- 1Department of Invertebrate Zoology and Hydrobiology, University of Łódz, Łódz, Poland
- 2Center for Development Cooperation in Fisheries, Institute of Marine Research, Bergen, Norway
The Gulf of Guinea belongs to the most scarcely sampled marine basins in the oceans of the world. We have analyzed diversity and distribution patterns of cumacean communities on the shelf and slope, along the coast of Ghana. The material was collected in October and November of 2012 using a van Veen grab (0.1 m2) on nine transects. Six stations were located at each transect (25, 50, 100, 250, 500, and 1,000 m). Sixty-three species of Cumacea were recorded with Leucon and Eocuma as the most speciose genera, with 12 and eight species, respectively. Comparisons of species richness with literature data pointed that the Ghanaian coast hosts very diverse communities. About 95% of species were new to science, and the number of cumacean species known from the West Africa increased by over 100%. Nevertheless, most of the species had extremely low abundance, 13 singletons and 15 doubletons were found. Mean density of cumaceans was estimated at only 1.5 ind./0.1 m2. Species accumulation curve did not reach the asymptotic level, suggesting undersampling, despite the fact that sampling effort was high (250 samples). The highest species richness was recorded in the inner shelf (25–50 m) and on the slope (1,000 m). Cluster analysis separated shallow water communities from deeper regions on the shelf and upper slope. The most unique species composition was found at 1,000 m. Principal component analysis showed the importance of oxygen, sediments, and human-related disturbance for distribution of cumacean communities. In the shallows, oxygen content and presence of gravel were the most important factors structuring communities. In the deeper bottom areas (250–1,000 m), cumacean fauna was affected by local pollution, mainly by higher concentration of barium, other heavy metals, and THC.
Introduction
Continental margins constitute about 11% of the oceans of the world and are shaped by a complex set of environmental factors that are dynamically changing along a depth gradient (Levin and Sibuet, 2012). They are characterized by high habitat heterogeneity and belong to the most important marine biodiversity hot spots (Danovaro et al., 2009; Menot et al., 2010; Levin and Sibuet, 2012). At the same time, continental margins belong to areas of special economic interests, such as fishery and oil industry (Menot et al., 2010). This makes them one of the most interesting natural laboratories for studies of biodiversity, ecosystem services, and environmental gradients as well as influence of human activities and climate change on marine biota (Levin and Sibuet, 2012; Birchenough et al., 2015). On the other hand, recent analysis based on over 10 million records obtained mostly from the Ocean Biogeographic Information System (OBIS), revealed a strong sampling bias in the marine biodiversity assessment. There is a large gap in the knowledge about marine fauna associated with tropical areas, and it is visible not only in the deep sea (bathyal and abyssal) but also on the shelf, with average number of sampling events an order of magnitude lower than in northern and southern mid latitudes (Menegotto and Rangel, 2018). The authors of this research pointed out a lack of scientific infrastructure and funding for marine research in developing tropical countries as the main reason of this situation. It is highly visible in the case of African marine fauna.
The West African continental margin belongs to the most scarcely sampled regions. Most of the available studies were focused on the shallows and based on low sampling effort (Buchanan, 1957; Longhurst, 1958, 1959; Bassindale, 1961; Le Loeuff and Intés, 1999; Bamikole et al., 2009). There is a particular lack of ecological research based on quantitative sampling and lack of detailed biodiversity inventories based on species level identification. The deep sea communities of the Gulf of Guinea are almost completely neglected in earlier research, with the exception of the areas affected by organic discharge from the Congo River (e.g., Gaever et al., 2009; Galéron et al., 2009; Menot et al., 2009) and most recent studies from Gabon (Friedlander et al., 2014) and Ghana (Pabis et al., 2020; Sobczyk et al., 2021). At the same time, the Gulf of Guinea is facing serious problems associated with various types of anthropogenic impacts, such as pollution events associated with the oil industry (Scheren et al., 2002; Ayamdoo, 2016), but those problems are only scarcely studied and need further research based on the analysis of various taxonomic groups (Pabis et al., 2020; Sobczyk et al., 2021). Influence of heavy metals, hydrocarbons, and other pollutants might have a substantial influence on the composition, diversity, and abundance of benthic communities (Olsgard and Gray, 1995; Gomez-Gesteira et al., 2003; Stark et al., 2020). However, there are no studies demonstrating the influence of anthropogenic disturbance on Cumacea.
Based on literature data, Le Loeuff and Cosel (1998) listed only 1,440 benthic species from the large part of the West African coast, starting from the Mauretania and ending in the Namibia (up to 200 m depth), although the study was focused mostly on megafauna (corals, echinoderms, and decapod crustaceans) as well as polychaetes and bivalves. Analysis of the same set of samples as in the cumacean study of the authors revealed 253 species of Polychaeta (Sobczyk et al., 2021), only from the small fragment of the Ghanaian coast, placing this area amongst the important biodiversity hot spots for those marine annelids. We can expect that similar hidden biodiversity can be encountered for many other taxonomic groups, especially so important like small peracarid crustaceans.
Cumacea are classified as one of the orders of Peracarida. With about 1,400 recognized species (Gerken, 2018), this order is on the third place in terms of species richness within Peracarida, after Ampipoda (9,500 species) and Isopoda (about 6,000 species), and together with Tanaidacea (about 1,400 species). Their true diversity is vastly underestimated, mostly because of taxonomic expertise bias (Appeltans et al., 2012). As all peracarids Cumacea are small brooders with no planktonic larvae, they borrow in the surface layer of the sediment (Pilar-Cornejol et al., 2004). They are often found in the first few centimeters of sediments and occur from the intertidal, down to abyssal depths (Watling and Gerken, 2021). Cumacea are significant element of benthic communities, that in particular regions or depth zones (e.g., deep sea and tropical areas) might be one of the most diverse groups of crustaceans (Jones and Sanders, 1972; Cartes et al., 2003; Doti et al., 2020). For example, at the upper slope off Portugal, Cumacea together with Isopoda reached the highest number of the species (Cunha et al., 1997); while in the Angola Basin off Namibia, they were the third most abundant group of Peracarida, after Isopoda and Tanaidacea (Brandt, 2005). Moreover, some species might reach locally high abundance, even up to 500 individuals per square meter, both on the shelf, and in the deep sea (Bishop, 1982; Swaileh and Adelung, 1995). Cumacea also play an important role in the trophic webs, especially as food source for fish and some macroinvertebrates, such as decapods (Cartes, 1993; Watling and Gerken, 2021). For example, Diastylis rathkei might constitute even 35% of the diet of flounder (Swaileh and Adelung, 1995).
Available studies demonstrated that cumaceans display preferences to particular grain size, which makes them a good indicator of sediment type (Dixon, 1944; Wieser, 1956; Jones, 1976). Some species are known to be sensitive to environmental stress. Two dominant species in Algeciras Bay (Cumella limicola and Nannastacus unguiculatus) were strongly influenced by hydrodynamism, sedimentation, and water turbidity (Alfonso et al., 1998). Some species of Cumacea are also good indicators of eutrophication, and have been proposed as organisms appropriate for biomonitoring (Corbera and Cardell, 1995; Ateş et al., 2014). Nevertheless, studies on biology and ecology of particular species or distribution patterns and structure of cumacean communities are still scarce, especially in the deep sea (e.g., Gage et al., 2004; Pabis and Błazewicz-Paszkowycz, 2011; Corbera and Sorbe, 2020 and references therein).
The knowledge about cumacean fauna of the Ghanian coast is highly scattered and based mostly on taxonomic publications (e.g., Băcescu, 1961, 1972; Day, 1975, 1978, 1980; Mühlenhardt-Siegel, 1996, 2000; Petrescu, 1998). So far, only 154 species of Cumacea are known from the whole African coast (Watling and Gerken, 2021), which makes 11% of the world fauna (Gerken, 2018). From West Africa, 59 species have been recorded, mostly from the continental shelf (Watling and Gerken, 2021), and only three were found on the Ghanaian coast (Jones, 1956; Petrescu, 2018). There are no quantitative studies on cumacean communities conducted on the African coast. Most earlier studies were focused on taxonomy.
Biodiversity assessments of the tropical continental margins are among the most important priorities of the current marine science (Menegotto and Rangel, 2018). Therefore, the main aim of this study was to assess cumacean diversity on the continental shelf and slope of the Gulf of Guinea (25–1,000 m depth, Ghanaian coast) and compare it with literature data. We hypothesize that the Ghanaian continental margin hosts speciose cumacean communities with many species new to science. We wanted also to analyse the influence of various natural (e.g., oxygen, sediment type, salinity, and temperature) and anthropogenic (heavy metals and hydrocarbons) factors on the diversity and distribution patterns of those crustaceans. We hypothesize that local pollution might lower the abundance and diversity of those small crustaceans with limited dispersal abilities.
Materials and Methods
Study Area
The Gulf of Guinea is a large open basin located in West Africa, influenced by a complex set of currents (Guinea Current, Benguela Current, South Equatorial Counter Current; Ukwe et al., 2003, 2006) and upwelling events (Nieto and Mélin, 2017). The north part of the Gulf of Guinea is influenced by seasonal upwelling, bringing nutrient-rich mid-depth waters to the surface and increasing the primary production (Binet and Marchal, 1993). The southern part depends rather on nutrient input from land drainage and river flood, mostly the Volta River, which is the only larger river system located along the almost 600-km long Ghanaian coast (Buchanan, 1957; Ukwe et al., 2003). The Gulf of Guinea is classified as a province in the Tropical Atlantic Realm, with rich fishery resources as well as large oil and gas reserves, and its sectors (e.g., north, central, and south) are considered a separate ecoregion (Spalding et al., 2007). The heterogeneity of habitats on continental margins has influence on high diversity of habitats for benthic fauna. At the same time, industrialization and the oil industry create numerous sources of disturbance that can potentially affect marine communities (Germain and Armengol, 1999; Owusu-Boadi and Kuitunen, 2002).
Sampling
The material was collected in October and November of 2012 from the board of RV Fridtjof Nansen. Nine transects were distributed along the whole coast of Ghana (Figure 1). Six stations were designated at each transect (25, 50, 100, 250, 500, and 1,000 m). Five replicate samples were collected at each station using a van Veen grab (0.1 m2) supported with the Video Assisted Multi Sampler (VAMS), allowing for appropriate sediment penetration. The samples were washed using 0.3 mm mesh size and preserved in 4% formaldehyde solution. The material was collected in the framework of the Oil for Development (OfD) program, and supported by the Food and Agriculture Organization of the United Nations (FAO).
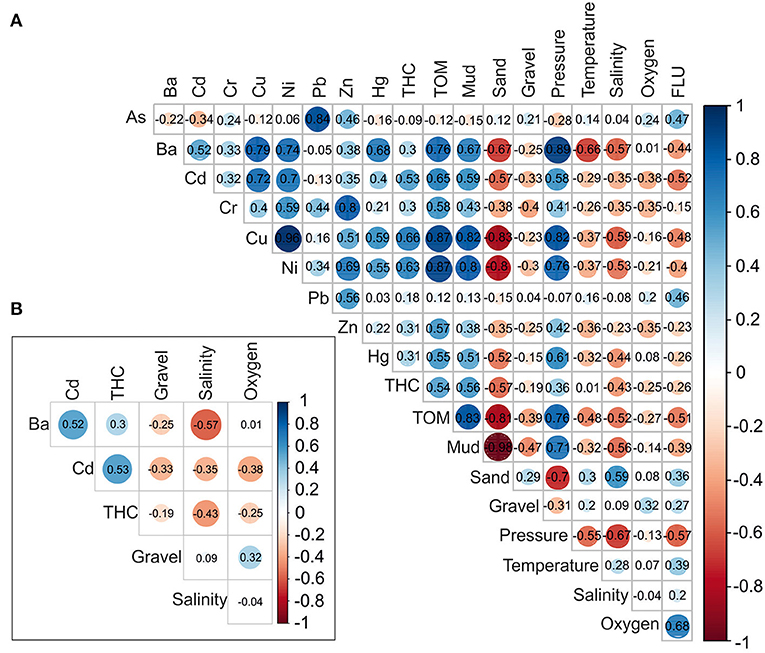
Figure 1. Correlation matrices with Pearson‘s r correlation coefficients calculated for: (A) all predictors and (B) the least correlated predictors. For full list of environmental variables please see Supplementary Table 1.
Environmental Factors
Physical and chemical properties of the sediment and water were also analyzed at each station. Temperature, conductivity, and oxygen level were measured using a Seabird 911 CTD Plus and SBE 21 Seacat thermosalinograph. Sediment structure (percentage content of gravel, sand, and silt) was also analyzed. Diameter of particles was calculated using the equations of Buchanan (1984), and Folk and Ward (1957). Level of total hydrocarbons (THC), toxic metals: arsenic (As), barium (Ba), cadmium (Cd), chromium (Cr), copper (Cu), nickel (Ni), lead (Pb), zinc (Zn), and content of total organic matter (TOM) were also measured. Total hydrocarbon content was analyzed using a gas chromatograph with a flame ionization detector (GC/FID), as outlined in the Intergovernmental Oceanographic Commission, Manuals and Guides No. 11, UNESCO Intergovernmental Oceanographic Commission (1982) while toxic metals contents were analyzed via inductively coupled plasma-atomic emission spectrometry (ICP-AES) (Jarvis and Jarvis, 1992; Elezz et al., 2018). Total organic matter was determined as the weight loss in a 2–3-g dried sample (dried at 105°C for 20 h) after 2 h of combustion at 480°C.
Analysis of Diversity and Abundance
Specimens were identified at the morphospecies level (Wägele, 2005). We have calculated species richness (S—number of species per sample), diversity (Shannon Index) and evenness (Pielou Index) (Magurran, 2012) as well as abundance [ind./0.1 m2] for each sample. Mean values with standard deviations (SD) of those indices were calculated for each depth zone and for the whole material. Statistical differences between the depth zones were assessed by non-parametric Kruskal–Wallis test. Post-hoc testing was done by Dunn's test. This part of the analysis was performed using a STATISTICA 13 package (StatSoft, 2006).
Species accumulation curves averaging over 999 permutations were created using the PRIMER package. The curve plotted the cumulative number of different species observed as each new sample was added (Clarke and Warwick, 2001).
We have also assessed the number of rare species recorded in the material. The number of singletons (species represented by only one individual in the whole material, in all collected samples) and doubletons (represented by two individuals), and the number of unique species (species found in one sample only) and duplicates (species found in two samples only) were also calculated. Additionally, we have calculated the number of species recorded only in a given depth zone or given transect as well as the number of species common to given depth zones and transects. Frequency of occurrence (F—percentage of samples where a species was found in total number of samples) was calculated for each species.
Multivariate Analysis
Hierarchical agglomerative clustering analysis, based on the Bray–Curtis formula, was performed to check for faunistic similarity among the stations. For the analysis, mean values of abundance of each species calculated for each station were used. Data were square root-transformed, and the group average method was used. A SIMPROF test with 1% significance level was performed to check the multivariate structure within groups. This part of the analysis was performed using a Primer package (Clarke and Warwick, 2001).
The R software was used for all analyses of environmental factors influence on cumacean communities (R Core Team, 2020). We used the Pearson correlation matrix (corrplot::corrplot; Wei and Simko, 2017) to assess pair-wise cross-correlation between each environmental variable. Based on strong correlation (r > 0.6), we included six variables from the initial set of 19 variables into further analysis, assuming they have an ecologically important role in explaining the richness of cumaceans.
Finally, six variables: Ba, Cd, THC, oxygen, gravel, and salinity were added into further analysis (Figure 2). For full list of environmental variables, please see Supplementary Table 1. Salinity was used in principal component analysis (PCA) only. Yeo-Johnson power transformation [caret::preProcess(); Kuhn, 2020] was used for reducing deviations linked with unequal ranges off selected factors (e.g., Ba). Next, PCA was performed to show dissimilarities in species composition among transects and stations [vegan::rda(); Oksanen et al., 2019]. Additionally, ranges of salinity were added to the PCA [vegan::ordisurf (); Oksanen et al., 2019] to demonstrate salt content relations in arrangement of stations in ordination space.
Species richness and PCA axis were used to fit generalized linear models (glm; for species richness) or linear models (lm; for PCA axis 1 and axis 2) with five environmental variables (Ba, Cd, THC, oxygen, and gravel) as fixed effects using the stats4 package [stats4::lm(), stats4::glm(); R Core Team, 2020]. Poisson distribution was used for species richness. To choose the best fitted models based on corrected Akaike Information Criterion (AICc), the dredge function was used (MuMIn::dredge; Bartoń, 2018). To calculate estimates of function slopes for the models with ΔAICc <2, model averaging was employed [MuMIn package model.avg(), confset95p(), and avgmod.95p()]. The RSquareAdj function (vegan::RSquareAdj; Oksanen et al., 2019) was computed to reveal how much variance was explained by averaged models for PCA axes 1 and 2.
Hierarchical partitioning function (hier.part::hier.part(); Walsh and Mac Nally, 2013) for species richness as well as PCA axes 1 and axis 2 was used for checking the independent effect (%) of each environmental variable and its joint contribution to all other predictors. To compute it, goodness-of-fit measures for all model combinations with all predictors, with Gaussian (for PCA axes 1 and 2) or Poisson distribution (for species richness) were used. Statistical significance of the relative contribution of each predictor were determined by randomization test [hier.part::rand.hp()] with implementing P-values and z-scores (confidence limit < 0.95).
Results
Diversity and Abundance
Altogether, 63 species (391 individuals) of Cumacea were identified. They represented 13 genera and six families (Table 1). It is assumed that 95% of species (60 species) are new to science. The most speciose genera were: Leucon (12 species), Eocuma (8), Iphinoe, and Diastaylis (both genera with seven species). The most abundant genera were: Eocuma (95 individuals), Bodotria (60 individuals), and Leucon (50 individuals). Together, they constituted over half of the material. The most speciose and abundant families were: Bodotridae (23 species, 235 individuals), Leuconidae (15 species, 66 individuals), Nannastacidea (10 species, 67 individuals), and Diastylidae (10 species, 20 individuals). A large number of rare species were also recorded. In the whole material, 13 singletons and 15 doubletons were found. Seventeen species were found only in one sample, and 14 in two samples only. Frequency of occurrence of species in the whole material was extremely low. Only five species had frequency higher than 4%. The species with the highest frequency of occurrence in the whole material was Eocuma sp. 7 that was found only in 7% of the samples (Table 1). The species accumulation curve did not reach the asymptotic level, suggesting undersampling of the studied area (Figure 3).
The mean density of cumaceans calculated for all collected samples equalled to only 1.5 ind./0.1 m2. General species richness and abundance decreased along a depth gradient. The highest number of species was found at 25 and 50 m with 17 and 28 species recorded, respectively (Table 2). Moreover, 15 species were common in those two depth zones (Table 3). On the outer shelf and upper slope, the number of species was lower and increased again to 19 species at 1,000 m stations (Table 2). It is also the depth zone with the most unique fauna, as 14 out of 19 species were recorded only here (Table 2). The general number of species was similar in most of the transects (Table 2). The highest number of species was found in a transect G6 (29 species), while the lowest species richness was recorded in transects G8 and G9 with seven and 10 species, respectively (Table 2). The highest number of species common with other transects was recorded in transect G6, but generally there was no clear pattern observed (Table 4).
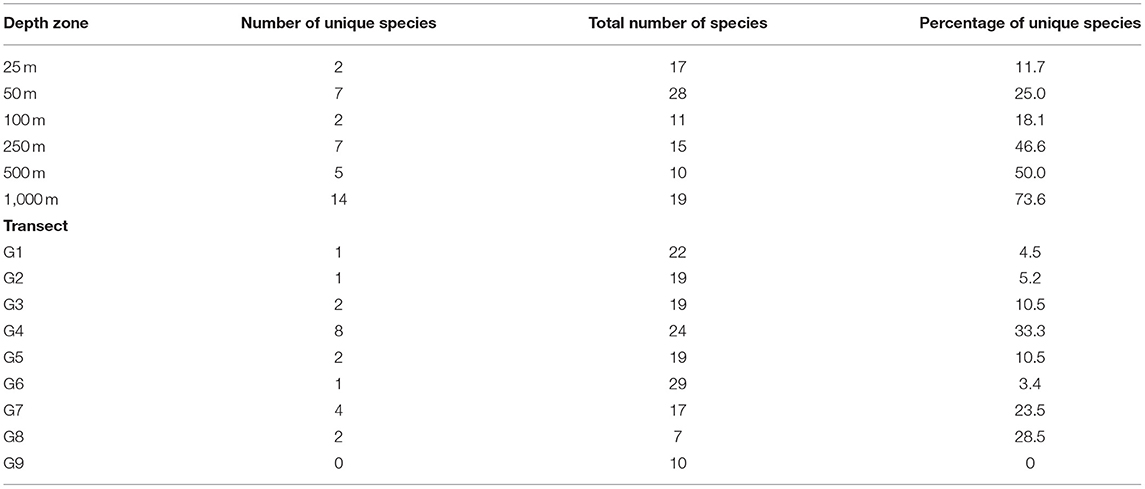
Table 2. Total number of species in each depth zone/transect and number of species recorded only in a given depth zone/transect.
Mean species richness and diversity per sample were the highest on the shallows (25 m – number of species per sample 1.04 ± 1; Shannon Index 0.2 ± 0.3, 50 m – number of species per sample 1.4 ± 1.2, Shannon Index 0.4 ± 0.4) and at 250 m (number of species per sample 1.1 ± 1, Shannon Index 0.3 ± 0.3) (Figure 4). Evenness was the highest at 1,000 m [0.9 ± 0.03 (Figure 4)]. Mean abundance changed along the depth gradient, and the highest values were observed at 50 (2.2 ± 2.2 ind./0.1 m2) and 250 m (1.8 ± 1.8 ind./0.1 m2). Below 250 m, it decreases with increasing depth (Figure 4).
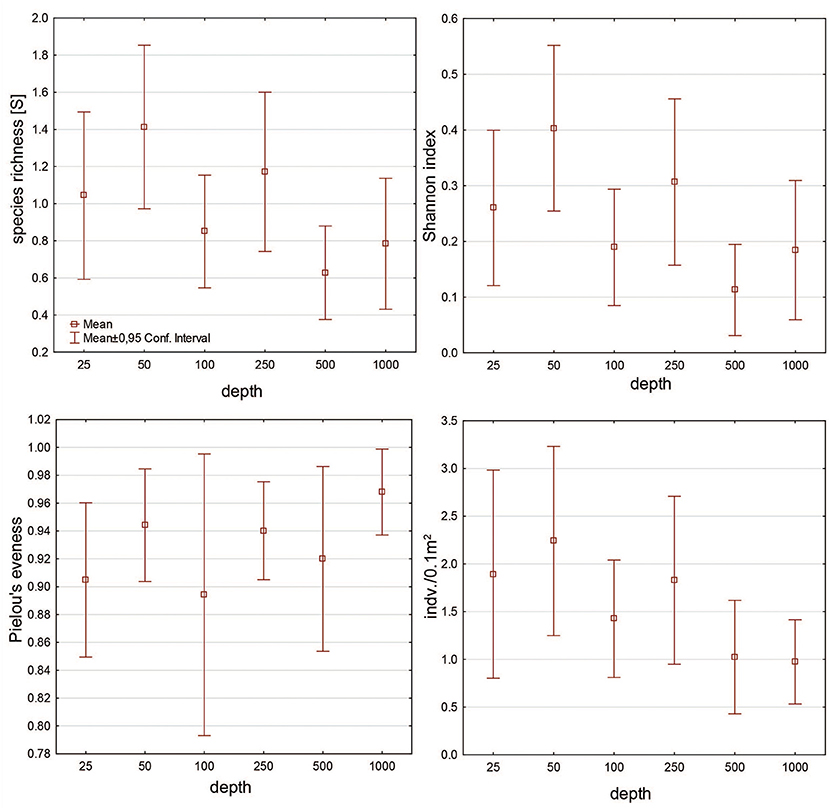
Figure 4. Mean value of species richness, diversity, evenness, and abundance calculated for cumacean fauna in each depth zone.
Cluster Analysis
Four groups were distinguished in the cluster analysis although at low similarity level (20% or less), but all were significantly differentiated by the SIMPROF (Figure 5). Inner shelf areas were clearly separated from the outer shelf and slope showing strong depth zonation of cumacean communities. Two clusters (B and C) of grouped samples were collected at depth 25–50 m. The next two clusters consist of samples collected at depth 100−500 (cluster A) and 500–1,000 m (cluster D). The clusters differ in family and genera composition, number of species, and frequency of the species.
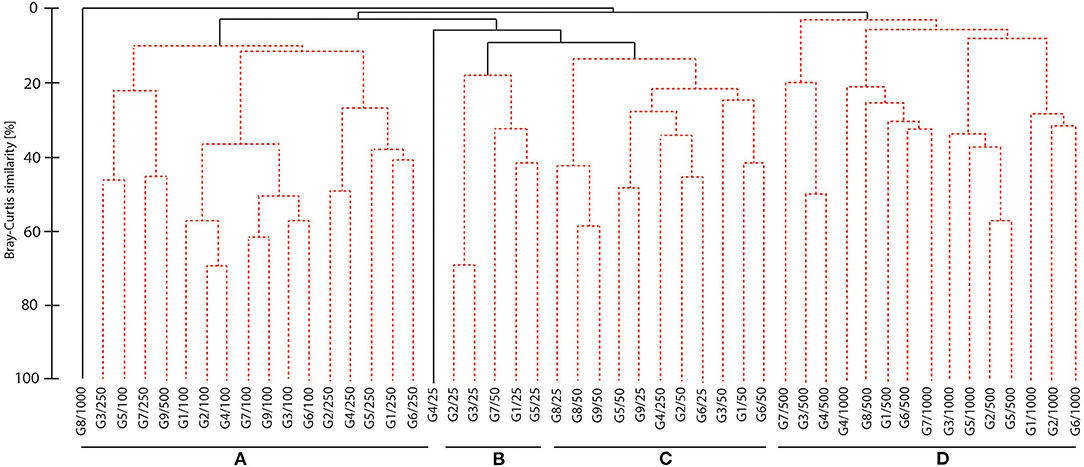
Figure 5. Dendrogram of samples, for Bray Curtis similarity, square root transformed data, and group average grouping method. (Spotted lines indicate the samples that cannot be significantly differentiated by SIMPROF).
In the samples grouped in cluster A, 22 species were found, and eight of them belong to family Bodotridae, and six to family Diastylidae. Genus Diastylis was represented by six species and genus Iphione and Eocuma by three species each. The highest frequency of occurrence (56%) was observed for Campylapsis sp 2 and Eocuma sp 7.
Eleven species were found in samples forming cluster B, and nine of them represent family Bodotridae. The most speciose genera were Bodotria with five species and Iphinoe with two species. Vaunthampsonia sp 1 was present in 80% of the samples, and Bodotria sp 2 and Eudorellopsis sp 1 were present in 60% of the samples.
Within cluster C, 28 species in total were observed, and 16 species belong to family Bodotridae. The most speciose genera were Eocuma with six species and Bodotria with five species. Iphinoe sp 2 and Eocuma sp 2 were characterized by the highest frequency of occurrence, which was 73 and 56%, respectively.
In the samples from cluster D, 25 species were recorded, and 10 belong to family Leuconidae and seven to Nannastacidae. Leucon was the most speciose genus (10 species), followed by Cumella (three species) and Campylapsis (three species). Nannastacidae sp. 1 had the highest frequency of occurrence, and it was present in 40% of the samples.
Influence of Physical and Chemical Factors on Cumacean Communities
PCA1 and PCA2 axes explained about 20% of variance. The first axis (10.7% variance explained) showed high dissimilarity between stations located at 100 m, and all other sites followed dissolved oxygen and salinity gradient. Three groups were established in the PCA mostly along the PCA2 axis (9% variance explained) (Figure 6). The first one (lower part of gradient) contained shallow water samples (25–50 m), characterized by higher concentration of oxygen and gravel. Here, a sandy type of substratum with relatively high contribution of gravel (depth zone 25–50 m) was noticed. Content of Ba, Cd, as well as THCs was significantly lower. The second group (higher part of gradient) contained samples from 250–1,000 m deep; and here, the samples were characterized by higher concentration of Ba, Cd, and THC. Lower concentration of oxygen and lower content of gravel and sand were noted here. Bottom deposits constituted mostly of silt. Salinity reached low to average values with high range (34.8–35.6%0). The third group contained samples from 100 m depth. The samples were distinguished from the other groups by high salinity content with low values range (35.7–35.8%0), and bottom substrate was dominated by sand and silt.
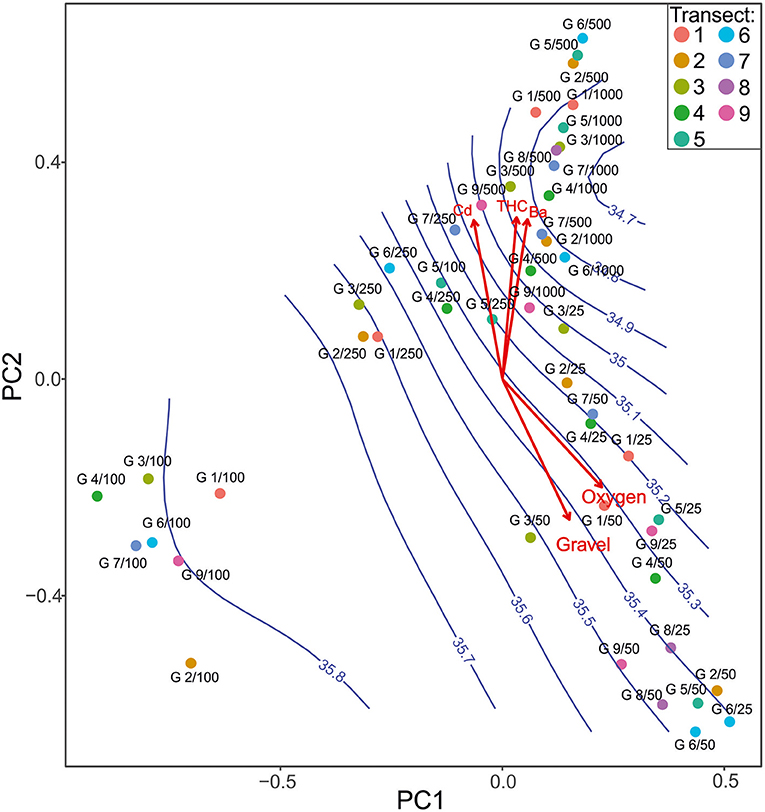
Figure 6. Principal component analysis showing species composition differences in each sampling site according to environmental factors with sampling sites (points) and ranges of salinity (blue isolines).
A set of two most parsimonious (with ΔAICc <2) linear models for PCA axis 1 revealed that high content of gravel (estimate slope: −0.30, p = 0.05) as well as oxygen (estimate slope: 0.25, p < 0.001) shaped species composition along the axis. The influence of gravel was negatively correlated with species composition along axis 1. However, higher concentration of oxygen dissolved in water had a positive influence on it (Table 5, Figure 7A, Supplementary Table 2). The model explained about 31% of total variance. Hierarchical partitioning revealed that only the influence of oxygen (relative contribution: 64.3%) was significant for PCA axis 1 (Figure 8).
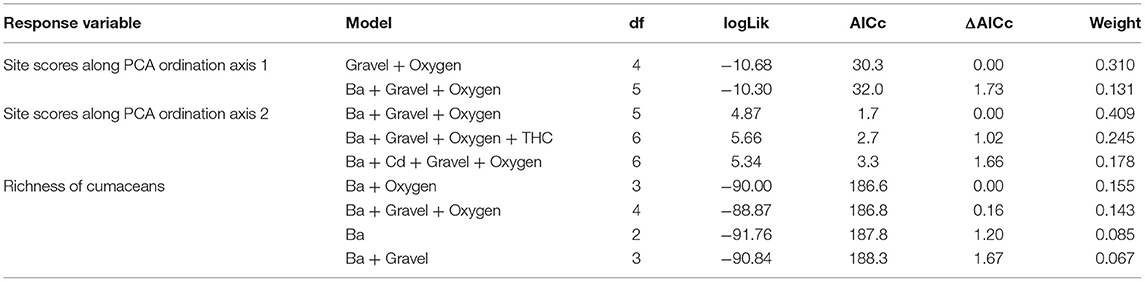
Table 5. Most supported (ΔAIC <2) models testing for impacts of environmental factors on species composition (site scores along PCA ordination axes 1 and 2, linear regression) and richness of cumaceans (generalized linear model with Poisson distribution).
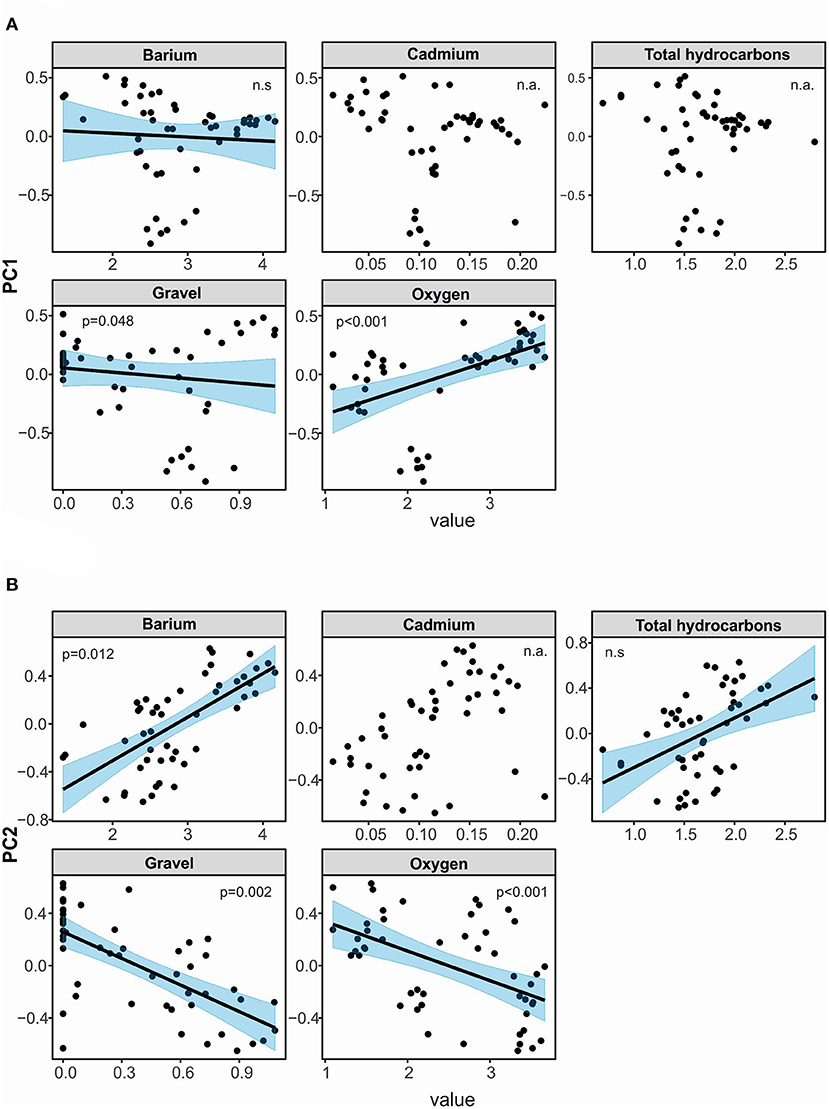
Figure 7. Visualization of linear models testing for effects of selected environmental factors on species composition of cumaceans, expressed as site scores along PCA ordination axis 1 (A) and axis 2 (B). Phrase “n.a.” means that environmental factor was not included in a set of the most parsimonious models. Phrase “n.s.” means its explanatory power was not significant despite the fact that means environmental factor was included in a set of the most parsimonious models.
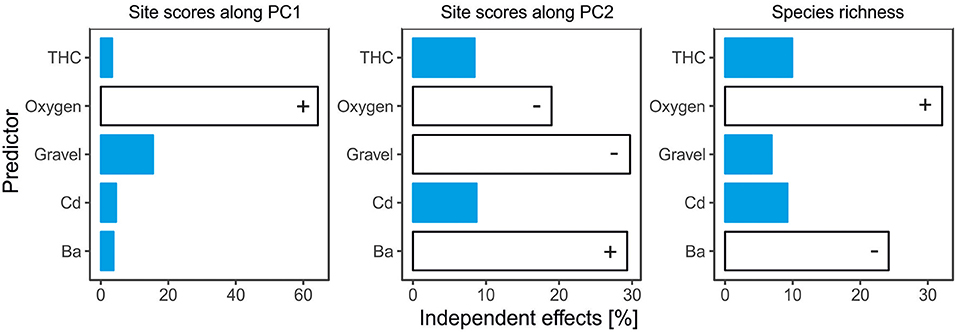
Figure 8. Relative contribution of each environmental factor to shared variability of full models testing for effects of environmental factors on species composition (expressed as site scores along PCA ordination axes (1 and 2) and richness of cumaceans. Predictors that had significant effect on response variables are given in white. Plus (+) signs express positive impact of predictors on response variables, and minus (-) signs express negative influences. For full predictor names, see Supplementary Table 1.
Form the three models best describing species composition along PCA axis 2 (containing Ba, THCs, oxygen, and gravel) we noted statistical significance of oxygen, gravel, and Ba. The higher content of oxygen (estimate slope: −0.16, p < 0.001) and gravel (estimate slope: −0.39, p = 0.002) had a negative influence on species composition along axis 2, while Ba (estimate slope: 0.21, p = 0.01) enhanced it (Table 5, Figure 7B, Supplementary Table 2). The model explained about 62% of total variance of cumaceans. The relative contribution of each factor supports the previous results. The influence of barium, gravel, and oxygen (independent effect: 29.3, 29.7, and 19%, respectively) was statistically significant (Figure 8).
A set of four most parsimonious models with ΔAICc <2 best explained richness of cumaceans species. Ba, gravel, and oxygen were included into best averaged model; however, only the adverse effect of Ba (estimate slope: −0.37, p = 0.04) was statistically significant and caused decrease in species richness (Table 5, Figure 9, Supplementary Table 2). Based on the results of hierarchical partitioning, we found that the influence of Ba and oxygen (relative contribution: 24 and 32.1%, respectively) was significantly correlated with species richness (Figure 8).
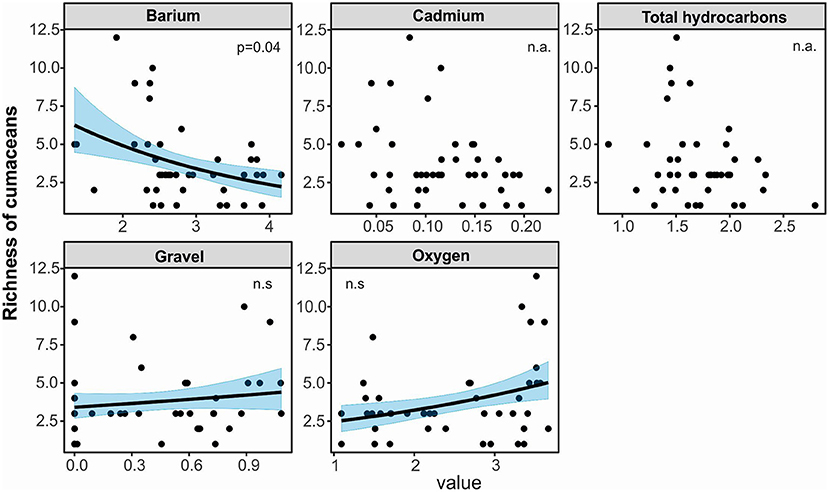
Figure 9. Visualization of generalized linear model testing for effects of environmental factors on species richness of cumaceans. Phrase “n.a.” means that environmental factor was not included in a set of the most parsimonious models. Phrase “n.s.” means its explanatory power was not significant despite the fact that means environmental factor was included in a set of the most parsimonious models.
Discussion
Species Richness
Despite very low total abundance, the cumacean species richness on the Ghanaian coast was very high, and the species accumulation curves still showed substantial undersampling (Figure 3). Taking into account generally high sampling effort (much larger than in most of other cumacean studies, see Table 6) and large number of sampled stations, it can be assumed that great species rarity was the main reason behind this result. Large number of singletons and doubletons as well as large number of species recorded in a very low number of samples was typical feature of cumacean communities along the Ghanaian coast (Table 1). Moreover, the primary analysis indicates that 95% of collected species are new to the science. The results demonstrated the highly underestimated diversity of those crustaceans in the Gulf of Guinea, even compared with the global diversity of Cumacea, which was estimated at about 1,400 species (Gerken, 2018). After this study, the list of known cumacea from the coast of Guinea increased from 3 to 66 species (Watling and Gerken, 2021), which is a significant result for about 500-km long part of the coastline.
Comparisons with other studies of cumacean species richness are difficult because of large discrepancies in type of gear used, scale of the sampling area, sampling effort, and studied depth range, not to mention the differences in local environmental conditions or geological history of various regions (Table 6). Nevertheless, the number of 63 species is comparable even with much larger areas that were sampled extensively for a very long time. For example, in the whole Antarctic waters, 86 species of cumacea were recorded (De Broyer and Danis, 2011). Extensive analysis of literature data resulted in the list of 172 species recorded from Iberian waters (Atlantic and Mediterranean) including 142 species found in bathyal (200–3,000 m) (Corbera, 1995). The current list of all Mediterranean cumaceans (such as Tyrrhenian, Adriatic, Aegena, and Levantine Seas) includes 99 species (Coll et al., 2010). Analysis of large set of 122 epibenthic sledge samples collected in the deep Atlantic (500–4,000 m depth) revealed the presence of 225 species, although from a large number of almost 56,000 individuals (Gage et al., 2004). On the other hand, only 29 species were recorded from a whole region of tropical Eastern Pacific (Jarquin-Gonzalez and Garcia-Madrigal Mdel, 2013) and only 34 species from the whole Chilean coast (Thiel et al., 2003). Even in the intensively sampled, large open system of the Bay of Biscay, the number of recorded cumacean species was lower than in this study. In the subtidal zone (up 63 m depth) 18 species were recorded in over 100 samples collected using the van Veen grab (Cacabelos et al., 2010; Corbera et al., 2013; Corbera and Galil, 2016). At the deeper areas of the bay in Kostarrenkala area, 42 species in total were collected (13 species were found at 170 m, six species at 300 m, nine species at 400 m, 18 species at 724 m, and 24 species at 1,000 m) (Frutos and Sorbe, 2014). We have analyzed sampling effort and cumacean species richness from 39 different sampling campaigns (Table 6). In majority of the studies, the number of species was lower than 35, even if the sampling effort was high, and even if an epibenthic sledge or other gears collecting large number of individuals and species were used. Nevertheless, it is worth mentioning that this study was conducted in a relatively wide depth range. Cumaceans have low dispersal potential (Jones and Sanders, 1972; Pilar-Cornejol et al., 2004), therefore, large depth range sample (25–1,000 m), together with large diversity of microhabitats and differences in environmental conditions, could result in recognition of a larger number of species. It is clearly visible in the analysis of species common in different depth zones (Table 3) and in the results of the cluster analysis (Figure 5).
Based on current data, we cannot postulate that the Gulf of Guinea is a hot spot of cumacean diversity, although that kind of assumptions is likely possible. There were previous suggestions that this region might be an important center of cumacean diversity. In the deeper parts of the Angola Bay (5,125–5,415 m), 45 species were recorded in just seven epibenthic sledge samples (Brandt, 2005; Mühlenhardt-Siegel, 2005), while Bochert and Zettler (2011) described 16 additional species from the shelf of the Angolan and Namibian waters. High diversity of Cumacea on the equator was already mentioned by Jones and Sanders (1972) and later supported by large scale latitudinal analysis of the deep Atlantic cumacean richness, although authors declare that it is difficult to say if this pattern is related to geological and evolutionary history (e.g., glaciation in the northern hemisphere) or differences in more recent changes in local ecological conditions (e.g., high productivity in tropical areas; Gage et al., 2004). Confrontation of those observed patterns with the knowledge about large sampling bias in the tropical marine waters (Menegotto and Rangel, 2018) demonstrates that many important questions regarding the distribution patterns and diversity are still open and need further comprehensive studies. Based on the current data, it is impossible to discuss about the dependencies between local (e.g., Ghanaian coast) and regional (e.g., whole Atlantic African coast, West Africa, and Gulf of Guinea) species pools (Witman et al., 2004) or provide any generalizations about factors influencing diversity on a larger scale. At the same time, we did not observe large differences in species composition on intermediate scale (between investigated transects). There were some transects with very low (G8 – 7 species) or very high (G6 – 29 species) total number of species, but at the same time the number of species unique to a given transect was very low (Table 2), and there was no clear spatial pattern in species common to different transects, even from opposite parts of the Ghanaian coast (Table 4). Those differences are rather not related to distance between the transects but are most probably due to the influence of local environmental conditions as shown in the PCA analysis.
Distribution Patterns and Diversity on a Background of Environmental Conditions
Oxygen content and sediment type (especially content of gravel) drive species composition and diversity especially in the 25–50 m stations. Well-oxygenated water and elevated primary production may increase species richness (Levin and Sibuet, 2012; McCallum et al., 2015). On the other hand, in previous studies, Pabis et al. (2020) reported low oxygen concentration at 250–500 m depth on the coast of Ghana, and those factors might also cause decrease in cumacean abundance and species richness in this depth zone, although the pattern is not clear, and visible only at 500 m. Lower oxygen concentrations might be caused by sinking organic matters resulting from seasonal upwelling at the Ghanaian coast (Nieto and Mélin, 2017).
There is no evidence that increased salinity may reduce the abundance and species richness of cumacea. We suspect that higher salinity values at 100 m were a result of seasonal and oceanographic factors such as upwelling events, bottom currents, temperature, or rainy seasons (Ukwe et al., 2003; Djagoua et al., 2011; Nieto and Mélin, 2017). Martin et al. (2010) showed that decrease in salinity may increase the activity of cumaceans in water column, although we have observed only slight differences in salinity along the coast of Ghana. Therefore, based on available data, we cannot speculate about its influence on cumacean communities.
Earlier studies support our result, pointing substrate grain size and organic matter content as the most important drivers of cumacean assemblages (Corbera and Cardell, 1995; Dos Santos and Pires-Vanin, 1999; Cristales and Pires-Vanin, 2014; Corbera and Sorbe, 2020). In the study of the shallow water communities of the Persian Gulf, the presence of gravel also had a positive influence on cumacean fauna (Martin et al., 2010). The positive effect of gravel on the shallow water communities was also confirmed in the study of polychaete functional diversity in the Ghanaian waters (Sobczyk et al., 2021). The presence of coarser sediment fractions might increase habitat complexity and heterogeneity for small infaunal invertebrates such as cumaceans or various groups of polychaetes, resulting in higher number of microhabitats and/or ecological niches and increased diversity (Sebens, 1991; Carvalho et al., 2017). We also have to take into account interactions with other benthic organisms occurring in the shallows. Generally, the abundance and diversity of benthic fauna of the Ghanaian coast were highest in the 25–50 m depth range (Pabis et al., 2020). This fact might increase the diversity of mutual interactions between various organisms, for example, because of higher level of sediment bioturbation, which could influence oxygenation of the sediment and food availability (Aller and Cochran, 2019). Such conclusions are supported by high abundance and diversity of burrowing polychaetes recorded in this depth zone along the Ghanaian coast (Sobczyk et al., 2021).
Sediment character might be crucial for cumacean survival, as it can be strictly related to the feeding strategy and respiratory mechanism (Dixon, 1944 in Dos Santos and Pires-Vanin, 1999). Cumacea feed on microorganism (especially diatoms) and/or detritus (Błazewicz-Paszkowycz and Ligowski,2002). It is assumed that mud-dwellers filter small particles of suspension, while sand dwellers scrub food from sand grains. However, studies on Cumella vaulgaris demonstrated that the attractiveness of a particular substratum depends on the amount and type of food (Wieser, 1956). The type of substrate is also suggested to have some impact on filter apparatus appearance in some cumaceans. Species that live in muddy sediment have the filter apparatus equipped with finely feathered bristles that allow easier water flow. For example, members of the Diastylis are known to have filter apparatus adapted to catching small particles of food from water (Dixon, 1944). Nevertheless, the knowledge on diet, habitat preferences, and other aspects of the cumacean biology is extremely scarce. We know nothing about the ecology and biology of majority of genera, and it is impossible to link the results with any data about the biology of particular species recorded in West Africa.
Slope communities were also affected by disturbance associated with the influence of barium, other heavy metals, and hydrocarbons that are associated mainly with increasing activities of petroleum companies. The oil industry (e.g., Jubilee Oil Field) combined with pollution from other sources such as the dyeing industry, leaks from crude oil storage, and inputs of polluted fresh water have an important influence on the Gulf of Guinea (Acquah, 1995; Owusu-Boadi and Kuitunen, 2002; Scheren et al., 2002; Ayamdoo, 2016; Hanson and Kwarteng, 2019). For example, between 2009 and 2011, there was a spill of oil-based mud in Ghanaian waters, and the control of pollution in this region remains poor and not well-documented, although it is considered to continuously increase (Ayamdoo, 2016). Moreover, Ghana is importing barite for the dyeing industry (Sobczyk et al., 2021). Larger concentration of Ba on the slope is also not surprising because of the influence of pressure on the solubility of barite (Neff, 2002). At the same time, elevated levels of barium were not visible in all slope stations, but only on part of the transects (Pabis et al., 2020), confirming that pollution has local anthropogenic origin. Despite the fact that cumacean abundance and species richness per sample were generally low along the whole depth range, we have noticed decreased values in the slope samples, where muddy sediments are characterized by higher content of barium, other toxic metals, and hydrocarbons (Pabis et al., 2020). Those factors might influence benthic communities (e.g., Olsgard and Gray, 1995; Gomez-Gesteira et al., 2003; Stark et al., 2020), and it is known that heavy metals might accumulate in cumacean bodies (Swaileh and Adelung, 1995). Ba and other heavy metals may affect development and cause decrease in abundance of benthic invertebrates (Lira et al., 2011), or influence embryos of crustaceans and bivalves (Macdonald et al., 1988). Similar effects were described for hydrocarbons (Main et al., 2015; Honda and Suzuki, 2020). Nevertheless, there are only scarce data about exact doses of various pollutants that could influence particular species or taxonomic groups of benthic organisms (Lira et al., 2011). We already noticed in the earlier study (Pabis et al., 2020) that levels of Ba and other toxic metals in the Gulf of Guinea were close to background levels according to OSPAR and KLIF (Norwegian Pollution Authority) guidelines (OSPAR, 2017), although literature data from other regions demonstrated that even low concentrations of Ba and other pollutants might influence benthic communities (Olsgard and Gray, 1995). The influence of local pollution associated with oil exploration in Ghanaian waters and dying industry was also visible in the study on polychaete functional diversity that was based on the same set of samples (Sobczyk et al., 2021). Patterns observed in cumacean study are very similar, although not that obvious and strong as in the case of polychaetes, which is most probably caused by generally very low abundance of those crustaceans. Moreover, polychaetes are considered perfect model organisms for various studies on ecosystem response to natural or anthropogenic changes and disturbances (Olsgard et al., 2003; Giangrande et al., 2005), and it is not surprising that they are good indicators of disturbance.
Cumaceans are small benthic brooders with limited dispersal potential. Therefore, they are considered to be sensitive to changes in environmental factors (Corbera and Cardell, 1995; Alfonso et al., 1998), although there are no data on influence of pollution on their communities, except those of one study showing decrease in abundance in the polluted site (de-la-Ossa-Carretero et al., 2012). However, studies on similar small peracarid crustaceans such as Tanaidacea demonstrated that they might be good indicators of disturbance processes (Guerra-García and García-Gómez, 2004). The influence of local pollution on the Ghanaian coast was visible even in the case of higher taxa, although the taxonomic level of phyla and orders is normally not sufficient for meaningful assessments of ecosystem health (Pabis et al., 2020). Moreover, we have to remember that despite the fact that Ba was a significant factor in the analysis, other variables such as hydrocarbons and other heavy metals such as Cd, Cu, and Ni could also be responsible for combined influence on cumacean communities (Sobczyk et al., 2021). In such cases, it is difficult to unequivocally assess the influence of one out of multiple stressors on benthic communities (Borja et al., 2011; Lenihan et al., 2018), even by advanced multivariate analysis and especially when we analyse communities of less abundant taxa-like cumaceans. The results of the PCA are not strong, since first PC axis explained only 10% of variance, which is due to very low abundance, large number of singletons, and highly patchy distribution of majority of species. Nevertheless, the results are supported by analysis based on the abundance of macrozoobenthic higher taxa and polychaete communities (Pabis et al., 2020; Sobczyk et al., 2021). Moreover, similar results of the PCA are sometimes sufficient for description of ecological patterns (Sarthou et al., 2010), although they have to be treated cautiously.
Nevertheless, it is, to some point, surprising that we have noticed two peaks in the general number of species, one in the shallows and one in the 1,000 m (Table 2), where the influence of Ba and hydrocarbons was the highest. Moreover, the cumacean fauna recorded at 1,000 m stations was also the most unique. Those facts might be associated with the general pattern showing that bathyal is the main biodiversity hot spot for benthic fauna due to higher habitat diversity (Danovaro et al., 2009; Rex and Etter, 2010). High diversity of bathyal cumacean communities was already demonstrated in many previous studies (e.g., Corbera, 1995; Gage et al., 2004; Corbera and Sorbe, 2020 and citations therein).
Distribution of genera and/or families along a depth gradient might also be at least partially explained by earlier studies on cumacean evolution and phylogeny, although we also know very little about those important problems (Gerken, 2018 and references therein). There are only scarce data about the possible origin of various families or genera and their affinities to given depth zones or regions. For example, Bodotriidae are classified as typical shallow water crustaceans (Day, 1978; Mühlenhardt-Siegel, 1996; Petrescu, 1998), while members of Leuconidae are more often recorded in the deep sea (Mühlenhardt-Siegel, 2005, 2011; Gerken, 2016). Diastylis, on the other hand, is considered to have relatively wide bathymetric distribution, from shallows to bathyal depths (Băcescu and Petrescu, 1991; Mühlenhardt-Siegel, 2005). On the other hand, those general patterns might be affected by some local conditions. For example, the presence of preferred sediment type might extend the bathymetric range of cumaceans, recognized as shallow water, even to upper bathyal depths (Corbera and Sorbe, 2020), demonstrating that similar generalizations are still far from being conclusive. Brandt et al. (2012) summarized the information about widely distributed peracarid crustaceans. According to this analysis, there are at least 48 eurybathic cumacean species in the deep sea, and at that least 25 have a very wide geographic distribution (two or more oceans), although we have to remember that those numbers could substantially change after detailed molecular studies.
The results suggest high level of undescribed cumacean diversity in West African waters. Future biodiversity studies should be focused on bathyal communities, especially in areas not affected by human related disturbance processes, and explore a wider depth range. The use of dredges or epibenthic sledge could also allow to collect a larger number of individuals than point scale samplers such as the van Veen grab. Probably, the most appropriate sampling strategy should include the use of both quantitative and semiquantitative methods, as it was already demonstrated in case of tanaidaceans (Józwiak et al., 2020). The hypothesis of the high diversity of cumacean fauna in tropical African waters still cannot be verified because of strong sampling bias. The great rarity, small population densities, and high level of patchiness in the distribution of particular species suggest the necessity of sampling at larger number of stations, allowing for more comprehensive biodiversity inventory of those small crustaceans. The high diversity of Cumacea observed in this study showed that small peracarids should be included in future research, especially since the pressure of human activities in large marine ecosystems such as the Gulf of Guinea could lead to substantial loss in marine diversity yet unknown. There is also a great need for further taxonomic studies on the region. They could help to accelerate the further analysis of ecological interactions occurring in West African seabed ecosystems, because they constitute an important base of any ecological research and biodiversity inventories.
Data Availability Statement
The original contributions presented in the study are included in the article/Supplementary Material, further inquiries can be directed to the corresponding author/s.
Author Contributions
AS: concept of the paper, taxonomic identification, statistical analysis, and manuscript writing. KP: concept of the paper, statistical analysis, writing of the manuscript, sampling design, and coordination of benthic ecology in the project. RS: statistical analysis. BS: sampling design, proofreading, and head of the FAO project. All authors contributed to the article and approved the submitted version.
Funding
The sampling cruise and the environmental data analysis within this program were funded by the Norwegian Agency for Development Cooperation (NORAD) (Oil for Development Program—OfD) and by the Food and Agriculture Organization of the United Nations (FAO). AS and KP were also supported by University of Lodz.
Conflict of Interest
The authors declare that the research was conducted in the absence of any commercial or financial relationships that could be construed as a potential conflict of interest.
Publisher's Note
All claims expressed in this article are solely those of the authors and do not necessarily represent those of their affiliated organizations, or those of the publisher, the editors and the reviewers. Any product that may be evaluated in this article, or claim that may be made by its manufacturer, is not guaranteed or endorsed by the publisher.
Acknowledgments
The authors want to thank Dr. Ute Mühlenhardt-Siegel for helping with identifying species.
Supplementary Material
The Supplementary Material for this article can be found online at: https://www.frontiersin.org/articles/10.3389/fmars.2021.703547/full#supplementary-material
References
Acquah, P. (1995). Natural Resources Management and Sustainable Development: The Case of the Gold Sector in Ghana. Geneva: United Nations Conference on Trade and Development (UNCTAD).
Alfonso, M. I., Bandera, M. E., López-González, P. J., and García-Gómez, J. C. (1998). The cumacean community associated with a seaweed as a bioindicator of environmental conditions in the Algeciras Bay (Strait of Gibraltar). Cah. Biol. Mar. 39, 197–205.
Aller, R. C., and Cochran, J. K. (2019). The critical role of bioturbation for particle dynamics, priming potential, and organic C remineralization in marine sediments: local and basin scales. Front. Earth Sci. 7:157. doi: 10.3389/feart.2019.00157
Appeltans, W., Ahyong, S. T., Anderson, G., Angel, M. V., Artois, T., Bailly, N., et al. (2012). The magnitude of global marine species diversity. Cur. Biol. 22, 2189–2202. doi: 10.1016/j.cub.2012.09.036
Ateş, A. S., Katagan, T., Sezgin, M., Özdilek, H. G., Berber, S., and Bulut, M. (2014). The effects of some domestic pollutants on the cumacean (Crustacea) community structure at the coastal waters of the Dardanelles, Turkey. Arthropods 3, 27–42. Available online at: http://www.iaees.org/publications/journals/arthropods/onlineversion.asp
Ayamdoo, N. A. (2016). Protecting the Gulf of Guinea in an oil boom: regulating offshore 618petroleum pollution in a divided world. J. World Energy Law Bus. 619, 219–232. doi: 10.1093/jwelb/jww007
Băcescu, M. (1961). Contribution à la connaissance du genere Makrokylindrus Stebbing (Crustacea: Cumacea). Rev. Biol. 6, 325–333.
Băcescu, M. (1972). Archaeocuma and Schizocuma, new genera of Cumacea from the American tropical waters. Rev. Roum. Biol. Academia RepubliciiSocialiste Romania 17, 241–250.
Băcescu, M., and Petrescu, I. (1991). New Cumacea (Crustacea, Peracarida) from the littoral waters of Brazil. Trav. Mus. Natl. Hist. Nat. 31, 327–340.
Bamikole, W., Ndubuisi, A., Ochuko, A. P., and Olamide-Olaronke, O. P. (2009). Macrobenthic faun aof Snake Island area of Lagos lagoon, Nigeria. Res. J. Biol. Sci. 4, 272–276. Available online at: https://medwelljournals.com/abstract/?doi=rjbsci.2009.272.276
Bartoń, K. (2018). MuMIn: Multi-model inference. R package version 1.42.1. Available online at: https://CRAN.R-project.org/package=MuMIn (accessed January 15, 2021).
Bassindale, R. (1961). On the marine fauna of Ghana. Proc. Zool. Soc. Lond. 137, 481–510. doi: 10.1111/j.1469-7998.1961.tb06086.x
Binet, D., and Marchal, E. (1993). The large marine ecosystem of shelf areas in the Gulf of Guinea: long-term variability induced by climatic changes, in Large Marine Ecosystems. Stress, Mitigation, and Sustainability, eds K. Sherman, L. D. Alexander and B. D. Gold (Washington, DC: AAAS Press), 104–118.
Birchenough, G. M. H., Johansson, M. E., Gustafsson, J. K., Bergström, J. H., and Hansson, G. C. (2015). New developments in goblet cell mucus secretion and function. Mucosal Immunol. 8, 712–719. doi: 10.1038/mi.2015.32
Bishop, J. D. D. (1982). Three new species of the genus Leucon Kröyer, 1846 (Crustacea: Cumacea) from the continental slope off Surinam. Zool. J. Linnean Soc. 74, 345–357. doi: 10.1111/j.1096-3642.1982.tb01157.x
Błazewicz-Paszkowycz, M., and Ligowski, R. (2002). Diatoms as food source indicator for some Antarctic Cumacea and Tanaidacea (Crustacea). Antarct. Sci. 14, 11–15. doi: 10.1017/S0954102002000524
Bochert, R., and Zettler, M. L. (2011). Cumacea from the continental shelf of Angola and Namibia with descriptions of new species. Zootaxa 2978, 1–33. doi: 10.11646/zootaxa.2978.1.1
Borja, A., Belzunce, M. J., Garmendia, J., Rodríguez, J. G., Solaun, O., and Zorita, I. (2011). Impact of pollutants on coastal and benthic marine communities, in Ecological Impacts of Toxic Chemicals, eds F. Sánchez-Bayo, P. J. van den Brink and R. M. Mann (Sharjah: Bentham Science Publishers), 165–186. doi: 10.2174/978160805121210165
Brandt, A. (1997). Abundance, diversity and community patterns of epibenthic- and benthic-boundary layer peracarid crustaceans at 75°N off East Greenland. Polar Biol. 17, 159–174. doi: 10.1007/s003000050118
Brandt, A. (2005). Diversity of peracarid crustaceans (Malacostraca) from the abyssal plain of the Angola Basin. Org. Divers. Evol. 5, 105–112. doi: 10.1016/j.ode.2004.10.007
Brandt, A., Błazewicz-Paszkowycz, M., Bamber, R. N., Mühlenhardt-Siegel, U., Malyutina, M. V., et al. (2012). Are there widespread peracarid species in the deep sea (Crustacea: Malacostraca)? Pol. Polar Res. 33. 139–162. doi: 10.2478/v10183-012-0012-5
Buchanan, J. B. (1957). The bottom fauna communities across the continental shelf off Accra, 571Ghana (Gold Coast). P. Zool. Soc. Lon. 130, 1–56. doi: 10.1111/j.1096-3642.1958.tb00562.x
Buchanan, J. B. (1984). Sediment analysis, in Methods for the Study of Marine Benthos, eds N. A. Holme and A. D McIntyre (Oxford: Blackwell Scientific Publications), 41–65.
Cacabelos, E., Lourido, A., and Troncoso, J. S. (2010). Composition and distribution of subtidal and intertidal crustacean assemblages in soft-bottoms of the Ria de Vigo (NW Spain). Sci. Mar. 74, 455–464. doi: 10.3989/scimar.2010.74n3455
Cartes, J. E. (1993). Diets of two deep-sea decapods: nematocarcinusexilis (Caridea: Nematocarcinidae) and munidatenuimana (Anomura: Galatheidae) on the Western Mediterranean slope. Ophelia 37, 213–229. doi: 10.1080/00785326.1993.10429919
Cartes, J. E., Jaume, D., and Madurell, T. (2003). Local changes in the composition and community structure of suprabenthic peracarid crustaceans on the bathyal Mediterranean: influence of environmental factors. Mar. Biol. 143, 745–758. doi: 10.1007/s00227-003-1090-z
Cartes, J. E., and Sorbe, J. C. (1997). Bathyal cumaceans of the Catalan Sea (North-western Mediterranean): faunistic composition, diversity and near-bottom distribution along the slope (between 389 and 1,859 m). J. Nat. Hist. 31, 1041–1054. doi: 10.1080/00222939700770541
Carvalho, L. R. S., Loiola, M., and Barros, F. (2017). Manipulating habitat complexity to understand its influence on benthic macrofauna. J. Exp. Mar. Biol. Ecol. 489, 48–57. doi: 10.1016/j.jembe.2017.01.014
Clarke, K. R., and Warwick, R. M. (2001). Change in Marine Communities: An Approach to Statistical Analysis and Interpretation. Plymouth: PRIMER-E.
Coll, M., Piroddi, C., Steenbeek, J., Kaschner, K., Ben RaisLasram, F., Aguzzi, J., et al. (2010). The biodiversity of the Mediterranean Sea: estimates, patterns, and threats. PLoS ONE 5:e11842. doi: 10.1371/journal.pone.0011842
Corbera, J. (2000). Systematics and distribution of cumaceans collected during BENTAR-95 cruise around South Shetland Islands (Antarctica). Sci. Mar. 64, 9–28. doi: 10.3989/scimar.2000.64n19
Corbera, J., and Cardell, M. J. (1995). Cumaceans as indicators of eutrophication on soft bottoms. Sci. Mar. 59, 63–69.
Corbera, J., and Galil, S. J. (2016). Cumacean assemblages on the Levantine shelf (Mediterranean Sea) – spatiotemporal trends between 2005 and 2012. Mar. Biol. Res. 12, 663–672. doi: 10.1080/17451000.2016.1169297
Corbera, J., Martin, D., Pinedo, S., and Sarda, R. (2013). Cumacean density, biomass and productivity in the sandy bottoms of the Bay of Blanes (western Mediterranean). Cah. Biol. Mar. 54, 63–70. Available online at: http://hdl.handle.net/10261/63729
Corbera, J., San Vicente, C., and Sorbe, J. C. (2008). Cumaceans (Crustacea) from the Bellingshausen Sea and off the western Antarctic Peninsula: a deep-water link with fauna of the surrounding oceans. Polar Biol. 32, 611–622. doi: 10.1007/s00300-008-0561-6
Corbera, J., and Sorbe, J. C. (2020). Bathyal cumacean assemblages from the southern margin of the Cap Ferret Canyon (SE Bay of Biscay). Sci. Mar. 84:7. doi: 10.3989/scimar.05031.07A
Cristales, P. A., and Pires-Vanin, A. M. S. (2014). Structuring factors of the Cumacean communities of the continental shelf of Southeastern Brazil. Braz. J. Oceanogr. 62, 103–116. doi: 10.1590/S1679-87592014056206202
Cunha, M. R., Sorbe, J. C., and Bernardes, C. (1997). On the structure of the neritic suprabenthic communities from the Portuguese continental margin. Mar. Ecol. Prog. Ser. 157, 119–137. doi: 10.3354/meps157119
Danovaro, R., Canals, M., Gambi, C., Heussner, S., Lampadariou, N., and Vanreusel, A. (2009). Exploring benthic biodiversity patterns and hotspots on European margin slopes. Oceanogr. 22, 16–25. doi: 10.5670/oceanog.2009.02
Day, J. (1975). Southern African Cumacea. Part 1. families Bodotriidae, subfamily Vaunthompsoniinae. Ann. S. Afr. Mus. 66, 177–220.
Day, J. (1978). South African Cumacea, Part 3: families lampropidae and ceratocumatidae. Ann. S. Afr. Mus. 76, 137–189.
Day, J. (1980). Southern African Cumacea. Part 4. families gynodiastylidae and diastylidae. Ann. S. Afr. Mus. 82, 187–292.
De Broyer, C., and Danis, B. (2011). How many species in the Southern Ocean? Towards a dynamic inventory of the Antarctic marine species. Deep Sea Res. Part II Top. Stud. Oceanogr. 58, 5–17. doi: 10.1016/j.dsr2.2010.10.007
de-la-Ossa-Carretero, J. A., Del-Pilar-Ruso, Y., Gimenez-Casalduero, F., and Sanchez-Lizaso, J. L. (2012). Assessing reliable indicators to sewage pollution in coastal soft-bottom communities. Environ. Monit. Assess. 184, 2133–2149. doi: 10.1007/s10661-011-2105-8
Dixon, A. Y. (1944). Notes on certain aspects of the biology of Cumopsisgoodsiri (Van Beneden) and some other cumaceans in relation to their environment. J. Mar. Biolog. Assoc. 26, 61–71. doi: 10.1017/S0025315400014454
Djagoua, E. V., Kassi, J. B., Mobia, B., Kouadio, J. M., Dro, C., Affian, K., et al. (2011). Ivorian and Ghanaian upwelling comparison: intensity and impact on phytoplankton biomass. AJSIR 5, 740–747. doi: 10.5251/ajsir.2011.2.5.740.747
Donath-Hernández, F. E. (1987). Distribution of the Cumacea (Crustacea, Peracarida) of the Bahia De Todos Santos, Baja California, Mexico. Cienc. Mar. 13, 35–52. doi: 10.7773/cm.v13i4.555
Dos Santos, M. F., and Pires-Vanin, S. A. M. (1999). The Cumacea community of the southeastern Brazilian Continental Shelf: structure and dynamics. Sci. Mar. 63, 15–25. doi: 10.3989/scimar.1999.63n115
Doti, B. L., Chiesa, I. L., and Roccatagliata, D. (2020). Biodiversity of isopoda and Cumacea (Peracarida, Crustacea) from the Marine Protected Area Namuncurá-Burdwood Bank, South-West Atlantic. Polar Biol. 43, 1519–1534. doi: 10.1007/s00300-020-02725-z
Elezz, A. A., Mustafa Hassan, H., Abdulla Alsaadi, H., Easa, A., Al-Meer, S., Elsaid, K., et al. (2018). Validation of total mercury in marine sediment and biological samples, using cold vapour atomic absorption spectrometry. Meth. Proth. 1:31. doi: 10.3390/mps1030031
Folk, R. L., and Ward, W. C. (1957). Brazos River bar [Texas]; a study in the significance of grain size parameters. J. Sediment. Res. 27, 3–26. doi: 10.1306/74D70646-2B21-11D7-8648000102C1865D
Friedlander, A. M., Ballesteros, E., Fay, M., and Sala, E. (2014). Marine communities on oil platforms in Gabon, West Africa: high biodiversity oases in a low biodiversity environment. PLoS One 9:e103709. doi: 10.1371/journal.pone.0103709
Frutos, I., and Sorbe, J. C. (2014). Bathyal suprabenthic assemblages from the southern margin of the Capbreton Canyon (“Kostarrenkala” area), SE Bay of Biscay. Deep Sea Res. II Top. Stud. Oceanogr. 104, 291–309. doi: 10.1016/j.dsr2.2013.09.010
Frutos, I., and Sorbe, J. C. (2017). Suprabenthic assemblages from the Capbreton area (SE Bay of Biscay). Faunal recovery after a canyon turbidity disturbance. Deep Sea Res. II Top. Stud. Oceanogr. 130, 36–46. doi: 10.1016/j.dsr.2017.10.007
Gaever, S., Olu, K., Derycke, S., and Vanreusel, A. (2009). Metazoan meiofaunal communities at cold 678 seeps along the Norwegian margin: influence of habitat heterogenity and evidence for connection with shallow-water habitats. Deep Sea Res. I Oceanogr. Res. Pap. 56, 772–785. doi: 10.1016/j.dsr.2008.12.015
Gage, J. D., Lambshead, P. J. D., Bishop, J. D. D., Stuart, C. T., and Jones, N. S. (2004). Large-scale biodiversity pattern of Cumacea (Peracarida: Crustacea) in the deep Atlantic. Mar. Ecol. Prog. Ser. 277, 181–196. doi: 10.3354/meps277181
Galéron, J., Menot, L., Renau, N., Crassous, P., Khripounoff, A., Treignier, C., et al. (2009). Spatial and temporal patterns of benthic macrofaunal communitites in the deep continental margin in the Gulf of Guinea. Deep Sea Res. II Top. Stud. Oceanogr. 56, 2299–2312. doi: 10.1016/j.dsr2.2009.04.011
Gerken, S. (2016). Leuconidae (Crustacea: Cumacea) from the collections of the Museum Victoria, Australia. Zootaxa 251, 251–292. doi: 10.11646/zootaxa.4067.3.1
Gerken, S. (2018). The Lampropidae (Crustacea: Cumacea) of the World. Zootaxa 4428, 1–192. doi: 10.11646/zootaxa.4428.1.1
Germain, J., and Armengol, C. (1999). Gulf of Guinea: a deep offshore oppotrunity. ICEED, 25, 87–95.
Giangrande, A., Licciano, M., and Musco, L. (2005). Polychaetes as environmental indicators revisited. Mar. Poll. Bull. 50, 1153–1162. doi: 10.1016/j.marpolbul.2005.08.003
Gladfelter, W. B. (1975). Quantitative distribution of shallow-water Cumacea from the vicinity of Dillon Beach, California, with descriptions of five new species. Crustaceana 29, 241–251. doi: 10.1163/156854075X00289
Gomez-Gesteira, J. L., Dauvin, J. C., and Salvande-Fraga, M. (2003). Taxonomic level for assessing oil spill effects on soft-bottom sublittoral benthic communities. Mar. Pollut. Bull. 46, 562–572. doi: 10.1016/S0025-326X(03)00034-1
Guerra-García, J., and García-Gómez, J. (2004). Crustacean assemblages and sediment pollution in an exceptional case study: a harbour with two opposing entrances. Crustaceana 77, 353–370. doi: 10.1163/1568540041181538
Hanson, R., and Kwarteng, T. (2019). Analysis of industrial effluent from some factories in Tema. EJEE 6, 19–29.
Honda, M., and Suzuki, N. (2020). Toxicities of polycyclic aromatic hydrocarbons for aquatic animals. Int. J. Environ. Res. Public Health 17:1363. doi: 10.3390/ijerph17041363
Jarquin-Gonzalez, J., and Garcia-Madrigal Mdel, S. (2013). Annotated checklist and keys for cumaceans (Crustacea: Peracarida) from the Tropical Eastern Pacific, with six new species from the Southern Mexican Pacific. Zootaxa 3721, 201–257. doi: 10.11646/zootaxa.3721.3.1
Jarvis, I., and Jarvis, K. E. (1992). Plasma spectrometry in the earth sciences: techniques, applications and future trends. Chem. Geol. 95, 1–33. doi: 10.1016/0009-2541(92)90041-3
Jones, N. S. (1976). British Cumaceans. Arthropoda: Crustacea. Keys and Notes for the Identification of the Species. Synopses of the British Fauna No. 7 by the Linnean Society of London. London; New York, NY: Academic Press.
Jones, N. S., and Sanders, H. L. (1972). Distribution of Cumacea in the deep Atlantic. Deep-Sea Res. 19, 737–745. doi: 10.1016/0011-7471(72)90066-6
Józwiak, P., Pabis, K., Brandt, A., and Błazewicz, M. (2020). Epibenthic sled versus giant box corer – comparison of sampling gears for tanaidacean species richness assessment in the abyssal benthic ecosystem. Prog. Oceanogr. 181:102255. doi: 10.1016/j.pocean.2019.102255
Kuhn, M. (2020). Caret: Classification and Regression Training. R PackageVersion 6.0–85. Available online at: https://CRAN.R-project.org/package=caret
Le Loeuff, P., and Cosel, R. (1998). Biodiversity patterns of the marine benthic fauna on the Atlantic coast of tropical Africa in relation to hydroclimatic conditions and paleogeographic events. ActaOecol. 19, 309–321. doi: 10.1016/S1146-609X(98)80035-0
Le Loeuff, P., and Intés, A. (1999). Macrobenthic communities in the continental shelf of Côted‘Ivoire. Seasonal and diel cycles in relation to hydroclimate. ActaOecol 22, 529–550. doi: 10.1016/S0399-1784(00)87685-9
Lenihan, H. S., Peterson, C. H., Miller, R. J., Kayal, M., and Potoski, M. (2018). Biotic disturbance mitigates effects of multiple stressors in a marine benthic community. Ecosphere 9:e02314. doi: 10.1002/ecs2.2314
Levin, L. A., and Sibuet, M. (2012). Understanding continental margin biodiversity: a new imperative. Ann. Rev. Mar. Sci. 4, 79–112. doi: 10.1146/annurev-marine-120709-142714
Lira, V. F., Santos, G. A. P., Derycke, S., Larrazabal, M. E. L., Fonsêca-Genevois, V. G., and andMoens, T. (2011). Effects of barium and cadmium on the population development of the marine nematode Rhabditis (Pellioditis) marina. Mar. Environ. Res. 72, 151–159. doi: 10.1016/j.marenvres.2011.07.003
Longhurst, A. R. (1958). An Ecological Survey of the West African MarineBenthos. London: Colonial Office Fisheries Publication, 1–102.
Longhurst, A. R. (1959). Benthos densities off tropical West Africa. Permanent International pour l‘Exploration de la Mer. J. deConseil/Coseil 25, 21–28. doi: 10.1093/icesjms/25.1.21
Lourido, A., Sorbe, J. C., and Troncoso, J. S. (2008). Inventario de los crustáceosbentónicos de sedimentosinfralitorales de la Ría de Aldán (Galicia, NO PenínsulaIbérica). Nova Acta Ci. Compostelana Biol. 17, 149–168. Available online at: http://hdl.handle.net/10347/6557
Macdonald, J. M., Shields, J. D., and Zimmer-Faust, R. K. (1988). Acute toxicities of eleven metals to early life-history stages of the yellow crab Cancer anthonyi. Mar. Biol. 98, 201–207. doi: 10.1007/BF00391195
Magurran, A. E. (2012). Biodiversity in the context of ecosystem function, in Marine Biodiversity and Ecosystem Functioning: Frameworks, Methodologies, and Integration, eds M. Solan, R. J. Aspden, and D. M. Paterson (Oxford: Oxford University Press), 16–23. doi: 10.1093/acprof:oso/9780199642250.003.0002
Main, C. E., Ruhl, H. A., Jones, D. O. B., Yool, A., Thornton, B., and Mayor, D. J. (2015). Hydrocarbon contamination affects deep-sea benthic oxygen uptake and microbial community composition. Deep Sea Res. I Oceanogr. Res. Pap. 100, 79–87. doi: 10.1016/j.dsr.2014.12.008
Marquiegui, M. A., and Sorbe, J. C. (1999). Influence of near-bottom environmental conditions on the structure of bathyal macrobenthic crustacean assemblages from the Capbreton canyon (Bay of Biscay, NE Atlantic). ActaOecol 20, 353–362. doi: 10.1016/S1146-609X(99)00131-9
Martin, D., Fernadez-Arcaya, U., Tirado, P., Dutrieux, É., and Corbera, J. (2010). Relationships between shallow-water cumacean assemblages and sediment characteristics facing the Iranian coast of the Persian Gulf. J. Mar. Biolog. Assoc. U. K. 90, 125–134. doi: 10.1017/S0025315409990695
McCallum, A. W., Woolley, S., Błazewicz-Paszkowycz, M., Browne, J., Gerken, S., Kloser, R., et al. (2015). Productivity enhances benthic species richness along an oligotrophic Indian Ocean continental margin. Glob. Ecol. Biogeogr. 24, 462–471 doi: 10.1111/geb.12255
Menegotto, A., and Rangel, T. F. (2018). Mapping knowledge gaps in marine diversity reveals a latitudinal gradient of missing species richness. Nat. Commun. 9:4713. doi: 10.1038/s41467-018-07217-7
Menot, L., Crassous, P., Desbruyeres, D., Galéron, J., Khriponoff, A., and Sibuet, M. (2009). Colonization patterns along the equatorial West African margin: implications for functioning and diversity maintenance of bathyal and abyssal communities. Deep Sea Res. II Top. Stud. Oceanogr. 82053, 2313–2325. doi: 10.1016/j.dsr2.2009.04.012
Menot, L., Sibuet, M., Carney, R. S., Levin, L. A., Rowe, G. T., Billett, D. S. M., et al. (2010). New perceptions of continental margin biodiversity, in Life in the World's Oceans, ed A. D. McIntyre (Singapore: Wiley-Blackwell), 79–102. doi: 10.1002/9781444325508.ch5
Modlin, R. F., and Dardeau, M. (1987). Seasonal and spatial distribution of cumaceans in the Mobile Bay estuarine system, Alabama. Estuaries 10, 291–297. doi: 10.2307/1351886
Monroy-Velázquez, L. V., Rodríguez-Martínez, R. E., and Alvarez, F. (2017). Taxonomic richness and abundance of cryptic peracarid crustaceans in the Puerto Morelos Reef National Park, Mexico. PeerJ 5:e3411. doi: 10.7717/peerj.3411
Mühlenhardt-Siegel, U. (1996). Cumacea (Crustacea) from the Red Sea and the Maldives (Indian Ocean) in the collection of the Zoological Museum, Hamburg, with the description of seven new species and a new genus. Beaufortia 46, 105–134.
Mühlenhardt-Siegel, U. (1999). On the biogeography of Cumacea (Crustacea, Malacostraca). A comparison between South America, the Subantarctic Islands and Antarctica: present state of the art. Sci. Mar. 63, 295–302. doi: 10.3989/scimar.1999.63s1295
Mühlenhardt-Siegel, U. (2000). Cumacea (Crustacea) from the Seychelles, Maldives, Sri Lanka (western Indian Ocean), and the Red Sea, with the description of six new species. Beaufortia 50, 197–222. Available online at: https://repository.naturalis.nl/pub/504899
Mühlenhardt-Siegel, U. (2005). Cumacea species (Crustacea: Peracarida) from the Deep-Sea Expedition DIVA-1 with RV “Meteor” to the Angola Basin in July 2000. Families Lampropidae, Bodotriidae. Org. Divers. Evol. 5, 113–130. doi: 10.1016/j.ode.2004.10.008
Mühlenhardt-Siegel, U. (2009). Cumacea (Crustacea, Peracarida) in the deep Mediterranean, with the description of one new species. Zootaxa 2096, 413–432. doi: 10.11646/zootaxa.2096.1.25
Mühlenhardt-Siegel, U. (2011). New and known species of the family Leuconidae (Cumacea, Peracarida) from Antarctic deep-sea basins. Zootaxa 3117, 1–68. doi: 10.11646/zootaxa.3117.1.1
Neff, J. M. (2002). Bioaccumulation in Marine Organisms: Effect of Contaminants From Oil Well Produced Water. Amsterdam: Elsevier. doi: 10.1016/B978-008043716-3/50002-6
Nieto, K., and Mélin, F. (2017). Variability of chlorophyll-a concentration in the Gulf of Guinea and its relation to physical oceanographic variables. Prog. Oceanogr. 151, 97–115. doi: 10.1016/j.pocean.2016.11.009
Oksanen, J., Blanchet, F. G., Friendly, M., Kindt, R., Legendre, P., McGlinn, D., et al. (2019). Vegan: Community Ecology Package. R package version 2. 5–4. Available online at: https://CRAN.R-project.org/package=vegan (accessed November 28, 2020).
Olsgard, F., Brattegard, T., and Holthe, T. (2003). Polychaetes as surrogates for marine biodiversity: 977 lower taxonomic resolution and indicator groups. Biodiv. Conserv. 12, 1033–1049. doi: 10.1023/A:1022800405253
Olsgard, F., and Gray, J. S. (1995). A comprehensive analysis of the effects of offshore oil and gas exploration and production on the benthic communities of the Norwegian continental shelf. Mar. Ecol. Prog. Ser. 122, 277–306. doi: 10.3354/meps122277
OSPAR (2017). Guidelines for Monitoring the Environmental Impact of Offshore Oil 981and Gas Activities. Agreement 2017-02. Cork: OSPARCommission.
Owusu-Boadi, K., and Kuitunen, M. (2002). Urban waste pollution in the Korle Lagoon, Accra, Ghana. Environmentalist 22, 301–309. doi: 10.1023/A:1020706728569
Pabis, K., and Błazewicz-Paszkowycz, M. (2011). Distribution and diversity of cumacean assemblages in Admiralty Bay, King George Island. Pol. Polar Res. 32, 341–354. doi: 10.2478/v10183-011-0024-6
Pabis, K., Sobczyk, R., Siciński, J., Ensrud, T., and Serigstadt, B. (2020). Natural and anthropogenic factors nfluencing abundance of the benthic macrofauna along the shelf and slope of the Gulf of Guinea, a large marine ecosystem off West Africa. Oceanologia 62, 83–100. doi: 10.1016/j.oceano.2019.08.003
Petrescu, I. (1998). Cumaceans (Crustacea: Cumacea) collected by the expedition of “GrigoreAntipa” Museum of Natural History from the coasts of Tanzania (1973-1974). Part I. Family Bodotriidae. Trav. Mus. Natl. Hist. Nat. GrigoreAntipa 40, 227–310.
Petrescu, I. (2018). On the family Nannastacidae (Crustacea, Cumacea) from the Australian Museum collection. Rec. Aust. Mus. 70, 1–111. doi: 10.3853/j.2201-4349.70.2018.1645
Petrescu, I., and Sterrer, W. (2001). Cumacea (Crustacea) from shallow waters of Bermuda. Ann. Naturhist. Mus. Wien B Bot. Zool. 103, 89–128. Available online at: https://www.jstor.org/stable/41767206
Pilar-Cornejol, M., Calderon, J., and Vincx, M. (2004). Spatial patterns of the surf zone hyperbenthic fauna of Valdivia Bay (Ecuador). Hydrobiologia 529, 205–224. doi: 10.1007/s10750-004-6417-3
R Core Team (2020). R: A Language and Environment for Statistical Computing. Vienna: R Foundation for Statistical Computing.
Rehm, P., Thatje, S., Mühlenhardt-Siegel, U., and Brandt, A. (2007). Composition and distribution of the peracarid crustacean fauna along a latitudinal transect off Victoria Land (Ross Sea, Antarctica) with special emphasis on the Cumacea. Polar Biol. 30, 871–881. doi: 10.1007/s00300-006-0247-x
Rex, M. A., and Etter, R. J. (2010). Deep-Sea Biodiversity; Patterns and Scale. Cambridge, MA: Harvard University Press.
Reyss, D. (1973). Distribution of cumacea in the deep mediterranean. Deep Sea Res. I Oceanogr. Res. Pap. 20, 1119–1123. doi: 10.1016/0011-7471(73)90025-9
San Vicente, C., Munilla, T., Corbera, J., Sorbe, J. C., and Ramos, A. (2009). Suprabenthic fauna from the Bellingshausen Sea and western Antarctic Peninsula: spatial distribution and community structure. Sci. Mar. 73, 357–368. doi: 10.3989/scimar.2009.73n2357
San Vicente, C., and Sorbe, J. C. (1999). Spatio-temporal structure of the suprabenthic community from Creixell beach (western Mediterranean). Acta Oecol. 20, 377–389. doi: 10.1016/S1146-609X(99)00129-0
San Vicente, C., and Sorbe, J. C. (2001). Temporal changes in the structure of the suprabenthic community from Hendaya beach (southern Bay of Biscay): a comparison with a northwesternmediterranean beach community. Bol. Inst. Esp. Oceanogr. 17, 107–120. Available online at: https://citeseerx.ist.psu.edu/viewdoc/download?doi=10.1.1.537.6244&rep=rep1&type=pdf
Sarthou, C., Larpin, D., Fonty, É., Pavoine, S., and andPonge, J.-F. (2010). Stability of plant communities along a tropical inselberg ecotone in French Guiana (South America). Flora 205, 682–694. doi: 10.1016/j.flora.2010.04.005
Scheren, P. A., Ibe, A. C., Janssen, F. J., and Lemmens, A. M. (2002). Environmental pollution in the Gulf of Guinea – a regional approach. Mar. Pollut. Bull. 44, 633–641. doi: 10.1016/S0025-326X(01)00305-8
Sebens, K. P. (1991). Habitat structure and community dynamics in marine benthic systems in Habitat Structure: The physical arrangement of objects in space,” eds S. S. Bell, E. D. McCoy, and H. R. Mushinsky (Dordrecht: Springer Netherlands), 211–234. doi: 10.1007/978-94-011-3076-9_11
Sobczyk, R., Czortek, P., Serigstad, B., and Pabis, K. (2021). Modelling of polychaete functional diversity: a Large Marine Ecosystem response tomultiple natural factors and human impacts on the West African continental margin. Sci. Total Environ. 792:148075. doi: 10.1016/j.scitotenv.2021.148075
Sorbe, J. C., and Elizalde, M. (2014). Temporal changes in the structure of a slope suprabenthic community from the Bay of Biscay (NE Atlantic Ocean). Deep Sea Res. II Top. Stud. Oceanogr. 106, 179–191. doi: 10.1016/j.dsr2.2013.09.041
Spalding, M. D., Fox, H. E., Allen, G. R., Davidson, N., Ferdaña, Z. A., Finlayson, M., et al. (2007). Marine ecoregions of the world: a bioregionalization of coastal and shelf areas. BioScience 57, 573–583. doi: 10.1641/B570707
Stark, J. S., Mohammad, M., McMinn, A., and Ingels, J. (2020). Diversity, abundance, spatial variation, and human impacts in marine meiobenthic Nematode and Copepod communities at Casey Station, East Antarctica. Front. Mar. Sci. 7:480. doi: 10.3389/fmars.2020.00480
StatSoft (2006). Elektroniczny Podrecznik Statystyki PL. Krakow. Available online at: http://www.statsoft.pl/textjournal/stathome.html (accessed May 10, 2021).
Swaileh, K. M., and Adelung, D. (1995). Effect of body size and season on the concentrations of Cu, Cd, Pb and Zn in Diastylisrathkei (Kröyer) (Crustacea: Cumacea) from Kiel Bay, Western Baltic. Mar. Pollut. Bull. 31, 103–107. doi: 10.1016/0025-326X(94)00258-B
Thiel, M., González, E. R., Balanda, M. J., Haye, P., Heard, R., and Watling, L. (2003). Diversity of Chilean peracarids (Crustacea: Malacostraca), in Contribuciones al Estudio de los Crustáceos del Pacífico Este, ed M. E. Hendrickx (Mexico: Instituto de Ciencias del Mar y Limnología, UNAM), 177–189.
Ukwe, C. N., Ibe, C. A., Alo, B. I., and Yumkella, K. K. (2003). Achieving a paradigm shift in environmental and living resources management in the Gulf of Guinea: the large marine ecosystem approach. Mar. Pollut. Bull. 47, 219–225. doi: 10.1016/S0025-326X(02)00473-3
Ukwe, C. N., Ibe, C. A., and Sherman, K. (2006). A sixteen-country mobilization for sustainable fisheries in the Guinea Current Large Marine Ecosystem. Ocean Coast. Manag. 49, 385–412. doi: 10.1016/j.ocecoaman.2006.04.006
UNESCO Intergovernmental Oceanographic Commission (1982). The Determination of Petroleum Hydrocarbons in Sediments (Manuals and Guides No. 11, Paris). Paris: UNESCO.
Walsh, C., and Mac Nally, R. (2013). hier.part: Hierarchical Partitioning. R package version 1.0-4. Available online at: https://CRAN.R-project.org/package=hier.part (accessed March 10, 2020).
Wang, Z., and Dauvin, J. C. (1994). The suprabenthic crustacean fauna of the infralittoral fine sand community from the Bay of Seine (Eastern English Channel): composition, swimming activity and diurnal variation. Cah. Biol. Mar. 35, 135–155.
Watling, L., and Gerken, S. (2021). World Cumacea Database. Available online at: http://www.marinespecies.org/cumaceaon~2021-04-27 (accessed July 7, 2020).
Wei, T., and Simko, V. (2017). R Package “Corrplot”: Visualization of a Correlation Matrix (Version 0.84). Available online at: https://guthub.com/taiyun/corrplot (accessed February 12, 2020).
Wieser, W. (1956). Factors influencing the choice of substratum in Cumella vulgaris Hart (Crustacea, Cumacea). Limnol. Oceanogr. 1, 274–285. doi: 10.4319/lo.1956.1.4.0274
Keywords: Cumacea, depth gradient, diversity, pollution, Gulf of Guinea
Citation: Stȩpień A, Pabis K, Sobczyk R and Serigstad B (2021) High Species Richness and Extremely Low Abundance of Cumacean Communities Along the Shelf and Slope of the Gulf of Guinea (West Africa). Front. Mar. Sci. 8:703547. doi: 10.3389/fmars.2021.703547
Received: 30 April 2021; Accepted: 29 June 2021;
Published: 04 August 2021.
Edited by:
Torben Riehl, Senckenberg Museum, GermanyReviewed by:
Mauricio Shimabukuro, University of Southern Denmark, DenmarkGustavo Fonseca, Federal University of São Paulo, Brazil
Copyright © 2021 Stȩpień, Pabis, Sobczyk and Serigstad. This is an open-access article distributed under the terms of the Creative Commons Attribution License (CC BY). The use, distribution or reproduction in other forums is permitted, provided the original author(s) and the copyright owner(s) are credited and that the original publication in this journal is cited, in accordance with accepted academic practice. No use, distribution or reproduction is permitted which does not comply with these terms.
*Correspondence: Anna Stȩpień, YW5uYS5zdGVwaWVuJiN4MDAwNDA7YmlvbC51bmkubG9kei5wbA==