- 1Institute for Terrestrial and Aquatic Wildlife Research (ITAW), University of Veterinary Medicine Hannover Foundation, Büsum, Germany
- 2Department of Animal Ecology, Federal Institute of Hydrology, Koblenz, Germany
- 3Institute for Integrated Natural Sciences, University of Koblenz-Landau, Koblenz, Germany
Microplastic ingestion by lower trophic level organisms is well known, whereas information on microplastic ingestion, egestion and accumulation by top predators such as cetaceans is still lacking. This study investigates microplastics in intestinal samples from harbour porpoises (Phocoena phocoena) found along the coastline of Schleswig-Holstein (Germany) between 2014 and 2018. Out of 30 individuals found along the North Sea (NS) and the Baltic Sea (BS) coast, 28 specimens contained microplastic. This study found a relationship between the nutritional status of cetaceans and the amount of found microplastics. Harbour porpoises with a good or moderate nutritional status contained a higher number of microplastics, when compared with specimens in a poor nutritional status. In addition, when individuals died accidently due to suspected bycatch in gillnets, where a feeding event is highly assumed or a pharyngeal entrapment happened, the microplastic burden was higher. In total, 401 microplastics (≥100 μm), including 202 fibres and 199 fragments were found. Intestines of the specimens of the BS contained more microplastics than the ones from the NS. Differences in the share of fibres could be revealed: for BS fibres constituted 51.44% and for NS, fibres constituted 47.97%. The polymers polyester, polyethylene, polypropylene, polyamide, acrylic (with nitrile component) and an acrylic/alkyd paint chip (with styrene and kaolin components) were identified. This is the first study investigating the occurrence of microplastics in harbour porpoises from German waters and will, thus, provide valuable information on the actual burden of microplastics in cetaceans from the North and Baltic Seas.
Introduction
The ubiquitous presence of marine litter, and especially the occurrence of small particles called microplastics (<5 mm) (Arthur et al., 2009) is already confirmed in different marine habitats and organisms (Fossi et al., 2014; Lusher et al., 2015b; Pereira et al., 2020). A trophic transfer of microplastic particles between species of different trophic levels can be assumed as this has previously been determined by other studies (Farrell and Nelson, 2013; Setälä et al., 2014; Nelms et al., 2018). In addition, the ingestion and presence of microplastics has been highly studied throughout the food web in recent years (Miller et al., 2020). Besides the unintentional uptake of microplastics by prey species, an intentional uptake by organisms caused by a burdened environment or due to accidental prey resemblance has already been shown (Ory et al., 2017; Roch et al., 2020).
When focusing on the study area, a microplastic burden in the North-East Atlantic area and its organisms occupying different trophic levels has also been verified (Lusher et al., 2013; Karlsson et al., 2017). Furthermore, it is assumed that microplastics and pollutants accumulate in marine top predator species (Fossi et al., 2014; Jepson et al., 2016; Garcia-Cegarra et al., 2021). The ingestion of marine litter is already confirmed by previous studies in the North Sea (NS) and Baltic Sea (BS) (Unger et al., 2017; van Franeker et al., 2018). This is not surprising, since the NS and adjacent waters are assumed to be highly affected by anthropogenic exploitations (Halpern et al., 2008). Nevertheless, the knowledge on the burden of microplastics in top predators, particularly in cetaceans of the eastern North Atlantic region, including the BS, is still lacking. Both seas are different in topography, salinity, hydrology (Frid et al., 2003; Sjöqvist et al., 2015), and the occurring ship traffic (OSPAR, 2021). Thus, differences in the presence and absence of marine litter and in particular of microplastics between both seas could be hypothesised.
For investigating this knowledge gap, this study focusses on intestinal samples of harbour porpoises (Phocoena phocoena) originating from both seas. This species is the only regularly occurring cetacean in the southern NS, and the only cetacean inhabiting the BS (Hammond et al., 2017). Therefore, this study aimed to gain knowledge regarding the following three aspects: (i) assessment of the general risk of microplastic accumulation in harbour porpoises, (ii) evaluation of potential health impacts, and (iii) the comparison between the individuals originating from the NS and the BS. The porpoises found along the German NS coast are part of the North-East Atlantic population, and the Baltic individuals belong to the subspecies of the western Baltic population (Gaskin, 1984; Andersen, 2003; Lah et al., 2016).
This is the first retrospective investigation of microplastics in relation to the health status of harbour porpoises from German waters to date, examining particles which are smaller in size than 1 mm. Based on the collected information on sex, age and health status during necropsies, this study enables to determine potential relationships between a suspected microplastic burden and different health aspects in harbour porpoises originating from the NS and the BS for the first time. In addition, since the polymer types of found particles are determined by micro-Fourier-transform infrared spectroscopy (μFTIR), possible microplastic sources could be hypothesised and discussed.
Materials and Methods
Based on the well-established stranding network in Schleswig-Holstein (Germany), carcasses of stranded and bycaught harbour porpoises from the NS and BS are collected in the course of a health monitoring (Siebert et al., 2001, 2020; Lehnert et al., 2005). This monitoring is established since 1990 at the Institute for Terrestrial and Aquatic Wildlife Research (ITAW), which regularly conducts necropsies of harbour porpoises using a standardised protocol (Siebert et al., 2001, 2020). Since 2014, intestinal samples of marine mammals, including harbour porpoises, were exclusively collected for microplastic analysis. Based on the necropsies, the age, sex, health status and the location in which each individual was found is assessed and recorded. Thus, this information is available for the investigated intestinal samples from harbour porpoises found between 2014 and 2018.
The following criteria were applied for choosing the most suitable samples: (i) the gastrointestinal tract (GIT) had to be intact, (ii) faeces were present, and (iii) the individual was already weaned. 30 individuals were chosen for analysis: 14 individuals from the NS and 16 individuals from the BS (Figure 1).
The intestinal samples were stored in pre-cleaned glass jars at −20°C until further processing. Then, each defrosted and opened intestinal sample was placed into a double-layered washing sachet made of nylon cloths. The inner bag of the washing sachet has a mesh size of 300 μm and the outer bag has a mesh size of 100 μm. Both cloths, including the sample, were sewn together with the help of a conventional sewing machine, resulting in a so-called washing sachet. These washing sachets were washed in a conventional washing machine at 60°C without spinning cycle. For the removal of biogenic matter, an enzyme based detergent and a conventional detergent were added for facilitating the washing procedure. Subsequently, a density separation, a vacuum filtration onto cellulose filters (Rotilabo®, Typ 11A, Ø 55 mm, retention 12–15 μm) and fluorescence microscopy enabled by Nile Red (diluted with chloroform) staining were conducted for microplastic isolation and identification. Subsequently, all potential microplastics found on the cellulose filters were photographed, counted, and measured in size. All steps of sample processing were conducted in a closed acrylic box to avoid airborne contamination. The whole implementation of sample handling and processing is described in detail in Philipp et al. (2020).
For polymer identification, selected microplastic particles were manually collected. In addition, a disinfectant step was conducted to exclude a passing on of bacterial or parasitical zoonosis. For this purpose, the cellulose filters containing the stained particles were sprayed with ethanol (70%). After evaporation, the particles showed the same fluorescence qualities as before. Thus, the potential microplastics were selected and manually collected with tweezers or needle pins and placed into a droplet of ethanol (70%) onto an aluminium oxide membrane filter (Anodisc, Ø 47 mm, 0.2 μm pore size, Whatman, Freiburg, Germany). The filter was kept still until the droplet was evaporated and the particles had attached to the filter. Since the transfer of particles was done manually, a loss of particles needs to be taken into account.
The polymer composition of 77 potential microplastics (incl. fragments and fibres) from intestinal sample were analysed by using a μFTIR spectroscope (Hyperion 2000, Bruker, Ettlingen, Germany). All measurements were conducted in transmission mode with 32 co-added scans (sometimes 100 scans for very thin fibres) and a spectral resolution of 4 cm–1 in a wavenumber range of 4,000–1,250 cm–1 as aluminium oxide membrane filters are infrared inactive between 3,800 and 1,250 cm–1. For background measurements, the blank aluminium oxide membrane filter was used. For thick particles, for which transmission mode was not suitable, the measurements were conducted in attenuated total reflectance (μATR) mode. Those μATR measurements were conducted between 4,000 and 600 cm–1.
Procedural blanks of the used detergents and materials, e.g., nylon sachets (n = 3), and the working environment (n = 10) were taken into account for avoiding an overestimation caused by secondary contamination. The analysed blank filters of the working environment accompanied the samples from time of collection until the staining procedure was finished.
On average, one fibre and seven particles were found in those procedural blanks and were finally subtracted from the microplastic counts in each parallel sample. Four of those potential microplastics could be collected, manually placed on the aluminium oxide membrane filter and were considered for μFTIR analysis. Moreover, the polymer composition of different equipment materials like the nitrile gloves and shavings of the used acrylic box were additionally determined by FTIR in ATR mode (Vertex 70; Bruker, Ettlingen, Germany) or by μFTIR to avoid an overestimation. For the Vertex measurements, ATR measurements were performed in a wavenumber range of 4,000–370 cm–1 with 8 co-added scans and a spectral resolution of 4 cm–1.
The quantity of found microplastics in comparable groups is given in mean ± standard deviation (M ± SD) to enable a comparison between findings. Moreover, the results were statistically analysed by determining the Cohen’s d and applying a paired t-test using the package “pwr” in the R software Version 4.0.2 (Champely et al., 2020; R Core Team, 2020). Thus, results were described as significant if p < 0.05. In addition, the Figures 2, 5–7 were visualised using the package “ggplot2” (Wickham, 2016).
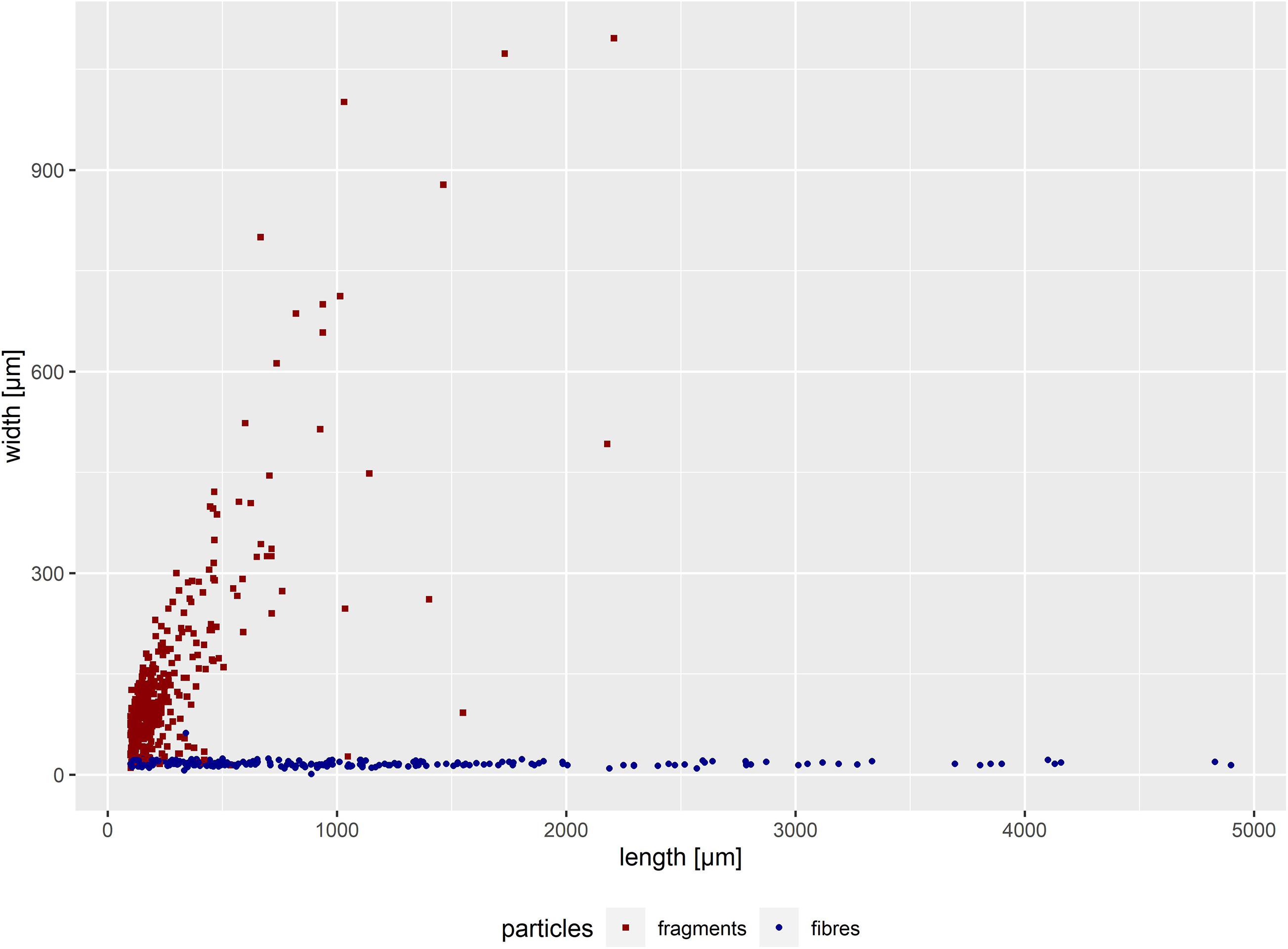
Figure 2. Width-length distribution of all suspected microplastic particles in intestine samples of 30 harbour porpoise in the size range of 100 μm up to 5,000 μm (n = 611). Secondary contamination was not considered.
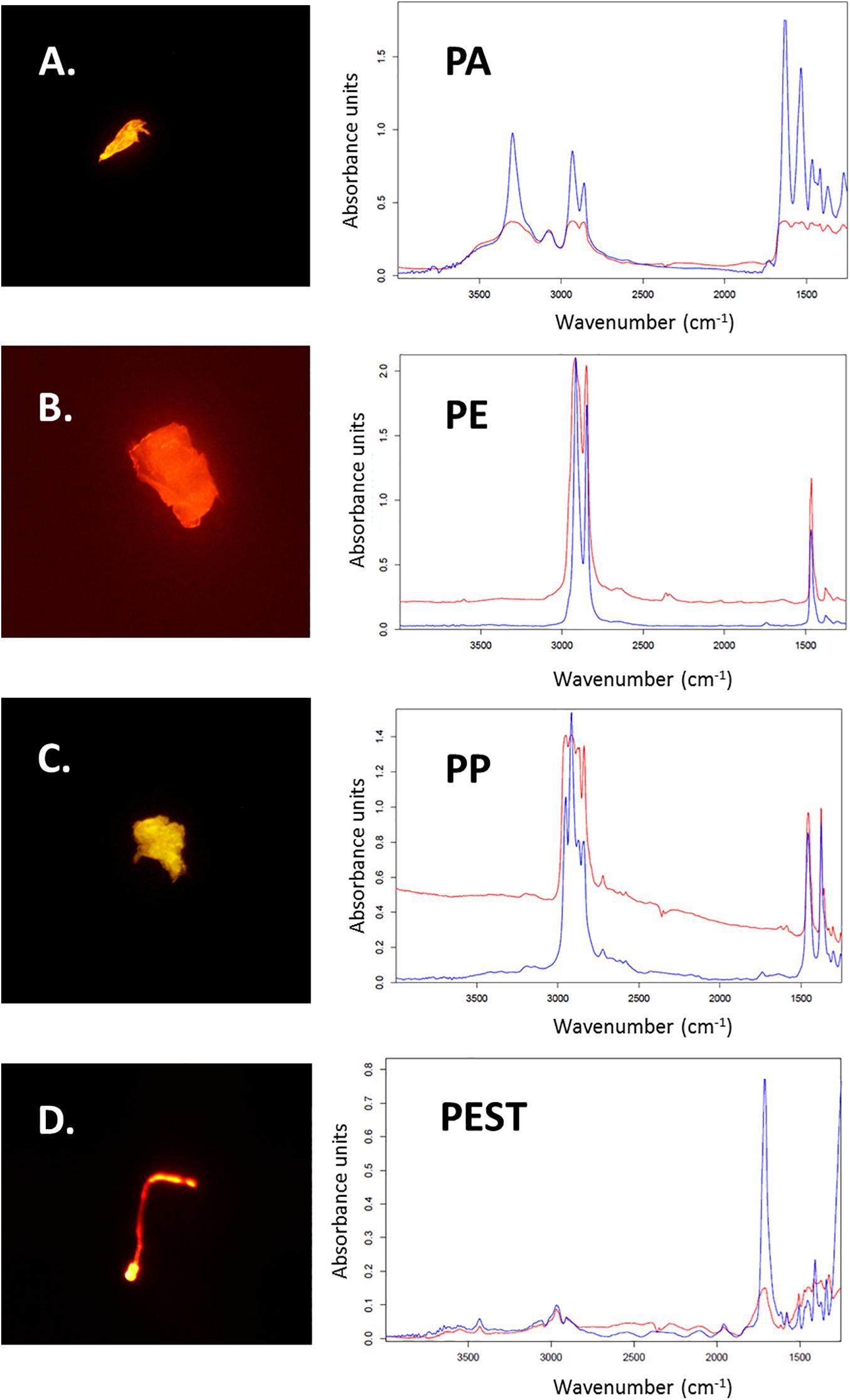
Figure 3. Microplastic particles stained with Nile Red (A–D) and the corresponding measured μFTIR spectra of found polymers (in red; Polyamide: PA; Polyethylene: PE; Polypropylene: PP and Polyester: PEST). Reference spectra from the OPUS software are blue (Bruker, Ettlingen, Germany).
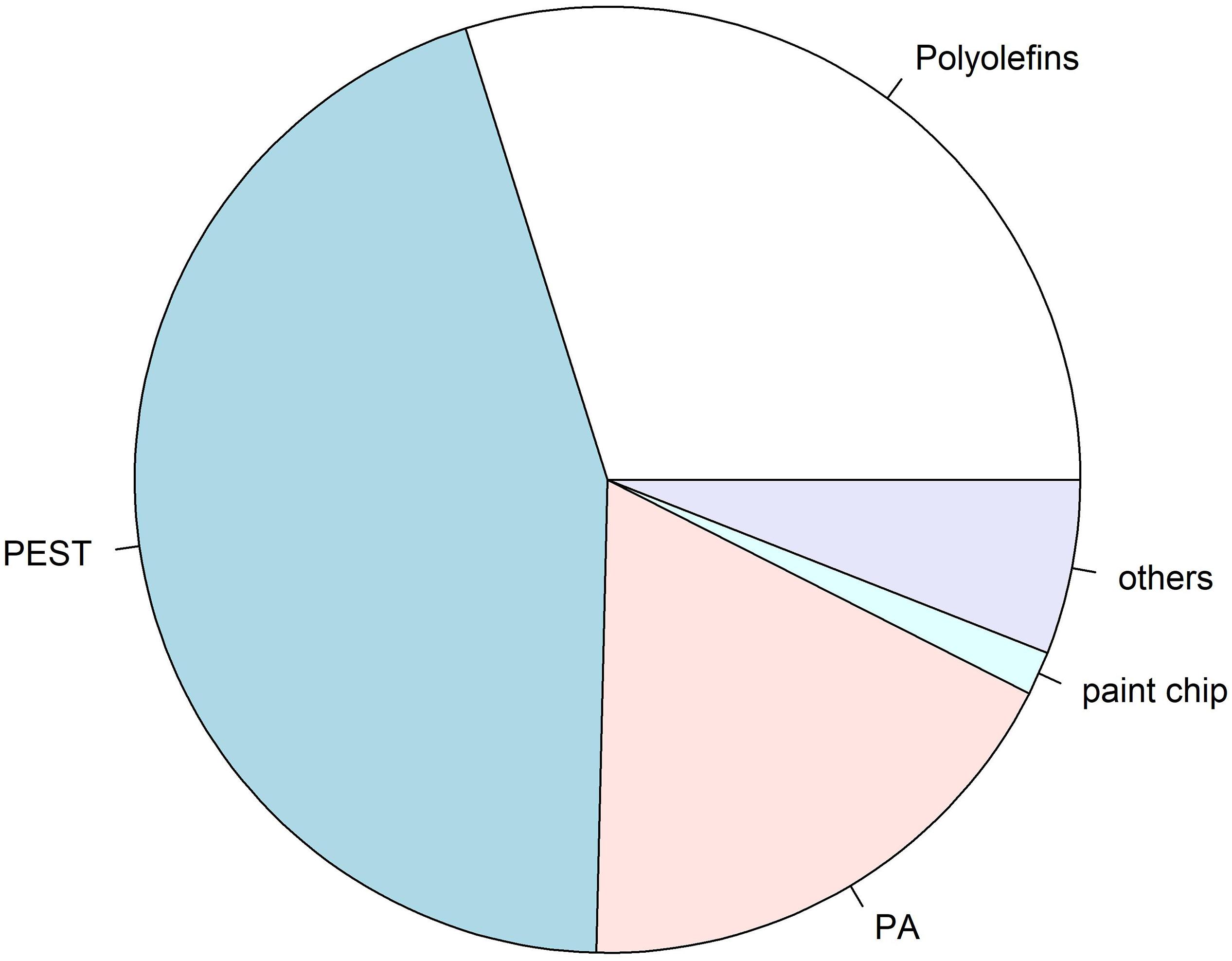
Figure 4. Overview of identified polymer compositions of microplastics (n = 67) found in intestinal samples analysed by analysed by μFTIR spectroscopy: PEST: 30%, polyolefine: 20% (incl. PE and PP), PA: 12%, paint chip: 1%, others: 4% (incl. acrylic with nitrile component, and cellulose acetate). Polymer composition of microplastics found in procedural blanks was not considered for this overview, albeit the coincidence of polymers in procedural blanks and samples were considered, and thus not taken into account.
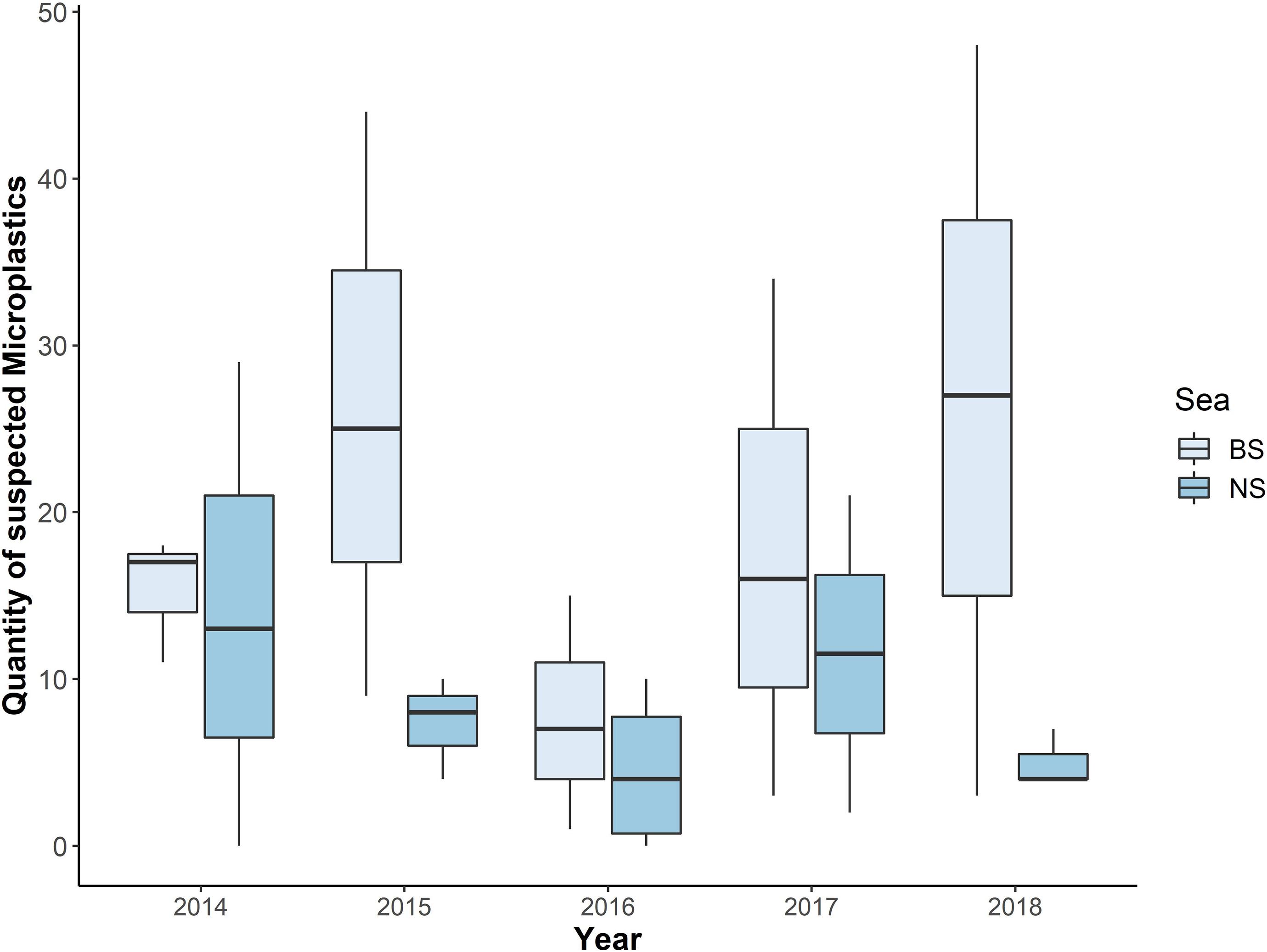
Figure 5. Quantity of suspected microplastics from each year, divided per sea (BS = Baltic Sea; NS = North Sea; n = 401).
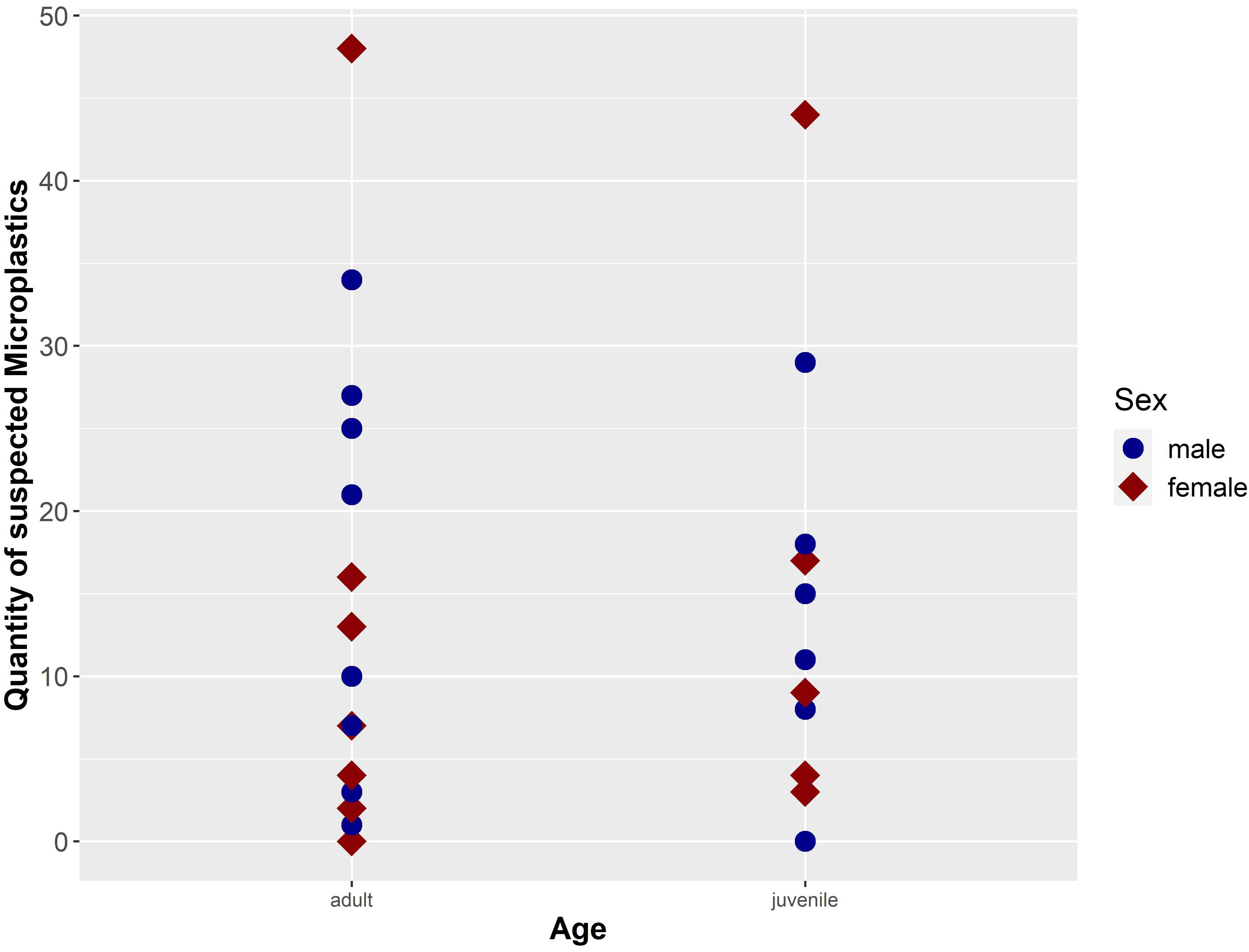
Figure 6. Quantity of suspected microplastic particles (n = 401; secondary contamination was considered and subtracted) separated by sex and age of the investigated harbour porpoises (n = 30) from German waters (NS and BS).
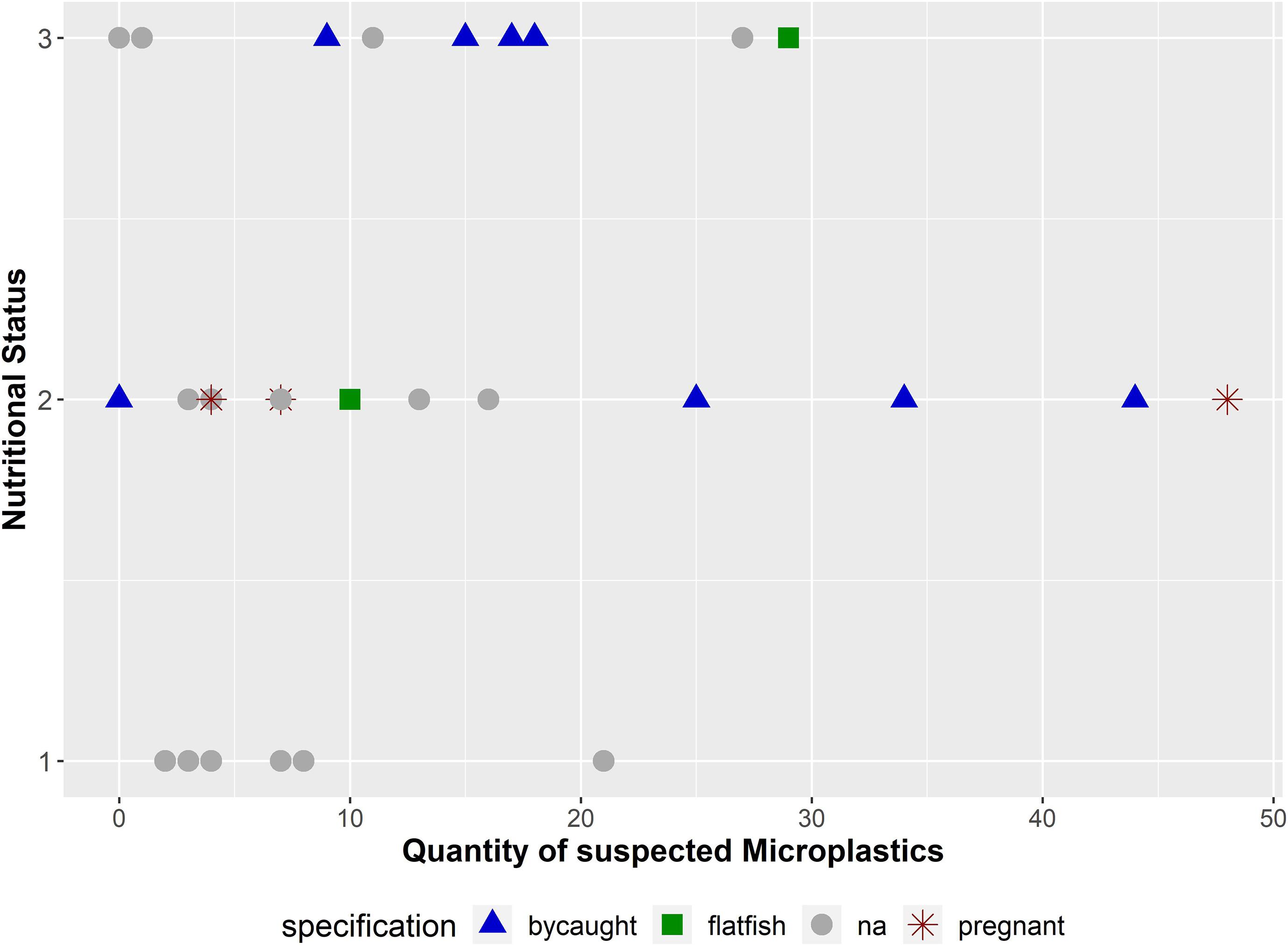
Figure 7. Quantity of suspected microplastics (n = 401) in combination with the nutritional status and further information about the carcasses (bycaught: the harbour porpoise was (suspected to be) bycaught by a fishing boat or in a gillnet; flatfish: the harbour porpoise was affected by a pharyngeal entrapment; na: no extraordinary finding could be revealed; pregnant: pregnancy in female was noticed). The nutritional status is coded as follows: (1): bad, (2): moderate, and (3): good.
Results
Quantity and Size
In total, 30 intestinal samples were available for analyses. An amount of 611 potential microplastics (incl. fragments and fibres, >100 μm) were found. A secondary contamination of one fibre and seven fragments were considered and subtracted from each sample. Thus, 401 microplastics were finally determined. This amount of microplastics was found in 28 intestinal samples, in the remaining an absence of microplastics was noticed. When categorising into particle type, 202 fibres and 199 fragments were found. Hence, only two intestines were free from microplastics. Most of the found fibres had a length between 100 and 2,000 μm (Figure 2).
Four additional fibres longer than 5,000 μm, thus defined as mesoplastics (Gregory and Andrady, 2003), were found. Three of them occurred in a sample of an adult male harbour porpoise found in 2017 (lengths: 8,450, 6,964, and 8,029 μm). The fourth fibre (7,365 μm in length) was found in a juvenile male stranded in 2014. Both carcasses were found in the BS. Based on the size, those four fibres were excluded from the results.
FTIR Results
Out of all 611 potential microplastics found in the 30 intestinal samples from the harbour porpoises, originally 94 particles (16%) were selected for polymer identification by μFTIR. Those fibres and fragments were manually collected and placed onto Anodisc membrane filters. Subsequently, 77 particles (12%, nfibres = 28, nfragments = 49) found in the intestinal samples were finally analysed by μFTIR. The remaining 17 microplastics (nfibres = 7, nfragments = 10) were either lost during the sample transport in closed petri dishes to the analysing site or could not be measured due to their small size. Polyester (PEST) was the most frequently found polymer in those investigated intestinal samples (nPEST = 30), followed by polyethylene (PE, n = 17) and polyamide (PA, n = 12) (Figure 3). Furthermore, two polypropylene (PP) particles, one paint chip (acrylic/alkyd with kaolin and styrene) (see Figure in the Supplementary Material), one none further identified polyolefin and one cellulose acetate fibre (which is a semi-synthetic cellulose) were determined. Three acrylic particles, including two with nitrile component, were additionally found. A visualisation of the found polymers (n = 67) is given in Figure 4.
Moreover, the polymer composition of two fragments (one fragment found in an intestine and one from a procedural blank) could not be identified. However, both showed strong similarities and were excluded from the analysis. Only four potential microplastic particles (nfibres = 2, nfragments = 2) were found on all procedural blank filters, and were additionally analysed by μFTIR. One fibre from the blanks was lost and one fragment could not be clearly spectroscopically identified. However, the other fibre was identified as PEST and the second fragment was determined as varnish with kaolin, styrene and calcium carbonate. Furthermore, two fragments from the intestinal samples had spectra which were highly similar to the varnish which was found in a procedural blank. Hence, those particles were excluded from the analysis. In five cases of potential microplastic particles, biogenic matter was identified and a sixth particle was clearly different from plastic. In addition, one particle could not be identified due to its small size, since it was broken during the manual collection and placement on the aluminium oxide filter.
Differences Between Seas
Comparing the samples based on their origin (NS or BS), there was a significantly higher amount of microplastics in individuals from the BS if compared to the NS (nBS = 278; M ± SD = 18.27 ± 14.54; nNS = 123; M ± SD = 8.2 ± 7.89; p-value = 0.03). Furthermore, the highest number of 48 microplastic particles was found in an adult female from the BS. When comparing the share of fibres in both seas, significant differences could be revealed (BS: 51.44%; NS: 47.97%; p-value = 0.02). The share of fragments, however, was similiar across locations (BS: 48.56%; NS: 52.03%; p-value = 0.1).
Differences Per Year
The annual mean values for each sea revealed a higher number of microplastic particles in harbour porpoises from the BS. Furthermore, the range of microplastics found in individuals from the NS was mostly between zero and up to 10 particles per individual in 2015, 2016, and 2018. Only in 2014 and 2017, more than 20 particles were found in the intestinal samples from the NS (2014: 29 and 2017: 21). However, in the BS samples more than 30 particles were found in 2015, 2017, and 2018. The years 2015 and 2018 were the ones with the highest number of findings per individual (44 particles in 2015 and 48 particles in 2018). In two cases from the NS, microplastics were not present (2014 and 2016). In comparison, in the BS microplastic was found in all samples. All this information is presented in Figure 5. However, no significant differences could be determined between the two sample sites (NS and BS; p-value = 0.21), mainly because of the low power of the statistical analysis, which resulted from the low sample size within each year and sea. Following the power analysis, a sample size of at least 12 individuals per year for each sea would be necessary for a reliable trend interpretation (power 80%, p-value = 0.05).
Differences in Age and Sex
This study investigates intestinal samples of 13 female and 17 male harbour porpoises (Figure 6). The microplastic burden in females is slightly higher (M ± SD = 13.38 ± 15.41), when compared to the amount of microplastics in males (M ± SD = 13.35 ± 10.56). Certainly, no significant difference in microplastic load could be revealed between sexes (p-value = 0.99). Moreover, no significant differences between adult harbour porpoises (n = 21; M ± SD = 13.82 ± 13.25) and juvenile ones (n = 9; M ± SD = 12.18 ± 12.31) were confirmed (p-value = 0.82), although the microplastic amount in adult ones seemed higher. The two unburdened samples from the NS originated from a juvenile male and an adult female. In both age classes the highest amount of microplastics was found in females (adult: 48 particles; juvenile: 44 particles; see Figure 6).
Health Status
The evaluation of the whole GIT revealed an absence of parasite specimens in all investigated intestines. A mild enteritis was found for seven individuals. In detail, in one of those harbour porpoises a mild diffuse eosinophilic enteritis was determined. Furthermore, in two cases (juveniles) a mild diffuse eosinophilic enteritis and a focal mural one were determined. A most likely parasitic etiology was observed in those two harbour porpoises. A fourth individual was affected by a diffuse mild lymphocytic-plasmacellular and eosinophilic infiltration of the lamina propria in combination with a moderate hyperplasia of the Peyer’s plaques. Three further porpoises showed evidence of gastritis (mild and high grade) and enteritis. Whereof two individuals suffered from a mild non-suppurative enteritis, and the third one was also affected by a diffuse moderate lymphocytic-plasmacellular and eosinophilic infiltration of the lamina propria. In total, parasite infestations of e.g. Pholeter gastrophilus and Anisakis simplex in the multi-chambered stomach was confirmed in 12 harbour porpoises.
Harbour porpoises investigated in this study, which were either accidentally bycaught (Siebert et al., 2020) or affected by a pharyngeal entrapment (Gross et al., 2020), showed a microplastic burden of 19.8 particles (SD = 12.77; n = 11) per individual. Compared to the remaining ones (n = 19), where no accidental death could be diagnosed (incl. the three pregnant females), a lower mean value of 10 (SD = 11.59) microplastics per porpoise was identified. Furthermore, if the individual was in a good (n = 9) or moderate (n = 14) nutritional status, the mean number of particles was significantly higher (Meangood = 14.11; Meanmoderate = 16.07) in contrast to a bad nutritional status (n = 7; Meanbad = 7) (Figure 7). This is also confirmed by the statistical analysis (p-value = 0.04).
Discussion
This study is the first to evaluate the microplastic burden in marine mammals inhabiting German waters focussing on particles smaller than 1 mm, in marine mammals inhabiting German waters. Furthermore, intestinal samples of harbour porpoises originating from the BS were investigated in microplastic research for the very first time. In total, 93% of all investigated samples from the NS and the BS show a burden of microplastic particles. Minor differences in the range of detected fibres, and no differences in the quantity of found fragments were revealed for both seas. Based on the loss of only 3% of hard parts during sample processing, and the considered secondary pollution, revealed by the preceding publication, the results are reliable and not overrated (Philipp et al., 2020).
Evaluation of the Method and Results
The Nile Red staining is a well-established method in microplastic pre-identification to preselect particles for further investigation (Erni-Cassola et al., 2017). Since some polymer types are melted or dissolved when stained with Nile Red diluted in chloroform (Tamminga et al., 2017), a loss of, e.g., polystyrene or cellulose acetate particles is highly likely. Hence, melted particles were excluded from pre-selection and further polymer identification based on their deficit in quality and the fragile consistency. Furthermore, based on the manual transferring of single particles onto the Anodisc filter, a loss of promising polymer particles has to be taken into account. For future analyses it is advisable to use the Anodisc filter straight away for filtering the samples, instead of transferring it manually after the filtration process.
Based on the fact that many potential microplastics (91%, 73 out of 80, incl. 77 analysed particles found in intestines and 3 analysed particles found on procedural blanks) could be identified as microplastics (incl. nintestine = 77 and nprocedural samples = 3) by μFTIR analysis, it is highly likely that most particles counted in our study were microplastic. Taken this evaluation and the validation of Philipp et al. (2020) into account (90%), a reliable number of 361 microplastics out of the 401 suspected particles is assumed. Thus, the results show the applicability of the protocol introduced by Philipp et al. (2020) for intestinal samples of cetaceans and determine the actual burden in a reliable way.
Comparison With Other Studies
The determined percentage of 93% (28 out of 30 examined intestines are burdened) coincides with the results of Lusher et al. (2018) investigating carcasses of cetaceans from Irish waters (98%), and Nelms et al. (2019) analysing marine mammals found along the coastline of Great Britain (100%). If focussing on harbour porpoises, the study by Lusher et al. (2018) determined a microplastic presence in only 6.25% of all investigated cetacean carcasses. One explanation for these differences in microplastic occurrences could be the chosen time period of the review by Lusher et al. (2018). It was conducted between 1990 and 2015. A second explanation could be the used mesh size of at least 300 μm, resulting in the loss of smaller particles, which are included in the study presented herein. Furthermore, no detailed information on the stranding site is given, which would be useful for comparison purpose, since differences in microplastic loads around Ireland (Irish Sea, Celtic Sea and the western coastline facing the open North Atlantic) were determined when the microplastic occurrence was compared at different prawn fishing grounds in 2016 (Hara et al., 2020). Furthermore, the study by Lusher et al. (2018), confirmed the microplastic burden in 21 individuals covering six different cetacean species summing up to 528 investigated GITs. In addition, microplastic (≥ 300 μm) was only found in Odontoceti species. The study of van Franeker et al. (2018) conducted on harbour porpoises stranded at the Dutch coast, revealed the presence of marine litter items [incl. macroplastic and microplastics (≥1 mm)] in 7% of all investigated stomachs. Van Franeker and colleagues are aware of the fact that due to the mesh size range of used sieves, particles smaller than 1 mm were lost and not considered during their study (van Franeker et al., 2018). As the here presented study confirmed that the main part of found microplastics are smaller than 1 mm (85%), the results of van Franeker et al. (2018) are not comparable with our study. In addition, the size limits of 100 μm and 5 mm, which are based on the used mesh sizes of the washing sachets (Philipp et al., 2020) and the definition of microplastics (Arthur et al., 2009), overlap with the size of zooplankton species, which a variety of invertebrates feed on (Devriese et al., 2015; Fischer, 2019). Thus, investigations in predatory fish or marine mammal species should also focus on these small-sized microplastic particles.
Reference studies of microplastics in marine mammals, especially from the BS, are scarce. Thus, studies investigating fish, sediment and water samples are considered for further discussion. The microplastic burden in different fish species is higher in the BS (11–22%) (Lenz et al., 2016; Beer et al., 2018) when compared to the southern NS (5.4%) (Foekema et al., 2013). In addition, studies investigating fish species inhabiting waters between Norway and Denmark determined a low risk of microplastic occurrence in fish (1.2%) (Foekema et al., 2013). In contrast, the microplastic concentrations in surface waters and sediment samples show higher concentration in the southern NS (Karlsson et al., 2017; Lorenz et al., 2019), compared to findings of the BS (Graca et al., 2017; Tamminga et al., 2018). Whereas, a model on the global fibre distribution in surface waters estimated a higher accumulation in the BS (∼1,760 ± 4,500 m–3), compared to the North Atlantic region (∼1,800 ± 1,720 m–3) (Lima et al., 2021). Nevertheless, an ubiquitous distribution of synthetic particles along the German BS coast is assumed (Stolte et al., 2015). Furthermore, the fact that marine mammals of the BS might be more exposed to marine litter than in the NS was already confirmed (Unger et al., 2017). Thus, a higher risk of microplastic burden in the BS could be hypothesised. The results obtained in this study underline the findings of marine litter and support the following hypothesis: Investigated harbour porpoises of the BS show a higher number of microplastic particles (incl. fibres and fragments), in contrast to individuals from the NS in each year. In particular, two females from the BS show a high amount of microplastics (44 and 48 particles). For avoiding an overassessment in future research, higher sample sizes per sea are highly recommended.
It will be worth to strive for a reference study investigating the area of the Baltic Proper, since this area is assumed to accumulate pollutants from the whole BS (Stolte et al., 2015), and the here occurring harbour porpoise subspecies is critically endangered (Carlén et al., 2018). Nevertheless, the time span of the available sample collection and the quantity of samples is still too low for identifying a trend in both seas. After statistical assessment with paired t-test and Cohen’s d, the samples size has to be increased if reliable comparisons in microplastic burden of individuals of both seas should be evaluated. Thus, a continuation of the herein presented approach is advisable and is intended.
Polymer Findings
In this study, the most frequently found polymer was PEST. Based on the fact that six fragments and 24 fibres were found in the intestinal samples and only one PEST fibre was identified in one of the procedural blanks, PEST microplastics were still taken into account and were not excluded from this study. Additionally, the procedural blanks show a low amount of fibres (Øfibres = 1 and Øfragments = 7 per procedural blank) and were already subtracted from the results presented here. To control for microplastic contamination, only cotton gloves and lab coats were worn while processing the samples (Philipp et al., 2020). Other studies investigating GIT samples of marine mammals from the North Atlantic found PEST particles, even though high protective measurements were used (Lusher et al., 2015a; Nelms et al., 2019). In addition, a high amount of synthetic fibres like PEST fibres were determined in the Northeast Atlantic region (Thompson, 2004; Lusher et al., 2014), and in inhabiting fish species (Lusher et al., 2013; McGoran et al., 2017; Pereira et al., 2020). The twelve found polyamide particles were not excluded from the analysis, since fibres of the used nylon cloth (PA) are obviously identifiable due to their unique fibre pattern (Philipp et al., 2020), and are clearly different from the PA particles found in the intestinal samples. Thus, those fibres were immediately excluded while pre-selecting, counting and collecting particles for microplastic analyses. Furthermore, not only PA fibres but also PA fragments were found. Previously, high numbers of PA microplastics were identified in GITs of marine mammals (Nelms et al., 2019) and in fish from the North-East Atlantic region (Lusher et al., 2013; McGoran et al., 2017).
Both, the NS and the BS are enclosed by highly populated countries (Halpern et al., 2008, 2015). The system of waste management is relatively sophisticated in European countries, but there is still a high amount of produced waste, thus the likelihood of litter input into the marine environment is increasing (Andreasi Bassi et al., 2017). Another source of microplastic particles, and in particular of fibres which originate from washed clothes are waste water treatment plants (WWTP) (Browne et al., 2011; Bretas Alvim et al., 2020). Moreover, it is well known that WWTP are not able to hold back microplastic fibres and particles (Dubaish and Liebezeit, 2013; Mani et al., 2016). Thus, it could be assumed that the found PA and PEST microplastics originated from waste water, which is transported by rivers and ends up in the marine environment (Browne et al., 2011; Dubaish and Liebezeit, 2013; Mani et al., 2016).
Besides land-based sources, which are responsible for 80% of marine litter inputs (Jambeck et al., 2015), sea-based sources like lost fishing gear or waste generated on ships or offshore installations need to be taken into account (Galgani et al., 2013). Furthermore, both seas are highly impacted by anthropogenic exploitations, such as fishing activities (Catchpole et al., 2005; Halpern et al., 2008, 2015). Thus, the fishing industry is a potential source of found microplastics such as PA and PEST, as well as PE and PP (Pruter, 1987; Deshpande et al., 2020).
Globally, the demand for PE is high (PlasticsEurope, 2020). Thus, it is not surprising that a high amount of PE microplastics was identified in this study. This result is comparable to findings in surface waters of the BS (Gewert et al., 2017). Studies investigating microplastics in marine mammals or fish from the North Atlantic found lower amounts of PE and PP (Lusher et al., 2013; Nelms et al., 2019). Due to the relatively low proportion of microplastics investigated with μFTIR spectroscopy, no final conclusions can be drawn on differences in polymer occurrences in the NS and the BS.
One of the microplastics found was identified as an acrylic or alkyd chip with evidence of kaolin and styrene. Such polymers and additives are commonly used for car (Yang et al., 2012) and ship paints (Lee et al., 2021). Hence, the microplastic chip that was found in the intestine of one harbour porpoise originating from the NS could have derived from abraded ship paint. It is well known that weathered paint from ship and boat surfaces is regularly released into the ocean (Song et al., 2014; Lacerda et al., 2019). Hence, paint fragments can be ingested by marine mammals such as cetaceans as also suggested by Lusher et al. (2018). Unfortunately, this pre-mentioned study did not provide information on paint polymer types. Furthermore, paint chips have already been found in Australian sea turtles (Caron et al., 2018) or in pelagic fish (Ory et al., 2017). Thus, an unintentional prey intake of paint chips by, e.g., fish while preying on zooplankton is assumed (Ory et al., 2017), and the transport through the food web could be considered.
Trophic Accumulation via the Food Web?
The study of van Franeker et al. (2018) pointed out, that the microplastic presence in harbour porpoises most likely occurred due to an unintentional intake while feeding on burdened fish specimens close to the sediment. Besides pelagic species like herring (Clupea harengus), gadoids (e.g., Merlangius merlangus) and sprat (Sprattus sprattus), different benthic sandeel species (e.g., Ammodytes marinus) and gobies (Gobiidae) form the main prey of harbour porpoises (Leopold, 2015). The linkage between burdened prey species and top predators was previously confirmed for grey seals preying on gadoids (Hernandez-Milian et al., 2019). Moreover, three of the here analysed harbour porpoises asphyxiated by pleuronectiforms (e.g., Solea solea) and two further individuals were bycaught in gillnets for benthic and demersal fish species. Those harbour porpoises showed a high mean microplastic amount per individual (M ± SD = 17.7 ± 12.42). Thus, a relationship between preying on benthic fish and the presence of microplastics in predator species is highly assumed. Furthermore, the bycaught or asphyxiated porpoises were in a better nutritional status in comparison to the remaining ones. However, a good nutritional status is not synonymous with a good health of a harbour porpoise (Siebert et al., 2020). Based on the results presented herein, it can be assumed that a harbour porpoise, which is not physically hampered in feeding or hunting, will accumulate more microplastics in the GIT than a diseased one. The results of this study are supported by the assumptions of Leopold (2015) and Rummel et al. (2016) who claimed that 5,000 individual gobies per day are needed for maintaining a harbour porpoise’s good physical condition, and the mean burden of 0.03 particles in each benthic fish may result in a daily intake of almost 150 microplastic particles by one adult harbour porpoise. Kastelein et al. (1997) detected a passage time of 143–196 min in captive harbour porpoises. If the assumption is that a full digestion takes ∼3 h, a harbour porpoise will approximately defaecate eight times a day. Thus, the potential accumulation of 150 microplastics has to be divided by eight, resulting in 18.75 particles per egestion. Certainly, this is just a rough estimate and many factors influence egestion and ingestion rates, but this calculation coincides with the findings of microplastics in samples from the BS (M ± SD = 18.27 ± 14.54).
Impact on the Health Status
Across all 30 individuals no intralesional parasites could be identified in the intestines. However, seven individuals showed an enteritis, whereof two are attributed to parasites and another one accompanied by a hyperplasia of Peyer’s plaques. These accumulations of follicles are known to adsorb different kinds of particles (biogenic and synthetic), and to transfer it into the lymph system when the particle is adequately small in size (Ensign et al., 2012). In total, only two juvenile harbour porpoises originating from the BS showed changes in the Peyer’s plaques, where a low number of suspected microplastics was found (n = 17 and n = 15), in comparison to the other 28 specimens. However, the stomachs were affected by parasites in 12 cases, whereof 11 suffered from a gastritis. Two harbour porpoises from the BS showed changes in the stomach tissue, including gastritis, with no evidence of parasite occurrence. Similar pathological findings were identified for individuals from the BS (Siebert et al., 2020). Thus, a relationship between the accumulation of microplastics and intestinal parasites could not be confirmed in harbour porpoises, as it was previously suggested for grey seals by Hernandez-Milian et al. (2019). In addition, a parasite infestation in the four-chambered stomach of harbour porpoises is more likely than an affection of the intestine (Lakemeyer et al., 2020). Furthermore, only 11 and 27 microplastic particles were found in the intestinal samples in those two cases. Hence, a relationship between tissue damage and microplastics seems to be unlikely. However, tissue damage and inflammations are assumed to be caused by micro- and nanoplastic occurrence (Carr et al., 2012; Stock et al., 2019). In addition, the occurrence of an enteritis in harbour porpoises seems to be rare, since only 9% of the Baltic individuals found in German waters seems to be affected (Siebert et al., 2020). Thus, it is still speculative if the presence of microplastics may cause the tissue damages found in the harbour porpoises as it has already been observed in beluga whales (Moore et al., 2020).
No positive or negative impacts could be revealed in pregnant females: (I) the observed quantity differs extremely between those three specimens (4, 7, and 48 microplastics were found per individual); (II) the number of examined pregnant specimens is, thus, too low. It has to be taken into account that on the one hand, the occurrence of solid particles (microplastics in this study) does not necessarily have to be accompanied by tissue damage. On the other hand, the observation of tissue damage does not absolutely indicate the presence of microplastics. Moreover, it was shown that synthetic materials adsorb pollutants and toxins, and serve as a vector (Yu et al., 2019) and, thus, most likely cause contaminant accumulation.
Conclusion
For analysing the microplastic burden in marine mammals most studies investigate the whole GIT (Lusher et al., 2015a; Nelms et al., 2019). The study presented herein revealed three benefits of focussing on only one part of the GIT: (1) avoidance of secondary contamination in smaller samples is easier, (2) the remaining carcass and GIT can be entirely evaluated for a health monitoring, and (3) the findings in the rectum and faeces confirm the egestion of microplastic particles. For evaluating the intake and egestion rate, further research is needed. Nevertheless, microplastic investigations and experiments in mammals, and especially in free-ranging marine mammals, are complicated based by field conditions. Furthermore, ethical concerns arise as indicated by Nelms et al. (2018). Thus, samples of carcasses and faecal samples of alive individuals are the most feasible approach to assess the microplastic burden in marine mammals.
This is the first study investigating harbour porpoises from different subpopulations for microplastics, and revealed differences in microplastic presence in the NS and the BS. A higher risk of exposure to microplastics was revealed for the western Baltic population, if compared to the North-East Atlantic population. Thus, a higher microplastic burden in the BS is assumed. Furthermore, evidence for the continuous accumulation of microplastics via the food web was given, but could not significantly be confirmed in adult individuals, compared to juvenile ones. Additionally, there is no significant difference in the quantity of synthetic particles in male or female harbour porpoises. To gain further knowledge on differences in sex or age, the quantity of samples has to be increased in future research.
An important relationship between a good or moderate nutritional status and the occurrence of microplastics is demonstrated in this study. Moreover, the egestion and thus, a discharge of microplastic particles out of the organism could be confirmed. No relationship between parasites, tissue damage and microplastic presence could be identified. Therefore, a histological investigation of cell damage or tissue damage localisation with the help of biomarkers would be advisable in future research. Further investigations are needed for evaluating the rate of accumulation and burden in harbour porpoises in the different seas. Indeed, this study outlines first evidence in retrospective microplastic burden. Nevertheless, a higher sample size, as well as a larger temporal coverage is needed to reliably estimate trends in the microplastic burden in harbour porpoises. Furthermore, this study supports the need for a comprehensive marine litter monitoring in predatory species to gain knowledge on accumulation processes and health assessment in apex species of the marine food web.
Data Availability Statement
The original contributions presented in the study are included in the article/Supplementary Material, further inquiries can be directed to the corresponding author/s.
Ethics Statement
Ethical review and approval was not required for the animal study because the investigations on marine mammals for scientific and conservation purpose were conducted in accordance with national and international regulations. During this study samples of dead found specimens were analysed and thus no invasive methods were used.
Author Contributions
CP, BU, and US conceptualized the study and acquired funding. CP conducted the laboratory analysis of processing the samples and isolate microplastics, assisted by BU. CP did the statistical analysis. JHEK provided the μFTIR and FTIR for polymer type analysis. SME conducted the polymer identification by μFTIR and FTIR. CP and SME generated the figures. The manuscript was prepared by CP, and contributed editing with perspectives and arguments was done by BU, SME, JHEK, and US. All authors approved the submitted version.
Funding
The stranding network and the health monitoring are partly funded by the Schleswig-Holstein Agency for Coastal Defence, National Park and Marine Conservation, Germany. The pathological investigations of the marine mammals are partly funded by the Ministry of Energy, Agriculture, Environment, Nature and Digitalisation of Schleswig-Holstein, Germany. The Federal Environment Agency (UBA) partly funded the analysis of samples within the project “Assessment and implementation for long-term monitoring of pollution of diverse marine compartments and biota with marine litter” (FKZ 3717252250).
Conflict of Interest
The authors declare that the research was conducted in the absence of any commercial or financial relationships that could be construed as a potential conflict of interest.
Acknowledgments
We would like to thank all seal rangers in Schleswig-Holstein, Germany for reporting and collecting carcasses. Further thanks go to our colleagues in the ITAW necropsy team for collecting and dissecting carcasses, as well as for collecting samples for our purpose continuously throughout the year. Special thanks go to Dieter Steinhagen for improving this study with his valuable comments. Besides, we would like to thank the reviewers for their valuable comments and advice, which helped to improve the manuscript.
Supplementary Material
The Supplementary Material for this article can be found online at: https://www.frontiersin.org/articles/10.3389/fmars.2021.682532/full#supplementary-material
References
Andersen, L. W. (2003). Harbour porpoises (Phocoena phocoena) in the north atlantic: distribution and genetic population structure. NAMMCO Sci. Publ. 5, 11–29.
Andreasi Bassi, S., Christensen, T. H., and Damgaard, A. (2017). Environmental performance of household waste management in europe - An example of 7 countries. Waste Manag. 69, 545–557. doi: 10.1016/j.wasman.2017.07.042
Arthur, C., Baker, J., and Bamford, H. (2009). Proceedings of the international research workshop on the occurrence, effects, and fate of microplastic marine debris. Sept 9-11-2008.
Beer, S., Garm, A., Huwer, B., Dierking, J., and Nielsen, T. G. (2018). No increase in marine microplastic concentration over the last three decades – a case study from the baltic sea. Sci. Total Environ. 621, 1272–1279. doi: 10.1016/j.scitotenv.2017.10.101
Bretas Alvim, C., Mendoza-Roca, J. A., and Bes-Piá, A. (2020). Wastewater treatment plant as microplastics release source – quantification and identification techniques. J. Environ. Manage. 255:109739. doi: 10.1016/j.jenvman.2019.109739
Browne, M. A., Crump, P., Niven, S. J., Teuten, E., Tonkin, A., Galloway, T., et al. (2011). Accumulation of microplastic on shorelines woldwide: sources and sinks. Environ. Sci. Technol. 45, 9175–9179. doi: 10.1021/es201811s
Carlén, I., Thomas, L., Carlström, J., Amundin, M., Teilmann, J., Tregenza, N., et al. (2018). Basin-scale distribution of harbour porpoises in the baltic sea provides basis for effective conservation actions. Biol. Conserv. 226, 42–53. doi: 10.1016/j.biocon.2018.06.031
Caron, A. G. M., Thomas, C. R., Berry, K. L. E., Motti, C. A., Ariel, E., and Brodie, J. E. (2018). Ingestion of microplastic debris by green sea turtles (Chelonia mydas) in the great barrier reef: validation of a sequential extraction protocol. Mar. Pollut. Bull. 127, 743–751. doi: 10.1016/j.marpolbul.2017.12.062
Carr, K. E., Smyth, S. H., McCullough, M. T., Morris, J. F., and Moyes, S. M. (2012). Morphological aspects of interactions between microparticles and mammalian cells: intestinal uptake and onward movement. Prog. Histochem. Cytochem. 46, 185–252. doi: 10.1016/j.proghi.2011.11.001
Catchpole, T. L., Frid, C. L. J., and Gray, T. S. (2005). Discards in north sea fisheries: causes, consequences and solutions. Mar. Policy 29, 421–430. doi: 10.1016/j.marpol.2004.07.001
Champely, S., Ekstrom, C., Dalgaard, P., Gill, J., Weibelzahl, S., Anandkumar, A., et al. (2020). Package “pwr.”. Available Online at: https://github.com/heliosdrm/pwr.
Deshpande, P. C., Philis, G., Brattebø, H., and Fet, A. M. (2020). Using material flow analysis (MFA) to generate the evidence on plastic waste management from commercial fishing gears in norway. Resour. Conserv. Recycl. X 5:100024. doi: 10.1016/j.rcrx.2019.100024
Devriese, L. I., van der Meulen, M. D., Maes, T., Bekaert, K., Paul-Pont, I., Frère, L., et al. (2015). Microplastic contamination in brown shrimp (crangon crangon, linnaeus 1758) from coastal waters of the southern north sea and channel area. Mar. Pollut. Bull. 98, 179–187. doi: 10.1016/j.marpolbul.2015.06.051
Dubaish, F., and Liebezeit, G. (2013). Suspended microplastics and black carbon particles in the jade system, southern north sea. Water Air Soil Pollut. 224:1352. doi: 10.1007/s11270-012-1352-9
Ensign, L. M., Cone, R., and Hanes, J. (2012). Oral drug delivery with polymeric nanoparticles: the gastrointestinal mucus barriers. Adv. Drug Deliv. Rev. 64, 557–570. doi: 10.1016/j.addr.2011.12.009
Erni-Cassola, G., Gibson, M. I., Thompson, R. C., and Christie-Oleza, J. A. (2017). Lost, but Found with nile red: a novel method for detecting and quantifying small microplastics (1 mm to 20 μm) in environmental samples. Environ. Sci. Technol. 51, 13641–13648. doi: 10.1021/acs.est.7b04512
Farrell, P., and Nelson, K. (2013). Trophic level transfer of microplastic: mytilus edulis (L.) to carcinus maenas (L.). Environ. Pollut. 177, 1–3. doi: 10.1016/j.envpol.2013.01.046
Fischer, E. (2019). Distribution of microplastics in marine species of the Wadden Sea along the coastline of Schleswig-Holstein, Germany. Hamburg, Germany: Hamburg University.
Foekema, E. M., De Gruijter, C., Mergia, M. T., van Franeker, J. A., Murk, A. J., and Koelmans, A. A. (2013). Plastic in north sea fish. Environ. Sci. Technol. 47, 8818–8824. doi: 10.1021/es400931b
Fossi, M. C., Coppola, D., Baini, M., Giannetti, M., Guerranti, C., Marsili, L., et al. (2014). Large filter feeding marine organisms as indicators of microplastic in the pelagic environment: the case studies of the mediterranean basking shark (cetorhinus maximus) and fin whale (balaenoptera physalus). Mar. Environ. Res. 100, 17–24. doi: 10.1016/j.marenvres.2014.02.002
Frid, C., Hammer, C., Law, R., Loeng, H., Pawlak, J. F., Reid, P. C., et al. (2003). Environmental Status of the European Seas. Available online at: https://archimer.ifremer.fr/doc/00040/15135/12473.pdf.
Galgani, F., Hanke, G., Werner, S., and De Vrees, L. (2013). Marine litter within the european marine strategy framework directive. ICES J. Mar. Sci. 70, 1055–1064. doi: 10.1093/icesjms/fst122
Garcia-Cegarra, A. M., Jung, J.-L., Orrego, R., Padilha, J., de, A., Malm, O., et al. (2021). Persistence, bioaccumulation and vertical transfer of pollutants in long-finned pilot whales stranded in chilean patagonia. Sci. Total Environ. 770:145259. doi: 10.1016/j.scitotenv.2021.145259
Gaskin, D. E. (1984). The harbour porpoise Phocoena phocoena (L.) regional populations, status, and infromation on direct and indirect catches. Rep. Int. Whal. Comm. 24:18.
Gewert, B., Ogonowski, M., Barth, A., and MacLeod, M. (2017). Abundance and composition of near surface microplastics and plastic debris in the stockholm archipelago, baltic sea. Mar. Pollut. Bull. 120, 292–302. doi: 10.1016/j.marpolbul.2017.04.062
Graca, B., Szewc, K., Zakrzewska, D., Dołȩga, A., and Szczerbowska-Boruchowska, M. (2017). Sources and fate of microplastics in marine and beach sediments of the southern baltic sea—a preliminary study. Environ. Sci. Pollut. Res. 24, 7650–7661. doi: 10.1007/s11356-017-8419-5
Gregory, M. R., and Andrady, A. L. (2003). “Plastics in the marine environment,” in Plastics and the Environment, ed. A. L. Andrady (New Jersey: John Wiley & Sons, Inc.), 389–390.
Gross, S., Roller, M., Haslob, H., Grilo, M., Lakemeyer, J., Reckendorf, A., et al. (2020). Spatiotemporal accumulation of fatal pharyngeal entrapment of flatfish in harbour porpoises (Phocoena phocoena) in the german north sea. PeerJ 8:e10160. doi: 10.7717/peerj.10160
Halpern, B. S., Frazier, M., Potapenko, J., Casey, K. S., Koenig, K., Longo, C., et al. (2015). Spatial and temporal changes in cumulative human impacts on the world’s ocean. Nat. Commun. 6:7615. doi: 10.1038/ncomms8615
Halpern, B. S., Walbridge, S., Selkoe, K. A., Kappel, C. V., Micheli, F., D’Agrosa, C., et al. (2008). A global map of human impact on marine ecosystems. Science 319, 948–952. doi: 10.1126/science.1149345
Hammond, P. S., Lacey, C., Gilles, A., Viquerat, S., Börjesson, P., Herr, H., et al. (2017). Estimates of cetacean abundance in European Atlantic waters in summer 2016 from the SCANS-III aerial and shipboard surveys. St Andrews: Sea Mammal Research Unit, 39.
Hara, J., Frias, J., and Nash, R. (2020). Quantification of microplastic ingestion by the decapod crustacean Nephrops norvegicus from Irish waters. Mar. Pollut. Bull. 152:110905. doi: 10.1016/j.marpolbul.2020.110905
Hernandez-Milian, G., Lusher, A., MacGabban, S., and Rogan, E. (2019). Microplastics in grey seal (Halichoerus grypus) intestines: are they associated with parasite aggregations? Mar. Pollut. Bull. 146, 349–354. doi: 10.1016/j.marpolbul.2019.06.014
Jambeck, J. R., Geyer, R., Wilcox, C., Siegler, T. R., Perryman, M., Andrady, A., et al. (2015). Plastic waste inputs from land into the ocean. Science 347, 768–771. doi: 10.1126/science.1260352
Jepson, P. D., Deaville, R., Barber, J. L., Aguilar, À, Borrell, A., Murphy, S., et al. (2016). PCB pollution continues to impact populations of orcas and other dolphins in European waters. Sci. Rep. 6:18573. doi: 10.1038/srep18573
Karlsson, T. M., Vethaak, A. D., Almroth, B. C., Ariese, F., van Velzen, M., Hassellöv, M., et al. (2017). Screening for microplastics in sediment, water, marine invertebrates and fish: method development and microplastic accumulation. Mar. Pollut. Bull. 122, 403–408. doi: 10.1016/j.marpolbul.2017.06.081
Kastelein, R. A., Nieuwstraten, S. H., and Verstegen, M. W. A. (1997). “Passage time of carmine red dye through the digestive tract of Harbour Porpoises (Phocoena phocoena),” in The biology of the harbour porpoise, (Woerden: De Spil Publishers), 235–245.
Lacerda, A. L. D. F., Rodrigues, L., dos, S., van Sebille, E., Rodrigues, F. L., Ribeiro, L., et al. (2019). Plastics in sea surface waters around the antarctic peninsula. Sci. Rep. 9:3977. doi: 10.1038/s41598-019-40311-4
Lah, L., Trense, D., Benke, H., Berggren, P., Gunnlaugsson, T., Lockyer, C., et al. (2016). Spatially explicit analysis of genome-wide SNPs detects subtle population structure in a mobile marine mammal, the harbor porpoise. PLoS One 11:e0162792. doi: 10.1371/journal.pone.0162792
Lakemeyer, J., Siebert, U., Abdulmawjood, A., Ryeng, K. A., IJsseldijk, L. L., and Lehnert, K. (2020). Anisakid nematode species identification in harbour porpoises (Phocoena phocoena) from the north sea, baltic sea and north atlantic using RFLP analysis. Int. J. Parasitol. Parasites Wildl. 12, 93–98. doi: 10.1016/j.ijppaw.2020.05.004
Lee, H., Lee, D., and Seo, J. M. (2021). Analysis of paint traces to determine the ship responsible for a collision. Sci. Rep. 11:134. doi: 10.1038/s41598-020-80088-5
Lehnert, K., Raga, J., and Siebert, U. (2005). Macroparasites in stranded and bycaught harbour porpoises from german and norwegian waters. Dis. Aquat. Organ. 64, 265–269. doi: 10.3354/dao064265
Lenz, R., Enders, K., Beer, S., Sørensen, T. K., Stedmon, C. A., and Reeh, L. (2016). Analysis of microplastic in the stomachs of herring and cod from the North Sea and Baltic Sea. Technical University of Denmark: DTU Aqua National Institute of Aquatic Resources.
Leopold, M. F. (2015). Eat and be eaten - Porpoise diet studies. Available Online at: https://www.wur.nl/upload_mm/6/a/a/8ba64cfe-3209-4f3f-a6dc-08d2647b11c4_Leopold 2015 PhD thesis Eat and be eaten-Porpoise diet studies.pdf
Lima, A. R. A., Ferreira, G. V. B., Barrows, A. P. W., Christiansen, K. S., Treinish, G., and Toshack, M. C. (2021). Global patterns for the spatial distribution of floating microfibers: arctic ocean as a potential accumulation zone. J. Hazard. Mater. 403:123796. doi: 10.1016/j.jhazmat.2020.123796
Lorenz, C., Roscher, L., Meyer, M. S., Hildebrandt, L., Prume, J., Löder, M. G. J., et al. (2019). Spatial distribution of microplastics in sediments and surface waters of the southern north sea. Environ. Pollut. 252, 1719–1729. doi: 10.1016/j.envpol.2019.06.093
Lusher, A. L., Burke, A., O’Connor, I., and Officer, R. (2014). Microplastic pollution in the northeast atlantic ocean: validated and opportunistic sampling. Mar. Pollut. Bull. 88, 325–333. doi: 10.1016/j.marpolbul.2014.08.023
Lusher, A. L., Hernandez-Milian, G., Berrow, S., Rogan, E., and O’Connor, I. (2018). Incidence of marine debris in cetaceans stranded and bycaught in Ireland: recent findings and a review of historical knowledge. Environ. Pollut. 232, 467–476. doi: 10.1016/j.envpol.2017.09.070
Lusher, A. L., Hernandez-Milian, G., O’Brien, J., Berrow, S., O’Connor, I., and Officer, R. (2015a). Microplastic and macroplastic ingestion by a deep diving, oceanic cetacean: the true’s beaked whale mesoplodon mirus. Environ. Pollut. 199, 185–191. doi: 10.1016/j.envpol.2015.01.023
Lusher, A. L., Tirelli, V., O’Connor, I., and Officer, R. (2015b). Microplastics in arctic polar waters: the first reported values of particles in surface and sub-surface samples. Sci. Rep. 5:14947. doi: 10.1038/srep14947
Lusher, A. L., McHugh, M., and Thompson, R. C. (2013). Occurence of microplastics in the gastrointestinal tract of pelagic and demersal fish from the english channel. Mar. Pollut. Bull. 67, 94–99. doi: 10.1016/j.marpolbul.2012.11.028
Mani, T., Hauk, A., Walter, U., and Burkhardt-Holm, P. (2016). Microplastics profile along the rhine river. Sci. Rep. 5:17988. doi: 10.1038/srep17988
McGoran, A. R., Clark, P. F., and Morritt, D. (2017). Presence of microplastic in the digestive tracts of european flounder, platichthys flesus, and european smelt, osmerus eperlanus, from the river thames. Environ. Pollut. 220, 744–751. doi: 10.1016/j.envpol.2016.09.078
Miller, M. E., Hamann, M., and Kroon, F. J. (2020). Bioaccumulation and biomagnification of microplastics in marine organisms: a review and meta-analysis of current data. PLoS One 15:e0240792. doi: 10.1371/journal.pone.0240792
Moore, R. C., Loseto, L., Noel, M., Etemadifar, A., Brewster, J. D., MacPhee, S., et al. (2020). Microplastics in beluga whales (Delphinapterus leucas) from the eastern beaufort sea. Mar. Pollut. Bull. 150:110723. doi: 10.1016/j.marpolbul.2019.110723
Nelms, S. E., Barnett, J., Brownlow, A., Davison, N. J., Deaville, R., Galloway, T. S., et al. (2019). Microplastics in marine mammals stranded around the british coast: ubiquitous but transitory? Sci. Rep. 9:1075. doi: 10.1038/s41598-018-37428-3
Nelms, S. E., Galloway, T. S., Godley, B. J., Jarvis, D. S., and Lindeque, P. K. (2018). Investigating microplastic trophic transfer in marine top predators. Environ. Pollut. 238, 999–1007. doi: 10.1016/j.envpol.2018.02.016
Ory, N. C., Sobral, P., Ferreira, J. L., and Thiel, M. (2017). Amberstripe scad decapterus muroadsi (carangidae) fish ingest blue microplastics resembling their copepod prey along the coast of rapa nui (easter island) in the south pacific subtropical gyre. Sci. Total Environ. 586, 430–437. doi: 10.1016/j.scitotenv.2017.01.175
OSPAR. (2021). Shipping and Ports. Available Online at: https://oap.ospar.org/en/versions/transport-shipping-and-ports-en-0-1-0/ [Accessed February 18, 2021].
Pereira, J. M., Rodríguez, Y., Blasco-Monleon, S., Porter, A., Lewis, C., and Pham, C. K. (2020). Microplastic in the stomachs of open-ocean and deep-sea fishes of the north-east atlantic. Environ. Pollut. 265:115060. doi: 10.1016/j.envpol.2020.115060
Philipp, C., Unger, B., Fischer, E. K., Schnitzler, J. G., and Siebert, U. (2020). Handle with care—microplastic particles in intestine samples of seals from german waters. Sustainability 12:10424. doi: 10.3390/su122410424
PlasticsEurope. (2020). Plastics – the Facts 2020. Available Online at: https://www.plasticseurope.org/en/resources/publications/4312-plastics-facts-2020
Pruter, A. T. (1987). Sources, quantities and distribution of persistent plastics in the marine environment. Mar. Pollut. Bull. 18, 305–310. doi: 10.1016/S0025-326X(87)80016-4
R Core Team. (2020). R: a language and environment for statistical computing. Vienna, Austria: R Foundation for Statistical Computing.
Roch, S., Friedrich, C., and Brinker, A. (2020). Uptake routes of microplastics in fishes: practical and theoretical approaches to test existing theories. Sci. Rep. 10:3896. doi: 10.1038/s41598-020-60630-1
Rummel, C. D., Löder, M. G. J., Fricke, N. F., Lang, T., Griebeler, E.-M., Janke, M., et al. (2016). Plastic ingestion by pelagic and demersal fish from the north sea and baltic sea. Mar. Pollut. Bull. 102, 134–141. doi: 10.1016/j.marpolbul.2015.11.043
Setälä, O., Fleming-Lehtinen, V., and Lehtiniemi, M. (2014). Ingestion and transfer of microplastics in the planktonic food web. Environ. Pollut. 185, 77–83. doi: 10.1016/j.envpol.2013.10.013
Siebert, U., Pawliczka, I., Benke, H., von Vietinghoff, V., Wolf, P., Pilāts, V., et al. (2020). Health assessment of harbour porpoises (PHOCOENA PHOCOENA) from baltic area of denmark, germany, poland and latvia. Environ. Int. 143:105904. doi: 10.1016/j.envint.2020.105904
Siebert, U., Wünschmann, A., Weiss, R., Frank, H., Benke, H., and Frese, K. (2001). Post-mortem findings in harbour porpoises (Phocoena phocoena) from the german north and baltic seas. J. Comp. Pathol. 124, 102–114. doi: 10.1053/jcpa.2000.0436
Sjöqvist, C., Godhe, A., Jonsson, P. R., Sundqvist, L., and Kremp, A. (2015). Local adaptation and oceanographic connectivity patterns explain genetic differentiation of a marine diatom across the north sea–baltic sea salinity gradient. Mol. Ecol. 24, 2871–2885. doi: 10.1111/mec.13208
Song, Y. K., Hong, S. H., Jang, M., Kang, J.-H., Kwon, O. Y., Han, G. M., et al. (2014). Large accumulation of micro-sized synthetic polymer particles in the sea surface microlayer. Environ. Sci. Technol. 48, 9014–9021. doi: 10.1021/es501757s
Stock, V., Böhmert, L., Lisicki, E., Block, R., Cara-Carmona, J., Pack, L. K., et al. (2019). Uptake and effects of orally ingested polystyrene microplastic particles in vitro and in vivo. Arch. Toxicol. 93, 1817–1833. doi: 10.1007/s00204-019-02478-7
Stolte, A., Forster, S., Gerdts, G., and Schubert, H. (2015). Microplastic concentrations in beach sediments along the german baltic coast. Mar. Pollut. Bull. 99, 216–229. doi: 10.1016/j.marpolbul.2015.07.022
Tamminga, M., Hengstmann, E., and Fischer, E. K. (2017). Nile red staining as a subsidiary method for microplastic quantification: a comparison of three solvents and factors influencing application reliability. SDRP J. Earth Sci. Environ. Stud. 2, doi: 10.15436/JESES.2.2.1
Tamminga, M., Hengstmann, E., and Fischer, E. K. (2018). Microplastic analysis in the south funen archipelago, baltic sea, implementing manta trawling and bulk sampling. Mar. Pollut. Bull. 128, 601–608. doi: 10.1016/j.marpolbul.2018.01.066
Thompson, R. C. (2004). Lost at sea: where is all the plastic? Science 304, 838–838. doi: 10.1126/science.1094559
Unger, B., Herr, H., Benke, H., Böhmert, M., Burkhardt-Holm, P., Dähne, M., et al. (2017). Marine debris in harbour porpoises and seals from german waters. Mar. Environ. Res. 130, 77–84. doi: 10.1016/j.marenvres.2017.07.009
van Franeker, J. A., Bravo Rebolledo, E. L., Hesse, E., IJsseldijk, L. L., Kühn, S., Leopold, M., et al. (2018). Plastic ingestion by harbour porpoises Phocoena phocoena in the netherlands: establishing a standardised method. Ambio 47, 387–397. doi: 10.1007/s13280-017-1002-y
Yang, S.-H., Shen, J. Y., Chang, M. S., and Wu, G. J. (2012). Quantification of vehicle paint components containing polystyrene using pyrolysis-gas chromatography/mass spectrometry. Anal. Methods 4:1989. doi: 10.1039/c2ay05809j
Keywords: microplastic burden, FTIR, marine mammals, cetacean, North Sea, Baltic Sea, nutritional status, health
Citation: Philipp C, Unger B, Ehlers SM, Koop JHE and Siebert U (2021) First Evidence of Retrospective Findings of Microplastics in Harbour Porpoises (Phocoena phocoena) From German Waters. Front. Mar. Sci. 8:682532. doi: 10.3389/fmars.2021.682532
Received: 18 March 2021; Accepted: 14 April 2021;
Published: 10 May 2021.
Edited by:
Robson G. Santos, Federal University of Alagoas, BrazilReviewed by:
Anish Kumar Warrier, Manipal Academy of Higher Education, IndiaAndré Ricardo Araújo Lima, Center for Marine and Environmental Sciences (MARE), Portugal
Copyright © 2021 Philipp, Unger, Ehlers, Koop and Siebert. This is an open-access article distributed under the terms of the Creative Commons Attribution License (CC BY). The use, distribution or reproduction in other forums is permitted, provided the original author(s) and the copyright owner(s) are credited and that the original publication in this journal is cited, in accordance with accepted academic practice. No use, distribution or reproduction is permitted which does not comply with these terms.
*Correspondence: Ursula Siebert, dXJzdWxhLnNpZWJlcnRAdGloby1oYW5ub3Zlci5kZQ==