- 1Biodiversity Unit, Archipelago Research Institute, University of Turku, Turku, Finland
- 2Department of Biological and Environmental Science, University of Jyväskylä, Jyväskylä, Finland
- 3Pyhäjärvi Institute, Kauttua, Finland
In fish, the lipid resources of the female form a link between the environment and progeny, contributing to the contents of the egg yolk. Variation of the environmental conditions is therefore expected to affect the egg quality via maternal pathways, reflecting the female’s response to the environmental factors before spawning. We investigated the content of lipids and thyroid hormones in the ovary and eggs of the Baltic herring during 1988–2019, when salinity of the Baltic Sea first declined and then stabilized to a lower level, and winters varied between severe and mild. The total lipid resources of spawning females decreased by 40–50% during the study, and the ovarian lipid concentration followed this trend resulting in a decrease of the lipid content of eggs. The concentration of thyroid hormones in the ovary suggested a hormonal response in females to salinity and winter temperature and was observable also in the content of thyroid hormones in the eggs. A change in the egg lipid content took place approximately around 1998 (SE ± 3 years) suggesting that in declining salinity, egg quality was associated with salinity and intrinsic factors in the female, i.e., fecundity and amount of lipids deposited into the ovary. However, during the period of stable salinity, egg quality was mainly limited by the female’s lipid resources. As also the body size of the females decreased over the years, it is suggested that small body size can be a key phenotypic trait to reduce the environmental impact on egg quality in variable environmental conditions.
Introduction
The variability of climate is a major factor contributing to the fluctuation of fish populations and stocks affecting especially the recruitment of small-sized pelagic fish like anchovies, sardines and herring (Lehodey et al., 2006; Peck et al., 2021). In these species, environmental factors modify the reproductive success when the eggs and sperm are released into the water, but they act also through the parent population by affecting the females’ capacities to produce viable offspring. The influence from the maternal environment originates from various sources depending on the reproductive mode of the species (e.g., Green, 2008), but ultimately, the resources used for the build-up of gonadal products are derived from the female’s body.
The energy budget of the female depends on the incoming energy, i.e., the availability and quality of food, but abiotic factors like salinity and temperature matter as well, since they impact directly on the neuroendocrine system that regulates the metabolism and energy expenditure of the fish via hormonal pathways. Abiotic factors, if non-optimal for the species, may change the partitioning of energy reserves between different body functions, as the hormonal system responds to the environmental stress, which complicates the linkage of the environment to the offspring (Zera and Harshman, 2001; Schulte, 2014). In small pelagic fish, the lipid reserves of the female form a relatively direct link between the environment and progeny (Brosset et al., 2016), since during oogenesis, the female deposits lipids into the ovary, where they form the main constituent of the egg yolk and act as carriers of hormones and vitamins to the eggs and larvae (e.g., Reading et al., 2018). Being maternally derived, the thyroid hormones (THs) are the mediator of maternal effects in all vertebrates (Ruuskanen and Hsu, 2018), and in fish, they contribute to the development of the embryo and larvae in various ways (Ayson and Lam, 1993; Yoo et al., 2000; Power et al., 2001; Liu and Chan, 2002; Castillo et al., 2015; Deal and Volkoff, 2020).
The Baltic herring (Clupea harengus membras) is a small-sized pelagic species that has a Baltic-wide distribution, a high but variable stock biomass and a central position in the ecosystem and fisheries of the Baltic Sea. For a marine fish, the Baltic Sea is a challenging environment because of its low and unstable salinity, which varies irregularly as a consequence of the freshwater discharge and inflows of high-saline water from the Atlantic Ocean (Hänninen et al., 2000). Under these conditions, the maternal influence on offspring quality is expected to increase (Green, 2008; Kuijper and Hoyle, 2015), since the alternating periods of high and low salinity put the fish under physiological stress induced by abiotic or biotic factors, or both. During the latest high-salinity period from the late 1970s onwards, the growth rate of herring increased, but as the period lasted for only a few years, growth decreased resulting in a reduction of the body size and fish condition (Rönkkönen et al., 2004; Casini et al., 2011). With the salinity decline, also the lipid content of spawning females decreased indicating intra-specific food competition and a response to salinity (Rajasilta et al., 2018).
Against this background, our aim was to examine the impact of salinity and winter temperature on the egg quality of the Baltic herring, when also the female’s own characteristics change. The fish samples were collected from the Archipelago Sea, northern Baltic, where the spawning herring population has been monitored by annual samplings since 1984 (Rajasilta et al., 2006). The data analyzed consisted of determinations of the lipid concentration of the herring ovary from 1988 to 2019 in addition to the muscle lipids and other traits of the spawning fish. The concentration of THs in the ovary was determined from preserved samples as another key trait of egg quality, and the amounts of lipids and THs per one egg were estimated using the fecundity-ovary weight-relationship and the monitoring data. Generalized Additive Mixed Models (GAMMs) were used to find out how the abiotic factors (salinity and temperature) and female traits (length and lipid reserves) influenced the content of lipids in the ovary and in the eggs.
Materials and Methods
Herring and Environmental Conditions in the Study Area
The herring of the Archipelago Sea (referred as AS; Figure 1) are capital-breeders, which acquire their energy resources during the summer and autumn, store them as lipids in the muscle tissue and develop their gonads during winter, when lipids are transported into the ovary (Vuorela et al., 1979; Rajasilta, 1992). The AS herring reproduce from May to mid-July on shallow bottoms, and show a constant pattern in the use of the spawning beds in our study area (Rajasilta et al., 1993). During 1984–2019, the mean length of the spawning population decreased from 21 to 16 cm due to the reduction of their growth rate (Figure 1). The herring mainly feed and overwinter in the outer archipelago and the Bothnian Sea (BS; Figure 1), which is about a 374,000 km2 wide sea area north of the Baltic Proper. The BS is relatively shallow, with a mean depth of 58 m, and during most winters, is totally or partly ice-covered. Since the late 1980s, water temperature has been increasing during the winter months (Figure 1). After the major inflow of oceanic water in 1976/1977, the mean annual salinity reached its maximum at 6.1 PSU in the upper water column (0–50 m) in 1983 but decreased to its present level (∼5.0 PSU) during approximately 1994–1996 (Figure 1). The oxygen concentration is high, even in the deep bottoms, due to the efficient vertical mixing of the water.
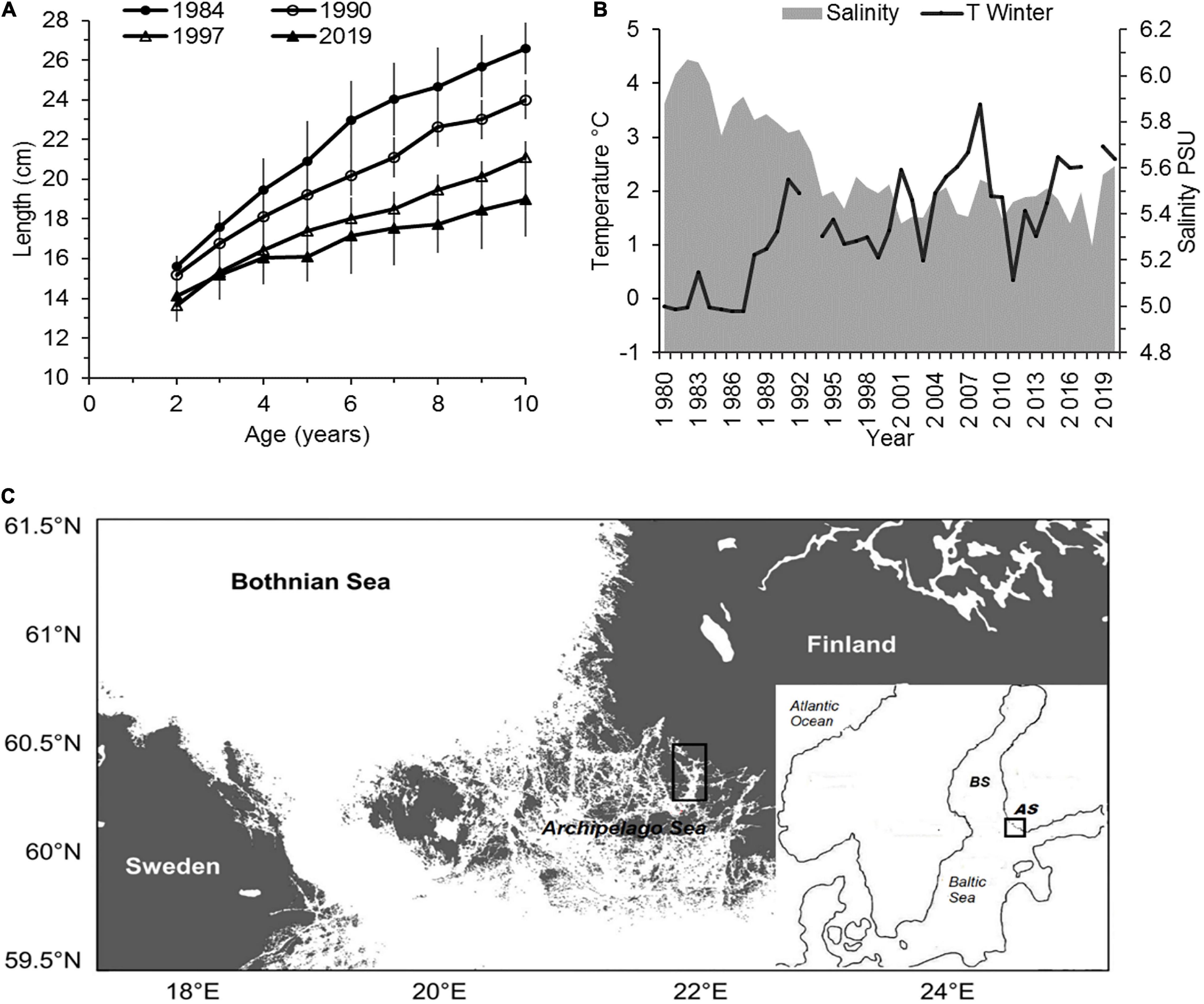
Figure 1. (A) Length-at-age (cm) of spawning Baltic herring population in the Archipelago Sea in 1984, 1990, 1997, and 2019 at ages 2–10 years (mean with SD; own data); (B) salinity (PSU) and winter temperature (°C, mean in January–April at 0–50 m depth) in the Bothnian Sea during 1980–2019 (data source: https://www.ices.dk/data/data-portals/Pages/ocean.aspx); (C) the study area in the Archipelago Sea ( = AS; BS = the Bothnian Sea).
Fish Samples
Herring samples were collected annually from commercial trap nets set on the herring spawning grounds in AS in early spring to monitor the characteristics of the spawning population. The annual sampling aimed at covering the whole spawning season, and all fish were treated following the same procedure (for a detailed description; see Rajasilta et al., 2006). A random sample of about 100–150 fish per sampling date was taken from the trap net catch and brought to the laboratory; the length of fish was measured with up to a 0.1 cm precision, and the sex and maturity stages of the gonads were determined visually using a maturity scale, where 2 = virgin, 3–4 = developing, 5 = ripe, 6 = spawning with no substantial loss of eggs observable, 7 = spawning/spent and 8 = spent. Total body weight and gonad weight were determined as wet weight (WW) at a 0.1 and a 0.01 g precision, respectively, and otoliths were removed for reading the fish age (Eklund et al., 2000; Peltonen et al., 2002). In the present study in 1988–2019, only the early spawning fish were included with a spawning time in May to the middle of June, as this represents the peak spawning time of herring in the study area (Rajasilta et al., 1993). A subsample consisting of 10–30 females at maturity stage 6 was taken randomly from the population samples for the lipid and thyroid hormone analyses (see Supplementary Tables 1,2), wrapped individually in aluminium foil and stored in plastic bags at −25°C for further analyses.
Lipid Analyses
The lipid analyses were made within some weeks or months after sampling with the standard method used for the extraction of storage lipids from fish tissues (e.g., Srigley and Mossoba, 2017). First, fish were thawed at room temperature; the skin was removed and a 3–5 g piece of the dorsal muscle was dissected between the dorsal fin and the tail. For the analyses of the ovarian lipids, both ovaries were taken. The samples’ wet mass was determined (0.1 mg precision), and they were dried to constant weight in a freeze-drier for 48–72 h. Next, samples were homogenized, mixed with a small amount of anhydrous sodium sulfate (Merck KGaA, Darmstadt, Germany) to remove excess moisture, transferred to cellulose tubes and extracted in a Soxhlet apparatus for 6 hours with 150 ml diethyl ether (Merck KGaA, Darmstadt, Germany). The ether was evaporated in a vacuum, and the lipid residue was weighed to the nearest 0.1 mg. Lipid concentration of the ovary and muscle tissue was expressed as a percentage of the sample’s wet mass (% WW), which was recorded for all samples in 1988–2019. The sample’s dry mass (DW at 0.1 mg precision) was available for a shorter period only (1992–2019); therefore, in order to extend the time series, the lipid concentrations on WW were calibrated against the DW data. As they were found to be equal (ovary: R2 = 0.96; p < 0.001; df = 125; muscle: R2 = 0.99; p < 0.001; df = 93), the total amount of lipids in the ovary was calculated from the wet mass of the ovary (mg WW) and the respective values of the lipid concentration (% WW). To determine the total amount of lipids in the muscle tissue, the amount of muscle tissue in the female was first calculated using the formula Total muscle mass (g WW) = 0.303 ∗ Female total weight (g WW) + 1.187 (R2 = 0.96; Rajasilta et al., 2015) and then the lipid content (% WW) of the muscle tissue was applied to these values. For the ovarian lipid analyses, 32 samples were obtained (total number of females analyzed = 380, of which muscle lipids were determined from 17 samples (n = 291).
Thyroid Hormone Concentration in the Ovary
The concentration of thyroid hormones, T4 (thyroxin, tetraiodothyronine) and its active form T3 (triiodothyronine), in the ovary (maturity stage 6) was determined retrospectively from randomly taken samples collected in 1990 (n = 15), 1992 (n = 3), 1994 (n = 3), 1999 (n = 5), 2006 (n = 9), 2010 (n = 10), 2014 (n = 12), 2017 (n = 6), and 2019 (n = 12), and stored at -25°C until analyzed in 2020. Thyroid hormones were analyzed using validated methods following Ruuskanen et al. (2018) assuming only a minor effect of the storage time (Männistö et al., 2007). A small sample of the ovarian tissue (ca. 50 mg) was weighed, and samples were homogenized in methanol using a tissue lyser (Qiagen, Retsch GmbH, Haan, Germany). As an internal recovery tracer, a known amount of 13C12-T4 (Larodan, Sweden) was added to each sample. This allowed us to control for the variation in recovery (i.e., extraction efficiency) for each sample. Next, 600 μl of chloroform was added to sample. After centrifugation (15 min, 1,900 g, +4°C), the supernatant was collected, and the pellet was re-extracted in a mixture of chloroform and methanol (2:1). Back-extraction into an aqueous phase (0.05% CaCl2) was followed by a re-extraction with a mixture of chloroform:methanol: 0.05% CaCl2 (3:49:48) and this phase was further purified in-house on Bio-Rad AG 1-X2 (United States) resin columns. The iodothyronines were eluted with 70% acetic acid and evaporated under N2. Blanks (plain reagents without any sample) were analyzed in each extraction batch to detect any contamination. Samples from different years were equally distributed across the extraction batches. T3 and T4 were quantified using a nanoflow liquid chromatography-mass spectrometry (nano-LC-MS/MS) method, which was developed and validated by Ruuskanen et al. (2018, 2020). Before the analysis, the dry samples were diluted in ammonium (NH3). Internal standards 13C6-T3 and 13C6-T4 (Sigma-Adrich, St. Louis, United States) were added to each sample to identify and quantify the THs. A triple quadrupole mass spectrometer (TSQ Vantage, Thermo Scientific, San Jose, CA) was used to analyze the samples. For the chromatographic separation of hormones, a nanoflow HPLC system Easy-nLC (Thermo Fisher Scientific) was applied. On-column quantification limits were 10.6 amol for T4 and 17.9 amol for T3 (Ruuskanen et al., 2018). MS data was acquired automatically using Thermo Xcalibur software (Thermo Fisher Scientific) and analyzed using Skyline (MacLean et al., 2010). For the analyses, peak-area ratios of sample to internal standard were calculated. For calculating water content and dry mass, another sample of the same ovarian tissue was weighed and dried for 24 h at 60°C. We analyzed pg T3 and total TH (T4+T3) per dry weight, as both hormones (via conversion of T4 to T3) can influence the development of the eggs.
Lipids and Thyroid Hormones in Eggs
The content of lipid and THs per one herring egg was estimated calculating first the total content in the whole ovarian mass, and then dividing the values with the estimated total number of eggs in the ovary (Fecundity, F). Fecundity was calculated from the ovarian weight (maturity stage 6; full ovaries with no observable ovulation) with the equation F = (1542.7 × Ovarian weight g WW) + 330.75 (R2 = 0.94). The equation was formulated from egg number data, determined gravimetrically by counting the number of eggs in subsamples taken from Gilson’s fluid-preserved whole ovaries during 1988–1991 (n = 128) and from subsamples taken during later years (1993–1994; n = 27), containing some hundreds of eggs, which were counted and dried to a constant dry weight (0.01 mg precision) (Laine and Rajasilta, 1998). The calculations of the egg lipid content were made on a wet-mass basis and the egg thyroid hormone (total TH or T3) content was determined using the dry mass values of the ovary to account for the potential effect of storage time on the wet mass.
Environmental Data
Data on seawater salinity and temperature in the Bothnian Sea were obtained from the HELCOM monitoring station SR5 [61°05.00′ N, 19°34.78′ E] (ICES Baltic Sea monitoring data, 2020; https://www.ices.dk/data/data-portals/Pages/ocean.aspx) consisting of samples taken from the water column at different depths. In the present study, values from the depths of 0–50 m were selected as the representative depths of where herring mostly live (Peltonen et al., 2004). Salinity was expressed as the annual means and temperature as the mean values during the winter months preceding the spawning period (mean T°C in January–April).
Statistical Analyses
Three Generalized Additive Mixed Models (GAMMs) were fitted to assess the effects of abiotic factors and female traits on ovarian lipid concentration (% WW) and egg lipid content (μg WW), because they allow one to study the associations between the response variable and predictors without defining a particular curve order a priori, while also accounting for temporal correlation (Wood, 2017). The GAMMs were calculated with the function gamm in the mgcv package version 1.8–28 in the R software version 3.6.1 (R Core Team, 2020). The GAM model A included the arcsine-transformed ovarian lipid concentration (%) as the response variable and salinity, winter temperature and length as the explanatory variables. Length was added as a response variable to the model because in some years the fish length exceeded the population mean length (Figure 2). Model B examined the effect of abiotic factors on the egg lipid content by using the log-transformed egg lipid content as the response variable and salinity and winter temperature as the explanatory variables. Finally, with model C, we studied the effect of female traits (lipid reserves and length) on the log-transformed egg lipid content. In spawning Baltic herring, lipids are found in the muscle and ovarian tissue only, as the visceral lipid depositions formed during the feeding period are depleted before the reproduction (Rajasilta, 1992). The female’s total lipid reserves were therefore expressed as the sum of the total content of lipids in muscle and ovary (Fish Total, mg), and the percentage of ovarian lipids of the total (index OL, %) was calculated to indicate the partitioning of lipids between the ovarian and somatic production (e.g., Calow, 1985; Zera and Harshman, 2001). The model C thus included female length, total lipid content (mg) and the index OL (%) as the explanatory variables. Before fitting the model, the potential interrelationship between the egg lipid content, the index OL and the total lipid content of the females in the annual samples was examined from a correlation matrix and found to be insignificant (p > 0.05) in the total dataset. In all models A–C, the best fit was achieved with a Gaussian distribution and identity link function. Temporal autocorrelation in the time series data was fitted using continuous-time autoregressive of order 1 (corCAR1) structure. In all models, the data analyses were performed on individual basis. Also, if the number of samples was > 1/year they were pooled over each year after testing the group means (p > 0.05 in all cases; see Supplementary Table 1).
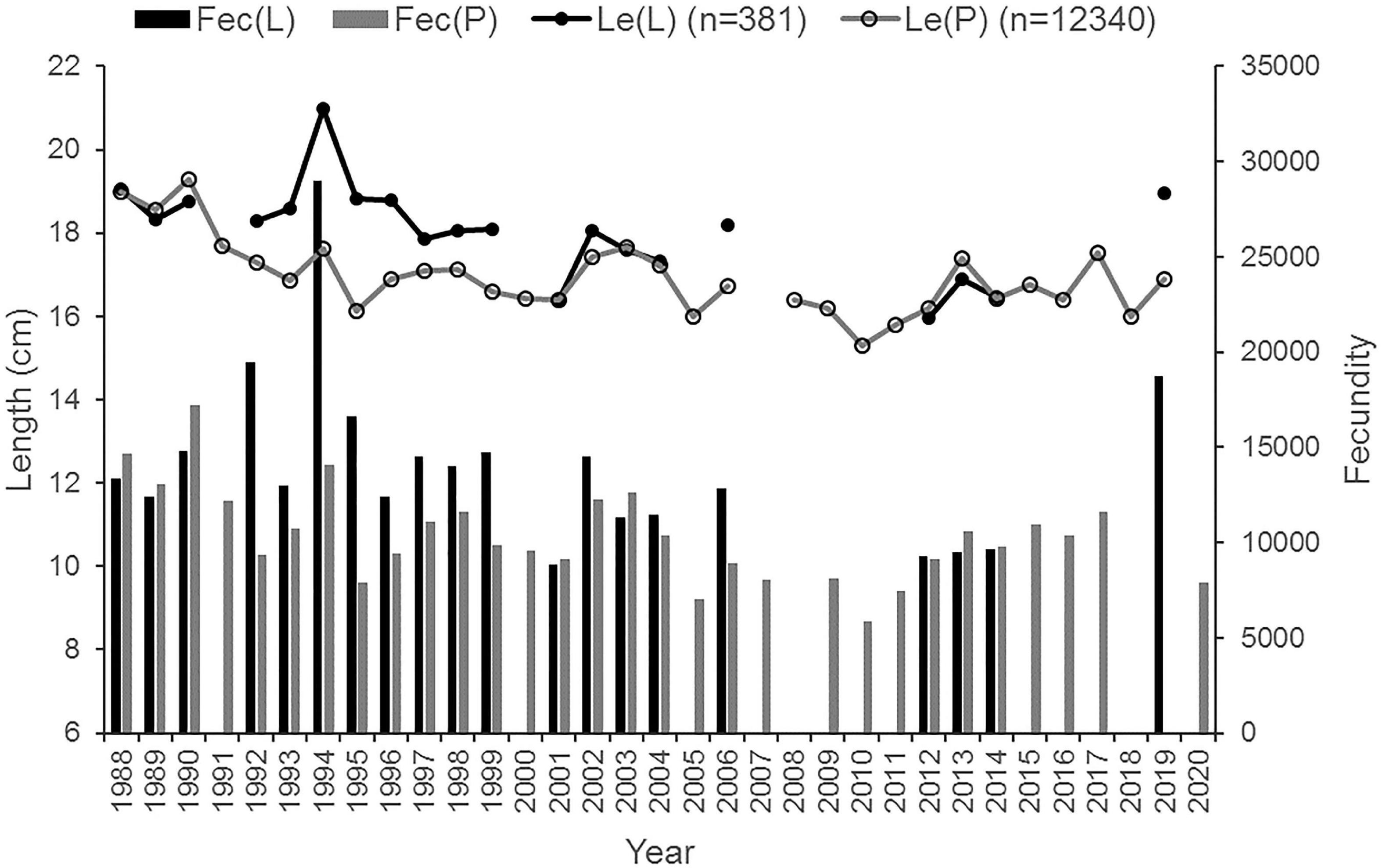
Figure 2. Mean length (cm) of the Baltic herring females in the lipid samples (LeL) and in the spawning population (LeP), and the mean number of eggs in the females in the lipid [Fecundity = Fec(L)] and population samples [Fec(P)] in different years (n = number of females in the samples; for the data; see Supplementary Table 2).
To study if there was a breakpoint in the time-series of the lipid content of the eggs, we conducted a segmented regression model by fitting piecewise linear regression lines with significantly different slopes to subsequent parts of the time series (segmented function in the R package segmented version 1.3.1) (Muggeo, 2003, 2008). In the analysis, annually averaged egg lipid content was set as the response variable and year as the predictor.
Comparisons of the group means (i.e., in the concentrations of THs and T3 in the ovary and in the amount of lipids, THs and T3 per egg) were performed with the non-parametric Kruskall-Wallis test, as the data mostly failed to meet the requirements of the parametric tests even after transformations. The association between different variables was computed with the Pearson moment-product correlation test, if the assumptions for normality were met after log-transformation of the data, otherwise, the Spearman rank correlation test was used. In the analyses, the SPSS statistical package was used (IBM Corp. Relased, 2015).
Results
Concentration of Lipids and Thyroid Hormones in the Ovarian Tissue
The mean concentration of lipids in the ovary varied between 1.4 and 2.0% WW in the beginning of the time-series (1988–1990), but decreased thereafter, being at its lowest in 1998–2001 (<1.0% WW) and then slightly increasing again (Figure 3). In 1988–1990, the between-individual variation was high (min.–max. = 0.4–6.9% WW), but the concentration had no correlation with female length or age which varied between 14 and 26 cm and 2–7 years, respectively (p > 0.1 in both cases). Thereafter, the highest concentrations disappeared from the samples that leveled off the differences among the females during the 2000s (min.–max. = 0.3–2.1% WW). The mean length of females analyzed for the lipid concentration decreased over the years, although somewhat less than in the spawning population, where their average length decreased from 19 cm to about 16 cm during 1988–2019 (Figure 2).
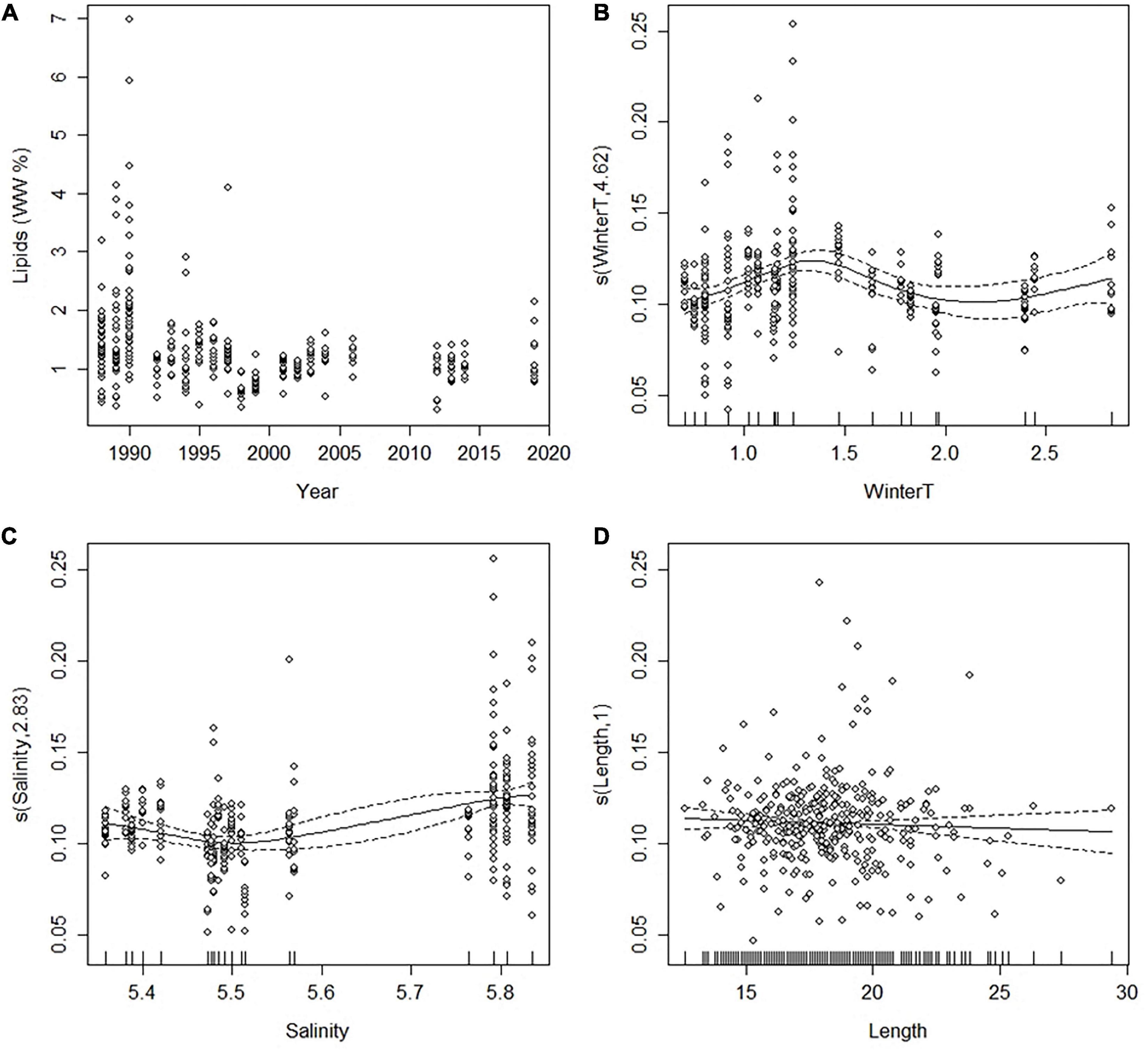
Figure 3. (A) The concentration of lipids (% WW) in the ovarian tissue of the Baltic herring in 1988–2019 (original data); and (B–D) generalized additive mixed model (A) plots showing the partial effects of selected explanatory variables on the arcsine-transformed concentration of lipids. The tick marks on the x-axis are observed data points. The y-axis represents the partial effect of each variable. The dotted lines indicate the 95% confidence intervals. WinterT = water temperature (°C) in January-April. See text for further details.
The lipid content of the whole fish, including muscle and ovary, was determined annually during 1988–1999, when herring females contained on average 670 mg lipids (SD ± 685; n = 223; Figure 4). During 2000–2019, lipids were mostly determined from the ovary only, but when results on both the muscle and ovary were obtained, they showed a decrease of fish total lipids by about 50% (mean = 288 mg SD ± 404; n = 68). The data series also indicated a high variation among the females and a variable pattern in the partitioning of lipids between the female and ovary (index OL; Figure 4). The mean OL varied between 20 and 40%, but the variation among the females was high in all samples (Figure 4). The relation between the total lipids and OL was negative and significant (r = -0.56, p < 0.001; n = 291) indicating a higher proportion of the female’s lipid reserves in the ovary at low total lipid reserves and vice versa (Figure 4).
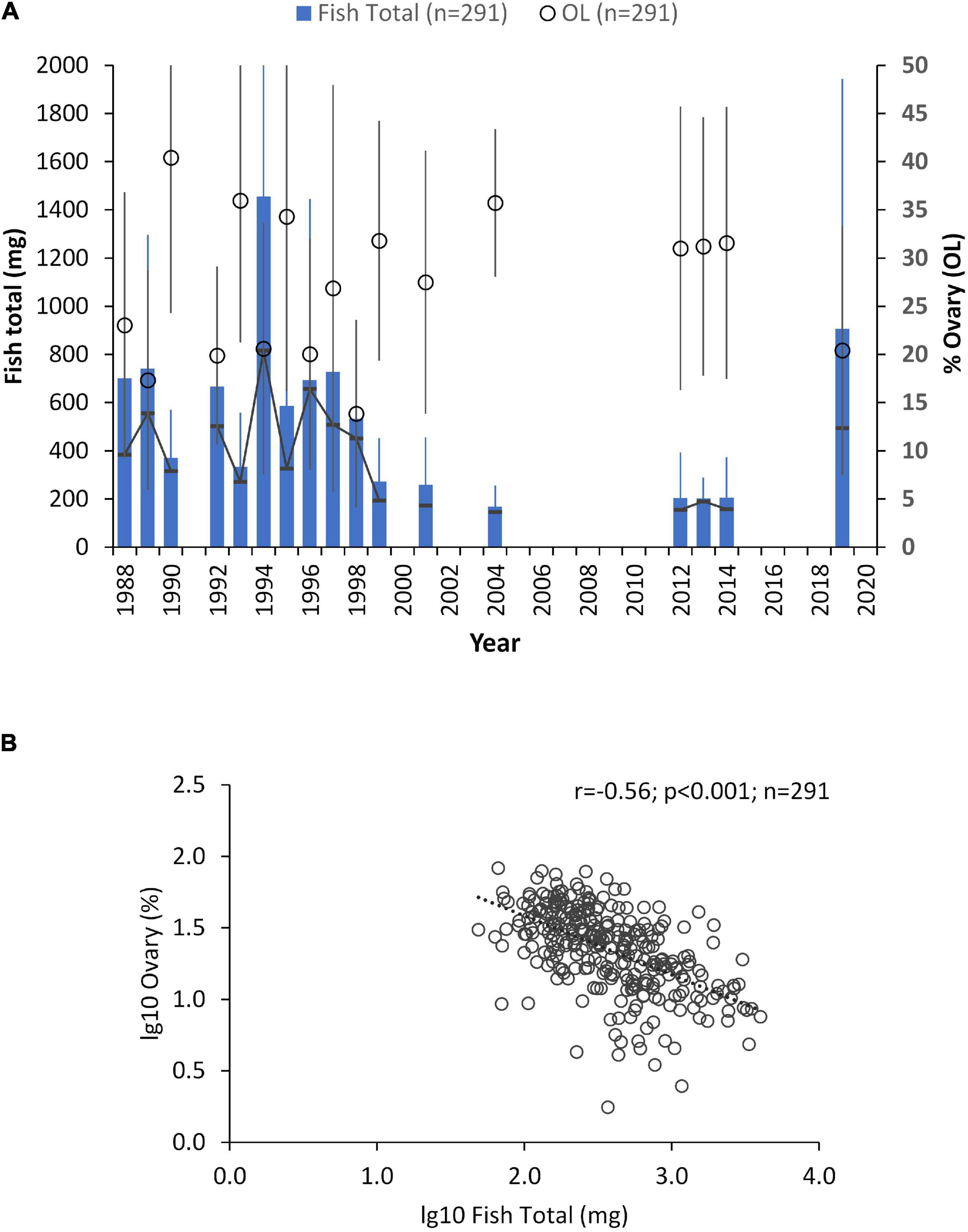
Figure 4. (A) The total amount of lipids in spawning Baltic herring females (Fish Total, mg; columns) and the proportion of lipids in their ovary as % of total lipids in fish (% Ovary (OL); open circles) during 1988–2019. Mean and standard deviation in the samples; the line with (-) -symbols indicates the median value of Fish Total. The empty spaces between the columns = no data; (B) relationship between the lg10-transformed values of the total lipids in fish (lg10Fish Total, mg) and OL (lg10Ovary %); r = Pearson product-moment correlation coefficient; p = risk level; n = number of females analyzed.
The GAM model (A) examining the effects of salinity, winter temperature and female length on the ovarian lipid concentration (Table 1) showed that female length, which varied between 12.6 and 29.4 cm in the data, had no effect on the lipid concentration (p > 0.10), but instead, the model indicated a significant association between the lipid concentration and the abiotic factors (Table 1). The partial effect plots of the two environmental variables suggested that the lipid concentration of the ovary was highest at salinity of ∼5.8 PSU (years 1988–1992; Figure 3), and when the winter temperature before the spawning season was approximately 1.0–1.5° (years 1990, 1994–1998, and 2013; Figure 3).
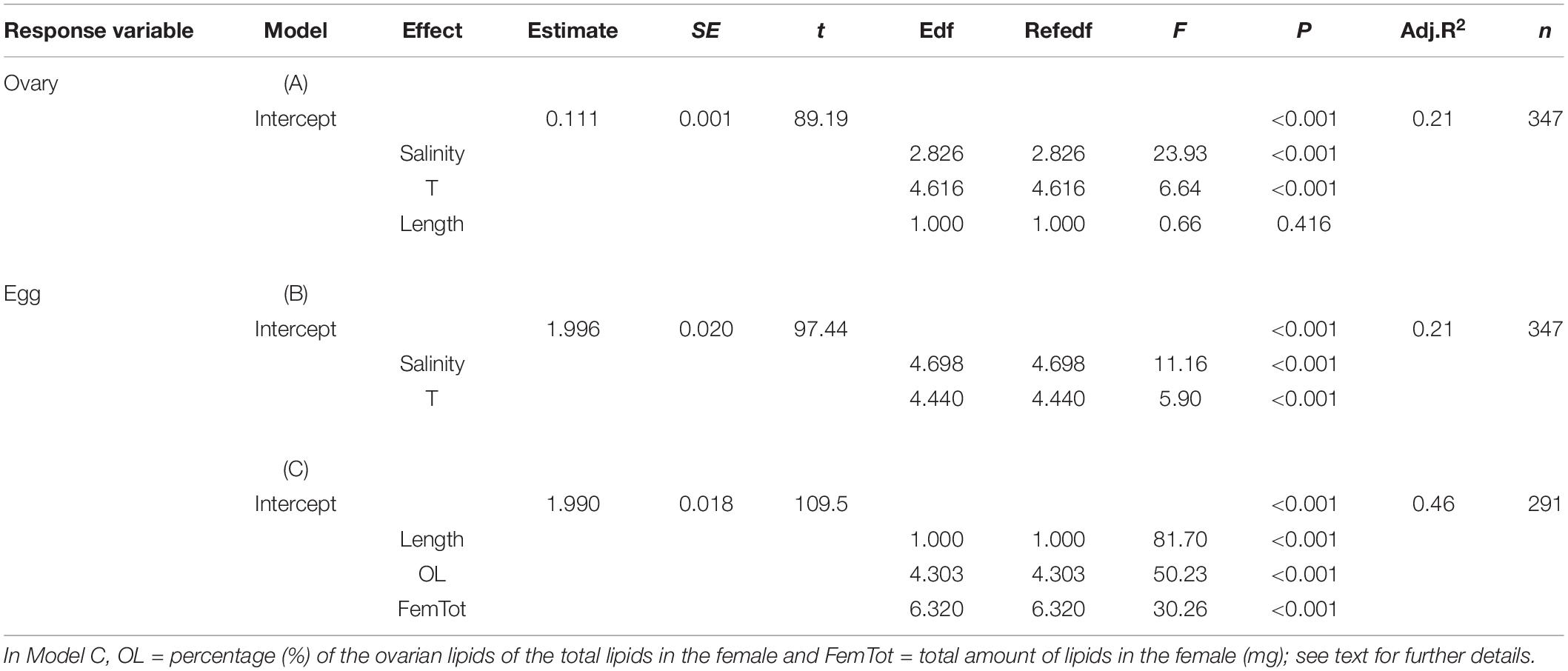
Table 1. Parameter estimates from the Generalized Additive Mixed-effect Model analyzing the effect of salinity, winter temperature (T) and female length on the concentration of lipids in the ovary (% WW) of the Baltic herring in 1988–2019 ( = Model A), and the effect of the abiotic factors ( = Model B) and female traits ( = Model C) on the lipid content of eggs.
The mean concentration of thyroid hormones (THs) was 97 pg/mg DW (SD ± 32) in the first study year 1990 (Figure 5), but was slightly lower during the later years varying among the individuals and between the samples (41 ± 9 to 80 ± 18 pg/mg DW). Likewise, the mean concentration of T3 was highest in 1990 (44 ± 17 pg/mg DW) decreasing thereafter to 26 ± 10 pg/mg DW in 2019 (Figure 5). There was a significant among-year variation both in average THs and average T3 (χ2 = 33.227, p < 0.001 and χ2 = 23.726, p < 0.01, respectively). Both had a significant and positive correlation with salinity of the sampling year (THs: p < 0.001, T3: p < 0.001; Table 2) but a negative correlation with winter temperature (THs: p < 0.01, T3: p < 0.01).
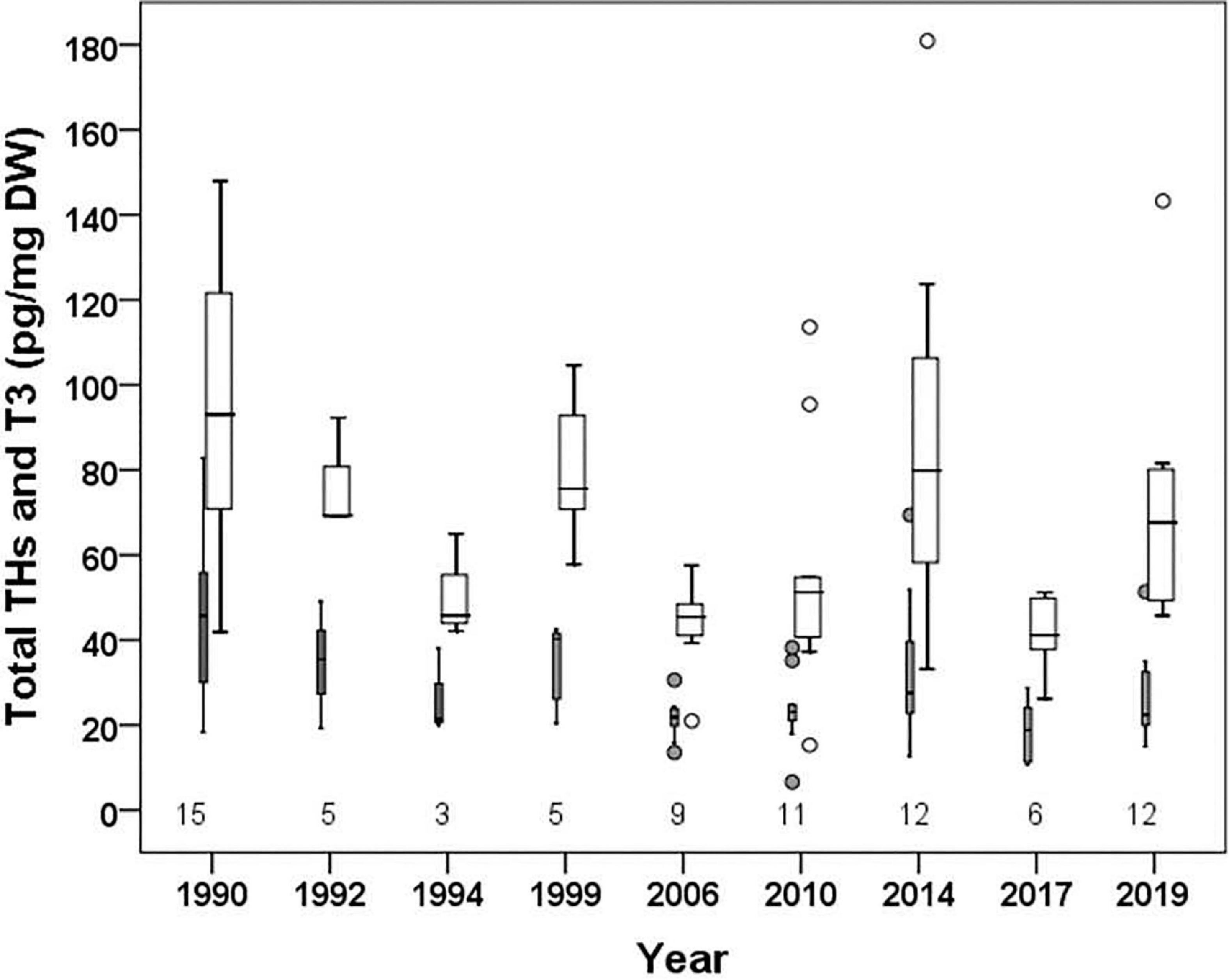
Figure 5. Box-plots showing the mean concentration of thyroid hormones (Total THs, pg mg–1 DW; broad white box) and triiodothyrenine (T3, pg mg–1 DW; narrow gray box) in the ovarian tissue of the spawning Baltic herring during 1990–2019. The number of females analyzed per year is indicated under the box-plots in the panel.
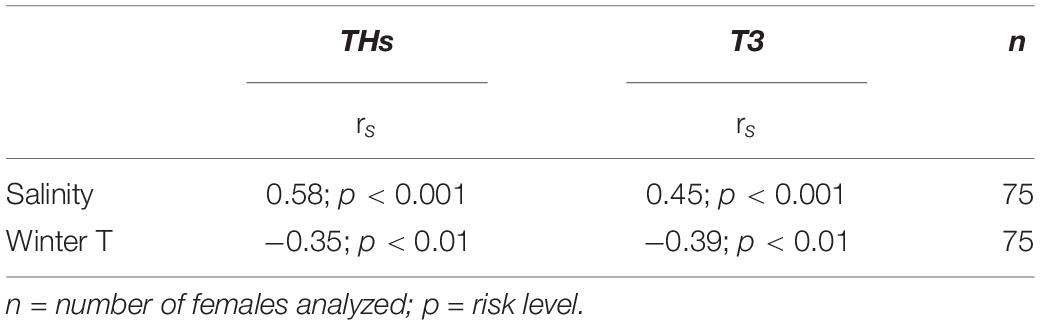
Table 2. Spearman rank correlation coefficient (rs) examining the relation between salinity, winter temperature (T) and concentration of thyroid hormones (THs and T3) in the ovarian tissue of the Baltic herring in 1990–2019.
Lipid and T3 Content of the Eggs
The mean lipid content of eggs differed between the years (χ2 = 141.62, p < 0.001; n = 19), decreasing from the average 9–10 μg/egg from the first study years to 6–7 μg/egg in the 2000s (Figure 6). Likewise, the mean content of T3 in eggs varied among the years (χ2 = 22.60, p < 0.01; n = 8), indicating a higher content in the eggs in 1990 (mean = 6.3 pg/egg, SD ± 2.5) than for instance in 2019 (mean = 3.9 pg/egg, SD ± 1.4; Figure 6).
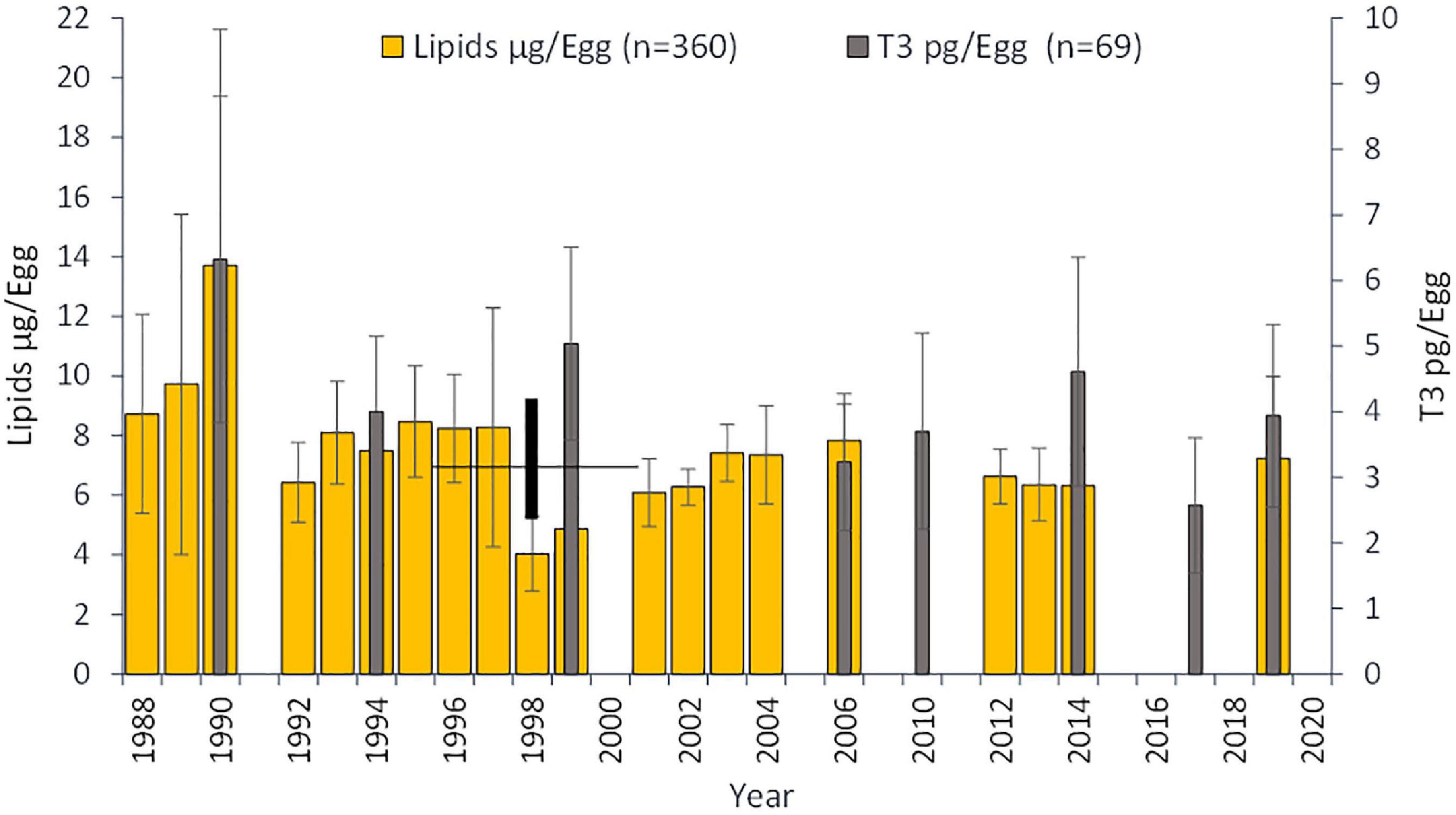
Figure 6. The mean amount of lipids (μg) and triiodothyronine (T3 pg) per one egg (±SD) in the Baltic herring in the Archipelago Sea, northern Baltic in different years. Black vertical bar indicates the breakpoint year (1998) with standard error (±3 years) in the timeseries of the egg lipid content; see text.
The GAM model (B) indicated a significant association between the egg lipids, salinity and winter temperature (Table 1), suggesting that similar to the ovarian lipid concentration, the lipid content of eggs was higher in years of the highest salinity (∼5.8 PSU; Figure 7) and when winter temperature was between 1.0 and 1.5°C. Concluding from the adjusted R2-values, the best model (C; Table 1) included the female’s length, total lipid resources and OL; i.e., the proportion of lipids in the ovary as % of the female’s total lipid resources (R2 = 0.46). According to the parameter estimates (Figure 7), the lipid content of eggs was greatest in small-sized females (length ∼15–17 cm) which had rich lipid reserves in their body and which invested approximately 20–40% of the body lipids into the ovary.
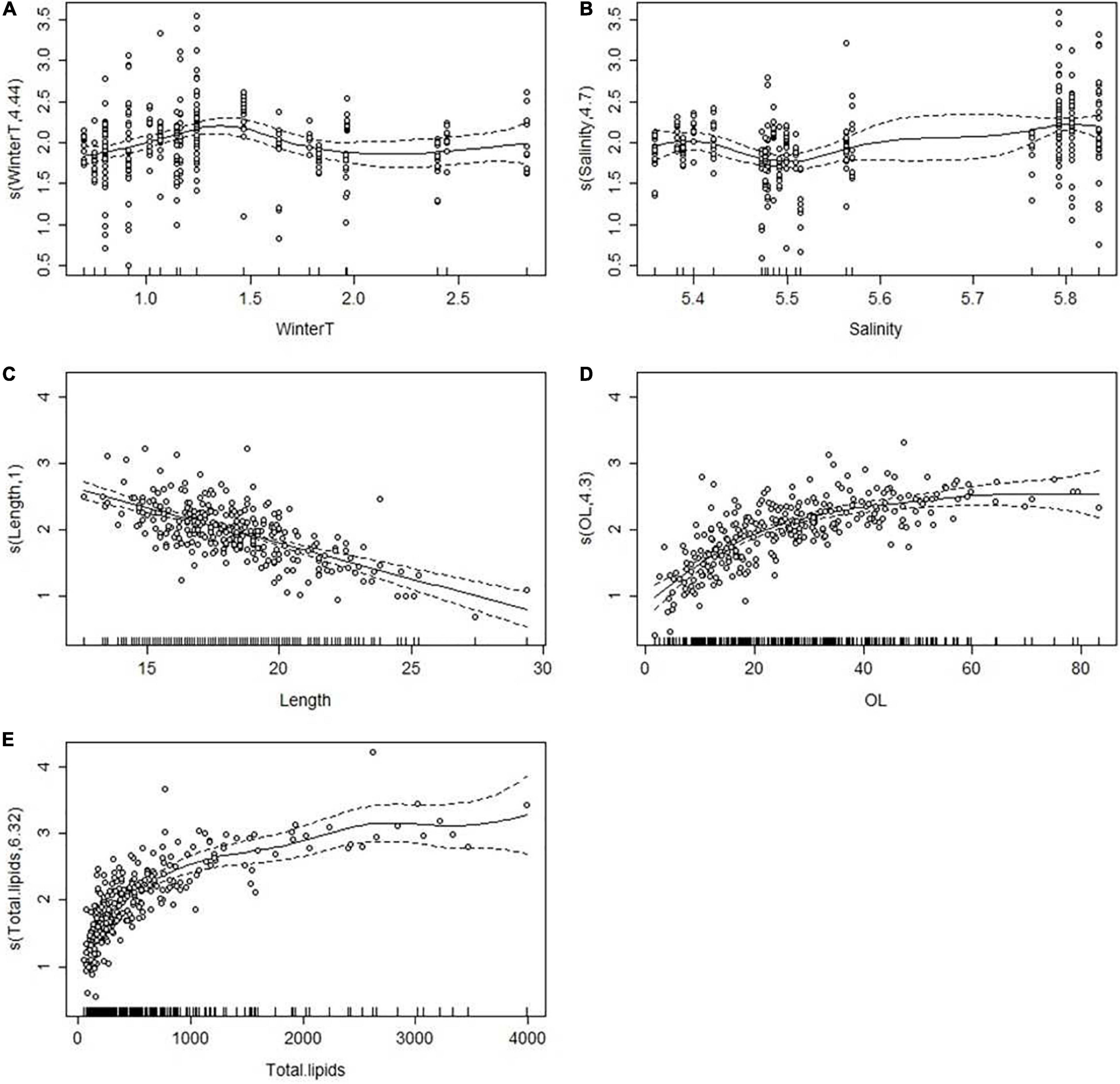
Figure 7. Generalized additive mixed models B (A,B) and C (C–E) plots showing the partial effects of selected explanatory variables on the log-transformed content of lipids in the eggs (μg/egg). The tick marks on the x-axis are observed data points. The y-axis represents the partial effect of each variable. The dotted lines indicate the 95% confidence intervals. WinterT = water temperature (°C) in January-April, OL = percentage (%) of ovarian lipids of the total lipids of the female, Total lipids = total content of lipids (mg) in herring females. See text for further details.
The segmented regression analysis applied on the egg lipid data indicated a breakpoint in the time series in approximately 1998 (SE ± 3 years, p < 0.02). The data were therefore examined further with a Pearson correlation test in two periods before (1988–1997) and after (1999–2019) the break-point year (Table 3). In the first period, the egg lipid content was positively correlated with OL (p < 0.001) and negatively correlated with fecundity (p < 0.05), but during the later period, egg lipids correlated positively with the total amount of lipids in the female (p < 0.01; Table 3). In addition, the egg lipids had a positive correlation with salinity in the first period, but not anymore during the later one, whereas the correlations with winter temperature showed the opposite pattern (Table 3).
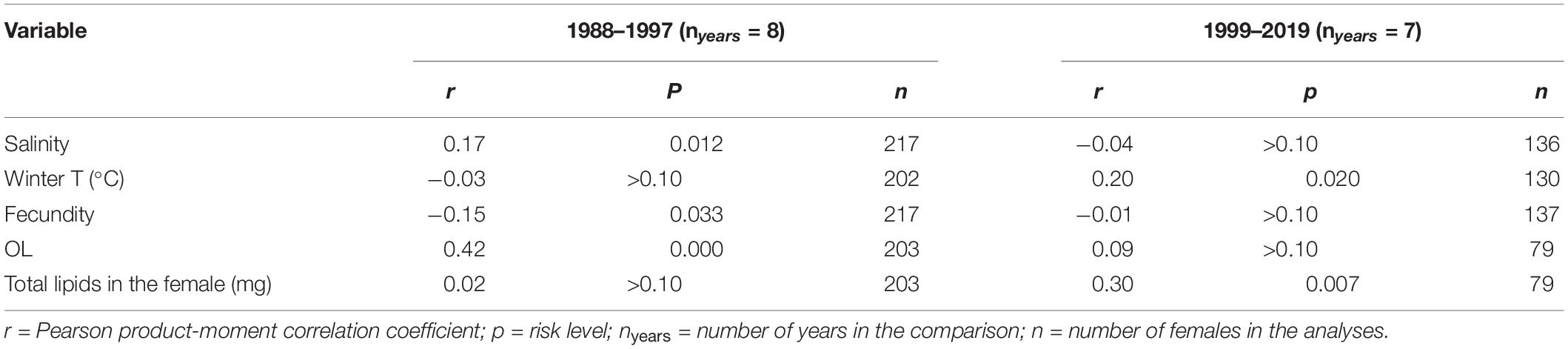
Table 3. Correlations between the egg lipid content (μg/egg) and abiotic factors (salinity and winter temperature) and different female traits during the periods of declining (1988–1997) and stable salinity (1999–2019) in the northern Baltic Sea.
Discussion
Ovarian Lipids
In the Baltic Sea, the variations of salinity are connected to the atmospheric conditions in the Northern Atlantic (e.g., Hänninen et al., 2000), which have caused the present low-salinity phase by decreasing the inflows of the North Sea water and by increasing the freshwater runoff from the catchment area. As a consequence of the global climate change, also the winters have become milder especially in the northern sea area, where water temperature shows an increasing trend by approximately 1°C per decade (HELCOM, 2013). Our study was made during the period when the Baltic salinity first clearly decreased and then stabilized to the current level, and the mean winter months’ water temperature in the overwintering areas of the herring fluctuated.
According to our time-series data, the amount of lipids decreased significantly both in the ovarian tissue and also in the whole fish, when measured as the sum of muscle and ovarian lipids. In all, salinity and winter temperature showed a significant relation with the ovarian lipid concentration during 1988–2019, which indicates a similar type of connection to abiotic factors as previously found for herring muscle lipids (Rajasilta et al., 2018). Female length had no association with the ovarian lipid concentration that follows the general pattern found in many other fish species, which also show a high variation of the lipid storage but no connection between the storage and fish size (Martin et al., 2017).
The role of food resources in the formation of the lipid reserves of the herring is obvious, but the available information on the abundance of the herring’s prey species is scarce or even contradictory in the study area. For instance, the zooplankton biomass in its main feeding area, the Bothnian Sea, has increased since the late 1980s (Postel et al., 2011), but in spite of this, the lipid content of the herring females decreased during the same period. This could be a consequence of intraspecific food competition among the herring stock (Lindegren et al., 2011; Rajasilta et al., 2018), but it also suggests that zooplankton is of less importance as a source of the lipid reserves. In fact, the accumulation of lipids takes place during autumn, when herring mainly feed on macroscopic prey such as amphipods and mysid shrimps (Aneer, 1980; Rajasilta et al., 2014). These nektobenthic species form an important link between the benthic and pelagic system in the Bothnian Sea (Kiljunen et al., 2020), but only little is known about their temporal and local variations.
In herring females, lipids are first stored in the mesenteries and muscle tissue and then deposited into the ovaries during oogenesis, reaching the maximum concentration at the midway to maturation in the winter (Vuorela et al., 1979). During the overwintering period, temperature can thus have an impact on the ovarian lipids changing the energy expenditure of the female or the deposition rate from the female to the ovary. In the present study, the winter temperature ranged between 0.7 and 2.8°C, meaning that the effect of temperature on herring females may have been higher in the warmest winters in comparison with the years of colder overwintering conditions.
The GAMM (A) detected a close association between temperature and ovarian lipid concentration, but the effect was non-linear, as the highest lipid concentrations were found at overwintering temperatures 1.0–1.5°C decreasing toward the lower and higher temperatures. The reason for this result cannot be confirmed in our study, but it may be an indication of lipid quality, since the fluidity of lipids through cell membranes is temperature dependent and specific to the fatty acid composition (Farkas et al., 2001; Malekar et al., 2018). Most likely, also the distribution of the annual samples across the temperature range affected the results. The majority of the samples represent cold winter seasons, and as the warm winters, being those with a mean temperature of greater than 2.0°, were still few during the study, the uncertainty of the model inevitably increased at this end of the data series. It seems, however, that the ovarian lipid content will not remain unchanged if the winter temperature increases in the future, especially when also the salinity of the Baltic water continues to decline as predicted (HELCOM, 2013).
Salinity is the major factor influencing the internal homeostasis in fish, which is controlled by several hormones including THs (Deal and Volkoff, 2020). The thyroid gland of the Baltic herring is known to react to the salinity conditions in the environment (Lahti, 1989). The thyroid hormone content of the ovary, being maternally derived, is likely to follow the production of TH in the female at least to some extent (e.g., Ruuskanen and Hsu, 2018).
During 1990–2019, the mean concentration of T3 in the Baltic herring ovary varied between 21 and 51 ng/g DW, being somewhat higher than, for instance, in mature eggs of the Pacific herring, Clupea pallasi, (10 ng/g WW; Tagawa et al., 1990), probably due to the different study units of the analyses (i.e., DW vs. WW). In our time series, the different storage time might be argued to influence the results, but as shown by Männistö et al. (2007), the thyroid hormone content in frozen tissue samples remains stable for years. In the present study, the concentration of THs was in any case higher in the samples stored for 24 years than in those from the recent years suggesting that storage impact on the concentration was none or insignificant. THs and lipids in the ovarian tissue were both high in 1990 probably illustrating the means of transportation of THs with lipids into the ovary. As thyroid hormones play a central role in the mobilization of lipids (e.g., Sheridan, 1994), the changes found in the muscle and ovarian lipids may actually illustrate a change in the activity of the thyroid in variable salinity. A somewhat weaker, but significant correlation was found between THs and winter temperature, which tells that the hormonal system of the herring responded to the variations of the winter conditions as well, possibly regulating the process of temperature acclimation (Little et al., 2013).
Egg Quality
The amount of lipids and THs in the eggs suggested an overall decrease of egg quality over the years being related to salinity and winter temperature, but the GAMM results indicated an even stronger relation between the egg lipid content and the total lipid reserves of the female. However, the role of the abiotic factors and those intrinsic of the female was different in the two periods indicated by the break-point analysis applied to the time-series. During the salinity decline, the lipid content of eggs was associated with the index OL, suggesting that the egg lipids depended on the allocation of lipids between somatic and reproductive functions in the female. At this phase, however, females also had better lipid reserves than from the year 1999 onwards, when egg lipid content was mainly associated with the (low) lipid reserves of the female.
Most likely, all herring females tend to maintain the egg quality as good as possible, but how this is made seems to depend on the female’s resources. As an iteroparous species, the Baltic herring reproduces repeatedly over its lifetime, which can be 15–20 years in our study area (Peltonen et al., 2002). The environmental conditions determine the female’s condition in different years, but if the condition is good, the female can expect to reproduce also later in life and therefore, part of its lipid resources is spared for further reproductions; a female in poor condition, instead, invests most into the current reproduction as the probability of further reproductions is low. The difference between the two periods found in the herring may thus indicate a change of the reproductive strategy, also found, for instance, in the lake whitefish, Coregonus clupeaformis (Muir et al., 2014).
Lipids are just one component of the yolk contents, since yolk contains also proteins. Both can be used as a fuel by the developing embryo, but they also provide building material for the formation of tissues and organs (e.g., Kamler, 2005; Reading et al., 2018). At the early developmental phases when cell division is rapid, the fatty acid composition of lipids is probably more important than the quantity alone (Brooks et al., 1997; Pickova et al., 1997; Tocher, 2003). In the Baltic herring, a high amount of triacylglycerol in the eggs increased early post-fertilization mortality, indicating that an excess amount of storage lipids can be even harmful (Laine and Rajasilta, 1999). For the hatching larvae, instead, lipids of the egg yolk are of crucial importance as a source of energy before the onset of external feeding. At time of hatching, temperature conditions impact on the energy consumption of larvae and thus determine the time window for optimal hatching conditions (Peck et al., 2012). A low lipid content of the yolk may therefore increase larval mortality in the Baltic herring in warm springs if the consumption of energy increases, causing fast depletion of lipids in the egg yolk. The link between rising winter temperature and reduction of the herring’s reproductive success has already been established in the southern Baltic Sea (Polte et al., 2021). In the northern Baltic, no respective findings have been made this far, but here, also salinity may contribute to the reproductive success of the species in addition to temperature as both show an effect on the females.
It is obvious that the lipid reserves of the female form an important mechanism mediating the parent-progeny effects in the Baltic herring, and being maternally derived, thyroid hormones are involved in this process as well. The exact role of THs in fish eggs is not known, as the hormonal treatment of females or eggs may result in accelerated development of the embryo and improved hatching rates, but also, in larval deformities, depending on the dose given (Brown et al., 2014). Liu and Chan (2002) showed that in zebrafish, Danio rerio, THs are important especially at the transition phase from yolk-sac stage to free-swimming larvae coordinating organogenesis during the switch from endogenous to exogenous feeding. In addition to this, THs may regulate the metabolism of lipids in the yolk in the same way as in adult fish (Sheridan, 1994). For instance, a low amount of THs in the yolk could reduce the catabolic processes consuming lipids and thus prolong the time between hatching and onset of exogenous feeding. As this is the critical period in the larval life (e.g., Yúfera and Darias, 2007), the decrease of THs in the yolk would compensate for the adverse effect of the low lipid content at the larval phase and be an essential component of egg quality in the interaction with the yolk lipids.
According to the GAMM, the greatest lipid content in the eggs was achieved at female lengths approximately 15–17 cm. This suggests that also female size was connected to the quality of eggs; possibly by a trade-off relation between egg quality and female growth, which is found, for example, in the Atka mackerel, Pleurogrammus monopterygius (McDermott et al., 2011). Alternatively, the gradual change in the herring population toward a smaller body size may be an indication of an adaptive process favouring slow-growing individuals, which are able to increase their fitness due to better egg quality. As fish tend to scale their energy budget to the body size (Svendsen et al., 2017), this perspective should also be taken into account as an alternative explanation in further studies, since it means that small body size could be the phenotypic trait selected for in the variable environmental conditions of the Baltic Sea.
Data Availability Statement
The original contributions presented in the study are included in the article/Supplementary Material, further inquiries can be directed to the corresponding author/s.
Ethics Statement
Ethical review and approval was not required for the animal study because no living fish were used in the study. The fish samples were obtained from the professional fishermen who catch the Baltic herring under the control of the state authorities; the herring fishery follows the instructions by the ICES and the regulations of the European Union.
Author Contributions
MR designed the study, made most lipid analyses over the years, wrote the first draft, and finished the final version. KM performed the statistical analyses, wrote the respective texts in mat and met, and participated in writing the text. SR was responsible for the hormonal analyses and the text in mat and met and participated in writing the text. PL determined the fecundity, made part of the lipid analyses, and participated in writing the text. JH participated in the project management and writing the final version. All authors contributed to the article and approved the submitted version.
Funding
This study has received financial support from the European Union (project ref. No. 96-068), the Sakari Alhopuro Foundation (project ref. No. 20200084) and Academy of Finland (project ref. No. 286278). This study has utilized the research infrastructure facilities provided by FINMARI (the Finnish Marine Research Infrastructure network).
Conflict of Interest
The authors declare that the research was conducted in the absence of any commercial or financial relationships that could be construed as a potential conflict of interest.
Publisher’s Note
All claims expressed in this article are solely those of the authors and do not necessarily represent those of their affiliated organizations, or those of the publisher, the editors and the reviewers. Any product that may be evaluated in this article, or claim that may be made by its manufacturer, is not guaranteed or endorsed by the publisher.
Acknowledgments
We thank the project workers for assistance in the laboratory and field, and the local fishermen for providing us the fish samples over many years. Robert M. Badeau, M.Sc., Ph.D., of Aura Professional English Consulting, Ltd. (www.auraenglish.com) performed the English language checking service for this manuscript.
Supplementary Material
The Supplementary Material for this article can be found online at: https://www.frontiersin.org/articles/10.3389/fmars.2021.698480/full#supplementary-material
References
Aneer, G. (1980). Estimates of feeding pressure on pelagic and benthic organisms by Baltic herring (Clupea harengus v. membras L.). Ophelia Suppl. 1, 265–275.
Ayson, F. G., and Lam, T. J. (1993). Thyroxine injection of female rabbitfish (Siganus guttatus) brookstock: changes in thyroid hormone levels in plasma, eggs, and yolk-sac larvae, and its effect on larval growth and survival. Aquaculture 109, 83–93. doi: 10.1016/0044-8486(93)90488-k
Brooks, S., Tyler, C. R., and Sumpter, J. P. (1997). Egg quality in fish: what makes a good egg? Rev. Fish Biol. Fish. 7, 387–416.
Brosset, B., Lloret, J., Muñoz, M., Fauvel, C., Van Beveren, E., Marques, V., et al. (2016). Body reserves mediate trade-offs between life-history traits: new insights from small pelagic fish reproduction. R. Soc. Open Sci. 3, 1–15. doi: 10.1098/rsos.160202
Brown, C. L., Criscuolo Urbinati, E., Zhang, W., Brown, S. B., and McComb-Kobza, M. (2014). Maternal thyroid and glucocorticoid hormone interactions in larval fish development, and their applications in aquaculture. Rev. Fish. Sci. Aquac. 22, 207–220. doi: 10.1080/23308249.2014.918086
Calow, P. (1985). “Adaptive aspects of energy allocation,” in Fish Energetics. New Perspectives, eds P. Tytler and P. Calow (London: Croom Helm Ltd), 13–31. doi: 10.1007/978-94-011-7918-8_1
Casini, M., Kornilovs, G., Cardinale, M., Möllmann, C., Grygiel, W., Jonsson, P., et al. (2011). Spatial and temporal density dependence regulates the condition of Central Baltic Sea clupeids: compelling evidence using an extensive international acoustic survey. Pop. Ecol 53, 511–523. doi: 10.1007/s10144-011-0269-2
Castillo, S., Bollfrass, K., Mendoza, R., Fontenont, Q., Lazo, J. P., Aguilera, C., et al. (2015). Stimulatory effect of thyroid hormones improves larval development and reproductive performance in alligator gar (Atractosteus spatula) and spotted gar (Lepisosteus oculatus). Aquac. Res. 2013, 1–10. doi: 10.1111/are.12363
Deal, C. K., and Volkoff, H. (2020). The role of thyroid axis in fish. Front. Endocrinol. 11:596585. doi: 10.3389/fendo.2020.596585
Eklund, J., Parmanne, R., and Aneer, G. (2000). Between-reader variation in herring otolith ages and effects on estimated population parameters. Fish. Res. 46, 147–154. doi: 10.1016/s0165-7836(00)00141-7
Farkas, T., Fodor, E., Kitajka, K., and Halver, J. E. (2001). Response of fish membranes to environmental temperature. Aquac. Res. 32, 645–655. doi: 10.1046/j.1365-2109.2001.00600.x
Green, B. S. (2008). Maternal effects in fish populations. Adv. Mar. Biol. 54, 1–105. doi: 10.1016/S0065-2881(08)0000-1
Hänninen, J., Vuorinen, I., and Hjelt, P. (2000). Climatic factors in the Atlantic control the oceanographic and ecological changes in the Baltic Sea. Limnol. Oceanogr. 45, 703–710. doi: 10.4319/lo.2000.45.3.0703
HELCOM (2013). Climate Change in the Baltic Sea Area: HELCOM Thematic Assessment In 2013. Balt. Sea Environ. Proc. No. 137. Helsink: HELCOM.
Kamler, E. (2005). Parent-egg-progeny relationships in teleost fishes: and energetics perspective. Rev. Fish Biol. Fish. 15, 399–421. doi: 10.1007/s11160-006-0002-y
Kiljunen, M., Peltonen, H., Lehtiniemi, M., Uusitalo, L., Sinisalo, T., Norkko, J., et al. (2020). Benthic-pelagic coupling and trophic relationships in the northern Baltic Sea food webs. Limnol. Oceanogr. 65, 1706–1722. doi: 10.1002/lno.11413
Kuijper, B., and Hoyle, R. B. (2015). When to rely on maternal effects and when on phenotypic plasticity? Evolution 69, 950–968. doi: 10.1111/evo.12635
Lahti, E. (1989). The thyroid status of Baltic herring (Clupea harengus L.) in the Gulf of Bothnia (Baltic Sea) in relation to ambient salinity and iodine. Arch. Hydrobiol. 117, 117–125.
Laine, P., and Rajasilta, M. (1998). Changes in the reproductive properties of Baltic herring females during the spawning season. Fish. Res. 36, 67–73. doi: 10.1016/s0165-7836(98)00087-3
Laine, P., and Rajasilta, M. (1999). The hatching success of Baltic herring eggs and its relation to female condition. J. Exp. Mar. Biol. Ecol. 237, 61–73. doi: 10.1016/s0022-0981(98)00213-5
Lehodey, P., Alheit, J., Barange, M., Baumgartner, T., Beaugrand, G., Drinkwater, K., et al. (2006). Climate variability, fish and fisheries. J. Clim. 19, 5009–5030.
Lindegren, M., and Östman, Ö, and Gårdmark, A. (2011). Interacting trophic forcing and the population dynamics of herring. Ecology 92, 1407–1413. doi: 10.1890/10-2229.1
Little, A. G., Kunisue, T., Kannan, K., and Seebacher, F. (2013). Thyroid hormone actions are temperature-specific and regulate thermal acclimation in zebrafish (Danio rerio). BMC Biol. 11:26. doi: 10.1186/1741-7007-11-26
Liu, Y.-W., and Chan, W.-K. (2002). Thyroid hormones are important for embryonic to larval transitory phase in zebrafish. Differentiation 70, 36–45. doi: 10.1046/j.1432-0436.2002.700104.x
MacLean, B., Tomazela, D. M., Shulman, N., Chambers, M., Finney, G. L., Frewen, B., et al. (2010). Skyline: an open source document editor for creating and analyzing targeted proteomics experiments. Bioinformatics 26, 966–968. doi: 10.1093/bioinformatics/btq054
Malekar, V. C., Morton, J. D., Hider, R. N., Cruickshank, R. H., Hodge, S., and Metcalf, V. J. (2018). Effect of elevated temperature on membrane lipid saturation in Antarctic notothenioid fish. PeerJ 6:e4765. doi: 10.7717/peerj.4765
Männistö, T., Surcel, H.-M., Bloigu, A., Ruokonen, A., Hartikainen, A.-L., Järvelin, M.-R., et al. (2007). The effect of freezing, thawing, and short- and long-term storage on serum thyrotropin, thyroid hormones, and thyroid autoantibodies: implications for analyzing samples stored in serum banks. Clin. Chem. 53, 1986–1987. doi: 10.1373/clinchem.2007.091371
Martin, B. T., Heintz, R., Danner, E. M., and Nisbet, R. M. (2017). Integrating lipid storage into general representations of fish energetics. J. Anim. Ecol. 86, 812–825. doi: 10.1111/1365-2656.12667
McDermott, S. F., Cooper, D. W., Guthridge, J. L., Spies, I. B., Canino, M. F., Woods, P., et al. (2011). Effects of maternal growth on fecundity and egg quality of wild and captive Atka mackerel. Mar. Coast. Fish. 3, 324–335. doi: 10.1080/19425120.2011.608592
Muggeo, V. M. R. (2003). Estimating regression models with unknown break-points. Stat. Med. 22, 3055–3071. doi: 10.1002/sim.1545
Muggeo, V. M. R. (2008). Segmented: a R package to fit regression models with broken-line relationships. R News 8, 20–25.
Muir, A. M., Arts, M. T., Koops, M. A., Johnson, T. B., Krueger, C. C., and Sutton, T. M. (2014). Reproductive life-history strategies in lake whitefish (Coregonus clupeaformis) from the Laurentian Great Lakes. Can. J. Fish. Aquat. Sci 71, 1256–1269. doi: 10.1139/cjfas-2013-0254
Peck, M. A., Alheit, J., Bertrand, A., Catalán, I. A., Garrido, S., Moyano, M., et al. (2021). Small pelagic fish in the new millennium: a bottom-up view of global research effort. Prog. Oceanogr. 191:102494. doi: 10.1016/j.pocean.2020.102494
Peck, M. A., Kanstinger, P., Holste, L., and Martin, M. (2012). Thermal window supporting survival of the earliest life stages of Baltic herring (Clupea harengus). ICES J. Mar. Sci. 69, 529–536. doi: 10.1093/icesjms/fss038
Peltonen, H., Raitaniemi, J., Parmanne, R., Eklund, J., Nyberg, K., and Halling, F. (2002). Age determination of Baltic herring from whole otoliths and from neutral red stained otolith cross sections. ICES J. Mar. Sci. 59, 323–332. doi: 10.1006/jmsc.2001.1156
Peltonen, H., Vinni, M., Lappalainen, A., and Pönni, J. (2004). Spatial feeding patterns of herring (Clupea harengus L.), sprat (Sprattus sprattus L.), and the three-spined stickleback (Gasterosteus aculeatus L.) in the Gulf of Finland, Baltic Sea. ICES J. Mar. Sci. 61, 966–971.
Pickova, J., Dutta, P. C., Larsson, P.-O., and Kissling, A. (1997). Early embryonic cleavage pattern, hatching success, and egg-lipid fatty acid composition: comparison between two cod (Gadus morhua) stocks. Can. J. Fish. Aquat. Sci. 54, 2410–2416.
Polte, P., Gröhsler, T., Kotterba, P., von Nordheim, L., Moll, D., Santos, J., et al. (2021). Reduced reproductive success of Western Baltic herring (Clupea harengus) as a response to warming winters. Front. Mar. Sci. 8:589242. doi: 10.3389/fmars.2021.589242
Postel, L., Margonski, P., Lehtiniemi, M., Flinkman, J., Pollumäe, A., Põllupuu, M., et al. (2011). ICES Zooplankton Status Report 2008/2009. ICES Cooperative Research Report No. 307. Denmark: International Council for the Exploration of the Sea.
Power, D. M., Llewellyn, L., Faustino, M., Nowell, M. A., Björnsson, B. T., Einarsdottir, I. E., et al. (2001). Thyroid hormones in growth and development of fish. Comp. Biochem. Physiol. C 130, 447–459.
R Core Team (2020). R: A Language And Environment For Statistical Computing. Vienna: R Foundation for Statistical Computing.
Rajasilta, M. (1992). Relationship between food, fat, sexual maturation, and spawning time of Baltic herring (Clupea harengus membras) in the Archipelago Sea. Can. J. Fish. Aquat. Sci. 49, 644–654. doi: 10.1139/f92-073
Rajasilta, M., Eklund, J., Hänninen, J., Kurkilahti, M., Kääriä, J., Rannikko, P., et al. (1993). Spawning of herring (Clupea harengus membras L.) in the Archipelago Sea. ICES J. Mar. Sci. 50, 233–246. doi: 10.1006/jmsc.1993.1026
Rajasilta, M., Eklund, J., Hänninen, J., Vuorinen, I., and Laine, P. (2015). Female Baltic herring Clupea harengus allocate resources from growth to reproduction in poor feeding conditions. J. Fish Biol. 86, 575–591. doi: 10.1111/jfb.12577
Rajasilta, M., Eklund, J., Laine, P., Jönsson, N., and Lorenz, T. (2006). Intensive Monitoring Of Spawning Populations Of The Baltic Herring (Clupea harengus membras L.). Turku: Seili Archipelago Research Institute Publications.
Rajasilta, M., Hänninen, J., and Vuorinen, I. (2014). Decreasing salinity improves the feeding conditions of the Baltic herring (Clupea harengus membras) in spring in the Bothnian Sea, northern Baltic. ICES J. Mar. Sci. 71, 1148–1152. doi: 10.1093/icesjms/fsu047
Rajasilta, M., Hänninen, J., Laaksonen, L., Laine, P., Suomela, J.-P., Vuorinen, I., et al. (2018). Influence of environmental conditions, population density, and prey type on the lipid content in Baltic herring (Clupea harengus membras) from the northern Baltic Sea. Can. J. Fish. Aquat. Sci. 76, 576–585. doi: 10.1139/cjfas-2017-0504
Reading, B. J., Andersen, L. K., Ryu, Y.-W., Mushiroba, Y., Todo, T., and Hiramatsu, N. (2018). Oogenesis and egg quality in finfish: yolk formation and other factors influencing female fertility. Fishes 3:45. doi: 10.3390/fishes3040045
Rönkkönen, S., Ojaveer, E., Raid, T., and Viitasalo, M. (2004). Long-term changes in Baltic herring (Clupea harengus membras) growth in the Gulf of Finland. Can. J. Fish. Aquat. Sci. 61, 219–229. doi: 10.1139/f03-167
Ruuskanen, S., and Hsu, B.-Y. (2018). Maternal thyroid hormones: an unexplored mechanism underlying maternal effects in an ecological framework. Physiol. Biochem. Zool. 91, 904–916. doi: 10.1086/697380
Ruuskanen, S., Hsu, B.-Y., Heinonen, A., Darras, V. M., Vainio, M., Sarraude, T., et al. (2018). A new method for measuring thyroid hormones using nano-LC-MS/MS. J. Chromatogr. B 1093–1094, 24–30. doi: 10.1016/j.jchromb.2018.06.052
Ruuskanen, S., Mottola, G., and Anttila, K. (2020). Experimental copper exposure, but not heat stress, leads to elevated intraovarian thyroid hormone levels in three-spined sticklebacks (Gasterosteus aculeatus). Ecotoxicology 29, 1431–1440. doi: 10.1007/s10646-020-02278-1
Schulte, P. M. (2014). What is environmental stress? Insights from fish living in a variable environment. J. Exp. Biol. 217, 23–34. doi: 10.1242/jeb.089722
Sheridan, M. A. (1994). Regulation of lipid metabolism in poikilothermic vertebrates. Comp. Biochem. Physiol. 107B, 495–508. doi: 10.1016/0305-0491(94)90176-7
Srigley, C. T., and Mossoba, M. M. (2017). Current Analytical Techniques For Food Lipids. Food and Drug Administration Papers 7. Available online at: http://digitalcommons.unl.edu/usfda/7 (accessed September 14, 2021).
Svendsen, M. B. S., Christensen, E. A. F., and Steffensen, J. F. (2017). To scale or not to scale: a perspective on describing fish energy budgeting. Conserv. Physiol. 5:cox056. doi: 10.1093/conphys/cox056
Tagawa, M., Tanaka, M., Matsumoto, S., and Hirano, T. (1990). Thyroid hormones in eggs of various freshwater, marine and diadromous teleosts and their changes during egg development. Fish Physiol. Biochem. 8, 515–520. doi: 10.1007/bf00003409
Tocher, D. R. (2003). Metabolism and functions of lipids and fatty acids in teleost fish. Rev. Fish. Sci. 11, 107–184. doi: 10.1080/713610925
Vuorela, R., Kaitaranta, J., and Linko, R. R. (1979). Proximate composition of fish roe in relation to maturity. Can. Inst. Food Technol. J. 12, 186–188.
Yoo, J. H., Takeuchi, T., Tagawa, M., and Seikai, T. (2000). Effect of thyroid hormones on the stage-specific pigmentation of the Japanese flounder Paralichthys olivaceus. Zool. Sci. 17, 1101–1106. doi: 10.2108/zsj.17.1101
Yúfera, M., and Darias, M. J. (2007). The onset of exogenous feeding in marine fish larvae. Aquaculture 268, 53–63. doi: 10.1016/j.aquaculture.2007.04.050
Keywords: lipids, thyroid hormones, ovary, egg, salinity, temperature, Baltic herring
Citation: Rajasilta M, Mäkinen K, Ruuskanen S, Hänninen J and Laine P (2021) Long-Term Data Reveal the Associations of the Egg Quality With Abiotic Factors and Female Traits in the Baltic Herring Under Variable Environmental Conditions. Front. Mar. Sci. 8:698480. doi: 10.3389/fmars.2021.698480
Received: 21 April 2021; Accepted: 27 September 2021;
Published: 22 October 2021.
Edited by:
Jose Luis Iriarte, Austral University of Chile, ChileReviewed by:
Guðmundur Óskarsson, Marine and Freshwater Research Institute, IcelandPatrick Polte, Institut für Ostseefischerei, Thünen-Institut, Germany
Copyright © 2021 Rajasilta, Mäkinen, Ruuskanen, Hänninen and Laine. This is an open-access article distributed under the terms of the Creative Commons Attribution License (CC BY). The use, distribution or reproduction in other forums is permitted, provided the original author(s) and the copyright owner(s) are credited and that the original publication in this journal is cited, in accordance with accepted academic practice. No use, distribution or reproduction is permitted which does not comply with these terms.
*Correspondence: Marjut Rajasilta, marjut.rajasilta@utu.fi
†These authors have contributed equally to this work and share first authorship