- CSIR–National Institute of Oceanography, Goa, India
Marginal reefs are known for severe stress-inducible perturbations such as high sedimentation, eutrophication, ocean warming, and acidification from anthropogenic climate change. The corals striving in such stressful environments develop physiological adaptations induced by differential genomic expressions or association with thermal stress-tolerant algal symbionts (Symbiodiniaceae). Despite such adaptations, corals are threatened by other space competitors such as algae and sponges. Coral-eroding sponges belonging to the Cliona viridis complex are one such space competitors that also associate with Symbiodiniaceae algal photosymbiont. The diversity of Symbiodiniaceae associates with the coral and sponge from the same ecosystems is scarcely known. In the present study, Symbiodiniaceae community structure in the coral Turbinaria mesenterina, a newly described coral-eroding sponge Cliona thomasi, and their surrounding seawater was determined from the nearshore marginal reef along the central west coast of India. The results revealed a significantly higher relative abundance of Durusdinium and Gerakladium than Symbiodinium and Cladocopium in the seawater. Interestingly, both investigated host species showed differential Symbiodiniaceae association with significantly higher abundance of Durusdinium in coral and Gerakladium in sponge. The beta diversity analysis by Permutational multivariate analysis of variance (PERMANOVA) confirmed significant differences in Symbiodiniaceae profiles between sponge and coral. Durusdinium and Gerakladium are thermotolerant genera known to associate with different hosts in suboptimal conditions. Our field surveys suggested the bleaching resistance of the coral T. mesenterina despite the fact that the sea surface temperature reached the coral thermal threshold of 31°C during different periods of the years 2015, 2016, 2017, 2018, and 2019. Therefore, the thermal tolerance of the investigated coral and sponge species may be attributed to their respective thermotolerant photosymbiont associations. Furthermore, the results also indicated the host-specific photosymbiont selection from the local environment. Although these observations provide valuable biological insight, more research is needed to understand the tripartite association of sponge-coral-symbiont together to evaluate the competitive fitness of holobionts.
Introduction
Coral reefs harbor the most dynamic and complex plethora of microbial communities, including bacteria, fungi, viruses, archaea, and dinoflagellates (Mies et al., 2017; Osman et al., 2020; Pernice et al., 2020). Endosymbiotic dinoflagellates of the Symbiodiniaceae family have gained considerable attention as an indicator for the well-being of the coral reef health and ecosystem functioning (Silverstein et al., 2012; Osman et al., 2020). Symbiodiniaceae are present in the coral’s ambient environment (seawater and sediment) as free-living form as well as in association with different host organisms like sponges, foraminifera, clams, etc. (Takabayashi et al., 2012; Granados-Cifuentes et al., 2015). Free-living Symbiodiniaceae are crucial pool of photosymbiont for host organisms, as they acquired new symbiont from the ambient environment at different life stages (Granados-Cifuentes et al., 2015; Nitschke et al., 2016). Endosymbionts of reef-building corals contribute to most of the energy requirement of coral through photosynthesis, enabling calcium carbonate accretion and growth (Al-Horani, 2016). In return, coral supports Symbiodiniaceae by providing a protective environment and necessary components required for photosynthesis (Williams and Patterson, 2020). The dynamics in coral-Symbiodiniaceae interaction influences the ability of corals to function under different environmental conditions (Jones et al., 2008; Ziegler et al., 2017). The association of coral with different Symbiodiniaceae is regulated by local environmental conditions (Thornhill et al., 2017; Baker et al., 2018). So far, obligatory symbiosis of corals with different Symbiodiniaceae genera has been documented from a broad geographical and environmental regimes, that include Symbiodinium, Breviolum, Cladocopium, Durusdinium and, occasionally Fugacium and Gerakladium (formerly clades A, B, C, D, F, and G, respectively) (LaJeunesse et al., 2018; Osman et al., 2020). Each symbiont genus provides a different degree of support to the host coral (Stat et al., 2008; Camp et al., 2020; Pernice et al., 2020). For instance, Symbiodiniaceae diversity in Leptoria phrygia, experiencing thermal instability showed an exclusive association with Durusdinium for coping with thermal stress (Carballo-Bolaños et al., 2019). Furthermore, D’Angelo et al. (2015) confirmed the specific association of different Porites spp. with Cladocopium (C3) type symbiont in the warm and hypersaline waters of the Persian/Arabian Gulf.
Recent studies have confirmed that some corals (e.g., Montastraea cavernosa, Pocillopora damicornis, Stylophora pistillata, and Porites lutea) can adapt, sustain, and survive against local environmental stressors by switching or shuffling their associated photosymbionts (Silverstein et al., 2015; Boulotte et al., 2016; Osman et al., 2018; Tan et al., 2020). Response to environmental stressors varies among coral species and endosymbiont communities (van Woesik et al., 2011; Cunning et al., 2017). The local environment exerts selection pressure which eliminates the sensitive Symbiodiniaceae taxa and results in the dominance of stress-resilient species (Cunning et al., 2015; Claar et al., 2020). This differential abundance of specific thermotolerant Symbiodiniaceae taxa affects the functional characteristics of their host (LaJeunesse et al., 2010a; Carballo-Bolaños et al., 2019), eventually leading to the emergence of a particular “space winner” genotype (LaJeunesse et al., 2010a; Stat and Gates, 2011).
Globally, coral reefs are under threat due to climate change induced thermal stress and anthropogenic impacts, which disrupt delicate coral-Symbiodiniaceae interaction, causing mass coral bleaching, mortality, and rapid health deterioration of reef ecosystems (Hughes et al., 2017, 2018). Moreover, increasing disturbance may contribute to the overgrowth of benthic space competitors like cyanobacterial mats, macroalgae, and sponges, which pose additional threats to stressed reef-building corals (Horwitz et al., 2017). Among these benthic space competitors, bioeroding sponges are one of the most aggressive competitors that are known to erode reef-building corals and destruct the reef (Schönberg, 2000; Zundelevich et al., 2007; Fang et al., 2018b). For instance, species belonging to Cliona viridis species complex accounts for significant erosion of corals at the rate of >10 kg/m sponge area/year (Schönberg, 2000; Achlatis et al., 2019). The cosmopolitan sponge genus Cliona consists of 86 species (Van Soest et al., 2020), and among those, only about 25% of species are known to harbor symbiotic dinoflagellate communities (Achlatis, 2018; Mote et al., 2019). Studies have shown that algal symbionts of Cliona species provide their host with energetic inputs needed for excavating calcium carbonate through investment in photosynthates and/or photosynthesis by-products (Marlow et al., 2018; Achlatis et al., 2019). These symbionts occur in high densities in Cliona viridis species complex and display remarkably rapid lateral expansion and high bioerosion rates (Schönberg et al., 2008; Hill et al., 2011). Therefore, Symbiodiniaceae family members support their bioeroding host in space competition as well as in coping with the fluctuating environmental conditions (Fang et al., 2017, 2018b).
A few studies have shown that some sponges are associated with algal symbionts from Symbiodinium, Breviolum, and Gerakladium genera (formerly clades A, B, and G) (Hill et al., 2011; Ramsby et al., 2017). Moreover, these Symbiodiniaceae diversity analyses were mainly based on specific and/or multiple gene amplification followed by sequencing (Hill et al., 2011; Ramsby et al., 2017). However, the application of the next-generation sequencing (NGS) approach to dissect the taxonomic diversity of Symbiodiniaceae family associated with sponges is sparsely studied compared with other invertebrates (Riesgo et al., 2014; Ramsby et al., 2018). In order to gain insights into the role of Symbiodiniaceae members in the reef ecosystem, it is essential to study the multifaceted association between coral-symbiont, sponge-symbiont, and sponge-coral.
Determination of the composition and variability of symbiont community in reef-building corals and space-competing sponges is crucial to get an insight into how the symbiont compositional change influences the host’s physiology and competitive abilities in response to suboptimal environmental conditions. Although previous studies significantly contributed to our understanding of coral-symbiont diversity and the environmental-induced plasticity, the understanding of symbiosis between bioeroding sponges and Symbiodiniaceae is still sparse. Therefore, the present study employed a deep sequencing approach to elucidate the Symbiodiniaceae diversity associated with the coral Turbinaria mesenterina and the bioeroding sponge Cliona thomasi, both competing in a marginal coral reef environment on the west coast of India. Shallow water nearshore coral reefs in this region are subjected to a broad range of chronic and acute stressors, which are considered suboptimal for coral reef development, including thermal stress-driven recurrent coral bleaching episodes (De et al., 2015, 2021; Hussain et al., 2016), coral diseases (Hussain et al., 2016), monsoonal freshwater influx, and anthropogenic perturbations like, siltation, turbidity, nutrient input, fishing, and intensive tourism-mediated damage (De et al., 2020). C. thomasi investigated herein is a newly discovered ecologically important sponge species, abundant in the Indian Ocean coral reefs (Mote et al., 2019). Therefore, aggressive growth of this species on live corals raises serious concern about reefs health (Mote et al., 2019).
Materials and Methods
Study Site and Field Surveys
The study was conducted at the nearshore shallow water patch coral reef in the Grande Island archipelago, Central West Coast of India (15° 21′ 14.2″ N, 73° 45′ 57.8″ E). This nearshore patch coral reef on the Eastern Arabian Sea is highly disturbed by a wide range of stressors due to ongoing frequent thermal stress, low pH, monsoonal dilution of coastal seawater, higher sedimentation, and turbidity as two major rivers (Zuari and Mandovi rivers) discharge large amount of terrigenous sediment runoff and organic matter enrichment (Purnachandra Rao et al., 2011; Shynu et al., 2015; Veerasingam et al., 2015; Manikandan et al., 2016; Hussain and Ingole, 2020). The coral community in this reef habitat is mainly dominated by stress-resilient genera like Porites, Siderastrea, Pseudosiderastrea, Coscinarea, Turbinaria, and Goniopora; notably, sensitive branching taxa like Acropora are absent in this region (Hussain and Ingole, 2020). In recent years, corals in this nearshore habitat experienced an adverse impact from mass coral bleaching event (∼50%) during 2014–2017 (Hussain and Ingole, 2020), along with overgrowth of coral-eroding Cliona sponges (Manikandan et al., 2016; Mote et al., 2019), algal turf, macroalgae (Manikandan et al., 2016), coral diseases like white plaque and trematodiasis (Manikandan et al., 2016), and bioinvasion of alien octocoral Carijoa riisei (Patro et al., 2015). The study area is under continuous monitoring for the coral reef health assessment program since 2015. The field surveys showed a massive bleaching of corals in 2015. These bleaching events were continuously observed under subsequent field surveys. Furthermore, an increase in the sponge prevalence was recorded during 2015–2017. The details of survey times, methodology applied to monitor and the prevalence of coral-eroding sponges, and coral bleaching from the study site are given in Supplementary Information.
Sample Collection
The samples of the bioeroding sponge C. thomasi (n = 4) growing over the coral (T. mesenterina) colonies and healthy coral species T. mesenterina (n = 4) were collected in January 2019 from the depth of 6–8m. Massive growth forms of sponge species C. thomasi over different individual coral species are shown in Figures 1a–d. Utmost care was taken while collecting the samples, wherein each sample was distinct and separated from the other by at least 5 m. The coral and sponge tissue samples of ∼1–2 cm2 in diameter and 0.5–1 cm in thickness were collected using precleaned hammers and chisels. To compare the community with the ambient environment, surrounding seawater samples (n = 4) were collected from a distance of 2–4 m away from sponge-coral colonies. Samples were immediately brought on board, fixed in liquid nitrogen, and transported to the laboratory for further processing. The sponge species was identified by spicule morphology and molecular analysis following the established protocol (Mote et al., 2019).
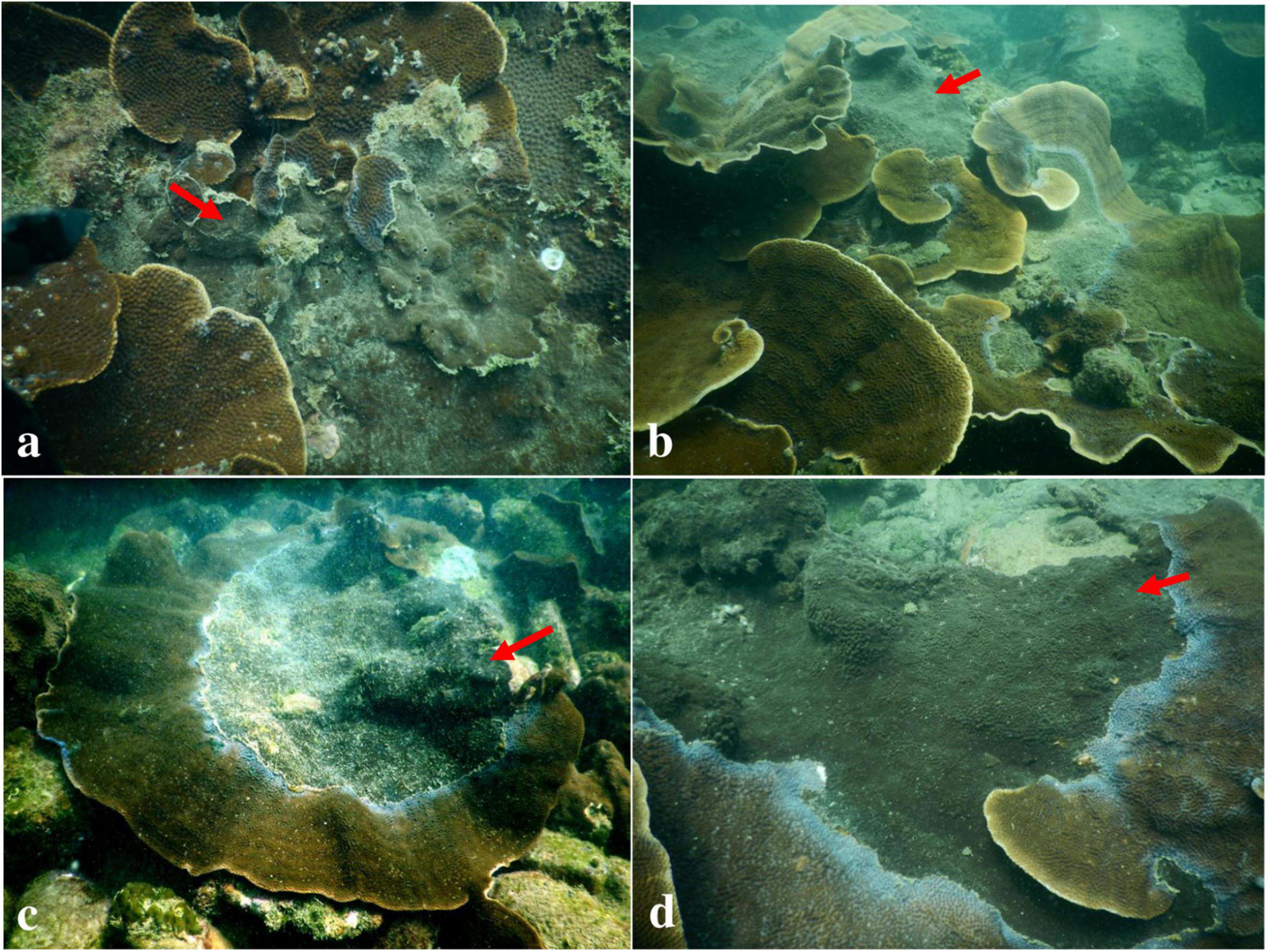
Figure 1. Massive growth forms of Cliona thomasi observed over different live coral colonies of Turbinaria mesenterina (a–d) from the studied site Grande Island.
DNA Extraction and Sequencing
The total DNA from each collected sample was extracted using the DNeasy Plant Mini Kit (Qiagen, Hilden, Germany) according to the manufacturer’s protocol. About 500 ml of seawater for each sample was filtered using 0.22 μm polycarbonate membrane filter (Whatman), and the filter paper was used for DNA isolation. For the identification of the Symbiodiniaceae community, the internal transcribed spacer 2 (ITS2) region was selected for amplification and sequencing using the primer set as forward 5′-GTGAATTGCAGAACTCCGTG-3′ and reverse 5′-CCTCCGCTTACTTATATGCTT-3′ (Boulotte et al., 2016). PCR amplifications were carried out in a 50-μl reaction volume containing ∼50 ng of DNA, 25 μl of 2×Taq Platinum PCR Master, 200nM of each primer, and ddH2O to make up the final volume. Reactions were performed at 95°C for 5 min, followed by 35 cycles of 95°C for 30 s, 51°C for 30 s, and 72°C for 30 s, and a final extension at 72°C for 4 min using a Mastercycler (Applied Biosystems, Foster City, United States). Illumina MiSeq libraries for the ITS2 region were generated and validated using 2100 Bioanalyzer (Agilent Technologies) for quality, and samples were sequenced using the 2 × 250 paired−end chemistry according to the manufacturer’s specification (HiSeq Reagent Kit). Sequencing was done on the MiSeq Illumina platform and produced 4,525,076 paired-end raw reads with an average read length of ∼350 to ∼420 bp.
Bioinformatic Analysis
Sequence reads generated through the Illumina Miseq platform were demultiplexed, and primers were trimmed using Cutadapt (Martin, 2011). DADA2 pipeline recommended by Callahan et al. (2016) for ITS analysis based amplicon sequence variant (ASVs) generation was followed. The determined ITS2 ASVs were assigned a taxonomic identity using the RDP classifier (Wang et al., 2007) against reference database sequences. This study employed three different databases for taxonomic classification of ASVs: (i) Db1 (Claar et al., 2020), (ii) Db2 (Shi et al., 2021), and (iii) Db31. To aid in the removal of non−Symbiodiniaceae sequences (e.g., non-target dinoflagellates), default RDP bootstrap value of 50 was used. The rank was set “unassigned” below this threshold, and these were hence removed from further analysis. The defined Symbiodiniaceae ASV table was utilized for downstream analysis, and abundance variations of Symbiodiniaceae genera and subtypes were analyzed.
The intragenomic variations (IGVs) among ITS2 regions challenged the Symbiodiniaceae diversity estimation. It is therefore important to determine the IGV profiles. The IGV profile for the Symbiodiniaceae diversity from the investigated samples was determined by SymPortal (Hume et al., 2019). For the SymPortal analysis, all sets of sequences were remotely submitted to SymPortal.org. SymPortal identify the specific sets of defining intragenomic variants (DIVs) that are used to characterize ITS2-type profiles (Camp et al., 2020; Hume et al., 2020).
Statistical Analysis
The vegan package v.2.5-6 in R (Oksanen et al., 2018) was used to calculate the alpha-diversity indices such as ASV richness, Shannon, Chao, and Simpson indices and to prepare a rarefaction curve for the investigated samples. The significant differences among the diversity indices values were determined by the Kruskal-Wallis test (with Dunn’s post hoc test). Furthermore, the beta-diversity measures to test the statistical difference of Symbiodiniaceae community composition among the samples were determined by applying permutational multivariate analysis of variance (PERMANOVA) with 999 permutations using Bray-Curtis dissimilarity matrix in PRIMER v7 (Clarke and Gorley, 2015). Symbiodiniaceae community composition was ordinated using non-metric multidimensional scaling (nMDS), as implemented in vegan package in R using metaMDS function (Oksanen et al., 2018). Similarity percentage (SIMPER) analysis was performed to calculate the contribution of each ITS2 subtype to the dissimilarity within and between samples in the PRIMER v7. Analysis of similarity (ANOSIM) was carried out using 999 permutations with a Bray-Curtis distance in vegan package R using Anosim function to test if there is a statistical difference between the Symbiodiniaceae communities of samples.
Results
Diversity and Composition of Symbiodiniaceae Based on ITS2 Sequence Analysis
Deep sequencing analysis of ITS2 region recovered a total of 4,534,038 raw sequence reads from three compartment of the coral reef habitat, i.e., reef-building coral, bioeroding sponge, and seawater (Supplementary File 1 and Supplementary Table 1). The raw amplicon sequencing dataset is available at the NCBI sequence read archive (SRA) database (accession PRJNA636097). After processing through DADA2 pipeline, the filtered sequence reads (3, 647, 450) were assigned to 1,008 unique ASVs (Supplementary File 1 and Supplementary Table 1). The taxonomic classification of these ASVs showed high degree of variations when assigned against different Symbiodiniaceae databases investigated in this study. The ASVs with more than 50% confidence for their taxonomic assignments were 613, 594, 253 for the databases Db1, Db2, and Db3, respectively. The variations obtained at the genera level for the ASVs from different databases are shown in Supplementary File 2 (Supplementary Table 1). The databases Db1 and Db2 showed similar results of taxonomic distribution and determined six Symbiodiniaceae genera (Durusdinium, Gerakladium, Symbiodinium, Cladocopium, Breviolum, and Fugacium) (Supplementary File 2 and Supplementary Table 1). However, Db3 showed the lowest ASVs (253), which were mainly assigned only to Durusdinium, Gerakladium, and Symbiodinium (Supplementary File 2 and Supplementary Table 1). At ITS2-subtype level, Db1 and Db2 identified more Symbiodiniaceae diversity than Db3 (Supplementary File 2 and Supplementary Figures 1–3). Based on the higher diversity determination, we followed the Db1 database sequences to represent Symbiodiniaceae diversity in our samples.
The assigned 613 Symbiodiniaceae ASVs further distributed as 174 for coral, 180 for sponge, and 464 in seawater. The overlap of these ASVs between coral, sponge, and seawater is shown in Figure 2. The distribution of these ASVs among the samples with sequencing reads was investigated with a rarefaction curve (Supplementary File 1 and Supplementary Figure 1). Shannon’s diversity index determined for the studied samples was in the order of seawater (3.24 ± 0.29) > T. mesenterina (2.46 ± 0.23) > C. thomasi (2.03 ± 0.40) (Supplementary File 1 and Supplementary Table 2). A similar, trend was observed in the Simpsons index as seawater (0.92 ± 0.02) > T. mesenterina (0.85 ± 0.03) > C. thomasi (0.78 ± 0.06) (Supplementary File 1 and Supplementary Table 2). The alpha-diversity indices determined were found significantly different only between coral and seawater based on the Kruskal-Wallis test, followed by Dunn’s post hoc test (p < 0.05). The beta-diversity measures for the investigated samples showed significant differences among the composition of the Symbiodiniaceae community associated with the coral species T. mesenterina and the sponge species C. thomasi (PERMANOVA, p = 0.04; ANOSIM, R = 0.854, p = 0.04, see Supplementary Tables 3, 4). The Symbiodiniaceae communities between sponge-seawater and coral-seawater were also found to be significantly different (PERMANOVA and ANOSIM, p < 0.05, Supplementary File 1 and Supplementary Tables 3, 4). The significant differences in beta-diversity between investigated coral and sponge species are mainly contributed by Symbiodiniaceae genus Durusdinium and Gerakladium. The results from the SIMPER analysis further confirmed higher dissimilarity in the Symbiodiniaceae community association between T. mesenterina and C. thomasi (average dissimilarity 58.3%) than T. mesenterina-seawater (average dissimilarity 42.4%) and C. thomasi-seawater (average dissimilarity 40%) (Supplementary File 1 and Supplementary Table 5). The differential Symbiodiniaceae association was further confirmed by the clustering pattern obtained from nMDS analysis. The investigated host types were clustered distinctly and separately based on their associated Symbiodiniaceae community (Figure 3). The observed distinct variation among the Symbiodiniaceae species assemblage in bioeroding sponge and coral indicates the symbiont selectivity among two different hosts within the same reef habitat.
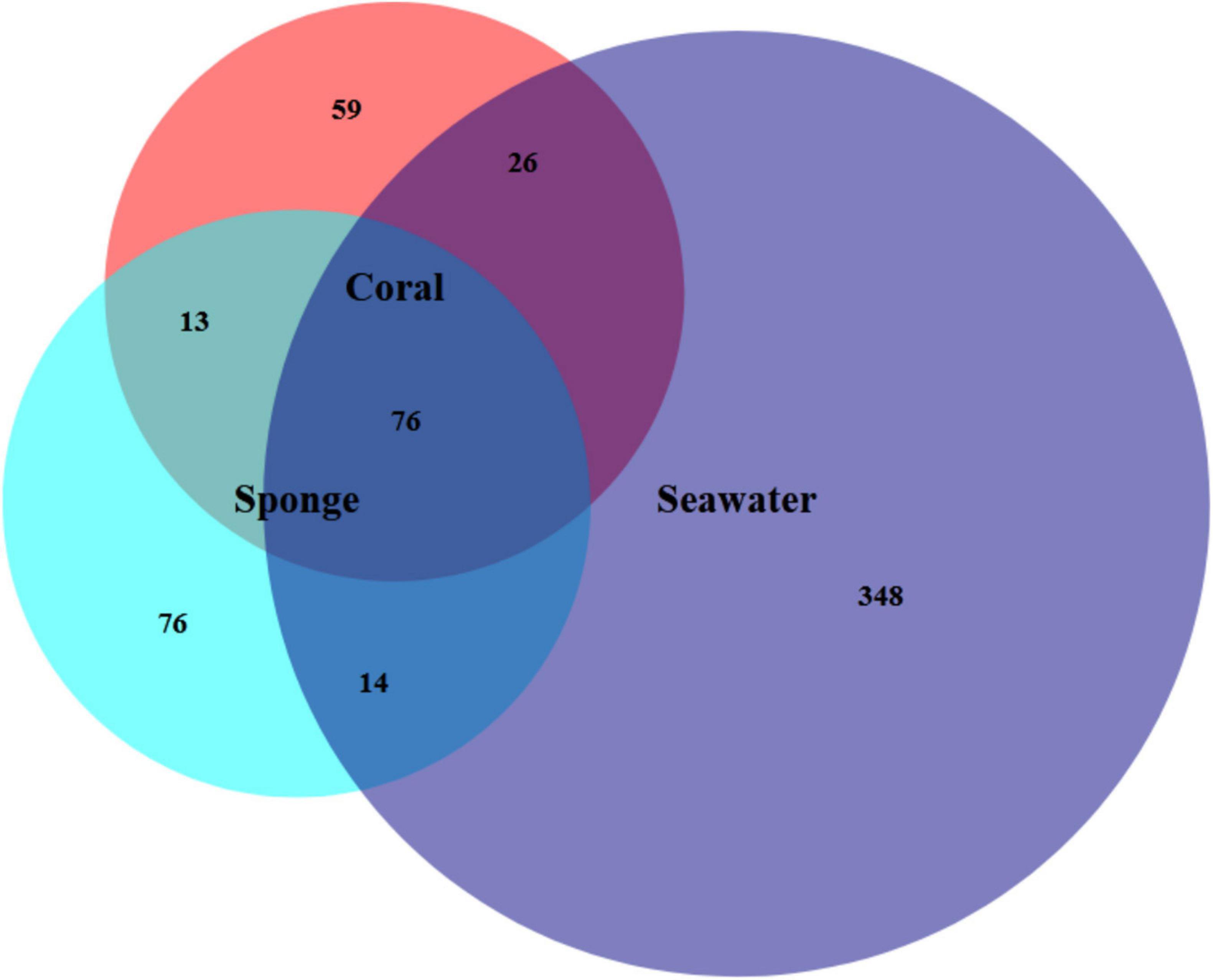
Figure 2. Venn diagram shows the number of Symbiodiniaceae amplicon sequence variants (ASVs) present in each reef habitat compartment (coral, sponge, and seawater), as well as the amount of overlap between and among samples.
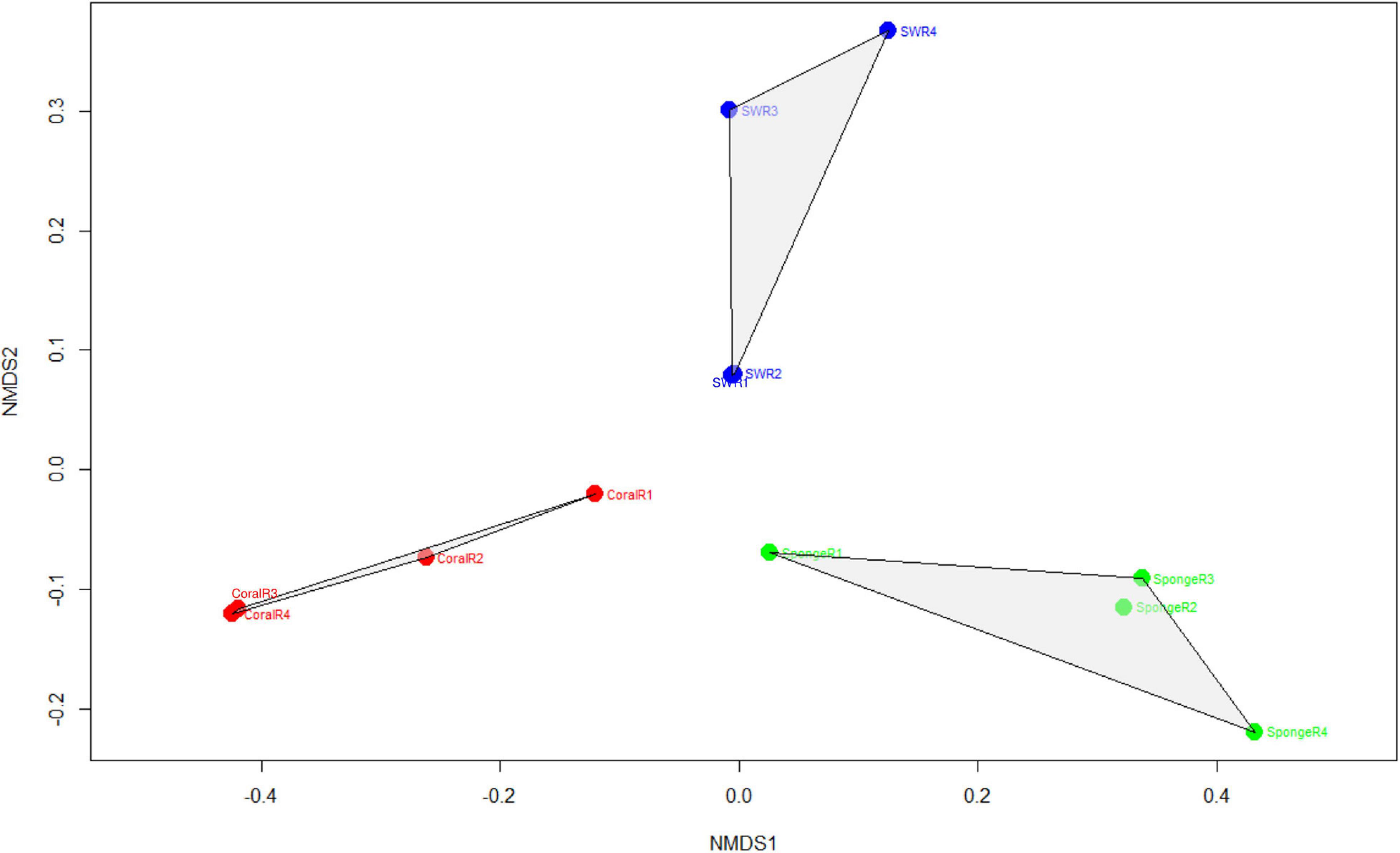
Figure 3. Non-metric multidimensional scaling plots showing the pattern of Symbiodiniaceae communities recovered from the coral samples, sponge samples, and seawater (stress value: 0.12). Stress value <0.05 shows an excellent representation in nMDS analysis.
The most abundant Symbiodiniaceae genus found in the investigated coral species was Durusdinium (89.8 ± 11.7%), followed by Gerakladium (10.2 ± 11.7%) (Figure 4A). At ITS2 subtype level, Durusdinium sp. (89.1 ± 13.4%) was found to be the dominant, followed by G3.3 (9.9 ± 12.9%) in the coral species T. mesenterina (Figure 4D). The other types of Durusdinium, i.e., D1.8, D105, D106, D120, D124, and D2 were detected in lower abundance (Figure 4D). On the contrary, the sponge species C. thomasi contained a higher abundance of Gerakladium (83.3 ± 23.7%), followed by Durusdinium (16.6 ± 23.8%) (Figure 4B). Among Gerakladium types, the highest dominance determined was for Gerakladium G3.3 (78.5 ± 21.2%), followed by Durusdinium D1 (16.6 ± 23.7%) (Figure 4E). The other types of Gerakladium G100, G101, G3.1, and G3.2 were detected in lower abundance (Figure 4E). The most abundant Symbiodiniaceae taxa in seawater samples were Durusdinium (51.0 ± 10.1%) and Gerakladium (40.3 ± 3.3%), followed by Symbiodinium (3.5 ± 5.5%), Fugacium (3.0 ± 3.1%), and Cladocopium in (2.0 ± 3.5%) (Figure 4C). Breviolum was also detected in seawater in a very low abundance (<1%). Furthermore, at the ITS2 type level, seawater contained Durusdinium sp. (50.6 ± 13.9%) and Gerakladium G3.3 (36.3 ± 5.1%) (Figure 4F).
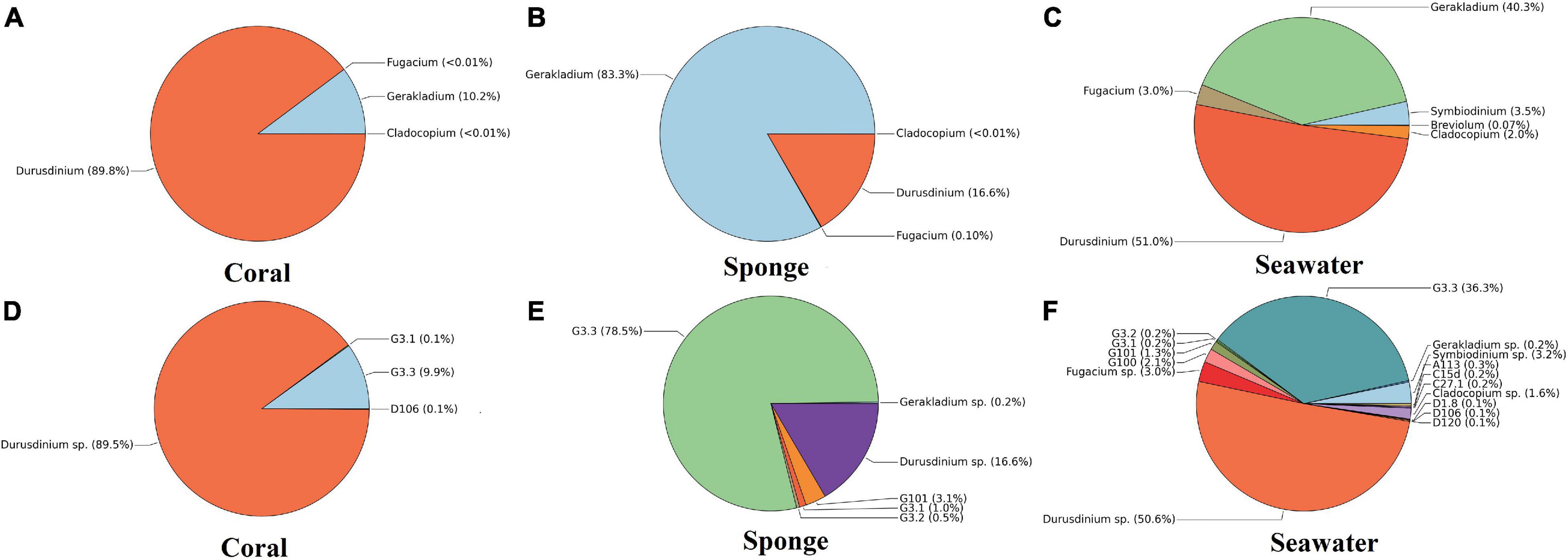
Figure 4. Symbiodiniaceae community composition at Grande Island. (A–C) Relative abundances of Symbiodiniaceae genera in host (coral and sponge) and free living in seawater. (D–F) Relative abundances of Symbiodiniaceae at subtypes level in host (coral and sponge) and free living in seawater. Values represent mean relative abundances across group samples.
In order to define the intragenomic variants for the ITS2 region, SymPortal DIV profiles for the Symbiodiniaceae community in corals, sponge, and free-living habitats were determined. SymPortal analysis provided the complete ITS2 profile only for coral samples, which showed the dominance of ITS2 type profile D1 and lower dominance of types D4-D4c-D1c-D2-D6 (Supplementary File 2 and Supplementary Figure 4). However, seawater and sponge samples could not show the complete ITS2 type profiles (Supplementary File 2 and Supplementary Figure 5).
Discussion
This study determined the Symbiodiniaceae diversity associated with the two space competitors (i.e., coral and sponge) in the same reef habitat using high-throughput sequencing of the ITS2 region. ITS2 marker is commonly used to analyze Symbiodiniaceae (Thornhill et al., 2007; Stat et al., 2011; Shi et al., 2021). The present study followed the ASV-based approach for defining the Symbiodiniaceae diversity over the conventional operational taxonomic units (OTUs) using 97% similarity clustering threshold to collapse sequence diversity into OTUs. The redundancy in ITS2 delineation has been well documented based on the reference database employed (Tonk et al., 2013; Shi et al., 2021). This study confirms the variations in ITS2 delineation and taxonomic assignment through three different reference databases. The difference in the results obtained may be attributed to the sequence length and ITS2 boundary variations in the databases. Furthermore, this study confirms the IGV level delineation called as DIVs for ITS2 subtypes using SymPortal. The DIV profiles to search for Symbiodiniaceae community of coral showed strong overlap with the sponge and free-living habitat due to the high relative abundance of Durusdinium (D1) (Supplementary File 2 and Figure 3).
Symbiodiniaceae Community Associated With the Coral T. mesenterina
The investigated coral T. mesenterina showed a higher abundance of the genus Durusdinium. Interestingly, Durusdinium genus is widely known to be associated with scleractinian corals from the Caribbean and Indo Pacific reefs (Knowlton and Rohwer, 2003; Jones et al., 2008; Brener-Raffalli et al., 2018). Previous studies have suggested that the association of coral with Durusdinium may indicate local environmental stressors, such as high temperature, sedimentation, and light intensity (D’Angelo et al., 2015; Osman et al., 2020; Wall et al., 2020). Studies from different global reefs attribute the increased association of Durusdinium with corals to the adaptation against thermal stress (Oladi et al., 2019; Chankong et al., 2020; Williams and Patterson, 2020). Durusdinium communities in corals from the Andaman Sea with high host specificity were attributed to higher turbidity and high nutrient concentrations in the reef (LaJeunesse et al., 2010b). In general, the studies have confirmed that the Durusdinium type D1a or D1-4 (Durusdinium trenchii) are more frequently associated with corals in thermally challenged marginal habitats (Cooper et al., 2009; LaJeunesse et al., 2010a; Stat et al., 2013; Oladi et al., 2019; Qin et al., 2019; Williams and Patterson, 2020). D. trenchii is often referred to as an opportunistic symbiont which makes their association heterologous (Smith et al., 2017; Matthews et al., 2018).
The marginal coral reef of the present study was subjected to severe thermal stress-driven coral-bleaching events from 2014 to 2016. Sea surface temperature (SST) data of NOAA Coral Reef Watch (NOAA-CRW) platform revealed that the SST exceeded the thermal bleaching thresholds throughout the years 2015 to 2019 (Supplementary File 1 and Supplementary Figure 2). The underwater field survey confirmed in situ coral bleaching during October 2014, April 2015, October 2015, and April 2016 (Hussain and Ingole, 2020). The years 2014–2017 were the warmest years in history, leading to mass coral mortalities globally (Eakin et al., 2019; Skirving et al., 2019). There have been reports of coral bleaching in all major reefs of India during this period (Hussain and Ingole, 2020). Such warming events in the last few decades have significantly altered the coral population and led to the emergence of non-reef-forming communities (Hughes et al., 2017; Lough et al., 2018). This clearly implies that temperature and local stressors remain important drivers of coral decline; therefore, the response of Symbiodiniaceae to stressors is crucial for predicting the response of the reef community as a whole.
Our in situ surveys confirmed high bleaching resilience in T. mesenterina (Figures 5a–c) while other coral genera such as Coscinaria, Goniopora, and Porites were susceptible to thermal bleaching (Supplementary File 1 and Supplementary Figure 3). The determined association of stress-tolerant Symbiodiniaceae members belonging to Durusdinium with the coral T. mesenterina may be attributed to its stress tolerance and bleaching resistance. A detailed analysis of Symbiodiniaceae association with other coral species though is needed to confirm the thermal-tolerance adaptation of T. mesenterina. Increased stress on marginal reefs tends to shift the coral community toward low species heterogeneity and to increase the dominance of species well adapted to increasing temperature regime (van Woesik et al., 2011; Hoegh-Guldberg et al., 2019). The switching of endosymbiont from Cladocopium to Durusdinium has been reported in adults of Acropora millepora in response to heat stress and, more recently, in Acropora spp. from Palk Bay (India) after the bleaching event in 2016 (Thinesh et al., 2019). In the disturbed reefs in Singapore, the higher bleaching resilience of Pocillopora was attributed to its association with thermotolerant Durusdinium (Guest et al., 2016). The coral Galaxea fascicularis from the South China Sea was found to be more resistant to thermal stress because of its association with Durusdinium D1a (Zhou et al., 2017). In another report, Pocillopora verrucosa associated with Durusdinium D1a was found to be more resistant to thermal stress than Pocillopora lutea associated with Cladocopium (Qin et al., 2019). Interestingly, a decadal study with higher temperature variability in the Kenyan reef found that Pavona and Pocillopora were resistant to bleaching due to their association with Durusdinium (McClanahan et al., 2015). Similar results of higher temperature regime tolerance were reported for scleractinian coral Leptoria phrygia from Southern Taiwan (Carballo-Bolaños et al., 2019). Studies revealed that under high-temperature stress, Durusdinium D1a increases the coral bleaching thresholds by 1.0 to 1.5°C (Berkelmans and Van Oppen, 2006; Silverstein et al., 2015) by maintaining a high photochemical efficiency compared with Breviolum and Cladocopium (Cunning et al., 2017; Klueter et al., 2017). Therefore, our observations suggest that T. mesenterina is tolerant against the thermal bleaching events in the studied marginal reef habitat. Our finding implies that the higher thermal resilience in T. mesenterina was possibly due to the association with Durusdinium. A similar association of Durusdinium with T. mesenterina has been reported from the stressed marginal habitat of Taiwan (Chen et al., 2005).
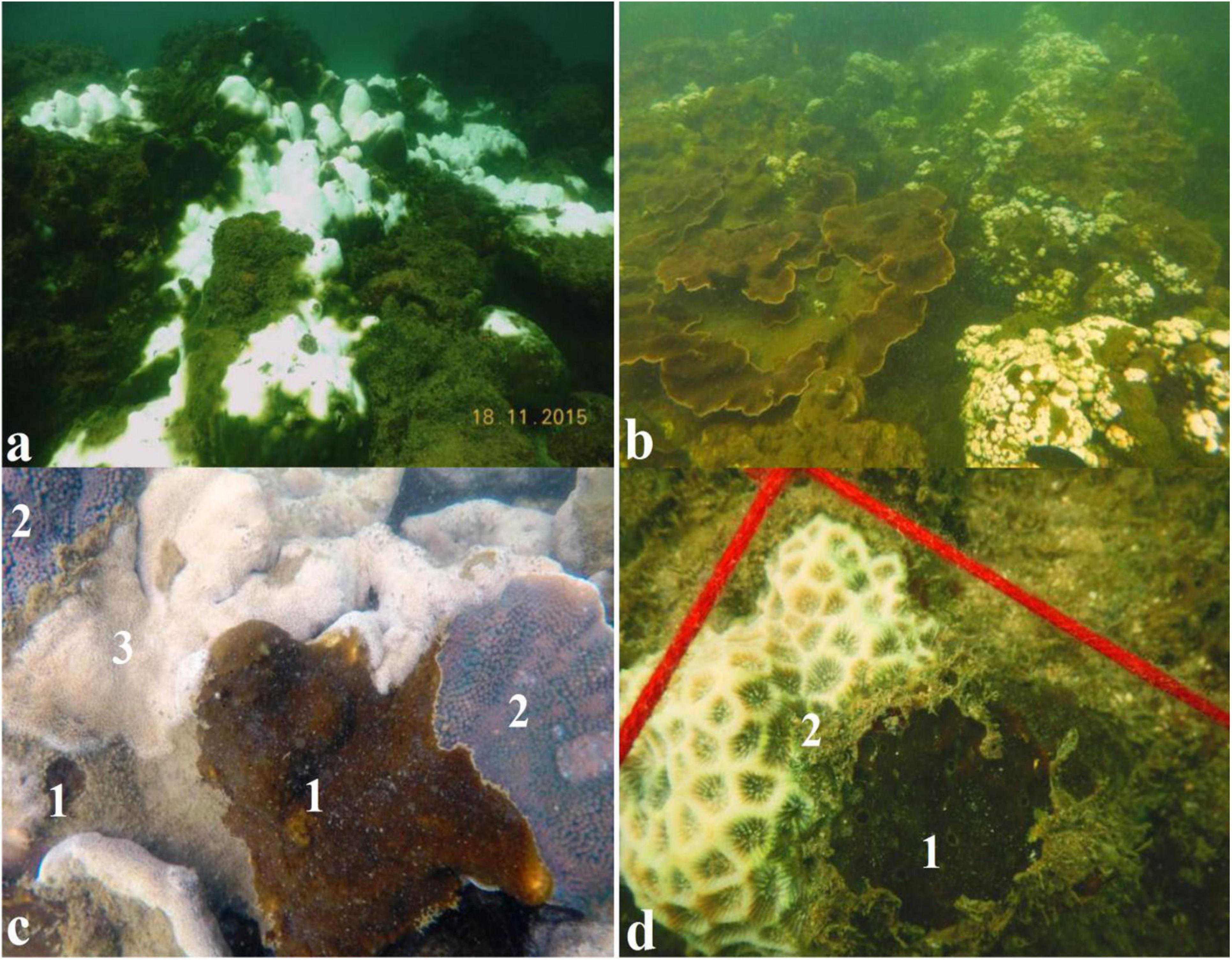
Figure 5. Mass bleaching event observed during November 2015: (a) In situ observation of coral bleaching in 2015 [Photograph provided by Hussain and Ingole (2020)]. (b) In situ observation for bleaching resistance of T. mesenterina than the other coral species observed. (c) Thermal bleaching resistance observed in coral boring sponge C. thomasi and T. mesenterina: (1) healthy C. thomasi, (2) healthy T. mesenterina, and (3) partially bleached Porites sp. colony. (d) (1) Healthy C. thomasi and (2) partially bleached Favites sp.
Along with Durusdinium, Gerakladium (10.2%) was the subdominant genera in T. mesenterina. The association of Gerakladium with scleractinian coral is rare though a few studies reported its association with Porites lobata in Hawaii (Stat et al., 2013), Orbicella annularis from Virgin Islands (Edmunds et al., 2014; Pochon et al., 2014a), Acropora sp. from Western Australia (Thomas et al., 2014), and Diploastrea heliopora from the Great Barrier Reef (Chakravarti and van Oppen, 2018). Gerakladium is mostly associated with the specific group of sponges (Schönberg and Loh, 2005; Hill et al., 2011), soft corals (Van Oppen et al., 2005), and foraminifera (Pochon et al., 2014b). The simultaneous occurrence of Durusdinium and Gerakladium was reported in thermally tolerant Porites lutea, from the South China Sea (Qin et al., 2019). However, the Gerakladium association with T. mensenterina needs to be ascertained by increasing the range of study sites from different geographical locations.
Symbiodiniaceae Community Associated With the Sponge C. thomasi
The present study confirmed the dominance of Gerakladium in the encrusting sponge species C. thomasi unlike the dominance of Durusdinium in host coral T. mesenterina. The results indicated the host-specific association of Symbiodiniaceae members. The species-specificity and flexibility in the Symbiodiniaceae association have also been reported in some sponges (Hill et al., 2011; Ramsby et al., 2017). For instance, Caribbean clionaid species C. aprica, C. laticavicola, and C. tenuis are known to associate with Symbiodinium and Breviolum, while the other member of the family C. caribbaea, C. varians, and C. tumula are known for their multi symbiont association with Gerakladium, Symbiodinium, or Breviolum (Granados et al., 2008; Hill et al., 2011; Ramsby et al., 2017). The Indo-Pacific Cliona orientalis is known to harbor only Gerakladium (Hill et al., 2011).
The Symbiodiniaceae of sponges were studied earlier using Symbiodiniaceae-specific markers based on Sanger sequencing approach or denaturing gradient gel electrophoresis (DGGE) methods (Schönberg and Loh, 2005; Granados et al., 2008; Hill et al., 2011; Pochon et al., 2014b; Ramsby et al., 2017, 2018) and a study by Ramsby et al. (2018) by NGS. The available literature suggests the wide occurrence of Gerakladium genus in C. viridis species complex, but there is less information about cryptic species or subtypes of Symbiodiniaceae (Supplementary File 1 and Supplementary Table 6). At the subtype level, only G2.1 was reported from the sponge C. orientalis. In the present study, we have utilized the high-throughput sequencing of the ITS2 region, which improved the detection of different Symbiodiniaceae subtypes from mixed populations and also determined community composition in the sponge. Within sponge C. thomasi samples, type G3.3 had the highest relative abundance (78.5%) followed by G101 (3.0%). In addition, the results detected type G3.1 and G3.2 at lower abundance (<1%), which indicates genetically distinct Symbiodiniaceae taxa in sponges. The advancement in detecting relatively rare Symbiodiniaceae subtypes reveals that sponge symbiont association can be flexible or specific within the same genus at the subtype level. The lower abundance of Symbiodiniaceae subtypes is known to play a significant role in coral holobiont resilience and response to perturbation (Ziegler et al., 2018). Therefore, the findings presented here indicate that there are additional sponge-associated species within Gerakladium genus which prove the great importance of the way that ITS2 sequence data are analyzed and interpreted in studies concerning the biological diversity. The knowledge of the dinoflagellate communities associated with bioeroding sponges is limited at the species level, with only two known species (Gerakladium endoclionum from C. orientalis, and Gerakladium spongiolum from C. varians) from sponges (Ramsby et al., 2017). Our ITS2 rDNA based analysis could not confirm the species identity of Symbiodiniaceae associated with C. thomasi due to lack of species-specific subtypes information in the revised taxonomy of Gerakladium (LaJeunesse et al., 2018).
Different studies have confirmed the ecological significance of Gerakladium in the bioerosion capabilities, survival, and growth rate of Clionaid sponges (Fang et al., 2016, 2018a; Achlatis et al., 2019). Furthermore, Chakravarti and van Oppen (2018) showed the Gerakladium genus as thermotolerant by ex situ analysis. Our in situ observations indicated the increased prevalence of C. thomasi from 6% in 2015 to 11% in 2017 (Supplementary File 1 and Supplementary Figure 4) and bleaching resistance during mass bleaching events (Figures 5c,d). The thermal tolerance of sponges during mass bleaching events may be attributed to its association with Gerakladium.
Environmental Acquisition of Symbiodiniaceae
Symbiodiniaceae communities of the ambient seawater were predominantly represented by Durusdinium and Gerakladium, while Symbiodinium, Fugacium, and Cladocopium in were less dominant in the present study. Water column Symbiodiniaceae community may be exclusively free-living or symbiotic with different benthic hosts (Yamashita and Koike, 2013; Nitschke et al., 2016; Claar et al., 2020). Most of corals (∼80–85%) tend to acquire different symbionts from the surrounding environment at different life stages (Stat et al., 2008; Boulotte et al., 2016). The determined higher abundance of Durusdinium sp. in T. mesenterina and in ambient seawater (equal to Gerakladium) from the thermally challenged marginal sites indicates two possibilities of their association: (1) that the symbiont population in coral may have been retrieved from seawater or (2) the symbiont is typically associated with the coral hosts (Claar et al., 2020). Since horizontal acquisition is well established in corals, the former possibility appears a better fit. Unlike corals, vertical transmission of Symbiodiniaceae has been suggested in few sponges (Mariani et al., 2000, 2001). However, a few studies also suggested the environmental acquisition of free-living photosymbiont in sponges (Strehlow et al., 2016; Francis and Cleary, 2019). Also, it has been experimentally demonstrated that C. varians acquire symbiont from the ambient environment (Riesgo et al., 2014). The present study revealed the dominance of Gerakladium G3.3 in the sponge samples and also in ambient seawater.
Therefore, it can be inferred that there is a possibility for the acquisition of free-living symbiont from the ambient environment by C. thomasi, although detailed studies are required to understand their mode of acquisition. Our results support the possibility of host-specific acquisition of photosymbionts as Durusdinium in coral and Gerakladium in the sponge (Figure 6). Host-specific Symbiodiniaceae association in coral is well noted from different bioregions (Bernasconi et al., 2019; Howe-Kerr et al., 2020; Wall et al., 2020). The differential acquisition of symbionts by different hosts from the same environment can be attributed to various factors such as (1) cellular signaling to and from host and symbiont (Davy et al., 2012), (2) metabolic requisites of both the host and symbiont (Suggett et al., 2017), (3) microhabitat conditions of the host, (4) mode of transmission of the symbiont, i.e., horizontal or vertical (Stat et al., 2008), and (5) selection mediated reshuffling and/or re-switching of symbiont (Boulotte et al., 2016). Nevertheless, physiological, molecular, genetical, and signaling related detailed studies are needed to confirm the mode of host-specific Symbiodiniaceae association.
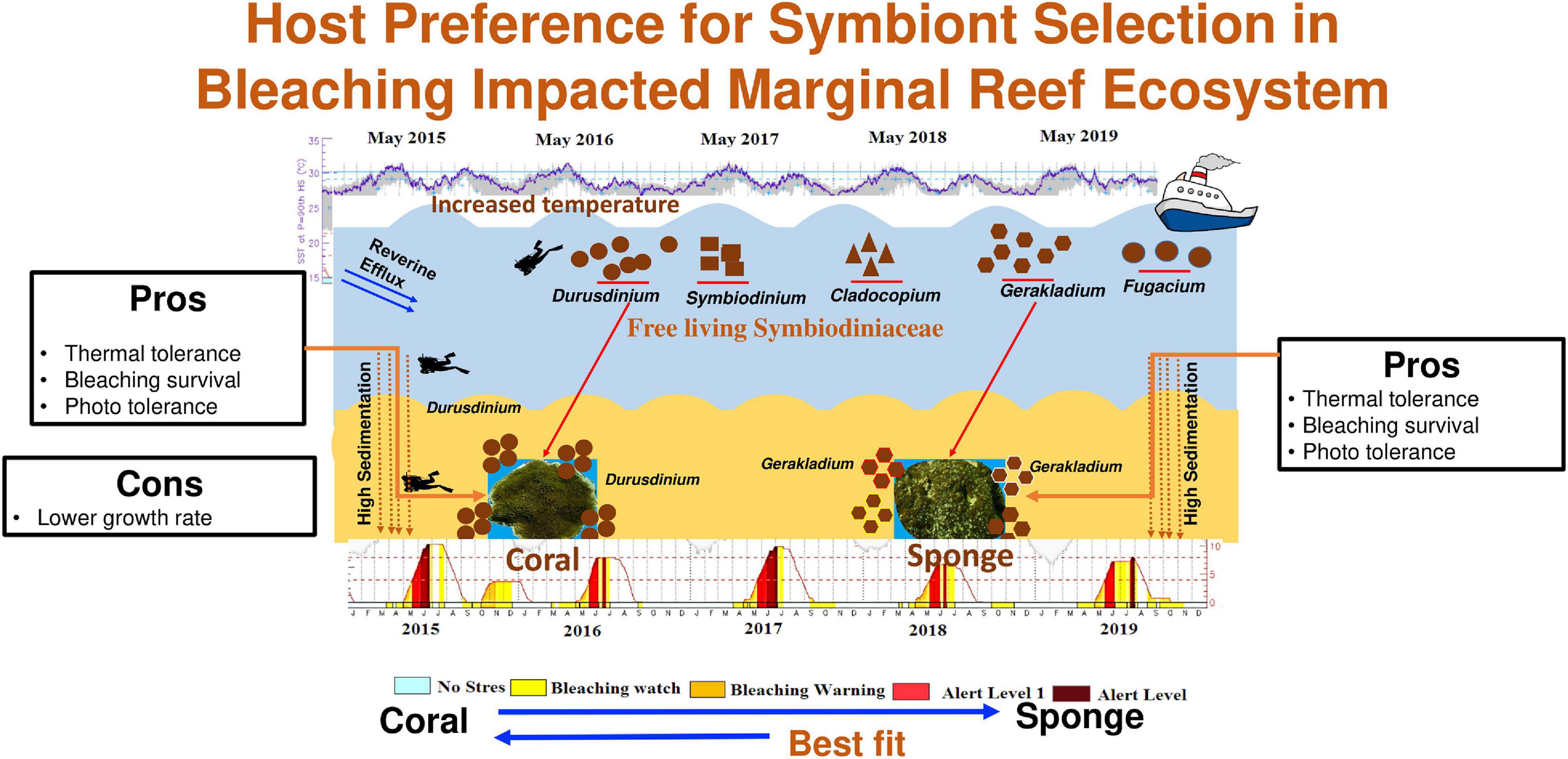
Figure 6. Graphical representation of host-specific acquisition of Symbiodiniaceae and its benefit to the host survival in bleaching impacted marginal coral reef ecosystem.
Conclusion
The present study provides an important insight into the coral-Symbiodiniaceae-sponge symbiosis in a data-deficient marginal coral reef habitat. Our results unveil a high abundance of thermal stress-tolerant Symbiodiniaceae Durusdinium and Gerakladium in an ambient environment experiencing severe temperature anomalies for the last 5 years. Furthermore, the study showed differential associations of Symbiodiniaceae between two hosts indicating a host-specific mode of selection. Since both the dominant Symbiodiniaceae members are well known for their thermotolerance, the determined thermal resistance by both the hosts in this habitat may be attributed to their association with the resistant Symbiodiniaceae Durusdinium and Gerakladium. Hence, the study highlights the influence of Durusdinium and Gerakladium and their possible role in enhanced acclimatization capacity of coral T. mesenterina and bioeroding sponge C. thomasi in a suboptimal environmental regime. At large, the stress-tolerant symbiont association may increase the resistance of few species and aid their survival, thereby increasing their dominance in space competition. Further studies on physiological, metabolic, and transcriptomic insights will help confirm the energetic tradeoffs by symbiont communities in the success of their respective hosts.
Sampling and Field Studies
The authors have obtained all necessary permits for sampling and field observation from the competent authorities.
Data Availability Statement
The datasets presented in this study can be found in online repositories. The names of the repository/repositories and accession number(s) can be found below: Nucleotide sequences have been submitted to the NCBI database under Bio project ID- PRJNA636097.
Author Contributions
SM and VG conceptualized the study, designed the experiment, and analyzed the sequenced data. SM and KD performed sampling. SM performed DNA extraction experiments. KM performed the nMDS. AH did the field survey for bleaching and coral health assessment. MN, BI, and VG supervised the project and acquired the fund for the study. VG, SM, KD, and MN wrote the manuscript. KM and BI proofread the manuscript. All authors contributed to the article and approved the submitted version.
Funding
The financial support received through “Rajiv Gandhi Science and Technology Commission (RGSTC),” Government of Maharashtra for the Maharashtra Gene Bank project (GAP2871) is acknowledged. SM acknowledges the CSIR-Senior Research Fellowship (CSIR-SRF). AH and KD acknowledge the DST-INSPIRE fellowship, respectively. This study is a part of the Ph.D. thesis of SM.
Conflict of Interest
The authors declare that the research was conducted in the absence of any commercial or financial relationships that could be construed as a potential conflict of interest.
Supplementary Material
The Supplementary Material for this article can be found online at: https://www.frontiersin.org/articles/10.3389/fmars.2021.666825/full#supplementary-material
Footnotes
- ^ https://raw.githubusercontent.com/nitschkematthew/Symbiodatabaceae/master/Combining_databases/ITS2dbn1_Dinophy_Symbio.fasta
References
Achlatis, M. (2018). Bioeroding Sponges in a Time of Change: Insights into the Physiology and Cellbiology of a Photosymbiotic Coral-Eroding Sponge. Ph.D. thesis. St Lucia, QLD: The University of Queensland. doi: 10.14264/uql.2018.806
Achlatis, M., Schönberg, C. H. L., van der Zande, R. M., LaJeunesse, T. C., Hoegh-Guldberg, O., and Dove, S. (2019). Photosynthesis by symbiotic sponges enhances their ability to erode calcium carbonate. J. Exp. Mar. Bio. Ecol. 516, 140–149. doi: 10.1016/j.jembe.2019.04.010
Al-Horani, F. A. (2016). “Physiology of Skeletogenesis in Scleractinian Coral,” in Diseases of Coral, eds C. M. Woodley, C. A. Downs, A. W. Bruckner, J. W. Porter, and S. B. Galloway (Hoboken, NJ: John Wiley and Sons Inc), 192–205. doi: 10.1002/9781118828502.ch12
Baker, D. M., Freeman, C. J., Wong, J. C. Y., Fogel, M. L., and Knowlton, N. (2018). Climate change promotes parasitism in a coral symbiosis. ISME J. 12, 921–930. doi: 10.1038/s41396-018-0046-8
Berkelmans, R., and Van Oppen, M. J. H. (2006). The role of zooxanthellae in the thermal tolerance of corals: a “nugget of hope” for coral reefs in an era of climate change. Proc. R. Soc. B Biol. Sci. 273, 2305–2312. doi: 10.1098/rspb.2006.3567
Bernasconi, R., Stat, M., Koenders, A., and Huggett, M. J. (2019). Global networks of symbiodinium-bacteria within the coral holobiont. Microb. Ecol. 77, 794–807. doi: 10.1007/s00248-018-1255-4
Boulotte, N. M., Dalton, S. J., Carroll, A. G., Harrison, P. L., Putnam, H. M., Peplow, L. M., et al. (2016). Exploring the Symbiodinium rare biosphere provides evidence for symbiont switching in reef-building corals. ISME J. 10, 2693–2701. doi: 10.1038/ismej.2016.54
Brener-Raffalli, K., Clerissi, C., Vidal-Dupiol, J., Adjeroud, M., Bonhomme, F., Pratlong, M., et al. (2018). Thermal regime and host clade, rather than geography, drive Symbiodinium and bacterial assemblages in the scleractinian coral Pocillopora damicornis sensu lato. Microbiome 6, 2–13. doi: 10.1186/s40168-018-0423-6
Camp, E. F., Kahlke, T., Nitschke, M. R., Varkey, D., Fisher, N. L., Fujise, L., et al. (2020). Revealing changes in the microbiome of Symbiodiniaceae under thermal stress. Environ. Microbiol. 22, 1294–1309. doi: 10.1111/1462-2920.14935
Carballo-Bolaños, R., Denis Id, V., Huang, Y.-Y., Keshavmurthy Id, S., Allen, C., and Id, C. (2019). Temporal variation and photochemical efficiency of species in Symbiodinaceae associated with coral Leptoria phrygia (Scleractinia; Merulinidae) exposed to contrasting temperature regimes. PLoS One 14:e0218801. doi: 10.1371/journal.pone.0218801
Callahan, B. J., Mcmurdie, P. J., Rosen, M. J., Han, A. W., Johnson, A. J. A., and Holmes, S. P. (2016). DADA2: high-resolution sample inference from Illumina amplicon data. Nat. Methods 13, 581–583. doi: 10.1038/nmeth.3869.
Chakravarti, L. J., and van Oppen, M. J. H. (2018). Experimental evolution in coral photosymbionts as a tool to increase thermal tolerance. Front. Mar. Sci. 5:227. doi: 10.3389/fmars.2018.00227
Chankong, A., Kongjandtre, N., Senanan, W., and Manthachitra, V. (2020). Community composition of Symbiodiniaceae among four scleractinian corals in the eastern Gulf of Thailand. Reg. Stud. Mar. Sci. 33:100918. doi: 10.1016/j.rsma.2019.100918
Chen, C. A., Yang, Y. W., Wei, N. V., Tsai, W. S., and Fang, L. S. (2005). Symbiont diversity in scleractinian corals from tropical reefs and subtropical non-reef communities in Taiwan. Coral Reefs 24, 11–22. doi: 10.1007/s00338-004-0389-7
Claar, D. C., Tietjen, K. L., Cox, K. D., Gates, R. D., and Baum, J. K. (2020). Chronic disturbance modulates symbiont (Symbiodiniaceae) beta diversity on a coral reef. Sci. Rep. 10:4492. doi: 10.1038/s41598-020-60929-z
Clarke, K. R., and Gorley, R. N. (2015). PRIMER v7 Plymouth Routines In Multivariate Ecological Research. Available online at: www.primer-e.com (accessed February 15, 2020).
Cooper, T. F., Gilmour, A. J. P., and Fabricius, A. K. E. (2009). Bioindicators of changes in water quality on coral reefs: review and recommendations for monitoring programmes. Coral Reefs 28, 589–606. doi: 10.1007/s00338-009-0512-x
Cunning, R., Gates, R. D., and Edmunds, P. J. (2017). Using high-throughput sequencing of ITS2 to describe Symbiodinium metacommunities in St. John, U.S. Virgin Islands. PeerJ 5:e3472. doi: 10.7287/peerj.preprints.2925v1
Cunning, R., Yost, D. M., Guarinello, M. L., Putnam, H. M., and Gates, R. D. (2015). Variability of symbiodinium communities in waters, sediments, and corals of thermally distinct reef pools in American Samoa. PLoS One 10:e0145099. doi: 10.1371/journal.pone.0145099
D’Angelo, C., Hume, B. C. C., Burt, J., Smith, E. G., Achterberg, E. P., and Wiedenmann, J. (2015). Local adaptation constrains the distribution potential of heat-tolerant Symbiodinium from the Persian/Arabian Gulf. ISME J. 9, 2551–2560. doi: 10.1038/ismej.2015.80
Davy, S. K., Allemand, D., and Weis, V. M. (2012). Cell Biology of Cnidarian-Dinoflagellate Symbiosis. Microbiol. Mol. Biol. Rev. 76, 229–261. doi: 10.1128/mmbr.05014-11
De, K., Nanajkar, M., Arora, M., Nithyanandan, M., Mote, S., and Ingole, B. (2021). Application of remotely sensed sea surface temperature for assessment of recurrent coral bleaching (2014-2019) impact on a marginal coral ecosystem. Geocarto Int. 1–22. doi: 10.1080/10106049.2021.1886345
De, K., Nanajkar, M., Mote, S., and Ingole, B. (2020). Coral damage by recreational diving activities in a Marine Protected Area of India: unaccountability leading to ‘tragedy of the not so commons.’. Mar. Pollut. Bull. 155:111190. doi: 10.1016/j.marpolbul.2020.111190
De, K., Sautya, S., Mote, S., Tsering, L., Patil, V., Nagesh, R., et al. (2015). Is climate change triggering coral bleaching in tropical reef? Curr. Sci. 109, 1379–1880.
Eakin, C. M., Sweatman, H. P. A., and Brainard, R. E. (2019). The 2014–2017 global-scale coral bleaching event: insights and impacts. Coral Reefs 38, 539–545. doi: 10.1007/s00338-019-01844-2
Edmunds, P., Pochon, X., Levitan, D., Yost, D., Belcaid, M., Putnam, H., et al. (2014). Long-term changes in Symbiodinium communities in Orbicella annularis in St. John, US Virgin Islands. Mar. Ecol. Prog. Ser. 506, 129–144. doi: 10.3354/meps10808
Fang, J. K. H., Schönberg, C. H. L., and Rupert, O. F. G. (2018b). Are some photosymbiotic bioeroding sponges more bleaching-tolerant than hard corals? J Mar Biol Ocean. 7:1000187. doi: 10.4172/2324-8661.1000187
Fang, J. K. H., Schönberg, C. H. L., Hoegh-Guldberg, O., and Dove, S. (2016). Day–night ecophysiology of the photosymbiotic bioeroding sponge Cliona orientalis Thiele, 1900. Mar. Biol. 163:100. doi: 10.1007/s00227-016-2848-4
Fang, J. K. H., Schönberg, C. H. L., Hoegh-Guldberg, O., and Dove, S. (2017). Symbiotic plasticity of Symbiodinium in a common excavating sponge. Mar. Biol. 164, 1–11. doi: 10.1007/s00227-017-3088-y
Fang, J. K. H., Schönberg, C. H. L., Mello-Athayde, M. A., Achlatis, M., Hoegh-Guldberg, O., and Dove, S. (2018a). Bleaching and mortality of a photosymbiotic bioeroding sponge under future carbon dioxide emission scenarios. Oecologia 187, 25–35. doi: 10.1007/s00442-018-4105-7
Francis, D., and Cleary, R. (2019). A comparison of microeukaryote communities inhabiting sponges and seawater in a Taiwanese coral reef system. Ann. Microbiol. 69, 861–866. doi: 10.1007/s13213-019-01476-5
Granados, C., Camargo, C., Zea, S., and Sánchez, J. A. (2008). Phylogenetic relationships among zooxanthellae (Symbiodinium) associated to excavating sponges (Cliona spp.) reveal an unexpected lineage in the Caribbean. Mol. Phylogenet. Evol. 49, 554–560. doi: 10.1016/j.ympev.2008.07.023
Granados-Cifuentes, C., Neigel, J., Leberg, P., and Rodriguez-Lanetty, M. (2015). Genetic diversity of free-living Symbiodinium in the Caribbean: the importance of habitats and seasons. Coral Reefs 34, 927–939. doi: 10.1007/s00338-015-1291-1
Guest, J. R., Low, J., Tun, K., Wilson, B., Ng, C., Raingeard, D., et al. (2016). Coral community response to bleaching on a highly disturbed reef. Sci. Rep. 6:20717. doi: 10.1038/srep20717
Hill, M., Allenby, A., Ramsby, B., Schönberg, C., and Hill, A. (2011). Molecular phylogenetics and evolution Symbiodinium diversity among host clionaid sponges from Caribbean and Pacific reefs?: evidence of heteroplasmy and putative host-specific symbiont lineages. Mol. Phylogenet. Evol. 59, 81–88. doi: 10.1016/j.ympev.2011.01.006
Hoegh-Guldberg, O., Pendleton, L., and Kaup, A. (2019). People and the changing nature of coral reefs. Reg. Stud. Mar. Sci. 30:100699. doi: 10.1016/j.rsma.2019.100699
Horwitz, R., Hoogenboom, M. O., and Fine, M. (2017). Spatial competition dynamics between reef corals under ocean acidification. Sci. Rep. 7:40288. doi: 10.1038/srep40288
Howe-Kerr, L. I., Bachelot, B., Wright, R. M., Kenkel, C. D., Bay, L. K., and Correa, A. M. S. (2020). Symbiont community diversity is more variable in corals that respond poorly to stress. Glob. Chang. Biol. 26, 2220–2234. doi: 10.1111/gcb.14999
Hughes, T. P., Anderson, K. D., Connolly, S. R., Heron, S. F., Kerry, J. T., Lough, J. M., et al. (2018). Spatial and temporal patterns of mass bleaching of corals in the Anthropocene. Science 359, 80–83. doi: 10.1126/science.aan8048
Hughes, T. P., Kerry, J. T., Álvarez-Noriega, M., Álvarez-Romero, J. G., Anderson, K. D., Baird, A. H., et al. (2017). Global warming and recurrent mass bleaching of corals. Nature 543, 373–377. doi: 10.1038/nature21707
Hume, B. C. C., Mejia-Restrepo, A., Voolstra, C. R., and Berumen, M. L. (2020). Fine-scale delineation of Symbiodiniaceae genotypes on a previously bleached central Red Sea reef system demonstrates a prevalence of coral host-specific associations. Coral Reefs 39, 583–601. doi: 10.1007/s00338-020-01917-7
Hume, B. C. C., Smith, E. G., Ziegler, M., Warrington, H. J. M., Burt, J. A., LaJeunesse, T. C., et al. (2019). SymPortal: a novel analytical framework and platform for coral algal symbiont next-generation sequencing ITS2 profiling. Mol. Ecol. Resour. 19, 1063–1080. doi: 10.1111/1755-0998.13004
Hussain, A., and Ingole, B. (2020). Massive coral bleaching in the patchy reef of Grande Island, along the eastern Arabian Sea during the 2015/16 global bleaching event. Reg. Stud. Mar. Sci. 39:101410. doi: 10.1016/j.rsma.2020.101410
Hussain, A., De, K., Thomas, L., Nagesh, R., Mote, S., and Ingole, B. (2016). Prevalence of skeletal tissue growth anomalies in a scleractinian coral: Turbinaria mesenterina of Malvan Marine Sanctuary, Eastern Arabian Sea. Dis. Aquat. Organ. 121, 79–83. doi: 10.3354/dao03038
Jones, A. M., Berkelmans, R., Van Oppen, M. J. H., Mieog, J. C., and Sinclair, W. (2008). A community change in the algal endosymbionts of a scleractinian coral following a natural bleaching event: field evidence of acclimatization. Proc. R. Soc. B Biol. Sci. 275, 1359–1365. doi: 10.1098/rspb.2008.0069
Klueter, A., Trapani, J., Archer, F. I., McIlroy, S. E., and Coffroth, M. A. (2017). Comparative growth rates of cultured marine dinoflagellates in the genus Symbiodinium and the effects of temperature and light. PLoS One 12:e0187707. doi: 10.1371/journal.pone.0187707
Knowlton, N., and Rohwer, F. (2003). Multispecies microbial mutualisms on coral reefs: the host as a habitat. Am. Nat. 162, S51–S62. doi: 10.1086/378684
LaJeunesse, T. C., Everett Parkinson, J., Gabrielson, P. W., Jin Jeong, H., Davis Reimer, J., Voolstra, C. R., et al. (2018). Systematic revision of symbiodiniaceae highlights the antiquity and diversity of coral endosymbionts. Curr. Biol. 28, 2570–2580.e6. doi: 10.1016/j.cub.2018.07.008
LaJeunesse, T. C., Pettay, D. T., Sampayo, E. M., Phongsuwan, N., Brown, B., Obura, D. O., et al. (2010a). Long-standing environmental conditions, geographic isolation and host-symbiont specificity influence the relative ecological dominance and genetic diversification of coral endosymbionts in the genus Symbiodinium. J. Biogeogr. 37, 785–800. doi: 10.1111/j.1365-2699.2010.02273.x
LaJeunesse, T. C., Smith, R., Walther, M., Pinzón, J., Pettay, D. T., McGinley, M., et al. (2010b). Host-symbiont recombination versus natural selection in the response of coral-dinoflagellate symbioses to environmental disturbance. Proc. R. Soc. B Biol. Sci. 2925–2934. doi: 10.1098/rspb.2010.0385
Lough, J. M., Anderson, K. D., and Hughes, T. P. (2018). Increasing thermal stress for tropical coral reefs: 1871–2017. Sci. Rep. 8:6079. doi: 10.1038/s41598-018-24530-9
Manikandan, B., Ravindran, J., Mohan, H., Periasamy, R., ManiMurali, R., and Ingole, B. S. (2016). Community structure and coral health status across the depth gradients of Grande Island, Central west coast of India. Reg. Stud. Mar. Sci. 7, 150–158. doi: 10.1016/j.rsma.2016.05.013
Mariani, S., Piscitelli, M. P., and Uriz, M. J. (2001). Temporal and spatial co-occurrence in spawning and larval release of Cliona viridis (Porifera: Hadromerida). J. Mar. Biol. Assoc. United Kingdom 81, 565–567. doi: 10.1017/S0025315401004246
Mariani, S., Uriz, M. J., and Turon, X. (2000). Larval bloom of the oviparous sponge Cliona viridis: coupling of larval abundance and adult distribution. Mar. Biol. 137, 783–790. doi: 10.1007/s002270000400
Marlow, J., Davy, S. K., Shaffer, M., Haris, A., and Bell, J. J. (2018). Bleaching and recovery of a phototrophic bioeroding sponge. Coral Reefs 37, 565–570. doi: 10.1007/s00338-018-1680-3
Martin, M. (2011). Cutadapt removes adapter sequences from high-throughput sequencing reads. EMBnet. J. 17:10. doi: 10.14806/ej.17.1.200
Matthews, J. L., Oakley, C. A., Lutz, A., Hillyer, K. E., Roessner, U., Grossman, A. R., et al. (2018). Partner switching and metabolic flux in a model cnidarian–dinoflagellate symbiosis. Proc. R. Soc. B Biol. Sci. 285:20182336. doi: 10.1098/rspb.2018.2336
McClanahan, T. R., Maina, J., and Ateweberhan, M. (2015). Regional coral responses to climate disturbances and warming is predicted by multivariate stress model and not temperature threshold metrics. Clim. Change 131, 607–620. doi: 10.1007/s10584-015-1399-x
Mies, M., Sumida, P. Y. G., Rädecker, N., and Voolstra, C. R. (2017). Marine invertebrate larvae associated with Symbiodinium: a mutualism from the start? Front. Ecol. Evol. 5:56. doi: 10.3389/fevo.2017.00056
Mote, S., Schönberg, C. H. L., Samaai, T., Gupta, V., and Ingole, B. (2019). A new clionaid sponge infests live corals on the west coast of India (Porifera, Demospongiae, Clionaida). Syst. Biodivers. 17, 190–206. doi: 10.1080/14772000.2018.1513430
Nitschke, M. R., Davy, S. K., and Ward, S. (2016). Horizontal transmission of Symbiodinium cells between adult and juvenile corals is aided by benthic sediment. Coral Reefs 35, 335–344. doi: 10.1007/s00338-015-1349-0
Oksanen, J., Eduard Szoecs, E., and Mhh, W. H. (2018). Package “vegan” Title Community Ecology Package Version 2.5-6. Available online at: https://cran.r-project.org/package=vegan (accessed February 15, 2020).
Oladi, M., Shokri, M. R., and Rajabi-Maham, H. (2019). Extremophile symbionts in extreme environments; a contribution to the diversity of Symbiodiniaceae across the northern Persian Gulf and Gulf of Oman. J. Sea Res. 144, 105–111. doi: 10.1016/j.seares.2018.11.010
Osman, E. O., Smith, D. J., Ziegler, M., Kürten, B., Conrad, C., El-Haddad, K. M., et al. (2018). Thermal refugia against coral bleaching throughout the northern Red Sea. Glob. Chang. Biol. 24, e474–e484. doi: 10.1111/gcb.13895
Osman, E. O., Suggett, D. J., Voolstra, C. R., Pettay, D. T., Clark, D. R., Pogoreutz, C., et al. (2020). Coral microbiome composition along the northern Red Sea suggests high plasticity of bacterial and specificity of endosymbiotic dinoflagellate communities. Microbiome 8:8. doi: 10.1186/s40168-019-0776-5
Patro, S., Krishnan, P., Gopi, M., Raja, S., Sreeraj, C. R., Ramachandran, P., et al. (2015). Snowflake coral, Carijoa riisei from Grand Island, Goa: a case of invasion of an alien species or re-establishment of a native species? Curr. Sci. 109, 1028–1030. doi: 10.18520/cs/v109/i6/1028-1030
Pernice, M., Raina, J. B., Rädecker, N., Cárdenas, A., Pogoreutz, C., and Voolstra, C. R. (2020). Down to the bone: the role of overlooked endolithic microbiomes in reef coral health. ISME J. 14, 325–334. doi: 10.1038/s41396-019-0548-z
Pochon, X., Gates, R. D., Vik, D., and Edmunds, P. J. (2014a). Molecular characterization of symbiotic algae (Symbiodinium spp.) in soritid foraminifera (Sorites orbiculus) and a scleractinian coral (Orbicella annularis) from St John, US Virgin Islands. Mar. Biol. 161, 2307–2318. doi: 10.1007/s00227-014-2507-6
Pochon, X., Putnam, H. M., and Gates, R. D. (2014b). Multi-gene analysis of Symbiodinium dinoflagellates: a perspective on rarity, symbiosis, and evolution. PeerJ 2014:e394. doi: 10.7717/peerj.394
Purnachandra Rao, V., Shynu, R., Kessarkar, P. M., Sundar, D., Michael, G. S., Narvekar, T., et al. (2011). Suspended sediment dynamics on a seasonal scale in the Mandovi and Zuari estuaries, central west coast of India. Estuar. Coast. Shelf Sci. 91, 78–86. doi: 10.1016/j.ecss.2010.10.007
Qin, Z., Yu, K., Chen, B., Wang, Y., Liang, J., Luo, W., et al. (2019). Diversity of symbiodiniaceae in 15 coral species from the Southern South China sea: potential relationship with coral thermal adaptability. Front. Microbiol. 10:2343. doi: 10.3389/fmicb.2019.02343
Ramsby, B. D., Hill, M. S., Thornhill, D. J., Steenhuizen, S. F., Achlatis, M., Lewis, A. M., et al. (2017). Sibling species of mutualistic Symbiodinium clade G from bioeroding sponges in the western Pacific and western Atlantic oceans. J. Phycol. 53, 951–960. doi: 10.1111/jpy.12576
Ramsby, B. D., Hoogenboom, M. O., Whalan, S., and Webster, N. S. (2018). Elevated seawater temperature disrupts the microbiome of an ecologically important bioeroding sponge. Mol. Ecol. 27, 2124–2137. doi: 10.1111/mec.14544
Riesgo, A., Peterson, K., Richardson, C., Heist, T., Strehlow, B., McCauley, M., et al. (2014). Transcriptomic analysis of differential host gene expression upon uptake of symbionts: a case study with Symbiodinium and the major bioeroding sponge Cliona varians. BMC Genomics 15:376. doi: 10.1186/1471-2164-15-376
Schönberg, C. H. L. (2000). “Sponges of the ’Cliona viridis complex’-a key for species identification,” in 9th International Coral Reef Symposium, Bali, 295–300.
Schönberg, C. H. L., and Loh, W. K. W. (2005). Molecular identity of the unique symbiotic dinoflagellates found in the bioeroding demosponge Cliona orientalis. Mar. Ecol. Prog. Ser. 299, 157–166. doi: 10.3354/meps299157
Schönberg, C. H. L., Suwa, R., Hidaka, M., and Loh, W. K. W. (2008). Sponge and coral zooxanthellae in heat and light: preliminary results of photochemical efficiency monitored with pulse amplitude modulated fluorometry. Mar. Ecol. 29, 247–258. doi: 10.1111/j.1439-0485.2007.00216.x
Shi, T., Niu, G., Kvitt, H., Zheng, X., Qin, Q., Sun, D., et al. (2021). Untangling ITS2 genotypes of algal symbionts in zooxanthellate corals. Mol. Ecol. Resour. 21, 137–152. doi: 10.1111/1755-0998.13250
Shynu, R., Rao, P. C., Sarma, V. V. S. S., Kessarkar, P. M., and ManiMurali, R. (2015). Sources and fate of organic matter in suspended and bottom sediments of the Mandovi and Zuari estuaries, western India on JSTOR. Curr. Sci. 108, 226–238.
Silverstein, R. N., Correa, A. M. S., and Baker, A. C. (2012). Specificity is rarely absolute in coral–algal symbiosis: implications for coral response to climate change. Proc. R. Soc. B Biol. Sci. 279, 2609–2618. doi: 10.1098/rspb.2012.0055
Silverstein, R. N., Cunning, R., and Baker, A. C. (2015). Change in algal symbiont communities after bleaching, not prior heat exposure, increases heat tolerance of reef corals. Glob. Chang. Biol. 21, 236–249. doi: 10.1111/gcb.12706
Skirving, W. J., Heron, S. F., Marsh, B. L., Liu, G., De La Cour, J. L., Geiger, E. F., et al. (2019). The relentless march of mass coral bleaching: a global perspective of changing heat stress. Coral Reefs 38, 547–557. doi: 10.1007/s00338-019-01799-4
Smith, E. G., Hume, B. C. C., Delaney, P., Wiedenmann, J., and Burt, J. A. (2017). Genetic structure of coral-Symbiodinium symbioses on the world’s warmest reefs. PLoS One 12:0180169. doi: 10.1371/journal.pone.0180169
Stat, M., and Gates, R. D. (2011). Clade D symbiodinium in scleractinian corals: a “Nugget” of hope, a selfish opportunist, an ominous sign, or all of the above? J. Mar. Biol. 2011, 1–9. doi: 10.1155/2011/730715
Stat, M., Bird, C. E., Pochon, X., Chasqui, L., Chauka, L. J., Concepcion, G. T., et al. (2011). Variation in Symbiodinium ITS2 sequence assemblages among coral colonies. PLoS One 6:e15854. doi: 10.1371/journal.pone.0015854
Stat, M., Morris, E., Gates, R. D., and Karl, D. M. (2008). Functional diversity in coral-dinoflagellate symbiosis. Proc. Natl. Acad. Sci. U.S.A. 105, 9256–9261. doi: 10.1073/pnas.0801328105
Stat, M., Pochon, X., Franklin, E. C., Bruno, J. F., Casey, K. S., Selig, E. R., et al. (2013). The distribution of the thermally tolerant symbiont lineage (Symbiodinium clade D) in corals from Hawaii: correlations with host and the history of ocean thermal stress. Ecol. Evol. 3, 1317–1329. doi: 10.1002/ece3.556
Strehlow, B., Friday, S., McCauley, M., and Hill, M. (2016). The potential of azooxanthellate poriferan hosts to assess the fundamental and realized Symbiodinium niche: evaluating a novel method to initiate Symbiodinium associations. Coral Reefs 35, 1201–1212. doi: 10.1007/s00338-016-1465-5
Suggett, D. J., Warner, M. E., and Leggat, W. (2017). Symbiotic dinoflagellate functional diversity mediates coral survival under ecological crisis. Trends Ecol. Evol. 32, 735–745. doi: 10.1016/j.tree.2017.07.013
Takabayashi, M., Adams, L. M., Pochon, X., and Gates, R. D. (2012). Genetic diversity of free-living Symbiodinium in surface water and sediment of Hawai’i and Florida. Coral Reefs 31, 157–167. doi: 10.1007/s00338-011-0832-5
Tan, Y. T. R., Wainwright, B. J., Afiq-Rosli, L., Ip, Y. C. A., Lee, J. N., Nguyen, N. T. H., et al. (2020). Endosymbiont diversity and community structure in Porites lutea from Southeast Asia are driven by a suite of environmental variables. Symbiosis 80, 269–277. doi: 10.1007/s13199-020-00671-2
Thinesh, T., Meenatchi, R., Jose, P. A., Kiran, G. S., and Selvin, J. (2019). Differential bleaching and recovery pattern of southeast Indian coral reef to 2016 global mass bleaching event: occurrence of stress-tolerant symbiont Durusdinium (Clade D) in corals of Palk Bay. Mar. Pollut. Bull. 145, 287–294. doi: 10.1016/j.marpolbul.2019.05.033
Thomas, L., Kendrick, G. A., Kennington, W. J., Richards, Z. T., and Stat, M. (2014). Exploring Symbiodinium diversity and host specificity in Acropora corals from geographical extremes of Western Australia with 454 amplicon pyrosequencing. Mol. Ecol. 23, 3113–3126. doi: 10.1111/mec.12801
Thornhill, D. J., Howells, E. J., Wham, D. C., Steury, T. D., and Santos, S. R. (2017). Population genetics of reef coral endosymbionts (Symbiodinium, Dinophyceae). Mol. Ecol. 26, 2640–2659. doi: 10.1111/mec.14055
Thornhill, D. J., Lajeunesse, T. C., and Santos, S. R. (2007). Measuring rDNA diversity in eukaryotic microbial systems: how intragenomic variation, pseudogenes, and PCR artifacts confound biodiversity estimates. Mol. Ecol. 16, 5326–5340. doi: 10.1111/j.1365-294X.2007.03576.x
Tonk, L., Bongaerts, P., Sampayo, E. M., and Hoegh-Guldberg, O. (2013). SymbioGBR: a web-based database of Symbiodinium associated with cnidarian hosts on the Great Barrier Reef. BMC Ecol. 13:7. doi: 10.1186/1472-6785-13-7
Van Oppen, M. J. H., Mieog, J. C., Sánchez, C. A., and Fabricius, K. E. (2005). Diversity of algal endosymbionts (zooxanthellae) in octocorals: the roles of geography and host relationships. Mol. Ecol. 14, 2403–2417. doi: 10.1111/j.1365-294X.2005.02545.x
Van Soest, R. W. M., Boury-Esnault, N., Hooper, J. N. A., Rützler, K., de Voogd, N. J., Alvarez, B., et al. (2020). World Porifera Database. Available online at: http://www.marinespecies.org/porifera/index.php (accessed August 18, 2020)
van Woesik, R., Sakai, K., Ganase, A., and Loya, Y. (2011). Revisiting the winners and the losers a decade after coral bleaching. Mar. Ecol. Prog. Ser. 434, 67–76. doi: 10.3354/meps09203
Veerasingam, S., Vethamony, P., Mani Murali, R., and Fernandes, B. (2015). Depositional record of trace metals and degree of contamination in core sediments from the Mandovi estuarine mangrove ecosystem, west coast of India. Mar. Pollut. Bull. 91, 362–367. doi: 10.1016/j.marpolbul.2014.11.045
Wall, C. B., Kaluhiokalani, M., Popp, B. N., Donahue, M. J., and Gates, R. D. (2020). Divergent symbiont communities determine the physiology and nutrition of a reef coral across a light-availability gradient. ISME J. 1–14. doi: 10.1038/s41396-019-0570-1
Wang, Q., Garrity, G. M., Tiedje, J. M., and Cole, J. R. (2007). Naive Bayesian classifier for rapid assignment of rRNA sequences into the new bacterial taxonomy. Appl. Environ. Microbiol. 73, 5261–5267. doi: 10.1128/AEM.00062-07
Williams, S. D., and Patterson, M. R. (2020). Resistance and robustness of the global coral–symbiont network. Ecology 101:e02990. doi: 10.1002/ecy.2990
Yamashita, H., and Koike, K. (2013). Genetic identity of free-living Symbiodinium obtained over a broad latitudinal range in the Japanese coast. Phycol. Res. 61, 68–80. doi: 10.1111/pre.12004
Zhou, G., Cai, L., Li, Y., Tong, H., Jiang, L., Zhang, Y., et al. (2017). Temperature-Driven Local Acclimatization of Symbiodnium Hosted by the Coral Galaxea fascicularis at Hainan Island, China. Front. Microbiol. 8:2487. doi: 10.3389/fmicb.2017.02487
Ziegler, M., Arif, C., Burt, J. A., Dobretsov, S., Roder, C., LaJeunesse, T. C., et al. (2017). Biogeography and molecular diversity of coral symbionts in the genus Symbiodinium around the Arabian Peninsula. J. Biogeogr. 44, 674–686. doi: 10.1111/jbi.12913
Ziegler, M., Eguíluz, V. M., Duarte, C. M., and Voolstra, C. R. (2018). Rare symbionts may contribute to the resilience of coral-algal assemblages. ISME J. 12, 161–172. doi: 10.1038/ismej.2017.151
Keywords: coral, sponge, Cliona, Turbinaria, Symbiodiniaceae, symbiont, ITS2, bioerosion
Citation: Mote S, Gupta V, De K, Hussain A, More K, Nanajkar M and Ingole B (2021) Differential Symbiodiniaceae Association With Coral and Coral-Eroding Sponge in a Bleaching Impacted Marginal Coral Reef Environment. Front. Mar. Sci. 8:666825. doi: 10.3389/fmars.2021.666825
Received: 11 February 2021; Accepted: 01 April 2021;
Published: 08 June 2021.
Edited by:
Jin Sun, Ocean University of China, ChinaReviewed by:
Manoj Kumar, University of Technology Sydney, AustraliaMahshid Oladi, Shahid Beheshti University, Iran
Copyright © 2021 Mote, Gupta, De, Hussain, More, Nanajkar and Ingole. This is an open-access article distributed under the terms of the Creative Commons Attribution License (CC BY). The use, distribution or reproduction in other forums is permitted, provided the original author(s) and the copyright owner(s) are credited and that the original publication in this journal is cited, in accordance with accepted academic practice. No use, distribution or reproduction is permitted which does not comply with these terms.
*Correspondence: Vishal Gupta, v.gupta@bham.ac.uk; Mandar Nanajkar, mandar@nio.org
†Present address: Vishal Gupta, School of Biosciences, University of Birmingham, Birmingham, United Kingdom