- 1Institute of Fisheries Science, National Taiwan University, Taipei, Taiwan
- 2Fisheries Research Institute, Council of Agriculture, Keelung, Taiwan
- 3National Synchrotron Radiation Research Center, Hsinchu, Taiwan
- 4Institute of Marine Affairs and Resources Management, National Taiwan Ocean University, Keelung, Taiwan
- 5Department of Life Science, National Taiwan University, Taipei, Taiwan
- 6Department of Biochemical Science and Technology, National Taiwan University, Taipei, Taiwan
Cephalopods play an important role in both ecology and fisheries. Variations in the dietary compositions of squids with large populations further promote interactions between different trophic levels in marine ecosystems. Moreover, due to marine pollution, squids are also at risks of artifact ingestion, and there is limited understanding about the influence of artifacts on the feeding behavior of squids. We examined 296 stomachs of the Argentine shortfin squid Illex argentinus collected through commercial catches across the Southwest Atlantic from February to April of 2018 and 2019 to establish the monthly dietary compositions and risks of artifact ingestion. The results supported typical observations that the Argentine shortfin squids switched from a diet dominated by crustaceans at small sizes to consuming larger prey, predominantly fishes and/or cephalopods, at large sizes during their growth and southward migration. Significantly higher consumption of fishes was observed in recent years compared with that observed in other studies since 1992. The ingested artifacts examined were composed of plastic and non-plastic materials. Artifacts were observed in 19.9% of the total number of stomachs, with 20.5% of the empty stomachs containing artifact remains. The results indicate that although the main dietary compositions of the Argentine shortfin squid maintain the taxonomic groups of prey compositions, the dietary structure, i.e., composition percentage, is varying. The detection of artifacts suggests that environmental monitoring is needed in this region in order to manage and conserve the squid and safeguard aquatic food safety.
Introduction
Cephalopods, accounting for approximately 4% of the total capture fisheries and 5% of total global fisheries production, contribute significantly to both ecology and fisheries due to their large populations (Doubleday et al., 2016). Squids are cephalopods in the superorder Decapodiformes and in the middle level of the food chain, existing as an insatiable predator feeding opportunistically on variable diets (Ivanovic and Brunetti, 1994; Rodhouse and Nigmatullin, 1996) and as prey for the top predators (Klages, 1996; Smale, 1996), importantly linking various trophic levels and forming food webs that map the interactions among marine organisms in the oceans. These characteristics make squids a key linkage between different trophic levels in marine ecosystems (Piatkowski et al., 2001). However, compared with other marine animals at similar trophic levels, squids are more susceptible to environmental changes because of their short lifespans (Rodhouse and Hatfield, 1990; Rodhouse and Nigmatullin, 1996). Furthermore, the increasing seawater temperature might expand the distribution ranges of squid populations and influence their predator-prey interactions with other species, unbalancing the entire food chain (Zeidberg and Robison, 2007; Hoving et al., 2013; Golikov et al., 2014; Doubleday et al., 2016).
Argentine shortfin squid Illex argentinus is one of the major resources in squid fisheries globally, accounting for about 10% of the total catch of cephalopods (FAO, 2019). The I. argentinus engages in horizontal and vertical migrations in the daytime and early night following prey availability during its short lifespan (Arkhipkin, 1993, 2000; Santos and Haimovici, 1997; Haimovici et al., 1998). In addition, the I. argentinus is a migratory species: its higher concentrations are found at 46°S in January–February, then it migrates southwards to the Falkland Islands (with peak concentrations in March–May). At the end of this period, it migrates again northwards to northern Argentina, Uruguay, and Brazil, to spawn and die around July–August (Wang et al., 2018). Multiple factors have demonstrated effects on the patterns in the dietary compositions of I. argentinus. For example, regardless of season, the I. argentinus switched from a diet dominated by crustaceans at small sizes to consuming larger prey, predominantly fishes and/or cephalopods, at large sizes, but the contribution of dietary compositions varied (Ivanovic and Brunetti, 1994; Mouat et al., 2001). Spatiotemporal variations in the diet have indicated that the importance of crustaceans is the highest in the early morning and declines through night toward the morning (Ivanovic and Brunetti, 1994; Mouat et al., 2001). With climate change resulting in the alteration of prey availability and compositions in the Antarctic, the I. argentinus have been shown fluctuating diets in recent years (Ivanovic and Brunetti, 1994; Mouat et al., 2001; Jansen et al., 2007; Rosas-Luis et al., 2014). The changes in the sea surface temperature and chlorophyll-a concentrations cause the reduction of krill populations, which is later replaced by other crustaceans (Murphy et al., 2007; Atkinson et al., 2012), and there is evidence that squids have shifted their diets when the population of crustaceans changed (Rodhouse and Nigmatullin, 1996; Rodhouse, 2013). However, few studies have been conducted on understanding the dietary compositions of I. argentinus for consecutive months during growth and southward migration, and also, estimated potential dietary shifts both among and within the taxonomic groups for the squids under climate change conditions.
Stomach content analysis provides important insight into feeding patterns and quantitative assessments of food habits, which are important aspects of fisheries management (Hyslop, 1980; Zacharia, 2017). Accurate descriptions of the diets and feeding habits of marine organisms based on the stomach content analysis can reveal the basis for understanding trophic interactions in food webs, representing an integration of many important ecological components (including behavior, energy intake, and inter/intraspecies interactions), distinguishing between multiple functional food components (nutrients), and linking physiology, behavior, and ecology, all of which can enable a better understanding of the responses of marine animals to their environments (Raubenheimer et al., 2009). Furthermore, the dietary results can be used to determine the most frequently consumed prey and the relative importance of different food types to species nutrition and quantify the consumption rates of individual prey types. All of the above information could help us to examine past ecosystem changes, speculate about future changes, and establish or modify the current strategies for fisheries management and marine ecological conservation.
In addition, marine pollution has grown to become a major problem and threatens marine lives throughout the nested ecological hierarchy and the habitats they depend on (van Sebille et al., 2015). Particularly, among the main pollutants, plastics make up approximately 80% of all marine pollution, being found from water surface to deep-sea sediments (PlasticsEurope, 2018). The accumulation and ingestion of microplastics have been increasingly reported to cause diverse and detrimental effects on marine organisms such as mussels and copepods (Avio et al., 2015; Cole et al., 2015; van Sebille et al., 2015). On the other hand, synthetic fabrics, which are human-made textiles used to substitute natural fibers, are ranked as the second largest contributor to marine pollution, and their manufacturing process involves carcinogenic chemicals that lead to a potential health risk in both humans and other organisms (Acuner and Dilek, 2004). The I. argentinus consumes a great amount of marine food throughout their life cycle. The ingestion of artifacts, including both plastics and synthetic fabrics, can become a risk owing to not only impacts on individual organisms but also bioaccumulation in the food web, as well as causing challenges for food safety in human society. Nevertheless, unlike commercial mollusks and jumbo squid Dosidicus gigas, which have received more attention regarding the influences of marine pollution (Rosas-Luis, 2016; Abidli et al., 2019), the potential amount of artifacts ingested by I. argentinus has not yet been considered.
Here, we identified and quantified predation to understand feeding habits and potential dietary shifts during the life cycle, and artifact ingestion risks for the I. argentinus collected during the months of February to April in 2018 and 2019 in the Southwest Atlantic. The objectives of this study were to: (1) establish the monthly dietary compositions and key prey species of the I. argentinus, (2) examine relationships between squid size and the prey consumed, (3) explore risks of artifact ingestion by the I. argentinus, and (4) compare whether the dietary compositions of I. argentinus influence their risks of artifact ingestion.
Materials and Methods
Study Area and Squid Stomach Sampling
The squid samples were collected from catches of Taiwanese jigging vessels at 11 sites across the open sea east of Argentina and north of the exclusive economic zones of the Falkland Islands from February to April in 2018 and 2019 (Table 1 and Figure 1A). All squid samples were quickly frozen and preserved after capture. The dorsal mantle length (ML), sex, and stomach fullness were measured and characterized in the laboratory. Stomach fullness was rated by a scale from 0 to 5, which was separately represented as empty, scarce, few, half full, full, and full to distended (Breiby and Jobling, 1985). Each stomach without cecum was then extracted and frozen at −20 °C for further analyses. Due to the low occurrences in the catches for male individuals, only female individuals in each sampling site were used for the following analyses.
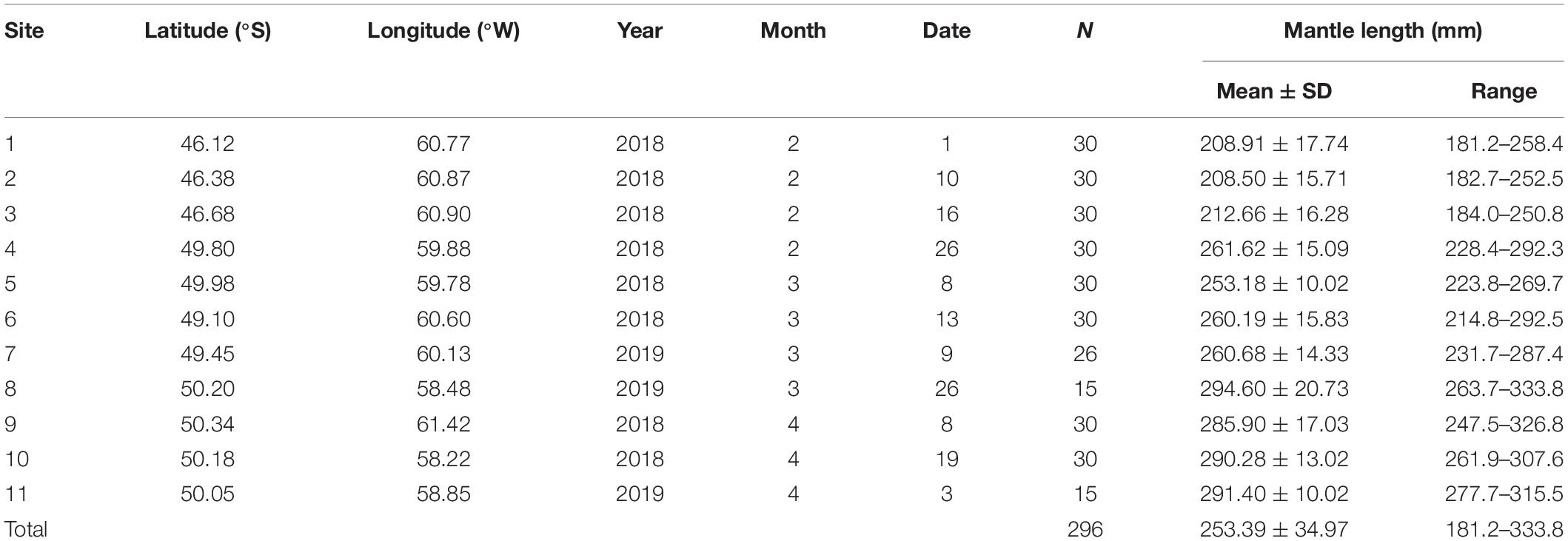
Table 1. Summary data for the Argentine shortfin squid Illex argentinus collected in the Southwest Atlantic during 2018–2019.
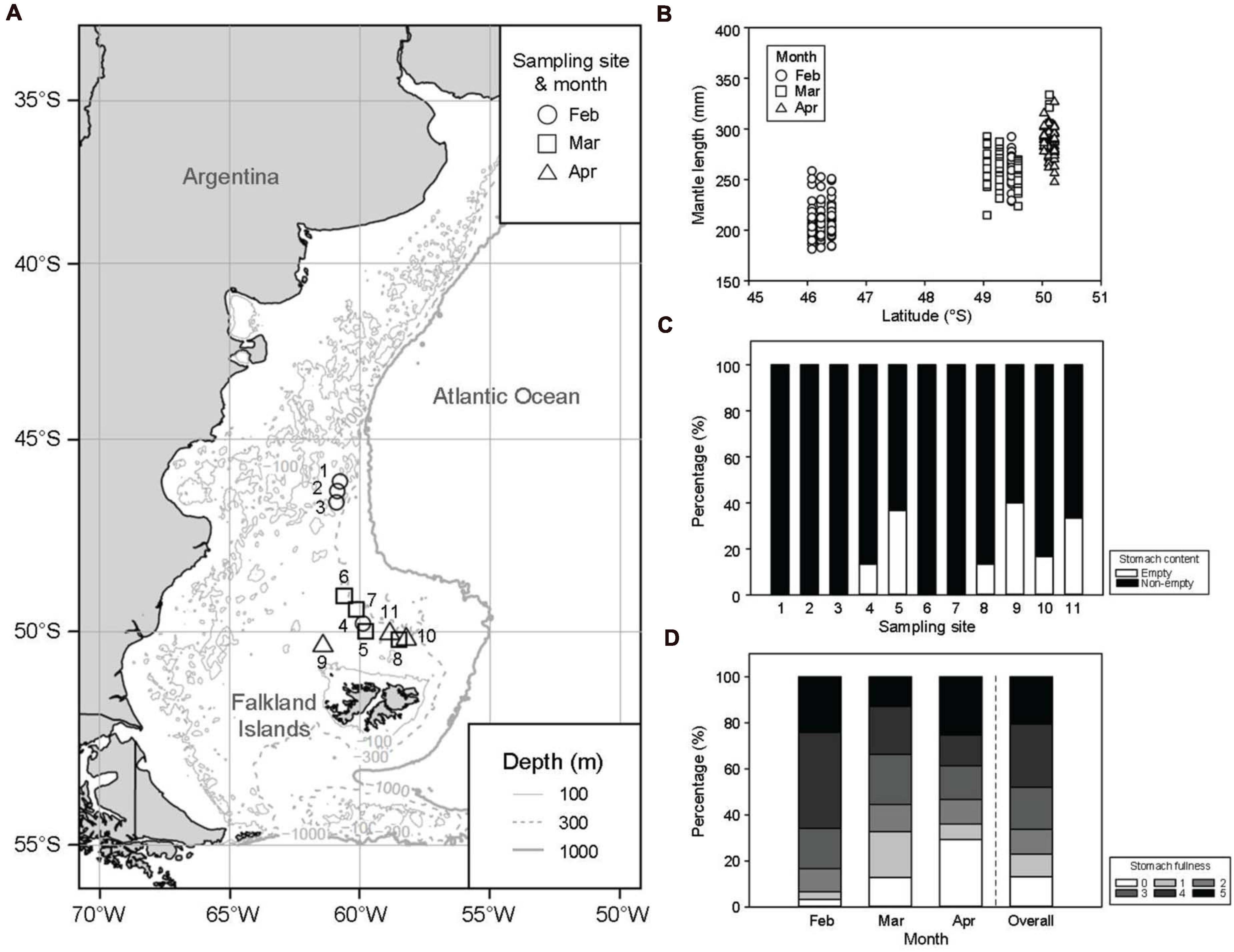
Figure 1. (A) Map of the Southwest Atlantic and sampling sites for the Argentine shortfin squids Illex argentinus captured from February to April in 2018 and 2019 in the study area. Gray thin solid, dashed, and thick solid lines represent depths of 100, 300, and 1000 m, respectively. (B) The correlation between mantle length of squids and latitudes of sampling sites. (C) The percentage of condition of stomach contents and stomach fullness shows the distribution of non-empty (black) and empty (white) stomachs at each site, and (D) shows the monthly distributions. Scores from 0 to 5 are separately indicated as empty, scarce, few, half full, full, and full to distended.
Dietary Analysis
Each stomach of the squid samples was exposed, and the contents were rinsed with deionized water over 200 and 330 μm mesh sieves. The identification and quantification of prey contents in each stomach were determined by a stereomicroscope (Olympus SZ 61, Japan). To identify all fragments of prey to the lowest taxon, sagittal otoliths and lenses were used for fish identification (Lombarte et al., 2006), statoliths, lenses, and beaks for cephalopod identification (Clarke, 1986; Xavier and Cherel, 2009), and exoskeletons for crustacean identification (Boschi et al., 1992; Chapman, 2007). Subsequently, seven dietary indices were calculated to quantify dietary compositions, including the frequency of occurrence (FO), the relative frequency of occurrence (FO %), percentage occurrence (PO %), number (N), number percentage (N %), the average number per squid (Ave N), and the individual number percentage in each squid sample (Ind N %; Mouat et al., 2001; Markaida and Sosa-Nishizaki, 2003; Markaida et al., 2008). The equations were as follows:
To summarize, the former six dietary indices were calculated to represent the overall estimation, while the latter represented the individual analysis. In addition, it was difficult to distinguish whether multiple structures belonged to the same single prey individual in this study, the amount of fish prey in each stomach was counted by the maximum number of otoliths and lenses, while the cephalopod prey was enumerated by the maximum number of statoliths, lenses, and beaks. In addition, although tentacle fragments were observed in some stomachs, they were excluded in counting the number of cephalopod prey. This was because high cannibalism of I. argentinus might occur during harvest operations when they were highly aggregated onboard. On the other hand, due to the typical feeding behaviors of squid, the number of crustaceans was hard to precisely assess. Therefore, the occurrences of crustacean prey were represented based on presence or absence (Portner et al., 2019).
Artifact Detection
After examining the dietary compositions, the entire stomach contents of individual squids were dissolved in 10% KOH in a 60°C water bath for 48 h (Lusher et al., 2017; Jamieson et al., 2019), and the volume of KOH that was added as three times the volume of the stomach contents (Foekema et al., 2013) to digest the biological particles on the surfaces of artifacts remaining in the stomach contents (Dehaut et al., 2016; Kühn et al., 2017). Each sample was shaken for 30 s every day to promote digestion, and the dissolved contents were then filtered through Whatman Grade 541 filter papers and finally moved to a petri dish to detect potential artifacts under a stereomicroscope (Jamieson et al., 2019). Once the artifacts were found, each was photographed and scaled using ImageJ software (v 1.52, NIH, Bethesda, MD, United States).
The qualitative analysis of artifacts found in each stomach was additionally recorded using a Fourier transform infrared spectrometer (FTIR; INVENIO R, Bruker, Germany). An IR microscope (Hyperion 3000, Bruker, Germany) was integrated with the spectrometer for FTIR imaging at TLS 14A1 of National Synchrotron Radiation Research Center, and a single element mercury-cadmium-telluride (MCT) photodiode cooled by liquid nitrogen was also equipped. The spectral range of the apparatus was 650–4000 cm–1 at a resolution of 4 cm–1 in 64 scans. The spectral results of the artifacts were compared with the spectra library of the software OPUS (v.8.1, Bruker, Germany) and confirmed by inspection of peak matching.
To quantify the relative compositions of artifacts ingested by the I. argentinus, the dietary indices were calculated as in the aforementioned dietary analysis, with all stomachs, i.e., non-empty and empty, included.
Statistical Analysis
Thirty-nine empty stomachs were excluded from the dietary analysis, whereas both empty and non-empty stomachs were analyzed for artifact ingestion to better evaluate the latent risk. The relationship between ML and latitude was estimated using Spearman’s correlation. To compare differences among dietary compositions based on prey types, the Kruskal–Wallis test was used with Dunn’s post hoc multiple comparisons. Linear regressions were used to determine relationships between ML and dietary/artifact compositions. All analyses were performed in R 4.0.1 (R Core Team, 2020) and SigmaPlot 14 (SYSTAT, NA) softwares.
Results
Of the 296 squid samples, their ML ranged from 181.2 to 333.8 mm, with an average of 253.4 ± 35.0 mm (Table 1). The ML significantly increased with the month, with average ML of 222.9 ± 27.6 mm, 263.3 ± 19.9 mm, and 288.8 ± 14.3 mm in February, March, and April, respectively. There was a positive correlation (r = 0.825, p < 0.01) between the latitudes of sampling sites and the ML of squids (Figure 1B), indicating that the growth of the I. argentinus was determined both by location and month. Most squid samples (60.0–100%) had food in the stomachs at each sampling site (Figure 1C), and over 53.3% of squid samples showed more than half-full stomachs in each month (Figure 1D).
Dietary Composition
The dietary compositions of I. argentinus were mainly composed of fishes, cephalopods, and crustaceans (Table 2). Among prey groups, the identifiable fish species included Dolichopteryx sp., Rhynchohyalus sp., Gymnoscopelus nicholsi, Notophycis marginata, and Micromesistius australis, while the identifiable cephalopod species were Loligo gahi, Histioteuthis atlantica, and I. argentinus, which further showed with the latter showing their cannibalistic behavior in the study area (Figure 2). For the crustaceans, mostly the Amphipoda, Decapoda, and Euphausiacea orders were observed (Figure 2). Unlike the fishes and crustaceans that were consistently as the prey species of I. argentinus, cephalopods were observed in the stomachs only in March and April.
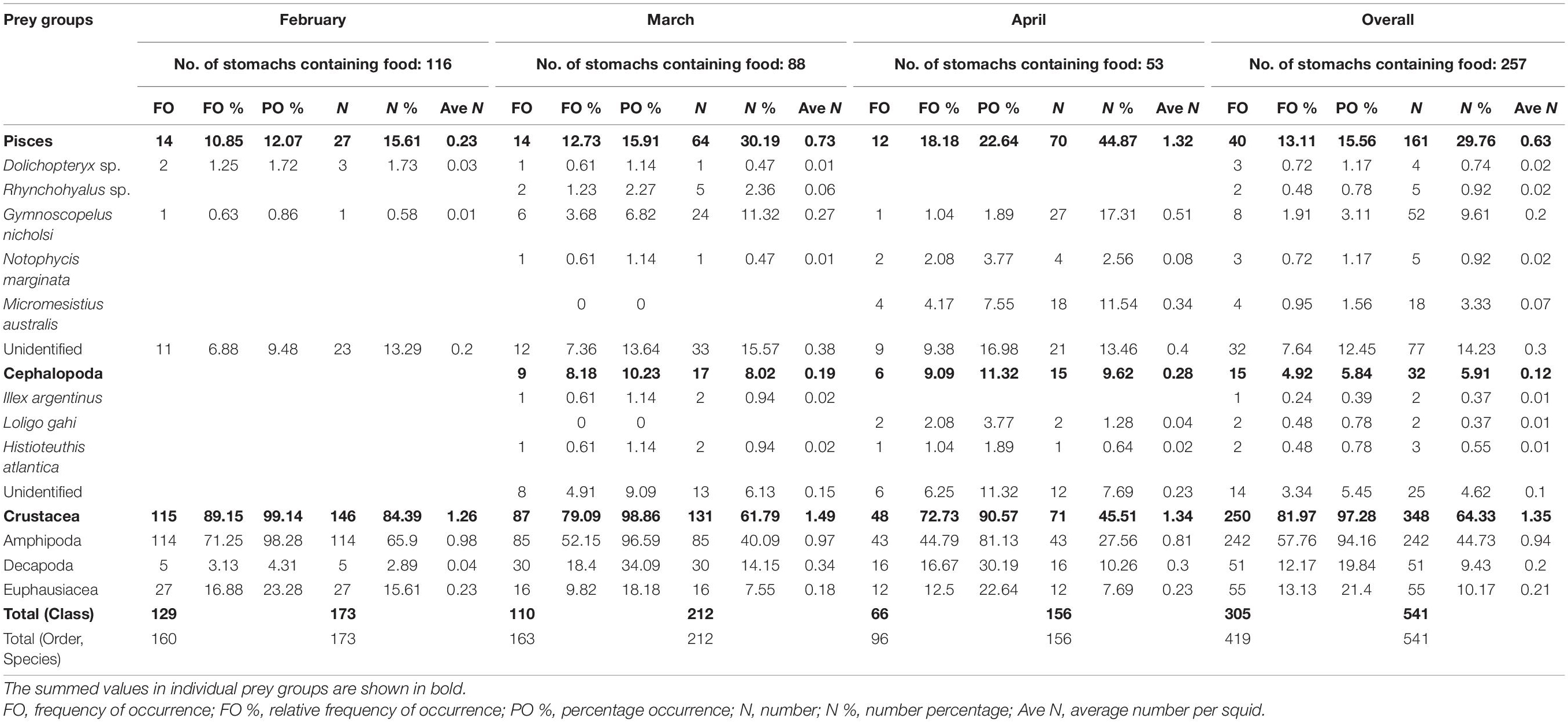
Table 2. Dietary compositions of prey taxonomic groups, identified to the lowest possible taxonomic levels, from the stomach content analysis of Argentine shortfin squid Illex argentinus in the Southwest Atlantic.
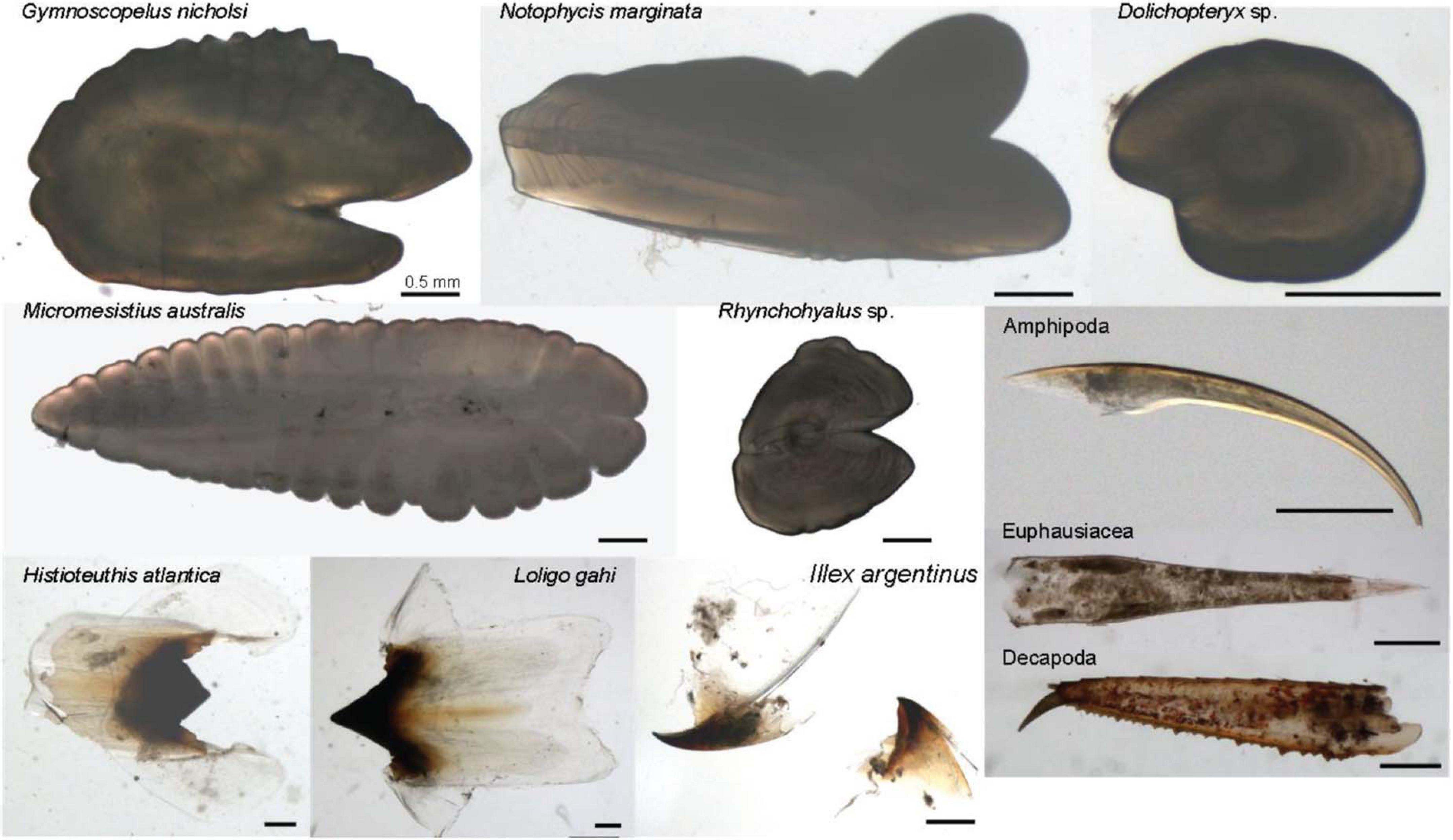
Figure 2. Photographs showing examples of identifiable prey, including fishes (Dolichopteryx sp., Rhynchohyalus sp., Gymnoscopelus nicholsi, Notophycis marginata, and Micromesistius australis), cephalopods (Illex argentinus, Loligo gahi, and Histioteuthis atlantica), and crustaceans (the Amphipoda, Decapoda, and Euphausiacea orders). The inset scale bars denote 0.5 mm for each photo.
For the quantification of prey types consumed by the I. argentinus, overall, crustaceans were the most dominant and important food source among the three prey taxonomic groups in each month, showing the highest values in all dietary indices (Table 2). When exploring the three dietary indices that were expressed as percentages of the dietary compositions, i.e., FO %, PO %, and N %, the consumption of fishes by the I. argentinus increased with the month (FO %: from 10.85 to 18.18%; PO %: from 12.07 to 22.64%; N %: from 15.61 to 44.87%), whereas the dietary requirements of crustaceans decreased with the month (FO %: from 89.15 to 72.73%; PO %: from 99.14 to 90.57%; N %: from 84.39 to 45.51%). The percentage of cephalopod consumption remained within 8–12% whether considering in FO %, PO %, and N %. Similar patterns were also observed through the estimation based on the individual analysis, and the consumption of crustaceans was significantly higher than that of fishes and cephalopods in each month (all p < 0.001; Figure 3). The average Ind N % (87.6%) of predation of crustaceans was slightly higher than overall N % (64.3%), again indicating a high demand for crustaceans as food, particularly at the individual level. The proportion of fish and cephalopod fragments ingested by individual squids increased with months from 7.1 to 15.1% and from 0 to 7.6%, respectively, whereas that of ingested crustacean fragments decreased with months, from 87.6 to 77.3%. Moreover, the highest variation among individual squids existed consistently in April for the three prey taxonomic groups. When further considering the relationships between the dietary compositions and ML of the I. argentinus, the consumption of both fishes and cephalopods showed an increasing trend with ML, while the consumption of crustaceans displayed an opposite decreasing pattern (Figure 4 and Supplementary Figure 1).
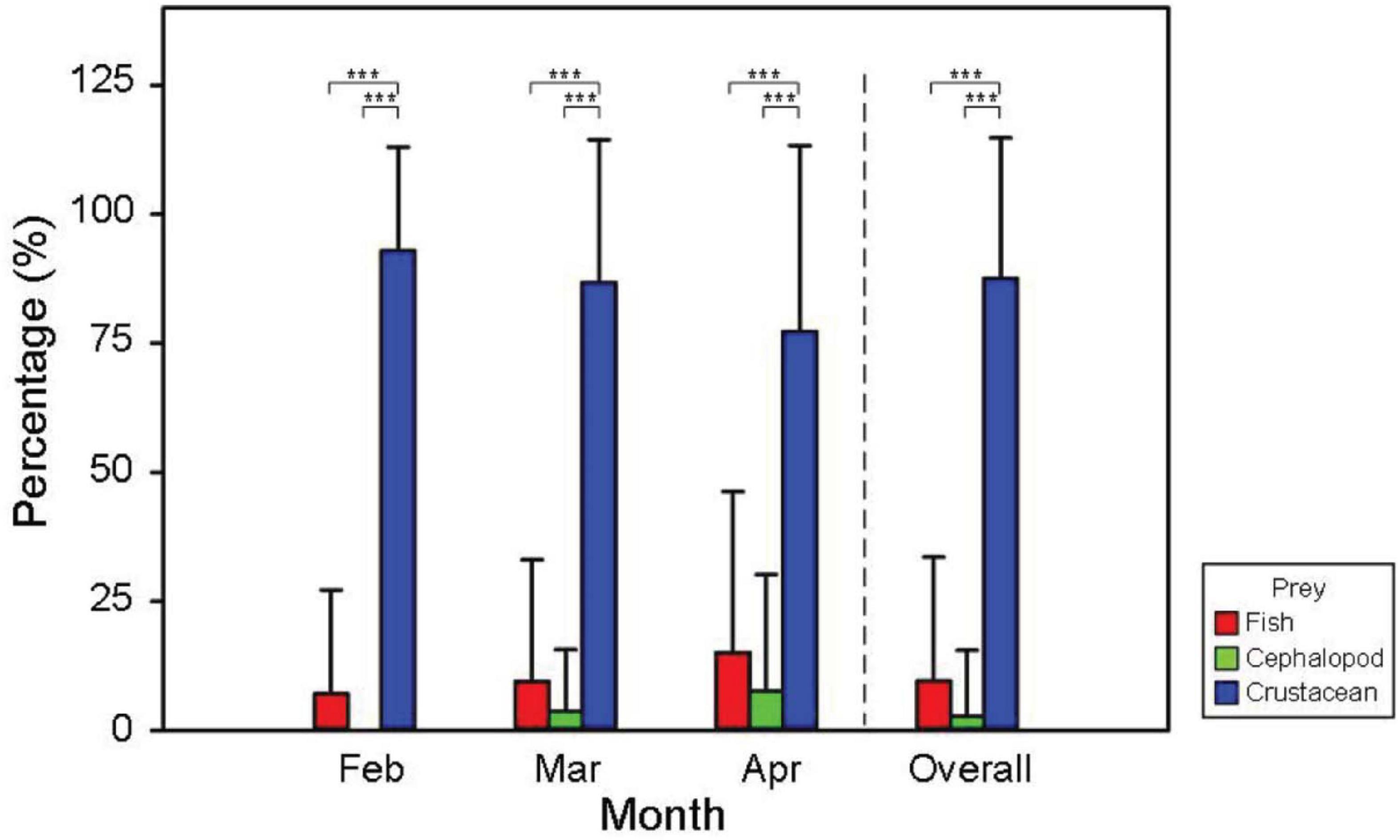
Figure 3. Bar plots of the means (± SD) of the individual number percentage of three main prey taxonomic types of the Argentine shortfin squid Illex argentinus in each month. Asterisks indicate significant differences between prey taxonomic types in Dunn’s post hoc tests (***p < 0.001).
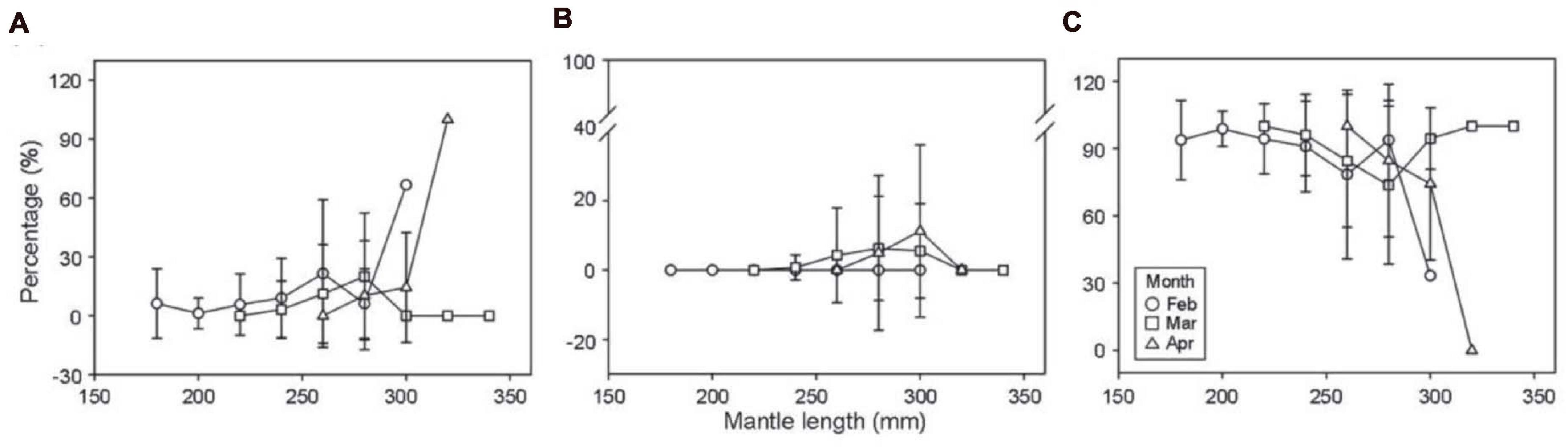
Figure 4. Percentage distributions of the Argentine shortfin squid Illex argentinus with consumed (A) fishes, (B) cephalopods, and (C) crustaceans based on the individual number percentage (mean ± SD) by mantle length for each month in the study area. Squid sizes, ranging between 150 and 350 mm mantle length, are grouped into 20 mm intervals.
These results imply that the dietary compositions of I. argentinus shifted by month, location, and ML. The squids may switch their prey preference from mostly crustaceans to still mostly crustaceans, but with an increasing proportion of fishes and cephalopods over time during their life cycle.
Artifact Ingestion
A total of 75 artifacts, with 24, 27, and 24 artifacts in February, March, and April, respectively, were found in the squid stomachs, showing that the average detection was 0.2 artifact items per squid in February, 0.27 in March, and 0.32 in April (Table 3 and Figure 5). Ingested artifacts occupied 19.9% of the total number of all stomachs, indicating that artifacts might be a considerable threat to the I. argentinus. The material components of most artifacts could not be identified, and only six artifacts were identified as plastic-like artifacts, including three made by nylon 66, one made by polyethylene terephthalate (PET), and the remaining two composed of unknown plastics.
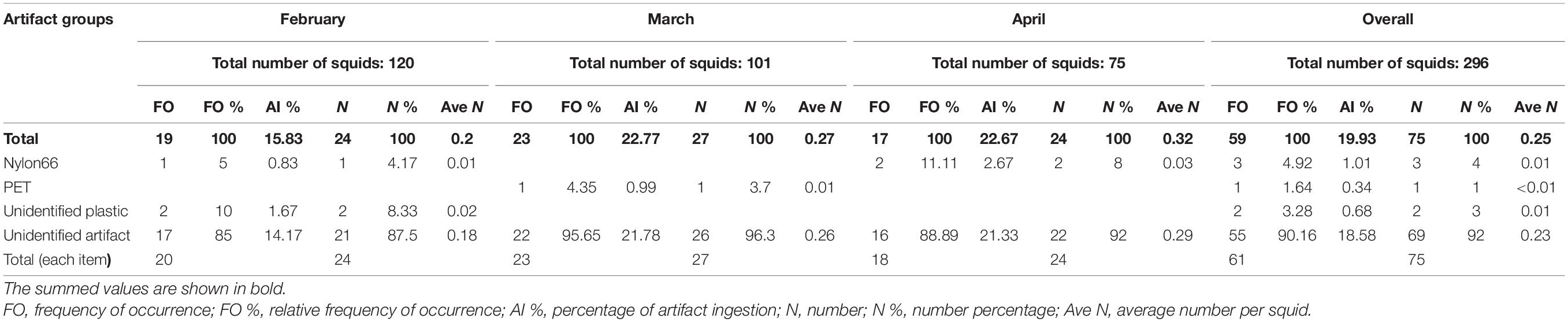
Table 3. Artifact ingestion from the stomach content analysis of Argentine shortfin squid Illex argentinus in the Southwest Atlantic.
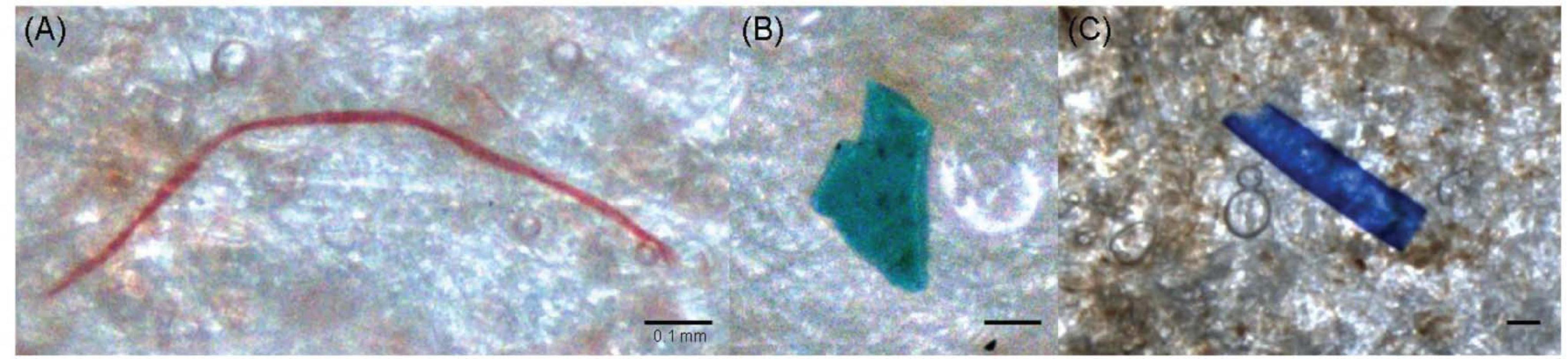
Figure 5. (A–C) Photographs showing examples of different colors, sizes, and shapes of artifacts found in the stomachs of the Argentine shortfin squid Illex argentinus from the Southwest Atlantic. The inset scale bars denote 0.1 mm for each photo.
The spatial distributions of the I. argentinus with ingested artifacts were widely dispersed and evenly observed in each sampling site, despite a slightly higher opportunity to ingest artifacts at the sites close to coastal areas, e.g., Sites 5, 8, 10, and 11, than at other sites (Figure 6A). The average percentage, i.e., AI %, of stomachs with ingested artifacts increased from February to March and April (from 15.8 to 22.8 and 22.7%, respectively) (Figure 6B). The number of ingested artifacts increased with the ML of squids (Figure 6C and Supplementary Figure 2), suggesting that the I. argentinus with larger size might have a higher risk of artifact ingestion than those with smaller size.
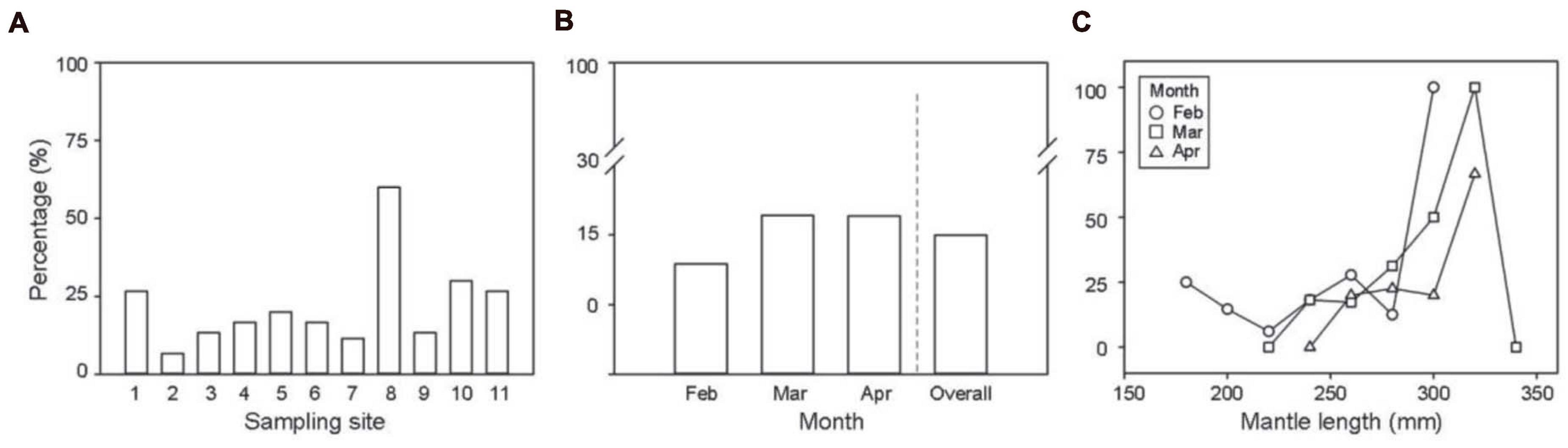
Figure 6. Risks of artifact ingestion for the Argentine shortfin squid Illex argentinus in the Southwest Atlantic. Percentage distributions of artifact ingestion by (A) sampling site, (B) month, and (C) mantle length. Squid sizes, ranging between 150 and 350 mm mantle length, are grouped into 20 mm intervals.
Discussion
We have provided recent detailed evidence of the monthly variations, particularly from February to April, in dietary compositions of the I. argentinus in the Southwest Atlantic in this study. The results revealed the importance of crustacean, fish, and cephalopod prey resources, particularly crustaceans, and how dietary compositions may vary with squid size, month, and migration. The decadal changes in diets of the I. argentinus potentially related to the changing environments and climate over the years, simultaneously resulting in changing structures of species compositions and food webs in the Southwest Atlantic (Hoving et al., 2013; Rosas-Luis et al., 2014). Our additional estimation of artifact ingestion also enabled us to bring attention to the risks of marine artifact pollution, a contemporary global environmental problem, in areas where the I. argentinus forages and migrates. These findings improve our understandings on growth, feeding, and distribution ecology of the I. argentinus and provide basic information on potential threats of ingested artifacts to both ecosystems and humans, which play a critical role in ecological and food security risk assessments.
Stomach content analysis provides a unique and important opportunity to understand the diets of marine predators with high taxonomic resolution (Young et al., 2015). As the first of its kind, our monthly dietary assessments for subadults and adults of the I. argentinus in the high seas east of Argentina and north of the exclusive economic zones of the Falkland Islands revealed that teleosts, particularly deep-sea Nichol’s lanternfish (G. nicholsi) but also an array of pelagic species, were the main prey consumed by the squids in this region. A similar pattern has also been observed in subadult and adult I. argentinus (120–350 mm ML) in the Bonaerensis region, where teleost prey consisted predominantly of deep-sea lanternfish, including G. nicholsi, Protomyctophum tensioni, and Lampichthys procerus, and potentially included a partial diversity of pelagic species, such as Engraulidae, suggesting regular feeding on non-pelagic prey sources by the I. argentinus. Our findings also supported that the diet of I. argentinus throughout their early life stages was characterized by a low diversity of prey items, including crustaceans, fishes, and cephalopods (Ivanovic and Brunetti, 1994), and we demonstrated that the dietary compositions of I. argentinus varied with the migration route, month, and growth of squids. At lower latitudes, the squids mainly consume crustaceans, and during their southward migration, they increase the proportion of ingested fishes and cephalopods. In addition, the dietary shifts with increasing ML showed that the squids undergo ontogenetic dietary shifts, similar to diverse organisms, such as insects, amphibians, and fishes, and such physiological adaptation usually accompanies variable habitat conditions and prey availability (Nakazawa, 2015). On the other hand, an increase in body size also changes metabolism, swimming ability, and predation risk (Nakazawa, 2015; Sánchez-Hernández et al., 2019) and thus may affect the need to switch their feeding selection.
A comparison of the long-term consistency in dietary compositions over about three decades showed that although crustaceans had usually been the main diet of I. argentinus, the PO % of fishes in this study, i.e., during 2018–2019, was significantly higher than those observed in 1992 and 1998 at similar latitudes but significantly lower than those observed between 50 and 52°S from March to April in 2012, which might have been as high as 72% (Table 4). Although slight differences in sampling area and sampling squid size existed among studies, such large differences in percentage compositions of the three main dietary taxonomic types might imply changing structures of species compositions and food webs in the Southwest Atlantic.
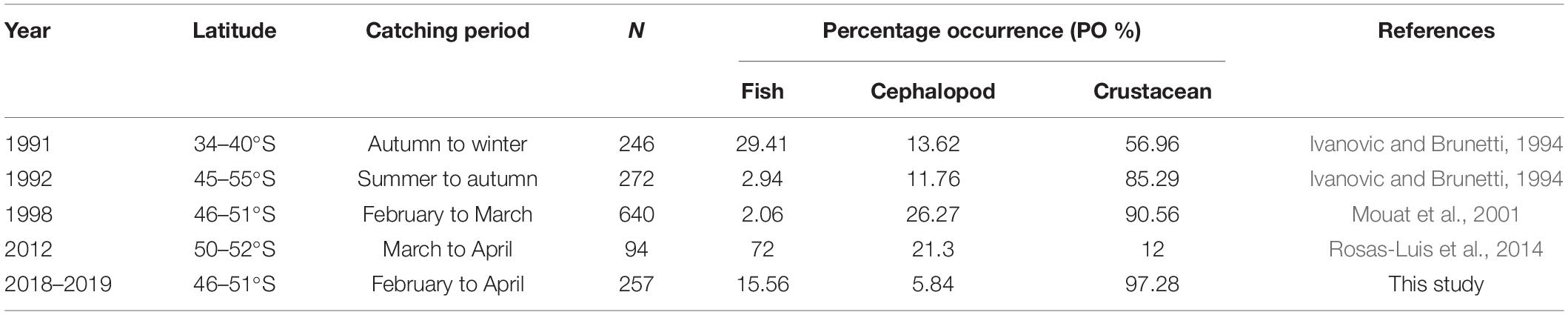
Table 4. Comparison of dietary compositions of the Argentine shortfin squid Illex argentinus in the Southwest Atlantic over decades.
The increasing proportion of predation of fishes by the I. argentinus in recent years may result from the overfishing of large fishes (Srinivasan et al., 2010). Due to the lack of potential predators or competitors, the squids can consume more fishes without limitations. Furthermore, contemporary anthropogenic influences, such as global warming, overfishing, oil and gas exploration, aquaculture expansion, ocean acidification, pollution, and so on, have significantly affected the marine ecosystems, causing changes in food web structure, decreases in biodiversity, and even marine defaunation (Merino et al., 2010; Tuomainen and Candolin, 2011; McCauley et al., 2015). The reduction in biodiversity facilitates trophic cascades accompanying shifts in diet and limits on the food chain length in marine ecosystems (Poloczanska et al., 2013). This can further influence not only the distributions of primary production through bottom-up controls (Hays et al., 2005; Frederiksen et al., 2006), but also the distributions of high trophic-level organisms through predator-prey interactions (Hazen et al., 2013; Green and Côté, 2014). Our results bring attention to the dietary shifts in the middle of the food chain, e.g., the I. argentinus, that may further cause unexpected consequences on the community structure, energy flow, and stability of the marine ecosystems (Jansen et al., 2007; Murphy et al., 2007; Atkinson et al., 2012; Sánchez-Hernández et al., 2019). On the other hand, the availability of resources and predation pressure affect the size of prey and potentially feed back to the size of the predator. For example, most apex predators, such as tunas, compete for prey in a specific size range (Ménard et al., 2006), and immature and mature striped dolphins show trophic overlaps when a decrease in small pelagic fishes is caused by overfishing (Gómez-Campos et al., 2011). When considering changes in dietary compositions and relationships between prey preference and size of predators in the long-term period, the quantity and quality of food resources in month or year may lead to a restructuring of fisheries and marine ecosystems.
Notably, although stomach content analysis is a universal technique for understanding dietary compositions for each species, detection, recognition, and counting the detached components of prey in the stomach contents remain critical challenges and unresolved weaknesses in this widely used and accepted methodology (Hyslop, 1980; Brown et al., 2012; Zacharia, 2017; Ibáñez et al., 2021). Particularly, I. argentinus crushes food with their beaks, and their digestion is fast, both resulting in a higher number of empty stomachs and unidentified fragments in the stomachs. Because unpaired otoliths, lenses, and beaks were highly observed in our samples and difficulties to define variations in thresholds within individuals based on size, the maximum number of otoliths, lenses, and beaks as the amount of different prey was used in this study, which may overestimate prey compositions. Moreover, the crystalline lens are fundamental and a key component for identifying the diet of squids. However, we found that the appearances of crystalline lenses of cephalopods and fish were quite similar in the stomach contents of the I. argentinus, and thus, the lenses in the stomachs was differentiated by soft stabbing with tweezers in this study. In contrast to fishes, whose lenses are usually spherical and hard to separate, the lenses of cephalopods can be easily separated into two parts. We also observed the lack of statoliths of cephalopods found in the stomachs. In addition to the small size of the cephalopods’ statoliths being difficult to observe, cephalopods’ statoliths may be more susceptible than fishes’ otoliths to the gastric acid of the I. argentinus (Clarke, 1978). Furthermore, considering abundance estimation, unbiased indices, and phases of digestion to better characterize diets of abundant, data-poor species to avoid faculty or incomplete conclusion about their trophic roles is further necessary as a reasonable measures for dietary studies in cephalopods (Brown et al., 2012; Ibáñez et al., 2021).
Considering both the dietary compositions and artifact ingestion, importantly, artifacts were observed in 20.5% of the empty stomachs, demonstrating the risk of persistent and indigestible artifacts in the stomachs of the squids (Table 5). More interestingly, the squids that consumed large prey, such as fishes, had a higher proportion of artifact ingestion than those that consumed crustaceans, implying that when the squids selected larger prey during their growth, this might simultaneously result in a higher risk of artifact ingestion. Approximately one-fifth of the stomachs were observed to have ingested artifacts in this study, which may be because the diet of the squids in this area was mainly composed of crustaceans; the serious artifact ingestion by crustaceans in the surrounding areas has been previously addressed (Jones-Williams et al., 2020). Furthermore, the larger I. argentinus, which usually obtain the ability and opportunity to consume larger prey such as fishes or cephalopods, showed a higher proportion of artifact ingestion, indicating that the effects of bioaccumulation and biomagnification of artifacts may exist through the food chain. Surprisingly, 20.5% of the empty stomachs were observed to contain artifacts, suggesting that the ingested artifacts may remain longer than food in stomachs and have difficulties being eliminated by the squids. Furthermore, considering other species at higher trophic levels in surrounding oceans, we found that the numbers of ingested artifacts by the southern pelagic opah fish Lampris immaculatus (one average prey item per stomach) in 1993 and 1994 in the Southwest Atlantic and gentoo penguins Pygoscelis papua (0.23 ± 0.53 items individual–1 scat) in 2009 in the Antarctic were slightly higher and close to those observed in the I. argentinus, respectively, indicating that the issue of artifact pollution may have existed for several years and consecutively affected local species and other organisms in the marine food webs (Jackson et al., 2000; Bessa et al., 2019). Regarding the ecological features and behavior, artifact ingestion occupied a higher percentage of the I. argentinus (19.9%) than of the jumbo squid (12%; Rosas-Luis, 2016), suggesting the potential for serious threats of marine pollution in the Southwest Atlantic although differences of feeding habits and prey compositions exist between two species, and further studies on the impacts of artifact debris on the I. argentinus are undoubtedly needed.
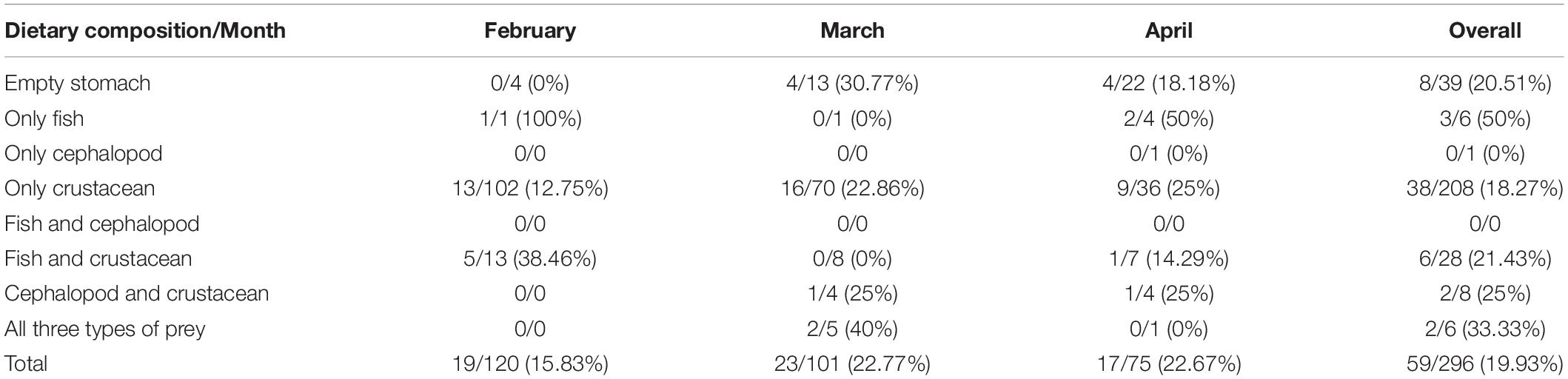
Table 5. Comparison of dietary compositions and artifact ingestion of the Argentine shortfin squid Illex argentinus sampled in this study, shown as the number of stomachs with artifact ingestion/number of total stomachs, including empty and non-empty, and the calculated percentages are shown in parentheses.
The considerable proportion of unidentified artifacts in the study was because most artifacts observed were not plastic but made of processed natural materials such as cotton or rayon. The first basis for identifying artifacts was their unusual colors in the stomachs, then the tough texture after dissolution by potassium hydroxide, and finally comparing the peaks at 2800–3000 cm–1 of the absorbance spectrum to plastic with a C–H bond. Although artifacts from natural materials often appear, the peak representing pentose in the spectrum often combines with other chemical structures and produces noisy spectra in frequency bands, making the results unidentifiable.
Through a combination of stomach content analysis and detection of artifact ingestion, we provided the first monthly dietary assessment for subadult and adult I. argentinus in the Southwest Atlantic and new insights into this species’ feeding ecology, particularly dietary changes over short- and long-term periods, i.e., monthly and decadal, respectively. Consistent with previous studies, the diets of the I. argentinus in the Southwest Atlantic were predominantly crustaceans, with dietary inclusion of fishes and cephalopods also observed during the juvenile stage. There was evidence for ML-based dietary variations with an increase in fish and cephalopod consumption with increasing ML. The dietary changes over decades bring attention to the changing structures of the marine food webs in the area. Estimated artifact ingestion appears similar for sites, months, and squid sizes, which might raise concerns locally about the major environmental problem of micro- and macro-plastics in seas. Further exploration of the interactions between the I. argentinus dietary requirements and the dynamics of prey quality and availability as well as constant risks of artifact ingestion for the squids will make a substantial contribution to the local levels of management and conservation strategies.
Data Availability Statement
The raw data supporting the conclusion of this article will be made available by the authors, without undue reservation.
Ethics Statement
The animal study was reviewed and approved by Institute of Fisheries Science, National Taiwan University.
Author Contributions
S-WC, R-GC, T-SC, and C-YK designed and performed the research. R-GC contributed data on field sampling. S-WC, T-HL, and C-YK analyzed data, participated in conceptualizing the study and design of graphics, and wrote the manuscript. Y-CL and C-SC provided technical support and revised the manuscript. All authors contributed to the article and approved the submitted version.
Funding
This research was funded by the Fisheries Agency and the Fisheries Research Institute, Council of Agriculture, Executive Yuan, Taiwan, under grants, 108AS-9.1.4-FA-F1, 109AS-9.1.4-FA-F1, and 110AS-6.1.2-AI-A2.
Conflict of Interest
The authors declare that the research was conducted in the absence of any commercial or financial relationships that could be construed as a potential conflict of interest.
Acknowledgments
We thank the Taiwanese jigging vessels, the Overseas Fisheries Development Council of the Republic of China, and the Taiwan Squid Fishery Association for providing squid samples and the consults.
Supplementary Material
The Supplementary Material for this article can be found online at: https://www.frontiersin.org/articles/10.3389/fmars.2021.675560/full#supplementary-material
Supplementary Figure 1 | Percentage distributions of the Argentine shortfin squid Illex argentinus with consumed (A) fishes (R2 = 0.053, p < 0.01), (B) cephalopods (R2 = 0.052, p < 0.01), and (C) crustaceans (R2 = 0.095, p < 0.01) based on the individual number percentage by mantle length for each month in the study area.
Supplementary Figure 2 | Relationship between the number of ingested artifacts and mantle length of the Argentine shortfin squid Illex argentinus captured in each month in the study area (R2 = 0.028, p < 0.01).
References
Abidli, S., Lahbib, Y., and Menif, N. T. E. (2019). Microplastics in commercial molluscs from the lagoon of Bizerte (Northern Tunisia). Mar. Pollut. Bull. 142, 243–252. doi: 10.1016/j.marpolbul.2019.03.048
Acuner, E., and Dilek, F. (2004). Treatment of tectilon yellow 2G by Chlorella vulgaris. Process, Biochem. 39, 623–631. doi: 10.1016/s0032-9592(03)00138-9
Arkhipkin, A. (1993). Age, growth, stock structure and migratory rate of pre-spawning short-finned squid Illex argentinus based on statolith ageing investigations. Fish. Res. 16, 313–338. doi: 10.1016/0165-7836(93)90144-v
Arkhipkin, A. (2000). Intrapopulation structure of winter-spawned Argentina shortfin squid, Illex argentinus (Cephalopoda, Ommastrephidae), during its feeding period over the Patagonian Shelf. Fish. Bull. 98:1.
Atkinson, A., Schmidt, K., Fielding, S., Kawaguchi, S., and Geissler, P. (2012). Variable food absorption by Antarctic krill: relationships between diet, egestion rate and the composition and sinking rates of their fecal pellets. Deep Sea Res. Part II Top. Stud. Oceanogr. 59, 147–158. doi: 10.1016/j.dsr2.2011.06.008
Avio, C. G., Gorbi, S., Milan, M., Benedetti, M., Fattorini, D., d’Errico, G., et al. (2015). Pollutants bioavailability and toxicological risk from microplastics to marine mussels. Environ. Pollut. 198, 211–222. doi: 10.1016/j.envpol.2014.12.021
Bessa, F., Ratcliffe, N., Otero, V., Sobral, P., Marques, J. C., Waluda, C. M., et al. (2019). Microplastics in gentoo penguins from the Antarctic region. Sci. Rep. 9:14191.
Boschi, E. E., Fischbach, C. E., and Iorio, M. I. (1992). Catálogo ilustrado de los crustáceos estomatópodos y decápodos marinos de Argentina. Frente Marítimo, Argentina Uruguay 10A, 7–94.
Breiby, A., and Jobling, M. (1985). Predatory role of the flying squid (Todarodes sagittatus) in north Norwegian waters. NAFO Sci. Counc. Stud. 9, 125–132.
Brown, S. C., Bizzarro, J. J., Cailliet, G. M., and Ebert, D. A. (2012). Breaking with tradition: redefining measures for diet description with a case study of the Aleutian skate Bathyraja aleutica (Gilbert 1896). Environ. Biol. Fish. 95, 3–20. doi: 10.1007/s10641-011-9959-z
Chapman, J. W. (2007). Amphipoda: chapter 39 of the light and smith manual: intertidal invertebrates from Central California to Oregon. Completely Revised and Expanded. Corvallis OR: Oregon State Univcorvallisersity.
Clarke, M. R. (1978). The cephalopod statolith – An introduction to its form. J. Mar. Biolog. Assoc. U.K. 58, 701–712. doi: 10.1017/s0025315400041345
Clarke, M. R. (1986). A Handbook for the Identification of Cephalopod Beaks. Oxford: Clarendon Press.
Cole, M., Lindeque, P., Fileman, E., Halsband, C., and Galloway, T. S. (2015). The impact of polystyrene microplastics on feeding, function and fecundity in the marine copepod Calanus helgolandicus. Environ. Sci. Technol. 49, 1130–1137. doi: 10.1021/es504525u
Dehaut, A., Cassone, A.-L., Frère, L., Hermabessiere, L., Himber, C., Rinnert, E., et al. (2016). Microplastics in seafood: benchmark protocol for their extraction and characterization. Environ. Pollut. 215, 223–233. doi: 10.1016/j.envpol.2016.05.018
Doubleday, Z. A., Prowse, T. A., Arkhipkin, A., Pierce, G. J., Semmens, J., Steer, M., et al. (2016). Global proliferation of cephalopods. Curr. Biol. 26, R406–R407.
FAO (2019). FAO Yearbook. Fishery and Aquaculture Statistics 2017/FAO Annuaire. Statistiques des Pêches et de L’aquaculture 2017/FAO Anuario. Estadísticas de Pesca y acuicultura 2017. Rome: FAO.
Foekema, E. M., De Gruijter, C., Mergia, M. T., van Franeker, J. A., Murk, A. J., and Koelmans, A. A. (2013). Plastic in north sea fish. Environ. Sci. Technol. 47, 8818–8824. doi: 10.1021/es400931b
Frederiksen, M., Edwards, M., Richardson, A. J., Halliday, N. C., and Wanless, S. (2006). From plankton to top predators: bottom-up control of a marine food web across four trophic levels. J. Anim. Ecol. 75, 1259–1268. doi: 10.1111/j.1365-2656.2006.01148.x
Golikov, A. V., Sabirov, R. M., Lubin, P. A., Jørgensen, L. L., and Beck, I.-M. (2014). The northernmost record of Sepietta oweniana (Cephalopoda: Sepiolidae) and comments on boreo-subtropical cephalopod species occurrence in the Arctic. Mar. Biodivers. Rec. 7:e58.
Gómez-Campos, E., Borrell, A., Cardona, L., Forcada, J., and Aguilar, A. (2011). Overfishing of small pelagic fishes increases trophic overlap between immature and mature striped dolphins in the mediterranean sea. PLoS One 6:e24554. doi: 10.1371/journal.pone.0024554
Green, S. J., and Côté, I. M. (2014). Trait-based diet selection: prey behaviour and morphology predict vulnerability to predation in reef fish communities. J. Anim. Ecol. 83, 1451–1460. doi: 10.1111/1365-2656.12250
Haimovici, M., Brunetti, N. E., Rodhouse, P. G., Csirke, J., and Leta, R. H. (1998). Illex argentinus. FAO Fish. Tech. Pap. 27-58. Rome: FAO.
Hays, G. C., Richardson, A. J., and Robinson, C. (2005). Climate change and marine plankton. Trends Ecol. Evol. 20, 337–344. doi: 10.1016/j.tree.2005.03.004
Hazen, E. L., Jorgensen, S., Rykaczewski, R. R., Bograd, S. J., Foley, D. G., Jonsen, I. D., et al. (2013). Predicted habitat shifts of Pacific top predators in a changing climate. Nat. Clim. Chang. 3, 234–238. doi: 10.1038/nclimate1686
Hoving, H. J. T., Gilly, W. F., Markaida, U., Benoit Bird, K. J., Brown, Z. W., Daniel, P., et al. (2013). Extreme plasticity in life-history strategy allows a migratory predator (jumbo squid) to cope with a changing climate. Glob. Chang. Biol. 19, 2089–2103. doi: 10.1111/gcb.12198
Hyslop, E. J. (1980). Stomach contents analysis – a review of methods and their application. J. Fish Biol. 17, 411–429. doi: 10.1111/j.1095-8649.1980.tb02775.x
Ibáñez, C. M., Riera, R., Leite, T., Díaz-Santana-Iturrios, M., Rosa, R., and Pardo-Gandarillas, M. C. (2021). Stomach content analysis in cephalopods: past research, current challenges, and future directions. Rev. Fish Biol. Fish. doi: 10.1007/s11160-021-09653-z
Ivanovic, M. L., and Brunetti, N. E. (1994). Food and feeding of Illex argentinus. Antarct. Sci. 6, 185–193. doi: 10.1017/s0954102094000295
Jackson, G. D., Buxton, N. G., and George, M. J. (2000). Diet of the southern opah Lampris immaculatus on the Patagonian Shelf; the significance of the squid Moroteuthis ingens and anthropogenic plastic. Mar. Ecol. Prog. Ser. 206, 261–271. doi: 10.3354/meps206261
Jamieson, A., Brooks, L., Reid, W., Piertney, S., Narayanaswamy, B., and Linley, T. (2019). Microplastics and synthetic particles ingested by deep-sea amphipods in six of the deepest marine ecosystems on Earth. Royal Soc. Open Sci. 6:180667. doi: 10.1098/rsos.180667
Jansen, E., Overpeck, J., Briffa, K., Duplessy, J., Joos, F., Masson-Delmotte, V., et al. (2007). Paleoclimate. Climate change : The physical science basis contribution of Working Group I to the Fourth Assessment Report of the Intergovernmental Panel on Climate Change. Geneva: IPCC.
Jones-Williams, K., Galloway, T., Cole, M., Stowasser, G., Waluda, C., and Manno, C. (2020). Close encounters-microplastic availability to pelagic amphipods in sub-antarctic and antarctic surface waters. Environ. Int. 140, 105792. doi: 10.1016/j.envint.2020.105792
Klages, N. T. (1996). Cephalopods as prey. II. Seals. Philos. Trans. R. Soc. Lond. B, Biol. Sci. 351, 1045–1052. doi: 10.1098/rstb.1996.0092
Kühn, S., Van Werven, B., Van Oyen, A., Meijboom, A., Rebolledo, E. L. B., and van Franeker, J. A. (2017). The use of potassium hydroxide (KOH) solution as a suitable approach to isolate plastics ingested by marine organisms. Mar. Pollut. Bull. 115, 86–90. doi: 10.1016/j.marpolbul.2016.11.034
Lombarte, A., Chic, Ò, Parisi-Baradad, V., Olivella, R., Piera, J., and García-Ladona, E. (2006). A web-based environment for shape analysis of fish otoliths. The AFORO database. Sci. Mar. 70, 147–152. doi: 10.3989/scimar.2006.70n1147
Lusher, A., Welden, N., Sobral, P., and Cole, M. (2017). Sampling, isolating and identifying microplastics ingested by fish and invertebrates. Anal. Methods 9, 1346–1360. doi: 10.1039/c6ay02415g
Markaida, U., Gilly, W. F., Salinas-Zavala, C. A., Rosas-Luis, R., and Booth, J. A. T. (2008). Food and feeding of jumbo squid Dosidicus gigas in the central Gulf of California during 2005-2007. Calcofi Rep. 49, 90–103.
Markaida, U., and Sosa-Nishizaki, O. (2003). Food and feeding habits of jumbo squid Dosidicus gigas (Cephalopoda: Ommastrephidae) from the Gulf of California. Mexico J. Mar. Biolog. Assoc. U.K. 83, 507–522. doi: 10.1017/s0025315403007434h
McCauley, D. J., Pinsky, M. L., Palumbi, S. R., Estes, J. A., Joyce, F. H., and Warner, R. R. (2015). Marine defaunation: animal loss in the global ocean. Science 347, 1255641. doi: 10.1126/science.1255641
Ménard, F., Labrune, C., Shin, Y.-J., Asine, A.-S., and Bard, F.-X. (2006). Opportunistic predation in tuna: a size-based approach. Mar. Ecol. Prog. Ser. 323, 223–231. doi: 10.3354/meps323223
Merino, G., Barange, M., Mullon, C., and Rodwell, L. (2010). Impacts of global environmental change and aquaculture expansion on marine ecosystems. Glob. Environ. Change 20, 586–596. doi: 10.1016/j.gloenvcha.2010.07.008
Mouat, B., Collins, M. A., and Pompert, J. (2001). Patterns in the diet of Illex argentinus (Cephalopoda: Ommastrephidae) from the Falkland Islands jigging fishery. Fish. Res. 52, 41–49. doi: 10.1016/s0165-7836(01)00229-6
Murphy, E., Watkins, J., Trathan, P., Reid, K., Meredith, M., Thorpe, S., et al. (2007). Spatial and temporal operation of the Scotia Sea ecosystem: a review of large-scale links in a krill centred food web. Philos. Trans. R. Soc. Lond., B, Biol. Sci. 362, 113–148. doi: 10.1098/rstb.2006.1957
Nakazawa, T. (2015). Ontogenetic niche shifts matter in community ecology: a review and future perspectives. Popul. Ecol. 57, 347–354. doi: 10.1007/s10144-014-0448-z
Piatkowski, U., Pierce, G. J., and da Cunha, M. M. (2001). Impact of cephalopods in the food chain and their interaction with the environment. Fish. Res. 52, 1–142.
PlasticsEurope (2018). “Annual review 2017–2018,” in PlasticsEurope AISBL Brussels (Belgium: PlasticsEurope).
Poloczanska, E. S., Brown, C. J., Sydeman, W. J., Kiessling, W., Schoeman, D. S., Moore, P. J., et al. (2013). Global imprint of climate change on marine life. Nat. Clim. Chang. 3, 919–925.
Portner, E. J., Markaida, U., Robinson, C. J., and Gilly, W. F. (2019). Trophic ecology of Humboldt squid, Dosidicus gigas, in conjunction with body size and climatic variability in the Gulf of California, Mexico. Limnol. Oceanogr. 65, 732–748. doi: 10.1002/lno.11343
R Core Team (2020). ). R: A Language and Environment for Statistical Computing. Vienna: R Foundation for Statistical Computing.
Raubenheimer, D., Simpson, S. J., and Mayntz, D. (2009). Nutrition, ecology and nutritional ecology: toward an integrated framework. Funct. Ecol. 23, 4–16. doi: 10.1111/j.1365-2435.2009.01522.x
Rodhouse, P. G. (2013). Role of squid in the Southern Ocean pelagic ecosystem and the possible consequences of climate change. Deep Sea Res. Part II Top. Stud. Oceanogr. 95, 129–138. doi: 10.1016/j.dsr2.2012.07.001
Rodhouse, P. G., and Hatfield, E. M. C. (1990). Dynamics of growth and maturation in the cephalopod Illex argentinus de Castellanos, 1960 (Teuthoidea: Ommastrephidae). Philos. Trans. R. Soc. Lond., B, Biol. Sci. 329, 229–241. doi: 10.1098/rstb.1990.0167
Rodhouse, P. G., and Nigmatullin, C. M. (1996). Role as consumers. Philos. Trans. R. Soc. Lond., B, Biol. Sci. 351, 1003–1022.
Rosas-Luis, R. (2016). Description of plastic remains found in the stomach contents of the jumbo squid Dosidicus gigas landed in Ecuador during 2014. Mar. Pollut. Bull. 113, 302–305. doi: 10.1016/j.marpolbul.2016.09.060
Rosas-Luis, R., Sánchez, P., Portela, J. M., and Del Rio, J. L. (2014). Feeding habits and trophic interactions of Doryteuthis gahi, Illex argentinus and Onykia ingens in the marine ecosystem off the Patagonian Shelf. Fish. Res. 152, 37–44. doi: 10.1016/j.fishres.2013.11.004
Santos, R. A., and Haimovici, M. (1997). Food and feeding of the short-finned squid Illex argentinus (Cephalopoda: Ommastrephidae) off southern Brazil. Fish. Res. 33, 139–147. doi: 10.1016/s0165-7836(97)00071-4
Smale, M. (1996). Cephalopods as prey. IV. Fishes. Philos. Trans. R. Soc. Lond., B, Biol. Sci. 351, 1067–1081. doi: 10.1098/rstb.1996.0094
Srinivasan, U. T., Cheung, W. W. L., Watson, R., and Sumaila, U. R. (2010). Food security implications of global marine catch losses due to overfishing. J. Bioeconomics 12, 183–200. doi: 10.1007/s10818-010-9090-9
Sánchez-Hernández, J., Nunn, A. D., Adams, C. E., and Amundsen, P. A. (2019). Causes and consequences of ontogenetic dietary shifts: a global synthesis using fish models. Biol. Rev. 94, 539–554. doi: 10.1111/brv.12468
Tuomainen, U., and Candolin, U. (2011). Behavioural responses to human-induced environmental change. Biol. Rev. 86, 640–657. doi: 10.1111/j.1469-185x.2010.00164.x
van Sebille, E., Wilcox, C., Lebreton, L., Maximenko, N., Hardesty, B. D., Van Franeker, J. A., et al. (2015). A global inventory of small floating plastic debris. Environ. Res. Lett. 10:124006. doi: 10.1088/1748-9326/10/12/124006
Wang, J., Chen, X., and Chen, Y. (2018). Projecting distributions of Argentine shortfin squid (Illex argentinus) in the Southwest Atlantic using a complex integrated model. Acta Oceanol. Sin. 37, 31–37. doi: 10.1007/s13131-018-1231-3
Xavier, J. C., and Cherel, Y. (2009). Cephalopod Beak Guide for the Southern Ocean: Jose Xavier. Cambridge: British Antarctic Survey.
Young, J. W., Hunt, B. P. V., Cook, T. R., Llopiz, J. K., Hazen, E. L., Pethybridge, H. R., et al. (2015). The trophodynamics of marine top predators: current knowledge, recent advances and challenges. Deep Sea Res. Part II Top. Stud. Oceanogr. 113, 170–187. doi: 10.1016/j.dsr2.2014.05.015
Zacharia, P. U. (2017). Trophic Levels and Methods for Stomach Content Analysis of Fishes. In: Course Manual Summer School on Advanced Methods for Fish Stock Assessment and Fisheries Management. Lecture Note Series No. 2/2017. Kochi: CMFRI, 278–288.
Keywords: stomach contents, cephalopod, Argentine shortfin squid, Illex argentinus, artifact ingestion, squid diet
Citation: Chang S-W, Chen R-G, Liu T-H, Lee Y-C, Chen C-S, Chiu T-S and Ko C-Y (2021) Dietary Shifts and Risks of Artifact Ingestion for Argentine Shortfin Squid Illex argentinus in the Southwest Atlantic. Front. Mar. Sci. 8:675560. doi: 10.3389/fmars.2021.675560
Received: 03 March 2021; Accepted: 07 June 2021;
Published: 21 July 2021.
Edited by:
Pierluigi Carbonara, COISPA Tecnologia & Ricerca, ItalyReviewed by:
Patrick Polte, Thünen-Institut, GermanyAntonello Mulas, University of Cagliari, Italy
Daniel Oesterwind, Thünen-Institut, Germany
Copyright © 2021 Chang, Chen, Liu, Lee, Chen, Chiu and Ko. This is an open-access article distributed under the terms of the Creative Commons Attribution License (CC BY). The use, distribution or reproduction in other forums is permitted, provided the original author(s) and the copyright owner(s) are credited and that the original publication in this journal is cited, in accordance with accepted academic practice. No use, distribution or reproduction is permitted which does not comply with these terms.
*Correspondence: Chia-Ying Ko, cyko235@ntu.edu.tw
†These authors have contributed equally to this work