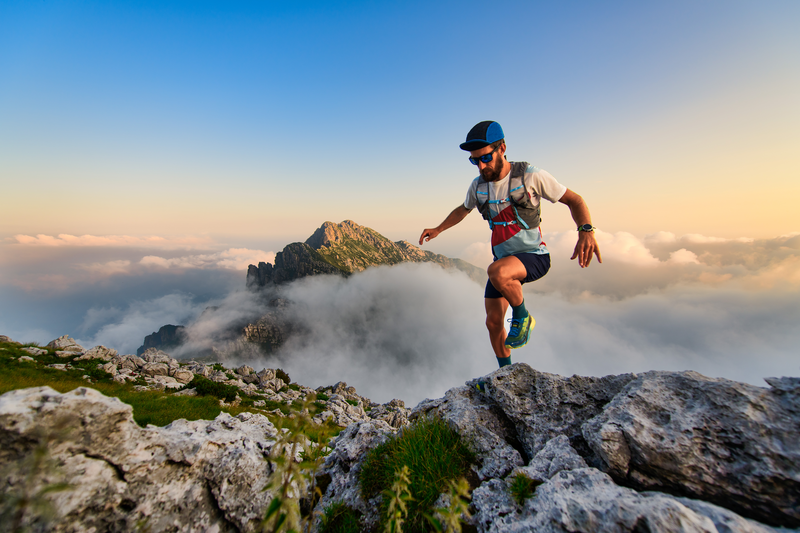
95% of researchers rate our articles as excellent or good
Learn more about the work of our research integrity team to safeguard the quality of each article we publish.
Find out more
BRIEF RESEARCH REPORT article
Front. Mar. Sci. , 05 August 2021
Sec. Marine Megafauna
Volume 8 - 2021 | https://doi.org/10.3389/fmars.2021.631449
Subsea infrastructure of the oil and gas industry attracts commercial fish species as well as megafauna including sea lions, turtles, sharks and whales. Potential impacts of this attraction, whether positive or negative, are unknown. As part of a pilot study, we deployed acoustic telemetry equipment around offshore infrastructure to assess its effectiveness in detecting tagged marine animals and to gain insights into patterns of megafauna occurrence around these structures. Acoustic receivers were placed around four oil and gas platforms and on two remotely operated vehicles (ROVs) on Australia’s North West Shelf. Two whale sharks (Rhincodon typus) tagged in the World Heritage Ningaloo Reef Marine Park were detected at two platforms, North Rankin A and Pluto, located up to 340 km to the northeast. The shark at North Rankin A was detected infrequently and only 15 times over ∼6 weeks. The shark at Pluto was detected each day of the 24-day deployment, in total 4,894 times. Detections at Pluto platform were highest during the day, with peaks at dusk and dawn. Our study indicates that acoustic telemetry around platforms may be an effective method for understanding how marine megafauna utilise these structures. We recommend collaborating with industry to undertake receiver detection range testing to understand the effectiveness of the method. Furthermore, future studies should co-occur with tagging programs at sites like Ningaloo Reef and around the structures themselves to maximise the probability of detecting animals at these sites, thereby improving our understanding of how marine megafauna interact with these structures.
Offshore oil and gas (O&G) infrastructure such as pipelines and platforms have proliferated along continental margins and in the deeper oceans worldwide (Ars and Rios, 2017). These structures are colonised by sessile and motile epibiota, which in turn attract fish and larger fauna (van der Stap et al., 2016; Bond et al., 2018; Thomson et al., 2018; McLean et al., 2019a; Todd et al., 2019). The role these structures play as artificial reefs has attracted greater attention worldwide as they age and as industry considers decommissioning options (Macreadie et al., 2011; Bull and Love, 2019). In the Gulf of Mexico, this interest culminated in the ‘Rigs to Reef’ program in the 1980s, where obsolete oil platforms were left in place, forming additional habitat that has ultimately increased local fish abundance (Kaiser and Pulsipher, 2005; Downey et al., 2018). While clearly being sites of enhanced biomass of important fish species (McLean et al., 2018, 2019b), megafauna such as sharks, manta rays, sea turtles, seals, dolphins and porpoises have also been recorded around platforms, pipelines, manifolds and other structures (Robinson et al., 2013; Todd et al., 2016, 2020). However, few studies have focused on the role of these structures in relation to habitat use and movement by these taxa. Such studies are important as species such as whale sharks (Rhincodon typus) are listed as Endangered by the International Union for the Conservation of Nature (IUCN) (Pierce and Norman, 2016) and included on Appendix II of the Convention on the International Trade in Endangered Species of Wild Fauna and Flora (CITES).1
On Australia’s North West Shelf (NWS), amidst Australia’s largest oil and gas producing area, studies indicate that whale sharks and other megafauna are frequent visitors to subsea infrastructure (Pradella et al., 2013; McLean et al., 2019b, 2020). However, little is known of the nature of interactions between marine megafauna and these structures. The NWS and its several thousand kilometres of pipelines, >1000 wells, >20 monopods and large platforms, lies to the north of the World Heritage-listed Ningaloo Reef where whale sharks aggregate annually (Meekan et al., 2006; Wilson et al., 2006). This region has been the focus of research programs into the movement patterns of marine megafauna such as the Commonwealth Scientific and Industrial Research Organisation’s (CSIRO) Ningaloo Outlook Program2 and the Australian Institute of Marine Science’s North West Shoals to Shore Research Program (Parsons et al., 2018). Numerous marine species, including humpback whales (Megaptera novaeangliae), whale sharks, manta rays (Mobula alfredi and M. birostris) and important fishes have been documented to move widely across the NWS (Jenner et al., 2001; Pillans et al., 2014, 2017; Norman et al., 2016; Reynolds et al., 2017; Vanderklift et al., 2020). Thus, this presents a unique opportunity to determine whether subsea structures are visited frequently by these species and gain insights into how they may use these artificial habitats. Understanding megafauna habitat use around these structures may help inform future decommissioning options, aid in the understanding of marine connectivity between natural and artificial reefs, and ultimately aid in conservation and management efforts of endangered species such as the whale shark.
Passive acoustic telemetry is a widely recognised tool for tracking marine species over short and long distances (Heupel and Webber, 2012; Donaldson et al., 2014; Hussey et al., 2015). It involves tagging target species with a uniquely coded transmitter that can be detected through its low frequency (69 kHz) acoustic transmission by receivers strategically deployed underwater at sites of importance or along migratory pathways (Voegeli, 1988; Heupel et al., 2006, 2019). At Ningaloo Reef, acoustic telemetry has been used to track species including lemon sharks (Negaprion acutidens), grey reef sharks (Carcharhinus amblyrhynchos) and black tip reef sharks (C. melanopterus) (Vanderklift et al., 2020 and references therein) and has been used on fish aggregation devices (FADs) to help track and understand the behaviour of tuna (Robert et al., 2013a,b). It has also been used around O&G platforms off the coast of California and Louisiana to examine site fidelity of resident fish species (Lowe et al., 1996; Topping and Szedlmayer, 2011; Everett et al., 2020). In this pilot study, we trialled acoustic telemetry around O&G infrastructure to assess its efficacy in (a) identifying the timing and duration of visitations by tagged species; (b) understanding how megafauna may use these habitats; and (c) assessing connectivity between these structures and natural habitats in the region. Here, we successfully document the detection of two endangered whale sharks around O&G platforms on the NWS for the first time.
This study was undertaken on Australia’s NWS located to the northeast of Ningaloo Reef Marine Park (Figure 1A). Since 2010, a total of 40 Innovasea V16 69 kHz acoustic transmitters (Innovasea, Bedford, NS, Canada) have been externally attached to whale sharks at Ningaloo Reef, as part of BHP-CSIRO Ningaloo Outlook Marine Research Partnership (n = 16) and the Ecocean Whale Shark Research Program (n = 24). These transmitters are typically programmed to transmit their unique identifier every few minutes, which is logged by underwater receiver hydrophones whenever the tagged animal swims within 200–800 m of a receiver. The V16-4H transmitters deployed by both programs had a power output of 158 dB and were programmed to transmit every 120 s. Each tag deployed on the whale sharks was attached to a titanium tag anchor with a 30 cm length of stainless steel wire. The tag anchor was inserted below the second dorsal fin using a rubber-powered spear gun (Figure 2B).
Figure 1. (A) Location of Ningaloo Reef and positions of platforms Pluto, North Rankin complex (A&B) and Goodwyn Alpha, and the approximate pipelines survey locations of the Angel infield and Vincent fields on the North West Shelf. Also shown are last known positions of two whale sharks tagged with acoustic transmitters at the World Heritage Ningaloo Reef before being detected again at North Rankin A and Pluto platforms. (B) Acoustic receiver stations of the IMOS/CSIRO Ningaloo Reef Ecosystem Tracking Array and detection locations of the two tagged whale sharks later detected at Pluto and North Rankin A platforms. Shark 19503 was detected 26 times over 2 days in the area where it was tagged on the receiver north line and was not detected again until Pluto platform. Shark 19499 was detected 182 times within the Ningaloo region over the next 12 months at 10 receiver stations between the north and south extent of the deployed receivers. Note that all shark 19503 detections that occurred within a small area cannot be shown due to map scale.
Figure 2. (A) A stylised platform jacket showing approximate placement of a VR2W receiver (in red) on a mudmat at the base of the jacket and (B) a whale shark tagged with a V16 69 kHz acoustic transmitter (within dashed circle) pictured at Ningaloo Reef (picture by R. Pillans).
In a collaboration with Woodside Energy, we deployed Innovasea VR2W 69 kHz acoustic receivers during pipeline surveys in the Angel and Vincent infield areas and at the base of four platforms including North Rankin A and B (NRA and NRB, linked by a 100 m bridge), Pluto A and Goodwyn Alpha (GWA) (Figure 1A). One receiver was deployed at each platform at depths up to 130 m for periods of up to 90 days in 2017 and 2018 (Supplementary Table 1). During the pipeline surveys, a receiver was attached upright on the vertical frame of an ROV. Around the platforms, a receiver was deployed to the seafloor during a jacket inspection campaign using a platform-tethered ROV. A small weight was attached to the base of the receiver as an anchor and a small float on a line was attached to maintain the receiver in an upright orientation. The receivers were placed at a platform leg on mudmats used to prevent sinking of equipment into soft substrate (Figure 2A). During these opportunistic deployments, operational constraints severely limited our ability to deploy more than one receiver around each platform and there was no opportunity to conduct range testing of the receivers during any of the deployments.
Upon receiver recovery, data were downloaded using the manufacturer software, merged into a database and analysed in the R programming language (R Core Team, 2021). Data from receivers deployed as part of the Integrated Marine Observing System (IMOS)3 Animal Tracking Facility and CSIRO Ningaloo Reef Ecosystem Tracking Array (NRETA) project were also accessed via the national IMOS Animal Acoustic Telemetry Database4. In this region, a total of 205 receivers have been deployed in discrete receiver arrays and curtains at distinct locations along the Ningaloo coastline since 2009 (Figure 1B). We then searched the IMOS database to match each detected transmitter ID to the tagged animals. As the detected transmitters were programmed with identical ping frequencies of 120 s, standardisations between the transmitters was unnecessary.
Both datasets were analysed using the Animal Tracking Toolbox (ATT) in the VTrack package in R (Campbell et al., 2012; Udyawer et al., 2018). After false detections were identified and removed (Simpfendorfer et al., 2015), we used the ATT to summarise detection metrics such as number of detections, number of days detected and, in the case of the Ningaloo receivers, the number of receivers on which the transmitters were detected.
Two different whale sharks were detected at two separate platforms, Pluto A and NRA. Both sharks were tagged with 69 kHz acoustic transmitters at Ningaloo Reef as part of the Ningaloo Outlook project2. There were no detections from the ROV pipeline surveys within the Angel and Vincent fields, or at the platforms NRB and GWA.
Transmitter A69-9001-19499 was attached to a whale shark on the 2 June 2016 at -21.83782°S, 113.96828°E along the north acoustic receiver line at Ningaloo Reef, hereafter called shark 19499 (Figures 1A,B and Table 1). After being tagged, shark 19499 was detected within 2 h and 6 km away (Figure 1B). The shark was detected another 182 times at 10 receiver stations on 21 unique days along the Ningaloo coastline between North West Cape in the north and Coral Bay in the south between 2 June 2016 and 13 May 2017 (Figure 1B). Most detections were on receivers in water depths between 50 and 100 m close to the reef front. Shark 19499 was last detected in the Ningaloo region along the acoustic curtain of the north line (Figures 1A,B). This shark was not detected again along the Western Australian coastline until almost 1.5 years later on 22 October 2018 when the tag was heard at the NRA platform, approximately 340 km to the northeast of North West Cape.
Table 1. Deployment and detection details for the V16 69 kHz acoustic transmitters deployed on whale sharks at Ningaloo Reef and detected at the oil and gas platforms on the North West Shelf.
At NRA platform, shark 19499 was detected 15 times over the 90-day deployment period between the 22 October 2018 and 10 January 2019 (Figure 3A and Table 1). These detections occurred infrequently on 6 unique days of the deployment. The initial detections from the 28 November 2018 were sparse but detections increased in frequency between the 13 and 19 December 2018 (Figure 3A). However, there were only 1–4 detections occurring per detection day and the period between detections varied widely, with a maximum gap of 126 h. All detections occurred during the day, being 05:44–18:23 h at the start of the deployment in October 2018 and 05:49–19:04 h in January 2019 (Figure 3C).
Figure 3. The number of acoustic detections of whale sharks 19499 and 19503 by deployment day [(A,B), respectively] and by local hour of day (C,D) at North Rankin A (maroon) and Pluto (blue) platforms, respectively, during the night (shaded) and the day (unshaded). Note the different y-axis scales for detection number on all plots where detections at Pluto were up 100 times those at North Rankin A.
Transmitter A69-9001-19503 was attached to another whale shark, shark 19503. Shark 19503 was also tagged on 2 June 2016 and at a location close to that of shark 19499 (Table 1A and Figure 1B). Shark 19503 was detected 26 times in total in the same area over the next 2 days but was not detected again along the Western Australian coastline until more than 2 years later at Pluto A platform on 16 June 2018.
At Pluto, shark 19503 was detected between 130 and 580 times each day of the 24-day deployment period (excluding part days) between 16 June 2018 and 10 July 2018 (Table 1 and Figure 3B). Overall, shark 19503 was detected 4,894 times, with a maximum gap between detections of 4.9 h. Fifty nine percent of detections occurred during the day (05:50 to 17:50 h) with 41% occurring at night (Figure 3D).
Marine megafauna are frequently observed around subsurface infrastructure by crew sightings, underwater inspectional footage and studies using surface observations (Delefosse et al., 2017; Todd et al., 2020). However, we have very little understanding of how these taxa use these structures, the role of connectivity these structures play along coastlines, nor their impact on migratory species transiting to and from feeding and/or breeding grounds. Satellite tracking of marine megafauna can help answer some of these questions but is poorly suited to answering questions on subsurface behaviour and movement as positions are only sent when the saltwater switch on the tag is dry and the antenna has broken the water surface. Conversely, our acoustic detections at platforms feature only limited locations but provide data on interactions with the platforms, and acoustic tags can stay affixed to animals for up to several years. While our pilot study does not presume to answer the above questions, it does provide insight into how these questions may be addressed using proven acoustic telemetry technology in collaboration with the O&G industry. Furthermore, our results may have wider implications for the use of acoustic telemetry on other subsea structures such as shipwrecks, artificial reefs and FADs, which can be instrumented and used as scientific platforms (Moreno et al., 2016).
We detected two whale sharks at two separate platforms. However, despite both detected sharks having the same type of acoustic tag attached in identical positions, with identical power and ping frequency parameters, detection patterns were very different. The whale shark at Pluto platform was detected almost continually every day of the 24-day deployment, with the longest period between detections being approximately 5 h. This suggests a strong affinity to Pluto or the surrounding area and that shark 19503 spent its time in close proximity to the platform, within the theoretical maximum range of the receiver of 200 – 800 m. At NRA, shark 19499 was detected only infrequently (between 1 and 6 times) on 6 days over a 23-day time period, although the deployment totalled 90 days. This detection pattern may indicate a low affinity or even a repulsion to the platform, with the shark infrequently approaching and/or remaining on the edge of detection whilst in the general area over 23 days. However, the few and infrequent detections at the NRA platform were uninformative. We could not determine whether they were a result of the shark’s behaviour around the platform, seasonal influences affecting movement more broadly or alternatively, factors that affected transmitter signal strength and/or the detection performance of the instruments that are discussed further below.
What factors may have influenced the limited detection patterns we did record? Studies have shown that artificial lights emitted by platforms at night may act as ‘light traps’ for plankton, enhancing local food supplies (Keenan et al., 2007) and attracting predators such as harbour porpoises (Todd et al., 2009). However, our data suggests that both whale sharks we detected moved away from the platform receivers at night. Diel movements of fish species driven mostly by feeding and reproduction are well known and the abundance of some fish around offshore infrastructure has been shown to decrease at night (Baggerman, 1980; Lowry and Suthers, 1998; Nagelkerken et al., 2000; Bond et al., 2018). In the Gulf of Mexico, Barker and Cowan (2018) found fish communities in surface waters around lit versus unlit platforms were most abundant during daylight hours, suggesting they utilised the structure during the day for shelter and had an aversion to the lights at night, potentially to avoid predation. On the NWS, predatory fish species that sheltered along pipelines during the day were absent at night, thought to be foraging in adjacent habitats (Bond et al., 2018). As the world’s largest fish, the whale sharks we detected may be undertaking similar, nocturnal movements away from the platform, possibly using them as a refuge during the daylight hours. Alternatively, our fewer detections at night might be due to increased background noise from vertical plankton migrations and/or the whale sharks themselves moving to shallower depths above the platform receivers at this time.
Our results indicate there is a degree of connectivity between the platforms and Ningaloo Reef Marine Park, exhibited by the movement of these whale sharks. This highlights the importance of understanding the interactions between the whale sharks and the platforms as the NWS subsea infrastructure lies along known whale shark tracks from Ningaloo Reef to the northeast and the northwest (Wilson et al., 2006; Sleeman et al., 2010; Vanderklift et al., 2020). However, it is difficult to determine if there are advantages or impacts associated with this connectivity or the attraction to offshore infrastructure. While there are support vessels within the 500 m exclusion zone around these structures, their speed is limited to four knots and they are unlikely to cause strikes on whale sharks as seen at tourism locations such as Ningaloo Reef and Oslob in the Philippines (Lester et al., 2020; Penketh et al., 2020). In fact, this exclusion zone around operating platforms in Western Australia may provide pinpoints of protection. However, this is unlikely to be significant to highly migratory species like whale sharks, which have been recorded moving thousands of kilometres with a high degree of individual variability (Vanderklift et al., 2020). Continued detection around offshore platforms presumably implies that the animals are remaining in the vicinity of these structures for a purpose. Given the sharks we detected were not sexually mature, feeding on the increased plankton abundance around the platforms is the most likely explanation. A better understanding of plankton biomass around platforms and how this relates to whale shark occurrence patterns is required.
Worldwide, the offshore O&G industry is estimated to be supported by >12,000 platforms and around 180,000 km of pipelines, mostly located in depths up to 150 m on continental shelves (CNCP, 2015; Ars and Rios, 2017; Kaiser, 2018; Jouffray et al., 2020). Combining acoustic telemetry with this infrastructure could vastly increase our ability to track and understand migratory movements of megafauna, especially in areas that are ecologically significant, remote and difficult to access. For example, Ningaloo Reef has a history of marine animal tagging and a long established acoustic detection array but very few listening stations to its north. A dedicated receiver deployment program around O&G structures on the NWS combined with a tagging campaign of migratory species would greatly assist in understanding broad scale movement patterns and how infrastructure may be used by these species. Furthermore, this would vastly extend the IMOS Animal Tracking Network along this remote coastline, enhancing monitoring opportunities for species of national interest (i.e., recreationally and commercially important species and threatened, endangered or protected species). As migratory species cross international boundaries, an enhanced network along the Western Australian coast may also foster collaborations and knowledge leading to significant conservation outcomes. These outcomes would reflect positively on offshore industry and enhance their social licence to operate.
Our few detections are most likely due to several key factors. Firstly, the deployments were opportunistic, determined by inspection schedules and not biological imperatives, over a relatively short time periods and at five different locations. In addition, few highly mobile animals have been tagged with acoustic transmitters in North-Western Australia prior to this study. Based on records in the IMOS database, only 108 individuals likely to move across the NWS, including whale sharks, green turtles (Chelonia mydas) and tiger sharks (Galeocerdo cuvier) have been tagged in the region since 2009. Forty of these were whale sharks and only 24 individuals were tagged within 3 years of our receiver deployments. Such low numbers of recently tagged animals limited the probability of detection at our sites and this number was reduced further by the longevity of tag attachment to the shark. For example, of the 10 external acoustic tags attached to whale sharks by CSIRO in 2016, five tags were confirmed as detached from sharks from Ningaloo tourist industry photo identifications the following year. That two of the tagged whale sharks were detected at the platforms over a year later in 2018 hints at an underestimation of numbers visiting platforms we have recorded here. This is further illustrated by visual sightings of four individual, untagged whale sharks at GWA during the same time period our receiver was deployed at this site (McLean et al., 2019a). Two of these individuals were positively identified from photographs previously taken at Ningaloo Reef and submitted to the Wildbook for Whale Sharks.5
Finally, we have a very poor understanding of the detection range of acoustic receivers around O&G structures and especially receivers attached to ROVs, with their relatively loud, hydraulically operated parts. Several studies stress the importance of range testing for acoustic tags and receivers as signal strength and probability of detection can be impacted by many variables. These include deployment duration, receiver depth, receiver orientation and environmental factors such as sea state, ambient noise, current strength, wind speed, and physical properties of the water column such as thermocline depth (Simpfendorfer et al., 2008; Kessel et al., 2014; Huveneers et al., 2016; Loher et al., 2017). Therefore, it is likely that each of these factors, either separately or in combination, may have impacted our ability to detect tagged animals.
Our experience leads us to several recommendations. An essential first step prior to further studies is range testing of the receivers to understand their effectiveness around O&G infrastructure and while attached to ROVs. Range testing may reveal that it is necessary to deploy more than one receiver per platform to overcome limitations, along with stationary reference tags to understand changes in detection probability with time and to refine detection range (Kessel et al., 2014; Huveneers et al., 2016). Furthermore, any collaboration with industry to deploy acoustic receivers around subsea infrastructure must be combined with an intensive animal tagging campaign nearby to enhance possible detections. Such campaigns should consider the rapidly evolving range of tags carrying sensors that can measure essential ocean variables (Harcourt et al., 2019). For example, acoustic tags should at least include a pressure sensor to help us understand how megafauna may interact with the infrastructure over depth.
Subsea infrastructure is well known to form artificial reefs that attract commercially important fishes and a range of megafauna. However, little is known about how marine megafauna may use these artificial reefs and how these structures may affect the movement of species such as whale sharks. This is particularly relevant on Australia’s NWS where large O&G fields lay along a north-south movement corridor for whales and whale sharks. In this study, the use of acoustic receivers around O&G platforms led to the detection and identification of two endangered whale sharks previously tagged in the nearby World Heritage Ningaloo Reef Marine Park. While these sharks showed different degrees of attraction to the platforms, our study confirmed that acoustic telemetry may be a useful tool in helping understand habitat use of O&G artificial reefs by megafauna. Furthermore, our experience allows us to offer recommendations for future studies. These include collaborations with industry to conduct range testing of the acoustic receivers around infrastructure and large-scale tagging campaigns to coincide with receiver deployments to optimise success and the collection of scientific data on endangered or regionally relevant species.
The datasets presented in this study can be found in online repositories. The names of the repository/repositories and accession number(s) can be found below: https://animaltracking.aodn.org.au/.
The animal study was reviewed and approved by the Western Australian Department of Parks and Wildlife Regulation 17 and a CSIRO Animal Ethics Permit (Permit number CSIRO AEC 2016-06).
PT conceived the idea, performed the data analysis, and prepared the initial manuscript draft and secured the Oceanworks prototyping grant. RP secured funding for tagging and tagged the whale sharks at Ningaloo Reef. DM and MT secured the project with Woodside Energy. FJ and RH contributed the acoustic receivers and provided guidance for receiver deployments and data analysis. All authors contributed ideas to improve the manuscript and helped in its editing and preparation.
This work was partially funded by an Oceanworks Prototyping Grant (PRO21) from The University of Western Australia.
The authors declare that the research was conducted in the absence of any commercial or financial relationships that could be construed as a potential conflict of interest.
All claims expressed in this article are solely those of the authors and do not necessarily represent those of their affiliated organizations, or those of the publisher, the editors and the reviewers. Any product that may be evaluated in this article, or claim that may be made by its manufacturer, is not guaranteed or endorsed by the publisher.
We would like to acknowledge Luke Smith and James Eu at Woodside Energy Ltd., for enabling this project and assistance onsite by Daniel Clarkson, Colin Murray, Mark Jones, Justin Economis and the ROV pilots and engineers. Deployment of acoustic receivers was supported by the Woodside-operated Pluto LNG and North West Shelf Projects. This study was initiated as part of the SEA-SERPENT (South-East Asia Scientific and Environmental ROV Partnership using Existing Industrial Technology) project. Tagging of whale sharks at Ningaloo was carried out by CSIRO Oceans and Atmosphere under the Ningaloo Outlook project, a BHP-CSIRO Industry-Science Marine Research Partnership investing A$5.4 million over 5 years to gather new knowledge on the Ningaloo reef and its important ecological values. Data was sourced from the Animal Acoustic Telemetry Database (https://animaltracking.aodn.org.au/) of the Integrated Marine Observing System (IMOS, http://www.imos.org.au/). IMOS is enabled by the National Collaborative Research Infrastructure Strategy (NCRIS). It is operated by a consortium of institutions as an unincorporated joint venture, with the University of Tasmania as Lead Agent.
The Supplementary Material for this article can be found online at: https://www.frontiersin.org/articles/10.3389/fmars.2021.631449/full#supplementary-material
Ars, F., and Rios, R. (2017). “Decommissioning: a call for a new approach,” in Offshore Technology Conference, Houston, TX, 7.
Baggerman, B. (1980). “Photoperiodic and endogenous control of the annual reproductive cycle in teleost fishes,” in Environmental Physiology of Fishes, ed. M. A. Ali (Boston, MA: Springer US), 533–567. doi: 10.1007/978-1-4899-3659-2_21
Barker, V. A., and Cowan, J. H. (2018). The effect of artificial light on the community structure of reef-associated fishes at oil and gas platforms in the northern Gulf of Mexico. Environ. Biol. Fish. 101, 153–166. doi: 10.1007/s10641-017-0688-9
Bond, T., Langlois, T. J., Partridge, J. C., Birt, M. J., Malseed, B. E., Smith, L., et al. (2018). Diel shifts and habitat associations of fish assemblages on a subsea pipeline. Fish. Res. 206, 220–234. doi: 10.1016/j.fishres.2018.05.011
Bull, A. S., and Love, M. S. (2019). Worldwide oil and gas platform decommissioning: a review of practices and reefing options. Ocean Coast. Manag. 168, 274–306. doi: 10.1016/j.ocecoaman.2018.10.024
Campbell, H., Watts, M., Dwyer, R., and Franklin, C. (2012). V-Track: software for analysing and visualising animal movement from acoustic telemetry detections. Mar. Freshw. Res. 63, 815–820. doi: 10.1071/mf12194
CNCP (2015). Submarine Oil and Gas Pipeline Technology. Beijing: China National Petroleum Corporation.
Delefosse, M., Rahbek, M., Roesen, L., and Clausen, K. (2017). Marine mammal sightings around oil and gas installations in the central North Sea. J. Mar. Biol. Assoc. U. K. 98, 993–1001.
Donaldson, M., Hinch, S., Suski, C., Fisk, A., Heupel, M., and Cooke, S. (2014). Making connections in aquatic ecosystems with acoustic telemetry monitoring. Front. Ecol. Environ. 12:565–573. doi: 10.1890/130283
Downey, C. H., Streich, M. K., Brewton, R. A., Ajemian, M. J., Wetz, J. J., and Stunz, G. W. (2018). Habitat-specific reproductive potential of red snapper: a comparison of artificial and natural reefs in the Western Gulf of Mexico. Trans. Am. Fish. Soc. 147, 1030–1041. doi: 10.1002/tafs.10104
Everett, A. G., Szedlmayer, S. T., and Gallaway, B. J. (2020). Movement patterns of red snapper Lutjanus campechanus based on acoustic telemetry around oil and gas platforms in the northern Gulf of Mexico. Mar. Ecol. Prog. Ser. 649, 155–173. doi: 10.3354/meps13448
Harcourt, R., Sequeira, A. M. M., Zhang, X., Roquet, F., Komatsu, K., Heupel, M., et al. (2019). Animal-borne telemetry: an integral component of the ocean observing toolkit. Front. Mar. Sci. 6:326. doi: 10.3389/fmars.2019.00326
Heupel, M., Ledee, E., Udywader, V., and Harcourt, R. (2019). “Acoustic telemetry: an essential sensor in ocean observing systems,” in Challenges and Innovations in Ocean In-Situ Sensors: Measuring Inner Ocean Processes and Health in the Digital Age, eds E. Delory and J. Pearlman (Amsterdam: Elsevier), 135–147. doi: 10.1007/978-3-319-78352-9_8
Heupel, M., Semmens, J., and Hobday, A. (2006). Automated animal tracking: scales, design and deployment of listening station arrays. Mar. Freshw. Res. 57, 1–13. doi: 10.1071/mf05091
Heupel, M., and Webber, D. M. (2012). Trends in acoustic tracking: where are the fish going and how will we follow them? Am. Fish. Soc. Symp. 76, 219–231.
Hussey, N. E., Kessel, S. T., Aarestrup, K., Cooke, S. J., Cowley, P. D., Fisk, A. T., et al. (2015). Aquatic animal telemetry: a panoramic window into the underwater world. Science 348:1255642. doi: 10.1126/science.1255642
Huveneers, C., Simpfendorfer, C. A., Kim, S., Semmens, J. M., Hobday, A. J., Pederson, H., et al. (2016). The influence of environmental parameters on the performance and detection range of acoustic receivers. Methods Ecol. Evol. 7, 825–835. doi: 10.1111/2041-210x.12520
Jenner, K. C. S., Jenner, M.-N. M., and McCabe, K. A. (2001). Geographical and temporal movements of humpback whales in Western Australian Waters. he App. J. 41, 749–765. doi: 10.1071/aj00044
Jouffray, J.-B., Blasiak, R., Norström, A. V., Österblom, H., and Nyström, M. (2020). The blue acceleration: the trajectory of human expansion into the ocean. One Earth 2, 43–54. doi: 10.1016/j.oneear.2019.12.016
Kaiser, M. J. (2018). The global offshore pipeline construction service market 2017 – Part I. Ships Offshore Struct. 13, 65–95. doi: 10.1080/17445302.2017.1342923
Kaiser, M. J., and Pulsipher, A. G. (2005). Rigs-to-reef programs in the gulf of Mexico. Ocean Dev. Int. Law 36, 119–134. doi: 10.1080/00908320590943990
Keenan, S., Benfield, M. C., and Blackburn, J. (2007). Importance of the artificial light field around offshore petroleum platforms for the associated fish community. Mar. Ecol. Prog. Ser. 331, 219–231. doi: 10.3354/meps331219
Kessel, S. T., Cooke, S. J., Heupel, M. R., Hussey, N. E., Simpfendorfer, C. A., Vagle, S., et al. (2014). A review of detection range testing in aquatic passive acoustic telemetry studies. Rev. Fish Biol. Fish. 24, 199–218. doi: 10.1007/s11160-013-9328-4
Lester, E., Meekan, M. G., Barnes, P., Raudino, H., Rob, D., Waples, K., et al. (2020). Multi-year patterns in scarring, survival and residency of whale sharks in Ningaloo Marine Park. Western Australia. Mar. Ecol. Prog. Ser. 634, 115–125. doi: 10.3354/meps13173
Loher, T., Webster, R. A., and Carlile, D. (2017). A test of the detection range of acoustic transmitters and receivers deployed in deep waters of Southeast Alaska, USA. Anim. Biotelemetry 5:27.
Lowe, C. G., Wetherbee, B. M., Crow, G. L., and Tester, A. L. (1996). Ontogenetic dietary shifts and feeding behavior of the tiger shark, Galeocerdo cuvier, in Hawaiian waters. Environ. Biol. Fishes 47, 203–211.
Lowry, M. B., and Suthers, I. M. (1998). Home range, activity and distribution patterns of a temperate rocky-reef fish, Cheilodactylus fuscus. Mar. Biol. 132, 569–578. doi: 10.1007/s002270050422
Macreadie, P. I., Fowler, A. M., and Booth, D. J. (2011). Rigs-to-reefs: will the deep sea benefit from artificial habitat? Front. Ecol. Environ. 9:455–461. doi: 10.1890/100112
McLean, D. L., Taylor, M. D., Giraldo Ospina, A., and Partridge, J. C. (2019a). An assessment of fish and marine growth associated with an oil and gas platform jacket using an augmented remotely operated vehicle. Cont. Shelf Res. 179, 66–84. doi: 10.1016/j.csr.2019.04.006
McLean, D. L., Vaughan, B. I., Malseed, B. E., and Taylor, M. D. (2019b). Fish-habitat associations on a subsea pipeline within an Australian Marine Park. Mar. Environ. Res. 153:104813. doi: 10.1016/j.marenvres.2019.104813
McLean, D. L., Taylor, M. D., Partridge, J. C., Gibbons, B., Langlois, T. J., Malseed, B. E., et al. (2018). Fish and habitats on wellhead infrastructure on the north west shelf of Western Australia. Cont. Shelf Res. 164, 10–27. doi: 10.1016/j.csr.2018.05.007
McLean, D. L., Vaughan, B. I., Malseed, B. E., and Taylor, M. D. (2020). Fish-habitat associations on a subsea pipeline within an Australian Marine Park. Mar. Environ. Res. 153:104813.
Meekan, M., Bradshaw, C., Press, M., McLean, C., Richards, A., Quasnichka, S., et al. (2006). Population size and structure of whale sharks Rhincodon typus at Ningaloo Reef, Western Australia. Mar. Ecol. Prog. Ser. 319, 275–285. doi: 10.3354/meps319275
Moreno, G., Dagorn, L., Capello, M., Lopez, J., Filmalter, J., Forget, F., et al. (2016). Fish aggregating devices (FADs) as scientific platforms. Fish. Res. 178, 122–129. doi: 10.1016/j.fishres.2015.09.021
Nagelkerken, I., Dorenbosch, M., Verberk, W. C. E. P., de la Morinière, E. C., and Van der Velde, G. (2000). Day-night shifts of fishes between shallow-water biotopes of a Caribbean bay, with emphasis on the nocturnal feeding of Haemulidae and Lutjanidae. Mar. Ecol. Prog. Ser. 194, 55–64. doi: 10.3354/meps194055
Norman, B., Reynolds, S., and Morgan, D. (2016). Does the whale shark aggregate along the Western Australian coastline beyond Ningaloo Reef? Pacific Conserv. Biol. 22, 72–80. doi: 10.1071/pc15045
Parsons, M. J. G., Meekan, M. G., Miller, K. J., Thums, M., and Gilmour, J. P. (2018). Innovation and technology in marine science: AIMS’ North West Shoals to shore research program. APPEA J. 58, 578–581. doi: 10.1071/aj17234
Penketh, L., Schleimer, A., Labaja, J., Snow, S., Ponzo, A., and Araujo, G. (2020). Scarring patterns of whale sharks, Rhincodon typus, at a provisioning site in the Philippines. Aquat. Conserv. 31, 99–111.
Pierce, S. J., and Norman, B. (2016). Rhincodon Typus. The IUCN Red List of Threatened Species 2016: e.T19488A2365291. Gland: International Union for Conservation of Nature and Natural Resources.
Pillans, R. D., Babcock, R. C., Thomson, D. P., Haywood, M. D. E., Downie, R. A., Vanderklift, M. A., et al. (2017). Habitat effects on home range and schooling behaviour in a herbivorous fish (Kyphosus bigibbus) revealed by acoustic tracking. Mar. Freshw. Res. 68, 1454–1467. doi: 10.1071/mf16199
Pillans, R. D., Bearham, D., Boomer, A., Downie, R., Patterson, T. A., Thomson, D. P., et al. (2014). Multi year observations reveal variability in residence of a tropical demersal fish, lethrinus nebulosus: implications for spatial management. PLoS One 9:e105507. doi: 10.1371/journal.pone.0105507
Pradella, N., Fowler, A. M., Booth, D. J., and Macreadie, P. I. (2013). Fish assemblages associated with oil industry structures on the continental shelf of north-western Australia. J. Fish Biol. 84, 247–255. doi: 10.1111/jfb.12274
R Core Team (2021). R: A Language and Environment for Statistical Computing. Vienna: R Foundation for Statistical Computing.
Reynolds, S. D., Norman, B. M., Beger, M., Franklin, C. E., and Dwyer, R. G. (2017). Movement, distribution and marine reserve use by an endangered migratory giant. Divers. Distrib. 23, 1268–1279. doi: 10.1111/ddi.12618
Robert, M., Dagorn, L., Filmalter, J. D., Deneubourg, J.-L., Itano, D., and Holland, K. (2013a). Intra-individual behavioral variability displayed by tuna at fish aggregating devices (FADs). Mar. Ecol. Prog. Ser. 484, 239–247. doi: 10.3354/meps10303
Robert, M., Dagorn, L., Lopez, J., Moreno, G., and Deneubourg, J.-L. (2013b). Does social behavior influence the dynamics of aggregations formed by tropical tunas around floating objects? An experimental approach. J. Exp. Mar. Biol. Ecol. 440, 238–243. doi: 10.1016/j.jembe.2013.01.005
Robinson, D. P., Jaidah, M. Y., Jabado, R. W., Lee-Brooks, K., Nour El-Din, N. M., Malki, A. A. A., et al. (2013). Whale sharks, Rhincodon typus, aggregate around offshore platforms in qatari waters of the Arabian Gulf to Feed on Fish Spawn. PLoS One 8:e58255. doi: 10.1371/journal.pone.0058255
Simpfendorfer, C. A., Heupel, M. R., and Collins, A. B. (2008). Variation in the performance of acoustic receivers and its implication for positioning algorithms in a riverine setting. Can. J. Fish. Aquat. Sci. 65, 482–492. doi: 10.1139/f07-180
Simpfendorfer, C. A., Huveneers, C., Steckenreuter, A., Tattersall, K., Hoenner, X., Harcourt, R., et al. (2015). Ghosts in the data: false detections in VEMCO pulse position modulation acoustic telemetry monitoring equipment. Anim. Biotelemetry 3:55.
Sleeman, J., Meekan, M., Wilson, S., Polovina, J., Stevens, J., Boggs, G., et al. (2010). To go or not to go with the flow: environmental influences on whale shark movement patterns. J. Exp. Mar. Biol. Ecol. 390, 84–98. doi: 10.1016/j.jembe.2010.05.009
Thomson, P. G., Fowler, A. M., Davis, A. R., Pattiaratchi, C. B., and Booth, D. J. (2018). Some old movies become classics – a case study determining the scientific value of ROV Inspection Footage on a Platform on Australia’s North West Shelf. Front. Mar. Sci. 5:471. doi: 10.3389/fmars.2018.00471
Todd, V. L. G., Lazar, L., Williamson, L. D., Peters, I. T., Hoover, A. L., Cox, S. E., et al. (2020). Underwater visual records of marine megafauna around offshore anthropogenic structures. Front. Mar. Sci. 7:230. doi: 10.3389/fmars.2020.00230
Todd, V. L. G., Pearse, W. D., Tregenza, N. C., Lepper, P. A., and Todd, I. B. (2009). Diel echolocation activity of harbour porpoises (Phocoena phocoena) around North Sea offshore gas installations. ICES J. Mar. Sci. 66, 734–745. doi: 10.1093/icesjms/fsp035
Todd, V. L. G., Warley, J. C., and Todd, I. B. (2016). Meals on Wheels? A decade of megafaunal visual and acoustic observations from offshore oil & gas rigs and platforms in the North and Irish Seas. PLoS One 11:e0153320. doi: 10.1371/journal.pone.0153320
Todd, V. L. G., Williamson, L. D., Cox, S. E. I, Todd, B., and Macreadie, P. I. (2019). Characterizing the first wave of fish and invertebrate colonization on a new offshore petroleum platform. ICES J. Mar. Sci. 77, 1127–1136. doi: 10.1093/icesjms/fsz077
Topping, D., and Szedlmayer, S. (2011). Site fidelity, residence time and movements of red snapper Lutjanus campechanus estimated with long-term acoustic monitoring. Mar. Ecol. Prog. Ser. 437, 183–200. doi: 10.3354/meps09293
Udyawer, V., Dwyer, R., Hoenner, X., Babcock, R., Brodie, S., Campbell, H., et al. (2018). A standardised framework for analysing animal detections from automated tracking arrays. Anim. Biotelemetry 6:17.
van der Stap, T., Coolen, J. W. P., and Lindeboom, H. J. (2016). Marine fouling assemblages on offshore gas platforms in the Southern North Sea: effects of depth and distance from shore on biodiversity. PLoS One 11:e0146324. doi: 10.1371/journal.pone.0146324
Vanderklift, M. A., Rc, B., Barnes, P. B., Cresswell, A. C., Feng, M., Haywood, M. D. E., et al. (2020). The oceanography and marine ecology of Ningaloo, a world heritage area. Oceanogr. Mar. Biol. 58, 143–178. doi: 10.1201/9780429351495-4
Voegeli, F. A. (1988). “Ultrasonic tracking, position monitoring and data telemetry systems,” in Proceedings of the 10th International Symposium on Biotelemetry, eds A. Uchiyama and C. J. Amlaner Tokyo: Waseda University Press.
Keywords: acoustic telemetry, decommissioning, Ningaloo Reef, North West Shelf, oil and gas infrastructure, whale shark, marine megafauna, connectivity
Citation: Thomson PG, Pillans R, Jaine FRA, Harcourt RG, Taylor MD, Pattiaratchi CB and McLean DL (2021) Acoustic Telemetry Around Western Australia’s Oil and Gas Infrastructure Helps Detect the Presence of an Elusive and Endangered Migratory Giant. Front. Mar. Sci. 8:631449. doi: 10.3389/fmars.2021.631449
Received: 20 November 2020; Accepted: 16 July 2021;
Published: 05 August 2021.
Edited by:
Adrian C. Gleiss, Murdoch University, AustraliaReviewed by:
Gonzalo Araujo, Large Marine Vertebrates Research Institute Philippines (LAMAVE), PhilippinesCopyright © 2021 Thomson, Pillans, Jaine, Harcourt, Taylor, Pattiaratchi and McLean. This is an open-access article distributed under the terms of the Creative Commons Attribution License (CC BY). The use, distribution or reproduction in other forums is permitted, provided the original author(s) and the copyright owner(s) are credited and that the original publication in this journal is cited, in accordance with accepted academic practice. No use, distribution or reproduction is permitted which does not comply with these terms.
*Correspondence: Paul G. Thomson, cGF1bC50aG9tc29uQHV3YS5lZHUuYXU=
†ORCID: Fabrice R. A. Jaine, orcid.org/0000-0002-9304-5034
Disclaimer: All claims expressed in this article are solely those of the authors and do not necessarily represent those of their affiliated organizations, or those of the publisher, the editors and the reviewers. Any product that may be evaluated in this article or claim that may be made by its manufacturer is not guaranteed or endorsed by the publisher.
Research integrity at Frontiers
Learn more about the work of our research integrity team to safeguard the quality of each article we publish.