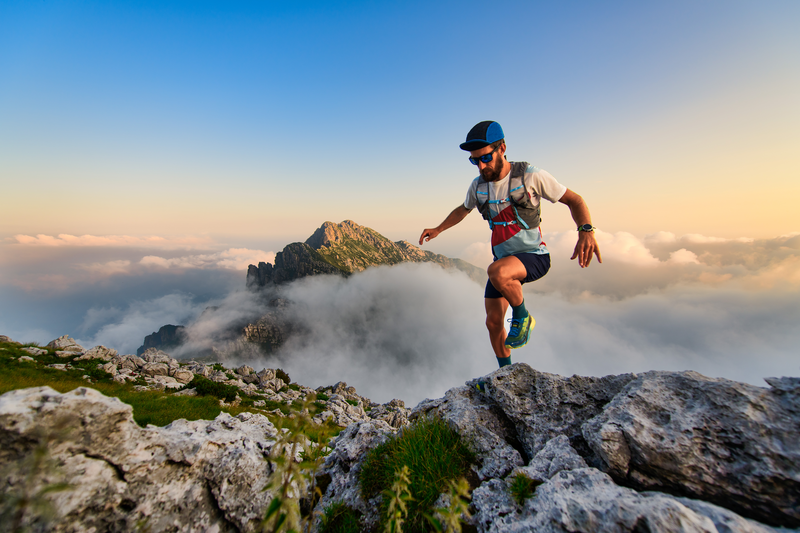
94% of researchers rate our articles as excellent or good
Learn more about the work of our research integrity team to safeguard the quality of each article we publish.
Find out more
BRIEF RESEARCH REPORT article
Front. Mar. Sci. , 06 April 2021
Sec. Marine Molecular Biology and Ecology
Volume 8 - 2021 | https://doi.org/10.3389/fmars.2021.642872
The history of elasmobranch ageing highlights the difficulty of age estimation in animals lacking ossified structures. Ageing techniques are numerous, yet all are limited by difficulties of validation and verification and inherent subjectivity. Sample scarcity further hinders progress as limited samples cannot be risked on exploratory techniques. This study explored the use of genetic analysis of telomeres as a method of age determination in a deep-sea shark, Etmopterus granulosus, a representative of a poorly studied taxon with regard to ageing due to sample scarcity. Telomeres were amplified in 39 individuals with qPCR, compared across samples, and were found to differ across size classes. More work is needed to determine whether telomeres consistently correlate with size class across elasmobranchs. However, telomeres offer easily obtainable data that can be combined with traditional methods to provide additional points of comparison for elasmobranch biology. Our results underscore the importance of expanding current ageing methods and highlight a necessary distinction between chronological and biological ages when describing elasmobranch populations.
Age assessment in elasmobranchs has a nearly century-long history. Over this period of time, the majority of ageing studies have relied on calcified hard structures, despite inter- and intraspecific variability prohibitive to confident age estimates. Verification and validation of age are still under-used, despite the development and availability of several methods. Limitations, particularly sample size and the subjective nature of ageing itself, add to potential error. Age and growth estimates are fundamental metrics by which species assessments and management policies are developed, but for effective management the chronological age of the organism cannot be divorced from its biology. Therefore, alternative methods are needed to accurately and reliably age elasmobranchs that allow for consideration of individual variability.
Ridewood (1921) was the first to introduce the idea of ageing elasmobranchs using vertebral calcification patterns. Within the same paper it was concluded that using vertebrae for taxonomic clarification or for ageing was likely not useful, adding that calcified masses within vertebrae were widely variable and related to the physiological role of the vertebral column (expanded on by Porter et al., 2006). Despite this early observation, vertebrae are still the most common structure used for elasmobranch ageing, with later studies focusing on methods of band enhancement (Table 1) such as histology (Ishiyama,1951a,b) to improve their utility.
The increased use of vertebral bands as age estimates brought about the need for validation and verification of the banding patterns (Cailliet et al., 1986; Cailliet, 1990, 2015; Cailliet and Goldman, 2004). Oxytetracycline injection coupled with tag-recapture demonstrated validity of banding patterns for some species, but only if specimens could be recaptured (Holden and Vince, 1973), while methods such as bomb radiocarbon dating (Kalish, 1993) have continued to gain popularity (Table 2). Even with several available methods, validated ageing studies remain rare (Harry, 2018), with several studies demonstrating vertebral banding patterns to be independent of chronological age (e.g., Squatina californica, the Pacific Angel shark (Cailliet et al., 1983; Natanson, 1984; Natanson and Cailliet, 1986, 1990), and Cetorhinus maximus, the Basking Shark (Natanson et al., 2008). These species highlight potential errors with current methods of ageing and the danger of assuming validation of one species applies to any other.
Deep-sea species pose additional challenges to ageing due to deficiencies in vertebral calcification, with near or complete absences of banding rendering vertebrae useless for ageing. Alternatively, dorsal fin spines are commonly used as an ageing structure for deep-sea species since Kaganovskaya (1933) established the method using the Spiny dogfish (Squalus acanthias). Much like use of vertebrae, this method suffers from confounding factors such as spine erosion—breakage or wearing down—and discrepancies between internal and external band counts (Irvine et al., 2006), further complicating age estimates with current practices.
The two most popular ageing methods—vertebrae and dorsal fin spines—are inherently subjective measurements affected by interpretation of those ageing the organisms. Studies have shown that reader experience, computer screen, imageing program selection, and more contribute to reader interpretation contributing to variability in estimates, even when ageing the same structure (Officer et al., 1996; Natanson et al., 2018). Advancements in the field are limited and primarily computational, such as the use of multiple growth models to find the best fit (Pardo et al., 2013; Smart et al., 2016). The shortcomings of ageing are well-known, with a clear need for new ageing methods that are objective, replicable, and informative in new ways for elasmobranchs.
Telomeres, DNA sequences at the ends of chromosomes, are known to shorten with age in diverse groups of eukaryotes (e.g., Haussmann et al., 2003). Rocco et al. (2002) assessed karyotypes and verified that the standard eukaryotic telomeric repeat (TTAGGG) is present in four cartilaginous species; Small-spotted catshark (Scyliorhinus canicula), Nursehound (Scyliorhinus stellaris), Starry skate (Raja asterias), and Bluespotted fantail ray (Taeniura lymma). Izzo et al. (2010), examined telomere length as a proxy for age, but found no correlation between telomere length and size. Yet prior estimates of elasmobranch ageing make it clear that metrics are rarely consistent across all species. Given that the maximum lifespan, or longevity, of most sharks is unknown, elasmobranchs represent a unique system to investigate telomere dynamics.
Telomeres are known to shorten with ageing, but other factors can influence telomeric changes. Environment and habitat are known to impact telomere length (Haussmann and Heidinger, 2015), and telomere length can affect an organism’s reproductive potential (Heidingerm et al., 2016). However, overall trends in telomere length in elasmobranchs must be determined before examination of environmental or reproductive effects are possible. If telomeres shorten with age in a predictable manner i.e., a consistent rate, telomere length may provide an additional measurement of longevity, or biological age, shifting the focus away from chronological dependence to a more biologically relevant metric.
In the present study, we examined relative telomere lengths in a deep-sea squaloid shark Etmopterus granulosus (Chondrichthyes:Squaliformes:Etmopteridae), the Southern Lanternshark. E. granulosus is common and abundant in the Southern Oceans, inhabiting waters from 220 to 1,500 m on continental shelves and slopes either on or near the bottom (Compagno et al., 1989; Daley et al., 2002; Ebert et al., 2013). E. granulosus feeds mainly on cephalopods, and bony fish, as well as penaeid shrimps and myctophids (Ebert et al., 1992; Hallett and Daley, 2010), has bioluminescent markings (Straube et al., 2010; Mallefet et al., 2021), and is known to be viviparous and engage in polyandry (Nehmens et al., 2020). Age estimates in E. granulosus have been made from dorsal fin spines and vertebrae. However, depending on the method used, the maximum age differs by an order of magnitude (Irvine et al., 2006). Intriguingly, some evidence suggests tooth characteristics may vary in male sharks with age (Straube et al., 2008). Various arguments could be made in support of either, however without proper validation, vulnerability risk assessments of the species would be based in speculation. Additionally, E. granulosus is a species that is commonly caught in large numbers as bycatch in commercial fisheries in the Southern Oceans, making it an ideal species for this study.
The objectives of this study were to determine (1) if there is any evidence of telomere shortening with size and (2) if the degree of shortening correlates with the most common rough estimate for age (body length).
Etmopterus granulosus specimens were obtained as bycatch from benthic and midwater trawls by the F/V “Will Watch,” a Cook Islands flagged commercial fishing vessel that operates out of Port Louis, Republic of Mauritius. The fishing grounds are in the southwestern Indian Ocean along the Madagascar Ridge, Walter’s Shoal, and Southwest Indian Ridge. The specimens obtained were collected during two separate trips from March to May in 2012 and April to June in 2014.
Specimens were frozen upon capture and brought to Albion Fisheries Center, Albion, Mauritius where they were thawed and processed. Total length (TL), measured to the nearest millimeter (mm), and overall body condition were recorded. Muscle tissue samples (stored in 95% EtOH) were taken from 39 individuals, from the post-dorsal when possible or, in the case of embryos, the entire post-cloacal region. For gravid females, the left and right uteri were extracted, the number, TL, position, and sex (when possible) of each pup was recorded from each uterus. The resulting number of individuals were five gravid females with three pups from each litter, and 19 population individuals (males n = 9, females n = 10) that were used for telomere analysis.
The nine males ranged from 360 to 630 mm, including subadult and adult size classes. Non-gravid females ranged from 490 to 910 mm including subadult and adult size classes. Gravid females ranged from 695 to 805 mm. The “pups” are comprised of individuals collected in utero at different developmental stages. The mean TL for each of the five litters are 30, 65, 102, 158, and 202 mm.
DNA was extracted using a Qiagen DNeasy Blood and Tissue Kit (Qiagen, Valencia CA). We ran genomic DNA samples on a gel to verify that samples had similar qualities and that none were degraded more than others as a result of ethanol storage. DNA purity was assessed via a NanoDrop2000 (all had 260:280 between 1.5 and 1.8 and 260:230 > 1.8). DNA quantification was carried out via a Qubit Broad Range DNA Kit (Qubit, Thermo Fisher Scientific, Waltham MA). All sample concentrations were standardized to 0.5 ng/μL and were stored at −20°C until analysis. Primers standard for deuterostomes that are designed to prevent amplification of primer dimer (problematic with purely repeat-based priming) were used to measure telomere length via qPCR: F 5′CGGTTTGTTTGGGTTTGGG TTTGGGTTTGGGTTTGGGTT3′, R 5′GCGTTGCCTTACCC TTACCCTTACCCTTACCCTTACCCT3′ (Farzaneh-Far et al., 2008). Assays were run on a StepOnePlus Real-time PCR System (ABI). Each 20 μL reaction consisted of 10 μL Power SYBR Green PCR Master Mix (Thermo Fisher Scientific, Waltham MA), 2 μL forward primer (1 μM), 4 μL reverse primer (1 μM), 3 μL purified water and 1 μL template DNA (0.5 ng/μL). Negative controls substituted 1 μL of template DNA for an additional 1 μL of purified water. All samples were run in triplicate and resulting Cycle Threshold (Ct) values were averaged. The qPCR program was 10 min 95°C, 40 cycles of 15 s 95°C, 1 min 56°C. qPCR efficiency was verified via a standard curve of DNA concentration.
Ct values were plotted against TL in a linear regression to test for initial relationships between the two factors. One female outlier was calculated using Grubb’s test (p = < 0.01) (Grubbs, 1950) and was removed from subsequent analyses as the reason for such a large difference was unknown and unattributable to the sample or to laboratory errors. Pup’s Ct values were plotted in a linear regression against their TL, with individual litters having a unique shape identifier.
Sex categories, male (M), female (F), and unknown including all pups (Unk), were compared to Ct using an ANOVA, with a Student’s t post-hoc test. Type categories of All (individuals not in the Gravid or Pup categories), Gravid, and Pup, were compared to Ct values using an ANOVA, with a Student’s t post-hoc.
The linear regression including the female outlier showed a positive significant relationship between Ct value and TL (R2 = 0.18, p = 0.008). The linear regression was re-run without the female outlier, and showed an increased relationship (R2 = 0.27 p = 0.007) (Figure 1).
Figure 1. Relationship between Ct and total length with outlier removed showing a significant relationship between the two factors (R2 = 0.27; p = 0.007). Male , Female
, Gravid
, Pups
. Pink = female, blue = male, and green = unknown.
ANOVA results testing Type compared to Ct values was significant (F = 8.27, df = 2, p = 0.001). A post-hoc Student’s t shows that All (mean = 23.92) and Pups (mean = 22.63) are significantly different (< p = 0.001) and Gravid (mean = 24.25) and Pups are significantly different (p = 0.004) from one another. However, All and Gravid are not significantly different from one another (p = 0.53).
ANOVA results testing Sex and Ct values, were significant (F = 8.18, p = 0.001) (Figure 2). The post-hoc Student’s t showed that the means between Females (23.90) and Males (24.13) were not significantly different from one another (p = 0.60), but are still significantly different from the Pups (22.63) (m to unk p = 0.001; f to unk p = 0.002).
Figure 2. Sex compared to Ct value (F = 8.18, df = 2, p = 0.001) shows a significant relationship. A post-hoc Student’s t, showed that the means between Females (23.90) and Males (24.13) were not significantly different from one another (p = 0.60), but both are significantly different from the unknown Pups (22.63) (M to Unk p = 0.001; F to Unk p = 0.002). Male , Female
, Pups
. Pink = female, blue = male, and green = unknown.
All pups were plotted in a linear regression comparing total length of Ct value. A significant relationship (R2 = 0.43, p = 0.008) was found within the pups, as was found with all individuals analyzed (Figure 3). The three pups from one of the litters has a larger spread of Ct values compared to the other litters. This litter happened to be the only litter analyzed that had pups that did not share a father and were cross-referenced from paternity data (Nehmens et al., 2020). Paternity data showed that the two pups that clustered together shared a sire, while the other pup from the same litter had a different sire.
Figure 3. A significant relationship between pup Ct value and length (R2 = 0.43, p = 0.008). Each shape defines a different litter. Pups from the litter depicted in circles have a larger spread in Ct values than other litters. The two pups circled in yellow share a sire, while the single individual circled in purple has a different sire.
The relationship comparing TL to Ct value (p = 0.007) provides evidence for the shortening of telomeres over the course of an Etmopterus granulosus individual’s lifetime. Shortening of telomeres is a phenomenon seen in organisms across many taxa including zebrafish (a teleost) (Kishi et al., 2003), birds (Vleck et al., 2003), mammals (Haussmann et al., 2003), and primates (Herbig et al., 2006).
The significant difference between individuals, gravid females and pups supports telomerase activity during gamete formation. In many organisms, offspring (e.g., Mantell and Greider, 1994; Wright et al., 1996; Betts and King, 1999; Xu and Yang, 2000) or regenerated body parts (Varney et al., 2017) activate telomerase to lengthen telomeres, making each generation or regeneration genetically younger than the previous (Schaetzlein et al., 2004). The significant relationship between pup length and Ct value provides support that telomerase activity may be mediated throughout development. As pups reach time at parturition, telomerase may no longer be active, and may continue to remain inactive leading to the shortening of telomeres throughout the lifespan of an individual (Tricola et al., 2018).
The ageing process, while often thought of as a visible progression, is controlled by cellular activity. As cells continuously divide, telomere length slowly shortens until the cell senesces and undergoes apoptosis, or cell death (Blackburn, 1991). If an organism is able to maintain or even lengthen its telomeres, then cell death does not occur, and the organism would perceivably not age. Izzo et al. (2010) found there was no trend between telomere length and individuals of different size classes, showing no positive or negative correlation for the Port Jackson’s Shark (Heterodontus portusjacksoni) that inhabits shallow waters. In this study, the deep-sea shark (E. granulosus) showed statistically significant differences between size classes and telomere length, with a positive correlation between TL and Ct value. Though the R2 explanatory variable explains approximately one-third of the relationship, the significance is size class telomere length changes show telomere length shortens over time and the rate of shortening, a key aspect of understanding longevity, needs to be quantified (Tricola et al., 2018). Additionally, the contrast between these two species from vastly different habitats is intriguing and warrants investigation into telomere dynamics across elasmobranch taxa and habitats beyond the ageing process (Bize et al., 2009). Attention should be given to environmental conditions (Angelier et al., 2013) and stressors, such as contaminants (Mizutani et al., 2013), that are known to affect telomere length. Exploring how environment affects elasmobranch telomere length may provide a better understand the resiliency of this group of fishes.
The potential signal of multiple paternity within the pups examined does not provide strong proof of an observable trend. Relative paternal and maternal contributions to offspring telomere length is not yet well-established, with predominantly avian studies producing contradictory results (Heidingerm et al., 2016; Johnsen et al., 2017). Therefore, further investigation of additional litters and corroboration with traditional methods using other genetic markers (Nehmens et al., 2020) is needed, but the implications of a potential fitness-related effect of multiple paternity, and thus genetic variability, merits attention.
This study concluded that telomeres shorten in relation to size (age) in this species. Although the sample size was small, these findings lay the groundwork for future studies of telomeres in relation to elasmobranch life history. Benefits from genetic studies like this one include non-destructive sampling that allows for continuous sampling throughout an organism’s lifetime and a deeper understanding of how telomeres may change over time, within and among individuals. With the information from this study providing a proof of concept, effort can be put into studies to validate the method and create a baseline of the rate of telomere shortening, known to be linked to species’ life span (Whittemore et al., 2019). Using this technique on captive animals, particularly those of known age allows for an establishment of a baseline that can then be applied organisms in situ allowing for better preservation of an already vulnerable group of animals due non-lethality of the method. Additionally, a shift from chronological age to a more biologically relevant perspective may be necessary to deepen our understanding of the paradigm of “ageing,” (Blackburn, 2000; Bize et al., 2009). Even if telomere shortening does not correlate directly with chronological age, it may provide other valuable information (Bateson, 2015) related to reproductive fitness, embryonic development, contaminant effects, and more.
The raw data supporting the conclusions of this article will be made available by the authors, without undue reservation, to any qualified researcher.
The animal study was reviewed and approved by the IACUC San Jose State University IACUC permit #801 and IACUC permit #2014-D.
DE and MN conceptualized the project and collected specimens and samples. MN and RV extracted the DNA. RV ran laboratory analyses with assistance from AJ. MN completed data analysis. All authors contributed to writing and formatting of the final manuscript, have given final approval of the manuscript, and have agreed to be listed for publication.
This study was supported in part by the Earl H. Myers and Ethel M. Myers Oceanographic and Marine Biology Trust, and Moss Landing Marine Laboratories. This study was also supported by the National Science Foundation’s Jaws and Backbone: Chondrichthyan Phylogeny and a Spine for the Vertebrate Tree of Life, Grant: DEB 01132229 to Gavin Naylor, University of Florida.
The authors declare that the research was conducted in the absence of any commercial or financial relationships that could be construed as a potential conflict of interest.
We would like to acknowledge Gregor Cailliet for encouragement in investigating alternative methods of ageing elasmobranchs. We thank the reviewers and editor for their time and contribution to the manuscript.
Aasen, O. (1963). Length and growth of the porbeagle (Lamna nasus Bonnaterre) in the northwest Atlantic. Fiskeridir. Skr. Ser. Havunder. 13, 20–37.
Angelier, F., Vleck, C. M., Holberton, R. L., and Marra, P. P. (2013). Telomere length nonbreeding habitat and return rate in male American redstarts. Funct. Ecol. 27, 342–350. doi: 10.1111/1365-2435.12041
Bateson, M. (2015). Cumulative stress in research animals: telomere attrition as a biomarker in a welfare context? Bioessays 38, 201–212. doi: 10.1002/bies.201500127
Betts, D. H., and King, W. A. (1999). Telomerase activity and telomere detection during early bovine development. Dev. Genet. 25, 397–403. doi: 10.1002/(sici)1520-6408(1999)25:4<397::aid-dvg13>3.0.co;2-j
Bize, P., Criscuolo, F., Metcalfe, N. B., Nasir, L., and Monaghan, P. (2009). Telomere dynamics rather than age predict life expectancy in the wild. Proc. R. Soc. B 276, 1679–1683. doi: 10.1098/rspb.2008.1817
Blackburn, E. H. (1991). Structure and function of telomeres. Nature 350, 569–573. doi: 10.1038/350569a0
Branstetter, S., and Stiles, R. (1987). Age and growth estimates of the bull shark, Carcharhinus leucas, from the northern Gulf of Mexico. Environ. BioI. Fishes 20, 169–181. doi: 10.1007/bf00004952
Brooks, J. L., Guttridge, T. L., Franks, B. R., Grubbs, R. D., Chapman, D. D., Gruber, S. H., et al. (2016). Using genetic inference to re-evaluate the minimum longevity of the lemon shark Negaprion brevirostris. J. Fish Biol. 88, 2067–2074. doi: 10.1111/jfb.12943
Cailliet, G. M. (1990). Elasmobranch Age Determination and Verification: An Updated Review In Elasmobranchs as Living Resources: Advances in the Biology Ecology Systematics and the Status of the Fisheries. Washington, DC: U.S. Department of Commerce, 157–165. NOAA Technical Report NMFS 90.
Cailliet, G. M. (2015). Perspectives on elasmobranch life-history studies: a focus on age validation and relevance to fishery management. J. Fish Biol. 87, 1271–1292. doi: 10.1111/jfb.12829
Cailliet, G. M., and Goldman, K. J. (2004). Age Determination and Validation in Chondrichthyan Fishes In: Biology of Sharks and their Relatives. Boca Raton FL: CRC Press, 399–447.
Cailliet, G. M., Martin, L. K., Harvey, J. T., Kusher, D., and Welden, B. A. (1983). Preliminary Studies on the Age and Growth of the Blue Prionace glauca, Common Thresher Alopias vulpinus, and Shortfin mako Isurus oxyrinchus Sharks From California waters. Washington, DC: U.S. Department of Commerce, 179–188. NOAA Technical Report NMFS 8.
Cailliet, G. M., Radtke, R. L., and Welden, B. A. (1986). Elasmobranch age determination and verification: a review In Indo-Pacific fish biology: proceedings of the second international conference on Indo-Pacific fishes. Ichthyol. Soc. Jpn. Tokyo 345–359.
Casey, J. G., Pratt, H. L. Jr., and Stillwell, C. E. (1985). Age and growth of the sandbar shark (Carcharhinus plumbeus) from the western North Atlantic. Can. J. Fish. Aquat. Sci. 42, 963–975. doi: 10.1139/f85-121
Casselman, J. M. (1983). Age and Growth Assessment of Fish From Their Calcified Structures-Techniques and Tools. Washington, DC: U.S. Department of Commerce, 1–17. NOAA Technical Report NMFS 8.
Compagno, L. J. V., Ebert, D. A., and Smale, M. J. (1989). The Sharks and Rays of Southern Africa. Cape Town: Struik Publishers, 1–160.
Correia, J. P., and Figueiredo, I. M. (1997). A modified decalcification technique for enhancing growth bands in deep-coned vertebrae of elasmobranchs. Environ. Biol. Fishes 50, 225–230. doi: 10.1023/a:1007368025164
Daiber, F. C. (1960). A technique for age determination in the skate. Raja eglanteria. Copeia 1960, 258–260. doi: 10.2307/1439680
Daley, R. K., Steven, J. D., Last, P. R., and Yearsley, G. K. (2002). Field Guide to Australian Sharks & Rays. Hobart: CSIRO Publishing, 1–84.
Ebert, D. A., Compagno, L. J. V., and Cowley, P. D. (1992). A preliminary investigation of the feeding ecology of squaloid sharks off the west coast of southern Africa. S. Afr. J. Mar. Sci. 12, 601–609. doi: 10.2989/02577619209504727
Ebert, D. A., Fowler, S., and Compagno, L. J. V. (2013). Sharks of the World. Plymouth: Wild Nature Press, 1–528.
Farzaneh-Far, R., Cawthon, R. M., Na, B., Browner, W. S., Schiller, N. B., and Whooley, M. A. (2008). Prognostic value of leukocyte telomere length in patients with stable coronary artery disease data from the heart and soul study. Arterioscler. Thromb. Vasc. Biol. 28, 1379–1384. doi: 10.1161/atvbaha.108.167049
Gelsleichter, J., Cortés, E., Manire, C. A., Hueter, R. E., and Musick, J. A. (1998). Evaluation of toxicity of oxytetracycline on growth of captive nurse sharks. Ginglymostoma cirratum. Fish. Bull. 96, 624–627.
Geraghty, P., Jones, A., Stewart, J., and Macbeth, W. (2012). Micro-computed tomography: an alternative method for shark ageing. J. Fish Biol. 80, 1292–1299. doi: 10.1111/j.1095-8649.2011.03188.x
Grubbs, F. E. (1950). Sample criteria for testing outlying observations. Ann. Math. Statt. 21, 27–58. doi: 10.1214/aoms/1177729885
Gruber, S. H., and Stout, R. G. (1983). Biological Materials for the Study of the Age and Growth in a Tropical Marine Elasmobranch, the Lemon Shark, Negaprion brevirostris (Poey). Washington, DC: U.S. Department of Commerce, 193–205. NOAA Technical Report NMFS 8.
Hallett, C. S., and Daley, R. K. (2010). Feeding ecology of the southern lanternshark (Etmopterus baxteri) and the brown lanternshark (E. unicolor) off southeastern Australia. ICES J. Mar. Sci. 68, 157–165. doi: 10.1093/icesjms/fsq143
Harry, A. V. (2018). Evidence for systemic age underestimation in shark and ray ageing studies. Fish Fish. 19, 185–200. doi: 10.1111/faf.12243
Haussmann, M. F., and Heidinger, B. J. (2015). Telomere dynamics may link stress exposure and ageing across generations. Biol. Lett. 11:20150396. doi: 10.1098/rsbl.2015.0396
Haussmann, M. F., Winkler, D. W., O’Reilly, K. M., Huntington, C. E., Nisbet, I. C. T., and Vleck, C. M. (2003). Telomeres shorten more slowly in long-lived birds and mammals than in shortlived ones. Proc. R. Soc. Lond. B 270, 1387–1392. doi: 10.1098/rspb.2003.2385
Heidingerm, B. J., Herborn, K. A., Granroth-Wilding, H. M. V., Boner, W., Burthe, S., Newell, M., et al. (2016). Parental age influences offspring telomere loss. Funct. Ecol. 30, 1531–1538. doi: 10.1111/1365-2435.12630
Herbig, U., Ferreira, M., Condel, L., Carey, D., and Sedivy, J. M. (2006). Cellular senescence in aging primates. Science 311:1257. doi: 10.1126/science.1122446
Hoenig, J., and Brown, C. (1988). A simple technique for staining growth bands in elasmobranch vertebrae. Bull. Mar. Sci. 42, 334–337.
Holden, M. J., and Vince, M. R. (1973). Age validation studies on the centra of Raja clavata using tetracycline. J. Conseil Int. Pour l’Exp. 35, 13–17. doi: 10.1093/icesjms/35.1.13
Irvine, S. B., Stevens, J., and Laurenson, L. (2006). Comparing external and internal dorsal-spine bands to interpret the age and growth of the giant lanternshark Etmopterus baxteri (Squaliformes: Etmopteridae). Environ. Bio Fishes 77, 253–264. doi: 10.1007/978-1-4020-5570-6_5
Ishiyama, R. (1951a). Age determination of Raja hollandi Jordan and Richardson chiefly inhabiting in thewaters of the EastChina Sea. Bull. Jpn.Soc. Sci. Fish. 16, 119–124.
Ishiyama, R. (1951b). On the age-determination of Japanese black-skate Raja fusca Garman (preliminary report) In: studies on the rays and skates belonging to the family Rajidae found in Japan and adjacent regions. Bull. Jpn.Soc. Sci. Fish. 16, 112–118. doi: 10.2331/suisan.16.12_112
Izzo, C., Bertozzi, T., Donnellan, S., and Gillanders, B. M. (2010). Telomere Length Varies Independently of Age in the Port Jackson Shark Heterdontus portusjacksoni: With a Commentary on Telomere Length Measurement Methods. PhD dissertation, The University of Adelaide, Adelaide, SA.
Johnsen, A., Pauliny, A., Lifjeld, J. T., and Blomqvist, D. (2017). Is telomere length associated with mate choice in a songbird with a high rate of extra-pair paternity? PLoS One 12:e0182446. doi: 10.1371/journal.pone.0182446
Johnson, A. G. (1979). A simple method for staining the centra of teleost vertebrae. Northeast Gulf Sci. 3, 113–115.
Jones, B. C., and Geen, G. H. (1977). Age determination of an elasmobranch (Squalus acanthias) by X-ray spectrometry. J. Fish. Res. Board Can. 34, 44–48. doi: 10.1139/f77-005
Kaganovskaya, S. I. (1933). A method of determining the age and the composition of the catches of the dogfish (Squalus acanthias L.). Bull. Far East Acad. Sci. USSR 1, 139–141.
Kalish, J. M. (1993). Pre and post bomb radiocarbon in fish otoliths. Earth Planet Sci. Lett. 114, 549–554. doi: 10.1016/0012-821x(93)90082-k
Kishi, S., Uchiyama, J., Baughman, A. M., Goto, T., Lin, M. C., and Tsai, S. B. (2003). The zebrafish as a vertebrate model of functional aging and very gradual senescence. Exp. Gerontol. 38, 777–786. doi: 10.1016/s0531-5565(03)00108-6
LaMarca, M. J. (1966). A simple technique for demonstrating calcified annuli in the vertebrae of large elasmobranchs. Copeia 1966, 351–352. doi: 10.2307/1441147
Mallefet, J., Stevens, D. W., and Duchatelet, L. (2021). Bioluminescence of the largest luminous vertebrate, the kitefin shark, dalatias licha: first insights and comparative aspects. Front. Mar. Sci. 8:633582. doi: 10.3389/fmars.2021.633582
Mantell, L. L., and Greider, C. W. (1994). Telomerase activity in germline and embryonic cells of Xenopus. EMBO J. 13, 3211–3217. doi: 10.1002/j.1460-2075.1994.tb06620.x
Mizutani, Y., Tomita, N., Niizuma, Y., and Yoda, K. (2013). Environmental perturbations influence telomere dynamics in long-lived birds in their natural habitat. Biol. Lett. 9:20130511. doi: 10.1098/rsbl.2013.0511
Natanson, L. J. (1984). Aspects of the Age Growth and Reproduction of the Pacific Angel Shark Squatina Californica Off Santa Barbara California. Master’s Thesis, San Jose State University, San Jose, CA.
Natanson, L. J., and Cailliet, G. M. (1986). Reproduction and development of the Pacific angel shark Squatina californica off Santa Barbara California. Copeia 4, 987–994. doi: 10.2307/1445296
Natanson, L. J., and Cailliet, G. M. (1990). Vertebral growth zone deposition in Pacific angel sharks. Copeia 4, 133–145.
Natanson, L. J., Skomale, G. B., Hoffmann, S. L., Porter, M. E., Goldman, K. J., and Serra, D. (2018). Age and growth of sharks: do vertebral band pairs record age? Mar. Freshw.Res. 69, 1440–1452. doi: 10.1071/mf17279
Natanson, L. J., Wintner, S. P., Johansson, F., Piercy, A., Campbell, P., De Maddalena, A., et al. (2008). Ontogenetic vertebral growth patterns in the basking shark Cetorhinus maximus. Mar. Eco. Prog. Series. 36, 267–278. doi: 10.3354/meps07399
Neer, J. A., and Cailliet, G. M. (2001). Aspects of the life history of the Pacific electric ray, Torpedo californica (Ayers). Copeia 2001, 842–847. doi: 10.1643/0045-8511(2001)001[0842:aotlho]2.0.co;2
Nehmens, M. C., Feldheim, K. A., and Ebert, D. A. (2020). Understanding what we cannot see: a genetic approach to the mating system of the Southern Lanternshark, Etmopterus granulosus. Mar. Biol. 167:139.
Officer, R. A., Day, R. W., Clement, J. G., and Brown, L. P. (1997). Captive gummy sharks, Mustelus antarcticus, form hypermineralised bands in their vertebrae during winter. Can. J Fish. Aquat. Sci. 54, 2677–2683. doi: 10.1139/f97-157
Officer, R. A., Gason, A. S., Walker, T. I., and Clement, J. G. (1996). Sources of variation in counts of growth increments in vertebrae from gummy shark Mustelus antarcticus and school shark Galeorhinus galeus: implications for age determination. Can.J. Fisher. Aquat. Sci. 53, 1765–1777. doi: 10.1139/f96-103
Pardo, S. A., Cooper, A. B., and Dulvy, N. K. (2013). Avoiding fishy growth curves. Methods Ecol. Evol. 4, 353–360. doi: 10.1111/2041-210x.12020
Parsons, G. R. (1983). An examination of the vertebral rings in the Atlantic sharpnose shark, Rhizoprionodon terraenovae. Northeast Gulf Sci. 6, 63–66.
Parsons, G. R. (1985). Growth and age estimation of the Atlantic sharpnose shark, Rhizoprionodon terraenovae: a comparison of techniques. Copeia 1985, 80–85. doi: 10.2307/1444793
Porter, M. E., Beltrán, J. L., Koob, T. J., and Summers, A. P. (2006). Material properties and biochemical composition of mineralized vertebral cartilage in seven elasmobranch species (Chondrichthyes). J. Exp. Biol. 209, 2920–2928. doi: 10.1242/jeb.02325
Raoult, V., Peddemors, V. M., Zahra, D., Howell, N., Howard, D. L., De Jonge, M. D., et al. (2016). Strontium mineralization of shark vertebrae. Sci. Rep. 6:29698.
Richards, S. W., Merriman, D., and Calhoun, L. H. (1963). Studies on the marine resources of southern New England. IX. The biology of the little skate, Raja erinacea. Bull. Bingham Oceanogr. Coll. Yale Univ. 18, 4–67.
Ridewood, W. G. (1921). On the calcification of the vertebral centra in sharks and rays. Philos. Trans. R. So. B 210, 311–407. doi: 10.1098/rstb.1921.0008
Rocco, L., Morescalchi, M. A., Costagliola, D., and Stingo, V. (2002). Karyotype and genome characterization in four cartilaginous fishes. Gene 295, 289–298. doi: 10.1016/s0378-1119(02)00730-8
Schaetzlein, S., Lucas-Hahn, A., Lemme, E., Kues, W. A., Dorsch, M., Manns, M. P., et al. (2004). Telomere length is reset during early mammalian embryogenesis. Proc. Natl. Acad. Sci. U.S.A. 101, 8034–8038. doi: 10.1073/pnas.0402400101
Schwartz, F. J. (1983). Shark Ageing Methods and Age Estimation of Scalloped hammerhead, Sphyrna lewini, and Dusky, Carcharhinus obscurus, Sharks Based on Vertebral Rings. Washington, DC: U.S. Department of Commerce, 167–174. NOAA Technical Report NMFS 8.
Simpfendorfer, C. A. (1993). Age and growth of the Australian sharpnose shark, Rhizoprionodon taylori, from north Queensland, Australia. Environ. Biol. Fish. 36, 233–241. doi: 10.1007/bf00001718
Smart, J. J., Chin, A., Tobin, A. J., and Simpfendorfer, C. A. (2016). Multimodel approaches in shark and ray growth studies: strengths weaknesses and the future. Fish Fish. 17, 955–971. doi: 10.1111/faf.12154
Stevens, J. D. (1975). Vertebral rings as a means of age determination in the blue shark (Prionace glatu:a L.). J. Mar. BioI. Assoc. U.K. 55, 657–665. doi: 10.1017/s0025315400017318
Straube, N., Iglesias, S. P., Sellows, D. Y., Kriwet, J., and Schliewen, U. K. (2010). Molecular phylogeny and node time estimation of bioluminescent Lantern Sharks (Elasmobranchii: Etmopteridae). Mol. Phyl. Evolu. 56, 905–917. doi: 10.1016/j.ympev.2010.04.042
Straube, N., Schliewen, U., and Kriwet, J. (2008). Dental structure of the Giant lantern shark Etmopterus baxteri (Chondrichthyes: Squaliformes) and its taxonomic implications. Environ. Biol. Fish. 82, 133–141. doi: 10.1007/s10641-007-9264-z
Tanaka, S., Chen, C. T., and Mizue, K. (1978). Studies on sharks. XVI Age and growth of Eiraku shark, Galeorhinus japonicus (Muller et Henle). Bull. Fac. Fish. Nagasaki Univ. 45, 12–28.
Tanaka, S., and Mizue, K. (1979). Studies on sharks – XV. Age and growth of Japanese dogfish, Mustelus manazo Bleeker in the East China Sea. Bull. Jpn. Soc. Sci. Fish. 45, 43–50.
Tricola, G. M., Simons, M. J. P., Atema, E., Boughton, R. K., Brown, J. L., Dearborn, D. C., et al. (2018). The rate of telomere loss is related to maximum lifespan in birds. Philos. Trans. R. Soc. Lond. B Biol. Sci. 373:20160445. doi: 10.1098/rstb.2016.0445
Varney, R. M., Pomory, C. M., and Janosik, A. M. (2017). Telomere elongation and telomerase expression in regenerating arms of the starfish Luidia clathrata (Asteroidea: Echinodermata). Mar. Biol. 164:195.
Vleck, C. M., Haussmann, M. F., and Vleck, D. (2003). The natural history of telomeres: tools for aging animals and exploring the aging process. Exp. Geront. 38, 791–795. doi: 10.1016/s0531-5565(03)00110-4
Welden, B. A. (1984). Radiometric Verification of Age Determination in Elasmobranch Fishes. M.S. thesis, California State University Hayward, California.
Welden, B. A., Cailliet, G. M., and Flegal, A. R. (1987). “Comparison of radiometric with vertebral band age estimates in four California elasmobranchs,” in The Age and Growth of Fish, eds R. C. Summerfelt and G. E. Hall (Ames: Iowa State Univ. Press), 301–315.
Whittemore, K., Vera, E., Martinez-Nevado, E., Sanpera, C., and Blasco, M. A. (2019). Telomere shortening rate predicts species life span. Proc. Natl. Acad. Sci. U.S.A. 116, 15122–15127. doi: 10.1073/pnas.1902452116
Wright, W. E., Piatyszek, M. A., Rainey, W. E., Byrd, W., and Shay, J. W. (1996). Telomerase activity in human germline and embryonic tissues and cells. Dev. Genet. 18, 173–179. doi: 10.1002/(sici)1520-6408(1996)18:2<173::aid-dvg10>3.0.co;2-3
Keywords: ageing, biology, elasmobranch, genetics, life history, Squaliformes, longevity
Citation: Nehmens MC, Varney RM, Janosik AM and Ebert DA (2021) An Exploratory Study of Telomere Length in the Deep-Sea Shark, Etmopterus granulosus. Front. Mar. Sci. 8:642872. doi: 10.3389/fmars.2021.642872
Received: 16 December 2020; Accepted: 02 March 2021;
Published: 06 April 2021.
Edited by:
Jürgen Kriwet, University of Vienna, AustriaReviewed by:
Shigehiro Kuraku, RIKEN Center for Biosystems Dynamics Research (BDR), JapanCopyright © 2021 Nehmens, Varney, Janosik and Ebert. This is an open-access article distributed under the terms of the Creative Commons Attribution License (CC BY). The use, distribution or reproduction in other forums is permitted, provided the original author(s) and the copyright owner(s) are credited and that the original publication in this journal is cited, in accordance with accepted academic practice. No use, distribution or reproduction is permitted which does not comply with these terms.
*Correspondence: Melissa C. Nehmens, bW5laG1lbnNAZ21haWwuY29t; bW5laG1lbnNAbWxtbC5jYWxzdGF0ZS5lZHU=
Disclaimer: All claims expressed in this article are solely those of the authors and do not necessarily represent those of their affiliated organizations, or those of the publisher, the editors and the reviewers. Any product that may be evaluated in this article or claim that may be made by its manufacturer is not guaranteed or endorsed by the publisher.
Research integrity at Frontiers
Learn more about the work of our research integrity team to safeguard the quality of each article we publish.