- 1Division of Biological Science, Faculty of Science, Prince of Songkla University, Songkhla, Thailand
- 2Biodiversity Research Center, Academia Sinica, Taipei, Taiwan
Barnacles are less common in rock pools of the rocky intertidal zone than on open rock surfaces adjacent to those pools. Rock pools on the Andaman coastlines of Thailand showed diurnal variations in water temperature, salinity, pH, and dissolved oxygen level, peaking in the afternoon. Multivariate analysis showed that water temperature and salinity (not pH and dissolved oxygen) can affect barnacle abundance in rock pools. The present study tests the hypothesis that a lack of recruitment or pool environmental conditions (continuous submergence, water temperature, and salinity extremes) affect the abundance of barnacles (Chthamalus malayensis and Amphibalanus amphitrite) in rock pools. During the recruitment season, recruits were found in a number of rock pools, but at significantly lower abundances than those in adjacent open rock surfaces. In a laboratory experiment, C. malayensis and A. amphitrite that were continuously submerged had a lower survival (80%) than the controls (simulated tide conditions; >90% survivals). Under different water temperature and salinities treatments, barnacles had low survival (<50%) in the low salinity (0‰) and high temperature treatment (40°C). The present study suggests that C. malayensis and A. amphitrite can recruit into rock pools, and the interplay of continuous submergence, high water temperature and low salinity in rock pools can bring about mortality in barnacles.
Introduction
The factors affecting species distribution constitute an interesting topic in ecological studies. The rocky intertidal zone can comprise a variety of microhabitats, including vertical rocks, horizontal rocks, shaded and exposed surfaces, rock pools, and crevices (Williams and Morritt, 1995; Seabra et al., 2011). These microhabitats differ mainly in their levels of heat stress, which affect species distribution (Williams and Morritt, 1995; Wang et al., 2020). Rock pools are depressions on rocky shores that retain water during low tide. Rock pools are foraging habitats and refugia from predation and physical stresses for many intertidal species (Moran, 1985; Delany et al., 1998; Laure et al., 2009). The physico-chemical environment of rock pools, comprising water temperature, salinity, pH, and dissolved oxygen levels, is largely regulated by the tides (Metaxas and Scheibling, 1993; Chan, 2000; Legrand et al., 2018). This environment also varies with the volume, surface area, depth, and tidal position (intertidal vs. supratidal) of pools (Metaxas and Scheibling, 1993; Chan, 2000). When pools are disconnected from the sea, temperature, salinity, pH, and dissolved oxygen concentration can be affected by solar radiation from direct sunlight and bedrocks, water evaporation, and the pool fauna and flora (Morris and Taylor, 1983). Salinity in tropical rock pools can be as low as 0‰ during heavy rain and as high as 40‰ during hot days (Chan, 2000; Firth and Williams, 2009). Intertidal pools on the mid-low shores experience less variation in physico-chemical parameters compared to supratidal pools. They often have higher species richness and biodiversity than their supratidal counterparts (Moschella et al., 2005; Firth et al., 2013, 2014; Evans et al., 2016). Sponges, ascidians, hydroids, and gobies are often found in intertidal pools (Evans et al., 2016).
Barnacles (Suborder Balanomorpha) are common inhabitants of intertidal rocky shores in Thailand (Pochai et al., 2017; Sukparangsi et al., 2019; Chan et al., 2020). They are habitat modifiers and can regulate the abundance and distribution of other organisms (Branch, 1976; Amnuaypon and Wangkulangkul, 2018). In the temperate Atlantic, barnacles are rarer in rock pools than on open rock surfaces (Singletary and Shadlou, 1983; Firth et al., 2014; Evans et al., 2016; Hall et al., 2019). Two studies have been conducted in temperate regions of the Atlantic Ocean to examine factors affecting the distribution of barnacles in rock pools. Magre (1974) revealed that Balanus (Semibalanus) balanoides settle in rock pools, and its settlement density decreases with pool depth. Singletary and Shadlou (1983) qualitatively monitored physical and biological parameters in rock pools and found that B. balanoides can settle in pools at high volumes, but these settlers soon die from hypoxia. Chemicals released by pool algae can also affect the survival of barnacle spats (Singletary and Shadlou, 1983). Predation by snails, which are common in pools, might also cause mortality (Singletary and Shadlou, 1983).
Chthamalus malayensis Pilsbry, 1916 (Chthamalidae) (Figure 1A) and Amphibalanus amphitrite (Darwin, 1854) (Balanidae) (Figure 1B) are the most common acorn barnacles on intertidal rocky shores off the tropical Andaman coast of Thailand (latitude ~6.4°-10°N) in the Indian Ocean (Tsang et al., 2012). C. malayensis is common on mid-high shores, while A. amphitrite occurs on low shores (Chen et al., 2014; Pochai et al., 2017). Rock pools are common in the intertidal zone of the Andaman coast. Barnacles (C. malayensis and A. amphitrite) are common on open intertidal rock surfaces, but rare inside rock pools (Figure 1C). Factors affecting the distribution of barnacles in tropical rock pools can be different from the results previously addressed in the temperate Atlantic Ocean. In the tropics, barnacles are rare in rock pools, which may be due to a lack of recruitment in pools. If barnacles can recruit in rock pools, then extreme variations in salinity and temperature inside the pool waters might affect barnacle survival. Marine invertebrates can tolerate a range of water temperatures (thermal windows) and salinities (Sherwood et al., 2012; Diaz et al., 2021). When barnacles experienced extremely high temperatures, heart beats increase, and they subsequently enter a coma state (opercular valves open without responses under stimulations). Barnacles will die if the coma condition persists for a long period (Chan et al., 2006). Gonads will also be impacted by high temperatures and result in a lower number of larvae produced (Fraser and Chan, 2019). Moreover, intertidal barnacles that live on rock surfaces experience tidal inundations, but those that live in pools experience continuous submergence. It is possible that continuous submergence is not the best living condition for intertidal barnacles.
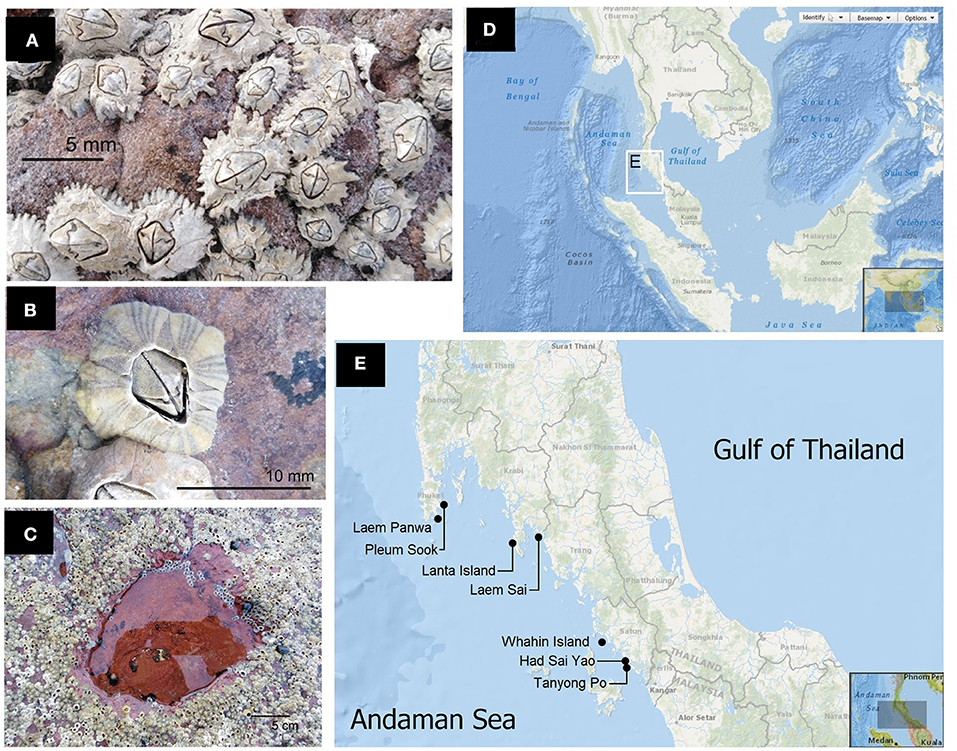
Figure 1. (A) Chthamalus malayensis is a common barnacle on mid shores of the Andaman rocky shores. (B) Amphibalanus amphitrite are common on low shores of the Andaman intertidal zone. (C) On the Andaman Coast of Thailand, barnacles are common on open rock surfaces adjacent to rock pools, but relatively rare inside pools. (D) Study region on the Andaman coast of Thailand, magnified in (E). (E) Seven study shores spanning from the northern to southern coasts on the Andaman coast of Thailand. (D,E) Courtesy of NOAA Bathymetric Data Viewer.
The present study aims to investigate if and how recruitment and the pool physico-chemical environment determine barnacle distribution in rock pools. We tested the hypothesis that barnacles are not common in rock pools on the Andaman rocky shores due to (1) a lack of recruitment, (2) continuous submergence, and (3) the effects of extreme salinity and temperature on barnacle survivals in pools was tested.
Materials and Methods
Study Shores
All rocky shores sampled were located in the Andaman Sea, Thailand (Figures 1D,E). The barnacle abundance and the physico-chemical environment of pools and the differences in barnacle abundance between pools and on rock, were examined on shores with a number of rock pools in Laem Panwa (7°47′57.7"N 98°24′30.1"E), Pleum Sook (7°53′13.7"N 98°26′31.7"E), Laem Sai (7°37′45.7"N 99°14′01.2"E), Whahin Island (6°47′14.0"N 99°45′38.3"E), and Tanyong Po (6°35′27.1"N 99°56′53.9"E). Lanta Island (7°35′23.2"N 99°01′50.2"E) was visited only to take diurnal measurements of the physico-chemical parameters of the rock pools. Lanta Island is suitable for such diurnal measurement because the accommodation site is very close to the field sites, which allows overnight monitoring possible. Recruitment surveys of barnacles on rocks and in pools were conducted in Tanyong Po and Had Sai Yao (6°37′13.5"N 99°57′10.2"E). All shores are wave-exposed or semi-exposed. Tide is semi-diurnal with a maximum tidal range of ~2.5 m. Shale was the predominant type of rock in the study areas.
Physico-Chemical Environment of Rock Pools and Barnacle Abundance
In September 2019, 20 pools were selected in each of the five sampled shores and their physico-chemical environments were surveyed. Pools were selected based on the following criteria: (1) no more than 12-cm deep (to reduce the effect of heterogeneity within the habitat), (2) no cobbles (cobbles can be stirred by water currents and create unstable habitat within the rock pool), and (3) in the intertidal zone (mid-shore level), at the same, tidal level as the barnacle zone.
The pool depths were measured using a ruler (±10 mm). Each pool was photographed from the top of the rock pools, then its surface area was calculated using the image analysis software ImageJ©. Temperature was measured using a glass thermometer (±0.1°C). Salinity was measured using a refractometer. pH was measured by a Portable Digital pH Meter (±0.1). Dissolved oxygen was measured by an OHAUS ST20 portable DO meter (±0.1 mgl−1). A quadrat (10 × 10 cm) was randomly placed inside each rock pool. The percentage cover of C. malayensis and A. amphitrite was scored for each quadrat.
The effect of physico-chemical parameters of rock pools (water temperature, salinity, pH, and dissolved oxygen) on the abundance of barnacles (C. malayensis and A. amphitrite) was analyzed using Multivariate Analysis (PRIMER 6.0 software). The relationship between barnacle abundance and physico-chemical parameters was assessed using the BEST routine in PRIMER (Clarke et al., 2008). The BIO-ENV function in the BEST routine was used to calculate the smallest subset of environmental variables that explained the greatest percentage of variation in the ordination patterns using the Spearman Rank Correlation method.
Diurnal Variation in Physico-Chemical Parameters in Rock Pools
To examine diurnal variation in the water temperature, salinity, dissolved oxygen, and pH of rock pools (methods for measuring physico-chemical parameters as in section Physico-Chemical Environment of Rock Pools and Barnacle Abundance), six rock pools (dimension criteria stated in section Physico-chemical Environment of Rock Pools and Barnacle Abundance) were selected from a rocky shore on Lanta Island on September 16–18, 2020 (late monsoon season). It rained during some periods of measurement. The water temperature, salinity, dissolved oxygen, and pH of pools were measured hourly during low tide (when pools were exposed to the air) and every 3 h during high tide (when pools were submerged).
Comparison of Barnacle Abundance Between Rock Pools and Adjacent Emergent Rocks
On each shore, whenever a rock pool within the selection criteria in section Physico-Chemical Environment of Rock Pools and Barnacle Abundance was found, a quadrat (10 × 10 cm) was randomly placed inside of that rock pools. A quadrat was established on the adjacent emergent rock surface (~20 cm from the rock pool). One quadrat was established for each pool due to pool size limitations. Ten to 20 pools were sampled from each shore. In each quadrat, the percentage cover of C. malayensis and A. amphitrite was scored.
Barnacle Recruitment in Rock Pools and on Adjacent Rock Surfaces
A quadrat (5 × 5 cm) was established to monitor recruitment in rock pools and their adjacent rock surfaces (~20 cm from the pools). Barnacle recruits (defined as having a rostral-carinal diameter of 0.5–1 mm) in each quadrat were scored. It is difficult to distinguish between recruits of C. malayensis and A. amphitrite, so recruits of both species were pooled. Recruitment monitoring was conducted monthly for 12 months, except from March to May 2020 because of logistical difficulties due to the COVID-19 lockdown.
Permutational Multivariate Analysis of Variance with 999 permutations was performed to test the effect of Habitat (two levels), Species (two levels), and Shore (five and three levels for C. malayensis and A. amphitrite, respectively) on the percentage cover of barnacles. As suggested by Anderson et al. (2008), Euclidean distance resemblance matrices (with a dummy variable added) were constructed from untransformed data for all univariate analyses. Data were pooled across the shores for the first analysis, and Habitat and Species were treated as fixed factors and crossed. For the second analysis, data from each species were analyzed separately and Habitat was crossed with Shore (random factor). C. malayensis was found on all shores, whereas A. amphitrite were not found in samples from Laem Panwa or Whahin Island. Hence, there was a difference in the number of shores between species. PERMANOVA was also performed to evaluate the effects of Habitats (fixed, two levels), Months (fixed, nine levels), and Shore (random, two levels) on the density of recruits. Pairwise comparisons were conducted on significant terms.
Effect of Continuous Submergence on Barnacle Survival
Small pieces of rock with barnacles attached were collected by a chisel and hammer. Sixty adult barnacles of each species with 4–6 mm (for C. malayensis) and 10–12 mm (for A. amphitrite) basal rostral-carinal diameter were collected from Tanyong Po. These sizes were determined to be small enough to represent young and vulnerable individuals but large enough to be handled in the experiment. Specimens were acclimated in aerated seawater for 48 h prior to the experiment. Each species of barnacle was maintained in two treatments: continuous submersion and control for 14 days. In the continuous submersion treatment, barnacles were continuously immersed in seawater. In the control treatment, barnacles were removed from the water for 4 h and immersed again in seawater for 8 h. This procedure was repeated two times a day to simulate the inundation period of the semidiurnal tide on the Andaman coast (a total of 8 h of emersion and 16 h of immersion per day). Each treatment had three replicates. For each replicate, 10 barnacles were kept in a 500 ml plastic container filled with continuously aerated 32‰ seawater prepared from artificial seawater (Aquarise©). Barnacles were fed with an ad libitum diet of Chaetoceros sp. (concentration: 3 × 105 cell/ml) daily. Lamps (120 W Phillips Par 38 Flood) were suspended ~30 cm above the containers and turned on for 12 h (06:00 to 18:00) per day. Heating from the lamps created an ambient temperature similar to the average shore temperature (~30°C). Seawater was changed every 2 days and the number of surviving barnacles was scored daily. Surviving barnacles were checked by stimulating their operculum using a wooden stick (Fraser and Chan, 2019).
The percentages of barnacles that survived were compared between the continuous submersion and control treatments on different days by two-way ANOVA. Treatment (two levels) and Day (15 levels) were treated as fixed factors. Treatment was crossed with Day. Tukey's test was performed on significant terms for post hoc multiple comparisons.
Effects of Temperature and Salinity on Barnacle Survival
Three hundred and sixty adult barnacles of C. malayensis and A. amphitrite were collected and acclimated as in section Effect of Continuous Submergence on Barnacle Survival. Barnacles were maintained in combinations of four different salinities (0, 33, 50, and 60‰) and three temperature levels (30, 40, and 50°C), with three replications per treatment. For each replication, 10 barnacles were kept in a 500 ml plastic container filled with continuously aerated solution prepared from artificial seawater. Containers were placed in a thermostatic heating water bath and the solution was heated from 27°C (ambient) at ~0.3°C min−1 until the target temperature was reached. Barnacles were exposed to the treatments for 5 h, after which the number of surviving barnacles was counted. Salinity was checked every 15 mins to ensure that the designated salinity levels were maintained. Distilled water was added in containers in which salinity had increased due to the heating. Barnacles were not fed.
Two-way ANOVA was performed to test the effect of temperature and salinity on the percentage of C. malayensis and A. amphitrite survival separately. Data was sqrt(x+1) transformed. Temperature (three levels) and salinity (four levels) were treated as fixed factors. Temperature was crossed with salinity. A Student–Newman–Keuls (SNK) test was performed on significant terms for post hoc multiple comparisons.
Results
Physico-Chemical Environment of Rock Pools and Barnacle Abundance
A total of 76 rock pools was measured for surface area, depth, water temperature, salinity, pH and dissolved oxygen, and the corresponding abundances of C. malayensis and A. amphitrite. There was no significant correlation detected between the size dimension of pools (surface area × depth) and any physico-chemical parameters measured (Figure 2). Water temperature from all the pools measured ranged from an approximate temperature of 27–37°C. Salinity ranged from ~5–46‰, pH ranged from ~6–9.7 and dissolved oxygen concentration ranged from ~0.9–11 mg L−1 (Figure 2 and Table 1).
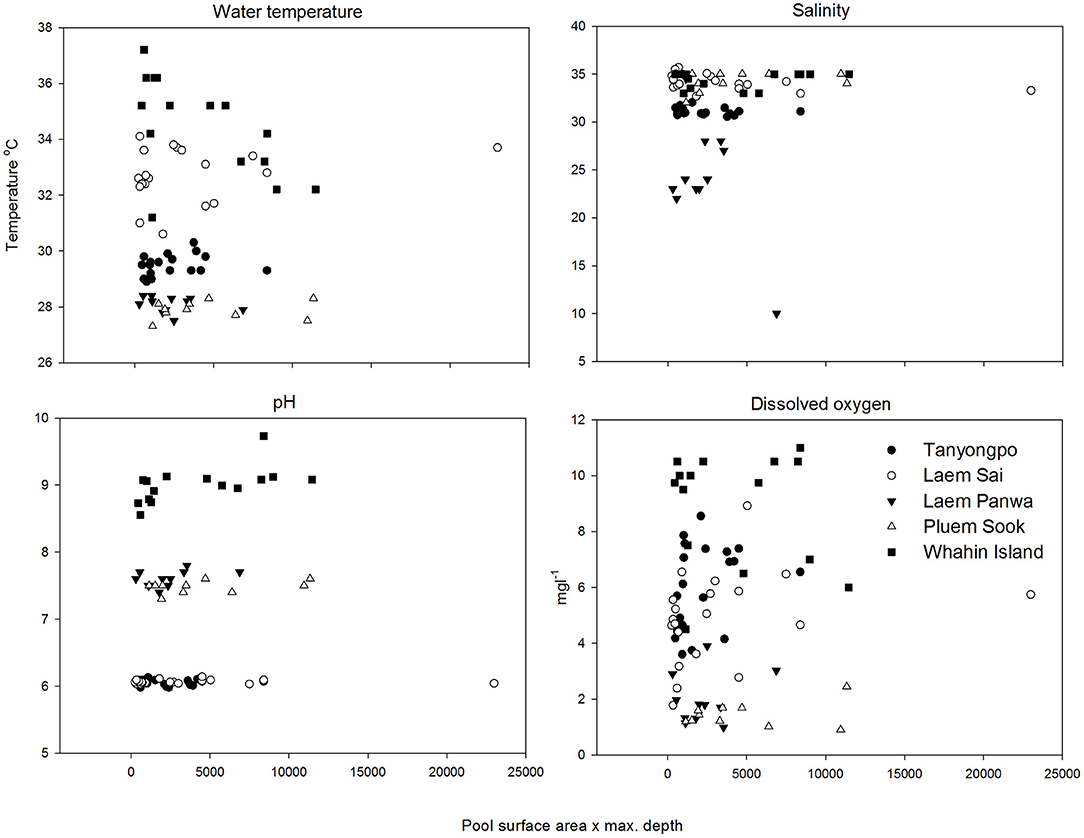
Figure 2. Variation in water temperature, salinity, pH, and dissolved oxygen with pool size dimensions (depth × surface area) from five study shores. Note that none of the physico-chemical parameters were significantly correlated with pool size dimensions.
Based on the Bio-Env multivariate analysis in the BEST (Bio-Env) routine, the correlation coefficients from a combination of different subsets of physico-chemical parameters to the barnacle abundance ranged from 0.14 to 0.30 (Table 2). The subset, including water temperature and salinity, yielded the highest correlation coefficient (0.30) to the barnacle abundance (Table 2). Having all the physico-chemical parameters in the analysis yielded a correlation coefficient of 0.16, lower than the subset containing water temperature and salinity (Table 2).
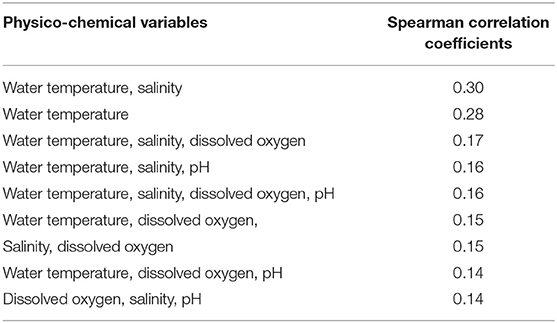
Table 2. Results of Bio-Env analysis in the BEST routine of PRIMER 6, showing the variation in the correlation coefficient with different subsets of physico-chemical variables.
Diurnal Variation in Physico-Chemical Parameters in Rock Pools
Water temperature, salinity, pH, and dissolved oxygen followed a diurnal pattern of variation. Pools were emersed and immersed two times daily. When the pools were immersed in seawater during high tides, all their physico-chemical parameters were similar to those of inshore seawater. When the pools were isolated during the daytime low tide, water temperature, salinity, pH, and dissolved oxygen started to increase gradually, peaking (water temperature at 33°C, salinity at 38‰, pH at 9.0 and dissolved oxygen at 15 mg L−1) in the late afternoon (at ~ 15:00) and then decreasing. When the pools were immersed again after the first low tide, the physico-chemical parameters became similar to those of inshore seawater again. The second low tides happened in the very early morning (03:00 to 07:00). During this period, when the pools were isolated, the water temperature, dissolved oxygen, and pH decreased and reached its lowest values (water temperature at ~27–28°C, pH at ~7.2 and dissolved oxygen at ~2 mg L−1) at around 05:00–06:00. In contrast to other physio-chemical parameters, salinity continued to increase during the early morning low tide. On the second conservative day of measurement, it rained during the afternoon low tides when the pools were emersed. Water temperature dropped (~27°C) and salinity plummeted to as low as 5‰, compared with 33‰ for the inshore seawater (Figure 3). The pH and dissolved oxygen followed previous trends of increase, peaking at around 17:00 and dropping again during high tides (Figure 3).
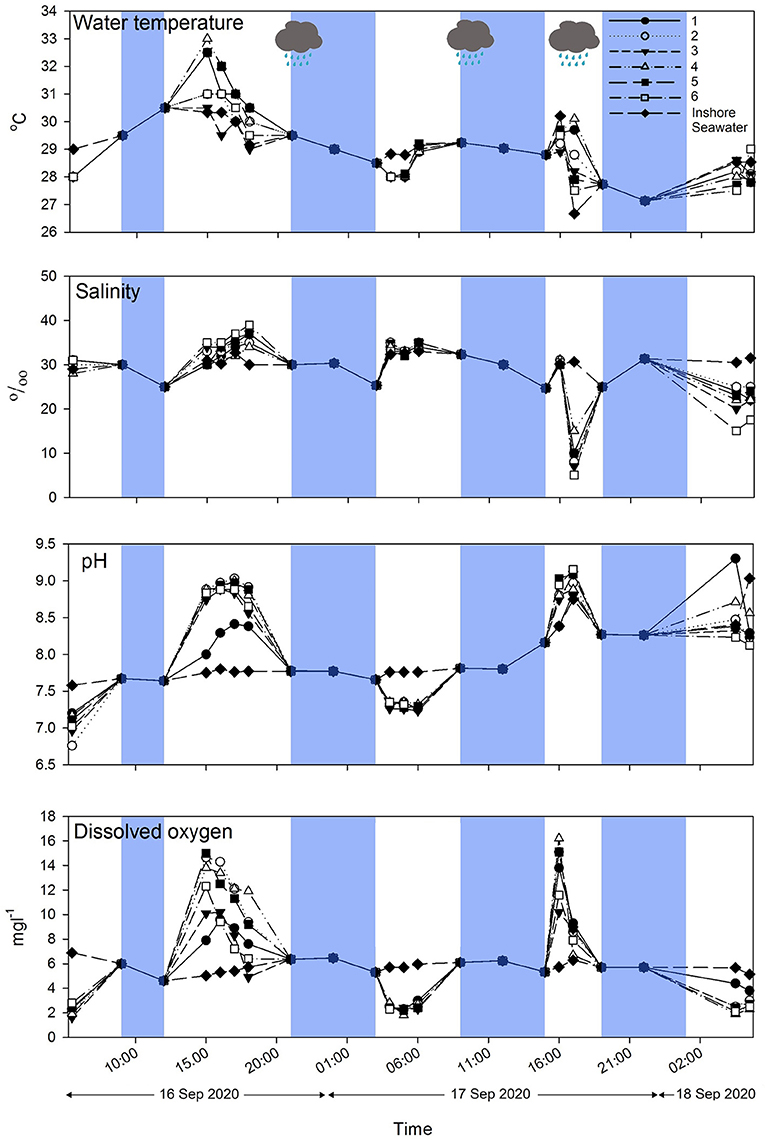
Figure 3. Diurnal variation in water temperature, salinity, pH, and dissolved oxygen in six intertidal pools and inshore seawater. Blue regions indicate pools that were immersed in high tides. White regions indicate pools that were isolated from the sea during low tides. Raining clouds indicate that rain occurred during sampling.
Abundance of Barnacles in Rock Pools and on Emergent Rock
Chthamalus malayensis had a higher abundance on the adjacent open rock surfaces than in pools on all shores (Figures 4A,B and Table 3). The abundance of A. amphitrite on adjacent open rock surfaces and in pools varied among shores (Figure 4C and Table 3). In Tanyong Po, A. amphitrite was more abundant on open rock surfaces than in pools, and this pattern was reversed in Pleum Sook (Figure 4C). Based on the pooled data from across all shores, there were no significant differences in A. amphitrite between the open rock surfaces and pools (Table 3). In general, A. amphitrite had a lower abundance than C. malayensis on open rock surfaces (Figure 4 and Table 3).
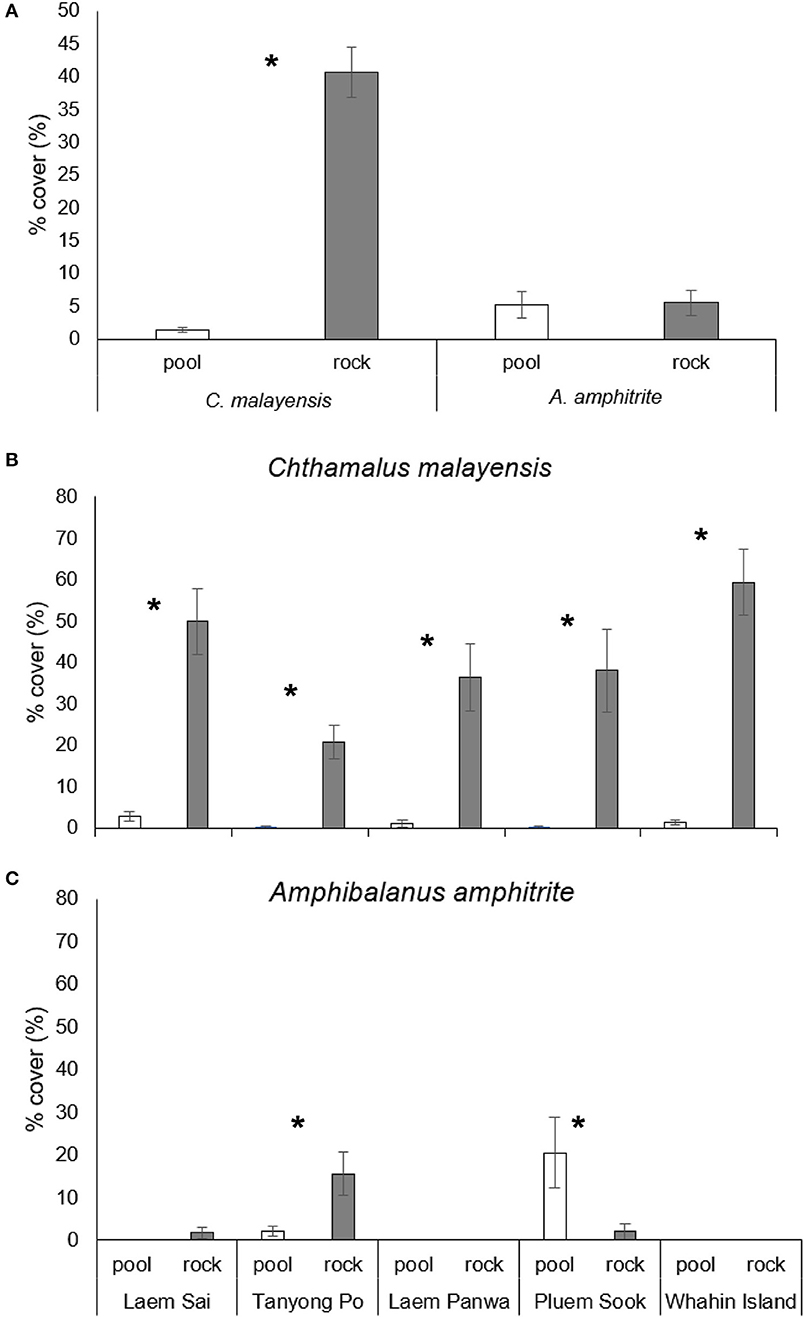
Figure 4. Percentage cover of barnacles in rock pools and on open rock surfaces adjacent to pool. (A) Percentage cover of barnacles (mean ± SE) pooled from all shores. (B,C) Percentage cover on five shores of C. malayensis and A. amphitrite, respectively. Icon: * = significant differences between habitats suggested by pairwise tests.
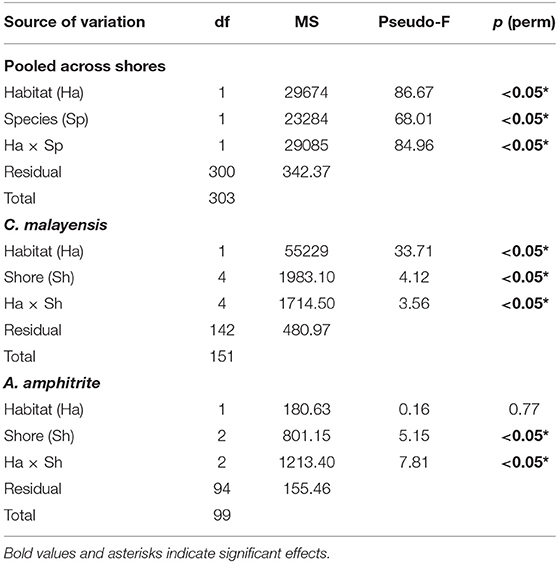
Table 3. Permutational multivariate ANOVA (PERMANOVA) for the effects of habitat (rock pools and adjacent open rock surfaces), species and shore on barnacle abundance.
Barnacle Recruitment in Rock Pools and on Emergent Rock
Barnacle recruitment was observed in October 2019 and from June to September 2020, when recruits could be seen on open rock surfaces (Figure 5). There was a significant difference in the density of recruits between the rock pools and adjacent open rock surfaces (Figure 5A and Table 4). Recruits were only recorded in pools at Had Sai Yao in June 2020 and at Tanyong Po in August 2020. No recruitment was found in pools in any other month on either shore (Figure 5). In Tanyong Po, the density of recruits was more than three times higher on adjacent open rock surfaces than inside pools (Figure 5A).
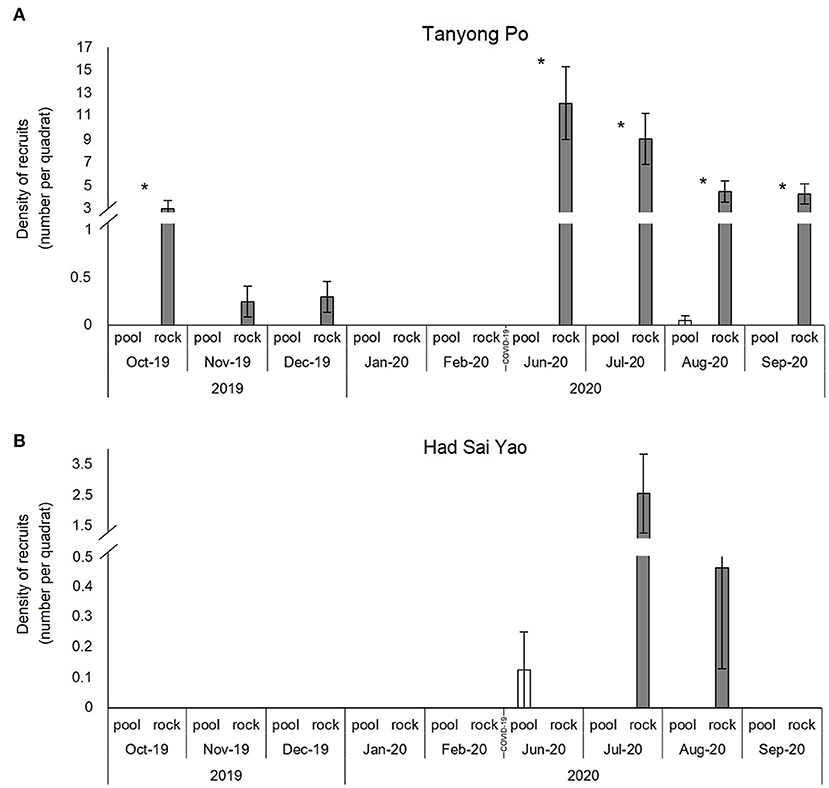
Figure 5. Density of recruits (mean ± SE) in rock pools and on emergent rock from October 2019 to September 2020. Samplings were not carried out from March 2020 to May 2020 due to logistical difficulties during COVID-19 lockdown. (A) and (B) Tanyong Po and Had Sai Yao, respectively. Icon: *significant differences between habitats as suggested by pairwise tests.
Submersion Experiment
Survivorship for C. malayensis differed between treatments (Figure 6A and Table 5). After 2 weeks, all C. malayensis survived well in the control treatment (> 90% survival) but had a survival of 80% under continuous submersion (Figure 6A). Survival of A. amphitrite was not different between the treatments (Figure 6B, Table 5).
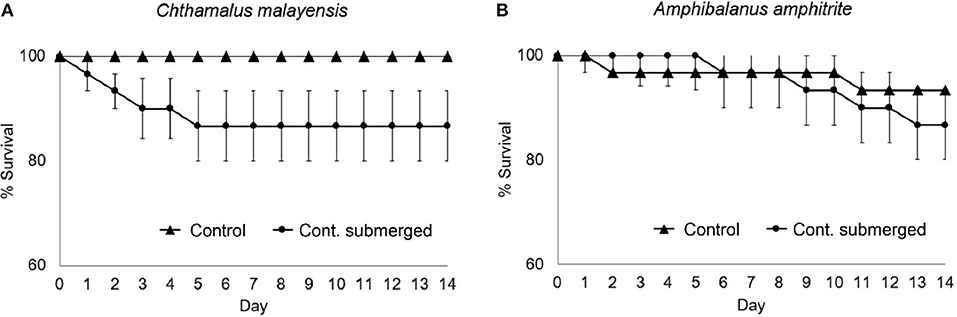
Figure 6. Percentage of survival (mean ± SE) of barnacles in submersion experiment. (A) C. malayensis and (B) A. amphitrite.
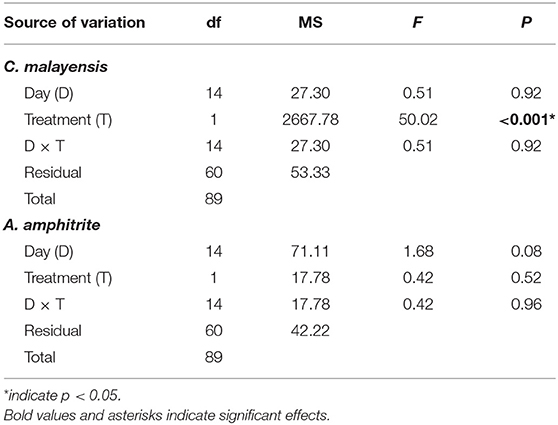
Table 5. Two-way ANOVA on the effects of treatment and day on the percentage of surviving barnacles in the submergence experiment.
Effect of Temperature and Salinity on Barnacle Survival
The C. malayensis and A. amphitrite had similar survival patterns at different temperatures and salinities (Figure 7). Based on the two-way ANOVA, the interaction between temperature × salinity in barnacle survivals was significant. At 50°C, none of the barnacles survived (0% survival), regardless of the salinity level. When barnacles were maintained at 40°C, ~40–50% survived at 0‰, whereas survival was up to 90% under 33, 50 and 60‰ salinities, with no significant difference among these top three salinities (SNK test; p < 0.05). At 30°C, there was no significant difference in survival among salinity treatments, and >90% of all barnacles survived (Figure 7 and Table 6).
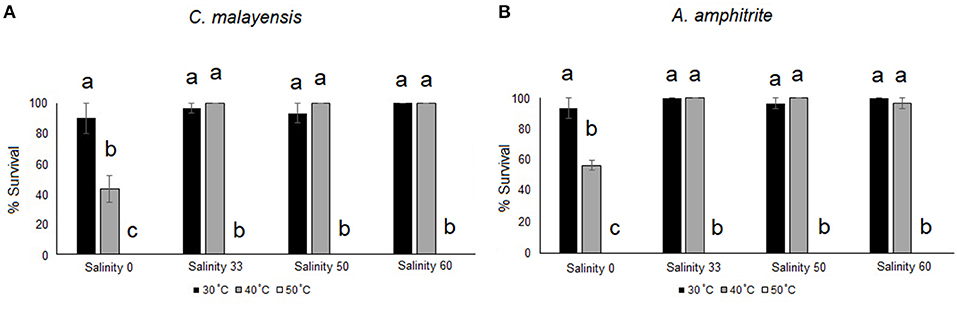
Figure 7. Percentage of barnacles, namely, (A) C. malayensis and (B) A. amphitrite (mean ± SE), that survived under different temperature and salinity treatments. Different small letters = significant difference between temperature within each salinity treatment. Note survival of barnacles is 0 in 50°C.
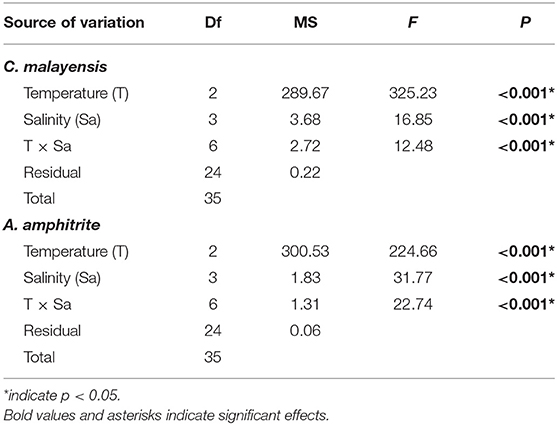
Table 6. Two-way ANOVA on the effects of Temperature and Salinity on the percentage of survival of two species of barnacles.
Discussion
Physico-Chemical Environment in Indian Ocean Rock Pools
The present study is the first to describe the physico-chemical environment of rock pools and its diurnal variation in the Indian Ocean. Water temperature, salinity, pH, and dissolved oxygen levels fluctuate across diurnal cycles, with one maximum and minimum period per day during the two low tides periods. The diurnal pattern of variations in physico-chemical parameters in the rock pools of the present study is similar to the patterns addressed in the subtropical Hong Kong, in the South China Sea of the Pacific region (Chan, 2000). Both regions have two periods of pool isolations from low tides, and the physico-chemical parameters of both peaked in the later afternoon. The range of variations in water temperature, salinity, pH, and dissolved oxygen is similar between Thailand and Hong Kong (Chan, 2000). Similar to the present study, the water temperature, salinity, pH and dissolved oxygen concentration in the NW coast of Brittany in the temperate region of the Atlantic had one maximum (later afternoon) and one minimum (early morning). However, rock pools in the Atlantic coast of France have a single low tide isolation per day. This isolation can last up to 10 h, much longer than the 6- to 7-h low tide isolation in the present study. Water temperature in the present study is higher than the summer water temperature in the Britany rock pools, but the range of salinity variation is similar between the present study and the Atlantic rock pools.
Distribution of Barnacles in Rock Pools and Adjacent Open Rock Surfaces
In the present study, it was found that Chthamalus malayensis was more abundant (>40%) on the adjacent rock surfaces than inside rock pools (<5%). This pattern is similar to the pattern addressed in Balanus balanoides in the temperate Atlantic (Singletary and Shadlou, 1983) that barnacles were more intense on rock surfaces than inside rock pools. High shore intertidal species often inhabit open rock surfaces instead of inside rock pools. The high shore limpet, Cellana grata, for example, are common on open rock surfaces and at rock pool interface (adjacent surface to pools) but never get submerged in rock pools. The limpets at pool interface had relatively lower heat stress when compared to open rock surfaces. The lower shore barnacle, Amphibalanus amphitrite, in contrast, had overall similar abundances (5–10%) in pools and also on the rock surfaces. The vertical distribution of A. amphitrite extends from lower intertidal to subtidal region. A. amphitrite often has intense settlement on shores and subtidal man-made objects (Desai and Anil, 2005). A. amphitrite is also one of the marine fouling species due to its intense settlement and high tolerance to environmental stresses (Desai and Anil, 2005). A. amphitrite had higher abundance in rock pools when compared with C. malayensis indicates this barnacle survives well inside the rock pools environment.
Factors Affecting Barnacle Survival in Rock Pools
In the present study, sparse recruitment of barnacles was recorded in some of the rock pools, suggesting that barnacle larvae can settle in the rock pools and grow into recruits. This does not support the first hypothesis, that barnacles are rare in pools because of a lack of recruitment. However, the density of recruits is much lower in pools than on adjacent open rock surfaces. This suggests that some factors may affect the post-settlement and post-recruitment mortality of barnacles recruited inside of rock pools. Rock pools in the Andaman Sea of Thailand are often inhabited by various species of gastropods including the Monodonta and Nerita snails (Sanpanich and Duangdee, 2013). Bulldozing effect of the settled cyprids by these mobile molluscan grazers can affect the success of the settlement (Chan and Williams, 2003). In the temperate Atlantic, the occurrence of some algae and biofilms might affect the settlement and post-settlement mortality of barnacles (Singletary and Shadlou, 1983). Algae are more abundant in rock pools than on open rock (Nylund and Pavia, 2003; Othmani et al., 2016).
Submergence experiments indicated that both C. malayensis and A. amphitrite can have at least 80% lower survival under submergence conditions compared to simulated tidal conditions (control) although barnacles suffer slight mortality under submerged conditions. Continuously submerged conditions in pools do not appear to be the major cause of barnacle mortality in rock pools. Compared to the high shore intertidal limpets, Cellana grata, which are common on rock surfaces but not found in rock pools, continuous submergence under laboratory conditions can result in 70% mortality after 96 h (Gray, 2010). This may explain continuous submergence can be a factor for the absences of C. grata inside rockpools.
Based on the multivariate analysis of rock pool physico-chemical environment and barnacle abundance, water temperature and salinity are the main factors controlling barnacle distribution. In the laboratory temperature and salinity experiment, barnacles did not survive in the high water temperature (50°C) environment. At 40°C, barnacles had greater mortality (~40–50%) only in the low salinity treatment (0‰), but not in the high salinity treatments. For the 30°C treatment, both barnacle species had high survivals in all salinity treatments. When barnacles were exposed to low salinity water, their opercular valves were closed to stop external water from getting inside the shell. As a result, mantle water and haemolymph can have higher osmolality than the external environment (Chan et al., 2001). However, at high temperatures, barnacles can enter a coma state and lose the ability to close their opercular valves. As a result, low salinity water can enter the mantle and affect the osmolality of the hemolymph, reducing survival. This suggests that the combination of high water temperature and low salinity (after rain) can lead to high barnacle mortality in rock pools. In natural rock pools, salinity can be as low as almost 0‰ during the heavy rain monsoon season on the Andaman coast (May to October), and intense solar radiation shortly after rains can heat up pool water. This condition can cause mortality in barnacles inside pools. This is probably a common phenomenon on tropical shores. During the wet season in Hong Kong, the limpet Cellana grata experienced heavy rainfall and subsequently, heat stresses had faster heart beat rates and lower haemolymph and mantle osmolalities when compared with limpets that were not stressed. Such patterns suggest that heat and raining can both impose different levels of stress and protein responses, but their combination had an interactive effect on the limpets' metabolism and survival (Williams et al., 2011). The brittle star, Ophiopholis mirabilis, is common in the temperate intertidal region of the West Pacific. The combination of temperature and salinity can affect its metabolism, ammonia excretion, and tissue moisture. Reduced salinity conditions in combination with temperature variations can result in mortality due to the tissue moisture beyond their regulatory capacity (Fang et al., 2015). In the cold Baltic Sea, the barnacle Balanus improvisus had increased mortality in low salinity (5 psu) and low temperature (12°C) because low water temperature narrowed the barnacle's tolerance toward low salinity (Nasrolahi et al., 2013). Salinity and water temperature can thus interplay together and impose interactive stress toward intertidal species and affect their mortality.
However, the present study has not tested the effect of dissolved oxygen on barnacle survival. On the Atlantic coast of the USA, pools may not be able to support high densities of settlers because such a condition would decrease the dissolved oxygen needed to support such densities of barnacles, thus resulting in hypoxia and high barnacle mortality (Singletary and Shadlou, 1983). In the present study, some of the rock pools had very low dissolved oxygen (1–3 mg. L−1). In such an environment, barnacles, if recruited in those pools, probably would not survive.
In conclusion, barnacles are rare in tropical Indian Ocean rock pools. This is a result of the interplay of continuous submergence, high water temperature, and low salinity causing barnacle mortality. Future research should investigate biological factors like predation (density of whelks in rock pools) or the grazing of molluscs, which bulldoze settlers (Chan and Williams, 2003).
Data Availability Statement
The original contributions generated for the study are included in the article/supplementary material, further inquiries can be directed to the corresponding authors.
Author Contributions
KW conceived the idea and designed the study. NB analyzed the data and wrote the first draft of the manuscript. BC involved in experiment design, manuscript writing and provided advice. All authors read and commented on the manuscript.
Funding
This study was supported by the Faculty of Science Research Fund, Prince of Songkla University (Contract No. 1-2562-02-006) and an Academia Sinica research grant.
Conflict of Interest
The authors declare that the research was conducted in the absence of any commercial or financial relationships that could be construed as a potential conflict of interest.
Publisher's Note
All claims expressed in this article are solely those of the authors and do not necessarily represent those of their affiliated organizations, or those of the publisher, the editors and the reviewers. Any product that may be evaluated in this article, or claim that may be made by its manufacturer, is not guaranteed or endorsed by the publisher.
Acknowledgments
We thank Lutfee Hayeewachi, Suphatsara Sangphuek, Vanyarat Kongsap, Apisara Nakpan, Phutita Wongwaiyut, Nattacha Khantasimachalerm, Kanyanat Kaewutai, Thitiya Choosun, Arisa Somprom, and Suntaree Karnchananiyom for their help with fieldwork. We also thank Thachanon Sinso for his help in setting up the experiment and Noah Last (third draft editing) for his English language editing.
References
Amnuaypon, P., and Wangkulangkul, K. (2018). Influence of habitat modification by rock oysters and barnacles on small-scale distribution of the tropical pulmonate limpet Siphonaria guamensis. Zool. Ecol. 28, 292–299. doi: 10.1080/21658005.2018.1520023
Anderson, M. J., Gorley, R. N., and Clarke, K. R. (2008). PERMANOVA + for PRIMER: Guide to Software and Statistical Methods. Plymouth: PRIMER-E, Ltd.
Branch, G. M. (1976). Interspecific competition experienced by south African Patella species. J. Anim. Ecol. 45, 507–530. doi: 10.2307/3888
Chan, B. K. K. (2000). Diurnal physico-chemical variations in Hong Kong rock pools. Asian Mar. Biol. 17, 43–54.
Chan, B. K. K., Morritt, D., and Williams, G. A. (2001). The effect of salinity and recruitment on the distribution of Tetraclita squamosa and Tetraclita japonica (Cirripedia; Balanomorpha) in Hong Kong. Mar. Biol. 138:999. doi: 10.1007/s002270000531
Chan, B. K. K., Morritt, D. M., Pirro, M. D., Leung, K. M. Y., and Williams, G. A. (2006). Summer mortality: effects on the distribution and abundance of the acorn barnacle Tetraclita japonica on tropical shores. Mar. Ecol. Prog. Ser. 328:195–204. doi: 10.3354/meps328195
Chan, B. K. K., Tsao, Y. F., and Ganmanee, M. (2020). Morphological and molecular evidence support the intertidal barnacle Octomeris intermedia Nilsson-Cantell, 1921 (Thoracica, Chthamalidae) as a valid species in Indo-Pacific waters. ZooKeys 914, 1–31. doi: 10.3897/zookeys.914.49328
Chan, B. K. K., and Williams, G. A. (2003). Effect of physical stress and mollusc grazing on the settlement and recruitment of Tetraclita species on a tropical shore. J. Exp. Mar. Biol. Ecol. 283, 1–23. doi: 10.1016/S0022-0981(02)00475-6
Chen, H. N., Tsang, L. M., Chong, V. C., and Chan, B. K. K. (2014). Worldwide genetic differentiation in the common fouling barnacle, Amphibalanus amphitrite. Biofouling 30, 1067–1078. doi: 10.1080/08927014.2014.967232
Clarke, K. R., Somerfield, P. J., and Gorley, R. N. (2008). Testing of null hypotheses in exploratory community analyses: similarity profiles and biota-environment linkage. J. Exp. Mar. Biol. Ecol. 366, 56–69. doi: 10.1016/j.jembe.2008.07.009
Delany, J., Myers, A. A., and McGrath, D. (1998). Recruitment, immigration and population structure of two coexisting limpet species in mid-shore tidepools, on the west coast of Ireland. J. Exp. Mar. Biol. Ecol. 221, 221–230. doi: 10.1016/S0022-0981(97)00129-9
Desai, D. V., and Anil, A. C. (2005). Recruitment of the barnacle Balanus amphitrite in a tropical estuary: implications of environmental perturbation, reproduction and larval ecology. J. Mar. Biol. Assoc. UK 85, 909–920. doi: 10.1017/S0025315405011884
Diaz, F., Re-Araujo, D., Carpizo-Ituarte, E., Garcia-Esquivel, Z., Larios-Soriano, E., Perez-Carrasco, L., et al. (2021). Thermal physiological performance and thermal metabolic scope of the whelk Kelletia kelletii (Forbes, 1850) (Gastropoda: Neptuneidae) acclimated to different temperatures. Zool. Stud. 60:44. doi: 10.6620/ZS.2021.60-44
Evans, A. J., Firth, L. B., Hawkins, S. J., Morris, E. S., Goudge, H., and Moore, P. J. (2016). Drill-cored rock pools: an effective method of ecological enhancement on artificial structures. Mar. Freshw. Res. 67, 123–130. doi: 10.1071/MF14244
Fang, J., Zhang, J., Liu, Y., Jiang, Z., Mao, Y., and Fang, J. (2015). Effects of temperature and salinity on mortality and metabolism of Ophiopholis mirabilis. Mar. Biol. Res. 11, 157–167. doi: 10.1080/17451000.2014.904884
Firth, L. B., Thompson, R. C., Bohn, K., Abbiati, M., Airoldi, L., Bouma, T. J., et al. (2014). Between a rock and a hard place: environmental and engineering considerations when designing coastal defence structures. Coast. Eng. 87, 122–135. doi: 10.1016/j.coastaleng.2013.10.015
Firth, L. B., Thompson, R. C., White, F. J., Schofield, M., Skov, M. W., Hoggart, S. P. G., et al. (2013). The importance of water-retaining features for biodiversity on artificial intertidal coastal defence structures. Divers. Distrib. 19, 1275–1283. doi: 10.1111/ddi.12079
Firth, L. B., and Williams, G. A. (2009). The influence of multiple environmental stressors on the limpet Cellana toreuma during the summer monsoon season in Hong Kong. J. Exp. Mar. Biol. Ecol. 375, 70–75. doi: 10.1016/j.jembe.2009.05.011
Fraser, C. M. L., and Chan, B. K. K. (2019). Too hot for sex: mating behaviour and fitness in the intertidal barnacle Fistulobalanus albicostatus under extreme heat stress. Mar. Ecol. Prog. Ser. 610, 99–108. doi: 10.3354/meps12848
Gray, D. R., and Williams, G. A. (2010). Knowing when to stop: rhythms of locomotor activity in the high-shore limpet, Cellana grata Gould. J. Exp. Mar. Biol. Ecol. 39, 125–130. doi: 10.1016/j.jembe.2010.06.021
Hall, A. E., Herbert, R. J., Britton, R. J., Boyd, I., and George, N. (2019). Shelving the coast with vertipools: retrofitting artificial rock pools on coastal structures as mitigation for coastal squeeze. Front. Mar. Sci. 6:456. doi: 10.3389/fmars.2019.00456
Laure, M. L. N., Hawkins, S. J., Jenkins, S. R., and Thompson, R. C. (2009). Grazing dynamics in intertidal rock pools: connectivity of microhabitats. J. Exp. Mar. Biol. Ecol. 370, 9–17. doi: 10.1016/j.jembe.2008.11.005
Legrand, E., Riera, P., Pouliquen, L., Boher, O., Carious, T., and Martin, S. (2018). Ecological characterization of intertidal rock pools: seasonal and diurnal monitoring of physico-chemical parameters. Reg. Stud. Mar. Sci. 17, 1–10. doi: 10.1016/j.rsma.2017.11.003
Magre, E. J. (1974). Population density of Balanus balanoides (L.) in relation to tide pool water level (Cirripedia Thoracica). Crustaceana 26, 139–142. doi: 10.1163/156854074X00497
Metaxas, A., and Scheibling, R. E. (1993). Community structure and organization of tidepools. Mar. Ecol. Prog. Ser. 98, 187–198. doi: 10.3354/meps098187
Moran, M. J. (1985). The timing and significance of sheltering and foraging behaviour of the predatory intertidal gastropod Morula marginalba Blainville (Muricidae). J. Exp. Mar. Biol. Ecol. 93, 103–114. doi: 10.1016/0022-0981(85)90152-2
Morris, S., and Taylor, A. C. (1983). Diurnal and seasonal variation in physico-chemical conditions within intertidal rock pools. Estuar. Coast. Shelf Sci. 17, 339–355. doi: 10.1016/0272-7714(83)90026-4
Moschella, P. S., Abbiati, M., Åberg, P., Airoldi, L., Anderson, J. M., Bacchiocchi, F., et al. (2005). Low-crested coastal defence structures as artificial habitats for marine life: using ecological criteria in design. Coast. Eng. 52, 1053–1071. doi: 10.1016/j.coastaleng.2005.09.014
Nasrolahi, A., Pansch, C., Lenz, M., and Wahl, M. (2013). Temperature and salinity interactively impact early juvenile development: a bottleneck in barnacle ontogeny. Mar. Biol. 160, 1109–1117. doi: 10.1007/s00227-012-2162-8
Nylund, G. M., and Pavia, H. (2003). Inhibitory effects of red algal extracts on larval settlement of the barnacle Balanus improvisus. Mar. Biol. 143, 875–882. doi: 10.1007/s00227-003-1093-9
Othmani, A., Bunet, R., Bonnefont, J. L., Briand, J. F., and Culioli, G. (2016). Settlement inhibition of marine biofilm bacteria and barnacle larvae by compounds isolated from the Mediterranean brown alga Taonia atomaria. J. Appl. Phycol. 28, 1975–1986. doi: 10.1007/s10811-015-0668-4
Pochai, A., Kingtong, S., Sukparangsi, W., and Khachonpisitsak, S. (2017). The diversity of acorn barnacles (Cirripedia, Balanomorpha) across Thailand's coasts: the Andaman Sea and the Gulf of Thailand. Zoosyst. Evol. 93, 13–34. doi: 10.3897/zse.93.10769
Sanpanich, K., and Duangdee, T. (2013). The biodiversity of marine gastropods of Thailand in the late decade. Malaysian J. Sci. 32, 47–64. doi: 10.22452/mjs.vol32no3.5
Seabra, R., Wethey, D. S., Santos, A. M., and Lima, F. P. (2011). Side matters: microhabitat influence on intertidal heat stress over a large geographical scale. J. Exp. Mar. Biol. Ecol. 400, 200–208. doi: 10.1016/j.jembe.2011.02.010
Sherwood, L., Klandorf, H., and Yancey, P. (2012). Animal Physiology: From Genes to Organisms. Belmont, CA: Cengage Learning.
Singletary, R. L., and Shadlou, R. (1983). Balanus balanoides in tide-pools: a question of maladaptation? Crustaceana 45, 53–70. doi: 10.1163/156854083X00190
Sukparangsi, W., Pochai, A., Wongkunanusorn, C., and Khachonpisitsak, S. (2019). Discovery of Neonrosella vitiata (Darwin) and Newmanella spinousus Chan and Cheang (Balanomorpha, Tetraclitidae) from the Andaman Sea, eastern Indian Ocean. Zookeys 833, 1–20. doi: 10.3897/zookeys.833.30689
Tsang, L. M., Wu, T. H., Shih, H. T., Williams, G. A., Chu, K. H., and Chan, B. K. K. (2012). Genetic and morphological differentiation of the Indo-West Pacific intertidal barnacle Chthamalus malayensis. Integr. Comp. Biol. 52, 388–409. doi: 10.1093/icb/ics044
Wang, H. Y., Tsang, L. M., Lima, F. P., Seabra, R., Ganmanee, M., Williams, G. A., et al. (2020). Spatial variation in thermal stress experienced by barnacles on rocky shores: the interplay between geographic variation, tidal cycles and microhabitat temperatures. Front. Mar. Sci. 7:553. doi: 10.3389/fmars.2020.00553
Williams, G. A., De Pirro, M., Cartwright, S., Khangura, K., Ng, W.-C., Leung, P. T. Y., et al. (2011). Come rain or shine: the combined effects of physical stresses on physiological and protein-level responses of an intertidal limpet in the monsoonal tropics. Func. Ecol. 25, 101–110. doi: 10.1111/j.1365-2435.2010.01760.x
Keywords: acorn barnacles, intertidals, rock pools, salinity, submergence, Thailand, Andaman Sea
Citation: Buasakaew N, Chan BKK and Wangkulangkul K (2021) Why Are Barnacles Common on Intertidal Rocks but Rare in Rock Pools? Effect of Water Temperature, Salinity, and Continuous Submergence on Barnacle Survival in Indian Ocean Rock Pools. Front. Mar. Sci. 8:688894. doi: 10.3389/fmars.2021.688894
Received: 31 March 2021; Accepted: 24 August 2021;
Published: 23 September 2021.
Edited by:
Mandar Nanajkar, Council of Scientific and Industrial Research (CSIR), IndiaReviewed by:
Guoyong Yan, Hong Kong University of Science and Technology, Hong Kong, SAR ChinaSumit Mandal, Presidency University, India
Copyright © 2021 Buasakaew, Chan and Wangkulangkul. This is an open-access article distributed under the terms of the Creative Commons Attribution License (CC BY). The use, distribution or reproduction in other forums is permitted, provided the original author(s) and the copyright owner(s) are credited and that the original publication in this journal is cited, in accordance with accepted academic practice. No use, distribution or reproduction is permitted which does not comply with these terms.
*Correspondence: Benny K. K. Chan, Y2hhbmtrQGdhdGUuc2luaWNhLmVkdS50dw==; Kringpaka Wangkulangkul, a3JpbmdwYWthLndAcHN1LmFjLnRo