- 1Community Ecology Laboratory, Marine Science Institute, University of the Philippines, Quezon City, Philippines
- 2UP Diliman Invertebrate Museum, Institute of Biology, University of the Philippines, Quezon City, Philippines
Philippine coral reefs have been on the decline since the 1970s, and this degradation has posed a risk to biodiversity, food security, and livelihood in the country. In an effort to arrest this degradation, marine protected areas (MPAs) were established across the country. MPAs are known to improve fish biomass, but their effect on live coral cover and other benthos is not yet well documented and understood. In this study, 28 MPAs across the Philippines were surveyed comparing benthic cover and indices between protected reefs and adjacent unprotected reefs. No consistent differences were found between reefs inside and outside MPAs through all the benthic categories and reef health indices considered that are indicative of protection effects or recovery within MPAs. However, there were notable site-specific differences in benthic cover across the study MPAs-suggesting that factors other than protection play important roles in influencing benthic cover inside and outside of MPAs. Storm frequency and proximity to rivers, as a proxy for siltation, were the strongest negative correlates to live coral cover. Also, high coastal population, a proxy for pollution, and occurrence of blast and poison fishing positively correlated with high dead coral cover. The lack of significant difference in benthic cover between reefs inside and outside MPAs suggests that protection does not necessarily guarantee immediate improvement in benthic condition. Correlations between benthic condition and storm frequency, siltation, and pollution suggest that it is necessary to augment MPAs with other management strategies that will address the multiple stressors that are usually indiscriminate of MPA boundaries. Supplementing long-term and systematic monitoring of benthic cover and biodiversity inside and outside of MPAs with data on other important environmental and human impact variables will help improve understanding of benthic cover and biodiversity dynamics inside and outside of MPA boundaries.
Introduction
The Philippine coral reefs have been on a decline since the first national assessment in the 1970s. At that time, Gomez and Alcala (1979) found only 30% of the reefs having more than 50% coral cover. Years of overfishing, destructive fishing methods, and siltation has brought this percentage even lower, where currently only 10% of the reefs had more than 50% coral cover (Wilkinson, 2008; Licuanan et al., 2017). With the demand for fish still increasing, destructive fishing methods are still rampant, and the effects of climate change now being realized, this decline is expected to continue unless more comprehensive actions are taken (Burke et al., 2011).
The Philippines, however, cannot risk further reef decline. The country is at the apex of the Coral Triangle and its reefs support the highest concentration of marine species per unit area (Licuanan and Gomez, 2000; Carpenter and Springer, 2005; Burke et al., 2011; Sanciangco et al., 2013). The demise of habitat forming species such as corals will bring about a cascade that will result in considerable marine biodiversity loss (Jones et al., 2004; Bruno and Selig, 2007). Furthermore, at least one-third of Filipinos directly utilize reef-derived food and services (Briones et al., 2004; SCTR-Philippines, 2012; Cabral et al., 2013).
The popular management action to arrest reef decline is the establishment of marine protected areas (MPAs) (Russ et al., 2004). These are areas of managed access where fishing and other activities are regulated (Alcala, 1998). MPAs have been shown to rapidly recover fish abundance and biomass after a few years of effective implementation (Alcala, 1998; Russ et al., 2004; Abesamis and Russ, 2005; Maliao et al., 2009). However, the effect of MPAs on benthic cover is not as straightforward. The immediate positive influence of protection on coral cover is not conclusive. Coral cover was found to increase inside the MPAs in the Bahamas (Mumby et al., 2007), in the Seychelles (Wilson et al., 2012), and even in the Philippines (Magdaong et al., 2013). The massive protection efforts in the Great Barrier Reef also led to the improvement in coral cover (McCook et al., 2010). In contrast, Selig and Bruno (2010) found no increase in coral cover within MPAs, although coral cover is declining in unprotected reefs. Declines in coral cover despite protection has been reported in another global study by Selig et al. (2012), in a regional scale studies in the Caribbean (Huntington et al., 2011), the Great Barrier Reef (De’ath et al., 2012), and in specific locations in the Florida Keys (Toth et al., 2014) and Papua New Guinea (Jones et al., 2004).
In this study, 28 MPAs (inside and adjacent) were evaluated to determine the effect of protection based on benthic cover attributes and reef health indices. The relative contribution of environmental factors other than protection was explored using multivariate analyses to determine their contribution to the observed the variation in benthic cover attributes. Insights from this study, will help reevaluate the effectiveness of MPAs in terms of metrics, and reorient management options toward increasing MPA management effectiveness and actions that will accelerate recovery of degraded reefs.
The main objectives of this paper are as follows: (1) To evaluate the differences in measured benthic cover variables and indices inside and outside MPAs across 28 study sites across the Philippines, and (2) To evaluate how variations in environmental variables across the study sites correlate with benthic cover attributes and reef health indices. The insights from these analyses may help inform expectations on how we define and improve impacts management interventions such as those of MPAs.
Materials and Methods
Study Sites
Benthic surveys were conducted inside and outside 28 MPAs across 12 provinces in the Philippines (Figure 1). The surveys on 15 MPAs were done from April to May 2012, while surveys on the other 13 MPAs were conducted from April to May 2014. The MPAs were all managed by their respective local government units and have different management levels at the time of survey. The ages of the MPAs at time of survey ranged from 0–21 years (mean = 8 years, median = 7 years) and core areas ranged from 9 to 453.25 ha (mean = 62.7 ha).
In each MPA, coral reef assessment was done using five replicate 50-m transects within the MPA no-take zone, and five replicate 50-m transects outside the MPA. Transects outside MPAs were at least 200 m away from the MPA boundaries. Depending on the extent of coral reef area, the actual number of transects laid within and outside MPAs were varied-, ranging from 2 to 7. But on average, the number of transects sampled is five inside and five outside MPAs. These transects were laid parallel to the shore, following the contour of the upper reef slope at depths ranging between 5 and 10 m.
Data Collection
Benthic cover was determined via the line intercept transect (LIT) method (English et al., 1997) on 50-m transects. The benthic categories were aggregated into five major benthic groupings (HC, live hard coral; DC/A, dead coral/with algae; AL, algae; OT, other benthic organisms; AB, abiotic components), and cover was expressed as percent cover.
The following reef health indices were calculated: Mortality Index (MI) (Gomez et al., 1994), Reef development index (DI), condition index (CI), and succession (by algae) index (SI) (Manthachitra, 1994), using the equations below. Note that the equation for the SI has been modified to take into consideration growing algae on dead coral, which are abundant in many sites:
To explore possible drivers of variation in benthic cover and reef health indices, the following environmental data were gathered: proximity to rivers, fisher density, coastal population, occurrence of poison fishing, occurrence of blast fishing, storm frequency, and relative exposure index. Proximity to rivers was chosen as a proxy for siltation as it is assumed that terrigenous materials are conveyed to the reef through river runoff (Gomez et al., 1994). Fisher density (number of fishers per square kilometer municipal water) was chosen as a proxy for overfishing which if unmanaged can translate to degradation of the reefs (Teh et al., 2013). Coastal population was chosen as a proxy for human activities and/or pollution (Gjertsen, 2005). Occurrences of poison and blast fishing were chosen as they led to coral mortality and destruction of the reef structure (Rubec, 1986; McManus et al., 1997). Storm frequency (number of storms from 1980 to 2000) and relative exposure index (to wind, factoring in wind speed and fetch, Villanoy et al., 2013) were also used to depict natural or climate-related factors (Brown, 1997).
As some of these data were discrete, while others had categorical measurement (e.g., occurrence of poison and blast fishing were from the surveys measured as low, moderate, and high), all environmental data were categorized from 1 to 3, with 1 being the lowest score and 3 being the highest.
Data Analyses
The biotic and environmental data obtained did not satisfy the assumptions of parametric tests despite transformation, hence comparisons and correlations were conducted through non-parametric univariate and multivariate data analyses.
Non-parametric Kruskal–Wallis test was implemented to compare transects inside and outside MPAs and across all study MPAs for various benthic variables (HC, AL, DCA, OT, and AB) and the reef health indices (development, condition, mortality, and succession indices) using SPSS Statistics 21 (IBM Corp, 2012).
Multivariate analyses approach (using PERMANOVA) was implemented to determine significant differences in benthic communities inside and outside the MPA, and across the 28 MPAs (Clarke and Gorley, 2006; Anderson et al., 2008). In running the PERMANOVA, Bray-Curtis resemblance matrix (using square root-transformed five categories of raw percent cover benthic data) was applied, and then using MPA locations and MPA status (inside/outside) as factors—P-values generated through 9,999 permutations. Pairwise PERMANOVA was also done to compare the individual MPAs, and see if there are significant differences inside and outside each MPAs in terms of the benthic community.
In addition, multivariate matrix correlation approach (BIO-ENV/BEST) in Primer 6 (Clarke and Gorley, 2006) was used to perform a Spearman correlation between the resemblance matrices of the benthic cover or reef health indices, and the environmental resemblance matrix, to determine which environmental factor best correlated to the biotic pattern observed.
Moreover, a Principal Coordinates analysis (PCO) plot was constructed to visualize the benthic variation across MPAs. This plot was overlaid with vectors that represent the Spearman correlation between benthic cover and environmental data, where the proximity of each data point (transect) to a certain vector (benthic cover or environmental factor) represents stronger correlation between that transect to the vector.
Results
No Clear Effects of Protection on Benthic Cover and Indices
We found no significant differences in benthic cover and reef health indices inside and outside the MPAs. Kruskal–Wallis tests did not show significant univariate differences in hard coral cover (P = 0.738), dead coral cover (P = 0.705), algal cover (P = 0.217), cover of other benthic organisms (P = 0.828), and abiotic components (P = 0.845). Likewise, no significant differences were observed inside and outside MPAs in terms of DI (P = 0.368), CI (P = 0.516), mortality index (P = 0.639), and succession index (P = 0.957).
Averaging the data (presented as mean ± SE) from the 267 transects surveyed (Figure 2), the live hard coral cover was at 41.9 ± 1.2% inside the MPAs and 42.7 ± 1.2% outside the MPAs. Reefs were generally dominated by massive and branching Porites, branching and tabular Acropora, and encrusting Montipora. The cover of dead coral was at 30.8 ± 1.1% inside the MPA and 30.3 ± 1.1% outside. Dead corals (except for a few recently killed colonies) were covered with short filamentous or matting algae. Larger fleshy macroalgae, calcifying algae (i.e., Halimeda), coralline algae, other turf algae, and indistinct algal assemblages collectively covered an average of 11.8 ± 1.1% of the reef bottom inside the MPA and 11.7 ± 1.0% outside. Benthic organisms other than corals and algae covered an average of 6.3 ± 0.5% both inside and outside the MPA. Abiotic components such as rock, rubble, and sand, made up 9.1 ± 0.7% and 9.0 ± 0.7% of the benthos inside and outside the MPA, respectively.
In terms of the reef health indices, we observed that the reefs were highly developed and relatively coral dominated, but with high mortality and a high proportion of algae. The average DI was within Manthachitra’s “very good” category at 2.03 ± 0.20 inside the MPA and 1.88 ± 0.18 outside, indicating that these reefs were well developed, with the spaces in the reefs being inhabited or are habitable by benthic organisms, in contrast to poorly developed reefs which were mostly sand or silt. The average CI was within Manthachitra’s “fair” category at −0.06 ± 0.04 inside the MPA and −0.70 ± 0.04 outside, implying that the reefs were fairly coral-dominated. On the other hand, the average mortality index was at 0.41 ± 0.02 inside the MPA and 0.42 ± 0.02 outside. Lastly, the average succession index was also within Manthachitra’s “very good” category, at 1.22 ± 0.10 inside the MPA and 1.29 ± 0.12 outside, suggesting substantial algal cover and that algae are likely to succeed corals in dominance among other benthic organisms.
PERMANOVA results comparing sites inside and outside the MPAs also did not yield significant differences (P = 0.4774), suggesting that cover of the five benthic categories were generally the same regardless of protection. The interaction term for the multivariate analysis (MPA location × MPA status) was significant (P = 0.0117). Pairwise tests revealed that the benthic cover multivariate varied significantly in five out of the 28 sites, which may be attributed to site-specific factors.
Site-Specific Variations in Environmental Variables That Correlated With Benthic Cover and Indices
Benthic cover and reef health indices varied; however, when compared across the 28 MPA locations (Figure 3). Kruskal–Wallis results yielded a P-value of less than 0.001 when MPA locations were univariately compared using each of the benthic categories and reef health indices tested. PERMANOVA results across MPA locations also gave a P-value of 0.001. As mentioned earlier, the interaction term (MPA location × MPA status) was also significant.
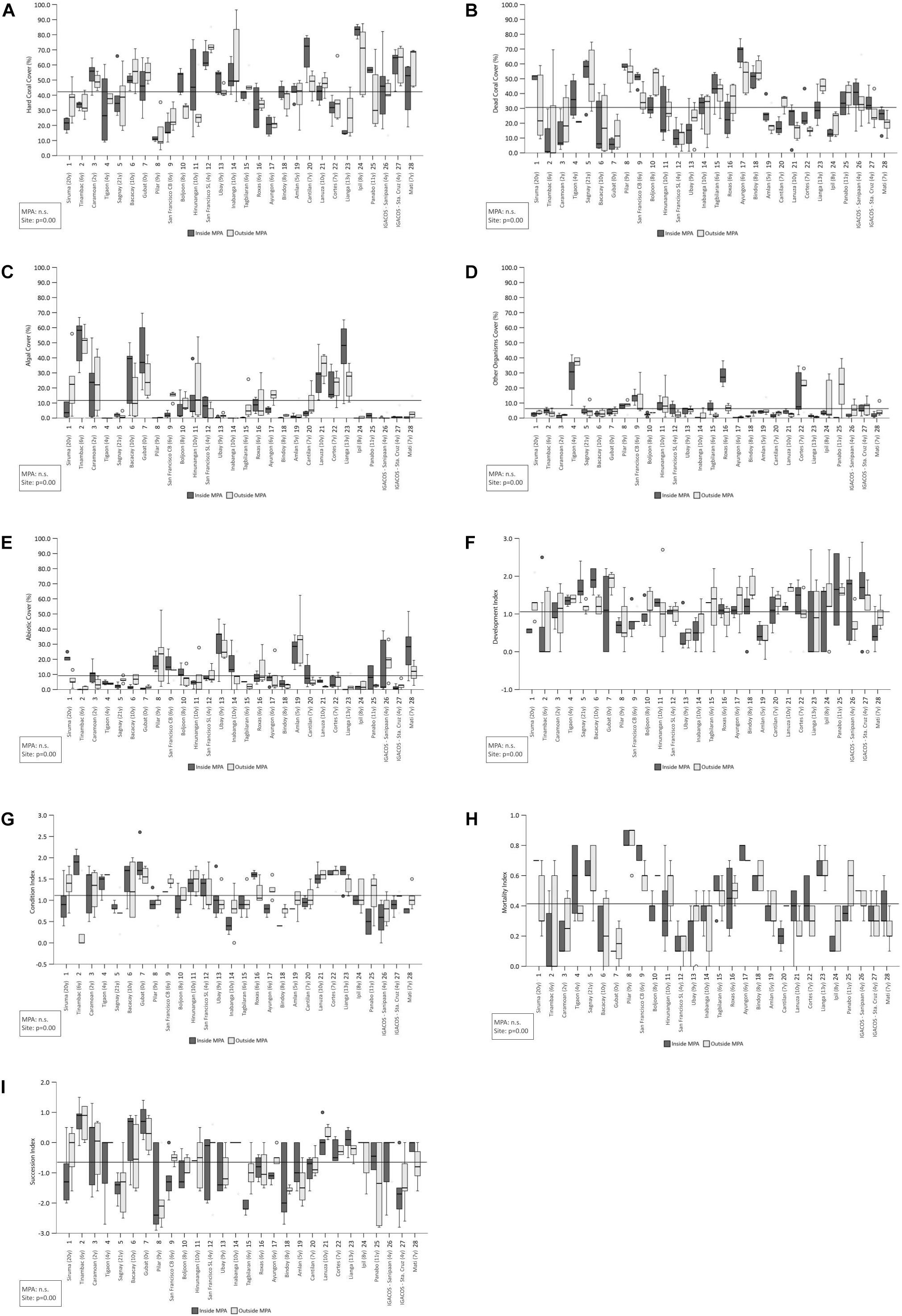
Figure 3. Variation of benthic condition across MPA locations in terms of (A) hard coral cover, (B) dead coral cover, (C) algal cover, (D) cover of other benthic organisms, (E) abiotic cover, (F) development index, (G) condition index, (H) mortality index, and (I) succession by algae index.
The resemblance matrix did not present a clear and consistent pattern in terms of benthic cover. The PCO plot (Figure 4) showed a wide data spread with no tight, distinct groupings either for MPA location or MPA status (inside/outside). The 70% similarity clusters showed overlaps and no clear spatial geographic patterns. The two axes of the PCO plot already jointly explain 76.7% of the variation, with a third axis (not illustrated) explaining a further 16.5%.
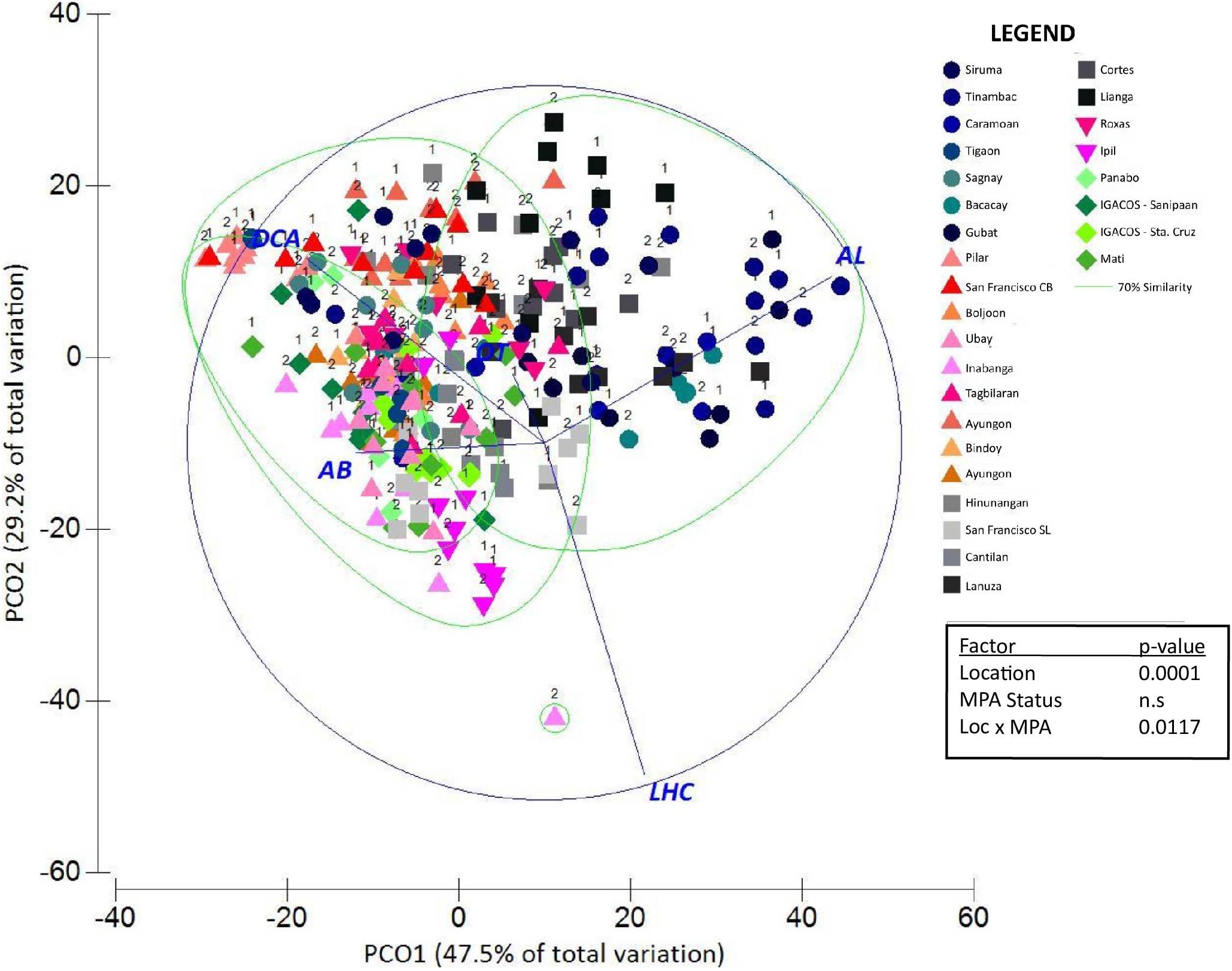
Figure 4. PCO Plot showing the distances between the locations according to differences in the multivariate benthic cover, with vectors depicting Spearman correlation with the different benthic groups. 1, inside MPA; 2, outside MPA.
The correlation results with the individual major benthic groups overlaid on the PCO plot showed a rough divide. Axis 1 separated the transects by algae (r = 0.806), while axis 2 showed gradients in dead coral (r = 0.516) and hard coral cover (r = −0.929). Most of the transects in the Pacific Seaboard (e.g., Tinambac, Gubat, Caramoan, Bacacay, Lianga, and Lanuza) were correlated with high algal cover, while the transects in internal seas (mostly in the Visayas Region) correlated with dead coral and abiotic components.
To explore if any of the environmental proxies may help explain spatial variation across MPA locations, we looked at the relationship of the resemblance matrices of benthic cover and reef health indices with that of environmental correlates identified. Bio-ENV results showed a weak yet significant correlation between the resemblance matrices of benthic cover and environmental factors (ρ = 0.151, P = 0.01). The same was also observed between the resemblance matrices of the reef health indices and the environmental factors (ρ = 0.097, P = 0.01).
Storm frequency was the strongest correlate among the environmental factors (Figure 5), followed by the combination of storm frequency and proximity to rivers. These two factors correlated more with transects in the Pacific Seaboard, which were also the sites with high algal cover. On the other hand, transects in the internal seas were less correlated with these two factors, but instead were more affected by anthropogenic factors such as high coastal population (which is a proxy for pollution and human activity), and high occurrences of blast and poison fishing. These were also the sites correlated with higher dead coral cover, which was rather intuitive, since these anthropogenic factors were often implicated in coral mortality.
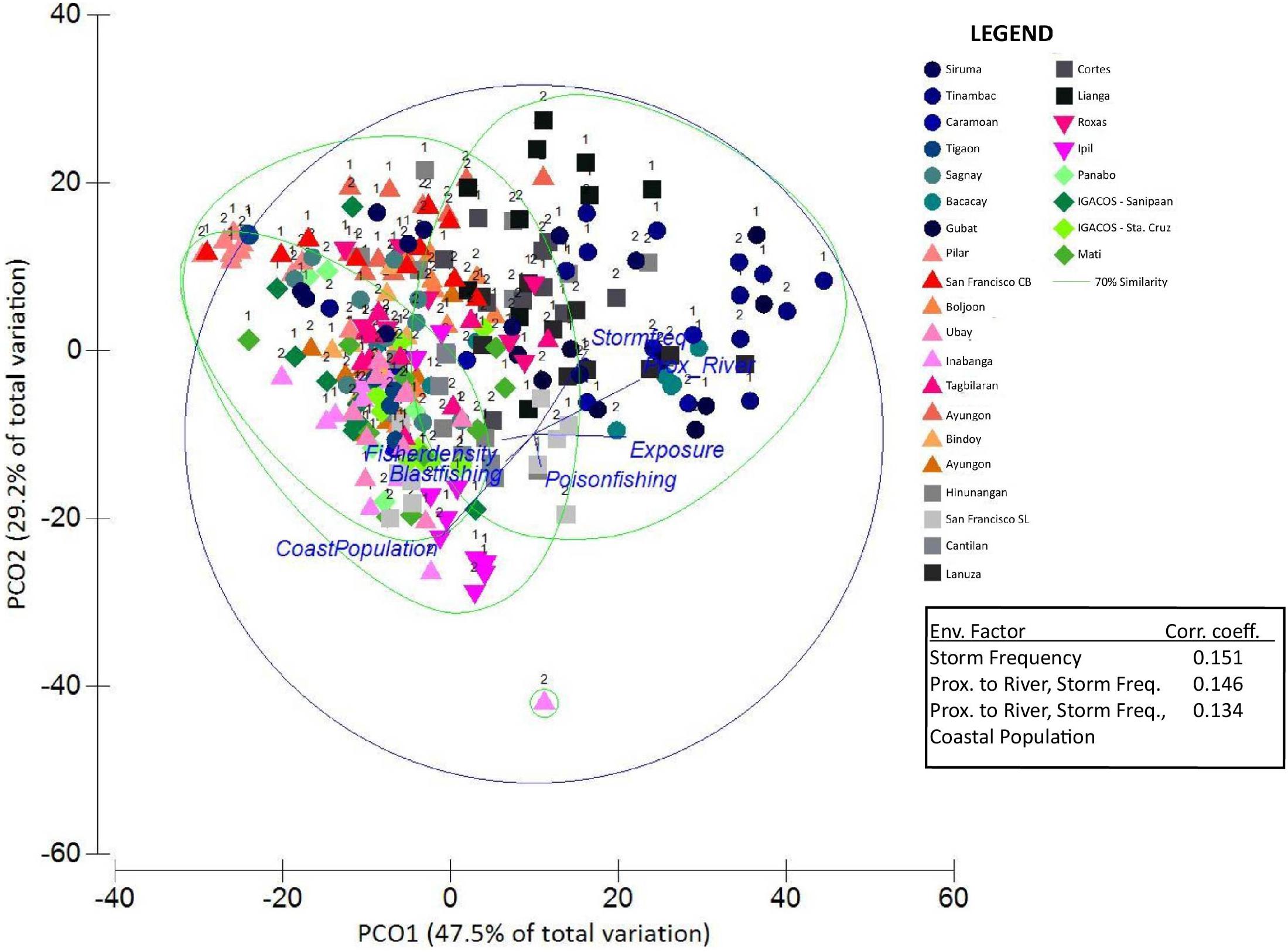
Figure 5. PCO Plot showing the distances between the locations according to differences in the multivariate benthic cover, with vectors depicting Spearman correlation with the environmental factors. 1, inside MPA; 2, outside MPA.
Discussion
The Status of Benthos and the Lack of Clear Protection Effects on Benthic Cover Across the MPA Study Sites
The average hard coral cover reported in this study is significantly higher (more than 40%) compared to what has been previously reported as national average (Magdaong et al., 2013; Licuanan et al., 2019). It is easy to assume that reefs are in better condition now, although care should be taken in saying this increase was a direct effect of conservation and management efforts. The transects surveyed here were within or adjacent to MPAs, in contrast to previous studies that were not as selective. More often, the reefs with good coral cover are chosen for protection as they fit the criteria recommended for MPA establishment (Roberts, 2000; Roberts et al., 2003), hence it is likely that we are averaging hard coral cover values from the best parts of the reefs in the surveyed areas. This may also explain why previous meta-analyses on the Philippine reefs were able to detect significantly higher coral cover inside MPAs than in reefs that were not protected (Magdaong et al., 2013). The non-protected areas surveyed here are adjacent to MPAs and may still have benefited from local management efforts (such as reduced destructive fishing methods), in contrast to areas that may not have any degree of management at all.
The average c oral mortality index is also higher in this study than previously reported. Despite transects in this study being within or adjacent to MPAs, the average mortality index was at 0.4 ± 0.01, while the national coral mortality index in 1994 was at 0.2 ± 0.03 (Gomez et al., 1994). Gomez et al. (1994) also indicated that the primary cause of coral mortality was siltation. Other causes of mortality include overfishing and destructive fishing methods (Burke et al., 2011; Magdaong et al., 2013). In many sites we surveyed, illegal and destructive fishing activities were still rampant (Muallil et al., 2014), and siltation was observed in varying degrees. Many of the reefs were also highly exposed to storms and waves, and without proper planning, these reefs are vulnerable, especially to the exacerbating effects of climate change. In fact, in 2010, a mass bleaching event occurred through many of the reefs in the Philippines (Philippine Coral Bleach Watch, unpublished), and though we have not looked into the bleaching history of each of the 28 MPAs, this event would have contributed to coral mortality in the reefs. It is, however, important to note that reef decline is a process (Bellwood et al., 2004). The extent of coral mortality is but a snapshot that indicates how much we have already lost, but a long-term closely-monitored study will be necessary to establish causal relationships.
The Site-Specific Variations in Environmental Variables That Correlated With Benthos Cover, and Their Implications
A commentary by Knowlton (2021a) on a paper by Donovan et al. (2021) on the ability of sea urchin and macroalgae abundance to amplify the impact of bleaching in corals, suggest and infer that local management can play an important role in mitigating climate impacts. Knowlton’s position, also related to her recent paper (Knowlton, 2021b), argues for optimism in marine conservation, despite the larger problems that the marine environment is facing—from large scale overfishing to climate change impacts. This is a call to change the narrative of gloom and doom in ocean conservation, similar to other offerings of hope for the ocean by 2050 if right actions are made (Duarte et al., 2020), as supported by increasing trends in numbers of established MPAs, and emerging efforts on coral restoration.
To date, however, the actual effects of MPAs on benthic and coral cover appear mixed and paradoxical (Howarth et al., 2015; Bates et al., 2019), and more often show coral reef benthos being greatly and negatively impacted by disturbances such as bleaching due to ocean warming (Huntington et al., 2011; Toth et al., 2014), reduced water quality—turbidity, siltation, nutrient loading, and pollution (Wenger et al., 2015), prior degradation before protection, and a lack of enforcement in many MPAs. In contrast, a global analysis of coral cover inside and outside MPAs (Selig and Bruno, 2010) showed that from 1969 to 2006, surveyed coral reefs showed more stable coral cover inside MPAs compared to declining coral cover in fished grounds, suggesting that in the long term, the MPAs may offer more benefits to coral reefs than no protection at all. Given these mixed results and a growing body of literature showing empirically that many benthic communities and corals failed to recover inside MPAs due to local stressors and larger disturbances ongoing despite the removal of fishing (Jones et al., 2004; Coelho and Manfrino, 2007; Carassou et al., 2013; Anderson et al., 2014; McClure et al., 2020), there is, indeed, a need to tease apart hierarchical or interacting effects of these multiple disturbances as well as management efforts inside and outside the MPAs, to understand ways to improve benthic and coral recovery.
The results of our study showed that, like majority of papers, coral reefs in the Philippines are not showing clear trends of recovery inside MPAs, but instead are somewhat related to the degrees of other disturbances such as siltation, possible nutrient loading, and impacts of storms that are causing coral degradation inside the MPA boundaries. Corals are slow-growing, sessile reef-building organisms, and as such, their growth and recovery rates could easily be overwhelmed and affected by stressors acting at a prolonged scale (Hughes and Connell, 1999; Carilli et al., 2009). What MPAs can directly do for corals is to prevent damage by excluding human activities such as trampling, anchoring, and fishing (Selig and Bruno, 2010). MPAs can also bring about a top-down trophic cascade, where protection will lead to an increase in abundance and biomass of fish, which in turn will help regulate algal growth, prevent outbreaks of coral predators, and lower prevalence of coral disease (Stockwell et al., 2000; Hughes et al., 2007; Mumby et al., 2007; Sweatman, 2008; Raymundo et al., 2009). However, the top-down effect of MPAs on coral communities is indirect and sometimes the mechanisms are not well established. The reduction of predator crown-of-thorns starfish, for example, has been greatly implicated in the improvement of coral cover in the protected Great Barrier Reef, although how protection actually reduces starfish outbreaks is still unclear (McCook et al., 2010). Furthermore, the stressors that drive coral decline such as frequent damage from storms, chronic siltation, and coral bleaching cannot be excluded by the boundaries of MPAs, hence these will continue to negatively affect the reefs unless they are addressed (Selig et al., 2012; Eakin et al., 2019).
In fact, half of the MPAs in this survey were about 5–10 years old, with two MPAs at least 20 years old, and still no consistent differences in benthic cover inside and outside the MPAs were observed. In some MPA locations, coral cover is high both inside and outside, suggesting that management efforts (such as constant patrolling) may be benefiting non-protected reefs as well. However, some of the MPA locations still suffer from poor water quality, pollution, and destructive fishing methods, problems that need other interventions such as water quality management, waste management, and fishery law enforcement, before coral conditions (regardless of protection) are expected to improve. Indeed, MPAs are not a “silver bullet” that can rapidly bring about an observable increase in coral cover and a decrease in algal cover (Gilby et al., 2015). The reef benthos will not be able to recover as rapidly so long as the threats that limit them in the first place are not eliminated (Burke et al., 2011). In addition, acute environmental disturbances can devastate reefs regardless of protection. The Apo Island No-Take Marine Reserve, for example, was not spared from back-to-back typhoons from 2010 to 2012, where coral cover dropped to ∼1%, a significant reduction compared to the more sheltered fished reefs outside the reserve (Russ et al., 2021).
MPAs as an approach addresses stressors at a single scale, while the different environmental factors such as those correlated in this study occur at different scales. The apparent spatial variation across MPA locations, despite the lack of significant difference in the MPA level (inside and outside) suggest that factors driving variation are in scales large enough to surpass the functional size of the MPA and thus affect the benthic communities protected and unprotected alike (Selig et al., 2012).
It is, indeed, important to match the scale of the intervention to the stressor. The Great Barrier Reef, for example, for the length of time it has been protected, the considerable management efforts, and the massiveness of the functional size of the protected area (especially when compared to locally-managed MPAs), have not been immune degradation, and what is being looked at as the “best bet” is really to manage the local stressors and to help improve local management in the face of large-scale disturbances that reefs and marine environment are experiencing beyond the impacts and removal of fishing (in the case of MPAs) (Knowlton, 2012).
This, however, does not reduce the need for MPAs for managing and conserving the reefs as they still function and provide valuable ecosystem goods and services (as safe refuge for fishes from fishing). The strong implementation of MPAs has been proven to be effective in improving fish abundance and biomass (Alcala, 1998; Maliao et al., 2009) important for food and livelihood security. Russ et al. (2004) have even shown a threefold increase in the biomass of commercially important fish inside the Apo Reef Marine Reserve. Even if the effects of MPAs on reef benthic communities are rather indirect, the establishment of MPAs is a good entry point in coastal resource management (Christie et al., 2002). The process of setting up and maintaining MPAs will guide the local management through the different considerations in conservation, such as the presence of local stressors, and threats to sustainability of management efforts (Roberts, 2000; Maypa et al., 2012). In this regard, MPAs can be considered as “canaries in the coal mine” (Tabor and Aguirre, 2004), where their deterioration despite strong protection will signal a need to reevaluate the MPA design, objectives or management strategies. Furthermore, excluding fishing can also assist coral reef recovery after disturbances such as coral bleaching (Wilson et al., 2012).
As most of the stressors that drive reef benthic variation occur in larger scales than the MPAs, there is need to augment MPAs with other management approaches that can address the stressors in their respective scales to “cover all bases” and provide a more holistic protection of the reefs (Agardy et al., 2011). This prevents stressors from overwhelming the benefits of protection (e.g., trophic cascades) and render the efforts in improving the reef benthic community in the MPAs futile (Huntington et al., 2011; Selig et al., 2012; Anderson et al., 2014). For example, MPAs that are often damaged because they are along the pathway of storms or other disturbances may be repositioned to more sheltered locations (Roberts et al., 2003) and need to be replicated in other areas for redundancy. MPAs that are degraded by heavy siltation from rivers may be moved farther from the river mouth, and activities upland such as agriculture are to be strictly managed to prevent terrigenous sedimentation from smothering the reefs (Gomez et al., 1994; Kroon et al., 2016; van Grieken et al., 2019). Regulations in areas overwhelmed by algae may be adjusted to control fishing of herbivores and reduce nutrient loading (Carassou et al., 2013). Indeed, the establishment and maintenance of MPAs should always be accompanied by adaptive measures to eliminate anthropogenic threats and keep the MPAs out of harm’s way (Roberts et al., 2003). These will ensure that the benthic communities are productive and resilient despite climate-related threats (Burke et al., 2011; Selig et al., 2012). Furthermore, networking of MPAs may be considered to provide ample representativeness, replication, and redundancy (Roberts et al., 2001; Eisma-Osorio et al., 2009) and evaluated as to their functions and multiple objectives.
We think that there is still a need to expand current analyses of the impacts of MPAs on sessile and benthic organisms, and explore the ongoing ecological processes inside and outside of MPA boundaries. While comparing biotic communities inside and outside the MPAs are necessary to monitor MPA health and effectiveness, this may be too simplistic, and should be analyzed within the frame of its environment and present stressors to provide clearer conditions, and management intervention needs. It may be interesting to also consider the morphology of the corals and algae, structural complexity, coral recruitment, and metrics other than benthic cover to gain more insights on reef condition. Consistent reef monitoring and analysis of trends will help investigate the effects of protection in decreasing coral loss (if not necessarily coral cover improvement), similar to what Selig and Bruno (2010) has found in their global analyses. These types of studies can further be aligned to the objectives of the MPAs to have a better handle of success (Edinger and Risk, 2000; Strain et al., 2019). Furthermore, an improved understanding of ecological interactions can also improve local management efforts in dealing with disturbances other than fishing. These in-depth analyses and management considerations will hopefully enhance local efforts and bring promising results, and contribute to the ocean conservation optimism that Knowlton and Duarte are proposing in the face of large-scale and long-term degradation and threats to marine biodiversity in the anthropocene.
Data Availability Statement
The raw data supporting the conclusions of this article will be made available by the authors, without undue reservation.
Author Contributions
FP collected and analyzed the data, discussed the results, and wrote the manuscript with support from all authors. JA contributed in data analysis and manuscript writing and revision. MQ contributed in data analysis, results discussion, and the manuscript revision. MA contributed in study design, data collection, results discussion, and the manuscript revision. PA contributed in study design, data analysis, and results discussion. All authors contributed to the article and approved the submitted version.
Funding
This study was made possible through funding from RARE Philippines and USAID, in collaboration with the Marine Environment and Resources Foundation (MERF).
Conflict of Interest
The authors declare that the research was conducted in the absence of any commercial or financial relationships that could be construed as a potential conflict of interest.
Publisher’s Note
All claims expressed in this article are solely those of the authors and do not necessarily represent those of their affiliated organizations, or those of the publisher, the editors and the reviewers. Any product that may be evaluated in this article, or claim that may be made by its manufacturer, is not guaranteed or endorsed by the publisher.
Acknowledgments
We would like to thank RARE Philippines and USAID, in collaboration with the Marine Environment and Resources Foundation (MERF), for research funding, coordination, and support in the execution of this research. We would also like to thank the RARE Conservation Fellows and Local Government Units for logistical support and coordination on all of the MPAs studied. We would also like to thank the Fisheries team of the MSI Community Laboratory for the municipal profile data, and the MSI Physical Oceanography Laboratory for the storm frequency and relative exposure index used in the environmental correlation. We would also like to thank the rest of the MERF-RARE Team/MSI Community Ecology Laboratory who joined and supported the many months of data gathering, encoding, and data analysis.
Supplementary Material
The Supplementary Material for this article can be found online at: https://www.frontiersin.org/articles/10.3389/fmars.2021.702071/full#supplementary-material
References
Abesamis, R. A., and Russ, G. R. (2005). Density-dependent spillover from a marine reserve: long-term evidence. Ecol. Appl. 15, 1798–1812. doi: 10.1890/05-0174
Agardy, T., di Sciara, G. N., and Christie, P. (2011). Mind the gap: addressing the shortcomings of marine protected areas through large scale marine spatial planning. Mar. Policy 35, 226–232. doi: 10.1016/j.marpol.2010.10.006
Alcala, A. C. (1998). Community-based coastal resource management in the Philippines: a case study. Ocean Coast. Manag. 38, 179–186. doi: 10.1016/s0964-5691(97)00072-0
Anderson, M. J., Gorley, R. N., and Clarke, K. R. (2008). PERMANOVA + for PRIMER: Guide to Software and Statistical Methods. Plymouth: PRIMER-E Ltd.
Anderson, R., Morrall, C., Jossart, J., Nimrod, S., Bolda, E., Musser, K., et al. (2014). Marine protected area monitoring in the nearshore waters of Grenada, Eastern Caribbean: benthic cover and fish populations. Int. J. Trop. Biol. 62, 273–286.
Bates, A. E., Cook, R. S. C., Duncan, M. I., Edgar, G. J., Bruno, J. F., Benedetti-Cecchi, L., et al. (2019). Climate resilience in marine protected areas and the ‘Protection Paradox’. Biol. Conserv. 236, 305–314.
Bellwood, D. R., Hughes, T. P., Folke, C., and Nystrom, M. (2004). Confronting the coral reef crisis. Nature 492, 827–833. doi: 10.1038/nature02691
Briones, M., Dey, M. M., and Ahmed, M. (2004). The future for fish in the food and livelihoods of the poor in Asia. NAGA WorldFish Center Quarterly 27(3–4), 48–50.
Brown, B. E. (1997). “Disturbances to reefs in recent times,” in Life and Death of Coral Reefs, ed. C. Birkeland (New York, NY: Chapman and Hall), 354–378. doi: 10.1007/978-1-4615-5995-5_15
Bruno, J. F., and Selig, E. R. (2007). Regional decline of coral cover in the Indo-Pacific: timing, extent, and subregional comparisons. PLoS One 2:e711. doi: 10.1371/journal.pone.0000711
Burke, L., Reytar, K., Spalding, M., and Perry, A. (2011). Reefs at Risk Revisited. Washington, DC: World Resources Institute.
Cabral, R., Cruz-Trinidad, A., Geronimo, R., Napitupulu, L., Lokani, P., Boso, D., et al. (2013). Crisis sentinel indicators: averting a potential meltdown in the Coral Triangle. Mar. Policy 39, 241–247. doi: 10.1016/j.marpol.2012.10.012
Carassou, L., Leopold, M., Guillemot, N., Wantiez, L., and Kulbicki, M. (2013). Does herbivorous fish protection really improve coral reef resilience? A case study from New Caledonia. PLoS One 8:e60564. doi: 10.1371/journal.pone.0060564
Carilli, J. E., Norris, R. D., Black, B. A., Walsh, S. M., and McField, M. (2009). Local stressors reduce coral resilience to bleaching. PLoS One 4:e6324. doi: 10.1371/journal.pone.0006324
Carpenter, K. E., and Springer, V. G. (2005). The center of the center of marine shore fish biodiversity: the Philippine Islands. Environ. Biol. Fish. 72, 467–480. doi: 10.1007/s10641-004-3154-4
Christie, P., White, A., and Deguit, E. (2002). Starting point or solution? Community-based marine protected areas in the Philippines. J. Environ. Manage. 66, 441–451. doi: 10.1006/jema.2002.0595
Coelho, V. R., and Manfrino, C. (2007). Coral community decline at a remote Carribean island: marine no-take reserves are not enough. Aquat. Conserv. 17, 666–685. doi: 10.1002/aqc.822
De’ath, G., Fabricius, K. E., Sweatman, H., and Puotinen, M. (2012). The 27-year decline of coral cover on the Great Barrier Reef and its causes. Proc. Natl. Acad. Sci. U. S. A. 109, 17995–17999. doi: 10.1073/pnas.1208909109
Donovan, M. K., Burkepile, D. E., Kratochwill, C., Shesinger, T., Sully, S., Oliver, T. A., et al. (2021). Local conditions magnify coral loss after marine heatwaves. Science 72, 977–980. doi: 10.1126/science.abd9464
Duarte, C. M., Agusti, S., Barbier, E., Britten, G. L., Castilla, J. C., Gattusso, J., et al. (2020). Rebuilding marine life. Nature 280, 39–51.
Eakin, C. M., Sweatman, H. P. A., and Brainard, R. E. (2019). The 2014–2017 global-scale coral bleaching event: insights and impacts. Coral Reefs 38, 539–545. doi: 10.1007/s00338-019-01844-2
Edinger, E. N., and Risk, M. J. (2000). Reef classification by coral morphology predicts coral reef conservation value. Biol. Conserv. 92, 1–13. doi: 10.1016/s0006-3207(99)00067-1
Eisma-Osorio, R.-L., Amolo, R. C., Maypa, A. P., White, A. T., and Christie, P. (2009). Scaling up local government initiatives toward ecosystem-based fisheries management in Southeast Cebu Island, Philippines. Coast. Manag. 37, 291–207. doi: 10.1080/08920750902851237
English, S., Wilkinson, C., and Baker, V. (1997). Survey Manual for Tropical Marine Resources. Townsville, QLD: Australian Institute of Marine Science.
Gilby, B. L., Maxwell, P. S., Tibbetts, I. R., and Stevens, T. (2015). Bottom-up factors for algal productivity outweigh no-fishing marine protected area effects in a marginal coral reef system. Ecosystems 18, 1056–1069. doi: 10.1007/s10021-015-9883-8
Gjertsen, H. (2005). Can habitat protection lead to improvements in human well-being? Evidence from marine protected areas in the Philippines. World Dev. 33, 199–217. doi: 10.1016/j.worlddev.2004.07.009
Gomez, E. D., and Alcala, A. C. (1979). “Status of Philippine Coral Reefs – 1978,” in Proceedings of the International Symposium on Marine Biogeography and Evolution in the Southern Hemisphere, Auckland, 663–669.
Gomez, E. D., Aliño, P. M., Yap, H. T., and Licuanan, W. Y. (1994). A review of the status of Philippine Reefs. Mar. Pollut. Bull. 29, 62–68. doi: 10.1016/0025-326x(94)90427-8
Howarth, L. M., Pickup, S. E., Evans, L. E., Cross, T. J., Hawkins, J. P., Roberts, C. M., et al. (2015). Sessile and mobile components of a benthic ecosystem display mixed trends within a temperate marine reserve. Mar. Environ. Res. 107:8e23.
Hughes, T. P., and Connell, J. H. (1999). Multiple stressors on coral reefs: a long-term perspective. Limnol. Oceanogr. 44, 932–940. doi: 10.4319/lo.1999.44.3_part_2.0932
Hughes, T. P., Rodrigues, M. J., Bellwood, D. R., Ceccarelli, D., Hoegh-Guldberg, O., McCook, L., et al. (2007). Phase shifts, herbivory, and the resilience of coral reefs to climate change. Curr. Biol. 17, 360–365. doi: 10.1016/j.cub.2006.12.049
Huntington, B. E., Karnauskas, M., and Lirman, D. (2011). Corals fail to recover at a Caribbean marine reserve despite ten years of reserve designation. Coral Reefs 30, 1077–1085. doi: 10.1007/s00338-011-0809-4
Jones, G. P., McCormick, M. I., Srinivasan, M., and Eagle, J. V. (2004). Coral decline threatens fish biodiversity in marine reserves. Proc. Natl. Acad. Sci. U. S. A. 101, 8251–8253. doi: 10.1073/pnas.0401277101
Knowlton, N. (2012). Iconic coral reef degraded despite substantial protection. Proc. Natl. Acad. Sci. U. S. A. 109, 17734–17735. doi: 10.1073/pnas.1215836109
Knowlton, N. (2021a). Local management matters for coral reefs. Science 372, 908–909. doi: 10.1126/science.abi7286
Knowlton, N. (2021b). Ocean optimism: moving beyond the obituaries in marine conservation. Annu. Rev. Mar. Sci. 13, 479–499. doi: 10.1146/annurev-marine-040220-101608
Kroon, F. J., Thorburn, P., Schaffelke, B., and Whitten, S. (2016). Towards protecting the Great Barrier Reef from land-based pollution. Glob. Change Biol. 22, 1985–2002. doi: 10.1111/gcb.13262
Licuanan, A. M., Reyes, M. Z., Luzon, K. S., Chan, M. A., and Licuanan, W. Y. (2017). Initial findings of the nationwide assessment of Philippine coral reefs. Philipp. J. Sci. 146, 177–185.
Licuanan, W. Y., and Gomez, E. D. (2000). Philippine Coral Reefs, Reef Fishes, and associated Fisheries: Status and Recommendations to Improve Their Management. Gland: Global Coral Reef Monitoring Network (GCRMN).
Licuanan, W. Y., Robles, R., and Reyes, M. (2019). Status and recent trends in coral reefs of the Philippines. Mari. Pollut. Bull. 142, 544–550. doi: 10.1016/j.marpolbul.2019.04.013
Magdaong, E. T., Fujii, M., Yamano, H., Licuanan, W. Y., Maypa, A., Campos, W. L., et al. (2013). Long-term change in coral cover and the effectiveness of marine protected areas in the Philippines: a meta-analysis. Hydrobiologia 733, 5–17. doi: 10.1007/s10750-013-1720-5
Maliao, R. J., White, A. T., Maypa, A. P., and Turingan, R. G. (2009). Trajectories and magnitude of change in coral reef fish populations in Philippine marine reserves: a meta-analysis. Coral Reefs 28, 809–822. doi: 10.1007/s00338-009-0532-6
Manthachitra, V. (1994). “Indices assessing the status of coral reef assemblage: formulated from benthic lifeform transect data,” in Proceedings of the Third ASEAN-Australia Symposium on Living Coastal Resources. 2: Research Papers, eds S. Sudara, C. R. Wilkinson, and L. M. Chou (Bangkok: Chulalongkorn University), 41–50.
Maypa, A. P., White, A. T., Canares, E., Martinez, R., Eisma-Osorio, R., Alino, P., et al. (2012). Marine protected area management effectiveness: progress and lessons in the Philippines. Coast. Manage. 40, 510–524. doi: 10.1080/08920753.2012.709465
McClure, E. C., Sieversa, K. T., Abesamis, R. A., Hoeyb, A. S., Alcala, A. C., and Russ, G. R. (2020). Higher fish biomass inside than outside marine protected areas despite typhoon impacts in a complex reefscape. Biol. Conserv. 241:108354. doi: 10.1016/j.biocon.2019.108354
McCook, L. J., Ayling, T., Cappo, M., Choat, J. H., Evans, R. D., De Freitas, D. M., et al. (2010). Adaptive management of the Great Barrier Reef: a globally significant demonstration of the benefits of networks of marine reserves. Proc. Natl. Acad. Sci. U. S. A. 107, 18278–18285. doi: 10.1073/pnas.0909335107
McManus, J. W., Reyes, R., and Nanola, C. (1997). Effects of some destructive fishing methods on coral cover and potential rates of recovery. Environ. Manage. 21, 69–78. doi: 10.1007/s002679900006
Muallil, R. N., Mamauag, S. S., Cabral, R. B., Celeste-Dizon, E. O., and Alino, P. M. (2014). Status, trends and challenges in the sustainability of small-scale fisheries in the Philippines: insights from FISHDA (Fishing Industries’ Support in Handling Decisions Application) Model. Mar. Policy 44, 212–221. doi: 10.1016/j.marpol.2013.08.026
Mumby, P. J., Harborne, A. R., Williams, J., Kappel, C. V., Brumbaugh, D. R., Micheli, F., et al. (2007). Trophic cascade facilitates coral recruitment in a marine reserve. Proc. Natl. Acad. Sci. U. S. A. 104, 8362–8367. doi: 10.1073/pnas.0702602104
Raymundo, L. J., Halford, A. R., Maypa, A. P., and Kerr, A. M. (2009). Functionally diverse reef-fish communities ameliorate coral disease. Proc. Natl. Acad. Sci. 106, 17067–17070. doi: 10.3354/meps08150
Roberts, C. M. (2000). Selecting marine reserve locations: optimality versus opportunism. Bull. Mar. Sci. 66, 581–592.
Roberts, C. M., Andelman, S., Branch, G., Bustamante, R. H., Castilla, J., Dugan, J., et al. (2003). Ecological criteria for evaluating candidate sites for marine reserves. Ecol. Appl. 13, S199–S214.
Roberts, C. M., Halpern, B., Palumbi, S. R., and Warner, R. R. (2001). Designing marine reserve networks: why small, isolated protected areas are not enough. Summer 2, 1–8. doi: 10.1002/9781119075806.ch1
Rubec, P. J. (1986). “The effects of sodium cyanide on coral reefs and marine fish in the Philippines,” in The First Asian Fisheries Forum, eds J. L. Maclean, L. B. Dizon, and L. V. Hosillos (Manila: Asian Fisheries Society), 297–302.
Russ, G. R., Alcala, A. C., Maypa, A. P., Calumpong, H. P., and White, A. T. (2004). Marine reserve benefits local fisheries. Ecol. Appl. 14, 587–606.
Russ, G. R., Rizzari, J. R., Abesamis, R. A., and Alcala, A. C. (2021). Coral cover a stronger driver of reef fish trophic biomass than fishing. Ecol. Appl. 31:e02224.
Sanciangco, J. C., Carpenter, K. E., Etnoyer, P. J., and Moretzsohn, F. (2013). Habitat availability and heterogeneity and the indo-pacific warm pool as predictors of marine species richness in the tropical indo-pacific. 8:e56245. doi: 10.1371/journal.pone.0056245
SCTR-Philippines (2012). State of the Coral Triangle Report – Philippines. Manado: Coral Triangle Initiative.
Selig, E. R., and Bruno, J. F. (2010). A global analysis of the effectiveness of marine protected areas in preventing coral loss. PLoS One 5:e9278. doi: 10.1371/journal.pone.00009278
Selig, E. R., Casey, K. S., and Bruno, J. F. (2012). Temperature-driven coral decline: the role of marine protected areas. Glob. Change Biol. 18, 1561–1570. doi: 10.1111/j.1365-2486.2012.02658.x
Stockwell, B., Jadloc, C. L., Abesamis, R. A., Alcala, A. C., and Russ, G. R. (2000). Trophic and benthic responses to no-take marine reserve protection in the Philippines. Mar. Ecol. Prog. Ser. 389, 1–15. doi: 10.3354/meps08150
Strain, E. M. A., Edgar, G. J., Ceccarelli, D., Stuart-Smith, R. D., Hosack, G. R., and Thomson, R. J. (2019). A global assessment of the direct and indirect benefits of marine protected areas for coral reef conservation. Divers. Distrib. 25, 9–20. doi: 10.1111/ddi.12838
Sweatman, H. (2008). No-take reserves protect coral reefs from predatory starfish. Curr. Biol. 18, R598–R599. doi: 10.1016/j.cub.2008.05.033
Tabor, G. M., and Aguirre, A. A. (2004). Ecosystem health and sentinel species: adding an ecological element to the proverbial “canary in the mineshaft”. EcoHealth 1, 226–228.
Teh, L. S., Teh, L. C., and Sumalia, R. U. (2013). A global estimate of the number of coral reef fishers. PLoS One 8:e65397. doi: 10.1371/journal.pone.0065397
Toth, L. T., van Woesik, R., Murdoch, T. T., Smith, S. R., Ogden, J. C., Precht, W. F., et al. (2014). Do no-take reserves benefit Florida’s corals? 14 years of change and stasis in the Florida Keys National Marine Sanctuary. Coral Reefs 33, 565–577. doi: 10.1007/s00338-014-1158-x
van Grieken, M. E., Roebeling, P. C., Bohnet, I. C., Whitten, S. M., Webster, A. J., Poggio, M., et al. (2019). Adoption of agricultural management for Great Barrier Reef water quality improvement in heterogeneous farming communities. Agric. Syst. 170, 1–8. doi: 10.1016/j.agsy.2018.12.003
Villanoy, C. L., Salamante, E., and Cabrera, O. (2013). “Chapter 3: exposure: waves and storm surges,” in MERF. (2013). Vulnerability Assessment Tools for Coastal Ecosystems: A Guidebook. R. C. Geronimo, N. M. G. Follosco, M. C. C. Quibilan, M. S. Samson, and M. G. J. P. Tiquio (Quezon City: Marine Environment and Resources Foundation, Inc.), 162.
Wenger, A. S., Williamson, D. H., da Silva, E. T., Ceccarelli, D. M., Browne, N. K., Petus, C., et al. (2015). Effects of reduced water quality on coral reefs in and out of no-take marine reserves. Conserv. Biol. 30, 142–153. doi: 10.1111/cobi.12576
Wilkinson, C. (2008). Status of Coral Reefs of the World: 2008. Townsville, QLD: Global Coral Reef Monitoring Network and Reef and Rainforest Research Centre.
Keywords: Philippines, marine protected areas, protection effects, health indices, coral reef benthos
Citation: Panga FM, Anticamara JA, Quibilan MCC, Atrigenio MP and Aliño PM (2021) Through the Boundaries: Environmental Factors Affecting Reef Benthic Cover in Marine Protected Areas in the Philippines. Front. Mar. Sci. 8:702071. doi: 10.3389/fmars.2021.702071
Received: 29 April 2021; Accepted: 14 July 2021;
Published: 18 August 2021.
Edited by:
Yehuda Benayahu, Tel Aviv University, IsraelReviewed by:
Susannah Leahy, Queensland Department of Agriculture and Fisheries, AustraliaGuillermo Diaz-Pulido, Griffith University, Australia
Copyright © 2021 Panga, Anticamara, Quibilan, Atrigenio and Aliño. This is an open-access article distributed under the terms of the Creative Commons Attribution License (CC BY). The use, distribution or reproduction in other forums is permitted, provided the original author(s) and the copyright owner(s) are credited and that the original publication in this journal is cited, in accordance with accepted academic practice. No use, distribution or reproduction is permitted which does not comply with these terms.
*Correspondence: Fleurdeliz M. Panga, fleurpanga@gmail.com