- 1Institute of Oceanology, Chinese Academy of Sciences, Qingdao, China
- 2University of Chinese Academy of Sciences, Beijing, China
- 3State Key Laboratory of Agricultural Microbiology, Huazhong Agricultural University, Wuhan, China
- 4Hubei Key Laboratory of Agricultural Bioinformatics, College of Informatics, Huazhong Agricultural University, Wuhan, China
- 5Center for Ocean Mega-Science, Chinese Academy of Sciences, Qingdao, China
- 6Function Laboratory of Marine Ecology and Environmental Sciences, Qingdao National Laboratory for Marine Science and Technology, Qingdao, China
The nitrogen cycle is an indispensable part of the biogeochemical cycle, and the reactions that occur in the ocean oxygen minimum zone (OMZ) mediate much of the loss of nitrogen from oceans worldwide. Here, nitrate-utilizing bacteria were isolated from the water column at 17 stations within the OMZ of the Changjiang (Yangtze River) Estuary using selective media and a culture-dependent method. The microbial diversity, nitrogen metabolism and nitrate reduction test of culturable heterotrophic bacteria were examined. A total of 164 isolates were obtained; they were mostly affiliated with Proteobacteria (81.1%), Actinobacteria (5.5%), Bacteroidetes (12.3%), and Firmicutes (0.6%). Pseudomonas aeruginosa, Sphingobium naphthae, and Zunongwangia profunda were found at most stations. Among 24 tested representative strains, 8 were positive for nitrate reduction; they belonged to genera Aurantimonas, Halomonas, Marinobacter, Pseudomonas, Thalassospira, and Vibrio. Pseudomonas aeruginosa contained the genes (napAB, norBC, nirS, and nosZ) for complete denitrification and may be responsible for mediating denitrification. 66% representative isolates (16/24) contained genes for reducing nitrate to nitrite (nasA, napAB, or narGHI) and 79% representative isolates (19/24) possessed genes for converting nitrite to ammonia (nirA or nirBD), suggesting that nitrate and nitrite could act as electron acceptors to generate ammonium, subsequently being utilized as a reduced nitrogen source. This study improves our understanding of the microbial diversity within the OMZ of Changjiang Estuary and may facilitate the cultivation and exploitation of bacteria involved in the nitrogen cycle.
Introduction
In the environment, the oxidation state of nitrogen ranges from -3 in ammonium (NH4+) to + 5 (NO3–), and is governed by several processes. The nitrogen cycle comprises the steps of nitrogen fixation, mineralization, nitrification, anammox, and denitrification (Hayatsu et al., 2008). After nitrogen fixation, nitrification and denitrification carry N return inorganic pool and return it to the atmosphere (Munn, 2011). Some regions experience an imbalance in the input and output of nitrogen: An example of this is the oxygen minimum zones (OMZs) of the world’s oceans, which are responsible for approximately 30–50% of the loss of nitrogen from these bodies of water (Codispoti et al., 2001).
Changjiang Estuary is located in the Western Pacific Ocean, East of China. A seasonal OMZ was discovered here at the end of the twentieth Century; its current range is from about 32°−30°N to the southern coastal sea (Chen et al., 2007). The main cause of this hypoxic zone is the inflow of a large amount of fresh water from the Changjiang River coupled with the upward invasion of the Taiwan Warm Current in summer, which leads to a seawater stratification that prevents the vertical transmission of oxygen (Zhang et al., 2007). Under suboxic or anoxic conditions, NO3– and NO2– are used as terminal electron acceptors by denitrifying bacteria in dissimilatory NO3– reduction (denitrification) (Wright et al., 2012). Because of the low oxygen concentration in the OMZ (dissolved oxygen < 2–3 mg/L) (Wang et al., 2012), denitrification that use inorganic nitrogen and release dinitrogen gas (Lam and Kuypers, 2011), are very active (Lam et al., 2009). The accumulation of NO2– within oceanic OMZs provides an ideal niche for microbes.
Most previous studies on the prokaryotes responsible for sustaining the nitrogen cycle in the OMZ of the Changjiang Estuary have focused on sedimentary organisms and used culture-independent methods (Dang et al., 2008; Li et al., 2009; Chen et al., 2014; He et al., 2016; Wu et al., 2019). Although important biogeochemical processes take place in the sediment, the metabolic activity and individual characteristics of microorganisms that mediate the loss of N are poorly known. Admittedly, there are limitations of culture method in the ecology exploration, but culture-based studies can allow microorganisms to be isolated from natural samples and enable researchers to perform physiological/experimental assays and elaborate metabolic activities during biogeochemical cycles, and thereby infer the functional and ecological roles of the microorganisms in detail (Mulla et al., 2018; Sanz-Saez et al., 2020). Here, we used a culture-dependent method to investigate microorganisms that participate in the nitrogen cycle in the seawater column of the Changjiang Estuary OMZ, aiming to: (1) explore the diversity of the culturable nitrate-utilizing bacteria; (2) explore nitrogen metabolism based on their genomes data; (3) test nitrate reduction of the isolated strains; (4) investigate the relationship between their distribution and environmental parameters (dissolved oxygen, nitrate, and nitrite).
Methodology
Sampling Areas and Sampling
Seawater samples were collected from the OMZ of Changjiang Estuary by the “Science III” scientific research ship in August 2020. A total of 61 seawater samples were collected using a CTD (conductivity–temperature–depth) apparatus from 17 stations at varying depths (2–6 depths per station). The stations were designated and located as follows (Figure 1): 3000-2 (30.00°N, 123.00°E), 3050-2 (30.49°N, 123.02°E), 3100-1 (30.90°N, 122.45°E), 3100-2 (30.98°N, 122.86°E), 3100-2a (31.01°N, 123.25°E), 3100-3 (31°N, 123.50°E), 3150-2 (31.40°N, 123.00°E), CJ-02 (31.79°N, 123.00°E), DH4-0 (29.63°N, 122.83°E), DH45 (29.36°N, 122.48°E), DH5-0 (29.14°N, 122.48°E), DH5-1a (29.02°N, 122.67°E), DH5-2 (28.90°N, 122.87°E), DH5-2a (28.78°N, 123.07°E), DH5-3 (28.65°N, 123.28°E), DH56 (28.81°N, 122.36°E), and DH6-1 (28.45°N, 122.18°E).
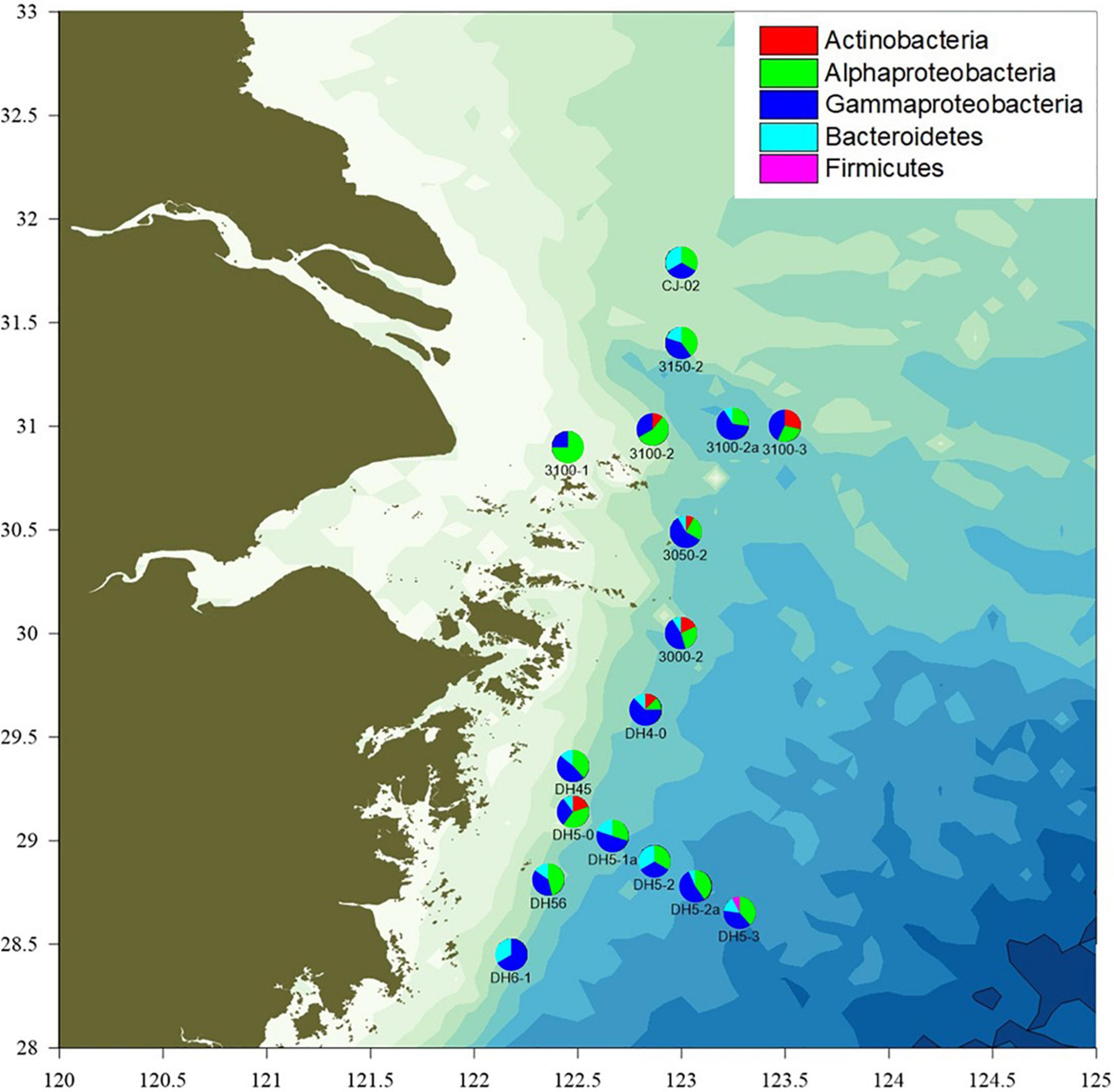
Figure 1. The sampling locations and proportion of isolates classified to each phylum and (partly) class in the Changjiang River Estuary OMZ.
Environmental Parameters
Each sample was placed in a 50-ml brown iodine flask and fixed with manganese sulfate and an alkaline potassium iodide solution, and dissolved oxygen (DO) was measured in situ by Winkler iodometry at a relative standard deviation of 2% (Ma et al., 2020). The sample was filtered through a glass-fiber membrane with a pore size of 0.7 μm (Whatman GF/F, United Kingdom), and frozen at −20°C. In the laboratory, the concentrations of nitrate (NO3-N) and nitrite (NO2-N) were measured using an automatic nutrient analyzer (SEAL QuAAtro, Germany). Their detection limits were 0.02 and 0.01 μmol/L, respectively (Ma et al., 2019, 2020).
Isolation, Culture, and Purification
On board the boat, the obtained seawater samples were immediately plated on nitrate agar medium (NAM; 0.2% KNO3, 0.02% MgSO4⋅7H2O, 0.08% K2HPO4⋅3H2O, 2% NaKC4H4O6, 1% NaCl, 2% agar) which allowed the growth of nitrate-utilizing bacteria using a clean bench. After incubation at 25°C in constant temperature incubator for 7–14 days, single colonies were selected and subcultured to achieve purity. The pure isolates were stored as a suspension in 20% (w/v) glycerol at -80°C.
DNA Extraction, 16SrDNA Amplification, and Genome Sequencing
Genomic DNA was extracted using a bacterial genomic DNA Mini kit (TaKaRa Bio) following the manufacturer’s protocol. The 16S rRNA gene was amplified by PCR with a pair of universal primers (Zhang et al., 2006). The reaction system contained 1 μl of forward and reverse oligonucleotide primers, 1 μl exacted DNA, 12.5 μl 2 × PCR Mix (100 mM KCl, 20 mM Tris-HCl, 3 mM MgCl2, 400 μM dNTPs, 0.1 U/μl Taq polymerase), and ddH2O to a final volume of 25 μl. The cycling conditions were: 94°C for 30 s, 55°C for 30 s, and 72°C for 1 min for 35 cycles, with a final extension at 72°C for 10 min. The PCR products was purified using a QIAquick PCR Purification kit (Qiagen). The purified 16S rDNA PCR product was sequenced using dideoxy chain termination/cycle sequencing, as applied with an ABI 3730XL sequencer (Applied Biosystems) and version 3.1 of the ABI Big Dye Terminator kit (Applied Biosystems). We compared the produced 16S rDNA sequences with those in the Ezbiocloud database to identify the isolates (Yoon et al., 2017). Strains having > 98% 16S rRNA gene sequence similarity to a given species and matching with the same species in a GenBank search were assigned to that species (Zhang et al., 2012).
Genome Sequencing, Assembly and Annotation
A paired-end library with an insert size of 350 bp was constructed for each genome, and sequenced using Illumina NovaSeq 6000 platform. The resulting 150 bp paired-end reads with about 200X, were quality checked and assembled using FastQC (v0.11.9) and SPAdes genome assembler v3.15.2 (Prjibelski et al., 2020). De Braiju graph-based assembly was tested with K-mers between 21 and 77, and the quality of the assemblies were evaluated by BUSCO (5.0.0) (Manni et al., 2021). Genes prediction and annotation of all genomes were generated using Prokka v1.14.6 (Seemann, 2014).
Phylogenetic Tree Construction
To clarify the taxonomic status and evolutionary relationship among strains, proteins in 24 genomes were divided into Orthologous groups using OrthoFinder version 2.5.2 (Emms and Kelly, 2017, 2018) and 197 single-copy genes were shared in all genomes. Briefly, an all-to-all Blast was set as E-value < 1 × 10–3, and the BLAST BIT scores were standardized based on gene length and phylogenetic distance. For each of these single-copy genes, all protein sequences were aligned using MAFFT v7.480 (Katoh and Standley, 2013). Each lineal homologous genome was selected to construct a gene tree by FastTree (Letunic and Bork, 2021) and constructed Species Tree using STRIDE algorithm. ITOL (Letunic and Bork, 2021) was used to visualize the phylogenetic tree.
Analysis of Nitrogen Metabolism
To identify genes for nitrogen metabolism, all of the annotated genes were searched against the KEEG database using KAAS BBH BLAST (GENES data set: prokaryotes) (Moriya et al., 2007). Genes belonging to different nitrogen metabolism types were classified by manual selection according to the results of KAAS.
Nitrate Reduction Test
Representative strains were tested for their ability to utilize nitrate. Each pure isolate was transferred to nitrate liquid medium (1 g/L KNO3, 10 g/L peptone, 1 L sterile seawater) and incubated at 25°C for 7 days. The presence of nitrite was measured in culture media using Griess reagents as previously described (Dong and Cai, 2001). Briefly, 2 drops of Griess A and Griess B reagents (Haibo Reagent, China) were sequentially added to the liquid medium. After incubation for 2 min, the test sample was compared with the control. If the test sample was red, pink, or orange, there was nitrite in the tube and the isolate was scored as positive for nitrate reduction and marked as “ + ” If no color appeared, zinc dust was added. The lack of any color change at this point was taken as indicating that there was no residual nitrate in the liquid medium, meaning that the strain could reduce nitrate completely; this was marked as “ ++ ” If the liquid medium changed from colorless to red, pink, or orange after the addition of the zinc dust (as did the control), indicating the continued lack of nitrite, the strain was considered to be unable to reduce nitrate, and was marked as“−”
Results and Discussion
Diversity of the Culturable Nitrate-Utilizing Bacteria
A total of 164 isolates were obtained from the different seawater layers sampled at 17 different stations. Most (63.4%) of the NAM-derived isolates belonged to Pseudomonas (57), Sphingobium (43), followed by Alteromonas (10), and Zunongwangia (21) (Supplementary Figure 1). Based on phenotypic characteristics (colony morphology and pigmentation) and 16S rRNA gene sequencing, 24/164 isolates were identified as potentially unique (<98% sequence similarity) and further studied (Supplementary Table 1). The majority of the potentially unique strains (81.1%) belonged to phylum Proteobacteria, with a predominance of Gammaproteobacteria (45.7%) and Alphaproteobacteria (35.4%). The other strains belonged to phyla Actinobacteria (5.5%), Bacteroidetes (12.3%), and Firmicutes (0.6%). These four phyla were previously isolated from surface seawater samples obtained from Changjiang Estuary and adjacent areas, as assessed using a culture-independent molecular approach (Wang et al., 2017). The Proteobacteria represent a major group (phylum) among the well-known and readily cultivable marine microorganisms, and hence are of biological significance (Rusch et al., 2007; Sunagawa et al., 2015; Fernandes et al., 2019). Studies on surface sediments of the hypoxic zone near the Changjiang Estuary and in the East China Sea also revealed that the bacterial community composition mainly comprised Gammaproteobacteria (Ye et al., 2016).
Vibrio spp. are distributed worldwide in coastal and ocean waters and sediments. In this study, we isolated some Vibrio spp. that are reported to be pathogenic, such as fish pathogenic Vibrio (Vibrio anguillarum) and crustacean pathogenic Vibrio campbellii (Faruque and Nair, 2006). A previous study found that Vibrio campbellii was dominant in Changjiang Estuary (Wang et al., 2020). The appearance of these Vibrio spp. in Changjiang Estuary is notable given that their abundance was previously found to relate to the plankton community and human disease (Vezzulli et al., 2016). Sphingobium soli appeared in a surface seawater sample (site 3100-2; 0 m); as this strain was originally isolated from soil (Du et al., 2015), we speculate that it may have been washed into sea by the Changjiang River. Six genera found in the OMZ water column of Changjiang Estuary were previously reported in other OMZs: Halomonas (Arabian Sea, South Pacific), Marinobacter (Arabian Sea, South Pacific), Pseudomonas (South Pacific), Vibrio (Arabian Sea, South Pacific, North Pacific), Marinomonas (South Pacific), and Marinobacter (Arabian Sea) (Stevens and Ulloa, 2008; Beman and Carolan, 2013; Mulla et al., 2018; Fernandes et al., 2020).
Numerous strains of Pseudomonas aeruginosa (52/164) were isolated in the present study, suggesting that Pseudomonas aeruginosa may be widespread in the seawater of Changjiang Estuary. As a well-known ubiquitous environmental bacterium, Pseudomonas aeruginosa is one of the top three causes of opportunistic human infections (Stover et al., 2000). Its presence in rivers and seawater is commonly attributed to contamination from domestic sewage (Devicente et al., 1991). Considering that Changjiang Estuary is adjacent to densely populated metropolises, such as Shanghai City and Hangzhou City, it is not surprising that Pseudomonas aeruginosa was found to be abundant in the water column. In recent years, the continuous expansion of the Changjiang Estuary’s summer OMZ has shown a close relationship with the input of nutrients from runoff of Changjiang River (Chi et al., 2017). These nutrients also lead to environmental problems, such as reduced water quality and red blooms, keeping the ecosystem of Changjiang Estuary in a sub-healthy state.
Ecology-Focused Statistical Analysis
The number of species (S), the number of isolates (N), the species richness (Margalef index, d), the species evenness (Pielou’s evenness, J’), and the species diversity (Shannon index, H’) are shown in Supplementary Table 2. The culturable bacteria diversity (H’) was the highest at station 3100_2a and lowest atstation 3100-1, where only two different species were isolated.
Denitrification Potential of Strains
Using a culture-dependent method has the advantage of enabling researchers to investigate the physiological and biochemical properties of microorganisms that are rare (even down to a single cell) in a sample. Here, we used the Griess test to analyze 24 representative strains from NAM for their ability to reduce nitrate. Of them, 8 strains were found to reduce nitrate: 4 strains (Vibrio natriegens, Vibrio campbellii, Halomonas meridiana, and Marinobacter nauticus) exhibited a strong positive reaction and could reduce nitrate completely during a 7-day incubation (Supplementary Table 3); other strains positive for nitrate reduction included Pseudomonas aeruginosa, Vibrio azureus, Aurantimonas coralicida, and Thalassospira povalilytica.
Most of the nitrate reduction-positive strains were not widely distributed in the OMZ. An exception was Pseudomonas aeruginosa, which could reduce nitrate and was found at almost all of the test stations (16/17); it was present in 37 water samples and represented 31.7% of the strains isolated from NAM. Although the representatives of Pseudomonas aeruginosa isolated in the present study did not reduce nitrate completely under the utilized aerobic conditions, the wide distribution of this microbe as a dominant isolate and its ability to utilize a denitrification mechanism under anaerobic conditions prompt us to speculate that Pseudomonas aeruginosa may be the mediator of the loss of nitrogen from the OMZ of Changjiang Estuary.
Nitrogen Metabolism of Representative Isolates
The genomes of 24 representative isolates were annotated for understanding the metabolic potentials based on the key genes of metabolic pathways of nitrogen. In the environment, nitrate levels are influenced by the microbially driven processes of nitrate reduction, whereby nitrate is reduced initially to nitrite, which subsequently can be reduced by denitrification or via assimilatory or dissimilatory nitrate reduction to ammonia (ANR or DNRA) (Smith et al., 2007). As shown in Figure 2, most representative isolates possessed genes involved in ANR or DNRA, and were lack of genes involved in final three steps in denitrification pathway (nirKS, norBC, and nosZ).
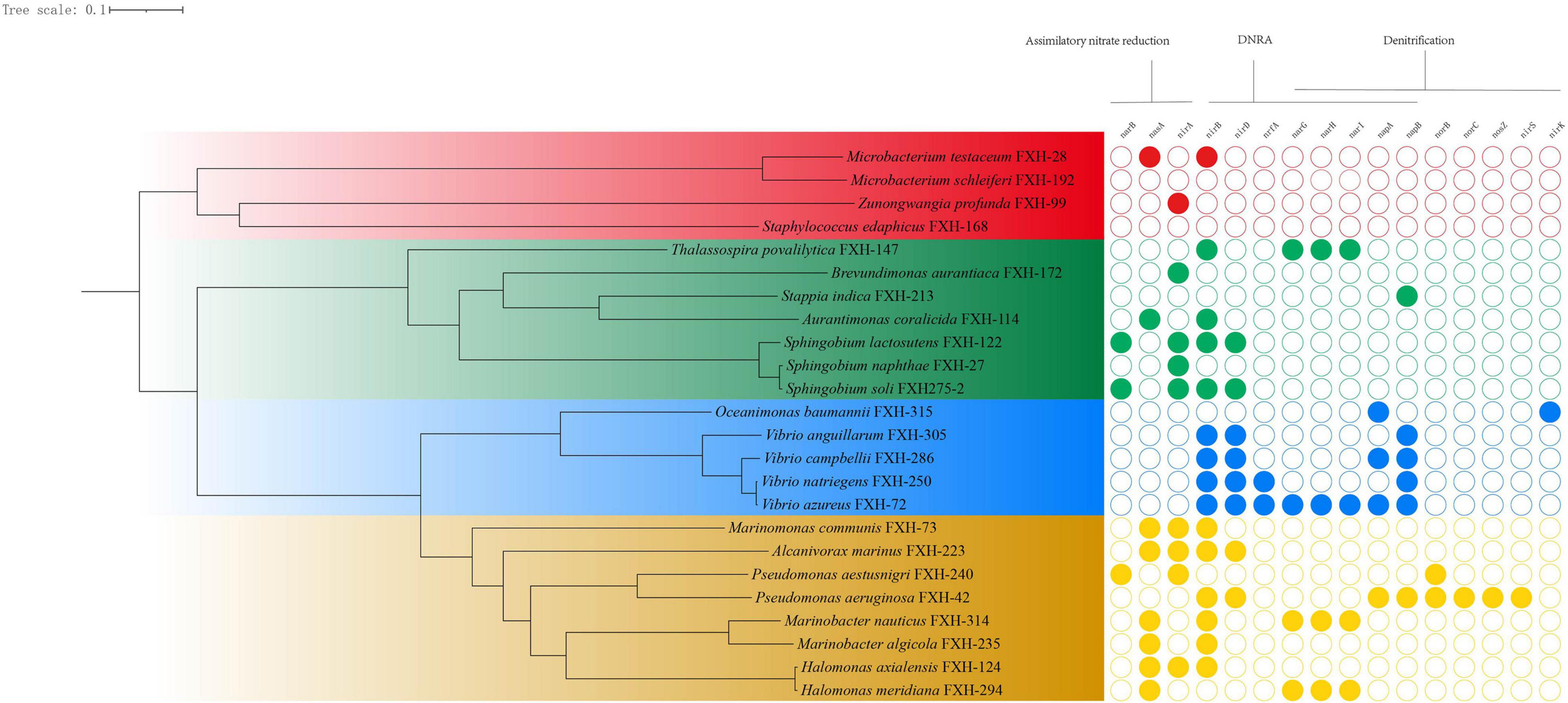
Figure 2. The genomic phylogenetic tree of representative isolates and corresponding genes involved in nitrogen metabolism.
Sixty six percent representative isolates (16/24) contained genes for reducing nitrate to nitrite (nasA, napAB, or narGHI) and 79% representative isolates (19/24) possesses genes for converting nitrite to ammonia (nirA or nirBD), suggesting that nitrate and nitrite could act as electron acceptors to generate ammonium, subsequently being utilized as a reduced nitrogen source. For example, all 4 Vibrio isolates contained the genes (nirBD and napB) for DNRA, suggesting that Vibrio isolates may be involved in nitrate reduction to ammonia.
Analysis of the genome sequence revealed Pseudomonas aeruginosa FXH-42 had a complete set of the denitrification enzymes (NapAB, NorBC, NirS, and NosZ) that reduce nitrate to molecular nitrogen via nitrite, nitric oxide (NO), and nitrous oxygen (NO2), which is consistent with the previous reports (Ye et al., 1995; Arai, 2011). Oceanimonas baumannii FXH-315 possessed the genes for nitrate reductase (napA) and nitrite reductase (nirK), but lack of the genes for nitrous oxide reductase (norBC) and nitrous oxide reductase (nosZ, functional for yielding N2), suggesting that it had the potential to release NO in the OMZ of the Changjiang Estuary.
The Relationship Between Bacterial Distribution and Dissolved Oxygen, NO2–-N, and NO3-N
By testing the DO, NO2–-N, and NO3–-N levels of different sites, we found that DO consistently decrease with increasing depth, whereas the levels of NO2–-N and NO3–-N varied with location. Across the sampled sites, NO2–-N ranged from 1.029 μmol/L (3150-2, 10 m) to 0 (DH5-3, 0 m) and NO3–-N from 27.96 μmol/L (3100-1, 3 m) to 0 μmol/L (DH5-2a, 3 m) (Figure 3). Water depth also had important effects on the composition of microorganisms. Especially in the OMZ of Changjiang Estuary, hypoxia is caused by water stratification, which didn’t affect creatures of the surface seawater, but causes the microbial structure in the lower (hypoxic) strata to be completely different from that at the water surface (Levin, 2003). The literature lacks a strict definition of hypoxic zone; 2 mg/L DO has been regarded as the limit of hypoxia in Changjiang Estuary (Guo et al., 2019), but a DO below 4∼6 mg/L will reportedly impact the survival of aquatic organisms (Gray et al., 2002).

Figure 3. Distribution of isolates at different levels of DO, NO2–-N, NO3–-N at the 17 different sampling stations.
A previous study used pyrosequencing of 16S rRNA genes to show that bacterial richness decreased with DO, and that it was maximal at the edge of the OMZ and decreased within it (Beman and Carolan, 2013). Pseudomonas aeruginosa, Sphingobium naphthae, and Zunongwangia profunda were found at most stations with different DO, NO3-N and NO2-N values. Pseudomonas aeruginosa, Sphingobium naphthae, Zunongwangia profunda, Microbacterium testaceum, and Halomonas axialensis were found in zones with DO values lower than 3 mg/L. Pseudomonas aeruginosa was unique at hypoxia zone (DO < 2 mg/L), while Marinobacter algicola, Sphingobium soli, Vibrio natriegens, Vibrio anguillarum, and Thalassospira povalilytica could only exist at oxygen-rich zones (DO > 7 mg/L) on the surface of the ocean. The numbers of species increased significantly in mid-oxygen zone (4 mg/L < DO < 6 mg/L).
Vibrio natriegens and Halomonas meridian which showed strong positive for nitrate reduction and contained genes for DNRA, were isolated from sites with high levels of nitrate (24.5 and 18.6 μmol/L) and nitrite (0.5 and 0.8 μmol/L), respectively. Microbacterium testaceum was only found in seawater where DO was lower than 4 mg/L, but the values of NO2–-N and NO3–-N were relatively high. Similarly, Stappia indica was located in seawater with low DO and high NO2–-N and NO3–-N values while Thalassospira povalilytica which contained genes (nirB and narGHI) for DNRA was distributed in seawater with high DO and low NO2–-N and NO3–-N values.
Conclusion
In the present research, a total of 24 representative strains were isolated. The majority of these strains belonged to phylum Proteobacteria, with a predominance of Gammaproteobacteria and Alphaproteobacteria. Of 24 representative isolates from NAM, 8 had the ability to reduce nitrate. Pseudomonas aeruginosa, Sphingobium naphthae, and Zunongwangia profunda were found at most stations. Genome analysis indicated that 66% representative isolates contained genes for reducing nitrate to nitrite (nasA, napAB, or narGHI) and 79% representative isolates possessed genes for converting nitrite to ammonia (nirA or nirBD), suggesting that nitrate and nitrite could act as electron acceptors to generate ammonium, subsequently being utilized as a reduced nitrogen source. Pseudomonas aeruginosa contained the genes (napAB, norBC, nirS, and nosZ) for complete denitrification and may be a mediator of denitrification within the OMZ of Changjiang Estuary. This study improves our understanding of the microbial diversity within the OMZ of Changjiang Estuary from the perspective of the cultivation and potential exploitation of culturable nitrate-utilizing bacteria involved in the nitrogen cycle.
Data Availability Statement
All sequences generated in this study have been deposited to GenBank. Representative isolates correspond to accession numbers MZ127529, MZ127532, MZ127533; MZ127538, MZ127545, MZ127546, MZ127551, MZ127556, MZ127560, MZ127562, MZ127564, MZ127565, MZ127566, MZ127567, MZ127569, MZ127571, MZ127572, MZ127573, MZ127576, MZ127578, MZ127579, MZ127580, MZ127582, and MZ127584. The genomes supporting the reported results have been deposited to GenBank under the BioProjectID PRJNA770179.
Author Contributions
XL and DZ designed this research. XL collected the seawater samples and tested environmental parameters. WH and ZJ isolated the strains and performed 16SrDNA amplification and DNA sequencing. SL and JZ performed the genome sequencing, assembly and annotation and analysis of the geomes and nitrogen metabolism. WH tested nitrate reduction of strains. WH and SL drafted the manuscript. XL and DZ supervised the study and contributed to text preparation and revised the manuscript. All authors read and approved the final manuscript.
Funding
This research work was supported by the National Natural Science Foundation of China (No. 91958103), the Strategic Priority Research Program of the Chinese Academy of Sciences (No. XDA23050501), and Shandong Provincial Natural Science Foundation (No. ZR2020YQ28).
Conflict of Interest
The authors declare that the research was conducted in the absence of any commercial or financial relationships that could be construed as a potential conflict of interest.
Publisher’s Note
All claims expressed in this article are solely those of the authors and do not necessarily represent those of their affiliated organizations, or those of the publisher, the editors and the reviewers. Any product that may be evaluated in this article, or claim that may be made by its manufacturer, is not guaranteed or endorsed by the publisher.
Supplementary Material
The Supplementary Material for this article can be found online at: https://www.frontiersin.org/articles/10.3389/fmars.2021.720413/full#supplementary-material
Supplementary Figure 1 | The phylum (A) and genus-level (B) composition of bacteria isolated from NAM.
References
Arai, H. (2011). Regulation and function of versatile aerobic and anaerobic respiratory metabolism in Pseudomonas aeruginosa. Front. Microbiol. 2:103. doi: 10.3389/fmicb.2011.00103
Beman, J. M., and Carolan, M. T. (2013). Deoxygenation alters bacterial diversity and community composition in the ocean’s largest oxygen minimum zone. Nat. Commun. 4:2705. doi: 10.1038/ncomms3705
Chen, C. C., Gong, G. C., and Shiah, F. K. (2007). Hypoxia in the East China Sea: one of the largest coastal low-oxygen areas in the world. Mar. Environ. Res. 644, 399–408. doi: 10.1016/j.marenvres.2007.01.007
Chen, Y., Zhen, Y., He, H., Lu, X., Mi, T., and Yu, Z. (2014). Diversity, abundance, and spatial distribution of ammonia-oxidizing beta-Proteobacteria in sediments from Changjiang Estuary and its adjacent area in East China Sea. Microb. Ecol. 67, 788–803. doi: 10.1007/s00248-013-0341-x
Chi, L., Song, X., Yuan, Y., Zhou, P., Cao, X., and Yu, Z. (2017). Distribution of dissolved oxygen in the Yellow Sea and East China Sea in summer and winter. Oceanol. Limnol. Sin. 48, 1337–1345.
Codispoti, L. A., Brandes, J. A., Christensen, J. P., Devol, A. H., Naqvi, S. W. A., Paerl, H. W., et al. (2001). The oceanic fixed nitrogen and nitrous oxide budgets: Moving targets as we enter the anthropocene? Sci. Mar. 65, 85–105. doi: 10.3989/scimar.2001.65s285
Dang, H., Zhang, X., Sun, J., Li, T., Zhang, Z., and Yang, G. (2008). Diversity and spatial distribution of sediment ammonia-oxidizing crenarchaeota in response to estuarine and environmental gradients in the Changjiang Estuary and East China Sea. Microbiology 154, 2084–2095. doi: 10.1099/mic.0.2007/013581-0
Devicente, A., Codina, J. C., and Romero, P. (1991). Relationship between Pseudomonas aeruginosa and bacterial indicators in polluted natural waters. Water Sci. Technol. 242, 121–124. doi: 10.2166/wst.1991.0041
Dong, X. Z., and Cai, M. Y. (eds) (2001). Determinative Manual for Routine Bacteriology. Beijing: Scientific Press.
Du, J., Singh, H., Yang, J. E., Yin, C. S., Kook, M., Yu, H., et al. (2015). Sphingobium soli sp nov isolated from rhizosphere soil of a rose. Antonie Van Leeuwenhoek 108, 1091–1097.
Emms, D. M., and Kelly, S. (2017). STRIDE: species tree root inference from gene duplication events. Mol. Biol. Evol. 34, 3267–3278. doi: 10.1093/molbev/msx259
Emms, D. M., and Kelly, S. (2018). STAG: species tree inference from all genes. bioRxiv [Preprint]. doi: 10.1101/267914
Faruque, S., and Nair, B. (2006). “Epidemiology,” in The Biology of Vibrios, eds F. L. Thompson, B. Austin, and J. Swings (Washington, DC: ASM Press), 385–398.
Fernandes, G. L., Shenoy, B. D., and Damare, S. R. (2020). Diversity of bacterial community in the oxygen minimum zones of Arabian sea and bay of Bengal as deduced by Illumina sequencing. Front. Microbiol. 10:3153. doi: 10.3389/fmicb.2019.03153
Fernandes, G. L., Shenoy, B. D., Menezes, L. D., Meena, R. M., and Damare, S. R. (2019). Prokaryotic diversity in oxygen depleted waters of the bay of Bengal inferred using culture-dependent and -independent methods. Indian J. Microbiol. 592, 193–199. doi: 10.1007/s12088-019-00786-1
Gray, J. S., Wu, R. S. S., and Or, Y. Y. (2002). Effects of hypoxia and organic enrichment on the coastal marine environment. Mar. Ecol. Prog. Ser. 238, 249–279. doi: 10.3354/meps238249
Guo, Y., Rong, Z., Li, B., Xu, Z., Li, P., and Li, X. (2019). Physical processes causing the formation of hypoxia off the Changjiang Estuary after Typhoon Chan-hom, 2015. J. Oceanol. Limnol. 371, 1–17. doi: 10.1007/s00343-019-7336-5
Hayatsu, M., Tago, K., and Saito, M. (2008). Various players in the nitrogen cycle: diversity and functions of the microorganisms involved in nitrification and denitrification. Soil Sci. Plant Nutr. 54, 33–45. doi: 10.1111/j.1747-0765.2007.00195.x
He, H., Zhen, Y., Mi, T., and Yu, Z. (2016). Community composition and abundance of ammonia-oxidizing archaea in sediments from the Changjiang Estuary and its adjacent area in the East China Sea. Geomicrobiol. J. 33, 416–425. doi: 10.1080/01490451.2014.986695
Katoh, K., and Standley, D. M. (2013). MAFFT multiple sequence alignment software version 7: improvements in performance and usability. Mol. Biol. Evol. 30, 772–780. doi: 10.1093/molbev/mst010
Lam, P., and Kuypers, M. M. (2011). Microbial nitrogen cycling processes in oxygen minimum zones. Annu. Rev. Mar. Sci. 3, 317–345.
Lam, P., Lavik, G., Jensen, M. M., van de Vossenberg, J., Schmid, M., Woebken, D., et al. (2009). Revising the nitrogen cycle in the Peruvian oxygen minimum zone. Proc. Natl. Acad. Sci. U.S.A. 106, 4752–4757. doi: 10.1073/pnas.0812444106
Letunic, I., and Bork, P. (2021). Interactive Tree Of Life (iTOL) v5: an online tool for phylogenetic tree display and annotation. Nucleic Acids Res. W293–W296. doi: 10.1093/nar/gkab301
Levin, L. A. (2003). “Oxygen minimum zone benthos: adaptation and community response to hypoxia,” in Oceanography and Marine Biology, Vol. 41, eds R. N. Gibson and R. J. A. Atkinson (London: Taylor & Francis Ltd), 1–45.
Li, J. L., Bai, J., Gao, H. W., Wang, X. D., Yu, J. H., and Zhang, G. L. (2009). Quantification of denitrifying bacteria and denitrification process in surface sediment at adjacent sea area of the Yangtze River Estuary in summer. China Environ. Sci. 29, 756–761.
Ma, J., Song, J., Li, X., Yuan, H., Li, N., Duan, L., et al. (2019). Environmental characteristics in three seamount areas of the Tropical Western Pacific Ocean: focusing on nutrients. Mar. Pollut. Bull. 143, 163–174. doi: 10.1016/j.marpolbul.2019.04.045
Ma, J., Song, J., Li, X., Yuan, H., Li, N., Duan, L., et al. (2020). Control factors of DIC in the Y3 seamount waters of the Western Pacific Ocean. J. Oceanol. Limnol. 38, 1215–1224. doi: 10.1007/s00343-020-9314-3
Manni, M., Berkeley, M. R., Seppey, M., Simão, F. A., and Zdobnov, E. M. (2021). BUSCO update: novel and streamlined workflows along with broader and deeper phylogenetic coverage for scoring of eukaryotic, prokaryotic, and viral genomes. Mol. Biol. Evol. 38, 4647–4654. doi: 10.1093/molbev/msab199
Moriya, Y., Itoh, M., Okuda, S., Yoshizawa, A. C., and Kanehisa, M. (2007). KAAS: an automatic genome annotation and pathway reconstruction server. Nucleic Acids Res. 35, W182–W185. doi: 10.1093/nar/gkm321
Mulla, A., Fernandes, G., Menezes, L., Meena, R. M., Naik, H., Gauns, M., et al. (2018). Diversity of culturable nitrate-reducing bacteria from the Arabian Sea oxygen minimum zone. Deep Sea Res. Part II Top. Stud. Oceanogr. 156, 27–33. doi: 10.1016/j.dsr2.2017.12.014
Munn, C. B. (2011). Marine Microbiology: Ecology and Applications. Garland Science, 2nd Edn. New York, NY: Taylor and Francis Group.
Prjibelski, A., Antipov, D., Meleshko, D., Lapidus, A., and Korobeynikov, A. (2020). Using SPAdes de novo assembler. Curr. Protoc. Bioinformatics 70:e102. doi: 10.1002/cpbi.102
Rusch, D. B., Halpern, A. L., Sutton, G., Heidelberg, K. B., Williamson, S., Yooseph, S., et al. (2007). The Sorcerer II global ocean sampling expedition: northwest Atlantic through eastern tropical pacific. PLoS Biol. 5:e77. doi: 10.1371/journal.pbio.0050077
Sanz-Saez, I., Salazar, G., Sanchez, P., Lara, E., Royo-Llonch, M., Sa, E. L., et al. (2020). Diversity and distribution of marine heterotrophic bacteria from a large culture collection. BMC Microbiol. 20:207. doi: 10.1186/s12866-020-01884-7
Seemann, T. (2014). Prokka: rapid prokaryotic genome annotation. Bioinformatics 2068–2069. doi: 10.1093/bioinformatics/btu153
Smith, C. J., Nedwell, D. B., Dong, L. F., and Mark Osborn, A. (2007). Diversity and abundance of nitrate reductase genes (narG and napA), nitrite reductase genes (nirS and nrfA), and their transcripts in estuarine sediments. Appl. Environ. Microbiol. 73, 3612–3622.
Stevens, H., and Ulloa, O. (2008). Bacterial diversity in the oxygen minimum zone of the eastern tropical South Pacific. Environ. Microbiol. 10, 1244–1259. doi: 10.1111/j.1462-2920.2007.01539.x
Stover, C. K., Pham, X. Q., Erwin, A. L., Mizoguchi, S. D., Warrener, P., Hickey, M. J., et al. (2000). Complete genome sequence of Pseudomonas aeruginosa PAO1, an opportunistic pathogen. Nature 406, 959–964.
Sunagawa, S., Coelho, L. P., Chaffron, S., Kultima, J. R., Labadie, K., Salazar, G., et al. (2015). Structure and function of the global ocean microbiome. Science 348:1261359. doi: 10.1126/science.1261359
Vezzulli, L., Grande, C., Reid, P. C., Helaouet, P., Edwards, M., Hofle, M. G., et al. (2016). Climate influence on Vibrio and associated human diseases during the past half-century in the coastal North Atlantic. Proc. Natl. Acad. Sci. U.S.A. 11334, E5062–E5071. doi: 10.1073/pnas.1609157113
Wang, B., Wei, Q., Chen, J., and Xie, L. (2012). Annual cycle of hypoxia off the Changjiang Yangtze River estuary. Mar. Environ. Res. 77, 1–5. doi: 10.1016/j.marenvres.2011.12.007
Wang, S. B., Wang, J. X., Liu, X. H., Fan, Y. P., Jiang, R., Liu, M. H., et al. (2017). Diversity and abundance of bacteria in the surface seawater of the Changjiang Estuary and its adjacent areas. Evol. Ecol. Res. 18, 515–530.
Wang, X. L., Liu, J. W., Liang, J. C., Sun, H., and Zhang, X. H. (2020). Spatiotemporal dynamics of the total and active Vibrio spp. populations throughout the Changjiang Estuary in China. Environ. Microbiol. 2210, 4438–4455. doi: 10.1111/1462-2920.15152
Wright, J. J., Konwar, K. M., and Hallam, S. J. (2012). Microbial ecology of expanding oxygen minimum zones. Nat. Rev. Microbiol. 106, 381–394. doi: 10.1038/nrmicro2778
Wu, D. M., Dai, Q. P., Liu, X. Z., Fan, Y. P., and Wang, J. X. (2019). Comparison of bacterial community structure and potential functions in hypoxic and non-hypoxic zones of the Changjiang Estuary. PLoS One 14:e0217431. doi: 10.1371/journal.pone.0217431
Ye, Q., Wu, Y., Zhu, Z. Y., Wang, X. N., Li, Z. Q., and Zhang, J. (2016). Bacterial diversity in the surface sediments of the hypoxic zone near the Changjiang Estuary and in the East China Sea. Microbiologyopen 52, 323–339. doi: 10.1002/mbo3.330
Ye, R. W., Haas, D., Ka, J. O., Krishnapillai, V., Zimmermann, A., Baird, C., et al. (1995). Anaerobic activation of the entire denitrification pathway in Pseudomonas aeruginosa requires Anr, an analog of Fnr. J. Bacteriol. 17712, 3606–3609. doi: 10.1128/jb.177.12.3606-3609.1995
Yoon, S. H., Ha, S. M., Kwon, S., Lim, J., Kim, Y., Seo, H., et al. (2017). Introducing EzBioCloud: a taxonomically united database of 16S rRNA and whole genome assemblies. Int. J. Syst. Evol. Microbiol. 67, 1613–1617. doi: 10.1099/ijsem.0.001755
Zhang, D. C., Mörtelmaier, C., and Margesin, R. (2012). Characterization of the bacterial archaeal diversity in hydrocarbon-contaminated soil. Sci. Total Environ. 421-422, 184–196. doi: 10.1016/j.scitotenv.2012.01.043
Zhang, D. C., Wang, H. X., Liu, H. C., Dong, X. Z., and Zhou, P. J. (2006). Flavobacterium glaciei sp. nov., a novel psychrophilic bacterium isolated from the China No.1 glacier. Int. J. Syst. Evol. Microbiol. 56, 2921–2925. doi: 10.1099/ijs.0.64564-0
Zhang, J., Wu, Y., Jennejahn, T. C., Ittekkot, V., and He, Q. (2007). Distribution of organic matter in the Changjiang Yangtze River Estuary and their stable carbon and nitrogen isotopic ratios: implications for source discrimination and sedimentary dynamics. Mar. Chem. 106, 111–126. doi: 10.1016/j.marchem.2007.02.003
Keywords: oxygen minimum zone, the Changjiang Estuary, culturable, nitrogen metabolism, nitrate-utilizing
Citation: He W, Liu S, Jiang Z, Zheng J, Li X and Zhang D (2021) The Diversity and Nitrogen Metabolism of Culturable Nitrate-Utilizing Bacteria Within the Oxygen Minimum Zone of the Changjiang (Yangtze River) Estuary. Front. Mar. Sci. 8:720413. doi: 10.3389/fmars.2021.720413
Received: 04 June 2021; Accepted: 19 October 2021;
Published: 26 November 2021.
Edited by:
Wen-Jun Li, Sun Yat-sen University, ChinaCopyright © 2021 He, Liu, Jiang, Zheng, Li and Zhang. This is an open-access article distributed under the terms of the Creative Commons Attribution License (CC BY). The use, distribution or reproduction in other forums is permitted, provided the original author(s) and the copyright owner(s) are credited and that the original publication in this journal is cited, in accordance with accepted academic practice. No use, distribution or reproduction is permitted which does not comply with these terms.
*Correspondence: Xuegang Li, bGl4dWVnYW5nQHFkaW8uYWMuY24=; Dechao Zhang, emhhbmdkZWNoYW9AcWRpby5hYy5jbg==
†These authors have contributed equally to this work