIS 3 is most likely related to the effective propagation of NADW to the BoB. This is in line with the previous results indicating an enhanced influence of NADW during the warm B/A event (Piotrowski et al., 2009). However, more research on high-resolution timescale is required to better understand the relative contributions of NADW and AAIW/AABW flow in modulating the deep ocean oxygenation history in BoB in concert with the D-O oscillations.
Conclusion
A high-resolution deep-sea benthic foraminiferal assemblage record obtained from a sediment core in the western BoB reveals millennial-scale variations during the last 45 ka. Temporal variations in faunal proxy records suggest a strong influence of surface primary productivity-related organic matter flux on the bottom water oxygen condition at the core site. Our records indicate oxygenated sea bottom condition during the Holocene, when primary productivity was significantly low as compared with the late glacial period between 21 and 45 ka, due to intensified summer monsoon resulting in high fluvial discharge to BoB. In addition to the surface productivity factor, changes in deep-water circulation at the millennial timescale might have also strongly influenced the benthic environment.
The relative abundance records of two broad groups of microhabitat categories (epifauna and infauna) and morphogroups (tapered/cylindrical and plano-convex) show major changes on glacial/interglacial and millennial timescales. We also used oxygen-sensitive benthic foraminiferal groups (low-oxygen and high-oxygen taxa) to better understand past changes in bottom water oxygen conditions. Our records reveal that the sea bottom oxygen condition in the western BoB varied in concert with the Northern Hemisphere Heinrich and D-O events. The benthic environment was oxygen depleted during the North Atlantic cold events (YD and Heinrich events) and the LGM (19–22 ka). We suggest that the bottom water was more ventilated by NADW during the warm B/A event, D-O interstadials (8, 9, and 11) of MIS 3, and during the Holocene.
Data Availability Statement
The original contributions presented in the study are included in the article/Supplementary Materials, further inquiries can be directed to the corresponding author/s.
Author Contributions
AS and PN conceptualized the study. KV carried out the sample analysis. AS supervised the study. AS and KV prepared the original draft of the manuscript with contributions from HS, PS, RS, and PN. All authors participated in reviewing, editing, and preparation of final draft of the manuscript.
Funding
AS acknowledges the financial support by the ISRO-GBP sponsored project (P-32-14) and IoE Incentive Grant BHU and KV for IOE Seed Grant (R/Dev/IOE/Seed grant/2020-21). HS acknowledges UGC for Senior Research Fellowship [419/(CSIR-UGC NET JUNE 2018)]. PS acknowledges the Climate Change Programme of DST (DST/CCP/CoE/80/2017-G) for Junior Research Fellowship. RS acknowledges the CSIR for Senior Research Fellowship.
Conflict of Interest
The authors declare that the research was conducted in the absence of any commercial or financial relationships that could be construed as a potential conflict of interest.
Publisher's Note
All claims expressed in this article are solely those of the authors and do not necessarily represent those of their affiliated organizations, or those of the publisher, the editors and the reviewers. Any product that may be evaluated in this article, or claim that may be made by its manufacturer, is not guaranteed or endorsed by the publisher.
Acknowledgments
The present study is part of the unpublished doctoral thesis of KV entitled Late quaternary oceanographic changes in the Bay of Bengal based on foraminiferal records, submitted to Banaras Hindu University and archived at http://hdl.handle.net/10603/268142. We thank the scientific party and crew of ORV Sagar Kanya for coring the samples in western BOB. We also thank two referees and editors for their constructive reviews that have helped to improve the manuscript.
Supplementary Material
The Supplementary Material for this article can be found online at: https://www.frontiersin.org/articles/10.3389/fmars.2021.733365/full#supplementary-material
Footnotes
1. ^Verma, K., Singh, A. D., Singh, P., Singh, H., Satpathy, R. K., Uddandam, P. R., et al. (under revision). Monsoon-related changes in surface hydrography and productivity in the Bay of Bengal over the last 45 kyr. Palaeogeogr. Palaeoclimatol. Palaeoecol.
References
Ahmad, S. M., and Labeyrie, L. (1994). Glacial to holocene delta 13 carbon variation in intermediate depth water masses of North Indian ocean. Geo. Mar. Lett. 14, 36–40. doi: 10.1007/BF01204469
Ahmad, S. M., Zheng, H., Raza, W., Zhou, B., Lone, M. A., Raza, T., et al. (2012). Glacial to holocene changes in the surface and deep waters of the northeast Indian Ocean. Mar. Geol. 329, 16–23. doi: 10.1016/j.margeo.2012.10.002
Almogi-Labin, A., Hemleben, C., Meischner, D., and Erlenkeuser, H. (1996). Response of red sea deep-water agglutinated foraminifera to water-mass changes during the late quaternary. Mar. Micropaleontol. 28, 283–297. doi: 10.1016/0377-8398(96)00005-9
Almogi-Labin, A., Schmiedl, G., Hemleben, C. H., Siman-Tov, R., Segl, M., and Meischner, D. (2000). The influence of the NE winter monsoon on productivity changes in the Gulf of Aden, NW Arabian sea, during the last 530 ka as recorded by foraminifera. Mar. Micropaleontol. 40, 295–319. doi: 10.1016/S0377-8398(00)00043-8
Altenbach, A. V., and Sarnthein, M. (1989). “Productivity record in benthic foraminifera,” in Productivity of the Ocean: Present and Past, ed W. H. Berger (New York, NY: John Wiley and Sons Ltd), 255–269.
Arumugm, Y., Gupta, A. K., and Panigrahi, M. K. (2014). Species diversity variations in Neogene deep-sea benthic foraminifera at ODP Hole 730A, western Arabian sea. J. Earth Syst. Sci. 123, 1671–1680. doi: 10.1007/s12040-014-0495-z
Barker, R. W. (1960). Taxonomic notes on the species figured by H. B. Brady in his “Report on the foraminifera dredged by H. M. S. Challenger during the years 1873-1876”. Soc. Econ. Paleontol. Mineral. 9, 1–238.
Bernhard, J. M. (1986). Characteristic assemblages and morphologies of benthic foraminifera from anoxic, organic-rich deposits: jurassic through holocene. J. Foraminiferal Res. 16, 207–215. doi: 10.2113/gsjfr.16.3.207
Bernhard, J. M. (1992). Benthic foraminiferal distribution and biomass related to pore water oxygen content: central California continental slope and rise. Deep Sea Res. I 39, 585–605.
Bharti, S. R. (2008). Late Quaternary Oceanographic and Climatic Variability in the Eastern Arabian Sea Based on Foraminiferal and Pteropod Records. (Ph. D. thesis), Banaras Hindu University, Varanasi (India).
Bharti, S. R., and Singh, A. D. (2013). Bulimina Arabiensis, a new species of benthic foraminifera from the Arabian Sea. J. Foraminiferal Res. 43, 255–261.
Bird, M. I., Robinson, R. A. J., Win Oo, N., Maung Aye, M., Lu, X. X., Higgitt, D. L., et al. (2008). A preliminary estimate of organic carbon transport by the ayeyarwady (irrawaddy) and thanlwin (salween) rivers of Myanmar. Q. Int. 186, 113–122. doi: 10.1016/j.quaint.2007.08.003
Brady, H. B. (1884). Report on the foraminifera dredged by H.M.S. Challenger during the Years 1873-1876. Report on the scientific results of the voyage of H.M.S. Challenger during the years 1873–76. Zoology 9 (part 22), i–xxi; 1–814.
Buzas, M. A., Culver, S. J., and Jorissen, F. J. (1993). A statistical evaluation of the microhabitats of living (stained) infaunal benthic foraminifera. Mar. Micropaleontol. 20, 311–320. doi: 10.1016/0377-8398(93)90040-5
Corliss, B. H. (1985). Microhabitats of benthic foraminifera within deep-sea sediments. Nature 314, 435–438. doi: 10.1038/314435a0
Corliss, B. H. (1986). Shape patterns of deep-sea benthic foraminifera and ecological implications. Eos. 67:974.
Corliss, B. H. (1991). Morphology and microhabitat preferences of benthic foraminifera from the northwest Atlantic Ocean. Mar. Micropaleontol. 17, 195–236.
Corliss, B. H., and Chen, C. (1988). Morphotype patterns of Norwegian sea deep-sea benthic foraminifera and ecological implications. Geology 16, 716–719. doi: 10.1130/0091-7613(1988)016<0716:MPONSD>2.3.CO;2
Corliss, B. H., and Emerson, S. (1990). Distribution of Rose Bengal stained deep-sea benthic foraminifera from the nova scotia continental margin and Gulf of Maine. Deep Sea Res. 37, 381–400. doi: 10.1016/0198-0149(90)90015-N
Corliss, B. H., and Fois, E. (1990). Morphotype analysis of deep-sea benthic foraminifera from the northwest Gulf of Mexico. Palaios 5, 589–605. doi: 10.2307/3514864
Da Silva, R., Mazumdar, A., Mapder, T., Peketi, A., Joshi, R. K., Shaji, A., et al. (2017). Salinity stratification controlled productivity variation over 300 ky in the Bay of Bengal. Sci. Rep. 7:14439. doi: 10.1038/s41598-017-14781-3
den Dulk, M., Reichart, G. J., Memon, G. M., Roelofs, E. M. P., Zachariasse, W. J., and Van der Zwaan, G. J. (1998). Benthic foraminiferal response to variations in surface water productivity and oxygenation in the northern Arabian sea. Mar. Micropaleontol. 35, 43–66. doi: 10.1016/S0377-8398(98)00015-2
den Dulk, M., Reichart, G. J., Van Heyst, S., Zachariasse, W. J., and Van der Zwaan, G. J. (2000). Benthic foraminifera as proxies of organic matter flux and bottom water oxygenation? A case history from the Northern Arabian sea. Palaeogeogr. Palaeoclimatol. Palaeoecol. 161, 337–359. doi: 10.1016/S0031-0182(00)00074-2
Denne, R. A., and Sengupta, B. K. (1989). Effects of taphonomy and habitat on the record of benthic foraminifera in modern sediments. Paleoceanography 4, 414–423.
Douglas, R. G. (1981). “Paleoecology of continental margin basins: a modern case history from the borderland of southern California,” in Depositional Systems of Active Continental Margin Basins, ed R. G. Douglas. Los Angeles, CA: Pacific Section, Society of Economic Paleontologists and Mineralogists, 121–156.
Douglas, R. G., and Heitman, H. L. (1979). “Slope and basin benthic foraminifera of the California borderland,” in Geology of Continental Slopes, Vol. 27, eds L. J. Doyle and O.H. Pilkey (SEPM special Publication), 231–246.
Drinia, H., Antonarakou, A., and Tsaparas, N. (2004). Diversity and abundance trends of benthic foraminifera from the southern part of the Iraklion basin, Central Crete. Bull. Geol. Soc. Greece 36, 772–781. doi: 10.12681/bgsg.16809
Gooday, A. J. (1986). Meiofaunal foraminiferans from the bathyal porcupine sea bight (northeast Atlantic): size structure, standing stock, taxonomic composition, species diversity and vertical distribution in the sediment. Deep Sea Res. 33, 1345-1373. doi: 10.1016/0198-0149(86)90040-3
Gooday, A. J. (1994). The biology of deep sea foraminifera: a review of some advances and their applications in paleoceanography. Palaios 9, 14–31. doi: 10.2307/3515075
Govil, P., and Naidu, P. D. (2011). Variations of Indian monsoon precipitation during the last 32 kyr reflected in the surface hydrography of the Western Bay of Bengal. Quat. Sci. Rev. 30, 3871–3879. doi: 10.1016/j.quascirev.2011.10.004
Guichard, S. (1997). Evolution de Lfenvironment Dans la Zone de Lfupwelling Maturitanien Pendant les Derniers 236,000 ans, le Temoignage de Lffecosysteme Benthique. (Thesis), l?fUniversite Bordeaux I, N. dfordre, Mauritania.
Gupta, A. K. (1987). Neogene Deep Water Benthic Foraminifera From the Northern Indian Ocean (DSDP LEG 22). (Ph.D. thesis), Banaras Hindu University, Varanasi (India).
Gupta, A. K., and Thomas, E. (1999). Latest miocene-pleistocene productivity and deep-sea ventilation in the Northwestern Indian Ocean (deep sea drilling project site 219). Paleoceanography 14, 62–73. doi: 10.1029/1998PA900006
Harman, R. A. (1964). Distribution of foraminifera in the Santa Barbara Basin, California. Micropaleontology 10, 81–96.
Hermelin, J. O. R., and Shimmield, G. B. (1990). The importance of the oxygen minimum zone and sediment geochemistry in the distribution of Recent benthic foraminifera in the northwest Indian Ocean. Mar. Geol. 91, 1–29. doi: 10.1016/0025-3227(90)90130-C
Jannink, N. T., Zachariasse, W. J., and Van der Zwaan, G. J. (1998). Living (rose bengal stained) benthic foraminifera from the Pakistan continental margin (Northern Arabian Sea). Deep Sea Res. 45, 1483–1513. doi: 10.1016/S0967-0637(98)00027-2
Joos, F., Plattner, G.-K., Stocker, T. F., Körtzinger, A., and Wallace, D. W. R. (2003). Trends in marine dissolved oxygen: Implications for ocean circulation changes and the carbon budget. EOS Trans. Am. Geophys. Union 84, 197–201. doi: 10.1029/2003EO210001
Jorissen, F. J. (1999). “Benthic foraminiferal microhabitats below the sediment-water interface,” in Modern Foraminifera, ed B. K. Sen Gupta (Dordrecht: Kluwer Academic Publishers), 161–179.
Jorissen, F. J., Barmawidjaja, D. M., Puskaric, S., and Van der Zwaan, G. J. (1992). Vertical distribution of benthic foraminifera in the northern Adriatic Sea: the relation with the organic flux. Mar. Micropaleontol. 19, 131–146. doi: 10.1016/0377-8398(92)90025-F
Jorissen, F. J., de Stigter, H. C., and Widmark, J. G. V. (1995). A conceptual model explaining benthic foraminiferal microhabitats. Mar. Micropaleontol. 26, 3–15. doi: 10.1016/0377-8398(95)00047-X
Jorissen, F. J., Fontanier, C., and Thomas, E. (2007). “Paleoceanographical proxies based on deep- sea benthic foraminiferal assemblage characteristics,” in Proxies in Late Cenozoic Paleoceanography, eds C. Hillaire-Marcel, and A. De Vernal (Elsevier Publishers), 277–340.
Jorissen, F. J., and Wittling, I. (1999). Ecological evidence from live-dead comparisons of benthic foraminiferal faunas off Cape Blanc (Northwest Africa). Palaeogeogr. Palaeoclimatol. Palaeoecol. 149, 151–170. doi: 10.1016/S0031-0182(98)00198-9
Jorissen, F. J., Wittling, I., Peypouquet, J. P., Rabouille, C., and Relexans, J. C. (1998). Live benthic foraminiferal faunas off Cap Blanc, NW Africa: community structure and microhabitats. Deep Sea Res. I 45, 2157–2188.
Kaiho, K. (1994). Benthic foraminiferal dissolved oxygen index and dissolved oxygen levels in the modern ocean. Geology 22, 719–722. doi: 10.1130/0091-7613(1994)022<0719:BFDOIA>2.3.CO;2
Kaiho, K. (1999). Effect of organic carbon flux and dissolved oxygen on the benthic foraminiferal oxygen index (BFOI). Mar. Micropaleontol. 37, 67–76. doi: 10.1016/S0377-8398(99)00008-0
Kay, S., Caesar, J., and Janes, T. (2018). “Marine dynamics and productivity in the Bay of Bengal,” in Ecosystem Services for Well-Being in Deltas, eds R. Nicholls, C. Hutton, W. Adger, S. Hanson, M. Rahman, M. Salehin. Cham: Palgrave Macmillan. doi: 10.1007/978-3-319-71093-8_14
Kuhnt, W., Hess, S., and Jian, Z. (1999). Quantitative composition of benthic foraminiferal assemblages as a proxy indicator for organic carbon rates in the South China sea. Mar. Geol. 156, 123–157. doi: 10.1016/S0025-3227(98)00176-5
Loeblich, A. R., and Tappan, H. (1988). Foraminiferal Genera and Their Classification. New York, NY: Van Nostrand Reinhold, 715. doi: 10.1007/978-1-4899-5760-3
Loeblich, A. R., and Tappan, H. (1990). “Present status of foraminiferal classification in Studies in Benthic foraminifera,” in Proceedings of the Fourth Symposium on benthic foraminifera, Sendai, eds Y. Takayanagi, and T. Saito. Tokyo, Tokai University Press, 93–102.
Lutze, G. F., Pflaumann, U., and Weinholz, P. (1986). Jungquartäre fluktuationen der benthischen foraminiferen faunen in tiefsee-sedimentenvor nw-afrika – eine reaktion auf productivitätsänderungenim oberfläschenwasser. Meteor Forsch. Ergebn. C40, 163–180.
Lynch-Stieglitz, J., Fairbanks, R. G., and Charles, C. D. (1994). Glacial-interglacial history of Antarctic intermediate water: relative strengths of antarctic versus Indian ocean sources. Paleoceanography 9, 7–29. doi: 10.1029/93PA02446
Ma, R., Sépulcre, S., Licari, L., Bassinot, F., Liu, Z., and Tisnérat-Laborde, N. (2019). Changes in intermediate circulation in the Bay of Bengal since the last glacial maximum as inferred from benthic foraminifera assemblages and geochemical proxies. Geochem. Geophys. Geosyst. 20, 1592–1608. doi: 10.1029/2018GC008179
Mackensen, A., and Douglas, R. G. (1989). Down-core distribution of live and dead deep-water benthic foraminifera in box cores from the Weddell Sea and the California continental borderland. Deep Sea Res. Part I Oceanogr. Res. Pap. 36, 879–900.
Martinson, D. G., Pisias, N. G., Hays, J. D., Imbrie, J., Moore, T. C. Jr, and Shackleton, N. J. (1987). Age dating and the orbital theory of the ice ages: development of a high-resolution 0 to 300,000-year chronostratigraphy. Q. Res. 27, 1–29. doi: 10.1016/0033-5894(87)90046-9
Mazumder, A., and Nigam, R. (2014). Bathymetric preference of four major genera of rectilinear benthic foraminifera within oxygen minimum zone in Arabian Sea off central west coast of India. J. Earth Syst. Sci. 123, 633–639. doi: 10.1007/s12040-014-0419-y
Naqvi, S. W. A., Jayakumar, D. A., Nair, M., Kumar, M. D., and George, M. D. (1994). Nitrous oxide in the western Bay of Bengal. Mar. Chem. 47, 269–278. doi: 10.1016/0304-4203(94)90025-6
Narvekar, J., and Prasanna Kumar, S. (2006). Seasonal variability of the mixed layer in the central Bay of Bengal and associated changes in nutrients and chlorophyll. Deep Sea Res. Part I Oceanogr. Res. Pape. 53, 820–835. doi: 10.1016/j.dsr.2006.01.012
Nigam, R., Mazumder, A., Henriques, P. J., and Saraswat, R. (2007). Benthic foraminifera as proxy for oxygen-depleted conditions off the central west coast of India. J, Geol. Soc. India 70, 1047–1054.
Ohkushi, K., Kennett, J. P., Zeleski, C. M., Moffitt, S. E., Hill, T. M., Robert, C., et al. (2013). Quantified intermediate water oxygenation history of the NE Pacific: A new benthic foraminiferal record from Santa Barbara basin. Paleoceanography 28, 453–467.
Otto, J., Hermelin, R., and Shimmield, G. B. (1995). Impact of productivity events on the benthic foraminiferal fauna in the Arabian sea over the last 150,000 years. Paleoceanography 10, 85–116. doi: 10.1029/94PA02514
Pattan, J. N., Mir, I. A., Parthiban, G., Karapurkar, S. G., Matta, V. M., Naidu, P. D., et al. (2013). Coupling between suboxic condition in sediments of the western Bay of Bengal and southwest monsoon intensification: a geochemical study. Chem. Geol. 343, 55–66. doi: 10.1016/j.chemgeo.2013.02.011
Phleger, F. B. (1951). Ecology of foraminifera, northwest Gulf of Mexico, part 1: foraminifera distribution. Geol. Soc. Am. Memoir 46, 1–88.
Phleger, F. B., and Soutar, A. (1973). Production of benthic foraminifera in three east pacific oxygen minima. Micropaleontology 19, 110–115. doi: 10.2307/1484973
Piotrowski, A. M., Banakar, V. K., Scrivner, A. E., Elderfield, H., Galy, A., and Dennis, A. (2009). Indian ocean circulation and productivity during the last glacial cycle. Earth Planet. Sci. Lett. 285, 179–189. doi: 10.1016/j.epsl.2009.06.007
Prasanna Kumar, S., Muraleedharan, P. M., Prasad, T. G., Gauns, M., Ramaiah, N., de Souza, S. N., et al. (2002). Why is the Bay of Bengal less productive during summer monsoon compared to the Arabian sea? Geophys. Res. Lett. 29, 22–35. doi: 10.1029/2002GL016013
Quinterno, P., and Gardner, J. V. (1987). Benthic foraminifers on the continental shelf and upper slope, Russian River area, northern California. J. Foraminiferal Res. 17, 132–152.
Raju, D. S. N., and Mishra, P. K. (1991). Miogypsinidae from the Andaman basin, India. J. Paleontol. Soc. India 36, 15–30.
Rathburn, A. E., and Corliss, B. H. (1994). The ecology of living (stained) deep-sea benthic foraminifera from the Sulu sea. Paleoceanography 9, 87–150. doi: 10.1029/93PA02327
Rathburn, A. E., Corliss, B. H., Tappa, K. D., and Lohmann, K. C. (1996). Comparison of the ecology and stable isotopic composition of living (stained) benthic foraminifera from the Sulu and South China Seas. Deep Sea Res. Part I 43, 1617–1646.
Rathburn, A. E., Willingham, J., Ziebis, W., Burkett, A. M., and Corliss, B. H. (2018). A new biological proxy for deep-sea paleo-oxygen: pores of epifaunal benthic foraminifera. Sci. Rep. 8:9456. doi: 10.1038/s41598-018-27793-4
Raza, T., Ahmad, S. M., Sahoo, M., Banerjee, B., Bal, I., Dash, S., et al. (2014). Hydrographic changes in the southern Bay of Bengal during the last ~65,000 y inferred from carbon and oxygen isotopes of foraminiferal fossil shells. Q. Int. 333, 77–85. doi: 10.1016/j.quaint.2014.02.010
Sarkar, S., and Gupta, A. K. (2014). Late quaternary productivity changes in the equatorial Indian ocean (ODP Hole 716A). Palaeogeogr. Palaeoclimatol. Palaeoecol. 397, 7–19. doi: 10.1016/j.palaeo.2013.12.002
Schlitzer, R. (2015). Ocean Data View. Available online at: http://odv.awi.de
Schmiedl, G., Hemleben, C., Keller, J., and Segl, M. (1998). Impact of climatic changes on the benthic foraminiferal fauna in the Ionian sea during the last 330, 0000 years. Paleoceanography 13, 447–458. doi: 10.1029/98PA01864
Schmiedl, G., and Leuschner, D. C. (2005). Oxygenation changes in the deep western Arabian sea during the last 190,000 years: productivity versus deepwater circulation. Paleoceanography 20:PA2008. doi: 10.1029/2004PA001044
Schmiedl, G., and Mackensen, A. (1997). Late quaternary paleoproductivity and deep water circulation in the eastern South Atlantic Ocean: Evidence from benthic foraminifera. Palaeogeogr. Palaeoclimatol. Palaeoecol. 130, 43–80.
Schnitker, D. (1994). “Deep-sea benthic foraminifers: food and bottom water masses,” in Carbon Cycling in the Glacial Ocean: Constraints on the Ocean's Role in Global Change. Berlin: Springer, 539–554. doi: 10.1007/978-3-642-78737-9_23
Schott, F., and McCreary, J. P. (2001). The monsoon circulation of the Indian ocean. Prog. Oceanogr. 51, 1–123. doi: 10.1016/S0079-6611(01)00083-0
Schwager, C. (1877). Quadro del proposto sistema di classificazione del foraminiferi con guscio. Boll. Reale Com. Geol. Italia 8, 18–27.
Sen Gupta, B. K. (2002). “Systematics of Modern Foraminifera,” in Modern Foraminifera, ed B. K. Sen Gupta (Dordrecht: Kluwer Academic Publishers), 7–36. doi: 10.1007/0-306-48104-9_2
Sharma, V. (1970). Studies in Late Tertiary Smaller Foraminifera of Car Nicobar Island, Bay of Bengal. (Ph.D. Thesis), Banaras Hindu University, Varanasi (India).
Singh, A. D., Jung, S. J. A., Anand, P., Kroon, D., and Ganeshram, R. S. (2018). Rapid switch in monsoon-wind induced surface hydrographic conditions of the eastern Arabian sea during the last deglaciation. Q. Int. 479, 3–11. doi: 10.1016/j.quaint.2018.03.027
Singh, A. D., Jung, S. J. A., Darling, K., Ganeshram, R., Ivanochko, T., and Kroon, D. (2011). Productivity collapses in the Arabian Sea during glacial cold phases. Paleoceanography 26(3). doi: 10.1029/2009PA001923
Singh, A. D., Rai, A. K., Verma, K., Das, S., and Bharti, S. K. (2015). Benthic foraminiferal diversity response to the climate induced changes in the eastern Arabian Sea oxygen minimum zone during the last 30 ka BP. Q. Int. 374, 118–125. doi: 10.1016/j.quaint.2014.11.052
Singh, D. N. (1979). Studies in Neogene Smaller Foraminifera of Little Andaman Island, Bay of Bengal. Ph. D. Thesis, Banaras Hindu University, (Varanasi), India.
Southon, J., Kashgarian, M., Fontugne, M., Metivier, B., and Yim, W. W.-S. (2002). Marine reservoir corrections for the Indian Ocean and Southeast Asia. Radiocarbon 44, 167–180.
Srinivasan, M. S., and Rajshekhar, C. (1981). New benthic foraminifera from the late cenozoic of ritchie's archipelago, Andaman Sea. Biovigyanam 7, 1–8.
Srinivasan, M. S., and Sharma, V. (1980). Schwager's Car Nicobar foraminifera in the Reports of the Novara Expedition a Revision. New Delhi: Today and Tomorrows Printers and Publishers.
Stuiver, M., and Reimer, P. J. (1993). Extended 14C data base and revised CALIB 3.0 14C age calibration program. Radiocarbon 35, 215–230.
Thomas, E., and Gooday, A. J. (1996). Cenozoic deep-sea benthic foraminifers: tracers for changes in oceanic productivity? Geology 24, 355–358. doi: 10.1130/0091-7613(1996)024<0355:CDSBFT>2.3.CO;2
Tomczak, M., and Godfrey, J. S. (2003). Regional Oceanography: An Introduction, 2nd Edn. Delhi: Daya Publishing House.
Verma, K., Bharti, S. K., and Singh, A. D. (2018). Late Glacial–Holocene record of benthic foraminiferal morphogroups from the eastern Arabian Sea OMZ: paleoenvironmental implications. J. Earth Syst. Sci. 127:21. doi: 10.1007/s12040-018-0920-9
Verma, K., and Singh, A. D. (2019). Genus cibicides from the western Bay of Bengal: relationship to deep sea changes. J. Palaeontol. Soc. India 64, 1–10.
von Rad, U., Schulz, H., Riech, V., den Dulk, M., Berner, U., and Sirocko, F. (1999). Multiple monsoon-controlled breakdown of oxygen-minimum conditions during the past 30,000 years documented in laminated sediments off Pakistan. Palaeogeogr. Palaeoclimatol. Palaeoecol. 152, 129–161. doi: 10.1016/S0031-0182(99)00042-5
Woodruff, F. (1985). Changes in Miocene deep sea benthic foraminiferal distribution in the miocene ocean: paleoceanography and biogeography. Geol. Soc. Am. 163, 131–176. doi: 10.1130/MEM163-p131
You, Y. (1998). Intermediate water circulation and ventilation of the Indian Ocean derived from water-mass contributions. J. Mar. Res. 56, 1029–1067. doi: 10.1357/002224098765173455
Keywords: oxygen minima zone, primary productivity, organic matter flux, Indian monsoon, ventilation, epifaunal and infaunal benthic foraminifera
Citation: Verma K, Singh H, Singh AD, Singh P, Satpathy RK and Naidu PD (2021) Benthic Foraminiferal Response to the Millennial-Scale Variations in Monsoon-Driven Productivity and Deep-Water Oxygenation in the Western Bay of Bengal During the Last 45 ka. Front. Mar. Sci. 8:733365. doi: 10.3389/fmars.2021.733365
Received: 30 June 2021; Accepted: 31 July 2021;
Published: 01 September 2021.
Edited by:
Neloy Khare, Ministry of Earth Sciences, IndiaReviewed by:
Abhi. M, Birbal Sahni Institute of Palaeosciences (BSIP), IndiaRaj K. Singh, Indian Institute of Technology Bhubaneswar, India
Copyright © 2021 Verma, Singh, Singh, Singh, Satpathy and Naidu. This is an open-access article distributed under the terms of the Creative Commons Attribution License (CC BY). The use, distribution or reproduction in other forums is permitted, provided the original author(s) and the copyright owner(s) are credited and that the original publication in this journal is cited, in accordance with accepted academic practice. No use, distribution or reproduction is permitted which does not comply with these terms.
*Correspondence: Arun Deo Singh, arundeosingh@yahoo.com