- 1College of Fisheries and Life Science, Shanghai Ocean University, Shanghai, China
- 2Tropical Aquaculture Research and Development Center, South China Sea Fisheries Research Institute, Chinese Academy of Fishery Sciences, Sanya, China
- 3Key Laboratory of South China Sea Fishery Resources Exploitation and Utilization, Ministry of Agriculture, Guangzhou, China
- 4Sanya Tropical Fisheries Research Institute, Sanya, China
- 5College of Science and Engineering, Flinders University, Adelaide, SA, Australia
To elucidate the possible molecular reaction of vertebral fusion in juvenile golden pompano at high temperature, we examined the changes in growth, vertebral fusion incidence, histological differences and transcription levels of genes related to bone development in juvenile golden pompano at 27 and 33°C for 30 days, respectively. The growth rate of juvenile fish was faster when the water temperature was 33°C, but the incidence of vertebral fusion was higher. Prolonged high water temperature reduced the osteogenic layer in the growth zone of the vertebral endplate and the elastic externa. The endplate growth areas of the fused vertebrae were transformed into cartilage tissue, which was then remodeled into bone. The intervertebral notochord tissue was transformed into bone and the intervertebral space disappeared. In normal phenotypes of vertebrae, short-term high temperature could promote the expression of genes related to cartilage differentiation and maturation, as well as genes related to osteoblastic differentiation. With the increase of culture time, the expression of genes related to cartilage and osteogenesis development was inhibited. In fused vertebrae, cartilage proliferation was enhanced, osteogenic differentiation was inhibited, and matrix mineralization may be enhanced. Genes associated with the development of chondrocytes and osteoblasts in the vertebrae of juvenile Trachinotus ovatus were significantly regulated by temperature and time. The results may contribute to further understanding of the occurrence of vertebral fusion at high temperature.
Introduction
The vertebral column, composed of vertebrae and joint tissues, is an important fundamental infrastructure unique to vertebrates (Fleming et al., 2015; Galbusera and Bassani, 2019) and could provide structural support and protection for the organism (Scaal, 2016). The abnormality of the vertebral column may bring serious consequences to the organism, and much attention has been focused on studying the structure, function, development and mechanism of the abnormality of the vertebral column. The abnormalities of the vertebral column are frequently observed in farmed fish, which is one of the important factors restricting the development of the aquaculture industry (Pedersen et al., 2011). Vertebral fusion is a common type of spinal abnormality. In farmed Atlantic salmon (Salmo salar), for example, fusion is the most common type of spinal deformity (Witten et al., 2009; Fjelldal et al., 2012). Fish with fusion phenotype will weigh less than fish with a normal phenotype (Davie et al., 2019). In addition, fusion involving more than six vertebrae may increase fish mortality (Hansen et al., 2010). The occurrence of vertebrae fusion in cultured fish may cause economic loss and lead to the welfare problems of fish. Existing research suggests that transformation, remodeling, and replacement of intervertebral tissue eventually lead to vertebral fusion, which involves alterations in extracellular matrix (ECM) components, metaplastic chondrogenesis and mineralization (Witten et al., 2006; Pedersen et al., 2011; Davie et al., 2019). Meanwhile, the occurrence of vertebral fusion is externally regulated by rearing temperature and nutritional factors (Ytteborg et al., 2010a; Wu et al., 2016; Munday et al., 2018).
With the development of science and technology, more and more methods, such as bony double staining, histology, X-ray and molecular tools, have been applied to the study of spinal development and deformity in bony fish (Sullivan et al., 2007; Ytteborg et al., 2010a, b; Pedersen et al., 2011; Cardeira et al., 2012; Perrott et al., 2018; Lovett et al., 2020). However, histological analysis of skeletal abnormalities caused by specific factors is rare (but see Nordvik et al., 2005; Ytteborg et al., 2010a; Fernandez et al., 2012; Wu et al., 2016), and the use of molecular tools in the study of skeletal development and abnormalities in bony fish is concentrated in zebrafish, medaka, and Atlantic salmon (Ytteborg et al., 2010a; Bensimon-Brito et al., 2012; Renn et al., 2013). It is noteworthy that, compared with tetrapods, the vertebral body of fish is formed through membranous ossification rather than endochondral ossification, and the process of membranous ossification varies among fish (Fleming et al., 2004). In contrast to mammals, there are more types of combinations of bone tissue in fish, including many types of bone and cartilage, as well as some types of tissue between connective tissue and bone, and between bone and cartilage (Boglione et al., 2013b). Moreover, different fish species may react differently to the same factors that induce malformations (Boglione et al., 2013a). These mean that the spinal abnormalities of bony fish may be different from those of mammals, and different species of bony fish may also show different histological and molecular responses under the influence of the same teratogenic factors. Therefore, the existing studies may not be enough to elucidate the determining mechanism and variation of the occurrence of vertebral fusion in bony fish.
Golden pompano (Trachinotus ovatus), a warm-water marine fish species belongs to the family Carangidae, is geographically distributed throughout tropical and temperate parts of the Indian Ocean, the Pacific Ocean and Atlantic Ocean (Ma et al., 2016b; Zhou et al., 2019). However, the skeletal deformity rate in T. ovatus can exceed 30%, and the incidence of vertebral fusion can reach 37.4%, which is the most frequent type of bone abnormality (Zheng et al., 2016; Sun et al., 2020). Abnormality of bone results in a bottleneck in the culture of T. ovatus. The effects of temperature and nutrition factors on the skeletal deformities of T. ovatus, and the expression of some genes related to skeletal development in T. ovatus has also been studied (Ma et al., 2016a, 2017a, 2017b, 2017c, 2018; Yang et al., 2016), but the regulatory mechanism of temperature on the occurrence of vertebral fusion in juvenile T. ovatus is still not clear, while histopathological studies on the skeleton of T. ovatus are lacking. The objective of the present study was to elucidate the possible molecular reaction of vertebral fusion in juvenile T. ovatus under high water temperature combined with the histopathology. Results from the present study could provide a further understanding of the occurrence of vertebral fusion and optimization of T. ovatus farming.
Materials and Methods
Experimental Fish
Trachinotus ovatus Juveniles from the same batch were produced by Tropical Fisheries Research and Development Center, South China Sea Fisheries Research Institute, Chinese Academy of Fishery Sciences, Xincun Town, Lingshui, Hainan Province. This research was approved by the Animal Care and Use Committee of South China Sea Fisheries Research Institute, Chinese Academy of Fishery Sciences (No. 2019CXTD418).
Experimental Design and Sample Collection
The experimental water temperature was set at two temperatures, 27°C as the low temperature (LT) group and 33°C as the high temperature (HT) group, with three replicates each. Before the experiment started, a total of 1,200 healthy fish were selected with similar weight (8.91 ± 0.36 g) and were randomly distributed in six 3,000-L fiberglass tanks (200 fish per tank, three tanks for LT group, other three tanks for HT group) for a week acclimation. All individuals were observed by X-Ray before being selected to ensure that there were no deformities. During this process, water temperature maintained at 27.0 ± 0.5°C. After acclimation, the water temperature of the LT group was kept constant, while that of the HT group was gradually increased to 33 ± 0.5°C (2°C per day). Then the experiment was performed and lasted 30 days. The juveniles were fed twice a day (at 08:00 and 16:00) with a commercial diet at a rate of 2% of total fish body weight. The feces were removed from the experimental tank daily by siphoning. During all period, the rearing water maintained at a salinity of dissolved oxygen >7.0 mg L–1, ammonia nitrogen <0.1 mg L–1, nitrite nitrogen <0.02 mg L–1, 32.0 ± 0.5‰ and pH 7.7 ± 0.3. The photoperiod was controlled at 14 h light and 10 h dark.
Fish were sampled on 10, 20, and 30 days. Fish were deprived of feed for 12 h before the sampling was conducted. After humanely euthanized by overdose with MS-222, 50 fish were randomly selected from each tank to count the incidence of vertebral fusion through X-ray. Afterward, 10 fish with normal phenotype were randomly selected from each tank for gene expression analysis. Meanwhile, fish with vertebral fusion in HT groups were also sampled for gene expression analysis. The sampling location was the spine 1–2 cm long below the dorsal fin. On the 30th day, all fish were weighed from each tank to determine specific growth rate (SGR = 100 × (ln (final body weight)− ln (initial body weight))/(ln (final body weight) − ln (initial body weight)) days. days). At the same time, 10 fish with normal phenotype under low and high water temperature and with fusion phenotype under high water temperature were, respectively, selected for histological analysis. Figure 1 shows radiographic images of normal and fused vertebral bodies.
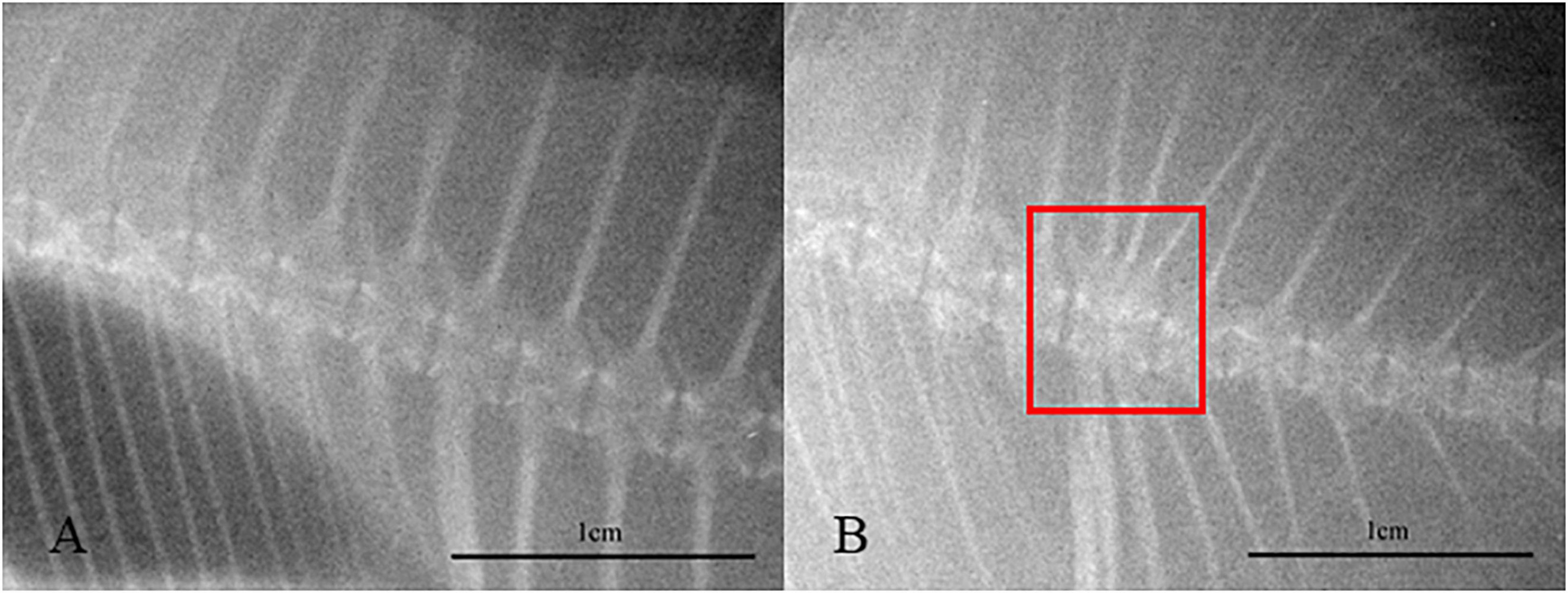
Figure 1. Radiographic images of normal (A) and fused (B) vertebral bodies. The red square area represents the vertebrae where fusion occurred.
Histology
Fish spine fixation and dehydration, paraffin embedding and hematoxylin-eosin staining was performed following standard protocols (Ofer et al., 2019). Each slide with spine sections was mounted permanently using neutral balsam. The sections were observed using a Nikon Eclipse Ni-U Upright Microscope (Nikon Instruments Inc., Japan), and ProgRes CapturePro (Jenoptik AG, Germany) was used for image collection and analysis.
Analysis of Bone Development Related Genes
The total RNA was isolated using TRIzol Reagent (Invitrogen, United States) according to the manufacturer’s instructions. The quantity of isolated RNA was later determined by measuring their absorbance at 260 and 280 nm using a Nano-300 Micro Spectrophotometer (Hangzhou Allsheng Instruments Co., Ltd., China), and the quality of total RNA was detected by electrophoresis on 1.0% agarose gel. Then the extracted RNA was reverse-transcribed into cDNA and removed gDNA using a EasyScript All-in-One First-Strand cDNA Synthesis SuperMix (TransGen Biotech Co., Ltd., China) and used for qPCR. The development related genes sequences were selected from the unpublished golden pompano transcriptome sequences (Illumina HiSeq2000, annotated by NR, KOG, KEGG, and Swissprot). Primers used for q-PCR for signaling molecules, transcription factors and ECM genes expression in pompano referred to the design of Han et al., 2020, Table 1) or designed by the Primer Premier 5 program.
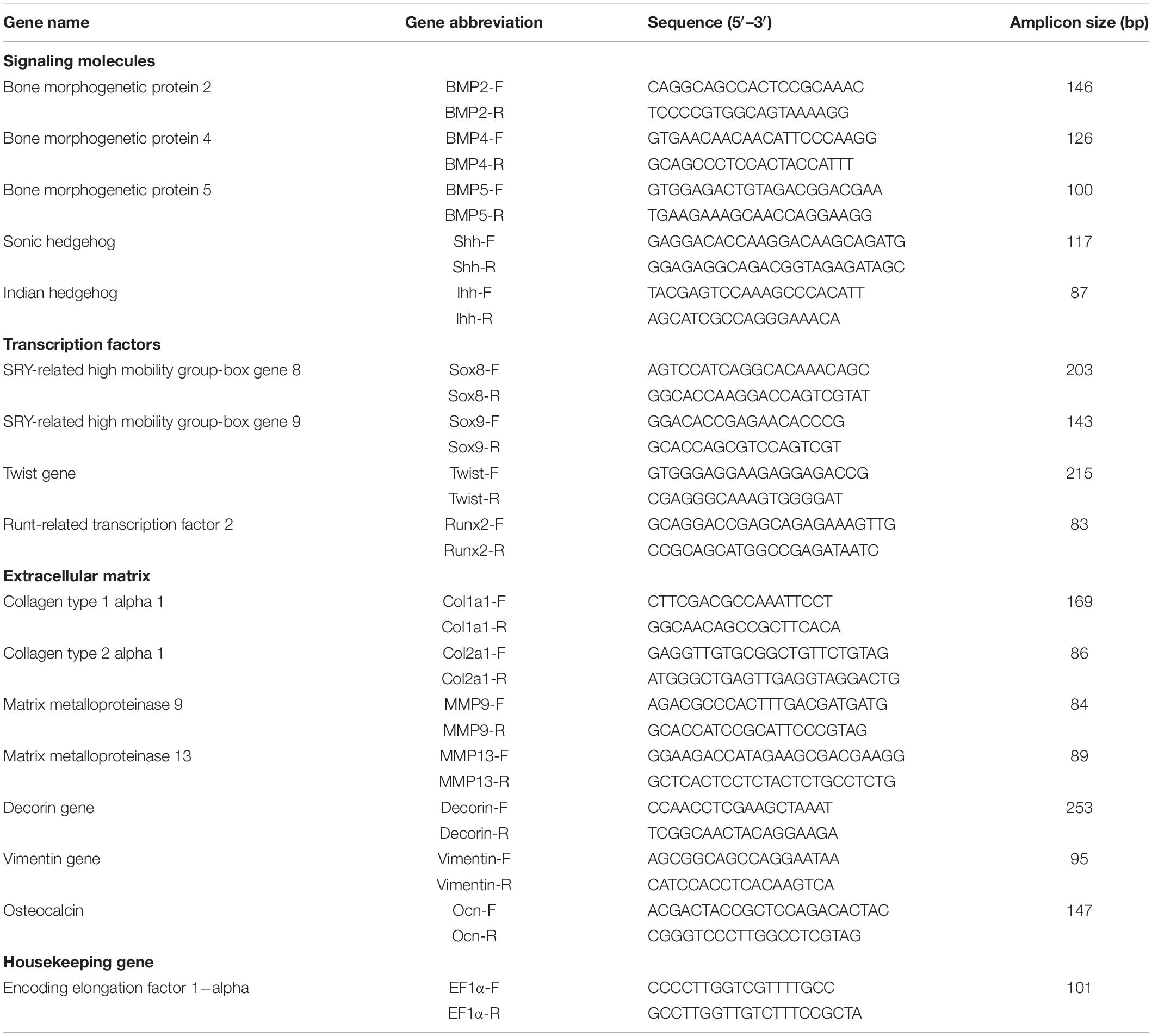
Table 1. Primers used for q-PCR for signaling molecules, transcription factors and extracellular matrix genes expression in juvenile Trachinotus ovatus.
The qPCR was conducted with the Real-time qPCR analysis (Analytik Jena GmbH, Germany) using SYBR Green (Tiangen Biotech Co., Ltd., China). The reaction conditions were as follows: initial denaturation at 95°C for 15 min, 40 cycles of denaturing at 95°C for 10 s, annealing at 60°C for 20 s, and extension at 72°C for 30 s. There were three repetitions of each test. At the end of PCR program, dissociation curves were analyzed to ensure that only specific products were obtained in each reaction. The relative mRNA expression levels of the target genes were determined by the 2–ΔΔCt method and were normalized based on the level of the housekeeping gene (EF1α). It has been verified that the reaction efficiency (E) was 90–110% and Pearson’s coefficients of determination (R2) > 0.97.
Statistical Analysis
The data were presented as the mean ± standard deviation (SD). Comparisons of SGR and the incidence of spinal abnormality between different groups were conducted by independent T-test (PASW Statistics 18.0, Chicago, SPSS Inc.), and the comparisons of mRNA transcription level were conducted by one-way ANOVA and LSD test. The significant difference was set at P < 0.05. All percentage data were transformed using square root to satisfy the assumptions of ANOVA.
Results
Growth and the Incidence of Spinal Abnormality of Golden Pompano
In the present study, the SGR of the juveniles in the HT group was significantly higher than that in the LT group (Figure 2, P < 0.05). On the 10th, 20th, and 30th day, the incidence of vertebral fusion in the HT group was significantly higher than that in the LT group (Figure 3, P < 0.05). With the change of time, the incidence of vertebral fusion in treatment groups all showed an increasing trend. On the 30th day, more than 40% of juveniles in the HT group showed a spinal abnormality.
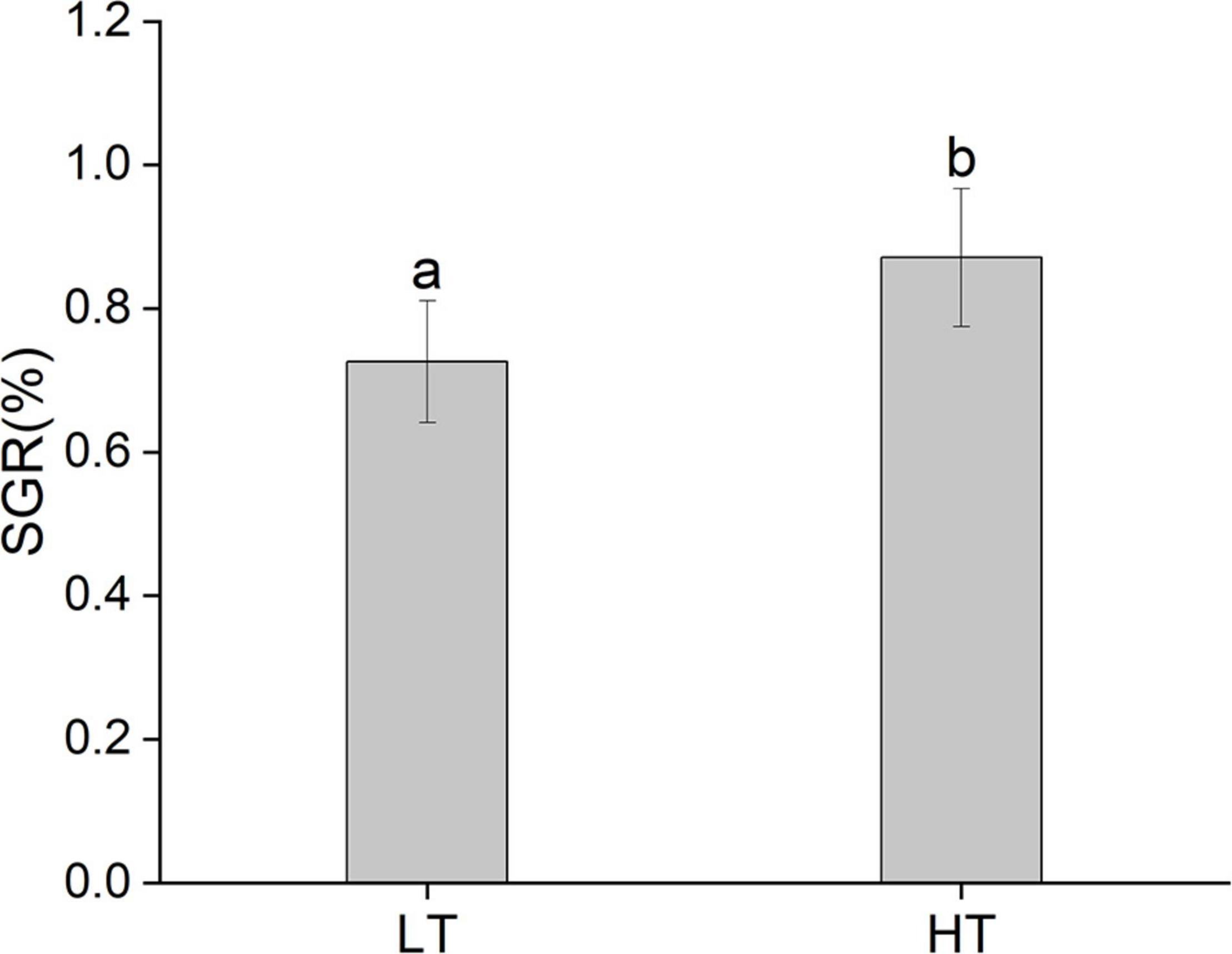
Figure 2. The SGR of juvenile Trachinotus ovatus (LT, low temperature group; HT, high temperature group).
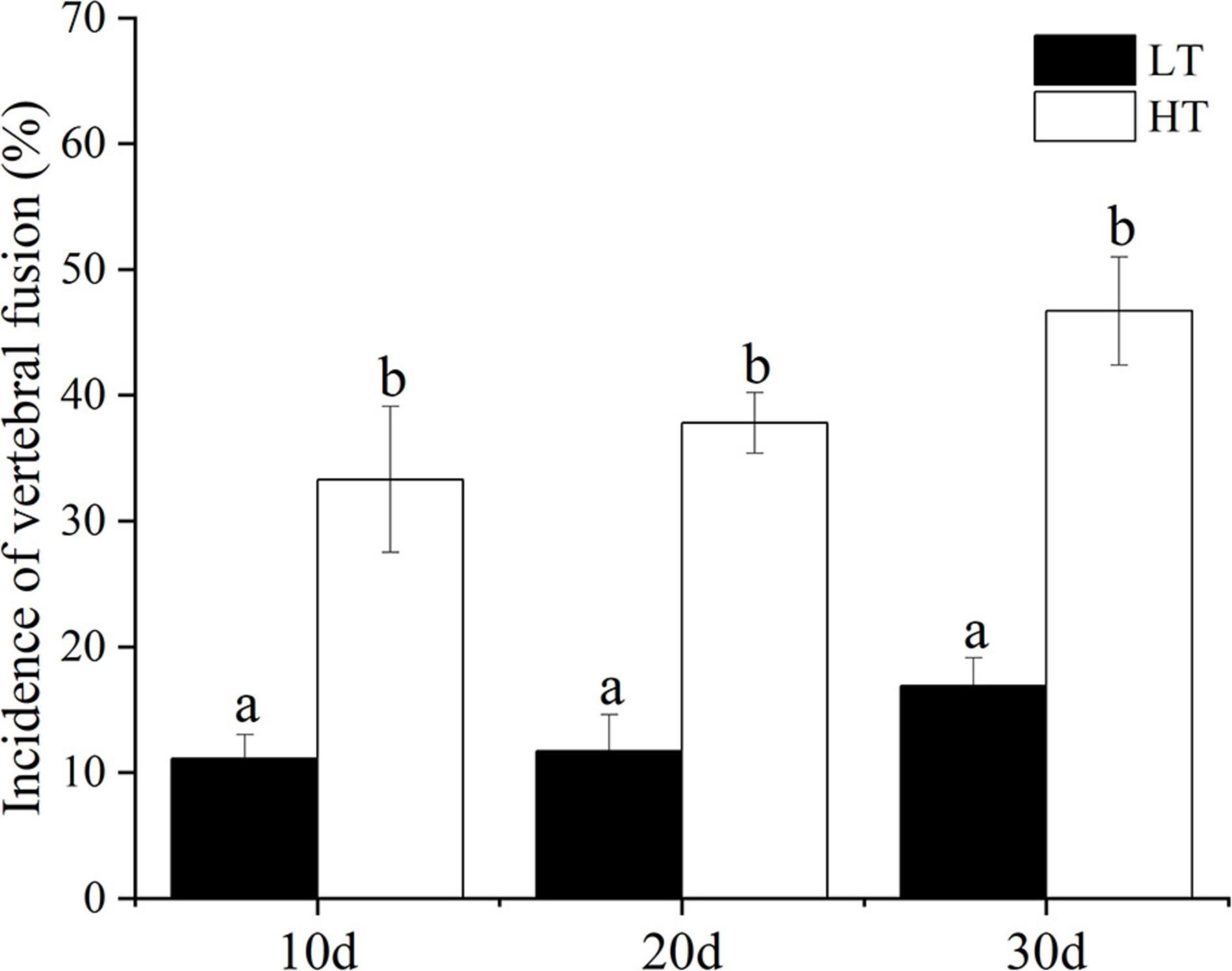
Figure 3. Incidence of vertebral fusion of juvenile T. ovatus (LT, low temperature group; HT, high temperature group).
Histopathology of the Vertebral Tissues
After 30 days of rearing, the vertebrae of the juvenile fish with normal phenotype in the LT group (Figures 4A,B) and the HT group (Figures 4C,D) were compared. We found that the osteogenic layer in the vertebral endplate growth zone in the HT group was thinner than that in the low temperature group (Figures 4B,D). Further, the thinner elastic externa of the vertebral body was observed in part of the samples of the HT group (Figure 4D).
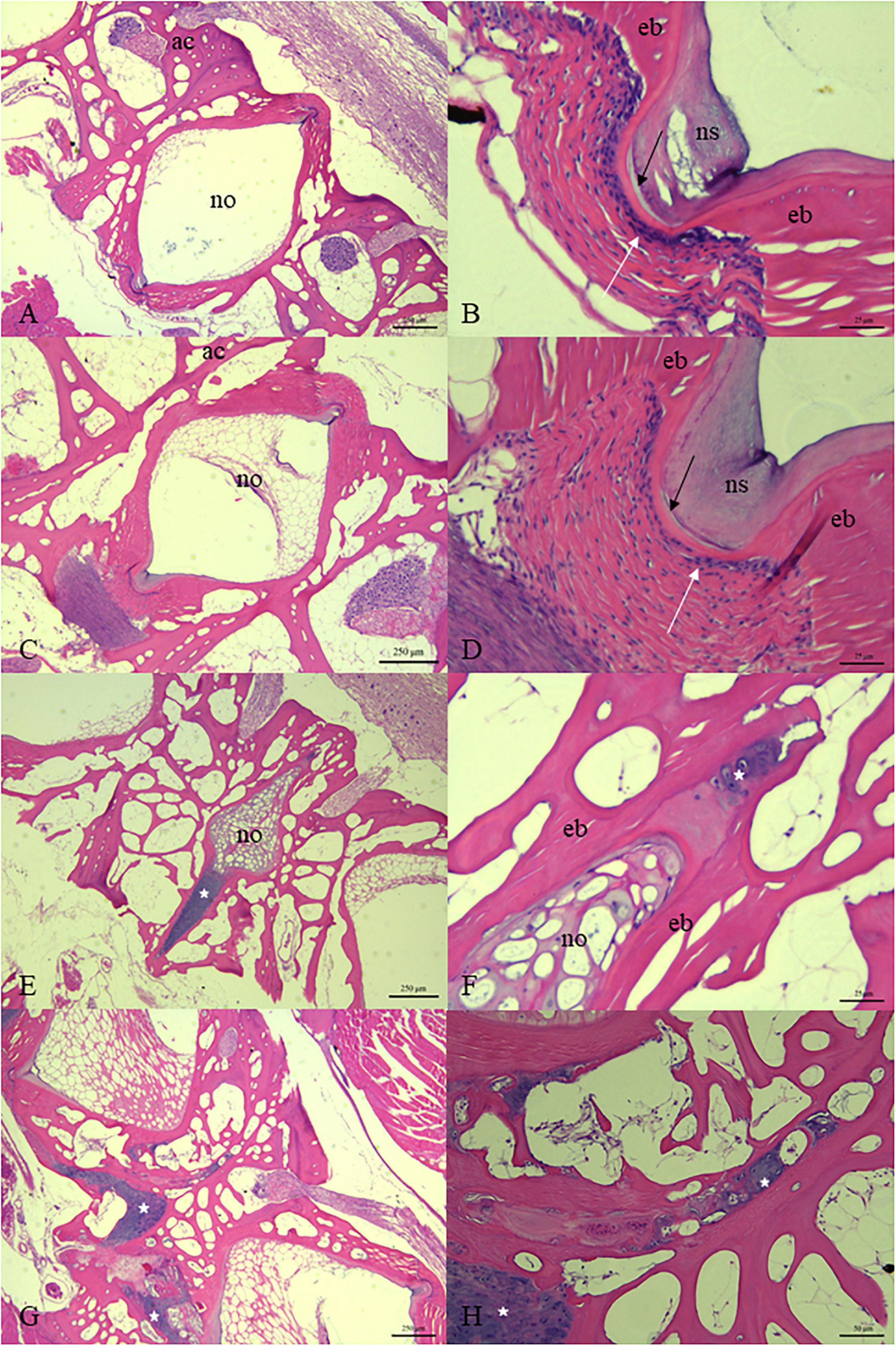
Figure 4. Histology of vertebral column of juvenile T. ovatus from LT group (A,B), HT group [(C–H) while (E–H) were samples of vertebrae with fusion]. The white arrow points to the osteogenic layer in the growth area of the vertebral endplate. The black arrow points to the elastic externa. White asterisk is located in the growth area of the vertebral endplate. ac, arch centra; no, notochord; ns, notochord sheath, ed, endbone. [(A,C,E,G) 50× magnification; (H) 200× magnification; (B,D,F) 400× magnification].
In the fused vertebral bodies, the intervertebral spaces between adjacent vertebral bodies gradually narrowed and then disappeared (Figures 4E–H). The notochordal sheath of the notochord basically disappeared. The basophilic staining of the ECM of the notochord was deepened (Figures 4E,F). Meanwhile, the area between the endplates of adjacent vertebral bodies was filled with a large number of chondrocytes. The proliferation of chondrocytes was observed in the center of the arch of some vertebral bodies (Figure 4G).
Genes Expression Levels in the Vertebral Tissues
The genes examined fall into three categories: signaling molecules, transcription factors and ECM components. The changes of transcriptional level of genes in the fish spine with normal phenotype in different temperature were compared. Among the ECM components gene, compared to the LT group, the level of Col1a1 in the HT group was significantly up-regulated on the 10th day, down-regulated on the 20th day, but no difference on the 30th day (Figure 5A). Col2a1 was significantly up-regulated on the 10th and 30th day, and down-regulated on the 20th day (Figure 5B). Decorin was significantly up-regulated at all three-time points (Figure 5C). Vimentin was up-regulated on 10th day and down-regulated on the 20th and 30th day (Figure 5D). MMP9 was significantly down-regulated at all three-time points (Figure 5E). MMP13 was significantly up-regulated on the 10th day, and down-regulated on the 20th and 30th day (Figure 5F). Ocn was significantly up-regulated on the 10th and 20th day, but there was no significant difference on the 30th day (Figure 5G). Among the signal molecules, BMP2 was significantly up-regulated on the 10th day, but there was no significant difference on the 20th and 30th days (Figure 6A). Both BMP4 and BMP5 were significantly up-regulated on the 10th and 20th day, and significantly down-regulated on the 30th day (Figures 6B,C). Shh was significantly up-regulated at three time points (Figure 6D). Ihh was significantly decreased on the 10th and 20th day, and there was no significant difference on the 30th day (Figure 6E). Among the transcription factors, Sox8 and Sox9 were significantly up-regulated at three time points, with the largest up-regulated extent on the 10th day (Figures 7A,B). Twist was significantly up-regulated on the 10th and 20th day, but there was no significant difference on the 30th day (Figure 7C). Runx2 was significantly up-regulated on the 10th and 20th day, and significantly down-regulated on the 30th day (Figure 7D).
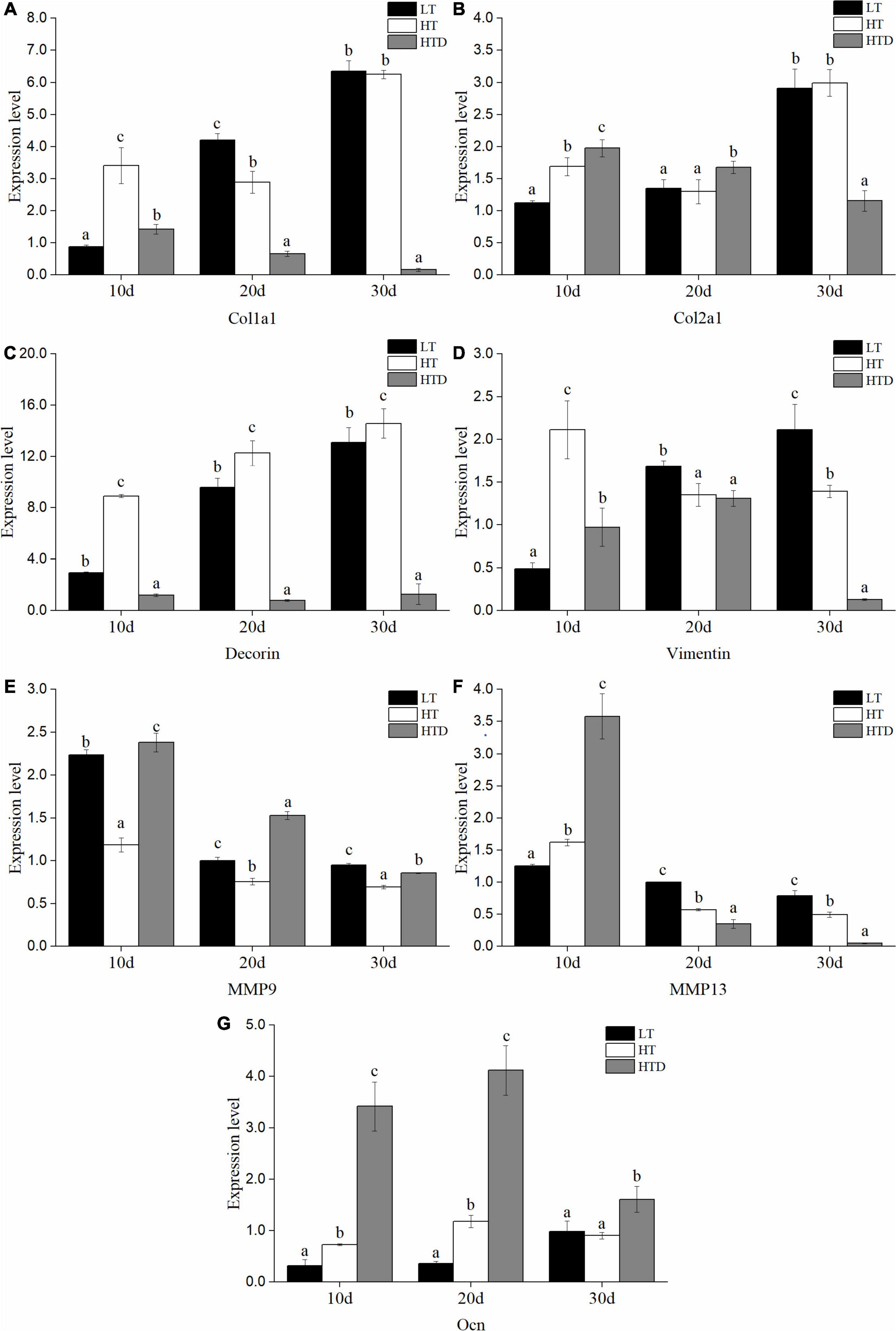
Figure 5. The expression levels of ECM components (A: Col1a1, B: Col2a1, C: Decorin, D: Vimentin, E: MMP9, F: MMP13, G: Ocn) in the spine of juvenile T. ovatus (LT, low temperature group; HT, high temperature group; HTD, high temperature deformity group).
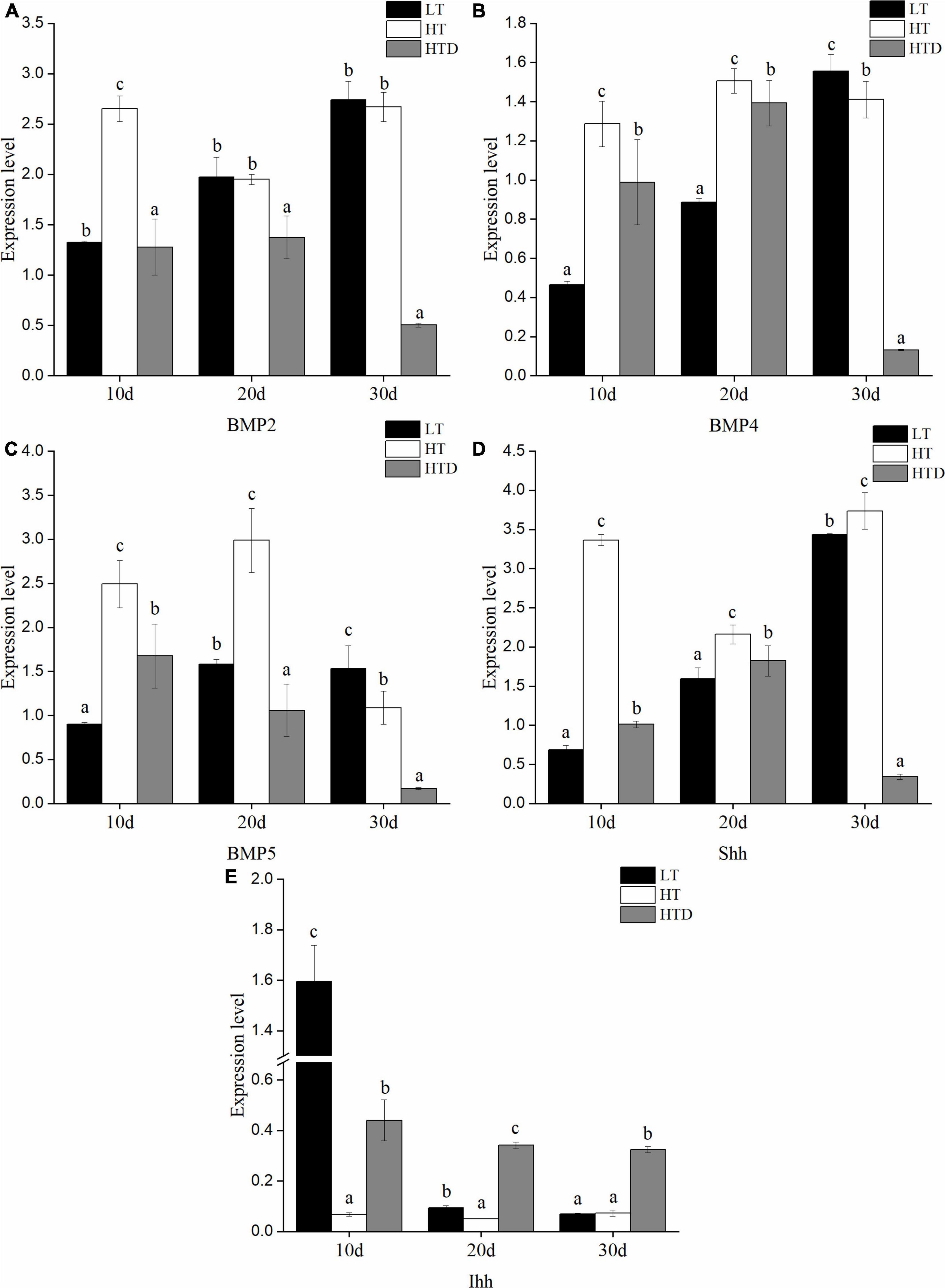
Figure 6. The expression levels of signaling molecules (A: BMP2, B: BMP4, C: BMP5, D: Shh, E: Ihh) in the spine of juvenile T. ovatus (LT, low temperature group; HT, high temperature group; HTD, high temperature deformity group).
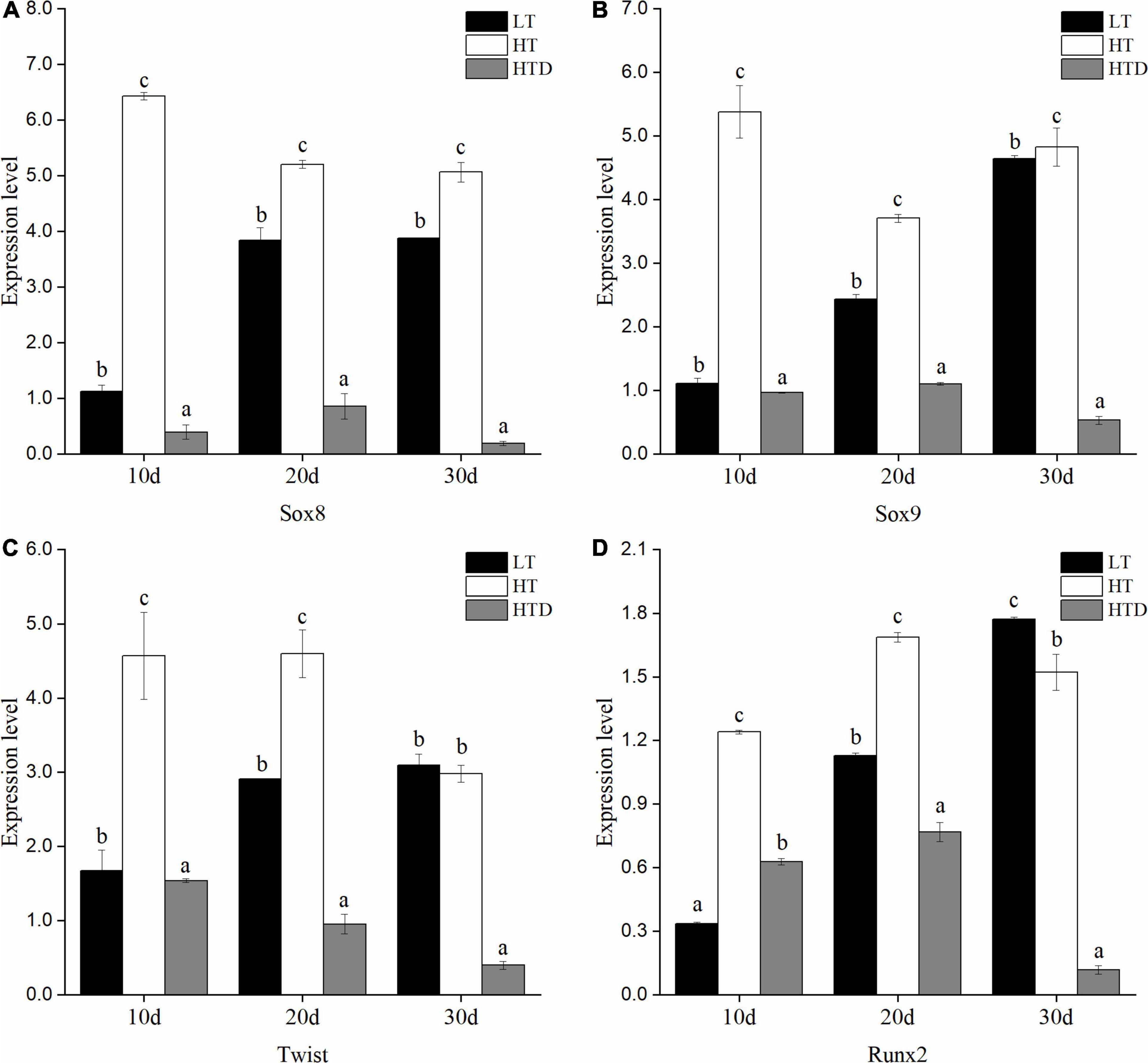
Figure 7. The expression levels of transcription factors (A: Sox8, B: Sox9, C: Twist, D: Runx2) in the spine of juvenile T. ovatus (LT, low temperature group; HT, high temperature group; HTD, high temperature deformity group).
The transcriptional levels of related genes in the spines of juvenile fish with the normal phenotypes and with vertebral fusion were compared in the HT group. The levels of Col1A1, decorin, BMP2, BMP4, BMP5, Shh, Sox8, Sox9, Twist, and Runx2 were significantly down-regulated at all three-time points in fish with vertebral fusion compared to fish with normal phenotypes. Col2a1 was significantly up-regulated on the 10th and 20th day, and significantly down-regulated on the 30th day (Figure 5B). Vimentin was significantly up-regulated on day 10 but significantly down-regulated on the 30th day (Figure 5D). MMP9 was significantly up-regulated on the 10th,20th and 30th day (Figure 5E). MMP13 was significantly up-regulated on the 10th day and down-regulated on day 20 and 30 (Figure 5F). Ocn was significantly up-regulated at all three time points (Figure 5G). Ihh was significantly up-regulated on the 10th, 20th, and 30th day (Figure 6E).
Discussion
Vertebral fusion is a type of spinal deformity with the highest incidence in T. ovatus rearing, and the relationship between the phenomenon and the water temperature is worthy of attention. Excessive water temperature has been proved to be an important cause of the occurrence of spinal deformity in T. ovatus larvae (Han et al., 2020). The results of this study showed that high water temperature could accelerate the growth of juvenile golden pompano, but it also led to the increase of vertebral fusion over time. However, the incidence of vertebral fusion showed an upward trend. However, Hainan Province is in the tropics, with a relatively high annual mean temperature. Sustained HT seems to be unavoidable, especially in summer. The aim of this study was to describe the effects of high rearing temperature on the occurrence of vertebrae fusion, as well as the histopathological and molecular pathological changes in the vertebrae of T. ovatus juveniles in the nursery phase.
Histopathological Analysis of a Vertebral Body of Juvenile T. ovatus
Histopathological analysis at the end of the experiment showed a thin osteogenic layer in the growth zone of the vertebral endplate in the normal vertebrae of the juvenile fish in the HT group. Some scholars believe that the narrow osteogenic layer in the vertebral endplate growth zone and the thickening of the fibrous layer may be a precursor of vertebral fusion (Fernandez et al., 2012). HT may have inhibited the osteogenic ability in the growth zone of vertebral endplate of juvenile T. ovatus. In addition, the elastic appearance of some normal vertebral bodies in the HT group has shown a tendency of becoming thinner in the present study. The intervertebral tissue of fish is mainly composed of a notochord, which provides flexibility for the vertebral body (Urban and Roberts, 2003). Notochord plays a role in regulating spinal development throughout the life of fish (Kaneko et al., 2016), and the integrity of the notochord is crucial for the development of the spine. In a study of Atlantic salmon, the elastic appearance became thinner during the fusion of the vertebral bodies (Ytteborg et al., 2010b). In mammals, the notochordal sheath is associated with nutrient transport (Corallo et al., 2015). Changes in the elastic appearance and notochord sheath may contribute to the development of vertebral fusion by leading to reduced notochord flexibility and possible nutrient transport (Ytteborg et al., 2010c). This also indicates that the change of the elastic membrane may be the precursor of fusion. HT may change the homeostasis of the intervertebral tissue, and the endplate growth zone and notochord tissue need to change to adapt to the rapid growth induced by HT.
The process of vertebral fusion has been described in Atlantic salmon. The osteoblasts in the growth area of the vertebral body are transformed into chondrocytes by trans-differentiation and then remolded into bone by chondrometaplasia. The chordal tissue becomes densified and eventually mineralized and remolded into bone (Witten et al., 2006; Ytteborg et al., 2012). In this study, the occurrence of vertebral fusion in juvenile golden pompano is consistent with the tissue changes in Atlantic salmon. This suggests that the pattern of vertebral fusion occurring in bony fish may be consistent. However, the disorder and proliferation of osteoblasts and choroidoblasts were not observed in this experiment, which may be related to the degree of fusion, so it is necessary to increase the number of samples for observation.
Effects of High Water Temperature on Genes Related to Chondrocyte Development
Our results showed that HT significantly altered the expression pattern of genes related to chondrogenesis. In the skeletal system, the ECMs provides rigidity, hardness and elasticity for bone or cartilage tissue, and the dynamic balance of ECMs affects the process of new bone formation and bone resorption (Bonnans et al., 2014; Alford et al., 2015; Lin et al., 2020). Col2a1 is one of the main components of the ECM of chondrocytes, mainly secreted by proliferating chondrocytes, so it is considered a marker of early chondrocyte differentiation (Castagnola et al., 1988; Ricard-Blum, 2011). Proper warming can stimulate the up-regulation of Col2a1 transcription level in cultured dedifferentiated chondrocytes in vitro and promote the redifferentiation of cartilage tissue (Ito et al., 2015). Studies in Atlantic salmon have shown that thermal stimulation during embryogenesis affects the expression of important developmental genes such as Col2a1, and this effect continues into subsequent development in fish (Clarkson et al., 2020). Ytteborg et al. (2010a) showed that Col2a1 transcription decreased in Atlantic salmon reared at HT. In this study, Col2a1 transcription in normal phenotypic vertebra of the HT group was significantly up-regulated on 10 days, indicating that chondrocyte proliferation is enhanced after a relatively short time of HT treatment. Vimentin plays an important role in the compression loading of chondrocytes and the regulation of cytoskeletal tension, which can maintain the homeostasis and hardness of chondrocytes (Blain et al., 2006; Haudenschild et al., 2011; Chen et al., 2016). HTs have been shown to enhance the upregulation of vitamin transcription in T. ovatus larvae (Han et al., 2020). In this experiment, the initial increase in vimentin indicates on 10 days that the vertebral body may face higher mechanical stress. The down-regulation of vimentin in the later period may indicate that long-term HT cultivation may lead to a decrease in the ability of the vertebral body to tolerate pressure, which may be a precursor to induce fusion. The levels of Shh, and Sox9 were significantly up-regulated in the normal phenotypic vertebra, while Ihh was significantly down-regulated. Sox9 can promote the early differentiation of chondrocytes and regulate the expression of Col2a1 in chondrocytes (Lefebvre, 2019). Shh and Ihh are upstream regulators of cartilage development (Kaneko et al., 2016). Shh induces early chondrogenic differentiation of spinal mesenchymal cells, which can stimulate the expression of col2a1 and sox9 (Warzecha et al., 2006). The up-regulation of these transcription factors and signaling molecule genes also enhanced proliferation and differentiation of cartilage. Ihh can inhibit the hypertrophy differentiation of chondrocytes (Ohba, 2020). On the 10th and 20th day, the down-regulation of Ihh of normal vertebral bodies in the HT group indicates that the hypertrophy and maturation of chondrocytes are also enhanced. The development and maturation of chondrocytes provide the condition for endochondral ossification. BMP2, BMP4, and BMP5 can induce the formation of ectopic bone and cartilage (Zhang et al., 2016; Kanjilal and Cottrell, 2019). Deficiency of BMP2 and BMP4 results in delayed endochondral ossification in mice (Bandyopadhyay et al., 2006). On 10 day, the expression of BMP2, BMP4, and BMP5 genes were all upregulated, also indicating that HT induced the development of vertebral cartilage. The studies of Ma et al. (2016a) also show that the increase of water temperature increased the expression of BMPs and resulted in an increase in the rate of jaw deformity in T. ovatus larvae. At the later stage of the experiment (30 days), the expressions of BMPs were relatively down-regulated in the HT group, which may be related to the adaptability of fish to water temperature. The development of cartilage may be affected not only by the water temperature of culture, but also by the time. Compared with the normal phenotypic vertebrae in the HT group, significantly up-regulation in col2a1 of fusion vertebrae was observed on the 10th and 20th day. Ihh was also significantly up-regulated in the fused vertebrae, which has been shown to promote chondrocyte proliferation (Ohba, 2020). As reported in Atlantic salmon, gradual replacement of the osteogenic layer in the growth zone of the notochord and vertebral endplate by chondrocytes is a key marker of fusion (Witten et al., 2006). In this study, the changes in the expression patterns of genes related to chondrogenesis in both the normal vertebral body and the previously fused vertebral body indicated the promoting effect of HT on the development of vertebral chondrogenesis.
The expression of MMP9 and MMP13 was inhibited in the HT group. Matrix metalloproteinases (MMPs) are the most important proteinases involved in ECM degradation and are essential for bone remodeling and endochondral ossification (Rajaram et al., 2016). MMP9 is the most abundant matrix metalloproteinase in osteoclasts, which can degrade denatured collagen and promote the apoptosis of hypertrophic chondrocytes (Vu et al., 1998; Zhang et al., 2017). MMP13 plays an important role in the remodeling of hypertrophic cartilage matrix (Kennedy et al., 2005). Studies in mice have shown that lack of MMP9 and MMP13 leads to inhibition of endochondral ossification (Stickens et al., 2004). The down-regulation of MMP9 and MMP13 indicates that the process of vertebral growth through endochondral ossification is inhibited under HT. The level of MMP9 in the fused vertebrae was significantly up-regulated. The relative expression level of MMP13 in the fused vertebrae on 10th day was also significantly higher than that in the normal vertebrae. Wargelius et al. (2010) showed that the up-regulation of MMP13 expression might be a signal of Atlantic salmon vertebral compression and increased remodeling of ECMs. Ytteborg et al. (2010a, b) demonstrated that MMP9 and MMP13 are significantly up-regulated in Atlantic salmon vertebrae under HT culture. This suggests that cartilage may also be remodeled into bone by means of endochondral ossification. Col2a1 and MMP13 in the fused vertebra were significantly down-regulated at the end of the experiment.
Effects of High Water Temperature on Genes Related to the Osteoblasts Development
At the early stage (10 days) of culture in the HT group, we also found that the transcriptional signals of normal vertebral osteoblasts were enhanced in the HT group. Col1a1 protein, mainly secreted by osteoblasts, is the most abundant organic component in bone matrix and plays an important role in bone strength and toughness (Mansour et al., 2017; Lin et al., 2020). The increased risk of fracture was associated with abnormalities of Col1a1 (Ammann and Rizzoli, 2003). As the most abundant non-collagenous protein in bone, Ocn is a marker of advanced osteoblasts and is associated with matrix mineralization (Ytteborg et al., 2010a; Tavakol and Vaughan, 2020). Runx2 is a major regulatory factor in osteoblastic development and is essential for osteoprogenitor cell proliferation and osteoblastic differentiation (Komori, 2020). The up-regulation of these genes indicates an accelerated differentiation and maturation of osteoblasts. Decorin is also secreted by osteoblasts, which can promote the formation of collagen fibers and act as an inhibitor of matrix mineralization (Mochida et al., 2009; Coulson-Thomas et al., 2015; Randilini et al., 2020). Sox8 is a negative regulator of osteoblast differentiation (Schmidt et al., 2005). As an osteogenic inhibitor, Twist can inhibit osteoblast differentiation by inhibiting Runx2 activity (Kronenberg, 2004). The vertebral body may attempt to maintain normal osteoblastic development through the up-regulation of Decorin and Sox8. However, the expression of Col1a1 in HT group was lower than that in LT group over time. On 30th day, Col1a1 and Ocn showed no significant difference between the two treatment groups. Compared with the normal phenotypic vertebrae in the HT group, the genes levels of early osteoblast development in the fusion vertebrae were also significantly down-regulated. The down-regulation of Twist did not lead to the up-regulation of Runx2. This is consistent with the transformation of osteoblasts into chondrocytes during fusion. However, the down-regulation of Decorin and the up-regulation of Ocn indicate the enhanced mineralization of the matrix. The changes of these genes confirmed the occurrence of transformation from the transcription level. Compared with the LT group, BMPs showed a downward trend in the HT group. These changes indicate that the differentiation and maturation of osteoblasts are also inhibited under high water temperature for a long time. This is consistent with histological observations.
It is worth noting that vertebral fusion can be performed by two pathways (the aggravation or the contained pathway), which have been demonstrated in Atlantic salmon and Chinook salmon (Witten et al., 2006; Perrott et al., 2018). Differences in the transcription patterns of osteogenic and cartilaginous genes in fused vertebrae at different times may also determine the progression of fusion and how much vertebrae are involved, but this needs to be further verified.
Conclusion
In summary, high rearing water temperature could promote the growth of juvenile T. ovatus but lead to an increase in the incidence of vertebral fusion. The homeostasis of the intervertebral tissue was altered by long-term HT cultivation. HT stress can up-regulate the transcription of genes related to bone and chondrocyte differentiation in the vertebrae of juvenile T. ovatus in a relatively short period of time. With the increase of stress time, transcription showed a downward trend. Genes associated with the development of chondrocytes and osteoblasts in the vertebrae were significantly regulated by rearing temperature and correlated with time. The results of this study improve our understanding of the effect of water temperature on bone development and the molecular reaction of vertebral fusion induced by high water temperature in bony fish.
Data Availability Statement
The raw data supporting the conclusions of this article will be made available by the authors, without undue reservation.
Ethics Statement
The animal study was reviewed and approved by Animal Care and Use Committee of South China Sea Fisheries Research Institute, Chinese Academy of Fishery Sciences.
Author Contributions
ZM conceptualized the study. MH was responsible for the experimental operation, prepared and wrote the original draft. ZF and RY were in charge of the field sampling. MH, ZF, and RY conducted the sample determination. ZM, ZF, and JQ reviewed, edited, and wrote the manuscript. All authors read and approved the final manuscript.
Funding
This study was funded by the Hainan Provincial Natural Science Foundation of China (2019CXTD418), Guangxi Innovation-Driven Development Projects (Guike AA18242031), and Central Public Interest Scientific Institution Basal Research Fund South China Sea Fisheries Research Institute CAFS (Nos. 2020TD55 and 2021SD09).
Conflict of Interest
The authors declare that the research was conducted in the absence of any commercial or financial relationships that could be construed as a potential conflict of interest.
References
Alford, A. I., Kozloff, K. M., and Hankenson, K. D. (2015). Extracellular matrix networks in bone remodeling. Int. J. Biochem. Cell Biol. 65, 20–31. doi: 10.1016/j.biocel.2015.05.008
Ammann, P., and Rizzoli, R. (2003). Bone strength and its determinants. Osteoporos. Int. 14, S13–S18.
Bandyopadhyay, A., Tsuji, K., Cox, K., Harfe, B. D., Rosen, V., and Tabin, C. J. (2006). Genetic analysis of the roles of BMP2, BMP4, and BMP7 in limb patterning and skeletogenesis. PLoS Genet. 2, 2116–2130.
Bensimon-Brito, A., Cardeira, J., Cancela, M. L., Huysseune, A., and Witten, P. E. (2012). Distinct patterns of notochord mineralization in zebrafish coincide with the localization of Osteocalcin isoform 1 during early vertebral centra formation. BMC Dev. Biol. 12:14.
Blain, E. J., Gilbert, S. J., Hayes, A. J., and Duance, V. C. (2006). Disassembly of the vimentin cytoskeleton disrupts articular cartilage chondrocyte homeostasis. Matrix Biol. 25, 398–408. doi: 10.1016/j.matbio.2006.06.002
Boglione, C., Gavaia, P., Koumoundouros, G., Gisbert, E., Moren, M., Fontagne, S., et al. (2013a). Skeletal anomalies in reared European fish larvae and juveniles. part 1: normal and anomalous skeletogenic processes. Rev. Aquac. 5, S99–S120.
Boglione, C., Gisbert, E., Gavaia, P., Witten, P. E., Moren, M., Fontagne, S., et al. (2013b). Skeletal anomalies in reared European fish larvae and juveniles. part 2: main typologies, occurrences and causative factors. Rev. Aquac. 5, S121–S167.
Bonnans, C., Chou, J., and Werb, Z. (2014). Remodelling the extracellular matrix in development and disease. Nat. Rev. Mol. Cell Biol. 15, 786–801. doi: 10.1038/nrm3904
Cardeira, J., Bensimon-Brito, A., Pousao-Ferreira, P., Cancela, M. L., and Gavaia, P. J. (2012). Lordotic-kyphotic vertebrae develop ectopic cartilage-like tissue in Senegalese sole (Solea senegalensis). J. Appl. Ichthyol. 28, 460–463. doi: 10.1111/j.1439-0426.2012.01978.x
Castagnola, P., Dozin, B., Moro, G., and Cancedda, R. (1988). Changes in the expression of collagen genes show two stages in chondrocyte differentiation in vitro. J. Cell Biol. 106, 461–467. doi: 10.1083/jcb.106.2.461
Chen, C., Yin, L., Song, X. B., Yang, H., Ren, X., Gong, X. Y., et al. (2016). Effects of vimentin disruption on the mechanoresponses of articular chondrocyte. Biochem. Biophys. Res. Commun. 469, 132–137. doi: 10.1016/j.bbrc.2015.11.083
Clarkson, M., Taylor, J. F., McStay, E., Palmer, M. J., Clokie, B. G. J., and Migaud, H. (2020). A temperature shift during embryogenesis impacts prevalence of deformity in diploid and triploid Atlantic salmon (Salmo salar L.). Aquac. Res. 52, 906–923. doi: 10.1111/are.14945
Corallo, D., Trapani, V., and Bonaldo, P. (2015). The notochord: structure and functions. Cell. Mol. Life Sci. 72, 2989–3008. doi: 10.1007/s00018-015-1897-z
Coulson-Thomas, Y. M., Coulson-Thomas, V. J., Norton, A. L., Gesteira, T. F., Cavalheiro, R. P., Meneghetti, M. C. Z., et al. (2015). The identification of proteoglycans and glycosaminoglycans in archaeological human bones and teeth. PLoS One 10:e0131105. doi: 10.1371/journal.pone.0131105
Davie, P. S., Walker, S. P., Perrott, M. R., Symonds, J. E., Preece, M., Lovett, B. A., et al. (2019). Vertebral fusions in farmed Chinook salmon (Oncorhynchus tshawytscha) in New Zealand. J. Fish Dis. 42, 965–974.
Fernandez, I., Ortiz-Delgado, J. B., Sarasquete, C., and Gisbert, E. (2012). Vitamin A effects on vertebral bone tissue homeostasis in gilthead sea bream (Sparus aurata) juveniles. J. Appl. Ichthyol. 28, 419–426. doi: 10.1111/j.1439-0426.2012.01997.x
Fjelldal, P. G., Hansen, T., Breck, O., Ornsrud, R., Lock, E. J., Waagbo, R., et al. (2012). Vertebral deformities in farmed Atlantic salmon (Salmo salar L.) - etiology and pathology. J. Appl. Ichthyol. 28, 433–440. doi: 10.1111/j.1439-0426.2012.01980.x
Fleming, A., Keynes, R., and Tannahill, D. (2004). A central role for the notochord in vertebral patterning. Development 131, 873–880. doi: 10.1242/dev.00952
Fleming, A., Kishida, M. G., Kimmel, C. B., and Keynes, R. J. (2015). Building the backbone: the development and evolution of vertebral patterning. Development 142, 1733–1744. doi: 10.1242/dev.118950
Galbusera, F., and Bassani, T. (2019). The Spine: a strong, stable, and flexible structure with biomimetics potential. Biomimetics 4:60. doi: 10.3390/biomimetics4030060
Han, M., Luo, M., Yang, R., Qin, J. G., and Ma, Z. (2020). Impact of temperature on survival and spinal development of golden pompano Trachinotus ovatus (Linnaeus 1758). Aquac. Rep. 18:100556. doi: 10.1016/j.aqrep.2020.100556
Hansen, T., Fjelldal, P. G., Yurtseva, A., and Berg, A. (2010). A possible relation between growth and number of deformed vertebrae in Atlantic salmon (Salmo salar L.). J. Appl. Ichthyol. 26, 355–359. doi: 10.1111/j.1439-0426.2010.01434.x
Haudenschild, D. R., Chen, J., Pang, N., Steklov, N., Grogan, S. P., Lotz, M. K., et al. (2011). Vimentin contributes to changes in chondrocyte stiffness in osteoarthritis. J. Orthop. Res. 29, 20–25. doi: 10.1002/jor.21198
Ito, A., Aoyama, T., Iijima, H., Tajino, J., Nagai, M., Yamaguchi, S., et al. (2015). Culture temperature affects redifferentiation and cartilaginous extracellular matrix formation in dedifferentiated human chondrocytes. J. Orthop. Res. 33, 633–639. doi: 10.1002/jor.22808
Kaneko, T., Freeha, K., Wu, X., Mogi, M., Uji, S., Yokoi, H., et al. (2016). Role of notochord cells and sclerotome-derived cells in vertebral column development in fugu, Takifugu rubripes: histological and gene expression analyses. Cell Tissue Res. 366, 37–49. doi: 10.1007/s00441-016-2404-z
Kanjilal, D., and Cottrell, J. A. (2019). Bone morphogenetic proteins (BMPs) and bone regeneration. Methods Mol. Biol. 1891, 235–245. doi: 10.1007/978-1-4939-8904-1_17
Kennedy, A. M., Inada, M., Krane, S. M., Christie, P. T., Harding, B., Lopez-Otin, C., et al. (2005). MMP13 mutation causes spondyloepimetaphyseal dysplasia, missouri type (SEMDMO). J. Clin. Investig. 115, 2832–2842. doi: 10.1172/jci22900
Kronenberg, H. M. (2004). Twist genes regulate Runx2 and bone formation. Dev. Cell 6, 317–318. doi: 10.1016/s1534-5807(04)00069-3
Lefebvre, V. (2019). “Roles and regulation of SOX transcription factors in skeletogenesis,” in Vertebrate Skeletal Development, ed. B. R. Olsen (San Diego: Elsevier), 171–193. doi: 10.1016/bs.ctdb.2019.01.007
Lin, X., Patil, S., Gao, Y. G., and Qian, A. R. (2020). The bone extracellular matrix in bone formation and regeneration. Front. Pharmacol. 11:15.
Lovett, B. A., Firth, E. C., Tuck, I. D., Symonds, J. E., Walker, S. P., Perrott, M. R., et al. (2020). Radiographic characterisation of spinal curvature development in farmed New Zealand Chinook salmon Oncorhynchus tshawytscha throughout seawater production. Sci. Rep. 10:20039.
Ma, Z., Hu, J., Yu, G., and Qin, J. G. (2017a). Gene expression of bone morphogenetic proteins and jaw malformation in golden pompano Trachinotus ovatus larvae in different feeding regimes. J. Appl. Anim. Res. 46, 164–177. doi: 10.1080/09712119.2017.1282371
Ma, Z. H., Fu, M. J., Hu, J., Yang, R., Wei, C. Y., Qin, J. G., et al. (2017b). Molecular cloning of Indian hedgehog gene and its expression in golden pompano Trachinotus ovatus (Linnaeus 1758) larvae at different water temperatures. Isr. J. Aquac. 69:13.
Ma, Z. H., Fu, M. J., Peng, X. Y., and Qin, J. G. (2017c). Expression of Sox5, Sox8 and Sox9 genes in golden pompano Trachinotus ovatus (Linnaeus 1758) larvae during ontogeny and in response to water temperature. Isr. J. Aquac. 69:9.
Ma, Z. H., Fu, M. J., Qin, J. G., Hu, J., Zhou, S. J., and Yang, R. (2018). Molecular cloning of twist gene and its expression in golden pompano Trachinotus ovatus (Linnaeus 1758) larvae at different water temperatures. Isr. J. Aquac. 70:11.
Ma, Z. H., Zheng, P. L., He, D., Jiang, S. G., and Qin, J. G. (2016b). Effect of feeding Artemia nauplii enriched with different enhancement products on larval performance of golden pompano Trachinotus ovatus (Linnaeus, 1758). Indian J. Fish. 63, 62–69.
Ma, Z., Zhang, N., Qin, J. G., Fu, M., and Jiang, S. (2016a). Water temperature induces jaw deformity and bone morphogenetic proteins (BMPs) gene expression in golden pompano Trachinotus ovatus larvae. Springerplus 5:1475.
Mansour, A., Mezour, M. A., Badran, Z., and Tamimi, F. (2017). Extracellular matrices for bone regeneration: a literature review. Tissue Eng. Part A 23, 1436–1451. doi: 10.1089/ten.tea.2017.0026
Mochida, Y., Parisuthiman, D., Pornprasertsuk-Damrongsri, S., Atsawasuwan, P., Sricholpech, M., Boskey, A. L., et al. (2009). Decorin modulates collagen matrix assembly and mineralization. Matrix Biol. 28, 44–52. doi: 10.1016/j.matbio.2008.11.003
Munday, J. S., Perrott, M. R., Symonds, J. E., Walker, S. P., Preece, M. A., and Davie, P. S. (2018). Prevalence of spinal abnormalities in Chinook salmon smolt and influence of early rearing temperature and growth rates. J. Fish Dis. 41, 1111–1116. doi: 10.1111/jfd.12804
Nordvik, K., Kryvi, H., Totland, G. K., and Grotmol, S. (2005). The salmon vertebral body develops through mineralization of two preformed tissues that are encompassed by two layers of bone. J. Anat. 206, 103–114. doi: 10.1111/j.1469-7580.2005.00372.x
Ofer, L., Dumont, M., Rack, A., Zaslansky, P., and Shahar, R. (2019). New insights into the process of osteogenesis of anosteocytic bone. Bone 125, 61–73. doi: 10.1016/j.bone.2019.05.013
Ohba, S. (2020). Hedgehog signaling in skeletal development: roles of Indian hedgehog and the mode of its action. Int. J. Mol. Sci. 21:6665. doi: 10.3390/ijms21186665
Pedersen, M. E., Takle, H., Ytteborg, E., Veiseth-Kent, E., Enersen, G., Faergestad, E., et al. (2011). Matrilin-1 expression is increased in the vertebral column of Atlantic salmon (Salmo salar L.) individuals displaying spinal fusions. Fish Physiol. Biochem. 37, 821–831. doi: 10.1007/s10695-011-9480-5
Perrott, M. R., Symonds, J. E., Walker, S. P., Hely, F. S., Wybourne, B., Preece, M. A., et al. (2018). Spinal curvatures and onset of vertebral deformities in farmed Chinook salmon, Oncorhynchus tshawytscha (Walbaum, 1792) in New Zealand. J. Appl. Ichthyol. 34, 501–511. doi: 10.1111/jai.13663
Rajaram, S., Murawala, H., Buch, P., Patel, S., and Balakrishnan, S. (2016). Inhibition of BMP signaling reduces MMP-2 and MMP-9 expression and obstructs wound healing in regenerating fin of teleost fish Poecilia latipinna. Fish Physiol. Biochem. 42, 787–794. doi: 10.1007/s10695-015-0175-1
Randilini, A., Fujikawa, K., and Shibata, S. (2020). An in situ hybridization study of decorin and biglycan mRNA in mouse osteoblasts in vivo. Anat. Sci. Int. 96, 265–272. doi: 10.1007/s12565-020-00588-2
Renn, J., Buettner, A., Thuy Thanh, T., Chan, S. J. H., and Winkler, C. (2013). A col10a1:nlGFP transgenic line displays putative osteoblast precursors at the medaka notochordal sheath prior to mineralization. Dev. Biol. 381, 134–143. doi: 10.1016/j.ydbio.2013.05.030
Scaal, M. (2016). Early development of the vertebral column. Semin. Cell Dev. Biol. 49, 83–91. doi: 10.1016/j.semcdb.2015.11.003
Schmidt, K., Schinke, T., Haberland, M., Priemel, M., Schilling, A. F., Mueldner, C., et al. (2005). The high mobility group transcription factor Sox8 is a negative regulator of osteoblast differentiation. J. Cell Biol. 168, 899–910. doi: 10.1083/jcb.200408013
Stickens, D., Behonick, D. J., Ortega, N., Heyer, B., Hartenstein, B., Yu, Y., et al. (2004). Altered endochondral bone development in matrix metalloproteinase 13-deficient mice. Development 131, 5883–5895. doi: 10.1242/dev.01461
Sullivan, M., Hammond, G., Roberts, R. J., and Manchester, N. J. (2007). Spinal deformation in commercially cultured Atlantic salmon, Salmo salar L.: a clinical and radiological study. J. Fish Dis. 30, 745–752. doi: 10.1111/j.1365-2761.2007.00889.x
Sun, J., Liu, G., Guo, H., Zhu, K., Guo, L., Liu, B., et al. (2020). Skeletal anomalies in cultured golden pompano Trachinotus ovatus at early stages of development. Dis. Aquat. Organ. 137, 195–204. doi: 10.3354/dao03436
Tavakol, M., and Vaughan, T. J. (2020). The structural role of osteocalcin in bone biomechanics and its alteration in Type-2 diabetes. Sci. Rep. 10:17321.
Urban, J. P. G., and Roberts, S. (2003). Degeneration of the intervertebral disc. Arthritis Res. Ther. 5, 120–130.
Vu, T. H., Shipley, J. M., Bergers, G., Berger, J. E., Helms, J. A., Hanahan, D., et al. (1998). MMP-9/gelatinase B is a key regulator of growth plate angiogenesis and apoptosis of hypertrophic chondrocytes. Cell 93, 411–422. doi: 10.1016/s0092-8674(00)81169-1
Wargelius, A., Fjelldal, P. G., Grini, A., Gil-Martens, L., Kvamme, B. O., and Hansen, T. (2010). MMP-13 (Matrix MetalloProteinase 13) expression might be an indicator for increased ECM remodeling and early signs of vertebral compression in farmed Atlantic salmon (Salmo salar L.). J. Appl. Ichthyol. 26, 366–371. doi: 10.1111/j.1439-0426.2010.01436.x
Warzecha, J., Goettig, S., Bruening, C., Lindhorst, E., Arabmothlagh, M., and Kurth, A. (2006). Sonic hedgehog protein promotes proliferation and chondrogenic differentiation of bone marrow-derived mesenchymal stem cells in vitro. J. Orthop. Sci. 11, 491–496. doi: 10.1007/s00776-006-1058-1
Witten, P. E., Gil-Martens, L., Huysseune, A., Takle, H., and Hjelde, K. (2009). Towards a classification and an understanding of developmental relationships of vertebral body malformations in Atlantic salmon (Salmo salar L.). Aquaculture 295, 6–14. doi: 10.1016/j.aquaculture.2009.06.037
Witten, P. E., Obach, A., Huysseune, A., and Baeverfjord, G. (2006). Vertebrae fusion in Atlantic salmon (Salmo salar): development, aggravation and pathways of containment. Aquaculture 258, 164–172. doi: 10.1016/j.aquaculture.2006.05.005
Wu, X. M., Chen, Q. R., Washio, Y., Yokoi, H., and Suzuki, T. (2016). Excess retinoic acid induces fusion of centra by degenerating intervertebral ligament cells in Japanese flounder, Paralichthys olivaceus. J. Exp. Zool. Part B Mol. Dev. Evol. 326, 464–473. doi: 10.1002/jez.b.22717
Yang, Q. B., Ma, Z. H., Zheng, P. L., Jiang, S. G., Qin, J. G., and Zhang, Q. (2016). Effect of temperature on growth, survival and occurrence of skeletal deformity in the golden pompano Trachinotus ovatus larvae. Indian J. Fish. 63, 74–82.
Ytteborg, E., Baeverfjord, G., Torgersen, J., Hjelde, K., and Takle, H. (2010a). Molecular pathology of vertebral deformities in hyperthermic Atlantic salmon (Salmo salar). BMC Physiol. 10:12. doi: 10.1186/1472-6793-10-12
Ytteborg, E., Torgersen, J., Baeverfjord, G., and Takle, H. (2010b). Morphological and molecular characterization of developing vertebral fusions using a teleost model. BMC Physiol. 10:13. doi: 10.1186/1472-6793-10-13
Ytteborg, E., Torgersen, J. S., Pedersen, M. E., Baeverfjord, G., Hannesson, K. O., and Takle, H. (2010c). Remodeling of the notochord during development of vertebral fusions in Atlantic salmon (Salmo salar). Cell Tissue Res. 342, 363–376. doi: 10.1007/s00441-010-1069-2
Ytteborg, E., Torgersen, J., Baeverfjord, G., and Takle, H. (2012). Four stages characterizing vertebral fusions in Atlantic salmon. J. Appl. Ichthyol. 28, 453–459. doi: 10.1111/j.1439-0426.2012.01984.x
Zhang, L., Ye, Y., Long, X., Xiao, P., Ren, X., and Yu, J. (2016). BMP signaling and its paradoxical effects in tumorigenesis and dissemination. Oncotarget 7, 78206–78218. doi: 10.18632/oncotarget.12151
Zhang, Y. H., Huang, H. G., Zhao, G. X., Yokoyama, T., Vega, H., Huang, Y., et al. (2017). ATP6V1H deficiency impairs bone development through activation of MMP9 and MMP13. PLoS Genet. 13:e1006481. doi: 10.1371/journal.pgen.1006481
Zheng, P. L., Ma, Z. H., Guo, H. Y., Zhang, D. C., Fu, M. J., Zhang, N., et al. (2016). Osteological ontogeny and malformations in larval and juvenile golden pompano Trachinotus ovatus (Linnaeus 1758). Aquac. Res. 47, 1421–1431. doi: 10.1111/are.12600
Keywords: high water temperature, histopathologic, molecular characterization, gene expression, vertebral fusion
Citation: Han M, Fu Z, Yang R, Qin JG and Ma Z (2021) Temperature Significantly Regulates Gene Expressions of Vertebrae Chondrocytes and Osteoblasts in Juvenile Golden Pompano (Trachinotus ovatus). Front. Mar. Sci. 8:668522. doi: 10.3389/fmars.2021.668522
Received: 16 February 2021; Accepted: 11 May 2021;
Published: 04 June 2021.
Edited by:
Lingling Wang, Dalian Ocean University, ChinaReviewed by:
Hongyu Ma, Shantou University, ChinaShina Wei, South China Agricultural University, China
Copyright © 2021 Han, Fu, Yang, Qin and Ma. This is an open-access article distributed under the terms of the Creative Commons Attribution License (CC BY). The use, distribution or reproduction in other forums is permitted, provided the original author(s) and the copyright owner(s) are credited and that the original publication in this journal is cited, in accordance with accepted academic practice. No use, distribution or reproduction is permitted which does not comply with these terms.
*Correspondence: Zhenhua Ma, zhenhua.ma@hotmail.com
†These authors have contributed equally to this work