- 1Institute of Marine Research, His, Norway
- 2Department of Natural Sciences, Centre for Coastal Research, University of Agder, Kristiansand, Norway
- 3Department of Ecology and Marine Resources, Instituto Mediterráneo de Estudios Avanzados (CSIC-UiB), Esporles, Spain
- 4Department of Ecology and Marine Resources, Instituto de Investigaciones Marinas (IIM-CSIC), Vigo, Spain
- 5Marine and Environmental Sciences Center (MARE), Funchal, Portugal
This article reviews a suite of studies conducted in a network of coastal Marine Protected Areas (MPAs) in Skagerrak, Southeast Norway. In 2006, Norway’s first lobster reserves were implemented, with the aim of protecting European lobster (Homarus gammarus) through a ban on fixed gear. A before–after control-impact paired series (BACIPS) monitoring program was initiated to evaluate effects of protection on depleted lobster populations. Experimental trapping and capture-recapture techniques were combined to track demography of populations, also including movement of individuals within and beyond MPAs and adjacent control areas. Further, population genetics and parentage studies were applied, allowing for estimation of gene flow, and novel work on sexual selection in lobsters. Additional studies have evaluated MPA effects on coastal cod (Gadus morhua), and on commercially harvested labrids (Ctenolabrus rupestris and Symphodus melops) and anadromous brown trout (Salmo trutta). Together, these studies reported effects of protection pertaining to increased population density, survival, body size and phenotypic diversity, changes in emigration and interaction with surrounding fisheries, and alteration of selection pressure on morphological- and behavioral traits. Designation of MPAs in close collaboration with fishers and managers, long-term monitoring, inclusion of citizen science and evolving research protocols—also including fisheries data—have revealed novel effects of protection and harvesting on marine populations, thus providing substantial contributions to conservation science. Moreover, knowledge of MPA effects on coastal species has impacted harvest regulations showing the utility of MPAs as empirically documented management tools in Norway.
Introduction
Fishing is well established as the driver having had the most impact on biodiversity in marine systems in the past 50 years (IPBES, 2019). Recognizing previous heavy exploitation as a reason for depressed levels of abundance is fundamental for taking appropriate management action. However, a lack of locally relevant scientific data on the potential contrast to the present-day depleted state of ecosystems has been considered an obstacle to set appropriate management targets for their recovery (Thurstan and Roberts, 2010). Chronic harvesting represents an impediment to observation of targeted species’ natural population dynamics, life-history patterns and -strategies. Marine Protected Areas (MPAs)—areas in the sea that forbid some or all extractive activities are highly useful tools to obtain valuable baseline information on marine populations (Lubchenco et al., 2003; Fenberg et al., 2012; Baskett and Barnett, 2015). Studies conducted in conjunction with the introduction of MPAs have been shown to be useful tools for research and management (Claudet et al., 2008; Babcock et al., 2010; Edgar et al., 2014), e.g., allowing modeling of possible outcomes of different harvest regulations for Atlantic cod Gadus morhua (Fernández-Chacón et al., 2015), and demonstrating benefits of protecting large bodied European lobster Homarus gammarus (Sørdalen et al., 2018).
Replicated control vs. impact study designs are powerful tools in environmental impact assessment, and since the early beginnings of the marine conservation science discipline, studies using before–after control-impact (BACI) have been suggested (Jones et al., 1992). The strength of BACI-type approaches in environmental impact assessment is the unequivocal detection of change in an “impacted” site from before impact to after, when compared to a control location where the impact persists. In a marine conservation setting, the “impact” will typically be represented by the removal of one or more anthropogenic disturbances, such as all or part of the harvesting occurring in an ecosystem. Modifications and refinement of the analytical framework associated with the BACI approach (Stewart-Oaten et al., 1986) were described by Underwood (1992, 1994) who expanded on asymmetrical designs with multiple controls to account for spatial heterogeneity and temporal variance that has nothing to do with the human disturbance (or its removal). Russ (2002) proposed a replicated BACI-approach as the “gold standard” in marine conservation science. As more time-series information became available from marine conservation sites (Babcock et al., 2010), it supported a notion that time is essential both for development and discovery of MPA effects (Claudet et al., 2008; Kaplan et al., 2019; Barceló et al., 2021). The BACI framework was thus expanded to include before-after control-impact paired series (BACIPS), involving time series data collected inside and outside in replicated sites (Osenberg et al., 2011; Thiault et al., 2019). Importantly, as highly valuable by-products of unequivocally demonstrated population effects from BACI-type approaches, studies using the same populations in the “after” state can contribute to novel insights regarding less obvious effects of the contrasting management regimes such as restoration of selection pressures (see Sørdalen et al., 2018, 2020).
In the early 2000s, European lobster (H. gammarus) census indicators reached an all-time low for southern Norway—and as assessment indicated a population size likely lower than 10% of the historical maximum, the species was red listed as “near threatened” according to the IUCN (International Union for Conservation of Nature) criteria (Oug et al., 2006). Motivated by the dire situation lamented by fishers in Norway’s south eastern Skagerrak region, and inspired by experiences gained from early protection trials in Lysekil, Sweden (Moland et al., 2013b), the first lobster reserves (as MPAs banning all gear types except hook-and-line) were established in Norway (Pettersen et al., 2009; Knutsen et al., unpublished). The objectives were to test whether spatial protection could indeed confer population benefits to lobster and provide information on the impact of the prevailing harvest pressure exerted on lobster in adjacent areas open to fishing. Additionally, it was proposed by participating scientists that partial protection imposed through the ban on fixed gear might confer quantifiable benefits to cod and potentially other harvested fish species. In 2004, population surveys began in proposed sites to evaluate their suitability, and from 2006 a replicated BACIPS design with paired lobster reserves and adjacent control areas have been monitored annually (see section “Study System and -Species”). Motivated by the early reported positive effects of MPAs on lobster, Tvedestrand municipality designated 15% of its coastal waters to protection in 2012 and became Norway’s first to implement a no-take zone providing full protection for the fish and crustaceans assemblages. Since publication of the first scientific paper documenting the initial effects of protection on European lobster (and Atlantic cod) in 2013 (Moland et al., 2013a), there has been a steady growth in designations of lobster reserves as local management tools in Norway with 54 areas covering 84 km2 implemented to date in total (Knutsen et al., unpublished).
Herein we review and summarize a suite of studies performed in conjunction with the designation of the first coastal MPAs in Southern Norway. Early effects of MPAs have been detected as indicated by population increases and shifts in age- and size-structure of protected populations. With time, the monitoring work has enabled (1) detection of longer-term demographic effects of protection, and (2) investigations into effects of the shifting selective “landscape” shaping individual traits disparately within protected and harvested populations. We discuss these findings considering MPA size and spacing in light of local population dynamics and -structure. We call for well managed MPAs as crucial to a holistic ecosystem approach to fisheries management, and as a nature-based solution to the challenge of restoring coastal populations and ecosystem function.
Study System and -Species
Study System
Skagerrak is characterized by harboring a portfolio of once productive local fish and shellfish stocks in depleted states (Kleiven et al., 2012; Rogers et al., 2014; Cardinale et al., 2017). At present, Skagerrak is under high pressure from intensive bottom trawling for northern shrimp Pandalus borealis which constitute the last economically viable fishery in this miniature deep sea (Knutsen et al., 2015; Kroodsma et al., 2018). In addition, environmental change influenced by land use in Skagerrak’s large catchment is exacerbated by climate change (Frigstad et al., 2020).
Although several definitions of MPA networks exist, they commonly refer to an integrated system of multiple protected areas, often designed to conserve regional biodiversity, and ecosystem function across habitats (Olsen et al., 2013). The studies reviewed herein were conducted in a network—in the sense of a collection of independent MPAs located on the Norwegian Skagerrak coast, mostly in the outer coastal seascape, potentially connected by gene flow (Figure 1). Capture of lobster has been effectively banned in the MPAs through gear restrictions, with only hook and line fishing allowed in the partially protected areas also alleviating harvest pressure on several fish species (Pettersen et al., 2009; Moland et al., 2013a; Fernández-Chacón et al., 2015; Halvorsen et al., 2017). The first designated MPAs (Figure 1 and Table 1, sites A–C, E) were established to generate knowledge on the development of lobster populations in areas unaffected by extractive fishing: Flødevigen in Agder county, the Bolærne archipelago at the mouth of the Oslo fjord in Vestfold and Telemark county, and the small island Kvernskjær in the Hvaler archipelago, in Viken county. Control areas are located adjacent to these and separated from MPAs by distances of 1,700, 850, and 2,250 m (from MPA center to control area center) in Flødevigen, Bolærne and Kvernskjær, respectively. A fourth MPA was established in Risør, in Agder county (Figure 1 and Table 1, site C).
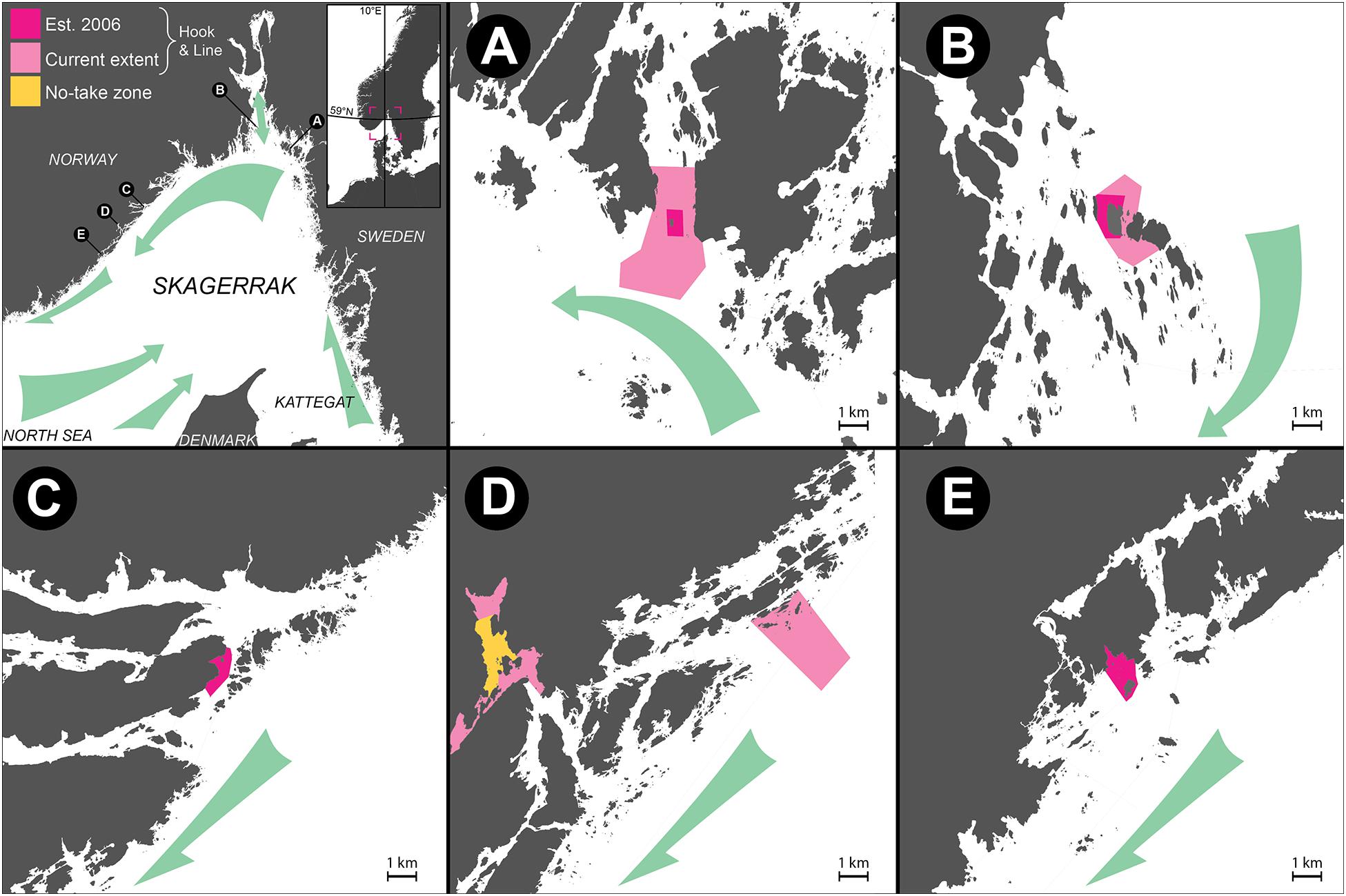
Figure 1. Maps of MPAs in Skagerrak reviewed herein, drawn on equal scale, presented in sequence according to position along the Norwegian Coastal Current: (A) Kvernskjær, (B) Bolaerne, (C) Risør, (D) Tvedestrand, (E) Flødevigen. Colored squares in upper left corner represent an area equivalent to 1 km2 in maps (A–E). Dark pink polygons represent the MPAs established in 2006 (e.g., in A–C,E); areas expanded or implemented at later dates (Table 1) are in light pink, while the no-take zone is shown in yellow. Prevailing ocean currents and implied larval drift/gene flow is represented by green arrows. For information on control areas, see the studies listed in Table 2.
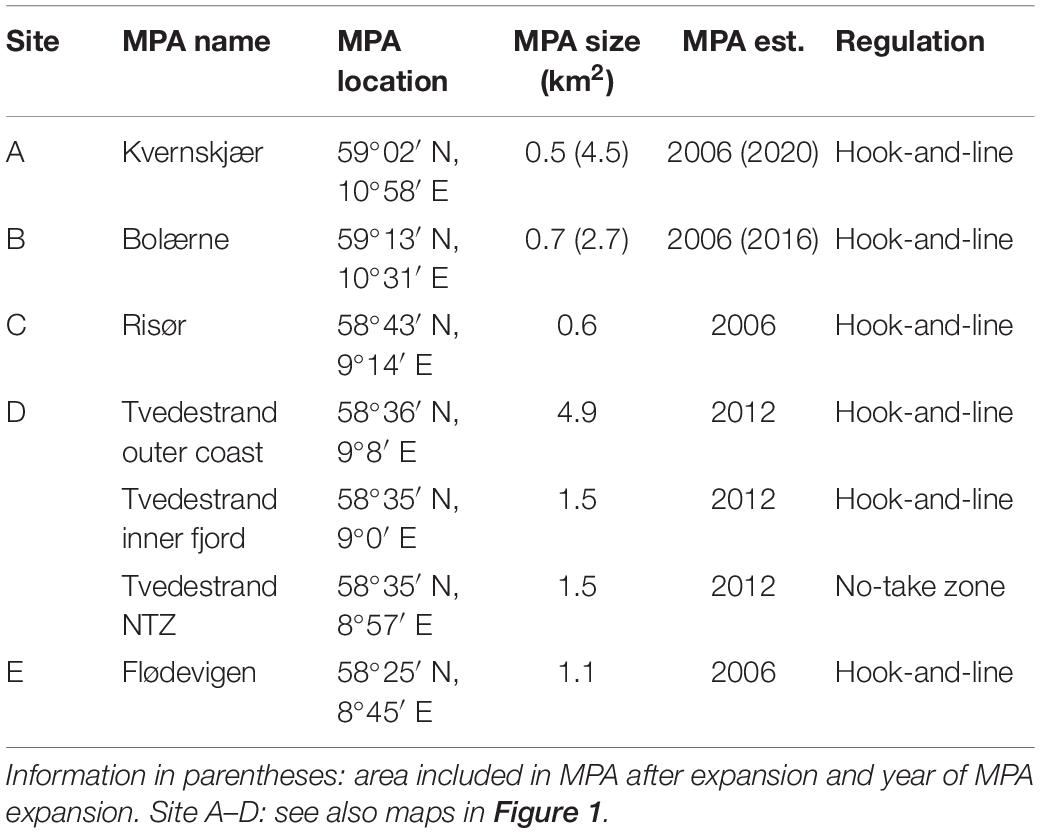
Table 1. Name and location of MPAs used as study sites in the reviewed literature listed from East to West in Skagerrak.
The fifth site covered by this review encompasses the sea areas of Tvedestrand municipality (Figure 1 and Table 1, site D), which initiated a zoning plan with MPAs implemented in 2012. A larger MPA (4.9 km2) was established in the municipality’s outer coastal zone, motivated by the early successes in small-scale experimental MPAs in Skagerrak (Nillos Kleiven et al., 2019). Also included in the zoning and covered by this review is the no-take zone in the Tvedestrand fjord, prohibiting all types of fishing. Surrounded by MPAs allowing hook-and-line-type fishing gear, the no-take zone enables studying effects of protection on fish (Figure 1 and Table 1). An acoustic telemetry array consisting of 56 acoustic receivers is deployed in the fjord, also including the no-take zone (for details, see Villegas-Ríos et al., 2017b; Thorbjørnsen et al., 2019; Freitas et al., 2021).
Since designation of the first set of MPAs, the areas have been subject to a range of studies designed to increase knowledge regarding the effects of protection for a collection of species that are targeted in Skagerrak fisheries (Table 2). Enforcement of MPAs is based on collaboration between the Directorate of Fisheries, the Coast Guard and local police. In these MPAs, only a few instances of poaching have been noted, mostly as a result of ignorance of MPA borders and regulations.
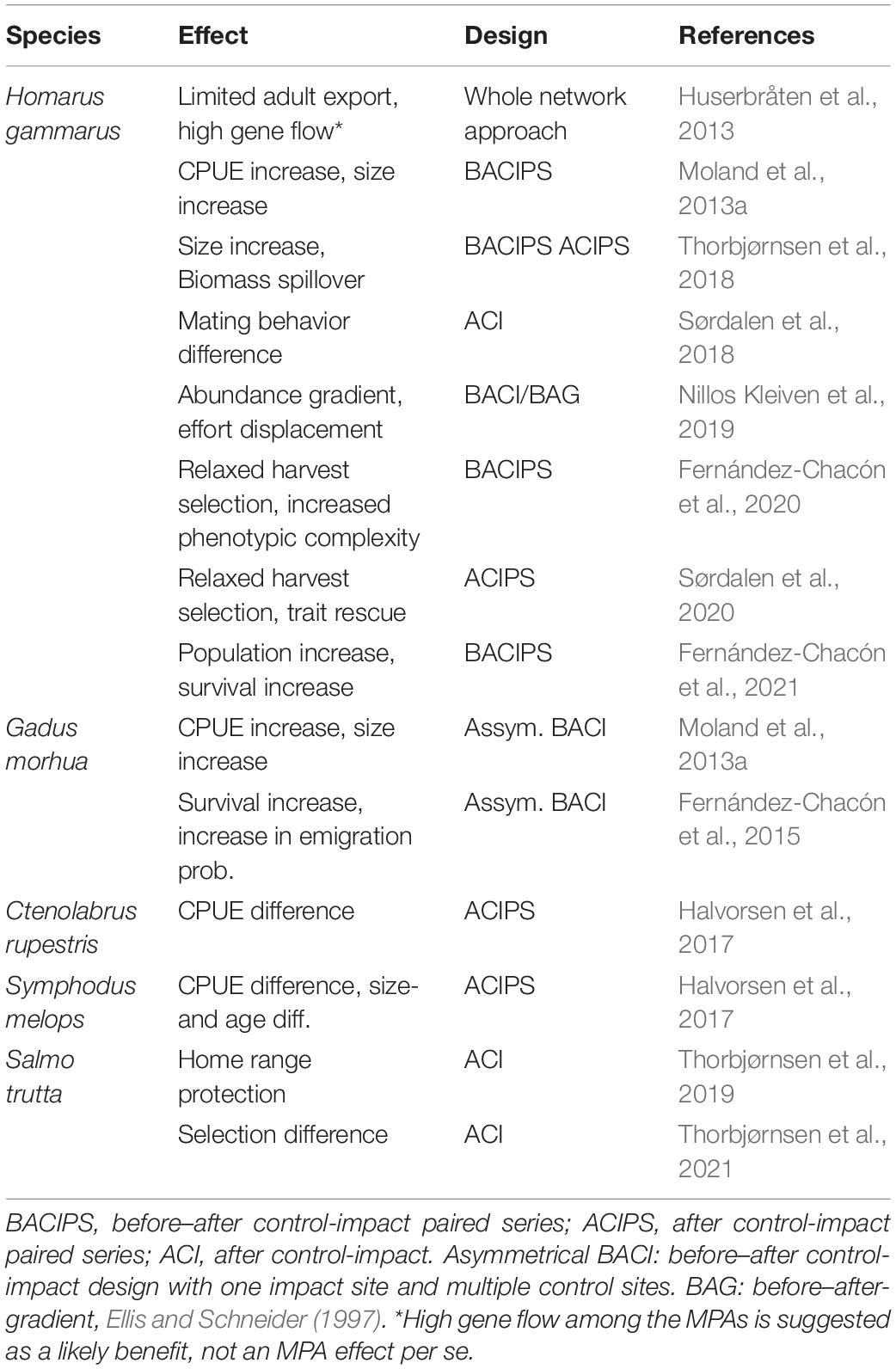
Table 2. Species and MPA effects reported in the reviewed literature based on BACI-derived study designs.
Study Species
European lobster (H. gammarus) (hereafter, lobster) is a large, long-lived sexually dimorphic crustacean and highly sought-after in Northern Europe’s commercial and recreational fisheries. In response to declining catches, a variety of management measures have been adopted such as closed season, gear restriction, and minimum (25 cm) and maximum (32 cm, in Skagerrak only) legal-size limits. Lobsters are solitary, territorial and typically live within limited shorter-term (<1 years) home ranges (∼0.02 km2) once they settle into a suitable habitat (Moland et al., 2011; Skerritt et al., 2015). Longevity may span several decades (Sheehy et al., 1999). Spatial genetic structure throughout the species’ range in Europe is limited, with only a weak differentiation between larger regions of the Swedish Skagerrak and the Atlantic areas to the west, with the population in Norway being a mix of these two (Ellis et al., 2017). In Skagerrak, the species is targeted in an intensive trap fishery, dominated by recreational fishers (Kleiven et al., 2012) exerting high levels of fishing mortality on legal lobsters (Wiig et al., 2013). Recent work combining an experimental evaluation of technological creep in the trap fishery with long-term CPUE time series suggest that coastal populations are in a severely depleted state compared to historical abundances (Kleiven et al., unpublished). Monitoring of lobster populations in MPAs and control areas (2005 to present, Figure 1 and Table 1, sites A, B, E) consisted of a standardized capture-recapture sampling program conducted annually in mid-August to September. Lobsters were caught using mackerel-baited parlor pots placed at the bottom at depths between 8 and 30 m, tagged with external tags for individual identification and released at the capture locations (for more details, see Moland et al., 2013a; Sørdalen et al., 2018).
Coastal Atlantic cod (G. morhua) (hereafter, cod) is a demersal generalist predator with severely depleted populations in Skagerrak (Olsen et al., 2009; Knutsen et al., 2018; Barth et al., 2019; Perälä et al., 2020). The coastal cod population complex in Skagerrak consists of two genetically and biologically distinct but coexisting ecotypes. They both occur in the outer sections of the coast but gene flow among them is limited. Coastal cod typically exhibit stationary movement behaviors compared to more mobile oceanic populations (Rogers et al., 2014; Villegas-Ríos et al., 2017b). The “fjord” ecotype seems to be more sedentary and stationary than the “North Sea” ecotype (Kristensen et al., 2021). Among these populations, age at 50% maturity varies from 2 to 4 years; whereas body length (BL) at 50% maturity varies from 35 to 60 cm (Olsen et al., 2004; Roney et al., 2016). In Southern Norway, coastal cod is legally caught when greater than or equal to 40 cm minimum legal size (MLS) by the full range of gear (Fernández-Chacón et al., 2017). For the studies reviewed herein, cod (size range: 16–97 cm) were captured, from April to July (2005–2013), using fyke nets (Danish type, designed for eel capture), tagged with external tags for individual identification and released at the capture location. Tagging and recaptures occurred every year simultaneously at the MPA (Figure 1 and Table 1, site E) and outside control areas (for more details, see Moland et al., 2013a; Fernández-Chacón et al., 2015).
Goldsinny wrasse (Ctenolabrus rupestris) and corkwing wrasse (Symphodus melops) are key mesopredators on rocky reef habitats and used as cleaner fish in salmonid aquaculture to control sea-lice infestations (Gjøsaeter, 2002; Halvorsen et al., 2020). The salmonid aquaculture industry demand for wild caught wrasse increased considerably from 2010, when salmon lice developed resistance to various delousing chemicals, and the fishery for wrasse as cleaner fish grew to its present size in absence of fundamental knowledge on demography and abundance of the targeted wrasse populations in Norwegian waters. To assess the consequences of harvesting, Halvorsen et al. (2017) sampled the wrasse populations in marine protected areas (Figure 1 and Table 1, sites C–E) and adjacent control areas on the Skagerrak coast in Southern Norway. The survey was conducted toward the end of the fishing season in 2013, with fyke nets and baited traps set at shallow depths, mimicking the fishing methods of the commercial wrasse fishery. Recent mark-recapture work shows that these labrids have very high site fidelity and the spill-over to adjacent fished areas is probably minimal (Halvorsen et al., 2021).
Brown trout (Salmo trutta) is a salmonid fish that either spends its whole life in fresh water or adopts an anadromous strategy largely motivated by access to more food (Olsen et al., 2006; Thorstad et al., 2016). Anadromous trout are commonly known as sea trout. Sea trout were caught around the center islands of the Tvedestrand fjord both inside and outside the no-take zone (2013–2016, Figure 1 and Table 1, site D). Individuals were captured in the fjord using a beach seine to minimize potential selective fishing of any behavioral type, and by electrofishing in the spawning river. Individuals were anaesthetized with clove oil before being tagged internally with an acoustic transmitter (Vemco V9P/V13P, for details, see Thorbjørnsen et al., 2019, 2021).
MPA Impacts
Population Density
The studies reviewed herein used catch-per-unit-effort (CPUE) as an indicator of abundance or population density in MPAs versus control areas (Table 2). Moland et al. (2013a) showed a 245% average increase in CPUE for lobster over the first 4 years of protection, versus 87% increase in control areas. Recently, Fernández-Chacón et al. (2021) applied a robust design model to the capture-recapture time series data to estimate annual abundances (corrected for imperfect detection) in the same areas (2006–2014). The results indicated that abundance levels increased after protection—albeit highly variable among MPAs. It also confirmed that the CPUE-based estimates for the first 4 years were indeed a realistic approximation of the average abundance increase.
A CPUE-based indicator also demonstrated an effect of partial protection on fish. After implementation, the Flødevigen MPA acquired and retained the highest probability of catching at least one adult cod per research trap compared to the three outside control areas open to harvesting (for details, see Moland et al., 2013a), indicating a survival benefit to cod protected from all but hook-and-line gear (see section “Survival Benefits”). For the two wrasse species commercially harvested as cleaner fish, C. rupestris and S. melops, Halvorsen et al. (2017) demonstrated higher CPUE in MPAs (banning fixed gear types) relative to adjacent controls, indicating that the fishery to some extent depletes local site-specific populations in areas open to harvesting. MPAs generally had higher abundance of the two wrasses, with the CPUE of goldsinny being 33–65% higher within MPAs, while ranging from 16% lower to 92% higher in MPAs for corkwing. This was the first study to assess the impact of this fishery and demonstrated the value of MPAs when coastal areas are faced with new, and unexpected stressors from profitable industries.
Although often used in fisheries research and capture-based monitoring studies, CPUE can be a problematic indicator as it relies on the catchability of the species in question (Maunder et al., 2006). In addition, it is difficult to estimate the rate at which change in CPUE is related to the real and unobserved change in abundance. However, in balanced designs with temporally overlapping sampling and standardized effort as used herein, CPUE should yield meaningful information on changes in abundance with time. Spatial heterogeneity in population responses was evident in the studies utilizing the long-term monitoring data collected in the original lobster reserves (Moland et al., 2013a; Fernández-Chacón et al., 2020, 2021). This underscores the utility of proper design choices and adequate replication in studies assessing MPA-effects.
Long-Term Effects on Demography
Survival Benefits
Using an array of acoustic receivers, Huserbråten et al. (2013) provided early demonstration of residency and high annual survival rate of acoustically tagged lobster in the Kvernskjaer MPA (0.5 km2). Population monitoring with capture-recapture allowed for modeling of vital rates in longer term studies. Moland et al. (2013b) found a linear positive trend in survival with body size in lobster in a Swedish MPA (Kåvra), with an additive effect of sex implying that females have lower natural mortality than males. Catchability (in research traps) was found to be higher in males in the same data, while the capture-recapture modeling applied to the data revealed that although caught less—and thus observed less than males in catches—the female population had higher survival, most likely due to lower growth rate and less risk prone behavior (Biro et al., 2014; Biro and Sampson, 2015). The same sex-specific differences in lobster survival and catchability were found in a recent study using capture-recapture data collected in the Norwegian Skagerrak (Fernández-Chacón et al., 2021), confirming this pattern for the species. In this case, they also reported, from one MPA that survival increased by 125.2 and 78.5% after protection for large-sized (>25 cm) males and females, respectively. Similarly, the capture-recapture data allowed Fernández-Chacón et al. (2015) to estimate the implicated survival increase conferred to cod, constituting 167 and 83% for small (<45 cm) and large cod (=45 cm), respectively (see Figure 2C) compared to “before,” with no change in the control areas.
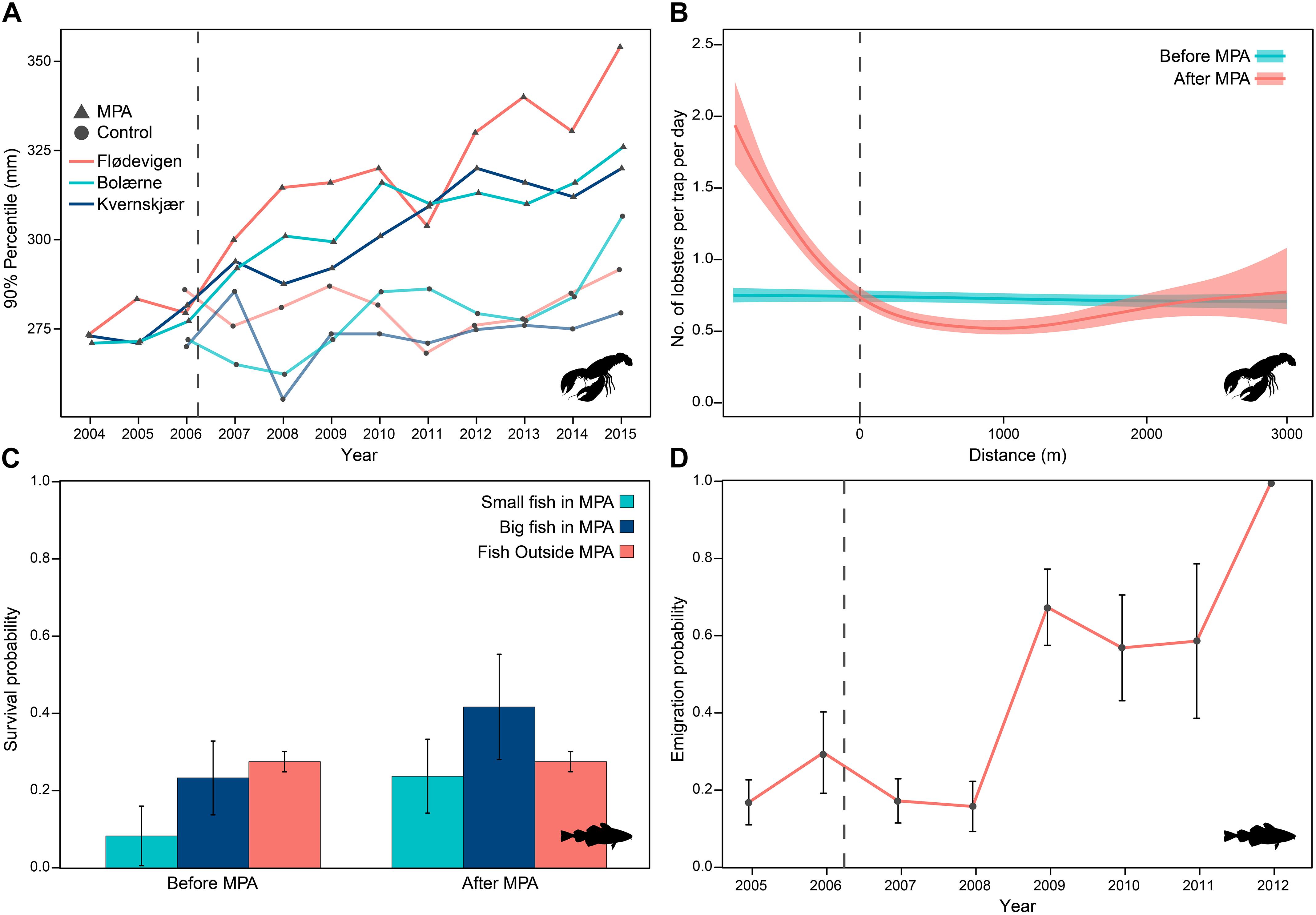
Figure 2. Population effects of MPAs before and after MPA implementation: (A) size-structure indicator (90 percentile) of H. gammarus in three MPAs (triangles) and three control areas (circles) in Skagerrak (modified from Fernández-Chacón et al., 2020); (B) cross-border abundance gradient in H. gammarus (modified from Nillos Kleiven et al., 2019); (C) average survival rates of G. morhua in a MPA and multiple control areas; and (D) development in emigration probability of G. morhua tagged inside a MPA (modified from Fernández-Chacón et al., 2015).
Recovery of Size Structure
Rebuilding of age- and size structure are hallmarks of lowered harvest pressure incurring lowered mortality in any harvested animal population (see Francis et al., 2007 and references therein). In southern Norway, at the onset of the monitoring time series, lobster was managed with a 24 cm total length (TL) minimum legal size (MLS). Harvested populations were thus characterized by few larger individuals. An increased average body size (13%) of lobster in MPAs relative to controls was evident after 4 years of protection from the annual lobstering season (Moland et al., 2013a), an effect somewhat dampened by introduction of a 25 cm MLS in 2008. Thorbjørnsen et al. (2018) presented “before” (2006) and “after” (2014) data on the demography of lobster in the three original MPAs, demonstrating a substantial widening of the size structure in the protected populations compared to the harvested before-state. By 2014, the average size increase in MPAs was 15% compared to 1% in control areas. More recently, Fernández-Chacón et al. (2020) analyzed body size data of tagged lobster sampled from 2004 to 2015 in all MPA-Control area pairs and reported a rapid change in the size structure characterized by an increase of the large-sized fraction of the population (see Figure 2A) with a subsequent increase in mean size. Overall, the diversity of sizes tended to increase inside protected areas. The study by Moland et al. (2013a) also detected a significant rebuilding of size-structure of cod inside one of the lobster reserves, where only hook and line fishing is allowed. Outside the reserves, both age- and size-structure of cod is severely truncated (see also, Olsen and Moland, 2011) as typically seen under intense harvest pressure (see “Harvest Selection on Phenotypes”).
Interaction With Surrounding Fisheries
Emigration
The initially implemented MPAs were small (∼0.5–1.1 km2), and since the onset of the research capture and tagging of lobster, recoveries have been reported from fishers operating outside of the MPAs or in adjacent control areas. Huserbråten et al. (2013) quantified the emigration from MPAs (Figure 1 and Table 1, sites A, B, D) and found that during the initial 6 years of protection 4.7% of tagged individuals emigrated from MPA sites. Both Huserbråten et al. (2013) and Thorbjørnsen et al. (2018) reported a highly left skewed distribution of movement behaviors, with the majority of individuals recaptured (in research traps) close to their tagging location. However, movement up to 24 km away from tagging location was reported (range: 34–24,670 m) by fishers recovering tagged lobsters. Whereas emigration by lobsters seemed stable over time at ∼4–5% of tagged individuals during the first 4–6 years of protection, the capture-recapture data on cod in the Flødevigen MPA analyzed by Fernández-Chacón et al. (2015) revealed an increasing tendency (annual probability) for cod to emigrate with time after MPA establishment (Figure 2D). This was attributed to ontogenetic and/or density dependent movement of tagged individuals.
Spillover of Biomass
Benefits of MPAs have been reported as net contribution to spiny lobster fishery catches through spillover (Goñi et al., 2010). Thorbjørnsen et al. (2018) compared cases of emigration and subsequent recovery by fishers for MPAs and control areas from 2006 to 2014. While there was no difference in movement rates between MPAs and control areas, the lobster emigrating from MPAs to fished areas were significantly larger (1.19 cm, p < 0.005) than lobsters emigrating from control areas.
Cross-Border Abundance Gradient
Nillos Kleiven et al. (2019) used experimental lobster fishing with randomized trap deployments in a wider area inside and around the outer Tvedestrand lobster MPA before and after implementation. The aim was to test for an effect gradient from inside- to far outside the MPA in a before-after-gradient (BAG) design (Ellis and Schneider, 1997). After 4 years, lobster abundance measured by CPUE increased significantly by a factor of 2.6 inside the MPA, with a decreasing gradient toward the border and into the fished areas outside. CPUE values in fished areas further away (3 km) remained similar to values before MPA establishment. However, a depression of population density developed at the MPA border compared to before data (Figure 2B). Using the effort data, the depression in density could be explained by the increased fishing effort close to the reserve border. The study thus demonstrated the effect of “fishing the line” (sensu Kellner et al., 2007)—possibly even penetrating into the MPA, and the need for effort control in areas surrounding MPAs.
Redistribution of Effort
In 2009, 3 years prior to implementation of the Tvedestrand zoning scheme, a study was carried out in which all traps deployed throughout the municipality seascape by commercial and recreational fishers during the first week of the lobstering season were registered. Repeating the same study in years 2, 3, and 4 after MPA implementation, Nillos Kleiven et al. (2019) were able to document a ∼80% increase of effort (trap numbers) in the total area, and pinpoint the appearance of fishing hotspots with a threefold increase in trap density. The highest increase was near the borders, indicating a shift in the preferred fishing areas.
Harvest Selection on Phenotypes
Body Size
Fernández-Chacón et al. (2020) provided size-dependent survival estimates of lobster in both MPA and control areas in Skagerrak, together with the shape of the relationship between survival and total body length. They documented significant negative relationships between survival and body size at the control areas but not in the MPAs, where the effect of body size was predominantly positive, and concluded that MPAs are a viable management approach for protecting against fisheries-induced selection, through the spatial refuge in both size-dependent and overall mortality.
Mating Patterns and Sexually Selected Traits
MPAs, in combination with control areas, can also be useful as field laboratories for understanding how fishing can affect mating systems of species. For instance, as lobsters have become more numerous and grown to larger sizes in the MPAs (Fernández-Chacón et al., 2020, 2021), the opportunity and scope for mate choice should also increase. In a parental assignment study conducted in Flødevigen, Sørdalen et al. (2018) found a female preference for males with larger body sizes, but that the relative size difference between females and males of mated pairs (the size-assortative mating pattern) were significantly larger in the reserve compared to mated pairs in the control area (Figure 3A). They also found positive selection differentials on male body- and claw size inside the MPA, but not in the control area, implying that size has less influence on male mating success in fished areas—most likely because fisheries-induced selection weakens female choice and the competition between males. Furthermore, estimations of sexual selection gradients on male traits uncovered selection to be acting strongest on relative claw size (claw size relative to body size), rather than on absolute claw and body size. This trait was later linked to an increased risk of being captured in the fishery (Moland et al., 2019), and the finding that male lobsters have up to 8% larger claws inside MPAs compared to similarly sized males in fished areas (Sørdalen et al., 2020). In sum, these findings strongly suggest that MPAs can be an effective means to preserve functional mating patterns, as well as restore and maintain diversity in sexually selected traits.
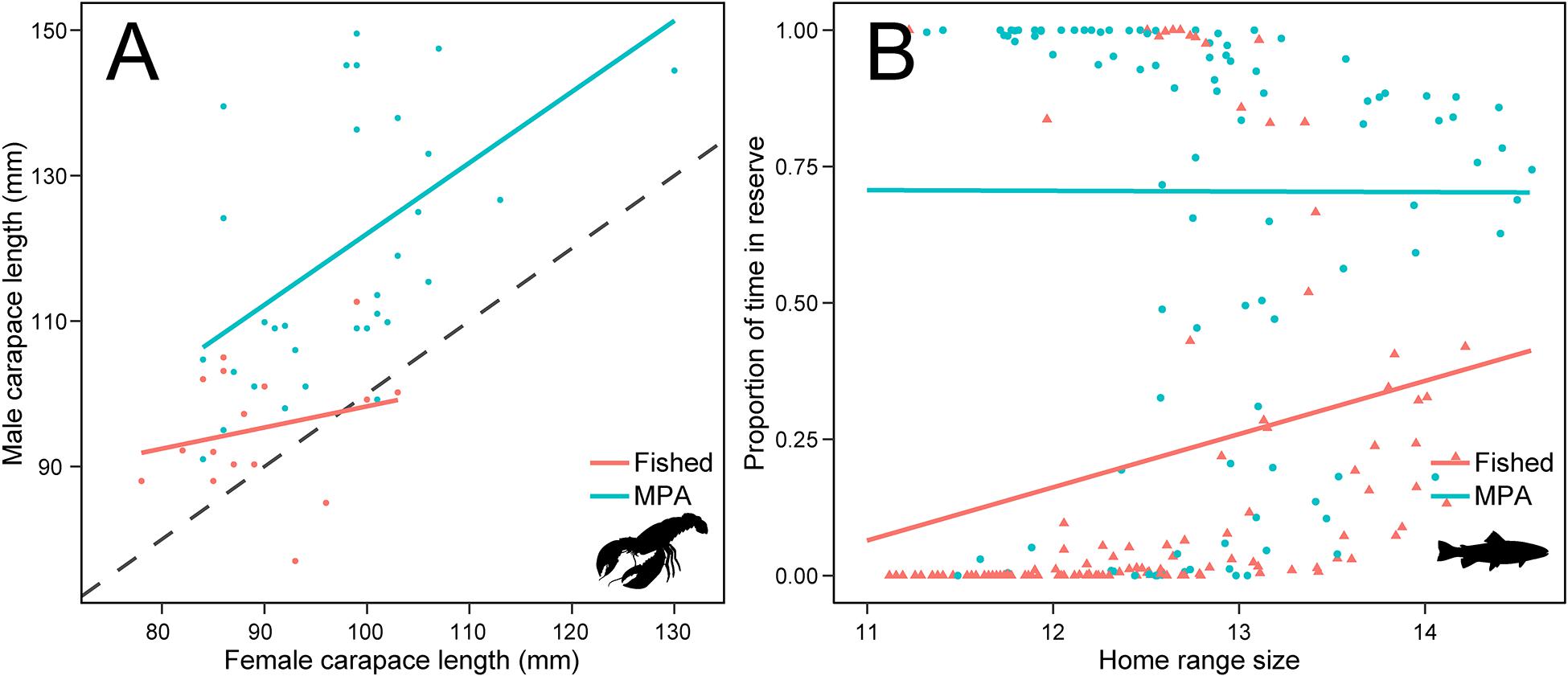
Figure 3. Evidence of altered selection landscapes in MPAs: (A) size-assortative mating in H. gammarus inside and outside a lobster reserve. Data points are mating confirmed by genetic parentage studies on lobster populations in protected (blue) and harvested (orange) states. Stippled line denote isometry (Y = X) where females and males are equal in size (modified from Sørdalen et al., 2018); (B) disparate protection potential (proportion of time spent in a no-take MPA) with increasing home range size in S. trutta tagged inside (blue, circles) and outside (orange, triangles) of the no-take zone in Tvedestrand fjord (modified from Thorbjørnsen et al., 2019).
Behavioral Traits
A series of studies conducted in conjunction with the MPAs aimed to investigate the effects of protection beyond demography and life-history traits. Villegas-Ríos et al. (2017a) suggested that protection might induce behavioral changes in favor of less mobile phenotypes in the protected population resulting from three processes/assumptions. First, individuals differ in their tendency to move, and therefore to leave the MPA and be exposed to the fishery outside. This idea is now widely recognized as several studies have demonstrated repeatability of movement traits such as dispersal tendency or home range size in several fish and aquatic invertebrates, including cod and lobster (Harrison et al., 2015; Villegas-Ríos et al., 2017b; Moland et al., 2019). Second, individuals with a smaller home range would spend more time under protection and thus increase their fitness, as shown by Villegas-Ríos et al. (unpublished). However, the potential for protection depends on the location of the home range. Thorbjørnsen et al. (2019) showed that the degree of potential protection conferred to sea trout by a no-take zone increased with home range size in sea trout initially tagged outside of the no-take zone (Figure 3B). Last, movement traits must be at least partially heritable to affect population behavioral composition over time, which seems to be the case across species and taxa according to a recent metanalysis by Dochtermann et al. (2019). Using sea trout as a model species, Thorbjørnsen et al. (2021) showed that home range size represented a personality trait that also affected survival differently depending on how much time the fish spent in the reserve. Having a larger home range led to a decrease in survival for individuals spending more time in the reserve, while having a larger home range led to an increase in survival for individuals spending less time in the reserve. To preserve behavioral variation in a population, a mosaic of marine protected areas and areas open to harvest was suggested as a management tool (Thorbjørnsen et al., 2021).
Discussion
Over the course of 16 years since the first before data were collected in designated experimental MPAs in Skagerrak, the network of MPAs described herein have allowed for substantial contributions to marine- and fisheries conservation science. Early effects of MPAs have been detected as population increases and positive shifts in age- and size-structure of protected populations. With time, the BACIPS-designed monitoring work has enabled detection of (1) longer-term demographic effects of protection, and (2) shifts in the selective landscape shaping phenotypic traits disparately within protected and harvested populations. Although the BACIPS-design has been used effectively in other MPA studies, e.g., in ecological evaluation of MPA networks (e.g., Grorud-Colvert et al., 2014; Thiault et al., 2019), they remain rare. In their seminal study, Claudet et al. (2008) showed that sufficient time (and size) is essential for MPA-effects to develop. Investment in long-term monitoring is thus a worthwhile priority for any entity tasked with evaluation of MPA-effects. In the Northern Channel Islands, California, there is evidence for trophic redundancy attained through restoration of predator abundance and -size structure, especially in older MPAs (Hamilton and Caselle, 2015; Caselle et al., 2018; Eisaguirre et al., 2020). However, using an extensive BACI time series, Malakhoff and Miller (2021) recently suggested that despite increased predator density, trophic cascades have yet to develop in California’s northern Channel Island marine reserves after 15 years of protection. They suggested that more time, and/or recovery of more effective urchin predators might be needed to induce kelp forest trophic cascades. Long-term BACIPS monitoring studies are helpful in producing realistic expectations regarding the development of population- and ecological effects of MPAs.
Clawed and spiny lobsters have shown unequivocal positive response to protection in MPAs elsewhere (Shears et al., 2006; Goñi et al., 2010; Hoskin et al., 2011). Considering the recent and historical harvest pressure on coastal European lobster populations in Skagerrak (Kleiven et al., 2012; Kleiven et al., unpublished), it is likely that the positive effects of cessation of harvesting reported by studies reviewed herein were enabled by the combination of long-term historical depletion and high pre-closure fishing pressure in the sites (Jaco and Steele, 2020). While rebuilding of local lobster populations was the main goal when implementing the MPAs, the decreased harvest pressure in these areas also conferred effects on several fish species. Striving to test hypotheses of general interest to conservation science, we deem the study of lobster and sympatric fish species as case studies with attributes that are transferable to other aquatic species using the marine environment for all or part of their life cycle (e.g., Sørdalen et al., 2018, 2020; Thorbjørnsen et al., 2021). Two wrasse species, goldsinny (Ctenolabrus rupestris) and corkwing wrasse (Symphodus melops), both harvested to provide cleaner fish services in the salmonid aquaculture industry, showed moderate positive responses to protection in MPAs reviewed herein. Wrasse species targeted by fisheries have shown positive responses in other studies conducted in temperate MPAs, e.g., California sheephead Semicossyphus pulcher (Hamilton and Caselle, 2015; Jaco and Steele, 2020) and the Mediterranean wrasses Coris julis and Symphodus doderleini (Claudet et al., 2006). There is scope for further studies on the utility of MPAs to alleviate harvesting pressure and ensure ecosystem function and recruitment of wrasse species currently being heavily targeted by intensive fisheries in Southern and Western Norway.
Optimal MPA size and spacing has been a subject of much debate and scientific inquiry (see e.g., Gaines et al., 2010; Costello and Connor, 2019), also suggesting MPAs designed to benefit highly migratory species with known migration routes, aggregating behavior, and philopatry (Boerder et al., 2019). Interestingly, the relatively small Tvedestrand no-take zone was large enough to protect individual sea trout (Thorbjørnsen et al., 2019), hereby showing protective effects for an anadromous species. European lobster is generally a resident species showing high site-fidelity in shorter-term studies, both inside and outside of MPAs (Moland et al., 2011; Huserbråten et al., 2013; Wiig et al., 2013). Due to a relatively long pelagic larval duration of 3–8 weeks, there is high potential for long distance dispersal (Manel et al., 2019). Using molecular techniques, Huserbråten et al. (2013) analyzed lobster tissue samples from the Skagerrak coastline spanning the MPA network (∼400 km, Figure 1). The work revealed high gene flow among populations which suggested a potential for downstream recruitment benefits from the MPAs in study. Although the initially implemented MPAs seemed to be of sufficient size to confer observable population effects, the recent trend has been establishment of somewhat larger MPAs in outer coastal areas of Skagerrak (Knutsen et al., unpublished). Two of the MPAs reviewed herein were subject to recent expansions (2016 and 2020) resulting from processes initiated by local management bodies to ensure management-relevant scaling of MPAs for lobster (see Figure 1). While Olsen and Moland (2011) demonstrated limited home range size of cod in a coastal seascape, the longer-term work by Fernández-Chacón et al. (2015) showed a tendency for increased annual emigration probability with time—thus indicating that the 1.1 km2 Flødevigen MPA was too small to harbor coastal cod home ranges throughout- and past ontogeny. An effect of density dependence was also suggested as a partial explanation for the increased emigration probability. The sympatric cod ecotypes inhabiting coastal habitats in Skagerrak (Knutsen et al., 2018) seem to harbor differences in habitat, feeding ecology and movement behavior, where the “fjord” ecotype is associated with fjord habitat and benthic feeding ecology while the “North Sea” ecotype is associated with pelagic feeding and an higher tendency to migrate (Barth et al., 2019; Kristensen et al., 2021). There are knowledge gaps considering optimal design of MPAs tailored to these ecotypes’ movement ecology. Commonly found occupying the outer coastal seascape, the “North Sea” ecotype is also overrepresented in cod samples from the bottom trawl fleet (Jorde et al., 2018). The more migratory behavior suggested for this ecotype might require larger, continuous fjord-to-deep-sea transect type MPAs to provide protection throughout the life cycle. Subject to intensive bottom trawling (Kroodsma et al., 2018), it is uncertain whether cod or other depleted demersal fish species might recover in Skagerrak under the present management regime (Cardinale et al., 2017). However, motivation to implement sufficiently large MPAs banning bottom trawling might be gleaned from Öresund, Sweden. Here, a ban on bottom trawling in effect since 1932 has allowed cod to prosper, also during periods of adverse environmental conditions, with size and age structure otherwise unseen at present in the Skagerrak-Kattegat neighborhood (Lindegren et al., 2010; Sundelöf et al., 2013).
Using data from the Tvedestrand fjord MPAs, Villegas-Ríos et al. (2017b) suggested that a small no-take zone could be maladaptive for fjord cod by favoring behavioral phenotypes characterized by small home ranges. While testing this hypothesis for sea trout in the Tvedestrand fjord, Thorbjørnsen et al. (2021) suggested a seascape mosaic of marine reserves, partially protected areas and areas open to harvest as a management tool to preserve behavioral variation in a sea trout population. Preserving behavioral variation is important to maintain a population’s resilience to environmental change (Dingemanse et al., 2004). Fishes captured using hook-and-line can experience behavior selection against more active individuals (Alós et al., 2016), and MPA networks can help oppose this selection. Utilizing the same acoustic telemetry array in Tvedestrand fjord, Freitas et al. (2021) showed that summer peaks in sea surface temperature represent challenges for cod residing in a fjord environment, while ballan wrasse (Labrus bergylta) and Atlantic pollack (Pollachius pollachius) retained their movement behavior. During such periods, cod were confined to deeper, cooler water masses—away from shallow habitats preferred during autumn and winter. Future MPAs designed to protect cod and other demersal cold water species would benefit from being placed in sites that might act as “climate refugia” (Davis et al., 2021), where access to cool and well oxygenated water masses is likely to prolong habitat suitability in the face of increased environmental variability.
The recovery of size-structure and survival benefits reported from the studies conducted in the Norwegian Skagerrak all respond to a shift in size-selective mortality occurring within MPAs as a result of protection from fisheries. Baskett and Barnett (2015) reviewed the ecological and evolutionary consequences of marine reserves and noted the theoretical potential for protection against fisheries-induced evolution. Two findings reviewed herein support this notion. Using parental assignment, Sørdalen et al. (2018) showed a pattern of size-assortative mating in lobster which was much more pronounced in an MPA due to the recovery of size-structure. Moreover, relative claw size was found to be the best predictor of male mating success. This trait—large relative claw size—is both under sexual selection in natural states, and subject to harvest selection due to correlated behavioral traits that confer catchability in traps (Moland et al., 2019). Sørdalen et al. (2020) later showed that this sexually selected trait is rescued by absence of harvesting in MPAs. These pioneering studies resulted in significant advancement of our understanding of the contrasting effects of harvesting and protection on a long-lived species with complex behavioral and reproductive biology and led to introduction of a maximum legal size limit in the Skagerrak lobster fishery as of 2017.
In conclusion, the MPAs reviewed herein conferred multiple population effects on several species inhabiting the Skagerrak coastal seascape. Importantly, BACI-designed monitoring of MPA-control area pairs provided unequivocal demonstrations of local effects of protection on lobster populations. Importantly, as highly valuable by-products of unequivocally demonstrated population effects from BACI-type approaches, studies using the same populations in the “after” state can contribute to novel insights regarding more subtle effects of protection. Effects of protection on two wrasse species harvested as cleaner fish in salmonid aquaculture underscore the value of MPAs when coastal areas are faced with new and unexpected stressors from profitable industries. Namely, the opportunity to better understand and measure cumulative effects on coastal ecosystems through control-impact studies which in turn enable managers to make knowledge based-management decisions.
Effects of protection on European lobster in terms of conspicuous size increase and density increase manifested as record breaking catches in research traps have created enthusiasm and optimism in the public eye, and thus provided an easily accessible entry to the concept of marine conservation and rebuilding potential in depleted coastal populations. This does not preclude the fact that allocation of areas in the coastal zone from fisheries to conservation purposes is still in an early phase in Norway and thus bound to be the subject of much debate among stakeholders. Nonetheless, MPAs in the form of small-scale lobster reserves—allowing hook and line fishing to continue—have provided a first introduction to the principles and potential benefits of marine conservation. Proper planning in close collaboration with fishers and managers, long-term scientific monitoring, inclusion of citizen science and evolving research protocols—also including fisheries data—have revealed novel effects of protection and harvesting on marine populations, with impact on harvest rules and the use of MPAs as empirically documented management tools in Norway.
Author Contributions
EM and JK contributed equally to the conception and outline of the review. EM, AF-C, ST, DV-R, TS, KH, MH, AK, and EO wrote the manuscript. PNK, HK, SE, CF, and JK commented on versions of the draft. All authors contributed to manuscript revision, read, and approved the submitted version.
Funding
Long term monitoring of the MPAs in Skagerrak was funded by the Institute of Marine Research coastal ecosystems research program. Preparation of the manuscript was supported by the University of Agder through a priority research grant awarded to the Centre of Coastal Research (CCR). EO received funding from the Marine Science Program within the Research Council of Norway, grant no. 294926 (CODSIZE). DV-R received funding from the European Union’s Horizon 2020 research and innovation programme under the Marie Sklodowska-Curie grant agreement No. 793627 (BEMAR) and from the programme IF_ERC from the Spanish National Research Council.
Conflict of Interest
The authors declare that the research was conducted in the absence of any commercial or financial relationships that could be construed as a potential conflict of interest.
Acknowledgments
We thank colleagues at the IMR Flødevigen marine research station for valuable assistance in field work and scientific cruises, as well as data management. We honor the late S.E. Enersen who worked side by side with JK in the early years of MPA site designation, implementation and monitoring. JK also trained a new generation of lobster researchers at IMR. EM and AK are indebted to their mentors G.P. Jones and G.R. Russ. We are thankful for the contributions from three referees who provided insightful reviews that improved the content of this review.
References
Alós, J., Palmer, M., Rosselló, R., and Arlinghaus, R. (2016). Fast and behavior-selective exploitation of a marine fish targeted by anglers. Sci. Rep. 6:e38093. doi: 10.1038/srep38093
Babcock, R. C., Shears, N. T., Alcala, A. C., Barrett, N. S., Edgar, G. J., Lafferty, K. D., et al. (2010). Decadal trends in marine reserves reveal differential rates of change in direct and indirect effects. Proc. Natl. Acad. Sci. U.S.A. 107, 18256–18261. doi: 10.1073/pnas.0908012107
Barceló, C., White, J. W., Botsford, L. W., and Hastings, A. (2021). Projecting the timescale of initial increase in fishery yield after implementation of marine protected areas. ICES J. Mar. Sci. fsaa233. doi: 10.1093/icesjms/fsaa233
Barth, J. M., Villegas-Ríos, D., Freitas, C., Moland, E., Star, B., André, C., et al. (2019). Disentangling structural genomic and behavioural barriers in a sea of connectivity. Mol. Ecol. 28, 1394–1411. doi: 10.1111/mec.15010
Baskett, M. L., and Barnett, L. A. K. (2015). The ecological and evolutionary consequences of marine reserves. Annu. Rev. Ecol. Evol. Syst. 46, 49–73. doi: 10.1146/annurev-ecolsys-112414-054424
Biro, P. A., Adriaenssens, B., and Sampson, P. (2014). Individual and sex-specific differences in intrinsic growth rate covary with consistent individual differences in behaviour. J. Anim. Ecol. 83, 1186–1195. doi: 10.1111/1365-2656.12210
Biro, P. A., and Sampson, P. (2015). Fishing directly selects on growth rate via behaviour: implications of growth-selection that is independent of size. Proc. Biol. Sci. 282:20142283. doi: 10.1098/rspb.2014.2283
Boerder, K., Schiller, L., and Worm, B. (2019). Not all who wander are lost: improving spatial protection for large pelagic fishes. Mar. Policy 105, 80–90. doi: 10.1016/j.marpol.2019.04.013
Caselle, J. E., Davis, K., and Marks, L. M. (2018). Marine management affects the invasion success of a non-native species in a temperate reef system in California,. USA. Ecol. Lett. 21, 43–53. doi: 10.1111/ele.12869
Cardinale, M., Svenson, A., and Hjelm, J. (2017). The “easy restriction” syndrome drive local fish stocks to extinction: the case of the management of Swedish coastal populations. Mar. Policy 83, 179–183. doi: 10.1016/j.marpol.2017.06.011
Claudet, J., Osenberg, C. W., Benedetti-Cecchi, L., Domenici, P., Garcia-Charton, J. A., Perez-Ruzafa, A., et al. (2008). Marine reserves: size and age do matter. Ecol. Lett. 11, 481–489. doi: 10.1111/j.1461-0248.2008.01166.x
Claudet, J., Pelletier, D., Jouvenel, J.-Y., Bachet, F., and Galzin, R. (2006). Assessing the effects of marine protected area (MPA) on a reef fish assemblage in a northwestern Mediterranean marine reserve: identifying community-based indicators. Biol. Conserv. 130, 349–369. doi: 10.1016/j.biocon.2005.12.030
Costello, M. J., and Connor, D. W. (2019). Connectivity is generally not important for marine reserve planning. Trends Ecol. Evol. 34, 686–688. doi: 10.1016/j.tree.2019.04.015
Davis, T. R., Champion, C., and Coleman, M. A. (2021). Climate refugia for kelp within an ocean warming hotspot revealed by stacked species distribution modelling. Mar. Environ. Res. 166:105267. doi: 10.1016/j.marenvres.2021.105267
Dingemanse, N. J., Both, C., Drent, P. J., and Tinbergen, J. M. (2004). Fitness consequences of avian personalities in a fluctuating environment. P. Roy. Soc. B. 271, 847–852. doi: 10.1098/rspb.2004.2680
Dochtermann, N. A., Schwab, T., Anderson Berdal, M., Dalos, J., and Royauté, R. (2019). The heritability of behavior: a meta-analysis. J. Hered. 110, 403–410. doi: 10.1093/jhered/esz023
Edgar, G., Stuart-Smith, R., Willis, T., Kininmonth, S., Baker, S. C., Banks, S., et al. (2014). Global conservation outcomes depend on marine protected areas with five key features. Nature 506, 216–220. doi: 10.1038/nature13022
Eisaguirre, J. H., Eisaguirre, J. M., Davis, K., Carlson, P. M., Gaines, S. D., and Caselle, J. E. (2020). Trophic redundancy and predator size class structure drive differences in kelp forest ecosystem dynamics. Ecology. 101:e02993. doi: 10.1002/ecy.2993
Ellis, C. D., Hodgson, D. J., Daniels, C. L., Collins, M., and Griffiths, A. G. F. (2017). Population genetic structure in European lobsters: implications for connectivity, diversity and hatchery stocking. Mar. Ecol. Prog. Ser. 563, 123–137.
Ellis, J. I., and Schneider, D. C. (1997). Evaluation of a gradient sampling design for environmental impact assessment. Environ. Monit. Assess. 48, 157–172.
Fenberg, P. B., Caselle, J. E., Claudet, J., Clemence, M., Gaines, S. D., Antonio García-Charton, J., et al. (2012). The science of European marine reserves: status, efficacy, and future needs. Mar. Policy 36, 1012–1021.
Fernández-Chacón, A., Buttay, L., Moland, E., Knutsen, H., and Olsen, E. M. (2021). Demographic responses to protection from harvesting in a long-lived marine species. Biol. Conserv. 257:109094. doi: 10.1016/j.biocon.2021.109094
Fernández-Chacón, A., Moland, E., Espeland, S. H., Kleiven, A. R., and Olsen, E. M. (2017). Causes of mortality in depleted populations of Atlantic cod estimated from multi-event modelling of mark–recapture and recovery data. Can. J. Fish. Aquat. Sci. 74, 116–126. doi: 10.1139/cjfas-2015-0313
Fernández-Chacón, A., Moland, E., Espeland, S. H., and Olsen, E. M. (2015). Demographic effects of full vs. partial protection from harvesting: inference from an empirical before-after control-impact study on Atlantic cod. J. Appl. Ecol. 52, 1206–1215.
Fernández-Chacón, A., Villegas-Ríos, D., Moland, E., Baskett, M. L., Olsen, E. M., and Carlson, S. M. (2020). Protected areas buffer against harvest selection and rebuild phenotypic complexity. Ecol. Appl. 30:e02108. doi: 10.1002/eap.2108
Francis, R. C., Hixon, M. A., Clarke, M. E., Murawski, S. A., and Ralston, S. (2007). Ten commandments for ecosystem-based fisheries scientists. Fisheries 32, 217–233.
Freitas, C., Villegas-Ríos, D., Moland, E., and Olsen, E. M. (2021). Sea temperature effects on depth use and habitat selection in a marine fish community. J. Anim. Ecol. doi: 10.1111/1365-2656.13497
Frigstad, H., Kaste, Ø., Deininger, A., Kvalsund, K., Christensen, G., Bellerby, R. G. J., et al. (2020). Influence of riverine input on Norwegian coastal systems. Front. Mar. Sci. 7:332. doi: 10.3389/fmars.2020.00332
Gaines, S. D., White, C., Carr, M. H., and Palumbi, S. R. (2010). Designing marine reserve networks for both conservation and fisheries management. Proc. Natl. Acad. Sci. U.S.A. 107, 18286–18293. doi: 10.1073/pnas.0906473107
Gjøsaeter, J. (2002). Fishery for goldsinny wrasse (Ctenolabrus rupestris) (Labridae) with pots along the Norwegian Skagerrak coast. Sarsia 87, 83–90.
Goñi, R., Hilborn, R., Diaz, D., Mallol, S., and Adlerstein, S. (2010). Net contribution of spillover from a marine reserve to fishery catches. Mar. Ecol. Prog. Ser. 400, 233–243.
Grorud-Colvert, K., Claudet, J., Tissot, B. N., Caselle, J. E., Carr, M. H., Day, J. C., et al. (2014). Marine protected area networks: assessing whether the whole is greater than the sum of its parts. PLoS One 9:e102298. doi: 10.1371/journal.pone.0102298
Halvorsen, K. T., Larsen, T., Browman, H. I., Durif, C., Aasen, N., Vøllestad, L. A., et al. (2021). Movement patterns of temperate wrasses (Labridae) within a small Marine Protected area. J. Fish Biol.
Halvorsen, K. T., Larsen, T., Sørdalen, T. K., Vøllestad, L. A., Knutsen, H., and Olsen, E. M. (2017). Impact of harvesting cleaner fish for salmonid aquaculture assessed from replicated coastal marine protected areas. Mar. Biol. Res. 13, 359–369. doi: 10.1080/17451000.2016.1262042
Halvorsen, K. T., Sørdalen, T. K., Larsen, T., Browman, H. I., Rafoss, T., Albretsen, J., et al. (2020). Mind the depth: the vertical dimension of a small-scale fishery shapes selection on species, size and sex in wrasses. Mar. Coast. Fish. 12, 404–422. doi: 10.1002/mcf2.10131
Hamilton, S. L., and Caselle, J. E. (2015). Exploitation and recovery of a sea urchin predator has implications for the resilience of southern California kelp forests. Proc. R. Soc. B 282:20141817. doi: 10.1098/rspb.2014.1817
Harrison, P. M., Gutowsky, L. F. G., Martins, E. G., Patterson, D. A., Cooke, S. J., and Power, M. (2015). Personality-dependent spatial ecology occurs independently from dispersal in wild burbot (Lota lota). Behav. Ecol. 26, 483–492. doi: 10.1093/beheco/aru216
Hoskin, M. G., Coleman, R. A., von Carlshausen, E., and Davis, C. M. (2011). Variable population responses to the establishment of a temperate no-take zone. Can. J. Fish. Aquat. Sci. 68, 185–200. doi: 10.1139/F10-143
Huserbråten, M. B. O., Moland, E., Knutsen, H., Olsen, E. M., André, C., and Stenseth, N. C. (2013). Conservation, spillover and gene flow within a network of Northern European marine protected areas. PLoS One 8:e73388. doi: 10.1371/journal.pone.0073388
IPBES (2019). Summary for Policymakers of the Global Assessment Report on Biodiversity and Ecosystem Services of the Intergovernmental Science-Policy Platform on Biodiversity and Ecosystem Services, eds S. Díaz, J. Settele, E. S. Brondízio, H. T. Ngo, M. Guèze, J. Agard, et al. (Bonn: IPBES secretariat), 56.
Jaco, E. M., and Steele, M. A. (2020). Pre-closure fishing pressure predicts effects of marine protected areas. J. Appl. Ecol. 57, 229–240. doi: 10.1111/1365-2664.13541
Jones, G. P., Cole, R. C., and Battershill, C. N. (1992). “Marine reserves: Do they work?,” in Proceedings of the Second International Temperate Reef Symposium, Auckland, 29–45.
Jorde, P. E., Kleiven, A. R., Sodeland, M., Olsen, E. M., Ferter, K., Jentoft, S., et al. (2018). Who is fishing on what stock: population-of-origin of individual cod (Gadus morhua) in commercial and recreational fisheries. ICES J. Mar. Sci. 75, 2153–2162. doi: 10.1093/icesjms/fsy080
Kaplan, K. A., Yamane, L., Botsford, L. W., Baskett, M. L., Hastings, A., Worden, S., et al. (2019). Setting expected timelines of fished population recovery for the adaptive management of a marine protected area network. Ecol. Appl. 29:e01949. doi: 10.1002/eap.1949
Kellner, J. B., Tetreault, I., Gaines, S. D., and Nisbet, R. M. (2007). Fishing the line near marine reserves in single and multispecies fisheries. Ecol. Appl. 17, 1039–1054. doi: 10.1890/05-1845
Kleiven, A. R., Olsen, E. M., and Vølstad, J. H. (2012). Total catch of a red-listed marine species is an order of magnitude higher than official data. PLoS One 7:e31216. doi: 10.1371/journal.pone.0031216
Knutsen, H., Jorde, P. E., Blanco Gonzalez, E., Eigaard, O. R., Pereyra, R. T., Sannaes, H., et al. (2015). Does population genetic structure support present management regulations of the northern shrimp (Pandalus borealis) in Skagerrak and the North Sea? ICES J. Mar. Sci. 72, 863–871. doi: 10.1093/icesjms/fsu204
Knutsen, H., Jorde, P. E., Hutchings, J. A., Hemmer-Hansen, J., Grønkjaer, P., Jørgensen, K. E. M., et al. (2018). Stable coexistence of genetically divergent Atlantic cod ecotypes at multiple spatial scales. Evol. Appl. 11, 1527–1539.
Kristensen, M. L., Moland, E., Koed, A., Källo, K, Olsen, E. M., Knutsen, H., et al. (2021). Disparate movement behaviour and feeding ecology in sympatric ecotypes of Atlantic cod. Ecol. Evol. accepted.
Kroodsma, D. A., Mayorga, J., Hochberg, T., Miller, N. A., Miller, K., Ferretti, F., et al. (2018). Tracking the global footprint of fisheries. Science 359, 904–908.
Lindegren, M., Diekmann, R., and Möllmann, C. (2010). Regime shifts, resilience and recovery of a cod stock. Mar. Ecol. Prog. Ser. 402, 239–253. doi: 10.3354/meps08454
Lubchenco, J., Palumbi, S. R., Gaines, S. D., and Andelman, S. (2003). Plugging a hole in the ocean: the emerging science of marine reserves. Ecol. Appl. 13, 3–7.
Malakhoff, K. D., and Miller, R. J. (2021). After 15 years, no evidence for trophic cascades in marine protected areas. Proc. Biol. Sci. 288:20203061. doi: 10.1098/rspb.2020.3061
Manel, S., Loiseau, N., Andrello, M., Fietz, K., Goñi, R., Forcada, A., et al. (2019). Long-distance benefits of marine reserves: Myth or Reality? Trends Ecol. Evol. 34, 342–354. doi: 10.1016/j.tree.2019.01.002
Maunder, M. N., Sibert, J. R., Fonteneau, A., Hampton, J., Kleiber, P., and Harley, S. J. (2006). Interpreting catch per unit effort data to assess the status of individual stocks and communities. ICES J. Mar. Sci. 63, 1373–1385. doi: 10.1016/j.icesjms.2006.05.008
Moland, E., Carlson, S. M., Villegas-Ríos, D., Wiig, J. R., and Olsen, E. M. (2019). Harvest selection on multiple traits in the wild revealed by aquatic animal telemetry. Ecol. Evol. 9, 6480–6491.
Moland, E., Olsen, E. M., Andvord, K., Knutsen, J. A., and Stenseth, N. C. (2011). Home range of European lobster (Homarus gammarus) in a marine reserve: implications for future reserve design. Can. J. Fish. Aquat. Sci. 68, 1197–1210.
Moland, E., Olsen, E. M., Knutsen, H., Garrigou, P., Espeland, S. H., Kleiven, A. R., et al. (2013a). Lobster and cod benefit from small-scale northern marine protected areas: inference from an empirical before-after control-impact study. Proc. Biol. Sci. 280:20122679.
Moland, E., Ulmestrand, M., Olsen, E. M., and Stenseth, N. C. (2013b). Long-term decrease in sex-specific natural mortality of European lobster within a marine protected area. Mar. Ecol. Prog. Ser. 491, 153–164.
Nillos Kleiven, P. J., Espeland, S. H., Olsen, E. M., Abesamis, R. A., Moland, E., and Kleiven, A. R. (2019). Fishing pressure impacts the abundance gradient of European lobsters across the borders of a newly established marine protected area. Proc. Biol. Sci. 286:20182455.
Olsen, E. M., Carlson, S. M., Gjøsaeter, J., and Stenseth, N. C. (2009). Nine decades of decreasing phenotypic variability in Atlantic cod. Ecol. Lett. 12, 622–631.
Olsen, E. M., Johnson, D., Weaver, P., Goñi, R., Ribeiro, M. C., Rabaut, M., et al. (2013). Achieving Ecologically Coherent MPA Networks in Europe: Science Needs and Priorities. Marine Board Position Paper 18, eds K. E. Larkin and N. McDonough (Ostend: European Marine Board).
Olsen, E. M., Knutsen, H., Gjøsaeter, J., Jorde, P. E., Knutsen, J. A., and Stenseth, N. C. (2004). Life history variation among local populations of Atlantic cod (Gadus morhua) from the Norwegian Skagerrak coast. J. Fish Biol. 64, 1725–1730. doi: 10.1111/j.1095-8649.2004.00402.x
Olsen, E. M., Knutsen, H., Simonsen, J. H., Jonsson, B., and Knutsen, J. A. (2006). Seasonal variation in marine growth of sea trout, Salmo trutta, in coastal Skagerrak. Ecol. Freshw. Fish 15, 446–452.
Olsen, E. M., and Moland, E. (2011). Fitness landscape of Atlantic cod shaped by harvest selection and natural selection. Evol. Ecol. 25, 695–710.
Osenberg, C. W., Shima, J. S., Miller, S. L., and Stier, A. C. (2011). “Ecology – Assessing effects of marine protected areas: confounding in space and possible solutions,” in Marine Protected Areas: A Multidisciplinary Approach, ed. J. Claudet (Cambridge: Cambridge University Press), 143–167.
Oug, E., Djursvoll, P., Aagaard, K., Brattegaard, T., et al. (2006). “Crustacea,” in Norwegian red list, eds J. A. Kålås, Å. Viken, and T. S. Bakken (Trondheim: Artsdatabanken), 197–206.
Perälä, T., Olsen, E. M., and Hutchings, J. A. (2020). Disentangling conditional effects of multiple regime shifts on Atlantic cod productivity. PLoS One 15:e0237414. doi: 10.1371/journal.pone.0237414
Pettersen, A. R., Moland, E., and Olsen, E. M. (2009). “Lobster reserves in coastal Skagerrak – an integrated analysis of the implementation process,” in Integrated Coastal Zone Management, eds E. Dahl, E. Moksness, and J. Støttrup (London: Wiley-Blackwell Publishing), 178–188.
Rogers, L. A., Olsen, E. M., Knutsen, H., and Stenseth, N. C. (2014). Habitat effects on population connectivity in a coastal seascape. Mar. Ecol. Prog. Ser. 511, 153–163.
Roney, N. E., Hutchings, J. A., Olsen, E. M., Knutsen, H., Albretsen, J., and Kuparinen, A. (2016). Fine-scale life-history structure in a highly mobile marine fish. Evol. Ecol. Res. 17, 95–109.
Russ, G. R. (2002). “Yet another review of marine reserves as reef fishery management tools,” in Coral Reef Fishes: Dynamics and Diversity in a Complex Ecosystem, ed. P. F. Sale (San Diego, CA: Academic Press), 421–443.
Shears, N. T., Grace, R. V., Usmar, N. R., Kerr, V., and Babcock, R. C. (2006). Long-term trends in lobster populations in a partially protected vs. no-take Marine Park. Biol. Conserv. 132, 222–231. doi: 10.1016/j.biocon.2006.04.001
Sheehy, M. R. J., Bannister, R. C. A., Wickins, J. F., and Shelton, P. M. J. (1999). New perspectives on the growth and longevity of the European lobster (Homarus gammarus). Can. J. Fish. Aquat. Sci. 56, 1904–1915.
Skerritt, D. J., Robertson, P. A., Mill, A. C., Polunin, N. V. C., and Fitzsimmons, C. (2015). Fine- scale movement, activity patterns and home-ranges of European lobster Homarus gammarus. Mar. Ecol. Prog. Ser. 536, 203–219.
Sørdalen, T. K., Halvorsen, K. T., Harrison, H. B., Ellis, C. D., Vøllestad, L. A., Knutsen, H., et al. (2018). Harvesting changes mating behaviour in European lobster. Evol. Appl. 11, 963–977.
Sørdalen, T. K., Halvorsen, K. T., Vøllestad, L. A., Moland, E., and Olsen, E. M. (2020). Marine protected areas rescue a sexually selected trait in European lobster. Evol. Appl. 13, 2222–2233. doi: 10.1111/eva.12992
Stewart-Oaten, A., Murdoch, W. W., and Parker, K. R. (1986). Environmental impact assessment: “Pseudoreplication” in time? Ecology 67, 929–940.
Sundelöf, A., Wennhage, H., and Svedäng, H. (2013). A red herring from the Öresund (ICES40G2): the apparent recovery of the Large Fish Indicator (LFI) in the North Sea hides a non-trawled area. ICES J. Mar. Sci. 70, 1081–1084. doi: 10.1093/icesjms/fst117
Thiault, L., Kernaléguen, L., Osenberg, C. W., Lison de Loma, T., Chancerelle, Y., et al. (2019). Ecological evaluation of a marine protected area network: a progressive-change BACIPS approach. Ecosphere. 10:e02576. doi: 10.1002/ecs2.2576
Thorbjørnsen, S. H., Moland, E., Huserbråten, M. B. O., Knutsen, J. A., Knutsen, H., and Olsen, E. M. (2018). Replicated marine protected areas (MPAs) support movement of larger, but not more, European lobsters to neighbouring fished areas. Mar. Ecol. Prog. Ser. 595, 123–133. doi: 10.3354/meps12546
Thorbjørnsen, S. H., Moland, E., Simpfendorfer, C., Heupel, M., Knutsen, H., and Olsen, E. M. (2019). Potential of a no-take marine reserve to protect home ranges of anadromous brown trout (Salmo trutta). Ecol. Evol. 9, 417–426. doi: 10.1002/ece3.4760
Thorbjørnsen, S. H., Moland, E., Villegas-Ríos, D., Bleeker, K., Knutsen, H., and Olsen, E. M. (2021). Selection on fish spatial personality differs between a no-take marine reserve and fished areas. Evol. Appl. doi: 10.1111/eva.13242
Thorstad, E. B., Todd, C. D., Uglem, I., Bjørn, P. A., Gargan, P. G., Vollset, K. W., et al. (2016). Marine life of the sea trout. Mar. Biol. 163:47. doi: 10.1007/s00227-016-2820-3
Thurstan, R. H., and Roberts, C. M. (2010). Ecological Meltdown in the Firth of Clyde, Scotland: two centuries of change in a coastal marine ecosystem. PLoS One 5:e11767. doi: 10.1371/journal.pone.0011767
Underwood, A. J. (1992). Beyond BACI: the detection of environmental impacts on populations in the real, but variable, world. J. Exp. Mar. Biol. Ecol. 161, 145–178. doi: 10.1016/0022-0981(92)90094-q
Underwood, A. J. (1994). On beyond BACI: sampling designs that might reliably detect environmental disturbances. Ecol. Appl. 4, 3–15. doi: 10.2307/1942110
Villegas-Ríos, D., Moland, E., and Olsen, E. M. (2017a). Potential of contemporary evolution to erode fishery benefits from marine reserves. Fish Fish. 18, 571–577. doi: 10.1111/faf.12188
Villegas-Ríos, D., Réale, D., Freitas, C., Moland, E., and Olsen, E. M. (2017b). Individual level consistency and correlations of fish spatial behaviour assessed from aquatic animal telemetry. Anim. Behav. 124, 83–94. doi: 10.1016/j.anbehav.2016.12.002
Keywords: acoustic telemetry, crustacean, displacement of effort, eco-evolutionary dynamics, fisheries management, marine reserve, rebuilding, spillover
Citation: Moland E, Fernández-Chacón A, SØrdalen TK, Villegas-Ríos D, ThorbjØrnsen SH, Halvorsen KT, Huserbråten M, Olsen EM, Nillos Kleiven PJ, Kleiven AR, Knutsen H, Espeland SH, Freitas C and Knutsen JA (2021) Restoration of Abundance and Dynamics of Coastal Fish and Lobster Within Northern Marine Protected Areas Across Two Decades. Front. Mar. Sci. 8:674756. doi: 10.3389/fmars.2021.674756
Received: 01 March 2021; Accepted: 15 June 2021;
Published: 08 July 2021.
Edited by:
Juan Jacobo Schmitter-Soto, The South Border College (ECOSUR), MexicoReviewed by:
Corey Morris, Department of Fisheries and Oceans, CanadaJennifer Caselle, University of California, Santa Barbara, United States
Hem Nalini Morzaria-Luna, Intercultural Center for the Study of Deserts and Oceans, United States
Copyright © 2021 Moland, Fernández-Chacón, Sørdalen, Villegas-Ríos, Thorbjørnsen, Halvorsen, Huserbråten, Olsen, Nillos Kleiven, Kleiven, Knutsen, Espeland, Freitas and Knutsen. This is an open-access article distributed under the terms of the Creative Commons Attribution License (CC BY). The use, distribution or reproduction in other forums is permitted, provided the original author(s) and the copyright owner(s) are credited and that the original publication in this journal is cited, in accordance with accepted academic practice. No use, distribution or reproduction is permitted which does not comply with these terms.
*Correspondence: Even Moland, ZXZlbi5tb2xhbmRAZ3JhZHVhdGVzLmpjdS5lZHUuYXU=