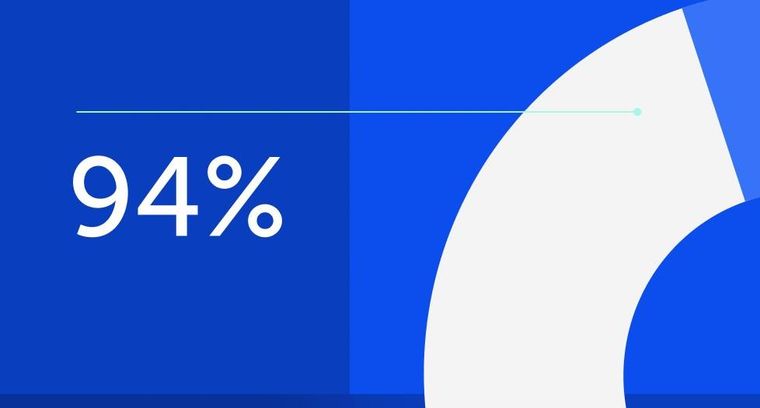
94% of researchers rate our articles as excellent or good
Learn more about the work of our research integrity team to safeguard the quality of each article we publish.
Find out more
ORIGINAL RESEARCH article
Front. Mar. Sci., 11 May 2021
Sec. Marine Fisheries, Aquaculture and Living Resources
Volume 8 - 2021 | https://doi.org/10.3389/fmars.2021.671545
Polychaetes can be successfully employed to recover essential fatty acids (EFA) from wasted uneaten aquafeeds present in aquaculture effluents. The optimization of the timeframe required to produce premium ragworms (Hediste diversicolor) biomass rich in EFA is paramount to make available to the aquafeeds industry another alternative ingredient to fish meal and fish oil. The present study aimed to evaluate the potential enrichment of ragworms fatty acid (FA) profile when fed a commercial aquafeed during 10, 20, and 40 days (D10, D20, and D40) under different combinations of water temperature (20 and 25°C) and salinity (15, 20, and 25). Total FA incremented progressively overtime, with D40 polychaetes exhibiting average values ranging between 70 and 90 μg mg–1 DW. The average values of n-6 FA ranged between 13 and 17 μg mg–1 DW, while that of n-3 FA varied between 17 and 19 μg mg–1 DW at D40. No significant differences were found in the FA profile of H. diversicolor cultured under different combinations of temperature and salinity. The FA profile of cultured polychaetes exhibited between 28 and 31% dissimilarity from that of wild conspecifics and displayed a higher content of two essential n-3 FA: eicosapentaenoic (20:5 n-3, EPA) and docosahexaenoic acids (22:6 n-3, DHA) (values ranging between 9.6–11.2% and 4.3–5.0% of total FA, respectively). A higher similarity in FA profile was recorded between D40 polychaetes and aquafeed than with initially stocked or wild specimens. Palmitic (16:0), oleic (18:1 n-9), linoleic (18:2 n-6), eicosadienoic (20:2 n-6), EPA (20:5 n-3), and DHA (22:6 n-3) were the FA whose concentration exhibited the highest increment. Evidence of de novo FA biosynthesis was observed through the formation of some FA that were neither present in the initially stocked biomass, nor in the aquafeed supplied, such as 5,11-eicosadienoate (Δ 5,1120:2), 7,13,16-docosatrienoate (Δ 7,13,1622:3), dihomo-gamma-linolenic (20:3 n-6), eicosatrienoic (20:3 n-3) and eicosatetraenoic (20:4 n-3) acids. A plateau of total FA, n-6, and n-3 FA was not reached over the study period. Overall, the present study highlights the potential of H. diversicolor as an extractive species for integrated multi-trophic aquaculture (IMTA) applications.
World aquaculture production reached an all-time record of 114.5 million tons in live weight in 2018 (USD 263.6 billion) (FAO, 2020). This figure includes the production of 54.2 million tons of fish (USD 139.7 billion) and 9.4 million tons of crustaceans (USD 69.3 billion), with 13 and 61% of these productions resulting from saltwater aquaculture (which includes marine and brackish water species), respectively (FAO, 2020). The majority of farmed species of both groups are produced using commercial aquafeeds (∼ 57 million tons in 2018) (FAO, 2020). Most marine teleosts (and other vertebrate species including humans) display a limited ability to perform de novo synthesis of n-3 highly unsaturated fatty acids (HUFA) due to the lack of desaturases Δ12 and Δ15, the enzymes responsible to produce polyunsaturated fatty acids (PUFA) from oleic acid (18:1 n-9) (Hastings et al., 2001, 2004; Bell and Tocher, 2009; Monroig and Kabeya, 2018). As such, it is paramount to include in aquafeeds a balanced profile of HUFA and not their FA precursors (Sargent et al., 2003; Tocher, 2015, 2003). Aquaculture of marine fishes and crustaceans thus depends on a rich supply of n-3 HUFA, including eicosapentaenoic (20:5 n-3, EPA) and docosahexaenoic (22:6 n-3, DHA) acids, through the formulation and supply of well-balanced aquafeeds commonly employing fishmeal and fish oil, two increasingly scarcer and costly resources for aquafeeds (Tacon and Metian, 2015, 2008; Cashion et al., 2017; FAO, 2020). The progressive reduction in the supply of these marine origin raw materials has been coupled with a surging demand driven by a fast-growing aquaculture industry (farmed aquatic animals grew on average 5.3% per year between 2001 and 2018) (FAO, 2020). There is a global requirement of approximately 0.4 million metric tons of n-3 HUFA per year (Tocher, 2015). Formulated aquafeeds have to both satisfy the nutritional needs of cultured species and safeguard that at the end of a productive cycle farmed species display an optimal biochemical profile for human nutrition.
Polychaetes display a significant commercial value for the culture of vertebrates and invertebrates (García-Alonso et al., 2008; Olive, 1999). According to reported values, approximately 121.000 tons of polychaetes (with an estimated value of USD 8.39 billion) were harvested globally in 2015, with these figures being comparable to some of the most important world fisheries (Watson et al., 2016). It has already been acknowledged that the collection of polychaetes from the wild is likely insufficient to satisfy global market demands and that this practice drives a multitude of negative environmental impacts (Fidalgo e Costa et al., 2006; Pombo et al., 2018; Xenarios et al., 2018). The polychaete Hediste diversicolor O.F. Müller, 1776, popularly known as ragworm, is a highly valued bait species for sports fishing (Fidalgo e Costa et al., 2006; Carvalho et al., 2013; Sá et al., 2017; Pombo et al., 2018). It also plays a key dietary role on the nutrition and production of some fish and crustacean species (e.g., soles, shrimps and crabs), being often used to trigger gonad maturation and spawning (Lytle et al., 1990; Dinis, 1992; Luis and Ponte, 1993; Dinis et al., 1999; Meunpol et al., 2005). This omnivorous species is an active predator (Fauchald and Jumars, 1979) that is also able to perform deposit-feeding (Reise, 1979; Scaps, 2002) and filter-feeding (Riisgård, 1994; Vedel et al., 1994). Their deposit-feeding behavior allows them to consume considerable amounts of organic matter present in the substrate where it burrows (Reise, 1979; Scaps, 2002). The biomass of ragworms contains essential ingredients that can act as important supplements for aquafeeds formulation, such as amino acids and other odorants that elicit a feeding response for several fish species (e.g., Senegalense sole) (Velez et al., 2007; Wang et al., 2019). Ragworms also display high proteic and lipidic contents (54–60% and 11–22% of DW biomass, respectively) (Wang et al., 2019; Yousefi-Garakouei et al., 2019). This species has already been shown to perform de novo biosynthesis of some essential fatty acids (EFA) from acetyl coenzyme A, by using several fatty acids (FA) desaturase and enlogase enzymes; as such, it is usual to detect higher concentrations of PUFA and HUFA in ragworms biomass than on their diet (Vásquez et al., 2014; Santos et al., 2016). The abundance of EFA and ragworms ability to recycle n-3 HUFA (such as EPA and DHA) from uneaten aquafeeds and sludge from aquaculture effluents, that would otherwise be lost to the environment, make H. diversicolor a key extractive species for integrated multi-trophic aquaculture (IMTA) applications (Bischoff et al., 2009; Santos et al., 2016; Marques et al., 2018; Wang et al., 2019; Yousefi-Garakouei et al., 2019). A large variability in the total pool of FA determined for H. diversicolor biomass has been reported to date, with values ranging between 50 and 280 μg mg–1 DW for specimens fed commercial aquafeeds (Fidalgo e Costa et al., 2000; García-Alonso et al., 2008; Santos et al., 2016; Pajand et al., 2017; Wang et al., 2019) or between 24 and 110 μg mg–1 DW when cultured using aquaculture effluents (García-Alonso et al., 2008; Bischoff et al., 2009; Pajand et al., 2017; Marques et al., 2018; Wang et al., 2019; Yousefi-Garakouei et al., 2019). Also, the proportion of n-3 and n-6 FA reported to date for this species is also highly variable, with the above-mentioned works reporting values ranging between 5 and 33% for n-3 FA and 9–27% to n-6 FA. This variability is a consequence of several factors, such as the duration of experimental trials, the maturation stage of polychaetes at the beginning and during experiments, the composition of the supplied diet and the abiotic conditions experienced during culture (e.g., temperature, salinity, photoperiod). The main source of EFA in IMTA designs will always be the aquafeed supplied to upstream species. However, the majority of studies performed to date including polychaetes as extractive species under IMTA frameworks fails to characterize the proportion of feces and uneaten aquafeed present in the particulate fraction of nutrients being supplied. Additionally, only a few studies have evaluated the initial FA profile of polychaetes in order to assess how these biomolecules evolve when ragworms are supplied a certain pool of nutrients (Bischoff et al., 2009; Marques et al., 2018; Wang et al., 2019). It also remains to be clarified how the enrichment in EFA occurs in ragworms biomass over time under different combinations of water temperature and salinity, and whether it is possible to reach a plateau for enrichment in total FA, n-6 and n-3 FA. All this information is paramount to optimize the timeframe required to produce a premium polychaete biomass rich in EFA.
In order to shed some light over the above-mentioned questions, the present study aimed to evaluate the evolution of the FA profile of H. diversicolor fed a commercial aquafeed (with a well-known FA composition) during 10, 20, and 40 days (D10, D20 and D40) under different combinations of water temperature (20 and 25−C) and salinity (15, 20, and 25). These are optimal conditions for the culture of ragworms, which are within the range of temperature and salinity commonly employed by warm-temperate aquaculture systems operating with brackish water. A comparison between cultured and wild polychaetes over time was also performed to confirm that the evolution of FA profile of polychaetes fed on aquafeeds was not influenced by natural cycles. The feeding and growth performances of H. diversicolor cultured under the different combinations of water temperature and salinity were evaluated over time as well.
To evaluate the evolution of FA profile of H. diversicolor, five independent replicates were considered for each of the six-temperature x salinity combinations, at each of the three-time sampling points considered (D10, D20, D40). Each replicate consisted of 10 polychaetes stocked on a glass flask (100 mm × 100 mm × 180 mm, ∼1-L volume) filled with 80 mm of sand (0.5–0.7 mm grain size) (approximately 1,000 ind. m –2) and aerated artificial sea water (see below for details). Five water baths were used for each of the temperatures being tested, with 100-W Eheim® thermocontrol 3612 aquarium heaters being used to control this parameter. Replicates of the different salinities were randomly distributed over these water baths. The different salinities were previously prepared using artificial sea water (prepared by mixing Red Sea® salt with tap water purified by a reverse osmosis unit), with the whole water volume of each glass flask being changed every 2 days. Constant water aeration was secured by air stones connected to an aeration pump (Hailea® vortex blower) and a 16:8 h light:dark photoperiod was employed using fluorescent white tubes connected to automatic timers. The schematic representation of the experimental set-up is represented in Figure 1.
Figure 1. Schematic representation of one of the five replicate water baths used to control temperatures (20 and 25°C) housing randomly distributed glass flasks stocked with 10 Hediste diversicolor at different salinities (S—15, 20, and 25) to evaluate the evolution of their fatty acid profiles after being fed a commercial aquafeed during 10, 20, and 40 days (D10, D20, and D40).
Polychaetes were fed, once a day, a commercial grow-out diet for flatfish that present 62% of crude protein, 18% of crude fat, and 0.3% of crude fiber (WINFlat–SPAROS). Aquafeed was supplied ad libitum until polychaetes lost interest in the pellets and these remained untouched at the top of the sand in the glass flasks. Uneaten feed was never removed from the glass flasks, with its presence or absence being used to adjust the feed being supplied the next day.
Water parameters, namely dissolved oxygen (DO), pH, temperature and salinity were monitored daily using a manual probe (pH/Cond 3320, WTW, Weilheim, Germany), with ammonia and nitrites being determined once a week using colorimetric tests (Salifert Profi Test).
Wild specimens of H. diversicolor were collected from Ria de Aveiro coastal lagoon (Portugal) at Espinheiro Channel (40°38′8.4′′N, 8° 39′41.8′′ W) at the end of February 2019 and transported to the laboratory. An acclimation period of 24 h to experimental conditions was carried out and no feed was supplied during this period, so as not to modify the FA profile of wild polychaetes. The polychaetes were randomly selected and weighed individually and distributed per each of the five replicates of the different experimental conditions being tested. At the end of each sampling point (D10, D20, D40), polychaetes were depurated for 24 h in aerated glass flaks holding pre-combusted sterilized sand and artificial seawater (prepared as detailed above) to safeguard empty guts and avoid potential bias when performing FA analysis. The same temperature x salinity combination was maintained for each condition during the depuration process. Following depuration, all samples were freeze-dried and stored at −80°C until further analysis.
Five composite samples of polychaetes (10 specimens per composite sample) were collected from the wild at the beginning of the experiment (February 2019) to characterize the FA profile of the initially stocked biomass (Initial). To clarify if the evolution of the FA profile of polychaetes being supplied with aquafeeds in the laboratory was not influenced by natural cycles, another five composite samples of wild specimens (10 specimens per composite sample) were collected in the same site at each sampling time point of the experiment (Wild10, Wild20, and Wild40) (February–March 2019). Depuration and storage processes for these samples were the same as detailed above.
Five composite samples of the aquafeed being used were also freeze dried and stored at −80°C for FA analysis.
The FA content of both polychaetes and aquafeeds was determined by screening FA methyl esters (FAME) analyzed by gas chromatography-mass spectrometry (GC-MS). Before analysis, all freeze-dried samples were powdered and homogenized. Lipid extraction was performed following the Bligh and Dyer (1959) method using an initial sample biomass of 20 mg. Each sample was transferred to a glass tube, homogenized with 2,500 μL methanol (MeOH) and 1,250 μL dichloromethane (CH2Cl2), sonicated for 1 min and then incubated on ice in an orbital shaker for 30 min. Afterward, each sample was centrifuged at 2,000 rpm for 10 min and the organic phase was collected. A volume of 1,250 μL CH2Cl2 and 1,250 μL of Mili Q water was added to the total organic phase to promote phase separation, followed by centrifugation for 10 min at 2,000 rpm. Organic phase aliquots of lipid extract (74 μL for polychaetes and 57 μL for aquafeeds; total lipid extract: 3,000 μL) were collected for a new tube, previously washed in n-hexane. In order to define the final aliquot volume, a previous study was performed to calculate the total amount of lipid extract present in the different aliquots and analyzing them in GC-MS. Lipid extract aliquots were dried under a nitrogen gas stream, and posteriorly used for methylation. FAME were prepared by adding 1 mL of the internal standard 19:0 FA (1.5 μg mL–1) in n-hexane (99%) to the tube containing lipids. Subsequently, 200 μL of methalonic (MeOH) KOH solution (2M) were added and vigorously vortexed for 2 min. Following this procedure, 2 mL of NaCl saturated solution was added to the tube, and then centrifuged for 5 min at 2,000 rpm. Then 600 μL of the organic phase contained FAME were collected. To remove cholesterol from FAME, the solution was cleaned by solid-phase extraction. A column containing 0.1 g of silica was activated with 3 mL of n-hexane (99%) and the aliquot containing FAME (600 μL) was applied in the column. Subsequently, 3 mL of n-hexane 99%:diethyl ether (95:5 by volume) were eluted to recover the FAME. This fraction was dried under a nitrogen stream and stored at −20°C until GC-MS analysis. FAME were then dissolved in 100 μL of n-hexane 99% and 2 μL of this solution were used for analysis on a GC-MS system (Agilent Technologies 5977 B GC/MSD) equipped with a DB-FFAP column (123-3232, J and W Scientific, Folsom, CA, United States) with the following specifications: 30 m length, an internal diameter of 0.32 mm and a film thickness of 0.25 μm. The system employed includes a Mass Selective Detector operating with an electron impact mode at 70 eV and scanning the mass range m/z 50–550 in a 1 s cycle in a full scan mode acquisition. The oven temperature program was as follows: (1) initial temperature of 80°C for 2 min; (2) linear increase to 160°C (25°C min–1); (3) linear increase to 210°C (2°C min–1); (4) linear increase to 225°C (20°C min–1); and (5) standing at 225°C for 20 min. Helium was used as the carrier gas (1 mL min–1). FA were integrated through Agilent’s Masshunter Solutions Quantitative Analysis v10.0 automatic integration. Identification of FA was performed considering retention time and analysis of MS spectra in comparison with MS spectra of FA standards (Supelco 37 Component FAME Mix, ref. 47885-U, Sigma-Aldrich) and comparison with chemical databases (Wiley 275 library, AOCS lipid library, and NIST 2014 Mass spectral library). The FA content (expressed as μg of FA mg–1 dry weight, DW) was determined using calibration curves obtained from FAME certified standard mixture (Supelco® 37 Component FAME Mix, Sigma-Aldrich) and 19:0 FA as internal standard.
In the present study, PUFA were defined as all FA with two or three double bonds, while HUFA refers to all FA with four or more double bonds.
FA increments between day 1–10, day 11–20, and day 21–40 (D1–D10, D11–D20, and D21–D40) were determined by calculating the differences in concentration between samples collected at the beginning of the experiment and D10, between samples from D10 and D20, and between samples from D20 and D40, respectively.
At each sampling point (D10, D20, D40) the whole sand of each glass flask was sieved, with polychaetes being sorted, counted and weighted individually. The specific growth rate (SGR), daily growth rate (DGR) and feeding rate (FR) were calculated as follows:
A two-way nested analysis of variance (ANOVA) was performed to determine the existence of significant differences in SGR, DGR and FR of H. diversicolor cultured under different combinations of water temperature and salinity. This analysis was performed for each independent group fed a commercial aquafeed during 10, 20, and 40 days (D10, D20, and D40) (n = 5). The factors being tested were as follows: “Temperature” (two fixed levels, 20 and 25°C) and “Salinity” (three fixed levels, 15, 20, and 25) nested within temperature. Data were previously checked for normality (Anderson-Darling test) and homogeneity of variances (Bartlett’s and Levene’s tests for normal and non-normal distribution, respectively). When normality was not verified, the hypotheses were tested using non-parametric Kruskal-Wallis test. Significant differences were always considered at p < 0.05. These statistical analyses were performed using MINITAB 18 Software (State College, PA).
To determine the existence of significant differences in the FA profile of cultured H. diversicolor under the different combinations of water temperature and salinity tested in the present work, a two-way nested analysis of similarities (ANOSIM) was performed on a resemblance matrix produced using Bray Curtis similarity coefficient of data previously transformed using the formula log (x + 1). This analysis was performed for each independent group at D10, D20, and D40 days (n = 5). The factors being tested were as follows: “Temperature” (two levels, 20°C and 25°C) and “Salinity” (three levels, 15, 20, and 25) nested within temperature. The differences between FA profile of cultured polychaetes under different combinations of water temperature and salinity and the FA profile of wild conspecifics collected in the same sampling period (Wild10, Wild20, and Wild40) (n = 5) were performed using a one-way ANOSIM. When significant differences were recorded, a similarity percentages (SIMPER) analysis was performed to evaluate which FA contributed the most to the dissimilarities being recorded between samples, until a total of 50% cumulative dissimilarity was achieved. A principal coordinates analysis (PCO) plot was also used to compare the results of total FA concentration, FA classes concentration (saturated FA [SFA], monounsaturated FA [MUFA], PUFA, and HUFA) and n-3 and n-6 FA concentration from D40 polychaetes cultured under different combinations of temperature and salinity, Wild40 polychaetes, initially stocked polychaetes (Initial) and the aquafeed supplied (resemblance matrix produced using Bray Curtis similarity used as input). These statistical analyses were performed using PRIMER v6 with the PERMANOVA + add-on. For a detailed description of all the statistical methods employed please see Anderson et al. (2008).
The average values of DO and pH inside the glass flask varied between 6.7–7.9 mg L–1 and 8.2–8.4 along the duration of the experiment, respectively. The average value of ammonia (NH4) and nitrites (NO2) varied between 0.4–1.2 and 0.5–1.4 mg L–1, respectively. Temperature and salinity were kept stable in the predefined conditions (see Supplementary Table 1).
The FR (%), SGR (% d–1), and DGR (g d–1) determined for polychaetes cultured under each combination of water temperature and salinity are summarized in Table 1 (complete data of biomass and aquafeed supplied summarized in Supplementary Table 2). No significant differences were found for FR, SGR, and DGR exhibited by polychaetes at D10, D20, and D40 (p > 0.05; Supplementary Table 3). The average values of polychaetes survival from the different treatments, D10, D20, and D40, varied between 66–78%, 48–70%, and 38–54%, respectively (Supplementary Table 2).
Table 1. Feeding Rate (FR), Specific Growth Rate (SGR), and Daily Growth Rate (DGR) of Hediste diversicolor fed during 10, 20, and 40 days (D10, D20, and D40) with a commercial aquafeed under different combinations of temperature (T—20 and 25°C) and salinity (S—15, 20, and 25). Average values (± SD) (n = 5).
A total of 16 FA were identified in the aquafeed supplied to polychaetes, with palmitic (16:0), oleic (18:1 n-9), linoleic (18:2 n-6, LA), EPA (20:5 n-3), myristic (14:0) and DHA (22:6 n-3) exhibiting the highest concentration (Table 2).
Table 2. Fatty acid (FA) profile (μg mg–1 DW) of the commercial aquafeed (WIN flat –SPAROS) supplied to Hediste diversicolor.
SFA and MUFA accounted for 33.4 and 33.5% of total FA, respectively, while PUFA and HUFA accounted for 16.5 and 16.7% of total FA, respectively. DHA and EPA accounted for 6.2 and 7.6% of total FA, respectively (accounting for 37.1% and 45.7 of total HUFA, respectively).
The FA profile of H. diversicolor from D40, along with that of initially stocked polychaetes (Initial) and conspecifics collected from the wild (Wild40) are detailed in Table 3 (FA profile of D10 and D20 polychaetes displayed in Supplementary Tables 4, 5, respectively). A total of 25 FA were identified in D40 polychaetes, with palmitic (16:0), EPA (20:5 n-3), oleic (18:1 n-9), LA (18:2 n-6), eicosadienoic (20:2 n-6), vaccenic (18:1 n-7), DHA (22:6 n-3), and stearic (18:0) FA displaying the highest concentrations. No significant differences were found in the FA profile of D10, D20, and D40 polychaetes (p > 0.05; Supplementary Table 6).
Table 3. Fatty acid profile (μg mg–1 DW) of Hediste diversicolor fed during 40 days with a commercial aquafeed under different combinations of temperature (T—20 and 25°C) and salinity (S—15, 20, and 25), along with conspecifics initially stocked (Initial) and conspecifics collected from the wild at the same time point (Wild40).
A progressive increment of total FA content over time was recorded in all combinations of water temperature and salinity evaluated in the present work, with values varying from 30 μg mg–1 DW (Initial) to 70–90 μg mg–1 DW (D40) (Figure 2). The proportion of PUFA incremented approximately 10% in the whole pool of FA over the 40 days of the experimental trial, with all FA classes (SFA, MUFA, PUFA, and HUFA) being present with a similar proportion at the end of the study (˜25% in all FA classes) (Supplementary Figure 1).
Figure 2. Evolution of the total amount of fatty acids (FA) of Hediste diversicolor fed a commercial aquafeed for 10, 20, and 40 days (D10, D20, and D40) under different combinations of water temperature (T—20 and 25°C) and salinity (S—15, 20, and 25) contrasted with that of initially stocked conspecifics (Initial) and conspecifics collected from the wild at the same time points (Wild10, Wild20, and Wild40). Average values (± SD) (n = 5).
The n-3 FA content in D40 polychaetes was approximately 3-times higher than that of Wild40 (values ranging between 16 and 19 μg mg–1 DW). The highest increment in n-3 FA was recorded between day 20 and 40 (comparing the values of Initial, D10, D20, and D40 polychaetes) (Figure 3A). The increment in n-6 FA content was progressive over the whole experimental period, with D40 polychaetes revealing a 2–3-times higher concentration than Wild40 (values between 13 and 17 μg mg–1 DW) (Figure 3B). A lower value of n-3/n-6 ratio was reported in D20 polychaetes (0.7–0.9), when compared to the ones observed in other sampling periods and wild conspecifics (1.0–1.2) (Figure 3C). DHA was not detected in Initial polychaetes, while values between 3.4 and 4.2 μg mg–1 DW were recorded for D40 specimens (Figure 3D). EPA concentration in D40 polychaetes was twice the one recorded on Initial ragworms, with values ranging between 7 and 9 μg mg–1 DW (Figure 3E). Both DHA and EPA experienced the highest increments between day 20 and 40 of the experimental trial. The DHA/EPA ratio was maintained over the sampling periods, with values averaging between 0.3 and 0.6 (Figure 3F). Palmitic, oleic, linoleic, eicosadienoic, EPA and DHA were the FA which exhibited the highest increment in concentration when comparing Initial polychaetes with conspecifics from D40 (Figure 4). The increment in concentration of the first three above mentioned FA (palmitic, oleic and linoleic) mainly occurred between D1 and D20 period, while the other FA (eicosadienoic, EPA and DHA) showed the highest increment between D21 and D40 period. From the above-mentioned FA, DHA was the only one that was not detected in wild polychaetes, while eicosadienoic was the only one that was not detected in the commercial aquafeed supplied. Dihomo-gamma-linolenic (20:3 n-6, DGLA), eicosatrienoic (20:3 n-3, ETE) and eicosatetraenoic (20:4 n-3, ETA) acids were only detected in D40 polychaetes and were not detected neither on Initial or Wild samples of polychaetes, nor in the aquafeed supplied. Significant differences were detected between the FA profile exhibited by D10, D20, and D40 polychaetes and the ones collected from the wild on the same periods (p < 0.05; Supplementary Table 7). SIMPER analysis (cut-off 50%) revealed the existence of dissimilarities between D10—Wild10 (15–20%), D20 -Wild20 (24–27%) and D40 -Wild40 (29–32%) (Supplementary Tables 8–10). DHA (22:6 n-3), LA (18:2 n-6), eicosadienoic (20:2 n-6), oleic (18:1 n-9), 5,11-eicosadienoate (Δ 5,1120:2), 11-docosenoate acid (22:1 n-11), DGLA (20:3 n-6) and methyl 10,13,16-docosatrienoate (22:3 n-6) are some of the FA that contributed the most to dissimilarities recorded between D40 and Wild40 (Supplementary Table 10).
Figure 3. Evolution of n-3 fatty acids (FA) (A), n-6 FA (B), n-3/n-6 ratio (C), docosahexaenoic acid (DHA) (D), eicosapentaenoic acid (EPA) (E) and DHA/EPA ratio (F) of Hediste diversicolor fed a commercial aquafeed for 10, 20, and 40 days (D10, D20, and D40) under different combinations of water temperature (T—20 and 25°C) and salinity (S—15, 20, and 25) contrasted with that of initially stocked conspecifics (Initial) and conspecifics collected from the wild at the same time points (Wild10, Wild20, and Wild40). Average values (± SD) (n = 5).
Figure 4. Evolution of the fatty acids (FA) in Hediste diversicolor fed a commercial aquafeed over time (D1–D10, D11–D20, and D21–D40) under different combinations of water temperature (T—20 and 25°C) and salinity (S—15, 20, and 25). Red diamond represents the final average increment recorded (± SD) (n = 5).
PCO plots revealed two well-separated groups, one formed by D40 polychaetes and aquafeed, and the other one formed by Initial and Wild40 polychaetes (Figures 5A–C). A similarity above 90% was recorded between D40 polychaetes and aquafeed for total FA concentration, FA classes concentration (SFA, MUFA, PUFA, and HUFA) and n-3 and n-6 FA concentration. The two PCO axis explained more than 99% of the variation recorded between samples from different group.
Figure 5. Principal coordinates analysis (PCO) of total fatty acids (FA) (A), sum of FA classes (SFA, MUFA, PUFA, and HUFA) (B), sum of n-3 and n-6 FA (C) of Hediste diversicolor fed a commercial aquafeed for 40 days under different combinations of water temperature (T—20 and 25°C) and salinity (S—15, 20, and 25) contrasted with that wild conspecifics initially stocked (Initial) and conspecifics collected on the same date (Wild40). Average values (± SD) (n = 5). Green and blue lines define the levels of similarity between samples. PUFA are defined as all FA with 2 or 3 double bonds and HUFA as all FA with ≥ 4 double bonds.
The amount of commercial aquafeed supplied to polychaetes stocked under the different treatments performed allowed to estimate an increment of 25.0–37.0 μg mg–1 DW in the total FA present in polychaetes biomass. Concerning n-3 and n-6 FA, the increments recorded were 5.6–8.0 and 4.8–7.7 μg mg–1 DW in polychaetes biomass, respectively (Table 4). DHA and EPA increased between 2.0–2.6 and 2.3–3.2 μg mg–1 DW in polychaetes biomass, respectively.
Table 4. Increment in polychaetes biomass (μg mg–1 DW) per gram of aquafeed supplied of total fatty acid, sum of n-3 (Σ n-3) and n-6 fatty acids (Σ n-6), docosahexaenoic acid (DHA) and eicosapentaenoic acid (EPA).
In the present study it was possible to verify that H. diversicolor fed a commercial aquafeed during 10, 20, and 40 days (D10, D20, and D40) under different combinations of water temperature (20 and 25°C) and salinity (15, 20, and 25) displayed similar SGR and DGR. This finding is in line with the ecophysiological traits of this species, which is very abundant in extreme environments, such as intertidal mudflats, exposed to major shifts in water temperature and salinity (Scaps, 2002; García-Alonso et al., 2008). The values of SGR reported in the present work were lower than those reported by previous studies testing commercial aquafeeds to culture this species (values ranging between 6.0 and 6.8% d–1) (Fidalgo e Costa et al., 2000; Santos et al., 2016). However, our values were higher than most of those reported when this species was fed on nutrients derived from aquaculture effluents (values ranging between 1.2 and 3.0% d–1) (Bischoff, 2007; Pajand et al., 2017; Wang et al., 2019). The values of DGR determined for D10 polychaetes were similar to those reported by Santos et al. (2016) for the same species fed a commercial aquafeed for 60 days. The progressive decrease of DGR over time and the lower SGR recorded for D40 polychaetes, may be related with their sexual maturation, as detailed below. It is worth highlighting that D40 polychaetes incremented their average weight up to 4-times, confirming the potential of this species to be produced under an IMTA framework and to generate a considerable biomass in a short period of time. The progressive mortality verified in D10, D20, and D40 samples, which reached values ∼50%, may have been related to the high stocking density employed at the beginning of the experiment (1,000 ind. m–2). This assumption is supported by the results reported by Nesto et al. (2012), who verified that H. diversicolor exhibited a higher growth and survival when cultured at a density of 300 ind. m–2 than at 1,000 and 3,000 ind. m–2.
Total FA reported for D40 polychaetes in the present work are among the highest values recorded to date for H. diversicolor (see Table 5). Geographic location, abiotic conditions, diet availability and composition, and maturation state contribute to the shaping of FA profiles in this species (Luis and Passos, 1995; García-Alonso et al., 2008; Santos et al., 2016; Marques et al., 2018). Therefore, the interpretation of contrasting FA profiles reported in different studies is a challenging task. Initially stocked polychaetes, as well as wild conspecifics Wild10, Wild20, and Wild40, were collected during February-March, a transition period between winter and spring in the sampling area. Wild specimens exhibited a reddish-brown coloration (Figure 6A), a characteristic feature of immature worms (Scaps, 2002). Two spawning periods, one in spring and the other in early autumn, were identified in wild populations of H. diversicolor monitored at the same sampling site of the specimens used in the present study (Abrantes et al., 1999). According to Luis and Passos (1995), during winter and early spring months, in the presence of a suitable diet, sexually immature worms accumulate lipids in their body. Immediately before reproduction, a bulk of FA is made available through triacylglycerol depletion, being these essential during the rapid growth of oocytes (Luis and Passos, 1995). This polychaete species is therefore well-known to reflect in its body composition the FA profile of its diet (Fidalgo e Costa et al., 2000; Santos et al., 2016; Pajand et al., 2017; Marques et al., 2018; Wang et al., 2019). This feature, along with maturation, are likely among the factors which most contributed for the differences in FA composition reported in previous studies testing the production of H. diversicolor using commercial aquafeeds or wasted nutrients derived from aquaculture effluents. In the present work it was possible to monitor the effect of diet over time (by comparing Initial, D10, D20, and D40 samples). The FA which exhibited higher increments in polychaete biomass were exactly the ones presented in higher levels in the commercial aquafeed employed. With only a few exceptions, these FA are also among the most well-represented ones reported in previous studies addressing the culture of this species (Fidalgo e Costa et al., 2000; García-Alonso et al., 2008; Bischoff et al., 2009; Santos et al., 2016; Pajand et al., 2017; Marques et al., 2018; Wang et al., 2019; Yousefi-Garakouei et al., 2019).
Table 5. Summary of the fatty acid (FA) profile of Hediste diversicolor reported from previous studies providing this species with a commercial aquafeed or wasted nutrients derived from aquaculture effluents.
Figure 6. Hediste diversicolor at the beginning of the experiment (A) and after being fed on a commercial aquafeed for 40 days under different combinations of water temperature (20 and 25°C) and salinity (15, 20, and 25) (B).
An advantage of the present study in relation to previous works is that it monitored the evolution of FA profile of ragworms over time. This issue is of paramount importance if producers aim to enhance the amount of EFA of cultured ragworms by using enriched diets for a short period of time at the end of the production cycle to obtain premium polychaetes. In addition, producers may also select to enrich wild polychaetes biomass, rather than culturing these organisms over their whole life cycle. A progressive increment of total FA was recorded over time. In the first 20 days (comparing Initial, D10 and D20 polychaetes), a predominant increment of SFA and MUFA was evidenced, while in the last 20 days of the trial (comparing D20 and D40 polychaetes) an increment of PUFA and HUFA prevailed. These results may well reflect an adaptation of polychaetes to the new diet being provided and/or the experimental conditions employed, latter followed by metabolic shifts triggered by maturation. According to Nesto et al. (2012), diets similar to the one used in the present work, with high-protein content, despite originating the best results of daily biomass production, also triggered an earlier onset of gametogenesis. Despite the increments recorded, it is worth highlighting that a plateau of total FA, n-6 FA and n-3 FA was not reached during the 40 days of the experimental trials. This finding allows us to infer that it may still be possible to further enhance H. diversicolor biomass in EFA if the study had been extended over a longer period. Nonetheless, one must acknowledge that when this species reaches adult size (similar to that recorded at D40 polychaetes) it starts to reproduce and die.
A comparative analysis of the FA profile of Initial, D10, D20, and D40 polychaetes also allowed to detect evidence suggesting de novo biosynthesis. A good example of this de novo biosynthesis is the 10–20% increment recorded in eicosadienoic (20:2 n-6), a FA present in Initial polychaetes but absent in the commercial aquafeed supplied. Other FA that were only detected in cultured polychaetes, being absent in initially stocked polychaetes and aquafeed, were 5,11-eicosadienoate (Δ 5,1120:2) (detected in D10, D20 and D40 polychaetes), 7,13,16-docosatrienoate (Δ 7,13,16 22:3) (detected in D20 and D40 polychaetes), and DGLA (20:3 n-6), ETE (20:3 n-3) and ETA (20:4 n-3) (only detected in D40 polychaetes). In de novo FA biosynthesis, three major enzymes are involved: namely methyl-end-desaturases (ω6–Δ12 and ω3–Δ15, Δ17, Δ19), front-end desaturases (Δ4, Δ5, Δ6, Δ8) and elongases (Elo); their pivotal roles in PUFA and HUFA biosynthesis in aquatic invertebrates have been reviewed by Monroig and Kabeya (2018) and are summarized in Figure 7. The detection of DGLA (20:3 n-6), ETE (20:3 n-3) and ETA (20:4 n-3) only in D40 polychaetes may have resulted from the activation of the respective alternative biosynthesis pathways (see Figure 7). Here it is important to clarify that gamma-linolenic (18:3 n-6) and stearidonic acid (18:4 n-3) from the main biosynthesis pathway were not detected. The fact that DGLA (20:3 n-6), ETE (20:3 n-3) and ETA (20:4 n-3) were only detected in D40 polychaetes, along with the fact that its precursors [LA (18:2 n-6) and ALA (18:3 n-3)] were already present in the initially stocked polychaetes and in the supplied aquafeed, may result from the activation of these biosynthesis pathways due to maturation. Indeed, at the end of the experimental period some polychaetes exhibited a bright grass-green color (see Figure 6B), a common feature of mature males (Dales, 1950; Scaps, 2002). To the authors best knowledge, this is the first time that the biosynthesis pathways mentioned above are suggested to occur in this species. In recent years, several studies have highlighted de novo biosynthesis in annelids, and for example the action of methyl-end desaturases has been demonstrated in studies addressing Alitta virens (a polychaete species of family Nereididae as H. diversicolor), which was able to perform de novo biosynthesis of ARA (20:4 n-6) and EPA (20:5 n-3) when supplied with an aquafeed supplemented with 13C-labeled palmitic acid (16:0) (Pairohakul, 2013). According to Monroig and Kabeya (2018), a comprehensive description of front-end-desaturases and elongase genes is still missing for annelids. However, there is enough evidence to state that these biosynthetic pathways exist in annelids, namely Ridge piscesae and Protis hydrothermica (Pond et al., 2002), Arenicola marina (Olive et al., 2009), A. virens (Pairohakul, 2013), and Perinereis aibuhitensis (Lv et al., 2017).
Figure 7. Schematic representation of putative biosynthetic pathways of some polyunsaturated fatty acids (PUFA) identified in Hediste diversicolor from the wild and after being fed on a commercial aquafeed for 40 days. Methyl-end desaturases (ω, green arrows); Front-end desaturases (red arrows); Elongation reactions (Elo, blue arrows). The “Δ” refers to the carbon position at which the incipient double bound (unsaturation) locates within the methyl and front ends of fatty acyl chains. AdA, adrenic acid; ALA, α-linolenic acid; ARA, arachidonic acid; DGLA, dihomo-gamma-linolenic acid; DHA, docosahexaenoic acid; DPA, Docosapentaenoic acid; EPA, eicosapentaenoic acid; ETA, eicosatetraenoic acid; ETE, eicosatrienoic acid; LA, linoleic acid. Scheme adapted from Monroig and Kabeya (2018).
The present study demonstrated how H. diversicolor biomass can be successfully enriched with n-6 and n-3 FA (including EPA and DHA) when provided a commercial aquafeed, even when exposed to different combinations of water temperature and salinity. This finding highlights the potential of using H. diversicolor as an extractive species in IMTA designs, thus allowing to recover valuable nutrients present in aquaculture effluents that would otherwise be wasted. The production of a ragworm biomass rich in EFA allows to provide the aquafeed industry with another alternative ingredient for fish meal and fish oil, at least for the formulation of premium maturation and finishing diets. The development of innovative aquaculture production models based on the integration of extractive species, such as polychaetes, to recover wasted nutrients derived from the aquaculture of fed species complies with sustainable aquaculture guidelines and is aligned with UN 2030 Sustainable Development Goals Agenda.
The original contributions presented in the study are included in the article/Supplementary Material, further inquiries can be directed to the corresponding author/s.
DJ and RC contributed to conceptualization and design of the study. DJ and HI performed the experiment culturing polychaetes under different conditions and collected the biomass samples. DJ, FR, and MD designed and performed the analytical work to evaluate fatty acid profile. DJ performed the statistical analysis and wrote the first draft of the manuscript. RC, AL, and MD contributed with supervision, funding and resources acquisition. All authors contributed to manuscript revision, read, and approved the submitted version.
This by work was supported by the project “VALORMAR—Full valorization of marine resources: potential, technological innovation and new applications” (24517, 10/SI/2016–Mobilizing R&TD Programs) co-funded by Compete 2020, Lisboa 2020, Algarve 2020, Portugal 2020 and European Union, through the European Regional Development Fund (ERDF), by project “AquaMMIn–Development and validation of a modular integrated multitrophic aquaculture system for marine and brackish water species” (MAR-02.01.01-FEAMP-0038) co-funded by Portugal 2020 and the European Union through Mar2020, the Operational Programme (OP) for the European Maritime and Fisheries Fund (EMFF) in Portugal and by the Integrated Programme of SR&TD “SmartBioR–Smart Valorization of Endogenous Marine Biological Resources Under a Changing Climate” (Centro-01-0145-FEDER-000018), and co-funded by Centro 2020 program, Portugal 2020, European Union, through the European Regional Development Fund. Daniel Jerónimo acknowledges FCT (Portuguese Foundation for Science and Technology) by financially supporting his PhD grant (PD/BD/127989/2016) and Felisa Rey by her Junior Researcher contract (reference CEECIND/00580/2017). Thanks for financial support are also due to CESAM (UIDB/50017/2020 + UIDP/50017/2020), to FCT/MEC through national funds, and the cofounding by the FEDER, within the PT2020 Partnership Agreement and Compete 2020.
The authors declare that the research was conducted in the absence of any commercial or financial relationships that could be construed as a potential conflict of interest.
We thanks to Tiago Conde and Daniela Couto (Departamento de Química–Universidade de Aveiro) for all the assistance provided in the lipid extraction and fatty acid analysis.
The Supplementary Material for this article can be found online at: https://www.frontiersin.org/articles/10.3389/fmars.2021.671545/full#supplementary-material
Abrantes, A., Pinto, F., and Moreira, M. H. (1999). Ecology of the polychaete Nereis diversicolor in the Canal de Mira (Ria de Aveiro, Portugal): population dynamics, production and oogenic cycle. Acta Oecol. 20, 267–283. doi: 10.1016/S1146-609X(99)00139-3
Anderson, M. J., Gorley, R. N., and Clarke, K. R. (2008). PERMANOVA+ for PRIMER: Guide to Software and Statistical Methods. (Plymouth: PRIMER.E_Ltd), 1–214.
Bell, M. V., and Tocher, D. R. (2009). “Biosynthesis of polyunsaturated fatty acids in aquatic ecosystems: general pathways and new directions,” in Lipids in Aquatic Ecosystems, eds M. T. Arts, M. T. Brett, and M. J. Kainz (New York, NY: Springer), 211–237. doi: 10.1093/plankt/fbp089
Bischoff, A. (2007). Solid Waste Reduction of Closed Recirculated Aquaculture Systems by Secondary Culture of Detritivorous Organisms. Ph.D. thesis. Kiel: Christian-Albrechts-Universität Zu Kiel.
Bischoff, A. A., Fink, P., and Waller, U. (2009). The fatty acid composition of Nereis diversicolor cultured in an integrated recirculated system: possible implications for aquaculture. Aquaculture 296, 271–276. doi: 10.1016/j.aquaculture.2009.09.002
Bligh, E. G., and Dyer, W. J. (1959). A rapid method of total lipid extraction and purification. Can. J. Biochem. Physiol. 37, 911–917. doi: 10.1139/y59-099
Carvalho, A. N., Vaz, A. S. L., Sérgio, T. I. B., and Santos, P. J. T. (2013). Sustainability of bait fishing harvesting in estuarine ecosystems – Case study in the Local Natural Reserve of Douro Estuary, Portugal. J. Integr. Coast. Zone Manage. 13, 157–168. doi: 10.5894/rgci393
Cashion, T., Le Manach, F., Zeller, D., and Pauly, D. (2017). Most fish destined for fishmeal production are food-grade fish. Fish Fish. 18, 837–844. doi: 10.1111/faf.12209
Dales, R. P. (1950). The reproduction and larval development of Nereis diversicolor O. F. Müller. J. Mar. Biol. Assoc. U.K. 29, 321–360. doi: 10.1017/S0025315400055405
Dinis, M. T. (1992). Aspects of the potential of Solea senegalensis Kaup for aquaculture: larval rearing and weaning to an artificial diet. Aquac. Res. 23, 515–520. doi: 10.1111/j.1365-2109.1992.tb00795.x
Dinis, M. T., Ribeiro, L., Soares, F., and Sarasquete, C. (1999). A review on the cultivation potential of Solea senegalensis in Spain and in Portugal. Aquaculture 176, 27–38. doi: 10.1016/S0044-8486(99)00047-2
FAO (2020). The State of World Fisheries and Aquaculture: Sustainability in Action. Rome: FAO. doi: 10.4060/ca9229en
Fauchald, K., and Jumars, P. A. (1979). The diet of worms: a study of polychaete feeding guilds. Oceanogr. Mar. Biol. Annu. Rev. 17, 193–284. doi: 10.12691/marine-1-1-6
Fidalgo e Costa, P., Gil, J., Passos, A. M., Pereira, P., Melo, P., Batista, F., et al. (2006). The market features of imported non-indigenous polychaetes in Portugal and consequent ecological concerns. Sci. Mar. 70, 287–292. doi: 10.3989/scimar.2006.70s3287
Fidalgo e Costa, P., Narciso, L., and Cancela da Fonseca, L. (2000). Growth, survival and fatty acid profile of Nereis diversicolor (O. F. Muller, 1776) fed on six different diets. Bull. Mar. Sci. 67, 337–343.
García-Alonso, J., Hardege, J. D., and Müller, C. T. (2008). Influence of food regimes and seasonality on fatty acid composition in the ragworm. Aquat. Biol. 4, 7–13. doi: 10.3354/ab00090
Hastings, N., Agaba, M., Tocher, D. R., Leaver, M. J., Dick, J. R., Sargent, J. R., et al. (2001). A vertebrate fatty acid desaturase with Δ5 and Δ6 activities. Proc. Natl. Acad. Sci. U.S.A. 98, 14304–14309. doi: 10.1073/pnas.251516598
Hastings, N., Agaba, M. K., Tocher, D. R., Zheng, X., Dickson, C. A., Dick, J. R., et al. (2004). Molecular cloning and functional characterization of fatty acyl desaturase and elongase cDNAs involved in the production of eicosapentaenoic and docosahexaenoic acids from α-linolenic acid in Atlantic salmon (Salmo salar). Mar. Biotechnol. 6, 463–474. doi: 10.1007/s10126-004-3002-8
Luis, O. J., and Passos, A. M. (1995). Seasonal changes in lipid content and composition of the polychaete Nereis (Hediste) diversicolor. Comp. Biochem. Physiol. B Biochem. Mol. Biol. 111, 579–586. doi: 10.1016/0305-0491(95)00029-8
Luis, O. J., and Ponte, A. C. (1993). Control of reproduction of the shrimp Penaeus kerathurus held in captivity. J. World Aquac. Soc. 24, 31–39. doi: 10.1111/j.1749-7345.1993.tb00147.x
Lv, F., Nie, Q., Wang, T., Wang, A., Yang, W., Liu, F., et al. (2017). The effects of five dietary lipid sources on growth, body composition and antioxidant parameters of the clamworm, Perinereis aibuhitensis. Aquac. Res. 48, 5472–5480. doi: 10.1111/are.13362
Lytle, J. S., Lytle, T. F., and Ogle, J. T. (1990). Polyunsaturated fatty acid profiles as a comparative tool in assessing maturation diets of Penaeus vannamei. Aquaculture 89, 287–299. doi: 10.1016/0044-8486(90)90133-8
Marques, B., Lillebø, A. I., Ricardo, F., Nunes, C., Coimbra, M. A., and Calado, R. (2018). Adding value to ragworms (Hediste diversicolor) through the bioremediation of a super-intensive marine fish farm. Aquac. Environ. Interact. 10, 79–88. doi: 10.3354/aei00255
Meunpol, O., Meejing, P., and Piyatiratitivorakul, S. (2005). Maturation diet based on fatty acid content for male Penaeus monodon (Fabricius) broodstock. Aquac. Res. 36, 1216–1225. doi: 10.1111/j.1365-2109.2005.01342.x
Monroig, Ó., and Kabeya, N. (2018). Desaturases and elongases involved in polyunsaturated fatty acid biosynthesis in aquatic invertebrates: a comprehensive review. Fish. Sci. 84, 911–928. doi: 10.1007/s12562-018-1254-x
Nesto, N., Simonini, R., Prevedelli, D., and Da Ros, L. (2012). Effects of diet and density on growth, survival and gametogenesis of Hediste diversicolor (O.F. Müller, 1776) (Nereididae, Polychaeta). Aquaculture 362–363, 1–9. doi: 10.1016/j.aquaculture.2012.07.025
Olive, P. J. W. (1999). Polychaete aquaculture and polychaete science: a mutual synergism. Hydrobiologia 402, 175–183. doi: 10.1023/A:1003744610012
Olive, P. J. W., Duangchinda, T., Ashforth, E., Craig, S., Ward, A. C., and Davies, S. J. (2009). Net gain of long-chain polyunsaturated fatty acids (PUFA) in a lugworm Arenicola marina bioturbated mesocosm. Mar. Ecol. Prog. Ser. 387, 223–239. doi: 10.3354/meps08088
Pairohakul, S. (2013). Evidence for Polyunsaturated Fatty Acid Biosynthesis in the Ragworm (Nereis virens) and the lugworm (Arenicola marina). Ph.D. thesis. Newcastle upon Tyne: Newcastle University.
Pajand, Z. O., Soltani, M., Bahmani, M., and Kamali, A. (2017). The role of polychaete Nereis diversicolor in bioremediation of wastewater and its growth performance and fatty acid composition in an integrated culture system with Huso huso (Linnaeus, 1758). Aquac. Res. 48, 5271–5279. doi: 10.1111/are.13340
Pombo, A., Baptista, T., Granada, L., Ferreira, S. M. F., Costa, L., and Fidalgo, P. (2018). Insight into aquaculture’s potential of marine annelid worms and ecological concerns: a review. Rev. Aquac. 12, 107–121. doi: 10.1111/raq.12307
Pond, D. W., Allen, C. E., Bell, M. V., Van Dover, C. L., Fallick, A. E., Dixon, D. R., et al. (2002). Origins of long-chain polyunsaturated fatty acids in the hydrothermal vent worms Ridgea piscesae and Protis hydrothermica. Mar. Ecol. Prog. Ser. 225, 219–226. doi: 10.3354/meps225219
Reise, K. (1979). Spatial configurations generated by motile benthic polychaetes. Helgoländer Wiss. Meeresunters. 32, 55–72. doi: 10.1007/BF02189892
Riisgård, H. U. (1994). Filter-feeding in the polychaete Nereis diversicolor: a review. Neth. J. Aquat. Ecol. 28, 453–458. doi: 10.1007/BF02334216
Sá, E., Fidalgo e Costa, P., Cancela da Fonseca, L., Alves, A. S., Castro, N., dos Santos Cabral, S., et al. (2017). Trade of live bait in Portugal and risks of introduction of non-indigenous species associated to importation. Ocean Coast. Manage. 146, 121–128. doi: 10.1016/j.ocecoaman.2017.06.016
Santos, A., Granada, L., Baptista, T., Anjos, C., Simões, T., Tecelão, C., et al. (2016). Effect of three diets on the growth and fatty acid profile of the common ragworm Hediste diversicolor (O.F. Müller, 1776). Aquaculture 465, 37–42. doi: 10.1016/j.aquaculture.2016.08.022
Sargent, J. R., Tocher, D. R., and Bell, J. G. (2003). “4 - The Lipids,” in Fish Nutrition, 3rd Edn, eds J. E. Halver and R. W. Hardy (San Diego, CA: Academic Press), 181–257. doi: 10.1016/B978-012319652-1/50005-7
Scaps, P. (2002). A review of the biology, ecology and potential use of the common ragworm Hediste diversicolor (O. F. Müller) (Annelida: Polychaeta). Hydrobiologia 470, 203–218. doi: 10.1023/A:1015681605656
Tacon, A. G. J., and Metian, M. (2008). Global overview on the use of fish meal and fish oil in industrially compounded aquafeeds: trends and future prospects. Aquaculture 285, 146–158. doi: 10.1016/j.aquaculture.2008.08.015
Tacon, A. G. J., and Metian, M. (2015). Feed matters: satisfying the feed demand of aquaculture. Rev. Fish. Sci. Aquac. 23, 1–10. doi: 10.1080/23308249.2014.987209
Tocher, D. R. (2003). Metabolism and functions of lipids and fatty acids in teleost fish. Rev. Fish. Sci. 11, 107–184. doi: 10.1080/713610925
Tocher, D. R. (2015). Omega-3 long-chain polyunsaturated fatty acids and aquaculture in perspective. Aquaculture 449, 94–107. doi: 10.1016/j.aquaculture.2015.01.010
Vásquez, V., Krieg, M., Lockhead, D., and Goodman, M. B. (2014). Phospholipids that contain polyunsaturated fatty acids enhance neuronal cell mechanics and touch sensation. Cell Rep. 6, 70–80. doi: 10.1016/j.celrep.2013.12.012
Vedel, A., Andersen, B. B., and Riisgard, H. U. (1994). Field investigations of pumping activity off the facultatively filter-feeding polychaete Nereis diversicolor using an improved infrared phototransducer system. Mar. Ecol. Prog. Ser. 103, 91–102. doi: 10.3354/meps103091
Velez, Z., Hubbard, P. C., Hardege, J. D., Barata, E. N., and Canário, A. V. M. (2007). The contribution of amino acids to the odour of a prey species in the Senegalese sole (Solea senegalensis). Aquaculture 265, 336–342. doi: 10.1016/j.aquaculture.2007.02.029
Wang, H., Seekamp, I., Malzahn, A., Hagemann, A., Carvajal, A. K., Slizyte, R., et al. (2019). Growth and nutritional composition of the polychaete Hediste diversicolor (OF Müller, 1776) cultivated on waste from land-based salmon smolt aquaculture. Aquaculture 502, 232–241. doi: 10.1016/j.aquaculture.2018.12.047
Watson, G. J., Murray, J. M., Schaefer, M., and Bonner, A. (2016). Bait worms: a valuable and important fishery with implications for fisheries and conservation management. Fish Fish. 18, 374–388. doi: 10.1111/faf.12178
Xenarios, S., Queiroga, H., Lillebø, A., and Aleixo, A. (2018). Introducing a regulatory policy framework of bait fishing in European coastal lagoons: the Case of Ria de Aveiro in Portugal. Fishes 3:2. doi: 10.3390/fishes3010002
Yousefi-Garakouei, M., Kamali, A., and Soltani, M. (2019). Effects of rearing density on growth, fatty acid profile and bioremediation ability of polychaete Nereis diversicolor in an integrated aquaculture system with rainbow trout (Oncorhynchus mykiss). Aquac. Res. 50, 725–735. doi: 10.1111/are.13918
Keywords: de novo biosynthesis, n-3 HUFA, POM bioremediation, ragworms, extractive species
Citation: Jerónimo D, Lillebø AI, Rey F, Ii HK, Domingues MRM and Calado R (2021) Optimizing the Timeframe to Produce Polychaetes (Hediste diversicolor) Enriched With Essential Fatty Acids Under Different Combinations of Temperature and Salinity. Front. Mar. Sci. 8:671545. doi: 10.3389/fmars.2021.671545
Received: 24 February 2021; Accepted: 12 April 2021;
Published: 11 May 2021.
Edited by:
Eduardo Almansa, Spanish Institute of Oceanography (IEO), SpainReviewed by:
Inmaculada Rasines, Spanish Institute of Oceanography (IEO), SpainCopyright © 2021 Jerónimo, Lillebø, Rey, Ii, Domingues and Calado. This is an open-access article distributed under the terms of the Creative Commons Attribution License (CC BY). The use, distribution or reproduction in other forums is permitted, provided the original author(s) and the copyright owner(s) are credited and that the original publication in this journal is cited, in accordance with accepted academic practice. No use, distribution or reproduction is permitted which does not comply with these terms.
*Correspondence: Daniel Jerónimo, ZGFuaWVsamVyb25pbW9AdWEucHQ=; Ricardo Calado, cmpjYWxhZG9AdWEucHQ=
Disclaimer: All claims expressed in this article are solely those of the authors and do not necessarily represent those of their affiliated organizations, or those of the publisher, the editors and the reviewers. Any product that may be evaluated in this article or claim that may be made by its manufacturer is not guaranteed or endorsed by the publisher.
Research integrity at Frontiers
Learn more about the work of our research integrity team to safeguard the quality of each article we publish.