- 1Department of Ocean Integrated Science, Chonnam National University, Yeosu, South Korea
- 2Department of Oceanography, Pukyong National University, Busan, South Korea
- 3Marine Eco-Technology Institute, Busan, South Korea
- 4School of Earth Sciences and Environmental Engineering, Gwangju Institute of Science and Technology, Gwangju, South Korea
We investigated long-term variations in the dominant phytoplankton groups with improvements in water quality over 11 years in the Yeongil Bay on the southeastern coast of Korea. River discharge declined during the study period but TN from river discharge remained stable, indicating the input of enriched nutrients to the bay was fairly consistent. NH4+ levels decreased with a decrease in TN from the POSCO industrial complex. While the study region was characterized by the P-limited and deficient environment, cryptophytes dominated with the intensified P-limitations. The relative abundance of cryptophytes declined from 70% in 2010 to 10% in 2016, but that of diatoms increased from 70% in 2009 to 90% in 2016. Correlation analysis showed a positive correlation of cryptophytes with NH4+ and a negative correlation with photic depth. Generalized additive models also exhibited an increase in diatom dominance and a decrease in cryptophyte dominance with an increase in water quality, indicating that a decrease in NH4+ and increase in light favored the diatom growth but suppressed the cryptophyte growth. Thus, water quality improvements shift the dominant group in the coastal ecological niche from cryptophytes to diatoms.
Introduction
Coastal ecosystems in bays and estuaries experience strong land-ocean interactions (Spatharis et al., 2007; Wang et al., 2020). Excessive nutrients from river discharge induce hypoxia or eutrophication in coastal ecosystems, causing coastal acidification (Paerl et al., 1998; Cai et al., 2011) and the development of harmful algal blooms (Glibert et al., 2006; Heisler et al., 2008). Phytoplankton biomass (i.e., chlorophyll a) is often utilized as an indicator of water quality conditions because of its strong interaction with environmental conditions (Boyer et al., 2009; Sathicq et al., 2017), and phytoplankton photopigments identifying the functional phytoplankton groups can be applied to assess coastal eutrophication (Paerl et al., 2003). Long-term water quality management often reduces nutrient inputs into coastal ecosystems (Zhang et al., 2020) while nutrient reduction leads to a decrease in the formation of toxic phytoplankton blooms (Marshall et al., 2006; Zhong et al., 2019). Cyanobacteria abundance can sharply increase with the degree of impaired water quality, whereas other phytoplankton groups such as diatoms and dinoflagellates perish (Marshall et al., 2006).
The ecological niche of phytoplankton is based on the nutrient strategies and functional differences among phytoplankton groups (Gobler et al., 2011; Alexander et al., 2015b). It is necessary to understand how the dominant group within a phytoplankton niche changes with variations in environmental conditions because it reveals the underlying mechanisms of phytoplankton bloom dynamics associated with group-specific or species-specific ecological and physiological features (Litchman et al., 2012; Brun et al., 2015). Functional group-specific or species-specific traits in resource partitioning and identification of the ecological and physiological traits of dominant phytoplankton have been investigated for various phytoplankton groups (Alexander et al., 2015a,b). Although cryptophytes are a cosmopolitan phytoplankton group found under a wide range of environmental conditions (Clay et al., 1999); previous studies on cryptophyte blooms have been confined to either the mixotrophic strategy or light conditions (Barone and Naselli-Flores, 2003; Adolf et al., 2008; Mendes et al., 2013; Johnson et al., 2018).
Most phytoplankton studies in Yeongil Bay are associated with the spatial and temporal distribution identified using morphological or pigment (e.g., chlorophyll a) characteristics (Kwak and Lee, 1977; Jo et al., 2000; Shim et al., 2013). Phytoplankton dynamics in the bay are largely influenced by P availability originating from Hyeongsan River (Shim et al., 2013) although most coastal bay ecosystems have seasonal-specific nutrient limitations due to seasonal variations in river discharge. Water quality in the bay mainly ascribes individual parameters such as dissolved inorganic nutrient levels and some physical parameters including DO and pH (Shim et al., 2013). Previously, the southern coastal estuarine ecosystem, Gwangyang Bay, the importance of nitrogen and suspended particulate matter (SPM) variations was suggested as the causal factors for the decline of phytoplankton biomass (Kang et al., 2020), emphasizing the role of NH4+ and light on controlling phytoplankton biomass in an anthropogenically eutrophic estuary. However, the role of those parameters in Yeongil Bay phytoplankton dynamics has not been investigated. Particularly, cryptophytes and diatoms are the dominant phytoplankton groups, historically occupying the ecological niche in Yeongil Bay (Kang et al., 2019b). To the best of our knowledge, long-term variations in the major phytoplankton groups in response to water quality improvement have not been evaluated previously in Korean coastal waters. In this study, we assessed (1) which phytoplankton groups dominated the pelagic habitat in response to water quality improvement and (2) how the major phytoplankton groups were affected by environmental variables.
Materials and Methods
Study Region
Yeongil Bay is a semi-enclosed shallow bay that interacts with both a river and the ocean. The bay is located at the southeastern tip of the Korean peninsula and is connected to the East Sea (Japan Sea). The geographical location of the bay characterizes its unique features, which are fed by various bodies of water. Yeongil Bay is distinct in that the East Korea Warm Current (EKWC) intrudes into the surface of the bay from offshore, the North Korean Cold Current (NKCC), which propagates southward along the coast of the East Sea, appears on the southern coast of Korea, and the cold East Sea-proper water exists in the middle or bottom layer outside the bay (Lie, 1989). Once the water enters the bay, it circulates counterclockwise and flows out, bringing the diluted pollutants offshore (Choi, 1993). Pollutants from the Hyeongsan River sink and move toward the inner bay, which serves as a nutrient trap (Yoon et al., 2003). The freshwater input from the Hyeongsan River largely influences the spatial and temporal variations in water quality of the Yeongil Bay (Kim and Kim, 2004). The fast-growing population in the land area (Pohang city) surrounding the bay generates pollutants from industrial complexes, sewage, and livestock wastes that cause the coastal eutrophication, which is the excessive nutrient enrichment (Kim et al., 2001). The pollutant loads from industrial complexes or sewage decreased the water quality in the bay in the early 2000s (Yoon et al., 2002, 2003). In the 2010s, a water quality improvement action plan was applied to the coastal waters in Korea to mitigate coastal pollution (Nam and Han, 2011).
Data Collection
Over 11 years from 2007 to 2017, field surveys were performed seasonally by the Marine Eco-Technology Institute at 13 stations for phytoplankton quantification in the winter (February), spring (May), summer (August), and fall (November) of each year (Figure 1). Seawater samples were collected in 1 L Nalgene polyethylene bottles using a Niskin water sampler (General Oceanics, Miami, FL, United States) from 1 m below the surface layer and 1 m above the bottom layer, and then preserved with Lugol’s iodine solution at a final concentration of 1%, and then wrapped with aluminum foil to prevent the samples from degrading due to light exposure. Samples that were brought to a laboratory immediately after collection were settled for 48 h to allow the particles to settle to the bottom of the bottles, and the supernatant was carefully removed. The residue was transferred into 200 mL glass tubes and allowed to settle for another 48 h to form the final 10 mL samples for the microscopic observations. The final sample (1 mL) was mounted on a Sedgewick Rafter counting chamber to enumerate phytoplankton individuals at the species level through microscopy with a magnification of 100× and 400×. The final cell density was presented in cells/L.
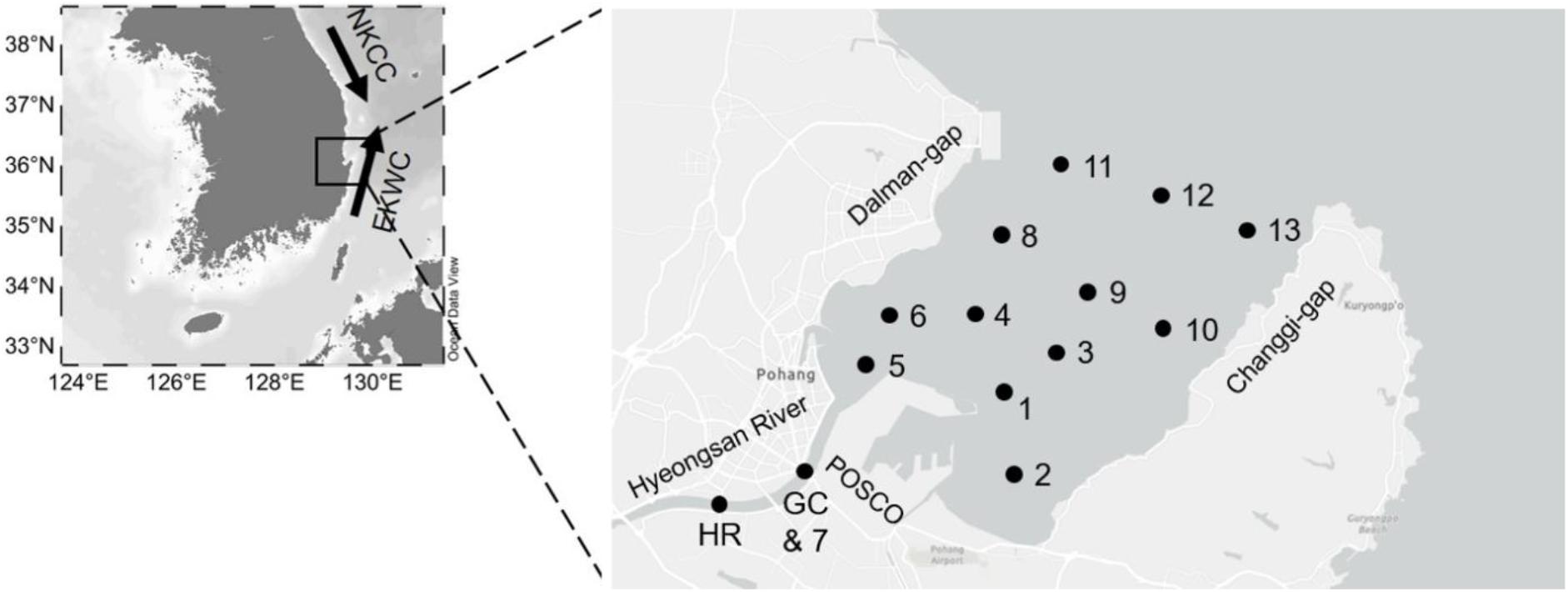
Figure 1. Station maps showing the location of the Yeongil Bay on the southeastern coast of Korea. Black dots indicate the sample stations. HR and GC are locations where total nitrogen samples were collected. HR, Hyeongsan River; GC, Gumu Creek; POSCO, Pohang Iron and Steel Company; NKCC, North Korea Cold Current; EKWC, East Korea Warm Current. Phytoplankton samples were collected by the Marine Eco-Technology Institute and environmental data were obtained from the Marine Environmental Information System (www.meis.go.kr).
Environmental data such as temperature, salinity, dissolved inorganic nitrogen (DIN; the sum of NO3–, NO2, and NH4+), dissolved inorganic phosphate (DIP; PO43–), SiO2, and water quality index (WQI) were obtained from the Marine Environment Information System1. WQI was calculated based on standard measurement suggested by the Ministry of Oceans and Fisheries of Korea (see footnote 1). Ecosystem-specific water quality measurement was applied because region-specific characteristics exist. Chl a (μg/L), DIN (μg/L), DIP (μg/L), DO saturation (%), and transparency (m) are utilized to calculate the WQI. Standardized scores of each environmental variable were applied to calculate the WQI.
Where DO is dissolved oxygen, chl a is chlorophyll a, DIN is dissolved inorganic nitrogen, and DIP is dissolved inorganic phosphorus.
River discharge data were acquired from the Water Resources Management Information System2 and total nitrogen (TN) data from the Hyeongsan River and Gumu Creek near the Pohang Iron and Steel Company (POSCO) were obtained from the Water Environment Information System3.
Data and Statistical Analyses
Nutrient data were analyzed after the DIN, DIP, and SiO2 values in mg/L were converted to μM and the difference in TN between the Gumu Creek (GC) and Hyeongsan River (HR) was considered to obtain the TN from the POSCO (TNPOSCO = TNGC—TNHR). Because a direct measure of TN from POSCO was not available and one end of GC was closed, we assumed that the difference between TNGC and TNHR can be representative of TN from POSCO. The mean annual variations in DIN, DIP, and SiO2 were calculated to access the annual variation from 2007 to 2017. The proportion of NH4+ in DIN was calculated to investigate the variation in the NH4+ ratio over the 11-year period. Macronutrient limitations (i.e., Si-limitation, N-limitation, and P-limitation) were determined based on the Redfield ratio (Tett et al., 1985): Si-limitation when Si:N < 1 and Si:P < 16, N-limitation when N:P < 16 and Si:N > 1, and P-limitation when N:P > 16 and Si:P > 16. Here, N denotes DIN.
Seasonal relative abundance represented the relative abundance of the identified phytoplankton groups, including cryptophytes, diatoms, dinoflagellates, euglenoids, green-algae, and silico-flagellates. Thereafter, the most abundant groups, which included cryptophytes, diatoms, and dinoflagellates, were further analyzed. The relative abundance of the three major phytoplankton groups was compared to assess the variation over the 11-year period. In addition, changes in the two most abundant groups, cryptophytes and diatoms, were compared with variations in environmental parameters such as WQI, proportion of NH4+ in DIN, and photic depth. The photic depth was calculated as 4.61/Kd (Cloern, 1987), where Kd is the light extinction coefficient, which was derived from Secchi depths (i.e., water transparency) using an equation applied to turbid waters, Kd = 1.44/Zs, where Zs is the Secchi depth measured using a Secchi disk (Holmes, 1970). The equation, Kd = 1.7/Zs was globally and locally applied to coastal waters (Poole and Atkins, 1929; Lee et al., 2017; Kang et al., 2020) but a modified coefficient, 1.44 was utilized for turbid waters (Holmes, 1970).
A correlation matrix was generated to investigate the relationship between the major phytoplankton groups, cryptophytes and diatoms, and environmental variables such as river discharge, photic depth, NO3–, NH4+, PO43–, and SiO2. The correlation matrix visualized the correlations among the analytic parameters, correlation coefficients, and statistical significance (∗p < 0.05, ∗∗p < 0.01, and ∗∗∗p < 0.001). Generalized additive models (GAMs) were performed using six main parameters (WQI, photic depth, NH4+, the proportion of NH4+ in DIN, PO43–, and the N:P ratio) to investigate the variation in dominance between cryptophytes and diatoms in response to water quality improvement, light availability, and nutrient dynamics. Log-transformation was applied to phytoplankton abundance data prior to performing GAMs. GAMs are non-parametric extensions of linear regression models with a linking function to predict the response of variables (dominance of cryptophytes and diatoms in this study) to a smoothed function of explanatory variables (the six parameters examined in this study), capturing the non-linearity between the responses and predictors (Kang et al., 2019a). The models were assembled as follows: log(Y) ∼ s(WQI) + s(Photic depth) + s(NH4+) + s(NH4+ ratio) + s(DIP) + s(N:P ratio), where Y is representative of either diatom abundance or cryptophyte abundance and s is a smooth function of each parameter. Statistical analyses were performed in R version 3.6.2 (R Foundation for Statistical Computing, Vienna, Austria) using the “PerformanceAnalytics” package for the correlation matrix (Peterson et al., 2018) and “mgcv” package for GAMs (Wood and Wood, 2015). Plots were generated using the package “ggplot2” (Wickham, 2016).
Results
River Discharge and Nutrient Dynamics
River discharge sharply declined from 92 m3/s in 2010 to 13 m3/s in 2017 after remaining stable for three 3 years from 2007 to 2009 (Figure 2A) while during the same period salinity increased from 33.3 to 33.7‰ in the surface layer and from 33.6 to 34.1‰ in the bottom layer (Figure 2B). The TN from the Hyeongsan River slightly decreased in the last 3 years but stayed consistent, and the TN from the POSCO declined sharply from 16.1 mg/L in 2010 to 4.1 mg/L in 2017, except a prompt increase in 2010 due to heavy rain (Figure 2C). Despite the dramatic decrease in river discharge, dissolved inorganic nutrients remained relatively stable (Figure 3). SiO2 remained consistent for 9 years, after which the level at the bottom increased to 5.2 μM (Figure 3A), while the levels of other dissolved nutrients except for NH4+ were consistent (Figures 3B,D). NH4+ gradually declined to 0.34 μM in 2017 (Figure 3C).
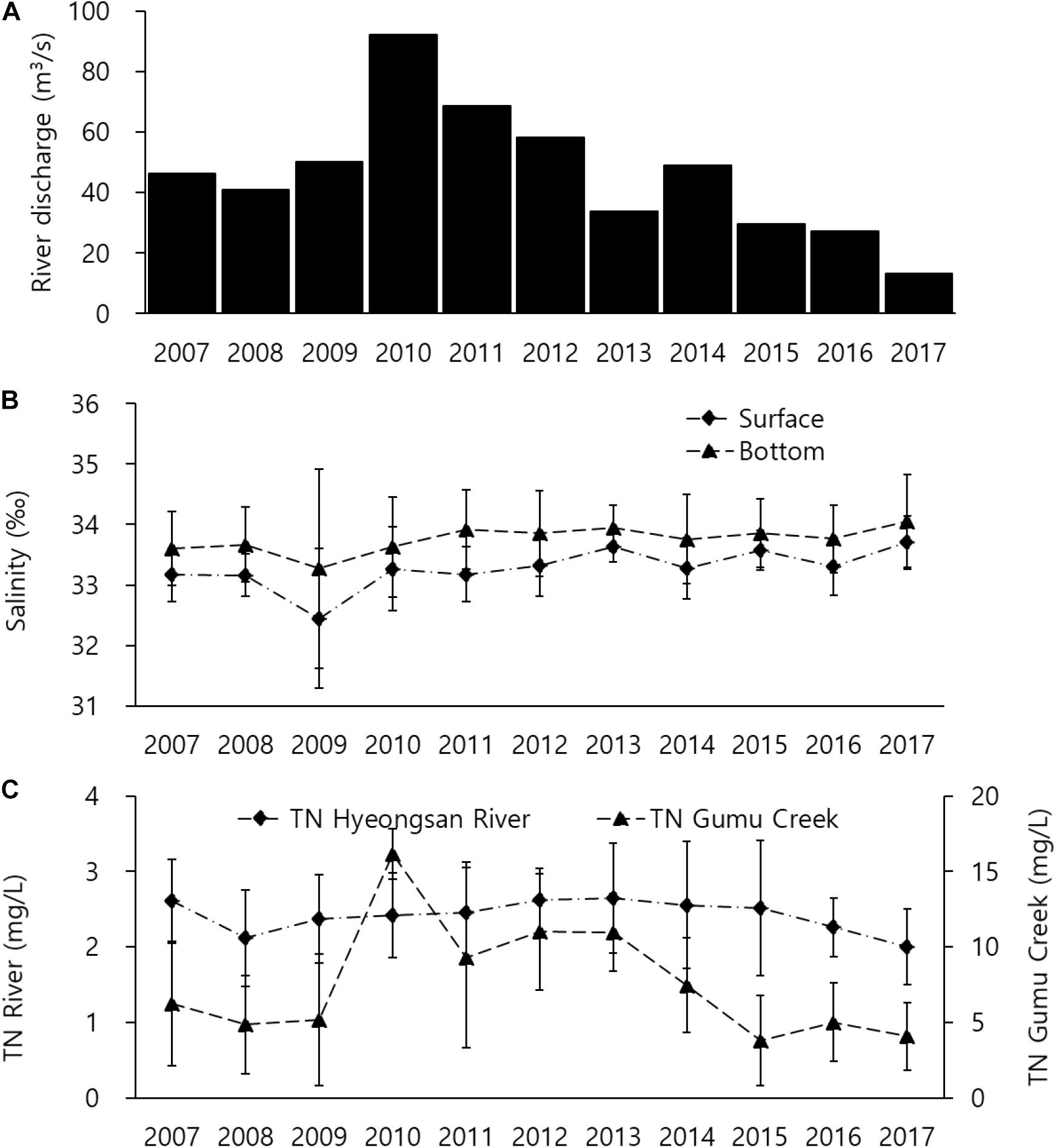
Figure 2. Long-term variation in river discharge (A), salinity (B), and total nitrogen (TN) from the Hyeongsan River (HR) and Gumu Creek (C), where the Pohang Iron and Steel Company (POSCO) is located. This company is mainly responsible for the TN discharge from the Gumu Creek.
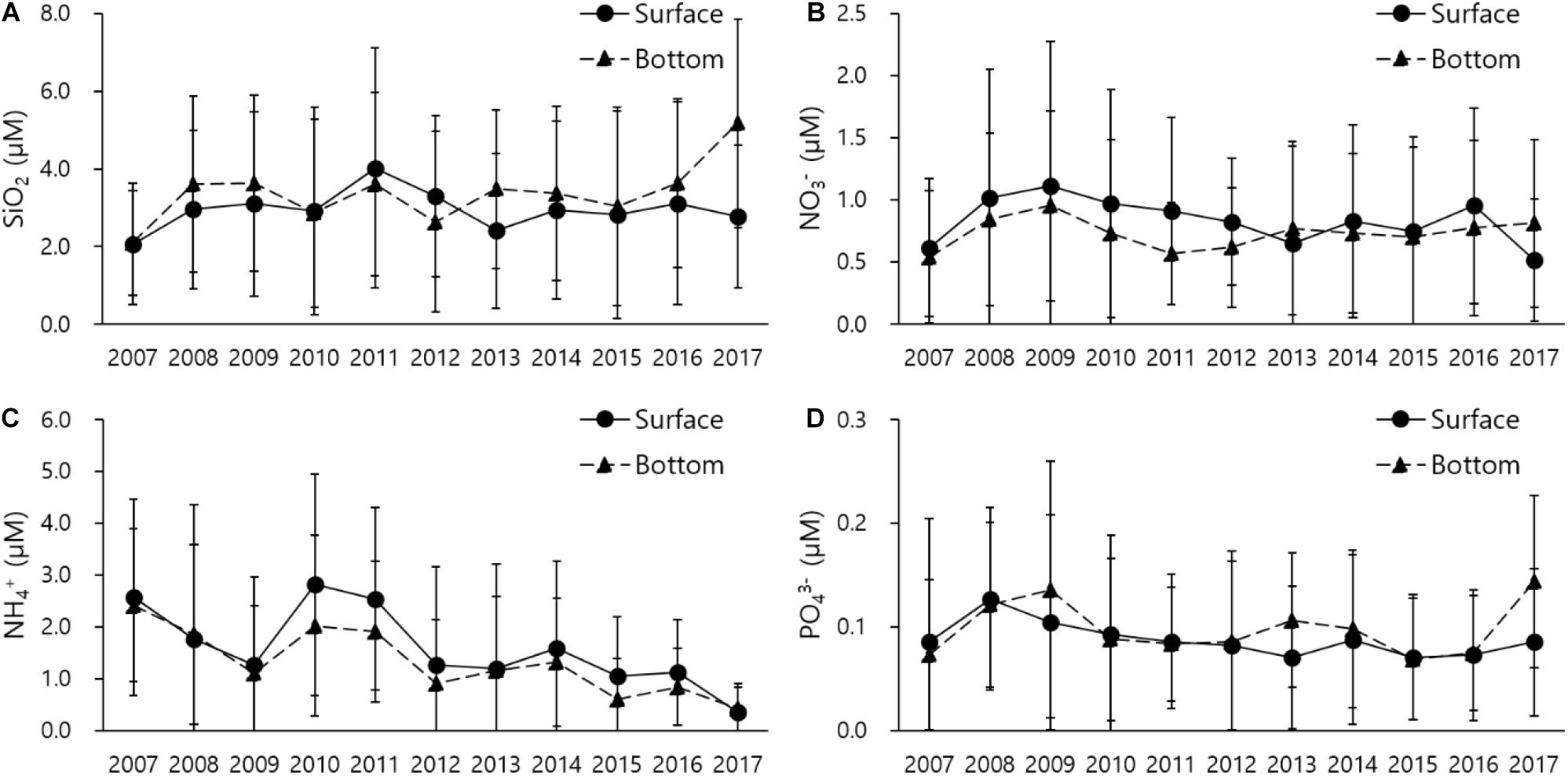
Figure 3. Long-term nutrient variation in the Yeongil Bay. (A) SiO2, (B) NO3–, (C) NH4+, and (D) PO43–.
Long-Term Variations in the Major Phytoplankton Groups
Cryptophytes, diatoms, and dinoflagellates were the three major phytoplankton groups in the Yeongil Bay while the dominant species varied for the last 11 years (Supplementary Table 1). The long-term variations in these three groups showed that cryptophytes varied significantly but diatoms and dinoflagellates remained relatively stable in both the surface and bottom layers (Figure 4). Cryptophyte dominance declined sharply, from 90 to 10% over the 11-year period, whereas diatom dominance increased from 70 to 90% and dinoflagellate dominance slightly decreased from 30 to 10% during the same period (Figure 4).
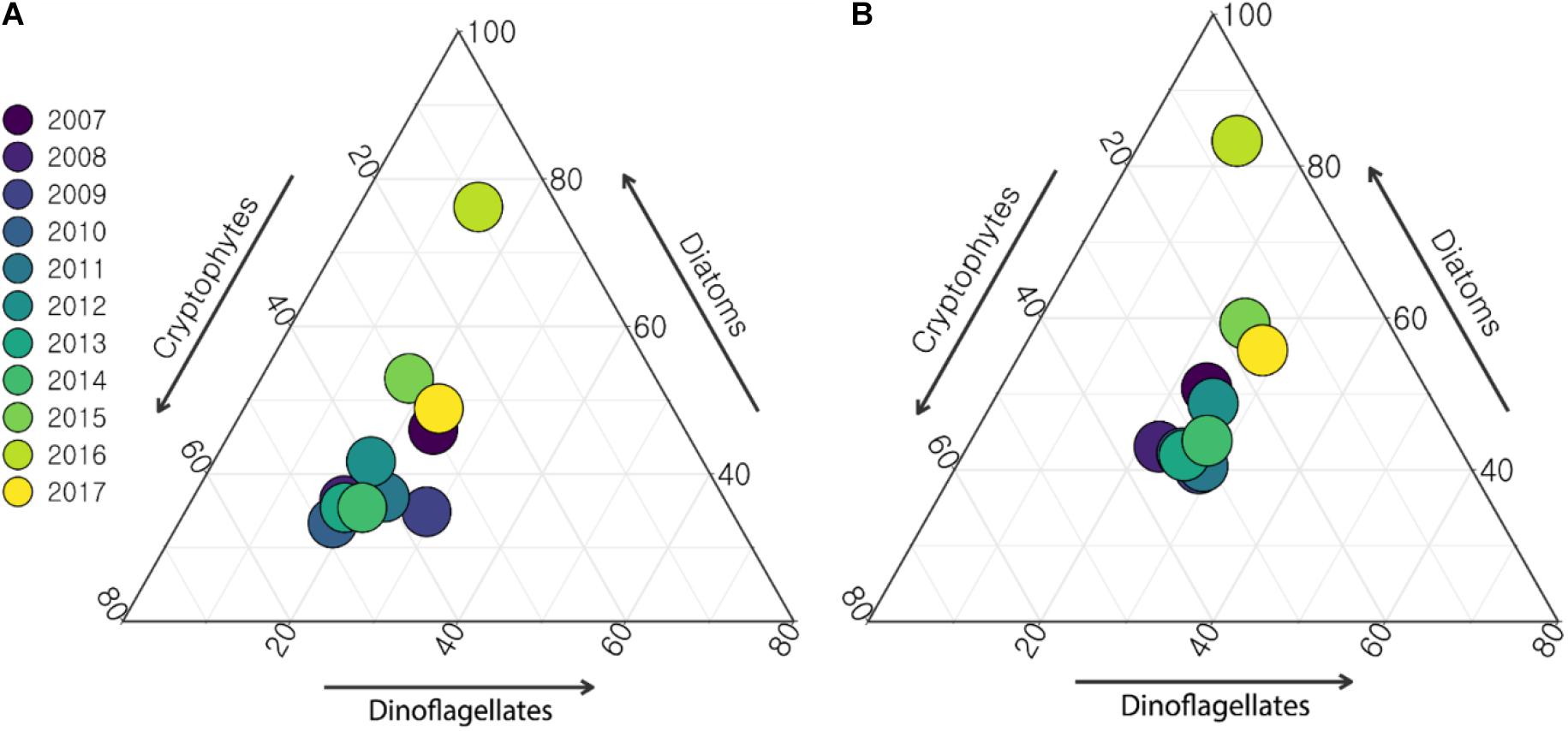
Figure 4. Relative abundance of the major phytoplankton groups including cryptophytes, diatoms, and dinoflagellates. Abundance in the surface layer (A) and abundance in the bottom layer (B).
The Redfield ratio, which was determined to be N:P:Si = 16:1:16, showed the macronutrient limitation in the bay. Silicate and nitrogen were not limited in most years (Figures 5A,B), whereas phosphate was limited during most periods except for 2007 and 2017 (Figure 5C). The P-limitation was more extreme on the surface layer where cryptophytes dominated, and the limitation was less intense in the bottom where diatoms dominated; the limitation varied annually and was more severe when cryptophytes dominated (Figure 5C).
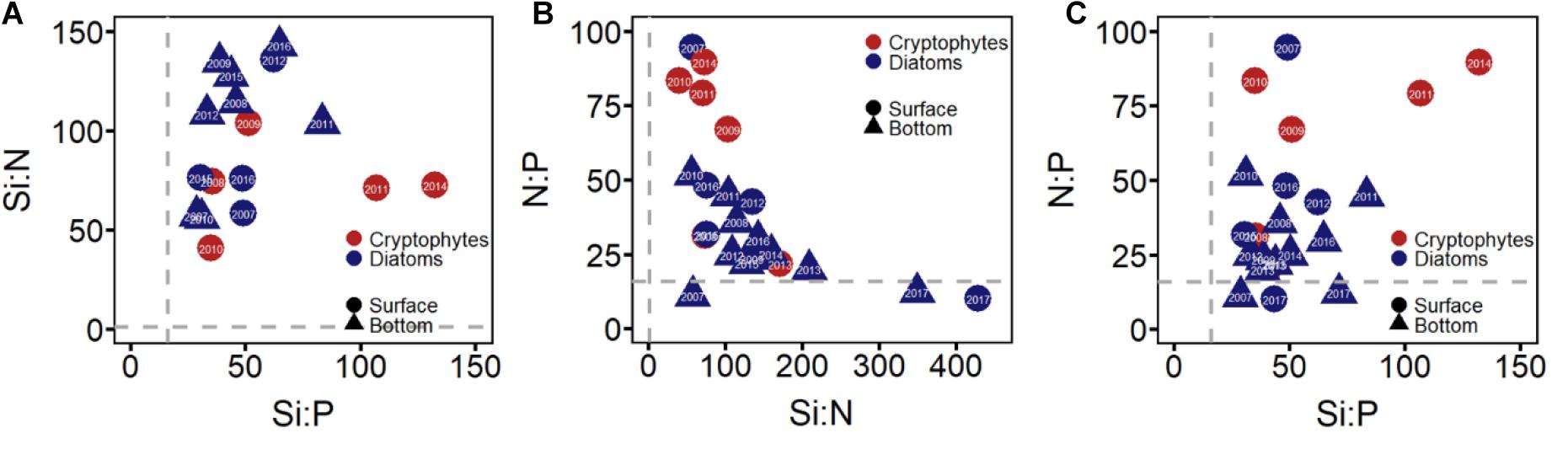
Figure 5. Redfield ratios depicting silicate limitation (A), nitrogen limitation (B), and phosphate limitation (C) in the Yeongil Bay. Different colors indicate the dominance of each group. Red indicate the dominance of cryptophytes and blue indicates the dominance of diatoms.
Responses of Cryptophytes and Diatoms to Environmental Parameters
The correlations between the two major phytoplankton groups, cryptophytes and diatoms, and the studied environmental parameters were investigated (Figure 6). Cryptophytes were negatively correlated with photic depth (correlation coefficient = −0.87; p < 0.001) whereas diatoms were not significantly related with the photic depth. Cryptophytes were positively correlated with NH4+ and PO43– (correlation coefficient = 0.45 and 0.42, respectively; p < 0.05). Diatoms were positively correlated with PO43– but negatively correlated with NH4+.
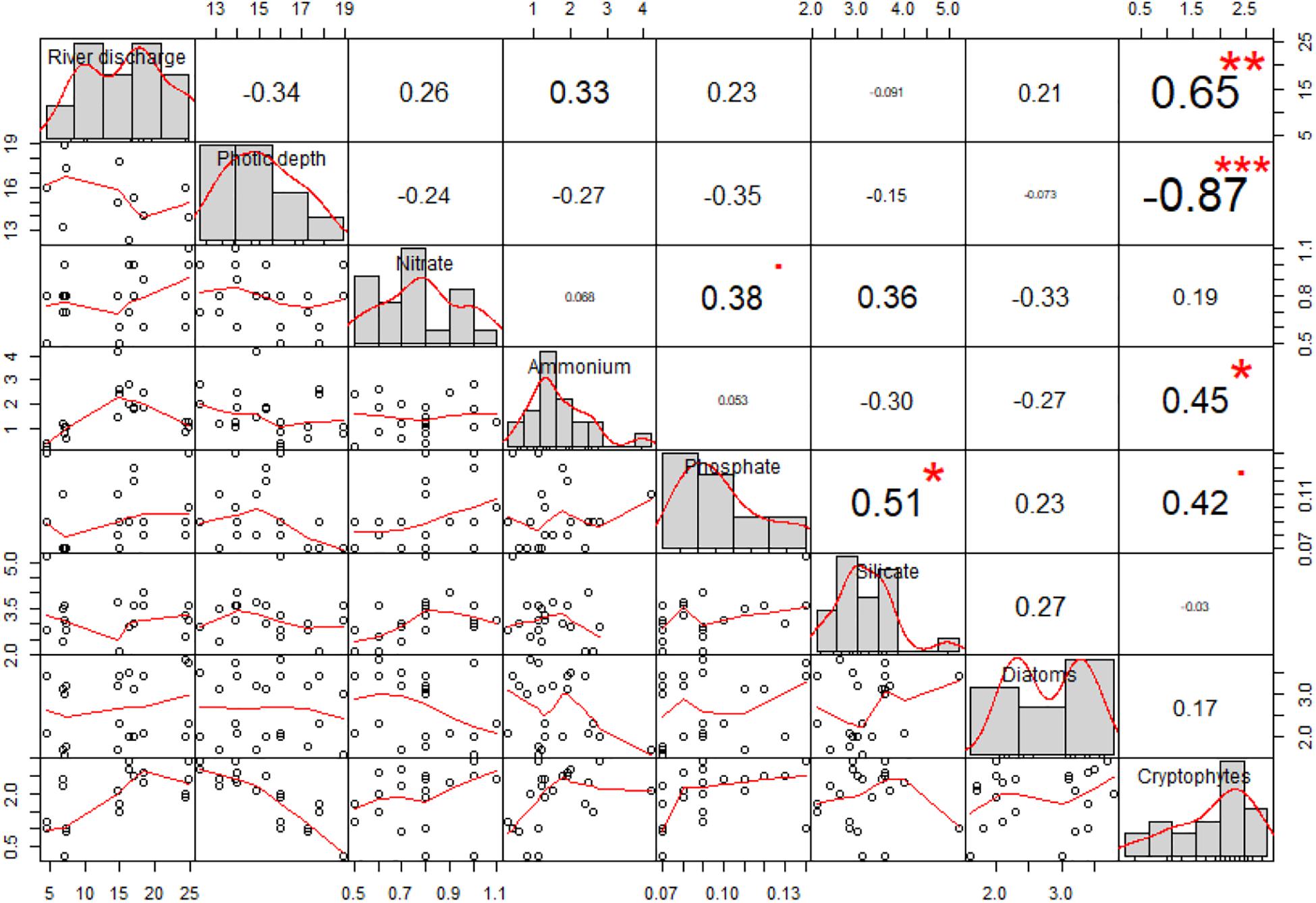
Figure 6. Correlation matrix illustrating the correlation coefficients and regressions between two major phytoplankton groups (cryptophytes and diatoms) and environmental variables including river discharge, photic depth, NH4+ and PO43–, and SiO2. The font size indicates the level of correlation coefficients and asterisks indicate the statistical significance (*p < 0.05; **p < 0.01, and ***p < 0.001).
Generalized additive models showed variations in the dominance of cryptophytes and diatoms in response to environmental parameters, and cryptophytes and diatoms mostly exhibited opposing patterns (Figure 7). The dominance of cryptophytes increased as water quality worsened, but diatom dominance declined with an increase in the WQI (Figure 7A). An increase in light penetration (i.e., increase in photic depth) decreased cryptophyte dominance and favored diatom growth (Figure 7B). NH4+ favored cryptophyte growth whereas it suppressed diatom growth, but the growth did not change when NH4+ levels exceeded 4 μM (Figure 7C). In addition, diatoms resisted up to approximately 50% of NH4+ in DIN whereas cryptophytes benefited more from increased NH4+ in the DIN (Figure 7D). Cryptophytes positively responded to DIP levels and were resistant to P-limitation whereas the dominance of diatoms declined with both DIP and P-limitation (Figures 7E,F).
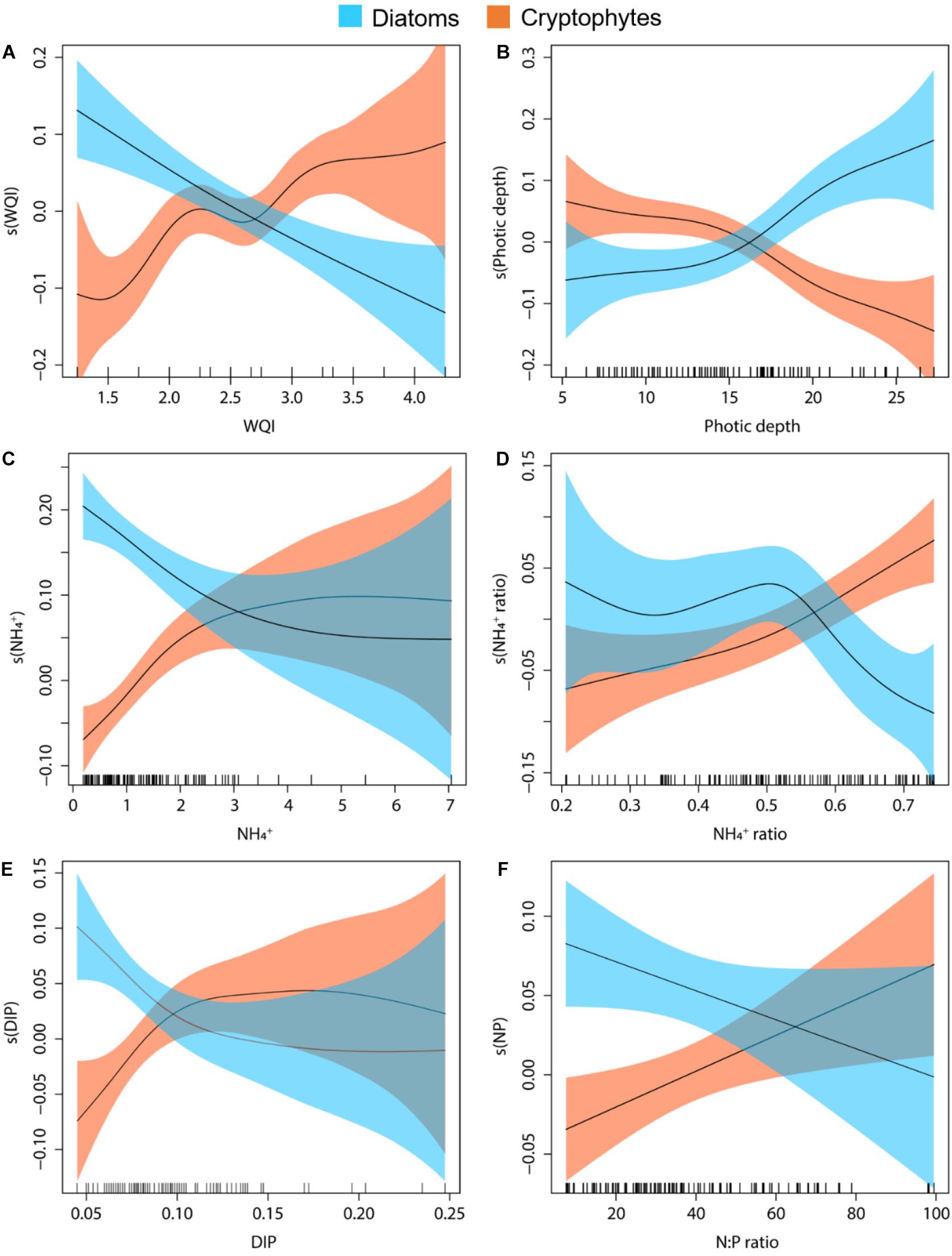
Figure 7. Generalized additive models illustrating variations in the dominance of cryptophytes and diatoms in response to multiple parameters. Responses of cryptophytes and diatoms to the water quality index (WQI) (A), responses to photic depth (B), responses to NH4+ (C), responses to the proportion of NH4+ in dissolved inorganic nitrogen (D), responses to dissolved inorganic phosphate (DIP) (E), and responses to the N:P ratio (F).
Discussion
Long-term phytoplankton dynamics in Yeongil Bay in response to environmental variations were investigated to assess the dominant groups, which are altered by water quality improvements. Although cryptophytes dominated in the surface layer and diatoms dominated in the bottom layer, cryptophyte abundance declined over the 11-year period and the diatom abundance relatively increased. An increase in photic depth and decrease in NH4+ indicating water quality improvement were strongly linked to a decrease in cryptophytes and increase in diatom dominance, while P-limitation and P-deficiency prevailed in this ecosystem. The GAMs depicted the variation in the cryptophyte and diatom dominances relative to environmental variations. Collectively, water quality improvement depressed the growth of cryptophytes but favored diatom dominance in the Yeongil Bay.
Nutrient Dynamics in a P-Limited and P-Deficient Environment
River discharge usually affects nutrient levels (Baek et al., 2015), the level of suspended particulate matter (SPM) controlling light availability (Acharyya et al., 2012), and the residence time (Kang et al., 2020), which are all associated with phytoplankton blooms in coastal waters. A reduction in river discharge often causes the dominance of small phytoplankton because less nutrients are available (Kim et al., 2019); however, in this study, the nutrient levels were fairly consistent despite the reduced river discharge. Interestingly, salinity slightly increased and the change was more apparent in the bottom layer, implying that seawater intrusion from the East Sea was enhanced with the river discharge reduction. TN from POSCO, which gradually declined, drove a decrease in NH4+ levels in Yeongil Bay, whereas the TN from the river was stable. This indicates that the amount of river discharge decreased but the concentration of dissolved inorganic nutrients was enriched, thereby inducing a stable input of nutrients via river discharge. The decadal increase in urban population and fertilizer usages and livestock near Yeongil Bay were potentially responsible for the remaining of enriched nutrient levels in the bay system.
Combined with the decline of NH4+ over the 11-year period, P-limitation became less intense but P-deficiency, indicated by DIP < 0.2 μM, prevailed during the same period. DIN and SiO2 levels exceeded the lower limit (i.e., DIN > 2 μM, SiO2 > 1 μM). This suggests that phytoplankton in Yeongil Bay needed to resist P-limitation and is adapted to P-deficient environments. Given that the ample amount of SiO2 presented throughout the bay and that Si:N ratios far surpassed 1, diatom growth might not have been limited, and diatoms were able to outcompete dinoflagellates, meaning that the ecological niche was dominated by diatoms.
Effects of Environmental Variables on the Dominance of Cryptophytes and Diatoms
The decline in river discharge meant that less SPM was introduced to the Yeongil Bay ecosystem, thereby leading to more light penetration. Since 2010, river discharge has sharply declined, and photic depth has gradually increased. The photic depth had an inverse relationship with the cryptophyte abundance; as the depth increased, the abundance decreased (Supplementary Figure 1). Cryptophytes can tolerate a wide range of salinity from freshwater to saline water (Clay et al., 1999) and can adapt well to high turbidity and low light availability (Barone and Naselli-Flores, 2003), conditions under which other phytoplankton (e.g., diatoms) are not usually able to experience optimal growth. Cryptophytes are opportunistic by proliferating under such conditions. Moreover, the photoprotective pigment which is enriched in cryptophytes also allows cryptophytes to thrive in surface water (Mendes et al., 2018).
Not only high turbidity but also high NH4+ can favor the growth of cryptophytes, whereas high levels of NH4+ suppress the growth of diatoms and dinoflagellates (Lomas and Glibert, 1999, 2000). In the Gwangyang Bay, which is located in the southern coastal waters of Korea, increases in NH4+ and SPM synergistically led to a decline in phytoplankton biomass (Kang et al., 2020), suggesting the dominance of small phytoplankton such as cryptophytes and picoplankton. The sharp decline in NH4+ might favor the growth of diatoms, steering the diatom dominance in the Yeongil Bay. However, the GAM results showed that 4 μM of NH4+ was the upper limit of cryptophyte resistance. Consistent with our results, in the San Francisco Bay, suppression of phytoplankton growth also occurred when NH4+ levels were above 4 μM as it inhibited the uptake of nitrate in diatoms but favored the growth of smaller primary producers (Dugdale et al., 2007, 2012). Slower river discharge and an increase in NH4+ supported cryptophyte blooms in the Krka River estuary in the eastern Adriatic Sea (Šupraha et al., 2014).
Although the DIP levels were lower than 0.2 μM, which is generally the lower limit for phytoplankton growth (Dortch and Whitledge, 1992), our results implied that the DIP levels were sufficient for phytoplankton growth, and particularly, high P-limitation triggered the cryptophyte dominance in the bay, whereas diatoms were favored by the weakened P-limitation. Consistent with this, Egge (1998) illustrated that diatoms are not the best competitors compared to small phytoplankton (nano or picoplankton) when DIP levels are low, and transcriptomic and proteomic responses of diatoms showed that DIP limitation drives multiple biochemical pathways in diatoms, including the utilization of dissolved organic phosphate, changes in cellular P allocation, and the generation of more P transport (Dyhrman et al., 2012). In addition, cryptophytes acquire nutrients via a mixotrophic mode, switching from utilizing dissolved nutrients to feeding on bacteria (Roberts and Laybourn-Parry, 1999; Yoo et al., 2017). This may be an alternative means of obtaining the required nutrients including N and P. Given that cryptophytes can tolerate harsher P-limited conditions, cryptophytes may express similar responses to diatoms; however, the transcriptomics or proteomics in response to P-limitation should be investigated to assess the role of the physiology of cryptophytes in their blooms.
Future Directions
A lack of hard exoskeleton structures and toxic metabolites enable cryptophytes to be nutritional food which contains high highly unsaturated fatty acid (HUFA) concentrations (Brett and Muller-Navarra, 1997), promoting high zooplankton growth and reproduction (Sterner and Schulz, 1998). However, cryptophyte blooms cause red coloration of bivalve tissue when bivalves feed on cryptophytes, resulting in unfavorable fishery products for commercial needs (Pastoureaud et al., 2003). In the meantime, the dominance of diatoms can drive efficient food webs, which are fundamentally based on larger phytoplankton while red pigments of phytoplankton that degrade the bivalve quality would no longer cause economic losses in bivalve mariculture.
In this study, bottom-up effects (i.e., nutrient effects) were investigated using data regarding the long-term variations in the major phytoplankton groups and nutrient dynamics. However, the interactive role of the top-down and bottom-up effects on phytoplankton communities should be considered to assess the variations in phytoplankton communities as zooplankton grazing is another factor that controls phytoplankton gains and losses in marine ecosystems (Kang et al., 2015). In addition, an advanced model which combines the biogeochemical model and hydrodynamic models, such as the Regional Ocean Modeling System, are required to understand how blooms of different phytoplankton groups are associated with biogeochemical cycles and hydrodynamics in the ecosystem. Given that the size of small phytoplankton (nanoplankton or picoplankton) limits the observation of phytoplankton groups, other identification techniques such as flow cytometry, high performance liquid chromatography, and environmental DNA metabarcoding should be applied to estimate phytoplankton communities including small phytoplankton. Finally, physiological responses such as enzyme activity and pigment change or transcriptional responses for functional genes to acquire the opened ecological niche with water quality improvement should be studied to better understand the ecological and physiological characteristics of phytoplankton dynamics in ecosystems.
Data Availability Statement
The original contributions presented in the study are included in the article/Supplementary Material, further inquiries can be directed to the corresponding author/s.
Author Contributions
YK: data acquisition and analysis and writing—original draft. H-JK: data analysis and reviewing. C-HM: supervision and funding acquisition. YY: reviewing and editing. C-KK: supervision and funding acquisition. All authors contributed to the article and approved the submitted version.
Funding
This study was supported by “Long-term change of structure and function in marine ecosystems of Korea” funded by the Ministry of Oceans and Fisheries, Korea, and by a grant from the Basic Science Research Program through the National Research Foundation of Korea funded by the Ministry of Education, Science and Technology (No. 2020R1F1A1076628).
Conflict of Interest
The authors declare that the research was conducted in the absence of any commercial or financial relationships that could be construed as a potential conflict of interest.
Publisher’s Note
All claims expressed in this article are solely those of the authors and do not necessarily represent those of their affiliated organizations, or those of the publisher, the editors and the reviewers. Any product that may be evaluated in this article, or claim that may be made by its manufacturer, is not guaranteed or endorsed by the publisher.
Acknowledgments
We would like to thank the students and researchers who participated in this study for their assistance with field samplings and laboratory analyses.
Supplementary Material
The Supplementary Material for this article can be found online at: https://www.frontiersin.org/articles/10.3389/fmars.2021.710891/full#supplementary-material
Supplementary Figure 1 | Long-term variation in cryptophytes and diatoms in response to variation in water quality parameters. Variations in cryptophytes and diatoms with the water quality index (A,B), variations in cryptophytes and diatoms with the proportion of NH4+ in the surface and bottom waters (C,D), and variations in cryptophytes and diatoms with the photic depth in the surface and bottom waters (E,F).
Supplementary Table 1 | Long-term variation in dominant species for the last 11 years in Yeongil Bay. Parenthesis illustrates dominance in%.
Footnotes
References
Acharyya, T., Sarma, V. V. S. S., Sridevi, B., Venkataramana, V., Bharathi, M. D., and Naidu, S. A., et al. (2012). Reduced river discharge intensifies phytoplankton bloom in Godavari estuary, India. Mar. Chem. 13, 15–22. doi: 10.1016/j.marchem.2012.01.005
Adolf, J. E., Bachvaroff, T., and Place, A. R. (2008). Can cryptophyte abundance trigger toxic Karlodinium veneficum blooms in eutrophic estuaries? Harmful Algae 8, 119–128. doi: 10.1016/j.hal.2008.08.003
Alexander, H., Jenkins, B. D., Rynearson, T. A., and Dyhrman, S. T. (2015a). Metatranscriptome analyses indicate resource partitioning between diatoms in the field. Proc. Natl. Acad. Sci. U.S.A. 112, E2182–E2190.
Alexander, H., Rouco, M., Haley, S. T., Wilson, S. T., Karl, D. M., and Dyhrman, S. T. (2015b). Functional group-specific traits drive phytoplankton dynamics in the oligotrophic ocean. Proc. Natl. Acad. Sci. U.S.A. 112, E5972–E5979.
Baek, S.-H., Kim, D., Son, M., Yun, S.-M., and Kim, Y.-O. (2015). Seasonal distribution of phytoplankton assemblages and nutrient-enriched bioassays as indicators of nutrient limitation of phytoplankton growth in Gwangyang Bay, Korea. Estuarine Coast. Shelf Sci. 163, 265–278. doi: 10.1016/j.ecss.2014.12.035
Barone, R., and Naselli-Flores, L. (2003). Distribution and seasonal dynamics of Cryptomonads in Sicilian water bodies. Hydrobiologia 502, 325–329. doi: 10.1023/b:hydr.0000004290.22289.c2
Boyer, J. N., Kelble, C. R., Ortner, P. B., and Rudnick, D. T. (2009). Phytoplankton bloom status: chlorophyll a biomass as an indicator of water quality condition in the southern estuaries of Florida, USA. Ecol. Indic. 9, S56–S67.
Brett, M., and Muller-Navarra, D. (1997). The role of highly unsaturated fatty acids in aquatic foodweb processes. Freshw. Biol. 38, 483–499. doi: 10.1046/j.1365-2427.1997.00220.x
Brun, P., Vogt, M., Payne, M. R., Gruber, N., O’brien, C. J., Buitenhuis, E. T., et al. (2015). Ecological niches of open ocean phytoplankton taxa. Limnol. Oceanogr. 60, 1020–1038. doi: 10.1002/lno.10074
Cai, W.-J., Hu, X., Huang, W.-J., Murrell, M. C., Lehrter, J. C., Lohrenz, S. E., et al. (2011). Acidification of subsurface coastal waters enhanced by eutrophication. Nat. Geosci. 4:766. doi: 10.1038/ngeo1297
Choi, K. (1993). Oceanographic Properties of the Yeongil Bay. Thesis. Busan Korea: Pukyong National University.
Clay, B. L., Kugrens, P., and Lee, R. E. (1999). A revised classification of Cryptophyta. Bot. J. Linn. Soc. 131, 131–151. doi: 10.1111/j.1095-8339.1999.tb01845.x
Cloern, J. E. (1987). Turbidity as a control on phytoplankton biomass and productivity in estuaries. Continent. Shelf Res. 7, 1367–1381. doi: 10.1016/0278-4343(87)90042-2
Dortch, Q., and Whitledge, T. E. (1992). Does nitrogen or silicon limit phytoplankton production in the Mississippi River plume and nearby regions? Continent. Shelf Res. 12, 1293–1309. doi: 10.1016/0278-4343(92)90065-r
Dugdale, R., Wilkerson, F., Parker, A. E., Marchi, A., and Taberski, K. (2012). River flow and ammonium discharge determine spring phytoplankton blooms in an urbanized estuary. Estuar. Coast. Shelf Sci. 115, 187–199. doi: 10.1016/j.ecss.2012.08.025
Dugdale, R. C., Wilkerson, F. P., Hogue, V. E., and Marchi, A. (2007). The role of ammonium and nitrate in spring bloom development in San Francisco Bay. Estuar. Coast. Shelf Sci. 73, 17–29. doi: 10.1016/j.ecss.2006.12.008
Dyhrman, S. T., Jenkins, B. D., Rynearson, T. A., Saito, M. A., Mercier, M. L., Alexander, H., et al. (2012). The Transcriptome and proteome of the diatom Thalassiosira pseudonana reveal a diverse phosphorus stress response. PLoS One 7:e33768. doi: 10.1371/journal.pone.0033768
Egge, J. K. (1998). Are diatoms poor competitors at low phosphate concentrations? J. Mar. Syst. 16, 191–198. doi: 10.1016/S0924-7963(97)00113-9
Glibert, P. M., Harrison, J., Heil, C., and Seitzinger, S. (2006). Escalating worldwide use of urea – a global change contributing to coastal eutrophication. Biogeochemistry 77, 441–463. doi: 10.1007/s10533-005-3070-5
Gobler, C. J., Berry, D. L., Dyhrman, S. T., Wilhelm, S. W., Salamov, A., Lobanov, A. V., et al. (2011). Niche of harmful alga Aureococcus anophagefferens revealed through ecogenomics. Proc. Natl. Acad. Sci. U.S.A. 108, 4352–4357. doi: 10.1073/pnas.1016106108
Heisler, J., Glibert, P. M., Burkholder, J. M., Anderson, D. M., Cochlan, W., Dennison, W. C., et al. (2008). Eutrophication and harmful algal blooms: a scientific consensus. Harmful Algae 8, 3–13. doi: 10.1016/j.hal.2008.08.006
Holmes, R. W. (1970). The secchi disk in turbid coastal waters. Limnol. Oceanogr. 15, 688–694. doi: 10.4319/lo.1970.15.5.0688
Jo, E.-Y., Cha, J.-H., Kim, M.-K., and Kim, K.-T. (2000). Studies on the ecosystem in the Yeong-Il Gulf of Korea: I. species composition and diversity of phytoplankton communities. ALGAE 15, 49–59.
Johnson, M. D., Beaudoin, D. J., Frada, M. J., Brownlee, E. F., and Stoecker, D. K. (2018). High grazing rates on cryptophyte algae in Chesapeake Bay. Front. Mar. Sci. 5:241. doi: 10.3389/fmars.2018.00241
Kang, Y., Kang, H.-Y., Kim, D., Lee, Y.-J., Kim, T.-I., and Kang, C.-K. (2019a). Temperature-dependent bifurcated seasonal shift of phytoplankton community composition in the coastal water off southwestern Korea. Ocean Sci. J. 54, 467–486. doi: 10.1007/s12601-019-0025-7
Kang, Y., Kang, Y.-H., Kim, J.-K., Kang, H. Y., and Kang, C.-K. (2020). Year-to-year variation in phytoplankton biomass in an anthropogenically polluted and complex estuary: a novel paradigm for river discharge influence. Mar. Pollut. Bull. 161:111756. doi: 10.1016/j.marpolbul.2020.111756
Kang, Y., Kim, H.-J., Kang, H. Y., Lee, K., Moon, C.-H., and Kang, C.-K. (2019b). “Responses of phytoplankton assemblages to environmental variability in Yeongil Bay on the southeastern coast of Korea,” in Proceedings of the Conference of the Korean Society of Oceanography: The Korean Society of Oceanography, Berlin.
Kang, Y., Koch, F., and Gobler, C. J. (2015). The interactive roles of nutrient loading and zooplankton grazing in facilitating the expansion of harmful algal blooms caused by the pelagophyte, Aureoumbra lagunensis, to the Indian River Lagoon, FL, USA. Harmful Algae 49, 162–173. doi: 10.1016/j.hal.2015.09.005
Kim, H.-D., Kim, J.-I., and Ryu, C.-R. (2001). Study on curent and water quality characteristics in Yongil Bay. J. Ocean Eng. Technol. 15, 28–37.
Kim, Y., Lee, J. H., Kang, J. J., Lee, J. H., Lee, H. W., Kang, C. K., et al. (2019). River discharge effects on the contribution of small-sized phytoplankton to the total biochemical composition of POM in the Gwangyang Bay, Korea. Estuar. Coast. Shelf Sci. 226:106293. doi: 10.1016/j.ecss.2019.106293
Kim, Y.-S., and Kim, Y.-S. (2004). The effect of freshwater inflow on the spatio-temporal variation of water quality of Yeongil Bay. Korean J. Environ. Biol. 22, 57–65.
Kwak, H.-S., and Lee, K.-R. (1977). Distribution of phytoplankton pigments in yeongil bay water of korea, Late October. J. Korean Soc. Oceanogr. 12, 57–66.
Lee, J.-H., Lee, D., Kang, J.-J., Joo, H.-T., Lee, J.-H., Lee, H.-W., et al. (2017). The effects of different environmental factors on the biochemical composition of particulate organic matter in Gwangyang Bay, South Korea. Biogeosciences 14:1903. doi: 10.5194/bg-14-1903-2017
Lie, H.-J. (1989). Observations of southeastward deep currents off the east coast of Korea. J. Oceanogr. Soc. Korea 24, 63–68.
Litchman, E., Edwards, K. F., Klausmeier, C. A., and Thomas, M. K. (2012). Phytoplankton niches, traits and eco-evolutionary responses to global environmental change. Mar. Ecol. Prog. Ser. 470, 235–248. doi: 10.3354/meps09912
Lomas, M., and Glibert, P. (1999). Interactions between NH4+ and NO3- uptake and assimilation: comparison of diatoms and dinoflagellates at several growth temperatures. Mar. Biol. 133, 541–551. doi: 10.1007/s002270050494
Lomas, M. W., and Glibert, P. M. (2000). Comparisons of nitrate uptake, storage, and reduction in marine diatoms and flagellates. J. Phycol. 36, 903–913. doi: 10.1046/j.1529-8817.2000.99029.x
Marshall, H. G., Lacouture, R. V., Buchanan, C., and Johnson, J. M. (2006). Phytoplankton assemblages associated with water quality and salinity regions in Chesapeake Bay, USA. Estuar. Coast. Shelf Sci. 69, 10–18. doi: 10.1016/j.ecss.2006.03.019
Mendes, C. R. B., Tavano, V. M., Dotto, T. S., Kerr, R., De Souza, M. S., Garcia, C. A. E., et al. (2018). New insights on the dominance of cryptophytes in Antarctic coastal waters: a case study in Gerlache Strait. Deep Sea Res. Part II Top. Stud. Oceanogr. 149, 161–170. doi: 10.1016/j.dsr2.2017.02.010
Mendes, C. R. B., Tavano, V. M., Leal, M. C., de Souza, M. S., Brotas, V., and Garcia, C. A. E. (2013). Shifts in the dominance between diatoms and cryptophytes during three late summers in the Bransfield Strait (Antarctic Peninsula). Polar Biol. 36, 537–547. doi: 10.1007/s00300-012-1282-4
Nam, Y.-W., and Han, K.-S. (2011). A study on the present state and improvement plan of domestic sewage sludge treatment. J. Korea Soc. Waste Manag. 28, 103–109.
Paerl, H. W., Pinckney, J. L., Fear, J. M., and Peierls, B. L. (1998). Ecosystem responses to internal and watershed organic matter loading: consequences for hypoxia in the eutrophying Neuse River Estuary, North Carolina, USA. Mar. Ecol. Prog. Ser. 166, 17–25. doi: 10.3354/meps166017
Paerl, H. W., Valdes, L. M., Pinckney, J. L., Piehler, M. F., Dyble, J., and Moisander, P. H. (2003). Phytoplankton photopigments as indicators of estuarine and coastal eutrophication. AIBS Bull. 53, 953–964. doi: 10.1641/0006-3568(2003)053[0953:ppaioe]2.0.co;2
Pastoureaud, A., Dupuy, C., Chrétiennot-Dinet, M., Lantoine, F., and Loret, P. (2003). Red coloration of oysters along the French Atlantic coast during the 1998 winter season: implication of nanoplanktonic cryptophytes. Aquaculture 228, 225–235. doi: 10.1016/s0044-8486(03)00266-7
Peterson, B. G., Carl, P., Boudt, K., Bennett, R., Ulrich, J., Zivot, E., et al. (2018). Package ‘performanceanalytics’. R Team Cooperation 3.
Poole, H., and Atkins, W. (1929). Photo-electric measurements of submarine illumination throughout the year. J. Mar. Biol. Assoc. U.K. 16, 297–324. doi: 10.1017/s0025315400029829
Roberts, E. C., and Laybourn-Parry, J. (1999). Mixotrophic cryptophytes and their predators in the Dry Valley lakes of Antarctica. Freshw. Biol. 41, 737–746. doi: 10.1046/j.1365-2427.1999.00401.x
Sathicq, M. B., Gómez, N., Bauer, D. E., and Donadelli, J. (2017). Use of phytoplankton assemblages to assess the quality of coastal waters of a transitional ecosystem: río de la Plata estuary. Continent. Shelf Res. 150, 10–17. doi: 10.1016/j.csr.2016.08.009
Shim, J.-M., Kwon, K.-Y., Jeong, H.-D., Choi, Y.-K., and Kim, S.-W. (2013). Spatial and temporal variability of phytoplankton in relation to environmental factors in Youngil Bay. J. Environ. Sci. Int. 22, 1683–1690. doi: 10.5322/jesi.2013.22.12.1683
Spatharis, S., Tsirtsis, G., Danielidis, D. B., Do Chi, T., and Mouillot, D. (2007). Effects of pulsed nutrient inputs on phytoplankton assemblage structure and blooms in an enclosed coastal area. Estuar. Coast. Shelf Sci. 73, 807–815. doi: 10.1016/j.ecss.2007.03.016
Sterner, R. W., and Schulz, K. L. (1998). Zooplankton nutrition: recent progress and a reality check. Aquat. Ecol. 32, 261–279.
Šupraha, L., Bosak, S., Ljubešić, Z., Mihanović, H., Olujić, G., Mikac, I., et al. (2014). Cryptophyte bloom in a Mediterranean estuary: high abundance of Plagioselmis cf. prolonga in the Krka River estuary (eastern Adriatic Sea). Sci. Mar. 78, 329–338. doi: 10.3989/scimar.03998.28c
Tett, P., Heaney, S., and Droop, M. (1985). The Redfield ratio and phytoplankton growth rate. J. Mar. Biol. Assoc. U.K. Plymouth 65, 487–504. doi: 10.1017/s0025315400050566
Wang, R., Liu, X., Wu, J., Wai, T.-C., Shen, P., and Lam, P. K. S. (2020). Long-term variations of phytoplankton community in relations to environmental factors in Deep Bay, China, from 1994 to 2016. Mar. Pollut. Bull. 153:111010. doi: 10.1016/j.marpolbul.2020.111010
Yoo, Y. D., Seong, K. A., Jeong, H. J., Yih, W., Rho, J.-R., Nam, S. W., et al. (2017). Mixotrophy in the marine red-tide cryptophyte Teleaulax amphioxeia and ingestion and grazing impact of cryptophytes on natural populations of bacteria in Korean coastal waters. Harmful Algae 68, 105–117. doi: 10.1016/j.hal.2017.07.012
Yoon, H.-S., Lee, I.-C., and Ryu, C.-R. (2003). Spatial and temporal variation characteristics between water quality and pollutant loads of Yeong-il Bay (1) - Seasonal variation of river discharge and inflowing pollutant loads -. J. Ocean Eng. Technol. 17, 23–30.
Yoon, H.-S., Lee, I.-C., Ryu, C.-R., and Park, J.-H. (2002). “Seasonal variation of pollutant load flowing into Yeong-Il bay,” in Proceedings of the Korea Committee for Ocean Resources and Engineering Conference: Korean Society of Ocean Engineers, Shanghai, 100–107.
Zhang, Q., Fisher, T. R., Trentacoste, E. M., Buchanan, C., Gustafson, A. B., Karrh, R., et al. (2020). Nutrient limitation of phytoplankton in Chesapeake Bay: development of an empirical approach for water-quality management. Water Res. 188:116407. doi: 10.1016/j.watres.2020.116407
Keywords: water quality, phytoplankton, Yeongil Bay, ammonium, photic depth, ecological niche
Citation: Kang Y, Moon C-H, Kim H-J, Yoon YH and Kang C-K (2021) Water Quality Improvement Shifts the Dominant Phytoplankton Group From Cryptophytes to Diatoms in a Coastal Ecosystem. Front. Mar. Sci. 8:710891. doi: 10.3389/fmars.2021.710891
Received: 17 May 2021; Accepted: 22 July 2021;
Published: 12 August 2021.
Edited by:
Gleyci A. O. Moser, Rio de Janeiro State University, BrazilReviewed by:
Maria Paches, Universitat Politècnica de València, SpainPriscila Kienteca Lange, Federal University of Rio de Janeiro, Brazil
Copyright © 2021 Kang, Moon, Kim, Yoon and Kang. This is an open-access article distributed under the terms of the Creative Commons Attribution License (CC BY). The use, distribution or reproduction in other forums is permitted, provided the original author(s) and the copyright owner(s) are credited and that the original publication in this journal is cited, in accordance with accepted academic practice. No use, distribution or reproduction is permitted which does not comply with these terms.
*Correspondence: Yoonja Kang, eW9vbmpha2FuZ0BqbnUuYWMua3I=