- 1Collecte Localisation Satellites, Ramonville Saint-Agne, France
- 2LEGOS, CNES, CNRS, UPS, IRD, Université de Toulouse, Toulouse, France
- 3Magellium, Ramonville Saint-Agne, France
- 4CNES, Toulouse, France
It is essential to monitor accurately current sea level changes to better understand and project future sea level rise (SLR). This is the basis to support the design of adaptation strategies to climate change. Altimeter sea level products are operationally produced and distributed by the E.U. Copernicus services dedicated to the marine environment (CMEMS) and climate change (C3S). The present article is a review paper that intends to explain why and to which extent the sea level monitoring indicators derived from these products are appropriate to develop adaptation strategies to SLR. We first present the main key scientific questions and challenges related to SLR monitoring. The different processing steps of the altimeter production system are presented including those ensuring the quality and the stability of the sea level record (starting in 1993). Due to the numerous altimeter algorithms required for the production, it is complex to ensure both the retrieval of high-resolution mesoscale signals and the stability of the large-scale wavelengths. This has led to the operational production of two different sea level datasets whose specificities are characterized. We present the corresponding indicators: the global mean sea level (GMSL) evolution and the regional map of sea level trends, with their respective uncertainties. We discuss how these products and associated indicators support adaptation to SLR, and we illustrate with an example of downstream application. The remaining gaps are analyzed and recommendations for the future are provided.
Introduction
Since the mid-nineteenth century, the increasing amount of greenhouse gases stored in the atmosphere has led to an imbalance at the top of the atmosphere between the incoming solar radiation and the outgoing infrared emission of the Earth (with a higher proportion of the former). The Earth system is forced to adapt to this excess of energy that is entering the system, with various consequences such as decreasing land ice, snow cover and glaciers and increasing ocean temperature, surface temperature and mean sea level rise (SLR), as well as coastal flooding and erosion, with also more extreme events in heat waves, precipitation and sea level. The Global Mean Sea Level (GMSL) rise is a precise indicator of this climate change since most of the energy in excess on the Earth is stored into the ocean and the cryosphere (von Schuckmann et al., 2020) and contributes directly to the SLR through thermal expansion and land ice melt (ice sheets and glaciers). Observing precisely current GMSL rise and understanding the different contributions to this rise (WCRP Global Sea Level Budget Group, 2018) allows to estimate the excess of energy in the climate system (Meyssignac et al., 2019) and the regions where this excess of energy is stored, leading to a better validation of climate models and their simulations of the past and future climate (Meyssignac et al., 2017; Loeb et al., 2018). This is one of the reasons that motivated space agencies to build up a continuous and accurate observing system of the sea level since 1993, with the constellation of satellite altimeters. Another reason that motivated space agencies was to provide the observational basis for the study of regional and coastal impacts of SLR through erosion and flooding (Cazenave and Le Cozannet, 2013) and also of changes in extreme events (Woodworth et al., 2011). In 2014, the sea-level variable was identified as an Essential Climate Variable (ECV, Bojinski et al., 2014) that should be monitored with enough accuracy and stability to report the GMSL variability at monthly to multidecadal time scales. In this context, the role of climate services (and marine services for ocean variables) is to use all information available (including the space data from satellite altimeters) to produce, validate and distribute sea level products that respond to the ECV requirements, and which can be used, in the end, by policy makers and stakeholders to elaborate adaptation and mitigation strategies.
The Copernicus program of the European Union provides Earth Observation information for the benefit of European citizens and consists of different services. The program plays a key role in the production and distribution of the different ECV products. The information delivered by the different Copernicus services include measurements of the sea level evolution on a global and regional scale and also added-value information that contribute to a better understanding of the SLR and to adapt to the associated impacts. The global strategy of the Copernicus program related to adaptation to sea level rise is presented in a dedicated article in this special issue (Melet et al., 2021).
Among the different services, the Copernicus Climate Change Service (C3S) and Copernicus Marine Environment Monitoring Service (CMEMS) provide a wide range of information related to the Earth system based on satellite measurements, in situ observations, model reanalyses and climate projections. The sea level evolution is one piece of this information. Within the CMEMS and C3S Copernicus services, the objectives of the production center in charge of the sea level satellite ECV product are to fit to the target requirements as described by the Global Climate Observing System (GCOS), to provide a high level of maturity of datasets and metadata and to answer the users needs (Copernicus users, meteo and climate organizations, data assimilation, research, climate model projections among others).
International cooperation has been at the origin of satellite altimetry with the joint effort of the NOAA and CNES French space agency together with the European Space Agency (ESA), leading to a 28-year long sea level time series starting in 1993 with the launch of the TOPEX-Poseidon mission and 14 other altimetry missions since then (see Figure 1). In Europe, the CNES/CLS DUACS (Data Unification and Altimeter Combination System) production system has processed the altimeter sea level measurements of the different missions since 20011 to provide multi-mission gridded sea surface heights and derived variables. The altimeter products have been distributed since 2003 through the AVISO CNES Satellite Altimetry Data portal.2 Since 2015, the whole processing, operational production and distribution of the DUACS along-track (Level 3, L3) and gridded (Level 4, L4) altimeter sea level products have been taken over by the European Copernicus Programme (with the support of EUMETSAT, ESA and CNES for the Sentinel missions). The AVISO portal still includes ocean monitoring indicators (such as the GMSL evolution), added value and pre-operational research products.3 In the current context of the Copernicus sea level data production, EUMETSAT is in charge of the production and distribution of the altimeter observations of the European Sentinel missions and CNES and ESA are responsible for the other altimeter missions in collaboration with NASA/NOAA as well as the Indian and Chinese space agencies for some of the missions (see Figure 1).
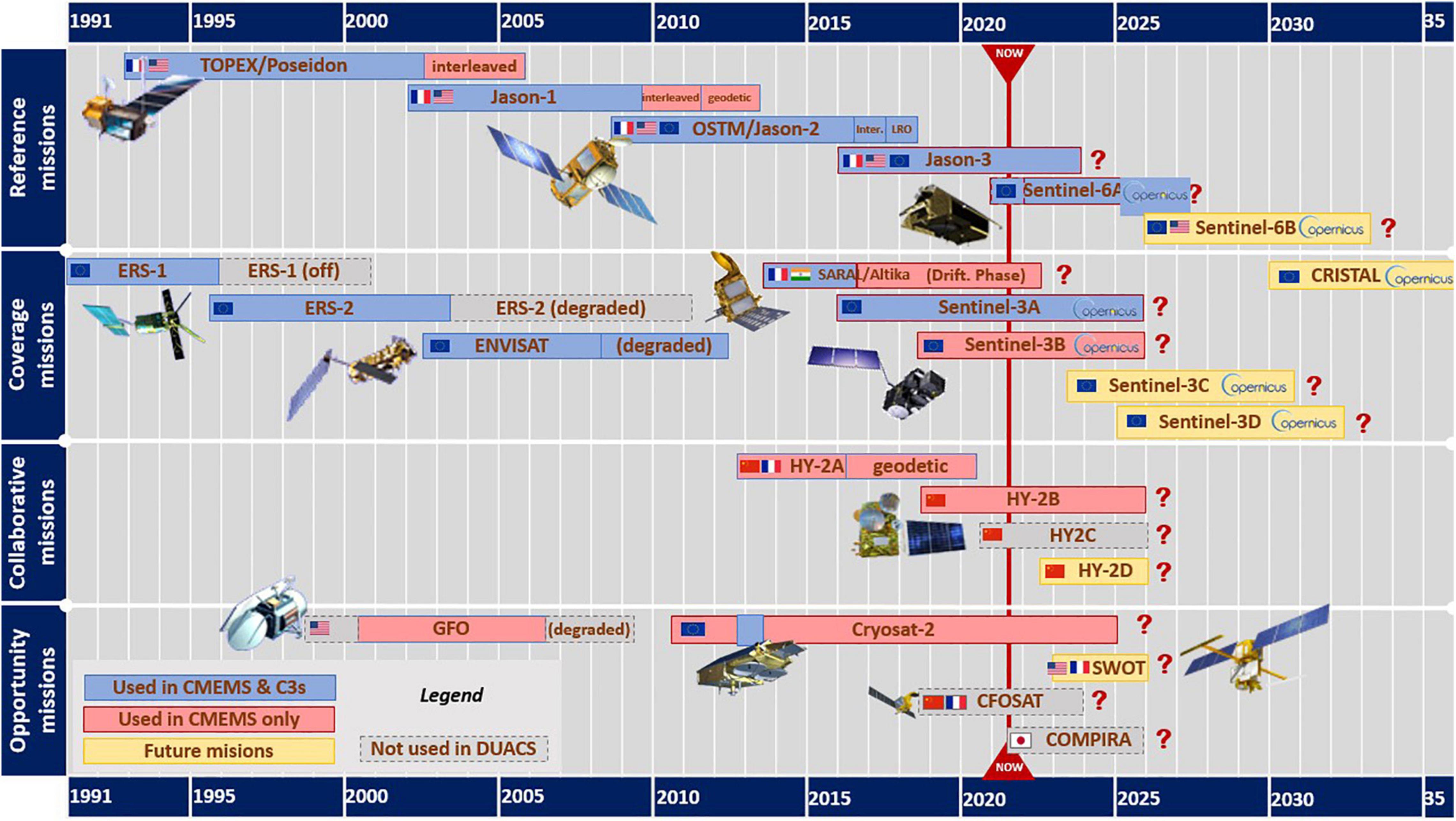
Figure 1. Timeline of modern radar altimetry missions. Reference missions are used to ensure the stability of the sea level products and are distinguished from the coverage, collaborative and opportunity missions. The color of the labels of the different missions depends on their use in the different Copernicus altimeter sea level products (see section “Building Ready to Use Altimeter Sea Level Products”).
These observational products build on the validated Level 2 Plus (L2P) altimeter datasets produced in-house by the different space agencies. They are also maintained up-to-date and at the highest level of quality thanks to the R&D activities funded by CNES and ESA. Figure 1 illustrates that a large number of new missions are expected to be included in the altimeter constellation during the next decade and they will contribute to ensure the long-term monitoring of sea level evolution.
The present paper is a review paper that intends to describe all the activities required to ensure the quality of the satellite-based sea level products developed by Copernicus services and to explain why and to which extent these products are appropriate to face the challenge of understanding and adapting to SLR. The paper does not aim at explaining the full methodology related to the sea level production system since the details are provided online with the datasets, together with the user manuals and the validation procedures. The interested readers are invited to access the documentation (see Table 1). The paper rather focuses on the presentation of the key processing steps that ensure the quality of the final product. These include the sea surface height computation, the calibration/validation activities, the use of homogeneous and validated along-track (L2P) altimeter standards from all missions, the role of the reference missions to ensure stability and the production of cross calibrated multi-mission L3 and gridded gap-free L4 sea level data (presented in section “Building Ready To Use Altimeter Sea Level Products”). All these activities contribute to the quality of the different Copernicus altimeter sea level products distributed to the users. A technical description of these products is provided, and the general maturity of the service is highlighted (section “The Copernicus Altimeter Sea Level Products and Ocean Monitoring Indicators”). These added-value products are then used to determine the mean sea level indicators and derive the sea level trend and acceleration at global and regional scales, which are key information to elaborate adaptation and mitigation strategies. At last, section “Remaining Gaps and Recommendations” provides discussion on the remaining gaps and recommendations related to the altimeter observing system and the associated uncertainties and it includes an illustration of a downstream project that contributes to define adaptation strategies to SLR.
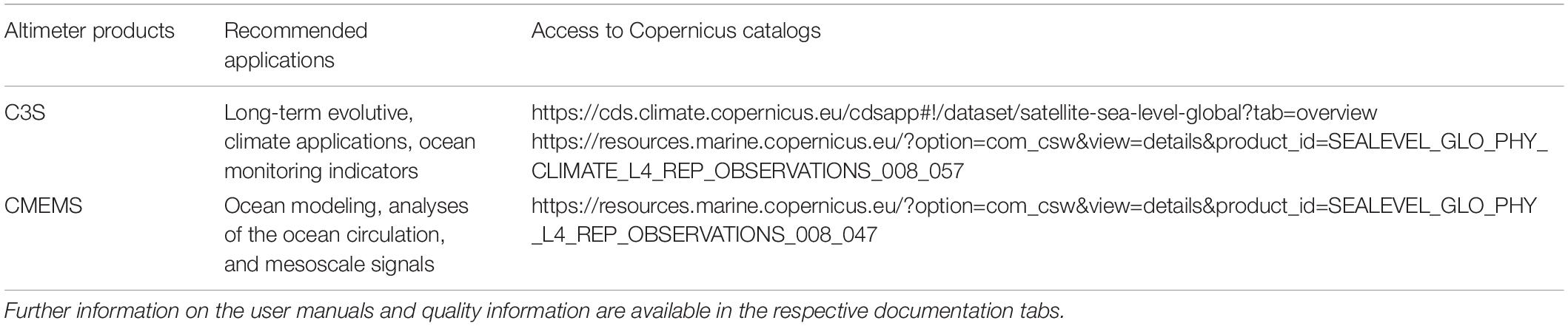
Table 1. Details of the delayed-time altimeter sea level products and their accesses in the Copernicus catalogs.
Building Ready to Use Altimeter Sea Level Products
In the following, we present the main processing steps required to ensure the high quality of the altimeter sea level products distributed to the users and especially the ones that contribute to the MSL stability.
Sea Surface Heights Computation
On the one hand, the raw sea level estimates are derived from the difference between the satellite altitude and the radar altimeter range. The former is derived from the Precise Orbit Determination (POD) which provides the altitude of the satellite above the reference ellipsoïd. The accuracy of this orbit determination directly affects the sea level estimates. The consolidated POD is made available in delayed-time for the different missions within 90 days in the geophysical data records. The multi-technique solution based on GPS, laser and DORIS techniques contribute to the accuracy of the satellite positioning and of the associated individual sea level estimates, which reaches about 1.5 cm (Escudier et al., 2017). However, at a decadal time scale, the POD errors remain one of the main contributors to the sea-level rise uncertainty at the global scale (e.g., Couhert et al., 2018; Ablain et al., 2019) and at the regional scale (Prandi et al., 2021).
On the second hand, the sea level estimates rely on the L2 ocean retracking of the radar altimeter waveforms, which provides the altimeter range (at the origin of the raw sea surface height), and other altimeter parameters such as the Sigma-0 roughness coefficient and Significant Wave Heights (Chelton et al., 2001). A stable retracking algorithm thus contributes to the stability of the mean sea level. The MLE4 retracking algorithm is the solution used in the Geophysical Data Records distributed to the users for each altimeter mission (Amarouche et al., 2004). The evolution of the instrumental characteristics (given by the mean of its Point Target Response, PTR) is continuously monitored and static Lookup Tables are used to correct range estimates from the differences between a true instrument PTR and the Gaussian approximation. The drift of the PTR (Internal Path Delay) is thus accounted for to produce the estimates of the range. However, the asymmetry of the PTR is not considered in the MLE4-based range nor the temporal evolution of this asymmetry. Note that recent work has led to the development of a numerical adaptive retracking solution for which the actual PTR is numerically introduced in the algorithm (Thibaut et al., 2020, 2021). With this solution, all drifts or instabilities of the PTR are thus « natively » accounted for (without any approximation), which further improves the mean sea level stability. The adaptive retracking is currently distributed in the Geophysical Data Records of the Jason-3 mission. Note that the impact of the retracking on the sea level uncertainties is relatively reduced and this source of error has not been specifically identified in the existing error budgets.
Calibration and Validation Activities
Calibration and validation (Cal/Val) activities (funded by the space agencies) are essential to ensure the quality of the Copernicus sea level products (previously known as “AVISO products”) and in particular, the sea-level stability strongly relies on these L2P activities. These activities include the assessment of the instrument performances in order to detect any anomaly that may affect the range estimates (Quartly et al., 2020). In addition, the monitoring of all geophysical and environmental altimeter corrections used to compute the sea level anomalies (Escudier et al., 2017) is a vital part of the Cal/Val activities so that any drift can be detected in the sea level observations. Indeed, some of the geophysical corrections are one of the main contributors to the altimeter sea-level rise uncertainty, such as the wet tropospheric correction derived from the microwave on-board radiometers (Legeais et al., 2014; Ablain et al., 2019). The Cal/Val activity is of particular importance for the missions currently in operation for which this activity is performed routinely.
Quality assessment of altimeter data can be performed thanks to internal comparisons (analyses of performances at crossover points between ascending and descending tracks) and cross-calibration with other altimeter missions flying at the same time. A third approach is to compare with external independent measurements derived from numerical models or in situ instruments. In particular, tide gages are extensively used (Mitchum, 1998; Valladeau et al., 2012; Watson et al., 2015) since they provide continuous high frequency sea level measurements over long periods although they are only located in the coastal ocean and the instruments are not homogeneously distributed over the coasts (hemispheric bias, see for example Prandi et al., 2015). Sea level dynamic heights derived from the in situ temperature and salinity profiles from the global Argo network are also of interest for Cal/Val activities (Valladeau et al., 2012; Legeais et al., 2016). Indeed, even if the physical content (steric height) cannot be directly compared to the altimeter observations of the total water column, the in situ observations can be used to detect geographically correlated altimetry errors (like hemispheric biases or other regional biases) and to assess the performances of orbit solutions at regional scale, as illustrated on Figure 2 with the impact of an updated orbit standard on the sea level regional consistency from Jason-1 mission (see also Couhert et al., 2015). At last, absolute calibration of the different altimeter missions is also performed in different sites located along a few tracks of the satellites (Watson et al., 2011; Mertikas et al., 2018; Bonnefond et al., 2019). This also contributes to the quality assessment of the altimeter MSL estimates.
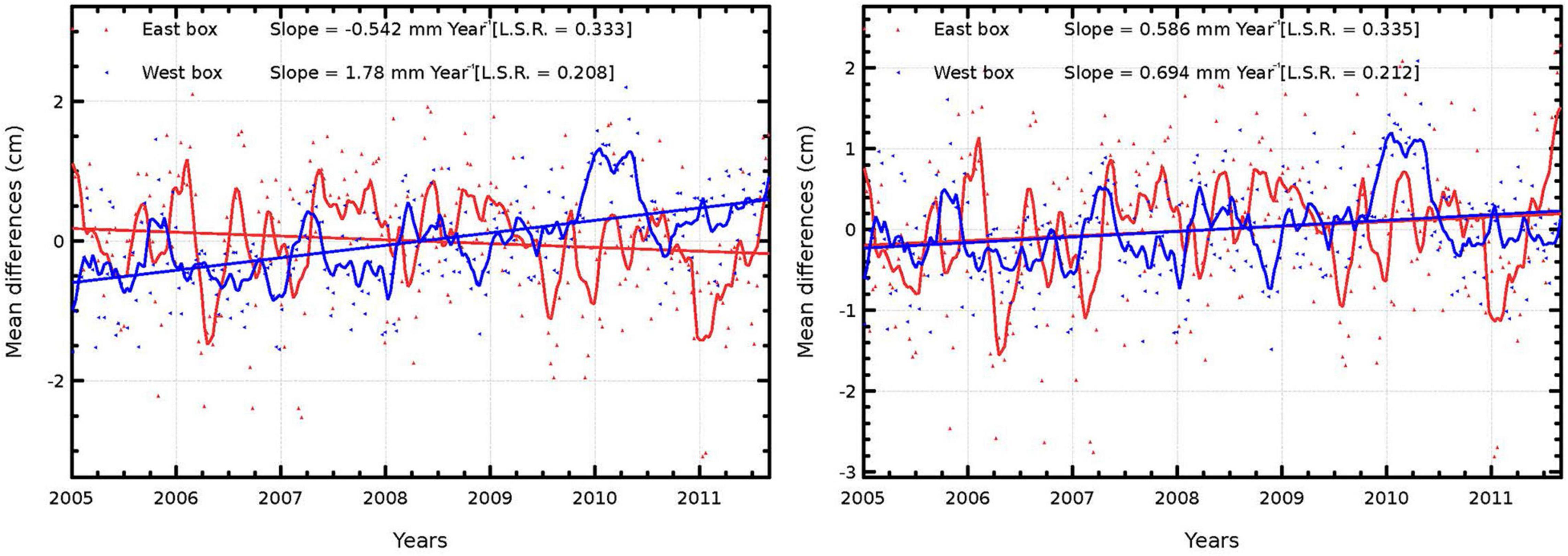
Figure 2. Sea Surface heights differences (cm) between Jason-1 altimeter data (cycles 1–355) and dynamic height anomalies derived from the Argo in situ measurements (referenced to 900 dbar) computed with GDR-D (left) and GDR-E orbit solution (right), separating east box (Long: 60°/120°, Lat: –30°/30°, in red) and west box (Long: –150°/–190°, Lat: –50°/10°, in blue). Corresponding annual and semi-annual signals are removed. Trends of raw data (dots) are indicated, and the 2-month filtered signal is added (curves). The hemispheric trend bias is reduced from 2.32 to 0.1 mm/yr. Adapted from Legeais et al. (2016).
L2P Altimeter Standards
The L2P altimeter data used as input to the DUACS production system are homogeneous along-track datasets of validated sea level anomalies for all past and present missions (see example on the top panel of Figure 3). These products also include all corrections used for the computation of these sea level anomalies as well as a validity flag (spurious measurements impacted by rain cells, sea ice or the coast are identified) and inter-mission biases (see next subsection). These datasets are key elements to ensure that the sea level ECV derived from the Copernicus products follows as much as possible the GCOS requirements.4 The L2P datasets used for the production of the current version (vDT2018) of the delayed-time Copernicus sea level products (Taburet et al., 2019) follow the recommendations made by the sea level project of the ESA Climate Change Initiative (SL_cci).5 Within this project, optimized altimeter algorithms have been developed, tested and selected through a dedicated “round robin” approach in order to improve the homogeneity and stability of the sea level record. This led to the production of a delayed-time gridded monthly sea level product covering the period 1993–2015 (Ablain et al., 2015; Quartly et al., 2017; Legeais et al., 2018). The L2P products benefit from regular full reprocessing from space agencies (CNES and EUMETSAT). They include the latest L2 reprocessing from individual missions and the latest geophysical and instrumental corrections recommended by the ocean science topography community, aiming at providing unified and up-to-date datasets for all past and present altimetry missions. The next version (vDT2021) of the Copernicus L4 sea level products is derived from the new 2021 release of the L2P product, produced by CNES/AVISO (Lievin et al., 2020).
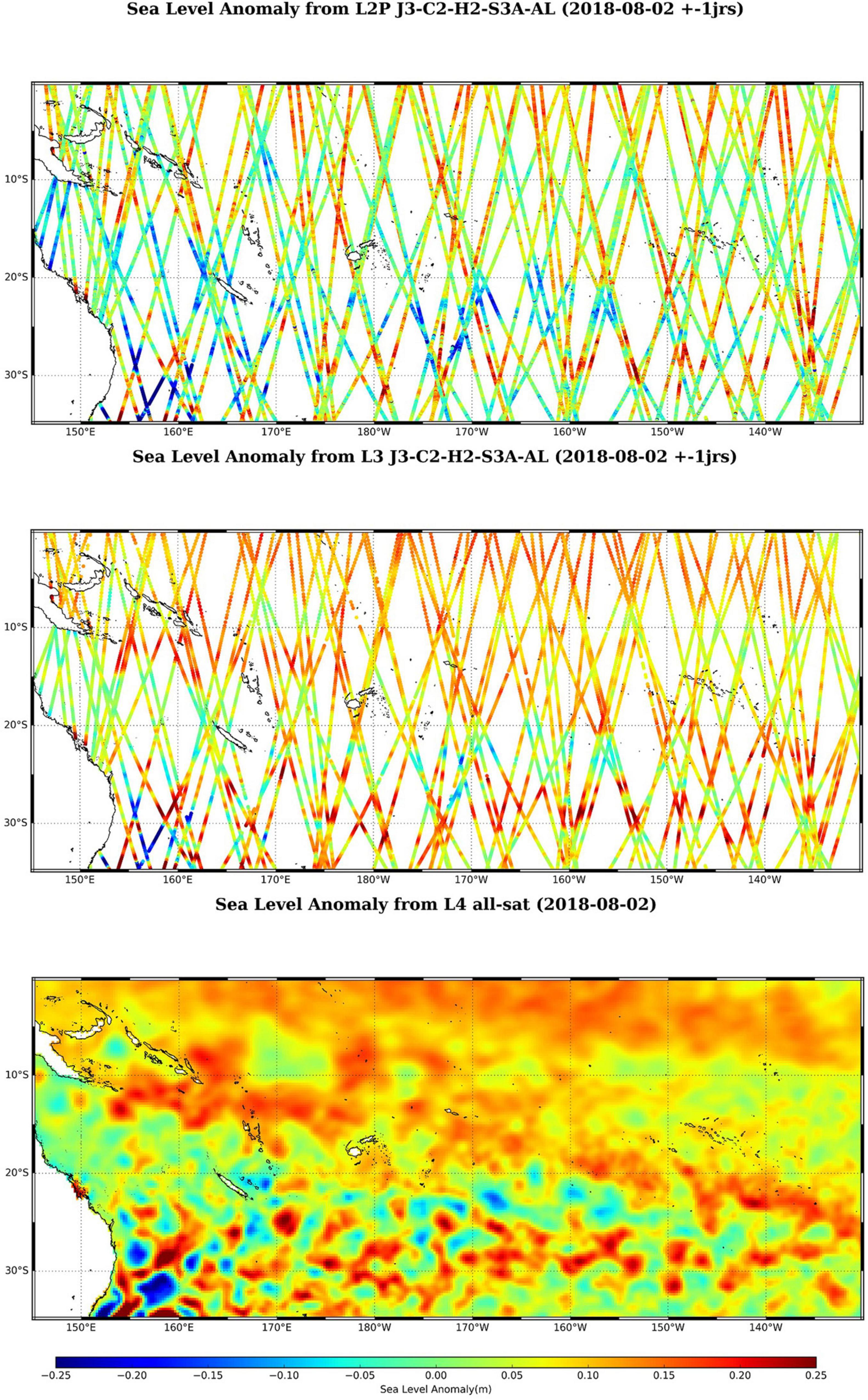
Figure 3. Sea level anomalies L2P input data processed by the DUACS production system from Jason-3, CryoSat-2, HY-2A, Sentinel-3A, and SARAL/AltiKa missions on 02/08/2028 (± 1 day) in the South-West Pacific Ocean (top). The corresponding along-track cross-calibrated L3 and merged L4 data are shown in the middle and bottom panels, respectively.
The Altimeter Reference Missions
The long-term stability of the sea level record is ensured by the TOPEX-Poseidon, Jason-1, Jason-2, and Jason-3 reference missions (and soon Jason-CS/Sentinel-6 MF mission). They are thus essential for the computation of the sea level trends (Ollivier et al., 2012; Ablain et al., 2015). They cover the ± 66° latitude band and have a repeat cycle of 10 days. Other complementary and opportunity missions (ERS-1, ERS-2, Envisat, SARAL/AltiKa, HY-2A and 2B, Sentinel-3A and 3B, and CryoSat-2) are homogenized with respect to the reference missions (with the reduction of the intermission sea surface heights differences at crossover locations) (Ducet et al., 2000; Pujol et al., 2016). These missions improve the geographical sampling of mesoscale processes by improving the spatiotemporal resolution, they provide high-latitude coverage (up to ± 82°) and increase the sea level accuracy.
Another crucial step required to compute the long-term sea level record is the reduction of the inter-mission biases at the transition between two successive missions. During these periods (e.g., between Jason-2 and Jason-3), calibration phases are planned for a few months during which the two successive satellites fly in tandem on the same ground track a few tens of seconds apart and thus observe the same ocean, allowing the computation of global and regional sea level biases between both missions. Despite the available measurements during the tandem phase, there are remaining uncertainties on these inter-mission biases, which directly affect the uncertainty of the trend of the GMSL evolution. The impact can reach 0.2 mm/yr of uncertainty during a 25-year-long period (SL_cci CECR, 2016; Zawadzki and Ablain, 2016; Guerou et al., 2020). Note that the estimates of the inter-mission biases strongly depend on the altimeter standards used in the sea level computation and thus, they have to be computed every time reprocessed sea level measurements are available.
L3 and L4 Production
In the context of the Copernicus activities, the DUACS system is used for the operational production of the inter-calibrated along-track (L3) mono-mission sea level products (ready to be assimilated in ocean models) and the multi-mission merged gridded 1/4° products (L4). After removing the biases between the different missions, long wavelength errors (e.g., orbit errors) are removed thanks to multi-mission crossover minimization (Dibarboure et al., 2011) and remaining aliased short scale signals are also filtered out (see middle of Figure 3). Measurements from all missions are merged in the gridded products with an objective analysis approach (Ducet et al., 2000; Le Traon et al., 2003; see bottom of Figure 3). The present paper does not aim at providing the details of the methodology related to the altimeter processing and production system. Readers interested in more information on the specification of the Copernicus altimeter sea level products are invited to consult the product user manuals of the respective Copernicus services (see Table 1 for the accesses to the documentation) as well as Pujol et al. (2016) and Taburet et al. (2019).
The delayed time DUACS products are regularly reprocessed (every ∼4 years) in order to consider the latest progress achievements in the upstream data (e.g., improved geophysical corrections, homogenization of the time series, new retrackings, etc.) and evolutions in the L3/L4 processing (e.g., improved parameters of the mapping technique). Since the beginning of the Copernicus service, the altimeter sea level Climate Data Record has benefited from three consecutive full reprocessing, named DUACS DT2010 (Dibarboure et al., 2011), DT2014 (Pujol et al., 2016), and DT2018 (Taburet et al., 2019). Each version benefits from regular temporal extensions (∼3 times per year) with the production of the Interim Climate Data Record, to keep the time series close to present time. This production is made possible thanks to the availability of the upstream input L2P datasets distributed by the different space agencies (see above).
The DUACS production system is regularly upgraded to ensure the best quality of the products. Implementation of a fully reprocessed version usually requires evolutions of the different processing parameters. System’s evolutions are also required to ensure the routine production and take into account the different changes in the constellation (see Figure 4): new missions are introduced in the system while past missions are deactivated, and specific evolutions may be required to avoid any impact of measurements with a reduced quality on the DUACS output products.
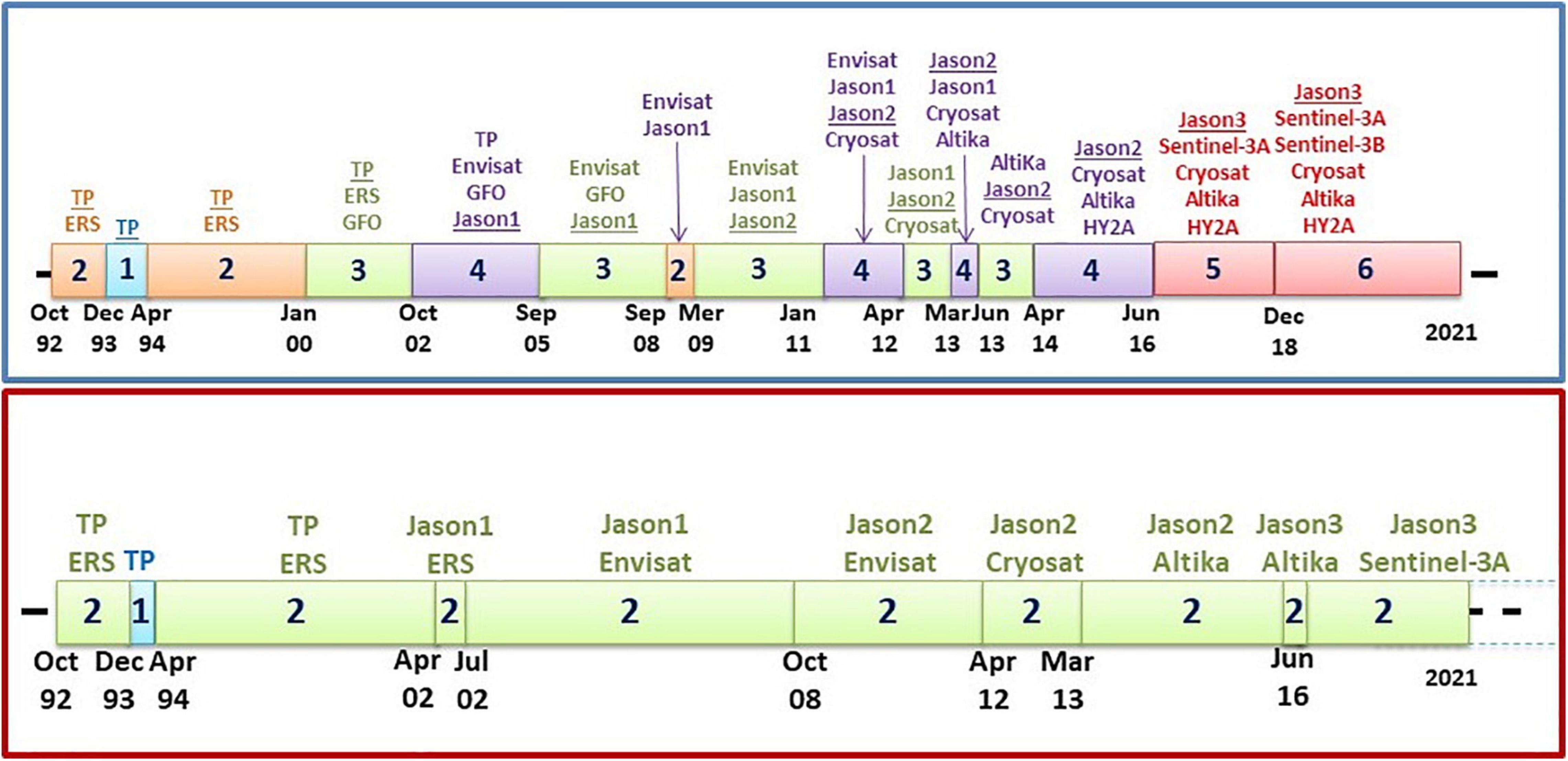
Figure 4. Number of altimeter missions considered in the constellation of both CMEMS and C3S altimeter sea level datasets.
An extensive quality assessment is performed before disseminating the products. A large spectrum of metrics are used to check the sea level time series integrity (no missing values; no corrupted file, etc.) as well as the quality of the various parameters delivered. The validation approach is based on different type of information, varying from the most simple (e.g., along-track statistics of the different variables) to more complex (e.g., statistics at altimeter cross-over positions), implying independent measurements (e.g., tide gauges, drifters, independent altimeter data not used in the processing) and other internal data for validation purpose (see Table 1 for the accesses to the quality information document and product quality assessment report distributed by the Copernicus services).
The Copernicus Altimeter Sea Level Products and Ocean Monitoring Indicators
The processing steps presented above are integrated in the sea level production center of the Copernicus program, and we present here the different sea level products available for the users and discuss their maturity. They allow the estimate of added-value ocean monitoring indicators (L4P) such as the mean sea level evolution. The associated trend and acceleration are presented, and we highlight the importance of the determination of the associated uncertainties.
Two Copernicus Altimeter Sea Level Products
The DUACS altimeter production system delivers two different types of delayed-time sea level datasets. In the first one, all the altimeter missions are processed to optimize the ocean sampling at each moment, allowing the retrieval of a large range of wavelengths, as required for ocean modeling and ocean circulation analyses. This dataset is produced by the Copernicus Marine Environment Monitoring Service (CMEMS).6 A second sea level dataset focuses on the monitoring of the long-term evolution of sea level for climate applications and the analysis of ocean/climate indicators. This climate-oriented dataset ensures the homogeneity and stability of the record (mainly based on the ESA SL_cci legacy) and it is produced by the Copernicus Climate Change Service (C3S).7 Numerous altimeter algorithms are required for the sea level production, and it is complex to ensure both the retrieval of high-resolution mesoscale signals and the stability of the large-scale wavelengths. This has led to the production and distribution of two altimeter sea level datasets available for the users in the climate (C3S) and marine (CMEMS) datastores (see Table 1 for accesses to the documentation and data; note that the C3S sea level dataset is also distributed in the CMEMS catalog). Both delayed-time datasets are available as daily 1/4° global gridded files derived from optimal interpolation and available from January 1993 with a ∼6 months timeliness. Temporal extensions are provided ∼3 times per year and a full reprocessing is performed every 4 years (the latest version vDT2021 will be released in 2021). The same L2P input data are used for both datasets and the main differences between the CMEMS and C3S altimeter sea level datasets concern the two following elements:
(i) The number of altimeters used in the satellite constellation: all available altimeters are considered in the CMEMS products whereas a steady number (currently two) of altimeters are included in the C3S products (see Figure 4). Previous studies (Pascual et al., 2006; Dibarboure et al., 2011) underscored the necessity of a minimum of a two-satellite constellation for the retrieval of mesoscale signals. The total number of satellites strongly varies during the altimetry era and this varying number has currently no impact on the global MSL estimate. However, when focusing on a regional/basin scale, the introduction of a new satellite in the constellation can be at the origin of some bias in the MSL time series, affecting the stability of the sea level record. To illustrate this, Figure 5 presents the impact of the change of the number of satellites in the constellation in the vDT2018 CMEMS dataset in terms of MSL averaged in the Black Sea area. The MSL derived from the all-satellite merged CMEMS product is compared to the MSL derived from the same product where only two satellites are used in the computation of the sea level anomalies, highlighting the impact of the number of satellites considered. A 1.5 cm increase of the MSL difference is observed in early 2012 (right axis, in red), corresponding to the change from Envisat to CryoSat-2 in the two-satellite merged dataset and to the Envisat mission termination (CryoSat-2 was already included) and the change of Jason-1 from a repeat to a drifting geodetic orbit in the all-satellite merged dataset (cf Figure 1). The observed MSL differences (red curve, of the order of ± 1 cm) contribute to the uncertainties in the MSL trend value [estimated here to be 0.3 mm/yr during the period (2009, 2016) considered for this figure].
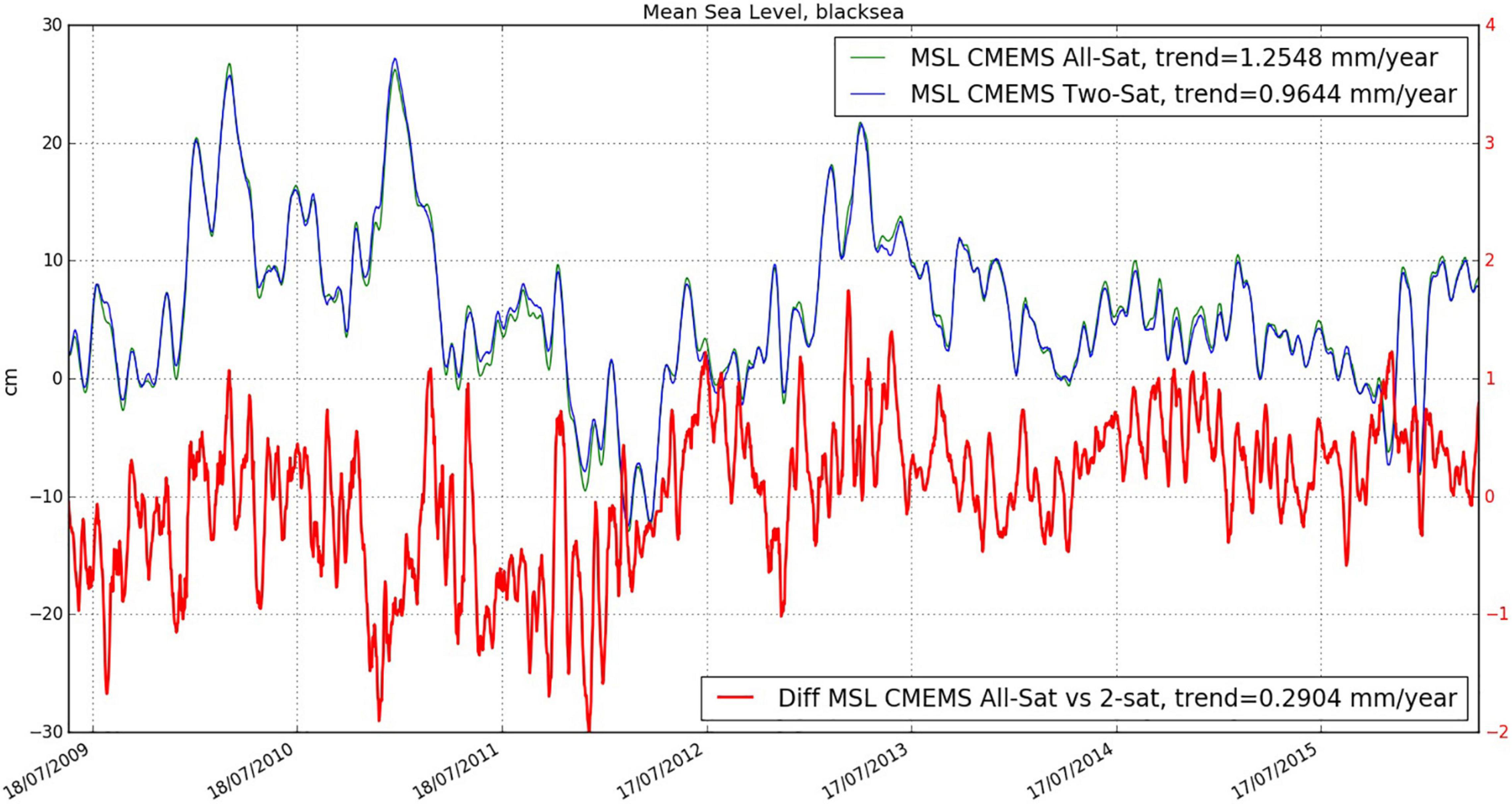
Figure 5. Area-averaged mean sea level in the Black Sea: impact of the difference of constellation between the all-satellite merged dataset (CMEMS vDT2018, in blue) and the corresponding dataset in a two-satellite merged configuration (in green), in terms of mean sea level in the Black Sea and their difference (in red, in cm).
The risk of introducing a bias remains with the integration of a future mission. This explains why a stable number of satellites (two) has been preferred for the design of the C3S product. Indeed, even if the spatial sampling is reduced with less satellites, such a choice contributes to the MSL stability (the bias introduction is more related to missions experiencing a change of orbit and these missions are usually not included in the C3S product). In the CMEMS product, the stability is provided by the reference missions and the mesoscale errors are reduced due to the improved ocean surface sampling thanks to the use of all satellites available in the constellation. Note that the varying number of satellites in the constellation also affects mesoscale signals, especially the number of eddy detections (Pegliasco et al., 2021). It is pointed out that both CMEMS and C3S sea level datasets are of interest for eddy detection and tracking application, depending on the final goal of the study: the C3S dataset rather ensures a stability in the retrieved mesoscale activity (EKE, number of detections through the time) whereas the CMEMS dataset provides a higher resolution and thus improved location and other characteristics (e.g., amplitude, size, shape) of the eddies.
(ii) The reference field used to compute sea level anomalies: an optimized reference field (mean along-track profiles of sea surface heights) is used for missions with a repetitive orbit in CMEMS to provide the best sea level estimates, especially in coastal areas. The use of a mean profile is, however, not possible for all missions (e.g., CryoSat-2, or more generally missions with a geodetic orbit). In these cases, a gridded Mean Sea Surface must be used and the merging of the sea level anomalies computed with the two different methods can introduce large scale errors affecting the MSL stability. Even if this has no impact on a global scale (with respect to the uncertainty on an interannual timescale), the MSL stability can be impacted on a regional scale. In the C3S sea level product, the gridded mean sea surface is used for all missions (as in the ESA SL_cci product), which can introduce errors at short wavelengths but ensures optimal estimates of the large-scale signals.
Figure 6 shows the difference of MSL in the Black Sea area between two altimeter datasets: they are both based on a stable two-satellite merged constellation but one is computed with a homogeneous mean sea surface reference field for all missions (i.e., the C3S product) whereas a mix of reference fields is used in the other dataset. Some jumps of up to 1 cm are observed in the difference of area-averaged sea-level in the Black Sea, corresponding to changes from a mission using a mean profile of sea surface heights or a mean sea surface (introduction of CryoSat-2 in 2011 and SARAL/AltiKa in 2013 and the change of this latter mission to a drifting orbit in 2015). This illustrates some remaining inhomogeneities between these two types of mean references, which should be reduced in the next version of the product (vDT2021). The associated impact in terms of MSL trend reaches almost 0.5 mm/yr during a 15-year long period [2002, 2016] (note that this impact varies according to the period considered and the date of the events within this period). Thus, the systematic use of a homogeneous reference field for the computation of sea level anomalies of all missions contributes to ensure the stability of the altimeter regional sea-level in the C3S dataset. On the other hand, the use of mean sea surface height profiles for repetitive missions remains of interest to increase the accuracy of the product at short scales (as requested for the CMEMS dataset).
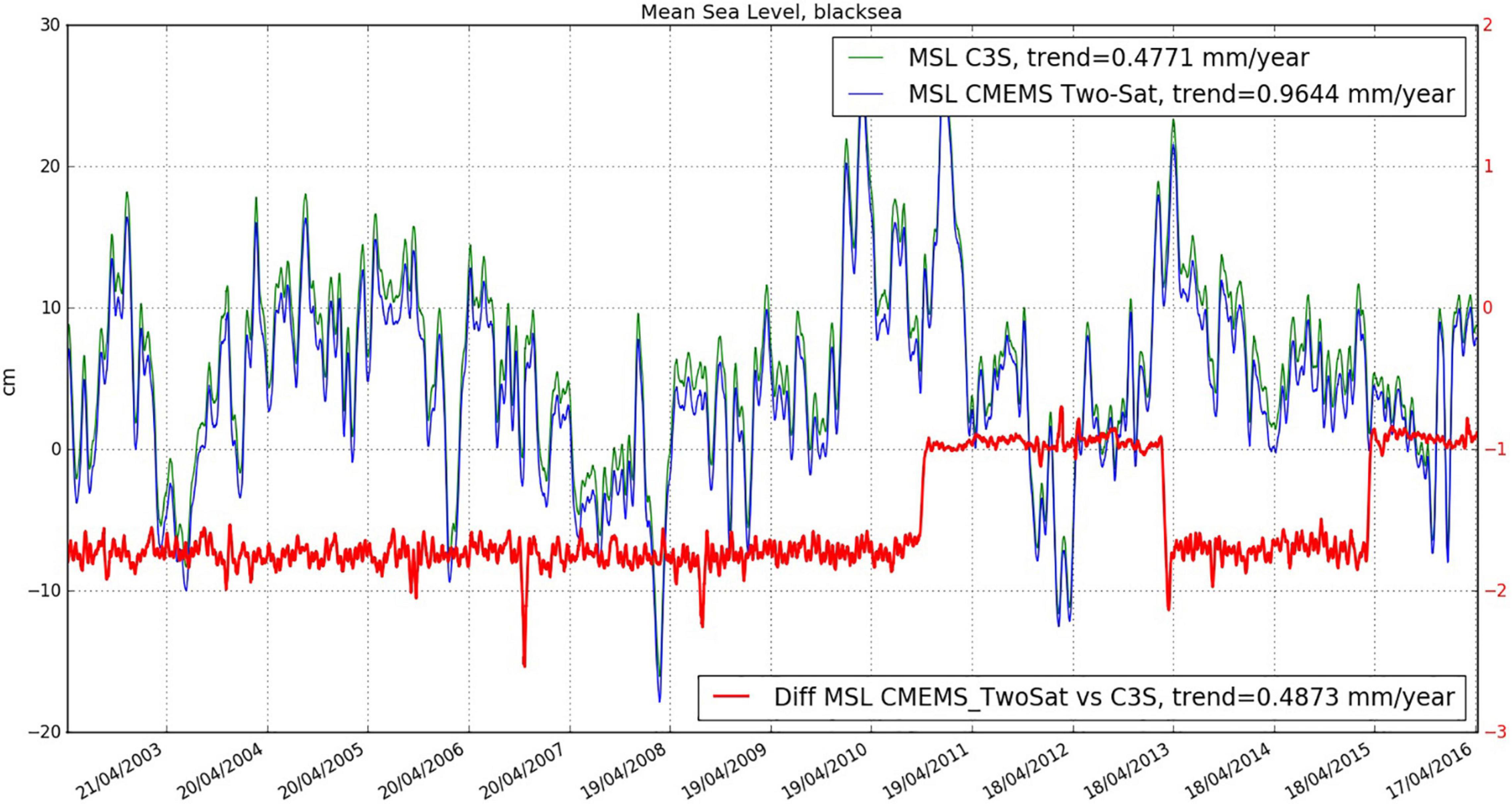
Figure 6. Area-averaged mean sea level in the Black Sea computed with two-satellite merged gridded global datasets including a homogeneous mean sea surface reference field for all missions (in green) and a combined use of a mean sea surface and mean profile of sea surface heights for missions with a drifting or repeat orbit, respectively (in blue). The difference between both regional mean sea level is shown in red (y-axis on the right, in cm).
Product Maturity
The production of these sea level datasets (previously known as “AVISO products”) is mature enough to be implemented operationally within the E.U. Copernicus services. However, the operational production requires some good practices in order to ensure the quality of the service for a vast range of users. Among its wider activities, the World Meteorological Organisation (WMO) fosters collaboration to develop technical guidance and standards for the collection, processing and management of data and forecast products. Part of this process aims at providing a tool that enables data providers to assess and rate their datasets quantifiably based on internationally validated data stewardship best practices. A dedicated stewardship maturity matrix is used to evaluate if the production of a data record follows best practices for specific aspects and facilitation of usage (with a maximum grade of 5). The tool is an essential part of ensuring and improving the way datasets are documented, preserved, and disseminated to users (Peng et al., 2019; Dunn et al., 2021). It also enables data providers to determine a roadmap for future development and improvement, as well as compare their process against other data providers. Note that the maturity assessment is only related to aspects of process maturity and does not guarantee the scientific reliability of a given climate data record.
The WMO stewardship maturity matrix has been used to assess the altimeter sea level Climate Data Record produced and distributed by C3S (Dunn et al., 2021). In terms of “Data Access” (ability to find and obtain the dataset), the grade is 5 for the “Discoverability” aspect since the dataset is searchable and easily available through the online institutional C3S catalog and 4.5 for “Accessibility” due to the Climate Data Store interface and the associated toolbox (no spatial subsetting is possible when downloading the data and all variables have to be downloaded together). Regarding the “Usability and Usage” (easy to use and impact of its usage), the attributed grade for “Data Portability” is 4.5 since the data are distributed as NetCDF (Network Common Data Form) files, compliant with the Climate and Forecast (CF) convention, but no other format is available. A grade of 5 is given for “Documentation” and “Usage” aspects since the dataset is fully documented and has been referenced in international climate assessment and published reports. In terms of “Quality Management,” the quality assurance procedure is fully documented with an additional independent evaluation and quality control performed by the Copernicus service. Target requirements and a detailed gap analysis are available, and details of the error budget have been published in peer-reviewed journals, leading to a grade of 5 for both “Quality Assurance and Assessment” aspects. The same grade of 5 is given for “Data Integrity,” which is systematically verified with a standard approach to ensure that the distributed data are the same as the initial data files. Finally, regarding the “Data Management” category, a grade of 4 is attributed for “Data Preservation” since the data are distributed on an institutionally maintained platform and are archived following a defined and implemented procedure which agrees with community standards. A grade of 5 is given for both “Metadata” and “Governance” aspects since the dataset is distributed with comprehensive metadata, detailed documentation and versioning system, and governance aspects are well-defined within the E.U. Copernicus programme and are compliant with international standards.
Mean Sea Level Indicators: Key Information for Adaptation and Mitigation Strategies
As presented earlier, the GMSL is a key ocean monitoring indicator that can be derived from satellite altimetry. It can either be computed by directly averaging the validated along-track data of the reference missions (as distributed by AVISO; see also Ablain et al., 2017)8 or averaging the multi-mission merged gridded Copernicus datasets (as distributed by Copernicus).9 The associated GMSLs are considered to be identical since the same altimeter standards are used to compute the sea level anomalies, and the long-term stability is ensured by the same reference missions. The remaining observed GMSL differences are not significant given the uncertainty considered on different scales (Ablain et al., 2015, 2019; Prandi et al., 2021).
The GMSL shows a significant rise over the 26-years of altimetry data record (1993–2021) of + 3.1 ± 0.4 mm/yr (at the 90% confidence level) (Figure 7; WCRP Global Sea Level Budget Group, 2018; Ablain et al., 2019). This means that over these 26 years, the sea level has risen by ∼8 cm on average, globally. At the regional scale, the SLR distribution ranges between 0 and 6 mm/yr (Figure 8), with uncertainties ranging from ± 0.8 to ± 1.2 mm/yr depending on the location. On the overall, sea level is rising almost everywhere over the globe (Prandi et al., 2021). Not only is the sea level rising, but it is accelerating (Dieng et al., 2017; Nerem et al., 2018; Ablain et al., 2019). This acceleration has been quantified at 0.12 ± 0.06 mm/yr2 (90% confidence level) at the global scale (Ablain et al., 2019) once the TOPEX/Poseidon drift is empirically corrected for (WCRP Global Sea Level Budget Group, 2018). At regional scales, the sea-level accelerations range between −1 mm/yr2 and + 1 mm/yr2 with uncertainties between 0.057 and 0.12 mm/yr2 (Prandi et al., 2021). A strong added value to the sea-level ECV is the provision of its uncertainties. They are essential to characterize the reliability of the observing systems and avoid misinterpretation of signals that could potentially come from observational errors. Quantifying the error at each level of the observational systems (instrumental, processing) is a difficult task and has been addressed by any group. An alternative approach based on error budgets has been proposed by Ablain et al. (2019) at the global scale, and recently adapted to the regional scale by Prandi et al. (2021). Such approach allows to specify a posteriori the different sources of errors contained in the data records. Different types of errors are provided such as inter-mission biases, correlated errors at different time scales (typically 2-months, 1 and 5 years), and linear drifts (orbits, ITRF, GIA). A variance-covariance matrix has been derived from this error budget and, combined with an ordinary least square estimation method to derive uncertainties on the trend and acceleration of sea level (see Ablain et al., 2019; Prandi et al., 2021 for more details).
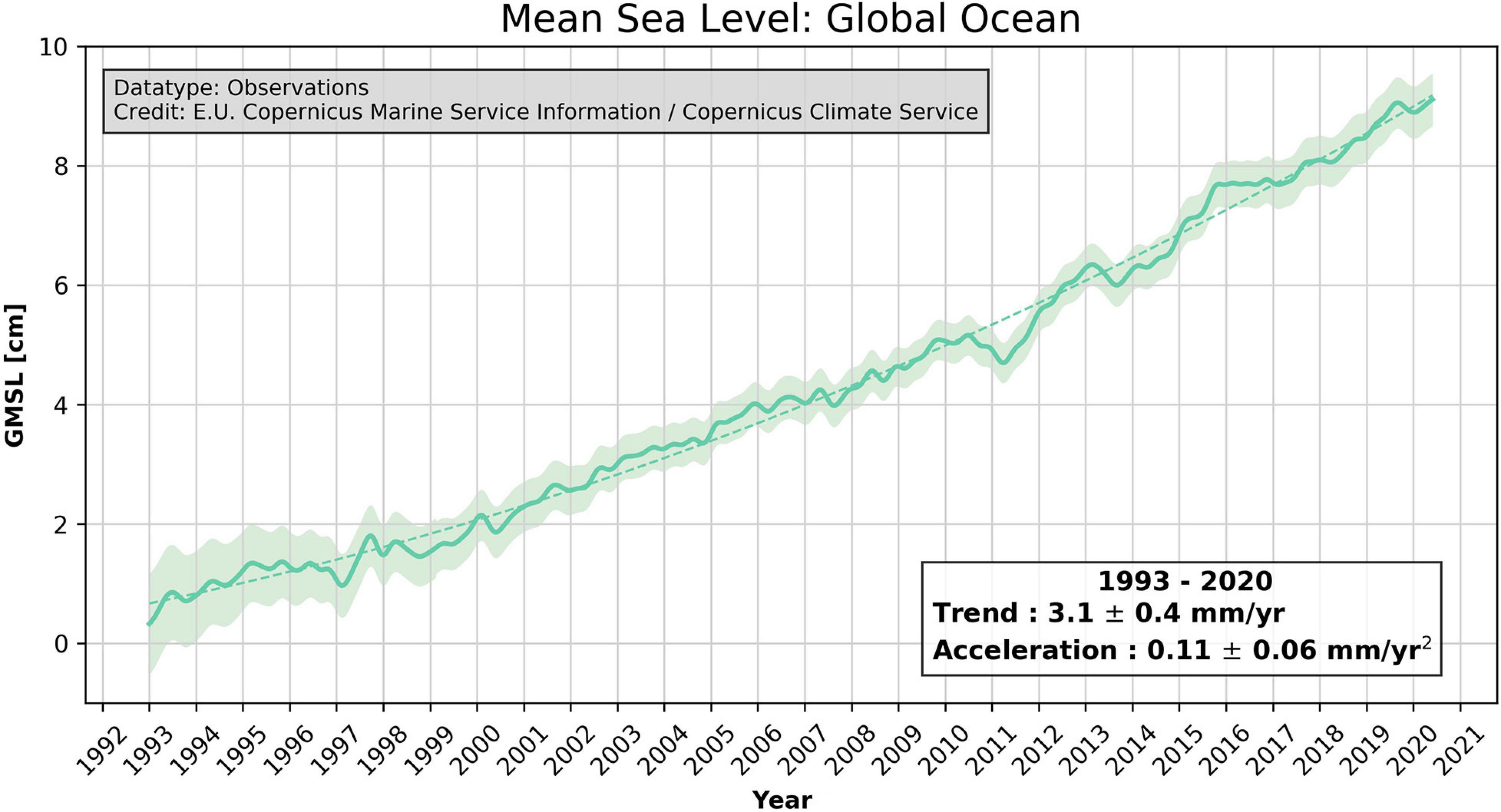
Figure 7. Global Mean Sea Level derived from the altimeter C3S multi-mission gridded sea level product (DUACS vDT2018) from January 1993 to June 2020. The global average is derived from box-averaged gridded sea level maps weighted by the cosine of the latitude. The timeseries is low-pass filtered, the annual and semi-annual periodic signals are adjusted. The curve is corrected for TOPEX-A instrumental drift during 1993–1998 (WCRP Global Sea Level Budget Group, 2018) and is corrected for the GIA effect. Quoted uncertainties are within a 90% confidence level (adapted from Ablain et al., 2019).
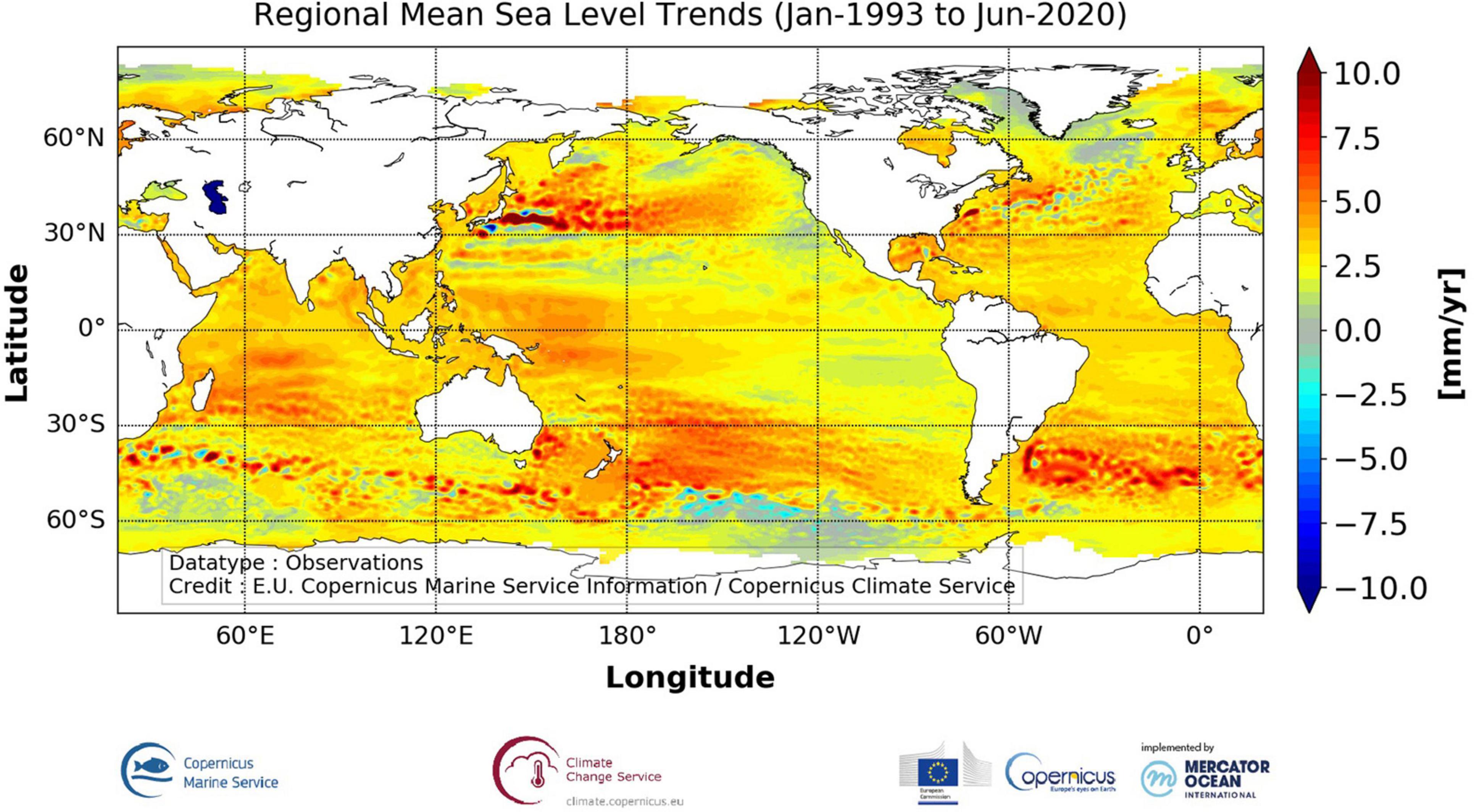
Figure 8. Regional distribution of the trends of the satellite altimeter sea level observations (in mm/yr) from January 1993 to June 2020. The trends are derived from the altimeter C3S multi-mission gridded sea level product (DUACS vDT2018). The trends are not corrected for the TOPEX-A instrumental drift nor for the GIA effect.
Remaining Gaps and Recommendations
As mentioned in the last IPCC report (chapter 4 of IPCC, 2019), the knowledge of the GMSL estimate as well as the determination of the regional and coastal sea level changes are of major importance for understanding SLR, validating climate model simulations of past and future SLR and for societal applications. In this section we identify the remaining gaps so that the sea level ECV products keep on answering the users needs and contributes to support public policies to adapt to and mitigate SLR. The first key element is to ensure the continuity of the sea level record. In terms of observing system, the long-term production of the Copernicus altimeter sea level products relies on the continuity of the Copernicus satellite missions. This continuity of altimeter measurements is essential for climatology, oceanography, hydrology and also marine glaciology. Sentinel-6A MF will be soon used as a reference mission (ensuring the stability of the record) and in the future, Sentinel-3C, 3D and Sentinel-6B as well as the SWOT prototype will be launched before 2025. However, most other missions are expected to reach their end of life before this date (CryoSat-2, HY-2A, B and C, Jason-3, Sentinel-3 A and B, CFOSat), which makes the future of the altimeter time series uncertain. Only Sentinel-3 Next Generation (NG) mission (after 2025) and Sentinel-6 NG mission (after 2030) are planned to be launched (and probably the HPCM Cristal mission, providing an improved resolution and spatial coverage at high latitudes). It is thus of major importance that these future Sentinel NG missions are launched to guarantee the long-term continuity of the sea level measurements and that Sentinel-6 NG flies on the same orbit as its predecessors so that the climate continuity is provided. A second major objective is to maintain the homogeneity and consistency of the altimeter sea level record. This requires the reprocessing of the past missions so that they remain up-to-date by space agencies (e.g., the on-going TOPEX-Poseidon L2 reprocessing by CNES/JPL) and the use of homogeneous algorithms for all missions (e.g., ERA-5 forcing for atmospheric altimeter corrections in the CNES 2021 L2P reprocessing) with adequate inter-mission calibration.
Another crucial element is to reduce altimeter sea level errors and to better characterize the associated uncertainties. Indeed, the GMSL estimate provides an essential source of information for several key questions of the World Climate Research Programme such as the detection and attribution of the sea level forced response to climate change, the detection of changes in the contributions to SLR (e.g., acceleration in ice mass loss, terrestrial water storage evolution, etc.; see IPCC, 2019), the detection of potential new contributions to SLR (e.g., the permafrost thawing, decrease of persistent snow cover or the increase of water vapor content, etc.; see WCRP Global Sea Level Budget Group, 2018), or the estimates of the energy imbalance at the top of the atmosphere that is responsible for climate change (Meyssignac et al., 2019). Different amplitudes and time scales are involved in these different physical processes. The current GCOS requirement on the stability of the GMSL (of ± 0.3 mm/yr over a decade) is probably enough to detect the current total GMSL rise and acceleration due to greenhouse gases (GHG) emissions but it is not enough to detect a potential contribution of the permafrost thawing or to help understand the change of Earth energy imbalance during Hiatus periods (of the order of 0.1 W.m–2 over 15 years; see Hedemann et al., 2017), which will rather require a ± 0.1 mm/yr accuracy over a decade (Meyssignac et al., 2019). On time scales longer than a decade, the acceleration and the trend in forced sea level may change in response to climate change mitigation policies. These evolutions are slow and may not exceed a few tenth of mm/yr over 20-year windows (see the difference in SLR between the RCP2.6 scenario and the RCP 8.5 scenario along the twenty-first century in IPCC, 2019). If we want to monitor these changes to support mitigation policies we will need an accuracy of the order of ± 0.1 mm/yr over 20-year windows which is beyond the current GCOS requirements. Reaching such a level of accuracy is a major challenge and we will have to make the most of future altimeter missions to go in this direction.
At regional scale, the accuracy in the altimeter SLR estimates is above ± 1 mm/yr over 10 years for regions of 1,000 square km and larger (Prandi et al., 2021). This is clearly not sufficient because the expected sea level signal forced by the anthropogenic GHG emissions is at the level of [0.5–0.8] mm/yr over 10 year and longer time scales for regions of 1 000 square km and larger (see for example Palanisamy et al., 2015; Fasullo and Nerem, 2018). Thus, the detection of the regionally forced signal requires the reduction of all sources of uncertainty in sea level estimates below this level of 0.5 mm/yr over regions of 1,000 square km. In order to reduce the uncertainty due to the altimeter instrument, Ablain et al. (2020) have suggested to plan a second 2 to 3 month tandem calibration phase (approximately 1.5–2 years after the first tandem phase) between Jason-3 and Sentinel-6A. Simulations show that this second tandem phase would allow linking the MSL from both missions with an accuracy below ± 0.5 mm/yr regionally and thus identify any issues or error between missions that lead to higher signals.
Another remaining gap is related to the improvement of the sea level estimates in some key regions. In ice-covered areas at the poles, the uncertainty on the sea level estimates remains relatively high due to the deteriorated conditions of retroflexion of the radar echo. The efforts made to better retrieve the sea level evolution in these regions should be encouraged to better understand its contribution to the global SLR. In coastal areas, it remains unclear if the sea level increases at the same rate as the SLR as seen by altimetry in the open ocean. The Climate Change Initiative Coastal Sea Level Team (2020) suggests that global gridded sea level products provide enough information to assess coastal sea level trends but this is not the case in some specific sites. More studies are required on the altimeter sea level products to understand the different processes involved in coastal SLR. At the coast, the altimeter observing system provides the sea surface height of the total water column whereas the information of interest is rather the sea level relative to the shore (Wöppelmann and Marcos, 2016). Indeed, vertical land motions remain the main source of uncertainty in the relative sea level and efforts should be made on their improved characterization involving available information (tide gauges and GNSS measurements, InSAR technique). The risk assessment at the coast related to SLR is also made more difficult due to the complexity to determine a common reference level between digital elevation models over land and the mean sea surface reference level. The relative sea level is a combination of the global MSL, the regional variability and the result of small-scale processes (shelf currents, changes in wind and waves, modification of the sea water density due to freshwater input from estuaries etc.). Additional observations (from in situ networks) and high-resolution models would be a great asset to contribute to the understanding of all these processes and their impact on the coastal sea level changes.
Working on solutions to face the rising MSL challenges a wide range of disciplines (e.g., climate science, geosciences, social sciences). Scientists are working on estimating the local impacts of sea-level rise at the shore to help policy makers define mitigation and/or adaptation strategies. As the impacts and adaptation needs are a response to relative SLR including vertical land movements (rather than global SLR), they require the knowledge of all processes involved at the coast, and the potential of all techniques (as the ones listed earlier) should be optimized in interaction with other expert communities. Understanding the processes and assessing the societal consequences remains difficult due to the partitioning of the research community and an integrated and interdisciplinary approach is thus needed (see for instance the JPI 2020 Knowledge Hub on SLR,10 and the Copernicus support to public policies).11 In addition, engagement with coastal stakeholders and showcasing the already existing Earth observation products and associated services should be strengthened to enhance these products and further improve decision making (see for instance the CEOS Coastal Observations, Applications, Services, and Tools).12 Satellite measurements are of major interest to provide concrete evaluation of the impact of climate change on coastal territories in various areas such as flooding, bathymetry and coastline mapping, coastal eutrophication, turbidity and sedimentation. Today, these satellite Earth observations provide the framework that further allow the elaboration of adaptation strategies and efforts should be made so that adaptation decisions can be directly supported by these observations. This is one of the objectives of the CLS/CNES Space Climate Observatory “Littoscope” project, which investigates the capacity of satellite-derived high resolution Digital Elevation Model (DEM) to be used in climate modeling studies or early warning tools, in combination with the Copernicus altimeter regional sea level trends to help in the anticipation and mitigation of coastal flood hazards. The risk assessment is made further possible by crossing the hazard intensity with social, economic, natural and cultural exposures derived from a multi-sources approach (datasets from geographical information systems combined with land use information derived from HR optical satellite imagery). In the near future (∼5 years), depending on the accuracy of the satellite-derived information (DEM, regional sea level changes, etc.) and considering the associated uncertainties, such an approach will contribute to the development of adaptation strategies to SLR.
Data Availability Statement
The datasets presented in this study can be found in online repositories. The names of the repository/repositories and accession number(s) can be found below: cds.climate.copernicus.eu/cdsapp#!/dataset/satellite-sea-level-global?tab=overview; https://resources.marine.copernicus.eu/?option=com_csw&view=details&product_id=SEALEVEL_GLO_PHY_L4_REP_OBSERVATIONS_008_047.
Author Contributions
J-FL has written the article with contributions provided by AG (on “Ocean Monitoring Indicators”), M-IP (on “L3 and L4 production”) and CD (Littoscope project description). BM, YF, MA, and GD contributed to the improvement and finalization of the article. All authors contributed to the article and approved the submitted version.
Funding
The development of the DUACS altimeter production system has been supported by CNES since 2001 and the operational production has been funded by Copernicus since 2015 (CMEMS SL-TAC, C3S_312a_Lot2 and C3S_312b_Lot3 contracts).
Conflict of Interest
The authors declare that the research was conducted in the absence of any commercial or financial relationships that could be construed as a potential conflict of interest.
Publisher’s Note
All claims expressed in this article are solely those of the authors and do not necessarily represent those of their affiliated organizations, or those of the publisher, the editors and the reviewers. Any product that may be evaluated in this article, or claim that may be made by its manufacturer, is not guaranteed or endorsed by the publisher.
Acknowledgments
The authors thank Guillaume Taburet for his contribution to the preparation of a figure.
Footnotes
- ^ duacs.cls.fr
- ^ www.aviso.altimetry.fr/en
- ^ www.aviso.altimetry.fr/en/data/products
- ^ gcos.wmo.int/en/essential-climate-variables/about/requirements
- ^ climate.esa.int/en/projects/sea-level/
- ^ marine.copernicus.eu/
- ^ climate.copernicus.eu/
- ^ www.aviso.altimetry.fr/en/data/products/ocean-indicators-products/mean-sea-level.html
- ^ marine.copernicus.eu/science-learning/ocean-monitoring-indicators/catalogue/
- ^ www.jpi-climate.eu/joint-activities/sealevelrise.knowledgehub
- ^ marine.copernicus.eu/support-public-policies/
- ^ earthobservations.org/geo_blog_obs.php?id=521
References
Ablain, M., Cazenave, A., Larnicol, G., Balmaseda, M., Cipollini, P., Faugère, Y., et al. (2015). Improved sea level record over the satellite altimetry era (1993–2010) from the climate change initiative project. Ocean Sci. 11, 67–82. doi: 10.5194/os-11-67-2015
Ablain, M., Jugier, R., Marti, F., Dibarboure, G., Couhert, A., Meyssignac, B., et al. (2020). Benefit of a second calibration phase to estimate the relative global and regional mean sea level drifts between Jason-3 and Sentinel-6a. Earth Space Sci. Open Arch. 1, 1–10 doi: 10.1002/essoar.10502856.2
Ablain, M., Legeais, J. F., Prandi, P., Fenoglio-Marc, L., Marcos, M., Dieng, H. B., et al. (2017). Satellite altimetry-based Sea level at global and regional scales. Surv. Geophys. 38, 7–31. doi: 10.1007/s10712-016-9389-8
Ablain, M., Meyssignac, B., Zawadzki, L., Jugier, R., Ribes, A., Cazenave, A., et al. (2019). Uncertainty in satellite estimate of global mean sea level changes, trend and acceleration. Earth Syst. Sci. Data 11, 1189–1202. doi: 10.5194/essd-11-1189-2019
Amarouche, L., Thibaut, P., Zanife, O. Z., Dumont, J.-P., Vincent, P., and Steunou, N. (2004). Improving the Jason-1 ground retracking to better account for attitude effects. Mar. Geod. 27, 171–197. doi: 10.1080/01490410490465210
Bojinski, S., Verstraete, M., Peterson, T. C., Richter, C., Simmons, A., and Zemp, M. (2014). The concept of essential climate variables in support of climate research, applications, and policy. Bull. Am. Meteor. Soc. 95, 1431–1443. doi: 10.1175/BAMS-D-13-00047.1
Bonnefond, P., Exertier, P., Laurain, O., Guinle, T., and Féménias, P. (2019). Corsica: a 20-Yr multi-mission absolute altimeter calibration site. Adv. Space Res. 68, 1171–1186. doi: 10.1016/j.asr.2019.09.049
Cazenave, A., and Le Cozannet, G. (2013). Sea Level rise and its coastal impacts. Earths Future 2, 15–34. doi: 10.1002/2013EF000188
Chelton, D. B., Ries, J. C., Haines, B. J., Fu, L.-L., and Callahan, P. S. (2001). “Chapter 1: satellite altimetry,” in Satellite Altimetry and Earth Sciences – A Handbook of Techniques and Applications International Geophysics, Vol. 69, eds L.-L. Fu and A. Cazenave (San Diego, CA: Academic Press), 1–131. doi: 10.1016/s0074-6142(01)80146-7
Couhert, A., Cerri, L., Legeais, J. F., Ablain, M., Zelensky, N., Haines, B., et al. (2015). Towards the 1 mm/y stability of the radial orbit error at regional scales. Adv. Space Res. 55, 2–23. doi: 10.1016/j.asr.2014.06.041
Couhert, A., Mercier, F., Moyard, J., and Biancale, R. (2018). Systematic error mitigation in DORIS-derived geocenter motion. JGR Solid Earth 123, 10142–11061. doi: 10.1029/2018JB015453
Dibarboure, G., Pujol, M.-I., Briol, F., Le Traon, P. Y., Larnicol, G., Picot, N., et al. (2011). Jason-2 in DUACS: updated system description, first tandem results and impact on processing and products. Mar. Geod. 34, 214–241.
Dieng, H. B., Cazenave, A., Meyssignac, B., and Ablain, M. (2017). New estimate of the current rate of sea level rise from a sea level budget approach. Geophys. Res. Lett. 44, 3744–3751, https://doi.org/10.1002/2017GL073308, 2017.
Ducet, N., Le Traon, P. Y., and Reverdin, G. (2000). Global high resolution mapping of ocean circulation from the combination of TOPEX/POSEIDON and ERS-1/2. J. Geophys. Res. 105, 19477–19498.
Dunn, R., Lief, C., Peng, G., Wright, W., Baddour, O., Donat, M., et al. (2021). Stewardship maturity assessment tools for modernization of climate data management. Data Sci. J. 20:1. doi: 10.5334/dsj-2021-007
Escudier, P., Couhert, A., Mercier, F., Mallet, A., Thibaut, P., Tran, N., et al. (2017). “Satellite radar altimetry: principle, geophysical correction and orbit, accuracy and precision,” in Satellite Altimetry Over Oceans and Land Surfaces, eds D. Stammer and A. Cazenave (Boca Raton, FL: CRC Press).
Fasullo, J. T., and Nerem, R. S. (2018). Altimeter-era emergence of the patterns of forced sea-level rise in climate models and implications for the future. Proc. Natl. Acad. Sci. U.S.A. 115, 12944–12949. doi: 10.1073/pnas.1813233115
Guerou, A., Zawadzki, L., Raynal, M., Ablain, M., and Jugier, R. (2020). Revised Uncertainties of the Global Mean Sea Level Biases Between the TOPEX and Jasons Reference Mission. OSTST. Available online at: meetings.aviso.altimetry.fr/fileadmin//user_upload/tx_ausyclsseminar/files/OS TST2020_GMSL_Bias_Uncertainties_01.pdf (accessed Nov 04, 2020).
Hedemann, C., Mauritsen, T., Jungclaus, J., and Marotzke, J. (2017). The subtle origins of surface-warming hiatuses. Nat. Clim. Change 7, 336–339. doi: 10.1038/nclimate3274
IPCC. (2019). Special Report on the Ocean and Cryosphere in a Changing Climate, eds H.-O. Pörtner, D. C. Roberts, V. Masson-Delmotte, P. Zhai, M. Tignor, E. Poloczanska, et al. (Geneva: IPCC).
Le Traon, P. Y., Faugère, Y., Hernandez, F., Dorandeu, J., Mertz, F., and Ablain, M. (2003). Can we merge GEOSAT follow-on with TOPEX/Poseidon and ERS-2 for an improved description of the ocean circulation? J. Atmos. Ocean. Technol. 20, 889–895.
Legeais, J.-F., Ablain, M., and Thao, S. (2014). Evaluation of wet troposphere path delays from atmospheric reanalyses and radiometers and their impact on the altimeter sea level. Ocean Sci. 10, 893–905. doi: 10.5194/os-10-893-2014
Legeais, J.-F., Ablain, M., Zawadzki, L., Zuo, H., Johannessen, J. A., Scharffenberg, M. G., et al. (2018). An improved and homogeneous altimeter sea level record from the ESA Climate Change Initiative. Earth Syst. Sci. Data 10, 281–301. doi: 10.5194/essd-10-281-2018
Legeais, J.-F., Prandi, P., and Guinehut, S. (2016). Analyses of altimetry errors using Argo and GRACE data. Ocean Sci. 12, 647–662. doi: 10.5194/os-12-647-2016
Lievin, M., Kocha, C., Courcol, B., Philipps, S., Denis, I., Guinle, T., et al. (2020). Reprocessing of Sea Level L2P Products for 28 Years of Altimetry Missions. OSTST. Available online at: meetings.aviso.altimetry.fr/fileadmin//user_upload/tx_ausyclsseminar/files/OS TST2020_Reprocessing_L2P_2020.pdf (accessed Nov 04, 2020).
Loeb, N. G., Thorsen, T. J., Norris, J. R., Wang, H., and Su, W. (2018). Changes in Earth’s energy budget during and after the “Pause” in global warming: an observational perspective. Climate 6:62. doi: 10.3390/cli6030062
Melet, A., Buontempo, C., Mattiuzzi, M., Salamon, P., Bahurel, P., Breyiannis, G., et al. (2021). European Copernicus services to inform on sea-level rise adaptation – current status and perspectives. In this research topic issue: climate services for adaptation to sea level rise. Front. Mar. Sci. 8:703425. doi: 10.3389/fmars.2021.703425
Mertikas, S. P., Donlon, C., Féménias, P., Mavrocordatos, C., Galanakis, D., Tripolitsiotis, A., et al. (2018). Fifteen years of Cal/Val service to reference altimetry missions: calibration of satellite altimetry at the permanent facilities in Gavdos and Crete, Greece. Remote Sens. 10:1557. doi: 10.3390/rs10101557
Meyssignac, B., Boyer, T., Zhao, Z., Hakuba, M. Z., Landerer, F. W., Stammer, D., et al. (2019). Measuring global ocean heat content to estimate the earth energy imbalance. Front. Mar. Sci. 6:432. doi: 10.3389/fmars.2019.00432
Meyssignac, B., Slangen, A. B. A., Melet, A., Church, J. A., Fettweis, X., Marzeion, B., et al. (2017). Evaluating model simulations of twentieth-century sea-level rise. Part II: regional sea-level changes. J. Clim. 30, 8565–8593. doi: 10.1175/JCLI-D-17-0112.1
Mitchum, G. T. (1998). Monitoring the stability of satellite altimeters with tide gauges. J. Atmos. Ocean. Tech. 15, 721–730.
Nerem, R. S., Beckley, B. D., Fasullo, J., Hamlington, B. D., Masters, D., and Mitchum, G. T. (2018). Climate change driven accelerated sea level rise detected in the altimeter era. Proc. Natl. Acad. Sci. U.S.A. 115, 2022–2025. doi: 10.1073/pnas.1717312115, 2018
Ollivier, A., Faugere, Y., Picot, N., Ablain, M., Femenias, P., and Benveniste, J. (2012). Envisat ocean altimeter becoming relevant for mean sea level trend studies. Mar. Geod. 35 (Supp. 1), 118–136, doi: 10.1080/01490419.2012.721632.
Palanisamy, H., Meyssignac, B., Cazenave, A., and Delcroix, T. (2015). Is anthropogenic sea level fingerprint already detectable in the Pacific Ocean? Environ. Res. Lett. 10:8. doi: 10.1088/1748-9326/10/8/084024
Pascual, A., Faugere, Y., Larnicol, G., and Le Traon, P.-Y. (2006). Improved description of the ocean mesoscale variability by combining four satellite altimeters. Geophys. Res. Lett. 33, L02611. doi: 10.1029/2005GL024633
Pegliasco, C., Delepoulle, A., Morrow, R., Faugère, Y., and Dibarboure, G. (2021). META3.1exp : a new Global Mesoscale Eddy trajectories atlas derived from altimetry. Earth. Syst. Sci. Data Discuss. [preprint]. doi: 10.5194/essd-2021-300.
Peng, G., Wright, W., Baddour, O., Lief, C., and The SMM-CD Work Group (2019). The guidance booklet on the WMO-Wide Stewardship Maturity Matrix for Climate Data. Figshare. 1:1. doi: 10.6084/m9.figshare.7002482
Prandi, P., Meyssignac, B., Ablain, M., Spada, G., Ribes, A., and Benveniste, J. (2021). Local sea level trends, accelerations and uncertainties over 1993-2019. Sci. Data 8:1. doi: 10.1038/s41597-020-00786-7
Prandi, P., Valladeau, G., Ablain, M., Picot, N., and Desjonquères, J.-D. (2015). Desjonquères: Accuracy of global Comparisons Between Altimetry and Tide Gauges. OSTST. Available online at: meetings.aviso.altimetry.fr/fileadmin/user_upload/tx_ausyclsseminar/files/OS TST2015/SC1-03-Pres_OSTST2015_AltiTgComparisons_Prandi.pdf (accessed Nov 03, 2020).
Pujol, M.-I., Faugère, Y., Taburet, G., Dupuy, S., Pelloquin, C., Ablain, M., et al. (2016). DUACS DT2014: the new multi-mission altimeter data set reprocessed over 20 years. Ocean Sci. 12, 1067–1090. doi: 10.5194/os-12-1067-2016
Quartly, G. D., Legeais, J. F., Ablain, M., Zawadzki, L., Fernandes, M. J., Rudenko, S., et al. (2017). A new phase in the production of quality-controlled sea level data. Earth Syst. Sci. Data 9, 557–572. doi: 10.5194/essd-9-557-2017
Quartly, G. D., Nencioli, F., Raynal, M., Bonnefond, P., Nilo Garcia, P., Garcia-Mondéjar, A., et al. (2020). The roles of the S3MPC: monitoring, validation and evolution of sentinel-3 altimetry observations. Remote Sens. 12:1763. doi: 10.3390/rs12111763
SL_cci CECR (2016). Comprehensive Error Characterisation Report, CLS-DOS-NT-13-100, SLCCI-ErrorReport-030. Available online at: https://climate.esa.int/media/documents/SLCCI-ErrorReport-030-2-2.pdf (accessed Nov 04, 2020).
Taburet, G., Sanchez-Roman, A., Ballarotta, M., Pujol, M.-I., Legeais, J.-F., Fournier, F., et al. (2019). DUACS DT2018: 25 years of reprocessed sea level altimetry products. Ocean Sci. 15, 1207–1224. doi: 10.5194/os-15-1207-2019
The Climate Change Initiative Coastal Sea Level Team (2020). Coastal sea level anomalies and associated trends from Jason satellite altimetry over 2002–2018. Sci. Data 7:357. doi: 10.1038/s41597-020-00694-w
Thibaut, P., Piras, F., Roinard, H., Guerou, A., Boy, F., Maraldi, C., et al. (2021). “Benefits of the “Adaptive retracking solution” for the Jason-3 GDR-F reprocessing campaign,” in Proceedings of the 2021 IEEE International Geoscience and Remote Sensing Symposium IGARSS, Brussels.
Thibaut, P., Roinard, H., Piras, F., Guerou, A., Bignalet-Cazalet, F., Dibarboure, G., et al. (2020). Benefit of the Adaptive Retracker for Improving Jason-3 GMSL Estimations. OSTST. Available online at: meetings.aviso.altimetry.fr/fileadmin//user_upload/tx_ausyclsseminar/files/Th ibaut_Benefits_of_Adaptive_For_GMSL_01.pdf (accessed October, 2020).
Valladeau, G., Legeais, J. F., Ablain, M., Guinehut, S., and Picot, N. (2012). Comparing altimetry with tide gauges and argo profiling floats for data quality assessment and mean sea level studies. Mar. Geod. 35(Suppl. 1), 42–60. doi: 10.1080/01490419.2012.718226
von Schuckmann, K., Cheng, L., Palmer, M. D., Hansen, J., Tassone, C., Aich, V., et al. (2020). Heat stored in the Earth system: where does the energy go? Earth Syst. Sci. Data 12, 2013–2041. doi: 10.5194/essd-12-2013-2020
Watson, C., White, N., Church, J., Burgette, R., Tregoning, P., and Coleman, R. (2011). Absolute calibration in Bass Strait, Australia: TOPEX, Jason-1 and OSTM/Jason-2. Mar. Geod. 34, 242–260. doi: 10.1080/01490419.2011.584834
Watson, C. S., White, N. J., Church, J. A., King, M. A., Burgette, R. J., and Legresy, B. (2015). Unabated global mean sea-level rise over the satellite altimeter era. Nat. Clim. Chang. 5, 565–568. doi: 10.1038/NCLIMATE2635
WCRP Global Sea Level Budget Group. (2018). Global sea-level budget 1993–present. Earth Syst. Sci. Data 10, 1551–1590. doi: 10.5194/essd-10-1551-2018
Woodworth, P. L., Menéndez, M., and Roland Gehrels, W. (2011). Evidence for century-timescale acceleration in mean sea levels and for recent changes in extreme sea levels. Surv. Geophys. 32, 603–618. doi: 10.1007/s10712-011-9112-8
Wöppelmann, G., and Marcos, M. (2016). Vertical land motion as a key to understanding sea level change and variability. Rev. Geophys. 54, 64–92. doi: 10.1002/2015RG000502
Keywords: Copernicus, sea level rise (SLR), satellite altimetry, adaptation strategies to climate change, ocean monitoring indicators
Citation: Legeais J-F, Meyssignac B, Faugère Y, Guerou A, Ablain M, Pujol M-I, Dufau C and Dibarboure G (2021) Copernicus Sea Level Space Observations: A Basis for Assessing Mitigation and Developing Adaptation Strategies to Sea Level Rise. Front. Mar. Sci. 8:704721. doi: 10.3389/fmars.2021.704721
Received: 03 May 2021; Accepted: 19 October 2021;
Published: 18 November 2021.
Edited by:
Michael Dylan Sparrow, World Meteorological Organization, SwitzerlandReviewed by:
Giovanni Besio, University of Genoa, ItalyRobert James Nicholls, University of East Anglia, United Kingdom
Copyright © 2021 Legeais, Meyssignac, Faugère, Guerou, Ablain, Pujol, Dufau and Dibarboure. This is an open-access article distributed under the terms of the Creative Commons Attribution License (CC BY). The use, distribution or reproduction in other forums is permitted, provided the original author(s) and the copyright owner(s) are credited and that the original publication in this journal is cited, in accordance with accepted academic practice. No use, distribution or reproduction is permitted which does not comply with these terms.
*Correspondence: Jean-François Legeais, jlegeais@groupcls.com