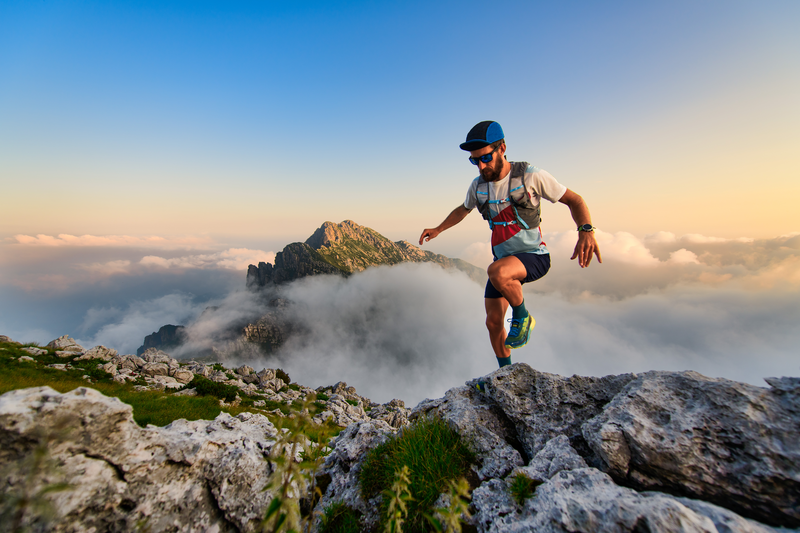
94% of researchers rate our articles as excellent or good
Learn more about the work of our research integrity team to safeguard the quality of each article we publish.
Find out more
ORIGINAL RESEARCH article
Front. Mar. Sci. , 22 July 2021
Sec. Marine Biotechnology and Bioproducts
Volume 8 - 2021 | https://doi.org/10.3389/fmars.2021.687701
This article is part of the Research Topic Marine Biotechnology, Revealing an Ocean of Opportunities View all 26 articles
Marine ecosystems are unique and rich reservoirs of biodiversity with high potential toward improving the quality of human life. The extreme physical–chemical conditions of the oceans have favored marine organisms to produce a great variety of new molecules as a mechanism to ensure their survival, and such compounds possess great biopharmaceutical interest. In particular, marine microbiota represent a promising and inexhaustible source for the development of new drugs. This work presents the taxonomic study of the samples obtained from the underwater volcano Tagoro, which has allowed us to develop a collection of 182 marine bacterial strains. On October 10th, 2011, at La Restinga–El Mar de Las Calmas Marine Reserve, an underwater eruption gave rise to a novel shallow submarine volcano at 1.8 km south of the island of El Hierro, Canary Islands, Spain. During the first 6 months, extreme physical–chemical perturbations, comprising thermal changes, water acidification, deoxygenation, and metal enrichment, resulted in significant alterations of the marine ecosystem. After March 2012, the submarine volcano Tagoro entered an active hydrothermal phase that involved a release of heat, gases, metals, and micronutrients that continues till our present. During 2016, our research team had the opportunity to participate in one of the monitoring oceanographic cruises carried out in the area in order to isolate microorganisms associated with both rock samples and deep-sea invertebrates over Tagoro submarine volcano. In this study, Proteobacteria revealed as the most abundant Phylum with 70.2% among all isolated strains, followed by Firmicutes 19%, Actinobacteria 9.5%, and Bacteroidetes 1.2%. Furthermore, we present the results of the antiproliferative assays of the extracts obtained from small-scale cultures of selected bacterial strains. An analysis of the effects of culture conditions in the antiproliferative activity showed that strains grown in Marine Broth (MB) presented lower GI50 values than those cultured in a modified medium (MM1). This effect is improved when the strains are incubated under agitation conditions. The antiproliferative potential of genera such as Halobacillus, Kangiella, Photobacterium, and Halomonas is revealed. Their biotechnological development provides an excellent starting point to access novel secondary metabolites and enzymes with potential for pharmaceutical and industrial applications.
Marine environments represent more than 70% of the earth’s surface with the deep sea comprising the largest part of the oceans, which is characterized by extreme conditions of pressure, temperature, light, salinity, and nutrient availability. Deep-sea benthic environments are predominantly occupied by microbial organisms (Hoshino et al., 2020), which are strongly influenced by the singular physical–chemical characteristics in the marine habitats (Poli et al., 2017). As a mechanism to ensure their survival, marine microbiota, such as bacteria, cyanobacteria, yeasts, fungi, and microalgae, respond by biosynthesizing a great variety of molecules. These compounds represent a unique reservoir of new bioactive substances of great biopharmaceutical potential for the development of new drugs (Carroll et al., 2021).
Microbial richness of marine environmental microbiomes is estimated to range between 104 and 106 prokaryotes/mL (Whitman et al., 1998; Hoshino et al., 2020). Despite this huge biodiversity, most of microorganisms that inhabit the marine environment remain uncultured under laboratory conditions (Kaeberlein et al., 2002; Mu et al., 2021), an essential requisite for biodiscovery and the access to new chemical entities. Nonetheless, innovative approaches for isolation and culture marine microbes represent a growing research field (Joint et al., 2010; Mu et al., 2021). For these reasons, the study of singular marine ecosystems is considered a very interesting source for biodiscovery (Rotter et al., 2021) that allow to access novel microorganisms with unique metabolic and physiological capabilities to colonize extreme habitats and biosynthesize compounds as an adaptive mechanism to the environmental conditions (Nikapitiya, 2012; Silva et al., 2020). This particularity has led to a constant description of bioactive metabolites from marine bacteria (Buijs et al., 2019; Puglisi et al., 2019). Marine compounds display high cytotoxicity; since the 1990s it was suggested that over 1% of the marine samples analyzed possesses antitumoral activity while the same is 0.2% of terrestrial samples (Wang et al., 2020).
Among singular marine ecosystems, deep-sea hydrothermal vents and submarine volcanoes constitute unique environments for bioprospection studies with significant emission of gases and solutes that react with seawater leading to physical–chemical changes that impact consequently the marine ecosystem (Fraile-Nuez et al., 2012). Thus, hydrothermal vents have been recognized as “natural hot spots” of bacterial strains that have developed multiple resistances as a result of the adaptive phenotypic and genotypic responses to environmental conditions (Farias et al., 2015).
On October 10th, 2011, at La Restinga–El Mar de Las Calmas Marine Reserve, an underwater eruption gave rise to a novel shallow submarine volcano at 1.8 km south of the island of El Hierro, Canary Island, Spain (27°37.116′N, 017°59.466′W). During the first 6 months (October 2011–March 2012), extreme physical–chemical perturbations caused by this event, comprising thermal changes, water acidification, deoxygenation, and metal enrichment, resulted in significant alterations of the marine ecosystem. After March 2012, the submarine volcano Tagoro entered an active hydrothermal phase that involved a release of heat, gases, metals, and micronutrients that continues till our present. In this way, volcano Tagoro became one of the newest and shallowest active submarine volcanoes in Europe, representing an excellent natural laboratory to study how the extreme physical–chemical and biological anomalies caused by this eruptive process have transformed one of the richest marine ecosystems of the Spanish coast (Fraile-Nuez et al., 2012, 2018).
Although Tagoro submarine volcano dataset has turned into one of the longest and more complete multidisciplinary time-series for the study of how physical–chemical anomalies impacted over the marine ecosystem (Fraile-Nuez et al., 2012, 2018; Rivera et al., 2013; Santana-Casiano et al., 2013, 2016, 2018; Ariza et al., 2014; Coca et al., 2014; Eugenio et al., 2014; Blanco et al., 2015; Danovaro et al., 2017; Santana-González et al., 2017; Álvarez-Valero et al., 2018; Sotomayor-García et al., 2019, 2020; González-Vega et al., 2020), just a few studies have shed light in the characterization of the microbial communities with geologically active marine environments, partly due to the difficulty in collecting samples as well as the eruption prediction and monitoring, usually in remote locations (Ferrera et al., 2015). These previous studies have exhibited the effects of the eruption on the survival and colonization by benthic and demersal species (Sotomayor-García et al., 2019) as well as microorganism communities (Ferrera et al., 2015).
In October 2016, our research team participated in one of the monitoring oceanographic cruises carried out in the area with the aim to explore the microbial biodiversity associated with both rock samples and deep-sea invertebrates over Tagoro submarine volcano. This work reports on the results of the taxonomic study of the samples obtained from this singular marine ecosystem which has allowed us to obtain a collection of 182 strains of marine bacteria. Furthermore, the results of the antiproliferative assays of the extracts from small-scale cultures of selected bacterial strains are showed. This collection represents an excellent starting point to access novel secondary metabolites and enzymes with potential for pharmaceutical and industrial applications.
In October 2016, 5 years after the completion of the magmatic activity, a multidisciplinary oceanographic cruise, on board R/V Ángeles Alvariño of the Spanish Institute of Oceanography, was carried out in the context of the VULCANO-II project in order to characterize the physical–chemical and biological anomalies of the underwater volcano in a degassing stage (Figure 1).
Figure 1. Location map. (A) Location of the island of El Hierro in the Canary Archipelago. (B) Location of Tagoro submarine volcano south of El Hierro island. (C) Rock dredge sampling over the lava flow of Tagoro submarine volcano and control area northwest of the submarine volcano. Shaded triangle represents the domain of La Restinga - Mar de Las Calmas Marine Reserve.
Multibeam echosounder datasets were acquired using a Kongsberg-Simrad EM710 during VULCANO-II-1016, which operates at sonar frequencies in the 70–100 kHz range and were processed using CARIS HIPS and SIPS yielding bathymetric grid resolution of 5 m with 100% coverage.
Benthic organisms were sampled using a benthic dredge with a horizontal opening of 1 m and a net mesh size of 1 cm. Although samplings were mainly performed to carry out a geological characterization of substrate types, samples of benthic organisms were also collected: in the base of Tagoro volcanic complex (DA01, Figure 1), around the main edifice and secondary and primary craters of Tagoro submarine volcano (DA02–06), and in the unaffected area in the interior of La Restinga-Mar de Las Calmas Marine Reserve (DA08). In that sense, a total of 30 samples were obtained from seven different benthic dredges (DA01–06, DA08) in a ranging depth of 160–800 m. The samples (Supplementary Table 1), which included deep-sea algae, invertebrates, and sediments occluded into pores of volcanic rocks, were washed with sterile sea water, stored in sterile tubes and kept at 4°C on board until processed in the laboratory.
A SeaBird 911-plus CTD instrument, equipped with dual temperature and conductivity sensors, with accuracies of 0.001°C and 0.0003 S/m, respectively, was used to collect temperature, conductivity, salinity, pressure, dissolved oxygen, fluorescence, and pH data over the study area. The CTD continuously recording data with a sampling interval of 24 Hz and the sensors were calibrated at the SeaBird laboratory before and after the cruises. Discrete water samples for inorganic nutrient analysis were obtained using a rosette of 24–12-L Niskin bottles.
During the cruise, 16 vertical profiles of CTD were carried out around the main volcano edifice together with 48 tow-yo transect, which involve continuously lowering and raising the rosette between 1 and 40 m above the seafloor, with the ship moving at 0.2–0.4 kn in Dynamic Positioning, obtaining a sawtooth-shaped dataset with high spatial resolution and closer to the source of the emissions. Physical–chemical parameters at sampling stations, including pH (ΔpH) and temperature (Δθ) anomalies around the main and secondary crater (DA04–06) are summarized in Supplementary Table 1.
The macroscopic samples (Figure 2) were surface sterilized with sterile seawater, followed by immersion in ethanol three times and processed according to their nature through two different methods: (a) by chopping, and (b) by homogenization with sterile seawater and vortexed for 5 min. Sediments were diluted in sterile seawater (50 mg/mL) and vortexed for 5 min. After sample settling, sequential dilutions of the supernatant were performed, and transferred in duplicate into Petri dishes containing two solid media: MA (Marine Agar 2216, Panreac) and MM1 [Yeast extract (4 g/L); starch (10 g/L); bacteriological peptone (2 g/L); agar (15 g/L); and filtered seawater (90%)]. Inoculated Petri dishes were incubated at 20°C and microbial growth was monitored periodically over 6 months. The microbial colonies observed were isolated in pure culture by repeated transfer from a single colony, until strains were established. All isolates were grown in liquid media and cryopreserved at −80°C in 20% glycerol and belong to the Marine Microbial Collection of IUBO-ULL.
Figure 2. (A) Hydrozoan Sertularella sp. (Depht: –170 to 270 m) from DA04. (B) Environmental samples AR19 and AR10–AR14 in sterile containers. (C) Microbial diversity observed in processed samples.
DNA extraction, 16S rRNA gene amplification and sequencing was carried out at the Genomic Service of the General Research Support Services of Universidad de La Laguna. DNA was extracted from liquid cultures after centrifugation (5 min at 18,000 × g) and the cell pellet was recovered by re-suspending in 200 μL of lysis buffer [40 mM Tris–HCl pH 7.8, 20 mM sodium acetate, 1 mM EDTA, 1% SDS, 0.04 μg/μL RNase-A (Promega), 0.04 μg/μL lysozyme (Thermo Fisher Scientific)]. Samples were incubated for 5 min at room, and 5 M NaCl and chloroform sequentially added. After centrifugation (18 000 × g, 2 min), the aqueous phase was recovered, DNA was precipitated with cold absolute ethanol and washed twice with 70% ethanol. DNA pellets were dried at room temperature and then suspended in 50 μL of H2O. 16S rRNA gene was amplified using universal primers E8F (5′-TAGAGTTTGATCMTGGCTCAG-3′) and 1492R (5′-TACGGYTACCTTGTTACGACTT-3′) (Weisburg et al., 1990). Commercial PCR kit AmpONE Taq DNA polymerase (GeneAll Biotech) was performed using a PTC200 MJ Research Thermal Cycler with the following conditions: initial denaturation at 95°C for 2 min, 35 cycles of 95°C for 20 s, 55°C for 20 s, and 72°C for 1.5 min, followed by a final extension at 72°C for 7 and 5 min at 16°C. The PCR-amplified products were checked for the presence of a single band of the expected size by standard agarose electrophoresis. PCR products were purified using the EXO-SAP-IT kit (Affymetrix-USB) following manufacturer’s instructions in a total volume of 7 μL and incubated at 35°C for 15 min, followed by heating inactivation to 80°C for 15 min. Sanger Sequencing was carried out using the BigDyeTM Terminator v3.1 Cycle Sequencing Kit (Thermo Fisher Scientific) and 3500 Series Genetic Analyzer automatic sequencer (Thermo Fisher Scientific). The SeqScape (Thermo Fisher Scientific) software was used for the analysis of sequencing data and filtering out low quality reads.
A total of 84 selected sequences were analyzed using Chromas (Chromas software 2.6.6, Technelysium, Pty. Ltd.1) and Seaview 5 (Gouy et al., 2010) programs. Consensus sequences were created and compared to the GenBank database by the Blast(n) algorithm to establish their closest relatives. Using Mothur program (Schloss et al., 2009), sequences were grouped by operational taxonomic units (OTUS) at 97% identity using the command classify.otu (Chappidi et al., 2019). Once identified the hits with high maximum identity, reference sequences were used to be aligned using MAFFT v7 (Katoh and Standley, 2013) with G-INS-i strategy (recommended for <200 sequences with global homology). The alignment obtained was used to construct the phylogenetic tree using PhyML v3.1 (Guindon and Gascuel, 2003; Anisimova and Gascuel, 2006; Dereeper et al., 2008, 2010) under the GTR model with bootstrap 1000 replicates value. Thermogymnomonas acidicola was used as outgroup. Tree manipulation and editing were done using FigTree v14.0 program.2 The 16S rRNA gene sequences were submitted to the GenBank database under the following accession numbers: MW826671–MW826754.
Bacterial isolates were cultured in four low-nutrient experimental conditions in 500 mL Erlenmeyer flasks containing 300 mL of 1:5 dilution ratio of marine broth (MB/5, Marine Broth 2216, Panreac) and MM1 medium (MM1/5). The flasks were incubated both under static conditions and in an orbital shaker (IKA KS501, Labortechnik) at 100 rpm, at 20°C in the dark for 20–30 days. After the incubation process, cultures were extracted three times with ethyl acetate (3 × 100 mL EtOAc). The organic layer was dried in a rotary evaporator and the resulting extracts stored at −20°C until required for biological tests.
Weighted dried organic extracts were dissolved in dimethyl sulfoxide to prepare a stock solution of 50 mg/mL for bioactivity assays. To evaluate the antiproliferative activity of the samples we selected the cancer cell lines A549 and SW1573 (non-small cell lung), HBL-100 and T-47D (breast), HeLa (cervix), and WiDr (colon). The tests were performed in 96-well plates using the SRB assay (Orellana and Kasinski, 2016) with the following specifications. Cell seeding densities were 2500 cells/well for A549, HBL-100, HeLa and SW1573, and 5000 cells/well for T-47D and WiDr. Drug incubation times were 48 h. The optical density of each well was measured at 530 (primary) and 620 (secondary) nm. The antiproliferative activity, expressed as 50% growth inhibition (GI50), was calculated according to NCI formulas (Monks et al., 1991).
Figure 3 shows the physical–chemical anomalies over the submarine volcano Tagoro with the use of a CTD and tow-yos close to the seabed. Although the pH anomalies are quite visible in a wide area around the volcano edifice (400–500 m radius around sta56), close to the main crater (sta60), and secondary crater (sta56) the pH anomalies reach a maximum value of −0.5. The anomaly plume is centered around 127 meters depth that correspond with the depth of the main crater (sta60) and could transport with the local currents for hundreds of meters (Santana-Casiano et al., 2016).
Figure 3. Physical–chemical anomalies over Tagoro submarine volcano. (A) High resolution bathymetry of Tagoro submarine volcano. CTD stations are marked with dots from 50 to 60. Blank lines represent the CTD tow-yo transects over the main volcanic edifice. (B) pH anomaly for every tow-yo transect with latitude. (C) Vertical mean pH anomaly (bold line) with its standard deviation (gray) and the minimum values (blue line). (D) Vertical mean potential temperature anomaly (bold line) with its standard deviation (gray) and the maximum values (blue line).
Moreover, in the interior of the main crater, not only a pH anomaly is found but also a thermal increase of up to +2.37°C, a salinity decrease of −1.02, and a density decrease of −1.43 (kg m–3). The combined effect of these anomalies is generating a buoyant plume over the volcano that returns downward waters by diffusion to close the circulation cells generating a horizontal flow by this vertical. Theoretically, this vortex is of sufficient magnitude to trap water in a local recirculation that could have a crucial effect on the expected dispersal of properties, including minerals, tracers, and organisms (Speer, 1989).
From the analysis and bioprospection of the microbiota of this unique and extreme habitat, a total of 182 bacterial strains were isolated from volcanic sediments clogged into rocks and as endophytes from the inner tissues of algae and deep-sea invertebrates sampled in the range of depths of −160 to −800 m (Figure 1 and Supplementary Table 1) for over 6 months. Marine samples were incubated in duplicates into two different solid media, MA and modified marine agar (MM1) as described in section “Sample Collection.” No fungal growing was observed. Among all isolated bacterial strains, 95 and 87 grew on MA and MM1, respectively. Those strains obtained from the same environmental sample which showed similar morphological characteristics were grouped and a representative strain was selected to continue the taxonomic and phylogenetic studies.
Thus, the 16S rRNA gene of 84 selected strains were sequenced and identified by its similarity with 16S rRNA gene sequences deposited in the GenBank database from the National Center for Biotechnology Information, NCBI (Table 1). Sequences were grouped into 80 OTUs comprising 28 genera classified on 4 Phyla. Proteobacteria is the most abundant Phylum and represents 70.2% of all isolated strains (18 genera, 59 strains), followed by Firmicutes 19% (3 genera, 16 strains), Actinobacteria 9.5% (6 genera, 8 strains), and Bacteroidetes 1.2% (1 genus, 1 strain), as represented in Figure 4.
Table 1. Summary of the bacterial isolates and phylogenetically closely related species from GenBank according to 16S rRNA gene sequence similarity.
Figure 4. Genera (external ring) and classes (internal ring) distribution of the marine bacterial collection isolated from volcano Tagoro.
The evolutionary relationships between the isolated bacterial strains are shown in the 16S rRNA phylogenetic tree (Figure 5). From the 84 isolates, 66 out strains exhibited more than 99% similarity compared to 16S rRNA gene sequences deposited in GenBank and 6 isolates exhibited <97% similarity; lower sequence similarities were observed mainly for three strains of Vibrio (AR30-01, AR30-05, and AR32-02) isolated from invertebrates collected at depths ranging 200–300 m (DA06 and DA08), Supplementary Table 2.
Figure 5. Circular maximum-likelihood phylogenetic tree of isolated bacterial strains and their closest NCBI (BLAST) relatives based on 16S rRNA gene sequences. Bootstrap values calculated from 1000 replicates using maximum likelihood are shown the most representatives at their representative node. Colors indicate distribution by Classes: Epsilonproteobacteria (gray), Gammaproteobacteria (purple), Alphaproteobacteria (yellow), Actinobacteria (green), and Bacilli (blue).
There are diverse studies focused on the diversity of culturable bacteria from marine sources such as sediment, invertebrates, and water samples, among other marine samples, within Proteobacteria representing an important proportion of the isolates (Buijs et al., 2019). In the present study, Proteobacteria were isolated from all the seven depths analyzed and, interestingly, strains isolated of samples from the crater (DA06), especially of inner sediment volcanic rocks, comprises the four phyla identified in this work. Although it is noticeable that some groups of samples are represented by a certain class, such as sponges from DA08, since type and number of samples are different for each dredge, no tendencies were observed in relation to the sample and depth.
Previous studies that show the pharmacological relevance of marine microorganisms prompted us to evaluate the antiproliferative properties of bacterial strains isolated from volcano Tagoro against A549, HBL-100, HeLa, SW1573, T-47D, and WiDr cell lines.
A selection of 33 bacterial isolates representing 21 different phylogenetic classes were cultured in 300 mL liquid media under low-nutrient conditions, using two different media, under static and agitation conditions, for a period of 30 days for each strain. The use of oligotrophic media and large fermentation time was intended to induce stress conditions that stimulate the activation of secondary-metabolism biogenetic gene clusters following an one strain many compounds (OSMAC)-based strategy (Bode et al., 2002). This strategy has revealed as a simple and effective approach to induce the activation of the biosynthetic mechanisms of microorganisms to produce new secondary metabolites by changing culture conditions (Reen et al., 2015). Successful examples include the addition of enzyme inhibitors, addition of signaling molecules, co-cultivation with other microbial strain, modification of medium composition, or changes of physical parameters, among others (Romano et al., 2018; Pan et al., 2019).
Microbial cultures were extracted with EtOAc and the solvent dried. The antiproliferative analysis of the obtained extracts (Table 2 and Supplementary Table 3) revealed that one actinobacteria, two Proteobacteria, and two Firmicutes showed GI50 < 20 μg/mL against at least one cell line: Micromonospora AR22-02, Kangiella AR14-04, Pseudoalteromonas AR04-01, and Halobacillus AR14-06 and AR01-01. Interestingly, besides the lowest GI50 values, the two Halobacillus strains were the most active bacteria evaluated, since they exhibited antiproliferative properties for at least five out from the six cell lines assayed. Also, 11 bacterial strains showed GI50 values ranging from 20 to 39 μg/mL against at least one cell line, the Bacteroidetes Salinimicrobium AR29-06; two Actinobacteria: Micrococcus AR07-11A and Mycobacterium AR05-15C; three Firmicutes comprising the genus Bacillus: AR13-02, AR14-07B, AR19-02; and five Proteobacteria: Limimaricola AR29-03, Aliivibrio AR26-02, Kangiella AR14-04, Photobacterium AR27-02 and Vibrio AR05-02. Overall, strains comprising the genera Halobacillus, Salinimicrobium, Micrococcus, Aliivibrio, Kangiella, Photobacterium, and Pseudoalteromonas showed GI50 < 40 μg/mL against at least four out from the six cell lines tested. Additionally, it was observed that 24 strains comprising 5 Actinobacteria, 5 Firmicutes and 14 Proteobacteria exhibited 40 ≤ GI50 ≤ 60 μg/mL.
Table 2. Antiproliferative activity of 33 selected bacterial strains isolated from environmental samples of Tagoro submarine volcano against human solid tumor cell lines.
Among the most active strains, the genus Halobacillus showed the best antiproliferative activities in MB, particularly when grown under agitation conditions. On the other hand, in the case of Pseudoalteromonas sp. strain AR04-01 the best results of activity were observed when grown under static culture in MM1 medium. Overall, comparison of the strains that exhibited GI50 < 40 μg/mL, the most sensible cells were A549, HeLa, and SW1573, whereas HBL-100 was the most resistant.
An analysis of the effects of culture conditions in the antiproliferative activity of the tested strains is showed in Figure 6. The radar charts reveal that strains grown in MB medium (1:5 diluted) showed lower GI50 values than the same strains cultured in a MM1 medium (1:5 diluted), with higher content in nutrients than the former. This effect is improved when the strains are incubated under agitation conditions compared to those grown in static cultures and is maintained in all cell lines tested (Supplementary Figure 1).
Figure 6. Radar chart of the effect of culture conditions in the antiproliferative activity (Min and Max GI50, μg/mL). MB and MM1 media were diluted in a 1:5 ratio for oligotrophic conditions. A, agitation conditions; S, static cultures.
Tagoro submarine volcano is considered a natural laboratory which has brought the opportunity to carry out a regular multidisciplinary monitoring from the beginning of the unrest. The monitoring has exhibited that the changes produced by the eruption were temporal and all microbial parameters analyzed indicated that the microbial community returned to normal levels (Ferrera et al., 2015). However, there is still an enormous potential for the bioprospection of this unique ecosystem. To date, Tagoro submarine volcano represents the last hydrothermal system reported in the Canary Islands, and a hypothetical modern analog of a seafloor under iron formation in genesis (González et al., 2020).
Some studies on the analysis of microbial communities surrounding submarine volcanoes, such as the Kolumbo volcano and the Solfatara Crater, point out Proteobacteria as the most abundant phylum followed by other taxa represented into Firmicutes, Bacteroidetes, and Acidobacteria (Glamoclija et al., 2004; Christakis et al., 2018).
In this study it is showed that, among Proteobacteria, Vibrio was the most abundant genus, with 20% of the isolates among Gammaproteobacteria, whereas Bacillus was the most abundant genus representing 75% of the isolated strains within Firmicutes. In this context and taking into consideration the high metabolic flexibility and genome plasticity of Vibrio spp. (Wang et al., 2019), and in order to determine its phylogenetic diversity, our results suggest continuing with the investigation of the Vibrio community isolated from volcano Tagoro.
All Firmicutes strains correspond to order Bacillales, considered as fast-growing bacteria. These genera are represented among the most abundant cultured and widely distributed species (Al-Amoudi et al., 2016). Overall, evidence suggest that Gram-positive bacteria constitute a significant proportion of deep-sea communities. Among them, members of order Bacillales are known for their versatility and ability to form endospores that can survive as resistant forms (Gontang et al., 2007; Ettoumi et al., 2009, 2013). Despite their ability to survive under varied conditions and their marked phylogenetic diversity, marine Bacillus strains have attracted less interest with respect to their terrestrial counterparts (Ettoumi et al., 2009).
The phylogenetic analysis (Figure 5) agrees with a previous study carried out by Ferrera et al. (2015) that monitored the waters surrounding the volcano in El Hierro during the eruptive and post-eruptive phases by culture-independent methods. The analysis by flow cytometry and 454-pyrosequencing of 16S rRNA gene amplicons, exhibited that most sequences were related to the phyla Proteobacteria (68.4%), with Alphaproteobacteria (54.8%), and Gammaproteobacteria (10.0%) as the most abundant classes. Subsequently, González et al. (2020) characterized and identified the microbial biomineralization in the hydrothermal vent of volcano Tagoro which are involved in the iron, sulfur, and methane cycles. Most of the ubiquitous bacterial taxa were those commonly found in the water column. Around 50% of the bacterial DNA relative abundance is related to Marinomonas sp. and Pseudomonas sp., both corresponding to the Gammaproteobacteria class. Other taxa detected were Acidobacteria and Bacteroidetes. Even though we did not use specific media nor the addition of antibiotics, our results show an important proportion of the common bacterial community in the marine environment and reinforces the findings reported by Sanz-Sáez et al. (2020), who developed a marine culture collection of heterotrophic bacteria covering a variety of oceanographic regions from different seasons and years, using a standard marine medium with the aim to describe the fraction of the bacterioplankton community that can be commonly isolated under laboratory conditions. The strains were mainly affiliated with the classes Alphaproteobacteria (35.9%), Gammaproteobacteria (38.6%), and phylum Bacteroidetes (16.5%).
Natural products have been an important source of pharmacologically active compounds and more than 10% of them have a microbial origin. However, even when rediscovery of known compounds led to reduced interest in natural sources, currently, in the light of an increased appreciation for the exceeding rich and unique biodiversity in the oceans, has been a renewed interest on marine natural products (Lyu et al., 2021). Nonetheless, the supply limitation, which is the well-known bottleneck on natural products, led scientists to put the view on microbial resources supported by the special characteristics of marine environment (Jensen and Fenical, 2000; Desbois, 2014; Rotter et al., 2021).
During the last decades, active compounds has been tested and discontinued, meanwhile other antitumor drugs has been successfully completed either obtained by synthesis, cultivation or by production in other organism such as microorganisms (Stonik, 2009). Especially, anticancer compounds represent more than one half of the new marine natural products discovered from 1985 to 2012. In this sense, marine microbes have been considered a hot spot in natural products research and among them actinomycetes (47.01%) and bacteria (46.38%) represents the highest proportion of bioactive compounds (Hu et al., 2015).
Our results reveal the extracts of Micromonospora AR22-02 (Actinobacteria), Kangiella AR14-04 and Pseudoalteromonas AR04-01 (Gammaproteobacteria), and Halobacillus, AR14-06 and AR01-01 (Firmicutes) as the most active under the studied conditions, and support the previous suggestion that most anticancer molecules produced by marine bacteria are derived from Actinobacteria, Proteobacteria (Gammaproteobacteria), and Firmicutes (Gavriilidou et al., 2021). In this sense, a large group of anticancer peptides and some small molecules have been obtained from bacteria occurring in marine sediments comprising genera as Bacillus, Halobacillus, and Paenibacillus (Karpiñski and Adamczak, 2018).
Marine actinobacteria are well-known to have the potential to produce biomolecules with interesting novel bioactivities and it is assumed that each strain has the genetic potential to produce among 15–25 secondary metabolites (Ul Hassan et al., 2017). Within this phylum, Micromonospora and Streptomyces are considered the most prolific producers of secondary metabolites. Nevertheless, properties of each strain can be widely diverse. For example, Davies-Bolorunduro et al. (2019) evaluated the anticancer activity of 32 actinomycetes strains against a panel of cancer cell lines and the isolates of genera Micromonospora and Streptomyces exhibited cytotoxicity with IC50 values ranging from 0.03 to 4.4 mg/mL. Among other Phyla, some extracts of Halomonas and Bacillus have showed cytotoxicity with IC50 < 50 μg/mL (Aboul-Ela et al., 2012; Sagar et al., 2013; Chen et al., 2019). It is noticeable that many secondary metabolites have been isolated from Bacillus, including peptides, terpenoids, polyketides, and isocumarins, however, a few marine Bacillus strains have been examined for pharmaceutical activities (Chen et al., 2019).
Furthermore, it has been possible to establish a correlation between the effects of the use of different culture conditions and the antiproliferative activity for each single strain. In this case, lower GI50 values were observed when strains are grown under low nutrient conditions (1:5 diluted MB medium). Aside from nutrient requirements, the importance of agitation, which allows the renewal of nutrients (Bonnet et al., 2020) and a higher diffusion coefficient for oxygen into water (Somerville and Proctor, 2013) may explain the observed effects. These results reinforce the implementation of strategies that stimulate the production of secondary metabolism with potential biological activity (Pan et al., 2019). Once the experimental conditions have been established for the strains of interest, dereplication studies, and scale-up cultures will be developed to characterize the chemical entities responsible for the antiproliferative activity observed.
During the history of marine natural products research, several metabolites extremely toxic against tumor cells have been described, many of them belonging to new structural series of antitumor substances that opened new prospects for synthetic modeling. Until those substances were discovered, all the natural products applied in chemotherapy were referred to not more than 4–5 structural types (Stonik, 2009; Khalifa et al., 2019). For this reasons, bioprospection studies of singular marine ecosystems will open new opportunities for biodiscovery of novel specimens as well as novel lead compounds and its potential biological applications based on a sustainable supply.
Bioprospecting studies of marine microorganisms have been focused on certain phyla or classes and several microbial genera had been minimized, in part because of the expectation to the discovery novel strains that produce different metabolites and mechanisms of action compared to terrestrial microorganisms (Martín-Rodríguez et al., 2014; de Vera et al., 2018). Nonetheless, our results exhibit the antiproliferative potential of genus such as Halobacillus, Kangiella, Photobacterium, Halomonas, among others, isolated from a singular ecosystem as Tagoro submarine volcano. Their biotechnological development provides an excellent starting point to access novel secondary metabolites and enzymes with potential for pharmaceutical and industrial applications.
The data presented in the study are deposited in the GenBank (https://www.ncbi.nlm.nih.gov/genbank) databank, accession numbers MW826671–MW826754.
AD-M and JF designed, conceived and supervised the research study, and experiments. SG-D and AD-M developed the experimental work of isolation and taxonomic identification of bacteria, culture, and extraction of bacterial strains. SG-D and CR performed the phylogenetic analysis. IL and JP achieved the antiproliferative analysis. EF-N was responsible for oceanographic cruise design. JF, AD-M, EF-N, and JP were project administration and funding acquisition. All authors performed the analysis and data compilation, contributed to the article and approved the submitted version.
This work was supported by project BIOALGRI (PID2019-109476RB-C21) funded by Ministerio de Ciencia e Innovación del Gobierno de España (MICINN). The oceanography cruise and data samples used in this work were collected in the framework of two main projects: (1) VULCANO-II (CTM2014-51837-R), funded by the Ministerio de Economía y Competitividad del Gobierno de España (MINECO) and FEDER and (2) VULCANA-I (IEO-2015–2017), funded by the Spanish Institute of Oceanography (IEO). JP thanks the Spanish Government for financial support through project PGC2018-094503-B-C22 (MCIU/AEI/FEDER, UE). AD-M obtained support from funds of Agustín de Betancourt Program (Cabildo de Tenerife, TF Innova Program supported by MEDI and FDCAN funds). SG-D (grant 740689) and IL thank CONACYT for a postdoctoral fellowship.
The authors declare that the research was conducted in the absence of any commercial or financial relationships that could be construed as a potential conflict of interest.
We would like to thank the Spanish Institute of Oceanography (IEO) for let us participate in the VULCANO-II-1016 cruise on board R/V Ángeles Alvariño and of course, we would like to thank the officers and crew of the R/V for their help at sea. We would also like to thank support of Genomic Service of General Research Support Services (SEGAI-ULL), and O. Monterroso and T. Cruz for assistance in taxonomic classification of marine sponges.
The Supplementary Material for this article can be found online at: https://www.frontiersin.org/articles/10.3389/fmars.2021.687701/full#supplementary-material
Supplementary Figure 1 | Radar chart of the antiproliferative activity (GI50) expressed in μg/mL for each cancer cell line.
Supplementary Table 1 | Physical–chemical parameters at sampling stations, including pH (ΔpH) and temperature (Δθ) anomalies around the main and secondary crater (DA04–06), sampling data, and identification.
Supplementary Table 2 | Summary of the bacterial isolates and phylogenetically closely related species from GenBank according to 16S rRNA gene sequence similarity organized by sampling location.
Supplementary Table 3 | Antiproliferative activity (GI50, μg/mL) of the marine bacterial extracts against human solid tumor cell lines.
Aboul-Ela, H. M., Shreadah, M. A., Abdel-Monem, N. M., Yakout, G. A., and van Soest, R. W. M. (2012). Isolation, cytotoxic activity and phylogenetic analysis of Bacillus sp. bacteria associated with the red sea sponge Amphimedon ochracea. Adv. Biosci. Biotechnol. 3, 815–823. doi: 10.4236/abb.2012.37101
Al-Amoudi, A., Essack, M., Simões, M. F., Bougouffa, S., Soloviev, I., Archer, J. A. C., et al. (2016). Bioprospecting red sea coastal ecosystems for culturable microorganisms and their antimicrobial potential. Mar. Drugs 14:165. doi: 10.3390/md14090165
Álvarez-Valero, A. M., Burgess, R., Recio, C., de Matos, V., Sánchez-Guillamón, O., Gómez-Ballesteros, M., et al. (2018). Noble gas signals in corals predict submarine volcanic eruptions. Chem. Geol. 480, 28–34. doi: 10.1016/j.chemgeo.2017.05.013
Anisimova, M., and Gascuel, O. (2006). Approximate likelihood-ratio test for branches: a fast, accurate, and powerful alternative. Syst. Biol. 55, 539–552. doi: 10.1080/10635150600755453
Ariza, A., Kaartvedt, S., Røstad, A., Garijo, J. C., Arístegui, J., Fraile-Nuez, E., et al. (2014). The submarine volcano eruption off El Hierro Island: effects on the scattering migrant biota and the evolution of the pelagic communities. PLoS One 9:e102354. doi: 10.1371/journal.pone.0102354
Blanco, M. J., Fraile-Nuez, E., Felpeto, A., Santana-Casiano, J. M., Abella, R., Fernandez-Salas, L. M., et al. (2015). Comment on “Evidence from acoustic imaging for submarine volcanic activity in 2012 off the west coast of El Hierro (Canary Islands, Spain)” by Pérez NM, Somoza L, Hernández PA, González de Vallejo L, León R, Sagiya T, Biain A, González FJ, Medialdea T, Barrancos J, Ibáñez J, Sumino H, Nogami K and Romero C [Bull Volcanol (2014) 76:882-896]. Bull Volc. 77:62. doi: 10.1007/s00445-015-0947-6
Bode, H. B., Bethe, B., Höfs, R., and Zeeck, A. (2002). Big effects from small changes: possible ways to explore nature’s chemical diversity. ChemBioChem 3, 619–627. doi: 10.1002/1439-7633(20020703)3:7<619::AID-CBIC619<3.0.CO;2-9
Bonnet, M., Lagier, J. C., Raoult, D., and Khelaifia, S. (2020). Bacterial culture through selective and non-selective conditions: the evolution of culture media in clinical microbiology. N. Microb. N. Infect 34:100622. doi: 10.1016/j.nmni.2019.100622
Buijs, Y., Bech, P. K., Vazquez-Albacete, D., Bentzon-Tilia, M., Sonnenschein, E. C., Gram, L., et al. (2019). Marine Proteobacteria as a source of natural products: advances in molecular tools and strategies. Nat. Prod. Rep. 36, 1333–1350. doi: 10.1039/c9np00020h
Carroll, A. R., Copp, B. R., Davis, R. A., Keyzers, R. A., and Prinsep, M. R. (2021). Marine natural products. Nat. Prod. Rep 38, 362–413. doi: 10.1039/d0np00089b
Chappidi, S., Villa, E. C., and Cantarel, B. L. (2019). Using mothur to determine bacterial community composition and structure in 16S ribosomal RNA datasets. Curr. Protoc. Bioinform. 67:e83. doi: 10.1002/cpbi.83
Chen, L., Wang, X.-N., Fu, C.-M., and Wang, G. Y. (2019). Phylogenetic analysis and screening of antimicrobial and antiproliferative activities of culturable bacteria associated with the ascidian Styela clava from the Yellow Sea, China. BioMed. Res. Int. 2019:1. doi: 10.1155/2019/7851251
Christakis, C. A., Polymenakou, P. N., Mandalakis, M., Nomikou, P., Kristoffersen, J. B., Lampridou, D., et al. (2018). Microbial community differentiation between active and inactive sulfide chimneys of the Kolumbo submarine volcano, Hellenic Volcanic Arc. Extremophiles 22, 13–27. doi: 10.1007/s00792-017-0971-x
Coca, J., Ohde, T., Redondo, A., García-Weil, L., Santana-Casiano, M., González-Dávila, M., et al. (2014). Remote sensing of the El Hierro submarine volcanic eruption plume. Int. J. Remote Sens. 35, 6573–6598. doi: 10.1080/01431161.2014.960613
Danovaro, R., Canals, M., Tangherlini, M., Dell’Anno, A., Gambi, C., Lastras, G., et al. (2017). A submarine volcanic eruption leads to a novel microbial habitat. Nat. Ecol. Evol 1, 0144. doi: 10.1038/s41559-017-0144
Davies-Bolorunduro, O. F., Adeleye, I. A., Akinleye, M. O., and Wang, P. G. (2019). Anticancer potential of metabolic compounds from marine actinomycetes isolated from lagos lagoon sediment. J. Pharm. Anal. 9, 201–208. doi: 10.1016/j.jpha.2019.03.004
de Vera, C. R., Díaz Crespín, G., Hernández Daranas, A., Montalvão Looga, S., Lillsunde, K.-E., Tammela, P., et al. (2018). Marine microalgae: promising source for new bioactive compounds. Mar. Drugs 16:317. doi: 10.3390/md16090317
Dereeper, A., Audic, S., Claverie, J. M., and Blanc, G. (2010). BLAST-EXPLORER helps you building datasets for phylogenetic analysis. BMC Evol. Biol. 10:8. doi: 10.1186/1471-2148-10-8
Dereeper, A., Guignon, V., Blanc, G., Audic, S., Buffet, S., Chevenet, F., et al. (2008). Phylogeny.fr: robust phylogenetic analysis for the non-specialist. Nucleic Acids Res. 36(Suppl.2), W465–W469. doi: 10.1093/nar/gkn180
Desbois, A. P. (2014). How might we increase success in marine-based drug discovery? Expert Opin. Drug Discov. 9, 985–990. doi: 10.1517/17460441.2014.927863
Ettoumi, B., Guesmi, A., Brusetti, L., Borini, S., Najjari, A., Boudabous, A., et al. (2013). Microdiversity of deep-sea Bacillales isolated from Tyrrhenian Sea sediments as revealed by ARISA, 16S rRNA gene sequencing and BOX-PCR fingerprinting. Microb. Environ. 28, 361–369. doi: 10.1264/jsme2.ME13013
Ettoumi, B., Raddadi, N., Borin, S., Daffonchuio, D., Boudabous, A., and Cherif, A. (2009). Diversity and phylogeny of culturable spore-forming Bacilli isolated from marine sediments. J. Basic Microbiol. 49(Suppl.1), S13–S23. doi: 10.1002/jobm.200800306
Eugenio, F., Martin, J., Marcello, J., and Fraile-Nuez, E. (2014). Environmental monitoring of El Hierro Island submarine volcano, by combining low and high resolution satellite imagery. Int. J. Appl. Earth Obs. Geoinf. 29, 53–66. doi: 10.1016/j.jag.2013.12.009
Farias, P., Espírito Santo, C., Branco, R., Francisco, R., Santos, S., Hansen, L., et al. (2015). Natural hot spots for gain of multiple resistances: arsenic and antibiotic resistances in heterotrophic, aerobic bacteria from marine hydrothermal vent fields. Appl. Environ. Microbiol. 81, 2534–2543. doi: 10.1128/AEM.03240-14
Ferrera, I., Arístegui, J., González, J. M., Montero, M. F., Fraile-Nuez, E., and Gasol, J. M. (2015). Transient changes in bacterioplankton communities induced by the submarine volcanic eruption of El Hierro (Canary Islands). PLoS One 10:e0118136. doi: 10.1371/journal.pone.0118136
Fraile-Nuez, E., González-Dávila, M., Santana-Casiano, J. M., Arístegui, J., Alonso-González, I. J., Hernández-León, S., et al. (2012). The submarine volcano eruption at the island of El Hierro: physical-chemical perturbation and biological response. Sci. Rep. 2:486. doi: 10.1038/srep00486
Fraile-Nuez, E., Santana-Casiano, J. M., González-Dávila, M., Vázquez, J. T., Fernández-Salas, L. M., Sánchez-Guillamón, O., et al. (2018). Cyclic behavior associated with the degassing process at the shallow submarine Volcano Tagoro, Canary Islands, Spain. Geosciences 8:457. doi: 10.3390/geosciences8120457
Gavriilidou, A., Mackenzie, T. A., Sánchez, P., Tormo, J. R., Ingham, C., Smidt, H., et al. (2021). Bioactivity screening and gene-trait matching across marine sponge-associated bacteria. Mar. Drugs 19:75. doi: 10.3390/md19020075
Glamoclija, M., Garrel, L., Berthon, J., and López-García, P. (2004). Biosignatures and bacterial diversity in hydrothermal deposits of Solfatara Crater. Italy. Geomicrobiol. J. 21, 529–541. doi: 10.1080/01490450490888235
Gontang, E. A., Fenical, W., and Jensen, P. R. (2007). Phylogenetic diversity of gram-positive bacteria cultured from marine sediments. Appl. Environ. Microbiol. 73, 3272–3282. doi: 10.1128/AEM.02811-06
González, F. J., Rincón-Tomás, B., Somoza, L., Santofimia, E., Medialdea, T., Madureira, P., et al. (2020). Low-temperature, shallow-water hydrothermal vent mineralization following the recent submarine eruption of Tagoro volcano (El Hierro, Canary Islands). Mar. Geol. 430:106333. doi: 10.1016/j.margeo.2020.106333
González-Vega, A., Fraile-Nuez, E., Santana-Casiano, J. M., González-Dávila, M., Escánez-Pérez, J., Gómez-Ballesteros, M., et al. (2020). Significant release of dissolved inorganic nutrients from the shallow submarine volcano Tagoro (Canary Islands) based on seven-year monitoring. Front. Mar. Sci. 6:829. doi: 10.3389/fmars.2019.00829
Gouy, M., Guindon, S., and Gascuel, O. (2010). SeaView version 4: a multiplatform graphical user interface for sequence alignment and phylogenetic tree building. Mol. Biol. Evol. 27, 221–224. doi: 10.1093/molbev/msp259
Guindon, S., and Gascuel, O. (2003). A simple, fast, and accurate algorithm to estimate large phylogenies by maximum likelihood. Syst. Biol. 52, 696–704. doi: 10.1080/10635150390235520
Hoshino, T., Doi, H., Uramoto, G. I., Wörmer, L., Adhikari, R. R., Xiao, N., et al. (2020). Global diversity of microbial communities in marine sediment. Proc. Natl. Acad. Sci. U.S.A. 117, 27587–27597. doi: 10.1073/pnas.1919139117
Hu, Y., Chen, J., Hu, G., Yu, J., Zhu, X., Lin, Y., et al. (2015). Statistical research on the bioactivity of new marine natural products discovered during the 28 years from 1985 to 2012. Mar. Drugs 13, 202–221. doi: 10.3390/md13010202
Jensen, P. R., and Fenical, W. (2000). “Marine microorganisms and drug discovery: current status and future potential,” in Drugs from the sea, ed. N. Fusetani (Basel: Karger), 6–29. doi: 10.1159/000062490
Joint, I., Mühling, M., and Querellou, J. (2010). Culturing marine bacteria – an essential prerequisite for biodiscovery. Microb. Biotechnol. 3, 564–575. doi: 10.1111/j.1751-7915.2010.00188.x
Kaeberlein, T., Lewis, K., and Epstein, S. S. (2002). Isolating “uncultivable” microorganisms in pure culture in a simulated natural environment. Science (New York, N.Y.) 296, 1127–1129. doi: 10.1126/science.1070633
Karpiñski, T. M., and Adamczak, A. (2018). Anticancer activity of bacterial proteins and peptides. Pharmaceutics 10:54. doi: 10.3390/pharmaceutics10020054
Katoh, K., and Standley, D. M. (2013). MAFFT multiple sequence alignment software version 7: improvements in performance and usability. Mol. Biol. Evol 30, 772–780. doi: 10.1093/molbev/mst010
Khalifa, S. A. M., Elias, N., Farag, M. A., Chen, L., Saeed, A., Hegazy, M.-E. F., et al. (2019). Marine natural products: a source of novel anticancer drugs. Mar. Drugs 17, 491. doi: 10.3390/md17090491
Lyu, C., Chen, T., Qiang, B., Liu, N., Wang, H., Zhang, L., et al. (2021). CMNPD: a comprehensive marine natural products database towards facilitating drug discovery from the ocean. Nucleic Acids Res. 49, 509–515. doi: 10.1093/nar/gkaa763
Martín-Rodríguez, A. J., Reyes, F., Martín, J., Pérez-Yépez, J., León-Barrios, M., Couttolenc, A., et al. (2014). Inhibition of bacterial quorum sensing by extracts from aquatic fungi: first report from marine endophytes. Mar. Drugs 12, 5503–5526. doi: 10.3390/md12115503
Monks, A., Scudiero, D., Skehan, P., Shoemaker, R., Paull, K., Vistica, D., et al. (1991). Feasibility of a high-flux anticancer drug screen using a diverse panel of cultured human tumor cell lines. J. Nat. Cancer Inst. 83, 757–766. doi: 10.1093/jnci/83.11.757
Mu, D. S., Ouyang, Y., Chen, G. J., and Du, Z-J. (2021). Strategies for culturing active/dormant marine microbes. Mar. Life Sci. Technol. 3, 121–131. doi: 10.1007/s42995-020-00053-z
Nikapitiya, C. (2012). Bioactive secondary metabolites from marine microbes for drug discovery. Adv. Food Nutr. Res. 65, 363–387. doi: 10.1016/B978-0-12-416003-3.00024-X
Orellana, E. A., and Kasinski, A. L. (2016). Sulforhodamine B (SRB) assay in cell culture to investigate cell proliferation. Bio. Protoc. 6:e1984. doi: 10.21769/BioProtoc.1984
Pan, R., Bai, X., Chen, J., Zhang, H., and Wang, H. (2019). Exploring structural diversity of microbe secondary metabolites using OSMAC strategy: a literature review. Front. Microbiol. 10:294. doi: 10.3389/fmicb.2019.00294
Poli, A., Finore, I., Romano, I., Gioiello, A., Lama, L., and Nicolaus, B. (2017). Microbial diversity in extreme marine habitats and their biomolecules. Microorganisms 5:25. doi: 10.3390/microorganisms5020025
Puglisi, M. P., Sneed, J. M., Ritson-Williams, R., and Young, R. (2019). Marine chemical ecology in benthic environments. Nat. Prod. Rep. 36, 410–429. doi: 10.1039/C8NP00061A
Reen, F. J., Romano, S., Dobson, A. D. W., and O’Gara, F. (2015). The sound of silence: activating silent biosynthetic gene clusters in marine microorganisms. Mar. Drugs 13, 4754–4783. doi: 10.3390/md13084754
Rivera, J., Lastras, G., Canals, M., Acosta, J., Arrese, B., Hermida, N., et al. (2013). Construction of an oceanic island: In-sights from the El Hierro (Canary islands) 2011–2012 submarine volcanic eruption. Geology 41, 355–358. doi: 10.1130/G33863.1
Romano, S., Jackson, S., Patry, S., and Dobson, A. (2018). Extending the “One Strain Many Compounds” (OSMAC) principle to marine microorganisms. Marine Drugs 16:244. doi: 10.3390/md16070244
Rotter, A., Barbier, M., Bertoni, F., Bones, A. M., Cancela, M. L., Carlsson, J., et al. (2021). The essentials of marine biotechnology. Front. Mar. Sci. 8:629629. doi: 10.3389/fmars.2021.629629
Sagar, S., Esau, L., Hikmawan, T., Antunes, A., Holtermann, K., Stingl, U., et al. (2013). Cytotoxic and apoptotic evaluations of marine bacteria isolated from brine-seawater interface of the Red Sea. BMC Complement. Altern. Med. 13:29. doi: 10.1186/1472-6882-13-29
Santana-Casiano, J., Fraile-Nuez, E., González-Dávila, M., Baker, E. T., Resing, J. A., and Walker, S. L. (2016). Significant discharge of CO2 from hydrothermalism associated with the submarine volcano of El Hierro Island. Sci. Rep. 6:25686. doi: 10.1038/srep25686
Santana-Casiano, J., González-Dávila, M., and Fraile-Nuez, E. (2018). “The emissions of the Tagoro submarine volcano (Canary Islands, Atlantic Ocean): Effects on the physical and chemical properties of the seawater,” in Volcanoes – Geological and geophysical settings, theoretical aspects and numerical modeling, applications to industry and their impact in the human health, ed. G. Aiello (IntechOpen), London, UK, 53–72. doi: 10.5772/intechopen.70422
Santana-Casiano, J., González-Dávila, M., Fraile-Nuez, E., de Armas, D., González, A. G., Domínguez-Yanes, J. F., et al. (2013). The natural ocean acidification and fertilization event caused by the submarine eruption of El Hierro. Sci. Rep. 3:1140. doi: 10.1038/srep01140
Santana-González, C., Santana-Casiano, J. M., González-Dávila, M., and Fraile-Nuez, E. (2017). Emissions of Fe(II) and its kinetic of oxidation at Tagoro submarine volcano. El Hierro Mar. Chem. 195, 129–137. doi: 10.1016/j.marchem.2017.02
Sanz-Sáez, I., Salazar, G., Sánchez, P., Lara, E., Royo-Llonch, M., Sà, E. L., et al. (2020). Diversity and distribution of marine heterotrophic bacteria from a large culture collection. BMC Microbiol. 20:207. doi: 10.1186/s12866-020-01884-7
Schloss, P. D., Westcott, S. L., Ryabin, T., Hall, J. R., Hartmann, M., Hollister, E. B., et al. (2009). Introducing mothur: open-source, platform-independent, community-supported software for describing and comparing microbial communities. Appl. Environ. Microbiol. 75, 7537–7541. doi: 10.1128/AEM.01541-09
Silva, L. J., Crevelin, E. J., Souza, D. T., Lacerda-Júnior, G. V., de Oliveira, V. M., Ruiz, A. L. T. G., et al. (2020). Actinobacteria from Antarctica as a source for anticancer discovery. Sci. Rep. 10:13870. doi: 10.1038/s41598-020-69786-2
Somerville, G. A., and Proctor, R. A. (2013). Cultivation conditions and the diffusion of oxygen into culture media: The rationale for the flask-to-medium ratio in microbiology. BMC Microbiol. 13:9. doi: 10.1186/1471-2180-13-9
Sotomayor-García, A., Rueda, J. L., Sánchez-Guillamón, O., Urra, J., Vázquez, J. T., Palomino, D., et al. (2019). First macro-colonizers and survivors around Tagoro submarine volcano. Canary Islands, Spain. Geosciences 9:52. doi: 10.3390/geosciences9010052
Sotomayor-García, A., Rueda, J. L., Sánchez-Guillamón, O., Vázquez, J. T., Palomino, D., Fernández-Salas, L. M., et al. (2020). “Geomorphic features, main habitats and associated biota on and around the newly formed Tagoro submarine volcano, Canary Islands,” in Seafloor Geomorphology as Benthic Habitat, eds T. Peter and E. B. Harris (Amsterdam: Elsevier), 835–846. doi: 10.1016/B978-0-12-814960-7.00051-8
Speer, K. G. (1989). A forced baroclinic vortex around a hydrothermal plume. Geophys. Res. Lett. 16, 461–464. doi: 10.1029/GL016i005p00461
Stonik, V. A. (2009). Marine natural products: a way to new drugs. Acta Nat. 1, 15–25. doi: 10.32607/20758251-2009-1-2-15-25
Ul Hassan, S. S., Anjum, K., Abbas, S. Q., Akhter, N., Shagufta, B. I., Shah, S. A. A., et al. (2017). Emerging biopharmaceuticals from marine actinobacteria. Environ. Toxicol. Pharmacol. 49, 34–47. doi: 10.1016/j.etap.2016.11.015
Wang, E., Sorolla, M. A., Krishnan, P. D. G., and Sorolla, A. (2020). From seabed to bedside: a review on promising marine anticancer compounds. Biomolecules 10:248. doi: 10.3390/biom10020248
Wang, X., Liu, J., Li, B., Liang, J., Sun, H., Zhou, S., et al. (2019). Spatial heterogeneity of Vibrio spp. in sediments of Chinese marginal seas. Appl. Environ. Microbiol. 85, e3064–e3018. doi: 10.1128/AEM.03064-18
Weisburg, W. G., Barns, S. M., Pelletier, D. A., and Lane, D. J. (1990). 16S Ribosomal DNA Amplification for Phylogenetic Study. Framingham, MA: GENE-TRAK Systems.
Keywords: bioprospection, microbial biodiversity, marine bacteria, OSMAC, antiproliferative activity, Tagoro, singular ecosystem, submarine volcanoes and hydrothermal vents
Citation: García-Davis S, Reyes CP, Lagunes I, Padrón JM, Fraile-Nuez E, Fernández JJ and Díaz-Marrero AR (2021) Bioprospecting Antiproliferative Marine Microbiota From Submarine Volcano Tagoro. Front. Mar. Sci. 8:687701. doi: 10.3389/fmars.2021.687701
Received: 29 March 2021; Accepted: 30 June 2021;
Published: 22 July 2021.
Edited by:
Marlen Ines Vasquez, Cyprus University of Technology, CyprusReviewed by:
Masteria Yunovilsa Putra, Indonesian Institute of Sciences, IndonesiaCopyright © 2021 García-Davis, Reyes, Lagunes, Padrón, Fraile-Nuez, Fernández and Díaz-Marrero. This is an open-access article distributed under the terms of the Creative Commons Attribution License (CC BY). The use, distribution or reproduction in other forums is permitted, provided the original author(s) and the copyright owner(s) are credited and that the original publication in this journal is cited, in accordance with accepted academic practice. No use, distribution or reproduction is permitted which does not comply with these terms.
*Correspondence: Ana R. Díaz-Marrero, YWRpYXptYXJAdWxsLmVkdS5lcw==; José J. Fernández, ampmZXJjYXNAdWxsLmVkdS5lcw==
Disclaimer: All claims expressed in this article are solely those of the authors and do not necessarily represent those of their affiliated organizations, or those of the publisher, the editors and the reviewers. Any product that may be evaluated in this article or claim that may be made by its manufacturer is not guaranteed or endorsed by the publisher.
Research integrity at Frontiers
Learn more about the work of our research integrity team to safeguard the quality of each article we publish.