- 1Institute for Crop Science and Plant Breeding, Department of Grass and Forage Science/Organic Agriculture, Christian Albrechts University, Kiel, Germany
- 2Marine Biology Station Piran, National Institute of Biology, Piran, Slovenia
The supply of nutrients for agricultural production faces enormous challenges as food security and sustainability goals have to be ensured. Processing of marine biomass has high potential to provide nutrients for agricultural purposes in coastal areas. One underexplored resource are jellyfish, which occur as blooms and by-catch of the fishing industry. In this context, a pot experiment investigated the effects of jellyfish as a fertilizer on biomass accumulation of annual ryegrass (Lolium multiflorum), and its effect on the important greenhouse gas N2O as a sustainability indicator of novel fertilizers. Dried and ground jellyfish was applied [3 species: Aurelia aurita (AA), Cyanea capillata (CC), Periphylla periphylla (PP)] and compared with an unfertilized and a mineral fertilized (calcium-ammonium-nitrate, CAN) treatment. Dried jellyfish and CAN were applied at equal N rates of 5 g N per m2. The N2O-fluxes from soil were measured over 56 days after fertilizer application. Grass dry matter yields, when using CC and PP treatments, were not significantly different to the CAN treatment (p > 0.05). After reducing its salinity, AA also showed no differences to CAN on plant growth and the lowest coefficient of variation for dry matter yield as an indicator for yield stability. Accumulated N2O-emissions were lowest in the control and were 3-times higher in AA and CC compared to CAN (p < 0.05). If salinity levels are moderate, jellyfish application to soil can compete with artificial mineral fertilizers in terms of N-supply for above- and belowground yield response, regardless of jellyfish species used. However, elevated N2O-emissions are likely to affect its suitability for large-scale application. Nevertheless, if energy-efficient methods of drying and desalination of jellyfish can be developed, in coastal areas dried jellyfish is a valuable fertilizer in coastal areas, particularly in situations where nutrient supplies for agriculture are limited.
Introduction
The FAO anticipates that global agricultural production will need to increase by approximately 50% by 2050, compared with the 2017 output, to enable enough food resources as a consequence of global population growth (FAO, 2017). A major challenge will be to achieve the necessary yield increases while also adhering to the United Nations sustainable development goal 2 (Zero Hunger) (UN, 2015).
In agricultural production, adequate nutrient levels are needed to provide favorable plant growth conditions to ensure optimum crop and forage yields. In intensive agricultural production, this usually requires inputs of high levels of mineral fertilizers, which is in particular observed in developed countries, although increasing levels of fertilizer use are observed worldwide (FAO, 2020). However, the use of mineral fertilizers is energy intensive and, particularly in the case of phosphorus, global resources are limited, which makes efficient use and recycling strategies for plant nutrients necessary (Withers et al., 2015). In terms of agricultural production, it is widely advocated that organic fertilizers should be used more efficiently, as they are often available naturally and locally, either as a renewable resource (i.e., green manures and biologically fixed nitrogen from legume-based swards in forage crop rotations) or as a by-product from agricultural production chains (i.e., manures from animal housing units). However, there are some disadvantages associated with organic fertilizers. The nutrients they contain are organically bound and become available erratically during their decomposition in the soil, with a nutrient-use efficiency often below 70% (Berzsenyi et al., 2000). Thus, new and innovative ways of making nutrients available for plant growth without depleting natural resources and provoking additional environmental threats are still part of the current debate around future perspectives for more sustainable agricultural production.
One of these innovative approaches is to utilize organic fertilizers from marine sources (Blue-green innovation) as an alternative way of ensuring sustainable terrestrial food production, without disrupting the ecosystem or depleting the resource (Council, 2002; Selvam and Sivakumar, 2014; Sivasangari Ramya et al., 2015; Emadodin et al., 2020a). On one hand, marine organic materials can provide nutrients for plant production; on the other hand, nutrients that have been lost as aquatic pollutants can be recycled for agricultural purposes. The main focus during recent decades has been on the use of seagrasses and seaweeds because they occur in large amounts along coastlines (Mossbauer et al., 2012; Franzén et al., 2019) and their use is attractive as a source of fertilizers (Selvam and Sivakumar, 2014; Thompson et al., 2020), biostimulants (El Boukhari et al., 2020) and for their role in supporting other ecosystem services (Acksel et al., 2017). However, there are many other potential marine resources that remain largely unexplored. For instance, in many coastal regions around the world, jellyfish blooms cause problems because of their negative impacts on marine ecosystems by competing with fish, while also feeding on zooplankton and ichthyoplankton (Purcell et al., 2007), and affecting built infrastructure (such as coastal power plants) or tourism (Ghermandi et al., 2015). A recent review by Emadodin et al. (2020a) provided evidence for the potential of jellyfish to provide a source of nutrients for terrestrial agriculture and thereby partly replace mineral fertilizers in a more sustainable way. Jellyfish can contain significant amounts of macro elements such as nitrogen (N), phosphorus (P) and potassium (K), comparable to other organic fertilizers (Emadodin et al., 2020a). For instance, positive effects on growth and yield were observed with jellyfish suspensions in the cultivation of pak choi (Brassica rapa), green soybeans (Glycine max), perilla (Perilla frutescens), spinach (Spinacia oleracea) and cherry tomatoes (Solanum lycopersicum) (Fukushi et al., 2004, 2005, 2017). The plant growth response can be further improved by desalination (Fukushi et al., 2006), i.e., desalinated suspensions were successfully tested in ice weed (Mesembryanthemum crystallinum) (Hattori et al., 2014). In extensive land-use systems and restoration projects, commercially available jellyfish chips in South Korea are often used to grow or establish trees such as oaks (e.g., Quercus glauca), pines (e.g., Pinus thunbergii), cypresses (Chamaecyparis obtusa) and Shorea macrophylla (Ezaki et al., 2008; Chun et al., 2011, 2012, 2015; Ezaki et al., 2011; Kohno et al., 2012; Seo et al., 2014; Kun Woo et al., 2019; Perumal et al., 2019). Soil amendments with jellyfish lead to a significant improvement of the trees’ morphological quality characteristics, such as number of leaves, length, root diameter and dry matter. These observations are attributed to the nutritional effect of the fertilizers as well as to an increased microbiological activity and increased water retention capacity of the soil. Cheol et al. (2019) further demonstrated an increase in photosynthetic activity in sawtooth oak (Quercus acutissima) treated with jellyfish. Moreover, in combination with other organic residues (sawdust, bamboo pieces, fungal residues), soil improvement mixtures were developed (Inamoto et al., 2013; Chun et al., 2014; Kohno et al., 2014, 2015).
The influence of jellyfish in the soil-plant-system can be manifold. The application of jellyfish biomass can lead to a change in the bacterial community structure in the soil. A phylogenetic analysis by Beck et al. (2012) showed a substantial increase of sphingobacteria from the bacteroidetes phylum. At the same time, Alpha- and Beta-proteobacteria disappeared completely. Bacteroidetes inhabit the rhizosphere of various plant species in a symbiotic relationship and promote their growth by degrading protein-containing matter (Green et al., 2006; Haichar et al., 2008; Marques et al., 2010). The results are consistent with various studies on the decomposition of jellyfish carcasses in the sea, which also affect the bacterial community composition (Tinta et al., 2010, 2016). It has been demonstrated that a homogenate from the tissue of Periphylla periphylla can be used by some bacteria as a substrate, while other bacteria are inhibited (Titelman et al., 2006). Moreover, as a consequence of the decomposition of jellyfish in the sea, a decrease in pH and anoxic conditions have been observed in the immediate vicinity (Qu et al., 2015; Chelsky et al., 2016).
Beyond the questions of agronomic suitability, novel organic fertilizers may also be associated with negative environmental impacts including increased greenhouse gas (GHG) emissions from agricultural soils. This is currently an important topic in the fertilizer industry as soils are responsible for more than 60% of the global nitrous oxide (N2O) emissions (Thomson et al., 2012). Nitrous oxide is considered to be one of the most important GHGs from the agricultural sector, and climate change linked to increased atmospheric GHG concentrations has emerged as one of the top societal challenges (WEF, 2020). It is produced as a by-product of nitrification and denitrification in the soil, whereby it is assumed that the majority of emissions are produced during the denitrification process (Senbayram et al., 2009). The bacteria responsible for denitrification have a heterotrophic metabolic process using nitrogen as electron acceptors when oxygen is limiting (Wrage et al., 2001). Thus, N2O emissions may increase when high contents of easily available carbon (C) from applied organic fertilizers coincide with high N contents and low oxygen availability in the soil. Accordingly, many studies have reported higher N2O emissions from organic fertilizers in comparison with mineral fertilizers, particularly when the C:N ratio is narrow (Biernat et al., 2020). For marine resources it is known that the C:N ratio can show a high variation depending on the biomass used (Emadodin et al., 2020a). Jellyfish has a narrow C:N ratio in comparison with other types of marine biomass (i.e., seagrass and seaweed), leading potentially to its rapid decay in the soil and thereby making associated N2O emissions more likely. As mentioned above, it is likely that jellyfish have the potential to alter the soil microbial communities. However, there is currently no evidence to which extent this can alter the N2O producing communities in the soil and the impacts on the sustainability of a jellyfish-derived plant fertilizer. Moreover, the use of jellyfish on a large scale would present problems as it contains large quantities of sodium (Na), potentially inhibiting plant growth at particular thresholds (Kronzucker et al., 2013). Its low dry matter content also limits its efficiency of use if transport and processing are required, although its transportability and nutrient value relative to its volume can be greatly improved by drying directly in an oven or by lyophilization (Alhamid et al., 2012). However, both these drying methods are energy intensive and unsuitable for large-scale use. Other methods, based on those used by the Asian food industry, include the gradual removal of water with a mixture of common salt (NaCl) and aluminum. During this process, the jellyfish are stored in containers with a lower salt concentration for a period of 20–40 days. The yield is about 7–10% of the wet material and consists of 16–25% salt (Peggy Hsieh et al., 2001). However, this process adapted from the food industry is not suitable for providing a fertilizer product as Al is a plant-toxic element and salinity of the dried material is still high. As an alternative to drying with metal salts, drying with alcohol has been developed (Pedersen et al., 2017). In this method the jellyfish are dipped into 96% ethanol and this is evaporated overnight at room temperature. The process can be described with the help of polyelectrolyte theory (Yuferova, 2017). However, the desalination effect of the alcohol drying method on the use of jellyfish as fertilizer is not well explored.
These agronomic benefits and environmental burdens associated with processing have to be considered when assessing marine biomass, and specifically jellyfish, as a potential source of fertilizers for ensuring sustainable development goals and plant growth responses. There are only a few studies that have explored the effects on plant growth (Emadodin et al., 2020a) and none have estimated the effect of relevant GHG emissions from soils after application of jellyfish, which are a key indicator for the efficiency of climate-smart fertilizers. In order to attain the first evidence-based research in this topic we conducted an experiment to evaluate the: (i) effect on plant growth after jellyfish application and (ii) the effect on N2O emissions from soil. Accordingly, and based on the available literature results, we had the following hypotheses:
• Jellyfish dry matter is rich in N and P and thus increases plant growth.
• However, due to high expected Na contents, plant growth is inhibited. If salinity is not reduced during pre-processing, it leads to a lower plant biomass compared to artificial mineral fertilizers.
• Jellyfish may inhibit soil microbial activity, which is shown in lower N2O emissions from soils after their application, in comparison with using mineral fertilizers, making them a potential climate-smart fertilizer resource for coastal areas.
Materials and Methods
Jellyfish Processing and Analysis
Three jellyfish species Aurelia aurita (AA), Cyanea capillata (CC), and Periphylla periphylla (PP) were obtained from different locations around the Norwegian- and Baltic Sea. Jellyfish were captured by vessels during cruises or in the harbor and along the coastline of fjords. They were frozen at –20°C directly after harvesting and shipped to the facilities of the Christian-Albrechts-University in Kiel, Institute for Crop Science and Plant Breeding.
Prior to further processing, jellyfish samples were dried until constant weight. For drying, two different processes were used: (1) samples were oven-dried at 50°C for 48–72 h, depending on the species and the time needed to reach constant dry weight. Dried materials were weighed, put in laboratory glass bottles and kept in a desiccator because of their sensitivity to humidity. (2) The alcohol-dried method of Pedersen et al. (2017) was applied in which the fresh or frozen jellyfish were submerged in ethanol (70%) for 24 h. Jellyfish were then removed and placed in distilled water for 30 min, after which they were dried at room temperature (around 21°C).
The jellyfish dry matter (DM) was homogenized in a porcelain mortar before chemical analyses. The C and N content was measured via dry combustion (Vario Max CN, Elementar Analysensysteme GmbH, Hanau, Germany). For further elemental analysis, 200 mg DM of ground jellyfish material was digested with 10 mL 15.6 M HNO3 at 190°C for 45 min in an 1,800 W microwave oven (MARS 6, Xpress, CEM, Matthews, MC, United States). After digestion, the concentrations of Ca, K, Mg, and Na were quantified with an atomic absorption spectrometer (AAS 5EA Thermo Electron S, Carl Zeiss, Jena, Germany). P concentrations in all extracts were determined photometrically with a continuous flow analyzer (Skalar Analytical B.V., Breda, Netherlands) by using the modified molybdenum–ascorbic acid blue method (Murphy and Riley, 1962). The nutrient content of different jellyfish species used for plant growth experiments are given in Table 1. With regards to species PP, only oven-dried material was used for plant growth experiments. The coefficient of variation across the different parameters measured were 1.1% C, 0.03% N, 4.3% P, 1.5% K, 2.1% Na, 1.6% Ca and 1.6% for Mg.
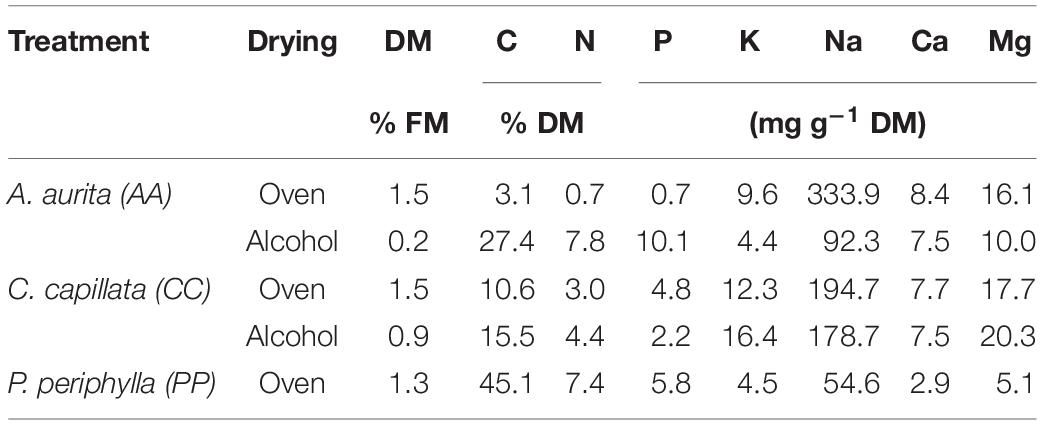
Table 1. Chemical analysis of the dry matter (DM) of the different jellyfish species and different processing methods (oven- and alcohol drying).
Plant Experiments
Plant experiments were conducted under controlled conditions in a greenhouse at the facility of Kiel University. Cylindrical pots (12 cm diameter × 10 cm height) were filled with sieved and dried soil (86% sand, 10% silt, 4% clay) to a bulk density of 1.6 g/cm3 collected from fields in the Baltic Sea Region [Eckernförde Bay (54°27N, 9°57E)]. The nutrient content per 100 g dry-soil was 20 mg P2O5, 7 mg K2O and 7 mg Mg. The pH-value was 6.9. Seeds of Lolium multiflorum Lam. Var. westerwoldicum were sown at 0.5 cm soil depth at a sowing density equivalent to 8 g per m2. Good growing conditions were maintained due to the constant water filled pore space of 50–60% using capillary tubes (Ortmann, Votloh, Germany). Temperature was kept constant at 22 ± 1°C. Illumination was provided by vapor-lamps (Hortilux Schreder, HPS 400W) for 10 h per day. After germination, the plants were grown until flowering stage and cut with scissors at a height of 5 cm above the soil surface. This was repeated 5 times during 2 months to obtain a fully established grass sward in each pot and scarcity of nutrients in the soil. Dried jellyfish and mineral fertilizer were applied after the fifth cut at rates equivalent to 5 g N per m2. The fertilizer treatments comprised dried material from the species AA and CC, obtained by both oven- and alcohol-dried methods, and that of PP, oven-dried only. These were compared with a mineral fertilizer [Calcium-Ammonium-Nitrate (CAN)] and a non-fertilized control (CT). Thus, there were seven treatments including the non-fertilized control, with five replicates of each, and the pots were arranged in a fully randomized design (Figure 1).
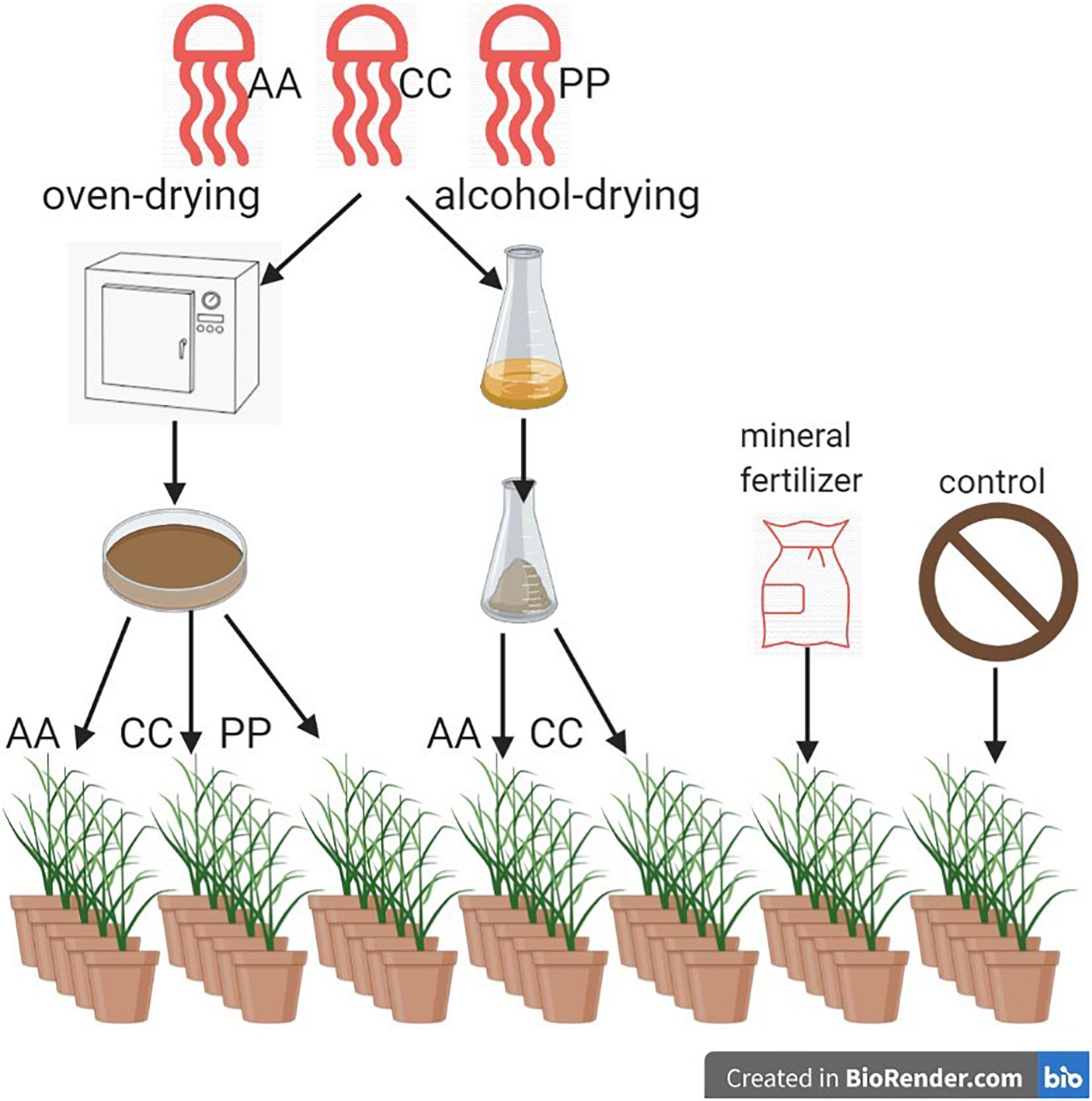
Figure 1. Schematic representation of the experimental set-up and jellyfish treatments (AA, A. aurita; CC, C. capillata; PP, P. periphylla).
There was no additional supplementation of other trace elements. Thus, the amount of other nutrients applied per treatment differed according to the chemical composition of the different sources of fertilizer. Total amounts of the major nutrients supplied to all treatments is given in Table 2.
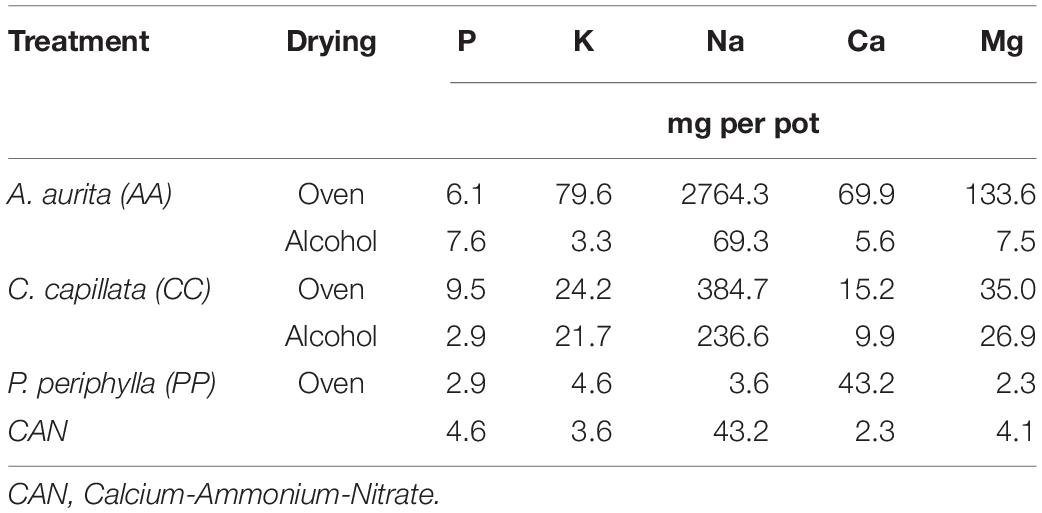
Table 2. Nutrients applied per pot in each treatment according to the controlled fertilization rate of 58.4 mg N per pot (50 kg N ha–1).
For application to the pots, the dried and ground jellyfish material was mixed with soil to a volume of 9 mL and inserted into three small holes (0.6 cm × 0.6 cm) below the soil surface. The same procedure was used for the CAN treatment. Afterward, two more cycles of grass regrowth after cutting were performed over a 56-day period. After the second cut, following fertilizer application, all the aboveground biomass (AGB) was removed at soil-surface level and divided into leaves, stalks and stubble. The soil inside the pots was processed in a hydropneumatic elutriation system (Smucker et al., 1982) to separate roots with a 2-mm sieve, which is referred to as belowground biomass (BGB). The AGB (leaves and stalks from the first and second cut after fertilization + stubble) and BGB were oven-dried at 54°C and the samples were then milled to pass a 2 mm sieve. For the C:N-ratio, ABG and BGB were analyzed by dry combustion (Elementar, Vario Cube, Hanau, Germany). In order to calculate the apparent nitrogen recovery (ANR) of the tested treatments the N-yield of the non-fertilized control was deduced from the N-yield (g N) of the respective fertilized treatment and divided by the total N applied.
Nitrous Oxide Measurements
After application of the different fertilizers, the pots were sealed inside a gastight chamber (d:12.5 cm, h:19.0 cm) twice a week for 60 min. Each chamber was equipped with a vent (diameter: 0.5 mm, length: 110 mm) to allow balanced air pressure between the inside and outside chamber. Gas sampling measurements were taken over a period of 2 months. To allow gas sampling, the chambers were equipped with a rubber septum on the top. Gas samples were taken at 20-min intervals (0, 20, 40, and 60 min) and stored in 12 ml pre-evacuated septum-capped vials (Labco, High Wycombe, United Kingdom) using a 30 ml syringe. The gas samples were analyzed for N2O through a gas chromatograph (SCION 456-GC, Bruker, Leiderdorp, Netherlands), equipped with a 63Ni electron-capture detector using helium as carrier gas and argon-methane as make-up gas. Samples were injected using an autosampler (model 271 LH, Gilson Inc., Middleton, WI, United States). Data were processed using the software Compass CDS (Version 3.0.1). Fluxes were calculated for each treatment and replicate by linear regression between measured N2O concentrations and time. The accumulated N2O emissions were calculated by linear interpolation between the measured daily fluxes for each replication.
Soil Analysis
Soil salinity was measured conductometrically during four consecutive days after AGB removal at the end of the experiment using an electrode (Decagon Devices Inc., WA, United States). Prior to BGB analyses, soil sub-samples were taken from each pot. Soil samples were oven-dried (100°C for 48 h) and ground to mesh size of 1 mm (Modell RMO 100, Retsch GmbH & Co., KG, Haan, Germany). Chemical soil properties (C, N, P, K, Mg) were measured using ICP-OES.
Statistical Analysis
For statistical computing and graphical support the statistical Software R (2020) was used to evaluate the data. Generalized least squares were used to evaluate the AGB, BGB and accumulated N2O emissions depending on the treatment (Laird and Ware, 1982; Verbeke and Molenberghs, 2000). The data were normally distributed and heteroscedastic due to the different fertilizer treatments. All pair comparisons according to Tukey (1953) and Hothorn et al. (2008) were conducted. Significance of the tested factor and comparisons of means were declared when p < 0.05. The coefficient of variation (CV) was used as a parameter for yield stability (Sanderson, 2010).
Results
Aboveground and Belowground Plant Biomass
The contents of macro- and micronutrients in the DM of the different jellyfish species are shown in Table 1. The C content in the DM varied from 3.1 to 45.1% and N content from 0.7 to 7.8% among the tested jellyfish treatments. Differences in nutrient values were mainly affected by different salt contents. The presence of salts was predominately NaCl (>90%) (data not shown). This is reflected in the high Na contents in the jellyfish DM. Thus, Na contents correlated negatively with C due to a dilution effect of organic matter by NaCl. However, Na contents showed wide variation (55–334 mg Na g–1 DM) among the different treatments and the highest values were found for oven-dried AA. Accordingly, the oven-dried material from AA used as fertilizer led to significantly lower grass biomass yields, and this treatment had the lowest biomass accumulation for both AGB and BGB (p < 0.05). All other jellyfish treatments showed higher DM yields of AGB, compared with the control, with no differences compared with the CAN treatment (p > 0.05). The highest DM yields, on average, were obtained in the alcohol-dried AA treatment. Thus, the range of total DM yields in all treatments were in the order: AA oven-dried [0.59 g DM (SE 0.07)] < control [1.47 g DM (SE 0.21)] < PP oven-dried [2.13 g DM (SE 0.50)] < CC alcohol-dried [2.40 g DM (SE 0.40)] < CC oven-dried [2.8 g DM (SE 0.31)] < CAN [2.98 g DM (SE 0.24)] < AA alcohol-dried [3.22 g DM (SE0.26)] (Figure 2A). The highest yield stability was observed for alcohol-dried AA and CAN (CV 0.18). Oven-dried AA (CV 0.24) and CC (CV 0.25) were comparable to the non-fertilized control (CV 0.32). The highest yield variability was observed for alcohol-dried CC (CV 0.40) and oven-dried PP (CV 0.54).
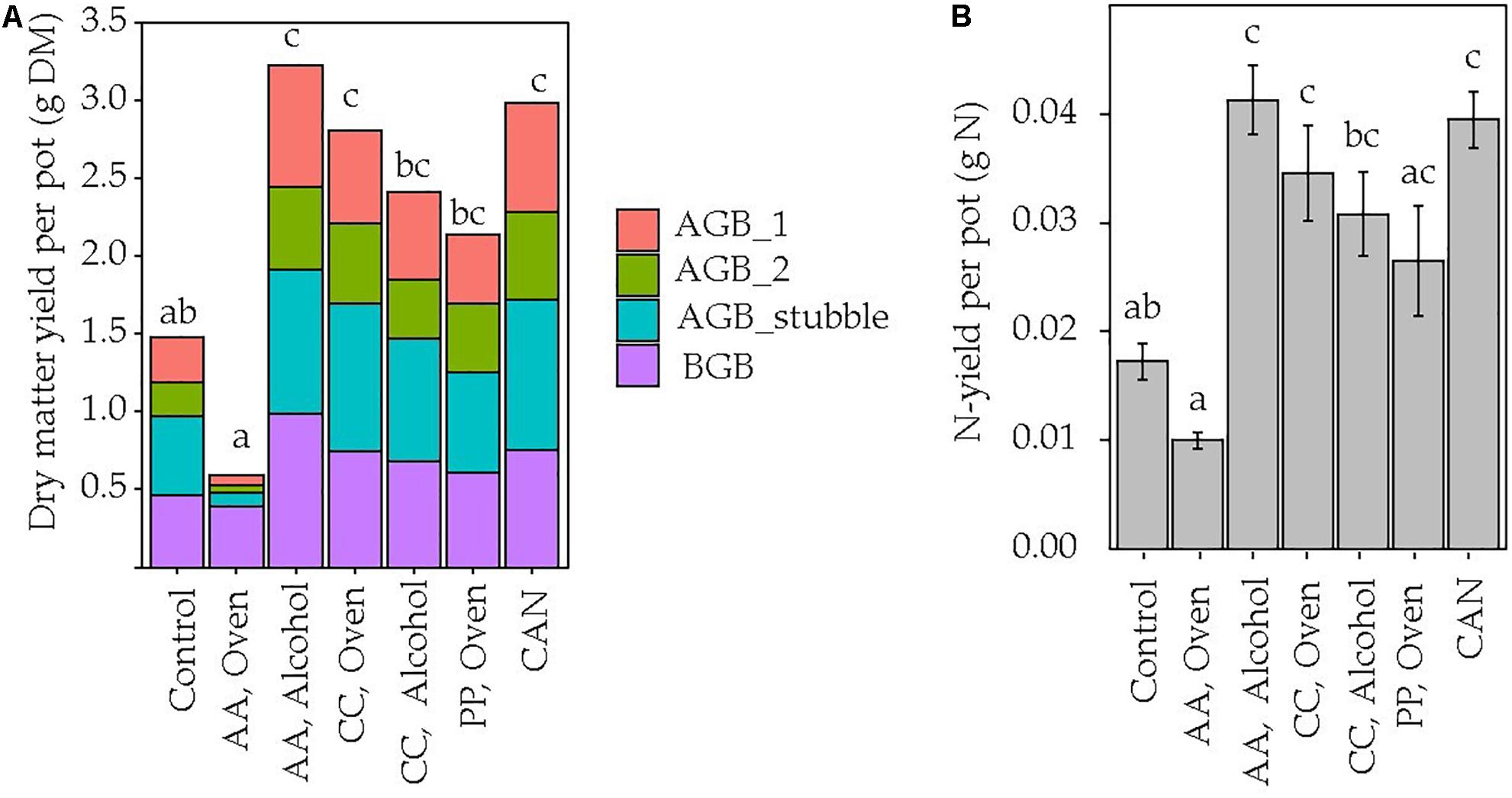
Figure 2. (A) Sum of the above- (AGB) and belowground biomass (BGB) after the two grass cuts. Yield increments of the first [AGB_1 (red)] and second cut [AGB_2 (green)], stubble biomass [AGB_stubble (blue)] and roots [BGB (purple)] are presented. (B) Nitrogen yield per pot. Mean values (A) and means plus standard error (B) are shown (n = 5). Jellyfish-derived fertilizers [A. aurita (AA), C. capillata (CC), P. periphylla (PP)] were applied as dry matter in amounts equivalent to an N rate of 50 kg N ha− 1 to an established pot-grown grass sward. No common lower-case letters indicate significant (p < 0.05) differences between treatments.
The N-yields followed the same patterns as the DM yields (Figure 2B). The ANR between the treatments was negative in the oven-dried AA treatment and lowest, yet positive, in the oven-dried PP (0.15) treatment. The highest ANR values were observed for alcohol-dried (0.23) and oven-dried (0.29) CC, CAN (0.38) and alcohol-dried AA (0.41).
Nitrous Oxide Emissions From Soil
After application of fertilizers, N2O-fluxes reached their maximum within 2 weeks after application, with lowest fluxes for the non-fertilized control and CAN. The highest fluxes were measured in CC and AA alcohol-dried (Figure 3). However, for oven-dried AA and CC the fluxes were maintained over a longer period than all other treatments. As a result, the accumulated N2O emissions during the study period were lowest in the control and highest in the AA and CC treatments irrespective of drying method used. With the exception of oven-dried PP and alcohol-dried AA, all jellyfish species treatments showed significantly higher (p < 0.05) accumulated N2O-emissions compared with that of CAN (Figure 4).
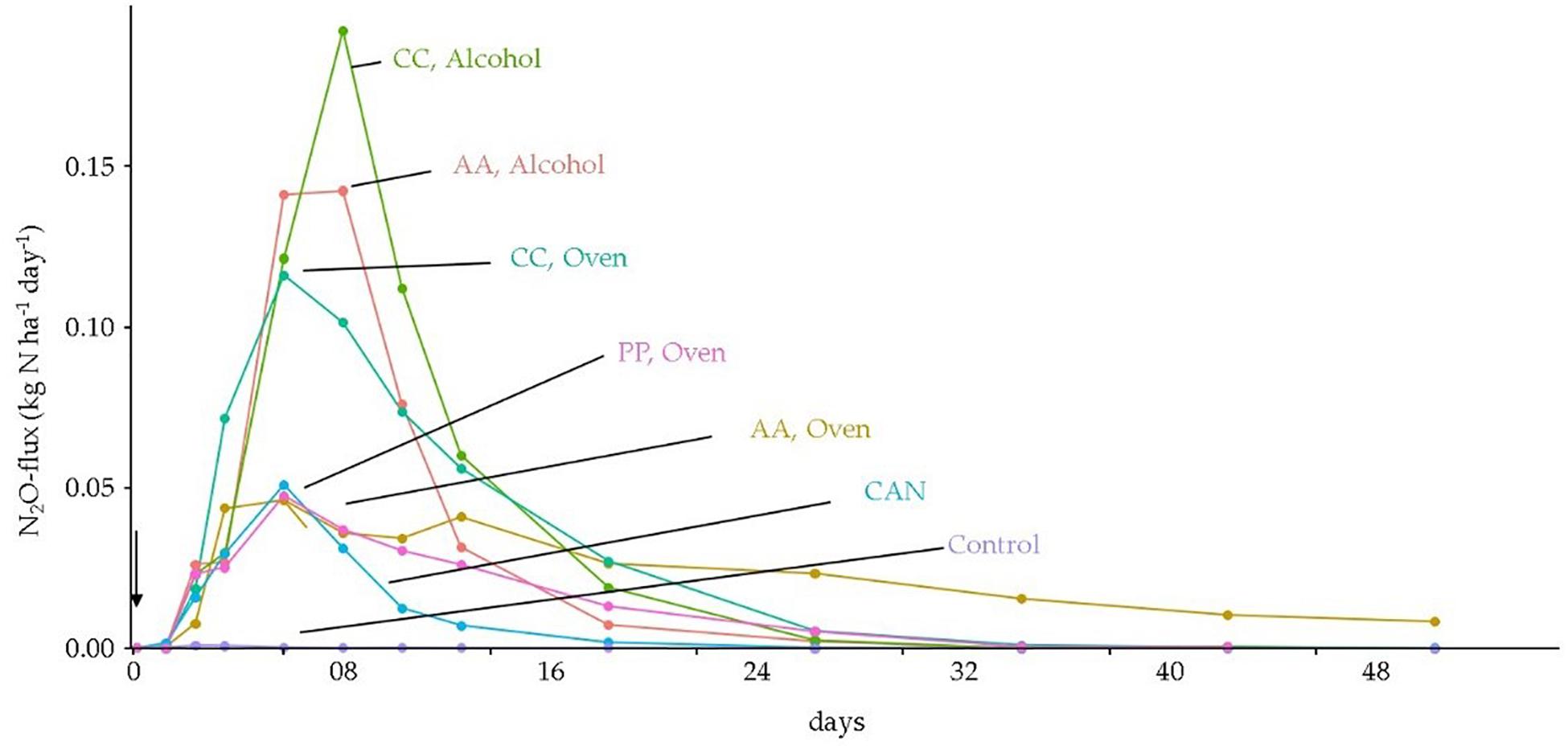
Figure 3. Daily N2O fluxes during the 56-day period after soil application of jellyfish differing in species [A. aurita (AA), C. capillata (CC), P. periphylla (PP)] and pre-treatment (oven-dried and alcohol-dried). The arrow indicates the fertilizer application event. Mean values are shown (n = 5).
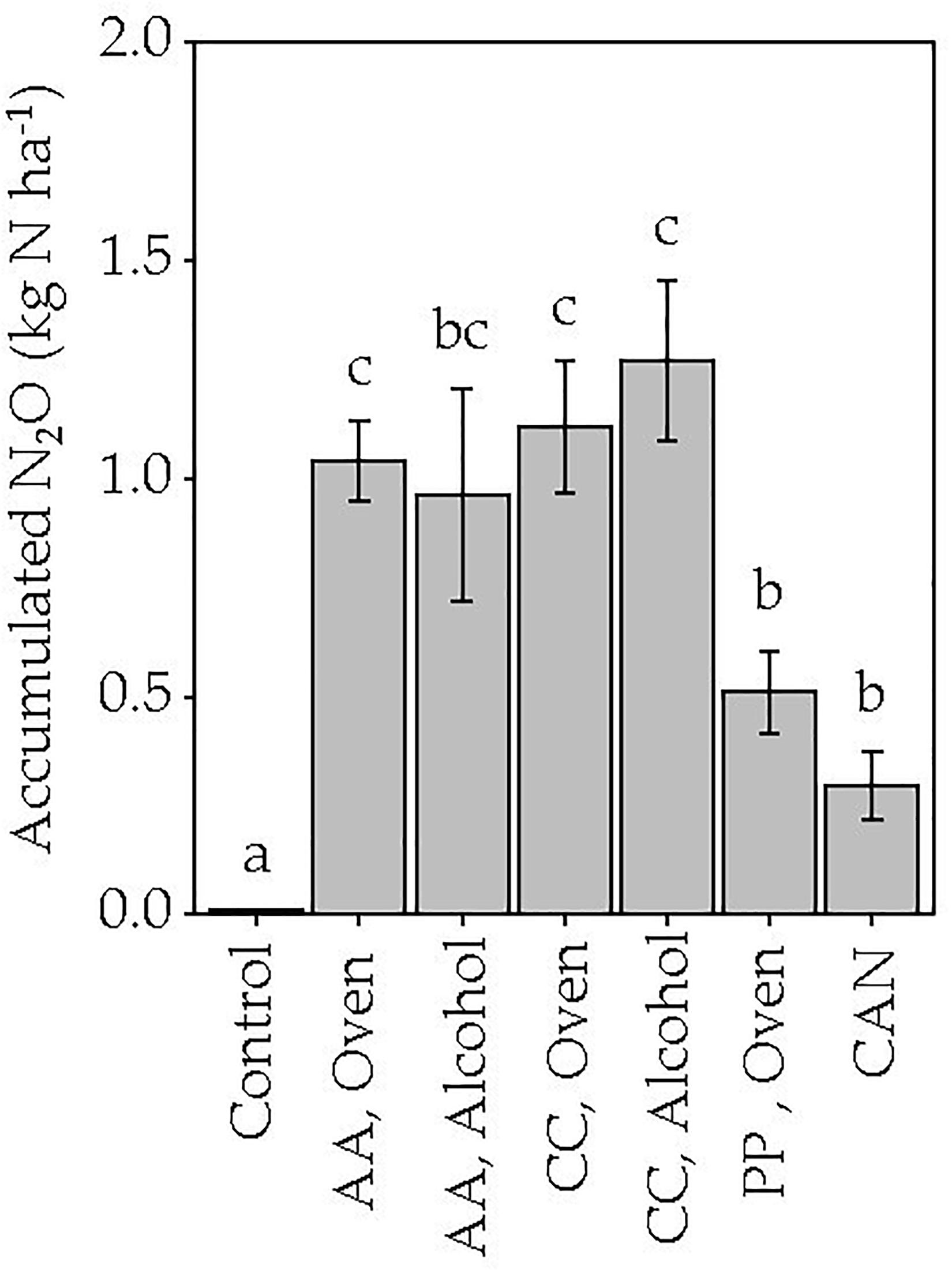
Figure 4. Accumulated N2O-fluxes during the study period (56 days) after application of different jellyfish species [A. aurita (AA), C. capillata (CC), P. periphylla (PP)] and pre-treatment (oven-dried and alcohol dried). No common lowercase letters indicated significant differences between the treatments (p < 0.05).
Soil Analysis
Soil chemical properties after the experiment showed lowest pH values (7.2) in the PP oven-dried treatment (p < 0.05); however, this difference was of a low extent. The pH value of all other treatments did not differ and showed an average pH of 7.4 (Table 3). Soil C:N ratio did not show any differences between treatments at the end of the experiments. Soil nutrient contents showed no differences for P; however, for K, the amounts were lowest for AA alcohol-dried and highest for Mg and K in the AA oven-dried among all other treatments. Salinity was highest in the oven-dried AA and CC treatments; however, with more than threefold higher amounts of Na in AA oven-dried in comparison to all other treatments.
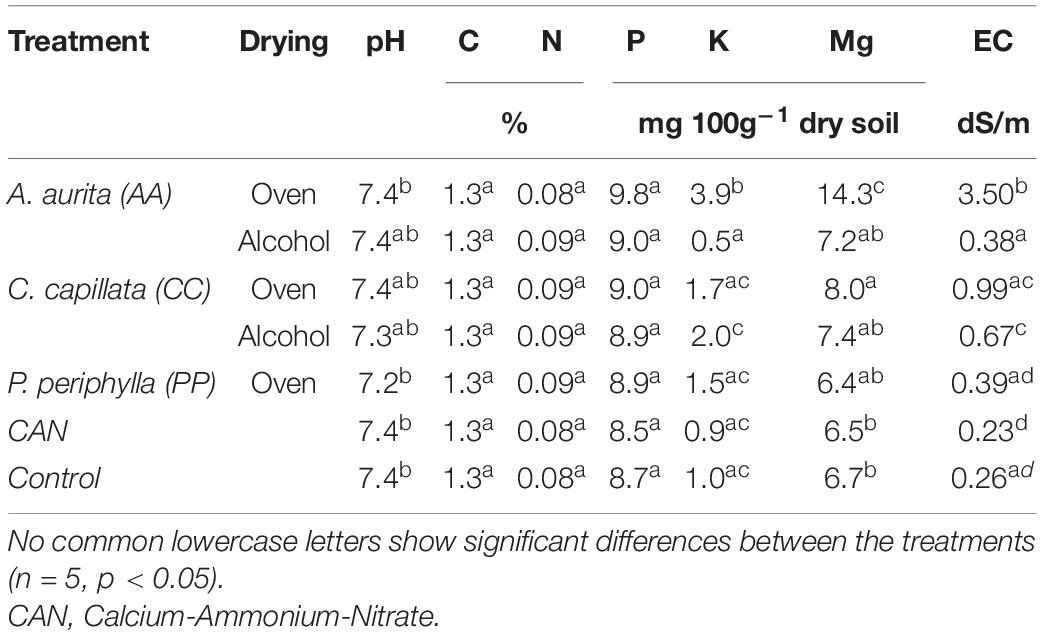
Table 3. Soil chemical properties after the experimental duration among the different treatments. Salinity measured as electrical conductivity (EC).
Discussion
Aboveground and Belowground Plant Biomass
The analysis of nutrient contents in the jellyfish DM showed comparable amounts of N to that of other organic fertilizers derived from animal husbandry (Table 4), but P contents were slightly lower and Ca contents were 2-times lower, whereas K content was 6–10-fold lower and Mg contents up to 2-fold higher (LWK-SH, 2019). The C:N ratio was narrower compared with that of most organic manures leading to a potentially higher turnover rate in the soil after application and thus a high plant availability of applied nutrients in the very short-term. Microelements such as Mn, Cu, and Zn were present in variable amounts (data not shown) and generally sufficient for plant nutrition, but mainly below the amounts commonly expected in other types of organic manure, such as digestates from biogas plants (Möller and Schultheiß, 2014; Emadodin et al., 2020a). The results for heavy metals such as Al and Fe were low (data not shown), however, other toxic elements were not analyzed in this study even though marine biomass can show a high potential for accumulating anthropogenic emissions from water, which was e.g., shown for pelagic Sargassum along the Mexican Caribbean (see Rodríguez-Martínez et al., 2020).
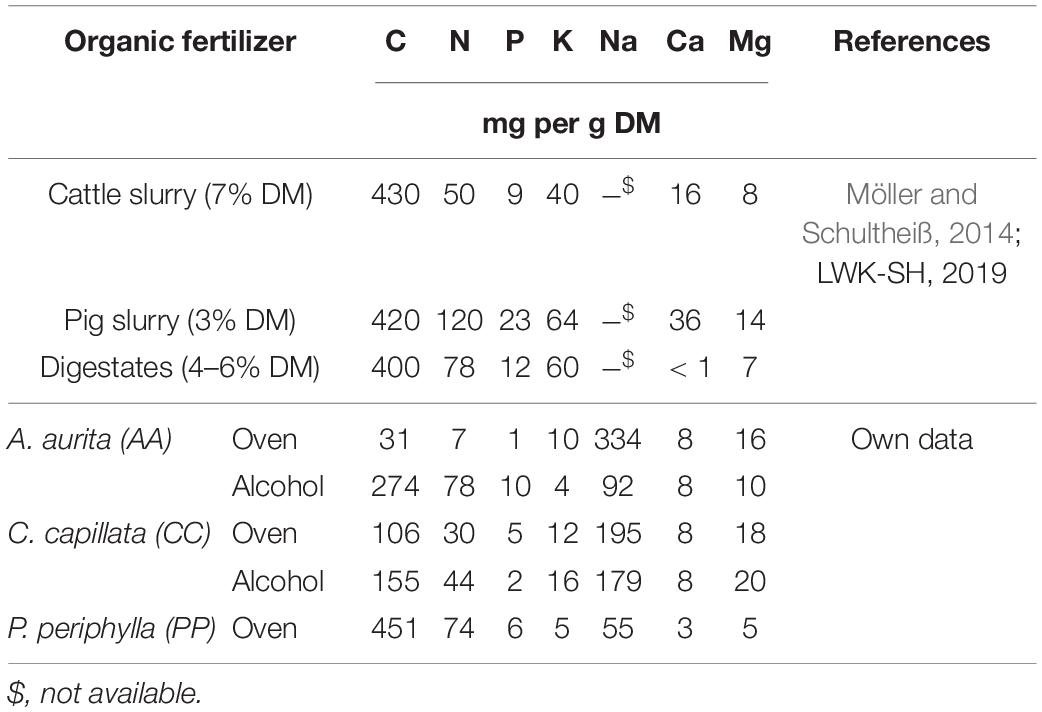
Table 4. Nutrient contents as in dry matter (DM) from reference values reported for selected organic manures from housed livestock in comparison with that of jellyfish in the present study.
The different drying methods affected the nutrient contents in the jellyfish DM. This was mainly due to differing salt levels after drying, and means that high NaCl levels result in a lower content of organic matter and thus lead to lower measured nutrient contents in the dry matter. During the alcohol-drying, NaCl became dissolved and hence its content in the jellyfish DM was reduced. This assumption was supported by the different measured Na levels and C contents of the dried materials, with lower Na contents and higher C contents in the alcohol-dried treatment (Table 1). However, the desalination effect of this drying method was different between the different jellyfish species, with a lower success for CC. This leads to the conclusion that alcohol-drying should not be universally applied for desalination among different jellyfish species, and other techniques, such as dialysis, might be more appropriate (Kogovšek et al., 2014). However, it should be mentioned that the fresh matter of CC already showed 10-times lower initial Na values compared to AA, indicating a lower success of desalination during the alcohol-drying as the Na content were already on a lower level.
The plant growth in our study was negatively affected by high Na levels only in the treatment with oven-dried AA. However, if Na can be successfully reduced, as was shown for AA, jellyfish as an organic fertilizer showed similar plant growth responses to that of CAN when supplied at the same level of N input. Moreover, after reducing salinity, the AA treatment gave higher biomass yields, on average, than CAN, which may be explained by additional nutritional effect from P, K and other microelements (Table 2). The plant growth is dependent mainly on the availability of the macro-nutrients such as N, P, and K. However, micro-nutrients might also inhibit or promote plant growth, if their supply is below/higher the necessary minimum/maximum nutrient supply. Organic biomass is usually rich in many nutrients. Thus, even though in this experiment we accounted for N, we did not control for other elements, probably causing differences in the N-yield response of the different treatments. In contradiction to that, the calculated ANR in this study was below the expected values given in the literature for mineral and other organic fertilizers, which usually exceeds 50% (Nannen et al., 2011). This can be explained by the high frequency of cutting intervals without excessive fertilizer amendments prior to the application of jellyfish. The scarcity of nitrogen, which is indicated by a high C:N ratio (15:1) at the end of the experiment in the soil of all treatments, potentially provoked an intensive N-immobilization (Kuzyakov et al., 2000). Moreover, nutrient deficits led to a shift in plant functional traits of grass, allocating more energy to roots rather than the shoot (Chen et al., 2016), suppressing the AGB allocation of N during the weeks after fertilization. However, a comparison of the different treatments shows that the AA alcohol-dried treatment revealed a similar ANR to the mineral fertilizer (CAN), indicating a fast decomposition of material due to the narrow C:N ratios. This is indeed an advantage relative to other types of organic fertilizer, where a high ratio of N is bound in the organic matter (Norg) and only available in mid-term (Gutser et al., 2005). These facts might potentially open possibilities in extensive land use systems, where soil nutrient deficits are present and plant available nutrients are needed in the short-term after application.
The results presented here are the first of several consecutive experiments. The amount of jellyfish, which can be dried at lab scale is limited by the size of equipment available. In this study, after drying and desalination, the processed material was only sufficient for five replicated pots per treatment. As there are large differences among different jellyfish species, a higher number of replicates should be considered in any further experiments. However, the effects of the two pre-processing methods were significant relative to the control, thereby presenting evidence of the potential of jellyfish as a source of fertilizer and of its positive effect on plant growth.
Nitrous Oxide Emissions From Soil and Sustainability Goals
Several other studies have indicated elevated N2O fluxes after application of C- and N-rich organic matter in comparison with mineral fertilizers, when water-filled pore space exceeds 50% (Senbayram et al., 2009). This elevation is mainly attributed to a higher activity of soil denitrifiers using C-rich material as an electron source and N as an electron acceptor, when oxygen is limiting (Davidson et al., 1986). However, this is also heavily dependent on the plant-N demand, environmental circumstances and experimental design. The experimental comparisons in plant experiments on N2O emissions are often related to either the mineral phase of N (NH4 + NO3) in the organic matter or the total N amount (NH4 + NO3 + Norg). For the latter, other experiments have also found lower N2O emissions from organic fertilizers in comparison with mineral fertilizers (Hansen et al., 2014) as Norg is not available for soil N2O-producing bacteria in the short-term. However, increased N2O-fluxes from soil can be triggered additionally when the C:N ratio is narrow (Biernat et al., 2020). The C:N ratio of jellyfish was below 5, which is a lower value than reported for other marine debris (Emadodin et al., 2020a), explaining the relatively high measured N2O-emissions even if the total amount of N applied is considered. Consequently, the hypothesized reduction of N2O-emissions from jellyfish-based fertilizers due to other metabolites (Yu et al., 2005, 2015) was not confirmed for AA and CC, although we observed significantly lower emissions from PP. However, recent observations have found that integrated fertilizer strategies, which combine mineral and organic fertilizer sources, could increase the production efficiency with only a minimum amount of N2O released, and this applies in tropical and temperate climates (Nyamadzawo et al., 2017). Thus, approaches using jellyfish biomass with a narrow C:N ratio together with other sources of nutrients that have a higher C:N ratio (e.g., seagrass or seaweed) could be considered for coastal areas in the context of increasing overall fertilization and nutrient-use efficiency, while additional N2O emissions from applied organic matter can be kept to a minimum.
Nevertheless, when considering marine biomass as an alternative resource for supplying plant nutrients with low environmental impact in terms of GHG emissions, the effect of N2O emissions from soils has to be considered in a full life-cycle-assessment (LCA), as on-farm emissions in agricultural production are responsible for the majority of emissions associated with agricultural commodities (Poore and Nemecek, 2018). From the perspective of LCA, marine biomass as a source of fertilizers may be beneficial in comparison with mineral fertilizer due to its relatively low energy use during production of raw material (e.g., harvest) (Seghetta et al., 2017; Halfdanarson et al., 2019). However, this advantage only applies when GHG emissions that result from land application (e.g., N2O), in addition to the emissions from the process chain, do not exceed those of mineral fertilizers (Christensen et al., 2014). There are additional expected GHG emissions associated with using jellyfish as a fertilizer arising from its transport, drying and desalination. Therefore, the results from measured N2O-emission can be crucial in evaluating the suitability of this raw material as a fertilizer in view of the very high global warming potential of N2O, at 265 times that of carbon dioxide (IPCC, 2014). Finally, an effective evaluation of the full potential and benefits of valorizing jellyfish into fertilizers and thus its contribution to the circular bioeconomy, requires three additional aspects to be taken into consideration. First, any of the pre-treatment methods, such as desalination, should be cost-efficient. Second, two levels of sustainability must be guaranteed: (i) sustainable supply of biomass regardless of its seasonal and geographical variability and (ii) sustainable transporting and storage methods which will not impact the quality and efficiency of the final product. Third, this study has shown a trend for jellyfish inter-species variability in terms of their nutrient contents, subsequent grass biomass yields after fertilization and N2O emissions. Therefore, to advance to a higher technological readiness in terms of sustainability goals, further studies will be obligatory. Unfortunately, our study was not designed to cover all these specifications; however, to provide a more holistic view on the jellyfish drying treatments used during this study, as well as lyophilization as an alternative to oven-drying, we constructed a decision matrix (Table 5). We selected six overall categories to help determine the two best drying methods in comparison with using CAN as the source of N fertilizer. The overall cost was selected as an important criterion, as it affects the competitiveness of fertilizers in terms of market value. The ranking included the known prices for CAN, ethanol and the estimated need for infrastructure and materials for other drying methods (oven-drying, lyophilization, dialysis, salt + aluminum). Energy demand was selected as the second selection criterion, as techniques that are highly demanding in terms of energy are not competitive in the market as they inflate the final fertilizer price. As salinity might be a plant growth-inhibiting factor, the potential salinity of the fertilizers (CAN in comparison to other jellyfish drying methods) was theoretically ranked based on the expected desalination capacity. The nutrient content, N2O emission potential and plant growth effect were ranked according to the findings in this study and on the assumed soil plant interaction controlled by inhibited/increased N2O emission due to increased/inhibited plant-N-uptake. In summary, for this matrix we found the lowest score and thus the highest efficiency for CAN. However, oven- and alcohol-drying turned out to show only three- and twofold higher values compared to CAN and lower values compared to more energy intensive procedures such like lyophilization. The salt + aluminum drying adapted from the food industry acted in this context as a negative example as it is completely unsuitable for fertilizer preparation due to elevated salt contents as confirmed by the decision matrix. The matrix also makes clear that a higher readiness for commercial use can be generated by the reduction of energy use and costs. For instance, the market price for 1 t calcium-ammonium-nitrate (27%N) is 173–225 € (0.64–0.83 € per kg N). We estimated operation costs of 20 € for drying one 1 kg jellyfish biomass to dry weight. Taking an average N-content of 2.6–7.2%N into account (Emadodin et al., 2020a, b) the price for 1 kg N accounts 769–278 €. Thus, at high N contents, the costs are comparable yet still higher compared to mineral fertilizers. For alcohol-drying we assume that costs are about threefold compared to oven-drying, however, but offering other advantages with regards to reduced salinity and consequently higher yields and potentially lower N-losses compared to oven-drying. Therefore, technical methods for cost efficient processing need further exploration.
Conclusion
Jellyfish as a by-catch, or its occurrence as beach wrack on coastal areas after blooms, are often treated as a waste product or debris. With regards to our introduced hypothesizes we made the following conclusions: (i) The dry matter of jellyfish contains adequate levels of nitrogen, phosphorus, calcium and magnesium for plant growth, and when applied to soil as an organic fertilizer it can compete with mineral nitrogen fertilizers in terms of supporting their above- and belowground productivity, thereby valorizing this side-stream material into a valuable product for agriculture. (ii) However, high salt levels can lead to a yield depression and high yield variability, once critical thresholds are reached. Future use of jellyfish-derived fertilizer in agriculture will require consideration of cost-efficient methods for its drying and desalination before use, as well as better understanding of associated negative implications, while at the same time recognizing that only a small quantity of dry matter is available after drying. Thus, sustainable processing strategies need to be developed to make jellyfish attractive as a product for use in agriculture. However, as those techniques are currently not available, its large-scale use seems to be challenging. (iii) The jelly fish application to soil increases N2O emission, which is similar to values associated with using mineral fertilizers. The reasons for differences between the different jellyfish species in terms of N2O losses after application to soil need further investigation.
Data Availability Statement
The original contributions presented in the study are included in the article/supplementary material, further inquiries can be directed to the corresponding author/s.
Author Contributions
TR designed the article concept and drafted the manuscript. All authors contributed in writing, read, edited, and approved of the final version of the manuscript.
Funding
The publication is part of a project that has received funding from the European Union’s Horizon 2020 Research and Innovation Programme under grant agreement No. 774499—GoJelly project. AR was funded by the Slovenian Research Agency (research core funding P1-0245).
Conflict of Interest
The authors declare that the research was conducted in the absence of any commercial or financial relationships that could be construed as a potential conflict of interest.
Publisher’s Note
All claims expressed in this article are solely those of the authors and do not necessarily represent those of their affiliated organizations, or those of the publisher, the editors and the reviewers. Any product that may be evaluated in this article, or claim that may be made by its manufacturer, is not guaranteed or endorsed by the publisher.
Acknowledgments
We appreciate the lab work linked to this project done by Rita Kopp. We acknowledge financial support by Land Schleswig-Holstein within the funding program. Open Access Publikationsfonds. Finally, we would like to thank the reviewers who gave valuable inputs for the improvement of this manuscript.
References
Acksel, A., Kappenberg, A., Kühn, P., and Leinweber, P. (2017). Human activity formed deep, dark topsoils around the Baltic Sea. Geoderma Region. 10, 93–101. doi: 10.1016/j.geodrs.2017.05.005
Alhamid, M. I., Yulianto, M., and Kosasih, E. A. (2012). Development of a Compact Vacuum Freeze Drying for Jelly Fish (Schypomedusae). J. Teknol. 8:1543.
Beck, B.-R., Choi, J.-H., Kim, Y.-R., Cha, H.-E., Do, H.-K., and Hwang, C.-W. (2012). Influences of Addition of Jellyfish Powder to Bed Soil and Bacterial Community Structure of Bed Soil. Korean J. Soil. Sci. Fert. 45, 227–234. doi: 10.7745/kjssf.2012.45.2.227
Berzsenyi, Z., Győrffy, B., and Lap, D. (2000). Effect of crop rotation and fertilisation on maize and wheat yields and yield stability in a long-term experiment. Eur. J. Agron. 13, 225–244. doi: 10.1016/s1161-0301(00)00076-9
Biernat, L., Taube, F., Loges, R., Kluß, C., and Reinsch, T. (2020). Nitrous Oxide Emissions and Methane Uptake from Organic and Conventionally Managed Arable Crop Rotations on Farms in Northwest Germany. Sustainability 12:3240. doi: 10.3390/su12083240
Chelsky, A., Pitt, K. A., Ferguson, A. J. P., Bennett, W. W., Teasdale, P. R., and Welsh, D. T. (2016). Decomposition of jellyfish carrion in situ. Short-term impacts on infauna, benthic nutrient fluxes and sediment redox conditions. Sci. Total Environ. 566-567, 929–937. doi: 10.1016/j.scitotenv.2016.05.011
Chen, S. M., Lin, S., Loges, R., Reinsch, T., Hasler, M., and Taube, F. (2016). Independence of seasonal patterns of root functional traits and rooting strategy of a grass-clover sward from sward age and slurry application. Grass Forage Sci. 71, 607–621. doi: 10.1111/gfs.12222
Cheol, L. K., Kun-Woo, C., Suk-Woo, K., Ju-Ung, Y., Rae, K. Y., Chang-Woo, L., et al. (2019). Effects of Jellyfish Fertilizer Treatment on the Photosynthetic Responses and Chlorophyll Contents of Quercus acutissima Carruth Seedling. J. Agric. Life Sci. 53, 109–119. doi: 10.14397/jals.2019.53.2.109
Christensen, B., Brentrup, F., Six, L., Pallière, C., and Hoxha, A. (2014). Assessing the carbon footprint of fertilisers at production and full life cycle. York: International Fertiliser Society.
Chun, K. W., Damdinsuren, E., Yeom, K., and Ezaki, T. (2011). Effect of Jellyfish Fertilizer Application on Seedling Growth. J. Jpn. Soc. Reveget. Tech. 37, 155–158. doi: 10.7211/jjsrt.37.155
Chun, K. W., Seo, J. I., Ezaki, T., and Kohno, S. (2014). Effect of soil amendment using bamboo and jellyfish chip for herbaceous plant. J. Jpn. Soc. Reveget. Tech. 40, 219–222. doi: 10.7211/jjsrt.40.219
Chun, K. W., Seo, J. I., Matsumoto, J., Doi, K., Inamoto, R., Ezaki, T., et al. (2015). Cultivation of the coastal forest for reducinng the tsunami damage. J. Jpn. Soc. Reveget. Tech. 41, 195–198. doi: 10.7211/jjsrt.41.195
Chun, K., Damdinsuren, W. E., Kim, Y.-R., and Ezaki, T. (2012). Effect of Jellyfish fertilizer on Seedling Growth and Soil Properties. J. Jpn. Soc. Reveget. Tech. 38, 192–195. doi: 10.7211/jjsrt.38.192
Council, N. R. (2002). Marine Biotechnology in the Twenty-First Century: Problems, Promise, and Products. Washington, DC: The National Academies Press.
Davidson, E. A., Swank, W. T., and Perry, T. O. (1986). Distinguishing between Nitrification and Denitrification as Sources of Gaseous Nitrogen-Production in Soil. Appl. Environ. Microbiol. 52, 1280–1286. doi: 10.1128/aem.52.6.1280-1286.1986
El Boukhari, M. E. M., Barakate, M., Bouhia, Y., and Lyamlouli, K. (2020). Trends in Seaweed Extract Based Biostimulants: Manufacturing Process and Beneficial Effect on Soil-Plant Systems. Plants 9:359. doi: 10.3390/plants9030359
Emadodin, I., Reinsch, T., Rotter, A., Orlando-Bonaca, M., Taube, F., and Javidpour, J. (2020a). A perspective on the potential of using marine organic fertilizers for the sustainable management of coastal ecosystem services. Environ. Sustain. 3, 105–115. doi: 10.1007/s42398-020-00097-y
Emadodin, I., Reinsch, T., Ockens, R.-R., and Taube, F. (2020b). Assessing the potential of jellyfish as an organic soil amendment to enhance seed germination and seedling establishment in sand dune restoration. Agronomy 10:863. doi: 10.3390/agronomy10060863
Ezaki, T., Kohno, S., Edashige, Y., Cha, D.-S., and Chun, K.-W. (2008). Development of greening materials utilizing Echizen jellyfish. J. Jpn. Soc. Reveget. Tech. 34, 195–198.
Ezaki, T., Kohno, S., Murakami, H., Ueno, T., Hyoudo, A., Oono, H., et al. (2011). Hillside planting works using jellyfish powder. J. Jpn. Soc. Reveget. Tech. 37, 151–154.
Franzén, D., Infantes, E., and Gröndahl, F. (2019). Beach-cast as biofertiliser in the Baltic Sea region-potential limitations due to cadmium-content. Ocean Coast. Manag. 169, 20–26. doi: 10.1016/j.ocecoaman.2018.11.015
Fukushi, K., Arita, T., Minami, S., Kitakata, M., Tsujimoto Tsujimoto, J.-I., and Yamashita, Y. (2006). Conversion of Nitrogen and Phosphorus in Jellyfish into an Inorganic Species and Processing Jellyfish as Fertilizer. Bull. Soc. Sea Water Sci. Jpn. 60, 173–180.
Fukushi, K., Hori, S., Yasumura, G., Mifune, K., Asai, T., and Tsujimoto, J.-I. (2017). Jellyfish (Aurelia aurita)Supernatant for Cherry Tomato (Lycopersicon esculentum Mill) and Tomato (Solanum lycopersicum) Cultivation. Bull. Soc. Sea Water Sci. Jpn. 71, 112–119.
Fukushi, K., Ishio, N., Tsujimoto, J.-I., Yokota, K., Hamatake, T., Sogabe, H., et al. (2004). Preliminary Study on the Potential Usefulness of Jellyfish as Fertilizer. Bull. Soc. Sea Water Sci. Jpn. 71, 112–119.
Fukushi, K., Tsjujimotot, J.-I., and Yokota, K. (2005). Determination of Inorganic Constituents in Jellyfish. Bunseki Kagaku 54, 175–178. doi: 10.2116/bunsekikagaku.54.175
Ghermandi, A., Galil, B., Gowdy, J., and Nunes, P. A. L. D. (2015). Jellyfish outbreak impacts on recreation in the Mediterranean Sea: welfare estimates from a socioeconomic pilot survey in Israel. Ecosyst. Serv. 11, 140–147. doi: 10.1016/j.ecoser.2014.12.004
Green, S. J., Inbar, E., Michel, F. C., Hadar, Y., and Minz, D. (2006). Succession of bacterial communities during early plant development. Transition from seed to root and effect of compost amendment. Appl. Environ. Microbiol. 72, 3975–3983. doi: 10.1128/aem.02771-05
Gutser, R., Ebertseder, T., Weber, A., Schraml, M., and Schmidhalter, U. (2005). Short-term and residual availability of nitrogen after long-term application of organic fertilizers on arable land. J. Plant. Nutr. Soil Sci. 168, 439–446. doi: 10.1002/jpln.200520510
Haichar, F. E. Z., Marol, C., Berge, O., Rangel-Castro, J. I., Prosser, J. I., Balesdent, J., et al. (2008). Plant host habitat and root exudates shape soil bacterial community structure. ISME J. 2, 1221–1230. doi: 10.1038/ismej.2008.80
Halfdanarson, J., Koesling, M., Kvadsheim, N. P., Emblemsvåg, J., and Rebours, C. (2019). “Configuring the Future Norwegian Macroalgae Industry Using Life Cycle Analysis,” in Advances in Production Management Systems. Towards Smart Production Management Systems. APMS 2019. IFIP Advances in Information and Communication Technology, Vol. 567, eds F. Ameri, K. Stecke, G. von Cieminski, and D. Kiritsis (Cham: Springer), 127–134. doi: 10.1007/978-3-030-29996-5_15
Hansen, S., Bernard, M.-E., Rochette, P., Whalen, J. K., and Dörsch, P. (2014). Nitrous oxide emissions from a fertile grassland in Western Norway following the application of inorganic and organic fertilizers. Nutr. Cycl. Agroecosyst. 98, 71–85. doi: 10.1007/s10705-014-9597-x
Hattori, T., Hidaka, Y., Fukushi, K., Hayakawa, M., Tsujimoto, J.-I., and Minato, T. (2014). Soilless Culture for the Common Ice Plant (Mesembryanthemum crystallinum L.) Using Supernatant Solutions of Jellyfish Suspensions. Bull. Soc. Sea Water Sci. Jpn. 68, 25–29.
Hothorn, T., Bretz, F., and Westfall, P. (2008). Simultaneous Inference in General Parametric Models. Biometr. J. 50, 346–363. doi: 10.1002/bimj.200810425
Inamoto, R., Tanaka, K., Takenami, N., Matsumoto, J., Doi, K., Fujishima, T., et al. (2013). Development of revegetation material using Nemopilema spp. J. Jpn. Soc. Reveget. Tech. 39, 162–165. doi: 10.7211/jjsrt.39.162
IPCC (2014). Climate Change 2014 - Climate Change. Mitigation of climate change. Contribution of working group III to the fifth assessment report of the intergovernmental panel on climate change. Geneva: IPCC.
Kogovšek, T., Tinta, T., Klun, K., and Malej, A. (2014). Jellyfish biochemical composition: importance of standardised sample processing. Mar. Ecol. Prog. Ser. 510, 275–288. doi: 10.3354/meps10959
Kohno, S., Ezaki, T., Inamoto, R., Matsumoto, J., Doi, K., Kimi, S. W., et al. (2015). Effect of application of soil amendment using bamboo and jellyfish chip. J. Jpn. Soc. Reveget. Tech. 41, 227–230. doi: 10.7211/jjsrt.41.227
Kohno, S., Ezaki, T., Kimi, S. W., and Chun, K. W. (2014). Development of soil amendment using bamboo and jellyfish chip. J. Jpn. Soc. Reveget. Tech. 40, 215–218. doi: 10.7211/jjsrt.40.215
Kohno, S., Ezaki, T., Yanagihara, S. W., Inamoto, A., Damdinsuren, R., and Chun, E. (2012). Cultivation of forest for coastal disaster prevention using jellyfish powder. J. Jpn. Soc. Reveget. Tech. 38, 188–191. doi: 10.7211/jjsrt.38.188
Kronzucker, H. J., Coskun, D., Schulze, L. M., Wong, J. R., and Britto, D. T. (2013). Sodium as nutrient and toxicant. Plant Soil 369, 1–23. doi: 10.1007/s11104-013-1801-2
Kun Woo, C., Suk Woo, K., Yukiyoshi, T., Junichi, M., Kanji, D. O. I., Shuichi, K., et al. (2019). Effect of application for woody plant of the Moon jellyfish chip. J. Jpn. Soc. Reveget. Tech. 45, 161–164. doi: 10.7211/jjsrt.45.161
Kuzyakov, Y., Friedel, J. K., and Stahr, K. (2000). Review of mechanisms and quantification of priming effects. Soil Biol. Biochem. 32, 1485–1498. doi: 10.1016/s0038-0717(00)00084-5
Laird, N. M., and Ware, J. H. (1982). Random-Effects Models for Longitudinal Data. Biometrics 38, 963–974.
LWK-SH (2019). Richtwerte für die Düngung 2019 (in German). Rendsburg: Landwirtschaftskammer Schleswig-Holstein.
Marques, A. P. G. C., Pires, C., Moreira, H., Rangel, A. O. S. S., and Castro, P. M. L. (2010). Assessment of the plant growth promotion abilities of six bacterial isolates using Zea mays as indicator plant. Soil Biol. Biochem. 42, 1229–1235. doi: 10.1016/j.soilbio.2010.04.014
Möller, K., and Schultheiß, U. (2014). Organische Handelsdüngemittel im ökologischen Landbau (in German). Bartningstraße: Kuratorium für Technik und Bauwesen in der Landwirtschaft e.V. (KTBL).
Mossbauer, M., Haller, I., Dahlke, S., and Schernewski, G. (2012). Management of stranded eelgrass and macroalgae along the German Baltic coastline. Ocean Coast. Manag. 57, 1–9. doi: 10.1016/j.ocecoaman.2011.10.012
Murphy, J., and Riley, J. P. (1962). A modified single solution method for the determination of phosphate in natural waters. Analyt. Chim. Acta 27, 31–36. doi: 10.1016/s0003-2670(00)88444-5
Nannen, D., Herrmann, A., Loges, R., Dittert, K., and Taube, F. (2011). Recovery of mineral fertiliser N and slurry N in continuous silage maize using the 15N and difference methods. Nutr. Cycl. Agroecosyst. 89, 269–280. doi: 10.1007/s10705-010-9392-2
Nyamadzawo, G., Shi, Y., Chirinda, N., Olesen, J. E., Mapanda, F., Wuta, M., et al. (2017). Combining organic and inorganic nitrogen fertilisation reduces N2O emissions from cereal crops: a comparative analysis of China and Zimbabwe. Mitig. Adapt. Strat. Glob. Change 22, 233–245. doi: 10.1007/s11027-014-9560-9
Pedersen, M. T., Brewer, J. R., Duelund, L., and Hansen, P. L. (2017). On the gastrophysics of jellyfish preparation. Int. J. Gastron Food Sci. 9, 34–38. doi: 10.1016/j.ijgfs.2017.04.001
Peggy Hsieh, Y.-H., Leong, F.-M., and Rudloe, J. (2001). Jellyfish_as_food. Hydrobiologia 451, 11–17. doi: 10.1007/978-94-010-0722-1_2
Perumal, M., Wasli, M. E., and Ying, H. S. (2019). Influences of inorganic and organic fertilizers to morphological quality attributes of Shorea macrophylla seedlings in a tropical nursery. Biodiversitas J. Biol. Diversity 20, 2110–2118.
Poore, J., and Nemecek, T. (2018). Reducing food’s environmental impacts through producers and consumers. Science 360, 987–992. doi: 10.1126/science.aaq0216
Purcell, J. E., Uye, S., and Lo, W. (2007). Anthropogenic causes of jellyfish blooms and their direct consequences for humans: a review. Mar. Ecol. Prog. Ser. 350, 153–174. doi: 10.3354/meps07093
Qu, C.-F., Song, J.-M., Li, N., Li, X.-G., Yuan, H.-M., Duan, L.-Q., et al. (2015). Jellyfish (Cyanea nozakii) decomposition and its potential influence on marine environments studied via simulation experiments. Mar. Pollut. Bull. 97, 199–208. doi: 10.1016/j.marpolbul.2015.06.016
Rodríguez-Martínez, R. E., Roy, P. D., Torrescano-Valle, N., Cabanillas-Terán, N., Carrillo-Domínguez, S., Collado-Vides, L., et al. (2020). Element concentrations in pelagic Sargassum along the Mexican Caribbean coast in 2018-2019. PeerJ 8:e8667. doi: 10.7717/peerj.8667
Sanderson, M. A. (2010). Stability of production and plant species diversity in managed grasslands: A retrospective study. Basic Appl. Ecol. 11, 216–224. doi: 10.1016/j.baae.2009.08.002
Seghetta, M., Romeo, D., D’Este, M., Alvarado-Morales, M., Angelidaki, I., Bastianoni, S., et al. (2017). Seaweed as innovative feedstock for energy and feed – Evaluating the impacts through a Life Cycle Assessment. J. Cleaner Prod. 150, 1–15. doi: 10.1016/j.jclepro.2017.02.022
Selvam, G. G., and Sivakumar, K. (2014). Influence of seaweed extract as an organic fertilizer on the growth and yield of Arachis hypogea L. and their elemental composition using SEM–Energy Dispersive Spectroscopic analysis. Asian Pac. J. Reprod. 3, 18–22. doi: 10.1016/s2305-0500(13)60179-7
Senbayram, M., Chen, R., Mühling, K. H., and Dittert, K. (2009). Contribution of nitrification and denitrification to nitrous oxide emissions from soils after application of biogas waste and other fertilizers. Rapid Comm. Mass Spectrom. 23, 2489–2498. doi: 10.1002/rcm.4067
Seo, J., Kim, Y., Otsuka, K., Kim, S., Yeom, K., Yi, J., et al. (2014). Contributions of Jellyfish Fertilizer to Survival and Growth of Seedlings Planted in a Recently Burned Forest, Republic of Korea. J. Faculty Agricult. Kyushu Univers. 59, 181–189. doi: 10.5109/1434410
Sivasangari Ramya, S., Vijayanand, N., and Rathinavels, S. (2015). Foiliar application of liquid biofertilizer of brown alga stoechospermi marginatumon growth, biochmical and yield of solanum melongena. Int. J. Recycl. Org. Waste Agric. 4, 96–90.
Smucker, A. J. M., McBurney, S. L., and Srivastava, A. K. (1982). Quantitative Separation of Roots from Compacted Soil Profiles by the Hydropneumatic Elutriation System. Agron. J. 74, 500–503. doi: 10.2134/agronj1982.00021962007400030023x
Thompson, T. M., Young, B. R., and Baroutian, S. (2020). Pelagic Sargassum for energy and fertiliser production in the Caribbean: A case study on Barbados. Renew. Sustain. Energy Rev. 118:109564. doi: 10.1016/j.rser.2019.109564
Thomson, A. J., Giannopoulos, G., Pretty, J., Baggs, E. M., and Richardson, D. J. (2012). Biological sources and sinks of nitrous oxide and strategies to mitigate emissions. Philosoph. Transact. R. Soc. B Biol. Sci. 367, 1157–1168. doi: 10.1098/rstb.2011.0415
Tinta, T., Kogovšek, T., Turk, V., Shiganova, T. A., Mikaelyan, A. S., and Malej, A. (2016). Microbial transformation of jellyfish organic matter affects the nitrogen cycle in the marine water column — A Black Sea case study. J. Exp. Mar. Biol. Ecol. 475, 19–30. doi: 10.1016/j.jembe.2015.10.018
Tinta, T., Malej, A., Kos, M., and Turk, V. (2010). Degradation of the Adriatic medusa Aurelia sp. by ambient bacteria. Hydrobiologia 645, 179–191. doi: 10.1007/978-90-481-9541-1_14
Titelman, J., Riemann, L., Sørnes, T. A., Nilsen, T., Griekspoor, P., and Båmstedt, U. (2006). Turnover of dead jellyfish. Stimulation and retardation of microbial activity. Mar. Ecol. Prog. 325, 43–58. doi: 10.3354/meps325043
Verbeke, G., and Molenberghs, G. (2000). Linear Mixed Models for Longitudinal Data. New York: Springer.
Withers, P. J. A., van Dijk, K. C., Neset, T.-S. S., Nesme, T., Oenema, O., Rubæk, G. H., et al. (2015). Stewardship to tackle global phosphorus inefficiency: The case of Europe. AMBIO 44, 193–206. doi: 10.1007/s13280-014-0614-8
Wrage, N., Velthof, G. L., van Beusichem, M. L., and Oenema, O. (2001). Role of nitrifier denitrification in the production of nitrous oxide. Soil Biol. Biochem. 33, 1723–1732. doi: 10.1016/s0038-0717(01)00096-7
Yu, H., Li, R., Chen, X., Yue, Y., Xing, R., Liu, S., et al. (2015). Effect of Venom from the Jellyfish Nemopilema nomurai on the Silkworm Bombyx mori L. Toxins 7, 3876–3886. doi: 10.3390/toxins7103876
Yu, H., Liu, X., Dong, X., Li, C., Xing, R., Liu, S., et al. (2005). Insecticidal activity of proteinous venom from tentacle of jellyfish Rhopilema esculentum Kishinouye. Bioorg. Med. Chem. Lett. 15, 4949–4952. doi: 10.1016/j.bmcl.2005.08.015
Yuferova, A. A. (2017). The Impact of Different Drying Modes of Scyphozoan Jellyfish Rhopilema Esculentum and Aurelia Aurita on the Protein and Carbohydrate Components in their Composition and the Possibility of Their Use as Dried Prepared Food. J. Food Process Eng. 40:e12326. doi: 10.1111/jfpe.12326
Keywords: organic fertilizer, marine biomass, circular economy, blue growth, organic agriculture
Citation: Borchert F, Emadodin I, Kluß C, Rotter A and Reinsch T (2021) Grass Growth and N2O Emissions From Soil After Application of Jellyfish in Coastal Areas. Front. Mar. Sci. 8:711601. doi: 10.3389/fmars.2021.711601
Received: 18 May 2021; Accepted: 08 July 2021;
Published: 03 August 2021.
Edited by:
Bin Wu, Zhejiang University, ChinaReviewed by:
Yueying Li, Texas A&M University, United StatesEdwin Cruz-Rivera, University of the Virgin Islands, US Virgin Islands
Copyright © 2021 Borchert, Emadodin, Kluß, Rotter and Reinsch. This is an open-access article distributed under the terms of the Creative Commons Attribution License (CC BY). The use, distribution or reproduction in other forums is permitted, provided the original author(s) and the copyright owner(s) are credited and that the original publication in this journal is cited, in accordance with accepted academic practice. No use, distribution or reproduction is permitted which does not comply with these terms.
*Correspondence: Thorsten Reinsch, dHJlaW5zY2hAZ2ZvLnVuaS1raWVsLmRl