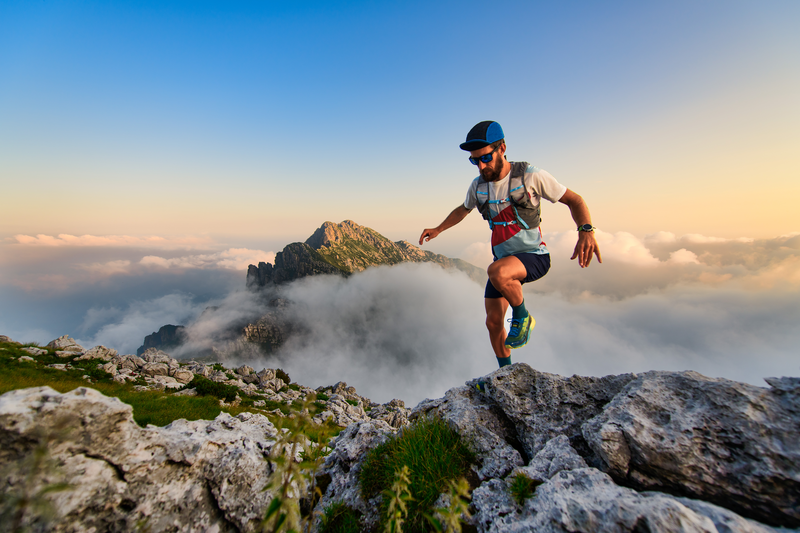
95% of researchers rate our articles as excellent or good
Learn more about the work of our research integrity team to safeguard the quality of each article we publish.
Find out more
ORIGINAL RESEARCH article
Front. Mar. Sci. , 24 May 2021
Sec. Marine Ecosystem Ecology
Volume 8 - 2021 | https://doi.org/10.3389/fmars.2021.656772
Until recently, polar night constituted truly a “mare incognitum” of our times. Yet, the first records from this very little-explored period showcased a surprisingly rich and active ecosystem. This investigation aims to reveal the level of scavenger activity during both Arctic polar night and day. It compares the shallow-water scavenging fauna observed during two contrasting seasons (winter vs. summer) in a high Arctic fjord (Kongsfjorden, 79° N, Spitsbergen, Svalbard Archipelago). In each of January and July 2015, two different bait types – Atlantic cod (Gadus morhua) and a bird carcass (chicken meat) were deployed at a depth of 12 m. Fauna were monitored remotely using time-lapse cameras equipped with bait traps, with photographs taken every 15 min over a period of 4 days. Thirty taxa were recorded at baits, dominated by lysianassid amphipods (Onisimus sp. 88%, Anonyx sp. 2%, but only during winter), and buccinid gastropods (B. undatum 5%, B. glaciale 1%, Buccinum sp. 3%, in both seasons). In most cases, buccinids were the first animals to appear at bait. The total number of recorded taxa, mean species richness per sampling unit, total abundance and associations among taxa were higher, on average, in winter than in summer deployments, while Pielou’s evenness index showed the opposite pattern. Scavenger assemblages differed significantly between the two seasons and also in response to the two different bait types, with seasonal effects being strongest. Contrary to expectations, bait consumption rates differed very little between the two seasons, being slow in general and only slightly faster in summer (0.05 g of cod bait consumed in 1 min) compared to winter (0.04 g min–1), yielding novel insights into ecological interactions and functions in shallow marine ecosystems during Arctic polar nights.
Scavengers play an important role in benthic nutrient and energy circulation essential for ecosystem functioning (Benbow et al., 2020). Their significance is emphasized in polar regions where this feeding type is a common strategy (Arnaud, 1977; Renaud et al., 2020). The relatively high level of disturbance, both physical (e.g., the forces of wind and ice, strong salinity gradients and iceberg seabed scouring close to glacier fronts) and biological (such as feeding by gray whales and walruses, or mass mortalities in dynamic populations) is advantageous to necrophages (Britton and Morton, 1994; Dunlop et al., 2014). Strong environmental seasonality with prolonged periods of total darkness (often more than 3 months) and ice cover in winter shapes the phenology of primary productivity. The fact that most organic carbon is produced during intense spring phytoplankton blooms, after the return of the sun, ice-cover breakdown and the stabilization of the upper water column (Sakshaug et al., 2009; Hodal et al., 2012) might suggest that benthic consumers in the Arctic are resource-controlled or food-limited and, hence, depend on seasonal vertical fluxes of phytodetritus to the sea floor (bottom-up control; Presler, 1986; see Piepenburg, 2005 and references therein). This classic perception of depleted life during the harsh polar night has been questioned recently, however, by Berge et al. (2015a, b) who documented not a dormant but a surprisingly rich and active winter ecosystem across nearly all of its components, including benthic invertebrates and scavengers, indicating a greater top-down influence on ecosystem structure and function during winter than was previously assumed. Undoubtedly in such a system, opportunistic omnivores capable of deriving nutrition from a variety of sources, especially those available year-round, are favored (Renaud et al., 2020).
There are few studies describing shallow-water assemblages of scavengers from higher latitudes, especially during the polar night (but see e.g., Legeżyńska et al., 2000, 2012; Smale et al., 2007; Nygård et al., 2012; Dunlop et al., 2020), which, until recently (Berge et al., 2015a,b), was one of the last unexplored areas in time and space for the marine realm, probably as a consequence of logistic constraints. Thus, our understanding of marine scavenging fauna comes primarily from lower latitudes and/or deep-sea ecosystems, as necrophagy becomes a more prominent strategy with increasing depth, due to declining food availability (Stockton and DeLaca, 1982; see also Dunlop et al., 2018 and references therein). Moreover, in contrast to Antarctica (Smale et al., 2007; Dunlop et al., 2014), studies on seasonality conducted in the Arctic (Legeżyńska et al., 2012; Nygård et al., 2012) have focused primarily on just one group of animals – amphipods – and only in the deep sea (Premke et al., 2006). Scavengers are comprised, however, of a very large and taxonomically diverse functional group. In addition, very few previous studies have used different types of bait. Scavenging may not be a purely random or opportunistic process; some scavengers may be selective in their food choice (Nygård et al., 2012). There are many potential sources of food in shallow marine systems, including carcasses of fish or birds. Both the Atlantic cod, Gadus morhua (Olsen et al., 2009; Renaud et al., 2012; Geoffroy and Priou, 2020), and some seabird species (Berge et al., 2015a; Ostaszewska et al., 2017) have recently shown increases in their relative abundances and spatial distribution in the Arctic polar night, due to rapid climatic warming.
Broadly, there are two methods generally used to study necrophagy: baited traps and remote photo-video apparatuses. Traps enable positive identifications of individual organisms caught (including potentially via consultation with taxonomic specialists), but they are selective – results do depend on the dimensions of the trap and the size of their opening(s). Cameras can be deployed either to monitor the bait (and its vicinity) continuously through time (via video footage), or at pre-programmed intervals (via time-lapse photography). In some cases, however, the identification of organisms might be difficult or even impossible to achieve directly from photographs or video footage. One possibility is to use a combination of methods (i.e., to deploy both baited traps and photo/video cameras) (Jones et al., 2003; Premke et al., 2006).
The objective of this study was to trace the decomposition caused by scavengers of two different bait types (Atlantic cod Gadus morhua, and bird meat) in each of two contrasting seasons (during polar night and polar day) in an Arctic shallow-water marine ecosystem. Underwater time-lapse photography combined with small baited traps were employed to investigate: (1) assemblage structure, (2) food consumption rate, and (3) feeding preferences of scavengers, as well as (4) inter-specific associations among scavenger species at bait. Due to known differences in overall food availability in winter (limited) vs. summer (more plentiful), we expected to see strong seasonal differences in (1)-(4) above. Specifically, we expected assemblages of scavengers would be richer and more abundant, show faster consumption rates and stronger inter-specific interactions in winter (when food is relatively scarce) compared to summer. Furthermore, we also expected assemblages of scavengers to change gradually over time, as the bait gradually decayed. In other words, we expected a decay in the similarity of species’ assemblages over time would correlate directly with a decay in the bait through time, over the 4-day period of our observations.
The study took place in the vicinity of Ny-Alesund in Kongsfjorden – an important Arctic monitoring site, and the best studied northerly fjord – located on the west coast of Spitsbergen, Svalbard Archipelago (Figure 1). Due to the lack of shallow sill at its entrance, and strong influx of Atlantic waters (mixed with Arctic waters) through the action of the West Spitsbergen Current, the conditions prevailing inside the fjord are more sub-Arctic than Arctic in character (Hop et al., 2002; Wiencke and Hop, 2016). Despite its high latitude and the proximity of three large recessing tidal glaciers (Van Pelt and Kohler, 2015), including the Kongsbreen – the most active one on the archipelago (Lefauconnier et al., 1994), only the inner parts of Kongsfjorden are strongly glacially influenced. Freshwater runoff, sediment deposition, and ice action thus decreases gradually along the axis of the fjord toward its mouth. In such a large fjordic system, benthic habitats are diverse, but due to sedimentation the seabed is generally muddy with overlying boulders and rocks. Fauna and flora represents a typical mix of boreal and Arctic species (Włodarska-Kowalczuk et al., 1998; Hop et al., 2002; Gulliksen and Svensen, 2004; Voronkov et al., 2016; Wiencke and Hop, 2016). Monthly mean air temperature in Ny-Alesund in January is −10°C, while in August it is ∼5°C above zero. Mean winter seawater temperature is 1°C, while in the summer it is ∼3.5–4°C. The salinity regime in shallow water varies between ca. 34.7–34.8 PSU in winter and 33.5–34 PSU in summer (Hop and Wiencke(eds), 2019). Surface water can freeze in winter for a few months; however, since 2005/2006, winter ice-cover is rare here (Cottier et al., 2007) and the sea did not freeze at the study locality during this study. In summer, the shallow seabed can be scoured by icebergs from tidal glaciers (Dowdesweell and Forsberg, 1992). Polar night begins 24 October and ends 18 February (light conditions can be characterized as polar twilight, civil polar night and nautical polar night at noon on winter solstice) while polar day (i.e., midnight sun) occurs 18 April to 24 August, with direct sun reaching Ny-Alesund 8 March to 8 October (Berge et al., 2020). During the civil polar night peak, surface irradiances are insufficient to support primary production (Cohen et al., 2015). Detailed physical characterization of the fjord is most recently presented in Hop et al. (2019). For more details regarding polar night, refer to Berge et al. (2020).
Two time-lapse camera systems, spaced 25 m apart, were deployed at a depth of 12 m in the vicinity of the Ny-Alesund harbor (Figure 1). Camera systems (Figure 2A), described in detail in Balazy et al. (2018), were secured on the soft-bottom seabed by divers with lead weights and rocks. Cameras were situated in front of two types of bait: Atlantic cod (Gadus morhua) and bird meat (chicken meat mimicking a bird food-fall item). Bait was anchored to the sediment with copper wire laden with small stones (Figure 2B). Each camera-system was also equipped with a cylindrical bait-trap (25 cm long and 10 cm in diameter) made of plastic with an elliptical opening (2.5 cm in diameter) at one end, and covered by a net (1 mm mesh) at the other end. Each trap contained a small piece (∼40 g) of bait that matched the bait situated outside the trap being photographed by the camera-system. The main aim of the trap was to support identification of organisms (particularly small-sized scavengers) that might prove difficult to identify in photographs. The camera system consisted of a Canon EOS 1110D camera with Canon EFS 18–55 mm lens set at its widest position. For lighting, a single 40W and 125° beam LED strobe firing for a second was used. The camera focus was set to manual mode and pointed at a distance of 30 cm, i.e., it was pointed at and focused to the exact position of the bait. The aperture was set to F14 to ensure a large depth of field. The baits were monitored over a period of 4 days, with each camera taking a photograph every 15 min. The traps and associated cameras were deployed for both types of bait in each of two seasons: winter (17–22nd January) and summer (5–9th August) in 2015 (see Table 1 for details). After that time, all the gear and bait remains were recovered, photographs downloaded, and animals from traps preserved in formalin. Organisms in traps and on photographs (located directly on bait and around it) were identified to the lowest possible level (typically species) and counted. Each combination of Season and Bait type is referred to, hereafter, as a “case.” Consumption rates were calculated as the difference in the weight of the bait from before deployment to after its recovery, divided by its period of submergence.
Figure 2. Time-lapse camera system, equipped with baited trap (on the left), in front of the bird bait (A), and a sample photographic image produced by the system (B).
Individual photographs taken at 15-min intervals were extremely variable in the identities of species they happened to contain, with some photographs containing very few species or no species at all. Thus, for subsequent analysis, we used a more consolidated measure of the scavenger community by combining (averaging) the data from 12 consecutive photographs (taken over a period of 180 min) into a single sampling unit (Anderson and Santana-Garcon, 2015). For each sampling unit, we calculated the mean abundance for each species as well as several summary metrics: species richness (S), evenness (J′), and log-transformed total abundance [log(Ntot)]. Variation in scavenger assemblages (mean abundance values per species) among sampling units was quantified using the Bray–Curtis dissimilarity measure after applying dispersion weighting (Clarke et al., 2006). Dispersion weighting ensured that species displaying highly erratic spatio-temporal clustering (i.e., amphipods) were suitably down-weighted for subsequent analyses.
Multivariate variation in community structure, encapsulated in the Bray–Curtis dissimilarity matrix, was then partitioned using permutational multivariate ANOVA (PERMANOVA, Anderson, 2001) in response to a fixed, 2-factor (Season, Bait type) design. As only one replicate camera system was deployed for each bait-type within each season, inferences are necessarily limited to these experimental units, with the primary aim being to characterize changes in the scavenger communities tracked over time in each case. In addition, individual sampling units (each drawing information from a period lasting 180 min) were also taken sequentially from individual cameras over a period of 4 days, so they were not independent through time. This was accounted for by including “Time” as an ordered sequential linear covariate in all formal PERMANOVA analyses. Significant effects detected by PERMANOVA were examined using appropriate post hoc pair-wise comparisons. A permutational test of multivariate dispersions (PERMDISP, Anderson et al., 2008), was used to compare temporal variation in scavenger assemblage structure across the four combinations of Season × Bait type. Similarity percentages (SIMPER) were used to identify species’ contributions toward within-group and between-group differences among groups. Patterns of change in scavenger communities through Time and in response to Season and/or Bait type were visualized using non-metric multi-dimensional scaling (MDS) on the distances among relevant centroids (e.g., Anderson, 2017) and metric MDS of bootstrap averages (Clarke et al., 2014). Temporal trajectories for different seasons and bait types were examined in separate ordination plots, with segmented bubbles showing S, log(Ntot) and J′. The univariate variables: S, log(Ntot), and J′, were also tested individually using the same ANCOVA design (2 factors, with Time as a covariate), but based on a Euclidean distance matrix (untransformed data, Clarke et al., 2006; Anderson et al., 2008). All statistical analyses were done using PRIMER 7 with the PERMANOVA + add-on (Anderson et al., 2008; Clarke and Gorley, 2015).
All permutation tests were done using 9999 permutations of residuals (Freedman and Lane, 1983). Interspecific relationships at bait were quantified using copulas (e.g., Mai and Scherer, 2017). First, the statistical significance of relationships was ascertained using permutation tests on the index of association (e.g., Somerfield and Clarke, 2013), then estimation of the size and direction of inter-species’ associations (positive or negative) was done using Gaussian copulas for discrete data (Anderson et al., 2019). Estimation was done separately for each of the four combinations of Season × Bait type. Correlation structures from the copulas were visualized using the ‘corrplot’ package (version 0.84; Wei and Simko, 2017) in the R software package (R Core Team, 2020).
A total of 30 taxa were identified from photographs (Table 2). Among these, 16 taxa were identified to species level, nine to genus, one to family, three to class and one to phylum. Twenty-six individuals remained unidentified. The lysianassid amphipod Onisimus sp. made up 88% of the total number of animals counted, however it did not occur frequently (appearing in 55% of the images, mostly in winter). The most frequently occurring species were buccinid gastropods (Buccinum undatum occurring in 93% of the images and accounting for 5% of the total number of animals counted; Buccinum sp. – 89% and 3%; and B. glaciale – 60% and 1%, respectively), the hermit crab Pagurus pubescens (40% occurrence and 0.5% of total abundance) and another amphipod, Anonyx sp. (31% occurrence and 2% of total abundance).
Table 2. List of taxa occurring on different bait types on photographs and in traps (W-Winter, S-Summer).
Only six species were found in all of the traps (Table 2). The most abundant of these was the gastropod B. undatum (10 individuals) followed by the amphipod Anonyx sarsi (six individuals) and another buccinid B. glaciale (three individuals). The other three species (Orchomenella minuta, Pagurus pubescens, Phyllodoce groenlandica) were represented by single specimens.
There were clear changes in the community of scavengers over time, which varied somewhat seasonally as well (Figure 3). In most cases, buccinids (B. undatum, B. glaciale, Bucinnum sp., Colus sp.) were the first species to arrive at the bait, generally occurring within the first 0–15 min. In the case of bird meat in winter, Anonyx sp. also appeared early. In the summer, Hyas araneus appeared at the bait after 45 min, then first B. undatum appeared.
The number of animals attracted to the bait over the course of deployment varied between bait types and seasons (Figures 4A,B). Due to the occasional occurrence of Onisimus sp. in huge numbers, reaching at times over 1500 individuals, two graphs with different scales are presented: one with a scale covering all the Onisimus sp. counted (Figure 4A) and one with a reduced scale to see other taxa more clearly (Figure 4B). Large quantities of amphipods were observed only in winter, on cod bait. On bird meat, no more than 380 individuals were observed at any single time. Despite being recorded almost right after bait placement (Figure 3), the sharp increase in Onisimus sp. numbers was observed only after 2 days, when the outer skin was already deteriorating and the initial scavengers had already started to leave. In the case of the cod bait in winter, the upward trend in Onisimus sp. abundance continued until the end of bait monitoring, while in the case of bird meat the numbers of this species started gradually to decrease after 84 h. Anonyx sp. was much less numerous. It occurred more often and in greater numbers on bird meat than on cod, and again mostly in winter. Maximum numbers (245 individuals at once) were recorded after 3 days but did not last long (i.e., only for 1.5 h). Buccinids (mostly B. undatum, B. glaciale, and/or Buccinum sp.) were the most numerous scavengers at bait in the summer (maximum 23 B. undatum individuals at once), and the second-most numerous in winter (maximum 26 B. undatum individuals at once). Apart from bird meat in winter, the temporal patterns in their occurrence and abundance at bait was similar across the bait types and season. Some fluctuations in Buccinum numbers might have been caused by these gastropods gaining access to soft tissues of the bait through external holes, after which they became invisible to the camera, so could no longer be counted. This was especially evident in the case of cod, which was denser than the chicken meat, so it was easy to observe the gastropods using the cod’s mouth or gill covers to get inside. Two crab species were recorded in every case: a small hermit crab Pagurus pubescens and the much larger spider crab Hyas araneus. However, they appeared sporadically without any clear pattern or trend. Only at cod bait in the summer were P. pubescens seen nearly throughout the whole monitoring period. Whenever Hyas araneus appeared at bait, amphipods disappeared. The remaining species (Table 2) constituted a small percentage of recorded necrophages. Their occurrence was more noticeable in winter, especially on bird bait.
Figure 4. Number of individuals observed at each bait type in each season: with scale including all the Onisimus sp. counted (A) with scale reduced to 30 individuals (allowing other taxa to be seen more clearly) (B). Gaps in the continuity of data over time are caused by lack of data due to obstruction of the camera’s lens by kelp being dragged in front of the camera by currents.
Due to the hindered access to the bird meat caused by dense kelp cover in the summer, the differences in bait consumption rate between the two seasons were analyzed only on cod bait (Table 1). Cod decomposition was slightly faster in summer (0.05 g min–1) than in winter (0.04 g min–1). Despite this, at the end of the monitoring in winter, the cod bait consisted only of bones, fin rays and some skin remains. Nevertheless, 1344 Onisimus sp. individuals and one Buccinum sp. were still present at it.
Figure 5 shows the number of taxa in each broader taxonomic group occurring in each of the four combinations of Season × Bait type. Among the recorded scavenger taxa, gastropods were the most species rich (six to ten taxa), while polychaetes and amphipods were represented by only one or two taxa. More taxa were recorded in winter (24 on cod, 25 on bird meat) than in summer (17 on cod, 15 on bird meat).
There was a significant interaction between Season and Bait type in their effects on scavenger assemblages (Table 3). Pair-wise comparisons indicated significant seasonal effects occurred for both types of bait, but the nature of these effects differed (Table 3 and Figures 3, 4, 6). Sizes of the (square-root transformed) components of variation (Sqrt, Table 3) were higher for Season (20.8) than for Bait type (16.9) indicating a greater effect of seasons over bait type. The three-way interaction (Time × Season × Bait type, 20.1) was also quite large relative to other components in the model, indicating that changes over time in the scavenger assemblages (i.e., the nature of the temporal trajectory of turnover) varied significantly across the four Season × Bait type groups. The greatest temporal variation occurred in summer for bird bait, followed by winter for either bird or cod (which did not differ significantly from one another in their temporal variation), followed by summer for cod, which showed significantly lower temporal variation than the other three groups (Table 4). The metric MDS ordination plot of bootstrap averages (Figure 6) displayed clear separation of the four cases (left-to-right corresponding to winter-summer differences, and top-to-bottom corresponding to cod-bird differences) and also displayed visually the greater variation in scavenger taxa (greater spread of bootstrap averages) observed through time for bird meat deployed in summer compared to the other cases. Taxa giving rise to observed differences between the seasons, on cod bait, were: amphipods Onisimus sp. and buccinid gastropods occurring in higher numbers in winter; and the hermit crab P. pubescens being more abundant in summer (Table 5). On bird meat, buccinids and the amphipod Anonyx sp. showed the highest contributions to the average dissimilarity between seasons. Different buccinid species were more abundant in different seasons, while Anonyx sp. occurred mainly in winter. The taxa contributing the most to observed differences between bait types included: Onisimus sp. and B. undatum (more abundant in winter on cod bait), and B. glaciale (more abundant on bird meat). In the summer, Buccinum sp. contributed the most to observed differences between the two bait types, and B. undatum and P. pubescens occurred in greater abundances on cod.
Table 3. Results of multivariate and univariate PERMANOVA analyses for differences in scavenger assemblages through time (as a linear covariate) and between seasons and bait types.
Table 4. Results of PERMDISP analyses comparing temporal variation in scavenger assemblage structure across four groups (combinations of Season × Bait type).
Table 5. Results of SIMPER analyses identifying taxa with the highest contributions to observed differences between the Seasons and Bait types.
Figure 6. Bootstrap average MDS plot of scavenger assemblages from different bait types (cod, bird) and seasons (winter, summer) calculated on the basis of Bray–Curtis dissimilarities after a transformation using dispersion weighting of average abundances (for n = 12 photographs).
The mean species richness of scavengers (S), was significantly higher in winter (8–9) than in summer (4–7), for either type of bait (Table 3 and Figure 7A). During the summer, mean S was higher at cod (7) than at bird bait (4). It is important to bear in mind, however, that cod bait was physically bigger in size (473 vs. 300 g, Table 1), and bird meat was often covered by algae. In winter, when both baits were of the same weight and not covered by algae, there was no significant difference in scavenger richness detected between the two bait types. Log-transformed total abundance was also much higher in winter (3.9–5.3) than in summer (2.0–2.7) (Table 3 and Figure 7B). During both seasons, log(Ntot), like richness, was higher on average at cod bait (2.7–5.3) than at bird bait (2.0–3.9, Figure 7B), but similar caveats (regarding differences in the amount of each bait type, etc.) apply. For evenness, however, the opposite trend was observed – mean J′ was higher in summer (0.7) than in winter (0.3–0.5) (Table 3 and Figure 7C). In addition, evenness was higher, on average, for bird meat (0.5–0.7) than for cod bait (0.3–0.7).
Figure 7. (A) Species richness (S), (B) log-transformed total abundance log(Ntot), and (C) Pielou’s evenness (J′) for different bait types in each of two seasons. Horizontal line in the middle of the inter-quartile range represents the median, bigger dots = average values, smaller dots = potential outliers.
The shape of temporal trajectories of scavenger assemblages varied for each of the four combinations of Season × Bait type (Figure 8). The scavenger assemblage for cod bait in winter (Figure 8A) showed effectively two distinct groups of sampling units. In the first 48 h, the bait was dominated by buccinid gastropods, but species evenness remained high. After that period (sample 18–19, Figure 8A; www1, 2021), the vast majority of the bait was already consumed and the first B. undatum and B. glaciale had started to leave the bait. At the same time, amphipods Onisimus sp. started coming to the bait in large numbers and to dominate it completely. This caused a dramatic rise in the total abundance of scavengers overall, with a concurrent drop in species evenness. Apart from samples 15 and 16 (which had either severely reduced numbers or no recorded species, when the camera viewfinder was covered with kelp), the decomposition of cod bait in the summer was more homogeneous. Although the hermit crab P. pubescens was attracted to the bait throughout the entire time, its highest numbers were recorded after 2 days. The temporal trajectory of changes in scavenger assemblages found on bird meat in winter was similar to that of cod, but without such a clear change happening before vs. after 48 h. As the resources of the bait were diminishing, buccinids diminished and amphipods increased (Onisimus sp. and Anonyx sp.), which was also reflected in successively increasing values for total abundance and concomitant decreasing species evenness over time. The decomposition of bird meat in summer was far less clearly successional; it did not follow a linear trend of turnover through time. Instead, a circular pattern was observed in the ordination plot (Figure 8D); this may be a reflection of extremely low frequencies and abundances in many sampling units (so very few taxa upon which to calculate similarities, particularly at later time points in the series), most probably due to the fact that the bait was repeatedly covered with kelp (Table 1). This also may explain the high temporal variation detected for this case (Table 4).
Figure 8. (A–D) Non-metric MDS plots of scavenger assemblages from different bait types (cod, bird) and seasons (winter, summer) calculated on the basis of Bray–Curtis dissimilarities after a transformation using dispersion weighting of average abundances (for n = 12 photographs), with temporal trajectory connecting the points through time (numbers show the sequential ordering from 1 up to ∼32 for the sampling units spanning 180 min) and overlying bubbles with data for S (yellow), log(Ntot) (orange), and J′ (pink). Each plot has a corresponding shade plot of the underlying data matrix. The larger the entry in a specific cell, the darker the shade plotted, white representing the absence of that species, and full black the largest entry in the whole matrix.
Correlation structures estimated using copulas indicated stronger measured associations occurred among taxa in winter than in summer, for both types of bait (Figure 9). The strongest negative relationships between taxa were recorded on cod bait in winter and concerned Onisimus sp. amphipods leaving the bait whenever Hyas sp. crabs appeared. Negative associations also were detected between amphipods and buccinid gastropods (B. undatum and B. glaciale), likely reflecting temporal turnover, with the increase in amphipods (as the bait decomposed) corresponding to times when the buccinids were leaving the bait. On bird meat in winter, Onisimus sp. also actively avoided and left the bait whenever it was approached by another potential predator: sculpin (Myoxocephalus scorpius).
Figure 9. (A–D) Correlations among pairs of species estimated using copulas for different bait types (cod, bird) and seasons (winter, summer).
This study, using both baited traps and photographic methods, revealed a highly abundant, taxonomically diverse, and active necrophagic community in both studied seasons. Many of the expected differences in scavenger assemblages (between seasons and bait types) were supported by our time-lapse observations of these experimental units. We documented more abundant and species-rich assemblages, having stronger and greater numbers of interspecific interactions in response to the winter deployments compared to the summer deployments, in direct accordance with a priori hypotheses. In contrast, we did not find significant seasonal differences in scavenger consumption rates. In addition, we documented successional turnover through time (over a period of 4 days), in both the identities and relative abundances of species, for a variety of scavengers belonging to a broad range of taxonomic groups.
Whelks (at least two species: Buccinum undatum and B. glaciale) were the most frequently observed necrophages in our study. These gastropods are primarily predators of bivalves and polychaetes, scavenging opportunistically (Nielsen, 1974; Taylor, 1978; Britton and Morton, 1994), with highest feeding activity from late autumn to early spring (i.e., May), when their breeding starts (Gulf of St. Lawrence, 50° N, Martel et al., 1986). In our study, we observed similar patterns of abundance for these species in winter and in summer cases, which does not support this phenology in the Arctic (79° N), but rather suggests a high level of physiological plasticity for B. undatum (UK 50° N, Iceland 65° N, Thatje et al., 2019). High densities of buccinids in the shallow waters of Kongsfjorden (up to 4 ind. per 0.023 m2, Kaczmarek et al., 2005; Włodarska-Kowalczuk, 2007) and their relatively rapid movement (15 cm per minute, Lapointe and Sainte-Marie, 1992) may explain why, in most cases, buccinids were the first species at the bait, appearing only 15 min after its placement on the seabed. Only when spider crabs (Hyas araneus) appeared first did B. undatum appear later (i.e., at 30 min, after crabs had left the bait). It has been suggested that these crabs can prevent or delay entry of whelks into baited traps, and that whelks typically avoid these crabs, which are their natural predators (see Lapointe and Sainte-Marie, 1992 and references therein). The fact that both species were observed at the bait simultaneously at a later state of bait decomposition might be explained by a highly mobile crab approaching the bait after it had already been colonized by the slower moving whelks. In a field experiment performed in Gronfjorden (Spitsbergen), Markowska et al. (2008) found no instances of aggressive behavior between H. araneus and Buccinum gastropods and stated that large whelks are not easily susceptible to predation. Size-related immunity from predation could explain the only slightly negative association observed here between spider crabs and Buccinum gastropods (Figures 9A,B). Interestingly, we observed crab in the vast majority of cases staying at the bait very briefly (for 15–30 min). Only on four occasions did it remain for longer periods of time (assuming the same individual was being seen in consecutive frames), i.e., for 45 (twice), 90 and 150 min (the last two occasions were observed at cod bait in winter). On one occasion, we also observed a crab trying to eat the small hagfish Myxine glutinosa. Perhaps these crabs treat the intact bait only as a place for feeding on other smaller necrophages, as has been observed for some deep-water fish that prey on amphipods consuming carrion (Jones et al., 1998).
In terms of numbers, lysianassid amphipods completely dominated scavenging assemblages in winter (Figures 4A,B), although these organisms (individually) are quite small in size. The large influx of amphipods was the main difference found between the seasons for cod bait, and one of the main seasonal differences observed for bird bait (Tables 3, 5). Their dominance at bait and food falls is typical both in the deep sea and in shallow coastal areas of polar seas and cold waters (Legeżyńska et al., 2000; Legeżyńska, 2001; Premke et al., 2006; Nygård et al., 2012). These highly mobile organisms with their well-developed chemoreceptors can locate carrion very quickly (Legeżyńska et al., 2000 and references therein). Anonyx sp. was the first species to appear in the case of bird meat, and Anonyx sp. and Onisimus sp. were the second species (but with higher abundance than the first buccinids) to appear at cod bait, in winter. Based on the identified animals found in baited traps and previous literature on lysianassid amphipod abundance and distribution in Kongsfjorden, we considered that two species were most likely to have been the species recorded in our photographs: A. sarsi (reported to occur mainly in shallower waters in contrast to A. nugax – a similar species occurring mainly deeper than 30 m), and O. edwardsii (peaking at 15 m depth) (Legeżyńska, 2001; Nygård et al., 2012). Both species are well known from the study region, utilizing necrophagy in their feeding repertoire and recorded throughout the year (Legeżyńska et al., 2000; Legeżyńska, 2008; Nygård et al., 2012). Dominance of Onisimus sp. at the study site (located in the middle of the glacial fjord with soft muddy sediments) is not a surprise, as Anonyx sp. occurs usually on coarser sediments, in the outer parts of Kongsfjorden where there are stronger currents (Legeżyńska, 2001).
Differences in the abundances of amphipods between winter and summer have been reported previously in Antarctica, where superabundant winter fauna were absent in summer (Smale et al., 2007), and in the Arctic. Nygård et al. (2012) observed at Spitsbergen much larger catches using traps in November/February than in July/August, when samples contained very few amphipods. Onisimus edwardsii is a markedly omnivorous feeder (Legeżyńska, 2001) that probably modifies its diet according to the changing accessibility of different kinds of food throughout the year (Legeżyńska, 2008). All Onisimus species exhibit seasonal shifts in feeding strategy. In the summer they typically feed close to glacial fronts, mainly on dead zooplankton (killed by freshwater discharge causing an osmotic shock at the surface waters, Zajaczkowski and Legezyńska, 2001; Legeżyńska, 2008). This might explain their almost complete absence at the bait deployment site in summer. In winter, when food resources are not that plentiful, and when glacial melt stops, the bait constitutes an easy alternative food source for these amphipods, which gather at it in large numbers (Nygård et al., 2012). On the other hand, amphipods are flexible with their feeding habits and do not rely exclusively on carrion (A. sarsi exhibits both scavenging and predatory behavior, Sainte-Marie, 1986; Legeżyńska, 2008). They may just be temporary passers-by, reacting to infrequent occurrences of carrion, with clusters of individuals varying randomly both spatially and temporally (Britton and Morton, 1994). The latter explanation suits better the patterns observed for Anonyx, a genus known for its rapid and efficient voracious feeding, and slow digestion, that can tolerate long periods of starvation (Sainte-Marie, 1986). Again, similar to the small buccinids observed early-on at the bait, when Hyas crabs were feeding at the bait, amphipods disappeared, or only appeared in small numbers (Figure 9A). An antagonistic response also appeared to happen (only in winter) after the appearance of sculpin Myoxocephalus scorpius in close proximity to the bait (Figure 9B).
In contrast to Antarctica, where phytodetritus produced in short seasonal blooms is stored in the sediment on the seabed and can serve as a “food-bank” throughout the year (Smith et al., 2012), benthic organisms in the Arctic typically utilize pelagic inputs very quickly (Renaud et al., 2007; Morata et al., 2015; but see Włodarska-Kowalczuk et al., 2016). Thus, the limited food resources available during Arctic polar night are most likely to be the reason for the occurrence of abundant, species-rich scavenging assemblages (Figures 7A,B), stronger interspecific interactions (Figure 9), higher overall numbers of species (Figure 5), and longer stays at bait (e.g., observed for the spider crabs Hyas spp.) recorded in winter compared to summer deployments. Kȩdra et al. (2011) also found slightly higher richness and abundance of shallow water (15 m) carnivorous and opportunistic species during the winter (March) in Kongsfjorden. However, from the pool of species that occurred at bait only in the winter, only Anonyx sp. and hagfish Myxine glutinosa are considered true opportunistic scavengers (Shelton, 1978; Sainte-Marie et al., 1989; Martini, 1998), with the latter known for its preference for fish (Leigh et al., 2016). The other species (e.g., Caprella sp., Coryphella verrucosa, Cryptonatica affinis/Euspira pallida, Dendronotus robustus, Harmothoe sp., Lebbeus polaris, Nemertea undet., Sclerocrangon sp., Table 2) occurred only occasionally, in very low numbers, and are not generally known as scavengers. Some of these were previously recorded in baited traps (single individuals of the skeleton shrimp C. septentrionalis and polar shrimp L. polaris, Legeżyńska et al., 2000), but their appearance at bait should be treated with caution and perhaps regarded as accidental. These results might also have been affected by water clarity; underwater visibility was generally greater in winter, enabling better detection of a greater number of organisms. We also consider that a time-lapse system’s strobe light could attract some animals (Smale et al., 2007), like cod and some plankton (e.g., Mertensia ovum, Sagittoidea). Potential artifacts caused by introducing artificial light would be much stronger during polar night (winter) sampling, when ambient light conditions are typically pitch black.
Differences in bait consumption rate between the two seasons were small (0.04 vs. 0.05 g min–1) despite different overall food availability in the ecosystem and seawater temperature. Our result supports earlier findings from Antarctica, where no significant differences between the seasons was detected (max. 0.04, mean 0.01–0.03 g min–1 in winter; 0.01–0.03 g min–1 in summer). Also, by comparison with lower latitudes in the deep sea, shallow-water (12 m depth) polar scavenging is slow (Smale et al., 2007). In a recent study performed in Kongsfjorden at 360 m, consumption rate was recorded to be an order of magnitude faster (i.e., 0.5 g min–1, Dunlop et al., 2020) than that recorded in our study.
Differences between bait types were slightly less pronounced than differences between the seasons (Sqrt, Table 3). Despite O. edwardsii being regarded as an omnivore with weak food preferences (Legeżyńska, 2008), we recorded many more Onisimus individuals on cod bait than on bird meat (Figures 4A,B and Table 5). Surprisingly, for Anonyx (most probably A. sarsi), which is considered to be a scavenger and predator of polychaetes and crustaceans (Oliver and Slattery, 1985), the opposite pattern occurred. Apart from lysianassids and hermit crabs Pagurus pubescens (more abundant at cod bait in the summer, Figure 4B and Table 5), other species did not exhibit any clear bait preferences. Many of the species are typical opportunists, like buccinid gastropods. For them, scavenging is only a subsidiary feeding mechanism utilized occasionally (Schembri, 1982). According to Britton and Morton (1994) predominance of facultative scavengers over specialized, obligate ones is a typical feature of benthic communities in shallow shelf seas. In terms of overall numbers of taxa in different groups, cod was slightly more attractive for various scavengers than bird meat in the summer deployment, but not in winter (Figure 5). At any particular time-point, the opposite pattern could be observed, and species richness in winter did not differ significantly between the two bait types (Table 3 and Figure 7A). Thousands of lysianassids invading the bait in winter (generating low measures of species evenness and higher total abundance; Figure 7C), suggest cod may be a more attractive bait type for many species. However, excluding Onisimus sp. amphipods, there was not a clear preference by scavengers for cod. Similarly, no tendency for scavengers to prefer autochthonous vs. allochthonous carrion was found by Morton and Jones (2003), but this is not always the case (Morton and Yuen, 2000). One possible explanation for the lack of evident preference for cod in our study may have been the presence of an intact skin cover on cod by comparison to only some skin leftovers being present on bird meat. As can be seen in time-lapse footage (www1, 2021), most of the scavengers are utilizing natural body holes such as gills, mouth and anus in order to get to the soft tissues of the cod, indicating that, for many of them, cod skin might be a difficult barrier to break through. Overall, these results suggest that increasing borealization of high-latitude fish communities anticipated in the near future (especially as the Barents Sea is considered a gateway to the Arctic Ocean; see Geoffroy and Priou, 2020 and references therein), could result in boreal gadids becoming one of the main food items for local scavengers in this region.
This study provides insights into the over-wintering strategies of bottom fauna, temporal turnover in scavengers present at carrion, scavenging community dynamics, and nutrient cycling and functioning of shallow-water ecosystems during polar night. Given the many limitations of the current study (e.g., a single study site, a single replicate camera system per bait type within each season, a relatively short physical distance of 25 m between the bait types), the general lack of such shallow-water seasonal data, the rapid change of Arctic systems due to climate warming (Overland et al., 2019), and the consequent modifications to carrion decomposition efficiency and energy flow (Dunlop et al., 2020), clearly more comprehensive investigations are needed. The high Arctic fjords of Svalbard Archipelago, which may represent an area similar in its environmental parameters to what might be expected for most Arctic coastal areas in the second half of this century (Piskozub, 2017), could serve as a potential model system. Future studies should be based on multiple sites across a broader geographic gradient, should have greater replication within seasons and across multiple years, should be mirrored/replicated in Antarctic systems, and should also include data collected in the absence of bait. Such future studies will enable a more complete understanding of marine scavengers’ essential role, as a community, in ocean nutrient cycling, with important implications for the far-reaching impacts anticipated under rapid climate change.
The raw data supporting the conclusions of this article will be made available by the authors, without undue reservation.
PB: conceptualization, fieldwork, image and data analysis, and writing-original draft. MA: data analysis and its concept, and writing-review and editing. MC: image analysis. MW-K: fieldwork and writing-review and editing. PK: funding acquisition and writing-review and editing. JB: funding acquisition and conceptualization. All authors contributed to the article and approved the submitted version.
Financial support was provided by Mare Incognitum (RIS no. 6575), the NFR Marine Night (no. 226417) and SPB Mare Incognitum (Ministry of Science and Higher Education, no. W157/Norway/2013) projects. Hardware modernizations were financed from the funds of the Leading National Research Centre (KNOW) received by the Centre for Polar Studies for the period 2014–2018. The study was finished thanks to the support of the National Science Centre, Poland project no. 2018/29/B/NZ8/02340.
MA is the Director of PRIMER-e (Quest Research Limited), Auckland, New Zealand.
The remaining authors declare that the research was conducted in the absence of any commercial or financial relationships that could be construed as a potential conflict of interest.
Among the people that offered advice and a helping hand while performing this study we would like to thank especially: Jakub Szuster for assistance in polar night diving and its challenging logistics, Daniel Vogedes for surface support, Mikołaj Mazurkiewicz for help with R programming for an earlier draft of the paper, and Joanna (Zosia) Legeżyńska for amphipod identification guidance and valuable comments regarding their habitat use and behavior. The manuscript was substantially improved thanks to the comments of the reviewers.
Anderson, M. J. (2001). A new method for nonparametric multivariate analysis of variance. Austral. Ecol. 26, 32–46. doi: 10.1111/j.1442-9993.2001.01070.pp.x
Anderson, M. J. (2017). Permutational Multivariate Analysis of Variance (PERMANOVA). Hoboken, NJ: Wiley.
Anderson, M. J., de Valpine, P., Punnett, A., and Miller, A. E. (2019). A pathway for multivariate analysis of ecological communities using copulas. Ecol. Evol. 9, 3276–3294. doi: 10.1002/ece3.4948
Anderson, M. J., Gorley, R. N., and Clarke, K. R. (2008). PERMANOVA+ for PRIMER: Guide to Software and Statistical Methods. Plymouth: PRIMER-E.
Anderson, M. J., and Santana-Garcon, J. (2015). Measures of precision for dissimilarity-based multivariate analysis of ecological communities. Ecol. Let. 18, 66–73. doi: 10.1111/ele.12385
Arnaud, P. M. (1977). “Adaptations within the Antarctic marine benthic ecosystem,” in Adaptations Within Antarctic Ecosystems, ed. G. A. Llano (Washington, DC: Smithsonian Institute), 135–158.
Balazy, P., Kuklinski, P., and Berge, J. (2018). Diver deployed autonomous time-lapse camera systems for ecological studies. J. Mar. Eng. Technol. 17, 137–142. doi: 10.1080/20464177.2017.1357164
Benbow, M. E., Receveur, J. P., and Lamberti, G. A. (2020). Death and decomposition in aquatic ecosystems. Front. Ecol. Evol. 8:17. doi: 10.3389/fevo.2020.00017
Berge, J., Daase, M., and Renaud, P. E. (2015a). Unexpected levels of biological activity during the polar night offer new perspectives on a warming arctic. Curr. Biol. 25, 2555–2561. doi: 10.1016/j.cub.2015.08.024
Berge, J., Renaud, P. E., Darnis, G., Cottier, F., Last, K., Gabrielsen, T. M., et al. (2015b). In the dark: a review of ecosystem processes during the Arctic polar night. Prog. Oceanogr. 139, 258–271. doi: 10.1016/j.pocean.2015.08.005
Berge, J., Johnsen, G., and Cohen, J. (2020). POLAR NIGHT Marine Ecology. Advances in Polar Ecology, vol 4. Cham: Springer.
Britton, J. C., and Morton, B. (1994). Marine carrion and scavengers. Oceanogr. Mar. Biol. An Annu. Rev. 32, 369–434.
Clarke, K. R., Chapman, M. G., Somerfield, P. J., and Needham, H. R. (2006). Dispersion-based weighting of species counts in assemblage analyses. Mar. Ecol. Prog. Ser. 320, 11–27. doi: 10.3354/meps320011
Clarke, K. R., Gorley, R. N., Somerfield, P. J., and Warwick, R. M. (2014). Change in Marine Communities: An Approach to Statistical Analysis and Interpretation, 3rd Edn. Plymouth: PRIMER-E.
Cohen, J. H., Berge, J., Moline, M. A., Sørensen, A. J., Last, K. S., Falk-Petersen, S., et al. (2015). Is ambient light during the high arctic polar night sufficient to act as a visual cue for zooplankton? PLoS One 10:e0126247. doi: 10.1371/journal.pone.0126247
Cottier, F. R., Nilsen, F., Inall, M. E., Gerland, S., Tverberg, V., and Svendsen, H. (2007). Wintertime warming of an Arctic shelf in response to large-scale atmospheric circulation. Geophys. Res. Lett. 34:L10607. doi: 10.1029/2007GL029948
Dowdesweell, J. A., and Forsberg, C. F. (1992). The size and frequency of icebergs and bergy bits derived from tidewater glaciers in Kongsfjorden, northwest Spitsbergen. Polar Res. 11, 81–91. doi: 10.3402/polar.v11i2.6719
Dunlop, K., Renaud, P. E., Berge, J., Jones, D. O. B., Harbour, R. P., Tandberg, A. H. S., et al. (2020). Benthic scavenger community composition and carrion removal in Arctic and Subarctic fjords. Polar Biol. 44, 31–43. doi: 10.1007/s00300-020-02773-5
Dunlop, K. M., Barnes, D. K. A., and Bailey, D. M. (2014). Variation of scavenger richness and abundance between sites of high and low iceberg scour frequency in Ryder Bay, west Antarctic Peninsula. Polar Biol. 37, 1741–1754. doi: 10.1007/s00300-014-1558-y
Dunlop, K. M., Jones, D. O. B., and Sweetman, A. K. (2018). Scavenging processes on jellyfish carcasses across a fjord depth gradient. Limnol. Oceanogr. 63, 1146–1155. doi: 10.1002/lno.10760
Freedman, D., and Lane, D. (1983). A nonstochastic interpretation of reported significance levels. J. Bus. Econ. Stat. 1, 292–298. doi: 10.2307/1391660
Geoffroy, M., and Priou, P. (2020). “Fish ecology during the polar night,” in POLAR NIGHT Marine Ecology. Advances in Polar Ecology, Vol. 4, eds J. Berge, G. Johnsen, and J. Cohen (Berlin: Springer), 181–217. doi: 10.1007/978-3-030-33208-2_7
Hodal, H., Falk-Petersen, S., Hop, H., Kristiansen, S., and Reigstad, M. (2012). Spring bloom dynamics in Kongsfjorden, Svalbard: nutrients, phytoplankton, protozoans and primary production. Polar Biol. 35, 191–203. doi: 10.1007/s00300-011-1053-7
Hop, H., Pearson, T., Hegseth, E. N., Kovacs, K. M., Wiencke, C., Kwasniewski, S., et al. (2002). The marine ecosystem of Kongsfjorden, Svalbard. Polar Res. 21, 167–208.
Hop, H., Pearson, T., and Hegseth, E. N. (2019). The Ecosystem of Kongsfjorden, Svalbard, eds H. Hop and C. Wiencke. Cham: Springer International Publishing.
Jones, E., Tselepides, A., Bagley, P., Collins, M., and Priede, I. (2003). Bathymetric distribution of some benthic and benthopelagic species attracted to baited cameras and traps in the deep eastern Mediterranean. Mar. Ecol. Prog. Ser. 251, 75–86. doi: 10.3354/meps251075
Jones, E. G., Collins, M. A., Bagley, P. M., Addison, S., and Priede, I. G. (1998). The fate of cetacean carcasses in the deep sea: observations on consumption rates and succession of scavenging species in the abyssal north-east Atlantic Ocean. Proc. R. Soc. Lond. Ser. B Biol. Sci. 265, 1119–1127. doi: 10.1098/rspb.1998.0407
Kaczmarek, H., Wlodarska-Kowalczuk, M., Legezynska, J., and Zajaczkowski, M. (2005). Shallow sublittoral macrozoobenthos in Kongsfjord, West Spitsbergen, Svalbard. Polish Polar Res. 26, 137–155.
Kȩdra, M., Legeżyńska, J., and Walkusz, W. (2011). Shallow winter and summer macrofauna in a high Arctic fjord (79° N, Spitsbergen). Mar. Biodivers. 41, 425–439. doi: 10.1007/s12526-010-0066-8
Lapointe, V., and Sainte-Marie, B. (1992). Currents, predators, and the aggregation of the gastropod Buccinum undatum around bait. Mar. Ecol. Prog. Ser. 85, 245–257. doi: 10.3354/meps085245
Lefauconnier, B., Hagen, J. O., and Rudant, J. P. (1994). Flow speed and calving rate of Kongsbreen glacier, Svalbard, using SPOT images. Polar Res. 13, 59–65. doi: 10.3402/polar.v13i1.6681
Legeżyńska, J. (2001). Distribution patterns and feeding strategies of lysianassoid amphipods in shallow waters of an Arctic fjord. Polish Polar Res. 22, 173–186.
Legeżyńska, J. (2008). Food resource partitioning among Arctic sublittoral lysianassoid amphipods in summer. Polar Biol. 31, 663–670. doi: 10.1007/s00300-008-0404-5
Legeżyńska, J., Kȩdra, M., and Walkusz, W. (2012). When season does not matter: summer and winter trophic ecology of Arctic amphipods. Hydrobiologia 684, 189–214. doi: 10.1007/s10750-011-0982-z
Legeżyńska, J., Wȩsławski, J., and Presler, P. (2000). Benthic scavengers collected by baited traps in the high Arctic. Polar Biol. 23, 539–544. doi: 10.1007/s003000000118
Leigh, K. L., Sparks, J. P., and Bemis, W. E. (2016). Food preferences of atlantic hagfish, myxine glutinosa, assessed by experimental baiting of traps. Copeia 104, 623–627. doi: 10.1643/ce-15-353
Mai, J. F., and Scherer, M. (2017). Simulating Copulas: Stochastic Models, Sampling Algorithms, and Applications, 2nd ed. Singapore. Singapore: World Scientific Publishing.
Markowska, M., Janecki, T., and Kidawa, A. (2008). Field observations of the spider crab, Hyas araneus (L., 1758): feeding behaviour in an Arctic fjord. Crustaceana 81, 1211–1217. doi: 10.1163/156854008x374531
Martel, A., Larrivée, D. H., Klein, K. R., and Himmelman, J. H. (1986). Reproductive cycle and seasonal feeding activity of the neogastropod Buccinum undatum. Mar. Biol. 92, 211–221. doi: 10.1007/bf00392838
Martini, F. H. (1998). “The ecology of hagfishes,” in The Biology of Hagfishes, eds J. P. Lomholt, H. Malte, R.E. Weber and J. M. Jørgensen (Berlin: Springer), 57–77. doi: 10.1007/978-94-011-5834-3_5
Morata, N., Michaud, E., and Włodarska-Kowalczuk, M. (2015). Impact of early food input on the Arctic benthos activities during the polar night. Polar Biol. 38, 99–114. doi: 10.1007/s00300-013-1414-5
Morton, B., and Jones, D. S. (2003). The dietary preferences of a suite of carrion-scavenging gastropods (Nassariidae, Buccinidae) in princess royal harbour, Albany, Western Australia. J. Molluscan Stud. 69, 151–156. doi: 10.1093/mollus/69.2.151
Morton, B., and Yuen, W. Y. (2000). The feeding behaviour and competition for carrion between two sympatric scavengers on a sandy shore in Hong Kong: the gastropod, Nassarius festivus (Powys) and the hermit crab, Diogenes edwardsii (De Haan). J. Exp. Mar. Bio. Ecol. 246, 1–29. doi: 10.1016/s0022-0981(99)00170-7
Nielsen, C. (1974). Observations on Buccinum undatum L. attacking bivalves and on prey responses, with a short review on attack methods of other prosobranchs. Ophelia 13, 87–108. doi: 10.1080/00785326.1974.10430593
Nygård, H., Berge, J., Søreide, J., Vihtakari, M., and Falk-Petersen, S. (2012). The amphipod scavenging guild in two Arctic fjords: seasonal variations, abundance and trophic interactions. Aquat. Biol. 14, 247–264. doi: 10.3354/ab00394
Oliver, J. S., and Slattery, P. N. (1985). Destruction and opportunity on the sea floor: effects of gray whale feeding. Ecology 66, 1965–1975. doi: 10.2307/2937392
Olsen, E., Aanes, S., Mehl, S., Holst, J. C., Aglen, A., and Gjøsæter, H. (2009). Cod, haddock, saithe, herring, and capelin in the Barents Sea and adjacent waters: a review of the biological value of the area. ICES J. Mar. Sci. 67, 87–101. doi: 10.1093/icesjms/fsp229
Ostaszewska, K., Balazy, P., Berge, J., Johnsen, G., and Staven, R. (2017). Seabirds during arctic polar night: underwater observations from Svalbard Archipelago, Norway. Waterbirds 40, 302–308. doi: 10.1675/063.040.0301
Overland, J., Dunlea, E., Box, J. E., Corell, R., Forsius, M., Kattsov, V., et al. (2019). The urgency of Arctic change. Polar Sci. 21, 6–13. doi: 10.1016/j.polar.2018.11.008
Piepenburg, D. (2005). Recent research on Arctic benthos: common notions need to be revised. Polar Biol. 28, 733–755. doi: 10.1007/s00300-005-0013-5
Piskozub, J. (2017). Svalbard as a study model of future High Arctic coastal environments in a warming world. Oceanologia 59, 612–619. doi: 10.1016/j.oceano.2017.06.005
Premke, K., Klages, M., and Arntz, W. E. (2006). Aggregations of Arctic deep-sea scavengers at large food falls: temporal distribution, consumption rates and population structure. Mar. Ecol. Prog. Ser. 325, 121–135. doi: 10.3354/meps325121
Presler, P. (1986). Necrophagous invertebrates of the Admiralty Bay of King George Island (South Shetland Islands, Antarctica). Polish Polar Res. 7, 25–61.
R Core Team (2020). R: A language and Environment for Statistical Computing. Vienna: R Foundation for Statistical Computing.
Renaud, P. E., Ambrose, W. G., and Wȩsławski, J. M. (2020). “Benthic communities in the polar night,” in POLAR NIGHT Marine Ecology. Advances in Polar Ecology, Vol. 4, eds J. Berge, G. Johnsen, and J. Cohen (Berlin: Springer), 161–179. doi: 10.1007/978-3-030-33208-2_6
Renaud, P. E., Berge, J., Varpe, Ø, Lønne, O. J., Nahrgang, J., Ottesen, C., et al. (2012). Is the poleward expansion by Atlantic cod and haddock threatening native polar cod, Boreogadus saida? Polar Biol. 35, 401–412. doi: 10.1007/s00300-011-1085-z
Renaud, P. E., Riedel, A., Michel, C., Morata, N., Gosselin, M., Juul-Pedersen, T., et al. (2007). Seasonal variation in benthic community oxygen demand: a response to an ice algal bloom in the Beaufort Sea, Canadian Arctic? J. Mar. Syst. 67, 1–12. doi: 10.1016/j.jmarsys.2006.07.006
Sainte-Marie, B. (1986). Feeding and swimming of lysianassid amphipods in a shallow cold-water bay. Mar. Biol. 91, 219–229. doi: 10.1007/bf00569437
Sainte-Marie, B., Percy, J. A., and Shea, J. R. (1989). A comparison of meal size and feeding rate of the lysianassid amphipods Anonyx nugax, Onisimus (=Pseudalibrotus) litoralis and Orchomenella pinguis. Mar. Biol. 102, 361–368. doi: 10.1007/bf00428488
Sakshaug, E., Johnsen, G., Kristiansen, S., von Quillfeldt, C. H., Rey, F., Slagstad, D., et al. (2009). “Phytoplankton and primary production,” in Ecosystem Barents Sea, eds E. Sakshaug, G. Johnsen, and K. M. Kovacs (Trondheim: Tapir Academic Press), 167–208.
Schembri, P. J. (1982). Feeding behaviour of fifteen species of hermit crabs (Crustacea: Decapoda: Anomura) from the Otago region, southeastern New Zealand. J. Nat. Hist. 16, 859–878. doi: 10.1080/00222938200770691
Shelton, R. G. J. (1978). On the feeding of the hagfish myxine glutinosa in the North Sea. J. Mar. Biol. Assoc. U.K. 58, 81–86. doi: 10.1017/s0025315400024413
Smale, D. A., Barnes, D. K. A., Fraser, K. P. P., Mann, P. J., and Brown, M. P. (2007). Scavenging in Antarctica: intense variation between sites and seasons in shallow benthic necrophagy. J. Exp. Mar. Bio. Ecol. 349, 405–417. doi: 10.1016/j.jembe.2007.06.002
Smith, C. R., De Master, D. J., Thomas, C., Sršen, P., Grange, L., Evrard, V., et al. (2012). Pelagic-benthic coupling, food banks, and climate change on the west Antarctic Peninsula shelf. Oceanography 25, 189–201.
Somerfield, P. J., and Clarke, K. R. (2013). Inverse analysis in non-parametric multivariate analyses: distinguishing groups of associated species which covary coherently across samples. J. Exp. Mar. Biol. Ecol. 449, 261–273. doi: 10.1016/j.jembe.2013.10.002
Stockton, W. L., and DeLaca, T. E. (1982). Food fall in the deep sea: occurence, quality and significance. Deep Sea Res. 29, 157–169. doi: 10.1016/0198-0149(82)90106-6
Taylor, J. D. (1978). The diet of Buccinum undatum and Neptunea antiqua (Gastropoda: Buccinidae). J. Conch, Lond. 3, 309–318.
Thatje, S., Dunbar, C. G., and Smith, K. E. (2019). Temperature-driven inter-annual variability in reproductive investment in the common whelk Buccinum undatum. J. Sea Res. 148–149, 17–22. doi: 10.1016/j.seares.2019.03.003
Van Pelt, W., and Kohler, J. (2015). Modelling the long-term mass balance and firn evolution of glaciers around Kongsfjorden, Svalbard. J. Glaciol. 61, 731–744. doi: 10.3189/2015jog14j223
Voronkov, A., Hop, H., and Gulliksen, B. (2016). Zoobenthic communities on hard-bottom habitats in Kongsfjorden. Svalbard. Polar Biol. 39, 1–19.
Wei, T., and Simko, V. (2017). corrplot: Visualization of a Correlation Matrix. Available online at: https://github.com/taiyun/corrplot (accessed March 1, 2021).
Wiencke, C., and Hop, H. (2016). Ecosystem Kongsfjorden: new views after more than a decade of research. Polar Biol. 39, 1679–1687. doi: 10.1007/s00300-016-2032-9
Włodarska-Kowalczuk, M. (2007). Molluscs in Kongsfjorden (Spitsbergen, Svalbard): a species list and patterns of distribution and diversity. Polar Res. 26, 48–63. doi: 10.1111/j.1751-8369.2007.00003.x
Włodarska-Kowalczuk, M., Górska, B., Deja, K., and Morata, N. (2016). Do benthic meiofaunal and macrofaunal communities respond to seasonality in pelagial processes in an Arctic fjord (Kongsfjorden, Spitsbergen)? Polar Biol. 39, 2115–2129. doi: 10.1007/s00300-016-1982-2
Włodarska-Kowalczuk, M., Wȩsławski, J. M., and Kotwicki, L. (1998). Spitsbergen glacial bays macrobenthos - a comparative study. Polar Biol. 20, 66–73. doi: 10.1007/s003000050277
www1 (2021). Atlantic Cod Decomposition – Winter. Available online at: https://youtu.be/AKWVvK0Z6Xg (accessed March 21, 2021).
Keywords: decomposition, carrion, necrophage, time lapse, image analysis, SCUBA
Citation: Balazy P, Anderson MJ, Chelchowski M, Włodarska-Kowalczuk M, Kuklinski P and Berge J (2021) Shallow-Water Scavengers of Polar Night and Day – An Arctic Time-Lapse Photography Study. Front. Mar. Sci. 8:656772. doi: 10.3389/fmars.2021.656772
Received: 21 January 2021; Accepted: 12 April 2021;
Published: 24 May 2021.
Edited by:
Paul Snelgrove, Memorial University of Newfoundland, CanadaReviewed by:
Neus Campanyà-Llovet, University of the Azores, PortugalCopyright © 2021 Balazy, Anderson, Chelchowski, Włodarska-Kowalczuk, Kuklinski and Berge. This is an open-access article distributed under the terms of the Creative Commons Attribution License (CC BY). The use, distribution or reproduction in other forums is permitted, provided the original author(s) and the copyright owner(s) are credited and that the original publication in this journal is cited, in accordance with accepted academic practice. No use, distribution or reproduction is permitted which does not comply with these terms.
*Correspondence: Piotr Balazy, YmFsYXp5QGlvcGFuLnBs
Disclaimer: All claims expressed in this article are solely those of the authors and do not necessarily represent those of their affiliated organizations, or those of the publisher, the editors and the reviewers. Any product that may be evaluated in this article or claim that may be made by its manufacturer is not guaranteed or endorsed by the publisher.
Research integrity at Frontiers
Learn more about the work of our research integrity team to safeguard the quality of each article we publish.