- Blue Biotechnology & Aquatic Bioproducts (B3Aqua), National Institute of Marine Sciences and Technologies (INSTM), University of Carthage, Tunis, Tunisia
Dictyotales, a common taxonomic group of brown seaweeds found in warm and temperate waters, are known for their richness in bioactive metabolites. In this study, six species of Dictyotales (Dictyopteris polypodioides, Dictyota dichotoma, Dictyota fasciola, Dictyota spiralis, Padina pavonica, and Taonia atomaria) collected from the Tunisian coasts were investigated for their antioxidant potentials, based on their contents of high added-value bioactive metabolites such as fucoxanthin and polyphenols. Fucoxanthin and polyphenols were analyzed quantitatively by high-performance liquid chromatography (HPLC) and UV spectrophotometer, respectively. The antioxidant property of extracts was also determined based on their ability to scavenge 2,2-Diphenyl-1-picrylhydrazyl (DPPH’s) free radical. Thus, the highest concentrations of fucoxanthin were obtained from T. atomaria and D. polypodioides (5.53 ± 1.2 and 3.43 ± 1.3 mg⋅g–1 dry weight, respectively), while the lowest amount was from D. spiralis (0.23 ± 0.1 m mg⋅g–1 dry weight). Dictyota dichotoma and T. atomaria gave the highest total phenol content (19.3 ± 0.4 and 15.2 ± 1.1 mg GAE⋅g–1 dry weight, respectively). In the second step, supercritical carbon dioxide (ScCO2) was used as a green and environmentally safe technique for the extraction of fucoxanthin from the most abundant species D. polypodioides. The extraction of fucoxanthin by ScCO2 allowed an extraction yield ranging from 0.50 ± 0.04% to 1.32 ± 0.02%, with 60°C temperature and 50-MPa pressure as the best extraction conditions. The maximum fucoxanthin and polyphenol recovery in the extract attained 15 and 64%, respectively. The results strengthen the possible use of Dictyotales from the Mediterranean Sea as a promising source of natural ingredients of health and economic interests contributing to Blue Growth in the region.
Introduction
Seaweeds are one of the main components of marine ecosystems; being primary producers in the food chain, they play a key ecological role in coastal ecosystems and are therefore a key element of the Blue Economy. In the marine environment, seaweeds are exposed to important ecological pressures such as light and temperature fluctuation, grazing, and fouling. Thus, in order to preserve their survival, algae developed various defense mechanisms including the production of secondary metabolites with biological properties (Da Gama et al., 2002; Ganesan et al., 2019). Such metabolites represented by a large number of potent antioxidant compounds including carotenoids, phlorotannins, and sulfated polysaccharides make algae valuable sources for exploitation (Corsetto et al., 2020).
Among these compounds, fucoxanthin, a natural carotenoid pigment, is commonly distributed in brown algae and diatoms. As a photosynthetic pigment (accessory pigment), fucoxanthin serves to harvest and transfer light energy to the photochemical reaction centers (Govindjee., 1999). In algae, carotenoids play also the important role of photoprotection, and this confers them various biological properties. As other carotenoids, fucoxanthin has interesting pharmacological properties.
Several studies reported its antiproliferative effects on cancer cells and cancer-preventive effects generated by modulating the expression of various cellular molecules and cellular signal transduction pathways (Takahashi et al., 2015; Satomi, 2017; Wang et al., 2019; Méresse et al., 2020). All of these findings gave fucoxanthin a good position as a natural cancer-preventive agent in the strategies to combat cancer. The recent study conducted by Pruteanu et al. (2020) strengthens the importance of nutraceutical supplementation of fucoxanthin in targeting challenging disease. In fact, the authors demonstrated that this pigment synergizes with the prototypic phosphatidylinositol 3-kinase (PI3K) inhibitor in producing growth arrest of glioblastoma cells, illustrating the potential of nutri-pharmaceutical combinations for cancer treatment.
Among other biological properties that make fucoxanthin of interest is its antiobesity property (Miyashita, 2014; Maeda, 2015; Dai and Kim, 2016). As overweight and obesity correspond to the fifth risk factor of deaths at a worldwide level, according to the World Health Organization (WHO, 2016), fucoxanthin represents a promising solution for antiobesity therapy. The low toxicity and the effective attenuation of body and white adipose tissue weight gain suggest that fucoxanthin and its derivatives can be used in human health supplement (Muradian et al., 2015). This is also supported by hyperglycemia described in several studies in genetically modified or normal mice under high-fat diets (Muradian et al., 2015). Gammone and D’Orazio (2015) reviewed the mechanism of action and metabolic pathway of fucoxanthin in the lipid metabolism, which has been well documented lately in animal model. Moreover, fucoxanthin displays an antidiabetic activity (Maeda, 2015; Kawee-Ai et al., 2019). Pangestuti et al. (2013) described its possible action in Alzheimer disease by attenuating inflammation and oxidative responses in microglia.
Additionally to fucoxanthin, brown algae are characterized by important levels of polyphenols, with high biological activities. Phenolic compounds from marine macroalgae vary from those of terrestrial plants, as they are usually derived from phloroglucinol and constitute often highly complex compounds called phlorotannins (Generalić Mekinić et al., 2019). Phlorotannins have exhibited protective effects against hyperglycemia, hyperlipidemia, inflammation, and oxidative stress, and several cardiovascular and diabetic complications, in cell culture, animal studies, and some human studies (Thomas and Kim, 2011; Murray et al., 2018a,b). Parada et al. (2019) reported the potential use of seaweed polyphenols to develop new low-glycemic-response foods through the inhibition of digestive enzymes related with the breakdown of glycemic carbohydrates.
The recovery of products from algal biomass is a matter of constant development and progress since among the modern world challenges, there is the need for compounds with a high-level bioactivity with no side effects obtained using sustainable and environmentally friendlier extraction methods (Sosa-Hernández et al., 2018). Conventional extraction of these metabolites by maceration in organic solvents can pose risk to health and the environment, with the most commonly used solvents being toluene, hexane, methanol, or petroleum ether (Quitain et al., 2013). The use of supercritical fluid extraction (SFE), considered as an environmentally safer and green extraction method, can be an alternative to solvent extraction for seaweed metabolites (Crampon et al., 2011; Chemat et al., 2012; Cikoš et al., 2018). Indeed, CO2 is a safe and non-flammable solvent with selectivity depending on pressure and temperature (Crampon et al., 2011). Due to its low viscosity and higher diffusion coefficient, increased mass transfer in supercritical carbon dioxide (ScCO2) extraction is a major advantage (Cikoš et al., 2018). Since carbon dioxide is a nonpolar solvent, ScCO2 is appropriate for nonpolar to low-polarity compound extraction, unless enhanced by a polar co-solvent (Shipeng et al., 2015; Saravana et al., 2017). Lourenço-Lopes et al. (2020) reviewed scientific approaches on extraction, purification, and stability of fucoxanthin, highlighting that most studies are still performed using conventional techniques; nevertheless, nonconventional techniques, such as SFE, are starting to gain popularity in the recovery of this compound.
The extraction of these bioactive compounds covered various brown algae from the genera Undaria, Laminaria, Eisenia, Alaria, Hijikia, and Sargassum (Lourenço-Lopes et al., 2020). However, except for Sargassum, the other most studied genera cannot be found in the Mediterranean Sea. On the other hand, Dictyotales (Ochrophyta, Phaeophyceae) are common in warm and temperate waters. A total of 317 species have been reported all over the world (Guiry and Guiry, 2020): 17 in the Mediterranean Sea (Ribera et al., 1992) and seven along the Tunisian coasts (Ben Maïz, 1995). Additionally, the Dictyotales order are known to produce a significant number of bioactive secondary metabolites, especially diterpenes (Ktari et al., 2010), polyphenols (Zubia et al., 2009), and sulfated polysaccharides (Teodosio Melo et al., 2013).
This work aimed to analyze fucoxanthin and the polyphenolic content of six Dictyotales collected from the Tunisian coasts. This work presents two parts: a comparative study of fucoxanthin, the polyphenol content, and DPPH antiradical-scavenging properties between six species of common brown algae collected from the Tunisian coasts; and secondly, the extraction procedure by a green method ScCO2 from the selected species, Dictyopteris polypodioides. Thus, this study may contribute to fulfill the gap of knowledge on the antioxidant properties of seaweeds collected from the southern Mediterranean coasts.
Materials and Methods
Algal Material
Six species from Dictyotales order were collected in shallow water (depth > 1.5 m) from April to July 2016 from the rocky shores of Cap Zebib (North of Tunisia) (37°15’49.66’N and 10°04’02.85”E): Dictyopteris polypodioides (De Candolle) Lamouroux, Dictyota dichotoma (Hudson) Lamouroux, Dictyota fasciola (Roth) Lamouroux, Dictyota spiralis Montagne, Padina pavonica (Linnaeus) Thivy, and Taonia atomaria (Woodward) Agardh. The whole thalli of macroalgae were collected randomly with several specimens per species. Seaweeds were identified based on morphological characteristics with the use of taxonomic keys (Hamel, 1975). To avoid mixture of species, a thorough verification was performed when cleaning the algae, and specimens of the same identified species were put in the same lot. Voucher specimens for each species were kept in 2% formaldehyde solution at the National Institute of Marine Sciences and Technologies. Algae were washed with seawater and then with tap water to remove epiphytes and excess salt and oven-dried at 40°C for 3 days. Dry algae were then weighted, grinded with an electrical grinder, and stored in glass jars sheltered from light and humidity.
Solvent and Reagents
All used solvents were of analytical grade except those used for high-performance liquid chromatography (HPLC), which were of HPLC grade. The Folin–Ciocalteu phenol reagent, 2,2-Diphenyl-1-picrylhydrazyl (DPPH), was purchased from Sigma-Aldrich Chemical Co. (Steinheim, Germany). Pure fucoxanthin was purchased from Merck as powder for reference.
Extraction Procedure
Phytochemical extraction has been done according to Terasaki et al. (2009), with slight modifications. Five grams of grinded seaweeds was extracted by maceration in 50 ml of MeOH overnight at room temperature, which was performed twice. Combined extracts were filtered, and MeOH was evaporated with a rotary evaporator. The extracts were weighed and kept at -20°C prior to analysis. Three extraction replicates were performed for each species.
Fucoxanthin Content
Extracts were re-dissolved in MeOH at 1 mg⋅ml–1 and filtered with a 0.22-μm membrane filter prior to HPLC injection. HPLC analyses were performed according to Steel and Keller (2000) and were carried out with an analytical KNAUER HPLC equipped with a S1000 pump and S2500 UV/Vis detector. The fucoxanthin content (FC) was determined by Eurospher 100-5 C18 reversed-phase column (5-μm particle size, 250 × 4.6 mm). Separation was performed with gradient elution of acetone/water (7:3, v/v) for 20 min followed by 100% acetone for the remaining 35 min at the flow rate of 1.0 ml⋅min–1 and the detection wavelength of 450 nm. A standard curve prepared using authentic standard was used for quantification of the FC in seaweed samples. The FC in seaweed samples was expressed as mg⋅g–1 dry weight (dw) of seaweed sample. The amount of fucoxanthin was quantified from the peak area using the standard calibration curve in the concentrations ranging from 0.01 to 1 mg⋅ml–1.
Total Phenolic Content
Total phenolic content (TPC) was determined by the Folin–Ciocalteu method in accordance with a protocol described by Dewanto et al. (2002). Gallic acid was used as a reference standard for plotting calibration curve. An aliquot of 125 μl of algae extract was mixed with 500 μl of distilled water and 125 μl of Folin–Ciocalteu reagent. Following agitation and 3-min incubation in the dark at room temperature, 1,250 μl of Na2CO3 (7%) was added, followed by 90-min incubation (in dark). The absorbance was measured using a spectrophotometer (JENWAY 6405 UV/Vis) at 760 nm. Results were expressed as mg gallic acid equivalent (GAE)⋅g–1 dw. The total polyphenol concentration is calculated by using the following equation:
with C as the total polyphenol content (mg GAE⋅g–1 dw), c as the concentration of gallic acid determined from the calibration curve (mg⋅L–1), V as the volume of the extract (L), and m as the mass of dry material used (g).
All tests were done in triplicate.
DPPH Radical-Scavenging Assay
The free-radical-scavenging capacity of each extracts was analyzed using the 2,2-Diphenyl-1-picrylhydrazyl (DPPH) test according to the method of Farasat et al. (2013). Briefly, 100 μl of sample extract with various dilutions was mixed with 100 μl of 0.16 mM DPPH solution. The solution was kept at room temperature for 30 min, and the absorbance was measured at 517 nm in an automated microplate reader. The DPPH-scavenging effect was calculated as follows:
Ac is the absorbance of the control (DPPH without sample), As is the absorbance of the test sample (the sample test and DPPH solution), and Ab is the absorbance of the sample blank (sample without the DPPH solution).
The half-maximal inhibitory concentration (IC50) was calculated based on trend lines obtained from DPPH% inhibition curves for each species. Furthermore, the lower the IC50, the stronger the antioxidant activity. Ascorbic acid was used as positive control. Extracts with an IC50 higher than 10 mg⋅ml–1 were considered as non-active extracts (Tanniou et al., 2013).
Supercritical CO2 Extraction From Dictyopteris polypodioides
The supercritical CO2 extraction was carried out in an apparatus of Supercritical Fluid Technologies Inc. (SFT-110). The schematic flow diagram is given in Figure 1. The apparatus includes a high-pressure pump for CO2 (SFT 10-CO2 pump), a heating chamber with a 100-ml stainless steel extraction vessel, and pressure regulator valves. In each experiment, 15 g of Dictyopteris polypodioides powder was loaded into the extraction vessel. A high pressure was applied to pump liquid CO2 into the extraction vessel to reach the desired pressure. Conditions of three temperatures (25, 40, and 60°C) and three pressures (30, 40, and 50 MPa) were tested. The CO2 flow rate was constant during the entire extraction period. Two flow rates were tested at 24 and 15 ml⋅min–1. The extract was collected at the end of the extraction process after at least 3 h or until the extract was colorless. Extracts were weighed to determine extraction yield and kept at −30°C before fucoxanthin, polyphenol, and DPPH analyses.
Statistical Analysis
All assays were performed in triplicate except for ScCO2 extraction (n=2). One-way ANOVA was performed, and significant differences (p < 0.05) were determined by Tukey’s test. Principal component analysis (PCA) was carried out to understand the correlations and identify relationships between the different variables. All statistical analyses were performed using XLSTAT 2016.1 by Addinsoft.
Results
Phytochemical Content of Dictyotales
Extraction Yield
In the first step, we studied the phytochemical content of the six Dictyotales collected on the Tunisian coasts.
Extraction yields in percentage, obtained for the different species, are given in Table 1. Among the six species, Dictyota dichotoma and Taonia atomaria extracts resulted in the highest and significantly different (p<0.05) extraction yields (4.34 ± 0.63 and 3.48 ± 0.38, respectively) followed by Dictyota fasciola and Dictyopteris polypodioides (2.15 ± 1.01 and 1.81 ± 0.09), while Padina pavonica and Dictyota spiralis gave the lowest yields (0.74 ± 0.38 and 0.73 ± 0.13), evidencing an inter-specific variation of secondary metabolites with different properties.
Fucoxanthin Content
The FC of Dictyotales extracts was obtained in the range of 0.19 ± 0.08 and 0.03 ± 0.02 mg⋅g–1 extract depending on the species (Table 1). Among the six species, D. polypodioides displayed the highest and significantly different (p<0.05) value of the FC followed by T. atomaria (0.16 ± 0.03 mg⋅g–1 extract), whereas D. fasciola and D. spiralis extracts showed the lowest amount of FC (0.04 ± 0.01 and 0.03 ± 0.02 mg⋅g–1 extract, respectively).
The estimation of the FC in dry weight, based on extraction yield for each species, resulted in T. atomaria presenting the highest significantly different amount (at 95% confidence level) (5.53 ± 1.2 mg⋅g–1 dw), followed by D. polypodioides (3.43 ± 1.3 mg⋅g–1 dw) (Table 1). Additionally, D. dichotoma gave a relatively high amount of fucoxanthin with 2.6 ± 1.0 mg⋅g–1 dw. The lowest FC was obtained for D. spiralis with 0.23 ± 0.1 mg⋅g–1 dw, while values obtained for D. fasciola (0.88 ± 0.3 mg⋅g–1 dw) and P. pavonica (0.71 ± 0.1 mg⋅g–1 dw) were not significantly different.
Total Phenolic Content
The TPC of the Dictyotales species ranged from 0.25 ± 0.02 to 0.54 ± 0.09 mg GAE⋅mg–1 extract (Table 1). The order of concentrations of total phenolic compounds in the six Dictyotales extracts was as follows: P. pavonica >T. atomaria = D. dichotoma >D. fasciola >D. spiralis >D. polypodioides. In fact, the higher significantly different TPC (p<0.05) was obtained for P.pavonica extract with 0.54±0.09mgGAE⋅mg–1 extract. Extracts of T. atomaria, D. dichotoma, and D. fasciola showed a lower TPC with no significant differences for the three species, whereas D. spiralis and Dictyopteris polypodioides gave lower significantly different TPCs (0.32±0.04 and 0.25±0.02mgGAE⋅mg–1 extract, respectively).
The estimation of the TPC in dry weight based on extraction yield for each species resulted in the TPC ranging between 19.3±0.4 and 2.5±0.1mg⋅g–1 dw. The order of concentrations of total phenolic compounds in dry algae was obtained as follows: D. dichotoma >T. atomaria >D. fasciola >D. polypodioides >P. pavonica >D. spiralis. Statistical analysis showed no significant differences (at 95% of confidence level) between D. dichotoma and T. atomaria, the two higher TPCs, and P. pavonica and D. spiralis, the two lower ones (Table 1).
DPPH Radical-Scavenging Capacity
Figure 2 represents the percentage of DPPH radical scavenging obtained for the six Dictyotales species at a concentration of 10 mg⋅ml–1 of extract. The higher significantly radical-scavenging capacity was obtained for P. pavonica and T. atomaria with 66.76 and 63.36%, respectively. Whereas, D. fasciola and D. spiralis gave significantly the lowest DPPH inhibition percentage (25.36 and 22.73%).
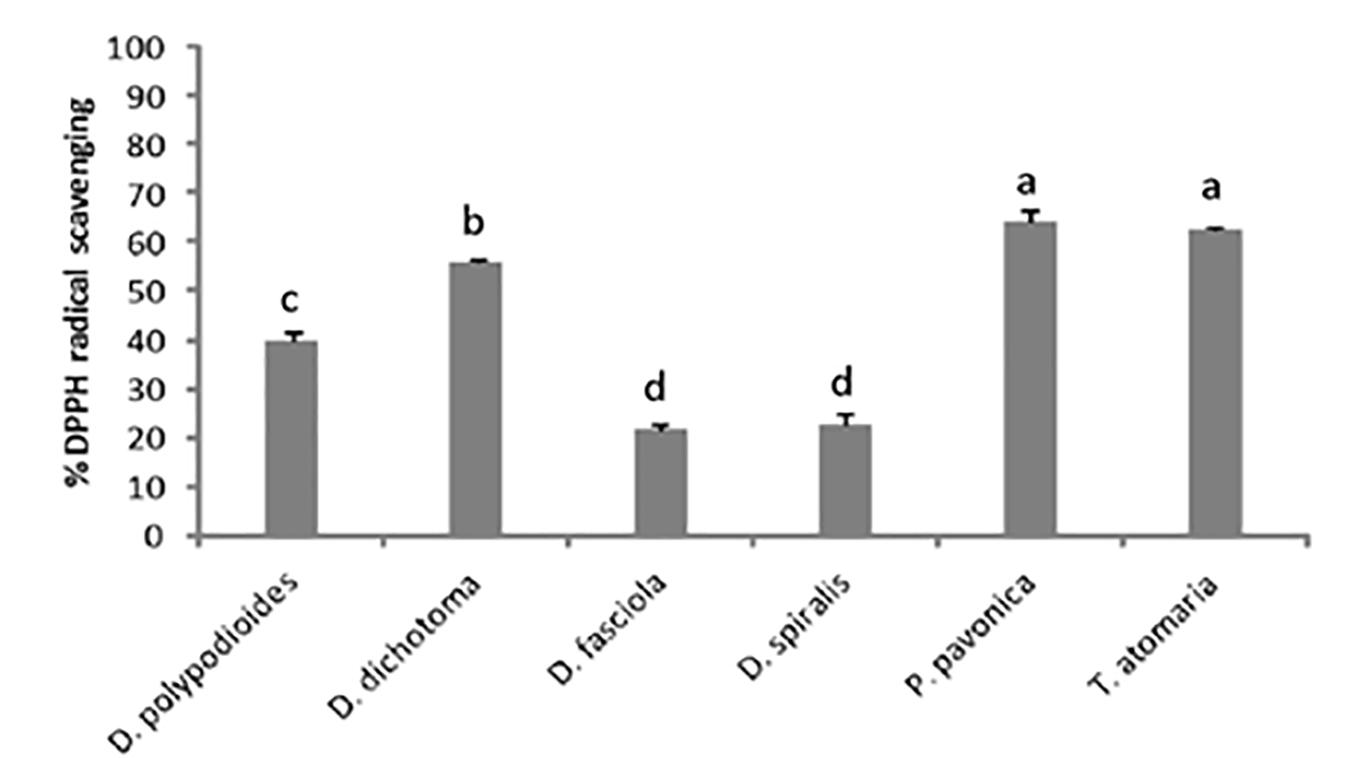
Figure 2. Percentage DPPH radical-scavenging capacity of Dictyotales MeOH extracts at a concentration of 10 mg⋅ml–1.
In Table 2 is presented the calculated IC50 based on trend lines obtained from DPPH% radical-scavenging curves of each species. P. pavonica extract had lower significantly different (p < 0.05) IC50 of 5.86 ± 0.4 mg⋅ml–1, thus presenting the most significant antiradical-scavenging capacity, followed by T. atomaria (7.58 ± 0.2 mg⋅ml–1). D. polypodioides and D. dichotoma gave higher IC50 values, therefore displaying lower antiradical-scavenging capacity, while it was not possible to calculate IC50 for both D. fasciola and D. spiralis, as no reliable trend line could be obtained from their DPPH% radical-scavenging curves (coefficient of determination R2 < 0.5). Thereby, no capacity of DPPH radical scavenging is obtained from the extracts of these two species.
The PCA of the FC, TPC, and DPPH radical-scavenging capacity (%) of the six seaweed extracts is presented in Figure 3. The first two principal components, F1 and F2, represent 97.2% of the total variance of the data set and explain it at 50.1 and 47.0%, respectively. The vectors represent a variant existing among factors (FC, TPC, and DPPH). The analysis allows to identify four groups of one or two species distributed according to two principal components. The first group is composed of T. atomaria, which represent the species with high FC TPC and high potential of DPPH scavenging, as it is on the positive side of both F1 and F2. D. polypodioides displays high FC but low values of the TPC and-scavenging potential compared with the cluster of P. pavonica and D. dichotoma, which are carried by the TPC vector. The last group is composed of D. spiralis and D. fasciola, which showed low FC and TPC and low DPPH-scavenging potential.
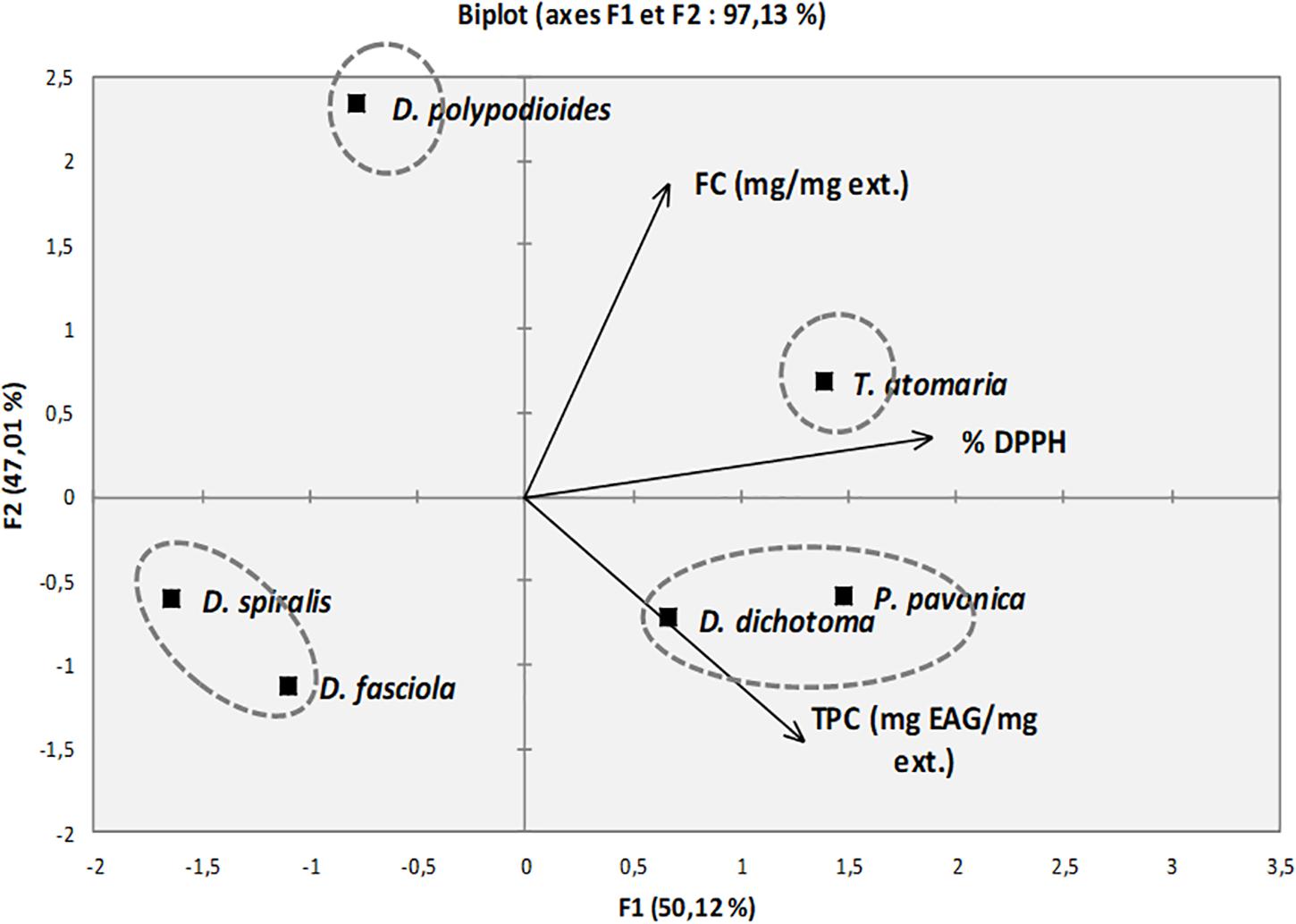
Figure 3. Principal component analysis (PCA) of fucoxanthin content (FC), total phenolic content (TPC), and DPPH radical-scavenging capacity (%) of extracts. Each species is distributed according to its variance.
Figure 4 represents the PCA performed for yield of extraction, the FC, and the TPC in the algae (dry weight) to examine their distribution among the species. The first two principal components, F1 and F2, represent 99.5% of the total variance of the data set explaining it at 82.8 and 16.7%, respectively. The vectors represent a variant existing among factors (Yield, FC, and TPC). A close loading of Yield and TPC, as both were closely located to each other, depicts a high correlation between them. The analysis allows to identify three groups of species distributed according to two principal components. The first group is composed of T. atomaria and D. polypodioides, which are both on the higher side of the second principal component carried by the FC and present high values of fucoxanthin. The second group contains D. dichotoma, which showed high yield of extraction and high phenolic content. The third cluster is composed of P. pavonica, D. spiralis, and D. fasciola, which represents the species with the lowest FC, TPC, and extraction yield as they are located on the negative side of both principal components. The analysis allowed to highlight the high potential of T. atomaria for extraction of both fucoxanthin and phenolic compounds, while D. polypodioides would be a good candidate for fucoxanthin extraction and D. dichotoma would be a good one for phenolic compound extraction.
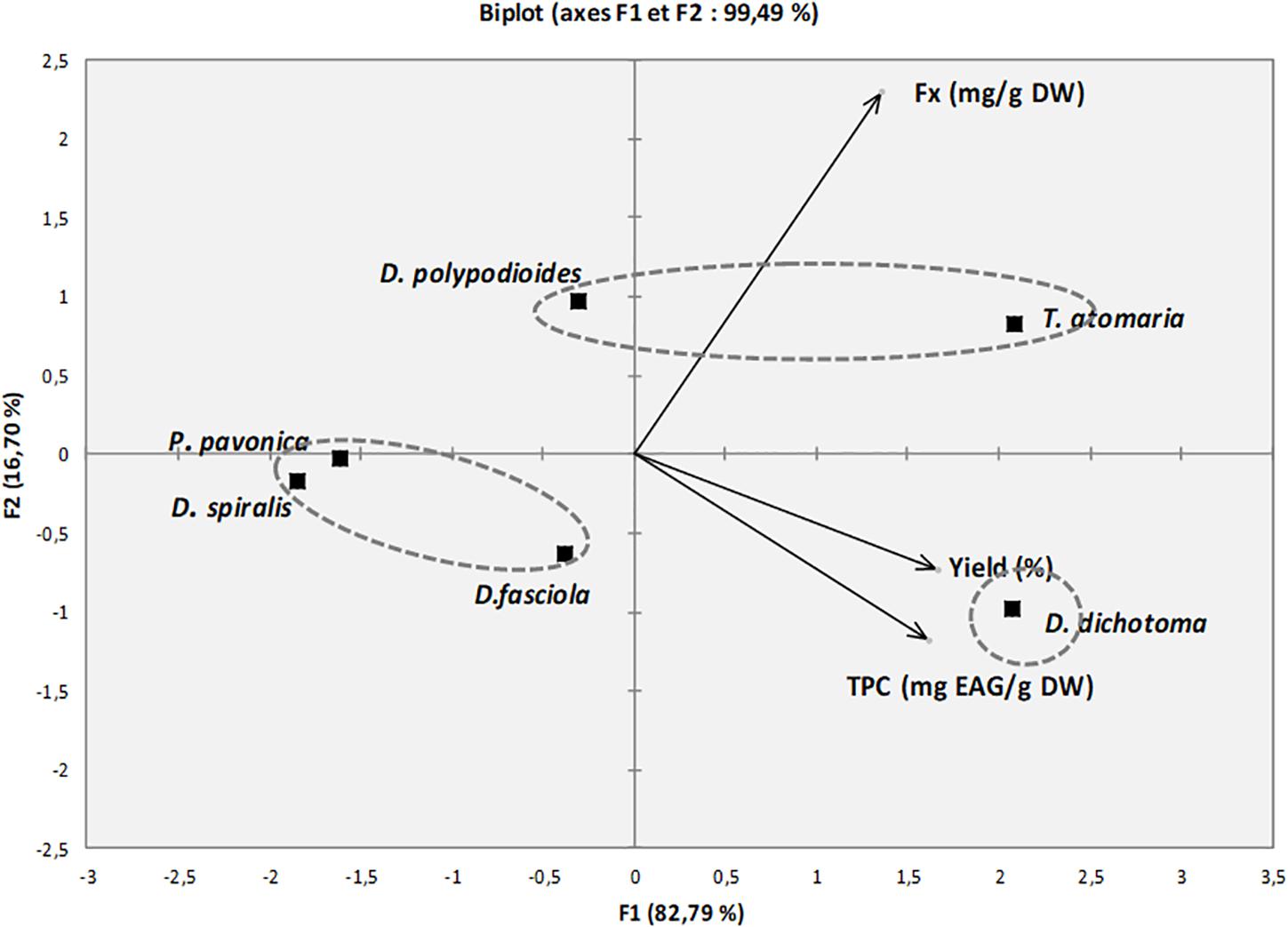
Figure 4. Principal component analysis of fucoxanthin content, total phenolic content, and yield of extraction of algae (dry weight).
Supercritical CO2 Extraction of Dictyopteris polypodioides
As fucoxanthin is of high added value and a promising future functional food, it was selected as the first criterion for choosing the species for ScCO2 extraction. Since D. polypodioides was considered as a potential candidate for fucoxanthin extraction (Figure 4) and considering its wide distribution on the Tunisian coasts and higher abundance than T. atomaria (unpublished work), this species has been selected for the ScCO2 extraction experiments. The extraction of oven-dried samples with different extraction parameters gave different yields (Table 3).
A higher yield was obtained for the following set of parameters: temperature (60°C), pressure (50 MPa), and flow rate (24 ml⋅min–1). The lower yield has been obtained for the set of parameters of 25°C, 40 MPa, and flow rate at 24 ml. Statistical analysis showed that there is a significant difference between yields obtained with temperature lower that 60°C and that obtained with an extraction temperature of 60°C. Contrarily, no significant difference has been obtained for extractions made at 60°C but with different pressures or flow rates.
Considering the fucoxanthin recovery, although the lower amount of fucoxanthin has been obtained by ScCO2 parameter set (T=60°C, P=30MPa, and F= 24 ml⋅min–1) and the higher obtained with parameter set (T=60°C, P=50MPa, and F= 24ml⋅min–1), no significance has been found between the extracts at a level of confidence of 95%. Besides, the estimation of the FC in dry algae based on extraction yield resulted in ScCO2 parameter set (T = 60°C, P=50MPa, and F= 24ml⋅min–1), allowing the highest significantly different value (at 95% confidence level) (Table 1).
The analysis of the TPC in ScCO2 extracts showed that phenolic compound extraction increased with the increase of pressure and temperature (Table 4). The highest significantly different TPCs (0.64 ± 0.04 mg GAE⋅mg–1 extract and 8.50 ± 0.52 mg GAE⋅g–1 dw) have been obtained for ScCO2 parameter set (T=60°C, P=50MPa, and F= 24ml⋅min–1).
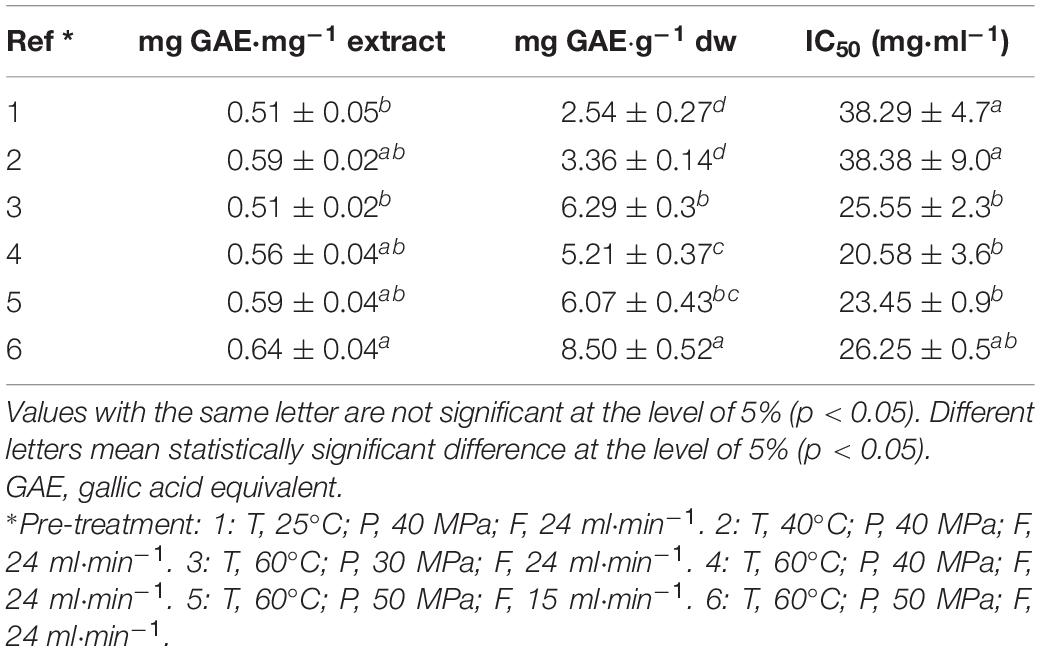
Table 4. Total phenol content and DPPH antiradical-scavenging capacity (IC50) of Dictyopteris ScCO2 extracts.
DPPH antiradical-scavenging capacity of ScCO2 extracts (IC50) ranged between 20.58 ± 3.6 and 38.38 ± 9.0 mg⋅ml–1. Extracts obtained with 60°C as extraction temperature displayed significantly lower different IC50 values, thus presenting higher antiradical-scavenging capacity than those obtained with a lower-temperature condition (25 and 40°C).
The results from the PCA of the phytochemical content (FC and TPC), DPPH antiradical capacity (IC50), and ScCO2 parameters (T° and pressure) of the six D. polypodioides extracts are presented in Figure 5. The first two principal components, F1 and F2, explained 93.9% of the total variance of the data set. The analysis of the first component (F1) highlighted strong negative correlations of the FC and TPC (on the right) with DPPH (on the left), revealing that the fraction with the highest DPPH radical-scavenging potentials (the lowest IC50 values, i.e., extracts 5 and 6) also showed the high fucoxanthin and TPC. Considering the ScCO2 parameter, the analysis depicted a high correlation between the FC, TPC, and pressure, as they were closely loaded on the positive side of first component (F1). However, there is a fair correlation of the FC and TPC with temperature as they were distantly loaded, but still all vectors are on the positive side of the F1 axis. The analysis confirms that the higher temperature and pressure conditions for SFE allowed higher fucoxanthin and phenolic compound contents.
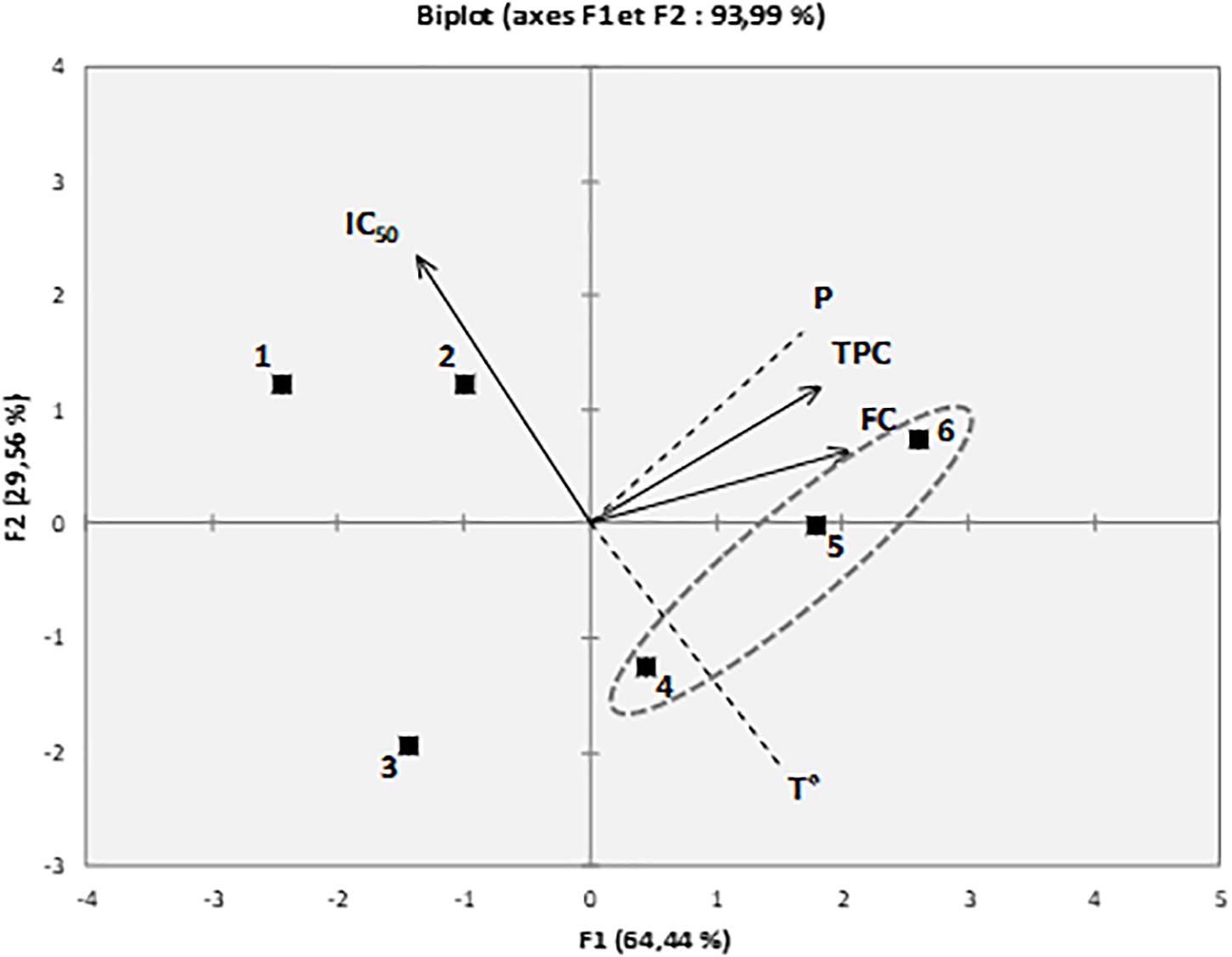
Figure 5. Principal component analysis (PCA) of fucoxanthin content (FC), total phenolic content (TPC), DPPH radical-scavenging capacity (IC50), and ScCO2 parameters [temperature (T) and pressure (P)] of D. polypodioides extracts.
Discussion
Fucoxanthin, Total Phenolic Content, and DPPH Radical-Scavenging Capacity of Dictyotales
Our results on extraction yield showed evidence of inter-specific variability. These finding are in accordance with those described by Rodrigues et al. (2020), who studied antioxidant properties of Dictyotales from Brazilian Tropical Reefs and obtained extraction yields varying up to four times from one species to another. El-Sheekh et al. (2021) also obtained significantly different yields of extraction depending on species and solvents. The morphological feature of species and environmental conditions can influence metabolites’ biosynthesis. Species with soft and thin thalli will have more easily extractible metabolites due to higher surface area and biomass ratio (Heriyanto et al., 2017). In addition, the low yield obtained for Padina pavonica can be attributed to the accumulation of CaCO3 in its tissue (Nunes et al., 2019).
Several studies showed that fucoxanthin concentration in species varies between taxonomic groups and among species of the same family or genus (Jaswir et al., 2013; Susanto et al., 2016; Koduvayur Habeebullah et al., 2018). Terasaki et al. (2009) evaluated the recoverable FC of 15 Phaeophyta species from Japanese coasts and obtained an FC that ranged from 0.1 ± 0.1 to 3.7 ± 1.6 mg⋅g–1 dw, with Sargassum horneri displaying the highest content. Additionally, the analysis of pigment composition of four brown seaweeds from the Philippine coasts showed that fucoxanthin concentrations ranged between 0.43 ± 0.11 and 4.11 ± 0.55 mg⋅g–1 dw, with Dictyota dentata (Dictyotales) presenting the highest content (Heriyanto et al., 2017). Our results demonstrated that within the Dictyotales order, there is a significant difference between species concerning their FC, highlighting two species as potential candidates for fucoxanthin extraction (Taonia atomaria and Dictyopteris polypodioides). Table 5 presents the FC of different species of Dictyotales found in the literature, showing that the amount of fucoxanthin varies according to various parameters, including species and the extraction procedure. Moreover, some contents are given on fresh weight basis and some other on dry weight basis, weakening the relevance of any comparison. However, our results highlight that Dictyotales from the Tunisian coasts present a significant amount of fucoxanthin.
The variation of the FC, as documented in different reports, depends on various abiotic and biotic factors such as season, geographical location, and life cycle (Saravana et al., 2015). The recovery of this pigment in the extract is also solvent dependent (Saravana et al., 2015; Kumar et al., 2020). Thereby, Saravana et al. (2015) reported significantly higher fucoxanthin recovery obtained for acetone–MeOH mix than with other solvents.
As reported by several studies, the concentration of phenolic compounds is highly variable among taxonomic groups and species (Dang et al., 2017; Generalić Mekinić et al., 2019). Our work highlighted the species of T. atomaria and Dictyota dichotoma being potential candidates for polyphenol extraction. These results are in agreement with those obtained by Zubia et al. (2009), who reported high values of the TPC for D. dichotoma collected from the French coasts (18.8 mg PGE⋅g–1 dw). Aly et al. (2016) reported high contents of phenolic compounds in T. atomaria extract such as vanillic acid, benzoic acid, and pyrogallol. The authors reported the relationship of phenolic and flavonoid composition with biological effects displayed by T. atomaria extracts such as antioxidant, anti-inflammatory, anti-Alzheimer, and anticancer activities.
Additionally, P. pavonica showed a high TPC in its extract. However, the extraction yield obtained for this species resulted in low phenolic content per dry weight. Abdelhamid et al. (2018) reported higher phenolic contents (7.06 mg PGE⋅g–1 dw) for P. pavonica collected from the eastern Tunisian coasts than those obtained for our sample (3.6 ± 0.2 mg GAE⋅g–1 dw). Given that this species showed the highest DPPH antiradical-scavenging capacity, several processes should be evaluated and optimized to obtain the highest yield possible. El-Sheekh et al. (2021) reported that the extraction yield of P. pavonica is doubled when using ethanol as an extraction solvent compared with methanol.
Contrary to our findings, Ben Aoun et al. (2010) obtained higher total phenolic compound values for D. polypodioides collected from the eastern Tunisian coasts. These authors reported values ranging between 33.8 and 84.96 mg GAE⋅g–1 dw, depending on the extraction solvent. These differences can be explained by the influence of several abiotic and biotic factors on the TPC, in addition to different extraction procedures from one study to another.
The genus Dictyota showed significant differences in the PCA (Figures 3, 4), particularly between Dictyota spiralis and Dictyota fasciola (formerly Dilophus genus) on the one side and D. dichotoma on the other side. Hörnig et al. (1992) merged Dilophus genus in Dictyota, based on morphological features; however, recent phylogenetic results presented by Küpper et al. (2019) argued in favor of a genus Dilophus, distinct from Dictyota. At the chemical level, since considerable variation has been observed between samples from the same Dictyota species taken at different localities and during different seasons, it is not possible to draw conclusions whether the chemical content is species-specific (Bogaert et al., 2020), though as fucoxanthin and polyphenols are dependent on environmental conditions including light exposure, the difference in their concentrations in closely related species might be due to differences among habitats (Heriyanto et al., 2017). Indeed, our specimens of D. spiralis and D. fasciola were collected in very shallow waters (less than 1 m) and light-exposed rocks, while D. dichotoma specimens were collected in shadowed rocks, as well as for D. polypodioides and T. atomaria.
ScCO2 Extraction of D. polypodioides
Several studies covered fucoxanthin extraction from seaweeds by ScCO2 technique (Roh et al., 2008; Quitain et al., 2013; Saravana et al., 2017). Quitain et al. (2013) analyzed the FC of ScCO2 extraction of Undaria pinnatifida (Laminariales). Conversely to our findings, these authors observed an influence of extraction temperature on fucoxanthin recovery in the extract, as they noticed a decrease in the FC with temperature increase, while in our study, no significant difference has been found in the fucoxanthin recovery regardless of the extraction parameter set used.
Our results showed that the optimum ScCO2 parameter set for maximum fucoxanthin recovery (2.02 ± 0.6 mg⋅g–1 dry algae) was obtained at a temperature of 60°C, pressure of 50 MPa, and a flow rate of 24 ml⋅min–1 CO2. Roh et al. (2008) obtained a maximum of fucoxanthin extracted from U. pinnatifida for a temperature of 323 K (50°C) and 200 bar (20 MPa). These authors demonstrated that fucoxanthin solubility is correlated to CO2 density fitting the Chrastil model.
For phenolic content, our results are in accordance with those achieved by Roh et al. (2008), who obtained an increase of polyphenol extraction with the increase of temperature and pressure in the ScCO2 process. Saravana et al. (2017) obtained the maximum phlorotannins extracted with the highest temperature and pressure used (55°C/300 bar). These authors prospected the effect of different co-solvent additions to ScCO2 on fucoxanthin and phenol extraction and obtained the best recovery with sunflower oil and water, respectively.
When comparing the values obtained for D. polypodioides for the FC, TPC, and DPPH IC50 depending on the extraction method, some points can be highlighted. No significant difference can be noticed between the recovery percentage of fucoxanthin in the extract regardless of the method. These findings are in agreement with those of Saravana et al. (2015), who determined that the ScCO2 process can extract a similar content of fucoxanthin similarly to solvent extraction procedure. However, the lower extraction yield registered for the ScCO2 extraction compared with the MeOH one is reflected in the FC per dry weight, which is lower for ScCO2 extraction. Several reports showed that the addition of ethanol, vegetable oils, or water as co-solvents significantly increased the efficiency of ScCO2 extraction yields (Roh et al., 2008; Saravana et al., 2017; Kumar et al., 2020).
Our results show that values of the TPC obtained by SCCO2 extraction are twice as high as those obtained by MeOH extraction. Cikoš et al. (2018), who reviewed modern methods for bioactive compound extraction from macroalgae, concluded that SFE is rarely used for the extraction of polar phenolic compounds, as this method is strongly dependent on nonpolar compound extraction unless a co-solvent is added. In accordance with these observations, Shipeng et al. (2015) reported a higher TPC in ScCO2 extract with ethanol as a co-solvent than other solvent extracted oils from S. horneri (Fucales). Besides, Tanniou et al. (2013) reported that NMR phenolic profiles of Sargassum muticum extracted by ScCO2 were different from those obtained from solvent extractions. Even though the Folin–Ciocalteu assay is the most commonly used procedure to determine the TPC, it is not specific for them, and the presence of reducing interferants (such as reducing sugars or ascorbic acid) can produce inaccurate estimations of TPC values (Sánchez-Rangel et al., 2013). Thus, deeper investigation of extract composition is needed.
About the antioxidant property of extracts, our results showed a lower DPPH antiradical-scavenging activity in ScCO2 extract compared with MeOH one. This is in agreement with the findings of Tanniou et al. (2013), who also obtained a DPPH antiradical-scavenging activity of the supercritical fluid extract much lower than that obtained by solvent extraction even though these authors used ethanol as a co-solvent in their ScCO2 procedure. Contrarily, Saravana et al. (2015) obtained a higher antioxidant property for Saccharina japonica and S. horneri ScCO2 extracts compared with the conventional solvent ones.
Thereby, the present study confirms the efficiency of ScCO2 extraction for fucoxanthin and phenolic compounds from D. polypodioides. Hattab et al. (2007), who extracted volatile compounds from Dictyopteris membranacea (syn. polypodioides) by ScCO2, demonstrated that this method takes a great importance also in the extraction of sulfur compounds, alkanes, and fatty acids, as at high pressure the fluid has low selectivity leading to the extraction of these chemical classes of compounds. Further chemical exploration of extracts is needed to determine their total composition.
Conclusion
Dictyotales are commonly distributed brown algae from the Mediterranean coasts. Among the six species investigated for high benefits in phytochemical extraction and antioxidant potential activity, three species resulted as promising candidates. Taonia atomaria and Dictyopteris polypodioides are potential candidates for fucoxanthin extraction, while Dictyota dichotoma and T. atomaria are good candidates for phenolic compound extraction. Additionally, Padina pavonica with a high DPPH radical-scavenging capacity and a high amount of phenolic compounds in its extract could be considered for antioxidant compound extraction, providing that the extraction efficiency is increased.
The use of green supercritical CO2 extraction of fucoxanthin and phenolic compounds from D. polypodioides showed that this method could give a similar content of fucoxanthin and higher phenolic content as when a solvent is used. These results are encouraging particularly for adapting an extraction method for the Mediterranean species. Such use of seaweeds, with respect to green extraction technology and sustainability of algal material supply, can represent valuable economic development perspective for the region.
Data Availability Statement
The raw data supporting the conclusions of this article will be made available by the authors, without undue reservation.
Author Contributions
LK and SS conceived and designed the experiments. CM and BA performed biochemical, ScCO2, and HPLC analyses. LK analyzed the data and prepared the original manuscript. SS and LC reviewed and edited the manuscript. All authors contributed to the manuscript and gave final approval for publication.
Funding
This work was supported by the BIOVecQ PS1.3_08 project “Biotechnologie Marine Vecteur d’Innovation et de Qualité” co-financed by the cross-border IEVP Italy Tunisia program and the Ministry of Higher Education and Scientific Research-Tunisia.
Conflict of Interest
The authors declare that the research was conducted in the absence of any commercial or financial relationships that could be construed as a potential conflict of interest.
References
Abdelhamid, A., Jouini, M., Bel Haj Amor, H., Mzoughi, Z., Dridi, D., Ben Said, R., et al. (2018). Phytochemical analysis and evaluation of the antioxidant, anti-inflammatory, and antinociceptive potential of phlorotannin-rich fractions from three mediterranean brown seaweeds. Mar. Biotechnol. 20, 60–74. doi: 10.1007/s10126-017-9787-z
Agatonovic-Kustrin, S., and Morton, D. W. (2017). High-performance thin-layer chromatography-direct bioautography as a method of choice for alpha-amylase and antioxidant activity evaluation in marine algae. J. Chromato. A. 1530, 197–203. doi: 10.1016/j.chroma.2017.11.024
Aly, H. H., Ibrahim, E. A., Abou Baker, D., and El-Baz, F. K. (2016). Evaluation of antioxidant, anti-inflammatory, anti-Alzheimer’s and anticancer activities of Taonia atomaria. Int. J. Pharma. Bio. Sci. 7, 421–429.
Ben Aoun, Z., Ben Said, R., and Farhat, F. (2010). Anti-inflammatory, antioxidant and antimicrobial activities of aqueous and organic extracts from Dictyopteris membranacea. Bot. Mar. 53, 259–264. doi: 10.1515/bot.2010.027
Ben Maïz (1995). Étude Nationale sur la Diversité Biologique de la Flore Marine et Aquatique en Tunisie. Projet MEAT/PNUE/GEF, Ministère de l’Environnement et de l’Aménagement du Territoire, Tunisie, 7.
Bogaert, K. A., Delva, S., and De Clerck, O. (2020). Concise review of the genus Dictyota J.V. Lamouroux. J Appl Phycol 32, 1521–1543. doi: 10.1007/s10811-020-02121-4
Chemat, F., Vian, A. M., and Cravotto, G. (2012). Green Extraction of natural products: concept and principles. Int. J. Mol. Sci. 13, 8615–8627. doi: 10.3390/ijms13078615
Cikoš, A.-M., Jokić, S., Šubarić, D., and Jerković, I. (2018). Overview on the application of modern methods for the extraction of bioactive compounds from marine macroalgae. Mar. Drugs 16:348. doi: 10.3390/md16100348
Corsetto, P. A., Montorfano, G., Zava, S., Colombo, I., Ingadottir, B., Jonsdottir, R., et al. (2020). Characterization of antioxidant potential of seaweed extracts for enrichment of convenience food. Antioxidants 9:249. doi: 10.3390/antiox9030249
Crampon, C., Boutin, O., and Badens, E. (2011). Supercritical carbon dioxide extraction of molecules of interest from microalgae and seaweeds. Ind. Eng. Chem. Res. 50, 8941–8953. doi: 10.1021/ie102297d
Da Gama, B. A. P., Pereira, R. C., Carvalho, A. G. V., Coutinho, R., and Yoneshigue-Valentin, Y. (2002). The effects of seaweed secondary metabolites on biofouling. Biofouling 18, 13–20. doi: 10.1080/08927010290017680
Dai, J., and Kim, J. C. (2016). In vivo anti-obesity efficacy of fucoxanthin-loaded emulsions stabilized with phospholipid. J. Pharm. Invest. 46, 669–675. doi: 10.1007/s40005-016-0270-z
Dang, T. T., Bowyer, M. C., Van Altena, I. A., and Scarlett, C. J. (2017). Comparison of chemical profile and antioxidant properties of the brown algae. Int. J. Food Sci. Tech. 53, 174–181. doi: 10.1111/ijfs.13571
Dewanto, V., Wu, X., Adom, K. K., and Liu, R. H. (2002). Thermal processing enhances the nutritional value of tomatoes by increasing total antioxidant activity. J. Agric. Food Chem. 50, 3010–3014. doi: 10.1021/jf0115589
Deyab, M. A., El-Katony, T. M., El-Adl, M. F., and Ward, F. M. (2017). Temporal variation in chemical composition of Dictyota dichotoma (Hudson) J.V. Lamouroux (Dictyotales, Phaeophyceae) from Red Sea coast, Egypt. J. Coast. Life Med. 5, 149–155. doi: 10.12980/jclm.5.2017j7-3
El-Sheekh, M. M., Abd El Khalek El-Shenody, R., Bases, E. A., and El Shafay, S. M. (2021). Comparative assessment of antioxidant activity and biochemical composition of four seaweeds, Rocky Bay of Abu Qir in Alexandria, Egypt. Food Sci. Tech. 41, 29–40. doi: 10.1590/fst.06120
Farasat, M., Khavari-Nejad, R.-A., Nabavi, S. M. B., and Namjooyan, F. (2013). Antioxidant properties of two edible green seaweeds from northern coasts of the Persian Gulf. Jundishapur. J. Nat. Pharm. Prod. 8, 47–52. doi: 10.5812/jjnpp.7736
Gammone, A., and D’Orazio, N. (2015). Anti-obesity activity of the marine carotenoid fucoxanthin. Mar. Drugs 13, 2196–2214. doi: 10.3390/md13042196
Ganesan, A. R., Tiwari, U., and Rajauria, G. (2019). Seaweed nutraceuticals and their therapeutic role in disease prevention. Food Sci. Hum. Well. 8, 252–263. doi: 10.1016/j.fshw.2019.08.001
Generalić Mekinić, I., Skroza, D., Šimat, V., Hamed, I., Čagalj, M., and Popović Perković, Z. (2019). Phenolic content of brown algae (Pheophyceae) species: extraction, identification, and quantification. Biomolecules 9:244. doi: 10.3390/biom9060244
Govindjee. (1999). “Carotenoids in photosynthesis: an historical perspective,” in The Photochemistry of Carotenoids, Advances in Photosynthesis and Respiration, eds H. A. Frank, A. J. Young, G. Britton, and R. J. Cogdell (Dordrecht: Springer), 1–19. doi: 10.1007/0-306-48209-6_1
Guiry, M. D., and Guiry, G. M. (2020). AlgaeBase. World-Wide Electronic Publication. Galway: National University of Ireland. https://www.algaebase.org; searched on (accessed November 15, 2020)
Hattab, M., Culioli, G., Piovetti, L., Chitour, S. E., and Valls, R. (2007). Comparison of various extraction methods for identification and determination of volatile metabolites from brown alga Dictyopteris membranacea. J. Chromatogr. A 1143, 1–7. doi: 10.1016/j.chroma.2006.12.057
Heriyanto, H., Juliadiningtyas, A. D., Shioi, Y., Limantara, L., and Brotosudarmo, T. H. P. (2017). Analysis of pigment composition of brown seaweeds collected from Panjang Island, Central Java, Indonesia. Philippine J. Sci. 146, 323–330.
Hörnig, I., Schnetter, R., and Prud’homme van Reine, W. F. (1992). The genus Dictyota (Phaeophyceae) in the North Atlantic. I. a new generic concept and new species. With contributions from Coppejans E., Aschenbach-Wege K., Over J.M. Nova Hedwigia 54, 45–62.
Jaswir, I., Noviendri, D., Salleh, H. M., Taher, M., Miyashita, K., and Ramli, N. (2013). Analysis of fucoxanthin content and purification of all-trans-fucoxanthin from Turbinaria turbinata and Sargassum plagyophyllum by SiO2 open column chromatography and reverse phase HPLC. J. Liq. Chrom. Rel. Tech. 36, 1340–1354. doi: 10.1080/10826076.2012.691435
Ktari, L., Ismail-Ben Ali, A., Ben Redjem, Y., Langar, H., and El Bour, M. (2010). Antifouling activity and chemical investigation of the brown alga Dictyota fasciola (Dictyotales) from Tunisian coast. Cahi. Biol. Mar. 51, 109–115.
Kawee-Ai, A., Kim, A. T., and Kim, S. M. (2019). Inhibitory activities of microalgal fucoxanthin against α-amylase, α-glucosidase, and glucose oxidase in 3T3-L1 cells linked to type 2 diabetes. J. Ocean. Limnol. 37, 928–937. doi: 10.1007/s00343-019-8098-9
Koduvayur Habeebullah, S. F., Surendraraj, A., and Jacobsen, C. (2018). Isolation of Fucoxanthin from brown algae and its antioxidant activity: in vitro and 5% fish oil-in-water emulsion. J. Am. Oil Chem. Soc. 95, 835–843. doi: 10.1002/aocs.12092
Kumar, L. R. G., Paul, P. T., Anas, K. K., Tejpal, C. S., Chatterjee, N. S., Anupama, T. K., et al. (2020). Screening of effective solvents for obtaining antioxidant-rich seaweed extracts using principal component analysis. J. Food Proces. Preserv. 44:e14716. doi: 10.1111/jfpp.14716
Küpper, F. C., Peters, A. F., Kytinou, E., Asensi, A. O., Vieira, C., Macaya, E. C., et al. (2019). Dictyota falklandica sp. nov. (Dictyotales, Phaeophyceae) from the Falkland Islands and southernmost South America. Phycologia 58, 640–647. doi: 10.1080/00318884.2019.1648990
Lourenço-Lopes, C., Garcia-Oliveira, P., Carpena, M., Fraga-Corral, M., Jimenez-Lopez, C., Pereira, A. G., et al. (2020). Scientific approaches on extraction, purification and stability for the commercialization of fucoxanthin recovered from brown algae. Foods 9:1113. doi: 10.3390/foods9081113
Maeda, H. (2015). Nutraceutical effects of fucoxanthin for obesity and diabetes therapy: a review. J. Oleo Sci. 64, 125–132. doi: 10.5650/jos.ess14226
Méresse, S., Fodil, M., Fleury, F., and Chénais, B. (2020). Fucoxanthin, a marine-derived carotenoid from brown seaweeds and microalgae: a promising bioactive compound for cancer therapy. Int. J. Mol. Sci. 21:9273. doi: 10.3390/ijms21239273
Miyashita, K. (2014). Anti-obesity therapy by food component: unique activity of marine carotenoid, fucoxanthin. Obes. Control. Ther. 1:4. doi: 10.15226/2374-8354/1/1/00103
Muradian, Kh, Vaiserman, A., Min, K. J., and Fraifeld, V. E. (2015). Fucoxanthin and lipid metabolism: a minireview. Nutr. Metab. Cardiovasc. Dis. 25, 891–897. doi: 10.1016/j.numecd.2015.05.010
Murray, M., Dordevic, A. L., Ryan, L., and Bonham, M. P. (2018a). An emerging trend in functional foods for the prevention of cardiovascular disease and diabetes: marine algal polyphenols. Crit. Rev. Food. Sci. Nutr. 58, 1342–1358. doi: 10.1080/10408398.2016.1259209
Murray, M., Dordevic, A. L., Ryan, L., and Bonham, M. P. (2018b). The impact of a single dose of a polyphenol-rich seaweed extract on postprandial glycaemic control in healthy adults: a randomised cross-over trial. Nutrients 10:270. doi: 10.3390/nu10030270
Nunes, N., Leça, J. M., Pereira, A. C., Pereira, V., Ferraz, S., Barreto, M. C., et al. (2019). Evaluation of fucoxanthin contents in seaweed biomass by vortex-assisted solid-liquid microextraction using high-performance liquid chromatography with photodiode array detection. Algal Res. 42:101603. doi: 10.1016/j.algal.2019.101603
Pangestuti, R., Vo, T. S., Ngo, D. H., and Kim, S. K. (2013). Fucoxanthin ameliorates inflammation and oxidative reponses in microglia. J. Agric. Food. Chem. 61, 3876–3883. doi: 10.1021/jf400015k
Panintingjati Brotosudarmo, T., Heriyanto, H., Shioi, Y., Indriatmoko, I., Adhiwibawa, M. A. S., Indrawati, R., et al. (2018). Composition of the main dominant pigments from potential two edible seaweeds. Philip. J. Sci. 147, 47–55.
Parada, J., Pérez-Correa, J. R., and Pérez-Jiménez, J. (2019). Design of low glycemic response foods using polyphenols from seaweed. J. Funct. Foods 56, 33–39. doi: 10.1016/j.jff.2019.03.004
Perez-Bermudez, P., Garcfa-Carrascosa, M., Comejo, M. J., and Segura, J. (1981). Water-depth effects in photosynthetic pigment content of the benthic algae Dictyota dichotoma and Udotea petiolata. Aquat. Bot. 11, 373—-377.
Pruteanu, L.-L., Kopanitsa, L., Módos, D., Kletnieks, E., Samarova, E., Bender, A., et al. (2020). Transcriptomics predicts compound synergy in drug and natural product treated glioblastoma cells. PLoS One 15:e0239551. doi: 10.1371/journal.pone.0239551
Quitain, A. T., Kai, T., Sasaki, M., and Goto, M. (2013). Supercritical carbon dioxide extraction of fucoxanthin from Undaria pinnatifida. J. Agric. Food. Chem. 61, 5792–5797. doi: 10.1021/jf400740p
Raguraman, V., MubarakAli, D., Narendrakumar, G., Thirugnanasambandam, R., Kirubagaran, R., and Thajuddin, N. (2018). Unravelling rapid extraction of fucoxanthin from Padina tetrastromatica: purification, characterization and biomedical application. Process. Biochem. 73, 211–219. doi: 10.1016/j.procbio.2018.08.006
Ribera, M. A., Gomez Garreta, A., Gallardo, T., Cormaci, M., Furnari, G., and Giaccone, G. (1992). Check-list of Mediterranean Seaweeds I. Fucophyceae (Warming, 1884). Bot. Mar. 35, 109–130. doi: 10.1515/botm.1992.35.2.109
Rodrigues, S., Harb, T. B., Falcão, E. P., da, S., Pereira, S. M. B., and Chow, F. (2020). Antioxidant activity of Dictyotales from tropical reefs of Brazil. App. Biochem. Biotech. 192, 665–679. doi: 10.1007/s12010-020-03344-4
Roh, M.-K., Uddin, Md.S, and Chun, B.-S. (2008). Extraction of fucoxanthin and polyphenol from Undaria pinnatifida using supercritical carbon dioxide with co-solvent. Biotech. Bioproc. Eng. 13, 724–729. doi: 10.1007/s12257-008-0104-6
Sánchez-Rangel, J. C., Benavides, J., Heredia, J. B., Cisneros-Zevallos, L., and Jacobo-Velázquez, D. A. (2013). The Folin–Ciocalteu assay revisited: improvement of its specificity for total phenolic content determination. Analytical Methods 5:5990. doi: 10.1039/c3ay41125g
Saravana, P. S., Getachew, A. T., Cho, Y. J., Choi, J. H., Park, Y. B., Woo, H. C., et al. (2017). Influence of co-solvents on fucoxanthin and phlorotannin recovery from brown seaweed using supercritical CO2. J. Supercrit. Fluids. 120, 295–303. doi: 10.1016/j.supflu.2016.05.037
Saravana, P. S., Yin, S., Choi, J. H., Park, Y. B., Woo, H. C., and Chun, B. S. (2015). Biological properties of fucoxanthin in oil recovered from two brown seaweeds using supercritical CO2 extraction. Mar. Drugs 13, 3422–3442. doi: 10.3390/md13063422
Satomi, Y. (2017). Antitumor and cancer-preventative function of fucoxanthin: a marine carotenoid. Anticancer Res. 37, 1557–1562. doi: 10.21873/anticanres.11484
Shipeng, Y., Woo, H.-C., Choi, J.-H., Park, Y.-B., and Chun, B.-S. (2015). Measurement of antioxidant activities and phenolic and flavonoid contents of the brown seaweed Sargassum horneri: comparison of supercritical CO2 and various solvent extractions. Fish. Aquat. Sci. 18, 123–130. doi: 10.5657/fas.2015.0123
Sosa-Hernández, J. E., Escobedo-Avellaneda, Z., Iqbal, H. M. N., and Welti-Chanes, J. (2018). State-of-the-art extraction methodologies for bioactive compounds from algal biome to meet bio-economy challenges and opportunities. Molecules 23:2953. doi: 10.3390/molecules23112953
Steel, C. C., and Keller, M. (2000). Influence of UV-B irradiation on the carotenoid content of Vitis vinifera tissues. Biochem. Soc. Trans. 28, 883–885. doi: 10.1042/bst0280883
Susanto, E., Fahmi, A. S., Abe, M., Hosokawa, M., and Miyashita, K. (2016). Lipids, fatty acids, and fucoxanthin content from temperate and tropical brown seaweeds. Aquatic Procedia. 7, 66–75. doi: 10.1016/j.aqpro.2016.07.009
Takahashi, K., Hosokawa, M., Kasajima, H., Hatanaka, K., Kudo, K., Shimoyama, N., et al. (2015). Anticancer effects of fucoxanthin and fucoxanthinol on colorectal cancer cell lines and colorectal cancer tissues. Oncology Lett. 10, 1463–1467. doi: 10.3892/ol.2015.3380
Tanniou, A., Esteban Serrano, L., Vandanjon, L., Ibanez, E., Mendiola, J. A., Cerantola, S., et al. (2013). Green improved processes to extract bioactive phenolic compounds from brown macroalgae using Sargassum muticum as model. Talanta 104, 44–52. doi: 10.1016/j.talanta.2012.10.088
Teodosio Melo, K., Gomes Camara, R., Queiroz, M., Jacome Vidal, A., Machado Lima, C., Melo-Silveira, R., et al. (2013). Evaluation of sulfated polysaccharides from the brown seaweed Dictyopteris Justii as antioxidant agents and as inhibitors of the formation of calcium oxalate crystals. Molecules 18, 14543–14563. doi: 10.3390/molecules181214543
Terasaki, M., Hirose, A., Narayan, B., Baba, Y., Kawagoe, C., Yasui, H., et al. (2009). Evaluation of recoverable functional lipid components of several brown seaweeds of Japan with special reference to fucoxanthin and fucosterol contents. J. Phycol. 45, 974–980. doi: 10.1111/j.1529-8817.2009.00706.x
Thomas, N. V., and Kim, S. K. (2011). Review: potential pharmacological applications of polyphenolic derivatives from marine brown algae. Environ. Toxicol. Pharmacol. 32, 325–335. doi: 10.1016/j.etap.2011.09.004
Wang, Z., Li, H., Dong, M., Zhu, P., and Cai, Y. (2019). The anticancer effects and mechanisms of fucoxanthin combined with other drugs. J Cancer Res Clin Oncol 145, 293–301. doi: 10.1007/s00432-019-02841-2
Keywords: antioxidant, fucoxanthin, polyphenols, Mediterranean, Dictyotales, supercritical carbon dioxide extraction
Citation: Ktari L, Mdallel C, Aoun B, Chebil Ajjabi L and Sadok S (2021) Fucoxanthin and Phenolic Contents of Six Dictyotales From the Tunisian Coasts With an Emphasis for a Green Extraction Using a Supercritical CO2 Method. Front. Mar. Sci. 8:647159. doi: 10.3389/fmars.2021.647159
Received: 29 December 2020; Accepted: 12 April 2021;
Published: 25 May 2021.
Edited by:
Susana P. Gaudêncio, New University of Lisbon, PortugalReviewed by:
Antje Labes, Fachhochschule Flensburg, GermanySaravana Periaswamy Sivagnanam, Teagasc Food Research Centre (Ireland), Ireland
Copyright © 2021 Ktari, Mdallel, Aoun, Chebil Ajjabi and Sadok. This is an open-access article distributed under the terms of the Creative Commons Attribution License (CC BY). The use, distribution or reproduction in other forums is permitted, provided the original author(s) and the copyright owner(s) are credited and that the original publication in this journal is cited, in accordance with accepted academic practice. No use, distribution or reproduction is permitted which does not comply with these terms.
*Correspondence: Leila Ktari, bGVpbGEua3RhcmlAaW5zdG0ucm5ydC50bg==