- 1Department of Earth and Environmental Sciences, University of Pavia, Pavia, Italy
- 2Smithsonian Environmental Research Center, Edgewater, MD, United States
- 3Department of Biology, Temple University, Philadelphia, PA, United States
Baseline port monitoring for fouling communities is an essential tool to assess non-indigenous species (NIS) introduction and spread, but a standardized and coordinated method among Mediterranean and European countries has not yet been adopted. In this context, it is important to test monitoring protocols that allow for the collection of standardized and directly comparable data, replicated across time and space. Here, for the first time in the Mediterranean Sea, we tested a standardized protocol developed by the Smithsonian Environmental Research Center (SERC) and used now in several countries. The 3-year monitoring survey (2018–2020) was conducted in the Gulf of La Spezia (Ligurian Sea, Italy), with the deployment of a total of 50 PVC panels per year in five different sites (a commercial harbor, three marinas and a site in the proximity of a shellfish farm). A total of 79 taxa were identified, including 11 NIS, ranging from zero to seven NIS for each panel. In comparison with previous surveys, new NIS arrivals were observed in the Gulf of La Spezia: Botrylloides cf. niger, Branchiomma sp., Branchiomma luctuosum, Paraleucilla magna, and Watersipora arcuata. At the end of the 3-year monitoring, mean richness? and percent cover of NIS were measured, and both measures differed across the monitoring sites, with higher values in two marinas and in the commercial harbor. Among years, richness of NIS was relatively stable at each monitoring site. The structure of the fouling was influenced more by native and cryptogenic species than by NIS. Moreover, among the monitoring sites, the density of artificial structures was not a reliable predictor or proxy for local NIS abundance. This first application of the SERC method in the Mediterranean Sea, demonstrates both pros and cons, including the detection of new NIS reported here. Further direct comparisons with other NIS monitoring tools are recommended, and additional tests to assess its effectiveness in this biogeographical area are encouraged. A broader application of this and other standard methods across temporal and spatial scales in the Mediterranean basin should be implemented, providing critical data needed to assess changes in the structure of fouling communities.
Introduction
The introduction of non-indigenous species (NIS) in marine environments is considered one of the most important factors contributing to global change (Ruiz et al., 1997; Occhipinti-Ambrogi, 2007; Galil et al., 2018a), including both ecological and economic impacts (Occhipinti-Ambrogi, 2007; Katsanevakis et al., 2014a; Ojaveer et al., 2015). The Mediterranean Sea is among the most invaded marine provinces (Bailey et al., 2020), with almost 800 multicellular NIS recorded (Galil et al., 2018b), and the main vectors of introduction include: the opening and enlargement of the Suez Canal, aquaculture and shipping (Ruiz et al., 2000; Galil et al., 2014, 2015; Katsanevakis et al., 2014b; Tsiamis et al., 2020). While most species introduced through the Suez Canal and by aquaculture vectors are generally confined in relatively restricted areas, vessels can further spread NIS throughout the whole Mediterranean Basin (Galil et al., 2018a). The maritime traffic involved in the introduction and spread of NIS includes both large commercial and small recreational vessels, which can carry species inside the ballast waters or as biofouling on vessel hulls (Ruiz et al., 2000; Clarke Murray et al., 2011; Canning-Clode et al., 2013; Zabin, 2014; Ferrario et al., 2017; Galil et al., 2018b). As defined by the International Maritime Organization, biofouling is “the accumulation of aquatic organisms such as microorganisms, plants and animals, on surfaces and structures immersed in or exposed to the aquatic environment” (IMO, 2012). The macrozoobenthic component of the fouling communities is usually composed of both sessile epibionts—sponges, serpulids, bivalves, barnacles, bryozoans, tunicates—and mobile marine invertebrates, like but not limited to flatworms, amphipods, isopods, sea spiders and brittle stars (Connell and Glasby, 1999; Glasby and Connell, 1999; Connell, 2001; Lord et al., 2015; Gavira-O’Neill et al., 2018; Culver et al., 2021).
Maritime traffic plays a key role in the introduction of fouling NIS, favoring their first settlement and establishment in ports and then creating hotspot areas for NIS. It has long been considered that large commercial ports act as primary hubs for fouling NIS, due to the transit of transoceanic cargo ships, while small boats are involved in their secondary spread in marinas and neighboring areas (Minchin et al., 2006; Floerl et al., 2009; Hulme, 2009; Ros et al., 2013; Zabin, 2014; Marchini et al., 2015). On the other hand, recent studies have suggested the importance of recreational boating in the primary introduction and spread of NIS (Ashton et al., 2014; Ferrario et al., 2017; Ulman et al., 2019a,b). After introduction to a port environment, the settlement of NIS can be facilitated both by the large abundance of available artificial substrates (Glasby et al., 2007) and the tolerance of these species to different environmental conditions, including polluted waters (Piola and Johnston, 2008; Piola et al., 2009).
For decades, fouling assemblages have served as a model to study bioinvasion processes (Ruiz et al., 2009; Canning-Clode et al., 2011; Marraffini et al., 2017; Leclerc et al., 2018; Marasinghe et al., 2018; Vieira et al., 2018; Ferrario et al., 2020). Furthermore, ports and marinas can be considered ideal sites for the early detection and monitoring of NIS presence and abundance, due to their importance as hotspot areas of NIS (Olenin et al., 2011; Ojaveer et al., 2014; Marraffini et al., 2017). However, no formal protocol has yet been adopted for the standardized assessment of fouling NIS in the European and Mediterranean marine regions. Monitoring of marine fouling communities is fundamental to track the introduction and spread of NIS, as well as to evaluate the power of legislation designed for limiting further introductions (Ruiz and Hewitt, 2002; Lehtiniemi et al., 2015; Marraffini et al., 2017). The implementation of long-term monitoring programs and a preventive approach can contribute to the assessment of the potential risks of NIS that have been demonstrated to negatively affect native communities—e.g., contributing to the decrease of vulnerable species (Marraffini et al., 2017; Occhipinti-Ambrogi, 2021). For these reasons, a standardized monitoring and a coordination among countries is highly recommended, in order to obtain comparable data replicated across time and space (Hewitt and Martin, 2001; Olenin et al., 2010, 2011; Latombe et al., 2017; Bailey et al., 2020). This is also in accordance with the Marine Strategy Framework Directive (MSFD 2008/56/EC), suggesting a development and application of standardized methods to detect NIS—a descriptor included in the achievement of the Good Environmental Status in European marine waters.
Several methodologies for sampling fouling communities in port habitats have been developed over the years. Most common techniques include (i) Rapid Assessment Surveys—RAS (e.g., Cohen et al., 2005; Campbell et al., 2007; Olenin et al., 2007; Minchin, 2012; Lehtiniemi et al., 2015; Marchini et al., 2015; Gewing and Shenkar, 2017; Ulman et al., 2017); (ii) net-assisted scraping (e.g., Ferrario et al., 2017; Ulman et al., 2017; Tempesti et al., 2020), including quadrat scraping during snorkeling or scuba diving (e.g., Neves et al., 2007; Awad et al., 2014; Ulman et al., 2019b); and artificial substrate units (ASU), such as (iii) two-dimensional settlement panels (e.g., Relini, 1977; Canning-Clode et al., 2013; Dos Santos Schwan et al., 2016; Lezzi et al., 2017; Leclerc and Viard, 2018; Marasinghe et al., 2018; Carlton, 2019; Leclerc et al., 2019); and (iv) three-dimensional artificial habitat collectors (e.g., Fowler et al., 2013; Gestoso et al., 2019; Outinen et al., 2019; Holmes and Callaway, 2020; Obst et al., 2020; Ros et al., 2020). Any of the above methods involves both advantages and disadvantages, and their success or suitability depend on the aim of the study. For example, quantitative methods (e.g., quadrats, settlement panels) are useful to thoroughly assess the entire fouling community but require a lot of fieldwork and laboratory effort. Conversely, qualitative methods like RAS allow for the collection of a lot of data on species distribution in a short timeframe, but the focus is typically only on target NIS already known and easily recognizable in the field. This method can limit the early detection of new arrivals, particularly of inconspicuous NIS. Net-assisted scraping probably ensures the most comprehensive picture of the fouling community, yet it requires long laboratory analyses and does not provide quantitative outputs (although semi-quantitative abundance estimates are possible; Ferrario et al., 2017). In addition, some methods are more suitable for sessile taxa (e.g., settlement panels), while other ones are better for mobile taxa (e.g., scrapes, 3-D ASU). Light exposure and orientation of the ASU also affects the ability of collecting the photosynthetic component of fouling communities. Therefore, the best practice for comprehensive sampling fouling communities should be a simultaneous multi-method approach in the same study area, as suggested by Tait et al. (2018) and Kakkonen et al. (2019).
In recent years, several sampling efforts focused along European and Mediterranean ports were conducted to monitor NIS presence. Using RAS, Ashton et al. (2006) and Nall et al. (2015) sampled in Scotland, while Bishop et al. (2015) sampled with the same method in the English Channel. In the Normandy region, Verlaque and Breton (2019) found macroalgal NIS through a RAS by scuba diving, involving citizen scientists after a multi-year field survey. In the ports of Gdynia and Gdańsk (Baltic Sea), Brzana et al. (2019) found a non-indigenous tanaid species using both settlement panels and artificial habitat collectors. In the subtropical island of Madeira (Atlantic Ocean), Canning-Clode et al. (2013) monitored NIS presence and abundance with a 6-year fouling survey through settlement panels. Regarding the Mediterranean Sea, Ferrario et al. (2017) sampled ports in the Western sector (Sardinian Sea, Ligurian Sea, and North Tyrrhenian Sea) by scraping the artificial hard substrates with a rigid hand-held net and Tempesti et al. (2020) sampled in the same way in the port area of Leghorn. In the Taranto Sea (Ionian Sea), Lezzi et al. (2017) carried out a 2-year macrofouling monitoring program using PVC settlement panels, while along the Slovenian coasts Fortič et al. (2019) combined different methods (RAS, scraping net and scuba dives) in their monitoring. In the Aegean Sea, Bariche et al. (2020) reported for the first time a non-indigenous nudibranch, using Autonomous Reef Monitoring Structures (ARMS) protocol, proposed by Obst et al. (2020). In the Southern Mediterranean Sea, Chebaane et al. (2019) sampled through RAS fishing ports and marinas in Monastir Bay, Tunisia. Lastly, the broadest investigation of non-indigenous invertebrates in Mediterranean marinas was conducted by Ulman et al. (2017) with both modified RAS and scrapes by hand-held rigid net. During the last few years, the metabarcoding approach has also started to be employed in NIS surveys with satisfying results (see also Borrell et al., 2017; Miralles et al., 2021; Pearman et al., 2021). Metabarcoding can be considered as an efficient tool to detect cryptic species or early life stages, but one of the main disadvantages is the incompleteness of genetic sequence reference libraries (Zaiko et al., 2018; Duarte et al., 2021). For this reason, an integrative approach should be considered as the gold standard (Padial et al., 2010; Cahill et al., 2018; Obst et al., 2020; Duarte et al., 2021).
In this study we applied for the first time in the Mediterranean Sea—namely in the Gulf of La Spezia (Ligurian Sea. Italy)—an international standard protocol for sessile fouling NIS monitoring in port habitats, with the aim to assess: (i) the fouling communities and NIS colonization in different harbor sites; (ii) the development of NIS colonization in the fouling communities over time; (iii) the effectiveness of this protocol for the early detection of newly introduced NIS in the Mediterranean; and (iv) the relationship between abundance of NIS and density of artificial structures in port habitats.
Materials and Methods
Study Area
The Gulf of La Spezia (44.09°N, 9.85°E) is one of the largest bays of the Ligurian Sea (Italy), characterized by the presence of several anthropogenic activities, including a commercial harbor and several marinas, and featuring intense anthropogenic influences such as urban runoff, naval industries, a military base, an electric power-plant and aquaculture facilities (Gasparini et al., 2009). The bay is about 5 km wide and 10 km long, and is separated in two parts by an artificial offshore dam about 2 km long (Gasparini et al., 2009).
The fouling monitoring program included five sites inside the gulf (Figure 1): in the marinas of Fezzano, Le Grazie and Porto Venere (FE, GR, and PV, respectively), near the commercial harbor of La Spezia (SP), and in a small marina near a mussel culture facility in Santa Teresa (ST). The trend of sea surface temperature (−0.915 m) from August 2018 to August 2020 was obtained by extrapolating data from loggers (ONSET HOBO WaterTemp Pro v2) deployed in the gulf for other experimental purposes by ENEA Marine Environment Research Centre (La Spezia).
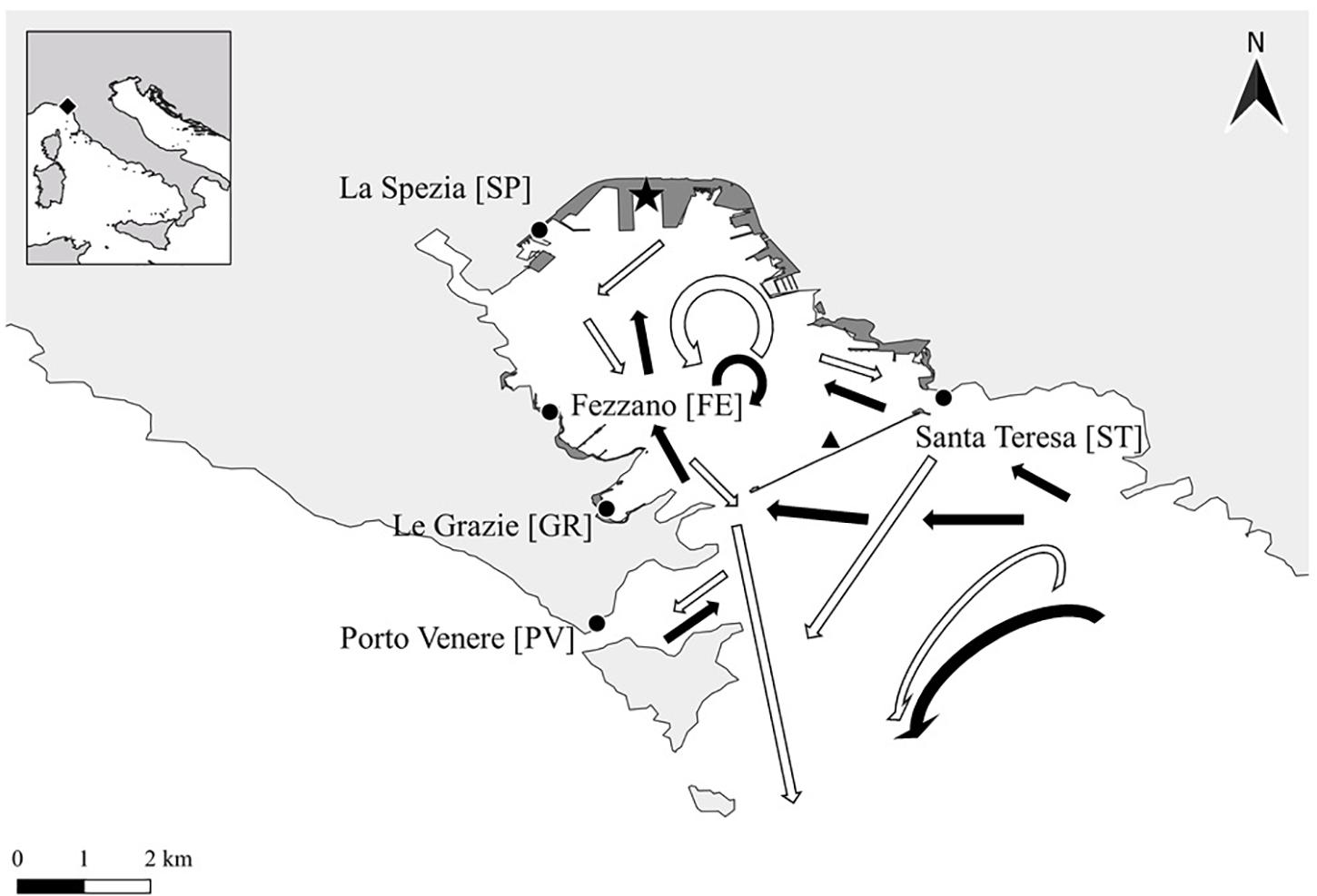
Figure 1. Map of monitoring sites in the Gulf of La Spezia (Ligurian Sea, Italy). Dark gray polygons indicate main port areas; black star indicates commercial harbor of La Spezia; black triangle indicates the artificial dam; white and black arrows indicate surface and deep main currents of the Gulf, respectively (adapted from Gasparini et al., 2009).
Monitoring Protocol
The monitoring was carried out for three consecutive years (2018–2020) by applying a standardized protocol developed by the Smithsonian Environmental Research Center (Edgewater, MD, United States) that is being used to detect and track NIS in the U.S as well as Canada, Ecuador, and Panama (see also de Rivera et al., 2005; Gartner et al., 2016; Simkanin et al., 2016; Marraffini et al., 2017; Tracy et al., 2017; Chang et al., 2018; Jurgens et al., 2018; Newcomer et al., 2018, 2019; Carlton, 2019; Torchin et al., 2021). A total of 50 PVC settlement panels (14 × 14 cm) were deployed in the summer of each year in the Gulf of La Spezia, allocating 10 panels in each site. Specifically, the passive collector (substrate) is composed of a settlement panel attached to a brick, with a sanded surface facing downward to minimize algal growth and facilitate invertebrate recruitment (Crooks et al., 2011; Marraffini et al., 2017; Jimenez et al., 2018). The experimental units were secured to docks or floating pontoons using rope, suspended 1 m below the sea surface, and immersed for 3 months during the summer season, in order to maximize the larval recruitment (Freestone et al., 2011; Marraffini et al., 2017). In 2018, the panels were immersed from July to October (87 days of deployment), in 2019 from April to July (89 days), and in 2020 from May to August (101 days). Differences in community assembly time across years were due to logistical constraints, in addition to mobility restrictions related to the health emergency of Coronavirus disease (COVID-19), and were considered during data analysis. At the end of the immersion period, all panels were retrieved, photographed and observed under dissecting microscopes for taxonomic identification of sessile invertebrates.
The percent cover of the whole fouling assemblage was assessed by point count method (n = 50 points; Chang et al., 2018), using a plastic grid (7 × 7 points, with an additional 50th point chosen randomly) positioned upon the plates observed under a dissecting microscope. With this method it is possible to observe more than one taxon in the same counting point, therefore percent cover for the whole community may be exceed 100%. Fouling communities on panels were retrieved and observed in vivo in 2018, while in 2019 and 2020 the panels were preserved in 70% ethanol upon retrieval and stored for later analysis.
Data Analysis
Species richness (S), NIS/native ratio and percent cover of each taxon were calculated for each panel (N = 10 per site). S and percent cover were assessed separately for all taxa, together with the assignment of biogeographic status sensu Chapman and Carlton (1991): NIS, native (including cryptogenic species—i.e., species that are not discernibly native or introduced, as defined by Carlton, 1996) and unresolved status (due to insufficient taxonomic resolution e.g., damaged specimens). Differences in mean values richness and percent cover of native and cryptogenic species and NIS were assessed by two-way ANOVA (Type III tests with two orthogonal fixed factors: “year,” three levels: 2018, 2019, 2020; and “site,” five levels: FE, GR, PV, SP, ST; unequal sample size). When pooling across sampling years, differences in mean richness and percent cover of NIS were assessed by one-way ANOVA (fixed factor “site,” five levels: FE, GR, PV, SP, ST; unequal sample size). In case of non-homogeneity of the variances, data were properly transformed. A more conservative critical value (α < 0.01) was chosen if the data transformation was not successful. Tukey’s tests were used for post hoc comparisons of means.
Percent cover data were analyzed using principal coordinate analysis (PCO) in order to visualize the pattern of clustering among fouling communities from each site during the years of monitoring. The ordination technique was carried out on centroids (year × site), based on Bray-Curtis similarity on fourth root transformed taxa percent cover data. PCO both for the whole community, and for NIS and native species component were applied separately. Distance-based test for homogeneity of multivariate dispersions (PERMDISP) and permutational multivariate analysis of variance (PERMANOVA) were carried out to test differences in NIS percent cover values of communities among sites (Anderson et al., 2008).
Correlation between NIS percent cover and density of immersed artificial structures (e.g., docks, pontoons) of each monitoring site was tested, as suggested by Susick et al. (2020). Density of each site was measured through satellite images as the linear length of mooring structures within a 200 m diameter circle of the potential marine habitat, centered on the spatial centroid of deployed panels. Length of artificial structure visible above water was measured using the path ruler tool in Google Earth Pro v. 7.3.3.7699 (Susick et al., 2020).
Analyses were carried out with the software Microsoft Excel, R (R Core Team., 2017), QGIS (QGIS Development Team., 2020) and PRIMER 6 with PERMANOVA + add-on package (Clarke and Gorley, 2006; Anderson et al., 2008).
Results
From August 2018 to August 2020, the range of surface temperature varied from 12 to 27°C (Supplementary Figure 1). Mean temperatures during the monitoring periods were 25 ± 1°C in 2018, 19 ± 3°C in 2019 and 21 ± 2°C in 2020.
Overall, we collected 126 out of the 150 panels initially deployed in the 3 years; the remaining panels (nine in 2018, four in 2019, and 11 in 2020) were lost due to external causes (e.g., coastal storms or vandalism).
After the 3-year monitoring, a total of 79 sessile taxa representing seven phyla were documented from the Gulf of La Spezia (Figure 2), including 11 NIS: four Bryozoa, four Annelida, one Porifera, and two Tunicata (Table 1). Among all the NIS observed, one has not been identified at species level (i.e., Branchiomma sp.). This species has been firstly assigned to Branchiomma boholense (Grube, 1878), but an accurate analysis of the specimens revealed divergent taxonomic characters, that cannot be attributed to the other Branchiomma spp. known to occur in the Mediterranean Sea, including the non-indigenous Branchiomma bairdi (McIntosh, 1885), B. boholense and Branchiomma luctuosum (Grube, 1870). Thus, further analyses are needed to confirm its identity, which could also be assigned to a fourth NIS belonging to the genus Branchiomma. The majority of the NIS were detected during all three monitoring campaigns, with the exception of Botrylloides cf. niger Herdman, 1886—found only in 2018—and B. luctuosum, found in 2018 and 2020. NIS were detected on all panels sampled over 3 years, except for a single panel at PV in 2019. Furthermore, nine panels exhibited only one NIS, seven of which were collected in PV from 2018 to 2020. The highest values of NIS richness (SNIS = 7) found on single panels were from FE 2018, GR 2020, and ST 2020.
For species richness, the lowest value of mean S at each site, i.e., Stot (± SD), was observed in SP in 2018 (11 ± 4), while the highest in ST in the same year (22 ± 2) (Figure 3). Regarding the mean SNIS, the lowest value was recorded in PV in 2019 (1 ± 1) and the highest in FE, GR and ST in 2018, as well as in FE and GR in 2020 (about five species on average). S of native and cryptogenic species (Snative) was the lowest in SP 2018 (7 ± 3) and the highest in PV 2020 (18 ± 3) (Figure 3). The mean NIS/(native + cryptogenic species) ratio showed a similar pattern, registering the lowest values in PV 2019 and 2020 (0.1 ± 0.04) and the highest in SP 2018 (0.6 ± 0.2). Snative resulted significantly different among sites, but not across years (F = 11.3172, p = 9.73 × 10–8; F = 1.6857, p = 0.19003, respectively). The site × year interaction resulted significant (F = 3.9884, p = 3.462 × 10–4) and post hoc test revealed that a single site (SP) contributed to this interaction (2018 vs. 2019 adjusted p = 2.913 × 10–4; 2018 vs. 2020 adjusted p = 7.745 × 10–4). Similarly, SNIS showed significative differences only among sites (F = 5.5382, p = 4.21 × 10–4) and not across years (F = 1.7775, p = 0.1738433), and no interaction was detected (F = 0.988, p = 0.4493411). Among the sites, PV showed significant differences with all the other four sites (adjusted p < 0.001).
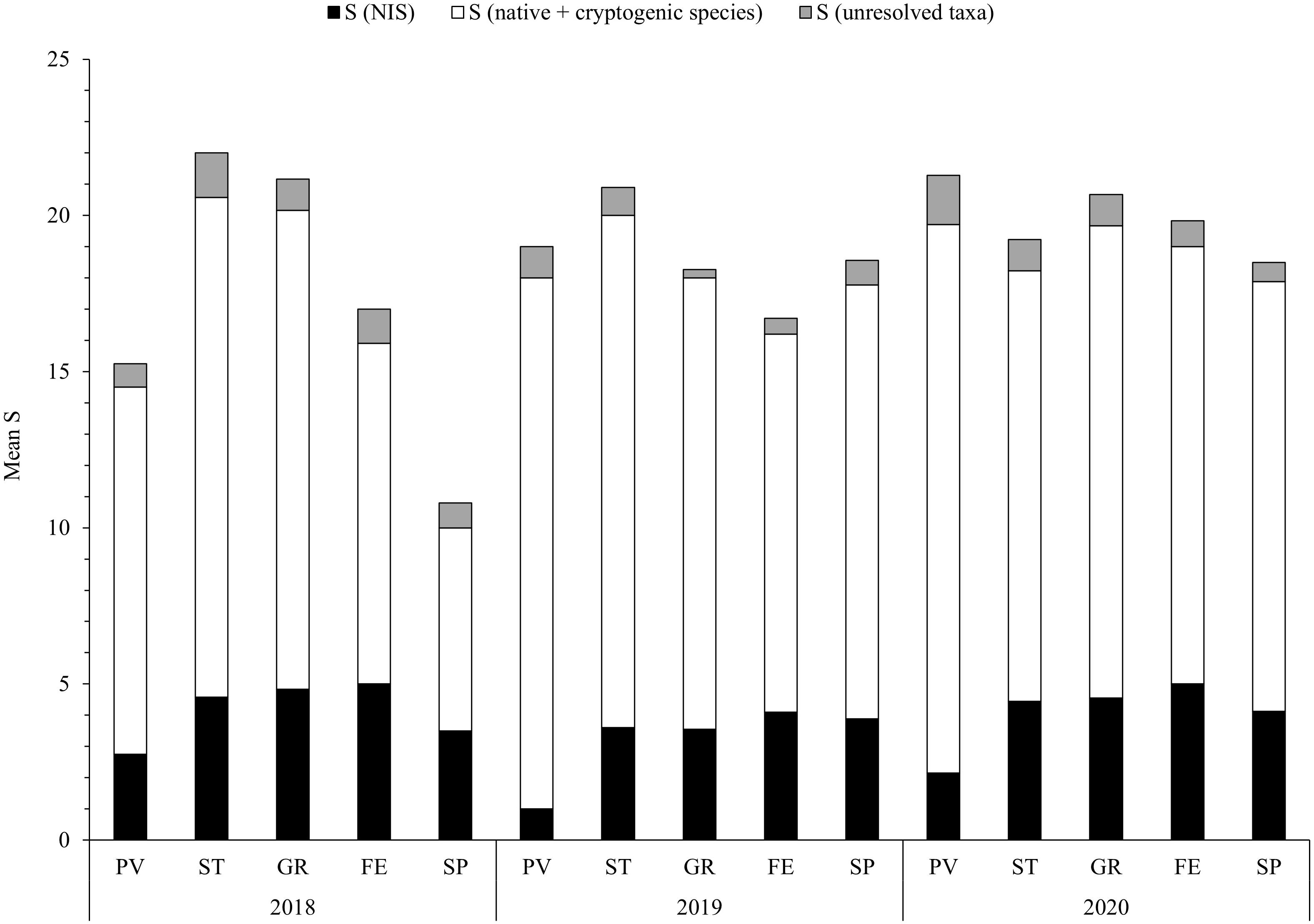
Figure 3. Mean species richness (S) of each monitoring site during the years of monitoring. Sites are showed at decreasing distance from La Spezia (SP). Abbreviations of sites are indicated in Figure 1.
Merging the data of the 3 years of monitoring, the sites with the highest and the lowest values of mean Stot were ST (21 ± 3) and SP (16 ± 5), respectively; while considering SNIS, the highest value was found in FE (5 ± 1) and the lowest in PV (2 ± 1). Most of the sites displayed higher values of mean Snative, namely GR (15 ± 4), PV (15 ± 4), and ST (15 ± 3); conversely, SP (11 ± 4) showed a slightly lower value (Table 2). During the 3 years of monitoring, mean percent cover of NIS was the lowest in PV (17 ± 23%) and the highest in SP (85 ± 50%).
Mean percent cover of native and cryptogenic species (Figure 4A) was highest value in GR 2020 (141 ± 32%) and lowest in SP 2018 (42 ± 35%). For NIS, mean percent cover (Figure 4B) was highest in SP 2018 (118 ± 38%) and lowest in PV 2019 (4 ± 5%). Percent cover of native species was significantly different across years (F = 9.0727, p = 2.241 × 10–4) and sites (F = 3.1586, p = 0.0168232), with no significant interaction (F = 1.5418, p = 0.1508879). Across years, the values observed in 2020 showed significant differences with 2018 and 2019 (adjusted p < 0.001); among sites, PV resulted significantly different from FE and SP (adjusted p < 0.01) (Figure 4A). Significant main effects were detected for NIS cover among sites (F = 7.5473, p = 2.05 × 10–05) and across years (F = 14.5618, p = 2.42E-06), but no interaction effect was detected (F = 1.2237, p = 0.2918). Post hoc tests revealed that percent cover of NIS was different between 2018 and the other 2 years (p < 0.001); between PV and the other four sites (p < 0.01); between ST and the other sites (p < 0.01) (Figure 4B).
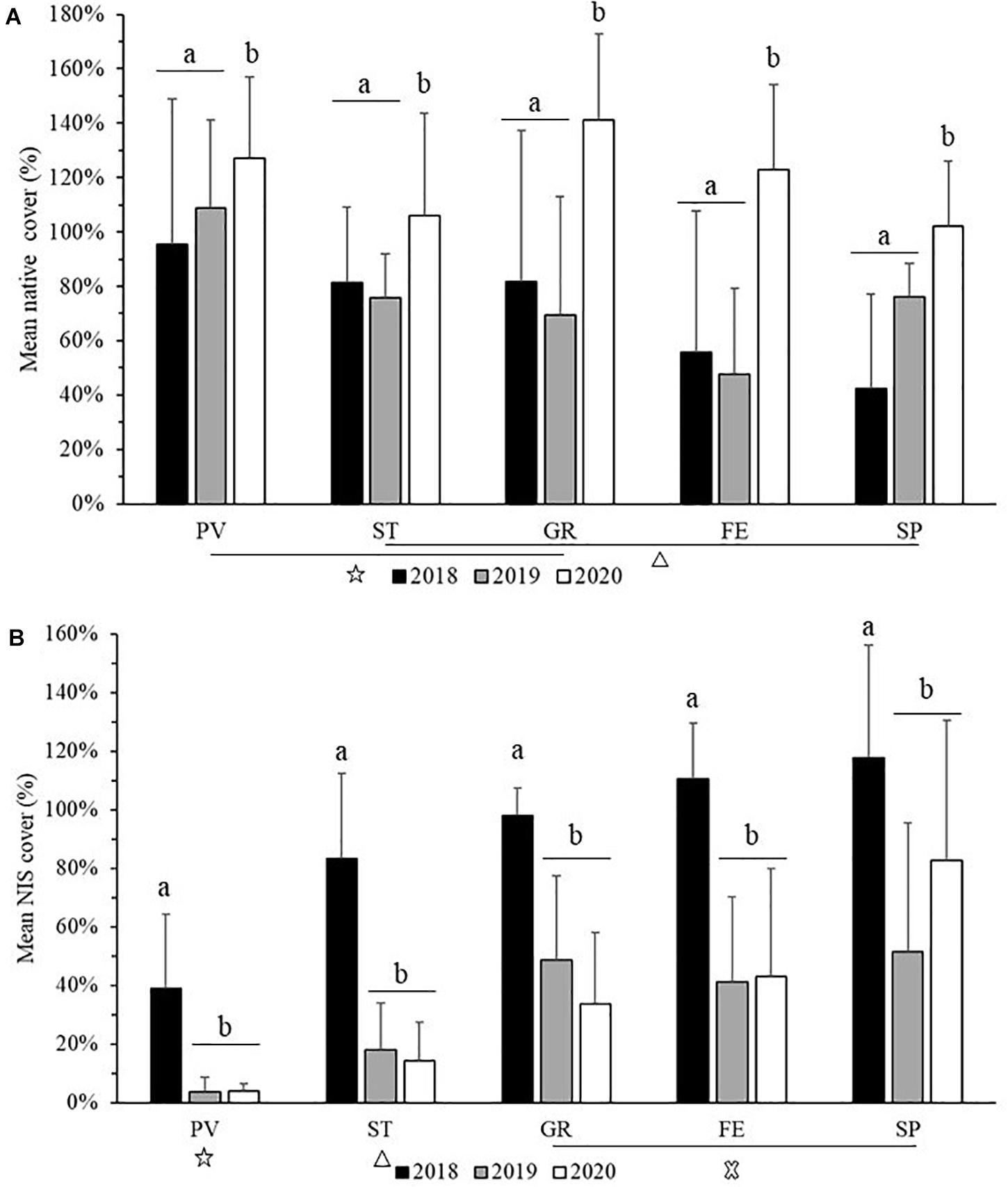
Figure 4. Mean (+ SD) percent cover of native and cryptogenic species (A), and NIS (B) in each monitoring site during the 3 years of monitoring. Letters (a, b) on the top of the two graphs indicate statistical divergence among years, while symbols on the bottom indicate statistical divergence among sites. Sites are showed at decreasing distance from La Spezia (SP) and abbreviations of sites are indicated in Figure 1.
The investigated sites were significantly different in terms of mean richness and percent cover of NIS, considering the 3 years of monitoring (F = 14.691, p = 8.257 × 10–10; F = 13.494, p = 4.00 × 10–9, respectively). Tukey’s tests showed a significant difference in richness between PV and all the other sites (p < 0.001) when considering SNIS. On the basis of NIS cover a significant difference came out between the sites PV-FE, PV-GR, and PV-SP (p < 0.001); ST-FE (p < 0.05) and ST-SP (p < 0.001). Relative abundance—i.e., percent cover data standardized to 100%—of unresolved taxa resulted very low in all sites (1–2%); while values for NIS and native species were similar in FE and SP (about 50% each); and in GR, PV, and ST the relative abundances were higher for the native species (63, 85, and 71%, respectively) in comparison with the NIS (36, 13, and 28%, respectively; Figure 5).
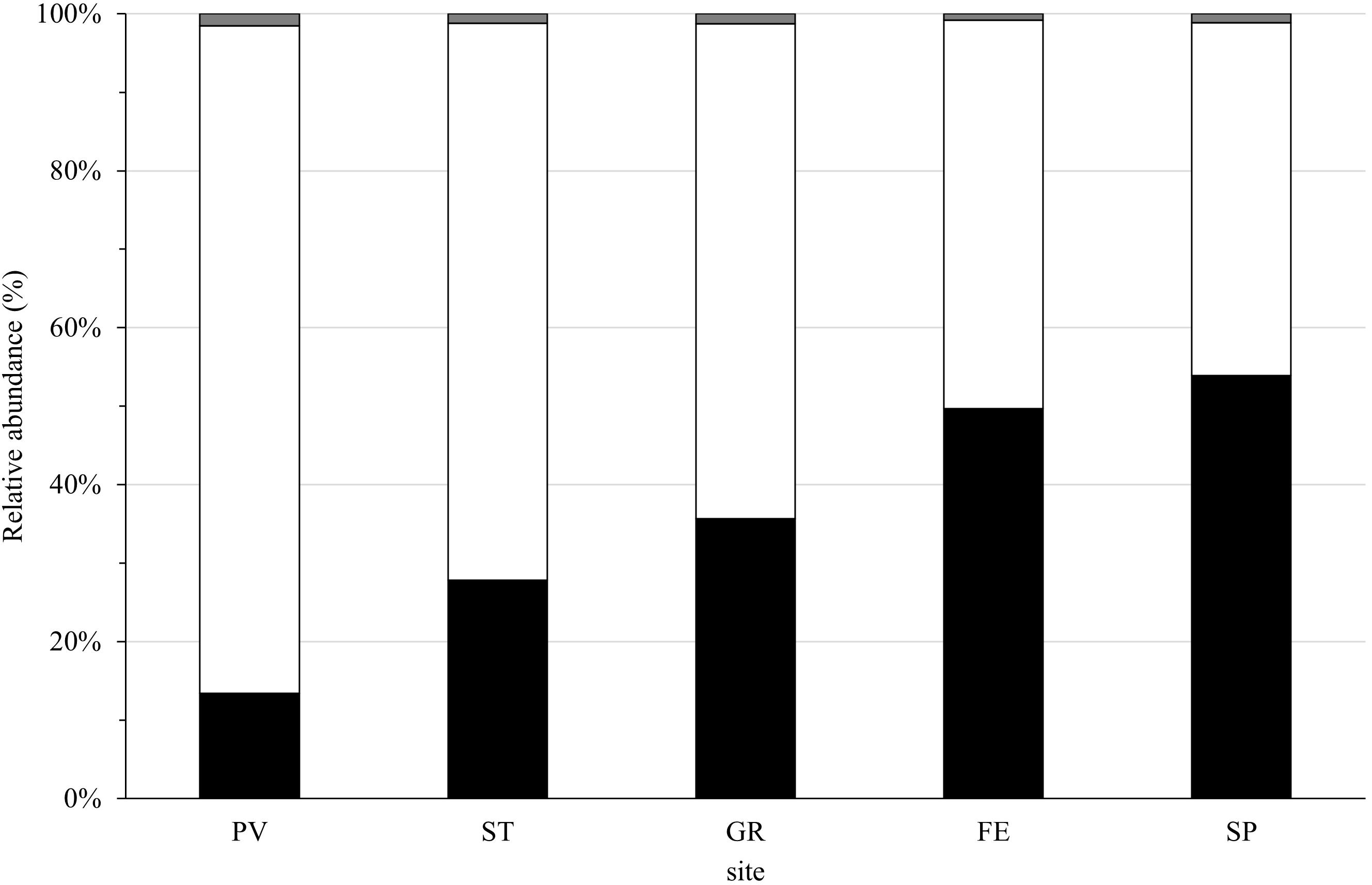
Figure 5. Relative abundance (%) of NIS percent cover (black bar), percent cover of native and cryptogenic species (white bar) and unresolved taxa percent cover (gray bar) in the monitoring sites in the 3-year monitoring. Sites are showed at decreasing distance from La Spezia (SP). Abbreviations of sites are indicated in Figure 1.
Regarding the structure of fouling communities, PCO showed slightly separated clusters per sampling year and the same distribution of sites within each year, with SP and PV being the most diversified sites (Figure 6). This pattern probably follows the percent cover of native species rather than NIS, which did not show a clear arrangement (Supplementary Figure 2). Furthermore, the values on percent cover of the non-indigenous component were more clumped than the native one (Supplementary Figure 2). PERMANOVA main test on NIS percent cover data of communities among monitoring sites (years merged) showed significant dissimilarity among community composition [pseudo-F = 13.138, p(perm) = 0.0001, unique permutations: 9915], while PERMDISP highlighted significant deviations of samples from centroid [F = 3.7702, p(perm) = 0.0116], rejecting the hypothesis of no difference in dispersion among the groups, i.e., “non-homogeneity” of samples dispersion. Moreover, pairwise PERMANOVA tests showed significant differences among sites, except for FE and GR [t = 1.3337, p(perm) = 0.1371, unique permutations: 9956], which therefore showed not-dissimilar NIS communities.
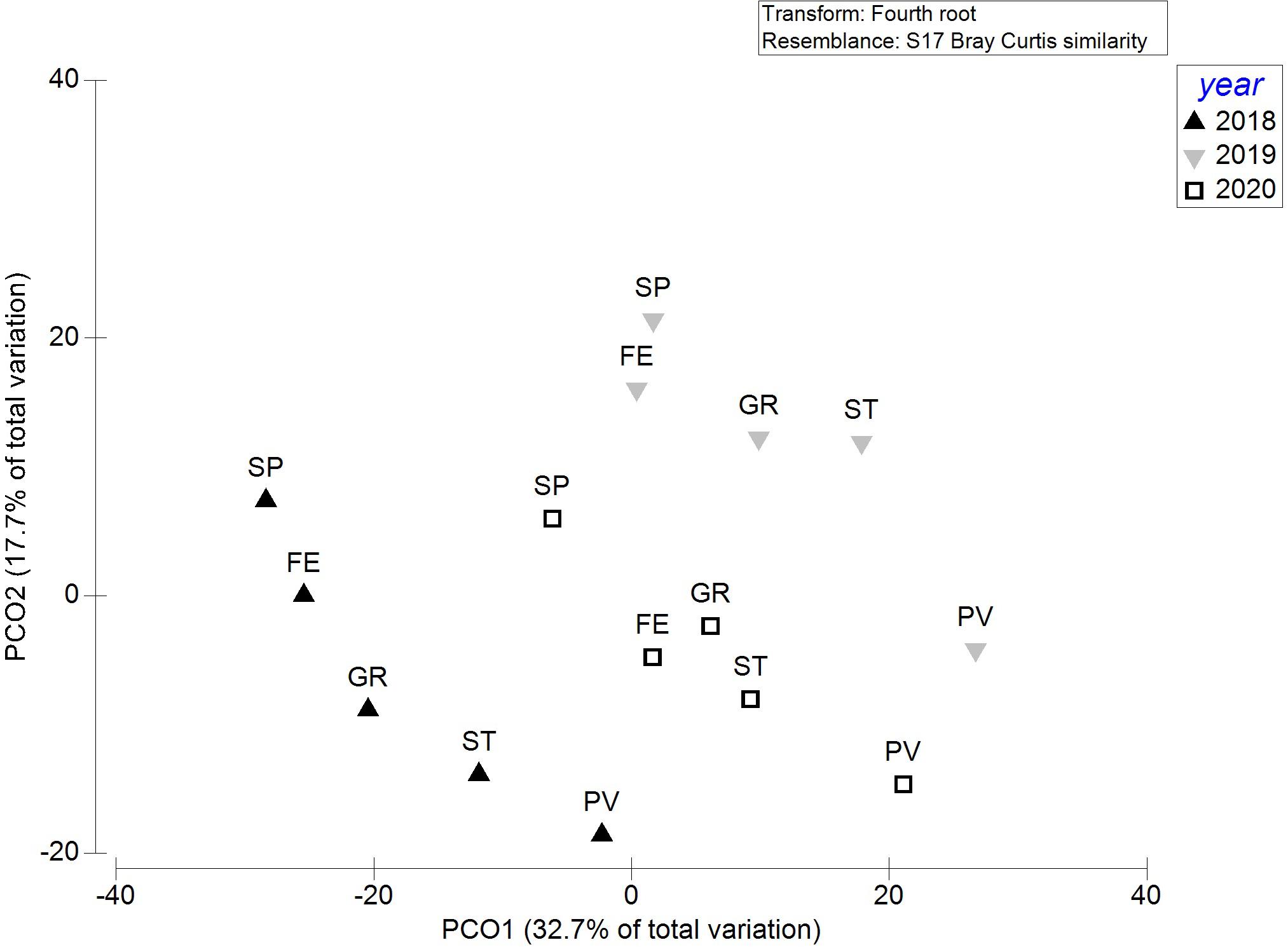
Figure 6. PCO (Principal Coordinate Analysis) based on distance among centroids (year × site) of whole fouling community percent cover data. Abbreviations of sites are indicated in Figure 1.
Density of artificial structures was the highest in SP (36.28 km × km–2) and the lowest in PV (13.74 km × km–2), with intermediate values in GR (29.04 km × km–2), FE (22.91 km × km–2), and ST (14.51 km × km–2). Correlation between NIS percent cover of samples (126) and these calculated densities resulted low (adjusted R2 = 0.2144, p = 2.876 × 10–8; Supplementary Figure 3).
Discussion
The 3-year monitoring on fouling communities in the Gulf of La Spezia allowed us to collect 79 taxa, half of which belong to Bryozoa and Tunicata, and to detect a total of 11 NIS. Seven NIS were already reported in the Gulf in 2010–2013 (Lodola et al., 2012, 2015; Ferrario et al., 2017; Katsanevakis et al., 2020), while five are new records, namely the sponge Paraleucilla magna Klautau, Monteiro and Borojevic, 2004, the sabellid polychaetes Branchiomma sp. and B. luctuosum, the bryozoan Watersipora arcuata Banta, 1969 and the ascidian Botrylloides cf. niger. Interestingly, in this study three NIS from Ferrario et al. (2017), observed in the harbor of La Spezia or in the marina of Lerici (near Santa Teresa site), were not recorded during the 3 years of monitoring, namely Amphibalanus improvisus (Darwin, 1854), Arcuatula senhousia (Benson, 1842), and Ficopomatus enigmaticus (Fauvel, 1923).
For the NIS observed in the present study, Paraleucilla magna is the only sponge reported as non-indigenous in the Mediterranean Sea (Longo et al., 2007). It was first described in Brazil and its putative native origin is the Indo-Pacific region and Australia, while its current distribution in the Mediterranean includes Italy, Spain, Malta, Croatia, Greece, Turkey, and Cyprus (Ulman et al., 2017). Although P. magna was already reported in the Ligurian Sea, namely along the Portofino coasts (Bertolino et al., 2013; Longobardi et al., 2017), this study provides a new record for the Gulf of La Spezia. The hypothesis expressed by Bertolino et al. (2013) and Longobardi et al. (2017) on the potential expansion of this species from the mussel farms of the Gulf of La Spezia to Portofino area, is strengthened by this last finding. The highest mean cover of P. magna was indeed found in Santa Teresa, the monitoring site near aquaculture facilities.
Regarding Branchiomma sp., we think this taxon must be further investigated, as this genus showed intraspecific variability that can lead to species misidentification (Capa et al., 2013). For example, a recent taxonomic study suggested that most of the Mediterranean specimens previously identified as B. bairdi should be probably be considered as B. boholense, with the exception of data reported by Ulman et al. (2019a) in different Mediterranean countries (Del Pasqua et al., 2018; Langeneck et al., 2020).
Branchiomma luctuosum, originally described from the Red Sea (Grube, 1870), was found for the first time in the Mediterranean Sea in Italy in 1978 (Knight-Jones et al., 1991). Then, B. luctuosum was recorded in several localities in the whole Mediterranean Sea, where it is considered established and able to colonize both artificial and natural substrates (Giangrande et al., 2012; Fernández-Romero et al., 2021). In the Western Mediterranean, B. luctuosum was found along the Spanish coasts since 2004 (El Haddad et al., 2012; López and Richter, 2017), in the Gulf of Genoa in 2011–2015 (Bianchi et al., 2018), in Sardinia in 2014 (Ferrario et al., 2017; Langeneck et al., 2020), and more recently in Leghorn in 2016 (Langeneck et al., 2020; Tempesti et al., 2020); the latter locality being about 37 nautical miles away from La Spezia.
The bryozoan Watersipora arcuata was reported for the first time in the Mediterranean Sea in 2013 in the Ligurian Sea (Ferrario et al., 2015). This species, likely originating from the Tropical Eastern Pacific, has now been recorded in most Mediterranean regions, namely Spain, Italy, Malta, and Turkey (Ulman et al., 2017; Ferrario et al., 2018). Furthermore, a recent revision of historical samples revealed its presence along the South Atlantic coast of Spain since 1990, suggesting its pathway of introduction in the Mediterranean Sea through the Gibraltar Strait (Reverter-Gil and Souto, 2019).
Finally, Botrylloides niger, a colonial ascidian native from the tropical Western Atlantic, is reported in several tropical and warm water regions across the world (Sheets et al., 2016), including Madeira island (Ramalhosa et al., 2021). In the Mediterranean Sea, it was first found in 2006 in Israel (Rubinstein et al., 2013) and erroneously identified as Botrylloides leachii (Savigny, 1816; Griggio et al., 2014). In general, species in the genus Botrylloides are easily confused (Brunetti, 2009; Viard et al., 2019), and in absence of molecular analyses, we use a conservative approach by considering the identification as tentative, pending further verification.
Our monitoring showed that mean NIS richness away varied from 1 to 5 species among sites and years, with similar values across the years of monitoring. For this reason, we did not find a clear increasing pattern in new NIS detections in the different sites, but continuation of this monitoring is required to identify more robust temporal patterns. On the other hand, native species richness significantly varied only in La Spezia among years, highlighting a stable number of native species during the monitoring. It should be highlighted that B. cf. niger was recorded only in 2018 in ST, but in the following years it did not disappear from the marina. Despite not being found on panel communities, it was still observed, even if in very low abundances, on other artificial substrates (e.g., on the supporting structure of the panels and on floating pontoons; authors personal observation). For this reason, B. cf. niger may be considered as an established NIS in the study area. After 3 years of monitoring, Porto Venere was found to be the least impacted site in terms of both richness and percent cover of NIS, while the other sites showed a higher impact by NIS, with values of mean NIS percent cover ranging from 34% (Santa Teresa) to 85% (La Spezia).
In general, the different submersion periods—mid-summer in 2018 and early spring in the following years—affected the development of fouling assemblages, mainly in terms of differences in taxa percent cover. NIS richness were indeed similar across the years of monitoring, while in 2018 definitely higher mean values of NIS cover were observed in all sites, compared to the other years of monitoring. The latter result is probably a consequence of the later submersion period of 2018, which led to the dominance on the panels of one NIS, namely Hydroides elegans (Haswell, 1883). A similar phenomenon with the dominance of one single species in fouling communities was also observed in marinas of South-eastern Brazil (Dos Santos Schwan et al., 2016), and could be related to the seasonal reproductive peaks (Reinhardt et al., 2013; Lezzi and Giangrande, 2018; Fortiè et al., 2021). These preliminary results suggest that maintaining the monitoring program between May and July is advisable. In fact, the number of detected NIS resulted independent from the submersion period, but during the period May-July it is possible to better capture the entire spectrum of potential colonizers, avoiding the dominance of a single species.
Considering the whole data set on NIS percent cover of the 3-year monitoring, higher values were found in La Spezia, with Fezzano, Le Grazie, Santa Teresa, and Porto Venere gradually showing lower values. The same gradient from La Spezia to Porto Venere was also observed when considering the structure of the entire community, and the gradient was probably more influenced by the composition of the native species than to the non-indigenous one. These patterns may reflect the different geographic features of the monitoring sites. In fact, the sites are located at an increasing distance from the main harbor of La Spezia, one of the largest commercial ports in Italy (accessed 1 March 2021)1. The harbor of La Spezia hosts international cargo ships and passengers’ vessels, as well as local ferries.
The distance of sites from the main harbor appears to be proportional to the mean NIS percent cover, while the proximity of Santa Teresa to the mussel farm showed higher abundances of P. magna, a NIS associated to aquaculture (Bertolino et al., 2013), as well as the exclusive presence of B. cf. niger in comparison with other sites (see figshare repository)2. In addition, the water circulation inside the Gulf is different in Porto Venere compared to the other sites, due to both the presence of the dam and the distance from the open sea (Figure 1; Gasparini et al., 2009). This circulation could advantage NIS larval dispersion and settlement inside the dam, where water exchange is surely lower than in Porto Venere (Gasparini et al., 2009). On the other hand, the density of artificial structures seems not to be a factor influencing NIS distribution in the area. The observed variability of NIS percent cover in the dataset can also be related to other features of sites, like the presence of floating pontoons, or the proximity to aquaculture facilities or to commercial harbors (Nall et al., 2015; Ulman et al., 2019a). All these aspects seem to affect the distribution of NIS and the composition of fouling communities in the Gulf of La Spezia. A regular data collection in the Gulf will allow us to examine in depth these results and better clarify the observed dynamics, but this first attempt should be considered in future management measures to prevent NIS introduction in the area, by implementing more rigid controls in sites closer to the harbor of La Spezia. Moreover, a longer data collection could also allow for obtaining limited statistical dispersion of samples within sites, in order to assess if difference in NIS percent cover in monitoring sites is purely due to the dissimilarity among sites and not to dispersion of samples (Anderson et al., 2008).
In conclusion, this monitoring allowed us to implement, for the first time in the Mediterranean Sea, an international standard protocol developed by the SERC to assess fouling communities and detect NIS. The number of detected NIS was in line with previous monitoring carried out in the Gulf of La Spezia and in other Ligurian port localities, but with additional new records for the area and three NIS no longer observed (Ferrario et al., 2017; Ulman et al., 2019a; Katsanevakis et al., 2020). These results are consistent with the role of commercial harbors and recreational marinas as hot-spot sites for the introduction of NIS (Clarke Murray et al., 2011; Ros et al., 2013; Ferrario et al., 2017; Verlaque and Breton, 2019), and the importance of continuous monitoring to detect changes in marine communities (Olenin et al., 2011; Ojaveer et al., 2014; Lehtiniemi et al., 2015). This monitoring protocol seems to be a strong and effective tool to control the presence and abundance of fouling NIS in the Mediterranean ports. Its main advantages are cost-effectiveness, ease of application and the capability to obtain quantitative results in a relatively short time. On the other hand, the effort for sampling and analyzing the panels is considerable. Finally, this protocol is predominantly focusing on the assessment of the sessile hard-bottom fauna, not providing complete quantitative data on the mobile component living on the panels (e.g., see Vicente et al., 2021), as well as on organisms dwelling in soft bottoms (e.g., see Chatzinikolaou et al., 2018), that should ideally be also considered for future monitoring programs on NIS in port habitats.
While this methodology provides a reliable option for sampling and monitoring fouling communities in the Mediterranean area, further analyses to test its effectiveness should be encouraged in other regional localities, together with a comparison to other fouling monitoring tools, including the use of genetic approaches (e.g., Ardura and Planes, 2017; Borrell et al., 2017; Marraffini et al., 2017; Cahill et al., 2018; Kakkonen et al., 2019; Obst et al., 2020Duarte et al., 2021). Similarly successful implementation in other locales could lead to the proposal of this method as an adopted standard for the assessment of marine bioinvasions in the Mediterranean. In fact, the application of the SERC method across the Mediterranean Sea could provide a simple option to gain standardized and quantitative data in a key invasion-prone habitat, allowing rapid assessment and one approach to evaluate changes in the structure of fouling communities, as it is being carried out along multiple coasts and countries in the Americas.
Data Availability Statement
The original contributions presented in the study are included in the article/Supplementary Material, further inquiries can be directed to the corresponding author/s.
Author Contributions
JF, EK, GMR, and AO-A conceived the study. MT, JF, EK, AM, and MFR performed the field work and laboratory analyses. MT carried out the statistics and wrote the first draft of the manuscript, with the contribution of JF. AO-A and GMR acquired funds. All the authors contributed to the manuscript revision, read and approved the submitted version.
Funding
Financial support to this project comes from MT Ph.D. scholarship (XXXIV Cycle), granted by the Department of Earth and Environmental Sciences of Pavia University, and by the crowdfunding project “A brick against alien species,” promoted by the “Universitiamo by UNIPV” platform at: https://universitiamo.eu/en/campaigns/un-mattone-contro-le-specie-aliene/. The authors declare that this study received funding from Boldarino S.P.A. and Curtiriso S.R.L., the main sponsors of the fundraising project “A brick against alien species”. These funders were not involved in the study design, collection, analysis, interpretation of data, the writing of this article or the decision to submit it for publication.
Conflict of Interest
The authors declare that the research was conducted in the absence of any commercial or financial relationships that could be construed as a potential conflict of interest.
Acknowledgments
We are grateful to: Municipality of Porto Venere, Port Authority of the Eastern Ligurian Sea, Scuola di Mare Santa Teresa, Cantiere Valdettaro, and Assonautica La Spezia for granting permissions for this study; Chiara Lombardi, Matteo Nannini and Andrea Bordone (ENEA La Spezia) for the logistic support and the temperature dataset; all the collaborators and students involved in logistic support during sampling surveys and laboratory activities (Alessandro Migliavacca, Alessandro Piazza, Alice Grioni, Chiara Mondaini, Cristina Cremonesi, Elisa Bigarella, Federica Gola, Gianmarco Riva, Giulia Quaini, Laura Francia, Lorenzo Pizzamiglio, and Marcella Lo Vullo) Alfonso Ángel Ramos Esplà (University of Alicante) and Joachim Langeneck (University of Pisa) for confirming the taxonomic identification of some ascidian and polychaete species, respectively, Ilaria Cabrini and the fundraising team “Universitiamo by UniPv”; Boldarino S.P.A and Curtiriso S.R.L and all the other donors and sponsors of the project; and Andrea Taccani (University of Pavia) for invaluable support in communication actions.
Supplementary Material
The Supplementary Material for this article can be found online at: https://www.frontiersin.org/articles/10.3389/fmars.2021.700730/full#supplementary-material
Footnotes
References
Anderson, M., Gorley, R. N., and Clarke, K. (2008). Permanova+ for Primer: Guide to Software and Statistical Methods. Plymouth: PRIMER-E.
Ardura, A., and Planes, S. (2017). Rapid assessment of non-indigenous species in the era of the eDNA barcoding: a Mediterranean case study. Estuar. Coast. Shelf Sci. 188, 81–87. doi: 10.1016/j.ecss.2017.02.004
Ashton, G., Boos, K., Shucksmith, R., and Cook, E. (2006). Rapid assessment of the distribution of marine non-native species in marinas in Scotland. Aquat. Invasions 1, 209–213. doi: 10.3391/ai.2006.1.4.3
Ashton, G., Davidson, I., and Ruiz, G. (2014). Transient small boats as a long-distance coastal vector for dispersal of biofouling organisms. Estuar. Coasts 37, 1572–1581. doi: 10.1007/s12237-014-9782-9
Awad, A., Haag, F., Anil, A. C., and Abdulla, A. (2014). Guidance on Port Biological Baseline Surveys GloBallast Monograph Series. London: GEF-UNDP-IMO GloBallast Partnerships.
Bailey, S. A., Brown, L., Campbell, M. L., Canning−Clode, J., Carlton, J. T., Castro, N., et al. (2020). Trends in the detection of aquatic non−indigenous species across global marine, estuarine and freshwater ecosystems: a 50−year perspective. Divers. Distrib. 26, 1780–1797. doi: 10.1111/ddi.13167
Bariche, M., Al-Mabruk, S., Ateş, M. A., Büyük, A., Crocetta, F., Dritsas, M., et al. (2020). New alien Mediterranean biodiversity records (march 2020). Mediterr. Mar. Sci. 21, 129–145. doi: 10.12681/mms.21987
Bertolino, M., Longo, C., Corriero, G., Pansini, M., and Bavestrello, G. (2013). “Prima segnalazione della specie tropicale Paraleucilla magna Klautau et al., 2004 (Porifera, Calcarea) nel Mar Ligure,” in Poster at the 74° Congresso Nazionale dell’Unione Zoologica Italiana, (Atti Società dei Naturalisti e Matematici di Modena).
Bianchi, C. N., Caroli, F., Guidetti, P., and Morri, C. (2018). Seawater warming at the northern reach for southern species: Gulf of Genoa, NW Mediterranean. J. Mar. Biol. Assoc. 98, 1–12. doi: 10.1017/S0025315417000819
Bishop, J. D., Wood, C. A., Leveque, L., Yunnie, A. L., and Viard, F. (2015). Repeated rapid assessment surveys reveal contrasting trends in occupancy of marinas by non-indigenous species on opposite sides of the western english channel. Mar. Pollut. Bull. 95, 699–706. doi: 10.1016/j.marpolbul.2014.11.043
Borrell, Y. J., Miralles, L., Do Huu, H., Mohammed-Geba, K., and Garcia-Vazquez, E. (2017). DNA in a bottle—rapid metabarcoding survey for early alerts of invasive species in ports. PLoS One 12:e0183347. doi: 10.1371/journal.pone.0183347
Brunetti, R. (2009). Botryllid species (Tunicata, Ascidiacea) from the Mediterranean coast of Israel, with some considerations on the systematics of Botryllinae. Zootaxa 2289, 18–32.
Brzana, R., Marszewska, L., Normant-Saremba, M., and Błażewicz, M. (2019). Non-indigenous tanaid sinelobus vanhaareni bamber, 2014 in the polish coastal waters – an example of a successful invader. Oceanol. Hydrobiol. Stud. 48, 76–84.
Cahill, A. E., Pearman, J. K., Borja, A., Carugati, L., Carvalho, S., Danovaro, R., et al. (2018). A comparative analysis of metabarcoding and morphology-based identification of benthic communities across different regional seas. Ecol. Evol. 8, 8908–8920. doi: 10.1002/ece3.4283
Campbell, M. L., Gould, B., and Hewitt, C. L. (2007). Survey evaluations to assess marine bioinvasions. Mar. Pollut. Bull. 55, 360–378.
Canning-Clode, J., Fofonoff, P., McCann, L., Carlton, J., and Ruiz, G. (2013). Marine invasions on a subtropical island: fouling studies and new records in a recent marina on Madeira Island (Eastern Atlantic Ocean). Aquat. Invasions 8, 261–270. doi: 10.3391/ai.2013.8.3.02
Canning-Clode, J., Fofonoff, P., Riedel, G. F., Torchin, M., and Ruiz, G. M. (2011). The effects of copper pollution on fouling assemblage diversity: a tropical-temperate comparison. Plos One 6:e18026. doi: 10.1371/journal.pone.0018026
Capa, M., Pons, J., and Hutchings, P. (2013). Cryptic diversity, intraspecific phenetic plasticity and recent geographical translocations in Branchiomma (Sabellidae, Annelida). Zool. Scr. 42, 637–655. doi: 10.1111/zsc.12028
Carlton, J. (2019). Assessing marine bioinvasions in the Galápagos islands: implications for conservation biology and marine protected areas. Aquat. Invasions 14, 1–20. doi: 10.3391/ai.2019.14.1.01
Chang, A. L., Brown, C. W., Crooks, J. A., and Ruiz, G. M. (2018). Dry and wet periods drive rapid shifts in community assembly in an estuarine ecosystem. Glob. Change Biol. 24, e627–e642. doi: 10.1111/gcb.13972
Chapman, J. W., and Carlton, J. T. (1991). A test of criteria for introduced species: the global invasion by the isopod Synidotea laevidorsalis (Miers, 1881). J. Crustac. Biol. 11, 386–400. doi: 10.2307/1548465
Chatzinikolaou, E., Mandalakis, M., Damianidis, P., Dailianis, T., Gambineri, S., Rossano, C., et al. (2018). Spatio-temporal benthic biodiversity patterns and pollution pressure in three Mediterranean touristic ports. Sci. Total Environ. 624, 648–660. doi: 10.1016/j.scitotenv.2017.12.111
Chebaane, S., Sempere-Valverde, J., Dorai, S., Kacem, A., and Ramzi Sghaier, Y. (2019). A preliminary inventory of alien and cryptogenic species in Monastir Bay, Tunisia: spatial distribution, introduction trends and pathway. Mediterr. Mar. Sci. 20, 616–626. doi: 10.12681/mms.20229
Clarke Murray, C., Pakhomov, E. A., and Therriault, T. W. (2011). Recreational boating: a large unregulated vector transporting marine invasive species. Divers. Distrib. 17, 1161–1172. doi: 10.1111/j.1472-4642.2011.00798.x
Cohen, A. N., Harris, L. H., Bingham, B. L., Carlton, J. T., Chapman, J. W., Lambert, C. C., et al. (2005). Rapid assessment survey for exotic organisms in southern California bays and harbors, and abundance in port and non-port areas. Biol. Invasions 7, 995–1002. doi: 10.1007/s10530-004-3121-1
Connell, S. D. (2001). Urban structures as marine habitats: an experimental comparison of the composition and abundance of subtidal epibiota among pilings, pontoons and rocky reefs. Mar. Environ. Res. 52, 115–125. doi: 10.1016/s0141-1136(00)00266-x
Connell, S. D., and Glasby, T. M. (1999). Do urban structures influence local abundance and diversity of subtidal epibiota? A case study from Sydney Harbour, Australia. Mar. Environ. Res. 47, 373–387. doi: 10.1016/s0141-1136(98)00126-3
Crooks, J. A., Chang, A. L., and Ruiz, G. M. (2011). Aquatic pollution increases the relative success of invasive species. Biol. Invasions 13, 165–176. doi: 10.1007/s10530-010-9799-3
Culver, C. S., Johnson, L. T., Page, H. M., Dugan, J. E., and Santschi, C. A. (2021). Integrated pest management for fouling organisms on boat hulls. N. Am. J. Fish. Manag. 41, 1–28. doi: 10.1002/nafm.10360
de Rivera, C. E., Ruiz, G., Crooks, J., Wasson, K., and Lonhart, S. (2005). Broad-scale non-indigenous species monitoring along the west coast in national marine sanctuaries and national estuarine research reserves. Environ. Sci. Manag. 76:2005.
Del Pasqua, M., Schulze, A., Tovar-Hernández, M. A., Keppel, E., Lezzi, M., Gambi, M. C., et al. (2018). Clarifying the taxonomic status of the alien species Branchiomma bairdi and Branchiomma boholense (Annelida: Sabellidae) using molecular and morphological evidence. PLoS One 13:e0197104. doi: 10.1371/journal.pone.0197104
Dos Santos Schwan, I., Dos Santos Brasil, A. C., Neves, D., and Dias, G. M. (2016). The invasive worm Hydroides elegans (Polychaeta – Serpulidae) in southeastern Brazil and its potential to dominate hard substrata. Mar. Biol. Res. 12, 96–103. doi: 10.1080/17451000.2015.1080370
Duarte, S., Vieira, P. E., Lavrador, A. S., and Costa, F. O. (2021). Status and prospects of marine NIS detection and monitoring through (e) DNA metabarcoding. Sci. Total Environ. 751:141729. doi: 10.1016/j.scitotenv.2020.141729
El Haddad, M., Azzati, R. C., and García-Carrascosa, A. M. (2012). Branchiomma luctuosum (Polychaeta: Sabellidae): a non-indigenous species at Valencia port (western Mediterranean Sea, Spain). Mar. Biodivers. Rec. 1:e6. doi: 10.1017/S1755267207006604
Fernández-Romero, A., Navarro-Barranco, C., Ros, M., Rodríguez, A. A., Moreira, J., and Guerra-García, J. M. (2021). To the Mediterranean and beyond: an integrative approach to evaluate the spreading of Branchiomma luctuosum (Annelida: Sabellidae). Estuar. Coast. Shelf Sci. 254:107357. doi: 10.1016/j.ecss.2021.107357
Ferrario, J., Caronni, S., Occhipinti-Ambrogi, A., and Marchini, A. (2017). Role of commercial harbours and recreational marinas in the spread of non-indigenous fouling species. Biofouling 33, 651–660. doi: 10.1080/08927014.2017.1351958
Ferrario, J., d’Hondt, J. L., Marchini, A., and Occhipinti-Ambrogi, A. (2015). From the Pacific Ocean to the Mediterranean Sea: Watersipora arcuata, a new non-indigenous bryozoan in Europe. Mar. Biol. Res. 11, 909–919. doi: 10.1080/17451000.2015.1041531
Ferrario, J., Gestoso, I., Ramalhosa, P., Cacabelos, E., Duarte, B., Caçador, I., et al. (2020). Marine fouling communities from artificial and natural habitats: comparison of resistance to chemical and physical disturbances. Aquat. Invasions 15, 196–216.
Ferrario, J., Rosso, A., Marchini, A., and Occhipinti-Ambrogi, A. (2018). Mediterranean non-indigenous bryozoans: an update and knowledge gaps. Biodivers. Conserv. 27, 2783–2794. doi: 10.1007/s10531-018-1566-2
Floerl, O., Inglis, G. J., Dey, K., and Smith, A. (2009). The importance of transport hubs in stepping-stone invasions. J. Appl. Ecol. 46, 37–45. doi: 10.1111/j.1365-2664.2008.01540.x
Fortič, A., Mavrič, B., Pitacco, V., and Lipej, L. (2021). Temporal changes of a fouling community: colonization patterns of the benthic epifauna in the shallow northern Adriatic Sea. Reg. Stud. Mar. Sci. 45:101818. doi: 10.1016/j.rsma.2021.101818
Fortič, A., Trkov, D., Mavrič, B., and Lipej, L. (2019). Assessment of bryozoan xenodiversity in the Slovenian coastal sea. Ser. Hist. Nat. 29, 173–186.
Fowler, A. E., Forsström, T., von Numers, M., and Vesakoski, O. (2013). The North American mud crab Rhithropanopeus harrisii (Gould, 1841) in newly colonized Northern Baltic Sea: distribution and ecology. Aquat. Invasions 8, 89–96.
Freestone, A. L., Osman, R. W., Ruiz, G. M., and Torchin, M. E. (2011). Stronger predation in the tropics shapes species richness patterns in marine communities. Ecology 92, 983–993. doi: 10.1890/09-2379.1
Galil, B. S., Boero, F., Campbell, M. L., Carlton, J. T., Cook, E., Fraschetti, S., et al. (2015). Double trouble’: the expansion of the Suez Canal and marine bioinvasions in the Mediterranean Sea. Biol. Invasions 17, 973–976. doi: 10.1007/s10530-014-0778-y
Galil, B. S., Marchini, A., and Occhipinti-Ambrogi, A. (2018b). “Mare nostrum, mare quod invaditur—the history of bioinvasions in the Mediterranean sea,” in Histories of Bioinvasions in the Mediterranean, eds A. I. Queiroz and S. Pooley (Cham: Springer International Publishing), 21–49.
Galil, B. S., Marchini, A., and Occhipinti-Ambrogi, A. (2018a). East is east and west is west? Management of marine bioinvasions in the Mediterranean sea. Estuar. Coast. Shelf Sci. 201, 7–16. doi: 10.1016/j.ecss.2015.12.021
Galil, B. S., Marchini, A., Occhipinti-Ambrogi, A., Minchin, D., Narscius, A., Ojaveer, H., et al. (2014). International arrivals: widespread bioinvasions in European seas. Ethol. Ecol. Evol. 26, 152–171. doi: 10.1080/03949370.2014.897651
Gartner, H. N., Murray, C. C., Frey, M. A., Nelson, J. C., Larson, K. J., Ruiz, G. M., et al. (2016). Non-indigenous invertebrate species in the marine fouling communities of British Columbia, Canada. BioInvasions Rec. 5, 205–212. doi: 10.3391/bir.2016.5.4.03
Gasparini, G. P., Abbate, M., Bordone, A., Cerrati, G., Galli, C., Lazzoni, E., et al. (2009). Circulation and biomass distribution during warm season in the Gulf of La Spezia (north-western Mediterranean). J. Mar. Syst. 78, S48–S62. doi: 10.1016/j.jmarsys.2009.01.010
Gavira-O’Neill, K., Guerra-García, J. M., Moreira, J., and Ros, M. (2018). Mobile epifauna of the invasive bryozoan Tricellaria inopinata: is there a potential invasional meltdown? Mar. Biodivers. 48, 1169–1178. doi: 10.1007/s12526-016-0563-5
Gestoso, I., Ramalhosa, P., Cacabelos, E., Ferrario, J., Castro, N., Monteiro, J., et al. (2019). “A new methodology for the study of biological invasions on coastal communities,” in Proceeding of the XX Iberian Symposium on Marine Biology Studies (SIEBM XX), (Lisbon).
Gewing, M. T., and Shenkar, N. (2017). Monitoring the magnitude of marine vessel infestation by non-indigenous ascidians in the Mediterranean. Mar. Pollut. Bull. 121, 52–59. doi: 10.1016/j.marpolbul.2017.05.041
Giangrande, A., Cosentino, A., Presti, C. L., and Licciano, M. (2012). Sabellidae (Annelida) from the Faro coastal lake (Messina, Ionian Sea), with the first record of the invasive species Branchiomma bairdi along the Italian coast. Mediterr. Mar. Sci. 13, 283–293. doi: 10.12681/mms.310
Glasby, T. M., Connell, S. D., Holloway, M. G., and Hewitt, C. L. (2007). Nonindigenous biota on artificial structures: could habitat creation facilitate biological invasions? Mar. Biol. 151, 887–895. doi: 10.1007/s00227-006-0552-5
Griggio, F., Voskoboynik, A., Iannelli, F., Justy, F., Tilak, M. K., Xavier, T., et al. (2014). Ascidian mitogenomics: comparison of evolutionary rates in closely related taxa provides evidence of ongoing speciation events. Genome Biol. Evol. 6, 591–605. doi: 10.1093/gbe/evu041
Grube, E. (1870). Beschreibungen neuer oder weniger bekannter von Hrn. Ehrenberg gesammelter anneliden des rothen meeres. Monatsberichte Akad Wiss Berlin 1869, 484–521.
Hewitt, C. L., and Martin, R. B. (2001). Revised protocols for baseline port surveys for introduced marine species: survey design, sampling protocols and specimen handling. Hobart 22:46.
Holmes, S., and Callaway, R. (2020). Design and implementation of two surveys targeted at describing fouling communities and identifying non-native species within active ports. J. Mar. Biol. Assoc. 100, 1191–1204. doi: 10.1017/S0025315420001150
Hulme, P. E. (2009). Trade, transport and trouble: managing invasive species pathways in an era of globalization. J. Appl. Ecol. 46, 10–18.
IMO. (2012). Guidance for Minimizing the Transfer of Invasive Aquatic Species as Biofouling (hullfouling) for Recreational Craft. London: International Maritime Organization.
Jimenez, H., Keppel, E., Chang, A. L., and Ruiz, G. M. (2018). Invasions in marine communities: contrasting species richness and community composition across habitats and salinity. Estuar. Coasts 41, 484–494. doi: 10.1007/s12237-017-0292-4
Jurgens, L. J., Bonfim, M., Lopez, D. P., Repetto, M. F., Freitag, G., McCann, L., et al. (2018). Poleward range expansion of a non-indigenous bryozoan and new occurrences of exotic ascidians in southeast Alaska. BioInvasions Rec. 7, 357–366. doi: 10.3391/bir.2018.7.4.02
Kakkonen, J. E., Worsfold, T. M., Ashelby, C. W., Taylor, A., and Beaton, K. (2019). The value of regular monitoring and diverse sampling techniques to assess aquatic non-native species: a case study from Orkney. Manag. Biol. Invasions 10, 46–79.
Katsanevakis, S., Poursanidis, D., Hoffman, R., Rizgalla, J., Bat-Sheva Rothman, S., and Levitt- Barmats, Y. (2020). Unpublished Mediterranean records of marine alien and cryptogenic species. BioInvasions Rec. 9, 165–182. doi: 10.3391/bir.2020.9.2.01
Katsanevakis, S., Coll, M., Piroddi, C., Steenbeek, J., Ben Rais Lasram, F., Zenetos, A., et al. (2014a). Invading the Mediterranean Sea: biodiversity patterns shaped by human activities. Front. Mar. Sci. 1:32. doi: 10.3389/fmars.2014.00032
Katsanevakis, S., Wallentinus, I., Zenetos, A., Leppakoski, E., Ozturk, M. B., Grabowski, M., et al. (2014b). Impacts of invasive alien marine species on ecosystem services and biodiversity: a pan-European review. Aquat. Invasions 9, 391–423. doi: 10.3391/ai.2014.9.4.01
Knight-Jones, P., Knight-Jones, W., and Ergen, Z. (1991). Sabelliform polychaetes, mostly from Turkey’s Aegean coast. J. Nat. Hist. 25, 837–858. doi: 10.1080/00222939100770561
Langeneck, J., Lezzi, M., Del Pasqua, M., Musco, L., Gambi, M. C., Castelli, A., et al. (2020). Non-indigenous polychaetes along the coasts of Italy: a critical review. Mediterr. Mar. Sci. 21, 238–275.
Latombe, G., Pyšek, P., Jeschke, J. M., Blackburn, T. M., Bacher, S., Capinha, C., et al. (2017). A vision for global monitoring of biological invasions. Biol. Conserv. 213, 295–308. doi: 10.1016/j.biocon.2016.06.013
Leclerc, C., Viard, F., Sepúlveda, E. G., Díaz, C., Hinojosa, J. N., Araneda, K. P., et al. (2019). Habitat type drives the distribution of non−indigenous species in fouling communities regardless of associated maritime traffic. Divers. Distrib. 26:75. doi: 10.1111/ddi.12997
Leclerc, J. C., and Viard, F. (2018). Habitat formation prevails over predation in influencing fouling communities. Ecol. Evol. 8, 477–492. doi: 10.1002/ece3.3654
Leclerc, J. C., Viard, F., González Sepúlveda, E., Díaz, C., Neira Hinojosa, J., Pérez Araneda, K., et al. (2018). Non-indigenous species contribute equally to biofouling communities in international vs local ports in the Biobío region, Chile. Biofouling 34, 784–799. doi: 10.1080/08927014.2018.1502276
Lehtiniemi, M., Ojaveer, H., David, M., Galil, B. S., Gollasch, S., McKenzie, C. H., et al. (2015). Dose of truth—monitoring marine non-indigenous species to serve legislative requirements. Mar. Policy 54, 26–35. doi: 10.1016/j.marpol.2014.12.015
Lezzi, M., Del Pasqua, M., Pierri, C., and Giangrande, A. (2017). Seasonal non-indigenous species succession in a marine macrofouling invertebrate community. Biol. Invasions 20, 937–961. doi: 10.1007/s10530-017-1601-3
Lezzi, M., and Giangrande, A. (2018). Seasonal and bathymetric effects on macrofouling invertebrates’ primary succession in a mediterraenan non-indigenous species hotspot area. Mediterr. Mar. Sci. 19, 568–584. doi: 10.12681/mms.14786
Lodola, A., Ferrario, J., and Occhipinti-Ambrogi, A. (2015). Further Mediterranean expansion of the non-indigenous bryozoan Celleporaria brunnea: multiple records along the Italian coasts. Sci. Mar. 79, 263–274.
Lodola, A., Savini, D., and Occhipinti-Ambrogi, A. (2012). First record of Tricellaria inopinata (Bryozoa: Candidae) in the harbours of La Spezia and Olbia, Western Mediterranean Sea (Italy). Mar. Biodivers. Rec. 5:e41. doi: 10.1017/S1755267212000309
Longo, C., Mastrototaro, F., and Corriero, G. (2007). Occurrence of Paraleucilla magna (Porifera: Calcarea) in the Mediterranean Sea. J. Mar. Biol. Assoc. 87, 1749–1755. doi: 10.1017/S0025315407057748
Longobardi, L., Bavestrello, G., Betti, F., and Cattaneo-Vietti, R. (2017). Long-term changes in a Ligurian infralittoral community (Mediterranean Sea): a warning signal? Reg. Stud. Mar. Sci. 14, 15–26. doi: 10.1016/j.rsma.2017.03.011
López, E., and Richter, A. (2017). Non-indigenous species (NIS) of polychaetes (Annelida: Polychaeta) from the Atlantic and Mediterranean coasts of the Iberian Peninsula: an annotated checklist. Helgol. Mar. Res. 71, 1–17. doi: 10.1186/s10152-017-0499-6
Lord, J. P., Calini, J. M., and Whitlatch, R. B. (2015). Influence of seawater temperature and shipping on the spread and establishment of marine fouling species. Mar. Biol. 162, 2481–2492. doi: 10.1007/s00227-015-2737-2
Marasinghe, M. M. K. I., Ranatunga, R. R. M. K. P., and Anil, A. C. (2018). Settlement of non-native Watersipora subtorquata (d’Orbigny, 1852) in artificial collectors deployed in Colombo Port. Sri Lanka. BioInvasions Rec. 7, 7–14.
Marchini, A., Ferrario, J., and Minchin, D. (2015). Marinas may act as hubs for the spread of the pseudo-indigenous bryozoan Amathia verticillata (Delle Chiaje, 1822) and its associates. Sci. Mar. 79, 355–365. doi: 10.3989/scimar.04238.03A
Marraffini, M. L., Ashton, G. V., Brown, C. W., Chang, A. L., and Ruiz, G. M. (2017). Settlement plates as monitoring devices for non-indigenous species in marine fouling communities. Manag. Biol. Invasions 8, 559–566. doi: 10.3391/mbi.2017.8.4.11
Minchin, D. (2012). Rapid assessment of the bryozoan, Zoobotryon verticillatum (Delle Chiaje, 1822) in marinas, Canary Islands. Mar. Pollut. Bull. 64, 2146–2150.
Minchin, D., Floerl, O., Savini, D., and Occhipinti-Ambrogi, A. (2006). “Small craft and the spread of exotic species,” in The Ecology of Transportation: Managing Mobility for the Environment, eds J. Davenport and J. L. Davenport (Netherlands: Springer), 99–118.
Miralles, L., Ibabe, A., González, M., García-Vázquez, E., and Borrell, Y. J. (2021). “If you know the enemy and know yourself”: addressing the problem of biological invasions in ports through a new NIS invasion threat score, routine monitoring, and preventive action plans. Front. Mar. Sci. 8:633118. doi: 10.3389/fmars.2021.633118
Nall, C., Guerin, A., and Cook, E. (2015). Rapid assessment of marine non-native species in northern Scotland and a synthesis of existing Scottish records. Aquat. Invasions 10, 107–121. doi: 10.3391/ai.2015.10.1.11
Neves, C. S., Rocha, R. M., Pitombo, F. B., and Roper, J. J. (2007). Use of artificial substrata by introduced and cryptogenic marine species in Paranagua Bay, southern Brazil. Biofouling 23, 319–330. doi: 10.1080/08927010701399174
Newcomer, K., Marraffini, M. L., and Chang, A. L. (2018). Distribution patterns of the introduced encrusting bryozoan Conopeum chesapeakensis (Osburn 1944; Banta et al., 1995) in an estuarine environment in upper San Francisco Bay. J. Exp. Mar. Biol. Ecol. 504, 20–31. doi: 10.1016/j.jembe.2018.04.001
Newcomer, K., Tracy, B. M., Chang, A. L., and Ruiz, G. M. (2019). Evaluating performance of photographs for marine citizen science applications. Front. Mar. Sci. 6:336. doi: 10.3389/fmars.2019.00336
Obst, M., Exter, K., Allcock, A. L., Arvanitidis, C., Axberg, A., Bustamante, M., et al. (2020). A marine biodiversity observation network for genetic monitoring of hard-bottom communities (ARMS-MBON). Front. Mar. Sci. 7:572680. doi: 10.3389/fmars.2020.572680
Occhipinti-Ambrogi, A. (2007). Global change and marine communities: alien species and climate change. Mar. Pollut. Bull. 55, 342–352. doi: 10.1016/j.marpolbul.2006.11.014
Occhipinti-Ambrogi, A. (2021). Biopollution by invasive marine non-indigenous species: a review of potential adverse ecological effects in a changing climate. Int. J. Environ. Res. Public Health 18:4268. doi: 10.3390/ijerph18084268
Ojaveer, H., Galil, B. S., Campbell, M. L., Carlton, J. T., Canning-Clode, J., Cook, E. J., et al. (2015). Classification of non-indigenous species based on their impacts: considerations for application in marine management. PLoS Biol. 13:e1002130. doi: 10.1371/journal.pbio.1002130
Ojaveer, H., Galil, B. S., Minchin, D., Olenin, S., Amorim, A., Canning-Clode, J., et al. (2014). Ten recommendations for advancing the assessment and management of non-indigenous species in marine ecosystems. Mar. Policy 44, 160–165. doi: 10.1016/j.marpol.2013.08.019
Olenin, S., Alemany, F., Cardoso, A. C., Gollasch, S., Goulletquer, P., Lehtiniemi, M., et al. (2010). Marine Strategy Framework Directive: Task Group 2 Report: Non-Indigenous Species, April 2010. Luxembourg: OPOCE.
Olenin, S., Elliott, M., Bysveen, I., Culverhouse, P. F., Daunys, D., Dubelaar, G. B., et al. (2011). Recommendations on methods for the detection and control of biological pollution in marine coastal waters. Mar. Pollut. Bull. 62, 2598–2604. doi: 10.1016/j.marpolbul.2011.08.011
Olenin, S., Minchin, D., and Daunys, D. (2007). Assessment of biopollution in aquatic ecosystems. Mar. Pollut. Bull. 55, 379–394.
Outinen, O., Forsström, T., Yli-Rosti, J., Vesakoski, O., and Lehtiniemi, M. (2019). Monitoring of sessile and mobile epifauna – considerations for non-indigenous species. Mar. Pollut. Bull. 141, 332–342. doi: 10.1016/j.marpolbul.2019.02.055
Padial, J. M., Miralles, A., De la Riva, I., and Vences, M. (2010). The integrative future of taxonomy. Front. Zool. 7:16. doi: 10.1186/1742-9994-7-16
Pearman, J. K., von Ammon, U., Laroche, O., Zaiko, A., Wood, S. A., Zubia, M., et al. (2021). Metabarcoding as a tool to enhance marine surveillance of nonindigenous species in tropical harbors: a case study in Tahiti. Environ. DNA 3, 173–189. doi: 10.1002/edn3.154
Piola, R. F., Dafforn, K. A., and Johnston, E. L. (2009). The influence of antifouling practices on marine invasions. Biofouling 25, 633–644. doi: 10.1080/08927010903063065
Piola, R. F., and Johnston, E. L. (2008). Pollution reduces native diversity and increases invader dominance in marine hard-substrate communities. Divers. Distrib. 14, 329–342. doi: 10.1111/j.1472-4642.2007.00430.x
QGIS Development Team. (2020). QGIS Geographic Information System. QGIS Association: QGIS Development Team.
R Core Team. (2017). R: A Language and Environment for Statistical Computing. R Foundation for Statistical Computing. Vienna: R Core Team.
Ramalhosa, P., Gestoso, I., Rocha, R. M., Lambert, G., and Canning-Clode, J. (2021). Ascidian biodiversity in the shallow waters of the Madeira Archipelago: fouling studies on artificial substrates and new records. Reg. Stud. Mar. Sci. 43:101672. doi: 10.1016/j.rsma.2021.101672
Reinhardt, J. F., Whitlatch, R. B., and Osman, R. W. (2013). Effects of temperature on the recruitment phenology and niche overlap of shallow epifaunal assemblages in southern New England. Mar. Ecol. Prog. Ser. 489, 61–74. doi: 10.3354/meps10423
Relini, G. (1977). Le metodologie per lo studio del fouling nell’indagine di alcuni ecosistemi marini. Boll. Zool. 44, 97–112. doi: 10.1080/11250007709430157
Reverter-Gil, O., and Souto, J. (2019). Watersiporidae (Bryozoa) in Iberian waters: an update on alien and native species. Mar. Biodiv. 49, 2735–2752.
Ros, M., Navarro-Barranco, C., González-Sánchez, M., Ostalé-Valriberas, E., Cervera-Currado, L., and Guerra-García, J. M. (2020). Starting the stowaway pathway: the role of dispersal behavior in the invasion success of low-mobile marine species. Biol. Invasions 22, 2797–2812. doi: 10.1007/s10530-020-02285-7
Ros, M., Vázquez-Luis, M., and Guerra-García, J. M. (2013). The role of marinas and recreational boating in the occurrence and distribution of exotic caprellids (Crustacea: Amphipoda) in the Western Mediterranean: Mallorca Island as a case study. J. Sea Res. 83, 94–103. doi: 10.1016/j.seares.2013.04.004
Rubinstein, N. D., Feldstein, T., Shenkar, N., Botero-Castro, F., Griggio, F., and Mastrototaro, F. (2013). Deep sequencing of mixed total DNA without barcodes allows efficient assembly of highly plastic ascidian mitochondrial genomes. Genome Biol. Evol. 5, 1185–1199. doi: 10.1093/gbe/evt081
Ruiz, G. M., Carlton, J. T., Grosholz, E. D., and HInes, A. H. (1997). Global invasions of marine and estuarine habitats by non-indigenous species: mechanisms, extent, and consequences. Am. Zool. 37, 621–632. doi: 10.1093/icb/37.6.621
Ruiz, G. M., Fofonoff, P. W., Carlton, J. T., Wonham, M. J., and Hines, A. H. (2000). Invasion of coastal marine communities in North America: apparent patterns, processes, and biases. Annu. Rev. Ecol. Evol. Syst. 31, 481–531. doi: 10.1146/annurev.ecolsys.31.1.481
Ruiz, G. M., Freestone, A. L., Fofonoff, P. W., and Simkanin, C. (2009). “Habitat distribution and heterogeneity in marine invasion dynamics: the importance of hard substrate and artificial structure,” in Marine Hard Bottom Communities. Ecological Studies, ed. M. Wahl (Berlin: Springer-Verlag), 321–332.
Ruiz, G. M., and Hewitt, C. L. (2002). “Toward understanding patterns of coastal marine invasions: a prospectus,” in Invasive aquatic species of Europe. Distribution, impacts and management, eds E. Leppäkoski, S. Gollasch, and S. Olenin (Dordrecht: Springer), 529–547.
Sheets, E. A., Cohen, C. S., Ruiz, G. M., and da Rocha, R. M. (2016). Investigating the widespread introduction of a tropical marine fouling species. Ecol. Evol. 6, 2453–2471. doi: 10.1002/ece3.2065
Simkanin, C., Fofonoff, P. W., Larson, K., Lambert, G., Dijkstra, J. A., and Ruiz, G. M. (2016). Spatial and temporal dynamics of ascidian invasions in the continental United States and Alaska. Mar. Biol. 163:163. doi: 10.1007/s00227-016-2924-9
Susick, K., Scianni, C., and Mackie, J. A. (2020). Artificial structure density predicts fouling community diversity on settlement panels. Biol. Invasions 22, 271–292. doi: 10.1007/s10530-019-02088-5
Tait, L., Inglis, G., and Seaward, K. (2018). Enhancing passive sampling tools for detecting marine bioinvasions. Mar. Pollut. Bull. 128, 41–50. doi: 10.1016/j.marpolbul.2018.01.015
Tempesti, J., Langeneck, J., Maltagliati, F., and Castelli, A. (2020). Macrobenthic fouling assemblages and NIS success in a Mediterranean port: the role of use destination. Mar. Pollut. Bull. 150:110768. doi: 10.1016/j.marpolbul.2019.110768
Torchin, M. E., Freestone, A. L., McCann, L., Larson, K., Schloder, C., Steves, B. P., et al. (2021). A symmetry of marine invasions across tropical oceans. Ecology e03434. Available online at: https://esajournals.onlinelibrary.wiley.com/doi/abs/10.1002/ecy.3434?casa _token=E0O-jLDrXKYAAAAA:Ki4CraFf38i2cRwSvG40ZOphgObF8flvtIqW4 vfiGX7CZYdS1Gm04dc7vozwO2hGrz9WiR1uzzmXiJ9n
Tracy, B., Larson, K. J., Ashton, G. V., Lambert, G., Chang, A. L., and Ruiz, G. M. (2017). Northward range expansion of three non-native ascidians on the west coast of North America. BioInvasions Rec. 6, 203–209. doi: 10.3391/bir.2017.6.3.04
Tsiamis, K., Azzurro, E., Bariche, M., Çinar, M. E., Crocetta, F., Clerck, O. D., et al. (2020). Prioritizing marine invasive alien species in the European Union through horizon scanning. Aquat. Conserv. 30, 794–845. doi: 10.1002/aqc.3267
Ulman, A., Ferrario, J., Forcada, A., Arvanitidis, C., Occhipinti-Ambrogi, A., and Marchini, A. (2019a). A hitchhiker’s guide to Mediterranean marina travel for alien species. J. Environ. Manag. 241, 328–339. doi: 10.1016/j.jenvman.2019.04.011
Ulman, A., Ferrario, J., Forcada, A., Seebens, H., Arvanitidis, C., Occhipinti−Ambrogi, A., et al. (2019b). Alien species spreading via biofouling on recreational vessels in the Mediterranean Sea. J. Appl. Ecol. 56, 2620–2629. doi: 10.1111/1365-2664.13502
Ulman, A., Ferrario, J., Occhipinti-Ambrogi, A., Arvanitidis, C., Bandi, A., Bertolino, M., et al. (2017). A massive update of non-indigenous species records in Mediterranean marinas. PeerJ 5:e3954. doi: 10.7717/peerj.3954
Verlaque, M., and Breton, G. (2019). Biological invasion: long term monitoring of the macroalgal flora of a major European harbor complex. Mar. Pollut. Bull. 143, 228–241. doi: 10.1016/j.marpolbul.2019.04.038
Viard, F., Roby, C., Turon, X., Bouchemousse, S., and Bishop, J. (2019). Cryptic diversity and database errors challenge non-indigenous species surveys: an illustration with Botrylloides spp. in the English Channel and Mediterranean sea. Front. Mar. Sci. 6:615. doi: 10.3389/fmars.2019.00615
Vicente, V. S., Ferreira, A. P., Peres, P. A., Siqueira, S. G., Leite, F. P., and Vieira, E. A. (2021). Succession of marine fouling community influences the associated mobile fauna via physical complexity increment. Mar. Freshw. Res. doi: 10.1071/MF21025 Available online at: https://www.publish.csiro.au/MF/MF21025
Vieira, E. A., Dias, G. M., and Flores, A. A. V. (2018). Adding early-stage engineering species affects advanced-stage organization of shallow-water fouling assemblages. Hydrobiologia 818, 211–222. doi: 10.1007/s10750-018-3612-1
Zabin, C. (2014). Small boats provide connectivity for nonindigenous marine species between a highly invaded international port and nearby coastal harbors. Manag. Biol. Invasions 5, 97–112. doi: 10.3391/mbi.2014.5.2.03
Keywords: macrozoobenthos, sessile fauna, settlement panels, marine bioinvasions, Ligurian Sea, fouling
Citation: Tamburini M, Keppel E, Marchini A, Repetto MF, Ruiz GM, Ferrario J and Occhipinti-Ambrogi A (2021) Monitoring Non-indigenous Species in Port Habitats: First Application of a Standardized North American Protocol in the Mediterranean Sea. Front. Mar. Sci. 8:700730. doi: 10.3389/fmars.2021.700730
Received: 26 April 2021; Accepted: 21 June 2021;
Published: 16 July 2021.
Edited by:
Periklis Kleitou, Marine and Environmental Research Lab (MER), CyprusReviewed by:
Thanos Dailianis, Hellenic Centre for Marine Research (HCMR), GreeceVasilis Gerovasileiou, Hellenic Centre for Marine Research (HCMR), Greece
Copyright © 2021 Tamburini, Keppel, Marchini, Repetto, Ruiz, Ferrario and Occhipinti-Ambrogi. This is an open-access article distributed under the terms of the Creative Commons Attribution License (CC BY). The use, distribution or reproduction in other forums is permitted, provided the original author(s) and the copyright owner(s) are credited and that the original publication in this journal is cited, in accordance with accepted academic practice. No use, distribution or reproduction is permitted which does not comply with these terms.
*Correspondence: Jasmine Ferrario, amFzbWluZS5mZXJyYXJpb0B1bmlwdi5pdA==
†These authors have contributed equally to this work and share senior authorship