- 1College of Marine and Environmental Sciences, Tianjin University of Science and Technology, Tianjin, China
- 2Tianjin Key Laboratory of Marine Resources and Chemistry, Tianjin University of Science and Technology, Tianjin, China
- 3Department of Chemistry, City University of Hong Kong, Hong Kong SAR, China
- 4State Key Laboratory in Marine Pollution, City University of Hong Kong, Hong Kong SAR, China
- 5College of Marine Life Science, Ocean University of China, Qingdao, China
Stable isotope tracer addition can enhance the isotopic differences of primary producers for a wider food-web resolution than the use of natural abundance stable isotopes (NASIs) alone, particularly in ecosystems where primary producers have similar NASI values. To investigate the food sources and the trophic status of benthic infauna in an intertidal area near a Halophila minor seagrass bed within inner Tai Tam Bay, Hong Kong, China, a 15N addition experiment was conducted, and the results were compared with those from NASI data. Only benthic microalgae (BMA) were labeled by applying 15N-enriched NH4Cl to the sediment daily for the first 7 days during a 21-day study. In contrast to the NASI results, Bayesian mixing models based on the isotope tracer experiment suggested a larger dietary contribution of BMA for nematode Daptonema sp. and copepods, whereas a higher reliance on phytoplankton and seagrass detritus was noted for polychaete Neanthes sp. However, both NASI and isotope tracer addition demonstrated that seagrass detritus was a major food source for nematode Spilophorella sp. The present isotope tracer experiment also revealed a contrasting result of the relatively lower contribution of meiofauna in the diets of carnivores/omnivores as compared to the results of NASIs. This finding suggested that the isotope values in these consumers may have not reached an equilibrium with the added isotope in the study period. Thus, there is a need for applying NASI coupled with isotope tracer addition in the investigation of ecosystems in which primary producers have similar isotope values, especially in ecosystems with lower tissue turnover rates, in order for a more accurate determination of dietary contribution and trophic status of consumers in the food-web study.
Introduction
Intertidal seagrass habitat offers a variety of primary producers that support secondary production. Apart from seagrass, benthic microalgae (BMA) and phytoplankton transported by incoming tides may also serve as food sources for a wide range of consumers (Lebreton et al., 2012; Ouisse et al., 2012). Being a primary consumer and serving as prey for larger animals such as fish, shellfish, and infauna (Gee, 1989), meiofauna acts as a key intermediate consumer linking the basal primary food sources to higher trophic levels within an ecosystem. Meiofauna also exhibits a range of feeding modes such as microvorous, deposit feeding, and epigrowth feeding (Wieser, 1953; Moens and Vincx, 1997), which allow them to exploit a variety of living or detrital primary producers from local sediment or imported by tides (Buffan-Dubau and Carman, 2000; Lebreton et al., 2012; Cnudde et al., 2015). As the food sources of seagrass ecosystems typically show large variations of quality and quantity depending on locations and seasons (Lebreton et al., 2009; Ha et al., 2014), such natural variability coupled with the small size of meiofauna has made it difficult to identify the food sources supporting the secondary production in seagrass ecosystems. However, the determination of food sources and trophic status of benthic infauna is important to understand the food-web linkage and ecology in seagrass habitat (Lebreton et al., 2012).
The use of multiple natural abundance stable isotopes (NASIs) has become an important tool in investigating the food sources and trophic interactions in marine benthic food webs (Herman et al., 2000; Vafeiadou et al., 2014; Braeckman et al., 2015; Qu et al., 2019; Yang et al., 2020). Based on such stable isotope data, new Bayesian mixing models (e.g., MixSIR, SIAR, and IsotopeR) have also been developed to overcome the limitation of the simple linear mixing models (e.g., IsoError, IsoConc, and IsoSource), allowing to determine the contributions of more than n + 1 food sources (n = number of elemental tracers) (Moore and Semmens, 2008; Jackson et al., 2009; Parnell et al., 2010; Solomon et al., 2011; Hopkins and Ferguson, 2012). These models can also incorporate uncertainty and variation in input parameters, such as changes in the consumers, food sources, and trophic discrimination factors, which often occur in natural ecosystems, and thus provide a more accurate assessment of consumer diets (Moore and Semmens, 2008; Swan et al., 2020). However, the application of NASI is most useful in ecosystems in which primary producers have distinct isotope values from their consumers (Moncreiff and Sullivan, 2001). The utility of NASI to identify dietary sources may be reduced within systems where primary producers have similar isotope values. One way of solving this problem is to use NASI coupled with isotope tracer addition (Carman and Fry, 2002; Levin et al., 2006; Mäkelä et al., 2017; Braeckman et al., 2018; Yorke et al., 2019). This is based on the rationale that isotope tracer addition can enhance the differences in the isotope values of the primary producers by creating a unique isotope label, and the label then enters the food web through consumption of the labeled primary producers. Hence, in combination with NASIs, isotope tracer addition allows for a better resolution of food source contribution and determination of the trophic status of consumers in marine food webs where primary producers have similar isotope values (Galván et al., 2011).
In this study, a 15N addition experiment was conducted in combination with the analysis of NASI data to reveal the contribution of primary producers to the diets of marine infauna and the meio-macrofaunal trophic linkage in a subtropical intertidal area adjacent to a Halophila minor seagrass bed. The NASI data have been published, showing that benthic microalgae (BMA) were a dominant food source for most consumers, except for nematode Spilophorella sp., which mainly relied on seagrass detritus (Xu et al., 2018). The isotope mixing model results based on the NASI data also indicated a higher contribution of nematodes and copepods to the diet of carnivorous polychaete Eunice sp. and a relatively lower proportion of meiofauna in the diets of omnivorous polychaete Neanthes sp. and carnivorous/omnivorous nematode Ditlevsenella sp. However, the similar NASI values of BMA and particulate organic matter (POM) in the study area may preclude an accurate identification of the diet sources from the isotope mixing model. Our aims of this study were to examine the importance of primary producers (i.e., from local sources and that transported by the incoming tide) to infauna and the dietary contribution in key predatory infauna, using the mixing model based on stable isotope tracer data, in an intertidal area near a seagrass bed. The results were then compared with the same model output from NASIs. As the study area is exposed at low tide and only has intermittent access to food resources imported by tides, we hypothesized that most infauna will have enriched isotope tracer values that more closely reflect the use of isotope-labeled BMA similar to the results from NASIs.
Materials and Methods
Experiment Setup
The investigation was carried out in an intertidal area (22°14′32.6″ N, 114°13′31.8′ E) within inner Tai Tam Bay, a semi-enclosed embayment on the southern side of Hong Kong Island, Hong Kong, China, during the summertime (Figure 1). Hong Kong experiences a subtropical climate with an average summer temperature of around 28°C, and the study area is pristine without much human disturbance, with mean dissolved oxygen of about 9.67 mg/L, pH 8.0, and salinity 32 (Chiu et al., 1994; Chan and Morton, 2001; Cheung et al., 2003; Liu et al., 2011). Inner Tai Tam Bay has been designated as a Site of Special Scientific Interest by the Hong Kong government, as the area covers a diversity of intertidal habitats with various plant and animal communities (Che and Morton, 1991). The investigation site chosen for this study was an intertidal area adjacent to an H. minor seagrass bed.
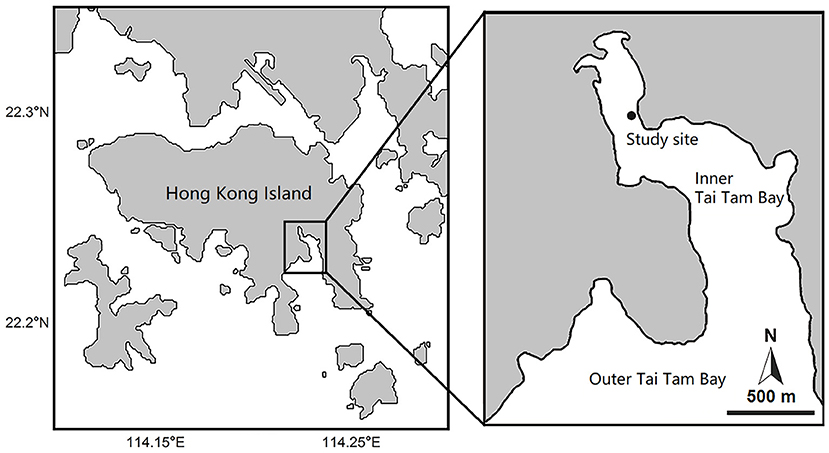
Figure 1. The study site at inner Tai Tam Bay on the southern coast of Hong Kong Island, Hong Kong SAR, China.
The field investigation was conducted during the summer (August), in which potential food sources including BMA, POM, and seagrass detritus of H. minor, as well as consumers including dominant meio- and macrofauna, were collected during the pre- and post-isotope tracer addition at the study site. Isotope tracer addition was carried out in three 1 × 1 m experimental plots in the first 7 days during a 21-day experiment. Each plot was separated by a linear distance of 10 m and was marked at the corners with PVC poles. Within each plot, the sediment was directly sprayed with 250 ml of 15NH4Cl solution (18.5 mg 15NH4Cl m−2, Carman and Fry, 2002) daily at low tide during the 7-day isotope tracer addition. 15NH4Cl (Sigma-Aldrich, St. Louis, USA) was dissolved in Whatman GF/F filtered seawater collected on site and applied in the field using a common garden sprayer. The tracer was unlikely to be absorbed by phytoplankton and seagrass detritus because of the dilution of the isotope addition by the tide, and there was no take-up of isotope label by the dead detritus as shown by Galván et al. (2008).
Samples of potential food sources and consumers were collected adjacent to the experimental plots prior to the daily isotope tracer spray, so as to determine the food sources of infauna by NASIs. Results from the NASIs in this experiment have been published (Xu et al., 2018). To determine the changes of 15N isotope values for primary producers and benthic infauna over the 7-day 15N tracer addition, triplicate samples were collected from each sprayed plot on Days 3 and 7. Samples were also collected on Days 14 and 21, 1 and 2 weeks after the tracer addition was stopped. To obtain a sufficient amount, BMA and infaunal samples collected from the three plots were pooled together for stable isotope analysis.
Benthic microalgae were collected following the method of Wainright et al. (2000). The sediment from an area of 20 × 20 cm in each experimental plot was first covered by a thin layer of pre-combusted silica sand, and then, a 63 μm nylon mesh was placed above the silica sand. Another thin layer of silica sand was applied to the mesh and moistened with seawater filtered by pre-combusted Whatman GF/F filters (0.7 μm porosity). The mesh together with the upper silica sand was retrieved after 2 h during which the microalgae would migrate onto the mesh. After returning to the laboratory, BMA and silica sand were rinsed from the mesh by distilled water and swirled in a beaker, and the suspended material (without sand) was filtered onto a pre-combusted Whatman GF/F filter with 0.7 μm retention. Filter papers were acidified in HCl vapor for 24 h to remove carbonates and dried at 60°C to a constant weight prior to stable isotope analysis. POM was collected as an indication of phytoplankton by filtering near-bottom seawater in situ on pre-combusted Whatman GF/F filter (0.7 μm porosity). Water samples were collected at high tide (± 1 h) by hand pumping near-bottom water into nine 2 L Nalgene bottles near the experimental area where isotope tracer addition was conducted. Samples were sieved by a 63 μm mesh to remove large zooplankton, and the residues were then examined microscopically. The portion <63 μm was dominated by phytoplankton with few zooplankton. Hence, POM could be used as a proxy for phytoplankton in our analysis. POM samples on filters were rinsed with distilled water to remove salt on the particle surface, then treated with HCl vapor for 24 h to remove carbonates, and dried at 60°C to a constant weight. Triplicate leaf samples of dead H. minor were manually collected, cleaned with distilled water, and then oven-dried and powdered using a grinder. The leaf samples were not acidified as suggested by Bunn et al. (1995) to avoid the effect on δ15N values.
For the collection of infauna, one large (20 × 20 cm) and one small (15 × 15 cm) area of sediment in each experimental plot was sampled randomly using a hand-held trowel at each sampling time. Macrofaunal samples were collected from sediment down to 20 cm depth in the large areas, while meiofaunal samples were obtained within the upper 8 cm sediment from the small areas. In the laboratory, sediment samples for macrofaunal analysis were sieved through a 0.5 mm screen. Polychaetes were handpicked from residues on the screen and identified to genus level. Adhered materials on the polychaete body were stripped off carefully using a needle and a pair of forceps, then rinsed in filtered seawater, and checked using a dissecting microscope to make sure all adhering materials were removed. The whole body of polychaetes was used for stable isotope analysis. At least five individuals were pooled, oven-dried at 60°C, and homogenized through grinding. Then, 1 mg of homogenized materials was transferred to a pre-combusted tin capsule for stable isotope analysis. Sediments for meiofaunal samples were processed according to Heip et al. (1985) by centrifugation with colloidal Ludox solution diluted to gravity of 1.15 with distilled water, in which the process would not significantly change the 13C and 15N isotope values of meiofauna (e.g., nematodes) as demonstrated by Moens et al. (2002). The supernatant containing meiofauna was then rinsed through a 38 μm screen with distilled water. The residues on the screen were washed onto a Petri dish, then, nematodes and copepods were handpicked and rinsed to remove adhering particles, and transferred to a drop of distilled water in pre-combusted tin capsules. Sorting of nematodes was conducted under a stereomicroscope only for those dominant and easily recognizable specimens that could be identified to genus level. Other small-sized nematodes that could not be identified to genus level were pooled together as bulk nematode samples. To obtain enough biomass for NASI analysis, for large-sized nematodes such as Ditlevsenella sp., at least 100 individuals were pooled to represent one replicate. For average-sized nematodes such as Daptonema sp. and Spilophorella sp., at least 1,000 individuals were pooled as one replicate. For harpacticoid copepods, at least 1,000 individuals were also pooled as one replicate. While for isotope-enriched samples, the number of individuals of the same meiofaunal species/group required per sample was half of that used for NASIs. Dried biomass of the same genus of polychaetes and nematodes and all the harpacticoid copepod individuals were pooled together to obtain adequate sample mass for stable isotope analysis and to homogenize spatial variability in the study area.
Stable Isotope Analysis
All the samples including NASIs and stable isotope tracer were analyzed in the Stable Isotope Facility, University of California, Davis, USA. Isotope values were reported in the standard δ-unit notation:
where X is 13C or 15N, and R is 13C:12C or 15N:14N, respectively. These values were reported relative to standards, the Vienna-PeeDee Belemnite (V-PDB), and air N2.
Data Analysis
The possible contributions of potential food sources to the consumers (nematodes, copepods, and polychaetes) from the NASI data before isotope tracer addition (published in Xu et al., 2018) and from the 15N addition experiment were determined using the Bayesian mixing model developed in the software IsotopeR 0.4 package (Hopkins and Ferguson, 2012) under the R 3.10 software (R Core Development Team, 2014). Trophic enrichment factors used for computations were based on the published values from various sources reported in Vander Zanden and Rasmussen (2001). For herbivores, the values adopted were −0.4‰ ± 1.1 (Mean ± SD) for δ13C and 2.5‰ ± 2.5 for δ15N. For carnivores/omnivores, the values used were 0.9‰ ± 1.0 for δ13C and 3.2‰ ± 0.4 for δ15N. The proportional contribution of food sources in the isotope tracer addition experiment was calculated following the method by Galván et al. (2008), in which δE (highest δ15N observed during the 21-day experiment minus natural abundance δ15N before isotope tracer addition) of BMA and benthic consumers were used instead of natural abundance δ15N in the Bayesian mixing model. The highest observed values were used because the tissue turnover rates of the consumers are assumed to be rapid and that all the fauna can reach an isotope steady-state equilibrium with the new labeled food sources. The interspecific differences in 15N addition during the 21-day experiment may be resulted from different tissue turnover times or different labeled and unlabeled food source contributions in their diets. Consumers with smaller body sizes are considered to reach their tissue equilibrium more quickly than larger ones and may assimilate more 15N-enriched isotopes over a short period of time. The duration of the present experiment was based on information from a previous stable isotope study with a tissue turnover time of about 3 days for meiofauna (Carman and Fry, 2002). For larger-sized consumers, such as polychaetes, the tissue turnover times were assumed in the range of 6–9 days, based on the findings by Doi et al. (2007) for stable isotopes in deposit-feeding chironomids with a body mass similar to polychaetes studied in the present experiment. Moreover, it tends to take a longer time for carnivores to reach tissue equilibrium with isotope addition than herbivores, due to their higher trophic levels. To determine the food source contribution of diets in the isotope tracer addition experiment, mean natural abundance δ13C values and highest δ15N values were used from the results of the 21-day study.
Results
Natural abundance stable isotope values of primary producers and consumers prior to the isotope tracer addition experiment are listed in Table 1, as extracted from Xu et al. (2018). Briefly, the NASI values of the food sources ranged from −23.6‰ (POM) to −10.0‰ (seagrass detritus) for δ13C and ranged from 5.5‰ (POM) to 6.4‰ (BMA) for δ15N before the isotope tracer addition commenced. BMA and POM had similar NASI values, while seagrass detritus showed more 13C enriched than other food sources. The δ13C signatures of the consumers ranged from −20.9‰ (copepods) to −9.8‰ (nematode Spilophorella sp.). The δ15N signatures of the consumers spanned 6.5‰ and their δ15N values were at least 3‰ higher than their food sources. The carnivorous polychaetes Eunice sp. showed an increase in δ15N compared with other consumers, which reflected a higher contribution of nematodes and copepods to their diets. However, the omnivorous polychaete Neanthes sp. and carnivorous/omnivorous nematode Ditlevsenella sp. showed relatively lower δ15N than polychaete Eunice sp., indicating a lower dependence of animals in their diets.
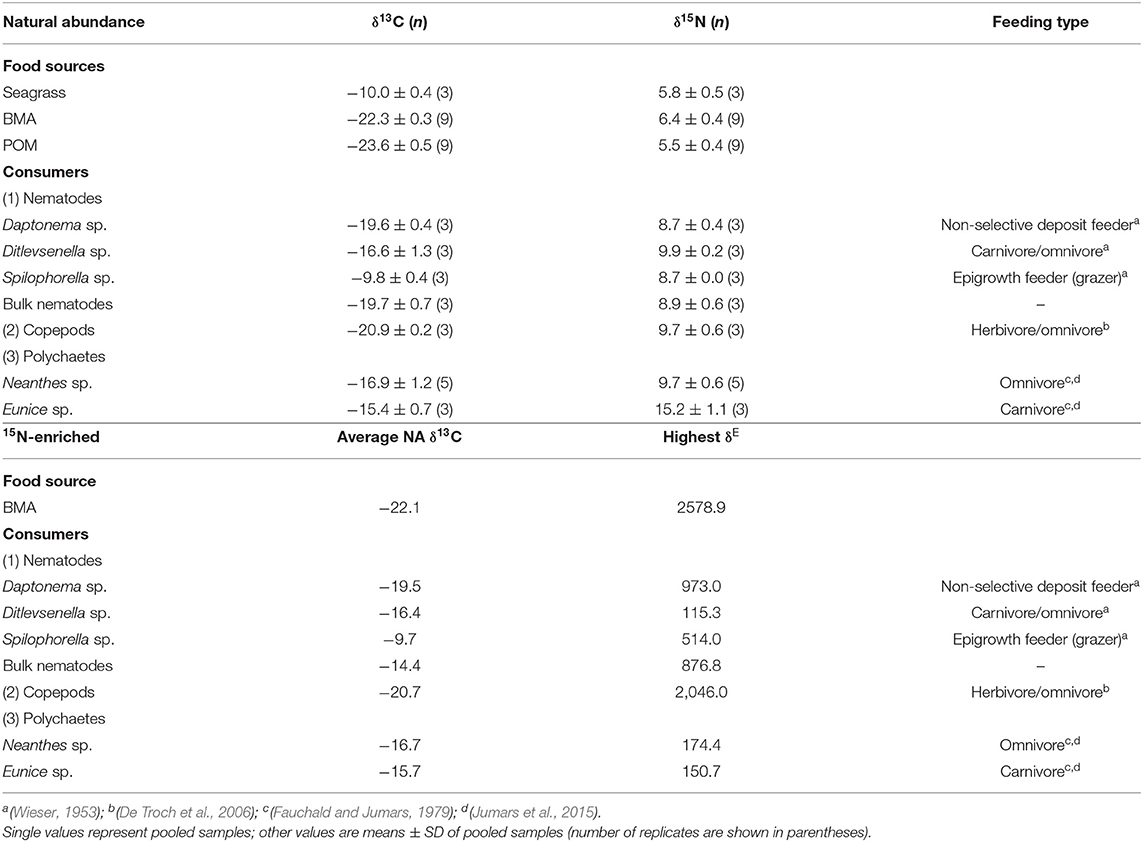
Table 1. Natural abundance stable isotope δ13C and δ15N values of primary producers and infauna (data from Xu et al., 2018), and δE (highest observed enrichment values minus natural abundance isotope values) over the 21-day experiment and average natural abundance (NA) δ13C values from the 21-day experiment.
For the results of the 15N addition experiment (Table 2), the 15N-enriched label was taken up by the BMA quickly, as the enrichment above NASI values was observed on Day 3, the earliest sampling time after the start of the tracer addition. The δ15N isotope values of BMA increased over the 7-day addition period, with a peak enrichment value of 2,578.9‰ higher than the NASI levels (Tables 1, 2). All the nematodes, copepods, and polychaetes investigated became 15N-enriched beyond the NASI values during the 21-day study (Table 2). Copepods were highly enriched and quickly reached a value of 2,046.0‰ on Day 7, the last day of isotope tracer addition, and the values decreased to 972.3 and 330.9‰, respectively, on 7 and 14 days after the addition of isotope tracer was stopped. Nematodes Daptonema sp. and Spilophorella sp. were enriched to 973.0 and 514.0‰ on Day 14 and Day 7, respectively. Polychaetes Eunice sp. and Neanthes sp. showed an increase in their enriched isotope values progressively and reached the highest values of 150.7 and 174.4‰, respectively, on Days 21 and 14, 2 weeks and 1 week after 15N addition was completed.
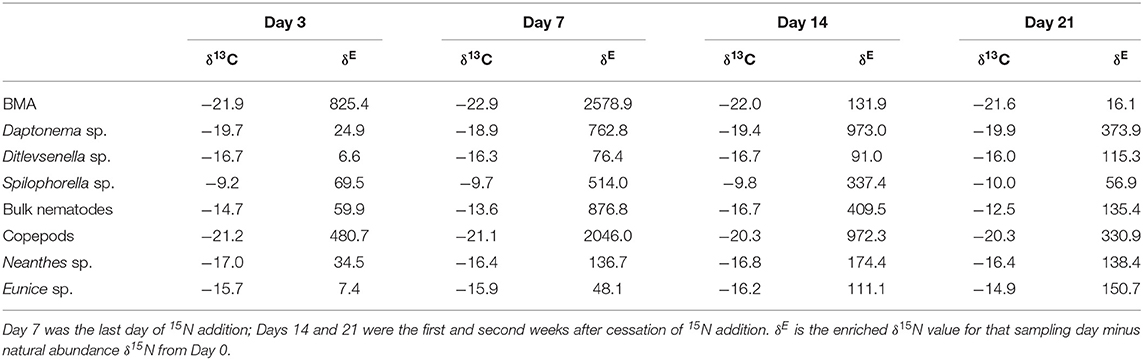
Table 2. 15N enrichment and natural abundance δ13C in primary producers and infauna over the 21-day isotope tracer study.
Results of the Bayesian mixing model from the isotope tracer addition data in Table 3 suggested a larger contribution of BMA in the diets of nematode Daptonema sp. and copepods than the model output from NASI data alone. For nematode Spilophorella sp., both model results from NASIs and isotope tracer addition data supported a dominant diet of seagrass detritus. However, for polychaete Neanthes sp., model results from the isotope tracer addition data did not reveal the importance of BMA in their diet, which contradicted the model output from the NASI values. Although data from isotope tracer addition indicated the importance of both POM (40.8%) and seagrass detritus (44.3%) to the diet of Neanthes sp., results of the mixing model based on NASI data revealed that POM contributed little to its diet. For polychaete Eunice sp., the model output from NASI data showed that nematodes and copepods supported more than 94% of its diet; however, model results from the isotope tracer addition study indicated that meiofaunal prey only constituted <38% of the Eunice diet in the study area.
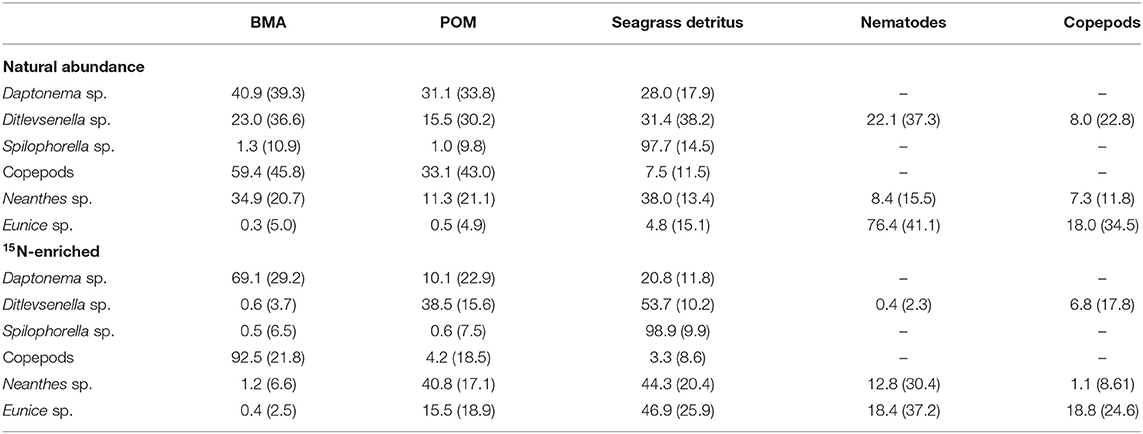
Table 3. Mixing model results from natural abundance stable isotopes (data from Xu et al., 2018) and isotope tracer addition data for mean percentage (SD) of food sources in the diet of animals (– = food source was not included).
Discussion
Food Sources for Benthic Consumers
As compared to NASI data, the addition of 15N tracer to the surface sediment and subsequent uptake by BMA appeared to widen the isotope signatures of primary producers (Figure 2), which could enhance the resolution of food sources and thus improve our understanding of the contribution of basal food resources to the benthic food web. This can be illustrated by the results of the isotope tracer addition that BMA was in fact an important diet for some infauna only. Hence, such isotope tracer addition experiments can allow for a wider food-web resolution than the use of NASIs alone, particularly in ecosystems where NASI values of primary producers are similar (Galván et al., 2008).
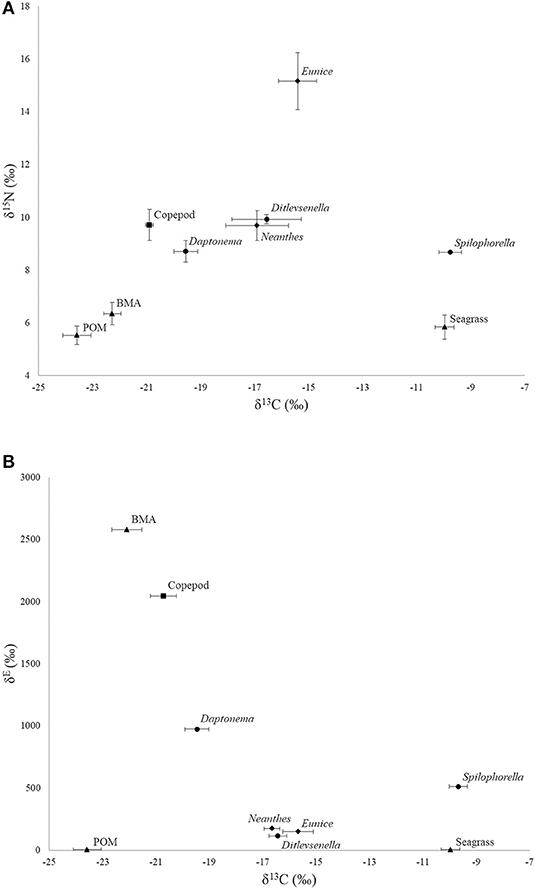
Figure 2. (A) Natural abundance stable isotope bi-plot (δ15N and δ13C; prior to isotope addition, data from Xu et al., 2018) and (B) 15N-addition (δE) stable isotope values of primary producers and consumers during the 21-day experiment plotted against average δ13C (±SD) values over the 21-day study. Nematodes are shown in circles, copepods in squares, polychaetes in diamonds, and primary producers in triangles.
A high 15N label uptake found in nematode Daptonema sp. and copepods confirmed the importance of BMA and indicated a higher contribution of BMA in their diets than based on NASI alone. The present findings agreed with many studies showing that grazing on diatoms is a common feeding strategy of harpacticoid copepods and nematode Daptonema sp. in shallow sediments (Moens and Vincx, 1997; Moens et al., 2005; De Troch et al., 2006; Cnudde et al., 2015; van der Heijden et al., 2019). The 15N label uptake by nematode Spilophorella sp. was found much lower compared with its counterpart nematode Daptonema sp. and copepods, suggesting a much lesser contribution of BMA and the importance of other isotope-unlabeled food sources in its diet. Mixing model outputs from both NASI and isotope tracer addition data also supported the finding that seagrass detritus contributed the most in the food source of nematode Spilophorella sp., with more than 97% in its dietary contribution (Table 3). However, with a heavily cuticularized mouthpart structure, nematode Ditlevsenella sp. is regarded as carnivorous/omnivorous in food-web ecology (Wieser, 1953). This can be reflected in the results of the present isotope tracer addition, as the 15N value of Ditlevsenella sp. only reached a maximum of 115.3‰ during the 21-day study (Table 2), suggesting that this species may prey on organisms that consumed isotope-labeled BMA.
Polychaete Neanthes sp. is classified as an omnivore (Fauchald and Jumars, 1979; Jumars et al., 2015), with the ability to utilize a variety of primary producers and to prey on small animals. The present isotope tracer addition experiment indicated that the contribution of 15N-labeled BMA to the diet of Neanthes sp. was minimal (circa 1%), implying a greater reliance on isotope-unlabeled food sources, such as POM and seagrass detritus in this study (Table 3). The model output from isotope tracer addition data also revealed a certain amount of nematodes and copepods in the diet of polychaete Neanthes sp. The progressive increase of the 15N-enriched values in the present isotope tracer addition experiment could be a result of its feeding on the 15N-enriched prey. Neanthes sp. is an opportunistic feeder. Its jawed, eversible proboscis is used primarily to ingest sediment deposits, but it is also capable of grazing some plant material and facultatively capturing small invertebrates (Craig et al., 2003). In agreement with our findings, phytoplankton was identified as the major contribution to the diet of Neanthes japonica in an estuarine environment (Kikuchi and Wada, 1996), while the same species also consumed decomposed macrophytes much faster than fresh materials (Tsuchiya and Kurihara, 1979). As reported in the literature, meiofauna, small crustaceans, and polychaetes are also found consumed by various Neanthes species [Kay and Brafield, 1973; Cammen, 1980; Pardo and Dauer, 2003; National Introduced Marine Pest Information System (NIMPIS), 2006]. The polychaete Eunice sp. is classified as a carnivore, which has a pair of mandibles and a complex set of maxillae with a strong muscular, eversible pharynx (Fauchald and Jumars, 1979; Jumars et al., 2015). The relatively high natural abundance δ15N in Eunice sp. also suggested a predatory feeding mode (Figure 2). During the isotope tracer addition experiment, Eunice sp. reached a maximum nitrogen value of 150.7‰ (Table 2), suggesting that it fed on 15N-enriched prey in the experimental plots.
Seagrass as a Food Source
Epigrowth and deposit-feeding infauna are widely distributed on shallow intertidal mudflats, regardless of whether they were proximate to macrophytes (Da Rocha et al., 2006; Galván et al., 2008). Epigrowth feeders, however, are characterized by the presence of a buccal armature, supposedly used to either scrape off particles from a substrate or to damage and open food items before emptying them (Moens and Vincx, 1997). Most published isotope studies showed that they mainly feed on BMA and phytoplankton (van Oevelen et al., 2006; Kanaya et al., 2008; Lebreton et al., 2011; Christianen et al., 2017). However, some studies have indicated macrophyte detritus in the diets of meiofauna as well as some polychaetes (Lebreton et al., 2012; Vafeiadou et al., 2013; Ha et al., 2014; Rossi et al., 2015). Most of these previous studies revealed limited assimilation of macrophyte detritus by the benthos. However, this study showed a very high contribution of seagrass detritus in the diet of nematode Spilophorella sp. from the model results of both NASI and isotope tracer addition data. The present observations, which are also indicated by other studies (Carman and Fry, 2002; Galván et al., 2008; Vafeiadou et al., 2014), suggested that infaunal organisms have flexible dietary needs and feeding strategies that may change spatially and temporally as well as vary among species, making a generalization of the food-web position of infauna difficult. Thus, the feeding ecology of benthic infauna should be interpreted with caution and cannot be relied on buccal morphology alone.
The refractory nature of macrophytes may make them difficult to serve as a food source for the infauna (Campanyà-Llovet et al., 2017). However, microbial activities are shown to be able to enhance nutritional quality (Holmer et al., 2004; Guy, 2010). Microbial decomposition of macrophyte detritus has been widely reported, and there are also studies illustrating the consumption of such bacteria by infauna through microbial grazing (Danovaro, 1996; Vafeiadou et al., 2013). Majdi et al. (2012) found that grazing on BMA only covered a small part of the energetic requirements for Chromadoridae nematode Chromadorina sp., suggesting that this species probably feeds on other biofilm food sources. Nevertheless, the isotope values of these bacteria which can decompose macrophyte detritus and are subsequently consumed by bacteria-feeding infauna should reflect the isotope values of the macrophyte detritus originally present in the sediment. Our mixing model results from both NASIs and isotope tracer addition also revealed that nematode Spilophorella sp., belonging to the same family Chromadoridae, consumed a large part of seagrass (>97%) in their diet. Hence, it is possible that Spilophorella may also graze on a large amount of bacteria associated with the decomposition of seagrass in the study area.
Comparison of Isotope Tracer Addition and Natural Abundance Stable Isotopes
For nematode Daptonema sp. and copepods, the model output from the NASI values overestimated the proportion of POM but underestimated the proportion of BMA in its diets comparing with the model results from isotope tracer addition data (Table 3). However, for polychaete Neanthes sp., only the addition of a 15N-enriched tracer elucidated the dietary importance of phytoplankton and seagrass detritus. These findings highlight the difficulty for the mixing model to output accurate assessments when food sources have similar isotope values and may lead to a false conclusion in the dietary composition if using NASIs alone. However, the isotope tracer addition method may also provide confusing information if it is not applied carefully. For nematode Ditlevsenella sp. and polychaete Eunice sp., the present isotope tracer data indicated a contrasting result of the relatively lower contribution of meiofauna in its diets as compared to the model results from NASIs (Table 3). An overestimation of POM and seagrass by the present isotope tracer addition experiment might be caused by the error that an equilibrium of isotopic signatures has not been reached in these consumers during the 21-day study period. There are also possibilities that the labeled BMA was not utilized by some consumers, and/or BMA produced before the application of 15N tracer addition contributed to the diet of some consumers. In the present experiment, overestimations of POM were found in the omnivorous/carnivorous species (nematode Ditlevsenella sp. and polychaete Eunice sp.), with relatively larger body size and lower tissue turnover rate. There is most likely a time lag in the isotope enrichment of their body tissues because they have not reached tissue equilibrium with 15N over a short-term (7-day) provision of the isotope-labeled food sources.
Overall, there are complementary strengths and weaknesses for the NASI and isotope tracer addition approaches. The isotope tracer addition is capable of distinguishing the importance of the isotope-labeled source in ecosystems where primary producers have similar isotope values. However, isotope tracer does not readily infiltrate in systems with lower tissue turnover rates, especially in short-term experiments. Hence, in this regard, the use of NASIs can provide a better solution to identify food sources and trophic pathways in such slow-turnover ecosystems (Fry, 2006). The application of NASIs also helps distinguish trophic levels in food-web ecology. A joint strategy of using NASIs coupled with isotope tracer addition is thus recommended in the investigation of ecosystems where primary producers have similar isotope values, especially in ecosystems in which lower tissue turnover rates are apparent.
Conclusion
This study demonstrated that the application of 15N-enriched NH4Cl to the surface sediment created distinct isotope values of primary producers, which increased the resolution for the determination of the dietary contribution of the consumers. However, it should be noted that isotope addition may also generate confusing information if the results are not interpreted carefully. One problem is the time lag of enrichment of stable isotope tracer, as shown in the findings of carnivorous/omnivorous infauna in this study, as tissue equilibrium with the isotope tracer addition may not have been reached over a short period of time. As such, a combination of NASIs coupled with isotope tracer addition would be a better approach to investigate dietary contributions and trophic status of consumers in ecosystems where primary producers have similar isotope values, especially in ecosystems with lower tissue turnover rates.
Data Availability Statement
The original contributions presented in the study are included in the article/supplementary material, further inquiries can be directed to the corresponding author/s.
Author Contributions
PS designed the experiment and revised and edited the manuscript. WX conducted the experiment with the help of AT and wrote the manuscript. YD, SC, ZZ, and JS gave constructive comments and revised the manuscript. All the authors approved the final manuscript.
Funding
This work was supported by the National Key Research and Development Project of China (No. 2019YFC1407803), the Natural Science Foundation of China (No. 41706184), and the General Research Fund (No. CityU 161009) from the Research Grants Council of the HKSAR Government.
Conflict of Interest
The authors declare that the research was conducted in the absence of any commercial or financial relationships that could be construed as a potential conflict of interest.
Publisher's Note
All claims expressed in this article are solely those of the authors and do not necessarily represent those of their affiliated organizations, or those of the publisher, the editors and the reviewers. Any product that may be evaluated in this article, or claim that may be made by its manufacturer, is not guaranteed or endorsed by the publisher.
Acknowledgments
We thank Prof. Xiaoshou Liu for his advice on meiofaunal sampling and Prof. Youji Wang for his technical assistance in the field and laboratory.
References
Braeckman, U., Janssen, F., Lavik, G., Elvert, M., Marchant, H., Buckner, C., et al. (2018). Carbon and nitrogen turnover in the Arctic deep sea: in situ benthic community response to diatom and coccolithophorid phytodetritus. Biogeosciences 15, 6537–6557. doi: 10.5194/bg-15-6537-2018
Braeckman, U., Provoost, P., Sabbe, K., Soetaert, K., Middelburg, J. J., Vincx, M., et al. (2015). Temporal dynamics in a shallow coastal benthic food web: insights from fatty acid biomarkers and their stable isotopes. Mar. Environ. Res. 108, 55–68. doi: 10.1016/j.marenvres.2015.04.010
Buffan-Dubau, E., and Carman, K. R. (2000). Diel feeding behavior of meiofauna and their relationships with microalgal resources. Limnol. Oceanogr. 45, 381–395. doi: 10.4319/lo.2000.45.2.0381
Bunn, S. E., Loneragan, N. R., and Kempster, M. A. (1995). Effects of acid washing on stable isotope ratios of C and N in penaeid shrimp and seagrass: implications for food-web studies using multiple stable isotopes. Limnol. Oceanogr. 40, 622–625. doi: 10.4319/lo.1995.40.3.0622
Cammen, L. M. (1980). The significance of microbial carbon in the nutrition of the deposit feeding polychaete Nereis succinea. Mar. Biol. 61, 9–20. doi: 10.1007/BF00410337
Campanyà-Llovet, N., Snelgrove, P. V. R., and Parrish, C. C. (2017). Rethinking the importance of food quality in marine benthic food webs. Prog. Oceanogr. 156, 240–251. doi: 10.1016/j.pocean.2017.07.006
Carman, K. R., and Fry, B. (2002). Small-sample methods for δ13C and δ15N analysis of the diets of marsh meiofaunal species using natural-abundance and tracer-addition isotope techniques. Mar. Ecol. Prog. Ser. 240, 85–92. doi: 10.3354/meps240085
Chan, K., and Morton, B. (2001). The distribution of Nassarius festivus in Hong Kong with a description and hydrographic analysis of three study sites at Starfish Bay, Tai Tam Bay and Tai Mong Tsai. Asian Mar. Biol. 18, 143–162.
Che, R. G. O., and Morton, B. (1991). Spatial and temporal variations in the subtidal microbenthic community of Tai Tam Bay, Hong Kong. Asian Mar. Biol. 8, 193–216.
Cheung, K. C., Wong, M. H., and Yung, Y. K. (2003). Toxicity assessment of sediments containing tributyltin around Hong Kong harbour. Toxicol. Lett. 137, 121–131. doi: 10.1016/s0378-4274(02)00386-7
Chiu, H. M. C., Hodgkiss, I. J., and Chan, B. S. S. (1994). Ecological studies of phytoplankton in Tai Tam Bay, Hong Kong. Hydrobiologia 273, 81–94. doi: 10.1007/bf00006850
Christianen, M. J. A., Middelburg, J. J., Holthuijsen, S. J., Jouta, J., Compton, T. J., van der Heide, T., et al. (2017). Benthic primary producers are key to sustain the Wadden Sea food web: stable carbon isotope analysis at landscape scale. Ecology 98, 1498–1512. doi: 10.1002/ecy.1837
Cnudde, C., Moens, T., Werbrouck, E., Lepoint, G., Van Gansbeke, D., and De Troch, M. (2015). Trophodynamics of estuarine intertidal harpacticoid copepods based on stable isotope composition and fatty acid profiles. Mar. Ecol. Prog. Ser. 524, 225–239. doi: 10.3354/meps11161
Craig, S. F., Thoney, D. A., Schlager, N., and Hutchins, M. (eds.), (2003). Grzimek's Animal Life Encyclopedia: vol. 2, Protostomes. Florence, KY: Cengage Gale.
Da Rocha, C. M. C., Venekey, V., Bezerra, T. N. C., and Souza, J. R. B. (2006). Phytal marine nematode assemblages and their relation with the macrophytes structural complexity in a Brazilian Tropical Rocky Beach. Hydrobiologia 553, 219–230. doi: 10.1007/s10750-005-0923-9
Danovaro, R. (1996). Detritus-Bacteria-Meiofauna interactions in a seagrass bed (Posidonia oceanica) of the NW Mediterranean. Mar. Biol. 127, 1–13. doi: 10.1007/BF00993638
De Troch, M., Chepurnov, V., Gheerardyn, H., Vanreusel, A., and Ólafsson, E. (2006). Is diatom size selection by harpacticoid copepods related to grazer body size? J. Exp. Mar. Biol. Ecol. 332, 1–11. doi: 10.1016/j.jembe.2005.10.017
Doi, H., Kikuchi, E., Takagi, S., and Shikano, S. (2007). Changes in carbon and nitrogen stable isotopes of chironomid larvae during growth, starvation and metamorphosis. Rapid Commun. Mass Spectrom. 21, 997–1002. doi: 10.1002/rcm.2925
Fauchald, K., and Jumars, P. A. (1979). The diet of worms: a study of polychaete feeding guilds. Oceanogr. Mar. Biol. Annu. Rev. 17, 193–284.
Galván, K., Fleeger, J. W., and Fry, B. (2008). Stable isotope addition reveals dietary importance of phytoplankton and microphytobenthos to saltmarsh infauna. Mar. Ecol. Prog. Ser. 359, 37–49. doi: 10.3354/meps07321
Galván, K., Fleeger, J. W., Peterson, B., Drake, D., Deegan, L. A., and Johnson, D. S. (2011). Natural abundance stable isotopes and dual isotope tracer additions help to resolve resources supporting a saltmarsh food web. J. Exp. Mar. Biol. Ecol. 410, 1–11. doi: 10.1016/j.jembe.2011.08.007
Gee, J. M. (1989). An ecological and economic review of meiofauna as food for fish. Zool. J. Linn. Soc. 96, 243–261. doi: 10.1111/j.1096-3642.1989.tb02259.x
Guy, H. (2010). The microbial role in carbon cycling within seagrass sediments. Plymouth Student Sci. 3, 234–244. Available online at: http://hdl.handle.net/10026.1/13905
Ha, S. Y., Min, W.-K., Kim, D.-S., and Shin, K.-H. (2014). Trophic importance of meiofauna to polychaetes in a seagrass (Zostera marina) bed as traced by stable isotopes. J. Mar. Biol. Assoc. U.K. 94, 121–127. doi: 10.1017/S0025315413001148
Heip, C., Vincx, M., and Vranken, G. (1985). The ecology of marine nematodes. Oceanogr. Mar. Bio. Ann. Rev. 23, 313–328.
Herman, P. M. J., Middelburg, J. J., Widdows, J., Lucas, C. H., and Heip, C. H. R. (2000). Stable isotopes as trophic tracers: combining field sampling and manipulative labelling of food resources for macrobenthos. Mar. Ecol. Prog. Ser. 204, 79–92. doi: 10.3354/Meps204079
Holmer, M., Duarte, C. M., Boschker, H. T. S., and Barrón, C. (2004). Carbon cycling and bacterial carbon sources in pristine and impacted Mediterranean seagrass sediments. Aquat. Microb. Ecol. 36, 227–237. doi: 10.3354/ame036227
Hopkins, I. I. I. J. B., and Ferguson, J. M. (2012). Estimating the diets of animals using stable isotopes and a comprehensive Bayesian mixing model. PloS ONE 7:e28478. doi: 10.1371/journal.pone.0028478
Jackson, A. L., Inger, R., Bearhop, S., and Parnell, A. (2009). Erroneous behaviour of MixSIR, a recently published Bayesian isotope mixing model: a discussion of Moore and Semmens (2008). Ecol. Lett. 12, E1–E5. doi: 10.1111/j.1461-0248.2008.01233.x
Jumars, P. A., Dorgan, K. M., and Lindsay, S. M. (2015). Diet of worms emended: an update of polychaete feeding guilds. Annu. Rev. Mar. Sci. 7, 497–520. doi: 10.1146/annurev-marine-010814-020007
Kanaya, G., Takagi, S., and Kikuchi, E. (2008). Dietary contribution of the microphytobenthos to infaunal deposit feeders in an estuarine mudflat in Japan. Mar. Biol. 155, 543–553. doi: 10.1007/s00227-008-1053-5
Kay, D. G., and Brafield, A. E. (1973). The Energy Relations of the Polychaete Neanthes (= Nereis) virens (Sars). J. Anim. Ecol. 42, 673–692.
Kikuchi, E., and Wada, E. (1996). Carbon and nitrogen stable isotope ratios of deposit-feeding polychaetes in the Nanakita River Estuary, Japan. Hydrobiologia 321, 69–75. doi: 10.1007/BF00018678
Lebreton, B., Richard, P., Galois, R., Radenac, G., Brahmia, A., Colli, G., et al. (2012). Food sources used by sediment meiofauna in an intertidal Zostera noltii seagrass bed: a seasonal stable isotope study. Mar. Biol. 159, 1537–1550. doi: 10.1007/s00227-012-1940-7
Lebreton, B., Richard, P., Galois, R., Radenac, G., Pfléger, C., Guillou, G., et al. (2011). Trophic importance of diatoms in an intertidal Zostera noltii seagrass bed: Evidence from stable isotope and fatty acid analyses. Estuar. Coast. Shelf Sci. 92, 140–153. doi: 10.1016/j.ecss.2010.12.027
Lebreton, B., Richard, P., Radenac, G., Bordes, M., Bréret, M., Arnaud, C., et al. (2009). Are epiphytes a significant component of intertidal Zostera noltii beds? Aquat. Bot. 91, 82–90. doi: 10.1016/j.aquabot.2009.03.003
Levin, L. A., Neira, C., and Grosholz, E. D. (2006). Invasive cordgrass modifies wetland trophic function. Ecology 87, 419–432. doi: 10.2307/20068956
Liu, X., Cheung, S. G., and Shin, P. K. S. (2011). Response of meiofaunal and nematode communities to sewage pollution abatement: a field transplantation experiment. Chin. J. Oceanol. Limnol. 29, 1174–1185. doi: 10.1007/s00343-011-0144-1
Majdi, N., Tackx, M., Traunspurger, W., and Buffan-Dubau, E. (2012). Feeding of biofilm-dwelling nematodes examined using HPLC-analysis of gut pigment contents. Hydrobiologia 680, 219–232. doi: 10.1007/s10750-011-0920-0
Mäkelä, A., Witte, U., and Archambault, P. (2017). Ice algae versus phytoplankton: resource utilization by Arctic deep sea macroinfauna revealed through isotope labelling experiments. Mar. Ecol. Prog. Ser. 572, 1–18. doi: 10.3354/meps12157
Moens, T., Bouillon, S., and Gallucci, F. (2005). Dual stable isotope abundances unravel trophic position of estuarine nematodes. J. Mar. Biol. Assoc. U.K. 85, 1401–1407. doi: 10.1017/S0025315405012580
Moens, T., Luyten, C., Middelburg, J. J., Herman, P. M. J., and Vincx, M. (2002). Tracing organic matter sources of estuarine tidal flat nematodes with stable carbon isotopes. Mar. Ecol. Prog. Ser. 234, 127–137. doi: 10.3354/meps234127
Moens, T., and Vincx, M. (1997). Observations on the feeding ecology of estuarine nematodes. J. Mar. Biol. Assoc. U.K. 77, 211–227. doi: 10.1017/s0025315400033889
Moncreiff, C. A., and Sullivan, M. J. (2001). Trophic importance of epiphytic algae in subtropical seagrass beds: evidence from multiple stable isotope analyses. Mar. Ecol. Prog. Ser. 215, 93–106. doi: 10.3354/meps215093
Moore, J. W., and Semmens, B. X. (2008). Incorporating uncertainty and prior information into stable isotope mixing models. Ecol. Lett. 11, 470–480. doi: 10.1111/j.1461-0248.2008.01163.x
National Introduced Marine Pest Information System (NIMPIS) (2006). Alitta Succina Information Page. NIMPIS
Ouisse, V., Riera, P., Migne, A., Leroux, C., and Davoult, D. (2012). Food web analysis in intertidal Zostera marina and Zostera noltii communities in winter and summer. Mar. Biol. 159, 165–175. doi: 10.1007/s00227-011-1796-2
Pardo, E. V., and Dauer, D. M. (2003). Particle size selection in individuals from epifaunal versus infaunal populations of the nereidid polychaete Neanthes succinea (Polychaeta: Nereididae). Hydrobiologia 496, 355–360. doi: 10.1023/A:1026181823273
Parnell, A. C., Inger, R., Bearhop, S., and Jackson, A. L. (2010). Source partitioning using stable isotopes: coping with too much variation. PLoS ONE 5:e9672. doi: 10.1371/journal.pone.0009672
Qu, P., Zhang, Z., Pang, M., Li, Z., Zhao, L., Zhou, X., et al. (2019). Stable isotope analysis of food sources sustaining the subtidal food web of the Yellow River Estuary. Ecol. Indic. 101, 303–312. doi: 10.1016/j.ecolind.2019.01.021
R Core Development Team. (2014). R: A Language and Environment for Statistical Computing. Vienna: R Foundation for Statistical Computing. Available online at: http://www.R-project.org/
Rossi, F., Baeta, A., and Marques, J. C. (2015). Stable isotopes reveal habitat-related diet shifts in facultative deposit-feeders. J. Sea Res. 95, 172–179. doi: 10.1016/j.seares.2014.07.004
Solomon, C. T., Carpenter, S. R., Clayton, M. K., Cole, J. J., Coloso, J. J., Pace, M. L., et al. (2011). Terrestrial, benthic, and pelagic resource use in lakes: results from a three-isotope Bayesian mixing model. Ecology 92, 1115–1125. doi: 10.1890/10-1185.1
Swan, G. J. F., Bearhop, S., Redpath, S. M., Silk, M. J., Goodwin, C. E. D., Inger, R., et al. (2020). Evaluating Bayesian stable isotope mixing models of wild animal diet and the effects of trophic discrimination factors and informative priors. Methods Ecol. Evol. 11, 139–149. doi: 10.1111/2041-210X.13311
Tsuchiya, M., and Kurihara, Y. (1979). The feeding habits and food sources of the deposit-feeding polychaete, Neanthes japonica (Izuka). J. Exp. Mar. Biol. Ecol. 36, 79–89. doi: 10.1016/0022-0981(79)90101-1
Vafeiadou, A. M., Materatski, P., Adão, H., De Troch, M., and Moens, T. (2013). Food sources of macrobenthos in an estuarine seagrass habitat (Zostera noltii) as revealed by dual stable isotope signatures. Mar. Biol. 160, 2517–2523. doi: 10.1007/s00227-013-2238-0
Vafeiadou, A. M., Materatski, P., Adão, H., De Troch, M., and Moens, T. (2014). Resource utilization and trophic position of nematodes and harpacticoid copepods in and adjacent to Zostera noltii beds. Biogeosciences 11, 4001–4014. doi: 10.5194/bg-11-4001-2014
van der Heijden, L. H., Graeve, M., Asmus, R., Rzeznik-Orignac, J., Niquil, N., Bernier, Q., et al. (2019). Trophic importance of microphytobenthos and bacteria to meiofauna in soft-bottom intertidal habitats: a combined trophic marker approach. Mar. Environ. Res. 149, 50–66. doi: 10.1016/j.marenvres.2019.05.014
van Oevelen, D., Soetaert, K., Middelburg, J. J., Herman, P. M. J., Moodley, L., Hamels, I., et al. (2006). Carbon flows through a benthic food web: Integrating biomass, isotope and tracer data. J. Mar. Res. 64, 453–482. doi: 10.1357/002224006778189581
Vander Zanden, M. J., and Rasmussen, J. B. (2001). Variation in δ15N and δ13C trophic fractionation: implications for aquatic food web studies. Limnol. Oceanogr. 46, 2061–2066. doi: 10.4319/lo.2001.46.8.2061
Wainright, S. C., Weinstein, M. P., Able, K. W., and Currin, C. A. (2000). Relative importance of benthic microalgae, phytoplankton and the detritus of smooth cordgrass Spartina alterniflora and the common reed Phragmites australis to brackish-marsh food webs. Mar. Ecol. Prog. Ser. 200, 77–91. doi: 10.3354/meps200077
Wieser, W. (1953). Die Beziehungen zwischen Mundhöhlengestalt, Ernährungsweise und Vorkommen bei freilebenden marinen Nematoden. Eine ökologisch-morphologische Studie. Ark. Zool. 4, 439–484.
Xu, W. Z., Cheung, S. G., Zhang, Z.-N., and Shin, P. K. S. (2018). Dual isotope assessment of trophic dynamics of an intertidal infaunal community with seasonal shifts in food sources. Mar. Biol. 165:221. doi: 10.1007/s00227-017-3278-7
Yang, Z., Chen, J., Wang, C., Zhang, D., Lu, B., Wang, K., et al. (2020). Food sources of benthic communities at the Caiwei Guyot and Yap Trench, Northwestern Pacific Ocean: inferences from carbon and nitrogen isotopes. J. Geophys. Res. Biogeosci. 125:e2019JG005432. doi: 10.1029/2019jg005432
Keywords: stable isotope, trophic study, meiofauna, nematodes, polychaetes, isotope tracer addition, infauna
Citation: Xu W, Dang Y, Cheung SG, Zhang Z, Sun J, Teng A and Shin PKS (2021) Stable Isotope Tracer Addition Reveals the Trophic Status of Benthic Infauna at an Intertidal Area Adjacent to a Seagrass Bed. Front. Mar. Sci. 8:657044. doi: 10.3389/fmars.2021.657044
Received: 22 January 2021; Accepted: 15 September 2021;
Published: 18 October 2021.
Edited by:
Punyasloke Bhadury, Indian Institute of Science Education and Research Kolkata, IndiaReviewed by:
Prasanta Sanyal, Indian Institute of Science Education and Research Kolkata, IndiaMenghong Hu, Shanghai Ocean University, China
Copyright © 2021 Xu, Dang, Cheung, Zhang, Sun, Teng and Shin. This is an open-access article distributed under the terms of the Creative Commons Attribution License (CC BY). The use, distribution or reproduction in other forums is permitted, provided the original author(s) and the copyright owner(s) are credited and that the original publication in this journal is cited, in accordance with accepted academic practice. No use, distribution or reproduction is permitted which does not comply with these terms.
*Correspondence: Wenzhe Xu, wenzhexu@126.com; Paul K. S. Shin, paulksshin@gmail.com