- 1Marine Ecology Consultant, Nelly Bay, QLD, Australia
- 2ARC Centre of Excellence for Coral Reef Studies, James Cook University, Townsville, QLD, Australia
- 3Oceania Regional Office, International Union for the Conservation of Nature, Suva, Fiji
- 4College of Science and Engineering, James Cook University, Townsville, QLD, Australia
- 5GRID-Arendal, Arendal, Norway
- 6Fisheries Consultant, Nuku'alofa, Tonga
- 7Secretariat of the Pacific Community, Noumea, New Caledonia
Networks of no-take marine protected areas (MPAs), where all extractive activities are prohibited, are the most effective tool to directly protect marine ecosystems from destructive and unsustainable human activities. No-take MPAs and MPA networks have been globally implemented in coastal seas, and their success has been significantly enhanced where science-based biophysical guidelines have informed their design. Increasingly, as human pressure on marine ecosystems is expanding further offshore, governments are establishing offshore MPAs—some very large—or MPA networks. Globally, there are growing calls from scientists, non-government organisations, and national governments to set global conservation targets upwards of 30%. Given that most of the ocean is found either in the high seas or offshore within national Exclusive Economic Zones, large offshore MPAs or networks of MPAs must be a major component of these global targets for ocean protection. However, without adequate design, these offshore MPAs risk being placed to minimise conflict with economic interests, rather than to maximise biodiversity protection. This paper describes detailed biophysical guidelines that managers can use to design effective networks of no-take MPAs in offshore environments. We conducted a systematic review of existing biophysical design guidelines for networks of MPAs in coastal seas, and found consistent elements relating to size, shape, connectivity, timeframes, and representation of biophysical features. However, few of the guidelines are tailored to offshore environments, and few of the large offshore MPAs currently in place were designed systematically. We discuss how the common inshore design guidelines should be revised to be responsive to the characteristics of offshore ecosystems, including giving consideration of issues of scale, data availability, and uncertainty. We propose 10 biophysical guidelines that can be used to systematically design offshore networks of MPAs which will also contribute to the global goal of at least 30% protection globally. Finally, we offer three priority guidelines that reflect the unique conservation needs of offshore ecosystems: emphasising the need for larger MPAs; maximising the inclusion of special features that are known and mapped; and representing minimum percentages of habitats, or, where mapped, bioregions. Ultimately, MPA guidelines need to be embedded within an adaptive management framework, and have the flexibility to respond to emerging knowledge and new challenges.
Introduction
Our oceans are immensely valuable, both intrinsically and to our economies, societies, and cultures. However, human pressures are causing significant and, in some cases, catastrophic declines in marine species (Duarte et al., 2020). Marine protected areas (MPAs), especially no-take MPAs that prohibit extractive use (Sala and Giakoumi, 2017), are considered among the best tools available to protect marine species and habitats from exploitation and damage, and to conserve marine biodiversity (Graham et al., 2011; Costello, 2014; Roberts et al., 2019). Common biophysical goals of MPAs are to maintain or restore native species diversity, habitat diversity and heterogeneity, keystone species, connectivity, and important ecological processes (McCook et al., 2010; Green et al., 2013, 2014). Usually, achievement of these biophysical and ecological goals allows the consequent achievement of socio-economic and cultural objectives, including, for example, the protection or restoration of fisheries, food security, and cultural landscapes (Gilman et al., 2011).
Whilst MPAs and MPA networks have been broadly established in the world's coastal seas, the application of spatial protection to offshore environments is much newer (Ban et al., 2014a). For the purposes of this paper, offshore waters (also referred to as the open ocean or deep sea) are defined as all marine areas (benthic and pelagic) beyond the seaward edge of the geomorphic continental shelf, which is often at a depth of ~200 m. Where there is no continental shelf (e.g., oceanic islands and atolls), offshore waters are understood to be marine areas beyond the 80 m depth contour, which is a generally accepted depth limit of light-dependent habitat-building organisms (Bongaerts et al., 2011; Bridge et al., 2011; Althaus et al., 2017; Lesser et al., 2019; Beger et al., 2020). We use the word “offshore” as an umbrella term to encompass benthic, demersal, and pelagic habitats both within the exclusive economic zones (EEZs) of nations and in areas beyond national jurisdiction (ABNJ), as long as they are beyond marine areas that are under the jurisdiction of local communities (i.e., beyond the scope of community-managed marine areas), beyond the continental shelf break or deeper than 80 m around oceanic islands. The legislative, economic, and practical requirements of establishing MPA networks by individual States within their EEZs are different from those of the international community when protecting ABNJ (Merrie et al., 2014). However, whilst important, those considerations are beyond the scope of this paper. This paper focuses on biophysical design guidelines only. These guidelines are not intended to replace existing design principles applied in coastal seas (e.g., Green et al., 2014), and they will most likely be tempered by socio-economic and cultural considerations, national legislation and international agreements.
The open ocean contains a wide variety of ecosystems and species assemblages, from the pelagic habitats at the surface to the deepest realms of the seabed. The view that the deep sea is physically and biologically homogeneous has been dispelled (Herring, 2002; Benoit-Bird et al., 2016), and the deep sea is now known to host levels of biodiversity that rival those of shallow-water coral reefs (Van den Hove et al., 2007).
Far from being resilient, the open ocean and the deep sea are home to some of the most long-lived and vulnerable marine animals, habitats and ecosystems on earth (Verity et al., 2002; Glover and Smith, 2003; Roberts et al., 2019). The open ocean is under increasing pressure from human impacts, especially overfishing, bycatch of non-target species, destructive fishing methods, noise, pollution and litter from land (including plastic), shipping (including cruise shipping), derelict fishing gear, deep sea mining for non-renewable resources and climate change (Verity et al., 2002; Halpern et al., 2008; Ramirez-Llodra et al., 2011; UN, 2015; UN Environment, 2017; Harris, 2020).
As coastal fisheries become depleted and technological improvements allow fishing vessels to venture further offshore, pelagic fish stocks and deepwater seabeds are more at risk of overexploitation than ever (Baum et al., 2003). Numerous heavily exploited offshore species are now of conservation concern, including some tuna, billfish, and sharks (Ferretti et al., 2010; Collette et al., 2011). In the open ocean, overfishing affects not just targeted stocks but also by-catch species, community composition, habitats, trophic functioning, and ecological linkages, in both the horizontal and vertical dimensions (Roberts, 2002; Worm and Tittensor, 2011; Ortuño Crespo and Dunn, 2017). The relatively low productivity, weaker governance, and data deficiency of the open ocean make it difficult to determine what level of fishing activity targeting pelagic and deep-sea species is sustainable (Collette et al., 2011; Norse et al., 2012; Ortuño Crespo and Dunn, 2017; Palomares et al., 2020; Pauly et al., 2020). Furthermore, the two-way coupling between offshore benthic and pelagic systems means that impacts in the upper parts of the open ocean, which are more commonly fished, cascade through the entire vertical span of offshore assemblages (Grober-Dunsmore et al., 2008).
While there are large gaps in knowledge (Palumbi, 2004; Claudet et al., 2010; Dunne et al., 2014), increasing evidence shows that no-take offshore MPAs can offer effective protection against human exploitation and damage (Mills and Carlton, 1998; Koldewey et al., 2010; Davies et al., 2012). Large offshore MPAs and MPA networks can protect pelagic ecosystems along with deep-sea benthic and demersal ecosystems that are highly fragile and closely inter-linked (Norse, 2005; Davies et al., 2007; Williams et al., 2010b; Huvenne et al., 2016). In fact, recent research suggests that offshore no-take MPAs can not only promote the recovery of highly mobile species (e.g., tuna) and protect large swathes of habitat, but also enhance fish stocks and help to stabilise catches outside MPA boundaries (Boerder et al., 2017). There is an increasing body of scientific research devoted to understanding the offshore environment (e.g., Schmidt Ocean Institute, 2020); much of this research identifies the need to define design guidelines for offshore networks of MPAs to achieve conservation and other management goals (Leathwick et al., 2008; Ban et al., 2011; Berglund et al., 2012; Chaniotis et al., 2020).
Currently, 2.7% of the global ocean is fully and/or highly protected within no-take MPAs; the proportion of countries' EEZs under MPA protection is higher (5.7%) than ABNJ (<1%; Marine Conservation Institute, 2020). In recent years, partly due to increased knowledge, the number of large-scale offshore MPAs has grown (Lewis et al., 2017; Duarte et al., 2020), and, worldwide, there are now over 30 no-take MPAs larger than 150,000 km2. Existing very large (>150,000 km2) offshore MPAs were shown to encompass at least 10% of the range of 26.9% of all species assessed worldwide; the remaining 73.1% of species fall short of a target of 10% coverage within these MPAs (Davies et al., 2017). The failure to meet species conservation targets is thought to be because, so far, very large MPAs have been opportunistic and placed mostly in remote areas to avoid interfering with commercial interests, rather than systematically designed to adequately protect the full range of habitats and species found within a given area (Leenhardt et al., 2013; OSCA, 2016; Devillers et al., 2020). The need to design offshore MPA networks according to robust biophysical guidelines is clear (Ban et al., 2014b; Davies et al., 2017; Lewis et al., 2017; IUCN-WCPA, 2018).
In 2011, the Convention for Biological Diversity (CBD) formulated the Aichi targets, of which Target 11 states that “By 2020, at least 17 per cent of terrestrial and inland water, and 10 per cent of coastal and marine areas, especially areas of particular importance for biodiversity and ecosystem services, are conserved through effectively and equitably managed, ecologically representative and well-connected systems of protected areas and other effective area-based conservation measures, and integrated into the wider landscapes and seascapes.” (CBD, 2011). This target has been reiterated, in 2015, by all United Nation members in the Sustainable Development Goals (specifically SDG14; UNDP, 2021). In 2016, members of the International Union for the Conservation of Nature (IUCN) at the World Conservation Congress approved new global target for MPAs, calling for 30% of each marine habitat to be set aside in highly protected MPAs and other effective area-based conservation measures by 2030 (IUCN, 2016a). Since then, this call has been echoed by various scientists, non-government organisations and national governments, including the UK Government who recently celebrated over 40 countries joining the UK-led “30 by 30” Global Ocean Alliance Initiative (UK Government, 2021), an international commitment to protect at least 30% of the global ocean in MPAs by 2030, through the UN Convention on Biodiversity in 2021 (O'Leary et al., 2019). In parallel, United Nations representatives are in the process of negotiating a treaty that would, among other things, create a mechanism to establish marine protected areas on the high seas (Gjerde, 2007). This mechanism includes an increasing expectation that global targets of 30% must be met in order to safeguard biodiversity, avoid fishery collapse and build ocean resistance to climate change (Partridge, 2009; O'Leary et al., 2019; Visalli et al., 2020). With most of the ocean found either in the high seas or offshore within national Exclusive Economic Zones, large offshore MPAs or networks of MPAs are integral parts of reaching global targets for ocean protection.
Generally, establishing an MPA or a network of MPAs consists of a series of steps that include defining objectives, planning, design, consultation, declaration, and management (Kelleher and Kenchington, 1992). This paper focuses upon the “design” step in the context of the global objectives referenced above. We describe detailed biophysical guidelines that managers can use to design effective networks of no-take MPAs in offshore waters. The systematic review that led to the definition of these guidelines sought to answer the following questions:
(1) What are the existing design guidelines for no-take MPA networks, largely applied to shallow coastal ecosystems?
(2) How do the differences between coastal and offshore ecosystems and species inform tailoring of those guidelines to offshore environments?
Materials and Methods
Existing literature that contained design principles or guidelines for MPAs were collated using online search engines (Web of Science Core Collection, Scopus), Google Scholar and the internal search functions of conservation organisation websites. The search term (“marine protected area*” OR “marine reserve*” OR “no-take”) AND (guideline* OR principle* OR criteria) was initially tested on 10 key documents (5 peer-reviewed and 5 “grey literature” reports), to ensure it was capable of detecting the relevant literature. Equal weight was given to grey literature in the literature search, in recognition that MPA principles or guidelines often appear in documents designed for use by management agencies, rather than for academic purposes. All results were uploaded to the online software Cadima (www.cadima.info), through which we specified the research question in terms of Population/Outcome, and which automatically detects duplicates and assists with screening and data extraction (O'Leary et al., 2016a). After the initial literature search and duplicate exclusion, the resulting 795 documents were screened for relevance first by title, then by abstract and lastly by full-text articles, resulting, initially, in 264 articles included for data extraction (Table 1, Supplementary Information 1). Of these, 177 documents contained information about design principles or guidelines for MPA design. The data extracted from these documents included the author(s), year, title, geographic location, specific MPAs, individual guidelines or principles, relevance to networks of MPAs (as opposed to individual MPAs) and relevance to offshore environments.
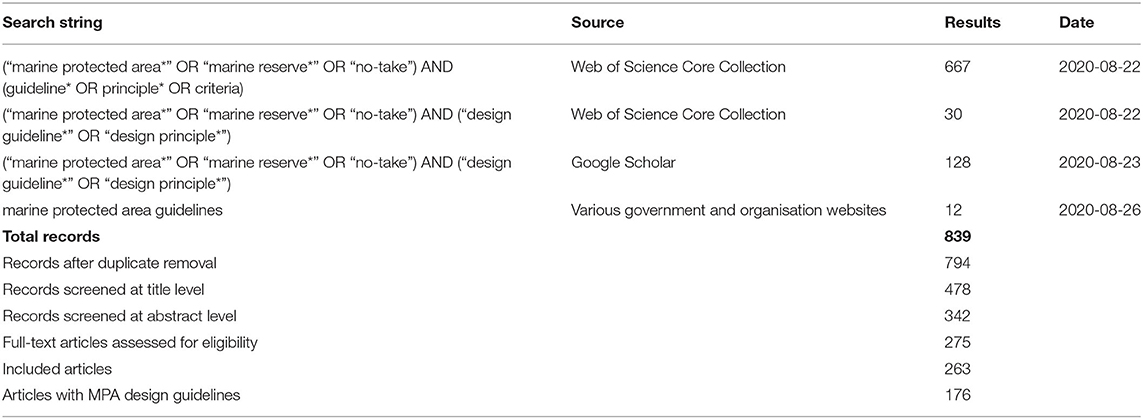
Table 1. Systematic review results of literature search per search string and database (see also Supplementary Information 1).
Each guideline or principle relevant to MPA network design was then assessed as to its applicability to an open ocean context and the guidelines tailored accordingly to be offshore-specific.
MPA Design Guidelines – Results of the Systematic Review
One hundred and seventy seven articles, published between 1992 and 2020, contained information about MPA design (Table 1). Of the 177 articles:
• 52 included a clear list of guidelines or principles, although 28 were more general in nature and did not provide explicit recommendations on, for example, the magnitude or percentage (of habitats, bioregions, etc.) to include in the MPA design;
• 23 included quantitative guidelines such as sizes, distances, and/or % protection targets;
• 49 were about MPAs in general and discussed or presented guidelines in a theoretical sense. The other 128 documents were about specific regions of the world, or specific MPAs;
• 129 (73%) were relevant to networks, rather than just individual MPAs;
• 89 (50%) had some direct relevance to offshore environments;
• 53 (30%) only considered one guideline or principle; adding those papers that considered only two principles brought the number to 77 (44%);
• the most commonly cited guidelines were representation of habitats (103 documents, 58%), connectivity (91 documents, 51%), and size (70 documents, 40%);
• Of the 52 studies (of the 177) that had a list of guidelines/principles, 25 possible guidelines or principles were presented in various combinations. Not all were biophysical, and this paper does not consider these non-biophysical guidelines further.
• 16 of the 23 papers that provided quantitative design guidelines referred to overarching % targets for no-take protection without specific targets for particular attributes of the environment (e.g., habitats, bioregions). Most others either referred to the Convention on Biological Diversity (CBD) 10% target (Arcos et al., 2012; Balbar and Metaxas, 2019), or used MARXAN (a conservation planning software tool to guide systematic MPA design) or a similar tool to explore ways in which to target a range of area percentages for conservation (e.g., Proudfoot et al., 2020). We note that papers referring to overarching percentage MPA targets recommend a range from 10 to 50% (e.g., Thomas and Shears, 2013; Dunn et al., 2018) and that, largely, the per habitat- or per bioregion-specific targets mentioned in other work, if implemented, would sum to these overarching targets.
Tailoring the Guidelines to Offshore Ecosystems
The applicability of guidelines found throughout the literature to offshore waters is detailed in the sections below. Guidelines are listed in order of priority (Table 2).
Offshore Guideline 1: Make MPAs Larger
Size is one of the most important design considerations when implementing MPAs, especially in data-poor areas (Halpern, 2003; Gilman et al., 2011). In coastal seas, the representation of habitats and/or bioregions tends to be prioritised over size. However, the ethos of “bigger is better” is one of the five characteristics that has led to the greatest realised benefits of no-take MPAs globally (Edgar et al., 2014), and often incidentally enhances connectivity (Álvarez-Romero et al., 2018; see Guideline 5). Very small, permanent, no-take MPAs can be effective in coastal seas, and especially when designed for the replenishment of fisheries target species through “spillover” (Russ, 2002; Jones et al., 2007; Fernandes et al., 2012; Harrison et al., 2012). However, larger areas can hold larger parts of (or entire) populations, and have a greater chance of including unknown habitats and species, bioregions, or special features, and tend to have a degree of biological integrity. Larger areas are more likely to be self-sustaining and therefore will persist over time (Gaines et al., 2010). Larger MPAs also reduce the edge effect, where human activities at the edges of an MPA, including illegal entry and take within MPA boundaries, can be intensive enough to undermine the MPA's overall effectiveness (Lester et al., 2009). The size of an MPA needs to be determined according to the extent and location of the species, features, bioregions, and ecological processes it is intended to protect (Green et al., 2014). Recent research has provided design guidelines for no-take MPAs based on known home ranges or distributions of shallow-water species of interest (Green et al., 2014). For instance, a no-take MPA designed to protect coral reef invertebrates and site-attached fishes could be as small as 400 to 1,000 m across, while an MPA of more than 20 km would be required for offshore pelagic species such as silvertip sharks (Carcharhinus albimarginatus) or trevallies (Carangidae; Jones et al., 2007; Green et al., 2014).
Coastal assemblages and sedentary oceanic species can benefit from smaller MPAs, but larger, more mobile and migratory species (as more often found offshore) require larger MPAs. In offshore environments, there is less information about habitats and bioregions. Additionally, habitats tend to be larger (e.g., deep-sea plains and plateaux compared to shallow reef systems) and many offshore species have greater home ranges and larval dispersal patterns (Herring, 2002). The larger information gaps and scale of habitats means that size becomes even more important for habitats protected within MPAs to have sufficient integrity (Shanks, 2009; UN, 2015; Lewis et al., 2017; Weeks et al., 2017). With ongoing and escalating discoveries of important new species in offshore environments, larger MPAs also provide greater insurance with regard to protecting that which remains to be discovered (Bridge et al., 2016). Huvenne et al. (2016) found that a deep-water (~1,000 m) no-take MPA of at least 30–40 km in diameter adequately protected deep-water coral communities, but where these corals were damaged, even these protected areas could not mediate recovery. Roberts et al. (2010) suggested that in English EEZ continental shelf waters beyond 12 nm, MPAs that are intended to protect commercial species should be at least 30 to 60 km in their minimum dimension. MPAs of >100–1000,000 km2 have been recommended for the protection of large sharks and rays whose home ranges extend beyond coastal areas (Rigby et al., 2019). In offshore pelagic and benthic habitats, the distributions of many soft-sediment (e.g., bivalves, elasipod holothurians) and pelagic taxa (e.g., tuna, lanternfishes) cover entire ocean basins, and many species are widely dispersed (McClain and Hardy, 2010; Reygondeau et al., 2012), even species with a sedentary adult phase and restricted habitat preferences, such as the mussel Bathymodiolus thermophilus at hydrothermal vents (Maas et al., 1999). Another benefit of larger MPAs is that in protecting the range, or part thereof, of a migratory or highly mobile species, they automatically also protect a large array of other species and features (Wilhelm et al., 2014).
The movement distance of marine organisms poses one of the greatest challenges to MPA design. The dispersive larval stage and sometimes far-ranging movements or migrations of juveniles or adults, differences in larval duration and metapopulation dynamics mean that it is highly unlikely for individual offshore MPAs to protect all life history stages of any one species, let alone all species (Gruss et al., 2011). In offshore environments, and especially in the deep sea, the difficulty of capturing species' ranges is compounded by the almost complete lack of data on larval duration and behaviour traits (Hilário et al., 2015). The dispersal of deep-sea organisms presents the added complexity of vertical swimming behaviour (Afonso et al., 2014), which can influence modelled dispersal distances by up to an order of magnitude (Gary et al., 2020; see also Guideline 5). If it is impossible to contain a species' entire range within one MPA, MPA networks that comply with connectivity guidelines (Guideline 5), replication (Guideline 7) and minimum percentage guidelines (Guidelines 3 and 4) can be combined to protect as many of the species' critical areas as possible, thereby achieving the best possible outcome for a species or population.
The potential mobility of species may conflict with their tendency for residency within a geographic location; many highly mobile species with the ability to travel 100s or 1000s of kms have smaller home ranges (10s of kms) once they settle. A tagging study of several pelagic species (tuna, billfishes, sharks) showed that most of them remained within the boundaries of the 450 km-radius British Indian Ocean Territory MPA (Carlisle et al., 2019). The evolutionary selection for behavioural polymorphism (Kaplan et al., 2014) is highlighted in the work of Mee et al. (2017). This genetic modelling research has shown an evolution of increased residency for highly mobile tuna species after the establishment of MPAs, as individuals that choose more sedentary behaviour pass on their genes to successive generations more frequently than those that move beyond MPA boundaries into fishing grounds (Mee et al., 2017). The model remains to be tested, but in a practical sense, this means that the benefits of offshore MPAs will grow over time, including over generations of the target species of interest.
Where documents provided MPA design guidelines with minimum size recommendations, these were highly variable, both for coastal and offshore environments (Table 3). In Edgar et al. (2014), the largest benefits were found in MPAs that were at least 100 km2. In coastal areas, the most common minimum size, and also the upper limit, was 20 km in diameter; no minimum size was found for offshore MPAs, except in Dunn et al. (2018), where the authors recommended 200 km. In practise, almost all existing offshore MPAs are larger than 2,500 km2 (Marine Conservation Institute, 2020), suggesting that a minimum diameter of 50 km is feasible.
In summary, the existing literature recommends that offshore MPAs (as part of a network of MPAs) be 50–200 km in minimum diameter.
Offshore Guideline 2: Include Special, Unique, Rare Features and/or Species
Sites may be selected for inclusion within an MPA according to criteria such as uniqueness, rarity, or special characteristics. These attributes include areas that are important for particular life stages of species, the presence of threatened, endangered or declining species or habitats, keystone species, distinctive habitat types, oceanographic or geological features, or places of especially high biological productivity or diversity (Salomon et al., 2006; Brock et al., 2012; Clark et al., 2014; Secretariat of the Convention on Biological Diversity, 2014; Table 4). For example, a site may be unique because there is a single population of an endemic species not found anywhere else. Special characteristics can be attributed to sites where key processes take place (e.g., spawning and feeding grounds, nurseries, migratory corridors, hotspots, etc.; Rigby et al., 2019). Sites can also be selected on the basis of hosting higher productivity than the surrounding areas; these “hotspots” can support high biodiversity, which is often also used as a criterion for selecting sites for inclusion into MPAs or MPA networks (Possingham and Wilson, 2005; Sydeman et al., 2006; Briscoe et al., 2016). Areas that host a large variety of species are important for the maintenance of resilience, evolutionary potential and ecosystem services (Worm et al., 2006).
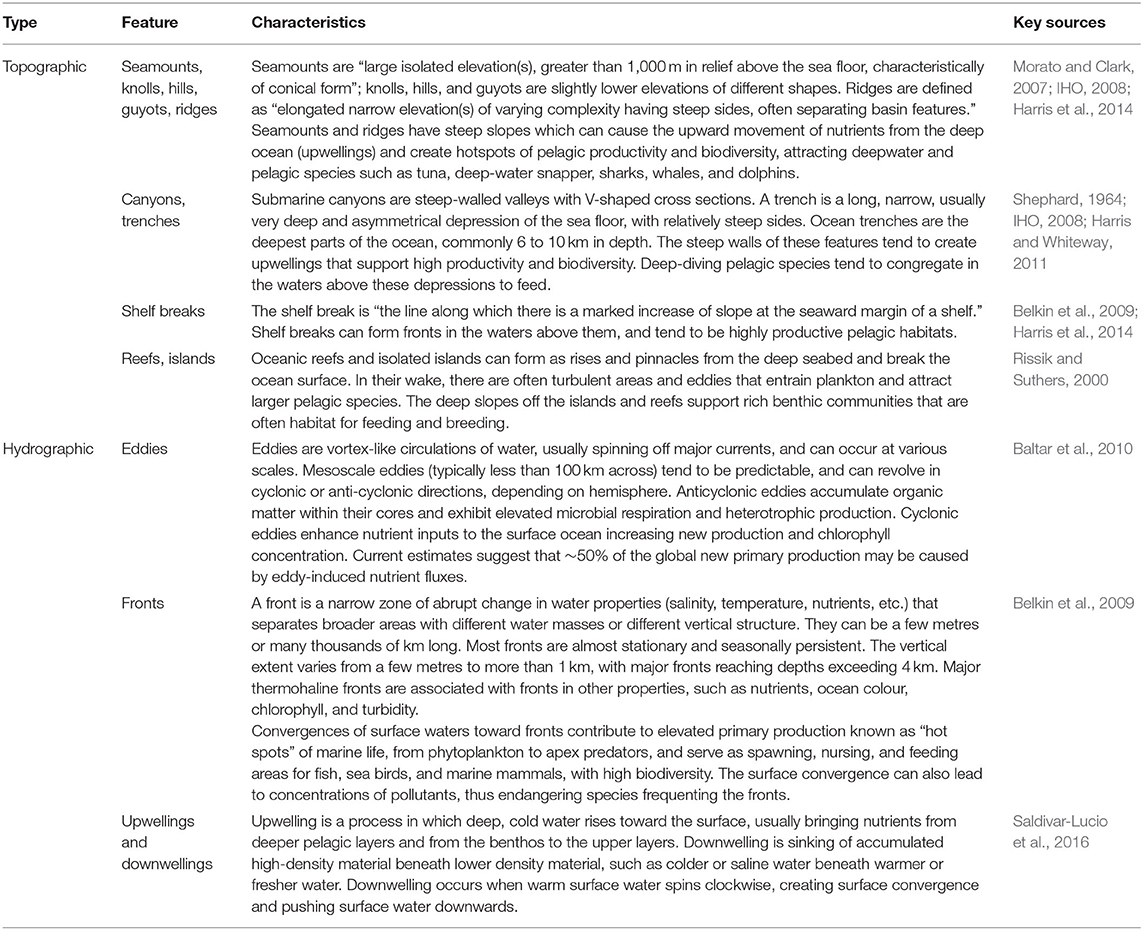
Table 4. Some potential topographically or hydrographically unique, special or rare features of the open ocean.
The inclusion of critical habitats and special or unique areas as a design guideline for MPAs stems from the biophysical operational principles for the Great Barrier Reef Marine Park Authority (2002) and the Convention for the Protection of the Marine Environment of the North-East Atlantic (OSPAR Convention) List (OSPAR Commission, 2008), and was included to ensure that special, unique areas were protected even if they were not captured by the protection of percentages of habitats or bioregions (see Guidelines 3 and 4; Great Barrier Reef Marine Park Authority, 2002). It has since been adopted throughout the literature for designing coastal MPAs (Fernandes et al., 2009; Green et al., 2014; Table 3); seven of the 23 documents (30.4%) that listed MPA design guidelines included this principle. Currently, it is listed as one of the steps for marine spatial planning processes adopted by multiple Pacific Island countries (Ceccarelli et al., 2018a).
Criteria for selecting Ecologically and Biologically Significant Areas (EBSAs) in offshore environments have already been developed for some regions, such as the Azores and the Southwest Pacific (CBD, 2009, 2014; Clark et al., 2014). Other initiatives that have established criteria for protecting marine environments specifically in offshore areas include the FAO's Vulnerable Marine Ecosystems, which seeks to identify and protect marine areas in the high seas that are vulnerable to deep-sea fisheries (FAO, 2019), and the IMO's Particularly Sensitive Sea Areas, which seeks to identify ecologically, socioeconomically or scientifically valuable areas vulnerable to damage by international shipping activities (IMO, 2006). Some countries have also decided to describe special, unique marine areas within their national boundaries using systematic criteria for their identification and definition (Ceccarelli et al., 2018d). The protection of special features was the first principle used in the creation of the UK's offshore MPA network (Chaniotis et al., 2020).
Prioritising special features provides some insurance against the declaration of very large offshore MPAs in areas that are of little value to commercial interests (Devillers et al., 2020). In fact, to safeguard against the declaration of large MPAs in areas of little value, this guideline could potentially be adopted first—the scale of many offshore features and migratory or mobile species ranges makes it highly likely that MPAs designed around Guideline 2 would automatically also be large. In offshore waters, uniqueness, rarity or special characteristics typically include current systems and fronts, upwellings, seamounts, trenches, deepwater coral or sponge assemblages, hydrothermal vents and fluid seeps (Hyrenbach et al., 2000; Graham et al., 2011; Hooker et al., 2011; Ban et al., 2014b; Lundquist et al., 2017; Barrie et al., 2020; Table 4). The Darwin Mounds in UK offshore waters, for example, is an area rich in the deep-water coral Lophelia pertusa which, once discovered, was deemed of special importance and protected from trawling, becoming the UK's first offshore MPA (De Santo and Jones, 2007). These features are usually unique to a certain area and isolated from other similar features or populations by sheer distance. The value of unique and/or special features or areas stems from the fact that they are not usually replicated elsewhere and therefore not replaceable (Salomon et al., 2006), and they contribute disproportionately to marine biodiversity and ecosystem function (Lundquist et al., 2017). Their loss results in a reduction in overall biodiversity or abundance of important species (Halpern et al., 2007; Palumbi et al., 2008). For special and/or unique sites or features that may be subject to particular stressors, it is important to understand the spatial distribution of potential stressors or impacts (Halpern et al., 2007; Brock et al., 2012). Any destructive activities taking place within the area should be prohibited (see also Guideline 10). The larger the spatial scale at which special or unique features typically occur, the greater the effect of their loss.
Unique or special species and populations in the open ocean have life histories and adaptations specific to the pelagic or deep benthic habitats they inhabit. Some large pelagic species may range very widely, while deep-dwelling species may have populations that are endemic or genetically disjointed due to the distance between suitable benthic habitats (e.g., seamounts or hydrothermal vents separated by large expanses of seafloor) (Richer de Forges et al., 2000). Despite the wide-ranging nature of many individuals within populations, large pelagic species of conservation interest regularly use particular sites and migration corridors that can be mapped, monitored, or predicted (Ceccarelli et al., 2017, 2018c,d).
Geomorphic features that are known to aggregate life could all be seen as special; mid-ocean ridges, seamounts, and submarine canyons cover only four percent of the seafloor, making them rare biodiversity hotspots within the vast extent of abyssal plains, hills, plateaus, basins, terraces, troughs, valleys, escarpments, and sedimented slopes that, according to current knowledge, tend to be more sparsely populated (Glover and Smith, 2003; Table 5). Many of these features are considered individual habitats or habitat types, and may be seen as covered by Guideline 3 (representation of habitats), which is useful when very little or nothing is known about a particular feature or habitat. For example, if a series of ridges are known to exist within an offshore area, with little or no information about their particular attributes, they would be protected under Guideline 3.
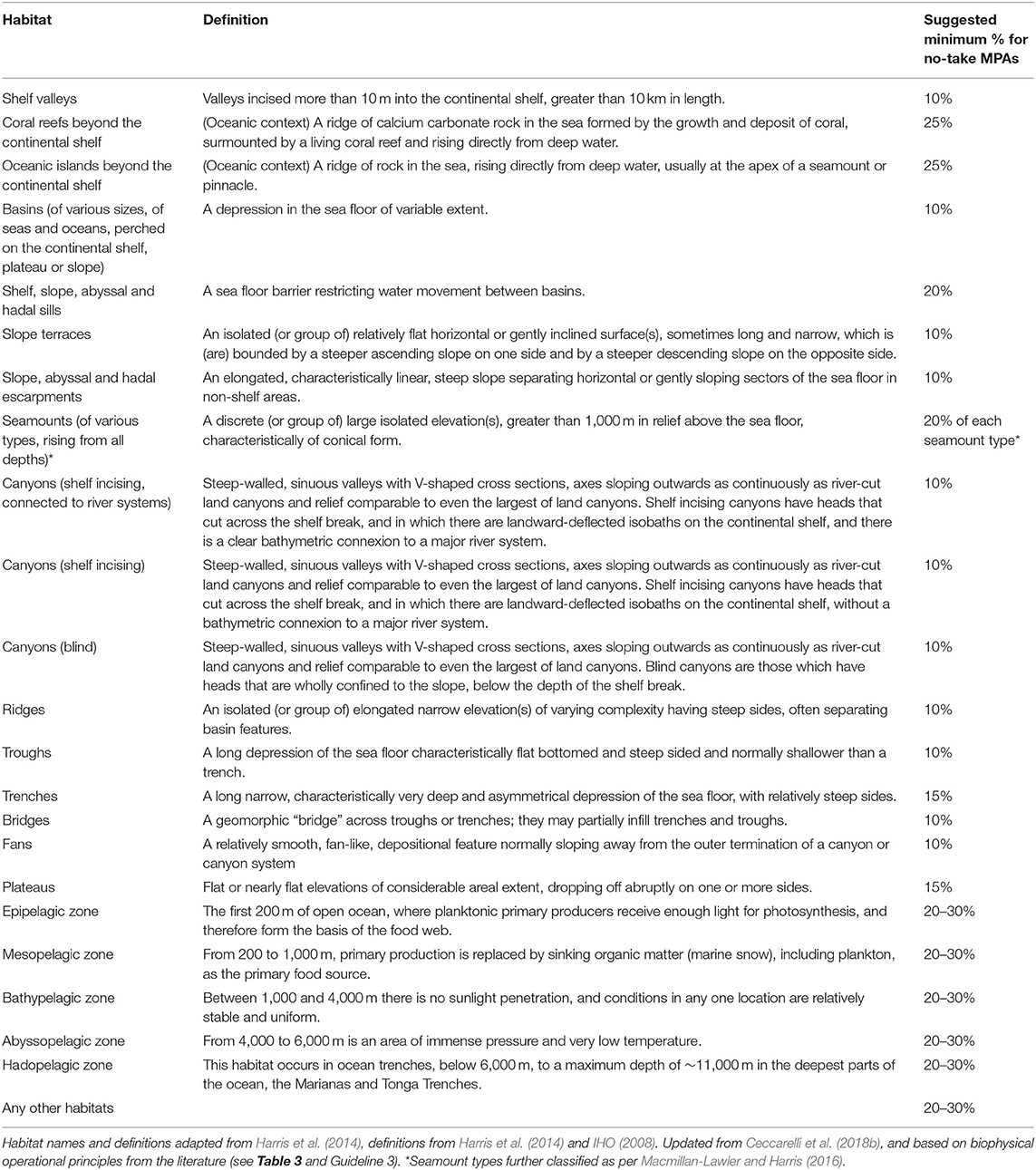
Table 5. Major habitats of open ocean environments and suggested minimum proportions for inclusion in no-take MPAs if Guideline 4 applies (representation of bioregions).
In sum, where knowledge exists about areas that contain special, unique, rare features and/or species in offshore environments, these areas should be included, in their entirety, in the MPA network (see Table 4).
Offshore Guideline 3: Representation of Habitats
Any network of no-take MPAs, inshore or offshore, should include representation of every known habitat type and bioregion (see Guideline 4) to ensure that as many species as possible are protected (Gilman et al., 2011; Day et al., 2012; Fernandes et al., 2012). For habitats, adequate representation requires that they are mapped and that habitat-specific “minimum amounts” of protection can be defined (see, for example, Great Barrier Reef Marine Park Authority, 2002). The concept of “representing” habitats (as opposed to bioregions or other ways of classifying the environment—see Guideline 4) is the most common specific principle or guideline in the MPA design literature, appearing in 44 of 52 papers (88%). Per-habitat protection percentage levels are often suggested to be 10–30% for coastal seas, and higher in the following areas: (1) areas with less existing management of activities outside the no-take MPA; (2) areas with more destructive activities; or (3) areas where marine bioregions are not defined (see Guideline 4).
Most documents that had percentage targets for coastal habitat protection agreed that including 20–30% of each habitat in no-take MPAs would be sufficient for biodiversity conservation and the protection of fisheries stocks (Table 3). Support for the 20–30% target was originally gleaned from reproductive theory, knowledge about the vulnerability of coral reef species to exploitation, analysis of fishery failures, empirical, and modelling studies of reserves and the precautionary principle (Bohnsack et al., 2000). These targets were then used and sometimes modified (down to 10% or up to 50%) for designing MPA networks in the Great Barrier Reef Marine Park (Fernandes et al., 2009), the California Channel Islands (Airamé et al., 2003), Honduras (Chollett, 2017), across the Mesoamerican Reef (Green et al., 2017), and in the Coral Triangle (Fernandes et al., 2012).
Global adherence to Guidelines 3 and 4 in the context of MPA design has been assessed by Fischer et al. (2019), who found that only 18 of 66 Large Marine Ecosystems (LMEs) contained greater than 10% of the marine geomorphic features and benthic habitats (listed in Table 5) within existing MPAs; hence MPAs in 48 out of 66 LMEs do not comply with the guidelines, even using this 10% requirement, which is at the lower end of the representation range. The OSPAR Convention Guidelines, used to designate deep-water MPAs in the UK's EEZ, include the principle of representation of habitats, and this led to the protection of representative examples of seamounts, canyons, deep-water coral mounds, and other features (Chaniotis et al., 2020). Representation of habitats was also one of three principles (along with comprehensiveness and adequacy) used for the designation of offshore MPAs in the Australian EEZ under the National Representative System of Marine Protected Areas Program (ANZECC, 1996; Commonwealth of Australia, 2003). There is therefore a strong precedent for the use of this guideline in offshore environments (see also Table 3).
Offshore environments have a multitude of static, recurring and ephemeral habitats, both benthic and pelagic, that can be mapped and used for spatial planning (Hyrenbach et al., 2000; Roberts et al., 2003; Belkin et al., 2009; Harris et al., 2014; Miller and Christodoulou, 2014). These habitats occur at a variety of scales and harbour different levels of diversity; for example, expanses of relatively homogeneous and low diversity basins or plains are very different from much smaller features, such as seamounts, which may nevertheless host higher concentrations of life. To a large extent, we still lack the knowledge to differentiate similar-looking open ocean habitats from one another. But we do know, for example, that not all seamounts are equally productive and diverse (Samadi et al., 2006). Identifying the location and mapping the extent of offshore habitats still largely relies on proxies; habitats may be identified by analysing the foraging distribution of higher predators (Hyrenbach et al., 2000; Patterson et al., 2016; Hobday et al., 2017; Queiroz et al., 2017), by making use of sophisticated real-time satellite imagery (Game et al., 2009), by using maps of seabed geomorphology (Harris and Baker, 2012; Harris et al., 2014; Beaman et al., 2016), oceanographic attributes or some combination of the above and other methods. In the context of spatial planning, lessons learned from general design guidelines are more difficult to apply to offshore waters, given the biophysically dynamic nature of pelagic seascapes (Kavanaugh et al., 2016); static geological habitats are more straightforward for MPA design (Table 5).
Given the relatively data-poor status of most offshore habitats, and because marine bioregions are not usually defined at a useful scale, (and therefore Guideline 4, below, cannot be applied), research suggests that representing 30–40% of each habitat in offshore no-take MPAs enhances the likelihood of capturing unknown, and therefore unmapped, within-habitat variability, and even unknown features (O'Leary et al., 2016b; Table 3). Where there is some knowledge about offshore marine bioregions at a scale useful within countries' EEZs and in ABNJ, Guideline 4 could be applied first, and subsequently 10–30% of each offshore habitat can additionally be represented in no-take MPAs as per Table 5.
In sum, the literature recommends that (a) where bioregions are not defined, 30% of each habitat should be included in no-take MPAs; and (b) where bioregions are defined, ensure that 10–30% of each offshore habitat is represented in no-take MPAs.
Offshore Guideline 4: Representation of Bioregions
Using surrogates for patterns of biodiversity during spatial planning allows for MPAs to capture close to 100% of the diversity of marine life within a given area, despite imperfect knowledge, and while requiring much less than 100% coverage of the geographic area (Foley et al., 2010; Bridge et al., 2016). Bioregions are commonly used surrogates that define areas with relatively similar assemblages of biological and physical characteristics, without requiring complete data on all species, habitats and processes (Spalding et al., 2007; Costello et al., 2017). Protecting an adequate proportion of bioregions within no-take MPAs helps to manage for the uncertainty associated with habitat and species distributions, and thus reduces the risk of overexploitation of marine populations in areas that remain open to extraction (Botsford et al., 2003; Gaines et al., 2010; Wilson et al., 2011; Day et al., 2012; Fernandes et al., 2012; Green et al., 2013; Ballantine, 2014).
Of the 52 studies that listed MPA design guidelines or principles, 15 (29%) included the representation of bioregions, indicating that habitats (included in 88% of studies) are more commonly understood than bioregions, even in coastal seas. Among studies that provided numeric guidance, only five included proportions of bioregions, and only in coastal areas, whilst 22 gave percentages of habitats to be included in no-take MPAs (Table 3). In coastal ecosystems, the best available evidence advises that at least 20–40% of each bioregion should be included in no-take MPAs or MPA networks to ensure that representative examples of marine biodiversity are captured (see also Guideline 3; Table 3). The percentage of each bioregion to be included in MPAs should be increased in areas experiencing less management generally (e.g., poor or absent fisheries management), or subject to more destructive activities.
The logic pertaining to bioregion guidelines is equally applicable to offshore environments. Management of the ocean from a biodiversity protection point of view is usually undertaken within the EEZ of individual countries, but most current bioregionalisations span many countries and are too coarse to undertake planning at a national level (UNESCO, 2009; Clark et al., 2011; O'Hara et al., 2011; Reygondeau et al., 2012; Watling et al., 2013; Proud et al., 2017; Sayre et al., 2017; Sutton et al., 2017). Finer-scale marine bioregions need to be described to support national planning processes (Etnoyer et al., 2004; Reygondeau et al., 2012; Mannocci et al., 2015; Proud et al., 2017). Recently, offshore marine bioregions have been defined at an appropriate scale in some parts of the global ocean; that is, they are described at a scale useful to the area being managed. For example, multiple offshore marine bioregions have been rigorously described within and beyond national jurisdictions within Southwestern Pacific Island countries (Wendt et al., 2018; Beger et al., 2020), Canada (e.g., Arafeh-Dalmau et al., 2017), and Australia (Fernandes et al., 2005; Department of the Environment and Heritage, 2006). Delineating bioregions at an appropriate scale allows for their use in ensuring representation of the range of offshore biodiversity in national-scale MPA design. Guideline 4 therefore applies only to jurisdictions or ABNJ where marine bioregions have been described at such an appropriate scale (Gilman et al., 2011); for other jurisdictions or ABNJ, see Guideline 3.
In sum, the literature recommends (a) the protection of 20–40% of each bioregion within no-take MPAs, or (b) where areas outside the MPA are subject to destructive activities or a lack of management, the percentage should increase.
Offshore Guideline 5: Space MPAs for Maximum Connectivity
Connectivity within a network of MPAs is important because it ensures that if a population vanishes or a habitat is damaged in one MPA, it can be restored through the movement of larvae or adults from another MPA, or an undamaged habitat upstream (Jones et al., 2007; Hilário et al., 2015). Genetic connectivity (genetic exchange among individuals within and between populations) depends on the absolute number of dispersers among populations, whereas demographic connectivity (exchange of individuals between spatially separate populations) depends on the relative contributions to population growth rates of dispersal vs. local recruitment (i.e., survival and reproduction of residents) (Lowe and Allendorf, 2010). Demographic connectivity, which influences recruitment levels, occurs over smaller scales than genetic connectivity. From a genetic standpoint, connectivity ensures genetic diversity within populations, which in turn ensures population persistence and evolutionary potential (Jones et al., 2007).
Connectivity and spacing of MPAs in a network are included in 28 (54%) of the 52 studies that explicitly discuss MPA design guidelines or principles. In a functioning marine ecosystem, populations or patches of similar habitat that are geographically separate are linked through the movement of organic and inorganic matter, nutrients, energy, larvae, juveniles and adults (Cowen et al., 2007; Brock et al., 2012; Worboys et al., 2016; Hillman et al., 2018). Larval connectivity within an MPA network can occur between MPAs that are from 1 to 200 km apart (Table 3), depending on the species, with inshore species generally connected over smaller scales than offshore species (Jones et al., 2007; Shanks, 2009; Gilman et al., 2011; Harrison et al., 2012; Green et al., 2014). Larval connectivity research on coastal coral reef fishes suggests that dispersal is a declining function with distance, with many larvae settling in or close to their natal reefs, and fewer travelling 10s to even 100s of kilometres away (Harrison et al., 2012; Almany et al., 2013, 2017; Williamson et al., 2016; Abesamis et al., 2017; Bode et al., 2019). On the Great Barrier Reef, reserves are commonly less than 15km apart, which is clearly well within the dispersal range for most coral reef organisms (Almany et al., 2009). A wide range of reserve spacings have been recommended, including <100 km apart (Sala et al., 2002), < 20 km (Shanks et al., 2003), 10–200 km (Palumbi, 2004), 40–80 km (Roberts et al., 2010), 1–50 km (Jones et al., 2009), 1–15 km (Green et al., 2014), and 50–200 km (Munguia-Vega et al., 2018). The accumulating empirical research suggests that connectivity levels in MPA networks will be robust to variation in reserve spacings in the ranges advocated, largely because most species appear to have a long tail to their dispersal kernels (Jones et al., 2007). While there is also clear evidence that some marine larvae disperse distances in excess of 1,000 km (Manel et al., 2019), it is questionable whether reserves spaced this distance apart would offer any demographically significant connectivity.
In offshore waters, larger distances between populations or habitats make connectivity more diffuse, but fewer barriers to dispersal means that some populations are more widely distributed than inshore (Maas et al., 1999). Migratory and wider-ranging species have populations that are connected over small scales as well as over 100s, and sometimes 1,000s of kilometres (Lam et al., 2016). It has been shown that designing MPAs with a focus on connectivity, rather than just for species or habitats on their own, is especially important and has a greater chance of success in pelagic ecosystems (Moffitt et al., 2011). The scales of dispersal and connectivity for MPA design in the deep sea are larger than those in shallow water, as suitable habitats tend to be more isolated (Baco et al., 2016). As for inshore and nearshore MPAs, offshore MPAs are likely to benefit from placement that takes into account adjacent inshore or nearshore MPAs, or areas with existing protection, such as areas in which tuna fishing or the killing of sharks is already banned (Jones et al., 2007). Furthermore, in offshore waters vertical connectivity is as important as horizontal connectivity, and occurs through the downward drift of organic matter (marine snow), deep-diving ocean predators, and the vertical migration of deep-dwelling species that move toward the surface to feed at night (Sutton, 2013; Afonso et al., 2014). MPA design needs to take into account potential connectivity pathways along benthic and demersal depth gradients (Papastamatiou et al., 2015). When designing MPAs in offshore waters, it may be necessary to include MPAs that serve as “stepping stones,” that play key roles in dispersal or migration, by providing resting or feeding points (e.g., the staging areas known in bird migrations). These may be otherwise unremarkable habitats, but crucial to the persistence of species of interest.
Movement occurs either passively with currents or actively, through active dispersal, movement and migration. Within networks of MPAs, movement ideally occurs between protected areas (Roberts et al., 2010), and also between protected and unprotected areas (Gaines et al., 2010). A study of larval dispersal across a number of different habitat types found that species in soft-bottom subtidal habitats have the greatest potential for extensive larval dispersal (Grantham et al., 2003). However, pelagic larval duration has been estimated for only 93 taxa that reside in depths over 200 m; deep-dwelling taxa have a range of larval durations from 2 to over 200 days (Hilário et al., 2015). The lack of knowledge about larval traits such as swimming ability (both horizontal and vertical) and larval duration is a serious impediment to predicting connectivity in offshore species (Gary et al., 2020).
Dispersal in deep-sea larvae has the added complexity of vertical swimming ability, which has a strong influence on horizontal dispersal because of the vertical layering of different currents (Gary et al., 2020). Dispersal strategies are also important; deep-sea sessile organisms such as corals can have either a dispersive larval stage or reproduce asexually, resulting in either highly connected or isolated populations, similarly to coastal species (Miller and Gunasekera, 2017; Strömberg and Larsson, 2017). However, in the deep sea isolated habitats, such as hydrothermal vents or deep-sea biogenic mussel reefs, are much more widely dispersed than inshore habitats, and are often largely self-seeding (Elsäßer et al., 2013). These discrete habitats can be captured through the application of Guidelines 2 and 3, and MPAs can therefore be sized to allow for self-replenishment and spaced at variable distances to allow for significant levels of connectivity. Greater benefits to the broader marine ecosystem are expected from MPAs that are self-replenishing, interconnected and/or important source areas for larvae (Krueck et al., 2017; Ross et al., 2017). The movement of larvae, juveniles and adults across MPA boundaries can be seen as negative because it implies a lower level of protection for individuals that move into areas where they can be exploited (e.g., Gruss et al., 2011). However, this “spillover” restores populations and target species and can therefore benefit fisheries and the broader ecosystem alike (Gell and Roberts, 2002; Harrison et al., 2012; Kerwath et al., 2013).
The maximum spacing recommended between MPAs in coastal networks is 200 km, with a wide range of distances depending on the geographic characteristics (Table 3). For offshore MPAs or in documents that included both coastal and offshore environments, the most common spacing recommendations were 20–200 or 50–100 km (Thomas and Shears, 2013; Lundquist et al., 2015; Burt et al., 2018). Based on these existing offshore guidelines and the current understanding of offshore connectivity, this guideline adopts the entire range (20–200 km) of existing spacing recommendations.
In summary, the existing literature recommends that offshore MPA spacing should be in the range of 20–200 km. This distance adequately encompasses the known range of dispersal distances for offshore marine species (Green et al., 2014) and acknowledges that network designs should be robust to a wide range of reserve spacings.
Offshore Guideline 6: Represent Whole Features
Some habitat areas and features (e.g., seamounts, submarine canyons, etc.) tend to function as complete entities and have a level of ecological integrity. The functioning of a habitat or feature depends on linked processes that may occur in different areas (e.g., the seamount summit vs. the slope), but are connected across the entire habitat or feature. It is therefore important to represent entire habitats or features within the same level of protection and avoid “split zoning” (Day et al., 2012; Fernandes et al., 2012; Rigby et al., 2019; Lecours et al., 2020). The concept of split zoning is not often encountered in the coastal MPA literature, as it is likely to reduce the ecological integrity of an MPA and lead to problems of public understanding and compliance (Day, 2002).
Representing whole features is equally important in coastal and offshore ecosystems. Using a seamount example, primary production and nutrient cycling that occur near the surface produce food which is then distributed to deeper areas; organisms from deeper areas may migrate vertically to feed at night (Clark et al., 2014). Therefore, protecting only part of a habitat or feature (such as a seamount) means that human impacts would still be affecting ecological communities adjacent to the no-take MPA, subjecting it to potential flow-on or indirect effects such as changes in the abundance or behaviour of organisms. Similarly, deeper parts of canyons are strongly dependent on processes from shallower areas, and vice versa. In the open ocean, habitats and features can be isolated by large expanses of deep open water (e.g., seamounts, canyons, ridges) or areas with hydrologically different characteristics (e.g., upwelling, fronts), and protecting them in their entirety becomes even more important than in inshore habitats for safeguarding ecological functions and processes.
Vertical zoning (applying different management rules to benthic and pelagic habitats of the same area) is also not recommended (Grober-Dunsmore et al., 2008; Lausche, 2011). Despite knowledge gaps around benthic-pelagic coupling (Day et al., 2012), emerging evidence suggests that it is stronger than previously thought (Grober-Dunsmore et al., 2008). Benthic communities, especially around prominent undersea features, provide food, shelter, and meeting points for pelagic species (Morato et al., 2010; Garrigue et al., 2015), which, in turn, also directly or indirectly regulate benthic communities. Passfield and Gilman (2010) show that the feeding of predators around seamounts affects seamount benthic ecology; vertical zoning would disturb this coupling. Some tuna aggregations may be present at an individual seamount for up to a period of weeks or months, resulting in a significant contribution to biological and ecological processes (Sibert et al., 2000). Similarly, bathypelagic fish assemblages have been found directly associated with ridge systems, where trophic linkages are likely to be bi-directional (Sutton et al., 2008).
The trophic influence of pelagic species on demersal and benthic communities may be largely indirect, such as large, mobile pelagic species preying on the predators of benthic prey, or preying on bentho-pelagic species (Allain et al., 2006). There is also an ontogenetic link between pelagic and benthic seamount habitats: most seamount benthic species have a pelagic stage, usually as larvae (Allain et al., 2006). Depletion of pelagic predators may therefore indirectly affect benthic communities through release from predation of certain functional groups, increasing prey species abundance and subsequently affecting their interactions with benthic species, such as occurs in trophic cascades (Estes et al., 2011). It could be argued that benthic communities become ever more dependent on pelagic species with increasing depth, as organisms in deeper waters become almost entirely dependent on marine snow and sinking carcasses of larger pelagic animals for food (Bochdansky et al., 2017).
Therefore, where possible, no-take MPAs should protect offshore features in their entirety, both horizontally and vertically.
Offshore Guideline 7: Replicate Protection of Bioregions or Habitats
The concept of replication in MPA design refers to representing each feature, bioregion, or habitat more than once, or placing multiple MPAs within a bioregion, geographic area or other feature of interest, at the scale of the area for which the MPA or MPA network is being designed. In the face of climate change, replication across environmental gradients increases the probability of survival, movement, regeneration, range shifts, or even adaptation of community assemblages and the species within them; this is just as relevant to the deep sea as to shallow-water habitats (Danovaro et al., 2017). Furthermore, representation of latitudinal or longitudinal gradients is important for capturing the range of habitat types and species compositions (Ministry of Fisheries and Department of Conservation, 2008), which are not usually organised into discrete areas, but blend into each other along such gradients.
The replication guideline is common throughout the literature (18 out of 23 documents with a list of guidelines, or 78.3%), and usually recommends protecting three of more examples of a habitat within no-take MPAs (Table 3). The primary goal is risk-spreading, to provide some redundancy to protect against unexpected disturbances or population collapse (e.g., Burt et al., 2018). Protecting several spatially separated examples of similar features (e.g., sites important for a population of a threatened species, patches of similar habitat, breeding sites), reduces the risk of losing the entire feature(s) of interest to disturbance, poaching or even random temporal variability (e.g., recruitment failure; cyclones; Gilman et al., 2011). Most destructive events are spatially patchy, allowing some areas or individuals to escape damage and provide a source of regeneration for damaged areas or depleted populations (Salm et al., 2006). However, while a number of papers provide modelled or empirical tests of the effectiveness of size and spacing guidelines (e.g., Edgar et al., 2014; Robb et al., 2015; Hargreaves-Allen et al., 2017), the replication guideline is yet to be explicitly tested, even in coastal MPAs.
Representing multiple examples of features or habitats in MPAs can be both easier and more problematic in the open ocean. On the one hand, larger MPAs are more feasible, which in turn increases the likelihood of encompassing multiple examples of a feature (e.g., multiple seamounts, canyons, hydrothermal vents, etc.). Also, bioregions tend to be large (e.g., O'Hara et al., 2011; Reygondeau et al., 2012), making it easier to include replicate no-take MPAs within a bioregion. On the other hand, and depending on scale, many features of interest in the open ocean are very large and some are unique (e.g., the Tonga Trench); in such cases, there are, effectively, no other features with exactly the same attributes in existence (Richer de Forges et al., 2000).
The replication guideline can also be used to protect populations of protected species along movement and migratory pathways. Migration pathways can cover entire ocean regions, making replication of the whole migration pathway impossible, but replicated sections of an individual pathway can be protected. Migratory and wide-ranging species may focus their routes over areas of high productivity, or they may rest or aggregate at particular locations (Block et al., 2011); these types of locations can also be replicated. Many populations of migratory species have only one main migration pathway (e.g., migratory seabirds, turtles that move between the western and eastern Pacific). MPA networks can therefore be designed to protect several points along each population's known migration route. Where the literature makes quantitative recommendations about replication in offshore MPAs, the numbers range from one to five replicates, but a minimum of three is the most common design guideline (Table 3).
In summary, where possible, including 3–5 examples of each feature, habitat or bioregion within the no-take MPA network is recommended.
Offshore Guideline 8: Use Simple Shapes
The boundaries of an MPA need to be determined according to the extent and location of the species, features, bioregions, and ecological processes they are intended to protect. Additionally, to maximise the ease of compliance, the boundaries of both inshore and offshore no-take MPAs are best placed according to parallel or perpendicular coordinates. Edges of MPAs can be subject to intense fishing pressure and fishing incursions, and therefore offer a weaker refuge than the core interior (Halpern, 2003; Halpern and Warner, 2003). Therefore, the ideal MPA shape is simple (Table 3) and minimises the edge effect by maximising the protected area to boundary ratio (Roberts et al., 2010; Rodríguez-Rodríguez et al., 2016). Squares or circles are considered to be the most favourable shapes to protect biodiversity; the former, or relatively “squat” rectangles, are preferable from a compliance point of view (Fernandes et al., 2012; White et al., 2012).
Simple, squarish shapes both minimise edge effects and simplify compliance.
Offshore Guideline 9: Choose Permanent Over Temporary Protection
The duration of no-take protection depends on the objectives of the MPA, but for biodiversity conservation objectives, permanent protection is recommended (Dudley, 2008), as the benefits of MPAs are known to increase measurably with age (Edgar et al., 2014). In addition, permanent protection provides time for the entire marine community to recover from human impacts as well as ensuring permanent fisheries benefit from “spillover” effects to be realised (IUCN-WCPA, 2008). Depending on the life cycle of protected species, it can take many years for populations to recover from exploitation (Russ, 2002); the re-establishment of balance and stability within a whole ecosystem can take 10 years or more even for shallow habitats (Johns et al., 2014). While seasonal, rotational or temporary closures may be beneficial for no-take areas designed for fisheries (Cinner, 2005; Kaplan et al., 2010; Sadovy et al., 2011), those benefits are quickly eroded or lost upon opening the area to fishing (Russell et al., 1998; Friedlander and DeMartini, 2002).
In the deep sea, recovery can take between three times and orders of magnitude longer (Huvenne et al., 2016; Fariñas-Franco et al., 2018; Girard et al., 2018). Large pelagic species of conservation interest and deep-water species tend to be long-lived, slow-growing and late-reproducing (K-selected life histories) compared to many of their coastal counterparts; therefore, these populations, once exploited, take longer to recover (Alcala et al., 2005; Hart, 2006). For example, the orange roughy (Hoplostethus atlanticus) is highly sought after by commercial deep-trawl fisheries, but its extraordinary lifespan (up to 150 years) makes it extremely vulnerable to overexploitation (Doonan et al., 2015). In the open ocean, recovery may also occur over the scale of decades, as seen, for example, in the case of the humpback whale populations after the cessation of widespread whaling (Pavanato et al., 2017). The rates of population increase of deep-sea elasmobranchs are less than half those of shelf and pelagic species; once a stock has been depleted, recovery is in the order of decades to centuries (Simpfendorfer and Kyne, 2009). Therefore, offshore ecosystems would especially benefit from permanent protection (Huvenne et al., 2016; Mee et al., 2017).
In sum, implement permanent protection of offshore networks of no-take MPAs.
Offshore Guideline 10: Minimise Threats Outside No-Take MPAs
The minimisation of threats to the marine environment as a component of MPA design was included in 12 of the 52 documents (23%) with a list of guidelines; in many cases, one of the tools proposed for threat minimisation was multiple-use zoning. Both stand-alone MPAs and networks of MPAs can allow for multiple-use zoning (Fraschetti et al., 2009), and for a proportion of each MPA to be designated as no-take (Bohnsack et al., 2004). This paper focuses on guidelines for no-take MPAs because of the conservation and compliance advantages they provide, but also due to the fact that most of the available science focusses on no-take MPAs (Edgar et al., 2014). Recognising, however, that other types of MPAs may also be useful for political, cultural or socio-economic reasons, some guidance is also given here for MPAs that allow some degree of human use. Definitions for a range of types of MPAs exist; the IUCN sets out categories for MPAs with different levels and types of permitted use (Dudley, 2008; Day et al., 2012). Zoning for different levels of use allows for the minimisation or exclusion of individual threats from a wider area (Day, 2002; Grantham and Possingham, 2011; Wilson et al., 2011).
Understanding the spatial distribution of potential stressors or impacts can provide additional guidance for the placement of other categories of MPAs (Halpern et al., 2007). The severity and extent of the stressors may also inform the percentage of an area to be included within MPAs of all categories, including no-take. MPA zoning should also be based on an understanding of cumulative impacts, which relies on the availability of both spatial and temporal data. This understanding can also help to assess the potential threats to future and existing MPAs, as well as the threats to unprotected areas. Any highly destructive activities should be prohibited within the area being managed or considered for inclusion within an MPA, regardless of zoning (Fernandes et al., 2012).
For the design of offshore MPA networks, a simplified version of the IUCN categories will be less confusing for stakeholders and easier for compliance monitoring and enforcement (Day et al., 2012). The rationale and guidelines applied to no-take MPAs should also apply, as much as possible, to other categories (Day et al., 2012). Reducing threats by the application of other categories of MPAs and other management to areas surrounding MPAs will enhance the effectiveness of no-take areas and enhance the ecological health of the management area as a whole. These threats may include shipping, fishing, mining, and other potentially destructive human uses (Halpern et al., 2007). Given the relatively data-poor nature of offshore waters, threat reduction in general can help secure areas, features, or species not yet identified as requiring spatial protection (Jessen et al., 2011).
In sum, apply management to minimise threats overall, and use globally accepted zoning categories that are recognisable to stakeholders.
Discussion and Conclusions
Many of the same design guidelines used to protect coastal regions apply in the open ocean (e.g., size, shape, distance, replication, percentages), with specific tailoring and prioritisation for the characteristics of oceanic ecosystems and species. However, designing MPAs and MPA networks in the open ocean requires a broader perspective than in coastal seas. The main differences between protecting inshore and oceanic areas are related to scale and distance, and are based on a lower level of knowledge and larger uncertainties associated with the open ocean (Table 2).
Next Steps: Applying the Design Guidelines
The guidelines developed in this paper are adapted for offshore environments from existing guidelines for the design and placement of inshore or coastal MPAs (Table 2). Whilst detailed, specific offshore MPA biophysical design guidelines have never been proposed before, the science about offshore marine environments and the effects of offshore MPAs has advanced enough for this initial set of guidelines to be developed. Additionally, some offshore MPAs have already been designed and implemented according to guidelines adapted from coastal MPA design, setting a precedent that indicates the need for a globally applicable set of offshore guidelines. These are guidelines based on current knowledge, however, and should not be interpreted as fixed targets (Agardy et al., 2003). Lessons learned from the first offshore MPAs in the UK reveal three key considerations: (1) offshore MPAs require a strong regulatory basis with integration of fisheries and conservation and a clear financial commitment to enforcement and monitoring; (2) uncertainty and the need for precautionary approaches increase with increasing distance offshore; and (3) transparency tends to be reduced, calling for greater stakeholder engagement (De Santo, 2013). The offshore MPA biophysical design guidelines in this paper have been prepared for the use of practitioners, and it is our hope that this paper may stimulate interest in further adaptations and refinements. We suggest that the next steps for operationalising the guidelines on an international level are (1) setting them into a systematic marine spatial planning framework; (2) prioritising guidelines for ease of application; (3) considering uncertainty; (4) emphasising the need for adaptive management; and (5) special considerations for monitoring in offshore environments.
Systematic Planning
Systematic marine spatial planning refers to a multi-step process that can be used to implement any network of MPAs, including offshore MPAs, and includes stakeholder consultation, application of design guidelines, strategies to incorporate uncertainty and adaptive management systems (Kelleher and Kenchington, 1992; Ehler, 2008; Ehler and Douvere, 2009; Ceccarelli et al., 2018a). This paper acknowledges that biophysical design principles for MPAs form only a small, albeit important, part of the overall marine spatial planning process. For example, the biophysical design guidelines presented here will need to be applied in concert with cultural, social, and economic considerations (Ehler and Douvere, 2009; Lewis et al., 2017). Introducing them into a broader marine spatial planning context will allow for their integrations into an existing regulatory context (De Santo, 2013). Ultimately, compliance with any MPA's restrictions will be the most important contributor to MPA success and an effective planning process will stimulate a higher degree of voluntary compliance (Edgar et al., 2014; Arias et al., 2016).
Systematic spatial planning for offshore waters has the same framework as in coastal ecosystems, but with larger areas, different scales of human operations and ecosystem functioning, higher uncertainty, and, in the case of ABNJ, international collaboration (O'Leary et al., 2012). In the absence of comprehensive information, it may be pragmatic to select some sites for MPAs based on fragmented knowledge, or scientific inference based on similar sites (O'Leary et al., 2012). Comprehensive MPA network design guidelines, such as those presented here, help counter and complement information limitations and high uncertainty by incorporating design features (e.g., minimum requirements, replication) that are robust to potential knowledge failures (Langford et al., 2009).
The first consideration identified from the UK experience suggests that the proposed biophysical guidelines need to be applied within a larger process of marine resource management, which may include other tools to managing large pelagic species, ecosystems and fisheries (Dulvy, 2013; Duarte et al., 2020). Part of broader toolbox may be multiple types of MPAs within a network within which different levels of activity can continue taking into account existing threats and endeavour to minimise them across the entire network (Day et al., 2012). Ultimately, no-take MPAs can only stop extractive uses, and must be used in conjunction with other sectoral resource management tools, pollution controls and actions to reduce greenhouse gas emissions (Hilborn, 2016; Duarte et al., 2020).
Prioritising the Guidelines
Depending on the information available, the guidelines may be more difficult to apply in different parts of the world, and especially in ABNJ where reaching international agreements can be a lengthy process. The challenge of wholly applying these guidelines in poorly understood offshore environments under pressure from unpredictable impacts (e.g., climate change) can be more easily met by prioritising at least some guidelines (Fernandes et al., 2009; Gilman et al., 2011; IUCN-WCPA, 2018). We prioritise the “top three” guidelines as follows:
1) Guideline 1 (size), because maximising the size of MPAs increases the likelihood of other guidelines being applied automatically. In data-poor systems, it also provides insurance against missing important, but as yet unknown, features (Rodrigues et al., 2004).
2) Guideline 2 (special areas), because this ensures that large-scale MPAs will not be placed in areas that are simply of less commercial interest, but actually include features or species that require protection (Devillers et al., 2020).
3) Guideline 3 (% representation of habitats) where bioregions are not defined or Guideline 4 (% representation of bioregions) where there are defined bioregions at an appropriate scale (Fernandes et al., 2009). Representativeness is prioritised because maximising the potential for representativeness also maximises the biodiversity and ecological processes that can be captured within no-take MPAs (Harris, 2007).
Guidelines 1 and 2 together offer the best precautionary approach to maximise the inclusion of offshore biodiversity in larger offshore MPAs, while capturing special features/areas and thus avoiding the protection of large areas of little commercial interest. When data are absent or limited for Guideline 2, Guidelines 3 or 4 become more important.
Dealing With Uncertainty
Uncertainty is a pervasive problem in both inshore and offshore marine resource management, because most marine areas are still data-poor. Observed patterns are often governed by multiple interacting factors at various spatial and temporal scales, many of which are poorly understood. Overcoming uncertainty and data challenges in offshore MPA design can include the use of remote measurements of environmental conditions as biological proxies, non-comprehensive data collected at different spatial scales, surrogate species, marine community classifications such as bioregionalisations, expert and stakeholder participatory decision-making, regional-scale remote sensing studies or a combination of these (Harris and Whiteway, 2009; Beger et al., 2020). Furthermore, to achieve most marine resource management goals in data-poor systems, it is prudent to be more reliant on the precautionary principle, where the burden of proof is shifted toward ecosystem protection first, followed by the proof of no environmental damage by human activities (Clark, 1996; Hooker et al., 2011).
A major source of uncertainty is the changing climate and its future impacts on the ocean. Natural disturbance regimes are a component of ecosystems that should also be considered in MPA design (Harris, 2014). Resistance and resilience to disturbance, or the ability to either absorb disturbance without change or to return to pre-disturbance conditions, are becoming more important as large-scale environmental impacts become more pervasive (Game et al., 2008; Palumbi et al., 2008). In fact, 12 (24%) of the documents identified during the systematic review that provided a list of design guidelines specified resilience or adaptation to climate change as a criterion for selecting MPAs. Identification of such areas can be difficult in data-poor systems, because ascertaining these qualities typically requires time-series data. Therefore, we have not included resilience as a specific guideline for offshore MPAs or MPA networks. It is possible, however, that the combination of guidelines, as presented, will contribute to building ecosystem resilience. Katsanevakis et al. (2020) suggest a risk assessment framework when implementing MPAs and MPA networks, which is an effective way to deal with uncertainty and is applicable in offshore ecosystems.
Adaptive Management
Other ways in which limited information and uncertainty can be acknowledged and accounted for is with an adaptive management approach (whereby management is altered if emerging information deems it necessary; Gormley et al., 2015; Weinert et al., 2021). Aside from knowledge gaps, management will need to occur in an uncertain future governed influenced by climate change, shifting distributions, home ranges or migration pathways; for these reasons also, MPA boundaries may require revision over time (Gruss et al., 2011; Brock et al., 2012; Nickols et al., 2019). The adaptive management cycle allows for flexibility and responsiveness to new and improved information as monitoring of the ecosystem reveals more information about an MPA's effectiveness. To allow for adaptive management offshore, MPA zoning could combine permanent protection with flexible approaches. Permanent protection is preferred (Guideline 9), but boundaries could define zones within which certain known destructive activities (e.g., industrial fishing, bottom trawling, deep-sea mining) are always prohibited, while the effects of other activities are monitored and their regulation tailored to new information.
Monitoring
Although monitoring is an integral part of MPA management, many offshore environments are lacking in even the most basic baseline data. However, to meet global conservation targets, it is impractical to wait until these data are collected before proceeding with marine spatial planning and MPA establishment. The guidelines set out in this paper are designed to optimise the placement of offshore MPAs and MPA networks using existing data, and allowing for the incorporation of data collected in the future.
To continue to improve the effectiveness of offshore networks of MPAs, especially within an adaptive management framework, effort must go into gathering and collating baseline data, followed by performance monitoring. This will include the use of remotely collected and centrally compiled biological and socio-economic data. Global datasets on fisheries and oceanic habitats are being compiled with ever increasing levels of spatial accuracy (Harris et al., 2014; Pauly et al., 2020; Global Fishing Watch, 2021). Regional or national datasets may be more available and appropriate to particular planning efforts and may inform baselines for monitoring; data from ABNJ may be less readily available. Information from monitoring can then feed into an adaptive management cycle for existing MPAs (e.g., Dunn et al., 2018) and broader offshore marine resource management efforts as well as help refine and improve the design guidelines listed here for new offshore MPAs.
Monitoring in the open ocean may rely more heavily on proxies or surrogates than in inshore areas, since data collection can be logistically challenging and expensive. Monitoring populations of some of the more wide-ranging species of interest in offshore MPAs will require a combination of methods, such as satellite technology, drifting baited stereo-videography, spotter planes, drones, horizontal acoustics, and vessel-based sampling (Jaine et al., 2014; Bouchet and Meeuwig, 2015; Letessier et al., 2017). Physical and chemical data can be easier to obtain, and, when available, can be a good predictor for the distribution of some open ocean species (Trebilco et al., 2011; Reygondeau et al., 2012; Hewitt et al., 2015; Stephenson et al., 2020). For example, Harris and Baker (2020) concluded that “Sediment grain size/composition was found to be the most useful surrogate for benthic communities in the most studies, followed by acoustic backscatter, water depth, slope, wave–current exposure, substrate type, seabed rugosity, and geomorphology/Topographic Position Index.”
Regions of the world where data have been or are being collected, and uncertainty is lower, can serve as testing grounds for MPA design. For example, a large portion of the southwest Pacific has defined bioregions, identified with a combination of empirical and modelled data and verified through participatory planning (Beger et al., 2020; Ceccarelli et al., 2021). Bioregions have also been defined in offshore Australian (Department of the Environment and Heritage, 2006), UK (Chaniotis et al., 2020), South African (Livingstone et al., 2018) and Canadian waters (Burt et al., 2018). Multiple national and international seabed mapping projects are underway, including efforts to map the entire seafloor by 2030 (Wölfl et al., 2019); technology innovations promise to deliver increasingly accurate biophysical data (e.g., Zhang et al., 2021). This ongoing global acquisition of data, together with sophisticated theoretical and modelling approaches, invites both a flexible and adaptive management approach that can incorporate new information within a systematic planning framework.
Contributing to a Global Effort
There is a global willingness to move toward effective ocean conservation, as indicated by the increasing number of large and very large MPAs (IUCN, 2016b; Lewis et al., 2017) and national Marine Spatial Planning efforts (Beger et al., 2020). The global target of “30 by 30” (protecting 30% of the global ocean within MPAs by 2030) is achievable, especially in the light of recent additions to the global MPA estate (Duarte et al., 2020). However, it will rely heavily on the protection of offshore waters, which make up over 90% of the global ocean (Harris et al., 2014; Inniss et al., 2016; Sala et al., 2018). Offshore guidelines, as presented in this paper, are an essential tool to further assist progress toward this global target. The biophysical MPA network guidelines developed here are equally applicable, in principle, within national jurisdictions, within ABNJ and, with international coordination and cooperation, across national boundaries or even across national and ABNJ boundaries. The United Nations Convention on Biological Diversity will convene in October 2021. One target that will be decided upon is a global commitment to 30% marine (and terrestrial) protected areas by 2030 (CBD, 2021). This paper provides a significant input to being able to make such a commitment real.
Data Availability Statement
The original contributions presented in the study are included in the article/Supplementary Information 2, further inquiries can be directed to the corresponding author/s.
Author Contributions
LF provided the original concept, overall guidance, contributed intellectual input to the development of the substance and substantial revisions of the paper. DC was the primary writer of the paper, conducted the systematic review, contributed intellectual input to the substance and revision of the paper, and editing. PH, KD, and GJ reviewed versions of the paper, contributed intellectual input to the content and contributed additional references. JR and SM reviewed and contributed to the substance of the original report upon which this paper is based and reviewed this manuscript. All authors contributed to the article and approved the submitted version.
Acknowledgements
This paper is adapted from a report prepared as part of the Marine and Coastal Biodiversity Management in Pacific Island Countries (MACBIO) project. MACBIO was funded by the German Federal Ministry for the Environment, Nature Conservation, Building and Nuclear Safety's (BMUB) International Climate Initiative (IKI), and implemented by the Deutsche Gesellschaft für Internationale Zusammenarbeit (GIZ) with the countries of Fiji, Kiribati, Solomon Islands, Tonga, and Vanuatu. MACBIO enjoyed technical support from the Oceania Regional Office of the International Union for the Conservation of Nature (IUCN) and worked in close collaboration with the Secretariat of the Pacific Regional Environment Program (SPREP). Permission from the copyright holder for the submission of this adaptation of the report Ceccarelli et al. (2018b) was given by the Marine Program Coordinator of IUCN-Oceania. We thank Craig Bohm, Kristina Gjerde, Jon Day, Daniel Dunn, Naushad Yakub, and Phil Gassner for comments on drafts of the report.
Funding
The original work for this paper was conducted under funding for the IUCN's MACBIO (Marine and Coastal Biodiversity Management in Pacific Island Countries) project by the Deutsche Gesellschaft für Internationale Zusammenarbeit (GIZ). Funding for the publication fees was provided by GJ, ARC Centre of Excellence for Coral Reef Studies (Grant number CE140100020) and PH, GRID-Arendal.
Conflict of Interest
The authors declare that the research was conducted in the absence of any commercial or financial relationships that could be construed as a potential conflict of interest.
Publisher's Note
All claims expressed in this article are solely those of the authors and do not necessarily represent those of their affiliated organizations, or those of the publisher, the editors and the reviewers. Any product that may be evaluated in this article, or claim that may be made by its manufacturer, is not guaranteed or endorsed by the publisher.
Supplementary Material
The Supplementary Material for this article can be found online at: https://www.frontiersin.org/articles/10.3389/fmars.2021.634574/full#supplementary-material
Supplementary Information 1. PRISMA 2020 flow diagram for systematic reviews of Marine Protected Area guidelines. Adapted from Page et al. (2021).
Supplementary Information 2. Literature search and data extraction results.
References
Abesamis, R. A., Green, A. L., Russ, G. R., and Jadloc, C. R. L. (2014). The intrinsic vulnerability to fishing of coral reef fishes and their differential recovery in fishery closures. Rev. Fish Biol. Fish 24, 1033–1063. doi: 10.1007/s11160-014-9362-x
Abesamis, R. A., Saenz-Agudelo, P., Berumen, M. L., Bode, M., Jadloc, C. R. L., Solera, L. A., et al. (2017). Reef-fish larval dispersal patterns validate no-take marine reserve network connectivity that links human communities. Coral Reefs 36, 791–801. doi: 10.1007/s00338-017-1570-0
Afonso, P., McGinty, N., Graça, G., Fontes, J., Inácio, M., Totland, A., et al. (2014). Vertical migrations of a deep-sea fish and its prey. PLoS ONE 9:e97884. doi: 10.1371/journal.pone.0097884
Agardy, T., Bridgewater, P., Crosby, M. P., Day, J., Dayton, P. K., Kenchington, R., et al. (2003). Dangerous targets? Unresolved issues and ideological clashes around marine protected areas. Aquat. Conserv. Mar. Freshw. Ecosyst. 13, 353–367. doi: 10.1002/aqc.583
Airamé, S., Dugan, J. E., Lafferty, K. D., Leslie, H., McArdle, D. A., and Warner, R. R. (2003). Applying ecological criteria to marine reserve design: a case study from the California Channel Islands. Ecol. Appl. 13, S170–S184. doi: 10.1890/1051-0761(2003)013[0170:AECTMR]2.0.CO;2
Alcala, A. C., Russ, G. R., Maypa, A. P., and Calumpong, H. P. (2005). A long-term, spatially replicated experimental test of the effect of marine reserves on local fish yields. Can. J. Fish. Aquat. Sci. 62, 98–108. doi: 10.1139/f04-176
Allain, V., Kirby, D., and Kerandel, J. (2006). Seamount Research Planning Workshop Final Report. Report of the Seamount Research Planning Workshop Held at the Secretariat of the Pacific Community, Noumea, New Caledonia, 20-21 March (2006). Secretariat of the Pacific Community, Noumea, New Caledonia.
Almany, G. R., Connolly, S. R., Heath, D. D., Hogan, J. D., Jones, G. P., McCook, L. J., et al. (2009). Connectivity, biodiversity conservation and the design of marine reserve networks for coral reefs. Coral Reefs 28, 339–351. doi: 10.1007/s00338-009-0484-x
Almany, G. R., Hamilton, R. J., Bode, M., Matawai, M., Potuku, T., Saenz-Agudelo, P., et al. (2013). Dispersal of grouper larvae drives local resource sharing in a coral reef fishery. Curr. Biol. 23, 626–630. doi: 10.1016/j.cub.2013.03.006
Almany, G. R., Planes, S., Thorrold, S. R., Berumen, M. L., Bode, M., Saenz-Agudelo, P., et al. (2017). Larval fish dispersal in a coral-reef seascape. Nat. Ecol. Evol. 1:e01482017. doi: 10.1038/s41559-017-0148
Alpine, J. E., and Hobday, A. J. (2007). Area requirements and pelagic protected areas: is size an impediment to implementation? Mar. Freshw. Res. 58, 558–569. doi: 10.1071/MF06214
Althaus, F., Williams, A., Alderslade, P., and Schlacher, T. A. (2017). Conservation of marine biodiversity on a very large deep continental margin: how representative is a very large offshore reserve network for deep-water octocorals? Divers. Distrib. 23, 90–103. doi: 10.1111/ddi.12501
Álvarez-Romero, J. G., Munguía-Vega, A., Beger, M., del Mar Mancha-Cisneros, M., Suárez-Castillo, A. N., Gurney, G. G., et al. (2018). Designing connected marine reserves in the face of global warming. Global Change Biol. 24, e671–e691. doi: 10.1111/gcb.13989
ANZECC (1996). The National Strategy for the Conservation of Australia's Biological Diversity. Canberra, ACT: Australian and New Zealand Environment and Conservation Council, Commonwealth Dept. of the Environment, Sport, and Territories.
Arafeh-Dalmau, N., Torres-Moye, G., Seingier, G., Montano-Moctezuma, G., and Micheli, F. (2017). Marine spatial planning in a transboundary context: linking baja california with california's network of marine protected areas. Front. Mar. Sci. 4:150. doi: 10.3389/fmars.2017.00150
Arcos, J. M., Becares, J., Villero, D., Brotons, L., Rodriguez, B., and Ruiz, A. (2012). Assessing the location and stability of foraging hotspots for pelagic seabirds: an approach to identify marine important bird areas (IBAs) in Spain. Biol. Conserv. 156, 30–42. doi: 10.1016/j.biocon.2011.12.011
Ardron, J. A., Gregr, E. J., Robinson, C. L. K., Coleman, H. M., Dearden, P., Sumaila, U. R., et al. (2015). Recommendations on Applying Canada-BC Marine Protected Area Network Principles in Canada's Northern Shelf Bioregion: Principles 6, 7, and 8. Produced by PacMARA for the British Columbia Marine Protected Areas Implementation Team, British Columbia, Canada.
Arias, A., Pressey, R. L., Jones, R. E., Alvarez-Romero, J. G., and Cinner, J. E. (2016). Optimizing enforcement and compliance in offshore marine protected areas: a case study from Cocos Island, Costa Rica. Oryx 50, 18–26. doi: 10.1017/S0030605314000337
Asaad, I., Lundquist, C. J., Erdmann, M. V., and Costello, M. J. (2017). Ecological criteria to identify areas for biodiversity conservation. Biol. Conserv. 213, 309–316. doi: 10.1016/j.biocon.2016.10.007
Baco, A. R., Etter, R. J., Ribeiro, P. A., Von Der Heyden, S., Beerli, P., and Kinlan, B. P. (2016). A synthesis of genetic connectivity in deep-sea fauna and implications for marine reserve design. Mol. Ecol. 25, 3276–3298. doi: 10.1111/mec.13689
Balbar, A. C., and Metaxas, A. (2019). The current application of ecological connectivity in the design of marine protected areas. Global Ecol. Conserv. 17:e00569. doi: 10.1016/j.gecco.2019.e00569
Ballantine, B. (2014). Fifty years on: lessons from marine reserves in New Zealand and principles for a worldwide network. Biol. Conserv. 176, 297–307. doi: 10.1016/j.biocon.2014.01.014
Baltar, F., Aristegui, J., Gasol, J. M., Lekunberri, I., and Herndl, G. J. (2010). Mesoscale eddies: hotspots of prokaryotic activity and differential community structure in the ocean. ISME J. 4, 975–988. doi: 10.1038/ismej.2010.33
Ban, N. C., Adams, V., Pressey, R. L., and Hicks, J. (2011). Promise and problems for estimating management costs of marine protected areas. Conserv. Lett. 4, 241–252. doi: 10.1111/j.1755-263X.2011.00171.x
Ban, N. C., Bax, N. J., Gjerde, K. M., Devillers, R., Dunn, D. C., Dunstan, P. K., et al. (2014a). Systematic conservation planning: a better recipe for managing the high seas for biodiversity conservation and sustainable use. Conserv. Lett. 7, 41–54. doi: 10.1111/conl.12010
Ban, N. C., Maxwell, S. M., Dunne, D. C., Hobday, A. J., Bax, N. J., Ardron, J., et al. (2014b). Better integration of sectoral planning and management approaches for the interlinked ecology of the open oceans. Mar. Policy 49, 127–136. doi: 10.1016/j.marpol.2013.11.024
Barrie, J. V., Greene, H. G., and Conway, K. W. (2020). “Chapter 50 - Benthic habitats of a mud volcano associated with the Queen Charlotte transform margin along northern British Columbia, Canada and Southern Alaska, United States,” in Seafloor Geomorphology as Benthic Habitat (Second Edition), eds P. T. Harris and E. Baker (Amsterdam: Elsevier), 825–834. doi: 10.1016/B978-0-12-814960-7.00050-6
Baum, J. K., Myers, R. A., Kehler, D. G., Worm, B., Harley, S. J., and Doherty, P. A. (2003). Collapse and conservation of shark populations in the Northwest Atlantic. Science 299, 389–392. doi: 10.1126/science.1079777
Beaman, R. J., Bridge, T. C. L., Lüter, C., Reitner, J., and Wörheide, G. (2016). Spatial patterns in the distribution of benthic assemblages across a large depth gradient in the Coral Sea, Australia. Mar. Biodivers. 46, 795–808. doi: 10.1007/s12526-015-0434-5
Beger, M., Wendt, H., Sullivan, J., Mason, C., LeGrand, J., Davey, K., et al. (2020). National-scale marine bioregions for the Southwest Pacific. Mar. Pollut. Bull. 150:110710. doi: 10.1016/j.marpolbul.2019.110710
Belkin, I. M., Cornillon, P. C., and Sherman, K. (2009). Fronts in large marine ecosystems. Prog. Oceanogr. 81, 223–236. doi: 10.1016/j.pocean.2009.04.015
Benoit-Bird, K. J., Southall, B. L., and Moline, M. A. (2016). Predator-guided sampling reveals biotic structure in the bathypelagic. Proc. R. Soc. B Biol. Sci. 283:20152457. doi: 10.1098/rspb.2015.2457
Berglund, M., Jacobi, M. N., and Jonsson, P. R. (2012). Optimal selection of marine protected areas based on connectivity and habitat quality. Ecol. Model. 240, 105–112. doi: 10.1016/j.ecolmodel.2012.04.011
Block, B. A., Jonsen, I. D., Jorgensen, S. J., Winship, A. J., Shaffer, S. A., Bograd, S. J., et al. (2011). Tracking apex marine predator movements in a dynamic ocean. Nature 475, 86–90. doi: 10.1038/nature10082
Bochdansky, A. B., Clouse, M. A., and Herndl, G. J. (2017). Eukaryotic microbes, principally fungi and labyrinthulomycetes, dominate biomass on bathypelagic marine snow. ISME J. 11, 362–373. doi: 10.1038/ismej.2016.113
Bode, M., Leis, J. M., Mason, L. B., Williamson, D. H., Harrison, H. B., Choukroun, S., et al. (2019). Successful validation of a larval dispersal model using genetic parentage data. PLoS Biol. 17:e3000380. doi: 10.1371/journal.pbio.3000380
Boerder, K., Bryndum-Buchholz, A., and Worm, B. (2017). Interactions of tuna fisheries with the Galápagos marine reserve. Mar. Ecol. Prog. Ser. 585, 1–15. doi: 10.3354/meps12399
Bohnsack, J. A., Ault, J. S., and Causey, B. (2004). Why have no-take marine protected areas? Am. Fish. Soc. Sympos. 42, 193–195.
Bohnsack, J. A., Causey, B., Crosby, M. P., Griffis, R. B., Hixon, M. A., Hourigan, T. F., et al. (2000). “A rationale for minimum 20-30% no-take protection,” in Proceedings of the 9th International Coral Reef Symposium (Bali).
Bongaerts, P., Kline, D. I., Hoegh-Guldberg, O., Bridge, T. C. L., Muir, P. R., Wallace, C. C., et al. (2011). Mesophotic coral ecosystems on the walls of coral sea atolls. Coral Reefs 30:335. doi: 10.1007/s00338-011-0725-7
Botsford, L. W., Micheli, F., and Hastings, A. (2003). Principles for the design of marine reserves. Ecol. Appl.13, S25–S31. doi: 10.1890/1051-0761(2003)013[0025:PFTDOM]2.0.CO;2
Bouchet, P. J., and Meeuwig, J. J. (2015). Drifting baited stereo-videography: a novel sampling tool for surveying pelagic wildlife in offshore marine reserves. Ecosphere 6, 1–29. doi: 10.1890/ES14-00380.1
Bridge, T. C. L., Done, T. J., Beaman, R. J., Friedman, A., Williams, S. B., Pizarro, O., et al. (2011). Topography, substratum and benthic macrofaunal relationships on a tropical mesophotic shelf margin, central Great Barrier Reef, Australia. Coral Reefs 30, 143–153. doi: 10.1007/s00338-010-0677-3
Bridge, T. C. L., Grech, A. M., and Pressey, R. L. (2016). Factors influencing incidental representation of previously unknown conservation features in marine protected areas. Conserv. Biol. 30, 154–165. doi: 10.1111/cobi.12557
Briscoe, D. K., Hobday, A. J., Carlisle, A. B., Scales, K. L., Eveson, J. P., Arrizabalaga, H., et al. (2017). Ecological bridges and barriers in pelagic ecosystems. Deep Sea Res. II 140, 182–192. doi: 10.1016/j.dsr2.2016.11.004
Briscoe, D. K., Maxwell, S. M., Kudela, R., Crowder, L. B., and Croll, D. (2016). Are we missing important areas in pelagic marine conservation? Redefining conservation hotspots in the ocean. Endanger. Spec. Res. 29, 229–237. doi: 10.3354/esr00710
Brock, R. J., Kenchington, E., and Martínez-Arroyo, A. (2012). Scientific Guidelines for Designing Resilient Marine Protected Area Networks in a Changing Climate. Montreal, QC: Commission for Environmental Cooperation.
Bromhead, D., Pepperell, J., Wise, B., and Findlay, J. (2004). Striped Marlin: Biology and Fisheries. Canberra, ACT: Bureau of Rural Science.
Burt, J. M., Akins, P., Latham, E., Beck, M., Salomon, A. K., and Ban, N. (2018). Marine Protected Area Network Design Features That Support Resilient Human-Ocean Systems: Applications for British Columbia, Canada. Simon Fraser University, British Columbia, Canada, 159. doi: 10.31230/osf.io/9tdhv
Carlisle, A. B., Tickler, D., Dale, J. J., Ferretti, F., Curnick, D. J., Chapple, T. K., et al. (2019). Estimating space use of mobile fishes in a large marine protected area with methodological considerations in acoustic array design. Front. Mar. Sci. 6:256. doi: 10.3389/fmars.2019.00256
CBD (2009). Azores Scientific Criteria and Guidance for Identifying Ecologically or Biologically Significant Marine Areas and Designing Representative Networks of Marine Protected Areas in Open Ocean Waters and Deep Sea Habitats. Montreal, QC: Secretariat of the Convention on Biological Diversity.
CBD (2011). Strategic Plan for Biodiversity 2011–2020 and the Aichi Targets. Montreal, QC: Secretariat of the Convention for Biological Diversity.
CBD (2014). Ecologically or Biologically Significant Marine Areas (EBSAs). Special Places in the World's Oceans. Volume 1: Western South Pacific Region. Montreal, QC: Secretariat of the Convention on Biological Diversity.
CBD (2021). Update of the Zero Draft of the Post-2020 Global Biodiversity Framework (CBD/POST2020/PREP/2/1). Available online at: https://www.cbd.int/doc/c/3064/749a/0f65ac7f9def86707f4eaefa/post2020-prep-02-01-en.pdf (accessed June13, 2021)
Ceccarelli, D. M., Davey, K., and Fernandes, L. (2018a). Developing a Marine Spatial Plan: A Toolkit for the Pacific. MACBIO (SPREP/IUCN/BMU), Suva, Fiji.
Ceccarelli, D. M., Matoto, V., Raubani, J., Jones, G. P., Harris, P. T., and Fernandes, L. (2018b). Biophysical Design Principles for Offshore Networks of Notake Marine Protected Areas. MACBIO (GIZ/IUCN/SPREP), Suva, Fiji.
Ceccarelli, D. M., Molisa, V., Wendt, H., Davey, K., Kaitu'u, J., and Fernandes, L. (2018c). Biophysically Special, Unique Marine Areas of Vanuatu. MACBIO (GIZ, IUCN, SPREP), Suva, Fiji.
Ceccarelli, D. M., Wendt, H., Kaitu'u, J., Bhurrah, M., Atonio, M., and Reddy, C. (2021). Marine Bioregions of Samoa. IUCN and MNRE, Samoa.
Ceccarelli, D. M., Wendt, H., Matoto, A. L., Fonua, E., and Fernandes, L. (2017). Biophysically Special, Unique Marine Areas of Tonga. MACBIO (GIZ, IUCN, SPREP), Suva, Fiji.
Ceccarelli, D. M., Wini-Simeon, L., Sullivan, J., Wendt, H., Vave-Karamui, A., Masu, R., et al. (2018d). Biophysically Special, Unique Marine Areas of the Solomon Islands. MACBIO (GIZ, IUCN, SPREP), Suva, Fiji.
Chaniotis, P. D., Robson, L. M., Lemasson, A. J., Cornthwaite, A. L., and Howell, K. L. (2020). UK deep-sea conservation: progress, lessons learned, and actions for the future. Aquat. Conserv. Mar. Freshw. Ecosyst. 30, 375–393. doi: 10.1002/aqc.3243
Chollett, I. (2017). Plan for a Network of Replenishment zones in Northern Honduras. Fort Pierce, FL: Smithsonian Institution.
Cinner, J. (2005). Socioeconomic factors influencing customary marine tenure in the Indo-Pacific. Ecol. Soc. 10, 36–50. doi: 10.5751/ES-01364-100136
Clark, C. W. (1996). Marine reserves and the precautionary management of fisheries. Ecol. Appl. 6, 369–370. doi: 10.2307/2269374
Clark, M. R., Rowden, A. A., Schlacher, T. A., Guinotte, J., Dunstan, P. K., Williams, A., et al. (2014). Identifying ecologically or biologically significant areas (EBSA): A systematic method and its application to seamounts in the South Pacific Ocean. Ocean Coast. Manag. 91, 65–79. doi: 10.1016/j.ocecoaman.2014.01.016
Clark, M. R., Watling, L., Rowden, A. A., Guinotte, J. M., and Smith, C. R. (2011). A global seamount classification to aid the scientific design of marine protected area networks. Ocean Coast. Manag. 54, 19–36. doi: 10.1016/j.ocecoaman.2010.10.006
Claudet, J., Osenberg, C. W., Domenici, P., Badalamenti, F., Milazzo, M., Falcon, J. M., et al. (2010). Marine reserves: fish life history and ecological traits matter. Ecol. Appl. 20, 830–839. doi: 10.1890/08-2131.1
Clear, N. P., Evans, K., Gunn, J., Hampton, J., Bestley, S., Hartmann, K., et al. (2005). Movement of Bigeye Tuna (Thunnus obesus) Determined From Archival Tag Light-Levels and Sea Surface Temperatures. Hobart, TAS: CSIRO, SPC, New Caledonia.
Collette, B. B., Carpenter, K. E., Polidoro, B. A., Juan-Jorda, M. J., Boustany, A., Die, D. J., et al. (2011). High value and long life— double jeopardy for tunas and billfishes. Science 333, 291–292. doi: 10.1126/science.1208730
Commonwealth of Australia (2003). Australia's South-east Marine Region: A User's Guide to Identifying Candidate Areas for a Regional Representative System of Marine Protected Areas. Canberra, ACT: Parks Australia South, Environment Australia.
Cosgrove, R., Arregi, I., Brophy, D., Arrizabalaga, H., Ortiz-de-Zárate-Vidal, V., and Griffin, N. (2010). A simulated archival tagging programme for albacore (Thunnus alalunga) in the Northeast Atlantic, including an analysis of factors affecting tag recovery. ICES J. Mar. Sci. 67, 1216–1221. doi: 10.1093/icesjms/fsq030
Costello, M. J. (2014). Long live marine reserves: a review of experiences and benefits. Biol. Conserv. 176, 289–296. doi: 10.1016/j.biocon.2014.04.023
Costello, M. J., Tsai, P., Wong, P. S., Cheung, A. K. L., Basher, Z., and Chaudhary, C. (2017). Marine biogeographic realms and species endemicity. Nat. Commun. 8:1057. doi: 10.1038/s41467-017-01121-2
Cowen, R. K., Gawarkiewic, G., Pineda, J., Thorrold, S. R., and Werner, F. E. (2007). Population connectivity in marine systems: an overview. Oceanography 20, 14–21. doi: 10.5670/oceanog.2007.26
Danovaro, R., Corinaldesi, C., Dell'Anno, A., and Snelgrove, P. V. R. (2017). The deep-sea under global change. Curr. Biol. 27, R461–R465. doi: 10.1016/j.cub.2017.02.046
Davies, A. J., Roberts, M., and Hall-Spencer, J. (2007). Preserving deep-sea natural heritage: emerging issues in offshore conservation and management. Biol. Conserv. 138, 299–312. doi: 10.1016/j.biocon.2007.05.011
Davies, T. E., Maxwell, S. M., Kaschner, K., Garilao, C., and Ban, N. C. (2017). Large marine protected areas represent biodiversity now and under climate change. Sci. Rep. 7:9569. doi: 10.1038/s41598-017-08758-5
Davies, T. K., Martin, S., Mees, C., Chassot, E., and Kaplan, D. M. (2012). A Review of the Conservation Benefits of Marine Protected Areas for Pelagic Species Associated With Fisheries. ISSF Technical Report 2012-02. International Seafood Sustainability Foundation, McLean, VA, Untied States.
Day, J., Dudley, N., Hockings, M., Holmes, G., Laffoley, D., Stolton, S., et al. (2012). Guidelines for Applying the IUCN Protected Area Management Categories to Marine Protected Areas. Gland: World Commission on Protected Areas - Marine.
Day, J. C. (2002). Zoning—lessons from the Great Barrier Reef Marine Park. Ocean Coast. Manage. 45, 139–156. doi: 10.1016/S0964-5691(02)00052-2
De Santo, E. M. (2013). The Darwin Mounds special area of conservation: implications for offshore marine governance. Mar. Policy 41, 25–32. doi: 10.1016/j.marpol.2013.01.007
De Santo, E. M., and Jones, P. J. S. (2007). The Darwin Mounds: from undiscovered coral to the development of an offshore marine protected area regime. Bull. Mar. Sci. 81, 147–156.
Della Pella, A., Koubbi, P., Bon, C., Bost, C.-A., and d'Ovidio, F. (2017). Lagrangian analysis of multi-satellite data in support of open ocean Marine Protected Area design. Deep Sea Res. II 140, 212–221. doi: 10.1016/j.dsr2.2016.12.014
Department of the Environment and Heritage (2006). A Guide to the Integrated Marine and Coastal Regionalisation of Australia – Version 4.0 June 2006. (IMCRA v4.0). Canberra, ACT: Commonwealth of Australia.
Devillers, R., Pressey, R., Ward, T., Grech, A., Kittinger, J., Edgar, G., et al. (2020). Residual marine protected areas five years on: are we still favouring ease of establishment over need for protection? Aquat. Conserv. Mar. Freshw. Ecosyst. 30, 1758–1764. doi: 10.1002/aqc.3374
Doonan, I. J., Fu, D., and Dunn, M. R. (2015). Harvest control rules for a sustainable orange roughy fishery. Deep Sea Res. Part I Oceanogr. Res. Pap. 98, 53–61. doi: 10.1016/j.dsr.2014.12.001
Duarte, C. M., Agusti, S., Barbier, E., Britten, G. L., Castilla, J. C., Gattuso, J.-P., et al. (2020). Rebuilding marine life. Nature 580, 39–51. doi: 10.1038/s41586-020-2146-7
Dudley, N., (ed.). (2008). Guidelines for Applying Protected Area Management Categories. Gland: International Union for Conservation of Nature and Natural Resources (IUCN).
Dulvy, N. K. (2013). Super-sized MPAs and the marginalization of species conservation. Aquat. Conserv. Mar. Freshw. Ecosyst. 23, 357–362. doi: 10.1002/aqc.2358
Dunn, D. C., Ardron, J., Ban, N. C., Bax, N. J., Bernal, P., Bograd, S. J., et al. (2011). Ecologically or Biologically Significant Areas in the Pelagic Realm: Examples & Guidelines – Workshop Report. Gland: IUCN.
Dunn, D. C., Van Dover, C. L., Etter, R. J., Smith, C. R., Levin, L. A., Morato, T., et al. (2018). A strategy for the conservation of biodiversity on mid-ocean ridges from deep-sea mining. Sci. Adv. 4:eaar4313. doi: 10.1126/sciadv.aar4313
Dunne, R. P., Polunin, N. V. C., Sand, P. H., and Johnson, M. L. (2014). The creation of the Chagos marine protected area: a fisheries perspective. Adv. Mar. Biol. 69, 79–127. doi: 10.1016/B978-0-12-800214-8.00003-7
Edgar, G. J., Stuart-Smith, R. D., Willis, T. J., Kininmonth, S., Baker, S. C., Banks, S., et al. (2014). Global conservation outcomes depend on marine protected areas with five key features. Nature 506, 216–228. doi: 10.1038/nature13022
Ehler, C. (2008). Conclusions: benefits, lessons learned, and future challenges of marine spatial planning. Mar. Policy 32, 840–842. doi: 10.1016/j.marpol.2008.03.014
Ehler, C., and Douvere, F. (2009). Marine Spatial Planning: A Step-by-Step Approach Toward Ecosystem-Based Management. Intergovernmental Oceanographic Commission and Man and the Biosphere Programme. IOC Manual and Guides No. 53, ICAM Dossier No. 6. Paris: UNESCO.
Elsäßer, B., Fariñas-Franco, J. M., Wilson, C. D., Kregting, L., and Roberts, D. (2013). Identifying optimal sites for natural recovery and restoration of impacted biogenic habitats in a special area of conservation using hydrodynamic and habitat suitability modelling. J. Sea Res. 77, 11–21. doi: 10.1016/j.seares.2012.12.006
Estes, J. A., Terborgh, J., Brashares, J. S., Power, M. E., Berger, J., Bond, W. J., et al. (2011). Trophic downgrading of planet earth. Science 333, 301–306. doi: 10.1126/science.1205106
Etnoyer, P., Canny, D., Mate, B., and Morgan, L. (2004). Persistent pelagic habitats in the Baja California to Bering Sea (B2B) ecoregion. Oceanography 17, 90–101. doi: 10.5670/oceanog.2004.71
FAO (2019). International Guidelines for the Management of Deep-Sea Fisheries in the High Seas. Rome: Food and Agriculture Organization.
Fariñas-Franco, J. M., Allcock, A. L., and Roberts, D. (2018). Protection alone may not promote natural recovery of biogenic habitats of high biodiversity damaged by mobile fishing gears. Mar. Environ. Res. 135, 18–28. doi: 10.1016/j.marenvres.2018.01.009
Fernandes, L., Day, J., Kerrigan, B., Breen, D., De'ath, G., Mapstone, B. D., et al. (2009). A process to design a network of marine no-take areas: Lessons from the Great Barrier Reef. Ocean Coast. Manag. 52, 439–447. doi: 10.1016/j.ocecoaman.2009.06.004
Fernandes, L., Day, J., Lewis, A., Slegers, S., Kerrigan, B., Breen, D., et al. (2005). Establishing representative no-take areas in the Great Barrier Reef: large scale implementation of theory on Marine Protected Areas. Conserv. Biol. 19, 1733–1744. doi: 10.1111/j.1523-1739.2005.00302.x
Fernandes, L., Green, A., Tanzer, J., White, A., Alino, P., Jompa, J., et al. (2012). Biophysical Principles for Designing Resilient Networks of Marine Protected Areas to Integrate Fisheries, Biodiversity and Climate Change Objectives in the Coral Triangle. Report prepared by The Nature Conservancy for the Coral Triangle Support Partnership.
Ferretti, F., Worm, B., Britten, G. L., Heithaus, M. R., and Lotze, H. K. (2010). Patterns and ecosystem consequences of shark declines in the ocean. Ecol. Lett. 13, 1055–1071. doi: 10.1111/j.1461-0248.2010.01489.x
Fischer, A., Bhakta, D., Macmillan-Lawler, M., and Harris, P. T. (2019). Existing global marine protected area network is not representative or comprehensive measured against seafloor geomorphic features and benthic habitats. Ocean Coast. Manag. 167, 176–187. doi: 10.1016/j.ocecoaman.2018.10.001
Foley, M. M., Halpern, B. S., Micheli, F., Armsby, M. H., Caldwell, M. R., Crain, C. M., et al. (2010). Guiding ecological principles for marine spatial planning. Mar. Policy 34, 955–966. doi: 10.1016/j.marpol.2010.02.001
Fraschetti, S., D'Ambrosio, P., Micheli, F., Pizzolante, F., Bussotti, S., and Terlizzi, A. (2009). Design of marine protected areas in a human- dominated seascape. Mar. Ecol. Prog. Ser. 375, 13–24. doi: 10.3354/meps07781
Friedlander, A. M., and DeMartini, E. E. (2002). Contrasts in density, size, and biomass of reef fishes between the northwestern and the main Hawaiian Islands: the effects of fishing down apex predators. Mar. Ecol. Prog. Ser. 230, 253–264. doi: 10.3354/meps230253
Gaines, S. D., White, C., Carr, M. H., and Palumbi, S. R. (2010). Designing marine reserve networks for both conservation and fisheries management. Proc. Natl. Acad. Sci. U.S.A. 107, 18286–18293. doi: 10.1073/pnas.0906473107
Game, E. T., Grantham, H. S., Hobday, A. J., Pressey, R. L., Lombard, A. T., Beckley, L. E., et al. (2009). Pelagic protected areas: the missing dimension in ocean conservation. Trends Ecol. Evol. 24, 360–369. doi: 10.1016/j.tree.2009.01.011
Game, E. T., McDonald-Madden, E. V. E., Puotinen, M. L., and Possingham, H. P. (2008). Should we protect the strong or the weak? Risk, resilience, and the selection of marine protected areas. Conserv. Biol. 22, 1619–1629. doi: 10.1111/j.1523-1739.2008.01037.x
Garrigue, C., Clapham, P. J., Geyer, Y., Kennedy, A. S., and Zerbini, A. N. (2015). Satellite tracking reveals novel migratory patterns and the importance of seamounts for endangered South Pacific humpback whales. R. Soc. Open Sci. 2:150489. doi: 10.1098/rsos.150489
Gary, S. F., Fox, A. D., Biastoch, A., Roberts, J. M., and Cunningham, S. A. (2020). Larval behaviour, dispersal and population connectivity in the deep sea. Sci. Rep. 10:10675. doi: 10.1038/s41598-020-67503-7
Gell, F. R., and Roberts, C. M. (2002). The Fishery Effects of Marine Reserves and Fishery Closures. Washington, DC: WWF-US.
Gilman, E., Dunn, D., Read, A., Hyrenbach, K. D., and Warner, R. (2011). Designing criteria suites to identify discrete and networked sites of high value across manifestations of biodiversity. Biodivers. Conserv. 20, 3363–3383. doi: 10.1007/s10531-011-0116-y
Girard, F., Shea, K., and Fisher, C. R. (2018). Projecting the recovery of a long-lived deep-sea coral species after the Deepwater Horizon oil spill using state-structured models. J. Appl. Ecol. 55, 1812–1822. doi: 10.1111/1365-2664.13141
Gjerde, K. M. (2007). High Seas Marine Protected Areas and Deep-Sea Fishing. Rome: Food and Agriculture Organization.
Global Fishing Watch (2021). Global Fishing Watch (www.globalfishingwatch.org). Global Washington, DC: Fishing Watch Inc.
Glover, A. G., and Smith, C. R. (2003). The deep-sea floor ecosystem: current status and prospects of anthropogenic change by the year (2025). Environ. Conserv. 30, 219–241. doi: 10.1017/S0376892903000225
Gormley, K. S. G., Hull, A. D., Porter, J. S., Bell, M. C., and Sanderson, W. G. (2015). Adaptive management, international co-operation and planning for marine conservation hotspots in a changing climate. Mar. Policy 53, 54–66. doi: 10.1016/j.marpol.2014.11.017
Graham, N. A. J., Ainsworth, T. D., Baird, A. H., Ban, N. C., Bay, L. K., Cinner, J. E., et al. (2011). From microbes to people: tractable benefits of no-take areas for coral reefs. Oceanogr. Mar. Biol. 49, 105–136. doi: 10.1201/b11009-4
Grantham, B. A., Eckert, G. L., and Shanks, A. L. (2003). Dispersal potential of marine invertebrates in diverse habitats. Ecol. Appl. 13, 108–116. doi: 10.1890/1051-0761(2003)013[0108:DPOMII]2.0.CO;2
Grantham, H. S., and Possingham, H. P. (2011). Zoning Marine Protected Areas for Biodiversity Conservation and Community Livelihoods: A Case Study From Raja Ampat, West Papua. Brisbane, QLD: Applied Environmental Decision Analysis, University of Queensland.
Great Barrier Reef Marine Park Authority (2002). Technical Information Sheet No 6: Biophysical Operational Principles as Recommended by the Scientific Steering Committee for the Representative Areas Program. Townsville, OLD: Great Barrier Reef Marine Park Authority.
Green, A., Chollett, I., Suárez, A., Dahlgren, C., Cruz, S., Zepeda, C., et al. (2017). Biophysical Principles for Designing a Network of Replenishment Zones for the Mesoamerican Reef System. Technical report produced by The Nature Conservancy, Comunidad y Biodiversidad, A.C., Smithsonian Institution, Perry Institute for Marine Science, Centro de Estudios Marinos, Healthy Reefs Initiative and Universidad Autónoma de Baja California Sur, 64.
Green, A., White, A., and Kilarski, S., (eds.). (2013). Designing Marine Protected Area Networks to Achieve Fisheries, Biodiversity, and Climate Change Objectives in Tropical Ecosystems: A Practitioner Guide. Cebu City: The Nature Conservancy, and the USAID Coral Triangle Support Partnership.
Green, A. L., Maypa, A. P., Almany, G. R., Rhodes, K. L., Weeks, R., Abesamis, R. A., et al. (2014). Larval dispersal and movement patterns of coral reef fishes, and implications for marine reserve network design. Biol. Rev. 90, 1215–1247. doi: 10.1111/brv.12155
Green, A. L., and Mous, P. J. (2007). Delineating the Coral Triangle, Its Ecoregions and Functional Seascapes. Brisbane, QLD: The Nature Conservancy.
Grober-Dunsmore, R., Wooninck, L., Field, J., Ainsworth, C., Beets, J., Berkeley, S., et al. (2008). Vertical zoning in Marine Protected Areas: ecological considerations for balancing pelagic fishing with conservation of benthic communities. Fisheries 33, 598–610. doi: 10.1577/1548-8446-33.12.598
Gruss, A., Kaplan, D. M., Guenette, S., Roberts, C. M., and Botsford, L. W. (2011). Consequences of adult and juvenile movement for marine protected areas. Biol. Conserv. 144, 692–702. doi: 10.1016/j.biocon.2010.12.015
Halpern, B. (2003). The impact of marine reserves: do reserves work and does size matter? Ecol. Appl. 13, S117–S137. doi: 10.1890/1051-0761(2003)013[0117:TIOMRD]2.0.CO;2
Halpern, B., Walbridge, S., Selkoe, K. A., Kappel, C. V., Micheli, F., and D'Agrosa, C. (2008). A global map of human impact on marine ecosystems. Science 319, 948–952. doi: 10.1126/science.1149345
Halpern, B. S., Selkoe, K. A., Micheli, F., and Kappel, C. V. (2007). Evaluating and ranking the vulnerability of global marine ecosystems to anthropogenic threats. Conserv. Biol. 21, 1301–1315. doi: 10.1111/j.1523-1739.2007.00752.x
Halpern, B. S., and Warner, R. R. (2003). Review paper. Matching Marine Reserve design to reserve objectives. Proc. Biol. Sci. 270, 1871–1878. doi: 10.1098/rspb.2003.2405
Hampton, J., and Gunn, J. (1998). Exploitation and movements of yellowfin tuna (Thunnus albacares) and bigeye tuna (T. obesus) tagged in the north-western Coral Sea. Mar. Freshw. Res. 49, 475–489. doi: 10.1071/MF97210
Hargreaves-Allen, V. A., Mourato, S., and Milner-Gulland, E. J. (2017). Drivers of coral reef marine protected area performance. PLoS ONE. 12:e0179394. doi: 10.1371/journal.pone.0179394
Harris, P. T. (2007). “Applications of geophysical information to the design of a representative system of marine protected areas in southeastern Australia,” in Mapping the Seafloor for Habitat Characterization Geological Association of Canada (Amsterdam), 463–481.
Harris, P. T. (2014). Shelf and deep-sea sedimentary environments and physical benthic disturbance regimes: a review and synthesis. Mar. Geol. 353, 169–184. doi: 10.1016/j.margeo.2014.03.023
Harris, P. T. (2020). “Chapter 3 - Anthropogenic threats to benthic habitats,” in Seafloor Geomorphology as Benthic Habitat (Second Edition), eds P. T. Harris and E. Baker (Amsterdam: Elsevier), 35–61. doi: 10.1016/B978-0-12-814960-7.00003-8
Harris, P. T., and Baker, E. K. (2012). Seafloor Geomorphology as Benthic Habitat: GeoHab Atlas of Seafloor Geomorphic Features and Benthic Habitats. Amsterdam: Elsevier. doi: 10.1016/B978-0-12-385140-6.00064-5
Harris, P. T., and Baker, E. K. (2020). “Chapter 60 - GeoHab Atlas of seafloor geomorphic features and benthic habitats–synthesis and lessons learned,” in Seafloor Geomorphology as Benthic Habitat (Second Edition), eds P. T. Harris and E. Baker (Elsevier), 969–990. doi: 10.1016/B978-0-12-814960-7.00060-9
Harris, P. T., Macmillan-Lawler, M., Rupp, J., and Baker, E. K. (2014). Geomorphology of the oceans. Mar. Geol. 352, 4–24. doi: 10.1016/j.margeo.2014.01.011
Harris, P. T., and Whiteway, T. (2009). High Seas Marine Protected Areas: benthic environmental conservation priorities from a GIS analysis of global ocean biophysical data. Ocean Coast. Manage. 52, 22–38. doi: 10.1016/j.ocecoaman.2008.09.009
Harris, P. T., and Whiteway, T. (2011). Global distribution of large submarine canyons: geomorphic differences between active and passive continental margins. Mar. Geol. 285, 69–86. doi: 10.1016/j.margeo.2011.05.008
Harrison, H. B., Williamson, D. H., Evans, R. D., Almany, G. R., Thorrold, S. R., Russ, G. R., et al. (2012). Larval export from marine reserves and the recruitment benefit for fish and fisheries. Curr. Biol. 22, 1023–1028. doi: 10.1016/j.cub.2012.04.008
Hart, D. R. (2006). When do marine reserves increase fishery yields? Can. J. Fish. Aquat. Sci. 63, 1445–1449. doi: 10.1139/f06-071
Hewitt, J. E., Wang, D., Francis, M., Lundquist, C., and Duffy, C. (2015). Evaluating demersal fish richness as a surrogate for epibenthic richness in management and conservation. Divers. Distrib. 21, 901–912. doi: 10.1111/ddi.12336
Hilário, A., Metaxas, A., Gaudron, S. M., Howell, K. L., Mercier, A., Mestre, N. C., et al. (2015). Estimating dispersal distance in the deep sea: challenges and applications to marine reserves. Front. Mar. Sci. 2:6. doi: 10.3389/fmars.2015.00006
Hilborn, R. (2016). Marine biodiversity needs more than protection. Nature 535, 224–226. doi: 10.1038/535224a
Hillman, J. R., Lundquist, C. J., and Thrush, S. F. (2018). The challenges associated with connectivity in ecosystem processes. Front. Mar. Sci. 5:364. doi: 10.3389/fmars.2018.00364
Hobday, A. J., Young, J., Moeseneder, C., and Dambacher, J. (2011). Defining dynamic pelagic habitats in oceanic waters off eastern Australia. Deep Sea Res. Part II Top. Stud. Oceanogr. 58, 734–745. doi: 10.1016/j.dsr2.2010.10.006
Hobday, A. J., Arrizabalaga, H., Evans, K., Scales, K. L., Senina, I., and Weng, K. C. (2017). International collaboration and comparative research on ocean top predators under CLIOTOP. Deep Sea Res. II 140, 1–8. doi: 10.1016/j.dsr2.2017.03.008
Holdsworth, J. C., Sippel, T. J., and Block, B. A. (2009). Near real time satellite tracking of striped marlin (Kajikia audax) movements in the Pacific Ocean. Mar. Biol. 156, 505–514. doi: 10.1007/s00227-008-1104-y
Hooker, S. K., Cañadas, A., Hyrenbach, K. D., Corrigan, C., Polovina, J. J., and Reeves, R. R. (2011). Making protected area networks effective for marine top predators. Endanger. Spec. Res. 13, 203–218. doi: 10.3354/esr00322
Howey, L. A., Tolentino, E. R., Papastamatiou, Y. P., Brooks, E. J., Abercrombie, D. L., Watanabe, Y. Y., et al. (2016). Into the deep: the functionality of mesopelagic excursions by an oceanic apex predator. Ecol. Evol. 6, 5290–5304. doi: 10.1002/ece3.2260
Huvenne, V. A. I., Bett, B. J., Masson, D. G., Le Bas, T. P., and Wheeler, A. J. (2016). Effectiveness of a deep-sea cold-water coral Marine Protected Area, following eight years of fisheries closure. Biol. Conserv. 200, 60–69. doi: 10.1016/j.biocon.2016.05.030
Hyrenbach, K. D., Forney, K. A., and Dayton, P. K. (2000). Marine protected areas and ocean basin management. Aquat. Conserv. Mar. Freshw. Ecosyst. 10, 437–458. doi: 10.1002/1099-0755(200011/12)10:6<437::AID-AQC425>3.0.CO;2-Q
IHO (2008). Standardization of Undersea Feature Names: Guidelines Proposal for Terminology. International Hydrographic Organisation and Intergovernmental Oceanographic Commission. Bathymetric Publication No. 6, Monaco.
IMO (2006). Revised Guidelines for the Identification and Designation of Particularly Sensitive Sea Areas. London: International Maritime Organization.
Inniss, L., Simcock, A., Ajawin, A. Y., Alcala, A. C., Bernal, P., Calumpong, H. I. P., et al. (2016). The First Global Integrated Marine Assessment: World Ocean Assessment I. New York, NY: United Nations.
IUCN (2016a). Increasing Marine Protected Area Coverage for Effective Marine Biodiversity Conservation. Gland: International Union for the Conservation of Nature.
IUCN (2016b). World Conservation Congress, Hawaii (2016). Resolution 050. Gland: International Union for the Conservation of Nature.
IUCN-WCPA (2008). Establishing Marine Protected Area Networks - Making It Happen. Washington, DC: IUCN World Commission on Protected Areas.
IUCN-WCPA (2018). Applying IUCN's Global Conservation Standards to Marine Protected Areas (MPA). Delivering Effective Conservation Action Through MPAs, to Secure Ocean Health & Sustainable Development. Version 1.0., Gland.
Jaine, F. R. A., Rohner, C. A., Weeks, S. J., Couturier, L. I. E., Bennett, M. B., Townsend, K. A., et al. (2014). Movements and habitat use of reef manta rays off eastern Australia: offshore excursions, deep diving and eddy affinity revealed by satellite telemetry. Mar. Ecol. Prog. Ser. 510, 73–86. doi: 10.3354/meps10910
Jessen, S., Chan, K., Dearden, P., De Santo, E., Fortin, M. J., et al. (2011). Science-Based Guidelines for MPAs and MPA Networks in Canada. Vancouver, BC: Canadian Parks and Wilderness Society.
Johns, K. A., Osborne, K. O., and Logan, M. (2014). Contrasting rates of coral recovery and reassembly in coral communities on the Great Barrier Reef. Coral Reefs 33, 553–563. doi: 10.1007/s00338-014-1148-z
Jones, G. P., Almany, G. R., Russ, G. R., Sale, P. F., Steneck, R. S., van Oppen, M. J. H., et al. (2009). Larval retention and connectivity among populations of corals and reef fishes: history, advances and challenges. Coral Reefs 28, 307–325. doi: 10.1007/s00338-009-0469-9
Jones, G. P., Srinivasan, M., and Almany, G. R. (2007). Population connectivity and conservation of marine biodiversity. Oceanography 20, 101–111. doi: 10.5670/oceanog.2007.33
Kahng, S. E., Garcia-Sias, J. R., Spalding, H. L., Brokovich, E., Wagner, D., Weil, E., et al. (2010). Community ecology of mesophotic coral reef ecosystems. Coral Reefs 29, 255–275. doi: 10.1007/s00338-010-0593-6
Kanaji, Y., Okazaki, M., and Miyashita, T. (2017). Spatial patterns of distribution, abundance, and species diversity of small odontocetes estimated using density surface modeling with line transect sampling. Deep Sea Res. II 140, 151–162. doi: 10.1016/j.dsr2.2016.05.014
Kaplan, D. M., Chassot, E., Dueri, S., Demarcq, H., Dagorn, L., et al. (2014). Spatial management of Indian Ocean tropical tuna fisheries: potential and perspectives. ICES J. Mar. Sci. 71, 1728–1749. doi: 10.1093/icesjms/fst233
Kaplan, D. M., Hart, D. R., and Botsford, L. W. (2010). Rotating spatial harvests and fishing effort displacement: a comment on Game et al. (2009). Ecol. Lett. 13, E10–E12. doi: 10.1111/j.1461-0248.2010.01499.x
Katsanevakis, S., Coll, M., Fraschetti, S., Giakoumi, S., Goldsborough, D., et al. (2020). Twelve recommendations for advancing marine conservation in European and contiguous seas. Front. Mar. Sci. 7:565968. doi: 10.3389/fmars.2020.565968
Kavanaugh, M. T., Oliver, M. J., Chavez, F. P., Letelier, R. M., Muller-Karger, F. E., and Doney, S. C. (2016). Seascapes as a new vernacular for pelagic ocean monitoring, management and conservation. ICES J. Mar. Sci. 73, 1839–1850. doi: 10.1093/icesjms/fsw086
Kelleher, G., and Kenchington, R. (1992). Guidelines for Establishing Marine Protected Areas. A Marine Conservation and Development Report. Gland: IUCN.
Kerwath, S. E., Winker, H., Götz, A., and Attwood, C. G. (2013). Marine protected area improves yield without disadvantaging fishers. Nat. Commun. 4:2347. doi: 10.1038/ncomms3347
Kingsford, M. J., and Defries, A. (1999). The ecology of and fishery for Coryphaena spp. in the waters around Australia and New Zealand. Sci. Mar. 63, 267–275. doi: 10.3989/scimar.1999.63n3-4277
Kohler, N. E., Turner, E. A., Hoey, J. J., Natanson, L. J., and Briggs, R. (2002). Tag and recapture data for three pelagic shark species: blue shark (Prionace glauca), shortfin mako (Isurus oxyrinchus), and porbeagle (Lamna nasus) in the north Atlantic Ocean. Collect. Sci. Pap. ICCAT 54, 1231–1260.
Koldewey, H. J., Curnick, D., Harding, S., Harrison, L. R., and Gollock, M. (2010). Potential benefits to fisheries and biodiversity of the Chagos Archipelago/British Indian Ocean Territory as a no-take marine reserve. Mar. Pollut. Bull. 60, 1906–1915. doi: 10.1016/j.marpolbul.2010.10.002
Krueck, N. C., Ahmadia, G. N., Green, A., Jones, G. P., Possingham, H. P., Riginos, C., et al. (2017). Incorporating larval dispersal into MPA design for both conservation and fisheries. Ecol. Appl. 27, 925–941. doi: 10.1002/eap.1495
Lam, C. H., Galuardi, B., Mendillo, A., Chandler, E., and Lutcavage, M. (2016). Sailfish migrations connect productive coastal areas in the West Atlantic Ocean. Sci. Rep. 6:38163. doi: 10.1038/srep38163
Langford, W. T., Gordon, A., and Bastin, L. (2009). When do conservation planning methods deliver? Quantifying the consequences of uncertainty. Ecol. Inform. 4, 123–135. doi: 10.1016/j.ecoinf.2009.04.002
Lauck, T., Clark, C. W., Mangel, M., and Munro, G. R. (1998). Implementing the precautionary principle in fisheries management through marine reserves. Ecol. Appl. 8, S72–S78. doi: 10.1890/1051-0761(1998)8[S72:ITPPIF]2.0.CO;2
Leathwick, J., Moilanen, A., Francis, M., Elith, J., Taylor, P., Julian, K., et al. (2008). Novel methods for the design and evaluation of marine protected areas in offshore waters. Conserv. Lett. 1, 91–102. doi: 10.1111/j.1755-263X.2008.00012.x
Lecours, V., Gabor, L., Edinger, E., and Devillers, R. (2020). “Fine-scale habitat characterization of The Gully, the Flemish Cap, and the Orphan Knoll, Northwest Atlantic, with a focus on cold-water corals,” in Seafloor Geomorphology as Benthic Habitat (Second Edition), eds P. T. Harris and E. Baker (Amsterdam: Elsevier), 735–751. doi: 10.1016/B978-0-12-814960-7.00044-0
Leenhardt, P., Cazalet, B., Salvat, B., Claudet, J., and Feral, F. (2013). The rise of large-scale marine protected areas: conservation or geopolitics? Ocean Coast. Manage. 85, 112–118. doi: 10.1016/j.ocecoaman.2013.08.013
Lesser, M. P., Slattery, M., Laverick, J. H., Macartney, K. J., and Bridge, T. C. (2019). Global community breaks at 60 m on mesophotic coral reefs. Global Ecol. Biogeogr. 28, 1403–1416. doi: 10.1111/geb.12940
Lester, S. E., Halpern, B. S., Grorud-Colvert, K., Lubchenco, J., Ruttenberg, B. I., Gaines, S. D., et al. (2009). Biological effects within no-take marine reserves: a global synthesis. Mar. Ecol. Prog. Ser. 384, 33–46. doi: 10.3354/meps08029
Letessier, T. B., Bouchet, P. J., and Meeuwig, J. J. (2017). Sampling mobile oceanic fishes and sharks: implications for fisheries and conservation planning. Biol. Rev. 92, 627–646. doi: 10.1111/brv.12246
Lewis, N., Day, J. C., Wagner, D., Gaymer, C., Friedlander, A., Parks, J., et al. (2017). Large-Scale Marine Protected Areas: Guidelines for design and management. Best Practice Protected Areas Guidelines Series, No. 26. IUCN, Gland, Switzerland. doi: 10.2305/IUCN.CH.2017.PAG.26.en
Livingstone, T. C., Harris, J. M., Lombard, A. T., Smit, A. J., and Schoeman, D. S. (2018). Classification of marine bioregions on the east coast of South Africa. Afr. J. Mar. Sci. 40, 51–65. doi: 10.2989/1814232X.2018.1438316
Long, M. H., Berg, P., de Beer, D., and Zieman, J. C. (2013). In situ coral reef oxygen metabolism: an eddy correlation study. PLoS ONE 8:e58581. doi: 10.1371/journal.pone.0058581
Lowe, W. H., and Allendorf, F. W. (2010). What can genetics tell us about population connectivity? Mol. Ecol. 19, 3038–3051. doi: 10.1111/j.1365-294X.2010.04688.x
Lundquist, C., Davies, K., and McCartain, L. (2015). Best Practice Guidelines for Marine Protected Area Network Design and Evaluation. Hamilton: National Institute of Water & Atmospheric Research Ltd.
Lundquist, C. J., Bulmer, R. H., Clark, M. R., Hillman, J. R., Nelson, W. A., Norrie, C. R., et al. (2017). Challenges for the conservation of marine small natural features. Biol. Conserv. 211, 69–79. doi: 10.1016/j.biocon.2016.12.027
Maas, P. A. Y., O'Mullan, G. D., Lutz, R. A., and Vrijenhoek, R. C. (1999). Genetic and morphometric characterization of mussels (Bivalvia: Mytilidae) from mid-Atlantic hydrothermal vents. Biol. Bull. 196, 265–272. doi: 10.2307/1542951
Macmillan-Lawler, M., and Harris, P. T. (2016). “Chapter 17: Multivariate classification of seamount morphology: assessing seamount morphotypes in relation to marine jurisdictions and bioregions,” in Ocean Solutions, Earth Solutions, ed D. J. Wright (Redlands, CA: ESRI Global), 312–355. doi: 10.17128/9781589484603_17d
Manel, S., Loiseau, N., Andrello, M., Fietz, K., Goñi, R., Forcada, A., et al. (2019). Long-distance benefits of marine reserves: myth or reality? Trends Ecol. Evol. 34, 342–354. doi: 10.1016/j.tree.2019.01.002
Mannocci, L., Monestiez, P., Spitz, J., and Ridoux, V. (2015). Extrapolating cetacean densities beyond surveyed regions: habitat-based predictions in the circumtropical belt. J. Biogeogr. 42, 1267–1280. doi: 10.1111/jbi.12530
Marine Conservation Institute (2020). Marine Protection Atlas (online). Available online at: https://mpatlas.org/ (accessed April 1, 2021).
Maxwell, S. M., Ban, N. C., and Morgan, L. E. (2014). Pragmatic approaches for effective management of pelagic marine protected areas. Endanger. Spec. Res. 26, 59–74. doi: 10.3354/esr00617
McClain, C. R., and Hardy, S. M. (2010). The dynamics of biogeographic ranges in the deep sea. Proc. R. Soc. B Biol. Sci. 277, 3533–3546. doi: 10.1098/rspb.2010.1057
McCook, L. J., Almany, G. R., Berumen, M. L., Day, J. C., Green, A. L., Jones, G. P., et al. (2009). Management under uncertainty: guide-lines for incorporating connectivity into the protection of coral reefs. Coral Reefs 28, 353–366. doi: 10.1007/s00338-008-0463-7
McCook, L. J., Ayling, A. L., Cappo, M., Choat, J. H., Evans, R. D., De Freitas, D. M., et al. (2010). Adaptive management of the Great Barrier Reef: a globally significant demonstration of the benefits of networks of marine reserves. Proc. Natl. Acad. Sci. U.S.A. 107, 18233–18742. doi: 10.1073/pnas.0909335107
McLeod, E., Salm, R., Green, A., and Almany, J. (2009). Designing marine protected area networks to address the impacts of climate change. Front. Ecol. Environ. 7, 362–370. doi: 10.1890/070211
Mee, J. A., Otto, S. P., and Pauly, D. (2017). Evolution of movement rate increases the effectiveness of marine reserves for the conservation of pelagic fishes. Evol. Appl. 10, 444–461. doi: 10.1111/eva.12460
Meester, G. A., Mehrotra, A., Ault, J. S., and Baker, E. K. (2004). Designing marine reserves for fishery management Management Science 50, 1031–1043. doi: 10.1287/mnsc.1040.0222
Merrie, A., Dunn, D. C., Metian, M., Boustany, A., Takei, Y., Elferink, A. O., et al. (2014). An ocean of surprises – Trends in human use, unexpected dynamics and governance challenges in areas beyond national jurisdiction. Global Environ. Change 27, 19–31. doi: 10.1016/j.gloenvcha.2014.04.012
Micheli, F., Halpern, B. S., Botsford, L. W., and Warner, R. R. (2004). Trajectories and correlates of community change in no-take marine reserves. Ecol. Appl. 14, 1709–1723. doi: 10.1890/03-5260
Miller, K. J., and Gunasekera, R. M. (2017). A comparison of genetic connectivity in two deep sea corals to examine whether seamounts are isolated islands or stepping stones for dispersal. Sci. Rep. 7:46103. doi: 10.1038/srep46103
Miller, P. I., and Christodoulou, S. (2014). Frequent locations of oceanic fronts as an indicator of pelagic diversity: application to marine protected areas and renewables. Mar. Policy 45, 318–329. doi: 10.1016/j.marpol.2013.09.009
Mills, C. E., and Carlton, J. T. (1998). Rationale for a system of international reserves for the open ocean. Conserv. Biol. 12, 244–247. doi: 10.1046/j.1523-1739.1998.96475.x
Ministry of Fisheries and Department of Conservation (2008). Marine Protected Areas: Classification, Protection Standard and Implementation Guidelines. Wellington: Ministry of Fisheries and Department of Conservation.
Moffitt, E. A., White, J. W., and Botsford, L. W. (2011). The utility and limitations of size and spacing guidelines for designing marine protected area (MPA) networks. Biol. Conserv. 144, 306–318. doi: 10.1016/j.biocon.2010.09.008
Morato, T., and Clark, M. R. (2007). “Seamount fishes: ecology and life histories,” in Seamounts: Ecology, Fisheries and Conservation: Blackwell Fisheries and Aquatic Resources Series, eds T. J. Pitcher et al. (Oxford: Blackwell Publishing), 170–188. doi: 10.1002/9780470691953.ch9
Morato, T., Hoyle, S. D., Allain, V., and Nicol, S. J. (2010). Seamounts are hotspots of pelagic biodiversity in the open ocean. Proc. Natl. Acad. Sci. U.S.A. 107:9707–9711. doi: 10.1073/pnas.0910290107
Munguia-Vega, A., Green, A. L., Suarez-Castillo, A., Espinosa-Romero, M., Aburto-Oropeza, O., Cisneros-Montemayor, A., et al. (2018). Ecological guidelines for designing networks of marine reserves in the unique biophysical environment of the Gulf of California. Rev. Fish Biol. Fish. 28, 749–776. doi: 10.1007/s11160-018-9529-y
Nickols, K. J., White, J. W., Malone, D., Carr, M. H., Starr, R. M., Baskett, M. L., et al. (2019). Setting ecological expectations for adaptive management of marine protected areas. J. Appl. Ecol. 56, 2376–2385. doi: 10.1111/1365-2664.13463
Norse, E. A. (2005). Pelagic protected areas: the greatest parks challenge of the 21st century. Parks 15, 32–39.
Norse, E. A., Brooke, S., Cheung, W. W. L., Clark, M. R., Ekeland, L., Froese, R., et al. (2012). Sustainability of deep-sea fisheries. Mar. Policy 36, 307–320. doi: 10.1016/j.marpol.2011.06.008
O'Hara, T. D., Rowden, A. A., and Bax, N. J. (2011). A southern hemisphere bathyal fauna is distributed in latitudinal bands. Curr. Biol. 21, 226–230. doi: 10.1016/j.cub.2011.01.002
O'Leary, B., Brown, R., Johnson, D., von Nordheim, H., Ardron, J., Packeiser, T., et al. (2012). The first network of marine protected areas (MPAs) in the high seas: the process, the challenges and where next. Mar. Policy 36, 598–605. doi: 10.1016/j.marpol.2011.11.003
O'Leary, B. C., Allen, H. L., Yates, K. L., Page, R. W., Tudhope, A. W., McClean, C., et al. (2019). 30 x 30: A Blueprint for Ocean Protection. Greenpeace: University of York and University of Oxford.
O'Leary, B. C., Kvist, K., Bayliss, H. R., Derroire, G., Healey, J. R., Hughes, K., et al. (2016a). The reliability of evidence review methodology in environmental science and conservation. Environ. Sci. Policy 64, 75–82. doi: 10.1016/j.envsci.2016.06.012
O'Leary, B. C., Winther-Janson, M., Bainbridge, J. M., Aitken, J., Hawkins, J. P., and Roberts, C. M. (2016b). Effective coverage targets for ocean protection. Conserv. Lett. 9, 398–404. doi: 10.1111/conl.12247
Ortuño Crespo, G., and Dunn, D. C. (2017). A review of the impacts of fisheries on open-ocean ecosystems. ICES J. Mar. Sci. 74, 2283–2297. doi: 10.1093/icesjms/fsx084
OSCA (2016). A Statement of Concern to the Australian Government: Review of the Commonwealth Network of Marine Reserves. Ocean Science Council of Australia.
OSPAR Commission (2008). Case Reports for the OSPAR List of Threatened and/or Declining Species and Habitats. OSPAR Commission.
Page, M. J., McKenzie, J. E., Bossuyt, P. M., Boutron, I., Hoffmann, T. C., Mulrow, C. D., et al. (2021). The PRISMA 2020 statement: an updated guideline for reporting systematic reviews. BMJ. 372:n71. doi: 10.1136/bmj.n71
Palomares, L. D., Froese, R., Derrick, B., Meeuwig, J. J., Nöel, S.-L., Tsui, G., et al. (2020). Fishery biomass trends of exploited fish populations in marine ecoregions, climatic zones and ocean basins. Estuar. Coast. Shelf Sci. 243:106896. doi: 10.1016/j.ecss.2020.106896
Palumbi, S. R. (2004). Marine reserves and ocean neighborhoods: the spatial scale of marine populations and their management. Annu. Rev. Environ. Resour. 29, 31–68. doi: 10.1146/annurev.energy.29.062403.102254
Palumbi, S. R., McLeod, K. L., and Gruenbaum, D. (2008). Ecosystems in action: lessons from marine ecology about recovery, resistance, and reversibility. Bioscience 58, 33–42. doi: 10.1641/B580108
Papastamatiou, Y. P., Meyer, C. G., Kosaki, R. K., Wallsgrove, N. J., and Popp, B. N. (2015). Movements and foraging of predators associated with mesophotic coral reefs and their potential for linking ecological habitats. Mar. Ecol. Prog. Ser. 521, 155–170. doi: 10.3354/meps11110
Partridge, E. (2009). High Seas Pacific Marine Reserves: A Case Study for the High Seas Enclaves. A Briefing to the CBD's Expert Workshop on Scientific and Technical Guidance on the Use of Biogeographic Classification Systems and Identification of Marine Areas Beyond National Jurisdiction in Need of Protection. Greenpeace.
Passfield, K., and Gilman, E. (2010). Effects of Pelagic Longline Fishing on Seamount Ecosystems Based on Interviews With Pacific Island Fishers. Suva: International Union for the Conservation of Nature, Oceania Regional Office.
Patterson, T., Sharples, R., Raymond, B., Welsford, D., Andrews-Goff, V., Lea, M. A., et al. (2016). Foraging distribution overlap and marine reserve usage amongst sub-Antarctic predators inferred from a multi-species satellite tagging experiment. Ecol. Indic. 70, 531–544. doi: 10.1016/j.ecolind.2016.05.049
Pauly, D., Zeller, D., and Palomares, M. L. D., (eds.). (2020). Sea Around Us Concepts, Design and Data. Available online at: seaaroundus.org (accessed April 23, 2021).
Pavanato, H. J., Wedekin, L. L., Guilherme-Silveira, F. R., Engel, M. H., and Kinas, P. G. (2017). Estimating humpback whale abundance using hierarchical distance sampling. Ecol. Model. 358, 10–18. doi: 10.1016/j.ecolmodel.2017.05.003
Possingham, H. P., and Wilson, K. (2005). Biodiversity: turning up the heat on hotspots. Nature 436, 919–920. doi: 10.1038/436919a
Proud, R., Cox, M. J., and Brierley, A. S. (2017). Biogeography of the global ocean's mesopelagic zone. Curr. Biol. 27, 113–119. doi: 10.1016/j.cub.2016.11.003
Proudfoot, B., Devillers, R., and Brown, C. J. (2020). Integrating fine-scale seafloor mapping and spatial pattern metrics into marine conservation prioritization. Aquat. Conserv. Mar. Freshw. Ecosyst. 30, 1613–1625. doi: 10.1002/aqc.3360
Queiroz, N., Vila-Pouca, C., Couto, A., Southall, E. J., Mucientes, G., Humphries, N. E., et al. (2017). Convergent foraging tactics of marine predators with different feeding strategies across heterogeneous ocean environments. Front. Mar. Sci. 4:239. doi: 10.3389/fmars.2017.00239
Rachor, E., Gunther, C. P., Kroncke, I., Turkay, M., and Sundermann, J., (eds.). (2001). “Concepts for offshore nature reserves in the southeastern North Sea. In: Burning issues of North Sea ecology,” in The 14th International Senckenberg Conference “North Sea 2000” (Wilhelmshaven).
Ramirez-Llodra, E., Tyler, P. A., Baker, M. C., Bergstad, O. A., Clark, M. R., Escobar, E., et al. (2011). Man and the last great wilderness: human impact on the deep sea. PLoS ONE 6:e22588. doi: 10.1371/journal.pone.0022588
Read, B. A., Kegel, J., Klute, M. J., Kuo, A., Lefebre, S. C., Maumus, F., et al. (2013). Pan genome of the phytoplankton Emiliania underpins its global distribution. Nature 499, 209–213. doi: 10.1038/nature12221
Reygondeau, G., Maury, O., Beaugrand, G., Fromentin, J. M., Fonteneau, A., and Cury, P. (2012). Biogeography of tuna and billfish communities. J. Biogeogr. 39, 114–129. doi: 10.1111/j.1365-2699.2011.02582.x
Richer de Forges, B., Koslow, J. A., and Poore, G. C. B. (2000). Diversity and endemism of the benthic seamount fauna in the southwest Pacific. Nature 405, 944–947. doi: 10.1038/35016066
Rigby, C. L., Simpfendorfer, C. A., and Cornish, A. (2019). A Practical Guide to Effective Design and Management of MPAs for Sharks and Rays. Gland: WWF.
Rissik, D., and Suthers, I. M. (2000). Enhanced feeding by pelagic juvenile myctophid fishes within a region of island-induced flow disturbance in the Coral Sea. Mar. Ecol. Prog. Ser. 203, 263–273. doi: 10.3354/meps203263
Robb, C. K., Bodtker, K. M., and Wright, K. (2015). Marine protected areas in the Canadian Pacific: do they fulfill network criteria? Coast. Manage. 43, 253–269. doi: 10.1080/08920753.2015.1030306
Roberts, C. M. (2002). Deep impact: the rising toll of fishing in the deep sea. Trends Ecol. Evol. 17, 242–245. doi: 10.1016/S0169-5347(02)02492-8
Roberts, C. M., Branch, G., Bustamante, R. H., Castilla, J. C., Dugan, J., Halpern, B. S., et al. (2003). Application of ecological criteria in selecting marine reserves and developing reserve networks. Ecol. Appl. 13, S215–S228. doi: 10.1890/1051-0761(2003)013[0215:AOECIS]2.0.CO;2
Roberts, C. M., Hawkins, J. P., Fletcher, J., Hands, S., Raab, K., and Ward, S. (2010). Guidance on the Size and Spacing of Marine Protected Areas in England. Natural England Commissioned Report NECR037, England.
Roberts, C. M., Page, R. W., O'Leary, B. C., Allen, H. L., Yates, K. L., Tudhope, A. W., et al. (2019). 30x30: A Blueprint for Ocean Protection. Greenpeace, University of York and University of Oxford.
Roberts, R. C., and Sargant, H. (2002). Fishery benefits of fully protected marine reserves: why habitat and behaviour are important. Nat. Resour. Model. 15, 487–507. doi: 10.1111/j.1939-7445.2002.tb00099.x
Robinson, N. J., Morreale, S. J., Nel, R., and Paladino, F. V. (2016). Coastal leatherback turtles reveal conservation hotspot. Sci. Rep. 6:37851. doi: 10.1038/srep37851
Rodrigues, A. S. L., Andelman, S. J., Bakarr, M. I., Boitani, L., Brooks, T. M., Cowling, R. M., et al. (2004). Effectiveness of the global protected area network in representing species diversity. Nature 428, 640–643. doi: 10.1038/nature02422
Rodríguez-Rodríguez, D., Rodríguez, J., Blanco, J. M., and Malak, D. A. (2016). Marine protected area design patterns in the Mediterranean Sea: implications for conservation. Mar. Pollut. Bull. 110, 335–342. doi: 10.1016/j.marpolbul.2016.06.044
Ross, R. E., Nimmo-Smith, W. A. M., and Howell, K. L. (2017). Towards ‘ecological coherence': assessing larval dispersal within a network of existing Marine Protected Areas. Deep Sea Res. Part I Oceanogr. Res. Pap. 126, 128–138. doi: 10.1016/j.dsr.2017.06.004
Russ, G. R. (2002). “Yet another review of marine reserves as reef fisheries management tools,” in Coral Reef Fishes: Dynamics and Diversity in a Complex Ecosystem, ed P. F. Sale (San Diego, CA: Academic Press), 421–443. doi: 10.1016/B978-012615185-5/50024-4
Russell, M., Greenwood, J. G., and Hall, N. J. (1998). Strategies for Reopening a Coral Reef to Fishing After Commercial Fishing Stocks Have Been Replenished (Using Bramble Reef Replenishment Area as an Example). Townsville, QLD: Great Barrier Reef Marine Park Authority.
Saarman, E., Gleason, M., Ugoretz, J., Airame, S., Carr, M., Fox, E., et al. (2013). The role of science in supporting marine protected area network planning and design in California. Ocean Coast. Manage. 74, 45–56. doi: 10.1016/j.ocecoaman.2012.08.021
Sadovy, Y. J., Clua, E., and Secretariat of the Pacific Community (2011). “The need for sustainable management of coral reef fish spawning aggregations,” in Seventh Heads of Fisheries Meeting, Secretariat of the Pacific Community (Noumea).
Sala, E., Aburto-Oropeza, O., Paredes, G., Parra, I., Barrera, J. C., and Dayton, P. K. (2002). A general model for designing networks of marine reserves. Science 298, 1991–1993. doi: 10.1126/science.1075284
Sala, E., and Giakoumi, S. (2017). No-take marine reserves are the most effective protected areas in the ocean. ICES J. Mar. Sci. 75, 1166–1168. doi: 10.1093/icesjms/fsx059
Sala, E., Lubchenco, J., Grorud-Colvert, K., Novelli, C., Roberts, C., and Sumaila, U. R. (2018). Assessing real progress towards effective ocean protection. Mar. Policy 91, 11–13. doi: 10.1016/j.marpol.2018.02.004
Saldivar-Lucio, R., Di Lorenzo, E., Nakamura, M., Villalobos, H., Lluch-Cota, D., and Del Monte-Luna, P. (2016). Macro-scale patterns in upwelling/downwelling activity at North American West Coast. PLoS ONE 11:e0166962. doi: 10.1371/journal.pone.0166962
Salm, R. V., Done, T., and McLeod, E. (2006). “Marine protected area planning in a changing climate,” in Coral Reefs and Climate Change: Science and Management (Washington, DC: American Geophysical Union). doi: 10.1029/61CE12
Salomon, A. K., Ruesink, J. L., and DeWreede, R. E. (2006). Population viability, ecological processes and biodiversity: valuing sites for reserve selection. Biol. Conserv. 128, 79–92. doi: 10.1016/j.biocon.2005.09.018
Samadi, S., Bottan, L., Macpherson, E., Richer de Forges, B., and Boisselier, M.-C. (2006). Seamount endemism questioned by the geographic distribution and population genetic structure of marine invertebrates. Mar. Biol. 149, 1463–1475. doi: 10.1007/s00227-006-0306-4
Sayre, R. G., Wright, D. J., Breyer, S. P., Butler, K. A., Van Graafeiland, K., Costello, M. J., et al. (2017). A three-dimensional mapping of the ocean based on environmental data. Oceanography 30, 90–103. doi: 10.4095/305925
Schaefer, K. M., Fuller, D. W., and Aldana, G. (2014). Movements, behavior, and habitat utilization of yellowfin tuna (Thunnus albacares) in waters surrounding the Revillagigedo Islands Archipelago Biosphere Reserve, Mexico. Fish. Oceanogr. 23, 65–82. doi: 10.1111/fog.12047
Schmidt Ocean Institute (2020). Visioning of the Coral Sea Marine Park 30-day Post Cruise Report. Palo Alto, CA.
Secretariat of the Convention on Biological Diversity (2014). Ecologically or Biologically Significant Marine Areas (EBSAs). Special Places in the World's Oceans. Volume 1: Western South Pacific Region. Secretariat of the Convention on Biological Diversity.
Sedberry, G. R., and Loefer, J. K. (2001). Satellite telemetry tracking of swordfish, Xiphias gladius, off the eastern United States. Mar. Biol. 139, 355–360. doi: 10.1007/s002270100593
Sepulveda, C. A., Knight, A., Nasby-Lucas, N., and Domeier, M. L. (2010). Fine-scale movements of the swordfish Xiphias gladius in the Southern California Bight. Fish. Oceanogr.19, 279–289. doi: 10.1111/j.1365-2419.2010.00543.x
Shanks, A. L. (2009). Pelagic larval duration and dispersal distance revisited. Biol. Bull. 216, 373–385. doi: 10.1086/BBLv216n3p373
Shanks, A. L., Grantham, B. A., and Carr, M. H. (2003). Propagule dispersal distances and the size and spacing of marine reserves. Ecol. Appl. 13, S159–S169. doi: 10.1890/1051-0761(2003)013[0159:PDDATS]2.0.CO;2
Sibert, J., and Hampton, J. (2002). Lifetime Displacements of Tropical Tunas: How Much Ocean Do You Need to Conserve “Your” Tuna? Pelagic Fisheries Program, University of Hawaii Secretariat of the Pacific Community, New Caledonia.
Sibert, J., and Hampton, J. (2003). Mobility of tropical tunas and the implications for fisheries management. Mar. Policy 27, 87–95. doi: 10.1016/S0308-597X(02)00057-X
Sibert, J., Holland, K. N., and Itano, D. G. (2000). Exchange rates of yellowfin and bigeye tunas and fishery interaction between Cross seamount and nears-shore FADs in Hawaii. Aquat. Liv. Resour. 13, 225–232. doi: 10.1016/S0990-7440(00)01057-3
Simpfendorfer, C. A., and Kyne, P. M. (2009). Limited potential to recover from overfishing raises concerns for deep-sea sharks, rays and chimaeras. Environ. Conserv. 36, 97–103. doi: 10.1017/S0376892909990191
Spalding, M. D., Fox, H. E., Halpern, B. S., McManus, M. A., Molnar, J., Allen, G. R., et al. (2007). Marine ecoregions of the world: a bioregionalization of coastal and shelf areas. Bioscience 57, 573–583. doi: 10.1641/B570707
Stephenson, F., Goetz, K., Sharp, B. R., Mouton, T. L., Beets, F. L., Roberts, J., et al. (2020). Modelling the spatial distribution of cetaceans in New Zealand waters. Divers. Distrib. 26, 495–516. doi: 10.1111/ddi.13035
Strömberg, S. M., and Larsson, A. I. (2017). Larval behavior and longevity in the cold-water coral Lophelia pertusa indicate potential for long distance dispersal. Front. Mar. Sci. 4:411 doi: 10.3389/fmars.2017.00411
Sutton, T., Porteiro, F., Heino, M., Byrkjedal, I., Langhelle, G., Anderson, C., et al. (2008). Vertical structure, biomass and topographic association of deep-pelagic fishes in relation to a mid-ocean ridge system. Deep Sea Res. Part II Top. Stud. Oceanogr. 55, 161–184. doi: 10.1016/j.dsr2.2007.09.013
Sutton, T. T. (2013). Vertical ecology of the pelagic ocean: classical patterns and new perspectives. J. Fish Biol. 83, 1508–1527. doi: 10.1111/jfb.12263
Sutton, T. T., Clark, M. R., Dunn, D. C., Halpin, P. N., Rogers, A. D., Guinotte, J., et al. (2017). A global biogeographic classification of the mesopelagic zone. Deep Sea Res. Part I Oceanogr. Res. Pap. 126, 85–102. doi: 10.1016/j.dsr.2017.05.006
Sydeman, W. J., Brodeur, R. D., Grimes, C., Bychkov, A., and Mckinnell, S. (2006). Marine habitat ‘hotspots' and their use by migratory species and top predators in the North Pacific Ocean: introduction. Deep Sea Res. Part II Top. Stud. Oceanogr. 53, 247–249. doi: 10.1016/j.dsr2.2006.03.001
The Ecology Centre (2009). Scientific Principles for Design of Marine Protected Areas in Australia: A Guidance Statement. Brisbane, OLD: The University of Queensland.
Theisen, T. C., Bowen, B. W., Lanier, W., and Baldwin, J. D. (2008). High connectivity on a global scale in the pelagic wahoo, Acanthocybium solandri (tuna family Scombridae). Mol. Ecol. 17, 4233–4247. doi: 10.1111/j.1365-294X.2008.03913.x
Thomas, H., and Shears, N. (2013). Marine Protected Areas: A Comparison of Approaches the Royal Forest and Bird Protection Society of New Zealand. Wellington.
Trebilco, R., Halpern, B. S., Flemming, J. M., Field, C., Blanchard, W., and Worm, B. (2011). Mapping species richness and human impact drivers to inform global pelagic conservation prioritisation. Biol. Conserv. 144, 1758–1766. doi: 10.1016/j.biocon.2011.02.024
UK Government (2021). Global Ocean Alliance: 30by30 Initiative. Available online at: https://www.gov.uk/government/topical-events/global-ocean-alliance-30by30-initiative (accessed April 10, 2021).
UN Environment (2017). Combating Marine Plastic Litter and Microplastics: An Assessment of the Effectiveness of Relevant International, Regional and Subregional Governance Strategies and Approaches. New York, NY: UN.
UNDP (2021). Sustainable Development Goals. Available online at: https://www.undp.org/content/undp/en/home/sustainable-development-goals.html (accessed April 10, 2021).
UNESCO (2009). Global Open Oceans and Deep Seabeds (GOODS) - Biogeographic Classification. IOC Technical Series, 84. UNESCO-IOC, Paris.
Van den Hove, S., Moreau, V., and UNEP-WCMC HERMES Project (2007). Deep-Sea Biodiversity and Ecosystems - A Scoping Report on Their Socio-economy, Management and Governance. UNEP-WCMC Biodiversity Series 28. UNEP-WCMC and HERMES Project, Cambridge.
Verity, P. G., Smetacek, V., and Smayda, T. J. (2002). Status, trends and the future of the marine pelagic ecosystem. Environ. Conserv. 29, 207–237. doi: 10.1017/S0376892902000139
Visalli, M. E., Best, B. D., Cabral, R. B., Cheung, W. W. L., Clark, N. A., Garilao, C., et al. (2020). Data-driven approach for highlighting priority areas for protection in marine areas beyond national jurisdiction. Mar. Policy122:103927. doi: 10.1016/j.marpol.2020.103927
Watling, L., Guinotte, J., Clark, M. R., and Smith, C. R. (2013). A proposed biogeography of the deep ocean floor. Prog. Oceanogr. 111, 91–112. doi: 10.1016/j.pocean.2012.11.003
Weeks, R., Green, A., Joseph, E., Peterson, N., and Terk, E. (2017). Using reef fish movement to inform marine reserve design. J. Appl. Ecol. 54, 145–152. doi: 10.1111/1365-2664.12736
Weinert, M., Mathis, M., Kröncke, I., Pohlmann, T., and Reiss, H. (2021). Climate change effects on marine protected areas: projected decline of benthic species in the North Sea. Mar. Environ. Res. 163:105230. doi: 10.1016/j.marenvres.2020.105230
Wendt, H., Beger, M., Sullivan, J., LeGrand, J., Davey, K., Yakub, N., et al. (2018). Marine bioregions in the Southwest Pacific. MACBIO (GIZ, IUCN, SPREP), Suva, Fiji.
White, C., Halpern, B. S., and Kappel, C. V. (2012). Ecosystem service tradeoff analysis reveals the value of marine spatial planning for multiple ocean uses. Proc. Natl. Acad. Sci. U.S.A. 109, 4696–4701. doi: 10.1073/pnas.1114215109
Wilhelm, T. A., Sheppard, C. R. C., Sheppard, A. L. S., Gaymer, C. F., Parks, J., Wagner, D., et al. (2014). Large marine protected areas - advantages and challenges of going big. Aquat. Conserv. Mar. Freshw. Ecosyst. 24, 24–30. doi: 10.1002/aqc.2499
Williams, A., Althaus, F., Dunstan, P. K., Poore, G. C. B., Bax, N. J., Kloser, R. J., et al. (2010a). Scales of habitat heterogeneity and megabenthos biodiversity on an extensive Australian continental margin (100–1100 m depths). Mar. Ecol. 31, 222–236. doi: 10.1111/j.1439-0485.2009.00355.x
Williams, A., Schlacher, T. A., Rowden, A. A., Althaus, F., Clark, M. R., Bowden, D. A., et al. (2010b). Seamount megabenthic assemblages fail to recover from trawling impacts. Mar. Ecol. 31, 183–199. doi: 10.1111/j.1439-0485.2010.00385.x
Williamson, D. H., Harrison, H. B., Almany, G. R., Berumen, M. L., Bode, M., Bonin, M. C., et al. (2016). Large-scale, multidirectional larval connectivity among coral reef fish populations in the Great Barrier Reef Marine Park. Mol. Ecol. 25, 6039–6054. doi: 10.1111/mec.13908
Wilson, J., Darmawan, A., Subijanto, J., Green, A., and Sheppard, S. (2011). Scientific Design of a Resilient Network of Marine Protected Areas. Lesser Sunda Ecoregion, Coral Triangle. Asia-Pacific Marine Program, The Nature Conservancy, Sanur, Bali.
Wölfl, A.-C., Snaith, H., Amirebrahimi, S., Devey, C. W., Dorschel, B., Ferrini, V., et al. (2019). Seafloor Mapping – The challenge of a truly global ocean bathymetry. Front. Mar. Sci. 6:283 doi: 10.3389/fmars.2019.00283
Worboys, G. L., Ament, R., Day, J. C., Lausche, B., Locke, H., McClure, M., et al. (2016). Advanced Draft, Connectivity Conservation Area Guidelines. Gland: IUCN.
Worm, B., Barbier, E. B., Beaumont, N., Duffy, J. E., Folke, C., Halpern, B. S., et al. (2006). Impacts of biodiversity loss on ocean ecosystem services. Science 314, 787–790. doi: 10.1126/science.1132294
Worm, B., Lotze, H. K., and Myers, R. A. (2003). Predator diversity hotspots in the blue ocean. Proc. Natl. Acad. Sci.U.S.A. 100, 9884–9888. doi: 10.1073/pnas.1333941100
Worm, B., and Tittensor, D. P. (2011). Range contraction in large pelagic predators. Proc. Natl. Acad. Sci. U.S.A. 108, 11942–11947. doi: 10.1073/pnas.1102353108
Keywords: marine reserves, oceanic, pelagic, marine conservation, ecological principles, marine protected areas, design, guidelines
Citation: Ceccarelli DM, Davey K, Jones GP, Harris PT, Matoto SV, Raubani J and Fernandes L (2021) How to Meet New Global Targets in the Offshore Realms: Biophysical Guidelines for Offshore Networks of No-Take Marine Protected Areas. Front. Mar. Sci. 8:634574. doi: 10.3389/fmars.2021.634574
Received: 09 December 2020; Accepted: 22 June 2021;
Published: 26 July 2021.
Edited by:
Pierre Failler, University of Portsmouth, United KingdomReviewed by:
Jose M. Fariñas-Franco, Galway-Mayo Institute of Technology, IrelandAnna Metaxas, Dalhousie University, Canada
Copyright © 2021 Ceccarelli, Davey, Jones, Harris, Matoto, Raubani and Fernandes. This is an open-access article distributed under the terms of the Creative Commons Attribution License (CC BY). The use, distribution or reproduction in other forums is permitted, provided the original author(s) and the copyright owner(s) are credited and that the original publication in this journal is cited, in accordance with accepted academic practice. No use, distribution or reproduction is permitted which does not comply with these terms.
*Correspondence: Daniela M. Ceccarelli, dmcecca@gmail.com