- 1Centre for Ecology and Conservation, University of Exeter, Penryn, United Kingdom
- 2School of Life and Environmental Sciences, Centre for Integrative Ecology, Deakin University, Geelong, VIC, Australia
- 3College of Life and Environmental Sciences, University of Exeter, Exeter, United Kingdom
- 4College of Science and Engineering, James Cook University, Townsville, QLD, Australia
- 5Department of Environment and Science, Threatened Species Unit, Brisbane, QLD, Australia
- 6Marine Ecology and Biodiversity, Plymouth Marine Laboratory, Plymouth, United Kingdom
- 7Greenpeace Research Laboratories, University of Exeter, Exeter, United Kingdom
- 8Department of Biodiversity Conservation and Attractions, Perth, WA, Australia
- 9Conservation Medicine Program, School of Veterinary Science, Murdoch University, Perth, WA, Australia
The ingestion of plastic by marine turtles is now reported for all species. Small juvenile turtles (including post-hatchling and oceanic juveniles) are thought to be most at risk, due to feeding preferences and overlap with areas of high plastic abundance. Their remote and dispersed life stage, however, results in limited access and assessments. Here, stranded and bycaught specimens from Queensland Australia, Pacific Ocean (PO; n = 65; 1993–2019) and Western Australia, Indian Ocean (IO; n = 56; 2015–2019) provide a unique opportunity to assess the extent of plastic (> 1mm) ingestion in five species [green (Chelonia mydas), loggerhead (Caretta caretta), hawksbill (Eretmochelys imbricata), olive ridley (Lepidochelys olivacea), and flatback turtles (Natator depressus)]. In the Pacific Ocean, high incidence of ingestion occurred in green (83%; n = 36), loggerhead (86%; n = 7), flatback (80%; n = 10) and olive ridley turtles (29%; n = 7). There was an overall lower incidence in IO; highest being in the flatback (28%; n = 18), the loggerhead (21%; n = 14) and green (9%; n = 22). No macroplastic debris ingestion was documented for hawksbill turtles in either site although sample sizes were smaller for this species (PO n = 5; IO n = 2). In the Pacific Ocean, the majority of ingested debris was made up of hard fragments (mean of all species 52%; species averages 46–97%), whereas for the Indian Ocean these were filamentous plastics (52%; 43–77%). The most abundant colour for both sites across all species was clear (PO: 36%; IO: 39%), followed by white for PO (36%) then green and blue for IO (16%; 16%). The polymers most commonly ingested by turtles in both oceans were polyethylene (PE; PO-58%; IO-39%) and polypropylene (PP; PO-20.2%; IO-23.5%). We frame the high occurrence of ingested plastic present in this marine turtle life stage as a potential evolutionary trap as they undertake their development in what are now some of the most polluted areas of the global oceans.
Introduction
Plastic debris is now one of the most ubiquitous and long-lasting pressures to marine systems (Barnes et al., 2009; Jambeck et al., 2015) and it has now been estimated to interact with over 700 species (Gall and Thompson, 2015; Kühn and Van Franeker, 2020). Plastic presents a threat through ingestion, entanglement, the degradation of key habitats and wider ecosystem effects. Plastic ingestion is now widely recognised to occur in all species of marine turtle (Schuyler et al., 2014; Nelms et al., 2016; Lynch, 2018), however, population-scale impacts have not been demonstrated (Senko et al., 2020).
Records of plastic ingestion (> 1 mm) by marine turtles have been reported in the Atlantic (Musick and Limpus, 1997; Witherington, 2002; Mascarenhas et al., 2004; Di Beneditto and Awabdi, 2014; Santos et al., 2016; Colferai et al., 2017; Pham et al., 2017; Rizzi et al., 2019; Eastman et al., 2020; Machovsky-Capuska et al., 2020; Rice et al., 2021), the Pacific (Wedemeyer-Strombel et al., 2015; Ng et al., 2016; Clukey et al., 2017a; Godoy and Stockin, 2018; Jung et al., 2018a), the Indian Ocean (Hoarau et al., 2014; Yaghmour et al., 2018) and the Mediterranean Sea (Tomas et al., 2002; Campani et al., 2013; Camedda et al., 2014; Matiddi et al., 2017; Domènech et al., 2019; Digka et al., 2020). Drivers behind marine debris ingestion have included visual selectivity (Schuyler et al., 2012; Fukuoka et al., 2016; Duncan et al., 2019a), odour (Pfaller et al., 2020), or failure of discrimination when mixed with normal dietary items (Di Beneditto and Awabdi, 2014). In addition, synthetic particles (<1 mm) have also been isolated from marine turtle gut content; however, ingestion pathways remain poorly understood (Caron et al., 2018; Duncan et al., 2019b).
While plastic ingestion has been linked to morbidity and mortality (Stamper et al., 2009; Poppi et al., 2012; Santos et al., 2015; Orós et al., 2016; Wilcox et al., 2018), it is particularly difficult to attribute mortality (Bjorndal et al., 1994) especially when few studies corroborated marine debris ingestion with associated pathology. Moreover, the effects on sea turtles, including sub-lethal impacts such as exposure to persistent organic pollutants (POPs) via marine plastics are not well-understood (Clukey et al., 2017b; Marn et al., 2020).
Habitat use and behaviours may make marine turtles particularly vulnerable to impacts of plastic pollution in the environment at certain points in their life history (Nelms et al., 2016). For all species other than the flatback turtle (Natator depressus), post-hatchlings enter the oceanic zone where they generally occur at low densities over vast areas (Wildermann et al., 2017; Vandeperre et al., 2019). For flatback turtles, the post hatchlings forage in the neritic waters over the Australian continental shelf instead of dispersing into oceanic waters (Limpus, 2007; Wildermann et al., 2017). This life stage is surface dwelling, only occasionally being more demersal when feeding in the vicinity of seamounts, oceanic banks and ridges that come close to the surface in the oceanic realm (Bolten, 2003). They exhibit an opportunistic epipelagic feeding strategy; their omnivorous diets being largely determined by the combination of relative abundance of potential food objects in the environment and residency in convergence zones (Boyle, 2006; Frick et al., 2009, Ryan et al., 2016). In most species and populations, this life history stage continues until the animals return to coastal waters, where this oceanic stage transitions to a benthic foraging, neritic juvenile stage (Bolten, 2003). The logistics of working in the open ocean makes this life stage particularly challenging to monitor and to assess the impacts of anthropogenic threats (Boyle, 2006; Campani et al., 2013; Schuyler et al., 2014; Nelms et al., 2016).
Small juveniles (including post-hatchlings and oceanic juveniles) are hypothesised as the life stage most likely to be highly impacted by plastic pollution as this life stage is particularly vulnerable to entanglement in floating marine plastic debris (Wilcox et al., 2015; Duncan et al., 2017), damaging effects of ingestion (Clukey et al., 2017a; Pham et al., 2017; Vélez-Rubio et al., 2018; White et al., 2018; Duncan et al., 2019a; Eastman et al., 2020) and sensitive to potential population-level effects (Rice et al., 2021). The consumption of non-nutritional items can lead to dietary dilution resulting in reduced energy and growth (McCauley and Bjorndal, 1999; Nelms et al., 2016), and has been shown to cause damage and blockage to the gastrointestinal tract, cloaca and bladder in some specimen (Bugoni et al., 2001; Lazar et al., 2011; Ryan et al., 2016; Wilcox et al., 2018). Australia has some of the most significant marine turtle nesting areas in the Indo-Pacific region, with nesting sites of green (Chelonia mydas), loggerhead (Caretta caretta), hawksbill (Eretmochelys imbricata), and flatback (Natator depressus). The olive ridley turtle (Lepidochelys olivacea) is a common foraging species in the deeper sub-tidal waters across northern Australia (Limpus, 2009; Boyle et al., 2017). Post-hatchlings of most of these species, are largely absent from coastal waters and the characteristic oceanic juvenile stage occurs in the southwest Pacific or eastern Indian Ocean (Boyle and Limpus, 2008; Limpus, 2009). The endemic flatback turtle is the exception; its post-hatchlings do not leave coastal waters to disperse beyond the continental shelf (Limpus, 2007; Wildermann et al., 2017). Stranded and bycaught specimens from the western Pacific Ocean (PO) and eastern Indian Ocean (IO) provide a unique opportunity to assess the extent of the exposure of plastic ingestion for five species of marine turtle at these critical life stages. Here we aim to 1. Assess the occurrence, type and colour of plastic ingested by this life stage across multiple species 2. Identify the main polymers of ingested plastic.
Materials and Methods
Study Area and Sample Collection
This study was conducted using specimens from Queensland, Pacific Ocean (PO) and Western Australia, Indian Ocean (IO) collected and frozen as part of the ongoing monitoring of marine wildlife strandings including sea turtles by the Queensland Department of Environment and Science (DES) and the Department of Biodiversity, Conservation and Attractions (DBCA) and Murdoch University; and were made available to the author team for necropsy (Supplementary Table 1 and Supplementary Figure 1).
“Small juveniles” used in this study for the PO included stranded post-hatchlings and bycaught oceanic juveniles from the longline fisheries in the Coral Sea and for the IO consisted of stranded post-hatchlings and juveniles including those that were likely to have recently undertaken recruitment into inshore areas (Supplementary Table 1).
Data recorded for each turtle included species, date and location of capture or stranding (dead) and a series of morphometric measurements. A total of 121 small juveniles were sampled. Animals sampled from the PO spanned a period of 26 years (1993–2019) and five species (green, n = 36; loggerhead, n = 7; flatback, n = 10; hawksbill, n = 5, olive ridley, n = 7) (Supplementary Table 1). Stranded turtles from IO were sampled from 2015 to 2019 with data from four species (green, n = 22; loggerhead, n = 14; flatback, n = 18; hawksbill, n = 2) (Supplementary Table 1).
All animals were subject to post-mortem assessments during which the sex was determined (where possible) by visual examination of gross gonadal morphology. During necropsy of PO turtles the entire gastrointestinal tract was removed and initial contents weighed. The contents were grossly examined then rinsed through a 1 mm mesh sieve to separate and remove the plastic for classification and diet items for storage and analysis. For IO turtles, the entire GI content was examined in detail. Furthermore, due to reports of plastic presence within sea turtle bladders (Ryan et al., 2016), the bladder of each turtle in the current study was thoroughly inspected for plastic.
To normalise for turtle size (for those individuals where turtle mass was recorded) the body burden of ingested plastic (g plastic/kg turtle) was calculated following calculations outlined in Clukey et al. (2017a) and Lynch (2018). Body condition index (turtle mass kg/SCL^3) was also calculated following indices described in Bjorndal et al. (2000a) and Rice et al. (2021) to compare with measures of plastic burdens. Curved Carapace Length (CCL) to Straight Carapace Length (SCL) conversions were taken from Lynch (2018).
Plastic Classification
Ingested plastic was classified using a system outlined in Duncan et al. (2019a) which builds upon the Fulmar Protocol and MSFD (Marine Strategy Framework Directive) Marine Litter Report 2011 (Descriptor 10) “toolkits” (van Franeker et al., 2011; Galgani et al., 2014). This involves recording the type of plastic debris: industrial plastic pellets or nurdles (IND) and user plastics (USE) which can be split into several sub-categories; sheetlike plastic (SHE) e.g., plastic bags, threadlike/filamentous plastic (THR), e.g., remains of rope, foamed plastics (FOAM), e.g., polystyrene, fragments (FRAG), e.g., hard plastic pieces and other (POTH), e.g., rubber, elastics, items that are ‘plastic-like’ items that do not clearly fit into another category. Dry weight (mg) was recorded for each individual piece isolated. Additional recordings of colour and three-dimensional measurements of each individual piece were also taken. Colour was recorded within 11 categories; Clear, White, Pink/Purple, Red, Orange, Yellow, Green, Blue, Brown, Black, Grey. Width: Length ratios were calculated (W/L) for all pieces ingested. A ratio close to 1 indicated a square or round piece of debris with ratios <1 leading to rectangular and progressively more linear shapes with decreasing ratio.
Polymer Identification
The polymer make-up of marine plastic debris may aid in identifying possible sources of the material. A sub-sample of retained items was subject to analysis using a PerkinElmer Frontier Fourier-transform infrared (FT-IR) spectrometer. This offers a simple, efficient non-destructive method for identifying and distinguishing polymers, based on infrared absorption bands representing distinct chemical functionalities present in the material (Jung et al., 2018b). Analysis was carried out using a PerkinElmer Spotlight 400 universal diamond –ATR (attenuated total reflection) attachment, placing each fragment or fibre onto the diamond surface (after precleaning the surface with analytical grade ethanol) and applying a consistent force using the sample clamp. Spectra were collected over a broad spectral range (630–4,000 cm–1) from an average of four sample scans with a resolution setting of 4 cm–1. Spectra were corrected for background variation. The infrared spectra were acquired, processed and analysed using PerkinElmer Spectrum software (version 10.5.4.738) and compared against a total of eight commercially available polymer libraries (adhes.dlb, Atrpolym.dlb, ATRSPE∼1.DLB, fibres.dlb, IntPoly.spl, poly1.dlb, polyadd1.dlb and POLYMER.DLB, as supplied by PerkinElmer), checking also against an additional library compiled at the Greenpeace Research Laboratories in order to exclude contaminants arising from materials commonly used in the laboratory. A subsample of 293 plastic pieces were tested. Spectrum software allowed for the comparison of spectra obtained for each sample against these nine libraries, reporting the 10 most likely matches. In each case, matches were then checked by the analyst to verify the quality of the match and the reliability of the identification. Match quality scores were generated for each spectrum, and only scores with >70% match similarity and/or reliable spectra were accepted.
Results
Plastic Ingestion
The presence of plastic was only found in the gastrointestinal tract, and not the cloaca or bladder during examination. A large proportion of the small juveniles sampled had ingested plastic (>1mm) although this varied by species and by ocean (Figure 1) with none of the smaller number of hawksbill turtles in either ocean showing evidence of plastic ingestion. The highest number of plastic pieces ingested occurred in green turtles, with the maximum number of pieces ingested by a single animal being 144 and 343 pieces, in samples from the PO and IO, respectively.
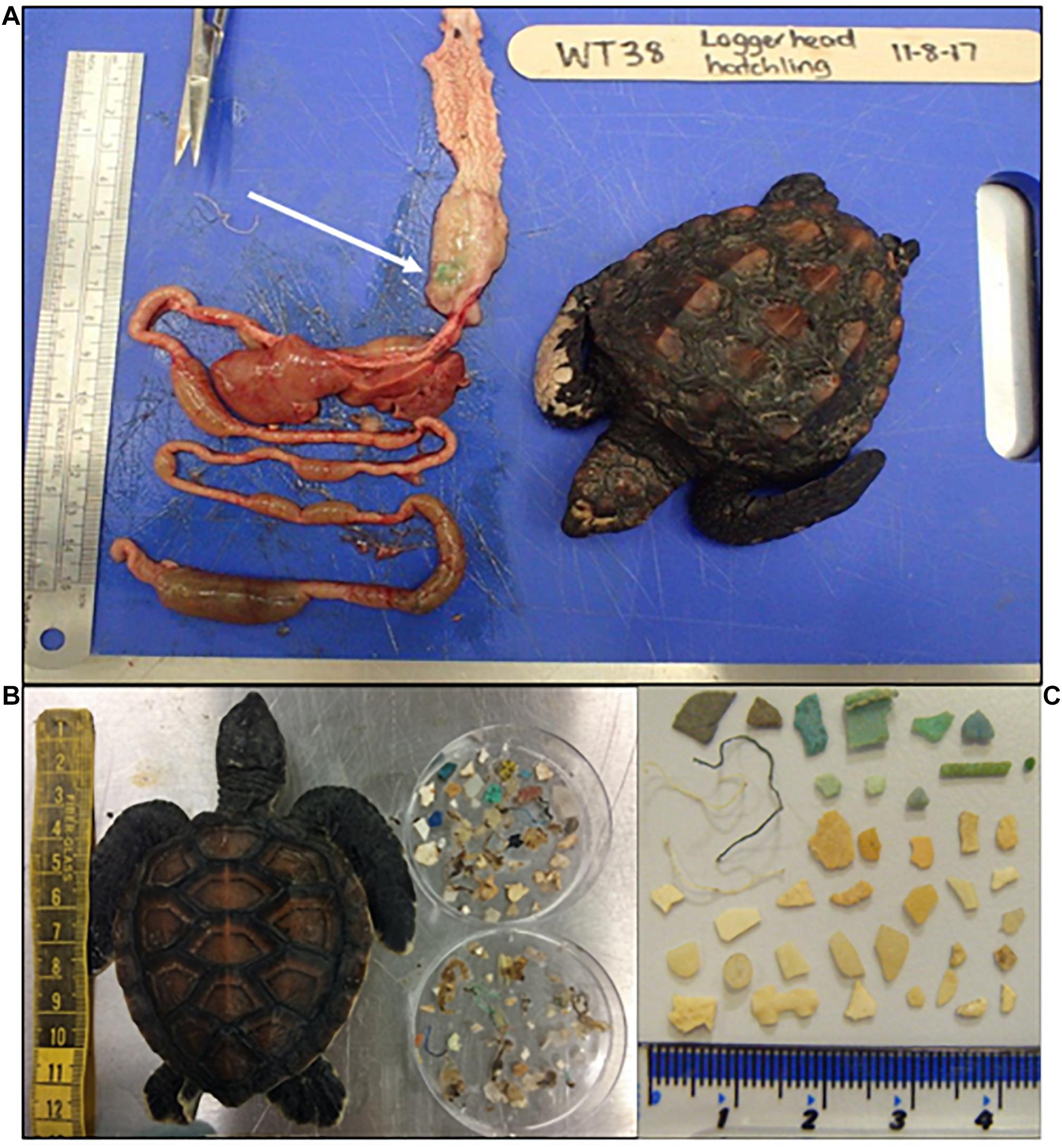
Figure 1. Case studies of plastic ingestion in sea turtles in the Pacific and Indian Ocean (A) Post-hatchling loggerhead turtle found in the Great Southern region of Western Australia in winter (August 2017) and was a suspect cold-stunned case. Twelve pieces of plastic were found on post-mortem including one piece of hard plastic that had caused an impaction in the distal large intestine and likely contributed to morbidity, but was not the primary cause of death (i.e., severe gastrointestinal parasitoses identified on histopathology) (B) stranded post-hatchling green turtle (8.4 cm CCL) from PO with all plastic present in GI tract at necropsy (C) example of ingested plastic from green turtle in PO illustrating that majority hard fragments, scale in cm.
For PO species the incidence of ingestion was generally high [green: 83%, mean number of pieces (mnp) ± SE 18 ± 5, n = 36; loggerhead: 86%, 3 ± 2, n = 7; flatback: 80%, 9 ± 3, n = 10; olive ridley: 29%, 4 ± 26, n = 7]. There was a significant difference in the number of plastic pieces ingested among species [Kruskal-Wallis (KW): χ2 = 15.98, df = 4, p = 0.003] and mass (g) of plastic ingested (KW-χ2 = 13.14, df = 4, p = 0.01). Pairwise comparisons using Dunn’s test indicated that green turtle scores were observed to be significantly different from those of hawksbill turtles for both number of pieces (p = 0.01) and mass of ingested plastic (p = 0.01). No other differences were statistically significant. There was a significant difference in body burden index among species (KW-χ2 = 16.5, df = 4, p = 0.002). Dunn’s test indicated that there was a significant difference between hawksbill/green (p = 0.01) and also hawksbill/flatback (p = 0.02) turtles, additionally there was a significant difference between olive ridley/flatback (p = 0.04) and olive ridley/green (p = 0.03) turtles (Supplementary Figure 2 and Supplementary Table 2). For IO the incidence of ingestion was generally lower, where the flatback ranked highest (28%, 3 ± 0.6, n = 18) followed by the loggerhead (21%, 44 ± 38, n = 14) and green turtle (9%; 0.8 ± 0.76, excluding the case which ingested 343 pieces; n = 21). There was, however, no significant difference among species on the number of pieces ingested (KW- χ2 = 3.89, df = 3, p = 0.27), the mass (g) of plastic ingested (KW- χ2 = 3.80, df = 3, p = 0.28) or body burden index (KW- χ2 = 2.51, df = 3, p = 0.47), (Supplementary Figure 2 and Supplementary Table 2).
For PO green turtles where the largest sample exists; n = 35) body burdens were highly variable, particularly at smaller sizes (CCL) (1.61 ± 0.39 mg/g; range: 0–9.2 mg/g), there was no significant correlation between turtle size and body burden index (Spearman’s Correlation: R = −0.30, p = 0.11) (Figure 2). For body condition index (BCI) there was no significant correlation between BCI and number of pieces (R = 0.24, p = 0.20) or body burden index (R = -0.06, p = 0.76). However, there was a significant positive correlation between BCI and mass of ingested plastic (g) (R = 0.47, p = 0.01) (Supplementary Figure 3 and Supplementary Table 2). Plastic incidence in IO flatback turtles (IO) (n = 15) were also highly variable, particularly at smaller sizes (0.16 ± 0.13 mg/g; range: 0–2.04 mg/g) with significant negative relationship between size of turtle (CCL) and body burden (mg/g) (R = -0.52, p = 0.04) (Supplementary Figure 4 and Supplementary Table 2). For body condition index (BCI) there was no significant correlation with number of pieces (R = 0.01 p = 0.97) or body burden index(R = -0.18, p = 0.50) or mass of ingested plastic (g) (R = -0.14, p = 0.61).
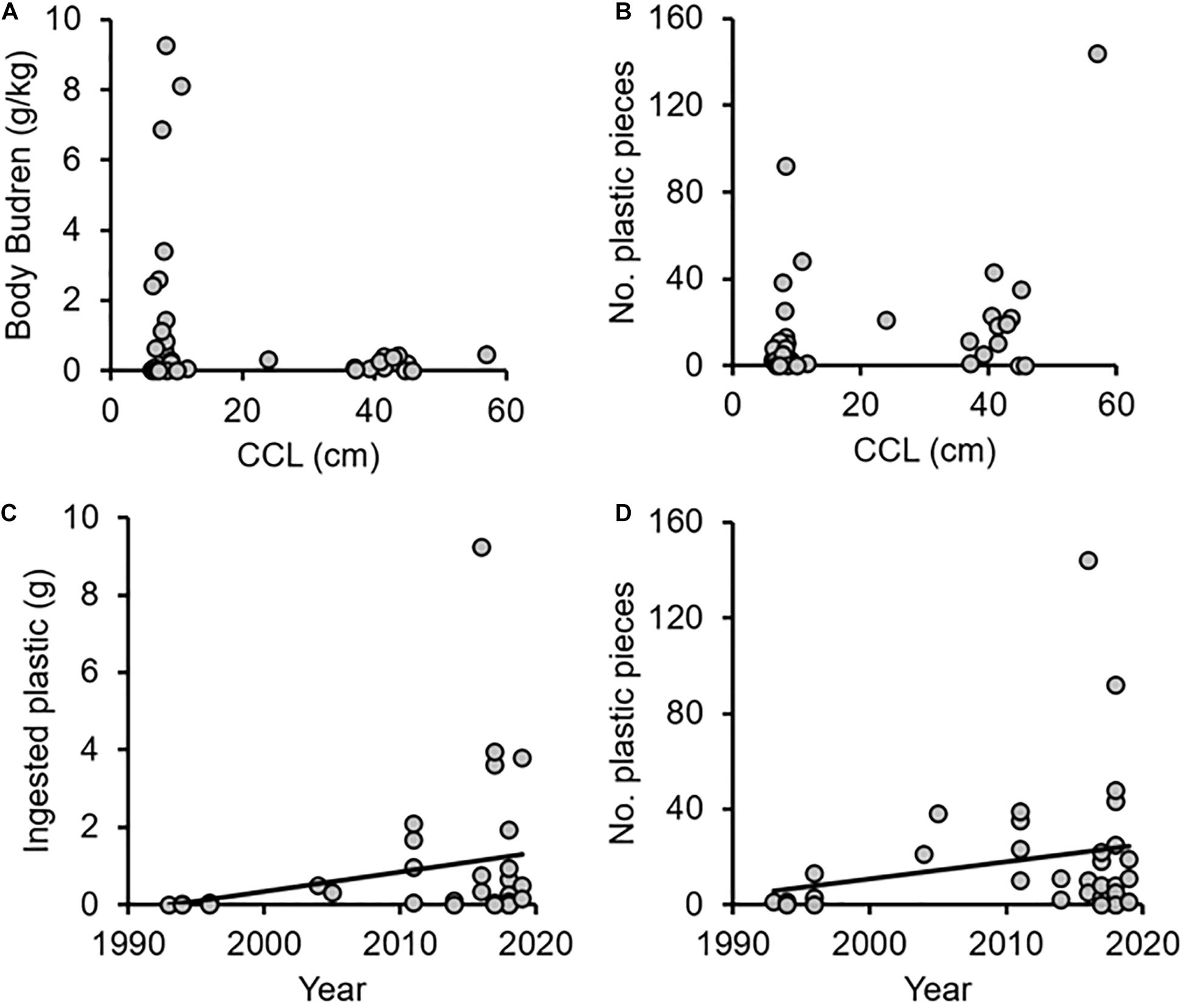
Figure 2. Turtle length and temporal incidence of plastic in green turtle (Chelonia mydas) post-hatchlings in the Pacific (n = 35). Relationship between curved carapace length and (A) plastic debris body burden index (mg plastic/g turtle) and (B) number of ingested pieces of plastic debris. Relationship between year of stranding and (C) mass of ingested plastic pieces (g) (D) number of ingested plastic pieces found at necropsy.
For PO green turtles, there were sufficient samples to compare stranded and bycaught turtles statistically. Of bycaught green turtles (n = 14) 87% had ingested plastic and had on average ingested 26 ± 10 pieces (range: 0–144) compared to stranded conspecifics (n = 22) of which 81% had ingested on average 13 ± 5 (range: 0–92). These two groups were not significantly different in terms number of ingested pieces (Wilcoxon Rank-Sum: W = 106.5, p = 0.126; Supplementary Table 2). However, there was a significant difference in the mass of ingested plastic (g) with stranded turtles (mean ± SE: 0.14 ± 0.05 g; range: 0–0.92 g) presenting with lower masses than those that were by caught (2.07 ± 0.67 g; 0–9.23g; W = 54, p = 0.001). In addition there was a significant difference in body burden index score between stranded turtles (1.75 ± 0.61 g/kg; 0–9.25 g/kg; W = 153, p < 0.001) presenting higher body burden index values than bycaught turtles (0.45 ± 0.19 g/kg; 0–0.45 g/kg). The significantly smaller size, thus GI tract volume, of the stranded post-hatchlings (mean ± SD: 8.9 ± 13.1 cm) compared bycaught oceanic juveniles (41.7 ± 2.9; T-test, t = -21, p < 0.001; Supplementary Table 2) potentially accounting for this.
There was a significant difference between green turtles in the PO and IO with regard to number of ingested pieces (Wilcoxon Rank-Sum: W = 673, p < 0.001), mass (g) of ingested pieces (PO: 0.89 ± 0.3, IO: 0.4 ± 0.36 g; W = 670, p < 0.001) and body burden index scores (W = 489, p < 0.001). For flatback turtles significant differences between oceans were observed for number of ingested pieces (PO: 9.6 ± 0.5, IO: 0.7 ± 0.3 pieces; W = 153.5, p = 0.001), mass (g) of ingested pieces (PO: 0.14 ± 0.49 g, IO: 0.01 ± 0.01 g; W = 152, p = 0.001) and body burden index scores (W = 147, p = 0.002). For both species all values being higher in PO turtles than IO turtles. In other species sample size prevented statistically testing means (Supplementary Table 2).
Ingested Plastic Description
The most abundant type of plastic (for all species) differed between PO and IO; in PO the majority of debris ingested by turtles were hard fragments (FRAG; 52%) whereas for IO these were filamentous (THR; 52%). In both sites this was followed by sheet plastic (SHE; PO: 38%; IO: 35%) (Figure 3 and Supplementary Table 1). Debris ingested spanned all 11 colour categories, the most numerous colour for both sites over all species was clear (PO: 36%; IO: 39%), followed by white for PO (36%) and green and blue for IO (16%; 16%) (Figure 3 and Supplementary Table 1). Larger width to length ratio (W/L ratio) (more square or circular shapes) being the most prevalent in PO samples (0.61–0.8; 29.8%) whereas the large majority of items W/L ratio in IO were thin and long (0.0–0.2; 56.3%). The majority of ingested plastics were >5 mm (PO: 78%; IO: 95%) (Supplementary Table 1).
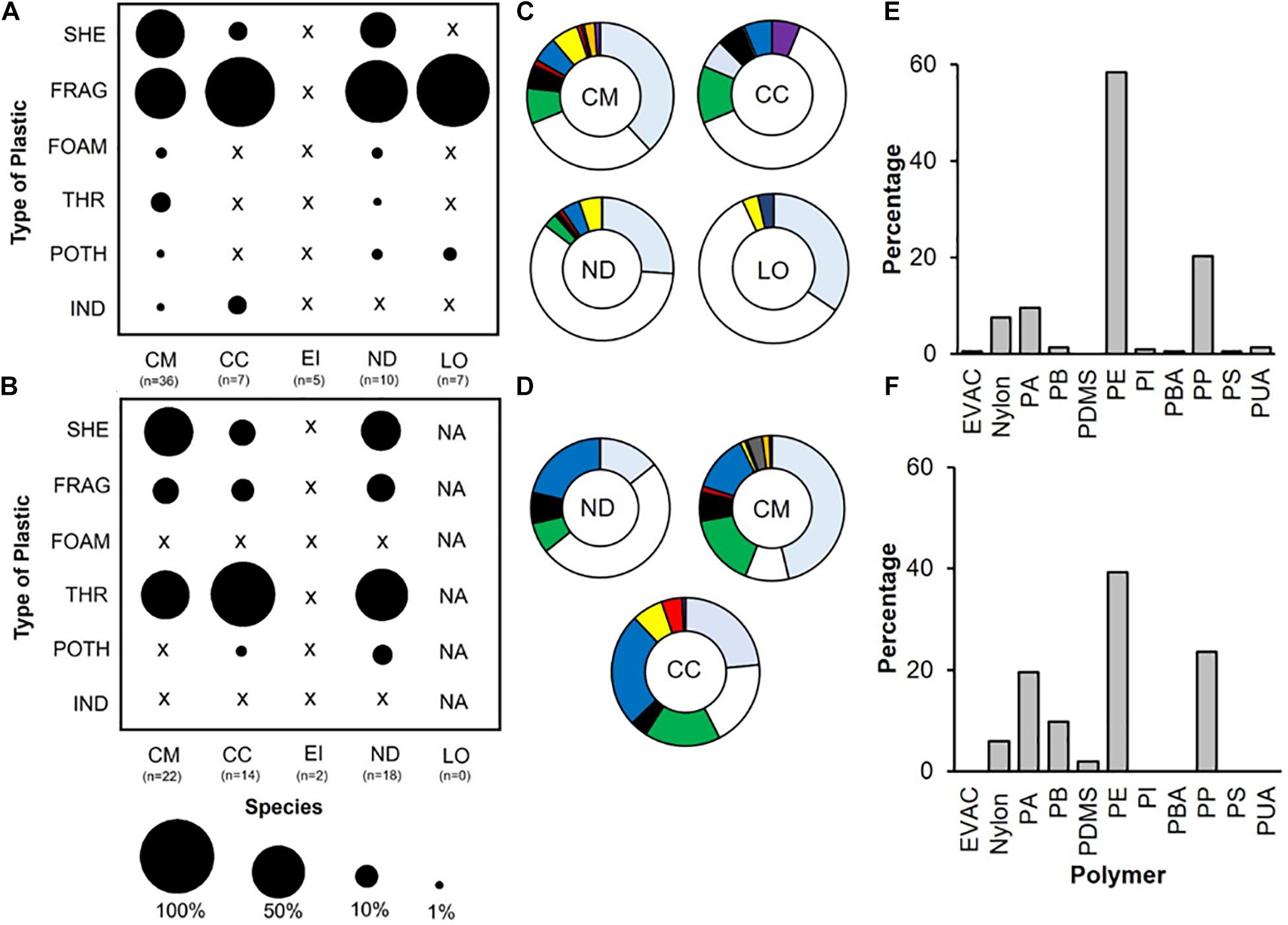
Figure 3. Type and colour of ingested plastics from post-hatchling gut content; (A) proportion (%) of type of plastic pieces ingested by post-hatchling and oceanic juveniles for PO and (B) for IO. (C) Proportional makeup of plastics by colour in PO samples (D) IO samples. For all parts CM, Green (Chelonia mydas); CC, loggerhead (Caretta caretta); EI, hawksbill (Eretmochelys imbricata); ND, flatback turtles (Natator depressus); LO, olive ridley (Lepidochelys olivacea) For parts (A and B), black circle scaled accordingly, x = no presence of particular type of plastic. SHE, sheetlike plastics; THR, threadlike plastics; FOAM, foamed plastics; FRAG, hard plastics; POTH, other ‘plastic like’ items; IND, industrial nurdles. For parts (C and D) grey shading = transparent materials and colours represent colours of the plastic (E) percentage (%) of ingested pieces by polymer for PO and (F) for IO turtles. EVAC, Ethylene/Vinyl Acetate Copolymer, Nylon; PA, Polyamide; PB, Polybutene; PDMS, Polydimethylisiloxane (silcone); PE, Polyethylene; PI, Polyisoprene; PBA, Polyoctylacrylate; PP, Polyproplene; PS, Polystyrene; PAU, Polyundecanoamide.
Polymer Identification
The most common polymers identified were polyethylene (PE; PO-58%; IO-39%) and polypropylene (PP; PO-20.2%; IO-23.5%). Other polymers identified were ethylene vinyl acetate (EVA), polyamide (PA), polybutene (PB), polyisoprene (PI) and polystyrene (PS) (Figure 3, Supplementary Figure 5, and Supplementary Table 1).
Ingestion Over Time
There was an indication of a positive, however, not statistically significant, relationship between the number of pieces ingested (R = 0.16; p = 0.36), mass of ingested pieces (g) (R = 0.25, p = 0.15) or body burden index (R = 0.12, p = 0.50) and the year of stranding for green turtles in PO (n = 35) (Figure 2). Variance was high in the other species but small sample sizes precluded analysis.
Discussion
Plastic Ingestion in Post-hatchling Marine Turtles
This study demonstrates that small juvenile marine turtles from the Pacific and Indian Ocean had high incidence of plastic ingestion (>1 mm). We highlight the prevalence of this in a number of species and make additions to the limited knowledge regarding plastic ingestion in relation to the ecology of the flatback turtle. We also highlight variability of body burden especially at smaller sizes. Ingestion occurrence appears higher in samples from the Pacific than the Indian Ocean and may indicate different vulnerability levels between oceans. However, owing to limited access to samples from this life stage deeper analysis was precluded, therefore collaborative combined datasets to achieve larger samples sizes and longer time series over multiple ocean basins to assess contributing factors (such as species, turtle size) will be crucial in the future. Furthermore frequencies of reported ingestion in this study would likely be higher with the inclusion of data on plastics < 1 mm, which has been previously been reported to be ubiquitous in marine turtles (Duncan et al., 2019b).
In contrast to other studies on this life stage, samples from the Indian Ocean were dominated by filamentous pieces that were green and blue in colour and were polyethylene, polypropylene and nylon in composition. One of the key sources of marine debris in this area, and indeed in similar studies of turtles in Northern Australia, is that of abandoned, lost or otherwise discarded fishing gear (ALDFG) often comprised of plastic fibres (Kiessling et al., 2003; Gunn et al., 2010; Wilcox et al., 2013; Van Der Mheen et al., 2020; Nelms et al., 2021; Pattiaratchi et al., 2021). Evidence from regionally relevant riverine inputs show polyethylene, polypropylene and nylon fishing gear can contribute to ALDFG in the environment (Nelms et al., 2021). This ghost gear has the capacity to breakdown into bioavailable pieces following long-term degradation (Cole et al., 2011; Jâms et al., 2020). Juvenile turtles are known to seek refuge under rafts of sargassum and other floating debris (Witherington et al., 2012) which may increase their chances of consuming rope fibres if foraged food is attached to drifting ALDFG. Results from Pacific Ocean are in concordance with those from other studies, with the recorded debris dominated by hard plastic fragments, being predominately clear and white in colour (Ryan et al., 2016; Clukey et al., 2017a; Pham et al., 2017; White et al., 2018; Eastman et al., 2020). Smaller post-hatchlings appear to experience the highest body burden of plastic debris which was also seen in the North Pacific (Clukey et al., 2017a). Taken together, these findings underline the potential utility of marine turtles as bio-indicators of marine litter regionally (Matiddi et al., 2017). For example the stark difference between the frequency occurrence ingestion ranges of hawksbills in this study (0%) compared to 100% reported in the Northwest Atlantic and Central Pacific (Lynch, 2018).
In both oceans, ingested plastics were composed primarily of polyethylene (PE) and polypropylene (PP) as seen in previous sea turtle studies (Pham et al., 2017; Jung et al., 2018b; White et al., 2018). Polyethylene and PP are low density polymers typically used in single-use, disposable packaging material and constitute significant proportions of the waste stream leaking into the ocean, e.g., larval fish nursey grounds (Gago et al., 2018; Gove et al., 2019). Lower density polymers, such as polypropylene and polyethylene, dominate sea surface samples but decrease in abundance through the water column and a different polymer mix will be found again on the seafloor due to chemical densities (Brignac et al., 2019; Erni-Cassola et al., 2019). With regards to the other polymers identified in this study there is also a profound lack of knowledge regarding the modifications that plastics undergo once subject to the environment and how this impacts analytical chemical results, e.g., multi-layer composites (Silva et al., 2018).
High ingestion abundances suggest significant negative health consequences are possible. Loggerhead post-hatchling turtles stranded in Florida had ingested up to 287 pieces and their initial health condition was reported as poor; animals being categorised as thin to emaciated and covered in epibiota (Eastman et al., 2020). While plastic ingestion has been linked to morbidity and mortality this is often difficult to accurately attribute, due to the lack of associated pathology (Bjorndal et al., 1994; Stamper et al., 2009; Poppi et al., 2012; Santos et al., 2015; Orós et al., 2016). Detailed veterinary diagnostic testing is infrequently used to support a diagnosis of morbidity or mortality by marine debris. Furthermore, working with stranded animals has been suggested to lead to biases (Casale et al., 2016). Surveying plastics in animals that die from fisheries interactions, however, might be more likely to exhibit normal healthy body condition than those that die of other causes (Barco et al., 2016; Casale et al., 2016). Consequently, longline bycaught turtles used in this study provide a unique data source to assess the plastic burden in what may be relatively healthy individuals. In this study an emaciated green turtle from IO having ingested 343 pieces of plastic had evidence of gastrointestinal ulceration on necropsy (adjacent to blockage), along with histopathology and ante-mortem blood results. However, bycaught green turtles from PO in this study had ingested maximum values exceeding 100 pieces and presented good body condition. Therefore, in the future it would be advantageous to work alongside veterinarians and other specialists, i.e., veterinary pathologists for access to veterinary diagnostic testing services.
Additionally it will be important to use this expertise to explore the relationship between Body Condition Index (BCI) and plastic ingestion amounts. In this study PO green turtles with higher BCI were correlated with a higher mass of ingested plastic, which has also been observed in other green turtle populations; BCI positively correlated with higher number of ingested pieces of plastic (Clukey et al., 2017a). This is contrary to potential sub-lethal effects reported due to plastic ingestion for example dietary dilution and weight loss (McCauley and Bjorndal, 1999).
Flatback Turtles
The Australian flatback turtle, endemic to the continental shelf shared by Australia and New Guinea, lacking an oceanic development phase, still displayed plastic ingestion; with some of the highest occurrence in both Pacific Ocean and Indian Ocean. Therefore bioavailable-sized plastic must also be present in neritic waters (Critchell et al., 2019; Jâms et al., 2020). The presence of buoyant plastics indicates these turtles are likely to be foraging at the surface even though they are capable of benthic dives from an early stage (Salmon et al., 2010). This age class has been recorded frequently in the Great Barrier Reef waters, over the continental shelf, feeding on planktonic organisms. However, much more information is needed on their distribution and diet to be able to determine the possibility of selective plastic ingestion; as shown in other neritic stage turtles (Limpus, 2009; Duncan et al., 2019a).
Points of Vulnerability
The probability of interactions between marine turtles and plastic is directly linked to the feeding ecology and habitat use of the species and/or life stages, and to the spatial distribution of plastic in the marine environment (Pham et al., 2017). Fluid dynamic processes that aggregate organisms into these areas have also been found to concentrate buoyant, passively floating plastics (van Sebille et al., 2012, 2015; Critchell et al., 2015; Gove et al., 2019). Regionally, these include the East Australian Current (EAC), Indian Ocean South Equatorial Current (SEC) and the Leeuwen Current (Limpus and Nicholls, 1988; Whiting et al., 2008; Boyle et al., 2009; Feng et al., 2011; Robson et al., 2017; Wildermann et al., 2017; Pattiaratchi et al., 2021). Knowledge indicates that small juveniles are associated within these currents or sites of convergence (Ryan et al., 2016; Pham et al., 2017; Eastman et al., 2020; Rice et al., 2021). Modelling has suggested that active and directed swimming behaviour appears to play a major role in post-hatchling distribution during the first few months of their life (Putman et al., 2012; Scott et al., 2014; Wildermann et al., 2017; Wolanski, 2017) and behaviour therefore influences the adoption of oceanic lifestyles and potential fidelity to discrete oceanic regions (Polovina et al., 2004; Etnoyer et al., 2006; Mansfield et al., 2009; Barceló et al., 2013).
This active dispersal will be directed by a variety of environmental cues, such as temperature, resulting in occupying and residing in convergence zones, with many ocean animals preferentially feeding within these zones due to high prey abundance (Bjorndal et al., 2000b; Bost et al., 2009; Miller et al., 2015; Siegelman et al., 2019; Whitney et al., 2021). Floating material on the surface of these areas now contains potentially harmful levels of plastic pollution (Carr, 1987; Wolanski, 2017). This makes this life stage particularly susceptible to floating plastic ingestion because they feed at or near the ocean surface in ocean currents in search of planktonic prey (Witherington, 2002; Witherington et al., 2012; Ryan et al., 2016). In addition ingestion probability is higher due to feeding largely in a non-selective manner (Schuyler et al., 2014; Nelms et al., 2016; Eastman et al., 2020). Indeed, Witherington (2002) showed there were similar mean proportions of plastic debris items recovered from habitat and lavage samples of post-hatchling loggerhead turtles caught in Atlantic downwelling zones, providing evidence of plastic exposure within occupied habitat. This could be similar in currents such as the EAC or SEC currents that the turtles within this study occupy.
Juvenile Marine Turtles and Plastic Pollution in Context of Evolutionary Traps
Evolutionary traps occur when relatively poor-quality resource, e.g., food or habitat attracts a large number of individuals, having negative effects on their overall fitness. This phenomenon occurs when organisms rely on environmental cues to make life history decisions, however, due to modern anthropogenic disturbances the environment is being altered rapidly and former reliable cues may no longer be associated with conditions favouring adaptive outcomes (Kokko and Sutherland, 2001). Human-induced global environmental change is capable of rapidly creating a diverse array of ecologically novel conditions to which animals have not evolved (Robertson and Blumstein, 2019). Therefore, organisms become “trapped” by their evolutionary responses. This becomes a concern for vulnerable species with delayed maturity (such as marine turtles) when they do not have the behavioural plasticity to assess and respond to the evolutionarily novel situation (Kokko and Sutherland, 2001).
One-way traps can arise when the novel element introduced in the environment (in this case plastic), mimics a traditional cue from habitat choice, thereby misleading the organism. Post-hatchlings occupy habitats that in the past were ideal locations for growth, however, these are now accumulating high abundances of plastic debris; potentially lowering survivorship and causing sub-lethal impacts on growth and maturity (Marn et al., 2020). Indeed it has been shown that plastic ingestion can create change in relation to nutrient acquisition and fitness consequences in marine turtles (Machovsky-Capuska et al., 2020) and be associated with pathology (Ryan et al., 2016) and potentially morbidity or mortality (Stamper et al., 2009; Poppi et al., 2012; Santos et al., 2015; Orós et al., 2016). The elevated occurrence of ingested plastic debris in small juvenile turtles is of concern as overall mortality and implications for eventual recruitment into breeding populations are still unknown (Vandeperre et al., 2019). Indeed within this study, smaller stranded turtles displayed higher body burdens of plastic than large bycaught individuals. To further our understanding it will be crucial to identify methods to measure changes in vital rates specific to locations and life stage, e.g., changes in somatic growth or survivorship (Rice et al., 2021). This will increase knowledge of poorly understood population-level mortality rates (Senko et al., 2020). Investigating these concepts will be critical as evolutionary traps are rarely recognised by wildlife conservation practitioners as a significant conservation threat that should be integrated into conservation planning and management (Robertson and Blumstein, 2019).
Conclusion
Small juveniles from the Western Pacific and East Indian Ocean had high incidence of plastic ingestion. The anthropogenic addition of plastic into the environment could make formerly reliable cues of developmental habitat no longer adaptive. This life stage has the potential for being “trapped” by their past evolutionary responses to develop in what are now some of the most polluted areas of the global oceans. Future studies should aim to continue to document the prevalence of plastic. It is also imperative that methods to assess this in live, in situ specimens are developed to assess plastic burdens in these developmental life stages. In addition, understanding the dispersal and foraging ecology of this cryptic life stage is essential for understanding their vulnerability in the context of this novel presence of plastic pollution. In addition future studies to better understand the physiological and population-level impacts of plastic pollution on marine turtles.
Data Availability Statement
All data presented in the study are included in the Supplementary Material. Further inquiries can be directed to the corresponding author/s.
Ethics Statement
The animal study was reviewed and approved by the University of Exeter.
Author Contributions
ED, AB, TG, PL, KC, BG, CL, and MH contributed to the conception and design of study. ED, CL, MH, AT, SW, EY, and DS were involved in the sample collection and collected the data. ED performed the data analysis. All authors contributed to the review and preparation of the manuscript.
Funding
Roger de Freitas provided generous support for ED. The Sea Life Trust provided support for ED and funding to enable data collection. National Geographic Early Career Grant provided support for ED and funding to enable data collection. Natural Environment Research Council discovery grant (NE/L003988/1) supported PL. BG and ED received support from the NERC SE Asia Plastics (SEAP Program), Risks and Solutions: Marine Plastics in Southeast Asia (NE/V009354/1) and BG and AB received support from the Darwin Initiative. BG and PL were awarded a University of Exeter—Plymouth Marine Laboratory collaboration award which supported ED. Queensland Department of Environment and Science (DES) Threatened Species Operations supported CL. Department of Biodiversity, Conservation and Attractions supported SW and AT. Murdoch University Pathology Diagnostic Services, the Perth Zoo Veterinary Department supported EY. Open access fees were provided by the University of Exeter.
Conflict of Interest
The authors declare that the research was conducted in the absence of any commercial or financial relationships that could be construed as a potential conflict of interest.
Publisher’s Note
All claims expressed in this article are solely those of the authors and do not necessarily represent those of their affiliated organizations, or those of the publisher, the editors and the reviewers. Any product that may be evaluated in this article, or claim that may be made by its manufacturer, is not guaranteed or endorsed by the publisher.
Acknowledgments
We would like to thank Sea World and SeaLife on the Gold Coast and Sunshine Coast of Queensland, respectively, and the numerous volunteers who assisted with fieldwork in collaboration with Queensland Department of Environment and Science (DES) Threatened Species Operations. We would also like to thank the Department of Biodiversity, Conservation and Attractions including regional staff, Yawuru and Nyul Nyul Indigenous Ranger groups, Murdoch University Pathology Diagnostic Services, the Perth Zoo Veterinary Department, and the other dedicated sea turtle rehabilitators in Western Australia.
Supplementary Material
The Supplementary Material for this article can be found online at: https://www.frontiersin.org/articles/10.3389/fmars.2021.699521/full#supplementary-material
References
Barceló, C., Domingo, A., Miller, P., Ortega, L., Giffoni, B., Sales, G., et al. (2013). High-use areas, seasonal movements and dive patterns of juvenile loggerhead sea turtles in the southwestern Atlantic Ocean. Mar. Ecol. Prog. Ser. 479, 235–250. doi: 10.3354/meps10222
Barco, S., Law, M., Drummond, B., Koopman, H., Trapani, C., Reinheimer, S., et al. (2016). Loggerhead turtles killed by vessel and fishery interaction in Virginia. USA, are healthy prior to death. Mar. Ecol. Prog. Ser. 555, 221–234. doi: 10.3354/meps11823
Barnes, D. K. A., Galgani, F., Thompson, R. C., and Barlaz, M. (2009). Accumulation and fragmentation of plastic debris in global environments. Philos. Trans. R. Soc. London. Ser. B 364, 1985–1998. doi: 10.1098/rstb.2008.0205
Bjorndal, K. A., Bolten, A. B., and Chaloupka, M. Y. (2000a). Green turtle somatic growth model?: evidence fordensity dependence. Ecol. Appl. 10, 269–282.
Bjorndal, K. A., Bolten, A. B., and Lagueux, C. J. (1994). Ingestion of marine debris by juvenile sea turtles in coastal Florida habitats. Mar. Pollut. Bull. 28, 154–158. doi: 10.1016/0025-326X(94)90391-3
Bjorndal, K. A., Bolten, A., and Martins, H. (2000b). Somatic growth model of juvenile loggerhead sea turtles Caretta caretta: duration of pelagic stage. Mar. Ecol. Prog. Ser. 202, 265–272. doi: 10.3354/meps202265
Bolten, A. B. (2003). “Variation in sea turtle life history patterns: neritic vs. oceanic developmental stages,” in The Biology of Sea Turtles, Vol. 2, eds J. A. Musick, J. Wyneken, and L. Lutz (Boca Raton, FL: CRC Press), 243–257.
Bost, C. A., Cotté, C., Bailleul, F., Cherel, Y., Charrassin, J. B., Guinet, C., et al. (2009). The importance of oceanographic fronts to marine birds and mammals of the southern oceans. J. Mar. Syst. 78, 363–376. doi: 10.1016/j.jmarsys.2008.11.022
Boyle, M. C. (2006). Post-Hatchling Sea Turtle Biology. Ph.D. thesis. Brisbane, QLD: James Cook University.
Boyle, M. C., and Limpus, C. J. (2008). The stomach contents of post-hatchling green and loggerhead sea turtles in the southwest Pacific: an insight into habitat association. Mar. Biol. 155, 233–241. doi: 10.1007/s00227-008-1022-z
Boyle, M. C., Fitzsimmons, N. N., Limpus, C. J., Kelez, S., Velez-Zuazo, X., and Waycott, M. (2009). Evidence for transoceanic migrations by loggerhead sea turtles in the southern Pacific Ocean. Proc. Biol. Sci. 276, 1993–1999. doi: 10.1098/rspb.2008.1931
Boyle, M., FitzSimmons, N., and van de Merwe, J. (2017). A Recovery Plan for Marine Turtles. (Brisbane, QLD: Department of environment and Energy).
Brignac, K. C., Jung, M. R., King, C., Royer, S., Blickley, L., Lamson, M. R., et al. (2019). Marine debris polymers on main Hawaiian Island Beaches, sea surface, and sea floor. Environ. Sci. Technol. 53, 12218–12226. doi: 10.1021/acs.est.9b03561
Bugoni, L., Krause, L., and Virgiânia, M. (2001). Marine debris and human impacts on sea turtles in southern Brazil. Mar. Pollut. Bull. 42, 1330–1334.
Camedda, A., Marra, S., Matiddi, M., Massaro, G., Coppa, S., Perilli, A., et al. (2014). Interaction between loggerhead sea turtles (Caretta caretta) and marine litter in Sardinia (Western Mediterranean Sea). Mar. Environ. Res. 100, 25–32. doi: 10.1016/j.marenvres.2013.12.004
Campani, T., Baini, M., Giannetti, M., Cancelli, F., Mancusi, C., Serena, F., et al. (2013). Presence of plastic debris in loggerhead turtle stranded along the tuscany coasts of the pelagos sanctuary for Mediterranean Marine Mammals (Italy). Mar. Pollut. Bull. 74, 225–230. doi: 10.1016/j.marpolbul.2013.06.053
Caron, A. G. M., Thomas, C. R., Berry, K. L. E., Motti, C. A., Ariel, E., and Brodie, J. E. (2018). Ingestion of microplastic debris by green sea turtles (Chelonia mydas) in the great barrier reef: validation of a sequential extraction protocol. Mar. Pollut. Bull. 127, 743–751. doi: 10.1016/J.MARPOLBUL.2017.12.062
Carr, A. (1987). New perspectives on the pelagic stage of sea turtle development. Conserv. Biol. 1, 103–121. doi: 10.1111/j.1523-1739.1987.tb00020.x
Casale, P., Freggi, D., Paduano, V., and Oliverio, M. (2016). Biases and best approaches for assessing debris ingestion in sea turtles, with a case study in the Mediterranean. Mar. Pollut. Bull. 110, 238–249. doi: 10.1016/j.marpolbul.2016.06.057
Cassola, G., Zadjelovic, V., Gibson, M. I., and Christie-oleza, J. A. (2019). Distribution of plastic polymer types in the marine environment; A meta- analysis. J. Hazard. Mater. 369, 691–698. doi: 10.1016/j.jhazmat.2019.02.067
Clukey, K. E., Lepczyk, C. A., Balazs, G. H., Work, T. M., and Lynch, J. M. (2017a). Investigation of plastic debris ingestion by four species of sea turtles collected as bycatch in pelagic Pacific longline fisheries. Mar. Pollut. Bull. 120, 117–125. doi: 10.1016/j.marpolbul.2017.04.064
Clukey, K. E., Lepczyk, C. A., Balazs, G. H., Work, T. M., Li, Q. X., Bachman, M. J., et al. (2017b). Persistent organic pollutants in fat of three species of Pacific pelagic longline caught sea turtles: accumulation in relation to ingested plastic marine debris. Sci. Total Environ. 610–611, 402–411. doi: 10.1016/j.scitotenv.2017.07.242
Cole, M., Lindeque, P., Halsband, C., and Galloway, T. S. (2011). Microplastics as contaminants in the marine environment: a review. Mar. Pollut. Bull. 62, 2588–2597. doi: 10.1016/j.marpolbul.2011.09.025
Colferai, A. S., Pinho Silva-Filho, R., Moreira Martins, A., and Bugoni, L. (2017). Distribution pattern of anthropogenic marine debris along the gastrointestinal tract of green turtles (Chelonia mydas) as implications for rehabilitation. Mar. Pollut. Bull. 119, 231–237. doi: 10.1016/j.marpolbul.2017.03.053
Critchell, K., Grech, A., Schlaefer, J., Andutta, F. P., Lambrechts, J., Wolanski, E., et al. (2015). Modelling the fate of marine debris along a complex shoreline: lessons from the great barrier reef. Estuar. Coast. Shelf Sci. 167, 414–426. doi: 10.1016/J.ECSS.2015.10.018
Critchell, K., Hamann, M., Wildermann, N., and Grech, A. (2019). Predicting the exposure of coastal species to plastic pollution in a complex island archipelago. Environ. Pollut. 252, 982–991. doi: 10.1016/j.envpol.2019.06.031
Di Beneditto, A. P. M., and Awabdi, D. R. (2014). How marine debris ingestion differs among megafauna species in a tropical coastal area. Mar. Pollut. Bull. 88, 86–90. doi: 10.1016/j.marpolbul.2014.09.020
Digka, N., Bray, L., Tsangaris, C., Andreanidou, K., Kasimati, E., Kofidou, E., et al. (2020). Evidence of ingested plastics in stranded loggerhead sea turtles along the Greek coastline, East Mediterranean Sea. Environ. Pollut. 263:114596. doi: 10.1016/j.envpol.2020.114596
Domènech, F., Aznar, F. J., Raga, J. A., and Tomás, J. (2019). Two decades of monitoring in marine debris ingestion in loggerhead sea turtle. Caretta caretta, from the western Mediterranean. Environ. Pollut. 244, 367–378. doi: 10.1016/j.envpol.2018.10.047
Duncan, E. M., Arrowsmith, J. A., Bain, C. E., Bowdery, H., Broderick, A. C., Chalmers, T., et al. (2019a). Diet-related selectivity of macroplastic ingestion in green turtles (Chelonia mydas) in the eastern Mediterranean. Sci. Rep. 9, 1–8. doi: 10.1038/s41598-019-48086-4
Duncan, E. M., Broderick, A. C., Fuller, W. J., Galloway, T. S., Godfrey, M. H., Hamann, M., et al. (2019b). Microplastic ingestion ubiquitous in marine turtles. Glob. Change Biol. 25, 744–752. doi: 10.1111/gcb.14519
Duncan, E., Botterell, Z., Broderick, A., Galloway, T., Lindeque, P., Nuno, A., et al. (2017). A global review of marine turtle entanglement in anthropogenic debris: a baseline for further action. Endanger. Species Res. 34, 431–448. doi: 10.3354/esr00865
Eastman, C. B., Farrell, J. A., Whitmore, L., Ramia, D. R. R., Thomas, R. S., Prine, J., et al. (2020). Plastic Ingestion in post-hatchling sea turtles?: assessing a major threat in Florida near shore waters. Front. Mar. Sci. 7, 1–11. doi: 10.3389/fmars.2020.00693
Etnoyer, P., Canny, D., Mate, B. R., Morgan, L. E., Ortega-Ortiz, J. G., and Nichols, W. J. (2006). Sea-surface temperature gradients across blue whale and sea turtle foraging trajectories off the Baja California Peninsula, Mexico. Deep. Res. Part II Top. Stud. Oceanogr. 53, 340–358. doi: 10.1016/j.dsr2.2006.01.010
Feng, M., Böning, C., Biastoch, A., Behrens, E., Weller, E., and Masumoto, Y. (2011). The reversal of the multi-decadal trends of the equatorial Pacific easterly winds, and the Indonesian throughflow and Leeuwin Current transports. Geophys. Res. Lett. 38, 1–6. doi: 10.1029/2011GL047291
Frick, M., Williams, K., Bolten, A., Bjorndal, K., and Martins, H. (2009). Foraging ecology of oceanic-stage loggerhead turtles Caretta caretta. Endanger. Species Res. 9, 91–97. doi: 10.3354/esr00227
Fukuoka, T., Yamane, M., Kinoshita, C., Narazaki, T., Marshall, G. J., Abernathy, K. J., et al. (2016). The feeding habit of sea turtles influences their reaction to artificial marine debris. Sci. Rep. 6:28015. doi: 10.1038/srep28015
Gago, J., Carretero, O., Filgueiras, A. V., and Viñas, L. (2018). Synthetic microfibers in the marine environment: a review on their occurrence in seawater and sediments. Mar. Pollut. Bull. 127, 365–376. doi: 10.1016/J.MARPOLBUL.2017.11.070
Galgani, F., Claro, F., Depledge, M., and Fossi, C. (2014). Monitoring the impact of litter in large vertebrates in the Mediterranean Sea within the European Marine Strategy Framework Directive (MSFD): constraints, specificities and recommendations. Mar. Environ. Res. 100, 3–9. doi: 10.1016/j.marenvres.2014.02.003
Gall, S. C., and Thompson, R. C. (2015). The impact of debris on marine life. Mar. Pollut. Bull. 92, 170–179. doi: 10.1016/j.marpolbul.2014.12.041
Godoy, D., and Stockin, K. (2018). Anthropogenic impacts on green turtles Chelonia mydas in New Zealand. Endanger. Species Res. 37, 1–9. doi: 10.3354/esr00908
Gove, J. M., Whitney, J. L., McManus, M. A., Lecky, J., Carvalho, F. C., Lynch, J. M., et al. (2019). Prey-size plastics are invading larval fish nurseries. Proc. Natl. Acad. Sci. U.S.A. 116, 24143–24149. doi: 10.1073/pnas.1907496116
Gunn, R., Hardesty, B. D., and Butler, J. (2010). Tackling “ghost nets”: local solutions to a global issue in northern Australia. Ecol. Manag. Restor. 11, 88–98. doi: 10.1111/j.1442-8903.2010.00525.x
Hoarau, L., Ainley, L., Jean, C., and Ciccione, S. (2014). Ingestion and defecation of marine debris by loggerhead sea turtles, Caretta caretta, from by-catches in the South-West Indian Ocean. Mar. Pollut. Bull. 84, 90–96. doi: 10.1016/j.marpolbul.2014.05.031
Jambeck, J. R., Geyer, R., Wilcox, C., Siegler, T. R., Perryman, M., Andrady, A., et al. (2015). Plastic waste inputs from land into the ocean. Science 347, 768–771. doi: 10.1126/science.1260352
Jâms, I. B., Windsor, F. M., Poudevigne-Durance, T., Ormerod, S. J., and Durance, I. (2020). Estimating the size distribution of plastics ingested by animals. Nat. Commun. 11, 1–7. doi: 10.1038/s41467-020-15406-6
Jung, M. R., Balazs, G. H., Work, T. M., Jones, T. T., Orski, S. V., and Rodriguez, C. V. (2018a). Polymer identification of plastic debris ingested by pelagic-phase sea turtles in the central Pacific. Environ. Sci. Technol. 52, 11535–11544. doi: 10.1021/acs.est.8b03118
Jung, M. R., Horgen, F. D., Orski, S. V., Rodriguez, V., Beers, K. L., Balazs, G. H., et al. (2018b). Validation of ATR FT-IR to identify polymers of plastic marine debris, including those ingested by marine organisms. Mar. Pollut. Bull. 127, 704–716. doi: 10.1016/j.marpolbul.2017.12.061
Kiessling, I., Office, O., Geeves, W., Szabo, S., Cuttriss, L., and Wwf, D. W. (2003). Derelicy Fishing Gear and Other Marine Debris in Northern Australia. Hobart, TAS: National Oceans Office (Australia).
Kokko, H., and Sutherland, W. J. (2001). Evolutionary Ecology Research. Evolutionary Ecology. Available online at: http://www.evolutionary-ecology.com/abstracts/v03/1308.html (accessed March 15, 2018).
Kühn, S., and Van Franeker, J. A. (2020). Quantitative overview of marine debris ingested by marine megafauna. Mar. Pollut. Bull. 151:110858. doi: 10.1016/j.marpolbul.2019.110858
Lazar, B., Maslov, L., Romani, S. H., Graèan, R., Krauthacker, B., Holcer, D., et al. (2011). Accumulation of organochlorine contaminants in loggerhead sea turtles, Caretta caretta, from the eastern Adriatic Sea. Chemosphere 82, 121–129. doi: 10.1016/j.chemosphere.2010.09.015
Limpus, C. J. (2007). A Biological Review of Australian Marine Turtles 5. Flatback turtle Nator Depressus (Garman). Brisbane, QLD: Queensland Environmental Protection Agency
Limpus, C. J. (2009). A Biological Review of Australian Marine Turtles. Brisbane, QLD: Queensland Environmental Protection Agency.
Limpus, C. J., and Nicholls, N. (1988). The southern oscillation regulates the annual numbers of green turtles (Chelonia mydas) breeding around Northern Australia. Wildl. Res. 15, 157–161. doi: 10.1071/WR9880157
Lynch, J. M. (2018). Quantities of marine debris ingested by sea turtles: global meta- analysis highlights need for standardized data reporting methods and reveals relative risk. Environ. Sci. Technol. 52, 12026–12038. doi: 10.1021/acs.est.8b02848
Machovsky-Capuska, G. E., Andrades, R., and Santos, R. G. (2020). Debris ingestion and nutritional niches in estuarine and reef green turtles. Mar. Pollut. Bull. 153:110943. doi: 10.1016/j.marpolbul.2020.110943
Mansfield, K. L., Saba, V. S., Keinath, J. A., and Musick, J. A. (2009). Satellite tracking reveals a dichotomy in migration strategies among juvenile loggerhead turtles in the Northwest Atlantic. Mar. Biol. 156, 2555–2570. doi: 10.1007/s00227-009-1279-x
Marn, N., Jusup, M., Kooijman, S. A. L. M., and Klanjscek, T. (2020). Quantifying impacts of plastic debris on marine wildlife identifies ecological breakpoints. Ecol. Lett. 23, 1479–1487. doi: 10.1111/ele.13574
Mascarenhas, R., Santos, R., and Zeppelini, D. (2004). Plastic debris ingestion by sea turtle in Paraíba, Brazil. Mar. Pollut. Bull. 49, 354–355. doi: 10.1016/j.marpolbul.2004.05.006
Matiddi, M., Hochsheid, S., Camedda, A., Baini, M., Cocumelli, C., Serena, F., et al. (2017). Loggerhead sea turtles (Caretta caretta): a target species for monitoring litter ingested by marine organisms in the Mediterranean Sea. Environ. Pollut. 230, 199–209. doi: 10.1016/j.envpol.2017.06.054
McCauley, S. J., and Bjorndal, K. A. (1999). Conservation implications of dietary dilution from debris ingestion: sublethal effects in post-hatchling loggerhead sea turtles. Conserv. Biol. 13, 925–929. doi: 10.1046/j.1523-1739.1999.98264.x
Miller, P. I., Scales, K. L., Ingram, S. N., Southall, E. J., and Sims, D. W. (2015). Basking sharks and oceanographic fronts: quantifying associations in the north−east Atlantic. Funct. Ecol. 29, 1099–1109. doi: 10.1111/1365-2435.12423
Musick, J. A., and Limpus, C. J. (1997). “Habitat utilization and migration in juvenile sea turtles,” in The Biology of Sea Turtles, eds P. L. Lutz, J. A. Musick, and J. Wyneken (Boca Raton, BR: CRC Press).
Nelms, S. E., Duncan, E. M., Broderick, A. C., Galloway, T. S., Godfrey, M. H., Hamann, M., et al. (2016). Plastic and marine turtles: a review and call for research. ICES J. Mar. Sci. 73, 165–181. doi: 10.1093/icesjms/fsv165
Nelms, S. E., Duncan, E. M., Patel, S., Badola, R., Bhola, S., Chakma, S., et al. (2021). Riverine plastic pollution from fisheries?: insights from the ganges river system. Sci. Total Environ. 756:143305. doi: 10.1016/j.scitotenv.2020.143305
Ng, C. K. Y., Ang, P. O., Russell, D. J., Balazs, G. H., and Murphy, M. B. (2016). Marine macrophytes and plastics consumed by green turtles (Chelonia mydas) in Hong Kong, South China sea region. Chelonian Conserv. Biol. 15, 289–292. doi: 10.2744/CCB-1210.1
Orós, J., Montesdeoca, N., Camacho, M., Arencibia, A., and Calabuig, P. (2016). Causes of stranding and mortality, and final disposition of loggerhead sea turtles (Caretta caretta) admitted to a wildlife rehabilitation center in Gran Canaria Island, Spain (1998-2014): a long-term retrospective study. PLoS One 11:e0149398. doi: 10.1371/journal.pone.0149398
Pattiaratchi, C., Van Der Mheen, M., Schlundt, C., Narayanaswamy, B. E., Sura, A., Hajbane, S., et al. (2021). Plastics in the Indian Ocean–sources, fate, distribution and impacts. Ocean Sci. [Preprint]. doi: 10.5194/os-2020-127
Pfaller, J. B., Goforth, K. M., Gil, M. A., Savoca, M. S., and Lohmann, K. J. (2020). Current biology odors from marine plastic debris elicit foraging behavior in sea turtles. Curr. Biol. 30, 191–214. doi: 10.1016/j.cub.2020.01.071
Pham, C. K., Rodríguez, Y., Dauphin, A., Carriço, R., Frias, J. P. G. L., Vandeperre, F., et al. (2017). Plastic ingestion in oceanic-stage loggerhead sea turtles (Caretta caretta) off the North Atlantic subtropical gyre. Mar. Pollut. Bull. 121, 222–229. doi: 10.1016/j.marpolbul.2017.06.008
Polovina, J. J., Balazs, G. H., Howell, E. A., Parker, D. M., Seki, M. P., and Dutton, P. H. (2004). Forage and migration habitat of loggerhead (Caretta caretta) and olive ridley (Lepidochelys olivacea) sea turtles in the central North Pacific Ocean. Fish. Oceanogr. 13, 36–51. doi: 10.1046/j.1365-2419.2003.00270.x
Poppi, L., Zaccaroni, A., Pasotto, D., Dotto, G., Marcer, F., Scaravelli, D., et al. (2012). Post-mortem investigations on a leatherback turtle Dermochelys coriacea stranded along the Northern Adriatic coastline. Dis. Aquat. Organ. 100, 71–76. doi: 10.3354/dao02479
Putman, N. F., Verley, P., Shay, T. J., and Lohmann, K. J. (2012). Simulating transoceanic migrations of young loggerhead sea turtles: merging magnetic navigation behavior with an ocean circulation model. J. Exp. Biol. 215, 1863–1870. doi: 10.1242/jeb.067587
Rice, N., Hirama, S., and Witherington, B. (2021). High frequency of micro- and meso-plastics ingestion in a sample of neonate sea turtles from a major rookery. Mar. Pollut. Bull. 167:112363. doi: 10.1016/j.marpolbul.2021.112363
Rizzi, M., Rodrigues, F. L., Medeiros, L., Ortega, I., Rodrigues, L., Monteiro, D. S., et al. (2019). Ingestion of plastic marine litter by sea turtles in southern Brazil: abundance, characteristics and potential selectivity. Mar. Pollut. Bull. 140, 536–548. doi: 10.1016/j.marpolbul.2019.01.054
Robertson, B. A., and Blumstein, D. T. (2019). How to disarm an evolutionary trap. Conserv. Sci. Pract. 1:e116. doi: 10.1111/csp2.116
Robson, N. A., Hetzel, Y., Whiting, S., Wijeratne, S., Pattiaratchi, C. B., Withers, P., et al. (2017). Use of particle tracking to determine optimal release dates and locations for rehabilitated neonate sea turtles. Front. Mar. Sci. 4:173 doi: 10.3389/fmars.2017.00173
Ryan, P. G., Cole, G., Spiby, K., Nel, R., Osborne, A., and Perold, V. (2016). Impacts of plastic ingestion on post-hatchling loggerhead turtles off South Africa. Mar. Pollut. Bull. 107, 155–160. doi: 10.1016/j.marpolbul.2016.04.005
Salmon, M., Hamann, M., and Wyneken, J. (2010). The development of early diving behavior by juvenile flatback sea turtles (Natator depressus). Chelonian Conserv. Biol. 9, 8–17. doi: 10.2744/ccb-0803.1
Santos, R. G., Andrades, R., Boldrini, M. A., and Martins, A. S. (2015). Debris ingestion by juvenile marine turtles: an underestimated problem. Mar. Pollut. Bull. 93, 37–43. doi: 10.1016/j.marpolbul.2015.02.022
Santos, R. G., Andrades, R., Fardim, L. M., and Martins, A. S. (2016). Marine debris ingestion and Thayer’s law–The importance of plastic color. Environ. Pollut. 214, 585–588. doi: 10.1016/j.envpol.2016.04.024
Schuyler, Q., Hardesty, B. D., Wilcox, C., and Townsend, K. (2012). To eat or not to eat? debris selectivity by marine turtles. PLoS One 7:e40884. doi: 10.1371/journal.pone.0040884
Schuyler, Q., Hardesty, B. D., Wilcox, C., and Townsend, K. (2014). Global analysis of anthropogenic debris ingestion by sea turtles. Conserv. Biol. 28, 129–139. doi: 10.1111/cobi.12126
Scott, R., Biastoch, A., Roder, C., Stiebens, V. A., and Eizaguirre, C. (2014). Nano-tags for neonates and ocean- mediated swimming behaviours linked to rapid dispersal of hatchling sea turtles. Proc. R. Soc. B Biol. Sci. 281:20141209.
Senko, J., Nelms, S., Reavis, J., Witherington, B., Godley, B., and Wallace, B. (2020). Understanding individual and population-level effects of plastic pollution on marine megafauna. Endanger. Species Res. 43, 234–252. doi: 10.3354/esr01064
Siegelman, L., O’Toole, M., Flexas, M., Rivière, P., and Klein, P. (2019). Submesoscale ocean fronts act as biological hotspot for southern elephant seal. Sci. Rep. 9, 1–13. doi: 10.1038/s41598-019-42117-w
Silva, A. B., Bastos, A. S., Justino, C. I. L., da Costa, J. P., Duarte, A. C., and Rocha-Santos, T. A. P. (2018). Microplastics in the environment: challenges in analytical chemistry–A review. Anal. Chim. Acta 1017, 1–19. doi: 10.1016/J.ACA.2018.02.043
Stamper, M. A., Spicer, C. W., Neiffer, D. L., Mathews, K. S., and Fleming, G. J. (2009). Morbidity in a juvenile green sea turtle (Chelonia mydas) due to ocean-borne plastic. J. Zoo Wildl. Med. 40, 196–198. doi: 10.1638/2007-0101.1
Tomas, J., Guitart, R., Mateo, R., and Raga, J. (2002). Marine debris ingestion in loggerhead sea turtles, from the Western Mediterranean. Mar. Pollut. Bull 44, 211–216. doi: 10.1016/S0025-326X(01)00236-3
Van Der Mheen, M., Van Sebille, E., and Pattiaratchi, C. (2020). Beaching patterns of plastic debris along the Indian Ocean rim. Ocean Sci. 16, 1317–1336. doi: 10.5194/os-16-1317-2020
van Franeker, J. A., Blaize, C., Danielsen, J., Fairclough, K., Gollan, J., Guse, N., et al. (2011). Monitoring plastic ingestion by the northern fulmar Fulmarus glacialis in the North Sea. Environ. Pollut. 159, 2609–2615. doi: 10.1016/j.envpol.2011.06.008
van Sebille, E., England, M. H., and Froyland, G. (2012). Origin, dynamics and evolution of ocean garbage patches from observed surface drifters. Environ. Res. Lett. 7:044040. doi: 10.1088/1748-9326/7/4/044040
van Sebille, E., Wilcox, C., Lebreton, L., Maximenko, N., Hardesty, B. D., van Franeker, J. A., et al. (2015). A global inventory of small floating plastic debris. Environ. Res. Lett. 10:124006. doi: 10.1088/1748-9326/10/12/124006
Vandeperre, F., Parra, H., Pham, C. K., Machete, M., Santos, M., Bjorndal, K. A., et al. (2019). Relative abundance of oceanic juvenile loggerhead sea turtles in relation to nest production at source rookeries: implications for recruitment dynamics. Sci. Rep. 9, 0–12. doi: 10.1038/s41598-019-49434-0
Vélez-Rubio, G. M., Teryda, N., Asaroff, P. E., Estrades, A., Rodriguez, D., and Tomás, J. (2018). Differential impact of marine debris ingestion during ontogenetic dietary shift of green turtles in Uruguayan waters. Mar. Pollut. Bull. 127, 603–611. doi: 10.1016/j.marpolbul.2017.12.053
Wedemeyer-Strombel, K. R., Balazs, G. H., Johnson, J. B., Peterson, T. D., Wicksten, M. K., and Plotkin, P. T. (2015). High frequency of occurrence of anthropogenic debris ingestion by sea turtles in the North Pacific Ocean. Mar. Biol. 162, 2079–2091. doi: 10.1007/s00227-015-2738-1
White, E., Clark, S., Manire, C. A., Crawford, B., Wang, S., Locklin, J., et al. (2018). Ingested micronizing plastic particle compositions and size distributions within stranded post-hatchling sea turtles. Environ. Sci. Technol 52, 10307–10316. doi: 10.1021/acs.est.8b02776
Whiting, A. U., Thomson, A., Chaloupka, M., and Limpus, C. J. (2008). Seasonality, abundance and breeding biology of one of the largest populations of nesting flatback turtles, Natator depressus: cape domett, Western Australia. Aust. J. Zool. 56:297. doi: 10.1071/ZO08038
Whitney, J. L., Gove, J. M., McManus, M. A., Smith, K. A., Lecky, J., Neubauer, P., et al. (2021). Surface slicks are pelagic nurseries for diverse ocean fauna. Sci. Rep. 11, 1–18. doi: 10.1038/s41598-021-81407-0
Wilcox, C., Hardesty, B. D., Sharples, R., Griffin, D. A., Lawson, T. J., and Gunn, R. (2013). Ghostnet impacts on globally threatened turtles, a spatial risk analysis for northern Australia. Conserv. Lett. 6, 247–254. doi: 10.1111/conl.12001
Wilcox, C., Heathcote, G., Goldberg, J., Gunn, R., Peel, D., and Hardesty, B. D. (2015). Understanding the sources and effects of abandoned, lost, and discarded fishing gear on marine turtles in northern Australia. Conserv. Biol. 29, 198–206. doi: 10.1111/cobi.12355
Wilcox, C., Puckridge, M., Schuyler, Q. A., Townsend, K., and Hardesty, B. D. (2018). A quantitative analysis linking sea turtle mortality and plastic debris ingestion. Sci. Rep. 8, 1–11. doi: 10.1038/s41598-018-30038-z
Wildermann, N., Critchell, K., Fuentes, M. M. P. B., Limpus, C. J., Wolanski, E., and Hamann, M. (2017). Does behaviour affect the dispersal of flatback post-hatchlings in the great barrier reef? R. Soc. Open Sci. 4:170164. doi: 10.1098/rsos.170164
Witherington, B. E. (2002). Ecology of neonate loggerhead turtles inhabiting lines of downwelling near a Gulf Stream front. Mar. Biol. 140, 843–853. doi: 10.1007/s00227-001-0737-x
Witherington, B., Hirama, S., and Hardy, R. (2012). Young sea turtles of the pelagic Sargassum-dominated drift community: habitat use, population density, and threats. Mar. Ecol. Prog. Ser. 463, 1–22. doi: 10.3354/meps09970
Wolanski, E. (2017). Bounded and unbounded boundaries–Untangling mechanisms for estuarine-marine ecological connectivity: scales of m to 10,000 km–A review. Estuar. Coast. Shelf Sci. 198, 378–392. doi: 10.1016/J.ECSS.2016.06.022
Yaghmour, F., Al Bousi, M., Whittington-Jones, B., Pereira, J., García-Nuñez, S., and Budd, J. (2018). Marine debris ingestion of green sea turtles. Chelonia mydas, (Linnaeus, 1758) from the eastern coast of the United Arab Emirates. Mar. Pollut. Bull. 135, 55–61. doi: 10.1016/J.MARPOLBUL.2018.07.013
Keywords: plastic, marine turtle, pollution, marine debris, evolutionary trap
Citation: Duncan EM, Broderick AC, Critchell K, Galloway TS, Hamann M, Limpus CJ, Lindeque PK, Santillo D, Tucker AD, Whiting S, Young EJ and Godley BJ (2021) Plastic Pollution and Small Juvenile Marine Turtles: A Potential Evolutionary Trap. Front. Mar. Sci. 8:699521. doi: 10.3389/fmars.2021.699521
Received: 23 April 2021; Accepted: 28 June 2021;
Published: 02 August 2021.
Edited by:
Robson G. Santos, Federal University of Alagoas, BrazilReviewed by:
Blair Witherington, Inwater Research Group, Inc., United StatesJennifer Lynch, National Institute of Standards and Technology (NIST), United States
Copyright © 2021 Duncan, Broderick, Critchell, Galloway, Hamann, Limpus, Lindeque, Santillo, Tucker, Whiting, Young and Godley. This is an open-access article distributed under the terms of the Creative Commons Attribution License (CC BY). The use, distribution or reproduction in other forums is permitted, provided the original author(s) and the copyright owner(s) are credited and that the original publication in this journal is cited, in accordance with accepted academic practice. No use, distribution or reproduction is permitted which does not comply with these terms.
*Correspondence: Emily M. Duncan, ed291@exeter.ac.uk; Brendan J. Godley, B.J.Godley@exeter.ac.uk