- 1Institute of Paleobiology, Polish Academy of Sciences, Warsaw, Poland
- 2Hellenic Centre for Marine Research (HCMR), Institute of Marine Biology, Biotechnology and Aquaculture (IMBBC), Heraklion, Greece
Desmas-bearing demosponges known as lithistids have heavily silicified skeleton and occur typically in bathyal environments of warm and tropical areas but may be found in certain shallow marine caves. Here we report, for the first time two lithistid species, i.e., Neophrissospongia endoumensis, and N. cf. nana, that were earlier known from Western Mediterranean marine caves, from four marine caves in the north-eastern Mediterranean, and their congener Neophrissospongia nolitangere from deep waters (ca. 300 m) of the Aegean Sea. All marine caves, and sections within these caves, where lithistids occur, have freshwater springs. We interpret this surprising association between lithistids and freshwater input by elevated concentration of silica in water in cave sections where such springs occur, being 8–11 times higher in comparison with shallow water outside caves, and comparable to that of deep waters, that promoted lithistids’ development. One of the studied caves harbored an abundant population of N. endoumensis which formed large masses. The age estimation of these lithistids, based on known growth rate of related deep-water sponges, suggest that they could be approximately 769–909 years old in the case of the largest specimen observed, about 100 cm large. These sponges could have colonized the caves from adjacent deep-water areas not earlier than 7,000–3,000 years ago, after the last glaciation, because earlier they were emerged. High variability of spicules, especially microscleres, and underdevelopment of megascleres may be related to silicic acid concentration.
Introduction
Lithistids are those demosponges of various systematic position with “rocky” choanosomal skeleton [hence their name derives from the Greek word “λθoς” (lithos) meaning stone], that consist of articulated, more or less regular and usually strongly sculptured spicules called desmas. They inhabit mostly waters deeper than 100 m to about 2000 m, but some species occur also in shallow-water settings (Zea, 1987), including coral reefs (Vacelet and Vasseur, 1965; Hall et al., 2014), and marine caves (Pisera and Vacelet, 2011; Gerovasileiou and Voultsiadou, 2012).
The Mediterranean lithistid fauna includes 13 species of which 10 have been recorded in marine caves. These are Aciculites mediterranea Manconi et al., 2006, Desmanthus incrustans (Topsent, 1889), Discodermia polymorpha Pisera and Vacelet, 2011, Gastrophanella phoeniciensis Perez et al., 2004, Microscleroderma lamina Perez et al., 2004, Neophrissospongia endoumensis Pisera and Vacelet, 2011, N. nana Manconi and Serusi, 2008, Neophrissospongia nolitangere (Schmidt, 1870), Neophrissospongia radjae Pisera and Vacelet, 2011, and Neoschrammeniella bowerbankii (Johnson, 1863). The remaining three species that have not been reported from marine caves, but mostly from deep waters, are: Leiodermatium lynceus Schmidt, 1870, Leiodermatium pfeifferae (Carter, 1873), and Siphonidium ramosum (Schmidt, 1870). Therefore, the marine cave environment seems to be particularly favorable for lithistids, harboring 77% of the Mediterranean lithistid diversity, including six endemic species which are known only from a single marine cave but not from other habitats – at least so far – and could be considered cave-exclusive sensu lato (Gerovasileiou and Voultsiadou, 2012).
The problem with specific attribution of many lithistid sponges is the fact that numerous species are known only from a single, or two specimens and no systematic studies of intraspecific variability or/and environmental variability in their morphology exist. Even more it concerns their spiculation, that, in the case when more specimens are known, good SEM photos are available only for one specimen. Atop of these problems is the fact that particular regions in the same specimen, that differ in structure and function, differ also in details of desma morphology and ectosomal spicules types (see for example Theonella wrightae in Pisera and Pomponi, 2015; A. Pisera unpublished observations). Thus, one may suspect that some lithistid species may in fact be only environmental variants of earlier described species. Another problem is that only well oriented samples showing the surface of desma skeleton can be compared, and random cut surface of choanosomal skeleton shows apparently chaotic desma articulation (what is not true). A separate question concerns microscleres which often exhibit high variability that should be assessed independently.
Mediterranean lithistid records are generally few and geographically scattered (Van Soest et al., 2020), mostly from the northern section of the basin, with some exceptions (e.g., Perez et al., 2004). Although the sponge fauna of the Aegean Sea (north-eastern Mediterranean), and particularly its Greek waters, is the most comprehensively studied in the eastern Mediterranean basin (Voultsiadou, 2005a, b, 2009; Voultsiadou et al., 2016), only two lithistids have been recorded in this area. These are Discodermia polymorpha, reported asDiscodermia polydiscus (Bowerbank, 1869) from several locations, at a depth range of 10–360 m, including marine caves (Voultsiadou, 2005a, b; Pisera and Vacelet, 2011), and Leiodermatium lynceus, collected at 207 m depth, off Epidaurus (Peloponnese, southern Aegean Sea) by Vamvakas (1970). More recently, another lithistid, belonging to the genus Neophrissospongia was reported from a semi-submerged marine cave in Agios Efstratios Island, northern Aegean Sea (Dimarchopoulou et al., 2018).
During the last decade, numerous marine caves have been studied in the north-eastern Mediterranean Sea, shedding light on new records and new sponge species (Gerovasileiou et al., 2015; Lage et al., 2018, 2019). The discovery of lithistids from marine caves with freshwater input coupled with the acquisition of material from deep waters constitute the basis of this work. This study aims at (a) describing for the first time lithistid demosponges collected from shallow marine caves of the north-eastern Mediterranean Sea; (b) investigating environmental factors that potentially control their distribution and occurrence; and (c) comparing congeneric lithistids from marine caves and deep waters of the north-eastern Mediterranean Sea.
Materials and Methods
During the last decade, approximately 40 marine caves and cave groups (i.e., sites with several caves and tunnels, sometimes connected) have been surveyed in the north-eastern Mediterranean Sea, and mostly in the island-dominated Aegean Archipelago, within different expeditions (Gerovasileiou et al., 2015; Sini et al., 2017; Galani et al., 2019). Lithistid sponges were found and collected from three marine caves (Figure 1) located on both northern and southern coasts of Crete (Aegean Sea: Blue Cave; Libyan Sea: Lithistid and Italian caves) and one cave (Trypia Spilia) in the isolated Agios Efstratios Island, northern Aegean Sea. The fact that the common feature between these marine caves was the presence of internal freshwater springs led us to collect water samples from the entrance zone and the internal freshwater springs in two of these caves (three replicates from each zone) in order to measure silicate concentration. In addition, the acquisition of a lithistid sample collected by the MEDITS Survey from deep waters of the south-eastern Aegean Sea, provided additional material allowing for comparisons between congeneric lithistids from marine caves and deep waters in the same ecoregion. Descriptions for each sampling site and event are provided below.
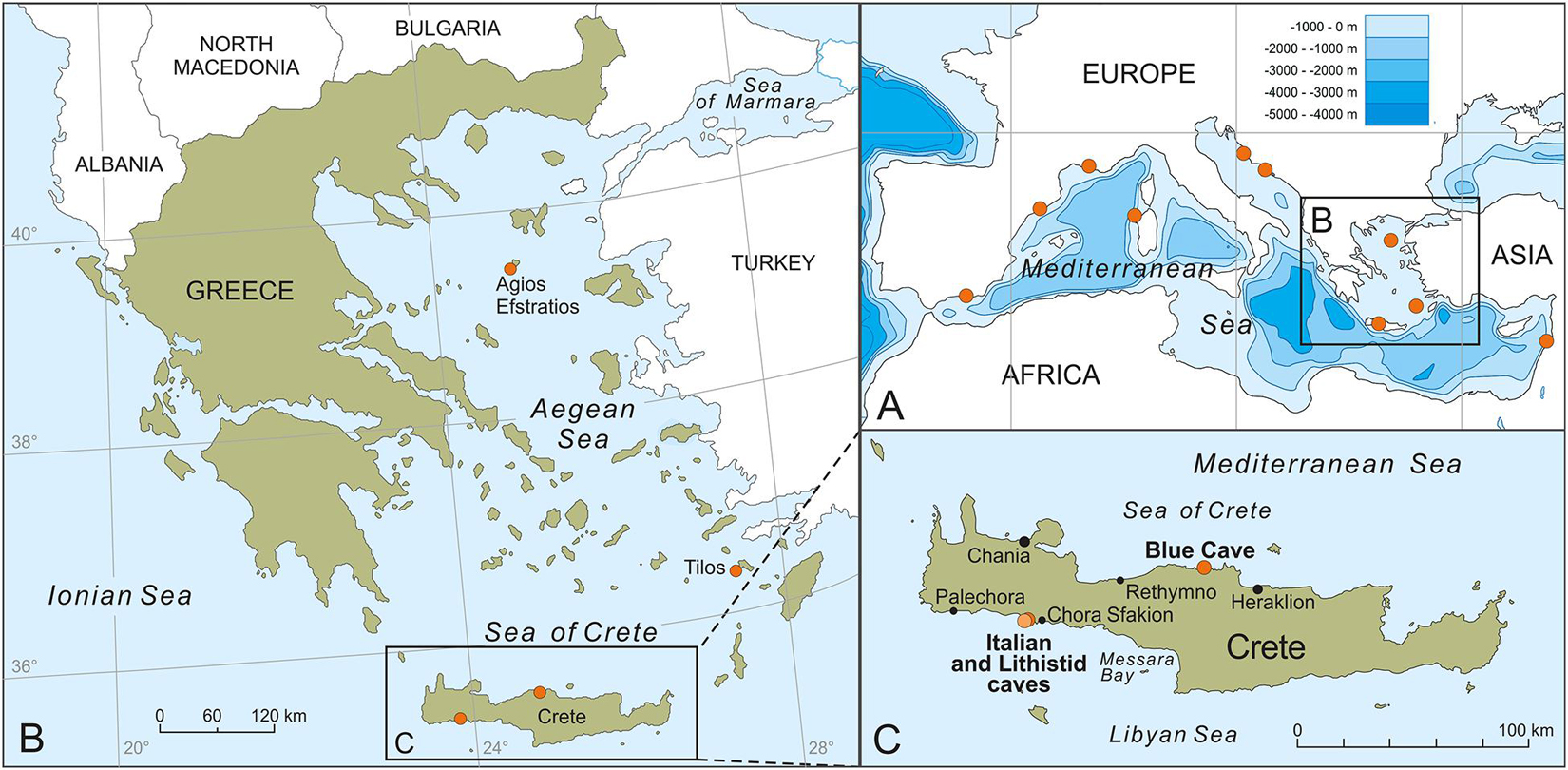
Figure 1. Locations of the studied specimens in the north-eastern Mediterranean Sea (A–C) and other discussed occurrences of lithistid demosponges in Mediterranean marine caves (A).
Two caves are located on the south coast of Crete (Libyan Sea), close to each other (ca. 80 m away), within the marine area of the National Park of Samaria, in Sfakia area: the “Lithistid Cave” (named after this work) and the Italian Cave. The Lithistid Cave (35.2008°N, 24.1191°E) is a large, semi-submerged tunnel cave (0–6 m deep). Lithistids develop in the semi-dark and dark zones of the cave, where freshwater springs are present, at a distance of 15–40 m from the main semi-submerged entrance and 5 m from the second submerged entrance of the tunnel. The highest lithistid abundance and biggest specimens were observed in areas with freshwater mixing and close to freshwater springs. Mean silicate concentration at the internal cave zone was 7.170 μM ± 1.244 (SD) versus 0.663 μM ± 0.042 (SD) at the cave entrance. The cave harbors a rich sessile benthic community (high sponge abundance and cover) that is highly diversified from other marine caves of the north-eastern Mediterranean, possibly due to the influence of freshwater influx (Galani et al., 2019). The Italian Cave (35.2013°N, 24.1198°E) is a large, submerged cave with an internal air pocket. Its entrance is located at 8–12 m depth and leads to a narrow corridor, descending to 18 m depth, which separates the entrance zone from the internal cave chamber that is filled with cool fresh water. While this chamber is seemingly azoic, the cave entrance hosts rich benthic fauna, with a few small-sized lithistids developing on the overhanging walls and ceiling of the entrance, in proximity to small crevices of freshwater influx. No silicate measurements were made due to the small size of crevices and rapid water mixing. The water temperature in both Italian and Lithistid caves was 25–26°C (measured with a SUUNTO dive computer in July 2016 and August 2017) while it was ten degrees lower (16°C) in the internal freshwater chamber of the Italian Cave.
The Blue Cave is a large semi-submerged cave (0–17 m) located at Agia Pelagia area (35.4198°N, 24.9794°E), on the north coast of Crete (Figure 1). The cave hosts a rich sessile benthic community, including one new homoscleromorph sponge species that was recently described by Lage et al. (2018) from a dark sub-vertical tunnel descending from the cave bed. Few lithistids developed in a narrow crevice with freshwater influx, in the terminal section of the cave, at a depth of 3–4 m (Figure 2A). Mean silicate concentration at the opening to this crevice was 3.280 μM ± 1.365 (SD) versus 0.427 μM ± 0.137 (SD) at the cave entrance. Water temperature inside the cave was 25°C (measured with a SUUNTO dive computer in July 2017).
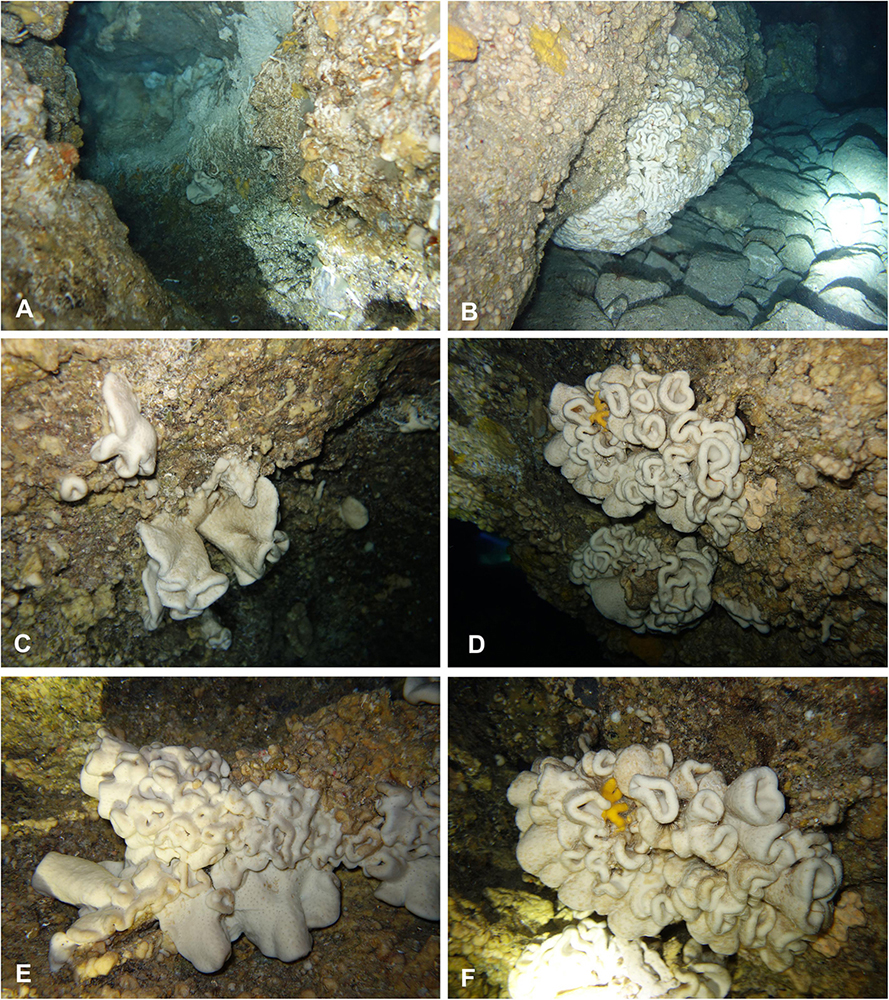
Figure 2. In situ underwater photos of Neophrissospongia endoumensis Pisera and Vacelet, 2011. (A) Blue Cave, northern Crete; (B–F) Lithistid Cave, southern Crete. Photos by V. Gerovasileiou.
Trypia Spilia (meaning hollow cave in Greek) is a largesemi-submerged tunnel on the western coasts of Agios Efstratios Island (39.5349°N, 24.9777°E), northern Aegean Sea. The cave is characterized by a complex geomorphology and hosts a rich sessile benthic community that have been described in detail byDimarchopoulou et al. (2018). The presence of freshwater input dropping from theemerged ceiling and influx from springs in the shallow cave bed (1–2 m deep) was observed during sampling in July 2011, but not in earlier expeditions (August 2009), indicating a sporadic andephemeral character (V. Gerovasileiou, personal observation). Lithistids were found on the walls and floor of the semi-dark cave zone (10–30 m from the two entrances of the tunnel), where freshwater influx was observed. They had a mean surface cover of 0.4% throughout the cave and a maximum cover of 5.5%, count in 25 cm × 25 cm photoquadrats (Dimarchopoulou et al., 2018). The water temperature in the marine cave was 22–24°C (measured with a HOBO data logger in August 2009 and a SUUNTO dive computer in July 2011).
A deep-water lithistid was trawled during the 2018 MEDITS Survey in the frame of the Greek National Data Collection Project from a depth range of 313–352 m, off Tilos Island, in the Dodecanese Islands’ marine region, south-eastern Aegean Sea (Trawl start: 36.3534°N, 27.1804°E, depth: 313 m, Temp: 14.76°C; Trawl end: 36.3375°N, 27.1976°E, depth: 352 m, Temp: 14.72°C).
The sampled material was investigated morphologically under the binocular microscope, as well as under SEM. Preparation of samples followed that described by Pisera and Pomponi (2015). Samples for SEM analyses were sputter-coated with platinum and observed at 25 kV using a Philips XL-20 scanning electron microscope (Institute of Paleobiology, Warsaw). The investigated material is housed in the Institute of Paleobiology, Warszawa, under the collection number ZPAL Pf. 30.
Results
Systematic Description
Order TETRACTINELLIDA Marshall, 1876
Suborder ASTROPHORINA Sollas, 1887
Family CORALLISTIDAE Sollas, 1888
Genus Neophrissospongia Pisera and Lévi, 2002
Type species
Corallistes nolitangere Schmidt, 1870
Remarks
There are four Neophrissospongia species reported from the Mediterranean Sea: N. nolitangere (Schmidt, 1870), N. endoumensis Pisera and Vacelet, 2011, N. radjae Pisera and Vacelet, 2011, and N. nana Manconi and Serusi, 2008. Other two known species, i.e., Neophrissospongia microstylifera Lévi and Lévi 1983 and Neophrissospongia galapagoensis Schuster et al., 2018, are reported from the Pacific. The species N. nolitangere was earlier reported from marine caves in the western Mediterranean but occurs also in the northern Atlantic Ocean (Azores, Madeira, Selvagens, and Canary Islands) (Cruz, 2002; Pisera and Vacelet, 2011; Carvalho et al., 2020). The report of N. cf. nolitangere form the Caribbean Sea (Pomponi et al., 2001) concerns most probably a new, yet undescribed species (A. Pisera, unpublished data). On the other hand, Neophrissospongia tubulata (van Soest and Stentoft, 1988) does not belong to Neophrissospongia (it represents a new undescribed genus – A. Pisera, unpublished).
The species N. endoumensis is known from a single marine cave in the western Mediterranean Sea (Pisera and Vacelet, 2011); N. nana occurs in a single marine cave in Sardinia, western Mediterranean (Manconi and Serusi, 2008) while N. radjae is known from a single marine cave in the Adriatic Sea (Pisera and Vacelet, 2011). Among those known from the Mediterranean and the Atlantic, two species (N. radjae and N. nana) have different habitus, i.e., either massive club shaped (N. radjae) or encrusting (N. nana); thus they are clearly different from our material from the north-eastern Mediterranean Sea. N. nolitangere may be ear-shaped to forming large folded masses (exactly like to studied specimens) while N. endoumensis, when small (only such specimen is known), is cup-shaped and can be developed to folded masses when large (this study). Also, the characters of exhalant and inhalant surfaces are similar in those two species not allowing for easy differentiation. The situation is not simpler in the case of skeletal characters: all these species have very similar dicranoclone desmas, streptaster-spiraster ectosomal microscleres, and microtylostyle choanosomal microscleres; differences between these two species are in small details.
Neophrissospongia endoumensis Pisera and Vacelet, 2011, Figures 2, 3A–G, 4–9.
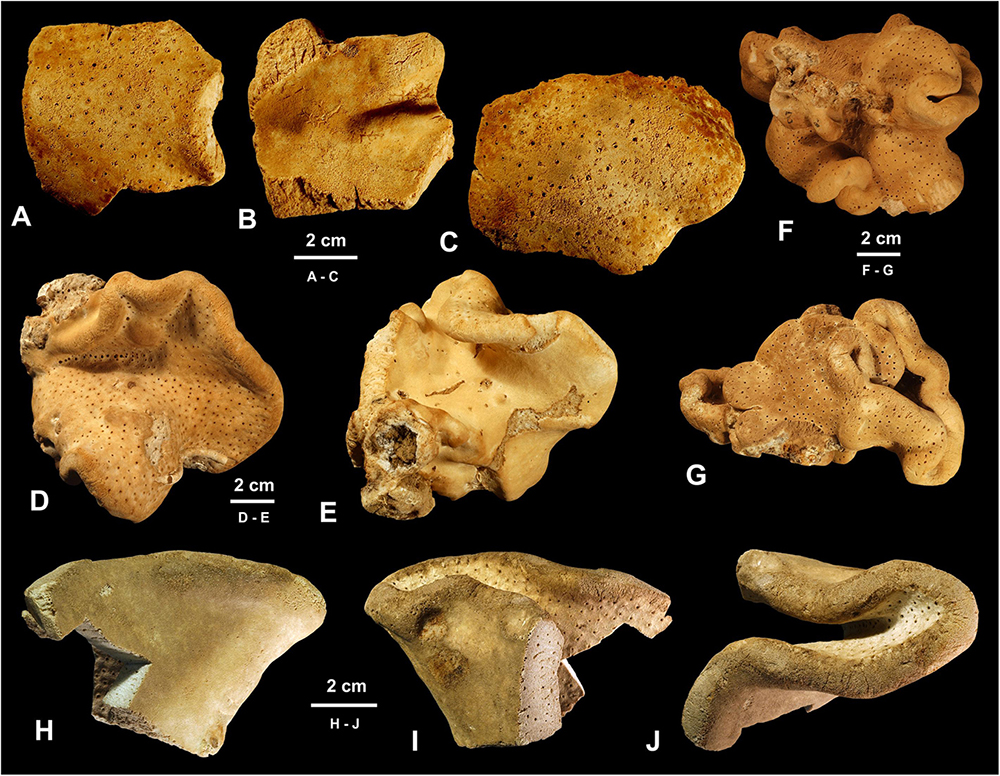
Figure 3. Morphology of studied specimens. (A–G) Neophrissospongia endoumensis Pisera and Vacelet, 2011. (A–C) Specimen ZPAL Pf. 30/1, fragments, Lithistid Cave, southern Crete. (A,C) Exhalant surface view; (B) Inhalant surface view; (D,E) Specimen ZPAL Pf. 30/2, Blue Cave, northern Crete; (D) Exhalant surface view; (E) Inhalant surface view; (F,G) Specimen ZPAL Pf. 30/3, Italian Cave, southern Crete complete contorted specimen in different views. (H–J) Neophrissospongia nolitangere (Schmidt, 1870), fragmentary specimen ZPAL Pf. 30/4, deep Aegean Sea, off Tilos Island [(H–J) inhalant surface views; (J) top view of a folded fragment].
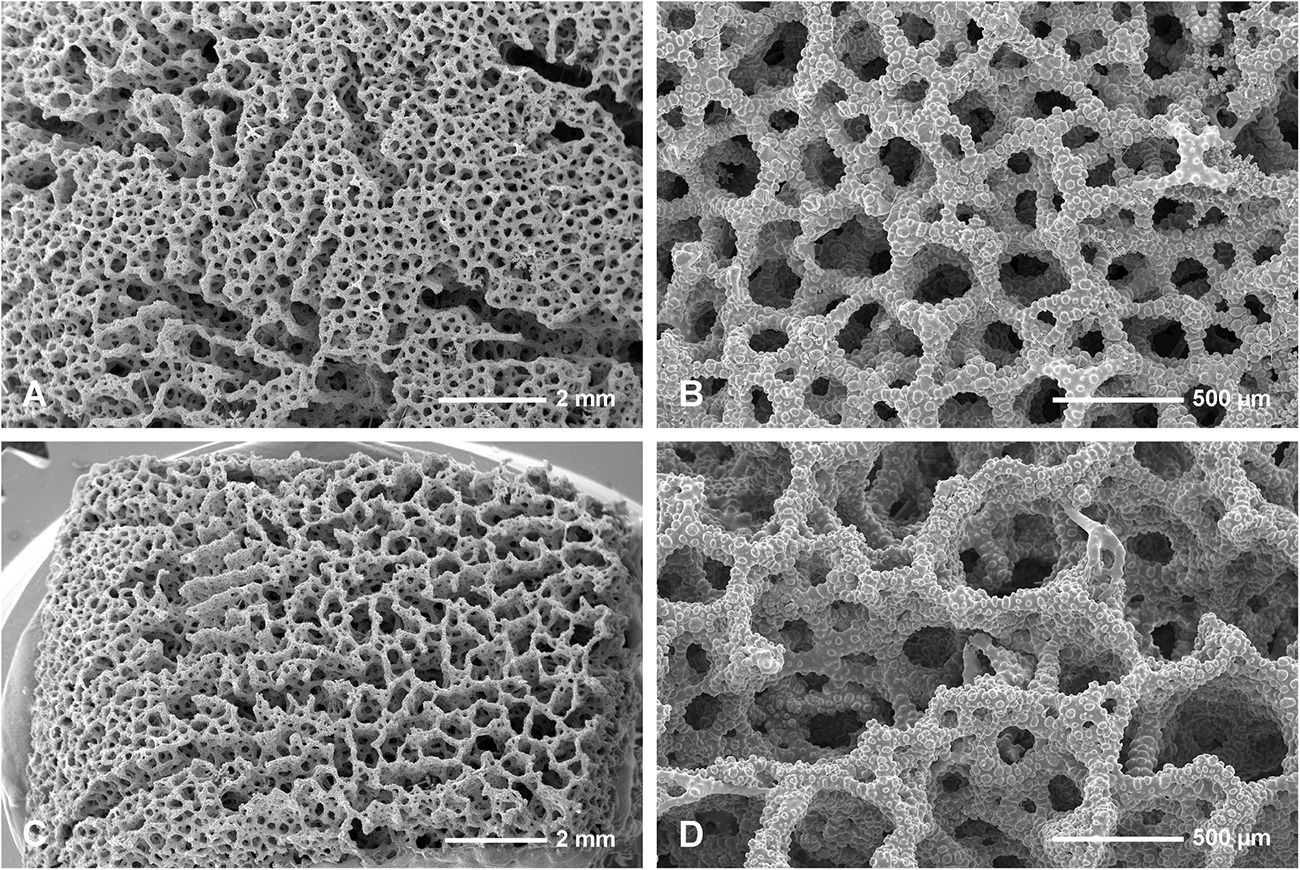
Figure 4. Neophrissospongia endoumensis, Pisera and Vacelet, 2011, Blue Cave, northern Crete. (A,B) Exhalant surface of the choanosomal skeleton of dicranoclone desmas, with system of canals corresponding to oscular openings (A); (C,D) Inhalant surface of the choanosomal skeleton of dicranoclone desmas; (C) General view showing net-like structure; (B) Details of articulated dicranoclones.
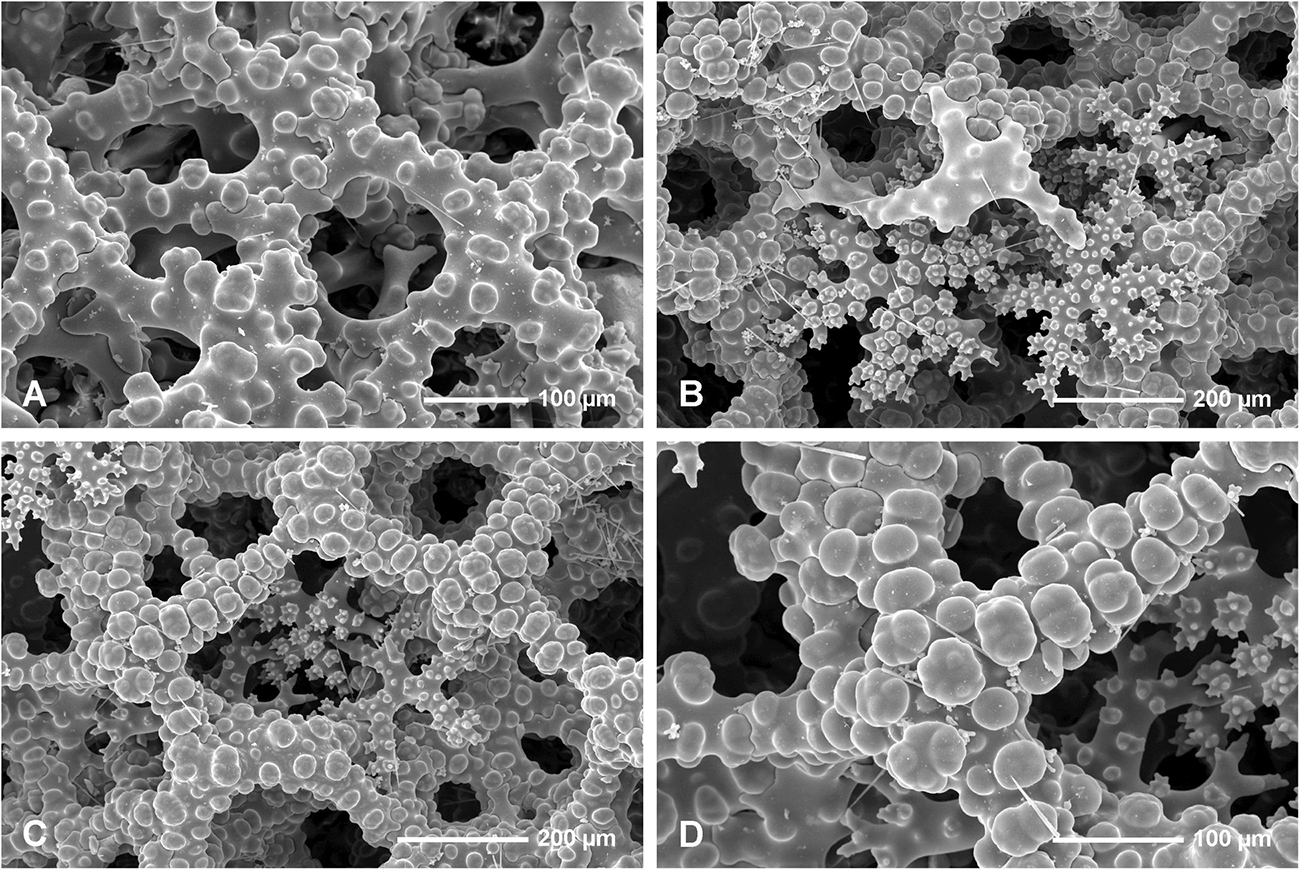
Figure 5. Neophrissospongia endoumensis Pisera and Vacelet, 2011, Blue Cave, northern Crete. (A) Sculpture of dicranoclone desmas of the inhalant surface; (B) Young dicranoclone and ectosomal dichotriaenes inserted among desmas of choanosomal skeleton, exhalant surface; (C,D) Sculpture of dicranoclone desmas of the exhalant surface.
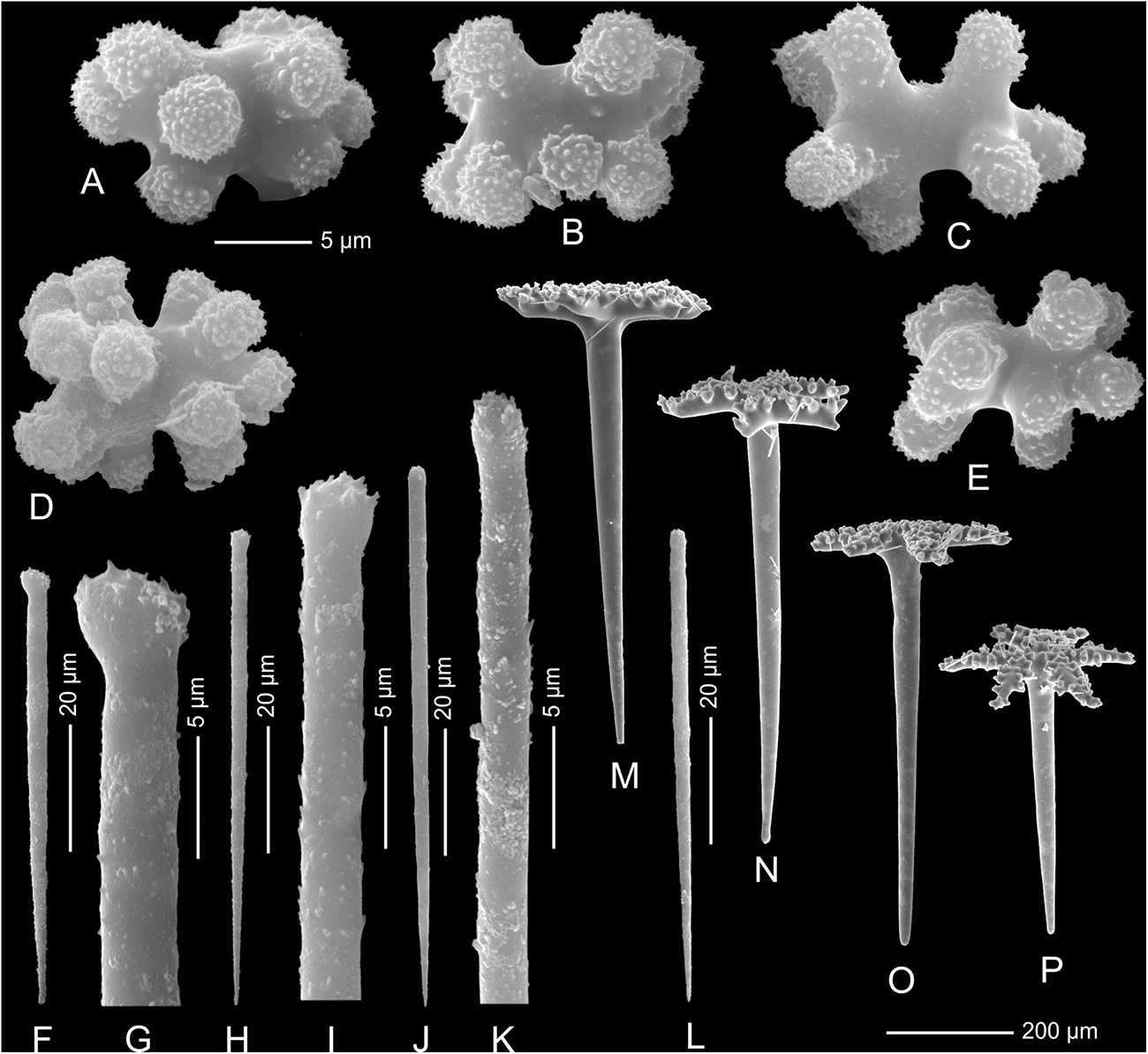
Figure 6. Neophrissospongia endoumensis Pisera and Vacelet, 2011, Blue Cave, northern Crete; (A–E) Streptasters-spirasters microscleres; (F–L) Choanosomal microtylostyles, general view (F,H,J,L), heads (G,I,K); Ectosomal dichotriaenes in side view (M,N,O) and oblique view (P).
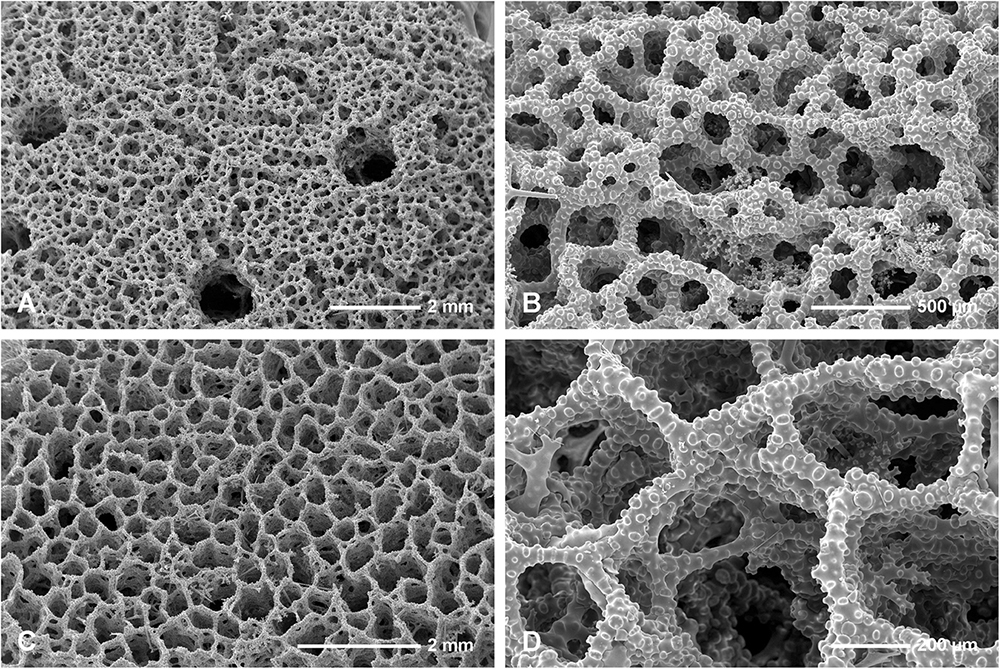
Figure 7. Neophrissospongia endoumensis Pisera and Vacelet, 2011, Lithistid Cave, southern Crete. (A,B) Choanosomal skeleton on the exhalant surface. (A) General view of choanosomal desma skeleton with canal openings corresponding to oscula. (B) Details of dicranoclones desmas articulation and sculpture on the surface; (C,D) Choanosomal skeleton of dicranoclone desmas on inhalant surface; (C) Net-like organization of dicranoclone desmas and (D) details of dicranoclone desmas.
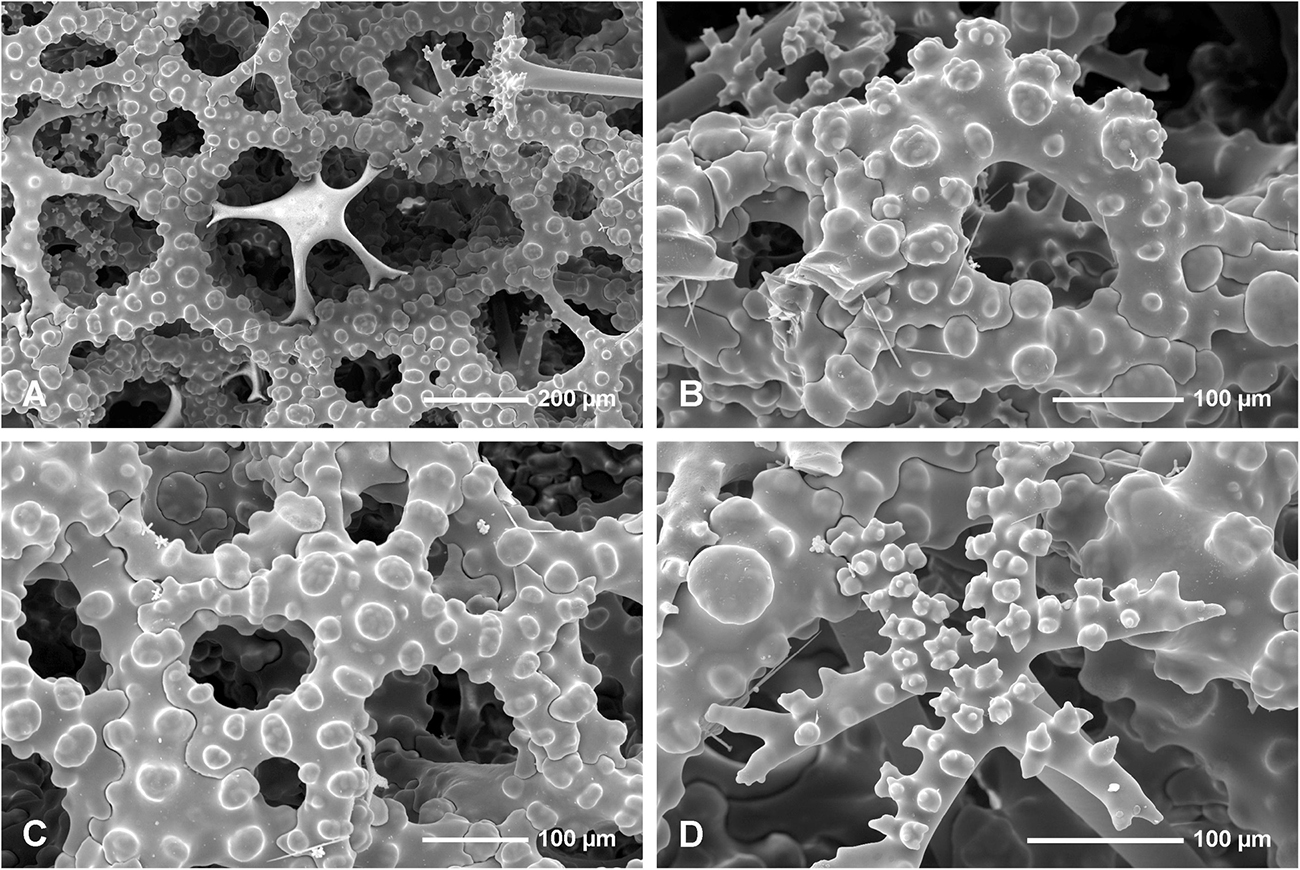
Figure 8. Neophrissospongia endoumensis Pisera and Vacelet, 2011, Lithistid Cave, southern Crete; Exhalant surface of the choanosomal skeleton. (A) Young desma inserted in a large mesh formed by fully developed desma; (B) Strongly arched and sculptured dicranoclone desma as seen in oblique view; (C) Strongly sculptured dicranoclone desmas as seen from the top; (D) Top surface of the cladome of the dichotriaene in situ; note that one arm is partly articulated with choanosomal skeleton of dicranoclones.
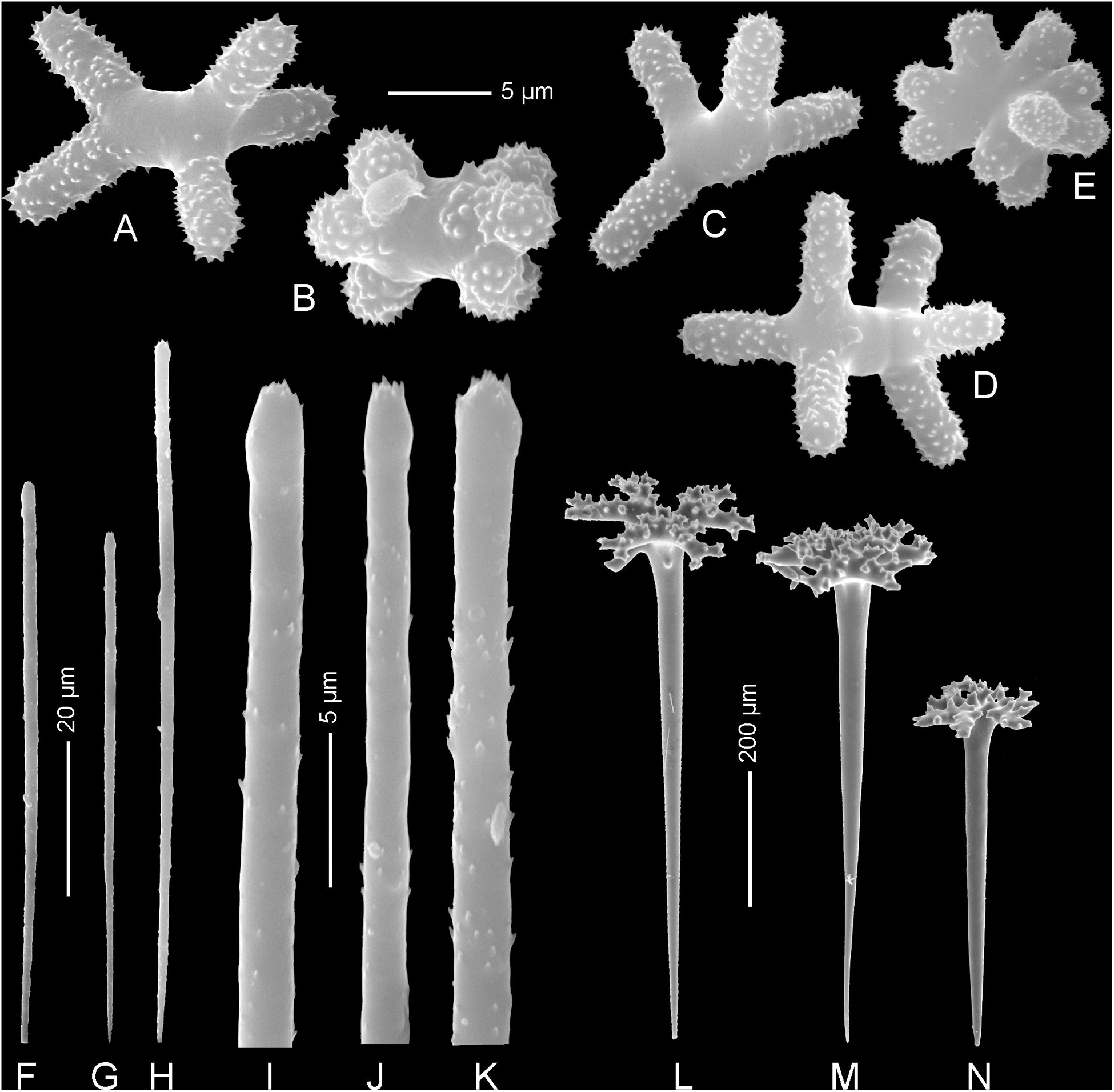
Figure 9. Neophrissospongia endoumensis Pisera and Vacelet, 2011, Lithistid cave, southern Crete. (A–E) Streptasters-spirasters microscleres; (F–K) Choanosomal microtylostyles, general view (F,G,H), and their heads (I,J,K); (L–N) Ectosomal dichotriaenes in slightly oblique view.
N. endoumensis Pisera and Vacelet, 2011: 27, Figures 2H–J, 13–15 and Table 1.
Material Examined
Blue Cave, Agia Pelagia area, north coast of Crete (one specimen – collected on 11 July 2017 by Vasilis Gerovasileiou and Guilherme Muricy); Italian Cave, Sfakia, south coast of Crete (one specimen – collected on 19 August 2017 by Vasilis Gerovasileiou); Lithistid Cave, south coast of Crete (three fragmentary specimens – collected on 11 July 2016 by Vasilis Gerovasileiou and Thanos Dailianis).
Description
In habitus all investigated specimens are very similar (observed in situ in the caves mostly) being cup- to ear-shaped when small (Figure 2C), later they form large (up to 100 cm × 40 cm) folded masses with some cup-trumpet-tubular elements (Figures 2B,D–F). The investigated specimen from the Blue Cave is a complete slightly folded plate about 12 cm × 10 cm; it has wall with rounded margins and is about 1.2 cm (Figures 3D,E). The other specimen from the Italian Cave, southern Crete, measuring 11 cm × 9 cm, is more irregularly folded and complex (Figures 3F,G). There are three fragmentary specimens (fragments of larger folded sponges) investigated from the nearby Lithistid Cave that measure about 6 cm × 5 cm, and 1.4 cm thick wall; 8 cm × 6 cm large and 1.5 cm thick wall and 5 cm × 5 cm large and 1.5 cm thick wall (Figures 3A–C). In all specimens one side (inhalant) is smooth (Figures 3A,E,F), except faint growth lines, and display small 30–50 μm in diameter ostia that are densely distributed over most of the surface. They are vaguely grouped above underlying meshes of choanosomal skeleton. The other surface (upper one) displays round, about 0.5–1 mm large openings (oscula) that are at the level with sponge surface or in some regions slightly raised (Figures 3A,C,D). In furrows of the folds these openings are slightly larger, up to 2 mm in size and much more densely located.
In all studied specimens choanosomal skeleton consists of strongly tuberculated and arched dicranoclones that are approximately (it is difficult to measure articulated spicules) (285 μm minimum) 333–446 μm on average in size (see Table 1 for differences in specimens from particular caves). Dicranoclones form dense irregular choanosomal skeleton on the exhalant side (Figures 4A,B, 7A,B) and less dense, in the form of less regular net, on the inhalant one (Figures 4C,D, 7C,D). On exhalant surface of choanosomal skeleton openings (about 1 mm in size) corresponding to oscula are visible; often they are surrounded by irregularly radial furrows in the surface of the skeleton (Figures 4A, 7A). Dicranoclones densely covered (especially on exhalant side) by large, mushroom shaped tubercles that may bear smaller low tubercles (Figure 5). Ectosomal dichotriaenes with massive clades, and strongly tuberculated upper surface of the cladome; they are covered with numerous densely packed, vertically pointed, tubercles that bear (are divided into) several spines on their top. Cladomes are between 181 and 349 μm in diameter (it varies between particular caves: see Table 1). Rhabdomes are 434–766 μm long (see Table 1) and 30.8–55.0 μm thick at the top and with rounded lower tip.
Microscleres are of two types: choanosomal spinose microtylostyles, and massive streptasters/spirasters with short and thick arms covered with triangular low spines, concentrated in the ectosome. Microtylostyles are from 64.4 to 99.2 μm long and 1.21 to 2.89 thick, while streptasters/spirasters size varies between 10.3–17.5 × 9.8–14.2 μm (see Table 1).
Remarks
The investigated specimens differ from the holotype, the only one earlier known specimen that is cup-shaped, by forming large folded masses. This difference follows, most probably, from the fact that the holotype is much smaller/younger. Otherwise, all characters of the specimens from Crete are the same as in the holotype. Dichotriaenes in our material have slightly larger cladomes and longer rhabdomes; on the contrary microtylostyles may be longer in the holotype, and slightly larger are streptasters, but in all cases their size overlaps in part with our material. In all the studied caves several motile invertebrates (e.g., crinoids, sea urchins, and gastropods) were sheltering in the cavities of the lithistids’ folded plates while small-sized sessile taxa (e.g., brachiopods attached to exposed dead fragments of choanosomal skeleton, and thinly encrusting sponges) developed on the lithistids’ hard surface. Therefore, Neophrissospongia seems to have an important ecosystem engineering role in the marine cave ecosystem, providing micro-habitats to various taxa.
This species is very similar to N. nolitangere, being identical with it in habitus. The difference is in details of spiculation, mostly character of streptasters/spirasters and ectosomal dichotriaenes. Streptasters are more massive, regular and with shorter arms and larger in N. endoumensis than in N. nolitangere. Dichotriaenes in N. endoumensis have more complex and massive cladomes while its desmas are more massive, often with swollen central part, while in N. nolitangere they are rather linear. Anyway, these differences are small and great intraspecific variability, as shown by specimens from different caves (Table 1), allows to raise the question if these two nominal species are not only ecophenotypic variants (cave versus deep-water) of the same species (see section “Discussion”). These aspects should be investigated in detail, especially by systematic study of spicules variability contrasted with silica contents of sea water, and by molecular methods. Finally, it is worth to mention that similar problems to interpret variations in morphology have been encountered in case of different populations (especially bathyal and shallow water cave populations) of the sphinctozoan sponge Vaceletia having calcareous skeleton (Vacelet et al., 1992; Wörheide and Reitner, 1996; Vacelet, 2002).
Neophrissospongia nolitangere (Schmidt, 1870), Figures 3H–J, 10–12 and Table 1.
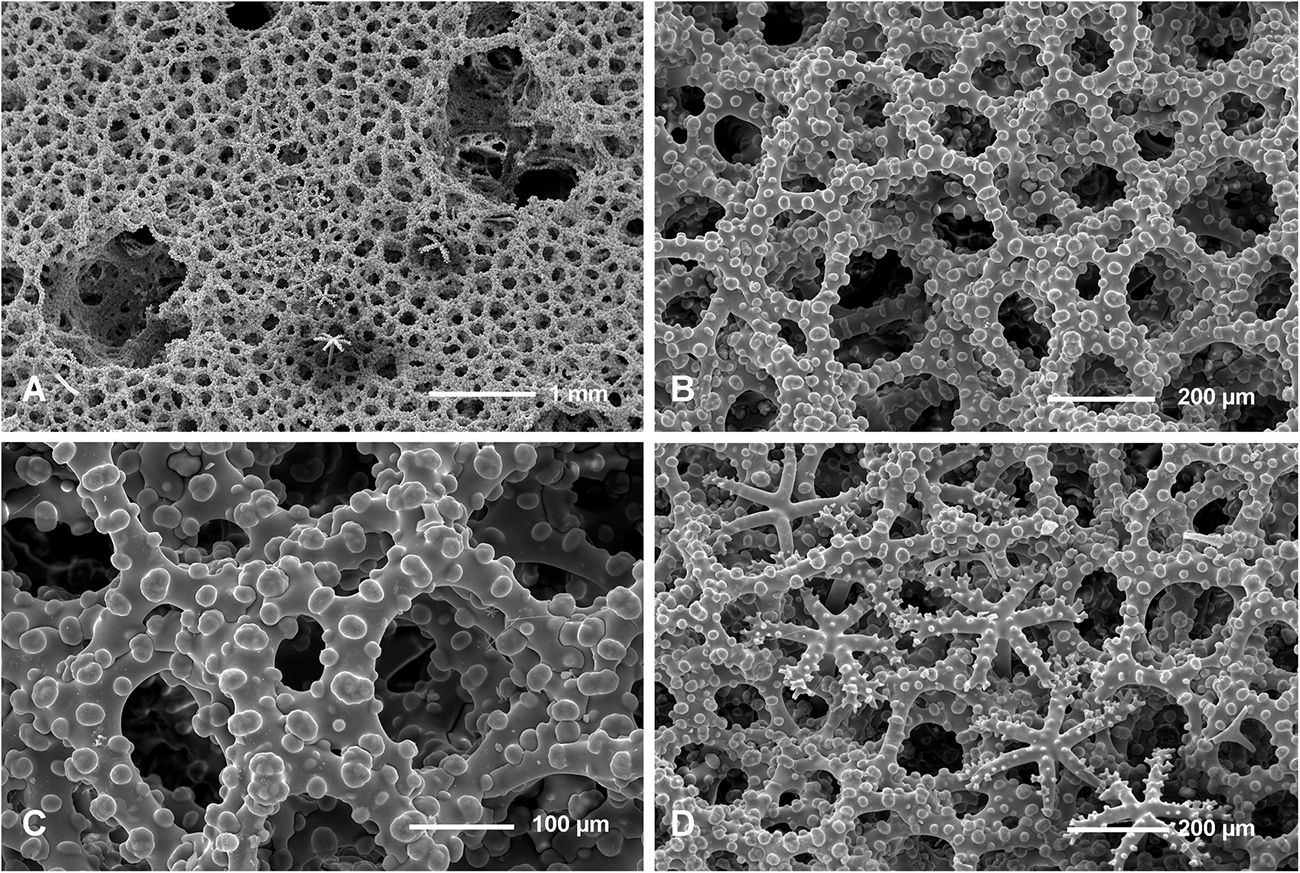
Figure 10. Neophrissospongia nolitangere (Schmidt, 1870), south-eastern Aegean Sea (deep-water), off Tilos Island; Exhalant surface of the choanosomal skeleton. (A) General view of choanosomal desma skeleton with canal openings corresponding to oscula; (B,C) Details of dicranoclone desmas articulation and sculpture; (D) Ectosomal dichotriaenes in situ.
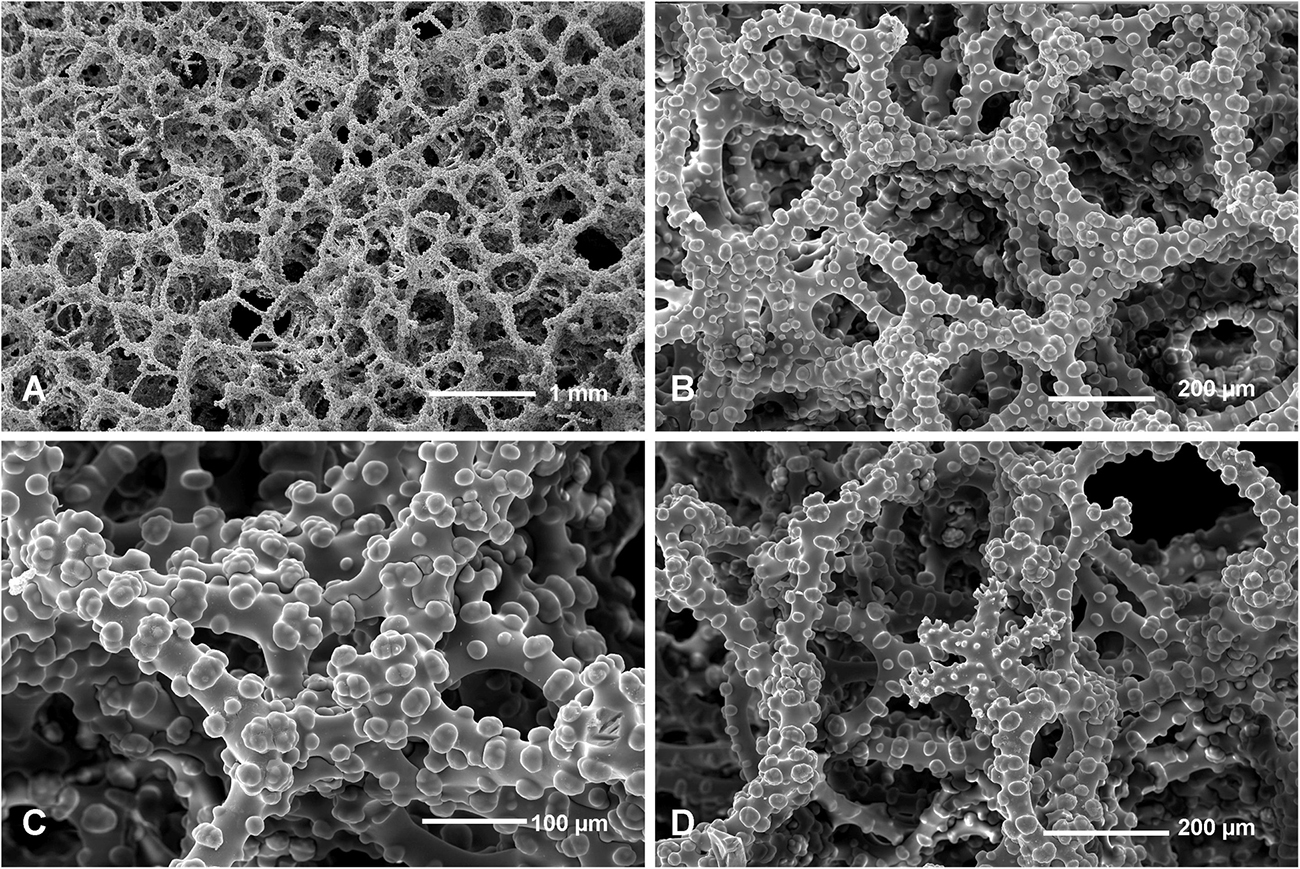
Figure 11. Neophrissospongia nolitangere (Schmidt, 1870), south-eastern Aegean Sea (deep-water), off Tilos Island. Inhalant surface of the choanosomal skeleton; (A) General view of choanosomal skeleton to show irregular net; (B,C) Details of dicranoclone desmas articulation and sculpture; (D) Ectosomal dichotriaene in situ, among desmas.
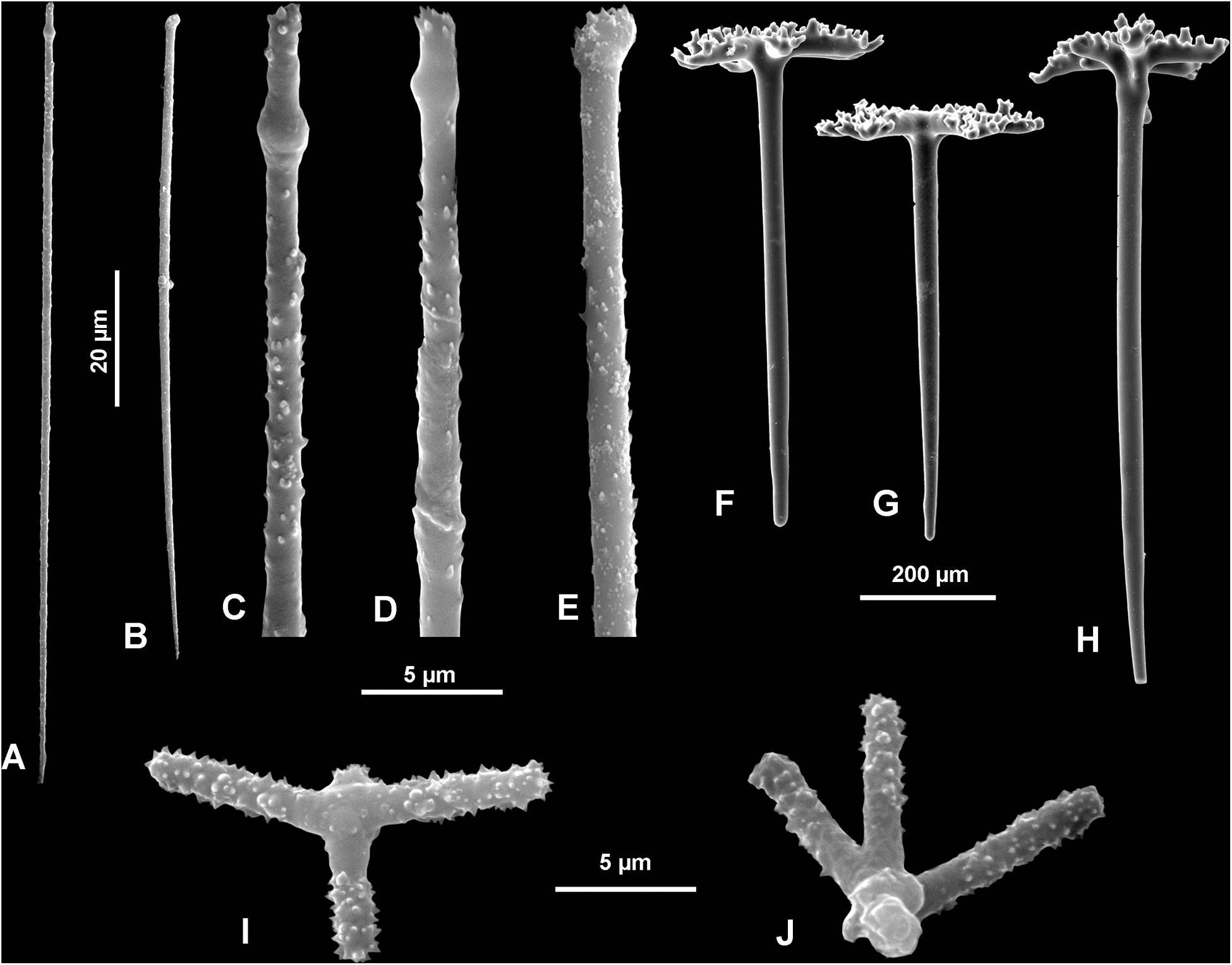
Figure 12. Neophrissospongia nolitangere (Schmidt, 1870), south-eastern Aegean Sea (deep-water), off Tilos Island. (A–E) Choanosomal microtylostyles, general view (A,B) and their heads (C–E); (F–H) Ectosomal dichotriaenes in lateral view; (I,J) Streptasters.
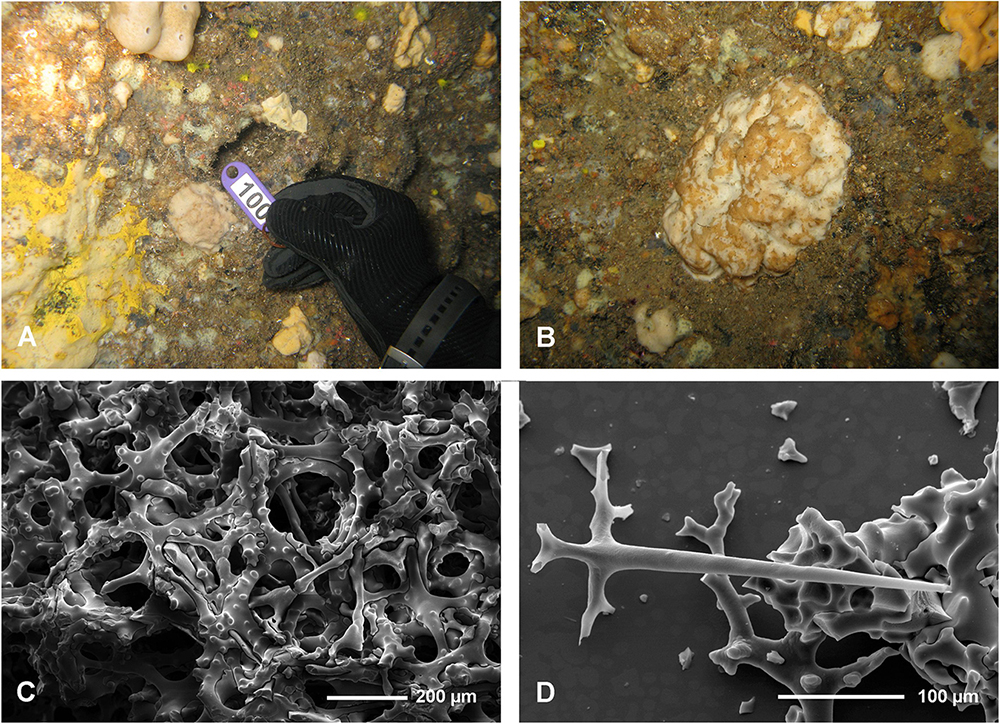
Figure 13. Neophrissospongia cf. nana Manconi and Serusi, 2008, (A,B) Habitus; underwater photo of sponges in situ; (C) Choanosomal skeleton of confused and malformed dicranoclone desmas; (D) Young (not completely formed; note open axial canals) dichotriaene. Photos (A,B) by V. Gerovasileiou.
Neophrissospongia nolitangere (Schmidt, 1870); Pisera and Vacelet, 2011: 26 cum syn., Figures 2C–G, 10–12 and Table 1; Carvalho et al., 2015: Figure 2B.
Material Examined
Aegean Sea; deep-water specimen from a depth of 313 to 352 m, off Tilos Island (Dodecanese Islands, southern Aegean Sea) (Figure 1). Collected on 12 July 2018 by Panagiota Peristeraki.
Description
The fragmentary specimen is about 9 cm × 6 cm large and represents a folded/contorted plate-like sponge with rounded margins; thickness of the wall is about 1.2 cm. One side smooth, with ostia, that are about 3–40 μm in diameters and organized in a vague field above underlying meshes of choanosomal skeleton. The opposite (exhalant) side with numerous oscula, about 0.7–1 mm in diameter, with slightly raised margins; they are evenly distributed over the entire surface.
Choanosomal desmas are strongly tuberculated dicranoclones (tubercles are mushroom-shaped, may be divided into low smaller tubercles) and arched, rather linear, and are 296–360 μm in size. They form irregular and dense net with small meshes on the exhalant surface, and irregular net with larger, 0.5–0.6 mm wide meshes on the inhalant surface.
Ectosomal dichotriaenes with regular and not branched (except slightly on tips) clades that bear on the upper surface numerous tubercles and spines (toward the tips) that may be divided into smaller spines. Cladomes are 299–411 μm in diameter, while rhabdomes are 543–904 μm long.
Microscleres are of two types: choanosomal spinose microtylostyles, and massive streptasters/spirasters with short and thick arms covered with triangular low spines, concentrated in the ectosome. Microtylostyles are from 90.8 to 116 μm long and 1.3–1.6 μm thick, while streptasters/spirasters size varies between 8–9 μm × 13–14 μm (see Table 1).
Remarks
This species is very similar in habitus to N. endoumensis described above. It differs from the investigated here specimens of N. endoumensis from Crete in details of spiculation. It has less massive clades/cladomes of the dichotriaenes that are more strongly tuberculated. Rhabdomes of the dichotriaenes are slightly longer in N. nolitangere. Meshes of choanosomal skeleton on the inhalant surface is less regular in N. nolitangere than in N. endoumensis; streptasters are smaller and less regularly developed, while microtylostyles are slightly longer and thinner than in N. endoumensis from Crete. See also remarks for N. endoumensis above.
Neophrissospongia cf. nana Manconi and Serusi, 2008, Figure 13.
cf. Neophrissospongia nana Manconi and Serusi, nov. sp.: p. 73–76, Figures 1–4 and Table 1.
Material Examined
Trypia Spilia Cave, Agios Efstratios Island, north Aegean Sea (fragmentary specimen ZPAL Pf. 30/5 – collected on 5 July 2011 by Vasilis Gerovasileiou and Elena Akritopoulou).
Description
The studied material consists of small fragments of a small encrusting sponge found in one cave (Trypia Spilia) in Agios Efstratios Island. An original sponge is about 6 cm in max diameter, low domical and rounded in shape (Figures 13A,B). Choanosomal skeleton consists of very irregular tuberculated desmas (dicranoclones) that form very irregular (confused) choanosomal skeleton. Desmas are so irregular that they can be interpreted as pathologic/not fully developed. No microscleres were observed. Only one young (not fully developed) dichotriaene with axial canals, still not closed, has been found.
Remarks
The investigated specimens lack important characters, i.e., microscleres, and well developed dichotriaenes that are either underdeveloped or pathologic, making precise determination impossible. In habitus and irregularity of the skeleton it resembles mostly the specimens from Sardinia described as N. nana (Manconi and Serusi, 2008). We are inclined to interpret our specimen as pathologic (possibly due to low silicate content of water). The same might concern species N. nana described by Manconi and Serusi (2008).
Discussion
The Provenance of the Lithistid Cave Fauna
A number of lithistid demosponges are known only from a single marine cave: Aciculites mediterranea from Grotta dei Laghi, Sardinia, Italy (Manconi et al., 2006), Gastrophanella phoeniciensis and Microscleroderma lamina from Lithistid Cave, Chak El Hatab, Lebanon (Perez et al., 2004), Neophrissospongia nana from Grotta delle Terrazze, Sardinia, Italy (Manconi and Serusi, 2008), N. endoumensis from Grotte d’Endoume, Marseille, France (Pisera and Vacelet, 2011), N. radjae from a cave in Korčula Island, Croatia (Pisera and Vacelet, 2011), and also some undescribed species from the Canary Islands (A. Pisera, unpublished data). In the context that cave lithistids fauna consist of species often not known outside particular caves, a question arises if these species are really restricted to this habitat and evolved within the cave system, representing cave-exclusive fauna, or are just vestiges of unrecognized deep-sea populations whose larvae just colonized suitable shallow caves from some yet unknown deep-water populations. The hypothesis of cave-exclusive (stygobiotic) fauna for lithistids should be rather rejected due to the fact that some of these species occur in geographically distant and fragmented marine caves; N. radjae in a single Adriatic cave and also in Spanish (Alboran Sea) caves (A. Pisera, unpublished data), and N. endoumensis in Marseille and now in caves of Crete. Thus, exchange of their propagules between these caves seems impossible, especially if we consider time available since cave colonization (see below).
A similar hypothesis has been suggested for the demospongeLycopodina hypogea (Vacelet and Boury-Esnault, 1996), belonging to the carnivorous deep-sea family Cladorhizidae, that was first described from a submarine cave with deep-sea affinities in Marseille (Trois Pépés Cave), then was recorded in two other caves in Marseille and the Adriatic Sea (Bakran-Petricioli et al., 2007) and was recently observed to thrive in several deep-water areas in the Mediterranean Sea (e.g., Aguilar et al., 2011; Bo et al., 2020) and in the north-eastern Atlantic (Chevaldonné et al., 2015). Another typical example of deep-sea sponges known from submarine caves is the hexactinellid Oopsacas minuta Topsent, 1927 that was known from the deep western Mediterranean Sea and Trois Pépés Cave in Marseille (Vacelet et al., 1994) and was later found in caves of the Adriatic Sea and several deep-water sites in the western and central Mediterranean basins (Bakran-Petricioli et al., 2007). The usual occurrence of several deep-water or “cryptobiotic” (i.e., preferring cryptic habitats) species in caves, which originate from external marine environments but become characteristic of this particular habitat only secondarily, has been also described as “secondary stygobiosis” (Gerovasileiou and Bianchi, in press, and references therein).
Dark marine caves present several analogies with deep-sea ecosystems and have been characterized as natural “mesocosms” of the bathyo-abyssal zone, providing an easily accessible model for the study of deep marine ecosystems (Vacelet, 1996; Harmelin and Vacelet, 1997). Themain ecological features shared between marine caves and deep-sea ecosystems include the lack of light (and primary production), oligotrophy and, in some cases, density-trapped seawater which remains cold and at a nearly constant temperature all year round (Vacelet et al., 1994; Harmelin, 1997), among others. Such conditions allow for the colonization of propagules of deep-sea species which are upwelled from adjacent deep-waters; for instance, this is the casefor many bathyal species in Trois Pépés Cave, whose likely source of propagules is the nearby Cassidaigne Canyon (Vacelet et al., 1994; Vacelet, 1996; Harmelin and Vacelet, 1997). Large populations of lithistid sponges have been recently described in the deep Mediterranean Sea, specifically aggregated clumps of Leiodermatium pfeifferae on the top of seamounts off the Balearic Islands (Maldonado et al., 2015). Indications that such unrecognized deep-water populations of lithistid sponges, which may be a source of propagules for shallow marine caves, exist in the deep north-eastern Mediterranean Sea, include the here described occurrence of N. nolitangere from the south-eastern Aegean Sea (313–352 m) and photos of tetractinellids on low outcropping reefs (473 m depth), possibly Neophrissospongia, taken by a Remotely Operated Vehicle in the central Aegean Sea (Figure 15A in Bo et al., 2020 and unpublished sources of V. Gerovasileiou). It is also worth noting that the steep southern margin of Crete, and specifically the marine area off Samaria, where two of the surveyed caves are located (Italian and Lithistid caves), is incised by numerous gullies and deep canyons. The occurrence of N. endoumensis in caves on both southern and northern coasts of Crete indicates that populations should exist in intermediate areas as well, which encompass extensive carbonate rocky coasts, with a high number of still unstudied marine caves, some of which have internal freshwater influx (V. Gerovasileiou unpublished data), but also deep waters. More specifically, the 200 m isobath is only ca. 2 km away from the Italian and Lithistid caves and ca. 3.5 km from the Blue Cave, while it is much closer to the coasts in intermediate locations between these caves (based on data from the EMODnet Bathymetry portal1). Agios Efstratios is one of the most isolated islands in the Aegean Archipelago, being approximately 30 km away from the nearest island (Lemnos), and is surrounded by waters deeper than 200 m (ca. 11 km from Trypia Spilia), standing in the center of the northern Aegean Sea (Dimarchopoulou et al., 2018).
It was also suggested that some submarine cave organisms may be “very old [even Mesozoic] elements” of relictual character and that “marine caves served as faunal refuges over very long periods of time” (Hart Jr., Manning and Iliffe, 1985). Lithistids from Lebanese caves have been characterized as “palaeoendemic” remnants of the subtropical/tropical assemblages, which thrived in the Mediterranean during warm periods of its history and survived only in the warmer south-eastern section of the basin during the subsequent cooling episodes (Perez et al., 2004). This hypothesis cannot be maintained in the case of the studied lithistids from submarine caves of Crete in particular, and the Mediterranean in general (but in fact it concerns any shallow water marine caves) because it is ahistorical, i.e., not accounting for physiographic changes leading to formation and appearance of caves, palaeogeography and sea level changes during long periods of time. In the Mediterranean case such events and sea level changes include, for example, Messinian salinity crisis (about 5.6 My ago) (Bianchi et al., 2012), and more recently last glaciation that is global and lasted between 115,000 and 11,700 years ago, causing sea level drop of about 145 m in the region of Crete about 20,000 years ago (Lambeck and Purcell, 2005).
Caves themselves cannot be of Mesozoic or even Paleogene in age. Most of the karstic caves in the Mediterranean regionoriginated subaerially, most probably during glaciations (especially last glaciation, i.e., during the period between 115,000 to 11,700 years ago) and were submerged after (Arko-Pijevac et al., 2001). Thus, cave invertebrates, including the studied here lithistid demosponges must have colonized the surveyed caves rather recently, after the last glaciation. During the last glaciation sea level around Crete was about 145 m lower than today, similarly as in the rest of the Mediterranean, and still about 10 m below the present sea level about 7,000 years ago and about 2 m lower about 3,000 years ago (Lambeck and Purcell, 2005). One has to remember that during Holocene relative sea level stands in western-central and eastern parts of Crete were considerably different due to tectonic movements (Mourtzas et al., 2016). Having in mind those values, the colonization of the studied caves that are 3–12 m deep could occur between about 7,000 and 3,000 years ago, but not earlier (depending on the cave).
The only relictual sponges known to date are fossilized lithistids (“mummies” – sponges preserved as complete specimens by early precipitation of calcium carbonate, see Matyja, 1978; Pisera, 1997) from Trois Pépés Cave (Pisera and Vacelet, 2011). They may be interpreted as relicts from previous submergence of the cave, about 8,000 years ago, or during earlier warm interglacial periods. Similar fossilized relictual sponges were observed in Sardinian caves (A. Pisera, unpublished) but were described also from deep Mediterranean waters (Allouc, 1987).
In the case of very old, relictual character of lithistid sponges, one should expect either much larger sponges to occur, or numerous fossilized (dead) sponges that should accumulate in a cave (lithistids due to their articulated skeleton preserve well after death). None of these is observed in all known to us caves (not only here reported). Very little is known about growth rate of lithistid demosponges. The only systematic study is the report by Ellwood et al. (2007) on growth rate of Corallistes undulatus Lévi and Lévi, 1983 (closely related genus to Neophrissospongia) from deep waters off New Caledonia, that was about 20 × 30 cm large, and revealed values between 1.1 and 1.3 mm/year, giving 135–160 years old. Should we apply these values to the largest specimen observed in the Lithistid Cave, that was 100 cm × 40 cm in size (Figure 2B), the estimated age of this specimen is between 769 and 909 years, if longer dimension is used. Although this is only a first approximate estimation, in the absence of other data for comparison, it equally indicates relatively new (in accordance with known geological history and sea level changes) colonization of the caves.
Silica Content of Sea Water and Occurrence of Lithistid Demosponges
Lithistid demosponges with their dense choanosomal skeleton consisting of articulated desmas are heavily silicified, being even called “hypersilicified” sponges. They occur mostly in deep waters below 100 m, being very common at several hundreds of meters, where silica concentration is much higher than in shallow water. Numerous species of lithistid demosponges, including Neophrissospongia, are known from Mediterranean marine caves (Pisera and Vacelet, 2011; Gerovasileiou and Voultsiadou, 2012), as well as from the Atlantic and Pacific oceans (A. Pisera, unpublished data). This is usually explained by the fact that the dark zones of marine caves are characterized by conditions similar to bathyal depths (see previous section) by being dark, oligotrophic and often with low temperature. Nevertheless, in the studied marine caves lithistids were not found exclusively in dark zones, but also close to entrances and in semi-dark zones, while water temperatures reached 22–26°C in summer versus 14.7°C in the deep Aegean Sea (313–352 m), where N. nolitangere was trawled.
Lithistid demosponges are found in some, but not all dark submarine caves. Their absence from some caves and presence in others may be caused by chance: lack/presence of deep-water sources of propagules, unfavorable/favorable physiographic conditions of the sea floor near the caves, and similar factors. We have identified, however, an interesting coincidence between the occurrence of lithistid sponges (in case of the Lithistid Cave of large masses of these sponges) in different marine caves of the oligotrophic eastern Mediterranean Sea; all caves – and sections within these caves – where lithistids were found have freshwater springs. In addition to our observations from the north-eastern Mediterranean, Perez et al. (2004) found dense populations of lithistids in a shallow (2–5 m deep) cave of Lebanon, Levantine Sea, including areas with freshwater mixing. Publications reporting lithistids from other Mediterranean marine caves do not provide specific information on freshwater input, except for caves with freshwater infiltrations in their inner and upper portions (Pouliquen, 1972). Such infiltrations (e.g., through cracks and percolations of rocks) are very common in Mediterranean marine caves but are usually sporadic and/or seasonal (Gerovasileiou and Bianchi, in press).
This association between caves with freshwater springs and lithistids is surprising and difficult to understand at the first moment due to the fact that lithistid demosponges are typical marine invertebrates. This situation is easier to understand if we consider that silicic acid concentration of water in such parts of the caves are 8–11 times higher (and comparable to deep seawater at several hundred meters) than its concentration in water near the cave entrance (and average of surface water in the area). In the case of Blue Cave silicic acid concentration is 3.280 μM ± 1.365 (SD) in the zone where lithistid sponges occur (similar values of about 2–3 μM characterized seawater at a depth of 200–300 m in the Cretan Sea, Souvermezoglou and Krasakopoulou, 2000) versus 0.427 μM ± 0.137 (SD) at the cave entrance. In the Lithistid Cave silicate concentration is 7.170 μM ± 1.244 (SD) in the zone where lithistid sponges thrive (6–11 μM for several hundred meters deep in the eastern Mediterranean Sea, Krom et al., 2014) versus 0.663 μM ± 0.042 (SD) at the cave entrance. In addition to the lithistid populations, other “hypersilicified” sponges (geodiids) also occur in high abundances and/or size in the Lithistid cave, but are uncommon in external environments of Crete, except for rocky areas with freshwater springs (V. Gerovasileiou, personal observations). The specimens of N. nolitangere found off Tilos Island, at about 300 m depth, occurred in waters characterized by values of 2.5–3 μM (Souvermezoglou and Krasakopoulou, 2000) that are still lower than those observed in the studied caves. Such differences may be significant for lithistids, with their heavily silicified skeleton as proven by desma-less theonellids occurring in shallow water (thus very low in silicic acid) of the Great Barrier Reef (Hall et al., 2014). Higher siliceous acid concentration, resulting from hydrothermal vents, is recalled as a factor promoting sponge growth in shallow waters of Indonesia (Bertolino et al., 2017). The importance of silicic acid concentration (however, much more extreme and under experimental conditions) for spiculogenesis in demosponges was demonstrated by Maldonado et al. (1999), while correlation between this concentration and disruption of spiculogenesis in natural conditions in other heavily silicified demosponges, i.e., geodiids was discussed by Cardenas and Rapp (2013). They have demonstrated that some spicules in shallower water characterized by lower silica concentration may become irregular and underdeveloped as it is the case of our N. cf. nana, for example. Also, high variability of spicules in other species described here may be of similar origin. Specifically, the difference between microscleres (that are the first to react to lower silica concentration – Cardenas and Rapp, 2013) between N. endoumensis and N. nolitangere could be the result of such dependence, and we should check if these two nominal species could be just ecomorphological variants. One has to remember that such correlation is not a simple one and not associated with particular level of silicic acid concentration alone, and probably depends on other associated factors such as food availability and temperature (Cardenas and Rapp, 2013). This possibility must be investigated more systematically in case of other marine caves with lithistid sponges with quantitative approaches and molecular methods.
Conclusions
All the three Neophrissospongia species described in this study are reported for the first time from the eastern Mediterranean Sea, revealing a broad geographic distribution, and are also new elements for the sponge fauna of Greece. The species N. endoumensis, previously known from only a single cave in Marseille (France), was described from three shallow marine caves on both southern and northern coasts of Crete. One of these caves harbored an abundant population of this lithistid that may reach large size (100 cm × 40 cm) and old age (approximate estimation between 769 and 909 years). Another species, i.e., N. nolitangere was described from deep waters (ca. 300 m) off Tilos Island (south-eastern Aegean Sea) and N. cf. nana from a shallow marine cave in Agios Efstratios Island (northern Aegean Sea). The studied caves in Crete had to be colonized by these sponges relatively recently, i.e., not earlier than 7 ky, and most probably 2–3 ky ago from yet unknown deep-water sources, as proven by the presence of N. nolitangere and other lithistids in the deep north-eastern Mediterranean Sea. To our opinion they are not relictual forms from the past that survived in marine caves from long time ago as sometime suggested.
Here we hypothesize that the presence of these lithistid sponges in specific marine caves of this marine area, out of the several tens of explored caves, was controlled not only, as postulated earlier, by the fact that dark zones of the studied caves resemble deep-water environment by lack of light and oligotrophy, but also because these parts are characterized by relatively high (as for the Mediterranean) concentration of silicic acid, delivered to the caves by freshwater sources occurring in these sections. This conclusion is also supported by the presence of other “hypersilicified” sponges in high abundances and size in these marine caves, which are uncommon in other habitats, and also from observations in other marine caves with freshwater springs.
We also hypothesize that high intraspecific variability of spiculation, as well as underdevelopment of spicules and/or pathologic development of the skeleton (our N. cf. nana) may be caused by great differences in, and/or low silicic acid concentration. If this is true then conspecificity of N. nolitangere and N. endoumensis must be seriously considered and investigated in a more rigorous way, as well as a possibility that N. cf. nana (and N. nana itself) is just an underdeveloped ecomorph of some other known species.
The occurrence of such slow-growing and ecosystem-engineering invertebrates of deep-water origin in shallow marine caves with particular characteristics, within an oligotrophic marine basin, highlight an urgent need for further study, appropriate management, and conservation actions.
Data Availability Statement
The original contributions presented in the study are included in the article. Further inquiries can be directed to the corresponding author.
Author Contributions
AP and VG conceived and wrote the manuscript, and acquired funding sources. AP examined the specimens and performed morphological lab work and taxonomic analysis. VG conducted sampling and provided collection data and underwater images. Both authors approved the submitted version of the manuscript.
Funding
This study was in part supported by the NSC Poland, grant no. 2016/21/B/ST10/02332 to AP who was also supported by statuary funds of the Institute of Paleobiology, Polish Academy of Sciences. Additional funds were provided to VG by the project “Centre for the study and sustainable exploitation of Marine Biological Resources (CMBR)” (MIS 5002670), which is implemented under the Action “Reinforcement of the Research and Innovation Infrastructure,” funded by the Operational Programme “Competitiveness, Entrepreneurship and Innovation” (NSRF 2014–2020) and co-financed by Greece and the EU (European Regional Development Fund). The studied deep-water sponge specimen was collected during the 2018 MEDITS Survey in the frame of the Greek National Data Collection Project.
Conflict of Interest
The authors declare that the research was conducted in the absence of any commercial or financial relationships that could be construed as a potential conflict of interest.
Acknowledgments
We acknowledge Snezana Zivanović, Eleni Dafnomili, and Manolis Tsapakis from the Hellenic Centre for Marine Research for measuring silicate concentration in water samples from the studied marine caves. We are grateful to Guilherme Muricy, Thanos Dailianis, and Elena Akritopoulou for their valuable help during fieldwork and Panagiota Peristeraki for providing the deep-water lithistid sample.
Footnotes
References
Aguilar, R., López Correa, M., Calcinai, B., Pastor, X., De La Torriente, A., and Garcia, S. (2011). First records of Asbestopluma hypogea Vacelet and Boury-Esnault, 1996 (Porifera, Demospongiae Cladorhizidae) on seamounts and in bathyal settings of the Mediterranean Sea. Zootaxa 2925, 33–40. doi: 10.11646/zootaxa.2925.1.3
Allouc, J. (1987). Les paléocommunautés profondes sur fond rocheux du Pléistocène méditerranéen. Description et essai d’interprétation paléoécologique. Geobios 20, 241–263. doi: 10.1016/S0016-6995(87)80036-0
Arko-Pijevac, M., Benac, Č, Kovačič, M., and Kirinčić, M. (2001). A submarine cave at the Island of Krk (North Adriatic Sea). Nat. Croat. 10, 163–184.
Bakran-Petricioli, T., Vacelet, J., Zibrowius, H., Petricioli, D., Chevaldonné, P., and Rađa, T. (2007). New data on the distribution of the ‘deep-sea’ sponges Asbestopluma hypogea and Oopsacas minuta in the Mediterranean Sea. Mar. Ecol. 28, 10–23. doi: 10.1111/j.1439-0485.2007.00179.x
Bertolino, M., Oprandi, A., Santini, C., Castellano, M., Pansini, M., Boyer, M., et al. (2017). Hydrothermal waters enriched in silica promote the development of a sponge community in North Sulawesi (Indonesia). Eur. Zool. J. 84, 128–135. doi: 10.1080/11250003.2016.1278475
Bianchi, C. N., Morri, C., Chiantore, M., Montefalcone, M., Parravicini, V., and Rovere, A. (2012). “Mediterranean Sea biodiversity between the legacy from the past and a future change,” in Life in the Mediterranean Sea: A Look at Habitat Changes, Chap. 1, ed. N. Stambler (New York, NY: Vova Science Publishers), 1–55.
Bo, M., Al Mabruk, S. A. A., Balistreri, P., Bariche, M., Batjakas, I. E., Betti, F., et al. (2020). New records of rare species in the Mediterranean Sea (October 2020). Mediterr. Mar. Sci. 21, 608–630. doi: 10.12681/mms.23674
Cardenas, P., and Rapp, T. T. (2013). Disrupted spiculogenesis in deep-water Geodiidae (Porifera, Demospongiae) growing in shallow waters. Invertebr. Biol. 132, 173–194. doi: 10.1111/ivb.12027
Carvalho, F. C., Cárdenas, P., Ríos, P., Cristobo, J., Rapp, H. T., and Xavier, J. R. (2020). Rock sponges (lithistid Demospongiae) of the Northeast Atlantic seamounts, with the description of ten new species. PeerJ 8:e8703. doi: 10.7717/peerj.8703
Carvalho, F. C., Pomponi, S. A., and Xavier, J. R. (2015). Lithistid sponges of the upper bathyal of Madeira, Selvagens and Canary Islands, with description of a new species of Isabella. J. Mar. Biol. Assoc. U. K. 95, 1287–1296. doi: 10.1017/s0025315414001179
Chevaldonné, P., Pérez, T., Crouzet, J.-M., Bay-Nouailhat, W., Bay-Nouailhat, A., Fourt, M., et al. (2015). Unexpected records of ‘deep-sea’ carnivorous sponges Asbestopluma hypogea in the shallow NE Atlantic shed light on new conservation issues. Mar. Ecol. 36, 475–484. doi: 10.1111/maec.12155
Cruz, T. (2002). Esponjas Marinas de Canarias. S/C Tenerife: Consejería de Política Territorial y Medio Ambiente del Gobierno de Canarias. doi: 10.1111/maec.12155
Dimarchopoulou, D., Gerovasileiou, V., and Voultsiadou, E. (2018). Spatial variability of sessile benthos in a semi-submerged marine cave of a remote Aegean Island (eastern Mediterranean Sea). Reg. Stud. Mar. Sci. 17, 102–111. doi: 10.1016/j.rsma.2017.11.015
Ellwood, M. J., Kelly, M., and Richer de Forges, B. (2007). Silica banding in the deep-sea lithistid sponge Corallistes undulatus: investigating the potential influence of diet and environment on growth. Limnol. Oceanogr. 52, 1865–1873. doi: 10.4319/lo.2007.52.5.1865
Galani, A., Dailianis, T., Sini, M., Katsanevakis, S., and Gerovasileiou, V. (2019). “Characterization of benthic communities in marine caves of the island-dominated Aegean ecoregion (Eastern Mediterranean Sea),” in Proceedings of the 2nd Mediterranean Symposium on the Conservation of Dark Habitats, eds H. Langar and A. Ouerghi (Tunis: SPA/RAC publi), 67–68.
Gerovasileiou, V., Chintiroglou, C., Vafidis, D., Koutsoubas, D., Sini, M., Dailianis, T., et al. (2015). Census of biodiversity in marine caves of the Eastern Mediterranean Sea. Mediterr. Mar. Sci. 16, 245–265. doi: 10.12681/mms.1069
Gerovasileiou, V., and Voultsiadou, E. (2012). Marine caves of the Mediterranean Sea: a sponge biodiversity reservoir within a biodiversity hotspot. PLoS One 7:e39873. doi: 10.1371/journal.pone.0039873
Gerovasileiou, V., and Bianchi, C. N. (in press). Mediterranean marine caves: a synthesis of current knowledge. Oceanogr. Mar. Biol. Annu. Rev. 59.
Hall, K. A., Ekins, M. G., and Hooper, J. N. A. (2014). Two new desma-less species of Theonella Gray, 1868 (Demospongiae: Astrophorida: Theonellidae), from the Great Barrier Reef, Australia, and a re-evaluation of one species assigned previously to Dercitus Gray, 1867. Zootaxa 3814, 451–477. doi: 10.11646/zootaxa.3814.4.1
Harmelin, J.-G. (1997). Diversity of bryozoans in a Mediterranean sublittoral cave with bathyal like conditions: role of dispersal processes and local factors. Mar. Ecol. Prog. Ser. 153 139–152. doi: 10.3354/meps153139
Harmelin, J.-G., and Vacelet, J. (1997). Clues to deep-sea biodiversity in a nearshore cave. Vie Milieu 47, 351–354.
Hart, C. W. Jr., Manning, R. B., and Iliffe, T. M. (1985). The fauna of Atlantic marine caves: evidence of dispersal by sea floor spreading while maintaining ties to deep waters. P. Biol. Soc. Wash. 98, 288–292.
Krom, M. D., Kress, N., and Fanning, K. (2014). Silica cycling in the ultra-oligotrophic eastern mediterranean sea. Biogeosciences 11, 4211–4223. doi: 10.5194/bg-11-4211-2014
Lage, A., Araújo, H. P. M., Gerovasileiou, V., and Muricy, G. (2018). A new cave-dwelling species of Plakina (Porifera: Homoscleromorpha) from Crete. Greece (Aegean Sea). Zootaxa 4466, 39–48. doi: 10.11646/zootaxa.4466.1.5
Lage, A., Gerovasileiou, V., Voultsiadou, E., and Muricy, G. (2019). Taxonomy of Plakina (Porifera: Homoscleromorpha) from Aegean submarine caves, with description of three new species and new characters for the genus. Mar. Biodivers. 49, 727–747. doi: 10.1007/s12526-018-0847-z
Lambeck, K., and Purcell, A. (2005). Sea-level change in the Mediterranean Sea since the LGM: model predictions for tectonically stable areas. Quat. Sci. Rev. 24, 1969–1988. doi: 10.1016/j.quascirev.2004.06.025
Maldonado, M., Aguilar, R., Blanco, J., García, S., Serrano, A., and Punzón, A. (2015). Aggregated clumps of lithistid sponges: a singular, reef-like bathyal habitat with relevant paleontological connections. PLoS One 10:e0125378. doi: 10.1371/journal.pone.0125378
Maldonado, M., Carmona, M. C., Uriz, M. J., and Cruzado, A. (1999). Decline in Mesozoic reef-building sponges explained by silicon limitation. Nature 401, 785–788. doi: 10.1038/44560
Manconi, R., and Serusi, A. (2008). Rare sponges from marine caves: discovery of Neophrissospongia nana nov. sp. (Demospongiae, Corallistidae) from Sardinia with an annotated checklist of Mediterranean lithistids. ZooKeys 4, 71–87. doi: 10.3897/zookeys.4.39
Manconi, R., Serusi, A., and Pisera, A. (2006). A new Mediterranean ‘lithistid’ sponge, Aciculites mediterranea sp. nov. (Porifera: Demospongiae) from a dark marine cave in Sardinia. J. Mar. Biol. Assoc. U. K. 86, 691–698. doi: 10.1017/S0025315406013580
Matyja, B. A. (1978). Struktury wczesnodiagenetyczne w wapiennych utworach oksfordu. Przeglqd Geol. 3, 156–158.
Mourtzas, N., Kolaiti, E., and Anzidei, M. (2016). Vertical land movements and sea level changes along the coast of Crete (Greece) since Late Holocene. Quat. Int. 401, 43–70. doi: 10.1016/j.quaint.2015.08.008
Perez, T., Vacelet, J., Bitar, G., and Zibrowius, H. (2004). Two new lithistids (Porifera: Demospongiae) from a shallow eastern Mediterranean cave (Lebanon). J. Mar. Biol. Ass. U. K. 84, 15–24. doi: 10.1017/S0025315404008859h
Pisera, A. (1997). Upper Jurassic siliceous sponge from the Swabian Alb: taxonomy and paleoecology. Palaeontol. Pol. 57, 1–216.
Pisera, A., and Lévi, C. (2002). “Family Corallistidae Sollas, 1888,” in Systema Porifera. A Guide to the Classification of Sponges, eds J. N. A. Hooper and R. W. M. Van Soest (New York, NY: Kluwer Academic/Plenum Publishers), 312–320. doi: 10.1007/978-1-4615-0747-5_35
Pisera, A., and Pomponi, S. A. (2015). New data on lithistid sponges from the deep Florida shelf with description of a new species of Theonella. J. Mar. Biol. Assoc. U. K. 95, 1297–1309. doi: 10.1017/S0025315414001477
Pisera, A., and Vacelet, J. (2011). Lithistid sponges from submarine caves in the Mediterranean: taxonomy and affinities. Sci. Mar. 75, 17–40. doi: 10.3989/scsimar.2011.75n1017
Pomponi, S. A., Kelly, M., Reed, J. K., and Wright, A. E. (2001). Diversity and bathymetric distribution of lithistid sponges in the tropical western Atlantic. Bull. Biol. Soc. Wash. 10, 344–353.
Pouliquen, L. (1972). Les spongiaires desgrottes sous-marines de la région de Marseille. Ecologie etsystématique. Téthys 3, 717–758.
Schmidt, O. (1870). Grundzüge einer Spongien-Fauna des Atlantischen Gebietes. Leipzig: Wilhelm Engelmann.
Schuster, A., Cárdenas, P., Pisera, A., Pomponi, S. A., Kelly, M., Wörheide, G., et al. (2018). Seven new deep-water Tetractinellida (Porifera: Demospongiae) from the Galápagos Islands – morphological descriptions and DNA barcodes. Zool. J. Linn. Soc. Lond. 184, 273–303. doi: 10.1093/zoolinnean/zlx110
Sini, M., Katsanevakis, S., Koukourouvli, N., Gerovasileiou, V., Dailianis, T., Buhl-Mortensen, L., et al. (2017). Assembling ecological pieces to reconstruct the conservation puzzle of the aegean sea. Front. Mar. Sci. 4:347. doi: 10.3389/fmars.2017.00347
Souvermezoglou, E., and Krasakopoulou, E. (2000). Chemical oceanography in the Cretan Sea: changes associated to the transient. Mediterr. Mar. Sci. 1, 91–103. doi: 10.12681/mms.292
Topsent, E. (1904). Spongiaires des Açores. Résultats des campagnes scientifiques accomplies par le Prince Albert I. Monaco. Résultats des campagnes scientifiques accomplies sur son yacht 25, 1–280.
Vacelet, J. (2002). “Recent ‘Sphinctozoa’, order verticillitida, family Verticillitidae Steinmann, 1882,” in Systema Porifera. A Guide to the Classification of Sponges, eds J. N. A. Hooper and R. W. M. Van Soest (New York, NY: Kluwer Academic/Plenum Publishers), 1097–1098. doi: 10.1007/978-1-4615-0747-5_113
Vacelet, J., and Boury-Esnault, N. (1996). A new species of carnivorous sponge (Demospongiae: Cladorhizidae) from a Mediterranean cave. Bull. Inst. R. Sci. Nat. Belg. 66, 109–115.
Vacelet, J., Boury-Esnault, N., and Harmelin, J.-G. (1994). Hexactinellid cave, a unique deep-sea habitat in the scuba zone. Deep Sea Res. Pt I 41, 965–973. doi: 10.1016/0967-0637(94)90013-2
Vacelet, J., Cuif, J.-P., Gautret, P., Massot, M., Richer de Forges, B., and Zibrowius, H. (1992). Un Spongiaire Sphinctozoaire colonial apparenté aux constructeurs de récifs triasiques survivant dans le bathyal de Nouvelle-Calédonie. C. R. Hebd. Séances Acad. sci. 314, 379–385.
Vacelet, J., and Vasseur, P. (1965). Spongiaires des grottes et surplombs des récifs de Tuléar. Recl. Trav. Stn. Mar. Endoume Marseille 4, 71–123.
Vamvakas, C. E. (1970). Peuplements Benthiques des Substrats Meubles du Sud de la Mer Egée. Tethys 2, 89–130.
Van Soest, R. W. M., Boury-Esnault, N., Hooper, J. N. A., Rützler, K., de Voogd, N. J., Alvarez, B., et al. (2020). World Porifera Database. Available online at: http://www.marinespecies.org/porifera (accessed 18 October, 2020).
Voultsiadou, E. (2005a). Demosponge distribution in the eastern Mediterranean: a NW–SE gradient. Helgoland Mar. Res. 59, 237–251. doi: 10.1007/s10152-005-0224-8
Voultsiadou, E. (2005b). Sponge diversity in the Aegean Sea: check list and new information. Ital. J. Zool. 72, 53–64. doi: 10.1080/11250000509356653
Voultsiadou, E. (2009). Reevaluating sponge diversity and distribution in the Mediterranean Sea. Hydrobiologia 628, 1–12. doi: 10.1007/s10750-009-9725-9
Voultsiadou, E., Gerovasileiou, V., and Bailly, N. (2016). Porifera of Greece: an updated checklist. BDJ 4:e7984. doi: 10.3897/BDJ.4.e7984
Wörheide, G., and Reitner, J. (1996). “‘Living fossil’ sphinctozoan coralline sponge colonies in shallow water caves of the Osprey Reef (Coral Sea) and the Astrolabe Reefs (Fiji Islands),” in Global and Regional Controls on Biogenic Sedimentation. I. Reef Evolution. Research Reports, eds J. Reitner, F. Neuweiler, and F. Gunkel (Göttinger Arb: Geol. Paläont), 145–148.
Keywords: Porifera, demosponges, lithistids, marine caves, deep sea, Mediterranean Sea, silicate
Citation: Pisera A and Gerovasileiou V (2021) Lithistid Demosponges of Deep-Water Origin in Marine Caves of the North-Eastern Mediterranean Sea. Front. Mar. Sci. 8:630900. doi: 10.3389/fmars.2021.630900
Received: 18 November 2020; Accepted: 07 January 2021;
Published: 04 February 2021.
Edited by:
Joana R. Xavier, University of Porto, PortugalReviewed by:
John Hooper, Queensland Museum, AustraliaVacelet Jean, UMR 7263 Institut Méditerranéen de Biodiversité et d’Ecologie Marine et Continentale (IMBE), France
Copyright © 2021 Pisera and Gerovasileiou. This is an open-access article distributed under the terms of the Creative Commons Attribution License (CC BY). The use, distribution or reproduction in other forums is permitted, provided the original author(s) and the copyright owner(s) are credited and that the original publication in this journal is cited, in accordance with accepted academic practice. No use, distribution or reproduction is permitted which does not comply with these terms.
*Correspondence: Andrzej Pisera, apis@twarda.pan.pl