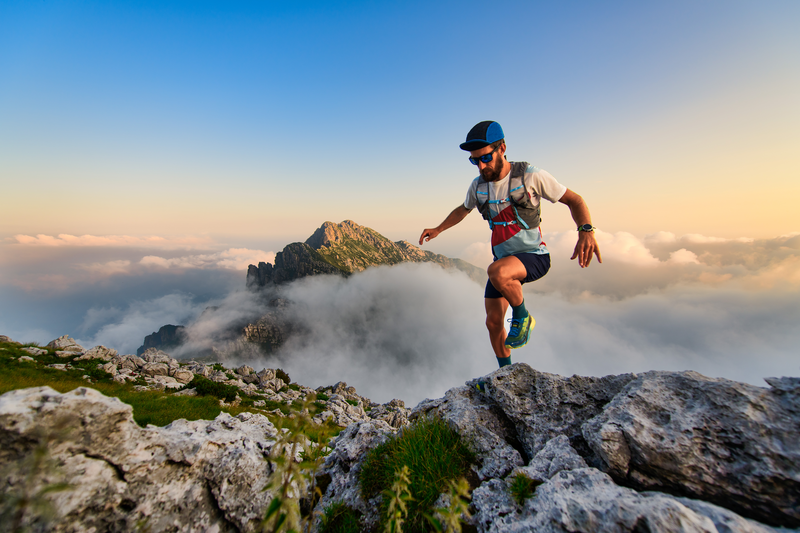
95% of researchers rate our articles as excellent or good
Learn more about the work of our research integrity team to safeguard the quality of each article we publish.
Find out more
ORIGINAL RESEARCH article
Front. Mar. Sci. , 13 October 2021
Sec. Marine Pollution
Volume 8 - 2021 | https://doi.org/10.3389/fmars.2021.728745
This article is part of the Research Topic Plastic Pollution in the Bay Areas View all 14 articles
Microplastics (MPs) contamination is widespread in the coral reef ecosystems leading to the exposure of both corals and other biotas. Knowledge gaps still exist concerning patterns in MPs abundance spatially. This work quantified the MPs abundance and characteristics in the seawater and corals in the Sanya Bay, Hainan Island. MPs abundance was detected in the seawater and coral samples ranging from 15.50 to 22.14 items L–1, and 0.01 to 3.60 items polyp–1, respectively. We found the predominant size and type of MPs in seawater and corals were smaller than 2 mm and fiber. Further analysis revealed that the characteristics of MPs in the corals were significantly different from those in the seawater environment, indicating that the MPs are selectively enriched in corals. Furthermore, the MPs particles ingested and retained in coral tissue may be related to the polyp size. This study shows that MPs are present in the whole coral reef region and the coral community structure would be potentially harmed by these contaminants.
Plastic debris was first noted as a potential coastal water problem in 1971 (Carpenter et al., 1972), and MPs (plastic fragments < 5 mm, MPs) are now a widespread form of contamination in marine ecosystems (Moore, 2008; Andrady, 2011), from the Arctic waters (Lusher et al., 2015) to the remote Islands (Ding et al., 2019; Saliu et al., 2019; Tan et al., 2020; Oldenburg et al., 2021) and the coastal waters (Sutton et al., 2016; Yan et al., 2019; Qi et al., 2020; Tsang et al., 2020; Xie et al., 2021). MPs could enter the marine environment via multiple ways, such as ship transportation, fisheries, and domestic sewage discharges (Lusher et al., 2015; Sutton et al., 2016). MPs can be ingested by diverse marine organisms and potentially be transferred through the food web (Setala et al., 2014; Rochman et al., 2015; Tanaka and Takada, 2016). It is a matter of great concern that the MPs may negatively affect the health of marine organisms (Moore, 2008) by blocking of the intestines (Carpenter et al., 1972), by acting as a vector for diseases (Lamb et al., 2018), and by acting as a carrier for the transport of heavy metals and chemical contaminants (Andrady, 2011; Saliu et al., 2019; Xie et al., 2021). Furthermore, colonization of MPs by sessile organisms provided carriers for the transport of alien species in the marine environment and may threaten the marine ecosystems (Moore, 2008).
Coral reefs are among the most biologically diverse region in the ocean, providing important ecosystem services to many millions of people, while coral reefs are degrading rapidly in response to various environmental and anthropogenic drivers (Hoegh-Guldberg et al., 2007; Hughes et al., 2017). Recently, high MPs abundance was reported in different coral reef ecosystems (Ding et al., 2019; Saliu et al., 2019; Oldenburg et al., 2021; Tang et al., 2021). MPs could be ingested by corals in the laboratory and natural habitats (Rotjan et al., 2019), which poses another threat to these vital organisms globally (Soares et al., 2020). Experimental study indicated that MPs could reduce growth performance, deteriorate health status and change the photosynthetic performance of corals (Reichert et al., 2019). Lamb et al. (2018) found the likelihood of disease increased when corals are in contact with plastic. Rotjan et al. (2019) found MPs ingestion could inhibit food intake of coral. In addition, there was evidence that MPs had negative impacts on their symbiotic association (Okubo et al., 2020; Su et al., 2020). All the research suggested that MPs ingestion might have negative physiological (Reichert et al., 2018; Su et al., 2020; Liao et al., 2021; Tang et al., 2021; Xiao et al., 2021) and ecological (Lamb et al., 2018; Saliu et al., 2019; Soares et al., 2020) consequences for corals.
As the MPs would potentially further magnify the effects of numerous other anthropogenic factors on the coral reefs, including overfishing, recreational activities, and water pollution, there is an urgent need to monitor the MPs in coral reef ecosystems. A recent United Nation Environment Program (UNEP) report also highlighted the critical need for monitoring MPs pollution patterns and ecological relevance in shallow water coral reefs (Sweet et al., 2019). With the MPs pollution in the South China Sea becoming an increasingly serious environmental problem, it is very important to understand the current status of MPs in coral reefs, especially in the fringing reefs. Fringing reefs, characterized by a long-term reef growth process from an attached shoreline, are always heavily impacted by human activities and natural causes.
The objective of this study was to investigate the abundance and characteristics of MPs in two reef-building corals (Acropora millepora, Galaxea fascicularis) and their living seawater environments in the Sanya Bay, Hainan Island. The coral G. fascicularis is one of the dominant species, while A. millepora is the common species within the study area (Sun et al., 2018). We sought to examine the difference and relationship of MPs contained in corals and seawater. This study would contribute to understand the potential impacts of MPs contamination on reef ecosystems and improve the strategies for local plastic waste management.
Sanya Bay is a typical tropical bay in China, located in the southern part (109°20′–109°30′E, 18°11′–18°18′N) of Hainan Island, with a sea area covers about 120 km2 and an average depth of 16 m. Xidao Island, Dongdao Island located in the middle of the bay with narrow fringing reefs surrounded, and Xiaozhou Island and Luhuitou Peninsula possess the typical fringing reef. The Sanya River located in the eastern part of the bay, characterized by a length of 31.3 km, drainage area of 337 km2 (Composed of Liuluo River, Shuijiao River, and Banling River. The mainstream Liuluo River originates from the southern foothill of the Zhongjian Mountain and flows from the north to the south.), and mean annual flow of 5.86 m3s–1. The annual mean seawater surface temperature (SST) and salinity in the bay are 26.9°C and 32.7‰ respectively (Lian et al., 2010).
The water and coral samples were collected from four sites in Sanya Bay, Hainan Island in November 2019, as showed in Figure 1. These sampling sites can be divided into two different regions. Sites Xidao (XD) and Dongdao (DD) were on the islets not far from the shore were treated as offshore sites (OS), while the sites Xiaozhoudao (XZD) and Luhuitou (LHT) were on the fringing reef along the shore were defined as inshore sites (IS). Both of the two types of sites suffered dense influence by human activities.
Figure 1. Sample sites in Sanya Bay, China. XD, Xidao; DD, Dongdao; XZD, Xiaozhoudao; LHT, Luhuitou.
All tools and containers were dealt with Milli-Q water before sampling. A total of 30 L seawater was collected from each site with a 5 L plexiglass water sampler for this study. Two replicates of 15 L of seawater were then filtered using a 45 μm pore size stainless steel sieve with a glass fiber membrane of 0.45 μm. The filtered residue on the sieves and membranes was washed with Milli-Q water and transferred into a 50 mL glass bottle as one sample. Samples were stored at −20°C before analysis.
Three coral colonies of Acropora millepora, Galaxea fascicularis in each sampling sites were collected using a hammer and a chisel by SCUBA diving, rinsed with pre-filtered seawater (filtered through a stainless sieve with 30 μm pore size with a glass fiber membrane of 0.45 μm), and preserved in a glass bottle with 95% ethanol immediately for transportation. All corals and seawater samples were collected at depths of 3–4 m at each sites.
The preparation of seawater and coral samples was performed with reference to previous studies (Hidalgo-Ruz et al., 2012; Catarino et al., 2017; Rotjan et al., 2019). In order to dissolve the organic matter in the seawater, samples were treated with 30 mL H2O2 (30%, V/V) for 24 h at room temperature (Nuelle et al., 2014; Yan et al., 2019). Then, the digested solution was filtered through 0.45 μm glass-fiber membrane (GF/F, 47 mm Ø, Whatman). The filters were transferred to covered glass petri dishes and air-dried for further analysis.
As the reef corals are colonial, meaning that the individuals called polyps are connected together by the coenosarc (a portion of continuous tissue that links polyps together). In the laboratory, fixed corals were removed from ethanol using metal forceps, cut into about 1 cm2 rectangle pieces (4–33 polyps), rinsed with Milli-Q water to remove surface debris, air-dried in a covered glass petri dish and weighed. Polyp number was recorded. Corals were then decalcified with a 1.0% HCl solution for 12 h (Rotjan et al., 2019), filtered using a glass-fiber membrane of 0.45 μm, and then back washed into a sterile glass vial (Rotjan et al., 2019; Oldenburg et al., 2021). After that, 150 ml NaOH (10%, V/V) was added to the vial to digest any organic tissue residue, and then the vials were placed in a 60 °C drying oven for at least 24 h to allow for digestion of organic material (Catarino et al., 2017). Following this incubation, all samples were rinsed thoroughly with Milli-Q water onto a 0.45 μm glass-fiber membrane to remove NaOH residue. Each cleaned sample was washed back into a glass vial, and then the contents of the vial were pipetted onto a glass microscope slide and placed in the drying oven at 60 °C until the sample was completely dried onto the slide.
Blank control samples were prepared similarly to the sample preparation protocol. For the seawater blank control samples, 15 L Milli-Q water and 30 mL H2O2 (30%, V/V) were filtered onto a 0.45 μm glass-fiber membrane, then the filtered residue on the sieves and membranes was washed with Milli-Q water and transferred into a 50 mL glass container as blank control. For the coral blank control samples, the sieve and membrane were washed with 100 mL Milli-Q water, added 10 mL 1.0% HCl solution and 150 mL NaOH (10%, V/V), filtered through a 0.45 μm glass-fiber membrane, rinsed and backwashed with Milli-Q water into a clean glass container, and then pipetted onto a glass microscope slide.
A stereomicroscope (M165 FC, Leica, Germany) was used for visually identifying and measuring the MPs according to their physical characteristics (Hidalgo-Ruz et al., 2012). The number, type (film, granule, fragment, fiber, fiber bundle), color (black, red, blue, green, transparent, other), and size of the plastics were recorded. According to the size, MPs are divided into five classifications: < 0.5, 0.5–1, 1–2, 2–3, and 3–5 mm.
For each sample and control, every MPs on the petri dish or slide was counted. Counts were conducted in triplicate for each sample. The mean value of controls was subtracted from the mean value of each sample. The unit of MPs abundance for seawater samples was reported as the number of particles per liter (items L–1), while for the coral samples, the values were divided by the polyp number of the sample to calculate the number of MPs per polyp (items polyp–1).
Additionally, a total of 121 representative particles from different types of samples were detected and identified by a micro-Raman spectrometer (Thermo Fisher Scientific DXR2, United States). Each measurement was performed with sixteen scans. All the suspected polymer types of the spectroscopy results were confirmed by comparison with the Raman polymer spectrum libraries that were applicable to the spectrometer. Only matches with the confidence levels of 70% at least was considered reliable and accepted (after visual inspection). Finally, the visual identification data were adjusted according to the Raman identification results before analysis.
In order to prevent potential pollution, all the containers were rinsed at least three times with Milli-Q water before being used. The microscope, lab bench, fume hood and drying oven were cleaned with 95% ethanol; meanwhile, the cotton lab coats and nitrile gloves were worn throughout the experiment. Prepared samples and the controls were stored in desiccator made of glass to reduce their exposure to the ambient environment. Furthermore, blank experiments without seawater, coral tissue were arranged simultaneously to correct and evaluate background MPs contamination during the experiment.
Means, standard error (SE) were calculated for each site or coral species. Prior to analysis, the raw data were checked for normality and homogeneity of variances by the Shapiro-Wilk test and Brown-Forsythe test, respectively, and data were log(x + +1)-transformed when necessary. The difference between the abundance of the four sample sites was analyzed with the one-way analysis of variance (one-way ANOVA). A two-way ANOVA was used to assess the effects of site and coral species on MPs abundance (Tables 1, 2). Tukey HSD post hoc tests were used to evaluate the significance of each pairwise comparison if factors were found to be significant (p < 0.05) in the ANOVA. Principal Coordinate analysis (PCO) was performed using Euclidean distance matrices to illustrate the clustering of the MPs characteristics between the seawater and coral samples. The MPs characteristics of the four sites in both seawater and corals were summarized using PCO combined with cluster analysis based on log(x + 1)-transformed/normalized of the raw data, including size, color and polymer type. Analyses were performed using Sigmaplot 14 and PRIMER 7 + PERMANOVA considering a 5% significance level (Anderson et al., 2008; Clarke and Gorley, 2015).
Table 1. Results of analysis of variance (ANOVA) to test the effect of coral species, site, and coral species: site on microfiber abundance in corals.
Table 2. Significant results of Tukey HSD test to assess the effect of coral species, site, and coral species: site on MPs abundance in corals.
MPs were detected in all the seawater and coral samples from the four coral reef stations in the Sanya Bay of Hainan Island (Figures 2A–D). Items classified as potential plastics were identified in all of the 32 samples. A total of 121 particles were identified, 81% were less than 5 mm in size classifying them as MPs. MPs particles abundance in the seawater ranging from 15.50 ± 1.50 items L–1 at DD to 22.14 ± 0.90 items L–1 at XZD, with an average of 18.37 ± 2.60 items L–1. The abundance at the XZD was significantly higher than that in the DD, and average MPs abundance in the seawater were higher in the IS (20.29 ± 1.16 items L–1) than in the OS (16.45 ± 0.85 items L–1) (p < 0.05) (Figures 2A,B).
Figure 2. MPs abundance in seawater (A,B) and corals (C,D). XD, Xidao; DD, Dongdao; XZD, Xiaozhoudao; LHT, Luhuitou. Shared letters above the bars indicate there were not statistically different as determined by Tukey’s HSD post hoc test.
The average MPs abundance in A. millepora was 0.27 ± 0.26 items polyp–1 whereas that in G. fascicularis was 2.32 ± 0.86 items polyp–1, and there was a significant difference between them (p < 0.001) (Table 1 and Figures 2C,D). There was no statistical difference was measured in coral A. millepora among the sample sites, and similar result in the coral G. fascicularis except between XZD and DD (Table 2). Within the four sample sites, coral G. fascicularis contained significantly more MPs than that in coral A. millepora, and the MPs abundance in the two corals differed significantly between each other at the same site (Figures 2C,D and Table 2). In addition, coral A. millepora in the IS and OS contained no different abundance of MPs while coral G. fascicularis differed from each other significantly (Figure 2D).
Both in the seawater and coral samples, more than 60 and 80% of the MPs were smaller than 1 and 2 mm, respectively, while those > 3 mm contributed the least to them (varied from 1.39 to 6.27%) (Figure 3A and Supplementary Table 3). MPs size of < 0.5 mm accounted for the largest proportion in seawater (42.83%), while the size of 0.5–1 mm accounted for the largest proportion in corals (A. millepora: 36.63%, G. fascicularis: 37.62%) (Figure 3A).
Figure 3. Proportion of MPs size (A), type (B), color (C) and composition (D) in seawater and coral samples. AM, A. millepora; GF, G. fascicularis.
Five types of MPs shapes were detected in the seawater and coral samples, including fiber, granule, fiber bundle, fragment and film, but the vast majority was fiber both in the seawater (77.18%) and corals (A. millepora: 92.46%, G. fascicularis: 90.34%) (Figure 3B).
The most common color of MPs detected in seawater was black, accounting for 58.63%, however, the most frequent color of MPs detected in corals was transparent, accounting for 66.82%, 69.94% in A. millepora, G. fascicularis, respectively (Figure 3C).
The identified MPs compounds included polyethylene terephthalate (PET), cellophane (CP), polyethylene (PE), polystyrene (PS), polyamide (PA), polypropylene (PP), and polypropylene–polyethylene (PP–PE). Results revealed that PET and CP were the dominant components of MPs both in the seawater and coral samples. In terms of the seawater samples, PET accounted for the highest proportion (47.02%), followed by CP (46.30%). As for the coral samples, CP component accounted for the majority (54.76%), followed by PET (35.12%) (Figure 3D).
The ordination biplot from PCO combined with cluster analysis clearly showed the separation between the characteristics of MPs contended in seawater and coral samples (Figure 4). The coral groups and seawater groups clustered separately first and then clustered with each other. The first two axes (PCO1 and PCO2) explained a total of 59.1% variance from the characteristics data of the seawater and coral samples. These findings implied that most of the tested seawater samples had similar MPs characteristics, and so did the corals. The characteristics of MPs in seawater had a close relationship except DD, whereas the features in the two coral species closed to each other within the same site.
Figure 4. PCO combined with cluster analysis showed the MPs distribution patterns in coral and seawater. XD, Xidao; DD, Dongdao; XZD, Xiaozhoudao; LHT, Luhuitou; AM, A. millepora; GF, G. fascicularis.
The present study carried out the investigation of MPs abundance and distribution characteristics in both seawater and corals among the coral reef systems of Sanya Bay of Hainan Island. The results provided evidence that the coral reef region in Sanya Bay is extensively affected by MPs pollution, which indicates that reef organisms in surrounding seawater are suffering extensively from MPs.
Our study observed the spatial differences in the extent of MPs contamination levels in the seawater of the Sanya Bay reef region. The average abundance of MPs in the four detected sites was 15.50 items L–1 which was greater in IS than in the OS. These variations could be linked to the sampling location and the impact degree of anthropogenic activities. The abundance of MPs in the XZD was significantly higher compared with the DD. This is because the XZD is located close to the Sanya River estuary and intensively affected by nearby human activities, while DD locates in relatively to the open-water site and under the control of the military. Another reason is that the rapid tourism industry development has driven the economic growth in Sanya since the late 1990s, and the increasing coastal development and urbanization have resulted in sewage discharge and increased levels of terrestrial runoff (Qiu, 2013). Additionally, due to the behavior of the visitors has not been effectively supervised (Haiying et al., 2018), visitors may discard plastics randomly or accidentally, such as bags, bottles and cutlery, directly into the sea.
The MPs abundance in the seawater were similar to that reported in the east coast of Hainan Island (14.9 items L–1) (Tang et al., 2021) and in the lagoon of the Xisha Islands (15.9 items L–1) (Ding et al., 2019). However, this was much higher than the other observations in the South China Sea, such as the Hong Kong coast, Haikou Bay, Pearl River Estuary, the Xisha Islands, and the Nansha Islands (Cai et al., 2018; Ding et al., 2019; Huang et al., 2019; Nie et al., 2019; Wang et al., 2019; Yan et al., 2019; Qi et al., 2020; Tan et al., 2020; Tsang et al., 2020; Xie et al., 2021).
Additionally, the difference in MPs abundance might due to the different sampling methods. In our study, the seawater were sampled by plexiglass water sampler and all the particles above 45 μm were collected, while the other collected by plankton net (153 μm) (Tsang et al., 2020) or nylon Neuston nets (333 μm) (Cai et al., 2018; Qi et al., 2020; Tan et al., 2020). The MPs with the size range of 153–333 μm could be easily lost when sampling. Therefore, this suggests the need to establish standardized methods to evaluate the MPs pollution status more efficiently and make the data obtained more comparable.
Our results showed that the MPs of smaller size (<1 mm), fibrous types, black, and PET and CP accounted for the largest proportion in the reef region. Similar results were also observed in other coral reef environment (Cai et al., 2018; Ding et al., 2019; Huang et al., 2019; Tan et al., 2020; Tang et al., 2021). Many studies reported that smaller size of MPs (<1 mm) were the most dominant in the human activity-intense areas, such as the Pearl River Estuary (Yan et al., 2019), Haikou Bay (Qi et al., 2020), and the east coast of Hainan Island (Tang et al., 2021). The higher proportion of fibers was also observed in Arctic polar water (Lusher et al., 2015), the San Francisco Bay (Sutton et al., 2016), and the Xisha Islands (Ding et al., 2019). Studies showed that the main sources of fibers may be related to intensive ship transportation, fisheries, and domestic sewage discharges (Lusher et al., 2015; Sutton et al., 2016; Forero-Lopez et al., 2021).
Additionally, PET and CP are widely used in the daily life as the packaging materials (Tang et al., 2021). Besides, the dominant color of black in the seawater was also observed in the Haikou Bay, the Bahía Blanca Estuary, and estuary in the Gulf of Mexico (Qi et al., 2020; Forero-Lopez et al., 2021; Sanchez-Hernandez et al., 2021). MPs fibers were mostly black, blue, and transparent in general, and high proportions of black MPs are mainly sourced from tires, abrasives and plastic bags (Lassen et al., 2015). Moreover, mechanical damage in some fibers (e.g., fractures and color fading) can be associated with the weathering of polymers in seawater, while the digestion process of some animals could aggravate the physical damage in fibers (Forero-Lopez et al., 2021). For example, a recent study reported there were 90% black and blue fibers in the abdominal muscle and digestive tract of the shrimp Pleoticus muelleri (Fernandez Severini et al., 2020).
Overall, our results revealed that MPs were widely distributed in the coral reef ecosystems of Sanya Bay. Spatially, the highest MPs abundance in seawater was found at XZD, and the high MPs concentrations may be associated with the local human activities, while the other reasons such as hydrological factors (water movement, etc.) require further assessment.
In the present investigation, the MPs abundance of the two corals differed significantly from each other among the sampling area. At each sampling site, the high MPs abundance in coral polyps corresponds to the high abundance in seawater, and the highest MPs concentration was found in G. fascicularis (3.34 items polyp–1) in XZD, of which the majority were fibers, consistent with the results by Tang et al. (2021). The higher feeding rates of G. fascicularis (Hii et al., 2009) probably resulted in the higher concentration in the tissue than that in the coral A. millepora, and the high MPs concentration in the seawater could increase the probability of corals ingesting the MPs particles.
Unlike the MPs in the seawater were dominantly black, most of those in coral tissue were transparent. Transparent MPs, as well as the size (20-200 μm) and shape (slender), are similar to many phytoplankton and microalgae species (Ding et al., 2019; Nie et al., 2019; Keshavarzifard et al., 2021). A recent study found the transparent MPs were the most abundant (43%) in tissues of shrimp Metapenaeus affinis, which could pretend to be their food sources (Keshavarzifard et al., 2021). Ding et al. (2019) reported that transparent MPs were preferentially selective in capturing prey by fish due to their size was closer to the diatoms. Besides, the transparent fiber with a slender shape was similar to little jellyfish or zooplankton might result in being easily mistakenly ingested by marine animals, and then enriched in their organs (Nie et al., 2019), because some low-nutrient organisms were more readily available and easily exposed to suspended microplastics (reviewed in Yang et al., 2021). Thus, the high proportion of transparent particles in coral tissue may be due to their higher heterotrophic ability and therefore such MPs were ingested by mistake.
Martin et al. (2019) found A. hemprichii with small-size polyps (∼0.5 mm) preferably ingested and retained smaller MPs particles, while no preference was observed for Goniastrea retiformis with large polyps (2–3 mm), and this result was further confirmed by our present study as the major size in the coral tissue was smaller than 1 mm. In our study, the polyp size of A. millepora and G. fascicularis is 0.4–1.6 mm and 4.0–10.2 mm, respectively. The size of MPs particles ingested and retained in corals most likely relates to the size of the polyp (Martin et al., 2019). Besides, MPs attached frequently on the corals surfaces were detected (Reichert et al., 2018; Ding et al., 2019; Martin et al., 2019), indicating that this phenomenon would serve as a potential sink for marine MPs (Martin et al., 2019). However, MPs ingestion can inhibit food intake (Rotjan et al., 2019), which in turn induce a variety of negative impacts on the health of corals (Soares et al., 2020), as well as on the coral-Symbiodiniaceae symbiosis (Okubo et al., 2020; Su et al., 2020). All these results revealed that MPs are an emerging threat to coral reefs globally and thus have a long-term effect on population dynamics.
The PCO combined with cluster analysis clearly separated the characteristics of MPs contended in seawater and coral samples implied that the characteristics of MPs in the two coral species differed from those in the seawater. An investigation along the east coast of Hainan Island revealed the characteristics of MPs enriched by two scleractinian coral differed from those in the environmental samples (Tang et al., 2021), and a study of Xisha Islands reported there was no apparent relationship between MPs distribution in seawater and corals (Ding et al., 2019).
The proportion of the MPs size of smaller than 2 mm, type of fragment, color of black, and the PET in the seawater samples were significant higher than those in coral samples, while the size of 0.5–1 mm, type of fiber, color of transparent, and the CP in the coral samples were significant higher than those in seawater samples. A previous study found MPs characteristics (size, shape and color) in reef-dwellers and the environments formed two distinct clusters, while variations were much smaller within the same group and mostly clustered together (Tang et al., 2021). Consistent with these previous studies, this study further revealed that MPs characteristics differed greatly in seawater and organisms, which was confirmed by the two distinct clusters between seawater and corals. DD was the outlier of the seawater cluster, and the MPs abundance in the seawater was the lowest among all the seawater sample sites due to the military control, while the proportion of the MPs size of smaller than 0.5 mm, type of fragment, and color of blue and green was the highest among all sites.
Although the MPs abundance was significantly different in the two corals, there were many overlaps within the coral cluster indicating that the MPs in the tissue of the two corals shared many similar characteristics, which highlights the conclusions that corals could selectively enrich MPs from the environment (Martin et al., 2019; Tang et al., 2021).
This is the first investigation to show the presence and distribution of MPs both in corals and seawater within the coral reef area of Sanya Bay, Hainan Island. The results showed widespread MPs contamination in the coral reef ecosystems in this research region. Difference in the spatial distribution of seawater may be related to the discharge from Sanya River and the intensive anthropogenic activities. The most frequently measured MPs we sampled were smaller than 2 mm, and shape of fiber, reiterating the strong presence of fiber as a contaminant in the ocean. Further analysis revealed that the MPs characteristics in the coral species were different from those in the seawater, indicating the potential selective enrichment in the corals. Furthermore, the size of MPs (0.5–1 mm) dominant in coral tissue suggests that the MPs particles ingested by corals most likely relates to the size of polyps. Corals are interacting with MPs in their natural habitats reflecting the coral communities to be potentially harmed by these contaminants. However, the origins and pathways bringing MPs to the Sanya Bay remain unclear. Further research is required to understand the effects of microplastic-coral interaction within this productive ecosystem. Ultimately, understanding the abundance, spatial distribution, and potential sources of MPs in coral reefs will help improve regional management of plastic waste in vital coastal ecosystems.
The original contributions presented in the study are included in the article/Supplementary Material, further inquiries can be directed to the corresponding author/s.
XL and HC collected the samples and analyzed the data. XL, HC, YL, YS, LJ, YZ, GZ, and HH wrote and revised the manuscript. All authors designed the experiment and collected the data.
This research was funded by the National Natural Science Foundation of China (Grant Nos. 41306144, 41876192, and 41976120), the Guangdong Basic and Applied Basic Research Foundation (Grant No. 2019A1515011532), the Key Research and Development Project of Hainan Province, China (Grant No. ZDYF2020200), the Science and Technology Planning Project of Guangdong Province, China (Grant No. 2020B1212060058), the Innovation Academy of South China Sea Ecology and Environmental Engineering, Chinese Academy of Sciences (Grant No. ISEE2021ZD03).
The authors declare that the research was conducted in the absence of any commercial or financial relationships that could be construed as a potential conflict of interest.
All claims expressed in this article are solely those of the authors and do not necessarily represent those of their affiliated organizations, or those of the publisher, the editors and the reviewers. Any product that may be evaluated in this article, or claim that may be made by its manufacturer, is not guaranteed or endorsed by the publisher.
The Supplementary Material for this article can be found online at: https://www.frontiersin.org/articles/10.3389/fmars.2021.728745/full#supplementary-material
Anderson, M. J., Gorley, R. N., and Clarke, K. R. (2008). PERMANOVA+ for PRIMER Guide To Software And Statistical Methods. Plymouth: PRIMER-E Ltd.
Andrady, A. L. (2011). Microplastics in the marine environment. Mar. Pollut. Bull. 62, 1596–1605. doi: 10.1016/j.marpolbul.2011.05.030
Cai, M. G., He, H. X., Liu, M. Y., Li, S. W., Tang, G. W., Wang, W. M., et al. (2018). Lost but can’t be neglected: huge quantities of small microplastics hide in the South China Sea. Sci. Total Envir. 633, 1206–1216. doi: 10.1016/j.scitotenv.2018.03.197
Carpenter, E. J., Anderson, S. J., Harvey, G. R., Miklas, H. P., and Peck, B. B. (1972). Polystyrene spherules in coastal waters. Science 178, 749–750. doi: 10.1126/science.178.4062.749
Catarino, A. I., Thompson, R., Sanderson, W., and Henry, T. B. (2017). Development and optimization of a standard method for extraction of microplastics in mussels by enzyme digestion of soft tissues. Environ. Toxicol. Chem. 36, 947–951. doi: 10.1002/etc.3608
Ding, J., Jiang, F., Li, J., Wang, Z., Sun, C., Wang, Z., et al. (2019). Microplastics in the coral reef systems from xisha islands of South China Sea. Environ. Sci. Technol. 53, 8036–8046. doi: 10.1021/acs.est.9b01452
Fernandez Severini, M. D., Buzzi, N. S., Forero Lopez, A. D., Colombo, C. V., Chatelain Sartor, G. L., Rimondino, G. N., et al. (2020). Chemical composition and abundance of microplastics in the muscle of commercial shrimp Pleoticus muelleri at an impacted coastal environment (Southwestern Atlantic). Mar. Pollut. Bull. 161(Pt A):111700. doi: 10.1016/j.marpolbul.2020.111700
Forero-Lopez, A. D., Rimondino, G. N., Truchet, D. M., Colombo, C. V., Buzzi, N. S., Malanca, F. E., et al. (2021). Occurrence, distribution, and characterization of suspended microplastics in a highly impacted estuarine wetland in Argentina. Sci. Total Environ. 785:147141. doi: 10.1016/j.scitotenv.2021.147141
Haiying, C., Linsi, H., Peng, L., Xiaohong, Z., and Changjiang, Y. (2018). Relationship of stakeholders in protected areas and tourism ecological compensation: a case study of sanya coral reef national nature reserve in China. J. Resour. Ecol. 9, 164–173. doi: 10.5814/j.issn.1674-764x.2018.02.006
Hidalgo-Ruz, V., Gutow, L., Thompson, R. C., and Thiel, M. (2012). Microplastics in the marine environment: a review of the methods used for identification and quantification. Environ. Sci. Technol. 46, 3060–3075. doi: 10.1021/es2031505
Hii, Y. S., Soo, C. L., and Liew, H. C. (2009). Feeding of scleractinian coral, Galaxea fascicularis, on Artemia salina nauplii in captivity. Aquac. Int. 17, 363–376. doi: 10.1007/s10499-008-9208-4
Hoegh-Guldberg, O., Mumby, P. J., Hooten, A. J., Steneck, R. S., Greenfield, P., Gomez, E., et al. (2007). Coral reefs under rapid climate change and ocean acidification. Science 318, 1737–1742. doi: 10.1126/science.1152509
Huang, Y., Yan, M., Xu, K., Nie, H., Gong, H., and Wang, J. (2019). Distribution characteristics of microplastics in Zhubi Reef from South China Sea. Environ. Pollut. 255(Pt 1):113133. doi: 10.1016/j.envpol.2019.113133
Hughes, T. P., Barnes, M. L., Bellwood, D. R., Cinner, J. E., Cumming, G. S., Jackson, J. B. C., et al. (2017). Coral reefs in the Anthropocene. Nature 546, 82–90. doi: 10.1038/nature22901
Keshavarzifard, M., Vazirzadeh, A., and Sharifinia, M. (2021). Occurrence and characterization of microplastics in white shrimp, Metapenaeus affinis, living in a habitat highly affected by anthropogenic pressures, northwest Persian Gulf. Mar. Pollut. Bull. 169:112581. doi: 10.1016/j.marpolbul.2021.112581
Lamb, J. B., Willis, B. L., Fiorenza, E. A., Couch, C. S., Howard, R., Rader, D. N., et al. (2018). Plastic waste associated with disease on coral reefs. Science 359, 460–462. doi: 10.1126/science.aar3320
Lassen, C., Hansen, S. F., Magnusson, K., Norén, F., Hartmann, N. I. B., Jensen, P. R., et al. (2015). Microplastics-Occurrence, Effects and Sources of Releases to the Environment in Denmark. Environmental Project No. 1793. Copenhagen K: The Danish Environmental Protection Agency. Available online at: https://www.tilogaard.dk/Miljostyrelsens_rapport_om_mikroplast_978-87-93352-80-3.pdf
Lian, J. S., Huang, H., Huang, L. M., and Wang, D. R. (2010). Coral Reef And Its Biodiversity Of Sanya. Beijing: Marine Press.
Liao, B., Wang, J., Xiao, B., Yang, X., Xie, Z., Li, D., et al. (2021). Effects of acute microplastic exposure on physiological parameters in Tubastrea aurea corals. Mar. Pollut. Bull. 165:112173. doi: 10.1016/j.marpolbul.2021.112173
Lusher, A. L., Tirelli, V., O’Connor, I., and Officer, R. (2015). Microplastics in Arctic polar waters: the first reported values of particles in surface and sub-surface samples. Sci. Rep. 5:14947. doi: 10.1038/srep14947
Martin, C., Corona, E., Mahadik, G. A., and Duarte, C. M. (2019). Adhesion to coral surface as a potential sink for marine microplastics. Environ. Pollut. 255(Pt 2):113281. doi: 10.1016/j.envpol.2019.113281
Moore, C. J. (2008). Synthetic polymers in the marine environment: a rapidly increasing, long-term threat. Environ. Res. 108, 131–139. doi: 10.1016/j.envres.2008.07.025
Nie, H., Wang, J., Xu, K., Huang, Y., and Yan, M. (2019). Microplastic pollution in water and fish samples around Nanxun Reef in Nansha Islands, South China Sea. Sci. Total Environ. 696:134022. doi: 10.1016/j.scitotenv.2019.134022
Nuelle, M. T., Dekiff, J. H., Remy, D., and Fries, E. (2014). A new analytical approach for monitoring microplastics in marine sediments. Environ. Pollut. 184, 161–169. doi: 10.1016/j.envpol.2013.07.027
Okubo, N., Tamura-Nakano, M., and Watanabe, T. (2020). Experimental observation of microplastics invading the endoderm of anthozoan polyps. Mar. Environ. Res. 162:105125. doi: 10.1016/j.marenvres.2020.105125
Oldenburg, K. S., Urban-Rich, J., Castillo, K. D., and Baumann, J. H. (2021). Microfiber abundance associated with coral tissue varies geographically on the Belize Mesoamerican Barrier Reef System. Mar. Pollut. Bull. 163:111938. doi: 10.1016/j.marpolbul.2020.111938
Qi, H. Y., Fu, D. D., Wang, Z. Z., Gao, M. Y., and Peng, L. C. (2020). Microplastics occurrence and spatial distribution in seawater and sediment of Haikou Bay in the northern South China Sea. Estuar. Coast. Shelf Sci. 239:106757. doi: 10.1016/j.ecss.2020.106757
Qiu, W. (2013). The sanya coral reef national marine nature reserve, China: a governance analysis. Mar. Policy 41, 50–56. doi: 10.1016/j.marpol.2012.12.030
Reichert, J., Arnold, A. L., Hoogenboom, M. O., Schubert, P., and Wilke, T. (2019). Impacts of microplastics on growth and health of hermatypic corals are species-specific. Environ. Pollut. 254(Pt B):113074. doi: 10.1016/j.envpol.2019.113074
Reichert, J., Schellenberg, J., Schubert, P., and Wilke, T. (2018). Responses of reef building corals to microplastic exposure. Environ. Pollut. 237, 955–960. doi: 10.1016/j.envpol.2017.11.006
Rochman, C. M., Tahir, A., Williams, S. L., Baxa, D. V., Lam, R., Miller, J. T., et al. (2015). Anthropogenic debris in seafood: plastic debris and fibers from textiles in fish and bivalves sold for human consumption. Sci. Rep. 5:14340. doi: 10.1038/srep14340
Rotjan, R. D., Sharp, K. H., Gauthier, A. E., Yelton, R., Lopez, E. M. B., Carilli, J., et al. (2019). Patterns, dynamics and consequences of microplastic ingestion by the temperate coral, Astrangia poculata. Proc. Biol. Sci. 286:20190726. doi: 10.1098/rspb.2019.0726
Saliu, F., Montano, S., Leoni, B., Lasagni, M., and Galli, P. (2019). Microplastics as a threat to coral reef environments: detection of phthalate esters in neuston and scleractinian corals from the Faafu Atoll, Maldives. Mar. Pollut. Bull. 142, 234–241. doi: 10.1016/j.marpolbul.2019.03.043
Sanchez-Hernandez, L. J., Ramirez-Romero, P., Rodriguez-Gonzalez, F., Ramos-Sanchez, V. H., Marquez Montes, R. A., Romero-Paredes Rubio, H., et al. (2021). Seasonal evidences of microplastics in environmental matrices of a tourist dominated urban estuary in Gulf of Mexico, Mexico. Chemosphere 277:130261. doi: 10.1016/j.chemosphere.2021.130261
Setala, O., Fleming-Lehtinen, V., and Lehtiniemi, M. (2014). Ingestion and transfer of microplastics in the planktonic food web. Environ. Pollut. 185, 77–83. doi: 10.1016/j.envpol.2013.10.013
Soares, M. O., Matos, E., Lucas, C., Rizzo, L., Allcock, L., and Rossi, S. (2020). Microplastics in corals: an emergent threat. Mar. Pollut. Bull. 161(Pt A):111810. doi: 10.1016/j.marpolbul.2020.111810
Su, Y., Zhang, K., Zhou, Z., Wang, J., Yang, X., Tang, J., et al. (2020). Microplastic exposure represses the growth of endosymbiotic dinoflagellate Cladocopium goreaui in culture through affecting its apoptosis and metabolism. Chemosphere 244:125485. doi: 10.1016/j.chemosphere.2019.125485
Sun, Y., Lei, X., Lian, J., Yang, J., Wu, Y., and Huang, H. (2018). Ecosystem status and health assessment of sanya coral reef national nature reserve. Biodivers. Sci. 26, 258–265. doi: 10.17520/biods.2017312
Sutton, R., Mason, S. A., Stanek, S. K., Willis-Norton, E., Wren, I. F., and Box, C. (2016). Microplastic contamination in the San Francisco Bay, California, USA. Mar. Pollut. Bull. 109, 230–235. doi: 10.1016/j.marpolbul.2016.05.077
Sweet, M., Stelfox, M., and Lamb, J. (2019). Plastics And Shallow Water Coral Reefs: Synthesis Of The Science For Policy-Makers. Nairobi: United Nations Environment Program.
Tan, F., Yang, H., Xu, X., Fang, Z., Xu, H., Shi, Q., et al. (2020). Microplastic pollution around remote uninhabited coral reefs of Nansha Islands, South China Sea. Sci. Total Environ. 725:138383. doi: 10.1016/j.scitotenv.2020.138383
Tanaka, K., and Takada, H. (2016). Microplastic fragments and microbeads in digestive tracts of planktivorous fish from urban coastal waters. Sci. Rep. 6:34351. doi: 10.1038/srep34351
Tang, J., Wu, Z., Wan, L., Cai, W., Chen, S., Wang, X., et al. (2021). Differential enrichment and physiological impacts of ingested microplastics in scleractinian corals in situ. J. Hazard. Mater. 404(Pt B):124205. doi: 10.1016/j.jhazmat.2020.124205
Tsang, Y. Y., Mak, C. W., Liebich, C., Lam, S. W., Sze, E. T. P., and Chan, K. M. (2020). Spatial and temporal variations of coastal microplastic pollution in Hong Kong. Mar. Pollut. Bull. 161(Pt B):111765. doi: 10.1016/j.marpolbul.2020.111765
Wang, T., Zou, X., Li, B., Yao, Y., Zang, Z., Li, Y., et al. (2019). Preliminary study of the source apportionment and diversity of microplastics: taking floating microplastics in the South China Sea as an example. Environ. Pollut. 245, 965–974. doi: 10.1016/j.envpol.2018.10.110
Xiao, B., Li, D., Liao, B., Zheng, H., Yang, X., Xie, Y., et al. (2021). Effects of Microplastics Exposure on the Acropora sp. Antioxidant, immunization and energy metabolism enzyme activities. Front. Microbiol. 12:666100. doi: 10.3389/fmicb.2021.666100
Xie, Q., Li, H. X., Lin, L., Li, Z. L., Huang, J. S., and Xu, X. R. (2021). Characteristics of expanded polystyrene microplastics on island beaches in the Pearl River Estuary: abundance, size, surface texture and their metals-carrying capacity. Ecotoxicology 30, 1632–1643. doi: 10.1007/s10646-020-02329-7
Yan, M., Nie, H., Xu, K., He, Y., Hu, Y., Huang, Y., et al. (2019). Microplastic abundance, distribution and composition in the Pearl River along Guangzhou city and Pearl River estuary, China. Chemosphere 217, 879–886. doi: 10.1016/j.chemosphere.2018.11.093
Keywords: microplastics, seawater, corals, Sanya Bay, distribution
Citation: Lei X, Cheng H, Luo Y, Zhang Y, Jiang L, Sun Y, Zhou G and Huang H (2021) Abundance and Characteristics of Microplastics in Seawater and Corals From Reef Region of Sanya Bay, China. Front. Mar. Sci. 8:728745. doi: 10.3389/fmars.2021.728745
Received: 22 June 2021; Accepted: 16 September 2021;
Published: 13 October 2021.
Edited by:
Xiaoshan Zhu, Tsinghua University, ChinaReviewed by:
Youji Wang, Shanghai Ocean University, ChinaCopyright © 2021 Lei, Cheng, Luo, Zhang, Jiang, Sun, Zhou and Huang. This is an open-access article distributed under the terms of the Creative Commons Attribution License (CC BY). The use, distribution or reproduction in other forums is permitted, provided the original author(s) and the copyright owner(s) are credited and that the original publication in this journal is cited, in accordance with accepted academic practice. No use, distribution or reproduction is permitted which does not comply with these terms.
*Correspondence: Hui Huang, aHVhbmdodWlAc2NzaW8uYWMuY24=
Disclaimer: All claims expressed in this article are solely those of the authors and do not necessarily represent those of their affiliated organizations, or those of the publisher, the editors and the reviewers. Any product that may be evaluated in this article or claim that may be made by its manufacturer is not guaranteed or endorsed by the publisher.
Research integrity at Frontiers
Learn more about the work of our research integrity team to safeguard the quality of each article we publish.