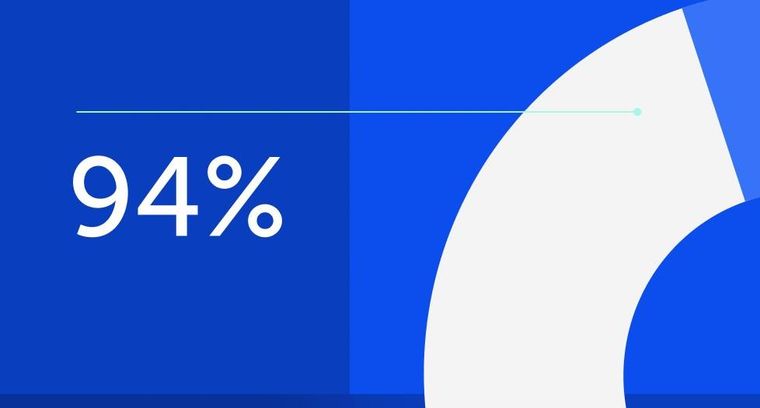
94% of researchers rate our articles as excellent or good
Learn more about the work of our research integrity team to safeguard the quality of each article we publish.
Find out more
REVIEW article
Front. Mar. Sci., 23 February 2021
Sec. Marine Biotechnology and Bioproducts
Volume 8 - 2021 | https://doi.org/10.3389/fmars.2021.636613
This article is part of the Research TopicMarine Biotechnology, Revealing an Ocean of OpportunitiesView all 26 articles
The benefits of the complex microscopic and industrially important group of microalgae such as diatoms is not hidden and have lately surprised the scientific community with their industrial potential. The ability to survive in harsh conditions and the presence of different pore structures and defined cell walls have made diatoms ideal cell machinery to produce a variety of industrial products. The prospect of using a diatom cell for industrial application has increased significantly in synch with the advances in microscopy, metabarcoding, analytical and genetic tools. Furthermore, it is well noted that the approach of industry and academia to the use of genetic tools has changed significantly, resulting in a well-defined characterization of various molecular components of diatoms. It is possible to conduct the primary culturing, harvesting, and further downstream processing of diatom culture in a cost-effective manner. Diatoms hold all the qualities to become the alternative raw material for pharmaceutical, nanotechnology, and energy sources leading to a sustainable economy. In this review, an attempt has been made to gather important progress in the different industrial applications of diatoms such as biotechnology, biomedical, nanotechnology, and environmental technologies.
The global trend of economy and society is shifting toward building a greener and more sustainable society to combat climate and health issues. This is a critical issue, which is being approached with various interdisciplinary strategies to produce a wide range of sustainable products. For instance, biotechnology research has invested a significant number of resources, time, and money in studying microorganisms to exploit them for human consumption in multiple ways. Furthermore, the decades of research and improvisation in cultivation strategies, extraction, and harvesting protocols strongly support a good return on investment in industrial applications of microbes. A pinch of soil and a drop of water contain a diversity of microbes that controls major biogeochemical cycles and subsequently have the potential of producing an abundance of sustainable products. Since the beginning of this century, a high amount of research work has been published on industrial applications of microbes such as bacteria, yeast, and microalgae (Figure 1). But, limited attention has been paid to diatoms which have the potential of becoming a robust sustainable industry because diatoms can continuously grow with an average annual yield of 132 MT dry diatoms ha–1 over almost 5 years (Wang and Seibert, 2017).
Figure 1. Approximate number of research articles indexed in Scopus database (September 14, 2020) in the area of industrial application of different microbes (bacteria, yeast, algae, and diatoms).
Diatoms are dynamic microorganisms with rich diversity and detailed membrane design. They are the most dominating phytoplankton with an overall number of around 200,000 species having complex variability in dimensions and shapes (Round et al., 1990; Smetacek, 1999; Mann and Vanormelingen, 2013). Diatoms’ distinctive characteristic compared to the phytoplankton community is their silica cell wall, known as a frustule. This innate ability to uptake silicon from the environment has made them an interesting community of microbes since the 19th century. Few studies have stated the role of frustule biosilicate as pH buffering material which facilitates shifting of bicarbonate to CO2 dissolved in cell fluids (the latter is readily metabolized by diatoms) (Milligan and Morel, 2002).
The access to advanced microscopes and modern genetic tools enabled us to study the detailed frustule structure and validate metabolic pathways involved in absorption, transportation, and polymerization of silicon and other biomolecules like lipids (Knight et al., 2016; Zulu et al., 2018). Furthermore, this advanced knowledge of metabolic pathways and validation of diatom structure can be applied to produce a wide range of renewable products such as optoelectronics, biofuels, nutritional supplements, ecology tools, etc. (Marella et al., 2020).
Other common factors that have shaped the evolution of diatoms are their ability to adapt and grow in various natural resources; fresh and marine water, wastewater, rivers, and oceans. Their abundance and adaptability in a wide range of climate and geographical areas make them suitable for different applications (Jin and Agustí, 2018). It was reported that diatoms are responsible to produce yearly, 40% of the organic carbon and 20% of oxygen (Tréguer et al., 1995; Falkowski et al., 1998; Afgan et al., 2016). Besides, these photoautotrophic organisms are involved in biogeochemical cycles, which play a significant role in global carbon fixation, carbon sequestration, and silicon cycle. They are also suitable candidates to capture nitrogen and carbon from various sources, which can be exploited by waste management and the biofuel industry to create carbon-neutral fuels (Singh et al., 2017). Furthermore, these algae are used to produce nutraceutical compounds, such as vegetarian proteins, omega, and other essential fatty acids for pharmaceutical industries (Wen and Chen, 2001a,b).
Multiple epidemiological, clinical, and pre-clinical studies have shown that omega fatty acids such as eicosapentaenoic acid (EPA) and docosahexaenoic acid (DHA) are useful in slowing down age-related diseases such as cardiovascular diseases and cancer (Cole et al., 2010; Dyall, 2015; Thomas et al., 2015; Wang and Daggy, 2017). The development of diatoms strains rich in omega fatty acids can replace the dependence on fish as a source of omega oils and reduce the problems associated with seasonal variations and ocean pollution which might affect the biochemical composition of fish oil (Alves Martins et al., 2013). Also, various marine diatoms are considered for the commercial production of antioxidant pigments such as fucoxanthin and other carotenoids. It has been reported that these pigments exhibit various protective effects such as strong antioxidant activities (Xia et al., 2013).
Thus, the flexible and complex nature of diatoms offers immense possibilities to develop a wide range of sustainable products and contributes to carbon neutrality. Because of its dimensions, pore distributions, and geometries, it is studied to develop tools for nanotechnology and biomedical industry such as nanofabrication techniques, chemo and biosensing, particle sorting, and control of particles in micro- and nano-fluidics (Mishra et al., 2017). Silica and biosilica can be used to develop advanced nanomaterial for electronic and optical technologies which can be employed for ultra-sensitive detection of biological compounds (Dolatabadi et al., 2011).
Recent accomplishment in diatoms metabarcoding, a reference database of the global population of diatoms, has advanced its use extensively in studying ecological problems such as climate change, acidification, and eutrophication (Nanjappa et al., 2014). Because of its robust nature and potential to inhabit different photic regions, from the equator to the poles, diatoms offer the potential to develop tools and products for all geographical regions (Medlin, 2016). The technological and infrastructure advancements of diatoms-based applications are at a new level. Besides, it requires different kinds of optimization either in laboratory or large-scale research such as energy utilization for different steps, financial modeling, and collaborating with different industries to make diatom-based products commercially successful. However, the standardization at various levels such as optimization of culture conditions, genetic tools, genome and transcriptome sequencing make diatoms based products commercially viable.
Therefore, this review aims to provide a better understanding of the potential of diatoms research at a laboratory scale. We have tried to provide comprehensive information on a variety of diatoms applications such as energy, biomedical products, and environment monitoring which are being investigated at different levels. All these applications have the potential to contribute toward a greener tomorrow. The purpose of the research is to increase the sustainable economy while reducing the dependence on non-renewable resources. Therefore, recovering and producing various sustainable products like biofuels, feed, bioactive molecules, and services like environment monitoring embedded in diatoms is a promising opportunity to be seized as shown in Figure 2.
Fast globalization and industrialization have impacted the ecosystem widely but shutting or slowing down the globalization is not the solution. At the moment, almost 95% of all the transportation industry is based on a non-renewable source of energy (Rodrigue and Notteboom, 2013). Therefore, developing sustainable and carbon-neutral fuels could reduce the existing dependence on fossil fuels and contribute to bringing back harmony in nature without disrupting the existing economic development. Few economic aspects of biofuel production from microalgae such as biodiesel productivity, land use, and oil yield support the use of microalgae for commercial production as compared to corn and other food crops. The oil yield for microalgae with high oil content is almost 15-fold more as compared to corn. Whereas, the land use for corn and maize is 66-fold more as compared to microalgae (Brocks et al., 2003).
The microalgae such as diatoms are the promising feedstock to replace non-renewable sources of energy. It has been proven by geochemists that algal lipids are the major feedstocks of petroleum and these lipids act as the biomarker remaining stable for millions of years (Brocks et al., 2003). The main biomarker for the diatoms is the ratio of C28 and C29 steranes and highly branched isoprenoid alkenes which are found in high-quality oil fields around the globe (Katz et al., 2004).
Moreover, targeting the diatom lipids by manipulating and optimizing the growth and culture conditions such as light, stress, and nutrients can provide an interesting alternative to help meet the existing demands of commercial production of biofuel. Knowing the potential of diatoms to accumulate high lipids and varied compositions of fatty acids, diatoms are an underexploited area of the biofuel industry. The most predominant saturated and unsaturated fatty acids in diatom species are 14:0; 16:0, 16:1, 16:2, 16:3, 18:1, 18:2, 18:3, 20:4, and 20:5 (Dunstan et al., 1993; Sharma et al., 2020). Various reports have been published on different species of diatoms regarding the lipid yield and triacylglycerol accumulation (TAG) under different treatments as shown in Table 1.
It is possible to improve the quality of biodiesel by optimizing the content of different fatty acids that impacts biodiesel properties; cetane number, level of emissions, cold flow, oxidative stability, viscosity, and lubricity (Knothe, 2005). Fatty acids with chain lengths from C16 to C18 should contribute the maximum amount in the final product (Knothe, 2009). Some researchers have reported that a high percentage of mono-unsaturation is also desirable for biodiesel (Knothe, 2012). Thus, optimizing the fatty acid profile along with increased biomass will significantly enhance their economic value.
Statistical analyses predicted that 100 mt/ha/year biomass of diatoms is required for commercial biofuel production (Gallagher, 2011). Over 10 years, productivity range was observed to be between 29 and 142 mt/ha/year (Sheehan et al., 1998; Huesemann and Benemann, 2009), these values motivate the researchers and industry experts to study diatom cell in-depth for the biofuel industry in both lab-scale and large scale.
Furthermore, the availability of advanced genetic tools can help to achieve the missing targets in developing diatoms cells as biofuel machinery (Radakovits et al., 2010; Tibocha-Bonilla et al., 2018). Based on theoretical calculations about the land area, lipid production, and photosynthetic energy conversion, the biofuel demand of the complete United States population could be met using only 5% of United States land (Levitan et al., 2014). Although various other factors that define the efficacy of biodiesel such as engine performance, that is based on (cylinder pressure, brake mean effective pressure, frictional mean effective pressure, power, torque, brake specific fuel combustion, brake thermal efficiency). The statistical data supports the use of microalgae-based biofuel but there are various limitations at a technological level for large-scale implementation of this project. Therefore, one of the alternatives is to use the blended form of biodiesel. It would be more efficient to make a blended version of petro-diesel and microalgae/diatoms based fuel for large-scale operation. The comparative studies of blended (20% microalgae fuel plus 80% petrodiesel) and 100% petrodiesel have no major performance variations. Furthermore, it was reported that there was a reduction in the CO, unburnt HC, and smoke emissions in blended form as compared to pure diesel (Soni et al., 2020).
The cost required to bring a new drug to the market has been estimated by the Tufts Centre for the Study of Drug Development at approximately 2.6 billion dollars (DiMasi et al., 2016). In addition, the current drug delivery systems have limited solubility, poor bio-distribution, lack of selectivity, premature degradation, and unfavorable pharmacokinetics (Aw et al., 2011a,b). Therefore, these limitations have motivated the research and development of alternative drug delivery systems to improve the performance of existing drugs (i.e., increasing bioavailability), while reducing undesirable effects. There is no doubt that existing biomedical technologies have increased the life span but the human society wants to improvise the quality of life further by adopting environment friendly methods. Therefore, we should speed up the process and conduct in-depth research on using diatom frustules, even other bio-inspired alternatives for biomedical applications.
Among the available drug delivery tools (liposomes, nanogels, carbon nanotubes), the intricate frustule characteristics of diatoms such as specific surface area, thermal stability, biocompatibility, and alterable surface chemistry, have attracted attention for its use in drug and gene delivery. It took million years of evolution for diatoms to manufacture this level of complex and delicate structure to protect from the unwanted conditions like high temperature and variable light fluctuations. 3-D section analyses of diatom frustules have shown the availability of multiple pore patterns that range from nanometer to micrometer (Chandrasekaran et al., 2014; Cicco et al., 2015; Ragni et al., 2017). These characteristics are sufficient to explore alternative and low-priced silica-based materials for the biomedical industry (Mishra et al., 2017; Terracciano et al., 2018). Diatoms’ frustule structure changes its homogenous nature, space, and intricate nature according to various environmental factors and silicon uptake efficiency (Knight et al., 2016). This ability can be used to change the frustule shape and pore size, which has multiple applications in the biomedical and nanotechnology industry. The process of biosilicification in diatoms is quite complex, it includes the role of silicic acid transporters, transportation of silica, and polymerization of silica monomers among other processes that have been extensively explained (Martin-Jézéquel et al., 2000; Knight et al., 2016). Moreover, a detailed investigation is being conducted to make the natural 3D porous structure an efficient substitute for delivery systems attributed to its chemical and mechanical features. For instance, some diatom species such as Coscinodiscus concinnus sp. (Gnanamoorthy et al., 2014), Thalassiosira weissflogii sp. (Aw et al., 2011a) are potential drug carriers candidates due to their amorphous nature and morphology. Additionally, various studies have shown that diatoms microcapsules are effective carriers for poorly soluble and water-soluble drugs, which can be applied in both oral and implant applications (Aw et al., 2011a; Ragni et al., 2017).
The defined structural architecture of diatoms, such as pore volume and controllable particle size, allows the synthesis of biomolecules at the micro- to nano-scale (Losic et al., 2005, 2010; Slowing et al., 2008). The growth of fibroblast and osteoblast has been observed on functionalized frustules supporting the idea of using biosilica from diatoms as smart support for cell growth (Ragni et al., 2017). Regarding modified diatoms, Losic et al. (2010) have designed the magnetically guided drug carrier via a functional surface of diatoms with dopamine-modified iron oxide. This modification has shown the capability of sustained release of poorly soluble drugs for 2 weeks, presenting an enhanced performance for drug delivery (Losic et al., 2010). Moreover, genetically modified biosilica has been used to selectively deliver anticancer drugs to tumor sites (Delalat et al., 2015). Overall, these findings have opened the doors to novel drug delivery systems using renewable material. Therefore, all properties of diatoms such as uniform pore structure, chemically inert and biocompatible, non-toxic, easy to transport, filtration efficiency, and specific drug delivery make it a potential model for drug delivery tools (Curnow et al., 2012; Milović et al., 2014; Rea et al., 2014; Vasani et al., 2015).
The controlled production of nanostructured silica is possible through chemical and mechanical treatment for a wide range of applications. This nanopore structure has a huge potential to attach the desired biomolecule (enzymes, DNA, antibodies) and develop label-free analytical tools or enhance the catalytic properties. It has also been shown that enzymes and DNA (oligonucleotides) can be conjugated to silica (Losic et al., 2005; Zamora et al., 2009). The encapsulation of enzymes in diatom biosilica exhibits improved enzymatic properties as compared to other immobilization technologies (Kato et al., 2020).
Additionally, luminescent nano- and micro-particles have gained the attention of the interdisciplinary scientific community (biology, chemistry, and physics). Current available fluorescent labeling agents are quantum dots, lanthanide-doped compounds, and organic fluorophore-tagged nanobeads, which offer good optical properties and a broad excitation spectrum. However, these agents have limitations in properties such as photobleaching and biocompatibility. For instance, De Stefano et al. (2009) studied diatoms’ potential to incorporate fluorophores with increased stability used to study the molecular event of antibody-antigen identification. Moreover, molecular recognition between antibody and antigen was observed in relation to the change in the photoluminescence spectrum of diatoms. Concluding that diatom’s frustules, due to their high sensitivity, low-cost, and availability are ideal alternative candidates for lab-on-particle applications (De Stefano et al., 2008, 2009).
There is no concrete evidence of diatoms’ presence in land animal bodies. Although, various studies showed the presence of diatoms in the internal organs and circulatory system of alive or dead animals in an aquatic environment (Ludes et al., 1996; Lunetta et al., 1998; Hürlimann et al., 2000; Lunetta and Modell, 2005; Horton et al., 2006; Levkov et al., 2017). The siliceous cell wall of this organism is resistant to degradation even under high acidic conditions for a long period (Lunetta and Modell, 2005). The investigation on the occurrence of these organisms inside dead bodies of aquatic environment that died from different causalities opened up a new possibility of forensic analysis through the examination of diatoms called ‘diatom axiom’ or ‘diatom test’(Lunetta et al., 1998). The diatom test is based on the hypothesis that the microalgae will not enter the systemic circulation and reach other internal organs and tissues such as bone marrow unless the circulation is functional. A forensic examiner can determine whether the individual was alive when it was entering the water by checking the presence of diatoms in various organs and tissues (Levkov et al., 2017). In addition, since diatoms are highly sensitive to environmental conditions, different water bodies have different diatom species abundance which allows forensics to identify the drowning site (Zhou et al., 2020).
Despite being a distinguishable method, the diatom test has limitations also. One of the major issues is the occurrence of diatoms in a drowning medium. The absence or low presence of diatoms in a water body can lead to a false positive or negative result. The presence of diatoms in different layers (water base, deeper, and surface) of the water body also can be varied (Levkov et al., 2017). Rapid death is another situation where the diatom test can be wrong. Instant death when an animal or human enters the water body for various reasons such as cold shock and cardiac diseases will give a negative result in the diatom test (Smol and Stoermer, 2010). The use of alcohol or drugs is another factor that can mislead in the diatom test (Ago et al., 2011). Recent advances in DNA Barcoding and pyrosequencing opened the possibility of increasing the accuracy of the diatom test by checking the presence of plankton specific genes (e.g., Rubisco gene) in animal tissue (Fang et al., 2019).
The advances in biotechnological tools have made it effective to characterize the frustules of diatoms for the fabrication of optoelectronics. The uptake of various elements such as zinc and germanium by diatom like Stephanodiscus hantzschii, Thalassiosira pseudonana, etc. to change the pore size, shape, and other characteristics which are being studied for a variety of functions such as paleolimnological indicator and photonic device application (Qin et al., 2008; Jaccard et al., 2009). It has been reported a relationship between the amount of Zn/Si (zinc/silicon) and free zinc ions which can be used as a proxy of paleolimnological indicators (Jaccard et al., 2009). The studies have raised intriguing questions about the uptake and the process of various elements which need detailed validations. Although, they have reported that they could only detect Zn and Fe as chemical elements. The analysis of various trace elements could be used as an environmental indicator which indeed will reduce the total workload needed to monitor large water bodies (Ellwood and Hunter, 2000).
The complex nanobiochemical machinery of diatoms can be exploited to fabricate a wide range of nanostructures with diverse optical and electronic properties (Rorrer et al., 2007). The ability to manufacture different pore size nanostructure molecules has inspired many research groups and industries to use diatoms in biosensing (De Tommasi, 2016). The incorporation of chemical elements such as germanium significantly affects the structure and size of frustule pores. A study tested the possibility of using Si-Germanium composite material in living diatoms in a two-stage photobioreactor cultivation process which reduced the pore size without disturbing the morphology (Rorrer et al., 2007). Another study reported that insertion of germanium in Nitzschia frustulum induces the nanocomb structure with blue photoluminescence (Qin et al., 2008). These nanostructure materials exhibit optical properties suitable for use in semiconductors and optoelectronics. Manufacturing of these materials combined with the silica frustule will improve the overall durability and range of applications in nanotechnology industries. These lab-scale scientific discoveries have shown that it is possible to create advanced nanomaterials in living diatoms.
The development of well-defined, advanced, and eco-friendly nanoparticles has attracted the attention of many researchers in the area of nanotechnology and its applications. Nanoparticles can be applied to study antimicrobial activity, catalyst, and filtering waste and chemical compounds. Biosynthesis of metallic nanoparticles in photoautotrophic organisms has gained the attention of nanotechnology researchers. Various approaches such as the sol-gel process, atomic layer deposition, chemical bath deposition, and inkjet printing process, have been used to modify the chemical composition of frustules. In this regard, an inexpensive chemical deposition technique was tested to deposit cadmium sulfide (CdS) on the surface on Pinnularia sp. without changing its morphology, since CdS has a wide range of applications in photodetectors and solar cells (Gutu et al., 2009).
Recently, it has been reported that diatoms can biosynthesize the nanoparticles such as gold and silver which has shown strong cytotoxicity against harmful microorganisms. Additionally, a highly ductile and malleable metal platinum (Pt) has been introduced in presence of dihydrogen hexachloroplatinate (IV) hexahydrate (DHH) in the living diatom Melosira nummuloides, without interfering the native morphology (Yamazaki et al., 2010). This is due to the platinum’s excellent resistance to corrosion and stability at high temperatures, hence having application in a broad spectrum of industries, besides biomedicine. Other various examples of the on-going investigation of diatoms silica-based materials and their applications in biomedicine are shown in Table 2.
We have discussed the major application of diatoms for established industries such as biofuels, nanomaterials, and biomedicine. However, diatoms also have other fascinating applications in environment monitoring, animal feed, and aquaculture, which indeed have a huge potential considering climate change and devastating impacts of globalization on ecology and environment.
Environment monitoring is an important aspect that is considered a necessity to deal with irregular changes or disturbances in our ecosystem. Therefore, researchers are developing tools using biotechnology and informatics to monitor the environment cost-effectively. Water resources are always under the influence of damaging anthropogenic pressures such as plastic waste and industrial sewage, which ultimately change or disturb the biogeochemical cycles and biodiversity. Besides, water is a universal solvent that holds the industries and economies together.
It is a well-established fact that diatoms hold the primary role in maintaining the aquatic ecosystem. Therefore, biodiversity assessment of diatom species in an environmental sample is one of the well-known strategies for biomonitoring. Presently, morphological assessment of the diatoms using microscopy is largely used which is time-consuming and requires special expertise (Larras et al., 2014). However, environmental metabarcoding has opened a quick way of analyzing the microbial DNA diversity in a natural environment such as flora and fauna (Bik et al., 2012; Taberlet et al., 2012). The metabarcoding approach is based on DNA sequencing a specific region (barcode) of the whole DNA extracted from an environmental sample (eDNA). For example, the sequencing data obtained from diatom metabarcoding are then used to assign precise taxonomic identification of the diatoms present in the eDNA sample, which are further compared with the conventional morphological database to confirm the efficacy of metabarcoding results. Diatoms metabarcoding tool has been optimized significantly to quantify the diversity of diatoms at the genus and species level (Vasselon et al., 2017; Kelly et al., 2018).
Currently, this approach is still in development, since various questions have been raised especially when deciding which are the most suitable barcodes. The barcodes that had been used are the ribosomal small subunit, cytochrome c, and the internal transcribed spacer region combined with the 5.8S rRNA gene (Zimmermann et al., 2011; Luddington et al., 2012).
Another main issue is processing the sequencing output data through computing. This method must be consistent with government policies for environmental regulation. For instance, MOTHUR is a comprehensive and efficient platform to study microbial diversity, but there are other bioinformatics software such as R, QIIME2 (Caporaso et al., 2010), LotuS (Hildebrand et al., 2014), and PIPITS (Gweon et al., 2015) that can be used to process a larger amount of data.
Additionally, various other research studies have supported the use of the diatoms metabarcoding approach as an alternative strategy to monitor river ecology on a timely basis. The results provide an estimated number of abundant and scarce species in samples obtained from different locations. Also, they give great insights into the fundamental status of the aquatic ecosystem (Larras et al., 2014). For instance, detailed evidence has been published by the Environmental Agency of the United Kingdom using diatoms indexes for river classification (Kelly et al., 2018). A similar study on detailed information on diatom biodiversity using metabarcoding has been conducted using environmental samples from Mayotte Island, France (Vasselon et al., 2017). Moreover, a recently published work studied the impact of treated effluents on benthic diatom communities that showed a systematic change in diatom community composition (Chonova et al., 2019). Concluding that detailed information about diatom diversity will give in-depth insights into climate change, micropollutants, and other organic pollutants, to study the disturbing effects of anthropogenic pressure on rivers. The use of metabarcoding for analyzing biodiversity is rapidly increasing and has been adopted by academic institutes and various companies/industries like Spygen (Canada), Naturemetrics (United Kingdom), IGAtech (Italy), Sinsoma (Austria), to name a few. This particular strategy has been adopted by public authorities as well and has shown the potential to be used as an additional screening tool to replace the existing methods, which require excessive infrastructure and human resources. It is indeed possible to make it a primary and permanent tool for river monitoring with advancements in sequencing, big data science, and artificial intelligence tools.
Besides the monitoring of river quality, water treatment is one of the major concerns for many countries around the world. In fact, human consumption has undoubtedly increased in the last few decades, subsequently, incrementing waste products presence in aquatic communities (Walker, 1983). Globally, almost 80% of the wastewater generated worldwide is discharged on rivers creating health and environmental hazards. The rise of nutrient accumulation in the aquatic system needs to be neutralized to maintain the balance in the environment. Increasing of pollution is disturbing the basic biogeochemical cycles, killing fish, depleting the dissolved oxygen, and producing different toxins, i.e., neurotoxins (Boyd, 1990). Hence, there is an urgent need to explore new ways and upscale the existing systems to test reports and mitigate pollution from rivers and lakes worldwide.
The use of microalgae for wastewater treatment has been a subject of research for a long period which could be applied in collaboration with small- and large-scale industries. The excess of industrial waste discharged in the aquatic system can be used as nutrient supply by diatoms. Different kinds of wastewater such as brewery (Choi, 2016), aquaculture (Tossavainen et al., 2019), and textile (El-Kassas and Mohamed, 2014) have been studied for phytoremediation capability and have shown interesting results. The published studies have established that diatoms and microalgae can treat the wastewater to an extent, therefore, it would be less damaging to treat the wastewater with microalgae/diatoms before discharging in water bodies. In addition, use the harvested biomass for different industrial products such as biofuel. It is safe to assume that it is possible to develop small scale business in collaboration with restaurants, breweries, textile industries, to name a few, to treat wastewater, and use the biomass for the production of valuable products such as fertilizers (Suleiman et al., 2020).
Heavy metal pollution is one of the major challenges which comes from the industries working with chemicals and dyes. Diatoms species are desirable organisms to study heavy metal pollution because of the simplicity of metal exposure, absorption, and detoxification of metal ions by single cells. This is a unique detoxification process of diatoms and microalgae due to metal-binding peptides known as phytochelatins (PCs) that protect photosynthetic organisms from heavy metals (Grill et al., 1985). Some intracellular PCs have been characterized in cultures of P. tricornutum exposed to different metals such as Cd, Pb, or Zn. Besides, they are used widely in waste degradation considering the unique structure of diatoms and their ability to respond to the changing environment (Glazer and Nikaido, 2007).
A study published in 2015 have reported a novel diatom Bacillariophyta sp. (BD1IITG) from petroleum biorefinery wastewater that can degrade phenol in a concentration range of 50−250 mg/L in Fog’s media (Das et al., 2016). Another example of the degradation of toxic molecules like phenylalanine hydroxylase into less toxic compounds using simple enzymatic oxidation has been identified in diatoms during the metabolism of phenanthrene and pyrene (Wang and Zhao, 2007). These results are relevant considering that around seven billion kg of phenol is produced for oil refining, pesticide production, and to use in the pharmaceutical industry. Traditional phenol removal techniques involve several steps including the generation of by-products, which increments the cost of the treatment (Senthilvelan et al., 2014). However, there are very few reports available on exploiting the potential of diatoms in biodegrading waste materials. It is interesting to note that the studies have shown interesting results but the field of algae biotechnology requires more entrepreneurs to join the pieces of industrial and academic research to build a successful circular economy. Furthermore, there are some upcoming and growing ventures and companies in microalgae working in diverse applications and producing valuable products such as healthcare, animal feed, water management, chocolates, etc. (Table 3).
Table 3. Different industries producing variety of products from microalgae and diatoms around the world.
Multiple epidemiological and clinical trials have shown the health benefits of omega fatty acids from fish oils and algae extracts (Cole et al., 2010; Cottin et al., 2011; Thomas et al., 2015; Wang and Daggy, 2017). Besides, there are few publications on cardio-protective and cognitive performance of omega fatty acids which have led to the commercial production of infant foods, infant formula, fortified snack bars, and other dairy products supplemented with omega fatty acids (Arterburn et al., 2007; Cottin et al., 2011).
Diatoms have an immense nutritional value that can be used to produce novel compounds such as antioxidants, vitamins, animal feed, and vegetarian protein supplements. Several photosynthetic pigments have been identified in diatoms including carotenoids such as fucoxanthin (Kuczynska et al., 2015). Additionally, Nitzschia laevis, Nitzschia inconspicua, Navicula saprophila, and Phaeodactylum tricornutum extracts have a noticeable amount of EPA and DHA that can be used as a nutritional feed in human diet and animal feed (Kitano et al., 1997; Wen and Chen, 2001a,b; Wah et al., 2015; Tocher et al., 2019).
Moreover, diatoms are known to have diverse defense mechanisms in form of chemical substances for them to be protected against pathogens. For instance, P. tricornutum has a high amount of omega-7 monounsaturated fatty acids such as palmitoleic acid (C16:1) and other bioactive compounds that are active against gram-positive pathogens (Desbois et al., 2009). Furthermore, the EPA-rich marine diatom, Odontella aurita, used as a dietary supplement has shown antioxidant effects in rats (Haimeur et al., 2012). O. aurita has been approved to be commercialized as food in France by following EC regulation 258/97 in 2002 (Pulz and Gross, 2004; Buono et al., 2014).
Increasing the content of these bioactive molecules in diatoms has attracted a large amount of research. Some studies have managed to enhance the production of flavonoid and polyphenol content by culture modifications, for instance, cultivation temperature and nutrient supplementation in Amphora sp. (Chtourou et al., 2015). The general tendency when changing the culture temperature is an increase in lipid content in most species, while the chemical composition varied between species (Renaud et al., 2002). For example, the total amount of saturated and monounsaturated fatty acids increases with temperature in Rhodomonas sp. (NT15) and Cryptomonas sp. (CRFI01). Whereas, there was a comparative decrease in polyunsaturated fatty acids in both Rhodomonas sp. (NT15) and Cryptomonas sp. (CRFI01) (Renaud et al., 2002).
The debate on using genetically modified microalgae and diatoms is on-going. However, it is a more controlled alternative for the production of recombinant proteins or any precursor molecules, considering the use of bioreactors for their production. The employment of genetic engineering tools in diatoms, to produce or increase the yield of compounds, allows the companies to optimize their use in the applications mentioned above. Therefore, genetic engineering is a promising method and an important branch to be used in the diatoms industry to further enhance the economic value of diatoms. However, it comes with two big challenges, firstly, to redesign the natural metabolic pathways in order to increase the production of desired endogenous compounds, and secondly, producing new heterologous compounds.
In the last 20 years, several projects have shown that these challenges can be solved at lab scale, by optimization of transformation methods, utilization of different gene promoters, expression of recombinant proteins, gene silencing, and genome editing methods; such as targeted mutagenesis techniques using meganucleases, gene knockouts, TALENS, and CRISPR/Cas9. Marketable bioproducts like lipids, pigments, nanomaterials, food supplements, fuel, syntheses of chemicals, drugs, and metabolites have been produced in P. tricornutum, T. pseudonana, and other diatoms species. While most of these analyses are related to lipid production for biofuel or bioenergy purposes, other studies showed that diatoms are biological factories that can generate a wide range of products from food to pharmaceutics biomaterial industry (Lauritano et al., 2016; Mishra et al., 2017; Slattery et al., 2018; Dhaouadi et al., 2020; Sharma et al., 2020). In addition, there are few companies such as Algenol Biofuels, Synthetic Genomics, which have reported the use of genetically modified microalgae for the production of biofuels.
Moreover, the approach of synthetic biology along with high throughput sequencing technologies open the doors to understanding the whole genome, the proteins that it encodes, and the regulatory elements of the cell during cellular growth and division (Hildebrand and Lerch, 2015; Huang and Daboussi, 2017). Several sequencing projects have been performed in P. tricornutum and T. pseudonana strains (Armbrust et al., 2004; Bowler et al., 2008; Koester et al., 2018; Rastogi et al., 2018), generating the transcriptomic and proteomic data sets that make possible precise reconstructions of metabolic networks (Fabris et al., 2012; Levering et al., 2016). Recently, the Synthetic Diatoms Project website has been launched as a platform to provide information to grow, transform, edit, and analyze P. tricornutum and T. pseudonana1 These projects have been used as a springboard to facilitate genome annotation for other diatoms species: T. oceanica, T. weissflogii, Fragilariopsis cylindrus, Pseudo-nitzschia multiseries, Pseudo-nitzschia multistriata, Seminavis robusta, Fistulifera solaris, Cyclotella cryptica (Table 4).
Diatoms are a robust model for genome editing and cell transformation. Optimized methods of DNA delivery have been developed using biolistic or via electroporation. In both techniques, the transgenes are randomly integrated into the genome, with multiple integration events, variable transgene copy numbers, and chromosomal positions. The biolistic gene transfer method affects genome integrity due to the break and repair of the DNA double-strand by non-homologous end joining (NHEJ) (Zaboikin et al., 2017). However, this method is needed if the aim is to transform the chloroplast genome. An alternative transformation technique is the extrachromosomal-based expression approach that depends on vectors containing a yeast-derived sequence, which can be delivered through bacterial conjugation using E. coli (Karas et al., 2015).
An important element for genetic engineering is the promoter. The most commonly used are the light-regulated promoters of the fucoxanthin chlorophyll a/c-binding protein genes fcpA/B/C/D (LHCF) (Zaslavskaia et al., 2000; Nymark et al., 2013). Alternatively, the elongation factor 2 (EF2) promoter sequence is a constitutive promoter (Seo et al., 2015). Recently, the most abundant secreted protein in P. tricornutum was identified, named “highly abundant secreted protein 1” (HASP1), and the activities of its promoter and the signal peptide were characterized using green fluorescent protein (GFP) as a reporter (Erdene-Ochir et al., 2019). A couple of inducible promoters have been reported: like nitrate reductase (NR) and alkaline phosphatase gene promoters in P. tricornutum, which are induced under nitrogen or phosphate starvation respectively (Slattery et al., 2018; Fabris et al., 2020) and glutamine synthetase gene promoter, induced by a blue light pulse (De Riso et al., 2009; Erdene-Ochir et al., 2016). In addition, promoter regions containing diatom-infecting viruses (DIVs) mediated a significantly higher level expression of the reporter gene in cells in the stationary phase compared to the exponential phase of growth (Kadono et al., 2015). Other elements needed for genetic engineering are reporter genes and selection markers. Among reporter genes, beta-glucuronidase uidA (GUS), fluorescent proteins like GFP/YFP/CFP, chloramphenicol acetyltransferase conferring resistance to chloramphenicol (CAT) and luciferase (LUC) are the most employed, other reporter proteins are listed in Table 5. The classic selection markers in diatoms are genes that confer resistance to zeocin, phleomycin, and nourseothricin, as shown in Table 5 are the most used. An alternative to using selective markers is the use of auxotrophic strains, such as uracil, histidine, and tryptophan auxotrophs (Sakaguchi et al., 2011; Slattery et al., 2020). Moreover, it is considered that the urease gene, either in an inactive or edited form, is an interesting tool for the selection of P. tricornutum and T. pseudonana strains (Weyman et al., 2015; Hopes et al., 2016; Slattery et al., 2018). An endogenous selectable marker in diatoms was generated by point mutations at a conserved residue Gly290 to Ser/Arg in the phytoene desaturase (PDS1) gene, which confers resistance to the herbicide norflurazon (Taparia et al., 2019).
Concerning heterologous recombinant protein expression, diatom gene codon optimization is required for optimal expression; to avoid silencing expression and better protein translation. Although it has not been reported in diatoms, different projects which were done in green algae, have shown that including introns in the expression cassette can increase transcript abundance (Baier et al., 2018, 2020; Kong et al., 2019). In addition, 5′-UTR and 3′-UTR of nitrate reductase (NR) allow the control of timing and level of transgene expression in C. fusiformis (Poulsen and Kröger, 2005). Down-regulation of gene expression can be achieved through silencing by expressing antisense repeat sequences of target genes (Table 5).
Industrial processes using diatoms are cost-effective and have performed well in large-scale cultures (Benedetti et al., 2018). This is supported by the plasticity to adapt to extreme environmental conditions of diatoms, making them great candidates for sustainable biofactories (Kung et al., 2012; Cho et al., 2015; d’Ippolito et al., 2015). Altogether, these developments in metabolic pathways and synthesis of heterologous compounds represent promising insights for the improvement of yield, quality of products, and sustainability in the use of diatoms as cell factories.
The documented studies stated the astounding nature and possible all-round use of diatoms. This is one of the approaches to increase human consumption of renewable products and contributes toward reducing carbon emissions. Although the commercial application of diatoms still needs improvements, it is indeed a crucial research area for human wellbeing. For example, developments in diatoms research can lead to innovative products in domains of drug delivery, sensing, and detection parts to build complex biomedical devices and nanoparticles for waste degradation. Moreover, recent advancements in sequencing technology and processing large biological datasets have made it possible to label and store the global biodiversity of diatoms in all geographical locations.
One of the major challenges in diatom-based industries is scaling up the process for large-scale manufacturing which is dependent on many micro and macro factors such as cultivation, harvesting, drying, genetic modification, lack of genomic, proteomic, and metabolic information, etc. However, it is possible to overcome these challenges in near future with advancements in genetic tools, bioreactors, and other infrastructure changes. In general, there are many challenges in bio-based industries at different levels; academic/industrial research, infrastructure, policies, education, and information gaps. The advancements in academic research and discoveries are consistent considering the publications but it requires support from other domains such as the development of infrastructure, reducing the knowledge gaps between scientific researcher and entrepreneurs, changes in the policies at both national and international level. And to conclude, the recent research phenomenon blasted in the last decade, which is diatoms’ industrial potential, still leaves many unsolved questions. Major questions will involve studying the extent of genetic or artificial manipulation without compromising its intact structure and delicate silica pattern. The unfolding of various missing links in genetic engineering, cultivation, and harvesting will make it possible to replicate complex plant pathways in diatoms. These tools have opened the door to study diatoms for eco-friendly processes.
Although the use of silica for food and agriculture has been approved by the FDA and is also labeled/classified as non-carcinogenic by the International Agency for Research on Cancer, this could be a big step toward accelerating its use at the biomedical level. It is not yet approved for biomedicine as it requires long-term evidence (Terracciano et al., 2018). All the biomedical inventions are scrutinized by multiple stakeholders like research leaders, public authorities such as provincial and federal government, before they reach the stage of commercial distribution. It is understandable considering that it will be used directly in the human body. Therefore, an innovative and different approach is required to bring in the academic researchers and bio-entrepreneurs to speed up the innovation rate in biomedical industry without harming the screening process set by public health authorities. The collaboration between entrepreneurs and researchers will allow thorough evaluation of the market for new inventions, manufacturing, investment, and globalization of the product. It seems plausible considering the rapid advancements in the biomedical infrastructure around the world. This has been demonstrated by the quick inventions in response to COVID-19 and should be adopted to be applied in other biotech based industries (Harris et al., 2020). The simultaneous advancements in the use of silica-based support system for drug delivery along with the change in infrastructure in pharmaceutical industries and hospitals to deliver these technologies to the users is possible in the near future. The other requirement is to join the gap of vast and complex scientific information and knowledge between entrepreneurs and academic researchers.
NS and ID-P conceived, designed, and led the study. NS, DS, AD-G, EF, and AM collected and analyzed the data, and prepared the figures and tables. NS, DS, AD-G, EF, AM, FM-M, HG, and ID-P authored and reviewed the drafts of the manuscript, and approved the final manuscript. All authors contributed to the article and approved the submitted version.
The authors declare that the research was conducted in the absence of any commercial or financial relationships that could be construed as a potential conflict of interest.
We acknowledge that financial support for this review was funded by the Canada Research Chair on plant specialized metabolism Award No. 950-232164 to ID-P. Thanks are extended to the Canadian taxpayers and to the Canadian Government for supporting the Canada Research Chairs Program. Additional support in the form of scholarships to NS, DS, AD-G, EF, and AM from Mitacs—Acceleration program grants nos. IT12310 and IT16463 to ID-P is also acknowledged.
Afgan, E., Baker, D., van den Beek, M., Blankenberg, D., Bouvier, D., Èech, M., et al. (2016). The Galaxy platform for accessible, reproducible and collaborative biomedical analyses: 2016 update. Nucleic Acids Res. 44, W3–W10.
Ago, K., Hayashi, T., Ago, M., and Ogata, M. (2011). The number of diatoms recovered from the lungs and other organs in drowning deaths in bathwater. Leg. Med. (Tokyo) 13, 186–190. doi: 10.1016/j.legalmed.2011.04.002
Alves Martins, D., Rocha, F., Castanheira, F., Mendes, A., Pousão-Ferreira, P., Bandarra, N., et al. (2013). Effects of dietary arachidonic acid on cortisol production and gene expression in stress response in Senegalese sole (Solea senegalensis) post-larvae. Fish Physiol. Biochem. 39, 1223–1238. doi: 10.1007/s10695-013-9778-6
Armbrust, E. V., Berges, J. A., Bowler, C., Green, B. R., Martinez, D., Putnam, N. H., et al. (2004). The genome of the diatom thalassiosira pseudonana : ecology, evolution, and metabolism. Science 306, 79–86. doi: 10.1126/science.1101156
Arterburn, L. M., Oken, H. A., Hoffman, J. P., Bailey-Hall, E., Chung, G., Rom, D., et al. (2007). Bioequivalence of docosahexaenoic acid from different algal oils in capsules and in a DHA-Fortified food. Lipids 42:1011. doi: 10.1007/s11745-007-3098-5
Aw, M. S., Simovic, S., Addai-Mensah, J., and Losic, D. (2011a). Polymeric micelles in porous and nanotubular implants as a new system for extended delivery of poorly soluble drugs. J. Mater. Chem. 21, 7082–7089. doi: 10.1039/c0jm04307a
Aw, M. S., Simovic, S., Addai-Mensah, J., and Losic, D. (2011b). Silica microcapsules from diatoms as new carrier for delivery of therapeutics. Nanomedicine (Lond) 6, 1159–1173. doi: 10.2217/nnm.11.29
Bai, X., Song, H., Lavoie, M., Zhu, K., Su, Y., Ye, H., et al. (2016). Proteomic analyses bring new insights into the effect of a dark stress on lipid biosynthesis in Phaeodactylum tricornutum. Sci. Rep. 6:25494.
Baier, T., Jacobebbinghaus, N., Einhaus, A., Lauersen, K. J., and Kruse, O. (2020). Introns mediate post-transcriptional enhancement of nuclear gene expression in the green microalga Chlamydomonas reinhardtii. PLoS Genet. 16:e1008944. doi: 10.1371/journal.pgen.1008944
Baier, T., Wichmann, J., Kruse, O., and Lauersen, K. J. (2018). Intron-containing algal transgenes mediate efficient recombinant gene expression in the green microalga Chlamydomonas reinhardtii. Nucleic Acids Res. 46, 6909–6919. doi: 10.1093/nar/gky532
Benedetti, M., Vecchi, V., Barera, S., and Dall’Osto, L. (2018). Biomass from microalgae: the potential of domestication towards sustainable biofactories. Microbial Cell Fact. 17:173.
Bik, H. M., Porazinska, D. L., Creer, S., Caporaso, J. G., Knight, R., and Thomas, W. K. (2012). Sequencing our way towards understanding global eukaryotic biodiversity. Trends Ecol. Evol. 27, 233–243. doi: 10.1016/j.tree.2011.11.010
Bowler, C., Allen, A. E., Badger, J. H., Grimwood, J., Jabbari, K., Kuo, A., et al. (2008). The phaeodactylum genome reveals the evolutionary history of diatom genomes. Nature 456, 239–244.
Boyd, C. E. (1990). Water Quality in Ponds for Aquaculture. Auburn StateAL: Alabama Agricultural Experiment Station.
Brocks, J. J., Buick, R., Logan, G. A., and Summons, R. E. (2003). Composition and syngeneity of molecular fossils from the 2.78 to 2.45 billion-year-old mount bruce supergroup, pilbara craton, Western Australia. Geochimica et Cosmochimica Acta 67, 4289–4319. doi: 10.1016/s0016-7037(03)00208-4
Buggé, J. A. (2015). Electroporation-Mediated Transformation and Post-Transcriptional Gene Regulation of Nitrate Reductase in the Marine Diatom Thalassiosira Pseudonana. Worcester, MA: Clark University.
Buono, S., Langellotti, A. L., Martello, A., Rinna, F., and Fogliano, V. (2014). Functional ingredients from microalgae. Food Funct. 5, 1669–1685. doi: 10.1039/c4fo00125g
Caporaso, J. G., Kuczynski, J., Stombaugh, J., Bittinger, K., Bushman, F. D., Costello, E. K., et al. (2010). QIIME allows analysis of high-throughput community sequencing data. Nat. Methods 7, 335–336.
Chandrasekaran, S., Sweetman, M. J., Kant, K., Skinner, W., Losic, D., Nann, T., et al. (2014). Silicon diatom frustules as nanostructured photoelectrodes. Chem. Commun. 50, 10441–10444. doi: 10.1039/c4cc04470c
Cho, C., Choi, S. Y., Luo, Z. W., and Lee, S. Y. (2015). Recent advances in microbial production of fuels and chemicals using tools and strategies of systems metabolic engineering. Biotechnol. Adv. 33, 1455–1466. doi: 10.1016/j.biotechadv.2014.11.006
Choi, H.-J. (2016). Parametric study of brewery wastewater effluent treatment using Chlorella vulgaris microalgae. Environ. Eng. Res. 21, 401–408. doi: 10.4491/eer.2016.024
Chonova, T., Kurmayer, R., Rimet, F., Labanowski, J., Vasselon, V., Keck, F., et al. (2019). Benthic diatom communities in an alpine river impacted by waste water treatment effluents as revealed using DNA metabarcoding. Front. Microbiol. 10:653. doi: 10.3389/fmicb.2019.00653
Chtourou, H., Dahmen, I., Jebali, A., Karray, F., Hassairi, I., Abdelkafi, S., et al. (2015). Characterization of Amphora sp., a newly isolated diatom wild strain, potentially usable for biodiesel production. Bioprocess Biosystems Eng. 38, 1381–1392. doi: 10.1007/s00449-015-1379-6
Cicco, S. R., Vona, D., De Giglio, E., Cometa, S., Mattioli-Belmonte, M., Palumbo, F., et al. (2015). Chemically modified diatoms biosilica for bone cell growth with combined drug-delivery and antioxidant properties. ChemPlusChem 80, 1104–1112. doi: 10.1002/cplu.201402398
Cole, G. M., Ma, Q.-L., and Frautschy, S. A. (2010). Dietary fatty acids and the aging brain. Nutr. Rev. 68, (Suppl 2), S102–S111.
Cottin, S. C., Sanders, T. A., and Hall, W. L. (2011). The differential effects of EPA and DHA on cardiovascular risk factors. Proc. Nutrition Soc. 70, 215–231. doi: 10.1017/s0029665111000061
Curnow, P., Senior, L., Knight, M. J., Thamatrakoln, K., Hildebrand, M., and Booth, P. J. (2012). Expression, purification, and reconstitution of a diatom silicon transporter. Biochemistry 51, 3776–3785. doi: 10.1021/bi3000484
d’Ippolito, G., Sardo, A., Paris, D., Vella, F. M., Adelfi, M. G., Botte, P., et al. (2015). Potential of lipid metabolism in marine diatoms for biofuel production. Biotechnol. Biofuels 8:28. doi: 10.1186/s13068-015-0212-4
Daboussi, F., Leduc, S., Maréchal, A., Dubois, G., Guyot, V., Perez-Michaut, C., et al. (2014). Genome engineering empowers the diatom Phaeodactylum tricornutum for biotechnology. Nat. Commun. 5:3831.
Das, B., Mandal, T. K., and Patra, S. (2016). Biodegradation of phenol by a novel diatom BD1IITG-kinetics and biochemical studies. Int. J. Environ. Sci. Technol. 13, 529–542. doi: 10.1007/s13762-015-0857-3
Davis, A., Crum, L. T., Corbeil, L. B., and Hildebrand, M. (2017). Expression of Histophilus somni IbpA DR2 protective antigen in the diatom Thalassiosira pseudonana. Appl. Microbiol. Biotechnol. 101, 5313–5324. doi: 10.1007/s00253-017-8267-8
De Riso, V., Raniello, R., Maumus, F., Rogato, A., Bowler, C., and Falciatore, A. (2009). Gene silencing in the marine diatom Phaeodactylum tricornutum. Nucleic Acids Res. 37:e96. doi: 10.1093/nar/gkp448
De Stefano, L., Lamberti, A., Rotiroti, L., and De Stefano, M. (2008). Interfacing the nanostructured biosilica microshells of the marine diatom Coscinodiscus wailesii with biological matter. Acta Biomaterialia 4, 126–130. doi: 10.1016/j.actbio.2007.09.003
De Stefano, L., Rotiroti, L., De Stefano, M., Lamberti, A., Lettieri, S., Setaro, A., et al. (2009). Marine diatoms as optical biosensors. Biosens. Bioelectron. 24, 1580–1584. doi: 10.1016/j.bios.2008.08.016
De Tommasi, E. (2016). Light manipulation by single cells: the case of diatoms. J. Spectroscopy 2016:2490128.
Delalat, B., Sheppard, V. C., Rasi Ghaemi, S., Rao, S., Prestidge, C. A., McPhee, G., et al. (2015). Targeted drug delivery using genetically engineered diatom biosilica. Nat. Commun. 6:8791.
Desbois, A. P., Mearns-Spragg, A., and Smith, V. J. (2009). A fatty acid from the diatom Phaeodactylum tricornutum is antibacterial against diverse bacteria including multi-resistant Staphylococcus aureus (MRSA). Mar. Biotechnol. 11, 45–52. doi: 10.1007/s10126-008-9118-5
Dhaouadi, F., Awwad, F., Diamond, A., and Desgagne-Penix, I. (2020). Diatoms’ breakthroughs in biotechnology: Phaeodactylum tricornutum as a model for producing high-added value molecules. Am. J. Plant Sci. 11, 1632–1670. doi: 10.4236/ajps.2020.1110118
DiMasi, J. A., Grabowski, H. G., and Hansen, R. W. (2016). Innovation in the pharmaceutical industry: new estimates of R&D costs. J. Health Econom. 47, 20–33. doi: 10.1016/j.jhealeco.2016.01.012
Dolatabadi, J. E. N., Mashinchian, O., Ayoubi, B., Jamali, A. A., Mobed, A., Losic, D., et al. (2011). Optical and electrochemical DNA nanobiosensors. TrAC Trends Anal. Chem. 30, 459–472. doi: 10.1016/j.trac.2010.11.010
Dunahay, T. G., Jarvis, E. E., and Roessler, P. G. (1995). Genetic transformation of the diatoms cyclotella cryptica and navicula saprophila. J. Phycol. 31, 1004–1012. doi: 10.1111/j.0022-3646.1995.01004.x
Dunstan, G. A., Volkman, J. K., Barrett, S. M., Leroi, J.-M., and Jeffrey, S. W. (1993). Essential polyunsaturated fatty acids from 14 species of diatom (Bacillariophyceae). Phytochemistry 35, 155–161. doi: 10.1016/s0031-9422(00)90525-9
Dyall, S. C. (2015). Long-chain omega-3 fatty acids and the brain: a review of the independent and shared effects of EPA, DPA and DHA. Front. Aging Neurosci. 7:52. doi: 10.3389/fnagi.2015.00052
Eilers, U., Bikoulis, A., Breitenbach, J., Büchel, C., and Sandmann, G. (2016). Limitations in the biosynthesis of fucoxanthin as targets for genetic engineering in Phaeodactylum tricornutum. J. Appl. Phycol. 28, 123–129. doi: 10.1007/s10811-015-0583-8
El-Kassas, H. Y., and Mohamed, L. A. (2014). Bioremediation of the textile waste effluent by Chlorella vulgaris. Egyptian J. Aquatic Res. 40, 301–308. doi: 10.1016/j.ejar.2014.08.003
Ellwood, M. J., and Hunter, K. A. (2000). The incorporation of zinc and iron into the frustule of the marine diatom Thalassiosira pseudonana. Limnol. Oceanography 45, 1517–1524. doi: 10.4319/lo.2000.45.7.1517
Erdene-Ochir, E., Shin, B.-K., Huda, M. N., Kim, D. H., Lee, E. H., Song, D.-G., et al. (2016). Cloning of a novel endogenous promoter for foreign gene expression in Phaeodactylum tricornutum. Appl. Biol. Chem. 59, 861–867. doi: 10.1007/s13765-016-0235-y
Erdene-Ochir, E., Shin, B.-K., Kwon, B., Jung, C., and Pan, C.-H. (2019). Identification and characterisation of the novel endogenous promoter HASP1 and its signal peptide from Phaeodactylum tricornutum. Sci. Rep. 9:9941.
Fabris, M., George, J., Kuzhiumparambil, U., Lawson, C. A., Jaramillo-Madrid, A. C., Abbriano, R. M., et al. (2020). Extrachromosomal genetic engineering of the marine diatom phaeodactylum tricornutum enables the heterologous production of monoterpenoids. ACS Synthetic Biol. 9, 598–612. doi: 10.1021/acssynbio.9b00455
Fabris, M., Matthijs, M., Rombauts, S., Vyverman, W., Goossens, A., and Baart, G. J. E. (2012). The metabolic blueprint of Phaeodactylum tricornutum reveals a eukaryotic Entner–Doudoroff glycolytic pathway. Plant J. 70, 1004–1014. doi: 10.1111/j.1365-313x.2012.04941.x
Falciatore, A., d’Alcalà, M. R., Croot, P., and Bowler, C. (2000). Perception of environmental signals by a marine diatom. Science 288, 2363–2366. doi: 10.1126/science.288.5475.2363
Falkowski, P. G., Barber, R. T., and Smetacek, V. V. (1998). Biogeochemical controls and feedbacks on ocean primary production. Science 281, 200–207. doi: 10.1126/science.281.5374.200
Fang, T., Liao, S., Chen, X., Zhao, Y., Zhu, Q., Cao, Y., et al. (2019). Forensic drowning site inference employing mixed pyrosequencing profile of DNA barcode gene (rbcL). Int. J. Legal Med. 133, 1351–1360. doi: 10.1007/s00414-019-02075-4
Ford, N. R., Hecht, K. A., Hu, D., Orr, G., Xiong, Y., Squier, T. C., et al. (2016). Antigen binding and site-directed labeling of biosilica-immobilized fusion proteins expressed in diatoms. ACS Synth Biol. 5, 193–199. doi: 10.1021/acssynbio.5b00191
Ford, N. R., Xiong, Y., Hecht, K. A., Squier, T. C., Rorrer, G. L., and Roesijadi, G. (2020). Optimizing the design of diatom biosilica-targeted fusion proteins in biosensor construction for bacillus anthracis detection. Biology 9:14. doi: 10.3390/biology9010014
Gallagher, B. J. (2011). The economics of producing biodiesel from algae. Renewable Energy 36, 158–162. doi: 10.1016/j.renene.2010.06.016
Gannavarapu, K. P., Ganesh, V., Thakkar, M., Mitra, S., and Dandamudi, R. B. (2019). Nanostructured Diatom-ZrO2 composite as a selective and highly sensitive enzyme free electrochemical sensor for detection of methyl parathion. Sensors Actuators B: Chem. 288, 611–617. doi: 10.1016/j.snb.2019.03.036
Glazer, A. N., and Nikaido, H. (2007). Microbial Biotechnology: Fundamentals of Applied Microbiology. Cambridge: Cambridge University Press.
Gnanamoorthy, P., Anandhan, S., and Prabu, V. A. (2014). Natural nanoporous silica frustules from marine diatom as a biocarrier for drug delivery. J. Porous Mater. 21, 789–796. doi: 10.1007/s10934-014-9827-2
Grill, E., Winnacker, E. L., and Zenk, M. H. (1985). Phytochelatins: the principal heavy-metal complexing peptides of higher plants. Science 230, 674–676. doi: 10.1126/science.230.4726.674
Gutu, T., Gale, D. K., Jeffryes, C., Wang, W., Chang, C.-H., Rorrer, G. L., et al. (2009). Electron microscopy and optical characterization of cadmium sulphide nanocrystals deposited on the patterned surface of diatom biosilica. J. Nanomater. 2009:860536.
Gweon, H. S., Oliver, A., Taylor, J., Booth, T., Gibbs, M., Read, D. S., et al. (2015). PIPITS: an automated pipeline for analyses of fungal internal transcribed spacer sequences from the illumina sequencing platform. Methods Ecol. Evol. 6, 973–980. doi: 10.1111/2041-210x.12399
Haimeur, A., Ulmann, L., Mimouni, V., Guéno, F., Pineau-Vincent, F., Meskini, N., et al. (2012). The role of Odontella aurita, a marine diatom rich in EPA, as a dietary supplement in dyslipidemia, platelet function and oxidative stress in high-fat fed rats. Lipids Health Dis. 11, 147–147. doi: 10.1186/1476-511x-11-147
Harada, H., Nakatsuma, D., Ishida, M., and Matsuda, Y. (2005). Regulation of the expression of intracellular β -Carbonic anhydrase in response to CO 2 and light in the marine diatom Phaeodactylum tricornutum. Plant Physiol. 139, 1041–1050. doi: 10.1104/pp.105.065185
Harris, M., Bhatti, Y., Buckley, J., and Sharma, D. (2020). Fast and frugal innovations in response to the COVID-19 pandemic. Nat. Med. 26, 814–817. doi: 10.1038/s41591-020-0889-1
Hempel, F., and Maier, U. G. (2012). An engineered diatom acting like a plasma cell secreting human IgG antibodies with high efficiency. Microbial Cell Factories 11:126. doi: 10.1186/1475-2859-11-126
Hempel, F., Bozarth, A. S., Lindenkamp, N., Klingl, A., Zauner, S., Linne, U., et al. (2011a). Microalgae as bioreactors for bioplastic production. Microbial Cell Factories 10:81. doi: 10.1186/1475-2859-10-81
Hempel, F., Lau, J., Klingl, A., and Maier, U. G. (2011b). Algae as protein factories: expression of a human antibody and the respective antigen in the diatom Phaeodactylum tricornutum. PLoS One 6:e28424. doi: 10.1371/journal.pone.0028424
Hildebrand, F., Tadeo, R., Voigt, A. Y., Bork, P., and Raes, J. (2014). LotuS: an efficient and user-friendly OTU processing pipeline. Microbiome 2:30. doi: 10.1186/2049-2618-2-30
Hildebrand, M., and Lerch, S. J. L. (2015). Diatom silica biomineralization: parallel development of approaches and understanding. Sem. Cell Dev. Biol. 46, 27–35. doi: 10.1016/j.semcdb.2015.06.007
Hopes, A., Nekrasov, V., Kamoun, S., and Mock, T. (2016). Editing of the urease gene by CRISPR-Cas in the diatom Thalassiosira pseudonana. Plant Methods 12:49.
Horton, B. P., Boreham, S., and Hillier, C. (2006). The development and application of a diatom-based quantitative reconstruction technique in forensic science. J. Forensic Sci. 51, 643–650. doi: 10.1111/j.1556-4029.2006.00120.x
Huang, W., and Daboussi, F. (2017). Genetic and metabolic engineering in diatoms. Philos. Trans. R. Soc. B: Biol. Sci. 372:20160411. doi: 10.1098/rstb.2016.0411
Huesemann, M. H., and Benemann, J. R. (2009). “Biofuels from microalgae: review of products, processes and potential, with special focus on Dunaliella sp,” in The Alga Dunaliella: Biodiversity, Physiology, Genomics and Biotechnology, eds A. Ben-Amotz, J. E. Polle, and D. V. W. Subba Rao (New Hampshire, NH: Science Publishers).
Hürlimann, J., Feer, P., Elber, F., Niederberger, K., Dirnhofer, R., and Wyler, D. (2000). Diatom detection in the diagnosis of death by drowning. Int. J. Legal Med. 114, 6–14. doi: 10.1007/s004149900122
Ifuku, K., Yan, D., Miyahara, M., Inoue-Kashino, N., Yamamoto, Y. Y., and Kashino, Y. (2015). A stable and efficient nuclear transformation system for the diatom Chaetoceros gracilis. Photosynth Res. 123, 203–211. doi: 10.1007/s11120-014-0048-y
Jaccard, T., Ariztegui, D., and Wilkinson, K. J. (2009). Incorporation of zinc into the frustule of the freshwater diatom Stephanodiscus hantzschii. Chem. Geol. 265, 381–386. doi: 10.1016/j.chemgeo.2009.04.016
Jensen, E. L., Yangüez, K., Carrière, F., and Gontero, B. (2020). Storage compound accumulation in diatoms as response to elevated CO2 concentration. Biology 9:5. doi: 10.3390/biology9010005
Jin, P., and Agustí, S. (2018). Fast adaptation of tropical diatoms to increased warming with trade-offs. Sci. Rep. 8:17771.
Kadono, T., Miyagawa-Yamaguchi, A., Kira, N., Tomaru, Y., Okami, T., Yoshimatsu, T., et al. (2015). Characterization of marine diatom-infecting virus promoters in the model diatom Phaeodactylum tricornutum. Sci. Rep. 5:18708.
Karaman, E. S., Wang, Z., Di Benedetto, G., Zunino, J. L., Meng, X., and Mitra, S. (2019). Fabrication of supercapacitors and flexible electrodes using biosilica from cultured diatoms. Mater. Today Energy 11, 166–173. doi: 10.1016/j.mtener.2018.11.004
Karas, B. J., Diner, R. E., Lefebvre, S. C., McQuaid, J., Phillips, A. P. R., Noddings, C. M., et al. (2015). Designer diatom episomes delivered by bacterial conjugation. Nat. Commun. 6:6925.
Kato, K., Lee, S., and Nagata, F. (2020). Efficient enzyme encapsulation inside sol-gel silica sheets prepared by poly-L-lysine as a catalyst. J. Asian Ceramic Soc. 8, 396–406. doi: 10.1080/21870764.2020.1747167
Katz, M. E., Finkel, Z. V., Grzebyk, D., Knoll, A. H., and Falkowski, P. G. (2004). Evolutionary trajectories and biogeochemical impacts of marine eukaryotic phytoplankton. Annual Rev. Ecol. Evol. Systemat. 35, 523–556. doi: 10.1146/annurev.ecolsys.35.112202.130137
Kelly, M., Boonham, N., Juggins, S., Kille, P., Mann, D., Pass, D., et al. (2018). A DNA Based Diatom Metabarcoding Approach for Water Framework Directive Classification of Rivers. Bristol: Environnment Agency.
Kitano, M., Matsukawa, R., and Karube, I. (1997). Changes in eicosapentaenoic acid content of Navicula saprophila, Rhodomonas salina and Nitzschia sp. under mixotrophic conditions. J. Appl. Phycol. 9, 559–563.
Knight, M. J., Senior, L., Nancolas, B., Ratcliffe, S., and Curnow, P. (2016). Direct evidence of the molecular basis for biological silicon transport. Nat. Commun. 7, 11926–11926.
Knothe, G. (2005). Dependence of biodiesel fuel properties on the structure of fatty acid alkyl esters. Fuel Process. Technol. 86, 1059–1070. doi: 10.1016/j.fuproc.2004.11.002
Knothe, G. (2009). Improving biodiesel fuel properties by modifying fatty ester composition. Energy Environ. Sci. 2, 759–766. doi: 10.1039/b903941d
Knothe, G. (2012). Fuel properties of highly polyunsaturated fatty acid methyl esters. prediction of fuel properties of algal biodiesel. Energy Fuels 26, 5265–5273. doi: 10.1021/ef300700v
Koester, J. A., Berthiaume, C. T., Hiranuma, N., Parker, M. S., Iverson, V., Morales, R., et al. (2018). Sexual ancestors generated an obligate asexual and globally dispersed clone within the model diatom species Thalassiosira pseudonana. Sci. Rep. 8:10492.
Kong, F., Yamaoka, Y., Ohama, T., Lee, Y., and Li-Beisson, Y. (2019). Molecular genetic tools and emerging synthetic biology strategies to increase cellular oil content in Chlamydomonas reinhardtii. Plant Cell Physiol. 60, 1184–1196. doi: 10.1093/pcp/pcz022
Kotzsch, A., Pawolski, D., Milentyev, A., Shevchenko, A., Scheffel, A., Poulsen, N., et al. (2016). Biochemical composition and assembly of biosilica-associated insoluble organic matrices from the diatom Thalassiosira pseudonana. J. Biol. Chem. 291, 4982–4997. doi: 10.1074/jbc.m115.706440
Kuczynska, P., Jemiola-Rzeminska, M., and Strzalka, K. (2015). Photosynthetic pigments in diatoms. Mar. Drugs 13, 5847–5881. doi: 10.3390/md13095847
Kung, Y., Runguphan, W., and Keasling, J. D. (2012). From fields to fuels: recent advances in the microbial production of biofuels. ACS Synthetic Biol. 1, 498–513. doi: 10.1021/sb300074k
Larras, F., Keck, F., Montuelle, B., Rimet, F., and Bouchez, A. (2014). Linking diatom sensitivity to herbicides to phylogeny: a step forward for biomonitoring? Environ. Sci. Technol. 48, 1921–1930. doi: 10.1021/es4045105
Lauritano, C., Andersen, J. H., Hansen, E., Albrigtsen, M., Escalera, L., Esposito, F., et al. (2016). Bioactivity screening of microalgae for antioxidant, anti-inflammatory, anticancer, anti-diabetes, and antibacterial activities. Front. Mar. Sci. 3:68. doi: 10.3389/fmars.2016.00068
Levering, J., Broddrick, J., Dupont, C. L., Peers, G., Beeri, K., Mayers, J., et al. (2016). Genome-Scale model reveals metabolic basis of biomass partitioning in a model diatom. PLoS One 11:e0155038. doi: 10.1371/journal.pone.0155038
Levitan, O., Dinamarca, J., Hochman, G., and Falkowski, P. G. (2014). Diatoms: a fossil fuel of the future. Trends Biotechnol. 32, 117–124. doi: 10.1016/j.tibtech.2014.01.004
Levkov, Z., Williams, D. M., Nikolovska, D., Tofilovska, S., Èakar, Z., Williams, M., et al. (2017). The Use of Diatoms in Forensic Science: Advantages and limitations of the diatom test in cases of drowning. The Archaeological and Forensic Applications of Microfossils: A Deeper Understanding of Human History. London: Geological Society of London,Google Scholar
Lin, G., Wang, Y., Guo, L., Ding, H., Hu, Y., Liang, S., et al. (2017). Verification of mutagen function of Zeocin in Nannochloropsis oceanica through transcriptome analysis. J. Ocean University country-regionChina 16, 501–508. doi: 10.1007/s11802-017-3231-x
Losic, D., Mitchell, J. G., and Voelcker, N. H. (2005). Complex gold nanostructures derived by templating from diatom frustules. Chem. Commun. 39, 4905–4907. doi: 10.1039/b508733c
Losic, D., Yu, Y., Aw, M. S., Simovic, S., Thierry, B., and Addai-Mensah, J. (2010). Surface functionalisation of diatoms with dopamine modified iron-oxide nanoparticles: toward magnetically guided drug microcarriers with biologically derived morphologies. Chem. Commun. 46, 6323–6325. doi: 10.1039/c0cc01305f
Luddington, I. A., Kaczmarska, I., and Lovejoy, C. (2012). Distance and character-based evaluation of the V4 region of the 18S rRNA Gene for the identification of diatoms (Bacillariophyceae). PLoS One 7:e45664. doi: 10.1371/journal.pone.0045664
Ludes, B., Coste, M., Tracqui, A., and Mangin, P. (1996). Continuous river monitoring of the diatoms in the diagnosis of drowning. J. Forensic Sci. 41, 425–428.
Lunetta, P., and Modell, J. H. (2005). “Macroscopical, microscopical, and laboratory findings in drowning victims,” in Forensic Pathology Reviews. Forensic Pathology Reviews, ed. M. Tsokos (Totowa, NJ: Humana Press).
Lunetta, P., Penttilä, A., and Hällfors, G. (1998). Scanning and transmission electron microscopical evidence of the capacity of diatoms to penetrate the alveolo-capillary barrier in drowning. Int. J. Legal Med. 111, 229–237. doi: 10.1007/s004140050159
Mann, D. G., and Vanormelingen, P. (2013). An inordinate fondness? the number, distributions, and origins of diatom species. J. Eukaryotic Microbiol. 60, 414–420. doi: 10.1111/jeu.12047
Marella, T. K., López-Pacheco, I. Y., Parra-Saldívar, R., Dixit, S., and Tiwari, A. (2020). Wealth from waste: diatoms as tools for phycoremediation of wastewater and for obtaining value from the biomass. Sci. Total Environ. 724:137960. doi: 10.1016/j.scitotenv.2020.137960
Martin-Jézéquel, V., Hildebrand, M., and Brzezinski, M. A. (2000). Silicon metabolism in diatoms: implications for growth. J. Phycol. 36, 821–840. doi: 10.1046/j.1529-8817.2000.00019.x
Medlin, L. K. (2016). Evolution of the diatoms: major steps in their evolution and a review of the supporting molecular and morphological evidence. Phycologia 55, 79–103. doi: 10.2216/15-105.1
Milligan, A. J., and Morel, F. M. M. (2002). A proton buffering role for silica in diatoms. Science 297, 1848–1850. doi: 10.1126/science.1074958
Milović, M., Simović, S., Lošić, D., Dashevskiy, A., and Ibrić, S. (2014). Solid self-emulsifying phospholipid suspension (SSEPS) with diatom as a drug carrier. Eur. J. Pharm. Sci. 63, 226–232. doi: 10.1016/j.ejps.2014.07.010
Mishra, M., Arukha, A. P., Bashir, T., Yadav, D., and Prasad, G. B. K. S. (2017). All new faces of diatoms: potential source of nanomaterials and beyond. Front. Microbiol. 8:1239. doi: 10.3389/fmicb.2017.01239
Muto, M., Tanaka, M., Liang, Y., Yoshino, T., Matsumoto, M., and Tanaka, T. (2015). Enhancement of glycerol metabolism in the oleaginous marine diatom Fistulifera solaris JPCC DA0580 to improve triacylglycerol productivity. Biotechnol. Biofuels 8:4. doi: 10.1186/s13068-014-0184-9
Nanjappa, D., Audic, S., Romac, S., Kooistra, W. H. C. F., and Zingone, A. (2014). Assessment of species diversity and distribution of an ancient diatom lineage using a DNA metabarcoding approach. PLoS One 9:e103810. doi: 10.1371/journal.pone.0103810
Niu, Y. F., Yang, Z. K., Zhang, M. H., Zhu, C. C., Yang, W. D., Liu, J. S., et al. (2012). Transformation of diatom Phaeodactylum tricornutum by electroporation and establishment of inducible selection marker. Biotechniques 52, 1–3.
Nowak, A. P., Sprynskyy, M., Brzozowska, W., and Lisowska-Oleksiak, A. (2019). Electrochemical behavior of a composite material containing 3D-structured diatom biosilica. Algal Res. 41:101538. doi: 10.1016/j.algal.2019.101538
Nymark, M., Sharma, A. K., Sparstad, T., Bones, A. M., and Winge, P. (2016). A CRISPR/Cas9 system adapted for gene editing in marine algae. Sci. Rep. 6:24951.
Nymark, M., Valle, K. C., Hancke, K., Winge, P., Andresen, K., Johnsen, G., et al. (2013). Molecular and photosynthetic responses to prolonged darkness and subsequent acclimation to re-illumination in the diatom Phaeodactylum tricornutum. PLoS One 8:e58722. doi: 10.1371/journal.pone.0058722
Poulsen, N., and Kröger, N. (2005). A new molecular tool for transgenic diatoms. FEBS J. 272, 3413–3423. doi: 10.1111/j.1742-4658.2005.04760.x
Poulsen, N., Chesley, P. M., and Kröger, N. (2006). Molecular genetic manipulation of the diatom thalassiosira Pseudonana (Bacillariophyceae)1. J. Phycol. 42, 1059–1065. doi: 10.1111/j.1529-8817.2006.00269.x
Pulz, O., and Gross, W. (2004). Valuable products from biotechnology of microalgae. Appl. Microbiol. Biotechnol. 65, 635–648. doi: 10.1007/s00253-004-1647-x
Qin, T., Gutu, T., Jiao, J., Chang, C.-H., and Rorrer, G. L. (2008). Biological fabrication of photoluminescent nanocomb structures by metabolic incorporation of germanium into the biosilica of the diatom Nitzschia frustulum. ACS Nano 2, 1296–1304. doi: 10.1021/nn800114q
Radakovits, R., Eduafo, P. M., and Posewitz, M. C. (2011). Genetic engineering of fatty acid chain length in Phaeodactylum tricornutum. Metab. Eng. 13, 89–95. doi: 10.1016/j.ymben.2010.10.003
Radakovits, R., Jinkerson, R. E., Darzins, A., and Posewitz, M. C. (2010). Genetic engineering of algae for enhanced biofuel production. Eukaryot. Cell 9, 486–501. doi: 10.1128/ec.00364-09
Ragni, R., Cicco, S., Vona, D., Leone, G., and Farinola, G. M. (2017). Biosilica from diatoms microalgae: smart materials from bio-medicine to photonics. J. Mater. Res. 32, 279–291. doi: 10.1557/jmr.2016.459
Rastogi, A., Maheswari, U., Dorrell, R. G., Vieira, F. R. J., Maumus, F., Kustka, A., et al. (2018). Integrative analysis of large scale transcriptome data draws a comprehensive landscape of Phaeodactylum tricornutum genome and evolutionary origin of diatoms. Sci. Rep. 8:4834.
Rea, I., Martucci, N. M., De Stefano, L., Ruggiero, I., Terracciano, M., Dardano, P., et al. (2014). Diatomite biosilica nanocarriers for siRNA transport inside cancer cells. Biochim. Biophys. Acta 1840, 3393–3403. doi: 10.1016/j.bbagen.2014.09.009
Renaud, S. M., Thinh, L.-V., Lambrinidis, G., and Parry, D. L. (2002). Effect of temperature on growth, chemical composition and fatty acid composition of tropical Australian microalgae grown in batch cultures. Aquaculture 211, 195–214. doi: 10.1016/s0044-8486(01)00875-4
Rodrigue, J.-P., and Notteboom, T. (2013). The geography of cruises: itineraries, not destinations. Appl. Geography 38, 31–42. doi: 10.1016/j.apgeog.2012.11.011
Rorrer, G., Jeffryes, C., Chang, C.-H., Lee, D.-H., Gutu, T., Jiao, J., et al. (2007). Biological Fabrication of Nanostructured Silicon-germanium Photonic Crystals Possessing Unique Photoluminescent and Electroluminescent Properties. Bellingham, DC: SPIE.
Round, F. E., Crawford, R. M., Mann, D. G., and Press, C. U. (1990). Diatoms: Biology and Morphology of the Genera. Cambridge: Cambridge University Press.
Sabatino, V., Russo, M. T., Patil, S., d’Ippolito, G., Fontana, A., and Ferrante, M. I. (2015). Establishment of genetic transformation in the sexually reproducing diatoms pseudo-nitzschia multistriata and pseudo-nitzschia arenysensis and inheritance of the transgene. Mar. Biotechnol. (NY) 17, 452–462. doi: 10.1007/s10126-015-9633-0
Sakaguchi, T., Nakajima, K., and Matsuda, Y. (2011). Identification of the UMP synthase gene by establishment of uracil auxotrophic mutants and the phenotypic complementation system in the marine diatom Phaeodactylum tricornutum. Plant Physiol. 156, 78–89. doi: 10.1104/pp.110.169631
Sakaue, K., Harada, H., and Matsuda, Y. (2008). Development of gene expression system in a marine diatom using viral promoters of a wide variety of origin. Physiol. Plant. 133, 59–67. doi: 10.1111/j.1399-3054.2008.01089.x
Schellenberger Costa, B., Jungandreas, A., Jakob, T., Weisheit, W., Mittag, M., and Wilhelm, C. (2012). Blue light is essential for high light acclimation and photoprotection in the diatom Phaeodactylum tricornutum. J. Exp. Bot. 64, 483–493. doi: 10.1093/jxb/ers340
Selvaraj, V., Muthukumar, A., Nagamony, P., and Chinnuswamy, V. (2018). Detection of typhoid fever by diatom-based optical biosensor. Environ. Sci. Poll. Res. 25, 20385–20390. doi: 10.1007/s11356-017-9362-1
Senthilvelan, T., Kanagaraj, J., Panda, R. C., and Mandal, A. B. (2014). Biodegradation of phenol by mixed microbial culture: an eco-friendly approach for the pollution reduction. Clean Technol. Environ. Pol. 16, 113–126. doi: 10.1007/s10098-013-0598-2
Seo, S., Jeon, H., Hwang, S., Jin, E., and Chang, K. S. (2015). Development of a new constitutive expression system for the transformation of the diatom Phaeodactylum tricornutum. Algal Res. 11, 50–54. doi: 10.1016/j.algal.2015.05.012
Sharma, N., Fleurent, G., Awwad, F., Cheng, M., Meddeb-Mouelhi, F., Budge, S., et al. (2020). Red light shift an effective alternative to boost biomass and lipids in Phaeodactylum tricornutum. Appl. Sci. 10:2531. doi: 10.3390/app10072531
Sheehan, J., Dunahay, T., Benemann, J., and Roessler, P. (1998). Look Back at the U.S. Department of Energy’s Aquatic Species Program: Biodiesel from Algae. Golden, CO: National Renewable Energy Laboratory.
Shrestha, R. P., and Hildebrand, M. (2015). Evidence for a regulatory role of diatom silicon transporters in cellular silicon responses. Eukaryot. Cell 14, 29–40. doi: 10.1128/ec.00209-14
Shrestha, R. P., and Hildebrand, M. (2017). Development of a silicon limitation inducible expression system for recombinant protein production in the centric diatoms Thalassiosira pseudonana and Cyclotella cryptica. Microbial Cell Factories 16:145.
Singh, A. K., Sharma, N., Farooqi, H., Abdin, M. Z., Mock, T., and Kumar, S. (2017). Phycoremediation of municipal wastewater by microalgae to produce biofuel. Int. J. Phytoremediation 19, 805–812. doi: 10.1080/15226514.2017.1284758
Singh, R., Khan, M. J., Rane, J., Gajbhiye, A., Vinayak, V., and Joshi, K. B. (2020). Biofabrication of diatom surface by tyrosine-metal complexes:smart microcontainers to inhibit bacterial growth. ChemistrySelect 5, 3091–3097. doi: 10.1002/slct.201904248
Slattery, S. S., Diamond, A., Wang, H., Therrien, J. A., Lant, J. T., Jazey, T., et al. (2018). An expanded plasmid-based genetic toolbox enables cas9 genome editing and stable maintenance of synthetic pathways in Phaeodactylum tricornutum. ACS Synthetic Biol. 7, 328–338. doi: 10.1021/acssynbio.7b00191
Slattery, S. S., Wang, H., Giguere, D. J., Kocsis, C., Urquhart, B. L., Karas, B. J., et al. (2020). Plasmid-based complementation of large deletions in Phaeodactylum tricornutum biosynthetic genes generated by Cas9 editing. Sci. Rep. 10:13879.
Slowing, I. I., Vivero-Escoto, J. L., Wu, C.-W., and Lin, V. S. Y. (2008). Mesoporous silica nanoparticles as controlled release drug delivery and gene transfection carriers. Adv. Drug Deliv. Rev. 60, 1278–1288. doi: 10.1016/j.addr.2008.03.012
Smetacek, V. (1999). Diatoms and the ocean carbon cycle. Protist 150, 25–32. doi: 10.1016/s1434-4610(99)70006-4
Smol, J. P., and Stoermer, E. F. (2010). The Diatoms: Applications for the Environmental and Earth Sciences. Cambridge: Cambridge Press.
Soni, A. K., Kumar, S., and Pandey, M. (2020). Performance comparison of microalgae biodiesel blends with petro–diesel on variable compression ratio engine. J. Instit. Eng. (India): Series E. https://doi.org/10.1007/s40034-020-00183-0
Suleiman, A. K. A., Lourenço, K. S., Clark, C., Luz, R. L., da Silva, G. H. R., Vet, L., et al. (2020). From toilet to agriculture: fertilization with microalgal biomass from wastewater impacts the soil and rhizosphere active microbiomes, greenhouse gas emissions and plant growth. Resources Conserv. Recycl. 161:104924. doi: 10.1016/j.resconrec.2020.104924
Taberlet, P., Coissac, E., Hajibabaei, M., and Rieseberg, L. H. Environmental DNA. Mol. Ecol. 21, 1789–1793.
Tanaka, A., Ohno, N., Nakajima, K., and Matsuda, Y. (2016). Light and CO 2 /cAMP signal cross talk on the promoter elements of chloroplastic β -carbonic anhydrase genes in the marine diatom Phaeodactylum tricornutum. Plant Physiol. 170, 1105–1116. doi: 10.1104/pp.15.01738
Taparia, Y., Zarka, A., Leu, S., Zarivach, R., Boussiba, S., and Khozin-Goldberg, I. (2019). A novel endogenous selection marker for the diatom Phaeodactylum tricornutum based on a unique mutation in phytoene desaturase 1. Sci. Rep. 9:8217.
Terracciano, M., De Stefano, L., and Rea, I. (2018). Diatoms green nanotechnology for biosilica-based drug delivery systems. Pharmaceutics 10:242. doi: 10.3390/pharmaceutics10040242
Thomas, J., Thomas, C. J., Radcliffe, J., and Itsiopoulos, C. (2015). Omega-3 fatty acids in early prevention of inflammatory neurodegenerative disease: a focus on Alzheimer’s Disease. BioMed. Res. Int. 2015:172801.
Tibocha-Bonilla, J. D., Zuñiga, C., Godoy-Silva, R. D., and Zengler, K. (2018). Advances in metabolic modeling of oleaginous microalgae. Biotechnol. Biofuels 11:241.
Tocher, D. R., Betancor, M. B., Sprague, M., Olsen, R. E., and Napier, J. A. (2019). Omega-3 long-chain polyunsaturated fatty acids, EPA and DHA: bridging the gap between supply and demand. Nutrients 11:89. doi: 10.3390/nu11010089
Tossavainen, M., Lahti, K., Edelmann, M., Eskola, R., Lampi, A.-M., Piironen, V., et al. (2019). Integrated utilization of microalgae cultured in aquaculture wastewater: wastewater treatment and production of valuable fatty acids and tocopherols. J. Appl. Phycol. 31, 1753–1763. doi: 10.1007/s10811-018-1689-6
Tréguer, P., Nelson, D. M., Van Bennekom, A. J., DeMaster, D. J., Leynaert, A., and Quéguiner, B. (1995). The silica balance in the world ocean: a reestimate. Science 268, 375–379. doi: 10.1126/science.268.5209.375
Trentacoste, E. M., Shrestha, R. P., Smith, S. R., Glé, C., Hartmann, A. C., Hildebrand, M., et al. (2013). Metabolic engineering of lipid catabolism increases microalgal lipid accumulation without compromising growth. Proc. Natl. Acad. Sci. U S A. 110, 19748–19753. doi: 10.1073/pnas.1309299110
Vasani, R. B., Losic, D., Cavallaro, A., and Voelcker, N. H. (2015). Fabrication of stimulus-responsive diatom biosilica microcapsules for antibiotic drug delivery. J. Mater. Chem. B 3, 4325–4329. doi: 10.1039/c5tb00648a
Vasselon, V., Rimet, F., Tapolczai, K., and Bouchez, A. (2017). Assessing ecological status with diatoms DNA metabarcoding: scaling-up on a WFD monitoring network (Mayotte island, France). Ecol. Indicat. 82, 1–12. doi: 10.1016/j.ecolind.2017.06.024
Wah, N. B., Ahmad, A. L. B., Chieh, D. C. J., and Hwai, A. T. S. (2015). Changes in lipid profiles of a tropical benthic diatom in different cultivation temperature. Asian J. Appl. Sci. Eng. 4, 91–101.
Walker, W. W. Jr. (1983). Significance of eutrophication in water supply reservoirs. J. AWWA 75, 38–42. doi: 10.1002/j.1551-8833.1983.tb05056.x
Wang, H., and Daggy, B. P. (2017). The role of fish oil in inflammatory eye diseases. Biomed. Hub. 2, 1–12. doi: 10.1159/000455818
Wang, J.-K., and Seibert, M. (2017). Prospects for commercial production of diatoms. Biotechnol. Biofuels 10:16.
Wang, X. C., and Zhao, H. M. (2007). Uptake and biodegradation of polycyclic aromatic hydrocarbons by marine seaweed. J. Coastal Res. SI50, 1056–1061.
Wen, Z. Y., and Chen, F. (2001a). A perfusion–cell bleeding culture strategy for enhancing the productivity of eicosapentaenoic acid by Nitzschia laevis. Appl. Microbiol. Biotechnol. 57, 316–322. doi: 10.1007/s002530100786
Wen, Z.-Y., and Chen, F. (2001b). Application of statistically-based experimental designs for the optimization of eicosapentaenoic acid production by the diatom Nitzschia laevis. Biotechnol. Bioeng. 75, 159–169. doi: 10.1002/bit.1175
Weyman, P. D., Beeri, K., Lefebvre, S. C., Rivera, J., McCarthy, J. K., Heuberger, A. L., et al. (2015). Inactivation of Phaeodactylum tricornutum urease gene using transcription activator-like effector nuclease-based targeted mutagenesis. Plant Biotechnol. J. 13, 460–470. doi: 10.1111/pbi.12254
Wu, S., Gu, W., Huang, A., Li, Y., Kumar, M., Lim, P. E., et al. (2019). Elevated CO2 improves both lipid accumulation and growth rate in the glucose-6-phosphate dehydrogenase engineered Phaeodactylum tricornutum. Microbial Cell Factories 18:161.
Xia, S., Wang, K., Wan, L., Li, A., Hu, Q., and Zhang, C. (2013). Production, characterization, and antioxidant activity of fucoxanthin from the marine diatom Odontella aurita. Mar. Drugs 11, 2667–2681. doi: 10.3390/md11072667
Xie, W. H., Zhu, C. C., Zhang, N. S., Li, D. W., Yang, W. D., Liu, J. S., et al. (2014). Construction of novel chloroplast expression vector and development of an efficient transformation system for the diatom Phaeodactylum tricornutum. Mar. Biotechnol. (NY) 16, 538–546. doi: 10.1007/s10126-014-9570-3
Yamazaki, T., Sasanuma, H., Mayama, S., and Umemura, K. (2010). Cultivation of melosira nummuloides cells in the presence of platinum: preparation of metal-containing frustules. Phys. Status Solidi c 7, 2759–2762. doi: 10.1002/pssc.200983808
Yoshinaga, R., Niwa-Kubota, M., Matsui, H., and Matsuda, Y. (2014). Characterization of iron-responsive promoters in the marine diatom Phaeodactylum tricornutum. Mar. Genom. 16, 55–62. doi: 10.1016/j.margen.2014.01.005
Zaboikin, M., Zaboikina, T., Freter, C., and Srinivasakumar, N. (2017). Non-Homologous end joining and homology directed DNA repair frequency of double-stranded breaks introduced by genome editing reagents. PLoS One 12:e0169931. doi: 10.1371/journal.pone.0169931
Zamora, P., Narváez, A., and Domínguez, E. (2009). Enzyme-modified nanoparticles using biomimetically synthesized silica. Bioelectrochemistry 76, 100–106. doi: 10.1016/j.bioelechem.2009.05.006
Zaslavskaia, L. A., Lippmeier, J. C., Kroth, P. G., Grossman, A. R., and Apt, K. E. (2000). Transformation of the diatom Phaeodactylum tricornutum (Bacillariophyceae) with a variety of selectable marker and reporter genes. J. Phycol. 36, 379–386. doi: 10.1046/j.1529-8817.2000.99164.x
Zhang, C., and Hu, H. (2014). High-efficiency nuclear transformation of the diatom Phaeodactylum tricornutum by electroporation. Mar. Genomics 16, 63–66. doi: 10.1016/j.margen.2013.10.003
Zhou, Y., Cao, Y., Huang, J., Deng, K., Ma, K., Zhang, T., et al. (2020). Research advances in forensic diatom testing. Forensic Sci. Res. 5, 98–105. doi: 10.1080/20961790.2020.1718901
Zimmermann, J., Jahn, R., and Gemeinholzer, B. (2011). Barcoding diatoms: evaluation of the V4 subregion on the 18S rRNA gene, including new primers and protocols. Organ. Divers. Evol. 11:173. doi: 10.1007/s13127-011-0050-6
Keywords: diatoms, microalgae biotechnology, metabolic engineering, metabarcoding, sustainable economy, biofuel, lipids
Citation: Sharma N, Simon DP, Diaz-Garza AM, Fantino E, Messaabi A, Meddeb-Mouelhi F, Germain H and Desgagné-Penix I (2021) Diatoms Biotechnology: Various Industrial Applications for a Greener Tomorrow. Front. Mar. Sci. 8:636613. doi: 10.3389/fmars.2021.636613
Received: 01 December 2020; Accepted: 26 January 2021;
Published: 23 February 2021.
Edited by:
Marlen Ines Vasquez, Cyprus University of Technology, CyprusReviewed by:
Gianluca Maria Farinola, University of Bari Aldo Moro, ItalyCopyright © 2021 Sharma, Simon, Diaz-Garza, Fantino, Messaabi, Meddeb-Mouelhi, Germain and Desgagné-Penix. This is an open-access article distributed under the terms of the Creative Commons Attribution License (CC BY). The use, distribution or reproduction in other forums is permitted, provided the original author(s) and the copyright owner(s) are credited and that the original publication in this journal is cited, in accordance with accepted academic practice. No use, distribution or reproduction is permitted which does not comply with these terms.
*Correspondence: Isabel Desgagné-Penix, SXNhYmVsLkRlc2dhZ25lLVBlbml4QHVxdHIuY2E=
Disclaimer: All claims expressed in this article are solely those of the authors and do not necessarily represent those of their affiliated organizations, or those of the publisher, the editors and the reviewers. Any product that may be evaluated in this article or claim that may be made by its manufacturer is not guaranteed or endorsed by the publisher.
Research integrity at Frontiers
Learn more about the work of our research integrity team to safeguard the quality of each article we publish.