- 1Department of Biotechnology and Life Sciences, University of Insubria, Varese, Italy
- 2Department of Biotechnology and Biosciences, University of Milano-Bicocca, Milan, Italy
- 3Institute of Molecular Bioimaging and Physiology (IBFM), National Research Council (CNR), Milan, Italy
Using a circular economy concept, the present study investigated the use of crude glycerol, a primary by-product of biodiesel production, as a low-priced nutrient source for heterotrophic cultivation of the fungus-like protist Schizochytrium limacinum SR21 strain. The whole biomass of this oleaginous microorganism, rich in docosahexaenoic acid (DHA) and high-quality proteins, was then paired with a vegetable oil (VO) source and used to replace fish oil (FO) in European sea bass (Dicentrarchus labrax) feeds. Four nutritionally balanced diets were formulated: diet FO (a FO-based diet), diet VO + 0 (a VO-based diet without S. limacinum), and diets VO + 5 and VO + 10 that were VO-based feeds supplemented with 5 and 10% of S. limacinum, respectively. After a 3-month feeding trial, fish of all dietary groups tripled their initial weight, but growth and feeding efficiencies of D. labrax were not significantly different among treatments. Although the formulated diets were balanced for polyunsaturated fatty acids (PUFAs), fish fed with feeds containing either VO or VO plus 5 and 10% of S. limacinum biomass had significantly higher levels of PUFAs in the flesh than fish fed the FO-based diet. Values of health-related lipid indexes, such as atherogenicity index, thrombogenicity index, and flesh lipid quality as well as n-6/n-3 and PUFAs/SFAs ratios confirmed the high nutritional value of sea bass filet, thus representing a healthy product for human consumption. Although the PUFAs/SFAs ratio showed a significantly higher value in fish fed with VO-based diets supplemented with S. limacinum than in those fed with FO diet, suggesting a better filet quality, the n-6/n-3 ratio clearly indicated that filet quality of dietary group FO was best (value of 0.55) and that of group VO + 10 second best (value of 0.98). We also evaluated the nutritional regulation of Δ6-desaturase (or fads2) gene expression in European sea bass liver. European sea bass fed the VO + 0 diet had the highest number of mRNA copies for Δ6-desaturase (or fads2), fish fed with diet VO + 10 the lowest. Our study adds to the growing body of literature concerning the use of thraustochytrid biomass as a valid alternative to marine-derived raw materials for European sea bass feeds.
Introduction
In the last few decades, the global demand for aquatic food products has been steadily increasing and aquaculture has been playing a major role in meeting this demand by boosting fish production. However, like all other food production practices, the aquaculture sector must expand in a sustainable way, as awareness of potential environmental issues of harvesting fish for feed has increased significantly. Therefore, most aqua farmers are continuously improving their production practices to make them more sustainable, efficient, and cost-effective. For an aquaculture system to develop in harmony with environmental goals, feed represents one limiting factor as it traditionally includes ocean-derived raw materials such as fishmeal (FM) and fish oil (FO) that provide high-quality proteins and lipids–mostly long-chain (C 20–C 22) polyunsaturated fatty acids (PUFAs)–to cultured fish (FAO, 2018). PUFAs, mainly eicosapentaenoic acid (EPA) (C 20:5n-3), and docosahexaenoic acid (DHA) (C 22:6n-3) have unique roles in controlling and regulating cellular metabolism and animal physiology. In fish, they are required early during ontogeny, principally for neural development, but also after this period, as they are involved in cell membrane fluidity, cellular metabolism, immune response, pigmentation, stress resistance, reproduction, and, finally, in schooling behavior (Sargent et al., 1997; Masuda and Tsukamoto, 1999; Montero et al., 2003; Tocher, 2003, 2010; Glencross, 2009). For these reasons, FO has constituted a major dietary lipid source in aquafeeds, but its limited availability and high cost have promoted the search for more affordable and sustainable alternatives.
Among alternative oils, those derived from seed plants [vegetable oils (VOs)], such as soybean, rapeseed, palm, linseed, and canola, have received much attention because of their competitive price, good catabolic potential to obtain dietary energy, and lower level of toxic pollutants, such as dioxins (Sales and Glencross, 2011; Carvalho et al., 2020). Little or no effects were reported on fish growth performance at low FO/VO replacement levels when minimum omega-3 and omega-6 intake requirements were met (Sargent et al., 1997; Martins et al., 2012), but reduced performance and decreased omega-3 content in fish filets were reported when high levels of VOs were included (Özşahinoğlu et al., 2013; Monge-Ortiz et al., 2018; Ayisi et al., 2019). Therefore, high FO/VO replacement levels may constrain production efficiency while also compromising many health benefits of fish consumption related to human diseases (Calder and Yaqoob, 2009; Siscovick et al., 2017; Layé et al., 2018). Human beings cannot synthesize EPA and DHA (humans are able to synthesize a small quantity of DHA, not de novo but from ingested α-linolenic acid (ALA, C 18:3n-3)) because they lack some of the enzymes needed for synthesis. This means that EPA and DHA are “essential” and should be taken through diet, principally by fish consumption (Erdman et al., 2012; Ross et al., 2012). Indeed, irrefutable evidence links an adequate dietary intake of fish (especially fatty fish) rich in EPA and DHA with a significant reduction in coronary risk (Innes and Calder, 2020).
A possible strategy to increase the content of PUFAs in fish feeds with no FO or low FO levels might be to include oleaginous microorganisms (bacteria, filamentous fungi, microalgae, yeast, and thraustochytrids) that are capable of accumulating high levels of lipids in their cellular compartments. Certain oleaginous species can accumulate up to 70% w/w of lipids on cell dry matter when cultivated in medium with a high carbon/nitrogen (C/N) ratio (Ratledge and Cohen, 2008; Beopoulos and Nicaud, 2012; Patel et al., 2020). These lipids, known as single-cell oils or microbial oils (MOs), are similar to VOs but it is easier to scale up their production (Becker, 2007; Moreira et al., 2011; Nasseri et al., 2011; Khot et al., 2012; Vanthoor-Koopmans et al., 2013). There are several advantages in using microorganisms to produce MOs. Indeed, microorganisms can be easily cultured in a controlled environment without depending on seasonal and climatic conditions can tolerate different cultivation conditions, can be genetically modified in order to obtain specific products and, finally, they do not compete with food crops for arable land and freshwater (Patel et al., 2020). However, the costs for producing MOs are still higher than for FOs, due to the nature of the substrates used for heterotrophic fermentation, the investment in lighting and electricity for phototrophic algae, and the refinement of products (Ratledge and Cohen, 2008; Sprague et al., 2015, 2017). Industrial wastes, which represent a threat to the environment, have been suggested as a solution to reduce such production costs; for instance, crude glycerol, which is the major by-product of the biodiesel industry, has been proposed (Mata et al., 2014; Katiyar et al., 2017).
Crude glycerol represents a burden for waste disposal in biodiesel production due to the different properties of this blend in comparison with pure glycerol (Johnson and Taconi, 2007; Checa et al., 2020). Therefore, different methods for valorizing crude glycerol have been developed; for instance, by using it as a nutrient source for the cultivation of oleaginous microorganisms (Athenaki et al., 2018; Patel et al., 2020). Among these, Thraustochytrids (heterotrophic fungus-like protists), such as Schizochytrium spp., are becoming increasingly important to produce oils rich in PUFAs. Schizochytrium is an excellent producer of DHA, when growing on glycerol. It synthesizes DHA through “anaerobic” polyketide synthases; therefore, low dissolved oxygen (DO) is used for DHA production. However, since low DO cannot facilitate cell proliferation, DHA concentration is improved by firstly optimizing cell proliferation at high DO and then shifting to low DO for lipid accumulation (Chang et al., 2013).
Although several studies have examined the replacement of dietary FO with MO from Schizochytrium spp. in fish and shrimp feeds (Harel et al., 2002; O’Grady and Morgan, 2011; Lung et al., 2016; Katiyar et al., 2017; Allen et al., 2019; Glencross et al., 2020b), only very few studies have reported using the whole of cell biomass of this strain in fish feeds (Kousoulaki et al., 2015; Katerina et al., 2020; Lee Chang et al., 2020; Hart et al., 2021). By using the harvested Schizochytrium spp. biomass as a whole in fish feeds, it is possible to take advantage of all major nutrients synthesized by this microorganism (e.g., lipids, proteins, minerals, vitamins, and carbohydrates), thus maximizing its nutritional value in fish feed. Moreover, in this way, from an economic standpoint, the cost related to extracting omega-3 from the biomass is spared, and these nutraceuticals are protected in whole cell form from possible oxidation during the extraction process.
In this view, the aim and novelty of the present study was to assess the effects of using whole-cell Schizochytrium limacinum biomass, cultivated on crude glycerol waste from a palm oil biodiesel plant, as nutritional supplement in European sea bass (Dicentrarchus labrax) feed. The investigation focused on fish growth performance, nutritional quality of filets, and expression levels of Δ6-desaturase (or fatty acid desaturase 2, fads2) gene. Four fish diets that included different percentages of S. limacinum biomass were tested in a 3-month feeding trial. The objective for including DHA-rich biomass in European sea bass feed was twofold: (1) to achieve optimal growth and development of the fish and (2) to provide a vector for the delivery of essential fatty acids to filets for human consumption.
Materials and Methods
Ethics Statement
All animal experiments were performed according to the European Union Directive (2010/63/EU) on the protection of animals for scientific purposes. The Italian Ministry of Health approved the animal experiments [REF: 483/2017-PR (response of Prot. Nr. 344C6.6 of 13/03/2017)] in accordance with Art.31 of D.lgs. 26/2014.
Schizochytrium limacinum Fermentation, Lipid Production, and Analysis
The ability of S. limacinum to accumulate PUFAs in a heterotrophic culture, using crude glycerol, was tested in 2-L bioreactors. S. limacinum SR21 was purchased from the American Type Culture Collection (ATCC, United States) and stored at −80°C in glycerol stocks (20% v/v). Starting cultures for bioreactor inoculation were obtained in 250-mL shake flasks with 50 mL of synthetic medium (glucose 20 g/L, peptone 20 g/L, and yeast extract 10 g/L): cultures were maintained in a shaker at 30°C and 160 rpm for 72 h. The exponential phase cultures were then used to inoculate bioreactors to reach a final optical density (OD600) of 0.5. Briefly, cells were centrifuged at 2621 × g for 10 min, washed twice with water, and finally resuspended in 10 mL of sterilized water. The batch experiments were conducted in 2 and 10 L stirred tank bioreactors (BIOSTAT® B plus, and BIOSTAT® C Sartorius Stedim Biotech GmbH, Göttingen, Germany) equipped with Visiferm DO ECS 225 for measuring pO2 and Easyferm Plus K8 200 for determining pH (both from Hamilton Bonaduz AG, Bonaduz, Switzerland). As growth medium for the batch fermentations By + medium (Chi et al., 2007) was modified as follows: 20 g/L glycerol, 1.5 g/L yeast extract, 1.5 g/L tryptone, and 30 g/L seawater salts (20.758 g/L NaCl, 0.587 g/L KCl, 0.17 g/L NaHCO3, 0.0746 g/L NaBr, 0.0225 g/L H3BO3, 0.0027 g/L NaF, 3.477 g/L Na2SO4, 9.395 g/L MgCl2 ⋅ 6 H2O, 1.316 g/L CaCl2 ⋅ 2 H2O, and 0.0214 g/L SrCl2 ⋅ 6 H2O). The medium was adjusted to pH 7 with HCl before sterilization, except for seawater salts, which were adjusted to pH 8 (using NaOH e HCl), filtrated with a 0.22-μm polytetrafluoroethylene (PTFE) filter, and added under sterile conditions to the final medium after bioreactor sterilization to prevent salt precipitation in the autoclave. After 28 h of growth, a crude glycerol supplement was added to the growth medium to reach a final concentration of 75 g/L, according to the method previously reported by Signori et al. (2016) for other oleaginous microbes, and the fermentation was carried out for 144 h to reach maximum lipid accumulation in the cells. Briefly, the crude extract consisted of 80% content of glycerol was centrifuged at 2621 × g for 20 min and then, the top dark red layer (mainly consisting of free fatty acids), was removed.
The parameters used during fermentation were 300 rpm of agitation in cascade to 25% of DO (to obtain a 1-vvm aeration rate) and a temperature of 30°C. A pH value of 7 was maintained during the entire fermentation process with NaOH 4 M and H2SO4 25%. All the reagents were purchased from Sigma-Aldrich Co. (St Louis, MO, United States), except for the yeast extract, which was from Biolife Italia S.r.l. (Milan, Italy). Crude glycerol was derived from industrial biodiesel production out of palm oil.
After fermentation, S. limacinum cells were harvested by centrifugation at 7885 × g for 20 min (Sorvall Evolution RC, Thermo Fisher Scientific, Waltham, MA, United States); then, cells were washed twice with distilled water. Dry cell weight (DCW) was determined gravimetrically by drying 1 mL of cell culture (Concentrator 5301, Eppendorf AG, Germany).
To determine the lipid content in the cells, lipids were extracted from 1 g of biomass, based on the method of Bligh and Dyer (1959). Briefly, after drying in an oven at 60°C for 12 h, cells were resuspended in 5 mL of HCl 2 M (1:1) and chemically disrupted in a thermostatic bath at 75°C for 40 min. Then, lipids were collected by adding 5 mL of n-hexane to the mixture. The organic phase at the bottom was collected and left overnight under a chemical hood to evaporate hexane and subsequently weighed. To assess the fatty acid profiles, methyl esters from fatty acids (FAMEs) were obtained by esterification: 5 mL of KOH-methanol 0.4 M were added to the lipids; the mixture was then vortexed and placed in a thermobath at 60°C for 1 h. Subsequently, 5 mL of BF3-methanol (14% w/w) (1:1) were added, mixed by vortex, and placed in a thermobath at 60°C for 1 h. Finally, 10 mL of n-hexane were added to extract FAMEs for gas chromatography (GC) analysis (Savi Laboratori and Service S.r.l., Italy) according to the following methods: UNI EN ISO 12966-1:2015 + UNI EN ISO 12966-2:2011 + UNI EN ISO 12966-4:2015.
To obtain S. limacinum biomass for producing fish feed after bioreactor fermentation, the broth culture was centrifuged in a Sorvall Evolution RC (Thermo Fisher Scientific, Waltham, MA, United States) at 7885 × g and 4°C for 10 min and the supernatant was discarded. The biomass was then frozen in liquid nitrogen and lyophilized (LIO5P, 5Pascal, Milan, Italy).
Experimental Diets
The four experimental diets, named FO, VO + 0, VO + 5, and VO + 10, were prepared at the University of Insubria (Varese, Italy), starting from a non-oil-based commercial feed manufactured by VRM S.r.l. Naturalleva feed company (Verona, Italy). Diets were formulated according to the nutritional requirements of D. labrax (Lupatsch, 2005; Tibaldi and Kaushik, 2005) and were isonitrogenous, isolipidic, and isoenergetic. In particular, diet FO was a FO-based diet, diet VO + 0 was a VO-based, whereas diets VO + 5 and VO + 10 were VO-based feeds supplemented with 5 and 10% of S. limacinum, respectively. Proximate composition of each feed is shown in Table 1. Feeds were prepared by using a food blender to mix and homogenize the ingredients that were commercial feed (without oil), soybean oil, and S. limacinum biomass at 5 and 10% for diets VO + 5 and VO + 10, respectively, and commercial feed (without oil) plus FO for diet FO or soybean oil for diet VO + 0. The final mixture was transferred to a meat grinder to obtain a 2 mm pellet. At the end of the process, the pellet was dried overnight in an oven at 50°C and then stored at room temperature until use (within a week). The feeds were prepared on a weekly basis. Amino acid and fatty acid composition of the four experimental diets are shown in Tables 2, 3, respectively.
Fish Feeding and Sampling
The fish feeding trial was conducted at the University of Insubria (Varese, Italy) in an indoor recirculating saltwater facility. At the beginning of the experiment, 280 European sea bass with an initial mean body weight of 22.38 ± 0.3 g and mean length of 10.13 ± 0.42 cm were randomly distributed in 8 rectangular tanks of 500 L in volume, with 35 fishes per tank (initial density: 1.56 kg/m3), and allowed to acclimatize for 1 week. The photoperiod was set at 12 h: 12 h light: dark cycle. The temperature (19 ± 1.5°C) and pH (8.3 ± 0.4) were continuously monitored during the trial. DO concentration (maintained over 85%), total ammonia nitrogen, and salinity were measured periodically. Fish mortality was recorded daily.
After acclimation, fish were fed for 21 weeks with the experimental diets and each of the experimental feeds was given to duplicate populations (2 tanks/diet). The fish-feeding rate was maintained at 1.5% of the total biomass. To calculate growth indexes, all the specimens were weighed and their length measured on a 3-weekly basis. Data were used to calculate the feed conversion ratio [FCR = (feed intake × dry matter)/(100 × wet weight gain)], specific growth rate [SGR = 100 × [ln (final body weight)–ln (initial body weight)]/days], condition factor [CF = 100 × (body weight/standard length3)], and feed efficiency [FE = wet weight gain/(feed intake × dry matter)].
At the end of the trial, fish were sacrificed to collect tissues and organs for subsequent analysis. For this, 12 fish/diet were euthanized with an overdose (320 mg/L at 22°C) of anesthetic (tricaine-methasulfonate MS-222, Sigma-Aldrich, Italy) and liver and white dorsal muscle were aseptically removed and then stored at −80°C until analysis.
Fatty Acid Extraction and Quantification
To assess the fatty acid profiles, six filets/dietary group and an aliquot from each diet were analyzed. The total fat content was extracted using acid hydrolysis (UNI 22605-1992). For analysis, fatty acids in methyl esters (FAMEs) were converted by esterification and determined by capillary GC, according to ISO 12966-2:2011 and ISO 12966-4:2015.
Indexes of Nutrition Quality of Filets
To evaluate the effects of replacing FO with VO and Schizochytrium biomass, the following indexes based on the ratios of constituent fatty acids were calculated (Ulbricht and Southgate, 1991; Abrami et al., 1992; Chen and Liu, 2020).
(1) Atherogenicity index [AI = (C 12:0 + (4 × C 14:0) + C 16:0)/(∑ Monounsaturated Fatty Acids (MUFAs) + n-6 PUFAs + n-3 PUFAs)];
(2) Thrombogenicity index [TI = (C 14:0 + C 16:0 + C 18:0)/(0.5 × ∑ MUFAs + 0.5 × n-6 PUFAs + 3 × n-3 PUFAs) + (n-3 PUFAs/n-6 PUFAs)];
(3) Flesh lipid quality [FLQ = C 20:5n-3 + C 22:6n-3/% Total FAs].
Gene Expression Analysis
The Δ6-desaturase transcript copies in fish liver were quantified using One-step TaqMan® quantitative real-time RT-PCR.
RNA Extraction and One-Step TaqMan® Real-Time RT-PCR
Total RNA was extracted from 100 mg of liver dissected out from each fish (6 fish/diet) using the kit Maxwell® 16 LEV simplyRNA Tissue (Promega, Italy) and Maxwell® 16 Instrument (Promega, Italy). The extracted RNA was quantified by measuring the absorbance at 260 nm using a NanoDropTM 2000c spectrophotometer (Thermo Scientific). One hundred nanograms of total RNA from each biological sample were then amplified via One-step TaqMan® quantitative real-time RT-PCR in the same plate with tenfold-diluted, defined amounts of standard mRNAs. The sequences of primers and TaqMan® probes used for target gene amplification are shown in Table 4. TaqMan® PCR reactions were performed on a Bio-Rad® CFX96TM System using the following run parameters: one cycle of 10 min at 50°C and 3 min at 95°C, followed by 40 cycles of 15 s at 95°C and 60 s at 60°C.

Table 4. Primers and probe sequences used for the real time PCR quantification of Δ6-desaturase (or fads2) gene transcripts.
Data from the TaqMan® PCR runs were collected with CFXTM Software. Cycle threshold (Ct) values corresponded to the number of cycles at which the fluorescence emission monitored in real time exceeded the threshold limit. The Ct values obtained by amplifying serial dilutions of the mRNAs were used to create a standard curve, which was then used to calculate the mRNA copies in the biologic samples.
Statistical Analysis
Data were analyzed using GraphPad Prism8 software (GraphPad Software, Inc., La Jolla, CA, United States). Statistical analyses for multiple comparisons were tested by one-way ANOVA followed by Tukey’s test. The differences were considered as statistically significant at p-value < 0.05.
Results
S. limacinum Culture
Schizochytrium limacinum biomass was obtained by fermentation in 2 and 10 L bioreactors. This microorganism was fed with crude glycerol as carbon and energy source to obtain a suitable biomass for subsequent use as a fish feed ingredient. The ratio between the carbon and the nitrogen source was chosen in order to trigger lipid accumulation. After 144 h of fermentation, a DCW of 8.0 ± 1.1 g/L was obtained with a biomass yield of 0.1 ± 0.09 g/gglycerol (10% w/w). The amount of lipids from the biomass was 4.8 ± 0.51 g/L, with a yield of 0.6 ± 0.41 g/L g/gbiomass (60% w/w). Table 5 shows the lipidogram of S. limacinum obtained from the fermentation. DHA constituted 22.82% of the total lipids in the biomass, being the second most represented after palmitic acid (56.35%). Therefore, the DHA titer was 1.1 ± 0.28 g/L with a yield of 0.13 ± 0.25 g/L g/gbiomass (13% w/w).
Fish Growth Performance and Feed Utilization
At the end of the trial, the total mortality of European sea bass was 2.14% and it was not correlated to a specific diet. Regarding the growth, after 21 weeks of feeding, fish of all dietary groups (Table 6) tripled their initial weight (from 22.38 ± 0.30 g to 78.11 ± 1.39 g final mean body weight). Moreover, nutrient intake was not affected by dietary treatment and fish appeared healthy throughout the feeding trial. Sea bass fed with diet VO + 5 achieved the highest performance not only for weight (82.02 ± 5.37 g) and length (16.71 ± 0.1 cm), but also for the growth indexes, such as SGR (0.93 ± 0.02) and FCR (1.50 ± 0.12) (please see the dedicated session in section “Materials and Methods” for the calculations). Nevertheless, no significant differences between dietary groups were recorded for any of the performance indexes considered (Table 6).
Assimilation of Fatty Acids in Fish Filets
The fatty acid compositions of fish filets from the four dietary groups are shown in Table 7 and Figure 1. Levels of different fatty acids were similar between dietary groups, ranging from 17.18 ± 0.02 to 18.62 ± 0.06. There were no significant differences in the total MUFAs and SFAs between fish dietary groups, whereas PUFAs were significantly higher in fish fed diets VO + 0, VO + 5, and VO + 10 than in fish fed the FO diet. In particular, fish fed with diet VO + 0 showed the highest level of n-6 fatty acids (20.81 ± 0.98), mostly constituted by linoleic acid (C 18:2n-6) (93%), but the lowest level of n-3 fatty acids (12.01 ± 0.52). Sea bass fed with diet VO + 10 showed significantly higher levels of n-3 fatty acids than fish fed diets VO + 0 and VO + 5 and similar levels in comparison to fish fed diet FO (Table 7 and Figure 1). If compared to dietary group FO, group VO + 10 showed significantly higher levels of n-6 fatty acids, but lower than group VO + 0 and similar to group VO + 5 (Figure 1).
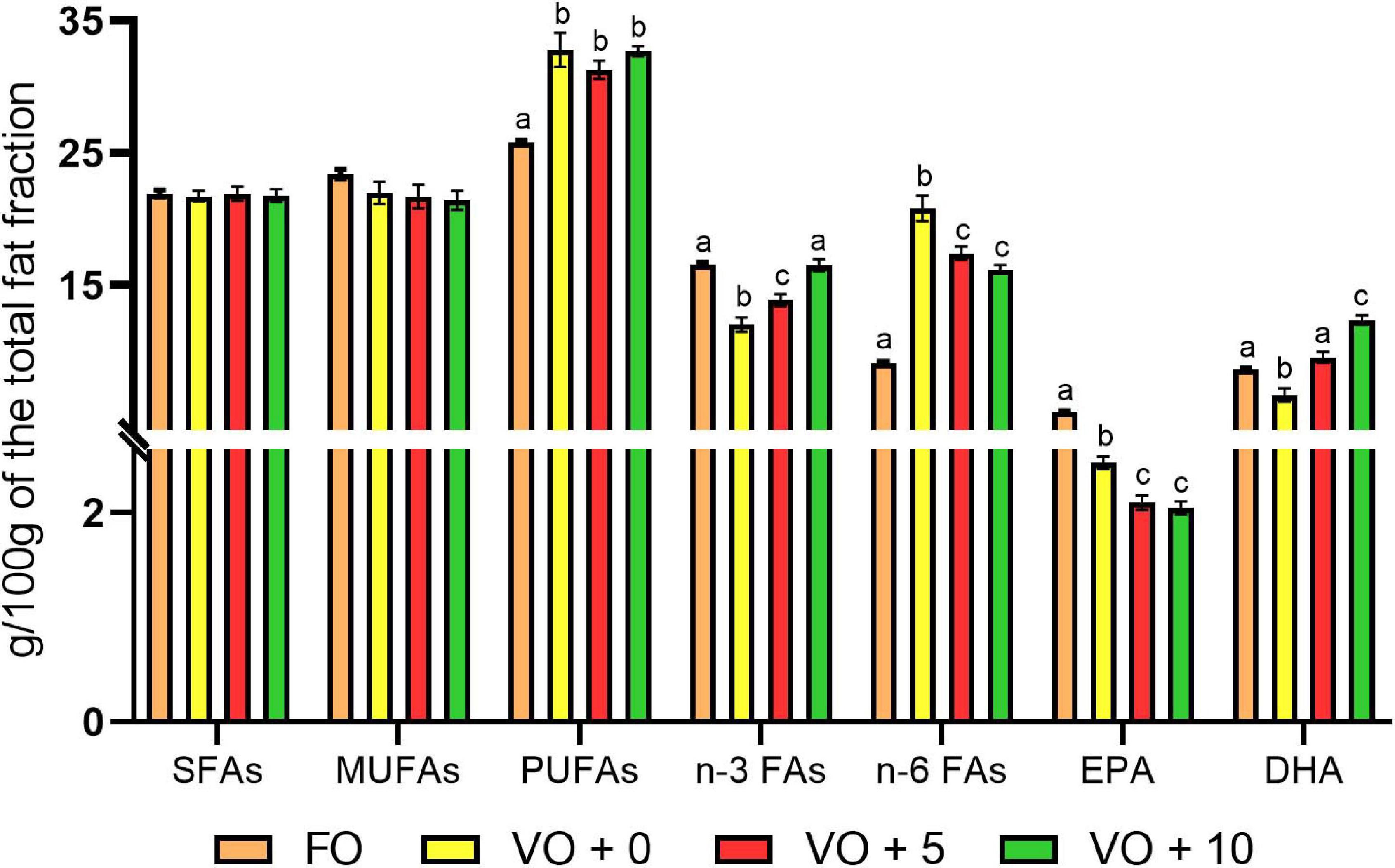
Figure 1. Profile of the main FAs in filets of fish fed with the four different experimental diets. The values are expressed as g/100g of the total fat fraction and are reported as mean ± SEM (n = 6). Different letters indicate significant differences between experimental diets as determined by one-way ANOVA with Tukey’s comparison test (p < 0.05).
With regard to EPA, filets of fish fed with diet FO had the highest level (5.43 ± 0.07), whereas fish fed with diets VO + 0, VO + 5, and VO + 10 had less than half of that level and groups VO + 5 and VO + 10 showed similar levels.
Concerning DHA content, although the percentage of DHA was higher in feed FO (Table 3), DHA levels in fish filets increased from 9.53 ± 0.4 in group VO + 5 to 12.34 ± 0.36 in VO + 10, reflecting the increase in S. limacinum inclusion levels from 5 to 10% (Table 7 and Figure 1). However, only DHA levels in group VO + 10 were significantly higher than those recorded in group FO.
Nutritional Quality of Filets
The ratios and the nutritional quality indexes of fish filets are shown in Table 8 and Figure 2. Although the PUFAs/SFAs ratio showed a significantly higher value in fish fed with VO + 0, VO + 5, and VO + 10 diets than in those fed with FO-based diet, suggesting a better filet quality, the n-6/n-3 ratio clearly indicated that the filet quality of group FO was the best (value of 0.55 ± 0.01) and that of group VO + 10 the second best, followed in a decreasing fashion by filets of groups VO + 5 and VO + 0.
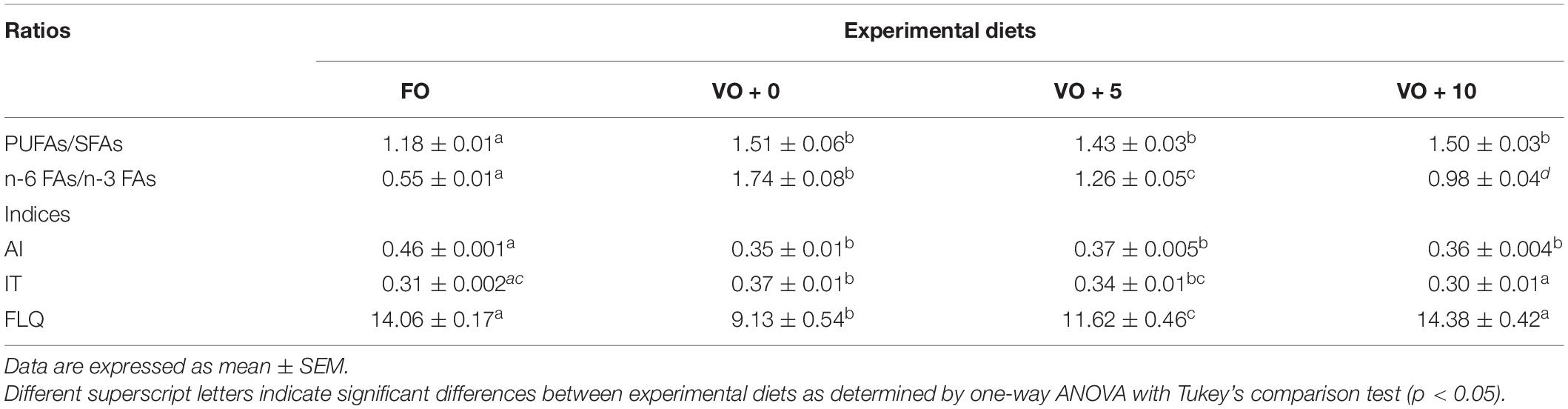
Table 8. Fatty acids ratios and nutritional indices of filets from fish fed with the four experimental diets.
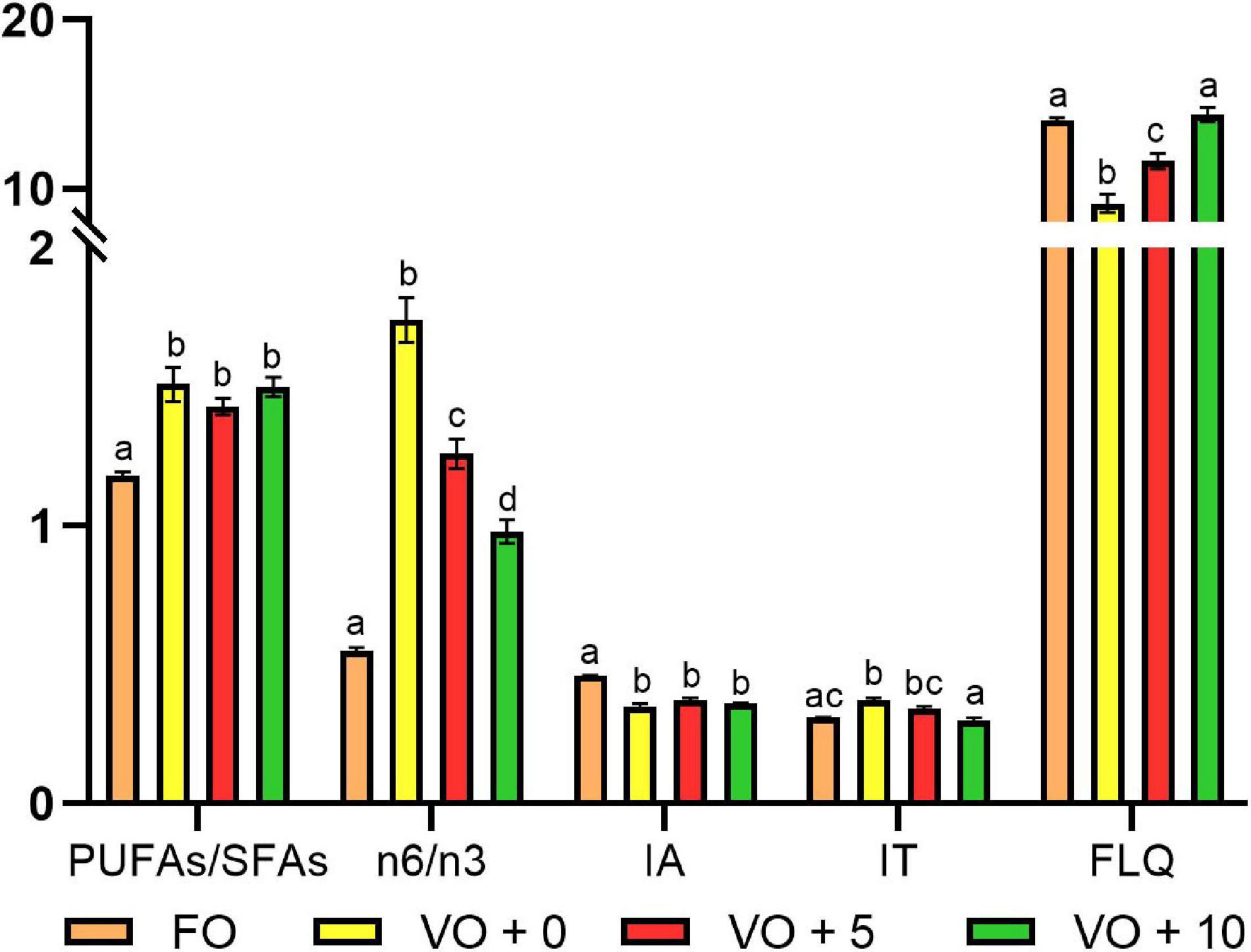
Figure 2. Fatty acids ratios and nutritional indices of filets from fish fed with the four experimental diets. Data are expressed as mean ± SEM. Different letters indicate significant differences between experimental diets as determined by one-way ANOVA with Tukey’s comparison test (p < 0.05).
The AI was significantly higher in fish fed with diet FO (0.46 ± 0.001) than in fish fed diets VO + 0, VO + 5, and VO + 10, with no differences between the latter three groups.
No significant differences in TI were shown between groups FO and VO + 10, which presented the lowest values (0.31 ± 0.002 and 0.30 ± 0.01, respectively), whereas there were significant differences between groups FO and VO + 0, VO + 0 and VO + 10, and VO + 5 and VO + 10.
The highest FLQ levels were recorded in groups FO and VO + 10 (14.06 ± 0.17 and 14.38 ± 0.42, respectively), followed in a decreasing fashion by groups VO + 5 and VO + 0. In the latter two, a significantly higher value was recorded in fish fed diet VO + 5 containing 5% of Schizochytrium biomass than in fish fed diet VO + 0, with only VO.
Gene Expression
As shown in Figure 3, the highest number of mRNA copies for Δ6-desaturase (Δ6D or fads2) were determined for fish fed with diet VO + 0, the lowest copy number for fish fed with diet VO + 10. There were no significant differences between expression levels found in fish fed with diet FO and diets VO + 5 and VO + 10 containing VO and 5 and 10% of Schizochytrium biomass, respectively.
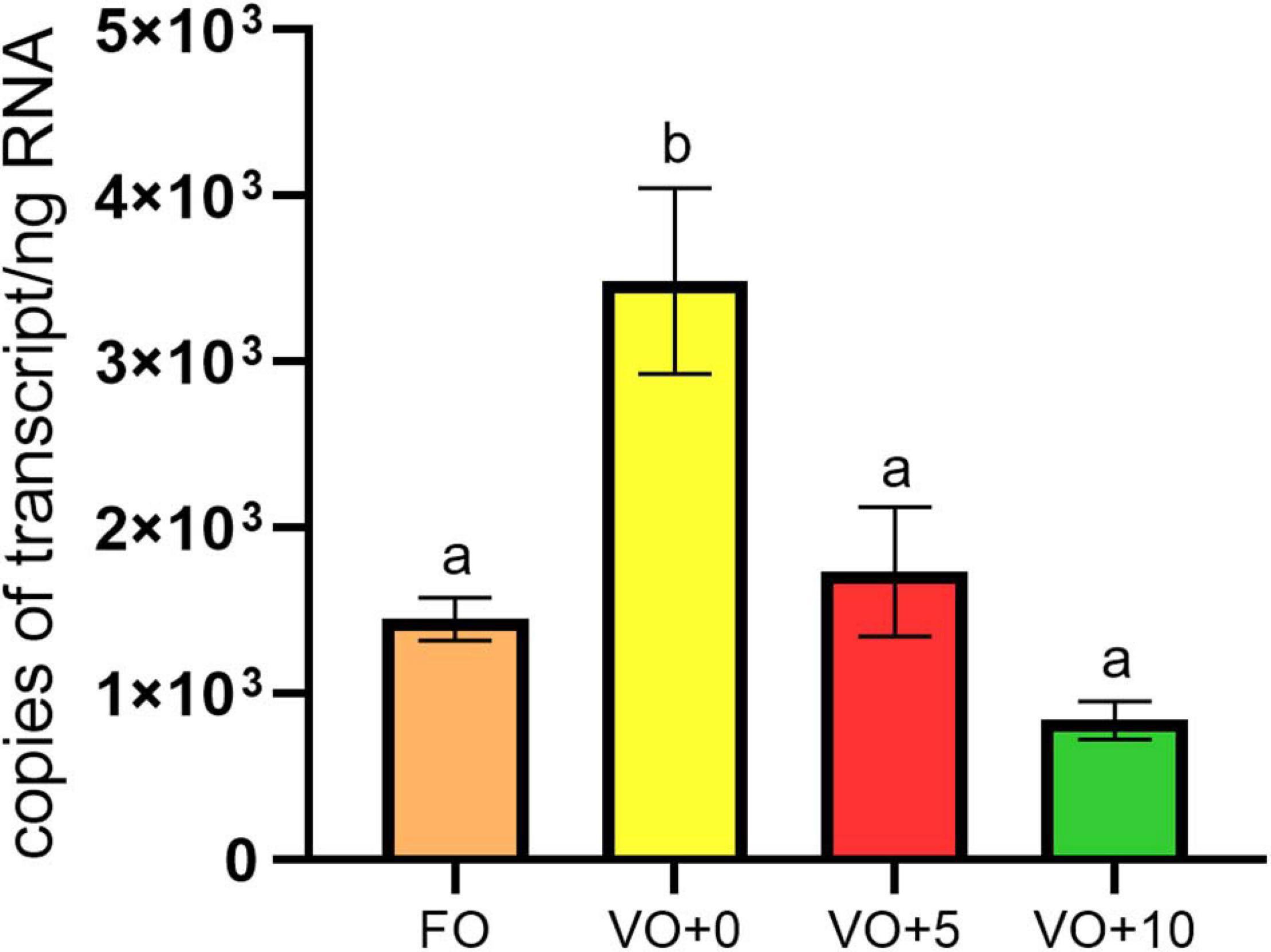
Figure 3. Expression levels of Δ6-desaturase gene in fish liver. Data are means ± SEM (n = 6). Different letters indicate significant differences between experimental diets as determined by one-way ANOVA with Tukey’s comparison test (p < 0.05).
Discussion
Unlike a linear economic model, which is based on a take-make-consume-dispose pattern, circular economy represents a model of production and consumption that implies reusing and recycling existing raw materials and products, thereby extending their life cycle as long as possible and reducing waste to a minimum (Yuan et al., 2006; Geissdoerfer et al., 2017; Sariatli, 2017). In a circular economy, products are not designed to be used once and then discarded, but are repeatedly reused, and those that cannot be reused are recycled by chemical or mechanical processes or through biological agents that convert wastes into value-added products, thereby providing alternative routes of exploitation in an environmentally friendly way.
The present research was framed in a circular economy, using crude glycerol derived from industrial biodiesel production as a low-cost carbon source for heterotrophic fermentation of the S. limacinum SR21 strain, which is a high-value biological product rich in DHA and high-quality proteins. For each ton of biodiesel produced, 100 kg of crude glycerol is generated as by-product (Pyle et al., 2008). Crude glycerol has a low economic value and discarding it can have negative consequences for the environment; therefore, biodiesel producers must seek new uses for this waste stream. A method of valorizing crude glycerol is to use it as a substrate for cultivating oleaginous microorganisms, such as marine protist Schizochytrium spp., which accumulate significant amounts of triacylglycerols with a high proportion of PUFAs. Producing DHA on crude glycerol represents a great opportunity to utilize a large quantity of this by-product, converting it into a value-added product.
In our study, S. limacinum SR21 strain was able to grow well on crude glycerol and to accumulate fatty acids for almost 60% of its DCW. This result is aligned with findings from Sun et al. (2014) and Bouras et al. (2020), who reported that this strain could accumulate lipids for up to 50% of its cell dry matter, in a short time, with a DHA output of 34% of the total lipid concentration. Our analysis of fatty acid profiles showed that palmitic acid and DHA were the major fatty acids produced by the strain. Similar results were obtained by Ethier et al. (2011), who described that S. limacinum had a relatively simple fatty acid profile, with palmitic acid and DHA being the major fatty acids and myristic, stearic, and docosapentaenoic acid (C 22:5) being the minor fatty acids. Data derived from GC are also in line with previous analyses of the fatty acid composition of S. limacinum biomass (Pyle et al., 2008; Abad and Turon, 2015), confirming that this strain is a promising high-lipid, DHA−rich ingredient for fish feed formulations.
The S. limacinum whole biomass paired with a terrestrial plant-derived oil source was then used to replace FO in European sea bass (D. labrax) feed. Four nutritionally balanced diets were formulated: diet FO (a FO-based diet), diet VO + 0 (a VO-based diet), and diets VO + 5 and VO + 10 that were VO-based feeds supplemented with 5 and 10% of S. limacinum, respectively. From an economic standpoint, S. limacinum whole cell biomass is preferable to extracted oil because extraction constitutes a costly processing step. Furthermore, lipids are less susceptible to oxidation in a whole-cell form and this can positively affect a product’s quality and shelf life (Hart et al., 2021).
All the feeds in our experiment were well accepted and there were no indications of feed rejection when sea bass were fed with diets without FO. After a 3-month feeding trial, fish of all dietary groups tripled their initial weight, but growth and feeding efficiencies of D. labrax were not significantly different among treatments. As is known, VOs contain some types of n-3 fatty acids, but they lack the n-3 PUFAs (EPA and DHA), which are found almost exclusively in FO and marine microalgae. Over the recent decades, VOs have replaced about half of the FO in fish feed formulations (Tacon and Metian, 2008). Although these levels of substitution have had no major effect on fish growth performance, they have detrimental effects to filet quality, reducing the proportions of health-beneficial fatty acids EPA and DHA in fish white muscle. Therefore, the challenge for aquaculture industry is to maintain healthful levels of EPA and DHA in cultured fish products as the percentage of FO in aquafeed formulations decreases (Glencross et al., 2020a). One of the ways this can be achieved is to add high DHA ingredients, such as products from thraustochytrids, to the VO-based diets.
So far, only few studies have reported the effects of whole S. limacinum cell biomass in fish diet on fish growth performance and most of them were focused on Atlantic salmon (Salmo salar) (Carter et al., 2003; Kousoulaki et al., 2015; Katerina et al., 2020; Lee Chang et al., 2020; Hart et al., 2021). In agreement with our data, results from those studies commonly indicated normal fish growth, demonstrating that S. limacinum is a source of highly digestible (98%) PUFAs and proteins for farmed fish. For instance, in the study of Lee Chang et al. (2020), no significant differences in specific growth rate, survival, feed consumption, and FCR were recorded after replacing FO (tuna oil) with 5% thraustochytrid biomass in salmon fingerlings diet. Using higher inclusion percentages, i.e., 10–13% of whole-cell thraustochytrids in canola oil-based diets for salmon, (Carter et al., 2003) reported similar data with no significant differences in weight gain, final weight, feed consumption, and feed efficiency ratio between the dietary groups. Nor did (Sprague et al., 2015) find any adverse effects on the growth of Atlantic salmon post-smolt fed for 133 days with diets in which 11 and 5.5% of FO was replaced with thraustochytrid biomass. Sea bass in our study behaved much like salmon in response to S. limacinum feeding, showing that feed formulations with this microbial biomass met the nutritional needs and growth potential of this farmed fish species, with no need for oil extraction or cell disruption.
Docosahexaenoic acid-rich biomass supplied in the diet ensured optimal fish growth, and also provided a vector for the accumulation of n-3 LC-PUFA in order to pass on to human consumers.
Fatty acid composition of the flesh generally mirrored the composition of the experimental diets, which is in accordance with results obtained in other fish species (Sprague et al., 2015; Katerina et al., 2020). Nevertheless, although the four formulated diets were balanced for PUFAs, fish fed with diets containing either VO (VO + 0) or VO plus 5 and 10% of S. limacinum biomass (diets VO + 5 and VO + 10) had significantly higher levels of PUFAs in the flesh than fish fed the FO diet. This could suggest that fatty acids are better accumulated and retained in fish muscle. Similar results were obtained using alternative lipids sources in different fish species, such as in salmon (Sargent et al., 1997), European sea bass (Monteiro et al., 2018), tilapia (Sarker et al., 2016), and greater amberjack (Monge-Ortiz et al., 2018).
To assess the nutritional value of sea bass filets, we used data on the fatty acid composition to calculate health-related lipid indexes, such as AI, TI, and FLQ, as well as n-6/n-3, and PUFAs/SFAs ratios. These indexes have become some of the most important markers for evaluating the potential effects of lipid consumption on human health and are used as a tool to compare the lipid fractions of different foods in terms of health outcome.
Atherogenicity index and TI consider the different effects that individual fatty acids might have on human health and, in particular, on the probability of increasing the incidence of cardiovascular diseases. It is widely recognized that dietary fatty acids can play an important role in increasing or preventing the risk of atherosclerosis and coronary thrombosis because of their effect on blood cholesterol and low-density lipoprotein concentrations (Siscovick et al., 2017).
Atherogenicity index indicates the relationship between the sum of the main SFAs and the sum of the main unsaturated fatty acids (UFAs). The main classes of SFAs, i.e., lauric (C12:0), myristic (C14:0), and palmitic (C16:0), but not stearic (C18:0), are considered proatherogenic, as they favor the retention of lipids in the walls of blood vessels and the subsequent plaque development (Chen and Liu, 2020). Moreover, myristic acid is considered four times more atherogenic than lauric and palmitic acids. UFAs (in particular, n-3 PUFAs, such as ALA, DHA, and EPA) are considered anti-atherogenic as they inhibit the accumulation of plaque and reduce the production of proinflammatory and adhesion proteins by the arterial wall, thereby providing primary protection against cardiovascular diseases (Siscovick et al., 2017; Abdelhamid et al., 2020). The IA values for sea bass white dorsal muscle were in the range of 0.35–0.46. In agreement with published reports reviewed by Chen and Liu (2020), these are to be considered as low levels, thus representing a healthy product with potential cardioprotective effects on human health.
The TI, defined as the ratio of the prothrombogenic (saturated) to anti-thrombogenic (unsaturated) fatty acids, indicates the tendency to form clots in the blood vessels. In particular, there is a very high correlation between the sum of three saturated acids, myristic, palmitic, and stearic acid, and thrombus formation (Ulbricht and Southgate, 1991). The TI value for fish ranges from 0.14 to 0.87 (Chen and Liu, 2020) and for D. labrax from 0.19 to 0.63 (Monteiro et al., 2018). In our study, the TI values were between 0.30 and 0.37, without showing any significant difference between the values for filets of sea bass fed with diets FO and VO + 10, which were lowest. While there were significant differences in the TI of filet from sea bass fed with the other diets, these values did not exceed 0.37. According to the scientific literature, potential beneficial health impact of filets with AI and TI values higher than 1.0 are minor (Monteiro et al., 2018); therefore, the AI and TI values in our study confirmed the high nutritional value of sea bass filet.
Flesh lipid quality calculates the sum of DHA and EPA as a percentage of total fatty acids. This index is suitable for marine products (fish or shellfish), which are rich in n-3 PUFA and, so far, it has only been used to assess the quality of lipid fractions in fish. The higher the value of this index, the better the quality of the dietary lipid source (Abrami et al., 1992). According to Chen and Liu (2020), in fish, the FLQ value ranges from 13.01 to 36.37. The FLQ value found in our study suggested a high nutritional value of D. labrax filet. The highest levels were recorded in fish fed with diets FO and VO + 10 (14.06 and 14.38, respectively), followed by fish fed with diets VO + 5 and VO + 0. In the latter two, a higher value was found in fish fed with diet VO + 5, containing VO and 5% of Schizochytrium biomass, than in fish fed with diet VO + 0, containing only VO.
The PUFAs/SFAs ratio is normally used to assess the impact of diet on cardiometabolic health. It hypothesizes that dietary PUFAs can reduce low-density lipoprotein (sometimes called “bad” cholesterol), and serum cholesterol levels, whereas all SFAs increase serum cholesterol. Thus, the higher this ratio, the more beneficial the effect. PUFAs/SFAs ratio of European sea bass filets was in the range of 1.18–1.51, which is much higher than the minimum suggested (0.45) for a human healthy diet (Elmadfa and Kornsteiner, 2009). This finding is also in agreement with the literature reviewed by Chen and Liu (2020) that reports fish PUFA/SFA ratios in the range of 0.5–1.79.
Although the PUFAs/SFAs ratio showed a significantly higher value in fish fed with VO + 0, VO + 5, and VO + 10 than in those fed with FO diet, suggesting a better filet quality, the n-6/n-3 ratio clearly indicated that the quality of filet for group FO was best (value of 0.55) and that of group VO + 10 fed with 10% of S. limacinum second best (value of 0.98). Evidence from intense scientific scrutiny suggests that beyond individual n-6 and n-3 intake, the n-6/n-3 PUFA ratio also affects human health. Although an optimum intake ratio has yet to be determined, it was recommended that the ratio should be about 4:1 to 1:1 (Kris-Etherton et al., 2000). In general, a close balance of the n-6:n-3 ratio in our diets may have protective cardiometabolic effects and reduce the risk of developing age-related cognitive decline and dementia (Simopoulos, 2002), whereas a ratio higher than 4:1 can lead to inflammatory disorders, which play a significant role in initiating atherosclerosis (Simopoulos, 2002). Thus, there is a potential to enrich the human diet with n-3 PUFAs by modifying fish feed composition to satisfy nutritional requirements in humans because both type and ratio of dietary oils affect the accumulation of fatty acids in fish filet.
The presence of VOs and S. limacinum in fish diets also prompted our interest in evaluating the nutritional regulation of Δ6-desaturase (or fads2) gene expression in European sea bass liver. FADS2 acts as the first enzyme in the biosynthesis of long-chain (≥C20) PUFAs (LC-PUFAs), such as EPA (C 20:5n-3), DHA (C 22:6n-3), and arachidonic acid (C 20:4n-6), starting from the shorter-chain PUFAs α-linolenic acid (ALA, C 18:3n-3) and linoleic acid (LA, C 18:2n-6) (Tocher, 2003; Izquierdo et al., 2008; Montero et al., 2012). In particular, Δ6-desaturase catalyzes the initial, rate−limiting desaturation of LA to γ−linolenic (C 18:3) and ALA to stearidonic acid (C 18:4) (Tocher, 2003). LC-PUFAs are considered as essential in fish and other vertebrates, as their endogenous production, if present, is insufficient to meet demand. However, unlike freshwater fish, which have maintained a certain degree of Δ6-desaturase and elongase activity and produce EPA and DHA if their precursors LA and ALA are present in the diet, marine fish do not produce LC-PUFA at a significant level as a consequence of living in LC-PUFA-rich marine environments (Izquierdo et al., 2008; Tocher et al., 2019). Vital to this process is providing LC-PUFA precursors LA and ALA, which cannot be synthesized de novo in hardly any living animal as their genomes do not encode for enzymes capable of synthesizing them (Bláhová et al., 2020). Hence, fish are usually dependent on the primary producers, such as phytoplankton, for providing the two major C18 PUFA precursors LA and ALA.
We noted that European sea bass fed the VO + 0 diet had the highest number of mRNA copies for Δ6-desaturase (or fads2), whereas fish fed with diet VO + 10 the lowest. A decreasing trend of expression was evident in fish that did not receive FO (diets VO + 0, VO + 5, and VO + 10). To assess these data, we first considered the fact that the content of DHA (C 22:6n-3), DPA (C 22:5n-3), and EPA (C 20:5n-3) in the flesh were higher than in the feeds, suggesting that these particular fatty acids accumulate and are retained in the muscle. Therefore, we assume that the DHA increase in filets of fish fed with S. limacinum (Table 7) could be related to the high content of this long-chain PUFA in S. limacinum, whereas the higher level of DHA found in the filet of fish fed with diet VO + 0 in comparison to the DHA level in the feed VO + 0 could be due to the over-expression of the Δ6-desaturase gene.
In vivo upregulation of fads2 has been previously reported in marine fish (including sea bass) fed with VO diets in comparison to fish fed FO diets (Izquierdo et al., 2008; Geay et al., 2010; Betancor et al., 2016; Houston et al., 2017; Carvalho et al., 2018). Although fads2 gene overexpression was shown to be correlated with an increase in FADS2 enzymatic activity in anadromous fish such as salmon fed with VO diets (Zheng et al., 2004, 2005), this is less straightforward and the results are controversial in marine fish. Previous studies, indeed, have shown that the partial or total substitution of FO with VO did not increase hepatic desaturase activity in sea bass (Geay et al., 2010), whereas a Δ6-desaturase increased activity in response to a deficiency in essential fatty acids has been suggested in gilthead sea bream (Sparus aurata) (Houston et al., 2017; Carvalho et al., 2021) as well as in meager (Argyrosomus regiu) (Carvalho et al., 2018). In these species, high retention of C 18:2n-6 and C 18:3n-6 and of chain-elongation products of LA and ALA, namely, C 20:2n-6 and C 20:3n-3, suggested that these PUFAs are endogenously synthesized through Δ6-desaturases and Elovl5 (fatty acid elongase 5), respectively.
Taken together our data suggest the value of microbial oils as a source of high value lipids, for use in aquaculture. Oil from thraustochytrid strains grown heterotrophically should be considered a sustainable alternative to partially replace dietary FO in marine fish diets contributing to a circular economy concept, as thraustochytrids can be reared on industrial by-products. Although further technological scale up of microbial oil production is needed, the experimental scenarios tested here provide different perspectives for the feed industry in the context of sustainable aquaculture development, as the environmental impacts connected with aquaculture and the increase in fish demand by a continuously growing global population require changes in fish feed formulations.
Conclusion
The present research was framed in a circular economy context, using crude glycerol derived from industrial biodiesel production as a low-cost carbon source for the heterotrophic fermentation of S. limacinum SR21 strain. This oleaginous microorganism was able to grow well on crude glycerol and to accumulate fatty acids for almost 60% of its DCW, with DHA being one of the major fatty acids produced by the strain. The S. limacinum whole biomass paired with a terrestrial plant-derived oil source was then successfully used to replace FO in European sea bass (D. labrax) feed. Based on fish filet fatty acid profiles and nutritional value indexes, S. limacinum whole-cell biomass appears to provide benefits for filet quality and n-3 LC-PUFA retention efficiency without any indications of negative impact on fish growth. Therefore, S. limacinum biomass can be used in feed for European sea bass as a good alternative source of marine-derived omega-3 fatty acids. However, considering the relatively high cost of Schizochytrium spp. biomass, future studies need to be carried out for the application of our protocol at larger scale, focusing into optimal ratios with VO to improve feed cost-effectiveness.
Data Availability Statement
The datasets presented in this study can be found in online repositories. The names of the repository/repositories and accession number(s) can be found in the article/supplementary material.
Ethics Statement
The animal study was reviewed and approved by the Italian Ministry of Health [REF: 483/2017-PR (response of Prot. Nr. 344C6.6 of 13/03/2017)] in accordance to the Art.31 of D.lgs. 26/2014.
Author Contributions
GT: conceptualization, experimental investigation, data curation, and writing. FM and MA: experimental investigation, methodology, data curation, and editing. SB and CP: experimental investigation, methodology, formal analysis, and editing. PB and ML: conceptualization and writing—review and editing. DP: review and editing. CC: experimental investigation and data curation. SR: experimental investigation, data curation, and writing—review and editing. All authors read and approved the final manuscript.
Funding
This work was funded by the CARIPLO Foundation grant number 2015-0395 (Mysushi) and co-funded by the EU Horizon 2020 AquaIMPACT project (Genomic and nutritional innovations for genetically superior farmed fish to improve efficiency in European aquaculture; 818367). This output reflects only the author’s view and the European Union cannot be held responsible for any use that may be made of the information contained herein. The funders had no role in study design, data collection and analysis, decision to publish, or preparation of the manuscript.
Conflict of Interest
The authors declare that the research was conducted in the absence of any commercial or financial relationships that could be construed as a potential conflict of interest.
Publisher’s Note
All claims expressed in this article are solely those of the authors and do not necessarily represent those of their affiliated organizations, or those of the publisher, the editors and the reviewers. Any product that may be evaluated in this article, or claim that may be made by its manufacturer, is not guaranteed or endorsed by the publisher.
Acknowledgments
Special thanks to Fabio Brambilla at the VRM Naturalleva s.r.l for his assistance with the feed formulation and manufacturing. Federico Moroni is a Ph.D. student of the “Dottorato in Scienze della Vita e Biotecnologie” at the “Università degli Studi dell’Insubria,” Varese, Italy.
References
Abad, S., and Turon, X. (2015). Biotechnological production of docosahexaenoic acid using aurantiochytrium limacinum: Carbon sources comparison and growth characterization. Mar. Drugs 13, 7275–7284. doi: 10.3390/md13127064
Abdelhamid, A. S., Brown, T. J., Brainard, J. S., Biswas, P., Thorpe, G. C., Moore, H. J., et al. (2020). Omega-3 fatty acids for the primary and secondary prevention of cardiovascular disease. Cochr. Database Syst. Rev. 2020:3177. doi: 10.1002/14651858.CD003177.pub5
Abrami, G., Natiello, F., Bronzi, P., McKenzie, D., Bolis, L., and Agradi, E. (1992). A comparison of highly unsaturated fatty acid levels in wild and farmed eels (Anguilla Anguilla). Compar. Biochem. Physiol. Biochem. 101, 79–81. doi: 10.1016/0305-0491(92)90161-J
Allen, K. M., Habte-Tsion, H. M., Thompson, K. R., Filer, K., Tidwell, J. H., and Kumar, V. (2019). Freshwater microalgae (Schizochytrium sp.) as a substitute to fish oil for shrimp feed. Sci. Rep. 9, 1–10. doi: 10.1038/s41598-019-41020-8
Athenaki, M., Gardeli, C., Diamantopoulou, P., Tchakouteu, S. S., Sarris, D., Philippoussis, A., et al. (2018). Lipids from yeasts and fungi: physiology, production and analytical considerations. J. Appl. Microbiol. 124, 336–367. doi: 10.1111/jam.13633
Ayisi, C., Zhao, J., and Apraku, A. (2019). Consequences of Replacing Fish Oil with Vegetable Oils in Fish. J. Anim. Res. Nutr. 4, 1–11. doi: 10.21767/2572-5459.100053
Becker, E. W. (2007). Micro-algae as a source of protein. Biotechnol. Adv. 25, 207–210. doi: 10.1016/j.biotechadv.2006.11.002
Beopoulos, A., and Nicaud, J. M. (2012). Yeast: A new oil producer? OCL - Oleagineux Corps Gras Lipides 19, 22–28. doi: 10.1684/ocl.2012.0426
Betancor, M. B., Sprague, M., Sayanova, O., Usher, S., Metochis, C., Campbell, P. J., et al. (2016). Nutritional Evaluation of an EPA-DHA Oil from Transgenic Camelina sativa in Feeds for Post-Smolt Atlantic Salmon (Salmo salar L.). PLoS One 11:e0159934. doi: 10.1371/journal.pone.0159934
Bláhová, Z., Harvey, T. N., Pšenièka, M., and Mráz, J. (2020). Assessment of fatty acid desaturase (Fads2) structure-function properties in fish in the context of environmental adaptations and as a target for genetic engineering. Biomolecules 10:20206. doi: 10.3390/biom10020206
Bligh, E. G., and Dyer, W. J. (1959). A rapid method of total lipid extraction and purification. Can. J. Biochem. Physiol. 37, 911–917. doi: 10.1139/y59-099
Bouras, S., Katsoulas, N., Antoniadis, D., and Karapanagiotidis, I. T. (2020). Use of biofuel industry wastes as alternative nutrient sources for DHA-yielding Schizochytrium limacinum production. Appl. Sci. 10, 1–16. doi: 10.3390/app10124398
Calder, P. C., and Yaqoob, P. (2009). Omega-3 polyunsaturated fatty acids and human health outcomes. BioFactors 35, 266–272. doi: 10.1002/biof.42
Carter, C. G., Bransden, M. P., Lewis, T. E., and Nichols, P. D. (2003). Potential of Thraustochytrids to Partially Replace Fish Oil in Atlantic Salmon Feeds. Mar. Biotechnol. 5, 480–492. doi: 10.1007/s10126-002-0096-8
Carvalho, M., Montero, D., Rosenlund, G., Fontanillas, R., Ginés, R., and Izquierdo, M. (2020). Effective complete replacement of fish oil by combining poultry and microalgae oils in practical diets for gilthead sea bream (Sparus aurata) fingerlings. Aquaculture 529:735696. doi: 10.1016/j.aquaculture.2020.735696
Carvalho, M., Montero, D., Torrecillas, S., Castro, P., Zamorano, M. J., and Izquierdo, M. (2021). Hepatic biochemical, morphological and molecular effects of feeding microalgae and poultry oils to gilthead sea bream (Sparus aurata). Aquaculture 532:736073. doi: 10.1016/j.aquaculture.2020.736073
Carvalho, M., Peres, H., Saleh, R., Fontanillas, R., Rosenlund, G., Oliva-Teles, A., et al. (2018). Dietary requirement for n-3 long-chain polyunsaturated fatty acids for fast growth of meagre (Argyrosomus regius, Asso 1801). fingerlings. Aquaculture 488, 105–113. doi: 10.1016/j.aquaculture.2018.01.028
Chang, G., Luo, Z., Gu, S., Wu, Q., Chang, M., and Wang, X. (2013). Fatty acid shifts and metabolic activity changes of Schizochytrium sp. S31 cultured on glycerol. Bioresour. Technol. 142, 255–260. doi: 10.1016/J.BIORTECH.2013.05.030
Checa, M., Nogales-Delgado, S., Montes, V., and Encinar, J. M. (2020). Recent advances in glycerol catalytic valorization: A review. Catalysts 10, 1–41. doi: 10.3390/catal10111279
Chen, J., and Liu, H. (2020). Nutritional indices for assessing fatty acids: A mini-review. Internat. J. Mole. Sci. 21, 1–24. doi: 10.3390/ijms21165695
Chi, Z., Pyle, D., Wen, Z., Frear, C., and Chen, S. (2007). A laboratory study of producing docosahexsaenoic acid from biodiesel-waste glycerol by microalgal fermentation. Proc. Biochem. 42, 1537–1545. doi: 10.1016/j.procbio.2007.08.008
Elmadfa, I., and Kornsteiner, M. (2009). Fats and fatty acid requirements for adults. Ann. Nutr. Metab 55, 56–75. doi: 10.1159/000228996
Erdman, J. W., MacDonald, I. A., and Zeisel, S. H. (2012). Present Knowledge in Nutrition: Tenth Edition. Hoboken, NJ: Wiley-Blackwell, doi: 10.1002/9781119946045
Ethier, S., Woisard, K., Vaughan, D., and Wen, Z. (2011). Continuous culture of the microalgae Schizochytrium limacinum on biodiesel-derived crude glycerol for producing docosahexaenoic acid. Bioresour. Technol. 102, 88–93. doi: 10.1016/j.biortech.2010.05.021
Geay, F., Santigosa, I., Culi, E., Corporeau, C., Boudry, P., Dreano, Y., et al. (2010). Regulation of FADS2 expression and activity in European sea bass (Dicentrarchus labrax, L.) fed a vegetable diet. Compar. Biochem. Physiol. B Biochem. Mole. Biol. 156, 237–243. doi: 10.1016/j.cbpb.2010.03.008
Geissdoerfer, M., Savaget, P., Bocken, N. M. P., and Hultink, E. J. (2017). The Circular Economy – A new sustainability paradigm? J. Clean. Product. 143, 757–768. doi: 10.1016/j.jclepro.2016.12.048
Glencross, B. D. (2009). Exploring the nutritional demand for essential fatty acids by aquaculture species. Rev. Aquacult. 1, 71–124. doi: 10.1111/j.1753-5131.2009.01006.x
Glencross, B. D., Baily, J., Berntssen, M. H. G., Hardy, R., MacKenzie, S., and Tocher, D. R. (2020a). Risk assessment of the use of alternative animal and plant raw material resources in aquaculture feeds. Rev. Aqua. 12, 703–758. doi: 10.1111/RAQ.12347
Glencross, B. D., Huyben, D., and Schrama, J. W. (2020b). The application of single-cell ingredients in aquaculture feeds—a review. Fishes 5, 1–39. doi: 10.3390/fishes5030022
Harel, Z., Gascon, G., Riggs, S., Vaz, R., Brown, W., and Exil, G. (2002). Supplementation with omega-3 polyunsaturated fatty acids in the management of recurrent migraines in adolescents. J. Adolesc. Health 31, 154–161. doi: 10.1016/S1054-139X(02)00349-X
Hart, B., Schurr, R., Narendranath, N., Kuehnle, A., and Colombo, S. M. (2021). Digestibility of Schizochytrium sp. whole cell biomass by Atlantic salmon (Salmo salar). Aquaculture 533:736156. doi: 10.1016/j.aquaculture.2020.736156
Houston, S. J. S., Karalazos, V., Tinsley, J., Betancor, M. B., Martin, S. A. M., Tocher, D. R., et al. (2017). The compositional and metabolic responses of gilthead seabream (Sparus aurata) to a gradient of dietary fish oil and associated n-3 long-chain PUFA content. Br. J. Nutr. 118, 1010–1022. doi: 10.1017/S0007114517002975
Innes, J. K., and Calder, P. C. (2020). Marine omega-3 (N-3) fatty acids for cardiovascular health: An update for 2020. Internat. J. Mole. Sci. 21, 1–21. doi: 10.3390/ijms21041362
Izquierdo, M. S., Robaina, L., Juárez-Carrillo, E., Oliva, V., Hernández-Cruz, C. M., and Afonso, J. M. (2008). Regulation of growth, fatty acid composition and delta 6 desaturase expression by dietary lipids in gilthead seabream larvae (Sparus aurata). Fish Physiol. Biochem. 34, 117–127. doi: 10.1007/s10695-007-9152-7
Johnson, D. T., and Taconi, K. A. (2007). The glycerin glut: Options for the value-added conversion of crude glycerol resulting from biodiesel production. Environ. Prog. 26, 338–348. doi: 10.1002/ep.10225
Katerina, K., Berge, G. M., Turid, M., Aleksei, K., Grete, B., Trine, Y., et al. (2020). Microalgal Schizochytrium limacinum Biomass Improves Growth and Filet Quality When Used Long-Term as a Replacement for Fish Oil, in Modern Salmon Diets. Front. Mar. Sci. 7:57. doi: 10.3389/fmars.2020.00057
Katiyar, R., Gurjar, B. R., Bharti, R. K., Kumar, A., Biswas, S., and Pruthi, V. (2017). Heterotrophic cultivation of microalgae in photobioreactor using low cost crude glycerol for enhanced biodiesel production. Renew. Ener. 113, 1359–1365. doi: 10.1016/j.renene.2017.06.100
Khot, M., Kamat, S., Zinjarde, S., Pant, A., Chopade, B., and Ravikumar, A. (2012). Single cell oil of oleaginous fungi from the tropical mangrove wetlands as a potential feedstock for biodiesel. Microb. Cell Fact. 11, 1–13. doi: 10.1186/1475-2859-11-71
Kousoulaki, K., Østbye, T. K. K., Krasnov, A., Torgersen, J. S., Mørkøre, T., and Sweetman, J. (2015). Metabolism, health and fillet nutritional quality in Atlantic salmon (Salmo salar) fed diets containing n-3-rich microalgae. J. Nutrit. Sci. 4:14. doi: 10.1017/jns.2015.14
Kris-Etherton, P. M., Taylor, D. S., Yu-Poth, S., Huth, P., Moriarty, K., Fishell, V., et al. (2000). Polyunsaturated fatty acids in the food chain in the United States. In: American Journal of Clinical Nutrition. Am. Soc. Nutr. 2000, 179S–188S. doi: 10.1093/ajcn/71.1.179s
Layé, S., Nadjar, A., Joffre, C., and Bazinet, R. P. (2018). Anti-inflammatory effects of omega-3 fatty acids in the brain: Physiological mechanisms and relevance to pharmacology. Pharm. Rev. 70, 12–38. doi: 10.1124/pr.117.014092
Lee Chang, K. J., Parrish, C. C., Simon, C. J., Revill, A. T., and Nichols, P. D. (2020). Feeding Whole Thraustochytrid Biomass to Cultured Atlantic Salmon (Salmo salar) Fingerlings: Culture Performance and Fatty Acid Incorporation. J. Mar. Sci. Eng. 8:207. doi: 10.3390/jmse8030207
Lung, Y. T., Tan, C. H., Show, P. L., Ling, T. C., Lan, J. C. W., Lam, H. L., et al. (2016). Docosahexaenoic acid production from crude glycerol by Schizochytrium limacinum SR21. Clean Technol. Env. Policy 18, 2209–2216. doi: 10.1007/s10098-016-1126-y
Lupatsch, I. (2005). Protein and energy requirements in Mediterranean species. Cahiers Option. Méditerrané. 63, 9–18.
Martins, D. A., Rocha, F., Martínez-Rodríguez, G., Bell, G., Morais, S., Castanheira, F., et al. (2012). Teleost fish larvae adapt to dietary arachidonic acid supply through modulation of the expression of lipid metabolism and stress response genes. Br. J. Nutr. 108, 864–874. doi: 10.1017/S0007114511006143
Masuda, R., and Tsukamoto, K. (1999). School formation and concurrent developmental changes in carangid fish with reference to dietary conditions. Environ. Biol. Fishes 56, 243–252. doi: 10.1023/A:1007565508398
Mata, T. M., Santos, J., Mendes, A. M., Caetano, N. S., and Martins, A. A. (2014). Sustainability Evaluation of Biodiesel Produced from Microalgae Chlamydomonas sp Grown in Brewery Wastewater. Chem. Eng. Trans. 37, 823–828. doi: 10.3303/CET1437138
Monge-Ortiz, R., Tomás-Vidal, A., Rodriguez-Barreto, D., Martínez-Llorens, S., Pérez, J. A., Jover-Cerdá, M., et al. (2018). Replacement of fish oil with vegetable oil blends in feeds for greater amberjack (Seriola dumerili) juveniles: Effect on growth performance, feed efficiency, tissue fatty acid composition and flesh nutritional value. Aqua. Nutr. 24, 605–615. doi: 10.1111/anu.12595
Monteiro, M., Matos, E., Ramos, R., Campos, I., and Valente, L. M. P. (2018). A blend of land animal fats can replace up to 75% fish oil without affecting growth and nutrient utilization of European seabass. Aquaculture 487, 22–31. doi: 10.1016/j.aquaculture.2017.12.043
Montero, D., Kalinowski, T., Obach, A., Robaina, L., Tort, L., and Caballero, M. J. (2003). Vegetable lipid sources for gilthead seabream (Sparus aurata): effects on fish health. Aquaculture 225, 353–370. doi: 10.1016/S0044-8486(03)00301-6
Montero, D., Terova, G., Rimoldi, S., Tort, L., Negrin, D., Zamorano, M. J., et al. (2012). Modulation of adrenocorticotrophin hormone (ACTH)-induced expression of stress-related genes by PUFA in inter-renal cells from European sea bass (Dicentrarchus labrax). J. Nutr. Sci. 4, 1–9. doi: 10.1017/jns.2012.2
Moreira, L. M., Da Silva, A., Rocha, R., Lúcia, C., Ribeiro, G., Da, R., et al. (2011). Nutritional evaluation of single-cell protein produced by Spirulina platensis. Afr. J. Food Sci. 5, 799–805. doi: 10.5897/AJFS11.184
Nasseri, A. T., Rasoul-Ami, S., Morowvat, M. H., and Ghasemi, Y. (2011). Single Cell Protein: Production and Process. Am. J. Food Technol. 6, 103–116. doi: 10.3923/ajft.2011.103.116
O’Grady, J., and Morgan, J. A. (2011). Heterotrophic growth and lipid production of Chlorella protothecoides on glycerol. Bioproc. Biosyst. Eng. 34, 121–125. doi: 10.1007/s00449-010-0474-y
Özşahinoğlu, I., Eroldogan, T., Mumoğullarinda, P., Dikel, S., Engin, K., Yílmaz, H. A., et al. (2013). Partial Replacement of Fish Oil with Vegetable Oils in Diets for European Seabass (Dicentrarchus labrax): Effects On Growth Performance and Fatty Acids Profile. Turkish J. Fish. Aquat. Sci. 2013, 819–825. doi: 10.4194/1303-2712-v13_3_05
Patel, A., Karageorgou, D., Rova, E., Katapodis, P., Rova, U., Christakopoulos, P., et al. (2020). An overview of potential oleaginous microorganisms and their role in biodiesel and omega-3 fatty acid-based industries. Microorganisms 8:8030434. doi: 10.3390/microorganisms8030434
Pyle, D. J., Garcia, R. A., and Wen, Z. (2008). Producing docosahexaenoic acid (DHA)-rich algae from biodiesel-derived crude glycerol: Effects of impurities on DHA production and algal biomass composition. J. Agr. Food Chem. 56, 3933–3939. doi: 10.1021/jf800602s
Ratledge, C., and Cohen, Z. (2008). Microbial and algal oils: Do they have a future for biodiesel or as commodity oils? Lipid Technol. 20, 155–160. doi: 10.1002/lite.200800044
Ross, A. C., Caballero, B., Cousins, R. J., Tucker, K. L., and Ziegler, T. R. (2012). Modern nutrition in health and disease: Eleventh edition. Netherlands: Wolters Kluwer Health Adis, doi: 10.1097/01.ccm.0000236502.51400.9f
Sales, J., and Glencross, B. (2011). A meta-analysis of the effects of dietary marine oil replacement with vegetable oils on growth, feed conversion and muscle fatty acid composition of fish species. Aqua. Nutr. 17:761. doi: 10.1111/j.1365-2095.2010.00761.x
Sargent, J. R., McEvoy, L. A., and Bell, J. G. (1997). Requirements, presentation and sources of polyunsaturated fatty acids in marine fish larval feeds. Aquaculture 155, 117–127. doi: 10.1016/S0044-8486(97)00122-1
Sariatli, F. (2017). Linear Economy Versus Circular Economy: A Comparative and Analyzer Study for Optimization of Economy for Sustainability. Visegr. J. Bioecon. Sust. Dev. 6, 31–34. doi: 10.1515/vjbsd-2017-0005
Sarker, P. K., Kapuscinski, A. R., Lanois, A. J., Livesey, Bernhard, K. P., and Coley, M. L. (2016). Towards sustainable aquafeeds: Complete substitution of fish oil with marine microalga Schizochytrium sp. improves growth and fatty acid deposition in juvenile Nile tilapia (Oreochromis niloticus). PLoS One 11, 1–17. doi: 10.1371/journal.pone.0156684
Signori, L., Ami, D., Posteri, R., Giuzzi, A., Mereghetti, P., Porro, D., et al. (2016). Assessing an effective feeding strategy to optimize crude glycerol utilization as sustainable carbon source for lipid accumulation in oleaginous yeasts. Microb. Cell Fact. 15:467. doi: 10.1186/s12934-016-0467-x
Simopoulos, A. P. (2002). The importance of the ratio of omega-6/omega-3 essential fatty acids. Biomed. Pharm. 56, 365–379. doi: 10.1016/S0753-3322(02)00253-6
Siscovick, D. S., Barringer, T. A., Fretts, A. M., Wu, J. H. Y., Lichtenstein, A. H., Costello, R. B., et al. (2017). Omega-3 Polyunsaturated Fatty Acid (Fish Oil) Supplementation and the Prevention of Clinical Cardiovascular Disease: A Science Advisory from the American Heart Association. Circulation 135, e867–e884. doi: 10.1161/CIR.0000000000000482
Sprague, M., Betancor, M. B., and Tocher, D. R. (2017). Microbial and genetically engineered oils as replacements for fish oil in aquaculture feeds. Biotechnol. Lett. 39, 1599–1609. doi: 10.1007/s10529-017-2402-6
Sprague, M., Walton, J., Campbell, P. J., Strachan, F., Dick, J. R., and Bell, J. G. (2015). Replacement of fish oil with a DHA-rich algal meal derived from Schizochytrium sp. on the fatty acid and persistent organic pollutant levels in diets and flesh of Atlantic salmon (Salmo salar, L.) post-smolts. Food Chem. 413–421. doi: 10.1016/j.foodchem.2015.03.150
Sun, L., Ren, L., Zhuang, X., Ji, X., Yan, J., and Huang, H. (2014). Differential effects of nutrient limitations on biochemical constituents and docosahexaenoic acid production of Schizochytrium sp. Bioresour. Technol. 159, 199–206. doi: 10.1016/j.biortech.2014.02.106
Tacon, A. G. J., and Metian, M. (2008). Global overview on the use of fish meal and fish oil in industrially compounded aquafeeds: Trends and future prospects. Aquaculture 285, 146–158. doi: 10.1016/j.aquaculture.2008.08.015
Tibaldi, E., and Kaushik, S. J. (2005). Amino acid requirements of Mediterranean fish species. Zaragoza: CIHEAM.
Tocher, D. R. (2003). Metabolism and functions of lipids and fatty acids in teleost fish. Rev. Fish. Sci. 11, 107–184. doi: 10.1080/713610925
Tocher, D. R. (2010). Fatty acid requirements in ontogeny of marine and freshwater fish. Aquacul. Res. 41, 717–732. doi: 10.1111/j.1365-2109.2008.02150.x
Tocher, D. R., Betancor, M. B., Sprague, M., Olsen, R. E., and Napier, J. A. (2019). Omega-3 long-chain polyunsaturated fatty acids, EPA and DHA: Bridging the gap between supply and demand. Nutrients 11:10089. doi: 10.3390/nu11010089
Ulbricht, T. L., and Southgate, D. A. (1991). Coronary heart disease: seven dietary factors. Lancet 338, 985–992. doi: 10.1016/0140-6736(91)91846-m
Vanthoor-Koopmans, M., Wijffels, R. H., Barbosa, M. J., and Eppink, M. H. M. (2013). Biorefinery of microalgae for food and fuel. Bioresour. Tech. 135, 142–149. doi: 10.1016/j.biortech.2012.10.135
Yuan, Z., Bi, J., and Moriguichi, Y. (2006). The circular economy: A new development strategy in China. J. Industr. Ecol. 10, 4–8. doi: 10.1162/108819806775545321
Zheng, X., Tocher, D. R., Dickson, C. A., Bell, J. G., and Teale, A. J. (2004). Effects of diets containing vegetable oil on expression of genes involved in highly unsaturated fatty acid biosynthesis in liver of Atlantic salmon (Salmo salar). Aquaculture 236, 467–483. doi: 10.1016/j.aquaculture.2004.02.003
Zheng, X., Torstensen, B. E., Tocher, D. R., Dick, J. R., Henderson, R. J., and Bell, J. G. (2005). Environmental and dietary influences on highly unsaturated fatty acid biosynthesis and expression of fatty acyl desaturase and elongase genes in liver of Atlantic salmon (Salmo salar). Biochim. Biophys. Acta Mole. Cell Biol. Lipids 1734, 13–24. doi: 10.1016/j.bbalip.2005.01.006
Keywords: aquaculture, aquafeed, Dicentrarchus labrax, Schizochytrium limacinum, Δ6-desaturase, gene expression, fish filet quality, fatty acid profile
Citation: Terova G, Moroni F, Antonini M, Bertacchi S, Pesciaroli C, Branduardi P, Labra M, Porro D, Ceccotti C and Rimoldi S (2021) Using Glycerol to Produce European Sea Bass Feed With Oleaginous Microbial Biomass: Effects on Growth Performance, Filet Fatty Acid Profile, and FADS2 Gene Expression. Front. Mar. Sci. 8:715078. doi: 10.3389/fmars.2021.715078
Received: 26 May 2021; Accepted: 21 July 2021;
Published: 10 August 2021.
Edited by:
Luisa M. P. Valente, University of Porto, PortugalReviewed by:
Ivan Viegas, University of Coimbra, PortugalCamilla Della Torre, University of Milan, Italy
Copyright © 2021 Terova, Moroni, Antonini, Bertacchi, Pesciaroli, Branduardi, Labra, Porro, Ceccotti and Rimoldi. This is an open-access article distributed under the terms of the Creative Commons Attribution License (CC BY). The use, distribution or reproduction in other forums is permitted, provided the original author(s) and the copyright owner(s) are credited and that the original publication in this journal is cited, in accordance with accepted academic practice. No use, distribution or reproduction is permitted which does not comply with these terms.
*Correspondence: Genciana Terova, Z2VuY2lhbmEudGVyb3ZhQHVuaW5zdWJyaWEuaXQ=