- 1IMAR – Inst. do Mar and Centro I&D Okeanos, University of Azores, Horta, Portugal
- 2University of Azores, Depto de Oceanografia e Pescas, Horta, Portugal
- 3MARE – Marine and Environmental Sciences Centre, Faculdade de Ciências, University of Lisbon, Lisbon, Portugal
- 4Depto de Biologia Animal, Faculdade de Ciências, University of Lisbon, Lisbon, Portugal
The deep ocean ecosystem hosts high biodiversity and plays a critical role for humans through the ecosystem services it provides, such as fisheries and climate regulation. However, high longevity, late reproduction, and low fecundity of many organisms living in the deep ocean make them particularly vulnerable to fishing and climate change. A better understanding of how exploitation and changing environmental conditions affect life-history parameters (e.g., growth) of commercially important fish species is crucial for their long-term sustainable management. To this end, we used otolith increment widths and a mixed-effects modeling approach to develop a 42-year growth chronology (1975–2016) of the commercially important deep-sea fish species blackspot seabream (Pagellus bogaraveo) among the three island groups of the Azores archipelago (Northeast Atlantic). Growth was related to intrinsic (age and age-at-capture) and extrinsic factors (capture location, temperature-at-depth, North Atlantic Oscillation (NAO), Eastern Atlantic Pattern (EAP), and proxy for exploitation (landings)). Over the four decades analyzed, annual growth patterns varied among the three island groups. Overall, temperature-at-depth was the best predictor of growth, with warmer water associated with slower growth, likely reflecting physiological conditions and food availability. Average population growth response to temperature was separated into among-individual variation and within-individual variation. The significant among-individual growth response to temperature was likely related to different individual-specific past experiences. Our results suggested that rising ocean temperature may have important repercussions on growth, and consequently on blackspot seabream fishery production. Identifying drivers of blackspot seabream growth variation can improve our understanding of past and present condition of the populations toward the sustainable management of the fishery.
Introduction
The deep ocean, below 200 m depth, hosts ecosystems of high biodiversity and plays a critical role for humans through the services it provides, such as fisheries (Van den Hove and Moreau, 2007; Thurber et al., 2014). Currently, seafood from the deep ocean is estimated to fall by as much as half in the next 100 years, mainly due to resource exploitation and impacts of climate change (Sweetman et al., 2017). Global environmental change and exploitation can have major consequences on marine ecosystems, including demographic and evolutionary changes in exploited populations, distribution range shifts, altered phenology, and marine productivity (e.g., Edwards et al., 2004; Cheung et al., 2010; Perry et al., 2014; Audzijonyte et al., 2016; Tu et al., 2018). The effects of these changes on ecosystems depend on species sensitivities, ecological functions and processes, and spatial scales that in turn make it difficult to detect and identify the species’ biological responses (Brown et al., 2010; Poloczanska et al., 2016).
For a better understanding of the fundamental mechanisms responsible for biological responses to environmental change, long-term biological data (continuous information, collected and archived over time) with the appropriate spatial-temporal resolution are needed. Such data can provide valuable and robust information on marine ecosystem changes and allow exploring species growth responses to environmental changes and exploitation, concerning their habitat, life history, and potential of adaptation (Thresher et al., 2007; Shelton et al., 2013; Coulson et al., 2014; Martino et al., 2019). Unfortunately, especially for deep-sea species, long-term data sets are scarce, however, samples containing valuable long-term information on growth variation are readily available from fish otoliths (Campana and Thorrold, 2001). These structures contain growth information, that allows the development of chronologies based on the variation in their growth increment widths and thus are a useful tool for understanding the effects of different extrinsic drivers on growth ([opetwcitep]B53,B51[clotwcitep]Morrongiello et al., 2012, 2019; Matta et al., 2018). Since environmental variables generally play a key role in driving growth variation and population dynamics in aquatic organisms (Morrongiello et al., 2012), this biochronological approach also allows the prediction of species’ responses to future climate change (Rountrey et al., 2014; Barrow et al., 2018).
Otolith increment-based growth chronologies have been successfully developed using linear mixed-effects models that allow analyzing intrinsic effects, such as age and age-at-capture, simultaneously with potential extrinsic sources of variation, including environmental drivers (e.g., temperature, SST, ENSO) (Weisberg et al., 2010; Helser et al., 2012; Rountrey et al., 2014; Morrongiello and Thresher, 2015). Furthermore, mixed-effect models allow the decomposition of population-level variation into its within- and among-individual components, and the inclusion of individual reaction norms allows exploring phenotypic plasticity that can provide valuable information about the adaptive potential of populations to deal with future environmental and exploitation changes (van de Pol and Wright, 2009; Morrongiello and Thresher, 2015; Fox et al., 2019). A better understanding of population resilience, adaptive potential, and individual phenotypic plasticity is key for deep-sea fish species as they are known to be vulnerable to changing environment and exploitation due to their high longevity, late reproduction, and low fecundity (Cheung, 2007; Thresher et al., 2007).
In this context, this work investigates long-term growth patterns of a deep-sea fish in the Azores archipelago (Northeast Atlantic), the blackspot seabream (Pagellus bogaraveo), a commercially highly valuable species with a complex life history (Pinho and Menezes, 2006; Higgins et al., 2015). Blackspot seabream is a protandrous hermaphrodite (Krug, 1990) that presents ontogenetic migrations, moving from island coastal waters to deeper island slopes or offshore seamounts (Menezes et al., 2006; Afonso et al., 2012) and exhibiting complex individual spatial behavior ([opetwcitep]B1,B2[clotwcitep]Afonso et al., 2012, 2014). The complex life history characteristics of this species (i.e., protandrous hermaphroditism and late maturity of females) make it highly sensitive to overfishing and climatic changes (Lorance, 2011; Gutiérrez-Estrada et al., 2017; Sanz-Fernández and Gutiérrez-Estrada, 2021).
Using archived otoliths of adult blackspot seabream collected in the three island groups of the Azores archipelago, this study aimed to evaluate how growth is affected by intrinsic (age-related variables) and extrinsic factors (environmental and fisheries-related variables) and to detect possible growth differences between island groups. The extrinsic factors driving growth variation were further investigated to estimate their importance in individual growth responses.
Materials and Methods
Area and Study Species
Azores archipelago has been selected as study area (Figure 1 and Table 1), which is part of the Macaronesian biogeographical region, located in the Northeast Atlantic Ocean (Friedlander et al., 2017). The Azores marine ecosystem has been defined as oceanic (Pinho and Menezes, 2009) characterized by an abundant abyssal area, narrow or absent coastal platform, pronounced slopes, and the existence of banks and seamounts (Menezes et al., 2013). The selected species, P. bogaraveo (Brünnich, 1768; blackspot seabream), is a deep-water sparid commonly found at depths of 100–600 m, both around coastal areas of the islands and offshore seamounts (Menezes et al., 2006; Pinho et al., 2014). Small individuals (<13 cm furcal length (FL)) live preferentially in coastal areas and shallow waters (nursery areas), pre-adults (<30 cm FL) in intermediate zones and larger individuals live at island slopes or offshore banks and deeper waters (Stockley et al., 2005; Pinho et al., 2014; Higgins et al., 2015).
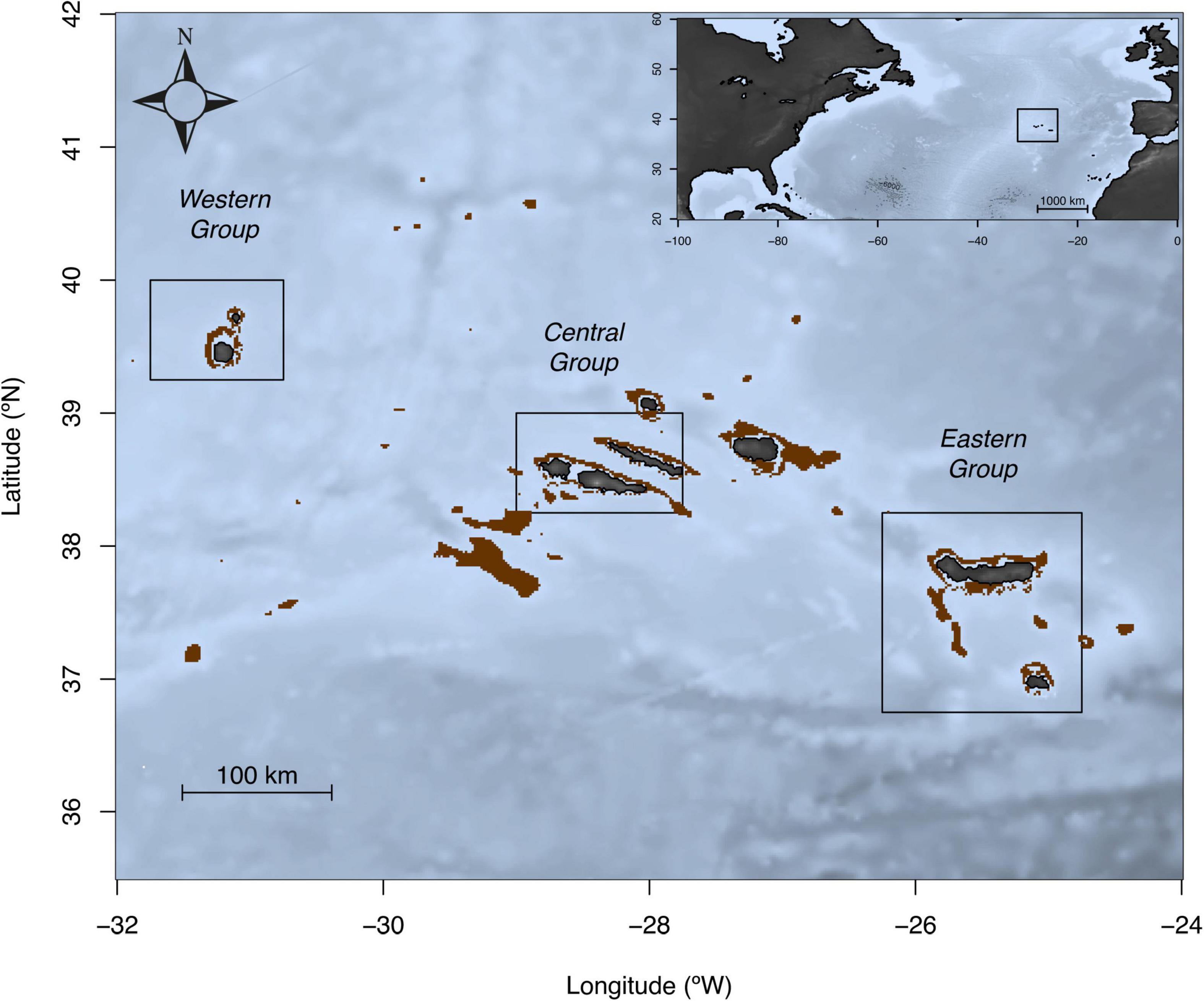
Figure 1. Sampling locations map of Pagellus bogaraveo in the Azores. Boxes in each sampling location indicate areas for which temperature-at-depth was obtained. Brown areas represent the depth range where the species generally occurs.
This species is a protandric hermaphrodite with primary females (females at birth, not changing sex during the life cycle), and males who can change sex during their life cycle, before maturity (c. 5 and 8 years of age, at around 28.2 and 33.9 cm FL, for males and females, respectively (Krug, 1998)). The maximum documented age and length are 15 years and 65 cm FL, respectively (Krug, 1989; Menezes et al., 2001).
Fish and Otolith Sampling
The otoliths of P. bogaraveo used in this study, archived at the Department of Oceanography and Fisheries (DOP) of the University of the Azores, located in Horta, Faial island, were collected during the annual monitoring surveys of demersal and deep-water fish abundance -ARQDAÇO surveys (e.g., [opetwcitep]B45,B46[clotwcitep]Melo et al., 2003, 2004; Rosa et al., 2017), conducted between 1995 and 2017, aboard the R/V Arquipélago. These surveys follow a stratified random sampling and use long-line gear, similar to those used by the local demersal fishing fleet, to sample island slopes and seamounts in the Azores (for methodological details see Menezes et al., 2006). Data on fishing effort and catches by species, and samples (length, sex, gonadal maturation stage, otoliths) are regularly collected.
For this study, adult female individuals sampled at island slopes were used. Selected individuals were aggregated by island groups (Eastern - São Miguel and Santa Maria islands; Central – Faial, Pico, and São Jorge islands; Western - Flores and Corvo islands) (Figure 1). Whenever possible, depending on sample availability, a similar number of individuals per capture year covering similar range of furcal length was selected (Supplementary Table 1).
Otolith Reading and Annual Growth Estimation/Increment Measurement
A total of 526 sagittal otolith pairs from fish ranging from 30 to 53 cm FL were selected (Supplementary Table 1). Otoliths were immersed in ethanol 72%, preferentially the left otolith was used, and immediately viewed through a Leica MZ16FA stereoscope to guarantee high quality increment visualization, and pictures were taken using a Leica MC190HD camera under reflected light against a dark background, and magnification between 7.11 and 24×. Annual growth increments or annuli were defined as consisting of one opaque and one translucent growth zone (Matta et al., 2018). Annuli were counted and measured using ImageJ software (version 1.52) with ObjectJ plugin (version 1.04) along an axis from the nucleus to the post-rostrum edge (Tanner et al., 2020). Increment measurements were marked at the border of each fully formed opaque growth zone. All otoliths were read twice by the same reader and only estimates with equal readings were included for further analysis.
The birth date of this species was assumed to be the 1st of January (Krug, 1989; Chilari et al., 2006). Due to the uncertainty that the first year of growth corresponds to a full year, measurements of the first increment were not included in the analysis (Morrongiello and Thresher, 2015; Tanner et al., 2020). Additionally, only increments up to age 20 were included in the data analysis to standardize the maximum age among island groups. The use of otolith increments as a proxy of fish growth relies on the premise of an allometric relationship between otolith and somatic growth. For each island group, this assumption was tested and confirmed in a subset of individuals (N = 75 individuals/location; Eastern: adjusted R2 = 0.692, slope = 7.21; Central: adjusted R2 = 0.681, slope = 6.38; Western: adjusted R2 = 0.639, slope = 5.94, all p < 0.0001) (Supplementary Figure 1).
Data Analysis
Inter-annual variation in the width of otolith growth increments was used as a proxy of somatic growth of blackspot seabream, and linear mixed-effects models were used to partition variance in otolith growth increment widths among intrinsic (age-related) and extrinsic (environmental-related and fishery-related) sources of growth variation using a statistical framework based on Morrongiello and Thresher (2015). Fixed effects (e.g., Age, Age at capture, Year, Temperature, and Abundance) describe the entire population and random effects (e.g., FishID, Cohort, and Area) are associated with randomly selected experimental units within the population (Morrongiello and Thresher, 2015; Lee and Punt, 2018). This approach explicitly quantifies age-related growth patterns and allows the exploration of environmental responses, thus making it the best approach for analyzing these hierarchical time series (otolith growth data). Increments were log-transformed to meet assumptions of normality and homoscedasticity, and both intrinsic and extrinsic predictor variables were mean-centered to facilitate model convergence and interpretation of interaction terms (Morrongiello et al., 2014).
Intrinsic Effects on Growth
A series of linear mixed-effect models with increased complexity, which included fixed intrinsic covariates and a series of random effects structures, were developed, and compared to determine the best baseline model describing fish otolith growth (Supplementary Table 2). First, the best random-effects model with a complete fixed-effect structure was determined for the data set. The random effect structures applied included random intercepts for individual fish (“FishID”), year of otolith increment formation (“Year”), and birth year (“Cohort”), to allow for correlations among growth increments within individual fish, calendar year and year class. Random “Age” slopes were also investigated for these covariates thus allowing individuals to have age-related growth trajectories. The capture location of the specimen (“IslandGroup”) was always included in combination with “Year” and “Cohort,” allowing for island group-specific year or cohort growth responses. The best random-effects model was then used to select the appropriate fixed-effect structure. Optimized fixed effects included the intrinsic (i.e., internal to the individual) terms “Age” and “Age at capture” (Supplementary Table 3) in interaction with the island group (Supplementary Table 2) which accounted for age-specific effects on growth that can vary between capture locations and location-specific differences in potential sampling bias or growth selectivity associated to certain phenotypes (Morrongiello and Thresher, 2015). Model selection was based on the comparison of the Akaike Information Criterion corrected for small sample sizes (AICc) (Burnham and Anderson, 2002). Variance in otolith growth explained by the combined fixed and random effects were calculated by the conditional R2 metric (Nakagawa and Schielzeth, 2013). Model parameters were estimated using restricted maximum likelihood (REML). For fixed effects optimization, models were fitted using maximum likelihood and the best model was subsequently refitted using REML to provide unbiased estimates (Zuur et al., 2009). The selected model with the best structure of intrinsic fixed effects was then extended in a stepwise procedure to determine if the addition of extrinsic fixed factors could further improve the model fit.
Extrinsic Variables Effects on Growth
Blackspot seabream growth variation was related to a series of extrinsic variables (i.e., environmental and exploitation) that potentially explain inter-annual variation in otolith growth (Supplementary Table 3). Monthly data of water temperature-at-depth in the approximate areas of fish sampling were obtained from Simple Ocean Data Assimilation (SODA3) reanalysis, version 3.4.2 (Carton et al., 2018). Based on the species’ preferential depth distribution (100–600 m depth) (Menezes et al., 2006), depth levels between 98 and 618 m were selected. Both climatic indices, North Atlantic Oscillation (NAO) and Eastern Atlantic Pattern (EAP) were obtained from the NOAA Climate Prediction Center1 since these large-scale phenomena can affect the local climate and flow of regional sea currents in the Azores and consequently the species dynamics (e.g., spatial distribution, reproduction, behavior, and feeding) (Pinho et al., 2011). Moreover, and specifically for P. bogaraveo, a lower abundance in the period 2010–2011 was associated with the negative phase of NAO during this period (Pinho et al., 2011). To explore potential fishery-dependent influences on growth variation, fish landings (tonnes) were used as a proxy for exploitation. Official data on landings were obtained from the public company managing Azores fish auction houses (Lotaçor S.A.). Data on temperature, NAO, EAP, and fishery proxy were available over the entire period of the chronologies (1975–2016) and so these four extrinsic variables were included in the optimized intrinsic model, using seasonal averages, and their influence was evaluated. Pairwise correlations among extrinsic variables were calculated (Pearson coefficient) and only variables with correlations <0.5 were simultaneously included as fixed effects into the models.
Average Thermal Reaction Norms
Within-subject centering was used to determine if the average population growth-temperature relationship and variation were driven by within-individual (representing evolutionary-fixed phenotypically plastic responses) or among-individual effects (representing individual differences in temperature response) (van de Pol and Wright, 2009; Morrongiello and Thresher, 2015; Martino et al., 2019; Morrongiello et al., 2019). This approach provides an estimate of the average growth phenotypic plasticity present within an individual (within-individual) and an estimate of how growth systematically varies across average lifetime conditions (among-individual) (Martino et al., 2019). For this purpose, two new variables were calculated, the average temperature experienced by each individual over its lifetime and the deviation of annual temperatures from this mean. The original temperature variable was replaced with the two new variables and resulting models were compared with the optimal extrinsic model using AICc (Supplementary Table 4). Differences in within-individual and among-individual effects were derived by replacing the within-individual term with the original temperature effect. The selected model also included a random within-individual reaction norm slope on FishID, representing the individual-specific differences in within-individual temperature slopes (Morrongiello and Thresher, 2015; Supplementary Table 4).
All analyses were conducted in R 3.6.1 (R Core Team, 2018) using the packages lme4 (Bates et al., 2014) for the linear mixed models.
Results
Blackspot seabream otolith growth chronologies spanned 42 years, from 1975 to 2016 (Supplementary Figure 2 and Supplementary Table 1). The individuals used in this study were aged between 6 and 28 years, and on average, the age at capture was 14.1 years (Supplementary Table 1). For a similar length range, the Western island group presented the oldest individuals (Table 1).
Intrinsic Effects
The optimal intrinsic random effect model contained a random intercept for FishID and IslandGroup:Year, and a random Age slope for FishID. For the fixed effects, the inclusion of Age and Age-at-capture as fixed effect terms was supported, both with IslandGroup interaction (Table 2). The otolith-derived growth chronologies for all island groups showed considerable inter-annual variation, with below and above average years of growth. The inter-annual variation pattern varied between locations, without a clear trend in any of the locations (Figure 2). Average growth in all locations declined with age (Figure 3A). Slight differences in age-related growth patterns of P. bogaraveo were observed among the three locations. Individuals from the Western island group showed the highest growth rate at the youngest age, whilst the growth rate at an older age in these individuals was lower than in the remaining groups (Figure 3A). For the Eastern group, a negative relationship between annual growth and Age-at-capture was identified, with individuals captured at younger age presenting higher growth rates than individuals captured at an older age (Figure 3B). On the other hand, no relationship between growth and Age-at-capture was found for the other islands’ groups (Figure 3B).
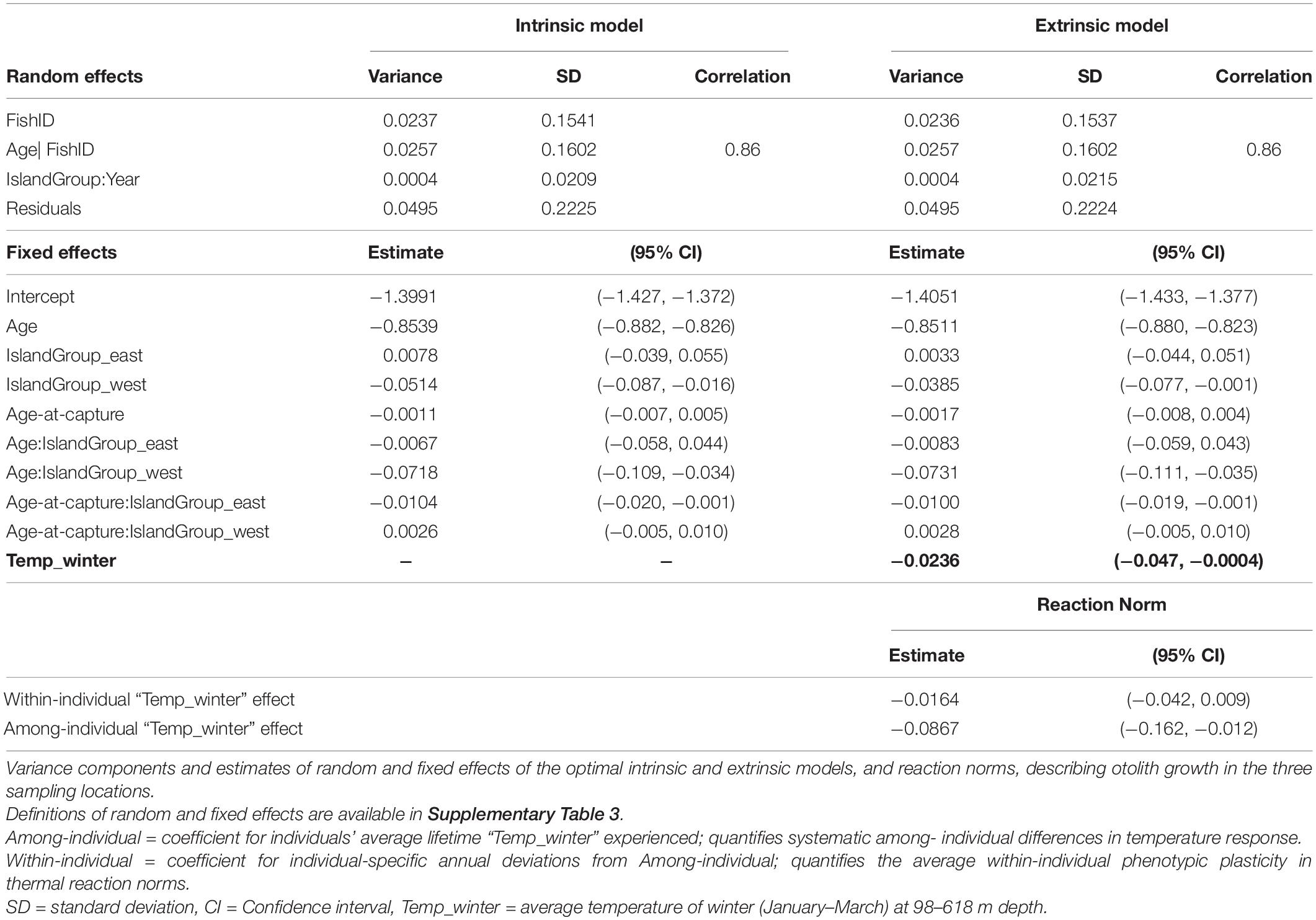
Table 2. Final Pagellus bogaraveo otolith growth model, with selected extrinsic fixed effects in bold.
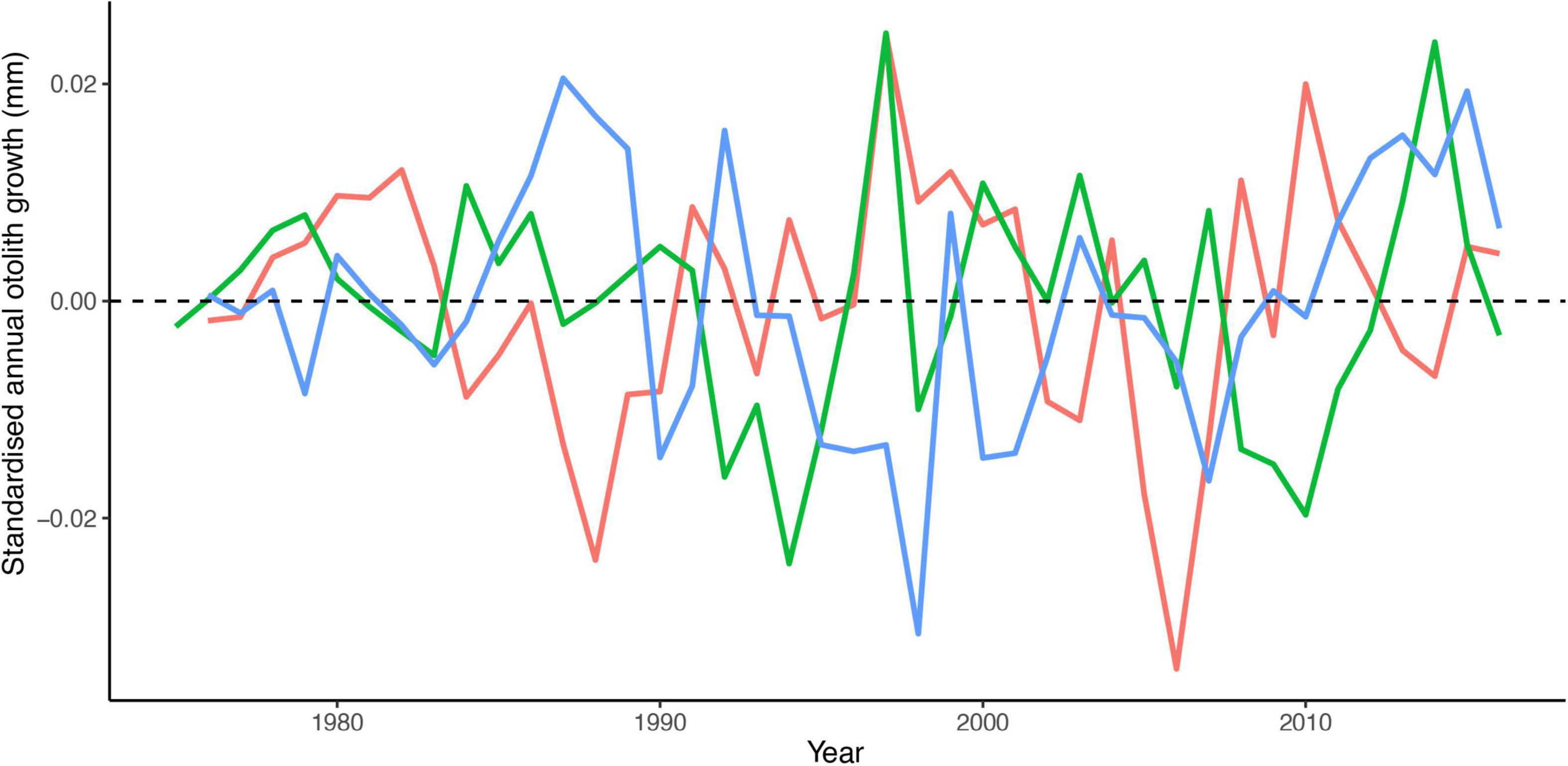
Figure 2. Pagellus bogaraveo predicted variations in annual otolith growth collected in Western (blue), Central (red), and Eastern (green) island groups.
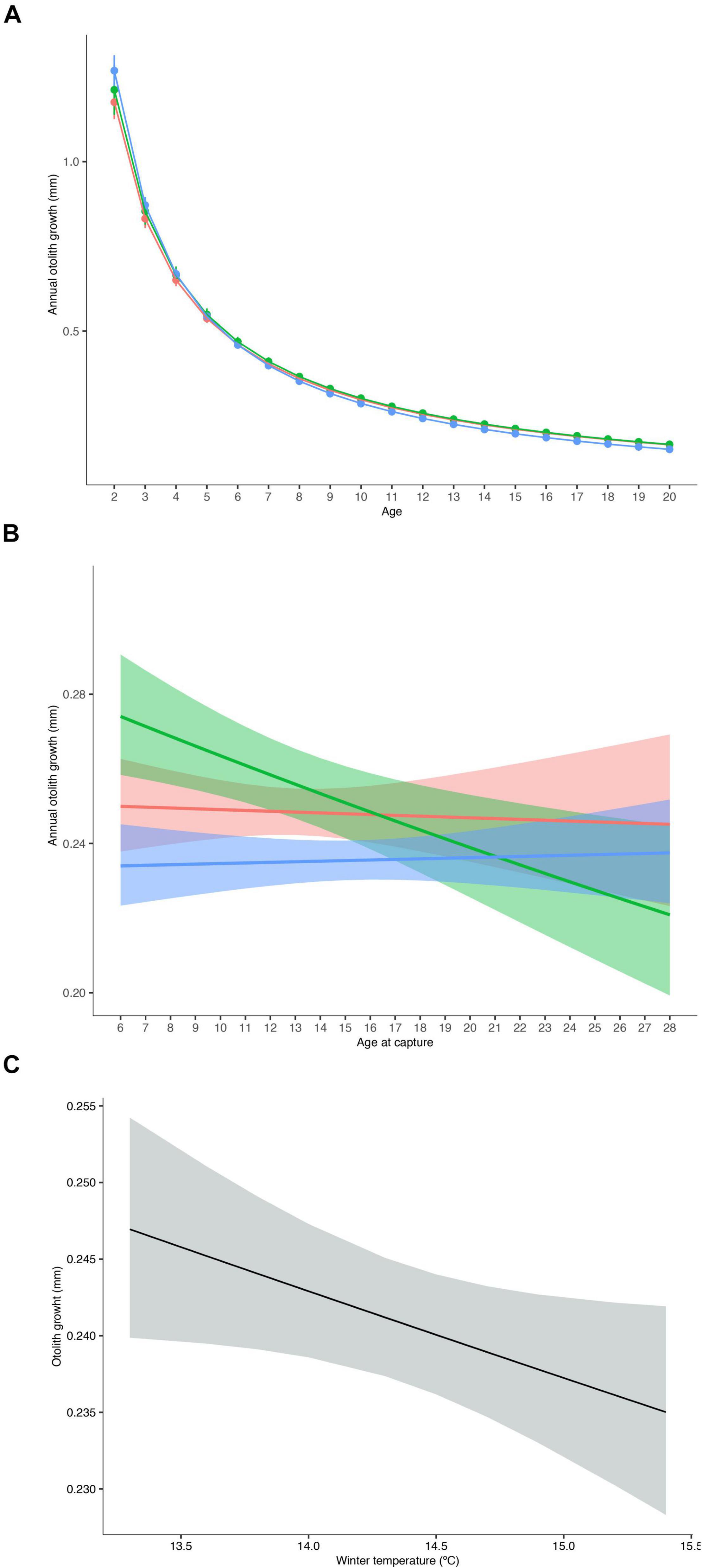
Figure 3. Annual mean otolith increments from Western (blue), Central (red), and Eastern (green) island groups by (A) age and (B) Age-at-capture; and (C) overall effect of average winter temperature (January–March) at depth (98–618 m) on Pagellus bogaraveo otolith growth. Vertical lines in (A) and shaded areas in (B,C) denote ±95% CI.
Extrinsic Variables Effects
Seasonal means of temperature-at-depth, NAO, EAP, and annual landings were added to the optimized intrinsic model. All these environmental variables were included simultaneously in the model, except for seasonal temperature estimates which were included separately due to the high correlation among means. Average winter temperature-at-depth was the only variable included in the final extrinsic model (Table 2). Overall, winter temperature had a negative effect on blackspot seabream growth (Figure 3C). Average winter temperature-at-depth was higher in the Western island group compared to the Central and Eastern groups (Supplementary Figure 3), however, these differences did not affect the overall response of growth in the different island groups.
Within Versus Among Individual Growth Variation
Average population growth response to winter temperature was separated into among-individual variation (representing facultative environmental responses) and within-individual variation (representing evolutionary-fixed, phenotypically plastic responses). We found significant among-individual growth response to temperature while the within-individual variation was weakly negative (Figure 4). The inclusion of within-individual temperature random slopes improved the model. Still, the effect was minimal, with high variation in individual response.
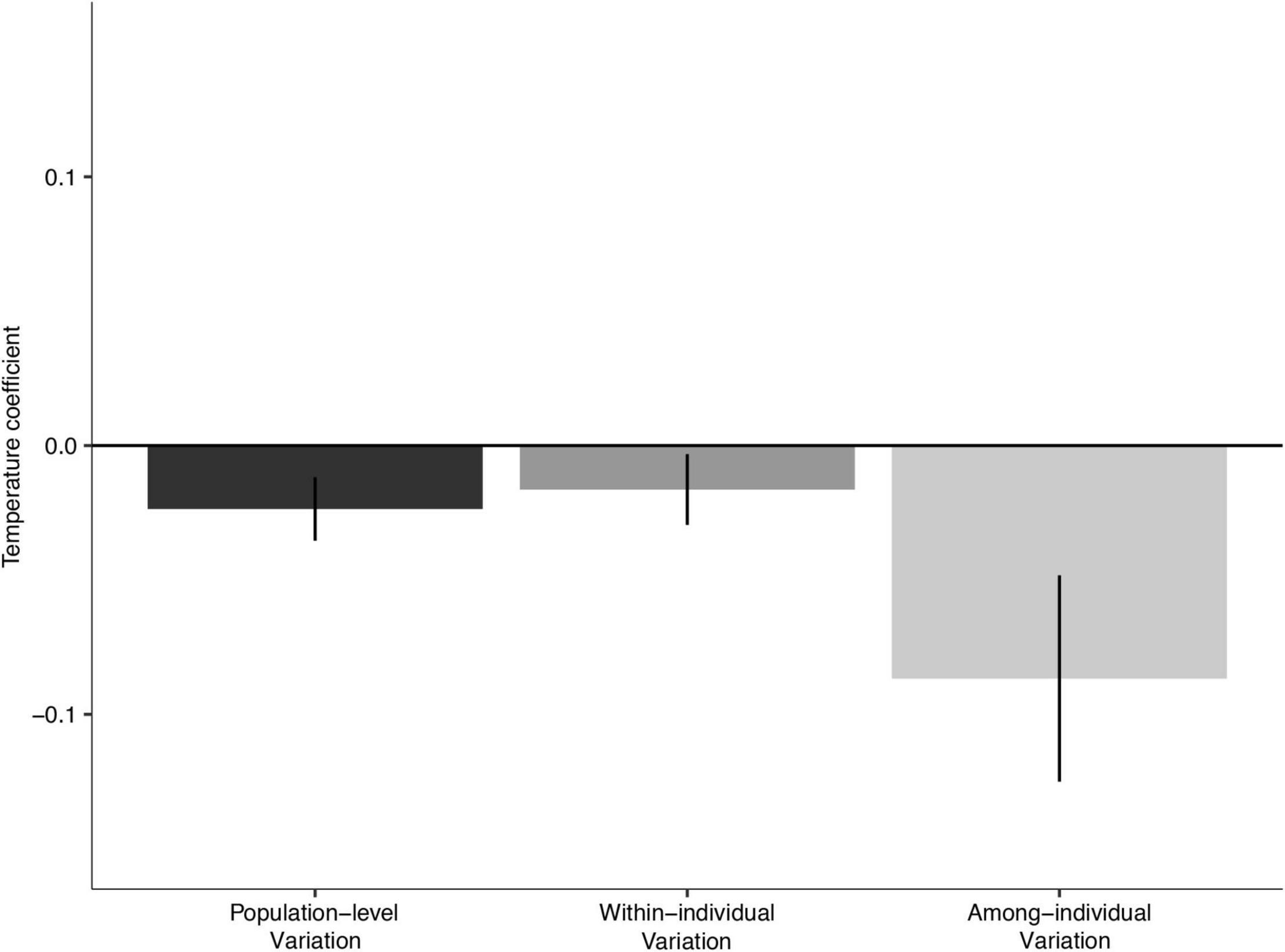
Figure 4. Winter temperature-related population-level variation, compared with within-individual (representing evolutionary-fixed phenotypically plastic responses), and among-individual (representing facultative environmental responses) variations.
Discussion
Otolith chronologies from this study reconstructed fish growth over 40 years based on individuals ranging from 6 to 28 years of age. We documented differences in inter-annual growth variation of blackspot seabream populations among the Azores island groups. These differences in growth patterns may be partly related to regional differences in habitat, oceanographic conditions, and exploitation patterns, which are known to induce fluctuations in populations dynamics (e.g., Cheal et al., 2007; Powney et al., 2010; Frank et al., 2016). Environmental seabed characteristics in the Azores archipelago show a clear dissimilarity between the Western island and the other two island groups (Amorim et al., 2017), likely related to the topographic discontinuity caused by the Mid-Atlantic Ridge and the associated oceanographic transition zone. This discontinuity may act as an ecological barrier potentially isolating western island fish populations relative to the central-eastern groups’ populations (e.g., Fontes et al., 2009) and may contribute to the distinct blackspot seabream growth patterns observed. On the other hand, regional-scale fishing pressure has varied among island groups over the last decades, with the Eastern island group subject to greater fishing pressure during the 1980s (Diogo et al., 2015), potentially adding further to the inter-island group growth variation documented.
Age was the best predictor of growth, with growth decreasing with age. Otolith growth was considered a proxy for somatic growth, a widely accepted assumption (Matta et al., 2018; Martino et al., 2019; [opetwcitep]B78,B77[clotwcitep]Tanner et al., 2019, 2020). This relationship and the age-at-length relationship have previously been validated for blackspot seabream ([opetwcitep]B37,B36[clotwcitep]Krug, 1989, 1998; Chilari et al., 2006; Higgins et al., 2015). In our study, this relationship is less robust in older specimens, which contributed with the oldest individuals to this study. Length-at-age and maximum age determined in this study differed from those previously documented for the region (Krug, 1989; Menezes et al., 2001). These differences may be attributed to the fact that the previous works were elaborated more than 20 years ago using different techniques and resolutions which likely led to distinct age determinations.
Regarding the Age-at-capture and annual growth relationship, significant differences were evident among the capture locations (island groups). Unequivocally in the Eastern group, a negative relationship between annual growth and Age-at-capture was identified, differentiating this group from the others. Individuals captured at a younger age presented higher growth rates than individuals captured at an older age. There are several possible explanations for the negative relationship between Age-at-capture and growth, such as life history trade-offs between growth rate, sexual maturity, and longevity; or higher vulnerability of fast growers to fishery activities (see e.g., Morrongiello and Thresher, 2015). For blackspot seabream from the Eastern island group, a fisheries-related mechanism appears to be the most plausible explanation since this group was subject to the highest exploitation rates in the archipelago resulting in local depletion of fish stocks (Diogo et al., 2015). Fast growers may be more vulnerable as they recruit to the fisheries earlier, or even showing a bolder behavior and consequently making them more vulnerable to fishing (Morrongiello and Thresher, 2015). Also, in fish populations under high fishing pressure, smaller fish tend to mature earlier, as a response to long-term, size-selective harvesting that removes the larger and faster-growing fish (Browman et al., 2000; Ernande et al., 2004; Olsen et al., 2009; Martino et al., 2019). Krug (1998) observed that female blackspot seabream matured at earlier ages and smaller size over a 10-year period which may have been a first indication of the effects of fishing pressure, environmental change, or an interaction of these factors on this species’ growth in the Azores. The presence of slow-growing individuals and the occurrence of the oldest individuals for the same length range in the Western island group may be the result of differential fishing pressure among island groups (Diogo et al., 2015). The relationship between growth and fishing pressure was formally explored in our model using as a proxy for exploitation, landings of blackspot seabream, the only exploitation-related variable available for the entire range of the chronology. However, this variable was not significant and consequently not included in the final model, which may be an indication of the lack of suitability of this proxy.
We identified temperature-at-depth during winter as the best predictor of growth across all locations, with higher values influencing the annual growth negatively. Water temperature may affect fish growth directly by influencing metabolism within the species-specific thermal optimum or indirectly by modulating other environmental variables such as dissolved oxygen concentration or food availability (Dutta, 1994; Pörtner and Farrell, 2008; Massie et al., 2020). Warmer winters in deep waters may lead to decreased dissolved oxygen concentration and higher metabolic rates, which require more energy (food) and oxygen (Pauly and Cheung, 2018). Physiological capacity disturbance, related to temperature and oxygen variations, can alter not only the growth but also affect other vital functions such as maturation or reproduction (Pörtner and Knust, 2007; Pauly, 2021). In the Azores archipelago, the reproduction of blackspot seabream occurs during the winter months (Krug, 1998; Estácio et al., 2001), coinciding with the temperature-at-depth used in our model, that negatively influenced somatic growth. The negative effect of warmer water on fish growth is expected in the context of Gill-oxygen limitation theory that states that lower relative oxygen supply induces sexual maturation and promotes a slowing of growth ([opethcitep]B57,B58,B59[clothcitep]Pauly, 2019a, b, 2021). Following this theory, blackspot seabream may invest more energy in reproduction and spawning, depriving the somatic growth, during winter periods with warmer water temperatures and potentially reduced oxygen availability, leading to the observed negative effect on growth. Temperature can also influence food availability which has been shown to play a considerable role in fish growth rates (Jones, 1986; Dutta, 1994; Anderson and Sabado, 1995; Cominassi et al., 2020). In the Azores archipelago, seasonal maximums of sea surface temperature (SST) have been shown to coincide with seasonal lows of chlorophyll (Caldeira and Reis, 2017), and winter temperature-at-depth during the study period were positively correlated with winter SST (r(df = 109) = 0.38, p < 0.001). Thus, warmer winters may result in lower primary productivity with potential effects on higher levels in the food chain. It has been previously recognized that the energy transfer rate from phytoplankton to mesopelagic fishes in oligotrophic regions (such as the Azores) is high, linking fishes’ biomass and primary production (Irigoien et al., 2014). Since mesopelagic fish (myctophids) and invertebrates are the main components in the diet of blackspot seabream (Morato et al., 2001) warmer winter resulting in lower primary production may lead to less food availability and energy intake, impacting growth negatively.
In addition to environmental and oceanographic factors, the genotype and physiological condition of the individual may also regulate growth (Dutta, 1994), and can promote different growth rates at the individual level. Exploring among-individual variation and individual thermal responses is particularly interesting in species with complex life histories, such as P. bogaraveo (Higgins et al., 2015), since these components differ in their evolutionary and ecological implications and thus may allow a better understanding of the species’ ability to respond to global change (Brommer et al., 2008). In this study, we found a negative among-individual variation in thermal response. Among-individual variation may result from genetic differences and individual-specific past experiences or a combination of both (Morrongiello and Thresher, 2015; Martino et al., 2019). For blackspot seabream, possible individual past experiences giving rise to among-individual variations in thermal response may be related to complex individual spatial feeding behavior, that includes vertical and horizontal migrations ([opetwcitep]B1,B2[clotwcitep]Afonso et al., 2012, 2014). As a consequence of genetic differences, it has been proposed that slower-growing individuals will be favored through long-term adaptation to higher temperatures (Martino et al., 2019). For blackspot seabream, such a genetic adaptation may also be possible although among-individual thermal responses were consistent for the three island groups. Furthermore, genetic differentiation in blackspot seabream in the Azores archipelago is low although a restriction of gene flow between the Western island group and the rest of the archipelago has been suggested (Stockley et al., 2005).
Our results showed differing growth patterns among Azorean island groups, with an overall negative temperature-at-depth effect on blackspot seabream growth which likely acted as an indirect factor affecting physiological condition, reproduction, and food availability. Also, the partitioning of the thermal population-level response into among-individual and within-individual variation is likely related to different individual-specific past experiences of blackspot seabream. Identifying drivers of blackspot seabream growth variation can promote an improved understanding of the present condition of the populations which represents essential information for the sustainable management of the fishery considering the future environmental change. In fact, the observed negative impact of warmer water on the growth of blackspot seabream may have important implications in the future as ocean temperature is forecasted to increase (Lyman et al., 2010) with potential repercussions for blackspot seabream fishery production. Thus, vulnerable species, such as blackspot seabream, may require more careful considerations in terms of management, and a better understanding of the factors involved in key life-history events. The long-term annually resolved growth information that can be derived from otoliths present significant potential to be used for this species from different locations, studying intra-regional differences in growth or even for other slow-growing species to obtain precise information on responses to biological and environmental effects. Considering the importance of age determination in fisheries assessment, long-term chronologies, such as developed in this study, can provide relevant contributions for the sustainable management of deep-sea resources.
Data Availability Statement
The raw data supporting the conclusions of this article will be made available by the authors, without undue reservation.
Author Contributions
ST and EG conceived and designed the study and secured funding. JN contributed to the design of the study. JN, EG, and GM collected sample information and organized the database. JN performed the laboratory processing and sample preparation, collected, and analyzed the otolith images. JN and ST performed the statistical analysis. JN wrote the first draft of the manuscript. All authors revised the manuscript and approved the submitted version.
Funding
This publication was financed by Portuguese national funds through FCT (Fundação para a Ciência e a Tecnologia) - IP under project reference UIDB/04292/2020 and by the European Union’s Horizon 2020 Research and Innovation Programme under grant agreement N810139: Project Portugal Twinning for Innovation and Excellence in Marine Science and Earth Observation – PORTWIMS. This study was funded by the FCT through PTDC/AAG-GLO/5849/2014. ST was supported by FCT, DL57/2016/CP1479/CT0022. EG was supported by the Azores Regional Government, CONDOR/DEMERSAIS projects. JF was supported by the research grant M3.1.a/F/062/2016 funded by Fundo Regional de Ciência e Tecnologia from Governo dos Açores. We also acknowledge funds and support from the FCT through the strategic project (UID/05634/2020) granted to OKEANOS.
Conflict of Interest
The authors declare that the research was conducted in the absence of any commercial or financial relationships that could be construed as a potential conflict of interest.
Publisher’s Note
All claims expressed in this article are solely those of the authors and do not necessarily represent those of their affiliated organizations, or those of the publisher, the editors and the reviewers. Any product that may be evaluated in this article, or claim that may be made by its manufacturer, is not guaranteed or endorsed by the publisher.
Acknowledgments
We thank Helena Krug and Domitilia Rosa for helpful suggestions regarding blackspot seabream otolith analysis, Alexandra Rosa for technical support, Ana Pabon for collecting species information, Robert Priester for the help in the interpretation of results and sharing knowledge on the metabolism and physiological performance of the species, and all technical staff that kindly help to the success of this work, contributing to sample collection. Otoliths used were collected as part of CONDOR/DEMERSAIS project campaigns, supported by the Azores Regional Government.
Supplementary Material
The Supplementary Material for this article can be found online at: https://www.frontiersin.org/articles/10.3389/fmars.2021.703820/full#supplementary-material
Footnotes
References
Afonso, P., Graça, G., Berke, G., and Fontes, J. (2012). First observations on seamount habitat use of blackspot seabream (Pagellus bogaraveo) using acoustic telemetry. J. Exp. Mar. Bio. Ecol. 436–437, 1–10. doi: 10.1016/j.jembe.2012.08.003
Afonso, P., McGinty, N., Graça, G., Fontes, J., Inácio, M., Totland, A., et al. (2014). Vertical migrations of a deep-sea fish and its prey. PLoS One 9:e97884. doi: 10.1371/journal.pone.0097884
Amorim, P., Perán, A. D., Pham, C. K., Juliano, M., Cardigos, F., Tempera, F., et al. (2017). Overview of the ocean climatology and its variability in the Azores region of the North Atlantic including environmental characteristics at the seabed. Front. Mar. Sci. 4, 1–16. doi: 10.3389/fmars.2017.00056
Anderson, T. W., and Sabado, B. D. (1995). Correspondence between food availability and growth of a planktivorous temperate reef fish. J. Exp. Mar. Bio. Ecol. 189, 65–76. doi: 10.1016/0022-0981(95)00011-F
Audzijonyte, A., Fulton, E., Haddon, M., Helidoniotis, F., Hobday, A. J., Kuparinen, A., et al. (2016). Trends and management implications of human-influenced life-history changes in marine ectotherms. Fish Fish. 17, 1005–1028. doi: 10.1111/faf.12156
Barrow, J., Ford, J., Day, R., and Morrongiello, J. (2018). Environmental drivers of growth and predicted effects of climate change on a commercially important fish, Platycephalus laevigatus. Mar. Ecol. Prog. Ser. 598, 201–212. doi: 10.3354/meps12234
Bates, D., Mächler, M., Bolker, B., and Walker, S. (2014). Fitting Linear Mixed-Effects Models Using lme4. Canada: McMaster University.
Brommer, J. E., Rattiste, K., and Wilson, A. J. (2008). Exploring plasticity in the wild: laying date-temperature reaction norms in the common gull Larus canus. Proc. Biol. Sci. 275, 687–693. doi: 10.1098/rspb.2007.0951
Browman, H. I., Browman, H. I., Hutchings, J. A., Conover, D. O., Stokes, K., Law, R., et al. (2000). Evolution’ of fisheries science. Mar. Ecol. Prog. Ser. 208, 301–309.
Brown, C. J., Fulton, E. A., Hobday, A. J., Matear, R. J., Possingham, H. P., Bulman, C., et al. (2010). Effects of climate-driven primary production change on marine food webs: implications for fisheries and conservation. Glob. Chang. Biol. 16, 1194–1212. doi: 10.1111/j.1365-2486.2009.02046.x
Brünnich, M. T. (1768). Ichthyologia Massiliensis: Sistens Piscium Descritpiones Eorumque Apud Incolas Nomina. Accedunt Spolia Maris Adriatica. Hafniae et Lipsiae, Apud Rothii Viduam et Proft. 110.
Burnham, K. P., and Anderson, D. R. (2002). A practical information-theoretic approach. Model Sel. multimodel inference, 2nd Edn. New York: Springer.
Caldeira, R. M. A., and Reis, J. C. (2017). The Azores confluence zone. Front. Mar. Sci. 4, 1–14. doi: 10.3389/fmars.2017.00037
Campana, S. E., and Thorrold, S. R. (2001). Otoliths, increments, and elements: keys to a comprehensive understanding of fish populations? Can. J. Fish. Aquat. Sci. 58, 30–38. doi: 10.1139/f00-177
Carton, J. A., Chepurin, G. A., and Chen, L. (2018). SODA3: a new ocean climate reanalysis. J. Clim. 31, 6967–6983. doi: 10.1175/jcli-d-18-0149.1
Cheal, A. J., Delean, S., Sweatman, H., and Thompson, A. A. (2007). Spatial synchrony in coral reef fish population and the influence of climate. Ecology 88, 158–169. doi: 10.1890/0012-9658
Cheung, W. W. L. (2007). Intrinsic vulnerability in the global fish catch. Mar. Ecol. Prog. Ser. 333, 1–12. doi: 10.3354/meps333001
Cheung, W. W. L., Lam, V. W. Y., Sarmiento, J. L., Kearney, K., Watson, R., Zeller, D., et al. (2010). Large-scale redistribution of maximum fisheries catch potential in the global ocean under climate change. Glob. Chang. Biol. 16, 24–35. doi: 10.1111/j.1365-2486.2009.01995.x
Chilari, A., Petrakis, G., and Tsamis, E. (2006). Aspects of the biology of blackspot seabream (Pagellus bogaraveo) in the Ionian Sea. Greece. Fish. Res. 77, 84–91. doi: 10.1016/j.fishres.2005.08.003
Cominassi, L., Moyano, M., Claireaux, G., Howald, S., Mark, F. C., Zambonino-Infante, J.-L., et al. (2020). Food availability modulates the combined effects of ocean acidification and warming on fish growth. Sci. Rep. 10:2338. doi: 10.1038/s41598-020-58846-2
Coulson, P. G., Black, B. A., Potter, I. C., and Hall, N. G. (2014). Sclerochronological studies reveal that patterns of otolith growth of adults of two co-occurring species of Platycephalidae are synchronised by water temperature variations. Mar. Biol. 161, 383–393. doi: 10.1007/s00227-013-2343-0
Diogo, H., Pereira, J. G., Higgins, R. M., and Canha, Â, and Reis, D. (2015). History, effort distribution and landings in an artisanal bottom longline fishery: an empirical study from the North Atlantic Ocean. Mar. Policy 51, 75–85. doi: 10.1016/j.marpol.2014.07.022
Edwards, M., Richardson, A. J., Martin, E., Anthony, J., and Richardson. (2004). Impact of climate change on marine pelagic phenology and trophic mismatch. Nature 430, 881–884. doi: 10.1038/nature02808
Ernande, B., Dieckmann, U., and Heino, M. (2004). Adaptive changes in harvested populations: plasticity and evolution of age and size at maturation. Proc. R. Soc. London. Ser. B Biol. Sci. 271, 415–423. doi: 10.1098/rspb.2003.2519
Estácio, S., Krug, H., Menezes, G. M., and Pinho, M. R. (2001). Aspects of the Reproduction of Six Exploited Demersal Fish Species in the Azores Archipelago. Arquipélago. Life Mar. Sci. 2, 83–94.
Fontes, J., Caselle, J. E., Sheehy, M. S., Santos, R. S., and Warner, R. R. (2009). Natal Signatures of Juvenile Coris julis in the Azores: investigating Connectivity Scenarios in an Oceanic Archipelago. Mar. Ecol. Prog. Ser. 387, 51–59. doi: 10.3354/meps08116
Fox, R. J., Donelson, J. M., Schunter, C., Ravasi, T., and Gaitán-Espitia, J. D. (2019). Beyond buying time: the role of plasticity in phenotypic adaptation to rapid environmental change. Philos. Trans. R. Soc. B Biol. Sci. 374:20180174. doi: 10.1098/rstb.2018.0174
Frank, K. T., Petrie, B., Leggett, W. C., and Boyce, D. G. (2016). Large scale, synchronous variability of marine fish populations driven by commercial exploitation. Proc. Natl. Acad. Sci. U. S. A. 113, 8248–8253. doi: 10.1073/pnas.1602325113
Friedlander, A. M., Ballesteros, E., Clemente, S., Gonçalves, E. J., Estep, A., Rose, P., et al. (2017). Contrasts in the marine ecosystem of two Macaronesian islands: a comparison between the remote Selvagens Reserve and Madeira Island. PLoS One 12:e0187935. doi: 10.1371/journal.pone.0187935
Gutiérrez-Estrada, J. C., Gil-Herrera, J., Pulido-Calvo, I., and Czerwinski, I. A. (2017). Is it possible to differentiate between environmental and fishery effects on abundance-biomass variation? A case study of blackspot seabream (Pagellus bogaraveo) in the Strait of Gibraltar. Fish. Oceanogr. 26, 455–475. doi: 10.1111/fog.12208
Helser, T. E., Lai, H. L., and Black, B. A. (2012). Bayesian hierarchical modeling of Pacific geoduck growth increment data and climate indices. Ecol. Modell. 247, 210–220. doi: 10.1016/j.ecolmodel.2012.08.024
Higgins, R. M., Diogo, H., and Isidro, E. J. (2015). Modelling growth in fish with complex life histories. Rev. Fish Biol. Fish. 25, 449–462. doi: 10.1007/s11160-015-9388-8
Irigoien, X., Klevjer, T. A., Røstad, A., Martinez, U., Boyra, G., Acuña, J. L., et al. (2014). Large mesopelagic fishes biomass and trophic efficiency in the open ocean. Nat. Commun. 5:3271. doi: 10.1038/ncomms4271
Jones, G. P. (1986). Food availability affects growth in a coral reef fish. Oecologia 70, 136–139. doi: 10.1007/BF00377123
Krug, H. (1998). Variation in the reproductive cycle of the blackspot seabream, Pagellus bogaraveo (Brünnich, 1768) in the Azores. Aquipelago. Life Mar. Sci. 16, 37–47.
Krug, H. M. (1989). The Azorean blackspot seabream, Pagellus bogaraveo (Brünnich, 1768) (Teleostei: sparidae): age and growth. Cybium 13, 347–355.
Krug, H. M. (1990). The azorean blackspot seabream, Pagellus bogaraveo (Brünnich, 1768) (Teleostei, Sparidae). Reproductive cycle, hermaphrodism, maturity and fecundity. Cybium 14, 151–159.
Lee, Q., and Punt, A. E. (2018). Extracting a time-varying climate-driven growth index from otoliths for use in stock assessment models. Fish. Res. 200, 93–103. doi: 10.1016/j.fishres.2017.12.014
Lorance, P. (2011). History and dynamics of the overexploitation of the blackspot sea bream (Pagellus bogaraveo) in the Bay of Biscay. ICES J. Mar. Sci. 68, 290–301. doi: 10.1093/icesjms/fsq072
Lyman, J. M., Good, S. A., Gouretski, V. V., Ishii, M., Johnson, G. C., Palmer, M. D., et al. (2010). Robust warming of the global upper ocean. Nature 465, 334–337. doi: 10.1038/nature09043
Martino, J. C., Fowler, A. J., Doubleday, Z. A., Grammer, G. L., and Gillanders, B. M. (2019). Using otolith chronologies to understand long-term trends and extrinsic drivers of growth in fisheries. Ecosphere 10:e02553. doi: 10.1002/ecs2.2553
Massie, D. L., Li, Y., and Wagner, T. (2020). A framework for assessing the ability to detect macroscale effects on fish growth. Can. J. Fish. Aquat. Sci. 78, 165–172. doi: 10.1139/cjfas-2019-0296
Matta, M. E., Helser, T. E., and Black, B. A. (2018). Intrinsic and environmental drivers of growth in an Alaskan rockfish: an otolith biochronology approach. Environ. Biol. Fishes 101, 1571–1587. doi: 10.1007/s10641-018-0801-8
Melo, O., Menezes, G. M., Pinho, M. R., Rosa, A., and Isidro, E. (2003). Relatório de Cruzeiro de Monitorização de Demersais realizado durante a Primavera de 2003 nos Açores (ARQDAÇO-17-P03). Arquivos do DOP. Série Cruzeiros 2003:23.
Melo, Octávio, Menezes, G. M., Pinho, M. R., and Rosa, A. (2004). Relatório dos Cruzeiros de Monitorização Anual de Demersais realizados nos Açores durante a Primavera dos anos de 2000 a 2002 (ARQDAÇO-13-P00, ARQDAÇO-15-P01 e ARQDAÇO-16-P02). Arquivos do DOP. Sér. Cruz. 2004:38.
Menezes, G. M., Giacomello, E., Menezes, G. M., and Bergstad, O. A. (2013). An integrated approach for studying seamounts: cONDOR observatory. Deep Sea Res. 2 Top. Stud. Oceanogr. 98, 1–6. doi: 10.1016/j.dsr2.2013.09.023
Menezes, G. M., Sigler, M. F., Silva, H. M., and Pinho, M. R. (2006). Structure and zonation of demersal fish assemblages off the Azores Archipelago (mid-Atlantic). Mar. Ecol. Prog. Ser. 324, 241–260. doi: 10.3354/meps324241
Menezes, G., Rogers, A. D., Krug, H., Mendonça, A., Stokley, B., Isidro, E. J., et al. (2001). Seasonal Changes in Biological and Ecological Traits of Demersal and Deep-water Fish Species in the Azores. Final report. DG XIV/C/1—study contract 97–081. Portugal: Universidade dos Açores.
Morato, T., Solà, E., Grós, M. P., and Menezes, G. (2001). Feeding habits of two congener species of seabreams, Pagellus bogaraveo and Pagellus acarne, off the Azores (northeastern Atlantic) during spring of 1996 and 1997. Bull. Mar. Sci. 69, 1073–1087.
Morrongiello, J. R., Sweetman, P. C., and Thresher, R. E. (2019). Fishing constrains phenotypic responses of marine fish to climate variability. J. Anim. Ecol. 88, 1645–1656. doi: 10.1111/1365-2656.12999
Morrongiello, J. R., and Thresher, R. E. (2015). A statistical framework to explore ontogenetic growth variation among individuals and populations: a marine fish example. Ecol. Monogr. 85, 93–115. doi: 10.1890/13-2355.1
Morrongiello, J. R., Thresher, R. E., and Smith, D. C. (2012). Aquatic biochronologies and climate change. Nat. Publ. Gr. 2, 849–857. doi: 10.1038/NCLIMATE1616
Morrongiello, J. R., Walsh, C. T., Gray, C. A., Stocks, J. R., and Crook, D. A. (2014). Environmental change drives long-term recruitment and growth variation in an estuarine fish. Glob. Chang. Biol. 20:12545. doi: 10.1111/gcb.12545
Nakagawa, S., and Schielzeth, H. (2013). A general and simple method for obtaining R2 from generalized linear mixed-effects models. Methods Ecol. Evol. 4, 133–142. doi: 10.1111/j.2041-210x.2012.00261.x
Olsen, E. M., Carlson, S. M., Gjøsæter, J., and Stenseth, N. C. (2009). Nine decades of decreasing phenotypic variability in Atlantic cod. Ecol. Lett. 12, 622–631. doi: 10.1111/j.1461-0248.2009.01311.x
Pauly, D. (2019a). A précis of gill-oxygen limitation theory (GOLT), with some emphasis on the eastern mediterranean. Mediterr. Mar. Sci. 20, 660–668. doi: 10.12681/mms.19285
Pauly, D. (2019b). Female fish grow bigger–let’s deal with it. Trends Ecol. Evol. 34, 181–182. doi: 10.1016/j.tree.2018.12.007
Pauly, D. (2021). The gill-oxygen limitation theory (GOLT) and its critics. Sci. Adv. 7:eabc6050. doi: 10.1126/sciadv.abc6050
Pauly, D., and Cheung, W. W. L. (2018). Sound physiological knowledge and principles in modeling shrinking of fishes under climate change. Glob. Chang. Biol. 24, e15–26. doi: 10.1111/gcb.13831
Perry, A. L., Low, P. J., Ellis, J. R., and Reynolds, J. D. (2014). Climate Change and Distribution Shifts in Marine Fishes. Science 308, 1912–1915. doi: 10.1126/science.1111322
Pinho, M. R., Bashmachnikov, I., and Martins, A. (2011). “The influence of the North Atlantic Oscillation on the abundance of Pagellus bogaraveo of the Azores, ICES Conference Proceedings” in The influence of the North Atlantic Oscillation on the Abundance of Pagellus Bogaraveo of the Azores, ICES Conference Proceedings. Portuga: University of the Azores. 1–15. doi: 10.1007/978-3-319-23534-9_1
Pinho, M. R., Diogo, H., Carvalho, J., and Pereira, J. G. (2014). Harvesting juveniles of Red (Blackspot) seabream (Pagellus bogaveo) in the Azores: biological implications, management and life cycle considerations. Ices J. Mar. Sci. 10. fsu089. doi: 10.1093/icesjms/fsu089
Pinho, M. R., and Menezes, G. (2006). Azorean Deepwater Fishery. in Deep Sea 2003: Conference Poster Papers and Workshop Papers. Canada: Food & Agriculture Org.
Pinho, M. R., and Menezes, G. (2009). Pescaria de demersais dos Açores. Bol. do Núcleo Cult. da Horta 18, 85–102.
Poloczanska, E. S., Burrows, M. T., Brown, C. J., García Molinos, J., Halpern, B. S., Hoegh-Guldberg, O., et al. (2016). Responses of Marine Organisms to Climate Change across Oceans. Front. Mar. Sci. 3:62. doi: 10.3389/fmars.2016.00062
Pörtner, H. O., and Farrell, A. P. (2008). Ecology. Physiology and climate change. Science 322, 690–692. doi: 10.1126/science.1163156
Pörtner, H. O., and Knust, R. (2007). Climate change affects marine fishes through the oxygen limitation of thermal tolerance. Science 315, 95–97. doi: 10.1126/science.1135471
Powney, G. D., Roy, D. B., Chapman, D., and Oliver, T. H. (2010). Synchrony of butterfly populations across species’ geographic ranges. Oikos 119, 1690–1696. doi: 10.1111/j.1600-0706.2010.18168.x
R Core Team (2018). R: a Language and Environment for Statistical Computing. R Foundation for Statistical Computing. Vienna. Available online at: URL http://www.R-project.org/ (accessed April 24, 2019).
Rosa, A., Catarino, D., De Girolamo, M., Pinho, M., Menezes, G., and Giacomello, E. (2017). Relatório do Cruzeiro de Monitorização de Demersais Realizado Durante a Primavera de 2016 nos Açores (ARQDAÇO-46-P16). Portugal: Universidade Dos Açores. doi: 10.13140/RG.2.2.23313.58722
Rountrey, A. N., Coulson, P. G., Meeuwig, J. J., and Meekan, M. (2014). Water temperature and fish growth: Otoliths predict growth patterns of a marine fish in a changing climate. Glob. Chang. Biol. 20, 2450–2458. doi: 10.1111/gcb.12617
Sanz-Fernández, V., and Gutiérrez-Estrada, J. C. (2021). Historical changes of Blackspot seabream (Pagellus bogaraveo) landing patterns in the Strait of Gibraltar from 1983 to 2016: environmental and legislation effects. Fish. Oceanogr. 30, 111–126. doi: 10.1111/fog.12506
Shelton, A. O., Satterthwaite, W. H., Beakes, M. P., Munch, S. B., Sogard, S. M., and Mangel, M. (2013). Separating Intrinsic and Environmental Contributions to Growth and Their Population Consequences. Am. Nat. 181, 799–814. doi: 10.5061/dryad.cj36j
Stockley, B., Menezes, G., Pinho, M. R., and Rogers, A. D. (2005). Genetic population structure in the black-spot sea bream (Pagellus bogaraveo Brünnich, 1768) from the NE Atlantic. Mar. Biol. 146, 793–804. doi: 10.1007/s00227-004-1479-3
Sweetman, A. K., Thurber, A. R., Smith, C. R., Levin, L. A., Mora, C., Wei, C.-L., et al. (2017). Major impacts of climate change on deep-sea benthic ecosystems. Elem. Sci. Anthr. 5:4.
Tanner, S. E., Giacomello, E., Menezes, G. M., Mirasole, A., Neves, J., Sequeira, V., et al. (2020). Marine regime shifts impact synchrony of deep-sea fish growth in the Northeast Atlantic. Oikos 129, 1781–1794. doi: 10.1111/oik.07332
Tanner, S. E., Vieira, A. R., Vasconcelos, R. P., Dores, S., Azevedo, M., Cabral, H. N., et al. (2019). Regional climate, primary productivity and fish biomass drive growth variation and population resilience in a small pelagic fish. Ecol. Indic. 103, 530–541. doi: 10.1016/j.ecolind.2019.04.056
Thresher, R. E., Koslow, J. A., Morison, A. K., and Smith, D. C. (2007). Depth-mediated reversal of the effects of climate change on long-term growth rates of exploited marine fish. Proc. Natl. Acad. Sci. 104, 7461–7465. doi: 10.1073/pnas.0610546104
Thurber, A. R., Sweetman, A. K., Narayanaswamy, B. E., Jones, D. O. B., Ingels, J., and Hansman, R. L. (2014). Ecosystem function and services provided by the deep sea. Biogeosciences 11, 3941–3963. doi: 10.5194/bg-11-3941-2014
Tu, C. Y., Chen, K. T., and Hsieh, C. H. (2018). Fishing and temperature effects on the size structure of exploited fish stocks. Sci. Rep. 8, 1–10. doi: 10.1038/s41598-018-25403-x
van de Pol, M., and Wright, J. (2009). A simple method for distinguishing within- versus between-subject effects using mixed models. Anim. Behav. 77, 753–758. doi: 10.1016/j.anbehav.2008.11.006
Van den Hove, S., and Moreau, V. (2007). Deep-sea Biodiversity and Ecosystems: a Scoping Report on Their Socio-economy, Management and Governanace. Kenya: United Nations Environment Programme.
Weisberg, S., Spangler, G., and Richmond, L. S. (2010). Mixed effects models for fish growth. Can. J. Fish. Aquat. Sci. 67, 269–277. doi: 10.1139/f09-181
Keywords: environmental change, mixed-effects modeling, otolith, blackspot seabream, reaction norms, sclerochronology
Citation: Neves J, Giacomello E, Menezes GM, Fontes J and Tanner SE (2021) Temperature-Driven Growth Variation in a Deep-Sea Fish: The Case of Pagellus bogaraveo (Brünnich, 1768) in the Azores Archipelago. Front. Mar. Sci. 8:703820. doi: 10.3389/fmars.2021.703820
Received: 30 April 2021; Accepted: 06 August 2021;
Published: 25 August 2021.
Edited by:
Janine Barbara Adams, Nelson Mandela University, South AfricaReviewed by:
R. P. Prabath K. Jayasinghe, National Aquatic Resources Research and Development Agency, Sri LankaAnna Maria Addamo, European Commission, Joint Research Centre (JRC), Italy
Copyright © 2021 Neves, Giacomello, Menezes, Fontes and Tanner. This is an open-access article distributed under the terms of the Creative Commons Attribution License (CC BY). The use, distribution or reproduction in other forums is permitted, provided the original author(s) and the copyright owner(s) are credited and that the original publication in this journal is cited, in accordance with accepted academic practice. No use, distribution or reproduction is permitted which does not comply with these terms.
*Correspondence: Susanne E. Tanner, c2V0YW5uZXJAZmMudWwucHQ=