- 1Department of Biology and Marine Program, Boston University, Boston, MA, United States
- 2College of Life and Environmental Sciences, University of Exeter, Penryn, United Kingdom
- 3Centre for Sustainable Ecosystem Solutions, School of Earth, Atmospheric and Life Sciences, University of Wollongong, Wollongong, NSW, Australia
Research on sociality in marine fishes is a vibrant field that is providing new insights into social evolution more generally. Here, we review the past two decades of research, identifying knowledge gaps and new directions. Two coral reef fishes, with social systems similar to other cooperative breeders, have emerged as models: the clown anemonefish Amphiprion percula and the emerald goby Paragobiodon xanthosoma. In these systems, non-breeders do not forgo their own reproduction to gain indirect genetic benefits. Rather, they do so because they stand to inherit the territory in the future and there are strong ecological and social constraints. The reasons why breeders tolerate non-breeders remain obscure, though it is plausibly a combination of weak kin selection, bet-hedging, and benefits mediated via mutualistic interactions with cnidarian hosts. The latter is particularly interesting, given the parallels with other social animals with mutualistic partners, such as acacia ants. Looking beyond the two model species, our attention is turning to species with more complex social organization, such as the damselfish Dascyllus aruanus. Here, variable group stability, conflict intensity, and reproductive skew provide opportunities to test theories of social evolution that have only been tested in a few taxa. New methods like social network analysis are enabling us to uncover more subtle effects of ecology on social interactions. More recently, comparative methods have yielded insights into the correlates of interspecific variation in sociality in the genera to which our model species belong. Phylogenetically controlled contrasts within the genus Gobiodon, have revealed the role of ecology, life history traits, and their interaction in sociality: smaller bodied species are more social than larger bodied species, which are only social on large corals. As climate change affects coral reefs, there is a pressing need to understand the many ways in which environmental disturbance influences these unique social systems. In sum, coral reef fishes have enabled us to test the robustness of current theories of social evolution in new taxa and environments, and they have generated new insights into social evolution that are applicable to a wider variety of taxa.
Introduction
A major transition in the evolution of life was animals beginning to live in groups (Szathmáry and Smith, 1995). Animal groups represent some of the most complex forms of life, and they exist on a continuum, from simple gatherings, which dilute the risk of predation, to complex societies with division of labor and reproduction (Sherman et al., 1995; Bourke, 2011). Complex societies, where some individuals forgo reproduction, have been a focus of evolutionary ecology ever since Darwin pointed out that such societies pose difficulties for his theory of natural selection (Darwin, 1859). Since Hamilton’s pivotal insight about kin selection (Hamilton, 1964), the field of social evolution has made significant advances in explaining eusocial societies in insects, and cooperative breeding in birds and mammals (e.g., Woolfenden and Fitzpatrick, 1984; Emlen and Wrege, 1988; Keller and Reeve, 1994; Kokko et al., 2002; Clutton-Brock, 2002; Griffin and West, 2003; Shen et al., 2017). Relatively few studies have attempted to explain similar societies in fishes, and marine fishes in particular have been overlooked (Buston and Balshine, 2007; Taborsky and Wong, 2017). This is likely because of the challenges of working in marine environments and because some criteria considered to be prerequisites for the evolution of complex societies are (presumably) unmet in most marine fishes. However, studying these taxa and their societies has the potential to test the robustness of current theories, generate new insights, and advance the field of social evolution.
Using Marine Systems to Test the Robustness of Current Theories in Social Evolution
The complex groups in which some marine fishes are organized bear many similarities with cooperatively breeding societies in mammals, birds, freshwater fishes and invertebrates (Taborsky and Limberger, 1981; Emlen, 1991; Sherman et al., 1995; Duffy et al., 2000; Bourke, 2011), but there are two key differences. First, alloparental care, where group members care for offspring other than their own, which is a feature of cooperative breeding in birds, mammals and freshwater fishes (Riedman, 1982; Wong and Balshine, 2011), has only very rarely been observed in marine fishes (see review in Wisenden, 1999; Phillips et al., 2020). However, cooperation in marine fishes may take other forms, such as subordinates modifying their growth to remain small and reduce conflict (Buston, 2003a; Wong et al., 2007), or defending and maintaining the territory (Mariscal, 1966; Iwata and Manbo, 2013). Second, the organization in family groups, which characterizes the social systems of most terrestrial species (Emlen, 1995), is lacking in marine systems. The vast majority of marine fishes have a dispersive larval phase, which was long presumed to prevent the formation of kin groups (Victor, 1984; Leis, 1991; Shanks, 2009). However, recent studies have shown that limited dispersal and other mechanisms may lead to subtle relatedness patterns in marine fishes (D’Aloia and Neubert, 2018; D’Aloia et al., 2018; Rueger et al., 2020, 2021), indicating that there is a possibility for weak kin selection to play a role in their social evolution.
Regardless of whether kin selection is operating or alloparental care is occurring in marine fishes, if we dismiss these species from the study of sociality on the grounds that they are different from other social vertebrates, then a great opportunity for expanding and transforming the field is missed. It has been argued that sociality should be viewed as a continuum, rather than falling in narrow categories (Sherman et al., 1995). Therefore, it is more useful for the field overall to study the remarkable behavioral convergence and distinctions between different taxa, rather than exclude large groups of animals from consideration on account of them not meeting specific criteria (Sherman et al., 1995; Hing et al., 2017).
Established Study Systems
The first two decades of social evolution research in marine fishes have focused on two coral reef fish species found in the Indo-Pacific: the clown anemonefish Amphiprion percula (Pomacentridae) and the emerald coral goby Paragobiodon xanthosoma (Gobiidae). The aim of using these fishes was to test the robustness of our current understanding of social evolution and generate new insights. These two fishes were chosen because they bear a striking resemblance to the simple eusocial societies of cooperatively breeding birds and mammals (Emlen, 1991; Sherman et al., 1995; Buston, 2002; Wong, 2007; Wong and Buston, 2013). In both A. percula and P. xanthosoma, groups of individuals are found in close association with cnidarian hosts (anemones or corals) that provide the fish with protection from predators, food and a place to lay their eggs (Lassig, 1976; Fautin, 1992). Each host contains one group of fish, which is typically composed of a breeding pair and a small number of subordinate non-breeders (Figure 1). Within each group there is a size-based dominance hierarchy: the largest two individuals are the breeders, and the non-breeders get progressively smaller (Buston, 2003a; Wong et al., 2007). These fishes, like many coral reef fishes, are hermaphroditic: clown anemonefish can change sex from male to female (Fricke and Fricke, 1977; Moyer and Nakazono, 1978); coral-dwelling gobies can change sex in both directions (Lassig, 1977; Kuwamura et al., 1994; Nakashima et al., 1996; Munday, 2002). Breeding occurs year-round and generally on a lunar cycle; for each egg clutch, the female lays several hundred eggs, which the male fertilizes and then takes care of until they hatch 1 week later (Buston, 2004b; Wong et al., 2008b).
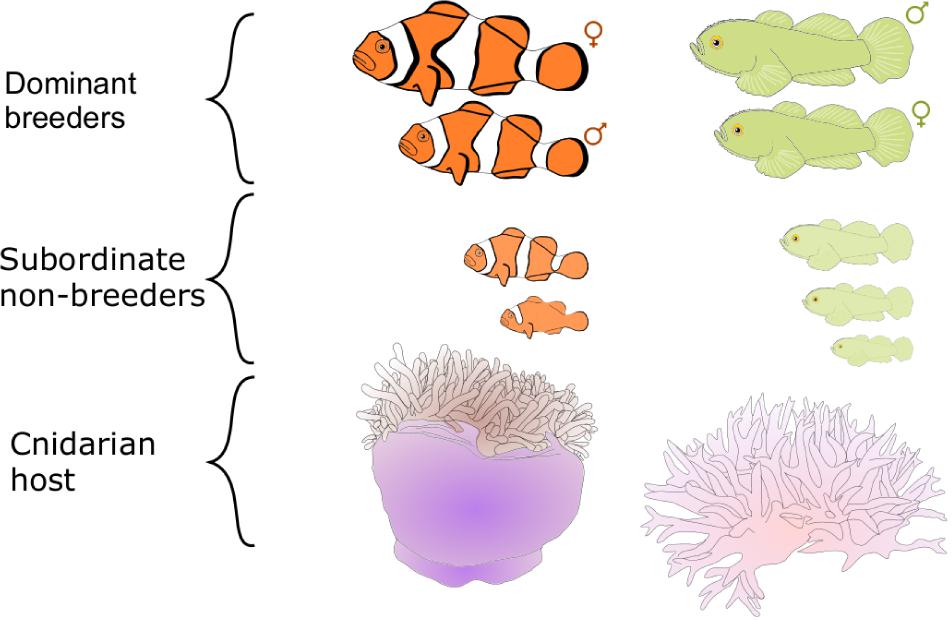
Figure 1. Schematic of the social organization of Amphiprion percula (left) and Paragobiodon xanthosoma (right).
While they have greatly improved our understanding of sociality in the marine realm, focusing solely on A. percula and P. xanthosoma presents some limitations. To broaden our understanding of social evolution, it is crucial to encompass model species with more variable social systems, reproductive skew, and individual mobility. Such species allow us to test the predictions of theoretical models by manipulating genetic, ecological, and social variables (Buston et al., 2007b).
Extending Research From Simple to Complex Social Systems
One good candidate that provides such opportunities is the humbug damselfish, Dascyllus aruanus (Pomacentridae). It is another coral reef fish that is widespread throughout the Indo-Pacific and lives in social groups in close association with branching corals (Sale, 1971; Forrester, 1991; Holbrook et al., 2000). Unlike A. percula and P. xanthosoma, D. aruanus sometimes have multiple coral hosts within their territories, and fish move between them, both on their own and in groups (Mann et al., 2014). Residents of each territory actively repel unfamiliar conspecifics (Schmitt and Holbrook, 1999; Jordan et al., 2010), limiting movement of individuals between territories (Forrester, 1991). Within each territory there is a single group of fish (Sale, 1971; Coates, 1980a; Forrester, 1991), composed of 1–2 breeding males, 3–4 breeding females and 2–4 subordinate non-breeders (Figure 2; Sale, 1972; Holbrook et al., 2000; Wong et al., 2012). A striking feature of humbug damselfish societies is that the mating system is plastic, shifting from monogamy to polygyny to polygynandry as group and coral size increases (Figure 2; Wong et al., 2012). The groups have weakly defined size-based dominance hierarchies: males tend to be the largest dominant individuals, females tend to be intermediate in size, and subordinate non-breeders tend to be the smallest individuals (Figure 2; Coates, 1980a; Cole, 2002; Asoh, 2003; Wong et al., 2012). These fish are generally protogynous hermaphrodites (Sale, 1970; Cole, 2002; Asoh, 2003): if the male of a focal group disappears, then a large female from the focal group or a nearby group changes sex and takes his place (Fricke and Holzberg, 1974; Coates, 1982); if the dominant female disappears, the next ranking male can revert back to being a female if no immigration occurs (Kuwamura et al., 2016). This species breeds on a lunar or semi-lunar cycle: females deposit eggs in a nest and males fertilize the eggs and care for them for 2–5 days until they hatch (Sale, 1970; Mizushima et al., 2000).
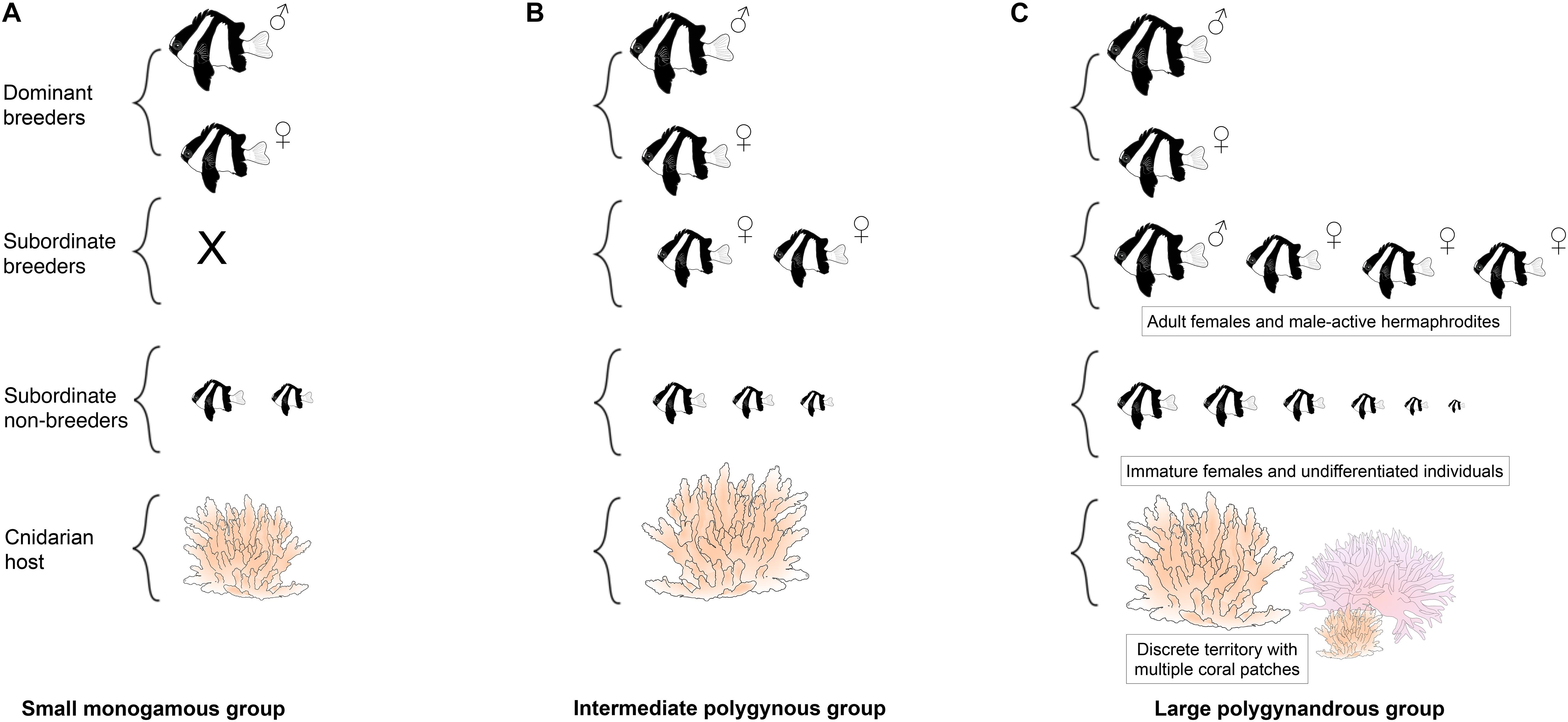
Figure 2. Schematic of the variable social organization (A–C) of Dascyllus aruanus. X denotes no individuals present at that rank.
In this review we synthesize the existing literature on A. percula, P. xanthosoma, and D. aruanus, highlighting how they have contributed to our understanding of sociality in the marine environment. We show that studying these and other marine fishes provides new insights into the evolution of sociality, and we uncover knowledge gaps and suggest future directions in the field.
Part 1: Why Do Non-Breeders Forgo Reproduction?
Most of the major hypotheses of social evolution that pertain to why subordinate non-breeders forgo their own reproduction have been tested in marine fishes using long-term monitoring, experimental manipulations, molecular tools and mathematical modeling throughout the past two decades (Table 1).
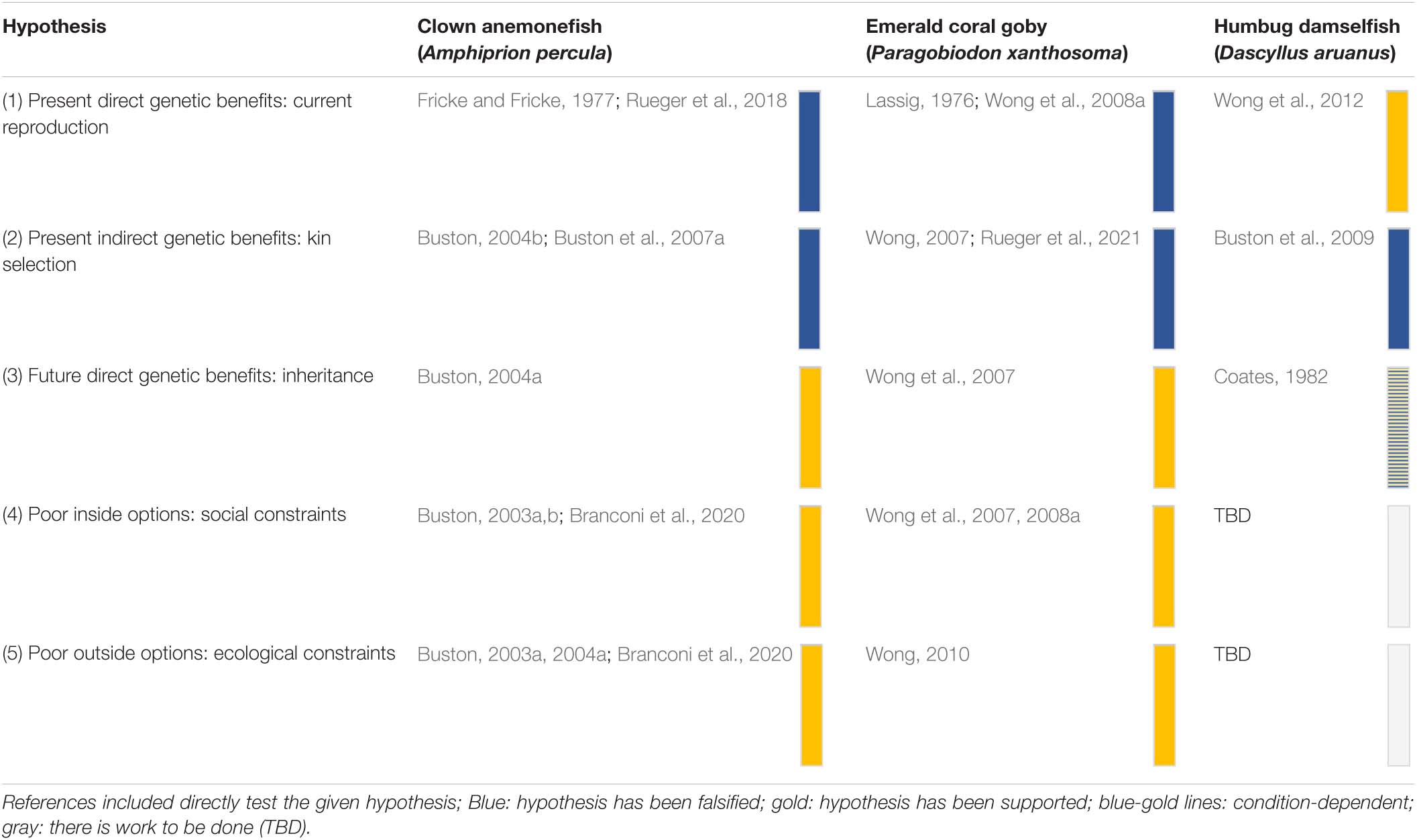
Table 1. Major hypotheses for why non-breeders forgo their own reproduction in three tractable systems within coral reefs: the clown anemonefish, the emerald coral goby and the Humbug damselfish.
Present Direct Genetic Benefits: Current Reproduction
The first question to address is whether subordinates in our model systems truly are non-breeders. Subordinates in A. percula and P. xanthosoma do not have functional gonads (Lassig, 1976; Fricke and Fricke, 1977; Moyer and Nakazono, 1978), and they do not develop functional gonads due to the threat of eviction (Wong et al., 2008a; Rueger et al., 2018). This confirms that the subordinates truly are non-breeders, and it demands further investigation as to why they choose to forgo reproduction and cooperate in social groups.
In contrast, for D. aruanus early evidence indicated that subordinates do have functional gonads (Asoh, 2003; Cole, 2002). A study combining field observations with genetic parentage analysis showed that high ranking and large individuals (dominants and high-ranking subordinates), from within the group as well as extra-group individuals, are more likely to breed and attain large reproductive shares than low-ranking and small individuals (Wong et al., 2012). However, low-ranking and small D. aruanus do reproduce, and reproductive skew is very variable compared to A. percula or P. xanthosoma.
Present Indirect Genetic Benefits: Kin Selection
The kin selection hypothesis makes two critical predictions: first, non-breeders enhance the fitness of breeders; second, non-breeders are closely related to breeders (Hamilton, 1964; Emlen and Wrege, 1988; Griffin and West, 2003). Both predictions have been tested using A. percula and P. xanthosoma. In A. percula, a removal experiment revealed that non-breeders had no direct effect on the survival or reproduction of breeders during a year-long study (Buston, 2004b). Similarly, there was no evidence that P. xanthosoma non-breeders engaged in behaviors that might enhance survival or reproduction of the breeders (Wong, 2007). Genetic analysis showed that subordinate non-breeders were not closely related to the breeders in A. percula (Buston et al., 2007a) or P. xanthosoma (Rueger et al., 2021).
Although the first prediction that non-breeders enhance the fitness of breeders has not been tested experimentally for D. aruanus, it seems likely to be supported in small groups where the subordinates are females, since the fitness of the dominant male is enhanced by having more potential mates. However, the answer may be more nuanced in large groups where some of the subordinates are males, since it is not clear how they impact the fitness of dominant breeders (Wong et al., 2012). The second prediction that non-breeders are closely related to breeders has been tested directly. The mean coefficient of relatedness among group members was close to zero, and any pairs of close relatives were small and similar in size, suggesting that siblings may recruit together but that kin associations break-up post recruitment (Buston et al., 2009).
Taken together, the results from all three species suggest that kin selection does not play a role in explaining why non-breeders tolerate their position in coral reef fishes. The limited role of kin selection in all three model species is interesting given that kin selection is thought to be the major driver of these types of social systems in other taxa.
Future Direct Genetic Benefits: Territory Inheritance
The territory inheritance hypothesis makes two critical predictions: first, non-breeders have the capacity to reproduce in the future; second, the probability of territory inheritance is high (Williams, 1966; Kokko and Johnstone, 1999). Both predictions were tested using A. percula and P. xanthosoma. In both species subordinate non-breeders have the capacity to reproduce in the future, as they filled breeding vacancies when dominant breeders were removed (Buston, 2004a; Wong et al., 2007). In all cases it was the highest ranked non-breeder (rank three in the group hierarchy) that inherited the breeding vacancy; in no cases did a smaller non-breeder from a lower rank in the same coral/anemone or a non-breeder from elsewhere usurp the breeding vacancy (Buston, 2004a; Wong et al., 2007). Taken together, these results are some of the clearest demonstrations (not confounded by kin selection) that individuals will adopt non-breeding positions because of the potential to reproduce in the future. These studies indicate that territory inheritance is a driving force behind the evolution of non-breeding strategies in coral reef fishes.
In D. aruanus, the first prediction that non-breeders have the capacity to reproduce in the future is likely less crucial, because parentage analyses indicate that subordinates gain some current reproduction (Wong et al., 2012). Regarding the second prediction that the probability of territory inheritance is high, dominant male-removal experiments showed that when the experimental corals were caged, it was the largest female of the group that changed sex and took his place (Coates, 1982). However, sexually mature individuals also have the potential to move from one territory to another (Figure 3; Sale, 1971; Asoh, 2003; Wong et al., 2012), which would reduce the probability of territory inheritance relative to A. percula and P. xanthosoma, because resident individuals can be usurped in some contexts.
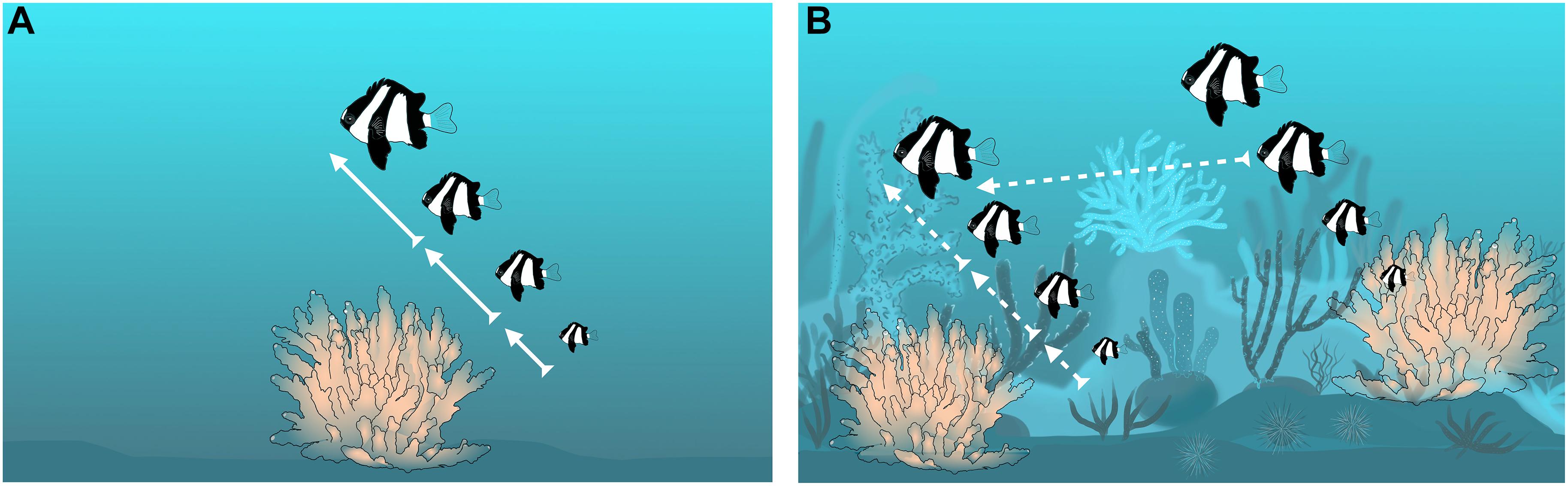
Figure 3. Relationship between ecological constraints and territory inheritance within groups of Dascyllus aruanus; arrows represent changes in rank via inheritance or movement (bold continuous arrows show high probability of territory inheritance in the group; dashed arrows show some lower probability of territory inheritance in the group and some probability of inheritance via movement from the neighboring group). (A) Patchy habitat with strong ecological constraints, little movement and high probability of territory inheritance; (B) More continuous habitat, with weak ecological constraints, lots of movement, and lower probability of territory inheritance.
Poor Inside Options: Social Constraints
The social constraints hypothesis makes two predictions: first, individuals will remain in groups and engage in cooperative actions when there is some social constraint; second, critically, the likelihood of individuals contesting to breed will increase when the social constraint is relaxed (Muthoo, 2000; Buston and Zink, 2009). In A. percula and P. xanthosoma, well-defined size differences are maintained between individuals adjacent in rank by precise regulation of subordinate growth (Buston, 2003b; Buston and Cant, 2006; Wong et al., 2007). In A. percula, higher ranked individuals evict or occasionally kill subordinates that are similar in size to themselves (Allen, 1972; Buston, 2003a). In both species, the likelihood of a subordinate winning a contest is zero when the pair’s size ratio matches that found under natural conditions (Wong et al., 2007, 2016), indicating that the size ratio represents a social constraint. In experimental settings, subordinates were more likely to contest and sometimes won a fight when the social constraints were relaxed (Wong et al., 2007; Branconi et al., 2020). These results demonstrate that strong social constraints are a driving force behind the evolution of non-breeding strategies in coral reef fishes.
To date, no experimental studies have been conducted to test the social constraints hypothesis in D. aruanus. However, multiple features of humbug damselfish societies point toward less social constraints operating than in other systems. In D. aruanus groups, there is no well-defined size ratio (sensu Buston and Cant, 2006) between individuals of different ranks. This suggests that social constraints over rank and reproduction may be more relaxed in this species, as shown by the frequent occurrence of reproduction by subordinates (Wong et al., 2012). Alternatively, other factors may influence subordinate reproduction. For example, the size of prey taken by individuals is not correlated with their absolute size but with their rank (Coates, 1980b); this could affect the amount of time spent by subordinates foraging for food, their energy budgets and, in turn, their fitness. Future research with experimental manipulation of inside options analogous to Branconi et al. (2020) is needed in D. aruanus.
Poor Outside Options: Ecological Constraints
The ecological constraints hypothesis makes two predictions: first, individuals will remain in groups and engage in cooperative actions when there is some ecological constraint; second, critically, the likelihood of individuals leaving to breed will increase when the ecological constraint is relaxed (Emlen, 1982; Cant and Johnstone, 2009). Both predictions have been tested using A. percula and P. xanthosoma. In both species, there are two types of ecological constraints: (i) it is risky to move between patches of habitat (Mariscal, 1970; Lassig, 1981; Elliott et al., 1995) and (ii) the alternative habitat is saturated (Lassig, 1977; Fautin, 1992; Elliott and Mariscal, 2001). In A. percula, non-breeders did not leave to breed elsewhere when habitat vacancies were created, showing that habitat saturation alone does not prevent them from dispersing (Buston, 2003a, 2004a). In both species, cross-factored experiments showed that the likelihood of dispersal increased as alternative habitats became less saturated and as risks of movement decreased (Wong, 2010; Branconi et al., 2020). These results demonstrate that individuals will adopt non-breeding positions because of the combination of habitat saturation and risks of movement, indicating that strong ecological constraints are a driving force behind the evolution of non-breeding strategies in coral reef fishes.
Observational evidence suggests that ecological constraints will also play a role in D. aruanus but may be condition-dependent. Small immature juveniles move more frequently between coral heads on continuous reef habitats (lower risk of movement) than in patchy reef habitats (higher risk of movement) (Nanami and Nishira, 2001). In addition, the survival rate was 3.3 times higher on continuous habitat than on patchy habitat, suggesting that there are real risks associated with living in patchy habitats (Nanami and Nishira, 2001). Large sexually mature individuals are known to move between groups to breed (Figure 3; Sale, 1971; Asoh, 2003). The reasons that large individuals might move more than small individuals are twofold: (i) large individuals are less likely to move forward in their resident queue than small individuals, because there are fewer individuals ahead of them to die; and, (ii) large individuals are less likely to be preyed upon while moving between groups than small individuals, because they are faster and exceed the gape limitation of more predators. To tease apart the relative effects of these factors and assess the validity of the ecological constraints hypothesis in D. aruanus, more experimental work analogous to Wong (2010) and Branconi et al. (2020) is needed.
Part 2: Why Do Breeders Tolerate Non-Breeders?
The major hypotheses looking at why dominant breeders would tolerate non-breeders that share their territories have received much less attention in marine fishes (Table 2).
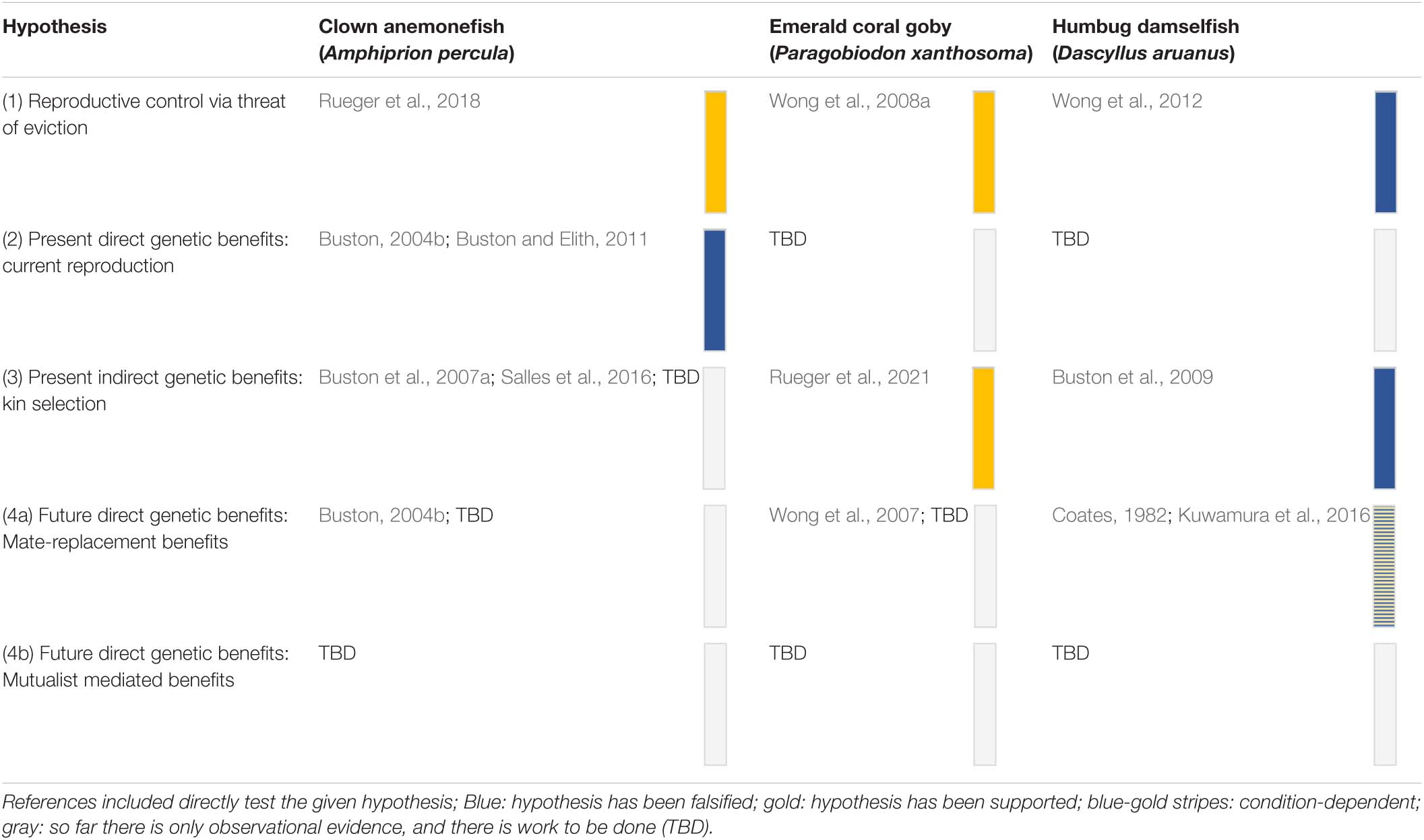
Table 2. Major hypotheses for why breeders tolerate non-breeders in their territories in three tractable systems within coral reefs: the clown anemonefish, the emerald coral goby and the Humbug damselfish.
Reproductive Control via Threat of Eviction
The reproductive control hypothesis makes two predictions: reproduction is resource-limited; and reproduction in subordinates is suppressed via the threat of eviction (Clutton-Brock et al., 2010). Both predictions have been tested using P. xanthosoma and A. percula. In both species, a feeding experiment revealed that reproduction by the dominant female is resource limited, providing an incentive for dominants to evict subordinates (Wong et al., 2008a; Rueger et al., 2018). Such evictions do indeed occur if subordinates are sexually mature (Wong et al., 2008a; Rueger et al., 2018). Overall, these studies provide clear evidence that dominants use the threat of eviction to keep subordinates from reproducing. The fact that dominants have the ability to evict subordinates suggests that subordinates are tolerated either because they are inconsequential or because they provide some benefit to dominant breeders.
In contrast, D. aruanus subordinate males and females can obtain large shares of total reproduction within their groups (Wong et al., 2012). While reproduction by males is limited by the number of females and eggs produced, no studies have tested whether dominants try to suppress the reproduction of same-sex subordinates or whether reproduction is resource-limited for females. Investigating how reproductive shares of both sexes are negotiated will be an interesting avenue of future research.
Present Direct Genetic Benefits: Current Reproduction
The present direct genetic benefits hypothesis predicts that the dominant breeders will accrue some immediate fitness advantages from the presence of non-breeders (Woolfenden and Fitzpatrick, 1984; Emlen and Wrege, 1988). In A. percula, this was tested using year-long observations of survival, growth and reproduction of 71 groups together with an experimental manipulation of 14 groups where subordinates were removed from breeding pairs (Buston, 2004b). Non-breeders had no effect on the survival, growth or reproduction of breeders, which rules out the possibility that present direct genetic benefits motivate breeders to tolerate non-breeders in their groups (Buston, 2004b; Buston and Elith, 2011). The generality of these findings has yet to be tested using P. xanthosoma.
For the dominant male in D. aruanus groups, having multiple breeding females may increase the number and genetic diversity of offspring he can sire, as long as he can provide sufficient parental care (Forsgren et al., 1996; Mizushima et al., 2000; Wong et al., 2012). For the dominant female, it is possible that the presence of subordinates enables her to feed more and produce more eggs because of the increased vigilance of the group and/or have higher survival due to predator dilution effects in larger groups (Rubenstein, 1978; Beauchamp, 2015). Future work is needed to experimentally test these hypotheses.
Present Indirect Genetic Benefits: Kin Selection
The kin selection hypothesis predicts that the dominants benefit from the presence of non-breeders because they are relatives that inherit the breeding territory. For such kin selection to operate, relatedness within groups does not need to be high but it does need to be higher than the population average (Hamilton, 1963, 1964; West-Eberhard, 1975). In A. percula, in a study using seven microsatellite markers to assess relatedness within nine groups, there was no evidence that these groups were on average composed of close relatives (r < 0.001; Buston et al., 2007a). However, low numbers of markers and samples may be insufficient to detect subtle relatedness patterns. A complete genealogy revealed that A. percula offspring often settle close to their parents and close relatives are sometimes found in the same group (Salles et al., 2016), suggesting there may be potential for weak kin selection. Recent research on P. xanthosoma, using a larger microsatellite panel and greater sample size (20 microsatellite markers and 16 groups), found evidence that groups are composed of distant relatives (r = 0.026; Rueger et al., 2021). This suggests that weak kin selection might tip the balance, motivating the dominants to tolerate subordinates within their territories even if they provide no other benefits. This and other recent examples of fine-scale relatedness patterns in marine fishes [likely caused by limited dispersal (Rueger et al., 2020)] underline the necessity to assess genetic relatedness when studying marine fishes, so that the potential for weak kin selection is not prematurely dismissed.
For D. aruanus, kin selection plays a role only for the early life stages, since siblings may be recruiting together but kin associations break up after settlement (see section “Present indirect genetic benefits: kin selection”, Buston et al., 2009). Accordingly, there are no present indirect genetic benefits conferred to the dominants by tolerating subordinates in D. aruanus.
Future Direct Genetic Benefits
Rapid Mate-Replacement Benefits
The mate-replacement hypothesis predicts that the dominants benefit from the presence of non-breeders because they serve as rapid mate replacements should one of the breeders perish (Fricke, 1979). This hypothesis was tested in A. percula, and the mean time taken for a widowed female to recommence breeding was only 2.3 lunar months less in the presence of non-breeders versus in their absence; this suggests that females who tolerated at least one subordinate non-breeder had just a 2% gain in relative fitness (Buston, 2004b). It is possible that instead, the major benefit comes in the form of reducing the variance rather than the mean in the time taken to recommence breeding (Rubenstein, 2011; Koenig and Walters, 2015), though this has not been tested in A. percula. When breeding females were removed from P. xanthosoma groups, non-breeders took their place within days and none of the breeding vacancies were taken over by an individual from another group (Wong et al., 2007). Mate-replacement benefits may be more important in P. xanthosoma than A. percula, because P. xanthosoma are estimated to have shorter breeding tenures than A. percula (Kuwamura et al., 1996; Buston and García, 2007) so that a single month of lost reproduction is a greater fraction of their total reproduction. The magnitude of rapid mate-replacement benefits and the generality of these findings are yet to be determined.
In D. aruanus, no studies have directly tested if subordinate non-breeders act as rapid mate replacements. However, the frequent occurrence of immigration by extra-group individuals (Fricke and Holzberg, 1974; Coates, 1982; Wong et al., 2012) suggests that sexually maturing subordinates may not represent the fastest mate-replacement option in this species, at least on continuous reef where ecological constraints are relaxed. Dominant male-removal experiments showed that another male or a large female (that can change sex to male) will frequently immigrate from another group to fill breeding vacancies (Coates, 1982). On the other hand, dominant female-removal experiments showed that small males can change sex back to female when no females or juveniles immigrate to their group (Kuwamura et al., 2016). The latter study suggests that, in isolated groups, subordinates may act as rapid mate replacements. Evidently, mate-replacement benefits will be context-dependent.
Mutualist Mediated Benefits
The mutualist mediated benefits hypothesis predicts that dominant breeders benefit from the presence of subordinate non-breeders because (i) non-breeders enhance the survival, growth, and size of the cnidarian hosts, and (ii) large cnidarian hosts enhance the survival, growth, and reproduction of the breeders. These synergistic effects, whereby the group achieves things that the breeders alone cannot (Bourke, 2011), have not been tested directly in any of the three focal species, but a range of evidence points toward the plausibility of the hypothesis.
There are positive correlations between the length of the dominant, the number of individuals in the group, and the size of the cnidarian host in A. percula and P. xanthosoma (Figure 4; Fautin, 1992; Elliott and Mariscal, 2001; Buston, 2003a; Wong, 2011; Chausson et al., 2018; Barbasch et al., 2020). These correlates could be caused by extrinsic factors, e.g., dominants, groups, and cnidarians may all flourish at good sites on the reef (the null hypothesis), or they could be caused by intrinsic factors, e.g., the number of fish in a group influencing host size. Indeed, the causality of some of these relationships has been determined: female size influences the number of fish in a group, due to the rules of the size hierarchy in both species (A. percula – Heteractis magnifica, Buston, 2003b; Buston and Cant, 2006; Branconi et al., 2020; P. xanthosoma – Seriatopora hystrix, Wong et al., 2007; Wong, 2011); and, at least in A. percula, anemone size is positively correlated with the growth of the fish, explaining why larger anemones are associated with larger females (Buston, 2002), and larger females lay more eggs, resulting in more parental care and higher embryo survival (Buston and Elith, 2011; Barbasch et al., 2020). Thereby larger anemones might have a positive influence on fish reproductive output. The latter results are likely explained by larger anemones providing greater foraging area, because foraging is confined to the anemone (Barbasch et al., 2020), and/or larger anemones providing more egesta, which may provide important nutrition (Verde et al., 2015). The critical experiment, manipulating cnidarian size and examining the effect on the breeders remains to be done.
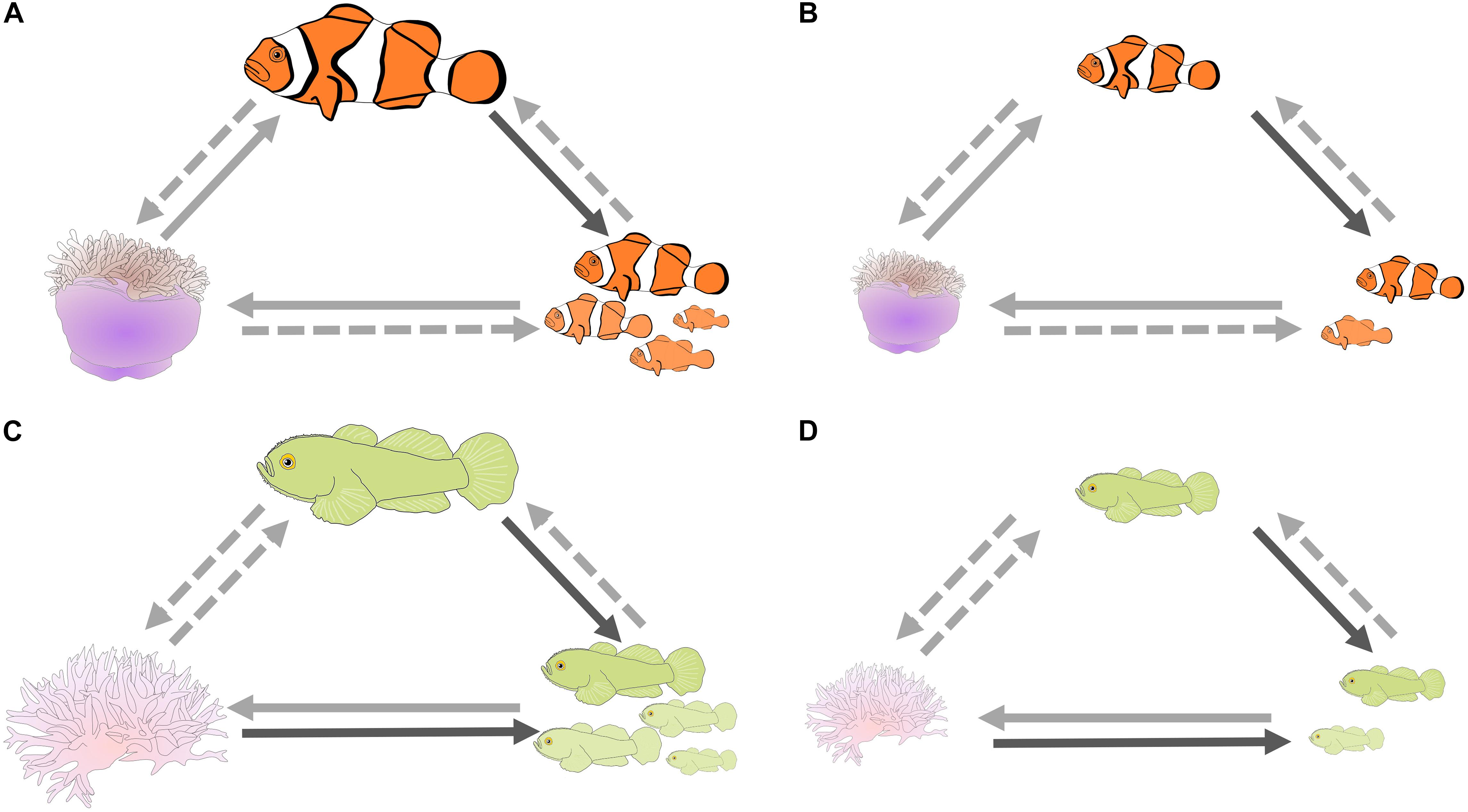
Figure 4. Potential synergistic effects between cnidarian hosts and social coral reef fishes that could help explain why breeders tolerate non-breeders; schematic of the relationships between length of the dominant individual, number of individuals in the group, and size of the cnidarian host for Amphiprion percula – Heteractis magnifica, and Paragobiodon xanthosoma – Seriatopora hystrix; (A,C) respectively, large host, large dominant breeder and large group, (B,D) small host, small dominant breeder and small group. Dark solid arrows: causality confirmed for the model species; light solid arrows: causality has been confirmed in closely related species; light dashed arrows: causality yet to be confirmed. Note the potential for positive feedback if all three arrows are confirmed clockwise or counterclockwise.
For both model species, we are also missing experimental tests of the first prediction, that non-breeders enhance the fitness of the cnidarian host. However, several mechanisms are plausible, and they have been experimentally demonstrated in other anemone-anemonefish interactions. The number of individuals in a group influences anemone size, due to effects on anemone growth and expansion behavior (A. bicinctus – Entacmaea quadricolor, Porat and Chadwick-Furman, 2004; A. chrysopterus – H. magnifica, Holbrook and Schmitt, 2005; and, A. melanopus – E. quadricolor, Frisch et al., 2016), and the presence of anemonefish facilitates recovery of anemones after bleaching events (A. akindynos – E. quadricolor, Pryor et al., 2020). A suite of cooperative behaviors directed toward the anemone have been observed in A. percula, including defensive behaviors toward anemone predators, and cleaning (Mariscal, 1966; Iwata and Manbo, 2013). It is plausible that similar behaviors beneficial to the host also occur in P. xanthosoma, because they have been found in closely related gobies. For example, Gobiodon histrio and P. echinocephalus trim competing seaweed and reduce coral damage in Acropora spp. (Dixson and Hay, 2012). In P. xanthosoma, the coral size has been shown to influence the number of individuals in a group in a manipulative experiment (Thompson et al., 2007).
In D. aruanus, there is also a correlation between fish group size and coral host size (Wong et al., 2012). Several studies suggest that the presence of fish might enhance the survival and growth of their coral hosts by: (i) providing aeration to the corals (Goldshmid et al., 2004); (ii) defending the coral from predation by corallivorous fish (Chase et al., 2014); (iii) enhancing coral bleaching resilience and recovery (Chase et al., 2018); and (iv) alleviating the impact of sediments on corals (Chase et al., 2020). Potentially, all of these mechanisms may be more pronounced and effective when groups are larger with multiple subordinates. While the effect of subordinate behaviors on the cnidarian hosts has yet to be measured directly, their occurrence in the group as a whole increases the plausibility of the hypothesis that non-breeders may have a positive effect on the host which, in turn, positively impacts breeders and provides incentives for breeders to tolerate non-breeders.
Conclusion of Parts 1 and 2
Why subordinates in coral reef fish societies forgo their own reproduction has been thoroughly investigated. Unlike cooperatively breeding birds and mammals, subordinates do not gain indirect genetic benefits. Rather, subordinates gain direct genetic benefits in the future, because they stand to inherit the breeding territory. They behave peacefully instead of contesting for a breeding position due to social constraints; they remain in the group instead of dispersing to breed elsewhere due to ecological constraints. This solves the paradox of why individuals forgo their own reproduction in marine fishes. More generally, it shows that non-breeding strategies can evolve, and complex groups can form, in the absence of kin selection.
Subordinate non-breeders in groups of A. percula and P. xanthosoma are tolerated, despite resource-limited reproduction and the demonstrated ability of dominant breeders to evict them. Why dominants tolerate subordinates may be explained by the effects of several factors that each confer small fitness advantages to the breeders. First, dominants might accrue some indirect genetic benefits from tolerating their distant relatives who go on to inherit the territory (Rueger et al., 2021). Second, dominants might accrue some future genetic benefits by tolerating subordinates who serve as rapid mate replacements (Buston, 2004b). Third, dominants might accrue some future genetic benefits by tolerating subordinates who contribute to the growth of the mutualistic host, which in turn helps breeders grow and reproduce more. The latter has yet to be tested directly in either model species, but some evidence indicates it may be a fruitful topic for future investigations.
Dascyllus aruanus has a much more variable social system and consequently their social group formation is more challenging to understand. In D. aruanus, present direct genetic benefits exist; subordinate males and females gain benefits by reproducing for themselves, and the dominants benefit either by reproducing with subordinates or gaining other fitness benefits due to the presence of subordinates. However, how their reproductive shares are determined remains to be seen. Kin selection only plays a limited role. The magnitude of future genetic benefits, in terms of territory inheritance for the subordinates and rapid mate replacement for the dominants, are dependent on the ecological context: individuals move readily between groups in continuous habitats when ecological constraints are weaker; individuals move less between groups in patchy habitats when ecological constraints are stronger. Future investigations, direct experiments, and the application of more innovative methods such as social network analysis (SNA), are essential to better understand why these fish live in such complex social groups (Box 1). The use of D. aruanus as a complex model system for the research of social evolution has the potential to generate new insights into the origin and maintenance of social systems in coral reef fishes and other marine taxa.
BOX 1. Complex methods for more complex groups.
While experimental approaches can help us understand some of the mechanisms involved in the origin and maintenance of social systems in coral reef fishes, the application of social network analysis (SNA) offers an opportunity to understand sociality on a finer scale. This is particularly crucial for species such as D. aruanus, that have larger groups and more variable dominance structures. SNA is one of the most powerful and effective methods used in evolutionary biology to characterize the temporary internal structure of social groups and their stability over time (Croft et al., 2008; Sueur et al., 2019; Romano et al., 2020; Sosa et al., 2021). In SNA, social entities (individuals) are considered nodes and their social relationships (interactions) are considered ties (Box Figure 1). This reveals the group network as a web of direct and indirect inter-relationships that provide a holistic perspective for the study of sociality and group dynamics (Croft et al., 2008; Wey et al., 2008).
With the ability to depict different levels of interactions within groups (dominant vs. subordinate individuals) and between groups (intra- vs. extra-group individuals), SNA could help us solve many open questions relating to D. aruanus sociality. In D. aruanus individuals show clearly defined aggressive and submissive interactions (Branconi et al., 2019a), making it possible to characterize social networks. For example, SNA, together with new tagging methods (Branconi et al., 2019b), may help evaluate group structure and stability across time, according to habitat quality and different degrees of social and ecological constraints.
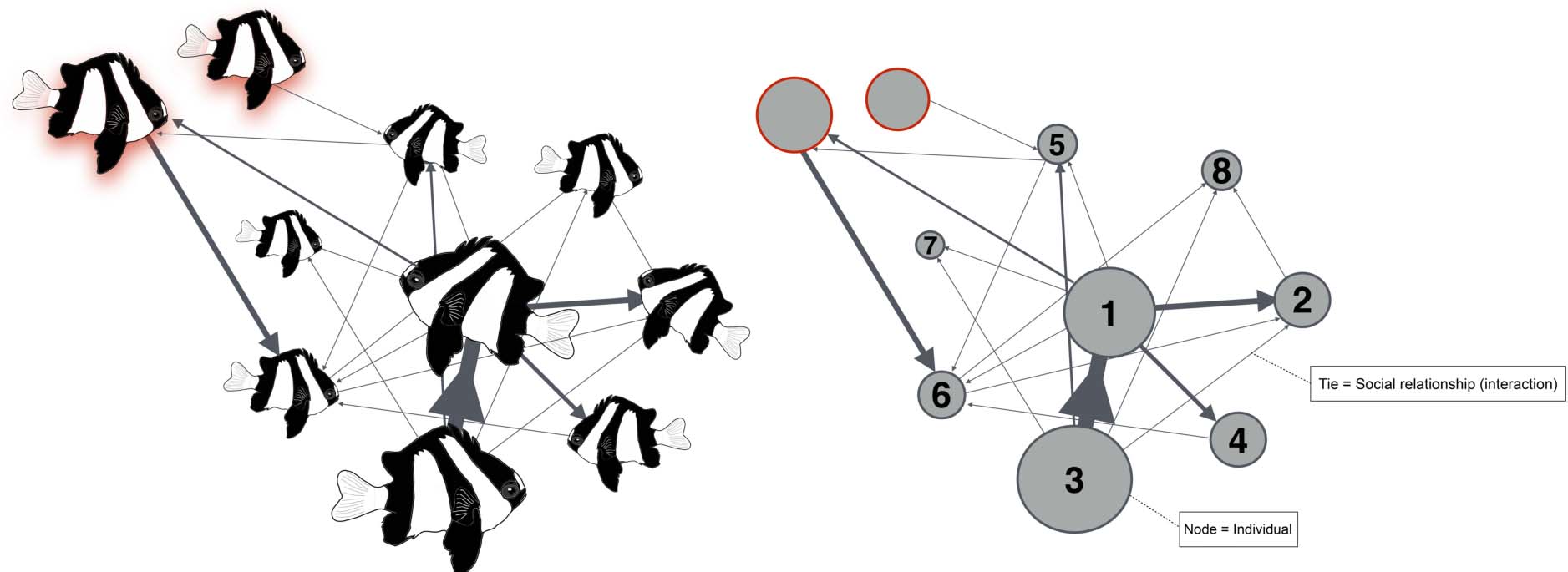
Box Figure 1. Hypothetical weighted and directed social network of a small group of Dascyllus aruanus. The thickness of the ties (interactions) is proportional to the strength of the connection between the individuals, and the size of the nodes (individuals) is proportional to their respective number of social interactions. Numbers denote individual ID. Red shaded individuals/nodes denote extra-group individuals.
Part 3: From Model Species to Model Genera
Amphiprion percula, P. xanthosoma, and D. aruanus have provided valuable insights into sociality in marine fishes. Moving beyond the investigation of these model species to comparative studies among congeners is an important next step, marking a transition from trying to understand what drives sociality within a species, to what drives variation in sociality among species. This next step is crucial for determining the extent to which variation in social systems across closely related species is explained by variation in key drivers of social evolution. In turn, this assists in discerning the conditions that likely gave rise to sociality (Brown, 1974; Kocher and Paxton, 2014; Hing et al., 2017).
The most important step in explaining drivers of interspecific social variation is to conduct phylogenetically controlled comparisons. Such a study has been conducted using the coral-dwelling gobies from the genus Gobiodon whose phylogeny is relatively well resolved (e.g., Harold et al., 2008; Herler et al., 2009; Duchene et al., 2013; Hing et al., 2019). Recent research on Gobiodon has investigated social variability by adopting an integrative approach that examines ecological factors and life-history traits together with phylogenetically controlled contrasts. Hing et al. (2019) used 15 Gobiodon species to measure the diversity and frequency of group sizes and calculated a sociality index for each species. The sociality index (outlined in Avilés and Harwood, 2012) accounts for the proportion of groups within the population, proportion of subordinates within groups (indicating propensity to join a group and be tolerated by dominants), and proportion of the life cycle spent in groups (indicating propensity for delayed dispersal). The sociality index for each Gobiodon species was used to determine the phylogenetic signal of sociality, and its link to factors previously identified as influencing fitness (ecological factors: host coral size and host coral generalization; and life-history trait: body size; Wong, 2011; Hing et al., 2018). While there was some evidence of a weak phylogenetic signal (i.e., ancestral basis) in the evolution of sociality in Gobiodon, ecological and life history factors were found to play more important roles (Hing et al., 2019). Larger-bodied species tended to be less social than smaller-bodied species (Hing et al., 2019). Furthermore, sociality depended more on coral size for larger species, likely due to the requirement for larger hosts in order for larger individuals to form groups (Figure 5; Hing et al., 2019). Interestingly, one of the few other comparative studies examining relationships between evolutionary history, ecological factors and life-history traits in marine fishes found that there was a strong ancestral basis for sociality (pair-forming versus solitary living) in Chaetodon butterflyfishes (Nowicki et al., 2018). Further, Hodge et al. (2018) identified the trade-off between morphological defense strategies and social organization as a crucial driver of butterflyfish evolution, highlighting the importance of employing broad phylogenetically informed approaches in studying reef fish sociality.
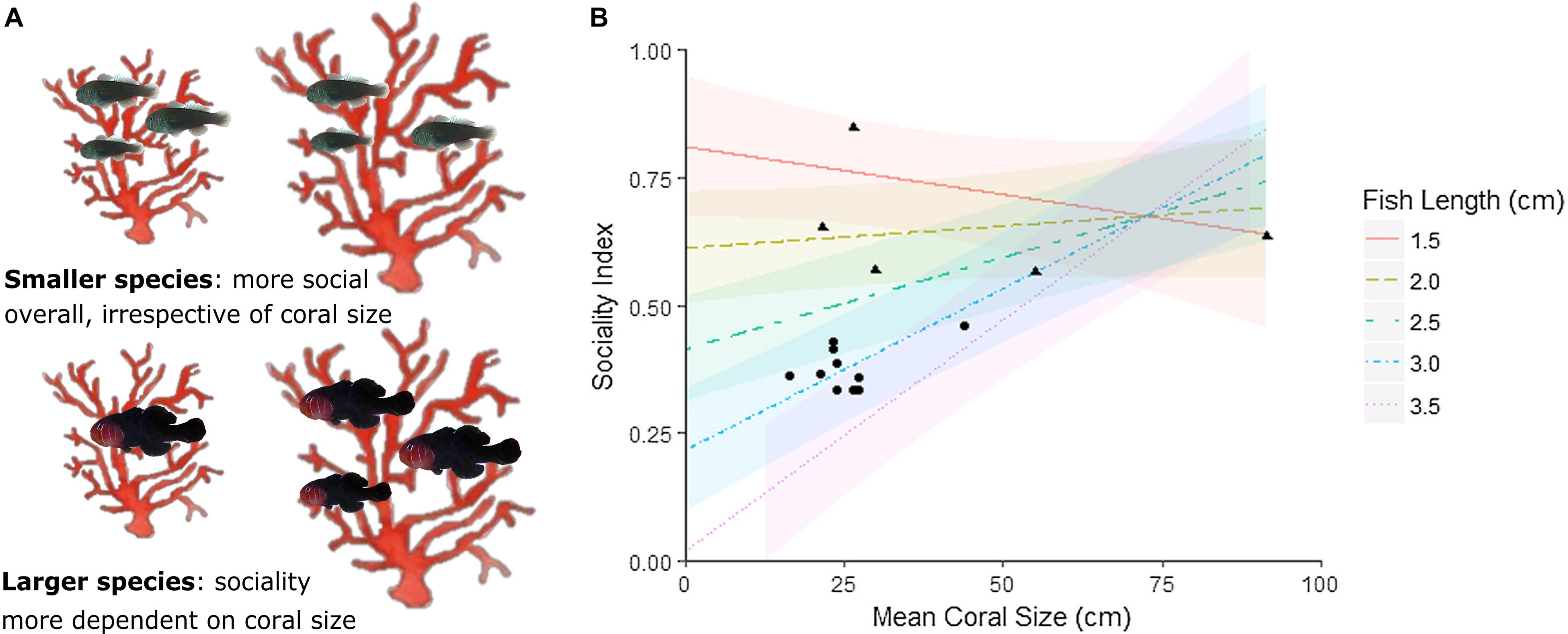
Figure 5. Relationships between social group size, host Acropora coral size, and body size for species in the genus Gobiodon. (A) Schematic depicting variation in group sizes between small and large species in relation to coral size; (B) modeled predictions for interactions between coral size and body size (fish length) and their effects on the sociality index (encompassing the proportion of groups within the population, proportion of subordinates within groups, and proportion of the life cycle spent in groups; see Avilés and Harwood, 2012) for Gobiodon species. Raw data are the black symbols where pair-forming species are represented by circles and group-forming species are represented with triangles. Modeled body sizes are represented by the colored lines shown in the legend (Figure modified from Hing et al., 2019). The figure is reproduced with permission from Elsevier.
In anemonefishes, there has been no formal investigation of interspecific variation in their social behavior using phylogenetically controlled comparisons, even though their phylogenetic relationships have been well resolved (e.g., Elliott et al., 1999; Santini and Polacco, 2006; Litsios et al., 2012; Litsios and Salamin, 2014; Li et al., 2015; Rolland et al., 2018). However, there is some observed social variation between species that are ecologically similar, which indicates that Amphiprion species are good candidates for comparative studies. Amphiprion ocellaris societies associated with the anemone Stichodactyla gigantea in Indonesia seem to function quite similarly to the A. percula societies associated with the anemone Heteractis magnifica in Papua New Guinea described above (Mitchell, 2003, 2005). In contrast, A. perideraion societies associated with H. magnifica in the same lagoons and bays as A. percula in Papua New Guinea seem to be subtly different (Allen, 1972; Fautin and Allen, 1992; Elliott and Mariscal, 2001; Buston and Cant, 2006; Rueger et al., 2018). The number of individuals in a group, the structure of the size hierarchy, aggression, and cooperative behaviors are traits that seem to vary across the genus (Allen, 1972; Moyer and Nakazono, 1978; Hattori, 1994; Srinivasan et al., 1999; Mitchell and Dill, 2005), raising the question: what causes this variation?
The strength of kin selection seems unlikely to vary greatly across the Amphiprion genus, because in all studies to date, their larvae have been shown to disperse from their natal anemone (A. polymnus, Jones et al., 2005; Saenz-Agudelo et al., 2011; A. percula, Almany et al., 2007; Planes et al., 2009; Buston et al., 2012; Almany et al., 2017; A. omanensis, Simpson et al., 2014; A. bicinctus, Nanninga et al., 2015; A. clarkii, Catalano et al., 2020). The strength of ecological constraints might vary across the genus, causing differences in movement analogous to the differences seen in D. aruanus in patchy and continuous habitats (see section “Poor inside options: social constraints” above; Figure 3). Ecological constraints can vary among species either because the ecology varies, e.g., A. clarkii moves more in sub-tropical waters than tropical waters perhaps due to reduced risks of predation and/or reduced habitat saturation (Hattori, 1994), or because the species traits vary while the ecology is constant, e.g., A. perideraion moves more than A. percula in the same waters (Rueger et al., 2018), perhaps due to being a better swimmer. A small reduction in the strength of ecological constraints is likely to have effects on territory inheritance because it creates the potential for individuals to have their position usurped (Figures 3, 6). This, in turn, could have knock-on effects for the size hierarchy, conflict, and aggression, as the incentives for subordinates to remain small are reduced (Figure 6). More work needs to be done to test these hypotheses and to understand the causes of interspecific variation in social behavior among closely related species of marine fishes.
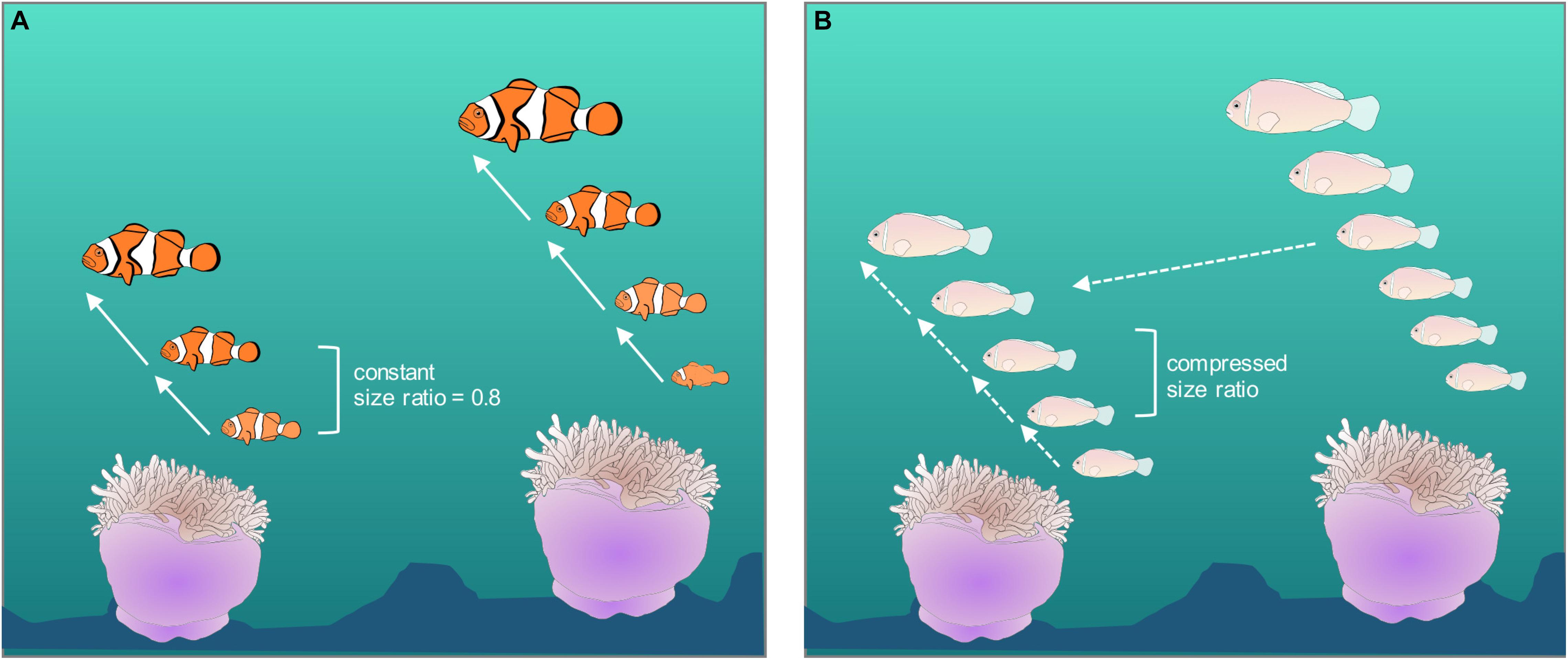
Figure 6. Possible relationship between ecological constraints, territory inheritance, and social organization and behaviors; (A) schematic of social organization in Amphiprion percula, where ecological constraints are high, movement between anemones does not occur, the probability of territory inheritance is high and the size hierarchy is strict with exact ratios; (B) social organization of Amphiprion perideraion where ecological constraints are relaxed, movement between anemones does occur, groups are less stable and the size hierarchy is more variable.
Conclusion of Part 3
Extending our investigations from model species to model genera is allowing us to gain new insights into social evolution in the marine realm. In Gobiodon/Paragobiodon (Gobiidae) and Amphiprion/Premnas (Pomacentridae), there is social variation among species that is unlikely to be explained by variation in the strength of kin selection across the genus. Instead, variation in life history traits might interact with the ecology (habitat patch size and risks of movement) to cause these differences. Comparative research on coral-dwelling gobies has highlighted the nuanced and complex nature of sociality within the taxon. Such integrative and quantitative approaches to the study of sociality can be applied to any taxa and provide a robust framework for future comparative work. Interestingly, whether or not there is a strong ancestral basis for sociality appears to differ depending on which reef fish family is examined. Thus, there may be different key drivers of social variation between different taxa. Further comparative studies in other marine fishes, using integrative approaches that incorporate sociality indices, will allow comparisons across diverse taxa to enhance our understanding of social evolution.
Part 4: Sociality and Environmental Change
It has long been recognized that environmental variation may alter the state of sociality within and amongst species (Rubenstein and Lovette, 2007; Duffy and Macdonald, 2009; Rubenstein, 2011; Shen et al., 2017). As environmental disturbances affect marine ecosystems with more frequency and intensity and environmental variability in these ecosystems increases (Turner, 2010; Hughes et al., 2018), a key question is: how will the sociality of coral reef fishes be influenced? Environmental disturbances can impact sociality in at least four distinct ways: (i) by influencing the relative population size and structure of the fish and their cnidarian hosts; (ii) by influencing the subordinate non-breeders’ payoffs associated with staying in or leaving groups; (iii) by influencing the dominant breeders’ payoffs associated with tolerating subordinates; and (iv) by negatively impacting the mechanisms that individuals use to engage in social interactions.
Environmental Disturbances Can Change Population Structure and Habitat Saturation
A recent study investigated the impacts of environmental disturbances using before and after disturbance data for populations of social and pair-forming Gobiodon species. Thirteen Gobiodon species and their mutually beneficial Acropora host coral species were monitored throughout two category 4 tropical storms in consecutive years to determine multi-species responses (Hing et al., 2018). After the two storms, the group size of group-forming species (n = 5) declined, while the group size of pair-forming species (n = 8) showed little variation. Group-forming species occupied larger corals than pair-forming species both before and after the storms, but as coral size decreased, so did the number of group members overall (Figures 7A,B). Interestingly, although the number of vacant corals did not change after disturbances, smaller corals became more saturated after disturbances and gobies occupied smaller corals. Thus, it appears that the benefits of group-living in this genus are affected by habitat size rather than habitat availability – both factors which can be strongly influenced by environmental disturbances (Hing et al., 2018).
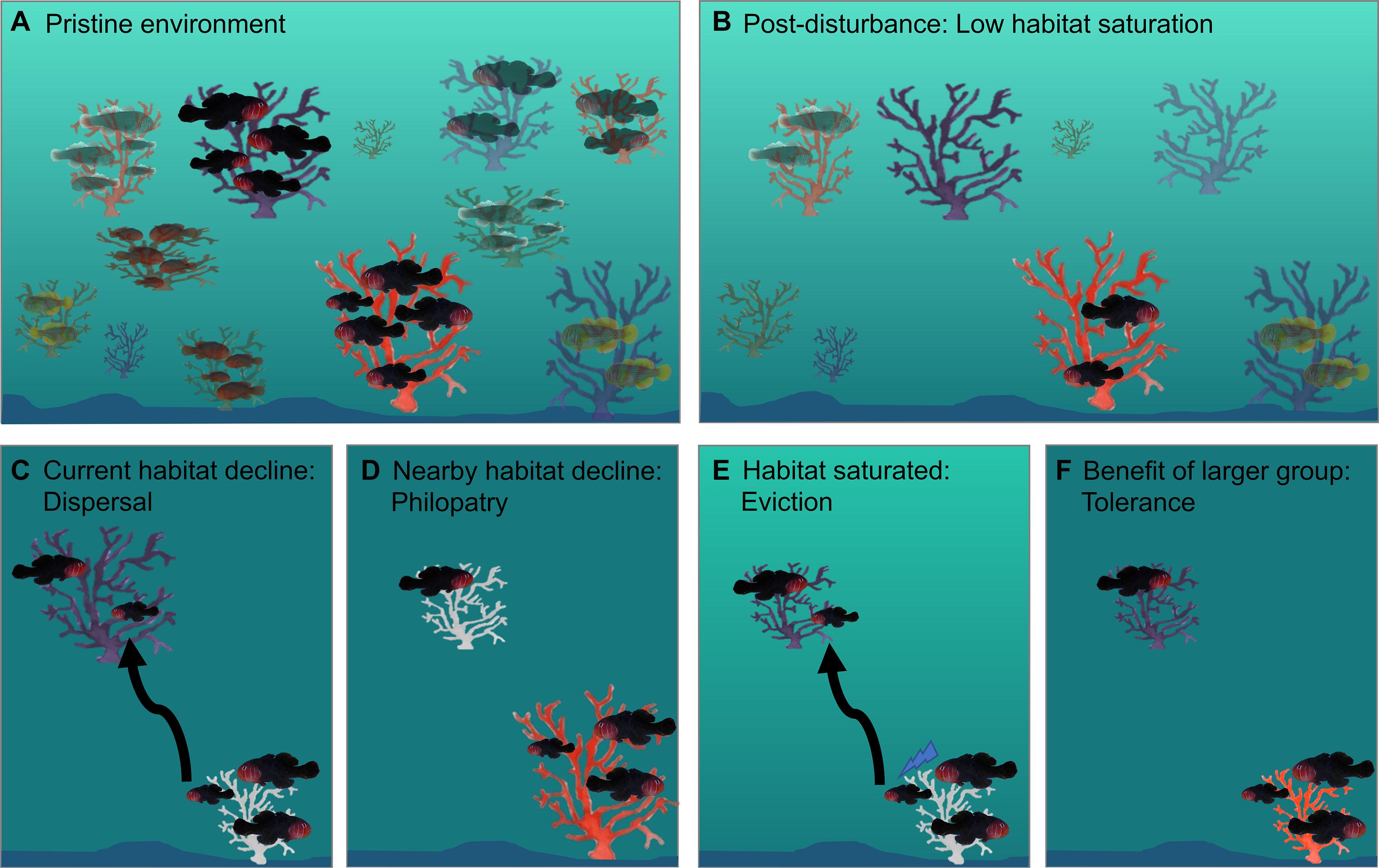
Figure 7. Possible effects of environmental disturbances on the sociality of reef fishes, using Gobiodon with Acropora coral hosts as examples. After disturbances, a pristine environment (A) can become less saturated if fish populations decline more than their habitat (B). If habitat declines (i.e., smaller and unhealthy corals) influence subordinates’ payoffs to stay or leave, then current coral patch quality (C) and nearby coral patch quality (D) will affect the subordinates’ decisions to stay or leave. If habitat declines influence payoffs of dominants tolerating subordinates, then subordinates in smaller patches will either be evicted (E) or be tolerated because of synergistic effects (F).
Environmental Disturbance Can Change Relative Payoffs Associated With Staying or Leaving
Some insights into how environmental conditions are driving sociality in marine fishes may be gained by studying the social consequences of recent disturbances (Hing et al., 2018). Climatic events and crown-of-thorns outbreaks are especially pertinent since they increase the frequency of bleaching and mortality of cnidarians (Cheal et al., 2017; Hughes et al., 2017, 2018, 2019; Pratchett et al., 2017), and social coral reef fishes such as gobies, damselfishes, and anemonefishes depend on live cnidarian hosts (Bonin et al., 2009; Saenz-Agudelo et al., 2011; Pratchett et al., 2020). Although there is a clear preference for healthy hosts, both gobies and anemonefishes are willing to settle and use bleached hosts as long as the hosts are alive (Bonin et al., 2009; Saenz-Agudelo et al., 2011). Individuals are however unwilling to remain in dead hosts (Bonin et al., 2009).
If cnidarian hosts die as a result of environmental disturbances, then dispersal of those occupying them to other habitats will be increasingly difficult (Figures 7C,D). After corals were heavily disturbed by crown-of-thorns starfish, D. aruanus and two other damselfish species declined substantially, but there was size-specific success in dispersing (Pratchett et al., 2020). Smaller and intermediate-sized individuals were more successful at relocating to a new group than larger individuals, likely because they represented less of a threat to bigger resident breeders (Coker et al., 2013; Pratchett et al., 2020). Dispersing individuals also risk finding uninhabited hosts, which are unattractive to damselfishes (Pratchett et al., 2020), gobies (Wong, 2010), and anemonefishes (Branconi et al., 2020). As the relative payoffs associated with staying and leaving shift under environmental disturbances, we anticipate they will continue to play a major role in governing sociality.
Environmental Disturbances Can Change the Payoffs Associated With Tolerating Subordinates
From the dominants’ perspectives, environmental disturbances might alter the payoffs associated with tolerating subordinates (Figures 7E,F). For example, larger-bodied goby species are typically only group-living when corals are large in size (Hing et al., 2019). Within our model species, group sizes tend to be larger when the cnidarians and the dominant breeders are larger (Buston and Cant, 2006; Wong, 2011). Thus, maintaining sociality may no longer be an option when the cnidarian hosts become smaller due to climate change (Pisapia et al., 2020), perhaps because there are simply not enough resources within a single host to support multiple individuals (Wong et al., 2008a; Rueger et al., 2018).
However, remaining in social groups may instead be especially important throughout disturbances, due to synergistic benefits of sociality. It is now known that fish inhabitants provide important services to their cnidarian hosts (Goldshmid et al., 2004; Chong-Seng et al., 2011; Dixson and Hay, 2012; Dirnwoeber and Herler, 2013; Garcia-Herrera et al., 2017), including the moderation of bleaching susceptibility and the promotion of host recovery through increasing water movement and nutrient cycling (Chase et al., 2018; Pryor et al., 2020). Larger group sizes could therefore lead to quicker recovery from bleaching. From a synergistic effects perspective, remaining social might then be beneficial for breeders and non-breeders alike.
Environmental Disturbances May Interfere With Proximate Mechanisms Essential for Sociality
Social group formation is not just dependent on the benefits associated with different social strategies, but it also depends on the ability of the fish to recognize these benefits (e.g., kin recognition), and to enact strategies that confer these benefits (e.g., growth regulation). If reef fishes lose important sensory and physiological abilities with environmental disturbances, as they seem to do (e.g., Munday et al., 2009, 2010; Dixson et al., 2010; Pankhurst and Munday, 2011; Donelson et al., 2016), then group formation and maintenance may be affected long-term. For example, ocean acidification can reduce the ability of coral reef fishes to detect predators, kin, and habitat (Munday et al., 2009, 2010; Dixson et al., 2010) and as a consequence, non-breeders may change their decisions to remain philopatric or disperse (Dixson et al., 2010). Additionally, new recruits may lose the ability to detect kin and habitat, which could result in changed levels of relatedness within groups and reduced fish replenishment, respectively (Munday et al., 2009, 2010). Finally, the stress levels of fish increase when their hosts bleach (Beldade et al., 2017), and juvenile fishes’ metabolism and behavior changes (Cortese et al., 2020), all of which could alter social decisions and group cohesion. Combined, there are several sensory and physiological effects of environmental disturbances that may impact the maintenance of sociality in coral reef fishes.
Conclusion of Part 4
As climate change causes disturbances on coral reefs, the decisions of non-breeders to forgo reproduction and breeders to tolerate non-breeders may be altered. From the point of view of the non-breeder, if habitat becomes less saturated as a result of disturbance, then leaving to breed elsewhere may become a better strategy to gain breeding status more quickly (Wong, 2010). If habitats are declining in quality, the decision to stay or leave will depend on how the current habitat is faring relative to the habitats around it. From the point of view of the breeder, if habitats become smaller as a result of continued disturbances (Pisapia et al., 2020), then tolerating non-breeders may not be a good strategy due to resource limitations (Wong et al., 2008a, b; Rueger et al., 2018; Hing et al., 2019). On the other hand, tolerating non-breeders might become more strongly favored because the synergistic benefits of sociality might enable the habitat to recover and grow quicker post-disturbance. Furthermore, environmental disturbances may negatively influence the proximate mechanisms that individuals require to engage in efficient social interactions. As we uncover more links between environmental effects and sociality, we will be able to assess whether sociality within species of coral reef fishes is stable or plastic in response to environmental change.
General Conclusion
In reviewing the past two decades of sociality research in coral reef fishes, we show that work on marine fishes can provide important insights into the evolution of sociality. Many of these insights are not just applicable to our model species, but to a wider range of taxa. First, even in taxa with dispersive larval phases, subtle relatedness patterns may help explain patterns of sociality (Buston et al., 2009; Rueger et al., 2021). However, in contrast to most terrestrial social animals, kin selection alone does not explain social evolution in marine fishes and does not play a central role in most cases. As we have laid out, relatedness may be only one of many factors working together to motivate dominant breeders to accept non-breeders, even if they provide no alloparental care. Second, we have come to understand that while alloparental care is absent, other forms of cooperative actions can be taken by subordinate group members, such as regulating their growth (Buston, 2003a; Buston and Cant, 2006; Wong et al., 2008a). The active regulation of subordinate growth is one of the most remarkable findings emerging from social evolution research in coral reef fishes and has since informed studies of growth regulation in other social vertebrates (Bender et al., 2005; Huchard et al., 2016). Third, coral reef fish studies have provided some of the only direct support for well-known social evolution hypotheses such as the mate-replacement hypothesis (Buston, 2004a) and the reproductive control hypothesis of reproductive skew (Rueger et al., 2018).
Future Directions
Recently recurring goals in the field of marine sociality research include expanding our focus from a few model species with simple social systems to fishes with more complex social systems and expanding to whole taxonomic groups to assess the variation of sociality. These goals will require the application of methods new to the field of marine fish research, such as social network analysis and the use of sociality indices and phylogenetically controlled models. Another crucial future research direction is what role the cnidarian host plays in the sociality of coral reef fish species. This is because (i) synergistic effects may be an important factor in motivating dominant breeders to tolerate subordinates that are not close relatives, and (ii) the host’s susceptibility to environmental disturbances may have severe consequences for the social organization of many marine species in the future, especially as we move further into the Anthropocene. Climate change and related stressors have the potential to alter sociality by influencing the physiology and behavior of animals directly or by altering their habitat and mutualistic partners. The applicability of these future studies will go well beyond coral reef fishes and extend to any social animal that lives in a mutualistic relationship with another organism.
Author Contributions
TR, MW, and PB conceived of the manuscript. TR, RB, CF, SH, MW, and PB reviewed the literature, contributed critical discussion, and wrote the manuscript. All the authors contributed to the article and approved the submitted version.
Funding
This project received funding from the European Union’s Horizon 2020 research and innovation programme under the Marie Sklodowska-Curie grant agreement No. 841263. TR was supported by a Marie Sklodowska-Curie Individual Global Fellowship (H2020-MSCA-IF-2018, Grant No. 841263). RB was supported by a Warren McLeod Fellowship from Boston University. CF was supported by a University Postgraduate Award. SH was supported by an Australian Government Research Training Program Scholarship, both administered through the University of Wollongong.
Conflict of Interest
The authors declare that the research was conducted in the absence of any commercial or financial relationships that could be construed as a potential conflict of interest.
Acknowledgments
We would like to thank two reviewers who helped us improve this manuscript.
References
Allen, G. R. (1972). The Anemonefishes: Their Classification and Biology. Neptune City, NJ: TFH Publ., Inc.
Almany, G. R., Berumen, M. L., Thorrold, S. R., Planes, S., and Jones, G. P. (2007). Local replenishment of coral reef fish populations in a marine reserve. Science 316, 742–744. doi: 10.1126/science.1140597
Almany, G. R., Planes, S., Thorrold, S. R., Berumen, M. L., Bode, M., Saenz-Agudelo, P., et al. (2017). Larval fish dispersal in a coral-reef seascape. Nat. Ecol. Evol. 1:148.
Asoh, K. (2003). Gonadal development and infrequent sex change in a population of the humbug damselfish, Dascyllus aruanus in continuous coral-cover habitat. Mar. Biol. 142, 1207–1218. doi: 10.1007/s00227-003-1051-6
Avilés, L., and Harwood, G. (2012). A quantitative index of sociality and its application to group-living spiders and other social organisms. Ethology 118, 1219–1229. doi: 10.1111/eth.12028
Barbasch, T. A., Rueger, T., Srinivasan, M., Wong, M. Y., Jones, G. P., and Buston, P. M. (2020). Substantial plasticity of reproduction and parental care in response to local resource availability in a wild clownfish population. Oikos 129, 1844–1855. doi: 10.1111/oik.07674
Beauchamp, G. (2015). Animal Vigilance: Monitoring Predators and Competitors. Cambridge, MA: Academic Press.
Beldade, R., Blandin, A., O’Donnell, R., and Mills, S. C. (2017). Cascading effects of thermally-induced anemone bleaching on associated anemonefish hormonal stress response and reproduction. Nat. Commun. 8:716. doi: 10.1038/s41467-017-00565-w
Bender, N., Hamilton, I. M., and Heg, D. (2005). Size differences within a dominance hierarchy influence conflict and help in a cooperatively breeding cichlid. Behaviour 142, 1591–1613. doi: 10.1163/156853905774831846
Bonin, M. C., Munday, P. L., McCormick, M. I., Srinivasan, M., and Jones, G. P. (2009). Coral-dwelling fishes resistant to bleaching but not to mortality of host corals. Mar. Ecol. Prog. Ser. 394, 215–222. doi: 10.3354/meps08294
Branconi, R., Barbasch, T. A., Francis, R. K., Srinivasan, M., Jones, G. P., and Buston, P. M. (2020). Ecological and social constraints combine to promote evolution of non-breeding strategies in clownfish. Commun. Biol. 3:649.
Branconi, R., Garner, J., Buston, P. M., and Wong, M. Y. (2019a). A new non-invasive technique for temporarily tagging reef fishes. Copeia 1, 85–91. doi: 10.1643/ot-18-057
Branconi, R., Wong, M. Y., and Buston, P. M. (2019b). Comparison of video and SCUBA diver observations for measuring coral reef fish behavior: a case study using the humbug damselfish Dascyllus aruanus. J. Fish Biol. 94, 490–498.
Brown, J. L. (1974). Alternate routes to sociality in jays – with a theory for the evolution of altruism and communal breeding. Am. Zool. 14, 63–80. doi: 10.1093/icb/14.1.63
Buston, P. M. (2002). Group Structure of the Clown Anemonefish, Amphiprion Percula. PhD thesis, Ithaca, N.Y: Cornell University.
Buston, P. M. (2003a). Forcible eviction and prevention of recruitment in the clown anemonefish. Behav. Ecol. 14, 576–582. doi: 10.1093/beheco/arg036
Buston, P. M. (2003b). Size and growth modification in clownfish. Nature 424, 145–146. doi: 10.1038/424145a
Buston, P. (2004a). Does the presence of non-breeders enhance the fitness of breeders? an experimental analysis in the clown anemonefish Amphiprion percula. Behav. Ecol. Sociob. 57, 23–31. doi: 10.1007/s00265-004-0833-2
Buston, P. M. (2004b). Territory inheritance in the clown anemonefish. Proc. R. Soc. B. 271, S252–S254.
Buston, P. M., and Balshine, S. (2007). Cooperating in the face of uncertainty: a consistent framework for understanding the evolution of cooperation. Behav. Process. 76, 152–159. doi: 10.1016/j.beproc.2007.01.020
Buston, P. M., Bogdanowicz, S. M., Wong, M. Y., and Harrison, R. G. (2007a). Are clownfish groups composed of close relatives? an analysis of microsatellite DNA variation in Amphiprion percula. Mol. Ecol. 16, 3671–3678. doi: 10.1111/j.1365-294x.2007.03421.x
Buston, P. M., Reeve, H. K., Cant, M. A., Vehrencamp, S. L., and Emlen, S. T. (2007b). Reproductive skew and the evolution of group dissolution tactics: a synthesis of concession and restraint models. Anim. Behav. 74, 1643–1654. doi: 10.1016/j.anbehav.2007.03.003
Buston, P. M., and Cant, M. A. (2006). A new perspective on size hierarchies in nature: patterns, causes, and consequences. Oecologia. 149, 362–372. doi: 10.1007/s00442-006-0442-z
Buston, P. M., and Elith, J. (2011). Determinants of reproductive success in dominant pairs of clownfish: a boosted regression tree analysis. J. Anim. Ecol. 80, 528–538. doi: 10.1111/j.1365-2656.2011.01803.x
Buston, P. M., Fauvelot, C., Wong, M. Y., and Planes, S. (2009). Genetic relatedness in groups of the humbug damselfish Dascyllus aruanus: small, similar-sized individuals may be close kin. Mol. Ecol. 18, 4707–4715. doi: 10.1111/j.1365-294x.2009.04383.x
Buston, P. M., and García, M. B. (2007). An extraordinary life span estimate for the clown anemonefish Amphiprion percula. J. Fish Biol. 70, 1710–1719. doi: 10.1111/j.1095-8649.2007.01445.x
Buston, P. M., Jones, G. P., Planes, S., and Thorrold, S. R. (2012). Probability of successful larval dispersal declines fivefold over 1 km in a coral reef fish. Proc. Biol. Sci. 279, 1883–1888. doi: 10.1098/rspb.2011.2041
Buston, P. M., and Zink, A. G. (2009). Reproductive skew and the evolution of conflict resolution: a synthesis of transactional and tug-of-war models. Behav. Ecol. 20, 672–684. doi: 10.1093/beheco/arp050
Cant, M. A., and Johnstone, R. A. (2009). How threats influence the evolutionary resolution of within-group conflict. Am. Nat. 173, 759–771. doi: 10.1086/598489
Catalano, K. A., Dedrick, A. G., Stuart, M. R., Puritz, J. B., Montes, H. R. Jr., and Pinsky, M. L. (2020). Quantifying dispersal variability among nearshore marine populations. Mol. Ecol. doi: 10.1111/mec.15732
Chase, T. J., Pratchett, M. S., Frank, G. E., and Hoogenboom, M. O. (2018). Coral-dwelling fish moderate bleaching susceptibility of coral hosts. PLoS One 13:e0208545. doi: 10.1371/journal.pone.0208545
Chase, T. J., Pratchett, M. S., McWilliam, M. J., Tebbett, S. B., and Hoogenboom, M. O. (2020). Damselfishes alleviate the impacts of sediments on host corals. R. Soc. Open Sci. 7:7192074.
Chase, T. J., Pratchett, M. S., Walker, S. P. W., and Hoogenboom, M. O. (2014). Small-scale environmental variation influences whether coral-dwelling fish promote or impede coral growth. Oecologia 176, 1009–1022. doi: 10.1007/s00442-014-3065-9
Chausson, J., Srinivasan, M., and Jones, G. P. (2018). Host anemone size as a determinant of social group size and structure in the orange clownfish (Amphiprion percula). PeerJ 6:e5841. doi: 10.7717/peerj.5841
Cheal, A. J., MacNeil, M. A., Emslie, M. J., and Sweatman, H. (2017). The threat to coral reefs from more intense cyclones under climate change. Glob. Change Biol. 23, 1511–1524. doi: 10.1111/gcb.13593
Chong-Seng, K. M., Cole, A. J., Pratchett, M. S., and Willis, B. L. (2011). Selective feeding by coral reef fishes on coral lesions associated with brown band and black band disease. Cor. Reef. 30, 473–481. doi: 10.1007/s00338-010-0707-1
Clutton-Brock, T. (2002). Breeding together: kin selection and mutualism in cooperative vertebrates. Science 296, 69–72. doi: 10.1126/science.296.5565.69
Clutton-Brock, T. H., Hodge, S. J., Flower, T. P., Spong, G. F., and Young, A. J. (2010). Adaptive suppression of subordinate reproduction in cooperative mammals. Am. Nat. 176, 664–673. doi: 10.1086/656492
Coates, D. (1980a). Prey-size intake in humbug Dacyllus aruanus (Pisces: Pomacentridae) living within social groups. J. Anim. Ecol. 49, 335–340. doi: 10.2307/4292
Coates, D. (1980b). The discrimination of and reactions towards predatory and non-predatory species of fish by humbug damselfish. Dascyllus aruanus (Pisces, Pomacentridae). Zeitschr. Tierpsy. 52, 347–354. doi: 10.1111/j.1439-0310.1980.tb00722.x
Coates, D. (1982). Some observations on the sexuality of the humbug damselfish Dascyllus aruanus (Pisces. Pomacentridae) in the field. Zeitschr. Tierpsy. 59, 7–18. doi: 10.1111/j.1439-0310.1982.tb00328.x
Coker, D. J., Walker, S. P. W., Munday, P. L., and Pratchett, M. S. (2013). Social group entry rules may limit population resilience to patchy habitat disturbance. Mar. Ecol. Prog. Ser. 493, 237–242. doi: 10.3354/meps10493
Cole, K. S. (2002). Gonad morphology, sexual development, and colony composition in the obligate coral-dwelling damselfish Dascyllus aruanus. Mar. Biol. 140, 151–163. doi: 10.1007/s002270100681
Cortese, D., Norin, T., Beldade, R., Crespel, A., Killen, S. S., and Mills, S. C. (2020). Physiological and behavioural effects of anemone bleaching on symbiont anemonefish in the wild. Funct. Ecol. 35, 663–674. doi: 10.1111/1365-2435.13729
Croft, D. P., James, R., and Krause, J. (2008). Exploring Animal Social Networks. Princeton, NJ: Princeton University Press.
D’Aloia, C. C., and Neubert, M. G. (2018). The formation of marine kin structure: effects of dispersal, larval cohesion, and variable reproductive success. Ecology 99, 2374–2384. doi: 10.1002/ecy.2480
D’Aloia, C. C., Xuereb, A., Fortin, M. J., Bogdanowicz, S. M., and Buston, P. M. (2018). Limited dispersal explains the spatial distribution of siblings in a reef fish population. Mar. Ecol. Prog. Ser. 607, 143–154. doi: 10.3354/meps12792
Dirnwoeber, M., and Herler, J. (2013). Toxic coral gobies reduce the feeding rate of a corallivorous butterflyfish on Acropora corals. Coral Reefs 32, 91–100. doi: 10.1007/s00338-012-0947-3
Dixson, D. L., and Hay, M. E. (2012). Corals chemically cue mutualistic fishes to remove competing seaweeds. Science 338, 804–807. doi: 10.1126/science.1225748
Dixson, D. L., Munday, P. L., and Jones, G. P. (2010). Ocean acidification disrupts the innate ability of fish to detect predator olfactory cues. Ecol. Lett. 13, 68–75. doi: 10.1111/j.1461-0248.2009.01400.x
Donelson, J. M., Wong, M., Booth, D. J., and Munday, P. L. (2016). Transgenerational plasticity of reproduction depends on rate of warming across generations. Evol. Appl. 9, 1072–1081. doi: 10.1111/eva.12386
Duchene, D., Klanten, S. O., Munday, P. L., Herler, J., and van Herwerden, L. (2013). Phylogenetic evidence for recent diversification of obligate coral-dwelling gobies compared with their host corals. Mol. Phylog. Evol. 69, 123–132. doi: 10.1016/j.ympev.2013.04.033
Duffy, J. E., and Macdonald, K. S. (2009). Kin structure, ecology and the evolution of social organization in shrimp: a comparative analysis. Proc. R. Soc. B. 277, 575–84. doi: 10.1098/rspb.2009.1483
Duffy, J. E., Morrison, C. L., and Ríos, R. N. (2000). Multiple origins of eusociality among sponge-dwelling shrimps (Synalpheus). Evolution 54, 503–516. doi: 10.1554/0014-3820(2000)054[0503:mooeas]2.0.co;2
Elliott, J. K., Elliott, J. M., and Mariscal, R. N. (1995). Host selection, location, and association behaviors of anemonefishes in field settlement experiments. Mar. Biol. 122, 377–389. doi: 10.1007/bf00350870
Elliott, J. K., Lougheed, S. C., Bateman, B., McPhee, L. K., and Boag, P. T. (1999). Molecular phylogenetic evidence for the evolution of specialization in anemonefishes. Proc. R. Soc. B. 266, 677–685. doi: 10.1098/rspb.1999.0689
Elliott, J. K., and Mariscal, R. N. (2001). Coexistence of nine anemonefish species: differential host and habitat utilization, size and recruitment. Mar. Biol. 138, 23–36. doi: 10.1007/s002270000441
Emlen, S. T. (1982). The evolution of helping. I. an ecological constraints model. Am. Nat. 119, 29–39. doi: 10.1086/283888
Emlen, S. T. (1991). “Evolution of cooperative breeding in birds and mammals,” in Behavioural Ecology: An Evolutionary Approach, 3rd Edn, eds J. R. Krebs and N. B. Davies (Oxford: Blackwell), 301–337.
Emlen, S. T., and Wrege, P. H. (1988). The role of kinship in helping decisions among white-fronted bee-eaters. Behav. Ecol. Sociob. 23, 305–315. doi: 10.1007/bf00300577
Fautin, D. G. (1992). Anemonefish recruitment: the roles of order and chance. Symbiosis 14, 143–160.
Fautin, D. G., and Allen, G. R. (1992). Field Guide to Anemonefishes and their Host Sea Anemones. Perth, WA: Western Australian Museum, 165.
Forrester, G. E. (1991). Social rank, individual size and group composition as determinants of food-consumption by humbug damselfish. Dascyllus aruanus. Anim. Behav. 42, 701–711. doi: 10.1016/s0003-3472(05)80116-2
Forsgren, E., Karlsson, A., and Kvarnemo, C. (1996). Female sand gobies gain direct benefits by choosing males with eggs in their nests. Behav. Ecol. Sociob. 39, 91–96. doi: 10.1007/s002650050270
Fricke, H., and Fricke, S. (1977). Monogamy and sex change by aggressive dominance in coral reef fish. Nature 266, 830–832. doi: 10.1038/266830a0
Fricke, H. W. (1979). Mating system, resource defence and sex change in the anemonefish Amphiprion akallopisos. Zeit. Tierpsychol. 50, 313–326. doi: 10.1111/j.1439-0310.1979.tb01034.x
Fricke, H. W., and Holzberg, S. (1974). Social units and hermaphroditism in a pomacentrid fish. Naturwissenschaften 61, 367–368. doi: 10.1007/bf00600312
Frisch, A. J., Rizzari, J. R., Munkres, K. P., and Hobbs, J. P. A. (2016). Anemonefish depletion reduces survival, growth, reproduction and fishery productivity of mutualistic anemone–anemonefish colonies. Coral Reefs 35, 375–386. doi: 10.1007/s00338-016-1401-8
Garcia-Herrera, N., Ferse, S. C. A., Kunzmann, A., and Genin, A. (2017). Mutualistic damselfish induce higher photosynthetic rates in their host coral. J. Exp. Biol. 220, 1803–1811. doi: 10.1242/jeb.152462
Goldshmid, R., Holzman, R., Weihs, D., and Genin, A. (2004). Aeration of corals by sleep-swimming fish. Limnol. Oceanogr. 49, 1832–1839. doi: 10.4319/lo.2004.49.5.1832
Griffin, A. S., and West, S. A. (2003). Kin discrimination and the benefit of helping in cooperatively breeding vertebrates. Science 302, 634–636. doi: 10.1126/science.1089402
Hamilton, W. D. (1964). The genetical evolution of social behaviour. II. J. Theor. Biol. 7, 17–52. doi: 10.1016/0022-5193(64)90039-6
Harold, A. S., Winterbottom, R., Munday, P. L., and Chapman, R. W. (2008). Phylogenetic relationships of Indo-Pacific coral gobies of the genus Gobiodon (Teleostei: Gobiidae), based on morphological and molecular data. Bull. Mar. Sci. 82, 119–136.
Hattori, A. (1994). Inter-group movement and mate acquisition tactics of the protandrous anemonefish Amphiprion clarkii, on a coral reef. Okinawa. Jap. J. Ichthy. 41, 159–165.
Herler, J., Koblmüller, S., and Sturmbauer, C. (2009). Phylogenetic relationships of coral-associated gobies (Teleostei, Gobiidae) from the Red Sea based on mitochondrial DNA data. Mar. Biol. 156, 725–739. doi: 10.1007/s00227-008-1124-7
Hing, M. L., Klanten, O. S., Dowton, M., Brown, K. R., and Wong, M. Y. (2018). Repeated cyclone events reveal potential causes of sociality in coral-dwelling Gobiodon fishes. PLoS One 13:e0202407. doi: 10.1371/journal.pone.0202407
Hing, M. L., Klanten, O. S., Dowton, M., and Wong, M. Y. (2017). The right tools for the job: cooperative breeding theory and an evaluation of the methodological approaches to understanding the evolution and maintenance of sociality. Front. Ecol. Evol. 5:100. doi: 10.3389/fevo.2017.00100
Hing, M. L., Klanten, O. S., Wong, M. Y., and Dowton, M. (2019). Drivers of sociality in Gobiodon fishes: an assessment of phylogeny, ecology and life-history. Mol. Phylo. Evol. 137, 263–273. doi: 10.1016/j.ympev.2019.05.020
Hodge, J. R., Alim, C., Bertrand, N. G., Lee, W., Price, S. A., Tran, B., et al. (2018). Ecology shapes the evolutionary trade-off between predator avoidance and defence in coral reef butterflyfishes. Ecol. Let. 21, 1033–1042. doi: 10.1111/ele.12969
Holbrook, S. J., Forrester, G. E., and Schmitt, R. J. (2000). Spatial patterns in abundance of damselfish reflects availability of suitable habitat. Oecologia 122, 109–120. doi: 10.1007/pl00008826
Holbrook, S. J., and Schmitt, R. J. (2005). Growth, reproduction and survival of a tropical sea anemone (Actiniaria): benefits of hosting anemonefish. Coral Reefs. 24, 67–73. doi: 10.1007/s00338-004-0432-8
Huchard, E., English, S., Bell, M. B., Thavarajah, N., and Clutton-Brock, T. (2016). Competitive growth in a cooperative mammal. Nature 533, 532–534. doi: 10.1038/nature17986
Hughes, T. P., Anderson, K. D., Connolly, S. R., Heron, S. F., Kerry, J. T., Lough, J. M., et al. (2018). Spatial and temporal patterns of mass bleaching of corals in the Anthropocene. Science 359, 80–83. doi: 10.1126/science.aan8048
Hughes, T. P., Kerry, J. T., Álvarez-Noriega, M., Álvarez-Romero, J. G., Anderson, K. D., Baird, A. H., et al. (2017). Global warming and recurrent mass bleaching of corals. Nature 543, 373–377.
Hughes, T. P., Kerry, J. T., Baird, A. H., Connolly, S. R., Chase, T. J., Dietzel, A., et al. (2019). Global warming impairs stock–recruitment dynamics of corals. Nature 568, 387–390. doi: 10.1038/s41586-019-1081-y
Iwata, E., and Manbo, J. (2013). Territorial behaviour reflects sexual status in groups of false clown anemonefish (Amphiprion ocellaris) under laboratory conditions. Acta Ethol. 16, 97–103. doi: 10.1007/s10211-012-0142-0
Jones, G. P., Planes, S., and Thorrold, S. R. (2005). Coral reef fish larvae settle close to home. Curr. Biol. 15, 1314–1318. doi: 10.1016/j.cub.2005.06.061
Jordan, L. A., Avolio, C., Herbert-Read, J. E., Krause, J., Rubenstein, D. I., and Ward, A. J. W. (2010). Group structure in a restricted entry system is mediated by both resident and joiner preferences. Behav. Ecol. Sociob. 64, 1099–1106. doi: 10.1007/s00265-010-0924-1
Keller, L., and Reeve, H. K. (1994). Partitioning of reproduction in animal societies. Tree 9, 98–102. doi: 10.1016/0169-5347(94)90204-6
Kocher, S. D., and Paxton, R. J. (2014). Comparative methods offer powerful insights into social evolution in bees. Apidologie 45, 289–305. doi: 10.1007/s13592-014-0268-3
Koenig, W. D., and Walters, E. L. (2015). Temporal variability and cooperative breeding: testing the bet-hedging hypothesis in the acorn woodpecker. Proc. Biol. Sci. B 282:20151742. doi: 10.1098/rspb.2015.1742
Kokko, H., Brooks, R., McNamara, J. M., and Houston, A. I. (2002). The sexual selection continuum. Proc. Biol. Sci. B. 269, 1331–1340.
Kokko, H., and Johnstone, R. A. (1999). Social queuing in animal societies: a dynamic model of reproductive skew. Proc. Biol. Sci. B. 266, 571–578. doi: 10.1098/rspb.1999.0674
Kuwamura, T., Nakashima, Y., and Yogo, Y. (1996). Plasticity in size and age at maturity in a monogamous fish: effect of host coral size and frequency dependence. Behav. Ecol. Sociobiol. 38, 365–370. doi: 10.1007/s002650050253
Kuwamura, T., Nakashimn, Y., and Yogo, Y. (1994). Sex change in either direction by growth-rate advantage in the monogamous coral goby, Paragobiodon echinocephalus. Behav. Ecol. 5, 434–438. doi: 10.1093/beheco/5.4.434
Kuwamura, T., Suzuki, S., and Kadota, T. (2016). Male-to-female sex change in widowed males of the protogynous damselfish Dascyllus aruanus. J. Ethol. 34, 85–88. doi: 10.1007/s10164-015-0450-8
Lassig, B. R. (1976). Field observations on the reproductive behaviour of Paragobiodon spp. (Osteichthyes: Gobiidae) at heron island great barrier reef. Mar. Freshw. Behav. Phy. 3, 283–293. doi: 10.1080/10236247609378517
Lassig, B. R. (1977). Socioecological strategies adopted by obligate coral dwelling fishes. Proc. 3rd Int. Symp. Coral Reefs. 1, 565–570.
Lassig, B. R. (1981). Significance of the epidermal ichthyotoxic secretion of coral-dwelling gobies. Toxicon 19, 729–735. doi: 10.1016/0041-0101(81)90068-4
Leis, J. M. (1991). “The pelagic stage of reef fishes: the larval biology of coral reef fishes,” in The Ecology of Fishes on Coral Reefs, ed. P. F. Sale (Cambridge, MA: Academic Press), 183–230. doi: 10.1016/b978-0-08-092551-6.50013-1
Li, J., Chen, X., Kang, B., and Liu, M. (2015). Mitochondrial DNA genomes organization and phylogenetic relationships analysis of eight anemonefishes (Pomacentridae: Amphiprioninae). PLoS One 10:e0123894. doi: 10.1371/journal.pone.0123894
Litsios, G., and Salamin, N. (2014). Hybridisation and diversification in the adaptive radiation of clownfishes. BMC Evol. Biol. 14:245. doi: 10.1186/s12862-014-0245-5
Litsios, G., Sims, C. A., Wüest, R. O., Pearman, P. B., Zimmermann, N. E., and Salamin, N. (2012). Mutualism with sea anemones triggered the adaptive radiation of clownfishes. BMC Evol. Biol. 12:212. doi: 10.1186/1471-2148-12-212
Mann, R. P., Herbert-Read, J. E., Ma, Q., Jordan, L. A., Sumpter, D. J. T., and Ward, A. J. W. (2014). A model comparison reveals dynamic social information drives the movements of humbug damselfish (Dascyllus aruanus). J. R. Soc. Interface 11:20130794. doi: 10.1098/rsif.2013.0794
Mariscal, R. N. (1966). “20 the symbiosis between tropical sea anemones and fishes: a review,” in Proceedings of the Galapagos: Proceedings of the Symposia of Galapagos International Scientific Project, (Berkeley, CA: Univ of California Press), 157.
Mariscal, R. N. (1970). The nature of the symbiosis between Indo-Pacific anemone fishes and sea anemones. Mar. Biol. 6, 58–65. doi: 10.1007/bf00352608
Mitchell, J. S. (2003). Mobility of Stichodactyla gigantea sea anemones and implications for resident false clown anemonefish. Amphiprion ocellaris. Environ. Biol. Fishes. 66, 85–90. doi: 10.1023/a:1023286009054
Mitchell, J. S. (2005). Queue selection and switching by false clown anemonefish. Amphiprion ocellaris. Anim. Behav. 69, 643–652. doi: 10.1016/j.anbehav.2004.05.017
Mitchell, J. S., and Dill, L. M. (2005). Why is group size correlated with the size of the host sea anemone in the false clown anemone fish? Canad. J. Zool. 83, 372–376. doi: 10.1139/z05-014
Mizushima, N., Nakashima, Y., and Kuwamura, T. (2000). Semilunar spawning cycle of the humbug damselfish Dascyllus aruanus. J. Ethol. 18, 105–108. doi: 10.1007/s101640070008
Moyer, J. T., and Nakazono, A. (1978). Protandrous hermaphroditism in six species of the anemonefish genus Amphiprion in Japan. Jap. J. Ichthyol. 25, 101–106.
Munday, P. L. (2002). Bi-directional sex change: testing the growth-rate advantage model. Behav. Ecol. Sociob. 52, 247–254. doi: 10.1007/s00265-002-0517-8
Munday, P. L., Dixson, D. L., Donelson, J. M., Jones, G. P., Pratchett, M. S., Devitsina, G. V., et al. (2009). Ocean acidification impairs olfactory discrimination and homing ability of a marine fish. PNAS 106, 1848–1852. doi: 10.1073/pnas.0809996106
Munday, P. L., Dixson, D. L., McCormick, M. I., Meekan, M., Ferrari, M. C. O., and Chivers, D. P. (2010). Replenishment of fish populations is threatened by ocean acidification. PNAS 107, 12930–12934. doi: 10.1073/pnas.1004519107
Nakashima, Y., Kuwamura, T., and Yogo, Y. (1996). Both-ways sex change in monogamous coral gobies, Gobiodon spp. Environ. Biol. Fish. 46, 281–288. doi: 10.1007/bf00005004
Nanami, A., and Nishira, M. (2001). Survival rates of juvenile coral reef fishes differ between patchy and continuous habitats. Bull. Mar. Sci. 69, 1209–1221.
Nanninga, G. B., Saenz-Agudelo, P., Zhan, P., Hoteit, I., and Berumen, M. L. (2015). Not finding Nemo: limited reef-scale retention in a coral reef fish. Coral Reefs 34, 383–392. doi: 10.1007/s00338-015-1266-2
Nowicki, J. P., O’Connell, L. A., Cowman, P. F., Walker, S. P. W., Coker, D. J., and Pratchett, M. S. (2018). Variation in social systems within Chaetodon butterflyfishes, with special reference to pair bonding. PLoS One 13:e0194465. doi: 10.1371/journal.pone.0194465
Pankhurst, N. W., and Munday, P. L. (2011). Effects of climate change on fish reproduction and early life history stages. Mar. Freshwater Res. 62, 1015–1026. doi: 10.1071/mf10269
Phillips, E., DeAngelis, R., Gogola, J. V., and Rhodes, J. S. (2020). Spontaneous alloparental care of unrelated offspring by non-breeding Amphiprion ocellaris in absence of the biological parents. Sci. Rep. 10:4610.
Pisapia, C., Edmunds, P. J., Moeller, H. V., Riegl, B. M., McWilliam, M., Wells, C. D., et al. (2020). Projected shifts in coral size structure in the Anthropocene. Adv. Mar. Biol. 87, 31–60. doi: 10.1016/bs.amb.2020.07.003
Planes, S., Jones, G. P., and Thorrold, S. R. (2009). Larval dispersal connects fish populations in a network of marine protected areas. PNAS 106, 5693–5697. doi: 10.1073/pnas.0808007106
Porat, D., and Chadwick-Furman, N. E. (2004). “Effects of anemonefish on giant sea anemones: expansion behavior, growth, and survival,” in Coelenterate Biology 2003, eds D. G. Fautin, J. A. Westfall, P. Cartwrigh, M. Daly, C. R. Wyttenbach (Dordrecht: Springer), 513–520. doi: 10.1007/978-1-4020-2762-8_58
Pratchett, M. S., Caballes, C. F., Wilmes, J. C., Matthews, S., Mellin, C., Sweatman, H. P. A., et al. (2017). Thirty years of research on crown-of-thorns starfish (1986–2016): scientific advances and emerging opportunities. Diversity 9:41. doi: 10.3390/d9040041
Pratchett, M. S., Messmer, V., and Wilson, S. K. (2020). Size-specific recolonization success by coral-dwelling damselfishes moderates resilience to habitat loss. Sci. Rep. 10:17016.
Pryor, S. H., Hill, R., Dixson, D. L., Fraser, N. J., Kelaher, B. P., and Scott, A. (2020). Anemonefish facilitate bleaching recovery in a host sea anemone. Sci. Rep. 10:18586.
Riedman, M. L. (1982). The evolution of alloparental care and adoption in mammals and birds. Quart. Rev Biol. 57, 405–435. doi: 10.1086/412936
Rolland, J., Silvestro, D., Litsios, G., Faye, L., and Salamin, N. (2018). Clownfishes evolution below and above the species level. Proc. R. Soc. B. 285:20171796. doi: 10.1098/rspb.2017.1796
Romano, V., Macintosh, A. J., and Sueur, C. (2020). Stemming the flow: information, infection, and social evolution. Tree 35, 849–853. doi: 10.1016/j.tree.2020.07.004
Rubenstein, D. I. (1978). “On predation, competition, and the advantages of group living,” in Social Behavior, eds P. P. G. Bateson and P. H. Klopfer (Boston, MA: Springer), 205–231. doi: 10.1007/978-1-4684-2901-5_9
Rubenstein, D. R. (2011). Spatiotemporal environmental variation, risk aversion, and the evolution of cooperative breeding as a bet-hedging strategy. PNAS 108, 10816–10822. doi: 10.1073/pnas.1100303108
Rubenstein, D. R., and Lovette, I. J. (2007). Temporal environmental variability drives the evolution of cooperative breeding in birds. Curr. Biol. 17, 1414–1419. doi: 10.1016/j.cub.2007.07.032
Rueger, T., Barbasch, T. A., Wong, M. Y., Srinivasan, M., Jones, G. P., and Buston, P. M. (2018). Reproductive control via the threat of eviction in the clown anemonefish. Proc. Biol. Sci. B. 285:20181295. doi: 10.1098/rspb.2018.1295
Rueger, T., Bogdanowicz, S. M., Buston, P. M., and Wong, M. Y. (2021). Potential for kin selection in groups of a social coral reef fish. Mol. Ecol. 18, 4707–4715.
Rueger, T., Harrison, H. B., Buston, P. M., Gardiner, N. M., Berumen, M. L., and Jones, G. P. (2020). Natal philopatry increases relatedness within groups of coral reef cardinalfish. Proc. Biol. Sci. B. 287:20201133. doi: 10.1098/rspb.2020.1133
Saenz-Agudelo, P., Jones, G. P., Thorrold, S. R., and Planes, S. (2011). Detrimental effects of host anemone bleaching on anemonefish populations. Coral Reefs 30, 497–506. doi: 10.1007/s00338-010-0716-0
Sale, P. F. (1971). Extremely limited home range in a coral reef fish. Dascyllus aruanus (Pisces, Pomacentridae). Copeia. 1971, 325–327.
Sale, P. F. (1972). Influence of corals on dispersion of the pomacentrid fish, Dascyllus aruanus. Ecology 53, 741–744. doi: 10.2307/1934795
Salles, O. C., Pujol, B., Maynard, J. A., Almany, G. R., Berumen, M. L., Jones, G. P., et al. (2016). First genealogy for a wild marine fish population reveals multigenerational philopatry. PNAS 113, 13245–13250. doi: 10.1073/pnas.1611797113
Santini, S., and Polacco, G. (2006). Finding nemo: molecular phylogeny and evolution of the unusual life style of anemonefish. Gene 385, 19–27. doi: 10.1016/j.gene.2006.03.028
Schmitt, R. J., and Holbrook, S. J. (1999). Mortality of juvenile damselfish: implications for assessing processes that determine abundance. Ecology 80, 35–50. doi: 10.1890/0012-9658(1999)080[0035:mojdif]2.0.co;2
Shanks, A. L. (2009). Pelagic larval duration and dispersal distance revisited. Biol. Bull. 216, 373–385. doi: 10.1086/bblv216n3p373
Shen, S. F., Emlen, S. T., Koenig, W. D., and Rubenstein, D. R. (2017). The ecology of cooperative breeding behaviour. Ecol. Lett. 20, 708–720.
Sherman, P. W., Lacey, E. A., Reeve, H. K., and Keller, L. (1995). Forum: the eusociality continuum. Behav. Ecol. 6, 102–108. doi: 10.1093/beheco/6.1.102
Simpson, S. D., Harrison, H. B., Claereboudt, M. R., and Planes, S. (2014). Long-distance dispersal via ocean currents connects Omani clownfish populations throughout entire species range. PLoS One 9:e107610. doi: 10.1371/journal.pone.0107610
Sosa, S., Jacoby, D. M. P., Lihoreau, M., and Sueur, C. (2021). Animal social networks: towards an integrative framework embedding social interactions, space and time. Meth. Ecol. Evol. 12, 4–9. doi: 10.1111/2041-210x.13539
Srinivasan, M., Jones, G. P., and Caley, M. J. (1999). Experimental evaluation of the roles of habitat selection and interspecific competition in determining patterns of host use by two anemonefishes. Mar. Ecol. Prog. Ser. 186, 283–292. doi: 10.3354/meps186283
Sueur, C., Romano, V., Sosa, S., and Puga-Gonzalez, I. (2019). Mechanisms of network evolution: a focus on socioecological factors, intermediary mechanisms, and selection pressures. Primat 60, 167–181. doi: 10.1007/s10329-018-0682-7
Taborsky, M., and Limberger, D. (1981). Helpers in fish. Behav. Ecol. Sociob. 8, 143–145. doi: 10.1007/bf00300826
Taborsky, M., and Wong, M. Y. (2017). “Sociality in fishes,” in Comparative Social Evolution, eds D. I. Rubenstein and P. Abbot (Cambridge: Cambridge University Press), 354. doi: 10.1017/9781107338319.013
Thompson, V. J., Munday, P. L., and Jones, G. P. (2007). Habitat patch size and mating system as determinants of social group size in coral-dwelling fishes. Coral Reefs 26, 165–174. doi: 10.1007/s00338-006-0181-y
Turner, M. G. (2010). Disturbance and landscape dynamics in a changing world. Ecology 91, 2833–2849. doi: 10.1890/10-0097.1
Verde, E. A., Cleveland, A., and Lee, R. W. (2015). Nutritional exchange in a tropical tripartite symbiosis II: direct evidence for the transfer of nutrients from host anemone and zooxanthellae to anemonefish. Mar. Biol. 162, 2409–2429. doi: 10.1007/s00227-015-2768-8
Victor, B. C. (1984). Coral reef fish larvae: patch size estimation and mixing in the plankton. Limnol. Oceanogr. 29, 1116–1119. doi: 10.4319/lo.1984.29.5.1116
West-Eberhard, M. J. (1975). The evolution of social behavior by kin selection. Quart. Rev. Biol. 50, 1–33. doi: 10.1086/408298
Wey, T., Blumstein, D. T., Shen, W., and Jordán, F. (2008). Social network analysis of animal behaviour: a promising tool for the study of sociality. Anim. Behav. 75, 333–344. doi: 10.1016/j.anbehav.2007.06.020
Williams, G. C. (1966). Natural selection, the costs of reproduction, and a refinement of Lack’s principle. Am. Nat. 100, 687–690. doi: 10.1086/282461
Wong, M., and Balshine, S. (2011). The evolution of cooperative breeding in the African cichlid fish, Neolamprologus pulcher. Biol. Rev. 86, 511–530. doi: 10.1111/j.1469-185x.2010.00158.x
Wong, M. Y. (2010). Ecological constraints and benefits of philopatry promote group-living in a social but non-cooperatively breeding fish. Proc. R. Soc. B. 277, 353–358. doi: 10.1098/rspb.2009.1453
Wong, M. Y., and Buston, P. M. (2013). Social systems in habitat-specialist reef fishes: key concepts in evolutionary ecology. Biosciences 63, 453–463. doi: 10.1525/bio.2013.63.6.7
Wong, M. Y., Buston, P. M., Munday, P. L., and Jones, G. P. (2007). The threat of punishment enforces peaceful cooperation and stabilizes queues in a coral-reef fish. Proc. R. Soc. B. 274, 1093–1099. doi: 10.1098/rspb.2006.0284
Wong, M. Y., Fauvelot, C., Planes, S., and Buston, P. M. (2012). Discrete and continuous reproductive tactics in a hermaphroditic society. An. Behav. 84, 897–906. doi: 10.1016/j.anbehav.2012.07.013
Wong, M. Y., Munday, P. L., Buston, P. M., and Jones, G. P. (2008a). Fasting or feasting in a fish social hierarchy. Curr. Biol. 18, R372–R373.
Wong, M. Y., Munday, P. L., Buston, P. M., and Jones, G. P. (2008b). Monogamy when there is potential for polygyny: tests of multiple hypotheses in a group-living fish. Behav. Ecol. 19, 353–361. doi: 10.1093/beheco/arm141
Wong, M. Y., Uppaluri, C., Medina, A., Seymour, J., and Buston, P. M. (2016). The four elements of within-group conflict in animal societies: an experimental test using the clown anemonefish, Amphiprion percula. Behav. Ecol. Soc. 70, 1467–1475. doi: 10.1007/s00265-016-2155-6
Wong, M. Y. L. (2011). Group size in animal societies: the potential role of social and ecological limitations in the group-living fish, Paragobiodon xanthosomus. Ethology 117, 638–644. doi: 10.1111/j.1439-0310.2011.01913.x
Wong, M. Y. (2007). The Evolution of Animal Societies: Monogamy, Group-living and Conflict in a Coral-dwelling Fish. PhD thesis, Douglas QLD: James Cook University.
Keywords: social evolution, eusociality, sociality, cooperative breeding, reproductive skew, coral reef fishes
Citation: Rueger T, Branconi R, Froehlich CYM, Heatwole SJ, Wong MYL and Buston PM (2021) The Next Frontier in Understanding the Evolution of Coral Reef Fish Societies. Front. Mar. Sci. 8:665780. doi: 10.3389/fmars.2021.665780
Received: 08 February 2021; Accepted: 01 April 2021;
Published: 05 May 2021.
Edited by:
David M. P. Jacoby, Zoological Society of London, United KingdomReviewed by:
Michael Gil, University of California, Davis, United StatesKátia de Meirelles Felizola Freire, Universidade Federal de Sergipe, Brazil
Copyright © 2021 Rueger, Branconi, Froehlich, Heatwole, Wong and Buston. This is an open-access article distributed under the terms of the Creative Commons Attribution License (CC BY). The use, distribution or reproduction in other forums is permitted, provided the original author(s) and the copyright owner(s) are credited and that the original publication in this journal is cited, in accordance with accepted academic practice. No use, distribution or reproduction is permitted which does not comply with these terms.
*Correspondence: Theresa Rueger, theresa.rueger@gmail.com