- 1Institute of Environmental Assessment and Water Research (IDAEA-CSIC), Barcelona, Spain
- 2Centro Oceanográfico de Murcia, Instituto Español de Oceanografía (IEO), Murcia, Spain
In this work a suspect-screening approach was employed to assess the polymers and plastic additives of micro(nano)plastics (NPL/MPLs) of size ranges from the nm range to 20 μm present in seawater from the top 5 cm of the Mar Menor lagoon during two sampling campaigns (summer and winter), as well of other potentially adsorbed compounds onto the plastic particles surfaces and suspended material. The identification of NPL/MPLs has been based on characteristic Kendrick Mass Defect analysis for each polymer type in mass spectra. The applied methodology allowed to identify NPLs/MPLs of polystyrene (PS), polyethylene (PE), polyisoprene (PI), polybutadiene (PBD), polypropylene (PP), polyamides (PA), polyvinylchloride (PVC), n-isopropylacrylamide (PNIPAm), and polydimethylsiloxanes. In addition, PS, PE, PI, PBD, PP, PA, and PVC were confirmed with standards, and the equivalent concentrations were quantified. The results of this study showed that most frequently found compounds were PP, PE, PA and PNIPAm, while the compound found at higher concentrations was by far PP reaching the 9,303 ± 366 ng/mL in one of the samples. A total number of 135 chemical compounds were tentatively identified, 74 of them plastic additives and compounds used in the polymers manufacture or coming from the polymer’s decomposition. In relation to plastic additives, the more frequently tentatively identified compounds were plasticizers such as phthalates group; stabilizers such as antioxidants (e.g., distearyl 3,3′-thiodipropionate, 2,5-di-tert-butylhydroquinone), and UV filters as benzotriazoles. Several flame retardants of the group of phosphates were as well detected. The other compounds tentatively identified in the samples were pharmaceuticals, pesticides, food additives, flavors and natural products that were attached onto the plastic particles and particulate matter from surrounding waters. In regards to the seasonal variation, during the summer a major number of compounds were tentatively detected, while de concentrations of polymers were slightly higher in winter. The spatial distribution showed higher contamination in the southern part of the coastal lagoon.
Introduction
Coastal areas and oceans are essential for the provision of food, green energy, economic growth, and coastal communities’ well-being. According to the EU blue economy Report 2020, in 2018 the economic sectors related to oceans and the coastal environment provided a turnover of €750 billion in the EU. However, at the same time, population growth in coastline areas increases the vulnerability of marine ecosystems. Of all marine litter and pollution in general, 80% originates from land-based sources that are primarily industrial, agricultural, and urban. Among them, plastic wastes constitute 83% of the total marine litter, and polymers that are the most widely identified are polypropylene (PP), polyethylene (PE), polystyrene (PS), polyethylene terephthalate (PET), and polyvinylchloride (PVC) (Andrady, 2011). Plastic wastes are accumulated in oceanic gyres, coastal areas, estuarine waters, semi-closed areas and marine lagoons (Llorca et al., 2020b).
Coastal pollution is composed of complex mixtures of legacy and emerging contaminants. Among the emerging groups of pollutants, microplastics (MPLs) are defined as plastic pieces smaller than 5 mm, including plastic particles at the nano range, called nanoplastics (NPLs). MPLs can have their origin in cosmetics, cleansing products, cloth fibers, tire wear (Fendall and Sewell, 2009) (classified as primary MPLs), and in the environmental fragmentation and erosion of plastic pieces and debris (Cole et al., 2011) (classified as secondary MPLs). Primary and secondary MPLs have become a global concern due to their persistency and widespread occurrence in water and sediments, and also as a consequence of their potential impact on the environment and living organisms. MPLs have been found in all environmental compartments (Shahul Hamid et al., 2018), in particular in marine water (Cózar et al., 2015; Suaria et al., 2016; Cincinelli et al., 2019; Llorca et al., 2021), sediments (Van Cauwenberghe et al., 2013; Vianello et al., 2013; Waller et al., 2017), and biota (Cole et al., 2013). The presence of MPLs in marine organisms has been reported during the last decades. Filter feeders such as mussels can consume small plastic particles (Van Cauwenberghe and Janssen, 2014; Dehaut et al., 2016) and it is hypothesized that the buoyancy, size (similar to plankton), and colors can be factors that attract their ingestion by fish (Wright et al., 2013; Pittura et al., 2018), including species at are used for human consumption (Hantoro et al., 2019; Neto et al., 2020; Schirinzi et al., 2020; Castelvetro et al., 2021). In this sense the occurrence of MPLs can be specially relevant in semiconfined areas, due to its limited dilution capacity such as Mar Menor lagoon (SE Spain). In fact, the prevalence of PE in beaches and sediment of this lagoon was confirmed in previous studies (Moreno-González et al., 2015; León et al., 2018; Filgueiras et al., 2021), however, no previous information is available about their occurrence in the water column.
Moreover, plastic polymers can adsorb and accumulate other contaminants from surrounding waters, facilitating their transfer to biota (Koelmans et al., 2016; León et al., 2018, 2019; Llorca et al., 2020a). On the other hand, plastics are composed of polymers and plastic additives that are not chemically bonded with the polymeric chains and for this reason they can easily leach and be transferred to marine biota (Koelmans et al., 2014; Schmidt et al., 2021; Zitouni et al., 2021).
Due to the higher active surface area of the smallest particles, these effects of accumulation and additive leaching can be hypothesized to be inversely proportional to the size of the particles. As mentioned above, a great effort has been made to assess pollution by MPLs in different compartments of coastal and marine environments. However, due to the limited number of analytical approaches that are able to assess the lowest range of particles, there is an important gap of information concerning the occurrence of MPLs with particles sizes inferior to 20 μm and also NPLs. The main analytical techniques that can be used to assess the low range of plastic particles in complex environmental samples are μ-Fourier-transform infrared spectroscopy (μ-FT-IR), μ-RAMAN, pyrolysis-gas chromatography–mass spectrometry (Pyr-GC-MS), and liquid chromatography coupled to high resolution mass spectrometry (LC-HRMS). μ-FT-IR informs about shape and polymeric composition of particles down to the limit of 20 μm, but it is not suitable for NPLs (Klein et al., 2018). Similarly, μ-RAMAN informs about shape and composition but can be within the nanometer range (Cai et al., 2021), and even 100 nm using RAMAN imaging (Sobhani et al., 2020). Another powerful technique is Pyr-GC–MS, which is not affected by the size of the plastic particles, and recently has been used for quantitative analysis (Fischer and Scholz-Böttcher, 2017; David et al., 2018; La Nasa et al., 2020). LC-HRMS has also been successfully used for the quantitative analysis of NPLs and MPLs (Schirinzi et al., 2019; Llorca et al., 2021), but the main limitation of this approach is the need to solubilize plastic particles in solvents that can be used in chromatography, such as toluene, but most of the commonly used polymers (polyethylene, polypropylene, polystyrene, polybutadiene, etc.) are toluene soluble.
In this work, we apply a double suspect screening based on LC-HRMS that was developed in our group (Llorca et al., 2021), in order to assess and quantify the main polymeric composition of NPLs/MPLs and the plastic additives in the Mar Menor lagoon. Therefore, the main goals of this work were: (i) To assess the main polymer composition of MPLs and NPLs ranging from 0.7 to 20 μm and plastic additives in surface waters (top 5 cm) in the Mar Menor Lagoon during two sampling campaigns that were carried out in summer and winter; (ii) to explore significant variations during the two seasons while considering the main anthropogenic factors.
Experimental Section
Chemicals and Reagents
Analytical standards for gel permeation chromatography/size exclusion chromatography (GPC/SEC) analyses were polyethylene (PE) and polystyrene (PS) (MW∼1,200 Da) supplied by Polymer Standard Service GmbH (PSS, Mainz, Germany), polybutadiene (PBD), polyisoprene (PI) STD KIT from Waters Cromatografía (Cerdanyola del Vallès, Barcelona, Spain) and polypropylene (PP) (MW∼1,200 Da) supplied by American Polymer Standards Corporation (Ohio, United States), polyvinyl chloride (PVC) was obtained from Sigma-Aldrich (Steinheim, Germany), and polyamides were based on ε-Caprolactam, and the analytical standard (analytical grade) was obtained from Supelco.
Toluene HPLC solvent with >99.7% purity and HPLC-water were acquired from J.T.Baker—VWR (Radnor, PA, United States) and nitrogen used as drying gas with an assessed 99.995% purity was supplied by Air Liquide (Barcelona, Catalonia, Spain). Toluene and methanol CHROMASOLV®Plus from Sigma-Aldrich were used during the instrumental analysis.
Glass microfiber filters of 0.70 μm GF/F were purchased from Whatman (Maidstone, United Kingdom).
Analytical Standards
The quantification of MPLs/NPLs was carried out with polymer analytical standards (MW≈1,000 Da). Quantification was undertaken by means of an external calibration curve. For this purpose, an individual standard solution of each polymer (PE, PS, PBD, PI, PP) was prepared in toluene (10 mg in 10 mL of toluene) and 5 min of ultrasonic assisted extraction (USAE). In the specific case of PA (Nylon), their identification was based on the presence of different polyamides including caprolactam and its cyclic dimer, trimer, and tetramer. The presence of all these polyamides indicates that their origin is from Nylon. Finally, the identification and quantification were based on comparison with the ε-caprolactam analytical standard by means of LC-(+ ES)-HRMS.
The different dilutions in toluene were done ranging from 1 μg/L to 1 mg/L (5 calibration points). The calibration curves were preserved at 4°C before analysis (within 1 week).
Study Area and Sampling Campaigns
The Mar Menor is one the largest hypersaline coastal lagoons in Europe, which is almost isolated from the Mediterranean Sea by La Manga del Mar Menor, which is a sand bar interrupted by a series of channels called golas, which allow a limited water flow between the Mar Menor and the Mediterranean. Consequently, this lagoon shows higher salinity and temperature range than Mediterranean, being particularly relevant seasonal variations. This geographical area is characterized by a dry Mediterranean climate that makes mild winters and hot summers with temperatures up to 40°C. Mar Menor is used for different water sports exploitations, tourism and traditional fishing exploitation. In addition, Mar Menor has other pressures including the clogging of the lagoon due to mining activity and deforestation debris that ends up in its waters, agricultural contamination, wastewater residues, human pressure (coastal cities and villages), and contamination due to fisheries and aquatic sports. It is also relevant the influence of flash flood events in the input of nutrients, pesticides (Moreno-González and León, 2017) and probably also for plastics and MPLs.
Two litter of seawater was taken at each sampling point from the upper water level (top 5 cm). Amber glass bottles, pre-rinsed with methanol and acetone in the laboratory and with seawater in each sampling point, were used for sampling. The bottles were transported under cool conditions to IEO (Murcia, Spain) laboratories and preserved at 4°C before shipment to IDAEA-CSIC (Barcelona, Spain) laboratories to perform the analytical process.
Two sampling campaigns were carried out in Mar Menor (Murcia, SE of Spain) during July 2018 (summer) in the middle of the peak season of vacation and tourism, and March 2019 (winter). Nine sampling points distributed along the whole lagoon in order to cover all of its extension were included in this study (Figure 1). The main physicochemical parameters of surface seawater (temperature, pH, conductivity, and dissolved oxygen) were measured in situ using a portable multiparametric meter (WTW, model Multi 340i/SET∗) during both campaigns are summarized in Table 1.
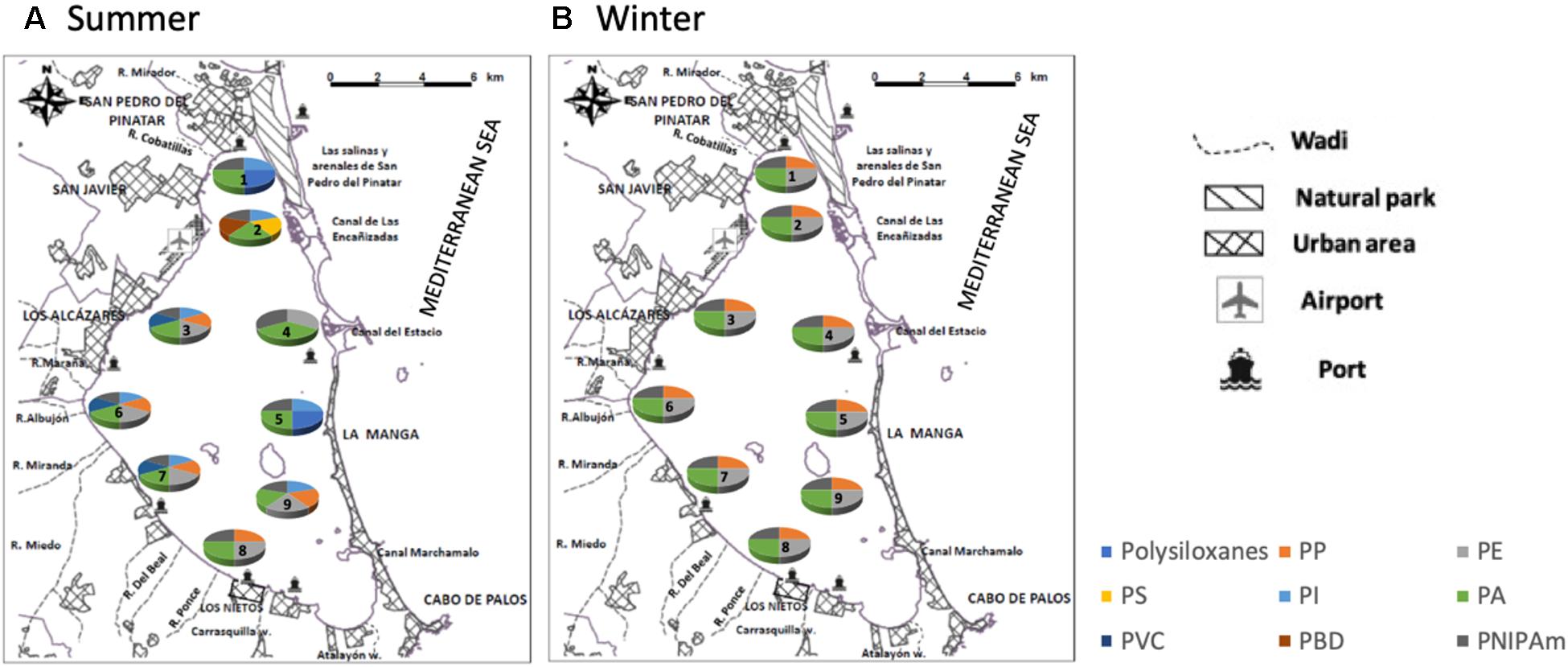
Figure 1. Occurrence of polymer micro(nano)plastics in Mar Menor during the 1st (A, Summer) and the 2nd (B, Winter) sampling periods of this study.
Sample Pre-treatment and Extraction
Initially water samples were sieved though a stainless sieve of 20 μm mesh to separate the larger MPLs. Then, according to Llorca et al. (2020c), 1.5 L of filtrate were passed through a 0.7 μm dry and tared fiber filter. The filter with particulate material was then dried overnight at 60°C until constant weight. The filters were preserved at –20°C before the extraction (within 1 week). The extraction of MPLs/NPLs from the filters was carried out with 10 mL of toluene by means of USAE for 10 min. After this time the 10 mL was collected with a Pasteur pipette and transferred to a 50 mL amber glass vial. This procedure was repeated two more times and then combined with the 10 mL used for the extraction, obtaining 30 mL of toluene. Finally, extracts were evaporated under a nitrogen stream to concentrate the samples near to 1.5 mL. The extracts were then vortexed and transferred to LC-vials and evaporated again to obtain 1.5 mL. This final extract was divided for: (i) MPLs/NPLs polymer analysis and plastic additives in toluene (1 mL) which was preserved at –20°C until chemical analysis; and (ii) plastic additives analysis in methanol (0.5 mL). This last extract was evaporated under a nitrogen stream until dryness and reconstituted in 0.5 mL of methanol. All the samples were analyzed in triplicates.
Analysis by Liquid Chromatography Coupled to High Resolution Mass Spectrometry (LC-HRMS)
Analytical Process of MPLs/NPLs’ Polymer Analyses by Size Exclusion Chromatography Coupled to High-Resolution Mass Spectrometry (SEC-HRMS)
The analysis was performed by LC-SEC-HRMS. The chromatographic separation was carried out using an Acquity LC (Waters, Milford, MA, United States) chromatographic system equipped with an advanced polymer chromatography column (Acquity APC XT45 1.7 μm) based on the work of Schirinzi et al. (2019). Twenty microliter were injected per sample with 100% toluene used as the mobile phase, in isocratic conditions and at a flow rate of 0.5 mL/min. The LC system was coupled to a Q-Exactive (Thermo Fisher Scientific, San Jose, CA, United States) hybrid quadrupole-Orbitrap mass spectrometer, equipped with an atmospheric pressure photoionization (APPI) source working in negative and positive conditions. Data acquisition was performed in full scan mode (m/z 500–3,000) with a resolution of 17,500 FWHM (Llorca et al., 2020c). The optimal parameters of the instrument can be seen elsewhere (Schirinzi et al., 2019).
Analysis of Plastic Additives and Potential Co-contaminants Adsorb to Plastic Particles Surfaces by LC-HRMS
The Acquity LC (Waters, Milford, MA, United States) chromatographic system was equipped with Purospher® STAR RP-18 endcapped column (5 μm, 2 × 125 mm; from Merck), one for the toluene mobile phase and the other for the methanol/water mobile phase. The extracts of toluene were separated by working with toluene under isocratic mode at 0.5 mL/min for 10 min, with 20 μL of sample. The extracts of methanol were separated working under gradient conditions with (A) methanol and (B) water. In this last case, the gradient started with 10% of (A) for 30 s and increased up to 90% (A) in 18 min. This percentage was maintained for 5 min and decreased again until 10% (A) in 1 min, and maintained for 30 s. The total run time was 25 min for the injection of 20 μL of sample. The chromatographic system was coupled to a QExactive (Thermo Fisher Scientific, San Jose, CA), equipped with an APPI source operating in negative and positive ionization modes for toluene extracts, while the ionization of methanolic extracts was done with an electrospray (ESI) operating in negative and positive ionization modes, in separate injections.
The instrumental process was controlled with Xcalibur 3.1 software for all LC-HRMS analysis.
Data Processing
Identification and quantification of polymers was carried out according to the methodology that was previously developed within our research group (Schirinzi et al., 2019; Llorca et al., 2020c). Very briefly, data processing was performed using the Xcalibur Qual Browser software (Thermo Fisher Scientific, San Jose, CA, United States) and Compound Discoverer 3.1 software (Thermo Fisher Scientific, San Jose, CA, United States), respectively. The total ion chromatograms (TIC) that were obtained by full-scan (FS) acquisition were processed in accordance the work of Llorca et al. (2020c). The first screening steps included peak picking and grouping. For each sample, the TIC chromatogram was interrogated every 30 s by intervals of 500 Da and peaks on which mass spectra showed repetitive mass losses were marked as suspected polymers.
Suspect profiles were tentatively identified using repetitive mass losses with a mass error below 3 ppm (identification confidence level 4) and they were subsequently filtered by comparing with a polymers’ homemade library (level 3). The tentative identification of polymers (level 2) was carried out by using Kendrick Mass Defect (KMD) analysis. The KMDs were calculated according to equations described elsewhere (Schirinzi et al., 2019) with the base unit being PolymerX/(round PolymerX-2) (Llorca et al., 2020c). Some examples can be seen in Supplementary Figures 1–3. Among the polymers that were tentatively identified, the confirmation (identification confidence level 1-confirmation) and the quantification of polymers was achieved by comparison with external curves of polymer standards based on equivalent concentration (Schirinzi et al., 2019).
Tentative Identification of Plastic Additives
Raw data from plastic additives analysis was processed using an automated screening with Compound Discoverer software version 3.1 (Thermo Fisher Scientific) as described elsewhere (Llorca et al., 2020c). This first list of suspected compounds was filtered by comparison with ChemSpider and mzCloud databases as well as the homemade database. Further filtering steps were the comparison of isotopic patterns, ionization efficiency, fragmentation patterns, and finally, the comparison of the product-ions obtained from the MS/MS spectrum of a suspected compound with the spectrum of a standard or a predicted fragmentation pattern using the information contained in the online databases. Unequivocal confirmation was only possible when a reference standard was available (identification at level 1) (Llorca et al., 2020c).
Quality Assurance and Quality Control
1.5 L of water for LC analysis was used as a blank and this was carried out during both sampling campaigns and extracted and analyzed together with the rest of the samples. On the other hand, the possible cross contamination coming from the instrumental analysis was monitored by injecting solvent blanks of toluene randomly with each injection samples list in the polymeric analysis or plastic additives in toluene as well as methanol for the methanolic fraction analysis.
Quality parameters including method limits of detection and quantification (MLOD and MLOQ, respectively), precision and recoveries were calculated by means of fortified seawater, previously filtrated by 0.2 μm fibber filters, with polymers of c.a. 1,000 Da of PP, PS, PE, PBD, PI, and PVC at three spiking levels (100, 500, and 1,000 ng/mL) according to Schirinzi et al. (2019). The values for MLOD were ranging from 2 to 11 pg/mL and MLOQ from 7 to 33 pg/mL. Intraday precision was calculated with 1,000 ng/mL spiked samples and was below 25% for all the polymers. Finally, recoveries ranged from 39% (PI) to 75% (PS).
Multivariate Analysis
Multivariate analyses using Principal Component Analysis (PCA) was carried out in order to further understand the variation among samples and the contribution of the several tentatively identified chemical compounds (Sanchís et al., 2020). PCA was performed by means of XLSTAT Excel data analysis add-on (Addinsoft, 2020). Correlation among variables and principal components (F) was quantified as the square cosine of their angle in the loading graph, which may range from 0 (α = 90°: Orthogonal, totally uncorrelated) to 1 (α = 0°: Parallel, perfectly correlated) (Sanchís et al., 2020).
Results and Discussion
Occurrence of MPLs/NPLs in the Seawater of the Mar Menor Lagoon
Two sampling campaigns were performed in Mar Menor lagoon during the summer and winter to identify seasonal differences. PE, PP, PA, and n-isopropylacrylamide (PNIPAm) were the more frequently found compounds in surface seawater, with PP being the polymer that was detected at higher concentrations, as shown in Supplementary Table 2 and Figure 2. The prevalence of PP instead of the most commonly used PE in surface seawaters can be consequence of its lower density which can favor their floatability and limit its deposition in sediments. Concerning PNIPAm and polydiemthylsiloxanes, they were only identified at confidence level 2, while the other polymers were confirmed by standards and the equivalent concentrations that were quantified. Polydiemethylsiloxanes were identified at level 2 based on their common repeating unit of (C2H6OSi)n (74.15394 uma), which was confirmed by Kendrick Mass Defect analysis. Regarding PA, which is commonly used in fishing nets, four different polymers were found: caprolactam, and the caprolactam cyclic dimer, trimer and tetramer, with the caprolactam being the polymer at the highest concentrations.
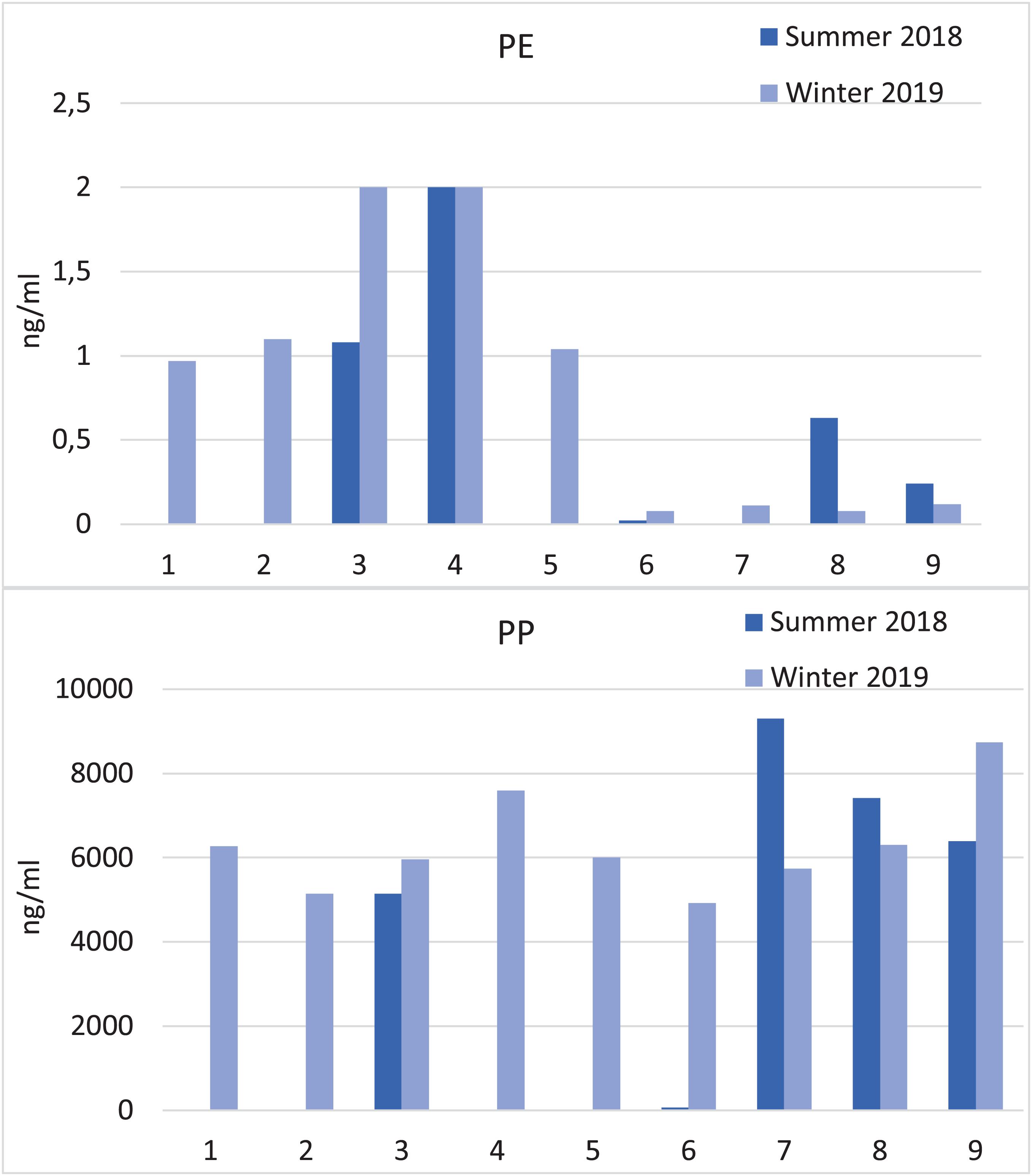
Figure 2. Concentration of PE (top graph) and PP (down graph) in samples during summer period (green color) and winter period (red color) of this study.
The main seasonal differences were observed in terms of polymer variety and concentrations. During the summer campaign, a wider variety of polymers was detected. Nine different groups were identified (PE, PI, PP, PS, PBS, PA, PVC, PNIPAm, and polysiloxanes), while only four of them (PE, PP, PA, and PNIPAm) were detected in winter. However, these four compounds were present in all of the samples that were collected in winter, showing more homogeneity along the lagoon. The total polymers were corresponding to MPLs/NPLs < 20 μm as quantified in the top 5 cm during the summer and were ranging between 0.13 and 9,303 ng/mL, while in the winter campaign the concentrations were between 4,918 and 8,744 ng/mL. Therefore, the maximum concentrations were similar, but in some sampling sites, higher concentrations were detected in winter than in summer. Nonetheless, it should be pointed out that these results are corresponding to punctual samplings, are not integrated in a period of time. Therefore, they are influenced by the hydrological conditions and punctual discharges.
At sample sites 1 (Lo Pagan) and 5 (La Manga) PI, PA, PNIPAm, and polysiloxanes were detected in summer, while PP, PE, PA, and PNIPAm were found in winter, but due to the concentrations of PP in the range of μg/mL the total concentration in both areas was higher in winter samples than in summer ones. In sampling site N°3, Los Alcazares, PP, PA, PE, and PNIPAm were found in both seasons but only polysiloxanes and PVC were present during the summer. As can be seen in these samples, the peak population in summer can influence the occurrence of some polymers through higher residues of personal care products, as is the case of polysiloxanes used in PCPs, water-repellent coatings (i.e., paints) and for inhibiting fouling on surfaces in contact with water, in cookware, electronics, among other uses (Llorca et al., 2020c), and it was identified in 77.8% of the samples of the first sampling campaign that was carried out in the summer. Furthermore, a similar situation was described for polysiloxanes in the Ebro River Delta on the east coast of Spain (Western Mediterranean Region) (Llorca et al., 2020c). In contrast, the continuous runoff from mulching, low tunnel or greenhouse films used in agricultural lands (mainly composed by PE; Loisi et al., 2017), fishing activities (harbor ropes, fishing nets made of PP and PE), and PA from fishing nets and wastewater textile fibers can influence the concentrations of these polymers throughout the year.
The central area of this lagoon (sampling points 3, 6, and 7), presented a wide variety of polymers in summer and is the most contaminated site of the northern part of the lagoon, probably as consequence of the influence of urban nuclei and El Albujón watercourse which covers the drainage of 83.4% of water-courses from land-use runoff and human activities including urbanization, tourism and fishing (Bayo et al., 2020).
The southern part of the lagoon presented the most contaminated sites and a large number of polymers in summer, in particular sites 7, 8, and 9 corresponding to Los Urritias, Los Nietos, and Isla del Baron, respectively. These results agree with previous works carried out in the same area in which the occurrence of larger-sized MPLs (León et al., 2018, 2019; de Haan et al., 2019; Bayo et al., 2020) was explored. For example, a previous study carried out by Bayo et al. (2020) investigating the presence of MPLs in the sand of natural and urban beaches of the lagoon, Los Urrutias and Los Narejos (near to the sampling point 3, Los Alcázares, in the present study) were the more contaminated sites, which is in agreement with our findings (see Figure 2 and Table 2). PE and PP were also identified in the sand of natural and urban beaches (Bayo et al., 2019, 2020), or in waters from the same lagoon. In these works, the presence of the same polymers was confirmed showing PE (up to 37%) and PP (up to 12.4%) among others (León et al., 2018), or in waters taken in 2016 in La Llana beach (near to sampling point 1 in our study). In another example, the PE was identified in MPLs up to 18%, PP 19.5%, PS 13%, and PA 45.5% (León et al., 2019). In another work carried out in waters from the Cartagena transect (outside Mar Menor lagoon), the proportion of polymer types identified in MPLs, as well as mesoplastics, was 47% of PE, 21% of PP, 5% of PS, 8% of paint chips (pure pigmented particles), among others, in all samples (de Haan et al., 2019).
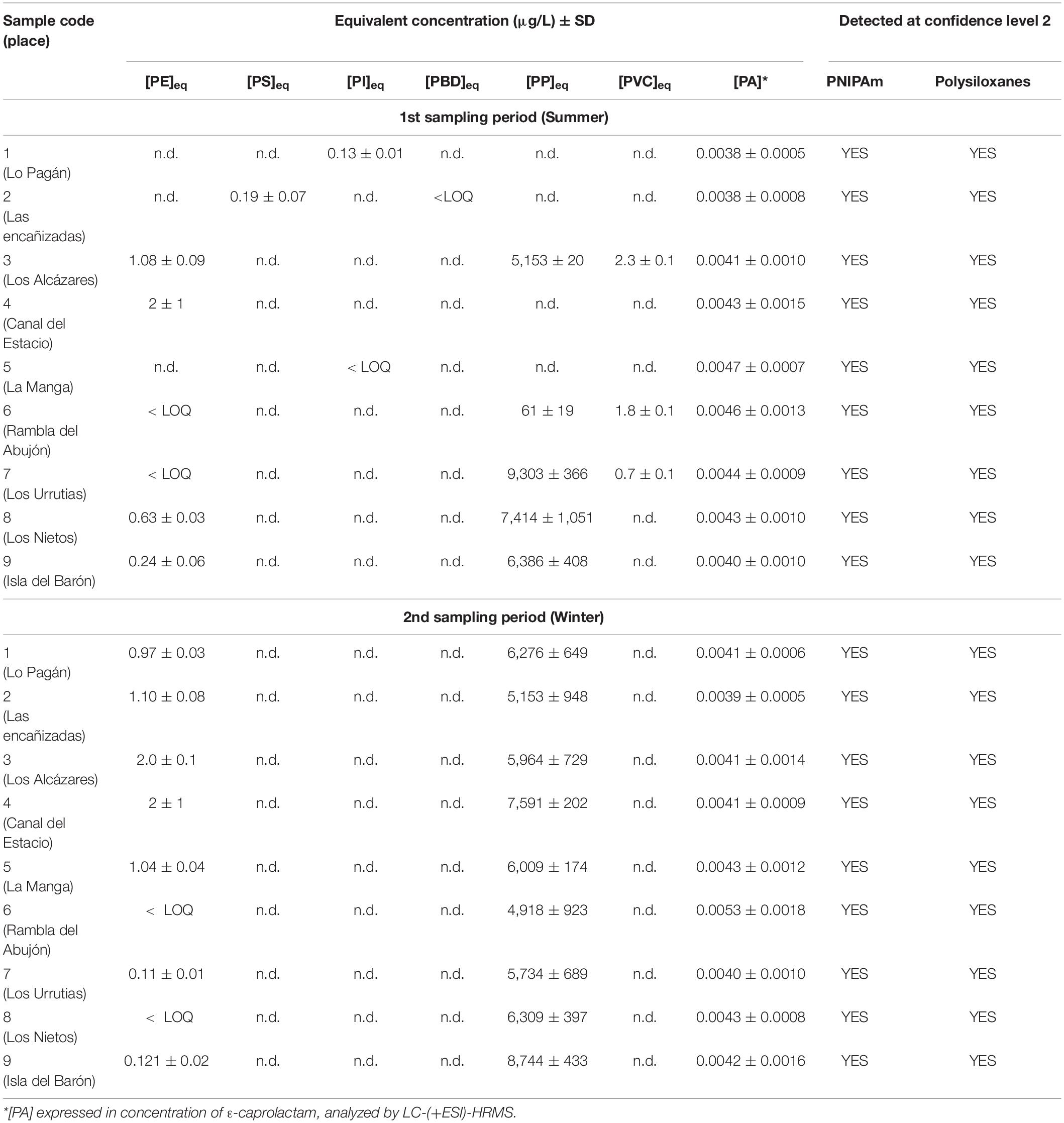
Table 2. Concentration of MPLs/NPLs polymers from suspended material samples expressed in equivalent concentration in μg/L (± SD).
In general trends, our results agree with the main classes of polymers identified in marine MPLs in other studies in the Mediterranean Sea, in which the most commonly found polymers were PE, PP, PS, PET, PVC, and PA (Gajšt et al., 2016; Munari et al., 2017; de Haan et al., 2019; Llorca et al., 2020b), and also in other regions (Cincinelli et al., 2017; Li et al., 2020; Ramírez-Álvarez et al., 2020). However, PE is the dominant compound in most of these studies, while the polymer found at much higher concentrations in the present work was PP. A possible explanation for this difference is the size of particles studied here (nm-20 μm), and the fact of high-density polyethylene is the dominant PE use in the applications in this area, being the buoyancy of PE particles lower than for PP, and this is shown especially notorious in the top 5 cm of the surface water in this hypersaline lagoon.
The suspect screening approach that was used here allowed identify the polymers that were solubilized in toluene and other related polymers which are inorganic (siloxanes) or others that are not considered to be plastics in the strict sense, but are a hybrid between synthetic plastic polymer and synthetic rubbers (Jubilant Performance Materials, 2020).
The detection of MNPLs in these samples is of special interest due to their small size but also because they can be another source of pollutants through the release of additives and plasticizers (Llorca et al., 2020b). In addition, MNPLs can accumulate organic and inorganic contaminants as well as pathogens from the environment (air, water or particulate matter), being an important vector for transport of those co-contaminants to aquatic organisms (Rios et al., 2007; Ashton et al., 2010; Cole et al., 2011; Llorca et al., 2014; Pittura et al., 2018). Due to their small size, similar to plankton, benthic protozoa and bacteria, MNPLs can enter into marine food web by their ingestion by aquatic organisms (Wright et al., 2013; Cole et al., 2014; Pittura et al., 2018; Llorca et al., 2020b). Therefore, it is necessary to consider the analysis of other integrative matrices (biota and/or sediments) and to evaluate the high spatial and temporary variability of concentrations.
Tentative Identification of Plastic Additives in Mar Menor Lagoon
According to the previously described process, the first screening step consisted of peak-picking, RT alignment and grouping of compounds, isotopes and adducts along with the samples. Signals below 1,000,000 were considered to be background and were discarded. The first list of suspected compounds with an error mass below 2 ppm was then filtered and compared with the ChemSpider and mzCloud databases, and 94,365 substances were included in this filtering. The next filtering steps, by limiting peak area (>750,000,000), generated a list of 11,673 potential compounds. This list was subsequently reduced, while considering the chromatographic peak factor resolution > 0.75. This process selected 3,876 candidates. Then, each compound was evaluated, and the final list included 135 chemicals among all of the samples. Of this list of chemical compounds, 43 were plastic additives and 31 more compounds are used in the production of polymers or are monomers coming from the decomposition of plastics. Other chemical compounds have diverse origins and were probably adsorbed to the outside of these particles and the particulate matter and are discussed in the next section (see Supplementary Tables 1, 2).
The major group of plastic additives detected (Supplementary Table 1) were plasticizers corresponding to the 56% of identified additives (level 2), and they were also the most frequently detected in the samples. Among them, seven different phthalates (dibutyl phthalate, diethyl phthalate, dimethyl phthalate, dioctyl phthalate (DNOP), dipropyl phthalate, mono (2-ethylhexyl) phthalate (MEHP), and monobutyl phthalate) were identified. This group was the most frequently detected in the samples in both sampling campaigns in 90 and 100% of the sampled sites in winter and summer, respectively. A recent study also evidenced the relevance of this group of contaminants because the occurrence of phthalates was confirmed in seawater from Mar Menor lagoon for 11–17% of samples, showing maximum concentrations of 560 and 1,270 ng/l in spring and autumn, respectively (Concha-Graña et al., 2021). Other examples of plasticizers detected in the samples were N-butylbenzenesulfonamide, tributyl citrate acetate (ATBC), the polyvinyl acetate, dimethyl sebacate (DMS), and the diethyl sebacate (DES), and these last two are very much used in thermoplastics as polyurethanes, and they are also used as UV stabilizers.
The second group of plastic additives identified were stabilizers that are used as antioxidants, such as distearyl 3, 3’-thiodipropionate, 2, 5-di-tert-butylhydroquinone and IRGANOX 3114, and the UV-filters such as the group of benzotriazoles and malonic acid used as UV filter, antioxidant, and corrosion inhibitor. Another relevant group of plastic additives present in the samples were flame retardants as dibutyl phosphate, diethylethyl phosphonate, triethylphosphonoacetate, and triethylphospate although this last example also has an application as plasticizer. Finally, other additives used, for example, for viscosity control, such as palmitamide were also found in some samples. Some of these plastic additives were previously found in the desorbed fraction to seawater (24 h) from plastic debris sampled in several Mar Menor beaches, being particularly relevant for tributyl acetyl citrate, tris-2chloropropyl phosphate and the UV filters (ethylhexhyl salicylate and benzophenone) (León et al., 2018).
Sites 3, 4, 5, 6, and 7 were those showing higher pollution of plastic additives in summer while 4, 5, 6, and 7 were those with the most compounds in winter, probably due to the influence of El Albujón watercourse discharges (groundwater, agriculture water irrigation excess, treated urban wastewaters, etc.). In the case of site 3, Los Alcázares is an urban area with important touristic activity and, as aforementioned, the higher level of contamination during summer can be attributed to the increase in population during the warmer period. Furthermore, it was one of the most contaminated sites with MPLs/NPLs (Figure 1). In the case of sampling point 4, Canal del Estacio, the contamination is related to harbor activities such as fishing, aquatic sports, and boat preservation materials. This point did not present a large variety of polymers but rather higher concentrations of PE in both sampling campaigns and PP during the winter.
In the case of monomers or other related compounds that are used during the synthesis of polymers, 2-ethylhexyl acrylate (2-EHA), indene, 10-hydroxy-2-decenoic acid and dibutyl sebacate have been the most detected examples during the first sampling period (summer), while 2-EHA, azelaic acid, indene and tripropylene glycol have been the most ubiquitous during the second sampling period (winter). The high ubiquity of 2-EHA could be explained because this compound and also butyl acrylate are the major base monomers for the preparation of acrylate adhesives, or are used in the copolymerization of vinyl acetate, methyl acrylate, and styrene to modify the properties of the resulting polymers. On the other hand, the presence of azelaic acid could be linked to the decomposition of nylon since this is one of the main monomers that are used during the synthesis of Nylon 6/9, which can be present in woven fabric and some fishing devices, among others. In the case of indene, this compound is used during the production of thermoplastic resins.
Other Compounds
Other compounds that were detected in this fraction of the samples are those that are potentially adsorbed onto plastic particles and the natural suspended material. These compounds were identified at confidence level 2 and most of them are medium to low polarity compounds, which tend to be accumulated from the surrounding waters onto the surfaces of buoyant plastic particles. The main groups of identified compounds were pharmaceuticals, PCPs and pesticides as can be seen in Supplementary Table 2 of the Supporting Information section. These results agree with previously reported studies of the same area (Moreno-González et al., 2014, 2015; Jiménez-Martínez et al., 2016; León et al., 2017), in which the same groups and contaminants were found. Moreover, León et al. (2018) confirmed the presence of some of the same group of contaminants adsorbed onto plastic debris sampled in beaches from the Mar Menor lagoon (León et al., 2018) as a consequence of their accumulation from air (Carratalá et al., 2017), freshwater, soil, etc. In Figure 3, the main results of the tentatively identified contaminants, grouped by families, are presented. As can be seen, a similar number of compounds were identified during both sampling campaigns, but only slightly higher in winter, probably due to the slower sun degradation of some compounds during the winter season.
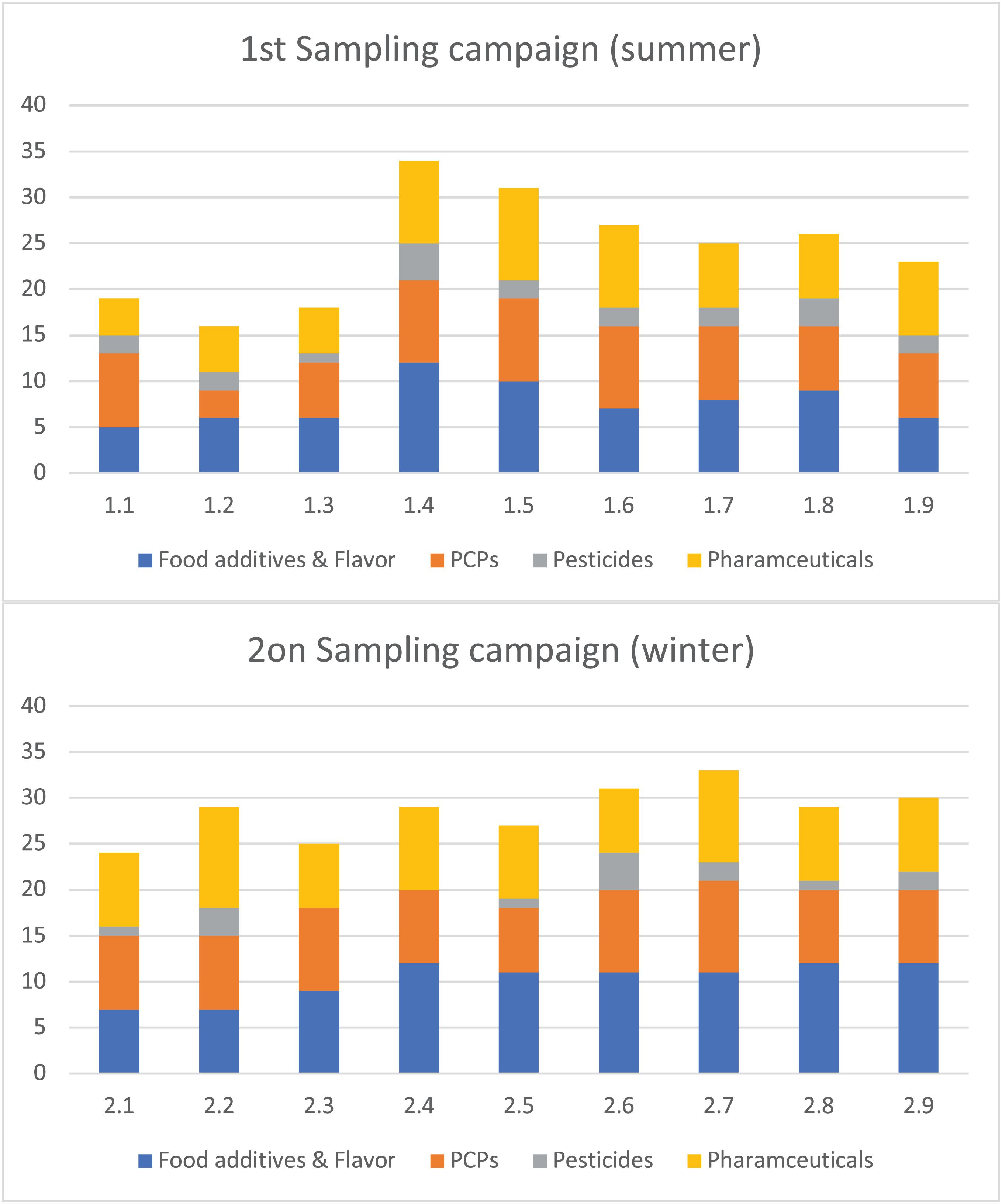
Figure 3. Number of compounds tentatively identified in both sampling periods of this study, distributed in families.
The main groups of compounds were those that were included in PCPs such as ethylhexyl ethylhexanoate which is used as a UV filter in sunscreens, as lauramide that is used in liquid shampoos, bubble bath preparations, dishwashing products and light-duty liquid detergents as a foam stabilizer, and organic acids such as myristic and steric which are very much used and incorporated into formulations such as lubricants, emollients, and emulsifiers. Another group of frequently detected compounds were pharmaceuticals, among which the most detected compounds were 1-hydroxy-4-methoxybenzene which is used in the treatment of solar lentigines and related hyperpigmented lesions resulting from chronic sun exposure; aceclidine which is used for glaucoma disease, and valpromide which is a carboxamide derivative of valproic acid that is used in the treatment of epilepsy and some affective disorders.
Another relevant group of compounds that is found attached to plastic particulates < 20 μm were food additives. Sixteen compounds were identified at level 2, being the flavoring agent 2-methylpropyl-3-o1obutanoate (isobutyl acetoacetate) which is the most frequently found in 100% of the samples in both sampling periods. Other food frequently found additives were pyruvic and hexanoic acids.
With regards to the geographical distribution, a higher number of compounds were found in the southern part of the lagoon (from 4 to 9) in both sampling periods. Sampling points 4 and 5, corresponding to Canal del Estacio and La Manga, respectively, are highly impacted by summer harbor activities and tourism, respectively, and this is reflected in the total chemical contamination in both sampling periods but particularly during summer. Sampling point 5 has been previously confirmed as depositional area subject to the influence el El Albujón watercourse discharges, particularly during flash flood events, showing higher concentrations for some contaminants in sediments than other surrounding points (Moreno-González and León, 2017).
Multivariate Analysis by PCA
To elucidate any trend among sampling sites and/or campaigns, PCA modeling has been performed. The first two principal components, F1 and F2, explain 29.64% of the data variance, thus indicating the high dimensionality and complexity of the data. Focusing on F1 and F2, the former is related to season changes among samples as observations that are effectively clustered in two separated groups (see Figure 4) with just 16.12% of total explained variance. F1 is mainly correlated to the variables 1,3-benzenediol, 2,6-Di-tert-butyl-4-methoxyphenol, 3-(3,5-di-tert-butyl-4-hydroxyphenyl) propanoic acid, 4-Tert-butylphenol, dipropyl phthalate and tetrahydrofurfuryl acrylate, all of them being plastic additives; 10-hydroxy-2-decenoic acid, 2-EHA and EDOT are products related to the synthesis of polymers that have been released to the media because of the weathering effects on bigger plastics; vanillin and non-ivamide can be used as food additive, flavoring agent, PCPs or pharmaceuticals; 1-benzosuberone and 2-(3H)-furanone are both from pharmaceutical usage; and 2-isobutoxynaphthalene is used as a food additive and flavoring agent. The latter, F2, does not have a clear interpretation but is related to some phthalates (benzyl butyl phthalate, dibutyl phthalate, diethyl phthalate) and tributyl citrate acetate plastic additives; 2-ethylhexanoic acid, azelaic acid, and N-methylpyrrolidone compounds are related to the synthesis of polymers; benzaldehyde is used as PCPs and pesticide; N-isopropylacrylamide and valpromide are from pharmaceutical use.
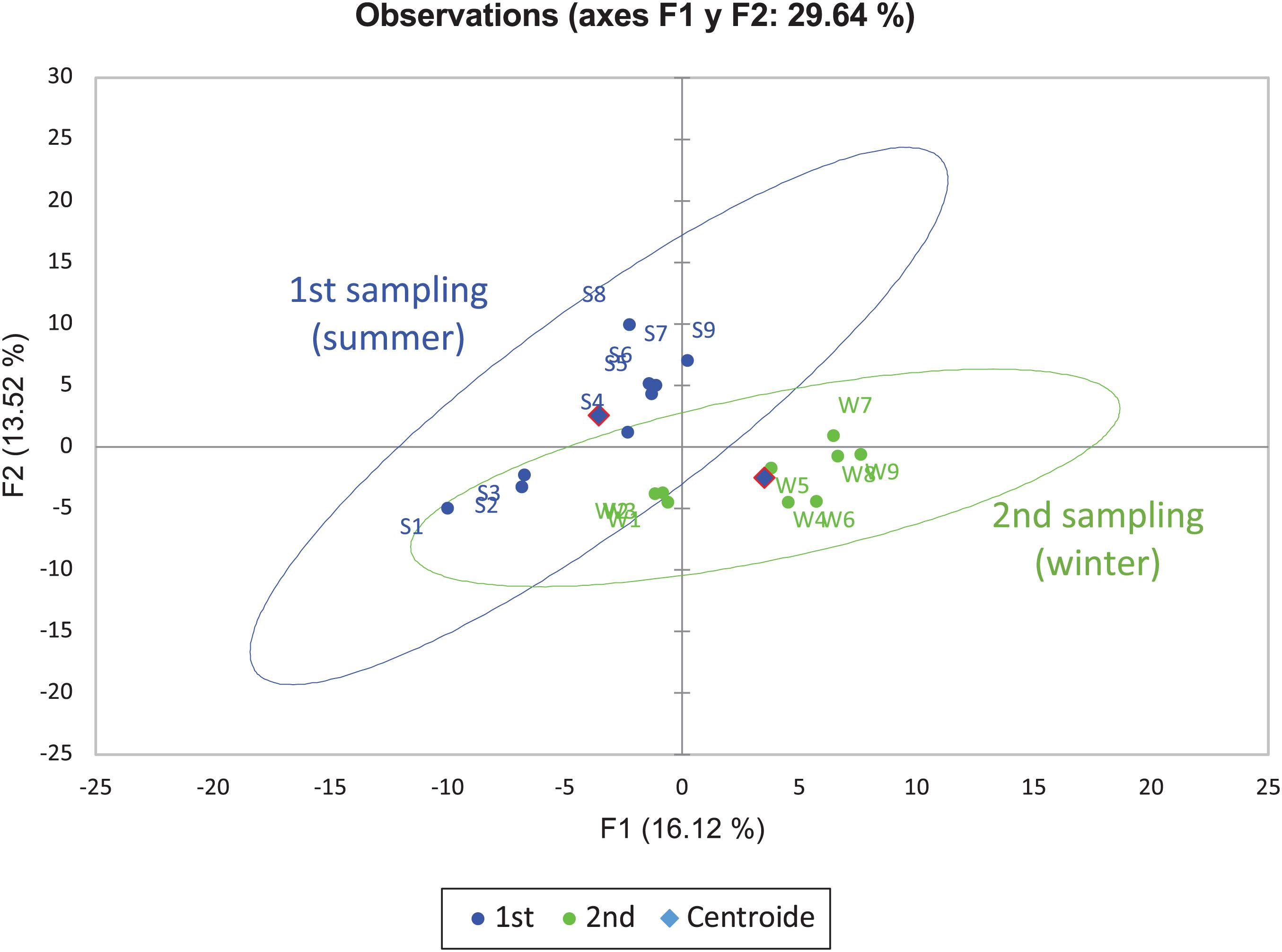
Figure 4. Score plot of PCA with scaled data, representing samples in F1 and F2 axes. Samples corresponding to 1st period (summer) of the study are presented in blue while the samples corresponding to the 2nd campaign (winter) are in green. The two ellipses were generated automatically thus highlighting an area with a 98% probability of containing samples of the same type.
Conclusion
This work reports on the presence of micro(nano)plastics in the top 5 cm of seawater from the Mar Menor lagoon through suspect-screening analysis. The methodology, based on a previously published work (Llorca et al., 2020c), consisted of USAE followed by SEC-(–/+)APPI-HRMS for the analysis of polymers and allowed the characterization of toluene soluble polymers, and LC-APPI-HRMS for the tentative identification of plastic additives and other contaminants that are potentially adsorbed to the surfaces of plastic particles.
The results confirmed the presence of nine polymers including PS, PE, PI, PBD, PP, PA, PVC, PNIPAm, and polysiloxanes. The more frequently detected polymers were PP, PE, PA, and PNIPAm, while the compound found at higher concentrations was PP probably due its lower density than other polymers. The presence of these plastic particles, in particular those in the nano-sized particle range, induces not only an environmental problem itself due to their low degradability but also the potential impact on biota that can bioaccumulate micro(nano)plastics in their organism and their potential transfer to the food webs. In this sense it is necessary to consider that additional studies are required for the determination of MPLs and NPLs using integrative matrices (biota and/or sediments) because water samples analysis only offer a snapshot for these contaminants occurrence with a high spatial and temporary variability of concentrations.
On the other hand, 74 of organic contaminants detected in these samples were plastic additives and compounds which are used in the manufacture of polymers or are coming from the decomposition of polymers, being plasticizers, the wider group of tentatively identified compounds. Finally, other pharmaceuticals, pesticides, food additives and flavors, and natural products were also identified in the samples. We hypothesize that these compounds are coming from the accumulation of surrounding waters their contribution to bioaccumulation as Trojan horse effect should be considered.
Data Availability Statement
The original contributions presented in the study are included in the article/Supplementary Material, further inquiries can be directed to the corresponding author/s.
Author Contributions
VL, EA, and MF contributed to the conception and design of the study. ML organized the database and performed the statistical analysis. AV-H and KS perform the experimental work. AV-H wrote the first draft of the manuscript. ML and MF wrote sections of the manuscript. All authors contributed to manuscript revision, read, and approved the submitted version.
Funding
This work was supported by the PLAS-MED project (CTM201 7-89701-C3-1-R and CTM2017-89701-C3-3-R) from the Spanish Ministry of Economy and Digital Transformation and by the European Union through the European Regional Development Fund (ERDF).
Conflict of Interest
The authors declare that the research was conducted in the absence of any commercial or financial relationships that could be construed as a potential conflict of interest.
Publisher’s Note
All claims expressed in this article are solely those of the authors and do not necessarily represent those of their affiliated organizations, or those of the publisher, the editors and the reviewers. Any product that may be evaluated in this article, or claim that may be made by its manufacturer, is not guaranteed or endorsed by the publisher.
Acknowledgments
AV-H thanks for his grant PRE2018-083989 from the Spanish Ministry of Science and Innovation.
Supplementary Material
The Supplementary Material for this article can be found online at: https://www.frontiersin.org/articles/10.3389/fmars.2021.697424/full#supplementary-material
References
Andrady, A. L. (2011). Microplastics in the marine environment. Mar. Pollut. Bull. 62, 1596–1605. doi: 10.1016/j.marpolbul.2011.05.030
Ashton, K., Holmes, L., and Turner, A. (2010). Association of metals with plastic production pellets in the marine environment. Mar. Pollut. Bull. 60, 2050–2055. doi: 10.1016/j.marpolbul.2010.07.014
Bayo, J., Rojo, D., and Olmos, S. (2019). Abundance, morphology and chemical composition of microplastics in sand and sediments from a protected coastal area: the mar menor lagoon (SE Spain). Environ. Pollut. 252, 1357–1366. doi: 10.1016/j.envpol.2019.06.024
Bayo, J., Rojo, D., Olmos, S., and López, M. (2020). Microplastic pollution on the strandline of urban and natural city beaches: the role of local activities. Int. J. Environ. Impacts 3, 155–167. doi: 10.2495/ei-v3-n2-155-167
Cai, H., Xu, E. G., Du, F., Li, R., Liu, J., and Shi, H. (2021). Analysis of environmental nanoplastics: progress and challenges. Chem. Eng. J. 410:128208. doi: 10.1016/j.cej.2020.128208
Carratalá, A., Moreno-González, R., and León, V. M. (2017). Occurrence and seasonal distribution of polycyclic aromatic hydrocarbons and legacy and current-use pesticides in air from a Mediterranean coastal lagoon (Mar Menor, SE Spain). Chemosphere 167, 382–395. doi: 10.1016/j.chemosphere.2016.09.157
Castelvetro, V., Corti, A., Bianchi, S., Giacomelli, G., Manariti, A., and Vinciguerra, V. (2021). Microplastics in fish meal: contamination level analyzed by polymer type, including polyester (PET), polyolefins, and polystyrene. Environ. Pollut. 273:115792. doi: 10.1016/j.envpol.2020.115792
Cincinelli, A., Martellini, T., Guerranti, C., Scopetani, C., Chelazzi, D., and Giarrizzo, T. (2019). A potpourri of microplastics in the sea surface and water column of the Mediterranean Sea. Trends Anal. Chem. 110, 321–326. doi: 10.1016/j.trac.2018.10.026
Cincinelli, A., Scopetani, C., Chelazzi, D., Lombardini, E., Martellini, T., Katsoyiannis, A., et al. (2017). Microplastic in the surface waters of the Ross Sea (Antarctica): occurrence, distribution and characterization by FTIR. Chemosphere 175, 391–400. doi: 10.1016/j.chemosphere.2017.02.024
Cole, M., Lindeque, P., Fileman, E., Halsband, C., Goodhead, R., Moger, J., et al. (2013). Microplastic ingestion by zooplankton. Environ. Sci. Technol. 47, 6646–6655. doi: 10.1021/es400663f
Cole, M., Lindeque, P., Halsband, C., and Galloway, T. S. (2011). Microplastics as contaminants in the marine environment: a review. Mar. Pollut. Bull. 62, 2588–2597. doi: 10.1016/j.marpolbul.2011.09.025
Cole, M., Webb, H., Lindeque, P. K., Fileman, E. S., Halsband, C., and Galloway, T. S. (2014). Isolation of microplastics in biota-rich seawater samples and marine organisms. Sci. Rep. 4:4528.
Concha-Graña, E., Moscoso-Pérez, C., Fernández-González, V., López-Mahía, P., Gago, J., León, V. M., et al. (2021). Phthalates, organotin compounds and per-polyfluoroalkyl substances in semiconfined areas of the Spanish coast: occurrence, sources and risk assessment. Sci. Total Environ. 780:146450. doi: 10.1016/j.scitotenv.2021.146450
Cózar, A., Sanz-Martín, M., Martí, E., González-Gordillo, J. I., Ubeda, B. Á, Gálvez, J., et al. (2015). Plastic accumulation in the mediterranean sea. PLoS One 10:e0121762. doi: 10.1371/journal.pone.0121762
David, J., Steinmetz, Z., Kuèerík, J., and Schaumann, G. E. (2018). Quantitative analysis of poly(ethylene terephthalate) microplastics in soil via thermogravimetry-mass spectrometry. Anal. Chem. 90, 8793–8799. doi: 10.1021/acs.analchem.8b00355
de Haan, W. P., Sanchez-Vidal, A., and Canals, M. (2019). Floating microplastics and aggregate formation in the Western Mediterranean Sea. Mar. Pollut. Bull. 140, 523–535. doi: 10.1016/j.marpolbul.2019.01.053
Dehaut, A., Cassone, A. L., Frère, L., Hermabessiere, L., Himber, C., Rinnert, E., et al. (2016). Microplastics in seafood: benchmark protocol for their extraction and characterization. Environ. Pollut. 215, 223–233. doi: 10.1016/j.envpol.2016.05.018
Fendall, L. S., and Sewell, M. A. (2009). Contributing to marine pollution by washing your face: microplastics in facial cleansers. Mar. Pollut. Bull. 58, 1225–1228. doi: 10.1016/j.marpolbul.2009.04.025
Filgueiras, A. V., Gago, J., García, I., León, V. M., and Viñas, L. (2021). Plackett burman design for microplastics quantification in marine sediments. Mar. Pollut. Bull. 162:111841. doi: 10.1016/j.marpolbul.2020.111841
Fischer, M., and Scholz-Böttcher, B. M. (2017). Simultaneous trace identification and quantification of common types of microplastics in environmental samples by pyrolysis-gas chromatography-mass spectrometry. Environ. Sci. Technol. 51, 5052–5060. doi: 10.1021/acs.est.6b06362
Gajšt, T., Bizjak, T., Palatinus, A., Liubartseva, S., and Kržan, A. (2016). Sea surface microplastics in slovenian part of the Northern Adriatic. Mar. Pollut. Bull. 113, 392–399. doi: 10.1016/j.marpolbul.2016.10.031
Hantoro, I., Löhr, A. J., Van Belleghem, F. G. A. J., Widianarko, B., and Ragas, A. M. J. (2019). Microplastics in coastal areas and seafood: implications for food safety. Food Addit. Contam. Part A Chem. Anal. Control Expo. Risk Assess. 36, 674–711. doi: 10.1080/19440049.2019.1585581
Jiménez-Martínez, J., García-Aróstegui, J. L., Hunink, J. E., Contreras, S., Baudron, P., and Candela, L. (2016). The role of groundwater in highly human-modified hydrosystems: a review of impacts and mitigation options in the Campo de Cartagena-Mar Menor coastal plain (SE Spain). Environ. Rev. 24, 377–392. doi: 10.1139/er-2015-0089
Jubilant Performance Materials (2020). Jubilant Performance Materials. Available online at: http://www.jubilant-material.com/ (accessed 2020).
Klein, S., Dimzon, I. K., Eubeler, J., and Knepper, T. P. (2018). “Analysis, occurrence, and degradation of microplastics in the aqueous environment,” in Handbook of Environmental Chemistry, eds M. Wagner and S. Lambert (Cham: Springer).
Koelmans, A. A., Bakir, A., Burton, G. A., and Janssen, C. R. (2016). Microplastic as a vector for chemicals in the aquatic environment: critical review and model-supported reinterpretation of empirical studies. Environ. Sci. Technol. 50, 3315–3326. doi: 10.1021/acs.est.5b06069
Koelmans, A. A., Besseling, E., and Foekema, E. M. (2014). Leaching of plastic additives to marine organisms. Environ. Pollut. 187, 49–54. doi: 10.1016/j.envpol.2013.12.013
La Nasa, J., Biale, G., Fabbri, D., and Modugno, F. (2020). A review on challenges and developments of analytical pyrolysis and other thermoanalytical techniques for the quali-quantitative determination of microplastics. J. Anal. Appl. Pyrolysis 149:104841. doi: 10.1016/j.jaap.2020.104841
León, V. M., García, I., González, E., Samper, R., Fernández-González, V., and Muniategui-Lorenzo, S. (2018). Potential transfer of organic pollutants from littoral plastics debris to the marine environment. Environ. Pollut. 236, 442–453. doi: 10.1016/j.envpol.2018.01.114
León, V. M., García-Agüera, I., Moltó, V., Fernández-González, V., Llorca-Pérez, L., Andrade, J. M., et al. (2019). PAHs, pesticides, personal care products and plastic additives in plastic debris from Spanish Mediterranean beaches. Science Total Environ. 670, 672–684. doi: 10.1016/j.scitotenv.2019.03.216
León, V. M., Moreno-González, R., García, V., and Campillo, J. A. (2017). Impact of flash flood events on the distribution of organic pollutants in surface sediments from a Mediterranean coastal lagoon (Mar Menor, SE Spain). Environ. Sci. Pollut. Res. 24, 4284–4300. doi: 10.1007/s11356-015-4628-y
Li, Y., Lu, Z., Zheng, H., Wang, J., and Chen, C. (2020). Microplastics in surface water and sediments of Chongming Island in the Yangtze Estuary. China. Environ. Sci. Eur. 32:15.
Llorca, M., Ábalos, M., Vega-Herrera, A., Adrados, M. A., Abad, E., and Farré, M. (2020a). Adsorption and desorption behaviour of polychlorinated biphenyls onto microplastics’ surfaces in water/sediment systems. Toxics 8:59. doi: 10.3390/toxics8030059
Llorca, M., Álvarez-Muñoz, D., Ábalos, M., Rodríguez-Mozaz, S., Santos, L. H. M. L. M., León, V. M., et al. (2020b). Microplastics in Mediterranean coastal area: toxicity and impact for the environment and human health. Trends Environ. Anal. Chem. 27:e00090. doi: 10.1016/j.teac.2020.e00090
Llorca, M., Farré, M., Karapanagioti, H. K., and Barceló, D. (2014). Levels and fate of perfluoroalkyl substances in beached plastic pellets and sediments collected from Greece. Mar. Pollut. Bull. 87, 286–291. doi: 10.1016/j.marpolbul.2014.07.036
Llorca, M., Vega-Herrera, A., Schirinzi, G., Savva, K., Abad, E., and Farré, M. (2020c). Screening of suspected micro (nano) plastics in the Ebro Delta (Mediterranean Sea). J. Hazard. Mater. 404:124022.
Llorca, M., Vega-Herrera, A., Schirinzi, G., Savva, K., Abad, E., and Farré, M. (2021). Screening of suspected micro(nano)plastics in the Ebro Delta (Mediterranean Sea). J. Hazard. Mater. 404:124022. doi: 10.1016/j.jhazmat.2020.124022
Loisi, R. V., Sica, C., Blanco, I., Schettini, E., Scarascia Mugnozza, G., and Vox, G. (2017). Land analysis of agricultural plastic waste production in a South Italy area by means of a geographical information system. Acta Hortic. 1170, 579–586. doi: 10.17660/actahortic.2017.1170.72
Moreno-González, R., and León, V. M. (2017). Presence and distribution of current-use pesticides in surface marine sediments from a Mediterranean coastal lagoon (SE Spain). Environ. Sci. Pollut. Res. 24, 8033–8048. doi: 10.1007/s11356-017-8456-0
Moreno-González, R., Rodriguez-Mozaz, S., Gros, M., Barceló, D., and León, V. M. (2015). Seasonal distribution of pharmaceuticals in marine water and sediment from a mediterranean coastal lagoon (SE Spain). Environ. Res. 138, 326–344. doi: 10.1016/j.envres.2015.02.016
Moreno-González, R., Rodríguez-Mozaz, S., Gros, M., Pérez-Cánovas, E., Barceló, D., and León, V. M. (2014). Input of pharmaceuticals through coastal surface watercourses into a Mediterranean lagoon (Mar Menor, SE Spain): sources and seasonal variations. Sci. Total Environ. 490, 59–72. doi: 10.1016/j.scitotenv.2014.04.097
Munari, C., Scoponi, M., and Mistri, M. (2017). Plastic debris in the Mediterranean Sea: types, occurrence and distribution along Adriatic shorelines. Waste Manag. 67, 385–391. doi: 10.1016/j.wasman.2017.05.020
Neto, J. G. B., Rodrigues, F. L., Ortega, I., Rodrigues, L. D. S., Lacerda, A. L. D. F., Coletto, J. L., et al. (2020). Ingestion of plastic debris by commercially important marine fish in southeast-south Brazil. Environ. Pollut. 267:115508. doi: 10.1016/j.envpol.2020.115508
Pittura, L., Avio, C. G., Giuliani, M. E., D’errico, G., Keiter, S. H., Cormier, B., et al. (2018). Microplastics as vehicles of environmental PAHs to marine organisms: combined chemical and physical hazards to the mediterranean mussels, Mytilus galloprovincialis. Front. Mar. Sci. 5:103. doi: 10.3389/fmars.2018.00103
Ramírez-Álvarez, N., Rios Mendoza, L. M., Macías-Zamora, J. V., Oregel-Vázquez, L., Alvarez-Aguilar, A., Hernández-Guzmán, F. A., et al. (2020). Microplastics: sources and distribution in surface waters and sediments of todos Santos Bay, Mexico. Sci. Total Environ. 703:134838. doi: 10.1016/j.scitotenv.2019.134838
Rios, L. M., Moore, C., and Jones, P. R. (2007). Persistent organic pollutants carried by synthetic polymers in the ocean environment. Mar. Pollut. Bull. 54, 1230–1237. doi: 10.1016/j.marpolbul.2007.03.022
Sanchís, J., Jaén-Gil, A., Gago-Ferrero, P., Munthali, E., and Farré, M. J. (2020). Characterization of organic matter by HRMS in surface waters: effects of chlorination on molecular fingerprints and correlation with DBP formation potential. Water Res. 176:115743. doi: 10.1016/j.watres.2020.115743
Schirinzi, G. F., Llorca, M., Seró, R., Moyano, E., Barceló, D., Abad, E., et al. (2019). Trace analysis of polystyrene microplastics in natural waters. Chemosphere 236:124321. doi: 10.1016/j.chemosphere.2019.07.052
Schirinzi, G. F., Pedà, C., Battaglia, P., Laface, F., Galli, M., Baini, M., et al. (2020). A new digestion approach for the extraction of microplastics from gastrointestinal tracts (GITs) of the common dolphinfish (Coryphaena hippurus) from the western Mediterranean Sea. J. Hazard. Mater. 397:122794. doi: 10.1016/j.jhazmat.2020.122794
Schmidt, N., Castro-Jiménez, J., Oursel, B., and Sempéré, R. (2021). Phthalates and organophosphate esters in surface water, sediments and zooplankton of the NW Mediterranean Sea: exploring links with microplastic abundance and accumulation in the marine food web. Environ. Pollut. 272:115970. doi: 10.1016/j.envpol.2020.115970
Shahul Hamid, F., Bhatti, M. S., Anuar, N., Anuar, N., Mohan, P., and Periathamby, A. (2018). Worldwide distribution and abundance of microplastic: how dire is the situation? Waste Manag. Res. 36, 873–897. doi: 10.1177/0734242x18785730
Sobhani, Z., Zhang, X., Gibson, C., Naidu, R., Megharaj, M., and Fang, C. (2020). Identification and visualisation of microplastics/nanoplastics by Raman imaging (i): down to 100 nm. Water Res. 174:115658. doi: 10.1016/j.watres.2020.115658
Suaria, G., Avio, C. G., Mineo, A., Lattin, G. L., Magaldi, M. G., Belmonte, G., et al. (2016). The Mediterranean plastic soup: synthetic polymers in Mediterranean surface waters. Sci. Rep. 6:37551.
Van Cauwenberghe, L., and Janssen, C. R. (2014). Microplastics in bivalves cultured for human consumption. Environ. Pollut. 193, 65–70. doi: 10.1016/j.envpol.2014.06.010
Van Cauwenberghe, L., Vanreusel, A., Mees, J., and Janssen, C. R. (2013). Microplastic pollution in deep-sea sediments. Environ. Pollut. 182, 495–499. doi: 10.1016/j.envpol.2013.08.013
Vianello, A., Boldrin, A., Guerriero, P., Moschino, V., Rella, R., Sturaro, A., et al. (2013). Microplastic particles in sediments of Lagoon of Venice, Italy: first observations on occurrence, spatial patterns and identification. Estuar. Coast. Shelf Sci. 130, 54–61. doi: 10.1016/j.ecss.2013.03.022
Waller, C. L., Griffiths, H. J., Waluda, C. M., Thorpe, S. E., Loaiza, I., Moreno, B., et al. (2017). Microplastics in the Antarctic marine system: an emerging area of research. Sci. Total Environ. 598, 220–227. doi: 10.1016/j.scitotenv.2017.03.283
Wright, S. L., Thompson, R. C., and Galloway, T. S. (2013). The physical impacts of microplastics on marine organisms: a review. Environ. Pollut. 178, 483–492. doi: 10.1016/j.envpol.2013.02.031
Keywords: microplastics, nanoplastics, LC-HRMS, suspected screening, additives
Citation: Vega-Herrera A, Llorca M, Savva K, León VM, Abad E and Farré M (2021) Screening and Quantification of Micro(Nano)Plastics and Plastic Additives in the Seawater of Mar Menor Lagoon. Front. Mar. Sci. 8:697424. doi: 10.3389/fmars.2021.697424
Received: 19 April 2021; Accepted: 16 July 2021;
Published: 10 September 2021.
Edited by:
Nunziacarla Spanò, University of Messina, ItalyReviewed by:
Pedro Neves Carvalho, Aarhus University, DenmarkXiaoyan Cao, Ocean University of China, China
Copyright © 2021 Vega-Herrera, Llorca, Savva, León, Abad and Farré. This is an open-access article distributed under the terms of the Creative Commons Attribution License (CC BY). The use, distribution or reproduction in other forums is permitted, provided the original author(s) and the copyright owner(s) are credited and that the original publication in this journal is cited, in accordance with accepted academic practice. No use, distribution or reproduction is permitted which does not comply with these terms.
*Correspondence: Marinella Farré, mfuqam@cid.csic.es