- 1Department of Fisheries Science, Faculty of Aquatic Biology, Chonnam National University, Jeonnam, South Korea
- 2Department of Fisheries Biology and Genetics, Faculty of Fisheries, Patuakhali Science and Technology University, Patuakhali, Bangladesh
- 3Department of Food Science and Technology, Sunchon National University, Jeonnam, South Korea
Temperature has crucial effects on gonadal development and reproduction of abalone. To understand the impact of thermal stress on molecular and physiological processes triggering the regulation of reproduction, changes in the mRNA expression of neuroendocrine genes encoding two abalone gonadotropin-releasing hormone (Hdh-GnRH, Hdh-GnRH-like peptide), GnRH receptor (Hdh-GnRH-R), Hdh-APGWamide, serotonin receptor (5-HThdh), and a heat shock protein HSP70 were examined in ganglia and testis of male Pacific abalone (Haliotis discus hannai). Abalone were exposed to low water temperature (LWT) and high water temperature (HWT) in early and peak breeding seasons for 7 days. Then, gonadosomatic index (GSI) was calculated, relative gene expression was measured by qRT-PCR, and levels of testosterone in hemolymph were also measured by ELISA during the peak breeding season. GSI did not show any significant changes during the early breeding season. However, it was significantly decreased in LWT- or HWT-exposed abalone compared to the normal water temperature (NWT) group during the peak breeding season. In the early breeding season, changes of mRNA expression of all five genes were significant between LWT and HWT groups on day-7. In the peak breeding season, compared to the NWT group, the mRNA expressions of different genes were significantly decreased in different tissues both in LWT and HWT groups of abalone, such as Hdh-GnRH-like peptide in the cerebral ganglion (CG) and testis; Hdh-GnRH in the pleuropedal ganglion (PPG) and branchial ganglion (BG); Hdh-GnRH-R in the CG, PPG, and testis; and Hdh-APGWamide in the PPG and testis. Interestingly, the expression of 5-HThdh was significantly increased in the HWT group but decreased in the LWT group. Expression of HSP70 was significantly increased in the testis after exposure to HWT. Hemolymph levels of testosterone were significantly decreased in the HWT group compared to those in the NWT group. Altogether, these results denote that thermal stress has a repressive effect on gonadal maturation and reproduction by regulating the expression of Hdh-GnRH-like peptide, Hdh-GnRH, Hdh-GnRH-R, Hdh-APGWamide, 5-HThdh, and HSP70 genes and levels of hemolymph testosterone.
Introduction
Pacific abalone (Haliotis discus hannai) is the most commercially important gastropod species in Korea as well as in Southeast Asian countries. It is widely used in commercial aquaculture because of its remarkable food value and high price (Park and Kim, 2013). Large-scale farming of Pacific abalone in sea cages has spread along small bays and islands of the southwest coast of Korea. There are more than 1,500 abalone hatcheries in Korea that are producing abalone seeds in conventional methods (Choi et al., 2015). Broodstock conditioning is the prime factor in conventional breeding of abalone that is practiced for early development of gonads by controlling exogenous factors (Kikuchi and Uki, 1974; Matsumoto and Maeda, 2021).
A number of exogenous and endogenous factors can control or coordinate the reproductive cycle of marine invertebrates (Nuurai et al., 2010). Among these exogenous factors, temperature plays a critical role in the regulation of reproductive parameters, including reproductive cycles, gametogenesis, and spawning of invertebrates (Giese and Kanatani, 1987; Mercier and Hamel, 2009). An increase in mean sea water temperature is known to cause changes in the annual reproductive cycle of marine invertebrates (Olive, 1995). Water temperature is an important environmental cue that triggers the onset of breeding of molluskan species (Wayne and Block, 1992). It is also considered a principal exogenous factor that regulates the reproductive cycle of many marine gastropod species (Hahn, 1989; Nuurai et al., 2010). Water temperature can influence the rate of gonad development and regulate the reproduction of most abalone species (Grubert and Ritar, 2004). In the sea, the peak spawning season of Pacific abalone is at a water temperature around 20°C (Koizumi and Tsuji, 2017). The increase of visual gonad index (VGI) of Pacific abalone is proportional to the increase of water temperature (Kikuchi and Uki, 1974; Fukazawa et al., 2007). High temperature is known to reduce gonadal development of red abalone (Rogers-Bennett et al., 2010). Both extremely high and low temperatures have adverse impacts on gonadal cells and developmental processes of clams (Lagade et al., 2019).
Increase or decrease of temperature from the ambient level not only can regulate reproductive parameters but also can affect endocrine functions (Pankhurst and King, 2010). Gonadotrophic and reproductive hormones seem to act as transducers between environmental parameters and the gamete, indicating that temperature can alter the levels of these hormones (Lawrence and Soame, 2004). Gonadal developmental activities of abalone are believed to be controlled by long-term endogenous rhythm that is influenced by exogenous environmental factors (Hahn, 1989). Abalone reproduction is largely regulated by a number of neurosecretory hormones synthesized in cerebral, pleuropedal, and visceral ganglia (Hahn, 1992). Secretion of neurohormones from cerebral and pleuropedal ganglia have been found to be correlated with gametogenesis and vitellogenesis and induction of spawning, respectively (Hahn, 1992). Gonadotropin-releasing hormone (GnRH)-like peptide, a neurohormone, plays a key role in the regulation of reproduction of molluskan species. It has been shown that octopus GnRH-like peptide (Oct-GnRH) might be involved in the regulation of reproduction (Minakata and Tsutsui, 2016). Scallop GnRH-like peptide (py-GnRH) can stimulate spermatogonial cell proliferation of Yesso scallop and Pacific oyster (Nakamura et al., 2007; Treen et al., 2012). Endogenous GnRH-like peptide can stimulate egg-laying of pond snail (Young et al., 1999). Pacific abalone GnRHs (Hdh-GnRH-like peptide, Hdh-GnRH) and GnRH receptor (Hdh-GnRH-R) have been reported to be involved in gonadal cell proliferation, gonadal development, and reproductive activities (Kim et al., 2017; Sharker et al.,2020a,c, 2021). Tropical abalone GnRH peptide (HasGnRH) has been shown to be involved in ovarian cell proliferation of H. asinina (Nuurai et al., 2016). It is evident that serotonin (5-HT), a neurohormone, is a potential regulator that controls the secretion of GnRH (Prasad et al., 2015). 5-HT is also known to play pivotal roles in invertebrates’ reproductive systems, including gonadal maturation and spawning (Hamida et al., 2004; Garnerot et al., 2006). 5-HT can induce gonadal maturation, egg release, and spawning of mollusks generally potentiated by sex steroids and may be regulated via the induction of serotonin receptor synthesis. Estrogens can promote 5-HT-induced spawning in scallops, Placopecten magellanicus, by synthesis of 5-HT receptors (Wang and Croll, 2006). In mussels, Mytilus edulis, following estradiol-17β (E2) treatment, the expression of 5-HT1-like receptor is decreased in the mature stage but increased in immature stages (Cubero-Leon et al., 2010). 5-HT receptors (5-HThdh) are also involved in the reproduction of Pacific abalone (Kim et al., 2019; Sharker et al., 2020b). Another neuropeptide, Ala-Pro-Gly-Trp-NH2 (APGWamide), can also control sexual maturation and reproduction of mollusks. APGWamide can function as a central and peripheral neuromediator in the control of male reproduction of Lymnaea stagnalis (Van Golen et al., 1995; De Boer et al., 1997) and Ilyanassa obsolete (Oberdorster et al., 2005). This neuropeptide is also induced during final maturation and spawning of both sexes of Pacific abalone and Sydney rock oyster (Van In et al., 2016; Kim et al., 2018). Induction of neuropeptides, GnRH, GnRH-like peptide, 5-HT, and APGWamide can induce sexual maturation and spawning both in vitro and in vivo, providing direct evidence that these signaling molecules are crucial for abalone reproduction (Chansela et al., 2008; Nuurai et al., 2010, 2016; Sharker et al., 2021). However, there is a scarcity of data on the effects of temperature on these neuroendocrine genes, and there is a need for a study to understand their effect on gonadal development and reproduction of abalone.
The effect of temperature on abalone reproduction and the relationship between temperature and biological processes during gonadal development have been studied extensively for several abalone species such as Pacific abalone (H. discus hannai) (Kikuchi and Uki, 1974; Hahn, 1994), New Zealand abalone (H. iris) (Kabir, 2001), austral abalone (H. australis) (Kabir, 2001), blacklip abalone (H. rubra) (Grubert and Ritar, 2004), and greenlip abalone (H. laevigata) (Grubert and Ritar, 2004). However, the effect of thermal stress on neuroendocrine and reproduction-associated genes during gonadal development and breeding season remains unclear. Therefore, the objective of the present study was to investigate the effects of low- and high-temperature stress on mRNA expression levels of neuroendocrine genes encoding two abalone gonadotropin-releasing hormones (Hdh-GnRH, Hdh-GnRH-like peptide), gonadotropin-releasing hormone receptor (Hdh-GnRH-R), Hdh-APGWamide, and a serotonin receptor (5-HThdh) in Pacific abalone (H. discus hannai) during breeding season. The expression of a heat shock protein HSP70 in testis and hemolymph level of testosterone were also examined.
Materials and Methods
Abalone Collection and Rearing
Pacific abalone (H. discus hannai) were collected from cages of abalone sea cage aquaculture of East sea of Wando-gun, Jeollanam-do, South Korea, in May and June for the first and second experiment during early and peak breeding seasons, respectively. The water temperature at the sampling site was about 18°C during the sampling period in May 2020 and 21°C in June 2020 (Supplementary Table 1). Collected abalone were transported to Tou-Jeong Soosan abalone hatchery in Dolsan-eup, Yeosu-si, Jeollanam-do, and conditioned in indoor tanks under natural photoperiod with recirculating sea water for 2 weeks. Water temperature during the conditioning period was similar to that at the sampling site.
Experimental Design
Two experiments under different temperature conditions were conducted in May and June 2020 with ambient temperature of about 18 and 21°C, respectively. In the first experiment during the early breeding season, abalone were exposed to a low-temperature (14°C), a normal-temperature (18°C), and a high-temperature (22°C) condition for 7 days. The second experiment was conducted during the peak breeding season in which abalone were exposed to a low temperature (16°C), a normal temperature (21°C), and a high temperature (26°C) for 7 days. Abalone was reared in the natural photoperiod: in May, 14:10 h, and in June, 14.5:9.5 h. For both experiments, to acclimatize abalone at low and high temperatures, temperature was gradually decreased or increased (Δ1°C per 24 h) from normal water temperature (NWT) to the target temperature. After reaching the target temperature, abalone were kept in the target water temperature for 3 and 7 days for day-3 and day-7 groups, respectively (n = 10 for each group). In addition, a set of abalone was sampled just before the start of the experiment for thermal stress. This group served as initial control (IC).
Abalone Husbandry and Monitoring
Water temperature of each experimental tank was maintained using an electric cooling unit (SunCool, DA-3000C, DAEIL, Korea), a heating unit (PB 51-1, HANIL, Korea), and a thermostat (DHE, South Korea) with a water flow rate at 1.5 L min–1. Water quality parameters such as dissolved oxygen, salinity, temperature, and pH were measured daily with YSI professional plus digital water quality meter (Pro 10102030; Xylem Inc., United States) (Supplementary Table 2). Abalone were fed daily to satiation with seaweed, Laminaria religosa. No abalone died during the experimental period.
Ethics Statement
Animal experiments were conducted in accordance with guidelines of the Institutional Animal Care and Use Committee of Chonnam National University (CNU IACUC) with a permission number of CNU IACUC-YS-2020-5 and according to Article 14th of the Korean Animal Protection Law of the Korean government. Animals were cared for in accordance with Guidelines for Animal Experiments of Chonnam National University.
Sample Collection
After temperature exposure, 10 abalone from each group were collected from the rearing tank on day-3 and day-7 to obtain tissue samples. Abalone were anesthetized and sacrificed before sample collection. Total length, width, and shell height were measured using a digimatic caliper (CD-P20S, Mitutoyo Corp., Japan) and body weight was taken using an electric balance (SPX223KR, OHAUS, United States) in nearest mm and g, respectively (Supplementary Table 3). Hemolymph samples were collected from the cephalic arterial sinus of abalone with 5-ml syringes as described previously (Donaghy et al., 2010). Collected hemolymphs were kept on ice and centrifuged at 5,000 rpm for 10 min at 4°C. Supernatants were carefully collected and then stored at –80°C until assay. For gonadosomatic index (GSI) calculation, gonads were removed after decapitation and weighted. Total body weight and soft-body weight were also measured. From each abalone, cerebral ganglion (CG), pleuropedal ganglion (PPG), branchial ganglion (BG), and a portion of gonad (testis) were collected, snap frozen in liquid nitrogen, and stored at –80°C until total RNA extraction. A portion of each gonad from individual abalone was fixed in 4% paraformaldehyde (PFA) for gonadal histology.
Calculation of Gonadosomatic Index
GSI was calculated as described previously (Litaay and De Silva, 2003; Onitsuka et al., 2007) using the following formula: GSI = (wet weight of gonad in grams/soft-body weight of abalone in grams) × 100.
Testicular Histology
After fixation in 4% PFA overnight, gonad samples were infiltrated with 30% sucrose, embedded in optimal cutting temperature (OCT) compound and cryo-sectioned at 7–8-μm thickness using a cryostat microtome (Leica CM 1860; Leica Biosystems, United States). Sections were stained with Mayer’s hematoxylin and counterstained with eosin Y (alcoholic) using H&E staining kit (ab245880; Abcam, United States). Stained slides were subsequently observed under a microscope (Eclipse E600; Nikon, Tokyo, Japan) to confirm testicular stages. Testicular stages of abalone were identified based on histological analysis following previously reported testicular stages (Kim et al., 2016).
Total RNA Extraction and cDNA Synthesis
Total RNA was extracted from all sampled tissues of Pacific abalone using an RNeasy mini kit (Qiagen, Hilden, Germany). Any possible contamination of genomic DNA was eliminated by treating with RNase-free DNase (Promega, Madison, WI, United States). Quality and quantity of total RNAs were evaluated by electrophoresis and spectrophotometry (UV Spectrophotometer; ASP-2680, ACTGene, United States). First-strand cDNAs were synthesized from total RNA using Oligo (dT) primer (OdT) (Sigma) and Superscript® III First-Strand cDNA synthesis kit (Invitrogen, United States). All steps of RNA extraction and cDNA synthesis were conducted following the manufacturer’s instructions.
Quantitative Real-Time PCR Analysis of Abalone Neuroendocrine Genes Encoding Hdh-GnRH, GnRH-like peptide, HdhGnRH-R, 5-HTHdh, Hdh-APGWamide, and HSP70
Quantitative real-time PCR (qRT-PCR) assay was carried out using 2x qPCRBIO SyGreen Mix Lo-Rox kit (PCR Biosystems Ltd., United Kingdom) as described previously (Sukhan et al., 2020). Briefly, the qRT-PCR reaction mixture was prepared with 1 μl of cDNA template, 1 μl (10 pmol) of both forward and reverse primers (Table 1), 10 μl of SYBR Green Mix, and dH2O with a final volume of 20 μl. Triplicate reactions were performed for target and reference genes in each tissue sample. PCR amplification conditions were: preincubation at 95°C for 5 min, followed by 40 cycles 95°C for 2 min, 60°C for 30 s, and 72°C for 30 s. The melting temperature was obtained using the default setting of the instrument. At the end of each cycle, a fluorescence reading was recorded for quantification. A LightCycler® 96 System (Roche, Germany) was used for amplification and data analysis. Specific amplification of each subtype cDNA was verified by melting curve analysis and gel electrophoresis of the product. Relative gene expression was quantified based on cycle threshold using the 2–ΔΔct method (Livak and Schmittgen, 2001) with β-actin gene (GenBank accession no. AY380809) as an internal reference.
Enzyme-Linked Immunosorbent Assay for Testosterone Analysis
Testosterone hormone was extracted from each hemolymph sample using diethyl ether as described previously (Sang et al., 2019) with slight modification and according to instruction of a testosterone ELISA kit (ADI-900-065; Enzo Life Sciences, United States). Briefly, 1 ml of diethyl ether was added to every milliliter of hemolymph and vortexed. The top ether layer was carefully taken by a pipette and placed into a clean test tube. This step was repeated twice more, and ether layers were combined. Ether was then evaporated to dryness under nitrogen. Extracted testosterone was then dissolved in an assay buffer provided in the kit. Levels of testosterone in hemolymph samples were measured using a species-independent testosterone ELISA kit (ADI-900-065) according to the manufacturer’s instructions. Testosterone concentration was measured at 405 nm using an EpochTM Microplate Spectrophotometer (BioTek Instruments Inc., United States). The amount of signal was indirectly proportional to the level of testosterone in the sample. A standard curve of testosterone was obtained using concentrations of standard ranging from 7.81 to 2,000 pg/ml.
Statistical Analysis
Values of GSI, mRNA expression, and testosterone level were analyzed statistically and expressed as mean ± standard deviation (SD). Changes in the GSI corresponding to different temperatures, relative mRNA expressions, and testosterone concentrations in different tissues were analyzed by non-parametric one-way analysis of variance (ANOVA) to generate graphs using GraphPad Prism 5.1 software1. Tukey’s post hoc test was performed to assess statistically significant differences among different temperature conditions. Statistical significance was set at p < 0.05. Different letters in figures showed significant differences between different temperatures. Changes in the relative mRNA expressions in different tissues across different thermal stress conditions were analyzed by non-parametric one-way ANOVA followed by post hoc Tukey’s homogeneity of variance test using statistical package IBM SPSS for Windows Ver. 25.0 and presented in Supplementary Tables 4–8.
Results
Changes in the Gonadosomatic Index During Early and Peak Breeding Seasons
In the early breeding season, the GSI of Pacific abalone showed no distinct changes when they were exposed to different water temperatures such as low (14°C), high (22°C), and normal (18°C) water temperatures on any exposure day (Figure 1A). On the other hand, during the peak breeding season, the GSI of abalone was significantly decreased in both low (16°C) and high (26°C) water temperature groups compared to that in the normal (21°C) water temperature group on day-7 (Figure 1B).
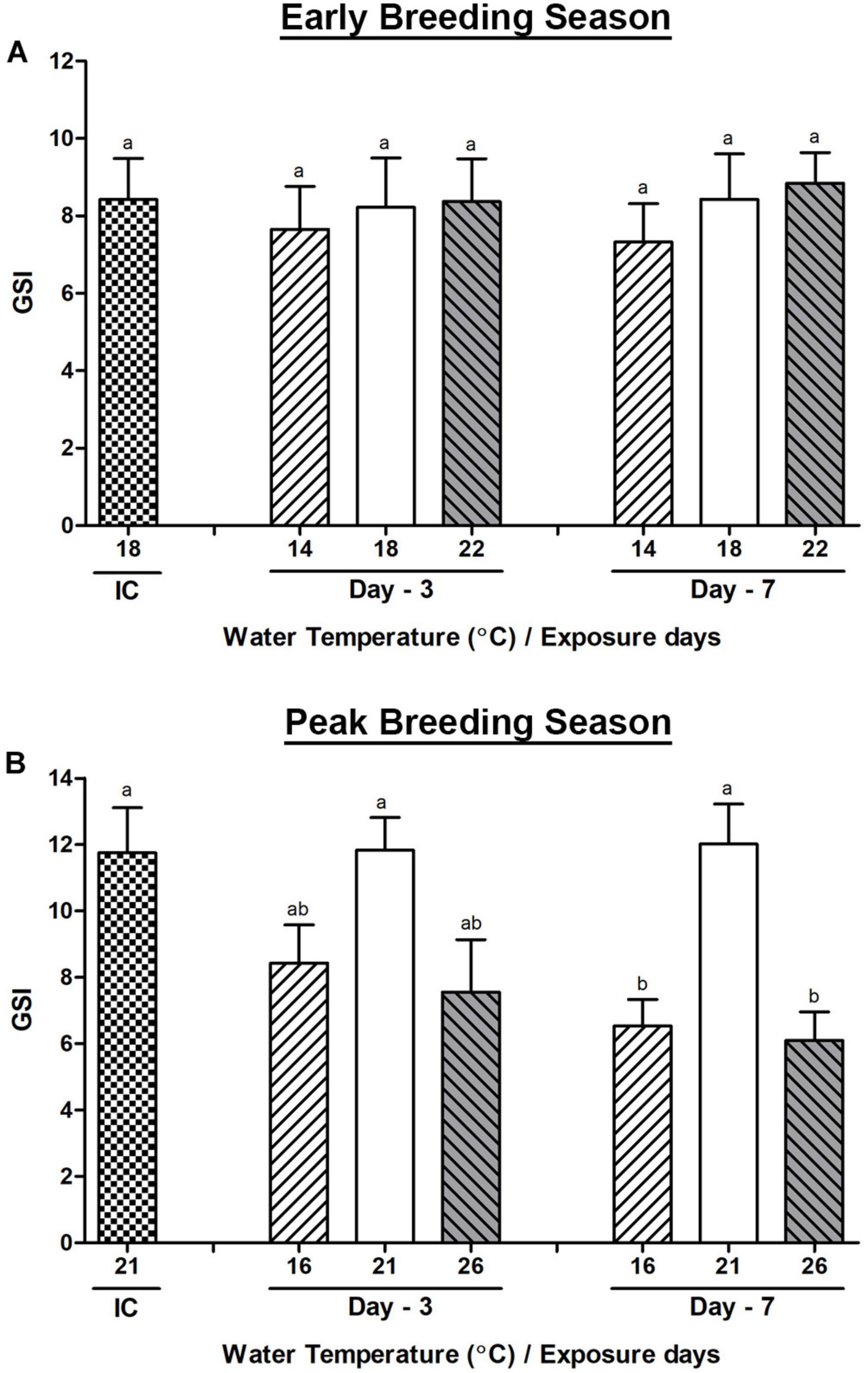
Figure 1. Changes in the gonadosomatic index (GSI) of male Pacific abalone during early (A) and peak (B) breeding seasons exposed to thermal stress for 7 days. Before exposure to the target temperature of thermal stress, abalone were sampled to obtain reference values that served as initial control (IC). Values represent the mean ± SD (n = 10). Different letters on the bar indicate significant differences among different temperature groups (p < 0.05).
Histological Changes in the Testicular Stages of Abalone During the Peak Breeding Season
Representative sections of testis tissues of abalone exposed to different temperatures (16, 21, and 26°C) during the peak breeding season were histologically analyzed and presented in Figure 2. Testicular stage was found in fully mature stage in NWT (21°C) group abalone characterized as full of sperm (Figure 2B). Both low (16°C) and high (26°C) temperature-exposed groups of abalone showed distinct changes in the histological stages compared to the NWT group of abalone (Figures 2A,C). In both groups of abalone, testicular stage seems to possess degenerative stage of testis.
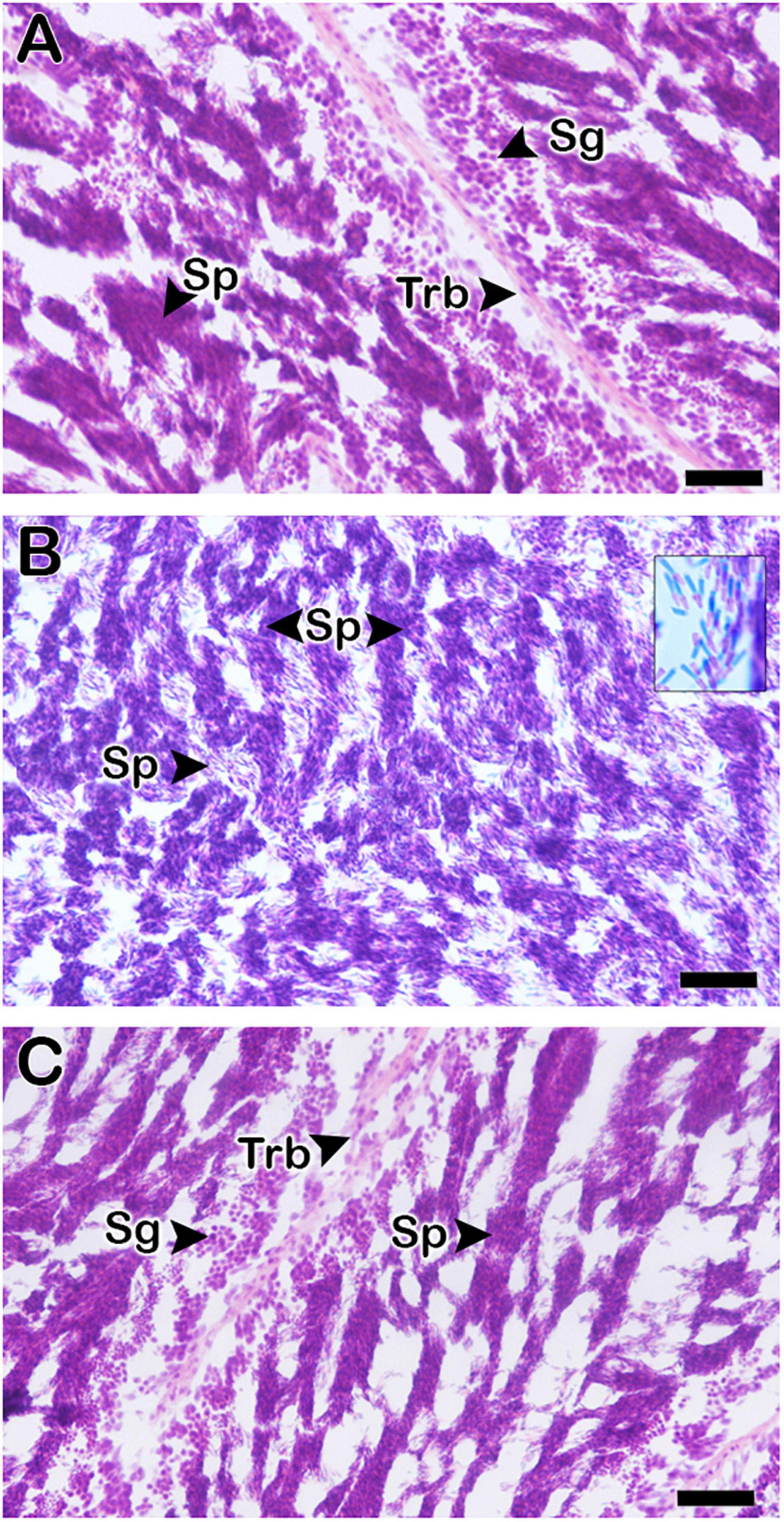
Figure 2. Histological analysis of testes in Pacific abalone (H. discus hannai) on day-7 in the second experiment during the peak breeding season after exposure to three temperature treatments (A: 16°C; B: 21°C; and C: 26°C) by hematoxylin–eosin staining. Low (A) and high (C) water temperature group of abalone showed distinct differences in mature sperm content compared to normal water temperature (B) group of abalone. The inset picture in (B) is at higher magnification of sperm. Sg, spermatogonia; Sp, sperm; Trb, trabeculae. Scale bar: 100 μm.
Changes in the Expression of Two Abalone Hdh-GnRHs and Hdh-GnRH-R Genes in Different Ganglia of Central Nervous System and Testis of Pacific Abalone During Early and Peak Breeding Seasons
The effects of thermal stress on mRNA expression levels of two abalone GnRHs (Hdh-GnRH and Hdh-GnRH-like peptide) and GnRH-R genes in CG, PPG, and BG of the central nervous system (CNS) and in testis tissues of Pacific abalone were examined in two experiments during early and peak breeding seasons.
Changes in the mRNA Expression in the Cerebral Ganglion
In the early breeding season, Hdh-GnRH-like peptide and GnRH-R genes showed no significant changes in the mRNA expression on day-3 among any temperature group, whereas significant changes in their expression were observed between low- and high-temperature groups of abalone on day-7 (Figures 3A,B). During the peak breeding season, both low and high water temperature (HWT) groups of abalone showed significantly lower expression of Hdh-GnRH-like peptide and GnRH-R gene compared to the NWT group on day-7 (Figures 3C,D). Hdh-GnRH gene did not show significant changes of expression in any breeding season (Supplementary Figure 1). Among these three genes, Hdh-GnRH-like peptide showed higher expression in CG than the other two genes (Supplementary Table 4).
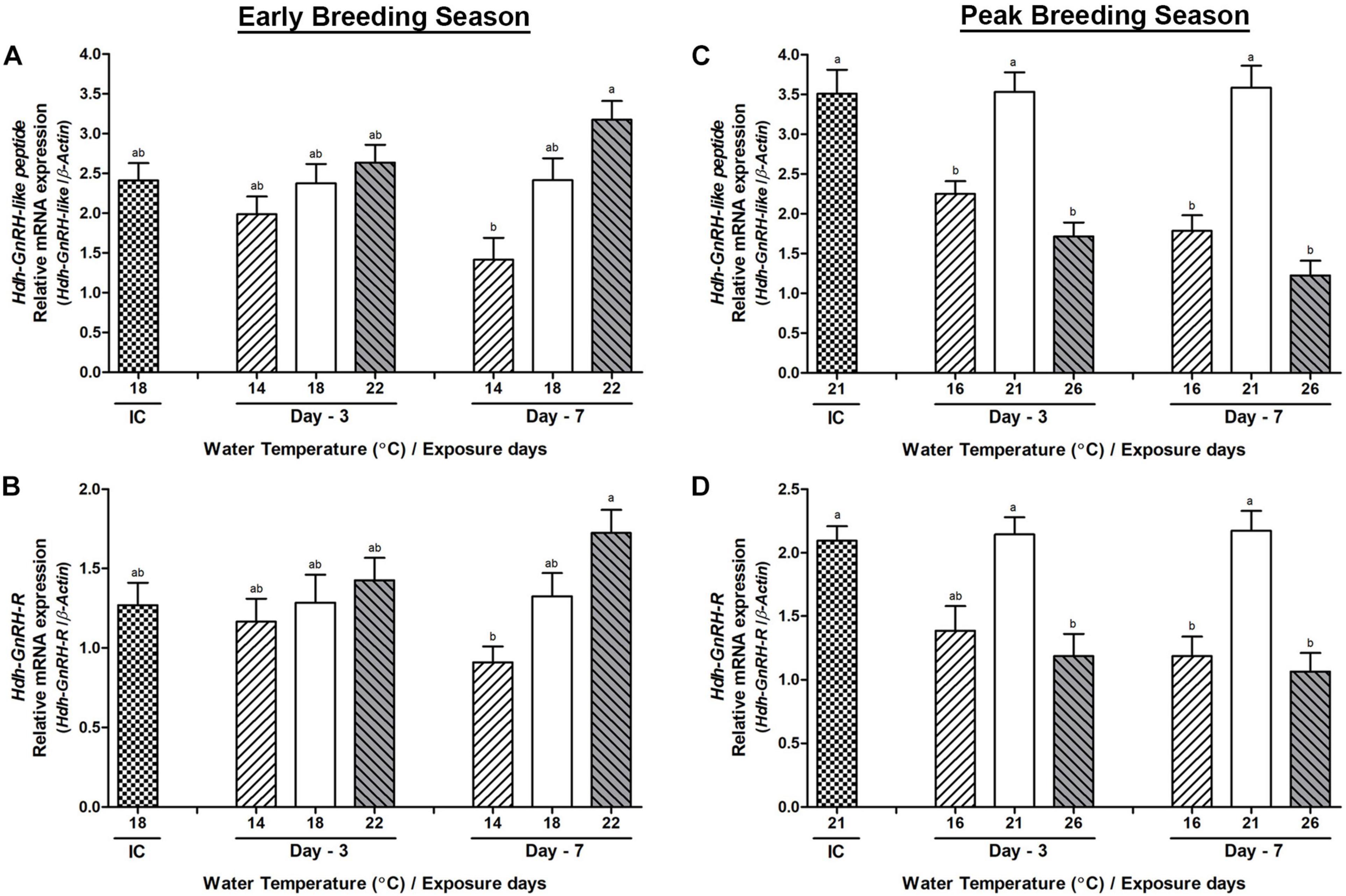
Figure 3. Changes in the relative mRNA expression of Hdh-GnRH-like peptide (A,C) and Hdh-GnRH-R (B,D) in the cerebral ganglion (CG) of male Pacific abalone during early and peak breeding seasons after exposure to thermal stress for 7 days. Before exposure to the target temperature of thermal stress, abalone were sampled to obtain reference values that served as initial control (IC). Values represent the mean ± SD (n = 10). Different letters on the bar indicate significant differences among different temperature groups (p < 0.05).
Changes in the mRNA Expression in the Pleuropedal Ganglion
During the early breeding season, changes in the mRNA expression of Hdh-GnRH and GnRH-R genes on day-3 were insignificant in any group of abalone, although significant changes in their expression were observed between low- and high-temperature groups on day-7 (Figures 4A,B). During the peak breeding season, changes in the mRNA expression of Hdh-GnRH gene were significantly lower in both high- and low-temperature groups of abalone compared to those in the NWT group on day-3 and day-7 (Figure 4C). Hdh-GnRH-R gene showed significantly lower expression in HWT group compared to that in the NWT group on day-7 (Figure 4D). Hdh-GnRH-like peptide expression did now show any significant changes in abalone of low, high, or NWT group on day-3 or day-7 in any breeding season (Supplementary Figure 2). In PPG, Hdh-GnRH gene showed higher expression than Hdh-GnRH-like peptide and Hdh-GnRH-R (Supplementary Table 5).
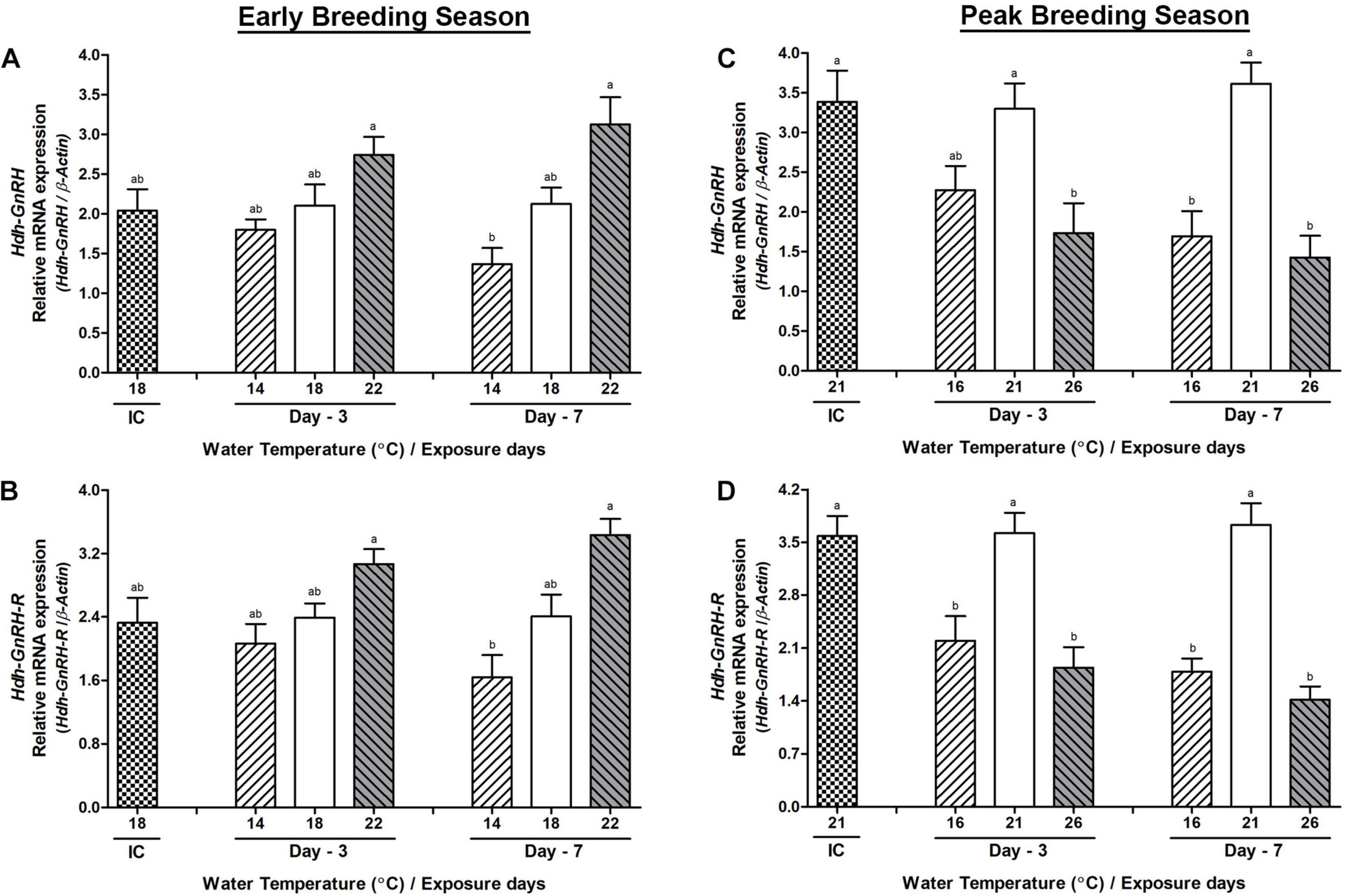
Figure 4. Changes in the relative mRNA expression of Hdh-GnRH (A,C) and Hdh-GnRH-R (B,D) in the pleuropedal ganglion (PPG) of male Pacific abalone during early and peak breeding seasons after exposure to thermal stress for 7 days. Before exposure to the target temperature of thermal stress, abalone were sampled to obtain reference values that served as initial control (IC). Values represent the mean ± SD (n = 10). Different letters on the bar indicate significant differences among different temperature groups (p < 0.05).
Changes in the mRNA Expression in the Branchial Ganglion
In BG, significant changes in the Hdh-GnRH mRNA expression were observed between low and HWT groups on day-7 during the early breeding season (Figure 5A). However, changes in its expression in abalone of HWT group were significantly lower compared to HWT group on day-3 and day-7 in the peak breeding season (Figure 5B). On the other hand, its expression levels in abalone of low water temperature (LWT) group were significantly lower than those in the NWT group on day-7. Expression levels of Hdh-GnRH-like peptide and Hdh-GnRH-R genes did not show any significant changes in any group of abalone on day-3 or day-7 in any breeding season (Supplementary Figure 3).
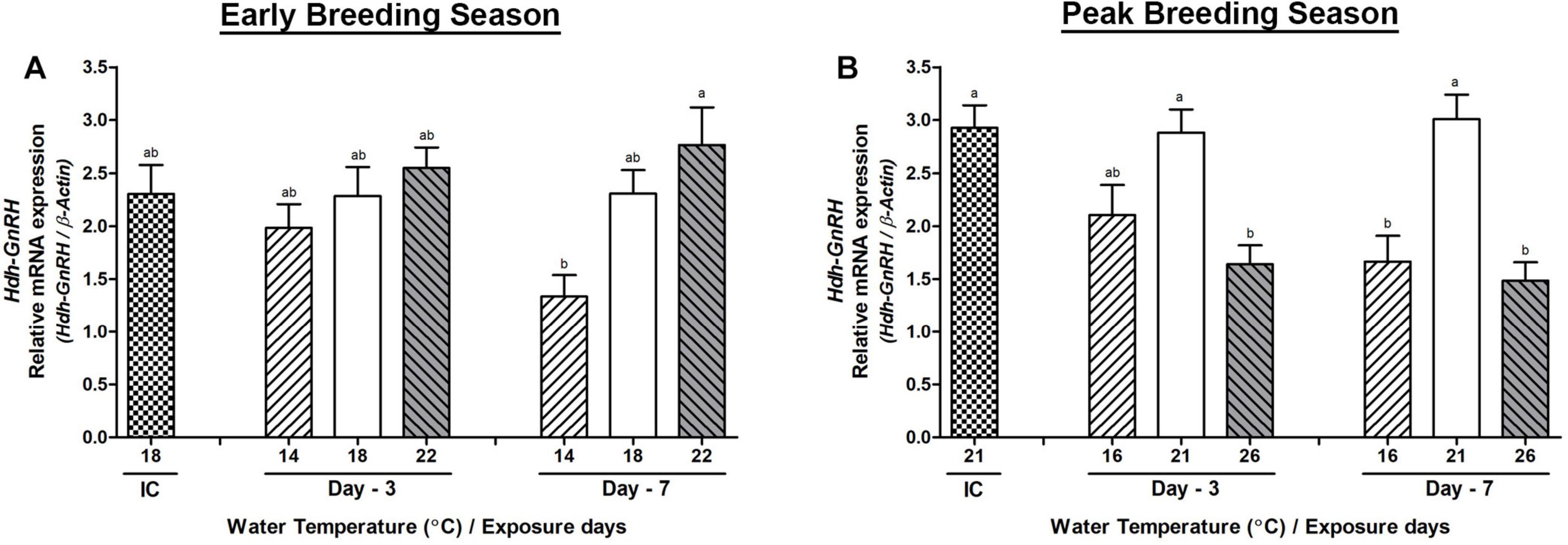
Figure 5. Changes in the relative mRNA expression of Hdh-GnRH (A,B) in the branchial ganglion (BG) of male Pacific abalone during early and peak breeding seasons after exposure to thermal stress for 7 days. Before exposure to the target temperature of thermal stress, abalone were sampled to obtain reference values that served as initial control (IC). Values represent the mean ± SD (n = 10). Different letters on the bar indicate significant differences among different temperature groups (p < 0.05).
Changes in the mRNA Expression in the Testis
The changes in the mRNA expression in the testis tissue of all three genes were not significant on day-3 during the early breeding season, whereas significant changes were observed between low and HWT groups of abalone on day-7 (Figures 6A–C). During the peak breeding season, on day-3, only Hdh-GnRH-like peptide showed significantly lower expression in HWT group of abalone compared to NWT group (Figure 6E). On day-7, changes in the expression of Hdh-GnRH-like peptide and Hdh-GnRH-R genes in both low and HWT groups of abalone showed significantly lower expression compared to NWT group (Figures 6E,F). However, the Hdh-GnRH gene showed significant changes only between high and NWT groups on day-7 (Figure 6D).
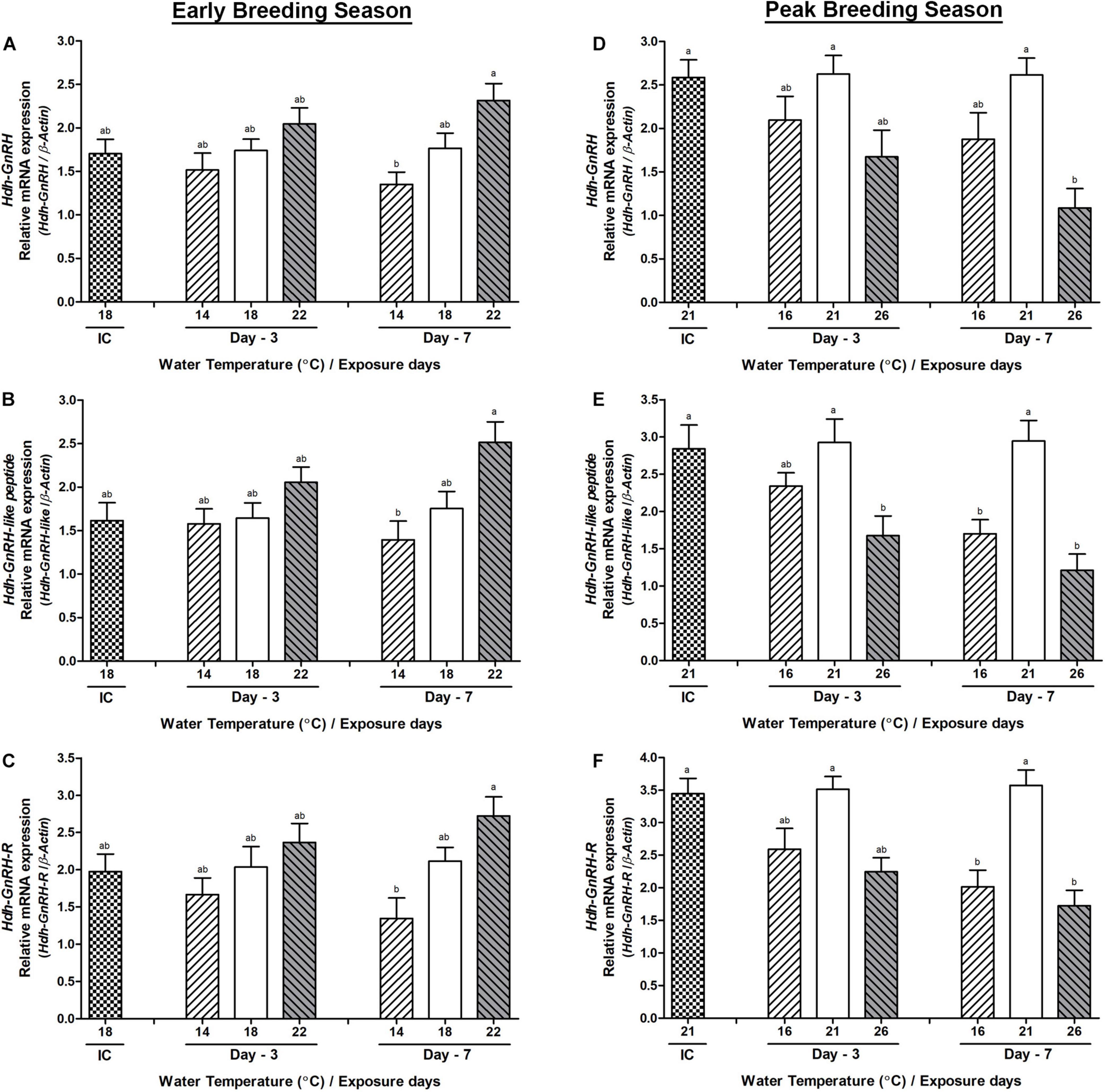
Figure 6. Changes in the relative mRNA expression of Hdh-GnRH (A,D), Hdh-GnRH-like peptide (B,E), and Hdh-GnRH-R (C,F) in the testis of male Pacific abalone during early and peak breeding seasons after exposure to thermal stress for 7 days. Before exposure to the target temperature of thermal stress, abalone were sampled to obtain reference values that served as initial control (IC). Values represent the mean ± SD (n = 10). Different letters on the bar indicate significant differences among different temperature groups (p < 0.05).
Changes in the Expression of Hdh-APGWamide and Serotonin Receptor (5-HThdh) Genes in Different Ganglia of the Central Nervous System and Testis of Pacific Abalone During the Early and Peak Breeding Seasons
Changes in the mRNA expression pattern of Hdh-APGWamide and 5-HThdh genes in CG, PPG, and BG of the CNS and testis of Pacific abalone were examined after exposed to low or HWT in two experiments during early and peak breeding seasons.
Changes in the mRNA Expression in the Cerebral Ganglion
In the CG, the mRNA expression of serotonin receptor (5-HThdh) interestingly showed an increased expression in the high-temperature group of abalone during the peak breeding season. The expression of 5-HThdh gene showed insignificant changes on day-3 or day-7 between any temperature groups of abalone during the early breeding season (Figure 7A). In the peak breeding season, the changes of expression between low- and high-temperature group abalone were significant on day-7, whereas no significant differences were observed on day-3 among any temperature groups (Figure 7B). The mRNA expression of Hdh-APGWamide gene did not show any significant differences in either the early or peak breeding season (Supplementary Figure 4).
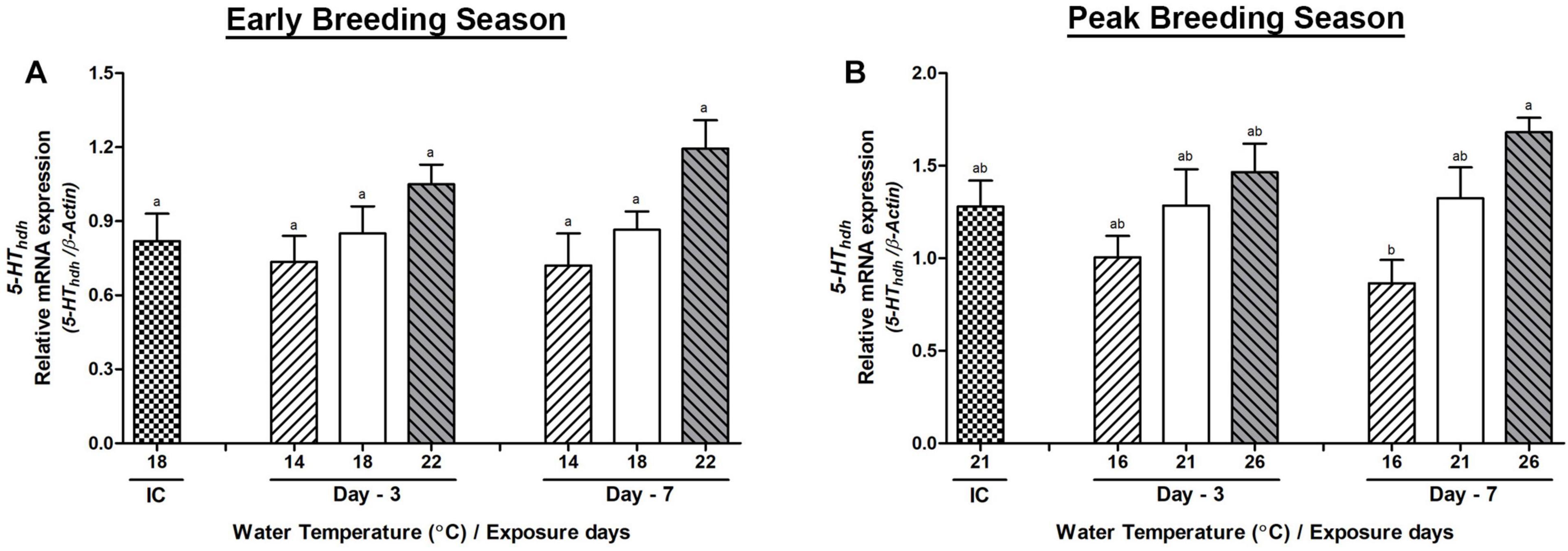
Figure 7. Changes in the relative mRNA expression of 5-HThdh (A,B) in the cerebral ganglion (CG) of male Pacific abalone during early and peak breeding seasons after exposure to thermal stress for 7 days. Before exposure to the target temperature of thermal stress, abalone were sampled to obtain reference values that served as initial control (IC). Values represent the mean ± SD (n = 10). Different letters on the bar indicate significant differences among different temperature groups (p < 0.05).
Changes in the mRNA Expression in the Pleuropedal Ganglion
The mRNA expression of Hdh-APGWamide gene did not show any significant difference in any temperature group during the early breeding season (Figure 8A). However, in the peak breeding season, it showed a significantly lower expression in the HWT group compared to that in the NWT group on day-7 (Figure 8C). Serotonin receptor (5-HThdh) gene showed a significantly higher mRNA expression in the HWT group of abalone than the LWT group in both early (Figure 8B) and peak (Figure 8D) breeding seasons on day-7. The expression was also significantly higher compared to that of the NWT group of abalone on day-7 during the peak breeding season.
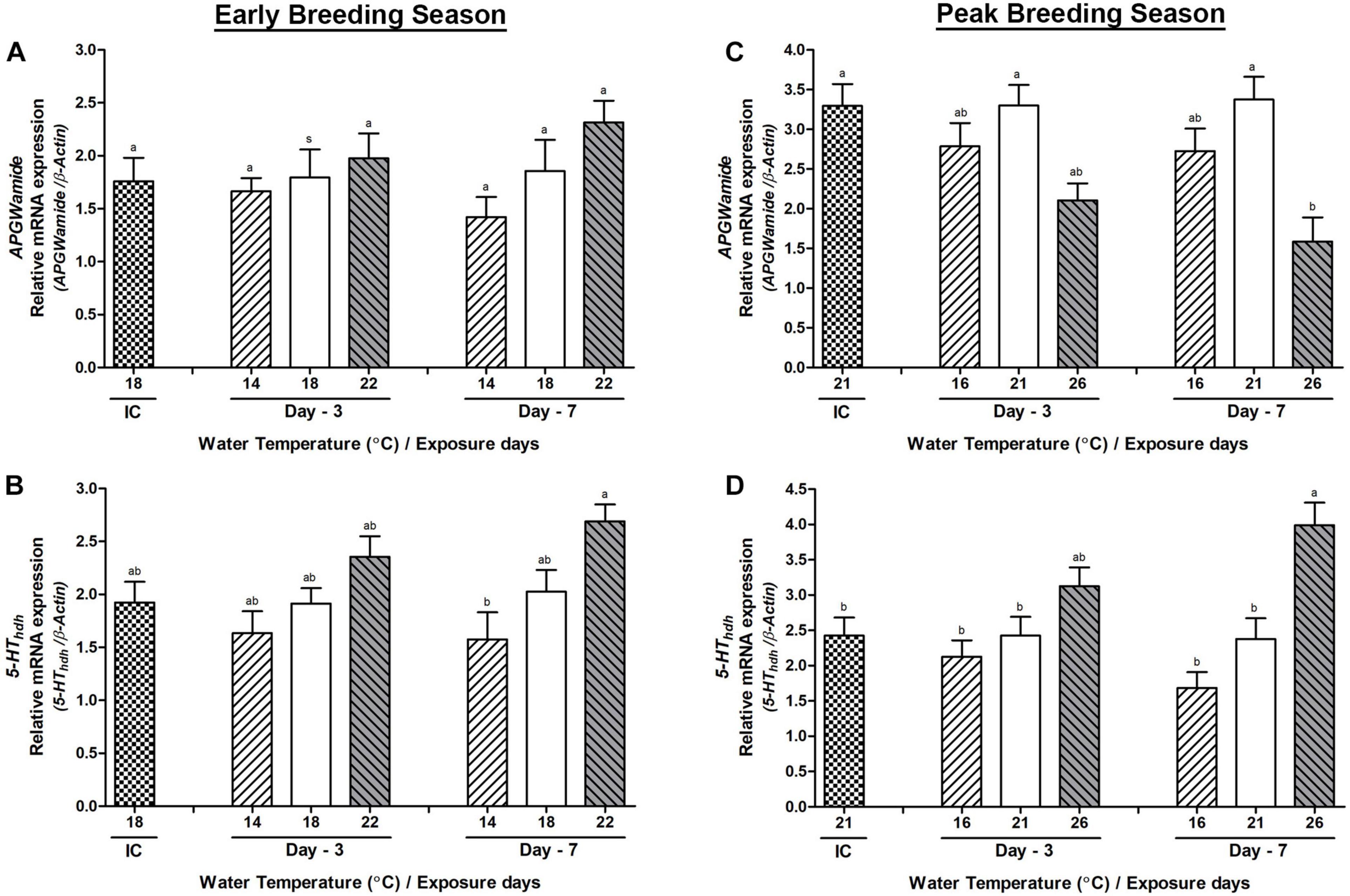
Figure 8. Changes in the relative mRNA expression of Hdh-APGWamide (A,C) and 5-HThdh (B,D) in the pleuropedal ganglion (PPG) of male Pacific abalone during early and peak breeding seasons after exposure to thermal stress for 7 days. Before exposure to the target temperature of thermal stress, abalone were sampled to obtain reference values that served as initial control (IC). Values represent the mean ± SD (n = 10). Different letters on the bar indicate significant differences among different temperature groups (p < 0.05).
Changes in the mRNA Expression in the Branchial Ganglion
In the BG, mRNA expression levels of Hdh-APGWamide and 5-HThdh genes did not show any significant differences among any temperature group of abalone during the early or peak breeding season (Supplementary Figure 5).
Changes in the mRNA Expression in the Testis
Hdh-APGWamide and 5-HThdh mRNA expression showed no significant changes among any temperature group on day-3 during the early breeding season. However, the HWT group of abalone showed a significantly higher expression of both genes compared to the low temperature group on day-7 (Figures 9A,B). During the peak breeding season, the HWT group of abalone showed a significantly lower Hdh-APGWamide mRNA expression than the NWT group (Figure 9C) on day-3 and day-7. Interestingly, serotonin receptor (5-HThdh) showed a different expression pattern compared to other examined genes. The HWT group of abalone showed significantly higher expression of 5-HThdh on day-7 compared to those of the low and NWT groups of abalone (Figure 9D).
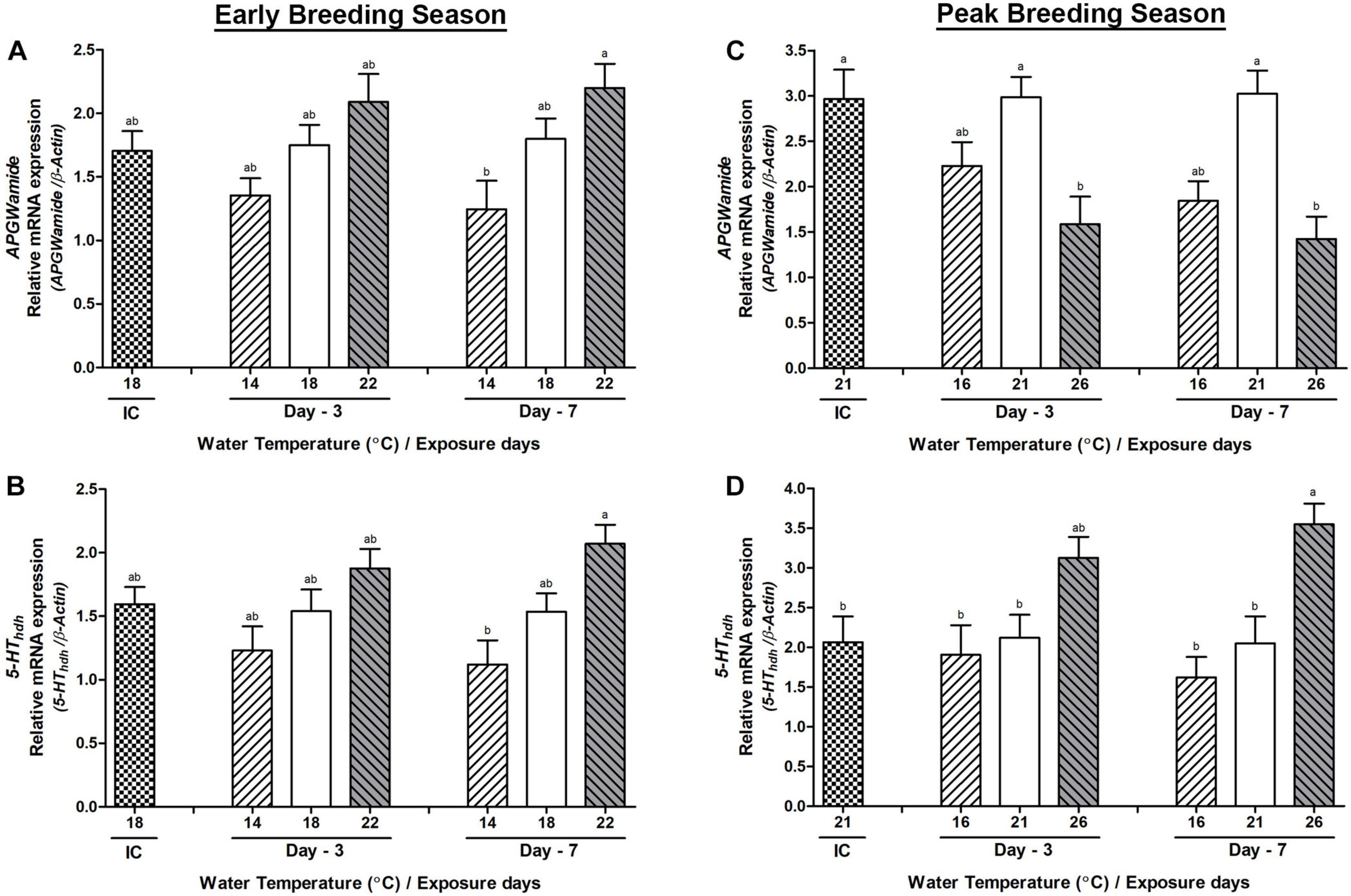
Figure 9. Changes in the relative mRNA expression of Hdh-APGWamide (A,C) and 5-HThdh (B,D) in the testis of male Pacific abalone during early and peak breeding seasons after exposure to thermal stress for 7 days. Before exposure to the target temperature of thermal stress, abalone were sampled to obtain reference values that served as initial control (IC). Values represent the mean ± SD (n = 10). Different letters on the bar indicate significant differences among different temperature groups (p < 0.05).
Changes in the mRNA Expression of Heat Shock Protein 70 (HSP70) Gene in the Testis of Pacific Abalone During the Peak Breeding Season
The mRNA expression levels of HSP70 were significantly increased in the testis of the HWT group of abalone compared to those of the low and NWT groups on day-3 and day-7 during the peak breeding season (Figure 10).
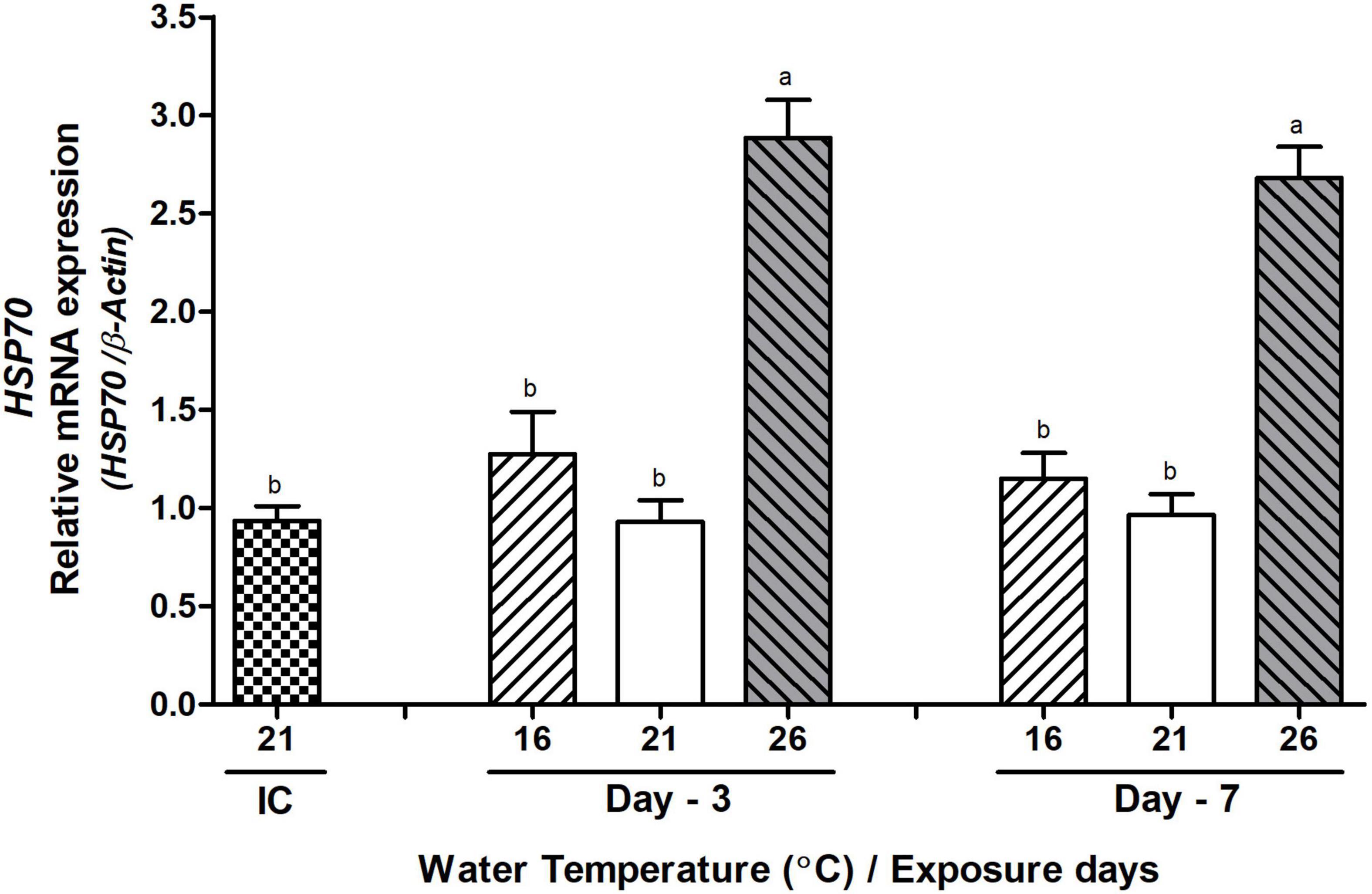
Figure 10. Changes in the relative mRNA expression of HSP70 in the testis of Pacific abalone during the peak breeding season after exposure to thermal stress for 7 days. Before exposure to the target temperature of thermal stress, abalone were sampled to obtain reference values that served as initial control (IC). Values represent the mean ± SD (n = 10). Different letters on the bar indicate significant differences among different temperature groups (p < 0.05).
Changes in the Hemolymph Testosterone During the Peak Breeding Season
Hemolymph levels of testosterone both in low and high temperature-exposed abalone showed decreasing trends. The levels of testosterone were significantly decreased in HWT -exposed abalone compared to those of the NWT group both on day-3 and day-7 during the peak breeding season. On the other hand, the changes of testosterone level were insignificant in the LWT-exposed abalone compared to those of the normal and HWT groups of abalone (Figure 11).
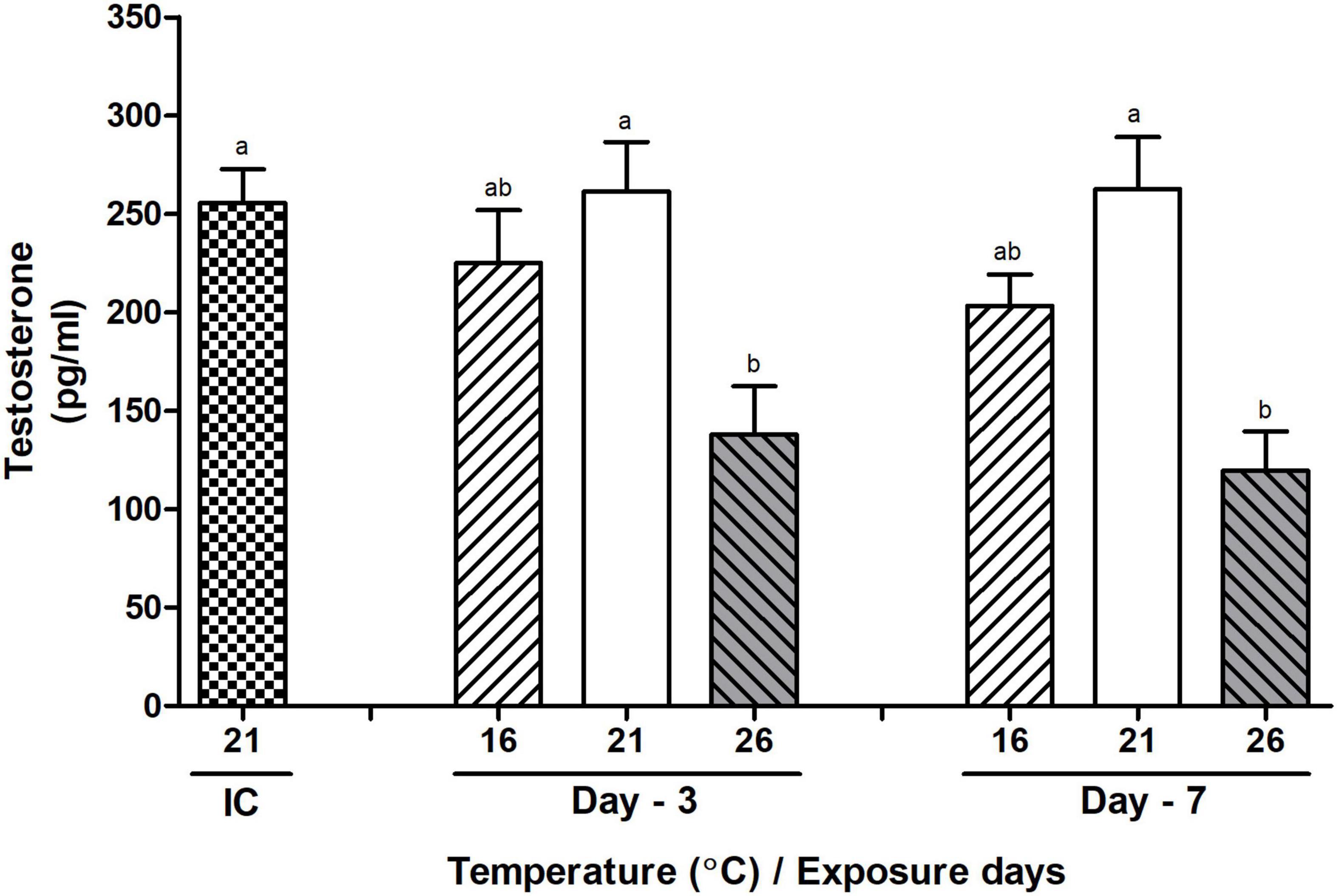
Figure 11. Changes in the levels of testosterone in the hemolymph of male Pacific abalone during the peak breeding season after exposure to thermal stress for 7 days. Before exposure to the target temperature of thermal stress, abalone were sampled to obtain reference values that served as initial control (IC). Values represent the mean ± SD (n = 10). Different letters on the bar indicate significant differences among different temperature groups (p < 0.05).
Discussion
Water temperature plays a crucial role in gonadal development and reproduction of abalone species. In the present experiment, to understand the role of temperature in gonadal development in Pacific abalone, the effects of low and HWT stress on the expression levels of abalone reproduction-associated genes encoding Hdh-GnRH, Hdh-GnRH-like peptide, Hdh-GnRH-R, Hdh-APGWamide, and 5-HThdh in the three ganglia (CG, PPG, and BG) of the CNS and testis tissues and a heat shock protein, HSP70 in the testis of mature male abalone were examined during early and peak breeding seasons. Examined reproduction-associated genes showed differential expression patterns in different ganglion tissues. Hdh-GnRH, Hdh-GnRH-R, APGWamide, and 5-HThdh genes showed higher expression levels in the PPG, whereas Hdh-GnRH-like peptide showed a higher expression level in the CG. Previously, it has been reported that Hdh-GnRH (Kim et al., 2017), Hdh-GnRH-R (Sharker et al., 2020c), APGWamide (Kim et al., 2018), and 5-HThdh (Sharker et al., 2020b) genes were synthesized from the PPG and Hdh-GnRH-like peptide (Sharker et al., 2020a) was synthesized from the CG in Pacific abalone. Besides its higher expression in the PPG, Hdh-GnRH also showed a higher expression in the BG. A similar kind of expression pattern of Hdh-GnRH has also been reported previously (Kim et al., 2017). The present study is the first one reporting the effects of low and HWT stress on the expression of reproduction-associated genes during the breeding season in a molluskan species.
The GSI of Pacific abalone did not show any significant differences among all temperature conditions in the early breeding season. In the peak breeding season when GSI was in a more advanced stage, it was significantly decreased in both low and HWT group of abalone compared to the NWT group. Reproduction activities of abalone can be severely affected by thermal stress. It has been shown that the gonad index of male red abalone is significantly decreased under acute thermal stress (Vilchis et al., 2005; Rogers-Bennett et al., 2010). Since the early breeding season of Pacific abalone starts in May when water temperature is about 18°C and the peak breeding season is in June when water temperature is about 20–21°C (Kim et al., 2016), the low temperature condition both in the first and the second experiments might have kept the abalone under less thermal stress. The high-temperature condition at 22°C in the early breeding season might be an acceptable temperature for abalone to progress gonadal maturation. At this condition, reproduction-associated genes showed an increasing trend of expression. On the other hand, in the peak breeding season, in the high-temperature condition at 26°C, the GSI tended to decrease. This high-temperature condition might have acted as an environmental signal to the CNS to terminate breeding activities. Expression levels of reproduction-associated genes such as Hdh-GnRH, Hdh-GnRH-like peptide, Hdh-GnRH-R, and APGWamide were also decreased significantly. Interestingly, 5-HThdh gene expression level was increased. Currently, there is no concrete evidence about the effect of thermal stress on the GSI of any invertebrate species other than Atlantic sea urchin. After exposure to a high temperature, percentages of gametes (eggs/sperm) in Atlantic sea urchin are decreased on day-7 (Johnstone et al., 2019). However, several experimental data on this issue have been published for different aquatic animals. For example, the GSI of mature male puffer fish is significantly decreased on day-7 after exposure to a high or low temperature (Shahjahan et al., 2017). The GSI of male blue gourami is reduced on day-9 after exposure to a low temperature (David and Degani, 2011). After exposure to a high temperature, the GSI of mature female red seabream is decreased on day-7 (Okuzawa and Gen, 2013). The GSI of mature female Atlantic salmon in a high-temperature condition is suppressed significantly (Pankhurst et al., 2011). These data indicate that like other aquatic animals, reproductive activities of abalone are also dependent on temperature and that under both low- and high-temperature stresses, the GSI of Pacific abalone is reduced significantly.
In the early breeding season, Hdh-GnRH, Hdh-GnRH-like peptide, Hdh-GnRH-R, APGWamide, and 5-HThdh genes in the CG did not show significant changes in the mRNA expression at low or HWT stress when each condition was compared to NWT. However, the expression levels of Hhd-GnRH, Hdh-GnRH-like peptide, and Hdh-GnRH-R genes were significantly different between low- and high-temperature stress conditions. In the PPG, Hhd-GnRH, Hdh-GnRH-R, and 5-HThdh but not Hdh-GnRH-like peptide and APGWamide showed an expression pattern similar to those observed in the CG. In the BG, only Hdh-GnRH gene showed significant differences on day-7 between low- and high-temperature groups of abalone.
In the peak breeding season, the expression of Hdh-GnRH-like peptide and Hdh-GnRH-R genes in CG showed significantly lower expression in both low- and high-temperature groups of abalone compared to NWT group with a synchronous decrease of GSI. The mRNA expression of Hdh-GnRH-like peptide was found significantly higher in CG than in other ganglion tissues (Supplementary Table 4). These results suggest that CG-synthesized Hdh-GnRH-like peptide might be involved in the reproductive process during the breeding season, and its expression could be downregulated in both low- and high-temperature stress. Previously, it has been reported that Hdh-GnRH-like peptide synthesized in CG might be involved in gonadal maturation and regulating the secretion of other reproductive hormones (Sharker et al., 2020a). In the PPG, Hdh-GnRH and Hdh-GnRH-R genes but not Hdh-GnRH-like peptide showed significantly lower expression in both low and HWT groups than those in the NWT group on day-7. Both Hdh-GnRH and Hdh-GnRH-R genes showed significantly higher expression in the PPG than in other ganglion tissues (Supplementary Tables 5,6). This result may suggest that these two genes that synthesized in PPG might be involved in the reproductive function, and both low- and high-temperature stresses can suppress their expression during the peak breeding season as well as gonadal maturation and GSI. Kim et al. (2017) reported that the Hdh-GnRH gene was synthesized from the PPG of Pacific abalone, and based on expression analysis, they have concluded that Hdh-GnRH synthesized in the PPG might be involved in reproductive activities. However, Sharker et al. (2020c) have isolated Hdh-GnRH-R gene from the PPG and suggested that PPG synthesizing Hdh-GnRH-R gene might be involved in the control of gonadal maturation and gametogenesis of Pacific abalone. In the BG, Hdh-GnRH-like peptide and Hdh-GnRH-R genes but not Hdh-GnRH showed no significant changes of expression in any temperature group of abalone. However, Hdh-GnRH gene showed significantly lower expression in both high- and low-temperature groups of abalone compared to that in the NWT group of abalone on day-7. This result may suggest that BG-synthesized Hdh-GnRH might also be involved in the gonadal developmental process, and both low- and high-temperature stress downregulated its mRNA expression in the BG. Kim et al. (2017) have reported that the expression of Hdh-GnRH gene that is synthesized in the BG and the PPG is tightly correlated with abalone reproductive activities.
Hdh-APGWamide gene expression showed no significant changes in the CG or BG in any temperature group of abalone in the peak breeding season. It showed significantly lower expression in the PPG on day-7 in HWT group of abalone compared to that in NWT group. A significantly higher expression of Hdh-APGWamide gene was observed in the PPG than in other ganglion tissues (Supplementary Table 7). This result suggests that Hdh-APGWamide that synthesized in the PPG might be involved in reproductive activities; however, HWT stress can suppress its expression. A previous study has concluded that APGWamide gene in Pacific abalone has a ganglion-specific expression, indicating that it may induce final maturation and spawning in both sexes (Kim et al., 2018). Interestingly, the expression of 5-HThdh gene was significantly higher in the high-temperature group of abalone in both the CG and PPG compared to those of the low- and normal-temperature groups of abalone. In the present experiment, the higher expression of 5-HThdh gene in the high-temperature group abalone is quite different from those of the other examined genes. In the LWT group of abalone, it showed significantly lower expression on day-7 in the PPG compared to the high-temperature group, but the changes were insignificant with NWT group. The 5-HThdh gene showed significantly higher expression in the PPG than those in the other ganglion tissues (Supplementary Table 8). These results may suggest that PPG-synthesized 5-HThdh gene might be involved in the regulation of reproduction. Furthermore, thermal stress can regulate the expression of 5-HThdh in Pacific abalone during the breeding season. Its expression in abalone is downregulated at low temperature but upregulated at high temperature. A similar result has been reported in two other molluskan species, blue and swan mussel. It has been shown that levels of serotonin (5-HT) in the CNS of Mytilus edulis and Anodonta cygnea are increased under high-temperature stress but decreased under cold-temperature stress (Stefano et al., 1978).
The mRNA expression levels of reproduction-associated genes were also examined in testis tissues. Genes encoding Hdh-GnRH, Hdh-GnRH-like peptide, Hdh-GnRH-R, Hdh-APGWamide, and 5-HThdh showed significantly higher expression during the early breeding season in the high-temperature group of abalone compared to those in the LWT group of abalone on day-7. However, in the peak breeding season, both low- and high-temperature groups of abalone showed significantly lower mRNA expression levels of Hdh-GnRH-like peptide and Hdh-GnRH-R compared to those in the NWT group on day-7. On the other hand, Hdh-GnRH and Hdh-APGWamide genes showed significantly lower expression only in the high-temperature group of abalone compared to NWT group on day-7. Interestingly, 5-HThdh gene showed significantly higher expression compared to normal and LWT group on day-7. Previously, it has been demonstrated that expression levels of these genes are increased in testis tissue during the peak breeding season (Kim et al., 2017, 2018, 2019; Sharker et al.,2020a,b,c). However, such data for either high or LWT-stressed abalone have not been published yet.
Furthermore, expression levels of HSP70 were significantly increased in the testis of HWT group of abalone compared to those of the NWT group both on day-3 and day-7 during the peak breeding season. This may lead the decrease of GSI and gonadal development. A number of animal research have reported that expression levels of HSP70 increased in the testis after thermal stress and affect gonadal maturation and reproduction. For example, the expression of HSP70 was increased after heat exposure in American oyster that leads to decreased testicular functions (Nash and Rahman, 2019). After exposure to heat stress, Atlantic sea urchins exhibited decreased percentages of gametes (eggs/sperm) as well as increased HSP70 (Johnstone et al., 2019). Levels of ot-HSP70 mRNA significantly increased in the testis of heat-treated Octopus tankahkeei (Long et al., 2015). Levels of HSP70 increased highly in the rabbit testis after exposure to thermal stress (Pei et al., 2012); with the increase of heating time, the HSP70 expression was markedly elevated in the testis of adult rat, which led to a decrease in sperm concentration (Cao et al., 2009).
In the present study, it was observed that levels of testosterone in hemolymph were significantly decreased in the HWT group of abalone compared to those in the NWT. Hemolymph levels of testosterone in the low-temperature group of abalone were also decreased, but the changes were insignificant. Decrease of hemolymph testosterone indicated the reduction of reproductive performance or shift of reproduction/gonadal stages. Several previous studies have reported that thermal stress downregulated the levels of hemolymph or plasma testosterone levels in different aquatic animals, which ultimately affects the gonadal development and reproduction, such as pejerrey (Elisio et al., 2012), Atlantic salmon (Pankhurst et al., 2011), Senegalese sole (García-López et al., 2006), European sea bass (Rodríguez et al., 2005), and common wolffish (Tveiten and Johnsen, 2001). Besides the gonadal regulation and reproduction function, plasma levels of testosterone are considered an indicator of stress during stress conditions. Increased cortisol, decreased testosterone, and the ratio between cortisol and testosterone levels have been widely used as endocrinological indicators of stress in animal studies (Henry, 1992; Von Holst, 1998; Choi et al., 2012). Several studies have reported that levels of plasma testosterone are reduced significantly under physical or environmental stress in various aquatic animals, such as mud snail (Gooding et al., 2003), mature sockeye salmon (Kubokawa et al., 1999), mature male brown trout (Pickering et al., 1987), and staghorn damselfish and spiny chromis damselfish (Demairé et al., 2020). In the present experiment, significantly lower levels of hemolymph testosterone in the high-temperature group of abalone may be indicating that a high-temperature condition might cause stress for Pacific abalone. Also, the significant increase of HSP70 during high-temperature stress coincides with the lower levels of testosterone. It is evident that HSP70 significantly increased in the testis of heat-treated mice along with a decrease in serum testosterone levels (Das et al., 2020).
In conclusion, both high- and low-temperature stress conditions showed significantly decreased mRNA expression levels of Hdh-GnRH, Hdh-GnRH-like peptide, Hdh-GnRH-R, and Hdh-APGWamide in the peak breeding season on day-7. However, 5-HThdh showed interestingly significantly increased expression in the high-temperature condition. Furthermore, HSP70 showed significantly higher expression in testis tissues in the peak breeding season on day-3 and day-7. Levels of hemolymph testosterone were significantly decreased under high-temperature stress, although their changes were insignificant under low-temperature stress. Altogether, these results indicate that thermal stress has an inhibitory effect on gonadal development and reproduction in male Pacific abalone by regulating the expression of Hdh-GnRH, Hdh-GnRH-like peptide, Hdh-GnRH-R, Hdh-APGWamide, 5-HThdh, and HSP70 in the CNS and testis tissues. These changes may occur due to physiological responses to thermal stress. Thus, downregulated expression of neuroendocrine genes in a high temperature might be an indicator for the termination of reproduction to the CNS at the end of the breeding season in Pacific abalone. These data could be useful for broodstock maintenance in abalone hatcheries.
Data Availability Statement
The datasets presented in this study can be found in online repositories. The names of the repository/repositories and accession number(s) can be found in the article/ Supplementary Material.
Ethics Statement
The animal study was reviewed and approved by the Institutional Animal Care and Use Committee of Chonnam National University (CNU IACUC).
Author Contributions
KK and ZS conceptualized, designed the experiments, and wrote the manuscript. ZS, MS, YC, SH, KC, and KK performed the experiments. ZS, MS, and KK analyzed the data. ZS prepared the figures and tables. YC, SH, and KC reviewed the draft manuscript. KK managed the research fund. All authors contributed to the article and approved the submitted version.
Funding
This research was a component of a project entitled “Development of technology for abalone aquaculture using sperm cryopreservation (Grant No. 2018- 2129)” funded by the Ministry of Oceans and Fisheries, Republic of Korea.
Conflict of Interest
The authors declare that the research was conducted in the absence of any commercial or financial relationships that could be construed as a potential conflict of interest.
Acknowledgments
We are grateful to Dongik Choi, President, Tou-Jeong Soosan abalone hatchery, Dolsan-eup, Yeosu-si, Jeollanam-do, Korea, for allowing the use of the hatchery facilities to conduct the field experiment.
Supplementary Material
The Supplementary Material for this article can be found online at: https://www.frontiersin.org/articles/10.3389/fmars.2021.664426/full#supplementary-material
Supplementary Figure 1 | Changes in relative mRNA expression of Hdh-GnRH in cerebral ganglion (CG) of male Pacific abalone during early (A) and peak (B) breeding seasons after exposure to thermal stress for 7 days. Before exposure to the target temperature of thermal stress, abalone were sampled to obtained reference values which served as initial control (IC). Values represent the mean ± SD (n = 10). Different letters on the bar indicate significant differences among different temperature groups (p < 0.05).
Supplementary Figure 2 | Changes in relative mRNA expression of Hdh-GnRH-like peptide in pleuropedal ganglion (PPG) of male Pacific abalone during early (A) and peak (B) breeding seasons after exposure to thermal stress for 7 days. Before exposure to the target temperature of thermal stress, abalone were sampled to obtained reference values which served as initial control (IC). Values represent the mean ± SD (n = 10). Different letters on the bar indicate significant differences among different temperature groups (p < 0.05).
Supplementary Figure 3 | Changes in relative mRNA expression of Hdh-GnRH-like peptide (A,C) and Hdh-GnRH-R (B,D) in branchial ganglion (BG) of male Pacific abalone during early and peak breeding seasons after exposure to thermal stress for 7 days. Before exposure to the target temperature of thermal stress, abalone were sampled to obtained reference values which served as initial control (IC). Values represent the mean ± SD (n = 10). Different letters on the bar indicate significant differences among different temperature groups (p < 0.05).
Supplementary Figure 4 | Changes in relative mRNA expression of APGWamide in cerebral ganglion (CG) of male Pacific abalone during early (A) and peak (B) breeding seasons after exposure to thermal stress for 7 days. Before exposure to the target temperature of thermal stress, abalone were sampled to obtained reference values which served as initial control (IC). Values represent the mean ± SD (n = 10). Different letters on the bar indicate significant differences among different temperature groups (p < 0.05).
Supplementary Figure 5 | Changes in relative mRNA expression of APGWamide (A,C) and 5-HThdh (B,D) in branchial ganglion (BG) of male Pacific abalone during early and peak breeding seasons after exposure to thermal stress for 7 days. Before exposure to the target temperature of thermal stress, abalone were sampled to obtained reference values which served as initial control (IC). Values represent the mean ± SD (n = 10). Different letters on the bar indicate significant differences among different temperature groups (p < 0.05).
Supplementary Table 1 | Mean sea water temperature of the sampling site of last week from March to June of 2020. Average temperature was calculated with the temperature of last 7 days of each month. Values represent the mean ± SD. Last week’s average temperature was shown as abalone samples were collected in the last week of May and June. Raw data were taken from real-time environment fishery information system overview of National Institute of Fisheries Sciences (NIFS), Korea (http://www.nifs.go.kr/risa/risaInfo.risa).
Supplementary Table 2 | Mean values of water quality parameter of rearing tanks during 1st and 2nd experimental period. Values represent the mean ± SD.
Supplementary Table 3 | Average shell length, shell height, and body weight of abalone used in the present study. Values represent the mean ± SD (n = 10).
Supplementary Table 4 | Changes in relative mRNA expression of Hdh-GnRH-like peptide in different ganglia of central nervous system and testis tissue of Pacific abalone exposed to different temperature for 3 and 7 days during early (experiment 1) and peak (experiment 2) breeding season. Values represent the mean ± SD (n = 3). Different letters in superscript indicates a significant difference among different temperature group (p < 0.05).
Supplementary Table 5 | Changes in relative mRNA expression of Hdh-GnRH gene in different ganglia of central nervous system and testis tissue of Pacific abalone exposed to different temperature for 3 and 7 days during early (experiment 1) and peak (experiment 2) breeding season. Values represent the mean ± SD (n = 3). Different letters in superscript indicates a significant difference among different temperature group (p < 0.05).
Supplementary Table 6 | Changes in relative mRNA expression of Hdh-GnRH-R gene in different ganglia of central nervous system and testis tissue of Pacific abalone exposed to different temperature for 3 and 7 days during early (experiment 1) and peak (experiment 2) breeding season. Values represent the mean ± SD (n = 3). Different letters in superscript indicates a significant difference among different temperature group (p < 0.05).
Supplementary Table 7 | Changes in relative mRNA expression of APGWamide gene in different ganglia of central nervous system and testis tissue of Pacific abalone exposed to different temperature for 3 and 7 days during early (experiment 1) and peak (experiment 2) breeding season. Values represent the mean ± SD (n = 3). Different letters in superscript indicates a significant difference among different temperature group (p < 0.05).
Supplementary Table 8 | Changes in relative mRNA expression of 5-HThdh gene in different ganglia of central nervous system and testis tissue of Pacific abalone exposed to different temperature for 3 and 7 days during early (experiment 1) and peak (experiment 2) breeding season. Values represent the mean ± SD (n = 3). Different letters in superscript indicates a significant difference among different temperature group (p < 0.05).
Footnotes
References
Cao, W., Huang, P., Zhang, L., Wu, H. Z., Zhang, J., and Shi, F. X. (2009). Acute heat stress increases HSP70 expression in the testis, epididymis and vas deferens of adult male mice. Nat. J. Androl. 15, 200–206.
Chansela, P., Saitongdee, P., Stewart, P., Soonklang, N., Stewart, M., Suphamungmee, W., et al. (2008). Existence of APGWamide in the testis and its induction of spermiation in Haliotis asinina Linnaeus. Aquaculture 279, 142–149. doi: 10.1016/j.aquaculture.2008.03.058
Choi, J. C., Chung, M., and Lee, Y. (2012). Modulation of pain sensation by stress-related testosterone and cortisol. Anaesthesia 67, 1146–1151. doi: 10.1111/j.1365-2044.2012.07267.x
Choi, K. S., Kim, B. H., and Lim, H. K. (2015). “Current status of abalone aquaculture in Korea,” in Proceedings of the Korean Society of Fisheries and Aquatic Science (KOSFAS) International Conference 2015 Proceedings. Available online at http://www.dbpia.co.kr/journal/articleDetail?nodeId=NODE06399684 (Accessed January 29, 2021)
Cubero-Leon, E., Ciocan, C. M., Hill, E. M., Osada, M., Kishida, M., and Itoh, N. (2010). Estrogens disrupt serotonin receptor and cyclooxygenase mRNA expression in the gonads of mussels (Mytilus edulis). Aquat. Toxicol. 98, 178–187. doi: 10.1016/j.aquatox.2010.02.007
Das, M., Marak, C. C., Jeremy, M., Gurusubramanian, G., and Roy, V. K. (2020). Heat-induced changes in the expression and localisation of PGC-1α in the mice testis. Andrologia 52:e13713.
David, D., and Degani, G. (2011). Temperature affects brain and pituitary gene expression related to reproduction and growth in the male blue gouramis, Trichogaster trichopterus. J. Exp. Zool. A Ecol. Genet. Physiol. 315, 203–214. doi: 10.1002/jez.663
De Boer, P., Ter Maat, A., Pieneman, A. W., Croll, R. P., Kurokawa, M., and Jansen, R. F. (1997). Functional role of peptidergic anterior lobe neurons in male sexual behavior of the snail Lymnaea stagnalis. J. Neurophysiol. 78, 2823–2833. doi: 10.1152/jn.1997.78.6.2823
Demairé, C., Triki, Z., Binning, S. A., Glauser, G., Roche, D. G., and Bshary, R. (2020). Reduced access to cleaner fish negatively impacts the physiological state of two resident reef fishes. Mar. Biol. 167, 1–10.
Donaghy, L., Hong, H. K., Lambert, C., Park, H. S., Shim, W. J., and Choi, K. S. (2010). First characterization of the populations and immune-related activities of hemocytes from two edible gastropod species, the disk abalone, Haliotis discus discus and the spiny topshell, Turbo cornutus. Fish. Shellfish Immunol. 28, 87–97. doi: 10.1016/j.fsi.2009.10.006
Elisio, M., Chalde, T., and Miranda, L. A. (2012). Effects of short periods of warm water fluctuations on reproductive endocrine axis of the pejerrey (Odontesthes bonariensis) spawning. Comp. Biochem. Physiol. A Mol. Integr. Physiol. 163, 47–55. doi: 10.1016/j.cbpa.2012.05.178
Fukazawa, H., Kawamura, T., Takami, H., and Watanabe, Y. (2007). Oogenesis and relevant changes in egg quality of abalone Haliotis discus hannai during a single spawning season. Aquaculture 270, 265–275. doi: 10.1016/j.aquaculture.2007.03.015
García-López, Á, Pascual, E., Sarasquete, C., and Martínez-Rodríguez, G. (2006). Disruption of gonadal maturation in cultured Senegalese sole Solea senegalensis Kaup by continuous light and/or constant temperature regimes. Aquaculture 261, 789–798. doi: 10.1016/j.aquaculture.2006.09.005
Garnerot, F., Pellerin, J., Blaise, C., and Mathieu, M. (2006). Immunohistochemical localization of serotonin (5-hydroxytryptamine) in the gonad and digestive gland of Mya arenaria (Mollusca: Bivalvia). Gen. Comp. Endocrinol. 149, 278–284 doi: 10.1016/j.ygcen.2006.06.008
Giese, A. C., and Kanatani, H. (1987). “Maturation and spawning,” in Reproduction of Marine Invertebrates, Vol 9, eds A. C. Giese, J. S. Pearse, and V. B. Pearse (California: The Boxwood Press), 251–329.
Gooding, M. P., Wilson, V. S., Folmar, L. C., Marcovich, D. T., and LeBlanc, G. A. (2003). The biocide tributyltin reduces the accumulation of testosterone as fatty acid esters in the mud snail (Ilyanassa obsoleta). Environ. Health Perspect. 111, 426–430. doi: 10.1289/ehp.5779
Grubert, M. A., and Ritar, A. J. (2004). Temperature effects on the dynamics of gonad and oocyte development in captive wild-caught blacklip (Haliotis rubra) and greenlip (H. laevigata) abalone. Invertebr. Reprod. Dev. 45, 185–196. doi: 10.1080/07924259.2004.9652590
Hahn, K. O. (1989). “Gonad reproductive cycles,” in Handbook of Culture of Abalone and Other Marine Gastropods, ed. K. O. Hahn (Boca Raton, FL: CRC Press Inc), 13–40.
Hahn, K. O. (1992). “Review of endocrine regulation of reproduction in abalone spp,” in Abalone of the Word: Biology, Fisheries and Culture, eds S. A. Shepherd, M. J. Tegner, and S. A. Guzman del Pro (Oxford: Blackwells), 49–58.
Hahn, K. O. (1994). Gametogenic cycle of the Japanese abalone (ezoawabi), Haliotis discus hannai, during conditioning with effective accumulative temperature. Aquaculture 122, 227–236. doi: 10.1016/0044-8486(94)90512-6
Hamida, L., Medhioub, M. N., Cochard, J. C., and Pennec, M. L. (2004). Evaluation of the effects of serotonin (5-HT) on oocyte competence in Ruditapes decussatus (Bivalvia, Veneridae). Aquaculture 239, 413–420. doi: 10.1016/j.aquaculture.2004.05.010
Henry, J. P. (1992). Biological basis of the stress response. Integr. Physiol. Behav. Sci. 27, 66–83.
Johnstone, J., Nash, S., Hernandez, E., and Rahman, M. S. (2019). Effects of elevated temperature on gonadal functions, cellular apoptosis, and oxidative stress in Atlantic sea urchin Arbacia punculata. Mar. Environ. Res. 149, 40–49. doi: 10.1016/j.marenvres.2019.05.017
Kabir, N. M. J. (2001). Environmental, Chemical and Hormonal Regulation of Reproduction in Two Commercially Important New Zealand Abalone, Haliotis iris and H. australis. Ph.D. Dissertation. Dunedin: University of Otago.
Kikuchi, S., and Uki, N. (1974). Technical study on artificial spawning of abalone, genus Haliotis. I. relation between water temperature and advancing sexual maturity of Haliotis discus hannai. Ino. Bull. Tohoku Reg. Fish. Res. Lab. 33, 69–78.
Kim, H., Kim, B. H., Son, M. H., Jeon, M. A., Lee, Y. G., and Lee, J. S. (2016). Gonadal development and reproductive cycle of cultured abalone, haliotis discus hannai (Gastropoda: Haliotidae) in Korea: implications for seed production. J. Shellfish Res. 35, 653–659. doi: 10.2983/035.035.0311
Kim, K. S., Kim, M. A., and Sohn, Y. C. (2019). Molecular characterization, expression analysis, and functional properties of multiple 5-hydroxytryptamine receptors in Pacific abalone (Haliotis discus hannai). Gen. Comp. Endocrinol. 276, 52–59. doi: 10.1016/j.ygcen.2019.03.001
Kim, K. S., Kim, T. H., Kim, M. A., Lee, J. S., and Sohn, Y. C. (2018). Expression profile and reproductive regulation of APGWamide in Pacific abalone (Haliotis discus hannai). Comp. Biochem. Physiol. A Mol. Integr. Physiol. 222, 26–35. doi: 10.1016/j.cbpa.2018.04.005
Kim, T. H., Kim, M. A., Kim, K. S., Kim, J. W., Lim, H. K., Lee, J. S., et al. (2017). Characterization and spatiotemporal expression of gonadotropin-releasing hormone in the Pacific abalone, Haliotis discus hannai. Comp. Biochem. Physiol. A Mol. Integr. Physiol. 209, 1–9. doi: 10.1016/j.cbpa.2017.04.001
Koizumi, Y., and Tsuji, Y. (2017). “Abalone Haliotis spp,” in Fisheries Science Series: Application of Recirculating Aquaculture Systems in Japan, ed. T. Takeuchi (Tokyo: Springer), 175–211. doi: 10.1007/978-4-431-56585-7_8
Kubokawa, K., Watanabe, T., Yoshioka, M., and Iwata, M. (1999). Effects of acute stress on plasma cortisol, sex steroid hormone and glucose levels in male and female sockeye salmon during the breeding season. Aquaculture 172, 335–349. doi: 10.1016/s0044-8486(98)00504-3
Lagade, V. M., Taware, S. S., Lagade, S. V., and Muley, D. V. (2019). Effect of temperature on cytological alterations in gonads of estuarine bivalves at Bhatye estuary, Ratnagiri coast. Asian J. Biol. Sci. 12, 860–868. doi: 10.3923/ajbs.2019.860.868
Lawrence, A. J., and Soame, J. M. (2004). The effects of climate change on the reproduction of coastal invertebrates. IBIS 146, 29–39. doi: 10.1111/j.1474-919x.2004.00325.x
Litaay, M., and De Silva, S. S. (2003). Spawning season, fecundity and proximate composition of the gonads of wild-caught blacklip abalone (Haliotis rubra) from Port Fairy waters, south eastern Australia. Aquat. Living Resour. 16, 353–361. doi: 10.1016/s0990-7440(03)00067-6
Livak, K. J., and Schmittgen, T. D. (2001). Analysis of relative gene expression data using real-time quantitative PCR and the 2-ΔΔCT method. Methods 25, 402–408. doi: 10.1006/meth.2001.1262
Long, L. L., Han, Y. L., Sheng, Z., Du, C., Wang, Y. F., and Zhu, J. Q. (2015). Expression analysis of HSP70 in the testis of Octopus tankahkeei under thermal stress. Comp. Biochem. Physiol. A Mol. Integr. Physiol. 187, 150–159. doi: 10.1016/j.cbpa.2015.05.022
Matsumoto, Y., and Maeda, T. (2021). Practical research on rearing broodstock abalone using a closed recirculating system. Janpan Agric. Res. Q. 55, 97–106. doi: 10.6090/jarq.55.97
Mercier, A., and Hamel, J. (2009). “Endogenous and exogenous control of gametogenesis and spawning in echinoderms,” in Advances in Marine Biology, ed. D. W. Sims (London: Academic Press, Inc)
Minakata, H., and Tsutsui, K. (2016). Oct-GnRH, the first protostomian gonadotropin-releasing hormone-like peptide and a critical mini-review of the presence of vertebrate sex steroids in molluscs. Gen. Comp. Endocrinol. 227, 109–114. doi: 10.1016/j.ygcen.2015.07.011
Nakamura, S., Osada, M., and Kijima, A. (2007). Involvement of GnRH neuron in the spermatogonial proliferation of the scallop, Patinopecten yessoensiss. Mol. Reprod. Dev. 74, 108–115. doi: 10.1002/mrd.20544
Nash, S., and Rahman, M. S. (2019). Short-term heat stress impairs testicular functions in the American oyster, Crassostrea virginica: molecular mechanisms and induction of oxidative stress and apoptosis in spermatogenic cells. Mol. Reprod. Dev. 86, 1444–1458. doi: 10.1002/mrd.23268
Nuurai, P., Cummins, S. F., Botwright, N. A., and Sobhon, P. (2016). Characterization of an abalone gonadotropin-releasing hormone and its effect on ovarian cell proliferation. Aquaculture 450, 116–122. doi: 10.1016/j.aquaculture.2015.07.008
Nuurai, P., Engsusophon, A., Poomtong, T., Sretarugsa, P., Hanna, P., Sobhon, P., et al. (2010). Stimulatory effects of egg laying hormone and gonadotropin releasing hormone on reproduction of the tropical abalone, Haliotis asinina Linnaeus. J. Shellfish Res. 29, 627–635. doi: 10.2983/035.029.0311
Oberdorster, E., Romano, J., and McClellan-Green, P. (2005). The neuropeptide APGWamide as a penis morphogenic factor (PMF) in gastropod mollusks. Integr. Comp. Biol. 45, 28–32. doi: 10.1093/icb/45.1.28
Okuzawa, K., and Gen, K. (2013). High water temperature impairs ovarian activity and gene expression in the brain-pituitary-gonadal axis in female red seabream during the spawning season. Gen. Comp. Endocrinol. 194, 24–30. doi: 10.1016/j.ygcen.2013.08.015
Olive, P. J. W. (1995). Annual breeding cycles in marine invertebrates and environmental temperature: probing the proximate and ultimate causes of reproductive synchrony. J. Therm. Biol. 20, 79–90. doi: 10.1016/0306-4565(94)00030-m
Onitsuka, T., Kawamura, T., Horii, T., Takiguchi, N., Takami, H., and Watanabe, Y. (2007). Synchronized spawning of abalone Haliotis diversicolor triggered by typhoon events in Sagami Bay, Japan. Mar. Ecol. Prog. Ser. 351, 129–138. doi: 10.3354/meps07150
Pankhurst, N. W., and King, H. R. (2010). Temperature and salmonid reproduction: implications for aquaculture. J. Fish Biol. 76, 69–85. doi: 10.1111/j.1095-8649.2009.02484.x
Pankhurst, N. W., King, H. R., Anderson, K., Elizur, A., Pankhurst, P. M., and Ruff, N. (2011). Thermal impairment of reproduction is differentially expressed in maiden and repeat spawning Atlantic salmon. Aquaculture 316, 77–87. doi: 10.1016/j.aquaculture.2011.03.009
Park, C. J., and Kim, S. Y. (2013). Abalone aquaculture in Korea. J. Shellfish Res. 32, 17–19. doi: 10.2983/035.032.0104
Pei, Y., Wu, Y., and Qin, Y. (2012). Effects of chronic heat stress on the expressions of heat shock proteins 60, 70, 90, A2, and HSC70 in the rabbit testis. Cell Stress Chaperones 17, 81–87. doi: 10.1007/s12192-011-0287-1
Pickering, A. D., Pottinger, T. G., Carragher, J., and Sumpter, J. P. (1987). The effects of acute and chronic stress on the levels of reproductive hormones in the plasma of mature male brown trout, Salmo trutta L. Gen. Comp. Endocrinol. 68, 249–259. doi: 10.1016/0016-6480(87)90036-0
Prasad, P., Ogawa, S., and Parhar, I. S. (2015). Role of serotonin in fish reproduction. Front. Neurosci. 9:195.
Rodríguez, L., Begtashi, I., Zanuy, S., and Carrillo, M. (2005). Long-term exposure to continuous light inhibits precocity in European male sea bass (Dicentrarchus labrax, L.): hormonal aspects. Gen. Comp. Endocrinol. 140, 116–125. doi: 10.1016/j.ygcen.2004.10.011
Rogers-Bennett, F., Dondanville, R. F., Moore, J. D., and Vilchis, L. I. (2010). Response of red abalone reproduction to warm water, starvation, and disease stressors: implications of ocean warming. J. Shellfish Res. 29, 599–611. doi: 10.2983/035.029.0308
Sang, H. M., Lam, H. S., Hy, L. H. K., Ky, P. X., and Minh-Thu, P. (2019). Changes in plasma and ovarian steroid hormone level in wild female blue tang fish paracanthurus hepatus during a reproductive cycle. Animals 9:889. doi: 10.3390/ani9110889
Shahjahan, M., Kitahashi, T., and Ando, H. (2017). Temperature affects sexual maturation through the control of kisspeptin, kisspeptin receptor, GnRH and GTH subunit gene expression in the grasspuffer during the spawning season. Gen. Comp. Endocrinol. 243, 138–145. doi: 10.1016/j.ygcen.2016.11.012
Sharker, M. R., Kim, S. C., Sumi, K. R., Sukhan, Z. P., Sohn, Y. C., Lee, W. K., et al. (2020a). Characterization and expression analysis of a GnRH-like peptide in the Pacific abalone, Haliotis discus hannai. Agri Gene 15:100099. doi: 10.1016/j.aggene.2019.100099
Sharker, M. R., Sukhan, Z. P., Kim, S. C., Hossen, S., Cho, Y., Choi, C. Y., et al. (2021). In vivo effects of GnRH peptide on gonadal proliferation and related gene expression of pacific abalone, Haliotis discus hannai. Aquaculture 531:735911. doi: 10.1016/j.aquaculture.2020.735911
Sharker, M. R., Sukhan, Z. P., Kim, S. C., Lee, W. K., and Kho, K. H. (2020b). Identification, characterization, and expression analysis of a serotonin receptor involved in the reproductive process of the Pacific abalone, Haliotis discus hannai. Mol. Biol. Rep. 47, 555–567. doi: 10.1007/s11033-019-05162-2
Sharker, M. R., Sukhan, Z. P., Kim, S. C., Lee, W. K., and Kho, K. H. (2020c). Molecular identification, characterization, and expression analysis of a gonadotropin-releasing hormone receptor (GnRH-R) in Pacific abalone, Haliotis discus hannai. Molecule 25:2733. doi: 10.3390/molecules25122733
Stefano, G. B., Hiripi, L., and Catapane, E. J. (1978). The effects of short and long term temperature stress on serotonin, dopamine and norepinephrine concentrations in molluscan ganglia. J. Therm. Biol. 3, 79–83. doi: 10.1016/0306-4565(78)90042-6
Sukhan, Z. P., Sharker, M. R., and Kho, K. H. (2020). Molecular cloning, in silico characterization and expression analysis of axonemal protein 66.0 in Pacific abalone, Haliotis discus hannai. Eur. Zool. J. 87, 648–658. doi: 10.1080/24750263.2020.1821800
Treen, N., Itoh, N., Miura, H., Kikuchi, I., Ueda, T., Takahashi, K. G., et al. (2012). Mollusc gonadotropin-releasing hormone directly regulates gonadal functions: a primitive endocrine system controlling reproduction. Gen. Comp. Endocrinol. 176, 167–117. doi: 10.1016/j.ygcen.2012.01.008
Tveiten, H., and Johnsen, H. K. (2001). Thermal influences on temporal changes in plasma testosterone and oestradiol-17β concentrations during gonadal recrudescence in female common wolffish. J. Fish Biol. 59, 175–178. doi: 10.1006/jfbi.2001.1624
Van Golen, F. A., Li, K. W., De Lange, R. P. J., Van Kesteren, R. E., Van Der Schors, R. C., and Geraerts, W. P. (1995). Co-localized neuropeptides conopressin and ALA-PRO-GLYTRP-NH2 have antagonistic effects on the vas deferens of Lymnaea. Neuroscience 69, 1275–1287. doi: 10.1016/0306-4522(95)00311-6
Van In, V., Ntalamagka, N., O’Connor, W., Wang, T., Powell, D., Cummins, S. F., et al. (2016). Reproductive neuropeptides that stimulate spawning in the Sydney Rock Oyster (Saccostrea glomerata). Peptides 82, 109–119. doi: 10.1016/j.peptides.2016.06.007
Vilchis, L. I., Tegner, M. J., Moore, J. D., Friedman, C. S., Riser, K. L., Robbins, T. T., et al. (2005). Ocean warming effects on growth, reproduction, and survivorship of southern California abalone. Ecol. Appl. 15, 469–480. doi: 10.1890/03-5326
Von Holst, D. (1998). The concept of stress and its relevance for animal behaviour. Adv. Study Behav. 27, 1–131. doi: 10.1016/s0065-3454(08)60362-9
Wang, C., and Croll, R. P. (2006). Effects of sex steroids on spawning in the sea scallop, Placopecten magellanicus. Aquaculture 256, 423–432. doi: 10.1016/j.aquaculture.2006.01.017
Wayne, N. L., and Block, G. D. (1992). Effects of photoperiod and temperature on egg-laying behavior in a marine mollusk, Aplysia californica. Biol. Bull. 182, 8–14. doi: 10.2307/1542176
Young, K. G., Chang, J. P., and Goldberg, J. I. (1999). Gonadotropin-releasing hormone neuronal system of the freshwater snails Helisoma trivolvis and Lymnaea stagnalis: possible involvement in reproduction. J. Comp. Neurol. 404, 427–437. doi: 10.1002/(sici)1096-9861(19990222)404:4<427::aid-cne1>3.0.co;2-r
Keywords: thermal stress, GnRH, GnRH-R, APGWamide, 5-HT, gonadal maturation, reproduction, Haliotis discus hannai
Citation: Sukhan ZP, Sharker MR, Cho Y, Hossen S, Choi KS and Kho KH (2021) Thermal Stress Affects Gonadal Maturation by Regulating GnRH, GnRH Receptor, APGWamide, and Serotonin Receptor Gene Expression in Male Pacific Abalone, Haliotis discus hannai During Breeding Season. Front. Mar. Sci. 8:664426. doi: 10.3389/fmars.2021.664426
Received: 05 February 2021; Accepted: 26 April 2021;
Published: 22 June 2021.
Edited by:
Vengatesen Thiyagarajan (Rajan), The University of Hong Kong, Hong Kong, SAR ChinaReviewed by:
Jose Fernando Lopez-Olmeda, University of Murcia, SpainRadha Chaube, Banaras Hindu University, India
Copyright © 2021 Sukhan, Sharker, Cho, Hossen, Choi and Kho. This is an open-access article distributed under the terms of the Creative Commons Attribution License (CC BY). The use, distribution or reproduction in other forums is permitted, provided the original author(s) and the copyright owner(s) are credited and that the original publication in this journal is cited, in accordance with accepted academic practice. No use, distribution or reproduction is permitted which does not comply with these terms.
*Correspondence: Kang Hee Kho, kkh@chonnam.ac.kr