- 1MARE – Marine and Environmental Sciences Centre, Faculdade de Ciências da Universidade de Lisboa, Lisbon, Portugal
- 2cE3c – Centre for Ecology, Evolution and Environmental Changes, Faculdade de Ciências da Universidade de Lisboa, Lisbon, Portugal
- 3Departamento de Biologia Vegetal, Faculdade de Ciências, Universidade de Lisboa, Lisbon, Portugal
- 4Biosystems and Applied Sciences Institute, Faculdade de Ciências da Universidade de Lisboa, Lisbon, Portugal
- 5Southern Seas Ecology Laboratories, School of Biological Sciences, The University of Adelaide, Adelaide, SA, Australia
- 6Departamento de Biologia Animal da Faculdade de Ciências da Universidade de Lisboa, Lisbon, Portugal
The invasion of natural communities by non-indigenous species represents one of the most serious threats to biodiversity. Understanding the ecophysiology of invasive species can provide insights into potential physiological handicaps relative to native species. By doing so, we can leverage the development of ecoengineering solutions for the removal of non-indigenous species, preferably using non-chemical methods. Spartina patens is a known invasive species of cordgrass aggressively proliferating in Mediterranean salt marshes, producing impenetrable monospecific stands. As its occurrence is delimited by the upper high tide water level, we hypothesized that S. patens is intolerant to waterlogging. Therefore, we developed a field experiment where strands of S. patens were kept waterlogged over the entire tidal cycle for 30 days. At the end of the experimental period, plants in the trial plots exhibited severe stress symptoms at different physiological levels compared with control plots (no intervention). At the photobiological level, intervened plants exhibited lower efficiency in producing chemical energy from light, whilst at the biochemical level waterlogging impaired the antioxidant system and increased lipid peroxidation products. Furthermore, the application of chlorophyll a pulse amplitude modulated (PAM) fluorometry, a non-invasive technique, allowed us to evaluate the effectiveness of the implemented measures, being the tool that provided the best separation between the control and intervened population. Considering the physiological traits observed here, ecoengineering solutions based on increased waterlogging of S. patens stands, can be a low-cost and efficient measure to reduce the spreading and growth of this invasive species in the Mediterranean and other salt marshes worldwide with little disturbance.
Introduction
Salt marshes provide a wide range of ecological services, including nursery habitats for many animals, protection against coastal erosion, water purification, having the considerable capacity to store and sequester carbon, and are key players in the ecosystem natural remediation capacity (Couto et al., 2013; Teixeira et al., 2014; Duarte et al., 2018, 2021), being these services valuated in several millions of euros per year (Duarte et al., 2021). Being preferred locations for human settlement and a profusion of anthropogenic activities, coastal, and transitional areas have been severely impacted in their health and functioning worldwide. Habitat loss and degradation, climate change and the introduction of invasive species are amongst the major threats to salt marsh ecosystems (Duarte et al., 2015, 2018; Repolho et al., 2017; Pérez-Romero et al., 2018). Therefore, in the context of increasing degradation rates, the need to restore salt marsh ecosystems has been recognized as a priority by managers, scientists, and general society. This is well-emphasized in the EU Biodiversity Strategy for 2030 and its EU Nature Restoration Plan (EU, 2020) and reinforced by the UN Decade on Ecosystem Restoration (UN, 2020).
Salt marshes have been widely affected by non-indigenous species (NIS) being a serious threat to wetland biodiversity (Heywood, 1989). Although many NIS plants were introduced long ago (more than a century), recent arrivals are of much concern (Aguiar and Ferreira, 2013; Ainouche and Gray, 2016; Martínez-Jauregui et al., 2018). The Spartina genus is highly successful amongst the halophyte plant group, being widespread across the globe. These plants have C4-type photosynthesis, in which a CO2 concentration mechanism at ribulose-1,5-bisphosphate carboxylase-oxygenase (Rubisco) level, involving the fixation of atmospheric CO2 by phosphoenol-pyruvate carboxylase (PEPC), increases Rubisco carboxylase activity and allows a faster growth rate (von Caemmerer, 2020). Spartina patens (Ait.) Muhl. (Gramineae) is a perennial grass distributed along a wide range of coastal habitats, aggressively competing with native species (Duarte et al., 2015). It was introduced in the Mediterranean Sea probably due to ship traffic from America, being used as packing material in ships boxes and crates (Hultén, 1958). Therefore, although being present for some time along the Western Mediterranean coasts, it had not been recorded on the Eastern Iberian coast until recently (Baumel et al., 2016).
Controlling invasive species is relevant for restoration efforts, and several approaches have been developed to address this issue, ranging from herbicide application to mowing and physical removal (Kerr et al., 2016). While the first appears as a simple and cost-effective method (Major et al., 2003; Patten et al., 2017), the long-term effects of herbicides may have serious impacts on the ecosystem (Cruz de Carvalho et al., 2020b). As for the other two methods, they will only prevent further spread needing to be constantly applied and being very time- and cost-consuming (Hedge et al., 2003). Therefore, we considered an alternative method underpinned on the physiology of the species, in particular the fact that S. patens has a low tolerance to waterlogging, due to poor aeration of the rhizosphere which consequently impairs its growth (Burdick, 1989; Bertness, 1991; Curado et al., 2020), and naturally limits this species distribution to the high tide border of salt marshes. Thus, in the present work, we tested the application of a physical barrier that would prolong waterlogging around the plants and evaluated the impact on its physiology.
Materials and Methods
Plant Material and Collection Site
Sampling occurred before the start of the growing season (February 2020) in the Hortas salt marsh (Alcochete; 38° 45.661’ N, 8° 56.116’ W), located in the middle estuary, adjacent to the Tagus Estuary Natural Reserve (Figure 1). This salt marsh is flooded twice per day, being dominated by the halophyte species Spartina maritima in the lower marsh (circa 12% coverage), Halimione portulacoides in the mid-upper marsh (circa 35% coverage), and Sarcocornia fruticosa in the upper marsh (circa 20% coverage) (Caçador et al., 2013). More recently, the halophyte invasive species S. patens has managed to establish itself in the margins, justifying the importance of the current study.
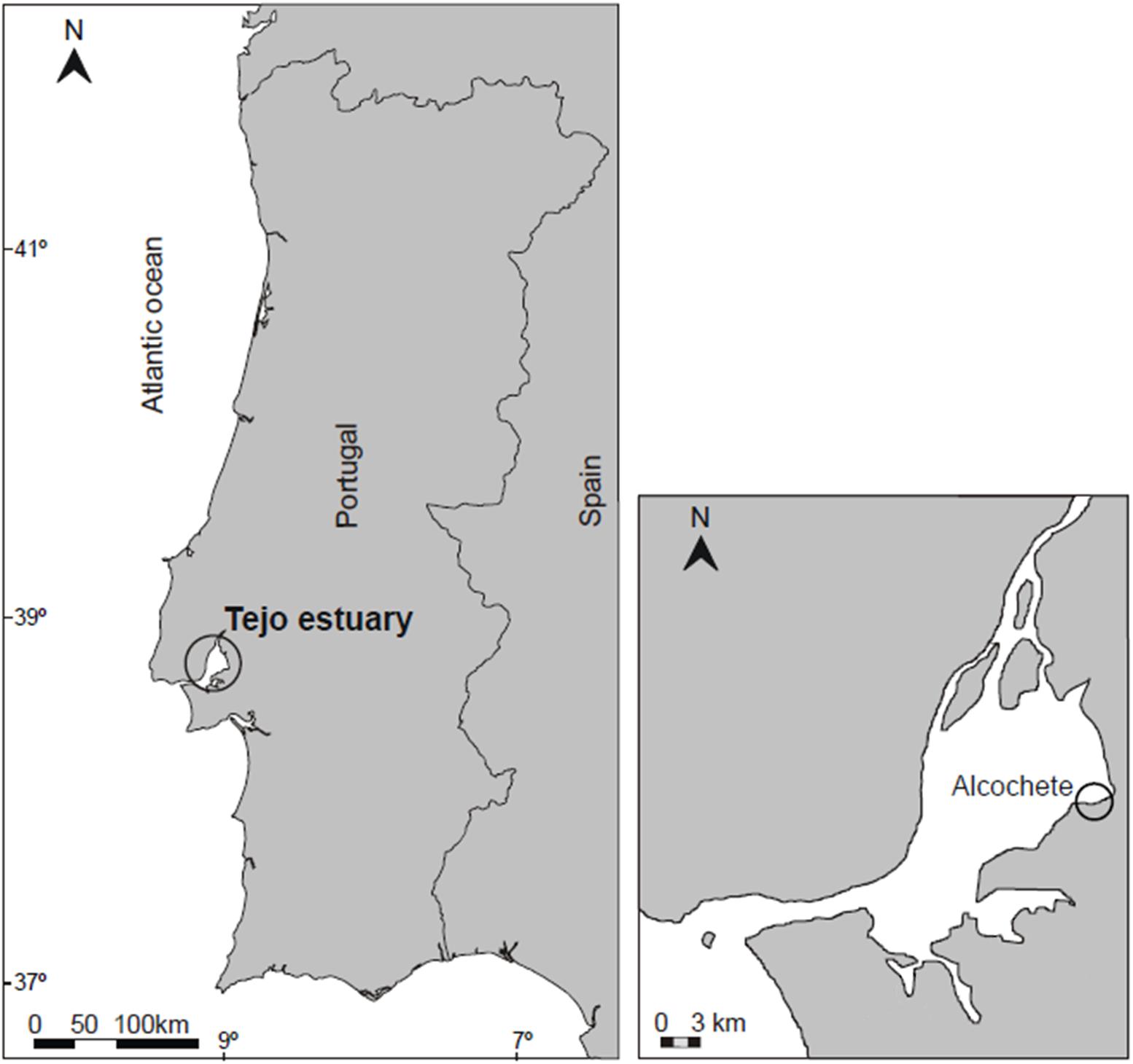
Figure 1. Map of the location of the sampling site in the Hortas salt marsh (Alcochete) in the Tagus estuary.
Applied Treatments
Spartina patens plants were subjected to two treatments: (1) control, where the plants did not undergo any intervention, and (2) the waterlogging treatment (waterlogged) where plant turfs filled the inside of a 50 cm long and 10 cm diameter PVC tube buried down into the sediments, leaving an 8 cm margin outside, which allowed the tidal water to enter the tube and remain longer inside and, thus, increase the waterlogging of the plants (n = 5 for each treatment). The experiment lasted for 30 days. Several field measurements were made, namely, in vivo chlorophyll a pulse amplitude modulated (PAM) fluorometry measurements. Moreover, 20 leaves from each treatment were also collected directly into individual tubes with liquid nitrogen (see sections below). Finally, whole plants of S. patens from both treatments were also collected, transported to the lab, washed, removed from excess water, weighted (fresh weight), and oven-dried at 60°C to constant weight (dry weight).
Chlorophyll a Pulse Amplitude Modulated Fluorometry
Ten leaves from plants from each treatment were dark-adapted for 15 min and PAM measurements were performed using a FluoroPen FP100 (Photo System Instruments, Czechia). For the analysis of chlorophyll transient light curves (Kautsky plot), and the derived fluorometric parameters (Table 1), the JIP-test was used (Duarte et al., 2017).
Leaf Infrared Thermography
Thermal images were obtained with a FLIR E50bx infrared camera (FLIR Systems, Inc., Wilsonville, OR, United States) producing images of 320 × 240 resolution with an accuracy of ±0.045°C. Ten leaves were randomly selected from plants of both treatments, having a water bottle at ambient temperature near the leaves as reference. The average temperature of each leaf was calculated on each image. All image processing and analysis were performed in FLIR Tools software (version 6.4.18039.1003, FLIR Systems, Inc.).
Root Respiration
Fresh fine roots of each treatment weighing approximately 100 mg were transferred to 10-mL reaction tubes, and 6 mL of TTC-solution [0.6% (w/v) triphenyl-tetrazolium chloride in 0.06 M Na2HPO4–KH2PO4 and 0.05% (v/v) Tween 20] was added to each tube (n = 5) (Brunner et al., 2002). In duplicate tubes, 0.15 mM KCN was added to determine the inhibited respiration. The samples were then incubated for 24 h at 25°C. After incubation, the TTC solution was decanted and triphenyl formazan (TPF) extraction was made by adding 2 mL of ethanol and boiling at 80°C for 15 min (Ruf and Brunner, 2003). After collecting the supernatant in new tubes, the absorbance of 1 mL was measured at 520 nm with a spectrophotometer (UV500 UV-Visible Spectrometer, Unicam, Waltham, MA, United States). The root residues in the test tubes were dried at 80°C for 72 h and weighed. Reduction of TTC was calculated as μg of TPF produced per hour per g dry weight (DW).
Proline Quantification
Proline content was determined according to Bates et al. (1973). For each treatment, plant leaves (n = 5) were homogenized in 3% aqueous sulfosalicylic acid and the homogenate centrifuged a 9,000 g for 15 min at 0°C (Sigma 2-16K, SIGMA Laborzentrifugen GmbH, Osterode am Harz, Germany). The supernatant was collected, and the reaction consisted of 2 mL of extract combined with 2 mL of glacial acetic acid and 2 mL of acid ninhydrin. The reaction occurred for 1 h at 100°C, after which the reaction was stopped in an ice bath. The reaction mixture was extracted with 4 mL of toluene and its absorbance read at 520 nm with a spectrophotometer (UV500 UV-Visible Spectrometer, Unicam, Waltham, MA, United States) and compared with a standard curve of proline, expressed in μmol g–1 fresh weight (FW).
C4-Photosynthetic Carboxylating Enzymes Activity and Pigment Analysis
Carboxylating enzymes, PEPC and Rubisco, were extracted from frozen leaf samples according to Carmo-Silva et al. (2008), except that 50 mM HEPES-KOH pH 7.3 was used and 0.5% (v/v) Triton X-100 added. Briefly, approximately 50 mg FW were extracted in a cold mortar containing quartz sand, 1% (w/v) insoluble polyvinylpyrrolidone (PVP) and 1 mL of ice-cold extraction medium [50 mM HEPES-KOH pH 7.3, 1 mM EDTA, 5% (w/v) PVP25000, 6% (w/v) polyethylene glycol (PEG4000), 10 mM dithiothreitol (DTT), 1% (v/v) protease cocktail inhibitor (Sigma, St Louis, MO, United States) and 0.5% (v/v) Triton X-100]. After taking aliquots for pigment analysis, the homogenate was centrifuged for 3 min at 16,800 g at 4°C (Sigma 2-16K, SIGMA Laborzentrifugen GmbH, Osterode am Harz, Germany) and the supernatant (crude extract) was kept at 4°C and immediately used for measuring the activities of Rubisco (EC 4.1.1.39) and PEPC (EC 4.1.1.31).
The activities of Rubisco were assayed at 25°C by 14CO2 incorporation into acid-stable products according to Parry et al. (1997) to with modifications (Correia et al., 2020). The assay medium (1 mL per sample) contained 50 mM Bicine-KOH pH 8.2, 40 mM MgCl2, 10 mM NaH14CO3 (7.4 kBq μM–1) and 0.4 mM ribulose-1,5-bisphosphate (RuBP). To measure Rubisco initial activity (Vi), 25 μL of crude extract was added to assay medium, and the reaction stopped after 1 min with the addition of 100 μL of 1 M HCl. To determine Rubisco total activity (Vt), 25 μL of crude extract was added to assay medium without RuBP for 3 min, to allow the carbamylation of enzyme catalytic sites. Rubisco Vt reaction was started by adding RuBP and stopped after 1 min with the addition of 100 μL of 1 M HCl. The mixture was completely dried at 60°C after which the residue was resuspended in 0.5 mL of distilled water and mixed with 5 mL of scintillation liquid (BioSafe LS Cocktail, Beckman, United States). Radioactivity of the 14C incorporated in the acid-stable products was measured by scintillation counting (LS 7800 spectrophotometer, Beckmann Instruments Inc., Fullerton, CA, United States). Rubisco activation state (%) was determined by the Vi/Vt ratio.
PEPC physiological (Vphysiol) and maximum (Vmax) activities were measured in a continuous assay at 340 nm and 25°C (UV500 UV-Visible spectrophotometer, Unicam, Cambridge, United Kingdom) according to Bakrim et al. (1992) with some modifications (Carmo-Silva et al., 2007). The reaction mixture for Vphysiol (1 mL) consisted of 50 mM HEPES-KOH pH 7.2, 10 mM MgCl2, 10 mM NaHCO3, 2.5 mM PEP (Sigma), 12 units of MDH (Sigma) and 20 μL of crude extract. For Vmax, the reaction mixture consisted of 50 mM HEPES-KOH pH 8.0, 10 mM MgCl2, 10 mM NaHCO3, 10 mM PEP (Sigma), 12 units of MDH (Sigma) and 20 μL of extract. In both cases, the reaction was started by the addition of 0.2 mM (final concentration) NADH (Sigma). Each measured activity is the mean of three replicate on the same extract. PEPC activation state (%) was calculated as Vphysiol/Vmax ratio.
For pigment analysis, each 20 μL aliquot previously retrieved from the leaf extract was diluted in 980 μL of methanol. After mixing in the vortex, the samples were left in the dark at 4°C overnight. After centrifuging for 1 min at 13,000 g at 4°C (Sigma 2-16K, SIGMA Laborzentrifugen GmbH, Osterode am Harz, Germany), the absorbance at 470, 652.4, 665.2, and 700 nm were measured in an EpochTM 2 Microplate Spectrophotometer (BioTek, Winooski, VT, United States). Pigment concentrations were determined according to the equations in Lichtenthaler and Buschmann (2001).
Antioxidant Enzyme Assays
To extract the soluble protein fraction, leaf samples were grinded in a cooled mortar with 0.5 mL of 50 mM sodium/potassium phosphate extraction buffer (with 0.1 mM Na-EDTA, 2 mM PVP, 10 mM DTT, 0.1 mM PMSF, and 24 μM NADP, pH 7.6). The homogenate was centrifuged at 13,000 g for 10 min at 4°C (Sigma 2-16K, SIGMA Laborzentrifugen GmbH, Osterode am Harz, Germany) and the supernatant was collected to a new tube. Protein concentration was determined according to Bradford (1976) in an EpochTM 2 Microplate Spectrophotometer (BioTek, Winooski, VT, United States).
Catalase (CAT; EC 1.11.1.6) activity was measured according to Teranishi et al. (1974), through H2O2 consumption monitoring and the decrease in absorbance at 240 nm (ε = 39.4 mM–1 cm–1). The reaction mixture contained 50 mM of sodium/potassium phosphate buffer (pH 7.0), and 30 mM of H2O2 with the reaction being started by the addition of 5 μL of extract. Ascorbate peroxidase (APx; EC 1.11.1.11) was assayed according to Tiryakioglu et al. (2006). The reaction mixture contained 50 mM of sodium/potassium phosphate buffer (pH 7.0), 0.1 mM of H2O2, and 0.25 mM L-ascorbate, and the reaction was also initiated with the addition of 5 μL of the extract. The activity was recorded as the decrease in absorbance at 290 nm and the amount of ascorbate oxidized calculated from the molar extinction coefficient (ε = 2.8 mM–1 cm–1). Guaiacol peroxidase (GOPx; EC 1.11.1.7) activity was assayed according to Mika and Lüthje (2003) through the monitorization of guaiacol oxidation at 470 nm (ε = 26.6 mM–1 cm–1). The reaction mixture contained 50 mM of sodium/potassium phosphate buffer (pH 7.0), 10 mM of H2O2, and 8 mM guaiacol, and the reaction was initiated with the addition of 5 μL of the extract. Superoxide dismutase (SOD; EC 1.15.1.1) activity was assayed according to Marklund and Marklund (1974) by measuring the reduction of pyrogallol at 325 nm. The reaction mixture contained 30 mM of sodium/potassium phosphate buffer (pH 7.0) and 0.24 mM of pyrogallol, with the reaction being started by the addition of 5 μL of extract. Glutathione reductase (GR; EC 1.8.1.7) activity was assayed according to Edwards et al. (1990) by measuring the fall in absorbance at 340 nm as NADPH was oxidized (ε = 6.22 mM–1 cm–1). The reaction mixture contained 25 mM of sodium/potassium phosphate extraction buffer (pH 7.6), 0.5 mM of oxidized glutathione and 0.2 mM NADPH, starting the reaction by the addition of 5 μL of extract. Control assays were done in the absence of substrate to evaluate the autoxidation of the substrates. All assays were performed in a total volume of 200 μL per well at 25°C in an EpochTM 2 Microplate Spectrophotometer (BioTek, Winooski, VT, United States).
Lipid Peroxidation Analysis
Lipid peroxidation products were determined as previously described (Heath and Packer, 1968). Leaves were homogenized briefly in 1.5 mL of 10% (v/v) trichloroacetic acid (TCA), containing 0.4% (w/v) thiobarbituric acid (TBA). The reaction was conducted at 100°C for 30 min, being halted through placement in ice. After centrifugation at 15,000 g for 10 min at 4°C (Sigma 2-16K, SIGMA Laborzentrifugen GmbH, Osterode am Harz, Germany), 1 mL of the supernatant was collected and mixed with 1 mL of 0.4% TBA and incubated again under the same conditions. The absorbance of the supernatant was recorded at 532 and 600 nm by spectrophotometry (UV500 UV-Visible Spectrometer, Unicam, Waltham, MA, United States). The concentration of malondialdehyde (MDA) was determined using the molar extinction coefficient (ε = 155 mM–1 cm–1).
Fatty Acid Profiles
The analysis of fatty acid was performed by direct trans-esterification of leaf samples, in freshly prepared methanol sulphuric acid (97.5:2.5, v/v), at 70°C for 60 min, using the internal standard pentadecanoic acid (C15:0) (Feijão et al., 2018). Fatty acid methyl esters (FAME) were recovered using petroleum ether, dried with an N2 flow, and re-suspended in an adequate amount of hexane. The FAME solution was analyzed through gas chromatography (Varian 430-GC gas chromatograph equipped with a hydrogen flame ionization detector set at 300°C, Middelburg, Netherlands), by addition of 1 μL, setting the injector temperature to 270°C, with a split ratio of 50. The fused-silica capillary column (50 m × 0.25 mm; WCOT Fused Silica, CP-Sil 88 for FAME; Varian, Middelburg, Netherlands) was maintained at a constant nitrogen flow of 2.0 mL min–1 and the oven set to 190°C. Fatty acids identification was performed by comparison of retention times with standards (Sigma-Aldrich) and chromatograms were analyzed by the peak surface method, using the Galaxy software. The double bond index (DBI) was calculated, to determine the membrane saturation levels, as previously described (Feijão et al., 2018):
Statistical Analysis
Since the data lacked normality and homogeneity, the statistical analysis was based on Mann-Whitney non-parametric tests (GraphPad Prism 8.4.2 for Windows, GraphPad Software, San Diego, CA, United States). Multivariate statistical analyses [SIMPER and Canonical Analysis of Principal Coordinates (CAP)] were performed using Primer 6 software (Clarke and Gorley, 2006). The data obtained from the Kautsky plots, thermography data, oxidative stress and fatty acids were used as the basis for the construction of the respective resemblance matrixes based on the Euclidean distances between samples. To evaluate the different metabolic datasets obtained as a whole (in opposition to univariate analysis), statistical multivariate models based on Kautsky plots, thermography data, oxidative stress and fatty acids were generated using Principal Coordinates Analysis (PCO) (Clarke and Gorley, 2006).
Results
Chlorophyll a PAM Analysis
Observing the Kautsky plots resultant from the in vivo PAM fluorometric analysis, lower fluorescence values could be observed in plants subjected to the treatment when compared with control plants (Figure 2).
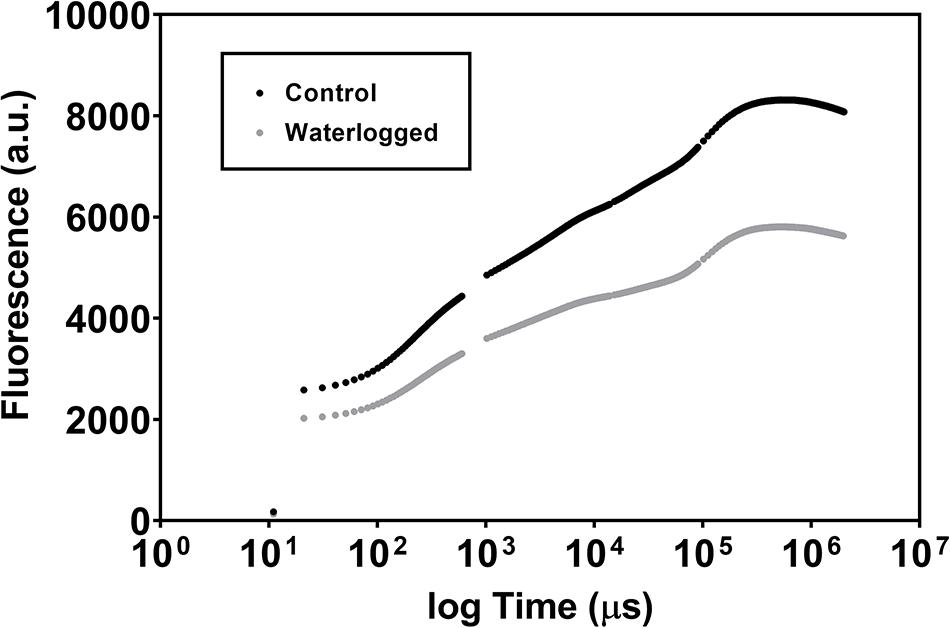
Figure 2. Chlorophyll transient kinetics (Kautsky plots) in leaves of control plants (dark gray) and waterlogged plants (light gray) of Spartina patens after 30 days (mean ± s.d., n = 10).
The four energy fluxes [Figure 3: A, energy absorbed by the photosystem II (PS II) antennae (ABS/CS); B, energy trapped inside the PS II (TR0/CS); C, energy transported within the electron transport chain (ETC) (ET0/CS); and D, the energy dissipation flux (DI0/CS)] showed the same pattern presenting lower values in the waterlogged plants, although that decrease was only statistically significant for TR0/CS and ET0/CS. It was also a similar reduction in the number of oxidized PS II reaction centers (RC/CS) (Figure 3E).
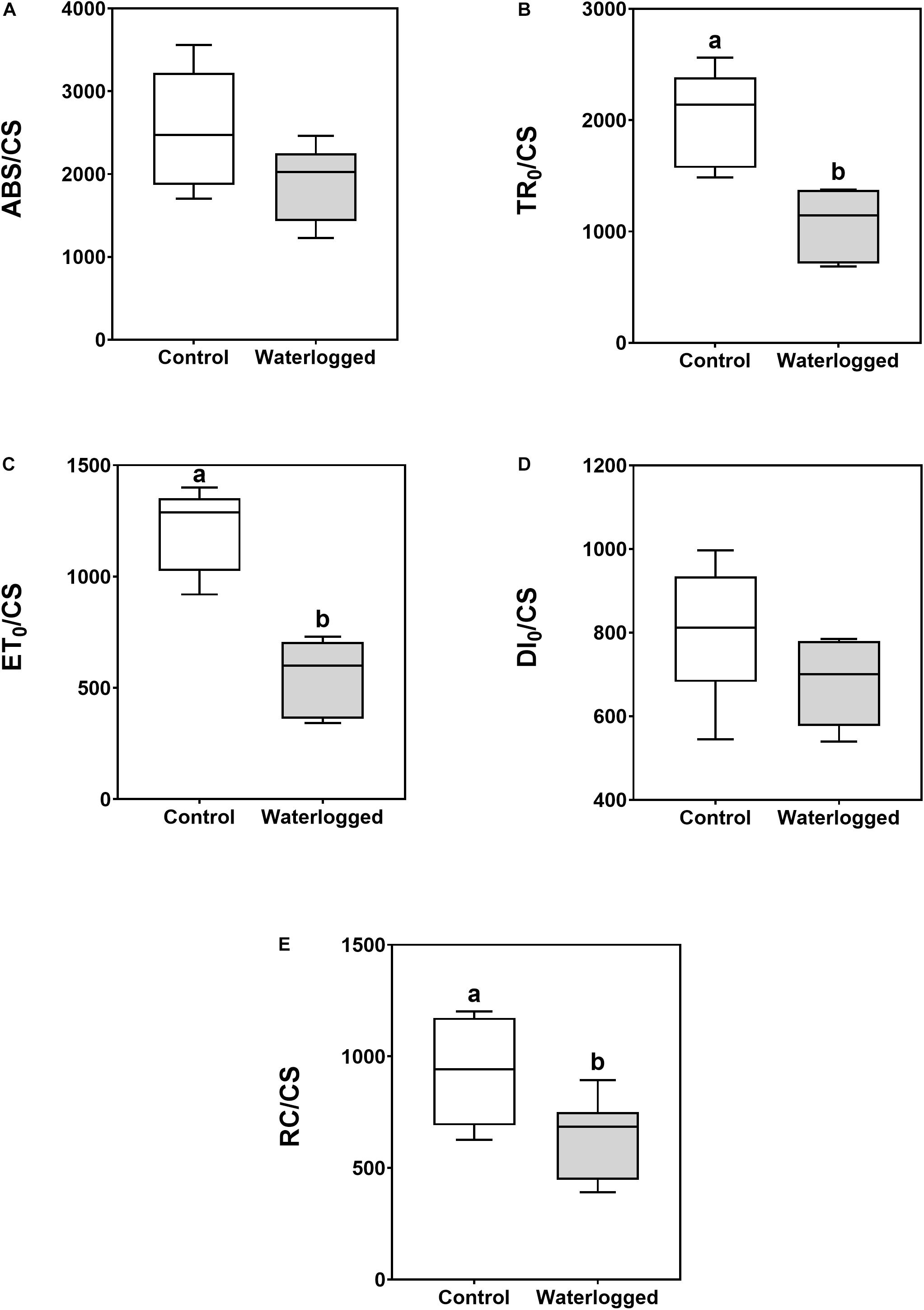
Figure 3. Boxplots of leaf energy fluxes [A, absorbed (ABS/CS); B, trapped (TR0/CS); C, transported (ET0/CS); D, dissipated (DI0/CS)] and (E) the number of available reaction centers per cross-section (RC/CS) in leaves of control plants (white boxes) and waterlogged plants (gray boxes) of Spartina patens after 30 days (n = 10, different letters indicate significant differences at p < 0.05).
Further analysis of the functioning of different components of the photosystems and ETC (Figure 4) showed a decrease in the oxidized quinone pool size in the waterlogged plants, followed by an enhancement in the number of QA redox turnovers until maximum fluorescence was reached (N). Although no significant changes were observed in the energy needed to close all RCs (SM), there was a decrease in the probability of a PS II chlorophyll molecule functioning as a RC (γRC) in the treated plants. However, no significant differences were observed in the QA reduction rate (M0).
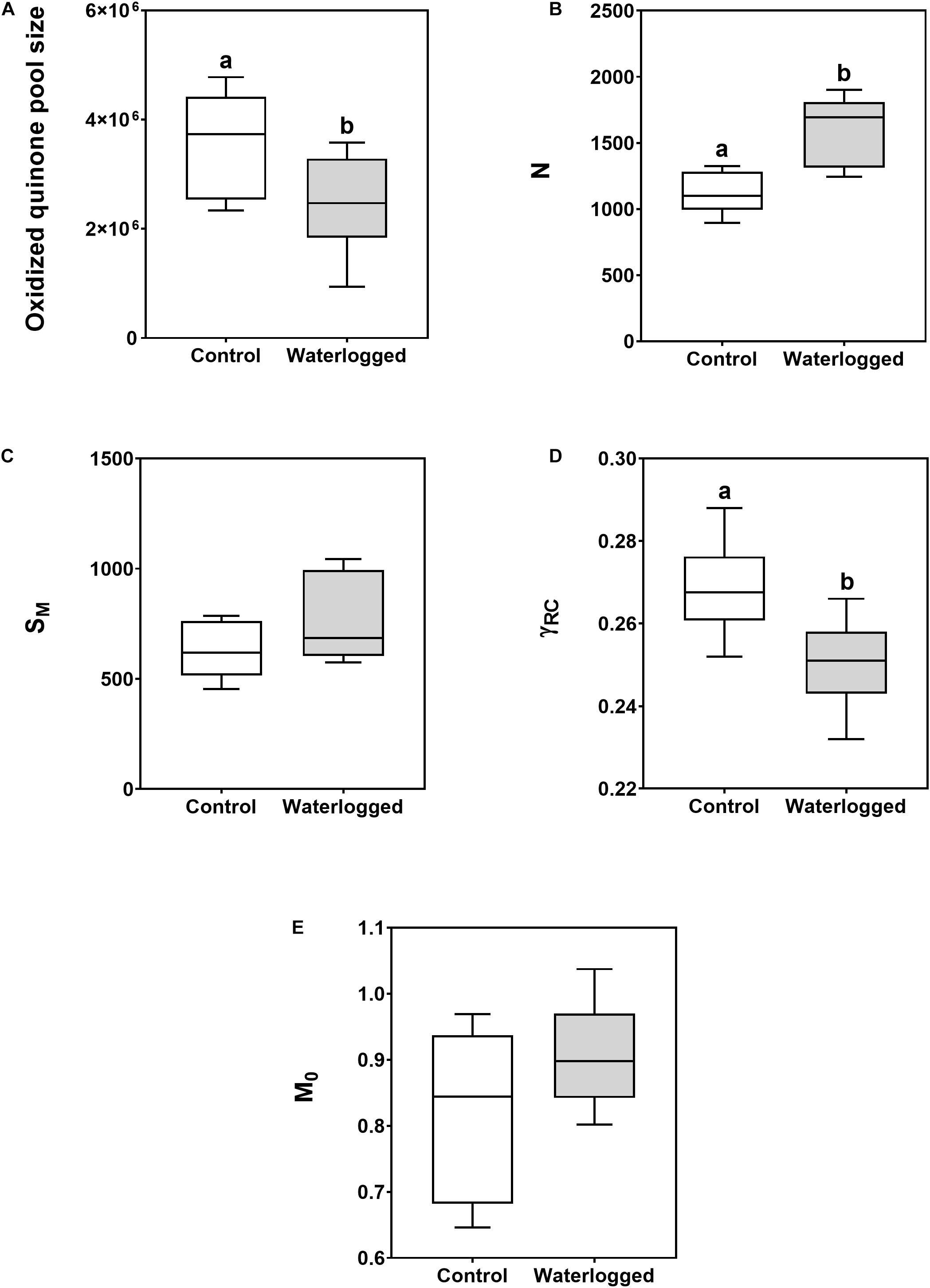
Figure 4. Boxplots of the photosystem II and ETC related photochemical traits [A, oxidized quinone pool; B, reaction center turnover rate (N); C, the energy needed to close all reaction centers (SM); D, the probability that a PSII chlorophyll molecule function as a RC (γRC); E, net rate of PS II RC closure (M0)], in leaves of control plants (white boxes) and waterlogged plants (gray boxes) of Spartina patens after 30 days (n = 10, different letters indicate significant differences at p < 0.05).
Although the active oxygen-evolving complexes (OEC) showed no differences between control and waterlogged plants (Figure 5A), the PG, the grouping probability that correlates with the disconnection between the two PS II units, increased in the later plant group (Figure 5B).
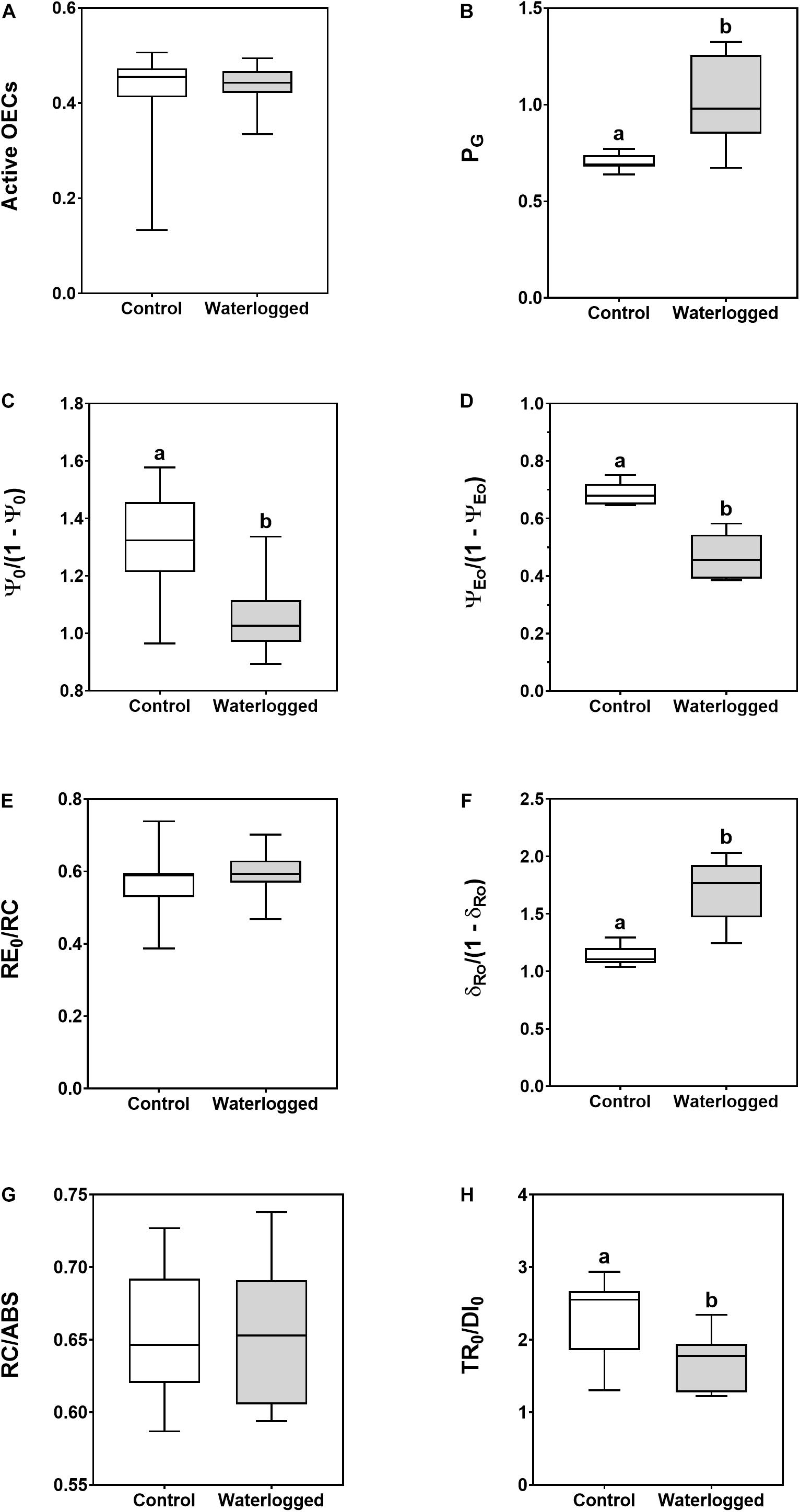
Figure 5. Boxplots of the photosystems I (PS I) and II (PS II) photochemical traits. (A) Active oxygen-evolving complexes (OECs); (B) grouping probability between the two PS II units (PG); (C) the contribution of the dark reactions from quinone A to plastoquinone [ψ0/(1 - ψ0)]; (D) the equilibrium constant for the redox reactions between PS II and PS I [ψE0/(1 - ψE0)]; (E) electron transport from PQH2 to the reduction of PS I end electron acceptors (RE0/RC); (F) the contribution of PS I reducing its end acceptors [δR0/(1- δR0)]; (G) reaction center II density within the antenna chlorophyll bed of PS II (RC/ABS); (H) contribution or partial performance due to the light reactions for primary photochemistry (TR0/DI0), in leaves of control plants (white boxes) and waterlogged plants (gray boxes) of Spartina patens after 30 days (n = 10, different letters indicate significant differences at p < 0.05).
Regarding PS II and PS I, waterlogged plants presented a significant decrease in photochemical processes, both in the contribution of light (TR0/DI0; Figure 5H) and dark [ψ0/(1 - ψ0); Figure 5C] reactions of the photochemical cycle. On the other hand, at the PS I level there was a significant increase in the activity of this photosystem [δR0/(1 - δR0); Figure 5F] in the intervened plants, although there was a decrease in the equilibrium constant for the redox reaction between both photosystems toward the PS II [ψE0/(1 - ψE0); Figure 5D]. Nevertheless, there were no significant changes in the reaction center density within the PS II antenna chlorophyll bed (RC/ABS; Figure 5G) or in the electron transport from PQH2 to the reduction of the PS I end acceptors (RE0/RC; Figure 5E).
In summary and observing the structure functional indexes, there was a decrease of the photochemical processes (Figure 6A) and an increase of the non-photochemical or dissipative processes (Figure 6B) in the waterlogged plants.
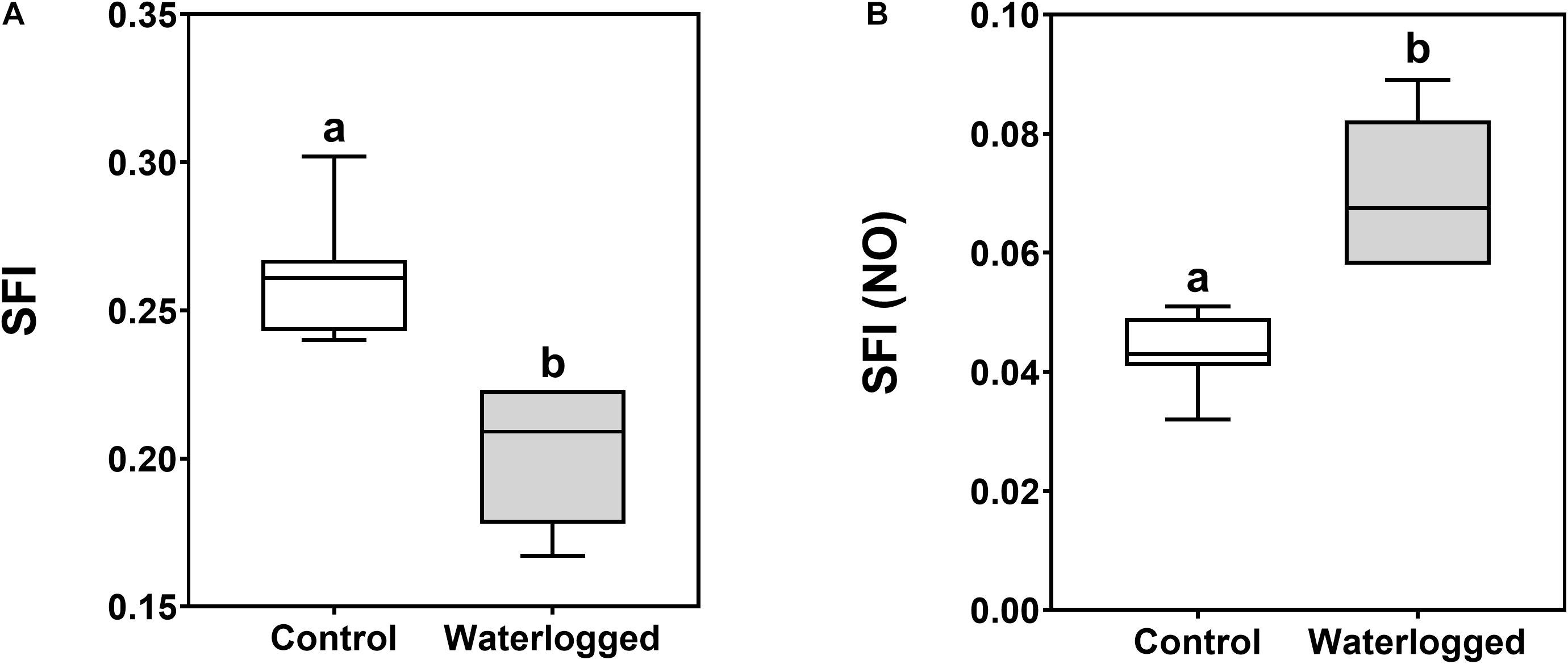
Figure 6. Boxplots of the structure functional indexes for photosynthesis (A, SFI) and non-photosynthetic or dissipation processes [B, SFI (NO)] in leaves of control plants (white boxes) and waterlogged plants (gray boxes) of Spartina patens after 30 days (n = 10, different letters indicate significant differences at p < 0.05).
Leaf Thermography
Regarding leaf surface temperature measured through infrared thermography, there was a statistically significant increase in temperature in the treated plants (11.06°C) relatively to control ones (10.77°C) (Figure 7).
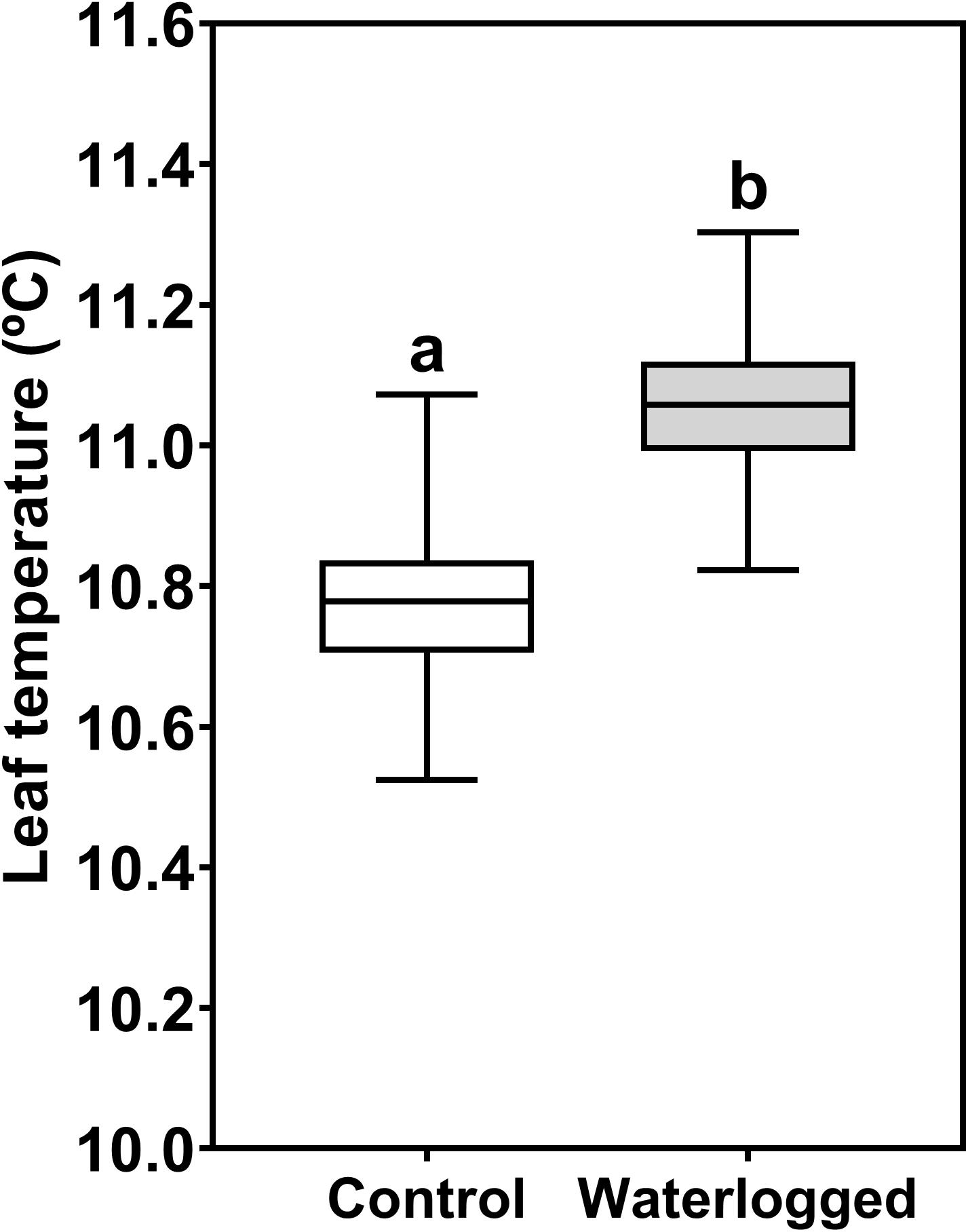
Figure 7. Boxplots of the leaf surface temperature measured through infrared thermography in leaves of control plants (white box) and waterlogged plants (gray box) of Spartina patens after 30 days (n = 10, different letters indicate significant differences at p < 0.05).
Root Respiration
An increase of root respiration in waterlogged plants was measured (Figure 8A), resulting not from changes in the alternative oxidase (AOX) activity (Figure 8B) but rather from a twofold increase in KCN-sensitive respiration (Figure 8C).
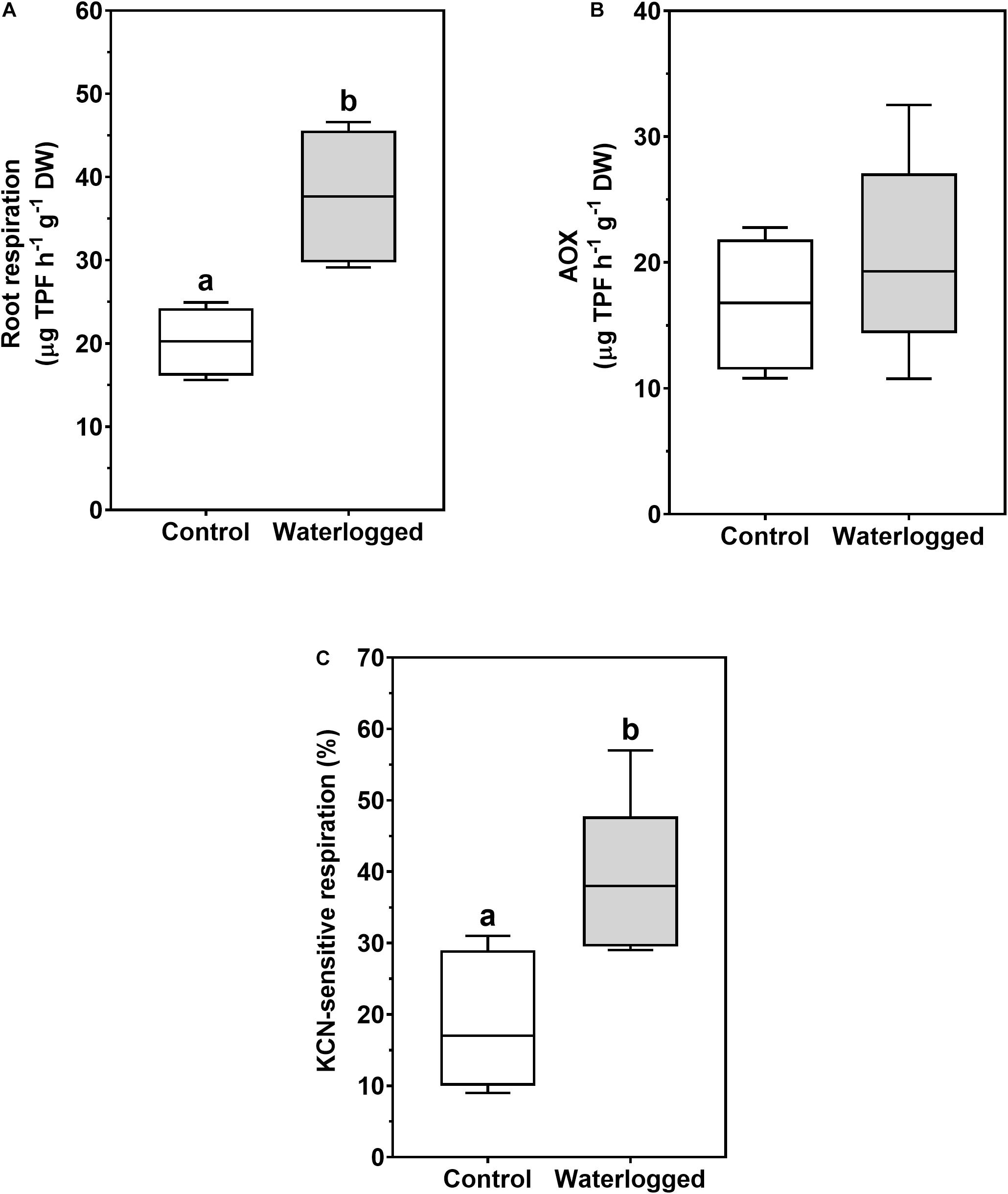
Figure 8. Boxplots of root respiration (A), alternative oxidase (AOX) activity (B), and percentage of KCN-sensitive respiration (C) in roots of control plants (white boxes) and waterlogged plants (gray boxes) of Spartina patens after 30 days (n = 5, different letters indicate significant differences at p < 0.05).
Proline
Leaves of waterlogged plants of S. patens experienced a fivefold increase in proline (Figure 9), to circa 20 μmol g–1 FW, contrasting with the leaves of control plants with circa 4 μmol g–1.
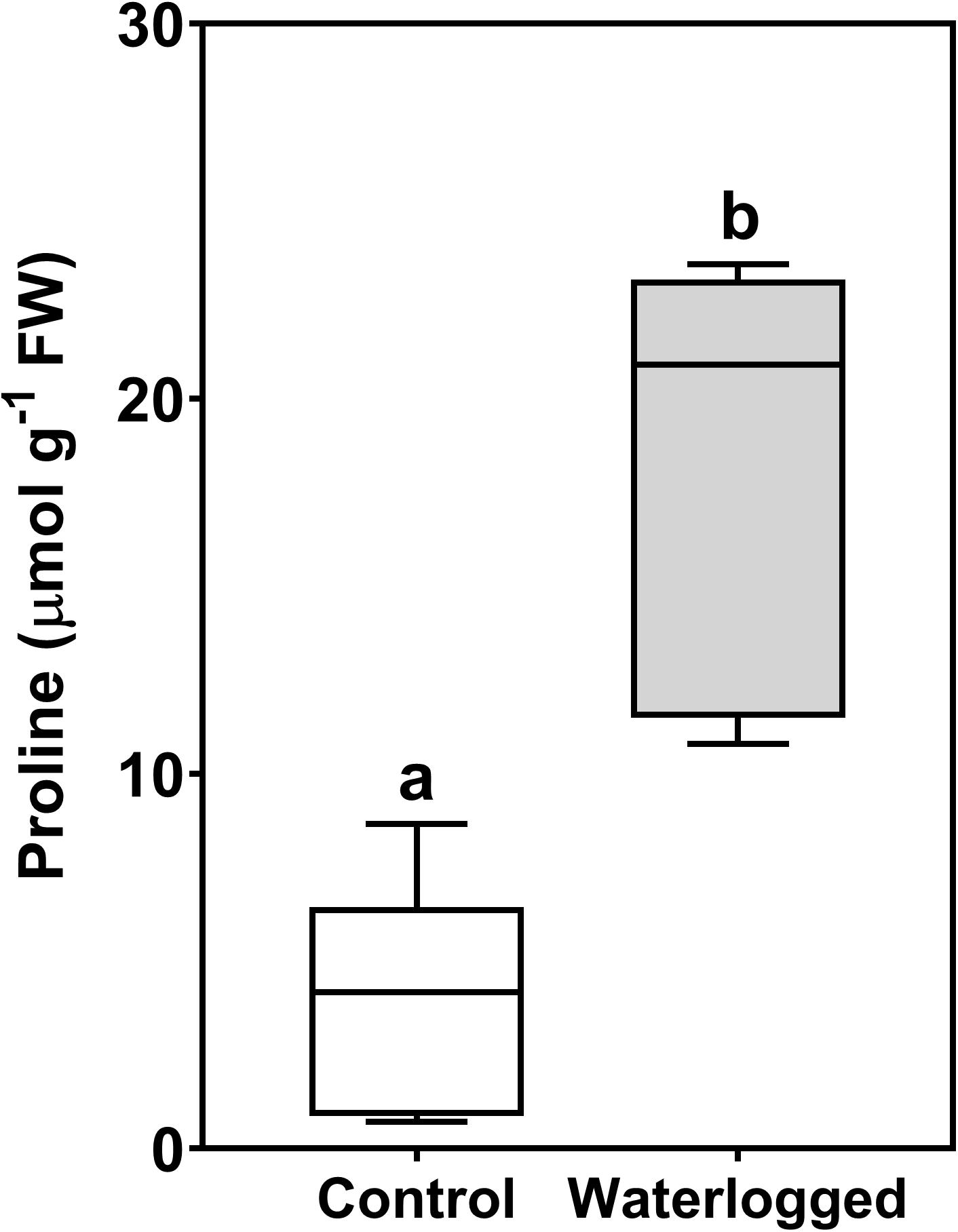
Figure 9. Boxplots of proline content in leaves of control plants (white box) and waterlogged plants (gray box) of Spartina patens after 30 days (n = 5, different letters indicate significant differences at p < 0.05).
C4-Photosynthetic Carboxylating Enzymes and Pigment Analysis
Relatively to the C4-photosynthetic carboxylating enzymes activities, no statistically significant changes were observed either in the activation state, or maximal (Vmax) and physiological (Vphysiol) rates of PEPC (Supplementary Figure 1), or the activation state, or initial (Vi) and total (Vt) rates of Rubisco (Supplementary Figure 2). The same was observed for pigments Chl a, Chl b, total chlorophyll, and carotenoids (Supplementary Figure 3).
Antioxidant Enzyme Analysis
Regarding the antioxidant enzymes CAT, APx, GOPx, SOD, and GR (Figure 10), only the latter two showed a significant decrease in activity on waterlogged plants, with a decrease of twofold in SOD and almost a threefold in GR.
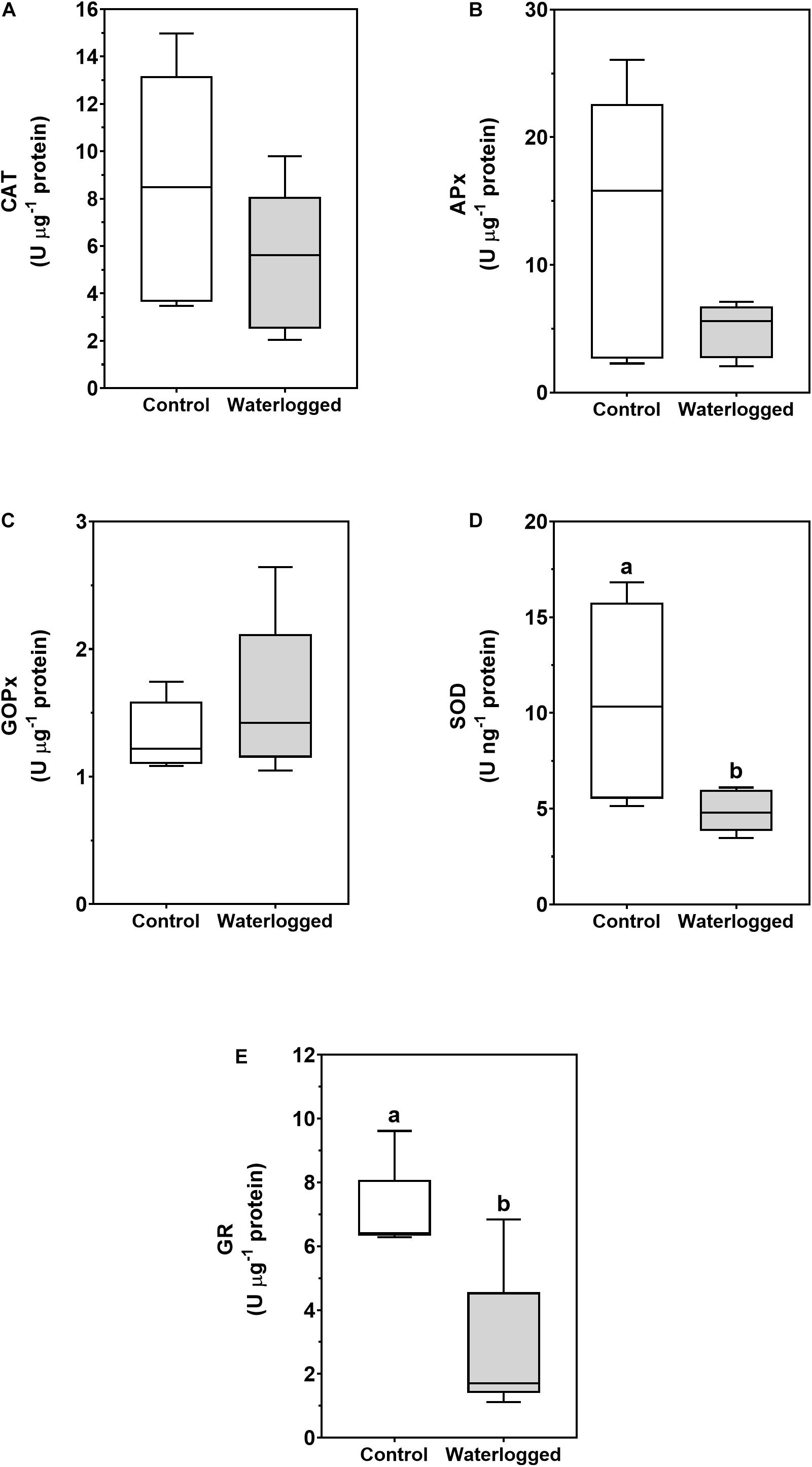
Figure 10. Boxplots of catalase (CAT, A), ascorbate peroxidase (APX, B), guaiacol peroxidase (GOPx, C), superoxide dismutase (SOD, D) and glutathione reductase (GR, E) enzymatic activities in leaves of control plants (white boxes) and waterlogged plants (gray boxes) of Spartina patens after 30 days (n = 5, different letters indicate significant differences at p < 0.05).
Lipid Peroxidation and Fatty Acid Profile
While lipid peroxidation products increased in waterlogged plants (Figure 11A), no changes occurred in total fatty acids content (Figure 11B).
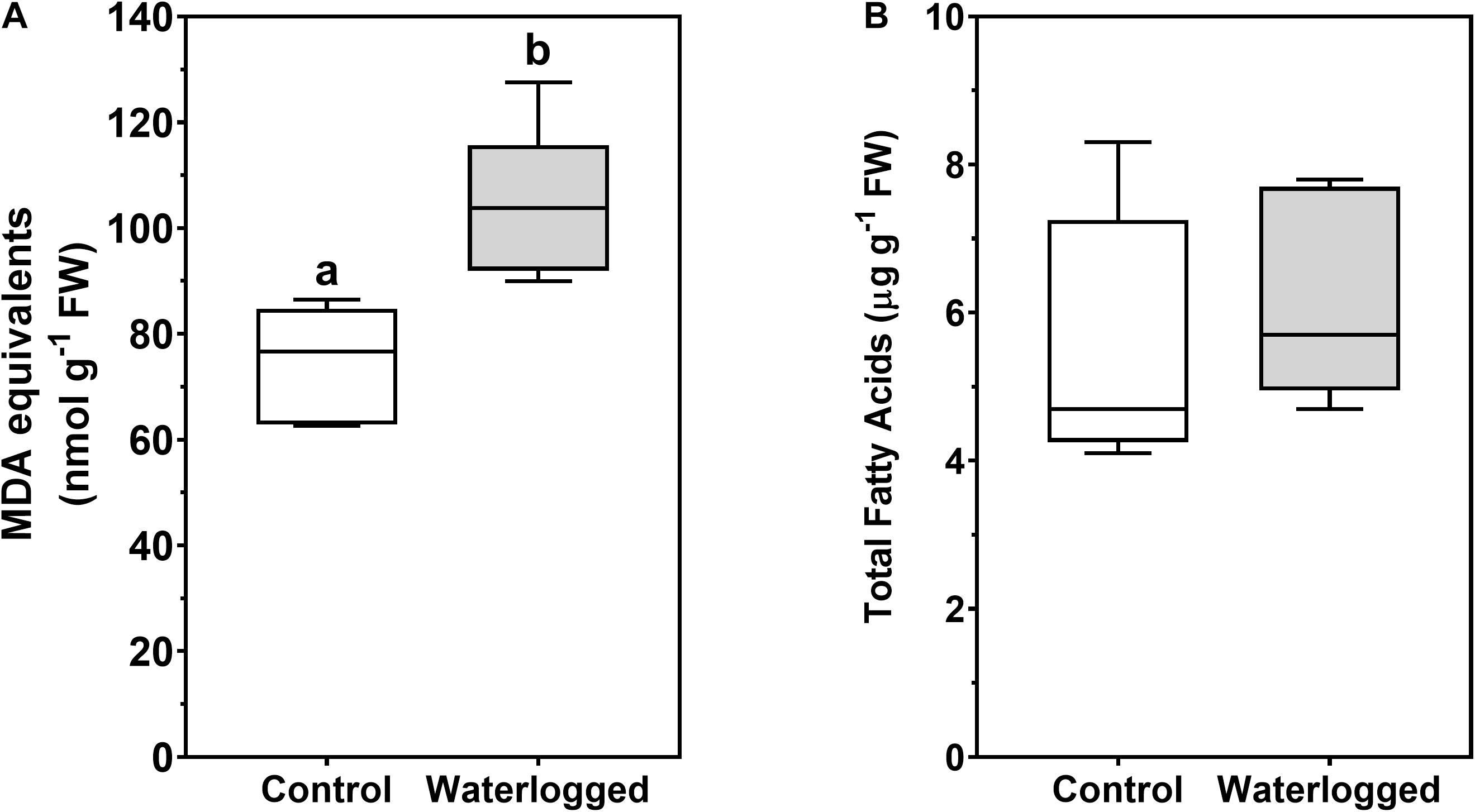
Figure 11. Boxplots of lipid peroxidation quantification measured as malondialdehyde (MDA) equivalents (A) and total fatty acid content (B) in leaves of control plants (white boxes) and waterlogged plants (gray boxes) of Spartina patens after 30 days (n = 5, different letters indicate significant differences at p < 0.05).
Regarding the relative abundance of fatty acids, there was a decrease in triunsaturated hexadecatrienoic acid (16:3), exclusively present in plastidial lipids, whilst there was an increase in stearic acid (C18:0) of waterlogged plants relative to controls (Figure 12). Although there were no significant changes in the major fatty acids classes (Supplementary Figure 4), the ratio of saturated to unsaturated fatty acids ratio (SFA/UFA) increased in waterlogged plants (Supplementary Figure 5).
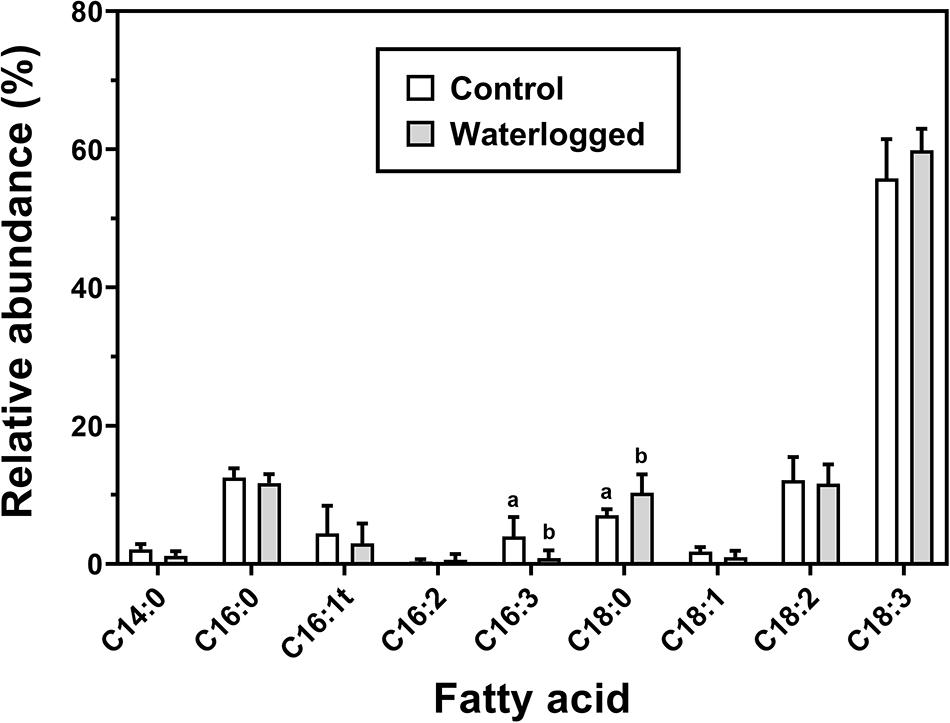
Figure 12. Fatty acid profile in leaves of control plants (white boxes) and waterlogged plants (gray boxes) of Spartina patens after 30 days (n = 5, different letters indicate significant differences at p < 0.05).
Biophysical and Biochemical Profiles
The multivariate PCO analysis allowed to distinguish the control from the waterlogged plants of S. patens using the Kautsky plot, leaf thermography, oxidative stress data and fatty acid profiles (Figures 13, 14), which explain the variables’ total variation with various degrees. Through the simple application of remote tools (Figure 13), the Kautsky plot first PCO axis explains 98.9% of the whole variation between the two evaluated groups (Figure 13A), while due to a higher dispersion along the second PCO axis, the thermography data PCO1 has a lower explanatory power (64.8% of total variation) (Figure 13B).
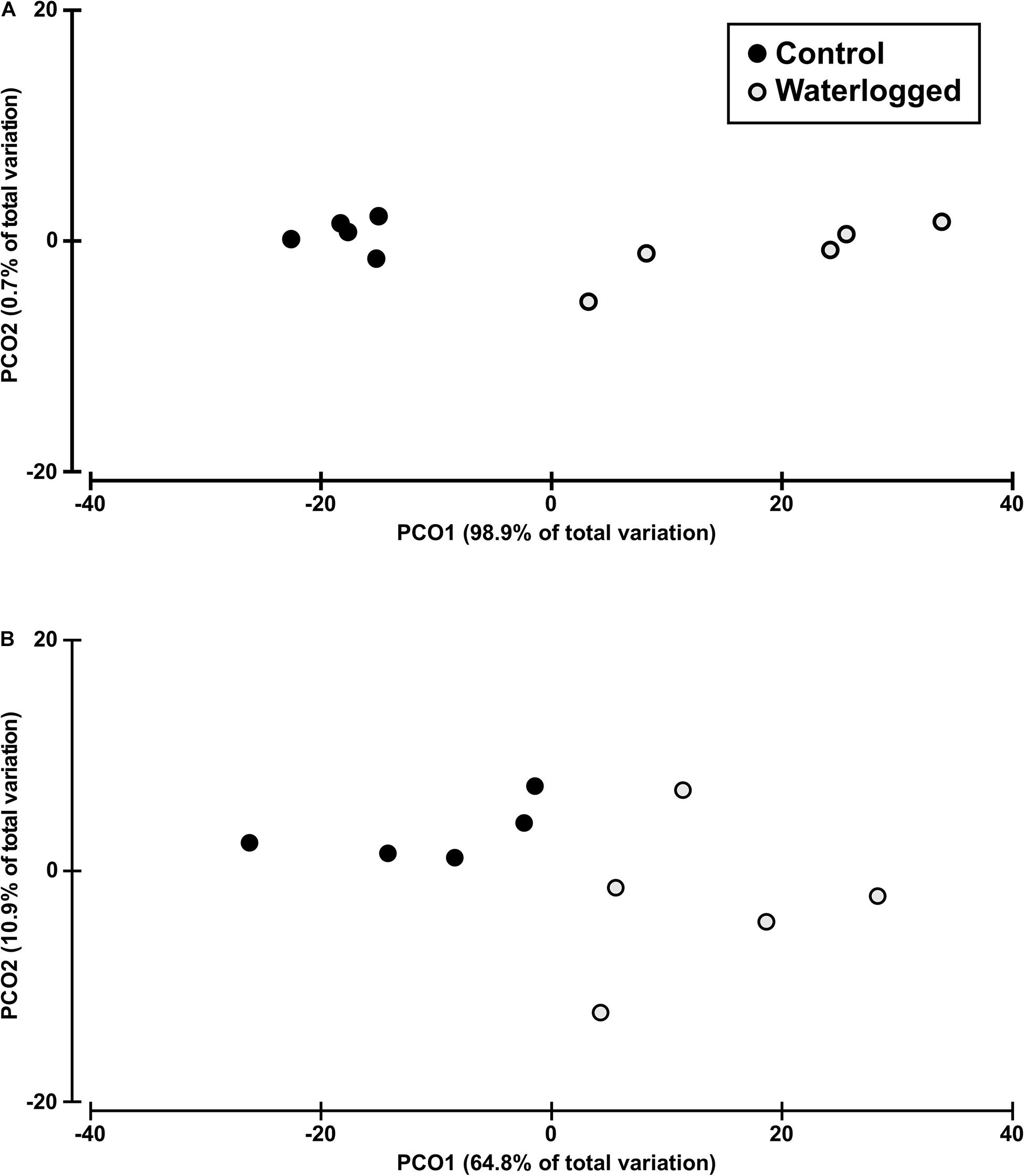
Figure 13. Canonical analysis plot based on the Kautsky plot (A), and thermography (B) in leaves of control plants (black circles) and waterlogged plants (gray circles) of Spartina patens after 30 days (n = 5, different letters indicate significant differences at p < 0.05).
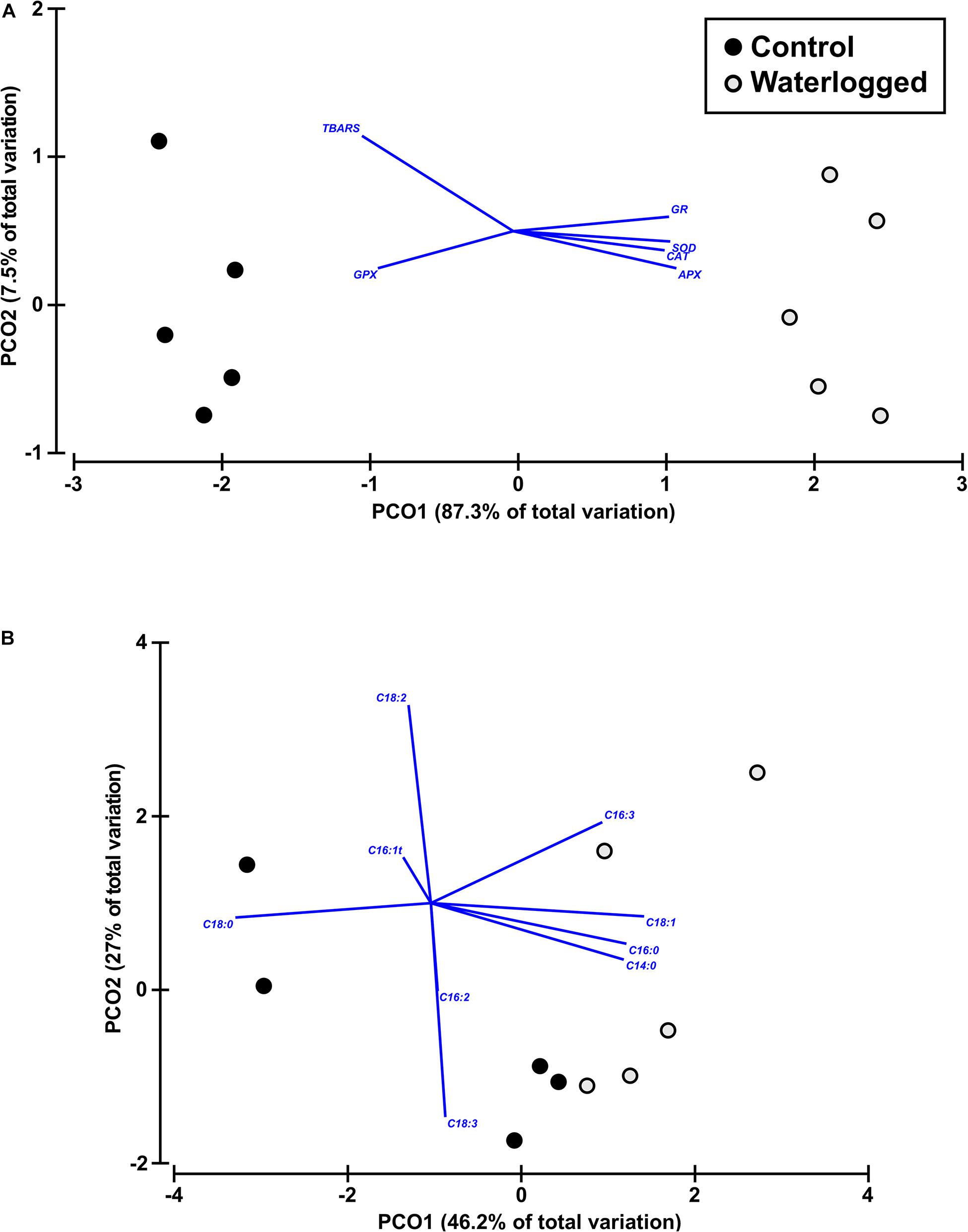
Figure 14. Canonical analysis plot based on oxidative stress (antioxidant enzymes and lipid peroxidation) data (A), and fatty acid profile (B) in leaves of control plants (black circles) and waterlogged plants (gray circles) of Spartina patens after 30 days (n = 5, different letters indicate significant differences at p < 0.05).
Observing the biochemical profiles, the oxidative stress measurements reveal good separation of the two groups (87.3% of total variation), along only one axis of the PCO (Figure 14A). On the other hand, when evaluating the samples fatty acid profiles, it was possible to observe a dispersion along the two PCO axis, indicative of a higher dispersion in the biochemical characteristics of the samples and lower explanatory power (46.2% of total variation) (Figure 14B).
Discussion
The impacts of a highly aggressive and invasive species, such as S. patens urges the need to develop measures, to counteract its spreading. Control measures should be directed to this species and are less harmful to the surrounding environment and co-occurring species. Our proposed alternative method for eliminating the invasive halophyte S. patens proved to be a very effective method to significantly increase the stress levels of the plants in 30 days, using the species physiology drawbacks. Results provide new insights for an ecoengineering solution to be applied in future S. patens control measures in intertidal systems. The fact that S. patens do not tolerate waterlogging is the only limiting factor that is currently preventing full occupation of the intertidal border (Burdick, 1989; Bertness, 1991; Curado et al., 2020), and fully replacing other halophyte native species. Furthermore, once the NIS is removed, indigenous species, such as H. portulacoides and S. fruticosa can reoccupy that area and restore habitat functionality (Curado et al., 2020). Thus, any tool that facilitates this NIS dieback, is of added value to recover the ecosystem floristic diversity.
The effect of waterlogging on S. patens was very clear in different photochemical-related variables and showed lower photosynthetic efficiency when compared with the non-intervened plants, although no significant changes were observed in C4 photosynthesis-related enzymes PEPC and Rubisco as well as in the pigment profile.
The observation of the two distinct treatments of S. patens showed a depression in the Kautsky curves of the waterlogged plants, presenting lower fluorescence values, a characteristic of stress effects (Duarte et al., 2015). Energy fluxes suffered a major impact at the level of electron transport (ET0/CS), with a reduction in the quinone pool size, given by the area above the transient curve (Strasser et al., 1995; Joliot and Joliot, 2002), and a decrease in the number of available reaction centers (RC/CS). This leads to an excessive energy accumulation (Kalaji et al., 2011) that can destroy the D1 protein, and eventually impair all photochemical machinery (Demmig-Adams and Adams, 1992; Qiu et al., 2003; Duarte et al., 2013). To prevent oxidative damage in the chloroplasts, this high energy accumulation needs to be dissipated, frequently through the xanthophyll cycle (Demmig-Adams and Adams, 1992). However, no changes were observed in the carotenoid pool, occurring a decrease in photochemical quenching and an increase in non-photochemical quenching (NPQ), leading to a decrease in the lumen pH, attributed to protonation of the light-harvesting complexes (LHC) proteins associated with the PSII (Cousins et al., 2002). This, in turn, can explain the increase in leaf temperature observed in the waterlogged plants (Zhang et al., 2019). Moreover, it was possible to observe that waterlogged plants had their trapped and transported energy fluxes reduced, but no increase in the energy dissipation. Under stress, a common feature observed is a maintenance of the absorbed and trapped energy flux, but a low energy use in the electron transport, leading to energy bursts inside the chloroplast that are normally diverted to dissipation in the form of heat or fluorescence (Duarte et al., 2016). In this case, only a reduction of the trapped and energy transport fluxes indicating a lower ability of the plants to harvest light, a condition that can promote leaf senescence and plant mortality.
With the roots under water for a more prolonged time, it would be expected to observe a decrease in root respiration (Pan et al., 2021). However, S. patens can develop aerenchyma, pumping atmospheric oxygen through the leaves to ventilate the roots and, thus, allowing it to increase its respiration (Burdick and Mendelssohn, 1987; Burdick, 1989). Nevertheless, and considering the lower energy harvested by waterlogged plants, increased root respiration and consequent consumption of energy reserves (such as carbohydrates), will inevitably increase the energetic stress of the plants. Spartina patens also presented an increased proline content in its leaves, increasing leaf water potential to counteract the differential osmotic pressures between soil and leaves (Briens and Larher, 1982; Koyro et al., 2006; Aziz et al., 2011). This mechanism also poses significant energetic costs to the individuals, that while trying to counteract the waterlogging osmotic constraints, end up increasing the degree of energetic stress of the plants (Iqbal et al., 2014).
Oxygen deprivation will lead inevitably to the increase of intracellular reactive oxygen species (ROS) due to metabolism impairment under stress (Bailey-Serres and Chang, 2005; Pucciariello et al., 2012), severely damaging, amongst others, proteins, and lipids of cells membranes (Sharma et al., 2012; Baxter et al., 2014). Nevertheless, under waterlogging no increase in the antioxidant enzymatic activity could be observed, thus contributing to the accumulation of ROS inside the cells of the waterlogged plants. In fact, two first-line defense enzymes (SOD and GR) had their activities severely reduced. All these impairments of the antioxidant enzymatic system are concomitant with the observed increase in lipid peroxidation. Once again, this will impose severe energetic stress, due to damage in the membranes of the energetic organelles and thus impacting the electronic transport systems and the cell energy budgets.
Additionally, exposure to waterlogging also induced changes in the fatty acid profile of S. patens, which increased the ratio between saturated and unsaturated fatty acids, mainly contributed by an increase in stearic acid (C18:0), which likely resulted in a decrease in membrane fluidity. Moreover, there was a significant decrease in the triunsaturated hexadecatrienoic acid (16:3), which is present in the major plastidial lipid monogalactosyldiacylglycerol and can be linked to lower photosynthetic efficiency measured in waterlogged plants (Kobayashi et al., 2016).
Using multivariate analysis of the assessed biophysical and biochemical traits it was possible to observe that techniques, such as PAM and infrared thermography, are the most efficient tools to evaluate in situ the success of the intervention and the stress levels imposed on the plants targeted to be removed. As in previous ecological studies in response to stress (Cruz de Carvalho et al., 2020a, b; Duarte et al., 2020), the use of bio-optical techniques (e.g., Kautsky plot) allowed an efficient identification of the waterlogged plants and, thus, provide efficient and reliable tools to monitor the success of control interventions, without disturbing the process itself and allowing repeated measures over the intervention period.
In terms, of the ecoengineering solution tested in the present work, the physiological changes observed indicate that the adopted strategy can be an eco-friendly control measure, increasing the plants stress levels significantly, although in the considered 30-day period there was no plant mortality, indicating that these control measures should be performed in more prolonged periods.
Conclusion
The application of a simple barrier to allow waterlogging proved to be an effective and low-cost solution to impair the physiology and biochemical pathways of the invasive halophyte S. patens. Although long-term studies need to be performed, this ecoengineering solution has the potential to control and eliminate this species from salt marshes and other intertidal systems in future ecosystem restoration programs. Since S. patens turfs are easily identified, the upscaling of this technique could involve the application of tubes with different diameters according to plant turfs density, allowing waterlogging to be prolonged in space and time. Furthermore, the application of a simple bio-optical tool will allow the stakeholders to easily follow the process of suppressing the species without the interference of the process and allowing repeated measures over the intervention period.
Data Availability Statement
The raw data supporting the conclusions of this article will be made available by the authors, without undue reservation.
Author Contributions
BD, VF, and PR-S: conceptualization. BD: methodology, supervision, project administration, and funding acquisition. RC and BD: formal analysis. RC, EF, ID, VP, MS, AS, and AM: investigation. RC: data curation and writing—original draft preparation. EF, ID, VP, MS, AM, AS, IC, PR-S, VF, and BD: writing—review and editing. All authors have read and agreed to the published version of the manuscript.
Funding
BD and VF were supported by investigation contracts (CEECIND/00511/2017 and DL57/2016/CP1479/CT0024). PR-S was supported by FCT through a postdoctoral grant (SFRH/BPD/95784/2013). We would like to thank the Fundação para a Ciência e a Tecnologia (FCT) for funding the research via project grants PTDC/CTA-AMB/30056/2017 (OPTOX), UID/MAR/04292/2019, and UIDB/04046/2020. We would also like to thank the MAR2020 program through the project RESTAURA2020 (16-01-04-FMP-0014).
Conflict of Interest
The authors declare that the research was conducted in the absence of any commercial or financial relationships that could be construed as a potential conflict of interest.
Publisher’s Note
All claims expressed in this article are solely those of the authors and do not necessarily represent those of their affiliated organizations, or those of the publisher, the editors and the reviewers. Any product that may be evaluated in this article, or claim that may be made by its manufacturer, is not guaranteed or endorsed by the publisher.
Supplementary Material
The Supplementary Material for this article can be found online at: https://www.frontiersin.org/articles/10.3389/fmars.2021.699528/full#supplementary-material
Abbreviations
APx, ascorbate peroxidase; CAP, canonical analysis of principal coordinates; CAT, catalase; DBI, double bond index; DTT, dithiothreitol; EDTA, ethylenediaminetetraacetic acid; ETC, electron transport chain; FAME, fatty acids methyl esters; GOPx, guaiacol peroxidase; GR, glutathione reductase; LC-PUFA, polyunsaturated fatty acids; MDA, malondialdehyde; NIS, non-indigenous species; PAM, pulse amplitude modulated; PCO, principal coordinates analysis; PEP, phosphoenol-pyruvate; PEPC, phosphoenol-pyruvate carboxylase; PMSF, phenylmethylsulfonyl fluoride; PS I, photosystem I; PS II, photosystem II; PVC, polyvinyl chloride; PVP, polyvinylpyrrolidone; RC, reaction centers; ROS, reactive oxygen species; Rubisco, ribulose-1,5-bisphosphate carboxylase-oxygenase; SOD, superoxide dismutase; TBA, thiobarbituric acid; TCA, trichloroacetic acid; TPF, triphenyl formazan; TTC, triphenyl-tetrazolium chloride.
References
Aguiar, F. C. F., and Ferreira, M. T. (2013). Plant invasions in the rivers of the Iberian Peninsula, south-western Europe: A review. Plant Biol. 147, 1107–1119. doi: 10.1080/11263504.2013.861539
Ainouche, M., and Gray, A. (2016). Invasive Spartina: lessons and challenges. Biol. Invas. 18, 2119–2122. doi: 10.1007/s10530-016-1201-7
Aziz, I., Gul, B., Gulzar, S., and Ajmal Khan, M. (2011). Seasonal variations in plant water status of four desert halophytes from semi-arid region of Karachi. Pak. J. Bot. 43, 587–594.
Bailey-Serres, J., and Chang, R. (2005). Sensing and signalling in response to oxygen deprivation in plants and other organisms. Ann. Bot. 96, 507–518. doi: 10.1093/aob/mci206
Bakrim, N., Echevarria, C., Cretin, C., Arrio-Dupont, M., Pierre, J. N., Vidal, J., et al. (1992). Regulatory phosphorylation of Sorghum leaf phosphoenolpyruvate carboxylase. Identification of the protein-serine kinase and some elements of the signal-transduction cascade. Eur. J. Biochem. 204, 821–830. doi: 10.1111/j.1432-1033.1992.tb16701.x
Bates, L. S., Waldren, R. P., and Teare, I. D. (1973). Rapid determination of free proline for water-stress studies. Plant Soil 39, 205–207. doi: 10.1007/BF00018060
Baumel, A., Rousseau-Gueutin, M., Sapienza-Bianchi, C., Gareil, A., Duong, N., Rousseau, H., et al. (2016). Spartina versicolor Fabre: Another case of Spartina trans-Atlantic introduction? Biol. Invasions 18, 2123–2135. doi: 10.1007/s10530-016-1128-z
Baxter, A., Mittler, R., and Suzuki, N. (2014). ROS as key players in plant stress signalling. J. Exp. Bot. 65, 1229–1240. doi: 10.1093/jxb/ert375
Bertness, M. D. (1991). Zonation of Spartina patens and Spartina alterniflora in New England Salt Marsh. Ecology 72, 138–148. doi: 10.2307/1938909
Bradford, M. M. (1976). A rapid and sensitive method for the quantitation of microgram quantities of protein utilizing the principle of protein-dye binding. Anal. Biochem. 72, 248–254. doi: 10.1016/0003-2697(76)90527-3
Briens, M., and Larher, F. (1982). Osmoregulation in halophytic higher plants: a comparative study of soluble carbohydrates, polyols, betaines and free proline. Plant Cell Environ. 5, 287–292. doi: 10.1111/1365-3040.ep11572682
Brunner, I., Brodbeck, S., and Walthert, L. (2002). Fine root chemistry, starch concentration, and “vitality” of subalpine conifer forests in relation to soil pH. For. Ecol. Manage 165, 75–84. doi: 10.1016/S0378-1127(01)00633-8
Burdick, D. M. (1989). Root aerenchyma development in Spartina patens in response to flooding. Am. J. Bot. 76, 777–780. doi: 10.2307/2444425
Burdick, D. M., and Mendelssohn, I. A. (1987). Waterlogging responses in dune, swale and marsh populations of Spartina patens under field conditions. Oecologia 74, 321–329. doi: 10.1007/BF00378924
Caçador, I., Neto, J. M., Duarte, B., Barroso, D. V., Pinto, M., and Marques, J. C. (2013). Development of an Angiosperm Quality Assessment Index (AQuA-Index) for ecological quality evaluation of Portuguese water bodies - A multi-metric approach. Ecol. Indic. 25, 141–148. doi: 10.1016/j.ecolind.2012.09.021
Carmo-Silva, A. E., Powers, S. J., Keys, A. J., Arrabaça, M. C., and Parry, M. A. J. (2008). Photorespiration in C4 grasses remains slow under drought conditions. Plant Cell Environ. 31, 925–940. doi: 10.1111/j.1365-3040.2008.01805.x
Carmo-Silva, A. E., Soares, A. S., Marques, da Silva, J., Bernardes da Silva, A., Keys, A. J., et al. (2007). Photosynthetic responses of three C4 grasses of different metabolic subtypes to water deficit. Funct. Plant Biol. 34, 204–213. doi: 10.1071/FP06278
Correia, P. M. P., Silva, A. B., Roitsch, T., Carmo−Silva, E., and Marques da Silva, J. (2020). Photoprotection and optimization of sucrose usage contribute to faster recovery of photosynthesis after water deficit at high temperatures in wheat. Physiol. Plant 2020:13227. doi: 10.1111/ppl.13227
Cousins, A. B., Adam, N. R., Wall, G. W., Kimball, B. A., Pinter, P. J., Ottman, M. J., et al. (2002). Photosystem II energy use, non-photochemical quenching and the xanthophyll cycle in Sorghum bicolor grown under drought and free-air CO2 enrichment (FACE) conditions. Plant. Cell Environ. 25, 1551–1559. doi: 10.1046/j.1365-3040.2002.00935.x
Couto, T., Duarte, B., Barroso, D., Caçador, I., and Marques, J. C. (2013). Halophytes as sources of metals in estuarine systems with low levels of contamination. Function. Plant Biol. 2013, 931–939. doi: 10.1071/FP12300
Cruz de Carvalho, R., Feijão, E., Kletschkus, E., Marques, J. C., Reis-Santos, P., Fonseca, V. F., et al. (2020a). Halophyte bio-optical phenotyping: A multivariate photochemical pressure index (Multi-PPI) to classify salt marsh anthropogenic pressures levels. Ecol. Indic. 119:106816. doi: 10.1016/j.ecolind.2020.106816
Cruz de Carvalho, R., Feijão, E., Matos, A. R., Cabrita, M. T., Novais, S. C., Lemos, M. F. L., et al. (2020b). Glyphosate-Based Herbicide Toxicophenomics in Marine Diatoms: Impacts on Primary Production and Physiological Fitness. Appl. Sci. 10:10217391. doi: 10.3390/app10217391
Curado, G., Gallego-Tévar, B., Figueroa, E., and Castillo, J. M. (2020). Effects of removal of alien Spartina densiflora and restoration of native S. maritima on succession and zonation in European salt marshes. Estuar. Coast. Shelf Sci. 244:105815. doi: 10.1016/j.ecss.2018.04.011
Demmig-Adams, B., and Adams, W. W. (1992). Photoprotection and other responses of plants to high light stress. Available online at: www.annualreviews.org (accessed April 23, 2021).
Duarte, B., Baeta, A., Rousseau-Gueutin, M., Ainouche, M., Marques, J. C., and Caçador, I. (2015). A tale of two spartinas: Climatic, photobiological and isotopic insights on the fitness of non-indigenous versus native species. Estuar. Coast. Shelf Sci. 167, 178–190. doi: 10.1016/j.ecss.2015.06.015
Duarte, B., Carreiras, J., and Caçador, I. (2021). Climate Change Impacts on Salt Marsh Blue Carbon, Nitrogen and Phosphorous Stocks and Ecosystem Services. Appl. Sci. 11, 1–18. doi: 10.3390/app11041969
Duarte, B., Couto, T., Freitas, J., Valentim, J., Silva, H., Marques, J. C., et al. (2013). Abiotic modulation of Spartina maritima photobiology in different latitudinal populations. Estuar. Coast. Shelf Sci. 130, 127–137. doi: 10.1016/j.ecss.2013.02.008
Duarte, B., Feijão, E., Cruz de Carvalho, R., Duarte, I. A., Silva, M., Matos, A. R., et al. (2020). Effects of Propranolol on Growth, Lipids and Energy Metabolism and Oxidative Stress Response of Phaeodactylum tricornutum. Biology 9:478. doi: 10.3390/biology9120478
Duarte, B., Marques, J. C., and Caçador, I. (2016). Ecophysiological response of native and invasive Spartina species to extreme temperature events in Mediterranean marshes. Biol. Invasions 18, 2189–2205. doi: 10.1007/s10530-015-0958-4
Duarte, B., Pedro, S. S., Marques, J. C., Adão, H., and Caçador, I. (2017). Zostera noltii development probing using chlorophyll a transient analysis ({JIP}-test) under field conditions: Integrating physiological insights into a photochemical stress index. Ecol. Indic. 76, 219–229. doi: 10.1016/j.ecolind.2017.01.023
Duarte, C. M., Poiner, I., and Gunn, J. (2018). Perspectives on a Global Observing System to Assess Ocean Health. Front. Mar. Sci. 5:265. doi: 10.3389/fmars.2018.00265
Edwards, E. A., Rawsthorne, S., and Mullineaux, P. M. (1990). Subcellular distribution of multiple forms of glutathione reductase in leaves of pea (Pisum sativum L.). Planta 180, 278–284. doi: 10.1007/BF00194008
EU (2020). Communication from the Commission to the European Parliament, the Council, the European Economic and Social Committee and the Committee of the Regions - EU Biodiversity Strategy for 2030. Available online at: https://eur-lex.europa.eu/legal-content/EN/TXT/HTML/?uri=CELEX:52020DC0380&from=EN. (accessed April 16, 2021).
Feijão, E., Gameiro, C., Franzitta, M., Duarte, B., Caçador, I., Cabrita, M. T., et al. (2018). Heat wave impacts on the model diatom Phaeodactylum tricornutum: Searching for photochemical and fatty acid biomarkers of thermal stress. Ecol. Indic. 95, 1026–1037. doi: 10.1016/j.ecolind.2017.07.058
Heath, R. L., and Packer, L. (1968). Photoperoxidation in isolated chloroplasts. I. Kinetics and stoichiometry of fatty acid peroxidation. Arch. Biochem. Biophys. 125, 189–198. doi: 10.1016/0003-9861(68)90654-1
Hedge, P., Kriwoken, L., and Patten, K. (2003). A Review of Spartina Management in Washington State. US. J. Aquat. Plant Manag. 41, 82–90.
Heywood, V. (1989). “Patterns, extents and modes of invasions by terrestrial plants,” in Biological invasions: a global perspective, eds M. Drake, J. A. Mooney, H. A. di Castri, F. Groves, R. H. Kruger, F. J. Rejmanek, et al. (Chichester: John Wiley), 31–60.
Hultén, E. (1958). he amphi-atlantic plants and their phytogeographical connections. Stockholm: Almquist & Wiksell.
Iqbal, N., Umar, S., and Nazar, R. (2014). “Manipulating Osmolytes for Breeding Salinity-Tolerant Plants,” in Emerging Technologies and Management of Crop Stress Tolerance, (Amsterdam: Elsevier Inc.), 385–404. doi: 10.1016/B978-0-12-800875-1.00016-8
Joliot, P., and Joliot, A. (2002). Cyclic electron transfer in plant leaf. Proc. Natl. Acad. Sci. U. S. A. 99, 10209–10214. doi: 10.1073/pnas.102306999
Kalaji, H. M., Bosa, K., and Ko, J. (2011). Effects of salt stress on photosystem II efficiency and CO 2 assimilation of two Syrian barley landraces. Environ. Exp. Bot. 73, 64–72. doi: 10.1016/j.envexpbot.2010.10.009
Kerr, D. W., Hogle, I. B., Ort, B. S., and Thornton, W. J. (2016). A review of 15 years of Spartina management in the San Francisco Estuary. Biol. Invasions 18, 2247–2266. doi: 10.1007/s10530-016-1178-2
Kobayashi, K., Endo, K., and Wada, H. (2016). “Roles of Lipids in Photosynthesis,” in Lipids in Plant and Algae Development, eds Y. Nakamura and Y. Li-Beisson (Cham: Springer International Publishing), 21–49. doi: 10.1007/978-3-319-25979-6_2
Koyro, H.-W., Geissler, N., Hussin, S., and Huchzermeyer, B. (2006). Mechanisms Of Cash Crop Halophytes To Maintain Yields And Reclaim Saline Soils In Arid Areas in Ecophysiology of High Salinity Tolerant Plants. Netherlands: Springer, 345–366. doi: 10.1007/1-4020-4018-0_22
Lichtenthaler, H. K., and Buschmann, C. (2001). Chlorophylls and Carotenoids: Measurement and Characterization by UV-VIS Spectroscopy. Curr. Protoc. Food Anal. Chem. 1, 1–.4. doi: 10.1002/0471142913.faf0403s01
Major, W., Grue, C., Grassley, J., and Conquest, L. (2003). Mechanical and Chemical Control of Smooth Cordgrass in Waillapa Bay, Washington. J. Aquat. Plant Manag. 41, 6–12.
Marklund, S., and Marklund, G. (1974). Involvement of the Superoxide Anion Radical in the Autoxidation of Pyrogallol and a Convenient Assay for Superoxide Dismutase. Eur. J. Biochem. 47, 469–474. doi: 10.1111/j.1432-1033.1974.tb03714.x
Martínez-Jauregui, M., Soliño, M., Martínez-Fernández, J., and Touza, J. (2018). Managing the Early Warning Systems of Invasive Species of Plants, Birds, and Mammals in Natural and Planted Pine Forests. Forests 9:9040170. doi: 10.3390/f9040170
Mika, A., and Lüthje, S. (2003). Properties of Guaiacol Peroxidase Activities Isolated from Corn Root Plasma Membranes. Plant Physiol. 132, 1489L–1498. doi: 10.1104/pp.103.020396
Pan, J., Sharif, R., Xu, X., and Chen, X. (2021). Mechanisms of Waterlogging Tolerance in Plants: Research Progress and Prospects. Front. Plant Sci. 11:2319. doi: 10.3389/fpls.2020.627331
Parry, M. A. J., Andralojc, P. J., Parmar, S., Keys, A. J., Habash, D., Paul, M. J., et al. (1997). Regulation of Rubisco by inhibitors in the light. Plant Cell Environ. 20, 528–534. doi: 10.1046/j.1365-3040.1997.d01-85.x
Patten, K., O’Casey, C., and Metzger, C. (2017). Large-Scale Chemical Control of Smooth Cordgrass (Spartina alterniflora) in Willapa Bay, WA: Towards Eradication and Ecological Restoration. Invasive Plant Sci. Manag. 10, 284–292. doi: 10.1017/inp.2017.25
Pérez-Romero, J. A., Idaszkin, Y. L., Duarte, B., Baeta, A., Marques, J. C., Redondo-Gómez, S., et al. (2018). Atmospheric CO2 enrichment effect on the Cu-tolerance of the C4 cordgrass Spartina densiflora. J. Plant Physiol. 220, 155–166. doi: 10.1016/j.jplph.2017.11.005
Pucciariello, C., Parlanti, S., Banti, V., Novi, G., and Perata, P. (2012). Reactive oxygen species-driven transcription in Arabidopsis under oxygen deprivation. Plant Physiol. 159, 184–196. doi: 10.1104/pp.111.191122
Qiu, N., Lu, Q., and Lu, C. (2003). Photosynthesis, photosystem II efficiency and the xanthophyll cycle in the salt−adapted halophyte Atriplex centralasiatica. New Phytol. 159, 479–486. doi: 10.1046/j.1469-8137.2003.00825.x
Repolho, T., Duarte, B., Dionísio, G., Paula, J. R., Lopes, A. R., Rosa, I. C., et al. (2017). Seagrass ecophysiological performance under ocean warming and acidification. Sci. Rep. 7:41443. doi: 10.1038/srep41443
Ruf, M., and Brunner, I. (2003). Vitality of tree fine roots: reevaluation of the tetrazolium test. Tree Physiol. 23, 257–263. doi: 10.1093/treephys/23.4.257
Sharma, P., Jha, A. B., Dubey, R. S., and Pessarakli, M. (2012). Reactive Oxygen Species, Oxidative Damage, and Antioxidative Defense Mechanism in Plants under Stressful Conditions. J. Bot. 2012:217037. doi: 10.1155/2012/217037
Strasser, R. J., Srivastava, A., and Govindjee. (1995). Polyphasic Chlorophyll a Fluorescence Transient in Plants and Cyanobacteria. Photochem. Photobiol. 61, 32–42. doi: 10.1111/j.1751-1097.1995.tb09240.x
Teixeira, A., Duarte, B., and Caçador, I. (2014). “Salt Marshes and Biodiversity,” in Sabkha Ecosystems: Volume IV: Cash Crop Halophyte and Biodiversity Conservation, eds M. A. Khan, B. Böer, M. Öztürk, T. Z. Al Abdessalaam, M. Clüsener-Godt, and B. Gul (Dordrecht: Springer), 283–298. doi: 10.1007/978-94-007-7411-7_20
Teranishi, Y., Tanaka, A., Osumi, M., and Fukui, S. (1974). Catalase activities of hydrocarbon-utilizing candida yeasts. Agric. Biol. Chem. 38, 1213–1220. doi: 10.1080/00021369.1974.10861301
Tiryakioglu, M., Eker, S., Ozkutlu, F., Husted, S., and Cakmak, I. (2006). Antioxidant defense system and cadmium uptake in barley genotypes differing in cadmium tolerance. J. Trace Elem. Med. Biol. 20, 181–189. doi: 10.1016/j.jtemb.2005.12.004
UN (2020). UN Decade on Ecosystem Restoration. Available online at: https://www.decadeonrestoration.org/. (accessed April 16, 2021).
von Caemmerer, S. (2020). Rubisco carboxylase/oxygenase: From the enzyme to the globe: A gas exchange perspective. J. Plant Physiol. 252:153240. doi: 10.1016/j.jplph.2020.153240
Keywords: ecological restoration, invasive species, salt marsh, remote sensing, halophytes
Citation: Cruz de Carvalho R, Feijão E, Duarte I, Pinto V, Silva M, Matos AR, da Silva AB, Caçador I, Reis-Santos P, Fonseca VF and Duarte B (2021) Ecoengineering Solutions for the Impairment of Spreading and Growth of Invasive Spartina patens in Mediterranean Salt Marshes. Front. Mar. Sci. 8:699528. doi: 10.3389/fmars.2021.699528
Received: 23 April 2021; Accepted: 09 August 2021;
Published: 30 August 2021.
Edited by:
Stelios Katsanevakis, University of the Aegean, GreeceReviewed by:
César Costa, Federal University of Rio Grande, BrazilJenneke Visser, University of Louisiana at Lafayette, United States
Copyright © 2021 Cruz de Carvalho, Feijão, Duarte, Pinto, Silva, Matos, da Silva, Caçador, Reis-Santos, Fonseca and Duarte. This is an open-access article distributed under the terms of the Creative Commons Attribution License (CC BY). The use, distribution or reproduction in other forums is permitted, provided the original author(s) and the copyright owner(s) are credited and that the original publication in this journal is cited, in accordance with accepted academic practice. No use, distribution or reproduction is permitted which does not comply with these terms.
*Correspondence: Ricardo Cruz de Carvalho, rfcruz@fc.ul.pt