- 1South China Sea Environmental Monitoring Center of State Oceanic Administration, Guangzhou, China
- 2School of Marine Engineering and Technology, Sun Yat-sen University, Guangzhou, China
- 3Southern Laboratory of Ocean Science and Engineering, Zhuhai, China
Nutrient enrichment and its quantitative cause-effect chains of the biogeochemical processes have scarcely been documented in the Pearl River Estuary (South China). Field investigations of nutrient samples taken between 1996 and 2018 showed significant differences in nitrogen and phosphorus with times and sites. The concentrations of DIN and DIP gradually increased over the past two decades, with good fitted linear curves (R2 = 0.31 for DIN, R2 = 0.92 for DIP); while the temporal variation in DSi was non-significant. Higher levels of nitrogen and silicate mainly appeared in the upper estuary because of the riverine influence. The phosphorus pollution was accumulated in the northeast (e.g., Shenzhen bay). The aquatic environment was highly sensitive to nutrient pollution and eutrophication risk, which accordingly corresponded to high phytoplankton production and biodiversity. Phosphorus was the limiting factor of phytoplankton growth in this estuary, and more frequently caused the eutrophication risks and blooms. The nutrient pollution was largely influenced by riverine inputs, quantified by PCA-generation, and the contributions of coastal emission and atmospheric deposition were followed. The two-end member mixing model differentiated the physical alterations from the biological activity and identified the dynamic source-sink patterns of nutrient species. Nitrogen and silicate had relatively conservative behaviors in the estuary and phosphate showed an active pattern.
1. Introduction
The aquatic environment in the estuary has been negatively affected by eutrophication risks and phytoplankton blooms, and the fundamental forcing is the accumulation of nutrient pollution (especially N and P) in the water column from the river to the sea (Bianchi et al., 2010; Guo et al., 2020; Potter et al., 2021). The biogeochemical properties of various nutrients vary spatially and temporally, related to the estuarine processes (Gan et al., 2014; Borges et al., 2020; Wang et al., 2021). These environmental factors and estuarine processes that influence the nutrient fluxes distribute over a large time-and-space scale. Understanding the nutrient status and their influencing factors is essential for managing the nutrient input into the estuarine coastal systems (Jiang et al., 2014; Geng et al., 2021).
Nutrients are important regulators of carbon cycling and primary production in an estuarine environment (Wang et al., 2021). Drastic increases in nutrients have posed a big threat to the ecological stability of the marine environment (Kim et al., 2014; Yuan et al., 2021), for instance, eutrophication, red tides, and dead fishes caused by hypoxia. These environmental pressures are closely correlated with the biogeochemical characteristics of nutrient source-sink patterns (Han et al., 2012; Li et al., 2017). Correspondingly, the roles of nutrient enrichment and estuarine processes have raised the necessity to understand the biological responses (Farrow et al., 2019; Mathew et al., 2021).
This study investigates nutrient enrichment and related environmental impacts in the Pearl River Estuary (PRE; Figure 1), located in the Guangdong province, south China. Nutrients in the PRE are different from those in the South China Sea and the Pearl River channels (Dai et al., 2008; Shi et al., 2019; Zeng et al., 2020). The Pearl River drainage basin discharges a large number of continuous pollutants (i.e., nutrients, PAHs, heavy metals) into the estuarine mixing zone. This estuary thus acts as a dynamic zone of source-sink exchange (Geeraert et al., 2021; Jia et al., 2021; Niu et al., 2021). The principal mechanisms of nutrient transportation in this estuary are complicated because of the strong mixing of freshwater and saline water (Cai et al., 2018; Tao et al., 2020). However, there are still few studies addressing the interacting effects of river discharge and marine current on nutrient status in the PRE.
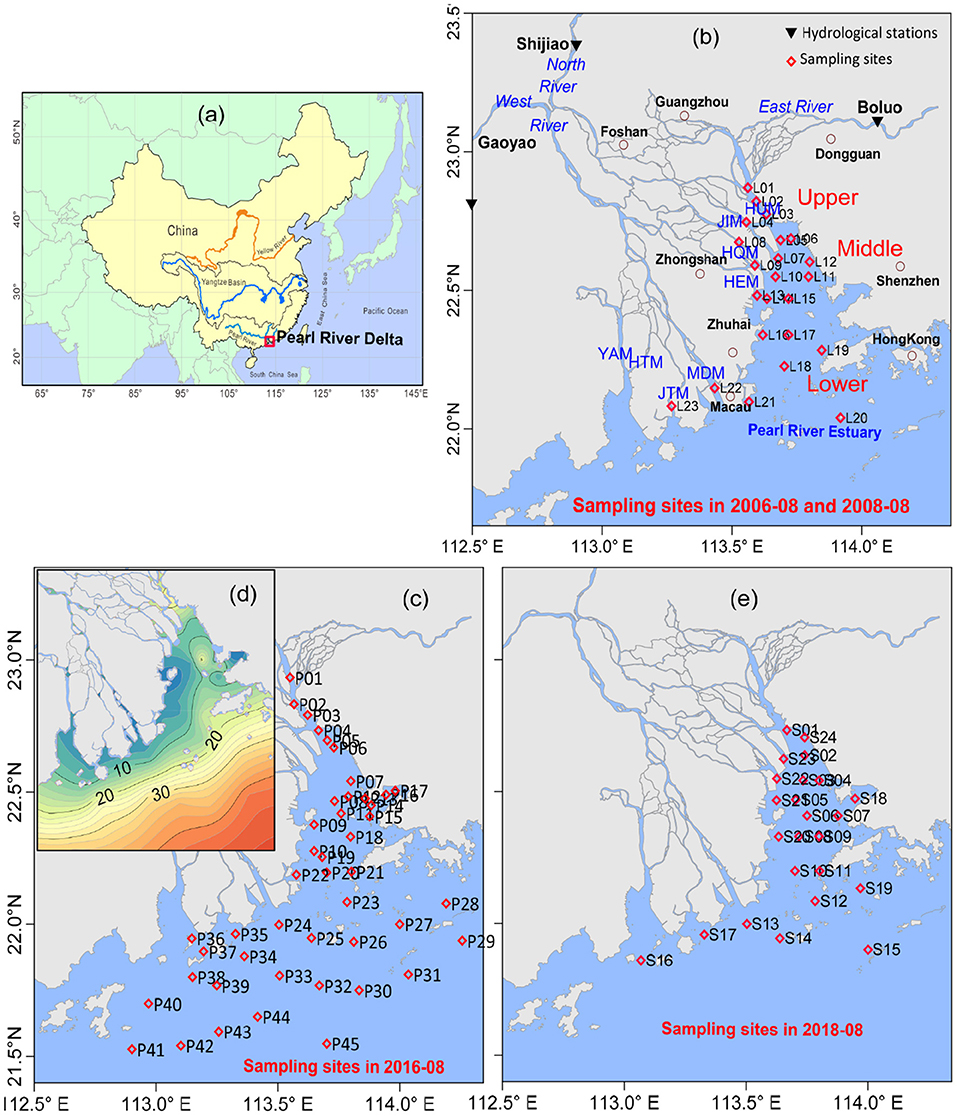
Figure 1. Sketch map of the Pearl River Estuary in China (a), sampling sites in 2006 and 2008 (b), 2016 (c), 2018 (e), and bathymetry in 2016 (d). The Pearl River system consists of river networks and estuaries entering into the South China Sea via eight outlets at Humen (HUM), Jiaomen (JIM), Hongqimen (HQM), Hengmen (HEM), Modaomen (MDM), Jitimen (JTM), Hutiaomen (HTM), and Yamen (YAM).
This field investigation, conducted between 1996 and 2018, considers the interplay between river discharge, nutrients, and biological response. It is critically important that we understand the quantitative cause-effect chains of biogeochemical properties of nutrient enrichment in the 1990s (as 1996, 1999), 2000s (as 2006, 2008), and 2010s (as 2016, 2018). Moreover, the period of 1990-2000 corresponded to the high intensity of human activity (e.g., land reclamation, channel dredging) in the Pearl River Delta (Niu et al., 2020). For this purpose, the objectives of this field investigation are designed to: (1) characterize the nutrient status and related ecosystem response over the past two decades in the PRE, spatially and temporally; (2) determine the driving factors (e.g., salinity, suspended sediment, DO, and COD) that influence nutrient accumulation; (3) differentiate the source-sink exchange of nutrients; and (4) evaluate the ecological significance for estuary management.
2. Materials and Methods
2.1. Description of the Study Area
This study is carried out in the PRE (112.9014°–114.2574° E, 21.5279°–22.9333° N). The PRE is a large-scale estuarine system in China. The Pearl River system consists of river networks (approximately a total of 322) and estuaries. The freshwater flows into the South China Sea via eight outlets (at Humen, Jiaomen, Hongqimen, Hengmen, Modaomen, Jitimen, Hutiaomen, and Yamen). Water masses are mainly influenced by the Pearl River Diluted Water, coastal current, and oceanic upwelling. The PRE receives the nutrient resources from the East and West rivers via the east four outlets. The Pearl River ranks at the second level in China regarding its freshwater discharge (3,338 × 108 m3/yr). It has a watershed area of 45.37 × 104 km2 and a mean annual runoff discharge of 336 billion m3/yr. The freshwater in the wet season (April-September, 80% of the total amount) is four times in the dry season (October-December, January-March, 20%) (Zhao, 1990).
The variation in the hydrology (freshwater, sediment) of the Pearl River is depicted in Supplementary Figure 1. Their values are the sum of Gaoyao (West River), Shijiao (North River), and Boluo (East River) hydrological stations. The annual water discharge and sediment over the past two decades (1996–2018) were 28.34 × 109 m3 and 0.35 × 106 t, respectively. Compared with that in 1996, the water discharge changed +17% in 1999, +9% in 2006, −16% in 2008, −11% in 2016, and +22% in 2018, with the changes of sediment load at −43% in 1999, −40% in 2006, −43% in 2008, −62% in 2016, and −86% in 2018. The long term variation in freshwater was non-significant; a significant decline in sediment load was detected because of the intense human activities such as dam constructions.
2.2. Sample Collection and Analysis
Four cruise surveys were conducted in the PRE in 2006-08 (as L01–23), 2008-08 (as L01–23), 2016-08 (as P01–45), and 2018-08 (as S01–S24). Data in 1996-08 were redrawn from Zhang et al. (1999), and in 1999-07 were extracted from Zhang and Li (2010) and Lin and Li (2002). Sampling sites in this estuary, presented in Figure 1, were designed based on their geographic importance and estuarine characteristics. These surveys aimed to explain the nutrient pollution in the 1990s, 2000s, and 2010s, and to identify their source-sink patterns under both the natural and anthropogenic influences. Environmental properties included suspended sediment (SS), salinity, chemical oxygen demand (COD), dissolved oxygen (DO), phosphorus (PO4), nitrite (NO2), nitrate (NO3), ammonium (NH4), and silicate (SiO4). Phytoplankton chlorophyll-a (Chla) was also measured. DIN is the sum of NO2, NO3, and NH4.
Water samples at different water layers (surface, middle, and bottom) were collected in the Niskin bottles. Here, we focused mostly on surface water samples. Salinity and water depth were measured in situ with a Conductivity-Temperature-Depth/Pressure Profiler (CTD, Sea-bird Electronics, USA). The concentrations of SS were weighed in the laboratory. Samples for various nutrients and chlorophyll-a were filtered in the field using a 0.45 μm cellulose acetate filter. These treated filtrates were stored at cool and were shipped back to the lab for further analysis. The concentrations of nutrients, DO, COD, and chlorophyll-a were determined according to the specification of marine monitoring (GB 17378-2007). The concentrations of dissolved nutrients were determined by colorimetry. The COD concentration was determined by the alkaline permanganate method. The DO concentration was determined by the iodometry method. The concentrations of chlorophyll-a were determined by UV spectrophotometry. Table 1 displays the summary of water quality variables and their ranges in the PRE.
2.3. Statistical Analysis
The case area is approximately divided into three zones according to the estuarine dynamics: upper-PRE (salinity <10; near the mouth), middle-PRE (10 <salinity <20; and lower-PRE (salinity >20). Spatial distributions of variables are plotted with Golden Software Surfer 13. The linkages between environmental factors (salinity, suspended sediment, DO, COD) and nutrients are discussed using the Pearson correlation analysis and regression analysis. The level of significance level less than 0.05 (p < 0.05) is defined as statistically significant. A principal component analysis (PCA) is applied to explore the associations between phytoplankton chlorophyll and environmental factors (Niu et al., 2015; Chai et al., 2016). Each variable is standardized to unit prior to PCA. The data preparations for Pearson correlation, linear regression, and PCA are conducted using the statistical package IBM SPSS Statistics 20 for Windows.
The two end-member mixing model is introduced to differentiate biological processes from the effects of physical mixing, as used in the earlier studies of Han et al. (2012), Wu et al. (2016), Li et al. (2017), and Niu et al. (2020). These two end members have different nutrient conditions. The freshwater end-member is dominated by physical forcing with high nutrient concentrations, and the sea end-member correlates with marine currents. The mixing model is based on nutrient-salinity relationships (Rowe and Chapman, 2002; Kim et al., 2020), described as:
where Sin−situ is the measured salinity; F1 is the freshwater fraction, and F2 is the seawater fraction; S1 and S2 denote the salinity levels of the two end-members.
The conservative nutrient concentrations of DIN (N*), DIP (P*), and DSi (Si*) calculated by the mixing model are given as:
where DIN1, DIN2, DIP1, DIP2, DSi1, and DSi2 are the corresponding concentrations of the two end-members for DIN, DIP, and DSi.
The difference/deviation between the model predictions and measurements is defined as Δ, which reflects the status of nutrient production (Δ <0) or uptake (Δ >0) associated with the biological response.
To evaluate water quality pollution in this estuary, classification of environmental quality assessment (EQA) based on the improved NCCR (EPA, 2008) and eutrophication risk assessment (Karydis et al., 1983; Zhang et al., 2009; Tao et al., 2020) are introduced to quantify the nutrient pollution in the PRE over the past two decades. The water bodies are classified as eutrophic when the eutrophication level is larger than 1. High eutrophication (>5) indicates a more severe environment.
3. Results and Discussion
3.1. Characteristics of Environmental Variables in the Estuary
The temporal distributions of DO, COD, suspended sediment (SS), and salinity in the PRE between 1996 and 2018 are presented in Supplementary Figure 2. DO showed a relatively declined trend between 1999 and 2018. The summer DO ranged from 5.57–7.51 mg L−1 in 1999, 0.83–6.73 mg L−1 in 2006, 3.41–7.24 mg L−1 in 2008, 1.90–10.84 mg L−1 in 2016, and 3.69–6.68 mg L−1 in 2018. Partial hypoxia (DO <3 mg L−1) occurred occasionally in the waters (as stations L01, L03, L05, L06, L10, L14, and L22 in 2006; and stations P01-P06 in 2016), thereby increasing the ecological risks to the aquatic microorganisms (Braga et al., 2000). Partial hypoxia closely correlated with the anthropogenic nutrient inputs to the estuary. The difference in annual COD was non-significant. The mean COD concentration in the entire estuary was 1.36 mg L−1 in 1999, 1.41 mg L−1 in 2008, 1.53 mg L−1 in 2016, and 1.12 mg L−1 in 2018.
The spatial distribution of salinity was displayed in Supplementary Figure 3. The mean salinity was 6.95 in 1996, 6.64 in 1999, 17.4 in 2006, 12.99 in 2008, 14.11 in 2016, and 13.13 in 2018. The average salinity in the entire estuary was less than 10 before 2000, while the level increased after 2000. These salinity results suggested that the saline water intruded into the upper estuary due to intense anthropogenic pressures (e.g., land reclamation, sand mining, channel dredging) after 2000. Horizontally, salinity increased southward, and also was relatively high in the eastern zone. The SS trend was inversely distributed in the estuary with the salinity. Temporally, the mean SS concentration was 17.42 mg L−1 in 1996, 23.07 mg L−1 in 1999, 32.55 mg L−1 in 2006, 16.98 mg L−1 in 2008, and 30.1 mg L−1 in 2018. The SS concentrations were relatively high in the western and northern PRE and decreased from the southwest to the southeast estuary (Zhang et al., 2019). Heavy rainfall during summer raised the water level, and large volumes of runoff then discharged more terrigenous sediments into the PRE, which also increased the SS concentrations in that area. Moreover, freshwater forcings in summer were strong and bottom sediments were potentially carried to the surface, resulting in additional re-suspended sediment in the water column (Zhang and Li, 2010).
3.2. Nutrient Enrichment in an Estuarine Environment
The nutrient levels (DIN, DIP, DSi) showed significant variability (p < 0.05) in the PRE, temporally and spatially. The concentrations of dissolved nutrients exhibited a clear decreasing trend from the river to the sea (Figures 2, 3). DIN in the entire estuary ranged from 0.07 mg L−1 to 1.21 mg L−1 (mean 0.81 mg L−1) in 1996, 0.49–1.62 mg L−1 (1.22 mg L−1) in 1999, 0.04–1.82 mg L−1 (0.80 mg L−1) in 2006, 0.24–1.80 mg L−1 (1.04 mg L−1) in 2008, 0.02–2.49 mg L−1 (1.23 mg L−1) in 2016, and 0.04–3.82 mg L−1 (1.67 mg L−1) in 2018. DIN levels in the surface waters were higher than that in the bottom layers, which was consistent with the previous studies (Yin et al., 2001; Li et al., 2017). Higher DIN values appeared in the upper-PRE, with the mean value of 1.33 mg L−1 in 1999, 1.54 mg L−1 in 2006, and 2.29 mg L−1 in 2016; the west zone of the estuary was more enriched in DIN than the east, implying the contributions of the river channels (i.e., Humen, Hengmen, Jiaomen, Hongqimen). These findings suggested that riverine input was one of the important DIN sources. Over time, DIN gradually increased from 1996 to 2018, with a fitted linear curve (R2= 0.31, N=6). Among the DIN compositions, NO3 was the most abundant form (Supplementary Figure 4), as reported from other studies in this estuary (Huang et al., 2003; Zhang et al., 2016; Niu et al., 2020; Tao et al., 2020). The proportions of NO2 and NH4 were followed. The mean value of NO3 was 0.63 mg/L in 2006, 0.89 mg/L in 2008, 0.99 mg/L in 2016, and 1.03 mg/L in 2018, accounting for 75, 83, 72, and 81% of DIN, accordingly. NO3 was mainly detected from the atmospheric deposition and agricultural applications (e.g., fertilizers, pesticides) in the Pearl River basin (Niu et al., 2020). The runoff transported the NO3 from the upper channel to the estuary.
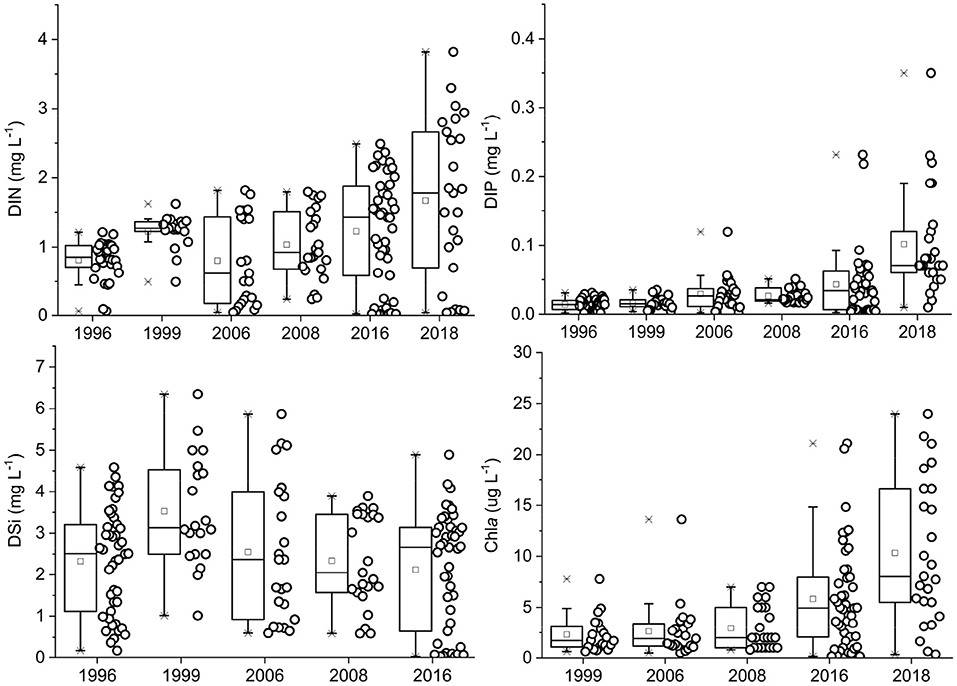
Figure 2. Boxplot graphics of DIN, DIP, DSi, and phytoplankton chlorophyll between 1996 and 2018 in the Pearl River Estuary.
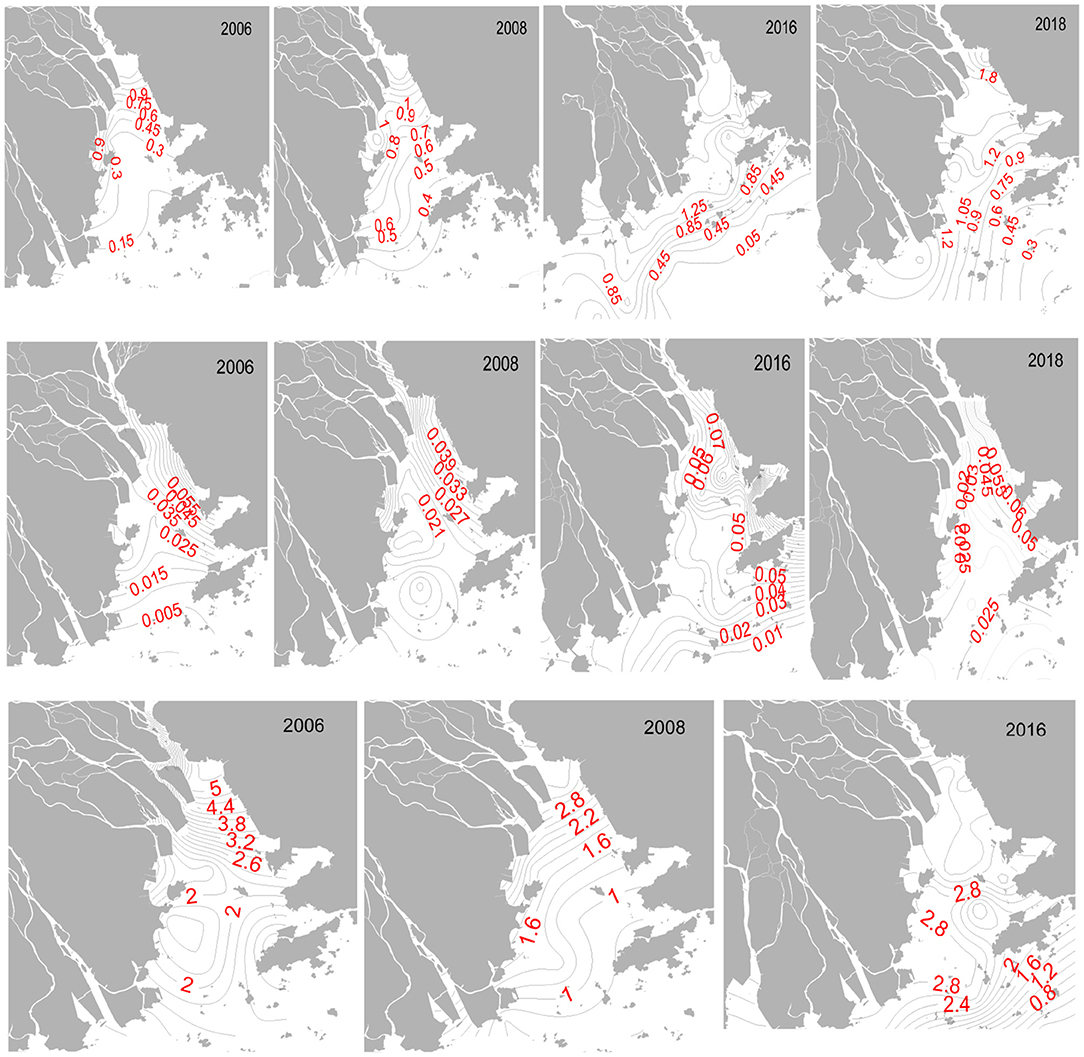
Figure 3. Spatial variations of DIN, DIP, and DSi (unit: mg L−1) in surface water of the Pearl River Estuary.
The DIP concentration in this estuary was relatively low. DIP significantly increased during the sampling periods, with a good fitted linear curve (R2 = 0.85, N = 6). The DIP concentrations in the total area varied from 0.002–0.029 mg L−1 in 1996, 0.004–0.035 mg L−1 in 1999, 0.002–0.119 mg L−1 in 2006, 0.016–0.051 mg L−1 in 2008, 0.003–0.231 mg L−1 in 2016, and 0.010–0.350 mg L−1 in 2018. The heavy contamination of DIP (>0.03 mg L−1; threshold value of severe pollution) mainly occurred in the northeast zone of the PRE (e.g., Shenzhen coast, Shenzhen Bay), declining to the southwest estuary. The DIP pattern in the entire estuary was different when compared with the spatial trend of DIN. These results of DIN and DIP implied that land-based pollutants from the cities of Shenzhen and Dongguan greatly contributed to the DIP levels, and riverine inputs were the main contributor of DIN.
The horizontal distribution of DSi (SiO4) was similar with DIN (Figures 3, 5). The DSi levels ranged from 0.183–4.59 mg L−1 in 1996, 1.023–6.35 mg L−1 in 1999, 0.603–5.86 mg L−1 in 2006, 0.593–3.89 mg L−1 in 2008, and 0.033–4.89 mg L−1 in 2016. DSi in the PRE exhibited a significant spatial variation and a non-significant temporal difference over the past two decades.
Compared with many estuarine systems in the world, as listed in Supplementary Table 1, the nutrient pollution in the PRE was classified at a severe level. The DIN level in this area was lower than that in the Tweed estuary (Uncles et al., 2003). However, the nutrient concentration in the PRE was still much higher than that in the Yellow River estuary (Wang et al., 2017), the Cochin estuary (Vipindas et al., 2018; Jabir et al., 2020), the Tagus River estuary (Borges et al., 2020), the Caete estuary (Monteiro et al., 2016), and the Razdolnaya River estuary (Shulkin et al., 2018). The DIP was at a comparable level. The DSi concentration in this estuary was lower than that in the Yangtze River estuary (Chai et al., 2006), but higher than that in the Yellow River estuary (Wang et al., 2017), the Tagus River estuary (Borges et al., 2020), the Razdolnaya River estuary (Shulkin et al., 2018), and the Tweed estuary (Uncles et al., 2003). Therefore, the water quality pollution caused by nitrogen and phosphorus species should be primarily concerned with avoiding more deterioration in the PRE ecosystems.
3.3. Phytoplankton Chlorophyll and Eutrophication Risk
A large amount of nutrients in summer were discharged from rivers into the PRE, which would potentially favor the phytoplankton growth. Chlorophyll-a levels in the entire estuary varied widely overtime periods (Supplementary Figure 5), ranging from 0.63–7.79 μg L−1 in 1999, 0.49–13.62 μg L−1 in 2006, 0.50–7.0 μg L−1 in 2008, 0.15–21.11 μg L−1 in 2016, and 0.42–10.15 μg L−1 in 2018. Spatial difference in chlorophyll was significant (p < 0.05). The high chlorophyll-a levels in 1999, 2006, and 2008 were mainly distributed in the middle and lower estuary, while the high values in 2016 and 2018 were near the river mouths and coasts (Dai et al., 2008; Wei and Huang, 2010; Li et al., 2014). The East River discharged the most pollutants from a major city group (i.e., Guangzhou, Dongguan, and Foshan) through the Humen mouth. Several channels of the West River and North River transported the riverine pollutants through Jiaomen, Hongqimen, and Hengmen. Vertically, chlorophyll-a levels decreased with the water depth because of the enrichment of nutrient resources in the surface layer.
The nutrient enrichment would induce the rapid growth of phytoplankton and high eutrophication risk. The eutrophication level in the PRE varied from 0.58 to 30.49 (mean 9.86) in 2008 and 0.003 to 184.71 (31.53) in 2016 (Supplementary Figure 6). The aquatic environment of the PRE in 2016 was more polluted than that in 2008, be related to the nutrient characteristics in that period. The total trend of eutrophication level in the estuary was similar to nitrogen and phosphorus, declining from the mouth to the sea. Most of the estuary was classified as eutrophic (E >1). The eutrophication level in the northeast PRE (E >5; e.g., Shenzhen and Dongguan coasts) was higher than that in the southwest. The pollution of eutrophication was negatively and significantly correlated with salinity (Niu et al., 2020; Zeng et al., 2020), demonstrated that the dilution-mixing process of marine waters strongly influenced the eutrophication risk. Based on the EQA, the overall water environment in the PRE was poor-general in 2006, general in 2008, general-poor in 2016, and poor in 2018 (Table 2). The aquatic environment in most zones of the PRE was classified as sub-healthy, mainly caused by DIN and DIP during these sampling periods.
3.4. Nutrient Distribution Shaping Phytoplankton Chlorophyll
3.4.1. Nutrient Limitation of Phytoplankton Growth
The stoichiometric requirement for phytoplankton growth suggested that the molar ratio of N:P ranged at 12–22 (mean 16) and Si: N was 1 (Redfield, 1958; Justic et al., 1995). Potential nutrient limitations were identified as follows (Justic et al., 1995): P was limiting if Si:P >22 and N:P >22, N was limiting if N:P <12 and Si: N >1, and Si was limiting if Si:P <12 and Si: N <1. Figure 4 plots the nutrient ratios (N:P, Si: N, and Si:P) for distinguishing the nutrient limitation of the phytoplankton growth. The average N:P ratio in the total estuary was 212 in 1999, 71 in 2006, 97 in 2008, and 79 in 2016. The average Si:N was 1.42 in 1999, 3.23 in 2006, 1.15 in 2008, and 0.89 in 2016. In 1999 and 2006, only P-limitation was predominantly detected in the whole estuary. Si-and N-limitation occurred occasionally in 2016. Low PO4 levels, relative to nitrogen and silicate, limited the phytoplankton production in most zones of the PRE. Similar results were also reported by the previous studies (Yin et al., 2004; Zhang et al., 2013; Gan et al., 2014; Li et al., 2017; Niu et al., 2020).
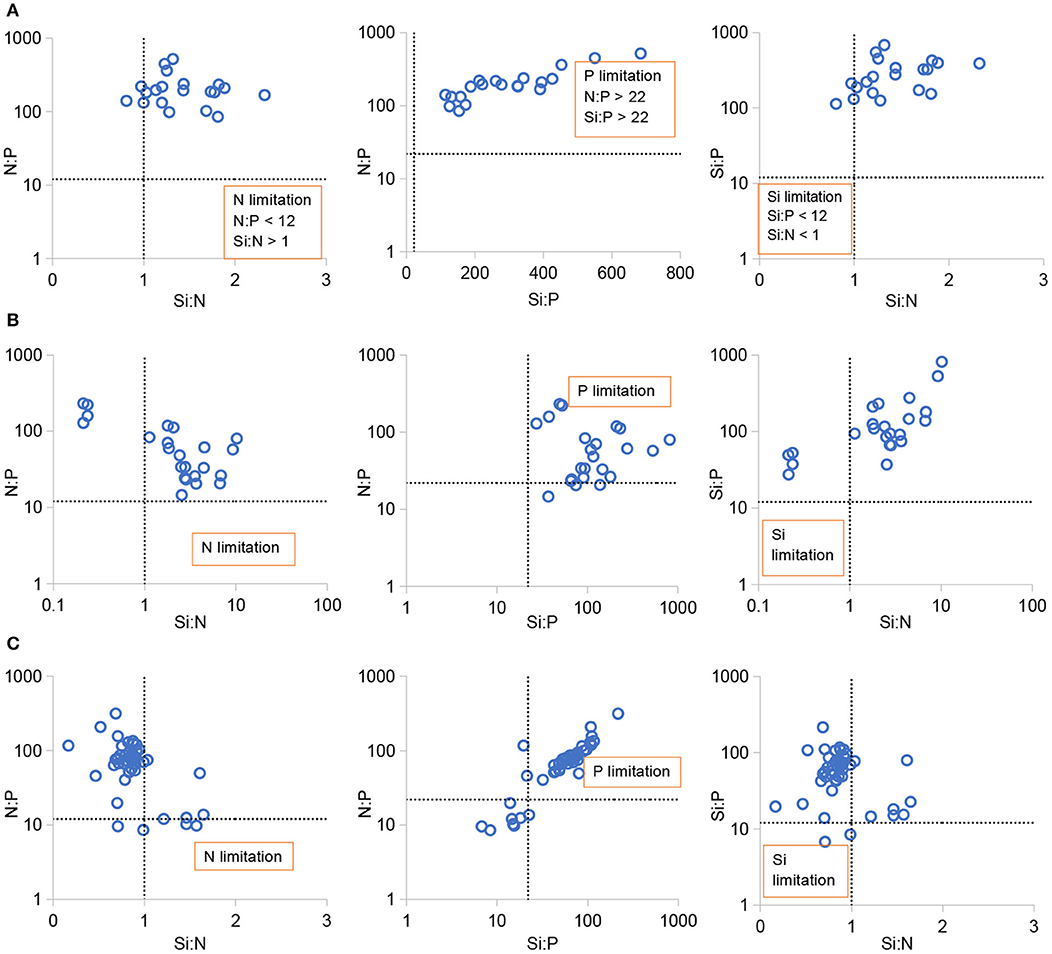
Figure 4. Scatter diagrams of atomic nutrient ratios (N:P, Si:N, and Si:P) during summer in the Pearl River estuary in 1999 (A), 2006 (B), and 2016 (C).
3.4.2. Correlation Between Chlorophyll-a and Environmental Factors
The source/sink exchange made the PRE more complex and played an important role in the estuarine ecosystems. Changes in the hydrological cycles affected the distribution of nutrient resources; these forces were the fundamental demand for phytoplankton growth. Principal component analysis (PCA) identified the impact-effect paths of phytoplankton chlorophyll and environmental factors and distinguished the major factors in each path. The first three components were extracted to represent most of the information (eigenvalue >1), shown in Table 3, with the cumulative proportion of 77% in 1999, 81% in 2006, and 83% in 2018. Figure 5 presents the first two-component loading.
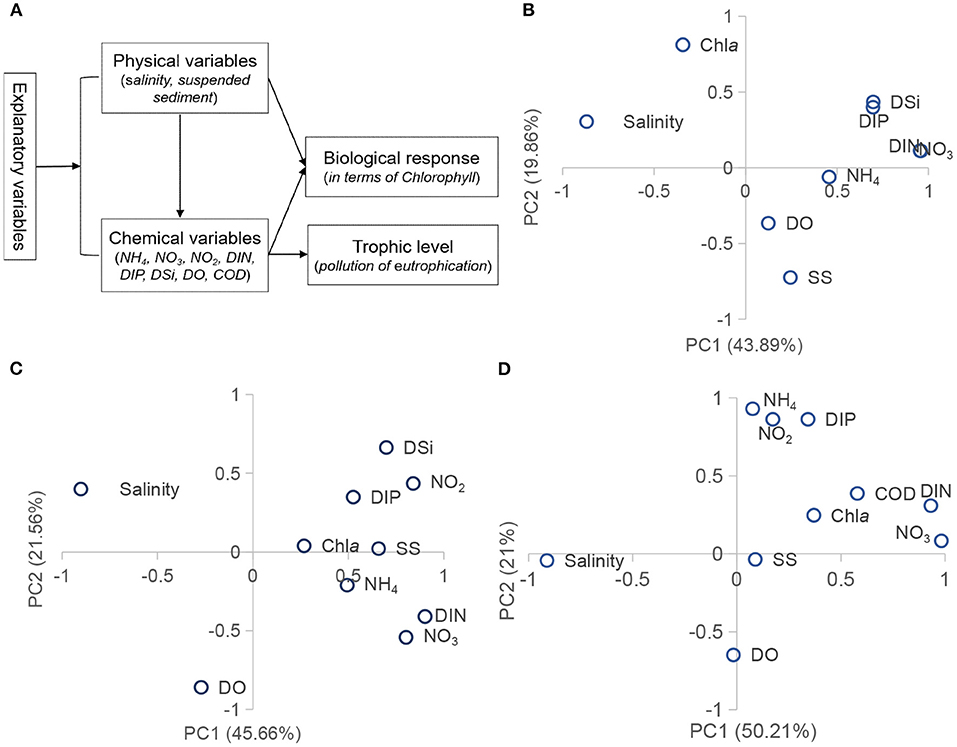
Figure 5. PCA results for associations of phytoplankton chlorophyll with factor scores during summer in the Pearl River estuary (A, theoretical model; B, 1999; C, 2006; D, 2018).
In 1999, PC1 with an eigenvalue of 3.95, accounting for 43.89% of the total variance, was heavily weighed by nutrients and salinity. In PC2 (19.86%), chlorophyll and suspended sediment were dominant. In which, nutrients positively contributed to phytoplankton chlorophyll, characterized by the uptake process; salinity and suspended sediment negatively influenced the chlorophyll level, characterized by the external driving force. In 2006, PC1 (46%) was dominated by salinity, suspended sediment, nitrogen, and silicate; PC2 (22%) was mainly represented by DO; chlorophyll-a and phosphorus were the main contributors in PC3 (13%). In 2018, salinity and nitrogen were the main contributors in PC1, phosphorus and DO in PC2, and suspended sediment and chlorophyll-a in PC3.
In summary, these PCA findings demonstrated the underlying mechanisms of nutrient enrichment, involved with the environmental stresses. Salinity, nitrogen, and silicate showed similar contributions to the chlorophyll-a during the time periods. In particular, salinity negatively correlated with phytoplankton chlorophyll, and the influence was transferred through the role of salinity on the nutrients. However, phosphorus and suspended sediment behaved differently. Phosphorus species were closely linked to NO3 and silicate in 1999, and to NH4 in 2006 and 2016. The suspended sediment displayed a negative role in 1999 and a positive effect in 2006 and 2016. The direct effects of salinity and suspended sediment on phytoplankton were less than that on the nutrient distributions, although riverine discharge would directly affect the phytoplankton production (Chou et al., 2012). Specifically, suspended sediment adsorbed the particulate phosphorus via humic acid and iron oxyhydroxide (Fe(OH)2), which would impact the P transformation (Lin et al., 2004).
The above findings also implied different sources for nitrogen-silicate and phosphorus. The human activities induced in the 1990s of the PRE were land reclamation and channel dredging, resulting in a declined water area. The estuary then became narrower and deeper, preventing the diffusion and transport of the pollutants (Li et al., 2014; Wu et al., 2016; Niu et al., 2020). Therefore, the nutrient pollution caused by nitrogen and silicate was detected in the upper estuary because of the riverine influence. The phosphorus pollution mainly appeared in the northeast (e.g., Shenzhen bay) and was transported with the salinity front to the sea. It was the reason why the high phytoplankton production appeared in the middle/lower estuary.
3.5. Factors Influencing Nutrient Source-Sink Patterns
3.5.1. Nutrient-Salinity Relationships
Hydrological factors controlled the distributions of nutrients and influenced the riverine inputs (Castillo, 2020; Geng et al., 2021; Zhang et al., 2021). Our study regions were within the contour 30 m, and the salinities were <34 (Supplementary Figure 3). The mixing process of freshwater and saline water in that area would influence the spatiotemporal distributions of dissolved nutrients. Figure 6 plots the nutrient-salinity correlations over the past two decades. The correlation coefficient (R2) between salinity and DIN was 0.86 in 1999 (N = 27, p <0.01), 0.98 in 2006 (N = 23, p <0.01), 0.90 in 2008 (N = 23, p <0.01), 0.92 in 2016 (N = 45, p <0.01), and 0.83 in 2018 (N = 25, p <0.01); the coefficient for DIP was 0.44 in 1999 (p <0.01), 0.22 in 2006 and 0.67 in 2016 (p <0.01); for DSi was 0.39 in 1999 (p <0.01), 0.89 in 2006 (p <0.01), 0.97 in 2008 (p <0.01), and 0.89 in 2016 (p <0.01). The slopes of DIN-salinity and DSi-salinity were similar during the sampling times. Off the river mouth, DIN and DSi concentrations continued to decrease as the salinity increased, while DIP concentrations were more variable, probably because of the external P sources such as P cycling and regeneration, biodegradation, emissions of coastal cities, and adsorption of sediment.
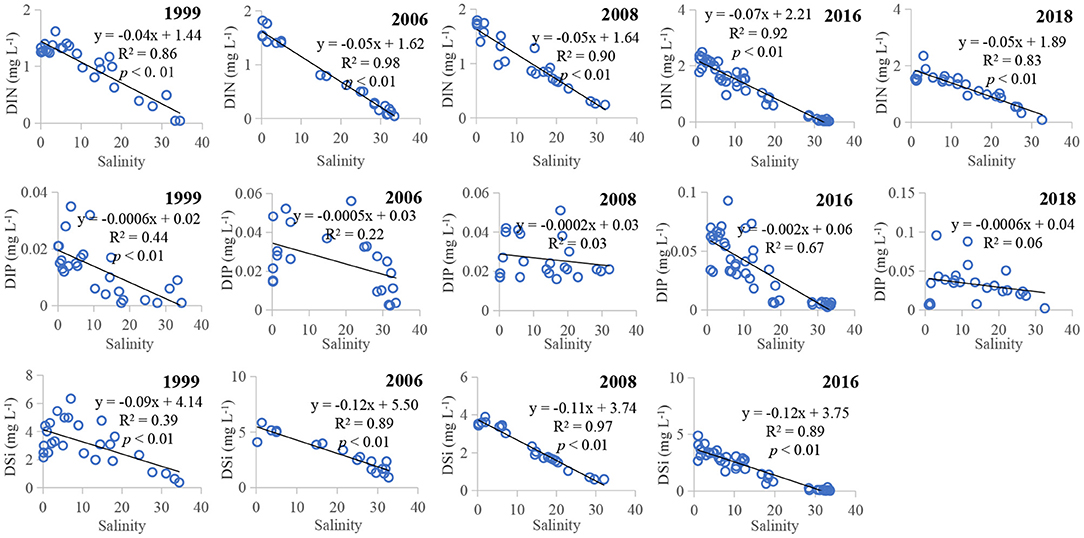
Figure 6. Plots showing the nutrient-salinity relationships during summer between 1999 and 2018 in the Pearl River Estuary.
Dissolved nutrients were negatively significantly correlated with salinity, corresponding well with the previous studies in this estuary (Zhang and Li, 2010; Shen et al., 2012; Shi et al., 2017; Niu et al., 2020; Tao et al., 2020). High-salinity marine currents, having fewer nutrients, mixed with the freshwater and diluted the estuarine nutrient concentrations. River inflows, carrying abundant nutrients, increased the nutrient levels in the estuarine mixing zone. These correlations results indicated that changes in nutrient concentrations were strongly associated with the tide-river mixing processes. The weak tidal forcing in summer resulted in a movement of salinity front to the sea. The nutrients were then transported farther and have provided the nutrient sources for the marine organisms. Therefore, the high phytoplankton production appeared around the salinity front (Supplementary Figure 5).
3.5.2. Effect of the Pearl River Discharge
The riverine inputs have provided enriched nutrient resources for the marine microorganisms. Considering the mixing degree of freshwater and seawater, the nutrient-salinity relationships also reflected the source and sink patterns besides the dilution process (Shulkin et al., 2018; Kim et al., 2020). Changes in nutrient concentrations were then considerably modulated by biological uptake processes associated with the physical mixing. We estimated the nutrient activities based on the nutrient-salinity relationships. The freshwater end-member was about 1.5–2.0 mg L−1 for DIN and 4–5 mg L−1 for DSi during our sampling times. The freshwater end-member for DIP was variable in this estuary because of the complexity of P-input. There was no difference in nutrient concentrations when salinities were >33 (as in 2016), thus the seawater end-member was 0.024 mg L−1 for DIN, 0.004 mg L−1 for DIP, and 0.07 mg L−1 for DSi. Figure 7 plots the nutrient deviation against salinity in the PRE in 1999, 2006, and 2016, identifying the effects of physical mixing and biological response. The dashed line in the figure represented no deviation (Δ = 0); the negative deviation (Δ <0) indicated a nutrient addition (e.g., emissions of coastal cities, decomposition of organic matter, regeneration, atmospheric deposition); and the positive deviation (Δ >0) represented the nutrient removal (e.g., biological uptake, transformation).
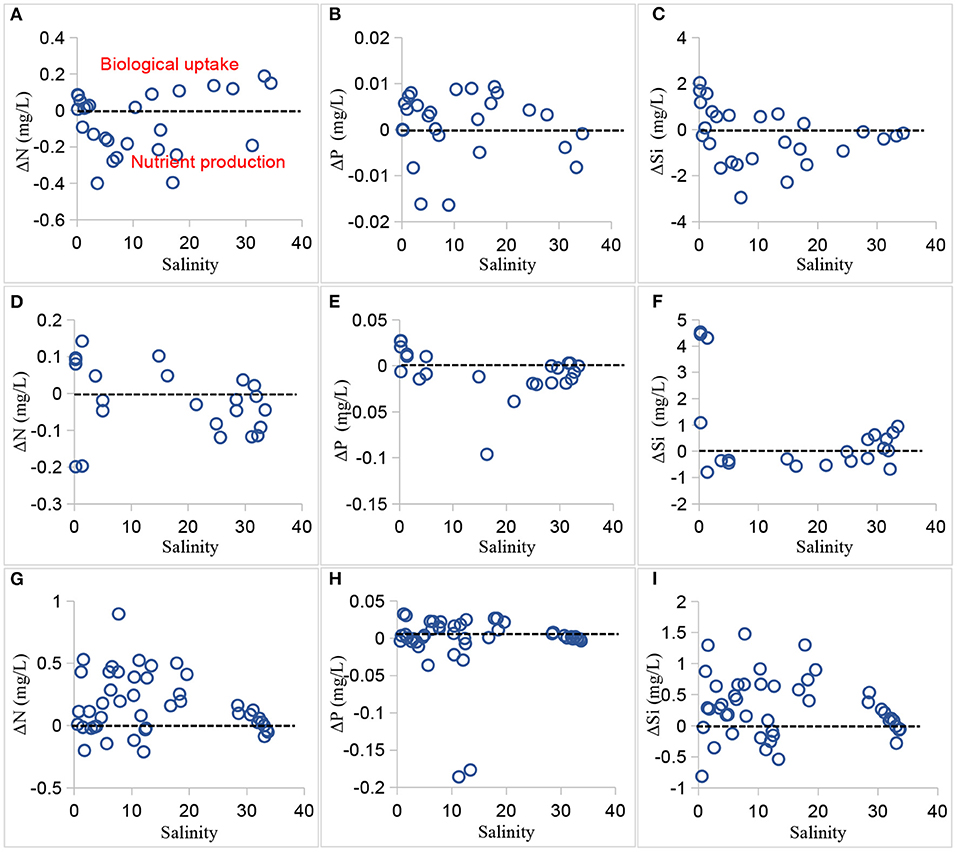
Figure 7. Nutrient deviation vs. salinity during summer in 1999 (A–C), 2006 (D–F), and 2016 (G–I), denoting the difference between observations and predictions based on the linear nutrient-salinity relationships. The dashed line represents no deviation (Δ = 0); the negative deviation (Δ <0) indicates a nutrient addition (e.g., emissions of coastal cities, cycling and regeneration, atmospheric deposition); the positive deviation (Δ >0) represents the nutrient removal (e.g., biological uptake, transformation).
In 1999, most of the deviations of nitrogen and silicate in the upper estuary (salinity <10) were less than 0, suggesting that N and Si in that area were mainly characterized by their productions. The likely cause of the riverine input (e.g., Humen, Jiaomen, Hongqimen, and Hengmen) contributed much to the addition of nitrogen and silicate. However, P activity in that area was controlled both by biological uptake and production. In the middle estuary, N, P, and Si activities were mostly characterized by biological uptake. The corresponding higher chlorophyll levels (mean 2.76 μg L−1) could also support the above discussion (Zhang and Li, 2010). Another possible reason was intended to the phosphorus concentrations because of its limiting role for phytoplankton growth. In the lower estuary (particulate at salinities > 33), the low nutrient concentrations could not favor the biological activities.
In 2006, the N activity was dominated by both biological consumption and production in the estuary. The degree of biological uptake of nitrogen was stronger than the addition. The possible N sources were from the river basin (upper estuary) and decomposition of organic matter and coastal zones near Zhuhai-Macau (lower estuary). The main P addition was mainly discovered on the Shenzhen coast (northeast estuary; Figure 3). The degrees of P and Si productions were stronger than their consumption processes.
In 2016, the biological uptake and production equally contributed to the nutrient activities. Moreover, the degree of biological uptake was stronger than the nutrient addition in the estuary, with the mean chlorophyll-a of 5.84 μg L−1 in 2016. In the lower estuary, the nutrient input was dominant. The main N and Si activities in the upper and middle estuary showed removal, probably caused by the biological uptake and sedimentation of phytoplankton debris (Han et al., 2012); these N and Si external sources were mainly detected in the upper and lower estuary, likely caused by the river discharge and land-based sources. Observed DIN had higher concentrations in the western estuary. These nitrogen deviations in the east and west PRE suggested different degrees of active internal N cycling, as discussed in other studies (Chai et al., 2006, 2009; Wu et al., 2016). The DIP removal was mainly induced by the biological response and adsorption on the suspended particles, while the P sink was likely due to the P replenishment (as in the middle estuary) and fertilizers in the agricultural applications (Li et al., 2017; Wang, 2018).
In summary, the two end-member mixing model has provided a useful tool to discern the dynamic source-sink patterns of the nutrient activities in the estuary. The DIN deviation results showed that the degree of N removal gradually increased between 1999 and 2016, corresponding to the increased nutrient and chlorophyll-a levels. The external N source was mainly detected in the middle and lower estuary, probably caused by the decay of organisms, transformation, atmospheric deposition, and wastewater of urban areas. The source-sink patterns of DSi activities were similar to DIN. Overall, the Si activity during the time periods was significantly changed from the dominated source addition to removal over the period. The degree of P removal gradually increased, and the P source input was mainly detected in the middle and lower estuary except that in 1999. Moreover, DIN was less particle active but may be removed by biological activities such as denitrification; DIP was strongly particle active and could be removed by suspended particulate matter. Additionally, factors of topography, residence time, and other environmental factors have also influenced the dynamic source-sink patterns of nutrient behaviors in the estuary.
3.5.3. Atmospheric Deposition
The atmospheric deposition is one of the potential nutrient sources and is important in regulating the phytoplankton biomass in an estuary (Yadav et al., 2016). Atmospheric deposition of TN and TP display annual cycles (Miyazako et al., 2015). Compared with other source paths of nutrients into the PRE, the contribution of atmospheric dry and wet deposition was relatively low (Supplementary Table 2; Li et al., 2011), accounting for 12.64% of TN and 1.14% of TP. The atmospheric deposition of TN in Guangzhou, Shenzhen, Zhuhai-Macau, Dongguan, Zhongshan, and Hongkong was 420, 5,600, 22,086, 930, 1,056, and 6789.6 t/a, respectively, with the corresponding values of 4.2, 63, 245.4, 9.15, and 12.32, and 74.52 t/a for TP.
Over the past two decades, estuarine coastal ecosystems have suffered a number of environmental problems that attributed to nutrient over-enrichment. For instance, surface runoff from agricultural land, atmospheric deposition, waste-water discharge from urban areas, and fuel combustion all added nutrients to the river inflow. Riverine input has provided a large number of nutrients and has contributed much to the dynamic source-sink pattern. In addition, the nutrient levels in summer were higher than other seasons because of the high inflows.
3.6. Ecological Significance in the PRE Ecosystem
Nutrient enrichment in the estuary has become a societal issue, as the excess anthropogenic nutrient input into the waters would trigger eutrophication and phytoplankton blooms (Howarth and Marino, 2006; Boyer et al., 2009; Srichandan et al., 2019). Nutrient input to an estuary can vary widely regarding its forms and sources, strongly correlated with population density and land use (Nedwell et al., 2002; Maier et al., 2009). High nutrient loads have induced disturbances in the phytoplankton species in the PRE. The phytoplankton community structure was changed over the past two decades, associated with the variation in the aquatic environment. The dominant species in the summer of 1999 were Skeletonema costatum, Nitzschia pongens, Nitzschia delicatissima, Asterionella japonica, and Leptocylindrus danicus (Huang et al., 2004). Skeletonema costatum, Nitzschia delicatissima, Chaetoceros curvisetus, Chaetoceros debilis, and Melosira granulata were the dominated phytoplankton species in 2006 (Li et al., 2011). In 2016, Skeletonema costatum, Nitzschia delicatissima, Chaetoceros decipiens, Coscinodiscus jonesianus, Chaetoceros affinis, Skeletonema tropicum, and Thalassiothrix frauenfeldii were dominant (our unpublished data).
The nutrient pollution in the PRE was classified as severely eutrophic due to the rapid economic development in the Pearl River Delta (e.g., industrialization). Excessive nutrient input related to human pressures has usually led to water quality problems and oxygen depletion for the majority of PRE ecosystems. Nitrogen and phosphorus have beneficial uses as fertilizer but excess applications of nutrients in agriculture (beyond crop needs) can lead to eutrophication in downstream estuaries, even the dead fishes. Once nutrient pollution issues have been identified, coastal managers need to understand the dynamic source-sink patterns in order to better control the nutrient enrichment.
4. Conclusions
The Pearl River Estuary was enriched in nutrients during the high inflows. The observed DIN and DIP during summer showed a gradual increase between 1996 and 2018, while the annual trend of DSi was non-significant during that period. Phosphorus limitation was detected in most of the PRE; it was also indicative of the relevance of anthropogenic pressures and potential contributions to eutrophication. The degrees of DIN and DIP removal/uptake gradually increased over the past decades, corresponding to the increased phytoplankton chlorophyll-a. DIN was less particle active but may be removed by biological activities. However, DIP was strongly particle active and might be removed by suspended matter and biological response. Overall, the dynamic source-sink patterns of nutrients were largely influenced by the biogeochemical characteristics of the estuary; these patterns would regulate the phytoplankton structure. This field investigation discussed how nutrient dynamics were involved with salinity regimes, biological uptake, riverine input, and atmospheric deposition. However, more studies related to nutrient forms and other influencing factors should be explored for a better understanding of the dynamic source-sink patterns of nutrients in the estuary in future.
Data Availability Statement
The original contributions presented in the study are included in the article/Supplementary Material, further inquiries can be directed to the corresponding author/s.
Author Contributions
WT: data curation and writing—original draft. LN: conceptualization, formal analysis, and writing—original draft. YD: investigation. TF: formal analysis. QL: supervision and project administration. All authors contributed to the article and approved the submitted version.
Funding
This research was financially supported by the National Key R&D Program of China (No. 2016YFC0402600) and National Natural Science Foundation of China (No. 51709289).
Conflict of Interest
The authors declare that the research was conducted in the absence of any commercial or financial relationships that could be construed as a potential conflict of interest.
Publisher's Note
All claims expressed in this article are solely those of the authors and do not necessarily represent those of their affiliated organizations, or those of the publisher, the editors and the reviewers. Any product that may be evaluated in this article, or claim that may be made by its manufacturer, is not guaranteed or endorsed by the publisher.
Acknowledgments
The authors would like to thank the editors and the reviewers for their valuable comments and suggestions on this paper.
Supplementary Material
The Supplementary Material for this article can be found online at: https://www.frontiersin.org/articles/10.3389/fmars.2021.713907/full#supplementary-material
References
Bianchi, T., DiMarco, S., Cowan, J., Hetland, R., Chapman, P., Day, J., et al. (2010). The science of hypoxia in the northern Gulf of Mexico: a review. Sci. Tot. Environ. 408, 1471–1484. doi: 10.1016/j.scitotenv.2009.11.047
Borges, C., Bettencourt, D. S. R., and Palma, C. (2020). Determination of river water composition trends with uncertainty: seasonal variation of nutrients concentration in Tagus river estuary in the dry 2017 year. Mar. Pollut. Bull. 158:111371. doi: 10.1016/j.marpolbul.2020.111371
Boyer, J., Kelble, C., Ortner, P., and Rudnick, D. (2009). Phytoplankton bloom status: chlorophyll a biomass as an indicator of water quality condition in the southern estuaries of Florida, USA. Ecol. Indicat. 9, s56–s67. doi: 10.1016/j.ecolind.2008.11.013
Braga, E., Bonetti, C., Burone, L., and Bonetti Filho, J. (2000). Eutrophication and bacterial pollution caused by industrial and domestic wastes at Baixada Santista estuarine system, Brazil. Mar. Pollut. Bull. 40, 165–173. doi: 10.1016/S0025-326X(99)00199-X
Cai, H., Huang, J., Niu, L., Ren, L., Liu, F., Ou, S., et al. (2018). Decadal variability of tidal dynamics in the Pearl river delta: spatial patterns, causes, and implications for estuarine water management. Hydrol. Process. 32, 3805–3819. doi: 10.1002/hyp.13291
Castillo, M. (2020). Suspended sediment, nutrients, and chlorophyll in tropical floodplain lakes with different patterns of hydrological connectivity. Limnologica 82:125767. doi: 10.1016/j.limno.2020.125767
Chai, C., Jiang, T., Cen, J., Ge, W., and Lu, S. (2016). Phytoplankton pigments and functional community structure in relation to environmental factors in the Pearl River Estuary. Oceanologia 58, 201–211. doi: 10.1016/j.oceano.2016.03.001
Chai, C., Yu, Z., Shen, Z., Song, X., Cao, X., and Yao, Y. (2009). Nutrient characteristics in the Yangtze river estuary and the adjacent east China sea before and after impoundment of the three Gorges Dam. Sci. Tot. Environ. 407, 4687–4695. doi: 10.1016/j.scitotenv.2009.05.011
Chai, C., Yu, Z., Song, X., and Cao, X. (2006). The status and characteristics of eutrophication in the Yangtze river (Changjiang) estuary and the adjacent east China Sea, China. Hydrobiologia 563, 313–328. doi: 10.1007/s10750-006-0021-7
Chou, W., Fang, L., Wang, W., and Tew, K. (2012). Environmental influence on coastal phytoplankton and zooplankton diversity: a multivariate statistical model analysis. Environ. Monitor. Assess. 184, 5679–5688. doi: 10.1007/s10661-011-2373-3
Dai, M., Zhai, W., Cai, W., Callahan, J., Huang, B., Shang, S., et al. (2008). Effects of an estuarine plume-associated bloom on the carbonate system in the lower reaches of the Pearl River Estuary and the coastal zone of the northern south China Sea. Continent. Shelf Res. 28, 1416–1423. doi: 10.1016/j.csr.2007.04.018
EPA (2008). National Coastal Condition Report III (EPA842-R-08-002). Environmental Protection Agency, Office of Water and Office of Research and Development, Washington, DC.
Farrow, C., Ackerman, J., Smith, R. H., and Snider, D. (2019). Riverine transport and nutrient inputs affect phytoplankton communities in a coastal embayment. Freshw. Biol. 65, 289–303. doi: 10.1111/fwb.13421
Gan, J., Lu, Z., Cheung, A., Dai, M., Liang, L., Harrison, P., et al. (2014). Assessing ecosystem response to phosphorus and nitrogen limitation in the Pearl river plume using the regional ocean modelling system (ROMs). J. Geophys. Res. Oceans 119, 8858–8877. doi: 10.1002/2014JC009951
Geeraert, N., Archana, A., Xu, M., Kao, S., Baker, D., and Thibodeau, B. (2021). Investigating the link between pearl river-induced eutrophication and hypoxia in Hong Kong shallow coastal waters. Sci. Tot. Environ. 772:145007. doi: 10.1016/j.scitotenv.2021.145007
Geng, M., Wang, K., Yang, N., Li, F., Zou, Y., Chen, X., et al. (2021). Spatiotemporal water quality variations and their relationship with hydrological conditions in Dongting lake after the operation of the three Gorges Dam, China. J. Clean. Product. 283:124644. doi: 10.1016/j.jclepro.2020.124644
Guo, C., Chen, Y., Xia, W., Qu, X., Yuan, H., Xie, S., et al. (2020). Eutrophication and heavy metal pollution patterns in the water suppling lakes of China's south-to-north water diversion project. Sci. Tot. Environ. 711:134543. doi: 10.1016/j.scitotenv.2019.134543
Han, A., Dai, M., Kao, S., Gan, J., Li, Q., Wang, L., et al. (2012). Nutrient dynamics and biological consumption in a large continental shelf system under the influence of both a river plume and coastal upwelling. Limnol. Oceanogr. 57, 486–502. doi: 10.4319/lo.2012.57.2.0486
Howarth, R., and Marino, R. (2006). Nitrogen as the limiting nutrient for eutrophication in coastal marine ecosystems: evolving views over three decades. Limnol. Oceanogr. 51, 364–376. doi: 10.4319/lo.2006.51.1_part_2.0364
Huang, L., Jian, W., Song, X., Huang, X., Liu, S., Qian, P., et al. (2004). Species diversity and distribution for phytoplankton of the Pearl River Estuary during rainy and dry seasons. Mar. Pollut. Bull. 49, 588–596. doi: 10.1016/j.marpolbul.2004.03.015
Huang, X., Huang, L., and Yue, W. (2003). The characteristics of nutrients and eutrophication in the Pearl River Estuary, south China. Mar. Pollut. Bull. 47, 30–36. doi: 10.1016/S0025-326X(02)00474-5
Jabir, T., Vipindas, P., Jesmi, Y., Valliyodan, S., Parambath, P., Singh, A., et al. (2020). Nutrient stoichiometry (N:P) controls nitrogen fixation and distribution of diazotrophs in a tropical eutrophic estuary. Mar. Pollut. Bull. 151:110799. doi: 10.1016/j.marpolbul.2019.110799
Jia, Z., Li, S., Liu, Q., Jiang, F., and Hu, J. (2021). Distribution and partitioning of heavy metals in water and sediments of a typical estuary (Modaomen, South China): the effect of water density stratification associated with salinity. Environ. Pollut. 287:117277. doi: 10.1016/j.envpol.2021.117277
Jiang, Z., Liu, J., Chen, J., Chen, Q., Yan, X., Xuan, J., et al. (2014). Responses of summer phytoplankton community to drastic environmental changes in the Changjiang (Yangtze River) estuary during the past 50 years. Water Res. 54, 1–11. doi: 10.1016/j.watres.2014.01.032
Justic, D., Rabalais, N., and Turner, R. (1995). Stoichiometric nutrient balance and origin of coastal eutrophication. Mar. Pollut. Bull. 30, 41–46. doi: 10.1016/0025-326X(94)00105-I
Karydis, M., Ignatiades, L., and Moschopoulou, N. (1983). An index associated with nutrient eutrophication in the marine environment. Estuar. Coast. Shelf Sci. 16, 339–344. doi: 10.1016/0272-7714(83)90151-8
Kim, J., Chapman, P., Rowe, G., and DiMarco, S. (2020). Categorizing zonal productivity on the continental shelf with nutrient-salinity ratios. J. Mar. Syst. 206:103336. doi: 10.1016/j.jmarsys.2020.103336
Kim, T., Lee, K., Duce, R., and Liss, P. (2014). Impact of atmospheric nitrogen deposition on phytoplankton productivity in the south China sea. Geophys. Res. Lett. 41, 3156–3162. doi: 10.1002/2014GL059665
Li, G., Lin, Q., Lin, J., Song, X., Tan, Y., and Huang, L. (2014). Environmental gradients regulate the spatial variations of phytoplankton biomass and community structure in surface water of the Pearl River Estuary. Acta Ecol. Sin. 34, 129–133. doi: 10.1016/j.chnaes.2014.01.002
Li, K., Chen, Z., and Jiang, G. (2011). Pollution Characteristics and Their Ecological Responses in the Pearl River Estuary and Adjacent Zones (in Chinese). Beijing: China Construction Industry Press.
Li, R., Xu, J., Li, X., Shi, Z., and Harrison, P. (2017). Spatiotemporal variability in phosphorus species in the Pearl River Estuary: influence of the river discharge. Sci. Rep. 7:13649. doi: 10.1038/s41598-017-13924-w
Lin, W., and Li, S. (2002). Vertical distribution of cod and do and its affecting factors in the Pearl River Estuary in summer (in Chinese with English abstract). Acta Sci. Nat. Univers. Sunyatseni 41, 82–86.
Lin, Y., Su, J., Hu, C., Zhang, M., Li, Y., Guan, W., et al. (2004). N and P in waters of the Zhujiang river estuary in summer (in Chinese with English abstract). Acta Oceanol. Sin. 26, 63–73.
Maier, G., Nimmo-Smith, R., Glegg, G., Tappin, A., and Worsfold, P. (2009). Estuarine eutrophication in the UK: current incidence and future trends. Aquat. Conserv. Mar. Freshw. Ecosyst. 19, 43–56. doi: 10.1002/aqc.982
Mathew, J., Singh, A., and Gopinath, A. (2021). Nutrient concentrations and distribution of phytoplankton pigments in recently deposited sediments of a positive tropical estuary. Mar. Pollut. Bull. 168:112454. doi: 10.1016/j.marpolbul.2021.112454
Miyazako, T., Kamiya, H., Godo, T., Koyama, Y., Nakashima, Y., Sato, S., et al. (2015). Long-term trends in nitrogen and phosphorus concentrations in the Hii river as influenced by atmospheric deposition from east Asia. Limnol. Oceanogr. 60, 629–640. doi: 10.1002/lno.10051
Monteiro, M., Jimeinez, J., and Pereira, L. (2016). Natural and human controls of water quality of an Amazon estuary (Caete-Pa, Brazil). Ocean Coast. Manage. 124, 42–52. doi: 10.1016/j.ocecoaman.2016.01.014
Nedwell, D., Dong, L., Sage, A., and Underwood, G. (2002). Variations of the nutrients loads to the mainland UK estuaries: correlation with catchment areas, urbanization and coastal eutrophication. Estuar. Coast. Shelf Sci. 54, 951–970. doi: 10.1006/ecss.2001.0867
Niu, L., Luo, X., Cai, H., Liu, F., Zhang, T., and Yang, Q. (2021). Seasonal dynamics of polycyclic aromatic hydrocarbons between water and sediment in a tide-dominated estuary and ecological risks for estuary management. Mar. Pollut. Bull. 162:111831. doi: 10.1016/j.marpolbul.2020.111831
Niu, L., Luo, X., Hu, S., Liu, F., Cai, H., Ren, L., et al. (2020). Impact of anthropogenic forcing on the environmental controls of phytoplankton dynamics between 1974 and 2017 in the Pearl River Estuary, China. Ecol. Indicat. 116:106484. doi: 10.1016/j.ecolind.2020.106484
Niu, L., Van Gelder, P., Zhang, C., Guan, Y., and Vrijling, J. (2015). Statistical analysis of phytoplankton biomass in coastal waters: case study of the Wadden sea near Lauwersoog (the Netherlands) from 2000 through 2009. Ecol. Informat. 30, 12–19. doi: 10.1016/j.ecoinf.2015.08.003
Potter, I., Rose, T., Huisman, J., Hall, N., Denham, A., and Tweedley, J. (2021). Large variations in eutrophication among estuaries reflect massive differences in composition and biomass of macroalgal drift. Mar. Pollut. Bull. 167:112330. doi: 10.1016/j.marpolbul.2021.112330
Redfield, A. (1958). The biological control of chemical factors in the environment. Am. Sci. 46, 205–221.
Rowe, G., and Chapman, P. (2002). Continental shelf hypoxia: some nagging questions. Gulf Mexico Sci. 20, 153–160. doi: 10.18785/goms.2002.08
Shen, P., Li, Y., Qi, Y., Zhang, L., Tan, Y., and Huang, L. (2012). Morphology and bloom dynamics of cochlodinium geminatum schutt in the Pearl Pearl River Estuary, south China Sea. Harmful Algae 13, 10–19. doi: 10.1016/j.hal.2011.09.009
Shi, Y., Zhao, H., Wang, X., Zhang, J., Sun, X., and Yang, G. (2019). Distribution characteristics of nutritive salts and chlorophyll a in the Pearl River Estuary. J. Guangdong Ocean Univers. 39, 56–65. doi: 10.3969/j.issn.1673-9159.2019.01.009
Shi, Z., Xu, J., Huang, X., Zhang, X., Jiang, Z., Ye, F., et al. (2017). Relationship between nutrients and plankton biomass in the turbidity maximum zone of the Pearl River Estuary. J. Environ. Sci. China 57, 72–84. doi: 10.1016/j.jes.2016.11.013
Shulkin, V., Tishchenko, P., Semkin, P., and Shvetsova, M. (2018). Influence of river discharge and phytoplankton on the distribution of nutrients and trace metals in Razdolnaya river estuary, Russia. Estuar. Coast. Shelf Sci. 211, 166–176. doi: 10.1016/j.ecss.2017.09.024
Srichandan, S., Baliarsingh, S., Prakash,1, S., Lotliker, A., Parida, C., and Sahu, K. (2019). Seasonal dynamics of phytoplankton in response to environmental variables in contrasting coastal ecosystems. Environ. Sci. Pollut. Res. 26, 12025–12041. doi: 10.1007/s11356-019-04569-5
Tao, W., Niu, L., Liu, F., Cai, H., Ou, S., Zeng, D., et al. (2020). Influence of river-tide dynamics on phytoplankton variability and their ecological implications in two Chinese tropical estuaries. Ecol. Indicat. 115:106458. doi: 10.1016/j.ecolind.2020.106458
Uncles, R., Frickers, P., and Harris, C. (2003). Dissolved nutrients in the Tweed estuary, UK: inputs, distributions and effects of residence time. Sci. Tot. Environ. 314–316, 727–736. doi: 10.1016/S0048-9697(03)00080-9
Vipindas, P., Anas, A., Jayalakshmy, K., Lallu, K., Benny, P., and Shanta, N. (2018). Impact of seasonal changes in nutrient loading on distribution and activity of nitrifiers in a tropical estuary. Continent. Shelf Res. 154, 37–45. doi: 10.1016/j.csr.2018.01.003
Wang, F., Cheng, P., Chen, N., and Kuo, Y. (2021). Tidal driven nutrient exchange between mangroves and estuary reveals a dynamic source-sink pattern. Chemosphere 270:128665. doi: 10.1016/j.chemosphere.2020.128665
Wang, Y. (2018). Actual costs of uneconomic growth of marine fisheries in China's Pearl river delta. Nat. Resour. 9, 297–312. doi: 10.4236/nr.2018.97018
Wang, Y., Liu, D., Lee, K., Dong, Z., Di, B., Wang, Y., et al. (2017). Impact of water-sediment regulation scheme on seasonal and spatial variations of biogeochemical factors in the Yellow river estuary. Estuar. Coast. Shelf Sci. 198, 92–105. doi: 10.1016/j.ecss.2017.09.005
Wei, P., and Huang, L. (2010). Water quality and eutrophication in the Guangzhou sea zone of the Pearl River Estuary. Chin. J. Oceanol. Limnol. 28, 113–121. doi: 10.1007/s00343-010-9032-3
Wu, M., Hong, Y., Yin, J., Dong, J., and Wang, Y. (2016). Evolution of the sink and source of dissolved inorganic nitrogen with salinity as a tracer during summer in the Pearl River Estuary. Sci. Rep. 6:36638. doi: 10.1038/srep36638
Yadav, K., Sarma, V., Rao, D., and Kumar, M. (2016). Influence of atmospheric dry deposition of inorganic nutrients on phytoplankton biomass in the coastal Bay of Bengal. Mar. Chem. 187, 25–34. doi: 10.1016/j.marchem.2016.10.004
Yin, K., Qian, P., Wu, M., Chen, J., Huang, L., Song, X., et al. (2001). Shift from P to N limitation of phytoplankton growth across the Pearl river estuarine plume during summer. Mar. Ecol. Prog. Ser. 221, 17–28. doi: 10.3354/meps221017
Yin, K., Song, S., Sun, J., and Wu, M. (2004). Potential P limitation leads to excess N in the Pearl river estuarine coastal plume. Continent. Shelf Res. 24, 1895–1907. doi: 10.1016/j.csr.2004.06.014
Yuan, X., Krom, M., Zhang, M., and Chen, N. (2021). Human disturbance on phosphorus sources, processes and riverine export in a subtropical watershed. Sci. Tot. Environ. 769:144658. doi: 10.1016/j.scitotenv.2020.144658
Zeng, D., Niu, L., Tao, W., Fu, L., and Yang, Q. (2020). Nutrient dynamics in Pearl River Estuary and their eutrophication evaluation (in Chinese with English abstract). J. Guangdong Ocean Univers. 40, 73–82.
Zhang, D., Qi, Q., Tong, S., Wang, J., Zhang, M., Zhu, G., et al. (2021). Effect of hydrological fluctuation on nutrient stoichiometry and trade-offs of Carex schmidtii. Ecol. Indicat. 120:106924. doi: 10.1016/j.ecolind.2020.106924
Zhang, G., Cheng, W., Chen, L., Zhang, H., and Gong, W. (2019). Transport of riverine sediment from different outlets in the Pearl River Estuary during the wet season. Mar. Geol. 415:105957. doi: 10.1016/j.margeo.2019.06.002
Zhang, H., and Li, S. (2010). Effects of physical and biochemical processes on the dissolved oxygen budget for the Pearl River Estuary during summer. J. Mar. Syst. 79, 65–88. doi: 10.1016/j.jmarsys.2009.07.002
Zhang, J., Huang, X., Jiang, Z., and Huang, D. (2009). Seasonal changes of eutrophication level in the Pearl River Estuary from 2006 to 2007 and its relationship with environmental factors (in Chinese with English abstract). Acta Ecol. Sin. 31, 113–120.
Zhang, J., Yu, Z., Wang, J., Ren, J., Chen, H., and Xiong, H. (1999). The subtropical Zhujiang (Pearl river) estuary: nutrient, trace species and their relationship to photosynthesis. Estuar. Coast. Shelf Sci. 49, 385–400. doi: 10.1006/ecss.1999.0500
Zhang, L., Shi, Z., Zhang, J., Jiang, Z., Huang, L., and Huang, X. (2016). Characteristics of nutrients and phytoplankton productivity in Guangdong coastal regions, south China. Mar. Pollut. Bull. 113, 572–578. doi: 10.1016/j.marpolbul.2016.08.081
Keywords: nutrient enrichment, source and sink, environmental fate, eutrophication risk, estuary
Citation: Tao W, Niu L, Dong Y, Fu T and Lou Q (2021) Nutrient Pollution and Its Dynamic Source-Sink Pattern in the Pearl River Estuary (South China). Front. Mar. Sci. 8:713907. doi: 10.3389/fmars.2021.713907
Received: 24 May 2021; Accepted: 24 August 2021;
Published: 16 September 2021.
Edited by:
Kenneth Mei Yee Leung, City University of Hong Kong, Hong Kong, SAR ChinaReviewed by:
Rajdeep Roy, Indian Space Research Organisation, IndiaRichard Brum, Australian Institute of Marine Science (AIMS), Australia
Copyright © 2021 Tao, Niu, Dong, Fu and Lou. This is an open-access article distributed under the terms of the Creative Commons Attribution License (CC BY). The use, distribution or reproduction in other forums is permitted, provided the original author(s) and the copyright owner(s) are credited and that the original publication in this journal is cited, in accordance with accepted academic practice. No use, distribution or reproduction is permitted which does not comply with these terms.
*Correspondence: Lixia Niu, bml1bGl4aWFAbWFpbC5zeXN1LmVkdS5jbg==