- 1School of Ocean and Earth Science, University of Liverpool, Liverpool, United Kingdom
- 2Marine Biological Association, Plymouth, United Kingdom
- 3Scottish Association for Marine Science, Oban, United Kingdom
- 4School of Ocean and Earth Science, University of Southampton, Southampton, United Kingdom
- 5School of Biological and Marine Sciences, University of Plymouth, Plymouth, United Kingdom
- 6The Dove Marine Laboratory, School of Natural and Environmental Sciences, Newcastle University, Newcastle, United Kingdom
Annual surveys of the abundance of intertidal invertebrates and macroalgae have been made at between 70 and 100 rocky intertidal time-series sites around the United Kingdom coastline since 2002 under the MarClim project. The data provide a unique opportunity to investigate the impacts of both pervasive climate change and their punctuation by extreme events on intertidal species. After the extreme storm events in the 2013/2014 winter season and the record heatwaves in the summers of 2018 and 2020, MarClim surveys recorded both physical and biological changes to rocky shore habitats. Subsequent surveys reassessed the effects on community structure via analysis of those species that resisted storm damage, those species that returned after the extreme storm events, and species that opportunistically occupied vacant habitat after storm-induced species loss. In addition, biannual storm damage surveys documenting communities recovery were carried out in the spring and winter of each year from 2014 to 2020 at three MarClim sites in north Cornwall (Crackington Haven, Trevone, and St. Ives), which experienced different types of abiotic and biotic damage resulting from these storms. Impacts of heatwaves and cold spells on the abundance of species were determined by regression on frequencies of event per year. Species of invertebrates and macroalgae generally declined in years of more frequent winter cold spells and summer heatwaves, while winter heatwaves and summer cold spells had similar numbers of positive and negative effects across species. Winter warm spells tended to have a more negative effect on cold-affinity species than on warm-affinity species. No abrupt shift was recorded after the 2013/2014 storms. Whilst a short-term change in some species was recorded in quantitative quadrat surveys, the biological communities returned to the long-term species composition and abundance within 2 years. The heatwave events caused sublethal heat damage in macroalgae, evidenced as dried areas of tissue on many individuals, with mortality-induced reductions in the abundance of only a few invertebrate species, recorded in Scotland and southwest England after the heatwave events in 2018 and 2020. MarClim and storm-damage surveys indicate that there have been no sustained impacts from either extreme thermal or storm events across the rocky intertidal communities, and biodiversity has not been significantly altered as a result. The abundance and biogeographical distributions of rocky intertidal species and communities around the United Kingdom are being driven by longer-term, large scale, pervasive change in environmental conditions, with a gradual shift towards dominance of Lusitanian species from the early 2000s in responses to warming of the marine climate.
Introduction
Storms and heatwaves are becoming more frequent as a result of climate warming (Oliver et al., 2018; IPCC, 2019) with significant impacts on the coastal ecosystems of the world (Smale et al., 2019). Long-term sustained observing programmes have shown evidence of community-wide shifts in composition from cold to warm affinity species (Simpson et al., 2011; Burrows et al., 2019) as long-term trends, but such studies neglect the short-term effects of extreme events.
The marine climate of the British Isles and Ireland has undergone oscillations throughout the 20th century, with the onset of global climate change first becoming evident in the late 1980s (Hawkins et al., 2003; Philippart et al., 2011). The warming trend has slowed down over the last decade (NOAA OISST; Reynolds et al., 2007) due to the climate-driven slowdown in the Atlantic Meridional Overturning Circulation (AMOC) causing a colder patch of surface water to the northwest of the United Kingdom1 This has been reflected in changes in intertidal communities (Burrows et al., 2020). Despite the recent slowing of the warming trend in the United Kingdom, the impacts of climate change are still being experienced, especially the punctuation of pervasive climate change by extreme events (Firth et al., 2015). There has been an increase in both winter and summer extreme events, with 2020 being recently announced as the warmest year on record (NOAA OISST; Reynolds et al., 2007; Madge, 2020; National Oceanic Atmospheric Administration, 2020). Thus it is important to improve understanding of the impacts of climate by extreme events, or climate-driven disturbances on the natural environment and the resultant persistence of the associated communities, rather than just focusing on average annual sea temperatures.
Biological responses to climate change in natural systems are variable, non-linear, and difficult to interpret (Harris et al., 2018). Climate change exerts complex, interacting influences on organismal physiology, with species-specific responses due to different functional traits, sensitivity, and adaptive and acclimatory capacities (Huey et al., 2012). Shifts in the temporal dynamics of recruitment and the abundance of ecosystem engineers in response to environmental changes can modify interaction networks and change the vulnerability of entire communities to disturbance, although the degree of potential change is poorly understood (Mrowicki et al., 2016). Biological responses include aphasic and idiosyncratic shifts in species distributions (Hawkins et al., 2009) that are likely to result in novel ecological conditions in future climates that have no previous analogue (Elith and Leathwick, 2009; Kuo and Sanford, 2009; Pearson et al., 2009). This results in non-analogous communities for which there is no contemporary equivalent in terms of species composition, abundance, community structure, and hence ecosystem processes. In the face of environmental changes in marine and terrestrial systems, there is a need for accurate forecasts of composition and structure of communities and likely consequences for functioning of ecosystems and hence delivery of services to society.
The effects of long-term trends in temperature on changes in the distribution and abundance of marine species are becoming better understood, with range limits shifting polewards (Poloczanska et al., 2013) and a shifting balance of species with colder thermal tolerance ranges (cold-affinity species) and species with warmer thermal tolerance ranges (warm affinity species) within communities (Burrows et al., 2019, 2020). Impacts of short-term variation in temperature, particularly marine heatwaves (Hobday et al., 2016), however, are less well understood. Positive and negative changes in abundance, as well as range expansions and contractions are associated with marine heatwaves (Smale et al., 2019), with species at locations in the warmer half of their distribution ranges tending to be more negatively impacted. Heatwaves are becoming more frequent, longer, and more intense (Oliver et al., 2018), with increasing ecological impacts expected for the rest of this century (Frölicher and Laufkötter, 2018; Oliver et al., 2018, 2019).
The British Isles and Ireland along with much of the North-East Atlantic have rich rocky intertidal habitats (Lewis, 1964; Hawkins et al., 2019b) due to a complex coastline with sharp wave exposure gradients, a broad spectrum of tidal ranges and diverse geologies contributing to differing topographic complexities. There are also mesoscale differences in the fetch and aspect of the coastline and gradients between oceanic and more neritic waters into the Irish and North Seas and along the English Channel. The complex coastline also contributes to differences in larval retention and dispersal. All the above occur across a broader environmental gradient of warmer waters to the south and west and colder waters to the north and east, creating a biogeographic boundary zone region where boreal, lusitanian, and invasive species with different thermal affinities mix (Forbes, 1858; Southward et al., 1995; Simkanin et al., 2005; Helmuth et al., 2006; Mieszkowska et al., 2006; Burrows et al., 2020).
Winter storm events are common, exerting both hydrodynamic pressure in the form of bottom currents, and wind driven storm disturbance (Birchenough et al., 2015). Storm events represent a natural disturbance event in United Kingdom intertidal habitats with impacts including smothering by sediment and displacement by hydrodynamic shear or scouring by wave driven cobbles and finer sediments (Sugden et al., 2008; Birchenough et al., 2015). There is differential adaptation of intertidal species to wave-generated disturbance along wave exposure gradients (Raffaelli and Hawkins, 1996). Changes to the frequency and intensity of extreme storm events may have a greater impact on species survival, communities composition to ephemeral early successional species (Sousa, 1979), with implications for stability, functioning, and resilience of rocky shore communities (Posey et al., 1996; Sugden et al., 2007; Machado et al., 2016; Dzwonkowski et al., 2020). Extreme events including storm events and heat waves are predicted to increase in frequency and intensity (IPCC, 2013) as a consequence of climate change (Machado et al., 2016), with an increase in North Atlantic storms already recorded since the end of the 20th Century (Wolf et al., 2020).
The 2013/2014 winter was meteorologically classified as “highly unusual” (Met Office, 2014), with “very severe” storms periods and associated wave conditions characterised. The combination of high cyclone frequency and above-average cyclone intensity resulted in exceptional storminess, with a succession of major storm events occurring between mid-December 2013 and January 2014. The sequence of storms affecting the United Kingdom was caused by a powerful jet stream driving a succession of low-pressure systems across the Atlantic. Twelve significant storms occurred between December 2013 and February 2014. These storm events reported in this winter period were said to be unmatched in terms of intensity and duration for over 50 years, causing this winter to be ranked as the stormiest on record for the Ireland–United Kingdom domain (Matthews et al., 2014). These conditions resulted in the bulk of the ocean wave energy from the North Atlantic being driven onto the coastlines of southwest England and Wales repeatedly between December 2013 and February 2014. The geomorphical storm response along the southwest coast of England displayed considerable spatial variability; this is mainly attributed to the embayed nature of the coastline and the associated variability in coastal orientation (Masselink et al., 2016). The size and intensity of the storms generated some of the largest waves ever recorded to hit land in Western Europe, reaching 16 m in height. These storms coincided with some of the highest spring tides of the year and record-breaking precipitation levels for the United Kingdom dating back 248 years, leading to saturated coastal ecosystems and an exacerbation of the physical impacts of storm wave forces. The “highly unusual” conditions reported in the 2013/2014 winter period were due to the intense storm events, whereas extreme storms did not occur the following two winters (2014/2015 and 2015/2016), however, these were two of the warmest on record thereby resulting in abnormally mild conditions.
Rocky shores provide an important role in the functioning of nearshore habitats and contribute a wide range of ecosystem services including natural erosion resilience, protection from coastal storms and flooding, habitat provision, economic value, aesthetic, and wellbeing provision as well as scientific research benefits (Hawkins et al., 2010; Corte et al., 2017). Despite their importance and accessibility, the response of these communities to extreme events remains poorly understood, partly due to a lack of long-term contextual data describing the ecological patterns of an area prior to the storm events. Extreme storm events are unpredictable and therefore studies in this area need to be responsive to an event (Harris et al., 2011). The MarClim time series provides a unique opportunity to investigate the impact of the two detailed extreme storm events on rocky shore communities by providing this contextual data, enabling the attribution of ecological patterns to the quantified disturbance event.
The rocky intertidal zone has long been used to examine relationships between abiotic stressors and biological interactions determining ecological (Raffaelli and Hawkins, 1996 for review; updated in chapters in Hawkins et al., 2019a, 2020) and biogeographical patterns (Fischer-Piette, 1934; Crisp and Southward, 1958; Forbes, 1858; Southward et al., 1995; Helmuth et al., 2006; Mieszkowska et al., 2006). High spatio-temporal variation in environmental and anthropogenic stressors occurs both at the local scale of the individual shore, leading to differential population structure, but also large latitudinal gradients in environmental conditions from the tropics to the poles (Mieszkowska and Sugden, 2016a). Rocky intertidal habitats around the United Kingdom coastline have been studied throughout the last century, providing a rich legacy of biological and biogeographical data unrivalled across the globe (see Southward et al., 2004 for review). The United Kingdom straddles the boreal-lusitanian biogeographic transition zone (Forbes, 1858; Lewis, 1964), providing a rich tapestry of habitats, communities and species with which to study the effects of pervasive climate change and extreme events on organisms from a range of evolutionary origins (Vye et al., 2020), including invasive non-native invasive species (NIS) (Mieszkowska et al., 2014a; Firth et al., 2020).
Understanding the spatio-temporal scales over which climate driven changes will impact marine systems is key to producing accurate forecasts of future changes and effective management actions to mitigate the effects of climate change where possible (United Nations, 2020). Here we examine the impacts of extreme events and pervasive climate change to better understand the effects of short-term extreme events and long-term pervasive climate change on rocky intertidal biodiversity. We tested the following hypotheses: (a) cold-affinity species with colder thermal tolerance ranges would be more negatively impacted by heatwaves than warm-affinity species with warmer thermal tolerance ranges, (b) cold spells would have a greater negative effect on warm-affinity species, and (c) summer heatwaves and winter cold spells would have greater impacts than winter warm spells and summer cold spells. Given the highly disturbed nature of rocky shores we also hypothesised that recovery from storm damage would be rapid by core foundation species such as mussels in the Mytilus species complex but would be prefaced by more ephemeral species. We have also considered important habitat-forming canopy seaweeds on, under and amongst which many invertebrates live (e.g., Thompson et al., 1996; Teagle et al., 2017).
Materials and Methods
United Kingdom Rocky Intertidal Long-Term Time-Series
The MarClim project annually surveys between 70 and 100 long-term time-series sites, with each site being surveyed in the same season of each year. These surveys record the abundance and geographic distribution of 82 species of invertebrates and macroalgae of boreal, lusitanian and invasive biogeographic origins, many of which have a distributional limit on, or close to the United Kingdom coastline. The original species list comprising 55 ecologically important and easy to identify species was developed from historical surveys carried out by Southward and Crisp in the 1950s (Crisp and Southward, 1958; Southward et al., 2004), Lewis and team from the 1970s (Lewis et al., 1982; Lewis, 1986) plus monitoring and experimental work by Hawkins and colleagues from the 1970s to the 2000s (Hawkins and Hartnoll, 1983; Hartnoll and Hawkins, 1985; Southward et al., 1995; all featured in Hawkins and Jones, 1992); these were added to over the years with the rise in number of NIS and lusitanian species colonizing rocky shores in the United Kingdom assisted by better field identification guides (e.g., Bunker et al., 2010). The surveys use the rank categorical SACFOR scale, derived from Crisp and Southward (1958) (amended by JNCC, 1990) to assess the abundance of all 82 species, combined with quadrat-based counts for limpets and barnacles, and timed searches for trochids.
Species Thermal Affinities
Thermal affinities for species in surveys were represented by the median temperatures of their known geographical ranges (Species Temperature Index, STI), obtained by overlaying distribution polygons on maps of average sea surface temperature (SST, see Burrows et al., 2020 for details). Average SST values from 1982 to 2011 were calculated using the same NOAA OISST dataset used to express heatwaves and cold spells in daily temperatures for within-range coastal 0.25° latitude/longitude grid cells.
Extreme Events
The RmarineHeatWaves package in R (Hobday et al., 2016; Smit et al., 2018) was used to detect abnormally hotter and colder events or spells in regional daily SSTs from the NOAA Optimal Interpolated Sea Surface Temperature dataset (OISSTv2 HR; Reynolds et al., 2007). Data were extracted and expressed as daily average temperatures for the whole of the region 49-53°N 6-0°W for the period January 1, 1982 to December 31, 2019. These regional average daily temperatures were used for hot and cold spell detection. The period from January 1, 1983 to December 31, 2012 was used as the reference period for calculation of day of the year temperature percentiles. Warm events were detected as those periods in which temperatures exceeded the 90th percentile temperature for that day of the year for five or more days (Hobday et al., 2016). Cold spells followed a similar definition; those periods where temperatures were below the 10th percentile for that day of the year for five or more days. We also obtained yearly maxima and minima and 5 and 95 percentile temperatures from the same daily time series. Warm and cold events were characterised by their intensity, as maximum and mean temperature above the 90th percentile, duration and cumulative intensity (days × mean intensity).
Changes in Average Annual Abundance and Annual Frequency of Heatwaves and Cold Spells
Species abundance time series were taken as average annual abundance grouped across all sites surveyed annually from 2002 to 2018 (Burrows et al., 2020). For each species, differences in abundance from the preceding year were related to the frequency of heatwaves and cold spells occurring in the winters and summers using trends derived from linear regression [lm () call in R 4.0.2] to test the hypotheses outlined in the introduction.
Heatwave Damage Surveys
Visual assessments of heat damage were recorded during MarClim annual surveys at all sites around the United Kingdom coastline, with digital photographic images taken of damaged organisms. The broadscale abundance of organisms was then recorded, using the SACFOR scale (Mieszkowska et al., 2014b), to place into a long-term context. Temporal patterns of abundance of intertidal communities were visualised using multidimensional scaling (MDS) ordination to show the recovery trajectories predicted for each site. MDS were based on Bray Curtis similarity coefficient calculated from non-standardised, square root transformed data. This biannual dataset is placed into context alongside the sustained time-series monitoring of MarClim intertidal community data at these locations dating back to the early 2000s.
Storm Damage Surveys
Biological impacts of the extreme storm events were initially surveyed at all MarClim sites in England 4 months afterwards, and at all MarClim sites in Wales 6 months after the storms during the annual surveys. Subsequent annual surveys have reassessed the effects on community structure following the 2013/2014 storms via analysis of: those species that were undamaged, those species that were able to return after the extreme storm events and invasive non-native species that opportunistically occupied vacant habitat after storm-induced species loss, colonising those shores affected by storm damage.
In addition, biannual surveys to document damage and potential recovery to rocky intertidal communities were carried out in the spring and winter of 2014 and 2020 at three sites on the north coast of Cornwall, Crackington Haven (50.7417N, −4.6405W), Trevone (50.5450N, −4.9850W) and St. Ives (50.2187N, −5.4751W) (Figure 3). Five replicate 50 cm × 50 cm quadrats were randomly placed in the lowshore and midshore regions at each of these sites (defined biologically) across a distance of approximately 50 m and the percentage cover of sessile species and the abundance of mobile species recorded within each quadrat. Storm damage was evident across large areas of these shores, and these quadrat surveys were carried out in addition to MarClim surveys to provide additional quantitative data specifically focusing on biological damage and recovery. Data were analysed using the PRIMER Version 7 Software and MDS ordination produced (as above) for each survey period and each site (Clarke and Gorley, 2015).
Results
Extreme Events
Several extreme weather events occurred in 2018. Between late February and early March, Britain experienced a severe spell of winter weather with notable extremenes in air temperature. Known as the “Beast from the East,” anticyclone Hartmut coincided with storm Emma to cause some of the worst weather conditions in decades. These events caused a mass mortality of subtidal biota along the east coast of England (Pinnegar et al., 2020), however, no observed changes in the abundance of any of the 50 species of invertebrate surveyed by MarClim were recorded at any long-term monitoring site along the North Sea coastline during 2018 (Mieszkowska et al., 2020). Repeat surveys at a frequently studied site, Heybrook Bay in southwest England found some damage to calcareous algae (crustose corallines and habitat forming Corallina officinalis) around the rim of rockpools, but no obvious signs of death of marine invertebrates including warm-water lusitanian species (e.g., Chthamalus species, Phorcus lineatus, Patella depressa).
Cold spells such as those caused by the extreme event of 2018 can occur at any time of the year, but are more frequent in the first half of the year in the region, with 30 cold spells occurring between January – June over the period of analysis (1982–2020) compared with 19 in the second half of the year between July – December around the southwest UK coastline (Table 1). Notably the cold spells of 2013, although short in duration, were the most intense event for 17 years and since this time there have been no cold spells for the past 7 years (Figure 1).
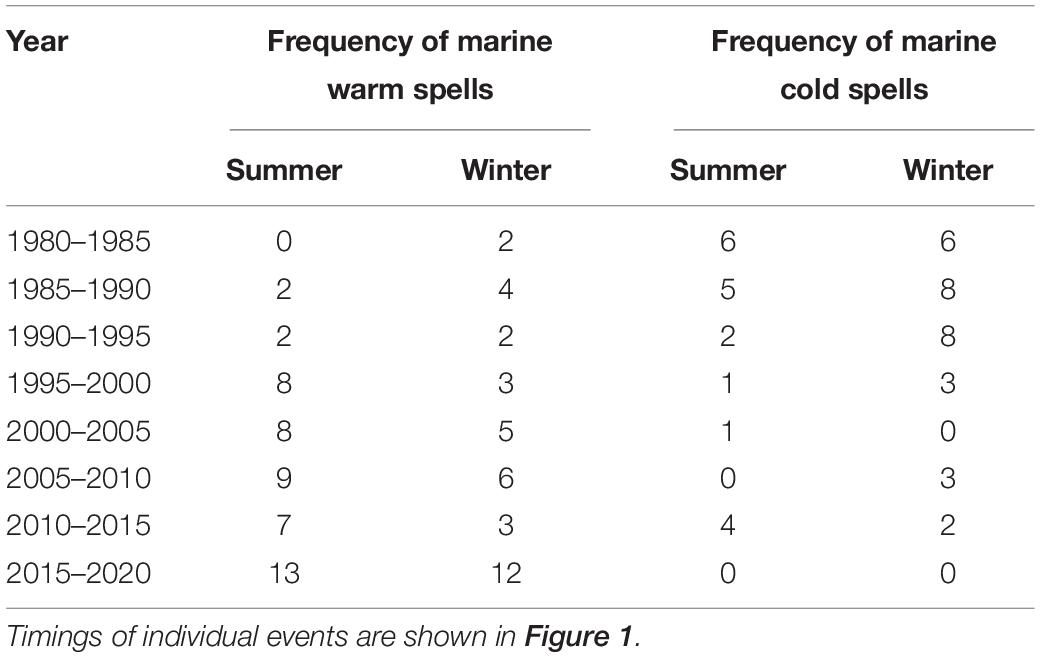
Table 1. Summary of frequencies of heatwaves and cold spells in the southwest Britain (49–53°N, 6–0°W) between 1980 and 2020.
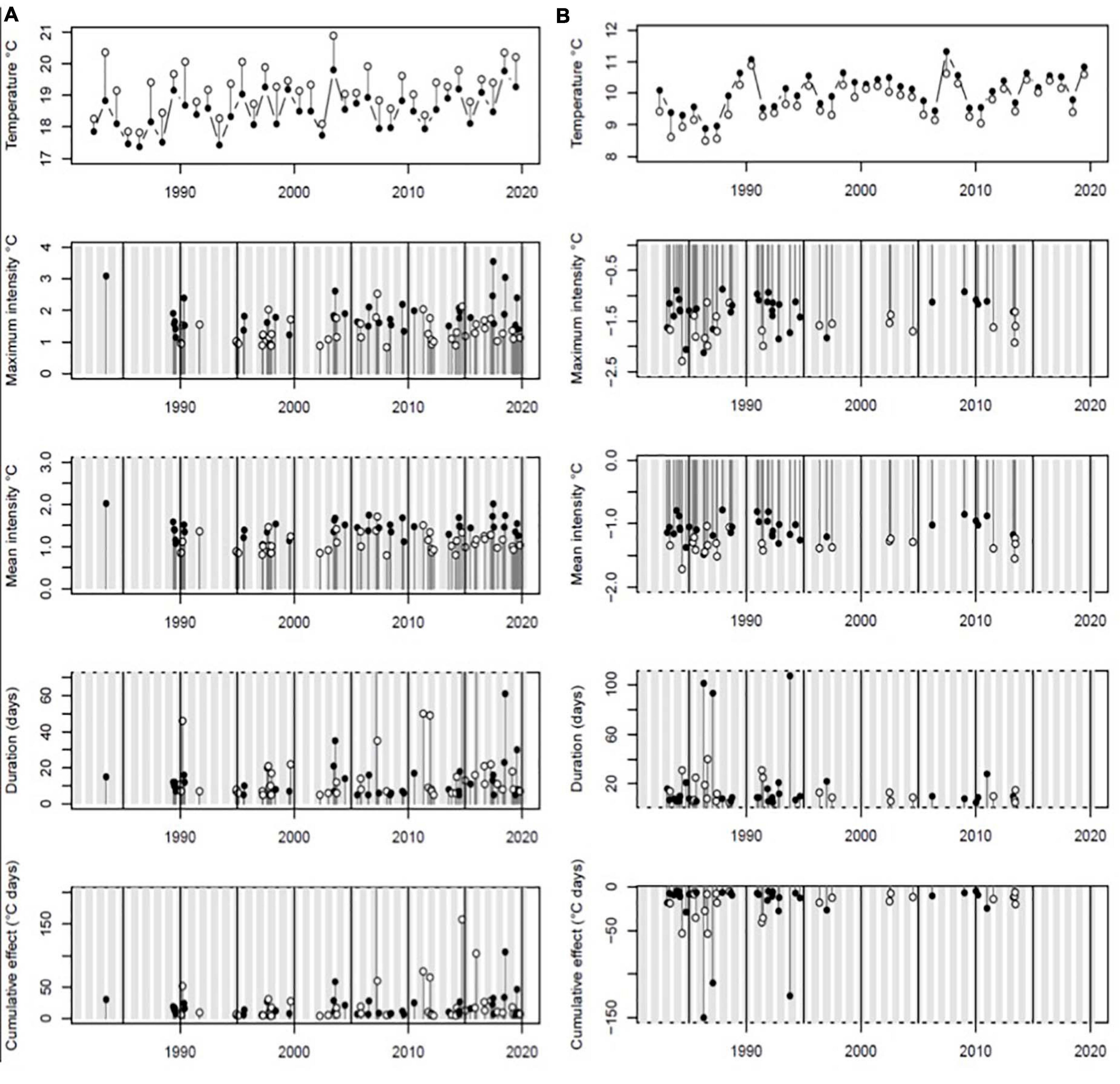
Figure 1. (A) Heatwaves, (B) cold spells from 1982 to 2020. Top plots show (A) 95th percentile (closed circles) and maximum values (open circles) of daily SSTs per year and (B) 5th percentile and minimum daily temperatures per year. Summer heatwaves (May to September) and winter cold spells are shown by filled circles, with winters and summers shown shaded and blank, respectively.
Marine warm spells have become more frequent in both summer (heatwaves) and winter (anomalously warm temperatures) since 1982, whereas cold spells are becoming less frequent in both summer and winter across the same period. This evidences a shift away from seasonal patterns of extreme cold temperature in the marine environment towards a more widespread set of warmer events throughout the year (Table 1).
In addition to the extreme cold weather, 2018 was one of the warmest years on record, with the joint hottest summers on record recorded in 2018 and 20202. Five of the warmest years ever recorded in the United Kingdom occurred since 2010. The Marine heatwaves are becoming more frequent since the 1980s, and possibly longer and more intense. The heatwave of 2018 stands out as being the longest (60 days) if not the most intense on record, and with the biggest cumulative degree × days score in excess of 19°C (Figure 2). Heatwaves occur most frequently in June (11 events between 1982 and 2020), July (13 events) and August (10 events) with the Met Office reporting an average air temperature of 1.5°C above the long-term average in 2018 (Met Office, 2020).
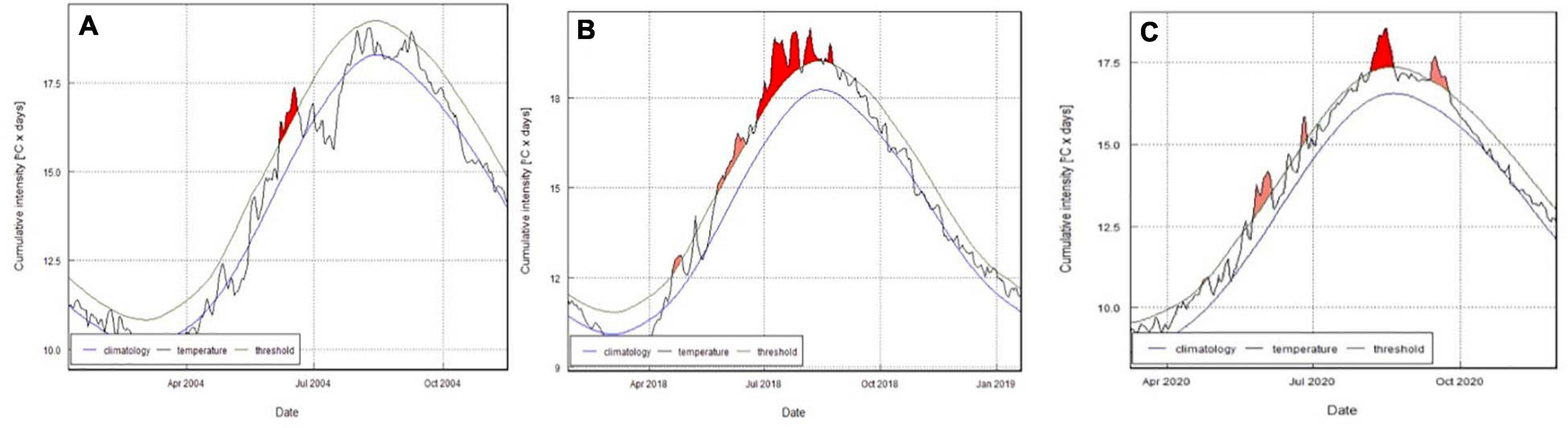
Figure 2. (A) 2004, (B) 2018, (C) 2020 heatwave events, durations, and cumulative intensities for SST.
MarClim surveys across the British Isles in 2018 and 2020 after hot weather in July and August found evidence of heat damage to the highshore habitat-forming fucoid species Pelvetia canaliculata, Fucus spiralis, and Fucus vesiculosus (Figure 3). This heat damage was probably caused by exposure to hot air temperatures during low tides. It did not, however, affect the overall abundance of these species at long-term sites compared to previous years, with no decrease in abundances recorded in surveys the following years, suggesting sub-lethal impacts of the heatwave at this time. The only evidence of negative impacts of heatwaves on invertebrates was recorded for the boreal barnacle Semibalanus balanoides, with patchy but total mortality recorded after the 2018 event at some sites in Oban, southwest Scotland, at the upper vertical limit of the species (Burrows, personal observation). A severe impact on invertebrates was also observed by SJH at Port Gaverne in July 2004 when amphipods (Ampithoe rubricata, and Hyale spp.) usually associated with fucoids, were observed at Port Gaverne, north Cornwall being baked red on the shore. This was not, however, observed during the heatwaves of 2018 or 2020.
Change in Abundance and Extreme Thermal Events
The annual time series for each species was analysed to investigate effects of extreme events on changes in abundance. Changes in abundance since the previous year were related to the number of summer and winter heatwaves in that year using linear regression, with the regression slope expressing the effect of as the response of abundance change to increases in annual frequencies of heatwaves and cold spells (Figures 4, 5). Declines across most species, regardless of thermal affinity, were found in years with more summer heatwaves (Figure 4A). The strength of response of abundance change to the frequency of summer heatwaves was not affected by the thermal affinity of the species (Figure 4A, P > 0.05 for the regression slope of species response on STI; P = 0.07 for the intercept). The increase in frequency of winter heatwaves has resulted in the cold-water, boreal species showing a decline in abundance for those years with more winter heatwaves. The impact of winter heatwaves on boreal species was much clearer than the impact of summer heatwaves on species with affinities for both warmer and colder thermal tolerance ranges (Figure 4B). Cold-water species clearly decline in years with more winter heatwaves, with a positive significant relationship with thermal affinity (P = 0.028).
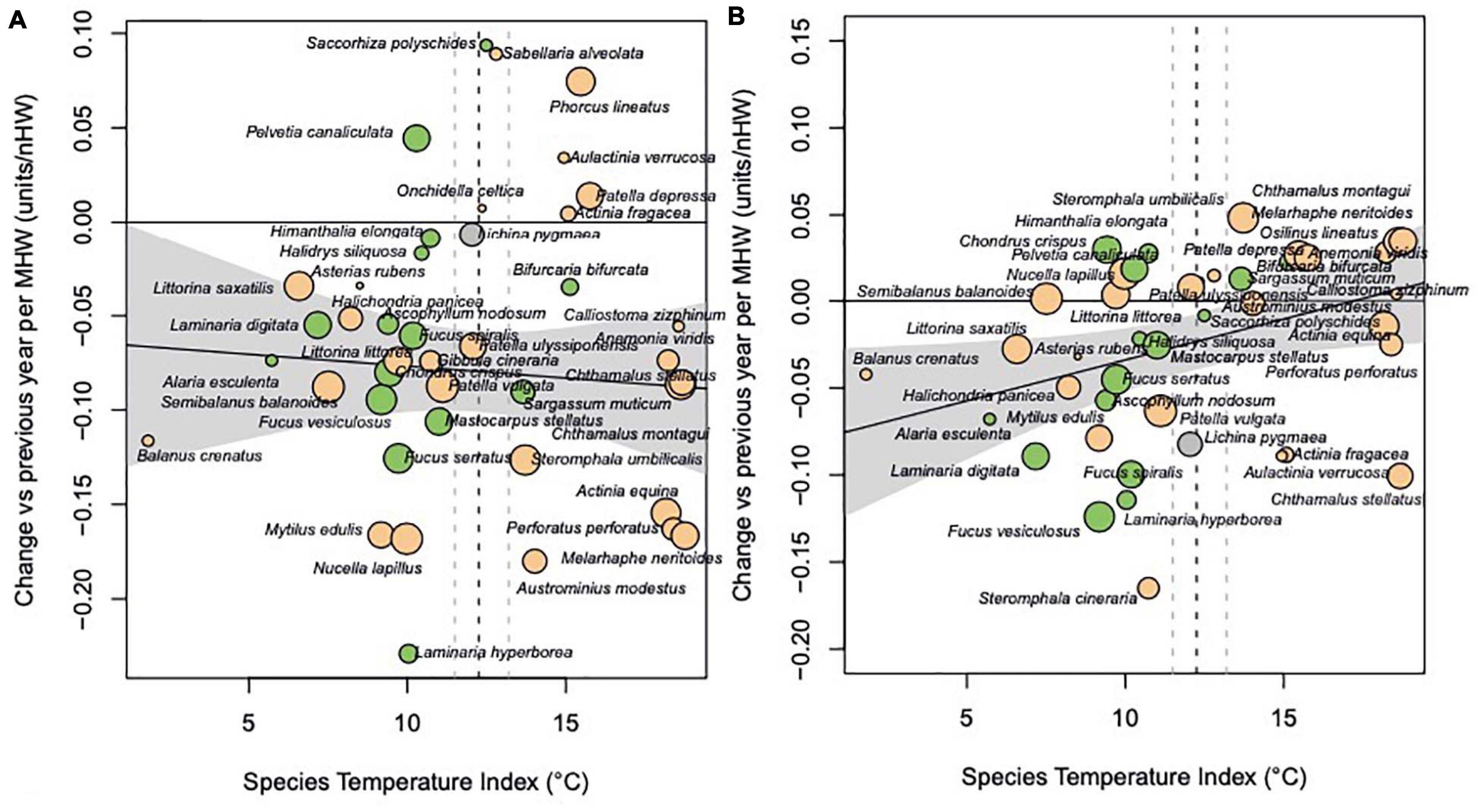
Figure 4. (A) Response to summer heatwaves, shown as the trend in annual abundance change relative to annual frequency of heatwaves. (B) Response to winter heatwaves. Regression slopes for (A) Y = –0.064 (±0.035 SE) – 0.0012.STI (±0.0027 SE), p = 0.65; (B) Y = –0.081 (±0.026 SE) + 0.0047.STI (±0.0021 SE), p = 0.028.
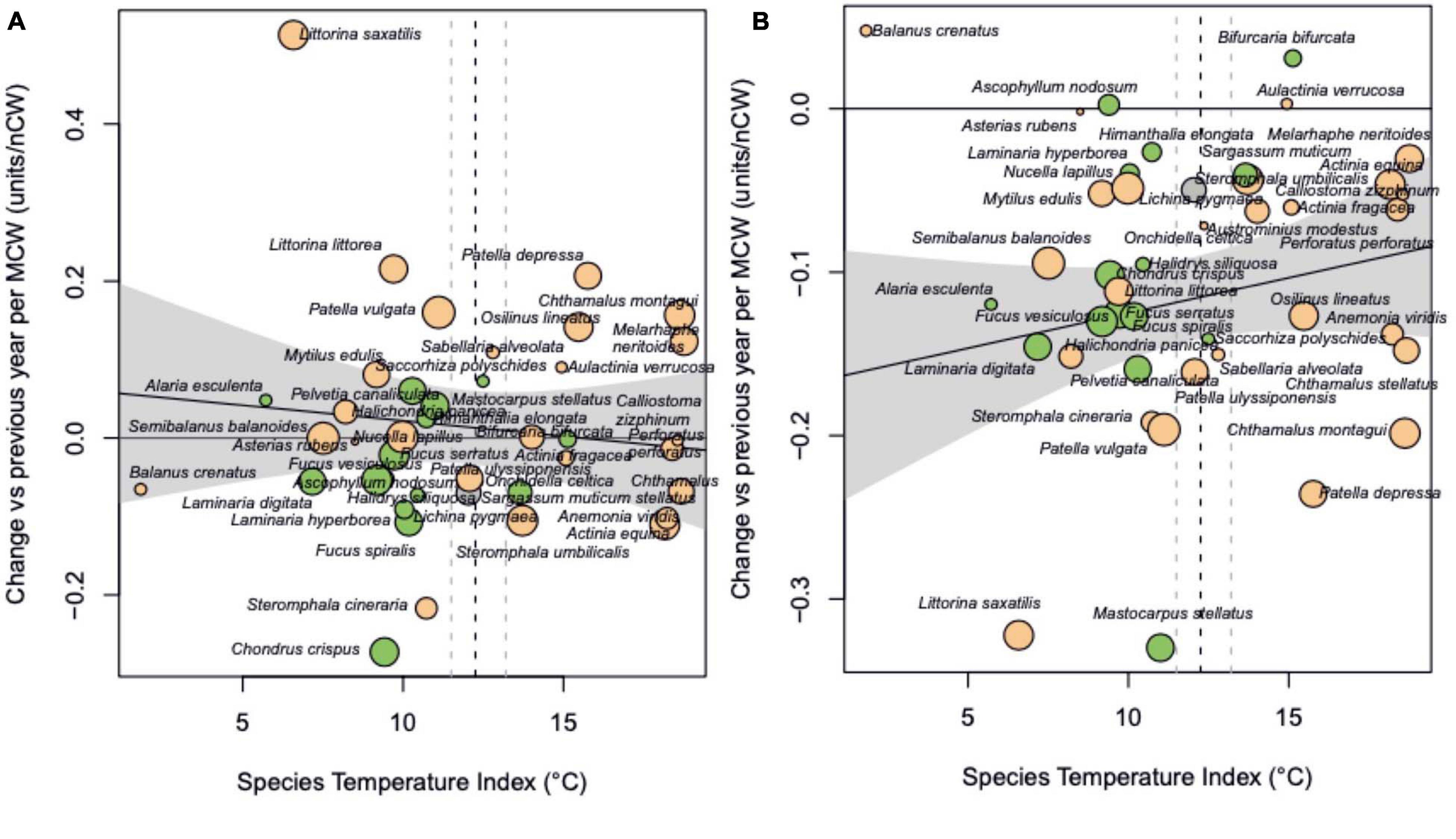
Figure 5. (A) Response to summer cold spells and (B) response to winter cold spells. Regression slopes for (A) Y = 0.049 (±0.076 SE) – 0.0024.STI (±0.0061 SE), p = 0.70; (B) Y = –0.160 (±0.043 SE) + 0.0047.STI (±0.0021 SE), p = 0.33. Regression intercept for (B) was significantly negative (p = 0.0005).
The negative slopes of abundance change on event frequencies shown in Figures 3A,B show that the change in abundance to the previous year becomes more negative with more heatwaves. Most species trends, as regression slope of change in abundance versus frequency of heatwaves per year, were negative (n = 35), showing greater decreases in abundance in years with more frequent heatwaves, with only nine species increasing in abundance due to an increase in the number of summer heatwaves. Summer heatwaves more frequently had negative effects but the magnitude of effects was not related to species thermal affinity (Figure 4A and Table 2).
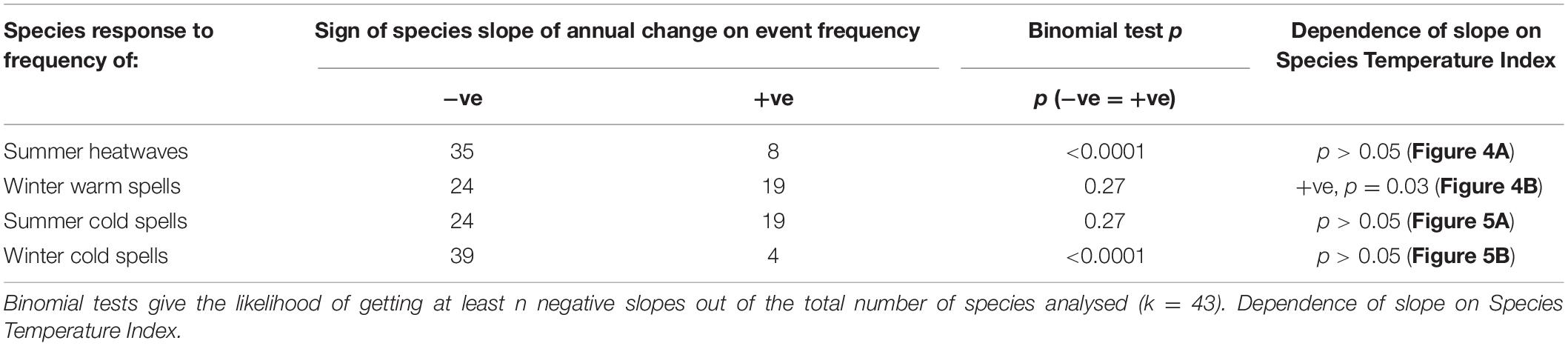
Table 2. Meta-analysis of species responses to frequencies of extreme climatic events, expressed as the sign of the slope of annual change in abundance to the number of extreme events, for time series in abundance of United Kingdom rocky shore species between 2002 and 2019.
Effects of summer cold spells on abundance were not consistently positive or negative (Figure 5A). There was a higher frequency of negative species responses to winter cold spells (Table 2), as expected, but no significant effect of species thermal affinity on responses (Figure 5B).
Storm Damage
Storm damage to intertidal habitats during the extreme events of the winter of 2013/2014 was site specific. Alterations to rock substrate were recorded at several sites; Lizard Point, Cornwall (49.9590, −5.2080), Renney Rocks, south Devon (50.3179, −4.1310), and Osmington Mills, Dorset (50.6330, −2.3760) experienced smothering by large amounts of soft sediment transported from nearby beaches, rocky habitat was uncovered by the loss of most of the sediment from the beach at Crackington Haven, north Cornwall (50.7417, −4.6405), a large boulderfield appeared on top of the soft sediment beach at Porth Neigwl, north Wales (52.7908, −4.5404), and large sections of rock cliff face were removed by the storms at Portland Bill (50.5130, −2.4600) (Figure 6). The smallest biological impacts of the storm events were seen at St. Ives (Figures 7A,B). Variation in the abundance and presence of species was evident between spring and winter each year, but this is in line with natural seasonal cycles at both the lower and mid eulittoral shore zones. No species were entirely removed from the community at this site by the storm events, and there was no change to the rocky habitat.
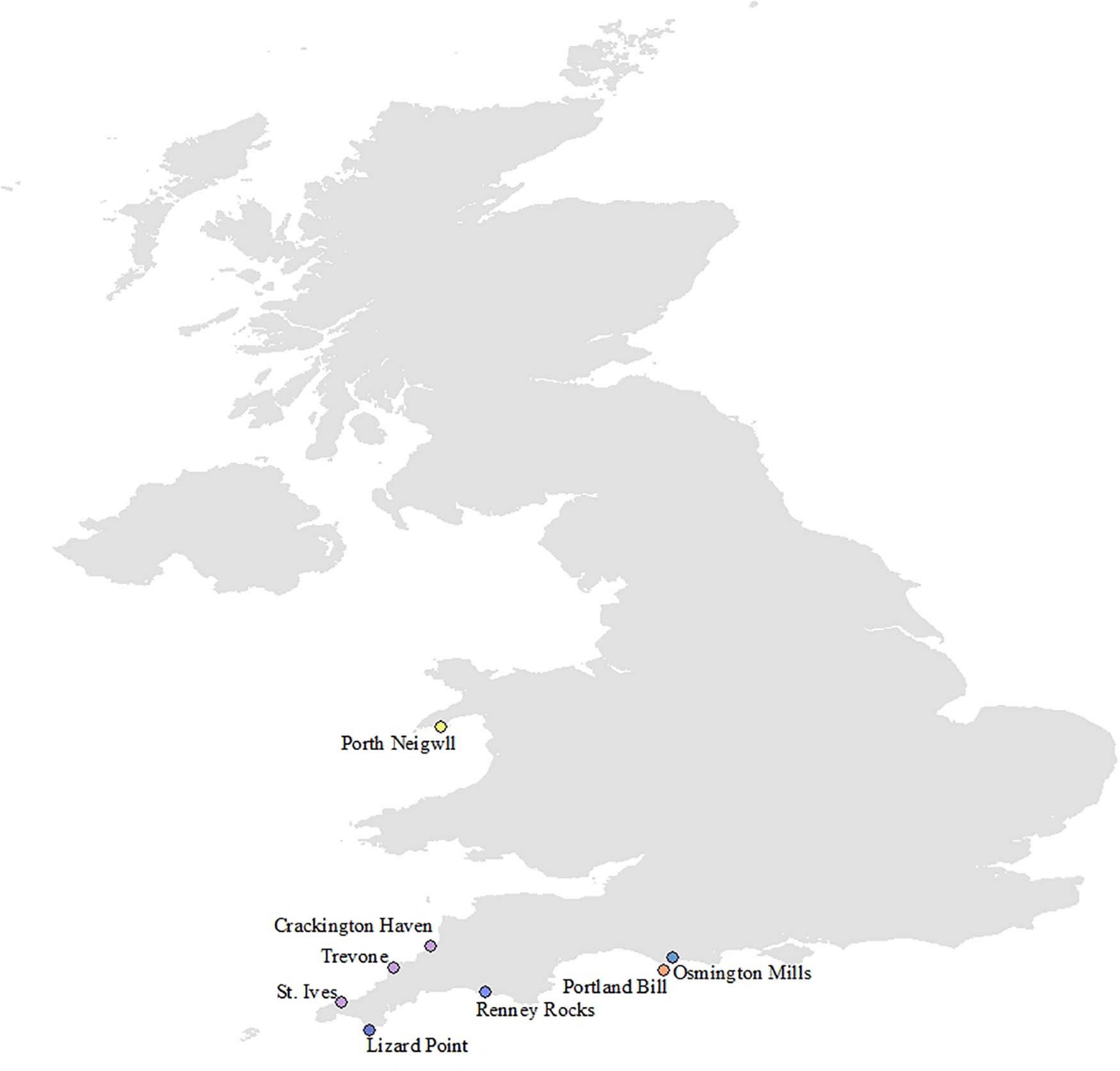
Figure 6. MarClim sites where storm damage was recorded in England and Wales, and biannual storm damage survey locations at Crackington Haven, Trevone, and St. Ives.
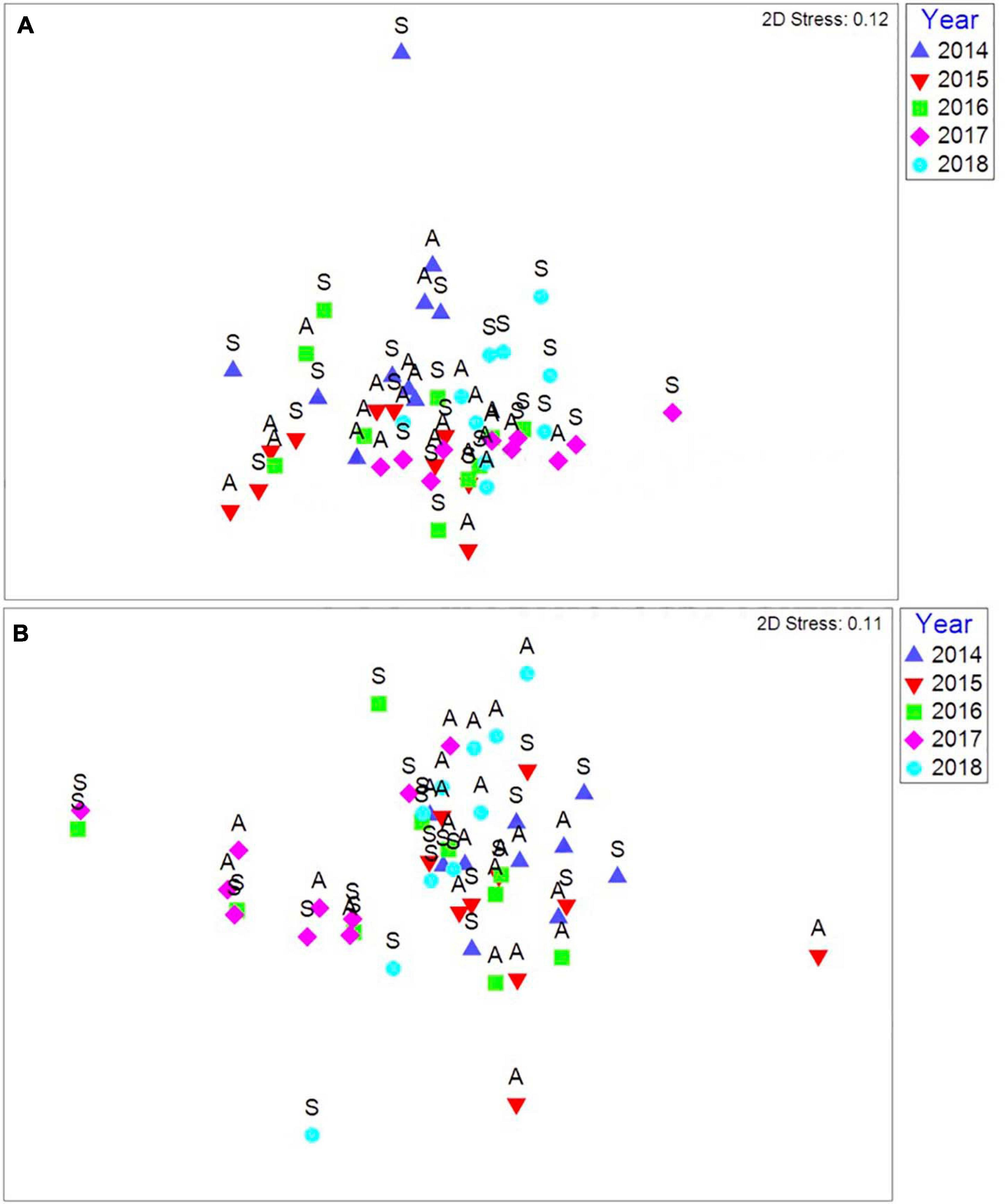
Figure 7. (A) Multi-dimensional scaling ordination of communities diversity per season (S, Spring; A, Autumn) from immediately after the storm events in spring 2014 until autumn 2018 in the low eulittoral at St. Ives, (B) Multi-dimensional scaling ordination of communities diversity per season (S, Spring; A, Autumn) from immediately after the storm events in spring 2014 until autumn 2018 in the mid eulittoral at St. Ives. Based on Bray Curtis similarity coefficient, non-standardised data and square root transformed abundances.
Crackington Haven had no species present on the rocky habitat that was uncovered by the storm events in the lower and eulittoral zones in 2014 and 2015. Species had begun to colonise these vacant rock habitats in 2016, with increasing species diversity in 2017 and 2018. There was a seasonal signal as for St. Ives which is related to natural seasonal cycles (Figures 8A,B).
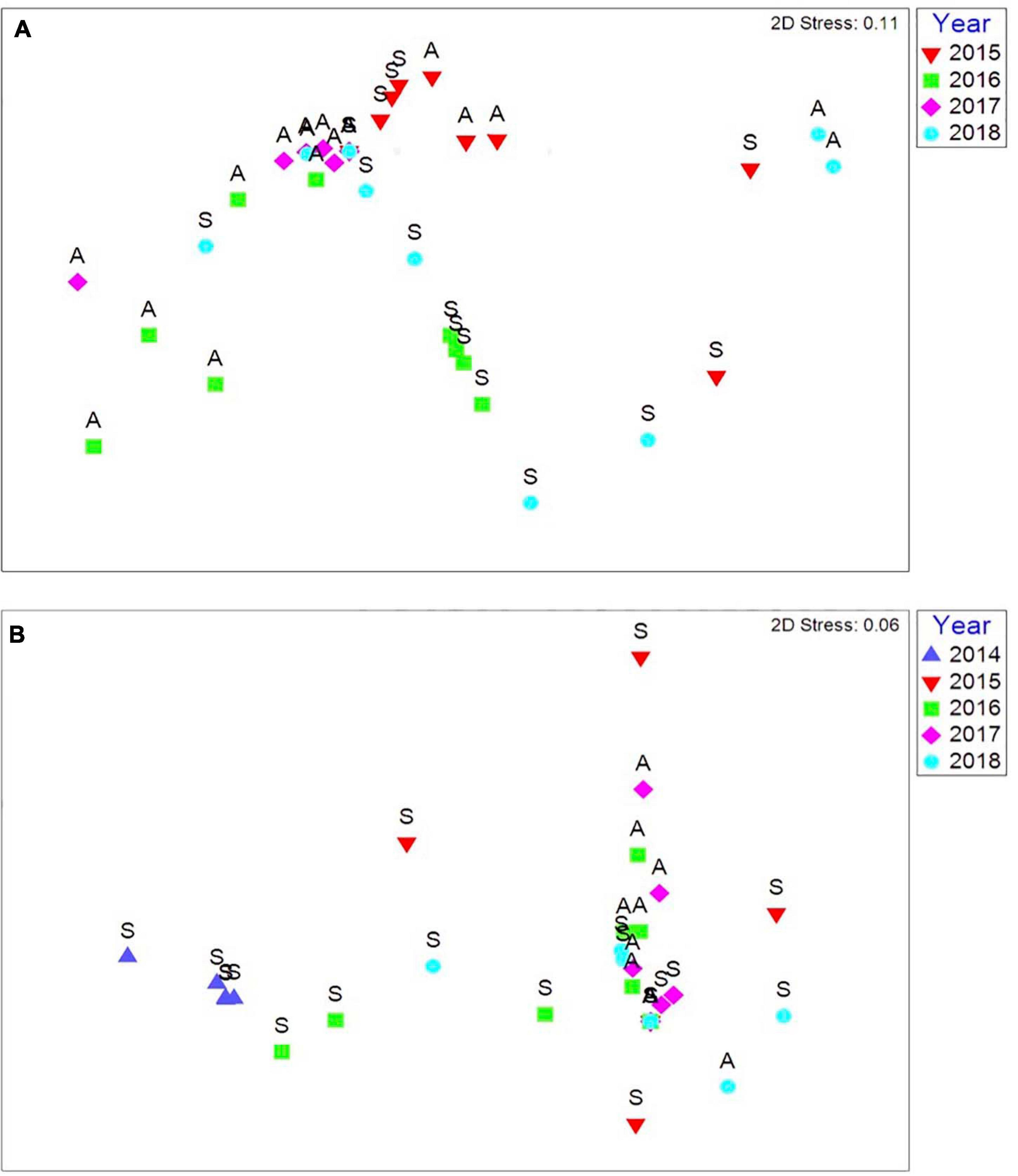
Figure 8. (A) Multi-dimensional scaling ordination of communities diversity per season (S, Spring; A, Autumn) from immediately after the storm events in 2015 until autumn 2018 in the low eulittoral at Crackington Haven, (B) Multi-dimensional scaling ordination of communities diversity per season (S, Spring; A, Autumn) from immediately after the storm events in spring 2014 until autumn 2018 in the mid eulittoral at Crackington Haven. Based on Bray Curtis similarity coefficient, non-standardised data and square root transformed abundances.
The largest biological responses were recorded at Trevone. At this site the complete removal of adult mussels (Mytilus edulis, Mytilus galloprovincialis and hybrids) from the lower eulittoral zone impacted the wider intertidal community (Figure 9A). Spring 2014 and spring and autumn 2015 were visually different to subsequent years, as biological recovery had not occurred. The low eulittoral took longer to stabilise than the mid eulittoral zone due to almost all adult mussels in the low eulittoral zone being removed by the storms (Figure 9B), and did not show signs of recovery until 2016, when juvenile M. edulis settlement had occurred in this zone. Similar to St. Ives and Crackington Haven, seasonal cycles were evident in the data.
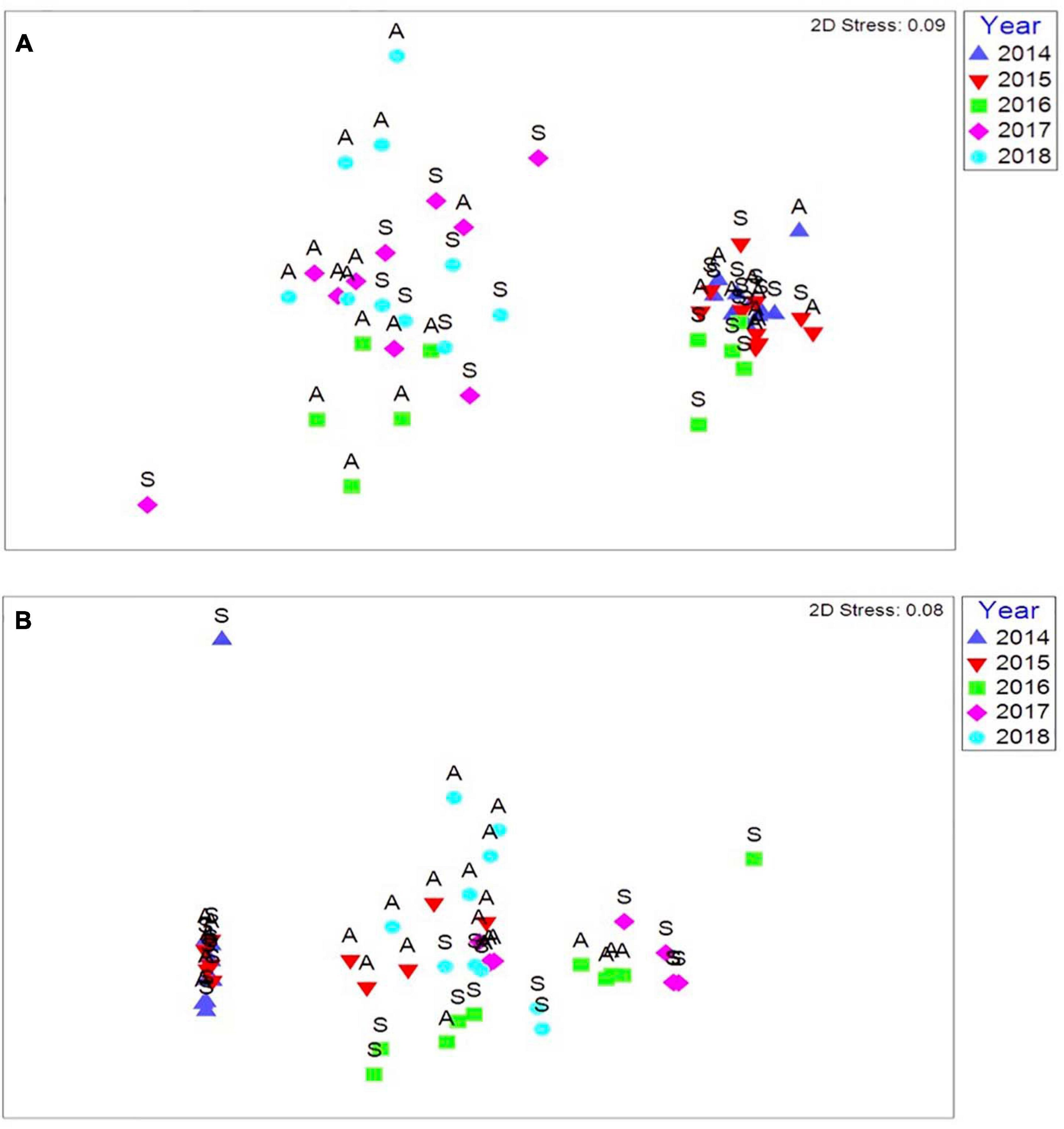
Figure 9. (A) Multi-dimensional scaling ordination of communities diversity per season (S, Spring; A, Autumn) from immediately after the storm events in spring 2014 until autumn 2018 in the low eulittoral at Trevone, (B) MDS plot showing communities diversity per season (S, Spring; A, Autumn) from immediately after the storm events in spring 2014 until autumn 2018 in the mid eulittoral at Trevone. Based on Bray Curtis similarity coefficient, non-standardised data and square root transformed abundances.
At all three sites where storm damage occurred, the community abundance and composition showed a gradual shift from the early 2000s, but no abrupt shift after the winter 2013/2014 storms (Figure 10). These sustained observations surveys demonstrate how the extreme storm events did not drive significant impacts across the rocky intertidal communities, with biodiversity not being significantly affected. Whilst a short-term change in some species was recorded in the quadrat surveys, the biological communities returned to the long-term composition within a few years as colonisation and succession occurred at all three sites.
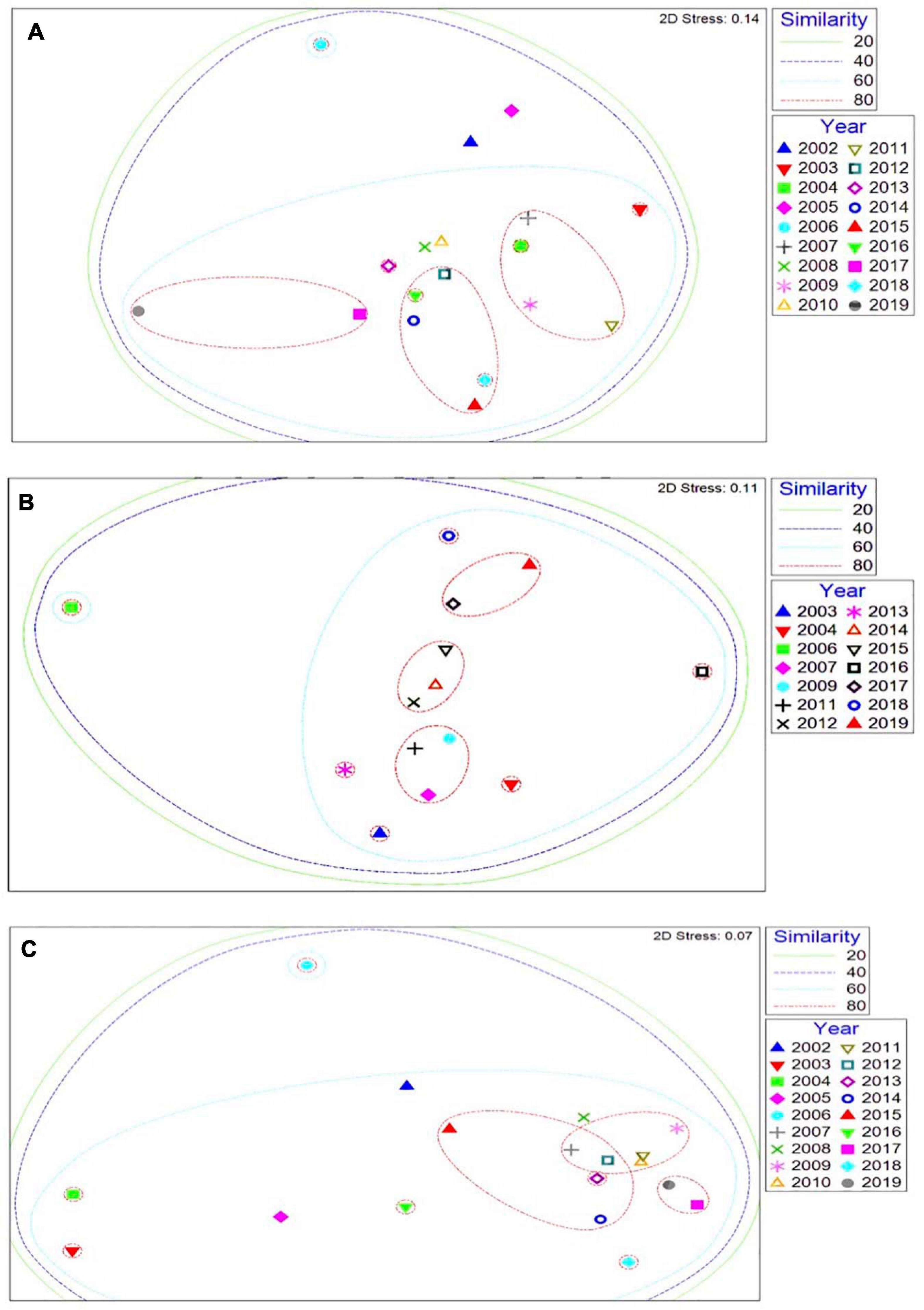
Figure 10. Multi-dimensional scaling ordination of MarClim community data at (A) St. Ives, (B) Crackington Haven, and (C) Trevone over the long-term time-series 2002–2019. Based on Bray Curtis similarity coefficient, non-standardised data and square root transformed abundances.
Discussion
Year-to-year changes in occupancy-derived measures of abundance for rocky intertidal species are a sensitive metric with which to track the vulnerability of species to extreme thermal events occurring in the marine climate. Declines in abundance were seen for the majority of invertebrates and macroalgae, regardless of their biogeographic origin, to anomalously warm conditions whenever they occurred during the year, with only responses to winter warm events influenced by thermal affinity. Thermal affinity of species also influenced responses to intense cold spells in winter, but not to the anomalous cooler spells in summer months, reflecting the seasonal acclimatory ability of temperate intertidal species (Vinagre et al., 2016), which are more cold-tolerant during the winter period where environmental temperatures are lower (Bourget, 2011). The negative responses of most species to summer heatwave frequencies again supports the occurrence of seasonal acclimation including drought hardening in upper shore algae (e.g., Schonbeck and Norton, 1979), and suggests that whilst temperate intertidal species are able to tolerate short periods of extreme heat or cold, they are more responsive to pervasive changes in the climate that occur across decadal timescales ultimately influencing performance in terms of growth and reproductive output leading to recruitment. The responses of intertidal species in the British Isles match global-scale analyses, indicating a general relationship between species thermal affinity and responses to marine climate change across the world (Webb et al., 2020), supporting the value of thermal affinity calculations in advancing the ability to accurately forecast population abundances and future distributional shifts (Sunday et al., 2012).
Our work has focused on analysing SST, rather than air temperature. In coastal waters, warm anomalies in summer sea temperatures are associated with heatwave summer air temperatures and very mild winter air temperatures; anomalously cold events in both winter and summer similarly follow air temperatures. Thus sea temperatures can indicate what is happening when the tide is out (e.g., Poloczanska et al., 2008) Extreme heat events, especially when the tide is out, will trim back the upper limits of species of both algae and animals, especially high on the shore (e.g., F. spiralis and P. canaliculata; Schonbeck and Norton, 1979; Hawkins and Hartnoll, 1985; see Figure 4), but do not seem to cause damage throughout the whole zone of a species. Those species that span the lower water mark such as Laminaria digitata are also highly susceptible. Interestingly as reported previously (Schonbeck and Norton, 1979; Hawkins and Hartnoll, 1985) mid and low shore fucoids showed much less damage than high shore fucoids, although in contrast to the 1970s and 1980s, some slight but not fatal damage was noticed in 2018–2020 across many locations in southwest England in F. vesiculosus, Ascophyllum nodosum and Fucus serratus at their upper zonal limits. Thus zones may be shifted downshore if physical factors act at the top of the zone and squeezed if biological interactions intensify at the bottom of a zone, as recently shown with a combination of observations of upper limit heat damage to Fucus guiryi (recently split from F. spiralis) in Azores, coupled with increased grazing damage lower in the zone due to spread of herbivorous fish from further south – showing a combination of direct and indirect effects of climate change (Martins et al., 2019).
The extreme storm events of winter 2013/2014 caused physical and ecological damage to intertidal ecosystems around the coastlines of England and Wales. These storms were unprecedented in terms of their wave height and magnitude in combination with high winds and heavy precipitation. They occurred during periods of large tides, exacerbating the impacts on intertidal habitats and ecosystems. The time-series surveys showed that the storm impacts were site-specific, with locations on the same stretch of coastline experiencing either biological removal, physical habitat damage, or the removal or deposition of soft sediment from neighbouring beaches. Some sites at exposed locations showed little biological variation from long-term trends in biodiversity and abundance, as species inhabiting these areas have evolved to tolerate rough sea conditions.
Biological impacts were site specific and species specific, with loss of the blue mussel M. edulis/M. galloprovincialis hybrids at Trevone, north Cornwall, with slow recovery due to new recruits taking a few years to attain the body size similar to those individuals removed during the storms. Damage to kelp beds was also recorded at many sites around southwest England, however, these species have evolved to survive in areas of high wave exposure and no reduction in SACFOR abundance of the six main species was recorded in subsequent years. Whilst declines in abundance in species of kelp were recorded immediately after the storm events (Smale and Vance, 2016), the lack of subsequent surveys by these authors meant that they did not record the recovery that occurred within a year or so of these storms as species abundance, composition and extent of kelp forests rapidly returned to pre-storm conditions (Mieszkowska and Sugden, 2016b, 2017, 2018). Rapid recovery has previously been found in experimental clearances of seaweed canopies in both the intertidal and shallow subtidal zone, with recovery within 2 years of laminarian (Hawkins and Harkin, 1985) and F. serratus canopies (Jenkins et al., 1999a), but not A. nodosum (Jenkins et al., 1999b, 2004) that recovers much more slowly. The biannual quadrat surveys demonstrated how species loss and subsequent recovery occurred at one site, Trevone. In contrast colonisation of “new” habitat after the beach sediments were washed away at Crackington Haven showed typical biological succession, with little biological impact recorded at St. Ives. These data echo the lack of significant long-term impacts recorded in the MarClim time-series surveys, and highlight the range of impacts occurring along the same stretch of coastline.
The site-specific effects of these events show how difficult it will be to accurately predict future impacts for intertidal ecosystems. Regardless of the type of impact, communities returned to their long-term species composition within a few years, although the biogenic habitat created by blue mussels will require several years for the newly settled organisms to grow and mature sufficiently for the communities to regain their previous structure and functional capacity. These findings support the importance of considering both the mean and variance when assessing climate change impacts (Benedetti-Cecchi, 2001, 2003; Bertocci et al., 2005; Vasseur et al., 2014) and the role of both weather and climate in shaping the distribution of species, as has also been shown for intertidal species across the Pacific (Harley and Paine, 2009; Mislan et al., 2009; Williams et al., 2016), Atlantic (Birchenough et al., 2015), and Mediterranean (Sarà et al., 2014). These studies support the hypothesis that heterogeneous performance is linked to latitude for intertidal systems around the world (Kingsolver et al., 2013; Mangano et al., 2020).
Long-term sustained observing of rocky intertidal species of macroalgae and invertebrates in the British Isles has put both the extreme temperatures and the storm damage with subsequent recovery into a longer-term context. Across the 2000s there was a species-specific increase in abundance of many Lusitanian species at many time-series sites around the United Kingdom, with extensions in the leading range edges of several gastropods and cirripedes (Mieszkowska et al., 2006; Mieszkowska and Sugden, 2016a). This general increasing trend stopped in 2010 when SST began to cool around the United Kingdom in response to the slowdown in the AMOC, but continued again after 2014; no obvious impacts of extreme thermal or storm events lasting more than a few years were apparent (Burrows et al., 2020). Our data show how pervasive climate change is ultimately driving the abundance and distribution of these species. In contrast short-term changes caused by proximate direct extreme events are much less long-lasting. These can, however, influence abundance along sharp local environmental gradients such as zonation patterns on rocky shores and could contribute to decreases in abundance and local extinctions – especially high on the shore should their frequency of occurrence increase and return time shorten (Benedetti-Cecchi, 2001; Bertocci et al., 2005). Moreover, climate change is more than just temperature and storm driven disturbance events can be important – especially in the North-east Atlantic where they tend to occur in anomalously warm, wet and windy NAO positive winters such as 2013/2014.
The data provide support for hypothesis (a), as cold-affinity species were more negatively impacted by heatwaves than warm-affinity species with warmer thermal tolerance ranges, and partial support for hypothesis (b), because cold spells in winters had greater negative effects on warm-affinity species, although this was not supported for summer cold spells. Summer heatwaves and winter cold spells did have greater impacts on species abundances, regardless of thermal affinity, than winter warm spells and summer cold spells, providing support for hypothesis (c).
Our study shows that the impacts of heatwaves, cold weather events, and extreme storm events can be seen in annual sustained observing spanning long timescales over large spatial scales. Time-series data such as these allow the impacts of extreme events to be placed into context of long-term pervasive trends in the environment and resultant impacts on biodiversity. With better understanding of the role of species traits and thermal tolerances in shaping responses to short-term events (Helmuth et al., 2015; Mieszkowska et al., 2019), we can make more robust predictions of future impacts of climate-driven change.
Data Availability Statement
The raw data supporting the conclusions of this article will be made available by the authors, without undue reservation.
Author Contributions
NM led the field data collection and manuscript writing. SH, MB, and HS contributed to the field data collection. MB led the statistical analysis. HS carried out the PRIMER analyses. All authors contributed to the article and approved the submitted version.
Funding
Natural Resources Wales, Natural England, NM was part-funded by a Marine Biological Association Fellowship.
Conflict of Interest
The authors declare that the research was conducted in the absence of any commercial or financial relationships that could be construed as a potential conflict of interest.
Acknowledgments
The authors would like to thank Natural Resources Wales, Natural England, Scottish Natural Heritage for funding the MarClim time-series, Louise Firth, Leoni Adams, Katrin Bohn, and Catherine Scott for assistance on fieldwork.
Footnotes
- ^ https://www.ncdc.noaa.gov/cdo-web/
- ^ https://www.metoffice.gov.uk/about-us/press-office/news/weather-and-climate/2019/2020-global-temperature-forecast
References
Benedetti-Cecchi, L. (2001). Variability in abundance of algae and invertebrates at different spatial scales on rocky sea shores. Mar. Ecol. Prog. Ser. 215, 79–92. doi: 10.3354/meps215079
Benedetti-Cecchi, L. (2003). The importance of the variance around the mean effect size of ecological processes. Ecology 84, 2335–2346. doi: 10.1890/02-8011
Bertocci, I., Maggi, E., Vaselli, S., and Benedetti-Cecchi, L. (2005). Contrasting effects of mean intensity and temporal variation of disturbance on a rocky seashore. Ecology 86, 2061–2067. doi: 10.1890/04-1698
Birchenough, S. N., Reiss, H., Degraer, S., Mieszkowska, N., and Borja, Á, and Buhl-Mortensen, L. (2015). Climate change and marine benthos: a review of existing research and future directions in the North Atlantic. Wiley interdisciplinary reviews: climate change. Wires Clim. Change 6, 203–223. doi: 10.1002/wcc.330
Bourget, E. (2011). Seasonal variations of cold tolerance in intertidal mollusks and relation to environmental conditions in the St. Lawrence Estuary. Can. J. Zool. 61, 1193–1201. doi: 10.1139/z83-162
Bunker, F. StP. D., Brodie, J. A., Maggs, C. A., and Bunker, A. R. (2010). Guide to Seaweeds of Britain and Ireland. Ross-on-Wye: Marine Conservation Society.
Burrows, M. T., Bates, A. E., Costello, M. J., Edwards, M., Edgar, G. J., Fox, C. J., et al. (2019). Ocean community warming responses explained by thermal affinities and temperature gradients. Nat. Clim. Chang. 9, 959–963. doi: 10.1038/s41558-019-0631-5
Burrows, M. T., Moore, J., Hawkins, S. J., Adams, L., Sugden, H., Firth, L., et al. (2020). Global-scale species distributions predict temperature-related changes in species composition of rocky shore communities in Britain. Glob. Change Biol. 25, 2093–2105. doi: 10.1111/gcb.14968
Corte, G. N., Schlacher, T. A., Checon, H. H., Barboza, C. A. M., Siegle, E., Coleman, R. A., et al. (2017). Storm effects on intertidal invertebrates: increased beta diversity of few individuals and species. PeerJ 5:e3360. doi: 10.7717/peerj.3360
Crisp, D. J., and Southward, A. J. (1958). The distribution of intertidal organisms along the coasts of the English channel. J. Mar. Biol. Assoc. UK 37, 157–208. doi: 10.1017/s0025315400014909
Dzwonkowski, B., Coogan, J., Fournier, S., Lockridge, G., Park, K., and Lee, T. (2020). Compounding impact of severe weather events fuels marine heatwave in the coastal ocean. Nat. Commun. 11:4623. doi: 10.1038/s41467-020-18339-2
Elith, J., and Leathwick, J. R. (2009). Species distribution models: ecological explanation and prediction across space and time. Annu. Rev. Ecol. Evol. Sci. 40, 677–697. doi: 10.1146/annurev.ecolsys.110308.120159
Firth, l. B., Duff, L., Gribben, P. E., and Knights, A. M. (2020). Do positive interactions between marine invaders increase likelihood of invasion into natural and artificial habitats? Oikos 130, 453–463. doi: 10.1111/oik.07862
Firth, L. B., Mieszkowska, N., Grant, L. M., Bush, L. E., Davies, A. J., and Frost, M. T. (2015). Historical comparisons reveal multiple drivers of decadal change of an ecosystem engineer at the range edge. Ecol. Evol. 5, 3210–3222. doi: 10.1002/ece3.1556
Fischer-Piette, E. (1934). Sur la distribution verticale des organismes fixes dans la zone de fluctuation des marees. CR Acad. Sci. Paris 198, 1721–1723.
Forbes, E. (1858). The Distribution of Marine Life, Illustrated Chiefly by Fishes and Molluscs and Radiata. Edinburgh: William Blackwood and Sons.
Frölicher, T. L., and Laufkötter, C. (2018). Emerging risks from marine heat waves. Nat. Commun. 9:650. doi: 10.1038/s41467-018-03163-6
Harley, C. D., and Paine, R. T. (2009). Contingencies and compounded rare perturbations dictate sudden distributional shifts during periods of gradual climate change. Proc. Natl. Acad. Sci. 106, 11172–11176. doi: 10.1073/pnas.0904946106
Harris, L., Nel, R., Smale, M., and Schoeman, D. (2011). Swashed away? Storm impacts on sandy beach macrofaunal communities. Estuar. Coast. Shelf Sci. 94, 210–221. doi: 10.1016/j.ecss.2011.06.013
Harris, R. M. B., Beaumont, L. J., Vance, T. R., Tozer, C. R., Remenyi, T. A., and Perkins-Kirkpatrick, S. E. (2018). Biological responses to the press and pulse of climate trends and extreme events. Nat. Clim. Chang. 8, 579–587. doi: 10.1038/s41558-018-0187-9
Hartnoll, R. G., and Hawkins, S. J. (1985). Patchiness and fluctuations on moderately exposed rocky shores. Ophelia 24, 53–64. doi: 10.1080/00785236.1985.10426619
Hawkins, S. J., and Harkin, E. (1985). Preliminary canopy removal experiments in algal dominated communities low on the shore and in the shallow subtidal on the Isle of Man. Bot. Mar. 28, 223–230. doi: 10.1515/botm.1985.28.6.223
Hawkins, S. J., and Hartnoll, R. G. (1983). Changes in a rocky shore community: an evaluation of monitoring. Mar. Environ. Res. 9, 131–181. doi: 10.1016/0141-1136(83)90051-x
Hawkins, S. J., and Hartnoll, R. G. (1985). Factors determining the upper limits of intertidal canopy-forming algae. Mar. Ecol. Prog. Ser. 20, 265–271. doi: 10.3354/meps020265
Hawkins, S. J., and Jones, H. D. (1992). Marine Conservation Society Marine Field Course Guide 1. London: Immel.
Hawkins, S. J., Bohn, K., Firth, L. B., and Williams, G. A. (2019a). Interactions in the Marine Benthos: Global Patterns and Processes. Cambridge: Cambridge University Press. doi: 10.1017/9781108235792
Hawkins, S. J., Pack, K. E., Firth, L. B., Mieszkowska, N., Evans, A. J., and Martins, G. M. (2019b). “The intertidal zone of the north-east atlantic region: pattern and process,” in Interactions in the Marine Benthos: Global Patterns and Processes, eds S. J. Hawkins, K. Bohn, L. B. Firth, and G. A. Williams (Cambridge: Cambridge University Press).
Hawkins, S. J., Pack, K. E., Hyder, K., Benedetti-Cecchi, L., and Jenkins, S. R. (2020). Rocky Shores as Tractable Test Systems for Experimental Ecology. Cambridge: Cambridge University Press. doi: 10.1017/S0025315420001046
Hawkins, S. J., Southward, A. J., and Genner, M. J. (2003). Detection of environmental change in a marine ecosystem – evidence from the western English Channel. Sci. Total Environ. 310, 245–256. doi: 10.1016/s0048-9697(02)00645-9
Hawkins, S. J., Sugden, H. E., Mieszkowska, N., Moore, P. J., Poloczanska, E., and Leaper, R. (2009). Consequences of climate-driven biodiversity changes for ecosystem functioning of North European rocky shores. Mar. Ecol. Prog. Ser. 396, 245–259. doi: 10.3354/meps08378
Hawkins, S. J., Sugden, H. E., Moschella, P. S., Mieszkowska, N., Thompson, R. C., and Burrows, M. T. (2010). “The Seashore”, in Silent Summer: The State of Wildlife in Britain and Ireland, ed. N. Maclean (Cambridge: Cambridge University Press), 591–614.
Helmuth, B. T., Russell, B. D., Connnell, S., Dong, Y., Harley, C. D. G., Lima, F. P., et al. (2015). Beyond long-term averages: making biological sense of long term averages in a changing world. Clim. Chang. Responses 1:6. doi: 10.1186/s40665-014-0006-0
Helmuth, B., Mieszkowska, N., Moore, P., and Hawkins, S. J. (2006). Living on the edge of two changing worlds: forecasting the impacts of climate change on rocky intertidal ecosystems. Annu. Rev. Ecol. Evol. Sci. 37, 373–404. doi: 10.1146/annurev.ecolsys.37.091305.110149
Hobday, A. J., Alexander, L. V., Perkins, S. E., Smale, D. A., Straub, S. C., and Oliver, E. C. J. (2016). A hierarchical approach to defining marine heatwaves. Prog. Oceanogr. 141, 227–238. doi: 10.1016/j.pocean.2015.12.014
Huey, R. B., Kearney, M. R., Krockenberger, A., Holtum, J. A. M., Jess, M., Stephen, E., et al. (2012). Predicting organismal vulnerability to climate warming: roles of behaviour, physiology and adaptation. Philos. Trans. R. Soc. B. 367, 1665–1679. doi: 10.1098/rstb.2012.0005
IPCC (2013). “Summary for policymakers,” in Climate Change 2013: the Physical Science Basis. Contribution of Working Group I to the Fifth Assessment Report of the Intergovernmental Panel on Climate Change, eds T. F. Stocker, D. Qin, and G.-K. Plattner (Cambridge: Cambridge University Press).
Jenkins, S., Hawkins, S., and Norton, T. (1999a). Interaction between a fucoid canopy and limpet grazing in structuring a low shore intertidal community. J. Exp. Mar. Biol. Ecol. 233, 41–63. doi: 10.1016/S0022-0981(98)00128-2
Jenkins, S., Hawkins, S., and Norton, T. (1999b). Direct and indirect effects of a macroalgal canopy and limpet grazing in structuring a sheltered inter-tidal community. Mar. Ecol. Prog. Ser. 188, 81–92.
Jenkins, S. R., Norton, T., and Hawkins, S. J. (2004). Long term effects of Ascophyllum nodosum canopy removal on mid shore community structure. J. Mar. Biol. Assoc. UK 84, 327–329. doi: 10.1017/S0025315404009221h
JNCC (1990). SACFOR Abundance Scale used for Both Littoral and Sublittoral Taxa from 1990 Onwards. Available online at: https://mhc.jncc.gov.uk/media/1009/sacfor.pdf [accessed December 2, 2020].
Kingsolver, J. G., Diamond, S. E., and Buckley, L. B. (2013). Heat stress and the fitness consequences of climate change for terrestrial ectotherms. Funct. Ecol. 27, 1415–1423. doi: 10.1111/1365-2435.12145
Kuo, E. S., and Sanford, E. (2009). Geographic variation in the upper thermal limits of an intertidal snail: implications for climate envelope models. Mar. Ecol. Prog. Ser. 388, 37–146. doi: 10.3354/meps08102
Lewis, J. R. (1986). Latitudinal trends in reproduction, recruitment and population characteristics of some rocky littoral molluscs and cirripedes. Hydrobiologia 142, 1–13. doi: 10.1007/978-94-009-4049-9_1
Lewis, J. R., Bowman, R. S., Kendall, M. A., and Williamson, P. (1982). Some geographical components in population dynamics: possibilities and realities in some littoral species. Neth. J. Sea Res. 16, 18–28. doi: 10.1016/0077-7579(82)90013-8
Machado, P. M., Costa, L. L., Suciu, M. C., Tavares, D. C., and Zalmon, I. R. (2016). Extreme storm wave influence on sandy beach macrofauna with distinct human pressures. Mar. Pollut. Bull. 107, 125–135. doi: 10.1016/j.marpolbul.2016.04.009
Madge, G. (2020). 2020 Set to Extend Series of Earth’s Warmest Years. Available online at: https://www.metoffice.gov.uk/about-us/press-office/news/weather-and-climate/2019/2020-global-temperature-forecast [accessed November 25, 2020]
Mangano, M. C., Mieszkowska, N., Helmuth, B., Domingos, T., Sousa, T., and Baiamonte, G. (2020). Moving towards a strategy for addressing climate displacement of marine resources: a proof-of-concept. Front. Mar. Sci. 7:408. doi: 10.3389/fmars.2020.00408
Martins, G. M., Harley, C. D. G., Faria, J., Vale, M., Hawkins, S. J., Neto, A. I., et al. (2019). Direct and indirect effects of climate change squeeze the local distribution of a habitat-forming seaweed. Mar. Ecol. Prog. Ser. 626, 43–52. doi: 10.3354/meps13080
Masselink, G., Scott, T., Poate, T., Russell, P., Davidson, M., and Conley, D. (2016). The extreme 2013/2014 winter storms: hydrodynamic forcing and coastal response along the southwest coast of England. Earth Surf. Proc. Land. 41, 378–391. doi: 10.1002/esp.3836
Matthews, T., Murphy, C., Wilby, R. L., and Harringan, S. (2014). Stormiest winter on record for Ireland and UK. Nat. Clim. Chang. 4, 738–740. doi: 10.1038/nclimate2336
Met Office (2014). Avaialble at: https://www.metoffice.gov.uk/binaries/content/assets/metofficegovuk/pdf/weather/learn-about/uk-past-events/interesting/2013/winter-storms-december-2013-to-january-2014—met-office.pdf (accessed November 25, 2020).
Met Office (2020). Available online at: https://www.metoffice.co.uk/about-us/press-office/news/weather-and-climate/2018/end-of-summer-stats (accessed November 25, 2020).
Mieszkowska, N., and Sugden, H. (2016a). Climate-driven range shifts within benthic habitats across a marine biogeographic transition zone. Adv. Ecol. Res. 55, 325–369. doi: 10.1016/bs.aecr.2016.08.007
Mieszkowska, N., and Sugden, H. (2016b). Marine Biodiversity and Climate Change Monitoring in the UK: Final Report to Natural England on the MarClim Annual Survey 2015. York: Natural England.
Mieszkowska, N., and Sugden, H. (2017). Marine Biodiversity and Climate Change Monitoring in the UK: Final Report to Natural England on the MarClim Annual Survey 2016. York: Natural England.
Mieszkowska, N., and Sugden, H. (2018). Marine Biodiversity and Climate Change Monitoring in the UK: Final Report to Natural England on the MarClim annual survey 2017. York: Natural England.
Mieszkowska, N., Benedetti-Cecchi, L., Burrows, M. T., Cristina Mangano, M., Queirós, A., Seuront, L., et al. (2019). Multinational, integrated approaches linking organismal to biogeographic impacts of climate change. Mar. Ecol. Prog. Ser. 613, 247–252.
Mieszkowska, N., Burrows, M. T., and Sugden, H. (2020). Impacts of climate change on intertidal habitats relevant to the coastal and marine environment around the UK. MCCIP Sci. Rev. 2020, 256–271.
Mieszkowska, N., Burrows, M., Pannacciulli, F., and Hawkins, S. J. (2014b). Multidecadal signals within co-occuring intertidal barnacles Semibalanus balanoides and Chthamalus spp. linked to the Atlantic Multidecadal Oscillation. J. Mar. Syst. 133, 70–76. doi: 10.1016/j.jmarsys.2012.11.008
Mieszkowska, N., Kendall, M. A., Hawkins, S. J., Leaper, R., Williamson, P., Hardman-Mountford, N. J., et al. (2006). Changes in the range of some common rocky shore species in Britain - a response to climate change? Hydrobiologia 555, 241–251. doi: 10.1007/1-4020-4697-9_20
Mieszkowska, N., Sugden, H., Firth, L., and Hawkins, S. J. (2014a). The role of sustained observations in tracking impacts of environmental change on marine biodiversity and ecosystems. Philos. Trans. R. Soc. A 372:20130339. doi: 10.1098/rsta.2013.0339
Mislan, K. A. S., Wethey, D. S., and Helmuth, B. (2009). When to worry about the weather: role of tidal cycle in determining patterns of risk in intertidal ecosystems. Glob. Change Biol. 15, 3056–3065. doi: 10.1111/j.1365-2486.2009.01936.x
Mrowicki, R. J., O’Connor, N. E., and Donohue, I. (2016). Temporal variability of a single population can determine the vulnerability of communities to perturbations. J. Ecol. 104, 887–897. doi: 10.1111/1365-2745.12533
National Oceanic Atmospheric Administration (2020). Available online at: https://www.ncdc.noaa.gov/cdo-web/ (accessed December 7, 2020).
Oliver, E. C. J., Burrows, M. T., Donat, M. G., Sen Gupta, A., Alexander, L. V., and Perkins-Kirkpatrick, K. (2019). Projected marine heatwaves in the 21st century and the potential for ecological impact. Front. Mar. Sci. 6:734. doi: 10.3389/fmars.2019.00734
Oliver, E. C. J., Donat, M. G., Burrows, M. T., Moore, P. J., Smale, D. A., and Alexander, L. V. (2018). Longer and more frequent marine heatwaves over the past century. Nat. Commun. 9:1324. doi: 10.1038/s41467-018-03732-9
Pearson, G. A., Lago-Leston, A., and Mota, C. (2009). Frayed at the edges: selective pressure and adaptive response to abiotic stressors are mismatched in low diversity edge populations. J. Ecol. 97, 450–462. doi: 10.1111/j.1365-2745.2009.01481.x
Philippart, C. J., Anadón, R., Danovaro, R., Dippner, J. W., Drinkwater, K. F., Hawkins, S. J., et al. (2011). Impacts of climate change on European marine ecosystems: observations, expectations and indicators. J. Exp. Mar. Biol. Ecol. 400, 52–69. doi: 10.1016/j.jembe.2011.02.023
Pinnegar, J. K., Wright, P. D., Maltby, K., and Garrett, A. (2020). The impact of climate change on fisheries, relevant to the coastal and marine environment around the UK. MCCIP Sci. Rev. 456–481.
Poloczanska, E. S., Brown, C. J., Sydeman, W. J., Kiessling, W., Schoeman, D. S., Moore, P. J., et al. (2013). Global imprint of climate change on marine life. Nat. Clim. Chang. 3, 919–925. doi: 10.1038/nclimate1958
Poloczanska, E. S., Hawkins, S. J., Southward, A. J., and Burrows, M. T. (2008). Modelling the response of populations of competing species to climate change. Ecology 89, 3138–3149. doi: 10.1890/07-1169.1
Posey, M., Lindberg, W., Alphin, T., and Vose, F. (1996). Influence of Storm Disturbance on an Offshore Benthic Community. Bull. Mar. Sci. 59, 523–529.
Raffaelli, D., and Hawkins, S. J. (1996). Intertidal Ecology. New York, NY: Kulwer Academic Publishers.
Reynolds, R. W., Smith, T. M., Lui, C., Chelton, D. B., Casey, K. S., and Schlax, M. G. (2007). Daily high-resolution-blended analysis for sea surface temperature. J. Clim. 20, 5473–5496. doi: 10.1175/2007jcli1824.1
Sarà, G., Milanese, M., Prusina, I., Sara, A., Angel, D. L., and Glamuzina, B. (2014). The impact of climate change on mediterranean intertidal communities: losses in coastal ecosystem integrity and services. Reg. Environ. Chang. 14, 5–17. doi: 10.1007/s10113-012-0360-z
Schonbeck, M. W., and Norton, T. A. (1979). Drought-hardening in the upper-shore seaweeds Fucus spiralis and Pelvetia canaliculata. J. Ecol. 67, 687–696. doi: 10.2307/2259120
Simkanin, C., Power, A.-M., Myers, A., McGrath, D., Southward, A. J., Mieszkowska, N., et al. (2005). Using historical data to detect temporal changes in the abundances of intertidal species on Irish shores. J. Mar. Biol. Assoc. U.K. 85, 1329–1340. doi: 10.1017/s0025315405012506
Simpson, S. D., Jennings, S., Johnson, M. P., Blanchard, J. L., Schön, P.-J., Sims, D. W., et al. (2011). Continental shelf-wide response of a fish communities to rapid warming of the sea. Curr. Biol. 21, 1565–1570. doi: 10.1016/j.cub.2011.08.016
Smale, D. A., and Vance, T. (2016). Climate-driven shifts in species’ distributions may exacerbate the impacts of storm disturbances on North-east Atlantic kelp forests. Mar. Freshw. Res. 67, 65–74. doi: 10.1071/MF14155
Smale, D. A., Wernberg, T., Oliver, E. C. J., Thomsen, M., Straub, S. C., and Burrows, M. T. (2019). Marine heatwaves threaten global biodiversity and the provision of ecosystem services. Nat. Clim. Chang. 9, 306–312. doi: 10.1038/s41558-019-0412-1
Smit, A. J., Hobday, A. J., Alexander, L. V., Perkins, S. E., Smale, D. A., and Straub, S. C. (2018). RmarineHeatWaves: Package for the Calculation of Marine Heat Waves Version 0.17.0. Bellville: South Africa: University of the Western Cape.
Sousa, W. P. (1979). Disturbance in marine intertidal boulder fields: the nonequilibrium maintenance of species diversity. Ecology 60, 1225–1239. doi: 10.2307/1936969
Southward, A. J., Hawkins, S. J., and Burrows, M. T. (1995). Seventy years’ observations of changes in distribution and abundance of zooplankton and intertidal organisms in the western English Channel in relation to rising sea temperature. J. Therm. Biol. 20, 127–155. doi: 10.1016/0306-4565(94)00043-i
Southward, A. J., Langmead, O., Hardman-Mountford, N. J., Aiken, J., Boalch, G. T., and Dando, P. R. (2004). Long-term biological and environmental researches in the western English Channel. Adv. Mar. Biol. 47, 1–105. doi: 10.1016/j.pocean.2014.07.004
Sugden, H., Lenz, M., Molis, M., Wahl, M., and Thomason, J. C. (2008). The interaction between nutrient availability and disturbance frequency on the diversity of benthic marine communities on the north-east coast of England. J. Anim. Ecol. 77, 24–31. doi: 10.1111/j.1365-2656.2007.01323.x
Sugden, H., Panusch, R., Lenz, M., Wahl, M., and Thomason, J. C. (2007). Temporal variability of disturbances: is this important for diversity and structure of marine fouling communitiess? Mar. Ecol. 28, 368–376. doi: 10.1111/j.1439-0485.2007.00184.x
Sunday, J. M., Bates, A. E., and Dulvy, N. K. (2012). Thermal tolerance and the global redistribution of animals. Nat. Clim. Chang. 2, 686–690. doi: 10.1038/nclimate1539
Teagle, H., Hawkins, S. J., Moore, P. J., and Smale, D. A. (2017). The role of kelp species as biogenic habitat formers in coastal marine ecosystems. J. Exp. Mar. Biol. Ecol. 492, 81–98. doi: 10.1016/j.jembe.2017.01.017
Thompson, R. C., Wilson, B. J., Tobin, M. L., Hill, A. S., and Hawkins, S. J. (1996). Biologically generated habitat provision and diversity of rocky shore organisms at a hierarchy of spatial scales. J. Exp. Mar. Biol. Ecol. 202, 73–84. doi: 10.1016/0022-0981(96)00032-9
United Nations (2020). Available online at: https://www.un.org/sustainabledevelopment/sustainable-development-goals/ (accessed November 21, 2020).
Vasseur, D. A., DeLong, J. P., Gilbert, B., Greig, H. S., Harley, C. D., McCann, K. S., et al. (2014). Increased temperature variation poses a greater risk to species than climate warming. Proc. R. Soc. B Biol. Sci. 281:20132612. doi: 10.1098/rspb.2013.2612
Vinagre, C., Leal, I., Mendonça, V., Madeira, D., Narciso, L., Diniz, M. S., et al. (2016). Vulnerability to climate warming and acclimation capacity of tropical and temperate coastal organisms. Ecol. Ind. 62, 317–327. doi: 10.1016/j.ecolind.2015.11.010
Vye, S. R., Dickens, S., Adams, L., Bohn, K., Chenery, J., Dobson, N., et al. (2020). Patterns of abundance across geographical ranges as a predictor for responses to climate change: evidence from UK rocky shores. Divers. Distrib. 26, 1357–1365. doi: 10.1111/ddi.13118
Webb, T. J., Lines, A., and Howarth, L. M. (2020). Occupancy-derived thermal affinities reflect known physiological thermal limits of marine species. Ecol. Evol. 10, 7050–7061. doi: 10.1002/ece3.6407
Williams, G. A., Helmuth, B., Russell, B. D., Dong, Y. W., Thiyagarajan, V., and Seuront, L. (2016). Meeting the climate change challenge: pressing issues in southern China and SE Asian coastal ecosystems. Reg. Stud. Mar. Sci. 8, 373–381. doi: 10.1016/j.rsma.2016.07.002
Keywords: climate change, intertidal, invertebrate, biogeography, range shift, time-series, Species Thermal Index, extreme event
Citation: Mieszkowska N, Burrows MT, Hawkins SJ and Sugden H (2021) Impacts of Pervasive Climate Change and Extreme Events on Rocky Intertidal Communities: Evidence From Long-Term Data. Front. Mar. Sci. 8:642764. doi: 10.3389/fmars.2021.642764
Received: 16 December 2020; Accepted: 12 March 2021;
Published: 31 May 2021.
Edited by:
Marcos Rubal, University of Porto, PortugalReviewed by:
Angus Jackson, Marine Conservation Society, United KingdomCamilla Bertolini, Ca’ Foscari University of Venice, Italy
Paul Robert Brooks, University College Dublin, Ireland
Copyright © 2021 Mieszkowska, Burrows, Hawkins and Sugden. This is an open-access article distributed under the terms of the Creative Commons Attribution License (CC BY). The use, distribution or reproduction in other forums is permitted, provided the original author(s) and the copyright owner(s) are credited and that the original publication in this journal is cited, in accordance with accepted academic practice. No use, distribution or reproduction is permitted which does not comply with these terms.
*Correspondence: Nova Mieszkowska, nova@liverpool.ac.uk; nova@MBA.ac.uk