- 1Instituto Español de Oceanografía, Centro Oceanográfico de A Coruña, A Coruña, Spain
- 2Instituto Español de Oceanografía, Centro Oceanográfico de Vigo, Vigo, Spain
The use of top-consumers as bioindicators of the health of food webs is hampered by uncertainties in their effective use of resources. In this study, the abundance of stable nitrogen isotopes in amino acids from homogenised eggs of the Yellow-legged Gull (Larus michahellis) allowed to identify variations in trophic resource exploitation between geographically adjacent nesting colonies in the Ria de Vigo (NW Spain) that exhibited marked differences in pollutants. Eggs from nests in the Cíes Islands (located in a National Park) showed a large variability in stable carbon and nitrogen isotopes in bulk egg content encompassing that of eggs from Vigo city (a major fishing harbour). However, both colonies differed in the relative concentration and abundance of nitrogen isotopes of lysine, an essential amino acid present in marine prey, but also extensively used in feed stocks for poultry and swine. Notwithstanding the similarity in trophic position for both colonies, gulls from Cíes Islands may have acquired a substantial fraction of lysine from garbage dump sites, while those of the urban colony relied on fish discards. This unexpected conclusion is partly supported by the large variability reported for gull’s diet in this region and calls for detailed estimations of diet when assessing the conservation status and pollution risks of marine ecosystems.
Introduction
Top consumers from oceanic food webs are good indicators of the biogeochemistry and conservation status of marine ecosystems. They integrate processes operating at lower food web levels and thus represent the result of ecosystem functioning at large spatial and temporal scales (Hebert et al., 2016; Ruiz-Cooley et al., 2017). Estimations of their trophic position (TP) are key to determine ecosystem properties as food-chain length (Vander Zanden and Fetzer, 2007; Reum et al., 2015) or the biomagnification of pollutants (Storr-Hansen et al., 1995; Dietz et al., 2000; Kelly et al., 2008). For instance, changes in TP are indicative of alterations in food web structure (Jenkins and Davoren, 2020) or in nutrient inputs (Ruiz-Cooley et al., 2017). Particularly, seabirds have been extensively used as biomonitors of persistent organic and trace metal contaminants (e.g., OSPAR, 1999), with biomagnification effects critically dependent on their TP (Ramos et al., 2013; Gatt et al., 2020). Methodological uncertainties, however, difficult the accurate determination of TP in these organisms.
Direct quantification of diets cannot be achieved for most field populations, as they may be inaccessible for long periods (e.g., Matias and Catry, 2010; Gatt et al., 2020) and the analysis of stomach contents, pellets or regurgitates cannot identify all the consumed prey (González-Solís, 2003; Abdennadher et al., 2010; Moreno et al., 2010; Calado et al., 2018; Méndez et al., 2020). Alternative determinations based on the progressive enrichment in heavy nitrogen isotopes (expressed as δ15N) through the food web allow for estimations of TP and diet composition integrated in space and time, but are highly dependent on factors as the appropriate selection of reference baselines (Post, 2002), the assumptions of the enrichment model (Hussey et al., 2014; Jennings and van der Molen, 2015) and the tissue analysed (Caut et al., 2009; Hebert et al., 2016). Nevertheless, these limitations have not impeded the extensive use of δ15N in bulk tissues to infer TP and biomagnification of pollutants in many studies (e.g., Abdennadher et al., 2010; Ramos et al., 2013). The application of compound-specific isotopic analyses (e.g., δ15N in amino acids) have reduced some of the uncertainties in TP estimations, because the changes in the nutrient sources at the base of the food web can be accounted for Chikaraishi et al. (2009). Even when there are still uncertainties in the variability of the isotopic enrichment along the food web (McMahon and McCarthy, 2016; Ohkouchi et al., 2017; Whiteman et al., 2019) the new estimations based on amino acids are being increasingly applied to marine birds (McMahon et al., 2015; Wu et al., 2018; Gatt et al., 2020).
Eggs of the Yellow-legged Gull (Larus michahellis) have been used to monitor organic and trace metal pollutants (Abdennadher et al., 2010; Ramos et al., 2013; Otero et al., 2018; Viñas et al., 2020). The large feeding plasticity reported for this species, however, difficult the identification of the sources of pollutants. For instance, gull colonies near urban areas have been shown to consume more terrestrial and anthropogenic resources than marine prey (Arizaga et al., 2013; Méndez et al., 2020; Zorrozua et al., 2020). A previous study of two colonies only 10 km apart in the Ria de Vigo (NW Spain) revealed significant differences in the load of pollutants in their eggs, pointing to a rapid adaptation to local feeding resources (Viñas et al., 2020). The colony nesting on the Cíes Islands (located in a marine protected area) showed lower concentrations of pollutants and a wider trophic niche than the colony nesting in the industrialised Vigo city. Furthermore, the estimated diet composition in the region, including colonies in the Cíes Islands, showed marked year to year variations related to the availability of marine vs. anthropogenic resources, the latter including mainly beef, pork, and chicken remains (Moreno et al., 2010; Calado et al., 2020). These findings suggest that major changes in the diet of the Yellow-legged Gull may affect TP and therefore TP can be used as an indicator of changes in the trophic structure of the food web. Alternatively, pollution loads could be caused by the differential exploitation of trophic resources without a substantial change in TP.
The objective of this study is to determine the trophic position of Yellow-legged Gull in two colonies differing in the accumulation of pollutants (Viñas et al., 2020). We applied for the first time estimations derived from the amino acid stable isotope composition of eggs, allowing to infer differences in trophic position and diet between the gulls in the two colonies. These estimations aimed to assist in the interpretation of the differential impact of contaminants in these colonies by revealing the sensitivity of TP estimations to diet variability.
Materials and Methods
Sampling
Yellow-legged Gull eggs were collected from nests in the Cíes Islands (11 eggs) and in the city of Vigo (7 eggs) during the breeding season of 2011 (April to early August). First laid eggs in each nest were selected for analysis, weighed and measured (length and width) before opening (Supplementary Table 1). Embryonated eggs were discarded and the remaining were individually homogenised, stored frozen (−20°C), and freeze-dried before analysis. Further details on sampling were provided in Viñas et al. (2020).
Analysis
Two types of isotopic determinations were made. First, C:N ratios and natural abundances of carbon (δ13C) and nitrogen (δ15N) stable isotopes were determined in bulk egg samples using an isotope ratio mass spectrometer coupled to an elemental analyzer. Egg samples were analysed in triplicate with precision < 0.05‰ for both isotopes. Due to the high lipid content of egg samples, δ13C values were corrected using the C:N ratio of each sample (Elliott and Elliott, 2016). These analyses were described in more detail in Viñas et al. (2020).
Second, 10 mg aliquots of the homogenised eggs were also used for determinations of δ15N in individual amino acids (Chikaraishi et al., 2009). Samples were hydrolysed with 6N HCl, esterified with acetyl chloride:2-propanol, and treated with a mixture of 3:1 diclomethane:trifluoracetic anhydride (McCarthy et al., 2013; Mompeán et al., 2016). Derivatised amino acids were purified by solvent extraction in 1:2 chloroform:phosphate buffer and centrifugation (Loick et al., 2019), evaporated at room temperature under N2, and stored at −20°C in 3:1 diclomethane:trifluoracetic anhydride for up to 6 months until isotope analysis. The individual amino acids were separated in a chromatography column (TraceGOLD TG-5MS, 60 m, 0.32 mm ID, 1.0 μm film) using a gas chromatograph (Trace1310GC, Thermo Fisher Scientific), and subsequently injected into a mass spectrometer (DeltaV Advantage, Thermo Fisher Scientific) via a continuous flow interface and combustion module (GC Isolink, Thermo Fisher Scientific). Amino acid δ15N values were calibrated with the values obtained for isolated standards (Shoko Science) analysed by combustion as described for bulk analysis, and further corrected using an internal L-norleucine standard (SIGMA) of known isotopic composition added to each sample. The molar fraction of each amino acid (% molar) was also determined in the same analytical run by calibration of the spectrometric signals with amino acid standards (McCarthy et al., 2013). Precision (±SE) of triplicate samples (two injections per sample) was <0.6‰ per individual amino acid.
Values of δ15N and %molar were obtained for trophic and source amino acids (McClelland and Montoya, 2002; McCarthy et al., 2013; McMahon and McCarthy, 2016). Trophic amino acids included alanine (Ala), leucine (Leu), isoleucine (Ile), proline (Pro), valine (Val), and the mixtures of glutamine (Gln) and glutamic acid (Glu), and of aspartamine (Asn) and aspartic acid (Asp). The latter mixtures were caused by the acid hydrolysis and were termed as Glx and Asx, respectively. Source amino acids included glycine (Gly), threonine (Thr), serine (Ser), methionine (Met), phenylalanine (Phe), and lysine (Lys). The representation of these amino acids in the bulk protein was assessed by correlation of their molar-weighted average δ15N (δ15NTHAA) with δ15N values for bulk samples (δ15Nbulk). Similarly, the correlations between molar-weighted averages of trophic (δ15Ntrp) or source amino acids (δ15Nsrc) with δ15Nbulk were used to determine the relative importance of variations in trophic vs. nitrogen source factors for the interpretation of δ15Nbulk.
Diet Estimations
The potential contribution of marine and anthropogenic food sources to the diet of the gulls of each colony was estimated from the δ15N signature of source amino acids. Reference values for marine sources were provided by sardines (Sardina pilchardus) and anchovies (Engraulis encrasicholus) collected in spring (April 2017 and April 2019) near the Ria de Vigo as part of a regular fish survey (see Bode et al., 2018). Anthropogenic sources were provided by samples of beef, pork and chicken meat obtained from local markets. Four individuals of each fish species and three replicates of each meat type were analysed as described for the gull eggs. The potential contribution of these sources to the gulls diet were estimated using the Mix SIAR Bayesian mixing model (SIAR v4.1.3) of Stock and Semmens (2013) by using only the δ15N values of individual amino acids that varied significantly between colonies and assuming no isotopic fractionation between sources and eggs. This simplified approach was intended only to highlight the potential implications of the marked differences in δ15N among the sources.
Trophic Position
Estimations of the trophic position of Yellow-legged Gull individuals were made using the δ15N values of representative trophic (Glx) and source (Phe) amino acids (TPAA) and a model considering two trophic discrimination factors (McMahon and McCarthy, 2016):
Where, β is the difference between Glx and Phe in primary producers, and TDFp and TDFb are the trophic discrimination factors for plankton and marine birds, respectively. Mean (±SE) values were 3.4 ± 0.9‰ for β (Chikaraishi et al., 2009), 7.6 ± 1.2‰ for TDFp and 3.5 ± 0.4‰ for TDFb (McMahon et al., 2015; Wu et al., 2018; Gatt et al., 2020). As there was isotopic fractionation between the different tissues, increments of 2.5‰ and 0.9‰ were applied to the measured values of δ15NGlx and δ15NPhe, respectively, to produce TP estimations equivalent to those obtained for the muscle of adult gulls (Hebert et al., 2016).
For comparative purposes, additional TP estimations were made using the measured δ15Nbulk in eggs using the classical model (Post, 2002):
where, δ15Nzoo was the value for zooplankton samples collected in the nearby shelf during spring 2011 (mean ± se = 5.97 ± 0.92‰, n = 14) as described in Bode et al. (2018), and TDFz = 3.4‰ (Post, 2002). The error for all TP estimations was computed by error propagation by taking into account the analytical errors in δ15N measurements in bulk or trophic and source amino acids, as well as the errors reported for TDF and β values employed (Post, 2002; Ohkouchi et al., 2017).
Further comparisons of TP were made with those reported in the literature for Yellow-legged Gull and similar species (Table 1). In the case of data reported in Lopezosa et al. (2019), TP was estimated from the reported δ15Nbulk in body feathers and diet data using the model described in Hebert et al. (2016) by considering the consumption of pelagic fish, demersal fish, and garbage.
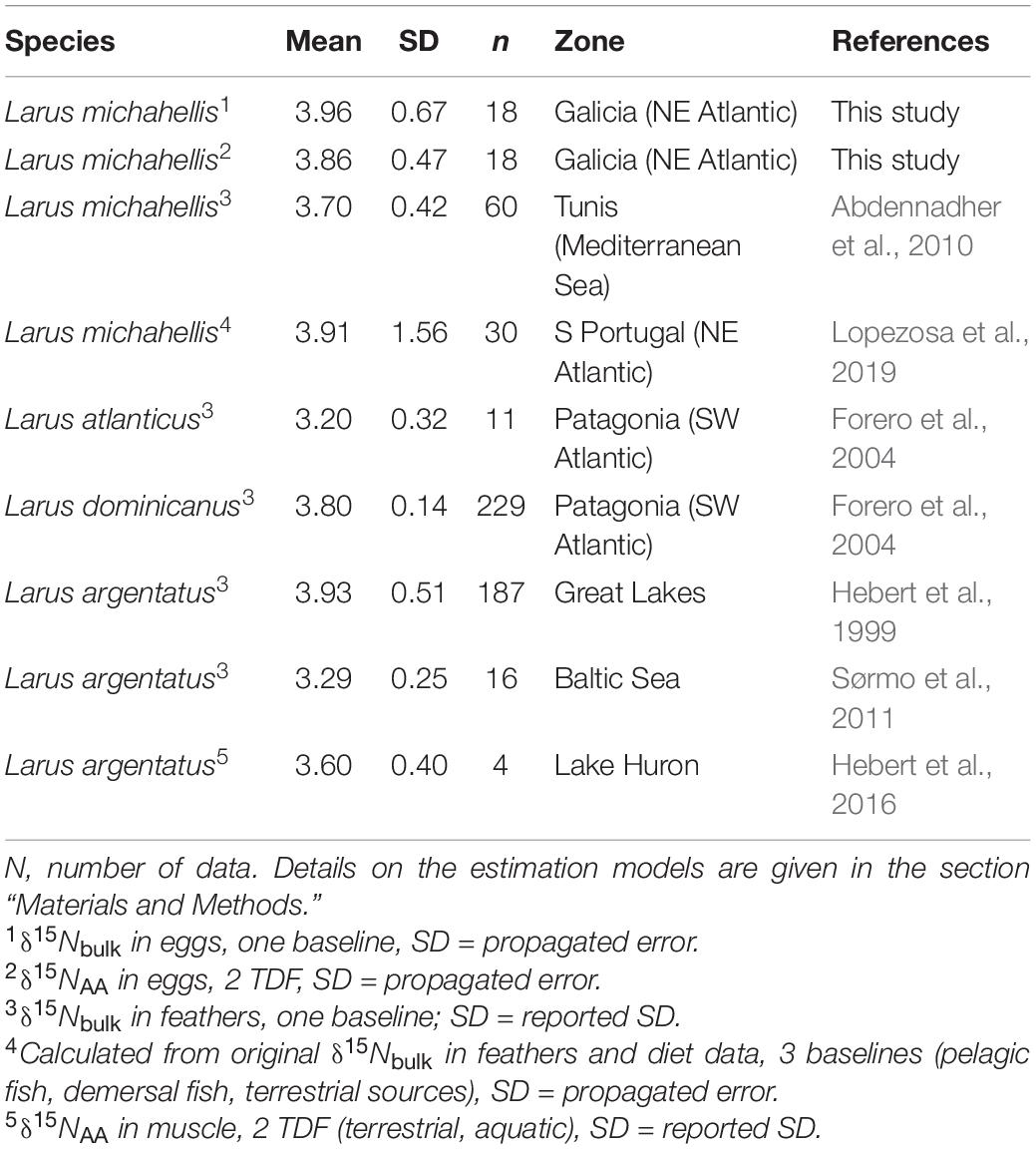
Table 1. Mean and sd trophic position values (TP) estimated for gull species using stable isotopes in this study and in the literature.
Statistics
Differences in isotopic measurements between eggs from Cíes and Vigo colonies were analysed by means of non-parametric ANOVA (Kruskal-Wallis). Regressions between variables were computed using reduced major axis regression, as there were large differences in the measurement error of the variables. Statistical analyses were performed using SPSS 17.0 (SPSS Inc.) and Past 4.0 (Hammer et al., 2001).
Results
There were no significant differences in median bulk δ15N or δ13C between both colonies, nor in averaged δ15N values for trophic or source amino acids (Kruskal-Wallis test, P > 0.05, Figure 1). However, the weighted means of δ15N for total amino acids (δ15NTHAA) and trophic amino acids (δ15Ntrp) were linearly correlated with bulk δ15N (P < 0.001, n = 16), while the means for source amino acids showed no correlation (P > 0.05, Supplementary Figure 1).
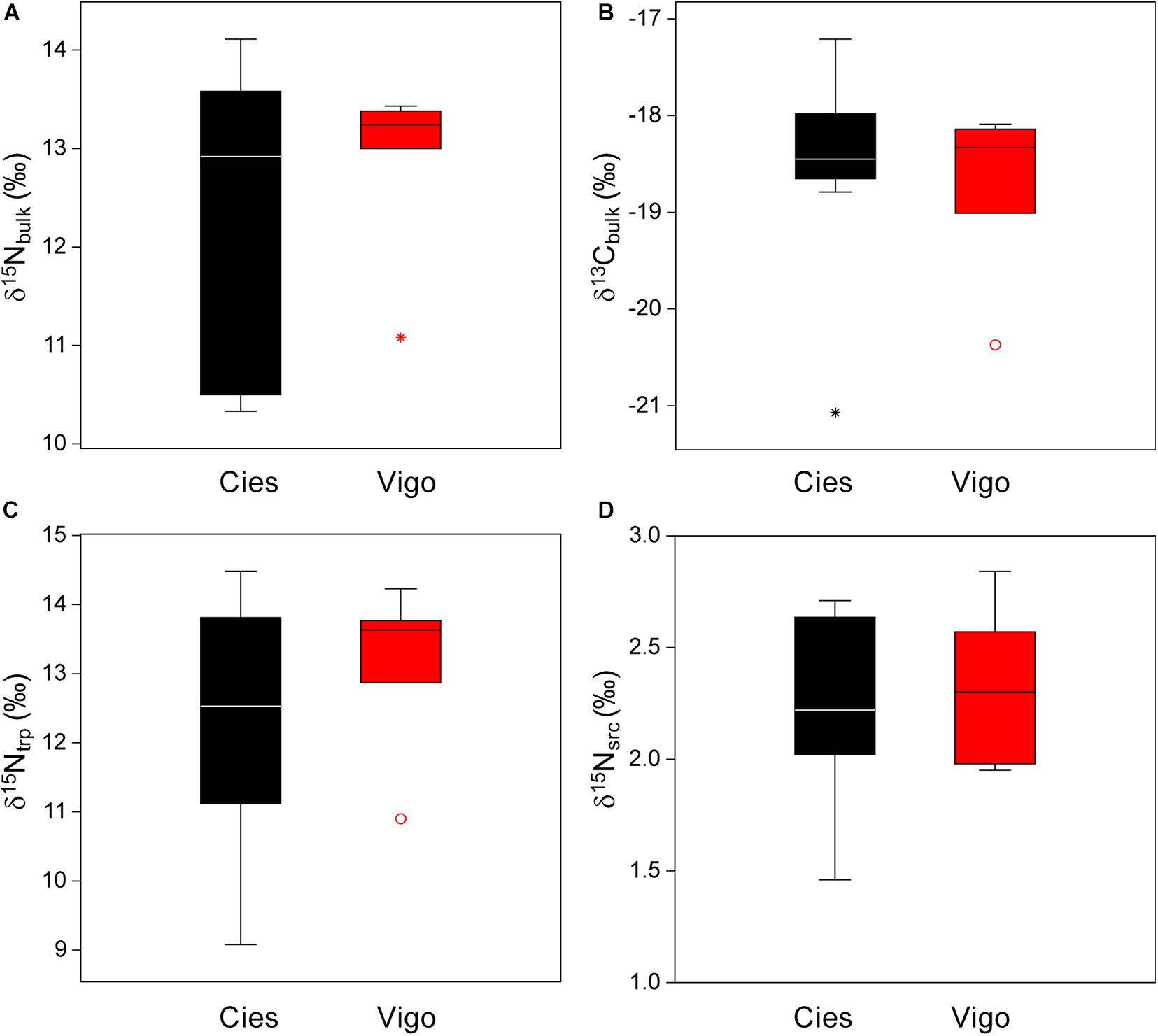
Figure 1. Box plot of (A) δ15Nbulk, (B) lipid-corrected δ13Cbulk, (C) δ15Ntrp, and (D) δ15Nsrc for Yellow-legged Gull eggs sampled in Cíes Islands (black) and Vigo (red). Each box encompasses the 25–75% percentiles, the median is indicated by the horizontal line, and the whiskers indicate the range excluding outliers exceeding 1.5 (circles) or 3 times (asterisks) the interquartile range.
Values of δ15N values for most amino acids were similar for the two colonies (Figure 2A), except in the case of lysine that showed lower values for the Vigo colony (Kruskal-Wallis test, P < 0.05). Mean ± SD δ15N values for lysine in eggs from the Cíes Islands were 1.50 ± 1.18‰ (n = 9) while in those from Vigo were 4.68 ± 0.77‰ (n = 7). Even when adjusted for variations in the δ15N of the canonical source amino acid phenylalanine, the differences in isotopic composition of Lys between colonies persisted, and were indicative of differences in the use of marine resources (Supplementary Table 2). The eggs from the Cíes Islands had also lower mean relative molar concentration of lysine and higher concentration of alanine than those from Vigo (Kruskal-Wallis tests, P < 0.05, Figure 2B).
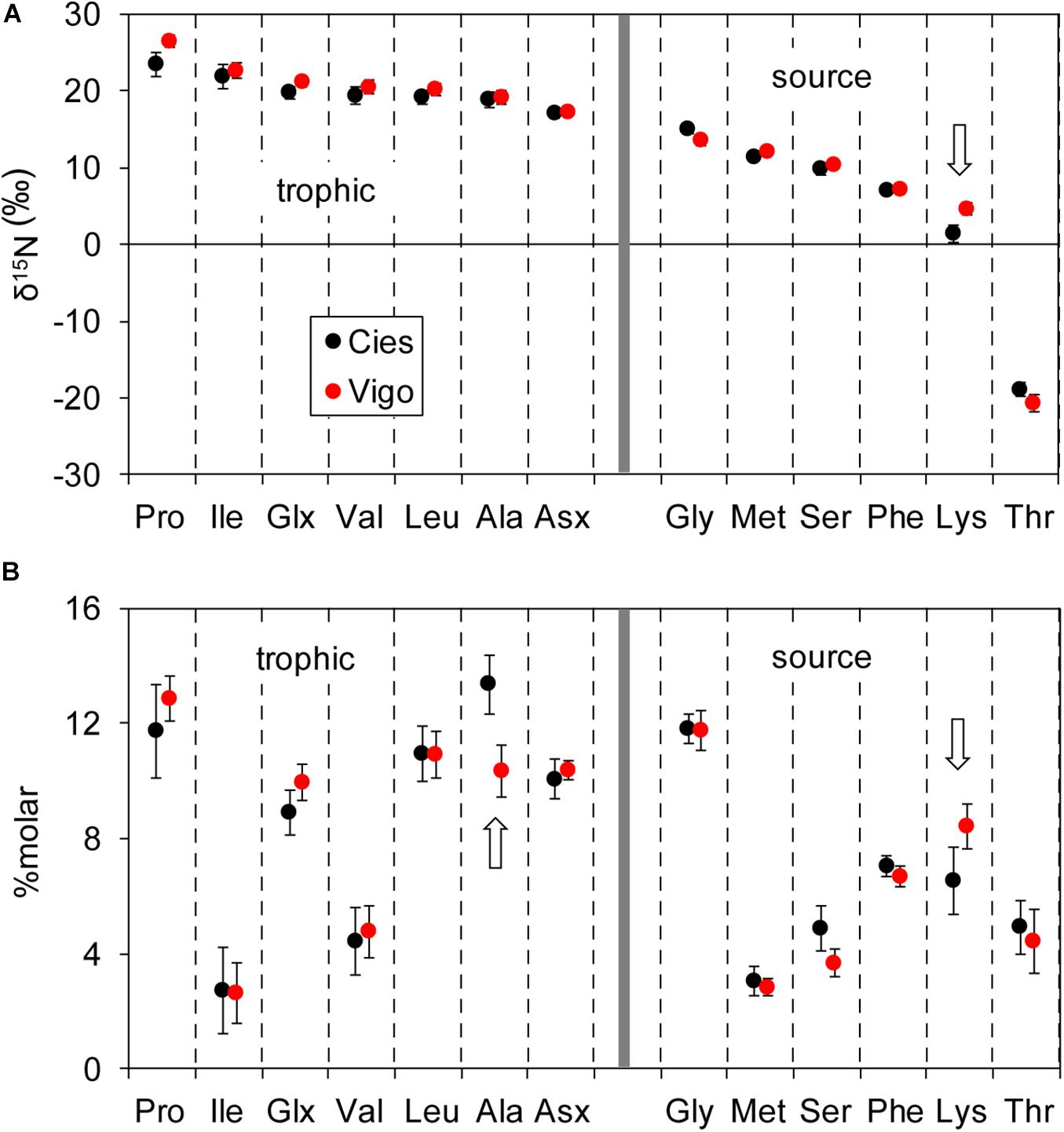
Figure 2. Mean ± SE (A) δ15N (‰) and (B) molar percent of trophic and source amino acids for Yellow-legged Gull eggs sampled in Cíes Islands (black dots) and Vigo (red dots). The arrows indicate significantly different medians (Kruskal-Wallis, P < 0.05).
As for gull eggs, there were also significant differences in Lys δ15N among food sources (Mann-Whitney test, P < 0.05, Supplementary Figure 2A). Using these values, the estimations of diet suggested that, notwithstanding marine sources had a major contribution potential for both colonies, the gulls from Cies had a higher contribution of anthropogenic sources than those from Vigo (Supplementary Figure 2B).
The estimations of TP (Table 1) indicated that the studied Yellow-legged Gulls were almost tertiary consumers (i.e., TP ca. 3) and revealed no significant differences using either bulk or amino acid δ15N (Kruskal-Wallis test, P > 0.05). Besides, there were no significant differences in TP between colonies (Figure 3, Kruskal-Wallis test, P > 0.05).
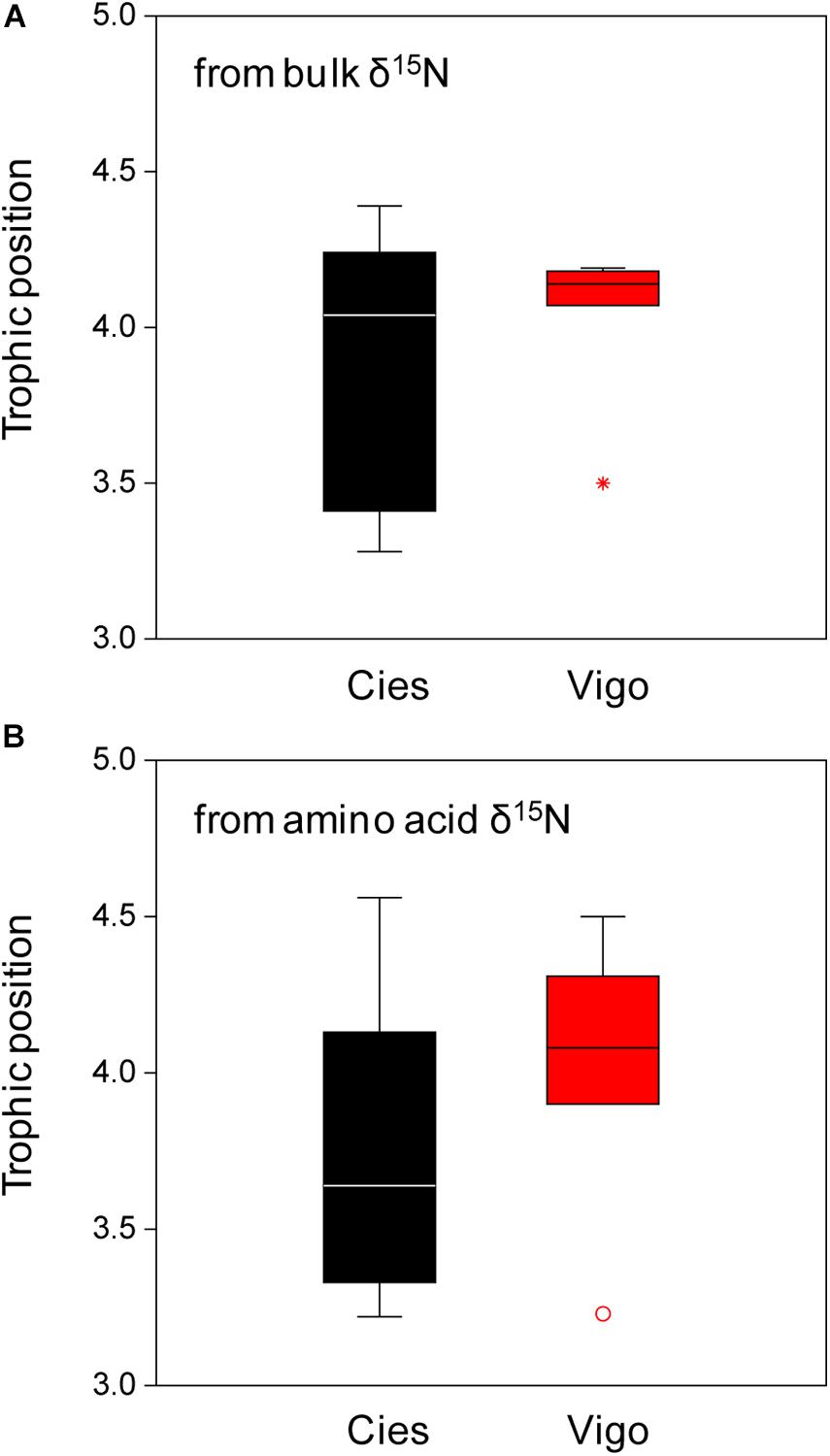
Figure 3. Box plot of trophic position of Yellow-legged Gull estimated from (A) bulk δ15N or (B) amino acid δ15N for samples from Cíes Islands (black) and Vigo (red). Boxes and symbols as in Figure 1.
Discussion
The results of this study represent the first estimation of Yellow-legged Gulls TP obtained with amino acid δ15N. These estimations are comparable to those obtained using bulk δ15N in this and previous studies in the same and related gull species (Table 1), and in other marine birds (Gatt et al., 2020). Furthermore, the significant correlation between δ15N of trophic amino acids and bulk δ15N (and the lack of correlation for δ15N in source amino acids) supports previous assumptions using bulk δ15N as an index of TP (Abdennadher et al., 2010; Muñoz-Arnanz et al., 2012; Pedro et al., 2013; Calado et al., 2018). However, the determination of δ15N in individual amino acids provided additional information on the variability of feeding among individuals that complements TP estimations. For instance, the similarity in TP between the studied colonies supports that the differences in pollution loads reported in Viñas et al. (2020) were caused by changes in diet unrelated to TP, even when detailed diet composition was not available for the individuals studied. Similar conclusions were reached in other studies where, diet and TP could be determined (Muñoz-Arnanz et al., 2012; Ramos et al., 2013).
The low δ15N in lysine found in eggs from the Cíes Island colony suggest the consumption of non-marine prey. First, because the mean difference between δ15N of Lys and Phe for the Cíes eggs was significantly lower than values typical of phytoplankton (Nielsen et al., 2015). Second, because the estimated contributions of anthropogenic sources to the diet in Cíes were generally higher than those in Vigo (Supplementary Figure 2). This is an unexpected result, as the gulls from the islands would have, in principle, more access to marine prey than those nesting in the city. Lysine is an essential amino acid in the diet of animals and is one of the major additives to feed stocks, notably for poultry and swine raising (Toride, 2002). It is produced industrially through fermentation with the addition of sugars and inorganic nitrogen (Ikeda, 2017). Industrial lysine is likely to have low δ15N, since ammonium derived from atmospheric nitrogen represents the most economic source for fertilizers (Smil, 2001). For instance, the values of Lys δ15N measured in beef, pork and chicken meat in this study were lower than those measured in fish. These results agree with those reported for bulk δ15N from chick gull regurgitates in the Bay of Biscay (Arizaga et al., 2013). Therefore, a substantial consumption of rearing animals remains by gulls from the Cíes Island colony cannot be discarded, as noted by previous studies (Moreno et al., 2010). Given the large variability reported in the diet of the Yellow-legged Gull, it would be not surprising that the Cíes Islands colony partly relied on garbage, at least during the time frame considered in this study. This is supported by the year-to-year reduction in the consumption of fish by this species along the NW Spanish coast and attributed to the decline in sardine populations (Calado et al., 2020). Furthermore, the reported mean composition of the gull diet in this region indicated the dominance of anthropogenic over marine food items during 2010, an exceptional contribution compared to the series for the whole recording period (Supplementary Table 3). In this regard, it must be noted that the isotopic composition of eggs is considered representative of both recent (ca. 1 month) and past (ca. 1 year) diet of the breeding adult females (Hebert et al., 2016). The high consumption of marine resources in Vigo agrees with the general pattern reported for the region and could be favoured by the proximity of the fishing harbour, one of the largest in Europe with 84,000 metric tons of fresh marine products landed in 2011 (Port Authority of Vigo, 2012). Fish offal would be a favoured resource for gulls nesting in Vigo, as reported for similar species elsewhere (Hebert et al., 1999; González-Solís, 2003; Calado et al., 2018).
Differences in diet, thus, were the likely cause of the high levels of pollutants in eggs from the Vigo colony (Viñas et al., 2020). Marine fishes of high TP were reported to bioaccumulate organic and inorganic pollutants. For instance, Hg compounds in tuna (e.g., Méndez et al., 2001) can reach concentrations equivalent to those found in the gull eggs from Vigo (Viñas et al., 2020). These results imply that biomagnification does not only depend on TP but also on the diet of the consumer species. Therefore, future assessments of pollution risks in food webs would require coupled studies of diet and TP. Gull diet can be reconstructed from observations of feeding remains or stomach contents, but also from instrumental techniques as the analysis of marker fatty acids (Hebert et al., 2016). The latter can be very sensitive when coupled to stable isotope determinations (Twining et al., 2020). The application of these techniques will help to determine the appropriate biomagnification factors when the monitored species, as the Yellow-legged Gull, feeds on different habitats or food webs.
Conclusion
Our study shows that reliable TP estimations for the yellow-legged gull can be obtained using δ15N either in bulk homogenised eggs or in individual source and trophic amino acids. However, the latter technique would be preferred because data or assumptions about the baseline reference values from other species are not required, and because it can provide valuable information on the variability in diet among individuals. Indeed, diet, rather than TP, appears as the dominant factor affecting the bioaccumulation of pollutants in the Yellow-legged Gull.
Data Availability Statement
The original contributions presented in the study are included in the article/Supplementary Material, further inquiries can be directed to the corresponding author.
Ethics Statement
Ethical review and approval was not required for the animal study because gull eggs for this study were collected as part of the pollution monitoring program of the IEO and the Ministerio para la Transición Ecológica (Spain).
Author Contributions
LV and VB designed the project. AB designed the research reported here, analysed the data, and wrote the manuscript with comments from coauthors. LV, BP-F, and AB analysed the samples. All authors contributed to the article and approved the submitted version.
Funding
This study was funded in part by a Fund Management Agreement between the IEO and the Ministerio para la Transición Ecológica (Spain) and by grant number IN607A2018/2 of the Axencia Galega de Innovación (GAIN, Xunta de Galicia, Spain).
Conflict of Interest
The authors declare that the research was conducted in the absence of any commercial or financial relationships that could be construed as a potential conflict of interest.
Acknowledgments
We acknowledge the National Park of the Atlantic Islands (Spain) for facilitating the sampling, and Maria Lema (Servicio de Análisis Instrumental, Universidad de A Coruña, Spain) for the isotopic determinations.
Supplementary Material
The Supplementary Material for this article can be found online at: https://www.frontiersin.org/articles/10.3389/fmars.2021.657131/full#supplementary-material
References
Abdennadher, A., Ramirez, F., Romdhane, M. S., Ruiz, X., Jover, L., and Sanpera, C. (2010). Biomonitoring of coastal areas in Tunisia: Stable isotope and trace element analysis in the yellow-legged gull. Mar. Pollut. Bull. 60, 440–447. doi: 10.1016/j.marpolbul.2009.10.003
Arizaga, J., Jover, L., Aldalur, A., Cuadrado, J. F., Herrero, A., and Sanpera, C. (2013). Trophic ecology of a resident yellow-legged gull (Larus michahellis) population in the Bay of Biscay. Mar. Environ. Res. 87-88, 19–25. doi: 10.1016/j.marenvres.2013.02.016
Bode, A., Carrera, P., González-Nuevo, G., Nogueira, E., Riveiro, I., and Santos, M. B. (2018). A trophic index for sardine (Sardina pilchardus) and its relationship to population abundance in the southern Bay of Biscay and adjacent waters of the NE Atlantic. Prog. Oceanogr. 166, 139–147. doi: 10.1016/j.pocean.2017.08.005
Calado, J. G., Matos, D. M., Ramos, J. A., Moniz, F., Ceia, F. R., Granadeiro, J. P., et al. (2018). Seasonal and annual differences in the foraging ecology of two gull species breeding in sympatry and their use of fishery discards. J. Avian Biol. 49, UNSe01463. doi: 10.1111/jav.01463
Calado, J. G., Paiva, V. H., Ramos, J. A., Velando, A., and Munilla, I. (2020). Anthropogenic food resources, sardine decline and environmental conditions have triggered a dietary shift of an opportunistic seabird over the last 30 years on the northwest coast of Spain. Reg. Environ. Change 20, 10. doi: 10.1007/s10113-10020-01609-10116
Caut, S., Angulo, E., and Courchamp, F. (2009). Variation in discrimination factors (Δ15N and Δ13C): the effect of diet isotopic values and applications for diet reconstruction. J. Appl. Ecol. 46, 443–453. doi: 10.1111/j.1365-2664.2009.01620.x
Chikaraishi, Y., Ogawa, N. O., Kashiyama, Y., Takano, Y., Suga, H., Tomitani, A., et al. (2009). Determination of aquatic food-web structure based on compound-specific nitrogen isotopic composition of amino acids. Limnol. Oceanogr. Methods 7, 740–750. doi: 10.4319/lom.2009.7.740
Dietz, R., Riget, F., Cleemann, M., Aarkrog, A., Johansen, P., and Hansen, J. C. (2000). Comparison of contaminants from different trophic levels and ecosystems. Sci. Total Environ. 245, 221–231. doi: 10.1016/S0048-9697(99)00447-7
Elliott, K. H., and Elliott, J. E. (2016). Lipid extraction techniques for stable isotope analysis of bird eggs: Chloroform–methanol leads to more enriched 13C values than extraction via petroleum ether. J. Exp. Mar. Biol. Ecol. 474, 54–57. doi: 10.1016/j.jembe.2015.09.017
Forero, M. G., Bortolotti, G. R., Hobson, K. A., Donazar, J. A., Bertelloti, M., and Blanco, G. (2004). High trophic overlap within the seabird community of Argentinean Patagonia: a multiscale approach. J. Anim. Ecol. 73, 789–801. doi: 10.1111/j.0021-8790.2004.00852.x
Gatt, M. C., Reis, B., Granadeiro, J. P., Pereira, E., and Catry, P. (2020). Generalist seabirds as biomonitors of ocean mercury: The importance of accurate trophic position assignment. Sci. Total Environ. 740, 140159. doi: 10.1016/j.scitotenv.2020.140159
González-Solís, J. (2003). Impact of fisheries on activity, diet and predatory interactions between Yellow-legged and Audouin’s gulls breeding at the Chafarinas Islands. Sci. Mar. 67(Suppl 2), 83–88. doi: 10.3989/scimar.2003.67s283
Hammer, Ø, Harper, D. A. T., and Ryan, P. D. (2001). PAST: Paleontological statistics software package for education and data analysis. Palaeontologia Electronica 4, 9.
Hebert, C. E., Popp, B. N., Fernie, K. J., Ka’apu-Lyons, C., Rattner, B. A., and Wallsgrove, N. (2016). Amino acid specific stable nitrogen isotope values in avian tissues: insights from captive american kestrels and wild herring gulls. Environ. Sci. Technol. 50, 12928–12937. doi: 10.1021/acs.est.6b04407
Hebert, C. E., Shutt, J. L., Hobson, K. A., and Weseloh, D. V. C. (1999). Spatial and temporal differences in the diet of Great Lakes Herring Gulls (Larus argentatus): evidence from stable isotope analysis. Can. J. Fish. Aquat. Sci. 56, 323–338. doi: 10.1139/f98-189
Hussey, N. E., MacNell, M. A., McMeans, B. C., Ollin, J. A., Dudley, S. F. J., Cliff, G., et al. (2014). Rescaling the trophic structure of marine food webs. Ecol. Lett. 17, 239–250. doi: 10.1111/ele.12226
Ikeda, M. (2017). Lysine Fermentation: History and Genome Breeding. Adv. Biochem. Eng. Biotechnol. 159, 73–102. doi: 10.1007/10_2016_27
Jenkins, E. J., and Davoren, G. K. (2020). Seabird species- and assemblage-level isotopic niche shifts associated with changing prey availability during breeding in coastal Newfoundland. Ibis 163, 183–196. doi: 10.1111/ibi.12873
Jennings, S., and van der Molen, J. (2015). Trophic levels of marine consumers from nitrogen stable isotope analysis: estimation and uncertainty. ICES J. Mar. Sci. 72, 2289–2300. doi: 10.1093/icesjms/fsv120
Kelly, B. C., Ikonomou, M. G., Blair, J. D., and Gobas, F. A. P. C. (2008). Bioaccumulation behaviour of polybrominated diphenyl ethers (PBDEs) in a Canadian Arctic marine food web. Sci. Total Environ. 401, 60–72. doi: 10.1016/j.scitotenv.2008.03.045
Loick, N., Fernández-Urruzola, I., Eglite, E., Liskow, I., Nausch, M., Schulz-Bull, D., et al. (2019). Stratification, nitrogen fixation, and cyanobacterial bloom stage regulate the planktonic food web structure. Glob. Change Biol. 25, 794–810. doi: 10.1111/gcb.14546
Lopezosa, P., Forero, M. G., Ramirez, F., and Navarro, J. (2019). Individuals within populations: No evidences of individual specialization in the trophic habits of an opportunistic predator. Estuar. Coast Shelf Sci. 229, 106427. doi: 10.1016/j.ecss.2019.106427
Matias, R., and Catry, P. (2010). The diet of Atlantic Yellow-legged Gulls (Larus michahellis atlantis) at an oceanic seabird colony: estimating predatory impact upon breeding petrels. Eur. J. Wildl. Res. 56, 861–869. doi: 10.1007/s10344-010-0384-y
McCarthy, M. D., Lehman, J., and Kudela, R. (2013). Compound-specific amino acid δ15N patterns in marine algae: Tracer potential for cyanobacterial vs. eukaryotic organic nitrogen sources in the ocean. Geochim. Cosmochim. Acta 103, 104–120. doi: 10.1016/j.gca.2012.10.037
McClelland, J. W., and Montoya, J. P. (2002). Trophic relationships and the nitrogen isotopic composition of amino acids in plankton. Ecology 83, 2173–2180.
McMahon, K. W., and McCarthy, M. D. (2016). Embracing variability in amino acid δ15N fractionation: mechanisms, implications, and applications for trophic ecology. Ecosphere 7, e01511.
McMahon, K. W., Polito, M. J., Abel, S., McCarthy, M. D., and Thorrold, S. R. (2015). Carbon and nitrogen isotope fractionation of amino acids in an avian marine predator, the gentoo penguin (Pygoscelis papua). Ecol. Evol. 5, 1278–1290. doi: 10.1002/ece3.1437
Méndez, A., Montalvo, T., Aymi, R., Carmona, M., Figuerola, J., and Navarro, J. (2020). Adapting to urban ecosystems: unravelling the foraging ecology of an opportunistic predator living in cities. Urban Ecosyst. 23, 1117–1126. doi: 10.1007/s11252-020-00995-3
Méndez, E., Giudice, H., Pereira, A., Inocente, G., and Medina, D. (2001). Total mercury content:fish weight relationship in swordfish (Xiphias gladius) caught in the southwest Atlantic Ocean. J. Food Compos. Anal. 14, 453–460. doi: 10.1006/jfca.2001.1005
Mompeán, C., Bode, A., Gier, E., and McCarthy, M. D. (2016). Bulk vs. aminoacid stable N isotope estimations of metabolic status and contributions of nitrogen fixation to size-fractionated zooplankton biomass in the subtropical N Atlantic. Deep Sea Res. 114, 137–148. doi: 10.1016/j.dsr.2016.05.005
Moreno, R., Jover, L., Munilla, I., Velando, A., and Sanpera, C. (2010). A three-isotope approach to disentangling the diet of a generalist consumer: the Yellow-legged Gull in northwest Spain. Mar. Biol. 157, 545–553. doi: 10.1007/s00227-009-1340-9
Muñoz-Arnanz, J., Roscales, J. L., Vicente, A., Aguirre, J. I., and Jiménez, B. (2012). Dechlorane Plus in eggs of two gull species (Larus michahellis and Larus audouinii) from the southwestern Mediterranean Sea. Anal. Bioanal. Chem. 404, 2765–2773. doi: 10.1007/s00216-012-6326-7
Nielsen, J. M., Popp, B. N., and Winder, M. (2015). Meta-analysis of amino acid stable nitrogen isotope ratios for estimating trophic position in marine organisms. Oecologia 178, 631–642. doi: 10.1007/s00442-015-3305-7
Ohkouchi, N., Chikaraishi, Y., Close, H. G., Fry, B., Larsen, T., Madigan, D. J., et al. (2017). Advances in the application of amino acid nitrogen isotopic analysis in ecological and biogeochemical studies. Org. Geochem. 113, 150–174. doi: 10.1016/j.orggeochem.2017.07.009
OSPAR. (1999). CEMP guidelines for monitoring contaminants in biota. Agreement 1999-02. London: OSPAR Commission. Coordinated Environmental Monitoring Programme (CEMP).
Otero, X. L., De La Pena-Lastra, S., Romero, D., Nobrega, G. N., Ferreira, T. O., and Perez-Alberti, A. (2018). Trace elements in biomaterials and soils from a Yellow-legged Gull (Larus michahellis) colony in the Atlantic Islands of Galicia National Park (NW Spain). Mar. Pollut. Bull. 133, 144–149. doi: 10.1016/j.marpolbul.2018.05.027
Pedro, P., Ramos, J., Neves, V., and Paiva, V. (2013). Past and present trophic position and decadal changes in diet of Yellow-legged Gull in the Azores Archipelago, NE Atlantic. Eur. J. Wild. Res. 59, 833–845. doi: 10.1007/s10344-013-0737-4
Port Authority of Vigo. (2012). Annual report 2011. Vigo: Autoridad Portuaria de Vigo. Puertos del Estado. Ministerio de Fomento.
Post, D. M. (2002). Using stable isotopes to estimate trophic position: models, methods, and assumptions. Ecology 83, 703–718.
Ramos, R., Ramirez, F., and Jover, L. (2013). Trophodynamics of inorganic pollutants in a wide-range feeder: The relevance of dietary inputs and biomagnification in the Yellow-legged Gull (Larus michahellis). Environ. Pollut. 172, 235–242. doi: 10.1016/j.envpol.2012.09.014
Reum, J. C. P., Jennings, S., and Hunsicker, M. E. (2015). Implications of scaled δ15N fractionation for community predator-prey body mass ratio estimates in size-structured food webs. J. Anim. Ecol. 84, 1618–1627. doi: 10.1111/1365-2656.12405
Ruiz-Cooley, R. I., Gerrodette, T., Fiedler, P. C., Chivers, S. J., Danil, K., and Ballance, L. T. (2017). Temporal variation in pelagic food chain length in response to environmental change. Sci. Adv. 3, e1701140. doi: 10.1126/sciadv.1701140
Smil, V. (2001). Enriching the earth. Fritz Haber, Carl Bosch, and the transformation of world food production. Cambridge, MA: The MIT Press.
Sørmo, E. G., Lie, E., Ruus, A., Gaustad, H., Skaare, J. U., and Jenssen, B. M. (2011). Trophic level determines levels of brominated flame-retardants in coastal herring gulls. Ecotoxicol. Environ. Safety 74, 2091–2098. doi: 10.1016/j.ecoenv.2011.06.012
Stock, B. C., and Semmens, B. X. (2013). MixSIAR GUI User Manual.Version 3.1. Available online at: https://github.com/brianstock/MixSIAR accessed date∗.
Storr-Hansen, E., Spliid, H., and Boon, J. P. (1995). Patterns of chlorinated biphenyl congeners in harbor seals (Phoca vitulina) and in their food: Statistical analysis. Arch. Environ. Contam. Toxicol. 28, 48–54. doi: 10.1007/BF00213968
Toride, Y. (2002). “Lysine and other amino acids for feed: production and contribution to protein utilization in animal feeding,” in Protein sources for the animal feed industry, ed. FAO (Roma: FAO), 161–168.
Twining, C. W., Taipale, S. J., Ruess, L., Bec, A., Martin-Creuzburg, D., and Kainz, M. J. (2020). Stable isotopes of fatty acids: current and future perspectives for advancing trophic ecology. Philos. Trans. R. Soc. B 375, 1804. doi: 10.1098/rstb.2019.0641
Vander Zanden, M. J., and Fetzer, W. (2007). Global patterns of aquatic food chain length. Oikos 116, 1378–1388. doi: 10.1111/j.2007.0030-1299.16036.x
Viñas, L., Besada, V., Pérez-Fernández, B., and Bode, A. (2020). Yellow-legged gull eggs (Larus michahellis) as persistent organic pollutants and trace metal bioindicator for two nearby areas with different human impact. Environ. Res. 190, 110026. doi: 10.1016/j.envres.2020.110026
Whiteman, J. P., Elliott Smith, E. A., Besser, A. C., and Newsome, S. D. (2019). A guide to using compound-specific stable isotope analysis to study the fates of molecules in organisms and ecosystems. Diversity 11, 8. doi: 10.3390/d11010008
Wu, L., Liu, X., Xu, L., Li, L., and Fu, P. (2018). Compound-specific 15N analysis of amino acids: A tool to estimate the trophic position of tropical seabirds in the South China Sea. Ecol. Evol. 8, 8853–8864. doi: 10.1002/ece3.4282
Keywords: bioindicator, stable isotopes, amino acids, seagull eggs, food web
Citation: Bode A, Besada V, Pérez-Fernández B and Viñas L (2021) Amino Acid δ15N Can Detect Diet Effects on Pollution Risks for Yellow-Legged Gulls Overlooked by Trophic Position. Front. Mar. Sci. 8:657131. doi: 10.3389/fmars.2021.657131
Received: 22 January 2021; Accepted: 19 April 2021;
Published: 14 May 2021.
Edited by:
Kyung-Hoon Shin, Hanyang University, South KoreaCopyright © 2021 Bode, Besada, Pérez-Fernández and Viñas. This is an open-access article distributed under the terms of the Creative Commons Attribution License (CC BY). The use, distribution or reproduction in other forums is permitted, provided the original author(s) and the copyright owner(s) are credited and that the original publication in this journal is cited, in accordance with accepted academic practice. No use, distribution or reproduction is permitted which does not comply with these terms.
*Correspondence: Antonio Bode, YW50b25pby5ib2RlQGllby5lcw==