- 1Department of Psychology, Centre for Research in Animal Behaviour, University of Exeter, Exeter, United Kingdom
- 2Center for Whale Research, Friday Harbor, WA, United States
Toothed whales (suborder Odontoceti) are highly social, large brained mammals with diverse social systems. In recent decades, a large body of work has begun investigating these dynamic, complex societies using a common set of analytical tools: social network analysis. The application of social network theory to toothed whales enables insight into the factors that underlie variation in social structure in this taxon, and the consequences of these structures for survival, reproduction, disease transmission, and culture. Here, we perform a systematic review of the literature regarding toothed whale social networks to identify broad patterns of social network structure across species, common drivers of individual social position, and the consequences of network structure for individuals and populations. We also identify key knowledge gaps and areas ripe for future research. We recommend that future studies attempt to expand the taxonomic breadth and focus on standardizing methods and reporting as much as possible to allow for comparative analyses to test evolutionary hypotheses. Furthermore, social networks analysis may provide key insights into population dynamics as indicators of population health, predictors of disease risk, and as direct drivers of survival and reproduction.
Introduction
The structure of social interactions between individuals is a fundamental feature of animal populations, with far reaching consequences (Kurvers et al., 2014). In recent decades, toothed whales (suborder Odontoceti) have emerged as a key subject of behavioural research into the diversity and function of social structure in animals. Highly social, large brained, and inhabiting a wide variety of marine and freshwater environments, these species exhibit a diverse array of social systems, some of which (such as lifelong bisexual social philopatry) are apparently unique among mammals (Connor et al., 1998).
Studying cetacean sociality presents a number of significant challenges. Social structure is defined by the pattern of repeated social interactions between individuals (Hinde, 1976). In cetaceans, the relevant social interactions typically occur underwater, and are therefore difficult to observe. In addition, individual cetaceans are highly mobile, often over large home ranges, which can make it challenging to conduct adequate repeated sampling of individuals to quantify their social interactions. The composition of toothed whale groups also tends to be highly dynamic, with individuals regularly joining and leaving temporary groupings (“fission-fusion dynamics”). Finally, toothed whale social relationships tend to be highly individualized, and thus a full accounting of social structure requires information at the individual and dyadic level, rather than groups or classes of individuals.
These characteristics make social network analysis the ideal framework to answer many questions about toothed whale societies. Social networks represent social actors (typically individuals) as nodes in a graph, connected by edges representing social relationships (Croft et al., 2008). In practice, these edges can be measured in many ways (Farine and Whitehead, 2015), however, in toothed whales they most often represent the rate of association between individuals. Association is typically defined as co-membership in the same group, as these individuals are assumed to have the opportunity to interact (“gambit of the group,” Whitehead and Dufault, 1999; Figure 1). This framework allows researchers to model individualized, dynamic social systems based on patterns of group membership or spatial occurrence.
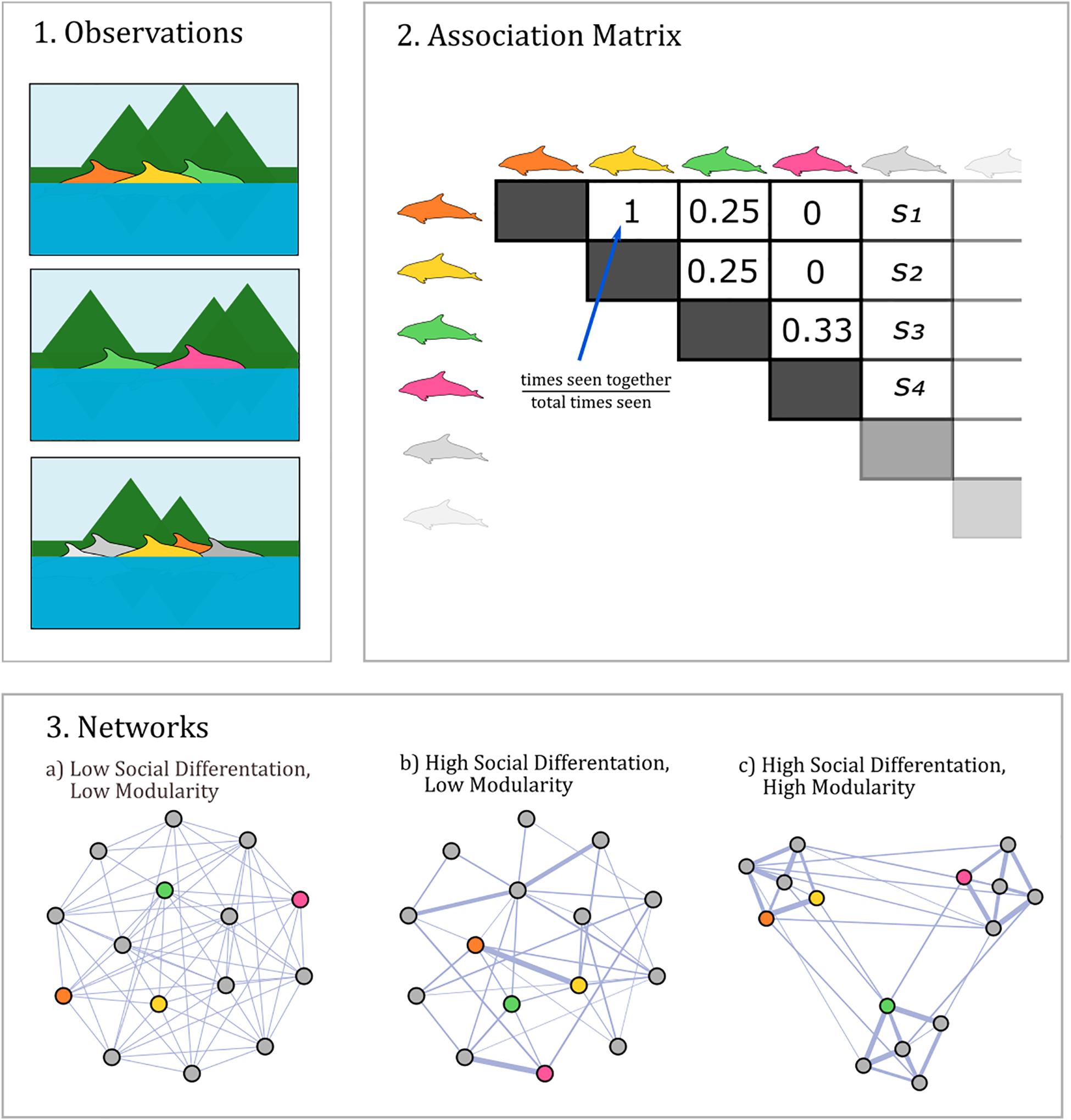
Figure 1. Measuring and analysing toothed whale social networks. Associations between individuals are typically derived from repeated photo-ID surveys (1). These samples are used to generate pairwise association indices between individuals and a corresponding association matrix (2). These matrices can be analysed as weighted social networks to quantify aspects of social structure, such as social differentiation and modularity (3). Dolphin silhouettes are by Chris Huh, re-used here with alteration under a Creative Commons Share-alike license (https://creativecommons.org/licenses/by-sa/3.0).
Several in-depth reviews exist discussing patterns of social structure in cetaceans (Connor et al., 1998; Gowans et al., 2007; Möller, 2011; Rendell et al., 2019) and the application of social network analysis to animals (Brent et al., 2011; Webber and Vander Wal, 2019), however, the widespread quantification of toothed whale social systems using network analysis warrants greater attention. This growing body of research provides the opportunity to study social structure in toothed whales in a comparative framework. Here, we perform a systematic review of the literature on the structure, function and consequences of toothed whale social networks. We extract network metrics and general results from these studies to point toward patterns across toothed whale species, and to evaluate and expand previously proposed models of cetacean sociality.
Review Methodology and Taxonomic Breadth
We used the Web of Science database (accessed March 27, 2020) to search for relevant literature. We searched for articles by pairing taxonomic terms (“cetacean,” “whale,” “dolphin,” or “porpoise”) with sociality terms (“social organisation,” “social structure,” or “social network”). We only retained peer-reviewed studies that generated or analysed matrices of social relationship measures between identified individuals; we did not include studies which analysed social behaviour without quantifying individual relationship. We further exclude studies that only analysed genetic networks without comparing them to behaviourally defined social networks; While kinship can be a basis for social relationships (see section 3.2), genetic relatedness does not necessarily indicate social affiliation, and thus genetic networks are not social networks. Furthermore, we do not include studies on captive groups of animals. We did not exclude studies developing methods for analysing animal social systems, however, we did exclude network science papers that used the Doubtful Sound bottlenose dolphin network (originally described by Lusseau, 2003) as a baseline for algorithm development without reference to the animals’ biology. Where data were available, we extracted measures of network modularity and social differentiation as general measures of global network structure, along with the results of statistical tests of network structure at the dyadic and nodal level. In some cases, a particular study did not report relevant social network measures from their data set, but these measures were reported in later studies that did not appear in our review. In these cases, we extracted measures from these later studies. Our initial search returned 732 studies, of which 179 were retained. Studies on bottlenose dolphins (Tursiops spp.) are by far the most common, representing a majority of all studies (51%), followed by killer whales (Orcinus orca, 13%) and sperm whales (Physeter macrocephalus, 9%). A single study on porpoises was found, which used animal-borne sensors to derive measures of synchrony between individuals (Sakai et al., 2011). While a valuable methodological study, these results are not broadly comparable with most studies of social network structure in toothed whales which are generally based on information of the co-occurence of individuals within groups. We found only one study on small pelagic dolphins, conducted on island associated spinner dolphins (Stenella longirostris, Karczmarski et al., 2005). Entirely absent from our review are studies of exclusively freshwater dolphin species, and members of Monodontidae (beluga whales Delphinapterus leucas and narwhals Monodon monoceros). We found 4 studies on members of the family Ziphiidae conducted on 4 species (northern bottlenose whale, Hyperoodon ampullatus, Baird’s beaked whale, Berardius bairdii, Blainville’s beaked whale, Mesoplodon densirostris, and Cuvier’s beaked whale, Ziphius cavirostris). Thus, our data for deriving cross-species patterns is limited to delphinids, sperm whales, and a small sampling of beaked whales (see Supplementary Table 1 for number of studies for each species, and the Supplementary Data for a dataset of all studies). Future studies focusing on beaked whales, porpoises, belugas, and narwhals, may be crucial for a broad comparative understanding of social structure in this taxon (see section 5).
The Structure of Toothed Whale Social Networks
The unifying feature of all studied toothed whale social networks is relatively densely connected population-level social networks and fairly rapid fission fusion dynamics. In contrast to most primate societies, where social interactions and fission-fusion dynamics typically occur within well-defined social units (Kappeler and van Schaik, 2002), most toothed whale populations exhibit open social networks, and thus the relevant level of analysis is typically the population. Even where stable social units are present, interactions between units are common (e.g., in killer whales, Parsons et al., 2009).
There are countless features of social network structure that could be examined. Here, we focus on those aspects of social network topology which are both commonly measured in toothed whale studies, and are potentially particularly relevant to the biology of these animals: social modularity and social differentiation, the role of kinship, sex, and behavioural phenotypes in shaping these networks, and what factors correlate with variation in social centrality.
Community Structure and Social Differentiation
Among the most common measures of global social structure in our review are social differentiation (28% of studies) and modularity (34% of studies). These measures describe easily interpretable aspects of social structure, and can be calculated from any association dataset, without the need for any information about individual attributes. For this reason, they serve as useful metrics to compare social structure between species and populations. However, some caution is needed in interpreting comparisons between species, as aspects of sampling (e.g., duration, intensity, environmental context) and network size can affect these measures. In addition, the methods used to collect network data, define associations, and calculate edge weights can have strong impacts on social network measures (Castles et al., 2014; Farine and Whitehead, 2015). Therefore, we restrict our review of social differentiation and modularity to studies using the most common sampling regime (association networks based on group membership, derived from photo identification, 94% of studies) and association index (the half-weight index, 79% of studies).
Social differentiation (abbreviated S) is an index of variation, or non-randomness, in association indices (see Box 1). Networks with high social differentiation have large variation in association strength, with individuals exhibiting strongly preferred and avoided associates. In practice, this is calculated by estimating the coefficient of variation of the underlying association probabilities using maximum likelihood (Whitehead, 2008), thus attempting to remove sampling noise from the estimate. This measure is strictly positive, with no natural upper bound. Typically, values of S below 0.5 indicate fairly homogenous associations, while values greater than 1 indicate extremely differentiated associations (Whitehead, 2008).
Box 1 | Glossary of social network terms
Adjacency matrix: Representation of a social network as an N x N matrix A, with the entries Aij indicating the relationship between individuals i and j.
Association: The occurrence of a pair of individuals in close enough proximity (often approximated membership in the same group) to engage in social interactions.
Association index: A measure of the frequency with which pairs of individuals associate, usually expressed as the probability of association in a given sampling period. Commonly used indices include the simple ratio index (SRI) and the half-weight index (HWI). Typically these take the general form X/D, where X is the number of sampling periods in which a given pair of individuals were seen together, and D is the number of sampling periods in which they could have been seen together (often with some form of correction).
Edge: A connection between nodes in a network. In social networks, edges represent some aspect of social relationships.
Group: A temporary collection of individuals in close physical proximity with some degree of coordination in behaviour.
Matrix correlation: The correlation (or regression coefficient) between the entries of a social adjacency matrix and a predictor matrix, with statistical significance determined through randomisations. Special cases include the Mantel test and multiple regression quadratic assignment procedure (MRQAP).
Modularity: Degree to which a social network is separated into social communities, ranging from 0 to 1. Usually represented by the letter Q. Mathematically, the modularity of a weighted network, for a particular community structure, is . Here, Aij is the edge weight between i and j, ki and kj are the weighted degree (sum of weights) for i and j, m is the sum of all edge weights in the network, and δ (ci,cj) is 1 if i and j are in the same community, and 0 otherwise.
Node: A point where edges in a network connect. In social networks, these represent social entities, typically individuals.
Social community: Subsets of individuals within a social network such that most associations or interactions occur within rather than between sets. These may or may not represent social units (see below).
Social differentiation: Estimated coefficient of variation of underlying association probabilities. High values indicate highly non-random associations. Usually estimated by fitting the parameters of a beta-binomial distribution to association index numerators and denominators using maximum likelihood.
Social network: A set of nodes and edges representing social entities and the social relationships between them, respectively.
Social unit: A stable set of individuals in near-constant association with one another.
In our review, we found strong variation in S across species. Reported values of S are lowest in the smaller dolphin species, with the lowest reported value being 0.24 in Sepetiba Bay Guiana dolphins (Sotalia guianensis, Beirao-Campos et al., 2016), and peaks in larger dolphins, particularly killer whales and pilot whales (Globicephala spp.), with values approaching S = 2 (Alves et al., 2013; Wierucka et al., 2014; Esteban et al., 2016a). Australian humpback dolphins (Sousa sahulensis) in Moreton Bay have been reported to have similarly high levels of social differentiation (S = 1.98, Hawkins et al., 2019). We did not find any reported population-level values of S for sperm whales, however, high within (S≈ 1) and between social unit values (S > 1) suggest that this species has similarly high social differentiation as the largest dolphins (Gero et al., 2015). Lying between these two extremes are the mid-sized dolphins, including spotted dolphins (Stenella frontalis, S≈ 0.7), bottlenose dolphins (S≈ 0.8), and most populations of humpback dolphins (Sousa spp., S≈ 1.1), as well as Baird’s beaked whales (Berardius bairdii, S = 0.56, Fedutin et al., 2015). For a complete dataset of average S values for each species and references, see Supplementary Table 1. We also find a great deal of between-population variance within species. In common bottlenose dolphins (T. truncatus), for example, reported values range from 0.29 in Bahia San Antonio (Vermeulen, 2018) to 1.08 in the northern Adriatic Sea (Genov et al., 2019). Some studies have also reported variations within populations between seasons, such as dusky dolphins (Lagenorhynchus obscurus) in Golfo Nuevo, where social differentiation is considerably higher during the winter (Degrati et al., 2018).
Social modularity (abbreviated Q) measures a slightly different aspect of social structure than social differentiation (see Box 1 and Figure 1). Modularity quantifies the degree of subgrouping in the network; values of Q very close to 1 indicate that the network is divided into extremely clear subgroups, while values close to 0 indicate little to no subgrouping (Newman, 2006). Networks with high modularity, by definition, have strong social differentiation, as associations cannot be both random and organized into subgroups, but socially differentiated networks are not necessarily modular (Whitehead, 2008; Figure 1). Because of this inherent correlation, cross-species patterns of social modularity correspond to those of social differentiation: smaller delphinids such as Guiana dolphins generally have lower modularity (Q < 0.3), followed by mid-sized dolphins and beaked whales (0.3 < Q < 0.5), with modularity peaking in the largest dolphins and sperm whales (Q > 0.5) (Supplementary Table 1). As with social differentiation, there is significant variance between population within species, again epitomized by differences across bottlenose dolphin populations.
What drives cross-species variation in modularity and social differentiation? Several hypotheses have been proposed to explain variations in toothed whale social systems, with implications relevant to social network structure. Bräger (1999) and Rendell et al. (2019) both point toward the importance of the mother-calf bond, suggesting that the extent of maternal investment and the need for cooperative care positively correlates with the stability of social groups (and thus the modularity and differentiation of social networks). Gowans et al. (2007) suggested that the predictability and distribution of resources may drive variation in social systems. Species feeding on locally abundant and predictable resources are predicted to have small home ranges, small groups, and fluid relationships (and thus less differentiated and modular networks). Finally, Möller (2011) suggested that the presence of stable social modules in the largest dolphins is a response to the increased threat of male harassment due to the strong sexual size dimorphism in these species.
We will attempt here to use the results from our review to very roughly evaluate these three hypotheses. In interpreting the patterns found in our review, we must again caution that differences in methodology between studies make solid comparisons difficult. In addition, data on individual species do not constitute independent data points, due to likely phylogenetic signal that must be accounted for in robust statistical tests. Nonetheless, the broad patterns between species may be useful for formulating hypotheses to test in future comparative analyses.
From our review, we find patterns that could support each of the three hypotheses outlined above. While the smaller dolphins that exhibit loose networks have calving intervals averaging around 2 years, the large dolphins and sperm whales have intervals in excess of 5 years, indicating greater maternal care (Ferguson and Higdon, 2013). In addition, the species with more modular social networks tend to exhibit greater sexual size dimorphism, with males 20 to 60% larger than females (Dines et al., 2015). Finally, while smaller dolphins tend to have home ranges spanning tens or perhaps hundreds of square kilometres, the species with the largest dolphins and sperm whales have ranges that may span many thousands or tens of thousands of square kilometres (Bräger and Bräger, 2019). Distinguishing which of these mechanisms is most important for determining social structure is difficult, as all three potential drivers are themselves correlated, primarily due to covariance with body size: larger species tend to have large home ranges, more extensive maternal care, and greater sexual dimorphism.
A potentially useful case study may be the social network structure of northern bottlenose whales (Figure 2). Recent evidence suggests that these whales, contrary to previous thought, exhibit prolonged maternal care, comparable to that of sperm and killer whales (Feyrer et al., 2020). Additionally, males are approximately 13% longer than females and have sex-specific weaponry (large melons used for headbutting; Gowans and Rendell, 1999), and thus under Möller’s sociosexual hypothesis males would pose a risk to females. These whales, however, exhibit very different social structure from the matrilineal whales, with undifferentiated relationships between females and weak community structure (Gowans et al., 2001; Whitehead and James, 2015). Aside from social structure, where bottlenose whales apparently differ from the matrilineal toothed whale species is in their ecology and ranging patterns. Northern bottlenose whales feed preferentially on relatively small squid (Gonatus spp., Hooker et al., 2001) within small home ranges (∼ 25 km2, Hooker et al., 2002). This contrast may point toward ecological factors, rather than sociosexual pressures or maternal investment, as a key determinant of toothed whale social modularity and differentiation. The convergence of northern bottlenose whales’ social network structure with that of the smaller coastal dolphins may therefore reflect some fundamental similarities in their ecology, with animals feeding on abundant, predictable resources, despite the extreme differences in their habitat. While instructive, this contrast is far from definitive evidence for a cross-species link between ecology and social network structure, and phylogenetically controlled comparative analyses are needed to address this hypothesis robustly.
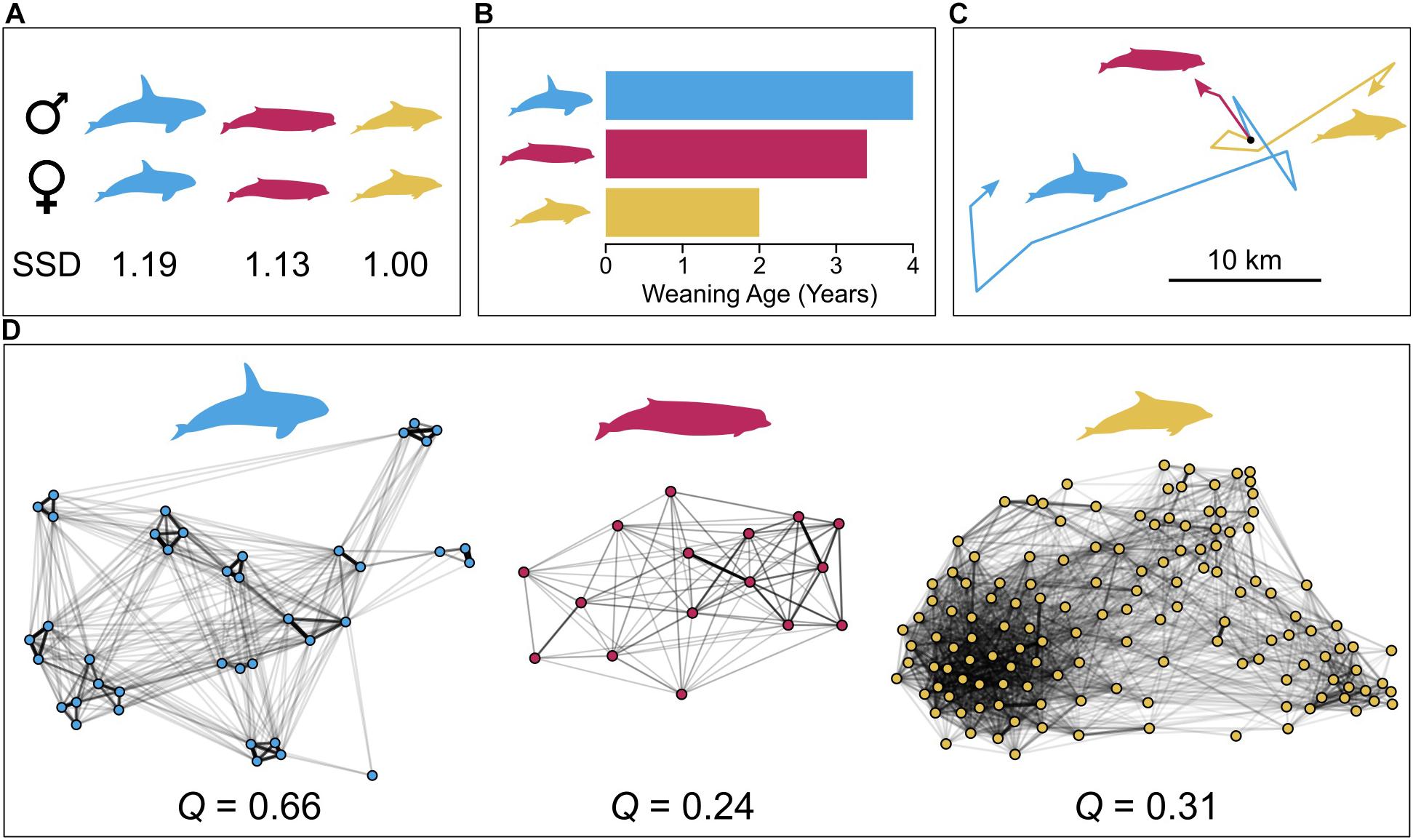
Figure 2. Comparison of network structure and hypothesized drivers in three species of toothed whale, killer whales (blue), northern bottlenose whales (red), and common bottlenose dolphins (yellow). Top panels indicate sexual size dimorphism (SSD) (A), maternal investment, approximated by weaning age (B), and the distribution of resources, as approximated by movement patterns (C). The bottom panel (D) contains plots of group-based half-weight index networks for each species with accompanying modularity estimate. Sexual size dimorphism estimates are the ratio of male to female lengths, and are taken from Dines et al. (2015). Weaning ages are based on stable isotope analysis of dental layers (killer whales: Newsome et al., 2009; bottlenose whales: Feyrer et al., 2020; bottlenose dolphins: Fruet et al., 2015). Movement data are tracks of single individuals obtained from animal-borne devices (radio tag for bottlenose whales, satellite tags for bottlenose dolphins and killer whales) over approximately 24 h, with a common initial point and tracks rotated for clarity. Movement and social data are taken from killer whales at the Prince Edward Islands, bottlenose whales at the Gully, Nova Scotia, and bottlenose dolphins off Georgia, United States. Movement data are replotted from Hooker et al. (2002); Reisinger et al. (2015), and Balmer et al. (2018), and social networks are replotted from Whitehead and James (2015); Reisinger et al. (2017), and Kovacs et al. (2017). Species silhouettes are by Chris Huh, re-used here with alteration under a Creative Commons Share-alike license (https://creativecommons.org/licenses/by-sa/3.0).
Why might the modularity and differentiation of social networks be linked to the distribution of resources in time and space? Sueur et al. (2019) suggested that when food resources are patchily distributed, individuals may limit their associations to kin and dominant individuals as they attempt to monopolize resources through contest competition, resulting in more modular networks. This mechanism could give rise to the pattern we’ve identified here, in which populations apparently feeding on more dispersed, patchy prey (manifesting in their more widespread movement patterns) have more modular, differentiated networks. Observations of cetaceans monopolizing prey patches, however, are sparse, and doing so in a three-dimensional environment is likely challenging. Alternatively, the presence of stable social bonds may relate to a need for cooperation in order to find and exploit large, unpredictable prey patches. Another possibility is that the distribution of resources modulates individuals’ dependence on different forms of social information. Theoretical studies suggest that dependence on social information during foraging is more likely to evolve when resources are unevenly distributed (Smolla et al., 2015), which may promote greater sociality in population relying on patchy resources. In toothed whales, the time-scale over which resources change may be key in modulating what type of social information is most beneficial. For example, in salmon-eating killer whales, the availability and distribution of prey changes over several decades, making vertical transmission of information from older females key (Brent et al., 2015), which may promote the formation of stable groups along maternal lines. In contrast, if the timescale of resource variation is shorter, individuals may rely on horizontal transmission, which may select for less exclusive social relationships as modularity is predicted to slow the spread of social information (Whitehead and Rendell, 2015). These potential mechanisms have yet to be thoroughly tested, and doing so will require new studies on poorly understood species, phylogenetically controlled statistical methods, and novel methods to correct for differences in methodology between studies.
Kinship Structure
While understanding the differentiation of social relationships and the degree of subgrouping gives us a general picture of social network structure, it does not provide any information about which individual and dyadic factors are associated with stronger social bonds or drive community structure. One factor that often drives social relationships in mammals generally (Smith, 2014) and toothed whales specifically (Möller, 2011; Rendell et al., 2019) is relatedness, or kinship. Studying variations in the patterns of kinship between associates is key for understanding the evolution of sociality in this taxon, as relatedness between social partners has profound implications for social evolution (Hamilton, 1964; Kay et al., 2020).
Measuring the correlation between social relationships and kinship requires either pedigrees derived from observed maternities, which take decades to estimate with confidence in long-lived mammals and only provides information about maternal relatedness, or genetic data which are often not available in cetacean populations. Studies of kin structuring in our review were limited to pilot whales (Alves et al., 2013; Van Cise et al., 2017), killer whales (e.g., Esteban et al., 2016a; Reisinger et al., 2017), sperm whales (e.g., Gero et al., 2008; Konrad et al., 2018a), and bottlenose dolphins (e.g., Louis et al., 2018; Diaz-Aguirre et al., 2019; Foroughirad et al., 2019). The lack of studies on smaller dolphins and beaked whales means our picture here is incomplete, and our knowledge is clearly taxonomically biased toward species with stable social units.
In the large dolphins, genetic studies and long-term observation suggest that social units are mixed sex groups of maternal kin (Pilot et al., 2010; Alves et al., 2013). In addition to determining social unit membership, kinship may determine associations between units in these populations. In pilot whales, between unit association rates correlate with genetic similarity (Alves et al., 2013). In killer whales, results are mixed with respect to the role of kinship in shaping between-unit social relationships, with some populations organized into semi-stable pods composed of genetically related matrilineal social units (Parsons et al., 2009; Pilot et al., 2010), while in other populations there does not appear to be a correlation between kinship and association between social units (Deecke et al., 2010; Reisinger et al., 2017).
Sperm whales, like the large dolphins, have primarily matrilineal societies. Unlike these species, however, sperm whale males disperse at maturity (Whitehead, 2003), and social units may contain multiple matrilines (Richard et al., 1996). Variations in kinship drive social association rates within units (Gero et al., 2008), however, kinship between units does not appear to predict cross-unit affiliation patterns (Konrad et al., 2018a).
In Indo-Pacific bottlenose dolphins, where males tend to form stable alliances, most studies have found that associations between females are structured according to kinship, however, bonds between males do not appear to be kin structured (Möller et al., 2001, 2006; Wiszniewski et al., 2010, 2012; Chabanne et al., 2017; Foroughirad et al., 2019). Studies on bottlenose dolphins in Coffin Bay, Australia (of the contested species T. australis), in contrast, suggest that male alliances are kin-biased, and the network is generally structured by genetic relatedness (Diaz-Aguirre et al., 2018, 2019). In our review, studies of common bottlenose dolphins almost universally found no correlation between genetic relatedness and association rates (Louis et al., 2018; Nykanen et al., 2018; Machado et al., 2019), with the exception of one study of male alliances in the Bahamas (Parsons et al., 2003).
In summary, it appears that kin-biased associations between adult males are rare in toothed whale social networks, perhaps only present in the largest dolphins and a few populations of bottlenose dolphins. In contrast, bonds between female kin are a fundamental aspect of many toothed whale societies. Rendell et al. (2019) suggested that maternal kinship structure is an important driver of modular social structure in cetaceans, with stronger maternal kin structure associated with greater modularity. While we do not have data on enough species to evaluate this hypothesis robustly, it allows us to make some predictions. If social modularity and kinship structure are inherently linked in cetaceans, we should expect to find strong kinship structuring in the social networks of highly modular systems, such as Australian humpback dolphins in Moreton Bay (Hawkins et al., 2019), and we expect to find little or no kinship structure in the beaked whales and small dolphins.
Sexual Segregation
In animal population, the sexes often differ in their nutritional needs and predation risk, and are under fundamentally different pressures. This often leads to the sexes segregating, either spatially or socially (Ruckstuhl, 2007). In our review, studies have reported social segregation between the sexes in both species of bottlenose dolphins (e.g., Kent et al., 2008; Mann et al., 2012), Australian humpback dolphins (Hawkins et al., 2019; Hunt et al., 2019), Risso’s dolphins (Hartman et al., 2007), Atlantic spotted dolphins (e.g., Herzing et al., 2017; Danaher-Garcia et al., 2019), and northern bottlenose whales (Gowans et al., 2001). In contrast, studies have found no evidence for sexual segregation in the association networks of killer whales (Baird and Whitehead, 2000; Williams and Lusseau, 2006; Tavares et al., 2017), pilot whales (Augusto et al., 2017), and spinner dolphins (Karczmarski et al., 2005). In addition, while not addressed directly by studies in our review, sperm whales are known to exhibit extreme spatial segregation between males and females (Whitehead, 2003). This has likely not been addressed directly using social network methods due to the extreme degree of segregation in this species meaning that individualized relationships need not be measured to identify sexual segregation. Importantly, in bottlenose dolphins, there appears to be intraspecific variation, with some populations showing no sexual segregation (Baker et al., 2018; Louis et al., 2018).
In terrestrial ungulates, sexual segregation is thought to be linked to sex differences in body size and weaponry leading to different energetic requirements and predation risk (Ruckstuhl and Neuhaus, 2002). This does not appear to be the case in toothed whales; while the most sexually segregated species, the sperm whale, does also have the most extreme sexual size dimorphism, the highly sexually dimorphic killer and pilot whales show no segregation, and many of the other sexually segregated species show almost no dimorphism. Instead, patterns of sexual segregation appear to be linked to species’ mating systems. Among the species that sexually segregate, there is evidence that males engage in direct contests when competing for females (Kato, 1984; Dines et al., 2015; Allen et al., 2017; Volker and Herzing, 2021), and coercion of females by groups of males has been observed in some of these species, particularly spotted dolphins (Herzing, 1996) and Indo-Pacific bottlenose dolphins (Connor and Krützen, 2015). With the notable exception of sperm whales, males in these sexually segregated species tend to have more stable intrasexual social bonds than females. It therefore seems likely that sexual segregation in these systems is driven by females avoiding harassment from males, while males form social bonds with each other in order to cooperatively monopolize females. Studies within bottlenose dolphin populations support this mechanism, suggesting that male social bonds are associated with variation in reproductive success (see below), and that sexual segregation is primarily driven by females avoiding males (Galezo et al., 2017).
Interesting variations and exceptions to this pattern are found in sperm whales and Risso’s dolphins. Sperm whales are highly sexually segregated generally, however, males regularly associate with units of females and offspring in mating grounds. While associated with females, males behave similarly to other group members, and do not exhibit aggression toward females or calves (Whitehead, 1993). It may be that the stable social units of females make coercion impossible, promoting alternative strategies. There is however, evidence that sperm whale males engage in aggressive contests (Kato, 1984), and relatively small testes suggest that males are able to monopolize access to females (Dines et al., 2015), however, direct observation of males defending groups of females from competitors are lacking. Importantly, the segregation between male and female sperm whales is not strictly social; for most of the year males inhabit higher latitudes than females, and males inhabit a different ecological niche than females (Whitehead, 2003). In Risso’s dolphins, males form stable long-term groups, while females exhibit fission-fusion sociality, a social structure with some similarities to those of some bottlenose dolphins (Hartman et al., 2007). However, anatomical evidence suggests strong post-copulatory competition, and therefore a lack of monopolisation of females (Dines et al., 2015). It is unclear, then, whether the stable male-male bonds of Risso’s dolphins provide benefits in terms of mating access or are instead useful for increasing foraging success or predator defense (Hartman et al., 2007).
Behavioural Assortment
Social bonds existing primarily between phenotypically similar individuals (“homophily”) is a common trait of social networks in humans (McPherson et al., 2001) and other animals (Croft et al., 2009). In toothed whales, several studies have found evidence that individuals preferentially associate with individuals that exhibit similar behaviours, such as foraging strategies and vocal repetoires. Hunting behaviour, particularly with respect to strategies that rely on human fisheries and aquaculture, have been of particular focus in social network studies.
Bottlenose dolphins often exhibit human-associated foraging, either in an opportunistic or cooperative context. In our systematic review, several studies report that bottlenose dolphins interacting with fisheries and aquaculture preferentially associate with each other (Chilvers and Corkeron, 2001; Kovacs et al., 2017; Genov et al., 2019; Machado et al., 2019; Methion and Diaz Lopez, 2020). This pattern is also present in Strait of Gibraltar killer whales, where social community structure perfectly correlates with degree of interaction with the local tuna fishery (Esteban et al., 2016a). Other evidence for assortment by foraging phenotype comes from stable isotope analysis. Studies in pilot whales, killer whales, and bottlenose dolphins have found that individuals with more similar stable isotope profiles, and thus likely have similar prey choice, have higher rates of association (de Stephanis et al., 2008; Esteban et al., 2016b; Louis et al., 2018).
An important caveat to these findings is that they are correlative, and the direction of causality, or even whether the causality is direct, is unclear. Correlations between behavioural similarity and association strength could be the result of social transmission of behaviour (see below). Associations and behavioural similarity could also be driven by a common underlying factor, such as kinship or space use (although many studies account for these confounds).
A study conducted on Moreton Bay bottlenose dolphins provides perhaps the strongest evidence that behavioural homophily can (directly or indirectly) drive social structure in toothed whales. In this population, a subset of individuals regularly interacted with the trawler fishery, and this set of individuals were socially segregated from non-interacting individuals (Chilvers and Corkeron, 2001). When the trawler fishery was reduced, the population’s social network became less differentiated and less modular, suggesting that interaction with the fishery drove the initial population split (Ansmann et al., 2012).
It is unclear if similar patterns are present in other species. In pilot whales and killer whales, social learning within maternal lineages may be a more parsimonious explanation for correlations between behaviour and association, however, some degree of behavioural homophily, perhaps based on acoustic cues, may be present.
Social Network Centrality
So far, we have focused on global aspects of social network structure. Also important, however, are individuals’ positions within their network. In social network analysis, the term “centrality” can have many meanings depending on the specific research question, but generally refers to how well connected or embedded individuals are in a social system, either directly or indirectly. Some centrality measures have clear parallels to individual behavioural phenotypes. For example, strength centrality (the sum of an individual’s connections) in association networks can be directly related to their typical group size, and thus their gregariousness (Whitehead, 2008). Other measures, particularly those that quantify an individual’s position within the broader network, are less clearly linked to individual behaviour, but can also be driven by relatively simple behavioural differences (Firth et al., 2017). Regardless of the behavioural substrate underlying variations in social position, social network centrality is often a key driver of individual fitness in social species (see below). In addition, variations in centrality between different age and sex classes can provide clues about the function of sociality in these species.
The correlates of social centrality are less well understood in toothed whales than global aspects of social network structure, but have been examined in several species. In our review, 25% of studies performed analyses of individual centrality, however, only 9% of studies investigated the relationship between network centrality and individual characteristics (such as age, sex, and behaviour).
One of the most commonly investigated correlates of centrality is sex. In Indo-Pacific bottlenose dolphins, spotted dolphins, and Hector’s dolphins, males tend to be more socially central than females (Slooten et al., 1993; Mann et al., 2012; Danaher-Garcia et al., 2019). In contrast, in common bottlenose dolphins and Australian humpback dolphins, females have been found to be more central, even if male-male bonds tend to be more stable than female-female bonds (Baker et al., 2018; Hawkins et al., 2019; Hunt et al., 2019). Age has also been found to be an important factor in shaping social centrality. In sperm whale social units, calves are the most central individuals, likely reflecting the function of social units in cooperative care (Gero et al., 2013). In both killer whales (Williams and Lusseau, 2006) and Indo Pacific bottlenose dolphins (Stanton et al., 2011), young individuals appear to be more socially explorative, having many social connections to diverse individuals that may not be connected to one another. In some populations of bottlenose dolphins, however, young individuals are less central in the networks (Louis et al., 2018). Reproductive state may also be an important determinant of social position; in both sperm whales and Indo Pacific bottlenose dolphins, females with infant offspring are more socially central than other females (Gero et al., 2013; Nishita et al., 2017). This suggests that social relationships may be partially driven by cooperative care in these systems.
Individual behavioural phenotypes can also play a role in determining centrality. Two studies have found correlations between centrality and interactions with human aquaculture and fisheries in bottlenose dolphins, however, the reported effects are in different directions (Pace et al., 2012; Methion and Diaz Lopez, 2020). In addition, levels of pollutants, likely reflecting differences in foraging and habitat use, correlate with centrality in bottlenose dolphins in the Indian River Lagoon (Titcomb et al., 2017).
The few studies of centrality in toothed whales, and the different methods used, precludes any robust interpretation of the drivers of centrality in a comparative context. There is no clear relationship between sexual segregation or mating systems and sex differences in centrality. While males apparently form stable bonds that are useful for gaining access to females in Indo-Pacific bottlenose dolphins (Connor et al., 2001) and potentially in Australian humpback dolphins (Allen et al., 2017) and Atlantic spotted dolphins (Elliser and Herzing, 2014), these three species do not show consistent effects of sex on network centrality. Young individuals apparently being socially explorative in killer whales and bottlenose dolphins resembles results in some terrestrial taxa (e.g., Goldenberg et al., 2016), and may reflect a strategy to establish important social bonds early in life. We recommend that more studies investigate the correlates of centrality in toothed whale social networks, particularly in terms of the relationship between centrality, age, and sex, and how these might relate to life history characteristics and mating systems.
Consequences of Social Network Structure
As discussed above, social network structure is predicted to have important implications for evolutionary and ecological processes, including variations in individual fitness (Snyder-Mackler et al., 2020), the spread of infectious disease (Craft, 2015), and the emergence of culture in animal populations (Cantor and Whitehead, 2013). Here, we’ll review the current state of our understanding of how these processes are influenced by social network structure in toothed whales.
Correlates of Survival and Reproduction
Social connections are vital for survival and health in humans and other social animals (Snyder-Mackler et al., 2020). Some of the key studies elucidating the link between social network structure and components of fitness have been conducted in toothed whale populations, particularly killer whales and bottlenose dolphins. There are multiple studies correlating survival with social network structure in these two species. In Indo-Pacific bottlenose dolphins, young males that are more central in their association networks are more likely to survive to adulthood, possibly due to increased protection from harassment by older males (Stanton and Mann, 2012). In the heavily studied southern resident killer whale population, both direct and indirect centrality within social communities correlated with increased survival in male, but not female, killer whales (Ellis et al., 2017). This effect was particularly important in years of low salmon, suggesting that social network position modulates individuals’ access to resources, either through providing food sharing opportunities or increasing access to social information. Similar results have been reported in sub-Antarctic killer whales (Busson et al., 2019).
Effects of social network centrality on reproduction are less well understood, with no study in our systematic review directly investigating this relationship. Some evidence for social centrality determining reproductive success comes from Shark Bay bottlenose dolphins, where males in larger and more stable alliances have greater mating success (Connor et al., 2001). Another study, which did not appear in our literature search, found that females in this population have correlated reproductive success with their social partners (Frère et al., 2010), however, this does not indicate whether more socially central females have enhanced fecundity or calf survival.
Social Information and Culture
Social learning, and the group specific, stable behavioural traditions that can emerge from it (“culture”) has been increasingly recognized as an important aspect of animal ecology. While culture was long considered to be a human-specific phenomenon, it has become clear that social transmission of information and behaviour, often resulting in multi-generation traditions, are likely present in many non-human animals (Schuppli and van Schaik, 2019). Much of the data fueling the early non-human culture debate was derived from studies of toothed whales. These species have a well-documented penchant for social learning, and the presence of group specific foraging tactics and vocal traditions in wild populations suggests that social learning is an important contributor to behavioural diversity in these species (Whitehead and Rendell, 2015).
Social network structure defines the opportunities that individuals have for social learning, and therefore is predicted to correlate with the occurrence, spread, and diversity of socially learned behaviours (Cantor and Whitehead, 2013). In toothed whales, correlations between behavioural similarity and association strength have often been used to test for the presence of cultural processes, particularly with respect to the acoustic repertoires of killer whales and sperm whales. In these species, results are mixed. While vocal similarity between matrilines correlates with association strength in killer whales, suggesting horizontal transmission (Deecke et al., 2010), there is no apparent correlation between association strength and vocal similarity within sperm whale clans (Konrad et al., 2018b).
Other evidence comes from analysis of foraging behaviour. Several species of toothed whale exhibit group or population specific foraging behaviours that are thought to be the result of cultural transmission, with perhaps the most notable example being the highly specialized foraging strategies found in killer whale populations (Riesch et al., 2012). Social network studies have born out the likelihood that foraging strategies are socially learned in several species. Similarity in foraging behaviour and stable isotope profiles correlate with association strength in pilot whales, killer whales, and bottlenose dolphins (de Stephanis et al., 2008; Esteban et al., 2016b; Louis et al., 2018). However, as discussed above, these results could be the result of either social learning or behavioural homophily. More solid evidence of social transmission within toothed whale social networks has been gained from diffusion modelling of novel foraging techniques in Shark Bay bottlenose dolphins. The “sponging” behaviour, where individuals utilize marine sponges to protect their rostrum while bottom feeding, has been found to socially spread along maternal lines (Wild et al., 2019). In contrast, the “shelling” behaviour, where individuals catch fish by chasing them into a shell before raising the shell to the surface, spreads horizontally between associates (Wild et al., 2020).
One of the key hypotheses linking social structure and social transmission is that more structured (i.e., modular and differentiated) social networks should have slower rates of social transmission and generate greater behavioural diversity than relatively random networks (Cantor and Whitehead, 2013). We found no studies investigating this hypothesis empirically in toothed whales, and we are unaware of any study investigating this question in natural systems. This question may be of particular interest in future comparative studies, perhaps using indices of diversity in foraging behaviour (such as isotopic niche widths) or vocal repertoires.
Disease Transmission
As in the case of information transmission, social network structure is a major factor shaping the pattern of disease transmission in animal populations, as social networks represent potential disease transmission pathways (Craft, 2015). Understanding disease transmission risk is toothed whale populations is crucial for evaluating the relative risk to populations and potentially informing strategies to manage outbreaks. Several unusual mortality events in toothed whale populations have been attributed to disease outbreaks, and the risk of these outbreaks may be increasing as oceans grow warmer (Sanderson and Alexander, 2020).
In our review, only three studies explicitly addressed the transmission of diseases in toothed whale social networks. Guimaraes Paulo et al. (2007) modelled the spread of a hypothetical pathogen over the social network of mammal eating killer whales, finding that the network was more vulnerable than random networks. The remaining two studies both analysed the impacts of observed disease outbreaks in relationship to social structure. Wierucka et al. (2014) found that increased mortality in Mediterranean long-finned pilot whales following a morbillivirus epizootic was limited to two social clusters, suggesting that sociality shaped the transmission of this disease. Similarily, Felix et al. (2019) found that the occurrence of lobomycosis-disease was linked to the structure of social communities in bottlenose dolphins. Interestingly, neither of these studies directly analysed the specific transmission pathway thought to be involved in the spread of these diseases (respiratory transmission and physical contact, respectively), but still found an influence of association network structure. This suggests that, at least in some cases, broad-scale associations can be a useful proxy for actual disease transmission pathways in toothed whales.
Future Research Directions
The application of social networks to animals has expanded greatly over the last 2 decades, and has been used to answer numerous biological questions (Webber and Vander Wal, 2019). In sync with this explosion of social network research, studies on toothed whale sociality has provided additional insight into the ecological and evolutionary forces shaping social structure (Rendell et al., 2019). Our review highlights some clear gaps in our current knowledge on toothed whale social networks, as well as some exciting opportunities for future research.
Most obviously, our review highlights severe taxonomic bias toward three genera, Tursiops, Orcinus, and Physeter. In particular, the majority of the studies in our review concerned bottlenose dolphins. Bottlenose dolphins are widespread, many populations inhabit coastal areas that are relatively easily accessible, and their relatively small home ranges of individuals mean that individuals can be reliably re-located, making them attractive research subjects. In addition, the presence of several forms of cooperation, social transmission, and multilevel alliances make this genus theoretically interesting. However, studies of a wider array of species will be important for understanding the origins and diversity of social structure in this taxon. In particular, further research on beaked whales, open ocean dolphins, river dolphins, beluga, and narwhals will faciliate more extensive comparative work to uncover the drivers and consequences of cetacean social structure. Studying these animals’ social systems comes with significant challenges. Many of them inhabit remote or challenging habitats, such as the open ocean, the high Arctic, and complex river systems. In the open ocean dolphins, the sheer size of groups makes identifying a reasonable portion of group members difficult, and the lack of dorsal fins in Monodonts and river dolphins makes traditional photo ID challenging. Developments in research technology, such as machine learning for individual identification (Kierdorf et al., 2020) and unoccupied aerial systems for observing submerged individuals and markings typically not visible from the surface (Torres et al., 2018) may begin to unravel the structure of these species’ social networks.
Our literature search also demonstrated a lack of studies investigating the consequences of social network centrality on reproductive success in toothed whales. Given the central role of cooperative calf care in many hypotheses about the evolution of social structure in this taxon, understanding how sociality influences female reproductive success is crucial. We would predict that, in the species where cooperative care is thought to be important, females with greater social centrality will have greater reproductive success due to increased calf survival.
As we have discussed throughout our review, the growing body of work in toothed whales has begun to provide the necessary data for comparative studies to investigate the drivers and consequences of social network structure. Such studies will require not only social metrics from a large number of species derived using the same methods, but will need to correct for effects of sampling intensity and network size. In addition, the observed features of social networks have inherent uncertainty (Lusseau et al., 2008), which will need to be incorporated into any such analyses. We recommend that all descriptive studies of toothed whale social systems report standard errors for global network metrics to allow for principled comparisons.
While this body of work studying association networks is undoubtedly valuable, it may be necessary for studies of toothed whales to begin to move past the gambit of the group and study social interactions themselves. The development of research technologies such as animal borne devices may again prove useful for these studies. Recent studies have demonstrated the usefulness of unoccupied aerial systems to observe sociality in greater detail in odontocetes (Hartman et al., 2020), and continued development of these methods have the potential to greatly expand our understanding of these systems.
Finally, there is an opportunity to incorporate social network structure more fully into the conservation of toothed whales. The application of social network theory to conservation problems generally has the potential to improve outcomes in endangered populations (Snijders et al., 2017), and social structure has been proposed as a key determinant of population dynamics in cetaceans (Wade et al., 2012). Several studies have utilized social networks to help define management units (Alves et al., 2013; Wang et al., 2015; Esteban et al., 2016b) or to better understand populations’ response to mortality and removal events (Williams and Lusseau, 2006; Herzing et al., 2017; Busson et al., 2019), however, we feel there are additional roles for social network analysis in conservation generally, and in toothed whales specifically. In many toothed whale populations, one large source of mortality is fisheries bycatch, which can simultaneously and suddenly remove entire social units. The effect of these removals on the stability of population-level social network structure, and the consequences of possible social disruption to population function, may be a vital area for further research.
Some studies have found that social network dynamics share common drivers with population dynamics (Parsons et al., 2009; Foster et al., 2012a; Herzing et al., 2017; Busson et al., 2019). This suggests that social network dynamics may serve as a useful behavioural indicator of population health. In other species, changes in behaviour have been used to detect novel stressors (e.g., Caro, 2005), indicate the success of management actions (e.g., Al-Shaer et al., 2018), and predict future population growth (van Gils et al., 2009). Given the apparently widespread link between ecological variables and social network dynamics in toothed whales, and the relative ease with which social network measures can be derived from photographic identification data, the application of social networks as a behavioural indicator in these populations bears further investigation.
In addition, social networks could help managers understand disease risk and target vaccinations. While our systematic review found only three studies explicitly linking social networks and disease, more recent work has expanded the application of social networks to disease management, by estimating age and sex specific risk (Leu et al., 2020), explicitly modelling the spread of specific pathogens along with possible vaccination strategies (Weiss et al., 2020), and using randomisation procedures to determine the relevance of association networks to observed disease outbreaks (Powell et al., 2020). Further work determining the impact of social network structure on population-level disease risk in a comparative context could further inform conservation efforts.
Disease mitigation is only one aspect of what Snijders et al. (2017) refer to as “relationship-based management strategies.” Understanding the relationships between individuals in threatened populations can additionally help maintain animal welfare, and potentially aid in predicting which animals are experiencing greater mortality risk. For example, in resident killer whales, the death of an individuals’ mother or grandmother increases their mortality risk, likely due to the loss of social benefits such as information and food sharing (Foster et al., 2012b; Nattrass et al., 2019). Social network methods may help identify other important social partners, the removal of which might cause increased stress or mortality.
Conclusion
The application of social network methods to free-ranging odontocetes has revealed a great diversity of social structures, and has elucidated some of the drivers and consequences of sociality in this taxon. Our review highlights both the vast body of knowledge generated through the applications of social network analysis to these interesting species, and the great potential of these methods for further study. We have attempted to summarize the current state of our knowledge, but as this is a young field, there is still a great deal of uncertainty, and some of the results we discuss here may be further confirmed or refuted by further study. Future research focusing on applying new methods, studying less well understood species, and applying this knowledge directly to conservation problems may provide important components of continued efforts to understand and conserve toothed whale populations worldwide.
Data Availability Statement
The database of studies generated for this study can be found in the Supplementary Material.
Author Contributions
MW conceived of the project and carried out the systematic review, with input from DC. MW wrote the initial draft of the manuscript, which was then developed with input from all authors. All authors contributed to the article and approved the submitted version.
Funding
This work was supported by a UK Natural Environmental Research Council Grant (NE/S010327/1) awarded to DC. SE was funded by a Leverhulme Early Career Research Fellowship.
Conflict of Interest
The authors declare that the research was conducted in the absence of any commercial or financial relationships that could be construed as a potential conflict of interest.
Acknowledgments
We thank our colleagues at the Centre for Research in Animal Behaviour, particularly the CRAB Social Network Club, for discussion and comments that helped shape this manuscript.
Supplementary Material
The Supplementary Material for this article can be found online at: https://www.frontiersin.org/articles/10.3389/fmars.2021.688842/full#supplementary-material
References
Allen, S. J., King, S. L., Krützen, M., and Brown, A. M. (2017). Multi-modal sexual displays in Australian humpback dolphins. Sci. Rep. 7:13644.
Al-Shaer, L., Bloch, A., Little, K., and Itzkowitz, M. (2018). Monitoring social behaviour as an assessment of translocation success in a reintroduced population of the endangered Leon Springs pupfish (Cyprinodon bovis). Aqu. Conserv. Mar. Freshw. Ecosyst. 28, 559–566. doi: 10.1002/aqc.2889
Alves, F., Querouil, S., Dinis, A., Nicolau, C., Ribeiro, C., Freitas, L., et al. (2013). Population structure of short-finned pilot whales in the oceanic archipelago of Madeira based on photo-identification and genetic analyses: implications for conservation. Aqu. Conserv. 23, 758–776.
Ansmann, I. C., Parra, G. J., Chilvers, B. L., and Lanyon, J. M. (2012). Dolphins restructure social system after reduction of commercial fisheries. Anim. Behav. 84, 575–581. doi: 10.1016/j.anbehav.2012.06.009
Augusto, J. F., Frasier, T. R., and Whitehead, H. (2017). Social structure of long-finned pilot whales (Globicephala melas) off northern Cape Breton Island, Nova Scotia. Behaviour 154, 509–540. doi: 10.1163/1568539x-00003432
Baird, R. W., and Whitehead, H. (2000). Social organization of mammal-eating killer whales: group stability and dispersal patterns. Can. J. Zool. 78, 2096–2105. doi: 10.1139/z00-155
Baker, I., O’Brien, J., McHugh, K., Ingram, S. N., and Berrow, S. (2018). Bottlenose dolphin (Tursiops truncatus) social structure in the Shannon Estuary, Ireland, is distinguished by age- and area-related associations. Mar. Mammal Sci. 34, 458–487. doi: 10.1111/mms.12462
Balmer, B., Zolman, E., Rowles, T., Smith, C., Townsend, F., Fauquier, D., et al. (2018). Ranging patterns, spatial overlap, and association with dolphin morbillivirus exposure in common bottlenose dolphins (Tursiops truncatus) along the Georgia, USA coast. Ecol. Evol. 8, 12890–12904. doi: 10.1002/ece3.4727
Beirao-Campos, L., Cantor, M., Flach, L., and Simoes-Lopes, P. C. (2016). Guiana dolphins form social modules in a large population with high ranging overlap and small demographic changes. Behav. Ecol. Sociobiol. 70, 1821–1830. doi: 10.1007/s00265-016-2188-x
Bräger, S. (1999). Association patterns in three populations of Hector’s Dolphin, Cephalorhynchus hectori. Can. J. Zool. 77, 13–18. doi: 10.1139/cjz-77-1-13
Bräger, S., and Bräger, Z. (2019). “Movement patterns of Odontocetes through space and time,” in Ethology and Behavioural Ecology of Odontocetes, ed. B. Würsig (Cham: Springer), 117–144. doi: 10.1007/978-3-030-16663-2_6
Brent, L. J., Lehmann, J., and Ramos-Fernandez, G. (2011). Social network analysis in the study of nonhuman primates: a historical perspective. Am. J. Primatol. 73, 720–730. doi: 10.1002/ajp.20949
Brent, L. J. N., Franks, D. W., Foster, E. A., Balcomb, K. C., Cant, M. A., and Croft, D. P. (2015). Ecological knowledge, leadership, and the evolution of menopause in killer whales. Curr. Biol. 25, 746–750. doi: 10.1016/j.cub.2015.01.037
Busson, M., Authier, M., Barbraud, B., Tixier, P., Reisinger, R. R., Janc, A., et al. (2019). Role of sociality in the response of killer whales to an additive mortality event. Proc. Natl. Acad. Sci. U.S.A. 116, 11812–11817.
Cantor, M., and Whitehead, H. (2013). The interplay between social networks and culture: theoretically and among whales and dolphins. Philos. Trans. R. Soc. B Biol. Sci. 368:20120340. doi: 10.1098/rstb.2012.0340
Caro, T. M. (2005). Behavioural indicators of exploitation. Ethol. Ecol. Evol. 17, 189–194. doi: 10.1080/08927014.2005.9522608
Castles, M., Heinsohn, R., Marshall, H. H., Lee, A. E. G., Cowlisha, G., and Carter, A. J. (2014). Social networks created with different techniques are not comparable. Anim. Behav. 96, 59–67. doi: 10.1016/j.anbehav.2014.07.023
Chabanne, D. B. H., Finn, H., and Bejder, L. (2017). Identifying the relevant local population for environmental impact assessments of mobile marine fauna. Front. Mar. Sci. 4:148. doi: 10.3389/fmars.2017.00148
Chilvers, B. L., and Corkeron, P. J. (2001). Trawling and bottlenose dolphins’ social structure. Proc. R. Soc. B Biol. Sci. 268, 1901–1905. doi: 10.1098/rspb.2001.1732
Connor, R. C., Heithaus, M. R., and Barre, L. M. (2001). Complex social structure, alliance stability and mating access in a bottlenose dolphin “super-alliance”. Proc. R. Soc. B Biol. Sci. 268, 263–267. doi: 10.1098/rspb.2000.1357
Connor, R. C., and Krützen, M. (2015). Male dolphin alliances in Shark Bay: changing perspectives in a 30-year study. Anim. Behav. 103, 223–235. doi: 10.1016/j.anbehav.2015.02.019
Connor, R. C., Mann, J., Tyack, P. L., and Whitehead, H. (1998). Social evolution in toothed whales. Trends Ecol. Evol. 13, 228–232. doi: 10.1016/s0169-5347(98)01326-3
Craft, M. E. (2015). Infectious disease transmission and contact networks in wildlife and livestock. Philos. Trans. R. Soc. B Biol. Sci. 370, 20140107. doi: 10.1098/rstb.2014.0107
Croft, D. P., James, R., and Krause, J. (2008). Exploring Animal Social Networks. Princeton, NJ: Princeton University Press.
Croft, D. P., Krause, J., Darden, S. K., Ramnarine, I. W., Faria, J. J., and James, R. (2009). Behavioural trait assortment in a social network: patterns and implications. Behav. Ecol. Sociobiol. 63, 1495–1503. doi: 10.1007/s00265-009-0802-x
Danaher-Garcia, N. A., Melillo-Sweeting, K., and Dudzinski, K. M. (2019). Social structure of Atlantic spotted dolphins (Stenella frontalis) off Bimini, The Bahamas (2003-2016): alternate reasons for preferential association in delphinids. Acta Ethol. 23, 9–21. doi: 10.1007/s10211-019-00329-3
de Stephanis, R., Garcia-Tiscar, S., Verborgh, P., Esteban-Pavo, R., Perez, S., Minvielle-Sebastia, L., et al. (2008). Diet of social groups of long-finned pilot whales (Globicephala melas) in the Strait of Gibraltar. Mar. Biol. 154, 603–612. doi: 10.1007/s00227-008-0953-8
Deecke, V. B., Barrett-Lennard, L. G., Spong, P., and Ford, J. K. B. (2010). The structure of stereotyped calls reflects kinship and social affiliation in resident killer whales (Orcinus orca). Naturwissenchaften 97, 513–518. doi: 10.1007/s00114-010-0657-z
Degrati, M., Coscarella, M. A., Crespo, E. A., and Dans, S. L. (2018). Dusky dolphin group dynamics and association patterns in Peninsula Valdes. Argentina. Mar. Mammal Sci. 35, 416–433. doi: 10.1111/mms.12536
Diaz-Aguirre, F., Parra, G. J., Passadore, C., and Möller, L. (2018). Kinship influences social bonds among male southern Australian bottlenose dolphins (Tursiops cf. australis). Behav. Ecol. Sociobiol. 72:190.
Diaz-Aguirre, F., Parra, G. J., Passadore, C., and Möller, L. (2019). Genetic relatedness delineates the social structure of southern Australian bottlenose dolphins. Behav. Ecol. 30, 948–959. doi: 10.1093/beheco/arz033
Dines, J. P., Mesnick, S. L., Ralls, K., May-Collado, L., Agnarsson, I., and Dean, M. D. (2015). A trade-off between precopulatory and postcopulatory trait investment in male cetaceans. Evolution 69, 1560–1572. doi: 10.1111/evo.12676
Elliser, C. R., and Herzing, D. L. (2014). Long-term social structure of a resident community of Atlantic spotted dolphins, Stenella Frontalis, in the Bahamas 1991–2002. Mar. Mammal Sci. 30, 308–328.
Ellis, S., Franks, D. W., Nattrass, S., Cant, M. A., Weiss, M. N., Giles, D., et al. (2017). Mortality risk and social network position in resident killer whales: sex differences and the importance of resource abundance. Proc. R. Soc. B 284:20171313. doi: 10.1098/rspb.2017.1313
Esteban, R., Verborgh, P., Gauffier, P., Gimenez, J., Foote, A. D., and de Stephanis, R. (2016a). Maternal kinship and fisheries interactions influence killer whale social structure. Behav. Ecol. Sociobiol. 70, 111–122. doi: 10.1007/s00265-015-2029-3
Esteban, R., Verborgh, P., Gauffier, P., Gimenez, J., Martin, V., Perez-Gil, M., et al. (2016b). Using a multi-disciplinary approach to identify a critically endangered killer whale management unit. Ecol. Indicat. 66, 291–300. doi: 10.1016/j.ecolind.2016.01.043
Farine, D., and Whitehead, H. (2015). Constructing, conducting, and interpreting animal social network analysis. J. Anim. Ecol. 84, 1144–1163.
Fedutin, I. D., Filatova, O. A., Mamaev, E. G., Burdin, A. M., and Hoyt, E. (2015). Occurrence and social structure of Baird’s beaked whales, Berardius bairdii, in the Commander Islands, Russia. Mar. Mammal Sci. 31, 853–865. doi: 10.1111/mms.12204
Felix, F., Van Bressem, M.-F., and Van Waerebeek, K. (2019). Role of social behaviour in the epidemiology of lobomycosis- like disease (LLD) in estuarine common bottlenose dolphins from Ecuador. Dis. Aqu. Organ. 134, 75–87. doi: 10.3354/dao03356
Ferguson, S. H., and Higdon, J. W. (2013). Grouping world cetaceans according to life-history characteristics indicates two divergent strategies. Can. Wildlife Biol. Manag. 2, 51–66.
Feyrer, L. J., Zhao, S., Whitehead, H., and Mattews, C. J. D. (2020). Prolonged maternal investment in northern bottlenose whales alters our understanding of beaked whale reproductive life history. PLoS One 15:e0235114. doi: 10.1371/journal.pone.0235114
Firth, J. A., Sheldon, B. C., and Brent, L. J. (2017). Indirectly connected: simple social differences can explain the causes and apparent consequences of complex social network positions. Proc. R. Soc. B Biol. Sci. 284:20171939.
Foroughirad, V., Levengood, A. L., Mann, J., and Frere, C. H. (2019). Quality and quantity of genetic relatedness data affect the analysis of social structure. Mol. Ecol. Resour. 19, 1181–1194. doi: 10.1111/1755-0998.13028
Foster, E. A., Franks, D. W., Mazzi, S., Darden, S. K., Balcomb, K. C., Ford, J. K., et al. (2012b). Adaptive prolonged postreproductive life span in killer whales. Science 337, 1313–1313. doi: 10.1126/science.1224198
Foster, E. A., Franks, D. W., Morrell, L. J., Balcomb, K. C., Parsons, K. M., van Ginneken, A., et al. (2012a). Social network correlates of food availability in an endangered population of killer whales, Orcinus orca. Anim. Behav. 83, 731–736. doi: 10.1016/j.anbehav.2011.12.021
Frère, C. H., Krützen, M., Mann, J., Connor, R. C., Bejder, L., and Sherwin, W. B. (2010). Social and genetic interactions drive fitness variation in a free-living dolphin population. Proc. Natl. Acad. Sci. U.S.A. 107, 19949–19954. doi: 10.1073/pnas.1007997107
Fruet, P. F., Genoves, R. C., Möller, L. M., Botta, S., and Secchi, E. R. (2015). Using mark-recapture and stranding data to estimate reproductive traits in female bottlenose dolphins (Tursiops truncatus) of the Southwestern Atlantic Ocean. Mar. Biol. 162, 661–673. doi: 10.1007/s00227-015-2613-0
Galezo, A. A., Krzyszczyk, E., and Mann, J. (2017). Sexual segregation in Indo-Pacific bottlenose dolphins is driven by female avoidance of males. Behav. Ecol. 29, 377–386. doi: 10.1093/beheco/arx177
Genov, T., Centrih, T., Kotnjek, P., and Hace, A. (2019). Behavioural and temporal partitioning of dolphin social groups in the northern Adriatic Sea. Mar. Biol. 166:11.
Gero, S., Engelhaupt, D., and Whitehead, H. (2008). Heterogeneous social associations within a sperm whale, Physeter macrocephalus, unit reflect pairwise relatedness. Behav. Ecol. Sociobiol. 63, 143–151. doi: 10.1007/s00265-008-0645-x
Gero, S., Gordon, J., and Whitehead, H. (2013). Calves as social hubs: dynamics of the social network within sperm whale units. Proc. R. Soc. B Biol. Sci. 280:20131113. doi: 10.1098/rspb.2013.1113
Gero, S., Gordon, J., and Whitehead, H. (2015). Individualized social preferences and long-term social fidelity between social units of sperm whales. Anim. Behav. 102, 15–23. doi: 10.1016/j.anbehav.2015.01.008
Goldenberg, S. Z., Douglas-Hamilton, I., and Wittemyer, G. (2016). Vertical transmission of social roles drives resilience to poaching in elephant networks. Curr. Biol. 26, 75–79. doi: 10.1016/j.cub.2015.11.005
Gowans, S., and Rendell, L. (1999). Head-butting in northern bottlenose whales (Hyperoodon ampullatus): A possible function for big heads? Mar. Mammal Sci. 15, 1342–1350. doi: 10.1111/j.1748-7692.1999.tb00896.x
Gowans, S., Whitehead, H., and Hooker, S. K. (2001). Social organization in northern bottlenose whales, Hyperoodon ampullatus: not driven by deep-water foraging? Anim. Behav. 62, 369–377. doi: 10.1006/anbe.2001.1756
Gowans, S., Würsig, B., and Karczmarski, L. (2007). The social structure and strategies of delphinids: predictions based on an ecological framework. Adv. Mar. Biol. 53, 195–294. doi: 10.1016/s0065-2881(07)53003-8
Guiamares, P. R. Jr., de Menezes, M. A., Baird, R. W., Lusseau, D., Guimaraes, P., and dos Reis, S. F. (2007). Vulnerability of a killer whale social network to disease outbreaks. Phys. Rev. E 76:042901.
Hamilton, W. D. (1964). The genetical evolution of social behaviour. J. Theor. Biol. 7, 1–16. doi: 10.1016/0022-5193(64)90038-4
Hartman, K., van der Harst, P., and Vilela, R. (2020). Continuous focal group follows operated by a drone enable analysis of the relation between sociality and position in a group of male Risso’s Dolphins (Grampus griseus). Front. Mar. Sci. 7:283. doi: 10.3389/fmars.2020.00283
Hartman, K. L., Visser, F., and Hendriks, A. J. E. (2007). Social structure of Risso’s dolphins (Grampus griseus) in the Azores: a stratified community based on highly associated units. Can. J. Zool. 86, 294–306. doi: 10.1139/z07-138
Hawkins, E. R., Pogson-Manning, L., Jaehnichen, C., and Meager, J. J. (2019). Social dynamics and sexual segregation of Australian humpback dolphins (Sousa sahulensis) in Moreton Bay, Queensland. Mar. Mammal Sci. 36, 500–521. doi: 10.1111/mms.12657
Herzing, D. (1996). Vocalizations and associated underwater behavior of free-ranging Atlantic spotted dolphins, Stenella frontalis and bottlenose dolphins, Tursiops truncatus. Aquatic Mammals 22, 61–79.
Herzing, D. L., Augliere, B. N., Elliser, C. R., Green, M. L., and Pack, A. A. (2017). Exodus! Large-scale displacement and social adjustments of resident Atlantic spotted dolphins (Stenella frontalis) in the Bahamas. PLoS One 12:e0180304. doi: 10.1371/journal.pone.0180304
Hinde, R. A. (1976). Interactions, relationships and social structure. Man 11, 1–17. doi: 10.2307/2800384
Hooker, S., Iverson, S., Ostrom, P., and Smith, S. (2001). Diet of northern bottlenose whales inferred from fatty-acid and stable-isotope analyses of biopsy samples. Can. J. Zool. 79, 1442–1454. doi: 10.1139/z01-096
Hooker, S. K., Whitehead, H., Gowans, S., and Baird, R. W. (2002). Fluctuations in distribution and patterns of individual range use of northern bottlenose whales. Mar. Ecol. Progress Ser. 225, 287–297. doi: 10.3354/meps225287
Hunt, T. N., Allen, S. J., Bejder, L., and Parra, G. J. (2019). Assortative interactions revealed in a fission-fusion society of Australian humpback dolphins. Behav. Ecol. 30, 914–927. doi: 10.1093/beheco/arz029
Kappeler, P. M., and van Schaik, C. P. (2002). Evolution of primate social systems. Int. J. Primatol. 23, 707–740.
Karczmarski, L., Würsig, B., Gailey, G., Larson, K. W., and Vanderlip, C. (2005). Spinner dolphins in a remote Hawaiian atoll: social grouping and population structure. Behav. Ecol. 16, 675–685. doi: 10.1093/beheco/ari028
Kato, H. (1984). Observation of tooth scars on the head of male sperm whale, as an indication of intra-sexual fightings. Sci. Rep. Whales Res. Inst. 35, 39–46.
Kay, T., Keller, L., and Lehmann, L. (2020). The evolution of altruism and the serial rediscovery of the role of relatedness. Proc. Natl. Acad. Sci. U.S.A. 117, 28894–28898. doi: 10.1073/pnas.2013596117
Kent, E. E., Mazzoil, M., McCulloch, S. D., and Defran, R. H. (2008). Group characteristics and social affiliation patterns of bottlenose dolphins (Tursiops truncatus) in the Indian River Lagoon, Florida. Florida Sci. 71, 149–168.
Kierdorf, J., Garcke, J., Behley, J., Cheeseman, T., and Roscher, R. (2020). Whate identified a whale by its fluke? On the benefit of interpretable machine learning for whale identification. ISPRS Ann. Photogr. Remote Sen. Spatial Inform. Sci. 2, 1005–1012. doi: 10.5194/isprs-annals-v-2-2020-1005-2020
Konrad, C. M., Frasier, T. R., Rendell, L., Whitehead, H., and Gero, S. (2018b). Kinship and association do not explain vocal repertoire variation among individual sperm whales or social units. Anim. Behav. 145, 131–140. doi: 10.1016/j.anbehav.2018.09.011
Konrad, C. M., Gero, S., Frasier, T., and Whitehead, H. (2018a). Kinship influences sperm whale social organization within, but generally not among, social units. R. Soc. Open Sci. 5:180914. doi: 10.1098/rsos.180914
Kovacs, C. J., Perrtree, R. M., and Cox, T. M. (2017). Social differentiation in common bottlenose dolphins (Tursiops truncatus) that engage in human-related foraging behaviors. PLoS One 12:e0170151. doi: 10.1371/journal.pone.0170151
Kurvers, R. H. J. M., Krause, J., Croft, D. P., Wilson, A. D. M., and Wolf, M. (2014). The evolutionary and ecological consequences of animal social networks: emerging issues. Trends Ecol. Evol. 29, 326–335. doi: 10.1016/j.tree.2014.04.002
Leu, S. T., Sah, P., Krzyszczyk, E., Jacoby, A., Mann, J., and Bansal, S. (2020). Sex, synchrony, and skin contact: integrating myultiple behaviours to assess pathogen transmission risk. Behav. Ecol. 31, 651–660.
Louis, M., Simon-Bouhet, B., Viricel, A., Lucas, T., Gally, F., Cherel, Y., et al. (2018). Evaluating the influence of ecology, sex and kinship on the social structure of resident coastal bottlenose dolphins. Mar. Biol. 165:80.
Lusseau, D. (2003). The emergent properties of a dolphin social network. Proc. R. Soc. B Biol. Sci. 270, S186–S188.
Lusseau, D., Whitehead, H., and Gero, S. (2008). Incorporating uncertainty into the study of animal social networks. Anim. Behav. 75, 1809–1815. doi: 10.1016/j.anbehav.2007.10.029
Machado, A. M. S., Cantor, M., Costa, A. P. B., Righetti, B. P. H., Bezamat, C., Valle-Pereira, J. V. S., et al. (2019). Homophily around specialized foraging underlies dolphin social preferences. Biol. Lett. 15:20180909. doi: 10.1098/rsbl.2018.0909
Mann, J., Stanton, M. A., Patterson, E. M., Bienenstock, E. J., and Singh, L. O. (2012). Social networks reveal cultural behaviour in tool-using using dolphins. Nat. Commun. 3:980.
McPherson, M., Smith-Lovin, L., and Cook, J. M. (2001). Birds of a feather: homophily in social networks. Ann. Rev. Sociol. 27, 415–444. doi: 10.1146/annurev.soc.27.1.415
Methion, S., and Diaz Lopez, B. (2020). Individual foraging variation drives social organizorganization in bottlenose dolphins. Behav. Ecol. 31, 97–106.
Möller, L. M. (2011). Sociogenetic structure, kin associations and bonding in delphinids. Mol. Ecol. 21, 745–764. doi: 10.1111/j.1365-294x.2011.05405.x
Möller, L. M., Beheregaray, L. B., Allen, S. J., and Harcourt, R. G. (2006). Association patterns and kinship in female Indo-Pacific bottlenose dolphins (Tursiops aduncus) of southeastern Australia. Behav. Ecol. Sociobiol. 61, 109–117. doi: 10.1007/s00265-006-0241-x
Möller, L. M., Beheregaray, L. B., Harcourt, R. G., and Krutzen, M. (2001). Alliance membership and kinship in wild male bottlenose dolphins (Tursiops aduncus) of southeastern Australia. Proc. R. Soc. B Biol. Sci. 268, 1941–1947. doi: 10.1098/rspb.2001.1756
Nattrass, S., Croft, D. P., Ellis, S., Cant, M. A., Weiss, M. N., Wright, B. M., et al. (2019). Postreproductive killer whale grandmothers improve the survival of their grandoffspring. Proc. Natl. Acad. Sci. U.S.A. 116, 26669–26673. doi: 10.1073/pnas.1903844116
Newman, M. E. J. (2006). Modularity and community structure in networks. Proc. Natl. Acad. Sci. U.S.A. 103, 8577–8582.
Newsome, S. D., Etnier, M. A., Monson, D. H., and Fogel, M. L. (2009). Retrospective characterization of ontogenetic shifts in killer whale diets via δ13C and δ15N analysis of teeth. Mar. Ecol. Progress Ser. 374, 229–242. doi: 10.3354/meps07747
Nishita, M., Shirakihara, M., and Amano, M. (2017). Patterns of association among female Indo-Pacific bottlenose dolphins (Tursiops aduncus) in a population forming large groups. Behaviour 154, 1013–1028. doi: 10.1163/1568539x-00003453
Nykanen, M., Dillane, E., Englund, A., Foote, A. D., Ingram, S. N., Louis, M., et al. (2018). Quantifying dispersal between marine protected areas by a highly mobile species, the bottlenose dolphin. Tursiops truncatus. Ecol. Evol. 8, 9241–9258. doi: 10.1002/ece3.4343
Pace, D. S., Pulcini, M., and Triossi, F. (2012). Anthropogenic food patches and association patterns of Tursiops truncatus at Lampedusa island. Italy. Behav. Ecol. 23, 254–264. doi: 10.1093/beheco/arr180
Parsons, K. M., Balcomb, K. C., Ford, J. K. B., and Durban, J. W. (2009). The social dynamics of southern resident killer whales and conservation implications for this endangered population. Anim. Behav. 77, 963–971. doi: 10.1016/j.anbehav.2009.01.018
Parsons, K. M., Durban, J. W., Claridge, D. E., Balcomb, K. C., Noble, L. R., and Thompson, P. M. (2003). Kinship as a basis for alliance formation between male bottlenose dolphins, Tursiops truncatus, in the Bahamas. Anim. Behav. 66, 185–194. doi: 10.1006/anbe.2003.2186
Pilot, M., Dahlheim, M. E., and Hoelzel, A. R. (2010). Social cohesion among kin, gene flow without dispersal and the evolution of population genetic structure in the killer whale (Orcinus orca). J. Evol. Biol. 23, 20–31. doi: 10.1111/j.1420-9101.2009.01887.x
Powell, S. N., Wallen, M. M., Miketa, M. L., Krzyszczyk, E., Foroughirad, V., Bansal, S., et al. (2020). Sociality and tattoo skin disease among bottlenose dolphins in Shark Bay. Australia. Behav. Ecol. 31, 459–466. doi: 10.1093/beheco/arz207
Reisinger, R. R., Keith, M., Andrews, R. D., and de Bruyn, N. (2015). Movement and diving of killer whales (Orcinus orca) at a Southern Ocean archipelago. J. Exp. Mar. Biol. Ecol. 473, 90–102. doi: 10.1016/j.jembe.2015.08.008
Reisinger, R. R., van Rensburge, C., Hoelzel, A. R., and de Bruyn, P. J. N. (2017). Kinship and association in a highly social apex predator population, killer whales at Marion Island. Behav. Ecol. 28, 750–759. doi: 10.1093/beheco/arx034
Rendell, L., Cantor, M., Gero, S., Whitehead, H., and Mann, J. (2019). Causes and consequences of female centrality in cetacean societies. Philos. Trans. R. Soc. B Biol. Sci. 374:20180066. doi: 10.1098/rstb.2018.0066
Richard, K. R., Dillon, M. C., Whitehead, H., and Wright, J. M. (1996). Patterns of kinship in groups of free-living sperm whales (Physeter macrocephalus) revealed by multiple molecular genetic analyses. Proc. Natl. Acad. Sci. U.S.A. 93, 8792–8795. doi: 10.1073/pnas.93.16.8792
Riesch, R., Barrett-Lennard, L. G., Ellis, G. M., Ford, J. K. B., and Deecke, V. B. (2012). Cultural traditions and the evolution of reproductive isolation: ecological speciation in killer whales? Biol. J. Linnean Soc. 106, 1–17. doi: 10.1111/j.1095-8312.2012.01872.x
Ruckstuhl, K. E. (2007). Sexual segregation in vertebrates: proximate and ultimate causes. Integr. Comparative Biol. 47, 245–257. doi: 10.1093/icb/icm030
Ruckstuhl, K. E., and Neuhaus, P. (2002). Sexual segregation in ungulates: a comparative test of three hypotheses. Biol. Rev. 77, 77–96. doi: 10.1017/s1464793101005814
Sakai, M., Wang, D., Wang, K., Li, S., and Akamatsu, T. (2011). Do porpoises choose their associates? A new method for analysing social relationships among cetaceans. PLoS One 6:e28836. doi: 10.1371/journal.pone.0028836
Sanderson, C. E., and Alexander, K. A. (2020). Unchartered waters: Climate change likely to intensify infectious disease outbreaks causing mass mortality events in marine mammals. Global Change Biol. 26, 4284–4301. doi: 10.1111/gcb.15163
Schuppli, C., and van Schaik, C. P. (2019). Animal cultures: how we’ve only seen the tip of the iceberg. Evol. Hum. Sci. 1:E2.
Slooten, E., Dawson, S. M., and Whitehead, H. (1993). Associations among photographically identified Hector’s dolphins. Can. J. Zool. 71, 2311–2318. doi: 10.1139/z93-324
Smith, J. E. (2014). Hamilton’s legacy: kinship, cooperation, and social tolerance in mammalian groups. Anim. Behav. 92, 291–304. doi: 10.1016/j.anbehav.2014.02.029
Smolla, M., Gilman, R. T., Galla, T., and Shultz, S. (2015). Competition for resources and explain patterns of social and individual learning in nature. Proc. R. Soc. B Biol. Sci. 282:20151405. doi: 10.1098/rspb.2015.1405
Snijders, L., Blumstein, D. T., Stanley, C. R., and Franks, D. W. (2017). Animal social network theory can help wildlife conservation. Trends Ecol. Evol. 32, 567–577. doi: 10.1016/j.tree.2017.05.005
Snyder-Mackler, N., Burger, J. R., Gaydosh, L., Belsky, D. W., Noppert, G. A., Campos, F. A., et al. (2020). Social determinants of health and survival in humans and other animals. Science 368:eaax9553. doi: 10.1126/science.aax9553
Stanton, M. A., Gibson, Q. A., and Mann, J. (2011). When mum’s away: a study of mother and calf ego networks during separations in wild bottlenose dolphins (Tursiops sp.). Anim. Behav. 82, 405–412. doi: 10.1016/j.anbehav.2011.05.026
Stanton, M. A., and Mann, J. (2012). Early social networks predict survival in wild bottlenose dolphins. PLoS One 7:e47508. doi: 10.1371/journal.pone.0047508
Sueur, C., Romano, V., Sosa, S., and Puga-Gonzalez, I. (2019). Mechanisms of network evolution: a focus on socioecological factors, intermediary mechanisms, and selection pressures. Primates 60, 167–181. doi: 10.1007/s10329-018-0682-7
Tavares, S. B., Samarra, F. I. P., and Miller, P. J. O. (2017). A multilevel society of herring-eating killer whales indicates adaptation to prey characteristics. Behav. Ecol. 28, 500–514. doi: 10.1093/beheco/arw179
Titcomb, E. M., Reif, J. S., Fair, P. A., Stavros, H.-C. W., Mazzoil, M., Bossart, G. D., et al. (2017). Blood mercury concentrations in common bottlenose dolphins from the Indian River Lagoon, Florida: patterns of social distribution. Mar. Mammal Sci. 33, 771–784. doi: 10.1111/mms.12390
Torres, L. G., Nieukirk, S. L., Lemos, L., and Chandler, T. E. (2018). Drone up! Quantifying whale behavior from a new perspective improves observational capacity. Front. Mar. Sci. 5:319. doi: 10.3389/fmars.2018.00319
Van Cise, A. M., Martien, K. K., Mahaffy, S. D., Baird, R. W., Webster, D. L., Fowler, J. H., et al. (2017). Familial social structure and socially driven genetic differentiation in Hawaiian short-finned pilot whales. Mol. Ecol. 26, 6730–6741. doi: 10.1111/mec.14397
van Gils, J. A., Kraan, C., Dekinga, A., Koolhaas, A., Drent, J., de Goeij, P., et al. (2009). Reversed optimality and predictive ecology: burrowing depth forecasts population change in a bivalve. Biol. Lett. 5, 5–8. doi: 10.1098/rsbl.2008.0452
Vermeulen, E. (2018). Association patterns of bottlenose dolphins (Tursiops truncatus) in Bahia San Antonio, Argentina. Mar. Mammal Sci. 34, 687–700. doi: 10.1111/mms.12481
Volker, C. L., and Herzing, D. L. (2021). Aggressive behaviors of adult male spotted dolphins: making signals count during intraspecific and interspecific conflicts. Anim. Behav. Cogn. 8, 36–51.
Wade, P. R., Reeves, R. R., and Mesnick, S. L. (2012). Social and behavioural factors in cetacean responses to overexploitation: Are Odontocetes less “resilient” than mysticetes? J. Mar. Sci. 2012:567276.
Wang, X., Wu, F., Turvey, S. T., Rosso, M., Tao, C., Ding, X., et al. (2015). Social organization and distribution patterns inform conservation management of a threatened Indo-Pacific humpback dolphin population. J. Mammol. 96, 964–971. doi: 10.1093/jmammal/gyv097
Webber, Q. M. R., and Vander Wal, E. (2019). Trends and perspectives on the use of animal social network analysis in behavioural ecology: a bibliometric approach. Anim. Behav. 149, 77–87. doi: 10.1016/j.anbehav.2019.01.010
Weiss, M. N., Franks, D. W., Balcomb, K. C., Ellifrit, D. K., Silk, M. J., Cant, M. A., et al. (2020). Modelling cetacean morbillivirus outbreaks in an endangered killer whale population. Biol. Conserv. 242:108398. doi: 10.1016/j.biocon.2019.108398
Whitehead, H. (1993). The behaviour of mature male sperm whales on the Galapagos Islands breeding grounds. Can. J. Zool. 71, 689–699. doi: 10.1139/z93-093
Whitehead, H. (2003). Sperm Whales: Social Evolution in the Ocean. Chicago, IL: The University of Chicago Press.
Whitehead, H. (2008). Precision and power in the analysis of social structure using associations. Anim. Behav. 75, 1093–1099. doi: 10.1016/j.anbehav.2007.08.022
Whitehead, H., and Dufault, S. (1999). Techniques for analyzing vertebrate social structure unsing identified individuals. Adv. Study Behav. 28, 33–74. doi: 10.1016/s0065-3454(08)60215-6
Whitehead, H., and James, R. (2015). Generalized affiliation indices extract affiliations from social network data. Methods Ecol. Evol. 6, 836–844. doi: 10.1111/2041-210x.12383
Whitehead, H., and Rendell, L. (2015). The Cultural Lives of Whales and Dolphins. Chicago, IL: The University of Chicago Press.
Wierucka, K., Verborgh, P., Meade, R., Colmant, L., Gauffier, P., Esteban, R., et al. (2014). Effects of a morbillivirus epizootic on long-finned pilot whales Globicephala melas in Spanish Mediterranean waters. Mar. Ecol. Progress Ser. 502, 1–10. doi: 10.3354/meps10769
Wild, S., Allen, S. J., Krutzen, M., King, S. L., Gerber, L., and Hoppitt, W. J. E. (2019). Multi-network-based diffusion analysis reveals vertical cultural transmission of sponge tool use within dolphin matrilines. Biol. Lett. 15:20190227. doi: 10.1098/rsbl.2019.0227
Wild, S., Hoppit, W. J. E., Allen, S. J., and Krützen, M. (2020). Integregating genetic, environmental, and social networks to reveal transmission pathways of a dolphin foraging innovation. Curr. Biol. 30, 3024–3030. doi: 10.1016/j.cub.2020.05.069
Williams, R., and Lusseau, D. (2006). A killer whale social network is vulnerable to targeted removals. Biol. Lett. 2, 497–500. doi: 10.1098/rsbl.2006.0510
Wiszniewski, J., Brown, C., and Moeller, L. M. (2012). Complex patterns of male alliance formation in a dolphin social network. J. Mammol. 93, 239–250. doi: 10.1644/10-mamm-a-366.1
Keywords: cetacea, Odontoceti, social structure, social evolution, socioecology
Citation: Weiss MN, Ellis S and Croft DP (2021) Diversity and Consequences of Social Network Structure in Toothed Whales. Front. Mar. Sci. 8:688842. doi: 10.3389/fmars.2021.688842
Received: 31 March 2021; Accepted: 22 June 2021;
Published: 14 July 2021.
Edited by:
Lyne Morissette, M – Expertise Marine, CanadaReviewed by:
Olivier Adam, Sorbonne Université, FranceJohn Gatesy, University of California, Riverside, United States
Copyright © 2021 Weiss, Ellis and Croft. This is an open-access article distributed under the terms of the Creative Commons Attribution License (CC BY). The use, distribution or reproduction in other forums is permitted, provided the original author(s) and the copyright owner(s) are credited and that the original publication in this journal is cited, in accordance with accepted academic practice. No use, distribution or reproduction is permitted which does not comply with these terms.
*Correspondence: Michael N. Weiss, bS53ZWlzc0BleGV0ZXIuYWMudWs=