- Smithsonian Marine Station, Fort Pierce, FL, United States
Brown tides formed by Aureoumbra lagunensis decrease light penetration in the water column and are often followed by hypoxic events that result in the loss of fish and shellfish. To understand the ability of bivalve filter feeders to control and prevent A. lagunensis blooms, we exposed eastern oysters (Crassostrea virginica), hooked mussels (Ischadium recurvum), and hard clams (Mercenaria mercenaria) to a naturally co-occurring brown tide in the Indian River Lagoon (IRL), Florida, United States. Bivalves were exposed in the laboratory to multiple concentrations (104 to 106 cells mL–1) of isotopically labeled (13C and 15N) A. lagunensis cells. The standard clearance rate (herein clearance rate) of each bivalve species was calculated using flow cytometry to quantify A. lagunensis cell removal. The highest clearance rates were at 104 cells mL–1, but values varied across bivalve species (2.16 ± 0.30, 3.03 ± 0.58, and 0.41 ± 0.12 L h–1 for C. virginica, I. recurvum, and M. mercenaria, respectively). Although clearance rates decreased with increasing bloom concentrations, bivalves were still consuming algal cells at all concentrations and were retaining and assimilating more cells at the highest concentrations, as revealed by δ13C and δ15N values. We highlight interspecific differences among bivalve species in the removal of A. lagunensis, supporting the importance of healthy and diverse filter feeding communities in estuaries, especially as threats of brown tides and other HABs are increasing in the Anthropocene.
Introduction
The proliferation of harmful microalgae in aquatic ecosystems, commonly known as Harmful Algal Blooms (HABs), have been recorded since ancient times (Moore, 1977; Hallegraeff, 2003). These HABs can be comprised of diverse microalgal species and may be toxic or non-toxic. Toxic blooms alter the health of or cause death in many organisms (Landsberg, 2002; Backer and McGillicuddyJr., 2006), while non-toxic HABs change abiotic conditions (Hallegraeff, 2003; Gobler and Sunda, 2012). These different mechanisms result in large ecosystem level effects. For instance, hypoxic events caused by bacteria degrading dead algal cells and depleting dissolved oxygen levels are often associated with non-toxic blooms and found in bottom waters that are isolated from surface waters by density gradients (Hallegraeff, 2003; Gobler et al., 2013). Brown tides are examples of HABs caused by two different species of marine microalgae from the class Pelagophyceae, Aureoumbra lagunensis and Aureococcus anophagefferens. These species are small (2–5 μm), spherical, non-motile cells with simple life cycles and contain the unique pigment 19′-butanoyloxyfucoxanthin (DeYoe et al., 1997). Both species have the ability to disrupt and damage coastal ecosystems but only A. anophagefferens may express toxicity (Bricelj and Lonsdale, 1997). However, the ecological effects caused by A. lagunensis cannot be underestimated.
The non-toxic brown tide, A. lagunensis, has been responsible for disrupting coastal lagoons and endangering their health and sustainability. These brown tides can substantially decrease light penetration in the water column, preventing adequate sunlight for seagrass growth and reducing seagrass abundance dramatically, with dangerous cascading effects for the ecosystem including fish and shellfish kills (Onuf, 1996; Gobler et al., 2013; Lapointe et al., 2020). Moreover, A. lagunensis blooms are often persistent within a system; for example, a brown tide persisted for over 8 years without interruption in the Laguna Madre, Texas (Buskey et al., 1998). In 2012, a brown tide (∼106 cells ml–1) was reported in the Indian River Lagoon, the first time outside Texas (Gobler et al., 2013), and reappeared in 2013, 2015, 2016, and 2018 (Galimany et al., 2017b; Phlips et al., 2019). Brown tides have also been reported in Guantánamo Bay, Cuba (Hall et al., 2018). The proliferation and recurrence of A. lagunensis may be related to eutrophication (Lapointe et al., 2015); however, other factors including extreme climatic conditions or suppression of grazer populations should not be ignored (Phlips et al., 2015).
Population dynamics of HABs are regulated by both “bottom-up” abiotic factors such as light and nutrients affecting their development, and “top-down” factors controlling their loss rates including pathogens and grazers (Buskey, 2008). For example, the lack of filter feeding grazers, such as bivalves, may have played an important part in brown tide formations in Laguna Madre, Texas (Montagna et al., 1993; Buskey et al., 1997). The ecosystem services offered by bivalves, including the maintenance of water quality through filtration and assimilation of phytoplankton, are key to sustaining coastal ecosystems. Nonetheless, bivalve populations are decreasing worldwide because of overharvesting and ecosystem degradation (Beck et al., 2011; FAO, 2018). When bivalve populations are depleted, instead of helping to mitigate bloom formation, they may be negatively affected by brown tides, further perpetuating water degradation. For example, hard clams (Mercenaria mercenaria) and eastern oysters (Crassostrea virginica) decreased their clearance rates when feeding during an A. lagunensis bloom in the Indian River Lagoon (Florida, United States) (Gobler et al., 2013; Galimany et al., 2017b). This altered feeding behavior may be a result of A. lagunensis cells producing exopolymer secretions that may affect the motility of cilia on the bivalves’ gills (Liu and Buskey, 2000) or, simply, a physiological response to an excess of organic matter clogging their gills (Ward and Shumway, 2004). However, both bivalve species (M. mercenaria and C. virginica) fed on A. lagunensis (Gobler et al., 2013; Galimany et al., 2017b, 2020), unlike other HABs, which generally cause bivalve closure and non-feeding (Shumway, 1990). Importantly, bivalves feed more efficiently on lower, pre-bloom densities of the brown tide, i.e., 104 cells mL–1, and the hooked mussel (Ischadium recurvum) even increased its feeding on A. lagunensis over time when the density was sustained at pre-bloom conditions (Galimany et al., 2020).
Bivalves provide important ecosystem services including water clarification through the removal of phytoplankton (Galimany et al., 2013, 2017b; Cranford, 2019). Previous studies in the IRL demonstrate that bivalves may remove A. lagunensis at low concentrations (Galimany et al., 2020) and some may even clear fully developed brown tides (Galimany et al., 2017b). However, there is a lack of information on the assimilation of this microalga by bivalves, including non-commercial species, to potentially ameliorate brown tides. To address this research gap we investigated how co-occurring bivalve species could potentially mitigate A. lagunensis cells from pre-bloom (the beginning of the bloom formation) to bloom concentrations (brown tide), conducting laboratory feeding assays with three bivalve species naturally found on the East coast of North America, including the Indian River Lagoon: eastern oysters (Crassostrea virginica), hooked mussels (Ischadium recurvum), and hard clams (Mercenaria mercenaria). The clearance rate of each bivalve species was calculated using flow cytometry as a proxy for algal cell removal. In addition, algal cells were isotopically (13C and 15N) labeled to verify that they were ingested, and algae-derived nutrients assimilated. We hypothesized that, although the clearance rates of these bivalve species would decrease with increasing concentrations of brown tide, all three species would be capable of continuing to feed on and assimilate A. lagunensis at bloom concentrations. This work aims to elucidate the important role of bivalves in coastal shallow ecosystems and highlight any species-specific differences in their potential to ameliorate A. lagunensis brown tides.
Materials and Methods
Brown Tide Collection
In December 2015, a brown tide caused by the pelagophyte Aureoumbra lagunensis D. A. Stockwell, DeYoe, Hargraves & P. W. Johnson, 1997, occurred in all the basins of the Indian River Lagoon and persisted for several months (Galimany et al., 2017b; Phlips et al., 2019). Water containing a natural brown tide (Aureoumbra lagunensis) was collected from the dock of the Banana River Resort, Indian River Lagoon (Florida, FL, United States) in Cocoa Beach (28° 16′20.54″ N; 80° 36′29.07″ W). The brown tide was immediately transported to the Smithsonian Marine Station at Fort Pierce (SMSFP) and kept in aerated buckets in the laboratory at 23°C. Aureoumbra lagunensis cells were isotopically labeled by incubation with inorganic tracer compounds enriched in the heavy isotopes of C and N (98 atom percent [at%] 13C or 15N. These compounds (NaH13CO3 [1.18 mM] and Na15NO3 [0.117 mM]) were added directly into buckets holding A. lagunensis 24 h prior to the start of experiments following methods in Freeman et al. (2013). We have shown previously that A. lagunensis assimilates Na15NO3 (Galimany et al., 2020), but dual labels (both 13C and 15N) were still used for these experiments to ensure that A. lagunensis cells were adequately enriched in at least one isotope to allow for evidence of trophic transfer (13C and/or 15N enrichment in bivalve tissue).
Brown Tide Identification
To characterize the phytoplankton bloom during the brown tide, we collected water samples from Cocoa Beach, FL. Water samples were taken on 25, 28 and 29 January and 5, 8 and 27 February 2016 to track the bloom in Banana River (FL). Water samples were analyzed through a C6 flow cytometer (BD Biosciences, San Jose, CA, United States) for 30 s on the slow flow rate setting (14 μL min–1) using plots contrasting side scatter (SSC) vs. red fluorescence (FL4) to identify and quantify the algal population. Plots from the bloom were compared with plots generated from a pure A. lagunensis culture provided by the University of Texas Marine Science Institute, which was isolated by the laboratory of Dr. Tracy Villareal, University of Texas, in April 1996 from the Laguna Madre (North Padre Island, Texas), as previously used in (Galimany et al., 2017b).
The presence of A. lagunensis was also confirmed by genetic identification using a fragment of the 16S ribosomal gene (16S rRNA) as a genetic marker. DNA was extracted from the cell pellet obtained by centrifuging a volume of 50 mL of water collected during the algal bloom. The cell pellet was freeze-dried and stored at −80°C for 48 h, transferred into a 1.5 mL tube, and powdered using a TissueLyser II (QIAGEN, DE). This powder was used to extract the total DNA using the extraction kit PowerPlant® Pro (Mo BIO, CA). The 16S rRNA fragment was amplified by PCR in 25 μL reactions with the following conditions: 10 mM Tris-HCl, 50 mM KCl, 2.5 mM MgCl2, 0.25 mM each dNTP, 0.8 μM of each primer. Primers used were CYA106F 5′ CGG ACG GGT GAG TAA CGC GTG A 3′ and equimolar mixture of CYA781R(a) 5′GAC TAC TGG GGT ATC TAA TCC CAT T 3′ and CYA781R(b) 5′ GAC TAC AGG GGT ATC TAA TCC CTT T 3′ (Nübel et al., 1997). The PCR product was sequenced at the Smithsonian Laboratories of Analytical Biology, the sequence was checked for base call and aligned to compare to reference homologous sequences using BLAST search tool in GenBank.
Experimental Bivalve Species
Three local bivalve species were used for the experiments: eastern oysters [Crassostrea virginica (Gmelin, 1791)], hooked mussels [Ischadium recurvum (Rafinesque, 1820)], and hard clams [Mercenaria mercenaria (Linnaeus, 1758)]. C. virginica and M. mercenaria were purchased from Aquagem Farms Inc. in Sebastian, Indian River Lagoon, FL (27° 48′ 25.00″ N; 80° 28′ 01.37″ W); I. recurvum was collected from Stuart, Indian River Lagoon, FL (27° 12′ 04.34″ N; 80° 15′ 19.67″ W). All bivalves were cleaned of epiphytes and other encrusting organisms and kept in the laboratory in a flowing seawater system feeding on a mix of natural water and Tetraselmis sp. for a week before the experiments. Adult individuals were used for the experiments with mean shell lengths (±SE) of 53.79 ± 2.70, 29.48 ± 0.60, and 49.89 ± 0.42 mm for C. virginica, I. recurvum, and M. mercenaria, respectively.
Bivalve Clearance Rates
To quantify the removal of A. lagunensis cells by the three studied bivalve species we conducted a depletion experiment. Five concentrations (cells mL–1) of A. lagunensis were tested: 104, 5 × 104, 105, 5 × 105, 106, encompassing pre-bloom to bloom concentrations of A. lagunensis. The natural brown tide was diluted using water at the same salinity (26 ppt) filtered through Millipore PVDF Membrane Filters (0.22 μm pore size). For each A. lagunensis concentration, five of each bivalve species were haphazardly selected from the acclimation tank containing previously collected bivalves and placed individually in an aerated 500 mL jar (N = 5 bivalves per species and algal density). One extra jar with an empty shell of each bivalve species at each algal concentration was left as a control. Bivalves were fed using a similar method described in Galimany et al. (2020). Briefly, bivalves were fed 13C- or 15N-enriched A. lagunensis twice a day for four days: (1) early morning, each bivalve was transferred to a jar containing filtered estuarine water (26 ppt). Meanwhile, the experimental jar was quickly emptied, washed and filled with the corresponding A. lagunensis concentration. The bivalves were placed back in the experimental jars in less than 30 s; (2) in the afternoon, the algal concentration remaining in each jar was calculated using a C6 flow cytometer (BD Biosciences, San Jose, CA, United States) as described below. Then, the amount of A. lagunensis remaining was calculated and, if needed, more was added to each jar to reach the initial concentrations.
On the last A. lagunensis feeding (day four), water samples from each jar were taken every 5 min, starting at time 0 and continuing for 30 min, after which samples were taken every 10 min until 1 h had passed. Each of these 10 samples was collected with a pipette and preserved with 1% formalin (final concentration) until analysis. Following the termination of feeding experiments, all bivalve individuals were held in fresh, filtered seawater for 12 h to ensure that their guts were empty from 13C or 15N labeled algal cells. Bivalves were then dissected and dried at 60°C for 48 h to determine individual dry tissue weight. Dried soft tissue from all individuals was used for stable isotope analyses.
To calculate A. lagunensis concentration in each jar, water samples were processed on a C6 flow cytometer (BD Biosciences, San Jose, CA, United States) for 2 min on the fast flow rate setting (35 μL min–1), as in Galimany et al. (2020). Algal cells were identified and quantified with plots contrasting side scatter (SSC) vs. red fluorescence (FL4) generated by the C6. Then, clearance rates (CR) were determined from the exponential decrease in algal cell concentration as a function of time using the formula (Riisgård, 2001):
where C0 and Ct are algal concentrations at time 0 and time t, and V is the volume of water (500 mL). All CR values were standardized (Ys) to 1 g of dried bivalve flesh using the following equation:
where, Ye is the experimentally determined rate, and We is the dry body mass measured for each bivalve. For the predetermined feeding rate constant b values, 0.73 was used for C. virginica (Riisgård, 1988), 0.8 was used for M. mercenaria (Riisgård, 1988), and 0.67 for I. recurvum (Galimany et al., 2020). These values differ among species as previously demonstrated by Riisgård (1988); thus, using specific b values provides more accurate calculations. All clearance rates reported are standardized and the unit for standard is L h–1 gDW–1 but, as commonly reported in feeding behavior studies, the units written will omit the weight.
To verify that bivalves filtered and cleared A. lagunensis cells from natural water samples, the percent decrease in A. lagunensis cells was calculated for each experimental jar. This was calculated as (Supplementary Figure 1):
Stable Isotopes
Dried whole bivalve tissue was ground to a fine powder using a mortar and pestle and acidified by exposure to 12 N HCl fumes in a closed environment for 12 h to remove inorganic carbon. After drying at 60°C to remove residual acid, samples were weighed to the nearest 0.001 mg into tared silver capsules. Isotope analysis was carried out at the Stable Isotope Facility at UC Davis using a PDZ Europa ANCA-GSL elemental analyzer coupled to a PDZ Europa 20-20 isotope ratio mass spectrometer (Sercon Ltd., Cheshire, United Kingdom). Isotope values are reported in δ notation in units of per mil (‰) following equations outlined in Fry (2006). The long term standard deviation for standards at the UC Davis isotope facility is 0.3‰ for 15N and 0.2‰ for 13C.
Statistical Analyses
Clearance rates and isotope values were compared using a two-way ANOVA with species and algal concentrations as factors. Data met assumptions for analysis; normality and homogeneity of variances were checked using Shapiro-Wilk and Levene’s test, respectively. Tukey post hoc tests determined differences within factors. When interactions were found, a one-way ANOVA was performed to find differences for each bivalve at the different brown tide concentrations. Only bivalves that were open and actively feeding were used for the statistical analyses (N = 4 for 104 cells mL–1 and 5 × 104 cells mL–1 for C. virginica; N = 5 in all other cases). The relationships between feeding and algal depletion, and between tissue isotope value and number of cells removed for each species were established with non-linear exponential regressions. The statistical software used was SPSS Statistics 23.0 (IBM).
Scaling Up Restoration Projects
Based on numbers calculated from the laboratory filter-feeding experiments, we were able to scale up minimum and maximum clearance rates for C. virginica and M. mercenaria at the population level, based on restoration projects carried out in the Indian River Lagoon. Scaling up clearance rates of I. recurvum to the population level was not possible because there are no estimates on the abundance of the species within the IRL nor any restoration projects with mussels. Examining mussel populations and their part in ecosystem health in the IRL is a potential next step in this research.
An oyster reef restoration project started in the Canaveral National Seashore (IRL) in 2007 to enhance the natural oyster population, which has severely decreased in the last decades1. The restored oysters measured 37.1 mm in length in 2019 (Walters et al., 2021). This reported measure was converted into soft tissue dry weight (0.35 g) using a weight/length relationship obtained with the collected data from the laboratory and previous field data (Galimany et al., 2017b). Oyster density in the restored reefs was variable and, when oysters reached adult sizes, the density ranged from about 500 oysters m–2 in 2017 to about 1,000 oysters m–2 in 2020. Estimates of the reported reef area, densities, and oyster lengths, allow us to estimate that in 2017, the reef had approximately 183,000 individuals of adult C. virginica, whereas, in 2020, the population increased to 367,000 adult individuals. Using these population calculations, the estimated oyster dry weights, and the calculated clearance rates we estimated the potential clearance of brown tide when feeding on the different concentrations of A. aureoumbra for the restored C. virginica reef. The IRL clam project emerged in 2019 with the goal to collect M. mercenaria that had survived consecutive brown tides. With the assumption that these would be resistant clams to new brown tides, the goal was to use these collected adults to obtain hatchery seed and release 2M 2.5 cm clams by summer 20212. Then, using a weight/length relationship obtained with the collected data from the laboratory and previous field data (Galimany et al., 2017b), 2.5 cm clams were estimated to weight 0.25 g. This measurement was used to estimate the clearance rates for a restored population of 2M individuals of M. mercenaria when feeding on the different brown tide concentrations.
Results
Brown Tide Identification
The presence of A. lagunensis in the algal bloom was confirmed by genetic identification. The alignment of the 16S rRNA gene partial sequence obtained from the water sample collected on February 27 of 2016 (GenBank accession number MH523586) to the chloroplast genome (cpDNA) of Aureoumbra lagunensis (GenBank accession number GQ231542.1) resulted in 100 % identity to homologous fragment at the 103 to 582 nucleotide positions of the cpDNA genome (Supplementary Table 1).
Bivalve Clearance Rates
Brown tide cells were removed by all three bivalve species as determined by % decrease in algal cells after 60 min (Figure 1), although % reduction decreased as algal concentrations increased. Clearance rates also showed decreases with increasing algal cell concentrations, and a significant interaction was found on clearance rates between bivalve species x brown tide concentration (F8,58 = 7.70, p < 0.001) (Table 1 and Figure 2). To elucidate differences within species, clearance rates were analyzed for each bivalve species with a one-way ANOVA comparing the different brown tide concentrations. Each species had significantly different clearance rates among the concentrations of brown tide (F4,18 = 21.68, p < 0.001 for oysters; F4,20 = 15.63, p < 0.001 for mussels; F4,20 = 4.77, p = 0.007 for clams). As expected, clearance rates at 104 cells mL–1 were the highest (Figure 2). Non-linear exponential regressions between clearance rates and A. lagunensis concentrations for each bivalve clarified these results and all bivalves had a negative relationship (Table 2). The slope of the function (number before e) indicates that C. virginica and I. recurvum both rapidly reduce their clearance rate as the density of brown tide increases, but M. mercenaria had a slower response and overall lower clearance rates at all concentrations (Figure 2).
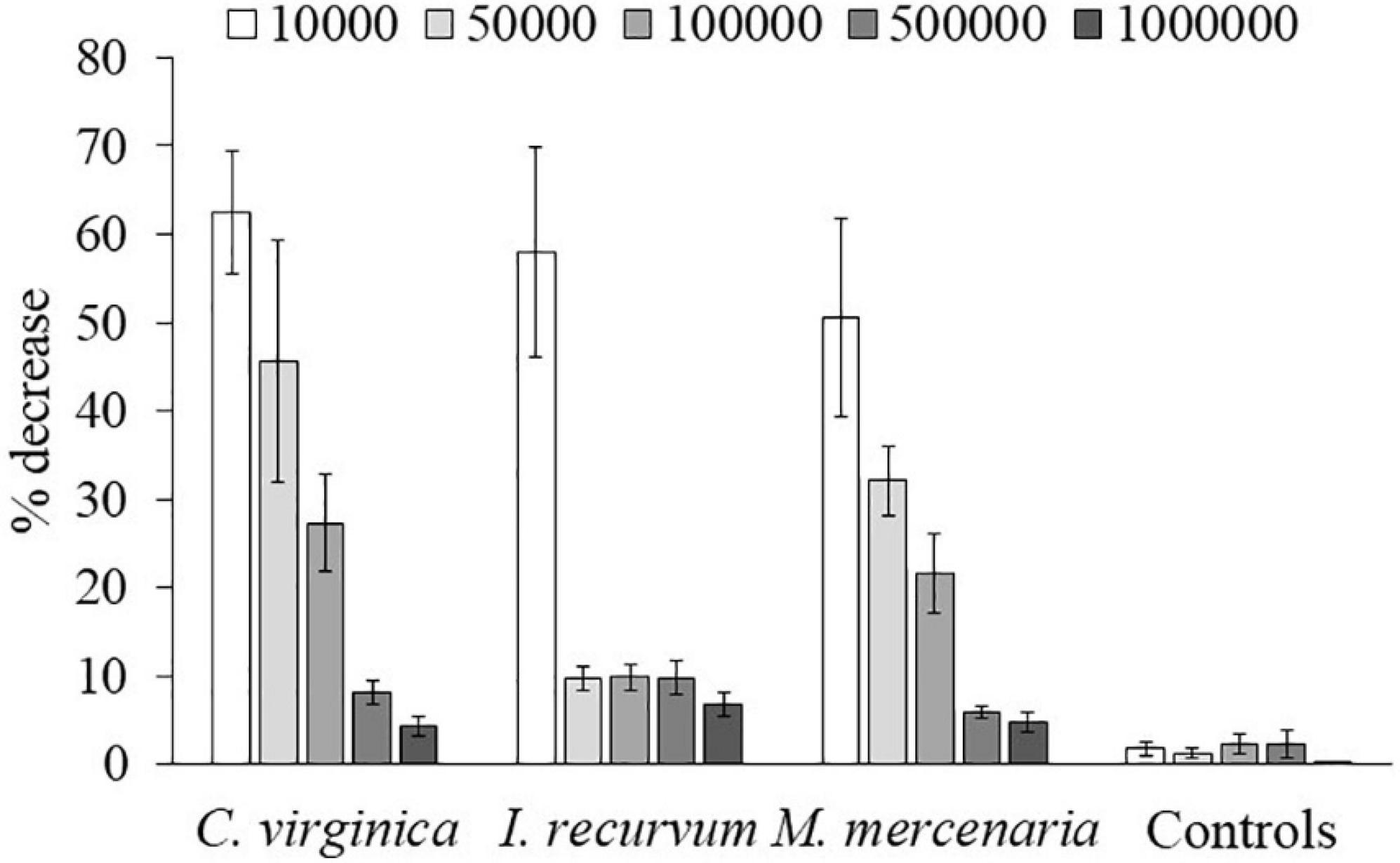
Figure 1. Mean values of percentage (±SE) of Aureoumbra lagunensis decrease at the different algal densities (cell mL− 1) for each bivalve species and the control jars.
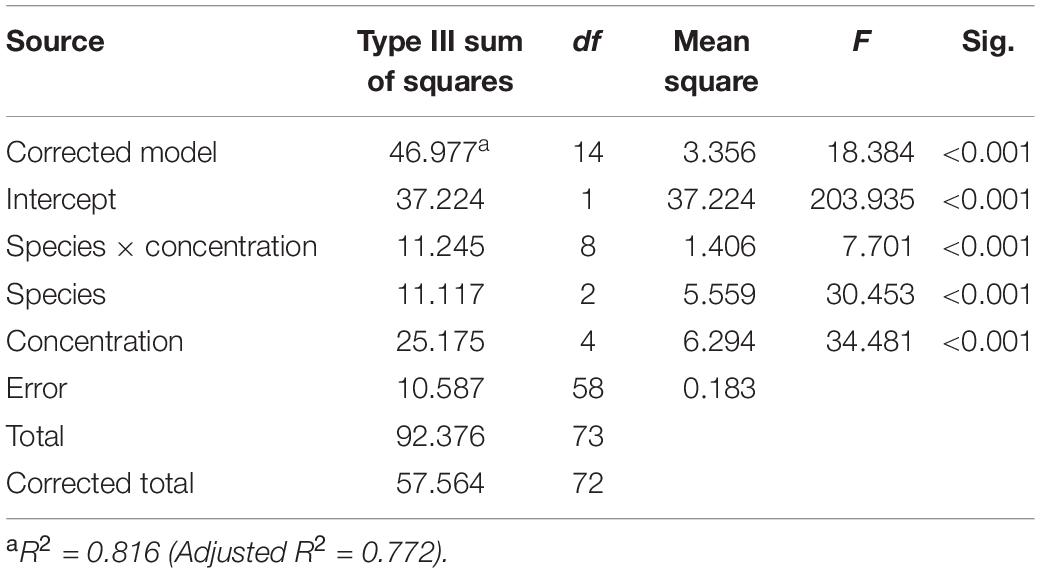
Table 1. Two-way ANOVA table analyzing the clearance rate of each studied species (Crassostrea virginica, Ischadium recurvum, and Mercenaria mercenaria) at each brown tide concentration.
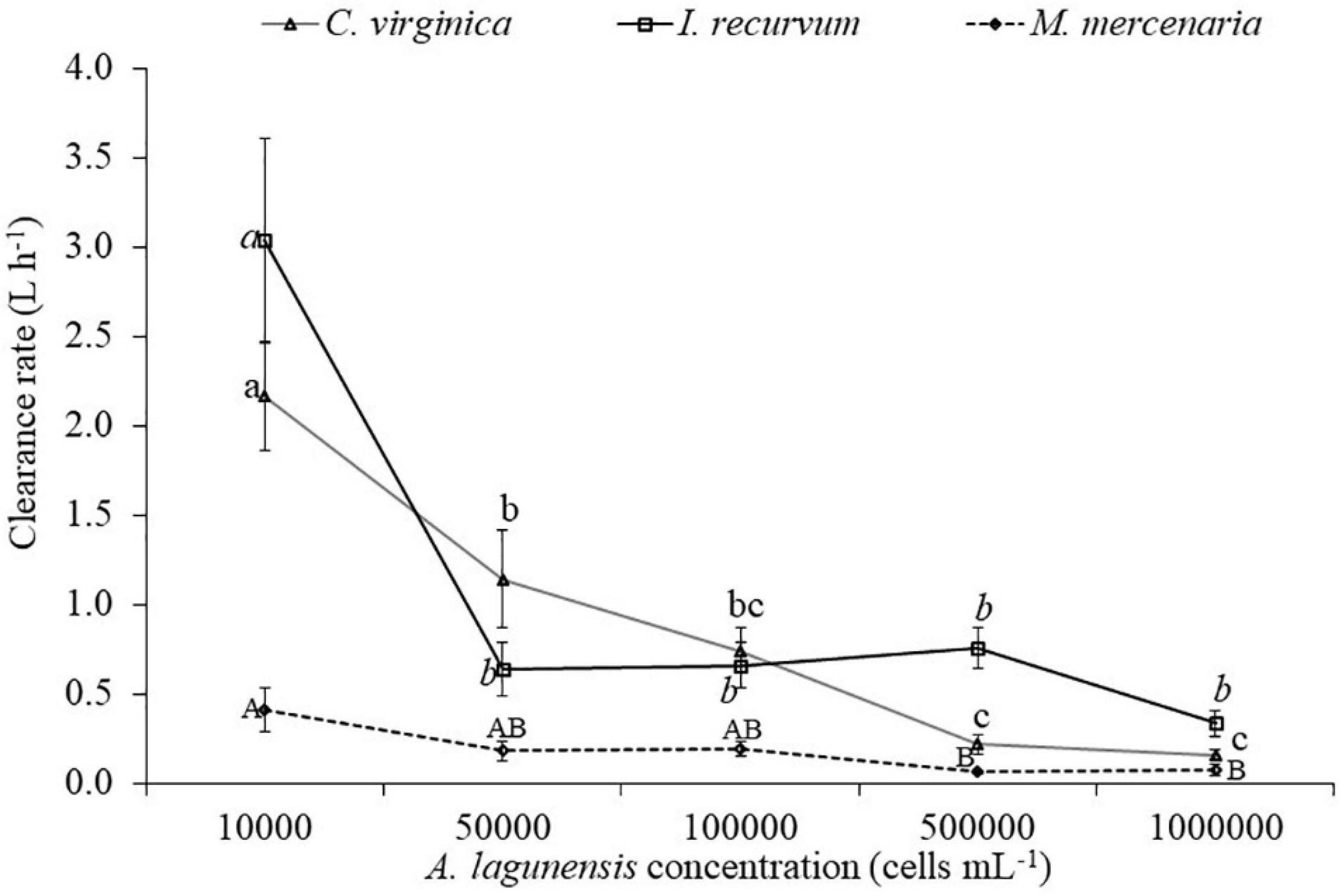
Figure 2. Mean values for the clearance rates (±SE) for each bivalve species (Crassostrea virginica, Ischadium recurvum, and Mercenaria mercenaria) at each brown tide concentration. Letters indicate significant differences for the one-way ANOVA for each species comparing the clearance rates at the different brown tide concentrations (lowercase for Crassostrea virginica, lowercase italics for Ischadium recurvum, capital letters for Mercenaria mercenaria).
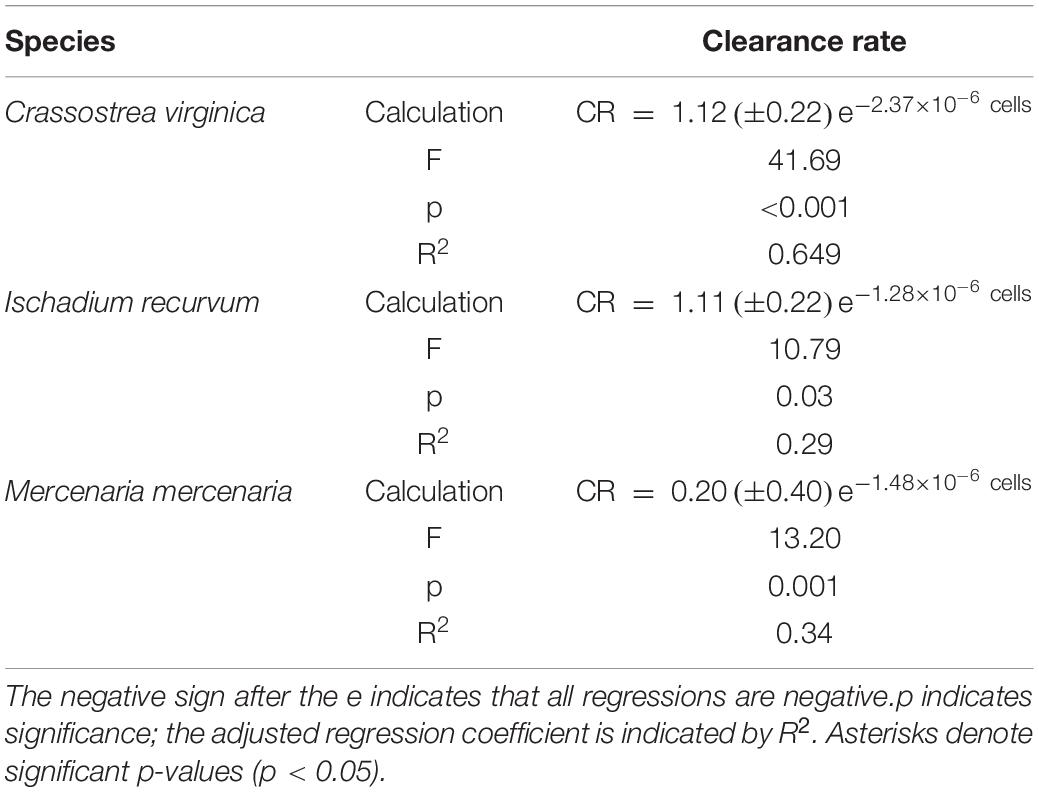
Table 2. Nonlinear exponential regressions between clearance rates and Aureoumbra lagunensis cell densities.
To elucidate differences between bivalve species, clearance rates including all brown tide concentrations were analyzed with a one-way ANOVA comparing the different bivalve species. The three species had significantly different clearance rates (F2,70 = 7.96, p = 0.001). Tukey post hoc tests showed that C. virginica and I. recurvum had higher clearance rates overall for brown tide than M. mercenaria. In detail, when studying differences among bivalve species at each algal concentration, we obtained significantly different clearance rates throughout (Table 3). Tukey post hoc tests elucidated the differences among each bivalve species, indicating that clearance rates between C. virginica and I. recurvum did not differ at most concentrations; however, clearance rates between these two species and M. mercenaria differed across many algal concentrations (Table 3). Interestingly, clearance rates between C. virginica and M. mercenaria did not differ at the two highest concentrations of algal cells, probably because the clearance rates were quite low for both bivalves at these bloom concentrations.
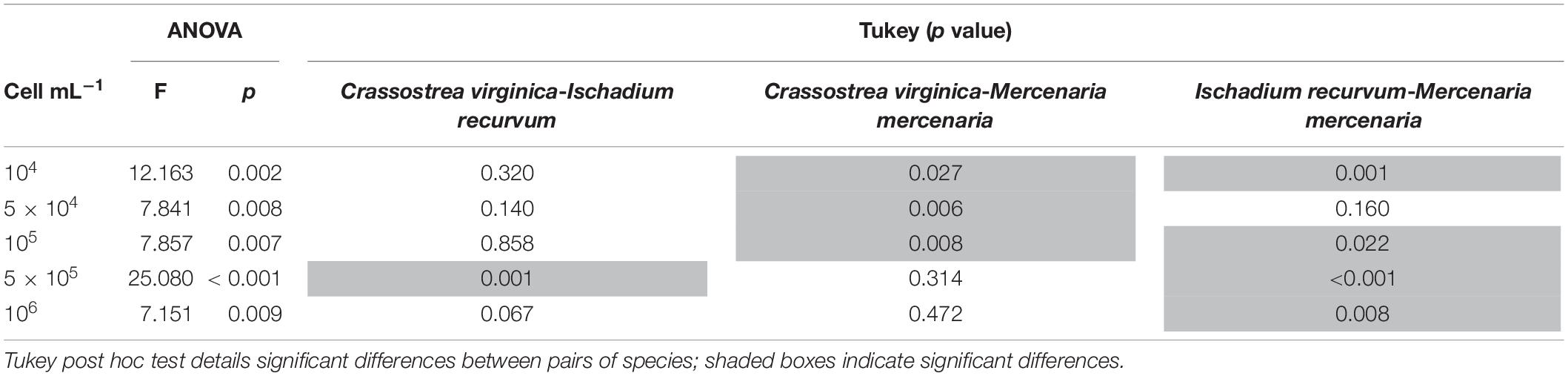
Table 3. One-way ANOVA table analyzing clearance rates differences among bivalve species at each brown tide concentration.
Stable Isotope Analysis
Bivalve tissue from these experiments was enriched in δ15N and δ13C compared to wild-caught bivalves from the IRL. For instance, average (across all treatments) δ15N values (±SE) from experimental samples were 357.41‰ ± 54.36, 317.63‰ ± 27.97, and 27.48‰ ± 2.66 for C. virginica, I. recurvum, and M. mercenaria, respectively), while mean δ15N values from wild-caught individuals were 6.75‰ for C. virginica and 8.62‰ for M. mercenaria. While we do not have natural abundance values for I. recurvum, other mussels from the IRL were also low: 6.22‰ for Geukensia demissa and 4.65‰ for Mytella charruana (Galimany et al., 2017a). Experimental δ13C values (±SE) were lower than δ15N values: 5.20‰ ± 4.54, −11.73‰ ± 1.31, and −15.77‰ ± 0.68 for C. virginica, I. recurvum, and M. mercenaria, respectively), but were still high compared to δ13C values of wild-caught bivalves: −19.32‰ for C. virginica, −21.22‰ for M. mercenaria, and −17.87 and −21.74‰ for the mussels Geukensia demissa and Mytella charruana, respectively (Galimany et al., 2017a).
A significant interaction was observed between bivalve species × brown tide concentration for both isotopes studied (F8,58 = 5.22, p < 0.001, and F8,58 = 9.40, p < 0.001, for δ13C and δ15N, respectively) (Table 4). To elucidate differences within species, isotope values were analyzed for each bivalve species with a one-way ANOVA comparing the different brown tide concentrations. C. virginica and M. mercenaria had significantly different isotope values among the concentrations of brown tide (δ13C F4,18 = 7.52, p = 0.001 and δ15N F4,18 = 17.12, p < 0.001 for oysters; δ13C F4,20 = 9.10, p = 0.001 and δ15N F4,20 = 7.27, p < 0.001 for clams). I. recurvum only had significantly different isotope values for δ13C (F4,20 = 14.44, p < 0.001) but not for δ15N (F4,20 = 2.14, p = 0.113) (Figure 3).
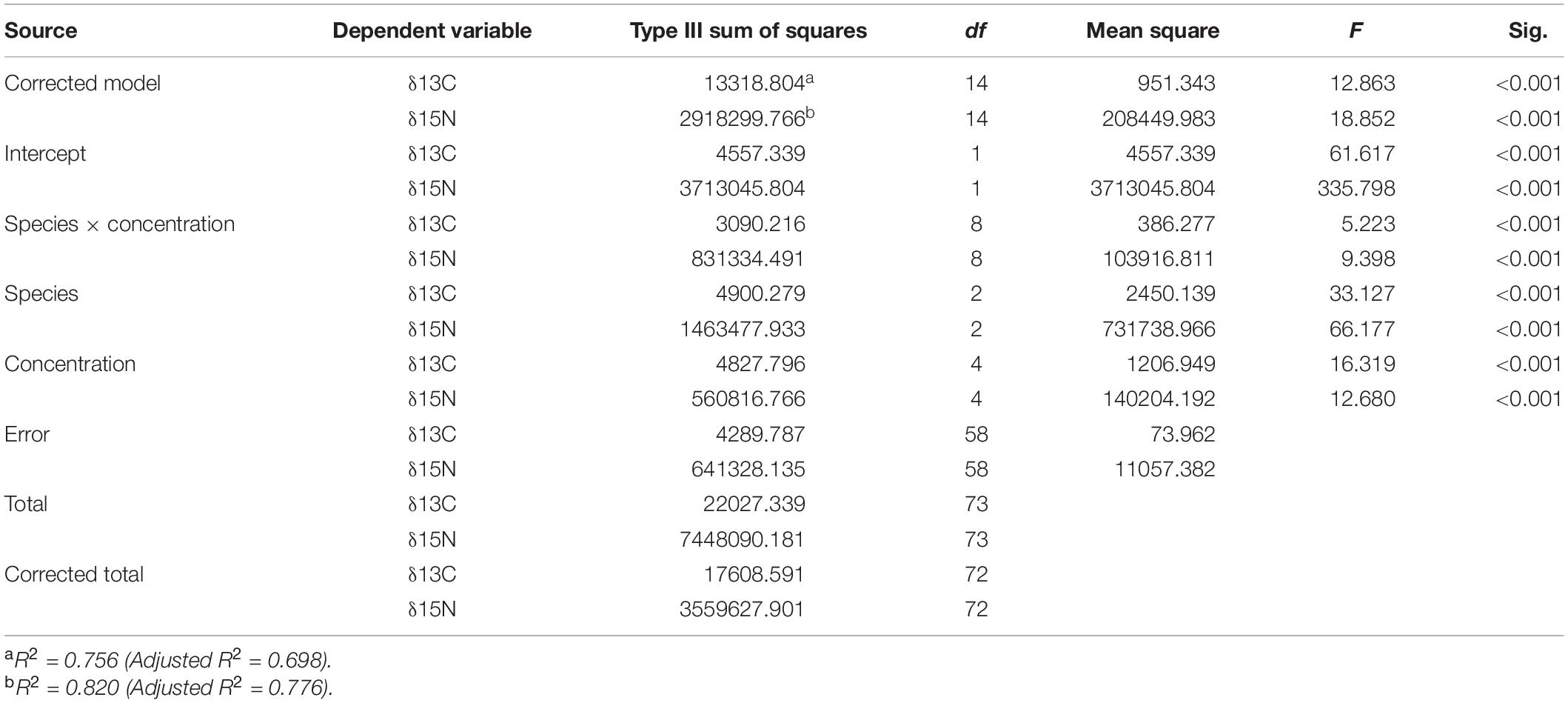
Table 4. Two-way ANOVA table analyzing the δ13C and δ15N isotope values of three different bivalve species studied (Crassostrea virginica, Ischadium recurvum, and Mercenaria mercenaria) at each brown tide concentration.
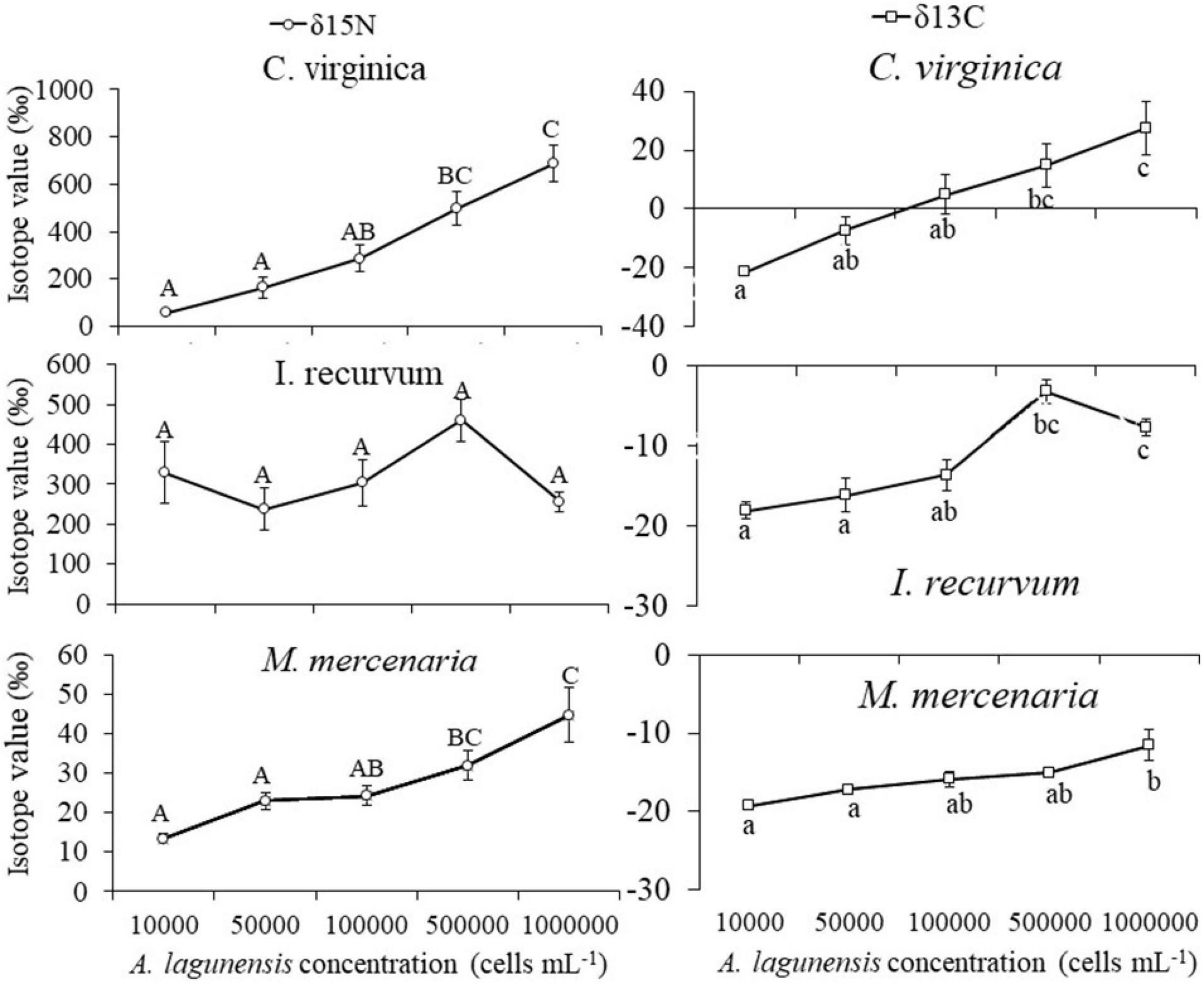
Figure 3. Mean isotope (±SE) value of δ15N (left) and δ13C (right) for each bivalve species (Crassostrea virginica, Ischadium recurvum, and Mercenaria mercenaria) at each brown tide concentration. Letters indicate significant differences among concentrations for the one-way ANOVA.
To elucidate differences between bivalve species, isotope values including all brown tide concentrations were analyzed with a one-way ANOVA comparing the different bivalve species. The three species had significantly different isotope values (F2,70 = 17.45, p < 0.001 for δ13C and F2,70 = 28.27, p < 0.001 for δ15N). I. recurvum and M. mercenaria had lower isotope values for δ13C than C. virginica. However, C. virginica and I. recurvum had higher δ15N isotope values than M. mercenaria.
After the algal exposures, δ13C isotope values for all three bivalves were positively related to the number of cells removed by the bivalves in one hour (Figure 4 and Table 4). For δ15N, only C. virginica and M. mercenaria had a significant positive relationship (Figure 5 and Table 5).
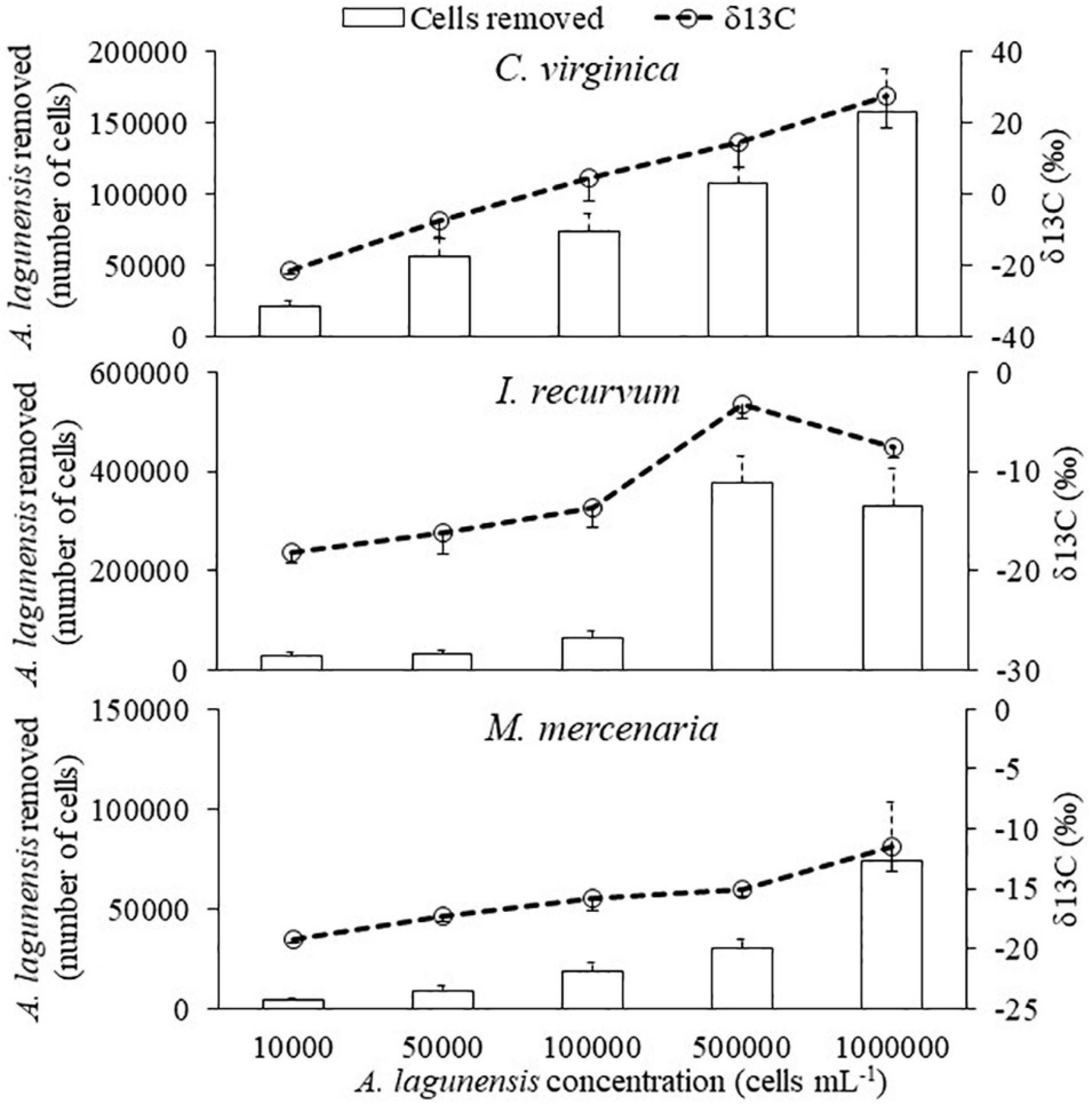
Figure 4. The relationship between the number of Aureoumbra lagunensis cells removed (+SE) by bivalves (bars) and δ13C isotope values (SE) (line) at each algal concentration in one hour.
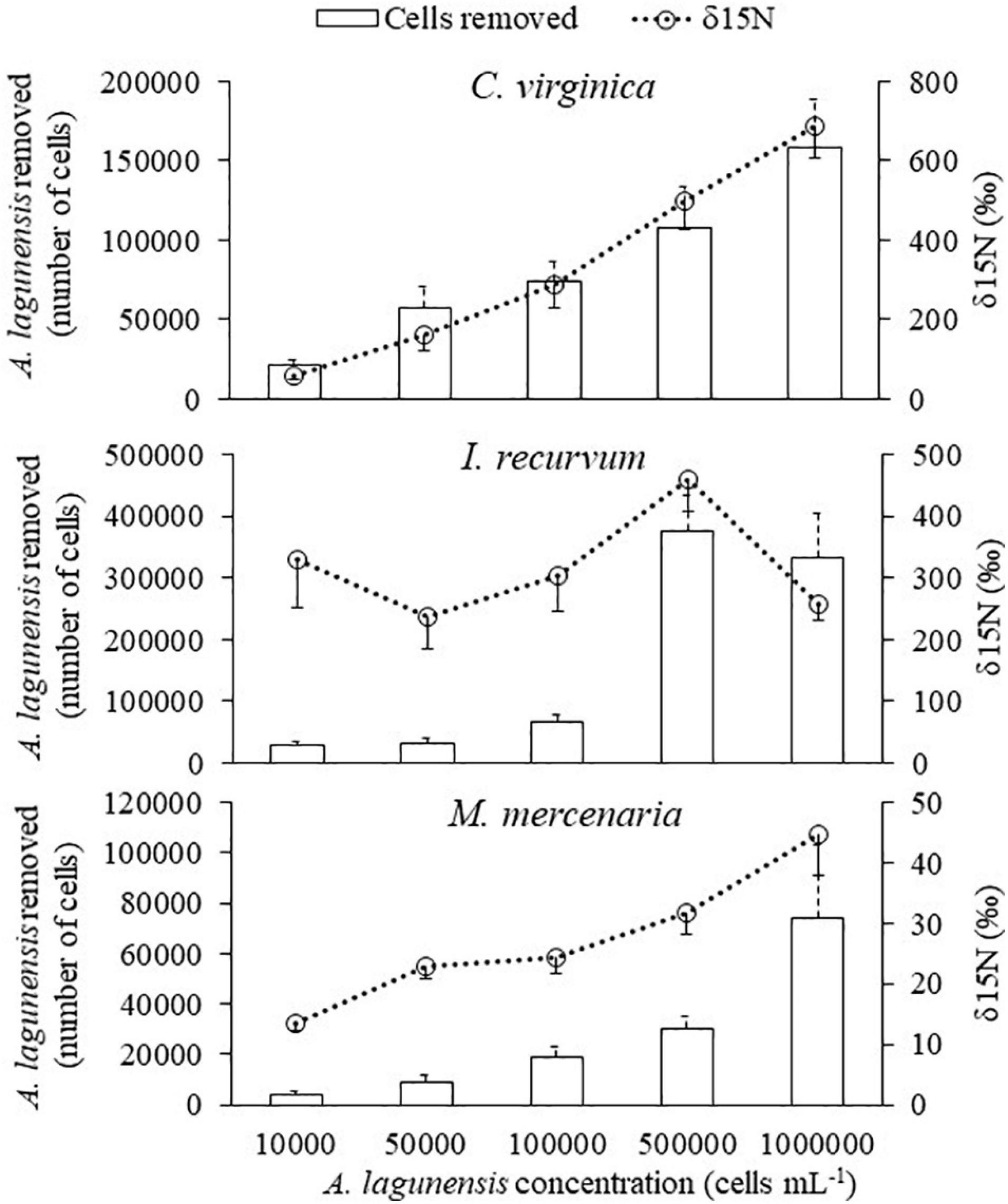
Figure 5. The relationship between the number of Aureoumbra lagunensis cells removed (+SE) by bivalves (bars) and the δ15N isotope values (SE) (line) at each algal concentration in one hour.
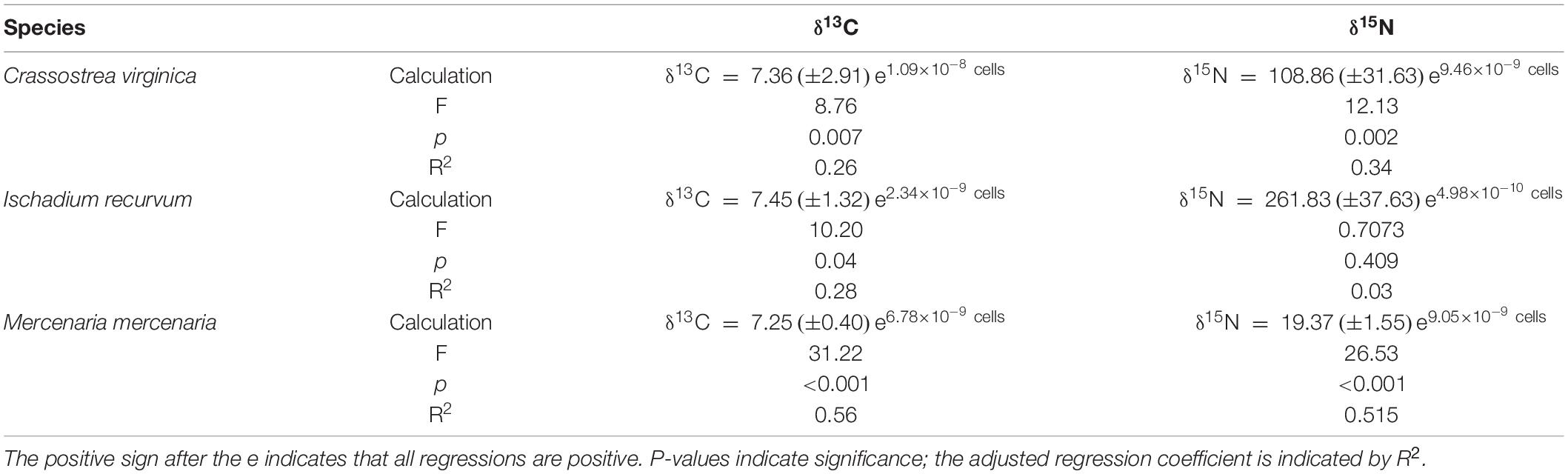
Table 5. Nonlinear exponential regressions between the number of Aureoumbra lagunensis cells removed by the bivalves from the water after 60 min exposed to the experimental algal concentrations and isotope values of the whole bivalve flesh.
Scaling Up
The scaling up estimates for C. virginica were based on both the low density restored reef from 2017 (500 oysters m–2) and the high density restored reef from 2020 (1,000 oysters m–2). Considering the low-density values, the potential of the oysters to clear the lowest concentration of brown tide would range between 8.2 106 and 107 L day–1 (Figure 6A). When feeding on the highest concentration of brown tide, these values would decrease to 5.7 105 and 8.3 105 L day–1 (Figure 6A).
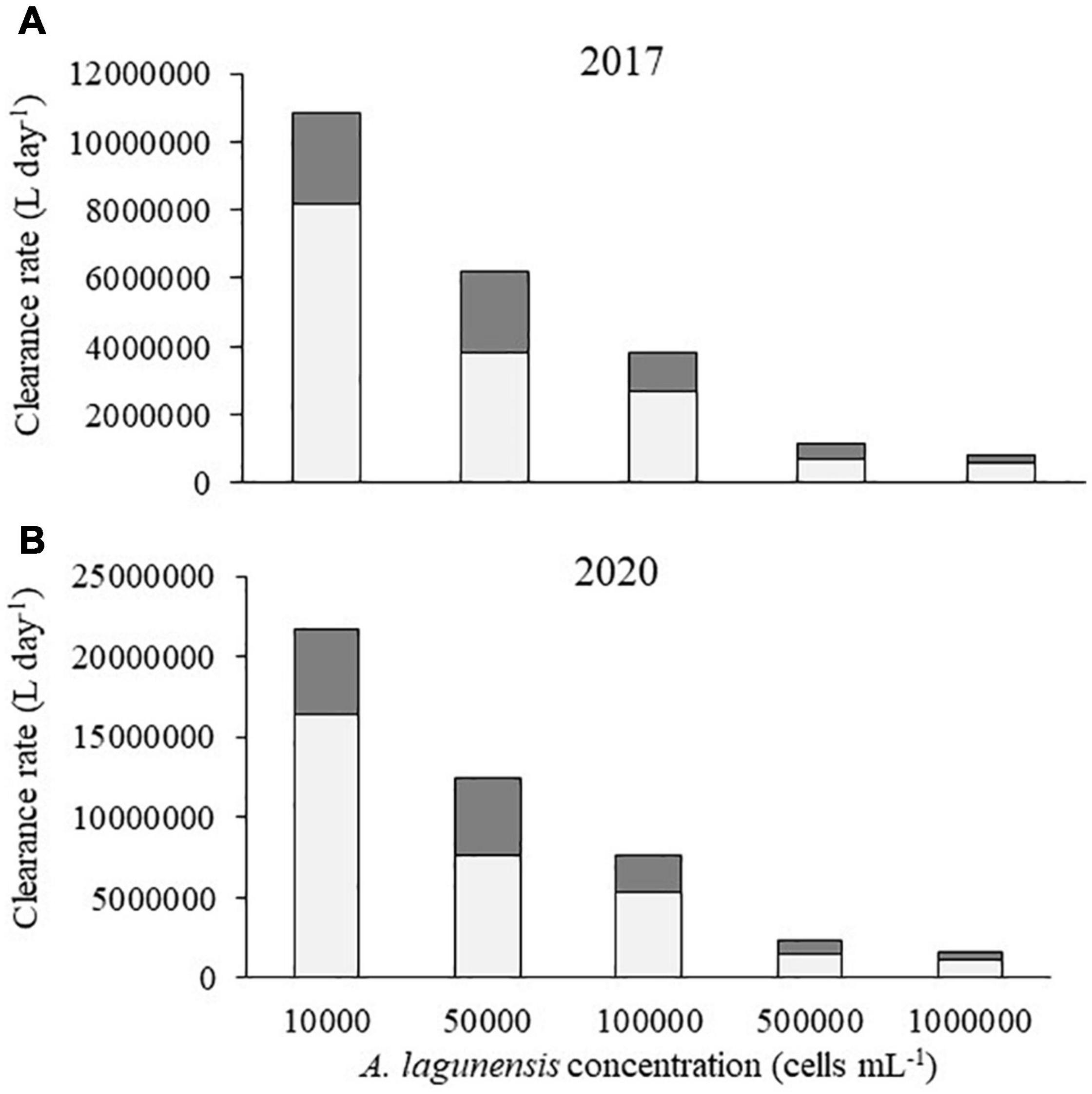
Figure 6. Minimum (light column) and maximum (dark column) values of the clearance rate for Crassostrea virginica estimated at each brown tide concentration for the restored reefs with the population density recorded in (A) 2017 (∼183,000 oysters) and (B) in 2020 (∼367,000 oysters).
Considering the reef with high density, the potential to clear the lowest concentration of brown tide would increase up to 16.4 106 to 21.7 106 L day–1 (Figure 6B). However, when feeding on the highest concentration of brown tide, these values would range between 1.1 106 and 1.7 106 L day–1 (Figure 6B).
The 2M individuals of restored M. mercenaria could filter an estimated maximum of 6.3 106 L day–1 when feeding on the lowest concentration of brown tide studied, whereas the minimum amount of A. lagunensis cleared from the water could be estimated in 540,000 L day–1 when feeding on bloom concentrations (Figure 7). Considering that M. mercenaria can reach lengths up to 13 cm,3 the potential for water quality sustainability of the 2M restored clams though filter-feeding could greatly increase, with rough estimates of 107 106 L day–1 when feeding at 104 cells mL–1, a value that would decrease to 19 106 L day–1 when feeding on a fully developed brown tide.
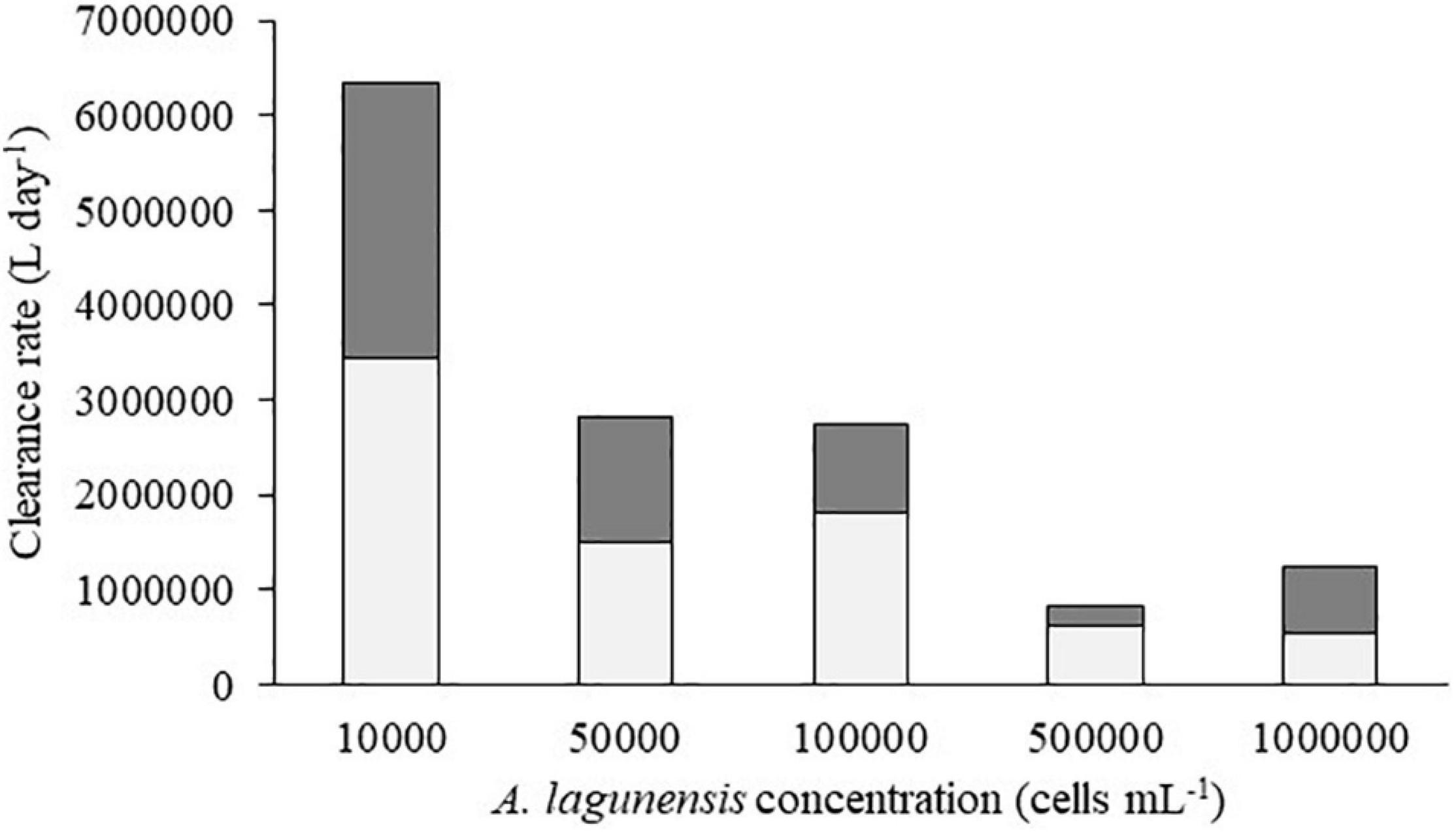
Figure 7. Graph plotting Mercenaria mercenaria clearance rate minimum (light column) and maximum (dark column) values estimated at each brown tide concentration for a population of 2M 1-inch individuals.
Discussion
All studied bivalve species cleared A. lagunensis. Clearance rates decreased with increasing microalgal concentrations, but in all cases, the number of cells removed increased with higher cell concentrations. Thus, although each species adjusts their feeding behavior to high loads of brown tide concentrations (Barillé et al., 1997; Velasco and Navarro, 2002), their utility as consumers of HAB cells remains high even at full bloom concentrations. C. virginica and I. recurvum were physiologically able to maintain active filtration at high concentrations of particulates (i.e., 104 cells mL–1), probably because when the material captured in the gills exceeds the ingestive capacity, the excess particles are eliminated as pseudofeces (Shumway et al., 1985; Hawkins et al., 1998). However, other bivalves, like M. mercenaria, are not as efficient at selecting particles prior to ingestion, therefore lowering their clearance rates as particle concentrations increase is their main strategy to cope with high seston loads (Prins et al., 1991; Hawkins et al., 1998; Galimany et al., 2017b). Similarly, in another study, when feeding on a A. lagunensis brown tide at 106 cells mL–1, clearance rates decreased to 0.06 L h–1 for C. virginica and 0.01 L h–1 for M. mercenaria (Gobler et al., 2013; Galimany et al., 2017b), allowing the bivalves to prevent gill collapse and filter the algal bloom. Behavioral differences may account for the low clearance rates recorded for M. mercenaria. While oysters and mussels attach to hard substrate and remain within the water column, hard clams bury within sediments to avoid predation and we did not provide any sediment for the clams to bury within, as resuspension could adversely influence our results. Therefore, our results may be underestimated. However, laboratory studies comparing the feeding of different clam species held without sediment report that M. mercenaria had lower clearance than the other clams thus it seems to be a bivalve with low feeding rates (Riisgård, 1988). Changes in clearance rates may disguise the fact that when feeding on high seston loads, such as an algal bloom, bivalves, not only increase pseudofeces production but may also pack undigested phytoplankton cells in their feces (Riisgård et al., 2011). Thus, the particles cleared from the water column and retained in the gills may not always be used as a food source (Kiørboe and Møhlenberg, 1981; Ward and Shumway, 2004).
Providing bivalves with algal cells enriched in the heavy atoms of carbon and nitrogen (13C and 15N) allows us to determine whether retained cells are actually consumed and assimilated into bivalve tissue (Galimany et al., 2020). Similar work labeling food items with 15N in situ has been carried out with bivalves in other systems (Raikow and Hamilton, 2001). Based on 15N enrichment, A. lagunensis cells were consumed and assimilated by all bivalves over the course of exposure to the bloom. Additionally, positive relationships between isotope values and cell removal indicate that while bivalves are altering their feeding behavior to accommodate bloom conditions, they are retaining and consuming more cells from the water column as cell concentrations increase. Therefore, while bivalves were most likely producing more pseudofeces, especially at the highest algal concentrations, they were also assimilating the brown tide at all concentrations. Although we used both 13C- and 15N-tracer compounds for this experiment, we found higher enrichment of 15N within bivalve tissue, suggesting that A. lagunensis cells assimilated exogenous nitrate more rapidly than they fixed carbon. A. lagunensis has an extremely high tolerance to phosphorus (P)-limited conditions, but an increase of nitrogen in the water seems to trigger the algal bloom (Liu et al., 2001), which might explain why the algae assimilated the 15N-labeled NO3 with a greater efficiency than fixing 13C.
Differences in clearance rates and 15N enrichment across bivalve species suggest that C. virginica and I. recurvum are more efficient at clearing and assimilating nutrients from A. lagunensis than M. mercenaria. Interestingly, the δ15N values of C. virginica and M. mercenaria tissue increased with the concentration of A. lagunensis, implying that, even with a reduced clearance rate, these bivalves were assimilating more algal cells as they were increasingly available at higher (up to bloom) concentrations. However, the δ15N values of I. recurvum were high but similar across treatments and were not related to the number of A. lagunensis cells removed. The clearance rate for this species was much higher at the lowest cell concentration but decreased and remained similar at concentrations higher than 104 cells mL–1. It is reasonable to suggest, then, that I. recurvum may utilize similar amounts of microalgae as a food source despite being exposed to higher concentrations. Thus, as brown tide concentration increased, the cells removed are most likely packed as undigested cells in feces instead, as observed for other bivalves when feeding on phytoplankton blooms (Hégaret et al., 2007). Taken together with the clearance rates, these data support the consumption of A. lagunensis cells at bloom concentrations, but also highlight that this removal varies across bivalve species.
The fact that bivalves consumed brown tide cells even at bloom concentrations supports the idea that bivalves play a key role in shallow ecosystems where brown tide blooms occur, such as Laguna Madre (TX) (Montagna et al., 1993) and the Indian River Lagoon (FL) (Galimany et al., 2020), potentially ameliorating A. lagunensis brown tides. Bivalves provide many ecosystem services including turbidity reduction by filtration (Dame, 2011). However, interspecific variation in filtration efficiency may determine their ability to feed during algal blooms. For instance, we found that clams had the lowest clearance rates and enrichment in 15N and 13C, suggesting that fewer cells are removed and assimilated compared to the other bivalve species. Clearance rates for C. virginica and M. mercenaria feeding on natural phytoplankton are about 1 to 3 L h–1 and 0.5 to 1 L h–1, respectively (Galimany et al., 2017b; Gray and Langdon, 2018), similar to our laboratory observations when the bivalves were exposed to pre-bloom concentrations (104 cells mL–1).
Understanding and quantifying the feeding of bivalves is important to estimate their use as a Nature-based solution to improve water quality in degraded ecosystems. It is important to consider, when using laboratory estimates, that factors such as predator interactions and changes in environmental parameters can affect feeding behaviors and reduce rates obtained in a controlled system (Ruesink, 2000). Nonetheless, while laboratory experiments may not fully represent field data, they are a good proxy to study the feeding behavior of bivalves (Velasco and Navarro, 2005). By using clearance rates found in the laboratory, we estimated the potential effect of restoration by scaling the laboratory values to actual restoration projects. Considering that the IRL has a water surface area of 914 km2 and an average depth of 1.22 m2, the two species of bivalves used in restoration projects mentioned herein may be clearing about 0.002% of the water volume when feeding on the lowest brown tide concentration. Several oyster restoration efforts have been undertaken in recent decades to improve bivalve populations and recover bivalve ecosystem services, including improved water quality (McClenachan et al., 2020). Monitoring restored oyster reefs is not a common a practice because of funding limitations, but if we use the densities reported in the Canaveral National Seashore, we can estimate that the oysters from the additional 89 restored reefs (McClenachan et al., 2020), only in one area of the IRL, could be clearing about 1% of the IRL volume daily. This estimate of total volume cleared is conservative, as it only takes into account the restoration projects within Mosquito Lagoon area and does not consider natural reefs, filtration of other species, or additional restoration projects throughout the IRL. Moreover, bivalve restoration actions tend to focus on commercial species, such as C. virginica and M. mercenaria, whereas non-commercial species (e.g., I. recurvum), are traditionally understudied (zu Ermgassen et al., 2020). Diversification of bivalve species may provide new opportunities to achieve greater outcomes for water quality and several bivalves are reported to filter water at rates that merit restoration attention (Kreeger et al., 2018), including I. recurvum based on our results. This study, and the population level estimates we have generated provide a working baseline to understand the potential role bivalves can play in mitigating harmful algal blooms both before and during a bloom.
In conclusion, this research provides evidence that the bivalves C. virginica, I. recurvum, and M. mercenaria can effectively consume A. lagunensis at different concentrations, from pre-bloom to bloom concentrations. In Florida, as many areas in the world, populations of bivalve mollusks of both recreational and commercial importance are stressed by a variety of factors, including habitat degradation, overfishing and development (Arnold, 2001). However, resident bivalve populations clear and assimilate bloom-forming algal species and may therefore play a role in the mitigation and prevention of HABs in coastal ecosystems (Edebo et al., 2000; Galimany et al., 2017b, 2020). Thus, the use of natural solutions, such as shellfish restoration and establishment of bioremediation strategies should be encouraged to help mitigate the effects of eutrophication (Galimany et al., 2017c; Locher et al., 2020) that may lead to A. lagunensis brown tides. We highlight the importance of suspension feeder biodiversity in the ecosystem function of coastal ecosystems, and suggest that diversification of bivalve restoration projects could be an important step in restoring ecosystem health.
Data Availability Statement
The datasets presented in this study can be found in online repositories. The names of the repository/repositories and accession number(s) can be found in the article/Supplementary Material.
Author Contributions
EG conceived and planned the experiments. EG, JL, CF, AD, and JH carried out the experiments. All coauthors contributed to sample preparation and analysis. EG, JL, CF, IS-G, JH, and VP contributed to the interpretation of the results. EG took the lead in writing the manuscript. All authors provided critical feedback and helped shape the research, analysis and manuscript.
Conflict of Interest
The authors declare that the research was conducted in the absence of any commercial or financial relationships that could be construed as a potential conflict of interest.
Publisher’s Note
All claims expressed in this article are solely those of the authors and do not necessarily represent those of their affiliated organizations, or those of the publisher, the editors and the reviewers. Any product that may be evaluated in this article, or claim that may be made by its manufacturer, is not guaranteed or endorsed by the publisher.
Acknowledgments
We would like to thank St. Johns River Water Management District for funding the project (Contract 27799) with additional support from Florida Fish and Wildlife Conservation Commission (FWC Grant No. 13051) and the Smithsonian Marine Station. We greatly appreciate the support of the staff at Banana River Resort, Cocoa Beach, for brown tide collection. This is Smithsonian Marine Station contribution #1164.
Supplementary Material
The Supplementary Material for this article can be found online at: https://www.frontiersin.org/articles/10.3389/fmars.2021.714816/full#supplementary-material
Footnotes
- ^ https://shuckandshare.org/projects.html
- ^ http://www.irlclamproject.com/
- ^ https://www.sealifebase.ca/summary/Mercenaria-mercenaria.html
References
Arnold, W. S. (2001). Bivalve enhancement and restoration strategies in Florida, U.S.A. Hydrobiologia 465, 7–19. doi: 10.1007/978-94-010-0434-3_2
Backer, L. C., and McGillicuddy, D. J. Jr. (2006). Harmful algal blooms: at the interface between coastal oceanography and human health. Oceanography 19, 94–106. doi: 10.5670/oceanog.2006.72
Barillé, L., Prou, J., Héral, M., and Razet, D. (1997). Effects of high natural seston concentrations on the feeding, selection, and absorption of the oyster Crassostrea gigas (Thunberg). J. Exp. Mar. Biol. Ecol. 212, 149–172. doi: 10.1016/s0022-0981(96)02756-6
Beck, M. W., Brumbaugh, R. D., Airoldi, L., Carranza, A., Coen, L. D., Crawford, C., et al. (2011). Oyster reefs at risk and recommendations for conservation, restoration, and management. Bioscience 61, 107–116. doi: 10.1525/bio.2011.61.2.5
Bricelj, V. M., and Lonsdale, D. J. (1997). Aureococcus anophagefferens: causes and ecological consequences of brown tides in U.S. mid-Atlantic coastal waters. Limnol. Oceanogr. 42, 1023–1038. doi: 10.4319/lo.1997.42.5_part_2.1023
Buskey, E. J. (2008). How does eutrophication affect the role of grazers in harmful algal bloom dynamics? Harmful Algae 8, 152–157. doi: 10.1016/j.hal.2008.08.009
Buskey, E. J., Montagna, P. A., Amos, A. F., and Whitledge, T. E. (1997). Disruption of grazer populations as a contributing factor to the initiation of the Texas brown tide algal bloom. Limnol. Oceanogr. 42, 1215–1222. doi: 10.4319/lo.1997.42.5_part_2.1215
Buskey, E. J., Wysor, B., and Hyatt, C. (1998). The role of hypersalinity in the persistence of the Texas ‘brown tide’ in the Laguna Madre. J. Plankton Res. 20, 1553–1565. doi: 10.1093/plankt/20.8.1553
Cranford, P. J. (2019). “Magnitude and extent of water clarification services provided by bivalve suspension feeding,” in Goods and Services of Marine Bivalves, eds A. C. Smaal, J. G. Ferreira, J. Grant, J. K. Petersen, and Ø Strand (Cham: Springer International Publishing), 119–141. doi: 10.1007/978-3-319-96776-9_8
Dame, R. F. (2011). Ecology of Marine Bivalves, an Ecosystem Approach, 2nd Edn. Boca Raton: CRC Press, 284.
DeYoe, H. R., Stockwell, D. A., Biolagare, R. R., Latasa, M., Johnson, P. W., Hargraves, P. E., et al. (1997). Description and characterization of the algal species Aureoumbra lagunensis gen. et sp. nov. and referral of Aureoumbra and Aureococcus to the Pelagophyceae. J. Phycol. 33, 1042–1048. doi: 10.1111/j.0022-3646.1997.01042.x
Edebo, L., Haamer, J., Lindahl, O., Loo, L. O., and Piriz, L. (2000). Recycling of macronutrients from sea to land using mussel cultivation. Int. J. Environ. Pollut. 13, 190–207. doi: 10.1504/ijep.2000.002315
Freeman, C. J., Thacker, R. W., Baker, D. M., and Fogel, M. (2013). Quality or quantity: is nutrient transfer driven more by symbiont identity and productivity than by symbiont abundance? ISME J. 7, 1116–1125. doi: 10.1038/ismej.2013.7
Galimany, E., Lunt, J., Freeman, C. J., Reed, S., Segura-García, I., and Paul, V. J. (2017b). Feeding behavior of eastern oysters Crassostrea virginica and hard clams Mercenaria mercenaria in shallow estuaries. Mar. Ecol. Prog. Ser. 567, 125–137. doi: 10.3354/meps12050
Galimany, E., Freeman, C. J., Lunt, J., Domingos, A., Sacks, P., and Walters, L. (2017a). Feeding competition between the native oyster Crassostrea virginica and the invasive mussel Mytella charruana. Mar. Ecol. Prog. Ser. 564, 57–66. doi: 10.3354/meps11976
Galimany, E., Wikfors, G. H., Dixon, M. S., Newell, C. R., Meseck, S. L., Henning, D., et al. (2017c). Cultivation of the ribbed mussel (Geukensia demissa) for nutrient bioextraction in an urban estuary. Environ. Sci. Technol. 51, 13311–13318. doi: 10.1021/acs.est.7b02838
Galimany, E., Lunt, J., Freeman, C. J., Houk, J., Sauvage, T., Santos, T., et al. (2020). Bivalve feeding responses to microalgal bloom species in the Indian River Lagoon: the potential for top-down control. Estuaries Coast. 43, 1519–1532. doi: 10.1007/s12237-020-00746-9
Galimany, E., Rose, J. M., Dixon, M. S., and Wikfors, G. H. (2013). Quantifying feeding behavior of ribbed mussels (Geukensia demissa) in two urban sites (Long Island Sound, USA) with different seston characteristics. Estuaries Coast. 36, 1265–1273. doi: 10.1007/s12237-013-9633-0
Gobler, C. J., Koch, F., Kang, Y., Berry, D. L., Tang, Y. Z., Lasi, M., et al. (2013). Expansion of harmful brown tides caused by the pelagophyte, Aureoumbra lagunensis DeYoe et Stockwell, to the US east coast. Harmful Algae 27, 29–41. doi: 10.1016/j.hal.2013.04.004
Gobler, C. J., and Sunda, W. G. (2012). Ecosystem disruptive algal blooms of the brown tide species, Aureococcus anophagefferens and Aureoumbra lagunensis. Harmful Algae 14, 36–45. doi: 10.1016/j.hal.2011.10.013
Gray, M. W., and Langdon, C. J. (2018). Ecophysiology of the olympia oyster, Ostrea lurida, and pacific oyster, Crassostrea gigas. Estuaries Coast. 41, 521–535. doi: 10.1007/s12237-017-0273-7
Hall, N. S., Litaker, R. W., Kenworthy, W. J., Vandersea, M. W., Sunda, W. G., Reid, J. P., et al. (2018). Consortial brown tide−picocyanobacteria blooms in Guantánamo Bay, Cuba. Harmful Algae 73, 30–43. doi: 10.1016/j.hal.2018.01.003
Hallegraeff, G. M. (2003). “Harmful algal blooms: a global overview,” in Manual on Harmful Marine Microalgae, eds G. M. Hallegraeff, D. M. Anderson, and A. D. Cembella (Paris: UNESCO), 25–49.
Hawkins, A. J. S., Bayne, B. L., Bougrier, S., Héral, M., Iglesias, J. I. P., Navarro, E., et al. (1998). Some general relationships in comparing the feeding physiology of suspension-feeding bivalve molluscs. J. Exp. Mar. Biol. Ecol. 219, 87–103. doi: 10.1016/s0022-0981(97)00176-7
Hégaret, H., Wikfors, G. H., and Shumway, S. E. (2007). Diverse feeding responses of five species of bivalve mollusc when exposed to three species of harmful algae. J. Shellfish Res. 26, 549–559. doi: 10.2983/0730-8000(2007)26[549:dfrofs]2.0.co;2
Kiørboe, T., and Møhlenberg, F. (1981). Particle selection in suspension-feeding bivalves. Mar. Ecol. Prog. Ser. 5, 291–296. doi: 10.3354/meps005291
Kreeger, D. A., Gatenby, C. M., and Bergstrom, P. W. (2018). Restoration potential of several native species of bivalve molluscs for water quality improvement in mid-Atlantic watersheds. J. Shellfish Res. 37, 1121–1157. doi: 10.2983/035.037.0524
Landsberg, J. H. (2002). The effects of harmful algal blooms on aquatic organisms. Rev. Fish. Sci. 10, 113–390. doi: 10.1080/20026491051695
Lapointe, B. E., Herren, L. W., Brewton, R. A., and Alderman, P. K. (2020). Nutrient over-enrichment and light limitation of seagrass communities in the Indian River Lagoon, an urbanized subtropical estuary. Sci. Total Environ. 699:134068. doi: 10.1016/j.scitotenv.2019.134068
Lapointe, B. E., Herren, L. W., Debortoli, D. D., and Vogel, M. A. (2015). Evidence of sewage-driven eutrophication and harmful algal blooms in Florida’s Indian River Lagoon. Harmful Algae 43, 82–102. doi: 10.1016/j.hal.2015.01.004
Liu, H., and Buskey, E. J. (2000). The exopolymer secretions (EPS) layer surrounding Aureoumbra lagunensis cells affects growth, grazing, and behavior of protozoa. Limnol. Oceanogr. 45, 1187–1191. doi: 10.4319/lo.2000.45.5.1187
Liu, H., Laws, E. A., Villareal, T. A., and Buskey, E. J. (2001). Nutrient-limited growth of Aureoumbra lagunensis (Pelagophyceae), with implications for its capability to outgrow other phytoplankton species in phosphate-limited environments. J. Phycol. 37, 500–508. doi: 10.1046/j.1529-8817.2001.037004500.x
Locher, B., Hurst, N. R., Walters, L. J., and Chambers, L. G. (2020). Juvenile oyster (Crassostrea virginica) biodeposits contribute to a rapid rise in sediment nutrients on restored intertidal oyster reefs (Mosquito Lagoon, FL, USA). Estuaries Coast. 44, 1363–1379. doi: 10.1007/s12237-020-00874-2
McClenachan, G. M., Donnelly, M. J., Shaffer, M. N., Sacks, P., and Walters, L. J. (2020). Does size matter? Quantifying the cumulative impact of small-scale living shoreline and oyster reef restoration projects on shoreline erosion. Rest. Ecol. 28, 1365–1371. doi: 10.1111/rec.13235
Montagna, P. A., Stockwell, D. A., and Kalke, R. D. (1993). Dwarf surfclam Mulinia lateralis (Say, 1822) populations and feeding during the Texas brown tide event. J. Shellfish Res. 12, 833–842.
Nübel, U., Garcia-Pichel, F., and Muyzer, G. (1997). PCR primers to amplify 16S rRNA genes from cyanobacteria. Appl. Environ. Microbiol. 63, 3327–3332. doi: 10.1128/aem.63.8.3327-3332.1997
Onuf, C. P. (1996). Seagrass responses to long-term light reduction by brown tide in upper Laguna Madre, Texas: distribution and biomass patterns. Mar. Ecol. Prog. Ser. 138, 219–231. doi: 10.3354/meps138219
Phlips, E. J., Badylak, S., Lasi, M. A., Chamberlain, R., Green, W. C., Hall, L. M., et al. (2015). From red tides to green and brown tides: bloom dynamics in a restricted subtropical lagoon under shifting climatic conditions. Estuaries Coast. 38, 886–904. doi: 10.1007/s12237-014-9874-6
Phlips, E. J., Badylak, S., and Nelson, N. (2019). Harmful Algal Blooms in the Indian River Lagoon, North. Annual report to the Indian RiverLagoon National Estuarine Program, contract 2018-03 Sebastian, Florida.
Prins, T. C., Smaal, A. C., and Pouwer, A. J. (1991). Selective ingestion of phytoplankton by the bivalves Mytilus edulis L. and Cerastoderma edule (L.). Hydrobiol. Bull. 25, 93–100.
Raikow, D. F., and Hamilton, S. K. (2001). Bivalve diets in a midwestern U.S. stream: a stable isotope enrichment study. Limmnol. Oceanogr. 46, 514–522. doi: 10.4319/lo.2001.46.3.0514
Riisgård, H. U. (1988). Efficiency of particle retention and filtration rate in 6 species of Northeast American bivalves. Mar. Ecol. Prog. Ser. 45, 217–223. doi: 10.3354/meps045217
Riisgård, H. U. (2001). On measurement of filtration rates in bivalves—the stony road to reliable data: review and interpretation. Mar. Ecol. Prog. Ser. 211, 275–291. doi: 10.3354/meps211275
Riisgård, H. U., Egede, P. P., and Barreiro Saavedra, I. (2011). Feeding behaviour of the mussel, Mytilus edulis : new observations, with a minireview of current knowledge. J. Mar. Biol. 312459, 1–13. doi: 10.1155/2011/312459
Ruesink, J. (2000). Intertidal mesograzers in field microcosm: linking laboratory feeding rates to community dynamics. J. Exp. Mar. Biol. Ecol. 248, 163–176. doi: 10.1016/s0022-0981(00)00170-2
Shumway, S. E. (1990). A review of the effects of algal blooms on shellfish and aquaculture. J. World Aquac. Soc. 21, 65–104. doi: 10.1111/j.1749-7345.1990.tb00529.x
Shumway, S. E., Cucci, T. L., Newell, R. C., and Yentsch, C. M. (1985). Particle selection, ingestion, and absorption in filter-feeding bivalves. J. Exp. Mar. Biol. Ecol. 91, 77–92. doi: 10.1016/0022-0981(85)90222-9
Velasco, L. A., and Navarro, J. M. (2002). Feeding physiology of infaunal (Mulinia edulis) and epifaunal (Mytilus chilensis) bivalves under a wide range of concentrations and qualities of seston. Mar. Ecol. Prog. Ser. 240, 143–155. doi: 10.3354/meps240143
Velasco, L. A., and Navarro, J. M. (2005). Feeding physiology of two bivalves under laboratory and field conditions in response to variable food concentrations. Mar. Ecol. Prog. Ser. 291, 115–124. doi: 10.3354/meps291115
Walters, L. J., Sacks, P. E., and Campbell, D. E. (2021). Boating impacts and boat-wake resilient restoration of the eastern oyster Crassostrea virginica in Mosquito Lagoon, Florida, USA. Florida Sci. 84, 173–199.
Ward, J. E., and Shumway, S. E. (2004). Separating the grain from the chaff: particle selection in suspension- and deposit-feeding bivalves. J. Exp. Mar. Biol. Ecol. 300, 83–130. doi: 10.1016/j.jembe.2004.03.002
Keywords: algal bloom, stable isotopes, clearance rate, Indian River Lagoon, filter feeding, bivalves, Aureoumbra lagunensis
Citation: Galimany E, Lunt J, Freeman CJ, Segura-García I, Mossop M, Domingos A, Houk J and Paul VJ (2021) Bivalve Feeding on the Brown Tide Aureoumbra lagunensis in a Shallow Coastal Environment. Front. Mar. Sci. 8:714816. doi: 10.3389/fmars.2021.714816
Received: 25 May 2021; Accepted: 09 September 2021;
Published: 07 October 2021.
Edited by:
Paul Snelgrove, Memorial University of Newfoundland, CanadaReviewed by:
Kenta Watanabe, Port and Airport Research Institute (PARI), JapanJan Vanaverbeke, Royal Belgian Institute of Natural Sciences, Belgium
Copyright © 2021 Galimany, Lunt, Freeman, Segura-García, Mossop, Domingos, Houk and Paul. This is an open-access article distributed under the terms of the Creative Commons Attribution License (CC BY). The use, distribution or reproduction in other forums is permitted, provided the original author(s) and the copyright owner(s) are credited and that the original publication in this journal is cited, in accordance with accepted academic practice. No use, distribution or reproduction is permitted which does not comply with these terms.
*Correspondence: Eve Galimany, Z2FsaW1hbnlAaWNtLmNzaWMuZXM=
†Present address: Eve Galimany, Institute of Marine Sciences ICM-CSIC, Barcelona, Spain; Jessica Lunt, Dauphin Island Sea Lab, Dauphin Island, AL, United States; Christopher J. Freeman, Department of Biology, College of Charleston, Charleston, SC, United States