- 1Department of Biology, Centre for Environmental and Marine Studies (CESAM), University of Aveiro, Aveiro, Portugal
- 2German Centre for Marine Biodiversity Research (DZMB), Senckenberg am Meer, Wilhelmshaven, Germany
Deep-sea mining in the Pacific Clarion-Clipperton Fracture Zone (CCZ), a low-energy sedimentary habitat with polymetallic nodules, is expected to have considerable and long-lasting environmental impact. The CCZ hosts extraordinarily high species diversity across representatives from all Domains of Life. Data on species biology and ecology remain scarce, however. The current study describes the reproductive biology of Ophiosphalma glabrum (Ophiosphalmidae) and Ophiacantha cosmica (Ophiacanthidae), two ophiuroids frequently found in the CCZ. Specimens collected in Spring 2015 and 2019 in four contract areas were examined morphologically and histologically. Size-class frequencies (disc diameter and oocytes feret diameters), sex ratios, gametogenic status, putative reproductive mode, and a simple proxy for fecundity are presented. Habitat use differs in each. While O. glabrum is epibenthic, occurring as single individuals, O. cosmica often forms size-stratified groups living on stalked sponges, suggesting gregarious settlement or retention of offspring (though no brooding individuals were found). Further molecular analyses are needed to establish whether O. cosmica groups are familial. In O. glabrum, for which sample sizes were larger, sex ratios approximated a 1:1 ratio with no size-structuring. In both species, individuals were at various stages of gametogenic maturity, but no ripe females were identified. Based on this, O. glabrum is most probably gonochoric. Reproductive mode remains inconclusive for O. cosmica. Both species are presumptively lecithotrophic, with vitellogenic-oocyte feret diameters exceeding 250 μm. Oocyte feret diameters at times exceeded 400 μm in O. glabrum, indicating substantial yolk reserves. Estimates of instantaneous fecundity (vitellogenic specimens of O. glabrum only) were confounded by interindividual variability in gonad characteristics. The well-furnished lecithotrophic larvae of O. glabrum would be capable of dispersing even under food-impoverished conditions. The current study examines ophiuroid reproductive biology over multiple localities in the CCZ concurrently for the first time, at sites characterised by differing productivity regimes. The reproductive biology of each species is thus discussed with reference to past evolutionary (habitat stability), contemporary (food supply), and future environmental drivers (potential impacts of deep-sea mining).
Introduction
The challenges of exploring remote deep-sea abyssal environments have, thus far, insulated the deep-sea benthos from the impacts of mineral resource extraction. However, the advent of the technological means to access and exploit the considerable mineral resources found in these environments (Ghosh and Mukhopadhyay, 2000), heralds imminent, unprecedented levels of disturbance in the deep-sea (Weaver et al., 2018). Fauna that have evolved under highly stable, food-limited environmental regimes are likely to be poorly adapted to large-scale disturbances that rapidly and/or irreversibly alter their environment (Stearns, 2000). The CCZ in the tropical Northeast (NE) Pacific exemplifies this scenario, where the largest known global reserve of polymetallic nodules is located, formed over geological timescales in abyssal soft sediments characterised by very low sedimentation rates (Hein et al., 2013). Recent studies that have sought to describe the benthic fauna that typify the CCZ have also revealed extraordinarily high taxonomic diversity across representatives from all Domains of Life (e.g., Amon et al., 2016; De Smet et al., 2017; Shulse et al., 2017; Wilson, 2017; Goineau and Gooday, 2019; Hauquier et al., 2019; Brix et al., 2020; Christodoulou et al., 2020), making the CCZ of critical importance for biodiversity conservation. In many of these studies, data (e.g., species-abundance curves) strongly suggest that many – arguably most – species remain unaccounted for, with high species turnover over relatively short spatial scales even in groups that are brooders like Isopoda (e.g., Wilson, 2017; Brix et al., 2020). Remoteness of habitat and spatial variability in species composition of this sort present challenges for performing robust ecological studies, as evidenced by the scarcity of ecologically meaningful data available for even the most conspicuous “common” epifaunal species in the CCZ (Danovaro et al., 2017), including many echinoderm species (Amon et al., 2016).
Members of the phylum Echinodermata represent some of the most biomass-dominant taxa found in deep-sea abyssal plains (Gage and Tyler, 1991). Echinoderms play a significant role in global marine carbon budget (Lebrato et al., 2010) and are abundant in many soft- and hard-substrate habitats globally (Gage and Tyler, 1991). The class Ophiuroidea are known to be particularly prevalent and diverse on bathyal slopes (e.g., O’Hara et al., 2008) but data concerning species diversity and abundances at abyssal depths are scarce by comparison (Stöhr et al., 2012). In the equatorial NE Pacific, such data is limited to select historic (e.g., HMS Challenger and Albatross expeditions, Lyman, 1878, 1879, 1882; Clark, 1911, 1949) and contemporary surveys (Amon et al., 2016; Vanreusel et al., 2016; Christodoulou et al., 2020). Drivers for large-scale regional variability in abyssal ophiuroid population densities are still poorly understood; however, in the CCZ it seems that local-scale patchiness in nodule substrate availability plays a key role in determining the distribution of many species. Under certain habitat conditions, ophiuroids and the echinoderms more generally, comprise one of the largest components of mobile epifauna at nodule-rich sites (Amon et al., 2016; Vanreusel et al., 2016: up to 15 individuals per 100 m2, with major contributions from ophiuroids). The presence of nodules appears particularly relevant to certain ophiuroid and echinoid species; when nodules are absent, mobile epifaunal densities fall sharply to one or two encounters in an equivalent 100 m2 area, largely due to much-reduced encounters with ophiuroid species (Vanreusel et al., 2016). Two recent studies published in 2020, one based on video-transect surveys and the other on specimens collected by remotely operated vehicle (ROV) and epibenthic sledge (EBS), have also identified ophiuroids in abundance and notably, at unexpectedly high levels of diversity in the easternmost regions of CCZ (Christodoulou et al., 2020; Simon-Lledó et al., 2020) with the discovery of previously unknown ancient lineages (Christodoulou et al., 2019, 2020).
Current knowledge of reproductive biology in abyssal ophiuroid species is limited to a few papers examining gametogenic and/or size-class patterns (e.g., Ophiomusa lymani in N Atlantic & NE Pacific, Gage, 1982; Gage and Tyler, 1982; Ophiocten hastatum in NE Atlantic, Gage et al., 2004; Ophiura bathybia, Amphilepis patens, Amphiura carchara, and Ophiacantha cosmica in NE Pacific, Booth et al., 2008; Ophiuroglypha irrorata loveni, Ophiura lienosa, Salix daleus, O. cosmica, Ophiernus quadrispinus, and Ophioplexa condita in S Indian Ocean, Billett et al., 2013), with no data available for specimens collected from polymetallic-nodule habitats. Two of the more frequently encountered ophiuroids within the eastern CCZ (Christodoulou et al., 2020) are the brittle stars Ophiosphalma glabrum (Lütken and Mortensen, 1899; Ophiosphalmidae) and Ophiacantha cosmica (Lyman, 1878; Ophiacanthidae). These two species make for an interesting comparative reproductive study due to their contrasting biology. Ophiosphalma glabrum is relatively large (35–40 mm maximum disc diameters, Clark, 1911, 1913), epifaunal on soft sediments (0–2 mm burial, Amon et al., 2016; Glover et al., 2016; Christodoulou et al., 2020) and likely a generalist deposit feeder, as is the case in the closely related genus Ophiomusa (Pearson and Gage, 1984, formerly Ophiomusium). Ophiacantha cosmica, by contrast, is considerably smaller (11–12 mm maximum disc diameters, Booth et al., 2008; Billett et al., 2013) and epizoic or epifaunal on hard substrata (Billett et al., 2013), where it filters feeds with its spinous arms (Pearson and Gage, 1984). Although reproductive data are currently not available for Ophiosphalma glabrum, a few data already exist for Ophiacantha cosmica in nodule-free habitats. These indicate that O. cosmica is probably both gonochoric and lecithotrophic (oocyte 30–560 μm in diameter in specimens from the Southern Indian Ocean, Billet et al., 2013), with seasonal fluctuations in body-size structure, suggesting that specimens spawn in response to peaks in particulate organic-carbon (POC) flux (Booth et al., 2008).
As part of a wider concerted effort to address significant knowledge gaps in our ecological understanding of nodule-rich seabeds while this habitat remains relatively pristine, the current study describes the reproductive biology of the brittle stars Ophiosphalma glabrum (Lütken and Mortensen, 1899; Ophiosphalmidae) and Ophiacantha cosmica (Lyman, 1878; Ophiacanthidae) in a nodule-rich environment, based on the histological analyses of specimens collected from several mining-contract areas within the eastern CCZ.
Methodology
Sample Collection, Fixation, Preservation, and Species Identification
Specimens were collected during ROV transects (ROV Kiel 6,000, GEOMAR, manipulator arm) undertaken over the course of two cruises in Spring 2015 and 2019 on the R/V Sonne in the eastern CCZ. Cruise SO239 (11 March–30 April 2015) visited four contract areas and one Area of Particular Environmental Interest (APEI, 400 × 400 square-km protected areas assigned to each of nine presumptive ecological subregions of the CCZ). The 2015 samples in the current study originate from three of these contract areas, where one or both of the species in the current study were found: BGR area (Federal Institute for Geosciences and Natural Resources, Germany), the southern IOM area (Interoceanmetal Joint Organization), and the easternmost GSR area (G-TEC Sea Mineral Resources NV, Belgium), where each area was located along a decreasing SE-to-NW POC gradient, concomitant with a decrease in surface primary productivity driven by basin-scale thermocline shoaling toward the N. Pacific subtropical gyre (Pennington et al., 2006). The 2019 samples from the follow-up cruise SO268 (18 February–21 May), originated from BGR (again) and the central GSR contract area (2015–2019 site separation: BGR ~ 50 km; GSR ~ 350 km). A site map is provided in Figure 1.
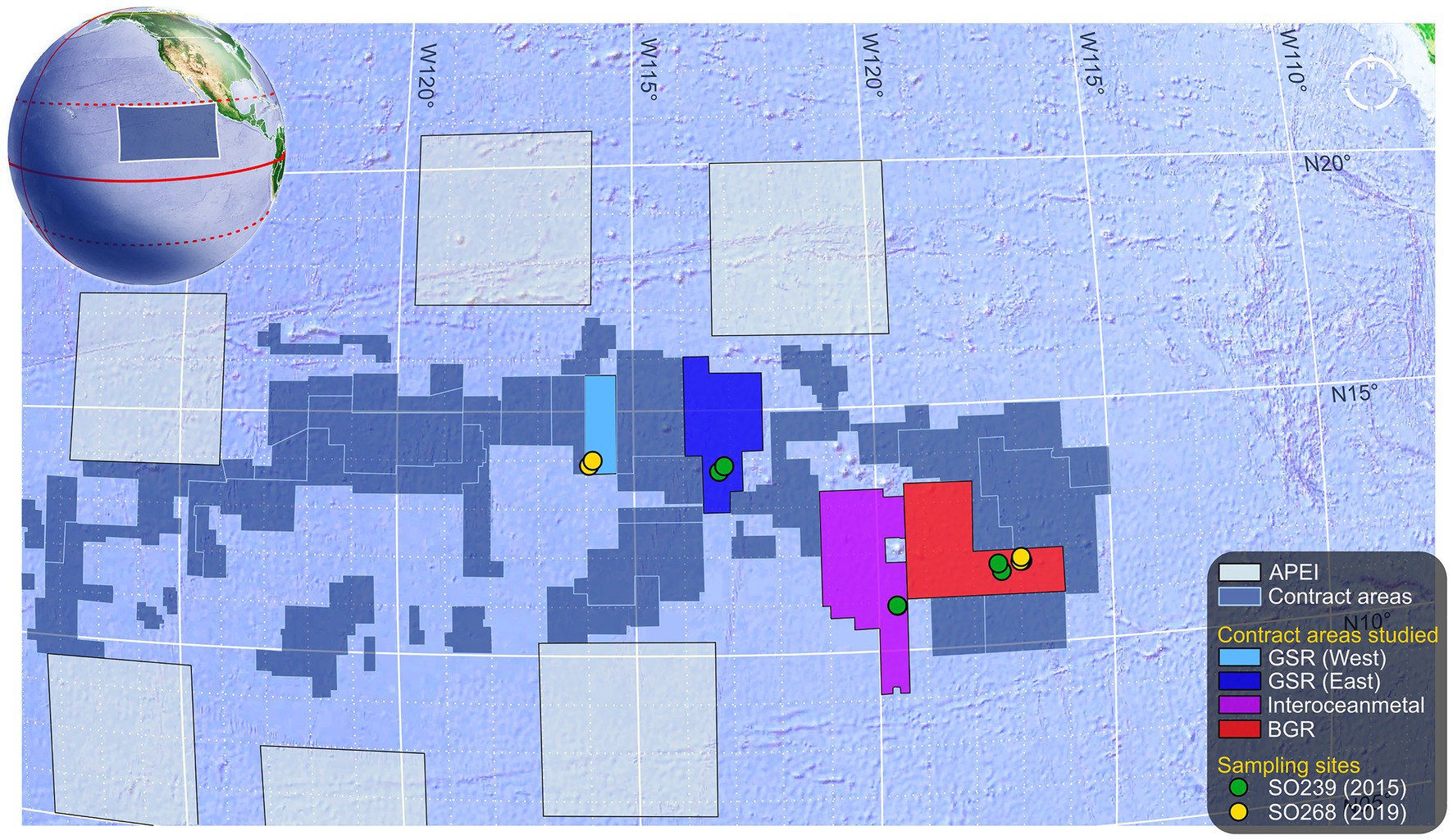
Figure 1. Map of eastern CCZ with sampling details. Map depicts the eastern half of the Area within the CCZ. Large 400 km × 400 km square regions are Areas of Particular Environmental Interest (APEI) that border numerous exploration contract areas in the centre (N.B. so-called “reserved” areas are not shown). The areas in which specimens were collected are identified along with site locations for each cruise. These were from the BGR area (Federal Institute for Geosciences and Natural Resources, Germany); the southern IOM area (Interoceanmetal Joint Organization); and both the central and the easternmost GSR areas (G-TEC Sea Mineral Resources NV, Belgium). Map and globe adapted from Google Earth projections.
Following collection, the arms of each specimen were removed for molecular species identification, while the central disc was fixed for reproductive histology. Arm fixation was in pre-cooled 96% EtOH, with replacement after 24 h (stored at −20°C thereafter); disc fixation was in 4% phosphate-buffered formaldehyde (48 h) following by serial transfer to 70% ethanol, stored at room temperature. Specimens were later identified to species level based on morphological characteristics (O. glabrum: Lütken and Mortensen, 1899; Baker, 2016; O. cosmica: Lyman, 1878; Paterson, 1985), supported by mtDNA COI analyses of genomic DNA extracted from arm tissues, described in detail in Christodoulou et al. (2020).
Size Measurements, Dissection, and Tissue Preparation for Microscopy
Micrographs of the oral and aboral faces of central discs were created from focus-stacks taken under a stereomicroscope (Leica M125 and DMC5400 camera, LAS-Leica Application Suite 3.7; focus-stacking in Combine ZP 1.0). Disc diameter – the length from the radial shield’s distal edge to the opposing interradial margin (Gage and Tyler, 1982) – was measured in LAS, to estimate size at first maturity and assess size-class frequencies as a function of sex. The aboral face, stomach, and bursa lining were then removed by dissection to reveal the bursal slits that border each arm base, along which the sac-like gonads are located, arranged in rows on each side. Intact gonad rows were dissected and then embedded either in paraffin wax or plastic resin, depending on tissue size. An additional decalcification step in 3% nitric acid in 70% ethanol (Wilkie, 2016) followed by a 70% ethanol rinse was required for smaller gonads, which were retained on a fragment of calcified tissue to facilitate tissue processing.
Larger gonad samples (all Ophiosphalma glabrum specimens and one Ophiacantha cosmica specimen with larger gonads) were infiltrated in molten paraffin at 60°C, following six-step serial transfer to 100% ethanol, four-step transfer to 100% MileGREEN™ (an iso-paraffin based solvent, Milestone) and final two-step transfer to paraffin in a mould to set. Blocks were trimmed and 5-μm sections cut, relaxed in a water bath, placed on Superfrost™+ slides, and dry-fixed by incubation at 50°C. Slides were stained in (Harris) Haematoxylin and Eosin(-Y) following standard protocols with dehydration by replacement using ethanol. Note that the O. cosmica specimen processed using paraffin wax was also processed with LR white resin (protocol below) using neighbouring gonads to identify any procedural biases in oocyte feret diameter.
Smaller tissue samples (all O. cosmica specimens) were blotted dry and then infiltrated directly in a hydrophilic methacrylate resin (LR white, London Resin Co.) at room temperature (three resin replacements by micropipette, 30-min. Each, fourth overnight) then transferred to a fresh resin-filled gelatine capsule (size 00, Electron Microscopy Sciences, United Kingdom), which was capped and polymerised at 50°C (20+ h). Having removed gelatine, LR-white resin pellets were trimmed and wet-sectioned on a Leica Ultracut UC6 Ultramicrotome (Germany), using a 45° glass knife. Sections 1-μm thick was transferred to individual aliquots arranged on Superfrost™+ slides. Periodic toluidine staining tracked progress through tissue. Standard H & E staining was modified by extending staining times and excluding ethanol, which distorts LR white. Slides were dried at 50°C with a desiccant (see Laming et al., 2020 for details). Regardless of protocol, sections were then cleared in MileGREEN™ and mounted with a cover slip (Omnimount™, Electron Microscopy Sciences).
Gametogenic Analyses
Stained serial sections were photographed under a compound microscope (Leica DM2500 with camera module ICC50W, LAS 3.7). Spermatogenesis was documented qualitatively only. Oogenesis was examined in more detail. Oocyte size frequencies were determined by image analysis of serial sections from ovaries, imported as image-stacks into Image-J (Schindelin et al., 2012) to track individual oocytes through the sample and prevent repeat counting. Up to 100 oocytes (with visible nuclei only) were counted per specimen, each measured along its longest axis (feret diameter) and classified into oogenic developmental stages, based on the presumption that germinal vesicle breakdown (GVBD) precedes oocyte release (Adams et al., 2019). Stages were: (1) Previtellogenic oocytes (Pv), smallest oocytes with no clear evidence of a germinal vesicle or vitellogenesis (i.e., eosinophilic granulated cytoplasm, rich in yolk proteins, has not yet developed); (2) Vitellogenic I (VI), “early” vitellogenic oocytes that are less than twice the diameter of previtellogenic oocytes, with the beginnings of an eosinophilic cytoplasm and a distinct germinal vesicle; (3) Vitellogenic II (VII), “mid-stage” vitellogenic oocytes that clearly possess an eosinophilic granulated cytoplasm, but whose diameter is less than double that of the germinal vesicle; (4) Vitellogenic III (VIII), “late” vitellogenic oocytes with large eosinophilic cytoplasmic reserves, whose diameter exceeds double that of the germinal vesicle; (5) Asymmetric (As), late vitellogenic oocytes with germinal vesicles located asymmetrically and in contact with the cell membrane, the precursor to germinal vesicle breakdown (GVBD) and onset of meiosis I; (6) Polar bodies (PB), oocytes that have undergone GVBD, with evidence of peripheral polar bodies, germinal vesicle lacking; and (7) Mature (M), fully mature oocytes, possessing only a small pronucleus (after Wessel et al., 2004).
Overall state of gonad maturity was assessed visually in terms of gonad aspect within the bursa and histological appearance. Gonad state was either defined as “immature” (no gonad), “no gametes” (gonad but no gametogenesis), and “developing” (gametogenesis at all the stages of development but with interstitial spaces remaining, equivalent to stages I and II in Tyler, 1977), “nearly ripe” (late-stage gametes dominate – spermatozoa in males or VIII-to-Mature, denoted here as “VIIIplus” in females – but gonad is not yet replete, equivalent to stage III in Tyler, 1977), “ripe” (fully mature gametes and no interstitial space, equivalent to stage IV in Tyler, 1977), and “post-spawning/recovery” (some residual near-mature gametes remain alongside degraded material, epithelial and myoepithelial layers are thickened, and nutritive phagocytes are typically present, equivalent to stages V in Tyler, 1977). For Ophiosphalma glabrum, the percentage area occupied by oocytes relative to gonad area and a maturity stage index (MSI) were also calculated, the latter using the fourth formula proposed from Doyle et al. (2012): Oocyte density × Size of individual−1 × Oocyte surface area × 0.01, where oocyte density in the current study refers to oocytes mm−2 of all gonads in cross section, size of individual is disc diameter in mm (whose reciprocal value compensates for any size bias) and oocyte surface area is derived from the mean feret diameter (¼πd2).
Statistical Analyses
Sex ratios for each species were assessed by χ2 test. The statistical significance of rank-based differences was tested by Kruskal-Wallis H test for: (1) disc diameter as a function of sex (including a juvenile category); (2) oocyte feret diameter as a function of year, and of disc diameter (for a given visual gonad state); and (3) percentage gonad occupied by oocytes, MSI and mean oocyte feret diameter each as a function of visual gonad state. Post-hoc pairwise comparisons were by Dunn test. To assess whether a trade-off exists between oocyte diameter and oocyte density (and thus, fecundity), a Spearman rank-order correlation was used to assess the strength of any proportional relationship between oocyte density and mean oocyte feret diameter. Expressions of variation around average values are standard deviation (sd., for means) and median absolute deviation (mad., for medians). All statistical and graphical analyses were performed in R 4.0.3 (R Core Team, 2020),1 using packages svglite, tidyverse, cowplot, ggExtra, and ggpubr (Attali and Baker, 2019; Wickham et al., 2019, 2020; Kassambara, 2020; Wilke, 2020), with figures prepared in Inkscape 1.0.2 (Inkscape Project).2
Results
Sex Ratios, Size-Class Frequencies, and Size at First Maturation
A total of 76 Ophiosphalma glabrum individuals (22 in 2015, 54 in 2019) and 23 Ophiacantha cosmica individuals (8 in 2015, and 15 in 2019) were processed. Molecular analyses of the arms of specimens in the current study used for species’ confirmation in a subset of individuals have been compiled into dedicated DOI-indexed dataset containing accession codes (Genbank), BOLD IDs and photos, trace files and collection data, and specimen metadata.3 Accession codes, BOLD IDs, and sample metadata are also provided in Supplementary Table 1. Of the two species, Ophiosphalma glabrum was larger (across entire sampling area, mean disc diameter 16.0 ± sd. 4.02 vs. Ophiacantha cosmica mean disc diameter 4.9 ± sd. 2.03, example specimens in Figure 2). Diameters ranged from 5.7–25.2 mm in Ophiosphalma glabrum and 2.2–10.0 mm in Ophiacantha cosmica. Although male Ophiosphalma glabrum specimens were numerically dominant (39 vs. 25 individuals), overall sex ratios did not differ significantly from a 1:1 ratio (χ21 = 3.06, p > 0.05). The three intermediate size classes for O. glabrum (together ranging from 12.5 to 20 mm) were characterised by equal numbers of males and females; however, smaller and larger mature O. glabrum individuals outside these size classes were exclusively male (Figure 3). Ranked-disc diameters did not significantly differ between sexes in O. glabrum, only between mature (male/female) and immature specimens, resulting in significant global differences across the three categories, when immature specimens were included in analyses (Kruskal-Wallis χ22 = 31.39, p < 0.01, Figure 3). Sample sizes were particularly small for specimens of Ophiacantha cosmica. Despite this, due to an over-whelming dominance of females (7:1), sex ratios were found to be significantly different (χ21 = 4.50, p < 0.05). The only male Ophiacantha cosmica collected was the largest individual of this species, making statistical comparisons of disc diameter between sexes impossible.
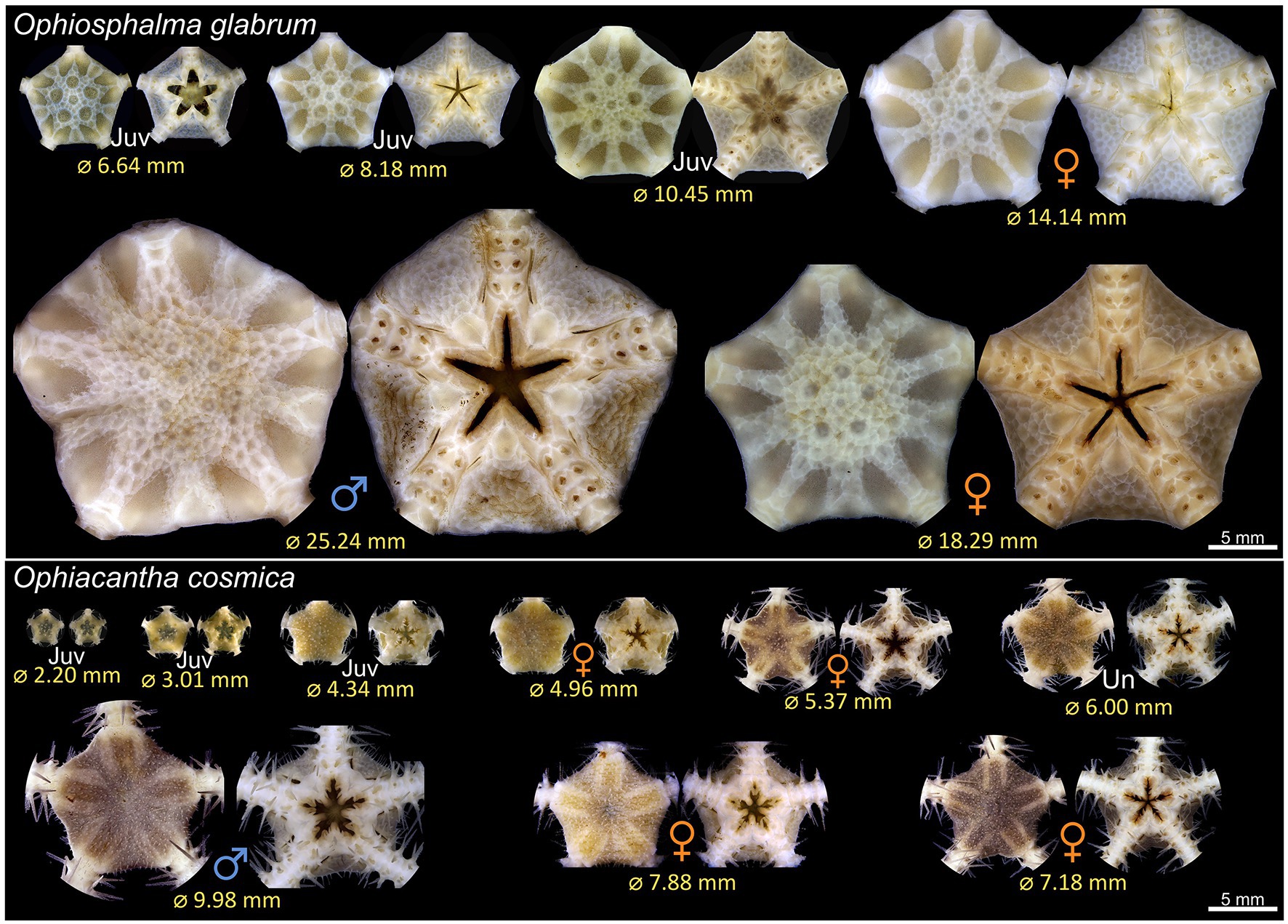
Figure 2. Example discs of O. glabrum and O. cosmica from the current study. The aboral and oral faces of a subset of specimens encountered during the SO268 cruise in 2019 are pictured, representative of the size ranges encountered. Abbreviations: Juv juvenile; Un undetermined sex (see main text).
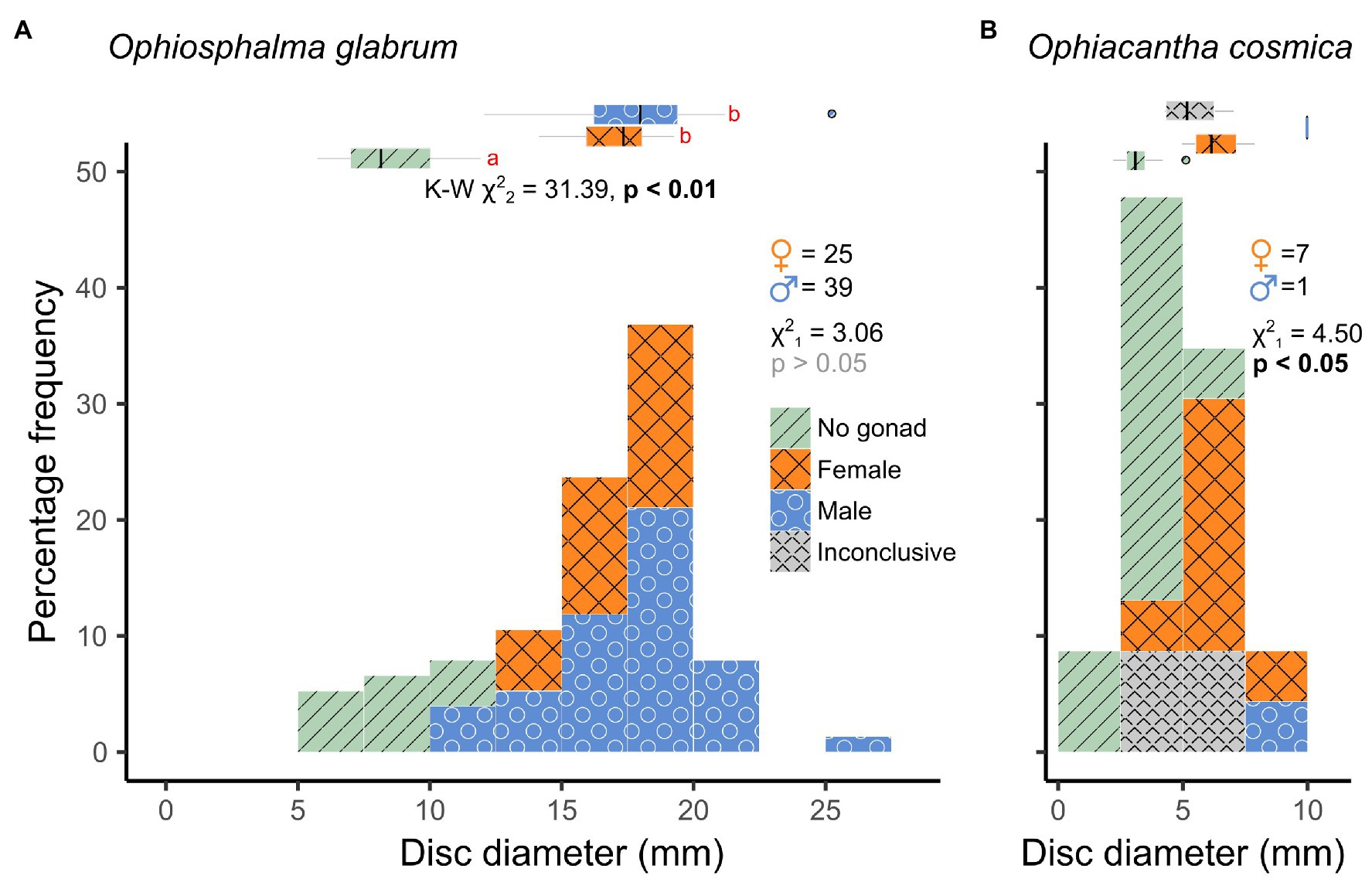
Figure 3. Size-class frequency distributions of O. glabrum and O. cosmica disc diameters. Frequency histograms displayed for (A) O. glabrum and (B) O. cosmica are colour-coded by sex (including a “No gonad” category for immature specimens and an “Inconclusive” category for specimens with gonads devoid of gametes). Main χ2 statistics relate to test for deviation from a 1:1 sex ratio (M vs. F only). Marginal box and whisker plots depict medians (vertical line), inter-quartile (box width), and 90% (whisker) ranges in disc diameter for each sex category. Proximate K-W χ2 statistic and a value of p in a) relate to Kruskal-Wallis test for ranked differences in disc diameter as a function of all sex categories in O. glabrum. Significant post-hoc pairwise comparisons (Dunn’s tests) are those with no letter annotations in common. Size-classes for each species are the same for comparative purposes.
For both species, several immature specimens were retrieved (Figures 2, 3), allowing rough estimates of size at first maturity to be made. In Ophiosphalma glabrum, the largest immature specimen (lacking discernible gonads) had an 11.93-mm wide disc; a further 11 smaller specimens also lacked gonads. The smallest O. glabrum individual that possessed a gonad was a male at 12.07 mm disc diameter, while the smallest female was 14.14 mm (gametogenesis observed in both), placing approximate sizes at first maturity in males at <12 mm disc diameter and at <14.2 mm disc diameter in females. In Ophiacantha cosmica, size at first the maturity is less concrete. The largest specimen with no discernible gonad had a disc diameter of 5.12 mm, with two smaller (4.28, 4.34 mm) and two larger (6.00, 7.04 mm) specimens each in possession of 1–2 very small gonad buds (ca. 100 μm diameter) not yet furnished with gametes. The smallest individual in which gametogenesis was identified was a female of 4.96 mm with six larger females with disc diameters of 5.37–7.88 mm (Figure 3; select specimens in Figure 2). The largest individual – the only O. cosmica male – had a 9.98-mm wide disc.
Gonad State and Gametogenesis
Gonad state and gametogenesis were assessed qualitatively in males (Figures 4, 5) and both qualitatively and semi-quantitatively in females (Figures 4–6). No evidence of simultaneous or sequential hermaphroditism (i.e., ovotestes/sex-specific size stratification) was identified in either species. Most Ophiosphalma glabrum males’ testes were developing (49%) with easily identifiable spermatogenic columns, composed of progressively more-advanced stages of spermatogenesis, extending from the inner-sac membrane into the central lumen (Figure 4A). Testes were somewhat angular (Figure 4G), lacking the creamy engorged appearance typical of ripe individuals (example in Figure 4H). A further 35% were considered nearly ripe, where testes aspect appears unchanged, but spermatogenic columns are no longer clearly visible, with roughly equal proportions of central mature spermatozoa, and surrounding tightly packed cells at earlier stages of spermatogenesis. Three specimens were ripe (8%), where gonads appear engorged, extensively spread throughout bursae and replete with spermatozoa (Figures 4B,H). Putative evidence of nutritive phagocytes was used as the basis for discriminating a minority of male specimens as being in a post-spawn/recovery state (8%, Figure 4C, with gonad aspect in Figure 4I). The single Ophiacantha cosmica male collected was at the developing stage, with evident spermatogenic columns (Figure 5A).
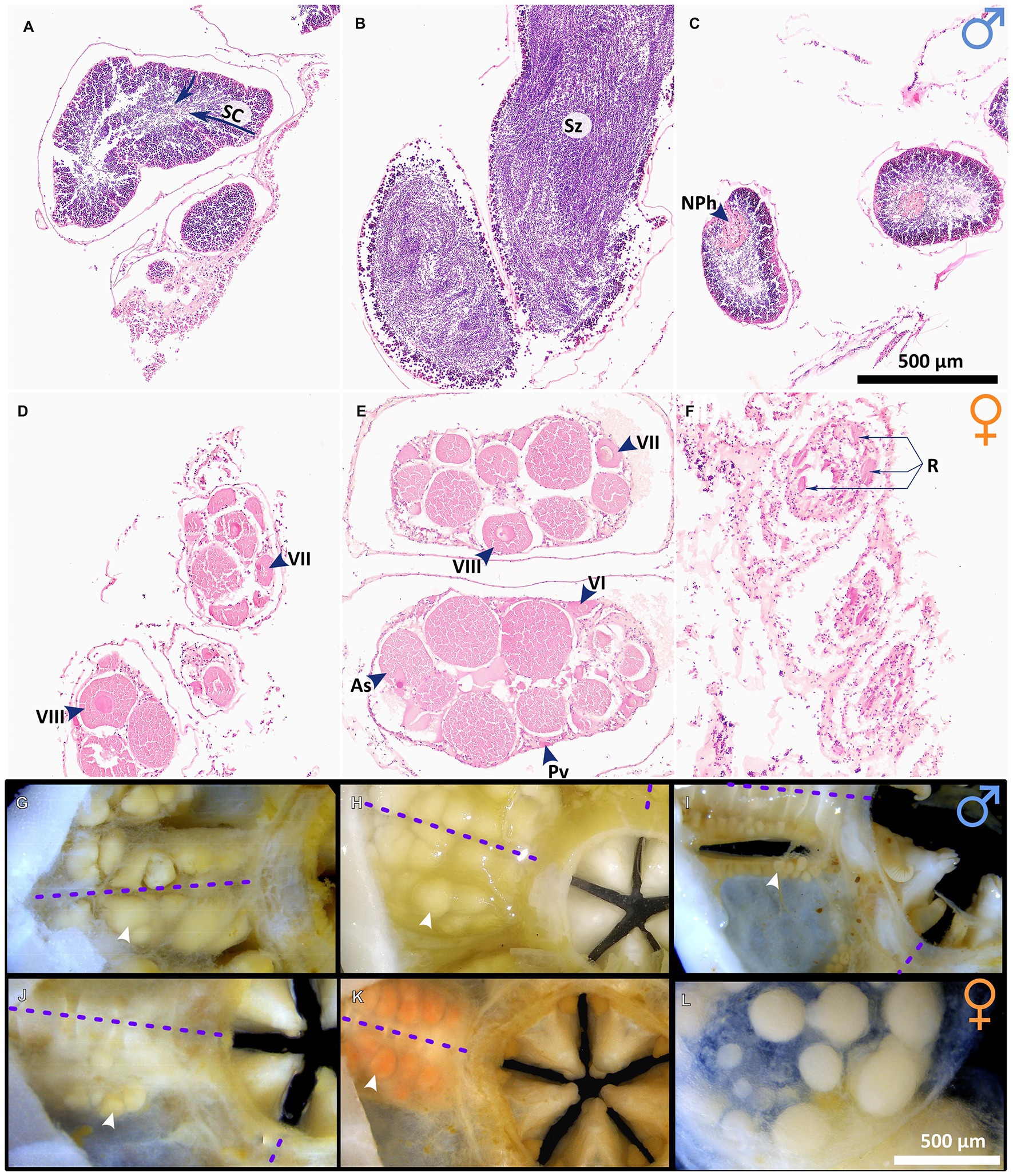
Figure 4. Gametogenesis and gonad development in O. glabrum. Gonads at various stages of development in O. glabrum, as follows: (A) developing testes in which spermatogenic columns (SC) are evident (indicated with arrows), (B) ripe testes and (C) post-spawn / recovery testes; (D) developing ovaries, (E) nearly ripe ovaries, and (F) post-spawn / recovery ovaries; visual aspect of G) developing testes (corresponding to A), (H) ripe testes (corresponding to B) and (I) post-spawn/recovery testes (corresponding to C) and finally, visual aspect of (J) developing ovaries (corresponding to D), (K) nearly ripe ovaries (corresponding to E) and (L) a single ovary viewed down the distal-proximal axis, where late-stage oocytes are located distally (foreground), having previously developed from the basal region, where early-stage oocytes are visible beneath. White arrowheads in (G-I) indicate single gonads, and purple dotted lines are midlines of arm bases. Other abbreviations: As Asymmetric oocytes, NPh Nutritive phagocytes, Pv Previtellogenic oocytes, R Residual oocytes, VI Vitellogenic I oocytes, VII Vitellogenic II oocytes, Sz Spermatazoa, VIII Vitellogenic III oocytes.
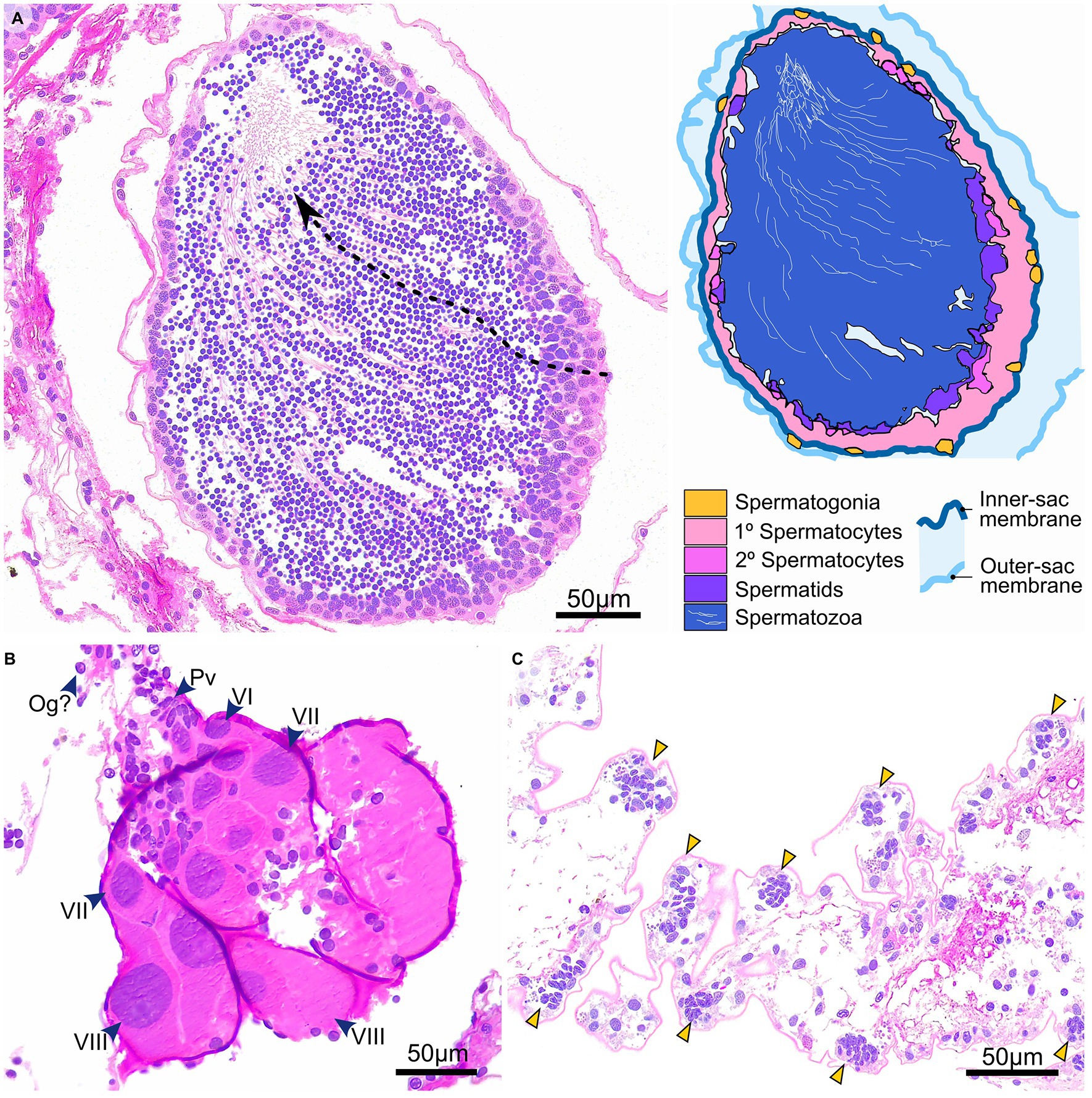
Figure 5. Gametogenesis and gonad development in O. cosmica. Gonads at different stages of development in O. cosmica: (A) developing testes, in which spermatogenic columns are evident (dotted arrow), where cells at progressive developmental stages form chains that extend from the inner-sac membrane into the central lumen; (B) developing ovaries, in which various oogenic stages co-occur (the largest oocytes are distal-most to region of gonad attachment); and (C) pre-gametogenic gonadal tissue, with densely arranged pockets of putative primordial germ cells with basophilic nuclei that appear to form node-like gonadal precursors. Abbreviations: Og Oogonia (putative), Pv Previtellogenic oocytes, VI Vitellogenic I oocytes, VII Vitellogenic II oocytes, VIII Vitellogenic III oocytes.
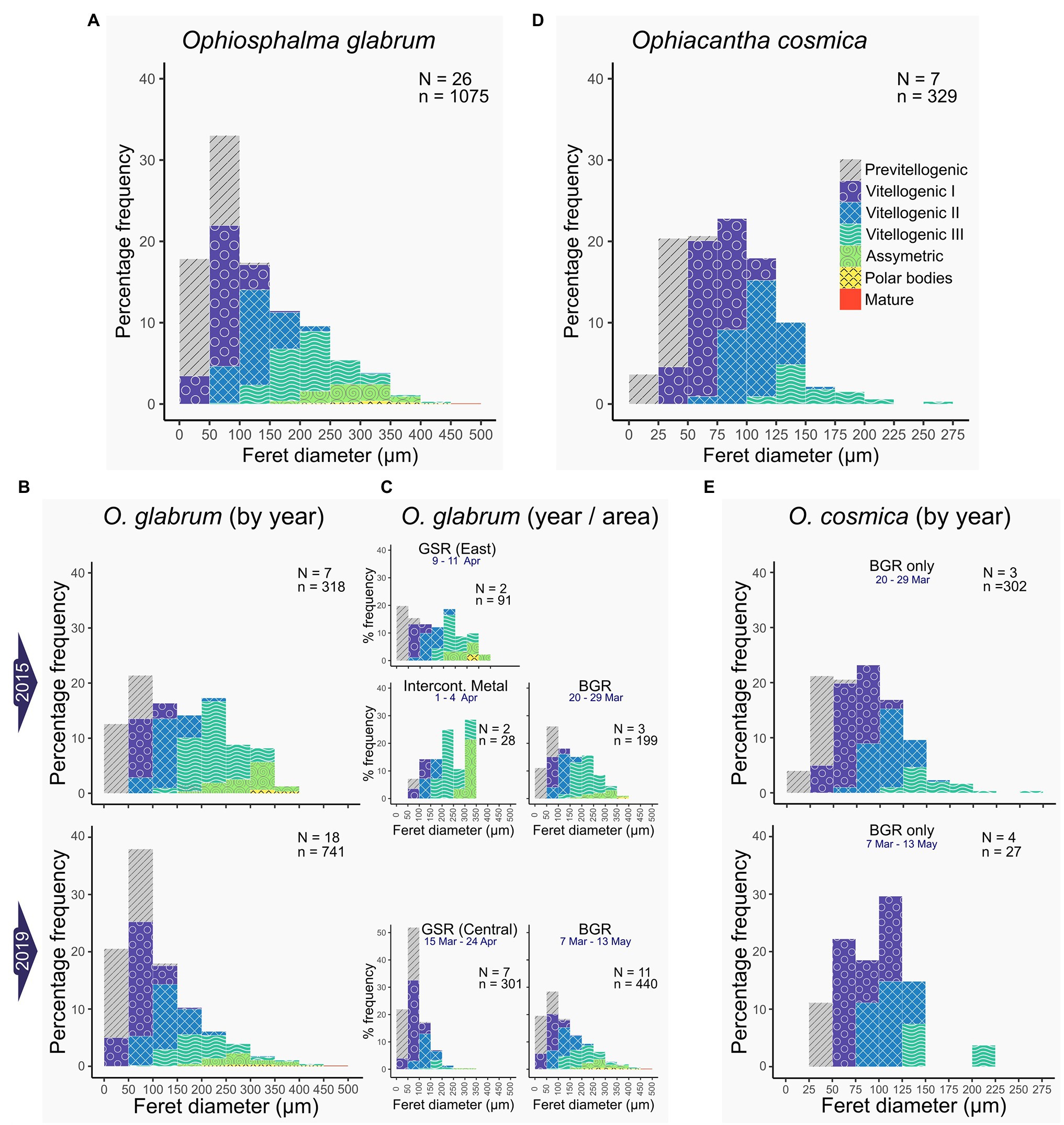
Figure 6. Oocyte size-class frequency distributions. Frequency histograms display the relative proportions of oocytes in each size-class for feret diameters measured in females of each species. Only oocytes with nuclei were considered. Colour coding relates to associated oogenic developmental stages, assessed on a case-by-case basis for each oocyte counted and measured. Data for O. glabrum are on the left (A–C) displaying; (A) whole O. glabrum dataset; (B) data by sampling year and; (C) data by site for each year. Data for O. cosmica are on the right (D,E), displaying; (D) whole O. cosmica dataset and (E) data by sampling year (this species was only recovered from the BGR contract area). N = total number of females, n = total number of oocytes pooled from N females.
No ripe females were identified in either species. However, most Ophiosphalma glabrum females had ovaries in a nearly ripe state (56% overall, 100% in 2015, and 39% in 2019), where VIIIplus oocytes dominate, but the ovary is not replete and has not yet spread far into the bursa (e.g., Figures 4E,K). A further 33% of individuals in post-spawn/recovery and 27% with developing ovaries (see Figures 4D,F) were also identified, all in 2019. All-but-one of the Ophiacantha cosmica females were developing (e.g., Figure 5B). The smallest female was in a post-spawn/recovery state (or possibly developing for the first time).
Oocytes at every stage of oogenesis were identified in Ophiosphalma glabrum (Figures 6A–C). Maximum oocyte feret diameters for O. glabrum and Ophiacantha cosmica were a mature oocyte of 453 μm and VIII oocyte of 273 μm, respectively. However, unlike Ophiosphalma glabrum, oocytes at stages more advanced than VIII were not observed in Ophiacantha cosmica generally (Figures 6D,E), so it is likely that maximum oocyte diameters in this species are underestimated. The higher proportion of nearly ripe Ophiosphalma glabrum females in 2015 vs. 2019, is reflected in significant differences in ranked oocyte feret diameters between 2015 and 2019 (Kruskal-Wallis χ21 = 63.5, p < 0.0001). These differences, echoed by mean and median oocyte feret diameters for each year (2015-mean 159.1 ± sd. 91.7, median 149.5 ± mad. 109.0 and 2019-mean 112.0 ± sd. 78.3, median 88.0 ± mad. 57.8), relate to higher frequencies of VIIIplus oocytes in the ovaries of specimens from 2015, clearly visible in the size-frequency data for both years (Figure 6B). Unfortunately, low sample sizes prohibit the quantitative comparison of oocyte frequency distributions as a function of contract area. That said, the proportion of large, late-stage oocytes found in specimens at the eastern-most sites appears higher (particularly for 2019, Figure 5C). No significant differences in ranked oocyte feret diameters between the two sampling years were found for Ophiacantha cosmica (Kruskal-Wallis χ21 = 2.34, p > 0.05), with similar oocyte size-class means, medians and distribution profiles identified for this species in both years (2015-mean 82.3 ± sd. 40.7, median 79.1 ± mad. 43.7; and 2019-mean 94.4 ± sd. 40.0, median 90.8 ± mad. 39.3; profiles in Figure 6E), though very low sampling sizes are likely undermining the statistical power of the test.
In addition to classifying oogenic stage and measuring oocyte feret diameters, the cross-sectional area occupied by oocytes relative to gonad area (inner-sac membrane) was calculated for Ophiosphalma glabrum – where tissue section quality allowed – as a proxy for the degree to which female gonads were full. A MSI previously demonstrated to be more sensitive to subtle changes in gonad maturation (Doyle et al., 2012) was also calculated for O. glabrum. Percentage of gonad occupied by oocytes (Kruskal-Wallis χ22 = 11.37, p < 0.01), mean oocyte feret diameter (Kruskal-Wallis χ22 = 10.55, p < 0.01) and MSI (Kruskal-Wallis χ22 = 9.13, p < 0.05) differed significantly as a function of O. glabrum gonad state (Figure 7). Evident differences existed in MSI and in the relative gonad area occupied by oocytes in nearly ripe females; however, these metrics both failed to resolve differences between developing and post-spawn females, with post-hoc pairwise comparisons revealing significant group differences only between nearly ripe females and the two other gonad states (Figure 7). Group differences in mean oocyte feret diameter were restricted to nearly ripe females and those classified as post-spawn/recovery (Figure 6), reflecting similar (non-significant) mean feret diameters between developing and nearly ripe O. glabrum females. Spearman-rank correlative analysis revealed oocyte density mm−2 of gonad cross-sectional area to be significantly, inversely related to mean oocyte feret diameter (coefficient R = −0.74, p < 0.0001, Figure 7), indicating a trade-off between investment per oocyte (i.e., size of yolk reserves) and the space available to house oocytes in each gonad.
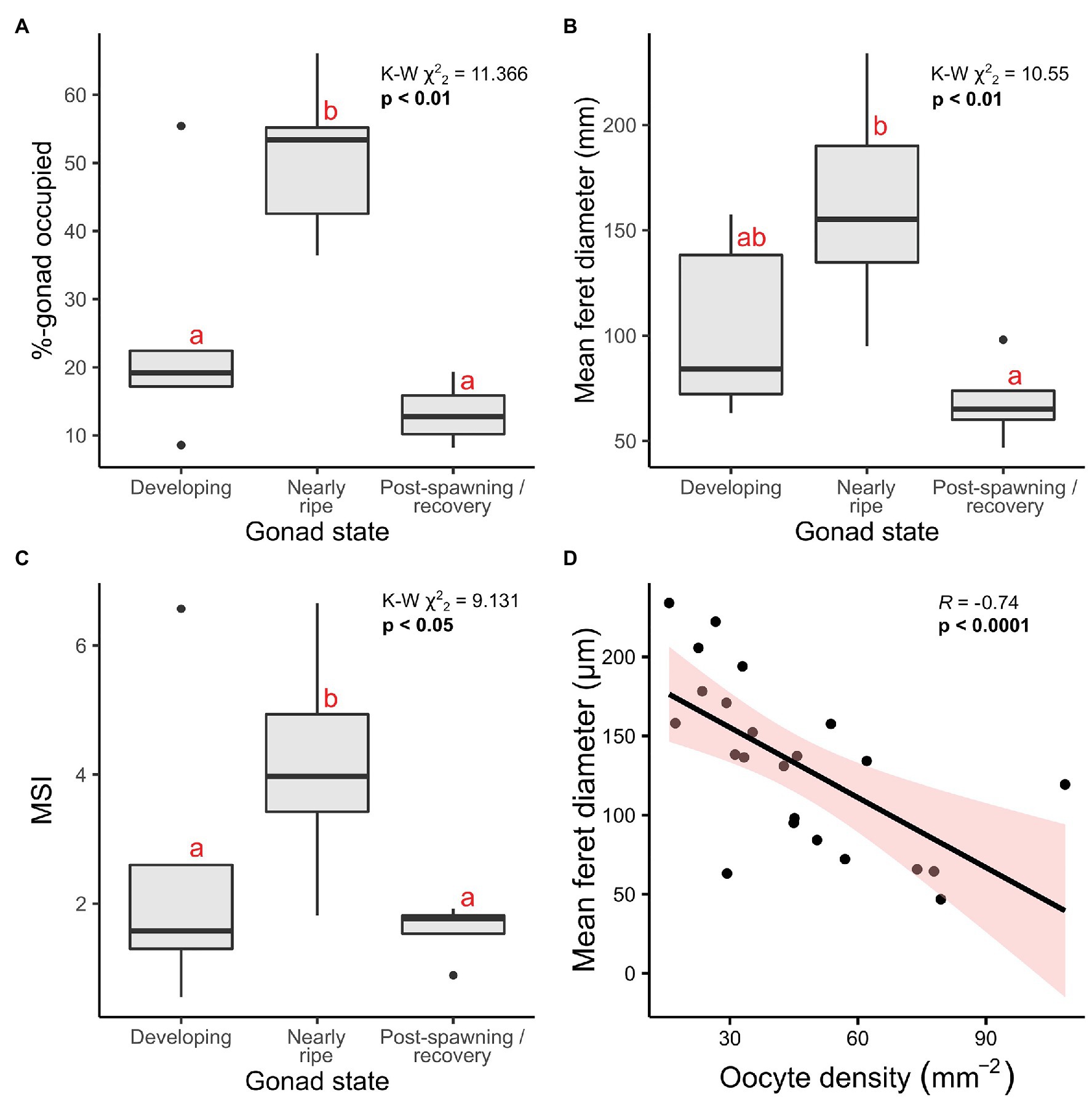
Figure 7. Reproductive metrics plotted as a function of gonad state and oocyte density. Analyses are based on 23 female specimens, of which 14 were nearly ripe, five were developing and four were in post-spawning/recovery. (A) Percentage-gonad occupied by oocytes, (B) overall mean feret diameter per individual, and (C) a MSI developed by Doyle et al. (2012) were each calculated and evaluated as a function of gonad state, using Kruskall-Wallis Chi-squared tests on ranked data, the results of which are included in the top right corners for each. Significant post-hoc pairwise comparisons (Dunn’s tests) are those with no letter annotations in common. Depicted in (D) is the Spearman rank-correlation analysis performed to identify any relationship between mean oocyte diameters and corresponding densities, with the resulting Rho statistic (R), a value of p and 95-% confidence intervals displayed.
Finally, intraindividual variation in the number and size of ovaries and oocyte densities per ovary in O. glabrum was considerable. Several developing females possessed neighbouring gonads at different stages of maturity based on visual aspect (e.g., Figure 4J). The presence of oocytes at various stages of oogenesis resulted in large variability in oocyte diameters (Figure 8), with large variances around median values (Supplementary Figure 1). Not unexpectedly – since one directly informed the other – the proportion of oocytes at each stage of oogenesis was relatively consistent in a given gonad state, irrespective of specimen size (Figure 8). However, overall ranked oocyte feret diameters were significantly higher in the largest nearly ripe disc-diameter size class, 17.5–20 mm, when compared with smaller size classes 12.5–15 mm and 15–17.5 mm (based on post-hoc comparisons, following a highly significant Kruskal-Wallis test for gonad state: Kruskal-Wallis χ22 = 18.94, p < 0.0001, Supplementary Figure 1). Significant post-hoc differences in ranked oocyte feret diameters were also identified across size classes in individuals in a state of post-spawn/recovery (Kruskal-Wallis χ22 = 12.63, p < 0.01), due to the relative dominance of previtellogenic oocytes in one individual from the intermediate 15–17.5 mm size category for this gonad state (Figure 8). No significant size-related differences were identified in the oocyte feret diameters of developing individuals of either species.
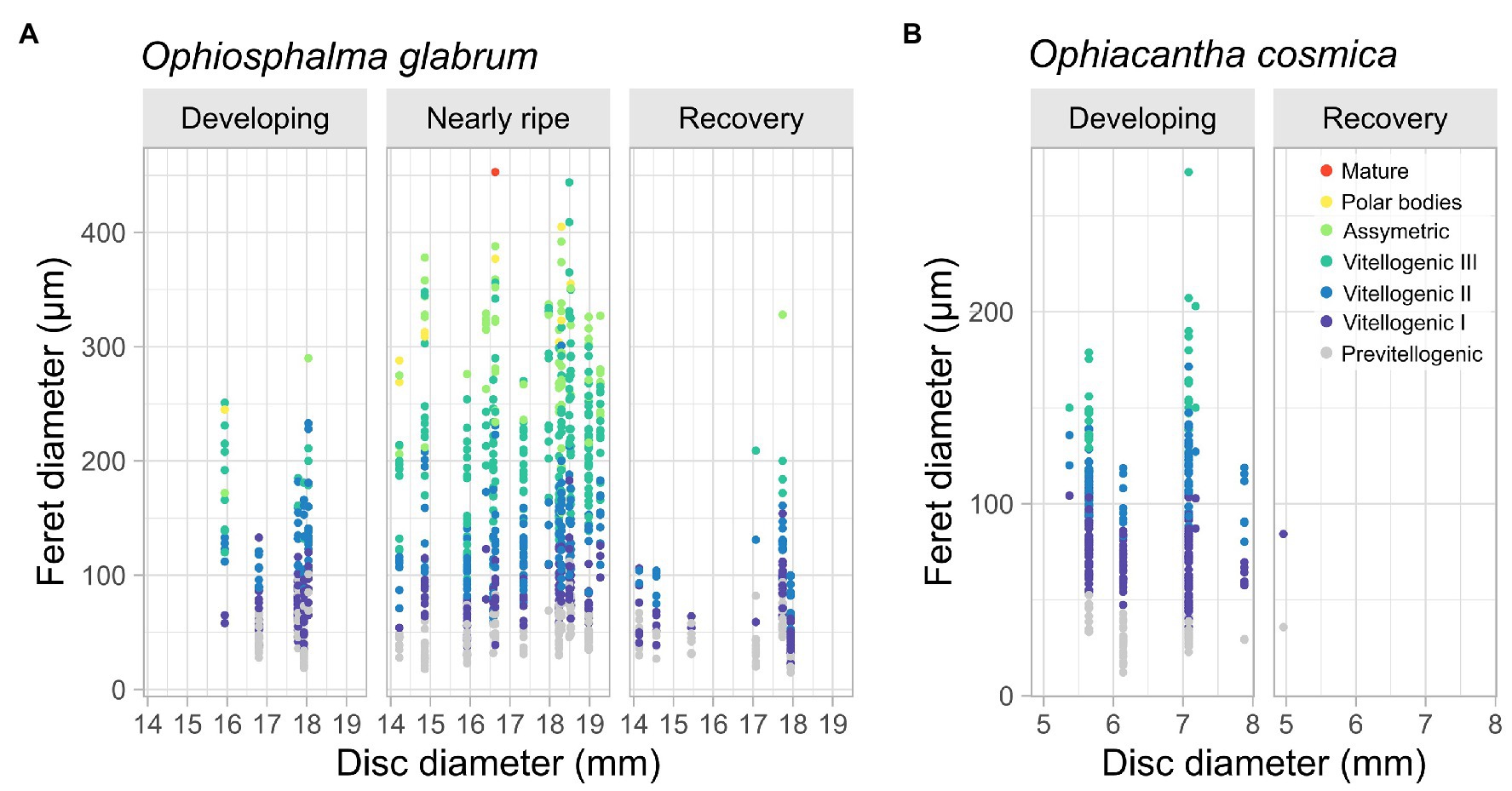
Figure 8. Variation in oocyte feret diameter in O. glabrum and O. cosmica with specimen size. Scatterplots display variation in oocyte feret diameter with specimen size (disc diameter mm) for female gonad states identified in (A) O. glabrum and (B) O. cosmica. Colour-coding depicts oogenic developmental stage of each oocyte. Analyses are based on 25 O. glabrum specimens, of which 14 were nearly ripe, five were developing, and six were in post-spawning/recovery and seven O. cosmica specimens, of which all but one was developing. Significant differences in oocyte feret diameter across specimen size classes were assessed for each gonad state (where possible), using Kruskall-Wallis Chi-squared tests on ranked data (see main text and Supplementary Figure 1 for Box and whisker plots of binned disc-diameter data).
As a result of intra- and inter-individual variability in gonad maturity overall, a reliable measure of fecundity proved impossible. However, extrapolating from 25 μm of tissue sectioned per individual, late-stage (VIIIplus) oocyte counts mm-sectioned−1 ovary −1 ranged from 1.3 to 12.0 oocytes in developing females (n = 4), 5.0–30.0 oocytes mm-sectioned−1 ovary −1 in nearly ripe females (n = 14), and 1.5–5.0 oocytes mm-sectioned−1 ovary −1 in post-spawn/recovery females (n = 2).
Discussion
The current study provides new ecologically relevant reproductive data for two species of ophiuroid living in an oligotrophic, highly stable low-energy environment in the NE equatorial abyss which, due to the presence of polymetallic nodules, is under future threat from deep-sea mining activities (Weaver et al., 2018). The current study documents the reproductive biology of O. glabrum for the first time. Equal sex ratios for intermediate modal disc-diameter size classes and the absence of any simultaneous hermaphrodites both point to gonochorism in O. glabrum. Unfortunately, the manner (and possibly timing) of sampling may have created biases in the size classes of each sex. Large (and ripe) females (i.e., >20-mm disc diameters) were conspicuously absent, suggesting either an unlikely upper limit on female disc diameter, or most probably, that large females were overlooked. This may simply be symptomatic of a patchy (or sex-specific) species distributions, where large communal echinoderm aggregations in the soft-sediment abyss only really occur during the episodic arrival of labile organic matter to the seafloor (Kuhnz et al., 2014; Smith et al., 2018) or during aggregative spawning events (Mercier and Hamel, 2009).
Oocyte feret diameters frequently exceeding 300 μm in the current study (max 453 μm in a mature oocyte) are indicative of a lecithotrophic (non-feeding) larval mode with substantial yolk-protein and lipid reserves (Sewell and Young, 1997; Young, 2003). Although this places an upper limit on transport time relative to planktotrophy, lecithotrophy releases larvae of nutritional constraints that likely exist in food-impoverished transport environments. In addition, if lecithotrophic dispersal occurs in deep waters, where temperatures remain relatively low, this can act to extend transport times through metabolic suppression, with capacities for dispersal equivalent to planktotrophy (Mercier et al., 2013). Planktotrophic (feeding) larvae, by contrast, would be confined to water masses with higher detrital input (Young et al., 1997). Lecithotrophy necessitates greater energy investment per oocyte than in planktotrophy. This likely places constraints on fecundity in a food-limiting environment (Ramirez Llodra, 2002), as evidenced by the inverse relationship between oocyte densities and feret diameter in the current study. Ovaries in the current study when nearly ripe, were classified as such based mainly on oogenic characteristics, since striking external indications of maturity were lacking in female specimens. Coloration was inconsistent and ovaries were not engorged, nor had they spread extensively into the bursae, as was witnessed in at least one ripe male. This indicates considerable scope remained for further reproductive investment in the females collected, under suitable environmental conditions. Without ripe females and intra- and inter-annual time-series sampling it is not possible to ascertain whether gametogenesis is seasonal (Tyler and Gage, 1980; Gage and Tyler, 1982) or aperiodic (“continuous”), though the presence of all oogenic stages in most specimens suggests aperiodic or semi-continuous spawning behaviour (Brogger et al., 2013). Oocyte size-class data also appear to suggest that slight differences exist between 2015 and 2019, with a higher proportion of females approaching maturity in 2015. This could reflect the slightly different timing of cruises, as no post-spawn recovery females were found in 2015 or site-related differences for each year since site locations were not the same. Further data are necessary to confirm this.
In an oligotrophic environment lacking strong seasonal fluctuations in POC flux, apparent aperiodic (or “continuous”) gametogenesis may arise in species that spawn (1) periodically, but histological studies fail to identify their cyclical nature (Mercier et al., 2007; Mercier and Hamel, 2008) or (2) opportunistically, occurring in rapid response to increased food supply related to episodic massive changes in surface productivity and as such, remain undetected (Mercier and Hamel, 2009). Coupled with lecithotrophy, aperiodic opportunistic spawning would be resource-driven, allowing a species to respond to fluctuating food availability by investing in gametogenesis only when suitable conditions arise (e.g., Booth et al., 2008). Responsive reproductive modes of this sort appear to be a feature of bathyal and abyssal ecosystems in the tropical NE Pacific (Kuhnz et al., 2014; Smith et al., 2018), where the influences of episodic disturbance events are of greater influence than seasonal changes in surface productivity. However, additional sampling from highly oligotrophic NW regions of the CCZ (e.g., APEI 3, Vanreusel et al., 2016; Christodoulou et al., 2020) would be needed to establish whether lower POC flux translates into reduced energetic investment in reproduction.
Maximum sizes recorded for O. glabrum vary considerably in the literature, but the largest attributed to this species is a 35 mm disc diameter (Clark, 1913), though there exists an Ophiomusium multispinum specimen – now synonymised with Ophiosphalma glabrum – with a 40-mm wide disc (Clark, 1911). In either case, the largest individual in the current study falls considerably short of these sizes, at 25.2 mm. Aside from some concerns around species assignation in historical samples and difficulties in collecting representative samples of species that occur at low densities in expansive and remote environments, these larger specimens also originate from shallower habitats (e.g., Cocos, Malpelo and Carnegie Ridge, Panama Basin, depths 800–3,200 m, Clark, 1911, 1913), where greater food availability arriving from productive coastal systems (Pennington et al., 2006) could enable larger maximum sizes. Although smaller specimens were undoubtedly underrepresented, enough immature O. glabrum were collected to establish a rough size at first maturity of <12 mm, around 30–35% maximum size. Without knowledge of growth rates, the time taken to reach these sizes remains unknown; however, if measures of growth (e.g., Gage et al., 2004) or temporal size-class frequency analyses can be compiled in future studies, this value could be easily converted to an estimate of age at first maturity, a highly relevant conservation metric for response to disturbance.
Sample sizes for the smaller species Ophiacantha cosmica limit any detailed interpretation of their reproductive biology. However, some data on size-class distributions and oocyte feret diameters already exist for this species from nodule-free habitats at Station M in the NE Pacific and around Crozet Island in the Southern Indian Ocean (Booth et al., 2008; Billett et al., 2013). Although sex ratios were significantly different in the current study, little stock can be placed in this result as the total number of mature specimens numbered less than 10 and maximum disc diameters for both sexes are markedly lower than in the literature. Evidence from other studies indicate that sex ratios follow a 1:1 ratio when sampled in larger numbers (Billett et al., 2013), with maximum disc diameters of 11–12 mm (Booth et al., 2008; Billett et al., 2013; M. Christodoulou, personal observations), although the disc diameter for this species’ holotype is unaccountably large, at 18 mm (Lyman, 1878). Maximum oocyte feret diameter in the current study (VIII oocyte at 273 μm) also appears to be underestimated when compared with those of specimens from the Crozet Plateau (Billett et al., 2013), which reached maximum feret diameters exceeding 500 μm, indicating lecithotrophy (or direct development, though no evidence of brooding has ever been recorded, Billett et al., 2013; this study). This is to be expected as females in the current study were relatively small by comparison and only in developing states, with no oocytes in the final stages of meiosis (i.e., asymmetric or later). The only individual classified as post-spawn/recovery was probably developing for the first time, particularly in light of its small disc diameter. Unlike the protandric hermaphrodite Ophiacantha fraterna (Tyler and Gage, 1982; species identification updated from Ophiacantha bidentata by Martynov and Litvinova, 2008), data from the current study – corroborated by that of Billett et al. (2013) – indicate that O. cosmica is gonochoric. However, the current study lacks sufficient data to confirm whether O. cosmica is also iteroparous, as is the case for O. fraterna (Tyler and Gage, 1982). Size at first maturity for O. cosmica was assessed in the current study but remains approximate at <4.96-mm disc diameter (in females), due to ambiguity around two relatively large specimens (6–7 μm disc diameters) that possessed tiny bud-like gonads in which no evidence for gametogenesis could be found. These gonads did possess nodes in which cell clumps with highly basophilic nuclei were located, which are believed to be gonadal precursors housing primordial germ cells (Figure 5C).
Ophiacantha spp. have been recorded from various hard substrata and vertically elevated fauna such as deep-sea corals (Tyler and Gage, 1980) and tube worms (Lauerman et al., 1996; Billett et al., 2013). In the current study, this species is recorded on stalked-sponge species Caulophacus sp. and Hyalonema spp. for the first time (Figure 9). Although alternative hard substrates are available in the CCZ (most obviously, polymetallic nodules), O. cosmica specimens here were exclusively epizoic; this species was not encountered elsewhere. Stalked sponges are some of the most elevated of fauna on the seafloor in the CCZ; colonising them at positions above the benthic boundary layer would mitigate the influences of shear on current speeds, which likely aids in a suspension-feeding lifestyle (Booth et al., 2008). The specimens in the current study either occurred as single individuals or, in certain instances, as size-stratified groups (Figure 9), suggestive of gregarious behaviour, offspring retention or fissiparity (though pentameral Ophiacantha spp. are not known to be fissiparous, Lee et al., 2019). A more detailed molecular work, beyond the scope of this study, would help to reveal common or distinct genetic origins for the members of these groups.
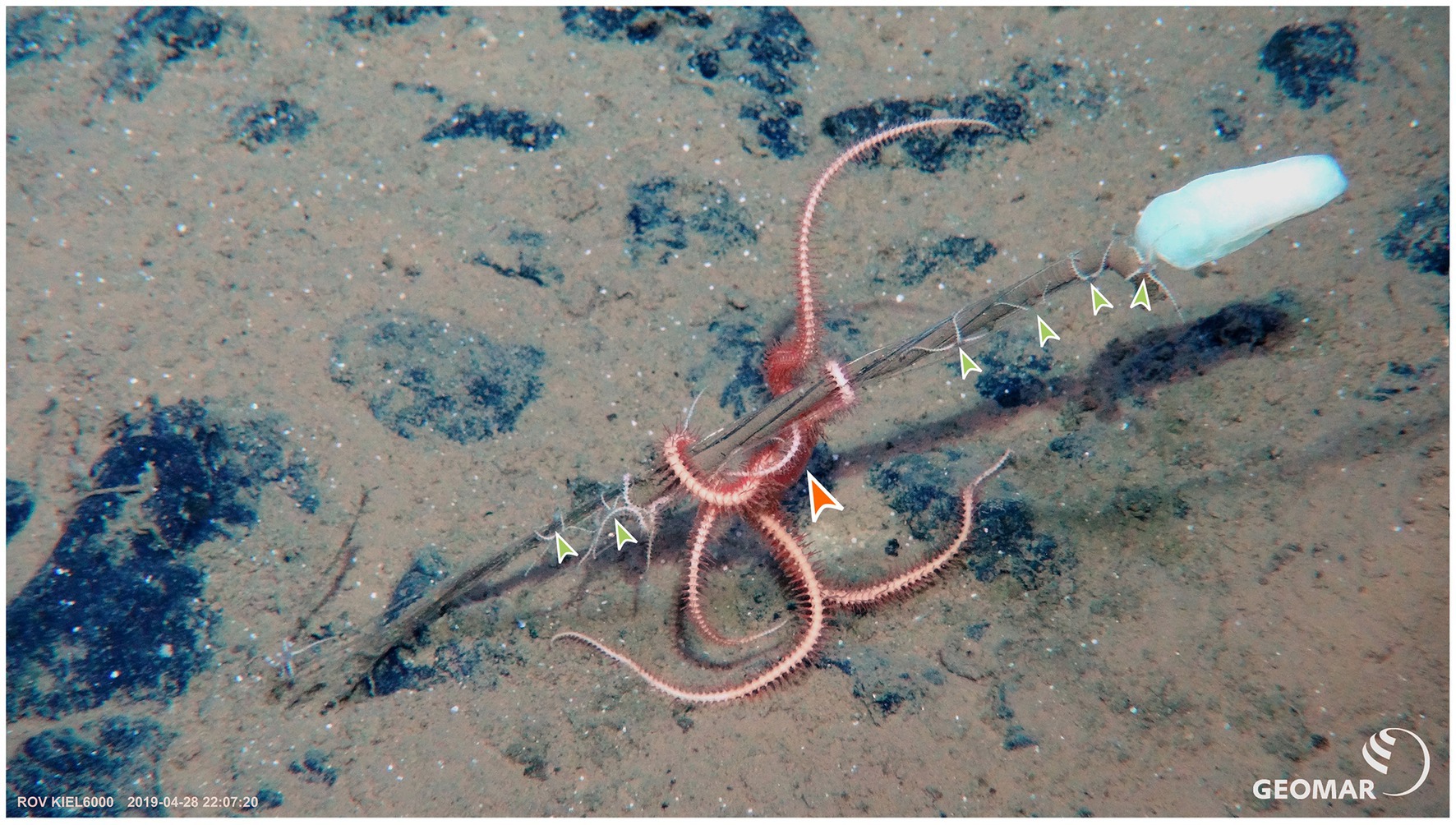
Figure 9. In-situ photograph of a group of O. cosmica on a stalked sponge. Pictured is a female (large orange arrowhead) and several juvenile specimens (small green arrowheads) of O. cosmica that were sampled in the BGR contract area in 2019 during the SO268 R/V Sonne cruise. NB. Dark rock-like deposits in sediment are polymetallic nodules. Photo credit: ROV Kiel 6000, GEOMAR.
In contrast, Ophiosphalma glabrum examined in the current study was epibenthic as found in previous studies both within the CCZ (Amon et al., 2016; Glover et al., 2016; Christodoulou et al., 2020) and more generally in the NE Pacific (Booth et al., 2008). Stomach contents of dissected specimens also indicate that O. glabrum is a deposit feeder, like members of the closely related genus Ophiomusa (Pearson and Gage, 1984). Single individuals generally occurred in the sediment around nodules, or under habitat-forming fauna, such as non-stalked sponges and xenophyophores – giant, deep-sea foraminifera with delicate agglutinated tests (Goineau and Gooday, 2019).
The limited availability of food in the deep-sea soft-sediment benthos is a principal driver in structuring deep-sea benthic communities characterised by low population densities but high levels of diversity (Hardy et al., 2015). The CCZ is a vast, food-limited ecosystem, reliant on the arrival of particulate organic matter (POM) to the seafloor, dictated largely by productivity at the surface (Pennington et al., 2006). While nodule substrate availability plays a role in determining species distributions (Vanreusel et al., 2016), food availability – largely dependent on POM flux – will ultimately determine population densities. Having evolved under these relatively stable conditions, communities in the abyssal deep-sea may be poorly adapted, and thus highly susceptible to future mining impacts. If, for example, elevated substrata provide a nursery-type habitat for Ophiacantha cosmica, then the ramifications for reproductive success following future mining disturbance events are cause for concern. In addition, filter-feeding organisms are expected to be severely impacted by plumes and settling aggregates generated by the resuspension of sea-floor sediments during nodule collections, resulting in the long-term loss of suspension feeding organisms (Simon-Lledó et al., 2019). As with all deposit feeders, changes in surface-sediment chemical and physical characteristics following mining activity (e.g., remobilisation of formerly sequestered heavy metals and sediment compaction) could increase the toxicity of ingested sediments and impact Ophiosphalma glabrum’s ability to forage for food.
Food availability (i.e., POC flux) in soft-sediment abyssal habitats is subject to spatial variability both regionally, due to an undulating seafloor topography and at ocean-basin scales, as its dependency on surface productivity (Smith et al., 2008). Such heterogeneity in POC flux could create habitat networks of richer or poorer food supply over spatial scales at which reproductive kinetics and larval dispersal become ecologically relevant (Hardy et al., 2015). The CCZ lies along a POC flux gradient: lowest in regions that underlie central oligotrophic ocean gyres and highest beneath or adjacent to coastal and equatorial regions characterised by productive upwelling zones (Smith et al., 2008). Since population densities decrease concomitantly with POC flux (Tittensor et al., 2011), species in food impoverished areas often occur at densities below the minimum needed for reproductive success (e.g., critically low encounter rates for mating), rendering them wholly dependent on larval supply from populations in higher POC-flux habitats (the oligotrophic sink hypothesis, Hardy et al., 2015). The two species examined in the current study are relatively common in the CCZ and beyond (Booth et al., 2008; Amon et al., 2016; Christodoulou et al., 2019, 2020; Simon-Lledó et al., 2019). In fact, it is for this reason that the current reproductive study was even possible. However, the relative densities at which they occur at regional scales (such as between contract areas/APEIs) varies considerably (e.g., Christodoulou et al., 2020). Should mining activities proceed in regions of higher-POC flux that play a formative role as larval sources, this may have wide-reaching detrimental effects on the most food-limited populations of these and other species that employ planktonic larval dispersal.
Reproductive kinetics plays a fundamental role in mediating biogeographic patterns, population dynamics, metapopulation connectivity, and ultimately, species survival (Ramirez Llodra, 2002). While molecular approaches can be used to examine both historical and contemporary connectivity, the temporal resolution of these data remains too poor to discriminate between sporadic regional genetic exchange that then spreads locally over successive generations, and regular regional genetic exchange that acts to supplement local populations to a biologically meaningful degree. Reproductive ecology remains vital in bridging gaps in our understanding. The current study sought to add to the paucity of reproductive data for deep-sea species in nodule environments. It also highlights an uncomfortable truth in deep-sea ecology: these classical reproductive studies of this sort, which necessitate large numbers of individuals, may not be practicable in habitats such as the CCZ, where the most susceptible species are typically the rarest. Support for more ambitious approaches that compensate for sampling constraints, such as temporal studies or permanent monitoring networks are urgently needed in order for conservation measures to be effective and informed.
Data Availability Statement
The original contributions presented in the study are included in the article/Supplementary Material, further inquiries can be directed to the corresponding author.
Author Contributions
AH conceived the reproductive study. AH, MC, and PM coordinated the sample collection, fixation, and preservation. MC and PM performed all aspects of molecular ID. SL performed the specimen imagery, dissections, sectioning, histological, graphical and statistical analyses, prepared figures, and wrote manuscript with scientific input from remaining authors. All authors contributed to the article and approved the submitted version.
Funding
Cruises SO239 and SO268 were financed by the German Ministry of Education and Science (BMBF) as a contribution to the European project JPI Oceans “Ecological Aspects of Deep-Sea Mining.” PM acknowledges funding from BMBF under contract 03F0707E and 03F0812E. SL and AH are supported by FCT/MCTES through CESAM (UIDP/50017/2020 + UIDB/50017/2020) funded by national funds, through the project REDEEM (PTDC/BIA-BMA/2986102/SAICT/2017) funded by FEDER within the framework of COMPETE2020 – Programa Operacional Competitividade e Internacionalização (POCI) and by Portuguese funds through FCT in special support of the JPIO pilot action “Ecological aspects of deep-sea mining” and the project MiningImpact2 (JPI Mining 2017, ref. Mining2/0002/2017), program 3599-PPCDT, Ciências do Mar – Sistemas Oceânicos e do Mar Profundo.
Conflict of Interest
The authors declare that the research was conducted in the absence of any commercial or financial relationships that could be construed as a potential conflict of interest.
The handling editor declared a past co-authorship with one of the authors PM.
Acknowledgments
The authors would like to acknowledge the captain and crew of the R/V Sonne and ROV Pilots for the Kiel 6000 (GEOMAR) and to the deep-sea scientists involved in cruise organisation as well as collection and processing of samples on board. The authors would also like to thank António and Sandra Calado for the use of an ultramicrotome. A pre-print of this manuscript is available on bioRxiv, Laming et al. (2020) at https://doi.org/10.1101/2021.02.06.428832.
Supplementary Material
The Supplementary Material for this article can be found online at: https://www.frontiersin.org/articles/10.3389/fmars.2021.663798/full#supplementary-material
Footnotes
References
Adams, N. L., Heyland, A., Rice, L. L., and Foltz, K. R. (2019). Procuring animals and culturing of eggs and embryos. Methods Cell Biol. 150, 3–46. doi: 10.1016/bs.mcb.2018.11.006
Amon, D. J., Ziegler, A. F., Dahlgren, T. G., Glover, A. G., Goineau, A., Gooday, A. J., et al. (2016). Insights into the abundance and diversity of abyssal megafauna in a polymetallic-nodule region in the eastern Clarion-Clipperton Zone. Sci. Rep. 6:30492. doi: 10.1038/srep30492
Attali, D., and Baker, C. (2019). ggExtra: Add Marginal Histograms to “ggplot2”, and More “ggplot2” Enhancements. Available at: https://CRAN.R-project.org/package=ggExtra
Baker, A. N. (2016). An illustrated catalogue of type specimens of the bathyal brittlestar genera Ophiomusium Lyman and Ophiosphalma HL Clark (Echinodermata: Ophiuroidea). Zootaxa 4097, 1–40. doi: 10.11646/zootaxa.4097.1.1
Billett, D. S. M., Bett, B. J., Evans, R., Cross, I., Tyler, P. A., and Wolff, G. A. (2013). The reproductive ecology of deep-sea ophiuroids around the Crozet plateau, Southern Indian ocean, under contrasting productivity regimes. Deep-Sea Res. II Top. Stud. Oceanogr. 92, 18–26. doi: 10.1016/j.dsr2.2013.03.002
Booth, J. A. T., Ruhl, H. A., Lovell, L. L., Bailey, D. M., and Smith, K. L. (2008). Size–frequency dynamics of NE Pacific abyssal ophiuroids (Echinodermata: Ophiuroidea). Mar. Biol. 154, 933–941. doi: 10.1007/s00227-008-0982-3
Brogger, M. I., Martinez, M. I., Zabala, S., and Penchaszadeh, P. E. (2013). Reproduction of Ophioplocus januarii (Echinodermata: Ophiuroidea): a continuous breeder in northern Patagonia, Argentina. Aquat. Biol. 19, 275–285. doi: 10.3354/ab00537
Brix, S., Osborn, K. J., Kaiser, S., Truskey, S. B., Schnurr, S. M., Brenke, N., et al. (2020). Adult life strategy affects distribution patterns in abyssal isopods–implications for conservation in Pacific nodule areas. Biogeosciences 17, 6163–6184. doi: 10.5194/bg-17-6163-2020
Christodoulou, M., O’Hara, T. D., Hugall, A. F., and Arbizu, P. M. (2020). Dark Ophiuroid biodiversity in a prospective abyssal mine field. Curr. Biol. 29, 3909–3912.e3. doi: 10.1016/j.cub.2019.09.012
Christodoulou, M., O’Hara, T., Hugall, A. F., Khodami, S., Rodrigues, C. F., Hilario, A., et al. (2020). Unexpected high abyssal ophiuroid diversity in polymetallic nodule fields of the Northeast Pacific Ocean and implications for conservation. Biogeosciences 17, 1845–1876. doi: 10.5194/bg-17-1845-2020
Clark, H. L. (1911). North Pacific Ophiurans in the Collection of the United States National Museum. Washington, DC: US Government Printing Office.
Clark, H. L. (1913). Echinoderms from Lower California, with descriptions of new species. ed. J. A. Allen (Joel Asaph) (New York: American Museum of Natural History).
Danovaro, R., Aguzzi, J., Fanelli, E., Billett, D., Gjerde, K., Jamieson, A., et al. (2017). An ecosystem-based deep-ocean strategy. Science 355, 452–454. doi: 10.1126/science.aah7178
De Smet, B., Pape, E., Riehl, T., Bonifácio, P., Colson, L., and Vanreusel, A. (2017). The community structure of deep-sea macrofauna associated with polymetallic nodules in the eastern part of the Clarion-Clipperton Fracture Zone. Front. Mar. Sci. 4:103. doi: 10.3389/fmars.2017.00103
Doyle, G. M., Hamel, J. -F., and Mercier, A. (2012). A new quantitative analysis of ovarian development in echinoderms: the maturity stage index. Mar. Biol. 159, 455–465. doi: 10.1007/s00227-011-1823-3
Gage, J. D. (1982). Age structure in populations of the deep-sea brittle star Ophiomusium lymani: a regional comparison. Deep Sea Res. A Oceano. Res. Papers 29, 1565–1586. doi: 10.1016/0198-0149(82)90044-9
Gage, J. D., Anderson, R. M., Tyler, P. A., Chapman, R., and Dolan, E. (2004). Growth, reproduction and possible recruitment variability in the abyssal brittle star Ophiocten hastatum (Ophiuroidea: Echinodermata) in the NE Atlantic. Deep-Sea Res. I Oceanogr. Res. Pap. 51, 849–864. doi: 10.1016/j.dsr.2004.01.007
Gage, J. D., and Tyler, P. A. (1982). Growth and reproduction of the deep-sea brittlestar Ophiomusium lymani Wyville Thomson. Oceanol. Acta 5, 73–83.
Gage, J. D., and Tyler, P. A. (1991). Deep-Sea Biology: A Natural History of Organisms at the Deep-Sea Floor. Cambridge, UK: Cambridge University Press.
Ghosh, A. K., and Mukhopadhyay, R. (2000). Mineral wealth of the ocean. Rotterdam, The Netherlands: AA Balkema, Rotterdam, 260.
Glover, A. G., Wiklund, H., Rabone, M., Amon, D. J., Smith, C. R., O’Hara, T., et al. (2016). Abyssal fauna of the UK-1 polymetallic nodule exploration claim, Clarion-Clipperton Zone, Central Pacific Ocean: Echinodermata. BDJ 4:e7251. doi: 10.3897/BDJ.4.e7251
Goineau, A., and Gooday, A. J. (2019). Diversity and spatial patterns of foraminiferal assemblages in the eastern Clarion–Clipperton Zone (abyssal eastern equatorial Pacific). Deep-Sea Res. I Oceanogr. Res. Pap. 149:103036. doi: 10.1016/j.dsr.2019.04.014
Hardy, S. M., Smith, C. R., and Thurnherr, A. M. (2015). Can the source–sink hypothesis explain macrofaunal abundance patterns in the abyss? A modelling test. Proc. R. Soc. B Biol. Sci. 282:20150193. doi: 10.1098/rspb.2015.0193
Hauquier, F., Macheriotou, L., Bezerra, T. N., Egho, G., Martínez Arbizu, P., and Vanreusel, A. (2019). Distribution of free-living marine nematodes in the Clarion–Clipperton Zone: implications for future deep-sea mining scenarios. Biogeo. 16, 3475–3489. doi: 10.5194/bg-16-3475-2019
Hein, J. R., Mizell, K., Koschinsky, A., and Conrad, T. A. (2013). Deep-ocean mineral deposits as a source of critical metals for high-and green-technology applications: comparison with land-based resources. Ore Geol. Rev. 51, 1–14. doi: 10.1016/j.oregeorev.2012.12.001
Kassambara, A. (2020). ggpubr: “ggplot2” Based Publication Ready Plots. Available at: https://CRAN.R-project.org/package=ggpubr
Kuhnz, L. A., Ruhl, H. A., Huffard, C. L., and Smith, K. L. (2014). Rapid changes and long-term cycles in the benthic megafaunal community observed over 24 years in the abyssal Northeast Pacific. Prog. Oceanogr. 124, 1–11. doi: 10.1016/j.pocean.2014.04.007
Laming, S. R., Hourdez, S., Cambon-Bonavita, M.-A., and Pradillon, F. (2020). Classical and computed tomographic anatomical analyses in a not-so-cryptic Alviniconcha species complex from hydrothermal vents in the SW Pacific. Front. Zool. 17:12. doi: 10.1186/s12983-020-00357-x
Lauerman, L. M. L., Kaufmann, R. S., and Smith, K. L. (1996). Distribution and abundance of epibenthic megafauna at a long time-series station in the abyssal Northeast Pacific. Deep-Sea Res. I Oceanogr. Res. Pap. 43, 1075–1103. doi: 10.1016/0967-0637(96)00045-3
Lebrato, M., Iglesias-Rodríguez, D., Feely, R. A., Greeley, D., Jones, D. O. B., Suarez-Bosche, N., et al. (2010). Global contribution of echinoderms to the marine carbon cycle: CaCO3 budget and benthic compartments. Ecol. Monogr. 80, 441–467. doi: 10.1890/09-0553.1
Lee, T., Stöhr, S., Bae, Y. J., and Shin, S. (2019). A new fissiparous brittle star, Ophiacantha scissionis sp. nov. (Echinodermata, Ophiuroidea, Ophiacanthida), from Jeju Island, Korea. Zool. Stud. 58, e8–e8. doi: 10.6620/ZS.2019.58-08
Lyman, T. (1878). Ophiuridae and Astrophytidae of the exploring voyage og HMS “Challenger” under Prof. Sir Wyville Thomson FRS. Part I. Bull. Mus. Comp. Zool. 5, 65–158.
Lyman, T. (1879). Ophiuridae and Astrophytidae of the exploring voyage og HMS “Challenger” under Prof. Sir Wyville Thomson FRS. Part II. Bull. Mus. Comp. Zool. 6, 17–83.
Lyman, T. (1882). Report on the Ophiuroidea dredged by HMS Challenger during the years 1873–1876. Report of the Scientific Results of the Voyage of HMS Challenger during 1873–1876, Zoology 5, 1–386.
Martynov, A. V., and Litvinova, N. M. (2008). Deep-water Ophiuroidea of the northern Atlantic with descriptions of three species and taxonomic remarks on certain genera and species. Mar. Biol. Res. 4, 76–111. doi: 10.1080/17451000701840066
Mercier, A., and Hamel, J.-F. (2008). Depth-related shift in life history strategies of a brooding and broadcasting deep-sea asteroid. Mar. Biol. 156, 205–223.
Mercier, A., and Hamel, J.-F. (2009). Advances in Marine Biology: Endogenous and Exogenous Control of Gametogenesis and Spawning in Echinoderms. Oxford, UK: Elsevier Science.
Mercier, A., Sewell, M. A., and Hamel, J.-F. (2013). Pelagic propagule duration and developmental mode: reassessment of a fading link. Glob. Ecol. Biogeogr. 22, 517–530. doi: 10.1111/geb.12018
Mercier, A., Ycaza, R. H., and Hamel, J.-F. (2007). Long-term study of gamete release in a broadcast-spawning holothurian: predictable lunar and diel periodicities. Mar. Ecol.: Prog. Ser. 329, 179–189. doi: 10.3354/meps329179
O’Hara, T. D., Rowden, A. A., and Williams, A. (2008). Cold-water coral habitats on seamounts: do they have a specialist fauna? Divers. Distrib. 14, 925–934. doi: 10.1111/j.1472-4642.2008.00495.x
Paterson, G. L. (1985). The deep-sea Ophiuroidea of the North Atlantic Ocean. Bull. Br. Mus. Nat. Hist. Zool. 49, 1–162.
Pearson, M., and Gage, J. D. (1984). Diets of some deep-sea brittle stars in the Rockall Trough. Mar. Biol. 82, 247–258. doi: 10.1007/BF00392406
Pennington, J. T., Mahoney, K. L., Kuwahara, V. S., Kolber, D. D., Calienes, R., and Chavez, F. P. (2006). Primary production in the eastern tropical Pacific: a review. Prog. Oceanogr. 69, 285–317. doi: 10.1016/j.pocean.2006.03.012
R Core Team (2020). R: A Language and Environment for Statistical Computing. Vienna, Austria: R Foundation for Statistical Computing. Available at: https://www.R-project.org/ (Accessed March 20, 2021).
Ramirez Llodra, E. (2002). Fecundity and life-history strategies in marine invertebrates. Adv. Mar. Biol. 43, 87–170. doi: 10.1016/S0065-2881(02)43004-0
Schindelin, J., Arganda-Carreras, I., Frise, E., Kaynig, V., Longair, M., Pietzsch, T., et al. (2012). Fiji: an open-source platform for biological-image analysis. Nat. Methods 9, 676–682. doi: 10.1038/nmeth.2019
Sewell, M. A., and Young, C. M. (1997). Are echinoderm egg size distributions bimodal? Biol. Bull. 193, 297–305. doi: 10.2307/1542932
Shulse, C. N., Maillot, B., Smith, C. R., and Church, M. J. (2017). Polymetallic nodules, sediments, and deep waters in the equatorial North Pacific exhibit highly diverse and distinct bacterial, archaeal, and microeukaryotic communities. MicrobiologyOpen 6:e00428. doi: 10.1002/mbo3.428
Simon-Lledó, E., Bett, B. J., Huvenne, V. A. I., Köser, K., Schoening, T., Greinert, J., et al. (2019). Biological effects 26 years after simulated deep-sea mining. Sci. Rep. 9:8040. doi: 10.1038/s41598-019-44492-w
Simon-Lledó, E., Pomee, C., Ahokava, A., Drazen, J. C., Leitner, A. B., Flynn, A., et al. (2020). Multi-scale variations in invertebrate and fish megafauna in the mid-eastern Clarion Clipperton Zone. Prog. Oceanogr. 187:102405. doi: 10.1016/j.pocean.2020.102405
Smith, C. R., De Leo, F. C., Bernardino, A. F., Sweetman, A. K., and Arbizu, P. M. (2008). Abyssal food limitation, ecosystem structure and climate change. Trends Ecol. Evol. 23, 518–528. doi: 10.1016/j.tree.2008.05.002
Smith, K. L., Ruhl, H. A., Huffard, C. L., Messié, M., and Kahru, M. (2018). Episodic organic carbon fluxes from surface ocean to abyssal depths during long-term monitoring in NE Pacific. Proc. Natl. Acad. Sci. U. S. A. 115, 12235–12240. doi: 10.1073/pnas.1814559115
Stearns, S. C. (2000). Life history evolution: successes, limitations, and prospects. Naturwissenschaften 87, 476–486. doi: 10.1007/s001140050763
Stöhr, S., O’Hara, T. D., and Thuy, B. (2012). Global diversity of brittle stars (Echinodermata: Ophiuroidea). PLoS One 7:e31940. doi: 10.1371/journal.pone.0031940
Tittensor, D. P., Rex, M. A., Stuart, C. T., McClain, C. R., and Smith, C. R. (2011). Species–energy relationships in deep-sea molluscs. Biol. Lett. 7, 718–722. doi: 10.1098/rsbl.2010.1174
Tyler, P. A. (1977). Seasonal variation and ecology of gametogenesis in the genus Ophiura (Ophiuroidea: Echinodermata) from the Bristol channel. J. Exp. Mar. Biol. Ecol. 30, 185–197. doi: 10.1016/0022-0981(77)90011-9
Tyler, P. A., and Gage, J. D. (1980). Reproduction and growth of the deep-sea brittlestar Ophiura ljungmani (Lyman). Oceanol. Acta 3, 177–185.
Tyler, P. A., and Gage, J. D. (1982). The reproductive biology of Ophiacantha bidentata (Echinodermata: Ophiuroidea) from the Rockall trough. J. Mar. Biol. Assoc. U. K. 62, 45–55. doi: 10.1017/S0025315400020099
Vanreusel, A., Hilario, A., Ribeiro, P. A., Menot, L., and Arbizu, P. M. (2016). Threatened by mining, polymetallic nodules are required to preserve abyssal epifauna. Sci. Rep. 6:26808. doi: 10.1038/srep26808
Weaver, P. P. E., Billett, D. S. M., and Van Dover, C. L. (2018). “Environmental risks of deep-sea mining,” in Handbook on Marine Environment Protection: Science, Impacts and Sustainable Management. eds. M. Salomon and T. Markus (Cham: Springer International Publishing), 215–245.
Wessel, G. M., Voronina, E., and Brooks, J. M. (2004). Obtaining and handling echinoderm oocytes. Methods Cell Biol. 74, 87–114. doi: 10.1016/S0091-679X(04)74005-4
Wickham, H., Averick, M., Bryan, J., Chang, W., McGowan, L. D., François, R., et al. (2019). Welcome to the Tidyverse. J. Open Source Softw. 4:1686. doi: 10.21105/joss.01686
Wickham, H., Henry, L., Pedersen, T. L., Luciani, T. J., Decorde, M., and Lise, V. (2020). svglite: An “SVG” Graphics Device. Available at: https://CRAN.R-project.org/package=svglite
Wilke, C. O. (2020). cowplot: Streamlined Plot Theme and Plot Annotations for “ggplot2.” Available at: https://CRAN.R-project.org/package=cowplot
Wilkie, I. C. (2016). Functional morphology of the arm spine joint and adjacent structures of the Brittlestar Ophiocomina nigra (Echinodermata: Ophiuroidea). PLoS One 11:e0167533. doi: 10.1371/journal.pone.0167533
Wilson, G. D. F. (2017). Macrofauna abundance, species diversity and turnover at three sites in the Clipperton-Clarion Fracture Zone. Mar. Biodivers. 47, 323–347. doi: 10.1007/s12526-016-0609-8
Young, C. M. (2003). “Reproduction, development and life-history traits,” in Ecosystems of the Deep Oceans. ed. P. A. Tyler (Amsterdam: Elsevier), 381–426.
Keywords: maturation, lecithotrophy, deep-sea mining, ecology, brittle stars, ophiuroidea, echinodermata, gonochoric
Citation: Laming SR, Christodoulou M, Martinez Arbizu P and Hilário A (2021) Comparative Reproductive Biology of Deep-Sea Ophiuroids Inhabiting Polymetallic-Nodule Fields in the Clarion-Clipperton Fracture Zone. Front. Mar. Sci. 8:663798. doi: 10.3389/fmars.2021.663798
Edited by:
Craig Randall Smith, University of Hawaii at Manoa, United StatesReviewed by:
Paul Alan Tyler, University of Southampton, United KingdomMary A. Sewell, The University of Auckland, New Zealand
Copyright © 2021 Laming, Christodoulou, Martinez Arbizu and Hilário. This is an open-access article distributed under the terms of the Creative Commons Attribution License (CC BY). The use, distribution or reproduction in other forums is permitted, provided the original author(s) and the copyright owner(s) are credited and that the original publication in this journal is cited, in accordance with accepted academic practice. No use, distribution or reproduction is permitted which does not comply with these terms.
*Correspondence: Sven R. Laming, c3ZlbmxhbWluZ0B1YS5wdA==