- 1DISTAV, Università degli Studi di Genova, Genova, Italy
- 2Centro de Investigación en Ecosistemas de la Patagonia (CIEP), Coyhaique, Chile
- 3Centro de Investigación Oceanográfica COPAS Sur-Austral, Universidad de Concepción, Concepción, Chile
Chilean Patagonia hosts one of the extensive fjord systems in the world, spanning more than 1,600 km between 41 and 55°S, and with a complex geomorphology and hydrography that supports rich and unique assemblages of marine fauna. The biodiversity of benthic organisms is possibly further enhanced by the geographic position of the region that extends far south into subantarctic waters. However, we currently lack an exhaustive picture of the zonation and ecological functioning of the benthic communities within Chilean fjords. The present study provides a detailed examination of the hard substrata megabenthic communities inhabiting the Puyuhuapi and Jacaf fjord system, in the Aysén Region of Chile. Fifty-nine stations scattered along these fjords were explored through SCUBA diving surveys, at depths between 5 and 30 m, and 16 stations were characterized in terms of benthic cover and diversity using replicated underwater photography. Ten hard bottom megabenthic communities were identified within the fjords, with some communities newly described for this region. Community composition varied both along-fjord, and with depth, and was apparently driven by variation in environmental properties. Our characterization of these fjord communities improves overall knowledge of the functioning of the fjords, and provides a useful baseline against which future anthropogenic pressures can be assessed. Future shifts in bathymetric and geographical distributions might indicate detrimental effects of climate changes, and we therefore propose that characteristic communities could be adopted as “sentinels” for overall environmental status of these unique fjord ecosystems. In this regard, detailed mapping of the distribution of megabenthic communities can provide a fundamental tool that assists in best management practices for these ecosystems.
Introduction
Fjords are deep, narrow incisions of high latitude coastlines derived from ice cap retreat and subsequent erosion by glaciers (e.g., Howe et al., 2010). Fjords represent some of the most biogeochemically dynamic environments on earth, and link terrestrial environments along coastlines to the open ocean (Walsh, 1991; Gattuso et al., 1998). The coastline of Chilean Patagonia represents one of the most extensive fjord regions in the world, extending for 1,600 linear km between 41 and 55°S, and characterized by highly heterogeneous geomorphological and hydrographical conditions (Silva and Palma, 2008; Pantoja et al., 2011; Sánchez et al., 2011). The water column in Patagonian fjords is estuarine in nature, driven by the interaction between intrusion of oceanic subantarctic waters and freshwater input derived from extremely high rainfall (annual mean varies between 1,500 and 4,500 mm) and melting of coastal glaciers (Lopez et al., 2010; Pantoja et al., 2011). Freshwater generates a buoyant brackish surface layer in the fjords, up to depths of 10 m. Salinity, temperature, and depth of this surface layer varies considerably during the year as a result of seasonal variation in freshwater input, whilst properties of deeper oceanic waters remain relatively stable. The interactions of oceanic water (rich in nitrogen and phosphorous) and freshwater (rich in silica) sustain high levels of primary production within the fjords that fuel high biomass and productivity of associated zooplankton and benthic organisms (Häussermann and Försterra, 2009; González et al., 2010). Moreover, the biogeographical importance of the Chilean fjord region is enhanced by its unique geographical position that provides an extensive transition area between the southern Antarctic coasts and the temperate northern Chilean waters (Brattström and Johanssen, 1983; Escribano et al., 2003).
This combination of geographical, geomorphological, and hydrological features in Patagonian fjords shape a rich and unique megabenthic community comprising about 1,650 species of identified megafaunal organisms (Häussermann and Försterra, 2009). These communities are characterized by a high diversity of endemic species (due to the relative isolation of the fjords and their peculiar environmental traits), deep-water species emergences (facilitated by the low penetration level of light through the turbid superficial layer), and the occurrence of species typically found in the Antarctic region (Brattström and Johanssen, 1983; Escribano et al., 2003; Häussermann and Försterra, 2009; Betti et al., 2017). Various zoological studies have been conducted on many fjord benthic taxa, both of soft and hard bottoms, including foraminiferans (Hromic et al., 2006), sponges (Bertolino et al., 2019, 2020), cnidarians (Försterra and Häussermann, 2003; Häussermann and Försterra, 2007; Sinniger and Häussermann, 2009), molluscs (Schwabe et al., 2006; Cárdenas et al., 2008), polychaetes (Cañete et al., 1999), pycnogonids (Melzer et al., 2006), and crustaceans (Mutschke and Gorny, 1999; Schmidt and Brandt, 2001; Betti et al., 2020).
Despite this extensive literature, a detailed overall picture of the zonation and environmental factors influencing the megabenthic communities of the Chilean fjords is yet to emerge. The megabenthic diversity of a temperate fjord has been investigated in a recent study (Villalobos et al., 2021); in addition, Betti et al. (2017) described the hard substrata infralittoral benthic communities and their variations inside the Seno Magdalena, a 14 km long and narrow indentation of the Isla Magdalena (44.656944°S, 7.80222°W). Five main communities were identified on the basis of 60 recorded Operative Taxonomic Units (OTUs), with distributions related to differences in salinity, turbidity and terrigenous input. More recently, the main soft-bottom communities of the Puyuhuapi Fjord have been identified in a study describing wide, previously unrecorded, aggregations of the squat lobster Munida gregaria (Fabricius, 1793) (Betti et al., 2020).
The present study aims to describe the hard-bottom megabenthic communities throughout the Puyuhuapi–Jacaf fjord system, attempting to identify the recurrent assemblages, their ecological role, their geographical variations, and the main environmental factors driving their distribution. This more localized information should help in understanding the dynamics of fjord ecosystems on the larger regional scale, and provide baseline data for their management and conservation.
Materials and Methods
Site Description
The Puyuhuapi–Jacaf fjord system is located in the Aysén Region of northern Chilean Patagonia (Figure 1A). The system is composed of two fjords, which delimit the Isla Magdalena, a large island located in the central portion of the Moraleda Channel and subjected to protection (Parque Nacional Isla Magdalena) (Figure 1B). The Puyuhuapi Fjord borders the southeastern coast of the Magdalena Island. It is about 90 km long and 7 km width, with a large mouth connected to the Moraleda Channel located in its southern portion. The Puyuhuapi Fjord runs approximately SW–NE, ending in a large bay nearby the Ventisquero Sound (Figure 1B). At around 15 km from the head of the Puyuhuapi Fjord, the Jacaf Fjord runs approximately NW for 55 km, delimiting the northern border of the Isla Magdalena before joining the Moraleda Channel.
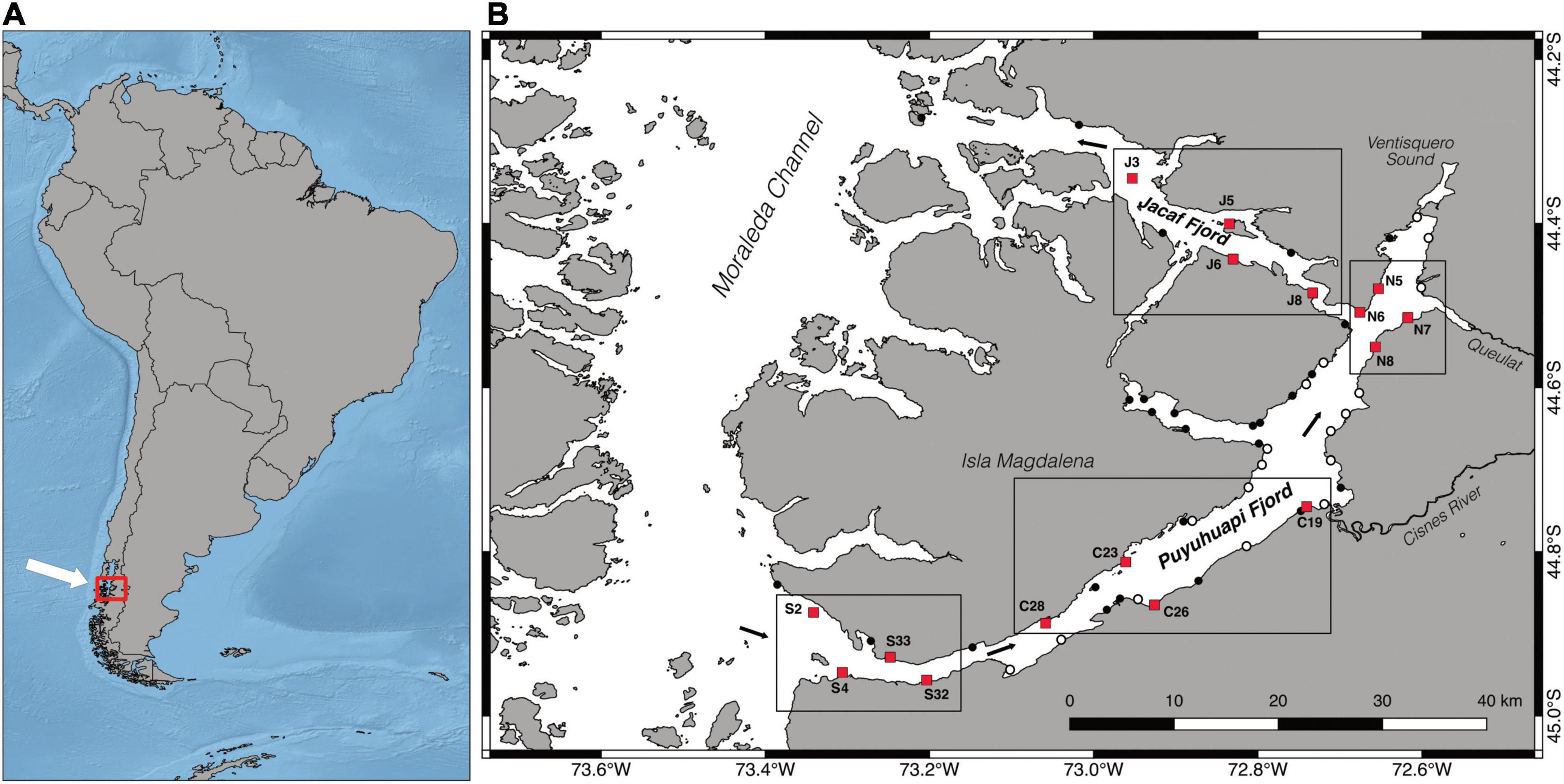
Figure 1. Location of the study area (A) and explored stations (B). White circles indicate non-cohesive seafloors, black circles indicate rocky bottoms, red squares indicate the stations selected for investigations, and black arrows indicate the main direction of water circulation. Frames and code letters identify the four macro-areas considered in this study: S, southern Puyuhuapi; C, central Puyuhuapi; N, northern Puyuhuapi; and J, Jacaf Fjord.
The main circulation of subsurface water is counter clockwise (Figure 1B): oceanic waters enter the Puyuhuapi Fjord from the South, flow NE, before entering the Jacaf Fjord and finally exiting into the Moraleda Channel (Schneider et al., 2014). Ten main rivers discharge into the Puyuhuapi Fjord, with the largest being the Cisnes River, with an average flow rate of 233 m3 s–1 (Prado-Fiedler and Castro, 2008), and with its mouth located in the central portion of the fjord (Figure 1B). River runoff, together with rainfall of up to 3,000 mm y–1 (DGA, 2003), contributes to create a brackish surface layer that is up to 7–10 m deep, highly stratified, and only partially mixed during winter months (González et al., 2011; Betti et al., 2017, 2020). Spatial variation in riverine input results in a fresher northern section and a more haline southern section to the Puyuhuapi Fjord, with the partial exception of winter months, when the intrusion of oceanic water via the Jacaf Fjord can locally reverse this pattern (Schneider et al., 2014). The sharp haline stratification in the fjord system reduces vertical mixing and results in a marked decrease in dissolved oxygen, from concentrations close to saturation near to surface, to 50% saturation at 50–100 m depth (Schneider et al., 2014).
Data Collection and Sampling Design
Between 2014 and 2019, a total of 59 stations were randomly chosen along the Puyuhuapi and Jacaf fjords (Figure 1B) and were subsequently explored by SCUBA diving. To avoid seasonality variations, all campaigns were conducted during the month of March. After a visual characterization, these stations were classified according to main seafloor type in rocky vs. soft bottoms (following Betti et al., 2020). Of the total stations, 18 were dominated by soft bottoms and were not considered in the present study, but instead analyzed using a different methodology [the results were published in Betti et al. (2020)]. Six stations characterized by hard substrata were localized in the small lateral inlet of Seno Magdalena and were reported in a different study (Betti et al., 2017). Among the remaining rocky sites, 16 were characterized by similar vertical or sub-vertical rocky cliffs and were used as representative sites for hard-bottoms megabenthic communities of the Puyuhuapi–Jacaf fjord system. The selected stations were located in four different “macro-areas” (four sites for each macro-area), corresponding to different sections of the Puyuhuapi and Jacaf fjords: S, including the stations scattered in the southern portion of the Puyuhuapi Fjord; C, including the stations in the central portion of the Puyuhuapi Fjord; N, representing the northern part of the fjord, and J, including the sites located in the Jacaf Fjord. Within each station, six different depths were investigated, namely 5, 10, 15, 20, 25, and 30 m. For each depth, five replicates photographs of 50 × 35 cm2 areas were taken, each representing the sampling unit (SU) adopted in this study. Images were shot using a Nikon D7000 camera and Tokina 10–17 mm lens in underwater housing and were later analyzed to identify the taxa present at up to the lowest possible taxonomic level. Voucher samples for those species for which identification through the video footage was difficult were manually collected and subsequently dried or fixed in absolute ethanol for further taxonomic identification. When specific identification was not possible, other OTUs were adopted.
Diversity Patterns
To characterize the rocky benthic communities of the Puyuhuapi–Jacaf fjord system, differences among macro-areas were investigated in terms of cover, species richness (SR), abundance of organisms, and diversity. The percentage cover of cyanobacteria, algae, and metazoans was calculated for each photographic area using the ImageJ64 software package: the standard frame of the pictures was used to calibrate the tools allowing the precise measures of each organism’s perimeter in each picture. SR was calculated as number of species per SU, equally considering metazoan organisms identified as morphospecies under different taxonomic levels (species, genus, or higher taxa). Local abundance of organisms was calculated considering the number of organisms per SU. Megabenthic diversity was estimated for each SU using two indices that give complementary information on the community and possess different statistical properties: (i) the Shannon’s diversity index (H′) applied to the densities of individuals and based on the natural logarithm, which provides an indication of community composition and frequency of species in a community (Shannon, 1948; Magurran, 2013), (ii) Simpson’s diversity index (1-D; Magurran, 2013) which ranges from 0 to 1 and represents the probability that two individuals drawn at random from the community belong to different species.
In order to detect differences in terms of cover of cyanobacteria, algae, and metazoans among the four investigated macro-areas, a one-way analysis of variance (ANOVA) was conducted considering the entire dataset [data normally distributed, sqrt(x) transformed, with n = 431]. In order to describe the community and to identify the effect of environmental predictors, a series of Generalized Linear Mixed Models (GLMMs) was built, on four different metrics: abundance, SR, and diversity indices. To model community metrics, depth, and distance from Puyuhuapi mouth were considered as predictors, SU as a random effect. Different error distributions and link functions were employed, on the basis of the response metrics: (i) Poisson error distribution and log link for count derived metrics (i.e., local abundance and SR; O’Hara and Kotze, 2010), (ii) gaussian distribution and identity link for Shannon diversity index (Leps et al., 2015), and (iii) binomial distribution and logit link for Simpson’s diversity index, using sample count as prior weight for the number of trials (Warton and Hui, 2011). Analyses were performed using Past 3.20 (Hammer et al., 2011) and package “Lme4” (Bates et al., 2007) within the R environment.
Community Analysis
To identify the major megabenthic communities of the Puyuhuapi and Jacaf fjords, a matrix of 431 observations (SU) per 95 variables (OUT abundance values) was created. Those species that accounted for fewer than three individuals throughout the whole dataset were considered rare and removed from the multivariate analyses. In addition, to reduce the background noise produced by the large number of samples with low abundance, only SUs with more than 10 individuals were included in the statistical analyses. Species associations were determined using the clustering algorithm Ward’s minimum variance method, constructed over square-root Bray–Curtis dissimilarity measures that were previously calculated from the square-root transformed abundance data. Clustering was performed using the function hclust of the package stats in the R environment. The optimal number of groups in which to split the dataset was determined using the silhouette function included in the cluster package. A distance-based permutational multivariate analysis of variance (PERMANOVA, number of permutations = 999) was run to determine statistically significant differences between groups, using the adonis function included in the vegan package (Oksanen et al., 2016). The indicator value (IndVal) was used to determine the relative importance of all taxa in each community, identifying those species that can be considered characteristic of each association (Dufrêne and Legendre, 1997; Dominguez-Carrió, 2018). IndVal measures were computed using the function indval included in the labdsv package (Roberts, 2016). Finally, the spatial distribution of all the identified megabenthic communities was mapped using the open-source software Quantum GIS.
Environmental Parameters
To better understand which factors contribute most significantly to variations in species composition among megabenthic communities, two environmental parameters were used to characterize each SU, namely water depth and distance from the fjord mouth. A distance-based redundancy analysis (dbRDA) was computed over the square-root of Bray–Curtis dissimilarity measures and the environmental matrix, with standardized values using the function capscale included in the vegan package (Oksanen et al., 2016).
Results
Diversity Patterns
The exploration of the 16 selected stations within the Puyuhuapi–Jacaf fjord system provided a total of 456 photographic quadrants, corresponding to a total explored area of 79.8 m2. Table 1 summarizes the main features of the macro-areas, while a detailed description of the stations is given in Supplementary Material 1.
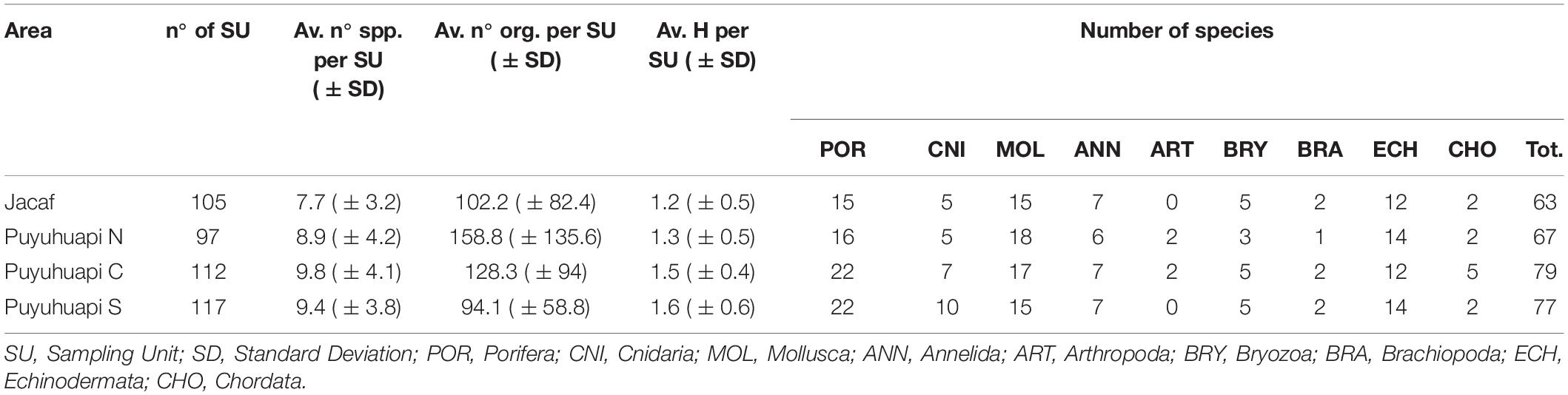
Table 1. Overall differences in species composition and diversity of the invertebrate megafauna between the four study macro-areas.
On average, 95% of substrata were covered by organisms, with macroalgae – always dominated by a coralline encrusting red alga (Order Corallinales) – the most important group, on average occupying 67% of the total biotic cover (Figure 2A). Cyanobacteria contributed on average 17% to total cover, with all the other components combined accounting for about 16% of total cover. Significant differences were shown among the four investigated macro-areas (J, N, C, and S) in terms of cyanobacteria (one-way ANOVA, df = 3, MS = 3386.59, F = 41.52, p < 0.001, and Tukey’s pairwise test J≠N≠C≠S) and algal cover (one-way ANOVA, df = 3, MS = 1003.69, F = 13.09, p < 0.001, and Tukey’s pairwise test J = N≠C≠S).
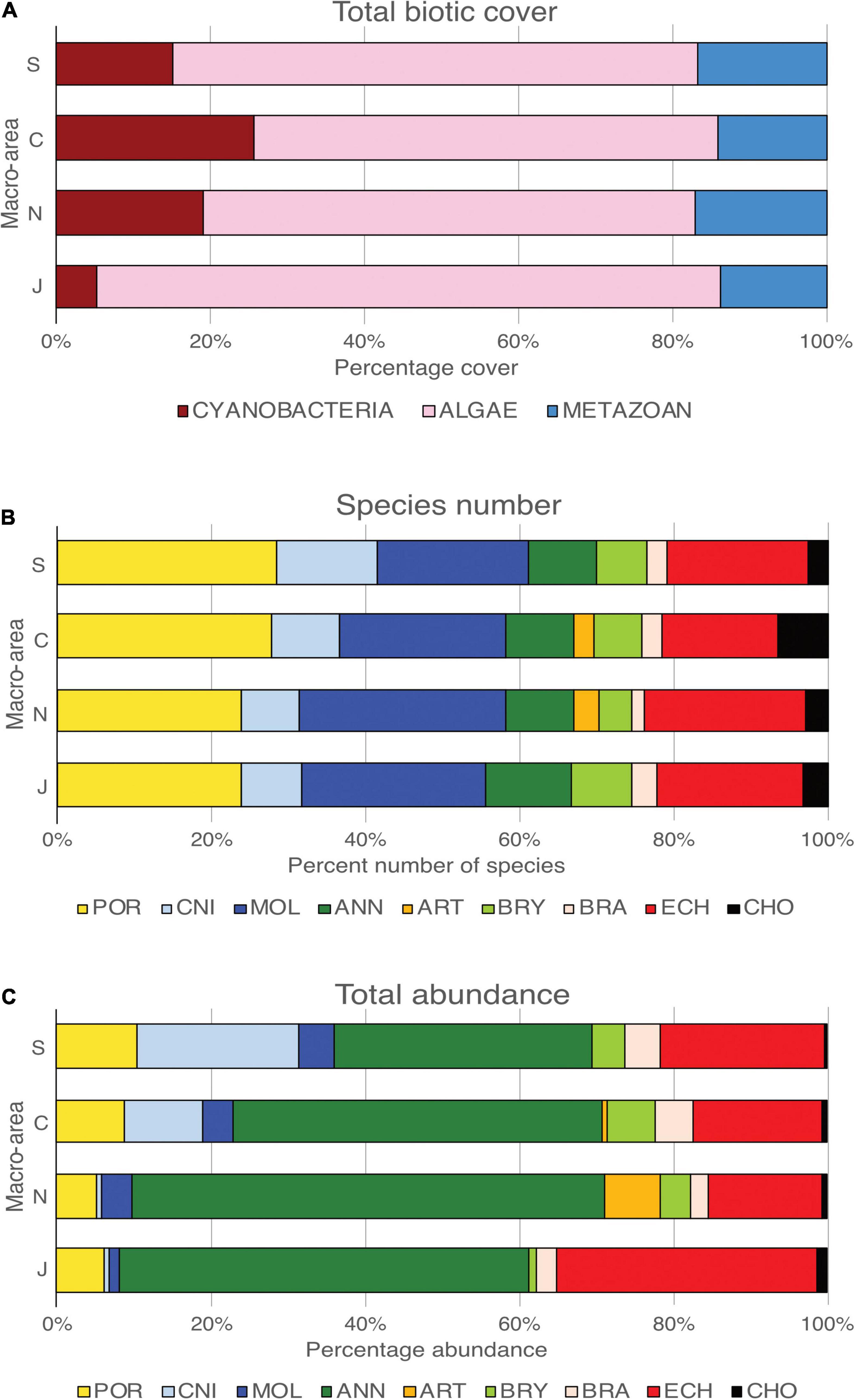
Figure 2. Diversity of megafauna species along the Puyuhuapi–Jacaf fjords. Total biotic cover of Cyanobacteria, algae, and metazoans (A), percentage of the number of species (B), and total abundance (C) of megafauna for each phylum in each of the four macro-areas.
A total of 51,517 individual metazoans – belonging to 137 OTUs – were identified in the photographic quadrants (Supplementary Material 2). Up to 67% of the taxa could be identified to species (51%) or genus level (16%). Porifera, with 35 OTUs (23% of the total identified taxa) represented the most diverse group, followed by Mollusca (22%), Echinodermata (16%), and Cnidaria (15%) (Figure 2B). Annelids were the most abundant group (50% of total abundance), followed by echinoderms (21%), poriferans (8%), and cnidarians (8%) (Figure 2C). The remaining phyla contributed 14% of the total number of organisms. The polychaete Spiochaetopterus sp., occurred in about 63% of the samples, and represented the most abundant organism with more than 12,500 individuals observed (Supplementary Material 2). Other abundant polychaetes were Spirorbinae (8,632 individuals, in 53% of SUs) and Chaetopterus variopedatus (Renier, 1804) (4,126 individuals, in 39% of SUs). The holothurian Psolus sp. (3,190 individuals, in 47% of SUs), the sea urchin Loxechinus albus (Molina, 1782) (2,901 individuals, in 25% of SUs) and the sea anemone Metridium cfr. Senile (Linnaeus, 1761) (2,710 individuals, in 10% of SUs) were also particularly abundant.
Generalized Linear Mixed Models conducted on local abundance showed a significant effect of distance from fjord mouth (Wald test: χ2 = 4.41, Df = 1, p < 0.05; Table 2), with the abundance decreasing with distance, while no significant effect of depth was observed (Wald test: χ2 = 2.29, Df = 1, p = 0.13; Table 2). SR was also negatively affected by distance from fjord mouth (Table 2; Wald test: χ2 = 23.55, Df = 1, and p < 0.001) and positively affected by sampling depth (Table 2 and Figure 3; Wald test: χ2 = 156.95, Df = 1, and p < 0.001). Finally, the same pattern has been observed for diversity indices, both Shannon’s (Figure 4) and Simpson’s diversity indices being affected significantly and positively by depth (Wald test Shannon’s H′: χ2 = 249.95, Df = 1, p < 0.001; Wald test Simpson’s 1-D: χ2 = 162.79, Df = 1, p < 0.001; Table 2 and Figure 3) while negatively by distance from Puyuhuapi mouth (Wald test Shannon’s H′: χ2 = 53.99, Df = 1, p < 0.001; Wald test Simpson’s 1-D: χ2 = 38.20, Df = 1, p < 0.001; Table 2).
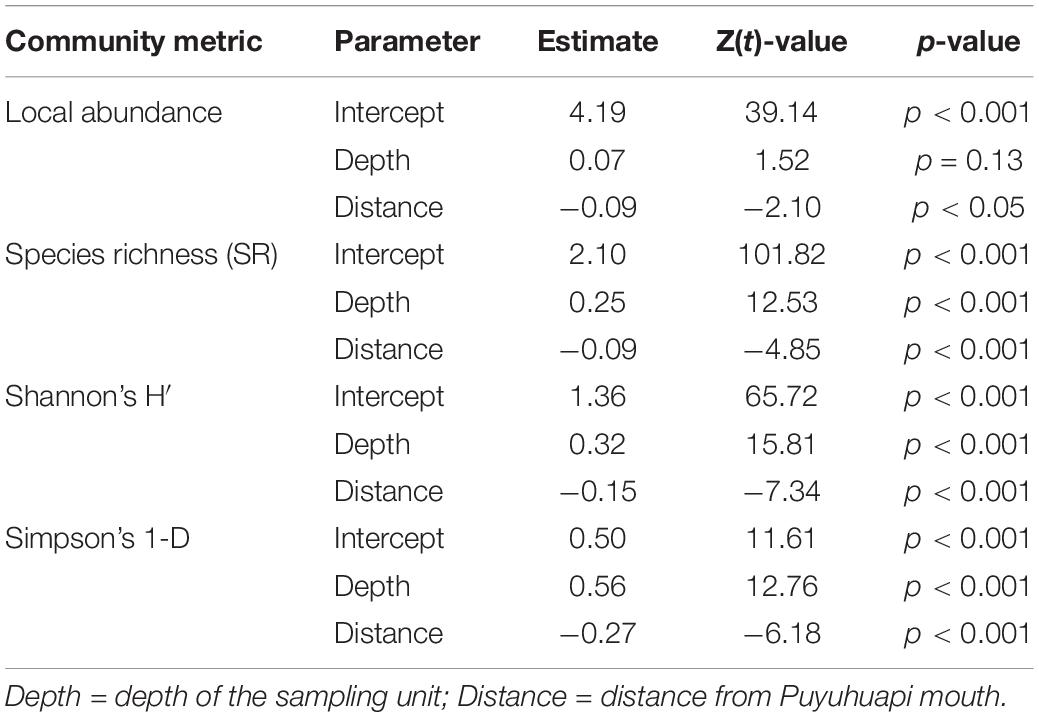
Table 2. Generalized Linear Mixed Models (GLMMs) parameter estimates for four community metrics: local abundance of organisms, species richness (SR), Shannon’s diversity index (H′), and Simpson’s diversity index (1-D).
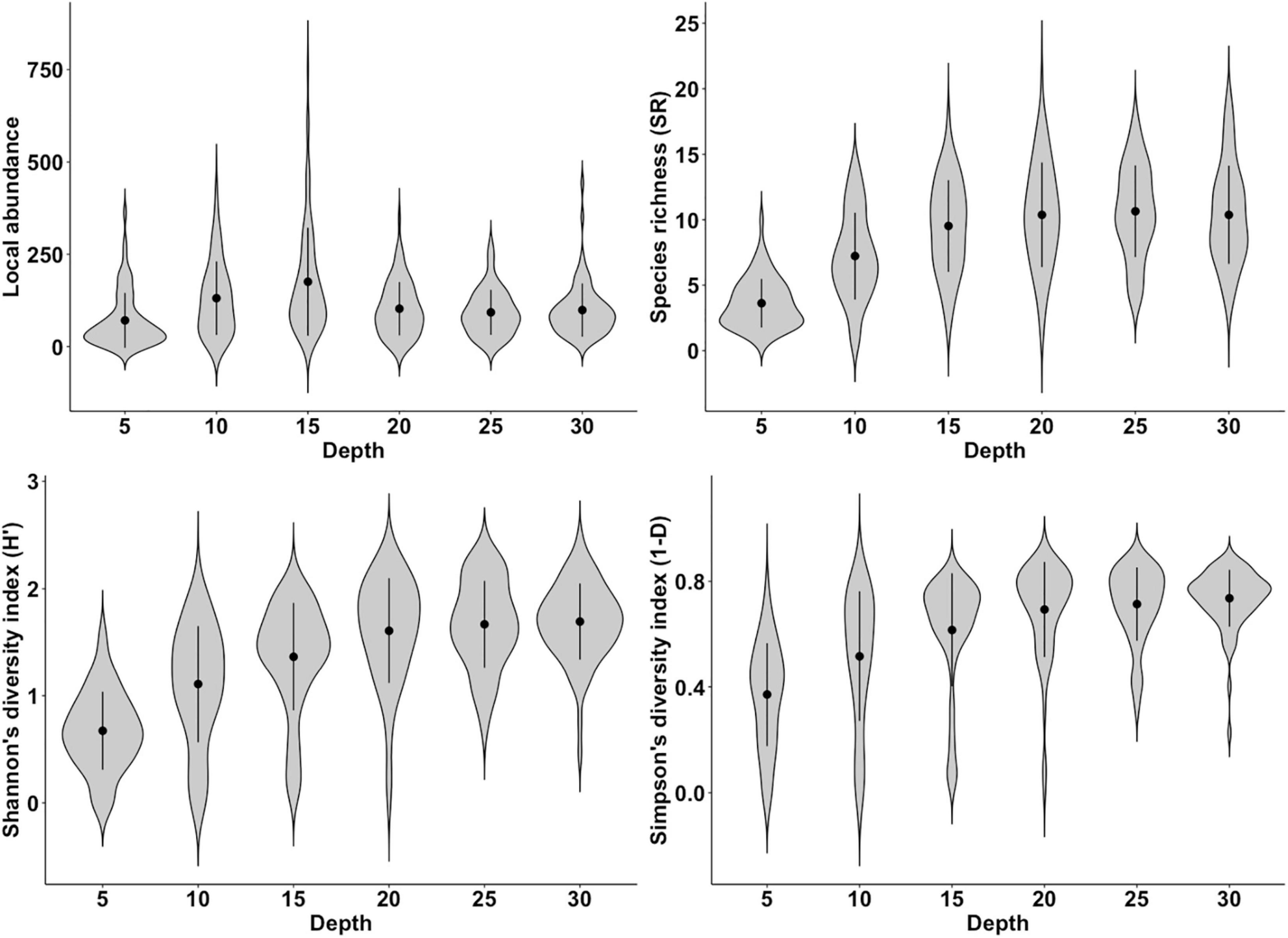
Figure 3. Variation of four community metrics [local abundance of organisms, species richness (SR), Shannon’s diversity index (H′), and Simpson’s diversity index (1-D)] in relation to depth of sampling, expressed in meters. Blacks dots and vertical lines represent, respectively the mean and the Standard Deviation for each violin-plot.
Megabenthic Communities
The clustering of 431 SUs was judged to be suitable for statistical analysis, and provided a total of 10 communities (Table 3 and Figure 5) that were significantly different from each other based upon species composition (PERMANOVA, df = 9, MS = 5.90, F = 33.62, and p < 0.001). The geographical distribution of the 10 megabenthic communities is shown in Figure 6, and a comprehensive list of the most important species characterizing each community (based on the IndVal index) is provided in Table 4. A description of each identified community follows:
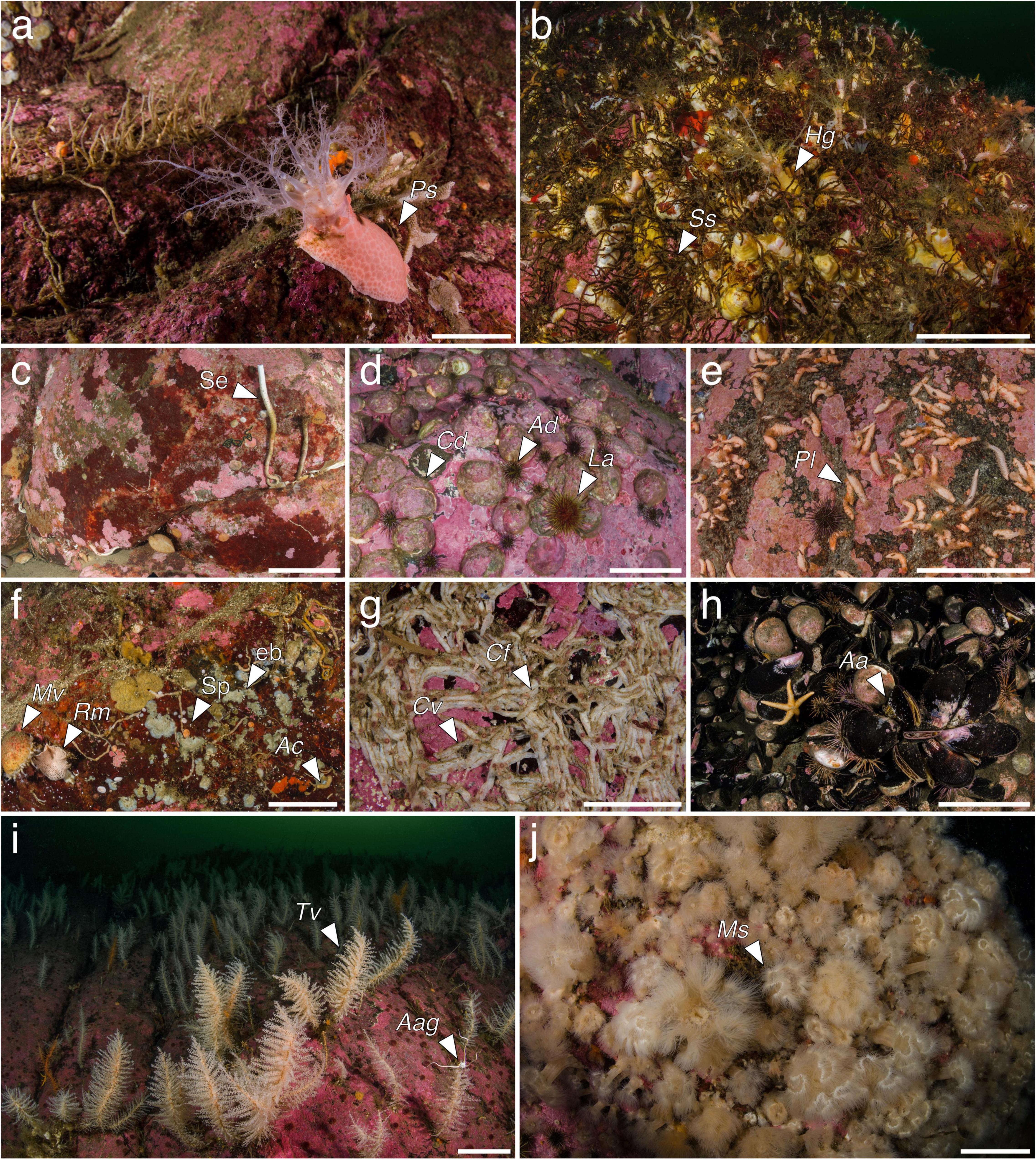
Figure 5. Megabenthic communities identified along the Puyuhuapi–Jacaf fiord system. Psolus sp. (Ps) aggregations (a); Spiochaetopterus sp. (Ss) and Heterocucumis godeffroyi (Hg) gardens (b); Serpulidae (Se) aggregations (c); Loxechinus albus (La) aggregations (d) including Arbacia dufresnii (Ad) and Crepipatella dilatata (Cd); Pentactella leonina (Pl) aggregations (e); community of deep walls and crevices (f) including Spirorbinae (Sp), encrusting bryozoans (eb), Axinella crinita (Ac), Reteporella magellensis (Rm), and Magellania venosa (Mv); Chaetopterus variopedatus (Cv) aggregations (g) including Clathrina fjordica (Cf); Aulacomya atra (Aa) belt (h), deep gorgonian forests (i) including Thouarella cfr. variabilis (Tv) and Astrotoma agassizii (Aag); Metridium cfr. senile (Ms) gardens (j). Scale bars: (a) 2 cm; (b–i) 10 cm; (j) 5 cm.
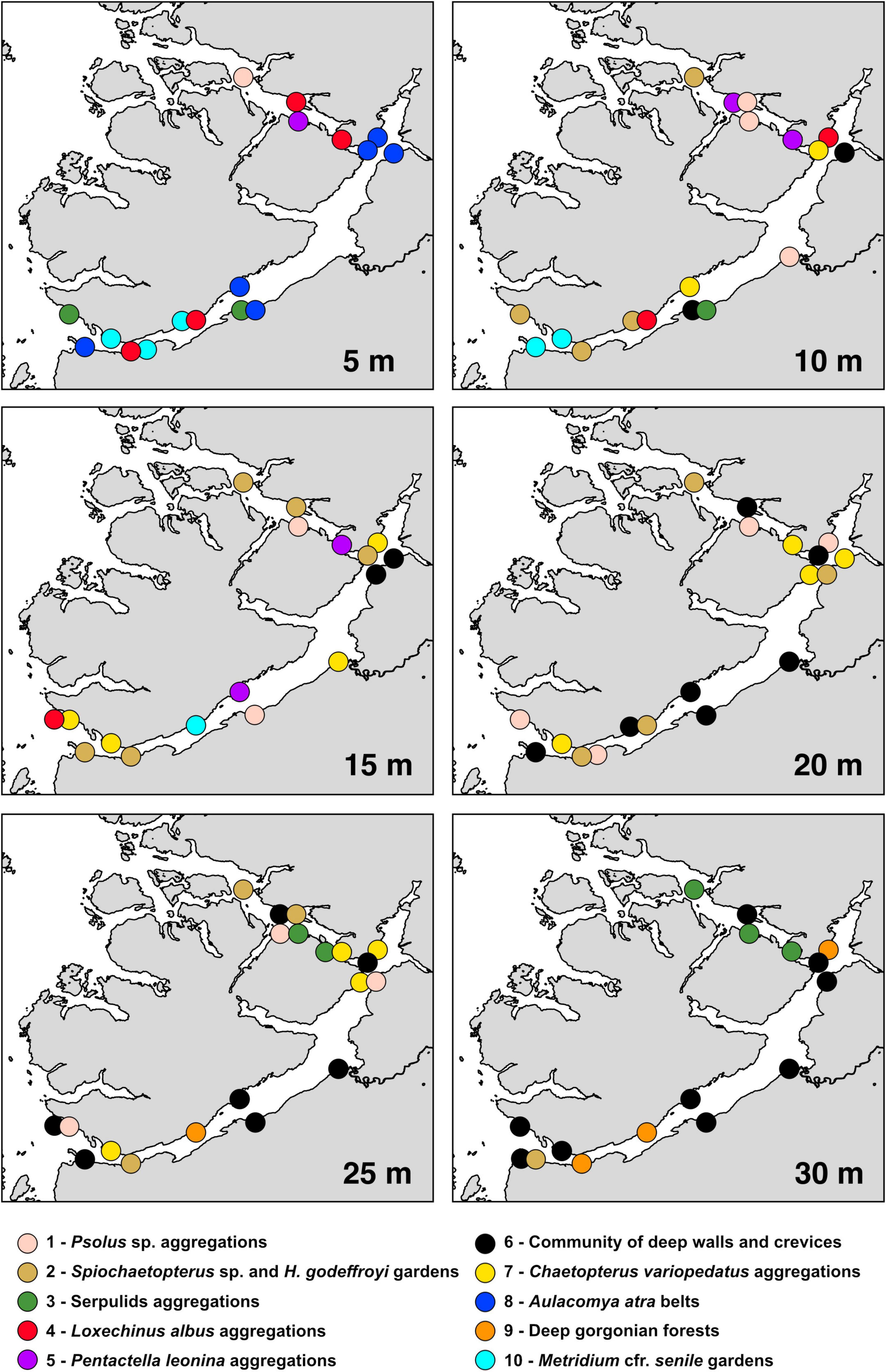
Figure 6. Distribution maps. Spatial distribution of the 10 megabenthic communities identified in the cluster analysis at the six analyzed depths along the Puyuhuapi–Jacaf fiord system.
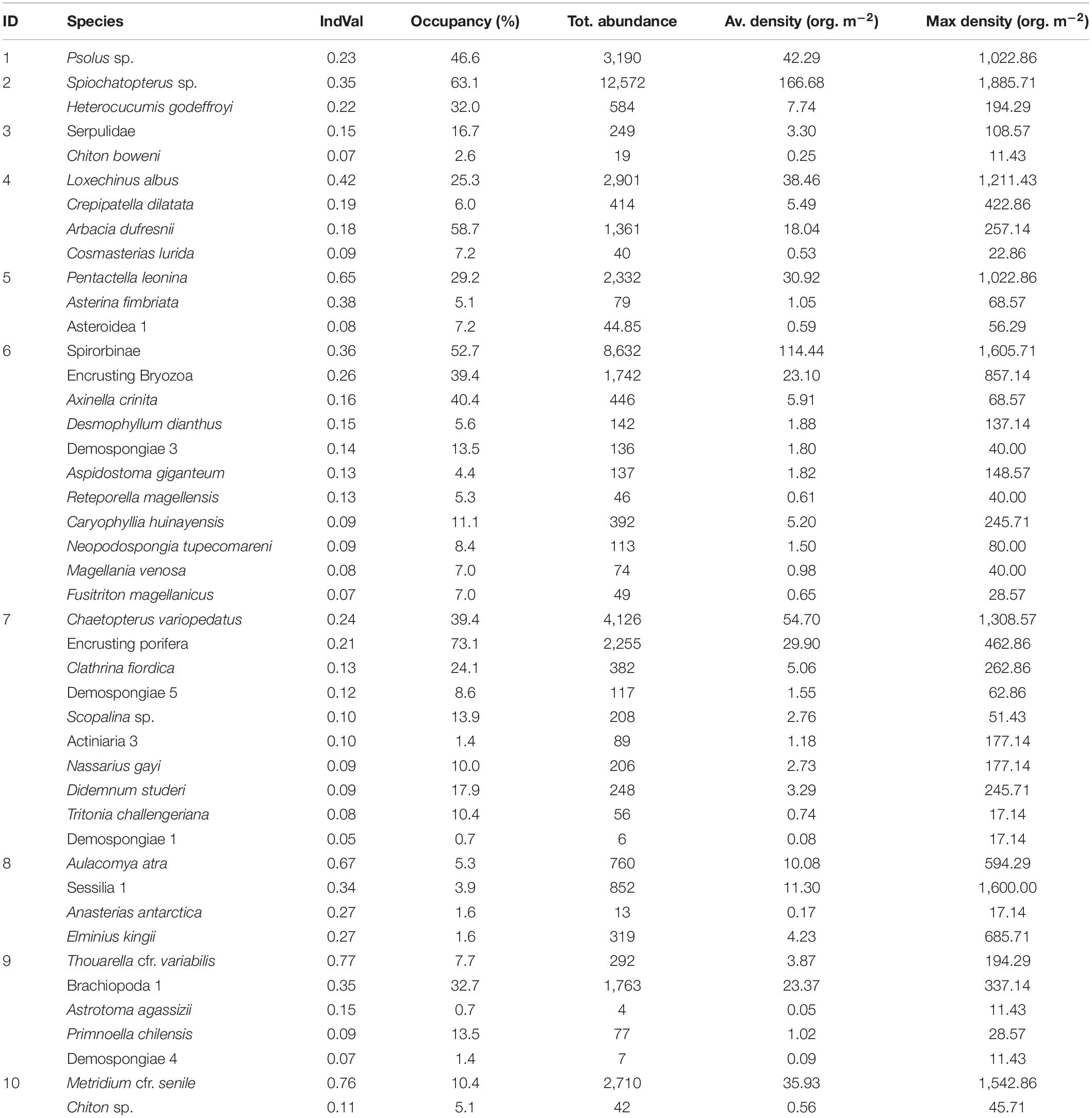
Table 4. Summary of the main species characterizing the 10 megabenthic communities identified in this study, with ecological information.
Community 1: Psolus sp. Aggregations
Small passive filter feeder holothurians belonging to the genus Psolus tend to aggregate in large numbers on vertical and sub-vertical walls, with a maximum recorded density of 1,023 individuals per m2 (ind. m–2). The aggregations are typically found between 5 and 25 m depth and are widespread along the Puyuhuapi and Jacaf fjords, being more common in the latter (Figure 5a).
Community 2: Spiochaetopterus sp. and Heterocucumis godeffroyi Gardens
This community occurred between 10 and 30 m depth and was more common in the Jacaf Fjord and the southern Puyuhuapi area. Polychaetes belonging to the genus Spiochaetopterus produce long and thin membranaceus tubes and can create wide and extremely dense aggregations (up to 1,886 ind. m–2) that protrude from rocky walls and overhangs. Using two long palps, these worms capture organic matter from the water column. The passive filter feeding holothurian H. godeffroyi (Semper, 1867) is associated with these polychaete gardens, living amongst the tubes and reaching a maximum density of 194 ind. m–2 (Figure 5b).
Community 3: Serpulidae Aggregations
This community is characterized by patches of bare rocks covered only with unidentified serpulid polychaetes, which reach a maximum density of 109 ind. m–2; the polyplacophoran Chiton boweni King 1832 is also often associated with these serpulids. This community is particularly frequent in the Jacaf Fjord, typically at depths of 25–30 m, but can also be occasionally found at depths of 5–10 m in the southern and central Puyuhuapi, mainly dominated by C. boweni (Figure 5c).
Community 4: Loxechinus albus Aggregations
The sea urchin L. albus, reaching maximum densities of 1,211 ind. m–2, is often associated with the gastropod Crepipatella dilatata (Lamarck, 1882) and another common sea urchin, Arbacia dufresnii (Blainville, 1825). The predatory sea star Cosmasterias lurida (Philippi, 1858) is also usually present. The community typically occurred at depths of 5–15 m in all studied areas (Figure 5d).
Community 5: Pentactella leonina Aggregations
This community was found almost exclusively along the Jacaf Fjord at depths of 5–15 m (Table 3). In the Puyuhuapi Fjord, the community was recorded only once at 15 m depth in the central portion of the fjord. The characteristic species was P. leonina (Semper, 1867), a holothurian that passively filters large particles and reached a maximum density of 1,023 ind. m–2. The small sea star Asterina fimbriata Perrier, 1875 was often found in the same environment (Figure 5e).
Community 6: Community of Deep Walls and Crevices
This was the most commonly observed community in the study area, and is widespread below 5 m depth in water more influenced by oceanic water (Table 3). The community was particularly notable in the central portion of the Puyuhuapi Fjord but was poorly represented in the Jacaf Fjord. The community is constituted mainly by spirorbin polychaetes, together with encrusting bryozoans. Other important taxa are the sponges Axinella crinita Thiele, 1905, and Neopodospongia tupecomareni Hajdu, Desqueyroux-Faúndez, Carvalho, Lôbo-Hajdu & Willenz, 2013, and an unidentified erect yellow sponge, the hard corals Desmophyllum dianthus (Esper, 1794), and Caryophyllia huinayensis Cairns, Häussermann & Försterra, 2005, the erect bryozoans Aspidostoma giganteum (Busk, 1854), and Reteporella magellensis (Busk, 1884), and the brachiopod Magellania venosa (Dixon, 1789). This brachiopod may occasionally form dense facies (Figure 5f).
Community 7: Chaetopterus variopedatus Aggregations
The principal representatives of this community are the polychaete C. variopedatus which produces membranous tubes attached to the rocks, and various species of encrusting sponges. The maximum recorded density of the polychaete was 1,309 tubes m–2. Other species typical of the community are the sponges Clathrina fiordica, Scopalina sp. and an unidentified massive yellow sponge, together with the encrusting ascidian Didemnum studeri. This community showed the highest average number of species per SU and the highest diversity H′ (Table 3) (Figure 5g).
Community 8: Aulacomya atra Belts
Reaching a maximum density of 594 ind. m–2, the ribbed mussel A. atra (Molina, 1782) created extensive large belts between the intertidal zone and the deepest extent of the brackish layer. This community occurred throughout the Puyuhuapi Fjord but was not observed in the Jacaf Fjord. Different species of barnacles were often observed associated with the mussels, together with the small sea star Anasterias antarctica (Lutken, 1857). This community showed the lowest average number of species per SU and the lowest H′ (Table 3) (Figure 5h).
Community 9: Deep Gorgonian Forests
This community is mainly constituted by large aggregations of the gorgonian Thouarella cfr. variabilis Wright & Studer, 1889, reaching a maximum density of 194 ind. m–2. Accessory species within the community are the whip gorgonian Primnoella chilensis (Phillipi, 1894), the epibiont ophiuroid Astrotoma agassizii Lyman, 1875 – sometimes living on branches of T. cfr. Variabilis – and an unidentified brachiopod. This community was present only at depths of 25–30 m in the central Puyuhuapi Fjord, and occurred only once in the northern portion. The community was absent from the Jacaf Fjord (Figure 5i).
Community 10: Metridium cfr. senile Gardens
The sea anemone M. cfr. senile creates large aggregations of up to more than 1,500 ind. m–2, representing the highest average number of individuals per SU observed in any of the communities (Table 3). The polyplacophoran Chiton sp. is often associated with this community. This community occurred only at depths of 5–15 m in the southern and central Puyuhuapi Fjord (Figure 5j).
Relationship With Environmental Parameters
Based on the dbRDA, the selected factors only partially explained total variability in species composition, with the first two axes explaining about 16% of the constrained variance (CAP1: 12.3%, CAP2: 3.6%; Figure 7A). Depth and distance from the fjord’s mouth represent good predictors in modeling the spatial distribution of benthic fauna (Figure 7B). The biplot shows that the shallow area at the mouth of the fjord, characterized by well-oxygenated waters with intermediate levels of salinity, is dominated by the sea anemone M. cfr. senile. The communities dominated by P. leonina and A. atra tend to prosper in shallow, low-salinity, and well-oxygenated waters of the central portion of the Puyuhuapi Fjord. With increasing salinity and depth, communities are dominated by Spiochaetopterus sp., H. godeffroyi, C. variopedatus, Psolus sp., C. dilatata, and echinoderms. In addition, Spiochaetopterus sp. results particularly abundant also at shallow depth in the Jacaf Fjord. Finally, spirorbin polychaetes, brachiopods and encrusting bryozoans are the most representative organisms in deeper water, where salinity levels are more stable and oxygen saturation is lower than in shallower water.
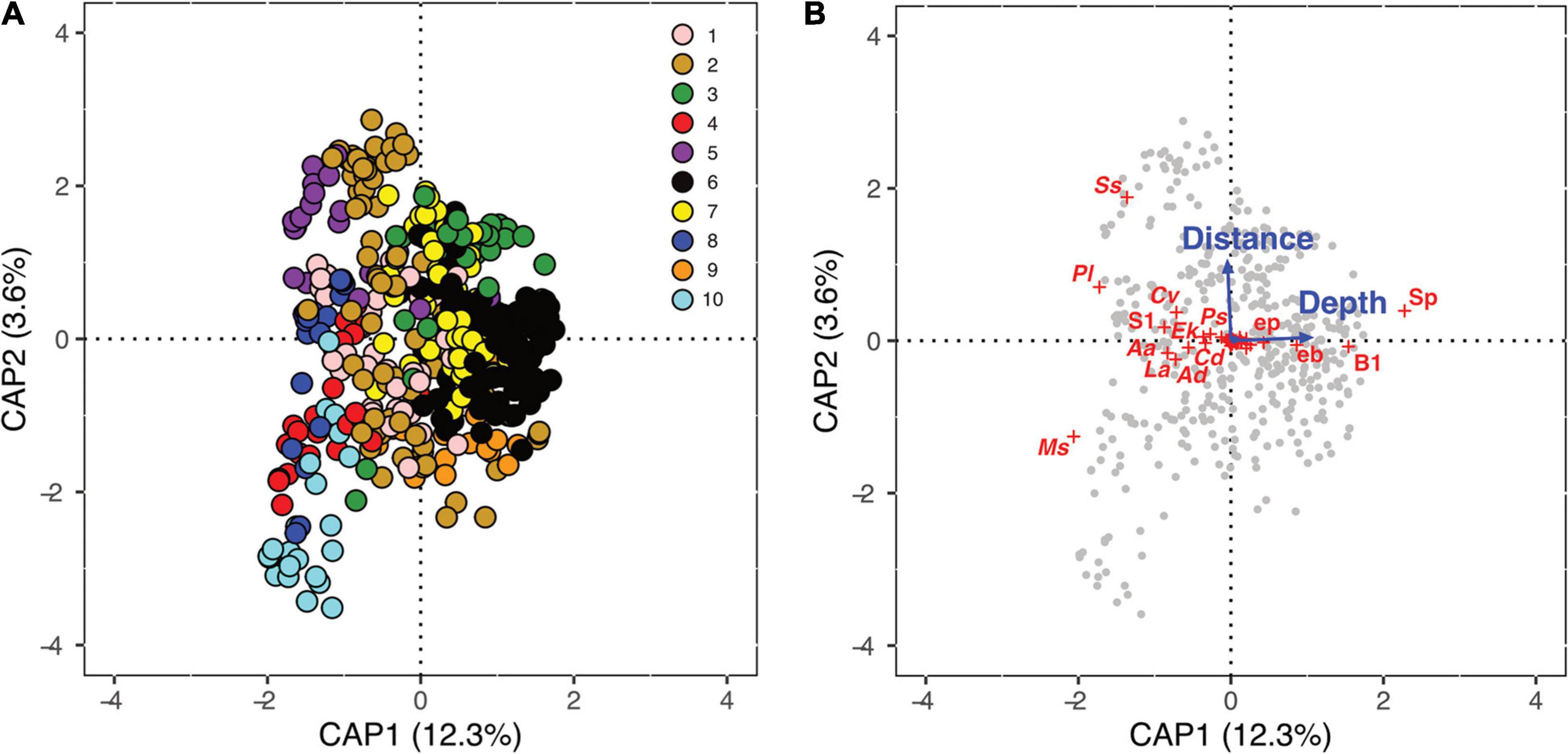
Figure 7. Distance-based redundancy analysis (dbRDA). Results of the dbRDA performed over Bray–Curtis dissimilarity matrix of square-root transformed density data. Samples are represented as colored [(A); color code is referred to Figure 6] or gray (B) dots, environmental variables represented as blue solid lines with arrows and species as red crosses. Names of the species with the highest scores are also shown in the biplot. Aa: Aulacomya atra, Ad: Arbacia dufresnii, B1: Brachiopoda 1, Cd: Crepipatella dilatata, Cv: Chaetopterus variopedatus, eb: encrusting bryozoans, Ek: Elminius kingii, ep: encrusting poriferans, La: Loxechinus albus, Ms: Metridium cfr. senile, Pl: Pentactella leonina, Ps: Psolus sp., S1: Sessilia 1, Sp: Spirorbinae, and Ss: Spiochaetopterus sp.
Discussion
A Diversity Hotspot
In recent years, the Puyuhuapi and Jacaf fjords have attracted an increasing number of studies, from oceanographic (e.g., Schneider et al., 2014; Pérez-Santos et al., 2018; Montero et al., 2021), to biochemical (e.g., Daneri et al., 2012), and zoological (e.g., Försterra et al., 2016; Betti et al., 2017, 2020; Bertolino et al., 2019, 2020) perspective. Therefore, our study of the benthic communities along the coastline of the two fjords complements this previous information to improve our understanding of how ecosystems function in this region. The Puyuhuapi and Jacaf fjord system can be considered very rich and complex because of the sheer number of megafauna species sustained, accounting for 137 identified metazoan taxa of hard bottoms. The high number of species observed is related to the large total sampled area (about 80 m–2 along a 150 km stretch; Supplementary Material 3), but also to the high diversification of the environmental conditions occurring along the rocky walls of the fjords. We identified ten distinct megabenthic communities, all typically dominated by heterotrophic suspension feeders, a functional group that plays a fundamental role in the transfer of matter and energy from the pelagic to the benthic realm. These communities are also structured to varying extents by conspicuous habitat-forming species, such as sponges, anthozoans, polychaetes, mussels, and bryozoans, all capable of modifying surrounding biotic and abiotic environmental properties. As a consequence, individuals within these communities build the so-called marine animal forests that are characterized by high complexity and biodiversity of benthic macrofauna (Försterra et al., 2016; Rossi et al., 2017).
Försterra et al. (2016) reported ten megabenthic aggregations structured by habitat-forming species throughout the whole region of Chilean Patagonian. Two of these aggregations, namely “mussel beds” and “gorgonian gardens,” closely overlap with communities 8 and 9 identified in the present study, and these were also recently reported in the small Seno Magdalena inlet that extends in an approximately NW direction about halfway along the Puyuhuapi Fjord (Betti et al., 2017). Most of the remaining aggregations discussed by Försterra et al. (2016) are also reported in the present study, but are present as separate mono or paucispecific facies within several other communities. For example, “sponge fields” were distributed within communities 6 and 7. The aggregation “polychaete fields” partially overlaps with community 7 (being both dominated by C. variopedatus) and, to a minor extent, with communities 2, 3, and 6. Similarly, aggregations of barnacles and ascidians may occur within communities 8 and 7, respectively. Community 6 consisted of deep walls and crevices and was more diversified. This included five of the aggregations proposed by Försterra et al. (2016), dominated by sponges, D. dianthus, polychaetes, bryozoans, and brachiopods, and the remaining four aggregations identified in Seno Magdalena by Betti et al. (2017). Community 4, represented by aggregations of the sea urchin L. albus, is widespread at the same depth range throughout northern Patagonia, with maximum densities comparable to those observed in the Puyuhuapi Fjord (Molinet et al., 2016; Contreras et al., 2019). Finally, three of the communities reported here are the first descriptions in the scientific literature for fjord ecosystems, and these were dominated by holothurians (1 and 5), and sea anemones (10).
From a wider perspective, the uniqueness of the fjords’ ecosystems with respect to megafaunal assemblages is confirmed by the striking structural differences of the assemblages observed along the northern Chilean coasts (outside the fjord region) where kelp forests dominate the coastal hard seafloors, and barrens grounds are common (Pérez-Matus et al., 2007; Stotz et al., 2016). In these areas macroalgae result more frequent and only very few species (i.e., L. albus) are in common with the Patagonian fjords.
Fjord Functioning
Benthic diversity is clearly unevenly distributed with regard to bathymetry: shallow environments within the surface brackish layer are subject to high variability in environmental properties (in particular, seasonal temperature oscillations are much more variable than in the oceanic layer), and a generally lower salinity, and are therefore less populated by benthos than the underlying layers influenced more by oceanic water. Similar patterns have already been described in the Seno Magdalena Inlet (Betti et al., 2017). Halocline and thermocline are known to greatly influence the distribution in the fjords of sessile and vagile species, as well as their larval stages (e.g., Landaeta and Castro, 2006; Bustos et al., 2007; Landaeta et al., 2007; Meerhoff et al., 2013; Villalobos et al., 2021). Salinity horizontal gradients (due to increasing distance from freshwater inputs, such as rivers) are also known to influence the distribution of the species in the fjords, both on hardgrounds (Kuklinski, 2013; Villalobos et al., 2021) and soft seafloors (Betti et al., 2020). The low light penetration through the turbid brackish layer may also partially explain our observations of poor algal biodiversity, as well as the dominance by red crustose coralline algae below the surface layer: these are adapted to low light conditions and find little competition within the layer influenced by oceanic water, thus potentially explaining their wide and consistent coverage below the halocline.
From the mouth of the Puyuhuapi Fjord to the seaward end of the Jacaf Fjord, the composition of hard bottom macrobenthic communities show a significant constant decrease in terms of organisms’ abundance, SR, Shannon, and Simpson indices. In particular, a slight decrease in abundance of passive predators (such as sea anemones) and active filter feeders (e.g., sponges, bryozoans, brachiopods, barnacles, the gastropod C. dilatata, and ascidians) is coupled with an increase in the diversity of passive filter feeders (e.g., other anthozoans, tubeworms, and holothurians). This is likely related to the variations in the characteristics of the water column throughout the fjord system. At the mouth of the Puyuhuapi Fjord, the oceanic waters from the Moraleda Channel are turbulent and rich in plankton, and thus favor passive predators such as sea anemones. Further along the fjord the amount of detritus increases, possibly favoring passive filter feeders such as holothurians that feed on larger particles. More extensive information is clearly required on dissolved and particulate organic matter cycling, local current regimes, turbidity, and other properties of the water column; these data might facilitate a better understanding of how the megabenthic communities are shaped along the fjords.
A Baseline for Further Studies
The analysis of the megabenthic communities in the present study represents a “snapshot” of the current status of the fjords. This provides an important baseline in an era of potentially significant shifts to these ecosystems caused both by direct anthropogenic stresses and the effects of climate change. Among the more direct impacts, both fishery and aquaculture activities are a constant threat to Patagonian fjord ecosystems; already some communities, such as L. albus aggregations, are declining as a result of intense fishing activities (Contreras et al., 2019). Moreover, a decreasing trend in hardgrounds megafaunal abundance due to aquaculture has been recorded (Häussermann et al., 2013). Regarding climate changes, increasing temperature, and sea levels will have relatively slow impacts on ecosystems, but other indirect effects are already evident. Recent studies have highlighted a historical decline in precipitation and streamflow in the fjords of northern Patagonia (down to 48° S) resulting from climate changes (i.e., Lara et al., 2008; Iriarte et al., 2016; Garreaud, 2018). This variation in freshwater inputs into fjords is likely to influence the volume and chemistry of the brackish surface layer, with consequences for phytoplankton communities that represent the base of the food chain for benthos. Clearly, fjords such as Puyuhuapi and Jacaf may act as a “sentinel” area that could highlight impacts of climate change if effectively monitored. A decrease in the influence of the brackish freshwater input could result in emergence of communities and species that characterize the oceanic layer, coupled with a reduction in communities typical of low salinity shallow waters, such as A. atra belts (found here only in the brackish layer), L. albus aggregations and M. cfr. senile gardens. The reduction of the typically turbid surface layer may cause an increase in light penetration through the water column, potentially increasing the presence and cover of non-Corallinales macroalgae. Reduced riverine input of organic material might induce a community shift in the Jacaf Fjord, particularly in communities characterized by large particle passive filter feeders such as P. leonina and Psolus sp. aggregations, and the communities dominated by gardens of Spiochaetopterus sp. and H. godeffroyi. Therefore, filter feeding Holothuroidea might represent a realistic and sensitive target species for monitoring activities, because a decrease in their abundance might provide an early warning of changes within fjord ecosystems.
A unique characteristic of Patagonian fjords is their latitudinal range; these fjords host many Antarctic species at the northern reach of their geographical distribution, and these species are clearly sensitive to global warming and associated effects of climate change. For instance, cold-water corals (CWCs) are known to be threatened both by warming and subsequent oxygen reduction within the water column (i.e., Jantzen et al., 2013). Therefore, the CWCs C. huinayensis and D. dianthus, both present in the Puyuhuapi Fjord, provide promising examples of potentially important sentinel species. On the contrary, brachiopods living in British Columbia fjords demonstrated a good tolerance to low oxygen concentrations (Tunnicliffe and Wilson, 1988); therefore, Chilean species such as M. venosa might be favored by an eventual oxygen reduction. Benthic macrofauna of European fjords showed an abrupted change in diversity, abundance and biomass at oxygen concentrations of approximately 2 mg O2 liter–1, with molluscs being the most tolerant species (Rosenberg, 1980).
Finally, ocean acidification, derived from CO2 emissions generated by anthropic activities, is considered one of the major threats to marine organisms all over the World (Fabry et al., 2008); nevertheless, the effects of acidification on benthic communities is still unclear, due to a high variability of processes influencing the small-scale phenomenon (e.g., river discharges, upwelling, ice melting, eutrophication, and pollution) (e.g., Salisbury et al., 2008; Vargas et al., 2017). Establishing a baseline can help in detecting early effects of acidification on fjords ecosystems; calcifiers organisms, such as gastropods and bivalves (e.g., Thomsen et al., 2012), and also CWCs (Turley et al., 2007) may represent valid sentinel species. Globally, brachiopods appear to be less impacted by ocean acidification (Cross et al., 2015, 2016), probably thanks to compensating mechanisms (Cross et al., 2019), but microstructural changes have been observed in the shell of the Chilean fjords’ species M. venosa (Ye et al., 2019). With warming and climate change seriously threatening these Chilean fjords benthic communities, it is imperative to reduce general stress levels on all organisms, and on the ecosystem itself. This can be achieved through effective management of other potential anthropogenic impacts, such as fishery and aquaculture activities. In order to protect benthic communities and fjord ecosystems from challenges brought about by climate change, comprehensive and continuous monitoring, and mapping activities are an important tool to verify the effectiveness of these management plans.
Data Availability Statement
The raw data supporting the conclusions of this article will be made available by the authors, without undue reservation.
Author Contributions
All authors greatly contributed to the manuscript. FB: project coordination, sampling activity, and manuscript writing. FE: sampling activity, data analysis, conceptualization, and manuscript writing. GB: conceptualization, project coordination, and manuscript reviewing. AC and MB: data analysis and manuscript reviewing. AM: data analysis. PO: manuscript reviewing. GD: project coordination and manuscript reviewing. All authors contributed to the article and approved the submitted version.
Conflict of Interest
The authors declare that the research was conducted in the absence of any commercial or financial relationships that could be construed as a potential conflict of interest.
Publisher’s Note
All claims expressed in this article are solely those of the authors and do not necessarily represent those of their affiliated organizations, or those of the publisher, the editors and the reviewers. Any product that may be evaluated in this article, or claim that may be made by its manufacturer, is not guaranteed or endorsed by the publisher.
Supplementary Material
The Supplementary Material for this article can be found online at: https://www.frontiersin.org/articles/10.3389/fmars.2021.635430/full#supplementary-material
Supplementary Material 1 | Biological characteristics of the 16 stations explored in this study.
Supplementary Material 2 | Comprehensive list of the species identified in this study.
Supplementary Material 3 | Species accumulation curves, built using the Mao Tau sample-based rarefaction method, and divided by macro-areas. S = southern portion of the Puyuhuapi Fjord, C = central portion of the Puyuhuapi Fjord; N = northern part of the Puyuhuapi Fjord, J = Jacaf Fjord. Shaded areas represent 95% confidence intervals.
References
Bates, D., Sarkar, D., Bates, M. D., and Matrix, L. (2007). The Lme4 Package. R Package Version 2.1.74.
Bertolino, M., Costa, G., Bavestrello, G., Pansini, M., and Daneri, G. (2020). New sponge species from seno magdalena, puyuhuapi fjord and jacaf canal (Chile). Eur. J. Taxon 715, 1–49.
Bertolino, M., Costa, G., Reboa, A., Bavestrello, G., Pansini, M., Betti, F., et al. (2019). The sponge fauna of the seno magdalena and puyuhuapi fjord (Chile), with a description of two new species. Zootaxa 4623, 306–320. doi: 10.11646/zootaxa.4623.2.5
Betti, F., Bavestrello, G., Bo, M., Enrichetti, F., Loi, A., Wanderlingh, A., et al. (2017). Benthic biodiversity and ecological gradients in the Seno Magdalena (Puyuhuapi Fjord, Chile). Estuar. Coast. Shelf Sci. 198, 269–278. doi: 10.1016/j.ecss.2017.09.018
Betti, F., Castro, L. R., Bavestrello, G., Enrichetti, F., and Daneri, G. (2020). Distribution, abundance and ecological requirements of the benthic phase of Munida gregaria (Anomura; Munididae) in the Puyuhuapi Fjord (Chilean Patagonia). Reg. Stud. Mar. Sci. 40:101534. doi: 10.1016/j.rsma.2020.101534
Brattström, H., and Johanssen, A. (1983). Ecological and regional zoogeography of the marine benthic fauna of Chile. Report No. 49 of the Lund University Chile Expedition 1948-49. Sarsia 68, 233–339.
Bustos, C. A., BalbontÍn, F., and Landaeta, M. F. (2007). Spawning of the southern hake Merluccius australis (Pisces: Merlucciidae) in Chilean fjords. Fish. Res. 83, 23–32. doi: 10.1016/j.fishres.2006.08.010
Cañete, J. I., Leighton, G. L., and Aguilera, F. F. (1999). Polychaetes from Aysén Fjord, Chile: distribution, abundance and biogeographical comparison with the shallow soft-bottom polychaete fauna from Antarctica and the Magellan Province. Sci. Mar. 63, 243–252. doi: 10.3989/scimar.1999.63s1243
Cárdenas, J., Aldea, C., and Valdovinos, C. (2008). Chilean marine Mollusca of northern Patagonia collected during the Cimar-10 fjords cruise. Gayana 72, 202–240.
Contreras, C., Niklitschek, E., Molinet, C., Díaz, P., and Díaz, M. (2019). Fishery-induced reductions in density and size truncation of sea urchin Loxechinus albus affects diversity and species composition in benthic communities. Estuar. Coast. Shelf Sci. 219, 409–419. doi: 10.1016/j.ecss.2019.02.030
Cross, E. L., Harper, E. M., and Peck, L. S. (2019). Thicker shells compensate extensive dissolution in brachiopods under future ocean acidification. Environ. Sci. Technol. 53, 5016–5026. doi: 10.1021/acs.est.9b00714
Cross, E. L., Peck, L. S., and Harper, E. M. (2015). Ocean acidification does not impact shell growth or repair of the Antarctic brachiopod Liothyrella uva (Broderip, 1833). J. Exp. Mar. Biol. Ecol. 426, 29–35. doi: 10.1016/j.jembe.2014.10.013
Cross, E. L., Peck, L. S., Lamare, M. D., and Harper, E. M. (2016). No ocean acidification effects on shell growth and repair in the New Zealand brachiopod Calloria inconspicua (Sowerby, 1846). ICES J. Mar. Sci. 73, 920–926. doi: 10.1093/icesjms/fsv031
Daneri, G., Montero, P., Lizárraga, L., Torres, R., Iriarte, J. L., Jacob, B., et al. (2012). Primary Productivity and heterotrophic activity in an enclosed marine area of central Patagonia (Puyuhuapi channel; 44° S, 73° W). Biogeosci. Discuss. 9, 5929–5968.
DGA. (2003). Dirección General de Aguas. Chile. Available online at: http://www.dga.cl.
Dominguez-Carrió, C. (2018). ROV-Based Ecological Study and Management Proposal for the Offshore Marine Protected Area of Cap de Creus (NW Mediterranean). Ph.D. thesis. Barcelona: Universitat de Barcelona.
Dufrêne, M., and Legendre, P. (1997). Species assemblages and indicator species: the need for a flexible asymmetrical approach. Ecol. Monogr. 67, 345–366. doi: 10.2307/2963459
Escribano, R., Fernández, M., and Aranís, A. (2003). Physical-chemical processes and patterns of diversity of the Chilean eastern boundary pelagic and benthic marine ecosystems: an overview. Gayana 67, 190–205.
Fabry, V. J., Seibel, B. A., Feely, R. A., and Orr, J. C. (2008). Impacts of ocean acidification on marine fauna and ecosystem processes. ICES J. Mar. Sci. 65, 414–432. doi: 10.1093/icesjms/fsn048
Försterra, G., Häussermann, V., and Laudien, J. (2016). “Animal forests in the Chilean fjords: discoveries, perspectives and threats in shallow and deep waters,” in Marine Animal Forests, eds S. Rossi, L. Bramanti, A. Gori, and C. Orejas (Cham: Springer).
Försterra, G., and Häussermann, V. (2003). First report on large scleractinian (Cnidaria: Anthozoa) accumulations in cold-temperate shallow water of south Chilean. Zool. Verh. 345, 117–128.
Garreaud, R. D. (2018). Record-breaking climate anomalies lead to severe drought and environmental disruption in western Patagonia in 2016. Clim. Res. 74, 217–229. doi: 10.3354/cr01505
Gattuso, J. P., Frankignoulle, M., and Wollast, R. (1998). Carbon and carbonate metabolism in coastal aquatic ecosystems. Ann. Rev. Ecol. Syst. 29, 405–434. doi: 10.1146/annurev.ecolsys.29.1.405
González, H. E., Calderón, M. J., Castro, L., Clement, A., Cuevas, L. A., Daneri, G., et al. (2010). Primary production and plankton dynamics in the Reloncaví fjord and the interior sea of Chiloé, Northern Patagonia, Chile. Mar. Ecol. Prog. Ser. 402, 13–30. doi: 10.3354/meps08360
González, H. E., Castro, L., Daneri, G., Iriarte, J. L., Silva, N., Vargas, C. A., et al. (2011). Seasonal plankton variability in Chilean Patagonia fjords: carbon flow through the pelagic food web of Aysen Fjord and plankton dynamics in the Moraleda Channel basin. Cont. Shelf Res. 31, 225–243. doi: 10.1016/j.csr.2010.08.010
Hammer, Ø, Harper, D. A. T., and Ryan, P. D. (2011). Reference Manual of PAST, Paleontological Statistics, Version 2.09. Natural History Museum. Oslo: University of Oslo.
Häussermann, V., and Försterra, G. (2007). Large assemblages of cold-water corals in Chile: a summary of recent findings and potential impacts. Bull. Mar. Sci. 81, 195–207.
Häussermann, V., and Försterra, G. (2009). Marine Benthic Fauna of Chilean Patagonia. Santiago: Nature in Focus.
Häussermann, V., Försterra, G., Melzer, R. R., and Meyer, R. (2013). Gradual changes of benthic biodiversity in Comau Fjord, Chilean Patagonia – lateral observations over a decade of taxonomic research. Spixiana 36, 161–171.
Howe, J. A., Austin, W. E. N., Forwick, M., Paetzel, M., Harland, R., and Cage, A. G. (2010). Fjord systems and archives: a review. Geol. Soc. Lond. Special Publ. 344, 5–15.
Hromic, T., Ishman, S., and Silva, N. (2006). Benthic foraminiferal distributions in Chilean fjords: 47 S to 54 S. Mar. Micropaleontol. 59, 115–134. doi: 10.1016/j.marmicro.2006.02.001
Iriarte, J., León-Muñoz, J., Marcé, R., Clément, A., and Lara, C. (2016). Influence of seasonal freshwater streamflow regimes on phytoplankton blooms in a Patagonian fjord. New Zeal. J. Mar. Freshw. Res. 51, 304–315. doi: 10.1080/00288330.2016.1220955
Jantzen, C., Häussermann, V., Försterra, G., Laudien, J., Ardelan, M., Maier, S., et al. (2013). Occurrence of a cold-water coral along natural pH gradients (Patagonia, Chile). Mar. Biol. 160, 2597–2607. doi: 10.1007/s00227-013-2254-0
Kuklinski, P. (2013). Biodiversity and abundance patterns of rock encrusting fauna in a temperate fjord. Mar. Environ. Res. 87-88, 61–72. doi: 10.1016/j.marenvres.2013.03.005
Landaeta, M. F., and Castro, L. R. (2006). Larval distribution and growth of the rockfish, Sebastes capensis (Sebastidae, Pisces), in the fjords of southern Chile. ICES J. Mar. Sci. 63, 714–724. doi: 10.1016/j.icesjms.2006.01.002
Landaeta, M. F., Martinez, R. A., Bustos, C. A., and Castro, L. R. (2007). Distribution of microplankton and fish larvae related to sharp clines in a Patagonian fjord. Rev. Biol. Mar. Oceanogr. 48, 401–407. doi: 10.4067/s0718-19572013000200020
Lara, A., Villalba, R., and Urrutia, R. A. (2008). 400-year tree-ring record of the Puelo River summer–fall streamflow in the Valdivian Rainforest eco-region, Chile. Clim. Change 86, 331–356. doi: 10.1007/s10584-007-9287-7
Leps, M., Tonkin, J. D., Dahm, V., Haase, P., and Sundermann, A. (2015). Disentangling environmental drivers of benthic invertebrate assemblages: the role of spatial scale and riverscape heterogeneity in a multiple stressor environment. Sci. Total Environ. 536, 546–556. doi: 10.1016/j.scitotenv.2015.07.083
Lopez, P., Chevallier, P., Favier, V., Pouyaud, B., Ordenes, F., and Oerlemans, J. (2010). A regional view of fluctuations in glacier length in southern South America. Glob. Planet. Change 71, 85–108. doi: 10.1016/j.gloplacha.2009.12.009
Meerhoff, E., Castro, L., and Tapia, F. (2013). Influence of freshwater discharges and tides on the 354 abundance and distribution of larval and juvenile Munida gregaria in the Baker river estuary, Chilean Patagonia. Cont. Shelf Res. 61, 1–11. doi: 10.1016/j.csr.2013.04.025
Melzer, R. R., Schrodl, M., Haussermann, V., Forsterra, G., and Bravo, M. F. M. (2006). Pycnogonids on cnidarians at fjord Comau, Southern Chile: a report on 2005 SCUBA collections. Spixiana 29, 237–242.
Molinet, C., Barahona, N., Díaz, M., Díaz, P. A., Millanao, M. O., Araya, P., et al. (2016). Using drift video transects and maximum likelihood geostatistics for quantifying and monitoring exploited subpopulations of Loxechinus albus at a mesoscale. Mar. Coast. Fish. 8, 70–80. doi: 10.1080/19425120.2015.1121939
Montero, P., Coppari, M., Betti, F., Bavestrello, G., and Daneri, G. (2021). Feeding of Aulacomya atra under different organic matter sources (autochthonous and allochthonous) in a Chilean Patagonia fjord ecosystem. Front. Mar. Sci. doi: 10.3389/fmars.2021.612406
Mutschke, E., and Gorny, M. (1999). The benthic decapod fauna in the channels and fjords along the South Patagonian Icefield, Southern Chile. Sci. Mar. 63, 315–319. doi: 10.3989/scimar.1999.63s1315
O’Hara, R., and Kotze, J. (2010). Do not log-transform count data. Met. Ecol. Evol. 1, 118–122. doi: 10.1111/j.2041-210x.2010.00021.x
Oksanen, J., Blanchet, F. G., Kindt, R., Legendre, P., Minchin, P. R., O’Hara, R. B., et al. (2016). Package “Vegan”, Version 2.3–5. CRAN Repository, 1-295.
Pantoja, S., Iriarte, J. L., and Daneri, G. (2011). Oceanography of the Chilean Patagonia. Cont. Shelf Res. 31, 149–153. doi: 10.1016/j.csr.2010.10.013
Pérez-Matus, A., Ferry-Graham, L. A., Cea, A., and Vásquez, A. (2007). Community structure of temperate reef fishes in kelp-dominated subtidal habitats of northern Chile. Mar. Freshw. Res. 58, 1069–1085. doi: 10.1071/mf06200
Pérez-Santos, I., Castro, L., Ross, L., Niklitschek, E., Mayorga, N., Cubillos, L., et al. (2018). Turbulence and hypoxia contribute to dense biological scattering layers in a Patagonian fjord system. Ocean Sci. 14, 1185–1206. doi: 10.5194/os-14-1185-2018
Prado-Fiedler, R., and Castro, J. S. (2008). Aporte fluvial y pluvial de nitrogeno y fosforo al fiordo Aysén y canales Jacaf-Ventisquero-Puyuhuapi, Ciencia y Tecnolog a del Mar. 31, 75–95.
Roberts, D. W. (2016). Labdsv: Ordination and Multivariate Analysis for Ecology. R Package Version 1.8–0.
Rosenberg, R. (1980). “Effect of oxygen deficiency on benthic macrofauna in fjords,” in Fjord Oceanography, eds Freeland et al. (New York, NY: Plenum Press), 499–514. doi: 10.1007/978-1-4613-3105-6_45
Rossi, S., Bramanti, L., Gori, A., and Orejas, C. (Eds.) Orejas (2017). Marine Animal Forests: the Ecology of Benthic Biodiversity Hotspots (pp. 1-1366). Cham: Springer International Publishing.
Salisbury, J., Green, M., Hunt, C. W., and Campbell, J. (2008). Coastal acidification by rivers: a threat to shellfish? Eos 89, 513–528. doi: 10.1029/2008eo500001
Sánchez, N., Gonzales, H. E., and Iriarte, J. L. (2011). Trophic interactions of pelagic crustaceans in Comau fjord (Chile): their role in the food web structure. J. Plankton Res. 33, 1212–1229. doi: 10.1093/plankt/fbr022
Schmidt, A., and Brandt, A. (2001). The tanaidacean fauna of the Beagle Channel (southern Chile) and its relationship to the fauna of the Antarctic continental shelf. Antarct. Sci. 13, 420–429. doi: 10.1017/s095410200100058x
Schneider, W., Pérez-Santos, I., Ross, L., Bravo, L., Seguel, R., and Hernández, F. (2014). On the hydrography of Puyuhuapi Channel, Chilean Patagonia. Prog. Oceanogr. 129, 8–18. doi: 10.1016/j.pocean.2014.03.007
Schwabe, E., Försterra, G., Häussermann, V., Melzer, R. R., and Schroedl, M. (2006). Chitons (Mollusca: Polyplacophora) from the southern Chilean Comau Fjord, with reinstatement of Tonicia calbucensis Plate, 1897. Zootaxa 1341, 1–27. doi: 10.11646/zootaxa.1341.1.1
Silva, N., and Palma, S. (2008). “The CIMAR program in the austral Chilean channels and fjords,” in Progress in the Oceanographic Knowledge of Chilean Interior Waters, From Puerto Montt to Cape Horn, eds N. Silva and S. Palma (Valparaíso: Comité Oceanográfico Nacional - Pontificia Universidad Católica de Valparaíso), 11–15.
Sinniger, F., and Häussermann, V. (2009). Zoanthids (Cnidaria: Hexacorallia: Zoantharia) from shallow waters of the southern Chilean fjord region, with descriptions of a new genus and two new species. Org. Divers. Evol. 9, 23–36. doi: 10.1016/j.ode.2008.10.003
Stotz, W. B., Aburto, J., Caillaux, L. M., and González, S. A. (2016). Vertical distribution of rocky subtidal assemblages along the exposed coast of north-central Chile. J. Sea Res. 107, 34–47. doi: 10.1016/j.seares.2015.11.006
Thomsen, J., Casties, I., Pansch, C., Körtzinger, A., and Melzner, F. (2012). Food availability outweighs ocean acidification effects in juvenile Mytilus edulis: laboratory and field experiments. Global Change Biol. 19, 1017–1027. doi: 10.1111/gcb.12109
Tunnicliffe, V., and Wilson, K. (1988). Brachiopod populations: distribution in fjords of British Columbia (Canada) and tolerance of low oxygen concentrations. Mar. Ecol. 47, 117–128. doi: 10.3354/meps047117
Turley, C. M., Roberts, J. M., and Guinotte, J. M. (2007). Corals in deep-water: will the unseen hand of ocean acidification destroy cold-water ecosystems? Coral Reefs 26, 445–448. doi: 10.1007/s00338-007-0247-5
Vargas, C. A., Lagos, N. A., Lardies, M. A., Duarte, C., Manriquez, P. H., Aguilera, V. M., et al. (2017). Species-specific responses to ocean acidification should account for local adaptation and adaptive plasticity. Nat. Ecol. Evol. 1:84.
Villalobos, V., Valdiviav, N., Försterra, G., Ballyram, S., Espinoza, J. P., Burgos-Andrade, K., et al. (2021). Depth-dependent diversity patterns of rocky subtidal macrobenthic communities along a temperate fjord in Northern Chilean Patagonia. Front. Mar. Sci. 8:635855.
Walsh, J. J. (1991). Importance of continental margins in the marine biogeochemical cycling of carbon and nitrogen. Nature 350, 53–55. doi: 10.1038/350053a0
Warton, D. I., and Hui, F. K. (2011). The arcsine is asinine: the analysis of proportions in ecology. Ecology 92, 3–10. doi: 10.1890/10-0340.1
Keywords: Chilean Patagonia, megabenthic diversity, global warming, sentinel species, cold water benthic communities
Citation: Betti F, Enrichetti F, Bavestrello G, Costa A, Moreni A, Bo M, Ortiz Saini P and Daneri G (2021) Hard-Bottom Megabenthic Communities of a Chilean Fjord System: Sentinels for Climate Change? Front. Mar. Sci. 8:635430. doi: 10.3389/fmars.2021.635430
Received: 30 November 2020; Accepted: 02 July 2021;
Published: 26 July 2021.
Edited by:
Susana Carvalho, King Abdullah University of Science and Technology, Saudi ArabiaReviewed by:
Alejandro Perez Matus, Pontificia Universidad Católica de Chile, ChileSimone Baldanzi, Universidad de Valparaiso, Chile
Copyright © 2021 Betti, Enrichetti, Bavestrello, Costa, Moreni, Bo, Ortiz Saini and Daneri. This is an open-access article distributed under the terms of the Creative Commons Attribution License (CC BY). The use, distribution or reproduction in other forums is permitted, provided the original author(s) and the copyright owner(s) are credited and that the original publication in this journal is cited, in accordance with accepted academic practice. No use, distribution or reproduction is permitted which does not comply with these terms.
*Correspondence: Giorgio Bavestrello, Z2lvcmdpby5iYXZlc3RyZWxsb0B1bmlnZS5pdA==