- 1Key Laboratory of Aquatic Nutrition and Feed Science of Jiangsu Province, College of Animal Science and Technology, Nanjing Agricultural University, Nanjing, China
- 2National Laboratory of Animal Science, Nanjing Agricultural University, Nanjing, China
- 3College of Veterinary Medicine, Nanjing Agricultural University, Nanjing, China
Although cortisol is considered the main glucocorticoid involved in regulating stress in fish by elevating the serum glucose, the presence of serum corticosterone and whether its level can be used as an indicator for fish stress activation and reflect the degree of fish stress remains to be determined. To comprehensively and accurately evaluate the performance of two glucocorticoids after stress, in this study, seven of the most common stressors (ammonia nitrogen, high-temperature, acid, alkali, Aeromonas hydrophila, and salinity) in aquaculture were selected. The levels of serum corticosterone, cortisol, and glucose were detected within 48 h, and then the dynamic changes were analyzed. In addition, the kidneys were collected to detect the expression of corresponding proteins involved in corticosterone and cortisol synthesis (3 β-HSD and CYP17A1) for further exploration. From the results obtained, the content of serum corticosterone was about 100 times higher than that of cortisol, and it was found that corticosterone and cortisol showed different dynamic changes within 48 h among the seven kinds of stress. In the subsequent detection of glucose, it was found that the dynamic shift in glucose was not consistent with the dynamic changes of corticosterone or cortisol entirely but seemed to be caused by the combination of the two. In the follow-up protein expression detection, we found that corticosterone seemed to respond to stress earlier. Therefore, it is concluded that the responses of corticosterone and cortisol to stress are independent, corticosterone may be more sensitive in the current seven acute stresses, and then they regulate glucose together to resist stress. The study aimed to fill the vacancy of corticosterone in fish research and provide a reference for the complete evaluation of fish stress degree.
Introduction
It is well known that stress can enhance the hypothalamus-pituitary-adrenal (HPI) axis, which causes the adrenal cortex to secrete corticosteroids to combat stress. As the two most important hormones, corticosterone and cortisol are widely used as the main biomarkers to reflect the degree of stress. Corticosterone is the main glucocorticoid in birds, herptiles, and rodents, while cortisol is the principal glucocorticoid in other mammals and most fish (Bebus et al., 2020). But Serum corticosterone was previously studied in numerous elasmobranch fishes (sharks, skates, and rays), but this steroid’s role, widespread throughout many taxa, has yet to be defined (Manire et al., 2007). Maybe in consideration of convenience and kit availability, researchers often detect the concentration of serum cortisol in fish as an indicator of stress, so cortisol is widely considered the main glucocorticoid involved in regulating stress responses in fish. Although cortisol is the principal glucocorticoid in serum, and related studies have shown that cortisol exhibits much higher glucocorticoid potency than corticosterone (Magiakou and Chrousos, 1994), the proportion of cortisol in the kidney, spleen, heart, and brain is higher than that of corticosterone (Fazekas and Fazekas, 1966). However, it has been found that corticosterone can play a physiological role independently of cortisol and has independent seasonal and circadian rhythms (Bottoms et al., 1972; Albers et al., 1985). Besides, cortisol and corticosterone are formed within the range of specific species, and their proportion varies according to the demands (Koren et al., 2012). Therefore, whether the stress degree of fish can be evaluated entirely by cortisol needs to be studied more deeply.
The FAO (2021), issued in June 2020, estimates that total fish production is set to increase to 204 million tons in 2030, which is up 15% from 2018. Aquaculture has been the fastest expanding food production sector globally for the last 50 years, growing at an average of 5.3% per year since the turn of the century. However, many factors (such as the water quality and transport) related to stress are affected by fish health (such as the water quality and transportation). Stress is one of the most critical factors affecting fish’s growth, development, and physiological function and may cause death in aquaculture. The related studies have even shown that fish in a state of stress are 20% more likely to be infected by exogenous bacteria than fish in a state of normal stress (Tort, 2011). The stress may bring about adverse physiological changes such as metabolic disruption and enzyme dysfunction and has become one of the issues in the aquaculture industry most worth investigating, which affected the production, and resulted in a tremendous economic loss of fish aquaculture (Nakayama et al., 1994; Olsson et al., 2003)
To sum up, the above results show that corticosterone and cortisol have different representativeness in fish under stress. Therefore, it is necessary to study the dynamic changes and correlation of corticosterone and cortisol under stress to develop a complete system to evaluate the stress status of fish accurately and then take timely measures to reduce the loss of farmers. Therefore, this study discussed the seven most common stresses in aquaculture. We focused on the dynamic changes of serum cortisol, corticosterone, and glucose in channel catfish within 48 h and detected the expression of proteins closely related to cortisol and corticosterone within 48 h of stress. And then profoundly explore its mechanism to provide a reference for the follow-up and complete evaluation of the stress degree of fish.
Materials and Methods
All experimental procedures involving animal care were conducted under the Care and Use of Laboratory Animals guidance in China. This present study was approved by the Animal Care and Use Committee of Nanjing Agricultural University (Nanjing, China) (permit number: SYXK (Su) 2011–0036).
Fish, Experimental Pre-treatment, and Sample
Juvenile channel catfish were taken from Yangzhou (Jiangsu hatchery, China) with a bodyweight of 57.2 ± 0.3 g, body length of 15.5 ± 0.5 cm, and an age of 5 months; we took 1,000 fish in total. Each experimental fish group was cultured in water tanks (1 × 0.7 × 0.7 m Length: Width: Height) for 2 weeks to adapt to transfer and stress caused by water quality. A total of 2 weeks later, when the fish condition was stable, they were fasted within 24 h before starting the experiment, and then the stress experiment began. A total of 420 fish were prepared for the stress test, with 35 water tanks (1 × 0.7 × 0.7 m Length: Width: Height). Each tank was filled with 30 L water, and the water quality was such that the temperature was 24 ± 0.5°C, the concentration of dissolved oxygen was 7.12 ± 0.2 mg/L, pH is 7 ± 0.2, the concentration of nitrite was less than 0.01 mg/kg, the total ammonia nitrogen was less than 0.4 mg/L, and we kept the natural light. The type of water quality analysis instrument for determining water quality was YSI ProPlus.
Experimental Scheme
During temporary cultivation, channel catfish were domesticated with commercial feed (protein 310 g/kg, fat 60.0 g/kg). The experiment was divided into seven stress groups, there were five water tanks in each stress group, 12 fish were put in each tank, ten fish were used for sampling, and the extra two fish are used as spares to deal with unexpected situations such as other stressors or unknown conditions, such as dying in the process of transfer. Experimental fish were sampled at 0 h before stress and 12, 24, 36, and 48 h after stress. Two experimental fish from each water tank were sampled at each sampling time point, a total of 10 fish were collected from each group at each time point, and each stress group was sampled according to the above method at each time point. The fish were anesthetized in 100 mg/L MS-222 diluent (trichloromethane sulfonate; Sigma). The serum samples were collected from the caudal vein by heparinization syringe and assembled into the anticoagulant tube of 2 ml. Then centrifuged the serum at 4°C and 12,000 revolutions for 10 min, the serum was taken into the 2 ML centrifuge tube and frozen at −80°C. In ammonia nitrogen stress, we collected three fish kidney tissues at 0, 12, 24, 36, and 48 h, respectively, at each time point for subsequent protein analysis. The kidney is collected into the frozen tube and put into the liquid nitrogen, then quickly divided into the cryopreservation box in the liquid nitrogen and transferred to −80°C for subsequent analysis.
Ammonia Nitrogen Stress
The pretest was determined by exposure to ammonia with graded doses of 10, 20, 50, and 100 mg/L of freshwater, and according to the result, we chose 100 mg/L of freshwater as the suitable ammonia level to stress the experiment fish. The desired ammonia levels (100 mg/L of freshwater) were elevated by gradually adding ammonium chloride (NH4CL) into the water, and the specific stress scheme refers to the experimental method of Zhang et al. (2014). Levels of ammonia were measured a day thrice using a spectrophotometric method described by Solorzano (1969).
High-Temperature Stress
Before the experiment, a water temperature of 25°C were selected. Then, it was transferred into the water tank with a water temperature of 34°C for heat stress treatment, maintaining the water temperature using an automatic heater and DO > 5 mg/L. The water temperature was measured every 2 h to ensure that the temperature was stable. The specific stress scheme refers to the experimental method of Ming et al. (2012).
Acid Stress
Before the experiment, we measured the water body’s PH value (7.2) and adjusted PH to 5 with dilute hydrochloric acid before transferring the experimental fish. The water body’s PH was measured once an hour to keep the PH value around 5. The specific stress scheme refers to the empirical method of Zahangir et al. (2015).
Alkali Stress
Before the experiment, we measured the PH value of the water was 7.2 and adjusted to 9.0 with sodium hydroxide before transferring this to the experimental fish. The water body’s PH was measured once an hour to keep the PH value around 5. The specific stress scheme refers to the empirical method of Zahangir et al. (2015).
Aeromonas Hydrophila Stress
In total, 0.1 mL (6.5 × 106 cfu/ml) of Aeromonas hydrophila was injected into the experimental fish intraperitoneally. The specific stress scheme refers to the experimental method of De Figueiredo and Plumb (1977).
Salinity Stress
Before the experiment, the water’s salinity was 3.2‰, and the salinity was adjusted to 10‰ with sea salt to stress the experimental fish. The salinity of the water was measured once an hour to keep the salinity around 10‰. The specific stress scheme refers to the experimental method of Boeuf and Harache (1982).
Hypoxia Stress
Each water tank was typically oxygenated (8.2 ± 0.2 mg/L). However, by continuously filling 100% nitrogen into the water to maintain the water’s hypoxia environment, the amount of dissolved oxygen in the water was reduced to 3.5 mg/L gradually. The specific stress scheme refers to the experimental method of Ni et al. (2014).
Serum Corticosterone, Cortisol, and Glucose
The blood samples were drawn using 2 mL syringes and collected in reaction vials (1.5 mL), then allowed to clot at room temperature for 60 min. The serum was collected after pooled blood samples was centrifuged at 3,000 × g at 4°C for 15 min and stored at −80°C until the serum stress indicators were carried out. The serum cortisol level (n = 10/time) was analyzed using a commercial EIA kit (Jiangsu, China, Meimian, sensitivity of 5 ng/ml) according to the manufacturer’s instruction. Serum glucose concentration (n = 10/time) was measured by using a commercial kit (Sysmex Wuxi Co., Let., China).
Western Blot Analysis
The western blot analysis process is taken from Coirini et al. (2002) and Cao et al. (2018). Kidney were homogenized in a lysing solution [1% sodium deoxycholate, 0.1% sodium dodecyl sulfate (SDS) and 0.8 mM (PBS)]. Homogenates were centrifugated at 1,500 × g for 15 min. Protein concentrations were assessed using a Bicinchoninic Acid Kit (Sigma). The following primary antibodies were used: mouse monoclonal 3β-HSD (1:1,000, #A8035, ABclonal), CYP17A1 (1:1,000, #A1373, ABclonal), and β-Actin (1:1,000, #SC-47778, Santa Gruz). The protein bands were detected by a chemiluminescent reagent (Pierce, Rockford, United States) with a luminescent image analyzer (Fujifilm LAS-3000, Japan). The protein levels were quantified using the Image J software (United States National Institutes of Health, Bethesda, MD, United States).
Data Analysis
All the stress groups had 10 repeats per time point in the serum data of cortisol and corticosterone. The protein data is triple. The data were presented as mean ± standard error (SEM) and were analyzed by one-way ANOVA followed by Duncan’s post-hot test with SPSS software. Differences were investigated statistically significant at P < 0.05.
Results
Analysis of Dynamic Changes of Corticosterone/Cortisol in Each Stress Groups
Ammonia Nitrogen Stress
Corticosterone
The concentration of corticosterone in the ammonia stress group at 36 h after stress was significantly different from that at 0 h before stress and 48 h after Stress (P < 0.05). The corticosterone concentration rose to between 0 and 36 h and returned to the average level at 48 h after stress.
Cortisol
The cortisol concentration in the 12 h stress group was significantly different from that in the 24 and 36 h stress group (P < 0.05). From 0 h before stress to 48 h after stress, the cortisol concentration increased at first, then decreased and then increased, and returned to the average level at 48 h. In general, the concentration of corticosterone in Channel Catfish serum in the stress group was about 30–45 μg/L, while cortisol concentration was about 2.0–2.6 μg/L. Thus, the concentration of corticosterone was about 10–20 times that of cortisol.
For details, see Figure 1A1 (corticosterone) and Figure 1A2 (cortisol).
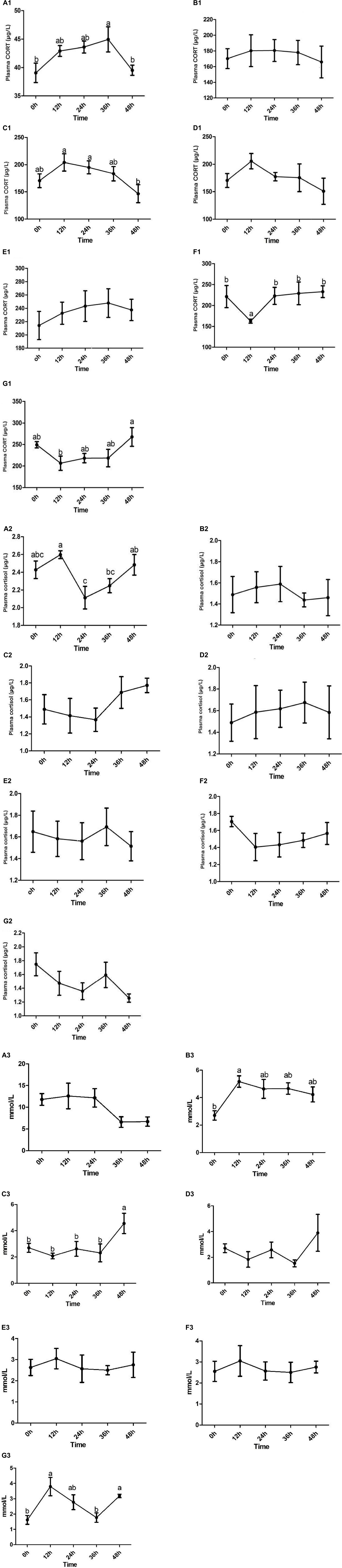
Figure 1. The concentration of serum corticosterone (A1–G1)/cortisol (A2–G2)/glucose (A3–G3) and the dynamic changes of Channel Catfish before and after stress were observed under seven stresses: ammonia nitrogen, high temperature, acid, alkali, Aeromonas hydrophila, high salinity, and hypoxia. Values are represented as mean ± SE (n = 10). Mean values with different letters (a–c) were significantly different (P < 0.05).
High-Temperature Stress
Corticosterone/cortisol
There was no significant difference between corticosterone and cortisol at each time point in the stress group. From 0 h before stress to 48 h after stress, the concentration of corticosterone increased at first and then decreased, which was stable as a whole. The concentration of cortisol increased at first, then decreased, and then increased. In general, the concentration of corticosterone in Channel Catfish serum in the stress group was about 140–200 μg/L, while the concentration of cortisol was about 1.2–1.8 μg/L, and the concentration of corticosterone was 100–200 times higher than that of cortisol.
For details, see Figure 1B1 (corticosterone) and Figure 1B2 (cortisol).
Acid Stress
Corticosterone
The concentration of corticosterone in the acid stress group at 12 and 24 h was significantly different from 48 h (P < 0.05). The corticosterone concentration increased at first and then decreased at 0 h before stress and 48 h after stress, and reached the lowest at 48 h.
Cortisol
There was no significant difference at each time point in the stress group. However, the cortisol concentration decreased at first and then increased from 0 h before stress to 48 h after stress and peaked at 48 h.
Generally speaking, in acid stress, the concentration of corticosterone in Channel Catfish serum in the stress group was about 100–250 μg/L, while the concentration of cortisol was about 1.0–2.0 μg/L, and the concentration of corticosterone was 100–200 times higher than that of cortisol.
For details, see Figure 1C1 (corticosterone) and Figure 1C2 (cortisol).
Alkali Stress
Corticosterone/cortisol
There was no significant difference between corticosterone and cortisol at each time point in this stress group. However, from 0 h before stress to 48 h after stress, the concentration of corticosterone increased at first and then decreased (from 12 to 48 h), and the concentration of cortisol also increased at first and then decreased (reached the maximum at 36 h and began to decrease). In alkali stress, the concentration of corticosterone in Channel Catfish serum was about 100–250 μg/L, while the concentration of cortisol was 1.2–2.0 μg/L. Thus, the concentration of corticosterone was 100–200 times higher than that of cortisol.
For details, see Figure 1D1 (corticosterone) and Figure 1D2 (cortisol).
Aeromonas Hydrophila Stress
Corticosterone/cortisol
There was no significant difference between corticosterone and cortisol at each time point in the stress group. However, the concentration of corticosterone first increased and then decreased from 0 h before stress to 48 h after stress (reached the maximum at 36 h and began to decrease), and the concentration of cortisol decreased at first and then increased and then decreased. Generally speaking, in the Aeromonas hydrophila stress, the concentration of corticosterone in the serum of Channel Catfish in the group was about 180–280 μg/L, while the concentration of cortisol was about 1.2–2.0 μg/L, and the concentration of corticosterone was 100–200 times higher than that of cortisol.
For details, see Figure 1E1 (corticosterone) and Figure 1E2 (cortisol).
Salinity Stress
Corticosterone
The concentration of corticosterone at 12 h in the stress group was significantly different from 0, 24, 36, and 48 h. In addition, the corticosterone concentration decreased at first and then increased at 0 h before stress and 48 h after stress.
Cortisol
There was no significant difference in cortisol concentration at each time point in the stress group, but it decreased at first and then increased in the period from 0 h before stress to 48 h after stress.
Generally speaking, in the high salt stress group, the concentration of corticosterone in Channel Catfish serum was about 150–300 μg/L, while the concentration of cortisol was 1.2–1.8 μg/L, and the concentration of corticosterone was about 100 times that of cortisol.
For details, see Figure 1F1 (corticosterone) and Figure 1F2 (cortisol).
Hypoxia Stress
Corticosterone
The concentration of corticosterone in the 12 h stress group was significantly different from that in the 48 h stress group (P < 0.05). The corticosterone concentration decreased at first and then increased during 0 h before stress and 48 h after stress.
Cortisol
In the stress group, the concentration of cortisol had no significant difference from 0 h before stress to 48 h after stress but decreased at first and then increased and then decreased as a whole.
Generally speaking, in hypoxic stress, the concentration of corticosterone in Channel Catfish serum was about 150–300 μg/L, while the concentration of cortisol was about 1.0–2.0 μg/L, and the concentration of corticosterone was about 100 times that of cortisol.
For details, see Figure 1G1 (corticosterone) and Figure 1G2 (cortisol).
Serum Glucose
The results showed that glucose has different dynamic changes in the seven stresses. The concentrations of glucose all showed a tendency to rise first at 12 h and then to fall and reached its maximum at 12 h in the ammonia nitrogen (Figure 1A3), high temperature (Figure 1B3), Aeromonas hydrophila (Figure 1E3), salinity (Figure 1F3), and hypoxia (Figure 1G3) stress. In addition, there was a significant difference in high temperature and hypoxia stress (P < 0.05). But there is a reverse trend in acid (Figure 1C3) and alkali (Figure 1D3) Stress, and there was a significant difference in acid stress (P < 0.05).
Protein Expression
The detection showed that the two essential target proteins involved in cholesterol production of corticosterone/cortisol at 0 h before stress and 12, 24, 36, and 48 h after stress were 3β-HSD CYP17A1, respectively. 3β-HSD was mainly involved in transforming PERG to PROG and then to corticosterone after a series of transformations. CYP17A1 was mainly involved in transforming PREG to 17α-OHPERG and PROG to 17α-OHPROG and finally to cortisol after a series of transformations. The results of protein detection are as follows.
As shown in the Figure 2, the protein level of 3β-HSD at 12 and 36 h after stress was significantly higher than that at 0 h before stress (P < 0.05) in the Figure 2A. However, the protein expression level at different time points after CYP17A1 stress was not significant as that before stress, but the protein expression level at 48 h after stress was significantly higher than that in 12 and 24 h groups (P < 0.05) in Figure 2B.
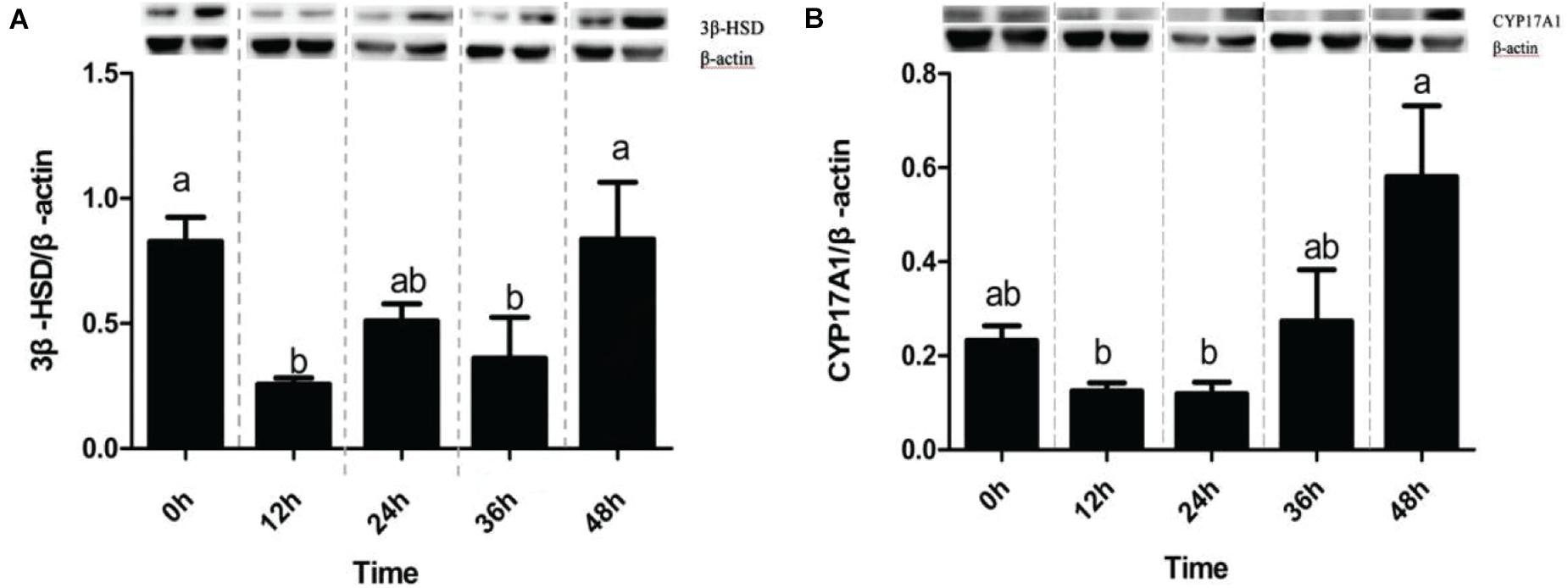
Figure 2. Expression profiles of tested proteins in Channel Catfish exposed to ammonia nitrogen stress. Western blot analysis of CYP17A1 and 3β-HSD in the kidney was at 0 h before ammonia nitrogen stress and 12, 24, 36, and 48 h after ammonia nitrogen stress. The protein relative expression levels of CYP17A1 and 3β-HSD were normalized to β-actin (A,B). Values are represented as mean ± SE (n = 3). Mean values with different letters (a–c) were significantly different (P < 0.05).
Discussion
From the results obtained in this study, it can be found that the changes of corticosterone in ammonia nitrogen, high temperature, acid, alkali, and Aeromonas hydrophila all increased at first and then decreased and finally returned to the average level within 48 h, while in ammonia nitrogen, high temperature, and alkali stress, cortisol showed the same dynamic changes. However, in salinity and hypoxia stress, corticosterone first decreased and then increased within 48 h, but cortisol showed this dynamic change in more stress types, such as acid, Aeromonas hydrophila, salinity, and hypoxia stress. And it is worth noting that the content of serum corticosterone is much higher than that of cortisol by about 100 times. In the follow-up glucose detection, it can be observed that the dynamic changes of glucose in each stress do not seem to be consistent with the changes of corticosterone or cortisol, which seems to be caused by the joint action of the two hormones. In the subsequent detection of corticosterone and cortisol-related proteins, it was observed that in ammonia nitrogen stress, the expression of 3 β-HSD decreased at 12 h but increased at 24 h and returned to average level at 48 h. The expression of CYP17A1 decreased at 12 and 24 h under stress, increased at 36 h, and reached the maximum within 48 h. It is concluded that corticosterone can respond to the activation of stress before cortisol and then take measures to combat stress. In general, the results of this experiment show that serum corticosterone plays an independent role after fish stress, and corticosterone should be taken into account when evaluating the degree of fish stress. Among the seven kinds of acute stress in this study, the evaluation of corticosterone as a stress index may be more accurate.
Stress is defined as a condition in which the dynamic equilibrium of animal organisms called homeostasis is threatened or disturbed due to the actions of intrinsic or extrinsic stimuli, commonly defined as stressors (Chrousos, 1992). The actions of stressors are twofold: they produce effects that threaten or disturb the homeostatic equilibrium. They elicit a coordinated set of behavioral and physiological responses thought to be compensatory and adaptive, enabling the animal to overcome the threat. The stress response of fish is similar to that of terrestrial vertebrates; the HPI axis occupies an important position. When fishes encounter stress, the HPI axis responds quickly. It exerts its function against stress and its functions, involving stimulation of oxygen uptake and transfer, mobilization of energy substrates, reallocation of energy away from growth and reproduction, and mainly suppressive effects on immune functions (Chrousos, 1992). There is also growing evidence for intensive interaction between the neuroendocrine system and the immune system in fish. Conspicuous differences, however, are present, and these are primarily related to the aquatic environment of fishes. For example, stressors increase the permeability of the surface epithelia, including the gills, to water and ions and thus induce systemic hydromineral disturbances. High circulating catecholamine levels and structural damage to the gills and perhaps the skin are prime causal factors. This is associated with increased cellular turnover in these organs (Wendelaar Bonga, 1997). In fish, cortisol combines glucocorticoid and mineralocorticoid actions, being essential for the restoration of homeostasis. Fish are exposed to aquatic pollutants via the extensive and delicate respiratory surface of the gills, together with the variety of susceptible perceptive mechanisms in the integument, explaining why the change of water body can cause a series of comprehensive stress responses fish (Wendelaar Bonga, 1997). The concentration of ammonium, temperature, pH, microorganism, salinity, dissolved oxygen in the water, and so on all have a significant impact on the intensity of the stressor.
Our study observed that cortisol levels significantly increased after 12 h of ammonia nitrogen stress, and from 0 to 24 h, they all showed a trend of increasing first and then decreasing. Similar results on the cortisol confirmed previous results on blunt snout bream (Zhang et al., 2014). In high-temperature stress, the cortisol level continued to rise in 0–12 h, which is in accord with Ming et al. (2012). But in the subsequent stress, which the study had not discussed, it as maintained up till 24 h and returned to normal levels in 48 h in the current study. Thus, high and low PH can cause different levels of stress on fish, which induced different dynamics in cortisol of the channel catfish observed in the present study. This difference could result from the different tolerance to the PH of the different species of fish. Grande et al. (1978) studied rainbow trout, and Kelso et al. (1986) studied in yellow perch had both confirmed it. However, cortisol concentration decreased first and then increased in 48 h under acid, Aeromonas hydrophila, salinity, and hypoxia stress, which may be due to the earlier response of cortisol to this type of stress than 12 h. And Iwama et al. (2006) stated that the cortisol reached the highest level 1 h after being stressed and returned to basal levels after 6 h in most fish.
Under stress, serum cortisol levels were the physiological responses most used as stress indicators in fish (Martínez-Porchas et al., 2009; Ellis et al., 2012). These agreed with the findings obtained in this study, which indicated that the seven different types of stress caused the cortisol levels varying degrees of change, especially after stress in 48 h. But among the seven kinds of stress in the current study, the cortisol level only had a significant difference in the ammonia nitrogen stress in this study, and just choosing the cortisol levels to determine whether the fish were stressed or not seemed to be inadequate.
Besides, using corticosterone reflects the negative impacts during the stress were reported on many studies on other animal species such as the rodent (Gong et al., 2015), rabbit (Kass et al., 1954; Ganjam et al., 1972), avian (Bebus et al., 2020), herptile (Bebus et al., 2020), and amphibian (Tornabene et al., 2020). But there are few studies on fish. Therefore, the corticosterone levels in the seven stressors had been measured in this study. The current study showed that compared with cortisol levels, corticosterone concentration after stress was significantly higher. In the ammonia nitrogen, acid, salinity, and hypoxia stress, the dynamic changes of corticosterone levels significantly differed in 48 h after stress. The changes of corticosterone in ammonia nitrogen, high temperature, acid, alkali, Aeromonas hydrophila, and all increased at first and then decreased and finally returned to the average level within 48 h, which was similar to the study in mice (Gong et al., 2015). Few types of stress show the opposite trend, which different species may cause.
Serum cortisol and glucose levels are the physiological responses most used as stress indicators in fish (Martínez-Porchas et al., 2009; Ellis et al., 2012) during the stress. These are in agreement with the findings obtained in this study, which indicated that all kinds of stress caused increased cortisol and glucose levels, especially after suffering stress directly. The elevation of cortisol stimulates gluconeogenesis and glycogenolysis pathways (Iwama et al., 1999), which caused the augmentation of glucose to cope with the energy demand produced by the stress. Similar results on cortisol and glucose confirmed previous results on juvenile tambaqui (Gomes et al., 2003), juvenile matrinxa (de Abreu et al., 2008), common carp (Dobšikova et al., 2009), zebrafish (Dhanasiri et al., 2013a,b), and Labeo rohita (Pakhira et al., 2015) subjected to stress. But in the current study, there also seemed to be similar trends between the corticosterone levels and glucose levels, such as in the ammonia nitrogen, high temperature and Aeromonas hydrophila stress. As to why corticosterone and cortisol levels did not correspond to changes in glucose, could it be because the glucocorticoids act synergistically with epinephrine or glucagon in enhancing and prolonging the increase in glucose? Over time, glucocorticoids inhibit insulin secretion. This fundamental antagonism to insulin is believed to be the metabolic role of glucocorticoids in stress (Munck et al., 1984). Hyperglycemia, attributable partly to suppressing insulin release by corticosterone, has been shown to occur in vitro (Barseghian and Levine, 1980). This explanation also considers our previous finding that corticosterone and cortisol levels did not correspond to changes in glucose.
Thus far, cortisol is the principal glucocorticoid in other mammals and most fish, while corticosterone is the main glucocorticoid in birds, herptiles, and rodents (Bebus et al., 2020). Prior studies have noted the importance of cortisol in assessing the stress degree in fish. However, very little was found in the literature on the question of corticosterone. We concluded that to evaluate the degree of stress entirely, corticosterone’s addition to evaluating stress is essential. Such connections likely exist between cortisol and corticosterone in assessing the stress degree together.
This discrepancy in the difference in cortisol and corticosterone could be attributed to the action and formation mechanism. As for the correlation and difference between corticosterone and cortisol, some studies have shown that cortisol is the primary stress hormone in higher mammals, including humans, and corticosterone is often used as a marker of stress in rodents and birds compared with cortisol in humans and other higher mammals (Nelson, 1995; Wasser et al., 2000). Although glucocorticoids are mainly cortisol and corticosterone, both act as glucocorticoids to regulate glucose balance. However, cortisol’s potency is higher than that of corticosterone (Magiakou and Chrousos, 1994). Nevertheless, corticosterone has the potential to signal independently of cortisol, can change independently with the change of pressure, and has independent seasonal and circadian rhythms (Bottoms et al., 1972; Albers et al., 1985). Therefore, the sensitivity levels of cortisol and corticosterone to ACTH may also be different in different environments (Vera et al., 2011). This fact was supported by the fact that corticosterone has an independent signal transmission and independent function.
Most of the available glucocorticoids bind to corticosteroid-binding protein (CBG) in a binding manner, but their concentration and binding ability vary significantly from species to species (Westphal, 1986; Boonstra, 2005; Romero et al., 2008). CBG usually shows higher specificity for major glucocorticoids, so if CBG binding sites are preferentially occupied by cortisol in species, it is easier to obtain free corticosterone in circulation and vice versa. According to the above discussion, there are differences in glucocorticoids’ main effects in different species and the same species. Whether it is cortisol or corticosterone can be found in previous studies. For example, cortisol has been studied as a significant marker of stress response in stress mice (Ayada et al., 2002; Chen et al., 2005; Podsevatkin et al., 2008; Zhang et al., 2010) and rats (Cheng et al., 2004; Bhat et al., 2007; Yin et al., 2007). However, after relevant dynamic analysis, it was found that corticosterone caused a higher stress response in mice with chronic Stress (Gong et al., 2015).
There are generally two pathways for synthesizing two stress hormones (mainly cholesterol to corticosterone through PROG, PREG, 17 deoxycorticosterone, or cholesterol) through PROG, PREG, 17 α-hydroxyprogesterone, 17α-progesterone, and 11-deoxycortisol (Luu-The, 2013). Two essential proteins, CYP17A1 and 3β-HSD, directly affect the synthesis of stress hormones (Guennoun et al., 1995). In the immunoblotting analysis of this experiment, rat adrenal 3 β-HSD antibodies and rat testicular CYP17A1 antibody were used to detect the expression of two proteins in channel catfish and participate in steroid metabolism in 42 and 57 kDa, respectively, which is the same as the study of Sakamoto et al. (2001) and Locke et al. (2009). It was found that there was a significant difference in the expression of 3β-HSD in the interrenal tissue of channel catfish before and after stress (12 and 36 h). However, there was no significant difference in CYP17A1 before and after stress, which may explain the overall concentration difference of serum cortisol/corticosterone before and after stress. In ammonia nitrogen stress, 3 β-HSD decreased at 12 h but increased at 24 h and returned to normal level at 48 h. The expression of CYP17A1 decreased at 12 and 24 h, increased at 36 h, and reached the maximum within 48 h. It is concluded that corticosterone should respond to stress activation before cortisol and then take measures against stress. A possible explanation for these results may be that channel catfish had a more robust tolerance to cortisol than corticosterone. In this respect, Strange (1980) indicated that channel catfish respond to stress more slowly because the cortisol levels did not show any significant changes. To explain the situation of the expression of 3 β-HSD and CYP17A1 decreased at 12 h after stress, and it may be since HSP70 played a role earlier than both. The related study also confirmed this point (Yamashita et al., 2010).
This finding, while preliminary, suggests that the theory of corticosterone as a stress indicator provides a valuable account of assessing the stress degree in fish, mainly in which is not impressible to cortisol entirely. Put differently, corticosterone can be a stress index in channel catfish.
But it is known that stress can activate the HPA axis and other pathways in the neuroendocrine system. Furthermore, the activation of different neuroendocrine pathways by different stressors may differentially affect the local regulation of glucocorticoid levels in different organs, leading to a different dynamic between cortisol and corticosterone under different stressful conditions (Gong et al., 2015). Therefore, the present results must be taken into account when choosing a biomarker to activate different stresses.
Conclusion
This study set out with the aim of assessing the importance of corticosterone in stress fish. The most prominent finding to emerge from the analysis is that cortisol and corticosterone’s dynamic changes in most stresses were different. Surprisingly, corticosterone concentration was significantly higher than that of cortisol after stress, and corticosterone can respond to the activation of stress before cortisol in acute stress. This finding was suggested that corticosterone can be the stress indicator in fish. This study lays the groundwork for future research into evaluating stress levels and providing a theoretical basis for improving stress.
Data Availability Statement
The original contributions presented in the study are included in the article/Supplementary Material, further inquiries can be directed to the corresponding author.
Ethics Statement
The studies involving human participants were reviewed and approved by Nanjing Agricultural University. The patients/participants provided their written informed consent to participate in this study. The animal study was reviewed and approved by Nanjing Agricultural University. Written informed consent was obtained from the owners for the participation of their animals in this study. Written informed consent was not obtained from the individual(s) for the publication of any potentially identifiable images or data included in this article.
Author Contributions
KX, G-ZJ, and W-BL contributed to the conception and design of the study. X-FL organized the database. KX performed the statistical analysis and wrote the first draft of the manuscript. XW, D-DZ, C-NZ, W-HC, and KA wrote the sections of the manuscript. All authors have taken part in the generation of ideas, experiment implementation, statistical analysis, and completion of the manuscript; contributed to manuscript revision; and read and approved the submitted version.
Funding
This research was supported by the National Technology System for Jiangsu Agricultural Industry Technology System [JATS (2020) 329] and the Natural Science Foundation (BK20201325).
Conflict of Interest
The authors declare that the research was conducted in the absence of any commercial or financial relationships that could be construed as a potential conflict of interest.
Publisher’s Note
All claims expressed in this article are solely those of the authors and do not necessarily represent those of their affiliated organizations, or those of the publisher, the editors and the reviewers. Any product that may be evaluated in this article, or claim that may be made by its manufacturer, is not guaranteed or endorsed by the publisher.
Supplementary Material
The Supplementary Material for this article can be found online at: https://www.frontiersin.org/articles/10.3389/fmars.2021.692726/full#supplementary-material
Supplementary Figure 1 | The standard curve of cortisol.
Supplementary Figure 2 | The standard curve of CORT.
References
Albers, H. E., Yogev, L., Todd, R. B., and Goldman, B. D. (1985). Adrenal corticoids in hamsters: role in circadian timing. Am. J. Physiol.-Regulatory Integrative Comp. Physiol. 248, R434–R438. doi: 10.1152/ajpregu.1985.248.4.r434
Ayada, K., Tadano, T., and Endo, Y. (2002). Gnawing behavior of a mouse in a narrow cylinder. Physiol. Behav. 77, 161–166. doi: 10.1016/S0031-9384(02)00844-2
Barseghian, G., and Levine, R. (1980). Effect of corticosterone on insulin and glucagon secretion by the isolated perfused rat pancreas∗. Endocrinology 106, 547–552. doi: 10.1210/endo-106-2-547
Bebus, S. E., Carlton Jones, B., and Anderson, R. C. (2020). Development of the corticosterone stress response differs among passerine species. Gen. Comp. Endocrinol. 291:113417. doi: 10.1016/j.ygcen.2020.113417
Bhat, M. S., Rao, G., Murthy, K. D., and Bhat, P. G. (2007). Housing in pyramid counteracts neuroendocrine and oxidative stress caused by chronic restraint in rats. Evidence-Based Complementary Alternat. Med. 4, 35–42. doi: 10.1093/ecam/nel049
Boeuf, G., and Harache, Y. (1982). Criteria for adaptation of salmonids to high salinity seawater in France. Aquaculture 28, 163–176. doi: 10.1016/0044-8486(82)90019-9
Boonstra, R. (2005). Equipped for life: the adaptive role of the stress axis in male mammals. J. Mammal. 86, 236–247. doi: 10.1644/bhe-001.1
Bottoms, G. D., Roesel, O. F., Rausch, F. D., and Akins, E. L. (1972). Circadian varia-tion in serum cortisol and corticosterone in pigs and mares. Am. J. Vet. Res. 33:785.
Cao, X.-F., Liu, W.-B., Zheng, X.-C., Yuan, X.-Y., Wang, C.-C., and Jiang, G.-Z. (2018). Effects of high-fat diets on growth performance, endoplasmic reticulum stress, and mitochondrial damage in blunt snout bream Megalobrama amblycephala. Aquacul. Nutrit. 25, 97–109. doi: 10.1111/anu.12834
Chen, Y., Kong, L.-D., Xia, X., Kung, H.-F., and Zhang, L. (2005). Behavioral and biochemical studies of total furocoumarins from seeds of Psoralea corylifolia in the forced swimming test in mice. J. Ethnopharmacol. 96, 451–459. doi: 10.1016/j.jep.2004.09.033
Cheng, Y., Wang, D., Li, S., and Geng, Z. (2004). Supplementation with micronutrients attenuating stress injuries in rats. Wei Sheng Yan Jiu 33, 179–182.
Chrousos, G. P. (1992). The concepts of stress and stress system disorders. JAMA 267:1244. doi: 10.1001/jama.1992.03480090092034
Coirini, H., Gouézou, M., Liere, P., Delespierre, B., Pianos, A., Eychenne, B., et al. (2002). 3β-Hydroxysteroid dehydrogenase expression in rat spinal cord. Neuroscience 113, 883–891. doi: 10.1016/s0306-4522(02)00224-5
de Abreu, J. S., Sanabria-Ochoa, A. I., Goncalves, F. D., and Urbinati, E. C. (2008). Stress responses of juvenile matrinxa (Brycon amazonicus) after transport in a closed system under different loading densities. Cienc. Rural 38, 1413–1417. doi: 10.1590/s0103-84782008000500034
De Figueiredo, J., and Plumb, J. A. (1977). Virulence of different isolates of Aeromonas hydrophila in channel catfish. Aquaculture 11, 349–354. doi: 10.1016/0044-8486(77)90084-9
Dhanasiri, A. K. S., Fernandes, J. M. O., and Kiron, V. (2013a). Acclimation of zebrafish to transport stress. Zebrafish 10, 87–91. doi: 10.1089/zeb.2012.0843
Dhanasiri, A. K. S., Fernandes, J. M. O., and Kiron, V. (2013b). Liver transcriptome changes in zebrafish during acclimation to transport-associated stress. PLoS One 8:e65028. doi: 10.1371/journal.pone.0065028
Dobšikova, R., Svobodova, Z., Blahova, J., Modra, H., and Velišek, J. (2009). The effect of transport on biochemical and haematological indices of common carp (Cyprinus carpio L.). Czeh J. Anim. Sci. 54, 510–518. doi: 10.17221/52/2009-cjas
Ellis, T., Yildiz, H. Y., López-Olmeda, J., Spedicato, M. T., Tort, L., Øverli, Ø, et al. (2012). Cortisol and finfish welfare. Fish Physiol. Biochem. 38, 163–188. doi: 10.1007/978-94-007-5383-9_11
Fazekas, I. G., and Fazekas, A. T. (1966). Papierchromatographischer nachweis der corticosteroidfunktion in kaninchenorganen und-geweben. Endokrinologie 50, 130–138.
Ganjam, V. K., Campbell, A. L., and Murphy, B. E. P. (1972). Changing patterns of circulating corticosteroids in rabbits following prolonged treatment with ACTH. Endocrinology 91, 607–611. doi: 10.1210/endo-91-2-607
Gomes, L. C., Araujo-Limrao, C. R. M., Oubach, D., Chippari-Gomeansd, A., Lopes, N., and Urbinati, E. C. (2003). Effect of fish density during transportation on stress and mortality of juvenile tambaqui Colossoma macropomum. J. World Aquacult. Soc. 34, 76–84. doi: 10.1111/j.1749-7345.2003.tb00041.x
Gong, S., Miao, Y.-L., Jiao, G.-Z., Sun, M.-J., Li, H., Lin, J., et al. (2015). Dynamics and correlation of serum cortisol and corticosterone under different physiological or stressful conditions in mice. PLoS One 10:e0117503. doi: 10.1371/journal.pone.0117503
Grande, H., Muniz, I. P., and Anderson, S. (1978). Relative tolerance of some salmonids to acid waters. Int. Assoc. Theor. Appl. Limn01. Proc. 20, 2076–2084.
Guennoun, R., Fiddes, R. J., Gouézou, M., Lombès, M., and Baulieu, E.-E. (1995). A key enzyme in the biosynthesis of neurosteroids, 3β-hydroxysteroid dehydrogenase/Δ5-Δ4-isomerase (3β-HSD) is expressed in rat brain. Mol. Brain Res. 30, 287–300. doi: 10.1016/0169-328x(95)00016-l
Iwama, G. K., Afonso, L. O. B., and Vijayan, M. M. (2006). “Stress in fishes,” in The Physiology of Fishes, 3rd Edn, eds D. H. Evans and J. B. Claiborne (Milton Park: Taylor and Francis), 319–342.
Iwama, G. K., Vijayan, M. M., Forsyth, R. B., and Ackerman, P. A. (1999). Heat shock proteins and physiological stress in fish. Am. Zool. 39, 901–909. doi: 10.1093/icb/39.6.901
Kass, E. H., Hechteb, O., Macchi, I. A., and Mou, T. W. (1954). Changes in patterns of secretion of corticosteroids in rabbits after prolonged treatment with ACTH. Exp. Biol. Med. 85, 583–587. doi: 10.3181/00379727-85-20959
Kelso, J. R. M., Minns, C. K., Gray, J. E., and Jones, M. L. (1986). Acidification of surface waters in eastern Canada and its relationship to aquatic biota. Can. Spec. Publ. Fish. Aquat. Sci. No. 87:42.
Koren, L., Whiteside, D., Fahlman, S., Ruckstuhl, K., Kutz, S., et al. (2012). Cortisol and corticosterone independence in cortisol-dominant wildlife. Gen. Comp. Endocrinol. 177, 113–119. doi: 10.1016/j.ygcen.2012.02.020
Locke, J. A., Fazli, L., Adomat, H., Smyl, J., Weins, K., Lubik, A. A., et al. (2009). A novel communication role for CYP17A1 in the progression of castration-resistant prostate cancer. Prostate 69, 928–937. doi: 10.1002/pros.20940
Luu-The, V. (2013). Assessment of steroidogenesis and steroidogenic enzyme functions. J. Steroid Biochem. Mol. Biol. 137, 176–182. doi: 10.1016/j.jsbmb.2013.05.017
Magiakou, M. A., and Chrousos, G. P. (1994). “Corticosteroid therapy, nonendocrine disease and corticosteroid withdrawal,” in Current Therapy in Endocrinology and Metabolism, 5th Edn, ed. C. W. Bardwin (Philadelphia, PA: Mosby Yearbook), 120–124.
Manire, C. A., Rasmussen, L. E. L., Maruska, K. P., and Tricas, T. C. (2007). Sex, seasonal, and stress-related variations in elasmobranch corticosterone concentrations. Comp. Biochem. Physiol. Part A: Mol. Integrative Physiol. 148, 926–935. doi: 10.1016/j.cbpa.2007.09.017
Martínez-Porchas, M., Martínez-Córdova, L. R., and Ramos-Enriquez, R. (2009). Cortisol and glucose: reliable indicators of fish stress? Pan-Am. J. Aquat. Sci. 4, 158–178.
Ming, J., Xie, J., Xu, P., Ge, X., Liu, W., and Ye, J. (2012). Effects of emodin and vitamin C on growth performance, biochemical parameters and two HSP70s mRNA expression of Wuchang bream (Megalobrama amblycephala Yih) under high temperature stress. Fish Shellfish Immunol. 32, 651–661. doi: 10.1016/j.fsi.2012.01.008
Munck, A., Guyre, P. M., and Holbrook, N. J. (1984). Physiological functions of glucocorticoids in stress and their relation to pharmacological actions. Endocrine Rev. 5, 25–44. doi: 10.1210/edrv-5-1-25
Nakayama, T., Toyoda, T., and Ooi, A. (1994). Physical property of carp muscle during rigor tension generation. Fish. Sci. 60, 717–721. doi: 10.2331/fishsci.60.717
Ni, M., Wen, H., Li, J., Chi, M., Bu, Y., Ren, Y., et al. (2014). The physiological performance and immune responses of juvenile Amur sturgeon (Acipenser schrenckii) to stocking density and hypoxia stress. Fish Shellfish Immunol. 36, 325–335. doi: 10.1016/j.fsi.2013.12.002
Olsson, G. B., Olsen, R. L., and Ofstad, R. (2003). Post-mortem structural characteristics and water-holding capacity in Atlantic halibut muscle. Lebenson. Wiss. Technol. 36, 125–133. doi: 10.1016/s0023-6438(02)00205-0
Pakhira, C., Nagesh, T. S., Abraham, T. J., Dash, G., and Behera, S. (2015). Stress responses in rohu, Labeo rohita transported at different densities. Aquac. Rep. 2, 39–45. doi: 10.1016/j.aqrep.2015.06.002
Podsevatkin, V. G., Kiriukhina, S. V., Podsevatkin, D. V., Podsevatkina, S. V., and Blinov, D. S. (2008). Dynamics of thebehavioral response and cortisol level caused by the combined action of mexidole, diazepam, thymogen, and hyperbaric oxygenation in mice under immobilization stress conditions. Eksp. Klin. Farmakol. 71, 22–25.
Romero, L. M., Meister, C. J., Cyr, N. E., Kenagy, G. J., and Wingfield, J. C. (2008). Seasonal glucocorticoid responses to capture in wild free-living mammals. Am. J. Physiol.-Regulatory Integrat. Comp. Physiol. 294, R614–R622. doi: 10.1152/ajpregu.00752.2007
Sakamoto, H., Ukena, K., and Tsutsui, K. (2001). Activity and localization of 3β-hydroxysteroid dehydrogenase/?5-?4-isomerase in the zebrafish central nervous system. J. Comp. Neurol. 439, 291–305. doi: 10.1002/cne.1351
Solorzano, L. (1969). Determination of ammonia in natural waters by the phenol hypochlorite method. Limnol. Oceanography 14, 799–801. doi: 10.4319/lo.1969.14.5.0799
Strange, R. J. (1980). Acclimation temperature influences cortisol and glucose concentrations in stressed channel catfish. Trans. Am. Fish. Soc. 109, 298–303. doi: 10.1577/1548-8659(1980)109<298:aticag>2.0.co;2
Tornabene, B. J., Breuner, C. W., and Hossack, B. R. (2020). Relative toxicity and sublethal effects of NaCl and energy-related saline wastewaters on prairie amphibians. Aquat. Toxicol. 228:105626. doi: 10.1016/j.aquatox.2020.105626
Tort, L. (2011). Stress and immune modulation in fish. Dev. Comp. Immunol. 35, 1366–1375. doi: 10.1016/j.dci.2011.07.002
Vera, F., Antenucci, C. D., and Zenuto, R. R. (2011). Cortisol and corticosterone exhibit different seasonal variation and responses to acute stress and captivity in tuco-tucos (Ctenomys talarum). Gen. Comp. Endocrinol. 170, 550–557. doi: 10.1016/j.ygcen.2010.11.012
Wasser, S. K., Hunt, K. E., Brown, J. L., Cooper, K., Crockett, C. M., Bechert, U., et al. (2000). A generalized fecal glucocorticoid assay for use in a diverse array of nondomestic mammalian and avian species. Gen. Comp. Endocrinol. 120, 260–275. doi: 10.1006/gcen.2000.7557
Wendelaar Bonga, S. E. (1997). The stress response in fish. Physiol. Rev. 77, 591–625. doi: 10.1152/physrev.1997.77.3.591
Westphal, U. (1986). “Steroid-Protein interactions revisited,” in Steroid-Protein Interactions II. Monographs on Endocrinology, (Berlin: Springer), doi: 10.1007/978-3-642-82486-9_1
Yamashita, M. T., Yamashita, M., and Ojim, N. (2010). Stress protein HSP70 in fish. Aqua-BioScience Monogr. 3, 111–141. doi: 10.5047/absm.2010.00304.0111
Yin, Y.-Y., Ming, L., Zheng, L.-F., Kan, H.-W., Li, C.-R., and Li, W.-P. (2007). Bioactive compounds from Paecilomyces tenuipes regulating the function of the hypothalamohypophyseal system axis in chronic unpredictable stress rats. Chin. Med. J. 120, 1088–1092. doi: 10.1097/00029330-200706020-00011
Zahangir, M. M., Haque, F., Mostakim, G. M., and Islam, M. S. (2015). Secondary stress responses of zebrafish to different pH: evaluation in a seasonal manner. Aquacul. Rep. 2, 91–96. doi: 10.1016/j.aqrep.2015.08.008
Zhang, C.-N., Li, X.-F., Tian, H.-Y., Zhang, D.-D., Jiang, G.-Z., Lu, K.-L., et al. (2014). Effects of fructooligosaccharide on immune response, antioxidant capability and HSP70 and HSP90 expressions of blunt snout bream (Megalobrama amblycephala) under high ammonia stress. Fish Physiol. Biochem. 41, 203–217. doi: 10.1007/s10695-014-0017-6
Keywords: stress, cortisol, corticosterone, CYP17A1, 3β-HSD, Ictalurus punctatus
Citation: Xiao K, Wang X, Liu W-B, Zhang D-D, Li X-F, Zhang C-N, Chen W-H, Abasubong KP and Jiang G-Z (2021) Corticosterone Can Be an Essential Stress Index in Channel Catfish (Ictalurus punctatus). Front. Mar. Sci. 8:692726. doi: 10.3389/fmars.2021.692726
Received: 09 April 2021; Accepted: 17 June 2021;
Published: 03 August 2021.
Edited by:
Shengming Sun, Shanghai Ocean University, ChinaCopyright © 2021 Xiao, Wang, Liu, Zhang, Li, Zhang, Chen, Abasubong and Jiang. This is an open-access article distributed under the terms of the Creative Commons Attribution License (CC BY). The use, distribution or reproduction in other forums is permitted, provided the original author(s) and the copyright owner(s) are credited and that the original publication in this journal is cited, in accordance with accepted academic practice. No use, distribution or reproduction is permitted which does not comply with these terms.
*Correspondence: Guang-Zhen Jiang, jianggz@njau.edu.cn